- 1Universidad de Buenos Aires (UBA), Facultad de Farmacia y Bioquímica, Departamento de Microbiología, Inmunología, Biotecnología y Genética, Cátedra de Inmunología, Buenos Aires, Argentina
- 2Consejo Nacional de Investigaciones Científicas y Técnicas (CONICET) Universidad de Buenos Aires, Instituto de Estudios de la Inmunidad Humoral “Prof. Ricardo A. Margni” (IDEHU), Buenos Aires, Argentina
- 3Universidad Nacional de Lujan, Departamento de Ciencias Básicas, Laboratorio de Genómica Computacional, Buenos Aires, Argentina
Introduction: Insulin and proinsulin autoantibodies (IAA/PAA) are usually the first markers to appear in patients with type 1 Diabetes Mellitus (T1DM) and their prevalence ranges from 10 to 60% in the child-adolescent population. The reference method for IAA/PAA detection is the Radioligand Binding Assay (RBA), a highly specific and sensitive technique, but expensive and polluting. The aim of this work was to develop a novel flow cytometric microsphere-based immunoassay (FloCMIA) for PAA detection, employing recombinant human proinsulin (PI), as an alternative method to RBA, less expensive and harmful to the environment.
Materials and Methods: Human PI was expressed as Thioredoxin fusion protein (TrxPI) in E. coli and a fraction was biotinylated. A double paratope model was used in which samples were incubated with TrxPI–biotin and microspheres adsorbed with TrxPI. The immune complexes were revealed using Streptavidin–Phycoerythrin. The geometric mean of the signals was analyzed, and the results were expressed as Standard Deviation scores (SDs). Sera from 100 normal human control and from 111 type 1 diabetic patients were evaluated by FloCMIA. To correlate the novel assay with RBA, 51 diabetic patients were selected, spanning a wide range of PAA reactivity by RBA.
Results: The study of ROC curves allowed choosing a cut-off value of 3.0 SDs and the AUC was 0.705, indicating that FloCMIA has fair ability to distinguish between samples from each group. A prevalence of 50% for PAA was obtained in the population of diabetic patients studied. The specificity was 96% and the analytical sensitivity (percentage of patients RBA positive, also positive by FloCMIA) was 69%. There was a substantial agreement between methods (kappa statistic=0.700).
Conclusions: A novel immunoassay based on flow cytometry that uses easy-to produce recombinant PI was developed. This assay constitutes an innovative and cost-effective alternative to RBA for the determination of PAA in patients’ sera. The method developed here, presents good performance and a wide dynamic range together with a small required sample volume. Furthermore, these results make it possible to develop multiplex immunoassays that allow the combined detection of autoantibodies present in T1DM and other related autoimmune diseases.
Introduction
Type 1 Diabetes Mellitus (T1DM) is an autoimmune disorder originated by the loss of tolerance towards components of insulin-producing pancreatic beta cells in genetically predisposed subjects. The destruction of beta cells is mediated by cytotoxic T lymphocytes and, due to this damage, several pancreatic autoantigens are released into the bloodstream and different autoantibodies are produced. The four main autoantibodies associated with risk of progression to clinical disease are: insulin/proinsulin autoantibodies (IAA/PAA), glutamic acid decarboxylase autoantibodies (GADA), insulinoma associated protein tyrosine phosphatase 2 autoantibodies (IA-2A) and zinc transporter isoform 8 autoantibodies (ZnT8A) (1–3). These markers can be detected in blood circulation long before the clinical symptoms are manifested (4) and, as this pathology is characterized by an asymptomatic period that can last within few months to more than ten years (5), the measurement of these autoantibodies can be very useful as risk predictors of T1DM in children and young adults (6).
IAA/PAA are often the first autoantibodies to appear during the natural history of the disease (7, 8) and their titer is remarkably high in young children, with an inverse correlation between their presence and the age of onset of the disease (9). Therefore, the prevalence of IAA/PAA varies widely according to patients’ age range (50–60% in subjects younger than 10 years and around 10% in post pubertal ones) (10) and regarding its ethnic origin (11).
The method historically considered as the reference one for IAA/PAA detection is the Radioligand Binding Assay (RBA) (12–15). This technique is widely used due its high specificity and sensitivity; however, as it uses radioactive tracers, is harmful to the environment, presents an elevated cost and is restricted only to authorized laboratories. Therefore, it is imperative to have alternative methods to the RBA and, in this sense, several research groups have worked on their development. Unlike what happens for the other markers of T1DM, until now it was not possible to develop an enzyme-linked immunosorbent assay (ELISA) with good performance for IAA/PAA detection. This may be due, in part, to the possible denaturation of the antigen (insulin/proinsulin) when it is immobilized on the solid phase and, besides, because of its low molecular weight critical epitopes may be hidden during immobilization, making them inaccessible to autoantibodies, contrary to what happens in fluid phase immunoassays (16). In RBA, antigen-antibody interaction occurs in a high dilution in the liquid phase, almost reaching the equilibrium state. Instead, in ELISA this interaction occurs in a solid phase where the amount of immunocomplexes formed is highly dependent on antibodies concentration. Taking this into account, and due to the really low concentration of autoantibodies in type 1 diabetic patients’ sera (in the order of 10-12 M), their detection employing solid phase assays is a real challenge. Likewise, multiple international workshops (Diabetes Antibody Standardization Program 2000, 2002, 2003 and 2005) (17) have reported the difficulty among different laboratories to achieve immunoassays with good performances for the detection of this marker. Over the last few years, the detection of these autoantibodies based on electrochemiluminescence has shown to achieve similar, and even better, performances than the RBA (18, 19). Nevertheless, this method requires MSD (Meso Scale Discovery) electrochemiluminescent instrument that is not available in mostly all medium complexity laboratories and hospitals of our country.
We have previously described the production of recombinant human proinsulin (PI) in Escherichia coli (E. coli) as a soluble and properly folded fusion protein with Thioredoxin (Trx). Also, it was possible to improve its yielding by recovering the chimera from inclusion bodies. This chimera retains full immunochemical reactivity (20) and can be easily produced and purified. Having a handy source of native PI, allowed us to develop a highly sensitive and quasi-quantitative protocol of flow cytometric microsphere-based immunoassay (FloCMIA) for PAA determination.
In this sense, we have previously designed alternative FloCMIA protocols for the detection of GADA, obtaining the best performance with the one based on the simultaneous interaction of GADA with TrxGAD65 immobilized on the surface of the microsphere and with TrxGAD65–biotin in the fluid phase (21).
So, the aim of the present work was to develop a FloCMIA protocol for the detection of PAA employing PI produced in E. coli, as an alternative method to RBA, less expensive and harmful to the environment.
Materials and Methods
Human Sera Collection
Serum samples were obtained from fasted individuals (8 hours) and then, stored at -20°C until assayed. The groups under study were:
Normal Human Control. Normal control sera (n = 100, mean age of 32 ± 8 years with median age of 30.5, range 21 to 60 and male/female: 38/62) were obtained from healthy subjects without personal or family history of Diabetes Mellitus or other autoimmune diseases. These sera were employed to calculate the cut-off value of the immunoassays. The collection of serum samples was approved by the Ethics Committee of the Clinical Hospital José de San Martín, University of Buenos Aires, Buenos Aires, Argentina. All subjects were informed about the purpose of the study, and a signed consent for study participation was obtained.
Type 1 Diabetic Patients. Serum samples (n = 111) were collected from children and adolescents admitted to the Nutrition Service at Gutierrez National Pediatric Hospital, Buenos Aires, Argentina, recently diagnosed according to WHO criteria (22). Samples were taken before or within 72 h of starting insulin treatment with the approval of the corresponding Ethical Committee and parental consent was obtained. In these samples, the other autoimmune markers GADA, IA-2A and ZnT8A, were determined by RBA (Supplementary Figure 1). To correlate the performance of FloCMIA with that of the reference method, out of these 111 samples, 51 were selected, spanning a wide range of PAA reactivity by RBA (mean age of 7.1 years at diagnosis, with median age of 8.0, range 0.1–6 years and male/female: 23/28).
PAA Detection by Radioligand Binding Assay
RBA for PAA was performed as previously described by our group (15). Briefly, following manufacturer instructions, cDNA coding for human PI was transcribed and translated using a rabbit reticulocyte lysate system in the presence of [35S]-cysteine (New England Nuclear, Boston, MA, USA). [35S]-PI was refolded by a disulfide reduction-reoxidation procedure, and finally, isolated by reverse phase HPLC.
Thirty microliters of sera were incubated with 1000 cpm of [35S]-PI in 90 μl of RBA buffer (50 mM sodium phosphate, 100 mM NaCl, pH 7, 0.1% Aprotinin and 0.1% bovine serum albumin -BSA-) for seven days at 4°C. Afterwards, to isolate the immunocomplexes, 50 μl of a 50% suspension of Protein G - Sepharose 4B FF (Amersham Biosciences, Piscataway, NJ, USA) in RBA buffer were added and the mixture incubated for 2 h at room temperature on an end-over-end shaker. Samples were centrifuged and supernatants were discarded. Pellets were washed four times with 200 μl of RBA buffer, and suspended in 100 μl of 1% sodium dodecyl sulphate (SDS). After a centrifugation of 5 minutes at 6000×g, supernatants were carefully transferred to appropriate vials for scintillation counting (5 min per tube). Results were calculated as Bound percentage (B%):
and expressed as Standard Deviation scores (SDs):
where Bc% was the mean B% of control sera and SDc its standard deviation. An assay was considered positive if SD score >3.0. Thirty normal human sera were included as controls and Bc% was normally distributed. As we have previously reported (15), the sensitivity of RBA using 35S-PI was 63.4%.
Expression of Proinsulin as a Recombinant Protein in E. coli, Purification and Biotinylation
Proinsulin Expression and E. coli Disruption
PI was expressed as a fusion protein with Trx (TrxPI) in E. coli as previously described (20). Briefly, E. coli strain GI724 was transformed with the recombinant vector pTrxPI. Bacteria were cultured in a medium containing 0.2% casein amino acids, 0.5% glucose, 1 mM MgCl2 and 100 μg/mL ampicillin at 30°C. Protein expression was induced with 100 μg/mL Tryptophan for 3 h at 37°C. In order to obtain total cell lysate, bacteria from 200 mL of culture were collected by centrifugation. Following a freezing and thawing cycle, the pellet was resuspended in 4 mL of lysis buffer (50 mM Tris–HCl, 100 mM NaCl, 1 mM EDTA, pH 7.0) and then sonicated in the presence of 1 mM 2-mercaptoethanol (2ME) and protease inhibitors (0.1% v/v aprotinin and 2 mM phenylmethylsulphonyl fluoride -PMSF-) over crushed ice. After sonication, Triton X- 100 was added to a final concentration of 0.1%, and the mixture was incubated for 10 min at 0°C. The intracellular soluble fraction (ISF) was separated from the inclusion bodies (IB) by centrifugation at 15000×g for 10 min at 4°C.
Protein Purification from ISF by Affinity Chromatography
TrxPI was purified from the ISF by affinity chromatography following the protocol previously described with minor modifications (20). The resin was based on an agarose support covalently modified with phenylarsine oxide, which permitted the binding of proteins containing vicinal dithiol residues as it occurs in Trx (23). ISF (≈ 4 mL) was added to the resin, previously equilibrated with lysis buffer, and activated with lysis buffer containing 20 mM 2ME; the resulting suspension was incubated for 1.5 h at 4°C. The resin was washed sequentially using lysis buffer with increasing concentrations of 2ME and, finally, the bounded proteins were eluted with several 2 mL aliquots of lysis buffer containing 100 mM 2ME. The protein concentration in purified fractions was determined using the Coomasie Plus (Bradford) Assay kit (Thermo Fisher Scientific, Waltham, MA, USA).
Protein Biotinylation
Two milliliters of the purified recombinant protein were subjected to buffer exchange to Phosphate Buffered Saline (PBS: 1.5 mM KH2PO4, 8.1 mM Na2HPO4, 140 mM NaCl, 2.7 mM KCl, pH 7.4) using a PD-10 desalting column (GE Healthcare, Chicago, IL, USA) according to the manufacturer’s instructions. The desalted protein was then incubated for 2 h at 0°C with 20-fold molar excess of sulfo-NHS-biotin (Pierce Biotechnology, Rockford, IL, USA) and free biotin was removed on a new PD-10 desalting column. The biotinylated protein was stored in a mixture containing 50% v/v glycerol, 50 mM 2ME and 0.1% w/v aprotinin.
Protein Isolation From IB
On the other hand, TrxPI was isolated from the IB by a first step of solubilization with 8 M urea in 0.1 M Tris, pH 8.5 followed by an oxidative refolding process through dialysis at 4°C against 0.5 M L-arginine, 50 mM Tris–HCl, 5 mM EDTA, 5 mM reduced glutathione and 0.5 mM oxidized glutathione, pH 9.5 (24). The refolded protein´s buffer was exchanged to PBS using a PD-10 desalting column and the protein was stored in fractions with 50 mM 2ME and 0.1% w/v aprotinin.
Sodium Dodecyl Sulphate-Polyacrylamide Gel Electrophoresis and Western Blot Analysis
Fractions at different steps of purification of TrxPI were collected, diluted in 0.2 mL of SDS–polyacrylamide gel electrophoresis (SDS-PAGE) sample buffer (50 mM Tris–HCl, 12.0% glycerol, 0.005% bromophenol blue, 4.0% SDS, 4.0% 2ME, pH 6.8) and boiled for 5 minutes for SDS-PAGE and western blotting (WB) analysis. For comparison, all SDS-PAGE lanes in each gel contained proteins recovered from the same number of cells. For WB, bands were transferred to nitrocellulose membranes. Unoccupied binding sites in the membrane were blocked with 3% skim milk in Tris buffer saline (TBS: 50 mM Tris–HCl, 150 mM NaCl, pH 7.5) by overnight incubation at 4°C. After three washing steps with TBS added with 0.05% Tween 20 (TBS-T), the membrane was incubated for 2 h at room temperature with purified polyclonal serum to Trx diluted 1:100 in 3% w/v BSA TBS-T and then washed five times with TBS-T. Bound antibodies were visualized by incubation with peroxidase-conjugated goat antibodies to rabbit IgG (Jackson ImmunoResearch Laboratories, Inc., West Grove, PA, USA) diluted 1/1500 in 3% w/v BSA–TBS-T followed by the addition of α-chloronaphthol (Sigma-Aldrich, Inc., St. Louis, MO, USA) and 10 vol H2O2.
PAA Detection by Flow Cytometric Microsphere-based Immunoassay (FloCMIA)
Passive Adsorption of Microspheres With TrxPI
This protocol was performed using the manufacturer’s instructions (Spherotech Inc., Lake Forest, IL, USA) with minor modifications. Thirty-three microliters of a suspension of 5 μm microspheres 5% w/v were mixed in a 0.5 mL Eppendorf tube with 30 μg TrxPI isolated from IB per 100 cm2 of microspheres in a final volume of 220 μL in Isotonic Buffered Saline (IBS: 2.3 mM NaH2PO4, 14.2 mM Na2HPO4, 140 mM NaCl, 3.8 mM KCl, pH 7.4) and incubated overnight at 4°C on an end-over-end shaker. The preparation was centrifuged at 12000×g for 10 min and the supernatant was discarded. In order to block microsphere’s free sites, the pellet was suspended with 220 μL of IBS-BSA 0.5% w/v and incubated for 1 h at room temperature on an end-over-end shaker. The preparation was centrifuged again at 12000×g for 10 min, the supernatant was discarded, and the pellet was washed with 400 μL of PBS. Finally, another 400 μL of PBS were added, resulting in 5x104 microspheres–TrxPI/μL and the suspension was stored at 4°C.
FloCMIA Protocol
The immunoassay was performed as previously described (21) with minor modifications. Except when otherwise indicated, all washing steps were performed with 200 μL of PBS-Tween 20 0.05% v/v (PBS–T) on a MultiscreenHTS vacuum manifold (Millipore Corporation, Billerica, MA, USA). Reagent dilutions were prepared using 0.5% w/v BSA in PBS–T.
Twenty microliters of pure human serum were mixed with 20 μL of a microsphere–TrxPI preparation (3.3x102 microspheres/μL) and with 3.6 ng of TrxPI–biotin in a final volume of 60 μL in 0.5 mL Eppendorf tubes. Incubation was performed in 2 steps: (i) 2 h at room temperature and (ii) 5 days at 4°C, both on an end-over-end shaker. After the incubation steps, mixtures were transferred to a MultiscreenHTS-HV 96-well filtration plate (Millipore Ireland BV) and washed 5 times. For detection of the bound antibody, 6.25 ng of Streptavidin-Phycoerythrin (MiltenyiBiotec, Germany) in a final volume of 50 μL were added in each well and incubated for 1 h at room temperature in the dark on a shaker. After that, plates were washed 4 times plus 1 final washing step with 200 μL of PBS and microspheres were suspended with another 200 μL of PBS. The suspension was transferred to Röhren tubes (3.5 mL, 55 x 12 mm, Sarstedt, Germany) and acquired on a PAS III PARTEC flow cytometer (PARTEC, Görlitz, Germany) or a Becton Dickinson FACSCalibur flow cytometer (Franklin Lakes, NJ, USA) equipped with a 488 nm argon laser. The data obtained for each sample were analyzed using Cyflogic software (CyFlo Ltd., Turku, Finland), identifying, firstly, the singlet population of microspheres by gating in a forward scatter (FSC) vs. side scatter (SSC) dot plot. Secondly, fluorescence signals were measured in an FL2 channel and reported as the Geometric Media of Fluorescence Intensity (GeoM) (Figure 1). Results were expressed as SDs:
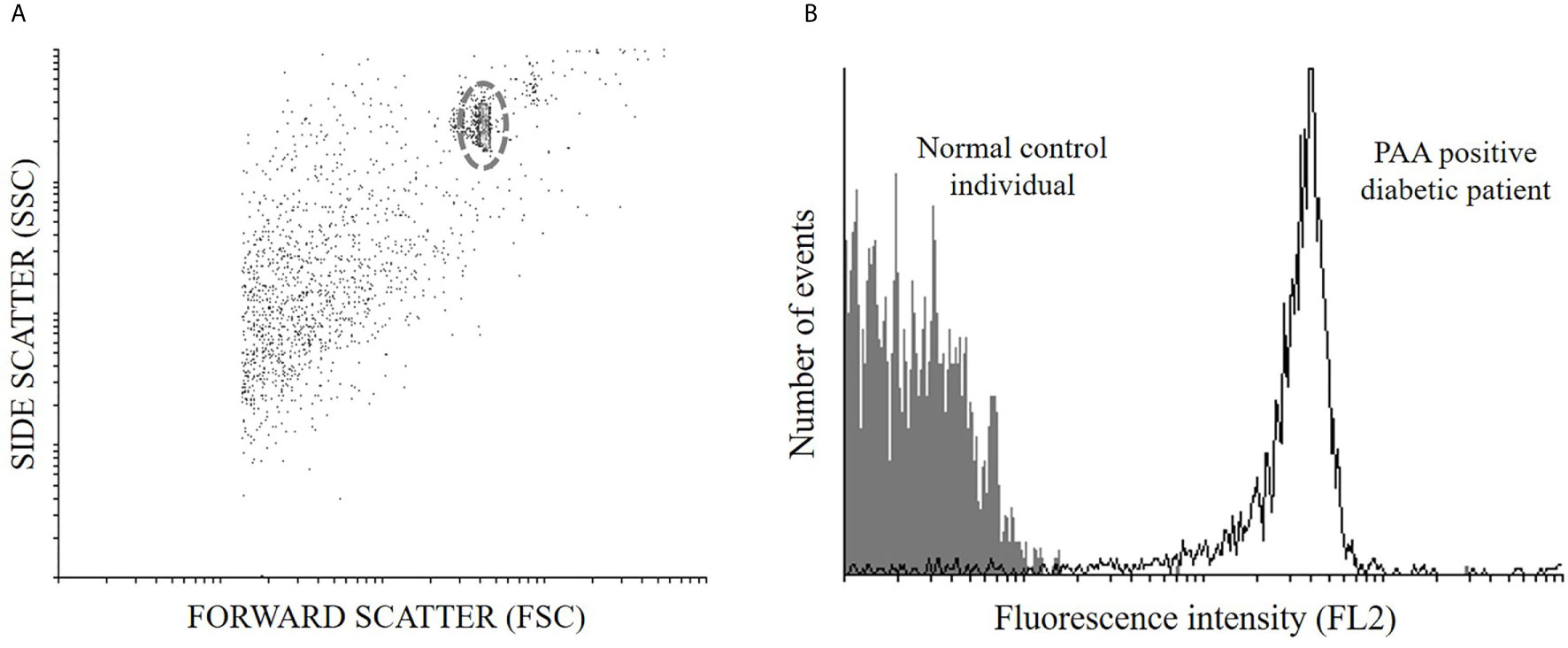
Figure 1 Signal processing. (A) Population of 5 μm microspheres adsorbed with TrxPI observed in a Dot plot graph of side scatter (SSC) vs. forward scatter (FSC) for purposes of gating (B) Histogram of number of events vs. Fluorescence intensity (FL2 channel) to measure the fluorescence associated with each sample; representative signals are shown for the sera from a normal control individual and a PAA positive diabetic patient.
where GeoMm is the mean GeoM of samples in duplicate, GeoMc is the mean GeoM of normal human control sera and SDc is its standard deviation.
Twenty-five human control sera were included in each assay and samples were considered positive when SDs >3.0.
Statistical Analysis
The normal distribution of the data was analyzed by the D’Agostino & Pearson Omnibus normality test. To select the cut-off with maximum sensitivity and specificity, ROC curves were made by plotting these parameters against the corresponding cut-off values. Statistical significance was assessed by parametric tests: Student’s t-test for unpaired samples with Welch correction; or non-parametric tests: Mann-Whitney U test for unpaired data, when applicable. Spearman coefficient of correlation (rs) was calculated to evaluate inter-assay correlation. The degree of agreement between RBA and FloCMIA was evaluated by calculating the kappa statistic. A kappa value of 0.01–0.20 was indicative of slight agreement; 0.21–0.40, fair agreement; 0.41-0.60, moderate agreement; 0.61–0.80, substantial agreement; and 0.81–1.00, almost perfect or perfect agreement (25–28). Calculations were performed using GraphPad Prism version 6.01 for Windows (GraphPad Software, San Diego California, USA, www.graphpad.com). A value of p <0.05 was considered statistically significant.
Results
Expression of Proinsulin as a Recombinant Protein in E. coli, Purification and Biotinylation
As previously described, recombinant fusion protein TrxPI was efficiently expressed in E. coli strain GI724 after 3 h induction. The ISF was analyzed by SDS-PAGE showing one band with the expected molecular weight (≈ 22 kDa) for the full-length engineered protein in the fractions obtained before and after purification (Figure 2A). A typical TrxPI preparation yielded ≈ 6.00 mg of TrxPI/L of culture medium. The identity of TrxPI was confirmed by WB analysis using a specific and purified rabbit polyclonal serum against Trx (Figure 2B).
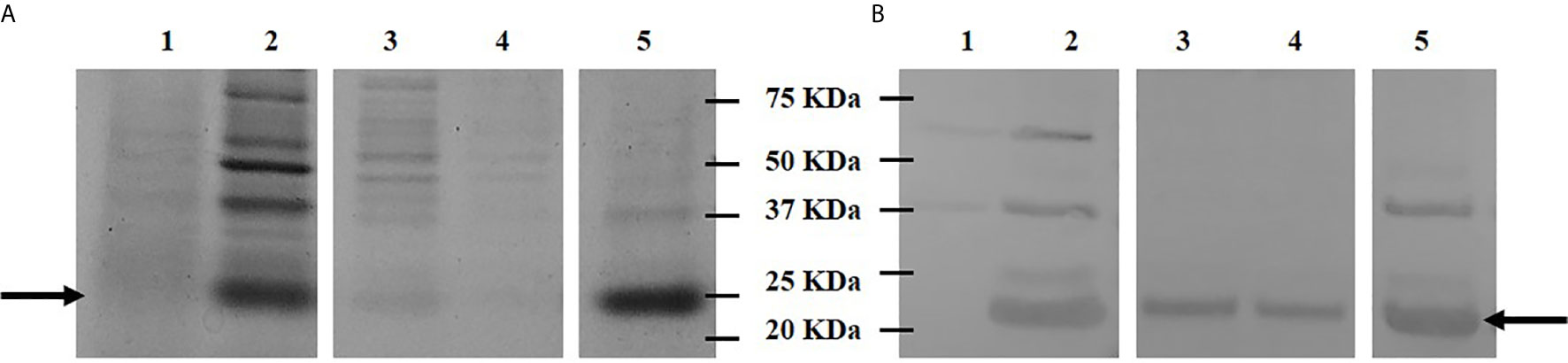
Figure 2 Purification of TrxPI from ISF by affinity chromatography and isolation from IB. Analysis of TrxPI fractions by (A) SDS-PAGE (12.1 % T, 6.0 % C, 1 mm, under reducing conditions, stained with Coomassie Brilliant Blue R-250) and (B) WB revealed with a rabbit polyclonal serum to Thioredoxin as primary antibody. In (A, B), lane 1 corresponds to total lysate of transformed E coli strain GI724 before induction, lane 2: total lysate of transformed E coli strain GI724 after induction, lane 3: purified TrxPI from ISF, lane 4: TrxPI biotinylated, lane 5: TrxPI from IB. Arrows indicate the electrophoretic mobility of TrxPI.
TrxPI isolated from the IB was also analyzed with SDS-PAGE and WB and, as it is shown in Figure 2, there was a band consistent with the expected molecular weight of the recombinant fusion protein that was also recognized by the purified anti Trx serum. Final yield of properly refolded 80–85% pure TrxPI isolated from de IB was 9 mg/L bacterial culture.
PAA Detection by Flow Cytometric Microsphere-Based Immunoassay (FloCMIA)
One hundred human control sera and 111 type 1 diabetic patients’ sera were analyzed by FloCMIA to determine the presence of PAA. ROC curves were performed to select the cut-off value with maximum sensitivity and specificity (Figure 3); the chosen one was 3.0 SDs. Moreover, the area under the ROC curve (AUC) was 0.705, indicating that the method has a fair ability to distinguish between samples from the two groups under study (29). A rabbit policlonal serum to proinsulin was used as the assay internal standard positive control. In order to calculate the coefficient variation a serum from a PAA positive patient was employed. The intra-assay coefficient variation was 1.73% (n = 4) and the inter-assay coefficient variation was 10.72% (n = 5).
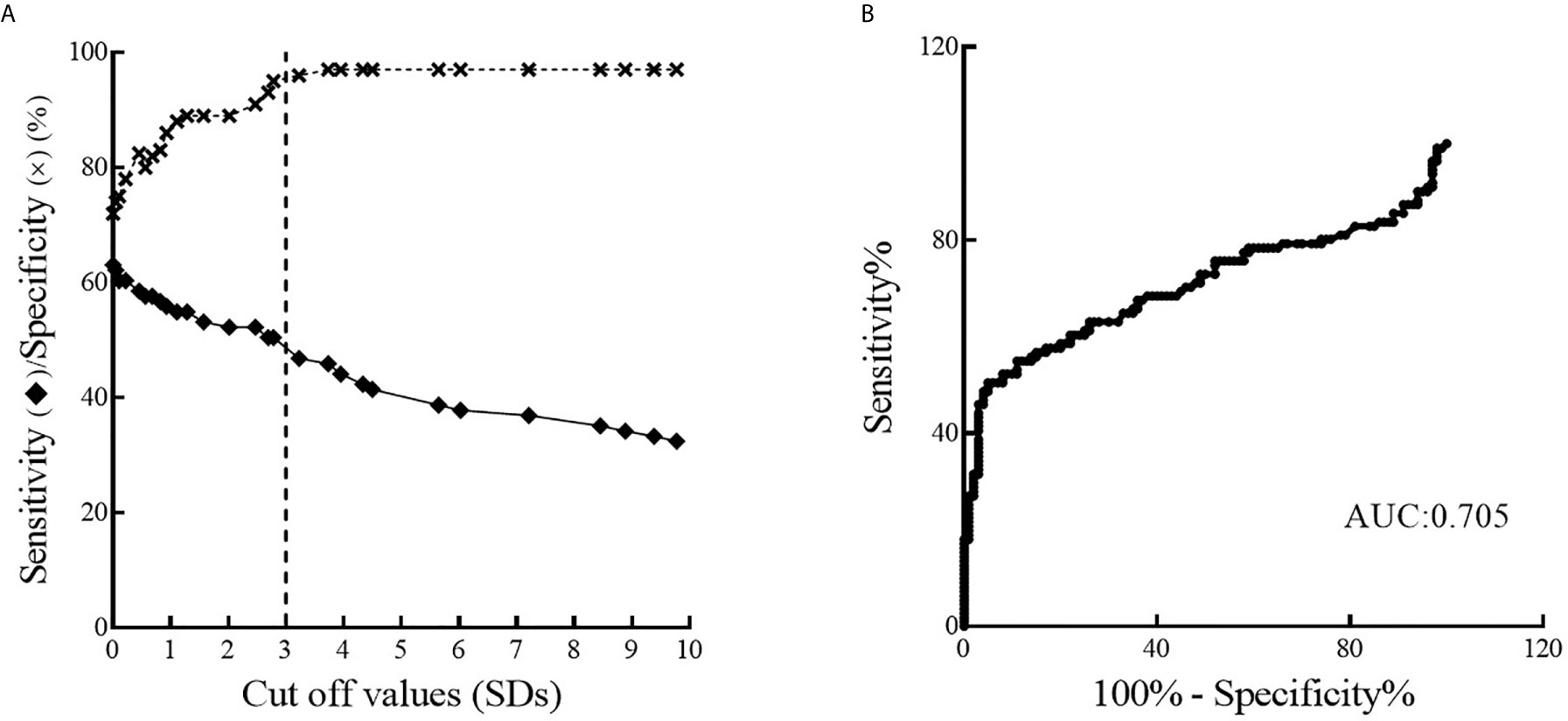
Figure 3 Analysis of the performance of FloCMIA resulting from the study of 100 sera from normal control individuals and 111 sera from infant-juvenile patients with type 1 Diabetes. (A) Sensitivity curve (solid line) and specificity (broken line) as function of the possible cut-off values. The vertical dashed line indicates the cut-off value with the optimized sensitivity and specificity parameters (cut-off = 3.0). (B) ROC curve analysis of FloCMIA, AUC is included.
The results obtained, expressed as SDs (range: -1.11 to 1650), are shown in Figure 4 and the Raw data is shown in Supplementary Table 1. Out of 111 type 1 diabetic patients analyzed, 55 (Sensitivity: 50%) were positive by FloCMIA. The specificity, calculated as 100 % minus the percentage of normal human sera detected as positive, was 96.0%. The median SDs of normal human controls (- 0.33) was significantly different from that of the patients (3.01), p< 0.0001. The clinical characteristics of the two subsets of diabetic patients positive or negative by FloCMIA, were summarized in the Table 1. No significant differences were found between both groups for any of the characteristics studied.
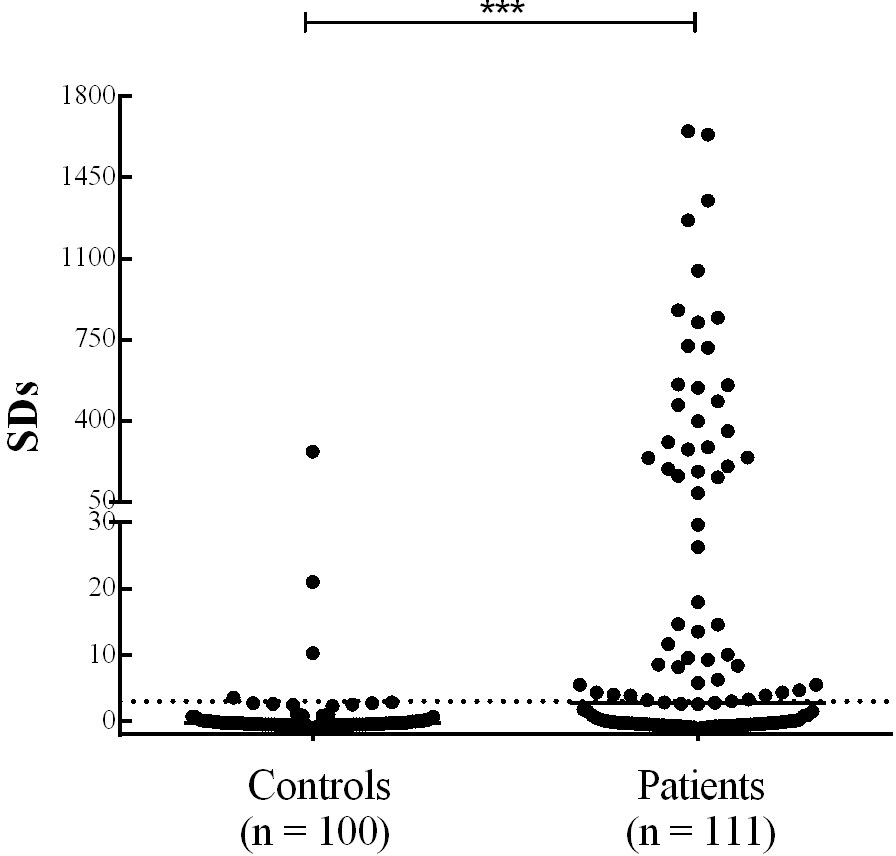
Figure 4 PAA results obtained by FloCMIA in sera from normal control individuals and sera from type 1 diabetic patients. Results are expressed as SDs. The cut-off value (SDs >3.0) is indicated by a dotted line and medians for each population are indicated as a full line (***p < 0.0001).
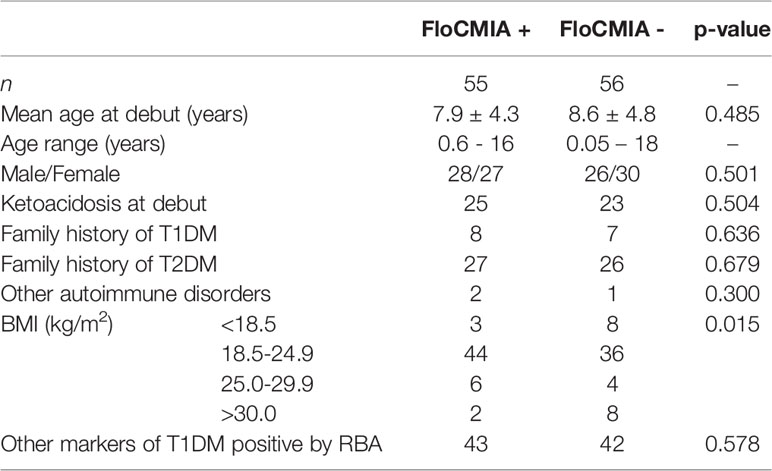
Table 1 Clinical characteristics for two subsets of diabetic patients positive (n=55) or negative (n=56) by FloCMIA.
Comparison of PAA detection by FloCMIA Using Recombinant TrxPI Produced in Bacteria and by RBA Using In Vitro Translated 35S-PI
For further evaluation of the ability of FloCMIA to detect PAA, 51 sera from type 1 diabetic patients that were positive by RBA, and 100 samples from normal human control sera were analyzed.
The antibodies levels employing RBA, expressed as SDs (range: -2.36 to 42.69) are shown in Figure 5A. The signals obtained from type 1 diabetic patients were characterized by a median of 7.07 and a mean of 9.18. On the other hand, the parameters observed for the normal human control sera were a median of 0.55 and a mean of 0.57. Furthermore, the specificity was 98% (Table 2).
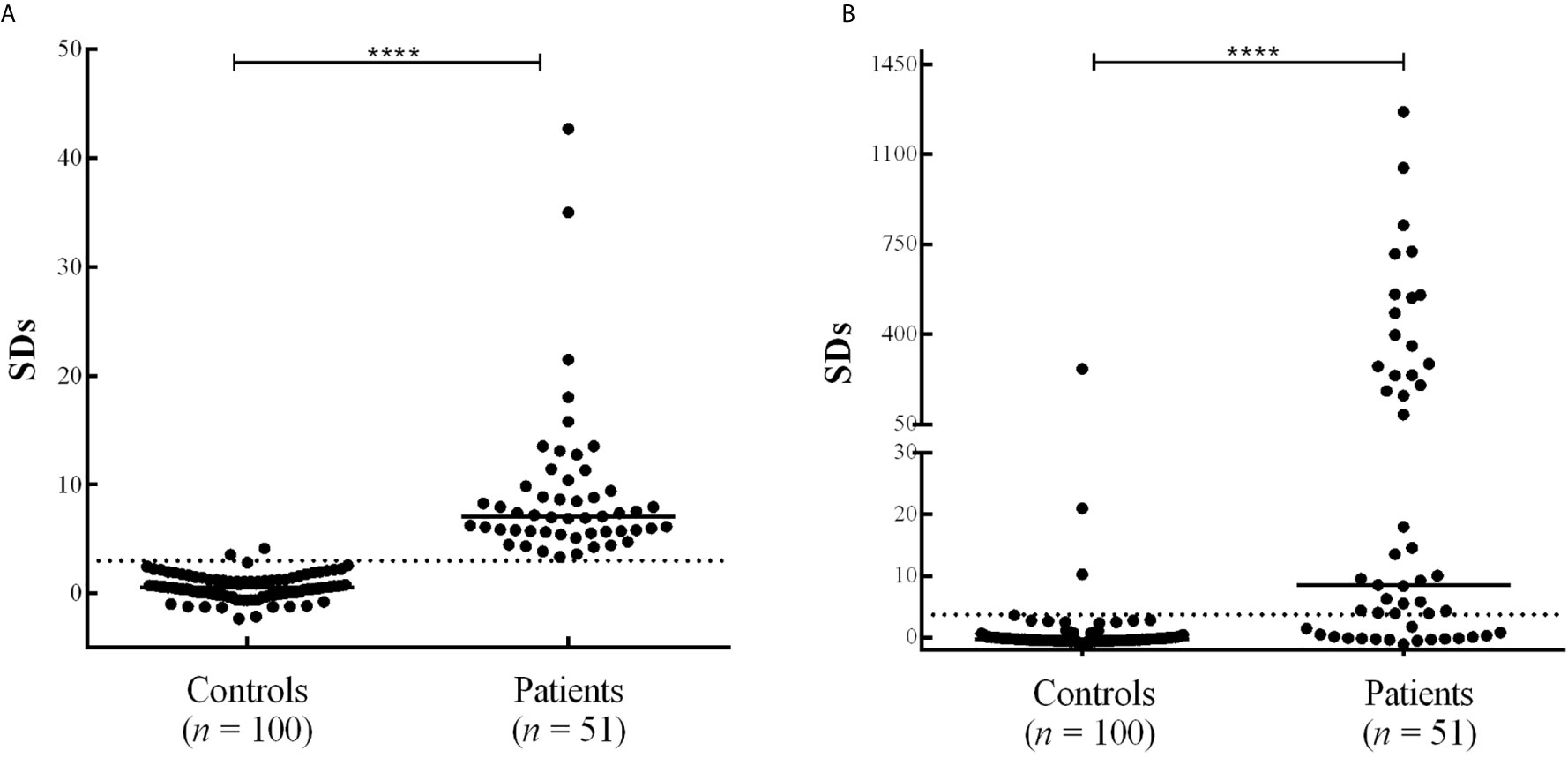
Figure 5 PAA results obtained by RBA (A) and FloCMIA (B) from 100 normal human sera (controls) and 51 type 1 diabetic patients’ sera. Results are expressed as SDs. The cut-off value (SDs >3.0) is indicated by a dotted line and medians for each population are indicated as a full line (****p < 0.0001).
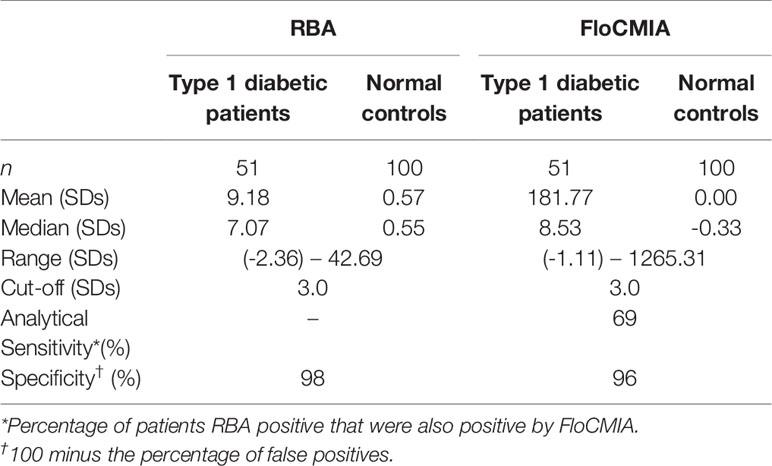
Table 2 Analytical parameters of RBA and FloCMIA from normal human controls and type 1 diabetic patients.
As it is shown in Figure 5B, FloCMIA had a wide range of SDs signals (from -1.11 to 1265.31). Besides, out of the 51 type 1 diabetic patients PAA positive by RBA evaluated, 35 scored positive by this novel assay, obtaining an Analytical Sensitivity of 69% (calculated as the percentage of patients RBA positive that were also positive by FloCMIA). This population was characterized by a median of 8.53 and a mean of 181.77. The specificity obtained was 96% and the median SDs of normal human controls (-0.33) was significantly different from that of the patients (8.53), p< 0.0001 (Table 2).
Integrated Results and Correlation Analysis
Figure 6 shows a statistical comparison of RBA and FloCMIA results from 100 normal human sera and 51 type 1 diabetic patients’ sera. Despite the differences in the physical–chemical principles of both compared techniques, a significant correlation was found between the levels of PAA measured by RBA and by FloCMIA (Spearman’s coefficient, rs=0.4662; p<0.0001). Moreover, the concordance between the two immunoassays was 96.7%, with a kappa statistic of 0.700, representing a substantial agreement between the two methods. Thus, the correlation between RBA and FloCMIA for the determination of PAA was real and not by random sampling.
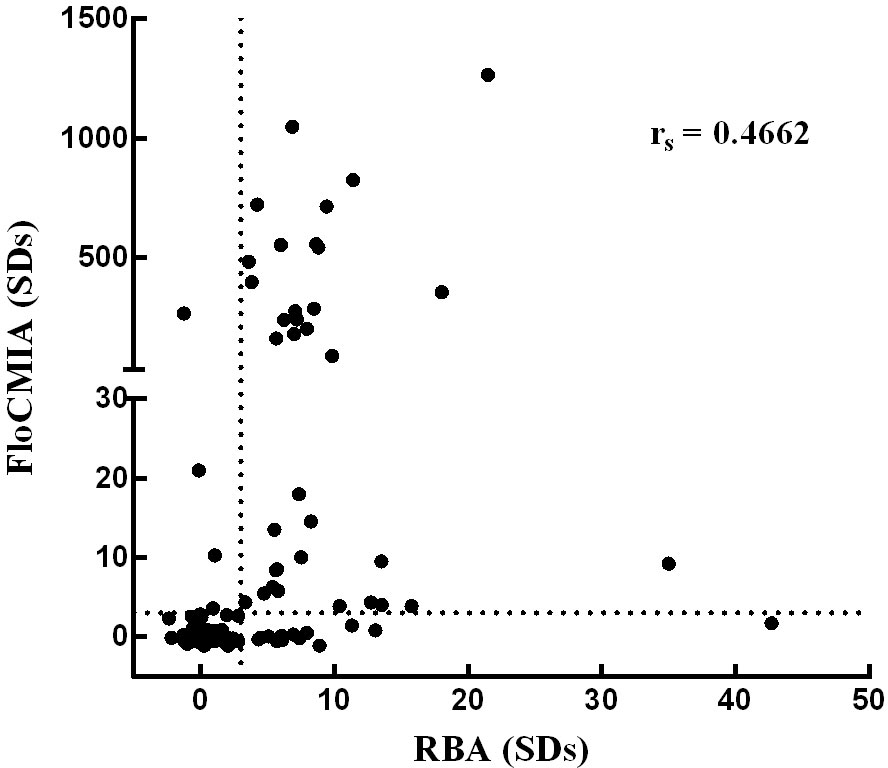
Figure 6 Comparison of PAA detection by FloCMIA using recombinant TrxPI produced in bacteria and by RBA using in vitro translated 35S-human PI. One hundred sera from normal control individuals and from 51 type 1 diabetic patients were tested. Results are expressed as SDs. Values above cut-off dotted lines were considered positive.
Discussion
Diabetes Mellitus is currently one of the main social health problems due to its prevalence, its specific complications and the presence of other pathologies that are usually linked with it. T1DM is a relatively common disorder that develops in genetically susceptible individuals and is associated with anti-islet autoantibodies, which can be found years before the clinical onset of the pathology. A series of well-characterized assays are currently available for the detection of the involved autoantibodies, evaluated through international quality control workshops, which are of utmost importance both for the prediction and diagnosis of T1DM, as well as for the design of trials for disease prevention.
In children followed prospectively from birth, IAA/PAA are frequently the first autoantibodies to appear before the clinical onset of T1DM (30). The methods to assess the presence of IAA/PAA are validated through workshops of the Diabetes Autoantibody Standardization Program (DASP) or, nowadays, the Islet Autoantibody Standardization Program (IASP) from the Center for Disease Control and Prevention (CDC) and the Immunology of Diabetes Society (17, 31). These workshops demonstrated that despite the ELISA assays are able to detect anti-insulin antibodies after subcutaneous injections of human insulin, the standard ELISA formats were not capable of detecting IAA present in recent onset diabetic patients or in individuals progressing to T1DM (16, 32, 33). These ELISA assays use immobilize insulin in the plate wells so there is a high chance that some essential insulin epitopes may be hidden.
Taking into account that there is evidence that describes that all IAA-positive patients react with PI in fluid phase radiometric assays (15, 33) and given the need to improve the assays for the detection of these autoantibodies, the TrxPI chimera was designed. The purpose of this design was to preserve the critical determinants in the specific recognition by IAA/PAA and, in addition, it is known that IAA/PAA appear in circulation as a consequence of the damage suffered by pancreatic beta cells, with the subsequent release of the precursors of insulin with autoantigenic potency stored in these cells. Therefore, the use of proinsulin representing the complete precursor of the insulin molecule could be an appropriate alternative for the measurement of IAA/PAA in sera from patients with autoimmune diabetes, allowing the detection of antibodies against insulin and C peptide.
Despite the good performance of RBA in the diagnosis of autoimmune diabetes, it is imperative to develop alternative non-radiometric assays since the use of radioactive materials is falling into disuse due to the multiple disadvantages that they present (high cost, environmental impact, low applicability in medium and low complexity laboratories, centralization of determinations, etc.). For this reason, some authors have developed autoantibody assays that do not require radioactivity. Yu et al. have described a highly sensitive and specific electrochemiluminescence assay for IAA determination (18). But since this assay requires an Imager SQ120 plate readers (MSD) which is not available in most laboratories and public hospitals in our country, we proposed a method that requires a flow cytometer, an equipment commonly used for the analysis and characterization of cell populations.
In this sense, human proinsulin fused to thioredoxin was expressed in E. coli as previously described (20), yielding approximately 6 mg of TrxPI/L culture medium from intracellular soluble fraction and 9 mg of 80–85% pure properly refolded recombinant protein isolated from inclusion bodies. This chimera was then used to develop a flow cytometric microsphere-based immunoassay for the detection of PAA in type 1 diabetic patients’ sera. For this purpose, microspheres were coated with TrxPI from IB and, on the other hand, TrxPI from ISF was biotinylated. Both reagents were then incubated with human sera as described in the Materials and Methods section.
To evaluate the FloCMIA performance, 111 sera from patients with recent diagnosis of T1DM according to clinical criteria and 100 sera from normal human controls were analyzed. The sensitivity, calculated as the percentage of patients that scored positive, was 50%, and the specificity, calculated as 100 minus the percentage of normal human sera detected as positive, was 96%. These results agree with those reported by other groups (11, 34–38).
For further evaluation of the novel assay, 51 sera from PAA-positive type 1 diabetic patients, previously assayed by RBA, were analyzed by FloCMIA, reaching 69% of Analytical Sensitivity regarding the radiometric method, with a wide range of SDs signals (from -1.11 to 1265.31 and a median of 8.53 as shown in Figure 5). Moreover, there was a substantial agreement between them as defined by the statistic kappa, demonstrating that the correlation between RBA and FloCMIA for PAA determination was real and not by random sampling.
In conclusion the microsphere-based immunoassay performed on Flow cytometers constitutes an innovative and cost-effective alternative to traditional determination of PAA by RBA and/or ECL. Its advantages can be listed as follows: (i) highly sensitive detection due to Fluorescent-labeling, (ii) the use of easy-to produce recombinant proinsulin from prokaryotic cells, (iii) intact immunoreactivity of the antigen after adsorption on microspheres, (iv) the combination of solid and fluid phase interactions, (v) a good specificity, (vi) a wide dynamic range, (vii) a small sample volume required, (viii) low cost and environmentally harmless, (ix) applicable in most medium-complexity laboratories and public hospitals, as it does not require special equipment or authorization by the Nuclear Regulatory Authority and (x) it does not require acid treatment of the samples, as with electrochemiluminescence-based assay, making it a simpler execution method.
Likewise, the results obtained for PAA detection by FloCMIA make it possible to develop multiplex immunoassays that allow the combined detection of autoantibodies present in T1DM and other related autoimmune diseases such as autoimmune thyroid disease and celiac disease, through the use of microspheres of different size or internal fluorescence easily distinguishable on the flow cytometer. The possibility of detecting multiple markers differentially in a single analytical act and with a small sample volume, has a direct impact on the requesting physicians, not only because they can know the autoimmunity profile of the patient with a single analytical determination, but also because it is possible to request the analysis of the combinatorial of markers appropriate according to the clinic and age of each patient.
Data Availability Statement
The raw data supporting the conclusions of this article will be made available by the authors, without undue reservation.
Ethics Statement
The studies involving human participants were reviewed and approved by Ethics Committee of the Clinical Hospital José de San Martín, University of Buenos Aires, Buenos Aires, Argentina and Ethics Committee of the Nutrition Service at Gutierrez National Pediatric Hospital, Buenos Aires, Argentina. Written informed consent to participate in this study was provided by the participants’ legal guardian/next of kin.
Author Contributions
AS, SB and SV conceived and designed the experiments. AS and SB performed the experiments. AS, SB and SV analyzed and interpreted the data. AT, RI, EP and SV contributed reagents/materials/analysis tools. AS, SB, AT and SV wrote the paper. AT, JM, LG, APS and NF contributed with the performance of experiments. EP, AT and SV supervised the research. All authors contributed to the article and approved the submitted version.
Funding
This work was supported in part by grants from FONCYT Program of the National Agency for Science and Technology Promotion (ANPCYT) PICT-2014-1928 and PICT-2017-1436, National Research Council (CONICET) PIP 11220120100256CO and PUE 22920160100017CO and the University of Buenos Aires (UBA 20020150100115BA).
Conflict of Interest
The authors declare that the research was conducted in the absence of any commercial or financial relationships that could be construed as a potential conflict of interest.
Acknowledgments
We thank L. Trifone and A. Roussos at the Gutierrez National Pediatrics Hospital (Buenos Aires, Argentina) for collecting and providing the sera of diabetic patients. We thank at the Hemoteraphy Division at the José de San Martín Clinical Hospital (Buenos Aires, Argentina) for collecting and providing the sera of healthy human individuals.
Supplementary Material
The Supplementary Material for this article can be found online at: https://www.frontiersin.org/articles/10.3389/fimmu.2021.648021/full#supplementary-material
Abbreviations
2ME, 2-mercaptoethanol; B%, Bound percentage; BSA, Bovine serum albumin; ELISA, Enzyme-linked immunosorbent assay; FloCMIA, Flow Cytometric Microsphere-based Immunoassay; GADA, Glutamic acid decarboxylase autoantibodies; GeoM, Geometric Media of Fluorescence Intensity; IA-2A, Insulinoma associated protein tyrosine phosphatase 2 autoantibodies; IAA/PAA, Insulin/Proinsulin autoantibodies; IB, Inclusion bodies; IBS, Isotonic Buffered Saline; ISF, Intracellular soluble fraction; PBS, Phosphate Buffered Saline; PBS-T, PBS with Tween 20 0.05% v/v; PI, Proinsulin; RBA, Radioligand Binding Assay; SDS, Sodium Dodecyl Sulphate; SDs, Standard Deviation scores; SDS-PAGE, SDS-polyacrylamide gel electrophoresis; T1DM, Type 1 Diabetes Mellitus; TBS, Tris buffer saline; TBS-T, TBS with 0.05% Tween 20; Trx, Thioredoxin; TrxPI, PI as a fusion protein with Thioredoxin; WB, Western blot; ZnT8A, Zinc transporter isoform 8 autoantibodies.
References
1. Eisenbarth GS, Jeffrey J. The Natural History of Type 1A Diabetes. Arquivos Brasileiros Endocrinol e Metabol (2008) 52(2):146–55. doi: 10.1590/S0004-27302008000200002
2. Knip M. Natural Course of Preclinical Type 1 Diabetes. Hormone Res Paediatr (2002) 57(1):6–11. doi: 10.1159/000053305
3. Knip M. Can We Predict Type 1 Diabetes in the General Population? Diabetes Care (2002) 25(3):623–5. doi: 10.2337/diacare.25.3.623
4. Atkinson MA. The Pathogenesis and Natural History of Type 1 Diabetes. Cold Spring Harb Perspect Med (2012) 2(11):1–18. doi: 10.1101/cshperspect.a007641
5. Knip M, Siljander H. Autoimmune Mechanisms in Type 1 Diabetes. Autoimmun Rev (2008) 7(7):550–7. doi: 10.1016/j.autrev.2008.04.008
6. Ziegler AG, Nepom GT. Prediction and Pathogenesis in Type 1 Diabetes. Immunity (2010) 32(4):468–78. doi: 10.1016/j.immuni.2010.03.018
7. Hummel M, Bonifacio E, Schmid S, Walter M, Knopff A, Ziegler AG. Brief Communication: Early Appearance of Islet Autoantibodies Predicts Childhood Type 1 Diabetes in Offspring of Diabetic Parents. Ann Internal Med (2004) 140(11):882–6. doi: 10.7326/0003-4819-140-11-200406010-00009
8. Ziegler AG, Hummel M, Schenker M, Bonifacio E. Autoantibody Appearance and Risk for Development of Childhood Diabetes in Offspring of Parents with Type 1 Diabetes: The 2-Year Analysis of the German BABYDIAB Study. Diabetes (1999) 48(3):460–8. doi: 10.2337/diabetes.48.3.460
9. Vardi P, Ziegler AG, Mathews JH, Dib S, Keller RJ, Ricker AT, et al. Concentration of Insulin Autoantibodies at Onset of Type I Diabetes: Inverse Log-Linear Correlation with Age. Diabetes Care (1988) 11(9):736–9. doi: 10.2337/diacare.11.9.736
10. Pihoker C, Gilliam LK, Hampe CS, Lernmark A. Autoantibodies in Diabetes. Diabetes (2005) 54:(SUPPL. 2). doi: 10.2337/diabetes.54.suppl_2.s52
11. Betterle C, Lazzarotto F, Fusari A, Zanchetta R, Benedini S, Pedini B, et al. Pancreatic Autoantibodies in Italian Patients with Newly Diagnosed Type 1 Diabetes Mellitus over the Age of 20 Years. Acta Diabetol (2006) 43(3):79–83. doi: 10.1007/s00592-006-0217-3
12. Palmer JP, Asplin CM, Clemons P, Lyen K, Tatpati O, Raghu PK, et al. Insulin Antibodies in Insulin-Dependent Diabetics before Insulin Treatment. Science (1983) 222(4630):1337–9. doi: 10.1126/science.6362005
13. Stumpo RR, Llera AS, Cardoso AI, Poskus E. Solid versus Liquid Phase Assays in Detection of Insulin Antibodies Influence of Iodination Site on Labelled Insulin Binding. J Immunol Methods (1994) 169(2):241–9. doi: 10.1016/0022-1759(94)90268-2
14. Kurtz AB, DiSilvio L, Bosi E. The Determination of Detection Limits for Insulin Antibody Assays. Diabetol: Clin Exp Diabetes Metab (1988) 31(6):395–9. doi: 10.1007/BF02341510
15. Valdez SN, Iacono RF, Villalba A, Cardoso Landaburu A, Ermácora MR, Poskus E. A Radioligand-Binding Assay for Detecting Antibodies Specific for Proinsulin and Insulin Using 35S-Proinsulin. J Immunol Methods (2003) 279(1–2):173–81. doi: 10.1016/S0022-1759(03)00197-2
16. Greenbaum CJ, Palmer JP, Kuglin B, Kolb H, Arnaiz-Villena A, De Beaufort CD, et al. Insulin Autoantibodies Measured by Radioimmunoassay Methodology Are More Related to Insulin-Dependent Diabetes Mellitus than Those Measured by Enzyme-Linked Immunosorbent Assay: Results of the Fourth International Workshop on the Standardization of Insulin Autoantibody Measurement. J Clin Endocrinol Metab (1992) 74(5):1040–4. doi: 10.1210/jc.74.5.1040
17. Schlosser M, Mueller PW, Törn C, Bonifacio E, Bingley PJ. Diabetes Antibody Standardization Program: Evaluation of Assays for Insulin Autoantibodies. Diabetologia (2010) 53(12):2611–20. doi: 10.1007/s00125-010-1915-5
18. Yu L, Miao D, Scrimgeour L, Johnson K, Rewers M, Eisenbarth GS. Distinguishing persistent insulin autoantibodies with differential risk: nonradioactive bivalent proinsulin/insulin autoantibody assay. Diabetes (2012) 61:179–86. doi: 10.2337/db11-0670
19. Gu Y, Zhao Z, Waugh K, Miao D, Jia X, Cheng J, et al. High-throughput multiplexed autoantibody detection to screen type 1 diabetes and multiple autoimmune diseases simultaneously. EBioMedicine (2019) 47:365–72. doi: 10.1016/j.ebiom.2019.08.036
20. Trabucchi A, Guerra LL, Faccinetti NI, Iacono RF, Poskus E, Valdez SN. Expression and Characterization of Human Proinsulin Fused to Thioredoxin in Escherichia Coli. Appl Microbiol Biotechnol (2012) 94(6):1565–76. doi: 10.1007/s00253-011-3721-5
21. Guerra LL, Trabucchi A, Faccinetti NI, Iacono RF, Ureta DB, Poskus E, et al. Flow Cytometric Microsphere-Based Immunoassay as a Novel Non-Radiometric Method for the Detection of Glutamic Acid Decarboxylase Autoantibodies in Type 1 Diabetes Mellitus. Analyst (2014) 139(12):3017–25. doi: 10.1039/c3an02243a
22. World Health Organization. Diabetes Mellitus. Report of a WHO Study Group (1985). Available at: http://www.ncbi.nlm.nih.gov/pubmed/3934850 ([Accessed May 15, 2020]).
23. Hoffman RD, Lane MD. Iodophenylarsine Oxide and Arsenical Affinity Chromatography: New Probes for Dithiol Proteins. Application to Tubulins and to Components of the Insulin Receptor-Glucose Transporter Signal Transduction Pathway. J Biol Chem (1992) 267(20):14005–11. doi: 10.1016/S0021-9258(19)49670-1
24. Faccinetti NI, Guerra LL, Sabljic AV, Bombicino SS, Rovitto BD, Iacono RF, et al. Prokaryotic Expression and Characterization of the Heterodimeric Construction of ZnT8 and Its Application for Autoantibodies Detection in Diabetes Mellitus. Microb Cell Factories (2017) 16(1):196. doi: 10.1186/s12934-017-0816-4
25. Cohen J. Weighted Kappa: Nominal Scale Agreement Provision for Scaled Disagreement or Partial Credit. psychol Bull (1968) 70(4):213–20. doi: 10.1037/h0026256
26. Landis JR, Koch GG. An Application of Hierarchical Kappa-Type Statistics in the Assessment of Majority Agreement among Multiple Observers. Biometrics (1977) 33(2):363–74. doi: 10.2307/2529786
27. Landis JR, Koch GG. The Measurement of Observer Agreement for Categorical Data. Biometrics (1977) 33(1):159–74. doi: 10.2307/2529310
28. Koch GG, Landis JR, Freeman JL, Freeman DH, Lehnen RG. A General Methodology for the Analysis of Experiments with Repeated Measurement of Categorical Data. Biometrics (1977) 33(1):133–58. doi: 10.2307/2529309
29. Carter JV, Jianmin P, Shesh NR, Galandiuk S. ROC-Ing along: Evaluation and Interpretation of Receiver Operating Characteristic Curves. Surgery (2016) 159(6):1638–45. doi: 10.1016/j.surg.2015.12.029
30. Achenbach P, Warncke K, Reiter J, Heike EN, Williams AJK, Bingley PJ, et al. Stratification of Type 1 Diabetes Risk on the Basis of Islet Autoantibody Characteristics. Diabetes (2004) 53(2):384–92. doi: 10.2337/diabetes.53.2.384
31. Törn C, Mueller PW, Schlosser M, Bonifaci E, Bingley J. Diabetes Antibody Standardization Program: Evaluation of Assays for Autoantibodies to Glutamic Acid Decarboxylase and Islet Antigen-2. Diabetologia (2008) 51(5):846–52. doi: 10.1007/s00125-008-0967-2
32. Greenbaum CJ, Wilkin TJ, Palmer JP, Agopian MS, Arnaiz-Villena A, Becker D, et al. Fifth International Serum Exchange Workshop for Insulin Autoantibody (IAA) Standardization. Diabetologia (1992) 35(8):798–800. doi: 10.1007/BF00429105
33. Castano L, Ziegler AG, Ziegler R, Shoelson S, Eisenbarth GS. Characterization of Insulin Autoantibodies in Relatives of Patients With Type I Diabetes. Diabetes (1993) 42(8):1202–9. doi: 10.2337/diabetes.42.8.1202
34. Bingley PJ, Bonifacio E, Williams AJK, Genovese S, Bottazzo GF, Gale EAM. Prediction of IDDM in the General Population: Strategies Based on Combinations of Autoantibody Markers. Diabetes (1997) 46(11):1701–10. doi: 10.2337/diabetes.46.11.1701
35. Kong YH, Kim MK, Lee DY. Comparison of the Prevalence of Islet Autoantibodies According to Age and Disease Duration in Patients with Type 1 Diabetes Mellitus. Ann Pediatr Endocrinol Metab (2013) 18(2):65–70. doi: 10.6065/apem.2013.18.2.65
36. Williams AJK, Bingley PJ, Bonifacio E, Palmer JP, Gale EAM. A Novel Micro-Assay for Insulin Autoantibodies. J Autoimmun (1997) 10(5):473–8. doi: 10.1006/jaut.1997.0154
37. Bingley PJ. Clinical Applications of Diabetes Antibody Testing. J Clin Endocrinol Metab (2010) 95(1):25–33. doi: 10.1210/jc.2009-1365
Keywords: diabetes mellitus, autoimmunity, autoantibodies, proinsulin, flow cytometry, immunoassay
Citation: Sabljic AV, Bombicino SS, Marfía JI, Guerra LL, Steinhardt AP, Faccinetti NI, Iacono RF, Poskus E, Trabucchi A and Valdez SN (2021) Novel Flow Cytometric Immunoassay for Detection of Proinsulin Autoantibodies in Diabetes Mellitus Employing a Recombinant Autoantigen Expressed in E. coli. Front. Immunol. 12:648021. doi: 10.3389/fimmu.2021.648021
Received: 31 December 2020; Accepted: 17 March 2021;
Published: 06 April 2021.
Edited by:
Kazumitsu Sugiura, Fujita Health University, JapanReviewed by:
Zhiguang Zhou, Central South University, ChinaAaron Walter Michels, University of Colorado Denver, United States
Copyright © 2021 Sabljic, Bombicino, Marfía, Guerra, Steinhardt, Faccinetti, Iacono, Poskus, Trabucchi and Valdez. This is an open-access article distributed under the terms of the Creative Commons Attribution License (CC BY). The use, distribution or reproduction in other forums is permitted, provided the original author(s) and the copyright owner(s) are credited and that the original publication in this journal is cited, in accordance with accepted academic practice. No use, distribution or reproduction is permitted which does not comply with these terms.
*Correspondence: Silvina Noemí Valdez, c2lsdmFsQGZmeWIudWJhLmFy
†These authors have contributed equally to this work