Corrigendum: LYG1 Deficiency Attenuates the Severity of Acute Graft-Versus-Host Disease via Skewing Allogeneic T Cells Polarization Towards Treg Cells
- Department of Hematology, Peking University First Hospital, Beijing, China
Acute graft-versus-host disease (aGVHD) is a lethal complication after allogeneic hematopoietic stem cell transplantation. The mechanism involves the recognition of host antigens by donor-derived T cells which induces augmented response of alloreactive T cells. In this study, we characterized the role of a previously identified novel classical secretory protein with antitumor function-LYG1 (Lysozyme G-like 1), in aGVHD. LYG1 deficiency reduced the activation of CD4+ T cells and Th1 ratio, but increased Treg ratio in vitro by MLR assay. By using major MHC mismatched aGVHD model, LYG1 deficiency in donor T cells or CD4+ T cells attenuated aGVHD severity, inhibited CD4+ T cells activation and IFN-γ expression, promoted FoxP3 expression, suppressed CXCL9 and CXCL10 expression, restrained allogeneic CD4+ T cells infiltrating in target organs. The function of LYG1 in aGVHD was also confirmed using haploidentical transplant model. Furthermore, administration of recombinant human LYG1 protein intraperitoneally aggravated aGVHD by promoting IFN-γ production and inhibiting FoxP3 expression. The effect of rhLYG1 could partially be abrogated with the absence of IFN-γ. Furthermore, LYG1 deficiency in donor T cells preserved graft-versus-tumor response. In summary, our results indicate LYG1 regulates aGVHD by the alloreactivity of CD4+ T cells and the balance of Th1 and Treg differentiation of allogeneic CD4+ T cells, targeting LYG1 maybe a novel therapeutic strategy for preventing aGVHD.
Introduction
Acute graft-versus-host disease (aGVHD) is medical complication which mainly destroy host tissues including the skin, liver, colon and the lung after allogeneic hematopoietic cell transplantation (allo-HSCT), representing a major cause for morbidity and non-relapse mortality (1, 2). Alloreactive T cells were the major detrimental factors during the pathogenesis of aGVHD (3, 4). In GVHD, the donor T cells recognize the host antigens, activate, differentiate and traffic to the target organs under guidance of cytokines and chemokines, and result in inflammatory damages in the target organs (5). IFN-γ is a central regulatory cytokine in the initiation and maintenance of aGVHD due to its crucial function for CD4+ Th1 cells differentiation and CD8+ T cells function during the priming and expansion phase (6). Regulatory T cells (Treg) which reduces the incidence and severity of aGVHD is one of the protective factors against aGVHD (7). Due to the inhibitory characteristics, Treg cells have been widely studied for GVHD treatment in pre-clinical models and clinical trials (8, 9).
Despite considerable achievements in the treatment of aGVHD, it remains a major clinical problem for the patients undergoing allo-HSCT. Approximately 40%-60% of recipients will develop aGVHD, imposing crucial risks for long term survival (10). Because the success of allo-HSCT relies on graft-versus-tumor (GVT) function mediated by T cells, immunosuppressive strategies are less attractive (5). Therefore, explorations on new mechanisms and novel therapeutic strategies for aGVHD with preserving GVT responses are important and necessary.
In our previous study, we have identified and characterized a novel classical secretory protein LYG1 (Lysozyme G-like 1) through immunogenomics strategy (11). Recombinant human LYG1 protein (rhLYG1) can inhibit tumor growth by promoting the activation and IFN-γ production of tumor antigen-specific CD4+ T cells (11). While LYG1 deficiency accelerated B16 and LLC1 tumor growth due to the inhibited T cell functions. However, the function of LYG1 in other immune diseases is unclear.
Given the enhanced T cell functions under rhLYG1 stimulation and the inhibited T cell functions with LYG1 deficiency, we hypothesized that LYG1 might participate in the development of GVHD. To verify the hypothesis, we explored the role and mechanisms of LYG1 during GVHD using aGVHD murine models in this study.
Materials and Methods
Mice
Six- to eight-week-old C57BL/6 (B6, H2Kb) and BALB/c (H2Kd) and (B6 × DBA/2) F1 (BDF1, H-2b–d) mice were purchased from Vital River Laboratories. The Lyg1 conventional knockout mice (C57BL/6 background, Lyg1-/-) were generous gifts from Prof. Wenling Han at Peking University Health Science Center (Beijing, China). IFN-γ-/-mice (B6.129S7-IFNgtm1Ts/J) were purchased from the Model Animal Research Center of Nanjing University. Homozygous knockout (Lyg1-/-) and the littermate wild-type (WT, Lyg1+/+) mice were used for all related experiments. All mice were bred at the center animal laboratory of Peking University First Hospital under specific pathogen-free conditions, and all experiments were approved by the Ethics Committee of Peking University First Hospital.
Mixed Lymphocyte Reaction (MLR)
Splenocytes derived from BALB/c mice were used as stimulator cells. CD3+ T cells were used as responder cells selected from splenocytes of Lyg1+/+ or Lyg1-/- mice using Mouse CD3+ T cell isolation kit (Biolegend, San Diego, US) according to the manufacturer’s instructions. The purity of CD3+ T cell was >90% assessed by flow cytometry. The responder cells (2 × 105 in 100 μl complete culture medium) labeled by CFSE were cultured with stimulator cells treated with mitomycin C (Selleck, Houston, US) for 30 minutes (5 × 105 in 100 μl complete culture medium) in 96 well plate. After 5 days of culture, the cells were analyzed by flow cytometry.
aGVHD Mouse Model
Bone marrow cells (BM) were collected by red blood cell lysis. Splenocytes were isolated by Ficoll gradient centrifugation. CD3+, CD4+ and CD8+ T cells were sorted from splenocytes of Lyg1+/+ or Lyg1-/- mice using Mouse CD3+, CD4+ and CD8+ T cell isolation kit (Biolegend, San Diego, US) according to the manufacturer’s instructions. The purities were >90% assessed by flow cytometry. Recipient (BALB/c, H2Kd) mice were conditioned with total body irradiation (TBI) at 750 cGy (60 Coγ source) on day 0 followed by allogeneic transplantation intravenously: 5 × 106 Lyg1+/+ B6 BM (H2Kb) and 3 × 106 CD3+ T cells (or 1.8 × 106 CD4+ T cells or 1.8 × 106 CD8+ T cells) from Lyg1+/+ or Lyg1-/- splenocytes (H2Kb) (12). BM control group were given 5 × 106 Lyg1+/+ B6 BM alone. Syngeneic transplant group (Syn) were given 5 × 106 BM (H2Kb) and 3 × 106 CD3+ T cells sorting from BALB/c splenocytes (H2Kd). Haploidentical (B6→BDF1) transplant model (Haplo-HSCT): recipient ((B6 × DBA/2) F1 (BDF1, H-2b–d)) mice were conditioned with TBI at 900 cGy on day 0 followed by allogeneic transplantation intravenously: 5 × 106 Lyg1+/+ B6 BM (H2Kb) and 2 × 107 cells from Lyg1+/+ or Lyg1-/- splenocytes (H2Kb) (13). For the experiments using rhLYG1 administration, recipient (BALB/c, H2Kd) mice were conditioned with TBI at 750 cGy on day 0 followed by allogeneic transplantation intravenously: 5 × 106 B6 BM (H2Kb) and 3 × 106 CD3+ B6 or IFN-γ-/- T cells (H2Kb). rhLYG1 were injected intraperitoneally in BALB/c recipients daily from day 1 to 7 after transplantation. Survival was monitored every day, recipient’s weight and GVHD score were measured every week. The scoring system to evaluate the severity of aGVHD includes five clinical parameters: weight, activity, skin, fur ruffling, and posture. Individual mice were scored 0-2 for each criterion (14). Representative tissues of aGVHD target organs (liver and lung) were excised from recipients on 28 days after transplantation and subjected to histopathological scoring (15, 16). Immunohistochemical (IHC) analysis for CD4+ and CD8+ (servicebio, China) were performed on the tissues of recipient mice at 7 days post-transplantation according to the manufacturer’s instructions.
GVT Mouse Model
2.5 × 104 mouse mastocytoma cell strain P815 (H2Kd) were injected intravenously into per aGVHD recipient on day 0 after allogeneic transplantation. Survival was monitored every day. The P815 was retrovirally transduced with a luc/neo plasmid using a protocol described previously (17). Mice that received P815-luc/neo were given intraperitoneal (200 mg/kg) D-Luciferin (Xenogen, Alameda, CA) and placed supine in the Xenogen IVIS bioluminescence imaging system under anesthesia with isofluorane. Pseudocolor images showing whole-body distribution of bioluminescent signal were superimposed on conventional grayscale photographs. Livers were excised from recipients died or 14 days after transplantation and tumor burden on the livers were analyzed.
Isolation of Cells and Flow Cytometry
Flow cytometry was performed using the following anti-mouse antibodies from Biolegend (Cal., US): H2Kb-FITC, CD3-APC/Cy7, CD4-PE/cy7, CD8-BV421, IFN-γ-PE, CD69-PE, CD44-FITC, CD62L-APC, T-bet-FITC. Spleens, livers and lungs were excised on day 7 after transplantation. Spleens and livers gently pressed through a cell strainer (70 µm). Livers infiltrating lymphocytes were isolated using Percoll (Living, Beijing, China). Perfused lungs were digested in RPMI-1640 medium containing type IV bovine pancreatic DNase (30 mg/ml; Sigma-Aldrich, US) and collagenase XI (0.7 mg/ml; Sigma-Aldrich, US) to obtain single-cell suspensions. Single-cell suspensions prepared from the above operation were kept on ice and blocked by incubation with anti-Fc receptor antibody. For membrane molecule analysis, cells were labeled with fluorescent conjugated antibodies at 4°C for 30 minutes followed by washes with cold PBS. For cytokine analysis, cells were stimulated with Cell Activation Cocktail (with Brefeldin A) (Biolegend, Cal., US) for 6 hours before cells were harvested for analysis. Cells were first stained with surface markers and then fixed and permeabilized with BD IntraSure Kit (BD Biosciences, NJ, US) according to the manufacturer’s instructions for intracellular staining. Foxp3 and T-bet were stained using a Foxp3 Fix/Perm Buffer Set (Biolegend, Cal., US), according to the manufacturer’s instructions. Flow cytometry analysis was performed on FACS Canto II (BD Biosciences, NJ, US) and analyzed with FlowJo software.
Measurements of Cytokines in Serum
The peripheral blood samples were obtained on day 7 after transplantation and clotted for 5 h at room temperature before centrifugation for 15 minutes at 2000g. The serums were collected and stored at -80°C. The serum concentrations of IFN-γ, TNF-α and IL-6 were quantitated using a mouse Th cytometric bead array kit (BD Biosciences, NJ, US) (Biolegend, Cal., US) according to the manufacturer’s instructions.
Real-Time Quantitative PCR (qPCR)
qPCR was performed for quantitative analyses in an ABI Prism 7000 Sequence Detection System (Applied Biosystems). Amplifications were performed using Power SYBR™ Green PCR Master Mix (Thermo Fisher Scientific, MA, US). The quantification data were analyzed with ABI Prism 7000 SDS software. The expression levels of the target genes were normalized to the internal standard gene GAPDH using the comparative Ct method (ddCt). Primers used in qPCR to examine the genes:
Foxp3:
Forward Primer (5’–3’) TTTCACCTATGCCACCCTTATC
Reverse primer (5’–3’) CATGCGAGTAAACCAATGGTAG
CCL5
Forward Primer (5’–3’) GTATTTCTACACCAGCAGCAAG
Reverse primer (5’–3’) TCTTGAACCCACTTCTTCTCTG
CXCL9
Forward Primer (5’–3’) AATCCCTCAAAGACCTCAAACA
Reverse primer (5’–3’) TCCCATTCTTTCATCAGCTTCT
CXCL10
Forward Primer (5’–3’) CAACTGCATCCATATCGATGAC
Reverse primer (5’–3’) GATTCCGGATTCAGACATCTCT
GAPDH
Forward Primer (5’–3’) CACCAACTGCTTAGCCCCC
Reverse primer (5’–3’) TCTTCTGGGTGGCAGTGATG
Statistical Analysis
Survival curve was analyzed using Kaplan-Meier method. Differences between groups in survival studies were determined using log-rank test. A student t test was applied for the other studies. Independent experiment was performed 3 times. The results in the repeats were similar in this study. p < 0.05 is considered statistically significant. *p < 0.05, **p < 0.01, and ***p < 0.001. Analyses were performed using GraphPad Prism 7.
Results
LYG1 Deficiency Inhibited Alloreactivity of CD4+ T Cells In Vitro
Firstly, we examined whether LYG1 affected the alloreactivity of CD4+ T cells in vitro by MLR assay. The expression of the activation maker CD69 on CD4+ T cells were decreased in Lyg1-/- mice compared with the Lyg1+/+ mice (Figure 1A), so was for the IFN-γ production (Figure 1B). While the percentages of Treg cells gated on CD4+ T cells were higher in Lyg1-/- group than Lyg1+/+ group (Figure 1C). The control group (without stimulating cells) had not response (Figure 1S). Whereas there were no differences in the expression of CD69, IFN-γ and Foxp3 between Lyg1+/+ and Lyg1-/- mice prior to the culture (Figure 1D). These results suggest that LYG1 deficiency restrains the alloreactivity of CD4+ T cells in vitro.
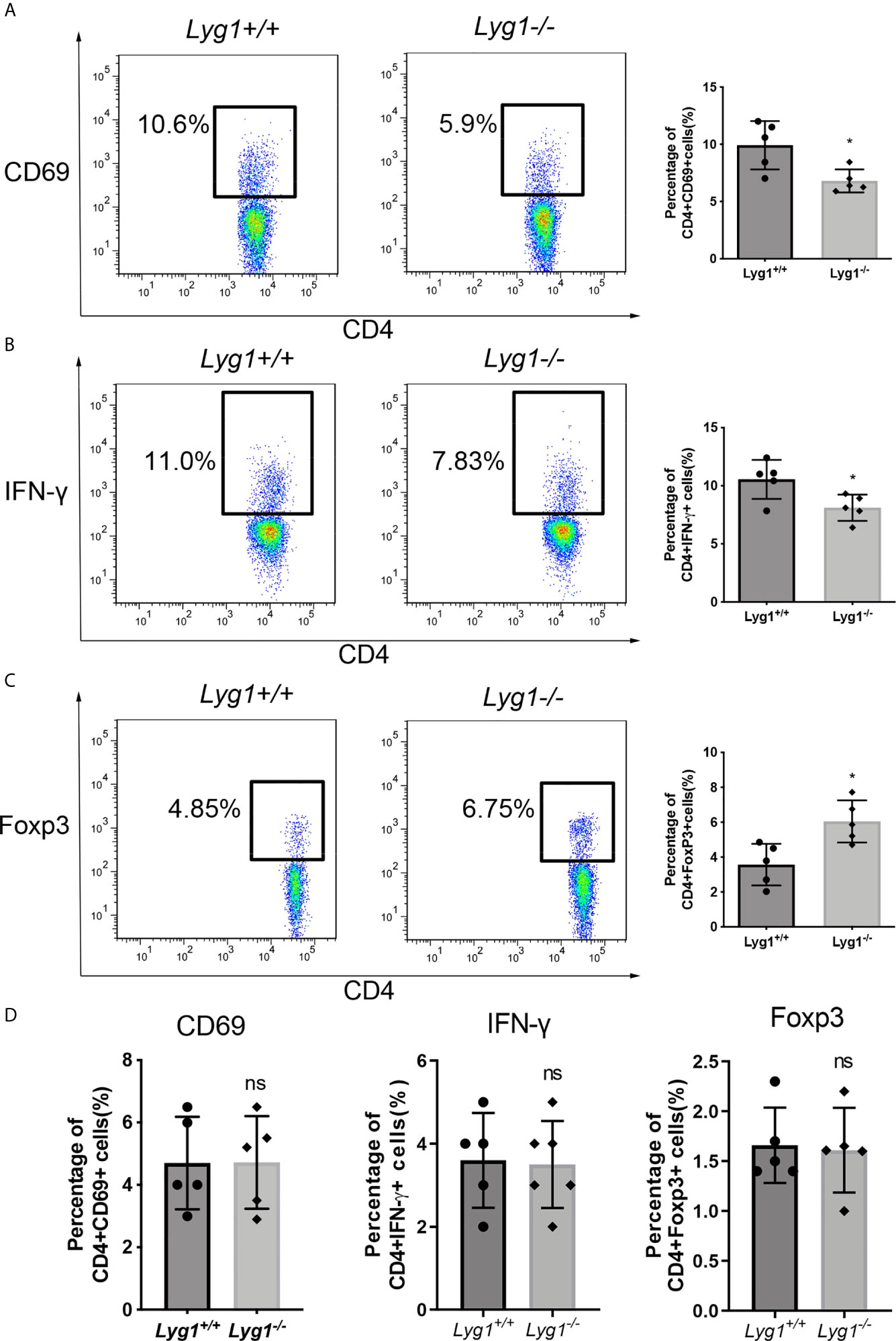
Figure 1 LYG1 deficiency inhibited alloreactivity of CD4+ T cells in vitro. In MLR assay, CD3+ T cells from Lyg1+/+ or Lyg1-/- mice as responder cells were cultured with mitomycin treated splenocytes from BALB/c mice as stimulator cells. After 5 days, CD69 expression (A), Th1 (B) and Treg (C) in the responder CD4+ T cells were detected by flow cytometry analysis. (D) The expression of CD69, IFN-γ and Foxp3 gated on Lyg1+/+ and Lyg1-/- CD4+ T cells prior to the culture. Independent experiment was performed 3 times. n = 5 per group. Representative plots gated on H2Kb+CD4+ T cells are shown and statistical results are expressed as the mean ± SD, *p < 0.05 compared with Lyg1+/+ group. ns, no significance.
LYG1 Deficiency in Donor T Cells Alleviated aGVHD
We adopted a major MHC mismatched aGVHD model to examine the role of LYG1 in the development of aGVHD (Figure 2A). There were no differences in distribution of T cells subsets, including naive (the most dominant subset), central memory and effector CD4+ T cells and CD8+ T cells, from Lyg1+/+ and Lyg1-/- mice before adoptive transfer (Figure S2). As shown in Figures 2B, C, the control mice in BM group (only transplantation of BM) and Syn group did not induce aGVHD. Comparing with recipients receiving Lyg1+/+ T cells, recipients receiving Lyg1-/- T cells showed significantly higher long-term survival rates (Figure 2B), less weight loss (Figure 2C), and lower aGVHD clinical scores (Figure 2D) after 3 weeks since the allogeneic transplantation. Pathology revealed that mice receiving Lyg1-/- T cells showed dramatically reduced inflammation in the livers and lungs compared with those receiving Lyg1+/+ T cells (Figure 2E). The histological grades of livers and lungs were significantly decreased in recipients receiving Lyg1-/- donor T cells (Figure 2F). There was no pathological lesion and inflammation in BM group and Syn group (Figures 2E, F). We also examined the LYG1 effect on aGVHD using haplo-HSCT model. The mice receiving Lyg1-/- T cells also exhibited a higher survival rate than did the control mice (Figure 2G). These results proved that LYG1 deficiency in donor T cells decreased aGVHD mortality and severity.
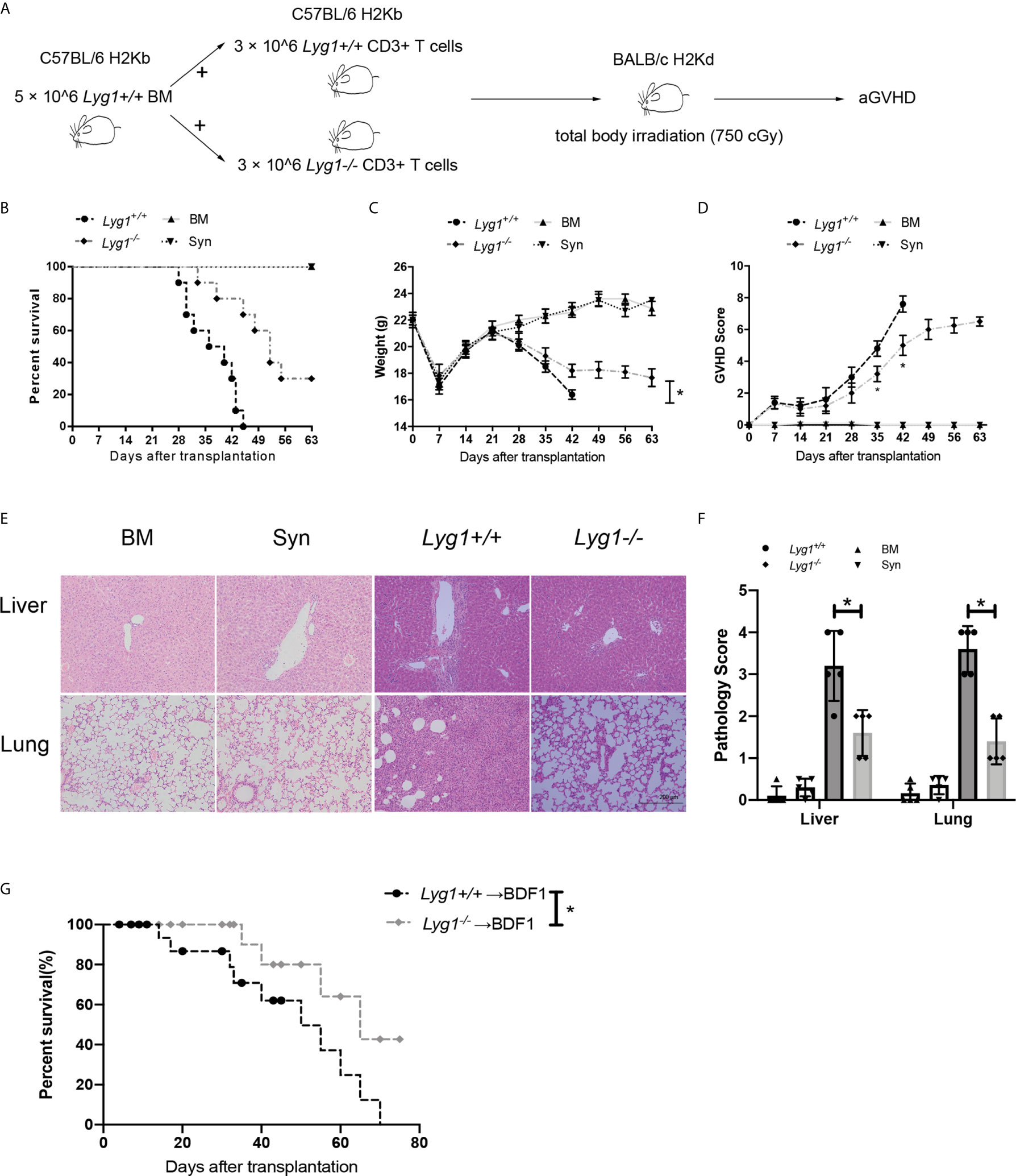
Figure 2 LYG1 deficiency in donor T cells alleviated aGVHD. Lethally irradiated BALB/c mice were reconstituted with 5 × 106 Lyg1+/+ BM and 3 × 106 T cells from Lyg1+/+ mice (Lyg1+/+ group) or Lyg1-/- mice (Lyg1-/- group). BM control (BM group) were given 5 × 106 Lyg1+/+ BM alone. (A) The diagram illustrating the experimental procedure. Survival (B), weight (C) and aGVHD scores (D) were monitored. (E) Histological examination (×200 magnification) of liver and lung in four groups were analyzed on day 28 after transplantation. (F) Histologic scores of liver and lung were shown. (G) Survival of BDF1 recipients given transplants with 5 × 106 Lyg1+/+ BM and 2 × 107 splenocytes from Lyg1+/+ mice (Lyg1+/+ group) or Lyg1-/- mice (Lyg1-/- group). Independent experiment was performed 3 times. Data pooled: 3 experiments (n = 10 for Lyg1+/+ group and Lyg1-/- group, n = 5 for BM group). Results are expressed as the mean ± SD, *p < 0.05 compared with Lyg1+/+ group.
LYG1 Deficiency in Donor T Cells Dampened the Function of Allogeneic CD4+ T Cells in Spleens
First, we examined the donor chimerism in the spleen of recipient mice on day 7 after transplantation. Nearly 98% of H2Kb+ donor cells can be observed in recipient spleens in Lyg1+/+ and Lyg1-/- aGVHD groups (Figure 3A), suggesting LYG1 deficiency in donor T cells did not affect the engraftment of donor cells. To explore potential regulation mechanisms for LYG1 in aGVHD, we investigated the activation and differentiation of H2Kb+ donor T cells from recipients receiving either Lyg1+/+ or Lyg1-/- T cells. We did not observe notable difference in the absolute number (data not shown) and ratio of CD4+ or CD8+ T cells between the two groups (Figure 3B); however, we noticed significant decrease of CD69 expression on CD4+ T in mice receiving Lyg1-/- T cells (Figures 3C, D), suggesting reduced activation of CD4+ T cells in these mice. The effector T cells (CD44hiCD62Llo) also decreased in mice receiving Lyg1-/- T cells (Figures 3E, F). The IFN-γ producing CD4+ (Th1) and IFN-γ producing CD8+ T cells (Tc1, T cytotoxic 1) were significantly reduced in recipients of Lyg1-/- T cells compared with those of Lyg1+/+ group (Figures 3G, H). T-bet is a master regulator for Th1 differentiation and IFN-γ production (18).Then we examined T-bet and found the percentages of T-bet on CD4+ T cells were lower in mice receiving Lyg1-/- T cells (Figure 3I).
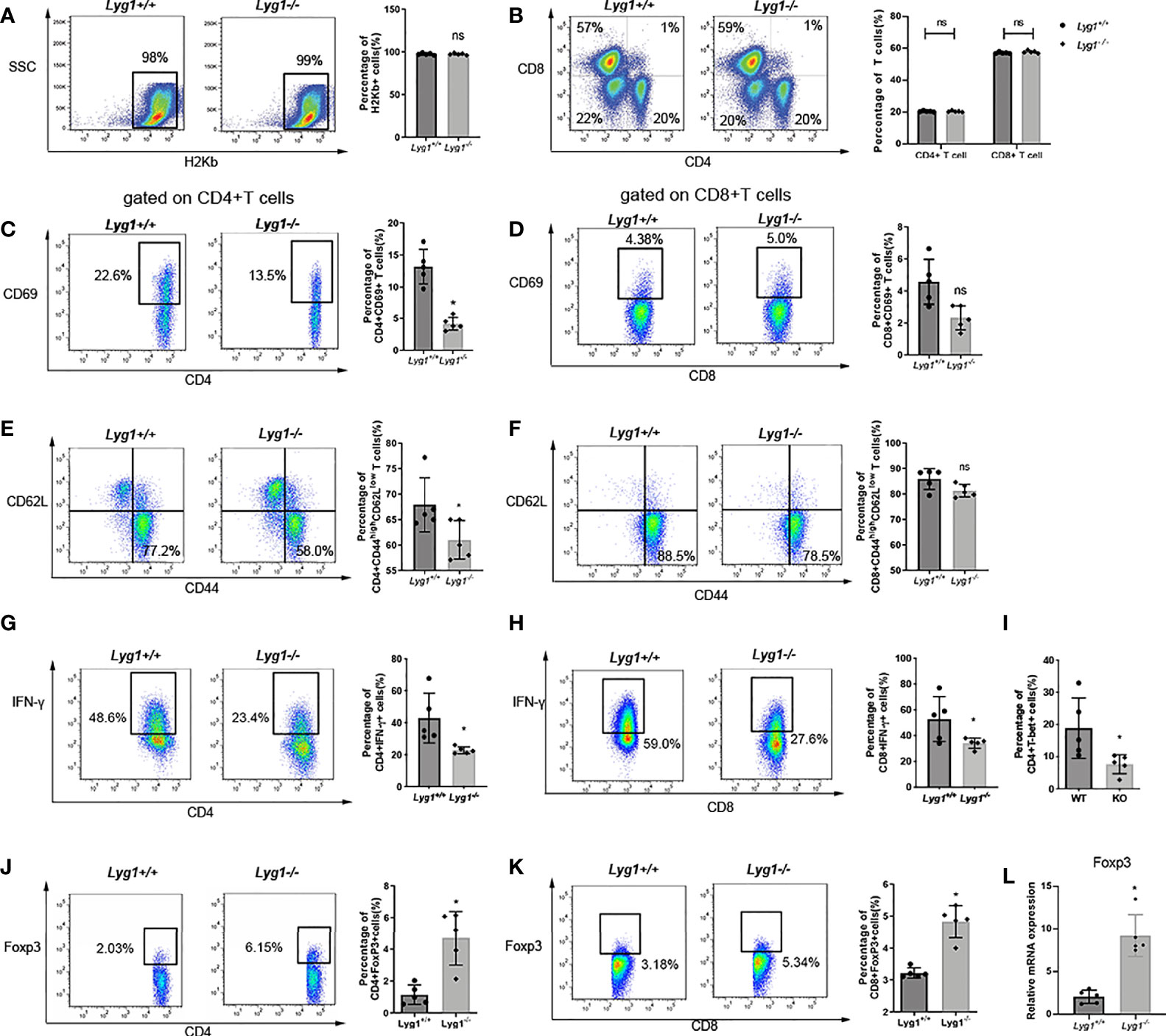
Figure 3 LYG1 deficiency reduced allogeneic T cells function in spleens. Splenocytes of recipient mice were isolated on day 7 after transplantation and analyzed by flow cytometry and qPCR. (A) The percentages of H2Kb+ cells in living splenocytes. (B) The percentages of CD4+ T and CD8+ T cells in H2Kb+ splenocytes. (C, D) The percentages of CD69 expression in CD4+ T cells and CD8+ T cells. (E, F) The expression of effector (CD44hiCD62Llo) phenotype gated on CD4+ T and CD8+ T cells. (G, H) The percentages of IFN-γ expression in CD4+ T cells and CD8+ T cells. (I) The percentages of T-bet expression in CD4+ T cells. (J, K) The percentages of Treg in CD4+ T cells and CD8+ T cells. The percentages of Figure 3 (C–K) were all gated on H2Kb+CD4+ cells or H2Kb+CD8+ cells. (L) Foxp3 expression of splenocytes were examined by qPCR. Independent experiment was performed 3 times. The results in the repeats were similar. n = 5 per group. Representative plots are shown and statistical results are expressed as the mean ± SD, *p < 0.05 compared with Lyg1+/+ group. ns, no significance.
Treg cells have been shown to be capable of reducing the severity of aGVHD by restraining immoderate immune activation and maintaining immune homeostasis (19). We found that the proportions of Treg cells (Foxp3+ gated on CD4+ T cells) in spleens were dramatically enhanced from recipients received Lyg1-/- donor T cells (Figure 3J), suggesting LYG1 deficiency in donor T cells promotes Treg differentiation. Interestingly, the proportions of Foxp3+ population gated on CD8+ T cells increased in spleens in Lyg1-/- group than Lyg1+/+ group (Figure 3K). The mRNA expression of Foxp3 in spleens were also higher in recipients received Lyg1-/- donor T cells (Figure 3L).
We found the similar results in the haplo-HSCT model, LYG1 deficiency in donor T cells decreased the expression of CD69 and IFN-γ, but increased the expression of FoxP3 on T cells (Figure S3). Whereas the BM and Syn control group had a lower T cells response (Figure S4).
LYG1 Deficiency in Donor T Cells Inhibited Allogeneic CD4+ T Cells Infiltration in aGVHD Target Organs
We also evaluated the lymphocytes in livers and lungs, the representative target organs of aGVHD. Similarly with spleens, the infiltrating lymphocytes were almost H2kb+ donor cells (data not shown). A significant reduction of CD4+ T cells in livers and lungs were observed in mice receiving Lyg1-/- donor T cells compared with the Lyg1+/+ group determined by IHC (Figure 4A). CD8+ T cells infiltration in aGVHD target organs also reduced slightly in Lyg1-/- group (Figure 4B). The T cells infiltration in livers and lungs were rarely detected in BM and Syn control group. The decrease of T cells infiltrating in livers and lungs suggested that LYG1 deficiency might change the expression of chemokines that recruited T cells. Therefore, we examined the expression of CCL5, CXCL9, CXCL10 in livers and lungs. LYG1 deficiency inhibited significantly the mRNA expression of CXCL9 and CXCL10, but had no obvious effect on CCL5 expression (Figures 4C, D).
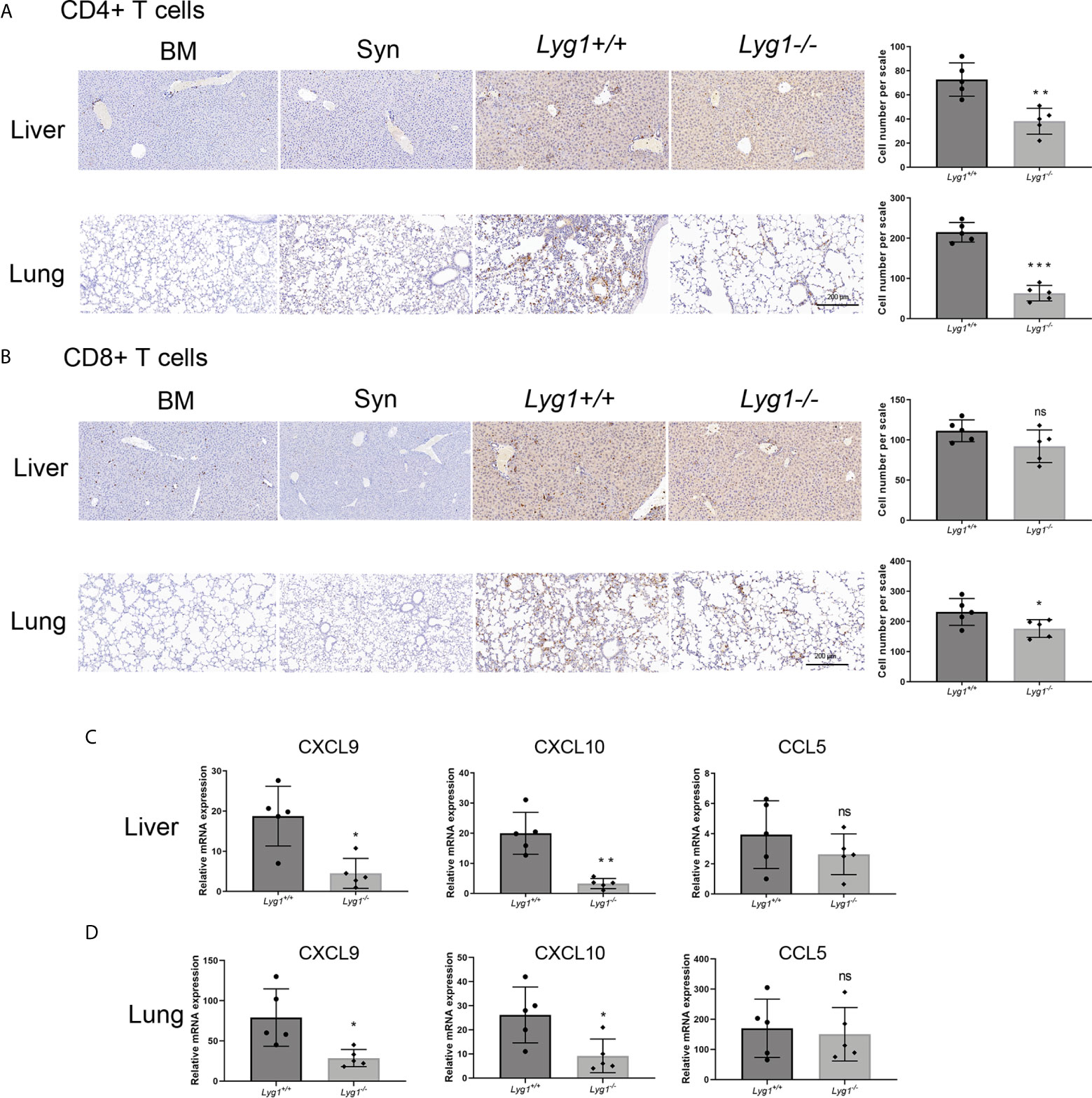
Figure 4 LYG1 deficiency inhibited allogeneic CD4+ T cells infiltration in aGVHD target organs. The samples of livers and lungs were excised at day 7 after transplantation and stained with antibodies CD4 and CD8. (A, B) The infiltration of CD4+ T cells and CD8+ T cells in livers and lungs in BM, Syn group, or mice receiving Lyg1+/+ and Lyg1-/- donor T cells determined by IHC assay (×200 magnification). The left is one representative section per group. The right is the number of CD4+ T cells and CD8+ T cells per scale in the livers and lungs. (C, D) Chemokines expression were examined by qPCR in lymphocytes isolated from livers and lungs. Independent experiment was performed 3 times. The results in the repeats were similar. n = 5 per group. Representative sections are shown and statistical results are expressed as the mean ± SD, *p < 0.05, **P < 0.01 and ***P < 0.001 compared with Lyg1+/+ group. ns, no significance.
LYG1 Deficiency in Donor T Cells Inhibited the Function of Allogeneic CD4+ T Cells in GVHD Target Organs
LYG1 deficiency reduced the number of T cells infiltrating in GVHD target organs, whether it affect allogeneic T cells function? Therefore, we investigated the activation and differentiation of donor T cells in livers and lungs from recipients receiving either Lyg1+/+ or Lyg1-/- T cells. Similarly, the CD69 and IFN-γ expression of CD4+ T cells and CD8+ T cells decreased in Lyg1-/- recipient livers and lungs compared with the Lyg1+/+ groups (Figures 5A–D). The percentages of Foxp3+ population gated on CD4+ T cells and CD8+ T cells were higher in livers and lungs in Lyg1-/- group than Lyg1+/+ group (Figures 5E, F). The expression of Foxp3 in mRNA level in livers and lungs also increased in recipients received Lyg1-/- donor T cells (Figures 5G, H).
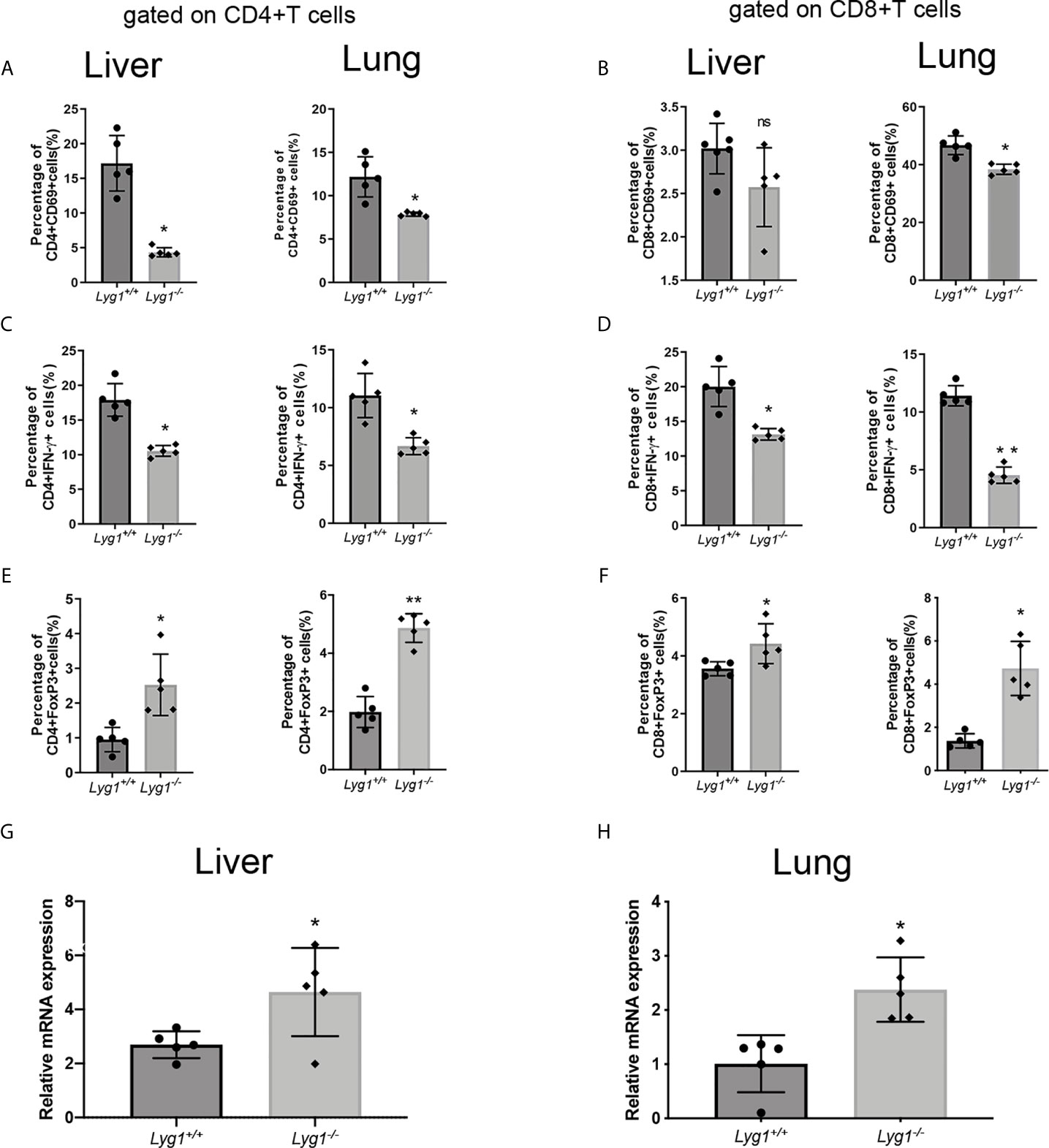
Figure 5 LYG1 deficiency inhibited allogeneic T cells function in livers and lungs. Lymphocytes were isolated from livers and lungs of recipient mice on day 7 after transplantation and analyzed by flow cytometry and qPCR. (A, B) The percentages of CD69 expression in CD4+ T cells and CD8+ T cells. (C, D) The percentages of IFN-γ expression in CD4+ T cells and CD8+ T cells. (E, F) The percentages of Treg in CD4+ T cells and CD8+ T cells. The percentages of Figure 5 (A–F) were all gated on H2Kb+CD4+ cells or H2Kb+CD8+ cells in lymphocytes isolated from livers and lungs. (G, H) Foxp3 expression of lymphocytes isolated from livers and lungs were examined by qPCR. Independent experiment was performed 3 times. The results in the repeats were similar. n=5 per group. Statistical results are expressed as the mean ± SD, *p < 0.05 and **P < 0.01 compared with Lyg1+/+ group. ns, no significance.
LYG1 Mediated GVHD Development Mainly Through CD4+ T Cells
To test whether the effects of LYG1 on GVHD mediated through CD4+ T cells or CD8+ T cells, we performed GVHD models using purified CD4+ T cells or CD8+ T cells as grafts, respectively. As illustrated in Figures 6A, F, the reduction of aGVHD lethality by LYG1 deficiency was observed in CD4+ T cells transplant, but not CD8+ T cells transplant. LYG1 deficiency in CD4+ T cells transplant significantly reduced the activation of CD4+ T cells and IFN-γ and T-bet expression, but increased Treg ratio (Figures 6B–E), but not in CD8+ T cells transplant (Figures 6G–I). Taken together, the results suggested that LYG1-mediated GVHD development mainly depended on CD4+ T cells, but not CD8+ T cells.
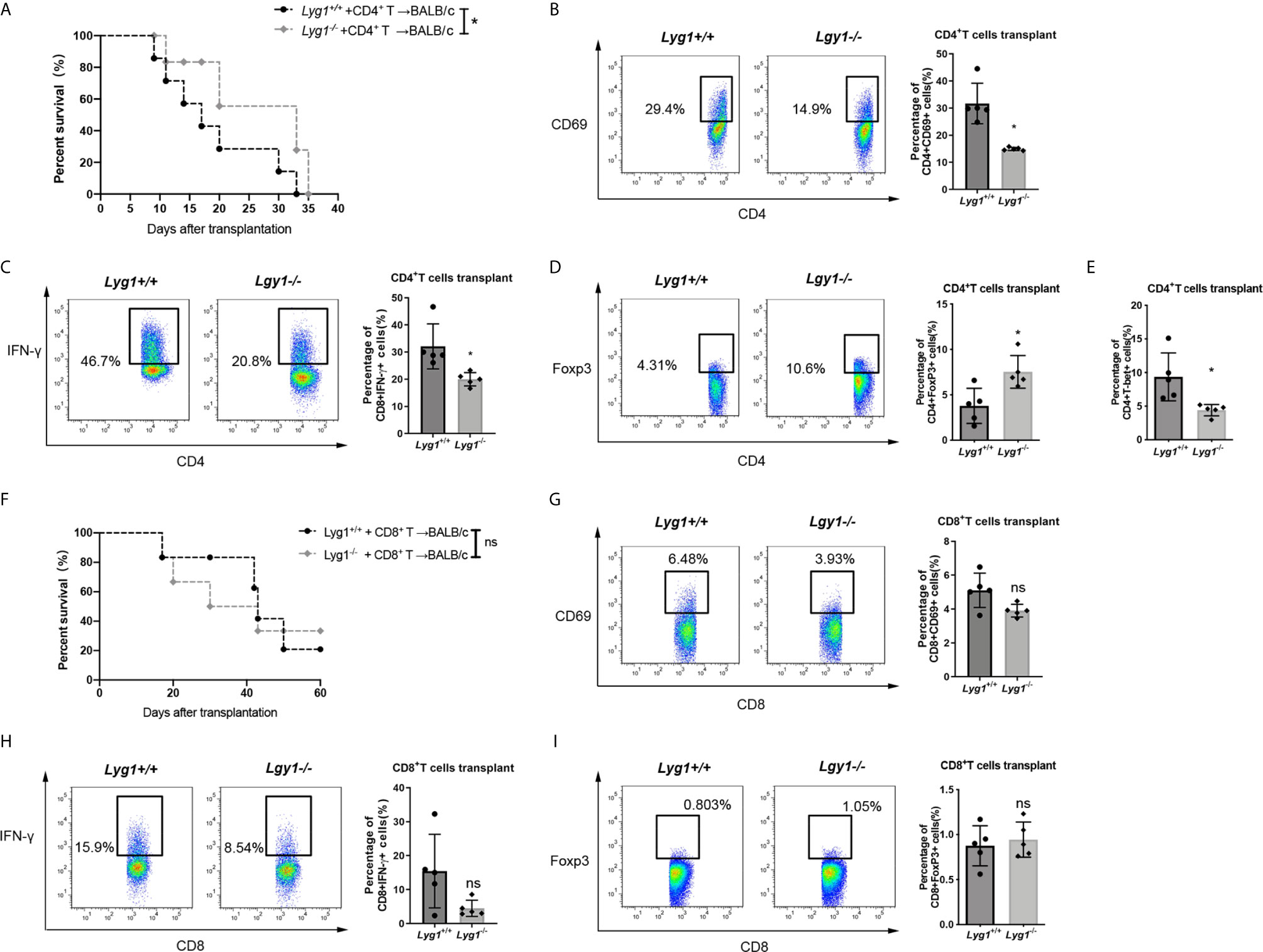
Figure 6 LYG1 mediated aGVHD development mainly through CD4+ T cells. Survival of mice receiving CD4+ (A) and CD8+ (F) donor T cells were monitored. Data pooled: n = 10 for Lyg1+/+ group and Lyg1-/- group (A, F). (B–E) The expression of CD69, IFN-γ, Foxp3 and T-bet gated on CD4+ T cells. (G–I) The expression of CD69, IFN-γ and Foxp3 gated on CD8+ T cells. n = 5 per group (B–E, G–I). Representative plots are shown and statistical results are expressed as the mean ± SD, *p < 0.05 compared with Lyg1+/+ group. ns, no significance.
rhLYG1 Aggravated aGVHD via Promoting IFN-γ Production and Inhibiting Foxp3 Expression
Furthermore, we used the purified rhLYG1 to evaluate the role of LYG1 in aGVHD model. As shown in Figures 7A, B, rhLYG1 significantly accelerated and exacerbated the death and weight loss compared with PBS control. Higher clinical aGVHD scores were seen in rhLYG1 group than in control group (Figure 7C). The IFN-γ production of CD4+ T cells and CD8+ T cells (Th1 and Tc1 cells) were significantly higher than PBS control in spleens (Figures 7D, E). The mRNA expression of FoxP3 in spleens decreased in mice treated with rhLYG1 compared with PBS (Figure 7F). The IFN-γ concentrations in serum from mice treated with rhLYG1 were higher than that from PBS group (Figure 7G). We also detected the IFN-γ, TNF-α and IL-6 in serum in the above GVHD models and found that the concentrations of IFN-γ, TNF-α and IL-6 reduced in recipients received Lyg1-/- donor T cells compared with recipients received Lyg1-/- donor T cells, especially for IFN-γ (Figure S5). Further we verified the role of IFN-γ in the effects of LYG1 on GVHD using IFN-γ-/- T cells as grafts. As showed in Figures 7H–J, with the deficiency of IFN-γ, the effect of rhLYG1 aggravating aGVHD was partially abrogated, which reconfirmed the crucial role of IFN-γ in LYG1-mediated GVHD development.
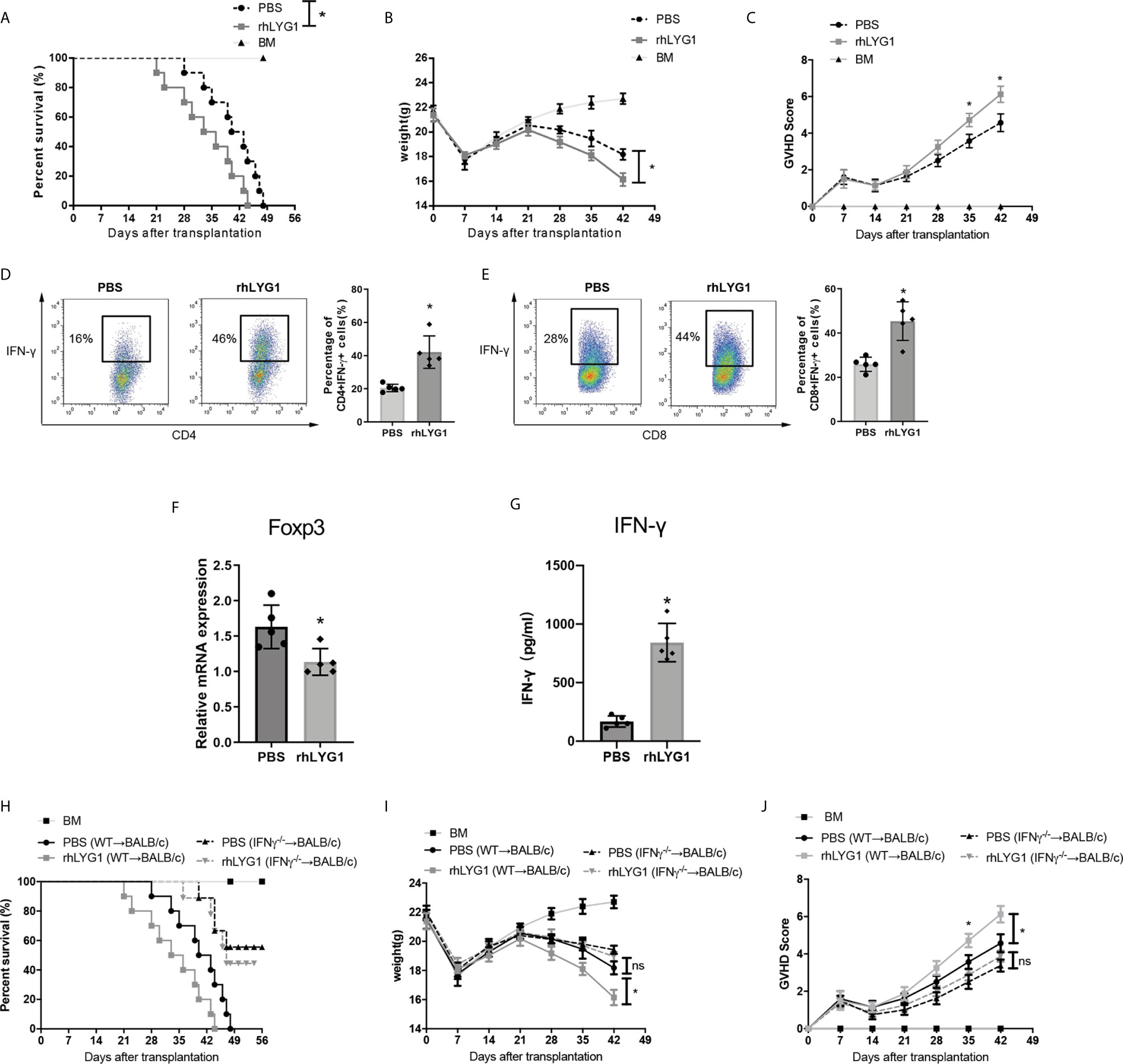
Figure 7 rhLYG1 aggravates aGVHD via promoting IFN-γ production and inhibiting Foxp3 expression. (A–C) Lethally irradiated BALB/c mice were reconstituted with 5 × 106 B6 BM and 3 × 106 B6 CD3+ T cells, rhLYG1 (20 μg per mice) or PBS was injected intraperitoneally (i.p.) each day on days 0 to +7 after transplantation. Survival (A), weight (B) and aGVHD scores (C) were monitored. (D, E) The percentages of IFN-γ positive gated on H2Kb+CD4+ T cells and H2Kb+CD8+ T cells in spleens at day 7 after transplantation. (F) Foxp3 expression of splenocytes were examined by qPCR at day 7 after transplantation. (G) The concentrations of IFN-γ in serum at day 7 after transplantation. (H–J) Lethally irradiated BALB/c mice were reconstituted with 5 × 106 B6 BM and 3 × 106 IFN-γ -/-CD3+ T cells, rhLYG1 (20 μg per mice) or PBS was injected intraperitoneally (i.p.) each day on days 0 to +7 after transplantation. Survival (H), weight (I) and aGVHD scores (J) were monitored. (A–C, H–J) Data pooled: n = 10 for PBS group and rhLYG1 group, n = 5 for BM group. (D–G) Independent experiment was performed 3 times. The results in the repeats were similar. n = 5 per group. Representative plots are shown and statistical results are expressed as the mean ± SD, *p < 0.05 compared with PBS group. ns, no significance.
LYG1 Deficiency in Donor T Cells Preserved GVT Response
To determine whether the reduction of aGVHD lethality by LYG1 deficiency would affect GVT activity, mouse mastocytoma cell strain P815 (H2Kd) were injected intravenously on day 0 to generate murine GVT model. The mice receiving Lyg1-/- T cells exhibited a higher survival rate, lower tumor signal and lower tumor burden than that of the mice receiving Lyg1+/+ T cells and BM cells (Figures 8A–C). Furthermore, there was no evident GVHD as the time of death in GVT model mice. The results suggested that targeting LYG1 might be an alternative to ameliorating aGVHD without impairing GVT function.
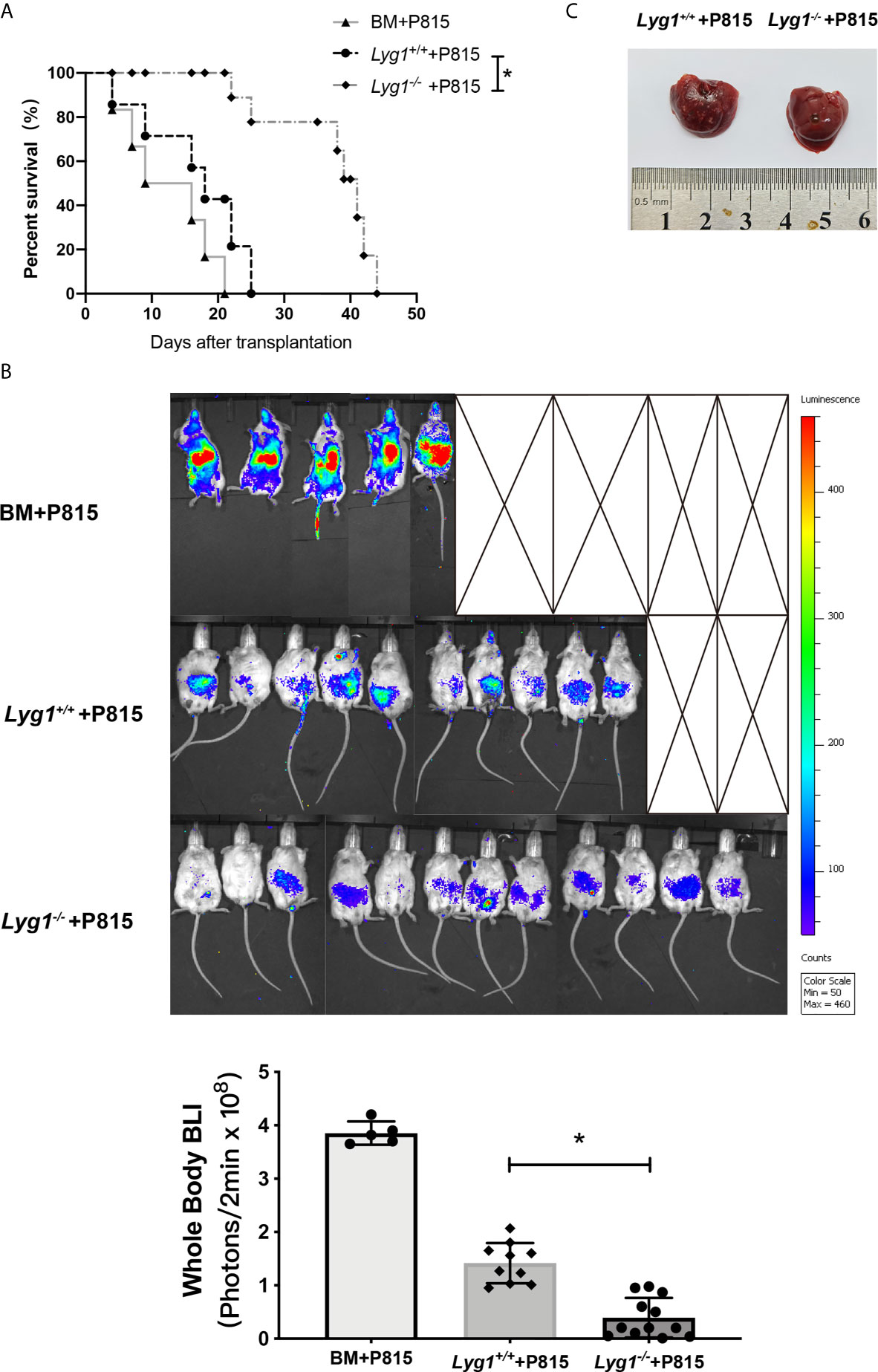
Figure 8 LYG1 deficiency in donor T cells preserved GVT response. Lethally irradiated BALB/c mice were reconstituted with 5 × 106 B6 BM with or without 3 × 106 T cells from Lyg1+/+ or Lyg1-/- mice, followed by 2.5 × 104 P815 cells (H2Kd) injected intravenously. (A) Survival after transplantation was monitored. (B) Tumor growth was monitored using bioluminescence imaging on day 14. Bioluminescence was quantified using whole body with Living Image software. Whole body images are shown and statistical results of average bioluminescence intensities are expressed as the mean ± SD, *p < 0.05 compared with Lyg1+/+ group. (C) Livers were excised when the mice died or on day 14 after transplantation. n = 12 for Lyg1+/+ or Lyg1-/- mice group, n = 10 for BM group. *p < 0.05 compared with Lyg1+/+ group.
Discussion
In this study, the role and mechanisms of LYG1 in aGVHD were explored. We demonstrated that mice receiving Lyg1-/- donor T cells alleviated aGVHD, increased long-term survival rates, showed less weight loss, lower GVHD clinical pathological scores and milder tissues damages, than mice receiving Lyg1+/+ donor T cells in CD3+ or CD4+ T cells transplanting-major MHC mismatched aGVHD model and in haplo-HSCT model. Additionally, rhLYG1 intraperitoneally administration aggravated aGVHD severity, which confirmed the results established in the Lyg1-/- mice. Furthermore, we discovered that LYG1 deficiency in donor T cells can decrease infiltration of alloreactive CD4+ T cells in aGVHD mice target organs, inhibit alloreactive of CD4+ T cells and Th1 differentiation, promote Treg differentiation of allogeneic CD4+ T cells in vitro and in vivo.
Donor-derived CD4+ T cells are particularly important in the pathogenesis of aGVHD. A large number of clinical trials have taken CD4+ T cells as a potential target for GVHD treatment (3). Firstly we proved that LYG1 mediated GVHD development mainly through CD4+ T cells, but not CD8+ T cells by using purified CD4+ and CD8+ T cells as grafts. In aGVHD, alloreactive CD4+T cells are directed by chemokines and migrate to target tissues and organs where they cause tissue injury (20–22). CXCL9, CXCL10-CXCR3 interactions has been linked to activated T cell trafficking to aGVHD target organs in humans and mice (16). Our previous study found that rhLYG1 administration in mice can enhance the expression of T cell chemokines, including CCL5, CXCL9 and CXCL10, and infiltration of T cells in tumors (11). In this study, the decreased infiltration of allogeneic CD4+ T cells in the livers and lungs of mice that received Lyg1-/- T cells, which maybe related that LYG1 deficiency inhibited the expression of CXCL9 and CXCL10, explained partially that LYG1 deficiency in donor T cells suppressed aGVHD.
Another mechanism for LYG1 deficiency alleviating GVHD was able to inhibit IFN-γ production of donor derived T cells. IFN-γ plays an important promoting role in the alloreactivity of donor derived T cells in aGVHD (23). However, other studies have found that IFN-γ played a protective role against aGVHD, which depended on the time phase of IFN-γ production in allo-HSCT (23–25). Exogenous injection of IL-12 or IL-18 increased the expression of IFN-γ, thereby inducing the expression of Fas in donor T cells, leading to activation-induc,ed cell death, reducing donor T cells responses to host antigens and finally attenuating aGVHD (26, 27). Our previous studies have shown that rhLYG1 can promote antigen specific activity and IFN-γ production of T lymphocytes in tumor models (11). In this study, we found inhibited activation of CD4+ T cells and IFN-γ expression of allogeneic T cells in mice receiving Lyg1-/- donor T cells, whereas rhLYG1 administration aggravated aGVHD severity through promoting IFN-γ production of allogeneic T cells, more importantly, the absence of IFN-γ in donor T cells could partially abrogate rhLYG1-induced GVHD development, corroborating that the effect of LYG1 on aGVHD were mainly mediated by IFN-γ.
Treg cells play a significant role in maintaining tolerance in aGVHD by limiting T cell function (28). Many studies have proven that therapeutic modulation or adoptive transfer of Treg can directly prevent GVHD (29). CD8+Foxp3+ T cells, a Treg subpopulation, can be induced and ameliorate GVHD in mouse models (30). In our study, LYG1 deficiency led to the enhanced proportions of Treg cells in vitro. Similarly, the absence of LYG1 in donor T cells increased the proportions of allogeneic Treg (CD4+Foxp3+ T cells and CD8+Foxp3+ T cells) in different GVHD models in vivo. These results provided another explanation that LYG1 deficiency in donor T cells alleviated GVHD. Importantly, CD8+Foxp3+ Treg cells display cytotoxic activity which can suppress tumor during GVHD (31). These results explained partially if not fully that LYG1 deficiency in donor T cells suppressing GVHD while preserving GVT effect.
Our study demonstrated that LYG1 deficiency in donor T cells suppressed Th1 cells and promoted Treg cells differentiation in aGVHD model. Th cell differentiation is regulated by multiple cytokines and transcription factors. In the absence of IL-6, TGF-β stimulates a transcriptional program in naive CD4+ T cells with Foxp3 up-regulation and leads the evolvement of Treg cells (32).TNF-α blockade was shown to increase Foxp3 expression in patients with RA (33, 34). In this study, we found that the absence of LYG1 in donor T cells reduced the production of IL-6 and TNF-α in different GVHD models. Therefore, we speculated that LYG1 deficiency promoted Treg cells differentiation by inhibiting IL-6 and TNF-α. T-bet is a transcriptional activator of IFN-γ and orchestrates the cell-migratory program by directly controlling expression of the chemokine receptors CXCR3 (18). We showed that the absence of LYG1 decreased the expression of T-bet and CXCL10 in GVHD models. It was supposed that LYG1 deficiency suppressed Th1 cells polarization via inhibiting T-bet pathway related with CXCL10-CXCR3 axis, which was consistent with this report (35).
As a secretory protein, the cell sources of LYG1 are unclear. In this study, we demonstrated the role of LYG1 in aGVHD using WT BM and Lyg1+/+ or Lyg1-/- T cells as the graft, but not KO BM and Lyg1+/+ or Lyg1-/- T cells as the graft. Because only transplantation of allogeneic BM did not induce aGVHD, we excluded the effects of LYG1 derived from of BM in aGVHD mouse model we used in this study. Second, the recipients and its irradiation conditions of WT and KO groups were all the same, so we excluded the impacts of LYG1 derived from recipients in aGVHD mouse model we used. More importantly, rhLYG1 aggravated the aGVHD severity by promoting IFN-γ production and inhibiting Foxp3 expression, providing orthogonal validation for the results established using the Lyg1-/- mice.
In summary, we demonstrate LYG1 regulates aGVHD via altering the alloreactivity of CD4+ T cells and the balance of Th1 and Treg differentiation of allogeneic CD4+ T cells. Our study indicates that LYG1 may be a novel target in aGVHD by mitigating aGVHD without impairing GVT effect. The therapeutic effect of targeting LYG1 is required in future investigations.
Data Availability Statement
The original contributions presented in the study are included in the article/Supplementary Material. Further inquiries can be directed to the corresponding authors.
Ethics Statement
The animal study was reviewed and approved by Ethics Committee of Peking University First Hospital. Written informed consent was obtained from the owners for the participation of their animals in this study.
Author Contributions
HL contributed conception and design of the study, performed the most experiments of this study and wrote the paper. ZY performed the MLR assay and part of aGVHD models. BT, SM, and CQ helped to establish aGVHD mouse models. YL, ZL, YS, and YZ performed part of flow cytometric analysis. QW, MY, and ZS performed part of qPCR experiments. HR and YD contributed conception and design of the study. All authors contributed to the article and approved the submitted version.
Funding
This study was supported by grant from The National Natural Science Foundation of China (NSFC) (Grant Number 81600144, 82071757 and 81970160) and grant from Beijing Natural Science Foundation (Grant Number 7212115).
Conflict of Interest
The authors declare that the research was conducted in the absence of any commercial or financial relationships that could be construed as a potential conflict of interest.
Acknowledgments
The authors would like to thank Prof. Wenling Han for insightful suggestions and providing Lyg1-/- mice and LYG1-expressing vector at Peking University Health Science Center (Beijing, China).
Supplementary Material
The Supplementary Material for this article can be found online at: https://www.frontiersin.org/articles/10.3389/fimmu.2021.647894/full#supplementary-material
Supplementary Figure 1 | Gating strategies for flow cytometry analyses presented in Figure 1. (A–C) The expression of CD69 (A), IFN-γ (B) and Foxp3 (C) in isotype (ISO) (left) and negative control without stimulating cells (right).
Supplementary Figure 2 | Gating strategies for flow cytometry analyses in T cells before adoptive transfer presented in Figure 2 (A). (B, C) Representative flow cytometry plots and frequencies of naive (CD44loCD62Lhi), central memory (CD44hiCD62Lhi), effector (CD44hiCD62Llo) in CD4+ T cells and CD8+ T cells respectively. n=5 per group. (D, E) The percentages of CD3+ T, CD4+ T and CD8+ T cells in BM cells from donor mice before adoptive transfer.
Supplementary Figure 3 | LYG1 deficiency reduced allogeneic T cells function in haploidentical transplant model. Splenocytes of recipient mice were isolated on day 14 after transplantation and analyzed by flow cytometry. (A, B) The percentages of CD69 expression in CD4+ T cells and CD8+ T cells. (C, D) The percentages of IFN-γ expression in CD4+ T cells and CD8+ T cells. (E, F) The percentages of Foxp3 expression in CD4+ T cells and CD8+ T cells. n=5 per group.
Supplementary Figure 4 | Gating strategies for flow cytometry analyses presented in Figure 3. The expression of CD69 (A, B), CD44 and CD62L (C, D), IFN-γ (E, F) and Foxp3 (G, H) in CD4+ T cells and CD8+ T cells respectively in ISO (left), BM control (middle) and Syn control (right).
Supplementary Figure 5 | The concentrations of IFN-γ, TNF-α and IL-6 in serum in aGVHD models. (A)The concentrations of IFN-γ, TNF-α and IL-6 in serum at day 7 after transplantation in major MHC mismatched aGVHD model. (B)The concentrations of IFN-γ, TNF-α and IL-6 in serum at day 14 after transplantation in haploidentical model. (C, D) The concentrations of IFN-γ, TNF-α and IL-6 in serum at day 7 after transplantation in purified CD4+ T cells or CD8+ T cells. n=4-5 per group. Statistical results are expressed as the mean ± SD, *p< 0.05 compared with Lyg1+/+ group.
Abbreviations
aGVHD, acute graft-versus-host disease; allo-HSCT, allogeneic allogeneic hematopoietic cell transplantation; BM, Bone marrow cells; Treg, Regulatory T cells; Th1, T helper 1 cells; Tc1, T cytotoxic 1 cells; rhLYG1, recombinant human LYG1 protein; LYG1, Lysozyme G-like 1; MLR, mixed lymphocyte reaction; TBI, total body irradiation; GVT, graft-versus-tumor.
References
1. Holtan SG, Pasquini M, Weisdorf DJ. Acute Graft-Versus-Host Disease: A Bench-To-Bedside Update. Blood (2014) 124(3):363–73. doi: 10.1182/blood-2014-01-514786
2. Ferrara JL, Levine JE, Reddy P, Holler E. Graft-Versus-Host Disease. Lancet (London England) (2009) 373(9674):1550–61. doi: 10.1016/s0140-6736(09)60237-3
3. Blazar BR, Murphy WJ, Abedi M. Advances in Graft-Versus-Host Disease Biology and Therapy. Nat Rev Immunol (2012) 12(6):443–58. doi: 10.1038/nri3212
4. Ichiki Y, Bowlus CL, Shimoda S, Ishibashi H, Vierling JM, Gershwin ME. T Cell Immunity and Graft-Versus-Host Disease (GVHD). Autoimmun Rev (2006) 5(1):1–9. doi: 10.1016/j.autrev.2005.02.006
5. Magenau J, Reddy P. Next Generation Treatment of Acute Graft-Versus-Host Disease. Leukemia (2014) 28(12):2283–91. doi: 10.1038/leu.2014.195
6. Henden AS, Hill GR. Cytokines in Graft-Versus-Host Disease. J Immunol (2015) 194(10):4604–12. doi: 10.4049/jimmunol.1500117
7. Romano M, Tung SL, Smyth LA, Lombardi G. Treg Therapy in Transplantation: A General Overview. Transpl Int (2017) 30(8):745–53. doi: 10.1111/tri.12909
8. Blazar BR, MacDonald KPA, Hill GR. Immune Regulatory Cell Infusion for Graft-Versus-Host Disease Prevention and Therapy. Blood (2018) 131(24):2651–60. doi: 10.1182/blood-2017-11-785865
9. Elias S, Rudensky AY. Therapeutic Use of Regulatory T Cells for Graft-Versus-Host Disease. Br J Haematol (2019) 187(1):25–38. doi: 10.1111/bjh.16157
10. Choi SW, Reddy P. Current and Emerging Strategies for the Prevention of Graft-Versus-Host Disease. Nat Rev Clin Oncol (2014) 11(9):536–47. doi: 10.1038/nrclinonc.2014.102
11. Liu H, Zhang Y, Liu Z, Wang P, Mo X, Fu W, et al. LYG1 Exerts Antitumor Function Through Promoting the Activation, Proliferation, and Function of CD4(+) T Cells. Oncoimmunology (2017) 6(4):e1292195. doi: 10.1080/2162402X.2017.1292195
12. Bader CS, Barreras H, Lightbourn CO, Copsel SN, Wolf D, Meng J, et al. STING Differentially Regulates Experimental GVHD Mediated by CD8 Versus CD4 T Cell Subsets. Sci Transl Med (2020) 12(552):eaay5006. doi: 10.1126/scitranslmed.aay5006
13. Zhang XH, Zhou Y, Zhang JM, Zhou SY, Wang M, Feng R, et al. IL-35 Inhibits Acute Graft-Versus-Host Disease in a Mouse Model. Int Immunopharmacol (2015) 29(2):383–92. doi: 10.1016/j.intimp.2015.10.025
14. Cooke KR, Kobzik L, Martin TR, Brewer J, Delmonte J Jr., Crawford JM, et al. An Experimental Model of Idiopathic Pneumonia Syndrome After Bone Marrow Transplantation: I. The Roles of Minor H Antigens and Endotoxin. Blood (1996) 88(8):3230–9. doi: 10.1182/blood.V88.8.3230.bloodjournal8883230
15. Fukui J, Inaba M, Ueda Y, Miyake T, Hosaka N, Kwon AH, et al. Prevention of Graft-Versus-Host Disease by Intra-Bone Marrow Injection of Donor T Cells. Stem Cells (2007) 25(6):1595–601. doi: 10.1634/stemcells.2006-0234
16. Hill GR, Crawford JM, Cooke KR, Brinson YS, Pan L, Ferrara JL. Total Body Irradiation and Acute Graft-Versus-Host Disease: The Role of Gastrointestinal Damage and Inflammatory Cytokines. Blood (1997) 90(8):3204–13. doi: 10.1182/blood.V90.8.3204
17. Edinger M, Hoffmann P, Ermann J, Drago K, Fathman CG, Strober S, et al. CD4+CD25+ Regulatory T Cells Preserve Graft-Versus-Tumor Activity While Inhibiting Graft-Versus-Host Disease After Bone Marrow Transplantation. Nat Med (2003) 9(9):1144–50. doi: 10.1038/nm915
18. Fu J, Wang D, Yu Y, Heinrichs J, Wu Y, Schutt S, et al. T-Bet is Critical for the Development of Acute Graft-Versus-Host Disease Through Controlling T Cell Differentiation and Function. J Immunol (2015) 194(1):388–97. doi: 10.4049/jimmunol.1401618
19. Ferreira LMR, Muller YD, Bluestone JA, Tang Q. Next-Generation Regulatory T Cell Therapy. Nat Rev Drug Discov (2019) 18(10):749–69. doi: 10.1038/s41573-019-0041-4
20. Moy RH, Huffman AP, Richman LP, Crisalli L, Wang XK, Hoxie JA, et al. Clinical and Immunologic Impact of CCR5 Blockade in Graft-Versus-Host Disease Prophylaxis. Blood (2017) 129(7):906–16. doi: 10.1182/blood-2016-08-735076
21. He S, Cao Q, Qiu Y, Mi J, Zhang JZ, Jin M, et al. A New Approach to the Blocking of Alloreactive T Cell-Mediated Graft-Versus-Host Disease by In Vivo Administration of Anti-CXCR3 Neutralizing Antibody. J Immunol (2008) 181(11):7581–92. doi: 10.4049/jimmunol.181.11.7581
22. Sackstein R. A Revision of Billingham’s Tenets: The Central Role of Lymphocyte Migration in Acute Graft-Versus-Host Disease. Biol Blood Marrow Transplant (2006) 12(1 Suppl 1):2–8. doi: 10.1016/j.bbmt.2005.09.015
23. Lu Y, Waller EK. Dichotomous Role of Interferon-Gamma in Allogeneic Bone Marrow Transplant. Biol Blood Marrow Transplant (2009) 15(11):1347–53. doi: 10.1016/j.bbmt.2009.07.015
24. Kappel LW, Goldberg GL, King CG, Suh DY, Smith OM, Ligh C, et al. Il-17 Contributes to CD4-Mediated Graft-Versus-Host Disease. Blood (2009) 113(4):945–52. doi: 10.1182/blood-2008-08-172155
25. Wang H, Yang YG. The Complex and Central Role of Interferon-γ in Graft-Versus-Host Disease and Graft-Versus-Tumor Activity. Immunol Rev (2014) 258(1):30–44. doi: 10.1111/imr.12151
26. Dey BR, Yang YG, Szot GL, Pearson DA, Sykes M. Interleukin-12 Inhibits Graft-Versus-Host Disease Through an Fas-mediated Mechanism Associated With Alterations in Donor T-cell Activation and Expansion. Blood (1998) 91(9):3315–22. doi: 10.1182/blood.V91.9.3315
27. Reddy P, Teshima T, Kukuruga M, Ordemann R, Liu C, Lowler K, et al. Interleukin-18 Regulates Acute Graft-Versus-Host Disease by Enhancing Fas-mediated Donor T Cell Apoptosis. J Exp Med (2001) 194(10):1433–40. doi: 10.1084/jem.194.10.1433
28. Vignali DA, Collison LW, Workman CJ. How Regulatory T Cells Work. Nat Rev Immunol (2008) 8(7):523–32. doi: 10.1038/nri2343
29. Edinger M. Regulatory T Cells for the Prevention of Graft-Versus-Host Disease: Professionals Defeat Amateurs. Eur J Immunol (2009) 39(11):2966–8. doi: 10.1002/eji.200940030
30. Robb RJ, Lineburg KE, Kuns RD, Wilson YA, Raffelt NC, Olver SD, et al. Identification and Expansion of Highly Suppressive CD8(+)FoxP3(+) Regulatory T Cells After Experimental Allogeneic Bone Marrow Transplantation. Blood (2012) 119(24):5898–908. doi: 10.1182/blood-2011-12-396119
31. Zheng J, Liu Y, Liu Y, Liu M, Xiang Z, Lam KT, et al. Human CD8+ Regulatory T Cells Inhibit GVHD and Preserve General Immunity in Humanized Mice. Sci Transl Med (2013) 5(168):168ra9. doi: 10.1126/scitranslmed.3004943
32. Fasching P, Stradner M, Graninger W, Dejaco C, Fessler J. Therapeutic Potential of Targeting the Th17/Treg Axis in Autoimmune Disorders. Molecules (2017) 22(1):134. doi: 10.3390/molecules22010134
33. Ehrenstein MR, Evans JG, Singh A, Moore S, Warnes G, Isenberg DA, et al. Compromised Function of Regulatory T Cells in Rheumatoid Arthritis and Reversal by anti-TNFalpha Therapy. J Exp Med (2004) 200(3):277–85. doi: 10.1084/jem.20040165
34. Aravena O, Pesce B, Soto L, Orrego N, Sabugo F, Wurmann P, et al. Anti-TNF Therapy in Patients With Rheumatoid Arthritis Decreases Th1 and Th17 Cell Populations and Expands IFN-γ-Producing NK Cell and Regulatory T Cell Subsets. Immunobiology (2011) 216(12):1256–63. doi: 10.1016/j.imbio.2011.07.006
Keywords: LYG1, aGVHD, allogeneic CD4+ T cells, alloreactivity, Th1 cells, Treg cells
Citation: Liu H, Yu Z, Tang B, Miao S, Qin C, Li Y, Liang Z, Shi Y, Zhang Y, Wang Q, Yan M, Song Z, Ren H and Dong Y (2021) LYG1 Deficiency Attenuates the Severity of Acute Graft-Versus-Host Disease via Skewing Allogeneic T Cells Polarization Towards Treg Cells. Front. Immunol. 12:647894. doi: 10.3389/fimmu.2021.647894
Received: 30 December 2020; Accepted: 11 June 2021;
Published: 28 June 2021.
Edited by:
Markus Y. Mapara, Columbia University, United StatesReviewed by:
Benny J. Chen, Duke University, United StatesFederico Simonetta, Geneva University Hospitals (HUG), Switzerland
Copyright © 2021 Liu, Yu, Tang, Miao, Qin, Li, Liang, Shi, Zhang, Wang, Yan, Song, Ren and Dong. This is an open-access article distributed under the terms of the Creative Commons Attribution License (CC BY). The use, distribution or reproduction in other forums is permitted, provided the original author(s) and the copyright owner(s) are credited and that the original publication in this journal is cited, in accordance with accepted academic practice. No use, distribution or reproduction is permitted which does not comply with these terms.
*Correspondence: Hanyun Ren, renhy0813@163.com; Yujun Dong, dongy@hsc.pku.edu.cn
†These authors have contributed equally to this work