- School of Medicine, Trinity College Dublin, Trinity Biomedical Sciences Institute, Dublin, Ireland
The transcription factor Related Orphan Receptor Alpha (RORα) plays an important role in regulating circadian rhythm, inflammation, metabolism and cellular development. Herein we show that in the absence of functional RORα in mice there is reduced susceptibility to LPS-induced endotoxic shock, with selective decreases in release of pro-inflammatory cytokines. Treatment of mice with a RORα selective synthetic inhibitor also reduced the severity of LPS-induced endotoxemia. The reduction in responses in Rora deficient mice was associated with an alterations in metabolic and pro-inflammatory functions of macrophages, both in vivo peritoneal macrophages and in vitro generated bone marrow derived macrophages. Using LysMCreRorafl/sg mice the reduced susceptibility to LPS was shown to be specific to Rora expression in the macrophages. This study identifies that Rora-mediated regulation of macrophages impacts on the pro-inflammatory responses elicited by LPS.
Introduction
The transcription factor retinoic acid receptor-related orphan receptor alpha (RORα) is a member of the nuclear hormone receptor superfamily, which provides a bridge between hormonal, nutritional, and pathophysiological signaling and gene regulation. In mammals there are three major isotypes, RORα, RORβ, and RORγ, with each of these able to form multiple variants through alternative splicing (1, 2). RORα itself has been identified as having roles in neural development, metabolism, cellular differentiation, immune regulation and circadian rhythm. Indeed, staggerer mice (Rorasg/sg), which express a truncated form of the RORα protein due to a spontaneous mutation in the Rora gene, show aberrant immune responses (3–6).
Indeed, studies on RORα in the context of metabolism have demonstrated the impact of RORα in signaling pathways associated with lipid and glucose metabolism, alongside influencing the low-grade chronic inflammation that is often a hallmark of metabolic disease (4, 7–9). We have previously demonstrated that Rora-expressing myeloid-derived macrophages play an integral role in metabolic dysfunction associated with obesity (8). While the expression of RORα in macrophage subsets is now widely observed, there is some disparity in the apparent role of RORα within these cells (10, 11). Many studies implicate RORα in the inflammatory response to the TLR agonist LPS through its ability to down-modulate NF-κB signaling and impair activation of the NLRP3 inflammasome (3, 6, 10). Furthermore, studies have shown Rora is required for the release of other pro-inflammatory cytokines, such as IL-6 and TNF, from macrophages upon activation (12), while in Kupffer cells, RORα drives the activation of alternatively activated macrophages (13), which collectively suggest an anti-inflammatory role for RORα. Conversely, other studies demonstrate a more pro-inflammatory role for RORα, with studies in the mouse retina demonstrating an upregulation in Socs3, and associated decrease in pro-inflammatory cytokines, in the absence of Rora (14). In addition, there is evidence that in the context of adipose tissue inflammation, as associated with obesity, RORα is capable of driving inflammation. Indeed, it has been demonstrated that RORα can drive endoplasmic reticulum stress, which promotes adipose tissue inflammation (11), and in the absence of Rora-expressing myeloid cells, there is a decrease in inflammatory macrophages accumulating in the adipose tissue in a model of obesity (8). These disparate roles for RORα in similar inflammatory context suggest that the impact of RORα may be modified by tissue- and cell-specific factors.
In this study the role for RORα in the genesis of LPS-induced endotoxic shock in mice was examined, with a focus on identifying the immune cells involved. Using Rorasg/sg, Rorasg/sg bone-marrow chimera (BMC) mice, synthetically blocking the action of RORα, and LysMCreRorafl/sg mice, modeling both ubiquitous and myeloid-cell specific deletion, we demonstrate that RORα can promote recruitment and activation of a myeloid-derived pro-inflammatory macrophage population, which impacts upon the severity and extent of LPS-induced endotoxic shock.
Materials and Methods
Animals
C57BL/6J, staggerer Rora spontaneous mutant (JAX strain number 002651; Rorasg/sg) and B6.SJL-PtprcaPepcb/BoyJ (JAX strain number 002014; CD45.1+) mice were purchased from Jackson Laboratories (Bar Harbor, MD, USA). Adult 6–10 weeks old female mice were used in all experiments. Conditional Rora floxed (Rorafl/fl; Lexicon Pharmaceuticals, USA) and Rorasg/sg mice were crossed with Lyz2tm1(cre)Ifo (JAX strain number: 004781; LysMCre) mice to generate LysMCreRorafl/sg animals with a conditional deletion of Rora in cells of a myeloid lineage. LysMCreRorafl/sg were used due to the variable levels of Lyz2 in different macrophage populations that may result in incomplete excision as seen in LysMCreIL-4Rαflfl mice (15). Animals were housed in a specific pathogen-free facility in individually ventilated and filtered cages under positive pressure. All animal experiments were performed in compliance with the Irish Medicines Board (Figures 1, 2) or Health Product's Regulatory Authority (Figures 3–5) and approved by the Trinity College Dublin's BioResources ethical review board.
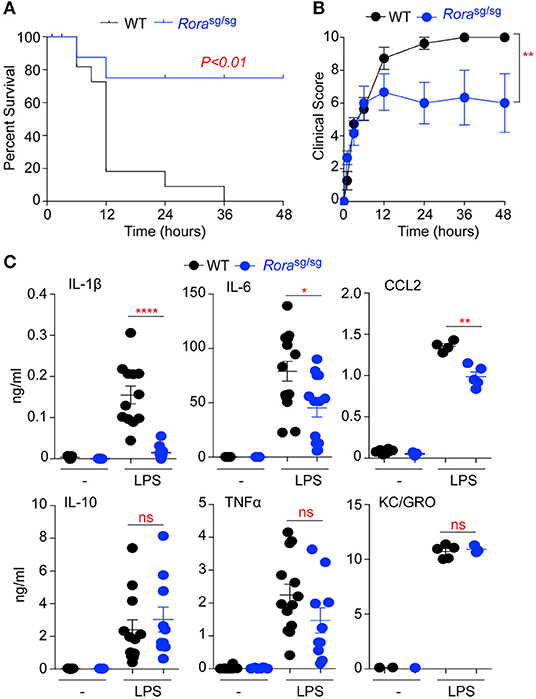
Figure 1. Rorasg/sg mice show decreased morbidity in LPS-induced endotoxic shock. WT C57BL/6 (n = 12) and Rorasg/sg C57BL/6 (n = 10) mice were challenged with 10 mg/kg UP-LPS i.p. Mice were monitored periodically for 48 h post LPS injection and death (A) and clinical score (B) recorded. Blood was collected from naïve animals (-) and those 3 h post LPS injection (LPS; n = 5 per strain), serum cytokines and chemokines were determined by ELISA (C). *P < 0.05, **P < 0.01, ****P < 0.00001.
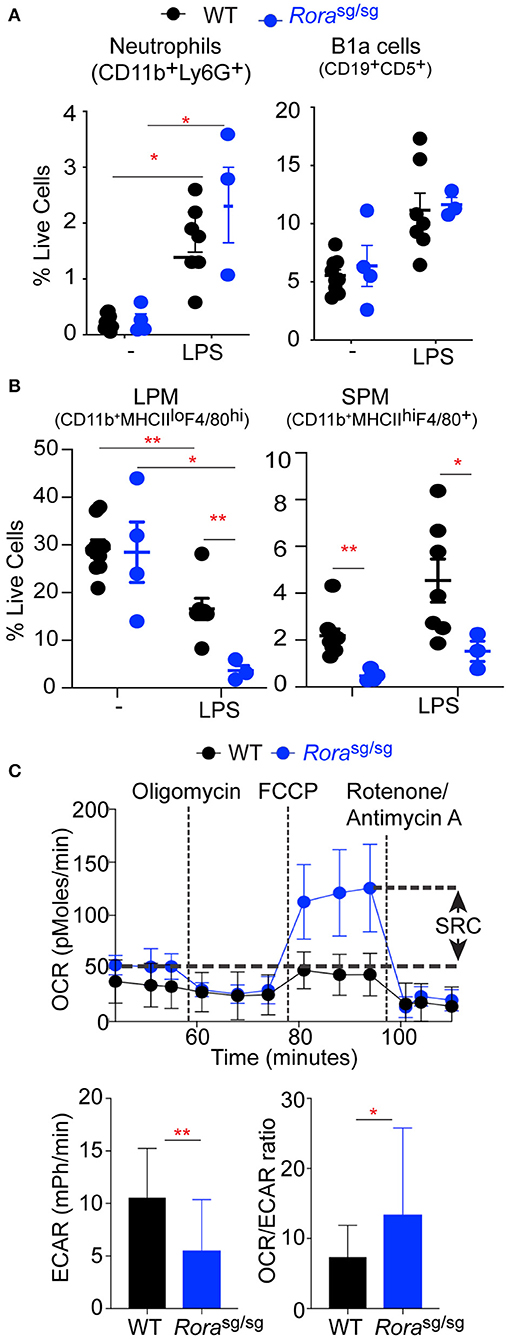
Figure 2. Rorasg/sg mice have an altered macrophage response in a naïve state and in response to LPS. Flow cytometric analysis of (A) neutrophils (CD11b+Ly6G+), B-1cells (CD19+CD5+) and (B) large peritoneal macrophages (LPM; CD11b+F4/80hiMHCIIlo) and small peritoneal macrophages (SPM; CD11b+F4/80+MHCIIhi) isolated from peritoneum of 3–9 naïve WT or Rorasg/sg mice and WT and Rorasg/sg mice 3 h post injection of 0.2 mg/kg UP-LPS. (C) Extracellular flux analysis of naïve peritoneal macrophages from WT (n = 3 mice, 8 replicates per mouse) and Rorasg/sg (n = 3 mice, 6 replicates per mouse) mice showing SRC, basal ECAR and OCR:ECAR ratio. *P < 0.05, **P < 0.01.
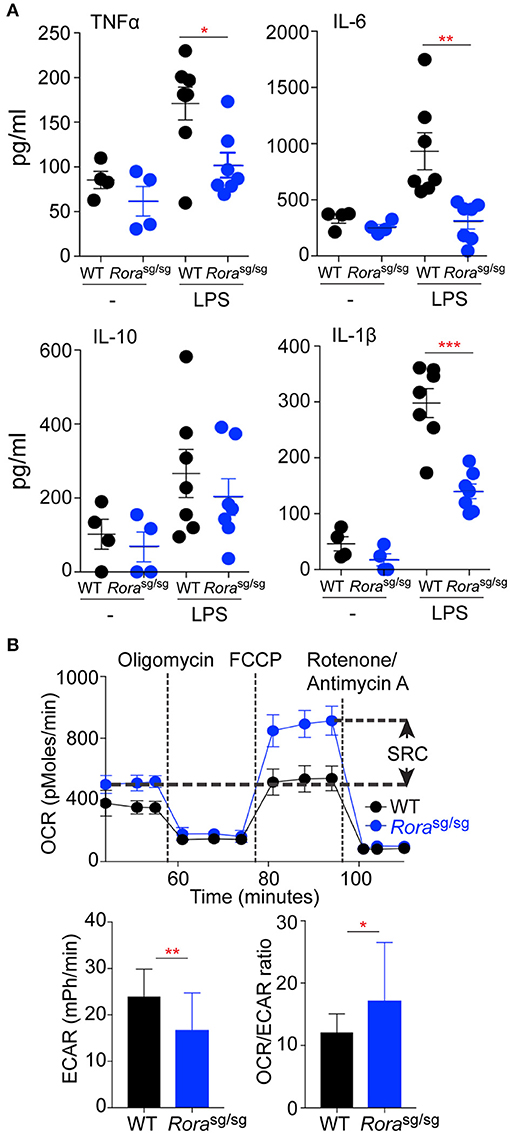
Figure 3. Macrophages derived from Rorasg/sg mice display a reduction in the in vitro inflammatory response to LPS. Peritoneal macrophages were isolated from naïve WT (n = 4, 1 replicate for media control, 2 replicates for LPS stimulated) and Rorasg/sg (n = 4, 1 replicate for media control, 2 replicates for LPS stimulated) mice and cultured with UP-LPS (200 ng/ml) for 6 h (A). (B) Extracellular flux analysis of BMDM from WT (n = 3 mice, 8 replicates per mouse) and Rorasg/sg (n = 3 mice, 6 replicates per mouse) mice showing SRC, basal ECAR and OCR:ECAR ratio. *P < 0.05, **P < 0.01, ***P < 0.0001.
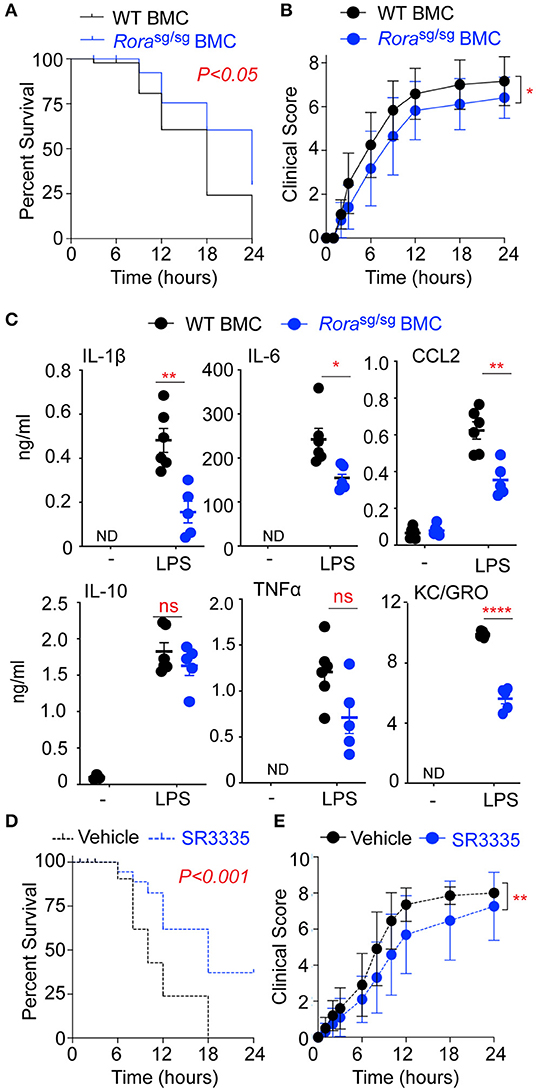
Figure 4. RORα BMC mice have decreased disease severity and inflammatory cytokine release in response to LPS. BMC mice were generated via transfer of bone marrow isolated from WT and Rorasg/sg mice into irradiated WT recipients (n = 12 and n = 17, respectively). Mice were challenged with 10 mg/kg UP-LPS i.p. and were monitored periodically for 24 h post LPS injection and death (A) and clinical score (B) recorded. Blood was collected from naïve BMC animals (-) and treated mice 3 h post LPS injection (LPS; n = 6 per group), serum cytokines and chemokines were determined by ELISA (C). WT mice were treated with vehicle control (n = 20) or SR3335 (n = 19) at a dose of 15 mg/kg for days i.p. On day 8 mice were challenged with 10 mg/kg ultra-pure LPS i.p. and were monitored periodically for 48 h post LPS injection and death (D) and clinical score (E) recorded. *P < 0.05, **P < 0.01, ****P < 0.00001.
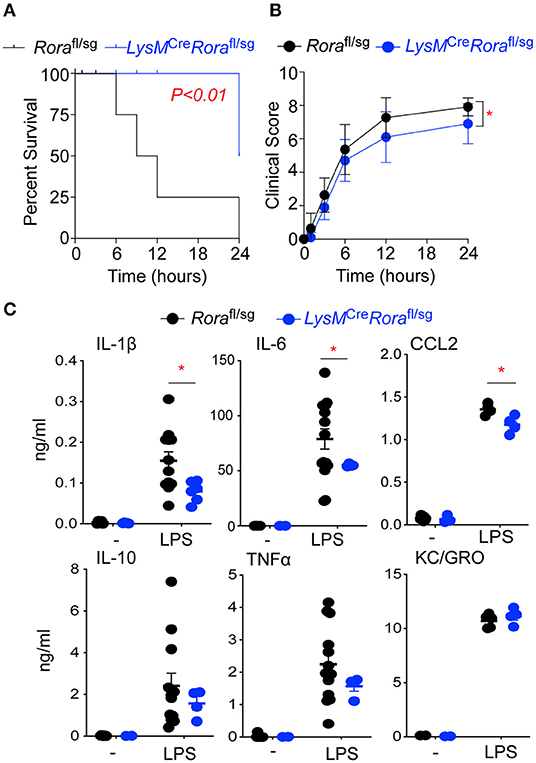
Figure 5. LysMCreRorafl/sg mice show decreased disease severity and an impaired ability to generate an inflammatory response in a model of endotoxic shock. Rorafl/sg (n = 11) and LysMCreRorafl/sg (n = 10) mice were challenged with 10 mg/kg ultra-pure LPS i.p. Mice were monitored periodically for 24 h post LPS injection and death (A) and clinical score (B) recorded. Blood was collected from naïve Rorafl/sg (n = 9) and LysMCreRorafl/sg (n = 5) mice (-) and Rorafl/sg (n = 12) and LysMCreRorafl/sg (n = 6) 3 h post LPS injection (LPS) and serum cytokines and chemokines determined by ELISA (C). *P < 0.05.
It is relevant that due to the importance of RORα in regulating circadian rhythm (16), and the corresponding circadian rhythm known to govern circulating monocytes and thus potentially impacting upon the inflammatory response (17), all experiments on mice and tissue isolations were performed at 10 a.m. to ensure no variations occurred due to alterations in the circadian rhythm due to time differences in experiments.
LPS-Induced Endotoxic Shock
Endotoxic shock was induced in age-matched male C57BL/6J, Rorasg/sg and LysMCreRorafl/sg mice by intraperitoneal injection of ultra-pure lipopolysaccharide (UP-LPS) isolated from Escherichia coli (Invivogen, France) at a dose of 10 mg/kg, as previously described (18). At 3 h post-injection, blood was collected via submandibular bleed for serum cytokine quantification. Temperature was monitored throughout using subcutaneously implanted temperature transponder chips (Bio Medic Data Systems; IPTT 300). Mice were visually scored, using a scale from 1 to 10, at regular intervals, with criteria dependent upon a combination of body temperature, appearance, condition, and behavioral characteristics. The scoring system was: Score 1, <1°C reduction in body temperature, no other change in condition; Score 2, <1°C reduction in body temperature accompanied by reduced interest in surroundings; Score 3, 1–2°C reduction in body temperature accompanied by a reduced interest in surroundings; Score 4, 1–2°C reduction in body temperature accompanied by piloerection; Score 5, 1–2°C reduction in body temperature accompanied by piloerection and reduced interest in surroundings; Score 6, 1–2°C reduction in body temperature accompanied by piloerection and reduced mobility; Score 7, >2°C reduction in body temperature accompanied by piloerection and reduced mobility; Score 8, >2°C reduction in body temperature accompanied by piloerection and nasal and ocular discharge and reduced mobility; Score 9, >2°C reduction in body temperature accompanied by piloerection and nasal and ocular discharge and vocalization; Score, 10 animals that are moribund. All mice were culled at humane end-points or after 48 h (Figures 1, 2) or 24 h (Figures 4, 5).
Clinical scores were performed blinded with individual mice tracked by the transponder chip code. Rorasg/sg mice are visually ataxic and stunted and therefore blinding was not practical. All further analysis of cells and serum was performed blinded.
In addition, a lower dose (0.2 mg/kg) of UP-LPS was used to induce cell trafficking into the peritoneal cavity. Groups of age-matched C57BL/6J and Rorasg/sg mice were injected intraperitoneally with 0.2 mg/kg UP-LPS and peritoneal cells collected by lavage after 3 h. Cell expression was quantified by flow cytometry.
Murine Macrophage Isolation and Culture
Peritoneal macrophages were cultured from naive C57BL/6J and Rorasg/sg mice as previously described (18). Briefly, the peritoneal cavity was lavaged with 5 ml ice-cold PBS and the resulting cells plated at 2 × 106 cells/ml in RPMI 1640 supplemented with 2 mM L-glutamine, 100 U/ml penicillin and 100 μg/ml streptomycin and incubated for 2 h at 37°C with 5% CO2, and any non-adherent cells removed. Adherent cells were stimulated with media-alone or UP-LPS (200 ng/ml) for 4 h, after which culture supernatants were collected for cytokine analysis.
Bone marrow-derived macrophages (BMDM) were cultured from the tibia and fibula of naive C57BL/6J and Rorasg/sg mice as previously described (18). Briefly, cells were flushed from the tibia and fibula to prepare a single cell suspension. After red blood cell lysis, the resultant cells were cultured at 3 × 106 cells/ml in RPMI 1640 supplemented with 2 mM L-glutamine, 100 U/ml penicillin, 100 μg/ml streptomycin and 10% fetal calf serum, macrophage colony-stimulating factor (M-CSF) from L929 mouse fibroblast supernatants at 37°C with 5% CO2 for 7 days. Cells were stimulated with media alone or UP-LPS (200 ng/ml) for 4 h, after which culture supernatants were collected for cytokine analysis.
Bone Marrow Chimera Generation
CD45.1+ C57BL/6 recipient mice were irradiated using an X-Ray irradiator (XStrahl CIX3), receiving 9 Gy in two doses (5 Gy and 4 Gy) 3 h apart. Mice were reconstituted with 1 × 107 BM cells from CD45.2+ C57BL/6 mice or Rorasg/sg mice. To ensure efficient irradiation and reconstitution, expression of CD45.1 vs. CD45.2 was assessed by flow cytometry of the spleen after 8 weeks (Supplementary Figure 1).
Chemical Inhibition of RORα
Mice were treated with SR3335 (N-[4-[2,2,2-trifluoro-1-hydroxy-1-(trifluoromethyl)ethyl]phenyl]-2-thiophenesulfonamide; Cayman) (Cambridge Biosciences, UK) a synthetic inverse agonist of RORα (19). C57BL/6 mice were treated with vehicle control, or SR3335 at a dose of 15 mg/kg per day i.p. for 7 days (19). On day 8 all animals were injected with UP-LPS i.p. as described above.
Metabolism Assays
Peritoneal macrophages and BMDM, prepared as above, were analyzed with an XF-24 Extracellular Flux Analyzer (Seahorse Biosciences, Agilent Technologies) to determine oxygen consumption rate (OCR) and extracellular acidification rate (ECAR). Cells cultured at a density of 5 × 105 cells/ml were incubated with non-buffered RPMI supplemented with 25 mM glucose with no CO2 for 1 h prior to analysis. Three consecutive measurements were taken at basal conditions and then after the sequential addition of 1 μM oligomycin, 1.5 μM FCCP (fluoro-carbonyl cyanide phenylhydrazone) and 1.25 μM rotenone plus 2.5 μM antimycin A. The OCR and ECAR were measured basally and after the addition of each inhibitor. The spare respiratory capacity (SRC) is determined as the difference between the basal OCR and maximal OCR, after the addition of FCCP (20).
Cytokine and Chemokine ELISA
Cytokine and chemokine levels were quantified in tissue culture supernatants and serum by sandwich ELISA. IL-6 was quantified using matched antibody pairs from BD Biosciences (Oxford, UK), IL-1β, TNFα, IL-10, GROα, and CCL2 were quantified using the DuoSet ELISA development system from R&D Systems (Abingdon, UK) following the manufacturer's protocol.
Flow Cytometry
Surface marker expression was assessed by flow cytometry with data collection on a CyAn ADP (Beckman Coulter, High Wycombe, UK) and data analyzed using FlowJo software (Tree Star, OR USA). Cells were isolated from the peritoneal cavity by lavage with ice-cold PBS. Cells were stained with BD Biosciences (Oxford, UK) mAbs; F4/80-FITC (BM8), CD45.2-PE-CF594 (104), SiglecF-AlexaFluor647 (E50-2440), CD3-PECF594 (145-2C11); eBiosciences (Dublin, Ireland) mAbs; CD11b-PerCP (M1/70), F4/80-eFluor450 (BM8), CD5-APC (53-7.3), MHC class II-eFluor 450 (MS/114.15.2); and BioLegend (London, UK) mAbs; Ly6G-APCCy7 (1A8), CD19-PerCP (6D5). Prior to surface staining, cells were incubated with LIVE/DEAD Fixable Aqua stain (Molecular probes, Invitrogen, Dublin, Ireland) to isolate dead cells. Using appropriate controls, quadrants were drawn and data were plotted on logarithmic scale density-plots.
Statistics
Statistical analysis was performed using GraphPad InStat®. Results are presented as mean +/- SEM. Differences, indicated as two-tailed P-value, were considered significant when P>0.05 as assessed by unpaired Student's t-test with Welch correction applied as necessary. Survival statistics were calculated using log-rank correlation. Area Under Curve (AUC) for the clinical scores of individual mice were determined and difference between group clinical score AUC were analyzed by Student's t-test.
Results
RORα Promotes Inflammation in a Model of LPS-Induced Endotoxic Shock
RORα has been widely implicated in the control of inflammatory signaling, with many studies demonstrating an anti-inflammatory role for RORα through suppression of IκB, a negative regulator of the NFκB pathway (3, 12). RORα is also integral in the generation of a functional type 2 immune response, through expression in ILC2 (21). To further explore roles for RORα in inflammation Rorasg/sg mutant mice, which produce a truncated form of the RORα protein, were challenged with LPS to induce endotoxic shock. Mutant mice had significantly improved survival (P < 0.01) after LPS challenge compared to WT C57BL/6 mice (Figure 1A), which was associated with a significant (P < 0.01) reduction in clinical signs of endotoxemia (Figure 1B).
Assessment of serum cytokines and chemokines in response to LPS treatment shows significantly (P < 0.05–0.0001) decreased levels of the pro-inflammatory IL-1β and IL-6 and the monocyte chemoattractant CCL2 in Rorasg/sg mice relative to WT animals (Figure 1C). Interestingly, there was no significant reduction in the serum expression of IL-10, TNFα or the neutrophil chemoattractant KC/GRO in Rorasg/sg mice compared to WT animals (Figure 1C). These data show RORα deficient mice have reduced susceptibility to LPS-induced endotoxic shock, with selective alteration in production of pro-inflammatory cytokines and chemokines.
The Macrophage Populations Are Altered in the Peritoneal Cavity of Rorasg/sg Mice
To further assess the in vivo cellular response to LPS, mice were administered a lower dose (0.2 mg/kg) to elicit local activation and cellular recruitment to the peritoneal cavity. In WT animals, the cellular profile in response to LPS can be characterized by an infiltration of neutrophils (CD11b+Ly6G+; Supplementary Figure 2B), B1a cells (CD19+CD5+; Supplementary Figure 2D) and small peritoneal macrophages (SPM; CD11b+F4/80+MHCIIhi; Supplementary Figure 2C) which temporarily replace the resident large peritoneal macrophage population (LPM; CD11b+F4/80hiMHCIIlo; Supplementary Figure 2C). There were no differences in neutrophils and B1a cells in the peritoneum of naive WT and Rorasg/sg mice, with comparable influx of both cells following LPS treatment (Figure 2A). However, while there was no differences in the number of resident LPM in naïve WT and Rorasg/sg mice, there were significantly (P < 0.01) fewer SPM in the peritoneal cavity of naïve Rorasg/sg mice (Figure 2B), in accordance with previously published studies (8). In response to LPS the influx of SPM in the peritoneal cavity was comparable between WT and Rorasg/sg animals (Figure 2B). Whereas, there was a significant decrease in the number of LPM in the peritoneal cavity of Rorasg/sg mice compared to WT animals after intraperitoneal LPS treatment (Figure 2B).
Alterations in recruited monocytes has also been observed in the adipose tissue, with myeloid-derived macrophages pro-inflammatory macrophages significantly decreased in the absence of RORα (8). In both the obesity model and in response to LPS, there is reduced response to the inflammatory stimuli in the absence of RORα. The response to inflammatory stimuli can be influenced by the metabolic state of the macrophage populations themselves, with inflammatory macrophages relying on aerobic glycolysis while anti-inflammatory macrophages, like utilizing fatty acid oxidation to fulfill their energy requirements, reflecting M1-like and M2-like cells, respectively (20, 22). To further examine the differences in the macrophage populations in Rorasg/sg mice, the metabolic status of peritoneal macrophages isolated from naive WT and Rorasg/sg mice were assessed by extracellular flux analysis. We compared the oxygen consumption by unstimulated peritoneal macrophages from WT and Rorasg/sg mice and show enhanced OCR and increased SRC in macrophages isolated from Rorasg/sg mice compared to WT macrophages (Figure 2C), which is indicative of increased oxidative phosphorylation, as associated with M2-like macrophages (20). Conversely, macrophages isolated from WT mice showed significantly (P < 0.01) increased ECAR, which is indicative of increased reliance upon anaerobic glycolysis and a switch toward an M1-like phenotype (Figure 2C). Additionally, comparison between the ratio of OCR to ECAR shows a significantly (P < 0.05) altered commitment toward oxidative phosphorylation and anaerobic glycolysis in cells from Rorasg/sg mice (Figure 2C). The characterization of the metabolic profile of peritoneal macrophages from Rorasg/sg mice suggests a reduction in an M1 phenotype in the absence of RORα.
These data indicate that in the absence of functional RORα mice develop alterations in the activation or recruitment of macrophages within the peritoneum. Resident LPM and monocytes recruited to the peritoneal cavity and SPM have different origins, with resident macrophages derived from an embryonic precursor and maintained by self-proliferation and renewal, while circulating monocytes are myeloid-derived (23–25). The observation that solely the myeloid-derived macrophage population are altered in naive Rorasg/sg mice suggests that during the embryonic stage where the resident cells are initially seeded, there is no apparent role for RORα, however, RORα does appear to impact on the recruitment and polarization of infiltrating monocytes into the peritoneal cavity. Indeed, circulating levels of CCL2, a chemokine well-defined as a recruitment signal for monocytes (26), are significantly decreased in Rorasg/sg mice (Figure 1C).
Macrophages Derived From Rorasg/sg Mice Display a Reduction in the Inflammatory Response to LPS in vitro
To further address the diminished inflammatory response in Rorasg/sg mice, in vitro assessment of macrophage populations was undertaken. There was a selective reduction in cytokine release from peritoneal macrophages isolated from naive Rorasg/sg mice (Figure 3A), which mirrored the in vivo cytokine responses. As the altered macrophage profile was shown in myeloid-derived macrophage populations in ex vivo conditions, the metabolic profile of BMDM from WT and Rorasg/sg mice was assessed. While macrophages from both strains display an OCR profile associated with oxidative phosphorylation as indicative of an alternatively activated phenotype—which would be expected as these macrophages were expanded in vitro with M-CSF—the BMDM from Rorasg/sg mice showed an increased SRC and decreased ECAR relative to cells from WT mice which is in agreement with a bias toward an anti-inflammatory phenotype in the absence of RORα (Figure 3B). This metabolic analysis suggesting an inherent bias toward an anti-inflammatory phenotype in the absence of Rora, is interesting supporting previous studies have focused on the anti-inflammatory role of RORα (3, 6, 10, 12, 27). While the signaling pathways elicited by RORα are anti-inflammatory, these data suggest that in the absence of functional RORα there is an alteration in the myeloid compartment that results in a reduction in pro-inflammatory macrophages, which results in the observed diminished pro-inflammatory response.
Deletion of Functional RORα in the Myeloid Compartment Reduces Inflammatory Cytokine Release and Mortality in a Model of LPS-Induced Endotoxic Shock
In mutant mice, the ubiquitous deletion of functional RORα results in an amelioration of inflammation and a reduction and delay in LPS-associated morbidity (Figure 1). However, due to the wide-ranging roles of RORα in cerebellar development and lipid and glucose metabolism, the mutant Rorasg/sg mice are stunted in growth, ataxic, and show increased mortality (28). To circumvent the impact of ubiquitous deletion of RORα, BMC mice were generated using bone marrow isolated from Rorasg/sg mice, with a control BMC reconstituted with bone marrow from WT mice. It should be noted that Rorasg/sg BMC and WT BMC were phenotypically comparable. Rorasg/sg BMC had significantly (P < 0.05) decreased mortality (Figure 4A) and improved clinical score (Figure 4B) in response to LPS-induced endotoxic shock relative to WT BMC. Furthermore, the reduced sensitivity to LPS was associated with a concomitant decrease in pro-inflammatory cytokines and chemokines in serum of Rorasg/sg BMC mice (Figure 4C).
To further explore roles for RORα endotoxic shock, mice were treated with SR3335, a synthetic RORα selective inverse agonist (19), for 7 days prior to the induction of LPS-induced endotoxic shock. Treatment of mice with SR3335 significantly improves mortality (Figure 4D) and clinical score (Figure 4E) from LPS-induced shock confirming that in this model blocking RORα reduces the severity of disease.
Deletion of RORα in Myeloid Cells Results in Decreased Inflammation in a Model of LPS-Induced Endotoxic Shock
To validate the role of Rora expressing macrophages in the inflammatory response, we generated LysMCreRorafl/sg mice, where the Rora gene is excised in cells expressing the Lyz2 gene, which includes macrophages. When Rorafl/sg or LysMCreRorafl/sg mice were injected with LPS, LysMCreRorafl/sg mice had significantly (P < 0.01) increased survival (Figure 5A), associated with improved clinical score (Figure 5B) when compared to Rorafl/sg animals. In addition, mice with myeloid deletion of Rora had significantly (P < 0.05) reduced elevations in serum levels of IL-1β, IL-6, and CCL2, but not IL-10, TNF-a or KC/GRO after LPS treatment (Figure 5C). These results are all comparable to the disease progression and severity observed following LPS treatment of Rorasg/sg and Rorasg/sg BMC mice, suggesting that it is macrophage expression of Rora that is responsible for the impact of RORα on the progression of inflammation in this acute model.
Discussion
RORα is a transcription factor functional in many aspects of neural function, cellular development, immune regulation, metabolism and circadian rhythm. In this study we demonstrate a novel role for RORα in pro-inflammatory response, through myeloid cell expression with the capacity to regulate initiation, recruitment and metabolism of macrophage populations, which impacts upon LPS-induced shock. Studies presented herein provide evidence supporting a role for RORα in promoting a pro-inflammatory macrophage phenotype, with a diminished response to LPS in vivo and in vitro in the absence of RORα. The pro-inflammatory macrophage specific functions for RORα is interesting considering previous studies have focused on the anti-inflammatory role of RORα through its ability to upregulate IκB and inhibit signaling through the NF-κB pathway (3, 12, 27). Indeed, a previous study has shown increased LPS-induced inflammation in Rorasg/sg mice in a model of acute lung injury (6). Furthermore, using the cecal ligation and puncture model of septic shock, an increase in RORα expression at both the mRNA and cytosolic protein level was observed in the heart tissue of septic mice, which was associated with the inhibition of NFκB induced by melatonin, suggesting that a functional RORα response is necessary for the initiation of an innate response against inflammation (10). In accordance with previous studies, we also note decreased expression of IκB in macrophages isolated from Rorasg/sg mice (data not shown). Indeed, while the signaling pathways impacted upon by RORα induces an anti-inflammatory role, the ability of RORα to alter the macrophage repertoire leads to the observed pro-inflammatory phenotype we have observed, as exemplified in reduced LPS responses in LysMCreRorafl/sg animals. Furthermore, while other studies have demonstrated an anti-inflammatory role for RORα in macrophages, it is important to note that this study focuses on primary macrophages isolated from animals deficient in functional RORα, while previous studies have focused on genetic manipulation of immortalized cells (6, 29, 30).
Rorasg/sg mice are associated with a stunted and ataxic phenotype, which may confound the response to inflammatory stimuli. BMC reconstituted with bone marrow of Rorasg/sg mice, have been used previously to generate ILC2-deficient animals (31). Rorasg/sg BMC mice were utilized to circumvent the associated phenotypic concerns with relying upon results derived from Rorasg/sg mice in isolation. Whilst Rorasg/sg BMC mice did indeed show significantly reduced mortality compared to WT BMC animals when exposed to LPS, the level of significance of this effect was reduced in these animals compared to the Rorasg/sg mice. Although we see limited impact of Rora expression in resident macrophages (8), we cannot rule out a potential role for these cells, or indeed other Rora-expressing stromal cells. Indeed, using a synthetic RORα inverse agonist, which targets RORα ubiquitously, we were able to recapitulate the reduction in mortality and decreased clinical response to LPS-induced endotoxic shock that was observed in both Rorasg/sg mice, with an increased level of significance to that observed in Rorasg/sg BMC mice. Interestingly we see a significant decrease in KC/GRO in LPS challenged Rorasg/sg BMC mice when compared to challenged WT BMC, which was not apparent in LPS challenged Rorasg/sg mice. This possibly occurs as a result of Rora-expressing hematopoietic cells impacting on neutrophil recruitment, in a manner that is not observed, or is compensated against, in Rorasg/sg animals. This reduction in KC/GRO may impact on the overall response to LPS, with the associated decrease in neutrophil recruitment in part responsible for the decreased protection against LPS-induced shock in these animals.
We have demonstrated that peritoneal macrophages express only low levels of Rora, with higher Rora expression in infiltrating monocyte-derived macrophages (8). Herein we demonstrate a reduction in pro-inflammatory cytokine release from macrophages deficient in functional RORα. Furthermore, we demonstrate a marked difference in the metabolism of macrophages isolated from Rora deficient mice, with both peritoneal macrophages from naive mice and BMDM from Rorasg/sg mice showing an increased reliance upon oxidative phosphorylation to meet their energy requirements. Further studies are warranted to explore in more detail the altered metabolic phenotype in Rora deficient macrophages. These data spanning characterization of macrophage by function and metabolic profile collectively suggest that Rora regulates the activation of macrophages to influence the pro-inflammatory response.
RORα has been identified as a transcription factor critical for the development of a number of immune cell populations, including Th17 cells, regulatory T cells, ILC2, ILC3, and macrophage populations (8, 21, 32–34). While RORα may promote intercellular anti-inflammatory signaling pathways, its ability to shape the immune cell repertoire and promote recruitment and activation of pro-inflammatory macrophages demonstrates that RORα also promotes inflammation. These data demonstrate new functions for RORα in macrophage activation and function that is relevant to the associations of RORA with inflammatory disease in man.
Data Availability Statement
The original contributions presented in the study are included in the article/Supplementary Materials, further inquiries can be directed to the corresponding author.
Ethics Statement
The animal study was reviewed and approved by Trinity College Dublin's BioResources Ethical Review Board.
Author Contributions
EH and PF: conceptualization and funding acquisition. EH, JR, and RB: methodology and investigation. EH: writing—original draft. All authors: writing—review and editing.
Funding
This work was funded by Science Foundation Ireland PI award to PF (10/IN.1/B3004) and a Science Foundation Ireland SIRG award (15/SIRG/3473) to EH. RB was supported by Molecular Medicine Ireland Clinical and Translational Research Scholars Programme, funded under PRTLI Cycle 5 and ERDF.
Conflict of Interest
The authors declare that the research was conducted in the absence of any commercial or financial relationships that could be construed as a potential conflict of interest.
Supplementary Material
The Supplementary Material for this article can be found online at: https://www.frontiersin.org/articles/10.3389/fimmu.2021.647329/full#supplementary-material
Supplementary Figure 1. Flow cytometry of blood of bone marrow chimeric (BMC) mice. Representative flow cytometry plots showing CD45.1+ or CD45.2+ cells in the spleen of a CD45.2+ Rorasg/sg donor mouse, a CD45.1+ recipient mouse and a Rorasg/sg BMC mouse.
Supplementary Figure 2. Generation of Gating strategy for cells isolated from the peritoneal cavity after LPS treatment. Peritoneal exudate cells were collected by lavage in sterile PBS 3 h after LPS treatment. (A) Initial gating strategy to remove dead cells, cell doublets and eosinophils (F4/80loSiglecFhi). (B) Identification of neutrophils (F4/80−Ly6G+FSChi). (C) Identification of small peritoneal macrophages (SPM; CD11b+F4/80+MHCIIhi), and large peritoneal macrophages (LPM; CD11b+F4/80hiMHCII+). (D) Identification of B1a cells (FSCloSSCloCD3−CD19+CD5+).
References
1. Giguere V, Tini M, Flock G, Ong E, Evans RM, Otulakowski G. Isoform-specific amino-terminal domains dictate DNA-binding properties of ROR alpha, a novel family of orphan hormone nuclear receptors. Genes Dev. (1994) 8:538–53. doi: 10.1101/gad.8.5.538
2. Hirose T, Smith RJ, Jetten AM. ROR gamma: the third member of ROR/RZR orphan receptor subfamily that is highly expressed in skeletal muscle. Biochem Biophys Res Commun. (1994) 205:1976–83. doi: 10.1006/bbrc.1994.2902
3. Delerive P, Monte D, Dubois G, Trottein F, Fruchart-Najib J, Mariani J, et al. The orphan nuclear receptor ROR alpha is a negative regulator of the inflammatory response. EMBO Rep. (2001) 2:42–8. doi: 10.1093/embo-reports/kve007
4. Kadiri S, Monnier C, Ganbold M, Ledent T, Capeau J, Antoine B. The nuclear retinoid-related orphan receptor-alpha regulates adipose tissue glyceroneogenesis in addition to hepatic gluconeogenesis. Am J Physiol Endocrinol Metab. (2015) 309:E105–14. doi: 10.1152/ajpendo.00518.2014
5. Lau P, Fitzsimmons RL, Raichur S, Wang SC, Lechtken A, Muscat GE. The orphan nuclear receptor, RORalpha, regulates gene expression that controls lipid metabolism: staggerer (SG/SG) mice are resistant to diet-induced obesity. J Biol Chem. (2008) 283:18411–21. doi: 10.1074/jbc.M710526200
6. Stapleton CM, Jaradat M, Dixon D, Kang HS, Kim SC, Liao G, et al. Enhanced susceptibility of staggerer (RORalphasg/sg) mice to lipopolysaccharide-induced lung inflammation. Am J Physiol Lung Cell Mol Physiol. (2005) 289:L144–52. doi: 10.1152/ajplung.00348.2004
7. Billon C, Sitaula S, Burris TP. Metabolic characterization of a Novel RORalpha knockout mouse model without ataxia. Front Endocrinol. (2017) 8:141. doi: 10.3389/fendo.2017.00141
8. Hams E, Roberts J, Bermingham R, Hogan AE, O'Shea D, O'Neill L, et al. Role for retinoic acid-related orphan receptor alpha (RORalpha) expressing macrophages in diet-induced obesity. Front Immunol. (2020) 11:1966. doi: 10.3389/fimmu.2020.01966
9. Kim K, Boo K, Yu YS, Oh SK, Kim H, Jeon Y, et al. RORalpha controls hepatic lipid homeostasis via negative regulation of PPARgamma transcriptional network. Nat Commun. (2017) 8:162. doi: 10.1038/s41467-017-00215-1
10. Garcia JA, Volt H, Venegas C, Doerrier C, Escames G, Lopez LC, et al. Disruption of the NF-kappaB/NLRP3 connection by melatonin requires retinoid-related orphan receptor-alpha and blocks the septic response in mice. FASEB J. (2015) 29:3863–75. doi: 10.1096/fj.15-273656
11. Liu Y, Chen Y, Zhang J, Liu Y, Zhang Y, Su Z. Retinoic acid receptor-related orphan receptor alpha stimulates adipose tissue inflammation by modulating endoplasmic reticulum stress. J Biol Chem. (2017) 292:13959–69. doi: 10.1074/jbc.M117.782391
12. Dzhagalov I, Giguere V, He YW. Lymphocyte development and function in the absence of retinoic acid-related orphan receptor alpha. J Immunol. (2004) 173:2952–9. doi: 10.4049/jimmunol.173.5.2952
13. Han YH, Kim HJ, Na H, Nam MW, Kim JY, Kim JS, et al. RORalpha induces KLF4-mediated M2 polarization in the liver macrophages that protect against nonalcoholic steatohepatitis. Cell Rep. (2017) 20:124–35. doi: 10.1016/j.celrep.2017.06.017
14. Sun Y, Liu CH, SanGiovanni JP, Evans LP, Tian KT, Zhang B, et al. Nuclear receptor RORalpha regulates pathologic retinal angiogenesis by modulating SOCS3-dependent inflammation. Proc Natl Acad Sci USA. (2015) 112:10401–6. doi: 10.1073/pnas.1504387112
15. Vannella KM, Barron L, Borthwick LA, Kindrachuk KN, Narasimhan PB, Hart KM, et al. Incomplete deletion of IL-4Ralpha by LysM(Cre) reveals distinct subsets of M2 macrophages controlling inflammation and fibrosis in chronic schistosomiasis. PLoS Pathog. (2014) 10:e1004372. doi: 10.1371/journal.ppat.1004372
16. Sato TK, Panda S, Miraglia LJ, Reyes TM, Rudic RD, McNamara P, et al. A functional genomics strategy reveals Rora as a component of the mammalian circadian clock. Neuron. (2004) 43:527–37. doi: 10.1016/j.neuron.2004.07.018
17. Nguyen KD, Fentress SJ, Qiu Y, Yun K, Cox JS, Chawla A. Circadian gene Bmal1 regulates diurnal oscillations of Ly6C(hi) inflammatory monocytes. Science. (2013) 341:1483–8. doi: 10.1126/science.1240636
18. Hams E, Saunders SP, Cummins EP, O'Connor A, Tambuwala MT, Gallagher WM, et al. The hydroxylase inhibitor dimethyloxallyl glycine attenuates endotoxic shock via alternative activation of macrophages and IL-10 production by B1 cells. Shock. (2011) 36:295–302. doi: 10.1097/SHK.0b013e318225ad7e
19. Kumar N, Kojetin DJ, Solt LA, Kumar KG, Nuhant P, Duckett DR, et al. Identification of SR3335 (ML-176): a synthetic RORalpha selective inverse agonist. ACS Chem Biol. (2011) 6:218–22. doi: 10.1021/cb1002762
20. Huang SC, Everts B, Ivanova Y, O'Sullivan D, Nascimento M, Smith AM, et al. Cell-intrinsic lysosomal lipolysis is essential for alternative activation of macrophages. Nat Immunol. (2014) 15:846–55. doi: 10.1038/ni.2956
21. Wong SH, Walker JA, Jolin HE, Drynan LF, Hams E, Camelo A, et al. Transcription factor RORalpha is critical for nuocyte development. Nat Immunol. (2012) 13:229–36. doi: 10.1038/ni.2208
22. Galvan-Pena S, O'Neill LA. Metabolic reprograming in macrophage polarization. Front Immunol. (2014) 5:420. doi: 10.3389/fimmu.2014.00420
23. Hashimoto D, Chow A, Noizat C, Teo P, Beasley MB, Leboeuf M, et al. Tissue-resident macrophages self-maintain locally throughout adult life with minimal contribution from circulating monocytes. Immunity. (2013) 38:792–804. doi: 10.1016/j.immuni.2013.04.004
24. Sieweke MH, Allen JE. Beyond stem cells: self-renewal of differentiated macrophages. Science. (2013) 342:1242974. doi: 10.1126/science.1242974
25. Yona S, Kim KW, Wolf Y, Mildner A, Varol D, Breker M, et al. Fate mapping reveals origins and dynamics of monocytes and tissue macrophages under homeostasis. Immunity. (2013) 38:79–91. doi: 10.1016/j.immuni.2012.12.001
26. Deshmane SL, Kremlev S, Amini S, Sawaya BE. Monocyte chemoattractant protein-1 (MCP-1): an overview. J Interferon Cytokine Res. (2009) 29:313–26. doi: 10.1089/jir.2008.0027
27. Kopmels B, Mariani J, Delhaye-Bouchaud N, Audibert F, Fradelizi D, Wollman EE. Evidence for a hyperexcitability state of staggerer mutant mice macrophages. J Neurochem. (1992) 58:192–9. doi: 10.1111/j.1471-4159.1992.tb09295.x
28. Dussault I, Fawcett D, Matthyssen A, Bader JA, Giguere V. Orphan nuclear receptor ROR alpha-deficient mice display the cerebellar defects of staggerer. Mech Dev. (1998) 70:147–53. doi: 10.1016/S0925-4773(97)00187-1
29. Han S, Li Z, Han F, Jia Y, Qi L, Wu G, et al. ROR alpha protects against LPS-induced inflammation by down-regulating SIRT1/NF-kappa B pathway. Arch Biochem Biophys. (2019) 668:1–8. doi: 10.1016/j.abb.2019.05.003
30. Nejati Moharrami N, Bjorkoy Tande E, Ryan L, Espevik T, Boyartchuk V. RORalpha controls inflammatory state of human macrophages. PLoS ONE. (2018) 13:e0207374. doi: 10.1371/journal.pone.0207374
31. Hams E, Locksley RM, McKenzie AN, Fallon PG. Cutting edge: IL-25 elicits innate lymphoid type 2 and type II NKT cells that regulate obesity in mice. J Immunol. (2013) 191:5349–53. doi: 10.4049/jimmunol.1301176
32. Lo BC, Gold MJ, Hughes MR, Antignano F, Valdez Y, Zaph C, et al. The orphan nuclear receptor ROR alpha and group 3 innate lymphoid cells drive fibrosis in a mouse model of Crohn's disease. Sci Immunol. (2016) 1:eaaf8864. doi: 10.1126/sciimmunol.aaf8864
33. Malhotra N, Leyva-Castillo JM, Jadhav U, Barreiro O, Kam C, O'Neill NK, et al. RORalpha-expressing T regulatory cells restrain allergic skin inflammation. Sci Immunol. (2018) 3:eaao6923. doi: 10.1126/sciimmunol.aao6923
Keywords: RORA, macrophage, inflammation, LPS, mice
Citation: Hams E, Roberts J, Bermingham R and Fallon PG (2021) Functions for Retinoic Acid-Related Orphan Receptor Alpha (RORα) in the Activation of Macrophages During Lipopolysaccharide-Induced Septic Shock. Front. Immunol. 12:647329. doi: 10.3389/fimmu.2021.647329
Received: 29 December 2020; Accepted: 16 February 2021;
Published: 09 March 2021.
Edited by:
Guo-Chang Fan, University of Cincinnati, United StatesReviewed by:
Thomas Burris, Washington University in St. Louis, United StatesMurugesan V. S. Rajaram, The Ohio State University, United States
Laura A. Solt, The Scripps Research Institute, United States
Copyright © 2021 Hams, Roberts, Bermingham and Fallon. This is an open-access article distributed under the terms of the Creative Commons Attribution License (CC BY). The use, distribution or reproduction in other forums is permitted, provided the original author(s) and the copyright owner(s) are credited and that the original publication in this journal is cited, in accordance with accepted academic practice. No use, distribution or reproduction is permitted which does not comply with these terms.
*Correspondence: Padraic G. Fallon, pfallon@tcd.ie