- 14th Department of Internal Medicine, Attikon University Hospital, National and Kapodistrian University of Athens, Athens, Greece
- 22nd Department of Pediatrics, P. & A. Kyriakou Children’s Hospital, National and Kapodistrian University of Athens, Athens, Greece
- 3Neonatal Intensive Care Unit, Department of Pediatrics and Neonatology, Saarland University Medical Center, Homburg, Germany
- 4Institute of Laboratory Medicine, Universities of Giessen and Marburg Lung Center (UGMLC), Philipps University Marburg, German Center for Lung Research (DZL), Marburg, Germany
The first 1000 days of life, including the intrauterine period, are regarded as a fundamental stepping stone for the development of a human. Unequivocally, nutrition during this period plays a key role on the proper development of a child, both directly through the intake of essential nutrients and indirectly by affecting the composition of the gut microbiota. The gut microbiota, including bacteria, viruses, fungi, protists and other microorganisms, is a highly modifiable and adaptive system that is influenced by diet, lifestyle, medicinal products and the environment. Reversely, it affects the immune system in multiple complex ways. Many noncommunicable diseases (NCDs) associated with dysbiosis are “programmed” during childhood. Nutrition is a potent determinant of the children’s microbiota composition and maturation and, therefore, a strong determinant of the NCDs’ programming. In this review we explore the interplay between nutrition during the first 1000 days of life, the gut microbiota, virome and mycobiome composition and the development of NCDs.
Introduction
Nutrition early in life, including the intrauterine stage when the fetus is exposed to nutrients through maternal diet, plays a pivotal role not only on the growth of children but also on mental development and the initiation of numerous noncommunicable diseases (NCDs), like cardiovascular diseases, cancers, respiratory diseases, diabetes, atopy and allergies (Supplementary Table 1), that are possibly programmed during this period (1, 2). Childhood nutritional programming of adult diseases, the so called “fetal and infant origin of disease” hypothesis, underlines the interrelation between malnutrition during early life and the susceptibility to the development of various diseases during adulthood (3, 4). Therefore, nutrition acts as an important environmental factor that accelerates or ameliorates the genetically programmed predisposition into several NCDs (5).
Even though the importance of nutrition during the pre- and postnatal period of life on the ontogenesis of healthy children and, consequently, of healthy adults, has been well understood, the pathogenetic mechanisms beyond this phenomenon are far more complex than just the consumption of essential nutrients for cell and tissue growth. An increasing body of data supports that early-life nutrition is a potent determinant of the children gut microbiome assembly and maturation (6, 7). The gut microbiota, including bacteria, viruses, fungi, protists and other microorganisms (Supplementary Table 1), is a highly modifiable and adaptive system (7, 8). Its formation starts early in life, during the intrauterine development (9). The gut microbiota can be influenced by multiple factors such as the type of delivery (8, 10), maternal diet and body mass index, as well as the maternal health status prior to conception, while other parameters like the geographical region, domestic environment and feeding modes are also significant contributors (2, 7, 11, 12). The interplay between postnatal nutrition, gut microbiota composition and abundance as well as their effects on children’s immune system and the development of NCDs is still poorly understood.
In this review we aim to present the effects of nutrition during the first 1000 days of life, including the stages of pregnancy, infancy and toddlerhood, on the children’s gut bacterial microbiota, virome and mycobiome, and the impact of these effects on the immune system and on the development of NCDs.
Effects of Maternal Nutrition During Pregnancy on the Infant’s Bacterial Microbiota
The impact of a “balanced” healthy maternal nutrition during pregnancy on fueling an efficient fetal development and organogenesis has long been recognized. However, the exact effects of maternal nutrition on the formation of fetal microbiota during intrauterine life are still being investigated (13). Although evidence is still limited, increasing data by studies exploring the interplay between fetal exposure to an array of different nutrients though mother’s dietary habits, and the offspring’s bacterial microbiota composition, support that a correlation actually exists (Table 1) (11, 14). Indeed, a study examining both human subjects and non-human primates in mother – offspring dyads, found that meconium microbiota composition altered according to maternal diet; in particular, a high-fat, rich in refined carbohydrates (like sugar and processed grains) and animal products and low in greens and vegetables diet, was associated with remarkable alterations in the offspring’s intestinal bacterial community a notable relative depletion of Bacteroides in the meconium exposed to a high-fat diet during gestation (14). These changes were evident for up to 6 weeks after birth in neonates born by mothers who followed a high-fat type of diet (14). A study aiming to delineate the effects of maternal artificially sweetened beverages (ASB) consumption during pregnancy on the infants’ body mass index (BMI) and on their gut microbiome, showed that ASB-exposed infants had a divergent microbiome maturation trajectory, with community-level shifts in the bacterial taxa and depletion of several Bacteroides spp (20). Additionally, ASB-exposed infants had higher BMI at the age of one-year-old, than their non-exposed peers (20). Another study attempted to evaluate the influence of prenatal maternal diet on infants’ microbiota by dividing the cases according to mode of delivery. Results showed three main microbial clusters: Bifidobacterium, Streptococcus and Clostridium, Bacteroides and Enterobacteriaceae in infants born vaginally. Intriguingly, high fruit consumption by mothers during pregnancy was associated with a higher presence of Clostridiaceae and a lower presence of Bifidobacterium in vaginally born children (6). On the other hand, a greater concentration of the Bifidobacterium spp. was detected in babies delivered with cesarean section by mothers who followed a diet rich in red processed meat (6). Additionally, in the cesarean section subgroup, increased dairy intake favored the colonies of Clostridium, while seafood and fish ingestion enabled the colonization of the gut with group B Streptococcus in both vaginally and non-vaginally delivered neonates (6). The KOALA Birth Cohort study, investigated the association between vitamin D exposure during intrauterine life and the prevalence or abundance of specific bacterial taxa in the infants’ gut microbiota; the researchers found a significant negative linear trend between the dose of maternal vitamin D supplementation and the counts of Bifidobacterium spp. and Clostridium difficile, underlining that the levels of vitamin D during pregnancy affect the offspring’s gut microbiota composition (18). In a subset of 1,157 mother-infant pairs of the CHILD Cohort study, maternal consumption of vitamin-D fortified milk was associated with reduced the likelihood of C. difficile colonization in infants (17).
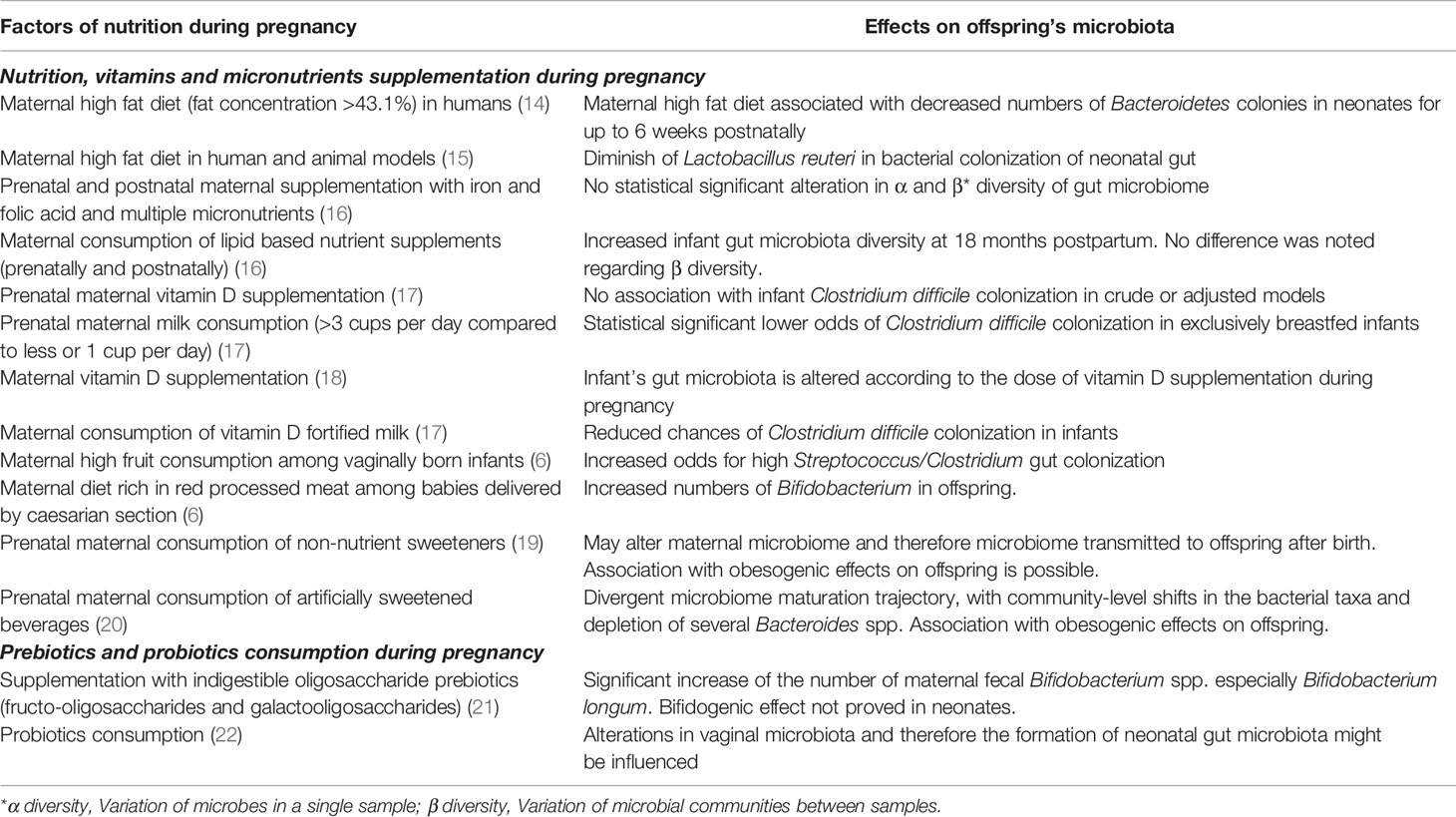
Table 1 Factors of maternal nutrition during pregnancy and associated modifications on the offspring’s microbiome.
Effects of Breastfeeding on Neonates’ Bacterial Microbiota
Breastfeeding is widely accepted as the nutritional gold standard for infants. The protective role of breastmilk against numerous diseases such as diabetes and obesity as well as its benefits on the intellectual development of the children has been well established (23, 24). Breastmilk is rich not only in proteins (immunoglobulins, cytokines etc.), lipids (free fatty acids, phospholipids etc.) and the human milk oligosaccharides (HMOs) but also in bacteria, viruses and fungi (9, 25–29).
The breastmilk’s endogenous content in bacterial species functions as the foundation for the formation of a rich gut microbiota, providing essential nutrients for its growth and expansion (9, 30, 31). Interestingly, certain bacterial strains (including Streptococcus spp. and Veillonella dispar) co-occur both in mothers’ milk and in their offspring’ stool, a phenomenon that is diminished in infants fed with pumped breastmilk (32). Undoubtedly, the infant’s gut microbiota is significantly influenced by the “breastfeeding exclusivity and duration” (32). Moreover, feeding of infants with pumped breastmilk has been associated with enrichment of the breastmilk microbiota with potential pathogens as well as depletion of bifidobacteria, a phenomenon that highlights the hypothesis of retrograde microbial inoculation of the milk by the infants’ oral cavity (30). Conversely, studies have shown evidence of a feedback relation between the maternal skin and milk on one side and the baby’s’ saliva on the other. This feedback loop manifests itself as an exchange of microbiota and pathogens from mother to offspring and vice versa. It is believed that this mechanism structures a biochemical pathway that assists innate immunity in the neonatal period (Figure 1). Hence, the composition of the breastmilk is adjusted to the needs of the newborn by changing over time in order to modulate the formation of the gut microbiota. For instance, colostrum has high concentrations of HMOs, while mature milk contains greater amounts of protein. HMOs are not only the main nutrient for the saccharolytic gut microbiota species, but are also crucial for the development of the Bifidocterium spp. colonies that dominate the gut of healthy breastfed infants (8, 9, 31). On the contrary, formula-fed infants show a greater alpha-diversity in gastrointestinal bacterial colonies and decreased numbers of Bifidobacterium spp. and higher numbers of Veillonella and Clostridioides (26, 31, 33, 34). A recent prospective observational study in preterm infants in a neonatal Intensive Care Unit, which attempted to reveal the effects of breastmilk in the gut microbiota, showed that preterm babies fed either with their mother’s own milk (MOM) or with pasteurized donor’s human milk (DHM) had closer microbiota profiles compared to the group of formula fed infants. On the other hand, MOM-fed neonates had a significantly greater presence of Bifidobacteriaceae and lower presence of Staphylococcaceae, Clostridiaceae, and Pasteurellaceae compared to preterm DHM-fed infants (35). A small cohort with 10 formula-fed and 10 MOM-fed preterm infants, found a similar alpha-diversity but a significantly different beta-diversity of the gut microbiota between the two groups; additionally, the Propionibacterium, Streptococcus, and Finegoldia genera and bacteria of the Clostridiales order had significantly higher relative abundance in the MOM group, while bacteria of the Enterobacteriaceae family, the Enterococcus and Veillonella genera, and Bacilli class were more abundant in the formula group (36).
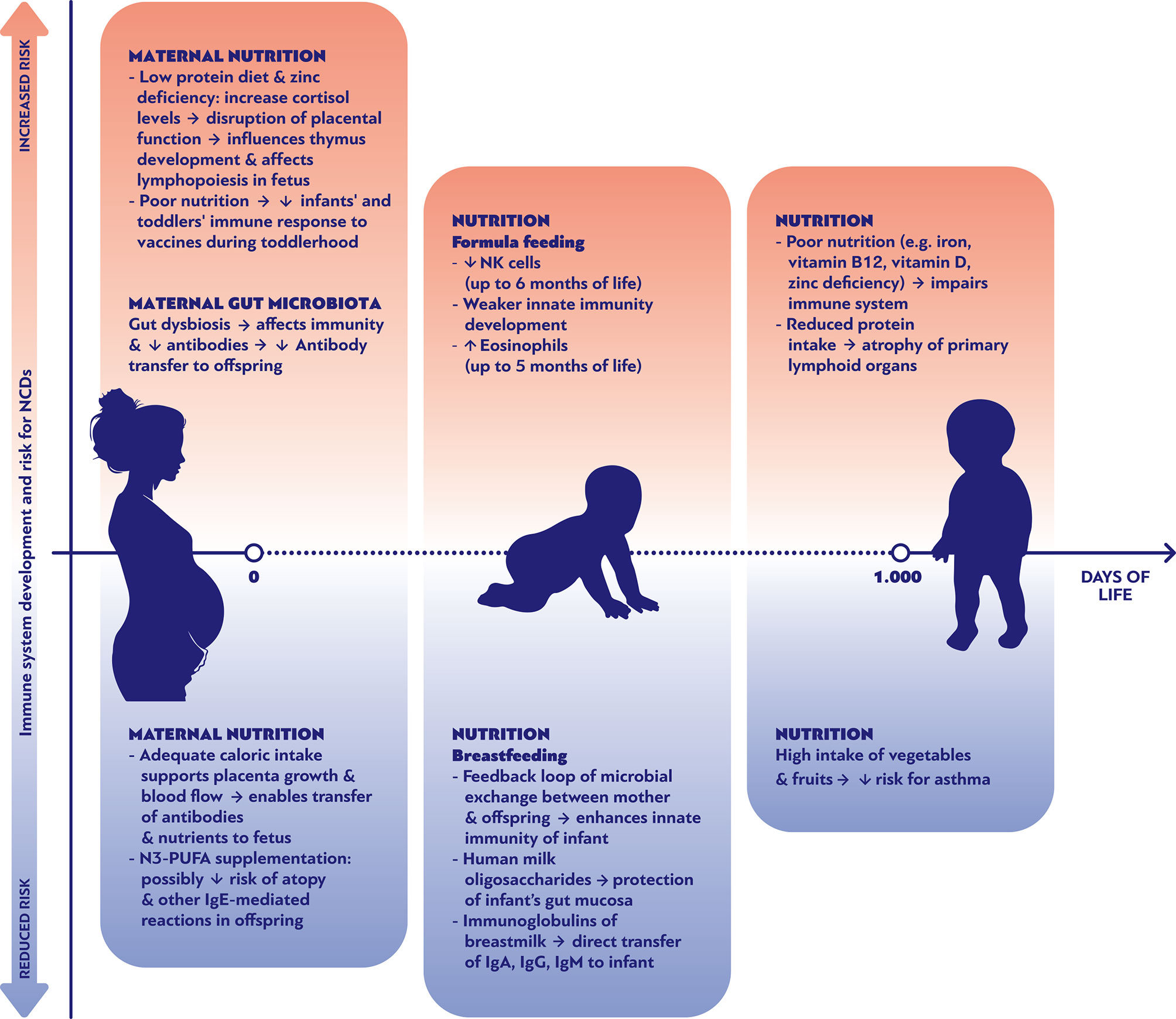
Figure 1 Nutritional parameters affecting positively or negatively the development of the immune system and the risk of noncommunicable diseases during the first 1000 days of life.
Effects of Introduction of Infant Formulas and Solid Food on Children’s Bacterial Microbiota
Although many studies have explored the short and long-term influence of the mode of birth and the type of milk (breastmilk versus formula) on the infants’ flora composition (33, 37–40), fewer studies have addressed the second phase of nutrition, which could be defined as the time after the introduction of solid food and prior to weaning, and the third phase of nutrition that begins with weaning.
The introduction of solid food leads to a significant diversification of nutrients from the relative homogeneous components of the breast or bovine milk towards plant and meat-derived meals, including the microbial content of these foods. Moreover, it coincides with the “oral stage” of development, which is associated with the uptake of a great variety of microbes through insertion of environmental objects into the mouth. Thus, the maturation of the “enterotypes” reflects a mixture of influences by food and multiple environmental exposures (41). Interestingly, this diversification of microbial exposure is associated with an inter-individual convergence of the microbiota, thus neonatal microbiota differ more from each other than the more mature microbiota of infants and children (42, 43). The introduction of solid foods is associated with a decrease in Bifodobacterium and an increase in Firmicutes phylum (42, 44). The introduction of soy formula milk was associated with an increase in Lachnospiraceae. This results in the enhancement of metabolic pathways suggesting a short-chain fatty acid (SCFA)-rich environment, including glycerol to 1-butanol fermentation which has been associated with dysbiosis (43). Intriguingly, Bifidobacteriaceae were reduced in infants prior to the introduction of soy formula. The introduction of soy formula could potentially reflect a reaction to gastrointestinal symptoms that can be interpreted as an intolerance to milk-based nutrition (43). This might be an early example for a microbiome-driven modification of nutritional habits which also may play a great role during later life. Moreover, the composition of the infant’s microbiome correlates with the domestic water source (tap water versus boiled/distilled water), probably due to differing microbial or chemical components (43).
Effects of Nutrition on Children Virome and Mycobiome
The virome of the human gastrointestinal tract consists mainly of eukaryotic viruses and bacteriophages (45). Bacteriophages are believed to play a fundamental role in enhancing the balance of microbiota and are probably responsible for the microbiome – virome interaction (46). The colonization of children’s intestine with viral species as well as its association with maternal diet has not been thoroughly studied so far and, therefore, a correlation between maternal nutritional profile and virome formation in neonates is yet to be established. A detailed analysis of fecal DNA virome from Malawian twins along with maternal stool samples suggested that age is the most determining factor that defines the composition of the gut virome (46). The same result was confirmed in a meta-analysis of individuals of Western background, whereby intestinal virome was found to be mainly dependent on age, even in members of the same family who shared the same external environment (47). The greatest viral diversity has been noticed in infants aged 0-3 years and in adults aged 18-65 years (48).
The postnatal diversification of the eucaryotic virome appears to be triggered by environmental exposure, in particular by the exposure to breastmilk or formula milk (47, 49). Recent studies have attempted to investigate the interrelation between breastfeeding and gut virome composition in neonates (29, 47, 49). In general, the virome and especially the presence of bacteriophages, is crucial for a balanced intestinal microenvironment (50). Intriguingly, the bacteriophage virome appears to be guided by the initial bacterial pioneer strains after birth. Moreover, an interesting study indicated that bacteriophages from milk are transferred to the neonate’s gut during breastfeeding (29). Additionally, the protective role of breastmilk against multiple viruses is a common ground, as supported by data showing an abundance of human viruses in fecal samples from formula-fed neonates compared to a weaker viral gut colonization in breastfed babies (49). The bacteriophage virome contracts during the first 2 years of life due to an increasing predominance of microviridae that reach 80% of the relative abundance at 24 months of age (47).
The human mycobiome consists of fewer strains than the bacterial microbiome (51). Preterm birth is associated with a predominance of saccharomyces, specifically Candida, in the meconium (52). Thus, the mycobiome may diversify during ontogeny paralleling the bacterial microbiome. However later in life, the human mycobiome is characterized by a relatively stable core mycobiome which is dominated by yeasts. A prospective study examining the mycobiota in mother-offspring dyads detected fungal colonies in the gut of newborns around the age of 2 weeks (53). The same study demonstrated traces of fungal DNA in the majority of both members of the same dyad, while maternal consumption of probiotics during pregnancy was associated with an increased concentration of maternal mycobiota (53). Another study reported that pre-, pro- and antibiotics dietary consumption have an effect on mycobiome formation in the infant’s oral cavity and gastrointestinal tract (54). Breastmilk is believed to be a transmitter of fungi, as studies have revealed the presence of Malassezia, Candida and Saccharomyces species in milk samples. A study examining the milk mycobiota from 271 mothers from the CHILD birth cohort, revealed that fungi were isolated in 21.4% of the mothers; the most dominant species were Candida, Alternaria, and Rhodotorula (25). Interestingly, the milk samples where fungi were isolated from, had lower concentrations of two HMOs (25). Data on the possible translocations of fungal species during breastfeeding between maternal skin and the infant’s oral cavity are scarce (55, 56). The transfer of fungal species from mother to infant and its significance to their health is increasingly being examined, although-unlike adult gut mycobiota data-the evidence is still limited. However, in a longitudinal study, the interindividual variations were as pronounced as the intraindividual variations over time (51). This may indicate that the mycobiome depends on environmental factors that are yet to be determined.
Early Life Microbiota Effects on Immunity and the Development of NCDs
The interaction between early life microbiota and the development of the immune system is quite complex. The exposure to microorganisms (either pathogenic or innocent bystanders, like normal flora) serves as an “immune-educator” that sets the foundations for sustainable immune responses which distinguish between self, non-self and pathogenic antigens (57). Nonpathogenic microbes evoke an immunosuppressive effect on intestinal epithelial cells by inhibiting the transcription factor nuclear factor (NF)-κB pathway, thus demonstrating a direct anti-inflammatory effect (58, 59). The key-role of “immune-education” is supported by data showing that probiotic bacteria may be protective against atopic disease by blunting a T helper (Th)2-skewed immune response (57). Moreover, the institution of antigen-tolerance bath in gut and systematically, can also be dependent on suppressive cytokines, such as IL-10 and transforming growth factor (TGF)-β produced by regulatory T cells, that control the activity of other cells like the Th1 and Th2 (58). The effect of gut microbial colonization on the development of the immune system, and its potential impact on programming of NCDs (such as atopy), was also supported by a study in newborns depicting that putative CD4+CD25+ regulatory T cells were expanded in infants colonized by toxin-producing Staphylococcus aureus early in life, compared to non-colonized infants or those who had a later colonization; children who developed allergy within the next 18 months, were significantly less often colonized by toxin-producing S. aureus during the first days after birth (57).
The link between the influences of inflammatory and metabolic pathways induced by different nutritionally-driven microbiome profiles and the determination of NCDs development has not been concretely established yet. NCDs are unequivocally multifactorial, and microbiome effects during childhood on their development later in life are only one piece of the puzzle, among others like genetic predisposition, environmental factors and lifestyle habits. The complexity of this puzzle is immense since it involves the interactions between the bacterial microbiome, virome, and mycobiome on one side, and virtually all organ systems of the host on the other (40). Notwithstanding, the advent of transcriptomics, microbial genomics and metabolomics has substantially contributed in deciphering the role of microbiome in modulating the immune system towards or away from the development of NCDs (60). For instance, animal studies have shown that gut microbiota of high fiber fed animals produce metabolites (short chain fatty acids, SCFAs) that induce T-regulatory lymphocyte populations and modulate bone marrow-derived antigen-presenting cell precursors, and these in turn, reduce dendritic cell proliferation and Th2 reactivation in the lungs, thus providing a possible protective mechanism against asthma development (60).
Understanding the link between early antigen exposures and programming of NCDs is a prerequisite for new therapeutic strategies. Specific diet recommendations might contribute in reducing the chances to develop specific types of NCDs. As shown in Figure 1, multiple nutritional factors can either promote or ameliorate the risk of NCDs through their effects on immune system development during pregnancy, early and late infancy period. For example, the maternal consumption of omega-3 fatty acid supplements during pregnancy has been associated with decreased risk of food allergies and other IgE mediated conditions during the first 12 months of life (Figure 1) (61). Genetic and epigenetic predispositions lead to a myriad of individual fates, indicating that there cannot be a “one size fits all” optimal microbiome, virome and mycobiome to prevent NCDs. However, large scale “omics” research has revealed distinct colonization patterns with prognostic relevance (40, 47, 62, 63). In general, it appears that shifts within the colonization often affect the risk of various NCDs in the same direction. Thus, it does not seem as if the implementation of preventive interventions against the development of one group of NCDs (such as allergies) would lead to an increased risk for another group of NCDs (such as obesity).
The central hypothesis may be that vaginal delivery, (long term) breastfeeding, early exposure to a wide variety of foods including potential allergens and a farm environment, as well as the avoidance of antibiotics and tobacco smoke are associated with “eubiosis” i.e. “a balanced host-microbe interaction (‘healthy’ microbiome)” (Supplementary Table 1) and a reduced risk of NCDs (9, 40, 64). Regarding the microbiome, “eubiosis” is characterized by a predominance of Bifidobacterium, Lactobacillus and Veillonella species during the time of exclusive breast feeding, whereas Bacteroides and Clostridiales dominate after the introduction of solid foods (40, 44). The gut microbiome of children born by caesarean section who are more prone to develop allergies later in life, is dominated by Enterobacteriaceae (38). The neonatal microbiome of infants that are born by caesarean section can be modified to resemble the microbiome after vaginal birth by postnatal inoculation with vaginal swabs from the mother (10). Although the long term effects are still under investigation, this study is regarded as a proof of principle that the neonatal microbiome can be modified successfully.
Little is known about the influence of the neonatal virome on the risk of NCDs. The virome may play multiple roles by either colonizing or infecting the host or by modifying the microbiome in the form of bacteriophages. The gut mycobiome regulates the host immunity and affects the course of chronic inflammatory diseases (65). However, it is still unclear whether the mycobiome has direct or indirect influences on the infant’s risk of developing NCDs.
Research Gaps and Future Research Needs
Although increasing evidence supports the association between pre- and postnatal nutrition of children and modifications of their gut microbial community, the interplay between nutrition and microbiome composition in other body sites remains obscure. Moreover, data regarding the effects of certain types of diet (like the vegetarian, the vegan, the ketogenic etc.) on the gut microbiome during gestation, during the phase of solid food introduction, as well as later in childhood are limited. Also, little is still known about the complex interactions between the specific modifications of microbiome during this period of life and its impact on immunobiological mechanisms that are associated with the development of NCDs later in life. Intriguingly, based on recent evidence that bacterial strains, once acquired, can be retained in gut microenvironment for a long time (66), research efforts should be made on exploring the reversibility of the microbiome-induced immune effects over time in children with nutritionally-induced dysbiosis. Finally, more data are needed regarding the nutritionally-driven alterations of virome and mycobiome during early life and how these are associated with morbidity phenotypes later in life.
Conclusions
Nutrition during the first 1000 days of life is unequivocally important for a prosperous developmental trajectory of children and for a healthier “programming” of adult life. Nutrition has a multidimensional impact on ontogenesis, including the modification of our microbiome, which in turn interacts with the immune system and promotes or halts numerous NCDs. The knowledge gained thus far, from adult and, to a lesser extent, from pediatric studies should guide further research and catalyze an attitude towards healthier and more balanced nutritional approaches, especially during the first years of life.
Author Contributions
CS and PF planned, structured and edited the manuscript. PF searched the literature and integrated all contributions. PF, DK and MZ participated in the writing of first draft of manuscript and subsequent revisions. All authors contributed to the article and approved the submitted version.
Funding
CS is supported by the Universities Giessen and Marburg Lung Center (UGMLC), the German Center for Lung Research (DZL), University Hospital Giessen and Marburg (UKGM) research funding according to article 2, section 3 cooperation agreement, and the Deutsche Forschungsgemeinschaft (DFG)-funded SFB 1021 (C04), KFO 309 (P10), and SK 317/1-1 (Project number 428518790) as well as by the Foundation for Pathobiochemistry and Molecular Diagnostics. PF is supported by Doctorate scholarship by the State Scholarships Foundation (IKY), Partnership Agreement (PA) 2014-2020, co-financed by Greece and the European Union (European Social Fund - ESF) through the Operational Programme “Human Resources Development, Education and Lifelong Learning 2014-2020”.
Conflict of Interest
For CS: Consultancy and research funding, Hycor Biomedical, BencardAllergie and Thermo Fisher Scientific; Research Funding, Mead Johnson Nutrition (MJN).
The remaining authors declare that the research was conducted in the absence of any commercial or financial relationships that could be construed as a potential conflict of interest.
Acknowledgments
Authors would like to thank the ESCMID Study Group on Respiratory Viruses (ESGREV) for providing a platform for interaction between authors.
Supplementary Material
The Supplementary Material for this article can be found online at: https://www.frontiersin.org/articles/10.3389/fimmu.2021.644269/full#supplementary-material
References
1. Schwarzenberg SJ, Georgieff MK. Advocacy for improving nutrition in the first 1000 days to support childhood development and adult health. Pediatrics (2018) 141:e20173716. doi: 10.1542/peds.2017-3716
2. García-Mantrana I, Bertua B, Martínez-Costa C, Collado MC. Perinatal nutrition: How to take care of the gut microbiota? Clin Nutr Exp (2016) 6:3–16. doi: 10.1016/j.yclnex.2016.02.002
3. Moore SE. Nutrition, immunity and the fetal and infant origins of disease hypothesis in developing countries. Proc Nutr Soc (1998) 57:241–7. doi: 10.1079/pns19980038
4. He Z, Sun Z, Liu S, Zhang Q, Tan Z. Effects of early malnutrition on mental system, metabolic syndrome, immunity and the gastrointestinal tract. J Vet Med Sci (2009) 71:1143–50. doi: 10.1292/jvms.71.1143
5. Delisle H, World Health Organization. WHO | Programming of chronic disease by impaired fetal nutrition (2018). WHO. Available at: http://www.who.int/nutrition/publications/obesity/WHO_NHD_02.3/en/ (Accessed November 13, 2020).
6. Lundgren SN, Madan JC, Emond JA, Morrison HG, Christensen BC, Karagas MR, et al. Maternal diet during pregnancy is related with the infant stool microbiome in a delivery mode-dependent manner. Microbiome (2018) 6:109. doi: 10.1186/s40168-018-0490-8
7. Lim ES, Wang D, Holtz LR. The Bacterial Microbiome and Virome Milestones of Infant Development. Trends Microbiol (2016) 24:801–10. doi: 10.1016/j.tim.2016.06.001
8. Subramanian S, Blanton LV, Frese SA, Charbonneau M, Mills DA, Gordon JI. Cultivating healthy growth and nutrition through the gut microbiota. Cell (2015) 161:36–48. doi: 10.1016/j.cell.2015.03.013
9. Mohammadkhah AI, Simpson EB, Patterson SG, Ferguson JF. Development of the Gut Microbiome in Children, and Lifetime Implications for Obesity and Cardiometabolic Disease. Children (2018) 5:160. doi: 10.3390/children5120160
10. Dominguez-Bello MG, Costello EK, Contreras M, Magris M, Hidalgo G, Fierer N, et al. Delivery mode shapes the acquisition and structure of the initial microbiota across multiple body habitats in newborns. Proc Natl Acad Sci U S A (2010) 107:11971–5. doi: 10.1073/pnas.1002601107
11. Ma J, Prince AL, Bader D, Hu M, Ganu R, Baquero K, et al. High-fat maternal diet during pregnancy persistently alters the offspring microbiome in a primate model. Nat Commun (2014) 5:3889. doi: 10.1038/ncomms4889
12. Bäckhed F, Roswall J, Peng Y, Feng Q, Jia H, Kovatcheva-Datchary P, et al. Erratum: Dynamics and Stabilization of the Human Gut Microbiome during the First Year of Life (Cell Host and Microbe (2015) 17(5) (690–703)). Cell Host Microbe (2015) 17:852. doi: 10.1016/j.chom.2015.05.012
13. Mirpuri J. Evidence for maternal diet-mediated effects on the offspring microbiome and immunity: implications for public health initiatives. Pediatr Res (2020) 89:301–6. doi: 10.1038/s41390-020-01121-x
14. Chu DM, Antony KM, Ma J, Prince AL, Showalter L, Moller M, et al. The early infant gut microbiome varies in association with a maternal high-fat diet. Genome Med (2016) 8:77. doi: 10.1186/s13073-016-0330-z
15. Al Rubaye H, Adamson CC, Jadavji NM. The role of maternal diet on offspring gut microbiota development: A review. J Neurosci Res (2021) 99:284–93. doi: 10.1002/jnr.24605
16. Kamng’Ona AW, Young R, Arnold CD, Patson N, Jorgensen JM, Kortekangas E, et al. Provision of Lipid-Based Nutrient Supplements to Mothers during Pregnancy and 6 Months Postpartum and to Their Infants from 6 to 18 Months Promotes Infant Gut Microbiota Diversity at 18 Months of Age but Not Microbiota Maturation in a Rural Malawian Setting: Secondary Outcomes of a Randomized Trial. J Nutr (2020) 150:918–28. doi: 10.1093/jn/nxz298
17. Drall KM, Field CJ, Haqq AM, de Souza RJ, Tun HM, Morales-Lizcano NP, et al. Vitamin D supplementation in pregnancy and early infancy in relation to gut microbiota composition and C. difficile colonization: implications for viral respiratory infections. Gut Microbes (2020) 12:1799734. doi: 10.1080/19490976.2020.1799734
18. Talsness CE, Penders J, Jansen EHJM, Damoiseaux J, Thijs C, Mommers M. Influence of vitamin D on key bacterial taxa in infant microbiota in the KOALA Birth Cohort Study. PLoS One (2017) 12:e0188011. doi: 10.1371/journal.pone.0188011
19. Azad MB, Archibald A, Tomczyk MM, Head A, Cheung KG, de Souza RJ, et al. Nonnutritive sweetener consumption during pregnancy, adiposity, and adipocyte differentiation in offspring: evidence from humans, mice, and cells. Int J Obes (2020) 44:2137–48. doi: 10.1038/s41366-020-0575-x
20. Laforest-Lapointe I, Becker AB, Mandhane PJ, Turvey SE, Moraes TJ, Sears MR, et al. Maternal consumption of artificially sweetened beverages during pregnancy is associated with infant gut microbiota and metabolic modifications and increased infant body mass index. Gut Microbes (2021) 13:1–15. doi: 10.1080/19490976.2020.1857513
21. Jinno S, Toshimitsu T, Nakamura Y, Kubota T, Igoshi Y, Ozawa N, et al. Maternal Prebiotic Ingestion Increased the Number of Fecal Bifidobacteria in Pregnant Women but Not in Their Neonates Aged One Month. Nutrients (2017) 9:196. doi: 10.3390/nu9030196
22. Swartwout B, Luo XM. Implications of probiotics on the maternal-neonatal interface: gut microbiota, immunomodulation, and autoimmunity. Front Immunol (2018) 9:2840. doi: 10.3389/fimmu.2018.02840
23. Horta BL, Victora CG, World Health Organization. WHO | Long-term effects of breastfeeding: a systematic review (2013). WHO. Available at: https://www.who.int/maternal_child_adolescent/documents/breastfeeding_long_term_effects/en/ (Accessed February 21, 2021).
24. Victora CG, Horta BL, de Mola CL, Quevedo L, Pinheiro RT, Gigante DP, et al. Association between breastfeeding and intelligence, educational attainment, and income at 30 years of age: A prospective birth cohort study from Brazil. Lancet Glob Heal (2015) 3:e199–205. doi: 10.1016/S2214-109X(15)70002-1
25. Moossavi S, Fehr K, Derakhshani H, Sbihi H, Robertson B, Bode L, et al. Human milk fungi: environmental determinants and inter-kingdom associations with milk bacteria in the CHILD Cohort Study. BMC Microbiol (2020) 20:146. doi: 10.1186/s12866-020-01829-0
26. van den Elsen LWJ, Garssen J, Burcelin R, Verhasselt V. Shaping the gut microbiota by breastfeeding: The gateway to allergy prevention? Front Pediatr (2019) 7:47. doi: 10.3389/fped.2019.00047
27. Gensollen T, Iyer SS, Kasper DL, Blumberg RS. How colonization by microbiota in early life shapes the immune system. Science (2016) 352:539–44. doi: 10.1126/science.aad9378
28. Ruiz L, García-Carral C, Rodriguez JM. Unfolding the human milk microbiome landscape in the omicsera. Front Microbiol (2019) 10:1378. doi: 10.3389/fmicb.2019.01378
29. Pannaraj PS, Ly M, Cerini C, Saavedra M, Aldrovandi GM, Saboory AA, et al. Shared and distinct features of human milk and infant stool viromes. Front Microbiol (2018) 9:1162. doi: 10.3389/fmicb.2018.01162
30. Moossavi S, Sepehri S, Robertson B, Bode L, Goruk S, Field CJ, et al. Composition and Variation of the Human Milk Microbiota Are Influenced by Maternal and Early-Life Factors. Cell Host Microbe (2019) 25:324–35.e4. doi: 10.1016/j.chom.2019.01.011
31. Gueimonde M, Laitinen K, Salminen S, Isolauri E. Breast Milk: A Source of Bifidobacteria for Infant Gut Development and Maturation? Neonatology (2007) 92:64–6. doi: 10.1159/000100088
32. Fehr K, Moossavi S, Sbihi H, Boutin RCT, Bode L, Robertson B, et al. Breastmilk Feeding Practices Are Associated with the Co-Occurrence of Bacteria in Mothers’ Milk and the Infant Gut: the CHILD Cohort Study. Cell Host Microbe (2020) 28:285–97.e4. doi: 10.1016/j.chom.2020.06.009
33. Ma J, Li Z, Zhang W, Zhang C, Zhang Y, Mei H, et al. Comparison of gut microbiota in exclusively breast-fed and formula-fed babies: a study of 91 term infants. Sci Rep (2020) 10:15792. doi: 10.1038/s41598-020-72635-x
34. Bezirtzoglou E, Tsiotsias A, Welling GW. Microbiota profile in feces of breast- and formula-fed newborns by using fluorescence in situ hybridization (FISH). Anaerobe (2011) 17:478–82. doi: 10.1016/j.anaerobe.2011.03.009
35. Parra-Llorca A, Gormaz M, Alcántara C, Cernada M, Nuñez-Ramiro A, Vento M, et al. Preterm gut microbiome depending on feeding type: Significance of donor human milk. Front Microbiol (2018) 9:1376. doi: 10.3389/fmicb.2018.01376
36. Wang Z, Neupane A, Vo R, White J, Wang X, Marzano SYL. Comparing Gut Microbiome in Mothers’ Own Breast Milk- and Formula-Fed Moderate-Late Preterm Infants. Front Microbiol (2020) 11:891. doi: 10.3389/fmicb.2020.00891
37. Dominguez-Bello MG, De Jesus-Laboy KM, Shen N, Cox LM, Amir A, Gonzalez A, et al. Partial restoration of the microbiota of cesarean-born infants via vaginal microbial transfer. Nat Med (2016) 22:250–3. doi: 10.1038/nm.4039
38. Shao Y, Forster SC, Tsaliki E, Vervier K, Strang A, Simpson N, et al. Stunted microbiota and opportunistic pathogen colonization in caesarean-section birth. Nature (2019) 574:117–21. doi: 10.1038/s41586-019-1560-1
39. Mesa MD, Loureiro B, Iglesia I, Gonzalez SF, Olivé EL, Algar OG, et al. The evolving microbiome from pregnancy to early infancy: A comprehensive review. Nutrients (2020) 12:133. doi: 10.3390/nu12010133
40. Renz H, Skevaki C. Early life microbial exposures and allergy risks: opportunities for prevention. Nat Rev Immunol (2020) 21:177–91. doi: 10.1038/s41577-020-00420-y Epub ahead of print
41. Arumugam M, Raes J, Pelletier E, Le Paslier D, Yamada T, Mende DR, et al. Enterotypes of the human gut microbiome. Nature (2011) 473:174–80. doi: 10.1038/nature09944
42. De Muinck EJ, Trosvik P. Individuality and convergence of the infant gut microbiota during the first year of life. Nat Commun (2018) 9:2233. doi: 10.1038/s41467-018-04641-7
43. Baumann-Dudenhoeffer AM, D’Souza AW, Tarr PI, Warner BB, Dantas G. Infant diet and maternal gestational weight gain predict early metabolic maturation of gut microbiomes. Nat Med (2018) 24:1822–9. doi: 10.1038/s41591-018-0216-2
44. Galazzo G, van Best N, Bervoets L, Dapaah IO, Savelkoul PH, Hornef MW, et al. Development of the Microbiota and Associations With Birth Mode, Diet, and Atopic Disorders in a Longitudinal Analysis of Stool Samples, Collected From Infancy Through Early Childhood. Gastroenterology (2020) 158:1584–96. doi: 10.1053/j.gastro.2020.01.024
45. Coffey MJ, Low I, Stelzer-Braid S, Wemheuer B, Garg M, Thomas T, et al. The intestinal virome in children with cystic fibrosis differs from healthy controls. PLoS One (2020) 15:e0233557. doi: 10.1371/journal.pone.0233557
46. Reyes A, Blanton LV, Cao S, Zhao G, Manary M, Trehan I, et al. Gut DNA viromes of Malawian twins discordant for severe acute malnutrition. Proc Natl Acad Sci U S A (2015) 112:11941–6. doi: 10.1073/pnas.1514285112
47. Lim ES, Zhou Y, Zhao G, Bauer IK, Droit L, Ndao IM, et al. Early life dynamics of the human gut virome and bacterial microbiome in infants. Nat Med (2015) 21:1228–34. doi: 10.1038/nm.3950
48. Gregory AC, Zablocki O, Zayed AA, Howell A, Bolduc B, Sullivan MB. The Gut Virome Database Reveals Age-Dependent Patterns of Virome Diversity in the Human Gut. Cell Host Microbe (2020) 28:724–40.e8. doi: 10.1016/j.chom.2020.08.003
49. Liang G, Zhao C, Zhang H, Mattei L, Sherrill-Mix S, Bittinger K, et al. The stepwise assembly of the neonatal virome is modulated by breastfeeding. Nature (2020) 581:470–4. doi: 10.1038/s41586-020-2192-1
50. Mukhopadhya I, Segal JP, Carding SR, Hart AL, Hold GL. The gut virome: the ‘missing link’ between gut bacteria and host immunity? Therap Adv Gastroenterol (2019) 12:1756284819836620. doi: 10.1177/1756284819836620
51. Nash AK, Auchtung TA, Wong MC, Smith DP, Gesell JR, Ross MC, et al. The gut mycobiome of the Human Microbiome Project healthy cohort. Microbiome (2017) 5:153. doi: 10.1186/s40168-017-0373-4
52. Willis KA, Purvis JH, Myers ED, Aziz MM, Karabayir I, Gomes CK, et al. Fungi form interkingdom microbial communities in the primordial human gut that develop with gestational age. FASEB J (2019) 33:12825–37. doi: 10.1096/fj.201901436RR
53. Schei K, Avershina E, Øien T, Rudi K, Follestad T, Salamati S, et al. Early gut mycobiota and mother-offspring transfer. Microbiome (2017) 5:107. doi: 10.1186/s40168-017-0319-x
54. Ward TL, Knights D, Gale CA. Infant fungal communities: Current knowledge and research opportunities. BMC Med (2017) 15:30. doi: 10.1186/s12916-017-0802-z
55. Boix-Amorós A, Puente-Sánchez F, du Toit E, Linderborg KM, Zhang Y, Yang B, et al. Mycobiome profiles in breast milk from healthy women depend on mode of delivery, geographic location, and interaction with bacteria. Appl Environ Microbiol (2019) 85:e02994–18. doi: 10.1128/AEM.02994-18
56. Boix-Amorós A, Martinez-Costa C, Querol A, Collado MC, Mira A. Multiple Approaches Detect the Presence of Fungi in Human Breastmilk Samples from Healthy Mothers. Sci Rep (2017) 7:13016. doi: 10.1038/s41598-017-13270-x
57. Calder PC, Krauss-Etschmann S, de Jong EC, Dupont C, Frick J-S, Frokiaer H, et al. Early nutrition and immunity - progress and perspectives. Br J Nutr (2006) 96:774–90.
58. Isolauri E. Dietary modification of atopic disease: Use of probiotics in the prevention of atopic dermatitis. Curr Allergy Asthma Rep (2004) 4:270–5. doi: 10.1007/s11882-004-0070-9
59. Neish AS, Gewirtz AT, Zeng H, Young AN, Hobert ME, Karmali V, et al. Prokaryotic regulation of epithelial responses by inhibition of IκB-α ubiquitination. Science (80 ) (2000) 289:1560–3. doi: 10.1126/science.289.5484.1560
60. Fujimura KE, Lynch SV. Microbiota in allergy and asthma and the emerging relationship with the gut microbiome. Cell Host Microbe (2015) 17:592–602. doi: 10.1016/j.chom.2015.04.007
61. Furuhjelm C, Warstedt K, Larsson J, Fredriksson M, Böttcher MF, Fälth-Magnusson K, et al. Fish oil supplementation in pregnancy and lactation may decrease the risk of infant allergy. Acta Paediatr Int J Paediatr (2009) 98:1461–7. doi: 10.1111/j.1651-2227.2009.01355.x
62. Stewart CJ, Ajami NJ, O’Brien JL, Hutchinson DS, Smith DP, Wong MC, et al. Temporal development of the gut microbiome in early childhood from the TEDDY study. Nature (2018) 562:583–8. doi: 10.1038/s41586-018-0617-x
63. Ege MJ, Mayer M, Normand A-C, Genuneit J, Cookson WOCM, Braun-Fahrländer C, et al. Exposure to Environmental Microorganisms and Childhood Asthma. N Engl J Med (2011) 364:701–9. doi: 10.1056/nejmoa1007302
64. Berg G, Rybakova D, Fischer D, Cernava T, Vergès MCC, Charles T, et al. Microbiome definition re-visited: old concepts and new challenges. Microbiome (2020) 8:103. doi: 10.1186/s40168-020-00875-0
65. Li XV, Leonardi I, Iliev ID. Gut Mycobiota in Immunity and Inflammatory Disease. Immunity (2019) 50:1365–79. doi: 10.1016/j.immuni.2019.05.023
Keywords: microbiome, microbiota, virome, mycobiome, nutrition, children, immune system, noncommunicable diseases
Citation: Fragkou PC, Karaviti D, Zemlin M and Skevaki C (2021) Impact of Early Life Nutrition on Children’s Immune System and Noncommunicable Diseases Through Its Effects on the Bacterial Microbiome, Virome and Mycobiome. Front. Immunol. 12:644269. doi: 10.3389/fimmu.2021.644269
Received: 20 December 2020; Accepted: 02 March 2021;
Published: 18 March 2021.
Edited by:
Kari Christine Nadeau, Stanford University, United StatesReviewed by:
Carina Venter, University of Colorado, United StatesJames Gern, University of Wisconsin-Madison, United States
Copyright © 2021 Fragkou, Karaviti, Zemlin and Skevaki. This is an open-access article distributed under the terms of the Creative Commons Attribution License (CC BY). The use, distribution or reproduction in other forums is permitted, provided the original author(s) and the copyright owner(s) are credited and that the original publication in this journal is cited, in accordance with accepted academic practice. No use, distribution or reproduction is permitted which does not comply with these terms.
*Correspondence: Chrysanthi Skevaki, Chrysanthi.Skevaki@uk-gm.de