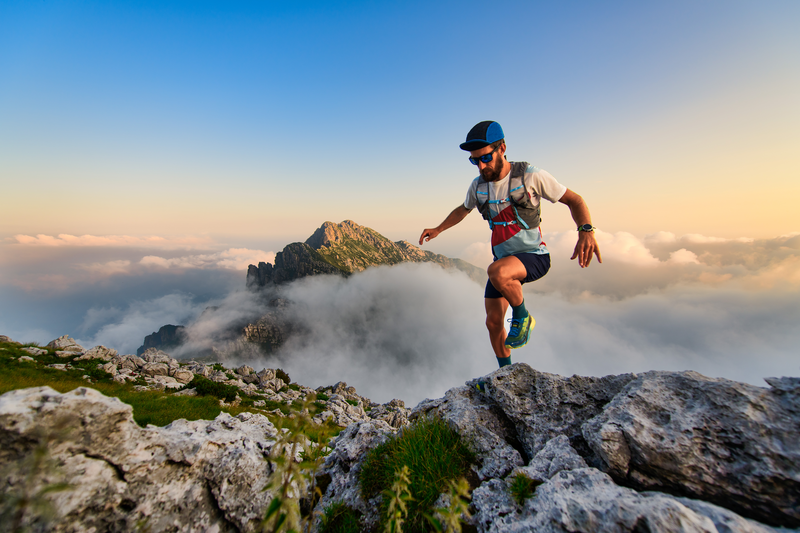
94% of researchers rate our articles as excellent or good
Learn more about the work of our research integrity team to safeguard the quality of each article we publish.
Find out more
ORIGINAL RESEARCH article
Front. Immunol. , 07 May 2021
Sec. Multiple Sclerosis and Neuroimmunology
Volume 12 - 2021 | https://doi.org/10.3389/fimmu.2021.642272
This article is part of the Research Topic Antibody-mediated autoimmune diseases of the CNS: Challenges and approaches to diagnosis and management View all 40 articles
Human antibodies against Myelin Oligodendrocyte Glycoprotein (MOG) from immunoglobulin-G subclasses (MOG-IgG) have been recently associated with a new subgroup of neurological autoimmune diseases with distinct clinical characteristics from multiple sclerosis and neuromyelitis optica spectrum disorders. The use of MOG-IgG as a biomarker is an essential tool to assist in the diagnosis and clinical prognosis. The cell-based assay (CBA) is a methodology that expresses high levels of natively folded human MOG protein in the cell membrane being the methodology most used for clinical MOG-IgG diagnosis. However, there is still no consensus about the best approach to perform CBA to improve the results. The CBA using flow cytometry (CBA-FC) is an automated technique with objective quantification, reducing the subject of human bias that occurred at CBA using immunofluorescence (CBA-IF). In this study, we compared the performance of CBA-IF and CBA-FC as an acquisition tool analysis. The sera of 104 patients diagnosed with inflammatory Central Nervous System diseases were tested in both CBA-IF and CBA-FC. We used the dilution of 1:128 for CBA-IF and three different dilutions (1:20, 1:100, and 1:640) for CBA-FC. The CBA-FC and CBA-IF results had 88.5% agreement between assays and the CBA-IF titers by endpoint-dilution correlated with the CBA-FC titers. The highest serum dilution resulted in an increased CBA-FC specificity, but there was a reduction in the CBA-FC sensitivity. Our study showed that CBA-FC can be used in clinical practice as a diagnostic technique for MOG-IgG. In addition, in some specific cases, the combination of both techniques could be used as a tool to discriminate unspecific binding and overcome single assay limitations.
Myelin Oligodendrocyte Glycoprotein (MOG) is a protein exclusively expressed in the Central Nervous System (CNS). It is present in the outer membrane of the myelin sheath and oligodendrocytes, being easily accessible to the immune-mediated response. The MOG has been used for decades as an antigen to produce experimental autoimmune encephalomyelitis (1, 2). The human antibodies against MOG from immunoglobulin-G subclasses (MOG-IgG) have been recently associated with a new subgroup of neurological autoimmune diseases, with distinct clinical characteristics and prognosis from multiple sclerosis (MS) and aquaporin-4-IgG positive neuromyelitis optica spectrum disorders (NMOSD). Our group proposed the acronym MONEM to facilitate the identification of suspected cases as most the MOG-IgG+ patients present with attacks of Optic Neuritis (ON), Encephalitis, and/or Myelitis (3), but some other groups have used the term MOG-associated disorder (MOGAD) (4). The ON is the most common clinical manifestation followed by transverse myelitis (TM) (5–7). The presence of MOG-IgG also occurs in some patients that can be clinically diagnosed with aquaporin-4-IgG seronegative NMOSD, acute disseminated encephalomyelitis (ADEM), and cortical encephalitis (8–10). The MONEM cases are found in Caucasian and non-Caucasian paediatric and adult patients with a slight female predominance (6, 11, 12).
The MOG-IgG were initially detected by enzyme-linked immunosorbent assay (ELISA) or Western Blot, which were later discarded for clinical practice mainly due to low specificity. These methods usually use unfold and/or denatured protein unable to distinguish specific antibodies against conformational sensitive MOG antibodies. These methodology analyses led to false-positive results in MS patients and also healthy individuals. Therefore, the development of techniques that discriminate MOG-IgG binding to conformational and non-conformational epitopes was essential for use in clinical practice (3, 13, 14). The cell-based assay (CBA) uses cell lines transfected with plasmids encoding the human MOG sequence. This methodology expresses high levels of natively folded MOG protein in the cell membrane. The detection of MOG-IgG by CBA has been recently used for clinical diagnoses (15–17). Commercial kits with pre-fixed cells can be used, however, there may have some loss of sensitivity and specificity compared to the cell-based assays with live transfected cells (15). Despite recent efforts to find the best methodology for detecting MOG-IgG in clinical practice, there is no standardization among MOG-IgG CBA protocols from different laboratories.
In terms of detection methods for MOG-IgG, the CBA can be performed by indirect immunofluorescence microscopy (CBA-IF) or flow cytometry (CBA-FC) (18). Currently, the CBA-IF is widely used for the detection of autoantibodies, but it is a semi-quantitative technique and subject to human bias, even if performed by experienced professionals. On the other hand, CBA-FC is an automated technique with objective quantification, reducing human error. Nevertheless, CBA-FC requires strict standardization of the flow-cytometry parameters, with variation between devices and days. In addition, the fluorescence signals may vary according to the MOG expression and secondary antibody binding in each assay batch (19). Concerning the analysis methods, the MOG-IgG by CBA-FC is usually evaluated by the ratio or the delta of mean fluorescence intensity (MFI) comparing transfected and untransfected cells (20).
Some MOG-IgG assays from distinct centers were compared in a recent study (15), as well a direct comparison of MOG-IgG assays detected by CBA-IF and CBA-FC developed in the same research laboratory (21). However, there is still no consensus on what is the most reliable way to detect MOG-IgG, or what is the best dilution of serum for use in clinical diagnosis. Therefore, in the present study, we compared the performance of CBA-IF and CBA-FC analysis, using the serum of patients diagnosed with inflammatory CNS diseases, suspecting positivity of MOG-IgG.
Sera from 104 patients diagnosed with CNS inflammatory diseases, suspecting MOG-IgG+ were included in the study. The blood samples and the clinical data were collected in the Neuroinflammation and Neuroimmunology Lab between 2015 and 2019. All samples were analyzed by a blind researcher who had no access to clinical information. The present study was approved by the Ethics Committee from the Pontifical Catholic University of Rio Grande do Sul (CAAE 03402818.4.0000.5336).
For live CBA, HEK293 cells (ATCC, LGC Standards GmbH, Wesel, Germany) were maintained in Dulbecco’s Modified Eagle’s Medium (DMEM) (Gibco, Life Technologies, NY, USA, Cat#12100-046) supplemented with 10% of Fetal Bovine Serum (FBS) (Gibco, Life Technologies, NY, USA, Cat#12657-029), 1% (100 U/mL) of penicillin/streptomycin (Gibco, Life Technologies, NY, USA, Cat#15140-122) and 0.1% (100 μg/mL) of gentamicin (Gibco, Life Technologies, NY, USA, Cat#15710-064) in the incubator (5% CO2; 37°C) until achieving 60% of confluence. Thereafter, the HEK293 cells were transfected with a plasmid containing full-length human MOG (FL-MOG) α1 isoform, using Fugene HD transfection reagent (Promega Corporation, WI, USA, Cat#E2311) according to manufacturer’s specifications. For live CBA-IF, HEK293 cells were transfected with a recombinant pIRES Ds-Red2 expression vector with full-length human MOG (FL-MOG). For live CBA-FC, HEK293 cells were transfected with a pEGFP-N1 plasmid containing FL-MOG fused to EGFP (MOG-EGFP). After 24 hours, the cells were trypsinized using 0.05% Trypsin-EDTA (Gibco, Life Technologies, NY, USA, Cat#15400-054), centrifuged, and resuspended in DMEM with geneticin G418 (Sigma-Aldrich, MO, USA, Cat#10131035) for selection, and maintenance of stably transfected cells.
For live CBA-IF, MOG-DsRed transfected cells were trypsinized, centrifuged, resuspended in DMEM, and transferred to glass slides, and maintained overnight in the incubator. Then, the cells were washed once with phosphate buffer saline (PBS) pH 7.4 and incubated with 20 µl of serum (dilution 1:128) for 30 min at room temperature. The cells were washed twice with PBS and added 15 µl of anti-Human IgG Fc-specific Cross-Adsorbed, DyLight 488 (Thermo Scientific, Life Technologies, MA, USA, Cat#SA5-10134) at 1:500 dilution for 30 min. After, cells were washed 3 times, fixed, and added mounting medium with DAPI (Fluoroshield™ with DAPI, Sigma-Aldrich, MO, USA, Cat#F6057). The slide was covered and analyzed on a confocal microscope. The cut-off value for seropositivity was 1:128 as previously described (10). For positive samples, MOG-IgG titers were determined using serial two-fold endpoint dilutions. The endpoint titer was defined as the highest dilution that gives a positive fluorescence signal.
For each sample, 1 × 105 MOG-EGFP transfected cells were harvested, washed twice with PBS pH 7.4, and incubated for 30 minutes at 4°C with patient serum. For the CBA-FC experiment, we used three different serum dilutions (1:20, 1:100, and 1:640). Thereafter, the cells were washed twice and incubated with Anti-Human IgG Fc-specific Cross-Adsorbed, DyLight 650 (Thermo Scientific, Life Technologies, MA, USA, Cat#SA5-10137) at 1:250 for 30 minutes at 4°C. The cells were washed three times, resuspended in PBS, and analyzed by flow cytometry (Attune NxT Flow Cytometer, Thermo Scientific, Life Technologies, MA, USA). Each sample analysis was performed in duplicate. For analysis, the optimal data acquisition gate was established and the binding was expressed as MFI. The MOG-IgG titers were analyzed by two methods: the ratio of MFI (rMFI), and the delta of MFI (ΔMFI) between MOG-expressing cells versus non-transfected cells. To establish the cut-off value, a threshold obtained from a cohort of negative MOG-IgG patients was used, determined by the mean of MFI plus four standard deviations of all the negative samples, both rMFI, and ΔMFI
Statistical analyses were performed using SPSS statistics 22.0 (IBM Corp. Armonk, NY, USA) and GraphPad Prism 5 (GraphPad Software, La Jolla, CA, USA). Flow cytometry data were analyzed using FlowJo™ Software 10 (Becton, Dickinson and Company, USA). We used Cohen’s kappa to evaluate the concordance between CBA-IF and CBA-FC analyses and Spearman’s nonparametric correlation for antibody titers by CBA-IF and CBA-FC. Receiver-Operating Characteristic (ROC) curve analysis was used to determine the performance of CBA-FC seropositivity using CBA-IF as a reference assay. A p-value < 0.05 was considered statistically significant.
Amongst the 104 patients with samples, 94.2% of them had available clinical data. Based on the CBA-IF results, the median age at disease onset was 20 years (± 15.4 years), and 42.2% were female in the MOG-IgG+ group. In the MOG-IgG- group, the median age at disease onset was 30 years (± 15.3 years), and 69.5% was female. About disease presentation, in the MOG-IgG+ group the majority of patients (65.9%) presented with ON, 14.7% had myelitis, 2.4% had both ON and myelitis, 7.3% were clinically diagnosed with seronegative NMOSD, 7.3% had ADEM, and 2.4% had epileptic seizures. The MOG-IgG- group had a clinical diagnosis as follows: 25.9% had ON, 27.6% had myelitis, 3.5% were clinically diagnosed with seronegative NMOSD, 5.2% were diagnosed with AQP4-NMOSD. In the MOG-IgG- group, 10.2% were aquaporin-4-IgG positive, with no aquaporin-4-IgG positive in the MOG-IgG+ group (for complete information see Supplementary Material Table 1).
The serostatus of 104 patients tested using CBA-IF was 56.7% (n=59) seronegative (Figure 1A) and 43.3% (n=45) seropositive (Figure 1B). The total IgG was evaluated and there was no difference between MOG-IgG+ and MOG-IgG- samples (Supplementary Material Table 2). Two negative samples had a weak fluorescence emission below the cut-off (1:8 and 1:64). The titers of the MOG-IgG+ group range from 1:128 to 1:65,556 (Figure 1C).
Figure 1 Comparison of MOG-IgG negative and positive samples by live cell-based assay immunofluorescence (CBA-IF). Representative fluorescence image of (A) negative and (B) positive samples. The green fluorescence DyLight 488 is an indicator of bound human serum MOG antibody. The blue fluorescence is a nuclear DAPI marker. Images were obtained using a confocal microscope with a 63× objective lens. (C) Represents the quantification of CBA-IF binding scores for negative and positive samples. The cut-off value for seropositivity (1:128 dilution) was represented by the dotted line. The titers of positive samples range from 1:128 to 1:65,556.
For the CBA-FC evaluation, we used three serum dilutions (1:20, 1:100, and 1:640) analyzed under two distinct conditions, both calculations based on mean fluorescent intensity (ΔMFI = MFI positive - negative cells, and rMFI = MFI positive/negative cells). The CBA-FC groups were named 1 to 6, according to respective acronyms: CBA-FC1 (1:20, using ΔMFI analysis); CBA-FC2 (1:20, using rMFI analysis); CBA-FC3 (1:100, using ΔMFI analysis); CBA-FC4 (1:100, using rMFI analysis); CBA-FC5 (1:640, using ΔMFI analysis); CBA-FC6 (1:640, using rMFI analysis).
The MOG-IgG+ group represented 45.2%, 46.2%, 45.2%, 46.2%, 37.5%, and 39.4% in the CBA-FC 1 to 6, respectively (see Figure 2 for an example of sample gate (2A), a seronegative (2B) and seropositive (2C) MOG-IgG by CBA-FC). In Table 1, we summarize the six CBA-FC analysis.
Figure 2 Scatter plots graphs of live cell-based assay flow cytometry (CBA-FC) analysis. (A) Representative flow cytometry strategy gating for HEK293 cells. The gate of flow cytometry data was based on forward scatter (FSC) versus side scatter (SSC), using a FlowJo™. Representative flow plots of (B) MOG-IgG-, and (C) MOG-IgG+ samples. The X-axis represents the MOG-EGFP transfection marker (488 nm) and the Y-axis represents the secondary anti-human Fc-IgG DyLight 650 nm antibody binding.
Comparing CBA-IF to CBA-FC, in all six analyses, 38 samples were seropositive and 54 were seronegative in both methods, corresponding to 88.5% of agreement. Only 12 patients (11.5%) had positive results only in one assay (CBA-FC or CBA-IF) and the data are summarized in Table 2. If the results were stratified into three subgroups, MOG-IgG-, MOG-IgG+ low-titer (1: 128 to 1: 512), and MOG-IgG+ high-titer (above 1:1024) based on CBA-IF, the agreement were 93.7%, 85.3%, and 95.7% respectively. Individually, the CBA-FC 5 and 6 had a better agreement in MOG-IgG negative samples (98.3%), CBA-FC 2 and 4 in the MOG-IgG positive samples with high-titer (100%), and CBA-FC1-4 in the MOG-IgG positive samples with low-titers (88.2%). It is very clear the reduction in agreement in MOG-IgG+ with low titer due to the proximity as the cut-off. The agreement data stratified into subgroups are summarized in Table 3.
Using the established CBA-IF as the reference standard the area under the curve (AUC) of the ROC curve was 0.959, 0.973, 0.968, 0.984, 0.931, and 0.946 in analyses CBA-FC 1 to 6 respectively (Table 4).The sensitivity analysis for CBA-FC1 to 6 were 93.3%, 95.6%, 93.3%, 95.6%, 84.4%, and 88.9%. The specificity was 91.5%, 91.5%, 91.5%, 91.5%, 98.3%, and 98.3% respectively. This data showed the high dilution (1:640) has greater specificity, but a slightly lower sensibility. The positive predictive value was 0.894, 0.896, 0.894, 0.896, 0.974, and 0.976 in analyses CBA-FC 1 to 6 respectively and the negative predictive value was 0.947, 0.964, 0.947, 0.964, 0.892, and 0.921. The kappa coefficient values used to assess the inter-method reliability were all above 0.8, indicating almost perfect agreement between methods (p<0.0001 for all analysis). In addition, the analysis of correlation comparing CBA-IF antibody titers to ΔMFI or rMFI used in CBA-FC analysis were 0.802, 0.824, 0.825, 0.844, 0.764 and 0.790 for CBA-FC1 to 6 respectively (Spearman’s rho; p<0.0001; Figure 3). The data demonstrate a strong positive correlation between the titers obtained in immunofluorescence microscopy and the analysis performed by cytometry.
Figure 3 Correlation of MOG-IgG titers and MFI values determined by CBA-IF and CBA-FC assays. The analysis were generated using CBA-IF and (A) CBA-FC1, (B) CBA-FC2, (C) CBA-FC3, (D) CBA-FC4, (E) CBA-FC5, and (F) CBA-FC6. The graphs are expressed as logarithmic scale, and the correlation values were calculated using the non-parametric Spearman correlation and correlation coefficient (r) are shown in the graph.
Comparing ΔMFI and rMFI analysis, the rMFI had better results with 10 inconsistent results contrasting with 12 inconsistent samples by ΔMFI, representing 90.4% of agreement. Looking at the inconsistent samples, the five negative samples in immunofluorescence, only one had positive data at CBA-FC6 (high dilution). This specific sample had a title of 1:64 at immunofluorescence microscopy analysis, a value below the cut-off. In the inconsistent positive samples analyzed CBA-IF, all of them were negative in CBA-FC5. These data indicate that CBA-FC5 has a high specificity, but lower sensitivity. Evaluating CBA-IF and CBA-FC4, the agreement increased to 93.2%, with the highest AUC value and Spearman’s coefficient. Five samples were positive by CBA-FC4 and negative by CBA-IF. On the other hand, only two samples were negative using CBA-FC4 analysis comparing to positive results by CBA-IF, both with low-titers (1:256). The CBA-FC4 data showed the highest specificity (91.5%) and very high sensibility (95.6%) among the CBA-FC analysis.
The MOGAD or MONEM is a new subgroup of neurological autoimmune disorders, distinct from MS and aquaporin-4-IgG+ NMOSD. The MOG-IgG has been increasingly used as a biomarker in inflammatory CNS disorders, helping to improve diagnostic accuracy and stratify prognosis, as well as promoting the development of new treatments targeting specific molecules (3, 17).
In this study, we examined the determination of MOG-IgG using live CBA performed by indirect immunofluorescence microscopy and multiple flow cytometry conditions. Using the CBA-IF as the standard, we compare the CBA-FC methodology using ΔMFI and rMFI in three different dilutions (1:20, 1:100, and 1:640). Our results indicate a high agreement between CBA-IF and CBA-FC with all kappa coefficient values close to 0.8. There were undoubtful MOG-IgG positive with high-titers and negative patients in all assays, with an agreement of 88.5% of the total of samples. However, twelve samples had discrepancies in the results from the CBA-IF and CBA-FC.
Further analyzing inconsistent samples, five of them were seronegative by CBA-IF while seropositive by CBA-FC1 to 4. The flow cytometry graphs have shown a distinct MOG-EGFP profile compared to strongly positive samples (data not shown). One of these patients was AQP4-IgG+ NMOSD with high background and less likely to be a case of double antigen positivity. There are very rare cases of double positivity, and most of these cases have a very high titer from one antibody and the other near the cut-off values (22). Evaluating the sample in question, we believe that it is not a case of double antigen positivity, but an unspecified fluorescence staining. Four positive samples that occurred in low-dilutions (1:20 and 1:100) were negative when tested in higher dilution (1:640). The presence of several antigens and proteins in the serum may contribute to nonspecific binding. Besides, the amount of sample added in the assay may contribute to this nonspecific effect, evidenced in the analysis by flow cytometry with smaller dilutions. In a previous study, concerns about the use of low serum dilutions were detected due to false seropositivity in healthy patients (14). Thus, specificity can be significantly increased by using higher dilutions. Studies have shown that intermediate dilutions, such as 1:200 (23, 24), 1:320 (20), could increase specificity without reducing sensitivity. Two patients were seropositive at CBA-IF and seronegative at CBA-FC, but these patients had MOG-IgG titers close to the cut-off. High dilutions (up to 1:640) in CBA-IF were used as cut-off values for seropositivity (21), so it might be possible that unspecific binding and increased slide background leading to reading errors in the CBA-IF that did not happen in the CBA-FC. Four other samples were seropositive at CBA-IF and CBA-FC1-4, but negative by CBA-FC 5 and 6 analysis, demonstrating that the sensitivity can be reduced using higher dilutions. Another sample was seropositive in the CBA-IF and CBA-FC 2 and 4 using rMFI but negative in other analysis. In this case, the high background in non-transfected cells reduced the ΔMFI below the cut-off limits, as the difference in the anti-human IgG secondary antibody binding between transfected cells and non-transfected cells is very small when there is a high background (25). In our study, the rMFI analysis had a slightly higher agreement with CBA-IF than ΔMFI analysis. However, the study by Tea et al. (2020) revealed that the ratio analysis also has the sensitivity reduced by high background binding (26). Therefore, in a few cases, we may need to perform combined analysis and review the flow-cytometry plots to increase the accuracy of the assay results.
All ROC curves from the six CBA-FC assays having the CBA-IF as a reference assay demonstrated high sensitivity and specificity. Amongst the six CBA-FC assays the CBA-FC 4 using a 1:100 dilution and rMFI resulted in the highest AUC index. The most sensitive CBA-FC were those evaluated by the ratio (CBA-FC 2 and 4), while the specificity was slightly higher in the analysis with the CBA-FC using higher serum dilutions (CBA-FC 5 and 6). This corroborates with our results that the rMFI analysis with higher dilutions results in high accuracy. In our study, CBA-FC provides a relatively good agreement with CBA-IF titers and CBA-FC values for all analyses, but other groups reported discrepant results (25, 27). Compared with CBA-IF, the CBA-FC4 yielded the highest accuracy among the CBA-FC analysis. In addition, the CBA-FC4 values provide a good agreement with the CBA-IF titers, suggesting to be the closest CBA-FC assay from the well-established CBA-IF.
his study has some limitations: (1) The sample size is relatively small, as the disorder caused by MOG-IgG is rare; (2) The cut-off point was performed with previously determined negative patients and not in a control group with healthy individuals; (3) A single cut-off point for CBA-FC was defined for all samples, while some authors recommend adjusting cut-off in every new experiment.
Both CBA-IF and CBA-FC are used in clinical practice, but each of them has different advantages and limitations. The CBA-IF is more commonly used for the detection of MOG-IgG in clinical practice, as it requires fluorescent microscopy available in most laboratories. The CBA-FC requires a multichannel flow cytometer which allows the simultaneous analysis of every single cell for the expression of MOG and the fluorescent intensity for anti-human IgG secondary antibody bound in the cell membrane in distinct channels. The antibody titers by CBA-FC can be calculated in the same data by different methods as described in this study. Furthermore, several samples can be analyzed on the same day and the data can be saved and re-analyzed by other statistical methodologies in the future.
In conclusion, our study showed that CBA-FC can be used in clinical practice as a diagnostic technique for MOG-IgG. The CBA-FC4 using a serum dilution of 1:100 and rMFI had a higher concordance with live CBA-IF. In addition, in some specific cases, the combination of both techniques could be used as a tool to discriminate unspecific binding and overcome single assay limitations.
The raw data supporting the conclusions of this article will be made available by the authors, without undue reservation.
The studies involving human participants were reviewed and approved by Ethics Committee of the Pontifical Catholic University of Rio Grande do Sul (CAAE 03402818.4.0000.5336). The patients/participants provided their written informed consent to participate in this study.
Conceptualization, AM and DKS Data curation, AM, GUA, and GH. Formal analysis, AM and GH. Funding acquisition, DKS. Investigation, AM, GUA, and GH. Methodology AM GH, and DKS. Project administration, AM, GH, and DKS. Resources, DKS. Supervision, DKS. Visualization, AM, GH, and DKS. Writing—original draft, AM. Writing—review and editing, GH and DKS. All authors contributed to the article and approved the submitted version.
This work was funded by grants by Coordenação de Aperfeiçoamento de Pessoal de Nivel Superior (CAPES)/Brazil– Finance Code 001. A.M. was supported by a scholarship from CAPES/Brazil. G.H. is a postdoctoral fellow supported by the CAPES-PRINT. D.K.S. received a scholarship from the Ministry of Education, Culture, Sports, Science and Technology (MEXT) of Japan, a Grants-in-Aid for Scientific Research from the Japan Society for the Promotion of Science (KAKENHI 15K19472), research support from CNPq/Brazil (425331/2016-4 and 308636/2019-8), TEVA, Merck, Biogen and Euroimmun AG for investigator-initiated studies, and speaker honoraria from Biogen, Novartis, Genzyme, TEVA, Merck, Roche and Bayer, and participated in advisory boards for Biogen, Roche, TEVA, and Merck.
The authors declare that the research was conducted in the absence of any commercial or financial relationships that could be construed as a potential conflict of interest.
AM would like to acknowledge CAPES/Brazil for Master’s Degree Scholarship support.
The Supplementary Material for this article can be found online at: https://www.frontiersin.org/articles/10.3389/fimmu.2021.642272/full#supplementary-material
Supplementary Table 1 | Demographic and Clinical Data.
Supplementary Table 2 | Total IgG analysis data.
1. Ramanathan S, Dale RC, Brilot F. Anti-MOG Antibody: The History, Clinical Phenotype, and Pathogenicity of a Serum Biomarker for Demyelination. Autoimmun Rev (2016) 15(4):307–24. doi: 10.1016/j.autrev.2015.12.004
2. Narayan R, Simpson A, Fritsche K, Salama S, Pardo S, Mealy M, et al. MOG Antibody Disease: A Review of MOG Antibody Seropositive Neuromyelitis Optica Spectrum Disorder. Mult Scler Relat Disord (2018) 25:66–72. doi: 10.1016/j.msard.2018.07.025
3. dos Passos GR, Oliveira LM, da Costa BK, Apostolos-Pereira SL, Callegaro D, Fujihara K, et al. Mog-IgG-associated Optic Neuritis, Encephalitis, and Myelitis: Lessons Learned From Neuromyelitis Optica Spectrum Disorder. Front Neurol (2018) 9:1–10. doi: 10.3389/fneur.2018.00217
4. Lee Y-J, Nam SO, Ko A, Kong J, Byun SY. Myelin Oligodendrocyte Glycoprotein Antibody-Associated Disorders: Clinical Spectrum, Diagnostic Evaluation, and Treatment Options. Clin Exp Pediatr (2020) 64(3):103–10. doi: 10.3345/cep.2019.01305
5. Oliveira LM, Apóstolos-Pereira SL, Pitombeira MS, Bruel Torretta PH, Callegaro D, Sato DK. Persistent MOG-IgG Positivity is a Predictor of Recurrence in MOG-IgG-associated Optic Neuritis, Encephalitis and Myelitis. Mult Scler J (2018) 25(14):1907–14. doi: 10.1177/1352458518811597
6. Jurynczyk M, Messina S, Woodhall MR, Raza N, Everett R, Roca-Fernandez A, et al. Clinical Presentation and Prognosis in MOG-antibody Disease: A UK Study. Brain (2017) 140(12):3128–38. doi: 10.1093/brain/awx276
7. Kitley J, Waters P, Woodhall M, Leite MI, Murchison A, George J, et al. Neuromyelitis Optica Spectrum Disorders With Aquaporin-4 and Myelin-Oligodendrocyte Glycoprotein Antibodies a Comparative Study. JAMA Neurol (2014) 71(3):276–83. doi: 10.1001/jamaneurol.2013.5857
8. Ogawa R, Nakashima I, Takahashi T, Kaneko K, Akaishi T, Takai Y, et al. MOG Antibody-Positive, Benign, Unilateral, Cerebral Cortical Encephalitis With Epilepsy. Neurol Neuroimmunol Neuroinflamm (2017) 4(2):1–10. doi: 10.1212/NXI.0000000000000322
9. O’Connor KC, McLaughlin KA, De Jager PL, Chitnis T, Bettelli E, Xu C, et al. Self-Antigen Tetramers Discriminate Between Myelin Autoantibodies to Native or Denatured Protein. Nat Med (2007) 13(2):211–7. doi: 10.1038/nm1488
10. Sato DK, Allegro D, Lana-Peixoto MA, Waters PJ, De Haidar Jorge FM, Takahashi T, et al. Distinction Between MOG Antibodypositive and AQP4 Antibody-Positive NMO Spectrum Disorders. Neurology (2014) 82(6):474–81. doi: 10.1212/WNL.0000000000000101
11. Tajfirouz DA, Bhatti MT, Chen JJ. Clinical Characteristics and Treatment of MOG-IgG–Associated Optic Neuritis. Curr Neurol Neurosci Rep (2019) Dec 2619(12):100. doi: 10.1007/s11910-019-1014-z
12. Jarius S, Ruprecht K, Kleiter I, Borisow N, Asgari N, Pitarokoili K, et al. Mog-IgG in NMO and Related Disorders: A Multicenter Study of 50 Patients. Part 2: Epidemiology, Clinical Presentation, Radiological and Laboratory Features, Treatment Responses, and Long-Term Outcome. J Neuroinflamm (2016) 13(1):280. doi: 10.1186/s12974-016-0718-0
13. Reindl M, Waters P. Myelin Oligodendrocyte Glycoprotein Antibodies in Neurological Disease. Nat Rev Neurol (2019) 15:89–102. doi: 10.1038/s41582-018-0112-x
14. Waters P, Woodhall M, O’Connor KC, Reindl M, Lang B, Sato DK, et al. MOG Cell-Based Assay Detects non-MS Patients With Inflammatory Neurologic Disease. Neurol Neuroimmunol Neuroinflamm (2015) 2(3):1–11. doi: 10.1212/NXI.0000000000000089
15. Waters PJ, Komorowski L, Woodhall M, Lederer S, Majed M, Fryer J, et al. A Multicenter Comparison of MOG-IgG Cell-Based Assays. Neurology (2019) 92(11):e1250–5. doi: 10.1212/WNL.0000000000007096
16. Lalive PH, Menge T, Delarasse C, Della Gaspera B, Pham-Dinh D, Villoslada P, et al. Antibodies to Native Myelin Oligodendrocyte Glycoprotein are Serologic Markers of Early Inflammation in Multiple Sclerosis. Proc Natl Acad Sci (2006) 103(7):2280–5. doi: 10.1073/pnas.0510672103
17. Molina RD, Conzatti LP, da Silva APB, Goi LDS, da Costa BK, Machado DC, et al. Detection of Autoantibodies in Central Nervous System Inflammatory Disorders: Clinical Application of Cell-Based Assays. Mult Scler Relat Disord (2020) 38:101858. doi: 10.1016/j.msard.2019.101858
18. Reindl M, Di Pauli F, Rostásy K, Berger T. The Spectrum of MOG Autoantibody-Associated Demyelinating Diseases. Nat Rev Neurol (2013) 9:455–61. doi: 10.1038/nrneurol.2013.118
19. Marchionatti A, Woodhall M, Waters PJ, Sato DK. Detection of MOG-IgG by Cell-Based Assay: Moving From Discovery to Clinical Practice. Neurol Sci (2020) 42(1)73–80. doi: 10.1007/s10072-020-04828-1
20. Cobo-Calvo A, Ruiz A, Maillart E, Audoin B, Zephir H, Bourre B, et al. Clinical Spectrum and Prognostic Value of CNS MOG Autoimmunity in Adults. Neurology (2018) 90(21):e1858–69. doi: 10.1212/WNL.0000000000005560
21. Reindl M, Schanda K, Woodhall M, Tea F, Ramanathan S, Sagen J, et al. An International Multicenter Examination of MOG Antibody Assays. Neuroimmunol Neuroinfllammation (2020) 7(2):1–12. doi: 10.1212/NXI.0000000000000674
22. Woodhall M, Çoban A, Waters P, Ekizoğlu E, Kürtüncü M, Shugaiv E, et al. Glycine Receptor and Myelin Oligodendrocyte Glycoprotein Antibodies in Turkish Patients With Neuromyelitis Optica. J Neurol Sci (2013) 335(1–2):221–3. doi: 10.1016/j.jns.2013.08.034
23. de Mol CL, Wong YYM, van Pelt ED, Wokke BHA, Siepman TAM, Neuteboom RF, et al. The Clinical Spectrum and Incidence of anti-MOG-associated Acquired Demyelinating Syndromes in Children and Adults. Mult Scler J (2019) 26(7):806–14. doi: 10.1177/1352458519845112
24. Spadaro M, Gerdes LA, Krumbholz M, Ertl-Wagner B, Thaler FS, Schuh E, et al. Autoantibodies to MOG in a Distinct Subgroup of Adult Multiple Sclerosis. Neurol - Neuroimmunol Neuroinflamm (2016) 3(5):e257. doi: 10.1212/NXI.0000000000000257
25. Kim Y, Hyun J-W, Woodhall MR, Oh Y-M, Lee J-E, Jung JY, et al. Refining Cell-Based Assay to Detect MOG-IgG in Patients With Central Nervous System Inflammatory Diseases. Mult Scler Relat Disord (2020) 40:1–9. doi: 10.1016/j.msard.2020.101939
26. Tea F, Pilli D, Ramanathan S, Lopez JA, Merheb V, Lee FXZ, et al. Effects of the Positive Threshold and Data Analysis on Human Mog Antibody Detection by Live Flow Cytometry. Front Immunol (2020) 11:119. doi: 10.3389/fimmu.2020.00119
27. Sugimoto K, Mori M, Liu J, Tanaka S, Kaneko K, Oji S, et al. The Accuracy of Flow Cytometric Cell-Based Assay to Detect Anti-Myelin Oligodendrocyte Glycoprotein (MOG) Antibodies Determining the Optimal Method for Positivity Judgement. J Neuroimmunol (2019) 336:1–9. doi: 10.1016/j.jneuroim.2019.577021
Keywords: myelin oligodendrocyte glycoprotein, cell-based assay, immunoglobulin-G, demyelinating disease, central nervous system
Citation: Marchionatti A, Hansel G, Avila GU and Sato DK (2021) Detection of MOG-IgG in Clinical Samples by Live Cell-Based Assays: Performance of Immunofluorescence Microscopy and Flow Cytometry. Front. Immunol. 12:642272. doi: 10.3389/fimmu.2021.642272
Received: 15 December 2020; Accepted: 19 April 2021;
Published: 07 May 2021.
Edited by:
Long-Jun Wu, Mayo Clinic, United StatesReviewed by:
Elisabetta Traggiai, Novartis, SwitzerlandCopyright © 2021 Marchionatti, Hansel, Avila and Sato. This is an open-access article distributed under the terms of the Creative Commons Attribution License (CC BY). The use, distribution or reproduction in other forums is permitted, provided the original author(s) and the copyright owner(s) are credited and that the original publication in this journal is cited, in accordance with accepted academic practice. No use, distribution or reproduction is permitted which does not comply with these terms.
*Correspondence: Douglas Kazutoshi Sato, ZG91Z2xhcy5zYXRvQHB1Y3JzLmJy
Disclaimer: All claims expressed in this article are solely those of the authors and do not necessarily represent those of their affiliated organizations, or those of the publisher, the editors and the reviewers. Any product that may be evaluated in this article or claim that may be made by its manufacturer is not guaranteed or endorsed by the publisher.
Research integrity at Frontiers
Learn more about the work of our research integrity team to safeguard the quality of each article we publish.