- 1Key Laboratory of Aquaculture Nutrition and Feed (Ministry of Agriculture and Rural Affairs), Key Laboratory of Mariculture (Ministry of Education), Ocean University of China, Qingdao, China
- 2Laboratory for Marine Fisheries Science and Food Production Processes, Qingdao National Laboratory for Marine Science and Technology, Qingdao, China
Marine fish larvae are vulnerable during the early life period. The early intervention using probiotics may be a promising method to improve growth of fish larvae. In this study, a 30-day feeding trial was conducted to evaluate the effects of early life intervention using probiotic Clostridium butyricum (CB) on growth performance, intestinal development, immune response and gut microbiota of large yellow croaker (Larimichthys crocea) larvae. Four isonitrogenous and isolipidic diets were formulated with the supplementation of four different levels of CB (5 × 109 CFU g−1), 0.00% (Control), 0.10% (CB1), 0.20% (CB2), and 0.40% (CB3). Results showed that larvae fed diets with CB had significant higher final length than the control group. Meanwhile, larvae fed the diet with 0.10% CB had significant higher final weight and specific growth rate (SGR) than the control group. However, no significant difference in survival rate was observed among dietary treatments. CB supplementation significantly increased the height of intestinal villus and the length of intestinal enterocyte. Similarly, CB supplementation significantly increased the expression of tight zonula occludens-2 (zo-2) and ornithine decarboxylase (odc) than the control group. Larvae fed the diet with 0.20% CB had significant higher lipase and leucine-aminopeptidase (LAP) activity than the control group. Moreover, CB supplementation significantly improved immune enzyme activities than the control group. Sequencing of bacterial 16S rRNA V4-5 region indicated that dietary CB altered intestinal microbiota profile and decreased intestinal microbial diversities of larvae. CB supplementation could effectively increase the abundance of CB, and decrease the abundance of some potential pathogenic bacteria in larval gut. These results revealed that early life intervention using 0.10–0.20% CB could promote growth of large yellow croaker larvae probably through promoting intestinal development, improving immune enzyme activities and modulating gut microbiota.
Introduction
Marine fish gut tract is not fully developed during the early stage of life (1). Fish larvae undergo major morphological, cellular and functional changes of gut tract during the first stage of life (2). The successful development of gut tract is important for fish larvae to digest and absorb food (3). Furthermore, gut microbiota is not fully assembled in marine fish digestive tract during the early stage of life (4). The microbes that reside in the gut tract have profound influence on host immune system priming, protection and development, as well as nutrient supplementation to the host (5, 6). The rate and trajectory of acquisition of gut microbiota have a considerable impact on later health outcomes (7).
In recent years, many studies have indicated that probiotic treatment in the early life is necessary for digestive physiology functions, gastrointestinal tract immune and microbiota development (8, 9). Clostridium butyricum (CB) is a gram-positive, obligate anaerobic and endospore-forming probiotic, which is part of the normal gut bacteria for both mammals and aquatic animals (10, 11). CB could improve gastrointestinal function, which has been used for the clinical treatment of newborn animals (12–14). In recent years, research studies in regard to CB were carried out in aquatic species, including tilapia (15), shrimp (16), and prawn (17). However, to our best knowledge, the role of dietary CB in marine fish larvae is still uncertain.
Large yellow croaker (Larimichthys crocea) is an economic important marine fish species in south China (18). Similarly to most marine fish species, large yellow croaker larvae had an undifferentiated and undeveloped gut and accessory organs (19). The production of large yellow croaker is often hampered by high mortality in larval stage (20). Therefore, the present study was conducted to investigate the effects of early life intervention using CB on the growth performance, immune response, intestinal development and gut microbiota of large yellow croaker (Larimichthys crocea) larvae. By using a marine fish larvae model, the present study might provide novel insights into probiotic treatment in early life intervention.
Materials and Methods
Animal Ethics
All procedures of this study were conducted on fish, and animal care was performed strictly followed the Management Rule of Laboratory Animals (Chinese Order No. 676 of the State Council, revised 1 March 2017).
Diet Formulation
Large yellow croaker larvae were fed with four isonitrogenous (52% crude protein) and isolipidic (19% crude lipid) diets, which were supplemented with different levels of CB (0.00% as the control group, 0.10% as CB1 group, 0.20% as CB2 group, and 0.40% as CB3 group) (Supplementary Table 1). The additive CB was purchased from Vland Biotech Co., Ltd., China, containing cells at 5 × 109 colony forming units (CFU) g−1. Micro-diet (MD) was manufactured by micro-bonding technology (20). The particle size of the formulated diets ranged from 250 to 380 μm for fish larvae between 14 and 24 days after hatch (DAH) and 380–500 μm for fish larvae between 25 and 47 DAH.
Experimental Procedure
The objective animal of this study were purchased from the Ningde Fufa Fishery Company Limited, Ningde, China, and were reared at the State Key Laboratory of Large Yellow Croaker, Ningde, China. All fish larvae in the hatchery were fed with rotifers, Brachionus plicatilis (0.5–2.0 × 104 individual L−1) from 3 to 7 DAH, Artemia nauplii (1.0–1.5 × 103 individual L−1) from 6 to 11 DAH, and Calanus sinicus from 10 to 14 DAH, and then the larvae were weaned onto experimental diets. Ten thousand larvae (17 DAH) were randomly allocated in 12 blue plastic tanks (water volume 1,000 L) to conduct the experiment. Different experimental diets were randomly allocated to triplicate groups of larvae. During the rearing period, water temperature, pH, and salinity were strictly controlled during the rearing period, ranging from 22 to 24°C, 7.9 to 8.3, 21 to 24‰, respectively. The water volume was renewed about 60–150% daily. Larvae were reared under 16h light:8h dark dial cycle photoperiod. All the larvae were manually fed to satiation with the experimental diets seven times daily (6:00, 8:00, 11:00, 14:00, 17:00, 20:00, and 22:00) during the whole experiment period (from 17 DAH to 47 DAH).
Sample and Dissection
Before the experiment was conducted, initial body weight and body length of 150 randomly collected larvae (17 DAH) were measured. At the end of the experiment, larvae were fasted for 24 h before sampling. Survival rate was calculated by comparing the remaining larvae in each tank to the initial number. Final body weight and final body length of 1,000 larvae randomly collected from each tank were measured. Thirty larvae were collected from each tank and preserved in 4% paraformaldehyde for 24 h and transferred to 75% alcohol for hematoxylin and eosin (H&E) staining. Ninety larvae from each tank were dissected on ice to obtain visceral mass, which contains a crude mixture of pancreas, liver, heart, spleen and intestine, for immune enzyme activity assay. Pancreatic and intestinal segments (PS and IS) of sixty larvae were separated on a glass plate maintained at 0°C under a dissecting microscope for digestive enzyme activity assay (20). The whole intestine of sixty larvae from each tank was separated aseptically under a dissecting microscope for the analysis of intestinal microflora and gene expression assays.
Analytical Methods
Digestive Enzyme Activities Assay
Four stages (22, 27, 37, and 47 DAH) of large yellow croaker larvae were chosen to reflect digestive enzyme activities during different growth stages (1). PS and IS (0.1–0.2 g) of 22, 27, 37, and 47 DAH larvae were weighed and homogenized in 2 ml 0°C normal saline, followed by centrifuging (2,500g, 10 min), then the supernatant was collected for further assay. Purified brush border membranes (BBMs) from homogenate of the intestinal segment were obtained according to a method described by published paper (21). Activities of leucine-aminopeptidase (LAP) were assayed in BBM according to published paper (22). Trypsin activity was assayed following published paper (23). Amylase activity was assayed with α-Amylase Assay Kit (Nanjing Jiancheng Bio-Engineering Institute, China). Lipase activity was assayed with Lipase Assay Kit (Nanjing Jiancheng Bio-Engineering Institute, China). Protein was determined by the Total protein quantitative assay kit (Nanjing Jiancheng Bio-Engineering Institute, China).
Immune Enzyme Activities Assay
The visceral mass was weighed and homogenized in phosphate-buffered saline. The proportion of tissue (g) and saline (ml) was 1:9. The homogenate of larvae was then centrifuged (4,000g, 15 min), and the supernatant was collected for the following assays. Immune enzymes kits (Nanjing Jiancheng Bio-Engineering Institute, China) were used to evaluate the activity of acid phosphatase (ACP), alkaline phosphatase (AKP), catalase (CAT), lysozyme (LZM), and superoxide dismutase (SOD) in the collected visceral mass.
Intestinal Histology Analysis
The intestinal micromorphology was determined based on the method described by published paper (19). Briefly, the whole larvae were washed and dehydrated with gradient alcohol, and then paraffin-embedded, sectioned, and stained with hematoxylin and eosin.
cDNA Synthesis and Real-Time Quantitative Polymerase Chain Reaction
Total RNA was extracted from the whole intestine of larvae using RNAiso Plus (Takara Biotech, Dalian, China) following the instructions of the manufacturer, and electrophoresed on a 1.2% denaturing agarose gel to detect the quality (20). And then extracted RNA was assessed by a Nano Drop®2000 spectrophotometer (Thermo Fisher Scientific, USA) to test the concentration. Then, RNA reverse was transcribed to cDNA by Prime Script-RT reagent Kit (Takara, Japan). The real-time quantitative polymerase chain reaction was carried out in a quantitative thermal cycler (CFX96TM Real-Time System, BIO-RAD, USA). The operational approach referred to Zuo et al. (24). The primer sequences for proliferating cell nuclear antigen (pcna), tight zonula occludens-1 (zo-1), tight zonula occludens-2 (zo-2), occludin, ornithine decarboxylase (odc), interleukin-1β (il-1β), interleukin-6 (il-6), interleukin-8 (il-8), interferon γ (ifnγ), cyclooxygenase-2 (cox-2) and β-actin were designed and synthesized based on the corresponding sequences in GenBank and published papers (Supplementary Table 2) (20, 25, 26). The fluorescence data acquired during the extension phase were normalized to β-actin via 2−ΔΔCT methods (27).
Gut Microbiota Collection and Bacterial Genomic DNA Extraction
Thirty fresh larvae gut of 47 DAH were mixed and stored at −80°C until DNA was extracted using the CTAB method (28). 1 ng of purified gut bacterial DNA was used to sequence and classify as previously described (29). The 515f/907r primer set amplified V4-5 region of the 16s rRNA gene was used to conduct PCR, using barcoded primers: Fwd5′-GTGCCAGCMGCCGCGGTAA-3′, Rev5′-CCGTCAATTCCTTTGAGTTT-3′. Subsequently, sequencing was performed on an Illumina MiSeq platform, provided by Beijing Novogene Genomics Technology Co. Ltd. (China). Complete data were submitted to the NCBI Sequence Read Archive (SRA) database under accession number PRJNA686247. FLASH (V1.2.7, http://ccb.jhu.edu/software/FLASH/) was used to merge the reads from the same original DNA (30). Uparse software (Uparse v7.0.1001, http://drive5.com/uparse/) was applied to cluster the unique sequences to acquire operational taxonomic units (OTUs) according to the similarity of sequence distance based up to 97% or greater (31). Subsequently, the representative OTUs were annotated through the RDP Classifier (Version 2.2, http://sourceforge.net/projects/rdp-classifier/) and GreenGene database (http://greengenes.lbl.gov/cgi-bin/nph-index.cgi) (32). QIIME (Quantitative Insights Into Microbial Ecology) V 1.7.0 software package (http://qiime.org/index.html) and the UPARSE (http://drive5.com/uparse/) pipeline were adopted to analyze the alpha and beta diversity. Principal component analysis (PCA) was conducted with Mothur and R software packages (http://www.R-project.org). Linear discriminant analysis (LDA) effect size (LefSe) analysis was used to identify the different abundant taxa between the control group and different CB groups (33).
Calculations and Statistical Analysis
The growth parameters were calculated as follows:
Where Nt is final number of larvae in each tank and Ni is initial number of larvae in each tank at the beginning of the experiment; Wt and Wi are the final and initial body weights, respectively; d is the experimental period in days.
Statistical analysis was performed in SPSS 16.0 (SPSS Inc., USA). Data from each treatment were subjected to one-way analysis of variance (ANOVA) followed by Tukey’s multiple-range test. For statistically significant differences, P <0.05 was applied. Results were presented as mean ± S.E. (Standard error of means).
Results
Early Life Intervention With CB Improved Growth of Fish Larvae
No significant difference in survival rate was observed among dietary treatments (P > 0.05) (Table 1), whereas the highest value was recorded in larvae fed diets with 0.10% CB, followed by 0.20% CB, 0.40% CB and 0.00% CB, respectively. With the increase of CB level in diets, the growth performance firstly increased and then decreased. CB supplementation significantly improved final body length than the control group (P < 0.05) (Table 1). Meanwhile, larvae fed the diet with 0.10% CB showed significant higher final body weight and SGR than the control group (P < 0.05) (Table 1).
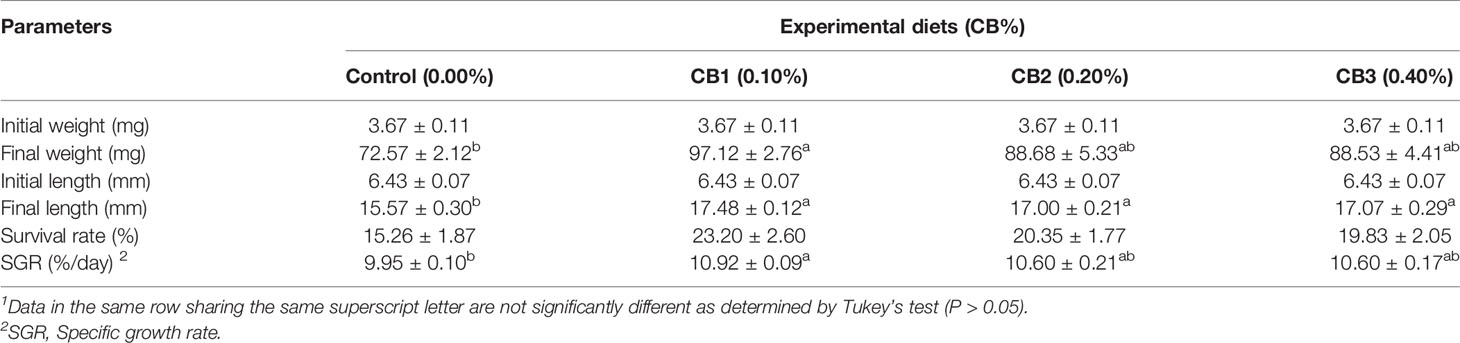
Table 1 Effects of early life intervention using Clostridium butyricum on growth performance of large yellow croaker larvae (Means ± S.E., n = 3)1.
Early Life Intervention With CB Improved Gut Tract Development
Early Life Intervention With CB Improved the Intestinal Morphology
CB supplementation effectively improved the intestinal morphology of large yellow croaker larvae (Supplementary Figure S1). Larvae fed diets with CB had significant higher villus height and enterocyte height than the control group (P < 0.05) (Table 2). Similarly, significant higher muscular thickness was observed in fish fed the diet with 0.10% CB than the control group (P < 0.05) (Table 2).

Table 2 Effects of early life intervention using Clostridium butyricum on morphology of the intestine of large yellow croaker larvae (Means ± S.E., n = 3)1.
Early Life Intervention With CB Increased Gene Expression of zo-2 and odc
The markers for epithelial proliferation and differentiation were selected (odc, pcna, zo-1, zo-2 and occludin) to compare intestinal development among larvae fed diets with different levels of CB. CB supplementation significantly increased mRNA expression of zo-2 and odc than the control group (P < 0.05) (Figure 1). However, no significant difference in mRNA expression of zo-1, occludin, and pcna was observed among dietary treatments (P > 0.05) (Figure 1).
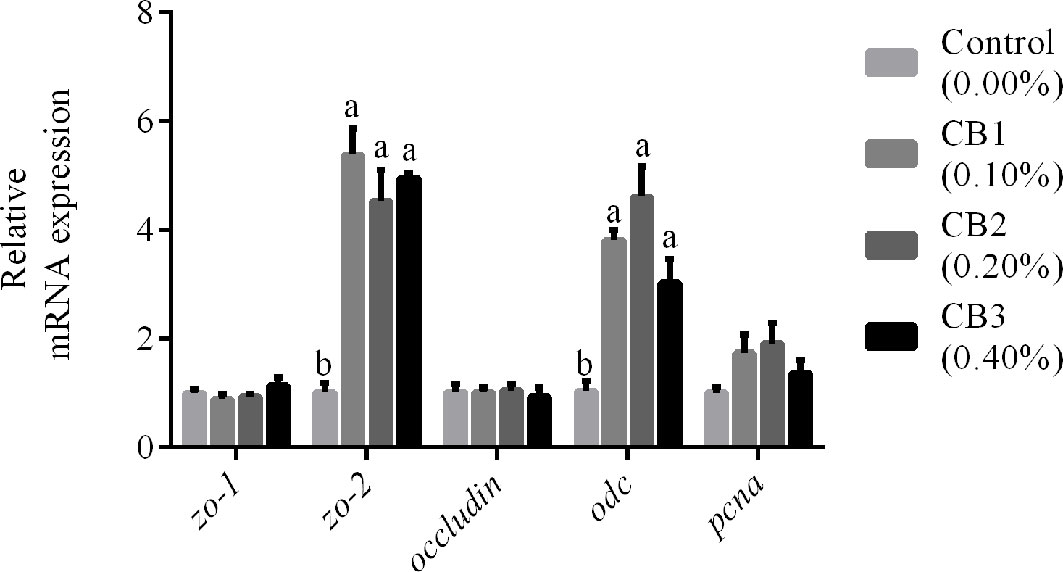
Figure 1 Effects of early life intervention using Clostridium butyricum on zo-1, zo-2, occludin, odc and pcna mRNA expression in whole intestine of large yellow croaker larvae. Values are means (n = 3), with their standard errors represented by vertical bars. Bars bearing the same letters were not significantly different (P > 0.05, Tukey’s test).
Early Life Intervention With CB Improved Lipase and LAP Activities
No significant difference in the activity of trypsin and amylase in IS and PS was observed among dietary treatments (P > 0.05) (Table 3). Compared with the control group, 0.20% CB supplementation not only increased lipase activity of 37 DAH larvae in PS and IS, but also increased lipase activity of 47 DAH larvae in IS (P < 0.05) (Table 3). Similarly, 0.20% CB supplementation significantly increased LAP activity of 47 DAH larvae in BBM than the control group (P < 0.05) (Table 3).
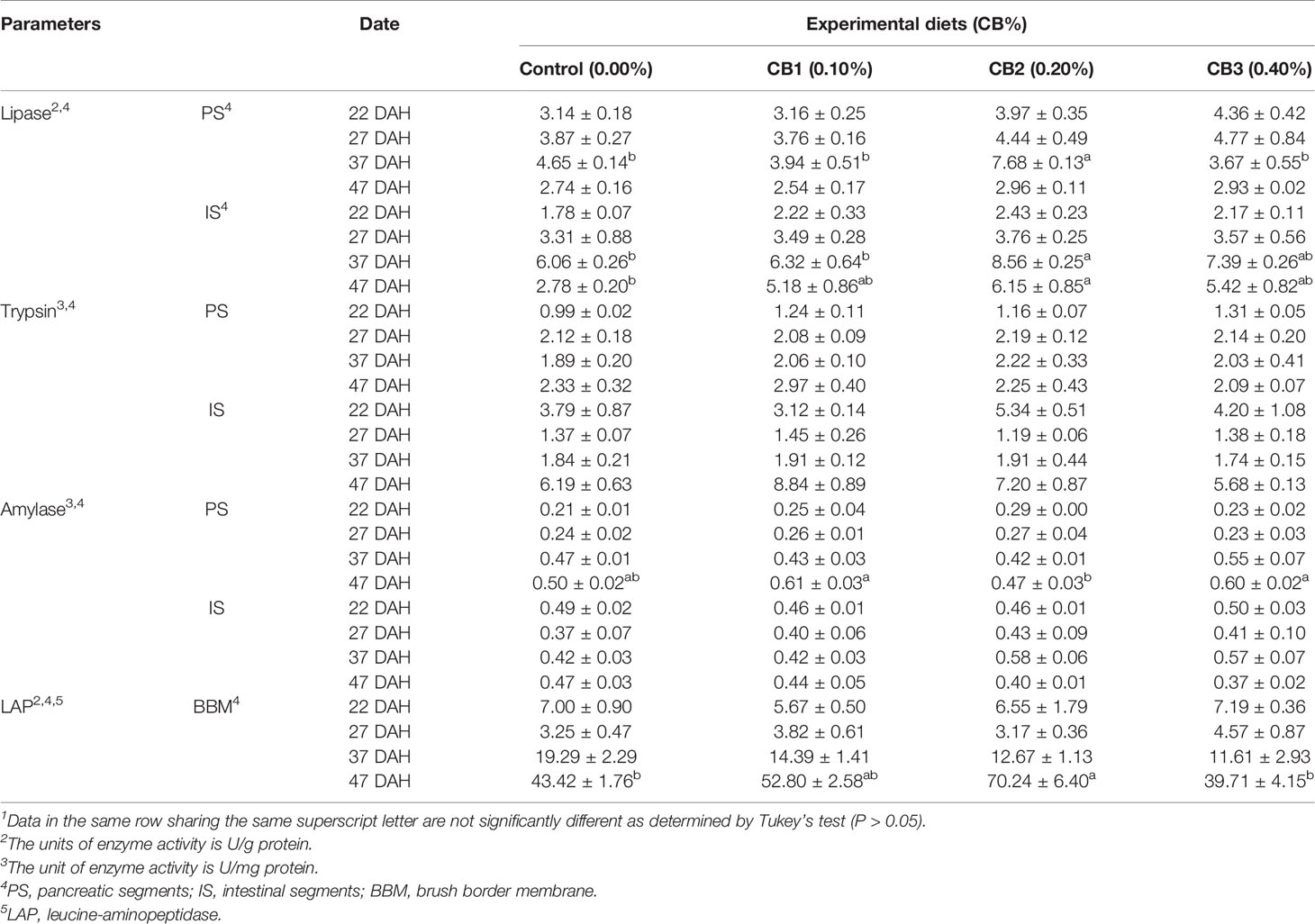
Table 3 Effects of early life intervention using Clostridium butyricum on digestive enzyme activity of large yellow croaker larvae (Means ± S.E., n = 3)1.
Early Life Intervention With CB Improved Immune Response
With the increase of CB level in diets, immune enzyme activities firstly increased and then decreased. CB supplementation significantly improved LZM activity than the control group (P < 0.05) (Table 4). Furthermore, 0.10 and 0.20% CB supplementation significantly increased AKP and CAT activities than the control group (P < 0.05) (Table 4). 0.10% CB supplementation significantly increased ACP activity than the control group (P < 0.05) (Table 4). However, no significant difference in the activity of SOD was observed among dietary treatments (P > 0.05) (Table 4). Similarly, no significant difference in mRNA expression of inflammatory factors (il-1β, il-6, il-8, ifnγ and cox-2) was observed among dietary treatments (P > 0.05) (Supplementary Figure 2).
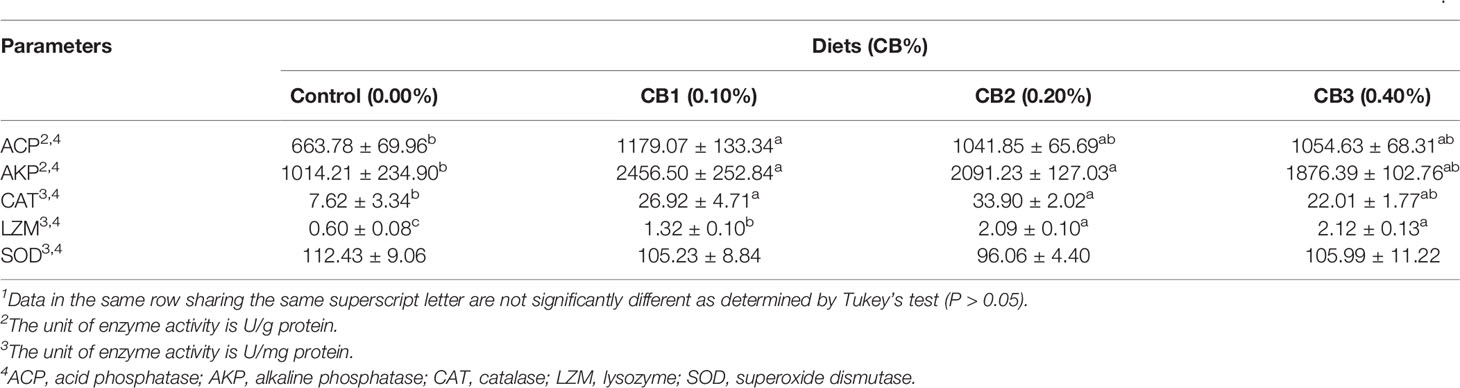
Table 4 Effects of early life intervention using Clostridium butyricum on immune enzyme activity in the visceral mass of large yellow croaker larvae (Means ± S.E., n = 3)1.
Early Life Intervention With CB Altered Gut Microbiota of Fish Larvae
Structural Changes of Gut Microbiota in Response to CB Supplementation
After assembled, quality screened and trimmed, a total of 768,414 high quality valid reads was obtained, ranging from 56,190 to 69,519, resulting in identification of 1,850 OTUs with 97% identity from 12 samples (data not shown). The OTUs were assigned to 326 species, 547 genera, 240 families, 117 orders, 49 class, and 30 phyla (data not shown). For all samples, the rarefied curves for OTU number tended to approach the saturation plateau, indicating complete sequencing efforts for all samples (Supplementary Figure 3).
A Venn diagram showed that 106 OTUs were shared by group Control, CB1, CB2 and CB3, and the number of unique OTUs in group Control, CB1, CB2 and CB3 was 312, 104, 80 and 121, respectively (Figure 2A). The alpha diversity index results indicated that the inclusion of dietary CB led to lower richness of observed species number in large yellow croaker larvae gut microbiota (P < 0.05) (Table 5). Alpha diversity including phylogenetic diversity whole tree (PD whole tree) index and abundance-based coverage estimator (ACE) index were markedly decreased in the gut microbiota of larvae in CB groups (P < 0.05) (Table 5). Meanwhile, larvae fed the diet with 0.20% CB showed significant lower Shannon index in the gut microbiota (P < 0.05) (Table 5). To analyze the extent of similarities in microbial communities, PCA was conducted to determine the beta diversity (Figure 2B). The PCA results showed that the intestine bacterial in the control group and CB groups were separated (Figure 2B). Similarly, the gut microbiota structure from groups CB1, CB2, and CB3 were similar and clustered within one higher branch, whereas the control group was distinct from the CB groups and formed another branch (Figure 2C).
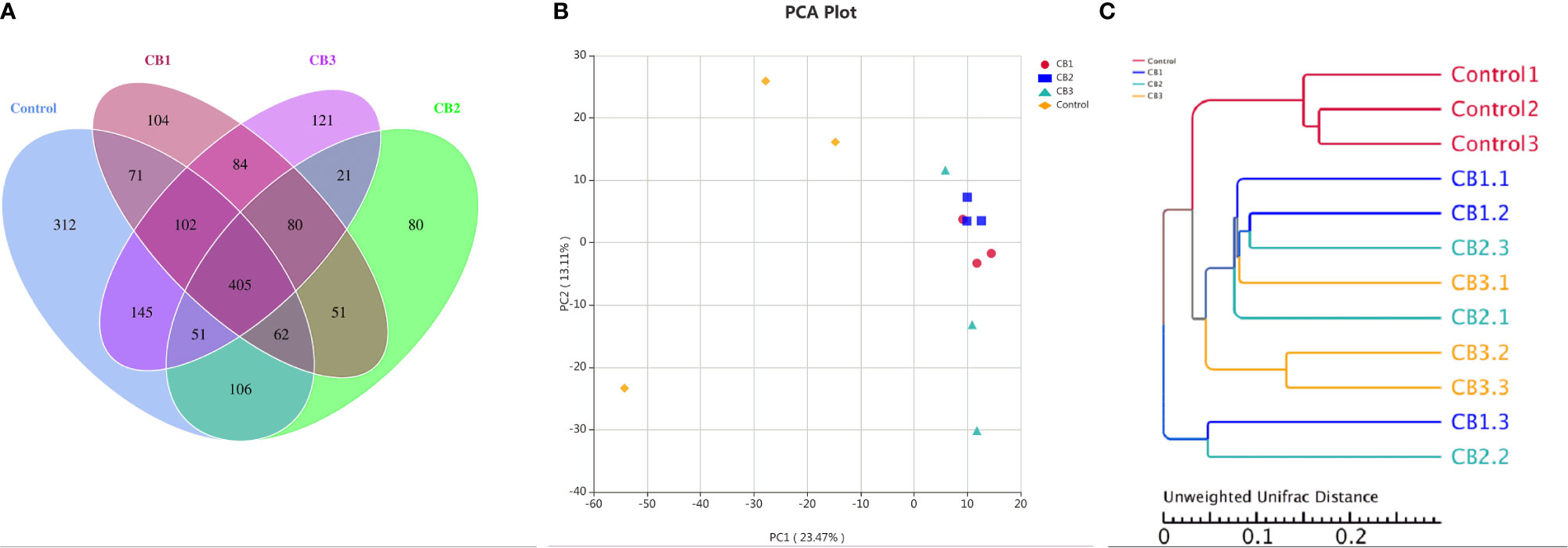
Figure 2 Effects of early life intervention using Clostridium butyricum on intestinal microbial structure of large yellow croaker larvae (n = 3/group). (A) Venn diagram; (B) principal component analysis (PCA); (C) unweighted uniFrac distance matrix.
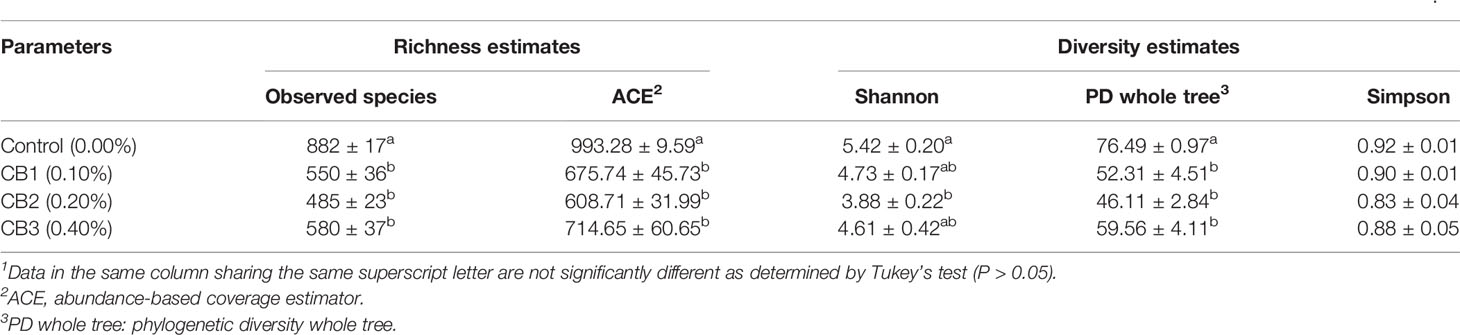
Table 5 Effects of early life intervention using Clostridium butyricum on alpha diversity index of intestinal microbiota of large yellow croaker larvae (Means ± S.E., n = 3)1.
Component Changes of Gut Microbiota in Response to CB Supplementation
At the phylum level, Proteobacteria, Firmicutes and Actinobacteria were detected as predominant bacterial phyla in the intestine of large yellow croaker from all groups (Figure 3A). At the genus level, Leisingera, Pseudomonas and unidentified Clostridiales were detected as predominant bacterial genera in the intestine of large yellow croaker larvae from all groups (Figure 3B). At the species level, Pseudomonas psychrotolerans, Clostridium butyricum, Alcaligenes faecalis composed dominant species of larval gut microbiota communities (Figure 3C).
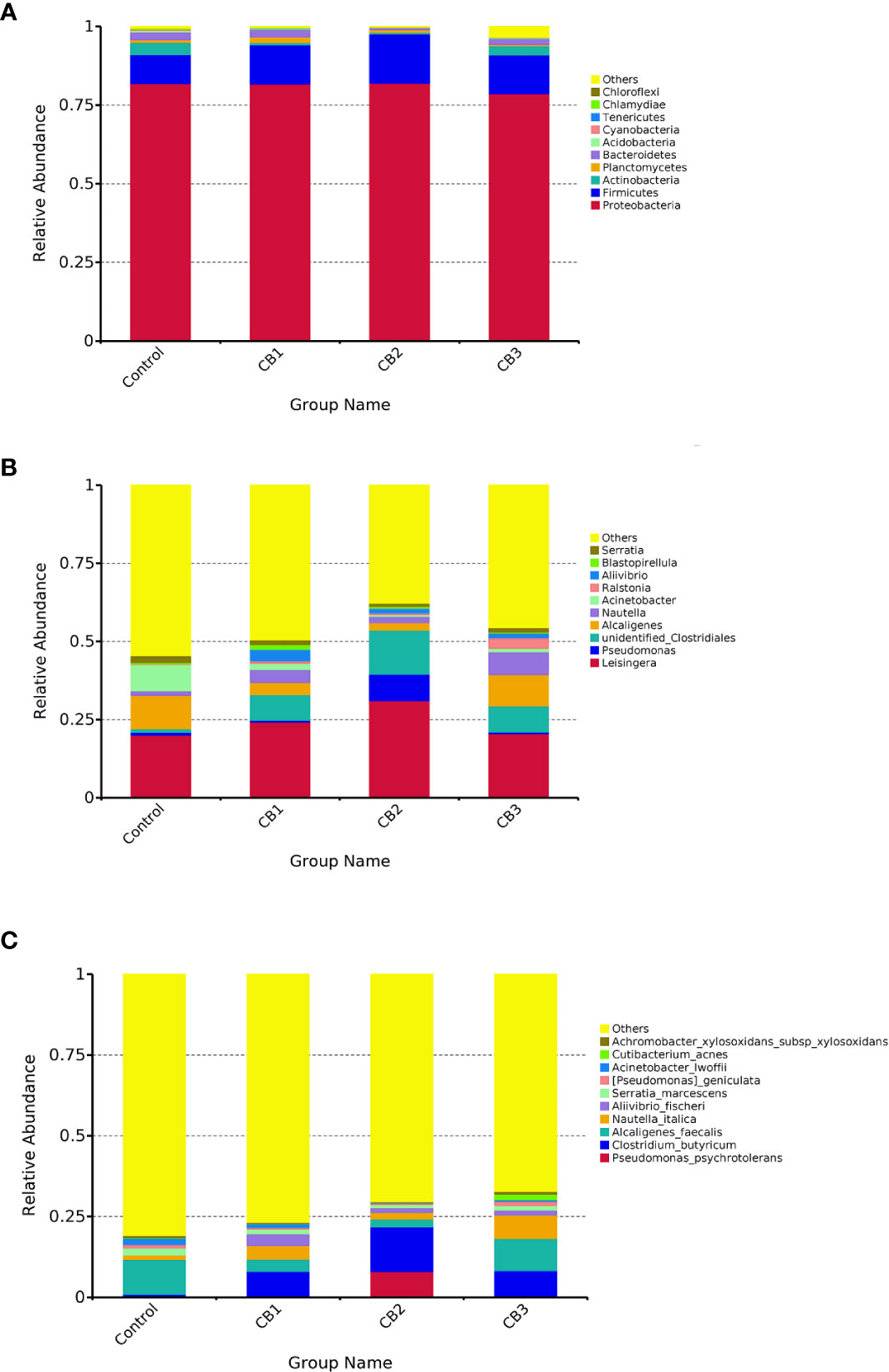
Figure 3 Taxonomy classification of reads at phylum (A), genus (B) and specie (C) taxonomic levels (n = 3/group). Only top 10 most abundant (based on relative abundance) bacterial phyla, genera and species were shown. Other phyla, genera and species were all assigned as ‘Others’.
To compare the differences in gut microbial community composition between the control group and CB groups, LEfSe analysis was performed (Figure 4). The results revealed that there were significant differences in taxonomic distribution of intestinal microbiota communities between the control group and CB groups. CB supplementation significantly increased the relative abundance of class Clostridia, order Closrtidiales, family unidentified Clostridiales, genus unidentified Clostridiales and specie Clostridium butyricum, while decreased the relative abundance of class Bacilli, family Moraxellaceae and genus Acinetobacter (P < 0.05) (Figures 4A–F). Furthermore, dietary CB at 0.10% level significantly increased the relative abundance of phylum Firmicutes, order Vibrionales, family Vibrionaceae, genus Aliivibrio and specie Aliivibrio fischeri, but significantly decreased the relative abundance of phylum Actinobacteria, class unidentified Actinobacteria, orders Bacillales and Pseudomonadales (P < 0.05) (Figures 4A, D). Dietary CB at 0.20% level significantly decreased the relative abundance of phylum Actinobacteria, class unidentified Actinobacteria, orders Bacillales, Lactobacillales and unidentified Gammaproteobacteria, family Burkholderiaceae, genus Alcaligenes and specie Alcaligenes faecalis (P < 0.05) (Figures 4B, E). Dietary CB at 0.40% level significantly increased the relative abundance of genera Ralstonia and Nautella and specie Nautella italica (P < 0.05) (Figures 4C, F).
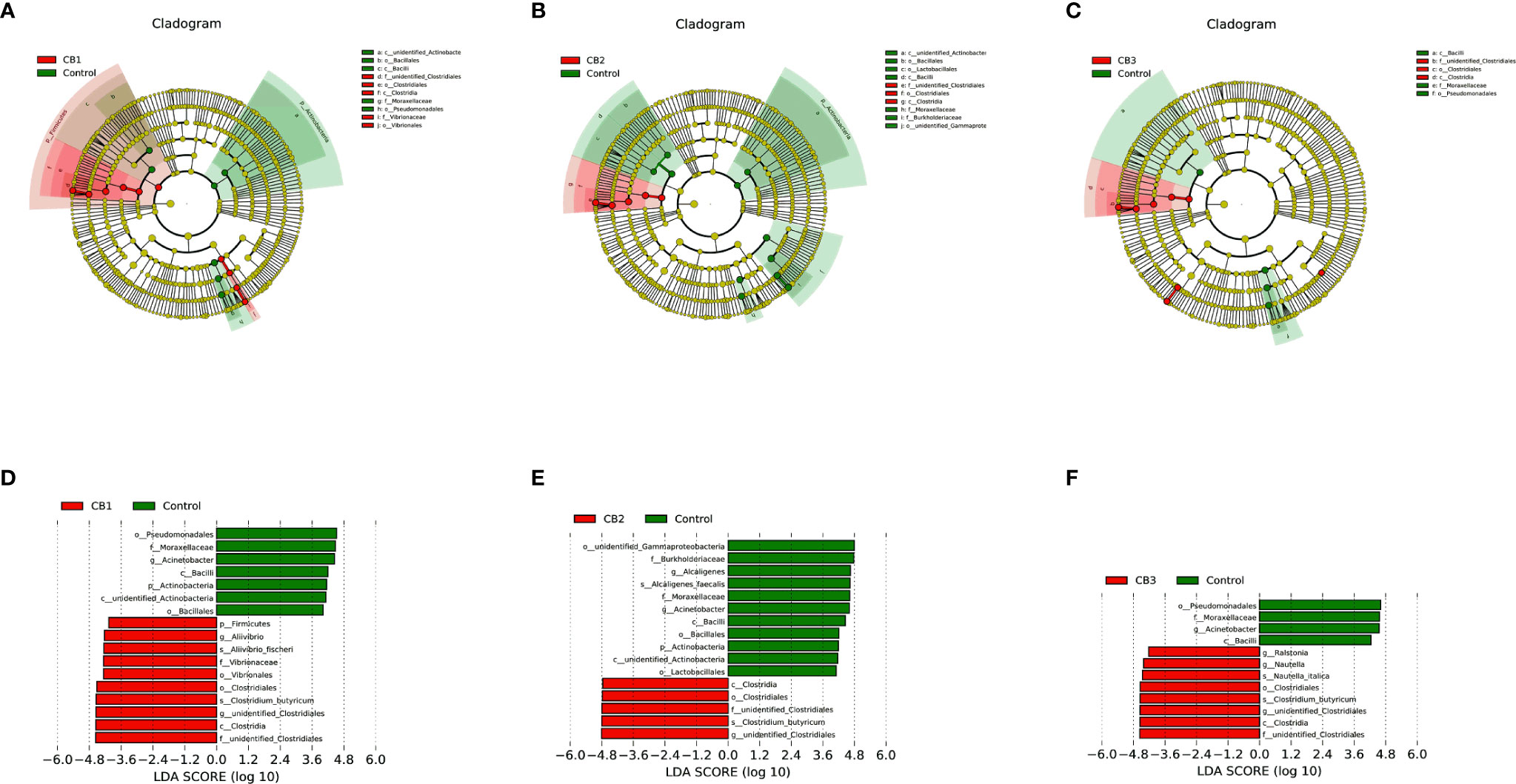
Figure 4 LEfSe analysis identified the most differentially abundant taxons between Control and Clostridium butyricum supplementation groups (n = 3/group). (A–C) Taxonomic representation of statistically and biologically consistent differences among intestinal microbiota of large yellow croaker fed with Control, CB1, CB2 and CB3 diets. Differences were represented by the color of the most abundant taxa (Green indicated one taxon with significantly higher relative abundance in Control treatment, red indicated one taxon significantly higher in CB treatment and yellow indicated no significant difference). (D–F) Histogram of linear discriminant analysis (LDA) scores for differentially abundant taxon. Cladogram was calculated by LefSe, and displayed according to effect size.
Discussion
There has been increasing evidence suggesting the maturation of intestinal tract, immune system and gut microbiota in the early life is significant for growth and development of marine fish larvae (34, 35). Early infancy is considered as an ideal window for gut microbial colonization (36, 37). Therefore, early intervention using probiotics during the critical period may be a promising method to enhance growth of fish larvae. In the present study, appropriate CB supplementation could enhance growth performance of large yellow croaker larvae, which were consistent with some previous findings in Miichthys miiuy (38), Penaeus monodon (39) and Litopenaeus vannamei (40).
The normal development of intestinal tract during the early life has a considerable impact on later health outcomes (1). The maturation process of gut tract can be enhanced, stopped or delayed depending on diet composition (19). In this study, CB supplementation could enlarge the villus height, enterocyte height, and muscular thickness of larval gut tract, which was consisted with some previous studies on tilapia (15), pacific white shrimp (40) and weaned piglets (41). Furthermore, the expression of zo-2 and odc was higher in larvae fed diets with CB than the control group. Odc plays important roles in the proliferation, migration, and differentiation of cells (42); zo-2 participates in tight junction structural integrity by binding to the actin cytoskeleton (43). These results suggested that CB could promote intestinal development of larvae by accelerating the proliferation of intestinal epithelial and maintaining the integrity of the intestinal barrier.
Moreover, digestive enzyme activities could be used as an indicator of development rate, food acceptance and digestive capacity (1). Previous research has indicated that CB could improve digestive function through producing a range of supplemental digestive enzymes (10, 39). In this study, 0.20% CB supplementation not only increased lipase in PS and IS of 37 DAH larvae, but also increased lipase in IS of 47 DAH larvae, which were consistent with the previous studies in broiler chickens (44), piglets (41), Macrobrachium rosenbergii (17) and Litopenaeus vannamei (40). Furthermore, the onset of BBM enzymes in intestine was considered as crucial in the maturation process of the digestive function (45). The present study found that 0.20% CB supplementation could improve activities of LAP in BBM, which demonstrated that appropriate dietary CB could promote the transition of larval digestive functions to adult mode (1).
The immune system during the early life is immature, which has been considered as a major restriction to healthy growth of fish larvae (46, 47). Probiotics can act as non-specific immune factors and have a positive effect on host immune system (35, 48) CB supplementation significantly affected nearly all immune enzyme activities (ACP, AKP, CAT and LZM), indicating CB supplementation could improve immune status of larvae. Intriguingly, no significant differences in the survival rate and mRNA expression of inflammatory factors were observed among dietary treatments. Zhao et al. (49) found CB could decrease cytokine levels (ifn-γ, il-1β, il-8, and tnf-α) in intestinal tissues of chickens with Salmonella infection, but no significant differences in cytokine levels were observed between CB treatment group and normal diet group (49). Above all, combining the results of the present study and current research, we speculated dietary CB could effectively decrease inflammatory factor level to protect host from pathogens.
Gut microbiota plays a crucial role in health and well-being of marine fish starting from hatch (4, 8). The current study indicated that modulation of intestine microbiota in large yellow croaker towards potentially more beneficial microbial community could be achieved through CB supplementation. The present study showed CB had a strong effect on the overall structure of intestinal microbiota in larvae. Similarly, compared with the control group, observed species number, PD whole tree index and ACE index were lower in the gut of larvae fed diets with CB supplementation. These results indicated that CB supplementation could regulate the structure of gut microbiota and decrease intestinal microbial diversity and richness, representing the positive effect of CB on gut microbiota development during the early life of larvae (35, 50).
Moreover, early life intervention using CB supplementation improved the composition of gut microbiota. CB supplementation at 0.10 and 0.20% levels significantly decreased the relative abundance of phylum Actinobacteria, indicating 0.10 and 0.20% CB may have a more profound effect on the intestinal community of larvae than 0.40% CB. Intriguingly, the present study found CB supplementation could increase the relative abundance of CB, which indicated larval intestine could be colonized by CB. Previous research has indicated that CB decreased the number of pathogens in the host gut tract by creating an oxygen-free environment, producing prebiotics and generating butyric acid (10). The present study found that CB significantly down-regulated the relative abundance of genus Acinetobacter, and CB at 0.20% significantly decreased the relative abundance of genus Alcaligenes and specie Alcaligenes faecalis. Acinetobacter was associated with bacteremia, pulmonary infections, meningitis, diarrhea, and nosocomial infections (51). And Alcaligenes faecalis was an opportunistic pathogen, which could be resistant to common antibiotics and closely related with nosocomial infections (52). These results revealed that CB could colonize in the fish larval gut tract, and play an inhibitory effect on opportunistic pathogens.
Conclusion
This study demonstrated that early life intervention using dietary CB could improve growth performance of large yellow croaker larvae probably through promoting intestinal development, improving immune enzyme activities, and modulating gut microbiota. The optimal CB (containing cells at 5 × 109 CFU g−1) supplementation dosage is 0.10–0.20%. Probiotic treatment in early life intervention may be a promising method to promote the maturation of the intestinal tract, immune system, and gut microbiota.
Data Availability Statement
The datasets presented in this study can be found in online repositories. The names of the repository/repositories and accession number(s) can be found below: NCBI BioProject, accession no: PRJNA686247.
Ethics Statement
The animal study was reviewed and approved by the Committee on the Ethics of Animal Experiments of Ocean University of China.
Author Contributions
KM, QA, and ZY designed the research. ZY, QL, and YH conducted the research. SG, WH, CY, and ZY analyzed the data. ZY wrote the manuscript. QA and YG provided language help. All authors contributed to the article and approved the submitted version.
Funding
This research was supported by the Agriculture Research System of China (Grant NO. CARS-47-11), the National Science Fund for Distinguished Young Scholars of China (Grant NO. 31525024), and the Key Program of National Natural Science Foundation of China (Grant NO. 31830103).
Conflict of Interest
The authors declare that the research was conducted in the absence of any commercial or financial relationships that could be construed as a potential conflict of interest.
Supplementary Material
The Supplementary Material for this article can be found online at: https://www.frontiersin.org/articles/10.3389/fimmu.2021.640767/full#supplementary-material
Supplementary Figure 1 | Effects of early life intervention using Clostridium butyricum on morphology of the intestine of large yellow croaker larvae (n = 3/group) (MT, muscular thickness; VH, villus height; EH, Enterocyte height). (A) Control group (0.00%); (B) CB1 group (0.10%); (C) CB2 group (0.20%); (D) CB3 group (0.40%); (HE staining; Scale bar = 100 mm).
Supplementary Figure 2 | Effects of early life intervention using Clostridium butyricum on il-1b, il-6, il-8, ifng and cox-2 mRNA expression in whole intestine of large yellow croaker larvae. Values are means (n = 3), with their standard errors represented by vertical bars. Bars bearing the same letters were not significantly different (P >0.05, Tukey’s test).
Supplementary Figure 3 | Rarefaction curve of gut microbiota of large yellow croaker larvae (n = 3/group).The rarefied curves for OTU number tended to approach the saturation plateau.
References
1. Zambonino Infante JL, Cahu CL. Ontogeny of the gastrointestinal tract of marine fish larvae. Comp Biochem Physiol Part C: Toxicol Pharmacol (2001) 130:477–87. doi: 10.1016/s1532-0456(01)00274-5
2. Sánchez-Hernández J, Nunn A, Adams C, Amundsen P-A. Causes and consequences of ontogenetic dietary shifts: a global synthesis using fish models. Biol Rev (2019) 94:539–54. doi: 10.1111/brv.12468
3. Comabella Y, Hernández Franyutti A, Hurtado A, Canabal J, García-Galano T. Ontogenetic development of the digestive tract in Cuban gar (Atractosteus tristoechus) larvae. Rev Fish Biol Fisheries (2012) 23:245–60. doi: 10.1007/s11160-012-9289-z
4. Stephens WZ, Burns AR, Stagaman K, Wong S, Rawls JF, Guillemin K, et al. The composition of the zebrafish intestinal microbial community varies across development. ISME J (2016) 10:644–54. doi: 10.1038/ismej.2015.140
5. Chung H, Pamp SünjeJ, Hill JA, Surana NK, Edelman SM, Troy EB, et al. Gut Immune Maturation Depends on Colonization with a Host-Specific Microbiota. Cell (2012) 149:1578–93. doi: 10.1016/j.cell.2012.04.037
6. Arrieta M-C, Stiemsma L, Amenyogbe N, Brown E, Finlay B. The intestinal microbiome in early life: health and disease. Front Immunol (2014) 5:427. doi: 10.3389/fimmu.2014.00427
7. Dogra SK, Sakwinska O, Soh S-E, Ngom-Bru C, Brück W, Berger B, et al. Dynamics of infant gut microbiota are influenced by delivery mode and gestational duration and are associated with subsequent adiposity. mBio (2015) 6:e02419–02414. doi: 10.1128/mBio.02419-14
8. Nguyen T, Chung H-J, Kim H-J, Hong S-T. Establishment of an ideal gut microbiota to boost healthy growth of neonates. Crit Rev Microbiol (2019) 45:1–12. doi: 10.1080/1040841X.2018.1561643
9. Roggero P, Liotto N, Pozzi C, Braga D, Troisi J, Menis C, et al. Analysis of immune, microbiota and metabolome maturation in infants in a clinical trial of Lactobacillus paracasei CBA L74-fermented formula. Nat Commun (2020) 11:2703. doi: 10.1038/s41467-020-16582-1
10. Duan Y, Wang Y, Dong H, Ding X, Liu Q, Li H, et al. Changes in the Intestine Microbial, Digestive, and Immune-Related Genes of Litopenaeus vannamei in Response to Dietary Probiotic Clostridium butyricum Supplementation. Front Microbiol (2018) 9:2191. doi: 10.3389/fmicb.2018.02191
11. Tran NT, Li Z, Ma H, Zhang Y, Zheng H, Gong Y, et al. Clostridium butyricum: a promising probiotic confers positive health benefits in aquatic animals. Rev Aquaculture (2020) 12:2573–89. doi: 10.1111/raq.12459
12. Ling Z, Liu X, Cheng Y, Luo Y, Yuan L, Li L, et al. Clostridium butyricum Combined with Bifidobacterium infantis Probiotic Mixture Restores Fecal Microbiota and Attenuates Systemic Inflammation in Mice with Antibiotic-Associated Diarrhea. BioMed Res Int (2015) 2015:582048. doi: 10.1155/2015/582048
13. Wang K, Cao G, Zhang H, Li Q, Yang C. Effects of Clostridium butyricum and Enterococcus faecalis on growth performance, immune function, intestinal morphology, volatile fatty acids, and intestinal flora in a piglet model. Food Funct (2019) 10:7844–54. doi: 10.1039/c9fo01650c
14. Xiang Q, Wu X, Pan Y, Wang L, Cui C, Guo Y, et al. Early-Life Intervention Using Fecal Microbiota Combined with Probiotics Promotes Gut Microbiota Maturation, Regulates Immune System Development, and Alleviates Weaning Stress in Piglets. Int J Mol Sci (2020) 21:503. doi: 10.3390/ijms21020503
15. Poolsawat L, Li X, He M, Ji D, Leng X. Clostridium butyricum as probiotic for promoting growth performance, feed utilization, gut health and microbiota community of tilapia (Oreochromis niloticus × O. aureus). Aquaculture Nutr (2019) 26:657–70. doi: 10.1111/anu.13025
16. Li H, Tian X, Zhao K, Jiang W, Dong S. Effect of Clostridium butyricum in different forms on growth performance, disease resistance, expression of genes involved in immune responses and mTOR signaling pathway of Litopenaeus vannamai. Fish Shellfish Immunol (2019) 87:13–21. doi: 10.1016/j.fsi.2018.12.069
17. Sumon MS, Ahmmed F, Khushi SS, Ahmmed MK, Rouf MA, Chisty MAH, et al. Growth performance, digestive enzyme activity and immune response of Macrobrachium rosenbergii fed with probiotic Clostridium butyricum incorporated diets. J King Saud Univ - Sci (2018) 30:21–8. doi: 10.1016/j.jksus.2016.11.003
18. Yang B, Zhou Y, Wu M, Li X, Ai Q. ω-6 Polyunsaturated fatty acids (linoleic acid) activate both autophagy and antioxidation in a synergistic feedback loop via TOR-dependent and TOR-independent signaling pathways. Cell Death Dis (2020) 11:607. doi: 10.1038/s41419-020-02750-0
19. Mai K, Yu H, Ma H, Duan Q, Gisbert E, Infante JLZ, et al. A histological study on the development of the digestive system of Pseudosciaena crocea larvae and juveniles. J Fish Biol (2005) 67:1094–106. doi: 10.1111/j.0022-1112.2005.00812.x
20. Liu Y, Miao Y, Xu N, Ding T, Cui K, Chen Q, et al. Effects of dietary Astragalus polysaccharides (APS) on survival, growth performance, activities of digestive enzyme, antioxidant responses and intestinal development of large yellow croaker (Larimichthys crocea) larvae. Aquaculture (2020) 517:734752. doi: 10.1016/j.aquaculture.2019.734752
21. Crane RK, Boge G, Rigal A. Isolation of brush border membranes in vesicular form from the intestinal spiral valve of the small dogfish (Scyliorhinus canicula). Biochim Biophys Acta (BBA) - Biomembranes (1979) 554:264–7. doi: 10.1016/0005-2736(79)90024-5
22. Maroux S, Louvard D, Baratti J. The aminopeptidase from hog intestinal brush border. Biochim Biophys Acta (BBA) - Enzymol (1973) 321:282–95. doi: 10.1016/0005-2744(73)90083-1
23. Holm H, Hanssen LE, Krogdahl Å., Florholmen J. High and Low Inhibitor Soybean Meals Affect Human Duodenal Proteinase Activity Differently: In Vivo Comparison with Bovine Serum Albumin. J Nutr (1988) 118:515–20. doi: 10.1093/jn/118.4.515
24. Zuo R, Ai Q, Mai K, Xu W, Wang J, Xu H, et al. Effects of dietary n-3 highly unsaturated fatty acids on growth, nonspecific immunity, expression of some immune related genes and disease resistance of large yellow croaker (Larmichthys crocea) following natural infestation of parasites (Cryptocaryon irritans). Fish Shellfish Immunol (2012) 32:249–58. doi: 10.1016/j.fsi.2011.11.005
25. Li Q, Cui K, Wu M, Xu D, Mai K, Ai Q. Polyunsaturated Fatty Acids Influence LPS-Induced Inflammation of Fish Macrophages Through Differential Modulation of Pathogen Recognition and p38 MAPK/NF-κB Signaling. Front Immunol (2020) 11:559332:559332. doi: 10.3389/fimmu.2020.559332
26. Zhang W, Zhang M, Cheng A, Hao E, Huang X, Chen X. Immunomodulatory and antioxidant effects of Astragalus polysaccharide liposome in large yellow croaker (Larimichthys crocea). Fish Shellfish Immunol (2020) 100:126–36. doi: 10.1016/j.fsi.2020.03.004
27. Livak KJ, Schmittgen TD. Analysis of relative gene expression data using real-time quantitative PCR and the 2(-Delta Delta C(T)) Method. Methods (2001) 25:402–8. doi: 10.1006/meth.2001.1262
28. Ge H, Wang Q, Chen H, Liu G, Pan Y, Chen J, et al. Effects of antimicrobial peptide APSH-07 on the growth performance, anti-oxidation responses, stress resistance and intestine microbiota in large yellow croaker Larimichthys crocea. Aquaculture Nutr (2019) 26:715–26. doi: 10.1111/anu.13031
29. Magoc T, Salzberg S. FLASH: fast length adjustment of short reads to improve genome assemblies. Bioinformatics (2011) 27:2957–63. doi: 10.1093/bioinformatics/btr507
30. Caporaso JG, Kuczynski J, Stombaugh J, Bittinger K, Bushman FD, Costello EK, et al. QIIME allows analysis of high-throughput community sequencing data. Nat Methods (2010) 7:335–6. doi: 10.1038/nmeth.f.303
31. Edgar RC. UPARSE: highly accurate OTU sequences from microbial amplicon reads. Nat Methods (2013) 10:996–8. doi: 10.1038/nmeth.2604
32. Desantis TZ, Hugenholtz P, Larsen N, Rojas M, Brodie EL, Keller K, et al. Greengenes, a chimera-checked 16S rRNA gene database and workbench compatible with ARB. Appl Environ Microbiol (2006) 72:5069–72. doi: 10.1128/aem.03006-05
33. Segata N, Izard J, Waldron L, Gevers D, Miropolsky L, Garrett WS, et al. Metagenomic biomarker discovery and explanation. Genome Biol (2011) 12:R60. doi: 10.1186/gb-2011-12-6-r60
34. Bentzon-Tilia M, Sonnenschein EC, Gram L. Monitoring and managing microbes in aquaculture - Towards a sustainable industry. Microbial Biotechnol (2016) 9:576–84. doi: 10.1111/1751-7915.12392
35. Borges N, Keller-Costa T, Sanches-Fernandes GMM, Louvado A, Gomes NCM, Costa R. Bacteriome Structure, Function, and Probiotics in Fish Larviculture: The Good, the Bad, and the Gaps. Annu Rev Anim Biosci (2021) 9:423–52. doi: 10.1146/annurev-animal-062920-113114
36. Gensollen T, Iyer S, Kasper D, Blumberg R. How colonization by microbiota in early life shapes the immune system. Science (2016) 352:539–44. doi: 10.1126/science.aad9378
37. Martínez I, Maldonado-Gómez M, Gomes-Neto J, Kittana H, Ding H, Schmaltz R, et al. Experimental evaluation of the importance of colonization history in early-life gut microbiota assembly. eLife (2018) 7:e36521. doi: 10.7554/eLife.36521
38. Song ZF, Wu TX, Cai LS, Zhang LJ, Zheng XD. Effects of dietary supplementation with Clostridium butyricum on the growth performance and humoral immune response in Miichthys miiuy. J Zhejiang Univ Sci B (2006) 7:596–602. doi: 10.1631/jzus.2006.B0596
39. Duan Y, Zhang J, Huang J, Jiang S. Effects of Dietary Clostridium butyricum on the Growth, Digestive Enzyme Activity, Antioxidant Capacity, and Resistance to Nitrite Stress of Penaeus monodon. Probiotics Antimicrob Proteins (2019) 11:938–45. doi: 10.1007/s12602-018-9421-z
40. Duan Y, Zhang Y, Dong H, Wang Y, Zheng X, Zhang J. Effect of dietary Clostridium butyricum on growth, intestine health status and resistance to ammonia stress in Pacific white shrimp Litopenaeus vannamei. Fish Shellfish Immunol (2017) 65:25–33. doi: 10.1016/j.fsi.2017.03.048
41. Hu X, Lin B, Luo M, Zheng X, Zhang H. The isolation, identification, physiological property of pig-isolate Clostridium butyricum LY33 using lactic acid and its effects on intestinal function of weaned piglets. Ital J Anim Sci (2019) 18:910–21. doi: 10.1080/1828051x.2019.1603089
42. Singh K, Coburn L, Asim M, Barry D, Allaman M, Shi C, et al. Ornithine Decarboxylase in Macrophages Exacerbates Colitis and Promotes Colitis-Associated Colon Carcinogenesis by Impairing M1 Immune Responses. Cancer Res (2018) 78:4303–15. doi: 10.1158/0008-5472.CAN-18-0116
43. Ou W, Hu H, Yang P, Dai J, Ai Q, Zhang W, et al. Dietary daidzein improved intestinal health of juvenile turbot in terms of intestinal mucosal barrier function and intestinal microbiota. Fish Shellfish Immunol (2019) 94:132–41. doi: 10.1016/j.fsi.2019.08.059
44. Zhang L, Zhang L, Zhan X, Zeng X, Zhou L, Cao G, et al. Effects of dietary supplementation of probiotic, Clostridium butyricum, on growth performance, immune response, intestinal barrier function, and digestive enzyme activity in broiler chickens challenged with Escherichia coli K88. J Anim Sci Biotechnol (2016) 7:3. doi: 10.1186/s40104-016-0061-4
45. Ma H, Cahu C, Zambonino J, Yu H, Duan Q, Le Gall MM, et al. Activities of selected digestive enzymes during larval development of large yellow croaker (Pseudosciaena crocea). Aquaculture (2005) 245:239–48. doi: 10.1016/j.aquaculture.2004.11.032
46. Georgountzou A, Papadopoulos NG. Postnatal innate immune development: From birth to adulthood. Front Immunol (2017) 8:957. doi: 10.3389/fimmu.2017.00957
47. López Nadal A, Ikeda-Ohtsubo W, Sipkema D, Peggs D, Mcgurk C, Forlenza M, et al. Feed, Microbiota, and Gut Immunity: Using the Zebrafish Model to Understand Fish Health. Front Immunol (2020) 11:114. doi: 10.3389/fimmu.2020.00114
48. Pérez-Sánchez T, Ruiz-Zarzuela I, De Blas I, Balcázar JL. Probiotics in aquaculture: a current assessment. Rev Aquaculture (2014) 6:133–46. doi: 10.1111/raq.12033
49. Zhao X, Yang J, Ju Z, Wu J, Wang L, Lin H, et al. Clostridium butyricum Ameliorates Salmonella Enteritis Induced Inflammation by Enhancing and Improving Immunity of the Intestinal Epithelial Barrier at the Intestinal Mucosal Level. Front Microbiol (2020) 11:299. doi: 10.3389/fmicb.2020.00299
50. Geraylou Z, Souffreau C, Rurangwa E, De Meester L, Courtin CM, Delcour JA, et al. Effects of dietary arabinoxylan-oligosaccharides (AXOS) and endogenous probiotics on the growth performance, non-specific immunity and gut microbiota of juvenile Siberian sturgeon (Acipenser baerii). Fish Shellfish Immunol (2013) 35:766–75. doi: 10.1016/j.fsi.2013.06.014
51. Hamuel JD, Ndakidemi P, Human I, Benade S. The Ecology, Biology and Pathogenesis of Acinetobacter spp.: An Overview. Microbes Eenviron (2011) 26:101–12. doi: 10.1264/jsme2.ME10179
Keywords: Clostridium butyricum, marine fish larvae, early life intervention, intestinal development, immune response, gut microbiota
Citation: Yin Z, Liu Q, Liu Y, Gao S, He Y, Yao C, Huang W, Gong Y, Mai K and Ai Q (2021) Early Life Intervention Using Probiotic Clostridium butyricum Improves Intestinal Development, Immune Response, and Gut Microbiota in Large Yellow Croaker (Larimichthys crocea) Larvae. Front. Immunol. 12:640767. doi: 10.3389/fimmu.2021.640767
Received: 12 December 2020; Accepted: 18 January 2021;
Published: 08 March 2021.
Edited by:
Jun Li, Lake Superior State University, United StatesReviewed by:
Qi Cun Zhou, Ningbo University, ChinaZhigang Zhou, Feed Research Institute (CAAS), China
Copyright © 2021 Yin, Liu, Liu, Gao, He, Yao, Huang, Gong, Mai and Ai. This is an open-access article distributed under the terms of the Creative Commons Attribution License (CC BY). The use, distribution or reproduction in other forums is permitted, provided the original author(s) and the copyright owner(s) are credited and that the original publication in this journal is cited, in accordance with accepted academic practice. No use, distribution or reproduction is permitted which does not comply with these terms.
*Correspondence: Qinghui Ai, cWhhaUBvdWMuZWR1LmNu