- 1Millennium Institute on Immunology and Immunotherapy, Departamento de Genética Molecular y Microbiología, Facultad de Ciencias Biológicas, Pontificia Universidad Católica de Chile, Santiago, Chile
- 2Servicio de Anatomía Patológica, Hospital Barros Luco Trudeau, Santiago, Chile
- 3Acute Lung Injury Center of Excellence, Division of Pulmonary, Allergy, and Critical Care Medicine, Department of Medicine, University of Pittsburgh, Pittsburgh, PA, United States
- 4Department of Pathology, Immunology and Laboratory Medicine, Center for Immunity and Inflammation, Rutgers New Jersey Medical School, Newark, NJ, United States
- 5Millennium Institute on Immunology and Immunotherapy, Departamento de Biología Celular, Facultad de Ciencias Biológicas y Facultad de Medicina, Universidad Andrés Bello, Santiago, Chile
- 6Departamento de Endocrinología, Facultad de Medicina, Pontificia Universidad Católica de Chile, Santiago, Chile
Neutrophils are immune cells classically defined as pro-inflammatory effector cells. However, current accumulated evidence indicates that neutrophils have more versatile immune-modulating properties. During acute lung infection with Streptococcus pneumoniae in mice, interleukin-10 (IL-10) production is required to temper an excessive lung injury and to improve survival, yet the cellular source of IL-10 and the immunomodulatory role of neutrophils during S. pneumoniae infection remain unknown. Here we show that neutrophils are the main myeloid cells that produce IL-10 in the lungs during the first 48 h of infection. Importantly, in vitro assays with bone-marrow derived neutrophils confirmed that IL-10 can be induced by these cells by the direct recognition of pneumococcal antigens. In vivo, we identified the recruitment of two neutrophil subpopulations in the lungs following infection, which exhibited clear morphological differences and a distinctive profile of IL-10 production at 48 h post-infection. Furthermore, adoptive transfer of neutrophils from WT mice into IL-10 knockout mice (Il10-/-) fully restored IL-10 production in the lungs and reduced lung histopathology. These results suggest that IL-10 production by neutrophils induced by S. pneumoniae limits lung injury and is important to mediate an effective immune response required for host survival.
Introduction
Pneumonia is defined as an inflammatory condition affecting the lower respiratory tract and is considered the leading cause of death due to infections in children under five years of age (1, 2). S. pneumoniae, a Gram-positive encapsulated bacterium, is a major cause of bacterial community-acquired pneumonia and one of the most important causative agents of invasive disease (3, 4). S. pneumoniae possesses numerous virulence factors required for a successful colonization of the nasopharynx and for immune evasion (5, 6). Once the immune response and the mucosal barriers of the host become compromised, S. pneumoniae disseminates to other organs, causing a wide spectrum of diseases, which range from mild as otitis and sinusitis, to severe such as Invasive Pneumococcal Disease (IPD) (7–9). IPD comprises bacteremic pneumonia, meningitis and sepsis and is considered an important cause of mortality in children and the elderly, representing approximately 12% of deaths every year worldwide (10). Although a consistent decrease in the prevalence of pneumonia in children under five years old has been reported due to immunization programs (11), still 3.7 millions of severe episodes of pneumococcal disease and more than 300,000 deaths in children were estimated worldwide in 2015 (4). Furthermore, lack of effective vaccination programs in developing countries, serotype replacement of those not included in current vaccines and the continuous emergence of antibiotic resistance make S. pneumoniae a worldwide concern (12–14).
During S. pneumoniae lung infection, the immune response of the host plays a critical role in the evolution of the infection (6, 15). The immune response against S. pneumoniae is characterized by the release of several cytokines and chemokines required for the recruitment of neutrophils, monocytes and lymphocytes to the lungs (5), being neutrophils essential for bacterial elimination (16–18). However, given that an appropriate lung function is critical to sustain life in mammals and humans, the immune response against S. pneumoniae in the lung must be tightly regulated to guarantee pathogen elimination and, at the same time, prevent excessive inflammation and tissue damage. To guarantee this requirement, anti-inflammatory cytokines, such as interleukin-10, are produced during infection to modulate the lung inflammatory response (19) and restore the host’s immune homeostasis (20, 21). Previous data published by our group showed that IL-10 is strongly induced in the lungs during the first 48 h after pneumococcal exposure (22). Results obtained in this study highlight that the prevention of excessive lung damage by IL-10 is critical to promote host survival, but it also might favor bacterial dissemination (22). Identification of the signals that trigger the early IL-10 production in pneumococcal pneumonia remains unaddressed. However, previous in vitro and ex vivo assays have shown that S. pneumoniae might be involved in the induction of an anti-inflammatory profile in macrophages and dendritic cells, which occurs through cell recognition of CpG motifs in bacterial DNA, pneumolysin, and cell wall components such as peptidoglycan and lipoteichoic acids (23–25). However, until now, it is not clear whether these stimuli and these myeloid cells are directly involved in IL-10 production during the first 48hpi with S. pneumoniae. In Klebsiella pneumoniae-induced pneumonia, macrophages, monocytic myeloid-derived suppressor cells (M-MDSCs) and neutrophils have been described as the main contributors to IL-10 production during bacterial pneumonia (19, 26, 27). In fact, using a GFP-reporter mouse during K. pneumoniae lung infection, neutrophils were identified as IL-10-producing cells in lungs during the first 48 h post-infection (19). These findings are in line with several studies, showing that neutrophils are able to produce IL-10 during infection, indicating that these cells might be playing a role beyond pathogen clearance in pneumonia (28–30).
Conventionally, neutrophils have been considered crucial players in pro-inflammatory processes and short-lived effector cells that react to infections and different inflammatory stimuli (31, 32). That view has changed over the last decade due to the observation that the half-life of neutrophils is on average 5.4 days in humans (33) and by the identification of immunomodulatory and suppressive properties in human and mouse neutrophils, such as IL-10 production (28, 34–36). In fact, there is evidence that anti-inflammatory neutrophils, expanded after exposure to bacterial components and suppressed the pro-inflammatory activity of macrophages, dendritic cells and T cells in vitro (30, 37). Based on the potential immunomodulatory role of neutrophils mediated by the production of IL-10, in the present study we evaluated whether the production of IL-10 by neutrophils recruited to the lungs in response to S. pneumoniae infection downmodulates inflammation of this tissue and promotes host survival. Using a mouse model of infection, our data shows that neutrophils contribute significantly to IL-10 production during the acute phase of S. pneumoniae infection in the lungs, displaying a regulatory role and influencing disease outcome. In addition, given the functional and morphological heterogeneity of neutrophils (38), we characterized the neutrophilic population recruited to the lungs in response to S. pneumoniae. Consistently with previously published data from our laboratory, we found two neutrophil subsets present in the lungs. We then characterized them in terms of morphology and their ability to produce IL-10, providing new insights on the functional heterogeneity of neutrophils and their roles during infection.
Materials and Methods
Mice
Adult (6-8 weeks) male C57BL/6 (WT), B6.129P2-Il10tm1Cgn/J (Il10-/-), B6(Cg)-Il10tm1.1Karp/J (IL-10::eGFP) and B6.SJL-Ptprca Pepcb/BoyJ (CD45.1) mice were obtained originally from the Jackson Laboratories (Bar Harbor, ME). All mice were co-housed and fed with the same chow for 12 generations in specific pathogen-free conditions in the animal facility (CIBEM) at the Pontificia Universidad Católica de Chile. All experimental protocols were reviewed and approved by the Scientific Ethical Committee for Animal and Environment Care and the Scientific Committee for Research Safety of the Pontificia Universidad Católica de Chile (protocol number 160822002). All animal experiments were performed by trained personnel according to the Guide for Care and Use of Laboratory Animals (39). A clinical score was used to supervise mouse wellness, which includes parameters such as weight loss, pain signs, and respiratory and neurologic changes (Supplementary Data and Table S1).
Bacterial Growth and Preparation
Streptococcus pneumoniae D39 (NCTC 7466/capsulated) and the non-capsulated derivative R6 (NCTC 13276) strains were grown in Todd Hewitt broth supplemented with 0.5% yeast extract (THYE), without agitation at 37°C/CO2 5%, until an OD600 0.5 was reached. Then, the bacterial suspension was stored in 10% glycerol at -80°C until use. Colony-forming units (CFUs) of bacterial suspensions were determined by serial dilutions on blood agar before the storage and after each assay (22, 40). To check the capsule presence in the D39 strain, we seeded D39 and R6, in order to compare the phenotype of colonies. Before use, bacterial suspensions were centrifuged at 4,700 g for 7 min at 4°C and washed with sterile PBS or 0.5% THYE broth. For infections, the bacterial pellet was resuspended in 0.5% THYE broth. For in vitro assays, bacteria were resuspended in sterile PBS and heat-inactivated at 95°C for 15 min.
Purification of Naïve Neutrophils From Bone-Marrow Cells
Femurs and tibias were obtained from WT and/or Il10-/- mice. Bones were cleaned from muscle and fat residues and bone marrow was flushed by perfusion with RPMI 1640 medium supplemented with 10% FBS and 2mM EDTA. Red blood cells were lysed with a hypotonic solution of NaCl 0.2% and lysis was stopped with an isotonic solution of NaCl 1.6%. Next, cells were counted and stained for negative selection by using a neutrophil purification kit (MACS, Miltenyi Biotech). The purity obtained after neutrophils purification was always ≥96% (Figure S1). After purification, neutrophils were diluted in sterile PBS. For in vitro assays, neutrophils were placed in HBSS containing Ca2+ and Mg2+ supplemented with 0.1% gelatin (Sigma- Aldrich G7765).
Adoptive Transfer Assays and Infections
Il10-/- mice received intranasally 50 μL of sterile PBS containing 1x106 purified neutrophils obtained from WT mice (Il10-/- + WT). Non-transferred Il10-/- mice (Il10-/-), non-transferred WT mice (WT) and Il10-/- mice transferred with Il10-/- neutrophils (Il10-/- + Il10-/-) were included as control groups. After 24 h, mice were intranasally infected with 3x107 CFUs of S. pneumoniae D39. The survival rate, weight loss and clinical score were monitored daily until 10 days post infection (dpi). Mice monitoring was assessed according to Table S1 and the score was recorded daily before mice processing. Additionally, adoptive transfer assays were also performed to evaluate disease severity parameters such as bacterial loads, lung infiltration by proinflammatory cells and tissue damage, at 48 h post infection (hpi). At least three independent assays were performed.
Tissue Preparation
Total blood was obtained by cardiac puncture in mice under deep terminal anesthesia. Then, dissection of the trachea was performed in euthanized mice, using sterile surgical material. A cannula was inserted through the trachea and serial washes were performed with 1,500 µl of sterile PBS to obtain bronchoalveolar lavage. Lungs, spleen and brain were extracted with sterile surgical instruments. Lungs were cut into small pieces with sterile scissors and digested in 7 mL of Collagenase IV (1mg/mL) for 1 h at 37°C with agitation. After the incubation period, 7 mL of 5 mM EDTA was added to each tube and mixed gently (this process was exclusively applied for lungs). Then, lungs and the remaining organs were homogenized and passed through a cell strainer (70 μm pore) and recovered in their original tube containing PBS. For bacterial load determination, serial dilutions of each organ were seeded in blood agar plates at 37°C with 5% CO2. Bacterial loads were calculated as CFUs per organ. Lungs and BALF pellets were separated by centrifugation during 5 min at 0.4 g. Each pellet was treated with a sterile solution of 1X ammonium chloride-potassium (ACK) for 5 min and then washed with PBS. Cells obtained after this process were stained with antibodies to characterize the inflammatory cell infiltrate by flow cytometry. Cytokine protein levels (IL-6, TNF-α, IFN-γ, IL-10, IL-12p40) were determined in BALF supernatants by ELISA (BD OptEIA).
Lung Histopathological Analyses
Histopathological analyses were performed in mice of each group at 48 hpi. Lungs were removed and fixed in 4% paraformaldehyde for 48 h, then embedded in paraffin wax blocks and sliced with a microtome in 5 μm sections. Slices were incubated at 60°C for 1 h and stained with Hematoxylin and Eosin (H&E staining). Images from each slide were obtained by using a Leica SCN400F slide scanner and analyzed with the Aperio ImageScope Software. Pathological changes in lungs were blindly evaluated by a pathologist and quantified following the parameters detailed in Table S2.
Flow Cytometry
Analyses of myeloid cells were performed by flow cytometry using the following markers: anti-CD45 (clone 30-F11, BD), anti-CD11b (clone M1/70, BD), anti-CD11c (clone HL3, BD), anti-Ly6C (clone AL-21, BD), anti-Ly6G (clone 1A8, Biolegend), anti-MHC-II (clone M5/114.15.2, BD), anti-Siglec-F (clone E50-2440, BD), anti-CD103 (clone M290, BD), anti-CD64 (clone 10.1, Biolegend), anti-CD45.1 (clone A20, BD), anti-CD31 (clone 390, Biolegend), anti-CD44 (clone IM7, BD), anti-CD62L (clone MEL-14, BD). Dead cells were excluded from analyses using the LIVE/DEAD Fixable Viability Stain 510 (BD). Data were acquired on a Fortessa X-20 flow cytometer (BD Bioscience, Oxford, UK) and analyzed using FACSDiva (BD Bioscience, UK) or FlowJo software (Tree Star, Ashland, Oregon). IL-10 intracellular staining was performed using an anti-IL-10 antibody (clone JES5-16E3, Biolegend). CountBright™ absolute counting beads (Invitrogen™ C36950) were added to samples before analyses and used to calculate the total number of cell populations per μL. Each organ was diluted in 1mL before the staining and 200 μL from each sample was mixed with 50 μL of beads solution. Quantification was performed according to manufacturer’s indications. Cell type identification was performed according to the gating strategy showed in the supplementary material (Figure S2). IL-10 expression data were calculated according to eGFP expression represented by fluorescence in the FITC channel. Additionally, the basal eGFP expression by each cell population was determined using an uninfected WT (eGFPneg) mice (Figure S3).
Neutrophil Characterization by Flow Cytometry and Transmission Electron Microscopy
IL-10::eGFP mice were intranasally instilled with vehicle (uninfected) or 3x107 CFUs of S. pneumoniae. Lungs and bronchoalveolar lavage (BALF) were obtained from both groups after 12, 24 and 48 hpi. Lungs were processed and stained for flow cytometry. Cells obtained from 24 hpi BALFs were stained and sorted by using a BD FACS Aria II (BD Biosciences, San Jose, USA). CD11b+CD11c-Ly6G+ cells were selected and subsequently separated by size (Forward scatter). FACS was conducted at the Flow Cytometry Facility of the Pontificia Universidad Católica de Chile. Sorted neutrophil subsets were fixed by immersion in 2.5% glutaraldehyde and subsequently treated and prepared at the Advanced Microscopy Facility of the Pontificia Universidad Católica de Chile. Sections of 80 nm of diameter were obtained using an ultramicrotome Leica Ultracut R. Grids were visualized and photographed at 8200X in a Phillips Tecnai 12 electron microscope at 80 kV. TEM microphotographs were analyzed with Image-J software. Morphological features evaluated for each neutrophil subtype included size, euchromatin fraction, quantification of cytoplasmic structures and cytoplasm electrodensity. Each cell characteristic was evaluated according to previously reported parameters (41). This assay was repeated twice.
In Vitro Assays for the Evaluation of IL-10 Production by Neutrophils
Bone marrow-derived neutrophils were incubated with different stimuli during 1 h in constant agitation at 37°C in 5% CO2 and subsequently incubated for 23 h. Cells were recovered for counting and viability evaluation by flow cytometry. Supernatants were stored at -80°C and then evaluated for IL-10 detection by ELISA. Bacteria used in these assays were inactivated by heat at 90°C for 15 min. Heat-killed bacteria were seeded in blood agar to confirm bacterial inactivation. The assays were performed with three different multiplicity of infection (MOIs) of heat-killed S. pneumoniae (MOI 25, 50, 100) to neutrophils. The experiments were also performed with BALFs obtained from mice intranasally infected or instilled with vehicle. BALF was obtained 24 h after the instillation. Subsequently, BALF was centrifugated and separated, and supernatants were stored at -80°C until use. Additionally, neutrophils were exposed to DNA obtained from S. pneumoniae D39 (MOI 25 and MOI 50). Some experiments were performed with pneumococcal purified cell wall obtained according to the protocol used by Fan and Jin1. At least three interdependent assays were performed.
Statistical Analyses
All the experiments contain data from two or three independent experiments and each assay was performed with at least 2 animals per group. D’agostino & Pearson Omnibus and Shapiro-Wilk normality tests were performed to confirm the normal distribution of data. Kruskal-Wallis tests with Dunn’s multiple comparison post-tests were performed to analyze the IL-10-producing cells at 24 and 48 hpi, to compare the phenotypical characteristics of FSClow and FSChigh subsets evaluated by TEM and data obtained from the IL-10 expression and production in the in vitro assays. One-way ANOVA with Sidak’s multiple comparison post-tests were also performed in adoptive transfer assays, in order to compare all the experimental groups with each other in analyses related to lung infiltration, bacterial loads in organs and cytokine determination in BALFs. Kruskal-Wallis with Dunn’s post-test were performed when the sample size was too small or with non-parametric data. Survival curves were compared using a log rank test. Two-way ANOVA and Sidak’s multiple comparisons post-test were performed to analyze the clinical score and the expression of IL-10 and surface markers by neutrophils. In all cases, a P value of <0.05 were considered statistically significant. Statistical analyses were performed using GraphPad Prism 5 for Mac, version 5.01. All data were expressed as means plus standard error (SEM).
Results
Neutrophils Contribute to IL-10 Production at Early Times During Pneumococcal Pneumonia
To assess which cells are involved in IL-10 production in the lungs during the acute phase of pneumococcal pneumonia, three experimental groups of IL-10::eGFP mice were either instilled with THYE or infected with S. pneumoniae for 24 h or 48 h. Lung cells were stained for flow cytometry characterization with an antibody panel designed to identify dendritic cells (DCs), alveolar macrophages, monocytes, interstitial macrophages, eosinophils and neutrophils (Figure S2). Furthermore, quantification of IL-10-production by each cell population was performed by analyzing the expression of eGFP (Figure 1). Our findings indicate that neutrophils are the most abundant cells that produced IL-10 either at 24 or 48 h post-infection (Figure 1A). Ly6Cneg monocytes and eosinophils showed a moderate IL-10 production at 48 h (Figures 1B, C). Interstitial macrophages also showed a non-significant upward trend of IL-10+ production (Figure 1D). Finally, resident alveolar macrophages, interstitial macrophages, monocyte-derived macrophages, Ly6C+ monocytes and DCs did not contribute to IL-10 production during S. pneumoniae infection (Figure S4). IL-10 expression in neutrophils following S. pneumoniae infection was further confirmed by intracellular staining with an anti-IL-10 antibody (Figure S5). Overall, our data show that neutrophils are the main source of IL-10 among myeloid cells in the lungs during S. pneumoniae infection.
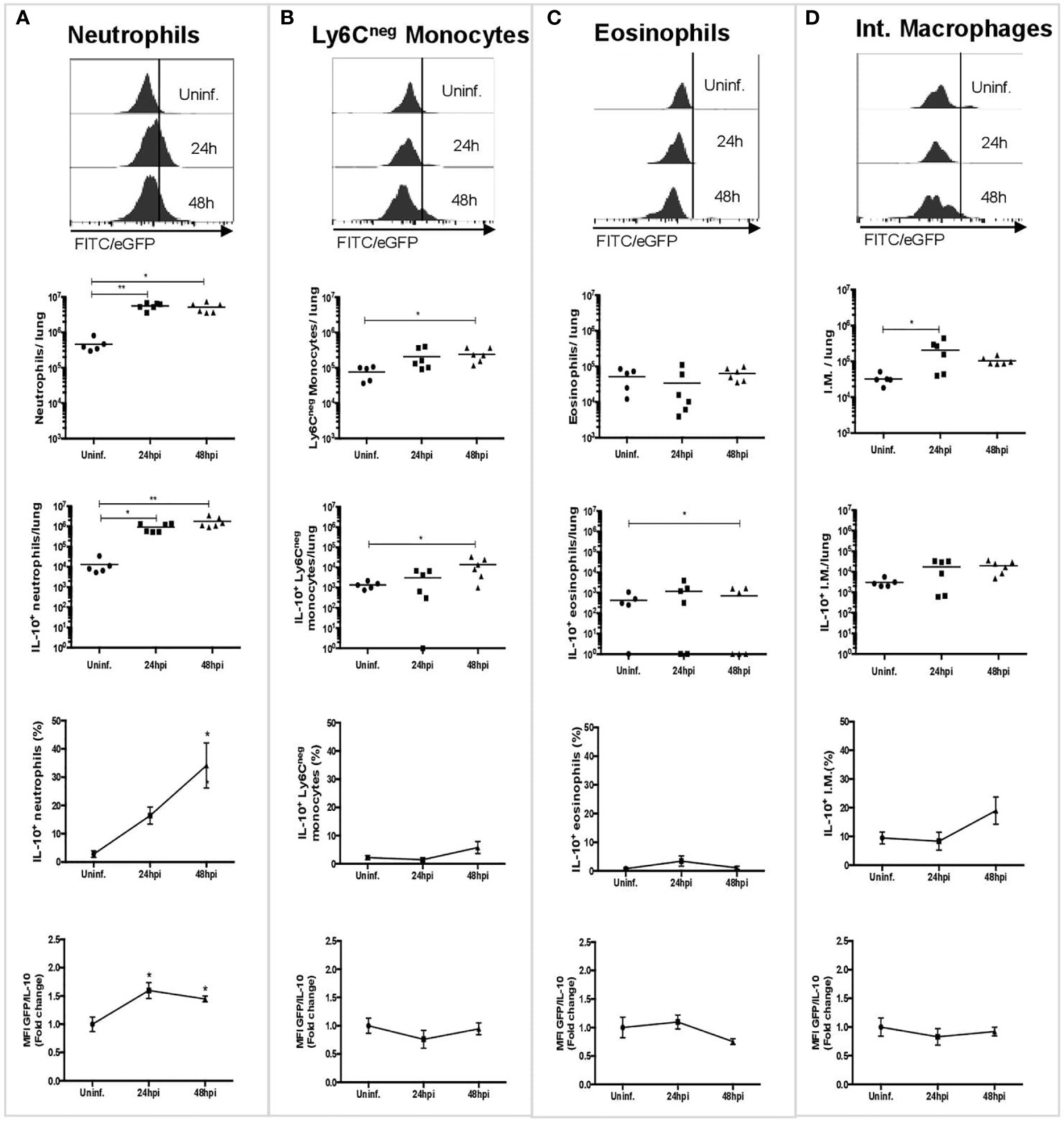
Figure 1 Neutrophils are the main source of IL-10 during the early phase of S. pneumoniae infection. Representative histograms and graphs showing the number of cells expressing IL-10 in lungs in uninfected mice (n = 5), and mice infected after 24 (n = 6) and 48 hpi (n = 6), and their corresponding MFI measurements. (A) Neutrophils. (B) Ly6Cneg monocytes. (C) Eosinophils. (D) Interstitial macrophages. WT uninfected mice were used to establish the baseline of GFP expression (Figure S3). Quantification of each cell population was performed using counting beads. Statistics: Kruskal-Wallis with Dunn’s multiple comparison post-test. *P < 0.05; **P < 0.01. Comparisons of the mean of infected and uninfected mice, 24 and 48 hpi. hpi, h post-infection; MFI, Median Fluorescence Intensity; I.M., Interstitial macrophages.
Neutrophils Detected in the Lungs During S. pneumoniae Infection Differ in Morphology and IL-10 Production
In a previous study, we described the presence of two neutrophil subsets in the lungs with marked differences in size (38). Both of these subsets were rapidly recruited to the lungs during pneumococcal infection, although their biological relevance or their morphological properties were not studied (38). Here, we confirmed the presence of these two subsets by flow cytometry in S. pneumoniae-infected and uninfected mice. We named these subsets as FSClow (N1: smaller size) and FSChigh (N2: larger size) (Figure 2A). Then, we aimed to determine whether these populations play different roles during pneumococcal infection. We first evaluated the expression of maturity and activation surface markers during the first 48 hpi, but no significant differences in the expression of surface markers in both populations were observed (Figure S6). Next, we evaluated the ability of each of these subsets to produce IL-10. We observed that FSChigh neutrophils significantly produced higher levels of IL-10 at 24 and 48 hpi as compared to FSClow neutrophils, which only showed a mild increase of IL-10 production at 24 hpi (Figures 2B, C). An additional morphological characterization of identified neutrophil subsets was performed by TEM in neutrophils sorted by size, using fluorescence activated cell sorting (FACS). Our results show that purified neutrophil populations have phenotypic differences in the shape of the nucleus and chromatin condensation, number of granules and autophagy-related structures (Figure 2D). Specifically, FSChigh neutrophils exhibited a nucleus with decondensed chromatin, while the FSClow subset had a nucleus predominantly constituted by heterochromatin. Moreover, we also observed differences related to the number of intracellular granules present in the cytoplasm as well as the presence of autophagy-related structures, particularly in the FSChigh population (Figure 2E). Together, these data show that the neutrophil population present in lungs in response to any stimulus is a heterogeneous population composed by two subsets that have major morphological differences and are characterized by a differential ability to produce IL-10 in vivo in response to S. pneumoniae.
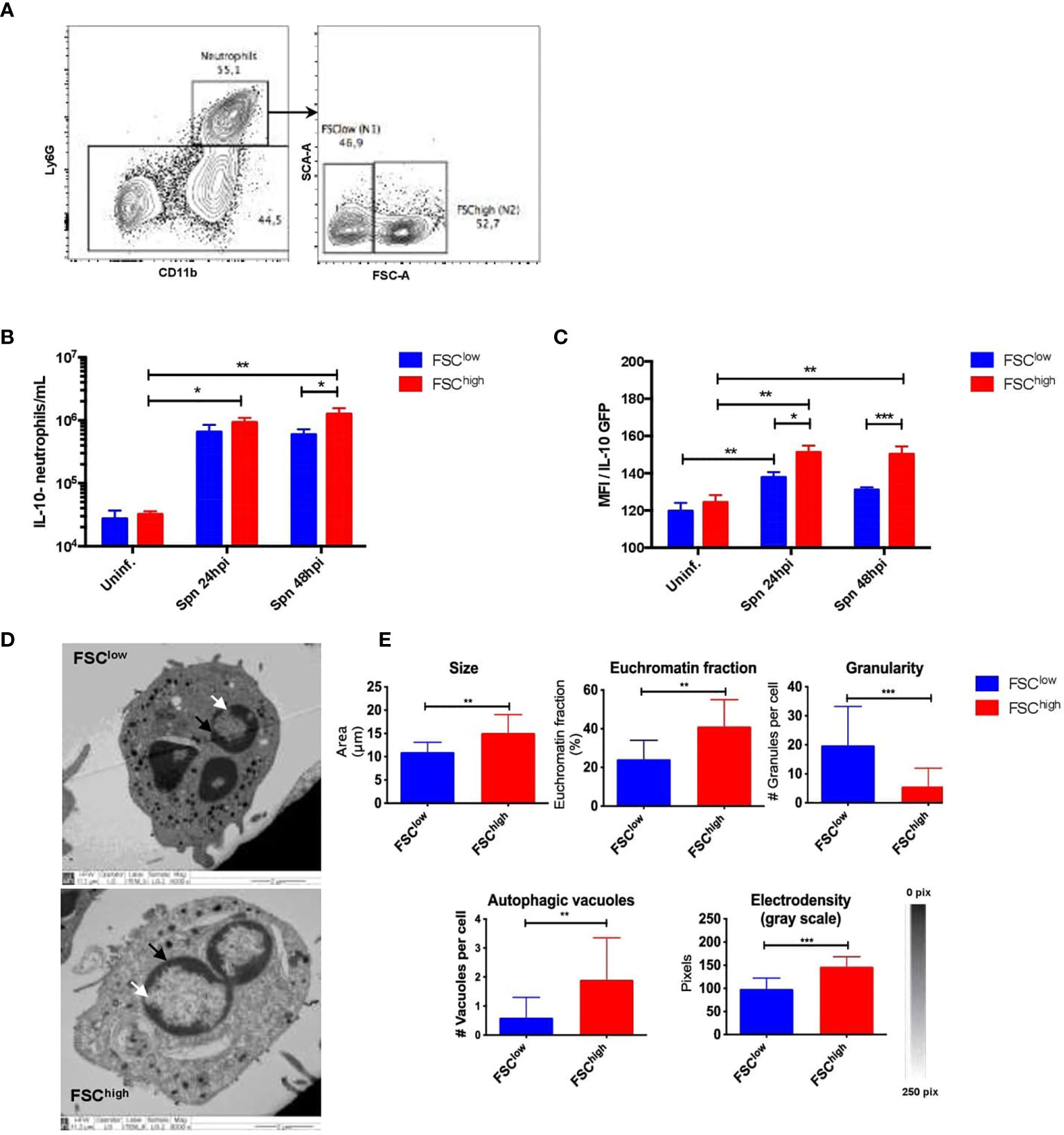
Figure 2 Neutrophil subsets isolated from infected mice exhibit structural and functional differences. (A) Representative flow cytometry plots of lung cells show neutrophils gated as CD11b+Ly6G+ cells. Neutrophil subsets were gated according to FSC parameters as FSClow and FSChigh. (B) Number of FSClow (blue bar) and FSChigh (red bar) neutrophils expressing IL-10 in uninfected mice together with the observed neutrophils at 24 and 48hpi. (C) IL-10 expression of neutrophils (from IL-10::eGFP mice subsets in lungs at 24 and 48hpi. (D) Representative TEM images of FSClow and FSChigh subsets obtained from 24h infected lungs. The white arrows point to the heterochromatin and the black arrows point to the euchromatin fraction. (E) Phenotypic parameters measured in FSClow and FSChigh populations with ImageJ software. Statistics: Tukey’s test following two-way ANOVA and a two-tailed T-test. *P < 0.05; **P < 0.01; ***P < 0.001. TEM, Transmission Electron Microscopy; hpi, hours post-infection; Uninf, Uninfected.
S. pneumoniae Directly Triggers IL-10 Production by Neutrophils in Vitro
In order to elucidate the nature of the stimuli that triggers IL-10 production in neutrophils, we performed in vitro assays with bone-marrow neutrophils obtained from IL-10::eGFP mice. These neutrophils were incubated with heat-killed bacteria (MOI 25, 50 and 100) and BALFs obtained from infected and non-infected WT mice. We included in these experiments two S. pneumoniae strains: capsulated and non-capsulated. Additionally, BALFs mixed with heat-killed bacteria were included as a treatment. After 12 h, eGFP fluorescence was measured in cells by flow cytometry and IL-10 production was measured by ELISA in the supernatant from cultured neutrophils after 6, 12 and 24 h post-treatment. Results showed that only heat-killed S. pneumoniae, but not a soluble factor present in the BALFs from either infected or uninfected mice, stimulated eGFP expression in neutrophils at 12 h post-treatment (Figure 3A). These results were confirmed by ELISA, where IL-10 in the supernatant was detected only in neutrophils stimulated with capsulated bacterium (Figure 3B). Additionally, we observed IL-10 production 24h post-treatment in neutrophils infected with the non-capsulated strain (MOI 25). However, at 24 h, no IL-10 production was observed in neutrophils infected with higher MOI of the non-capsulated strain (Figure 3B). In addition, we stimulated naïve neutrophils with DNA (data not shown) and cell wall extract (peptidoglycan) purified from S. pneumoniae D39. Our data show that cell wall extract did not induced IL-10 production in vitro (Figure 3B). These in vitro data suggest that IL-10 expression in neutrophils is directly induced by S. pneumoniae PAMPs present in the bacterial surface but not by soluble host or bacterial factors secreted to the airways during infection.
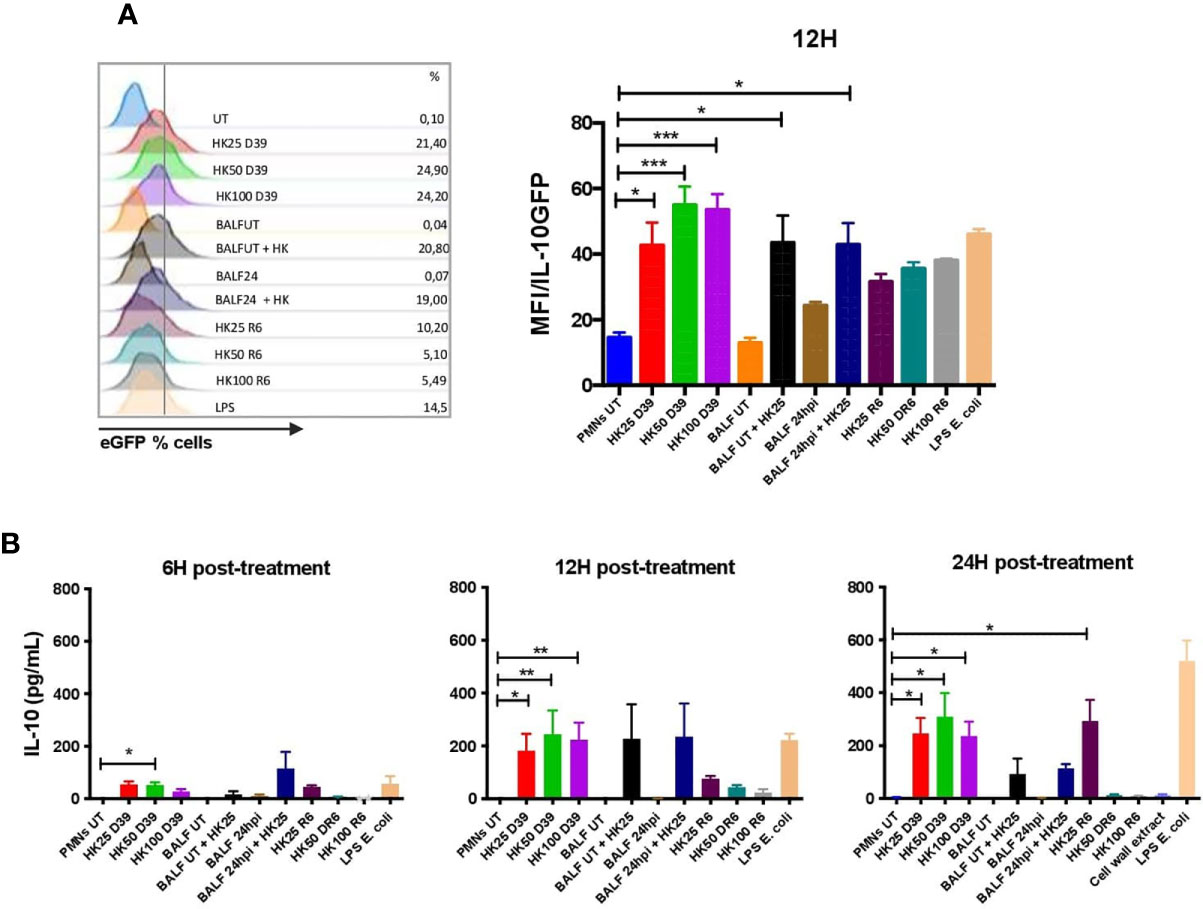
Figure 3 S. pneumoniae induces IL-10 production by neutrophils in vitro. Bone marrow-derived neutrophils were incubated with different MOIs of heat-killed S. pneumoniae (D39 and R6 strains) to determine IL-10 production in response to stimulus. (A) Histograms showing the percentage of IL-10 neutrophils by treatment, as well as the eGFP/MFI expression evaluated by flow cytometry at 12hpi. (B) Supernatants were recovered and evaluated for IL-10 detection by ELISA at 6, 12 and 24hpi. Kruskal-Wallis with Dunn’s multiple comparison post-tests were performed for statistical analyses. *P < 0.05; **P < 0.01; ***P < 0.001 in each treatment comparison with the untreated cells. Three independent experiments.
Adoptive Neutrophil Transfer Improves Survival and Clinical Signs of Il10-/- Mice, Independently of IL-10 Production by Transferred Cells
A previous study from our laboratory demonstrated that IL-10 plays a key role in mice survival during S. pneumoniae (22). Considering this, we aimed to perform neutrophil adoptive transfer assays into Il10-/- mice (highly susceptible to S. pneumoniae infection) to evaluate the impact of the IL-10 produced by neutrophils in mice survival. We transferred bone marrow-isolated neutrophils from WT or Il10-/- donor mice to Il10-/- recipient mice one day prior S. pneumoniae infection (day -1). Survival rate and clinical parameters were evaluated over the course of ten days after infection. Our data showed that whereas all non-transferred WT mice survived until the end of the experiment and had a low clinical score, non-transferred Il10-/- mice had the highest clinical score and 100% mortality at day 4 post-infection. Surprisingly, recipient Il10-/- mice transferred with either WT or Il10-/- neutrophils improved their survival rate up to 60% (Figure 4). The increased survival of individuals at the endpoint of the curve was similar in both groups of Il10-/- transferred mice and no significant differences were observed at any point in survival or score curves between these two groups. To further evaluate whether early transfer of neutrophils to Il10-/- mice improve their defense against S. pneumoniae, bone marrow neutrophils were isolated from IL-10::eGFP mice and subsequently stimulated for 12 h with heat-killed S. pneumoniae (MOI=25), to induce IL-10 production. Prior to adoptive transfer, we assessed IL-10 expression and viability by flow cytometry. At the time of transfer, 10-20% of neutrophils were IL-10+ with a viability of 80% (Figure S7A). The transfer of these cells at 12 hpi resulted in an outcome similar to the one observed in mice transferred with neutrophils previous infection (Figures S7B, S7C). Moreover, at the end of the experiment (day 10), we observed that Il10-/- mice that received Il10-/- neutrophils had higher numbers of neutrophils in the lungs as compared to Il10-/- mice that received IL-10::eGFP neutrophils (Figure S7D). These findings support the notion that early activation of neutrophils observed in recipient Il10-/- mice positively affect disease outcome and host survival, in a mechanism that is independent on IL-10 production.
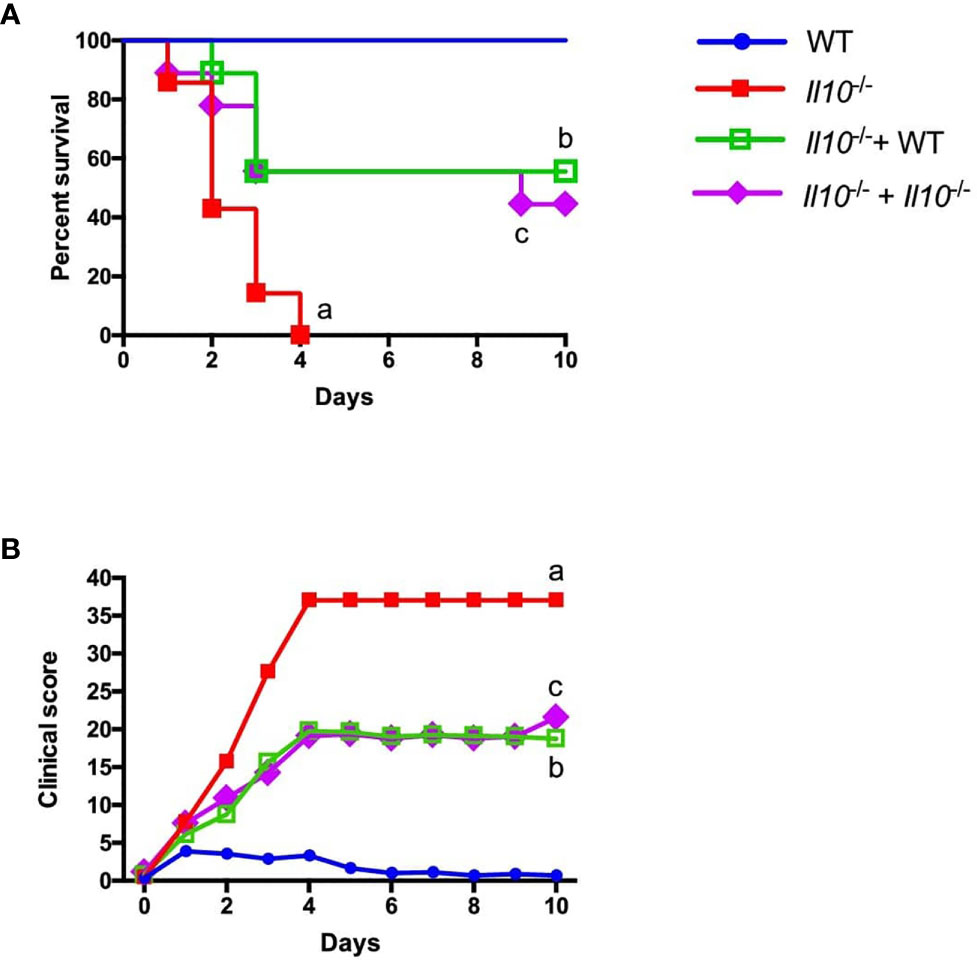
Figure 4 Adoptive transfer of neutrophil improves Il10-/- mice survival and clinical score independently of IL-10 production. Neutrophils obtained from WT and Il10-/- mice were intranasally transferred to Il10-/- mice and 24 h later infected with 3x107 cfu of S. pneumoniae (n = 7 to 9 for each group). This experimental design was performed to evaluate the survival and clinical parameters of mice in the different groups during 10 days. (A) Survival curve (B) Disease parameters were evaluated according to a clinical score guideline including activity, weight loss, body posture and general appearance (Table S1). Statistics: Log-rank (Mantel-Cox) test. (a) ****P < 0.0001 between WT and Il10-/-, (b) **P < 0.01 between Il10/- and Il10-/-+ WT, (c) *P < 0.05 between Il10-/- and Il10-/-+ Il10-/-. Two-way ANOVA with Tukey’s multiple comparisons post-test were performed. (a) ****P < 0.0001 between WT and Il10-/-, (b) **P < 0.01 between Il10/- and Il10-/-+ WT, (c) *P < 0.05 between Il10-/- and Il10-/-+ Il10-/-.
Adoptive Transfer of IL-10-Producing Neutrophils to Il10-/- Recipient Mice Reduced Lung Histopathology, but Impaired Bacterial Clearance of S. pneumoniae
We next evaluated the effect of neutrophil-produced IL-10 in monocyte and neutrophil recruitment, cytokine production, bacterial burden and histopathological changes in recipient mice at 48 hpi. The analyses of lung infiltration by flow cytometry showed no significant differences in the number of live neutrophils and monocytes in lungs at 48 hpi among the experimental groups (Figure 5A). However, our data shows that transfer of WT neutrophils fully restored the production of IL-10 in the BALF of Il10-/- recipient mice at 48 h, although it did not have a major effect in the production of important pro-inflammatory cytokines, such as IL-12, IFN-γ and TNF-α in the BALFs (Figures 5B–E). To determine that transferred neutrophils remained in the respiratory tract, 1 x 106 bone marrow-derived neutrophils from CD45.1 mouse were transferred into CD45.2 congenic recipient mice. Recipient mice were infected 1-day post transfer and at 24 hpi lungs, BALF, blood and spleen were harvested to determine neutrophil percentages from CD45.1 and CD45.2. As expected, transferred neutrophils in the BALF represented 10% of total neutrophils. In the lung tissue, this percentage was around 2-4% (Figure S8). We also evaluated the expression of some homing markers previously evaluated in lung infiltration (CD31, CD44 and CD62L) (42–44) (Figure S9). CD31 was significantly expressed in CD45.1 neutrophils obtained from lungs, particularly from infected mice, which is consistent with data previously reported for neutrophils in lung undergoing inflammation (44). CD44 is augmented in BALF, indicating that bone marrow-derived neutrophils change the expression of surface markers in response to infection, even when they are intranasally delivered and come from the bone marrow. Finally, the CD62L marker showed low expression in lungs and BALFs, while it was highly expressed in blood (Figure S9), which is in agreement with previous reports (42). These results show that intranasally transferred bone marrow-derived neutrophils remained in the lungs for at least 48 h after transfer. Further, homing data suggest that an important quantity of transferred neutrophils remained in the respiratory tract.
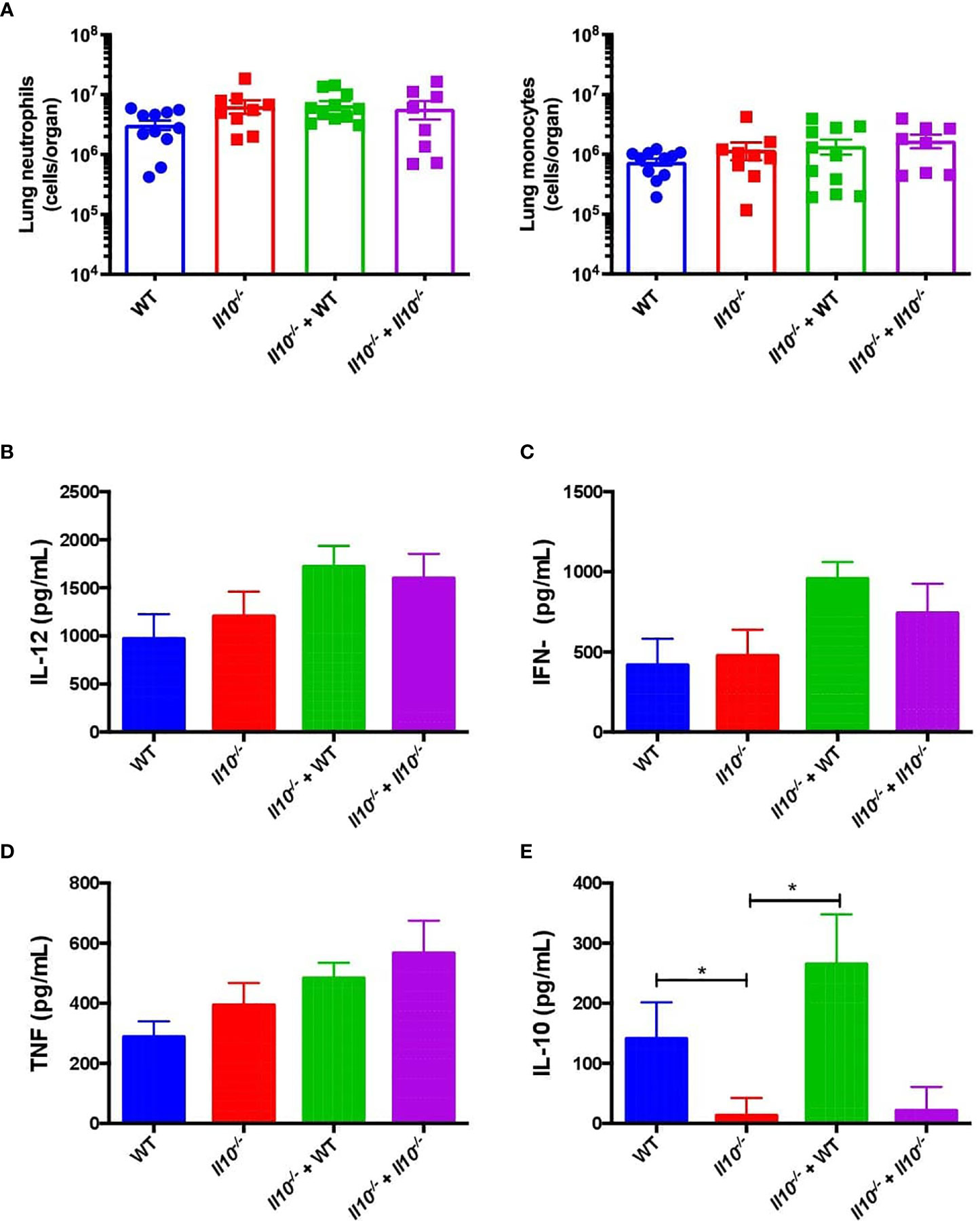
Figure 5 IL-10 produced by neutrophils does not have impact in reduction of pro-inflammatory cytokines or cells infiltration in Il10-/- mice. (A) Viable monocyte and neutrophil infiltration in mouse lungs of each group were measured at 48hpi by flow cytometry. Pro-inflammatory (B–D) and anti-inflammatory (E) cytokines obtained from BALFs at 48hpi were determined by ELISA in each control and transferred group. Statistics: One-way ANOVA with Sidak’s multiple comparison post-test. *P < 0.05. Comparisons of the mean of each group with the mean of every other groups. n = 9 to 12 per group. BALFs, Bronchoalveolar Lavages; hpi, hours post infection.
We also observed that, as compared to non-transferred Il10-/- or Il10-/- mice transferred with Il10-/- neutrophils, Il10-/- transferred with WT neutrophils showed significantly higher bacterial burden, which was equivalent to WT mice (Figure 6A). Moreover, a higher percentage of Il10-/- transferred mice with WT neutrophils presented bacterial dissemination, expressed as significantly higher bacterial burden in blood and spleen (Figures 6B, C). No differences among bacterial loads in mice brains were observed in any of the groups (Figure 6D). Importantly, our data show that transfer of WT neutrophils to Il10-/- mice reduced lung damage after 48 hpi and significantly protected the alveolar integrity from structural disruptions and hemorrhage, as compared to non-transferred Il10-/- mice (Figure 7A). Histopathological observations were scored according to the level of pro-inflammatory cell infiltration, hemorrhage incidence, loss of lung architecture and alveolar structure, and the extension of damage in both lungs (Supplementary data, Table S2). Results obtained from this analysis showed that Il10-/- mice transferred with WT neutrophils had significantly lesser total score as compared to the other Il10-/- groups (Figure 7B). Statistical analysis applied individually per parameter did not show significant differences among groups. However, it is possible to observe that the severity of alveolar wall swelling and lung damage in Il10-/-+ WT mice were similar to the observed in WT mice (Figure S10).
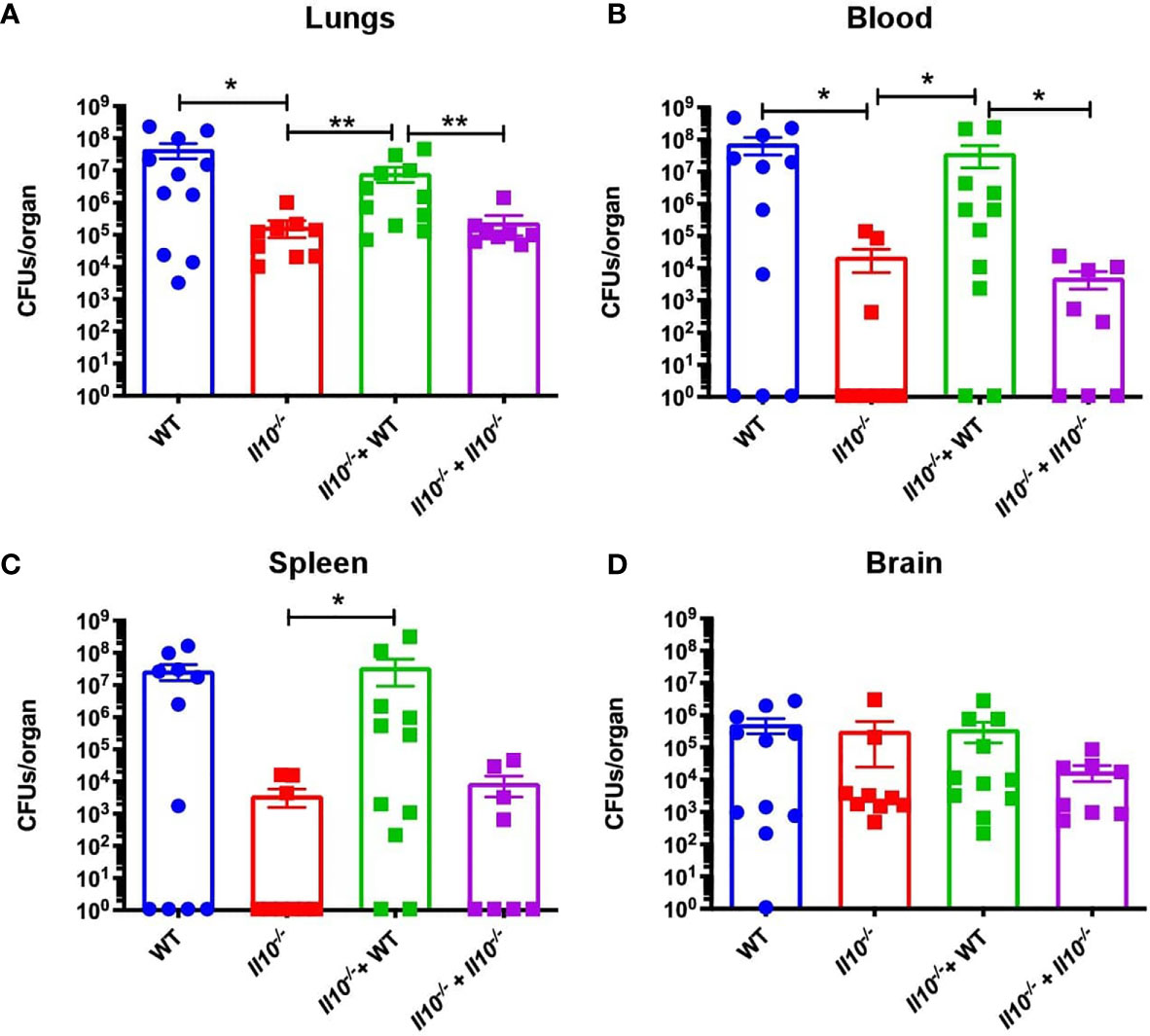
Figure 6 Transfer of WT neutrophils impairs bacterial clearance. Bacterial loads were measured at 48hpi in (A) lungs, (B) blood, (C) spleen and (D) brain. Data is shown as CFU per organ. Statistics: T-test with Mann-Whitney post-test. *P < 0.05; **P < 0.01. Comparisons of the mean of each group with the mean of all the other groups. n = 9 to 12 for each group. hpi, hours post-infection; CFUs, Colony Forming Units.
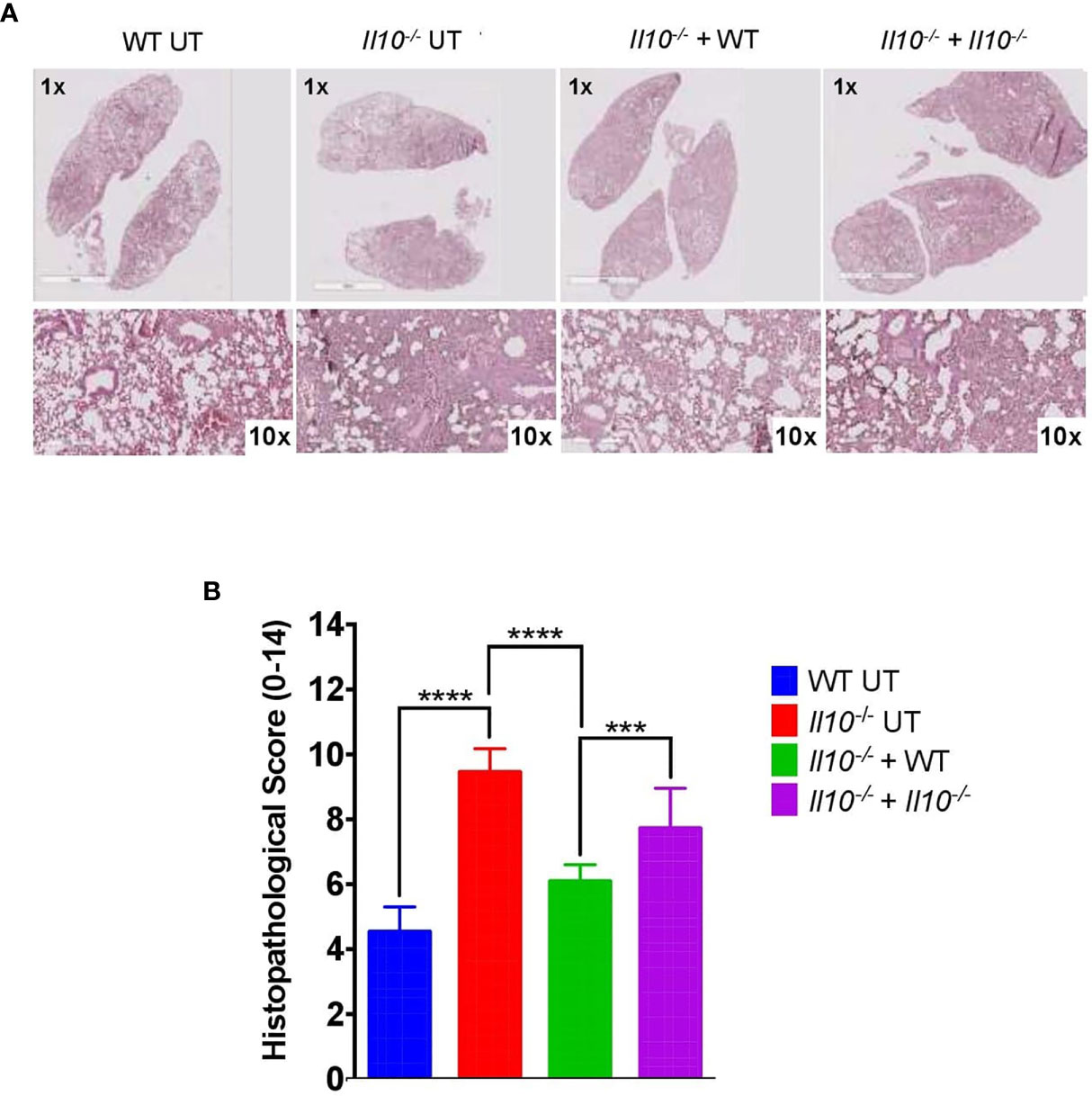
Figure 7 Adoptive transfer of neutrophils from WT to Il10-/- mice induces a decrease in the histopathological score at 48 hpi. (A) Representative lung tissue sections stained with H&E at 1X and 10X magnification. These images were used to evaluate histopathological changes after neutrophils adoptive transfer. (B) Histopathological score for lung damage. This score was used to quantify lung damage after neutrophils adoptive transfer. To capture photographs and analyze them, the Aperio ImageScope Software and GraphPad Prism Software were used. Statistics: Two-way ANOVA with Tukey’s multiple comparisons post-test were performed. ***P < 0.001; ****P < 0.0001.
Discussion
Neutrophils have been recently described as important actors of both innate and adaptive immunity due to their plasticity and immunomodulatory properties, including the ability to produce IL-10 during several inflammatory conditions (28, 45, 46). The modulatory role of neutrophils has been described during sepsis (47, 48) and in chronic infectious diseases, such as tuberculosis and Chagas (29, 49). In agreement with the literature, here we report the existence of heterogeneous population of neutrophils that produce IL-10 in the lungs during S. pneumoniae infection. We observed that among the myeloid cells recruited to the lungs, only neutrophils showed a significant production of IL-10 (Figure 1A). The high number of IL-10-producing neutrophils found at 24 and 48 hpi suggests that these cells are key components in the prevention of exacerbated inflammatory response during the early phase of S. pneumoniae lung infection. It is important to note that the involvement of neutrophils in IL-10 production is different among the broad range of pathogens able to cause pneumonia. For example, in viral pneumonia and tuberculosis, neutrophils are suppliers of IL-10 but the substantial production of this cytokine is produced by interstitial macrophages and T cells (50–52). In the case of lung infection caused by Klebsiella pneumoniae, M-MDSCs have the leading role in regulation mediated by IL-10 production (19). This background contrasts with our findings and lead us to propose that the role of neutrophils as the dominant source of IL-10 production is a distinctive feature of pneumococcal pneumonia.
Since S. pneumoniae induces a high influx of neutrophils into the lungs that subsequently would produce IL-10, this could be a host mechanism to prevent inflammatory damage activated by bacterial infection (21, 23, 53). Considering this possibility, a major aim of this study was to elucidate whether the production of IL-10 by neutrophils is triggered directly by S. pneumoniae or whether is mediated by host factors released in response to the infection. It has been reported that components of the cell wall of S. pneumoniae such as peptidoglycan, lipoteichoic acid and lipoproteins induce IL-10 production in macrophages and monocytes after their recognition by Toll-like receptors 2 (TLR2) and 4 (TLR4) (23, 53, 54). TLR2 and TLR4 are widely expressed in the surface of neutrophils and are important mediators of IL-10 production by these cells after ligand binding (28, 55, 56). Here, we provide evidence that neutrophils directly produce IL-10 after S. pneumoniae recognition. First, we observed that neutrophils significantly expressed IL-10 during S. pneumoniae lung infection in vivo, and then we confirmed these findings with in vitro assays. Our data show that IL-10 levels in the supernatant were significantly higher when neutrophils were stimulated with encapsulated S. pneumoniae as compared to neutrophils stimulated with the non-capsulated S. pneumoniae. The different abilities observed for these strains to induce IL-10 production by neutrophils are possible related to the capsule expression. Probably, non-capsulated strain expresses surface PAMPs that promote a pro-inflammatory profile in neutrophils, and higher bacterial loads could increase the exposure to these PAMPs. This effect could be favoring the establishment of an inflammatory response in neutrophils, blocking the pathways related to the production of IL-10. It is also possible that the capsule could be playing a role in coating the pro-inflammatory PAMPs, allowing only the exposition of molecules that might be stimulating IL-10 pathways in neutrophils due to the polysaccharides present in the pneumococcal capsule, which might be activating the paths necessary to activate an anti-inflammatory response. This point has not been evaluated in S. pneumoniae, but recently it has been reported for polysaccharide A present in the capsule of Bacteroides fragilis (57, 58). Until now, all of these hypotheses have not been addressed experimentally and further studies are required to clarify which bacterial components are the most relevant at inducing IL-10 production. However, based on our results, we suggest that molecular components of the capsule, such as lipoproteins and even capsule polysaccharides, might be potential inducers of IL-10 in neutrophils.
Recently, the discovery of novel roles for neutrophils has been accompanied by phenotypic and functional heterogeneity, which has led to the appearance of new subtypes of neutrophils (59). These subsets have been described mainly in cancer and some infectious diseases (37). However, data regarding neutrophil characterization during Streptococcus pneumoniae infection is still recent. After the evaluation of the contribution of neutrophils to IL-10-production during pneumococcal pneumonia, we characterized two neutrophil subsets based on their difference in size (FSClow: small or N1; FSChigh: large or N2). Further, flow cytometry analyses performed to evaluate differences in IL-10 production showed a significant difference in IL-10 expression between FSClow and FSChigh at 48 hpi only in infected mice. This result is in line with a recent study, where different neutrophils subsets were described in blood and BALFs obtained from children with viral pneumonia (36).
The characterization of neutrophil subtypes has been established based on differences in surface markers, or cytokine and chemokine profile (60). However, neutrophil plasticity is also supported by other phenotypical characteristics (41, 46). In this context, we performed analyses based on images obtained by transmission electron microscopy. We observed that FSClow cells are small, with a considerably high number of cytoplasmic granules, a low number of vacuoles and a nucleus mainly constituted by heterochromatin. On the other hand, FSChigh neutrophils are larger cells, have low presence -or absence- of granules and have presence of multiple vacuoles. Furthermore, FSChigh neutrophils present a significant euchromatin fraction, suggesting important changes in gene expression which might explain their differences in IL-10 production, as previously reported in macrophages (61). According to these morphological findings, FSClow and FSChigh subtypes display similar characteristics to the ones observed in neutrophil subtypes reported in a systemic inflammatory syndrome in response to intravenous Staphylococcus aureus infection (62). Tsuda and colleagues reported the presence of two neutrophil subtypes, the first defined as PMN-I which appeared as small cells with marked pro-inflammatory and cytotoxic properties, and the second named PMN-II, which looked larger and displayed anti-inflammatory properties, characterized by the expression of IL-10 (62). Similarly, FSClow and FSChigh subtypes have also been described in cancer, and have been associated with anti- and pro-tumor properties, respectively (34).
Noteworthy, in our study we describe that both neutrophil subsets have the ability to produce IL-10. In this line, our results could be controversial according to the research performed in cancer and systemic infection models, where FSClow like-cells show a very limited ability to secrete IL-10 (37). In this sense it is important to emphasize that experimental conditions used in our study are completely different to those present in a tumor environment or blood, where probably the neutrophil phenotype is conditioned by their anatomical location (46, 63, 64). Additionally, most studies characterizing neutrophil phenotypes are based in the results obtained from in vitro tests, which do not allow to evaluate the effect of the “cell-cell” and microenvironment interaction occurring in vivo, causing biases to the final phenotype observed (34, 65). On the contrary, here we provide new insights of neutrophil characteristics in the early stage of pneumococcal pneumonia, where IL-10-producing neutrophils have not been described previously. Moreover, according to the results obtained about the ability of both subsets to produce IL-10, we could speculate that FSChigh cells are not a different type of cell, but represent a late stage of FSClow cells, which already have the ability to produce IL-10 (66). This fact has been previously discussed, where other authors have reported that “bigger” neutrophils might correspond to cells experiencing an aging process or even might be cells going into apoptosis (30, 67). However, further complementary analyses based on single-cell sequencing would be required to confirm that FSClow and FSChigh are different cell subsets and to find whether they are playing differential roles during pneumococcal lung infection.
This study also comprised adoptive transfer assays to determine the impact of neutrophils able to produce IL-10 in a murine model of pneumonia. Neutrophil adoptive assays have previously been described in other inflammatory processes (45, 68), however our work is the first implementing this assay in pneumococcal pneumonia, based on a protocol previously carried out in our laboratory (19). Here we observed that transferred WT neutrophils restored IL-10 levels in BALF to similar levels observed in control WT mice, reinforcing the idea that neutrophils are an important source of IL-10 during pneumococcal pneumonia. Apparently, this IL-10 levels did not show a clear impact in lung infiltration with pro-inflammatory cells as observed in cytometry analyses. Regarding this point, the number of neutrophils in the lungs of untransferred Il10-/- mice is around 1x107 neutrophils per lung. Considering that the assay performed required 1x106 neutrophils per mouse, which represent around of 10% of the total neutrophils found in lungs during pneumococcal infection, it is possible that the amount of IL-10 producing neutrophils required to prevent inflammatory infiltration is higher. We confirmed this hypothesis by adoptively transferring 1x106 bone marrow-derived-neutrophils from CD45.1 mouse into CD45.2 congenic recipient mice. This assay confirmed that the proportion of donor neutrophils that remained in lungs corresponds to less than a 10% of the total neutrophils observed in lungs during pneumococcal infection and 48 h post-transfer.
Importantly, the IL-10 provided by WT neutrophils to Il10-/- mice had a negative effect in their ability to control bacterial growth and dissemination. Additionally, our results also indicate that IL-10 from WT neutrophils had a great impact in preventing alterations in lung tissue. Histopathological changes were evident in Il10-/- mice after WT neutrophil transfer, which exhibited less damage and better lung architecture as compared to the other Il10-/- experimental groups. These results indicate that the regulatory capacities of neutrophils related to IL-10 production seem to be determinant in the course of pneumococcal murine infection both at a local and systemic level, reinforcing the described dual role of IL-10 in infections (69). Next, to determine the impact of IL-10 provided by neutrophils in mouse survival, we performed adoptive transfer assays followed by a 10-days survival curve. In this experiment we obtained an increased survival in Il10-/- mice that received neutrophils, independent of their capacity to produce IL-10 (Figure 4). Our data suggest that the increased survival in Il10-/- mice transferred with WT or Il10-/- neutrophils is due by the sole addition of naïve bone marrow-derived neutrophils directly to the lungs. To evaluate whether the early transfer of neutrophils to Il10-/- mice improved their defense against S. pneumoniae independently on the production of IL-10, we changed the protocol and this time we transferred pre-stimulated IL-10-producing neutrophils 12 hpi. Taken together, these results suggest that the solely presence of naïve transferred neutrophils modulates the host response due to alternative mechanisms not evaluated in this study. These mechanisms may involve an improved microbicidal effect by transferred neutrophils or an improved activation of other cell types involved in S. pneumoniae clearance, triggered by transferred neutrophils. Further studies aimed at understanding how neutrophils interact and shape the early response in the lungs against S. pneumoniae are needed.
The reduced lung histopathology observed in Il10-/- mice transferred with WT neutrophils following S. pneumoniae caught our attention and may be an important indicative of the role of IL-10-producing neutrophils in response to this microbe. Several studies have shown that during lung bacterial infection, a balanced inflammatory response that promotes an efficient pathogen clearance with a limited tissue injury is required (19, 70, 71). In this context, we have previously shown that the production of IL-10 is critical to reduce lung inflammation and to improve host survival, but an early production of this cytokine impairs bacterial clearance (22). This fact has also been observed in a recent study showing that natural killer (NK) cells play a crucial role in pneumococcal pneumonia through IL-10 production, promoting bacterial growth and invasion to other host tissues after infection (72). We have extended this knowledge, providing evidence that neutrophils are the main myeloid cell source of IL-10 in response to S. pneumoniae after 48 h of infection. Here we also show that IL-10 production after infection is important to reduce lung histopathology but has negative impact on the ability of the mouse immune system to control bacterial burden. We also show that an early neutrophil recruitment is critical to ensure host survival in a mechanism that is apparently independent of IL-10 production. This finding highlights the plasticity and the complex biology of neutrophils that still remain largely unexplored. Unbiased techniques based on flow cytometry or single-cell RNA-seq may be useful to study the role of different neutrophil subsets in human and mice during infection. In fact, a recent study identified 10 different subsets/cluster of neutrophils in peripheral blood mononuclear cells (PBMCs) in COVID-19 patients, with potential implications in disease severity (73). These differential clusters identified showed significant differences in gene expression, showing a co-existence between anti-inflammatory/suppressive neutrophils and pro-inflammatory neutrophils, providing a more complete perspective of these cells, and also highlights the complexity of neutrophils during infection. Given the data available, it is possible that, in our model, neutrophils play a dual role in the lungs that, on the one side, protect the tissue from excessive immunopathology through the production of IL-10 but on the other side are required for bacterial clearance and pneumonia resolution. Based on our previous study (22) and the data provided here, we believe that the prevention of exacerbated lung inflammation by IL-10 production might be established following the bacterial clearance to guarantee an effective host defense. In this line and considering that neutrophils produce IL-10 before bacterial elimination, we might explain why WT mice and Il10-/- mice transferred with WT neutrophils present reduced lung inflammation and increased lung bacterial burden and dissemination.
The role of IL-10 production by human neutrophils is controversial. Conversely, there is an active debate about the capacity of human neutrophils to produce IL-10 (74). The origin of this discussion relies on one study that show that human neutrophils are unable to produce IL-10 due to an inactive chromatin configuration that impairs the Il10 transcription (75). However, recent studies incorporating analyses of circulating neutrophils, have successfully shown mRNA expression and increased levels of IL-10 in response to different pathogens and stimulus, and they also have described at least two different pathways that explain its production (30, 55, 76, 77). This evidence opens up the possibility that, to some extent, characterization data obtained in our work could be further studied in humans during pneumonia
In conclusion, our findings contribute to a better understanding of the modulatory role of neutrophils in response to lung bacterial infection. One of the main aims of this work was to provide new insights regarding the anti-inflammatory response of neutrophils during pneumococcal pneumonia during the first 48 h of the infection. Furthermore, here we provide new morphological and functional data of the different lung neutrophil subsets previously described in our laboratory. Additionally, our findings show that populations of neutrophils found in mouse lungs express IL-10 directly in response to S. pneumoniae, having a key role in the outcome of the pathological process. Finally, our in vitro and in vivo data determined the unrecognized role of IL-10-producing neutrophils in pneumococcal pneumonia. Several studies are needed to translate our findings to humans, although our data may constitute the first step that could eventually translate into novel therapeutic strategies that improve the management of patients with pneumococcal pneumonia and invasive pneumococcal disease.
Data Availability Statement
The raw data supporting the conclusions of this article will be made available by the authors, without undue reservation.
Ethics Statement
The animal study was reviewed and approved by Comité Ético Científico para el Cuidado de Animales y Ambiente, Pontificia Universidad Católica de Chile.
Author Contributions
LG, FM-G, VS, OV, LN, BS, and IS performed the experiments. LG, FM-G, and SB designed the experiments. LG, FM-G, and SB analyzed the data. LG, FM-G, HP, CR, PG, DP, AK, and SB contributed to writing the paper. AM, DP, OV, and JS performed histopathological analyses. All authors contributed to the article and approved the submitted version.
Funding
This study was supported by grants no. 1170964 (SB), 1190830 (AK), 1191300 (CR), and 1190864 (PG) from Fondo Nacional de Ciencia y Tecnología de Chile (FONDECYT), ANID-Millenium Science Initiative Program - ICN09_016: Millenium Institute on Immunology and Immunotherapy (ICN09_016; P09/016-F), grant no. 21150853 from the Agencia Nacional de Investigación y Desarrollo (LAG), grant no. 13CTI-21526 P5 from INNOVA-CORFO program of Chilean Ministry of Economy (SMB), the Innovation Fund for Competitiveness FIC- R 2017 (BIP Code: 30488811-0) a grant no. R01HL134870 from the NIH and Pilot Project Program in Hemostasis and Vascular Biology (P3HVB), University of Pittsburgh Vascular Medicine Institute, the Hemophilia Center of Western Pennsylvania, and the Institute for Transfusion Medicine (HFP).
Conflict of Interest
The authors declare that the research was conducted in the absence of any commercial or financial relationships that could be construed as a potential conflict of interest.
Acknowledgments
We are grateful of the Advance Microscopy Unit and the Flow Cytometry Unit from the Faculty of Biological Sciences, Pontificia Universidad Católica de Chile, and Daniela Moreno-Tapia, María José Altamirano and Pedro Silva from the Laboratory of Microbial Pathogenesis, Faculty of Biological Sciences, Pontificia Universidad Católica de Chile, for technical support.
Supplementary Material
The Supplementary Material for this article can be found online at: https://www.frontiersin.org/articles/10.3389/fimmu.2021.638917/full#supplementary-material
Footnotes
- ^ Yin, C & Fan C. (2012). Pneumococcal cell wall purification. http://www.bowdish.ca/lab/wp-content/uploads/2012/08/Pneumoncoccal-Cell-Wall-Purification-2DB-edits.pdf [Accessed August 5, 2020]
References
1. WHO. “Global Health Estimates 2016”, in: Disease Burden by Cause, Age, Sex, by Country and by Region, 2000-2016 (2018). Geneva: World Health Organization. Available at: https://www.who.int/healthinfo/global_burden_disease/estimates/en/ (Accessed June 28, 2019).
2. O’Brien KL, Baggett HC, Brooks WA, Feikin DR, Hammitt LL, Higdon MM, et al. Causes of Severe Pneumonia Requiring Hospital Admission in Children Without HIV Infection From Africa and Asia: The PERCH Multi-Country Case-Control Study. Lancet (2019) 394(10200):757–79. doi: 10.1016/S0140-6736(19)30721-4
3. Cillóniz C, Ewig S, Polverino E, Marcos MA, Prina E, Sellares J, et al. Community-Acquired Pneumonia in Outpatients: Aetiology and Outcomes. Eur Respir J (2012) 40(4):931–8. doi: 10.1183/09031936.00168811
4. Wahl B, O’Brien KL, Greenbaum A, Majumder A, Liu L, Chu Y, et al. Burden of Streptococcus Pneumoniae and Haemophilus Influenzae Type B Disease in Children in the Era of Conjugate Vaccines: Global, Regional, and National Estimates for 2000–15. Lancet Glob Heal (2018) 6(7):e744–57. doi: 10.1016/S2214-109X(18)30247-X
5. Nieto PA, Riquelme SA, Riedel CA, Kalergis AM, Bueno SM. Gene Elements That Regulate Streptococcus Pneumoniae Virulence and Immunity Evasion. Curr Gene Ther (2013) 13(1):51–64. doi: 10.2174/156652313804806615
6. Andre GO, Converso TR, Politano WR, Ferraz LFC, Ribeiro ML, Leite LCC, et al. Role of Streptococcus Pneumoniae Proteins in Evasion of Complement-Mediated Immunity. Front Microbiol (2017) 8:224. doi: 10.3389/fmicb.2017.00224
7. Mackenzie GA, Leach AJ, Carapetis JR, Fisher J, Morris PS. Epidemiology of Nasopharyngeal Carriage of Respiratory Bacterial Pathogens in Children and Adults: Cross-Sectional Surveys in a Population With High Rates of Pneumococcal Disease. BMC Infect Dis (2010) 10(1):304. doi: 10.1186/1471-2334-10–304
8. Simell B, Auranen K, Käyhty H, Goldblatt D, Dagan R, O’Brien KL. The Fundamental Link Between Pneumococcal Carriage and Disease. Expert Rev Vaccines (2012) 11(7):841–55. doi: 10.1586/erv.12.53
9. Adegbola RA, DeAntonio R, Hill PC, Roca A, Usuf E, Hoet B, et al. Carriage of Streptococcus Pneumoniae and Other Respiratory Bacterial Pathogens in Low and Lower-Middle Income Countries: A Systematic Review and Meta-Analysis. PloS One (2014) 9(8):e103293. doi: 10.1371/journal.pone.0103293
10. Kaplan W, Wirtz VJ, Mantel-Teeuwisse A, Stolk P, Duthey B, Laing R. Priority Medicines for Europe and the World 2013 Update (2013). Available at: https://www.who.int/medicines/areas/priority_medicines/MasterDocJune28_FINAL_Web.pdf?ua=1.
11. Whitney CG. Changing Epidemiology of Pneumococcal Disease in the Era of Conjugate Vaccines. Cur Epidemiol Rep (2016) 3(2):125–35. doi: 10.1007/s40471-016-0077–5
12. Chibuk TK, Robinson JL, Hartfield DS. Pediatric Complicated Pneumonia and Pneumococcal Serotype Replacement: Trends in Hospitalized Children Pre and Post Introduction of Routine Vaccination With Pneumococcal Conjugate Vaccine (Pcv7). Eur J Pediatr (2010) 169(9):1123–8. doi: 10.1007/s00431-010-1195–6
13. Darrieux M, Goulart C, Briles D, Leite LCDC. Current Status and Perspectives on Protein-Based Pneumococcal Vaccines. Crit Rev Microbiol (2015) 41(2):190–200. doi: 10.3109/1040841X.2013.813902
14. Balsells E, Guillot L, Nair H, Kyaw MH. Serotype Distribution of Streptococcus Pneumoniae Causing Invasive Disease in Children in the post-PCV Era: A Systematic Review and Meta-Analysis. PloS One (2017) 12(5):e0177113. doi: 10.1371/journal.pone.0177113
15. Chang AB, Ooi MH, Perera D, Grimwood K. Improving the Diagnosis, Management, and Outcomes of Children With Pneumonia: Where are the Gaps? Front Pediatr (2013) 1:29. doi: 10.3389/fped.2013.00029
16. Craig A, Mai J, Cai S, Jeyaseelan S. Neutrophil Recruitment to the Lungs During Bacterial Pneumonia. Infect Immun (2009) 77(2):568–75. doi: 10.1128/IAI.00832-08
17. Domon H, Nagai K, Maekawa T, Oda M, Yonezawa D, Takeda W, et al. Neutrophil Elastase Subverts the Immune Response by Cleaving Toll-Like Receptors and Cytokines in Pneumococcal Pneumonia. Front Immunol (2018) 9:732. doi: 10.3389/fimmu.2018.00732
18. Steck P, Ritzmann F, Honecker A, Vella G, Herr C, Gaupp R, et al. Interleukin 17 Receptor E (Il-17RE) and IL-17C Mediate the Recruitment of Neutrophils During Acute Streptococcus Pneumoniae Pneumonia. Infect Immun (2019) 87(11):e00329–19. doi: 10.1128/IAI.00329-19
19. Peñaloza HF, Noguera LP, Ahn D, Vallejos OP, Castellanos RM, Vazquez Y, et al. Interleukin-10 Produced by Myeloid-Derived Suppressor Cells Provides Protection to Carbapenem-Resistant Klebsiella Pneumoniae Sequence Type 258 by Enhancing its Clearance in the Airways. Infect Immun (2019) 87(5):e00665–18. doi: 10.1128/IAI.00665-18
20. Saraiva M, O’Garra A. The Regulation of IL-10 Production by Immune Cells. Nat Rev Immunol (2010) 10(3):170–81. doi: 10.1038/nri2711
21. Duell BL, Tan CK, Carey AJ, Wu F, Cripps AW, Ulett GC. Recent Insights Into Microbial Triggers of interleukin-10 Production in the Host and the Impact on Infectious Disease Pathogenesis. FEMS Immunol Med Microbiol (2012) 64(3):295–313. doi: 10.1111/j.1574-695X.2012.00931.x
22. Peñaloza HF, Nieto PA, Muñoz-Durango N, Salazar-Echegarai FJ, Torres J, Parga MJ, et al. Interleukin-10 Plays a Key Role in the Modulation of Neutrophils Recruitment and Lung Inflammation During Infection by Streptococcus Pneumoniae. Immunology (2015) 146(1):100–12. doi: 10.1111/imm.12486
23. Moreira LO, El Kasmi KC, Smith AM, Finkelstein D, Fillon S, Kim YG, et al. The TLR2-MyD88-NOD2-RIPK2 Signalling Axis Regulates a Balanced Pro-Inflammatory and IL-10-mediated Anti-Inflammatory Cytokine Response to Gram-positive Cell Walls. Cell Microbiol (2008) 10(10):2067–77. doi: 10.1111/j.1462-5822.2008.01189.x
24. Herta T, Bhattacharyya A, Bollensdorf C, Kabus C, García P, Suttorp N, et al. DNA-Release by Streptococcus Pneumoniae Autolysin LytA Induced Krueppel-like Factor 4 Expression in Macrophages. Sci Rep (2018) 8(1):1–14. doi: 10.1038/s41598-018-24152-1
25. Subramanian K, Neill DR, Malak HA, Spelmink L, Khandaker S, Dalla Libera Marchiori G, et al. Pneumolysin Binds to the Mannose Receptor C Type 1 (MRC-1) Leading to Anti-Inflammatory Responses and Enhanced Pneumococcal Survival. Nat Microbiol (2019) 4(1):62–70. doi: 10.1038/s41564-018-0280-x
26. Poe SL, Arora M, Oriss TB, Yarlagadda M, Isse K, Khare A, et al. STAT1-Regulated Lung MDSC-like Cells Produce IL-10 and Efferocytose Apoptotic Neutrophils With Relevance in Resolution of Bacterial Pneumonia. Mucosal Immunol (2013) 6(1):189–99. doi: 10.1038/mi.2012.62
27. Kang MJ, Jang AR, Park JY, Ahn JH, Lee TS, Kim DY, et al. Il-10 Protects Mice From the Lung Infection of Acinetobacter Baumannii and Contributes to Bacterial Clearance by Regulating Stat3-Mediated MARCO Expression in Macrophages. Front Immunol (2020) 11:270. doi: 10.3389/fimmu.2020.00270
28. Zhang X, Majlessi L, Deriaud E, Leclerc C, Lo-Man R. Coactivation of Syk Kinase and MyD88 Adaptor Protein Pathways by Bacteria Promotes Regulatory Properties of Neutrophils. Immunity (2009) 31(5):761–71. doi: 10.1016/j.immuni.2009.09.016
29. Boari JT, Vesely MCA, Bermejo DA, Ramello MC, Montes CL, Cejas H, et al. Il-17RA Signaling Reduces Inflammation and Mortality During Trypanosoma Cruzi Infection by Recruiting Suppressive IL-10-producing Neutrophils. PloS Pathog (2012) 8(4):e1002658. doi: 10.1371/journal.ppat.1002658
30. Lewkowicz N, Mycko MP, Przygodzka P, Ćwiklińska H, Cichalewska M, Matysiak M, et al. Induction of Human IL-10-producing Neutrophils by LPS-stimulated Treg Cells and IL-10. Mucosal Immunol (2016) 9(2):364–78. doi: 10.1038/mi.2015.66
31. Phillipson M, Kubes P. The Neutrophil in Vascular Inflammation. Nat Med (2011) 17(11):1381–90. doi: 10.1038/nm.2514
32. Döhrmann S, Cole JN, Nizet V. Conquering Neutrophils. PloS Pathog (2016) 12(7):e1005682. doi: 10.1371/journal.ppat.1005682
33. Pillay J, Den Braber I, Vrisekoop N, Kwast LM, De Boer RJ, Borghans JAM, et al. In Vivo Labeling With 2H2O Reveals a Human Neutrophil Lifespan of 5.4 Days. Blood (2010) 116(4):635–27. doi: 10.1182/blood-2010-01-259028
34. Sagiv JY, Michaeli J, Assi S, Mishalian I, Kisos H, Levy L, et al. Phenotypic Diversity and Plasticity in Circulating Neutrophil Subpopulations in Cancer. Cell Rep (2015) 10(4):562–73. doi: 10.1016/j.celrep.2014.12.039
35. Wéra O, Lancellotti P, Oury C. The Dual Role of Neutrophils in Inflammatory Bowel Diseases. J Clin Med (2016) 5(12):118. doi: 10.3390/jcm5120118
36. Cortjens B, Ingelse SA, Calis JC, Vlaar AP, Koenderman L, Bem RA, et al. Neutrophil Subset Responses in Infants With Severe Viral Respiratory Infection. Clin Immunol (2017) 176:100–6. doi: 10.1016/j.clim.2016.12.012
37. Perobelli SM, Galvani RG, Gonçalves-Silva T, Xavier CR, Nóbrega A, Bonomo A. Plasticity of Neutrophils Reveals Modulatory Capacity. Braz J Med Biol Res (2015) 48(8):665–75. doi: 10.1590/1414-431X20154524
38. Peñaloza HF, Salazar-Echegarai FJ, Bueno SM. Interleukin 10 Modulation of Neutrophil Subsets Infiltrating Lungs During Streptococcus Pneumoniae Infection. Biochem Biophys Rep (2018) 13:12–6. doi: 10.1016/j.bbrep.2017.11.004
39. Committee for the Update of the Guide for the Care and Use of Laboratory Animals, Institute for Laboratory Animal Research, Division on Earth and Life Studies, National Research Council. Guide for the care and use of laboratory animals: 8th edition. Washington, D.C., DC: National Academies Press (2011).
40. Nieto PA, Peñaloza HF, Salazar-Echegarai FJ, Castellanos RM, Opazo MC, Venegas L, et al. Gestational Hypothyroidism Improves the Ability of the Female Offspring to Clear Streptococcus Pneumoniae Infection and to Recover From Pneumococcal Pneumonia. Endocrinology (2016) 157(6):2217–28. doi: 10.1210/en.2015-1957
41. Borenstein A, Fine N, Hassanpour S, Sun C, Oveisi M, Tenenbaum HC, et al. Morphological Characterization of Para- and Proinflammatory Neutrophil Phenotypes Using Transmission Electron Microscopy. J Periodontal Res (2018) 53(6):972–82. doi: 10.1111/jre.12595
42. Hogg JC, Doerschuk CM. Leukocyte Traffic in the Lung. Annu Rev Physiol (1995) 57(1):97–114. doi: 10.1146/annurev.ph.57.030195.000525
43. Hyun Y, Hong C. Deep Insight Into Neutrophil Trafficking in Various Organs. J Leukoc Biol (2017) 102(3):617–29. doi: 10.1189/jlb.1ru1216-521r
44. Konrad FM, Wohlert J, Gamper-Tsigaras J, Ngamsri KC, Reutershan J. How Adhesion Molecule Patterns Change While Neutrophils Traffic Through the Lung During Inflammation. Mediators Inflamm (2019) 2019:1208086. doi: 10.1155/2019/1208086.j
45. Kasten KR, Muenzer JT, Caldwell CC. Neutrophils are Significant Producers of IL-10 During Sepsis. Biochem Biophys Res Commun (2010) 393(1):28–31. doi: 10.1016/j.bbrc.2010.01.066
46. Silvestre-Roig C, Fridlender ZG, Glogauer M, Scapini P. Neutrophil Diversity in Health and Disease. Trends Immunol (2019) 40(7):565–83. doi: 10.1016/j.it.2019.04.012
47. Ocuin LM, Bamboat ZM, Balachandran VP, Cavnar MJ, Obaid H, Plitas G, et al. Neutrophil IL-10 Suppresses Peritoneal Inflammatory Monocytes During Polymicrobial Sepsis. J Leukoc Biol (2011) 89(3):423–32. doi: 10.1189/jlb.0810479
48. Bouabe H, Liu Y, Moser M, Bösl MR, Heesemann J. Novel Highly Sensitive Il-10–β-Lactamase Reporter Mouse Reveals Cells of the Innate Immune System as a Substantial Source of IL-10 In Vivo. J Immunol (2011) 187(6):3165–76. doi: 10.4049/jimmunol.1101477
49. Moreira-Teixeira L, Redford PS, Stavropoulos E, Ghilardi N, Maynard CL, Weaver CT, et al. T Cell–Derived Il-10 Impairs Host Resistance to Mycobacterium Tuberculosis Infection. J Immunol (2017) 199(2):613–23. doi: 10.4049/jimmunol.1601340
50. Sun J, Madan R, Karp CL, Braciale TJ. Effector T Cells Control Lung Inflammation During Acute Influenza Virus Infection by Producing IL-10. Nat Med (2009) 15(3):277–84. doi: 10.1038/nm.1929
51. Sun J, Cardani A, Sharma AK, Laubach VE, Jack RS, Müller W, et al. Autocrine Regulation of Pulmonary Inflammation by Effector T-cell Derived IL-10 During Infection With Respiratory Syncytial Virus. PloS Pathog (2011) 7(8):e1002173. doi: 10.1371/journal.ppat.1002173
52. Doz E, Lombard R, Carreras F, Buzoni-Gatel D, Winter N. Mycobacteria-Infected Dendritic Cells Attract Neutrophils That Produce IL-10 and Specifically Shut Down Th17 Cd4 T Cells Through Their Il-10 Receptor. J Immunol (2013) 191(7):3818–26. doi: 10.4049/jimmunol.1300527
53. Griss K, Bertrams W, Sittka-Stark A, Seidel K, Stielow C, Hippenstiel S, et al. Micrornas Constitute a Negative Feedback Loop in Streptococcus Pneumoniae-Induced Macrophage Activation. J Infect Dis (2016) 214(2):288–99. doi: 10.1093/infdis/jiw109
54. Nguyen MT, Götz F. Lipoproteins of Gram-Positive Bacteria: Key Players in the Immune Response and Virulence. Microbiol Mol Biol Rev (2016) 80(3):891–903. doi: 10.1128/mmbr.00028-16
55. Balderramas HA, Penitenti M, Rodrigues DR, Bachiega TF, Fernandes RK, Ikoma MRV, et al. Human Neutrophils Produce IL-12, Il-10, PGE2 and LTB4 in Response to Paracoccidioides Brasiliensis. Involvement of TLR2, Mannose Receptor and Dectin-1. Cytokine (2014) 67(1):36–43. doi: 10.1016/j.cyto.2014.02.004
56. Gideon HP, Phuah J, Junecko BA, Mattila JT. Neutrophils Express Pro- and Anti-Inflammatory Cytokines in Granulomas From Mycobacterium Tuberculosis-Infected Cynomolgus Macaques. Mucosal Immunol (2019) 12(6):1370–81. doi: 10.1038/s41385-019-0195-8
57. Johnson JL, Jones MB, Cobb BA. Polysaccharide-Experienced Effector T Cells Induce IL-10 in FoxP3+ Regulatory T Cells to Prevent Pulmonary Inflammation. Glycobiology (2018) 28(1):50–8. doi: 10.1093/glycob/cwx093
58. Ramakrishna C, Kujawski M, Chu H, Li L, Mazmanian SK, Cantin EM. Bacteroides Fragilis Polysaccharide A Induces IL-10 Secreting B and T Cells That Prevent Viral Encephalitis. Nat Commun (2019) 10(1):1–13. doi: 10.1038/s41467-019-09884-6
59. Perobelli SM, Silva TG, Bonomo A. Neutrophils plasticity: the regulatory interface in various pathological conditions. In: Khajah M editor. Role of Neutrophils in Disease Pathogenesis. Intech Open Science Open Mind (2017). doi: 10.5772/68130
60. Kruger P, Saffarzadeh M, Weber ANR, Rieber N, Radsak M, von Bernuth H, et al. Neutrophils: Between Host Defence, Immune Modulation, and Tissue Injury. PloS Pathog (2015) 11(3):e1004651. doi: 10.1371/journal.ppat.1004651
61. Chen S, Yang J, Wei Y, Wei X. Epigenetic Regulation of Macrophages: From Homeostasis Maintenance to Host Defense. Cell Mol Immunol (2020) 17(1):36–49. doi: 10.1038/s41423-019-0315-0
62. Tsuda Y, Takahashi H, Kobayashi M, Hanafusa T, Herndon DN, Suzuki F. Three Different Neutrophil Subsets Exhibited in Mice With Different Susceptibilities to Infection by Methicillin-Resistant Staphylococcus Aureus. Immunity (2004) 21(2):215–26. doi: 10.1016/j.immuni.2004.07.006
63. Lok LSC, Dennison TW, Mahbubani KM, Saeb-Parsy K, Chilvers ER, Clatworthy MR. Phenotypically Distinct Neutrophils Patrol Uninfected Human and Mouse Lymph Nodes. Proc Natl Acad Sci USA (2019) 116(38):19083–9. doi: 10.1073/pnas.1905054116
64. Yang P, Li Y, Xie Y, Liu Y. Different Faces for Different Places: Heterogeneity of Neutrophil Phenotype and Function. J Immunol Res (2019) 2019:8016254. doi: 10.1155/2019/8016254
65. Ohms M, Möller S, Laskay T. An Attempt to Polarize Human Neutrophils Toward N1 and N2 Phenotypes In Vitro. Front Immunol (2020) 11:532. doi: 10.3389/fimmu.2020.00532
66. Christoffersson G, Phillipson M. The Neutrophil: One Cell on Many Missions or Many Cells With Different Agendas? Cell Tissue Res (2018) 371(3):415–23. doi: 10.1007/s00441-017-2780-z
67. Adrover JM, Nicolás-Ávila JA, Hidalgo A. Aging: A Temporal Dimension for Neutrophils. Trends Immunol (2016) 37(5):334–45. doi: 10.1016/j.it.2016.03.005
68. Hosoki K, Rajarathnam K, Sur S. Attenuation of Murine Allergic Airway Inflammation With a CXCR1/CXCR2 Chemokine Receptor Inhibitor. Clin Exp Allergy (2019) 49(1):130. doi: 10.1111/cea.13275
69. Peñaloza HF, Schultz BM, Nieto PA, Salazar GA, Suazo I, Gonzalez PA, et al. Opposing Roles of IL-10 in Acute Bacterial Infection. Cytokine Growth Factor Rev (2016) 32:17–30. doi: 10.1016/j.cytogfr.2016.07.003
70. Cicchese JM, Evans S, Hult C, Joslyn LR, Wessler T, Millar JA, et al. Dynamic Balance of Pro- and Anti-Inflammatory Signals Controls Disease and Limits Pathology. Immunol Rev (2018) 285(1):147–67. doi: 10.1111/imr.12671
71. Qu Y, Olonisakin T, Bain W, Zupetic J, Brown R, Hulver M, et al. Thrombospondin-1 Protects Against Pathogen-Induced Lung Injury by Limiting Extracellular Matrix Proteolysis. JCI Insight (2018) 3(3):e96914. doi: 10.1172/jci.insight.96914
72. Clark SE, Schmidt RL, Aguilera ER, Lenz LL. IL-10-Producing NK Cells Exacerbate Sublethal Streptococcus Pneumoniae Infection in the Lung. Transl Res (2020) 226:70–82. doi: 10.1016/j.trsl.2020.07.001
73. Schulte-Schrepping J, Reusch N, Paclik D, Baßler K, Schlickeiser S, Zhang B, et al. Severe COVID-19 is Marked by a Dysregulated Myeloid Cell Compartment. Cell (2020) 182(6):1419–40.e23. doi: 10.1016/j.cell.2020.08.001
74. Tamassia N, Bianchetto-Aguilera F, Arruda-Silva F, Gardiman E, Gasperini S, Calzetti F, et al. Cytokine Production by Human Neutrophils: Revisiting the “Dark Side of the Moon”. Eur J Clin Invest (2018) 48(Suppl 2):e12952. doi: 10.1111/eci.12952
75. Tamassia N, Zimmermann M, Castellucci M, Ostuni R, Bruderek K, Schilling B, et al. Cutting Edge: An Inactive Chromatin Configuration At the IL-10 Locus in Human Neutrophils. J Immunol (2013) 190(5):1921–5. doi: 10.4049/jimmunol.1203022
76. Sharma S, Srivastva S, Davis RE, Singh SS, Kumar R, Nylén S, et al. The Phenotype of Circulating Neutrophils During Visceral Leishmaniasis. Am J Trop Med Hyg (2017) 97(3):767–70. doi: 10.4269/ajtmh.16-0722
Keywords: pneumonia, Streptococcus pneumoniae, interleukin-10, IL-10-producing neutrophils, adoptive neutrophil transfer
Citation: González LA, Melo-González F, Sebastián VP, Vallejos OP, Noguera LP, Suazo ID, Schultz BM, Manosalva AH, Peñaloza HF, Soto JA, Parker D, Riedel CA, González PA, Kalergis AM and Bueno SM (2021) Characterization of the Anti-Inflammatory Capacity of IL-10-Producing Neutrophils in Response to Streptococcus pneumoniae Infection. Front. Immunol. 12:638917. doi: 10.3389/fimmu.2021.638917
Received: 07 December 2020; Accepted: 06 April 2021;
Published: 28 April 2021.
Edited by:
Laurel L. Lenz, University of Colorado, United StatesReviewed by:
Guillaume Sarrabayrouse, Université de Paris, FranceZhendong Cai, Ningbo University, China
Copyright © 2021 González, Melo-González, Sebastián, Vallejos, Noguera, Suazo, Schultz, Manosalva, Peñaloza, Soto, Parker, Riedel, González, Kalergis and Bueno. This is an open-access article distributed under the terms of the Creative Commons Attribution License (CC BY). The use, distribution or reproduction in other forums is permitted, provided the original author(s) and the copyright owner(s) are credited and that the original publication in this journal is cited, in accordance with accepted academic practice. No use, distribution or reproduction is permitted which does not comply with these terms.
*Correspondence: Susan M. Bueno, c2J1ZW5vQGJpby5wdWMuY2w=