- 1Rheumatology Section, Department of Health Promotion, Mother and Child Care, Internal Medicine and Medical Specialties, University Hospital “P. Giaccone”, Palermo, Italy
- 2Department of Precision Medicine, University of Campania “Luigi Vanvitelli”, Naples, Italy
Interleukin-23 (IL-23) is a pro-inflammatory cytokine composed of two subunits, IL-23A (p19) and IL-12/23B (p40), the latter shared with Interleukin-12 (IL-12). IL-23 is mainly produced by macrophages and dendritic cells, in response to exogenous or endogenous signals, and drives the differentiation and activation of T helper 17 (Th17) cells with subsequent production of IL-17A, IL-17F, IL-6, IL-22, and tumor necrosis factor α (TNF-α). Although IL-23 plays a pivotal role in the protective immune response to bacterial and fungal infections, its dysregulation has been shown to exacerbate chronic immune-mediated inflammation. Well-established experimental data support the concept that IL-23/IL-17 axis activation contributes to the development of several inflammatory diseases, such as PsA, Psoriasis, Psoriatic Arthritis; AS, Ankylosing Spondylitis; IBD, Inflammatory Bowel Disease; RA, Rheumatoid Arthritis; SS, Sjogren Syndrome; MS, Multiple Sclerosis. As a result, emerging clinical studies have focused on the blockade of this pathogenic axis as a promising therapeutic target in several autoimmune disorders; nevertheless, a greater understanding of its contribution still requires further investigation. This review aims to elucidate the most recent studies and literature data on the pathogenetic role of IL-23 and Th17 cells in inflammatory rheumatic diseases.
Review
Interleukin-23
Interleukin-23 (IL-23) is a member of the IL-12 cytokine family composed of the IL-23p19 subunit and the IL-12/23p40 subunit, the latter shared with IL-12, encoded by genes located on chromosomes 12q13.2 and 11q1.3, respectively (1–3).
IL- 23 is mainly secreted by activated macrophages and dendritic cells (DCs) located in peripheral tissues, such as skin, intestinal mucosa, joints and lungs (4–6).
Despite the protective role played by the IL-23/IL-17 axis against bacterial and fungal infections, extensive knowledge supports the contribution of its dysregulation in triggering chronic inflammation and autoimmunity, providing a solid substrate for the development of several autoimmune diseases like PsA, Psoriasis, Psoriatic Arthritis; AS, Ankylosing Spondylitis; IBD, Inflammatory Bowel Disease; RA, Rheumatoid Arthritis; SS, Sjogren Syndrome; MS, Multiple Sclerosis (3, 7–10) (Table 1).
The main role of IL-23 is to induce the differentiation of αβ T CD4+ naïve cells (Th0 cells) in T helper type 17 (Th17 cells) (11, 12), which are considered pivotal players in autoimmunity (1, 9).
Although IL-12 and IL-23 are both members of the IL-12 family and have a similar structure, the role of these two cytokines in Th0 differentiation is totally different (13, 14); indeed, unlike IL-23, IL-12 induces differentiation of Th0 cells into T helper type 1 (Th1 cells) rather than into Th17 (Figure 1) (15–19). Both cytokines are produced by DCs and the balance of IL-12 and IL-23 production is controlled by prostaglandin E2 (PGE2), which promotes inflammatory responses (20, 21).
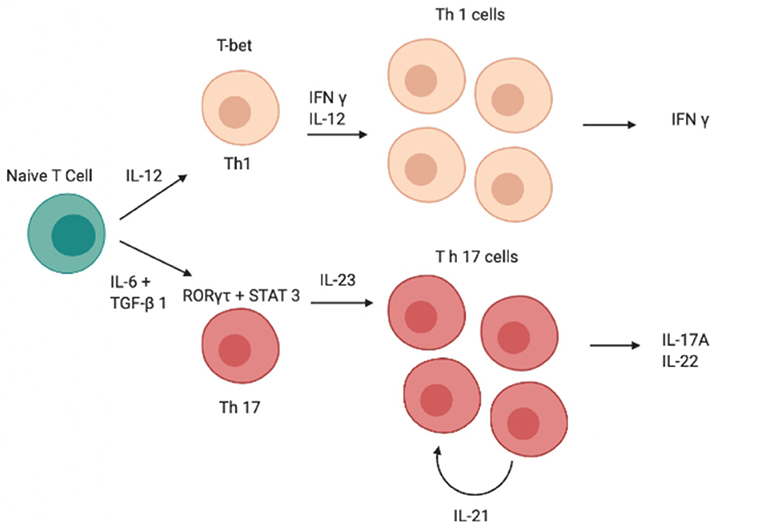
Figure 1. Schematic image of the cascade of cytokines and transcription factors involved in the differentiation of Th1 and Th17 cells. IFN-γ, interferon-γ; IL, interleukin; RORγt, retinoid-related orphan receptor γt, STAT, signal transducer and activator of transcription; GATA, GATA transcription factor; TGF-β, transforming growth factor β, Th, T helper.
IL-12 and IL-23 act as a bridge between the innate and adaptive arms of the immune response (22). IL-12, produced by antigen presenting cells (APCs), is essential for the optimal proliferation and production of cytokines by Th1 cells in response to antigens. Overall, IL-12-induced IFN-γ is an effective activator of the antimicrobial functions of phagocytes and plays a critical role in resistance to many pathogenic bacteria, fungi and intracellular parasites (23).
Regarding the IL-23/IL-17 axis, γδ T cells and innate lymphoid cells (ILCs) constitutively express the IL-23 receptor (IL-23R), suggesting their prompt first-line response to IL-23, followed by cytokine secretion and subsequent activation of the adaptive immune response. Moreover, since Th0 cells do not express IL-23R, they require prior stimulation with transforming growth factor β (TGF-β), IL-6 and IL-21 to become responsive to IL-23 (24–29).
In response to IL-23-mediated activation, αβ T cells, γδ T cells and ILCs produce IL-17, IL-22, TNF-α, and interferon-gamma (IFN-γ); in addition, IL-23-activated γδ T cells make αβ T cells refractory to the suppressive activity of regulatory T cells (Treg) and they also prevent the conversion of conventional T cells into FOXP3+ Treg cells in vivo (30).
IL-23 Receptor
The IL-23 receptor (IL-23R) is a heterodimeric receptor composed of 2 subunits: IL-12Rβ1, in common with the IL-12 receptor (IL-12R) and IL-23Rα, specific to IL-23 signaling (31).
Therefore, T cells lacking IL-12Rβ1 cannot respond to IL-12 nor IL-23. Conversely, IL-23Rα-deficient T cells cannot respond to IL-23, while maintaining IL-12 signaling capability (32). IL-23Rα and IL-12Rβ1 chains are expressed on T cells, natural killer (NK) T cells, monocytes/macrophage and DCs (33).
The intracellular pathways require different signaling proteins: JAK2, Janus kinase 2; TYK2, tyrosine kinase 2; STAT3, STAT4, signal transducer and activator of transcription 3 and 4 (34, 35).
Specifically, IL-12Rβ1 binds to TYK2 inducing STAT4 phosphorylation which is essential for increasing IFN-γ production and subsequent Th1 cells differentiation. Instead, IL-23Rα interacts with JAK2, inducing STAT3 phosphorylation and leading to the upregulation of retinoid-related orphan receptor gamma tau (RORγt), crucial for the development of Th17 cells (19, 31, 36, 37).
Once activated, STATs homodimers translocate into the nucleus, where they bind to DNA in the promoter region of target genes, acting as downstream effectors in the IL-23/IL-12 signaling pathway (38, 39).
Finally, the evaluation of IL-23 functions in vivo in different mouse models supports the hypothesis that IL-23 may act in both lymph nodes and peripheral tissues to drive terminal differentiation of effector Th17 cells in vivo, promoting Th-17-mediated inflammation. Confirming these observations, in the absence of Il-23, Th17 cells experience “arrested development” leading to impaired function (40).
Interleukin-17A
Interleukin-17A (IL-17A) is the first described member of the IL-17 cytokine family, which includes six members, IL-17A to IL-17F (41, 42).
Many IL-17A-producing cells have been reported, including T CD8+ cells (43), γδ T cells (44), and NK T cells (45); however, according to current knowledge, T CD4+ cells (Th17) are the major source of IL-17.
Although IL-23 has mainly been identified as the initiating factor for IL-17 expression from T cells (9, 46), Th0 cells do not constitutively express IL-23R, but they are still sensitive to IL-23 (47); in this context, it is reasonable to assume that IL-23R expression on these cells can be induced in the presence of other pro-inflammatory cytokines (48, 49).
Several findings clearly demonstrated that TGF-β and IL-6 are sufficient for Th17 differentiation in vitro and in vivo, in the absence of IL-23 (50–53).
Therefore, TGF-β, IL-6, and IL-21 seem to activate T lymphocytes and promote the initial differentiation of Th0 into Th17 cells, conferring responsiveness to IL-23 (50, 51, 54–60), which is a crucial step for Th17 cells stabilization and expansion (61).
Conversely, increased TGF-β levels coupled with the absence of inflammatory cytokines inhibit Th17 differentiation (62), as well as the common inhibitors of Th17 commitment (IFN-γ, IL-4, IL-25, IL-27) (54–56, 63–67).
Finally, IL-17-producing cells have been shown to express a wide range of heterogeneous cytokines such as IL-17A, IL-17F, IL-26 (62), and other proinflammatory mediators including IL-22, IL-21,IL-6, TNF-α, granulocyte colony-stimulating factors (GM-CSF), and chemokines (e.g., CCL20, CXCL8, CXCL1, CXCL10) (9).
IL-17 Receptor
IL-17 receptor (IL-17R) is expressed on many cell types, including epithelial cells, B and T cells, fibroblasts, monocytic cells, and bone marrow stroma (68).
IL-17 signaling activates nuclear factor κB (NFκB) activator adaptor protein (ACT1), which in turn acts on mitogen-activated protein kinases (MAPKs), including p38MAK (69), c-jun N-terminal kinase (JNK), extracellular signal-regulated kinase (ERK), Janus kinase (JAK), signal transducer and activator of transcription (STAT), phosphoinositol 3 kinase (PI3K) and induces several pro-inflammatory cytokines (IL-1β, IL-6, TNF-α, CCL2), antimicrobial peptides (β-defensin), and matrix metalloproteinases (69–71).
In health conditions, IL-17 is one of the main contributors to the host defense against microbial infections (68, 72–74). Of note, the IL-17 pathway regulates antifungal immunity, in human and mice, inducing upregulation of proinflammatory cytokines, antimicrobial peptides and neutrophil-recruiting chemokines, which lead to limit fungal overgrowth (75).
Historically, CD4+ T cells have been involved in protecting against Candida albicans infection were found in Human Immunodeficiency Virus (HIV) positive patients (76, 77).
Subsequently, Th17 subset was identified as CD4+ T cells with reactivity to Candida albicans (37, 78).
Moreover, ex vivo studies on human T cells demonstrated that Candida albicans triggers Th17 cells which produce IL-17 and IFN-γ, but not IL-10. On the contrary, Staphylococcus aureus–activated Th17 cells produce IL-10, which can limit the immune system responses. These different responses could derive from the presence, in the priming phase, of different cytokine environments induced by each microbe (79).
The specific role of IL-17 in protection against the fungus Candida albicans was confirmed by evidence that IL-17RA deficient mice or mice and humans with defects along the IL-17 signaling pathway, were susceptible to systemic Candida albicans infection (80–83).
Major roles of IL-17 include the promotion and initiation of chemotaxis and the recruitment and activation of neutrophils in inflamed tissues (71, 84, 85). Among its pleiotropic effects, the enhancement of angiogenesis (86) and the tissue remodeling through the production of angiogenic factors and matrix metalloproteases are worthy of note (87). IL-17 works in synergy with TNF-α causing release of the IL-6, TNF-α, and IL-1β, in order to amplify the multifaceted and complex inflammatory process (88).
Consistently, increased serum and tissue levels of IL-17 have been widely reported in inflammatory condition such as IBD, MS, and arthritis, compared to a non-pathological setting where IL-17A levels are extremely low or undetectable in human sera (Figure 2) (3, 10, 89–92).
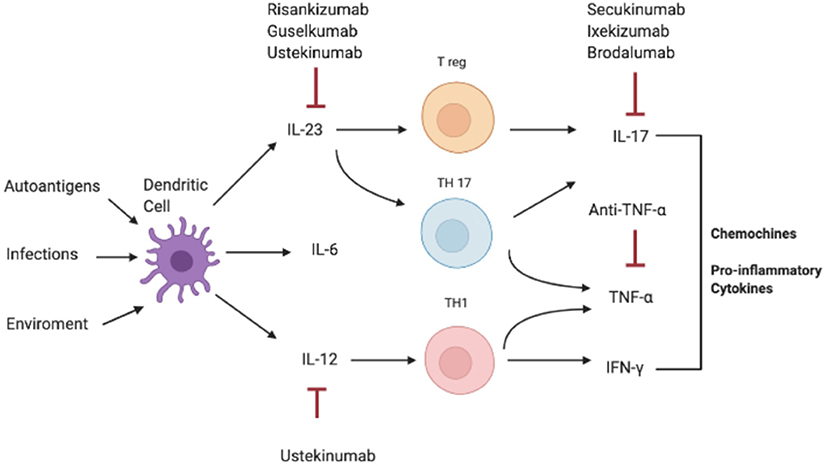
Figure 2. Pathogenesis of IL-17-correlated disease and different targets of therapy. IFN-γ, interferon-γ; IL, interleukin; Th, T helper; TNF-α, Tumor necrosis factor.
Ankylosing Spondylitis (AS)
Ankylosing Spondylitis (AS) is the prototypical subset of SpA characterized by a predominant axial involvement (93). As a result, sacroiliitis is the clinical hallmark of disease and its identification through the most sophisticated imaging techniques, such as high-field magnetic resonance imaging (MRI), is extremely important in order to achieve an early diagnosis that can prompt a rapid treatment administration. The new classification criteria published by the Assessment in Spondylo-Arthritis international Society (ASAS) in 2009 have included MRI as the gold standard technique to identify active sacroiliitis, consisting in bone marrow oedema and osteitis, even in patients that have not developed radiographical signs of disease (94). According to these criteria, the presence of alterations (both in standard radiography or MRI) coupled with clinical, genetic and laboratory data, allow the classification of AS patients into radiological axial SpA (r-axSpA) or non-radiological axial SpA (nr-axSpA) (95).
New bone formation, determined by chronic inflammation involving the spine, leads to vertebral ankylosis, severe chronic pain and disability as major consequences of disease progression.
The beginning of the inflammatory process in AS relies on a complex, multifactorial interplay between genetic, epigenetic and environmental factors associated with a dysregulated immune response. The current understanding of AS pathogenesis suggests that the IL-23/IL-17 axis acts as the major driver in disease development, even if type 17 response could not entirely elucidate the mechanisms behind this rheumatic disease (96).
Genetics and epigenetics play a pivotal role in the pathogenesis of AS; siblings of AS patients have a higher risk of developing the disease and a high degree of concordance in twins is observed, 50–63% in monozygotic and 13–20% in dizygotic twins, respectively (97). The strongest genetic association is with the allele human leukocyte antigen B27 (HLA-B27) of the Major Histocompatibility Complex-I (MHC-I) gene, located on chromosome 6 (98).
Several theories have emerged to explain the possible pathomechanism related to HLA-B27, AS starting, and subsequent dysregulated activation of the IL-23/IL-17 axis via Th17 cells (99). Modifications in the shape of HLA-B27 affect the protein binding domain impairing both the antigen presentation process and the correct folding of the HLA-B27 molecule (100). The altered binding domain may lead to the presentation of self-peptides to cytotoxic CD8+ T cells that in turn give rise to a pathological autoimmune response (101). On the other hand, the unfolded protein response (UPR) theory postulates that unconventional HLA-B27 variants homodimerize instead of heterodimerize; the misfolded proteins accumulate in the intracellular compartment triggering endoplasmic reticulum stress and increasing IL-23 production (102). In fact, heterodimers expressed on APC surface directly interact with cell receptors on a wide range of immune cells such as NK, monocytes, B cells and promote a significant impact on Th17 stimulation (103). In particular, the aberrant expression of the allele of the HLA B 27 in spondylitis, could act by binding cells that contain a natural killer receptor for HLA B 27 homodimer, named killer cell immunoglobulin-like receptor 3DL2 (KIR3DL2), determining IL-17 production (104).
HLA-B27 alone accounts for almost the 25% of AS heritability and genetic-wide association studies (GWAS) have identified multiple genetic loci linked to disease pathogenesis (105, 106). In particular, single nucleotide polymorphisms (SNPs) in genes coding for aminopeptidases expressed in the endoplasmic reticulum (ER), such as ERAP1 and ERAP2, were identified in the past decade. These proteins trim peptides in the ER so that these molecules get to the right length, usually between 8 and 10 amino acids, to be presented by MHC-I molecules (107). Mutations in ERAP enzymes are supposed to lead to the formation of the so called “arthritogenic peptide” which, through mechanisms related to molecular mimicry, triggers immune cells to react against self-antigens located at joint and enthesal sites (108). ERAP1 and HLA-B27 effects are linked in an epistatic way, meaning that ERAP1 mutation effects are only observed in HLA-B27 positive patients (109).
SNPs directly affecting the IL-23/IL-17 axis were described in AS and further stress the importance of this pathway. The most relevant are located in the genes coding for IL-23R and STAT3 and TYK2, which are downstream targets of IL-23 signaling (110, 111).
However, it is not entirely clear how this polymorphism is responsible for pathogenesis, but this probably alters the amino acid sequence of the intracellular portion of IL-23R, and it could have a secondary regulatory function; indeed, protective alleles have also been identified in IL-23R, and some studies have detected polymorphisms in the IL-23R as a strong protective genetic factor (112).
The fundamental role played by the IL-23/IL-17 axis comes from several lines of evidence depicting a clear increase in IL-23 and IL-17 levels in the sera of AS patients (113). This observation is coupled with the increased number of Th17 cells in peripheral blood from AS patients (114). In this regard, the most intriguing and recent theories suggest that the interface between environment and immune system in AS can be represented by the gut epithelial barrier, where IL-17A exerts its functions in maintaining mucosal immunity and barrier functions (115). The intimate relationship between the articular disease and the gut is underlined by the detection of subclinical inflammation in up to 70% of AS patients on endoscopic examination, as well as on histological samples (116) and in about 10% of these occurs a clinical IBD suggesting a pre-clinical stage of IBD (115). Even bone marrow oedema in sacroiliac joints was correlated to gut inflammation, as emerged from the analysis of the Ghent cohort (117).
At gut level the huge number of adherent and invading bacteria, known as human microbiota, may be perturbed leading to both quantitative and qualitative alterations that affect the integrity of the gut-epithelial and vascular barriers in accordance with the “joint-gut axis theory” (118). The “leaky gut” allows the translocation of bacteria-derived peptides and primed immune cells to the interstitium and then to the bloodstream, eliciting a systemic abnormal inflammatory response. The derangement of the gut interface has been related to the alteration of the tight junction system and an increase in zonulin level was retrieved both in gut epithelium and peripheral blood (119). In AS patients dysbiosis was evidenced in comparison to healthy individuals and increased level of IL-23 are found in patients' gut and in particular in the terminal ileum (120). Several cell populations involved in epithelial immunity and joint/enthesal inflammation, such as Th17, ILC, γδ T cells, and mucosa-associate invariant T (MAIT) cells, as well as cells involved in mucosal homeostasis, such as Paneth cells, produce IL-23 at intestinal level. ILC3 are expanded in gut and differentiate upon IL-17 and TNF-α stimulation becoming an important source of IL-23 and IL-17 (121). Among the intraepithelial lymphocyte (IEL) compartment γδ T cells are the most represented population, accounting for approximately the 50% of IEL; on the other hand, they represent only the 3–5% of circulating T cells. Once activated with IL-23 these cells produce IL-17 (44). Their number was found increased in gut and γδ T cells obtained from AS patients show hyper-responsiveness to IL-23, due to IL-23R hyper-expression, with consequent discharge of higher amount of IL-17 when stimulated (122).
According to the gut-joint axis theory the intestinal activation of different immune cell subsets, among the ones above described, followed by their recirculation in blood may lead to their final localization in joint and enthesis where the inflammatory process is carried out. Intestine seems to be the major site of IL-23 production and is also the site where it acts most. Even gut- derived IL-17 producing ILC3 were found expanded at bone marrow, joint and peripheral blood level. ILC3 are also characterized by a significant expression of an integrin that regulates intestinal T cells homing, called α4β7. Moreover, the receptor of this integrin (named MADCAM1) was found upregulated in the gut and in bone marrow of AS patients, suggesting a chemoattraction process of ILC3 at inflammation site (121). Cuthbert et al. described resident γδ T cells at spinal enthesis where they contribute to IL-17 production in both IL-23 dependent and independent ways. In fact, a subpopulation of these cells lacking IL-23R was proven to produce IL-17 (123). In addition, IL-17 producing MAIT cells were found elevated in AS patients both in blood and synovial fluid (124, 125). To add more pieces to the already complex puzzle of IL-17 producing cells, a new population of CD8+ T cells able to produce IL-17, named Tc17 cells, was described and was found increased in both peripheral blood and synovial fluid of AS patients (126). Other possible sources of IL-17 that deserve deeper investigation are tissue-resident memory T cells (TRM), mast cells and CD3−CD56+NK cells (127, 128). In axial tissues IL-23 producing cells are macrophages and DC while IL-17 sources are myeloid cells as neutrophils (129, 130).
Animal models have supported the actual knowledge on the role of the IL-23/IL-17 axis, even if human disease appears far more complex and no animal model can comprehensively elucidate it. Transgenic HLA-B27 rat model was used to demonstrate the importance of the HLA-B27, gut microbiota and Th17 cells in the pathogenesis of SpA (131). These rats, grown in germ free condition do not develop SpA (132). Several mice models even exist and have contributed to the unraveling of the pivotal role played by IL-23 and IL-17 in activating T cells and driving disease development via type 17 immunity (133).
Taken together, the above resumed evidence underlines the importance of this axis in inducing and sustaining the multifaceted inflammatory process depicted in SpA, making it a central therapeutic target.
Advances in understanding the pathogenesis of SpA have prompted the development of biologic drugs designed to inhibit the IL-23/IL-17 axis. In fact, for more than 15 years, TNF-α inhibitors were the only biologic treatment available and, despite an initial great success in SpA management, it came out clearly that almost 40% of patients failed to reach a significative response. The new therapeutic agents interfering with the IL-23/IL-17 axis can be divided into monoclonal antibodies directly targeting IL-17, IL-23 or their receptors and small molecules inhibiting the intracellular pathways triggered by these cytokines (134).
Among monoclonal antibodies targeting IL-17, Secukinumab and Ixekizumab, respectively a fully human monoclonal IgG1/k antibody and a humanized IgG4 monoclonal antibody, are the only two molecules already marketed for SpA.
The MEASURE trials demonstrated the superiority of Secukinumab against placebo in providing sustained efficacy in relieving signs and symptoms of AS as well as in granting a good retention rate, as demonstrated in the 5-years extension study (135, 136). In the 2-years follow up in the MEASURE 1 trial no radiographic progression was evidenced in the 80% of patients included (137).
Ixekizumab was licensed for r-axSpA treatment in 2019 and for nr-axSpA in 2020, the COAST-V trial demonstrated a superior response rate in ASAS40 score at week 16 over placebo (138).
Several trials aimed to assess the therapeutic value of other IL-17 inhibitors, such as Netakimab and Bimekizumab, are currently ongoing (139, 140). The randomized controlled trial on Brodalumab, a humanized IgG2 monoclonal antibodies that binds IL-17R was discontinued because of the occurrence of high suicide ideation in the active group (141).
Up to date, no IL-23 targeting drug has been proven effective for AS (142, 143).
New treatments for AS are small molecules inhibitors that target intracellular proinflammatory pathways, as those triggered by cytokine stimulation. Among targeted synthetic DMARDs (tsDMARDs), JAK-inhibitors stand out as the most promising treatment (144). In particular, Tofacitinib, a pan-JAK inhibitor, Filgotinib and Upadacitinib, both JAK1 inhibitors, were shown to be superior to placebo in axSpA (145–147).
The inhibition of PDE4 through the small molecule Apremilast failed to achieve the primary end-point in the specifically designed phase 2 trial including 490 AS patients (148).
Future perspectives to implement the therapeutic options for AS patients include broader anti-inflammatory approaches with multi-cytokine blockade to overcome the inhibition of a single pathway, for example targeting simultaneously TNF-α and IL-17, as in an ongoing study in PsA (149).
Psoriatic Arthritis
Psoriatic arthritis (PsA) is a chronic, immune-mediated, inflammatory disease dominated by a heterogeneous phenotype that mainly affects peripheral and axial joints, entheses, skin, and nails, leading to juxta-articular new bone formation, bone erosions and abnormal keratinocyte proliferation (31, 150).
Interactions between genes and environmental triggers, including infections, trauma, stress, obesity and smoking are recognized as crucial for the onset of the autoimmune process in PsA. Furthermore, the disruption of the gut microbiota composition in PsA patients is supported by consolidate evidence (151, 152).
The main effector cells of the inflammatory cascade, both in joints and in plaques of patients with PsA, are DCs, macrophages, NK cells (153, 154), mast cells, neutrophils (155, 156), γδ T cells (157), T CD4+ and T CD8 + cells.
All the ones above described have a predominant IL-17 secretory phenotype (41, 158), defined by the production of cytokines such us IL-17, IL-22, and TNFa; however, T CD4+ cells, as the major source of IL-17, are considered the cornerstone in the pathogenesis of psoriasis (157, 159).
Specifically, DC-derived cytokines, IL-23 and IL-12, drive the differentiation of distinct Th17 and Th1 cells, which are known to be implicated in the pathogenesis of PsA. Activated T cells move from the circulation to the target organs, and chronic inflammation occurs in the skin and joints.
Accordingly, increased numbers of Th17 cells were detected in the blood and affected skin of patients with psoriasis and in the blood and synovial fluid of patients with PsA. Furthermore, the assessment of the expression of IL-23, IL-17, and their related receptors in psoriatic skin lesions and inflamed synovium supports the concept of IL-23/IL-17 axis as a driving force of immune inflammation in psoriasis (160–164) PsA synovitis is characterized by significant infiltration of mononuclear cells, T and B cells, vascular proliferation and hyperplasia of synovial lining cells, similar to the pathological changes observed in RA (165). Additionally, ectopic lymphoid tissues were frequently found in PsA synovial membrane with microanatomical features for germinal center formation, capable of antibody production (166). The role of B cells in PsA is still elusive; a recent study reported that autoantibodies against a peptide sharing sequence homology with skin and entheseal autoantigens were detected in 85% of patients with PsA (167).
Several findings have clearly demonstrated the influence of IL-17 on bone metabolism. Despite the direct effects of IL-17 on osteoclasts, induction of osteoclastogenesis is mediated by the production of matrix metalloproteinases by macrophages and the activator of the NF-κB ligand receptor (RANKL) presented by osteoblasts (168–170).
Skin lesions from patients with psoriasis exhibit epidermal hyperplasia and infiltration with neutrophils, T CD4 + and T CD8 + cells, B cells, dendritic cells and mast cells, type 3 innate lymphoid cells (ILC3) and γδ T cells (171–174).
In contrast, IL-22, totally absent in synovial tissue, is highly expressed in entheses, where it promotes entheseal and periosteal bone formation through STAT3 activation, explaining the formation of enthesophyte and juxta-articular bone, hallmarks of PsA (175, 176). In skin lesions IL-22 drives keratinocyte hyperproliferation via STAT3 signaling (177) and prompts epithelial cells to release chemokines, such as IL-8 (178, 179), a key factor in neutrophil recruitment in psoriatic lesions and stimulates keratinocytes to secrete antimicrobial peptides (180, 181), preventing skin lesions from becoming infected (182).
In inflammation, Th17 cells also produce interleukin 9 (IL-9), which in turn induces the differentiation of Th17 cells and potentiates the suppressive effect of regulatory T lymphocytes via activation of STAT3 and STAT5. Our group demonstrated that IL-9 overexpression and T helper type 9 (Th9) polarization occur in the synovial tissue and peripheral blood of PsA patients. Furthermore, clinical improvement after treatment with TNFi and ustekinumab was associated with a significant reduction in circulating Th9 cells (183, 184).
Up to date, a wide range of therapeutic approaches have been proposed for PsA, depending on disease severity, including disease-modifying anti-rheumatic drugs (DMARDs) such as methotrexate; anti-tumor necrosis factor α (anti-TNF-α) agents, phosphodiesterase 4 (PDE4) inhibitors, IL-17 and IL-12/IL-23 inhibitors (185, 186).
The central contribution of IL-23/IL-17 axis in both PsA and psoriasis is confirmed by the efficacy of biologics neutralizing IL-17 or IL-23/IL-12 (187) as well as the effectiveness of TNF-α inhibition dependent on down-regulation of IL-17 pathway genes (188–190).
To date, several monoclonal antibodies have been approved for the treatment of PsA and can be introduce following the failure of non-steroidal anti-inflammatory drugs (NSAIDs) and/or conventional DMARDs, or an anti-TNF-α agent.
Ustekinumab is a human IgG1 monoclonal antibody that binds to the p40 subunit, shared with IL-12 and IL-23, and blocks downstream events of both the IL-12 and IL-23 signaling cascade through inhibition of IL-12Rβ1 binding (191). Ustekinumab has produced consistent and sustained clinical efficacy in two phase three clinical trials in PsA, PSUMMIT-1 and PSUMMIT-2, with data out to 52 weeks, and no new safety signals. PSUMMIT-1 included patients with active PsA despite conventional therapy who were all naïve to anti-TNF-α agents, whereas PSUMMIT-2 also included anti-TNF-α experienced patients (192).
Secukinumab, is a human IgG1κ monoclonal antibody that binds to IL-17A neutralizing its interaction with IL-17 receptors. It is currently approved in several countries for the treatment of PsA (193) and, in two phase 3 trials FUTURE1 and FUTURE 2, secukinumab has provided sustained improvements in disease signs and symptoms, assessing reduced radiographic progression in patients with active PsA through 2 years of therapy (194).
Ixekizumab is a humanized IgG4 monoclonal antibody targeting IL-17A, approved for the treatment of moderate-to-severe plaque psoriasis, active PsA, and active AS. Two phase three trials (SPIRIT-P1 and SPIRIT-P2) demonstrated that treatment with ixekizumab improved joint and skin disease compared to placebo (195, 196).
In addition, the SPIRIT-H2H study confirmed the superiority of ixekizumab over adalimumab in patients with Psa and inadequate response to csDMaRDs (197).
Over the past decade, the development of Janus kinase inhibitors (JAK inhibitors) has emerged as a new therapeutic option in autoimmune diseases, including PsA. The rationale of their use is suggested by the observation that the blockade of JAK receptor downregulates the production of the cytokines (TNF-α, IL-17, IL-6, IL-23) involved in the pathogenesis of PsA.
Tofacitinib is an orally administered inhibitor of predominantly JAK1 and JAK3, with functional selectivity to JAK2, used for the treatment of RA. Interestingly, its efficacy in managing treatment-resistant disease and ameliorating enthesitis, dactylitis, and radiographic progression has been reported. Consistently with previous observations, Tofacitinib could provide an alternative approach for PsA patients with inadequate response to DMARDs (198, 199).
Finally, Guselkumab, a monoclonal antibody targeting IL-23 via IL-23 p19 subunit, was recently approved for the treatment of PsA in adults with an inadequate response or intolerance to DMARDs therapy. Results for the use of Guselkumab derive from phase three clinical trials, DISCOVER-1 and DISCOVER-2, which demonstrated significantly better clinical and radiographic outcomes among PsA patients treated with the IL-23 inhibitor compared with placebo group.
Participants treated with Guselkumab achieved 20% improvement in American College of Rheumatology (ACR) response criteria at week 24 at rates of 52% in DISCOVER-1 and 64% in DISCOVER-2, whereas placebo-treated patients had rates of 22 and 33%, respectively (200, 201).
Rheumatoid Arthritis (RA)
Rheumatoid arthritis (RA) is a systemic autoimmune disease that primarily affects synovial joints, accompanied by systemic inflammation and production of autoantibodies (202).
The synovitis in RA is characterized by an inflammatory infiltrate, consisting of leukocytes such as T and B cells, macrophages, granulocytes and dendritic cells, together with a synovial milieu dominated by proinflammatory cytokines and chemokines (203).
For a long time, RA was considered a Th1 dependent disease, until a significant amount of research in RA patients and experimental mouse models suggested that Th17 cells may play a central role in the pathogenesis of RA.
IL-17 is involved in both early and established RA disease, promoting activation of fibroblast-like synoviocytes (FLS), osteoclastogenesis, recruitment and activation of neutrophils, macrophages and B cells (204).
Synergism between IL-17 and TNF-α has been shown to activate the production of pro-inflammatory mediators, such as IL-1β, IL-6, IL-8, PGE2, and matrix metalloproteinases (MMPs), promoting progression of early inflammation toward a chronic arthritis (205, 206).
The differentiation of osteoclasts is induced significantly in the presence of IL-17 either directly (207), or indirectly, through upregulation of RANKL.
By the late 1990s studies had already shown that IL-17 expression had increased in the joint of RA patients compared to healthy individuals or osteoarthritis (OA) patients (89).
More recently, an increased proportion of chemokine receptor CCR6+ Th17 cells has been described in the peripheral blood of treatment-naive patients with early RA (208), and higher frequencies of Th17 cells have been detected in the synovial compartment of RA patients, compared to OA patients (209).
Moreover, Th17 cells were associated with clinical parameters, such as disease activity score 28 (DAS28), C-reactive protein (CRP) levels and presence of anti-citrullinated protein antibodies (ACPAs), highly specific for RA (210, 211).
Direct evidence obtained from experimental mouse models confirms the critical role of the IL-23/IL-17 axis in the pathogenesis of arthritis. IL-23p19-deficient (Il23a−/−) mice were protected against the development of collagen-induced arthritis (CIA), a mouse model of RA. IL-17-producing CD4+ T cells were absent in the Il23a−/− mice despite normal induction of IFN-γ-producing CD4+ Th1 cells (212).
Interplay between IL-23 and IL-17 production may be a critical immune pathway and a potential therapeutic target for a range of inflammatory arthritis.
Antibodies against IL-17 (Ixekizumab and Secukinumab) or IL-17R (Brodalumab) have been tested in RA patients (213–216). In a phase I RCT of RA patients treated with oral DMARDs, the addition of Ixekizumab improved RA signs and symptoms and disease activity scores such as DAS28, compared to placebo (213). This improvement was confirmed in a phase II study in which Ixekizumab was administered to patients who were naive to biological therapy or resistant to TNF-α inhibitors (217).
In a phase II study enrolling RA patients with inadequate response to methotrexate, greater decreases in DAS28 were observed with Secukinumab than with placebo (214).
Furthermore, patients with active RA who did not respond to DMARDs showed improvements after long-term treatment (52 weeks) with 150 mg of Secukinumab, with ACR50 rates increased from 45% to 16 to 55% at week 16 at week 52 (215). Conversely, Brodalumab showed no evidence of clinical benefit in RA patients in a phase Ib study (213).
In addition, the efficacy of Ustekinumab, a human anti-IL-12/23 p40 monoclonal antibody and human anti-IL-23 monoclonal antibody, Guselkumab, has been evaluated in patients with active RA not responsive to methotrexate therapy. However, no significant clinical improvement was recorded compared to the control group (218).
Recently, the double blockade of IL-17 and TNF-α has been studied using ABT-122, a variable double domain Ig that targets human TNF-α and IL-17 (219). A phase II study showed that there was no significant difference in the ACR20 response at week 12 by the double inhibition of IL-17 and TNF-α compared to treatment with only anti-TNF-α (220). Lastly, double blockade of IL-17A and IL-17F using Bimekizumab in RA patients with inadequate TNF-α response resulted in a greater reduction in DAS28-CRP at week 20 compared to anti-TNF-α inadequate response plus placebo group (221).
Sjögren's Syndrome (SS)
Sjögren's syndrome (SS) is a systemic autoimmune disease characterized by the lymphocytic infiltration into the exocrine glands, mainly salivary and lacrimal glands, and other tissues (222).
The role of the adaptive immune system in the pathogenesis of SS is supported by the presence of ectopic germinal centers in almost 25% of the patients, which promote local expansion of antigen-specific B cells, production of autoantibodies and hypergammaglobulinemia, as well as increased risk of developing non-Hodgkin lymphoma (NHL) (223, 224).
In this regard, the interaction between CD4+ T cells and B cells appears to be a key step in the development of the disease (222).
Although SS has historically been considered a Th1-driven disease, subsequent studies have revealed that, in addition to Th1 cells, several subgroups of CD4+ T cells are involved, follicular T helper cells (Tfh) and Th17. In healthy subjects, Th17 cells play an important role at mucosal barriers and are involved in immune responses. In SS, Th17 cells may be activated by dendritic cells in lymph nodes draining the salivary and lacrimal glands by the production of cytokines as TGF-β and IL-23. In the later phases of the disease, naïve T cells can also be polarized locally in Th17 cells by APC and cytokines as IL-6 and TGF-β (225). IL-23 production by macrophages is also important for maintenance and expansion of Th17 cells by STAT3 activation (226). The main effector cytokines of Th17 cells are IL-17 and IL-22. Recent studies reported that IL-17 protein and mRNA are present within lymphocytic infiltrates of minor salivary gland (MSG) tissue of SS patients.
Furthermore, IL-17 mRNA levels in MSG biopsies were related to the degree of inflammation (226–228).
Along with IL-17, the expression of IL-23 and IL-22 was also increased in the inflamed salivary glands of SS patients. IL-17 and IL-22 secretion in the exocrine glands promote inflammation and the induction of matrix metalloproteinases which may cause acinar damage (229).
Moreover, the presence of Th17 cells in SS infiltrate has been hypothesized to be crucial in B cell activation and formation of germinal centers within glands (230).
The IL-23/IL-17 axis also plays a role in the symptoms development, as confirmed by the increased levels of IL-17 and IL-6 protein and mRNA in tears and saliva from SS patients compared to non-SS controls. Additionally, the expression of Th17-associated cytokines correlated with ocular surface parameters, such as Schirmer I Test, break up time (BUT) and corneal fluorescent staining (CFS) (231).
A recent study showed that serum IL-17F levels were significantly increased in SS patients and were associated with high levels of autoantibodies and increased EULAR SS disease activity index (ESSDAI), compared to IL-17A, suggesting the possibility of several pathogenetic roles played by different IL-17 family members (232).
The role of the IL-23/IL-17 pathway in the pathogenesis of SS has been also supported by data from animal models. In C57BL/6.NOD-Aec1Aec2 mouse, a model of spontaneous SS, genetic ablation of IL-17 reduced lymphocytic infiltration and restored glandular function, especially in female animals (233).
In addition, adoptive transfer of Th17 cells induced the development of experimental SS (ESS) in immunized IL-17 knockout mice (234).
Even if Th17 cells are the main source of IL-17 in SS, γδ T cells, NK cells, ILCs and CD8+ T lymphocytes can also produce IL-17 (68). However, recent evidence has shown that CD4− CD8− (double negative, DN) T cells and mast cells can also participate in local IL-17 production in SS (235).
Rituximab treatment demonstrated a significant reduction in IL-17 expression in the salivary glands of SS patients, while factors that are important for maintenance of Th17 cells, as STAT3 and IL-23, are likely not affected (228).
Despite the increase in Th17 cells in SS is well-established, data on the amount of T reg cells are still controversial. Th17 and Treg cells are counter-regulated. IL-6 promotes Th17 differentiation by inhibiting T reg generation (51), while IL-2 acts in the opposite way (236). Since reduced IL-2 levels could underline Th17 upregulation (237), the effects of administration of recombinant IL-2 have been studied in SS. Reduction of glucocorticoid and Hydroxychloroquine (HCQ) in patients treated with IL-2 and restored Th17/Treg balance have been reported (238).
Regarding IL-6 inhibition, as the IL-6 signaling is important for the IL-17 cells differentiation, an effect of tocilizumab on Th17 cells is probable. However, the results of a randomized placebo-controlled study showed that Tocilizumab had no impact on the main symptoms of SS, although improvements in joint involvement were observed (239).
Long-term efficacy of Ustekinumab, a human monoclonal antibody directed against the p40 protein subunit shared by IL-12 and IL-23, in a patient suffering from psoriasis and SS has been recorded on both the cutaneous and joint component (240). However, the inhibition of IL-17 achieved by systemic administration of Secukinumab did not affect the severity of the dry eye (241). Given the pilot role of the IL-23/IL-17 axis in SS pathogenesis, further investigations are needed to confirm the selective blockade of Th17-associated cytokines as a potential therapeutic target.
Systemic Lupus Erythematosus (SLE)
Systemic lupus erythematosus (SLE) is a prototypic systemic autoimmune disease with multiple immunological abnormalities including dysregulation of both T and B lymphocytes and production of autoreactive antibodies directed toward nuclear self-antigens, with immune complex formation and tissue damage (242). A wide number of cytokines is involved in disease pathogenesis. Recently, the role of the IL-23/IL-17 axis has emerged in SLE and has been investigated either in humans or mice. Higher serum levels of IL-17 and IL-23 as well as increased number of Th17 cells has been demonstrated in patients with SLE compared with healthy controls (243, 244). Moreover, T cells that express IL-17 were found within infiltrates in kidney biopsies of patients with active lupus nephritis and significant proportion of these cells are double negative (DN) T cells, which are also expanded in the peripheral blood of patients with SLE, produce significant amounts of IL-17 and contribute to the disease pathogenesis (245). In a murine model of lupus, Zhang et al. detected DN T cells expressing high levels of IL-17A in the kidneys of mice with active nephritis. Interestingly, the lymphocytes isolated from these lupus-prone mice progressively express higher levels of IL-23 receptor as their disease worsens. Treating these lymphocytes in vitro with IL-23, they induce nephritis when transferred to non-autoimmune, control mice. Additionally, the kidneys of these recipient affected mice showed significant Ig and complement deposition in the glomeruli. This finding suggests that IL-23 also promoted an autoimmune B cell response. These data added to previous evidence indicate that DN T cells provide excessive help to B cells, resulting in abnormal production of pathogenic autoantibodies in SLE (246).
Consistently with previous findings, Chen et al. found that circulating Th17 frequency correlated with SLE activity, in particular with Systemic Lupus Erythematosus Disease Activity Index (SLEDAI) and histological activity index in 24 lupus nephritis (LN) patients (247).
The link between IL-23/IL-17 axis and LN is also supported by the observation that high serum levels of IL-23 and IL-17 at baseline predict an unfavorable histopathological response and British Isles Lupus Assessment Group (BILAG)-non-responders had high IL-23, indicating that a number of LN-patients has a Th-17 phenotype that may influence response to treatment.
In addition to LN, high serum levels of IL-23 and Th17 cells are closely related to other SLE manifestations, including vasculitis, serositis, lymphopenia, central nervous system and cutaneous involvement and the production of autoantibodies (antinucleosome antibodies, antiphospholipid antibodies, and anti-SS-B/La antibodies) (244, 248–250).
Taken together, these findings suggest that Th17 cells are a promising therapeutic target for SLE.
Previous studies have shown that Hydroxychloroquine (HCQ), an essential drug for the treatment of SLE, can inhibit Th17 cell differentiation and production (251).
In a phase two trial of 102 adult patients with active SLE, the addition to standard-of-care treatment of Ustekinumab, a human IgG1k monoclonal antibody targeting both the IL-12 and IL-23 cytokines, resulted in better efficacy in clinical and laboratory parameters than placebo. In particular, 37 (62%) of 60 patients in the Ustekinumab group and 13 (33%) of 42 patients in the placebo group achieved a SLE disease activity index 2000 responder index-4 (SRI-4) response (difference 28%, p = 0.006) (252).
Few data are available in literature about the anti-IL-17A antibody (Secukinumab). A case of 62-year-old female who presented with psoriasis vulgaris and refractory lupus nephritis successfully treated with Secukinumab was reported. Further studies are needed to test the efficacy of drugs targeting.
Conclusion
This review provides an overview of the critical role played by the IL-23/IL-17 immune axis in a wide variety of inflammatory processes, and summarizes the current knowledge on cytokine milieu that regulates IL-17-producing cells. The growing body of evidence on the relevance of this intricate pathway in autoimmunity has allowed the implementation of treatment options in pathological conditions.
However, further studies are needed to clarify the complexity of IL-17 signaling, in order to allow the discovery of new potential therapeutic targets of inflammatory processes and the availability of cutting edge therapies designed on patients not responsive to standard treatments.
Author Contributions
CS and SF: conceptualization, methodology, formal analysis, investigation, resources, data curation, writing—original draft preparation, writing—review & editing, and visualization. GGr and FC: methodology, formal analysis, data curation, and writing—review & editing. CR: software, methodology, formal analysis, data curation, and writing—review & editing. LL: formal analysis, data curation, and writing—review & editing. GGu: conceptualization, methodology, formal analysis, investigation, resources, data curation, writing—original draft preparation, writing—review & editing, visualization, supervision, and project administration. All authors had access to the study data and reviewed and approved the final manuscript.
Conflict of Interest
The authors declare that the research was conducted in the absence of any commercial or financial relationships that could be construed as a potential conflict of interest.
References
1. Tang C, Chen S, Qian H, Huang W. Interleukin-23: as a drug target for autoimmune inflammatory diseases. Immunology. (2012) 135:112–24. doi: 10.1111/j.1365-2567.2011.03522.x
2. Kleinschek MA, Muller U, Brodie SJ, Stenzel W, Kohler G, Blumenschein WM, et al. IL-23 enhances the inflammatory cell response in Cryptococcus neoformans infection and induces a cytokine pattern distinct from IL-12. J Immunol. (2006) 176:1098–6. doi: 10.4049/jimmunol.176.2.1098
3. Oppmann B, Lesley R, Blom B, Timans JC, Xu Y, Hunte B, et al. Novel p19 protein engages IL-12p40 to form a cytokine, IL-23, with biological activities similar as well as distinct from IL-12. Immunity. (2000) 13:715–25. doi: 10.1016/S1074-7613(00)00070-4
4. McKenzie BS, Kastelein RA, Cua DJ. Understanding the IL-23/IL-17 immune pathway. Trends Immunol. (2006) 27:17–23. doi: 10.1016/j.it.2005.10.003
5. Goldberg M, Nadiv O, Luknar-Gabor N, Agar G, Beer Y, Katz Y. Synergism between tumor necrosis factor a and interleukin-17 to induce IL-23 p19 expression in fibroblast- like synoviocytes. Mol Immunol. (2009) 46:1854–9. doi: 10.1016/j.molimm.2009.01.004
6. Brentano F, Ospelt C, Stanczyk J, Gay RE, Gay S, Kyburz D. Abundant expression of the interleukin (IL)23 subunit p19, but low levels of bioactive IL23 in the rheumatoid synovium: differential expression and Toll-like receptor-(TLR) dependent regulation of the IL23 subunits, p19 and p40, in rheumatoid arthritis. Ann Rheum Dis. (2009) 68:143–50. doi: 10.1136/ard.2007.082081
7. Curtis MM, Way SS. Interleukin-17 in host defence against bacterial, mycobacterial and fungal pathogens. Immunology. (2009) 126:177–85. doi: 10.1111/j.1365-2567.2008.03017.x
8. Cua DJ, Sherlock J, Chen Y, Murphy CA, Joyce B, Seymour B, et al. Interleukin-23 rather than interleukin-12 is the critical cytokine for autoimmune inflammation of the brain. Nature. (2003) 421:744–8. doi: 10.1038/nature01355
9. Langrish CL, Chen Y, Blumenschein WM, Mattson J, Basham B, Sedgwick JD, et al. IL-23 drives a pathogenic T cell population that induces autoimmune inflammation. J Exp Med. (2005) 201:233–40. doi: 10.1084/jem.20041257
10. Kopp T, Lenz P, Bello-Fernandez C, Kastelein RA, Kupper TS, Stingl G. IL-23 production by cosecretion of endogenous p19 and transgenic p40 in keratin 14/p40 transgenic mice: evidence for enhanced cutaneous immunity. J Immunol. (2003) 170:5438–44. doi: 10.4049/jimmunol.170.11.5438
11. Belladonna ML, Renauld JC, Bianchi R, Vacca C, Fallarino F, Orabona C, et al. IL-23 and IL-12 have overlapping, but distinct, effects on murine dendritic cells. J Immunol. (2002) 168:5448–54. doi: 10.4049/jimmunol.168.11.5448
12. Bastos KR, de Deus Vieira de Moraes L, Zago CA, Marinho CR, Russo M, Alvarez JM, et al. Analysis of the activation profile of dendritic cells derived from the bone marrow of interleukin-12/interleukin-23-deficient mice. Immunology. (2005) 114:499–506. doi: 10.1111/j.1365-2567.2005.02118.x
13. Goriely S, Goldman M. The interleukin-12 family: new players in transplantation immunity? Am J Transplant. (2007) 7:278–84. doi: 10.1111/j.1600-6143.2006.01651.x
14. Goriely S, Neurath MF, Goldman M. How microorganisms tip the balance between interleukin-12 family members. Nat Rev Immunol. (2008) 8:81–6. doi: 10.1038/nri2225
15. Ferber IA, Brocke S, Taylor-Edwards C, Ridgway W, Dinisco C, Steinman L, Dalton D, et al. Mice with a disrupted IFN-c gene are susceptible to the induction of experimental autoimmune encephalomyelitis (EAE). J Immunol. (1996) 156:5–7.
16. Vermeire K, Heremans H, Vandeputte M, Huang S, Billiau A, Matthys P. Accelerated collagen-induced arthritis in IFN-c receptor-deficient mice. J Immunol. (1997) 158:5507–13.
17. Manoury-Schwartz B, Chiocchia G, Bessis N, Abehsira-Amar O, Batteux F, Muller S, et al. High susceptibility to collagen induced arthritis in mice lacking IFN-c receptors. J Immunol. (1997) 158:5501–6.
18. Schroder K, Hertzog PJ, Ravasi T, Hume DA. Interferon-c: an overview of signals,mechanisms and functions. J Leukoc Biol. (2004) 75:163–89. doi: 10.1189/jlb.0603252
19. Teng MWL, Bowman EP, McElwee JJ, Smyth MJ, Casanova JL, Cooper AM, et al. IL-12 and IL-23 cytokines: from discovery to targeted therapies for immune-mediated inflammatory diseases. Nat Med. (2015) 21:719–29. doi: 10.1038/nm.3895
20. Hayashi F, Yanagawa Y, Onoe K, Iwabuchi K. Dendritic cell differentiation with prostaglandin E results in selective attenuation of the extracellular signal-related kinase pathway and decreased interleukin-23 production. Immunology. (2010) 131:67–76. doi: 10.1111/j.1365-2567.2010.03275.x
21. Chizzolini C, Chicheportiche R, Alvarez M, de Rham C, Roux-Lombard P, Ferrari-Lacraz S, et al. Prostaglandin E2 synergistically with interleukin-23 favors human Th17 expansion. Blood. (2008) 112:3696–703. doi: 10.1182/blood-2008-05-155408
22. Langrish CL, McKenzie BS, Wilson NJ, de Waal Malefyt R, Kastelein RA, Cua DJ. IL-12 and IL-23: master regulators of innate and adaptive immunity. Immunol Rev. (2004) 202:96–105 doi: 10.1111/j.0105-2896.2004.00214.x
23. Trinchieri G. Interleukin-12 and the regulation of innate resistance and adaptive immunity. Nat Rev Immunol. (2003) 3:133–46. doi: 10.1038/nri1001
24. Kreymborg K, Etzensperger R, Dumoutier L, Haak S, Rebollo A, Buch T, et al. IL-22 is expressed by Th17 cells in an IL-23-dependent fashion, but not required for the development of autoimmune encephalomyelitis. J. Immunol. (2007) 179:8098–104. doi: 10.4049/jimmunol.179.12.8098
25. Meeks KD, Sieve AN, Kolls JK, Ghilardi N, Berg RE. IL-23 is required for protection against systemic infection with Listeria monocytogenes. J. Immunol. (2009) 183:8026–34. doi: 10.4049/jimmunol.0901588
26. Riol-Blanco L, Lazarevic V, Awasthi A, Mitsdoerffer M, Wilson BS, Croxford A, et al. IL-23 receptor regulates unconventional IL-17-producing T cells that control bacterial infections. J. Immunol. (2010) 184:1710–20. doi: 10.4049/jimmunol.0902796
27. Van Belle AB, de Heusch M, Lemaire MM, Hendrickx E, Warnier G, Dunussi-Joannopoulos K, et al. IL-22 is required for imiquimod-induced psoriasiform skin inflammation in mice. J. Immunol. (2012) 188:462–9. doi: 10.4049/jimmunol.1102224
28. Wakita D, Sumida K, Iwakura Y, Nishikawa H, Ohkuri T, Chamoto K, et al. Tumor-infiltrating IL-17-producing gammadelta T cells support the progression of tumor by promoting angiogenesis. Eur J Immunol. (2010) 40:1927–37. doi: 10.1002/eji.200940157
29. Sutton CE, Lalor SJ, Sweeney CM, Brereton CF, Lavelle EC, Mills KH. Interleukin-1 and IL-23 induce innate IL-17 production from gammadelta T cells, amplifying Th17 responses and autoimmunity. Immunity. (2009) 31:331–41. doi: 10.1016/j.immuni.2009.08.001
30. Petermann F, Rothhammer V, Claussen MC, Haas JD, Blanco LR, Heink S, Prinz I, et al. Gammadelta T cells enhance autoimmunity by restraining regulatory T cell responses via an interleukin-23-dependent mechanism. Immunity. (2010) 33:351–63. doi: 10.1016/j.immuni.2010.08.013
31. Parham C, Chirica M, Timans J, Vaisberg E, Travis M, Cheung J, et al. A receptor for the heterodimeric cytokine IL-23 is composed of IL-12Rbeta1 a novel cytokine receptor subunit, IL-23RJ. Immunol. (2002) 168:5699–708. doi: 10.4049/jimmunol.168.11.5699
32. Zhang GX, Yu S, Gran B, Li J, Siglienti I, Chen X, et al. Role of IL-12 receptor beta 1 in regulation of T cell response by APC in experimental autoimmune encephalomyelitis. J. Immunol. (2003) 171:4485–92. doi: 10.4049/jimmunol.171.9.4485
33. Pahan K, Jana M. Induction of lymphotoxin-a by interleukin-12 p40 homodimer, the so-called biologically inactive molecule, but not IL-12 p70. Immunology. (2009) 127:312–25. doi: 10.1111/j.1365-2567.2008.02985.x
34. Tato CM, Cua DJ. Reconciling id, ego, and superego within interleukin-23. Immunol Rev. (2008) 226:103–11. doi: 10.1111/j.1600-065X.2008.00715.x
35. Kastelein RA, Hunter CA, Cua DJ. Discovery and biology of IL-23 and IL-27: related but functionally distinct regulators of inflammation. Annu Rev Immunol. (2007) 25:221–42. doi: 10.1146/annurev.immunol.22.012703.104758
36. Zou J, Presky DH, Wu CY, Gubler U. Differential associations between the cytoplasmic regions of the interleukin-12 receptor subunits beta1 and beta2 and JAK kinases. J Biol Chem. (1997) 272:6073–7. doi: 10.1074/jbc.272.9.6073
37. Singh RP, Hasan S, Sharma S, Nagra S, Yamaguchi DT, Wong DT, et al. Th17 cells in inflammation and autoimmunity. Autoimmun Rev. (2014) 13:1174–81. doi: 10.1016/j.autrev.2014.08.019
38. Ghilardi N, Kljavin N, Chen Q, Lucas S, Gurney AL, De Sauvage FJ. Compromised humoral and delayed-type hypersensitivity responses in IL-23-deficient mice. J Immunol. (2004) 172:2827–33. doi: 10.4049/jimmunol.172.5.2827
39. Kikly K, Liu L, Na S, Sedgwick JD. The IL-23/Th(17) axis: therapeutic targets for autoimmune inflammation. Curr Opin Immunol. (2006) 18:670–5. doi: 10.1016/j.coi.2006.09.008
40. McGeachy MJ, Chen Y, Tato C M, Laurence A, Joyce-Shaikh B, et al. Interleukin 23 receptor is essential for terminal differentiation of effector T helper type 17 cells in vivo. Nat Immunol. (2009) 10:314–24. doi: 10.1038/ni.1698
41. Weaver CT, Hatton RD, Mangan PR, Harrington LE. IL-17 family cytokines and the expanding diversity of effector T cell lineages. Annu Rev Immunol. (2007) 25:821–52. doi: 10.1146/annurev.immunol.25.022106.141557
42. Gu C, Wu L, Li X. IL-17 family: cytokines, receptors and signaling. Cytokine. (2013) 64:477–85. doi: 10.1016/j.cyto.2013.07.022
43. Shin HC, Benbernou N, Esnault S, Guenounou M. Expression of IL-17 in human memory CD45RO+ T lymphocytes and its regulation by protein kinase A pathway. Cytokine. (1999) 11:257–66. doi: 10.1006/cyto.1998.0433
44. Roark CL, Simonian PL, Fontenot AP, Born WK, O'Brien RL. gammadelta T cells: an important source of IL-17. Curr Opin Immunol. (2008) 20:353–7. doi: 10.1016/j.coi.2008.03.006
45. Rachitskaya AV, Hansen AM, Horai R, Li Z, Villasmil R, Luger D, et al. Cutting edge: NKT cells constitutively express IL-23 receptor and RORgammat and rapidly produce IL-17 upon receptor ligation in an IL-6-independent fashion. J Immunol. (2008) 180:5167–71. doi: 10.4049/jimmunol.180.8.5167
46. Aggarwal S, Ghilardi N, Xie MH, de Sauvage FJ, Gurney AL. Interleukin-23 promotes a distinct CD4 T cell activation state characterized by the production of interleukin-17. J. Biol. Chem. (2003) 278:1910–14. doi: 10.1074/jbc.M207577200
47. Awasthi A, Riol-Blanco L, Jager A, Korn T, Pot C, Galileos G, et al. Cutting edge: IL-23 receptor gfp reporter mice reveal distinct populations of IL-17-producing cells. J. Immunol. (2009) 182:5904–8. doi: 10.4049/jimmunol.0900732
48. Nurieva R, Yang XO, Martinez G, Zhang Y, Panopoulos AD, Ma L, et al. Essential autocrine regulation by IL-21 in the generation of inflammatory T cells. Nature. (2007) 448:480–3. doi: 10.1038/nature05969
49. Zhou L, Ivanov II, Spolski R, Min R, Shenderov K, Egawa T, et al. IL-6 programs T(H)-17 cell differentiation by promoting sequential engagement of the IL-21 and IL-23 pathways. Nat. Immunol. (2007) 8:967–74. doi: 10.1038/ni1488
50. Bettelli E, Carrier Y, Gao W, Korn T, Strom TB, Oukka M, Weiner HL, et al. Reciprocal developmental pathways for the generation of pathogenic effector TH17 and regulatory T cells. Nature. (2006) 441:235–8. doi: 10.1038/nature04753
51. Veldhoen M, Hocking RJ, Atkins CJ, Locksley RM, Stockinger B. TGFbeta in the context of an inflammatory cytokine milieu supports de novo differentiation of IL-17-producing T cells. Immunity. (2006) 24:179–89. doi: 10.1016/j.immuni.2006.01.001
52. McGeachy MJ, Bak-Jensen KS, Chen Y, Tato CM, Blumenschein W, McClanahan T, et al. TGF-beta and IL-6 drive the production of IL-17 and IL-10 by T cells and restrain T(H)-17 cell-mediated pathology. Nat Immunol. (2007) 8:1390–7. doi: 10.1038/ni1539
53. Mangan PR, Harrington LE, O'Quinn DB, Helms WS, Bullard DC, Elson CO, Hatton RD, et al. Transforming growth factor-beta induces development of the T(H)17 lineage. Nature. (2006) 441:231–4. doi: 10.1038/nature04754
54. Chen Z, Tato CM, Muul L, Laurence A, O'Shea JJ. Distinct regulation of interleukin-17 in human T helper lymphocytes. Arthritis Rheum. (2007) 56:2936–46. doi: 10.1002/art.22866
55. Wilson NJ, Boniface K, Chan JR, McKenzie BS, Blumenschein WM, Mattson JD, et al. Development, cytokine profile and function of human interleukin 17-producing helper T cells. Nat Immunol. (2007) 8:950–7. doi: 10.1038/ni1497
56. Acosta-Rodriguez EV, Napolitani G, Lanzavecchia A, Sallusto F. Interleukins 1beta and 6 but not transforming growth factor-beta are essential for the differentiation of interleukin 17-producing human T helper cells. Nat Immunol. (2007) 8:942–9. doi: 10.1038/ni1496
57. Morishima N, Mizoguchi I, Takeda K, Mizuguchi J, Yoshimoto T. TGF-beta is necessary for induction of IL-23R and Th17 differentiation by IL-6 and IL-23. Biochem Biophys Res Commun. (2009) 386:105–10. doi: 10.1016/j.bbrc.2009.05.140
58. Korn T, Bettelli E, Gao W, Awasthi A, Jager A, Strom TB, et al. IL-21 initiates an alternative pathway to induce proinflammatory T(H)17 cells. Nature. (2007) 448:484–7. doi: 10.1038/nature05970
59. Huber M, Brustle A, Reinhard K, Guralnik A, Walter G, Mahiny A, von Low E, et al. IRF4 is essential for IL-21-mediated induction, amplification, and stabilization of the Th17 phenotype. Proc Natl Acad Sci USA. (2008) 105:20846–51. doi: 10.1073/pnas.0809077106
60. Wei L, Laurence A, Elias KM, O'Shea JJ. IL-21 is produced by Th17 cells and drives IL-17 production in a STAT3-dependent manner. J. Biol. Chem. (2007) 282:34605–10. doi: 10.1074/jbc.M705100200
61. Ivanov II, Zhou L, Littman DR. Transcriptional regulation of Th17 cell differentiation. Semin Immunol. (2007) 19:409–17. doi: 10.1016/j.smim.2007.10.011
62. Manel N, Unutmaz D, Littman DR. The differentiation of human T(H)- 17 cells requires transforming growth factor-beta and induction of the nuclear receptor RORgammat. Nat Immunol. (2008) 9:641–9. doi: 10.1038/ni.1610
63. Harrington LE, Hatton RD, Mangan PR, Turner H, Murphy TL, Murphy KM, et al. Interleukin 17-producing CD4+ effector T cells develop via a lineage distinct from the T helper type 1 and 2 lineages. Nat Immunol. (2005) 6:1123–32. doi: 10.1038/ni1254
64. Park H, Li Z, Yang XO, Chang SH, Nurieva R, Wang YH, et al. A distinct lineage of CD4 T cells regulates tissue inflammation by producing interleukin 17. Nat Immunol. (2005) 6:1133–4. doi: 10.1038/ni1261
65. Angkasekwinai P, Park H, Wang YH, Chang SH, Corry DB, Liu YJ, et al. Interleukin 25 promotes the initiation of proallergic type 2 responses. J Exp Med. (2007) 204:1509–17. doi: 10.1084/jem.20061675
66. Batten M, Li J, Yi S, Kljavin NM, Danilenko DM, Lucas S, et al. Interleukin 27 limits autoimmune encephalomyelitis by suppressing the development of interleukin 17-producing T cells. Nat Immunol. (2006) 7:929–36. doi: 10.1038/ni1375
67. Stumhofer JS, Laurence A, Wilson EH, Huang E, Tato CM, Johnson LM, et al. Interleukin 27 negatively regulates the development of interleukin 17-producing T helper cells during chronic inflammation of the central nervous system. Nat Immunol. (2006) 7:937–45. doi: 10.1038/ni1376
68. Patel DD, Kuchroo VK. Th17 cell pathway in human immunity: lessons from genetics and therapeutic interventions. Immunity. (2015) 43:1040–51. doi: 10.1016/j.immuni.2015.12.003
69. Shalom-Barak T, Quach J, Lotz M. Interleukin-17-induced gene expression in articular chondrocytes is associated with activation of mitogen-activated protein kinases and NF-kappaB. J Biol Chem. (1998) 273:27467–73. doi: 10.1074/jbc.273.42.27467
70. Adami S, Cavani A, Rossi F, Girolomoni G. The role of interleukin-17A in psoriatic disease. BioDrugs. (2014) 28:487–97. doi: 10.1007/s40259-014-0098-x
71. Laan M, Cui ZH, Hoshino H, Lötvall J, Sjöstrand M, Gruenert D. C, et al. Neutrophil recruitment by human IL-17 via C-X-C chemokine release in the airways. J Immunol. (1999) 162:2347–52.
72. Happel KI, Zheng M, Young E, Quinton LJ, Lockhart E, Ramsay AJ, et al. Cutting edge: roles of Toll-like receptor 4 and IL-23 in IL-17 expression in response to Klebsiella pneumoniae infection. J Immunol. (2003) 170:4432–6. doi: 10.4049/jimmunol.170.9.4432
73. Happel KI, Dubin PJ, Zheng M, Ghilardi N, Lockhart C, Quinton LJ, et al. Divergent roles of IL-23 and IL-12 in host defense against Klebsiella pneumoniae. J Exp Med. (2005) 202:761–9. doi: 10.1084/jem.20050193
74. Meller S, Di Domizio J, Voo KS, Friedrich HC, Chamilos G, Ganguly D, et al. T(H)17 cells promote microbial killing and innate immune sensing of DNA via interleukin 26. Nat Immunol. (2015) 16:970–9. doi: 10.1038/ni.3211
75. Conti HR, Gaffen SL. IL-17-mediated immunity to the opportunistic fungal pathogen candida albicans. J Immunol. (2015) 195:780–8. doi: 10.4049/jimmunol.1500909
77. Greenspan D, Greenspan JS. Oral mucosal manifestations of AIDS. Dermatol. Clin. (1987) 5:733–737. doi: 10.1016/S0733-8635(18)30717-4
78. Acosta-Rodriguez EV, Rivino L, Geginat J, Jarrossay D, Gattorno M, Lanzavecchia A, et al. Surface phenotype and antigenic specificity of human interleukin 17-producing T helper memory cells. Nat Immunol. (2007) 8:639–46. doi: 10.1038/ni1467
79. Zielinski CE, Mele F, Aschenbrenner D, Jarrossay D, Ronchi F, Gattorno M, et al. Pathogen-induced human TH17 cells produce IFN-γ or IL-10 and are regulated by IL-1β. Nature. (2012) 484:514–8. doi: 10.1038/nature10957
80. Huang W, Na L, Fidel PL, Schwarzenberger P. Requirement of interleukin-17A for systemic anti-Candida albicans host defense in mice. J Infect Dis. (2004) 190:624–31. doi: 10.1086/422329
81. Conti HR, Shen F, Nayyar N, Stocum E, Sun JN, Lindemann MJ, et al. Th17 cells and IL-17 receptor signaling are essential for mucosal host defense against oral candidiasis. J Exp Med. (2009) 206:299–311. doi: 10.1084/jem.20081463
82. Ferreira MC, Whibley N, Mamo AJ, Siebenlist U, Chan YR, Gaffen SL. Interleukin-17-induced protein lipocalin 2 is dispensable for immunity to oral candidiasis. Infect Immun. (2014) 82:1030–5. doi: 10.1128/IAI.01389-13
83. Gaffen SL, Jain R, Garg AV, Cua DJ. The IL-23-IL-17 immune axis: from mechanisms to therapeutic testing. Nat Rev Immunol. (2014) 14:585–600. doi: 10.1038/nri3707
84. Starnes T, Broxmeyer HE, Robertson MJ, Hromas R. Cutting edge: IL−17 D, a novel member of the IL-17 family, stimulates cytokine production and inhibits hemopoiesis. J Immunol. (2002) 169:642–6. doi: 10.4049/jimmunol.169.2.642
85. Dragon S, Saffar AS, Shan L, Gounni AS. IL-17 attenuates the anti-apoptotic effects of GM-CSF in human neutrophils. Mol Immunol. (2008) 45:160–8. doi: 10.1016/j.molimm.2007.04.027
86. Numasaki M, Fukushi J, Ono M, Narula SK, Zavodny PJ, Kudo T, et al. Interleukin-17 promotes angiogenesis and tumor growth. Blood. (2003) 101:2620–7 doi: 10.1182/blood-2002-05-1461
87. Jovanovic DV, Martel-Pelletier J, Di Battista JA, Mineau F, Jolicoeur F. C, Benderdour M, et al. Stimulation of 92-kd gelatinase (matrix metalloproteinase 9) production by interleukin-17 in human monocyte/macrophages: a possible role in rheumatoid arthritis. Arthritis Rheum. (2000) 43:1134–44. doi: 10.1002/1529-0131(200005)43:5<1134::AID-ANR24>3.0.CO;2-#
88. Ruddy MJ, Wong GC, Liu XK, Yamamoto H, Kasayama S, Kirkwood KL, et al. Functional cooperation between interleukin-17 and tumor necrosis factor-alpha is mediated by CCAAT/enhancer-binding protein family members. J Biol Chem. (2004) 279:2559–2567. doi: 10.1074/jbc.M308809200
89. Kotake S, Udagawa N, Takahashi N, Matsuzaki K, Itoh K, Ishiyama S, et al. IL-17 in synovial fluids from patients with rheumatoid arthritis is a potent stimulator of osteoclastogenesis. J Clin Invest. (1999) 103:1345–52. doi: 10.1172/JCI5703
90. Matusevicius D, Kivisakk P, He B, Kostulas N, Ozenci V, Fredrikson S, et al. Interleukin-17 mRNA expression in blood and CSF mononuclear cells is augmented in multiple sclerosis. Mult Scler. (1999) 5:101–4. doi: 10.1177/135245859900500206
91. Lock C, Hermans G, Pedotti R, Brendolan A, Schadt E, Garren H, et al. Gene-microarray analysis of multiple sclerosis lesions yields new targets validated in autoimmune encephalomyelitis. Nat Med. (2002) 8:500–8. doi: 10.1038/nm0502-500
92. Fujino S, Andoh A, Bamba S, Ogawa A, Hata K, Araki Y, et al. Increased expression of interleukin 17 in inflammatory bowel disease. Gut. (2003) 52:65–70. doi: 10.1136/gut.52.1.65
93. Taurog JD, Chhabra A, Colbert A. Ankylosing Spondylitis and Axial Spondyloarthritis. New Engl J Med. (2016) 374:2563–74. doi: 10.1056/NEJMra1406182
94. Rudwaleit M, Van der Heijde D, Landewé R, Listing J, Akkoc N, Brandt J, et al. The development of Assessment of SpondyloArthritis international Society classification criteria for axial spondyloarthritis (part II): validation and final selection. Ann Rheum Dis. (2009) 68:777–83. doi: 10.1136/ard.2009.108233
95. Van der Heijde D, Ramiro S, Landewé R, Baraliakos X, Van den Bosch F, Sepriano A, et al. 2016 Update of the ASAS-EULAR management recommendations for axial spondyloarthritis. Ann Rheum Dis. (2017) 76:978–91. doi: 10.1136/annrheumdis-2016-210770
96. Zhu W, He X, Cheng K, Zhang L, Di Chen Wang X, et al. Ankylosing spondylitis: etiology, pathogenesis, and treatments. Bone Res. (2019) 7:22. doi: 10.1038/s41413-019-0057-8
97. Brown MA, Kennedy LG, MacGregor AJ, Darke C, Duncan E, Shatford JL, et al. Susceptibility to ankylosing spondylitis in twins: the role of genes, HLA, and the environment. Arthritis Rheum. (1997) 40:1823–8. doi: 10.1002/art.1780401015
98. Chen B, Li J, He C, Li D, Tong W, Zou Y, et al. Role of HLA-B27 in the pathogenesis of ankylosing spondylitis. Mol Med Rep. (2017) 15:1943–51. doi: 10.3892/mmr.2017.6248
99. Brown MA, Kenna T, Wordsworth BP. Genetics of ankylosing spondylitis-insights into pathogenesis. Nat Rev Rheumatol. (2016) 12:81–91. doi: 10.1038/nrrheum.2015.133
100. Colbert RA, Tran MT, Layh-Schmitt G. HLA-B27 misfolding and ankylosing spondylitis. Mol Immunol. (2014) 57:44–51. doi: 10.1016/j.molimm.2013.07.013
101. Watad A, Bridgewood C, Russell T, Marzo-Ortega H, Cuthbert R, McGonagle D. The early phases of ankylosing spondylitis: emerging insights from clinical and basic science. Front Immunol. (2018) 9:2668. doi: 10.3389/fimmu.2018.02668
102. DeLay ML, Turner JM, Klenk EI, Smith JA, Sowders DP, Colbert RA. HLA-B27 misfolding and the unfolded protein response augment interleukin-23 production and are associated with Th17 activation in transgenic rats. Arthritis Rheum. (2009) 60:2633–43. doi: 10.1002/art.24763
103. Turner MJ, Sowders DP, DeLay ML, Mohapatra R, Bai S, Smith JA, et al. HLA-B27 misfolding in transgenic rats is associated with activation of the unfolded protein response. J Immunol. (2005) 175:2438–48. doi: 10.4049/jimmunol.175.4.2438
104. Chan AT, Kollnberger SD, Wedderburn LR, Bowness P. Expansion and enhanced survival of natural killer cells expressing the killer immunoglobulin-like receptor KIR3DL2 in spondylarthritis. Arthritis and Rhum. (2005) 54:3586–95. doi: 10.1002/art.21395
105. International Genetics of Ankylosing Spondylitis Consortium (IGAS) Australo-Anglo-American Spondyloarthritis Consortium (TASC); Groupe Française d'Etude Génétique des Spondylarthrites (GFEGS); Nord-Trøndelag Health Study (HUNT); Spondyloarthritis Research Consortium of Canada (SPARCC); Wellcome Trust Case Control Consortium 2 (WTCCC2). Identification of multiple risk variants for ankylosing spondylitis through high-density genotyping of immune-related loci. Nat Genet. (2013) 45:730–8. doi: 10.1038/ng.2667
106. Wellcome Trust Case Control Consortium Australo-Anglo-American Spondylitis Consortium (TASC) Biologics in RA Genetics and Genomics Study Syndicate (BRAGGS) Steering Committee Association scan of 14 500 nonsynonymous SNPs in four diseases identifies autoimmunity variants. Nat Genet. (2007) 39:1329–37. doi: 10.1038/ng.2007.17
107. York IA, Chang SC, Saric T, Keys JA, Favreau JM, Goldberg AL, et al. The ER aminopeptidase ERAP1 enhances or limits antigen presentation by trimming epitopes to 8–9 residues. Nat Immunol. (2002) 3:1177–84. doi: 10.1038/ni860
108. Guiliano DB, Fussell H, Lenart I, Tsao E, Nesbeth D, Fletcher AJ, et al. Endoplasmic reticulum degradation–enhancing α-mannosidase–like protein 1 targets misfolded hla–b27 dimers for endoplasmic reticulum–associated degradation. Arthritis Rheumatol. (2014) 66:2976–88. doi: 10.1002/art.38809
109. Cortes A, Pulit SL, Weisman MA, Pointon JJ, Robinson PC, Weisman MH, et al. Major histocompatibility complex associations of ankylosing spondylitis are complex and involve further epistasis with ERAP1. Nat Commun. (2015) 6:7146. doi: 10.1038/ncomms8146
110. Rahman P, Inman RD, Gladman DD, Reeve JP, Peddle L, Maksymowych WP. Association of interleukin-23 receptor variants with ankylosing spondylitis. Arthritis Rheum. (2008) 58:1020–5. doi: 10.1002/art.23389
111. Ellinghaus D, Jostins L, Spain SL, Cortes A, Bethune J, Han B, et al. Analysis of five chronic inflammatory diseases identifies 27 new associations and highlights disease-specific patterns at shared loci. Nature Genetics. (2016) 48:510–8. doi: 10.1038/ng.3528
112. Di Meglio P, Di Cesare A, Laggner U, Chu CC, Napolitano L, Villanova F, et al. The IL23R R381Q gene variant protects against immune-mediated diseases by impairing IL-23-induced Th17 effector response in humans. PLoS ONE. (2011) 6:e17160. doi: 10.1371/journal.pone.0017160
113. Mei Y, Pan F, Gao J, Ge R, Duan Z, Zeng Z, et al. Increased serum IL-17 and IL-23 in the patient with ankylosing spondylitis. Clin Rheum. (2011) 30:269–73. doi: 10.1007/s10067-010-1647-4
114. Jandus C, Jilles B, Rivals B, Rivals JP, Dudler J, Speiser D. Increased numbers of circulating polyfunctional Th17 memory cells in patients with seronegative spondylarthrities. Arthr Rheuma. (2008) 58:2307–17. doi: 10.1002/art.23655
115. Rizzo A, Guggino G, Ferrante A, Ciccia F. Role of subclinical gut inflammation in the pathogenesis of spondyloarthritis. Front Med. (2018) 1:5:63. doi: 10.3389/fmed.2018.00063
116. Van Praet L, Van den Bosch FE, Jacques P, Carron P, Jans L, Colman R, et al. Microscopic gut inflammation in axial spondyloarthritis: a multiparametric predictive model. Ann Rheum Dis. (2013). 72:414–7. doi: 10.1136/annrheumdis-2012-202135
117. Van Praet L, Jans L, Carron P, Jacques P, Glorieus R, Colman R, et al. Degree of bone marrow oedema in sacroiliac joints of patients with axial spondyloarthritis is linked to gut inflammation and male sex: results from the GIANT cohort. Ann Rheum Dis. (2014) 73:1186–9. doi: 10.1136/annrheumdis-2013-203854
118. Costello M A, Ciccia F, Dana W, Warrington W, Robinson PC, et al. Brief report: intestinal dysbiosis in ankylosing spondylitis. Arthritis Rheumatol. (2015) 67:686–91. doi: 10.1002/art.38967
119. Ciccia F, Guggino G, Rizzo A, Riccardo A, Luchetti MM, Milling S, et al. Dysbiosis and zonulin upregulation alter gut epithelial and vascular barriers in patients with ankylosing spondylitis. Ann Rheum Dis. (2017) 76:1123–32. doi: 10.1136/annrheumdis-2016-210000
120. Ciccia F, Bombardieri M, Principato A, Giardina A, Tripodo C, Porcasi R, et al. Overexpression of interleukin-23, but not interleukin-17, as an immunologic signature of subclinical intestinal inflammation in ankylosing spondylitis. Arthritis Rheum. (2009) 60:955–65. doi: 10.1002/art.24389
121. Ciccia F, Guggino G, Rizzo A, Saieva L, Peralta S, Giardina A, et al. Type 3 innate lymphoid cells producing IL-17 and IL-22 are expanded in the gut, in the peripheral blood, synovial fluid and bone marrow of patients with ankylosing spondylitis. Ann Rheum Dis. (2015) 74:1739–47. doi: 10.1136/annrheumdis-2014-206323
122. Kenna TJ, Davidson SI, Duan R, Bradbury LA, McFarlane J, Smith M, et al. Enrichment of circulating interleukin-17-secreting interleukin-23 receptor-positive γ/δ T cells in patients with active ankylosing spondylitis. Arthritis Rheum. (2012) 64:1420–9. doi: 10.1002/art.33507
123. Cuthbert RJ, Watad A, Fragkakis EM, Dunsmuir R, Loughenbury P, Khan A, et al. Evidence that tissue resident human enthesis γδT- cells can produce IL-17A independently of IL-23R transcript expression. Ann Rheum Dis. (2019) 78:1559–65. doi: 10.1136/annrheumdis-2019-215210
124. Toussirot E, Laheurte C, Gaugler B, Gabriel D, Saas P. Increased IL-22- and IL-17A-producing mucosal-associated invariant t cells in the peripheral blood of patients with ankylosing spondylitis. Front Immunol. (2018); 9:1610. doi: 10.3389/fimmu.2018.01610
125. Hayashi E, Chiba A, Tada K, Haga K, Kitagaichi M, Nakajima S, et al. Involvement of mucosal-associated invariant t cells in ankylosing spondylitis. J Rheumatology. (2016) 43:1695–703. doi: 10.3899/jrheum.151133
126. Wang C, Liao Q, Hu Y, Zhong D. T lymphocyte subset imbalances in patients contribute to ankylosing spondylitis. Chenggong Exp Therapeutic Med. (2014) 21:250–6. doi: 10.3892/etm.2014.2046
127. Chowdhury AC, Chaurasia S, Kumar S, Amita A, Misra R. IL-17 and IFN-γ producing NK and γδ-T cells are preferentially expanded in synovial fluid of patients with reactive arthritis and undifferentiated spondyloarthritis. Clin Immunol. (2017) 183:207–12. doi: 10.1016/j.clim.2017.03.016
128. Noordenbos T, Yeremenko N, Gofita I, Van de Sande M. Interleukin-17–positive mast cells contribute to synovial inflammation in spondylarthritis. Arthrt Rheum. (2011)54:99–109. doi: 10.1002/art.33396
129. N van Tok M, Na S, Lao CR, Alvi M, Pots D, Van de Sande MGH. The initiation, but not the persistence, of experimental spondyloarthritis is dependent on interleukin-23 signaling. Front Immunol. (2018) 9:1550. doi: 10.3389/fimmu.2018.01550
130. Ruiz de Morales JMG, Puig L, Daudén E, Cañete JD, Pablo JL, Olveira Martíng A. Critical role of interleukin (IL)-17 in inflammatory and immune disorders: an updated review of the evidence focusing in controversies. AutoimmRev. (2020) 19:102429. doi: 10.1016/j.autrev.2019.102429
131. Taurog JD, Maika s.D, Simmons WA, Breban M, Hammer RE. Susceptibility to inflammatory disease in HLA-B27 transgenic rat lines correlates with the level of B27 expression. J Immunol. (1993) 150:4168–78.
132. Taurog JD, Richardson JA, Croft JT, Simmons WA, Zhou M, Fernández-Sueiro LJ, et al. The germfree state prevents development of gut and joint inflammatory disease in HLA-B27 transgenic rats. J Exp Med. (1994) 180:2359–64. doi: 10.1084/jem.180.6.2359
133. Taurog JD. Animal models of spondyloarthritis. Adv Exp Med Biol. (2009) 649:245–54. doi: 10.1007/978-1-4419-0298-6_18
134. Poddubnyy D, Sieper J. treatment of axial spondyloarthritis: what does the future hold? Curr Rheumatol Rep. (2020) 22:47. doi: 10.1007/s11926-020-00924-5
135. Baeten D, Baraliakos X, Braun J, Sieper J, Emery P, Van der Heijde D, et al. Anti-interleukin-17A monoclonal antibody secukinumab in treatment of ankylosing spondylitis: a randomised, double-blind, placebo-controlled trial. Lancet. (2013) 382:1705–13. doi: 10.1016/S0140-6736(13)61134-4
136. Baeten D, Sieper J, Braun J, Baraliakos X, Dougados M, Emery P, et al. Secukinumab, an Interleukin-17A Inhibitor, in Ankylosing Spondylitis. Engl J Med. (2015) 373:2534–48. doi: 10.1056/NEJMoa1505066
137. Marzo-Ortega H, Sieper J, Kivitz A, Blanco R, Cohen M, Martin R, et al. Secukinumab and sustained improvement in signs and symptoms of patients with active ankylosing spondylitis through two years: results from a phase III study. Arthritis Care Res. (2017) 69:1020–9. doi: 10.1002/acr.23233
138. Deodhar A, Van der Heijde D, Gensler LS, Kim TH, Maksymowych W, Østergaard M, et al. Ixekizumab for patients with non-radiographic axial spondyloarthritis (COAST-X): a randomised, placebo-controlled trial. Lancet. (2020) 395:53–64. doi: 10.1016/S0140-6736(19)32971-X
139. Gaydukova I, Mazurov V, Erdes S, Dubinina T, Nesmeyanova O, Ilivanova E, et al. Netakimab reduces the disease activity of radiographic axial spondyloarthritis. Results of ASTERA study. Ann Rheum Dis. (2019) 78:193–4. doi: 10.1136/Annrheumdis-2019-eular.6633
140. Van der Heijde D, Gensler LS, Deodhar A, Baraliakos X, Poddubnyy D, Kivitz A, et al. Dual neutralisation of interleukin-17A and interleukin-17F with bimekizumab in patients with active ankylosing spondylitis: results from a 48-week phase IIb, randomised, double-blind, placebo-controlled, dose-ranging study. Ann Rheum Dis. (2020) 79:595–604. doi: 10.1136/annrheumdis-2020-216980
141. Wendling D, Verhoeven F, Prati C. Anti-IL-17 monoclonal antibodies for the treatment of ankylosing spondylitis. Taylor Francis Online. (2018) 19:55–64. doi: 10.1080/14712598.2019.1554053
142. Poddubnyy D, Hermann KGA, Callhoff J, Listing J, Sieper J. Ustekinumab for the treatment of patients with active ankylosing spondylitis: results of a 28-week, prospective, open-label, proof-of-concept study (TOPAS). Ann Rheum Dis. (2013) 73:817–23. doi: 10.1136/annrheumdis-2013-204248
143. Baeten D, Østergaard M, Cheng-Chung Wei J, Sieper J, Järvinen P, Tam LS, et al. Risankizumab, an IL-23 inhibitor, for ankylosing spondylitis: results of a randomised, double-blind, placebo-controlled, proof-of-concept, dose-finding phase 2 study. Ann Rheum Dis. (2018) 77:1295–302. doi: 10.1136/annrheumdis-2018-213328
144. Veale DJ, McGonagle D, McInnes IB, Krueger JG, Ritchlin CT, Elewaut E, et al. The rationale for Janus kinase inhibitors for the treatment of spondyloarthritis. Rheumatology. (2019) 58:197–205. doi: 10.1093/rheumatology/key070
145. Van der Heijde D, Deodhar A, Wei JC, Drescher E, Fleishaker E, Hendrikx T, et al. Tofacitinib in patients with ankylosing spondylitis: a phase II, 16-week, randomised, placebo-controlled, dose-ranging study. Ann Rheum Dis. (2016) 76:1340–7. doi: 10.1136/annrheumdis-2016-210322
146. Van der Heijde D, Baraliakos X, Lianne SG, Maksymowych W, Tseluyko V. Efficacy and safety of filgotinib, a selective Janus kinase 1 inhibitor, in patients with active ankylosing spondylitis (TORTUGA): results from a randomised, placebo-controlled, phase 2 trial Lancet. (2018) 392:2378–2387. doi: 10.1016/S0140-6736(18)32463-2
147. van der Heijde D, Song IH, Pangan AL, Deodhar A, van den Bosch F, Maksymowych WP, et al. Efficacy and safety of upadacitinib in patients with active ankylosing spondylitis (SELECT-AXIS 1): a multicentre, randomised, double-blind, placebo-controlled, phase 2/3 trial. Lancet. (2016) 394:2108–17. doi: 10.1016/S0140-6736(19)32534-6
148. Pathan E, Abraham S, Van Rossen E, Withrington R, Keat A, Charles PJ, et al. Efficacy and safety of Apremilast, an oral phosphodiesterase 4 inhibitor, in ankylosing spondylitis. Ann Rheum Dis. (2016) 72:1475–80. doi: 10.1136/annrheumdis-2012-201915
149. Mease PJ, Genovese MC, Weinblatt M, Peloso PM, Chen K, Othman AA, et al. Phase II study of ABT-122, a tumor necrosis factor– and interleukin-17a–targeted dual variable domain immunoglobulin, in patients with psoriatic arthritis with an inadequate response to methotrexate. Arthritis Rheumatol. (2018) 70:1778–89. doi: 10.1002/art.40579
151. Lowes MA, Bowcock AM, Krueger JG. Pathogenesis and therapy of psoriasis. Nature. (2007) 445:866–73. doi: 10.1038/nature05663
152. Veale DJ, Fearon U. The pathogenesis of psoriatic arthritis. Lancet. (2018) 391:2273–84. doi: 10.1016/S0140-6736(18)30830-4
153. Michel ML, Keller AC, Paget C, Fujio M, Trottein F, Savage PB, et al. Identification of an IL-17-producing NK1.1(neg) iNKT cell population involved in airway neutrophilia. J Exp Med. (2007) 204:995–1001. doi: 10.1084/jem.20061551
154. Doisne JM, Becourt C, Amniai L, Duarte N, Le Luduec JB, Eberl G, et al. Skin and peripheral lymph node invariant NKT cells are mainly retinoic acid receptor-related orphan receptor (gamma)t+ and respond preferentially under inflammatory conditions. J Immunol. (2009) 183:2142–9. doi: 10.4049/jimmunol.0901059
155. Brembilla NC, Stalder R, Senra L, Bohencke WH. IL-17A localizes in the exocytic compartment of mast cells in psoriatic skin. Br J Dermatol. (2017) 177:1458–60. doi: 10.1111/bjd.15358
156. Lin AM, Rubin CJ, Khandpur R, Wang JY, Riblett MB, Yalavarthi S, et al. Mast cells and neutrophils release IL-17 through extracellular trap formation in psoriasis. J Immunol. (2011) 187:490–500. doi: 10.4049/jimmunol.1100123
157. Di Cesare A, Di Meglio P, Nestle FO. The IL-23/Th17 axis in the immunopathogenesis of psoriasis. J Invest Dermatol. (2009) 129:1339–50. doi: 10.1038/jid.2009.59
158. Lowes MA, Suarez-Farinas M, Krueger JG. Immunology of psoriasis. Annu Rev Immunol. (2014) 32:227–55. doi: 10.1146/annurev-immunol-032713-120225
159. Blauvelt A, Chiricozzi A. The immunologic Role of IL-17 in psoriasis and psoriatic arthritis pathogenesis. Clin Rev Allergy Immunol. (2018) 55:379–90. doi: 10.1007/s12016-018-8702-3
160. Lee E, Trepicchio WL, Oestreicher JL, Pittman D, Wang F, Chamian F, et al. Increased expression of interleukin 23 p19 and p40 in lesional skin of patients with psoriasis vulgaris. J Exp Med. (2004) 199:125–30. doi: 10.1084/jem.20030451
161. Melis L, Vandooren B, Kruithof E, Jacques P, De Vos M, Mielants H, et al. Systemic levels of IL-23 are strongly associated with disease activity in rheumatoid arthritis but not spondyloarthritis. Ann Rheum Dis. (2010) 69:618–23. doi: 10.1136/ard.2009.107649
162. Van Baarsen LG, Lebre MC, van der Coelen D, Aarrass S, Tang MW, Ramwadhdoebe TH, et al. Heterogeneous expression pattern of interleukin 17A (IL-17A), IL-17F and their receptors in synovium of rheumatoid arthritis, psoriatic arthritis and oste-oarthritis: possible explanation for nonresponse to anti-IL-17 therapy? Arthritis Res Ther. (2014) 16:426. doi: 10.1186/s13075-014-0426-z
163. Tsukazaki H, Kaito T. The Role of the IL-23/IL-17 pathway in the pathogenesis of spondyloarthritis. Int J Mol Sci. (2020) 3:6401. doi: 10.3390/ijms21176401
164. Boutet MA, Nerviani A, Gallo Afflitto G, Pitzalis C. Role of the IL-23/IL-17 axis in psoriasis and psoriatic arthritis: the clinical importance of its divergence in skin and joints. Int J Mol Sci. (2018) 19:530. doi: 10.3390/ijms19020530
165. Veale D, Yanni G, Rogers S, Barnes L, Bresnihan B, FitzGerald O. Reduced synovial membrane macrophage numbers, ELAM-1 expression, and lining layer hyperplasia in psoriatic arthritis as compared with rheumatoid arthritis. Arthr Rheum. (1993) 36:893–900. doi: 10.1002/art.1780360705
166. Canete JD, Santiago B, Cantaert T, Sanmartí R, Palacin A, Celis R, et al. Ectopic lymphoid neogenesis in psoriatic arthritis. Ann Rheum Dis. (2007) 66:720–6. doi: 10.1136/ard.2006.062042
167. Dolcino M, Lunardi C, Ottria A, Tinazzi E, Patuzzo G, Puccetti A. Crossreactive autoantibodies directed against cutaneous and joint antigens are present in psoriatic arthritis. PLoS ONE. (2014) 6:e115424. doi: 10.1371/journal.pone.0115424
168. Mori G, Cantatore FP, Brunetti G, Oranger A, Colaianni G, Quarta L, et al. Synovial fluid fibroblasts and lymphocytes support the osteoclastogenesis in human psoriatic arthritis. Ann N Y Acad Sci. (2007) 1117:159–64. doi: 10.1196/annals.1402.013
169. Gottlieb A, Korman NJ, Gordon KB, Feldman SR, Lebwohl M, Koo JY.M, et al. Guidelines of care for the management of psoriasis and psoriatic arthritis. Section 2. Psoriatic arthritis: overview and guidelines of care for treatment with an emphasis on the biologics. J Am Acad Dermatol. (2008) 58:851–64. doi: 10.1016/j.jaad.2008.02.040
170. Pacifici R. The role of IL-17 TH17 cells in the bone catabolic activity of PTH. Front Immunol. (2016) 7:57. doi: 10.3389/fimmu.2016.00057
171. Zaba LC, Fuentes-Duculan J, Eungdamrong NJ, Abello MV, Novitskaya I, Pierson KC, et al. Psoriasis is characterized by accumulation of immunostimulatory and Th1/Th17 cell-polarizing myeloid dendritic cells. J Invest Dermatol. (2009) 129:79–88. doi: 10.1038/jid.2008.194
172. Teunissen MB, Munneke JM, Bernink JH, Spuls PI, Res PC, Te Velde A, et al. Composition of innate lymphoid cell subsets in the human skin: enrichment of NCR(+) ILC3 in lesional skin and blood of psoriasis patients. J Invest Dermatol. (2014) 134:2351–60. doi: 10.1038/jid.2014.146
173. Villanova F, Flutter B, Tosi I, Grys K, Sreeneebus H, Perera GK, et al. Characterization of innate lymphoid cells in human skin and blood demonstrates increase of NKp44+ ILC3 in psoriasis. J Invest Dermatol. (2014) 134:984–91. doi: 10.1038/jid.2013.477
174. Cai Y, Shen X, Ding C, Qi C, Li K, Li X, et al. Pivotal role of dermal IL-17-producing gammadelta T cells in skin inflammation. Immunity. (2011) 35:596–610. doi: 10.1016/j.immuni.2011.08.001
175. Sherlock JP, Joyce-Shaikh B, Turner SP, Chao CC, Sathe M, Grein J, et al. IL-23 induces spondyloarthropathy by acting on ROR-gammat + CD3 + CD4-CD8- entheseal resident T cells. Nat Med. (2012) 18:1069–76. doi: 10.1038/nm.2817
176. Benham H, Norris P, Goodall J, Wechalekar MD, FitzGerald O, Szentpetery A, et al. Th17 and Th22 cells in psoriatic arthritis and psoriasis. Arthritis Res Ther. (2013) 15:R136. doi: 10.1186/ar4317
177. Fitch E, Harper E, Skorcheva I, E Kurtz S, Blauvelt A. Pathophysiology of psoriasis: recent advances on IL-23 and Th17 cytokines. Curr Rheumatol Rep. (2007) 9:461–7. doi: 10.1007/s11926-007-0075-1
178. Liang SC, Tan XY, Luxenberg DP, Karim R, Dunussi-Joannopoulos K, Collins M, et al. Interleukin (IL)-22 and IL-17 are coexpressed by Th17 cells and cooperatively enhance expression of antimicrobial peptides. J Exp Med. (2006) 203:2271–9. doi: 10.1084/jem.20061308
179. Koga C, Kabashima K, Shiraishi N, Kobayashi M, Tokura Y. Possible pathogenic role of Th17 cells for atopic dermatitis. J Invest Dermatol. (2008) 128:2625–30. doi: 10.1038/jid.2008.111
180. Wolk K, Kunz S, Witte E, Friedrich M, Asadullah K, Sabat R. IL-22 increases the innate immunity of tissues. Immunity. (2004) 21:241–54. doi: 10.1016/j.immuni.2004.07.007
181. Wolk K, Witte E, Wallace E, Döcke WD, Kunz S, Asadullah K, et al. IL-22 regulates the expression of genes responsible for antimicrobial defense, cellular differentiation, and mobility in keratinocytes: a potential role in psoriasis. Eur J Immunol. (2006) 36:1309–23. doi: 10.1002/eji.200535503
182. Ong PY, Ohtake T, Brandt C, Strickland I, Boguniewicz M, Ganz T, et al. Endogenous antimicrobial peptides and skin infections in atopic dermatitis. N Engl J Med. (2002) 347:1151–60. doi: 10.1056/NEJMoa021481
183. Ciccia F, Guggino G, Ferrante A, Raimondo S, Bignone R, Rodolico R, et al. Interleukin-9 overexpression and th9 polarization characterize the inflamed gut, the synovial tissue, and the peripheral blood of patients with psoriatic arthritis. Arthritis Rheumatol. (2016) 68:1922–31. doi: 10.1002/art.39649
184. Elyaman W, Bradshaw EM, Uyttenhove C, Dardalhon V, Awasthi A, Imitola J, et al. IL-9 induces differentiation of TH17 cells and enhances function of FoxP3+ natural regulatory T cells. Proc Natl Acad Sci USA. (2009) 106:12885–90. doi: 10.1073/pnas.0812530106
185. Mease P, McInnes IB. Secukinumab: a new treatment option for psoriatic arthritis. Rheumatol Ther. (2016) 3:5–29. https://dx.doi.org/10.1007%2Fs40744-016-0031-5
186. Kavanaugh A, Mease PJ, Gomez-Reino JJ, Adebajo AO, Wollenhaupt J, Gladman DD, et al. Treatment of psoriatic arthritis in a phase 3 randomised,placebo-controlled trial with apremilast, an oral phosphodiesterase 4 inhibitor. Ann Rheum Dis. (2014) 73:1020–6. doi: 10.1136/annrheumdis-2013-205056
187. Sakkas LI, Bogdanos DP. Are psoriasis and psoriatic arthritis the same disease? The IL-23/IL-17 axis data. Autoimmun Rev. (2017) 16:10–15. doi: 10.1016/j.autrev.2016.09.015
188. Zaba LC, Suarez-Farinas M, Fuentes-Duculan J, Nograles KE, Guttman-Yassky E, Cardinale I, et al. Effective treatment of psoriasis with etanercept is linked to suppression of IL-17 signaling, not immediate response TNF genes. J Allergy Clin Immunol. (2009) 124:1022–10.e1-395. doi: 10.1016/j.jaci.2009.08.046
189. Chabaud M, Garnero P, Dayer JM, Guerne PA, Fossiez F, Miossec P. Contribution of interleukin 17 to synoviummatrix destruction in rheumatoid arthritis. Cytokine. (2000) 12:1092–99. doi: 10.1006/cyto.2000.0681
190. Yen D, Cheung J, Scheerens H, Poulet F, McClanahan T, McKenzie B, et al. IL-23 is essential for T cell-mediated colitis and promotes inflammation via IL-17 and IL-6. J. Clin. Invest. (2006) 116:1310–6. doi: 10.1172/JCI21404
191. Luo J, Wu SJ, Lacy ER, Orlovsky Y, Baker A, Teplyakov A, et al. Structural basis for the dual recognition of IL-12 and IL-23 by ustekinumab. J. Mol. Biol. (2010) 402:797–812. doi: 10.1016/j.jmb.2010.07.046
192. Dobbin-Sears I, Roberts J, O'Rielly DD, Rahman P. Ustekinumab in psoriatic arthritis and related phenotypes. Ther Adv Chronic Dis. (2018) 9:191–8. doi: 10.1177/2040622318781760
193. Patel NU, Vera NC, Shealy ER, Wetzel M, Feldman SR. A review of the use of secukinumab for psoriatic arthritis. Rheumatol Ther. (2017) 4:233–46. doi: 10.1007/s40744-017-0076-0
194. McInnes IB, Mease PJ, Ritchlin CT, Rahman P, Gottlieb AB, Kirkham B, et al. Secukinumab sustains improvement in signs and symptoms of psoriatic arthritis: 2 year results from the phase 3 FUTURE 2 study. Rheumatology. (2017) 56:1993–2003. doi: 10.1093/rheumatology/kex301
195. Van der Heijde D, Gladman DD, Kishimoto M, Masato Okada M, Rathmann SS, Moriarty SR, et al. Efficacy and safety of ixekizumab in patients with active psoriatic arthritis: 52-week results from a Phase III study (SPIRIT-P1). J Rheumatol. (2018) 45:1608. doi: 10.3899/jrheum.170429.C1
196. Nash P, Kirkham B, Okada M, Rahman P, Combe B, Burmester GR, et al. SPIRIT-P2 Study GroupIxekizumab for the treatment of patients with active psoriatic arthritis and an inadequate response to tumour necrosis factor inhibitors: results from the 24-week randomised, double-blind, placebo-controlled period of the SPIRIT-P2 phase 3 trial. Lancet. (2017) 389:2317–27. doi: 10.1136/annrheumdis-2017-eular.1576
197. Mease PJ, Smolen JS, Behrens F, Nash P, Leage LS, Li L, et al. A head-to-head comparison of the efficacy and safety of ixekizumab and adalimumab in biological-naïve patients with active psoriatic arthritis: 24-week results of a randomised, open-label, blinded-assessor trial. Ann Rheum Dis. (2020) 79:123–31. doi: 10.1136/annrheumdis-2019-215386
198. Ly K, Beck KM, Smith MP, Orbai AM, Liao W. Tofacitinib in the management of active psoriatic arthritis: patient selection and perspectives. Psoriasis. (2019) 9:97–107. doi: 10.2147/PTT.S161453
199. Chen M, Dai SM. A novel treatment for psoriatic arthritis: Janus kinase inhibitors. Chin Med J (Engl). (2020) 133:959–67. doi: 10.1097/CM9.0000000000000711
200. Deodhar A, Helliwell PS, Boehncke WH, Kollmeier AP, Hsia EC, Subramanian A, et al. Guselkumab in patients with active psoriatic arthritis who were biologic-naive or had previously received TNFα inhibitor treatment (DISCOVER-1): a double-blind, randomised, placebo-controlled phase 3 trial. Lancet. (2020) 395:1115–25. doi: 10.1016/S0140-6736(20)30265-8
201. Mease PJ, Rahman P, Gottlieb AB, Kollmeier AP, Hsia EC, Xu XL, et al. Guselkumab in biologic-naive patients with active psoriatic arthritis (DISCOVER-2): a double-blind, randomised, placebo-controlled phase. 3 trial. Lancet. (2020) 395:1126–36. doi: 10.1016/S0140-6736(20)30263-4
202. Scott DL, Wolfe F, Huizinga TWJ. Rheumatoid arthritis. Lancet. (2010) 376:1094–8. doi: 10.1016/S0140-6736(10)60826-4
203. McInnes I, Schett G. Cytokines in the pathogenesis of rheumatoid arthritis. Nat Rev Immunol. (2007) 7:429–42. doi: 10.1038/nri2094
204. Lubberts E. The IL-23–IL-17 axis in inflammatory arthritis. Nat Rev Rheumatol. (2015) 11:415–29. doi: 10.1038/nrrheum.2015.53
205. Van Hamburg JP, Asmawidjaja PS, Davelaar N, Mus AMC, Colin EM, Hazes JMW, et al. Th17 cells, but not Th1 cells, from patients with early rheumatoid arthritis are potent inducers of matrix metalloproteinases and proinflammatory cytokines upon synovial fibroblast interaction, including autocrine interleukin-17A production. Arthritis Rheum. (2011) 63:73–83. doi: 10.1002/art.30093
206. Paulissen SMJ, Van Hamburg JP, Davelaar N, Asmawidjaja PS, Hazes JMW, Lubberts E. synovial fibroblasts directly induce th17 pathogenicity via the cyclooxygenase/prostaglandin E2 pathway, independent of IL-23. J Immunology. (2013) 191:1364–72. doi: 10.4049/jimmunol.1300274
207. Yago T, Nanke Y, Ichikawa N, Kobashigawa T, Mogi M, Kamatani N, et al. IL-17 induces osteoclastogenesis from human monocytes alone in the absence of osteoblasts, which is potently inhibited by anti-TNF-α antibody: A novel mechanism of osteoclastogenesis by IL-17. J Cell Biochem. (2009) 108:947–55. doi: 10.1002/jcb.22326
208. Paulissen SM, Van Hamburg JP, Dankers W, Lubberts E. The role and modulation of CCR6+ Th17 cell populations in rheumatoid arthritis. Citokyne. (2015) 74:43–53. doi: 10.1016/j.cyto.2015.02.002
209. Penatti A, Facciotti F, De Matteis R, Larghi P, Paroni M, Murgo A, et al. Differences in serum and synovial CD4+ T cells and cytokine profiles to stratify patients with inflammatory osteoarthritis and rheumatoid arthritis. Arthritis Res Ther. (2017) 9:103. doi: 10.1186/s13075-017-1305-1
210. Paulissen SMJ, van Hamburg JP, Davelaar N, Vroman H, Hazes JMW, de Jong PHP, et al. CCR6+ Th cell populations distinguish ACPA positive from ACPA negative rheumatoid arthritis. Arthritis Res Ther. (2015) 17:344. doi: 10.1186/s13075-015-0800-5
211. Zizzo G, De Santis M, Bosello SL, Fedele AL, Peluso G, Gremese E, et al. Synovial fluid-derived T helper 17 cells correlate with inflammatory activity in arthritis, irrespectively of diagnosis. Clin Immunol. (2011) 138:107–16. doi: 10.1016/j.clim.2010.10.002
212. Murphy CA, Langrish CL, Chen Y, Blumenschein W, McClanahan T, Kastelein RA, et al. Divergent pro- and antiinflammatory roles for IL-23 and IL-12 in joint autoimmune inflammation. J Exp Med. (2003) 198:1951–7. doi: 10.1084/jem.20030896
213. Genovese MC, Van den Bosch F, Roberson SA, Bojin S, Biagini IM, Ryan P, et al. LY2439821, a humanized anti–interleukin-17 monoclonal antibody, in the treatment of patients with rheumatoid arthritis: A phase I randomized, double-blind, placebo-controlled, proof-of-concept study. Arthritis Rheum. (2010) 62:929–39. doi: 10.1002/art.27334
214. Genovese MC, Durez P, Richards HB, Supronik J, Dokoupilova E, Mazurov V, et al. Efficacy and safety of secukinumab in patients with rheumatoid arthritis: a phase II, dose-finding, double-blind, randomised, placebo controlled study. Ann Rheum Dis. (2013) 72:863–9. doi: 10.1136/annrheumdis-2012-201601
215. Genovese MC, Durez P, Richards HB, Supronik J, Dokoupilova E, Aelion JA, et al. One-year efficacy and safety results of secukinumab in patients with rheumatoid arthritis: phase ii, dose-finding, double-blind, randomized, placebo-controlled study. J Rheumatol. (2014) 41:414421. doi: 10.3899/jrheum.130637
216. Martin DA, Churchill M, Flores-Suarez LF, Cardiel MH, Wallace D, Martin R, et al. A phase Ib multiple ascending dose study evaluating safety, pharmacokinetics, and early clinical response of brodalumab, a human anti-IL-17R antibody, in methotrexate-resistant rheumatoid arthritis. Arthritis Res Ther. (2013) 15:R164. doi: 10.1186/ar4347
217. Genovese MC, Greenwald M, Cho C-S, Berman A, Jin L, Cameron GS, et al. A Phase II randomized study of subcutaneous ixekizumab, an anti–interleukin-17 monoclonal antibody, in rheumatoid arthritis patients who were naive to biologic agents or had an inadequate response to tumor necrosis factor inhibitors. Arthritis Rheumatol. (2014) 66:1693–704. doi: 10.1002/art.38617
218. Smolen JS, Agarwal SK, Ilivanova E, Xu XL, Miao Y, Zhuang Y, et al. A randomised phase II study evaluating the efficacy and safety of subcutaneously administered ustekinumab and guselkumab in patients with active rheumatoid arthritis despite treatment with methotrexate. Ann Rheum Dis. (2017) 76:83139. doi: 10.1136/annrheumdis-2016-209831
219. Fleischmann RM, Wagner F, Kivitz AJ, Mansikka HT, Khan N, Othman AA, et al. Safety, tolerability, and pharmacodynamics of ABT-122, a tumor necrosis factor– and interleukin-17–targeted dual variable domain immunoglobulin, in patients with rheumatoid arthritis. Arthritis Rheumatol. (2017) 69:2283–91. doi: 10.1002/art.40319
220. Genovese MC, Weinblatt ME, Aelion JA, Mansikka HT, Peloso PM, Chen K, et al. ABT-122, a bispecific dual variable domain immunoglobulin targeting tumor necrosis factor and interleukin-17a, in patients with rheumatoid arthritis with an inadequate response to methotrexate. Arthritis Rheumatol. (2018) 70:1710–20. doi: 10.1002/art.40580
221. Glatt S, Taylor PC, McInnes IB, Schett G, Landewé R, Baeten D, et al. Efficacy and safety of bimekizumab as add-on therapy for rheumatoid arthritis in patients with inadequate response to certolizumab pegol: a proof-of-concept study. Ann Rheum Dis. (2019) 78:1033–40. doi: 10.1136/annrheumdis-2018-214943
222. Voulgarelis M, Tzioufas A. Pathogenetic mechanisms in the initiation and perpetuation of Sjögren's syndrome. Nat Rev Rheumatol. (2010) 6:529–37. doi: 10.1038/nrrheum.2010.118
223. Salomonsson S, Jonsson MV, Skarstein K, Brokstad KA, Hjelmström P, Wahren-Herlenius M, et al. Cellular basis of ectopic germinal center formation and autoantibody production in the target organ of patients with Sjögren's syndrome. Arthritis Rheum. (2003) 48:3187–201. doi: 10.1002/art.11311
225. Yang XO, Pappu BP, Nurieva R, Akimzhanov A, Kang HS, Chung Y, et al. T helper 17 lineage differentiation is programmed by orphan nuclear receptors RORa and RORg. Immunity. (2007) 28:29–39. doi: 10.1016/j.immuni.2007.11.016
226. Nguyen CQ, Hu MH, Li Y, Stewart C, Peck AB. Salivary gland tissue expression of interleukin-23 and interleukin-17 in Sjögren's syndrome: findings in humans and mice. Arthritis Rheum. (2008) 58:734–43. doi: 10.1002/art.23214
227. Katsifis GE, Rekka S, Moutsopoulos NM, Pillemer S, Whal SM. Systemic and local interleukin-17 and linked cytokines associated with sjögren's syndrome immunopathogenesis. Am J Pathol. (2009) 175:1167–77. doi: 10.2353/ajpath.2009.090319
228. Ciccia F, Guggino G, Rizzo A, Alessandro R, Carubbi F, Giardina AR, et al. Rituximab modulates IL-17 expression in the salivary glands of patients with primary Sjögren's syndrome. Rheumatology. (2014) 53:1313–20. doi: 10.1093/rheumatology/keu004
229. Pérez P, Kwon YJ, Alliende C, Leyton L, Aguilera S, Molina C, et al. Increased acinar damage of salivary glands of patients with Sjögren's syndrome is paralleled by simultaneous imbalance of matrix metalloproteinase 3/tissue inhibitor of metalloproteinases 1 and matrix metalloproteinase 9/tissue inhibitor of metalloproteinases 1 ratios. Arthritis Rheum. (2005) 52:2751–60. doi: 10.1002/art.21265
230. Mitsdoerffer M, Lee Y, Jäger A, Kim H-J, Korn T, Kolls JK, et al. Proinflammatory T helper type 17 cells are effective B-cell helpers. Proc Natl Acad Sci USA. (2010) 107:14292–7. doi: 10.1073/pnas.1009234107
231. Liu R, Gao C, Chen H, Li Y, Jin Y, Qi H. Analysis of Th17-associated cytokines and clinical correlations in patients with dry eye disease. PLoS ONE. (2017) 12:e0173301. doi: 10.1371/journal.pone.0173301
232. Gan Y, Zhao X, He J, Liu X, Li Y, Sun X, et al. Increased interleukin-17F is associated with elevated autoantibody levels and more clinically relevant than Interleukin-17A in primary sjögren's syndrome. J Immunol Res. (2017) 2017:4768408. doi: 10.1155/2017/4768408
233. Voigt A, Esfandiary L, Wanchoo A, Glenton P, Donate A, Craft WF, et al. Sexual dimorphic function of IL-17 in salivary gland dysfunction of the C57BL/6.NOD-Aec1Aec2 model of Sjögren's syndrome. Sci Rep. (2016) 6:38717. doi: 10.1038/srep38717
234. Lin X, Rui K, Deng J, Wang X, Wang S, Ko KH, et al. Th17 cells play a critical role in the development of experimental Sjögren's syndrome. Ann Rheum Dis. (2015) 74:1302–10. doi: 10.1136/annrheumdis-2013-204584
235. Alunno A, Bistoni O, Bartoloni E, Caterbi S, Bigerna B, Tabarrini A, et al. IL-17-producing CD4–CD8– T cells are expanded in the peripheral blood, infiltrate salivary glands and are resistant to corticosteroids in patients with primary Sjögren's syndrome. Ann Rheum Dis. (2013) 72:286–92. doi: 10.1136/annrheumdis-2012-201511
236. Laurence A, Tato CM, Davidson TS, Kanno Y, Chen Z, Yao Z, et al. Interleukin-2 signaling via STAT5 constrains T helper 17 cell generation. Immunity. (2007) 26:371–81. doi: 10.1016/j.immuni.2007.02.009
237. Luo J, Ming B, Zhang C, Deng X, Li P, Wei Z, et al. IL-2 inhibition of Th17 generation rather than induction of treg cells is impaired in primary sjögren's syndrome patients. Front Immunol. (2018) 9:1755. doi: 10.3389/fimmu.2018.01755
238. Miao M, Hao Z, Guo Y, Zhang X, Zhang S, Luo J, et al. Short-term and low-dose IL-2 therapy restores the Th17/Treg balance in the peripheral blood of patients with primary Sjögren's syndrome. Ann Rheum Dis. (2018) 77:1838–1840. doi: 10.1136/annrheumdis-2018-213036
239. Felten R, Meyer N, Duffaut P, Saadoun D, Hachulla E, Hatron P, et al. Interleukin 6 receptor inhibition in primary Sjögren syndrome: a multicentre double-blind randomised placebo-controlled trial. Ann Rheum Dis. (2019) 2020:218467. doi: 10.1136/annrheumdis-2021-219882
240. Koutruba N, Emer J, Lebwohl M. Review of ustekinumab, an interleukin-12 and interleukin-23 inhibitor used for the treatment of plaque psoriasis. Ther Clin Risk Manag. (2010) 6:123–41. doi: 10.2147/tcrm.s5599
241. Grosskreutz CL, Hockey H-U, Serra D, Dryja TP. dry eye signs and symptoms persist during systemic neutralization of IL-1β by canakinumab or IL-17A by secukinumab, Cornea. (2015) 34:1551–6. doi: 10.1097/ICO.0000000000000627
242. Heinlen LD, McClain M.T, Merrill J, Akbarali YW, Edgerton CC, Harley JB, et al. Clinical criteria for systemic lupus erythematosus precede diagnosis, and associated autoantibodies are present before clinical symptoms. Arthritis Rheum. (2007) 56:2344–51. doi: 10.1002/art.22665
243. Vincent FB, Northcott M, Hoi A, Mackay F, Eric F, Morand EF. Clinical associations of serum interleukin-17 in systemic lupus erythematosus. Arthritis Res Ther. (2013) 15:R97. doi: 10.1186/ar4277
244. Wong CK, Lit LCW, Tam LS, Li EKM, Wong PTYW, Lam CWK. Hyperproduction of IL-23 and IL-17 in patients with systemic lupus erythematosus: implications for Th17-mediated inflammation in auto-immunity. Clin Immunol. (2008) 127:385–93. doi: 10.1016/j.clim.2008.01.019
245. Crispín JC, Oukka M, Bayliss G, Cohen RA, A Van Beek C, Stillman IE, Kyttaris VC, Juang YT, et al. Expanded double negative T cells in patients with systemic lupus erythematosus produce IL-17 and infiltrate the kidneys. J Immunol. (2008) 181:8761–6. doi: 10.4049/jimmunol.181.12.8761
246. Shivakumar JS, Tsokos GC, Datta SK. T cell receptor alpha/beta expressing double-negative (CD4-/CD8-) and CD4+ T helper cells in humans augment the production of pathogenic anti-DNA autoantibodies associated with lupus nephritis. Immunol. (1989) 143:103–12.
247. Chen DY, Chen YM, Wen MC, Hsieh TY, Hung WT, Lan JL. The potential role of Th17 cells and Th17-related cytokines in the pathogenesis of lupus nephritis Lupus. Lupus. (2012) 21:1385–96. doi: 10.1177/0961203312457718
248. Oke V, Brauner S, Larsson A, Gustafsson J, Zickert A, Gunnarsson I, Svenungsson E. IFN-λ1 with Th17 axis cytokines and IFN-α define different subsets in systemic lupus erythematosus (SLE). Arthritis Res Ther. (2017) 19:139. doi: 10.1186/s13075-017-1344-7
249. Fischer K, Przepiera-Bedzak H, Sawicki M, Walecka A, Brzosko I, Brzosko M. Serum interleukin-23 in polish patients with systemic lupus erythematosus: association with lupus nephritis, obesity, and peripheral vascular disease. Mediators Inflamm. (2017) 2017:9401432. doi: 10.1155/2017/9401432
250. Yang J, Chu Y, Yang X, Gao D, Zhu L, Yang X, et al. Th17 and natural Treg cell population dynamics in systemic lupus erythematosus. Arthritis Rheum. (2009) 60:1472–83. doi: 10.1002/art.24499
251. Yang J, Yang X, Yang J, Li M. Hydroxychloroquine inhibits the differentiation of th17 cells in systemic lupus erythematosus. J Rheumatol. (2018) 45:818–26. doi: 10.3899/jrheum.170737
252. van Vollenhoven RF, Hahn BH, Tsokos GC, Wagner CL, Lipsky P, Touma Z, et al. Efficacy and safety of ustekinumab, an IL-12 and IL-23 inhibitor, in patients with active systemic lupus erythematosus: results of a multicentre, double-blind, phase 2, randomised, controlled study. Lancet. (2018) 392:1330–9. doi: 10.1016/S0140-6736(18)32167-6
Keywords: IL-23, IL-17, IL-23/IL-17 axis, inflammatory diseases, autoimmune diseases
Citation: Schinocca C, Rizzo C, Fasano S, Grasso G, La Barbera L, Ciccia F and Guggino G (2021) Role of the IL-23/IL-17 Pathway in Rheumatic Diseases: An Overview. Front. Immunol. 12:637829. doi: 10.3389/fimmu.2021.637829
Received: 04 December 2020; Accepted: 25 January 2021;
Published: 22 February 2021.
Edited by:
Matteo Vecellio, University of Oxford, United KingdomReviewed by:
Saikat Majumder, University of Pittsburgh, United StatesJonilson Berlink Lima, Federal University of Western Bahia, Brazil
Copyright © 2021 Schinocca, Rizzo, Fasano, Grasso, La Barbera, Ciccia and Guggino. This is an open-access article distributed under the terms of the Creative Commons Attribution License (CC BY). The use, distribution or reproduction in other forums is permitted, provided the original author(s) and the copyright owner(s) are credited and that the original publication in this journal is cited, in accordance with accepted academic practice. No use, distribution or reproduction is permitted which does not comply with these terms.
*Correspondence: Giuliana Guggino, Z2l1bGlhbmEuZ3VnZ2lubyYjeDAwMDQwO3VuaXBhLml0