- 1Department of Pharmaceutical Chemistry, College of Pharmacy, Jazan University, Jazan, Saudi Arabia
- 2Substance Abuse and Toxicology Research Centre, Jazan University, Jazan, Saudi Arabia
- 3Department of Pharmacognosy, College of Pharmacy, Jazan University, Jazan, Saudi Arabia
- 4Department of Clinical Pharmacy, College of Pharmacy, Jazan University, Jazan, Saudi Arabia
Plants have been extensively studied since ancient times and numerous important chemical constituents with tremendous therapeutic potential are identified. Attacks of microorganisms including viruses and bacteria can be counteracted with an efficient immune system and therefore, stimulation of body’s defense mechanism against infections has been proven to be an effective approach. Polysaccharides, terpenoids, flavonoids, alkaloids, glycosides, and lactones are the important phytochemicals, reported to be primarily responsible for immunomodulation activity of the plants. These phytochemicals may act as lead molecules for the development of safe and effective immunomodulators as potential remedies for the prevention and cure of viral diseases. Natural products are known to primarily modulate the immune system in nonspecific ways. A number of plant-based principles have been identified and isolated with potential immunomodulation activity which justify their use in traditional folklore medicine and can form the basis of further specified research. The aim of the current review is to describe and highlight the immunomodulation potential of certain plants along with their bioactive chemical constituents. Relevant literatures of recent years were searched from commonly employed scientific databases on the basis of their ethnopharmacological use. Most of the plants displaying considerable immunomodulation activity are summarized along with their possible mechanisms. These discussions shall hopefully elicit the attention of researchers and encourage further studies on these plant-based immunomodulation products as potential therapy for the management of infectious diseases, including viral ones such as COVID-19.
Introduction
Severe acute respiratory syndrome coronavirus-2 (SARS-CoV-2) is a novel strain of coronavirus first isolated from humans in December 2019 in Wuhan city, China. It is highly contagious and produces a disease known as COVID-19, which has pneumonia-like symptoms and has resulted in a pandemic. COVID-19 can infect all age groups, showing relatively severe symptoms in people over 60 years of age (1) and currently no medications are approved for its therapy. For a disease without treatment, preventive medicines are prescribed and immunomodulators with antivirals can serve the purpose. To control such infections, vaccination is considered to be an effective method; however, it is known to prevent the incidence of acute respiratory infections in healthy individuals only to a certain extent (2). As seen in all viral infections including SARS-CoV-2, the virus-specific T cells responsible for cell-mediated immunity and B lymphocytes for humoral immunity, play important roles in the adaptive immune response by the body. There is an increase in neutrophils, interleukin (IL)-6 and C-reactive protein and a decrease in the total number of lymphocytes (3). The activation of T lymphocytes plays a role in exacerbation of inflammatory responses, whereas the B lymphocytes help in producing specific neutralizing antibodies for the virus (4, 5).
Herbal products are recognized as complementary approach to modern medication; consequently, immunomodulation agents from natural sources have proven to be safe and effective alternatives (6, 7). Immunomodulators stimulate the body’s natural defense against pathogens including viruses, thereby maintaining immune-system homeostasis and could be an effective way to prevent viral infections. Malfunctions or imbalances in the immune system are associated with a range of chronic diseases, including allergies, cancer, inflammatory bowel diseases, autoimmune disorders, viral infections, and many others. Carotenoids, terpenoids, flavonoids, polyphenols (e.g., stilbene derivatives), organosulfur-containing compounds (e.g., allyl isothiocyanate, hydrocinnamic acid derivatives) and polysaccharides are important phytocompounds with known chemical structures and considerable immunomodulating activities (8).
Among other popular therapeutic approaches, immunotherapy has been utilized indirectly for the treatment of cancer. Recently, immunotherapy demonstrated positive responses in cancer treatment with clinical successes by blocking antibodies at two immune checkpoints, namely, cytotoxic T-lymphocyte-associated protein 4 and planned cell death protein 1, in addition to chimeric antigen receptor-transduced T cells (9). Globally, around 180 million people are chronically infected with hepatitis C virus and owing to the quasispecies nature of the virus, the development of effective vaccination has long been a challenging task (10). Cerebral malaria (CM), often occurs in immunocompromised individuals along with the failure of antimalaria treatment. Plant-based natural products such as curcumin have demonstrated promising immunomodulation activity and can be used as an adjunctive therapy for CM (11). Dengue is another mosquito-borne viral disease causing high levels of pro-inflammatory cytokine production, leading to endothelial activation and vascular leakage. Antioxidants and natural immunomodulating agents from plant sources have demonstrated promising results in the treatment of a number of viral infections (12).
Dietary polysaccharides are the prebiotics which are known to affect gut microbial ecology. There has been a direct correlation between nutrients present in the diet and the immune function (13). A number of micronutrients, macronutrients and the gut microbiome mediate the immunological effects of body. The microbial transformation of dietary components to its metabolites has a considerable effect on the physiological and immunological processes. There is a close relationship between the bacterial metabolism and the efficacy of phytochemicals as it may increase or decrease upon metabolism (14). Following the advancements made in the field of microbial ecology, immunology and metabolomics, the impact of gut microbiota on host nutrition, immune modulation, resistance to pathogens, intestinal epithelial development and activity and energy metabolism has been recognized (15). Plant-based nutritional foods are known to improve the population of beneficial bacteria present in intestines which help and constitute up to 85% of the immune system (16). Spices used in the food and their secondary metabolites (17) also showed the immunostimulation potential and it was observed that the COVID-19 cases were more prevalent in countries with lesser gram spice supply per capita per day (18). Non-digestible nutrients that selectively promote the growth of normal intestinal bacterial flora are known to have beneficial effects on the large intestinal lining. After being hydrolyzed by gut bacteria, these nutrients may either bind to epithelia or get absorbed in the systemic circulation to stimulate the immune system (19, 20). Among studied immunomodulation factors (including recombinant interferon-gamma (rINF-γ), interferon-alpha (rINF-α), ampligen, and CL246), a total of 738 factors exhibited significant antiviral activity and were recommended for prophylactic or therapeutic use in early-stage infections of several species of viruses (21).
The aim of this review was to summarize the most important medicinal plants which displayed good immunomodulation activity from the recently published literature. It also covers the possible mechanism of immunomodulation for these plants and the role of natural products in the treatment and management of viral infections. Recently published articles from commonly used search engines such as Pubmed, ScienceDirect, Google Scholar, Microsoft Academic Research, etc. which were related to the scope of the review were included. A good number of reviews are present discussing various plants and their bioactive constituents demonstrating immunomodulation and antiviral activities, however, an updated review focusing on the immunomodulation activities of important medicinal plants and their isolated molecules along with their mechanisms is still warranted.
Immune System Responses to Viral Infection
Infection by pathogenic viruses causes a number of infectious diseases, such as influenza, smallpox, human immunodeficiency virus (HIV)/AIDS, measles, and newly identified COVID-19 (22). The body’s defense system recognizes the infecting organisms such as viruses and bacteria as foreign particles through innate or adaptive immunity systems. In case of innate immunity, foreign microbes are identified by pattern-recognition receptors (PRRs) situated on the surface of innate immune cells. Subsequently, the invading organisms are destroyed by certain defense processes, such as cytotoxin generation by natural killer (NK) cells and macrophage-mediated phagocytosis (23, 24). In adaptive immunity, microorganisms are recognized by T and B lymphocytes through cell surface-antigen-specific receptors (T cell and B cell receptors) and the invaders are eliminated through cytotoxic responses (e.g., neutrophils, CD45+ lymphocytes, CD8+ T cell, monocytes, and mature B lymphocytes) and antibody production (23, 25). However, pathogenic microbes often succeed in infecting the biological system despite the strong defense mechanisms even in immunocompetent individuals because of a number of immune-evasion tactics, including surpassing recognition by immune cells and immobilizing immune responses (26–28).
Owing to the high sequence variability of viruses, they can avoid immune responses via the variation and interruption of antigen generation from virus-infected cells (29, 30). Viruses can interfere with the antiviral signaling of the immune system by altering genetic materials, reducing cytokine generation (31), and hindering the expression of PRRs and their adapter (32). Viruses can also affect the immune response by reducing the cytotoxic function of T cells (33) and reducing NKG2D and MHC-1 molecules, thereby suppressing immune stimulation (34, 35). For example, dengue and West Nile viruses escape immune response through the blockage of IFN-α/IFN-β receptors (36), and influenza viruses achieve gene mutations involved in antigen binding through “antigen drift” (37). In another way of escaping immune responses, virus particles enter a host cell in the dormant state and subsequently are reactivated when the cell immunity weakens. Consequently, the detection of viruses becomes difficult because the infected cells start upregulating the expression of latency-related genes rather than lytic viral genes (38).
These viruses can be best exemplified by human papillomavirus (HPV), which can adopt a dormant state and after assuming dormancy, the migrating HIV-infected cells can be reactivated by differential responses to drugs (39, 40). These viruses can create a favorable environment for infection through alteration of various metabolic processes of host cells; such as enhanced fatty acid synthesis, change in aerobic glycolysis, and glutaminolysis (41, 42). Additionally, enhanced lactic acid production creates an acidic microenvironment that helps in virus pathogenesis as the fusion of an enveloped virus to the host cell membrane occurs more effectively at acidic pH (25, 43). For instance, Zika virus, hepatitis B virus (HBV), and HIV induce the dysregulation of glycolytic processes through enhanced glucose influx, lactic acid production, and GLUT1 expression in host cells (44). The short-term exposure of Ebola virus to an acidic environment may result in increased fusion rate to the host cell membrane (45).
Mechanism for Innate Antiviral Immunity
Innate immunity are the non-specific immunity which includes expression of factors resulting into inhibition of virus particles and slowing down the infections in a rapid manner without adaptation or generation of long-lasting memory for protection. The innate antiviral responses are critical in order to mobilize the protective immunity. Upon viral infection, the innate immunity cells get activated mainly through the germline-coded pattern recognition receptors (PRRs) which are present either on the cellular surfaces or within distinct intracellular compartments. The PRRs include the family of Toll-like receptors (TLRs), the retinoic acid-inducible gene-I-like receptors (RLRs), cytosolic DNA sensors and the nucleotide-oligomerization domain-like receptors (NLRs) (46). These receptors are mainly triggered by the exposure of viral nucleic acids and proteins. These PRRs present in a wide array of cellular components sense the presence of viral antigen and quickly respond to the viral infection and replication of these viruses in the cellular compartments.
Activation of family of TLRs including TLR-3, 7, 8 and 9 mediates the production of type-I interferons (IFN-Is) and pro-inflammatory cytokines. The cytokines are responsible for the production of a number of inflammatory mediators causing inflammation, fever and pain; whereas, the IFN-Is are the important mediators of antiviral immunity and are responsible for the antiviral activities (47). Similarly, RLRs sense and detect the viral RNA in the cytosol resulting into the induction of IFN-Is. The RLRs can generally differentiate between the viral RNA and the host RNA owing to the distinct features present in the viral RNA (48). The RLRs can detect the double-stranded viral RNA and activate NF-κB and interferon regulatory factors (IRFs) through the adaptive protein, mitochondrial antiviral signaling protein (MAVS), a protein that resides in the mitochondrial membrane and orchestrates host antiviral innate immune responses to the RNA-virus infection (49). Another important mechanism by which the host defense system neutralizes and kills the pathogens is via expression of natural antibodies (NAbs), which are innate-like serum antibodies (50). These NAbs play important role in early immune response against the viral infections and are crucial part of the host defense.
The activation of PRRs initiates signal transduction via a series of adaptor proteins leading to the expression of a number of transcription factors such as NF-κB, IRF-3, IRF-7 and several kinases; consequently the production of antiviral IFN-Is. However, certain viruses have evolved in such a way that they can block the innate immune-signaling and hinder the antiviral responses by host. They escape detection by PRRs, can block the activation of adaptor proteins and kinases and can disrupt the transcription factors; thereby successfully invade the host cells (51). Various components of innate immunity include macrophages/monocytes, neutrophils, granulocytes, natural killer (NK) cells and dendritic cells. These cells can identify and kill the viral pathogens by phagocytosis, cleavage and by cytotoxic activity (52).
Treatment of Viral Diseases Using Natural Immunomodulators
The treatment of infectious diseases involves either directly killing the infecting microbes or therapeutically modulating the body’s immune responses to eradicate pathogenic organisms from the body without causing injury to the host cells. The immunity to microbial attacks might involve nonspecific responses (innate immunity) to common antigens or acquired responses (adaptive immunity) to specific microbes (53, 54). Multiple potential targets have been identified to modulate the immune responses of body for the microbial attacks. Considering that viruses are obligate intracellular pathogens and entirely dependent on the host, direct therapy using pharmaceuticals for viral infection is difficult (55). The development of vaccine against a particular viral infection is a slow and tedious process and targeting immune responses becomes important for the intervention of these diseases (56). In recent years, immunotherapy has been developed as a promising therapy in form of complementary treatment for the diseases caused by viral infections (56–59).
Natural products are known to primarily stimulate the nonspecific immune responses (innate immunity), in which the mediators of immune system including cytokines, macrophages, neutrophils, acute-phase proteins and monocytes provide instant defense against pathogens (60). Pathogens express different factors known as pathogen-associated molecular patterns (PAMPs), which are recognized by the host to perceive the presence of pathogens. PAMPs are recognized by host sensors using PRRs triggering a sequence of immune reactions through the generation of type-1 IFNs, cytokines, and chemokines. PRR families include NOD-like receptors, Toll-like receptors (TLRs), RIG-I-like receptors and DNA receptors (60). TLR-3, -7,- 8, and -9 are extremely important in sensing viral infections as they transduce signals to the system, which then activates antiviral processes. Agents that nonspecifically stimulate TLR-mediated responses may be potential antiviral remedies (60). In nonspecific immunity, antigen-presenting cells (APCs) and macrophages play important roles in cytokine secretion, antibody-dependent cell-mediated cytotoxicity and antigen presentation, processing and phagocytosis. The stimulation of naïve B cells and naïve T cells occurs through dendritic cells. Natural killer cells are upregulated, leading to the production of granulocyte-macrophage colony stimulating factor, tumor necrosis factor (TNF)-α, and INF-γ (61–63) (Figure 1).
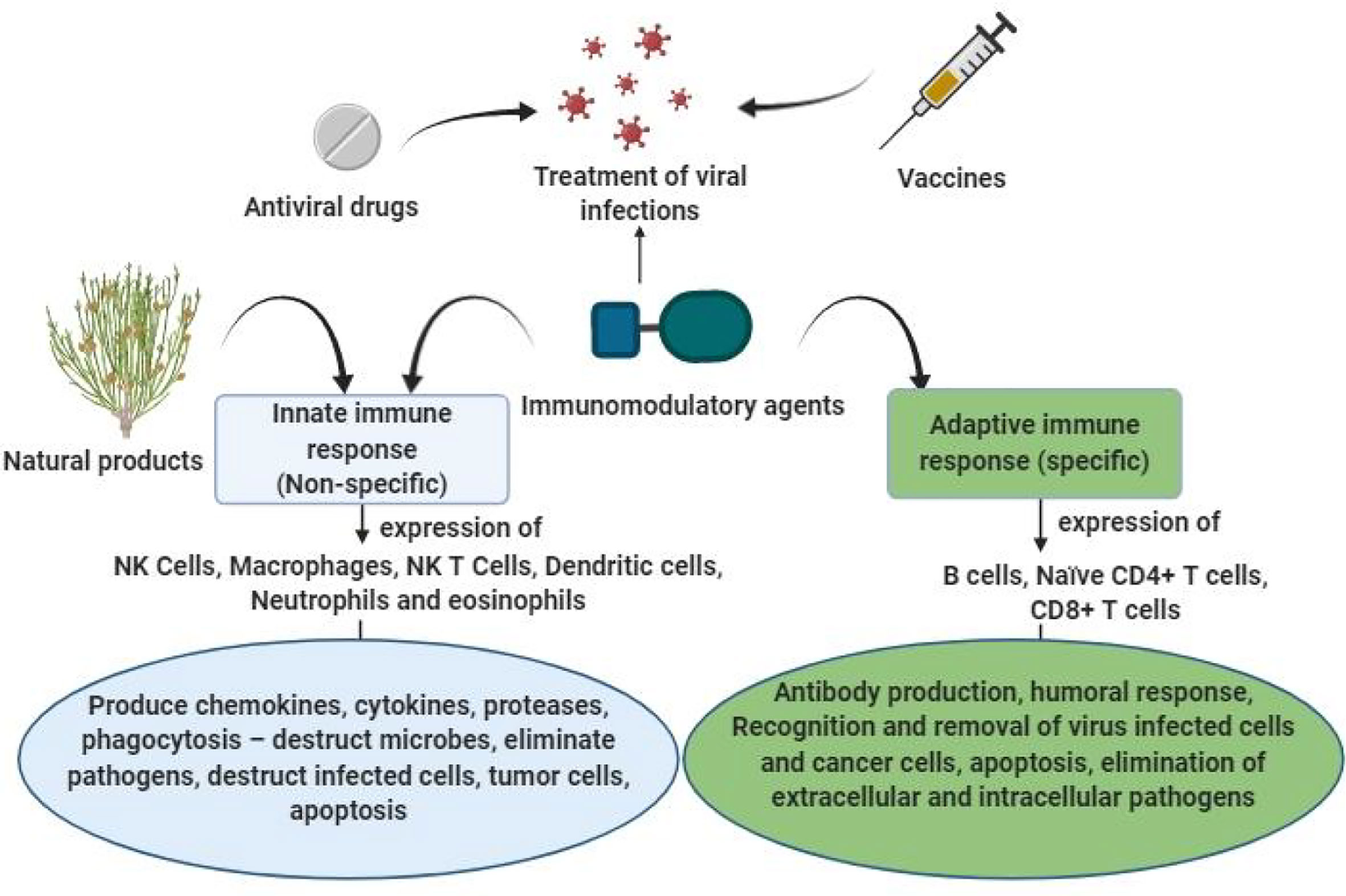
Figure 1 Possible role of natural immunomodulators for prevention and treatment of viral infections.
Natural Products as Immunomodulators
Normally, the body’s defense system maintains homeostasis; however, several endogenous or exogenous factors can alter its efficiency and effectiveness. Molecules from synthetic, natural, or biological origins can modify (suppress or stimulate) either the innate or adaptive component of immunity and are known as immunomodulators (63). Immunomodulators which improve the immune responses against infections either by activating or inducing the immune-system components are regarded as immunostimulants (64). In this review, the term immunomodulator refers to an immunostimulant. Some synthetic compounds and monoclonal antibodies are available as immunomodulators, but their use has been associated with a number of limitations, including side effects. For example, the use of recombinant cytokines as immunostimulants is associated with hypotension, myocardial infarction, cardiomyopathy and GI distress (63, 65). Thus, safe and effective agents are still required; indeed a significant amount of research has been conducted to identify natural products as immunomodulators. Immunomodulators from wide varieties of plants, their extracts, active plant ingredients and plant products have been investigated (66). Recently, plants containing polyphenolic compounds such as flavonoids in the form of fruit juice, extract, or isolated active constituents have been extensively studied for several beneficial effects, including their capability to modify the body’s defense system. Flavonoids are primarily antioxidant and exhibit inhibitory properties on inflammatory cytokine generation (67).
Several plant products have been investigated previously for direct treatment or as prophylaxis for infectious diseases (68, 69). Dietary supplements such as vitamins C, D and E are primarily antioxidants and are known to improve the immunity. The duration and severity of common cold has been shown to be reduced by a high dose of vitamin C and D supplements in patients (70, 71). Vitamin C has been included in at least 20 clinical studies to evaluate its efficacy in treating COVID-19 patients (72). Another ongoing trial is assessing the efficacy of vitamin A, B, C, D and E supplements on the improvement and mortality in COVID-19 patients (73). Vitamin E supplementation was found to improve cellular and humoral immunity and provides protection against infections (74); although, the degree of resistance is unclear. A clinical trial on elderly subjects revealed that long-term supplementation of vitamin E enhanced the resistance to mild respiratory viral infections, such as common cold (75). A large number of phytopharmaceuticals have been investigated for their beneficial effects in several diseases and many of them exerted significant immunomodulation properties. For example, resveratrol, an active constituent from grapevine, red wine, and peanuts, was found to show immunomodulation activity primarily through the inhibition of TNF-α or lipopolysaccharide (LPS)-mediated macrophages, NF-kB in phorbol 12-myristate acetate (PMA), dendritic cells, and myeloid (U-937) (63). Epigallocatechin-3-gallate (EGCG), a polyphenol constituent from green tea, reportedly exhibited immunomodulation activity primarily by blocking NF-kB activation. It also down-regulated nitric oxide (NO) production in macrophages and the expression of monocyte chemoattractant protein-1 (MCP-1), which is dependent on NF-kB inhibition (63). The squeezed sap of Echinacea purpurea showed nonspecific immunomodulation for use against mild to moderate respiratory infections, such as the common cold (70). Alkylamides are the principal active constituents, which act upon stimulation by the phagocytosis of macrophages and inhibition of inflammatory cytokine release such as TNF-α. It also increases circulating leukocytes, stimulates NK cells and possesses antiviral activity. Polysaccharide constituents were found to ameliorate the disease progress in mice infected with influenza (54) and were reported to improve the efficacy of influenza vaccine in immunosuppressed mice by activating the dendritic cells (76). Ginsenoside, the active component of ginseng, exhibited significant immunostimulant activity and was successfully used as an adjuvant with influenza vaccine (54, 77). Many of these phytochemicals have shown moderate to good immunomodulating activities in different ways in various in vitro and in vivo studies. Although, the results have been encouraging and favoring the use of these natural products as immunomodulators, high level systematic clinical studies are still warranted to establish their safety and efficacy. The structures of few important plant bioactive constituents exhibiting immunomodulation activity along with their sources are shown in Figure 2.
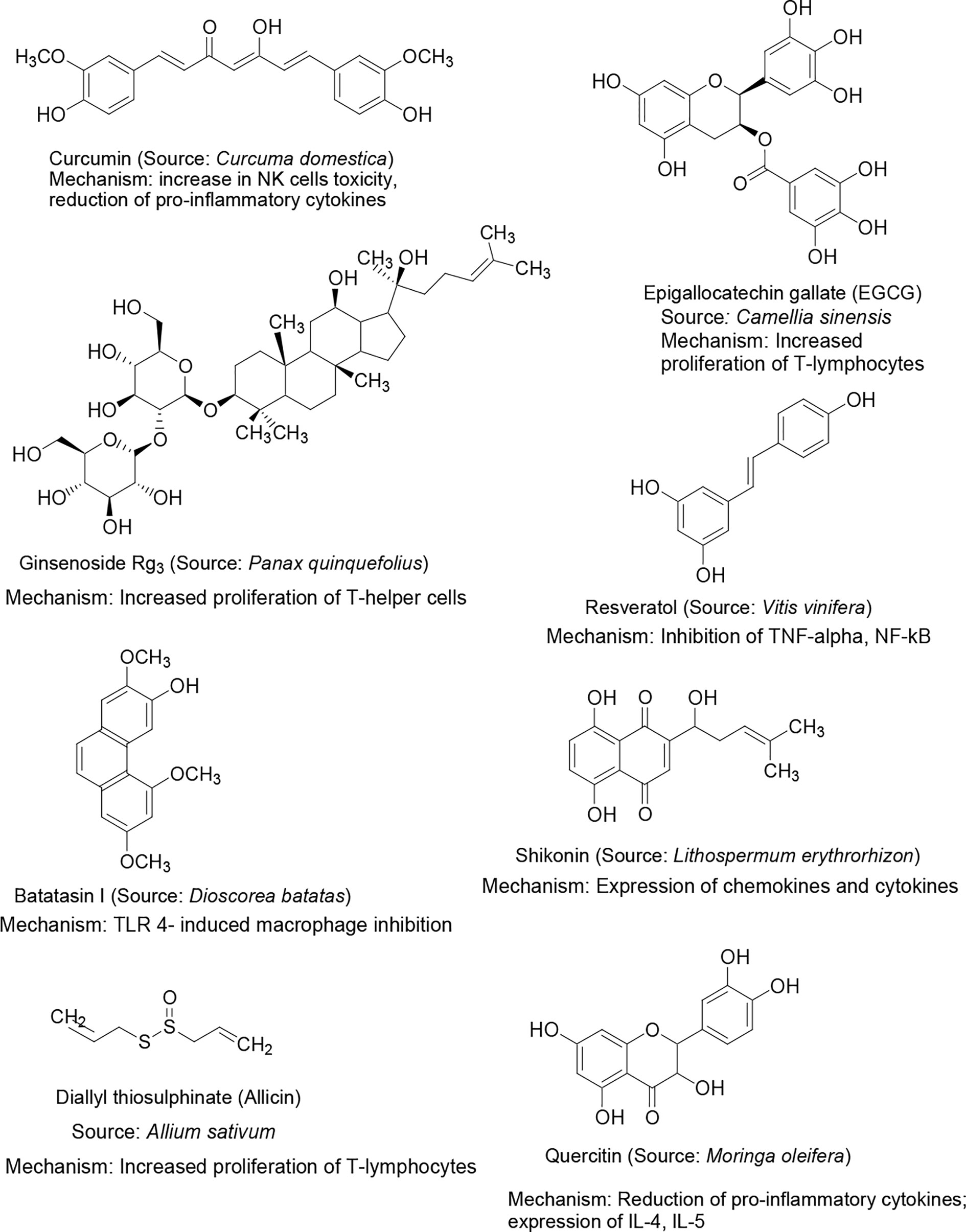
Figure 2 Chemical structure of selected natural bioactive compounds exhibiting immunomodulation activity, their major sources and mechanism of immunomodulation.
Medicinal Plants Exhibiting Immunomodulation Activity
The immunomodulating properties of medicinal plants have been studied much extensively during recent years due to the growing awareness on immune system modulation strategy to combat infectious diseases specifically the viral infections. A number of plants are already in use in folklore medicine for the treatment and prevention of viral infections either by directly affecting the pathogen or by stimulating the defense mechanism of human body in many ways. It is believed that the terpenoids present in these medicinal plants have promising efficacies in inhibiting the SARS-CoV-2 replications. Also, the alkaloidal structures such as lycorine, homoharringtonine and emetine have anti-coronaviral activities. Other isolated natural products such as emodin, baicalin, lguesterin, cryptotanshinone, silvestrol and sotetsuflavone showed inhibition of important viral replication enzymes inhibiting their growth (78). In this section, most important medicinal plants which have shown evidence based promising immunomodulation and antiviral activities in various in vitro and in vivo studies are summarized. In addition, other reported biological activities of the plant along with the mechanism by which they exert the immunomodulation activity are also discussed. Figure 3 shows the mechanisms by which medicinal plants demonstrate the immunomodulation activity.
Echinacea purpurea L.
E. purpurea L. is one of the most medicinally recognized plants and its products are commercially sold worldwide as a general health promoter and for its preventive actions on cold and flu. It shows effective antioxidant, anti-inflammatory, hypoglycemic, and antiproliferative activities. Root and above-ground parts extracts reportedly signal and stimulated immune responses by influencing macrophages, dendritic cells, monocytes, and NK cells and showed antiviral activity. The chemical constituents responsible for the antiviral activity and activating the body’s immune system include glycoproteins, soluble polysaccharides, phenolic compounds, caffeic acid derivatives, and alkylamides (79, 80). Other studies also showed that the preparations from plant extracts are among the most popular and high-demanding herbal product in USA as well as in other parts of the world due to its remarkable immunostimulatory activity and benefits in respiratory infections such as sore throat, cough, and cold (8, 81, 82). The oral administration of extract reportedly activates NK cells, polymorphic neutrophil immune cells, and macrophage phagocytic activity.
Dioscorea batatas Decne
D. batatas Decne is extensively used in traditional and modern medicines and the tuber of the plant contains a number of active components, including dioscorin, diosgenin, mucopolysaccharides, batatasins, and glycoproteins, exhibiting immunomodulation activities upon oral administration. Tuber protein and dioscorin displayed mucosal and systemic immunomodulation activity through TLR4-induced macrophage activation by stimulating signaling molecules such as NF-kB, JNK, p38, and ERK, as well as by expressing cytokines such as TNF-α and IL-6 (8, 83). The ethanolic extract of bark inhibited iNOS and COX-2 expression in RAW 264.7 cells via NF-kB and ERK1/2, conferring anti-inflammatory activity (84, 85). Tuber extract also exerted immunomodulation effects by remarkably enhancing the granulocyte-macrophage colony-stimulating factor (GM-CSF) promoter activity in inflamed and normal skin (86, 87).
Artemisia annua L
A. annua L. is a Chinese traditional medicine possessing artemisinin as the main active compound. Artemisinin is a sesquiterpene trioxane lactone primarily used as an antimalarial drug acting by disrupting the function of parasite mitochondria and by modulating host immunity. Artemisinin and its derivatives increase immune reconstitution and promote T cell activity; and are helpful in refurbishing immune function (88). In addition to antimalarial activity, it also showed beneficial effects in cancer treatment and inhibition of angiogenesis. Its tea infusion can be used for the treatment of malaria and HIV without any toxicity (89). Different phenolic compounds present in the extract showed activity against HIV and autoimmune diseases (8, 88) and was even found to be effective against the novel COVID-19 virus (90). Caffeic acid, quinic acid, artemisinin (antimalarial), scopoletin, melilotoside, casticin, artesunate, isovitexin, 3-caffeoyquinic acid, rosmarinic acid, and arteannuin B are some phytocompounds identified in tea infusion responsible for its activity (89, 91).
Lithospermum erythrorhizon
L. erythrorhizon (Gromwell), a Chinese traditional medicine used from ancient times to treat smallpox, measles, macular eruptions, eczema, burns, and carbucles, is extremely popular in China and Taiwan. This herb is well known for the treatment of abnormal skin conditions such as wounds, inflammation, and burns. Dextrose capsule with 1.5 g of root extract improved stratum corneum hydration in parallel with increased ceramides in the outermost layer of epidermis (92). The primary active compounds isolated from root of the plant are Shikonin and derivatives, which are extensively studied primarily because of their remarkable antitumor and anti-inflammatory activities (93). The components also exhibited other pharmacological properties, such as antioxidant, anti-platelet, anti-atherosclerosis and immunomodulation. Shikonin is reported to express approximately 50 genes, including chemokines (CCL4 and CCL8), cytokines (IL-1b, IL-4 and TNF-α), and inflammatory modulators (PTGS2 and NFATC3) and reduced the surface expression of a primary HIV-1 co-receptor, CCR5, which may lead to the development of potential anti-HIV agents (94). Overall, the findings on the activities of Shikonin and derivatives at the cellular and molecular levels strongly indicated its capability to induce specific chemokines and chemotaxis in various immune-response cell types (8, 95, 96).
Lycium barbarum
L. barbarum, characterized by bright orange red berries with yellow seeds, is rich in polysaccharides. Its glucosylated precursor, scopoletin, has carotenoids, flavonoids, and vitamins that are used as food supplements and therapeutic agents. Dietary polysaccharides can increase the ratio of probiotic bacteria, regulate the intestinal microenvironment such as decreasing the gut pH, and stimulating immunity such as macrophages or lymphocytes in intestinal mucosa. As a result, these polysaccharides are very helpful in fighting infections (97). Other studies reported that polysaccharides of the plant exhibit immunomodulation and antioxidant properties along with other various pharmacological activities (98). It activated local and systemic immune responses in H22 tumor-bearing mice, improved immune responses when used as an adjuvant with vaccines, and enhanced humoral immunity (99, 100)
Ganoderma lucidum
G. lucidum is a white root fungus also known as Reishi mushroom or Red Lingzhi and has been used mainly in China for a long time to promote good health. The antiaging effect of its extract is primarily due to its antioxidant and immunomodulation properties. It is also reported to have anti-breast cancer and anti-neurodegeneration properties (101). Its polysaccharide, one of the most studied components, has been reported as an effective immunomodulator and potential inhibitor of tumor growth (102). The immunomodulation effects of this polysaccharide are broad spectrum and regulate the functions of the mononuclear phagocyte system, antigen-presenting cells, and cellular and humoral immunities (103). The antitumor activities of G. lucidum are also believed to be due to immunomodulation processes (104). Approximately 400 bioactive principles including polysaccharides, nucleotides, sterols, triterpenoids, steroids, proteins and, fatty acids have been identified. Formulations containing G. lucidum are approved by the Chinese FDA for the treatment of a large number of health-related problems including physical weakness, chronic hepatitis, chronic bronchitis, coronary heart disease, and anemia. The polysaccharide preparation is used for its antitumor, antioxidant, immunomodulation, and hypoglycemic activities (105).
Eriobotrya japonica
E. japonica Lindl. is known as Loquat and used in Chinese folk medicine. The reported phytonutritional composition of its leaf and flower extracts is rich in phenolic and triterpenoid compounds, whereas the kernel is rich in proteins, minerals, starch, and tannins. It is cultivated commercially for its fruit, which is loaded with important nutrients such as carotenoids, flavonoids, vitamins, minerals, and antioxidants, known to have several health benefits (106). Loquat extract exhibited a wide range of medicinal properties, including those for the treatment of inflammation, diabetes, cancer, blood pressure regulation, immune-system improvement, viral infections, and has soothing effects on the respiratory system (107). The anti-inflammatory effects of Loquat are primarily due to its capability to reduce pro-inflammation mediators including iNOX, COX-2, IL-6, IL-8, TNF-α, and IL-1β and to enhance the generation of IL-10, an anti-inflammatory cytokine (106, 108).
Agaricus blazei
A. blazei Murrill. is an edible mushroom possessing significant medicinal values owing to a number of chemical and nutritional constituents, such as polysaccharides, glycoproteins, phytosterols, β-D-glucan, and saponins. The polysaccharide components of the fungus are thought to be the main active component responsible for its antihyperlipidemic, immunomodulator, antioxidant, and anti-inflammatory activities (109). Its polysaccharide proved to be a promising adjuvant for cancer immunotherapy, when integrated with tumor-specific antigen into animal bearing ovalbumin-expressing tumors (110). Host immune activity was considerably boosted with specific stimulation of type 1 T-helper responses (110). With respect to immunomodulation effects, water and ethanol extracts from medicinal mushroom including A. blazei exhibited opposite effects on NK cells. Water extract increased the cytotoxic activity of NK cells by stimulating the expression of cytotoxic proteins NKG2D cell surface receptors and ethanol extract reduced the expression of cytolytic and cell surface receptors (111, 112).
Dioscorea membranacea
D. membranacea Pirre is a Thai traditional medicine and one of the components for herbal formulation used to treat cancer and AIDS. Its rhizome extract enhances lymphocyte proliferation and NK cell activity, whereas the main constituent Dioscorealide B reduces these factors at high concentration (113). These findings indicated that the extract was an immunostimulant because of unknown compounds. It also exhibited antiallergy activity by suppressing β-hexosaminidase, TNF-α, and IL-4 in RBL-2H3 cells (114).
Berberis vulgaris
B. vulgaris contains benzodioxoloquinolizine alkaloid (berberine), which is shown to significantly induce interleukin (IL)-12 production in a dose-dependent manner to counter the effects of HCV infection. IL-12 consequently increased the IFN-γ production and decreased the IL-4 level in antigen primed CD4+ T cells. Berberine also stimulates the T helper lymphocytes subset 1 (Th1) cytokine synthesis and decreases the Th2 subset (10, 115). The fruit of the plant have medicinal importance due to its antioxidant, anticancer, anti-inflammatory, antidiabetic, antibacterial, and hepatoprotective properties (116).
Clerodendrum splendens
The polysaccharide obtained from the leaves of the plant C. splendens also exhibited potent immunomodulation activity. Its high-molecular-weight subfraction induced nitrous oxide and cytokine, TNF, granulocyte macrophage-colony stimulating factor, peripheral blood mononuclear cells, and monocytes with other wide-ranging agonist activities that considerably reduced disease severity (117, 118). Four clerodane diterpenes and phenyl propanoids from aerial parts showed antiproliferative activity (119).
Tinospora crispa
T. crispa is a herbaceous vine with more than 65 different compounds including flavonoids, lactones, furanoditerpenes, alkaloids, lignans, and steroids, which have been isolated and used traditionally to treat a wide range of ailments (120). Magnoflorin, syringin, cardioside, quercetin, eicosenoic acid, and boldine are some of the active constituents of the plant with higher antioxidant potential than ascorbic acid and also responsible for activating the immune system by increasing the expression of IL-6, IL-8, and INF-γ. The plant extract was shown to increase the chemotaxis and phagocytic activity of macrophages and augmented the production of NO, ROS and pro-inflammatory cytokines (120). Studies suggested that T. crispa is a rich source of nutrients and antioxidants and showed hypoglycemic effects in an in vivo experimental study (121). The stem extract of the plant also showed application in prolonged recurrent malaria treatment at 100 and 200 mg/kg doses (122).
Curcuma domestica
C. domestica (turmeric) is an Indian traditional medicine popular as dietary spice. Its polyphenolic compound, curcumin, is the main compound isolated from rhizome. Curcumin is available in various commercial preparations and effectively used in the treatment of several health problems. Curcumin has shown remarkable efficacy in the treatment of cerebral malaria through immunomodulation mechanisms (123). It is found to inhibit NF-κB activation and reduce pro-inflammatory cytokine production with expression of cytoadhesion molecules on endothelial cells (11, 124). Curcumin showed activity against various important human pathogens including influenza virus, HCV, HIV as well as SARS-CoV-2 (17). It can also block herpes simplex virus (HSV) type-2 (HSV-2) infection and abrogate the production of inflammatory cytokines and chemokines through genital epithelial cells in vitro (125, 126). The polyphenolic component also competently blocked Kaposi’s sarcoma-associated herpes virus replication and inhibited the pathogenic processes of angiogenesis and cell invasion (127). Different derivatives of curcumin were also reported to inhibit human influenza A viruses by blocking neuraminidase in a cellular and animal model (128).
Achyranthes bidentata
Pretreatment with the immunomodulatory polysaccharide constituents of A. bidentata inhibited the proliferation of malaria parasite through immunostimulant mechanism. It was shown to strongly enhance the Th1 immune responses in body against the malarial parasites (129). Its combination with cubosome polysaccharides is found to be effective in promoting lymphocyte augmentation and in triggering the transformation of T-lymphocytes into Th-cells (130). Saponins from the plant have potential cellular and humoral immune responses with slight hemolytic and specific antibody enhancement (131, 132).
Carica papaya
C. papaya Linn. seeds exhibited good antioxidant capacity and are proven to be beneficial in the treatment of type-2 diabetes mellitus by inhibiting α-amylase and α-glucosidase enzymes (133). The immunomodulation activity is shown by constituents pheophorbide A and saponin obtained from leaves, which exhibited cytotoxic activity on SCC25 cancer cells and stimulated cell- and antibody-mediated immunity, respectively (134, 135). Leaf juice of the plant has been shown to significantly increase the plasma CCL2/MCP-1 level to treat DEN-2 dengue virus (12). Aqueous fruit extract thereof improved the immune functions against acrylamide-induced oxidative stress (136).
Morinda citrifolia
M. citrifolia L. reportedly showed immunomodulation and cytotoxic effects due to the presence of nordamnacanthal, which is an anthraquinone derived from fruits and stem of the plant. Moreover, an increase in the production of T helper, cytotoxic T1, and NK cells with a decrease in the size of tumors have been observed through in vivo studies (137). The plant exhibited a number of therapeutic effects including immunostimulant, anticancer, and anti-inflammatory. The fermented fruit extract was shown to restore skin-barrier-related proteins including filaggrin, loricrin, involucrin, zonula occludens-1, and occludin, and minimize 2,4-dinitrochlorobenzene (DNCB)-induced atopic dermatitis-like lesions through the modulation of skin barrier function and immune balance (138).
Phyllanthus amarus
The methanol extract of roots of Phyllanthus amarus Schum. & Thonn. exhibited potent anticancer activity in MCF-7 cells through apoptosis induction mediated by improved intracellular reactive oxygen species (ROS) in conjugation with decreased mitochondrial membrane potential. Antioxidant, antidiabetic, antimicrobial, antiviral, anti-venom, and anti-inflammatory activities of the plant preparations have also been reported (139). Lignan-rich fractions from fruits showed potential to stimulate apoptotic cell death in cervical cancer cell lines via the commencement of P53 and P21 against DNA damage (140). Phytochemicals including corilagin, geraniin, gallic acid, phyllanthin, hypophyllanthin, ellagic acid, phyltetralin, niranthin, catechin, quercetin, astragalin, and chebulagic acid isolated from different plant parts are accountable for improving the innate and adaptive immune systems through different mechanisms (141).
Azadirachta indica
A. indica (Neem) is extremely popular in many parts of the world with more than 140 biologically active components from different parts of the tree. This plant is traditionally used for its diverse medicinal properties, including anti-infective, anti-inflammatory, immunomodulatory, antioxidant, antiulcer, antimutagenic, and anticancer (142). The chemical constituents are mainly of two types: isoprenoids (azadiractins, salanin, vilasinin, and nimbin) and non-isoprenoids (polyphenolics, flavonoids, coumarins, and sulfurous compounds). The compound hyperoside isolated from leaves showed excellent interactions with conserved residues of nucleoprotein and could be a future drug for influenza virus (143). Approximately 35 components have shown significant influence as tumor suppressors by interfering with the carcinogenesis process in cervical, ovarian, and breast cancers (144). An experiment performed on mice has revealed that neem oil showed nonspecific immune stimulant activity via cell-mediated immune process. It also showed enhanced leukocyte cells, phagocytosis, expression of MHC class II antigens, gamma interferons, and lymphocytic proliferation (145).
Chromolaena odorata
Polysaccharides isolated from the leaves of C. odorata (L.) showed significant antioxidant and immunostimulatory activities via the stimulation of peripheral blood mononuclear cells and production of IFN-γ in a dose-dependent manner. Apart from polysaccharides, the plant also contains alkaloids, sterol, polyterpenes, and flavonoids which exhibited a wide range of therapeutic profile, such as wound healing, cytotoxic, anti-inflammatory, and antioxidant (146). Flavonoids present in the plant may transform the appearance of glucagon-like peptide 1 and its release via Takeda-G-protein-receptor-5, thereby showing antidiabetic activity (147).
Spatholobus suberectus
S. suberectus Dunn is a widely used traditional medicine for its therapeutic potential. Flavonoids, the major bioactive compounds of the plant reportedly reduced the PCV2-infected oxidative stress and exhibited immunosuppression. They were found to enhance the spleen and thymus indices such as SOD activity, GSH level, and GSH to GSSG ratio, as well as the downregulation of XOD and MPO activities. This finding indicated that the plant might act as a good antioxidant for treating diseases associated with oxidative stress including viral infections (148, 149). The dried vine stem of the plant also showed good anti-inflammatory activity by reducing the mRNA expression of some pro-inflammatory cytokines such as TNF-α, iNOS, and COX-2 (150).
Quillaja saponaria
Q. saponaria contains saponins as its major component, which modulated immune responses in clinical and preclinical studies and is useful as a vaccine adjuvant. Its action is attributed to the activation of cytotoxic T lymphocyte (Th1) and cytokine (Th2) production. The bark of the plant also exhibited antitumor, hepatoprotective, antiviral, antibacterial, and antiparasitic potential (151). As hepatoprotective agent, bark saponin decreased the iron-induced elevation of ALT, AST, ALP, GGT, LDH, MDA, NOx, TC, and TG, as well as the total direct and indirect bilirubin and albumin levels (152).
Arisaema jacquemontii
A. jacquemontii Blume is mainly found in the Himalayan region and the hexane extract of the plant exhibited remarkable antioxidant and immunomodulation activity comprising both stimulation and suppression. Immune stimulation was based on humoral and DTH responses, and immunosuppression was due to the mitogen-induced proliferation of T and B cells (153). The phenolic and flavonoid compounds present in ethanol and methanol extract were responsible for a wide range of pharmacological activities, including antioxidant, antileishmanial, anti-infective, and protein-kinase inhibition, whereas n-hexane and chloroform extracts exhibited high cytotoxicity against prostate cancer (154–156).
Radix Astragali
R. Astragali reduced the immunosuppression induced by methotrexate in mouse spleen cells. The cell proliferation was suppressed by the plant extract, and the immunomodulation effect was demonstrated through the enhancement of IL-1α and IL-12p40 mRNA expression (157, 158). Plant products in combination with Radix Angelicae Sinensis exerted a synergistic effect on the immunological balance of T lymphocytes (159).
Nymphaea rubra
Purified polysaccharides of N. rubra Roxb showed immunomodulation activity with stimulating effect on immature dendritic cells and promotion of the secretion of TR1 cytokines (160, 161). Its young leaves, peduncle, and rhizomes were found to be rich in proteins (20.5%–43.5%) and carbohydrates (63.14%), making it valuable remedy to treat diarrhea, piles, and cough (162).
Dimocarpus longan
Longan polysaccharide 1 from fruit pulp of D. longan significantly stimulated the production of cytokine interferon-γ, enhanced the activity of murine macrophages, and improved B-and T-lymphocyte production to inhibit tumor formation combined with immunomodulation effect (163, 164). Moreover, the polysaccharide–protein complex from fruit pulp showed strong immunomodulation activity (165).
Camellia sinensis
C. sinensis, popularly known as green tea, has been extensively used worldwide as a traditional medicine since ancient times owing to its antioxidant property. Catechins including epigallocatechin gallate (EGCG), epigallocatechin (EGC) epicatechin (EC), and EC gallate (ECG) are the major bioactive molecules isolated from tea leaves and are responsible for its antioxidant, antiviral, anticancer, and antifungal activities. EGC and EGCG are reported to exhibit immunomodulation activity through the generation of cytokines and T lymphocyte proliferation. The extract also reportedly enhanced the production of lymphocyte, monocytes, IL-1α, and IL-1β (63, 166). Non-catechin flavonoids from seeds improved TNF-α-impaired insulin, stimulating glucose uptake and insulin signaling with anti-metabolic syndrome and anti-inflammatory properties (167). Sapogenins, glycosides, and organic acids are triterpenoid saponins from tea leaves that reportedly inhibit human ovarian cancer cells selectively by mediating apoptosis through the extrinsic pathway and initiating anti-angiogenesis (168). Conversely, aflavin polyphenol compounds obtained from black tea are anti-HSV-1 agents (169).
Aronia melanocarpa
A. melanocarpa contains polyphenolic compounds, procyanidins and anthocyanins in its berries and bark which exert immunomodulation and anti-inflammation activity by inhibiting nitric oxide production (170). Reduction in blood glucose level and antioxidant properties are observed through the inhibition of alpha-glucosidase and xanthine oxidase, respectively (171, 172). Along with polyphenols, flavonoids such as anthocyanins, proanthocyanidins, flavanols, flavonols, cynidin-3-galactoside, and cyaniding-3-arabinoside exhibited wide range of therapeutic benefits, including gastroprotective, hepatoprotective, antiproliferative, and cardiovascular-protective properties (173).
Chenopodium quinoa
Polysaccharides obtained from the seeds of C. quinoa Willd. are considered to be excellent dietary sources of natural antioxidants promoting human health through immune-system modification (174). Purified polysaccharides from the plant have been further reported to exhibit immunomodulation activity by increasing macrophage proliferation and suppression of NO production on inflammatory RAW264.7, in addition to antioxidant and anticancer properties (175). Quinoa saponins such as phytolaccagenic, oleanolic and serjanic acids, hederagenin, 3β,23,30 trihydroxy olean-12-en-28-oic acid, 3β-hydroxy-27-oxo-olean-12en-28-oic acid, and 3β,23,30 trihydroxy olean-12-en-28-oic acid exhibit molluscicidal, antifungal, anti-inflammatory, hemolytic, and cytotoxic activities (176).
Abrus cantoniensis
A. cantoniensis is a Chinese folk medicine and vegetable (Hance); the isolated polysaccharide fractions of which showed significant immunomodulation and antitumor properties. The fraction activated lymphocyte proliferation, stimulated the production NO of peritoneal macrophages, and exhibited inhibitory effects on the migration of MCF-7 cells (177). The whole plant showed considerable wound-healing capacity at a concentration of 5% (v/w) as evidenced by increased wound contraction, hydroxyproline content, and decreased epithelialization time compared with control (178).
Rhizoma gastrodiae
The rhizomes of R. gastrodiae have been used to treat convulsions, hemiplegia, neurasthenia, headache, ischemia, and dementia. Polysaccharides, namely, gastrodin, 4-hydroxybenzyl alcohol and parishin B are the main phenolic compounds present in its rhizomes. They were shown to stimulate the macrophages to release NO and enhance phagocytosis in a dose-dependent manner, producing immunological activity (179). The antidepressant effect of the drug is probably exhibited by the modulation and regulation of its monoamine oxidase (MAO) activity and monoamine concentration. Furthermore, its antioxidant activity, GABAergic system induction, neuroprotection and anti-inflammatory properties have also been reported (180, 181). Acidic polysaccharides reduced hypertension and improved serum lipid levels in rats fed with high-fat diet (182).
Hypoxis Hemerocallidea
African potato (H. Hemerocallidea) is one of the most popular medicinal plants in South African region as a remedy for several diseases. The plant is primarily used for immunomodulation activity to treat diseases occurring when the body immunity is weakened, such as in HIV/AIDS, cancer, flu, common cold, and rheumatoid arthritis. Gold nanoparticles prepared from aqueous extracts of the plant and its glycoside content hypoxoside showed immunomodulation effects via reduction in cytokine levels in macrophages and NK cells, indicating their potential as an anti-inflammatory agent (183). Hypoxoside isolated from the plants is frequently used as an immunity enhancer in South Africa. Rooperol, a hydrolytic product of hypoxoside, demonstrated antioxidant activity by inducing the production of NO and ROS and phagocytosis (184).
Panax quinquefolius
P. quinquefolius (American ginseng) plant extract exerted powerful immunomodulation effects on innate and adaptive immunity in healthy mice, apart from showing potential antioxidant activity to combat the oxidative stress (185, 186). The plant extract also showed reduced incidence, duration, and severity of flu and colds in controlled clinical trials involving healthy and infected individuals (187, 188). Among herbal supplements, ginseng is one of the most extensively studied for its beneficial effects and few toxic effects. Clinical substantiation showed its efficiency in specific disease treatments, such as dementia, diabetes mellitus, respiratory infections, and cancer (187, 189).
Macrocystis pyrifera
Fucoidans are polysaccharides in M. pyrifera containing sizable contents of L-fucose and sulfate ester moieties with wide-ranging biological activities, such as antiviral, antitumor, immunomodulation, and antioxidant. Fucoidans from M. pyrifera have potent immune-activating effects by influencing human neutrophils, NK cells, dendritic cells, and T cells to enhance antiviral and antitumor responses (190).
Orthosiphon stamineus
O. stamineus is an important medicinal plant containing a number of phytochemicals including terpenoids, flavonoids, and essential oils, which are responsible for a wide range of traditional and therapeutic uses of the plant (191). The leaf extract of the plant exhibited promising anti-infective potential by influencing the immune mechanism of the host cells by significantly restoring the suppressed lys-7 defense gene expression in nematodes infected with Staphylococcus aureus (192). In another study, O. stamineus exerted considerable antioxidant and immunomodulation effects and antibacterial potential against gram-positive bacteria. The leaf extract also exhibited stimulatory action on human peripheral blood mononuclear cells and could thus be used to increase immunity and avoid ROS related disorders (193).
Hedysarum polybotrys
H. polybotrys extract contain formononetin and proanthocyanidin as chemical constituents which showed remarkable immune stimulation activity. The proliferation of splenocytes and LPS-activated RAW 264.7 cells were stimulated and NO radical scavengering was increased, whereas NO-induced cytotoxicity was decreased. Conversely, the extract also inhibited PGE2, COX-2, iNOS, NO, LPS-activated 264.7 cells, and splenocytes (194). This finding suggested that the extract can be used when immunity stimulation and anti-inflammatory therapy is required (195). Furthermore, a wide range of chemical constituents are present in the plant, making it therapeutically important to treat several diseases, including viral infections and cancers (196).
Momordica charantia
M. charantia is a subtropical vegetable with remarkable medicinal value and is utilized as a traditional remedy for the treatment of various diseases. Polysaccharides from its fruits showed a significant increase in serum hemoysin production, NK cell cytotoxicity, carbolic particle clearance index, thymus index, and spleen index in cyclophosphamide-induced immunosuppression in mice (197). The proliferation of normal and concanavalin A-induced splenic lymphocytes was also stimulated, showing its potential as an immunotherapeutic adjuvant (197). The methanol and diethyl ether extracts of M. charantia leaves exhibited significant therapeutic potential against S. typhi infections owing to immunomodulation activity (198). The extract also increased the antibody production and leukocyte mobilization against the infection.
Ficus aurantiaca
Triterpenoids isolated from stem of F. aurantiaca Griff have been investigated for inhibitory action on polymorphonuclear leucocyte chemotaxis and the production of neutrophil and whole-blood ROS. These components might act as potential lead molecules for the development of new immunomodulation agents for the innate immune response of phagocytes (199).
Gynostemma pentaphyllum
In vivo studies on G. pentaphyllum showed that its polysaccharides effectively increased the spleen and thymus indices, activated macrophage phagocytosis and NK cells, and exhibited activity on Con A/LPS-stimulated splenocytes in a dose-dependent manner. It also increased the IL-2 levels in sera and spleen, as well as the SOD, GSH-Px, T-AOC, GSH, and CAT levels; conversely, MDA levels were decreased (200). Aerial parts showed promising prospects as purposeful food and nutraceuticals owing to their numerous biological activities including antioxidant, immunomodulation, and antitumor (201). Multiple mechanisms of action have been proposed regarding the anticancer activities of plants including immunomodulating action (202).
Astragalus membranaceus
A. membranaceus is used as traditional medicine to treat cold and flu and is also administered as health tonic. The plant reportedly boosted the immune system, which was attributed to its major constituents, polysaccharides, flavonoids, amino acids, and minerals (188, 203). Another study reported that the oral administration of polysaccharides from A. membranaceus dose-dependently inhibited tumor growth and protected the immune system by promoting macrophage pinocytosis. Thus, the lymphocyte subsets in the peripheral blood of tumor-bearing mice increased (204). Several other studies showed it as an anti-inflammatory and antioxidant remedy against inflammation and gastrointestinal diseases (205, 206). The plant was also found to reduce intestinal mucosal damage and promoted tissue repair by inhibiting the expression of inflammatory cytokines (207).
Zizania latifolia
Z. latifolia is a traditional medicine also known as Chinese wild rice. It is rich in proteins, minerals, vitamins, and bioactive substances, such flavonoids, polysaccharides, and saponins, exhibiting therapeutic potential including immunomodulation and antioxidant activities and shows effectiveness in treating various diseases. Water-extractable polysaccharides isolated from swollen culms reportedly exhibited potent immunostimulation by increasing the proliferation, phagocytosis, and NO production of murine macrophages RAW 264.7. The plant has the potential to be considered as a promising immunomodulator in the field of medicine and functional foods (208, 209).
Moringa oleifera
M. oleifera is extensively utilized as food and medicine because of its wide range of nutritional and therapeutic potential including immunostimulatory effects. These effects are attributed to a number of bioactive principles, such as vitamins, flavonoids, minerals, isothiocyonates, and polyphenols present in the leaf, pod, seed, and bark of the plant (210). Several studies reported that the leaf extract stimulated humoral and cell-mediated immunity and might be useful as an alternative remedy under immunosuppressed conditions (211). The extract showed an increase in lymphocyte, neutrophil, and WBC count in cyclophosphamide-immunosuppressed rats, in addition to enhanced hemagglutination antibody titer in sheep red blood cells (212, 213). The plant is widely consumed in Uganda as an immunity booster to treat several diseases (210, 213). The leaf extract inhibits NF-kB action and NF-kB-dependent downstream events, thereby reducing the inflammatory processes and restoring the antioxidant status in mice fed with high-fat diet (214).
Allium sativum
A. sativum L. (Garlic) is frequently consumed as a spice worldwide and as a traditional medicine in China and India owing to its diverse health benefits. The therapeutic effects of the plant is attributed to the presence of a number of bioactive constituents, including organosulfurs, saponins, and polysaccharides. The strong immunomodulation activity of garlic is primarily because of its polysaccharide contents, which regulate interferon-γ, TNF-α, IL-6, and IL-10 in macrophages (215). Garlic extracts regulated immune-system homeostasis and maintained immune responses mainly by the expression and proliferation of cytokine genes for instance; diallyl trisulfide content present in garlic increased the T lymphocyte proliferation in mice. The protein fraction of garlic was reported to enhance the cytotoxicity of macrophages and lymphocytes (216). The occurrence and severity of flu and cold is also considerably reduced by the intake of aged garlic extract through immune-system improvement (217).
Emblica officinalis
E. officinalis (Amla) was shown to restore the Cr-induced immunosuppression by re-establishing the proliferation of lymphocytes and the production of IL-2 and INF-α (141). Furthermore, the fruit extract restored the antioxidant status to normal by inhibiting the Cr-induced production of free radicals and reversing Cr-induced DNA fragmentation and apoptosis in an in vitro experiment (218). Amla is extremely rich in vitamin C (even more than orange or lemon) and has been traditionally used as a tonic to recover vigor and lost energy. It also contains other bioactive constituents such as alkaloids, phenolic compounds, and tannins and exhibit a wide range of therapeutic actions, including antidiabetic, antioxidant, and anticancer. Amla stimulated the NK cell activity and antibody-dependent cellular cytotoxicity, enhancing 35% life span of tumor-bearing mice (219, 220). The immunomodulation mechanism of amla in reducing arsenic-induced oxidative damage and apoptosis in mice was also reported (221).
Origanum vulgare
Peppermint oregano (O. vulgare) is a popular herb in the mint family known for its important medicinal qualities. Carvacrol, the main constituent of essential oil, offered good antiviral properties against murine norovirus in an in vitro study (222). It also exhibited antiviral properties against HSV type-1 (HSV-1), rotavirus, and respiratory syncytial virus (RSV) (223).
Salvia officinalis
Stems and leaves of sage (Salvia officinalis) contain safficinolide, α-pinene, and β-myrcene which were derived from its volatile oil and showed good antiviral activities against HIV type-1 (HIV-1), HSV-1, and Indiana vesiculovirus (224). Another ex vivo study on the leaf extract of the plant showed presence of triterpenic compounds and verbascoside which were responsible for its immunomodulation activity (225).
Osimum basilicum
Basil (O. basilicum) (Tulsi) is rich in compounds such as apigenin and ursolic acid, exhibiting potent antiviral activity against herpes, hepatitis B, and enterovirus. Aerial parts of Tulsi increased the levels of helper T cells and NK cells to boost the immune system (226, 227). Trans anethole from the plant has powerful antiviral effects against herpes viruses, as well as potent immunomodulation activity (228).
Other Plants
There are a good number of other medicinal plants which have shown good to moderate immunomodulation and antiviral activities in various in vitro and in vivo models. For instance, oleanolic acid in rosemary (Salvia rosmarinus) has been proven to have good effect against herpes, HIV, influnza, and hepatitis A viruses (229). Sambucus, licorice, ginger, ginseng, and dandelion showed dominant antiviral activities in many laboratory experiments. Glycyrrhizin and lycorine from G. glabra and Lycoris radiate, respectively, showed good activity against SARS-CoV (230). Glycyrrhizin has even showed good potential against the COVID-19 virus SARS-CoV-2 and may emerge as an alternative drug for its treatment (231). Based on these findings, a novel combination of vitamin C, curcumin and glycyrrhizic acid (VCG plus) was proposed for the treatment of coronavirus infections (232). This combination is believed to regulate the innate immune response. Many other plants such as Centella asiatica, Zingeber officinale, Andrographis paniculate, Panax ginseng, Trigonella foemnum graecum, Picrorhiza scrophulariiflora, Phyllanthus debilis, Baliospermum montanum, Tinospora cordifolia, Curcuma longa, and Pouteria cambodiana reportedly displayed either immunostimulant or immunosuppressive properties by modulating acquired or innate immune responses (199). A number of single and compound drugs are advised in the Unani medicine based on the medicinal plants which are claimed to be antiviral, antipyretic, blood purifier, cardioprotective and expectorant activities and can be effective in the treatment and management of viral infections including COVID-19 (233).
The immunomodulation potential exhibited by plant extracts or active compounds offered novel sources of lead compounds for the development of new molecules intended to be used for treating various diseases including viral infections through immunotherapy. Apart from the plants mentioned in this review, numerous other plants also exhibited immunomodulation properties which might prove effective in fighting viral infections as direct or prophylactic therapy. The important plants showing immunomodulation activity, along with their regional sources, bioactive constituents, and other reported biological activities, are listed in Table 1.
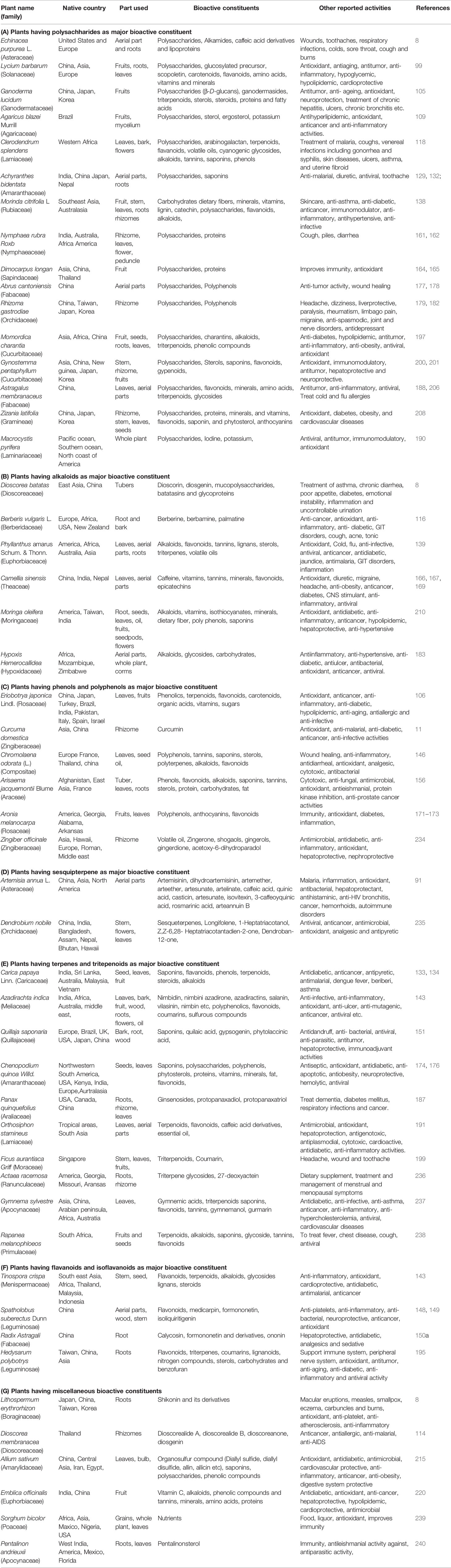
Table 1 List of selected plants demonstrating immunomodulation activity, source countries, parts used, their bioactive chemical constituents and other reported activities.
Traditional Chinese Medicines (TCM) for Prevention and Treatment of COVID-19
Traditional Chinese Medicine (TCM) is an ancient practice of therapy involving use of various forms of herbal medicines, exercise, acupuncture, dietary therapy and massage. It has been proven to be an effective approach to treat various health problems including viral infections. Recently, some reports were published regarding the effectiveness of the TCM in the prevention and treatment of COVID-19. According to the reports, TCM has been identified to be much effective in the treatment and prevention of COVID-19 and had played vital role in controlling the spread of the disease in China (241–243). Use of decoction based on the TCM principles among the medical professionals prevented the iatrogenic infection of the virus. TCM exhibited excellent curative potential; mainly responsible for early control over the spread of new viral infection and thought to be main reason for faster cure rate and discharge from the hospitals in China in comparison to other countries (1, 241, 244, 245). Treatment of COVID-19 based on TCM has also achieved good results as in one of the study (246), it was reported that out of 1016 diagnosed COVID-19 patients in Hunan province of China, 981 were treated with TCM and 95.76% (779 patients) of them were discharged. Moreover, out of the 233 hospitalized patients, all were cured using TCM with 100% recovery rate (246). In another study, 51 out of 52 COVID-19 patients receiving TCM treatment, showed either improvement or were cured and discharged, with one death (247). Decoction prepared from a mixture of Chinese herbs is the most popular therapy of COVID-19 in TCM and the herbs including Armeniacae Semen Amarum, Gypsum fibrosum, Glycyrrzhiae Radix et Rhizoma and Ephedra herba were the most commonly mentioned in Chinese guidelines (241, 248).
At molecular level, TCM products were reported to exhibit antiviral property either by directly inhibiting the viral particles by heat clearing and detoxification or indirectly through regulation of body’s immune response. In this regard, Gentiana, Astragalus, Acanthopaax senticosus and Salvia were found to induce Ig and IFN and produce antiviral activity (241). Chinese Multi herbal formula, Qing Fei Pai Du Tang (QFPDT) optimized for COVID-19 was recommended by government bodies contains quercetin, kaempferol, luteoline, isorrine and naringin as the first five active ingredients. QFPDT was found to act by modulation of body’s immune response, inhibition of inflammatory responses, protection of nerve and lung functions through regulation of MAPK1, MAPK3, MAPK8, IL-6, STA T1 and RELA and signaling pathways TNF and NF-kB (98, 249).
Future Perspectives
Dysfunction of immune system has been evidently implicated with the development of a number of diseases and modulating and maintaining an efficient immune system has proven to be a justified approach in the treatment of associated diseases. However, there are certain limitations to this approach owing to the complexity of immune system as well as the unavailability of expedient analytical tools to measure the immune functions. Future research on NK cells, cytokine receptors and markers and blood-borne particles are warranted. Despite these limitations, certain medicinal plants have been tested to offer novel avenues for the immune system modulation either alone or in combination and the results are very encouraging. In comparison to other immunity-targeted therapies such as the administration of monoclonal antibodies, plants and their derived products have shown important advantages including abundance and lesser adverse effects.
Nevertheless, these medicinal plants have to undergo standardization assessing both quality of the product and the methodology of research. Another scope of future research on medicinal plants is the chemical modification of active ingredients directed to enhance its efficacy and pharmacokinetic properties. For example, curcumin is not effective alone clinically owing to its absorption and metabolism issue, but chemically modified curcumin (demethoxycurcumin and bis-demoethoxycurcumin) have shown better druggability properties and are under clinical trials. Many of the active constituents of these medicinal plants face the pharmacokinetics related problems and suffer from poor absorption and rapid metabolism leading to poor bioavailability. Several alternate strategies are being adopted and researches are being focused on the development of nanotechnology-based formulations, changing the way of administration and changing the drug delivery technique.
Another promising approach is to test the combination of active constituents obtained from these medicinal plants which could achieve targeted therapy and have synergistic effects with reduced toxicities. Multitarget drugs can also be designed by linking the bioactive molecules by means of suitable spacers and fusion or amalgamation of the pharmacophoric elements which are essential for the activity to get the hybridized molecule. The mechanisms by which these medicinal plants and their bioactive molecules demonstrate immunomodulation are complex and involve multiple signal transduction pathways. Most of these products show extensive and multiple pharmacological effects and their targets and molecular mechanisms are not fully elucidated and understood. Most of the reported biological activities of these medicinal plants are based on cell based in vitro and animal based in vivo studies, but high level clinical studies are still required to establish their efficacies and toxicities in humans. It is therefore expected that with the advancements in the future molecular level studies, these medicinal plants and their derived products would demonstrate promising breakthroughs and constitute one of the important approaches for immunotherapy.
Conclusions
Respiratory diseases caused by viral infections including influenza and common cold are globally widespread apart from the repetitive viral outbreaks from SARS-CoV, MERS-CoV and SARS-CoV-2. Direct treatment of such viral infections by modern medicines are not very efficient, and although vaccination is considered to be effective in preventing viral infections, the incidence of acute respiratory infections could be reduced only to some extent. Consequently, alternative treatments for viral infections are necessary, and in this regard, the stimulation of the body’s immune defense system could be an efficient method of preventing these infections. A wide range of plant extracts, herbal products, and pure plant constituents have shown potential immunomodulation effects and used either as prophylactic or therapeutic agents for infectious diseases. Apart from infectious diseases, plant-based immunomodulators can help treat several other immunologic and inflammatory illnesses including cancer, rheumatoid arthritis, plaque psoriasis, Crohn’s disease, etc. These plant products and their bioactive molecules modulate immune responses through the stimulation and modification of lymphocytes, macrophages, and cytokine production. Many plant extracts contain a number of active principles, including polysaccharides, terpenoids, alkaloids, flavonoids, glycosides, and essential oils, which have tremendous capability to maintain and/or stimulate the immune system primarily through the modulation of nonspecific immune responses. However, these plant extracts may contain one or more of the active constituents and their relative contribution and mechanism in the generation of immunomodulation activity are difficult to understand. Isolated pure plant constituents may prove to be specific and effective immunomodulators capable of counteracting the high cost and adverse effects of modern medication. Nevertheless, the drawbacks associated with the application of plant products include the development of standard testing and quality control protocols need to be properly addressed. The present review highlights the importance of medicinal plants containing immunomodulating components of different chemistries with possible utilization in controlling the viral infections. This might stimulate the efforts of researchers towards developing alternative plant-based treatments for infectious diseases.
Author Contributions
HA and MA developed the concept of the manuscript. Literature survey was conducted by SM, HM and MT. Methodology and data curation were done by SS and WA. SS, SJ and AK wrote the draft manuscript, while writing-review and editing was performed by AN, AM and MA. HA supervised the work. All authors contributed to the article and approved the submitted version.
Conflict of Interest
The authors declare that the research was conducted in the absence of any commercial or financial relationships that could be construed as a potential conflict of interest.
References
1. Wang L, Wang Y, Ye D, Liu Q. Review of the 2019 Novel Coronavirus (SARS-CoV-2) Based on Current Evidence. Int J Antimicrob Agents (2020) 55(6):105948. doi: 10.1016/j.ijantimicag.2020.105948
2. Demicheli V, Jeerson T, Ferroni E, Rivetti A, Di Pietrantonj C. Vaccines for Preventing Influenza in Healthy Adults. Cochrane Database Syst Rev (2003) 2:CD001269. doi: 10.1002/14651858.CD001269.pub6
3. Qin C, Zhou L, Hu Z, Zhang S, Yang S, Tao Y, et al. Dysregulation of Immune Response in Patients With COVID-19 in Wuhan, China. Clin Infect Dis (2020) 12 pii:ciaa248. doi: 10.1093/cid/ciaa248
4. di Mauro G, Scavone C, Rafaniello C, Rossi F, Capuano A. Sars-CoV-2 Infection: Response of Human Immune System and Possible Implications for the Rapid Test and Treatment. Int Immunopharmacol (2020) 84:106519. doi: 10.1016/j.intimp.2020.106519
5. Shah VK, Firmal P, Alam A, Ganguly D, Chattopadhyay S. Overview of Immune Response During SARS-CoV-2 Infection: Lessons From the Past. Front Immunol (2020) 11:1949. doi: 10.3389/fimmu.2020.01949
6. Nugraha RV, Ridwansyah H, Ghozali M, Khairani AF, Atik N. Traditional Herbal Medicine Candidates as Complementary Treatments for COVID-19: A Review of Their Mechanisms, Pros and Cons. Evid Based Complement Altern Med (2020) 2020:2560645. doi: 10.1155/2020/2560645
7. Nahas R, Balla A. Complementary and Alternative Medicine for Prevention and Treatment of the Common Cold. Can Fam Phys (2011) 57:31–6.
8. Wen CC, Chen HM, Yang NS. Developing Phytocompounds From Medicinal Plants as Immunomodulators. Adv Bot Res (2012) 62:197–272. doi: 10.1016/B978-0-12-394591-4.00004-0
9. Drake CG, Lipson EJ, Brahmer JR. Breathing New Life Into Immunotherapy: Review of Melanoma, Lung and Kidney Cancer. Nat Rev Clin Oncol (2014) 11(1):24–37. doi: 10.1038/nrclinonc.2013.208
10. Ghareeb DA, Elwakeel EH, Khalil R, Aziz MS, El Demellawy MA. Investigation of the Immunomodulatory Effect of Berberis Vulgaris on Core-Pulsed Dendritic Cell Vaccine. BMC Complement Altern Med (2016) 16:325. doi: 10.1186/s12906-016-1327-2
11. Mimche PN, Taramelli D, Vivas L. The Plant-Based Immunomodulator Curcumin as a Potential Candidate for the Development of an Adjunctive Therapy for Cerebral Malaria. Malaria J (2011) 10(1):S10. doi: 10.1186/1475-2875-10-S1-S10
12. Norahmad NA, Razak MRMA, Misnan MN, Jelas NHM, Sastu UR, Muhammad A, et al. Effect of Freeze-Dried Carica Papaya Leaf Juice on Inflammatory Cytokines Production During Dengue Virus Infection in AG129 Mice. BMC Complement Med Ther (2019) 19:44. doi: 10.1186/s12906-019-2438-3
13. Childs CE, Calder P, Miles EA. Diet and Immune Function. Nutrients (2019) 11:1993. doi: 10.3390/nu11081933
14. Zhang LS, Davies SS. Microbial Metabolism of Dietary Components to Bioactive Metabolites: Opportunities for New Therapeutic Interventions. Genome Med (2016) 8(1):46. doi: 10.1186/s13073-016-0296-x
15. Calder PC, Carr AC, Gombart AF, Eggersdorfer M. Optimal Nutritional Status for a Well-Functioning Immune System is an Important Factor to Protect Against Viral Infections. Nutrients (2020) 12(4):1181. doi: 10.3390/nu12041181
16. Arshad MS, Khan U, Sadiq A, Khalid W, Hussain M, Yasmeen A, et al. Coronavirus Disease (COVID-19) and Immunity Booster Green Foods: A Mini Review. Food Sci Nutr (2020) 8(8):3971–6. doi: 10.1002/fsn3.1719
17. Gupta H, Gupta M, Bhargava S. Potential Use of Turmeric in COVID-19. Clin Exp Dermatol (2020) 45(7):902–3. doi: 10.1111/ced.14357
18. Elsayed Y, Khan NA. Immunity-Boosting Spices and the Novel Coronavirus. ACS Chem Neurosci (2020) 11(12):1696–8. doi: 10.1021/acschemneuro.0c00239
19. Alagawany M, Attia YA, Farag MR, Elnesr SS, Shafi M, Khafaga A, et al. The Strategy of Boosting the Immune System Under COVID-19 Pandemic. Front Vet Sci (2021) 7:570748. doi: 10.3389/fvets.2020.570748
20. Ferrara F, De Rosa F, Vitiello A. The Central Role of Clinical Nutrition in COVID-19 Patients During and After Hospitalization in Intensive Care Unit. SN Compr Clin Med (2020) 1–5. doi: 10.1007/s42399-020-00410-0
21. Pinto AJ, Morahan PS, Brinton MA. Comparative Study of Various Immunomodulators for Macrophage and Natural Killer Cell Activation and Antiviral Efficacy Against Exotic RNA Viruses. Int J Immunopharmacol (1988) 10(3):197–209. doi: 10.1016/0192-0561(88)90050-1
22. Pitlik SD. Covid-19 Compared to Other Pandemic Diseases. Rambam Maimonides Med J (2020) 11(3):e0027. doi: 10.5041/RMMJ.10418
23. Chaplin DD. Overview of the Immune Response. J Allergy Clan Immunol (2010) 125(Suppl. 2):S3–23. doi: 10.1016/j.jaci.2009.12.980
24. Vaure C, Liu Y. A Comparative Review of Toll-Like Receptor 4 Expression and Functionality in Different Animal Species. Front Immunol (2014) 5:316. doi: 10.3389/fimmu.2014.00316
25. Wong J, Choi SYC, Liu R, Xu E, Killam J, Gout PW, et al. Potential Therapies for Infectious Diseases Based on Targeting Immune Evasion Mechanisms That Pathogens Have in Common With Cancer Cells. Front Cell Infect Microbiol (2019) 9:25. doi: 10.3389/fcimb.2019.00025
26. Thakur A, Mikkelsen H, Jungersen G. Intracellular Pathogens: Host Immunity and Microbial Persistence Strategies. J Immunol Res (2019) 2019:1356540. doi: 10.1155/2019/1356540
27. Finlay BB, McFadden G. Anti-Immunology: Evasion of the Host Immune System by Bacterial and Viral Pathogens. Cell (2006) 124:767–82. doi: 10.1016/j.cell.2006.01.034
28. Reddick LE, Alto NM. Bacteria Fighting Back: How Pathogens Target and Subvert the Host Innate Immune System. Mol Cell (2014) 54:321–8. doi: 10.1016/j.molcel.2014.03.010
29. Dos Santos WG. Impact of Virus Genetic Variability and Host Immunity for the Success of COVID-19 Vaccines. BioMed Pharmacother (2021) 136:111272. doi: 10.1016/j.biopha.2021.111272
30. Balamurugan A, Claiborne D, Ng HL, Yang OO. HIV-1 Epitope Variability is Associated With T Cell Receptor Repertoire Instability and Breadth. J Virol (2017) 91:e00771–17. doi: 10.1128/JVI.00771-17
31. Zou ZQ, Wang L, Wang K, Yu JG. Innate Immune Targets of Hepatitis B Virus Infection. World J Hepatol (2016) 8:716–25. doi: 10.4254/wjh.v8.i17.716
32. Chan YK, Gack MU. Viral Evasion of Intracellular DNA and RNA Sensing. Nat Rev Microbiol (2016) 14:360–73. doi: 10.1038/nrmicro.2016.45
33. Wieland D, Hofmann M, Thimme R. Overcoming CD8+ T Cell Exhaustion in Viral Hepatitis: Lessons From the Mouse Model and Clinical Perspectives. Dig Dis (2017) 35:334–8. doi: 10.1159/000456584
34. Pymm P, Illing PT, Ramarathinam SH, O’Connor GM, Hughes VA, Hitchen C, et al. MHC-I Peptides Get Out of the Groove and Enable a Novel Mechanism of HIV-1 Escape. Nat Struct Mol Biol (2017) 24:387–94. doi: 10.1038/nsmb.3381
35. Schmiedel D, Mandelboim O. Disarming Cellular Alarm Systems Manipulation of Stress-Induced NKG2D Ligands by Human Herpesviruses. Front Immunol (2017) 8:390. doi: 10.3389/fimmu.2017.00390
36. Gack MU, Diamond MS. Innate Immune Escape by Dengue and West Nile Viruses. Curr Opin Virol (2016) 20:119–28. doi: 10.1016/j.coviro.2016.09.013
37. Wu NC, Wilson IA. A Perspective on the Structural and Functional Constraints for Immune Evasion: Insights From Influenza Virus. J Mol Biol (2017) 429:2694–709. doi: 10.1016/j.jmb.2017.06.015
38. Phelan D, Barrozo ER, Bloom DC. HSV1 Latent Transcription and non-Coding RNA: A Critical Retrospective. J Neuroimmunol (2017) 308:65–101. doi: 10.1016/j.jneuroim.2017.03.002
39. Hoppe-Seyler K, Bossler F, Lohrey C, Bulkescher J, Rösl F, Jansen L, et al. Induction of Dormancy in Hypoxic Human Papillomavirus Positive Cancer Cells. Proc Natl Acad Sci USA (2017) 114:E990–8. doi: 10.1073/pnas.1615758114
40. Bohn-Wippert K, Tevonian EN, Megaridis MR, Dar RD. Similarity in Viral and Host Promoters Couples Viral Reactivation With Host Cell Migration. Nat Commun (2017) 8:15006. doi: 10.1038/ncomms15006
41. Thaker SK, Ch’ng J, Christofk HR. Viral Hijacking of Cellular Metabolism. BMC Biol (2019) 17:59. doi: 10.1186/s12915-019-0678-9
42. Sanchez EL, Lagunoff M. Viral Activation of Cellular Metabolism. Virology (2015) 479:609–18. doi: 10.1016/j.virol.2015.02.038
43. Desai TM, Marin M, Mason C, Melikyan GB. Ph Regulation in Early Endosomes and Interferon-Inducible Transmembrane Proteins Control Avian Retrovirus Fusion. J Biol Chem (2017) 292:7817–27. doi: 10.1074/jbc.M117.783878
44. Masson JJ, Billings HW, Palmer CS. Metabolic Reprogramming During Hepatitis B Disease Progression Offers Novel Diagnostic and Therapeutic Opportunities. Antivir Chem Chemother (2017) 25:53–7. doi: 10.1177/2040206617701372
45. Markosyan RM, Miao C, Zheng YM, Melikyan GB, Liu SL, Cohen FS. Induction of Cell-Cell Fusion by Ebola Virus Glycoprotein: Low Ph is Not a Trigger. PloS Pathog (2016) 12:e1005373. doi: 10.1371/journal.ppat.1005373
46. Thompson MR, Kaminski JJ, Kurt-Jones EA, Fitzgerald KA. Pattern Recognition Receptors and the Innate Immune Response to Viral Infection. Viruses (2011) 3(6):920–40. doi: 10.3390/v3060920
47. Asami J, Shimizu T. Structural and Functional Understanding of the Toll-Like Receptors. Protein Sci (2021) 30(4):761–72. doi: 10.1002/pro.4043
48. Stok JE, Vega Quiroz ME, van der Veen AG. Self RNA Sensing by RIG-I-like Receptors in Viral Infection and Sterile Inflammation. J Immunol (2020) 205(4):883–91. doi: 10.4049/jimmunol.2000488
49. Seth R, Sun L, Chen Z. Antiviral Innate Immunity Pathways. Cell Res (2006) 16:141–7. doi: 10.1038/sj.cr.7310019
50. New JS, King RG, Kearney JF. Glycan Reactive Natural Antibodies and Viral Immunity. Viral Immunol (2020) 33(4):266–76. doi: 10.1089/vim.2019.0136
51. Zhang X, Paget M, Wang C, Zhu Z, Zheng H. Innate Immune Evasion by Picornaviruses. Eur J Immunol (2020) 50(9):1268–82. doi: 10.1002/eji.202048785
52. Weber F. Antiviral Innate Immunity: Introduction. Encyclopedia Virol (2021) 577–83. doi: 10.1016/B978-0-12-809633-8.21290-9
53. Brandes R, Lang F, Schmidt R. Physiologie Des Menschen: Mit Pathophysiologie. Berlin: Springer (2019).
54. Parnham MJ. Immunomodulatory Approaches to the Treatment of Infections. Croat J Infect (2011) 31:15–27.
55. Ramamurthy D, Nundalall T, Cingo S, Mungra N, Karaan M, Naran K, et al. Recent Advances in Immunotherapies Against Infectious Diseases. Immunother Adv (2021) 1(1):ltaa007. doi: 10.1093/immadv/ltaa007
56. Varadé J, Magadán S, González-Fernández Á. Human Immunology and Immunotherapy: Main Achievements and Challenges. Cell Mol Immunol (2021) 18(4):805–28. doi: 10.1038/s41423-020-00530-6
57. Rijckborst V, Sonneveld MJ, Janssen HL. Chronic Hepatitis B Anti-Viral or Immunomodulatory Therapy? Aliment Pharmacol Ther (2011) 33:501–13. doi: 10.1111/j.1365-2036.2010.04555.x
58. Darwish I, Mubareka S, Liles WC. Immunomodulatory Therapy for Severe Influenza. Expert Rev Anti Infect Ther (2011) 9:807–22. doi: 10.1586/eri.11.56
59. Pizzolla A, Smith JM, Brooks AG, Reading PC. Pattern Recognition Receptor Immunomodulation of Innate Immunity as a Strategy to Limit the Impact of Influenza Virus. J Leukoc Biol (2016) 101:851–61. doi: 10.1189/jlb.4MR0716-290R
60. Federico S, Pozzetti L, Papa A, Carullo G, Gemma S, Butini S, et al. Modulation of the Innate Immune Response by Targeting Toll-Like Receptors: A Perspective on Their Agonists and Antagonists. J Med Chem (2020) 63:13466–513. doi: 10.1021/acs.jmedchem.0c01049
61. Aram J, Frakich N, Morandi E, Alrouji M, Samaraweera A, Onion D, et al. Increased IL-2 and Reduced TGF-β Upon T-cell Stimulation are Associated With GM-CSF Upregulation in Multiple Immune Cell Types in Multiple Sclerosis. Biomedicines (2020) 8:226. doi: 10.3390/biomedicines8070226
62. Marischen L, Englert A, Schmitt AL, Einsele H, Loeffler J. Human NK Cells Adapt Their Immune Response Towards Increasing Multiplicities of Infection of Aspergillus Fumigatus. BMC Immunol (2018) 19(1):39. doi: 10.1186/s12865-018-0276-6
63. Jantan I, Ahmad W and Bukhari SNA. Plant-Derived Immunomodulators: An Insight on Their Preclinical Evaluation and Clinical Trials. Front Plant Sci (2015) 6:655. doi: 10.3389/fpls.2015.00655
64. Catanzaro M, Corsini E, Rosini M, Racchi M, Lanni C. Immunomodulators Inspired by Nature: A Review on Curcumin and Echinacea. Molecules (2018) 23(11):2778. doi: 10.3390/molecules23112778
65. Strayer DR, Carter WA. Recombinant and Natural Human Interferons: Analysis of the Incidence and Clinical Impact of Neutralizing Antibodies. J Interferon Cytokine Res (2012) 32:95–102. doi: 10.1089/jir.2011.0069
66. Divya M, Vijayakumar S, Chen J, Vaseeharan B, Durán-Lara EF. South Indian Medicinal Plant has the Ability to Combat Against Deadly Viruses Along With COVID-19? - A Review. Microb Pathog (2020) 148:104277. doi: 10.1016/j.micpath.2020.104277
67. Tungmunnithum D, Thongboonyou A, Pholboon A, Yangsabai A. Flavonoids and Other Phenolic Compounds From Medicinal Plants for Pharmaceutical and Medical Aspects: An Overview. Medicines (Basel) (2018) 5(3):93. doi: 10.3390/medicines5030093
68. Ganjhu RK, Mudgal PP, Maity H, Dowarha D, Devadiga S, Nag S, et al. Herbal Plants and Plant Preparations as Remedial Approach for Viral Diseases. Virus Dis (2015) 26:225–36. doi: 10.1007/s13337-015-0276-6
69. Hassan STS, Masarčíková R, Berchová K. Bioactive Natural Products With Anti-Herpes Simplex Virus Properties. J Pharm Pharmacol (2015) 67(10):1325–36. doi: 10.1111/jphp.12436
70. Rondanelli M, Miccono A, Lamburghini S, Avanzato I, Riva A, Allegrini P, et al. Self-Care for Common Colds: The Pivotal Role of Vitamin D, Vitamin C, Zinc, and Echinacea in Three Main Immune Interactive Clusters (Physical Barriers, Innate and Adaptive Immunity) Involved During an Episode of Common colds-Practical Advice on Dosages and on the Time to Take These Nutrients/Botanicals in Order to Prevent or Treat Common Colds. Evid Based Complement Alternat Med (2018) 2018:5813095. doi: 10.1155/2018/5813095
71. Ran L, Zhao W, Wang J, Wang H, Zhao Y, Tseng Y, et al. Extra Dose of Vitamin C Based on a Daily Supplementation Shortens the Common Cold: A Meta-Analysis of 9 Randomized Controlled Trials. BioMed Res Int (2018) 2018:1837634. doi: 10.1155/2018/1837634
72. Baladia E, Pizarro AB, Ortiz-Muñoz L, Rada G. Vitamin C for COVID-19: A Living Systematic Review. Medwave (2020) 20(6):e7978. doi: 10.5867/medwave.2020.06.7978
73. Beigmohammadi MT, Bitarafan S, Hoseindokht A, Abdollahi A, Amoozadeh L, Abadi MAM, et al. Impact of Vitamins A, B, C, D, and E Supplementation on Improvement and Mortality Rate in ICU Patients With Coronavirus-19: A Structured Summary of a Study Protocol for a Randomized Controlled Trial. Trials (2020) 21(1):614. doi: 10.1186/s13063-020-04547-0
74. Lee GY, Han SN. The Role of Vitamin E in Immunity. Nutrients (2018) 10(11):1614. doi: 10.3390/nu10111614
75. Meydani SN, Han SN, Hamer DH. Vitamin E and Respiratory Infection in the Elderly. Ann NYAcad Sci (2004) 1031:214–22. doi: 10.1196/annals.1331.021
76. Sun P, Kim Y, Lee H, Kim J, Han BK, Go E, et al. Carrot Pomace Polysaccharide (CPP) Improves Influenza Vaccine Efficacy in Immunosuppressed Mice Via Dendritic Cell Activation. Nutrients (2020) 12(9):2740. doi: 10.3390/nu12092740
77. Ratan ZA, Youn SH, Kwak YS, Han CK, Haidere MF, Kim JK, et al. Adaptogenic Effects of Panax Ginseng on Modulation of Immune Functions. J Ginseng Res (2021) 45(1):32–40. doi: 10.1016/j.jgr.2020.09.004
78. Boozari M, Hosseinzadeh H. Natural Products for COVID-19 Prevention and Treatment Regarding to Previous Coronavirus Infections and Novel Studies. Phytother Res (2021) 35(2):864–76. doi: 10.1002/ptr.6873
79. Dobrange E, Peshev D, Loedolff B, Van den Ende W. Fructans as Immunomodulatory and Antiviral Agents: The Case of Echinacea. Biomolecules (2019) 9(10):615. doi: 10.3390/biom9100615
80. Aarland RC, Bañuelos-Hernández AE, Fragoso-Serrano M, Sierra-Palacios ED, Díaz de León-Sánchez F, Pérez-Flores LJ, et al. Studies on Phytochemical, Antioxidant, Anti-Inflammatory, Hypoglycaemic and Antiproliferative Activities of Echinacea Purpurea and Echinacea Angustifolia Extracts. Pharm Biol (2017) 55(1):649–56. doi: 10.1080/13880209.2016.1265989
81. Manayi A, Vazirian M, Saeidnia S. Echinacea Purpurea: Pharmacology, Phytochemistry and Analysis Methods. Pharmacogn Rev (2015) 9(17):63–72. doi: 10.4103/0973-7847.156353
82. Karsch-Völk M, Barrett B, Kiefer D, Bauer R, Ardjomand-Woelkart K, Linde K. Echinacea for Preventing and Treating the Common Cold. Cochrane Database Syst Rev (2014) 2(2):CD000530. doi: 10.1002/14651858.CD000530.pub3
83. Huong PT, Jeon YJ. Macrophage Activation by Glycoprotein Isolated From Dioscorea Batatas. Toxicol Res (2011) 27(3):167–72. doi: 10.5487/TR.2011.27.3.167
84. Lim JS, Hahn D, Gu MJ, Oh J, Lee JS, Kim J-S. Anti-Inflammatory and Antioxidant Effects of 2, 7-dihydroxy-4, 6-Dimethoxy Phenanthrene Isolated From Dioscorea Batatas Decne. Appl Biol Chem (2019) 62:29. doi: 10.1186/s13765-019-0436-2
85. Jin M, Suh SJ, Yang JH, Lu Y, Kim SJ, Kwon S, et al. Antiinflammatory Activity of Bark of Dioscorea Batatas DECNE Through the Inhibition of iNOS and COX-2 Expressions in RAW264.7 Cells Via NFkappaB and ERK1/2 Inactivation. Food Chem Toxicol (2010) 48:3073–9. doi: 10.1016/j.fct.2010.07.048
86. Wen C-C, Shyur L-F, Jan J-T, Liang P-H, Kuo C-J, Arulselvan P, et al. Traditional Chinese Medicine Herbal Extracts of Cibotium Barometz, Gentiana Scabra, Dioscorea Batatas, Cassia Tora, and Taxillus Chinensis Inhibit SARS-CoV Replication. J Trad Complement Med (2011) 1(1):41–50. doi: 10.1016/S2225-4110(16)30055-4
87. Su PF, Staniforth V, Li CJ, Wang CY, Chiao MT, Wang SY, et al. Immunomodulatory Effects of Phytocompounds Characterized by In Vivo Transgenic Human GM-CSF Promoter Activity in Skin Tissues. J BioMed Sci (2008) 15:813–22. doi: 10.1007/s11373-008-9266-7
88. Septembre-Malaterre A, Lalarizo Rakoto M, Marodon C, Bedoui Y, Nakab J, Simon E, et al. Artemisia Annua, a Traditional Plant Brought to Light. Int J Mol Sci (2020) 21(14):4986. doi: 10.3390/ijms21144986
89. Namuli A, Bazira J, Casim TU. Engeu Po. A Review of Various Efforts to Increase Artemisinin and Other Antimalarial Compounds in Artemisia Annua L Plant. Cogent Biol (2018) 4:1. doi: 10.1080/23312025.2018.1513312
90. Adhikari B, Marasini BP, Rayamajhee B, Bhattarai BR, Lamichhane G, Khadayat K, et al. Potential Roles of Medicinal Plants for the Treatment of Viral Diseases Focusing on COVID-19: A Review. Phytother Res (2021) 35(3):1298–312. doi: 10.1002/ptr.6893
91. Suberu JO, Gorka AP, Jacobs L, Roepe PD, Sullivan N, Barker GC, et al. Anti-Plasmodial Polyvalent Interactions in Artemisia Annua L. Aqueous Extract- Possible Synergistic and Resistance Mechanisms. PloS One (2013) 8(11):e80790. doi: 10.1371/journal.pone.0080790
92. Cho HR, Cho Y, Kim J, Seo DB, Kim SH, Lee SJ, et al. The Effect of Gromwell (Lithospermum Erythrorhizon) Extract on the Stratum Corneum Hydration and Ceramides Content in Atopic Dermatitis Patients. Ann Dermatol (2008) 20(2):56–66. doi: 10.5021/ad.2008.20.2.56
93. Tatsumi K, Yano M, Kaminade K, Sugiyama A, Sato M, Toyooka K, et al. Characterization of Shikonin Derivative Secretion in Lithospermum Erythrorhizon Hairy Roots as a Model of Lipid-Soluble Metabolite Secretion From Plants. Front Plant Sci (2016) 7:1066. doi: 10.3389/fpls.2016.01066
94. Andújar I, Ríos JL, Giner RM, Recio MC. Pharmacological Properties of Shikonin - A Review of Literature Since 2002. Planta Med (2013) 79(18):1685–97. doi: 10.1055/s-0033-1350934
95. Chen X, Yang L, Zhang N, Turpin JA, Buckheit RW, Osterling C, et al. Shikonin, a Component of Chinese Herbal Medicine, Inhibits Chemokine Receptor Function and Suppresses Human Immunodeficiency Virus Type 1. Antimicrob Agents Chemother (2003) 47:2810–6. doi: 10.1128/aac.47.9.2810-2816.2003
96. Chiu SC, Tsao SW, Hwang PI, Vanisree S, Chen YA, Yang NS. Differential Functional Genomic Effects of Anti-Inflammatory Phytocompounds on Immune Signaling. BMC Genomics (2010) 11:513. doi: 10.1186/1471-2164-11-513
97. Zhu W, Zhou S, Liu J, McLean RJC, Chu W. Prebiotic, Immuno-Stimulating and Gut Microbiota-Modulating Effects of Lycium Barbarum Polysaccharide. BioMed Pharmacother (2020) 121:109591. doi: 10.1016/j.biopha.2019.109591
98. Feng L, Xiao X, Liu J, Wang J, Zhang N, Bing T, et al. Immunomodulatory Effects of Lycium Barbarum Polysaccharide Extract and its Uptake Behaviors At the Cellular Level. Molecules (2020) 25(6):1351. doi: 10.3390/molecules25061351
99. Cheng J, Zhou ZW, Sheng HP, He LJ, Fan XW, He ZX, et al. An Evidence-Based Update on the Pharmacological Activities and Possible Molecular Targets of Lycium Barbarum Polysaccharides. Drug Des Devel Ther (2015) 9:33–78. doi: 10.2147/DDDT.S72892eCollection2015
100. Deng X, Luo S, Luo X, Hu M, Ma F, Wang Y, et al. Polysaccharides From Chinese Herbal Lycium Barbarum Induced Systemic and Local Immune Responses in H22 Tumor-Bearing Mice. J Immunol Res (2018) 2018:3431782. doi: 10.1155/2018/3431782eCollection201
101. Wong JH, Ng TB, Chan HHL, Liu Q, Man GCW, Zhang CZ, et al. Mushroom Extracts and Compounds With Suppressive Action on Breast Cancer: Evidence From Studies Using Cultured Cancer Cells, Tumor-Bearing Animals, and Clinical Trials. Appl Microbiol Biotechnol (2020) 104(11):4675–703. doi: 10.1007/s00253-020-10476-4
102. Rubel R, Santa HSD, Dos Santos LF, Fernandes LC, Figueiredo BC, Soccol CR. Immunomodulatory and Antitumoral Properties of Ganoderma Lucidum and Agaricus Brasiliensis (Agaricomycetes) Medicinal Mushrooms. Int J Med Mushrooms (2018) 20(4):393–403. doi: 10.1615/IntJMedMushrooms.2018025979
103. Cizmarikova M. The Efficacy and Toxicity of Using the Lingzhi or Reishi Medicinal Mushroom, Ganoderma Lucidum (Agaricomycetes), and its Products in Chemotherapy (Review). Int J Med Mushrooms (2017) 19(10):861–77. doi: 10.1615/IntJMedMushrooms.2017024537
104. Lin ZB. Cellular and Molecular Mechanisms of Immuno-Modulation by Ganoderma Lucidum. J Pharmacol Sci (2005) 99(2):144–53. doi: 10.1254/jphs.crj05008x
105. Zeng P, Guo Z, Zeng X, Hao C, Zhang Y, Zhang M, et al. Chemical, Biochemical, Preclinical and Clinical Studies of Ganoderma Lucidum Polysaccharide as an Approved Drug for Treating Myopathy and Other Diseases in China. J Cell Mol Med (2018) 22(7):3278–97. doi: 10.1111/jcmm.13613
106. Liu Y, Zhang W, Xu C, Li X. Biological Activities of Extracts From Loquat (Eriobotrya Japonica Lindl.): A Review. Int J Mol Sci (2016) 17(12):1983. doi: 10.3390/ijms17121983
107. Kuraoka-Oliveira ÂM, Radai JAS, Leitão MM, Lima Cardoso CA, Silva-Filho SE, Leite Kassuya CA. Anti-Inflammatory and Anti-Arthritic Activity in Extract From the Leaves of Eriobotrya Japonica. J Ethnopharmacol (2020) 249:112418. doi: 10.1016/j.jep.2019.112418
108. Cai J, Chen T, Zhang Z, Li B, Qin G, Tian S. Metabolic Dynamics During Loquat Fruit Ripening and Postharvest Technologies. Front Plant Sci (2019) 10:619. doi: 10.3389/fpls.2019.00619
109. Li Y, Lu X, Li X, Guo X, Sheng Y, Li Y, et al. Effects of Agaricus Blazei Murrill Polysaccharides on Hyperlipidemic Rats by Regulation of Intestinal Microflora. Food Sci Nutr (2020) 8(6):2758–72. doi: 10.1002/fsn3.1568
110. Jiang L, Yu Z, Lin Y, Cui L, Yao S, Lv L, et al. Low-Molecular-Weight Polysaccharides From Agaricus Blazei Murrill Modulate the Th1 Response in Cancer Immunity. Oncol Lett (2018) 15(3):3429–36. doi: 10.3892/ol.2018.7794
111. Venturella G, Ferraro V, Cirlincione F, Gargano ML. Medicinal Mushrooms: Bioactive Compounds, Use, and Clinical Trials. Int J Mol Sci (2021) 22(2):634. doi: 10.3390/ijms22020634
112. Lu CC, Hsu YJ, Chang CJ, Lin CS, Martel J, Ojcius DM, et al. Immunomodulatory Properties of Medicinal Mushrooms: Differential Effects of Water and Ethanol Extracts on NK Cell-Mediated Cytotoxicity. Innate Immun (2016) 22(7):522–33. doi: 10.1177/1753425916661402
113. Saekoo J, Dechsukum C, Graidist P, Itharat A. Cytotoxic Effect and its Mechanism of Dioscorealide B From Dioscorea Membranacea Against Breast Cancer Cells. J Med Assoc Thai (2010) 93(Suppl 7):S277–82.
114. Panthong S, Ruangnoo S, Thongdeeying P, Sriwanthana B, Itharat A. Immunomodulatory Activity of Dioscorea Membranacea Pierre Rhizomes and of its Main Active Constituent Dioscorealide B. BMC Complement Altern Med (2014) 14:403. doi: 10.1186/1472-6882-14-403
115. Kalmarzi RN, Naleini SN, Ashtary-Larky D, Peluso I, Jouybari L, Rafi A, et al. Anti-Inflammatory and Immunomodulatory Effects of Barberry (Berberis Vulgaris) and its Main Compounds. Oxid Med Cell Longev (2019) 2019:6183965. doi: 10.1155/2019/6183965
116. Rahimi-Madiseh M, Lorigoini Z, Zamani-Gharaghoshi H, Rafieian-Kopaei M. Berberis Vulgaris: Specifications and Traditional Uses. Iran J Basic Med Sci (2017) 20(5):569–87. doi: 10.22038/IJBMS.2017.8690
117. Wang JH, Luan F, He XD, Wang Y, Li MX. Traditional Uses and Pharmacological Properties of Clerodendrum Phytochemicals. J Tradit Complement Med (2017) 8(1):24–38. doi: 10.1016/j.jtcme.2017.04.001
118. Kouakou K, Schepetkin IA, Jun S, Kirpotina LN, Yapi A, Khramova DS, et al. Immunomodulatory Activity of Polysaccharides Isolated From Clerodendrum Splendens: Beneficial Effects in Experimental Autoimmune Encephalomyelitis. BMC Complement Altern Med (2013) 13:149. doi: 10.1186/1472-6882-13-149
119. Faiella L, Temraz A, Cotugno R, De Tommasi N, Braca A. Diterpenes and Phenylpropanoids From Clerodendrum Splendens. Planta Med (2013) 79(14):1341–7. doi: 10.1055/s-0033-1350648
120. Ahmad W, Jantan I, Kumolosasi E, Haque MA, Bukhari SNA. Immunomodulatory Effects of Tinospora Crispa Extract and its Major Compounds on the Immune Functions of RAW 264.7 Macrophages. Int Immunopharmacol (2018) 60:141–51. doi: 10.1016/j.intimp.2018.04.046
121. Abood WN, Fahmi I, Abdulla MA, Ismail S. Immunomodulatory Effect of an Isolated Fraction From Tinospora Crispa on Intracellular Expression of INF-γ, IL-6 and IL-8. BMC Complement Altern Med (2014) 14:205. doi: 10.1186/1472-6882-14-205
122. Ounjaijean S, Chachiyo S. Somsak, Hypoglycemia Induced by Plasmodium Berghei Infection is Prevented by Treatment With Tinospora Crispa Stem Extract. Parasitol Int (2019) 68(1):57–9. doi: 10.1016/j.parint.2018.10.009
123. Panaro MA, Corrado A, Benameur T, Paolo CF, Cici D, Porro C. The Emerging Role of Curcumin in the Modulation of TLR-4 Signaling Pathway: Focus on Neuroprotective and Anti-Rheumatic Properties. Int J Mol Sci (2020) 21(7):2299. doi: 10.3390/ijms21072299
124. Yadav R, Jee B, Awasthi SK. Curcumin Suppresses the Production of Pro-Inflammatory Cytokine interleukin-18 in Lipopolysaccharide Stimulated Murine Macrophage-Like Cells. Indian J Clin Biochem (2015) 30(1):109–12. doi: 10.1007/s12291-014-0452-2
125. Praditya D, Kirchhoff L, Brüning J, Rachmawati H, Steinmann J, Steinmann E. Anti-Infective Properties of the Golden Spice Curcumin. Front Microbiol (2019) 10:912. doi: 10.3389/fmicb.2019.00912
126. Vitali D, Bagri P, Wessels JM, Arora M, Ganugula R, Parikh A, et al. Curcumin can Decrease Tissue Inflammation and the Severity of HSV-2 Infection in the Female Reproductive Mucosa. Int J Mol Sci (2020) 21(1):337. doi: 10.3390/ijms21010337
127. Li H, Zhong C, Wang Q, Chen W, Yuan Y. Curcumin is an APE1 Redox Inhibitor and Exhibits an Antiviral Activity Against KSHV Replication and Pathogenesis. Antiviral Res (2019) 167:98–103. doi: 10.1016/j.antiviral.2019.04.011
128. Lai Y, Yan Y, Liao S, Li Y, Ye Y, Liu N, et al. 3D-Quantitative Structure-Activity Relationship and Antiviral Effects of Curcumin Derivatives as Potent Inhibitors of Influenza H1N1 Neuraminidase. Arch Pharm Res (2020) 43(5):489–502. doi: 10.1007/s12272-020-01230-5
129. Zhu X, Pan Y, Zheng L, Cui L, Cao Y. Polysaccharides From the Chinese Medicinal Herb Achyranthes Bidentata Enhance Anti-Malarial Immunity During Plasmodium Yoelii 17XL Infection in Mice. Malar J (2012) 11:49. doi: 10.1186/1475-2875-11-49
130. Ou N, Sun Y, Zhou S, Gu P, Liu Z, Bo R, et al. Evaluation of Optimum Conditions for Achyranthes Bidentata Polysaccharides Encapsulated in Cubosomes and Immunological Activity In Vitro. Int J Biol Macromol (2018) 109:748–60. doi: 10.1016/j.ijbiomac.2017.11.064
131. He X, Wang X, Fang J, Chang Y, Ning N, Guo H, et al. The Genus Achyranthes: A Review on Traditional Uses, Phytochemistry, and Pharmacological Activities. J Ethnopharmacol (2017) 203:260–78. doi: 10.1016/j.jep.2017.03.035
132. Sun HX. Adjuvant Effect of Achyranthes Bidentata Saponins on Specific Antibody and Cellular Response to Ovalbumin in Mice. Vaccine (2006) 24(17):3432–9. doi: 10.1016/j.vaccine.2006.02.014
133. Agada R, Usman WA, Shehu S, Thagariki D. In Vitro and In Vivo Inhibitory Effects of Carica Papaya Seed on α-Amylase and α-Glucosidase Enzymes. Heliyon (2020) 6(3):e03618. doi: 10.1016/j.heliyon.2020.e03618
134. Nguyen TT, Parat MO, Shaw PN, Hewavitharana AK, Hodson MP. Traditional Aboriginal Preparation Alters the Chemical Profile of Carica Papaya Leaves and Impacts on Cytotoxicity Towards Human Squamous Cell Carcinoma. PloS One (2016) 11(2):e0147956. doi: 10.1371/journal.pone.0147956
135. Ojiako CM, Okoye EI, Oli AN, Ike CJ, Esimone CO, Attama AA. Preliminary Studies on the Formulation of Immune Stimulating Complexes Using Saponin From Carica Papaya Leaves. Heliyon (2019) 5(6):e01962. doi: 10.1016/j.heliyon.2019.e01962
136. Sadek KM. Antioxidant and Immunostimulant Effect of Carica Papaya Linn. Aqueous Extract in Acrylamide Intoxicated Rats. Acta Inform Med (2012) 20(3):180–5. doi: 10.5455/aim.2012.20.180-185
137. Abu N, Zamberi NR, Yeap SK, Nordin N, Mohamad NE, Romli MF, et al. Subchronic Toxicity, Immunoregulation and Anti-Breast Tumor Effect of Nordamnacantal, an Anthraquinone Extracted From the Stems of Morinda Citrifolia L. BMC Complement Altern Med (2018) 18(1):31. doi: 10.1186/s12906-018-2102-3
138. Kim SH, Seong GS, Choung SY. Fermented Morinda Citrifolia (Noni) Alleviates DNCB-induced Atopic Dermatitis in NC/Nga Mice Through Modulating Immune Balance and Skin Barrier Function. Nutrients (2020) 12(1):249. doi: 10.3390/nu12010249
139. Meena J, Sharma RA, Rolania R. A Review on Phytochemical and Pharmacological Properties of Phyllanthus Amarus Schum. and Thonn. Int J Pharm Sci Res (2018) 5:1377–86. doi: 10.13040/IJPSR.0975-8232.9(4
140. Paul S, Patra D, Kundu R. Lignan Enriched Fraction (LRF) of Phyllanthus Amarus Promotes Apoptotic Cell Death in Human Cervical Cancer Cells In Vitro. Sci Rep (2019) 9(1):14950. doi: 10.1038/s41598-019-51480-7
141. Jantan I, Haque MA, Ilangkovan M, Arshad L. An Insight Into the Modulatory Effects and Mechanisms of Action of Phyllanthus Species and Their Bioactive Metabolites on the Immune System. Front Pharmacol (2019) 10:878. doi: 10.3389/fphar.2019.00878
142. Alzohairy MA. Therapeutics Role of Azadirachta Indica (Neem) and Their Active Constituents in Diseases Prevention and Treatment. Evid Based Complement Alternat Med (2016) 2016:7382506. doi: 10.1155/2016/7382506
143. Ahmed W, Jantan I, Bukhari SNA. Tinospora Crispa (L.) Hook. F. & Thomson: A Review of its Ethnobotanical, Phytochemical, and Pharmacological Aspects. Front Pharmacol (2016) 7:59. doi: 10.3389/fphar.2016.00059
144. Moga MA, Bălan A, Anastasiu CV, Dimienescu OG, Neculoiu CD, Gavriş C. An Overview on the Anticancer Activity of Azadirachta Indica (Neem) in Gynecological Cancers. Int J Mol Sci (2018) 19(12):3898. doi: 10.3390/ijms19123898
145. Patel SM, Nagulapalli Venkata KC, Bhattacharyya P, Sethi G, Bishayee A. Potential of Neem (Azadirachta Indica L.) for Prevention and Treatment of Oncologic Diseases. Semin Cancer Biol (2016) 40-41:100–15. doi: 10.1016/j.semcancer.2016.03.002
146. Boudjeko T, Megnekou R, Woguia AL, Kegne FM, Ngomoyogoli JE, Tchapoum CD, et al. Antioxidant and Immunomodulatory Properties of Polysaccharides From Allanblackia Floribunda Oliv Stem Bark and Chromolaena Odorata (L.) King, and H.E. Robins Leaves. BMC Res Notes (2015) 8:759. doi: 10.1186/s13104-015-1703-x
147. Omotuyi OI, Nash O, Inyang OK, Ogidigo J, Enejoh O, Okpalefe O, et al. Flavonoid-Rich Extract of Chromolaena Odorata Modulate Circulating GLP-1 in Wistar Rats: Computational Evaluation of TGR5 Involvement. 3 Biotech (2018) 8(2):124. doi: 10.1007/s13205-018-1138-x
148. Chen HL, Yang J, Fu YF, Meng XN, Zhao WD, Hu TJ. Effect of Total Flavonoids of Spatholobus Suberectus Dunn on PCV2 Induced Oxidative Stress in RAW264.7 Cells. BMC Complement Altern Med (2017) 17(1):244. doi: 10.1186/s12906-017-1764-6
149. Fu YF, Jiang LH, Zhao WD, Xi-Nan M, Huang SQ, Yang J, et al. Immunomodulatory and Antioxidant Effects of Total Flavonoids of Spatholobus Suberectus Dunn on PCV2 Infected Mice. Sci Rep (2017) 7(1):8676. doi: 10.1038/s41598-017-09340-9
150. Liu J, Wei J, Wang C, Meng X, Chen H, Deng P, et al. The Combination of Radix Astragali and Radix Angelicae Sinensis Attenuates the IFN-γ-Induced Immune Destruction of Hematopoiesis in Bone Marrow Cells. BMC Complement Altern Med (2019) 19(1):356. doi: 10.1186/s12906-019-2781-4
151. Fleck JD, Betti AH, da Silva FP, Troian EA, Olivaro C, Ferreira F, et al. Saponins From Quillaja Saponaria and Quillaja Brasiliensis: Particular Chemical Characteristics and Biological Activities. Molecules (2019) 24(1):171. doi: 10.3390/molecules24010171
152. Ahmed Abdel-Reheim M, Messiha BAS, Abo-Saif AA. Quillaja Saponaria Bark Saponin Protects Wistar Rats Against Ferrous Sulphate-Induced Oxidative and Inflammatory Liver Damage. Pharm Biol (2017) 55(1):1972–83. doi: 10.1080/13880209.2017.1345950
153. Sudan R, Bhagat M, Gupta S, Singh J, Koul A. Iron (FeII) Chelation, Ferric Reducing Antioxidant Power, and Immune Modulating Potential of Arisaema Jacquemontii (Himalayan Cobra Lily). BioMed Res Int (2014) 2014:179865. doi: 10.1155/2014/179865
154. Ali H, Yaqoob U. Traditional Uses, Phytochemistry, Pharmacology and Toxicity of Arisaema (Areaceae): A Review. Bull Natl Res Cent (2021) 45:47. doi: 10.1186/s42269-021-00489-y
155. Kant K, Lal UR, Rawat R, Kumar A, Ghosh M. Genus Arisaema: A Review of Traditional Importance, Chemistry and Biological Activities. Comb Chem High Throughput Screen (2020) 23(7):624–48. doi: 10.2174/1386207323666200416150754
156. Tabassum S, Zia M, Carcahe de Blanco EJ, Batool R, Aslam R, Hussain S, et al. Phytochemical, in-Vitro Biological and Chemo-Preventive Profiling of Arisaema Jacquemontii Blume Tuber Extracts. BMC Complement Altern Med (2019) 19(1):256. doi: 10.1186/s12906-019-2668-4
157. Chen Z, Liu L, Gao C, Chen W, Vong CT, Yao P, et al. Astragali Radix (Huangqi): A Promising Edible Immunomodulatory Herbal Medicine. J Ethnopharmacol (2020) 258:112895. doi: 10.1016/j.jep.2020.112895
158. Lee YS, Han OK, Park CW, Suh SI, Shin SW, Yang CH, et al. Immunomodulatory Effects of Aqueous-Extracted Astragali Radix in Methotrexate-Treated Mouse Spleen Cells. J Ethnopharmacol (2003) 84(2-3):193–8. doi: 10.1016/s0378-8741(02)00298-2
159. Liu XY, Zhang YB, Yang XW, Yang YF, Xu W, Zhao W, et al. Anti-Inflammatory Activity of Some Characteristic Constituents From the Vine Stems of Spatholobus Suberectus. Molecules (2019) 24(20):3750. doi: 10.3390/molecules24203750
160. Kumar K, Sharma S, Kumar A, Bhardwaj P, Barhwal K, Hota SK. Acute and Sub-Acute Toxicological Evaluation of Lyophilized Nymphaea X Rubra Roxb. Ex Andrews Rhizome Extract. Regul Toxicol Pharmacol (2017) 88:12–21. doi: 10.1016/j.yrtph.2017.04.008
161. Cheng JH, Lee SY, Lien YY, Lee MS, Sheu SC. Immunomodulating Activity of Nymphaea Rubra Roxb. Extracts: Activation of Rat Dendritic Cells and Improvement of the T(H)1 Immune Response. Int J Mol Sci (2012) 13(9):10722–35. doi: 10.3390/ijms130910722
162. Devi SA, Thongam B, Handique PJ. Nymphaea Rubra Roxb. Ex Andrews Cultivated as an Ornamental, Food and Vegetable in the North Eastern Region of India. Genet Resour Crop Evol (2015) 62:315–20. doi: 10.1007/s10722-014-0177-3
163. Kunworarath N, Rangkadilok N, Suriyo T, Thiantanawat A, Satayavivad J. Longan (Dimocarpus Longan Lour.) Inhibits Lipopolysaccharide-Stimulated Nitric Oxide Production in Macrophages by Suppressing NF-κb and AP-1 Signaling Pathways. J Ethnopharmacol (2016) 179:156–61. doi: 10.1016/j.jep.2015.12.044
164. Meng FY, Ning YL, Qi J, He Z, Jie J, Lin JJ, et al. Structure and Antitumor and Immunomodulatory Activities of a Water-Soluble Polysaccharide From Dimocarpus Longan Pulp. Int J Mol Sci (2014) 15(3):5140–62. doi: 10.3390/ijms15035140
165. Yi Y, Liao ST, Zhang MW, Zhang RF, Deng YY, Yang B, et al. Immunomodulatory Activity of Polysaccharide-Protein Complex of Longan (Dimocarpus Longan Lour.) Pulp. Molecules (2011) 16(12):10324–36. doi: 10.3390/molecules161210324
166. Rahayu RP, Prasetyo RA, Purwanto DA, Kresnoadi U, Iskandar RPD, Rubianto M. The Immunomodulatory Effect of Green Tea (Camellia Sinensis) Leaves Extract on Immunocompromised Wistar Rats Infected by Candida Albicans. Vet World (2018) 11(6):765–70. doi: 10.14202/vetworld.2018.765-770
167. Chen FC, Shen KP, Ke LY, Lin HL, Wu CC, Shaw SY. Flavonoids From Camellia Sinensis (L.) O. Kuntze Seed Ameliorates Tnf-α Induced Insulin Resistance in HepG2 Cells. Saudi Pharm J (2019) 27(4):507–16. doi: 10.1016/j.jsps.2019.01.014
168. Jia LY, Wu XJ, Gao Y, Rankin GO, Pigliacampi A, Bucur H, et al. Inhibitory Effects of Total Triterpenoid Saponins Isolated From the Seeds of the Tea Plant (Camellia Sinensis) on Human Ovarian Cancer Cells. Molecules (2017) 22(10):1649. doi: 10.3390/molecules22101649
169. de Oliveira A, Prince D, Lo CY, Lee LH, Chu TC. Antiviral Activity of Theaflavin Digallate Against Herpes Simplex Virus Type 1. Antiviral Res (2015) 118:56–67. doi: 10.1016/j.antiviral.2015.03.009
170. Gajic D, Saksida T, Koprivica I, Vujicic M, Despotovic S, Savikin K, et al. Chokeberry (Aronia Melanocarpa) Fruit Extract Modulates Immune Response In Vivo and In Vitro. J Funct Foods (2020) 66:103836. doi: 10.1016/j.jff.2020.103836
171. Ho GTT, Bräunlich M, Austarheim I, Wangensteen H, Malterud KE, Slimestad R, et al. Immunomodulating Activity of Aronia Melanocarpa Polyphenols. Int J Mol Sci (2014) 15(7):11626–36. doi: 10.3390/ijms150711626
172. Bräunlich M, Slimestad R, Wangensteen H, Brede C, Malterud KE, Barsett H. Extracts, Anthocyanins and Procyanidins From Aronia Melanocarpa as Radical Scavengers and Enzyme Inhibitors. Nutrients (2013) 5(3):663–78. doi: 10.3390/nu5030663
173. Jurikova T, Mlcek J, Skrovankova S, Sumczynski D, Sochor J, Hlavacova I, et al. Fruits of Black Chokeberry Aronia Melanocarpa in the Prevention of Chronic Diseases. Molecules (2017) 22(6):944. doi: 10.3390/molecules22060944
174. Yao Y, Shi Z, Ren G. Antioxidant and Immunoregulatory Activity of Polysaccharides From Quinoa (Chenopodium Quinoa Willd.). Int J Mol Sci (2014) 15:19307–18. doi: 10.3390/ijms151019307
175. Hu Y, Zhang J, Zou L, Fu C, Li P, Zhao G. Chemical Characterization, Antioxidant, Immune-Regulating and Anticancer Activities of a Novel Bioactive Polysaccharide From Chenopodium Quinoa Seeds. Int J Biol Macromol (2017) 99:622–9. doi: 10.1016/j.ijbiomac.2017.03.019
176. El Hazzam K, Hafsa J, Sobeh M, Mhada M, Taourirte M, El Kacimi K, et al. An Insight Into Saponins From Quinoa (Chenopodium Quinoa Willd): A Review. Molecules (2020) 25(5):1059. doi: 10.3390/molecules25051059
177. Wu S, Fu X, You L, Abbasi AM, Meng H, Liu D, et al. Antioxidant, Antitumor and Immunomodulatory Activities of Water-Soluble Polysaccharides in Abrus Cantoniensis. Int J Biol Macromol (2016) 89:707–16. doi: 10.1016/j.ijbiomac.2016.04.005
178. Zeng Q, Xie H, Song H, Nie F, Wang J, Chen D, et al. In Vivo Wound Healing Activity of Abrus Cantoniensis Extract. Evid Based Complement Alternat Med (2016) 2016:6568528. doi: 10.1155/2016/6568528
179. Chen J, Tian S, Shu X, Du H, Li N, Wang J. Extraction, Characterization and Immunological Activity of Polysaccharides From Rhizoma Gastrodiae. Int J Mol Sci (2016) 17(7):1011. doi: 10.3390/ijms17071011
180. Liu Y, Gao J, Peng M, Meng H, Ma H, Cai P, et al. A Review on Central Nervous System Effects of Gastrodin. Front Pharmacol (2018) 9:24. doi: 10.3389/fphar.2018.00024
181. Chen PJ, Sheen LY. Gastrodiae Rhizoma (Tiān Má): A Review of Biological Activity and Antidepressant Mechanisms. J Tradit Complement Med (2011) 1(1):31–40. doi: 10.1016/s2225-4110(16)30054-2
182. Lee OH, Kim KI, Han CK, Kim YC, Hong HD. Effects of Acidic Polysaccharides From Gastrodia Rhizome on Systolic Blood Pressure and Serum Lipid Concentrations in Spontaneously Hypertensive Rats Fed a High-Fat Diet. Int J Mol Sci (2012) 13(1):698–709. doi: 10.3390/ijms13010698
183. Elbagory AM, Hussein AA, Meyer M. The In Vitro Immunomodulatory Effects of Gold Nanoparticles Synthesized From Hypoxis Hemerocallidea Aqueous Extract and Hypoxoside on Macrophage and Natural Killer Cells. Int J Nanomed (2019) 14:9007–18. doi: 10.2147/IJN.S216972
184. Boukes GJ, van de Venter M. Rooperol as an Antioxidant and its Role in the Innate Immune System: An In Vitro Study. J Ethnopharmacol (2012) 144(3):692–9. doi: 10.1016/j.jep.2012.10.014
185. Szczuka D, Nowak A, Zakłos-Szyda M, Kochan E, Szymańska G, Motyl I, et al. American Ginseng (Panax Quinquefolium L.) as a Source of Bioactive Phytochemicals With Pro-Health Properties. Nutrients (2019) 11(5):1041. doi: 10.3390/nu11051041
186. Yu Z-P, Xu D-D, Lu L-F, Zheng X-D, Chen W. Immunomodulatory Effect of a Formula Developed From American Ginseng and Chinese Jujube Extracts in Mice. J Zhejiang Univ Sci B (2016) 17(2):147–57. doi: 10.1631/jzus.B1500170
187. Mancuso C, Santangelo R. Panax Ginseng and Panax Quinquefolius: From Pharmacology to Toxicology. Food Chem Toxicol (2017) 107:362–72. doi: 10.1016/j.fct.2017.07.019
188. Roxas M, Jurenka J. Colds and Influenza: A Review of Diagnosis and Conventional, Botanical, and Nutritional Considerations. Altern Med Rev (2007) 12(1):25–48.
189. Vuksan V, Xu ZZ, Jovanovski E, Jenkins AL, Beljan-Zdravkovic U, Sievenpiper JL, et al. Efficacy and Safety of American Ginseng (Panax Quinquefolius L.) Extract on Glycemic Control and Cardiovascular Risk Factors in Individuals With Type 2 Diabetes: A Double-Blind, Randomized, Cross-Over Clinical Trial. Eur J Nutr (2019) 58(3):1237–45. doi: 10.1007/s00394-018-1642-0
190. Zhang W, Oda T, Yu Q, Jin JO. Fucoidan From Macrocystis Pyrifera has Powerful Immune-Modulatory Effects Compared to Three Other Fucoidans. Mar Drugs (2015) 13(3):1084–104. doi: 10.3390/md13031084
191. Ashraf K, Sultan S, Adam A. Orthosiphon Stamineus Benth. Is an Outstanding Food Medicine: Review of Phytochemical and Pharmacological Activities. J Pharm Bioallied Sci (2018) 10(3):109–18. doi: 10.4103/jpbs.JPBS_253_17
192. Kong C, Tan MW, Nathan S. Orthosiphon Stamineus Protects Caenorhabditis Elegans Against Staphylococcus Aureus Infection Through Immunomodulation. Biol Open (2014) 3(7):644–55. doi: 10.1242/bio.20148334
193. Alshawsh MA, Abdulla MA, Ismail S, Amin ZA, Qader SW, Hadi HA, et al. Free Radical Scavenging, Antimicrobial and Immunomodulatory Activities of Orthosiphon Stamineus. Molecules (2012) 17(5):5385–95. doi: 10.3390/molecules17055385
194. Zhao LG, Chen TQ, Feng DM, Xiao TG, Dang ZL, Feng SL, et al. Structural Characterization and Antioxidant Activity of a Heteropolysaccharide Isolated From Hedysarum Polybotrys. J Asian Nat Prod Res (2014) 16(6):677–84. doi: 10.1080/10286020.2014.893512
195. Huang GC, Lee CJ, Wang KT, Weng BC, Chien TY, Tseng SH, et al. Immunomodulatory Effects of Hedysarum Polybotrys Extract in Mice Macrophages, Splenocytes and Leucopenia. Molecules (2013) 18(12):14862–75. doi: 10.3390/molecules181214862
196. Dong Y, Tang D, Zhang N, Li Y, Zhang C, Li L, et al. Phytochemicals and Biological Studies of Plants in Genus Hedysarum. Chem Cent J (2013) 7(1):124. doi: 10.1186/1752-153X-7-124
197. Deng YY, Yi Y, Zhang LF, Zhang RF, Zhang Y, Wei ZC, et al. Immunomodulatory Activity and Partial Characterisation of Polysaccharides From Momordica Charantia. Molecules (2014) 19(9):13432–47. doi: 10.3390/molecules190913432
198. Mahamat O, Flora H, Tume C, Kamanyi A. Immunomodulatory Activity of Momordica Charantia L. (Cucurbitaceae) Leaf Diethyl Ether and Methanol Extracts on Salmonella Typhi-Infected Mice and LPS-induced Phagocytic Activities of Macrophages and Neutrophils. Evid Based Complement Alternat Med (2020) 2020:5248346. doi: 10.1155/2020/5248346
199. Mawa S, Jantan I, Husain K. Isolation of Terpenoids From the Stem of Ficus Aurantiaca Griff and Their Effects on Reactive Oxygen Species Production and Chemotactic Activity of Neutrophils. Molecules (2016) 21:9. doi: 10.3390/molecules21010009
200. Shang X, Chao Y, Zhang Y, Lu C, Xu C, Niu W. Immunomodulatory and Antioxidant Effects of Polysaccharides From Gynostemma Pentaphyllum Makino in Immunosuppressed Mice. Molecules (2016) 21(8):1085. doi: 10.3390/molecules21081085
201. Ji X, Shen Y, Guo X. Isolation, Structures, and Bioactivities of the Polysaccharides From Gynostemma Pentaphyllum (Thunb.) Makino: A Review. BioMed Res Int (2018) 2018:6285134. doi: 10.1155/2018/6285134
202. Li Y, Lin W, Huang J, Xie Y, Ma W. Anti-Cancer Effects of Gynostemma Pentaphyllum (Thunb.) Makino (Jiaogulan). Chin Med (2016) 11:43. doi: 10.1186/s13020-016-0114-9
203. Zheng Y, Ren W, Zhang L, Zhang Y, Liu D, Liu Y. A Review of the Pharmacological Action of Astragalus Polysaccharide. Front Pharmacol (2020) 11:349. doi: 10.3389/fphar.2020.00349
204. Liu AJ, Yu J, Ji HY, Zhang HC, Zhang Y, Liu HP. Extraction of a Novel Cold-Water-Soluble Polysaccharide From Astragalus Membranaceus and its Antitumor and Immunological Activities. Molecules (2017) 23(1):62. doi: 10.3390/molecules23010062
205. Auyeung KK, Han QB, Ko JK. Astragalus Membranaceus: A Review of its Protection Against Inflammation and Gastrointestinal Cancers. Am J Chin Med (2016) 44(1):1–22. doi: 10.1142/S0192415X16500014
206. Adesso S, Russo R, Quaroni A, Autore G, Marzocco S. Astragalus Membranaceus Extract Attenuates Inflammation and Oxidative Stress in Intestinal Epithelial Cells Via NF-κb Activation and Nrf2 Response. Int J Mol Sci (2018) 19(3):800. doi: 10.3390/ijms19030800
207. Cui Y, Wang Q, Sun R, Guo L, Wang M, Jia J, et al. Astragalus Membranaceus (Fisch.) Bunge Repairs Intestinal Mucosal Injury Induced by LPS in Mice. BMC Complement Altern Med (2018) 18(1):230. doi: 10.1186/s12906-018-2298-2
208. Yan N, Du Y, Liu X, Chu C, Shi J, Zhang H, et al. Morphological Characteristics, Nutrients, and Bioactive Compounds of Zizania Latifolia, and Health Benefits of its Seeds. Molecules (2018) 23(7):1561. doi: 10.3390/molecules23071561
209. Wang M, Zhao S, Zhu P, Nie C, Ma S, Wang N, et al. Purification, Characterization and Immunomodulatory Activity of Water Extractable Polysaccharides From the Swollen Culms of Zizania Latifolia. Int J Biol Macromol (2018) 107:882–90. doi: 10.1016/j.ijbiomac.2017.09.062
210. Vergara-Jimenez M, Almatrafi MM, Fernandez ML. Bioactive Components in Moringa Oleifera Leaves Protect Against Chronic Disease. Antioxid (Basel) (2017) 6(4):91. doi: 10.3390/antiox6040091
211. Obi A, Egwurugwu JN, Ojefa SO, Ohamaeme MC, Ekweogu CN, Ogunnaya FU. Immunomodulatory Effects of Hydromethanolic Extract of Moringa Oleifera Leaf on Male Wistar Rats. Niger J Exp Clin Biosci (2018) 6:26–32. doi: 10.4103/njecp.njecp_13_18
212. Gupta A, Gautam MK, Singh RK, Kumar MV, ChV R, RK G, et al. Immunomodulatory Effect of Moringa Oleifera Lam. Extract on Cyclophosphamide Induced Toxicity in Mice. Indian J Exp Biol (2010) 48(11):1157–60.
213. Nfambi J, Bbosa GS, Sembajwe LF, Gakunga J, Kasolo JN. Immunomodulatory Activity of Methanolic Leaf Extract of Moringa Oleifera in Wistar Albino Rats. J Basic Clin Physiol Pharmacol (2015) 26(6):603–11. doi: 10.1515/jbcpp-2014-0104
214. Das N, Sikder K, Ghosh S, Fromenty B, Dey S. Moringa Oleifera Lam. Leaf Extract Prevents Early Liver Injury and Restores Antioxidant Status in Mice Fed With High-Fat Diet. Indian J Exp Biol (2012) 50(6):404–12.
215. Shang A, Cao SY, Xu XY, Gan RY, Tang GY, Corke H, et al. Bioactive Compounds and Biological Functions of Garlic (Allium Sativum L). Foods (2019) 8(7):246. doi: 10.3390/foods8070246
216. Moutia M, Habti N, Badou A. In Vitro and In Vivo Immunomodulator Activities of Allium Sativum L. Evid Based Complement Alternat Med (2018) 2018:4984659. doi: 10.1155/2018/4984659
217. Percival SS. Aged Garlic Extract Modifies Human Immunity. J Nutr (2016) 146:433S–6S. doi: 10.3945/jn.115.210427
218. Ram MS, Neetu D, Yogesh B, Anju B, Dipti P, Pauline T, et al. Cyto-Protective and Immunomodulating Properties of Amla (Emblica Officinalis) on Lymphocytes: An in-Vitro Study. J Ethnopharmacol (2002) 81(1):5–10. doi: 10.1016/s0378-8741(01)00421-4
219. Variya BC, Bakrania AK, Patel SS. Emblica Officinalis (Amla): A Review for its Phytochemistry, Ethnomedicinal Uses and Medicinal Potentials With Respect to Molecular Mechanisms. Pharmacol Res (2016) 111:180–200. doi: 10.1016/j.phrs.2016.06.013
220. Bhandari PR, Kamdod MA. Emblica Officinalis (Amla): A Review of Potential Therapeutic Applications. Int J Green Pharm (2012) 6:257–69. doi: 10.4103/0973-8258.108204
221. Singh MK, Yadav SS, Gupta V, Khattri S. Immunomodulatory Role of Emblica Officinalis in Arsenic Induced Oxidative Damage and Apoptosis in Thymocytes of Mice. BMC Complement Altern Med (2013) 13:193. doi: 10.1186/1472-6882-13-193
222. Han F, Ma GQ, Yang M, Yan L, Xiong W, Shu JC, et al. Chemical Composition and Antioxidant Activities of Essential Oils From Different Parts of the Oregano. J Zhejiang Univ Sci B (2017) 18(1):79–84. doi: 10.1631/jzus.B1600377
223. Zhang XL, Guo YS, Wang CH, Li GQ, Xu JJ, Chung HY, et al. Phenolic Compounds From Origanum Vulgare and Their Antioxidant and Antiviral Activities. Food Chem (2014) 152:300–6. doi: 10.1016/j.foodchem.2013.11.153
224. Loizzo MR, Saab AM, Tundis R, Statti GA, Menichini F, Lampronti I, et al. Phytochemical Analysis and In Vitro Antiviral Activities of the Essential Oils of Seven Lebanon Species. Chem Biodivers (2008) 5(3):461–70. doi: 10.1002/cbdv.200890045
225. Salomón R, Firmino JP, Reyes-López FE, Andree KB, González-Silvera D, Esteban MA, et al. The Growth Promoting and Immunomodulatory Effects of a Medicinal Plant Leaf Extract Obtained From Salvia Officinalis and Lippia Citriodora in Gilthead Seabream (Sparus Aurata). Aquaculture (2020) 524:735291. doi: 10.1016/j.aquaculture.2020.735291
226. Eftekhar N, Moghimi A, Mohammadian Roshan N, Saadat S, Boskabady MH. Immunomodulatory and Anti-Inflammatory Effects of Hydro-Ethanolic Extract of Ocimum Basilicum Leaves and its Effect on Lung Pathological Changes in an Ovalbumin-Induced Rat Model of Asthma. BMC Complement Altern Med (2019) 19(1):349. doi: 10.1186/s12906-019-2765-4
227. Romeilah RM, Fayed SA, Mahmoud GI. Chemical Compositions, Antiviral and Antioxidant Activities of Seven Essential Oils. J Appl Sci Res (2010) 6(1):50–62.
228. Badgujar SB, Patel VV, Bandivdekar AH. Foeniculum Vulgare Mill: A Review of its Botany, Phytochemistry, Pharmacology, Contemporary Application, and Toxicology. BioMed Res Int (2014) 2014:842674. doi: 10.1155/2014/842674
229. Jiang RW, Lau KM, Hon PM, Mak TC, Woo KS, Fung KP. Chemistry and Biological Activities of Caffeic Acid Derivatives From Salvia Miltiorrhiza. Curr Med Chem (2005) 12(2):237–46. doi: 10.2174/0929867053363397
230. Mukherjee PK. Antiviral Evaluation of Herbal Drugs. Qual Control Eval Herbal Drugs (2019) 599–628. doi: 10.1016/B978-0-12-813374-3.00016-8
231. Bailly C, Vergoten G. Glycyrrhizin: An Alternative Drug for the Treatment of COVID-19 Infection and the Associated Respiratory Syndrome? Pharmacol Ther (2020) 214:107618. doi: 10.1016/j.pharmthera.2020.107618
232. Chen L, Hu C, Hood M, Zhang X, Zhang L, Kan J, et al. A Novel Combination of Vitamin C, Curcumin and Glycyrrhizic Acid Potentially Regulates Immune and Inflammatory Response Associated With Coronavirus Infections: A Perspective From System Biology Analysis. Nutrients (2020) 12(4):1193. doi: 10.3390/nu12041193
233. Fatima S, Haider N, Alam MA, Gani MA, Ahmad R, Taha M. Herbal Approach for the Management of C0VID-19: An Overview. Drug Metab Pers Ther (2020). doi: 10.1515/dmdi-2020-0150
234. An S, Guanzhong L, Guo X, An Y, Wang R. Ginger Extract Enhances Antioxidant Ability and Immunity of Layers. Anim Nutr (2019) 5(4):407–9. doi: 10.1016/j.aninu.2019.05.003
235. Meitei AL, Pamarthi RK, Kumar R, Bhutia NT, Rai D, Babu PK, et al. Dendrobium Nobile Orchid in Traditional Medicine - A Phytochemical Analysis. Indian J Hort (2019) 76(3):557–60. doi: 10.5958/0974-0112.2019.00090.2
236. Smith MJ, Germolec DR, Frawley RP, White KL Jr. Immunomodulatory Effects of Black Cohosh (Actaea Racemosa) Extract in Female B6C3F1/N Mice. Toxicology (2013) 308:146–57. doi: 10.1016/j.tox.2013.03.017
237. Khan F, Sarker M, Ming LC, Mohamed IN, Zhao C, Sheikh BY, et al. Comprehensive rReview on Phytochemicals, Pharmacological and Clinical Potentials of Gymnema Sylvestre. Front Pharmacol (2019) 10:1223. doi: 10.3389/fphar.2019.01223
238. Mehrbod P, Abdalla MA, Fotouhi F, Heidarzadeh M, Aro AO, Eloff JN, et al. Immunomodulatory Properties of Quercetin-3-O-α-L-rhamnopyranoside From Rapanea Melanophloeos Against Influenza A Virus. BMC Complement Altern Med (2018) 18(1):184. doi: 10.1186/s12906-018-2246-1
239. Benson KF. West African Sorghum Bicolor Leaf Sheaths Have Anti-Inflammatory and Immune-Modulating Properties In Vitro. J Med Food (2013) 16(3):230–8. doi: 10.1089/jmf.2012.0214
240. Oghumu S, Varikuti S, Saljoughian N, Terrazas C, Huntsman AC, Parinandi NL, et al. Pentalinonsterol, a Constituent of Pentalinon Andrieuxii, Possesses Potent Immunomodulatory Activity and Primes T Cell Immune Responses. J Nat Prod (2017) 80(9):251523. doi: 10.1021/acs.jnatprod.7b00445
241. Zhao Z, Li Y, Zhou L, Zhou X, Xie B, Zhang W, et al. Prevention and Treatment of COVID-19 Using Traditional Chinese Medicine: A Review. Phytomedicine (2020) 20:153308. doi: 10.1016/j.phymed.2020.153308
242. Deng JG, Hou XT, Zhang TJ, Bai G, Hao EW, Chu JJH, et al. Carry Forward Advantages of Traditional Medicines in Prevention and Control of Outbreak of COVID-19 Pandemic. Chin Herb Med (2020) 12(3):207–13. doi: 10.1016/j.chmed.2020.05.003
243. Ahsan W, Alhazmi HA, Patel KS, Mangla B, Al Bratty M, Javed S, et al. Recent Advancements in the Diagnosis, Prevention, and Prospective Drug Therapy of COVID-19. Front Public Health (2020) 8:384. doi: 10.3389/fpubh.2020.00384
244. Luo Y, Wang CZ, Hesse-Fong J, Lin JG, Yuan CS. Application of Chinese Medicine in Acute and Critical Medical Conditions. Am J Chin Med (2019) 47(6):1223–35. doi: 10.1142/S0192415X19500629
245. Li K, Qiu R. TCM Prevention Measures of Novel Coronavirus Pneumonia Based on the “Natural Factor” and the “Human Factor”. Acta Chin Med (2020).
246. Lou E, Zhang D, Lou H, Liu B, Zhao K, Zhao Y, et al. Treatment Efficacy Analysis of Traditional Chinese Medicine for Novel Coronavirus Pneumonia (COVID-19): An Empirical Study from Wuhan, Hubei Province, China. Chin Med (2020) 15:34. doi: 10.1186/s13020-020-00317-x
247. Chen R, Luo YP, Xu XH, Miao Q, Wang YG. Preliminary Exploration of TCM Syndrome and Analysis of Typical Cases Based on 52 Cases of New Coronavirus Pneumonia in Wuhan. J Traditional Chin Med (2020).
248. Ang L, Lee HW, Kim A, Lee MS. Herbal Medicine for the Management of COVID-19 During the Medical Observation Period: A Review of Guidelines. Integr Med Res (2020) 9(3):100465. doi: 10.1016/j.imr.2020.100465
Keywords: COVID-19, phytoconstituents, immunomodulation, cellular immunity, humoral, viral infections, traditional Chinese medicine
Citation: Alhazmi HA, Najmi A, Javed SA, Sultana S, Al Bratty M, Makeen HA, Meraya AM, Ahsan W, Mohan S, Taha MME and Khalid A (2021) Medicinal Plants and Isolated Molecules Demonstrating Immunomodulation Activity as Potential Alternative Therapies for Viral Diseases Including COVID-19. Front. Immunol. 12:637553. doi: 10.3389/fimmu.2021.637553
Received: 10 December 2020; Accepted: 26 April 2021;
Published: 13 May 2021.
Edited by:
Antonio Toniolo, University of Insubria, ItalyReviewed by:
Manojit Bhattacharya, Fakir Mohan University, IndiaMuhammad Bilal, Shanghai Jiao Tong University, China
Birbal Singh, Indian Veterinary Research Institute (IVRI), India
Copyright © 2021 Alhazmi, Najmi, Javed, Sultana, Al Bratty, Makeen, Meraya, Ahsan, Mohan, Taha and Khalid. This is an open-access article distributed under the terms of the Creative Commons Attribution License (CC BY). The use, distribution or reproduction in other forums is permitted, provided the original author(s) and the copyright owner(s) are credited and that the original publication in this journal is cited, in accordance with accepted academic practice. No use, distribution or reproduction is permitted which does not comply with these terms.
*Correspondence: Sadique A. Javed, sajaved@jazanu.edu.sa