- 1National Clinical Research Center for Geriatric Disorders, Xiangya Hospital, Central South University, Changsha, China
- 2Research Center of Carcinogenesis and Targeted Therapy, Xiangya Hospital, Central South University, Changsha, China
- 3The Higher Educational Key Laboratory for Cancer Proteomics and Translational Medicine of Hunan Province, Xiangya Hospital, Central South University, Changsha, China
- 4Hunan Key Laboratory of Viral Hepatitis, Xiangya Hospital, Central South University, Changsha, China
- 5Huaihua Key Laboratory of Research and Application of Novel Molecular Diagnostic Techniques, School of Public Health & Laboratory Medicine, Hunan University of Medicine, Huaihua, China
- 6Department of Hunan key laboratary of aging biology, Xiangya Hospital, Central South University, Changsha, China
- 7Department of Dermatology, Xiangya Hospital, Central South University, Changsha, China
The Na+/K+-ATPase (NKA), has been proposed as a signal transducer involving various pathobiological processes, including tumorigenesis. However, the clinical relevance of NKA in hepatocellular carcinoma (HCC) has not been well studied. This study revealed the upregulation of mRNA of ATP1A1, ATP1B1, and ATP1B3 in HCC using TCGA, ICGC, and GEO database. Subsequently, ATP1B3 was demonstrated as an independent prognostic factor of overall survival (OS) of HCC. To investigate the potential mechanisms of ATP1B3 in HCC, we analyzed the co-expression network using LinkedOmics and found that ATP1B3 co-expressed genes were associated with immune-related biological processes. Furthermore, we found that ATP1B3 was correlated immune cell infiltration and immune-related cytokines expression in HCC. The protein level of ATP1B3 was also validated as a prognostic significance and was correlated with immune infiltration in HCC using two proteomics datasets. Finally, functional analysis revealed that ATP1B3 was increased in HCC cells and tissues, silenced ATP1B3 repressed HCC cell proliferation, migration, and promoted HCC cell apoptosis and epithelial to mesenchymal transition (EMT). In conclusion, these findings proved that ATP1B3 could be an oncogene and it was demonstrated as an independent prognostic factor and correlated with immune infiltration in HCC, revealing new insights into the prognostic role and potential immune regulation of ATP1B3 in HCC progression and provide a novel possible therapeutic strategy for HCC.
Introduction
Hepatocellular carcinoma (HCC) is a primary liver cancer with high mortality and is the most common malignancy (1), which occurs frequently in Asia, Africa, southern Europe and China (2). Although early surgical resection and liver transplantation are effective treatments for HCC (3), the 5-year recurrence rate for HCC remains poor because of its high recurrence and metastasis rates (4). Therefore, useful prognostic and therapeutic indicators are urgently needed.
The ion transporter Na+/K+-ATPase (NKA) is a transmembrane protein that transports Na+ and K+ across cell membranes (5), which is essential for the cellular electrochemical gradient (6), ion homeostasis (7), cell adhesion (8), and intracellular signaling (9). The functional NKA consists of α subunits and β subunits. So far, 4 NKA α-subunits (α1, α2, α3, and α4) and 4 β-subunits (β1, β2, β3, and β4) have been identified. The abnormal NKA could lead to a variety of diseases, including hypokalaemic periodic paralysis and CNS symptoms (10), cardiovascular disorders (11), atherosclerosis (12), Alzheimer (13). Recent studies showed that NKA was dysregulated in multiple cancers and involved in the progression of these cancers (14). For example, Mathieu et al. (15) showed that the NKA α1 subunit is highly expressed in human melanoma and involved in cell migration and apoptosis. Lee et al. (16) reported that the NKA β1 subunit is low-expressed in medulloblastoma. Bechmann et al. (17) revealed that NKA α1, α3, and β1 subunits were highly expressed in colorectal cancers and associated with tumor metastases. Nevertheless, the clinical relevance of NKA in HCC remains unclear.
In this study, we investigated the expression of NKA α/β subunits in HCC using 6 independent public datasets. We demonstrated ATP1B3 as a prognostic factor which is correlated with immune infiltrating in HCC. Functional analysis revealed ATP1B3 as a potential oncogene of HCC, indicating that ATP1B3 as a diagnostic and potential therapeutic target in HCC.
Materials and Methods
NKA Expression in Different Datasets
The expression levels of NKA α/β subunits in HCC were identified from ICGC (https://icgc.org/daco) and TCGA (https://cancergenome.nih.gov/) datasets (18). Then, the expression levels of ATP1A1, ATP1B1, and ATP1B3 were verified in three independent GEO datasets (GSE45436, GSE76427 and GSE102079) download from https://www.ncbi.nlm.nih.gov/gds (19).
The transcription levels of NKA genes in various cancers were detected in the GEPIA database (http://gepia.cancer-pku.cn/) (20) and ONCOMINE database (https://www.oncomine.org/) (21). The thresholds were set as: logFC > 1 and p < 0.01.
Survival Analysis
The prognostic value of ATP1A1, ATP1B1, and ATP1B3 for HCC in the TCGA database were appraised by the Kaplan-Meier plotter database (http://kmplot.com/analysis/) (22) and then validated using the ICGC database using R software (version 3.5.2).
The Relationship Between ATP1B3 and Clinical Characteristics of HCC
The expression of ATP1B3 in HCC patients with different clinical characteristics was analyzed using R software and then validated using the UALCAN database (http://ualcan.path.uab.edu) (23). The significance of differential gene expression was assessed by t-test and one-way ANOVA. *, p < 0.05; **, p < 0.01; ***, p < 0.001.
LinkedOmics Database Analysis
The co-expressed genes of ATP1B3 in HCC was detected using LinkedOmics (http://www.linkedomics.org/login.php). Co-expressed genes can be analyzed statistically and displayed in the volcano, Heat maps. Gene set enrichment analysis (GSEA) can also be used in LinkedOmics functional modules to perform Gene Ontology (GO) term annotation, KEGG pathway analysis, and target enrichment of kinases, miRNAs, and transcription factors’ (TF) (24). Pearson test was used to evaluate the significant correlation of co-expressed genes. FDR < 0.01 was significant expression, p < 0.05 was significantly related genes.
Correlations of ATP1B3 Expression With Immune Infiltration in TIMER and GEPIA
The association between ATP1B3 and immune cells infiltration in HCC was confirmed using the TIMER database (http://cistrome.org/TIMER/). It provides the infiltration of 6 types of immune cells to assess the abundance of immune infiltration (25, 26). Furthermore, the expression of ATP1B3 in immune subtypes and molecular subtypes in HCC was identified using the TISIDB database (http://cis.hku.hk/TISIDB/). It integrates a large amount of tumor immunity-related data, including 988 genes related to anti-tumor immunity, and can analyze the data of 30 TCGA cancer types to calculate the gene expression of immune subtypes and molecular subtypes in different tumors (27).
Next, the correlations between ATP1B3 and immune markers expression in HCC was investigated using the TIMER and GEPIA databases. These immune markers have been referenced previously (28). The correlation between ATP1B3 and each immune gene markers was presented using scatterplots, Pearson test was used for statistical significance evaluation, and log2 RSEM was adopted to regulate gene expression levels. ATP1B3 was plotted on the y-axis, while marker genes are plotted on the x-axis.
Proteomics Database Analysis
The expression and prognosis of ATP1B3 protein were generated using the CPTAC proteomics database (https://cptac-data-portal.georgetown.edu/cptacPublic/). Moreover, the proteomics and phospho-proteomics data from 316 HCC patients were download from Gao’s work (29). These data can well verify the relationship between proteins and survival and clinical, and find candidate proteins that can be used as tumor biomarkers (30, 31).
Cell Lines and Culture
HCC cell lines (Huh7 and HCCLM3) and human normal liver cell (LO2) were obtained from American Type Culture Collection (ATCC, Manassas, VA, USA). Huh7 and HCCLM3 were cultured in RPMI-1640 medium (BI, Israel) containing 10% FBS (BI, Israel) at 37° C in 5% CO2. And LO2 cultured in DMEM (BI, Israel) medium containing 10% FBS (BI, Israel) at 37° C in 5% CO2.
qRT-PCR and Western Blot
The protein and mRNA expression levels of ATP1B3 in the HCC cells and normal liver cell were detected by Western blots and qRT-PCR, respectively, as described previously by us (32–34). The Anti-ATP1B3 antibody was purchased from Santa (sc-135998, 1:50), the Anti-Tubulin antibody was purchased from Elabscience (E-AB-20036, 1:2000), and the ATP1B3 primer used for the amplification was as follows: 5′-TGATCCAACTTCGTATGCAGGG-3′ and 5′-ACATGCAACATAAACTGGACCC-3′ (Sangon Biotech, China).
Transfection of siATP1B3
50 nM of siATP1B3 was transfected into HCC cells by using Lipofectamine™ 2000 (Invitrogen) according to the manufacturer’s instruction (35). The ATP1B3 siRNA was 5′-CUCAUAAUGGAAUGAUAGATT-3′ and 5′-UCUAUCAUUCCAUUAUGAGTT-3′ (TSINGKE, China).
Cell Migration Assay
Transwell migration assay and wound healing assay were performed as described previously (36, 37). The assay was performed three times in triplicate.
Plate Clone Formation and MTT Assay
Cell proliferation was monitored by Plate clone formation and MTT assay as described previously by us (38, 39). The assay was performed three times in triplicate.
Cell-Cycle and Cell Apoptosis Assay
Cell-cycle and cell apoptosis were performed by flow cytometry analysis as described previously (35, 40). The assay was performed three times in triplicate.
Clinical Samples and Immunohistochemistry (IHC)
Fifteen formalin-fixed, paraffin-embedded HCC and paired adjacent liver tissues were collected from Xiangya Hospital of Central South University from September 2019 to January 2020. Our study was approved by the ethics committee of Xiangya Hospital, Central South University.
According to our previously described (41, 42), IHC and an immunoreactive score of ATP1B3 (Anti-ATP1B3 antibody: 67554-1-Ig, proteintech, 1:1000) were conducted on the formalin-fixed and paraffin-embedded tissue sections.
Statistical Analysis
Statistical obtained from TCGA were all analyzed by R-3.6.1. The differential expression of the 8 NKA genes in the TCGA and ICGC cohort were evaluated using the “limma” and “vioplot” package, and the heat map was generated using the heatmap package of the R software. The survival package was used for the survival analysis of the sample from ICGC. The relationship of ATP1B3 expression and clinical characteristics were assessed applying logistic regression. Univariate and multivariate analysis revealed the relationship between ATP1B3 and the clinical factors, the immune cell infiltration with OS of HCC using the “survival” R package. The ROC curves, with AUC values quantified with the survival ROC package. Other data were calculated statistically using SPSS software ver20.0 (SPSS, Inc, Chicago, IL, USA) and GraphPad Prism 7.0 (GraphPad Software, La Jolla, CA, USA). p < 0.05 was considered statistically significant.
Results
NKA Genes Expression in HCC
We first analyzed the mRNA level of 8 NKA genes (ATP1A1-4, ATP1B1-4) in HCC using TCGA and ICGC (LIRI-JP) datasets. Among these, the mRNA expression of ATP1A1, ATP1B1, and ATP1B3 were evidently increased in HCC compared to normal tissue in TCGA with logFC >1 and p<0.01 (Figure 1A and Table S1). Although ATP1A2, ATP1A4, and ATP1B2 were differently expressed between HCC tissue and normal tissue, ATP1A2, ATP1B2 and ATP1A4 mRNA levels were very much low in both HCC and normal tissue, and ATP1B2 expression was slightly reduced in HCC compared to liver tissue with |log2FC|<0.5. Similar results were also observed in ICGC (LIRI-JP) datasets (Figure 1B and Table S2). Subsequently, the expression levels of three genes (namely ATP1A1, ATP1B1, and ATP1B3) were also validated in 3 independent GEO datasets (GSE45436, GSE76427, and GSE102079) (Figure 1C). Finally, the Oncomine database and GEPIA database showed that the mRNA expression of ATP1B1 and ATP1B3 are widely upregulated in various cancers, including Leukemia, Lung cancer, Lymphoma, Head and neck cancer and so on (Figures S1–S3).
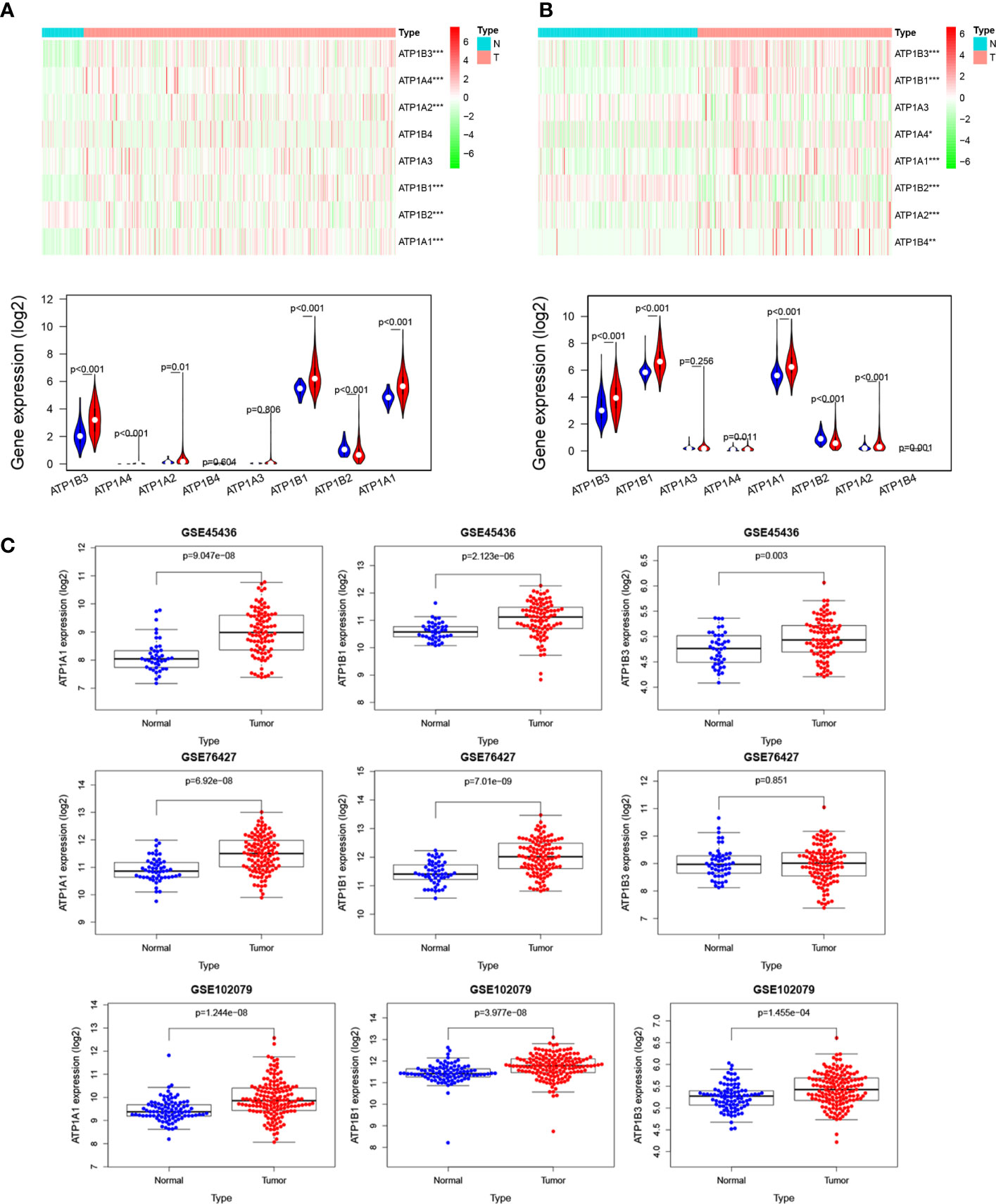
Figure 1 NKA genes expression in HCC. The mRNA levels of NKA genes in HCC from (A) TCGA database. Up, heatmap. Down, Violin plot, Red: HCC tissue; Blue: normal tissue. (B) ICGC database. Up, heatmap. Down, Violin plot, Red: HCC tissue; Blue: normal tissue. (C) The mRNA levels of ATP1A1, ATP1B1, and ATP1B3 in HCC from four independent GEO datasets (GSE45436, GSE76427, GSE64041, and GSE102079).
Prognostic Value of ATP1A1, ATP1B1, and ATP1B3 in HCC
We next investigated the prognostic value of ATP1A1, ATP1B1, and ATP1B3 for HCC using the Kaplan-Meier plotter. The HCC patients with high ATP1A1 showed worse overall Survival (OS: HR = 1.66 (1.14-2.4), p = 0.007), Progression-Free Survival (PFS: HR = 1.61 (1.13-2.31), p = 0.0082), Relapse Free Survival (RFS: HR = 1.73 (1.16-2.59), p = 0.007) and Disease Free Survival (DSS: HR = 1.92 (1.06-3.48), p = 0.029) in Figure 2A. High ATP1B3 was related to worse prognosis in HCC (OS: HR = 2.3 (1.59-3.34), p = 5.8E-6; PFS: HR = 1.39 (1.03-1.86), p = 0.029; RFS: HR = 1.43 (1.02-1.98), p = 0.034; DSS: HR = 2.01 (1.29-3.15), p = 0.0018). Similar results were also observed in the ICGC database (Figure 2B). Moreover, the univariate and multivariate analysis showed that only ATP1B3 was an independent prognostic factor for OS of HCC using both TCGA and ICGC database (Figures 2C, D). Finally, the AUC values of ATP1B3 for the OS model from TCGA and ICGC database were 0.684 and 0.732 respectively, which were more sensitivity and specificity than the clinical factors (Figures 2C, D, right). These results indicated that ATP1B3 was an independent prognostic biomarker for HCC.
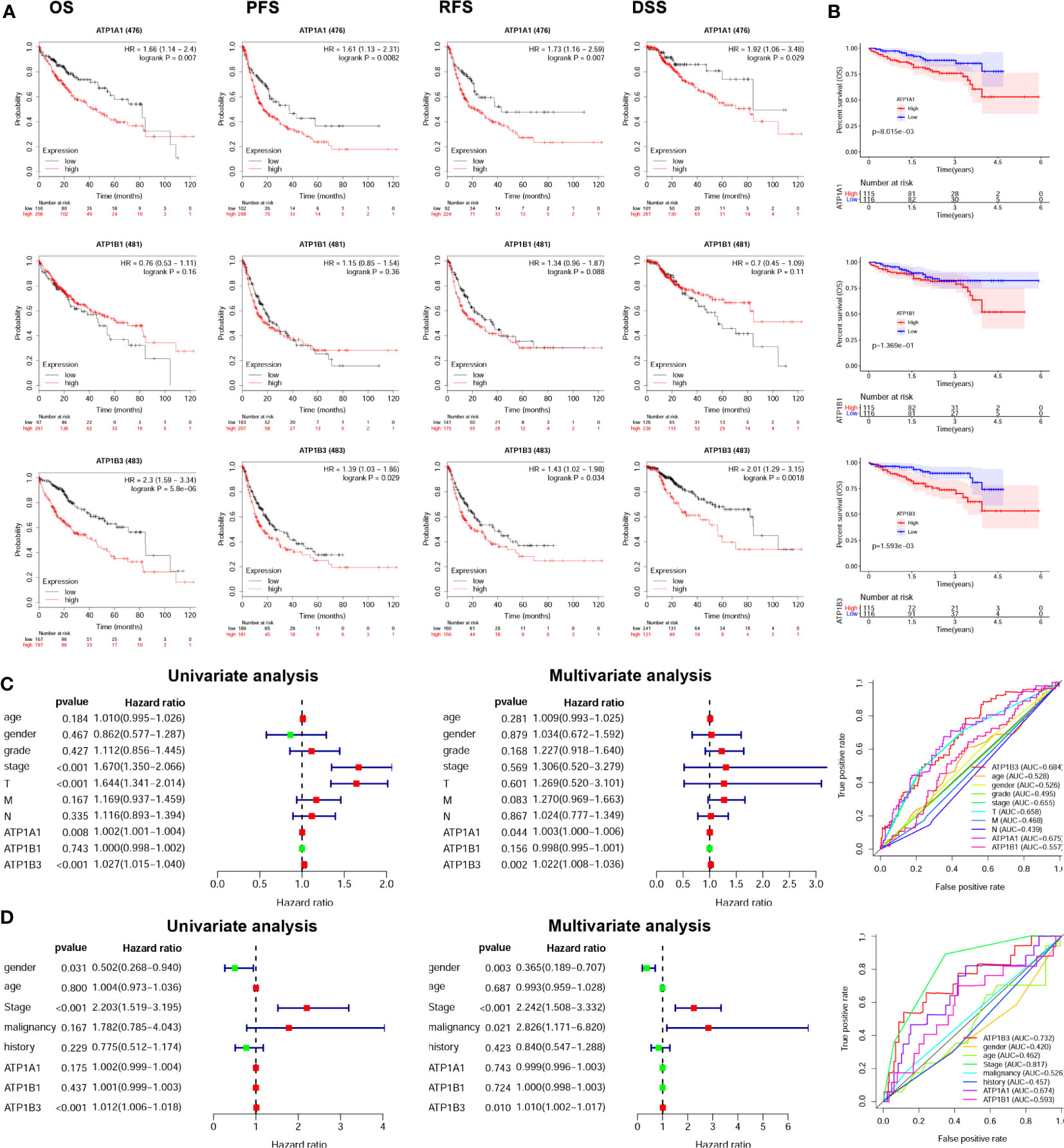
Figure 2 ATP1A1, ATP1B1, and ATP1B3 mRNA are associated with prognosis of HCC patients. (A) The survival curves of OS, PFS, RFS, and DSS with high/low ATP1A1, ATP1B1 and ATP1B3 in TCGA HCC cohorts using the Kaplan-Meier plotter (OS, n=364; RFS, n=316; PFS, n=370; DSS, n=362). The high and low mRNA expression is splitting by best cutoff. (B) The survival curves of OS with high/low ATP1A1, ATP1B1, and ATP1B3 in ICGC HCC cohorts, the high and low mRNA expression is splitting by median. Univariate and multivariate analysis and ROC curve revealed the relationship between ATP1A1, ATP1B1, ATP1B3, and the clinical factors with overall survival of HCC in (C) TCGA database and (D) ICGC database. (T, stage T; N, stage N; M, stage M).
ATP1B3 Is Correlated With Clinicopathological Characteristics in HCC
Based on the clinical data extracted from TCGA-LIHC, we found that high ATP1B3 was associated with higher stage, higher grade, and more dead (p=0.01, p=0.03, and p=0.008) (Figure 3A). Consistent with these results, high ATP1B3 was associated with higher stage, higher grade, and more dead in the ICGC database (Figure 3B). These results were also confirmed by the UALCAN (Figure S4).
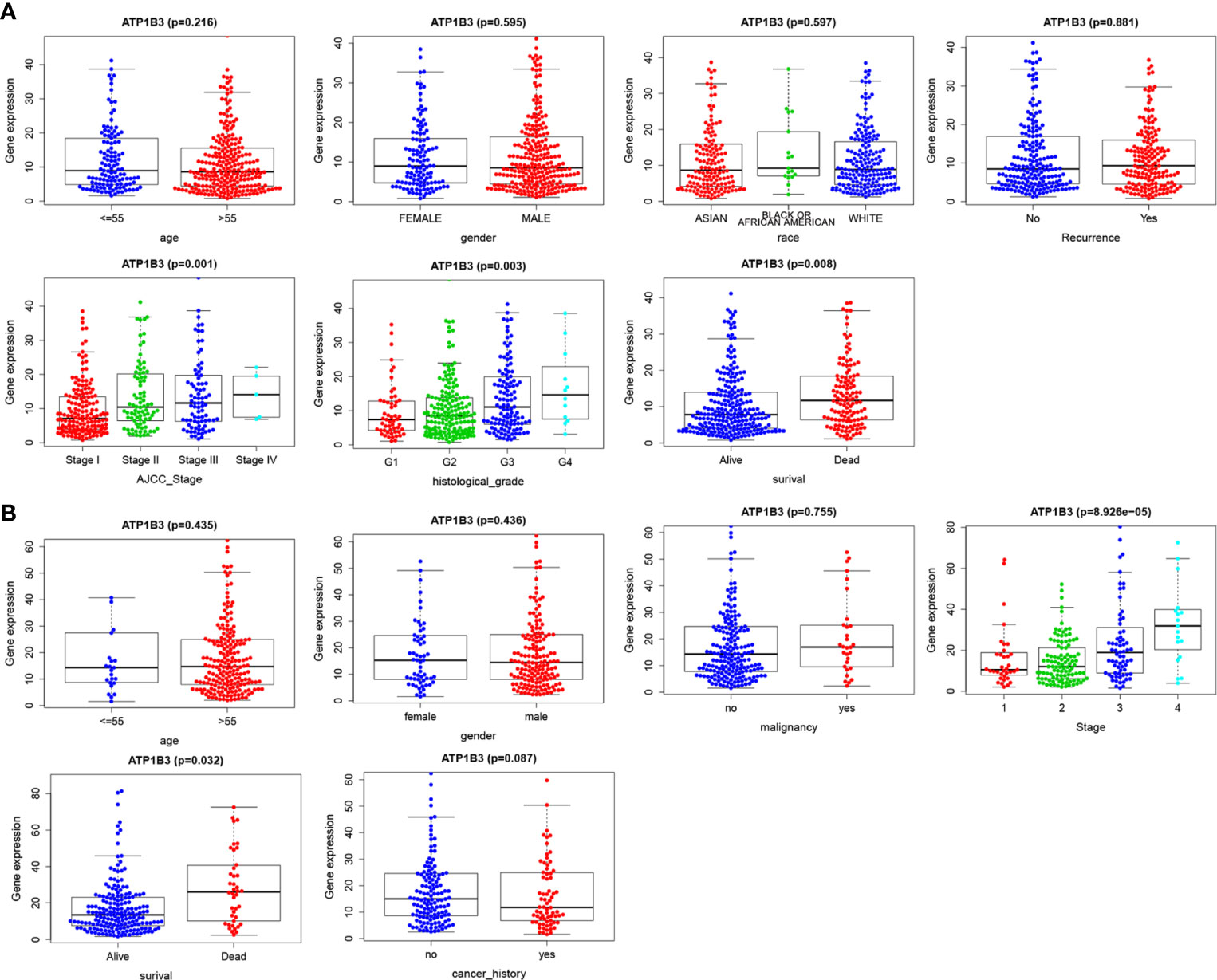
Figure 3 ATP1B3 is correlated with clinicopathological characteristics of HCC patients. (A) TCGA database. (B) ICGC database.
We next explored the association between ATP1B3 expression and the clinicopathological characteristics of HCC patients using Kaplan-Meier Plotter (Table 1). For OS, high expressed ATP1B3 related with poor OS in all stage, grade I/II/III, T 1/2/3, none-vascular invasion, grade (male/female), White and Asian race, no-Alcohol consumption, both with or without Hepatitis virus. For PFS, ATP1B3 expression was significantly hazardous to HCC patients with stage I, grade II, T 1, none-vascular invasion, female, Asian, and Hepatitis virus (Table 1).
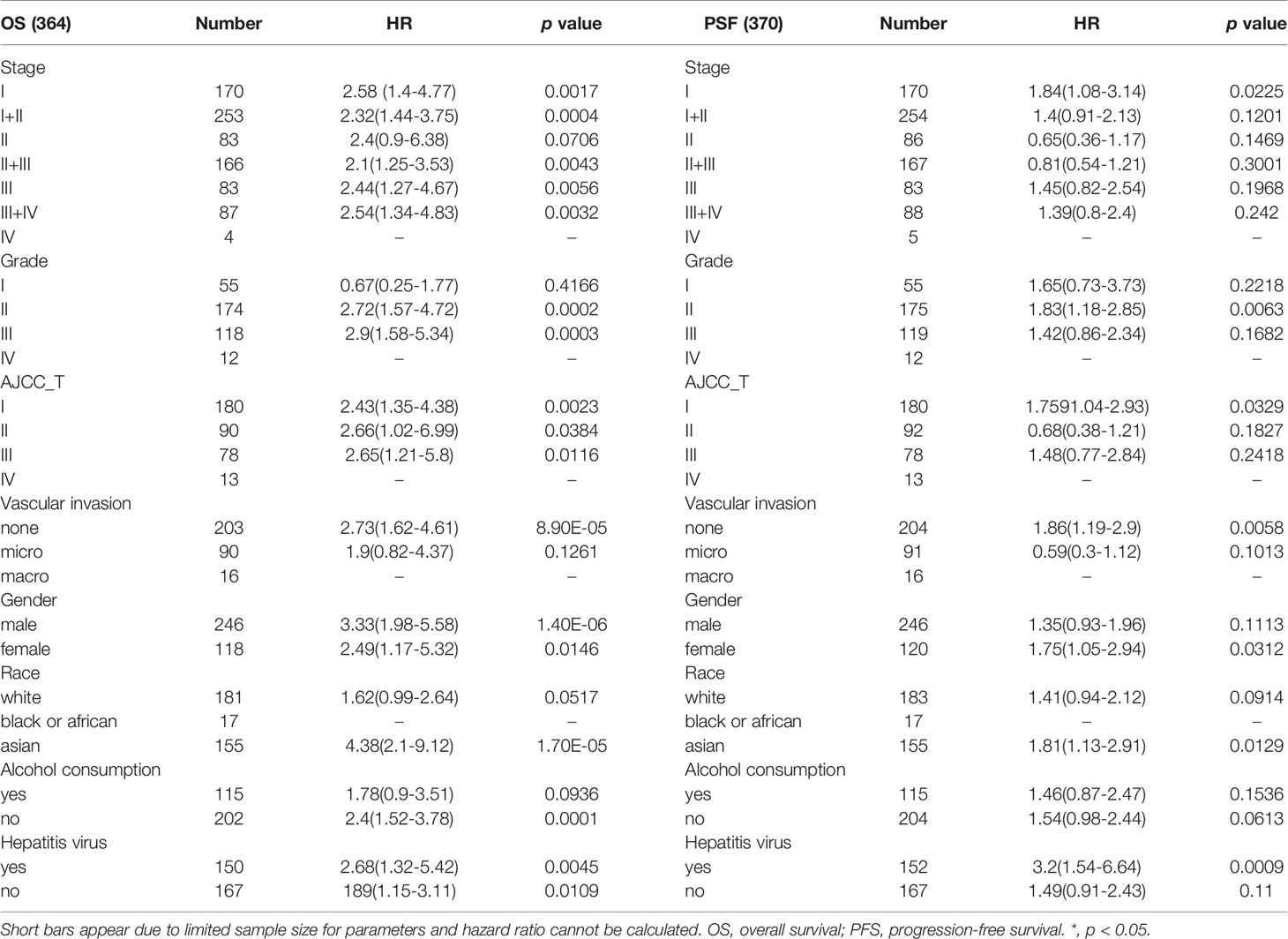
Table 1 Correlation of ATP1B3 mRNA expression with OS (n = 364) and PFS (n = 370) in liver hepatocellular carcinoma with different clinicopathological features.
ATP1B3 Co-Expression Networks in HCC
We analyzed the ATP1B3 co-expression networks in HCC using LinkedOmics. As shown in Figure 4A, a total of 9,531 genes expression were significant correlations with ATP1B3 expression (FDR < 0.01) with 2,564 (green dots) negatively correlated genes and 6,967 positively correlated genes (red dots). The top 50 positively and negatively co-expressed genes were shown in the heat map (Figures 4B, C and Table S3). Among these, 39 of 50 positive genes and 21 of 50 negative genes were associated with OS of HCC with a high/low hazard ratio (HR) (p < 0.05) (Figures 4D, E).
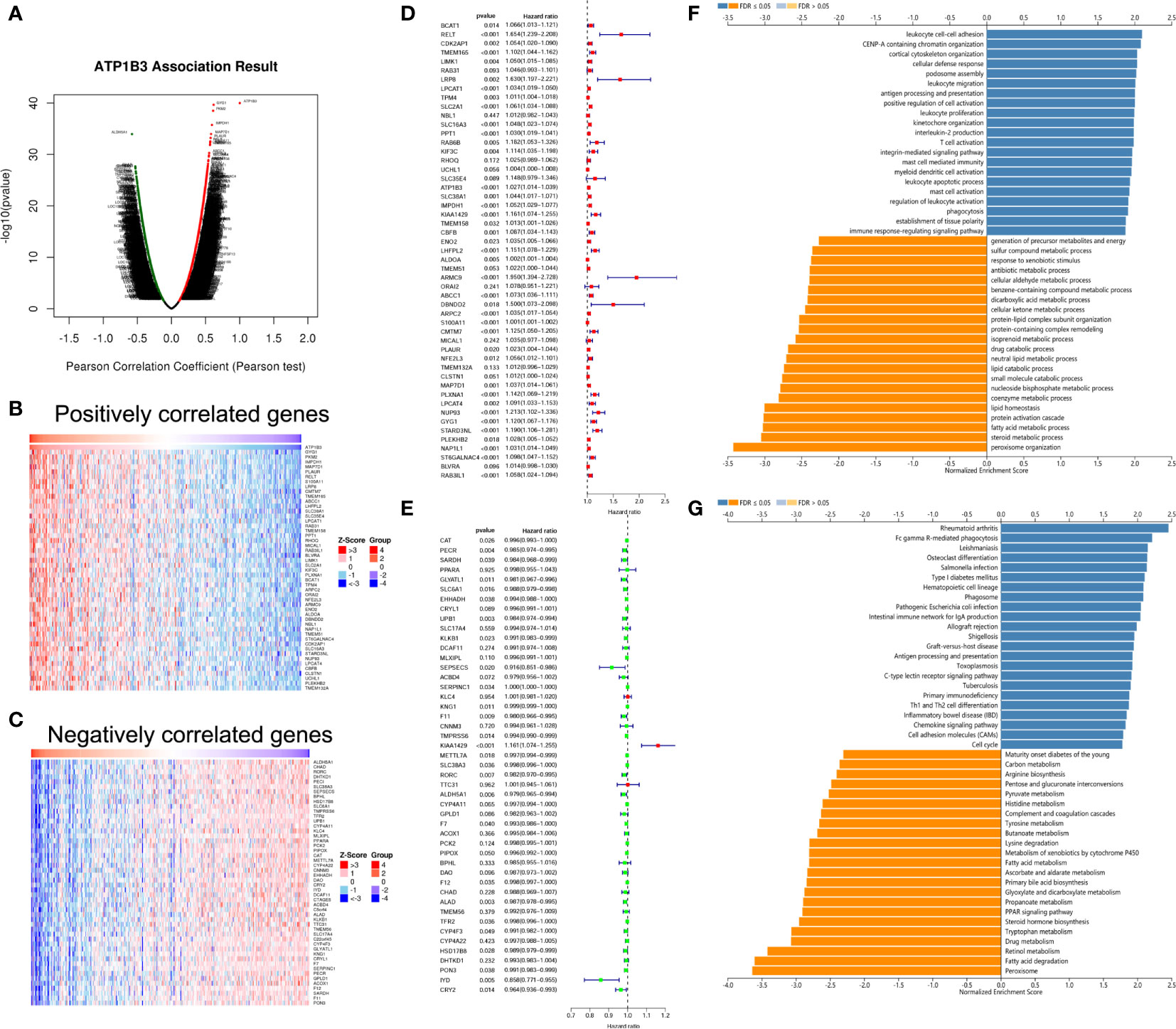
Figure 4 ATP1B3 co-expression genes in HCC using the LinkedOmics. (A) The volcano plot of ATP1B3 co-expression genes. Heat maps revealed the top 50 positively (B) and negatively (C) co-expressed genes of ATP1B3 in HCC. Cox analysis revealed the prognosis of 50 positively (D) and negatively (E) co-expressed genes of ATP1B3 in HCC. The GO enrichment (F) and KEGG pathways (G) of ATP1B3.
GO annotation revealed that these genes participate in various immune response, including leukocyte cell-cell adhesion, leukocyte migration, antigen processing and presentation, leukocyte proliferation. In contrast, various metabolic processes were inhibited, including steroid metabolic process, antibiotic metabolic process, fatty acid metabolic process, and dicarboxylic acid metabolic process (Figure 4F and Table S4). KEGG pathway analysis showed the enrichment in immune and metabolic pathways, including rheumatoid arthritis, Fc gamma R-mediated phagocytosis, Leishmaniasis, and so on (Figure 4G and Table S5). These findings demonstrated that ATP1B3 is involved in the immune response and the metabolic regulation of HCC.
ATP1B3-Related Networks in HCC
To address the ATP1B3-related network in HCC, we analyzed the transcription factors (TF), miRNAs, and kinases in ATP1B3 co-expressed genes. The top 3 most significant related kinases are LCK proto-oncogene (LCK), p21 (RAC1) activated kinase 1 (PAK1), LYN proto-oncogene (LYN) (Tables 2 and S6). No ATP1B3 co-expressed miRNA was enriched by GSEA (Table S7). The most significant ATP1B3 co-expressed TF was belong to the SRF transcription factor family (Table S8), including CFL1, CAP1, SUSD1, FOSL1, KCNMB1.
The Association of ATP1B3 and Immune Infiltration in HCC
Basing on the GO analysis, we next detected the correlations of ATP1B3 and immune cells in HCC using the TIMER. We found that ATP1B3 was correlated with tumor purity (r = -0.353, p = 1.33E-11) and the B cells infiltration (r =0.266, p = 5.52E-7), CD8+ T infiltration (r = 0.249, p = 3.25E-6), CD4+ T infiltration (r = 0.169, p = 1.65E-3), Macrophage infiltration (r = 0.356, p = 1.25E-11), Neutrophil infiltration(r = 0.301, p = 1.21E-8) and Dendritic cell infiltration (r = 0.328, p = 5.46E-10) (Figure 5). Particularly, ATP1B3 CNV has evidently correlated with immune infiltration including B cells, CD8+ T cells, macrophages and neutrophils (Figure 5). Moreover, Univariate analysis showed that ATP1B3, Neutrophil and Macrophage were significantly associated with OS in HCC, and multivariate analysis showed that ATP1B3 and CD8+ T cells were independent factors of OS in HCC (Figure 5). Furthermore, ATP1B3 was also observed differently expressed in immune subtypes (Figure 5) and molecular subtypes (Figure 5) in HCC using TISIDB database. In addition, Figure S5 showed that ATP1B3 was associated immune cells infiltration in pan-cancer.
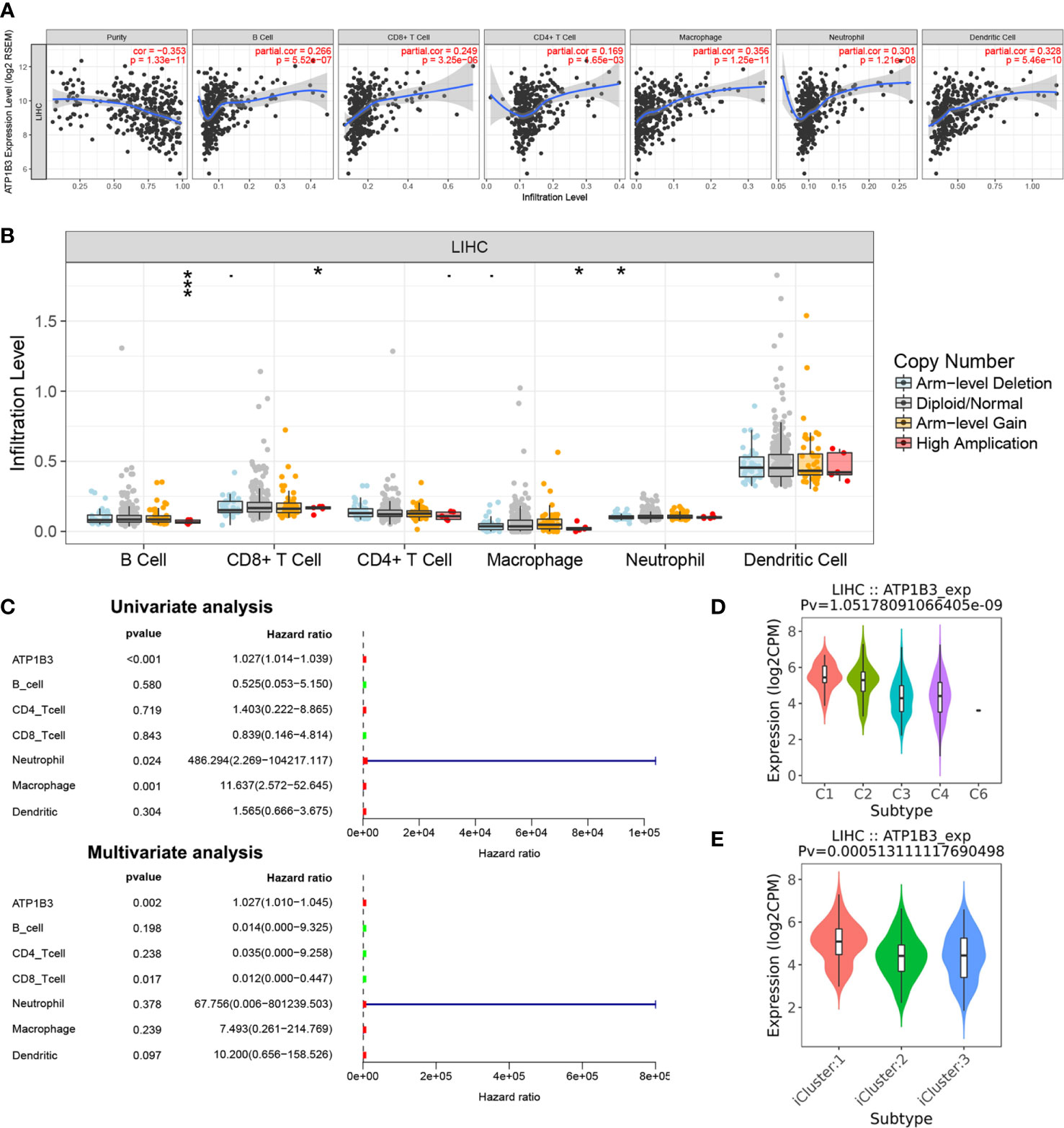
Figure 5 The association of ATP1B3 and immune infiltration level in HCC using the TIMER. (A) The correlation between ATP1B3 expression level and immune infiltration. (B) The relationship between ATP1B3 CNV and immune infiltration. *p < 0.05, **p < 0.01, ***p < 0.001. (C) The prognosis of ATP1B3 and immune cells for OS of HCC. (D) Associations between ATP1B3 expression and immune subtypes in HCC. C1 (wound healing); C2 (IFN-gamma dominant); C3 (inflammatory); C4 (lymphocyte depleted); C5 (immunologically quiet); C6 (TGF-b dominant). (E) Associations between ATP1B3 expression and molecular subtypes in HCC.
The Correlation Between ATP1B3 and Immune Markers and Immune-Related Cytokines in HCC
Next, we investigated the ATP1B3 crosstalk with immune cells, basing on the correlations between ATP1B3 and immune-related gene expression in HCC using the TIMER (Figure 6 and Table 3) and GEPIA databases (Table 4). The results revealed that ATP1B3 expression was positively correlated with the makers of CD8+ T, T cell, M1 Macrophage, B cell, TAM (tumor-associated macrophage), DCs, Th1 (T helper cell 1), Tfh (Follicular helper T cell), and T cell exhaustion. Moreover, ATP1B3 was also associated with HCC-related cytokines and chemokines. Our research shows that the expression of ATP1B3 is positively correlated with IL10, IL22, IL34 and negatively correlated with IL27 (Figure 6B). These findings revealed the potential association between ATP1B3 and immune cell infiltration in HCC. As HCC is associated a higher level of inflammation, it is relatively evident that every marker upregulated to such HCC initiation and progression will be correlated to inflammation markers. So further experiments are needed for this speculation.
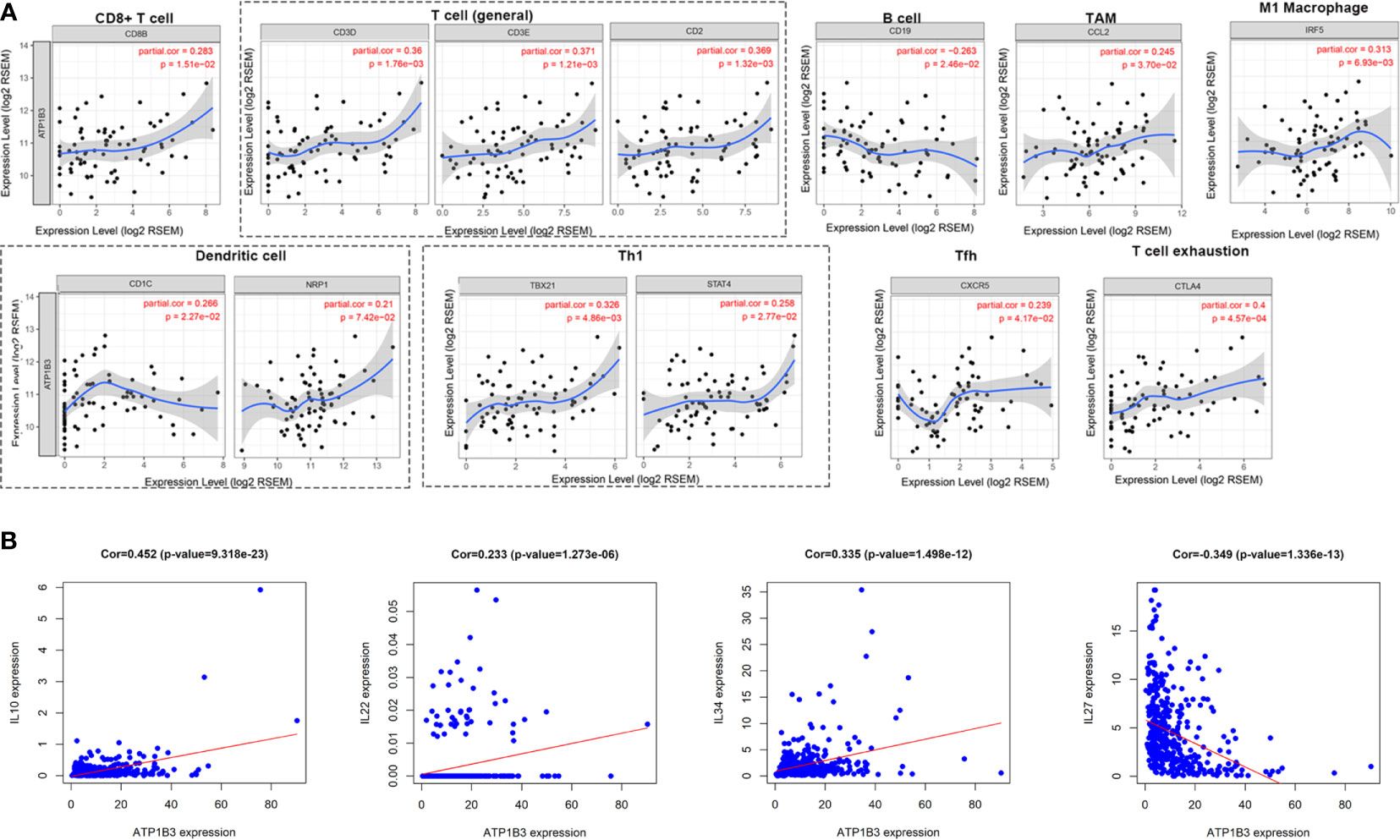
Figure 6 Correlation between ATP1B3 and immune markers and immune-related cytokines in HCC. (A) The correlation between ATP1B3 and immune-related marker genes in HCC (TIMER). (B) The correlation between ATP1B3 and HCC-related cytokines.
ATP1B3 Protein Expression and Prognosis in HCC
To further confirm the function of ATP1B3 in HCC, we analyzed the expression and prognosis of ATP1B3 protein levels using the CPTAC proteomics database. The ATP1B3 protein level was elevated in tumor tissue compared to normal tissues (Figure 7A), and its’ expression was associated with the high differentiated tumor and medical history of liver cirrhosis (Figure 7B). HCC patients with high-expressed ATP1B3 showed poor OS (p =0.07) (Figure 7C). Moreover, the univariate analysis proved that ATP1B3, tumor size, and differentiation were significantly associated with OS in HCC, and multivariate analysis showed that tumor size and differentiation were independent factors of OS in HCC using the CPTAC database (Figure 7D). The relationship between ATP1B3 and 50 top negative/positive co-expressed genes were confirmed using the CPTAC database in Figures S6 and S7. The correlation between ATP1B3 and immune gene was also confirmed using the CPTAC database in Figure S8. Moreover, the proteomics and phospho-proteomics levels of ATP1B3 of 316 HCC patients were analyzed using Gao’s data (29). As shown in Figure 7E, the protein level of ATP1B3 was elevated and the phosphorylation of ATP1B3 was downregulated in tumor tissue compared to paratumor tissues. And ATP1B3 protein level was associated with TNM stage (p= 0.06) (Figure 7F). HCC patients with high-expressed ATP1B3 shows worse OS (P=0.002) (Figure 7G). Moreover, the univariate analysis proved that ATP1B3, TNM, and age were significantly associated with OS in HCC, and multivariate analysis showed ATP1B3 was an independent factor of OS in HCC (Figure 7H).
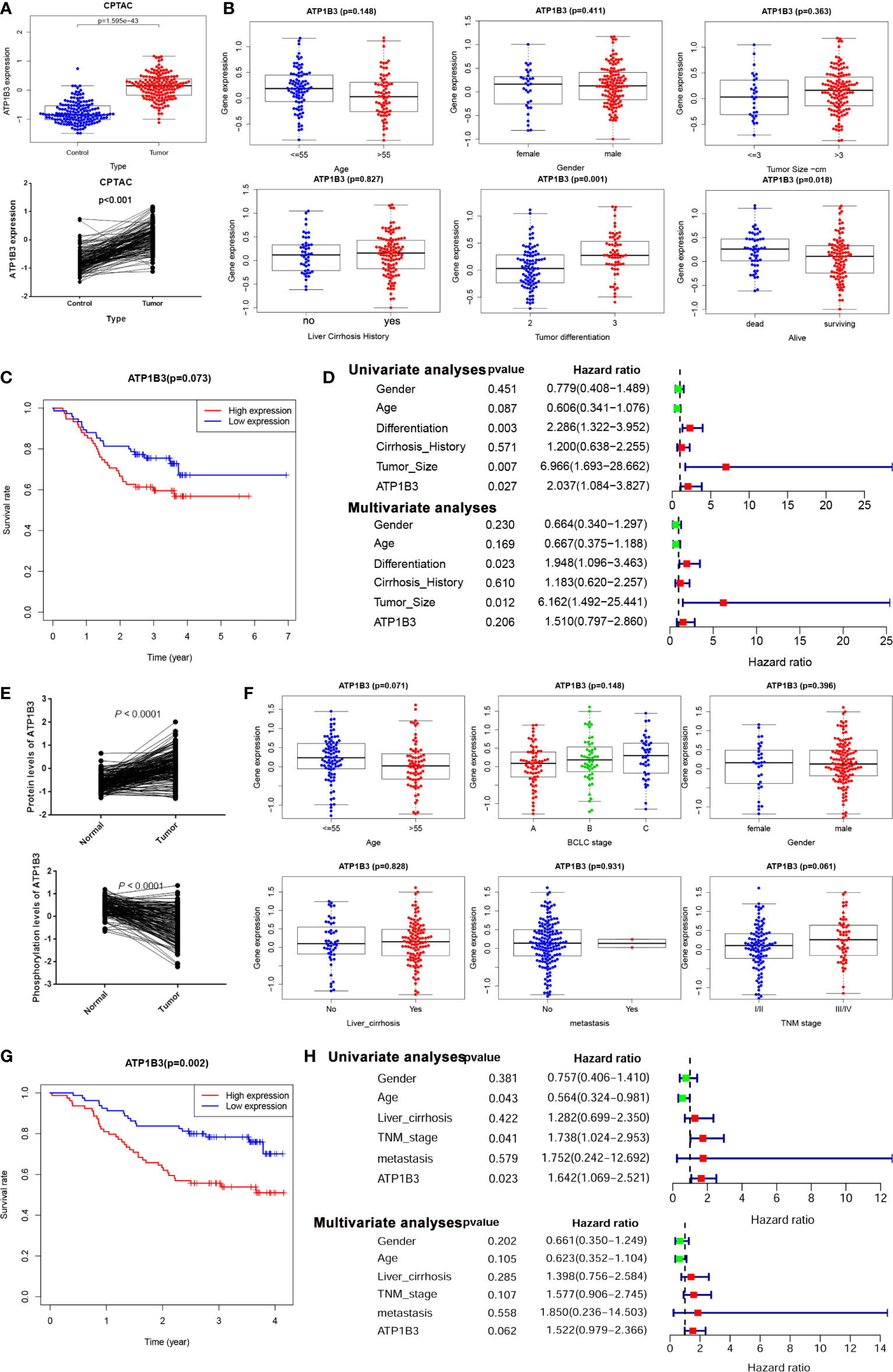
Figure 7 ATP1B3 protein expression and prognosis in HCC. (A) ATP1B3 protein levels in HCC using CPTAC proteomics database. (B) The association between ATP1B3 protein expression and clinical features in HCC using CPTAC proteomics database. (C) The survival curves of OS with high/low ATP1B3 in CPTAC HCC cohorts. (D) Univariate and multivariate analysis revealed the relationship between ATP1B3 and the clinical factors with OS of HCC in the CPTAC database. (E) The protein level and the phosphorylation level of ATP1B3 in the proteomics and phosphor-proteomics data. (F) The association between ATP1B3 protein expression and clinical features in the proteomics and phosphor-proteomics data. (G) The survival curves of OS with high/low ATP1B3 in the proteomics and phosphor-proteomics data. (H) Univariate and multivariate analysis revealed the relationship between ATP1B3 and the clinical factors with OS of HCC in the proteomics and phosphor-proteomics data.
ATP1B3 Related Potential Drug in HCC
Drug sensitivity plays a crucial role in HCC treatment. We next analyzed the correlation of ATP1B3 expression to sorafenib-therapy and PD-1 immunotherapy using GSE109211 and GSE120714 database. We found that HCC patients with sorafenib-resistant have higher ATP1B3 expression compared to HCC patients with sorafenib-sensitive (Figure 8). However, no significant difference in ATP1B3 expression was observed between with/without PD-1 immunotherapy in HCC patients (Figure 8B). To further investigated the potential drug for HCC patients with high ATP1B3 expression, we analyzed the role of 34 chemicals on ATP1B3 expression using GSE69844 (Table S9). We found that 10 μM and 100 μM Progesterone could slightly reduce ATP1B3 expression in HepaRG cells (Figure 8C). These results demonstrate that Progesterone may be an expected drug for the treatment of HCC patients with high-expressed ATP1B3. This needs to be further confirmed by experiments.
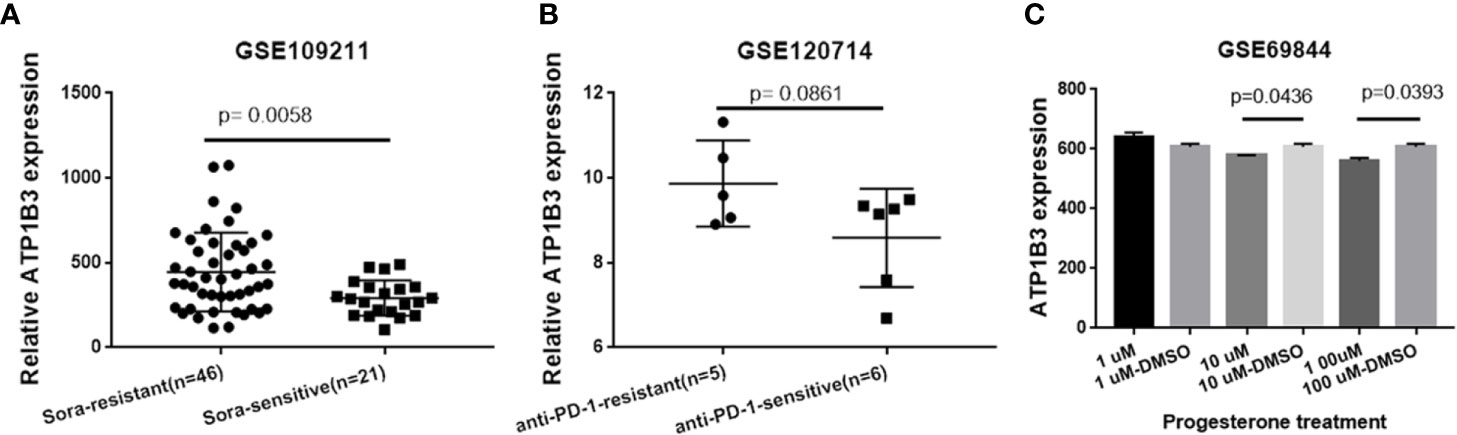
Figure 8 ATP1B3 related potential drug in HCC. (A) ATP1B3 expression in sorafenib-resistant/-sensitive HCC patients. (B) ATP1B3 expression in anti-PD1 immunotherapy-resistant/-sensitive HCC patients. (C) The GSE69844 dataset revealed that Progesterone could reduce ATP1B3 expression in HepaRG cells.
ATP1B3 Expression Is Increased in the HCC Cells and HCC Tissues
We further confirmed the expression of ATP1B3 in HCC cells and HCC tissues using qPCR, western blot and IHC. As shown in Figure 9A, ATP1B3 is upregulated in HCC tissues compared with paratumor tissues. Similarly, compared with human normal liver cells (LO2), both protein expression and mRNA expression levels of ATP1B3 were upregulated in HCC cells (Hhu7 and HCCLM3) (Figures 9B, C).
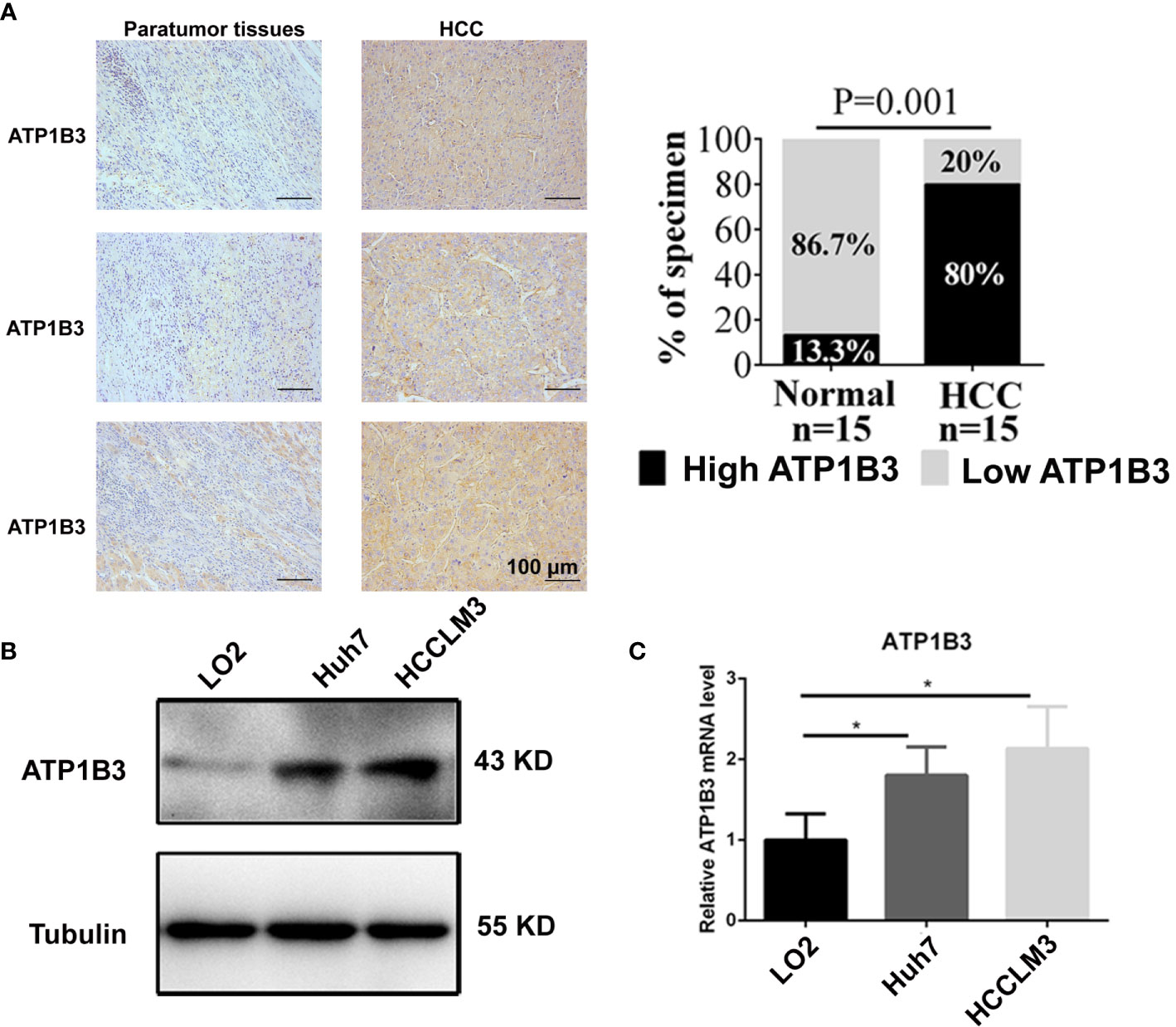
Figure 9 ATP1B3 expression in the HCC cells and HCC tissues. (A) The protein levels of ATP1B3 in HCC tissues and paratumor tissues were detected by IHC. Scale bars= 100 μm. (B) The protein expression level of ATP1B3 in HCC cells and normal liver cell were detected by Western Blot. (C) The mRNA expression level of ATP1B3 in HCC cells and normal liver cell were detected by qPCR. *p < 0.05.
Silenced ATP1B3 Represses HCC Cell Proliferation, Migration and Induces HCC Cell Apoptosis
To investigate the role of ATP1B3 in HCC, we transfected ATP1B3 siRNA (Hhu7-siATP1B3 and HCCLM3-siATP1B3) into Hhu7 and HCCLM3 cells to knockdown ATP1B3 expression (Figures 10A, B), and then analyzed the effects of silenced ATP1B3 on HCC cells proliferation, migration, invasion and cycle, apoptosis. MTT and plate clone formation assay suggested that silenced ATP1B3 significantly inhibited HCC cells proliferation (Figures 10C, D). Transwell migration assay and wound healing assay suggested that silenced ATP1B3 significantly inhibited HCC cells migration (Figures 10E, F). Flow analysis suggested that silenced ATP1B3 induced HCC cells apoptosis (Figure 10G) and blocked cell cycle in G0/G1 phase (Figure 10H). Moreover, we detected the EMT markers in ATP1B3 silenced HCC cells by western blot. The results showed that silenced ATP1B3 significantly upregulated E-cadherin expression, and downregulated N-cadherin and vimentin expression. These results proved that ATP1B3 promoted EMT in HCC (Figure 10I). Moreover, we have tried to detect the effects of ATP1B3 on cell proliferation in LO2 by MTT (Figure 10J) and plate clone formation (Figure 10K). We found that ATP1B3 silencing had no significant effect on the proliferation of healthy liver cells. In conclusion, these results proved that ATP1B3 could promote the tumorigenicity of HCC.
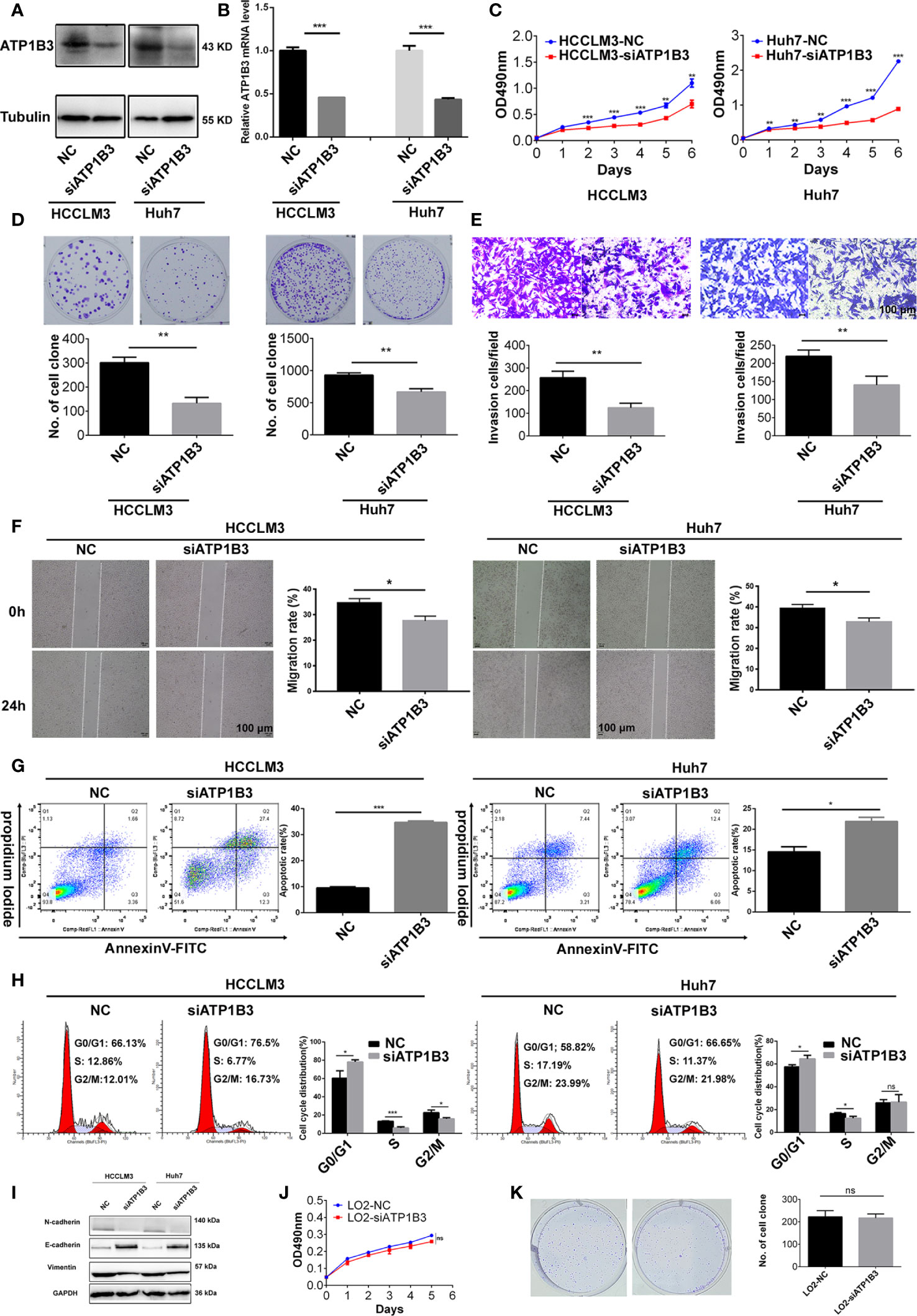
Figure 10 ATP1B3 promotes HCC cell proliferation, migration and inhibits HCC cell apoptosis. Western Blot (A) and qPCR (B) showed that the expression of ATP1B3 were silenced by siRNA in Hhu7 and HCCLM3, respectively. MTT (C) and plate clone formation (D) analysis revealed the cell proliferation regulated by ATP1B3. Transwell migration (E) and scratch wound healing (F) assay revealed the migration ability regulated by ATP1B3. Apoptosis (G) and Cell cycle (H) assay revealed the regulation of ATP1B3 on cell apoptosis and cell cycle using flow cytometry. (I) The EMT markers in ATP1B3 silenced HCC cells. The effects of ATP1B3 on cell proliferation in LO2 by MTT (J) and plate clone formation (K). Scale bars= 100 μm, *p < 0.05, **p < 0.01, ***p < 0.001.
Discussion
Na+/K+-ATPase (NKA) is a multifunctional transmembrane protein that plays a crucial role in cell adhesion (14), cell movement (43), cell proliferation and apoptosis (8), and signal transduction (44). Emerging studies have shown the abnormal expression (6) and the prognosis of NKA in various cancers (9). However, the clinical relevance of NKA in HCC remains limited. In this paper, multiple public databases are used for the first time to comprehensively analyze the expression of NKA subunits in HCC and its correlation with HCC prognosis and reveal its possible mechanism in HCC.
NKA was reported to be dysregulated in multiple cancers (14). For example, NKA α1 subunit (ATP1A1) is upregulated in non-small cell lung cancer (NSCLC) (45), esophageal squamous cell carcinoma (ESCC) (46), renal cell carcinoma (47), glioma (48), but downregulated in prostate cancer (49). NKA β1 subunit (ATP1B1) is downregulated in human epithelial cancer cells (50–52). A few studies report the abnormal expression of NKA in HCC. For example, Shibuya et al. (53) and Li et al. (54) pointed out that ATP1A3 overexpression in HCC is related to the antitumor activity of bufalin. It can be used as a therapeutic target for bufalin. L Zhuang et al. (4) showed that ATP1A1 was upregulated in HCC, and its function as an oncogene by promoting proliferation and migration of HCC cells. Whereas the potential prognostic role of NKA in HCC remains unclear. Consistent with previous study, we found that ATP1A1 and ATP1A3 were upregulated in HCC from TCGA database. Moreover, ATP1B3 were also significantly upregulated in HCC with logFC > 1 and p < 0.01 using TCGA, ICGC, and GEO datasets. The prognostic analysis revealed that ATP1B3 was an independent factor for the OS of HCC based on transcriptomic data from TCGA, ICGC, and GEO.
ATP1B3 encodes the β3 subunit of NKA and regulates cell adhesion (55). The study has displayed that ATP1B3 expression was increased in gastric cancer tissues and was closely related to related to gastric cancer patients’ clinical characteristics (51). Here, we found that ATP1B3 high expression was associated with clinical characteristics of HCC patients including stage and grade. Subsequently, the expression and prognosis of ATP1B3 protein in HCC were also confirmed using the CPTAC database and proteomics and phospho-proteomics data from Gao’s work. The results indicated that ATP1B3 is a useful biomarker for diagnosis and prognosis of HCC prognosis. Furthermore, we validated that ATP1B3 is increased in HCC cells and tissues. Meanwhile, we also proved that silenced ATP1B3 repressed HCC cell proliferation, migration and induced HCC cell apoptosis. In brief, these results suggest that ATP1B3 could be an oncogene and promote tumorigenicity of HCC.
To investigate the potential mechanism of ATP1B3 in HCC, we analyzed the co-expressed genes of ATP1B3. The results showed that they were mainly involved in various immune responses, simultaneously inhibiting the metabolism of steroids and fatty acids. At the same time, the ATP1B3 expression was positively related to kinase expression, including LCK and LYN, which have been reported to play a crucial part in regulating B cell receptor signaling (52, 56). As previously reported that NKA regulates Src family kinase activity (including FYN and LYN) (57). Recruitment of NKA-LYN complex in macrophages promotes atherosclerosis (12). The NKA α-1/Src complex activates a variety of pro-inflammatory factors/chemokines and mediates pro-inflammatory effects (58). Pieces of evidence have proven the involvement of NKA in the inflammatory response (59). Therefore, we speculated that ATP1B3 might be involved in the immune regulation of HCC.
Immune infiltration is a significant factor in the tumor microenvironment, which plays a crucial part in the development and prognosis of tumors (60). Various immune cells contributed to the immune microenvironment of HCC including macrophages, neutrophil, dendritic cell, adaptive immune CD4+, CD8+ T-lymphocytes, and NK cells (61). Studies showed that infiltrated macrophages were polarized M2-TAM (tumor-associated macrophages), which act as immune suppressor cells and lead to reduction and exhaustion of CD8+T cells in HCC (62). Tregs were proved to be increased in HCC and impede immune surveillance (63). Despite the effect of NKA on tumor immunity has not been widely reported, it is known that knockdown of NKA α1 in macrophages can inhibit cardiotonic steroid (CTS)-induced macrophage infiltration and the accumulation of immune cells in vivo (64). We found that ATP1B3 was significantly correlated with tumor purity and B cell infiltration, CD8+ T infiltration, CD4+ T infiltration, Macrophage infiltration, Neutrophil infiltration, and Dendritic cell infiltration. Also, ATP1B3 and CD8+ T cells were found to be independent factors of HCC. In addition, we also found that ATP1B3 expression was positively correlated with the makers of CD8+ T, T cell, B cell, TAM, M1 Macrophage, DCs, Th1, Tfh, and T cell exhaustion. These immune cells are regulated by various cytokines and chemokines in the tumor environment, leading to different functions (65). Our research shows that the expression of ATP1B3 is positively correlated with IL10, IL22, IL34, and negatively correlated with IL27. IL10 was reported to inhibit the cytotoxicity of NK cells through the STAT3 signaling pathway, thereby promoting the recurrence and metastasis of HCC (66). In addition, IL22 is highly expressed in HCC and is related to the growth and malignancy of HCC tumors (67). IL-34 promotes the proliferation and migration of HCC through CSF1-R and CD138 (68). In addition, the DC-derived cytokine IL27 can exert anti-tumor activity by activating NK cells (69). These results imply that ATP1B3 may involve in immune infiltration by regulating immune-related cytokine in HCC.
Basing on the potential therapeutic and prognostic role of ATP1B3 on HCC patients, we analyzed the drug sensitivity of HCC patients with different expressed ATP1B3. Our result revealed that HCC patients with sorafenib-resistant have higher ATP1B3 expression compared to HCC patients with sorafenib-sensitive, suggesting that ATP1B expression is associated with sorafenib-resistant in HCC patients. Subsequently, 34 chemicals analysis results showed that 10 μM and 100 μM Progesterone slightly reduced ATP1B3 expression in HepaRG cells, indicating that Progesterone may be a combined drug strategy for sorafenib in the treatment of HCC. Moreover, studies showed that Na/K-ATPase is a target for anticancer drugs perillyl alcohol (POH), and Cardioprotection drug DRRSAb, indicating their potential therapeutic effect for HCC (11, 70). However, the treatment effect of these drugs for HCC has yet to be proved.
Conclusions
In summary, our results indicate that ATP1B3 is upregulated and promote the tumorigenicity of HCC, and it is also an independent prognostic biomarker for the diagnosis of HCC with a potential immunomodulatory role, providing a novel prognostic biomarker and potential therapeutic target for HCC.
Data Availability Statement
The original contributions presented in the study are included in the article/Supplementary Material. Further inquiries can be directed to the corresponding author.
Ethics Statement
The studies involving human participants were reviewed and approved by The ethics committee of Xiangya Hospital, Central South University. Written informed consent for participation was not required for this study in accordance with the national legislation and the institutional requirements.
Author Contributions
Conceptualization: YZ and SL. Methodology: SL, SC and XP. Investigation: SL and XP. Writing – Original Draft: YX and SL. Writing – Review and Editing: YZ, SL and RC. Funding Acquisition: YZ, RC and XP. All authors contributed to the article and approved the submitted version.
Funding
This work was supported by the National Natural Science Foundation of China (81703149 and 81874251). This work was supported by the National Natural Sciences Foundation of Hunan province (2020JJ5950 and 2019JJ50417).
Conflict of Interest
The authors declare that the research was conducted in the absence of any commercial or financial relationships that could be construed as a potential conflict of interest.
Supplementary Material
The Supplementary Material for this article can be found online at: https://www.frontiersin.org/articles/10.3389/fimmu.2021.636614/full#supplementary-material
References
1. Gu Y, Li X, Bi Y, Zheng Y, Wang J, Li X, et al. CCL14 is a prognostic biomarker and correlates with immune infiltrates in hepatocellular carcinoma. Aging (Albany NY) (2020) 12:784–807. doi: 10.18632/aging.102656
2. Liao X, Zhu G, Huang R, Yang C, Wang X, Huang K, et al. Identification of potential prognostic microRNA biomarkers for predicting survival in patients with hepatocellular carcinoma. Cancer Manage Res (2018) 10:787. doi: 10.2147/CMAR.S161334
3. Schneider NFZ. Cytotoxic and cytostatic effects of digitoxigenin monodigitoxoside (DGX) in human lung cancer cells and its link to Na,K-ATPase. BioMed Pharmacother (2018) 97:684–96. doi: 10.1016/j.biopha.2017.10.128
4. Zhuang L, Xu L, Wang P, Jiang Y, Yong P, Zhang C, et al. Na+/K+-ATPase α1 subunit, a novel therapeutic target for hepatocellular carcinoma. Oncotarget (2015) 6:28183–93. doi: 10.18632/oncotarget.4726
5. Liu C-G, Xu K-Q, Xu X, Huang J-J, Xiao J-C, Zhang J-P, et al. 17β-oestradiol regulates the expression of Na+/K+-ATPase β1-subunit, sarcoplasmic reticulum Ca2+-ATPase and carbonic anhydrase IV in H9C2 cells. Clin Exp Pharmacol Physiol (2007) 34:998–1004. doi: 10.1111/j.1440-1681.2007.04675.x
6. Mijatovic T, Dufrasne F, Kiss R. Na+/K+-ATPase and cancer. Pharm Pat Anal (2012) 1:91–106. doi: 10.4155/ppa.12.3
7. Rajasekaran SA, Huynh TP, Wolle DG, Espineda CE, Inge LJ, Skay A, et al. Na,K-ATPase Subunits as Markers for Epithelial-Mesenchymal Transition in Cancer and Fibrosis. Mol Cancer Ther (2010) 9:1515–24. doi: 10.1158/1535-7163.MCT-09-0832
8. Felippe Gonçalves-de-Albuquerque C, Ribeiro Silva A, Ignácio da Silva C, Caire Castro-Faria-Neto H, Burth P. Na/K Pump and Beyond: Na/K-ATPase as a Modulator of Apoptosis and Autophagy. Molecules (2017) 22:578. doi: 10.3390/molecules22040578
9. Alevizopoulos K, Calogeropoulou T, Lang F, Stournaras C. Na+/K+ ATPase inhibitors in cancer. Curr Drug Targets (2014) 15:988–1000. doi: 10.2174/1389450115666140908125025
10. Castañeda MS, Zanoteli E, Scalco RS, Scaramuzzi V, Caldas VM, Reed UC, et al. A novel ATP1A2 mutation in a patient with hypokalaemic periodic paralysis and CNS symptoms. Brain (2018) 141:3308–18. doi: 10.1093/brain/awy283
11. Yan Y, Shapiro JI. The physiological and clinical importance of sodium potassium ATPase in cardiovascular diseases. Curr Opin Pharmacol (2016) 27:43–9. doi: 10.1016/j.coph.2016.01.009
12. Chen Y, Kennedy DJ, Ramakrishnan DP, Yang M, Huang W, Li Z, et al. Oxidized LDL-bound CD36 recruits a Na+/K+-ATPase-Lyn complex in macrophages that promotes atherosclerosis. Sci Signaling (2015) 8:ra91. doi: 10.1126/scisignal.aaa9623
13. Ohnishi T, Yanazawa M, Sasahara T, Kitamura Y, Hiroaki H, Fukazawa Y, et al. Na, K-ATPase α3 is a death target of Alzheimer patient amyloid-β assembly. Proc Natl Acad Sci U S A (2015) 112:E4465–74. doi: 10.1073/pnas.1421182112
14. Magpusao AN, Omolloh G, Johnson J, Gascón J, Peczuh MW, Fenteany G. Cardiac glycoside activities link Na(+)/K(+) ATPase ion-transport to breast cancer cell migration via correlative SAR. ACS Chem Biol (2015) 10:561–9. doi: 10.1021/cb500665r
15. Mathieu V, Pirker C, Martin de Lassalle E, Vernier M, Mijatovic T, DeNeve N, et al. The sodium pump α1 sub-unit: a disease progression–related target for metastatic melanoma treatment. J Cell Mol Med (2009) 13:3960. doi: 10.1111/j.1582-4934.2009.00708.x
16. Lee SJ, Litan A, Li Z, Graves B, Lindsey S, Barwe SP, et al. Na,K-ATPase β1-subunit is a target of sonic hedgehog signaling and enhances medulloblastoma tumorigenicity. Mol Cancer (2015) 14:159. doi: 10.1186/s12943-015-0430-1
17. Bechmann MB, Rotoli D, Morales M, Maeso MdC, García MdP, Ávila J, et al. Na,K-ATPase Isozymes in Colorectal Cancer and Liver Metastases. Front Physiol (2016) 7:9. doi: 10.3389/fphys.2016.00009
18. Liu Y, Yang Y, Luo Y, Wang J, Lu X, Yang Z, et al. Prognostic potential of PRPF3 in hepatocellular carcinoma. Aging (Albany NY) (2020) 12:912–30. doi: 10.18632/aging.102665
19. Xie L, Li H, Zhang L, Ma X, Dang Y, Guo J, et al. Autophagy-related gene P4HB: a novel diagnosis and prognosis marker for kidney renal clear cell carcinoma. aging (2020) 12:1828–42. doi: 10.18632/aging.102715
20. Tang Z, Li C, Kang B, Gao G, Li C, Zhang Z. GEPIA: a web server for cancer and normal gene expression profiling and interactive analyses. Nucleic Acids Res (2017) 45:W98. doi: 10.1093/nar/gkx247
21. Rhodes DR, Kalyana-Sundaram S, Mahavisno V, Varambally R, Yu J, Briggs BB, et al. Oncomine 3.0: Genes, Pathways, and Networks in a Collection of 18,000 Cancer Gene Expression Profiles. Neoplasia (New York NY) (2007) 9:166. doi: 10.1593/neo.07112
22. Yuan Q, Sun N, Zheng J, Wang Y, Yan X, Mai W, et al. Prognostic and Immunological Role of FUN14 Domain Containing 1 in Pan-Cancer: Friend or Foe? Front Oncol (2020) 9:1502. doi: 10.3389/fonc.2019.01502
23. Chandrashekar DS, Bashel B, Balasubramanya SAH, Creighton CJ, Ponce-Rodriguez I, Chakravarthi BVSK, et al. UALCAN: A Portal for Facilitating Tumor Subgroup Gene Expression and Survival Analyses. Neoplasia (New York NY) (2017) 19:649. doi: 10.1016/j.neo.2017.05.002
24. Vasaikar SV, Straub P, Wang J, Zhang B. LinkedOmics: analyzing multi-omics data within and across 32 cancer types. Nucleic Acids Res (2018) 46:D956. doi: 10.1093/nar/gkx1090
25. Li B, Severson E, Pignon J-C, Zhao H, Li T, Novak J, et al. Comprehensive analyses of tumor immunity: implications for cancer immunotherapy. Genome Biol (2016) 17:174. doi: 10.1186/s13059-016-1028-7
26. Li T, Fan J, Wang B, Traugh N, Chen Q, Liu JS, et al. TIMER: A web server for comprehensive analysis of tumor-infiltrating immune cells. Cancer Res (2017) 77:e108. doi: 10.1158/0008-5472.CAN-17-0307
27. Ru B, Wong CN, Tong Y, Zhong JY, Zhong SSW, Wu WC, et al. TISIDB: an integrated repository portal for tumor–immune system interactions. Bioinformatics (2019) 35:4200–2. doi: 10.1093/bioinformatics/btz210
28. Pan J, Zhou H, Cooper L, Huang J, Zhu S, Zhao X, et al. LAYN Is a Prognostic Biomarker and Correlated With Immune Infiltrates in Gastric and Colon Cancers. Front Immunol (2019) 10:6. doi: 10.3389/fimmu.2019.00006
29. Gao Q, Zhu H, Dong L, Shi W, Chen R, Song Z, et al. Integrated Proteogenomic Characterization of HBV-Related Hepatocellular Carcinoma. Cell (2019) 179:561–77.e22. doi: 10.1016/j.cell.2019.08.052
30. Deb B, Sengupta P, Sambath J, Kumar P. Bioinformatics Analysis of Global Proteomic and Phosphoproteomic Data Sets Revealed Activation of NEK2 and AURKA in Cancers. Biomolecules (2020) 10:237. doi: 10.3390/biom10020237
31. Tabb DL, Wang X, Carr SA, Clauser KR, Mertins P, Chambers MC, et al. Reproducibility of Differential Proteomic Technologies in CPTAC Fractionated Xenografts. J Proteome Res (2016) 15:691. doi: 10.1021/acs.jproteome.5b00859
32. Li J-Y, Xiao T, Yi H-M, Yi H, Feng J, Zhu J-F, et al. S897 phosphorylation of EphA2 is indispensable for EphA2-dependent nasopharyngeal carcinoma cell invasion, metastasis and stem properties. Cancer Lett (2019) 444:162–74. doi: 10.1016/j.canlet.2018.12.011
33. Feng J, Lu S-S, Xiao T, Huang W, Yi H, Zhu W, et al. ANXA1 Binds and Stabilizes EphA2 to Promote Nasopharyngeal Carcinoma Growth and Metastasis. Cancer Res (2020) 80:4386–98. doi: 10.1158/0008-5472.CAN-20-0560
34. Feng X-P, Yi H, Li M-Y, Li X-H, Yi B, Zhang P-F, et al. Identification of Biomarkers for Predicting Nasopharyngeal Carcinoma Response to Radiotherapy by Proteomics. Cancer Res (2010) 70:3450–62. doi: 10.1158/0008-5472.CAN-09-4099
35. Xiao T. RACK1 promotes tumorigenicity of colon cancer by inducing cell autophagy. Cell Death Dis (2018) 9:1148. doi: 10.1038/s41419-018-1113-9
36. Zhu J-F, Huang W, Yi H-M, Xiao T, Li J-Y, Feng J, et al. Annexin A1-suppressed autophagy promotes nasopharyngeal carcinoma cell invasion and metastasis by PI3K/AKT signaling activation. Cell Death Dis (2018) 9:1154. doi: 10.1038/s41419-018-1204-7
37. He Q-Y, Yi H-M, Yi H, Xiao T, Qu J-Q, Yuan L, et al. Reduction of RKIP expression promotes nasopharyngeal carcinoma invasion and metastasis by activating Stat3 signaling. Oncotarget (2015) 6:16422–36. doi: 10.18632/oncotarget.3847
38. Zeng G-Q, Yi H, Li X-H, Shi H-Y, Li C, Li M-Y, et al. Identification of the proteins related to p53-mediated radioresponse in nasopharyngeal carcinoma by proteomic analysis. J Proteomics (2011) 74:2723–33. doi: 10.1016/j.jprot.2011.02.012
39. Qu J-Q, Yi H-M, Ye X, Zhu J-F, Yi H, Li L-N, et al. MiRNA-203 Reduces Nasopharyngeal Carcinoma Radioresistance by Targeting IL8/AKT Signaling. Mol Cancer Ther (2015) 14:2653–64. doi: 10.1158/1535-7163.MCT-15-0461
40. Zheng Z. MiR-125b regulates proliferation and apoptosis of nasopharyngeal carcinoma by targeting A20/NF-κB signaling pathway. Cell Death Dis (2017) 8:e2855. doi: 10.1038/cddis.2017.211
41. Lu S, Yu Z, Xiao Z, Zhang Y. Gene Signatures and Prognostic Values of m6A Genes in Nasopharyngeal Carcinoma. Front Oncol (2020) 10:875. doi: 10.3389/fonc.2020.00875
42. Xiang Y-P, Xiao T, Li Q-G, Lu S-S, Zhu W, Liu Y-Y, et al. Y772 phosphorylation of EphA2 is responsible for EphA2-dependent NPC nasopharyngeal carcinoma growth by Shp2/Erk-1/2 signaling pathway. Cell Death Dis (2020) 11:709. doi: 10.1038/s41419-020-02831-0
43. Fujii T, Shimizu T. Crosstalk between Na+,K+-ATPase and a volume-regulated anion channel in membrane microdomains of human cancer cells. Biochim Biophys Acta (BBA) - Mol Basis Dis (2018) 1864:3792–804. doi: 10.1016/j.bbadis.2018.09.014
44. Wang H-YL, O’Doherty GA. Modulators of Na/K-ATPase: a patent review. Expert Opin Ther Pat (2012) 22:587–605. doi: 10.1517/13543776.2012.690033
45. Mijatovic T, Roland I, Van Quaquebeke E, Nilsson B, Mathieu A, Van Vynckt F, et al. The α1 subunit of the sodium pump could represent a novel target to combat non-small cell lung cancers. J Pathol (2007) 212:170–9. doi: 10.1002/path.2172
46. Wu I-C, Chen Y-K, Wu C-C, Cheng Y-J, Chen W-C, Ko H-J, et al. Overexpression of ATPase Na+/K+ transporting alpha 1 polypeptide, ATP1A1, correlates with clinical diagnosis and progression of esophageal squamous cell carcinoma. Oncotarget (2016) 7:85244. doi: 10.18632/oncotarget.13267
47. Seligson DB, Rajasekaran SA, Yu H, Liu X, Eeva M, Tze S, et al. Na,K-Adenosine Triphosphatase α1-Subunit Predicts Survival of Renal Clear Cell Carcinoma. J Urol (2008) 179:338–45. doi: 10.1016/j.juro.2007.08.094
48. Lefranc F, Mijatovic T, Kondo Y, Sauvage S, Roland I, Debeir O, et al. Targeting the α 1 subunit of the sodium pump to combat glioblastoma cells. Neurosurgery (2008) 62:211–22. doi: 10.1227/01.NEU.0000311080.43024.0E
49. Li Z, Zhang Z, Xie JX, Li X, Tian J, Cai T, et al. Na/K-ATPase Mimetic pNaKtide Peptide Inhibits the Growth of Human Cancer Cells. J Biol Chem (2011) 286:32394. doi: 10.1074/jbc.M110.207597
50. Rajasekaran SA. Multiple Functions of Na,K-ATPase in Epithelial Cells. Semin Nephrol (2005) 25:328–34. doi: 10.1016/j.semnephrol.2005.03.008
51. Li L, Feng R, Xu Q, Zhang F, Liu T, Cao J, et al. Expression of the β3 subunit of Na+/K+-ATPase is increased in gastric cancer and regulates gastric cancer cell progression and prognosis via the PI3/AKT pathway. Oncotarget (2017) 8:84285. doi: 10.18632/oncotarget.20894
52. Talab F, Allen JC, Thompson V, Lin K, Slupsky JR. LCK Is an Important Mediator of B-Cell Receptor Signaling in Chronic Lymphocytic Leukemia Cells. Mol Cancer Res (2013) 11:541–54. doi: 10.1158/1541-7786.MCR-12-0415-T
53. Shibuya K, Fukuoka J, Fujii T, Shimoda E, Shimizu T, Sakai H, et al. Increase in ouabain-sensitive K+-ATPase activity in hepatocellular carcinoma by overexpression of Na+,K+-ATPase α3-isoform. Eur J Pharmacol (2010) 638:42–6. doi: 10.1016/j.ejphar.2010.04.029
54. Li H, Wang P, Gao Y, Zhu X, Liu L, Cohen L, et al. Na+/K+-ATPase α3 mediates sensitivity of hepatocellular carcinoma cells to bufalin. Oncol Rep (2011) 25:825–30. doi: 10.3892/or.2010.1120
55. Rajasekaran SA, Palmer LG, Quan K, Harper JF, Ball WJ Jr, Bander NH, et al. Na,K-ATPase β-Subunit Is Required for Epithelial Polarization, Suppression of Invasion, and Cell Motility. Mol Biol Cell (2001) 12:279. doi: 10.1091/mbc.12.2.279
56. Pinato DJ, Guerra N, Fessas P, Murphy R, Mineo T, Mauri FA, et al. Immune-based therapies for hepatocellular carcinoma. Oncogene (2020) 14:1–18. doi: 10.1038/s41388-020-1249-9
57. Kennedy DJ, Chen Y, Huang W, Viterna J, Liu J, Westfall K, et al. CD36 and Na/K-ATPase-α1 Form a Pro-inflammatory Signaling Loop in Kidney. Hypertension (2013) 61:216. doi: 10.1161/HYPERTENSIONAHA.112.198770
58. Khalaf FK, Dube P, Kleinhenz AL, Malhotra D, Gohara A, Drummond CA, et al. Proinflammatory Effects of Cardiotonic Steroids Mediated by NKA α-1 (Na+/K+-ATPase α-1)/Src Complex in Renal Epithelial Cells and Immune Cells. Hypertension (2019) 74:73–82. doi: 10.1161/HYPERTENSIONAHA.118.12605
59. Wei X, Shao B, He Z, Ye T, Luo M, Sang Y, et al. Cationic nanocarriers induce cell necrosis through impairment of Na + /K + -ATPase and cause subsequent inflammatory response. Cell Res (2015) 25:237–53. doi: 10.1038/cr.2015.9
60. Fu Y, Liu S, Zeng S, Shen H. From bench to bed: the tumor immune microenvironment and current immunotherapeutic strategies for hepatocellular carcinoma. J Exp Clin Cancer Res (2019) 38:398. doi: 10.1186/s13046-019-1396-4
61. Cheng H, Sun G, Chen H, Li Y, Han Z, Li Y, et al. Trends in the treatment of advanced hepatocellular carcinoma: immune checkpoint blockade immunotherapy and related combination therapies. Am J Cancer Res (2019) 9:1536.
62. Krenkel O, Tacke F. Liver macrophages in tissue homeostasis and disease. Nat Rev Immunol (2017) 17:306–21. doi: 10.1038/nri.2017.11
63. Nakano S, Eso Y, Okada H, Takai A, Takahashi K, Seno H. Recent Advances in Immunotherapy for Hepatocellular Carcinoma. Cancers (2020) 12:775. doi: 10.3390/cancers12040775
64. Khalaf FK, Tassavvor I, Mohamed A, Chen Y, Malhotra D, Xie Z, et al. Epithelial and Endothelial Adhesion of Immune Cells Is Enhanced by Cardiotonic Steroid Signaling Through Na+/K+-ATPase-α-1. J Am Heart Assoc (2020) 9:e013933. doi: 10.1161/JAHA.119.013933
65. Gil M, Kim KE. Interleukin-18 Is a Prognostic Biomarker Correlated with CD8+ T Cell and Natural Killer Cell Infiltration in Skin Cutaneous Melanoma. J Clin Med (2019) 8:1993. doi: 10.3390/jcm8111993
66. Cui C, Fu K, Yang L, Wu S, Cen Z, Meng X, et al. Hypoxia-inducible gene 2 promotes the immune escape of hepatocellular carcinoma from nature killer cells through the interleukin-10-STAT3 signaling pathway. J Exp Clin Cancer Res (2019) 38:229. doi: 10.1186/s13046-019-1233-9
67. Qin S, Ma S, Huang X, Lu D, Zhou Y, Jiang H. Th22 cells are associated with hepatocellular carcinoma development and progression. Chin J Cancer Res (2014) 26:135–41. doi: 10.3978/j.issn.1000-9604.2014.02.14
68. Kong F, Zhou K, Zhu T, Lian Q, Tao Y, Li N, et al. Interleukin-34 mediated by hepatitis B virus X protein via CCAAT/enhancer-binding protein α contributes to the proliferation and migration of hepatoma cells. Cell Prolif (2019) 52:e12703. doi: 10.1111/cpr.12703
69. Hu P, Hu H-D, Chen M, Peng M-L, Tang L, Tang K-F, et al. Expression of interleukins-23 and 27 leads to successful gene therapy of hepatocellular carcinoma. Mol Immunol (2009) 46:1654–62. doi: 10.1016/j.molimm.2009.02.025
Keywords: Na+/K+-ATPase (NKA), hepatocellular carcinoma (HCC), ATP1B3, biomarker, immune
Citation: Lu S, Cai S, Peng X, Cheng R and Zhang Y (2021) Integrative Transcriptomic, Proteomic and Functional Analysis Reveals ATP1B3 as a Diagnostic and Potential Therapeutic Target in Hepatocellular Carcinoma. Front. Immunol. 12:636614. doi: 10.3389/fimmu.2021.636614
Received: 01 December 2020; Accepted: 12 March 2021;
Published: 02 April 2021.
Edited by:
Frank Tacke, Charité – Universitätsmedizin Berlin, GermanyReviewed by:
Isabella Lurje, Charité – Universitätsmedizin Berlin, GermanyJoeri Lambrecht, Charité – Universitätsmedizin Berlin, Germany
Copyright © 2021 Lu, Cai, Peng, Cheng and Zhang. This is an open-access article distributed under the terms of the Creative Commons Attribution License (CC BY). The use, distribution or reproduction in other forums is permitted, provided the original author(s) and the copyright owner(s) are credited and that the original publication in this journal is cited, in accordance with accepted academic practice. No use, distribution or reproduction is permitted which does not comply with these terms.
*Correspondence: Yiya Zhang, yiya0108@csu.edu.cn