- Division of Rheumatology, Departments of Medicine, Microbiology and Immunology, and Biochemistry and Molecular Biology, College of Medicine, State University of New York, Upstate Medical University, Syracuse, NY, United States
Interleukin-2 (IL-2) expands the depleted T regulatory (Treg) cell population, and it has emerged as a potential therapy in systemic lupus erythematosus (SLE). However, IL-2 administration may involve the risk of expanding unwanted pro-inflammatory cells. We herein studied the effects of IL-2 on pro-inflammatory cytokine production by CD4+ and CD8+ T cells in parallel with Treg development following CD3/CD28 co-stimulation. While Treg cells are depleted in SLE patients, their CD4+ T cells were poised to receive and activate IL-2 signaling as evidenced by upregulation of CD25 and enhanced IL-2-incued STAT5 phosphorylation during Treg differentiation. In patients with SLE, however, IL-2 also expanded CD8+ T cells capable of producing interleukin-5, interkeukin-13 (IL-13), and interferon-γ (IFN-γ) that occurred with enhanced expression of GATA-3 and phosphorylation of STAT6 but not STAT5. Our data pinpoint a safety signal for systemic administration of IL-2 and challenges a long-held conceptual platform of type 1 and 2 cytokine antagonism by newly documenting the IL-2-dependent development of IL-13 and IFN-γ double-positive (IL-13+IFNγ+) CD8+ T cells in SLE.
Introduction
Systemic lupus erythematosus (SLE) is an autoimmune disease characterized by aberrant T- and B-cell activation culminating in a production of antinuclear antibodies (1). Among numerous immune dysregulation pathways implicated in the pathogenesis, the depletion of T regulatory (Treg) cells has emerged as an important mediator of the disease (2–4). In this respect, interleukin (IL)-2 elicits T regulatory (Treg) cell differentiation in association with phosphorylation of STAT5 and its binding to the Foxp3 gene (5). These findings along with IL-2 deficiency in lupus patients (6) yielded the notion that supplementation of IL-2 could restore the immune tolerance by expanding the Treg cell population. Indeed, low dose IL-2 therapy was shown to expand Treg cells and ameliorate the lupus disease activity (7).
Non-selective administration of IL-2, however, poses a concern for potential expansion of unwanted pro-inflammatory cells in view of its pleiotropic functions, in particular, its role as a T-cell growth factor (8). In fact, IL-2 induces Th1 and Th2 differentiation in a STAT5-dependent manner (9, 10). In addition, IL-2 elicits the differentiation of naïve CD8+ T cells into effector and memory cytotoxic T cells along with the induction of interferon (IFN)-γ, perforin, and granzymes (11).
While extensive evidence underpins the essentiality of CD4+ and CD4-CD8- double-negative T cells in lupus pathogenesis (12–14), roles for CD8+ T cells have also been increasingly recognized. CD8+ T cells during a lupus flare exhibit more prominent cytotoxic phenotype and functions than during remission, and the frequency of such cells correlates with the SLE disease activity index (SLEDAI) score (15). Tubulointerstitial nephritis associated with CD8+ T cell infiltrates confers an increased risk for progressive lupus nephritis (16). With regard to the type of immune response mediated by T cells, it remains controversial whether SLE is driven by type 1 or type 2 immunity given the various animal models showing discrepant findings. In humans, some studies showed increased IL-4, but decreased IFN-γ in lupus patients (17), whereas others indicate the importance of IFN-γ in diffuse proliferative lupus nephritis (18). SLE patients with higher SLEDAI score have lower IFN-γ but higher IL-4 expression than those with lower SLEDAI score (19). Another type 2 cytokine, IL-13, shares many biological functions with IL-4, as exemplified by when human IL-13 elicits B-cell proliferation and its immunoglobulin production (20–22). In addition to the contribution of IL-13 to asthma and allergic disorders (23, 24), it is important to note that GATA-3-dependent IL-13 production by CD8+ T cells promotes fibrosis in systemic sclerosis (25, 26). While our understanding of lupus T cell biology has been rapidly evolving, it is unknown how IL-2 affects the lineage-specification of lupus T cells, and whether IL-13 plays a role in immune dysregulation in SLE.
In this study, we evaluated the effects of IL-2 on pro-inflammatory cytokine expression in SLE CD4+ and CD8+ T cells in comparison to those on Treg cell development. While Treg population is depleted in SLE patients, their CD4+ T cells were primed to receive and activate IL-2 signaling as evidenced by upregulation of CD25 and enhanced IL-2-induced STAT5 phosphorylation during Treg differentiation. On the other hand, SLE CD8+ T cells produced greater amount of IL-13 and IFN-γ than CD4+ T cells in an IL-2-dependent manner. In addition, IL-2 expanded the IL-13-producing lupus CD8+ T cells that also expressed IL-5 and IFN-γ in association with STAT6 phosphorylation and GATA-3 expression, but not with STAT5 phosphorylation. Our data conveys a clear safety signal in further pursuit of systemic administration of IL-2, and supports the rationale for Treg-cell-targeted delivery of IL-2 in the treatment of SLE.
Materials and Method
Human Subjects
In total, 33 patients with SLE fulfilling the American College of Rheumatology diagnostic criteria (27) were studied. In each experiment, peripheral blood was obtained from SLE patients (all female) and healthy control (HC) subjects who were matched to the patients by age (within 10 years), sex, and ethnic background. Age of study participants was 44.8 ± 2.0 (mean ± SD) years in SLE, and 44.2 ± 1.9 years in HC subjects. Disease activity was assessed by the SLEDAI scores (28), which ranged from 0 to 34 (mean ± SD: 6.24 ± 1.17). Mean daily prednisone dose was 6.52 ± 1.62 mg. Immunosuppressive drugs taken by the study subjects included hydroxychloroquine (N=31), methotrexate (N=1), mycophenolate mofetil (N=10), mycophenolic acid (N=1), azathioprine (N=5), cyclosporine (N=1), tacrolimus (N=1), belimumab (N=6), and abatacept (N=1). The study was approved by the Institutional Review Board at the SUNY Upstate Medical University.
Isolation of Untouched T Cells and Cell Culture
Peripheral blood mononuclear cells (PBMCs) were isolated by using Ficoll Histopaque gradient (GE Health Care Bio-Sciences). CD3+ T cells were isolated by negative selection using untouched human T cell isolation kit (Life technologies, Cat# 11344D). Purity of CD3+ T cells was confirmed to be above 97%. Cells were cultured in RPMI culture media with 10% FCS, 1% Penicillin/Streptomycin, and 1% L-glutamine (all from Corning CellGro except for FCS, which was from Gibco) for 3 days, and stained with PE Cy7-conjugated anti-CD4 (Clone: SK3, Cat# 557852, RRID : AB_396897) and phycoerythrine (PE)-conjugated anti-CD25 (Clone: M-A251, Cat# 555432, RRID : AB_395826, both from BD Biosciences). The cells were permeabilized as per the manufacturer’s instructions and stained with AF-647-conjugated anti-FoxP3 (Biolegend, Clone: 150D, Cat# 320014, RRID : AB_439750).
Isolation of CD4+ and CD8+ T Cells and Cell Culture
CD4+ and CD8+ T cells were isolated by negative selection using human CD4+ T cell enrichment (Cat# 15062) and CD8+ T cell isolation kits (Cat# 17953, both from STEMCELL), respectively. Cells were cultured for 3 days in the presence of plate-bound anti-CD3 (anti TCR ϵ hybridoma from ATCC) and soluble anti-CD28 (1 μg/ml, BD Biosciences, Clone: CD28.2, Cat# 555725, RRID : AB_396068) in the presence or absence of IL-2 (100 IU/ml, Peprotech, Cat# 200-02) or anti-IL-2 (100 or 1000 ng/ml, R&D Systems, Cat# MAB202, RRID : AB_2264789).
Intracellular Staining
Cells were pre-incubated with PMA (5 ng/ml) and ionomycin (500 ng/ml) for 6 h, and with brefeldin A for 5 h (10 μg/ml; all from Sigma-Aldrich). For cytokine detection, FITC-conjugated anti–IFN-γ (Clone: B27, Cat# 554700, RRID : AB_395517), PE-conjugated anti-IL-5 (Clone: JES1-39D10, Cat# 559332, RRID : AB_397229), or IL-17 (Clone: SCPL1362, Cat# 560436, RRID : AB_1645514), BV711 conjugated anti-IL-13 (Clone: JES10-5A2, Cat# 564288, RRID : AB_2738731), and allophycocyanin-conjugated anti-IL-4 (Clone: 8D4-8, Cat# 560671, RRID : AB_1727546) or IL-21 (Clone: 3A3-N2.1, Cat# 562043, RRID : AB_10896655, all from BD Biosciences) were used alone or together. Isotype control Abs included FITC-conjugated mouse IgG1 κ (Clone: MOPC-21, Cat# 551954, RRID : AB_394297), PE-conjugated rat IgG2a κ (Clone: R35-95, Cat# 559317, RRID : AB_10050484), BV711-conjugated rat IgG1 κ (Clone: R3-34, Cat# 563283, RRID : AB_2869482), and Alexa Fluor (AF)-647-conjugated mouse IgG1 κ (Clone: MOPC-21, Cat# 557732, RRID : AB_396840, all from BD Biosciences).
T Regulatory Cell Polarization
Naive CD4+ T cells were isolated from SLE and matched HC subjects by using Human Naive CD4+ T cell Enrichment Kit (STEMCELL, Cat# 19555). The purity of naive CD4+ T cells as defined by the proportion of CD4+CD45RA+CD62L+ cells was above 99%. Cells were cultured for 72 h in the presence of anti-CD3/CD28 and TGF-β (5 ng/ml, Peprotech, Cat# 100-21) with IL-2 (50 IU/ml) or anti-IL-2 (100 or 1000 ng/ml). Cells were stained with FITC-conjugated anti-CD25 (Clone: M-A251, Cat# 555431, RRID : AB_395825) and AF-647-conjugated anti-FoxP3 (Clone: 259D/C7, Cat# 560045, RRID : AB_1645411 both from BD Biosciences). Frequency of CD4+CD25+FOXP3+ cells was determined by flow cytometry. In other experiments, CD4+ T cells isolated from matched SLE and HC subjects were cultured for 3 days in the presence of anti-CD3/CD28 and TGF-β (20 ng/ml) with or without IL-2 (100 IU/ml) or anti-IL-2 (100 ng/ml).
Immunoblotting
Using lysates of CD4+ T cells cultured under Treg-polarizing conditions, total STAT5 (Clone: A-9, Cat# sc-74442, RRID : AB_1129711) and its phosphorylation at tyrosine 694 (pSTAT5Y694, Clone: C11C5, Cat# 9359, RRID : AB_823649) were detected by immunoblotting. Using lysates of CD8+ T cells cultured in the presence or absence of IL-2 or anti-IL-2, phosphorylation of STAT5 at tyrosine 694, phosphorylation of STAT6 at tyrosine 641 (pSTAT6Y641, Clone: C11A12, Cat# 9364, RRID : AB_2271227), and expression of GATA-3 (Clone: HG3-31, Cat# sc-268, RRID : AB_2108591) were determined by immunoblotting (anti-STAT5 and anti-GATA-3 were from Santa Cruz Biotechnology whereas the remainder was from Cell Signaling Technology). The signal intensity was normalized to that of actin (Millipore, Clone: C4, Cat# MAB1501, RRID : AB_2223041).
Statistical Analysis
Student t test was performed for comparison of phenotype between two groups with two-tailed p values < 0.05 considered significant. Two-way ANOVA was followed by Bonferroni’s posttest for multiple comparisons using Prism 8 software (GraphPad, La Jolla, CA). Association of two variables was determined by Pearson’s and Spearman’s correlation analyses.
Results
Systemic Lupus Erythematosus CD4+ T Cells Are Poised to Receive Interleukin-2 Signaling During T Regulatory Cell Differentiation
To confirm the essentiality of IL-2 signaling in Treg differentiation in SLE, naïve CD4+ T cells were cultured under Treg-polarizing conditions in the presence or absence of IL-2 or anti-IL-2. IL-2 blockade abrogated Treg differentiation both in SLE and healthy control subjects (Figure 1A). Of note, supplementation of IL-2 induced STAT5 phosphorylation in SLE CD4+ T cells, but not in healthy control CD4+ cells (Figure 1B). Such a heightened sensitivity to IL-2 prompted us to examine the expression of IL-2 receptor α chain (CD25) on SLE CD4+ T cells (29). Instead of immunophenotyping cells immediately after isolation, untouched CD3+ T cells were rested in culture media without anti-CD3/CD28 stimulation for 3 days to eliminate the effects of immunosuppressive drugs that the study subjects had received. Although CD4+CD25+FOXP3+ Treg population was depleted, CD25 was upregulated on lupus CD4+ T cells, resulting in diminished proportion of CD4+CD25+FOXP3+ Treg cells among the CD4+CD25+ cells in SLE (Figure 1C). Collectively, although SLE CD4+ T cells are primed to receive and activate IL-2 signaling as evidenced by the upregulation of CD25 and increased IL-2-induced STAT5 phosphorylation under Treg-polarizing conditions, Treg cells are depleted in SLE patients (Figure 1C). However, it is corrected once cells are stimulated with anti-CD3/CD28 (Figure 1A (12, 30),) likely because of a large amount of IL-2 produced by T cells (30). These findings point to the IL-2 deficiency underlying the SLE Treg depletion (6).
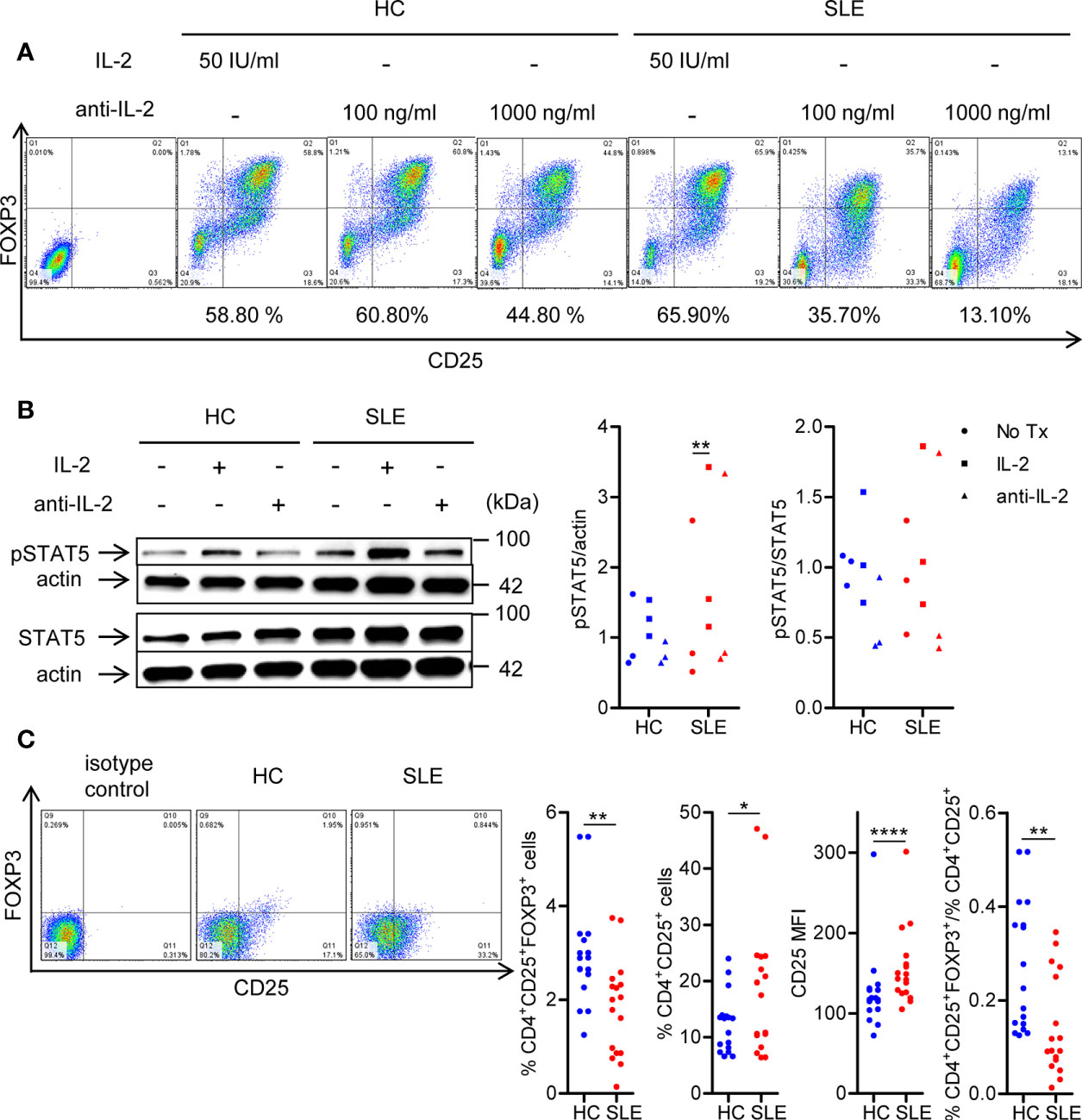
Figure 1 SLE CD4+ T cells are poised to activate IL-2 signaling during Treg differentiation. (A) Naïve CD4+ T cells were isolated from a systemic lupus erythematosus (SLE) patient and matched healthy control (HC) subject, and cultured for 3 days in the presence of anti-CD3/CD28 and TGF-β (5 ng/ml) with IL-2 (50 IU/ml) or anti-IL-2 (100 or 1,000 ng/ml). The frequency of CD4+CD25+FOXP3+ cells was determined by flow cytometry. Numbers below the plots represent the frequency of CD4+CD25+FOXP3+ Tregs. The dot plots on the left end represent isotype control staining. (B) CD4+ T cells isolated from matched SLE and HC subjects were cultured for 3 days in the presence of anti-CD3/CD28 and TGF-β (20 ng/ml) with or without IL-2 (100 IU/ml) or anti-IL-2 (100 ng/ml). Total STAT5 and its phosphorylation at tyrosine 694 were detected by immunoblotting. Representative immunoblot staining (left panel). The signal intensity of phospho-STAT5 and total STAT5 was normalized to that of actin. The normalized pSTAT5 signal intensity (middle panel) and the ratio of normalized pSTAT5 signal intensity over normalized STAT5 signal intensity (right panel) from 3 pairs of matched HC and SLE subjects. (C) Untouched T cells from matched SLE and HC subjects were cultured for 3 days without anti-CD3/CD28 stimulation. Expression of CD25 and FOXP3 in CD4+ cells were determined by flow cytometry. Representative flow cytometry dot plots are shown (left panel). Cumulative data of frequency of CD4+CD25+FOXP3+ and CD4+CD25+ cells, mean fluorescence intensity (MFI) of CD25 expression in CD4+ T cells, and the proportion of CD4+CD25+FOXP3+ cells among CD4+CD25+ cells from 17 pairs of matched SLE and HC subjects (right panel). Data were analyzed by a paired two-tailed t-test (*p<0.05, **p<0.01, ****p<0.0001).
Interleukin-2 Elicits the Expansion of Interleukin-13-Producing CD8+ T Cells That Also Express Interleukin-5 and Interferon-γ
To evaluate the roles for IL-2 in pro-inflammatory cytokine expression in SLE T cells, magnetically isolated CD4+ and CD8+ T cells were cultured in the presence or absence of IL-2 or anti-IL-2. IL-2 positively controls the expression of IL-5, IL-13, and IFN-γ, but not IL-4, in particular, in CD8+ T cells (Figures 2, 3, S1). CD4+ T cells appeared to be the predominant source of IL-21, and it was not IL-2 dependent (Figure S2). No meaningful IL-17 expression was observed (data not shown). Among these IL-2-dependennt cytokines, CD8+ T cells produced greater amount of IL-13 (HC: 30.41 ± 2.36%, 1.51 ± 0.14%, p<0.0001; SLE: 37.91 ± 2.27%, 1.53 ± 0.22%, p<0.0001) and IFN-γ (HC: 46.00 ± 3.22%, 25.07 ± 6.89%, p=ns; SLE: 48.04 ± 4.46%, 29.53 ± 2.83%, p<0.01) than CD4+ T cells (Figures 2 and 3). IL-13 expression in CD8+ T cells was greater than that in CD4+ T cells on a per cell basis as determined by the mean fluorescence intensity (MFI) (HC: 396.67 ± 26.54, 165.64 ± 6.10, p<0.0001; SLE: 470.42 ± 27.39, 158 ± 8.65, p<0.0001). Of note, SLE CD8+ T cells produced greater amount of IL-13 (% positive cells: SLE: 37.91 ± 2.27%, HC: 30.41 ± 2.36%, p<0.05; MFI: SLE: 470.42 ± 27.39, HC: 396.67 ± 26.54, p<0.05) than healthy control CD8+ T cells (Figures 2 and 3A).
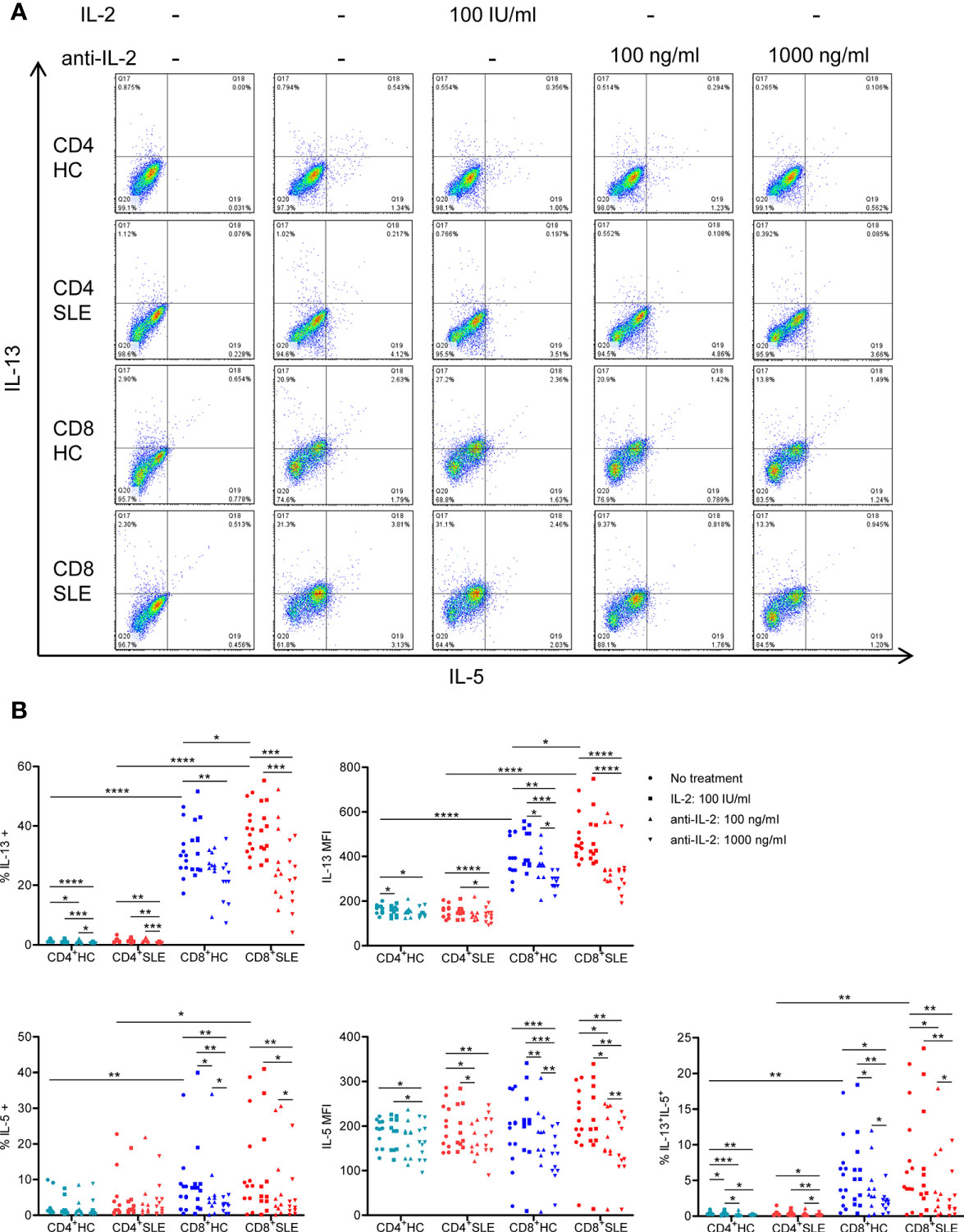
Figure 2 Systemic lupus erythematosus (SLE) CD8+ T cells produce increased IL-13 in an IL-2-dependent manner. (A) CD4+ and CD8+ T cells isolated from matched SLE and health control (HC) subjects were cultured for 3 days with anti-CD3/CD28 in the presence or absence of IL-2 (100 IU/ml) or anti-IL-2 (100 or 1,000 ng/ml). IL-5 and IL-13 expression was determined by flow cytometry. Representative flow cytometry dot plots are shown. The dot plots on the left end represent isotype control staining. (B) Cumulative data of MFI and the frequency of expression of individual cytokines from 12 pairs of matched SLE and HC subjects. Statistical analysis was made by two-way ANOVA followed by Bonferroni’s correction for multiple comparisons (*p<0.05, **p<0.01, ***p<0.001, ****p<0.0001).
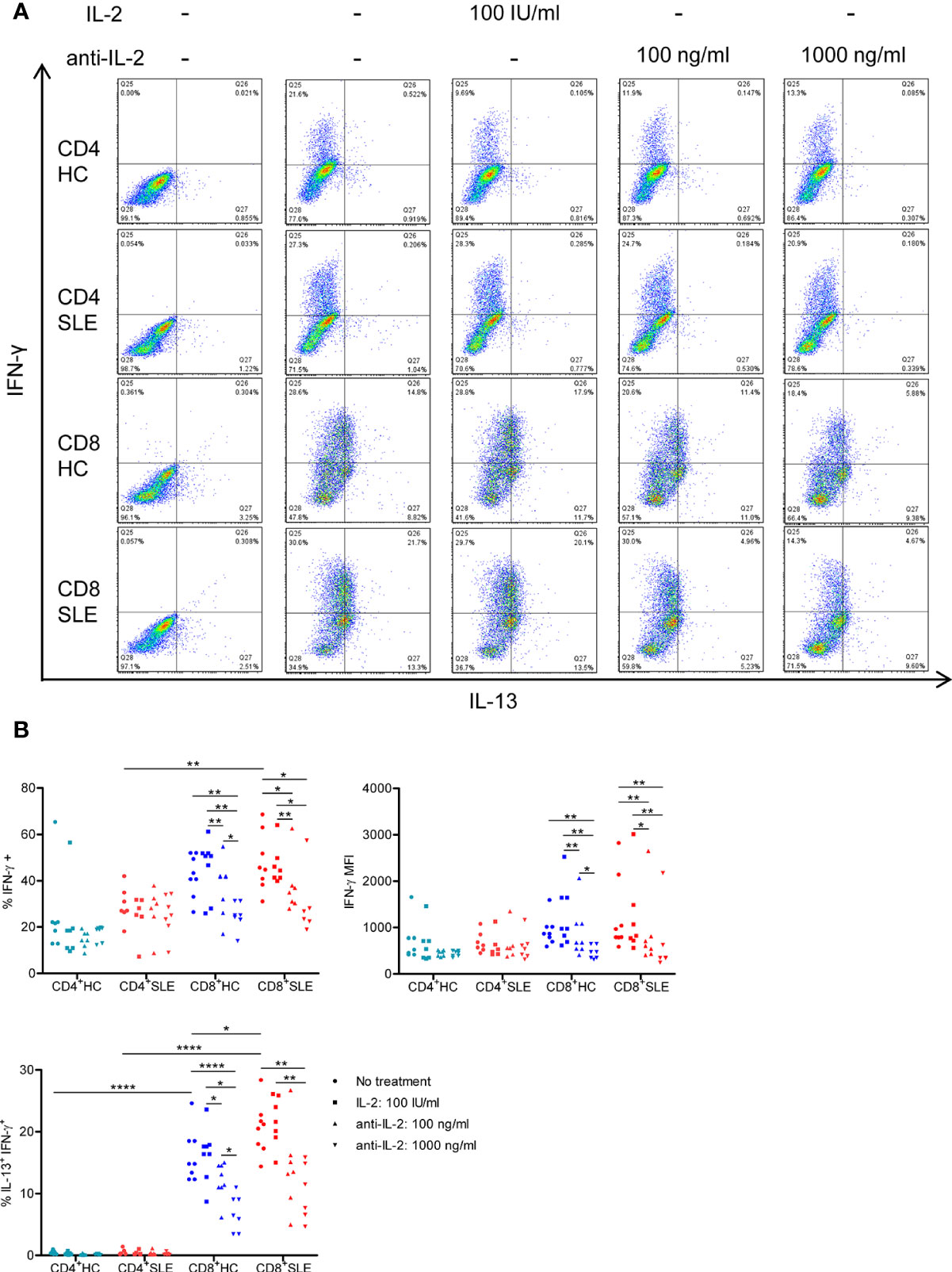
Figure 3 IL-2 expands IL-13+IFN-γ+ CD8+ T cells in systemic lupus erythematosus (SLE). (A) CD4+ and CD8+ T cells from matched SLE and health control (HC) subjects were cultured as described in Figure 2, and IL-13 and IFN-γ expression was determined by flow cytometry. (B) Cumulative data of mean fluorescence intensity (MFI) of IFN-γ and the frequency of IFN-γ+ and IL-13+IFN-γ+ cells. Statistical analysis was made by two-way ANOVA followed by Bonferroni’s correction for multiple comparisons (*p<0.05, **p<0.01, ****p<0.0001).
We next turned to the correlation analysis of type 1 and 2 cytokine expression. IL-13 expression was greater in IL-5-expressing cells than IL-5-non-expressing cells both in CD4+ and CD8+ T cells (Figure S3A). Accordingly, the expression of IL-13 and IL-5 was positively correlated in CD8+ T cells (Figure S3B), yielding a IL-13+ IL-5+ CD8+ T cell population that contracted in association with IL-2 blockade (Figure 2). In contrast, the expression of IL-13 and IL-4 appeared to be mutually exclusive (Figure S1A), and there was no difference of IL-13 expression between IL-4-expressing and -non-expressing cells (Figure S3A). Unexpectedly, IL-13 expression in IFN-γ-expressing CD8+ T cells was greater than that in IFN-γ-non-expressing CD8+ T cells (Figure S3A). Along this line, there was a positive correlation between the expression of IL-13 and IFN-γ (Figure S3B), resulting in the expansion of IL-13+ IFN-γ+ double-positive CD8+ T cells in SLE (SLE: 20.53 ± 1.48%, HC: 16.15 ± 1.48%, p<0.05, Figures 3A, B) which contracted in association with IL-2 blockade. Collectively, IL-2 expanded IL-13-expressing CD8+ T cells including an IL-13+ IFN-γ+ double-positive subset in SLE.
Interleukin-13 and Interleukin-5 Expression Are Associated with Interleukin-2-Induced STAT6 Phosphorylation and GATA-3 Expression in CD8+ T Cells
To understand the mechanisms by which IL-2 induces the expansion of IL-13- and IL-5-producing CD8+ T cells, phosphorylation of STAT5 and STAT6 and GATA-3 expression were determined in the lysates of CD8+ T cells cultured in the presence or absence of IL-2 or anti-IL-2. Neutralization of IL-2 profoundly diminished the phosphorylation of STAT6 and GATA-3 expression in SLE CD8+ T cells; however, with regard to the impact of IL-2 blockade on STAT5 phosphorylation, such dose-dependent abolishment of phospho-STAT5 was not observed (Figures 4A, B). Of note, the expression of IL-13 and IL-5 were positively correlated with STAT6 phosphorylation and GATA-3 expression, but negatively correlated with STAT5 phosphorylation in CD8+ T cells (Figure 4C). In this respect, we previously documented increased GATA-3 expression in SLE CD8+ T cells as compared with healthy control CD8+ T cells (12). In contrast to these type 2 cytokines, IL-4 expression was not correlated with STAT5 or STAT6 phosphorylation or GATA-3 expression (data not shown). Unexpectedly, there was a positive correlation between the expression of IFN-γ and GATA-3 in CD8+ T cells (Figure 4C), which may account for the concurrent expression of IL-13 and IFN-γ (Figure 3). Our data collectively suggests that IL-2 induces IL-13, IL-5, and IFN-γ expression in lupus CD8+ T cells via STAT6-GATA-3 dependent mechanisms in contrast to the Treg differentiation in which IL-2-STAT5 axis plays a more essential role (Figure 1).
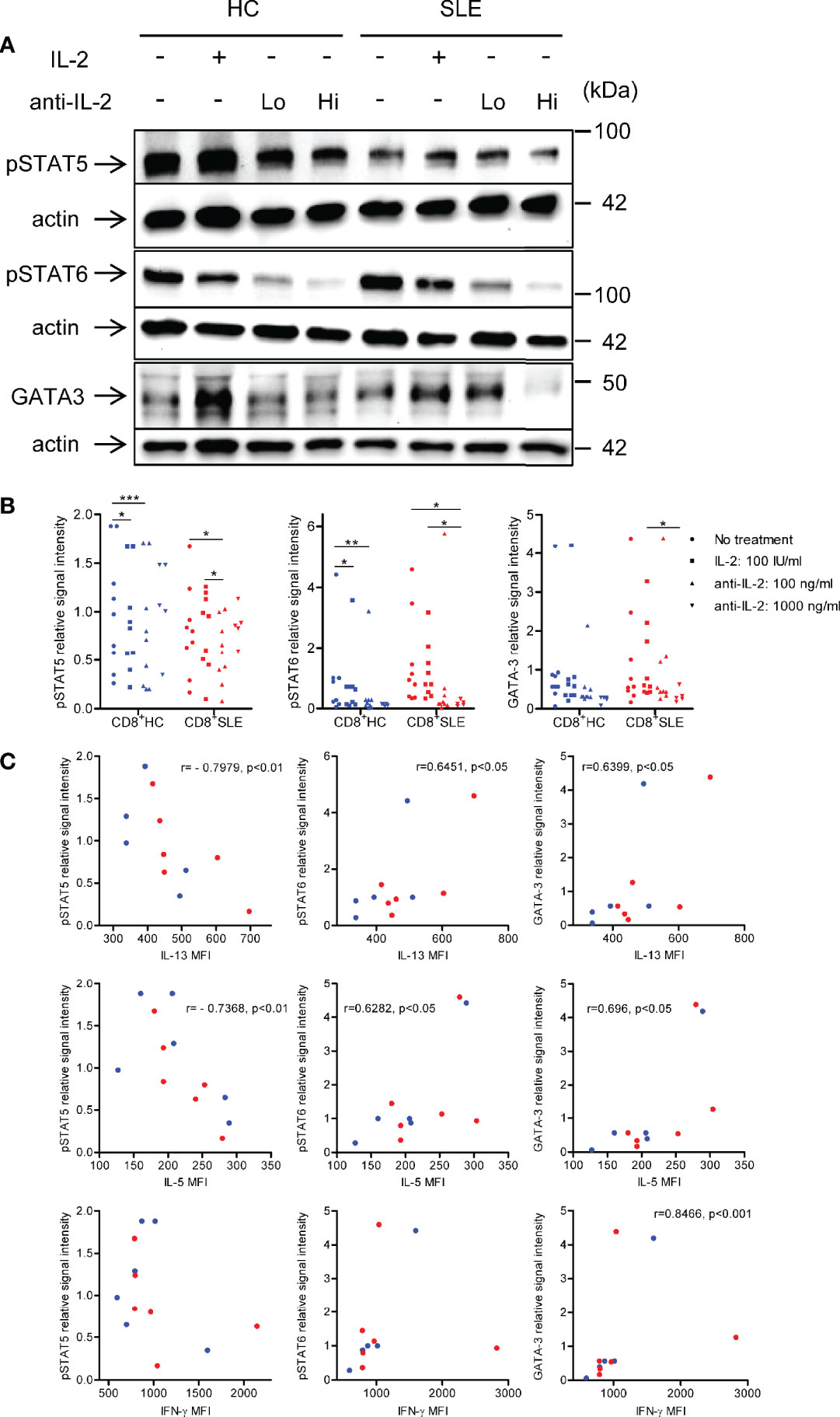
Figure 4 IL-2 induces STAT6 phosphorylation and GATA3 expression in systemic lupus erythematosus (SLE) CD8+ T cells. (A) CD8+ T cells from matched SLE and health control (HC) subjects were cultured as described in Figure 2. Expression of GATA-3 and phosphorylation of STAT5 at tyrosine 694 and STAT6 at tyrosine 641 were detected by immunoblotting. Representative immunoblot staining was presented. Lo and Hi concentrations of anti-IL-2 denote 100 and 1,000 ng/ml, respectively. (B) The signal intensity of phospho-STAT5, phospho-STAT6, and GATA-3 were normalized to that of actin. Cumulative data from 9 pairs of matched HC and SLE subjects. Data were analyzed by a two-tailed t-test (*p<0.05, **p<0.01, ***p<0.001). (C) Pearson’s and Spearman’s correlation analyses were performed to determine the association between the expression of cytokines (IL-13, IL-5, and IFN-γ) and transcription factors (phospho-STAT5, phospho-STAT6, and GATA-3). The blue and red plots represent data from HC and SLE patients, respectively. Spearman correlation coefficient was presented for the association between IL-13 and phospho-STAT5, IL-5 and phospho-STAT-5, and IFN-γ and GATA-3. Pearson correlation coefficient was presented for the remainder of associations.
Discussion
In this study, we documented that IL-2-STAT5 pathway was more critical to Treg differentiation in SLE than in health control subjects. On the other hand, IL-2 expanded IL-13-producing CD8+ T cells that also expressed IL-5 and IFN-γ in lupus patients via a signaling pathway likely involving the activation of STAT6-GATA-3 axis, but not STAT5. It is important to note that lupus CD4+ T cells were primed to receive and activate IL-2 signaling as evidenced by the upregulation of CD25 and enhanced IL-2-induced STAT5 phosphorylation during Treg differentiation even though the CD4+CD25+FOXP3+ Treg population was depleted in SLE patients. However, the Treg depletion is corrected by anti-CD3/CD28 stimulation in vitro (12, 30). A series of these findings pinpoint the IL-2 deficiency underlying the Treg depletion in SLE (6).
While it is not clear how IL-2 activates STAT6 and GATA-3 in CD8+ T cells, our data provides compelling evidence that this pathway is STAT5 independent. The neutralization of IL-2 did not have a robust impact on STAT5 phosphorylation unlike STAT6 phosphorylation and GATA-3 expression. In addition, the expression of IL-13 and IL-5 were negatively correlated with STAT5 phosphorylation in CD8+ T cells. It is worth noting here that IL-2-dependent STAT5 phosphorylation and GATA-3 expression are essential for IL-4-independent early IL-4 expression in CD4+ T cells (31). These observations suggest that IL-2 utilizes different signaling pathways in eliciting the activation and differentiation of CD4+ Treg cells, CD4+ non-Treg cells, and CD8+ T cells. Unlike the neutralization of IL-2, the supplementation of IL-2 had no effects on the expression of IL-5 and IL-13 and IFN-γ, which is attributed to a large amount of IL-2 produced by T cells in response to CD3/CD28 stimulation alone (30).
Although IL-2 activates the mechanistic target of rapamycin (mTOR), its blockade by sirolimus elicits Treg expansion both in vitro (30) and in vivo (32). This suggests that mTOR activation is not essential for IL-2-induced Treg expansion. IL-2 also activates the Akt-mTOR axis in CD8+ T cells (33). Our previous study showed that mTOR blockade restrained GATA-3 expression in FOXP3-CD4+ T cells (30). These findings suggest that the IL-2-Akt-mTOR axis may also affect GATA-3 expression in CD8+ T cells.
Among the three type 2 cytokines studied, there was a positive correlation between the expression of IL-13 and IL-5. IL-2 blockade abolished the expression of IL-13 and IL-5 in association with diminished STAT6 phosphorylation and GATA-3 expression. Additionally, the expression of these type 2 cytokines was positively correlated with STAT6 phosphorylation and GATA-3 expression. We previously reported increased GATA-3 expression in lupus CD8+ T cells (12). A series of these findings suggest that STAT6-GATA-3 axis drives the expression of IL-13 and IL-5 in CD8+ T cells. Conversely, the expression of IL-13 and IL-4 appeared mutually exclusive, and there was no appreciable size of IL-13+ IL-4+ population. Furthermore, IL-4 expression was resistant to IL-2-blockade-mediated abrogation of STAT6 phosphorylation and GATA-3 expression, and not correlated with these transcription factors. While our data does not exclude the involvement of STAT6-GATA-3 axis in the initial commitment to IL-4-expressing lineage as the study was not conducted on naïve CD8+ T cells, it suggests that CD8+ T cells maintain their IL-4 expression by STAT6-GATA-3-independent mechanisms.
While concurrent IFN-γ and IL-4 expression in CD4+ T cells has been reported (34), this is the first study documenting a dual expression of IL-13- and IFN-γ in CD8+ T cells. GATA-3 blocks IFN-γ expression (35, 36), whereas T-bet suppresses IL-4 and IL-5 expression in CD4+ T cells (37). Nonetheless, as such an antagonism has not been documented in CD8+ T cells, it is plausible that lupus CD8+ T cells employ a distinct molecular mechanism that allows for a dual IL-13- and IFN-γ expression. We identified a positive correlation between the expression of IFN-γ and GATA-3 in CD8+ T cells. Instead of proposing GATA-3 as a driver of IFN-γ expression in CD8+ T cells, we reason that IL-2 signaling activates a transcription factor upstream of both type 1 and type 2 programs. One such candidate is Notch as it was shown to concurrently regulate both Th1 and Th2 programs (38). Whether these cells in lupus patients are equipped with both helper- and cytotoxic functions would merit further investigation.
In conclusion, our data suggest that IL-2 supplementation may be a double-edged sword in the treatment of SLE, and supports the importance of Treg-cell-targeted delivery of IL-2. This study also challenged a long-held paradigm of type 1 and 2 cytokine antagonism by newly identifying IL-13+ IFN-γ+ CD8+ T cells that may potentially promote inflammation in SLE.
Data Availability Statement
The original contributions presented in the study are included in the article/Supplementary Material. Further inquiries can be directed to the corresponding author.
Ethics Statement
The studies involving human participants were reviewed and approved by SUNY UPSTATE IRB. The patients/participants provided their written informed consent to participate in this study.
Author Contributions
Both authors participated in the design and analysis of the experiments and writing of the manuscript. HK executed the experiments. All authors contributed to the article and approved the submitted version.
Funding
This work was supported by grants R01-AI072648 and AI122176 from the National Institutes of Health and the Department of Medicine at the SUNY Upstate Medical University.
Conflict of Interest
The authors declare that the research was conducted in the absence of any commercial or financial relationships that could be construed as a potential conflict of interest.
Acknowledgments
We thank all patients and healthy donors who contributed to this study.
Supplementary Material
The Supplementary Material for this article can be found online at: https://www.frontiersin.org/articles/10.3389/fimmu.2021.635531/full#supplementary-material
Supplementary Figure 1 | IL-2 does not control IL-4 expression. (A) CD4+ and CD8+ T cells from matched SLE and HC subjects were cultured as described in Figure 2 and IL-13 and IL-4 expression was determined by flow cytometry. (B) Cumulative data of MFI and frequency of IL-4+ cells. Statistical analysis was made by two-way ANOVA followed by Bonferroni’s correction for multiple comparisons (*p<0.05).
Supplementary Figure 2 | CD4+ T cells produce greater amount of IL-21 than CD8+ T cells in an IL-2-independent manner. (A) CD4+ and CD8+ T cells from matched SLE and HC subjects were cultured as described in Figure 2 and IL-13 and IL-21 expression was determined by flow cytometry. (B) Cumulative data of MFI and frequency of IL-21+ cells. Statistical analysis was made by two-way ANOVA followed by Bonferroni’s correction for multiple comparisons (*p<0.05, **p<0.01, ***p<0.001, ****p<0.0001).
Supplementary Figure 3 | Expression of IL-13 positively correlates with that of IL-5 and IFN-γ. (A) CD4+ and CD8+ T cells from matched SLE and HC subjects were cultured as described in Figure 2. The MFI of IL-13 was compared between IL-5+ and IL-5- cells, IFN-γ+ and IFN-γ- cells, and IL-4+ and IL-4- cells. Data were analyzed by a paired two-tailed t-test (*p<0.05, **p<0.01, ***p<0.001, ****p<0.0001). (B) Pearson’s and Spearman’s correlation analyses were performed to determine the association between IL-13 and IL-5 or IFN-γ. Pearson correlation coefficient was presented for the association of IL-13 with IL-5. Spearman correlation coefficient was presented for the association of IL-13 with IFN-γ.
References
1. Tsokos GC. Systemic lupus erythematosus. N Engl J Med (2011) 365:2110–21. doi: 10.1056/NEJMra1100359
2. Bonelli M, Savitskaya A, von Dalwigk K, Steiner CW, Aletaha D, Smolen JS, et al. Quantitative and qualitative deficiencies of regulatory T cells in patients with systemic lupus erythematosus (SLE). Int Immunol (2008) 20:861–8. doi: 10.1093/intimm/dxn044
3. Mellor-Pita S, Citores MJ, Castejon R, Tutor-Ureta P, Yebra-Bango M, Andreu JL, et al. Decrease of regulatory T cells in patients with systemic lupus erythematosus. Ann Rheum Dis (2006) 65:553–4. doi: 10.1136/ard.2005.044974
4. Miyara M, Amoura Z, Parizot C, Badoual C, Dorgham K, Trad S, et al. Global natural regulatory T cell depletion in active systemic lupus erythematosus. J Immunol (2005) 175:8392–400. doi: 10.4049/jimmunol.175.12.8392
5. Burchill MA, Yang J, Vogtenhuber C, Blazar BR, Farrar MA. IL-2 receptor beta-dependent STAT5 activation is required for the development of Foxp3+ regulatory T cells. J Immunol (2007) 178:280–90. doi: 10.4049/jimmunol.178.1.280
6. Linker-Israeli M, Bakke AC, Kitridou RC, Gendler S, Gillis S, Horwitz DA. Defective production of interleukin 1 and interleukin 2 in patients with systemic lupus erythematosus (SLE). J Immunol (1983) 130:2651–5.
7. He J, Zhang X, Wei Y, Sun X, Chen Y, Deng J, et al. Low-dose interleukin-2 treatment selectively modulates CD4(+) T cell subsets in patients with systemic lupus erythematosus. Nat Med (2016) 22:991–3. doi: 10.1038/nm.4148
8. Morgan DA, Ruscetti FW, Gallo R. Selective in vitro growth of T lymphocytes from normal human bone marrows. Science (1976) 193:1007–8. doi: 10.1126/science.181845
9. Liao W, Lin JX, Wang L, Li P, Leonard WJ. Modulation of cytokine receptors by IL-2 broadly regulates differentiation into helper T cell lineages. Nat Immunol (2011) 12:551–9. doi: 10.1038/ni.2030
10. Shi M, Lin TH, Appell KC, Berg LJ. Janus-kinase-3-dependent signals induce chromatin remodeling at the Ifng locus during T helper 1 cell differentiation. Immunity (2008) 28:763–73. doi: 10.1016/j.immuni.2008.04.016
11. Pipkin ME, Sacks JA, Cruz-Guilloty F, Lichtenheld MG, Bevan MJ, Rao A. Interleukin-2 and inflammation induce distinct transcriptional programs that promote the differentiation of effector cytolytic T cells. Immunity (2010) 32:79–90. doi: 10.1016/j.immuni.2009.11.012
12. Kato H, Perl A. Mechanistic target of rapamycin complex 1 expands Th17 and IL-4+ CD4-CD8- double-negative T cells and contracts regulatory T cells in systemic lupus erythematosus. J Immunol (2014) 192:4134–44. doi: 10.4049/jimmunol.1301859
13. Lai ZW, Borsuk R, Shadakshari A, Yu J, Dawood M, Garcia R, et al. Mechanistic target of rapamycin activation triggers IL-4 production and necrotic death of double-negative T cells in patients with systemic lupus erythematosus. J Immunol (2013) 191:2236–46. doi: 10.4049/jimmunol.1301005
14. Crispin JC, Oukka M, Bayliss G, Cohen RA, Van Beek CA, Stillman IE, et al. Expanded double negative T cells in patients with systemic lupus erythematosus produce IL-17 and infiltrate the kidneys. J Immunol (2008) 181:8761–6. doi: 10.4049/jimmunol.181.12.8761
15. Blanco P, Pitard V, Viallard JF, Taupin JL, Pellegrin JL, Moreau JF. Increase in activated CD8+ T lymphocytes expressing perforin and granzyme B correlates with disease activity in patients with systemic lupus erythematosus. Arthritis Rheum (2005) 52:201–11. doi: 10.1002/art.20745
16. Winchester R, Wiesendanger M, Zhang HZ, Steshenko V, Peterson K, Geraldino-Pardilla L, et al. Immunologic characteristics of intrarenal T cells: trafficking of expanded CD8+ T cell beta-chain clonotypes in progressive lupus nephritis. Arthritis Rheum (2012) 64:1589–600. doi: 10.1002/art.33488
17. Horwitz DA, Gray JD, Behrendsen SC, Kubin M, Rengaraju M, Ohtsuka K, et al. Decreased production of interleukin-12 and other Th1-type cytokines in patients with recent-onset systemic lupus erythematosus. Arthritis Rheum (1998) 41:838–44. doi: 10.1002/1529-0131(199805)41:5<838::AID-ART10>3.0.CO;2-S
18. Akahoshi M, Nakashima H, Tanaka Y, Kohsaka T, Nagano S, Ohgami E, et al. Th1/Th2 balance of peripheral T helper cells in systemic lupus erythematosus. Arthritis Rheum (1999) 42:1644–8. doi: 10.1002/1529-0131(199908)42:8<1644::AID-ANR12>3.0.CO;2-L
19. Min DJ, Cho ML, Cho CS, Min SY, Kim WU, Yang SY, et al. Decreased production of interleukin-12 and interferon-gamma is associated with renal involvement in systemic lupus erythematosus. Scand J Rheumatol (2001) 30:159–63. doi: 10.1080/030097401300162932
20. Aversa G, Punnonen J, Cocks BG, de Waal Malefyt R, Vega F Jr., Zurawski SM, et al. An interleukin 4 (IL-4) mutant protein inhibits both IL-4 or IL-13-induced human immunoglobulin G4 (IgG4) and IgE synthesis and B cell proliferation: support for a common component shared by IL-4 and IL-13 receptors. J Exp Med (1993) 178:2213–8. doi: 10.1084/jem.178.6.2213
21. Defrance T, Carayon P, Billian G, Guillemot JC, Minty A, Caput D, et al. Interleukin 13 is a B cell stimulating factor. J Exp Med (1994) 179:135–43. doi: 10.1084/jem.179.1.135
22. McKenzie AN, Culpepper JA, de Waal Malefyt R, Briere F, Punnonen J, Aversa G, et al. Interleukin 13, a T-cell-derived cytokine that regulates human monocyte and B-cell function. Proc Natl Acad Sci USA (1993) 90:3735–9. doi: 10.1073/pnas.90.8.3735
23. Miyahara N, Takeda K, Kodama T, Joetham A, Taube C, Park JW, et al. Contribution of antigen-primed CD8+ T cells to the development of airway hyperresponsiveness and inflammation is associated with IL-13. J Immunol (2004) 172:2549–58. doi: 10.4049/jimmunol.172.4.2549
24. Wills-Karp M, Luyimbazi J, Xu X, Schofield B, Neben TY, Karp CL, et al. Interleukin-13: central mediator of allergic asthma. Science (1998) 282:2258–61. doi: 10.1126/science.282.5397.2258
25. Fuschiotti P, Larregina AT, Ho J, Feghali-Bostwick C, Medsger TA Jr. Interleukin-13-producing CD8+ T cells mediate dermal fibrosis in patients with systemic sclerosis. Arthritis Rheum (2013) 65:236–46. doi: 10.1002/art.37706
26. Medsger TA Jr, Ivanco DE, Kardava L, Morel PA, Lucas MR, Fuschiotti P. GATA-3 up-regulation in CD8+ T cells as a biomarker of immune dysfunction in systemic sclerosis, resulting in excessive interleukin-13 production. Arthritis Rheum (2011) 63:1738–47. doi: 10.1002/art.30489
27. Tan EM, Cohen AS, Fries JF, Masi AT, McShane DJ, Rothfield NF, et al. The 1982 revised criteria for the classification of systemic lupus erythematosus. Arthritis Rheum (1982) 25:1271–7. doi: 10.1002/art.1780251101
28. Bombardier C, Gladman DD, Urowitz MB, Caron D, Chang CH. Derivation of the SLEDAI. A disease activity index for lupus patients. The Committee on Prognosis Studies in SLE. Arthritis Rheum (1992) 35:630–40. doi: 10.1002/art.1780350606
29. Sakaguchi S, Sakaguchi N, Asano M, Itoh M, Toda M. Immunologic self-tolerance maintained by activated T cells expressing IL-2 receptor alpha-chains (CD25). Breakdown of a single mechanism of self-tolerance causes various autoimmune diseases. J Immunol (1995) 155:1151–64.
30. Kato H, Perl A. Blockade of Treg Cell Differentiation and Function by the Interleukin-21-Mechanistic Target of Rapamycin Axis Via Suppression of Autophagy in Patients With Systemic Lupus Erythematosus. Arthritis Rheumatol (2018) 70:427–38. doi: 10.1002/art.40380
31. Yamane H, Zhu J, Paul WE. Independent roles for IL-2 and GATA-3 in stimulating naive CD4+ T cells to generate a Th2-inducing cytokine environment. J Exp Med (2005) 202:793–804. doi: 10.1084/jem.20051304
32. Lai ZW, Kelly R, Winans T, Marchena I, Shadakshari A, Yu J, et al. Sirolimus in patients with clinically active systemic lupus erythematosus resistant to, or intolerant of, conventional medications: a single-arm, open-label, phase 1/2 trial. Lancet (2018) 391:1186–96. doi: 10.1016/S0140-6736(18)30485-9
33. Kim EH, Sullivan JA, Plisch EH, Tejera MM, Jatzek A, Choi KY, et al. Signal integration by Akt regulates CD8 T cell effector and memory differentiation. J Immunol (2012) 188:4305–14. doi: 10.4049/jimmunol.1103568
34. Krawczyk CM, Shen H, Pearce EJ. Functional plasticity in memory T helper cell responses. J Immunol (2007) 178:4080–8. doi: 10.4049/jimmunol.178.7.4080
35. Zhu J, Min B, Hu-Li J, Watson CJ, Grinberg A, Wang Q, et al. Conditional deletion of Gata3 shows its essential function in T(H)1-T(H)2 responses. Nat Immunol (2004) 5:1157–65. doi: 10.1038/ni1128
36. Yagi R, Junttila IS, Wei G, Urban JF Jr., Zhao K, Paul WE, et al. The transcription factor GATA3 actively represses RUNX3 protein-regulated production of interferon-gamma. Immunity (2010) 32:507–17. doi: 10.1016/j.immuni.2010.04.004
37. Szabo SJ, Kim ST, Costa GL, Zhang X, Fathman CG, Glimcher LH. A novel transcription factor, T-bet, directs Th1 lineage commitment. Cell (2000) 100:655–69. doi: 10.1016/S0092-8674(00)80702-3
Keywords: systemic lupus erythematosus, interleukin-2 (IL-2), interleukin-13 (IL-13), interferon-γ (IFN-γ), T regulatory (Treg) cell, CD8+ T cells, STAT6, GATA-3
Citation: Kato H and Perl A (2021) Double-Edged Sword: Interleukin-2 Promotes T Regulatory Cell Differentiation but Also Expands Interleukin-13- and Interferon-γ-Producing CD8+ T Cells via STAT6-GATA-3 Axis in Systemic Lupus Erythematosus. Front. Immunol. 12:635531. doi: 10.3389/fimmu.2021.635531
Received: 30 November 2020; Accepted: 13 January 2021;
Published: 02 March 2021.
Edited by:
Antonio La Cava, University of California, Los Angeles, United StatesReviewed by:
José Carlos Crispín, Instituto Nacional de Ciencias Médicas y Nutrición Salvador Zubirán (INCMNSZ), MexicoSokratis A. Apostolidis, Hospital of the University of Pennsylvania, United States
Copyright © 2021 Kato and Perl. This is an open-access article distributed under the terms of the Creative Commons Attribution License (CC BY). The use, distribution or reproduction in other forums is permitted, provided the original author(s) and the copyright owner(s) are credited and that the original publication in this journal is cited, in accordance with accepted academic practice. No use, distribution or reproduction is permitted which does not comply with these terms.
*Correspondence: Andras Perl, perla@upstate.edu