- Department of Urology, Huashan Hospital, Fudan University, Shanghai, China
Kidney transplantation is a primary therapy for end-stage renal disease (ESRD) all the time. But it does not mean that we have fully unraveling the mystery of kidney transplantation and confer every patient favorable prognosis. Immune rejection has always been a stumbling block when we try to increase the success rate of kidney transplantation and improve long-term outcomes. Even if the immune rejection is effectively controlled in acute phase, there is a high possibility that the immune response mediated by chronically activated antibodies will trigger chronic rejection and ultimately lead to graft failure. At present, immunosuppressive agent prepared chemically is mainly used to prevent acute or chronic rejection, but it failed to increase the long-term survival rate of allografts or reduce the incidence of chronic rejection after acute rejection, and is accompanied by many adverse reactions. Therefore, many studies have begun to use immune cells to regulate the immune response in order to control allograft rejection. This article will focus on the latest study and prospects of more popular regulatory myeloid cells in the direction of renal transplantation immunotherapy and introduce their respective progress from experimental research to clinical research.
Introduction
In 1954, Dr. Merril and Murray of Harvard University completed the first successful kidney transplant between a pair of twins in order to avoid allograft immunity. The patient did not take any immunosuppressive drugs, and the transplanted kidney achieved long-term survival (1). But this is a rare condition after all, and there are certain genetic differences between most donors and recipients, mainly referring to human leukocyte antigen (HLA), which greatly ascends the possibility of allograft rejection (2). Based on the onset time, the rejections that occur after kidney transplantation can be classified into hyperacute rejection reaction, accelerated rejection reaction, acute rejection reaction, and chronic rejection reaction. The most important ones are acute rejection and chronic active antibody-mediated rejection (AMR). We are going to discuss the prevention and treatment of them (3). Thanks to preoperative histocompatibility tests, more reasonable and standardized surgical procedures, improved tissue typing, and especially the advent of securer and more efficient immunosuppressive drugs, the incidence of rejection (mostly acute rejection) during the first year has gradually decreased over the years (4). Clinical kidney transplant has become the gold standard for ESRD, which improves patients' quality of life within a certain period compared with continuous dialysis (3, 5). At present, the main clinical method of chemical immunosuppressive treatment routinely after kidney transplantation is calcineurin inhibitor (CNI), and other drugs including mycophenolic acid and corticosteroids are usually used in combination (6, 7). Immunosuppressive agents seem to have reached their limits, but many problems remain, such as leading to excessive suppression of immune system and increasing the rate of infectious diseases and the incidence of tumors (8). It can also cause side effects without an immunological relationship, including nephrotoxicity, making recipients susceptible to cardiovascular dysfunction, metabolic diseases, and complications of other organs (9). Unfortunately, due to the lack of immunological or antigen specificity, CNI based immunosuppressive can hinder alloresponses, but does not ultimately prevent late dysfunction or loss. And in fact, CNI treatment even causes inhibition of regulatory T-cell development, which leaves a major challenge (10). Therefore, although these drugs reduce the rate of acute rejection, they fail to significantly improve the long-term survival of transplanted kidney (11).
Given the concerns over chemical immunosuppressive agents and the disappointing long-term results of kidney transplants, it is clearly necessary to revisit our choice of immunomodulation methods. Although immune rejection is mainly mediated by effector T cells or antibodies secreted by B cells, some other types of white blood cells can promote a tolerant immune response and extend survival of the graft (12). In kidney transplant immunity, mechanisms of tolerance are crucial because whether an allograft is acceptable to the recipient depends largely on the balance between effector cells with alloantigen reactivity and regulatory immune cells (13). Regulatory immune cells are one such group of leukocyte populations that have the potential to specifically prevent acute rejection or graft-vs.-host disease (GVHD). They gradually acquire regulatory functions during development, and the immunosuppressive property is acquired in the local microenvironment of allografts or in lymphatic tissue drained by the graft. And this immunosuppressive property will cause the surrounding environment to change toward the long-term survival of the graft, thereby preventing the graft from being rejected or GVHD. Therefore, regulatory immune cells play a critical role in influencing long-term outcomes after kidney transplantation (14).
Regulatory Myeloid Cells
Currently, researchers working on organ transplantation are hoping to minimize the dependence of recipients on immunosuppressive drugs and increase donor-specific immune tolerance using immunomodulatory therapy. Many animal experiments and observations have shown that regulatory immune cells can promote transplant tolerance and reduce the infection rate after kidney transplantation (15). There are also considerable experiments confirming that they could prolong the survival of kidney allografts in non-human primate (NHP) models (16, 17). Most of these experiments focus on the role of regulatory T cells (Tregs). From basic experiments to clinical related research, Tregs have excellent immune regulation effect after transplantation of various organs, and they occupied an important position in transplantation immunity (13, 18, 19). There was some small-scale clinical trials which purify Tregs from patients' own blood and perform polyclonal expansion. Experimental results show that the project is safe and well-tolerated. The infused Tregs have a certain degree of durability and stability, proven to reduce the inflammation of the transplanted kidney through biopsy (20). Another group of cells with regulatory functions, regulatory myeloid cells, has excellent immunosuppressive effects in many transplant responses, in addition to being more diverse and complex. Some of these effects received widespread attention because they may be related to Tregs (Figure 1). The regulatory myeloid cells discussed in the previous articles generally include three types, which are regulatory macrophages, regulatory dendritic cells, and myeloid-derived suppressor cells (21). Due to the homology and excellent anti-rejection prospects, here we will broadly include bone marrow mesenchymal stromal cells. We will focus on the progress of kidney transplantation immunity of several regulatory myeloid cells, and their characteristics are shown (Table 1).
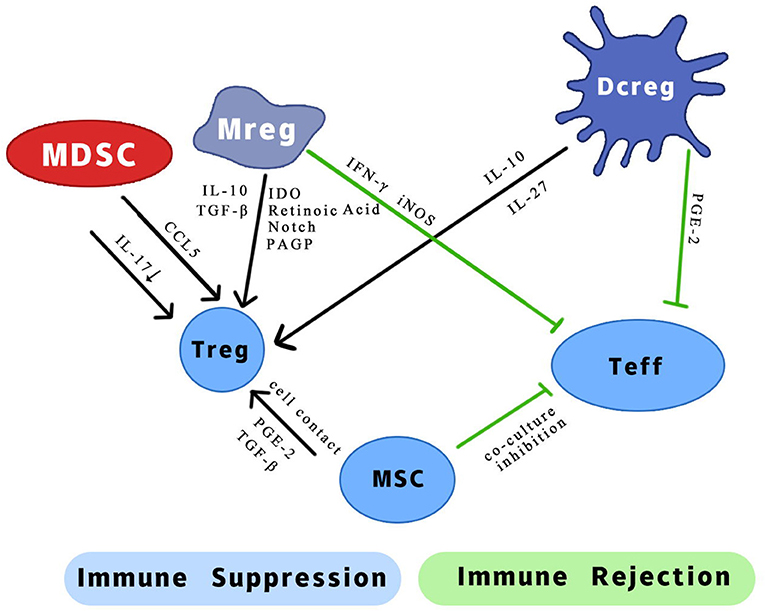
Figure 1. Possible mechanism of different effects of regulatory myeloid cells on two types of T cells. IL, interleukin; CCL5, chemotactic C-C motif 5; IFN-γ, interferon-gamma; iNOS, inducible nitric oxide synthase; TGF-β, transforming growth factor-β; IDO, indoleamine 2,3-dioxygenase; PAGP, progestagen-associated endometrial protein; PGE-2, prostaglandin E2.
Regulatory Macrophages
Macrophages are derived from monocytes and they are an important part of innate immunity. When the body is injured, monocytes in the blood vessels are transported to the inflamed tissue and become macrophages. The accumulation of macrophages in organs has been considered a feature of allograft rejection for many years (26). Depending on the microenvironment, immunogenic monocytes will infiltrate into the allograft early after transplantation, and be renamed macrophages, which will respond to the transplanted organ and trigger organ rejection (27). The classically activated macrophages' (M1) inflammatory response is characterized by high levels of pro-inflammatory cytokines in the surrounding environment and the promotion of the Th1 response. However, in addition to the role of M1 in promoting immune responses, alternatively activated macrophages (M2) are believed to be associated with the alleviation of tissue inflammation. The latest research found that when macrophage colony-stimulating factor (M-CSF) and interferon-γ (IFN-γ) act on monocytes, they will transform into a new type of inhibitory cells called regulatory macrophages (Mregs). Mregs are another uniquely characterized group of cells that can produce interleukin 10 (IL-10) but do not generate arginase-1, which can reduce the proinflammatory immune response (28). Recent studies have shown that Mregs promote successful long-term transplantation and may be necessary for inducing transplant tolerance (29). Hutchinson et al. administered Mregs to two living donor kidney transplant recipients. After transplantation, two patients were treated with the smallest dose of tacrolimus monotherapy (aiming for trough serum levels between 4 and 8 ng/ml) in 24 weeks and maintained favorable transplant function later. No adverse effects were observed in 2 patients, with 3 years follow-up (30). It is inferred that this phenomenon may be caused by Mregs inhibiting the proliferation of effector T cells by producing IFN-γ. During cell experiments, it was found that mouse Mregs can produce induced nitric oxide synthase (iNOS) to inhibit the activity of T cells in vitro and can kill and engulf co-cultured T cells subsequently (31). The latest research also shows that Mregs can convert allogeneic CD4 + T cells into regulatory T cells, thereby non-specifically inhibiting bystander T cells and the maturation of dendritic cells. Preoperative administration of donor-sourced Mreg to kidney transplant recipients can cause a sharp increase in circulating Treg, promoting the acceptance of allogeneic transplantation by rapidly inducing Tregs (32). In addition to conducting further clinical and in vitro experiments, some researchers have proposed the concept of macrophage isolation and characterization according to the tissue environment, and the function of macrophages according to different circumstances (33). Du et al. conducted in vitro and in vivo experiments and found that insulin-like growth factor 2 (IGF-2) can induce anti-inflammatory phenotype of mature macrophages by changing their mitochondrial metabolism, and transfer them to mice can alleviate their disease (34). Maybe using IGF-2 or other inducing factors can also actively induce macrophages to produce specific cells with Mreg function based on their phenotypes for application in immune regulation after kidney transplantation.
Regulatory Dendritic Cells
Dendritic cells (DCs) are another type of cells that are essential to elicit a specific T cell response to alloantigen, but they can also enhance a tolerance response in some cases (35). DCs are functionally classified as myeloid DCs (mDCs) and plasmacytoid DCs (pDCs). Since most of them belong to mDCs, they are generally classified as myeloid immune cells. DCs belong to important antigen presenting cells (APCs), which play an essential role in the activation and adjustment of non-specific and specific immunity and induction of immune tolerance to maintain homeostasis in steady state under inflammatory conditions. Although the main function of DCs is to trigger the immune system, it seems that both mDCs and pDCs can enhance the tolerance to the same antigen (36). The two-way role of DCs in coordinating this delicate balance has attracted widespread attention. Increasing evidence indicates that the coordinate ability of DCs is dependent upon their immature state and can be regulated by many factors such as immunosuppressive molecules, genetic mechanism, certain pathogenic stimulation, signals from immune cells or apoptotic cells, and tissue or tumor microenvironment (37). Regulatory DCs (DCregs) retain the ability to present antigens to specific T cells. However, at the same time, they descend the expression of costimulatory molecules (CD80, CD86, and CD40) and pro-inflammatory cytokines (IL-12), and ascend the expression of inhibitory molecules (PDL1, CD95L, and IDO) and anti-inflammatory cytokines (TGF-β and IL-10), and is resistant to signals that induce maturation (38). The mechanisms of DCregs promoting immune tolerance include inducing T cell non-responsiveness, generating Tregs, inhibiting T cell response and inducing T cell apoptosis (39). The result published by Marin E et al. shows that DCregs regulate immune responses through lactate synthesis, inhibition of T cell proliferation and expansion of Tregs by secreting factors. The high level of lactate shifts T cell response to tolerance, delaying graft-vs.-host disease (40). At the University of Nantes, as part of the ONE Study, autologous DCreg infusion 1 day before transplant, is under examination in renal transplantation with SOC triple immunosuppressive (azathroprine, steroid, tacrolimus) (41). Therefore, researchers have begun to consider how to efficiently prepare DCregs to facilitate future large-scale use. Cai et al. produced enough high-quality functional DCregs from induced pluripotent stem cells (iPSCs) derived from murine, and named them iPS-DCregs. Even under strong stimuli, this kind of DCreg can still be in a “stable immature stage,” and can induce permanent acceptance of mouse heart allografts (42). Some researchers have successfully used mouse DCregs to perform allogeneic transplantation of mouse skin and significantly prolonged graft survival (43). Pang et al. went a step further, purifying exosomes from DCregs and injecting them into allogeneic kidney transplantation model mice. After observation, it was determined that the exosomal group significantly improved survival, reduced the levels of (CD4+) T cell and cytokines related to rejection (IFN-γ, IL-2, and IL-17), and promoted the percentage of (Foxp3+) (CD4+)T cells in mice allograft, which indicates that exosomes of DCregs are involved in the induction of immune tolerance (44). Recently, Thomson et al. have produced Good Manufacturing Practice (GMP) grade DCregs for human organ transplantation. They briefly reviewed their experience in modulating immunotherapy in organ transplantation and the generation and characterization of human monocyte-derived DCregs. And they proposed a phase I/II safety study in which the effect of donor-derived DCregs combined with traditional immunosuppressive agent treatment on subclinical and clinical allograft rejection and adverse reactions will be observed in detail (45).
Myeloid-Derived Suppressor Cells
Myeloid derived suppressor cells (MDSCs) are a heterogeneous population of bone marrow-derived myeloid progenitors that suppress immune responses in different kinds of inflammatory environments, such as organ transplantation, malignant tumors, infectious diseases, and autoimmune diseases (46). They are functionally defined as a class because of phenotypically expression with characteristics related to precursors of hematopoietic cells that can stably differentiate into mature macrophages, DCs and granulocytes at all stages (47). The pivotal position of MDSCs was initially established in the field of tumor immunity (48). Since then, many researchers have begun to explore the role of MDSCs in the host's immune response during organ transplantation. Dugast et al. first reported the important role of MDSCs in inducing tolerance in rat kidney transplant models. By increasing the number of MDSCs, the proliferation of allogenic T cells can be inhibited in vivo, and MDSCs may participate in the phase of NO-dependent tolerance maintenance (49). After this groundbreaking study determined the importance of MDSCs in transplant immunity, research on the therapeutic potential and immunoregulatory effects of MDSCs has been extensively conducted. Currently, MDSC are divided in three subpopulations: polymorphonuclear (PMN), monocytic (M) and early-stage (e) MDSC, and each one has different immunosuppressive mechanism (22). Dilek et al. focused on the connection between MDSCs and Tregs in transplant immunity and discovered the importance of a chemotactic C-C motif 5 (CCL5) between the two. The results show that the gradient of CCL5 around the graft contributes to MDSC's enhancement of tolerance in kidney allograft recipients. This gradient controls the recruitment of Tregs to the graft, which may help maintain tolerance (50). Studies performed by Luan et al. revealed blood derived M-MDSCs were able to expand Treg in vitro and correlated with increased Treg numbers in vivo (51). There are also many studies focusing on the effect of using MDSCs to extend the survival time of transplants after allogeneic transplantation of organs such as heart and skin in mice (52–55). These studies have discovered pathways and mechanisms such as carbon monoxide that may be related to the immune suppression of MDSCs through animal experiments, but more teams have begun to turn more to clinical trials. Meng et al. compared the allogeneic function, severity of tissue damage, and long-term survival of patients with high and low MDSCs in vivo after kidney transplantation. Compared with the low-dose MDSCs group, the allograft function of the high-dose MDSCs group was significantly enhanced. In addition, his team also found that MDSCs isolated from transplant recipients can up-regulate Tregs and inhibit IL-17 production in vitro (56). Hock et al. found that the number of MDSCs in patients after kidney transplantation increased rapidly and reached a peak after the start of immunosuppression, but then fluctuated due to unknown reasons. Therefore, the purpose of establishing follow-up research is to explore the factors that regulate MDSC mobilization (57). Recent studies have shown that in patients with intestinal transplantation, MDSCs increase in vivo and restrain the response of effector T cells to the intestinal epithelium of the graft (58). This shows that due to the close relationship with T cells, MDSCs may have the prospect of immunosuppression for various organ transplants throughout the body. But some pessimistic points emerged, Lee et al. showed that adoptive MDSCs transfer after transplantation improves graft survival in mice, however their late depletion did not damage graft survival (59). Moreover, Utrero-Rico et al. found that higher maintained level of M-MDSC after transplantation in kidney recipients is associated with higher risk of cancer (60). These findings demonstrate together that MDSC could be beneficial early after transplantation to prolong graft survival, but, if maintained, it may promote tumoral development for patients due to excessive immunosuppression.
Mesenchymal Stromal Cells
Bone marrow-derived mesenchymal stromal cells (MSCs) are also a type of cells that have regulatory functions in transplantation immunity. Although MSCs are found in many tissues, most are derived from bone marrow, as we also include them in regulatory myeloid cells. MSCs are a kind of cells with multi-directional differentiation potential. They can induce differentiation into a variety of tissue cells under specific in vivo and in vitro environments (61). MSCs could also migrate to sites of inflammation and to transplanted organs. So, many researchers have put it into testing for autoimmune diseases and post-transplant immunomodulatory therapies (62, 63). In experiments aimed at post-transplant immunity, MSCs have shown unique, effective, and undisputed immune-modulating properties. MSCs can act on a wide range of immune cells such as T cells, B cells, macrophages, natural killer cells, dendritic cells, and granulocytes (64). The much profound impact of MSC on adaptive immune response may be closely related to its innate source, so myeloid cells may be the earliest affected cells. MSCs interact with innate and acquired immune cells and immunomodulate them to make them develop more stable (and occasionally more intense) (65, 66). They impede the differentiation of dendritic cells and inhibit their maturation, thereby downregulating the ability of antigen presenting cells in vivo and in vitro (67–69). MSCs reduce the cytotoxicity of T lymphocytes that respond to foreign antigens and prevent effector T cells from replicating at the G0/G1 phase, thereby reducing their ability to produce IFN-γ and IL-2 (70). And they also promoted T cells to differentiate into Tregs and induce graft tolerance (71, 72). In addition, MSCs alter the proliferation of natural killer cells and γδT cells (73), reduce cytotoxicity and IFN-γ production, and inhibit B cell activation and antibody secretion (74).
Although there are many studies in various fields, the exact mechanism of action of MSCs has not been obtained. However, since MSCs can be easily isolated from different tissues including bone marrow, umbilical cord or connective tissue, and based on the current advanced technology, MSCs can be expanded in vitro to achieve an applicable cell dose in a relatively short time for clinic (75). Many studies on the use of MSCs cell therapy to modulate immunity after kidney transplantation have been performed worldwide. The goal of these studies is usually to induce inhibition through various forms of treatment with MSCs, so that patients can maintain the effect of immune tolerance based on minimal doses of immunosuppressive drug therapy to get the long-time survival of graft (76).
Conclusion
From the above analysis, it can be found that the immune system has a natural balance. This balance consists of the coordination of pro-inflammatory and regulatory cells, which involves a large series of regulatory factors. This is a very complicated and delicate process. As an important part of the regulatory cell side and possessing capability of promoting Treg proliferation, regulatory myeloid cells are expected to enhance success of early immunosuppressive drug withdrawal and become one of the anti-rejection options for patients after renal transplantation soon. An interesting matter to remark would be the need of prospective studies regarding the regulation of myeloid regulatory cells on the long term and which is the effect of immunosuppressive regiments on these cells, as well as their relationship with clinical outcomes, in order to develop strategies to promote tolerance. Most pilot studies were highly variable in design, did not incorporate a parallel trial arm of patients. This requires us all to further carry out more clinical-based experiments based on researches that has been reproduced in order to prove the advantages of its efficacy and higher safety and operability. In addition, whether it can be made into the very promising and available cell-based medicinal products (CBMP) also requires a unified standard. The existing data is still very ambiguous on this point.
Author Contributions
JZ collected data, performed the analysis, and prepared the manuscript. JH conceived the article contents and endorsed the final draft submitted. Both authors contributed to the article and approved the submitted version.
Funding
Publication fees are covered by the National Natural Science Foundation of China (Grant No. 81771683).
Conflict of Interest
The authors declare that the research was conducted in the absence of any commercial or financial relationships that could be construed as a potential conflict of interest.
The reviewer RR declared shared affiliation, with no collaboration, with the authors to the handling editor at the time of review.
References
1. Murray JE. Reflections on the first successful kidney transplantation. World J Surg. (1982) 6:372–6. doi: 10.1007/BF01653562
2. Cornell LD, Smith RN, Colvin RB. Kidney transplantation: mechanisms of rejection and acceptance. Annu Rev Pathol. (2008) 3:189–220. doi: 10.1146/annurev.pathmechdis.3.121806.151508
3. Kidney Disease: Improving Global Outcomes Transplant Work G. KDIGO clinical practice guideline for the care of kidney transplant recipients. Am J Transplant. (2009) 9(Suppl. 3):S1–155. doi: 10.1111/j.1600-6143.2009.02834.x
4. Meier-Kriesche HU, Schold JD, Srinivas TR, Kaplan B. Lack of improvement in renal allograft survival despite a marked decrease in acute rejection rates over the most recent era. Am J Transplant. (2004) 4:378–83. doi: 10.1111/j.1600-6143.2004.00332.x
5. Fisher R, Gould D, Wainwright S, Fallon M. Quality of life after renal transplantation. J Clin Nurs. (1998) 7:553–63. doi: 10.1046/j.1365-2702.1998.00189.x
6. Tsipotis E, Gupta NR, Raman G, Zintzaras E, Jaber BL. Bioavailability, efficacy and safety of generic immunosuppressive drugs for kidney transplantation: a systematic review and meta-analysis. Am J Nephrol. (2016) 44:206–18. doi: 10.1159/000449020
7. Halloran PF. Immunosuppressive drugs for kidney transplantation. N Engl J Med. (2004) 351:2715–29. doi: 10.1056/NEJMra033540
8. Rizvi SMH, Aagnes B, Holdaas H, Gude E, Boberg KM, Bjortuft O, et al. Long-term change in the risk of skin cancer after organ transplantation: a population-based nationwide cohort study. JAMA Dermatol. (2017) 153:1270–7. doi: 10.1001/jamadermatol.2017.2984
9. Srinivas TR, Meier-Kriesche HU. Minimizing immunosuppression, an alternative approach to reducing side effects: objectives and interim result. Clin J Am Soc Nephrol. (2008) 3(Suppl. 2):S101–16. doi: 10.2215/CJN.03510807
10. Kirchhof J, Wilde B, Schmidt J, Mulling N, Petrakova L, Brinkhoff A, et al. Acute versus chronic administration of calcineurin-inhibitors differen-tially affect T-cell function. Endocr Metab Immune Disord Drug Targets. (2020). doi: 10.2174/1871530320999200831161710. [Epub ahead of print].
11. Xu QX, Qiu XY, Jiao Z, Zhang M, Zhong MK. FOXP3 rs3761549 polymorphism predicts long-term renal allograft function in patients receiving cyclosporine-based immunosuppressive regimen. Gene. (2018) 644:93–100. doi: 10.1016/j.gene.2017.10.081
12. Wood KJ, Goto R. Mechanisms of rejection: current perspectives. Transplantation. (2012) 93:1–10. doi: 10.1097/TP.0b013e31823cab44
13. Papp G, Boros P, Nakken B, Szodoray P, Zeher M. Regulatory immune cells and functions in autoimmunity and transplantation immunology. Autoimmun Rev. (2017) 16:435–44. doi: 10.1016/j.autrev.2017.03.011
14. Wood KJ, Bushell A, Hester J. Regulatory immune cells in transplantation. Nat Rev Immunol. (2012) 12:417–30. doi: 10.1038/nri3227
15. Sawitzki B, Harden PN, Reinke P, Moreau A, Hutchinson JA, Game DS, et al. Regulatory cell therapy in kidney transplantation (The ONE Study): a harmonised design and analysis of seven non-randomised, single-arm, phase 1/2A trials. Lancet. (2020) 395:1627–39. doi: 10.1016/S0140-6736(20)30167-7
16. Bashuda H, Kimikawa M, Seino K, Kato Y, Ono F, Shimizu A, et al. Renal allograft rejection is prevented by adoptive transfer of anergic T cells in nonhuman primates. J Clin Invest. (2005) 115:1896–902. doi: 10.1172/JCI23743
17. Ma A, Qi S, Song L, Hu Y, Dun H, Massicotte E, et al. Adoptive transfer of CD4+CD25+ regulatory cells combined with low-dose sirolimus and anti-thymocyte globulin delays acute rejection of renal allografts in Cynomolgus monkeys. Int Immunopharmacol. (2011) 11:618–29. doi: 10.1016/j.intimp.2010.11.001
18. Taylor PA, Lees CJ, Blazar BR. The infusion of ex vivo activated and expanded CD4(+)CD25(+) immune regulatory cells inhibits graft-versus-host disease lethality. Blood. (2002) 99:3493–9. doi: 10.1182/blood.V99.10.3493
19. Wood KJ, Sakaguchi S. Regulatory T cells in transplantation tolerance. Nat Rev Immunol. (2003) 3:199–210. doi: 10.1038/nri1027
20. Chandran S, Tang Q, Sarwal M, Laszik ZG, Putnam AL, Lee K, et al. Polyclonal Regulatory T Cell Therapy for Control of Inflammation in Kidney Transplants. Am J Transplant. (2017) 17:2945–54. doi: 10.1111/ajt.14415
21. Rosborough BR, Raich-Regue D, Turnquist HR, Thomson AW. Regulatory myeloid cells in transplantation. Transplantation. (2014) 97:367–79. doi: 10.1097/TP.0b013e3182a860de
22. Bronte V, Brandau S, Chen SH, Colombo MP, Frey AB, Greten TF, et al. Recommendations for myeloid-derived suppressor cell nomenclature and characterization standards. Nat Commun. (2016) 7:12150. doi: 10.1038/ncomms12150
23. Guo F, Hu M, Huang D, Zhao Y, Heng B, Guillemin G, et al. Human regulatory macrophages are potent in suppression of the xenoimmune response via indoleamine-2,3-dioxygenase-involved mechanism(s). Xenotransplantation. (2017) 24:e12326. doi: 10.1111/xen.12326
24. Moreau A, Varey E, Beriou G, Hill M, Bouchet-Delbos L, Segovia M, et al. Tolerogenic dendritic cells and negative vaccination in transplantation: from rodents to clinical trials. Front Immunol. (2012) 3:218. doi: 10.3389/fimmu.2012.00218
25. Pool M, Leuvenink H, Moers C. Reparative and regenerative effects of mesenchymal stromal cells-promising potential for kidney transplantation? Int J Mol Sci. (2019) 20:4614. doi: 10.3390/ijms20184614
26. Jose MD, Ikezumi Y, van Rooijen N, Atkins RC, Chadban SJ. Macrophages act as effectors of tissue damage in acute renal allograft rejection. Transplantation. (2003) 76:1015–22. doi: 10.1097/01.TP.0000083507.67995.13
27. Oberbarnscheidt MH, Zeng Q, Li Q, Dai H, Williams AL, Shlomchik WD, et al. Non-self recognition by monocytes initiates allograft rejection. J Clin Invest. (2014) 124:3579–89. doi: 10.1172/JCI74370
28. Fleming BD, Mosser DM. Regulatory macrophages: setting the threshold for therapy. Eur J Immunol. (2011) 41:2498–502. doi: 10.1002/eji.201141717
29. Conde P, Rodriguez M, van der Touw W, Jimenez A, Burns M, Miller J, et al. DC-SIGN(+) macrophages control the induction of transplantation tolerance. Immunity. (2015) 42:1143–58. doi: 10.1016/j.immuni.2015.05.009
30. Hutchinson JA, Riquelme P, Sawitzki B, Tomiuk S, Miqueu P, Zuhayra M, et al. Cutting edge: immunological consequences and trafficking of human regulatory macrophages administered to renal transplant recipients. J Immunol. (2011) 187:2072–8. doi: 10.4049/jimmunol.1100762
31. Riquelme P, Tomiuk S, Kammler A, Fandrich F, Schlitt HJ, Geissler EK, et al. IFN-gamma-induced iNOS expression in mouse regulatory macrophages prolongs allograft survival in fully immunocompetent recipients. Mol Ther. (2013) 21:409–22. doi: 10.1038/mt.2012.168
32. Riquelme P, Haarer J, Kammler A, Walter L, Tomiuk S, Ahrens N, et al. TIGIT(+) iTregs elicited by human regulatory macrophages control T cell immunity. Nat Commun. (2018) 9:2858. doi: 10.1038/s41467-018-05167-8
33. Ochando J, Conde P. Functional Characterization of Regulatory Macrophages That Inhibit Graft-reactive Immunity. J Vis Exp. (2017) (124). doi: 10.3791/54242
34. Du L, Lin L, Li Q, Liu K, Huang Y, Wang X, et al. IGF-2 preprograms maturing macrophages to acquire oxidative phosphorylation-dependent anti-inflammatory properties. Cell Metab. (2019) 29:1363–75 e8. doi: 10.1016/j.cmet.2019.01.006
35. Banchereau J, Steinman RM. Dendritic cells and the control of immunity. Nature. (1998) 392:245–52. doi: 10.1038/32588
36. van Kooten C, Lombardi G, Gelderman KA, Sagoo P, Buckland M, Lechler R, et al. Dendritic cells as a tool to induce transplantation tolerance: obstacles and opportunities. Transplantation. (2011) 91:2–7. doi: 10.1097/TP.0b013e31820263b3
37. Steinman RM, Banchereau J. Taking dendritic cells into medicine. Nature. (2007) 449:419–26. doi: 10.1038/nature06175
38. Morelli AE, Thomson AW. Tolerogenic dendritic cells and the quest for transplant tolerance. Nat Rev Immunol. (2007) 7:610–21. doi: 10.1038/nri2132
39. Manicassamy S, Pulendran B. Dendritic cell control of tolerogenic responses. Immunol Rev. (2011) 241:206–27. doi: 10.1111/j.1600-065X.2011.01015.x
40. Marin E, Bouchet-Delbos L, Renoult O, Louvet C, Nerriere-Daguin V, Managh AJ, et al. Human tolerogenic dendritic cells regulate immune responses through lactate synthesis. Cell Metab. (2019) 30:1075–90 e8. doi: 10.1016/j.cmet.2019.11.011
41. Thomson AW, Humar A, Lakkis FG, Metes DM. Regulatory dendritic cells for promotion of liver transplant operational tolerance: rationale for a clinical trial and accompanying mechanistic studies. Hum Immunol. (2018) 79:314–21. doi: 10.1016/j.humimm.2017.10.017
42. Cai S, Hou J, Fujino M, Zhang Q, Ichimaru N, Takahara S, et al. iPSC-derived regulatory dendritic cells inhibit allograft rejection by generating alloantigen-specific regulatory T cells. Stem Cell Reports. (2017) 8:1174–89. doi: 10.1016/j.stemcr.2017.03.020
43. Segovia M, Louvet C, Charnet P, Savina A, Tilly G, Gautreau L, et al. Autologous dendritic cells prolong allograft survival through Tmem176b-dependent antigen cross-presentation. Am J Transplant. (2014) 14:1021–31. doi: 10.1111/ajt.12708
44. Pang XL, Wang ZG, Liu L, Feng YH, Wang JX, Xie HC, et al. Immature dendritic cells derived exosomes promotes immune tolerance by regulating T cell differentiation in renal transplantation. Aging (Albany NY). (2019) 11:8911–24. doi: 10.18632/aging.102346
45. Thomson AW, Zahorchak AF, Ezzelarab MB, Butterfield LH, Lakkis FG, Metes DM. Prospective clinical testing of regulatory dendritic cells in organ transplantation. Front Immunol. (2016) 7:15. doi: 10.3389/fimmu.2016.00015
46. Almand B, Clark JI, Nikitina E, van Beynen J, English NR, Knight SC, et al. Increased production of immature myeloid cells in cancer patients: a mechanism of immunosuppression in cancer. J Immunol. (2001) 166:678–89. doi: 10.4049/jimmunol.166.1.678
47. Dilek N, van Rompaey N, Le Moine A, Vanhove B. Myeloid-derived suppressor cells in transplantation. Curr Opin Organ Transplant. (2010) 15:765–8. doi: 10.1097/MOT.0b013e3283401742
48. Pak AS, Wright MA, Matthews JP, Collins SL, Petruzzelli GJ, Young MR. Mechanisms of immune suppression in patients with head and neck cancer: presence of CD34(+) cells which suppress immune functions within cancers that secrete granulocyte-macrophage colony-stimulating factor. Clin Cancer Res. (1995) 1:95–103.
49. Dugast AS, Haudebourg T, Coulon F, Heslan M, Haspot F, Poirier N, et al. Myeloid-derived suppressor cells accumulate in kidney allograft tolerance and specifically suppress effector T cell expansion. J Immunol. (2008) 180:7898–906. doi: 10.4049/jimmunol.180.12.7898
50. Dilek N, Poirier N, Usal C, Martinet B, Blancho G, Vanhove B. Control of transplant tolerance and intragraft regulatory T cell localization by myeloid-derived suppressor cells and CCL5. J Immunol. (2012) 188:4209–16. doi: 10.4049/jimmunol.1101512
51. Luan Y, Mosheir E, Menon MC, Wilson D, Woytovich C, Ochando J, et al. Monocytic myeloid-derived suppressor cells accumulate in renal transplant patients and mediate CD4(+) Foxp3(+) Treg expansion. Am J Transplant. (2013) 13:3123–31. doi: 10.1111/ajt.12461
52. Liao J, Wang X, Bi Y, Shen B, Shao K, Yang H, et al. Dexamethasone potentiates myeloid-derived suppressor cell function in prolonging allograft survival through nitric oxide. J Leukoc Biol. (2014) 96:675–84. doi: 10.1189/jlb.2HI1113-611RR
53. Nakamura T, Nakao T, Ashihara E, Yoshimura N. Myeloid-derived suppressor cells recruit CD4(+)/Foxp3(+) regulatory T cells in a murine cardiac allograft. Transplant Proc. (2016) 48:1275–8. doi: 10.1016/j.transproceed.2015.10.060
54. Zhao Y, Shen XF, Cao K, Ding J, Kang X, Guan WX, et al. Dexamethasone-induced myeloid-derived suppressor cells prolong allo cardiac graft survival through iNOS- and glucocorticoid receptor-dependent mechanism. Front Immunol. (2018) 9:282. doi: 10.3389/fimmu.2018.00282
55. Nakao T, Nakamura T, Masuda K, Matsuyama T, Ushigome H, Ashihara E, et al. Dexamethasone prolongs cardiac allograft survival in a murine model through myeloid-derived suppressor cells. Transplant Proc. (2018) 50:299–304. doi: 10.1016/j.transproceed.2017.11.014
56. Meng F, Chen S, Guo X, Chen Z, Huang X, Lai Y, et al. Clinical significance of myeloid-derived suppressor cells in human renal transplantation with acute T cell-mediated rejection. Inflammation. (2014) 37:1799–805. doi: 10.1007/s10753-014-9910-5
57. Hock BD, McKenzie JL, Cross NB, Currie MJ. Dynamic changes in myeloid derived suppressor cell subsets following renal transplant: a prospective study. Transpl Immunol. (2015) 32:164–71. doi: 10.1016/j.trim.2015.05.001
58. Okano S, Abu-Elmagd K, Kish DD, Keslar K, Baldwin WM, 3rd, Fairchild RL, et al. Myeloid-derived suppressor cells increase and inhibit donor-reactive T cell responses to graft intestinal epithelium in intestinal transplant patients. Am J Transplant. (2018) 18:2544–58. doi: 10.1111/ajt.14718
59. Lee YS, Zhang T, Saxena V, Li L, Piao W, Bromberg JS, et al. Myeloid-derived suppressor cells expand after transplantation and their augmentation increases graft survival. Am J Transplant. (2020) 20:2343–55. doi: 10.1111/ajt.15879
60. Utrero-Rico A, Laguna-Goya R, Cano-Romero F, Chivite-Lacaba M, Gonzalez-Cuadrado C, Rodriguez-Sanchez E, et al. Early posttransplant mobilization of monocytic myeloid-derived suppressor cell correlates with increase in soluble immunosuppressive factors and predicts cancer in kidney recipients. Transplantation. (2020) 104:2599–608. doi: 10.1097/TP.0000000000003179
61. Casiraghi F, Perico N, Remuzzi G. Mesenchymal stromal cells for tolerance induction in organ transplantation. Hum Immunol. (2018) 79:304–13. doi: 10.1016/j.humimm.2017.12.008
62. Hashmi S, Ahmed M, Murad MH, Litzow MR, Adams RH, Ball LM, et al. Survival after mesenchymal stromal cell therapy in steroid-refractory acute graft-versus-host disease: systematic review and meta-analysis. Lancet Haematol. (2016) 3:e45–52. doi: 10.1016/S2352-3026(15)00224-0
63. Pistoia V, Raffaghello L. Mesenchymal stromal cells and autoimmunity. Int Immunol. (2017) 29:49–58. doi: 10.1093/intimm/dxx008
64. Le Blanc K, Mougiakakos D. Multipotent mesenchymal stromal cells and the innate immune system. Nat Rev Immunol. (2012) 12:383–96. doi: 10.1038/nri3209
65. Bernardo ME, Fibbe WE. Mesenchymal stromal cells: sensors and switchers of inflammation. Cell Stem Cell. (2013) 13:392–402. doi: 10.1016/j.stem.2013.09.006
66. Spaggiari GM, Moretta L. Cellular and molecular interactions of mesenchymal stem cells in innate immunity. Immunol Cell Biol. (2013) 91:27–31. doi: 10.1038/icb.2012.62
67. Jiang XX, Zhang Y, Liu B, Zhang SX, Wu Y, Yu XD, et al. Human mesenchymal stem cells inhibit differentiation and function of monocyte-derived dendritic cells. Blood. (2005) 105:4120–6. doi: 10.1182/blood-2004-02-0586
68. Nauta AJ, Kruisselbrink AB, Lurvink E, Willemze R, Fibbe WE. Mesenchymal stem cells inhibit generation and function of both CD34+-derived and monocyte-derived dendritic cells. J Immunol. (2006) 177:2080–7. doi: 10.4049/jimmunol.177.4.2080
69. Chiesa S, Morbelli S, Morando S, Massollo M, Marini C, Bertoni A, et al. Mesenchymal stem cells impair in vivo T-cell priming by dendritic cells. Proc Natl Acad Sci USA. (2011) 108:17384–9. doi: 10.1073/pnas.1103650108
70. Karlsson H, Samarasinghe S, Ball LM, Sundberg B, Lankester AC, Dazzi F, et al. Mesenchymal stem cells exert differential effects on alloantigen and virus-specific T-cell responses. Blood. (2008) 112:532–41. doi: 10.1182/blood-2007-10-119370
71. Luz-Crawford P, Kurte M, Bravo-Alegria J, Contreras R, Nova-Lamperti E, Tejedor G, et al. Mesenchymal stem cells generate a CD4+CD25+Foxp3+ regulatory T cell population during the differentiation process of Th1 and Th17 cells. Stem Cell Res Ther. (2013) 4:65. doi: 10.1186/scrt216
72. Hall BM. CD4+CD25+ T regulatory cells in transplantation tolerance: 25 years on. Transplantation. (2016) 100:2533–47. doi: 10.1097/TP.0000000000001436
73. Najar M, Fayyad-Kazan M, Merimi M, Burny A, Bron D, Fayyad-Kazan H, et al. Mesenchymal stromal cells and natural killer cells: a complex story of love and hate. Curr Stem Cell Res Ther. (2019) 14:14–21. doi: 10.2174/1574888X13666180912125736
74. Rosado MM, Bernardo ME, Scarsella M, Conforti A, Giorda E, Biagini S, et al. Inhibition of B-cell proliferation and antibody production by mesenchymal stromal cells is mediated by T cells. Stem Cells Dev. (2015) 24:93–103. doi: 10.1089/scd.2014.0155
75. Perico N, Casiraghi F, Gotti E, Introna M, Todeschini M, Cavinato RA, et al. Mesenchymal stromal cells and kidney transplantation: pretransplant infusion protects from graft dysfunction while fostering immunoregulation. Transpl Int. (2013) 26:867–78. doi: 10.1111/tri.12132
Keywords: kidney transplant, regulatory myeloid cell, allograft rejection, immunosuppression, prevention, end-stage renal disease
Citation: Zhuang J and Hou J (2021) The Role of Regulatory Myeloid Cell Therapy in Renal Allograft Rejection. Front. Immunol. 12:625998. doi: 10.3389/fimmu.2021.625998
Received: 04 November 2020; Accepted: 03 February 2021;
Published: 24 February 2021.
Edited by:
Bin Yang, University of Leicester, United KingdomCopyright © 2021 Zhuang and Hou. This is an open-access article distributed under the terms of the Creative Commons Attribution License (CC BY). The use, distribution or reproduction in other forums is permitted, provided the original author(s) and the copyright owner(s) are credited and that the original publication in this journal is cited, in accordance with accepted academic practice. No use, distribution or reproduction is permitted which does not comply with these terms.
*Correspondence: Jiangang Hou, aG91X2ppYW5nYW5nQDEyNi5jb20=