- 1Department of Immunology and Oncology, Spanish National Centre for Biotechnology (CNB), Consejo Superior de Investigaciones Científicas (CSIC), Madrid, Spain
- 2Mycobacteria Genetics Group, University of Zaragoza Medical School, IIS Aragón, CIBERES, Zaragoza, Spain
- 3Departament de Genètica i Microbiologia, Facultat de Biociències, Universitat Autònoma de Barcelona, Barcelona, Spain
- 4Custom Solutions Department, Bacteriology Division, Medicines and Healthcare Products Regulatory Agency – National Institute for Biological Standards and Control (MHRA-NIBSC), Potters Bar, United Kingdom
Natural Killer cell receptors allow this heterogeneous immune population to efficiently fight both tumors and infection, so their use as immunotherapy agents is an active field of research. Cytokine activation, particularly by myeloid cell-derived IL15, can induce potent NK anti-tumor responses. While studying the mechanism of action of intravesical instillations of Bacille Calmette-Guérin (BCG) as therapy for patients with high risk non-muscle invasive bladder cancer, we showed that BCG can activate a cytotoxic CD56bright NK cell population which efficiently recognized bladder cancer cells. This pioneer immunotherapy provides an invaluable model to understand the role of different immune populations in tumor elimination. However, during the propagation of BCG worldwide a large number of genetically diverse BCG substrains developed. Here, we investigated the capacity of different BCG substrains to promote NK cell activation and confirmed that they were able to activate lymphocytes. Tice, Connaught and Moreau were the substrains with a stronger NK activation effect as measured by CD56 upregulation. Surprisingly, dead mycobacteria also stimulated PBMC cultures and we further demonstrate here that subcellular fractions of BCG-Tice, in the absence of live mycobacteria, could also induce an NK cell response. Lipids from BCG-Tice, but not from Mycobacterium bovis, stimulated NK cell activation and degranulation, whereas the aqueous fraction of either bacteria did not activate lymphocytes. However, delipidated BCG-Tice bacteria were able to activate effector cells (CD3+CD56+ and NK, CD3-CD56+). These data demonstrate that different components of mycobacteria can stimulate different immune subpopulations resulting in phenotypes suitable for cancer elimination.
Introduction
Natural Killer cells express a large number of receptors recognizing both pathogen-infected and transformed cells which allow them to contribute to many arms of the immune response (1). The capacity of NK cells to recognize tumors has been long known, however, their role for cancer immunotherapy is currently the focus of active research (2). The contribution of NK cells in the elimination of human bladder cancer has been extensively reported in the context of local instillations with the tuberculosis vaccine Bacille Calmette-Guérin (BCG) (3, 4), which is the treatment of choice for T1G3 non-muscle invasive bladder cancer (NMIBC) appearing in the form of either papillary tumors or carcinoma in situ (CIS). Since the beginning of the use of this therapy several decades ago, the survival time of bladder cancer patients increased notably. However, survival rates have not changed in the last 30 years and many questions about the mechanism of action of the BCG against bladder cancer and about the optimal dose and recall instillations to be used in patients remain open. While studying the phenotypical changes of NK cells mediating tumor elimination in the context of BCG, we have recently reported that, after exposure of Peripheral Blood Mononuclear Cells (PBMCs) to BCG, NK cells proliferate leading to a CD56bright phenotype while keeping functional characteristics of mature NK cells including cytotoxic activity and a high capacity to mediate Antibody Dependent Cellular Cytotoxicity (ADCC) (5). This unconventional cytotoxic subpopulation of CD56bright NK cells is reminiscent of the potent anti-tumor NK cells described after blood cell IL15 priming that result in enhanced elimination of multiple myeloma (6). The anti-tumor BCG-stimulated CD56bright NK cell population that we previously described (5) can be distinguished from “classical” CD56bright NK cells normally found in a small percentage in peripheral blood, because they have markers of mature NK cells. Most express high levels of CD94 and are CD16+, and a subset is KIR2D+. Further, this population is able to mediate both degranulation and ADCC. The role of BCG in CD56 upregulation was consistent when using large numbers of different donors, however, the bacterial components involved were not studied.
BCG was generated in 1921, after 13 years of passage of Mycobacterium bovis (M. bovis) in vitro, during which this tuberculosis-causing agent acquired a number of mutations and gene modifications, with the loss of the RD1 region being the main cause for attenuation (7). Through this process, a new strain named BCG was generated. The new cultures of attenuated mycobacteria, perfectly able to reproduce, were distributed worldwide (8, 9). Since no freeze-drying methods were available until the 1960s, the different host laboratories kept different subcultures of the new bacilli growing and BCG acquired new genetic modifications thereby generating independent substrains which received different names, e.g. BCG-Pasteur, -Connaught, -Tokyo, -Danish, -Tice (10–12). Thus, a whole family of BCG substrains are included under the BCG denomination. Commercial preparations of lyophilized BCG of different substrains are used for intravesical instillations of bladder cancer patients and the viable bacteria content is highly variable ranging from 106–109 (10, 13).
BCG has been used for treatment of bladder cancer as intravesical instillations for decades, after the first successful trials in the 1970s (14–16) and FDA approval in 1989 for CIS, followed by an extension in 1998 to treat Ta/T1 papillary tumors. However, because of the way in which BCG was developed, many different regimes are used in the treatment of bladder cancer patients with BCG: different hospitals use different substrains, mycobacterial concentrations and schedules (13, 17, 18). The general regime of BCG treatment consists of an initial cycle of 6-weekly intravesical instillations, called the induction phase. The addition of a maintenance phase lasting one to three years, that consists in successive cycles of 3 weekly instillations separated by 3-month rest periods after the induction phase, has represented the main improvement of this therapy in the multiple decades in use. Importantly, clinical studies have directly addressed the effect of the different protocols and there are contradicting data on the influence of different substrains of BCG in patient outcome. Clinical trials to study recurrence free survival (RFS) using different substrains are difficult to compare since they also differ in the schedules of induction vs induction + maintenance (with different maintenance regimes), one-arm vs two to three-arms, 1–5 years follow-up, tens to thousands of patients (18). In this context, a trial including a large number of patients (2,451 patients with primary T1G3 tumors from 23 clinics) in a non-randomized retrospective comparison, found that BCG Connaught reduced the recurrence rate, overall progression and death compared to BCG-Tice when no maintenance was used, but the opposite was true when maintenance was given. However, for time to progression and overall survival, Connaught and Tice had a similar efficacy (19, 20). These data agree with a prospective randomized single-institution trial with treatment of 142 patients with BCG Connaught or Tice study in which Connaught had better RFS rate. However, the low number of cases did not allow statistical conclusions on progression-free survival, disease-specific survival or overall-survival. In the same report, Rentsch et al. (20) treated mice with the same BCG substrains and showed, in the animal model, that BCG Connaught induced a stronger Th1 response, greater priming of BCG-specific CD8+ T cells, and more robust T-cell recruitment to the bladder than BCG Tice. Other retrospective studies in BCG-treated bladder cancer patients conclude, however, that there is not much difference between BCG substrains in the treatment of bladder cancer on progression-free survival or disease-specific survival (21).
Since data in the literature point towards a high degree of heterogeneity between the different BCG preparations available for bladder cancer patients, we decided to compare the effect of different BCG substrains on the activation of PBMCs, with the hypothesis that both the intensity and the quality of the immune activation might vary between different BCG preparations. There is a strong Natural Killer (NK) cell component in the mechanism of action of BCG in bladder cancer (3, 22) evidenced by the proliferation and activation of BCG-stimulated CD56bright NK cells, hereafter, referred to as CD56bright NK cells (5). Here, we specifically characterized the generation of the anti-tumoral CD56bright NK cell subpopulations in vitro in response to different substrains of BCG. During these studies we discovered that, in addition to different numbers of viable bacilli, the different commercially available presentations of BCG can contain high ratios of dead mycobacteria accompanying the colony-forming units (CFU), information that cannot be inferred from the supplier leaflet. This finding led us to demonstrate that dead BCG also contribute to the activation of certain pathways of the immune response, in particular, NK cell anti-tumor activity. These data are consistent with, and may explain, previous in vivo findings in which autoclaved BCG inhibited tumor growth in mice with transplanted bladder cancer cells (23). Interestingly, in other models, NK and T cell recruitment to tumors was dependent on BCG viability (24, 25), suggesting that other immune cell types need to be activated for a complete response. Evaluating subcellular mycobacterial components from M. bovis and BCG-Tice, we determined that fragments from M. bovis could strongly provoke lymphocyte proliferation, but less skewed towards an NK cells response when compared with BCG-Tice fragments. Delipidated BCG-Tice was very efficient in stimulating CD56 upregulation, suggesting that non-covalently bound mycobacterial lipids and glycolipids are not strongly involved in NK activation.
Materials and Methods
Cells, BCG Substrains, and Reagents
Bladder cancer cell lines, T24 and RT112, and the erythroleukemia K562 cell line were previously described (5).
PBMCs from buffy coats of healthy donors were obtained from the Regional Transfusion Centre (Madrid) with ethical permission and experimental protocols approved by the institutional committees: Regional Transfusion Centre (PO-DIS-09) and assessed by the bioethics committee of CSIC. Informed consent was obtained at the Transfusion Centre from all participants. All methods were carried out in accordance with biosafety guidelines and regulations authorized by CNB-CSIC.
PBMCs were isolated by centrifugation on Ficoll-HyPaque and cultured for one week in RPMI-1640 (Biowest) supplemented with 5% FBS, 5% human male AB serum, 2 mM glutamine, 1 mM sodium pyruvate, 0.1 mM non-essential amino acids, 10 mM Hepes, 100 U/ml penicillin and 100 U/ml streptomycin (Biowest) at a concentration of 1 x 106 cells/ml in 96-well plates.
World Health Organization Reference Reagents of BCG-Russian (code 07/274) and BCG-Tokyo 172 (code 07/272) substrains were obtained from the National Institute for Biological Standards and Control (NIBSC, UK); BCG-Pasteur strain 1173P2 was originally obtained from Institut Pasteur Paris, BCG-Moreau was a gift from Dr Sylvia Leao, Federal University of Sao Paulo, Brazil); BCG-Tice (OncoTICE) and BCG-Connaught (ImmuCyst) were both commercially available.
Flow Cytometry
For flow cytometry, cells were washed with PBA [PBS supplemented with 0.5% bovine serum albumin (BSA), 1% FBS and 0.1% sodium azide] and incubated with antibodies against surface markers [CD3-CF (Immunostep); CD3-FITC, CD56-PC5, CD16-PE-Cy7, CD4-PC5.5, CD8-PC7 and LAMP1-APC (Biolegend)] at 4°C for 30 min in the dark. For intracellular staining, after surface labelling, cells were fixed with 1% p-formaldehyde for 10 min at RT, permeabilized with 0.2% saponin for 10 min at RT and stained with Perforin-PE, Granzyme B-PE (Biolegend) for 30 min. Cells were washed in PBA and analyzed using a Gallios Flow Cytometer (Beckman Coulter). Analysis of the experiments was performed using Kaluza software.
PBMC Stimulation With BCG
Aliquots of reconstituted BCG were prepared in RPMI with 10% DMSO and stored at −20°C. For BCG stimulation, experiments were performed as previously described (5). Briefly, 0.5 x 106 PBMCs/ml were incubated in 48-well plates with or without BCG at a 1:10 ratio (viable bacteria:PBMC). One week later, cells in suspension were recovered from the co-culture, centrifuged, analyzed by flow cytometry.
Live Versus Dead Mycobacteria Counting
Viable bacteria were determined by serial dilutions in Middlebrook 7H10 medium supplemented with Albumin Dextrose Catalase (ADC). To obtain heat-killed BCG, bacteria were washed in PBS and treated at 100°C for 20–30 min. The total number of BCG, including live and dead bacilli, was calculated by flow cytometry using a protocol optimized for this study as follows. A calibration curve was prepared, using a log-phase growth culture of BCG, in Middlebrook 7H9 medium supplemented with ADC, assuming that the whole culture was viable. Then, serial dilutions (1:2) were prepared and analyzed by flow cytometry. Bacterial viable units in the culture were calculated by plating in solid medium and counting colonies for each standard curve point. For these analyses, FSC and SSC parameters were set in the flow cytometer to visualize bacteria (Supplementary Figure 1). Samples were acquired, using a Beckman Coulter Gallios flow cytometer, at constant speed during 30 s and the number of events was defined as the total amount of bacteria. For analysis, weasel software was used. Data of the different dilutions were used to build the calibration curve and, the latter to interpolate and calculate the total amount of BCG present in different formulations.
Degranulation Experiments
Untreated or BCG-treated PBMCs were used as effector cells; bladder cancer cell lines or the erythroleukemia K562 (as positive control for NK degranulation) were used as target cells, always pretreated with HP1F7 antibody to block MHC-I. The percentage of NK cells was determined for each donor, so that equivalent effector NK cell ratios were set up for the different donors and conditions. NK cells were allowed to degranulate in co-cultures of PBMCs and targets for 2 h at an E:T ratio of 5:1 (which corresponds usually to 1:2 NK:target ratio), following the method published by Bryceson et al. (26). Surface expression of LAMP1 (CD107a) was analyzed by flow cytometry as shown in Supplementary Figure 3. Statistical analyses were performed using the Prism 6 software.
Mycobacterial Subcellular Fractions
The following reagents were obtained through the NIH Biodefense and Emerging Infections Research Resources Repository, NIAID, NIH: γ-irradiated whole cells M. bovis, Strain AF 2122/97 (ATCC® BAA-935™) Catalog No. NR-31210; Total Lipids, Catalog No. NR-44100, Cell Membrane Fraction, Catalog No. NR-31214 and Cytosol Fraction, Catalog No. NR-31215 from M. bovis; Trehalose-6,6-dimycolate (TDM), Catalog No. NR-14844 and Mycolic Acid, Catalog No. NR-14854 from M. tuberculosis, strain H37Rv.
To obtain BCG-Tice fragments, BCG-Tice was grown at 37°C on Middlebrook 7H10 medium supplemented with Oleic-Albumin-Dextrose-Catalase (OADC). After 4 weeks, cells were scraped from the surface and non-covalently attached cell wall lipids were extracted, first with chloroform/methanol (2:1, v/v), and then with chloroform/methanol (1:2, v/v) mixtures. Pooled organic extracts, containing the lipids, were combined, dried and partitioned with chloroform/methanol/water (8:4:2 v/v). Aqueous phase (“aqueous” antigen) and chloroform phase (“lipid” antigen) were then separated and evaporated to dryness. Delipidated bacteria were collected and stored in aliquots in sterile glass tubes (“delipidated” antigen).
Results
Differential Activation of CD56bright NK Cells by BCG Substrains Depends on the Number of Total Mycobacteria
To test whether the different BCG substrains used in the clinics could differently activate the immune response, experiments to study the variability of mycobacteria in the context of NK activation were initiated. We recently reported that stimulation with BCG, i.e., culture with the clinical grade preparation OncoTICE® at a ratio of 1:10 (105 live bacteria to 106 PBMC), led to the generation of an anti-tumoral NK cell population characterized by upregulation of the surface marker CD56 on NK cells together with expression of CD16 (5). Although we have described previously that this population of “BCG-stimulated CD56bright NK cells” differ both in phenotype and function capacities from “peripheral blood CD56bright NK cells”, for simplicity, NK cells expressing high vs low amounts of CD56 are called in this paper CD56bright and dim. However, this only refers to the level of expression of the receptor. Thus, to confirm the activation of an anti-tumor NK population, in this paper, we check the expression of high levels of CD56 together with CD16 and the degranulation capacity of these cells after incubation with bladder cancer cells.
Firstly, we compared the effect of two reference BCG vaccines with OncoTICE® on the upregulation of the surface marker CD56 on NK cells using the same ratio as previously. Incubation of PBMCs with both Tokyo and Russian substrains (lyophilized preparations obtained from NIBSC) also resulted in an increase in the percentage of CD56bright NK cells (Figure 1A). Since different commercial formulations contain different concentrations of BCG, laboratory grown cultures of the mentioned different substrains were subsequently used to plan new experiments using equal numbers of mycobacteria. When using laboratory grown substrains, upregulation of CD56 also could be observed, although surprisingly, the percentages of CD56bright cells obtained were much lower with the laboratory preparations than with the commercial BCG (Figure 1B). Since the pellet obtained after centrifugation of the suspensions from commercially available preparations was substantial, we hypothesized that a larger number of bacteria, presumably dead, were present in addition to the live BCG indicated in the leaflet. Thus, the numbers of live vs dead BCG were analyzed in each lyophilized sample. Live BCG were measured by counting CFU in Middlebrook 7H10 agar plates and the total number of BCG was estimated by flow cytometry, using a protocol optimized for the present study (see Materials and Methods and Supplementary Figure 1). These numbers were compared with the concentration range provided by the manufacturer. While the CFU range provided in the product insert agreed with the numbers that were obtained by the culture method, the number of total BCG obtained by flow cytometry was, in general, much higher. Table 1 summarizes the numbers obtained for the different BCG preparations. For example, OncoTICE® contains 2–8 x 108 viable BCG per vial, according to the manufacturer’s information but the total number of mycobacteria measured by flow cytometry was 5.95x 109, thus, only around 10% of mycobacteria present in the vial were alive after reconstitution in buffer. Similarly, only 38% of mycobacteria were alive in the BCG Tokyo lyophilized ampoule (NIBSC, WHO reference reagent, Japan BCG laboratory) (Table 1).
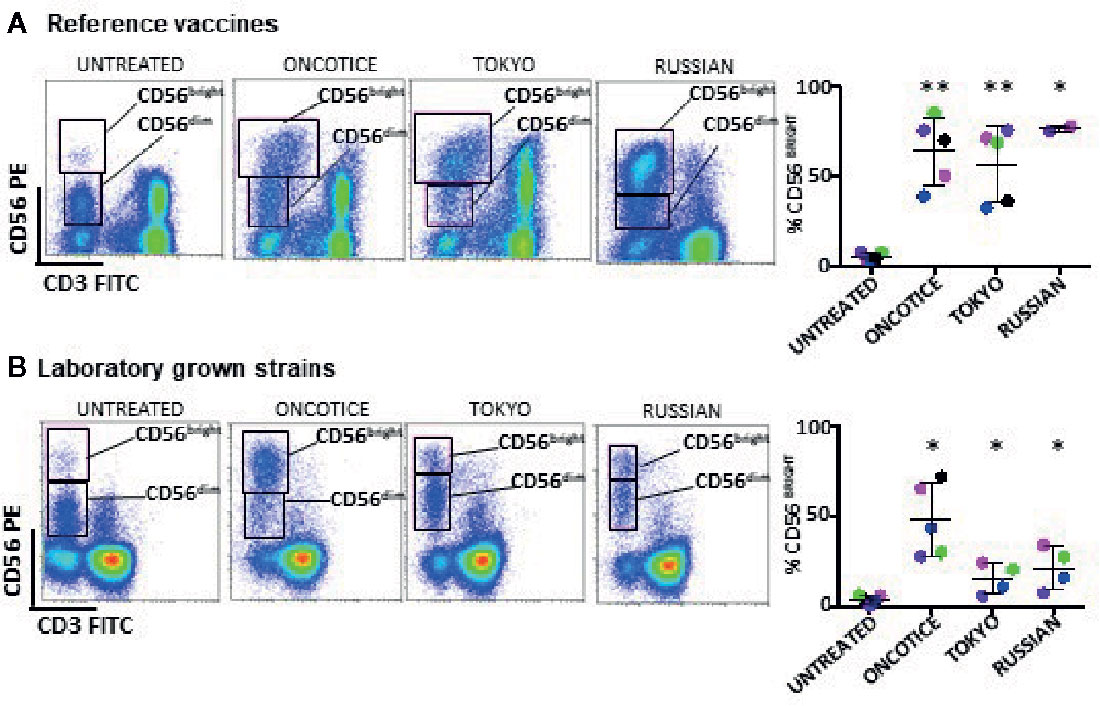
Figure 1 NK cell activation, based on the increase of CD56bright cells, occurs after incubation with several substrains of BCG. PBMCs from healthy donors were incubated with or without the indicated substrains of BCG at a 1:10 ratio (viable bacteria to PBMC). Commercial OncoTice was used for comparison. At day 7, cells in suspension were recovered from the co-culture, centrifuged and analyzed by flow cytometry. The percentage of the CD56bright and CD56dim populations within NK cells was obtained for each donor and plotted in a bar graph. Statistical analysis was performed using T-student test to compare each substrain with OncoTice (*p < 0.05; **p < 0.01). (A) Comparison of reference vaccines with Oncotice. (B) Comparison of laboratory-grown substrains with Oncotice.
Thus, the differences observed in BCG-NK activation between the commercial lyophilized product (OncoTice) and the laboratory cultures might be due to the presence of large numbers of dead bacteria in the former.
Dead Mycobacteria Can Activate Anti-Tumor CD56bright NK Cells to Degranulate Against Bladder Cancer Cell Lines
To test whether dead BCG could contribute to the activation of CD56bright NK cells, the capacities of clinical grade Tice and several laboratory-cultured BCG preparations to stimulate PBMCs were compared using either viable or heat-inactivated BCG. In order to avoid heterogeneity due to culture/production conditions, the different substrains were grown in laboratory using the same culture medium and method. BCG added to PBMCs were either mostly viable (at log phase), completely inactivated or mixed 50% dead/50% alive. In all the cases, PBMC activation was observed as shown by the emergence of lymphoblast cells in the FCShigh/SSChigh region (Figure 2A, Supplementary Figure 2). The percentage of activated lymphocytes was plotted for the different donors showing very similar percentages with viable and heat-killed bacteria. The commercial product, OncoTICE, was used as a positive control for comparison, since it was the one used for our initial description of NK cell activation. CD56bright NK cells were present in all the cases (Figure 2B). The percentage of CD56bright NK cells increased significantly with respect to untreated cells even when PBMCs were incubated with 100% heat-inactivated BCG, suggesting that the activation of NK cells in these cultures does not require interaction with live mycobacteria.
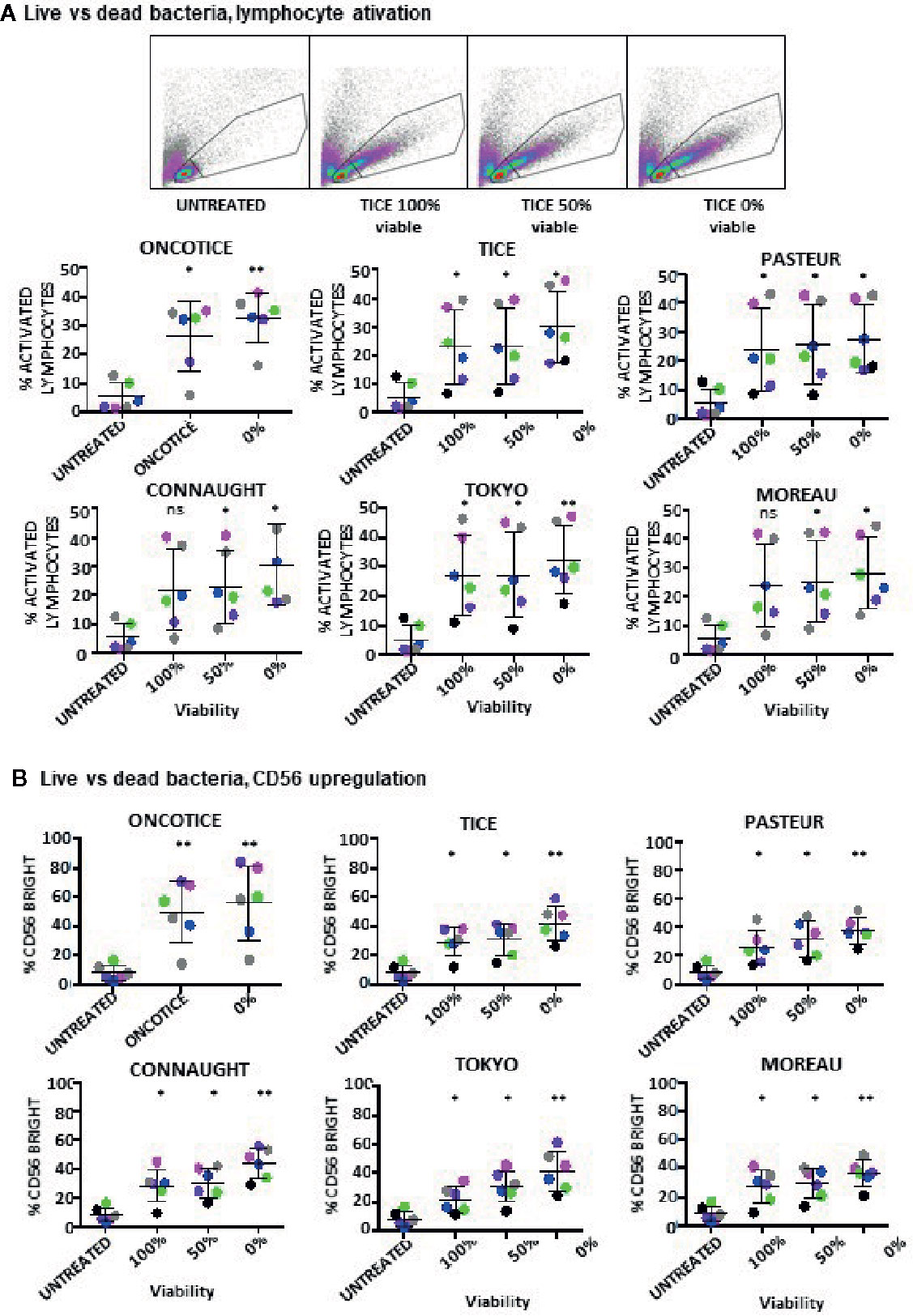
Figure 2 Lymphocyte activation occurs in co-cultures using either live or dead BCG. PBMCs from healthy donors were incubated with the indicated BCG substrains. The ratio was calculated to mimic the amount of total bacteria in the commercial vaccine, 6:1 ratio (total bacteria to PBMC) from cultures either 100% viable, 50% viable or completely inactivated by 20 min at 100°C. At day 7, cells in suspension were recovered from the co-culture, centrifuged and analyzed by flow cytometry. (A) The percentage of activation was determined by identifying the resting and activating lymphocyte regions by FSC vs SSC. The percentage of activated lymphocytes was plotted for each donor (different colors) and viability condition. Statistical analysis was performed using one-way ANOVA comparing each condition with the untreated culture (*p < 0.05; **p < 0.01; ns, non-significant). (B) The percentage of the CD56bright population was obtained within the NK cell region for each donor and analyzed as in (A).
These experiments show that NK cells can be activated when non-viable BCG is co-cultured with PBMCs. To further confirm this hypothesis, the capacity of different laboratory-grown BCG substrains using approximately 10% of live bacteria (proportion found in OncoTICE) to activate PBMCs was tested showing lymphocyte activation once again (Figure 3A, left panel). Although the final amount of different subtypes of lymphocytes varied slightly, all the BCG substrains tested gave rise to the upregulation of CD56 on NK cells (Figure 3A, right panel) and increased degranulation against bladder cancer cells (Figure 3B). Indeed, upregulation of CD56 was significant for all seven donors tested, compared to untreated PBMCs, and the percentage of BCG-stimulated CD56bright cells was very consistent when comparing between the different substrains. In parallel, the capacity of these activated NK cells to degranulate after recognition of two bladder cancer cell lines [T24, a grade 3 cell line recognized by activated NK cells from most donors, and RT112, a low grade cell line usually poorly recognized by activated NK cells (22)], was tested. Supplementary Figure 3 shows a gating strategy for this type of experiments. These experiments demonstrate an increase in degranulation of BCG-stimulated CD56bright NK cells when using different BCG substrains containing approximately 10% of viable bacteria (Figure 3B). In these experiments, the regions corresponding to T cells could also be analyzed, confirming that after BCG exposure the main granule mobilization activity was observed for CD56bright cells. CD3 degranulation against bladder cancer cells did not occur and it was not different to that seen for CD3 cells in the absence of target. To sum up, these results suggest that dead mycobacteria in co-culture with PBMCs contribute to the activation of NK cells for an enhanced anti-tumoral activity.
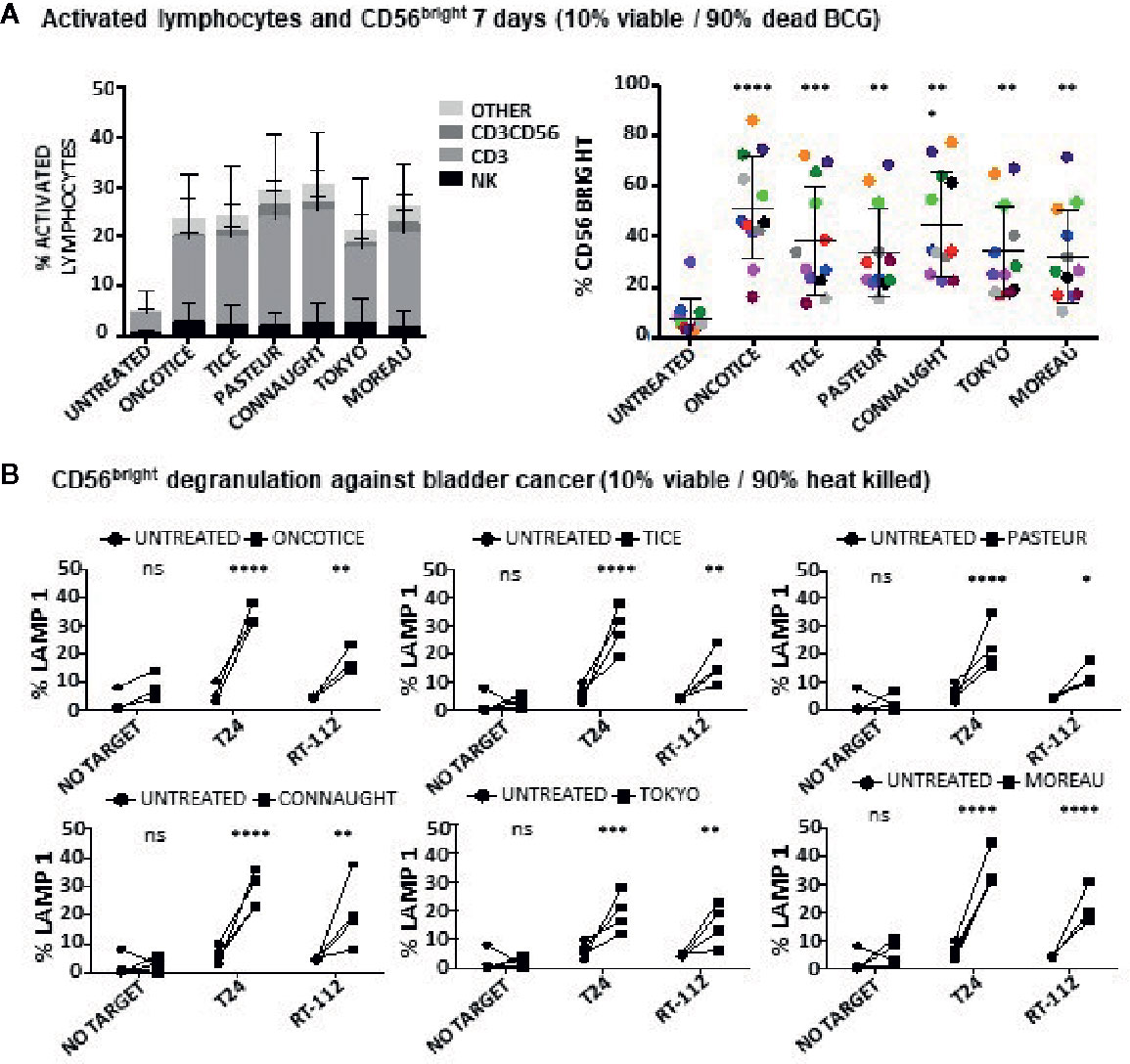
Figure 3 Lymphocyte populations and NK degranulation after co-cultures with 10% viable BCG. PBMCs from healthy donors were incubated with the indicated substrains of BCG at a 1:10 ratio (live bacteria to PBMC), using cultures containing approximately 10% viable bacteria plus 90% inactivated bacteria by 20-30 min at 100°C. OncoTice resuspended from the commercial vial was used as a control. At day 7, cells in suspension were recovered from the co-culture, centrifuged and analyzed by flow cytometry. (A) The percentage of activated lymphocytes was determined by identifying the resting and activating lymphocyte regions by FSC vs SSC and further analyzed to distinguish T cells from NK cells by staining with antibodies against population markers (CD3, CD56) (left). The percentage of activated lymphocytes was plotted for each donor (different populations are displayed in different colors). The percentage of the CD56bright population within the NK cell region was obtained for each donor (different colors) and analyzed by one-way ANOVA (**p < 0.01; ***p < 0.001; ****< 0.0001) (right). (B) Degranulation of CD56bright NK cells against bladder cancer cells (T24, RT-112) was measured by analyzing surface LAMP-1 (CD107a) within the CD3-CD56+ region. Different donors are represented by symbols and compared with the untreated culture. Statistical analysis was performed using one-way ANOVA (**p < 0.01; ***p < 0.001; ****p < 0.0001; ns, non-significant).
Different Mycobacterial Fragments Lead to the Activation of Different Immune Populations
Since dead BCG contributed to the activation of BCG-stimulated CD56bright NK cells, experiments were designed to study their effect on different PBMC subpopulations. Different subcellular fractions of mycobacterial extracts from M. bovis and BCG-Tice were also used in co-culture experiments with PBMCs to explore the idea that the use of fragments of bacteria or different strains (including non-pathogenic bacteria) might avoid toxicity in bladder cancer patients, as has been suggested previously (28–30). Whole irradiated M. bovis, and different fractions, membrane, cytosolic, total lipids and individual lipids [Trehalose-6,6-dimycolate (TDM) and mycolic acid] were incubated at different concentrations in co-culture with PBMCs (7 days) and the percentage of activated lymphocytes and NK subpopulations was analyzed by flow cytometry (Supplementary Figure 4A). Since most of the compounds tested could activate lymphocytes, experiments to define the relative activation of each lymphocyte subpopulation were performed (Figure 4A). Whole irradiated M. bovis and membrane fractions led to an increase in lymphocyte activation and, although an increase in CD56bright cells could be observed, the population of NK cells did not proliferate as much as CD3 lymphocytes. In particular, the percentage of CD4 cells was higher in cultures where the membrane fraction was added (Figure 4B). Data in this figure show the relative percentage of CD4 compared to CD8 within the CD3 lymphocyte region, indicating that in general the two subpopulations of T lymphocytes maintain the same ratio in the co-cultures, except when the membrane fractions of M. bovis were used. In this case, the proportion of CD4 cells increased significantly. In agreement with a moderate activation of NK cells, low degranulation capacity of NK cells against the two bladder cancer cell lines, T24 and RT112, was found in these cultures (Figure 4C). These experiments demonstrated that, although OncoTICE led to a lower percentage of activated lymphocytes than M. bovis, the composition of activated subpopulations was more skewed towards NK cells in the former leading to higher percentage of CD56bright upregulation and NK degranulation capacity. On the other hand, CD4 cells were activated more efficiently by M. bovis fragments or irradiated cells. The cytosolic fraction of M. bovis only stimulated significant NK cell degranulation against the T24 cell line although an upward trend, that did not become significant, could be observed for RT112. The lipidic fractions of M. bovis, in the concentrations used for these experiments, did not stimulate the PBMC culture.
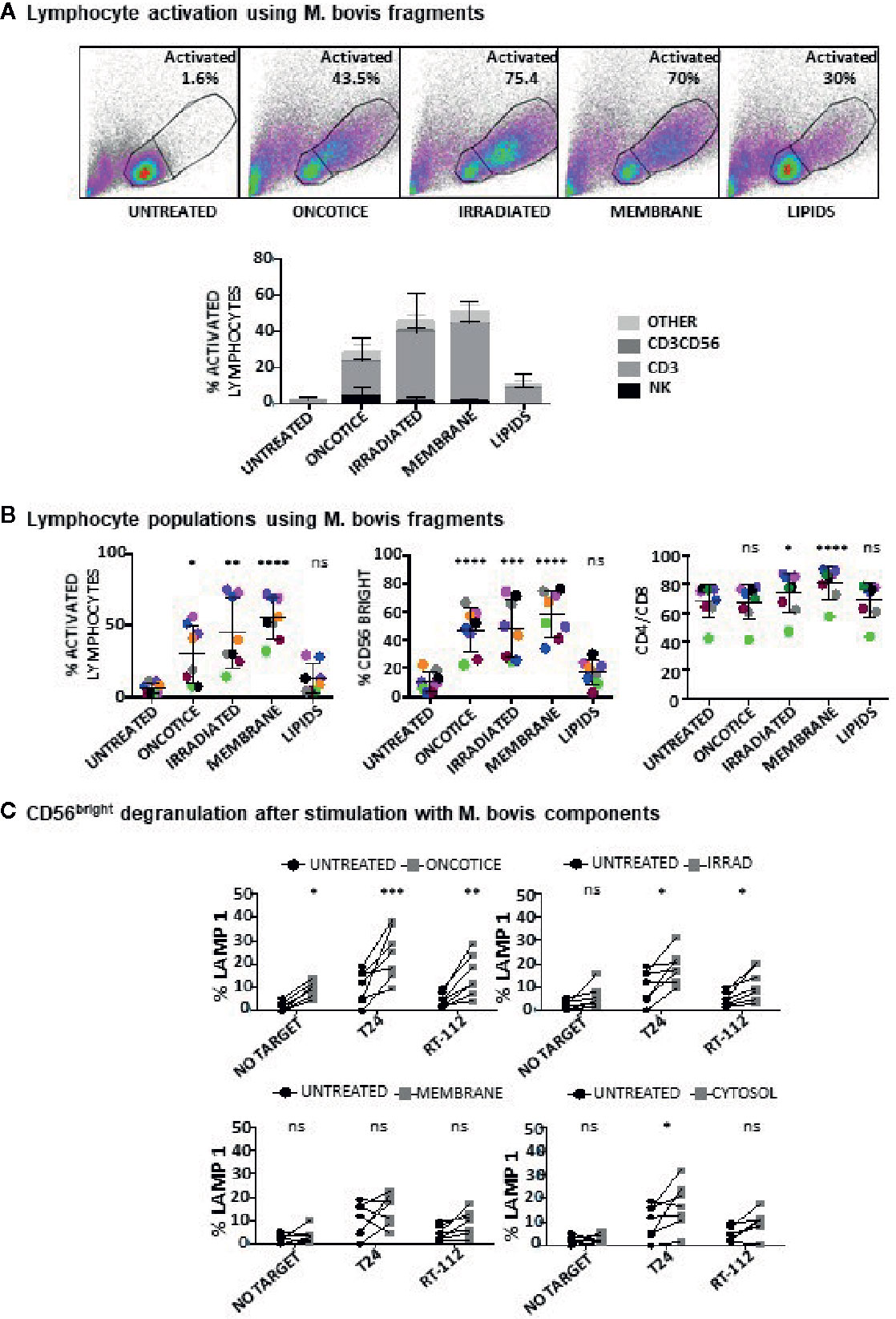
Figure 4 Lymphocyte activation using M. bovis and its subcellular fractions. PBMCs from healthy donors were incubated with the indicated fractions of M. bovis obtained through BEI Resources, NIAID, NIH. At day 7, cells in suspension were recovered from the co-culture, centrifuged and analyzed by flow cytometry. (A) The percentage of activation was determined by identifying the resting and activating lymphocyte regions by FSC vs SSC and further analyzed to distinguish T cells from NK cells by staining with antibodies against population markers (CD3, CD56) (top). The percentage of activated lymphocytes was plotted for each donor (different populations are displayed in different shades of grey/black) (bottom). (B) The percentage of the CD56bright population was obtained within the NK cell region and the percentage of CD4 vs CD8 was obtained within the CD3+ region (labelled as CD4/CD8). Data are displayed as different colors for each donor. Statistical analysis was performed using one-way ANOVA comparing each condition with the untreated culture (*p < 0.05; **p < 0.01; ***p < 0.001; ****p < 0.0001; ns, non-significant). (C) Degranulation of CD56bright NK cells against bladder cancer cells (T24, RT-112), was measured by analyzing surface LAMP-1 (CD107a) within the CD3-CD56+ region. Different donors are represented by symbols and compared with the untreated culture. Statistical analysis was performed using one-way ANOVA (*p < 0.05; **p < 0.01; ***p < 0.001; ns, non-significant).
The same type of experiments were also performed to evaluate NK activation using BCG-Tice fragments (Supplementary Figure 4B; Figure 5). In this case, delipidated-BCG, as well as the lipidic and the aqueous fractions were used. In contrast to M. bovis fragments, Tice lipids, mildly activated PBMC cultures while the aqueous phase did not activate lymphocytes at all (Figure 5A). The more activated cultures were obtained upon incubation with delipidated BCG. No fragment was as efficient as intact OncoTICE at stimulating NK CD56 upregulation, however the percentage of activated lymphocytes was higher in cultures with both delipidated-BCG and lipid fragments than in untreated PBMCs (Figure 5B). Both the lipid and the delipidated, but not the aqueous fraction, led to only low level of CD56bright activation, but when present, these cells were able to recognize bladder cancer cells in degranulation assays (Figure 5C). In fact, the amounts of intracellular granzyme B and perforin in BCG-stimulated CD56bright NK cells were clearly increased when PBMCs were treated with delipidated-BCG in a comparable manner to cultures stimulated with BCG-Tice (Figure 6). Although NK cells became activated by BCG lipid, CD3 lymphocytes did not show degranulation when exposed to bladder cancer cells after stimulation with BCG components (not shown). These data suggest that different mycobacteria fractions can activate immune populations differently and that, depending on the intended use of BCG, different fragments could be used to produce specific effects.
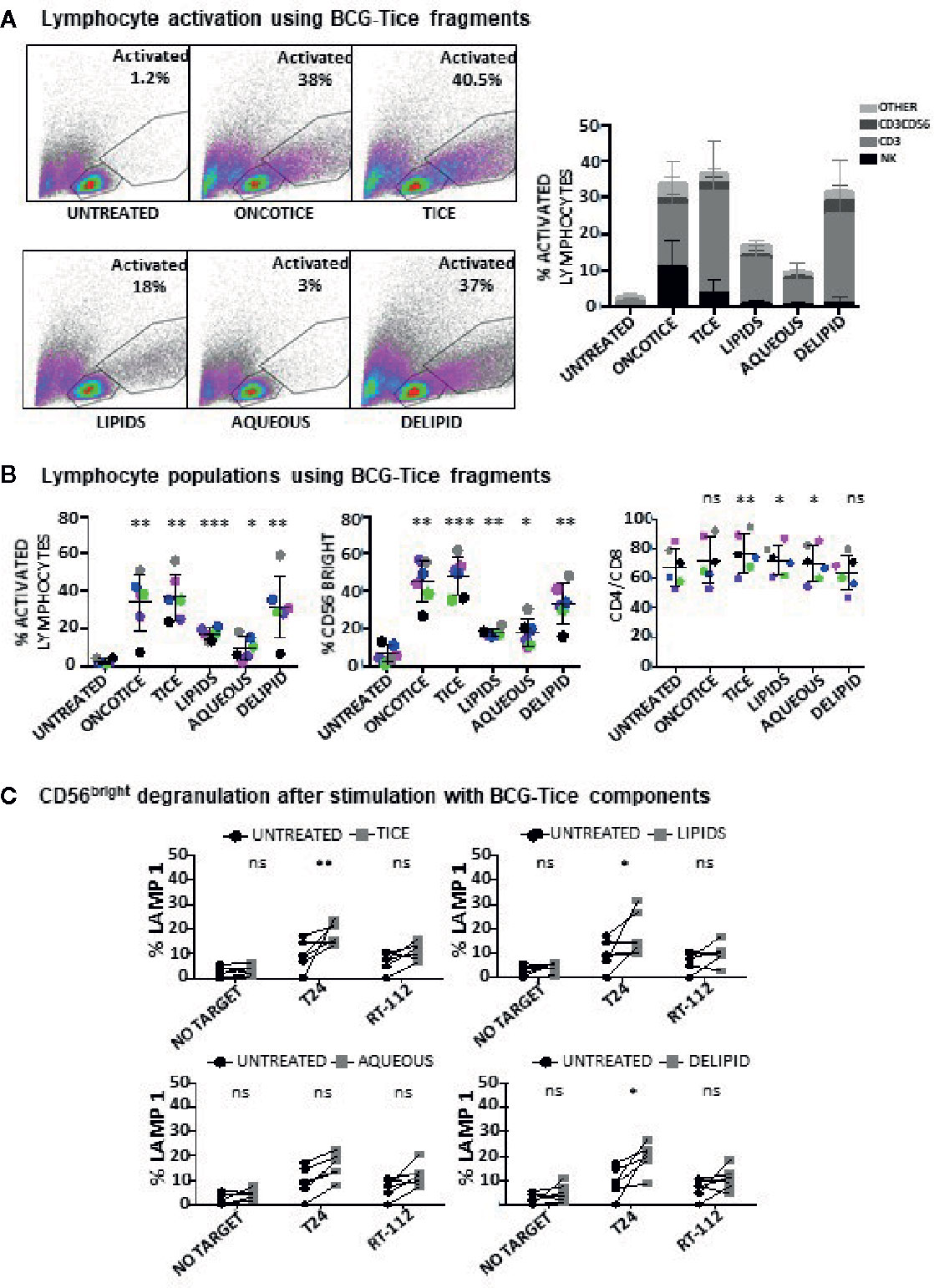
Figure 5 Lymphocyte activation using Tice BCG subcellular fractions. PBMCs from healthy donors were incubated with the indicated fractions of Tice BCG (see Materials and Methods). At day 7, cells in suspension were recovered from the co-culture, centrifuged and analyzed by flow cytometry. (A) The percentage of activation was determined by identifying the resting and activating lymphocyte regions by FSC vs SSC and further analyzed to distinguish T cells from NK cells by staining with antibodies against population markers (CD3, CD56). The percentage of activated lymphocytes was plotted for each donor (different populations are displayed in different shades of grey/black) (bottom). (B) The percentage of the CD56bright population was obtained within the NK cell region and percentage of CD4 vs CD8 was obtained within the CD3+ region (labelled as CD4/CD8). Data are displayed as different colors for each donor. Statistical analysis was performed using one-way ANOVA comparing each condition with the untreated culture (*p < 0.05; **p < 0.01; ***p < 0.001; ns, non-significant). (C) Degranulation of CD56bright NK cells against bladder cancer cells (T24, RT-112), was measured by analyzing surface LAMP-1 (CD107a) within the CD3-CD56+ region. Different donors are represented by symbols and compared with the untreated culture. Statistical analysis was performed using one-way ANOVA (*p < 0.05; **p < 0.01; ns, non-significant).
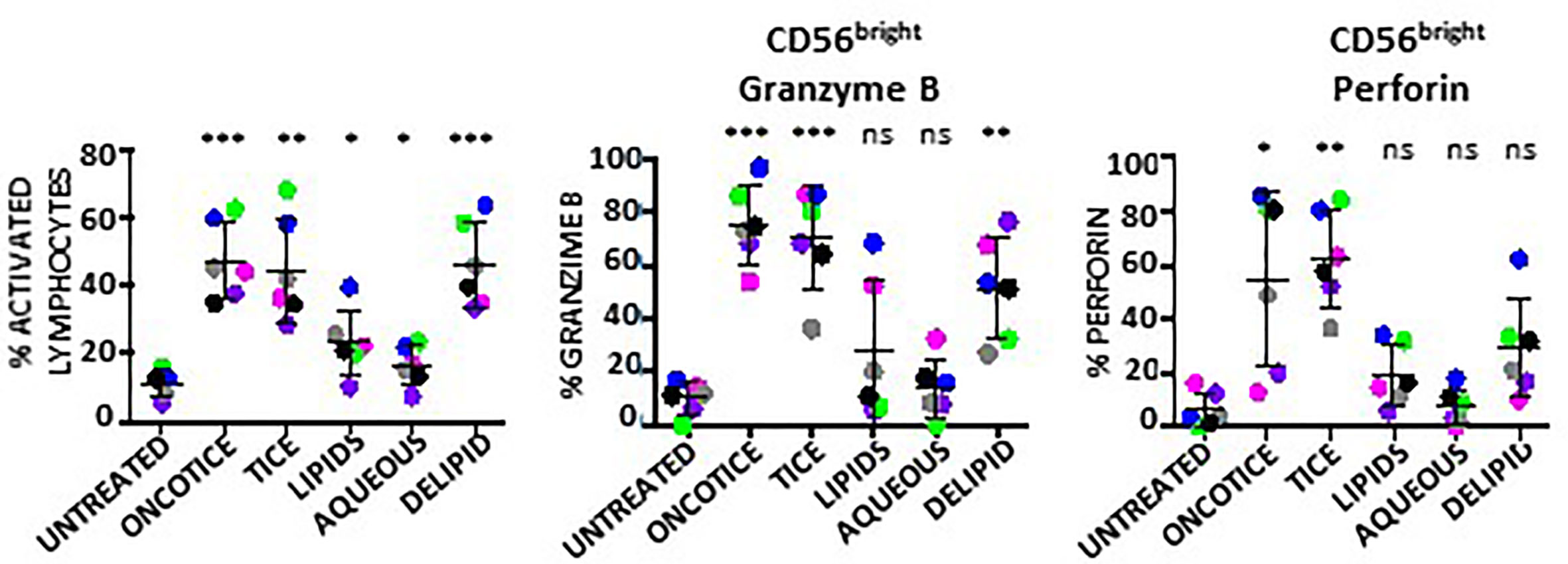
Figure 6 Granzyme B and perforin within NK cells activated using BCG-Tice subcellular fractions. PBMCs from healthy donors were incubated with the indicated fractions of BCG-Tice (see Materials and Methods). At day 7, cells in suspension were recovered from the co-culture, centrifuged and analyzed by flow cytometry. The percentage of activation was determined by identifying the resting and activating lymphocyte regions by FSC vs SSC and further analyzed to distinguish T cells from NK cells by staining with antibodies against population markers (CD3, CD56). The percentage of activated lymphocytes was plotted for each donor (different colors) (left). The percentage of the CD56bright population was obtained within the NK cell region and the content of Granzyme B (middle) and perforin (right) were determined by intracellular staining. Statistical analysis was performed using one-way ANOVA (*p < 0.05; **p < 0.01; ***p < 0.001; ns, non-significant) and compared with the untreated culture.
Discussion
BCG has been used both as TB vaccine and to treat bladder cancer for many decades, however, the heterogeneity of action and the existence of many different substrains still poses many unresolved questions. This study evaluates the effect of BCG heterogeneity in the immune response exerted by NK cells against bladder cancer cells, as a model of the successful immunotherapy used routinely in NMIBC patients. The data presented here confirm that the different mycobacterial strains have different capacities to stimulate leukocytes and that the subpopulations of lymphocytes stimulated by different bacterial compounds diverge.
A key discovery reported here is that, although all BCG substrains can stimulate NK cell proliferation and response against high grade bladder cancer, clearly some substrains provoke NK cell degranulation more strongly than others. Different BCG substrains were generated from 1921 when the original culture was continuously grown separately in different countries (13, 17). Several genetic groups of BCG appeared subsequently differing in regions of difference (RD) that have particular deletions, insertions and mutations. RD1 deletion was the first main genetic change that occurred between M. bovis and BCG. Interestingly, in our degranulation experiments, the stronger increase in response against T24 bladder cells occurred using as stimulation of PBMCs Tice, Connaught and Moreau. The genetic branch of the Moreau substrain separated early, in 1924, before the loss of IS6110, and belongs to the same group as Tokyo, 1925, which stimulated a more discrete NK response. Moreau and Tokyo differ in the regions, RD16 and ΔRv3405c, while Tice (1934) and Connaught (1948) belong to the same genetic group [tandem duplication 2 (DU2) group 4] and their main difference is the loss of RD15 that occurred in Connaught. Whether these genes are involved in the production of a stronger or milder NK cytotoxic response was not explored here, but could contribute to the functional differences found among the different BCG substrains.
A second finding from this work is that vaccine preparations contain, in addition to the CFU specified, a large number of dead bacteria and this prompted a series of experiments to evaluate the effect of dead mycobacteria on stimulation of leukocytes. Here, we confirm that the capacity of dead bacteria to stimulate lymphocyte proliferation and NK cell activation in co-cultures of PBMCs was similar to the stimulation caused by live bacteria. In certain strains, dead bacteria were even more potent. Early animal model experiments already concluded that dead BCG could mediate an anti-tumor response when bacteria were applied by intradermal injection (31) and, since then, several approaches have been investigated in order to eliminate the use of live bacteria in bladder cancer treatment to avoid toxicity, for example using bacterial cell wall and nucleic acids that could benefit patients failing other therapies (32). This study also provides a first evaluation of the impact of different mycobacterial components in the activation of NK cells to recognize bladder cancer cells. The experiments using M. bovis and BCG-Tice subcellular components confirmed that CD56bright NK cells respond strongly against bladder cancer and that this activation might be modulated by different mycobacterial compounds, but again, experiments showed differences between the two mycobacteria strains. While the lipidic fraction of M. bovis did not stimulate any immune population, the lipids from Tice mainly stimulated T cell proliferation, increasing the proportion of CD4 vs CD8. However, these lymphocytes did not degranulate in the presence of bladder cancer cells. On the other hand, delipidated BCG-Tice did not change the proportion of CD4/CD8, but caused an increase in effector cells including both CD3+CD56+ and NK cells. All these data support the idea that new inactivated BCG-based preparations could be possible both for cancer treatment and other situations in which NK cell stimulation could be beneficial. In fact, new approaches using intravenous BCG to stimulate certain immune populations, such as Th1/Th17 CD4 or CD8 T cells, proposed to increase TB protection in youngster or adults could benefit from the use of fractions and not viable bacteria (33). Data presented here show that different substrains induce differential proliferation of T vs NK cells. Connaught and Pasteur provoke a strong lymphocyte proliferation and a greater percentage of CD3+ cells than after co-culture of PBMCs with Tice. Culture with these substrains also led to an increase in the proportion of effector lymphocytes CD3+CD56+. Thus, it is tempting to propose that a particular immune population could be stimulated selectively by choosing the right BCG substrain or component. In this line, more research should be conducted to comprehensively identify the immune cell subpopulations responding to specific mycobacterial products. The use of NK cells as a therapeutic agent in cancer has been long discussed and nowadays, a large body of research is available on the use of NK cells for treatment of cancer, both autologous or allogeneic after ex vivo stimulation. Recent research and clinical trials are starting to generate promising results (34–37). The work presented here could open a new manner for NK stimulation with therapeutic purposes.
In conclusion, here we demonstrate that BCG stimulates the activation of NK cells through the interaction with mycobacteria subcellular components and this type of stimulation could be of interest beyond bladder cancer treatment. The presence of dead mycobacteria in lyophilized BCG preparations suggest that live bacilli could be less important than initially thought for cancer elimination and this opens new possibilities for a desirable decrease of unwanted effects caused by live BCG.
Data Availability Statement
The raw data supporting the conclusions of this article will be made available by the authors, without undue reservation.
Author Contributions
GE and NA: acquired, analyzed, and interpreted data. EJ, OA, and MH: generated reagents. NA, CM, and MV-G: provided material support and supervised the study. All authors contributed to the article and approved the submitted version.
Funding
This work was supported by grants from the Spanish Ministry of Science and Innovation (RTC-2017-6379-1, RTI2018-093569-B-I00 (MCIU/AEI/FEDER, EU) (MV-G) and the regional government of Madrid (S2017/BMD-3733-2); Generalitat of Catalunya (2017SGR-229) (EJ). The group of MV-G belongs to the research network TENTACLES (RED2018-102411-T) funded by the Spanish Ministry of Science.
Conflict of Interest
The authors declare that the research was conducted in the absence of any commercial or financial relationships that could be construed as a potential conflict of interest.
Acknowledgments
The authors would like to thank Dr Sylvia Leao, for the gift of BCG. The following reagents were obtained through BEI Resources, NIAID, NIH: γ-irradiated whole cells Mycobacterium bovis, Strain AF 2122/97 (ATCC® BAA-935™) Catalog No. NR-31210; Total Lipids, Catalog No. NR-44100, Cell Membrane Fraction, Catalog No. NR-31214 and Cytosol Fraction, Catalog No. NR-31215 from M. bovis; Trehalose-6,6-dimycolate (TDM), Catalog No. NR-14844 and Mycolic Acid, Catalog No. NR-14854 from Mycobacterium tuberculosis, Strain H37Rv. Data appeared online as a preprint in BioRxiv (BIORXIV/2020/129361). We acknowledge support of the publication fee by the CSIC Open Access Publication Support Initiative through its Unit of Information Resources for Research (URICI).
Supplementary Material
The Supplementary Material for this article can be found online at: https://www.frontiersin.org/articles/10.3389/fimmu.2021.622995/full#supplementary-material
References
1. Shimasaki N, Jain A, Campana D. NK cells for cancer immunotherapy. Nat Rev Drug Discovery (2020) 19:200–18. doi: 10.1038/s41573-019-0052-1
2. Morvan MG, Lanier LL. NK cells and cancer: you can teach innate cells new tricks. Nat Rev Cancer (2016) 16:7–19. doi: 10.1038/nrc.2015.5
3. Brandau S, Riemensberger J, Jacobsen M, Kemp D, Zhao W, Zhao X, et al. NK cells are essential for effective BCG immunotherapy. Int J Cancer (2001) 92:697–702. doi: 10.1002/1097-0215(20010601)92:5<697::AID-IJC1245>3.0.CO;2-Z
4. Suttmann H, Jacobsen M, Reiss K, Jocham D, Bohle A, Brandau S. Mechanisms of bacillus Calmette-Guerin mediated natural killer cell activation. J Urol (2004) 172:1490–5. doi: 10.1097/01.ju.0000131944.52354.63
5. Garcia-Cuesta EM, Esteso G, Ashiru O, Lopez-Cobo S, Alvarez-Maestro M, Linares A, et al. Characterization of a human anti-tumoral NK cell population expanded after BCG treatment of leukocytes. Oncoimmunology (2017) 6:e1293212. doi: 10.1080/2162402X.2017.1293212
6. Wagner JA, Rosario M, Romee R, Berrien-Elliott MM, Schneider SE, Leong JW, et al. CD56bright NK cells exhibit potent antitumor responses following IL-15 priming. J Clin Invest (2017) 127:4042–58. doi: 10.1172/JCI90387
7. Pym AS, Brodin P, Brosch R, Huerre M, Cole ST. Loss of RD1 contributed to the attenuation of the live tuberculosis vaccines Mycobacterium bovis BCG and Mycobacterium microti. Mol Microbiol (2002) 46:709–17. doi: 10.1046/j.1365-2958.2002.03237.x
8. Calmette A, Bocquet A, Negre L. Contribution a l’etude du bacilli tuberculeux bilie. Ann Inst Pasteur (1921) 9:561–70.
9. Gandhi NM, Morales A, Lamm DL. Bacillus Calmette-Guerin immunotherapy for genitourinary cancer. BJU Int (2013) 112:288–97. doi: 10.1111/j.1464-410X.2012.11754.x
10. Brosch R, Gordon SV, Garnier T, Eiglmeier K, Frigui W, Valenti P, et al. Genome plasticity of BCG and impact on vaccine efficacy. Proc Natl Acad Sci U.S.A. (2007) 104:5596–601. doi: 10.1073/pnas.0700869104
11. Ritz N, Curtis N. Mapping the global use of different BCG vaccine strains. Tuberculosis (Edinb) (2009) 89:248–51. doi: 10.1016/j.tube.2009.03.002
12. Zwerling A, Behr MA, Verma A, Brewer TF, Menzies D, Pai M. The BCG World Atlas: a database of global BCG vaccination policies and practices. PloS Med (2011) 8:e1001012. doi: 10.1371/journal.pmed.1001012
13. Gan C, Mostafid H, Khan MS, Lewis DJ. BCG immunotherapy for bladder cancer–the effects of substrain differences. Nat Rev Urol (2013) 10:580–8. doi: 10.1038/nrurol.2013.194
14. Morales A, Eidinger D, Bruce AW. Intracavitary Bacillus Calmette-Guerin in the treatment of superficial bladder tumors. J Urol (1976) 116:180–3. doi: 10.1016/S0022-5347(17)58737-6
15. Martinez-Pineiro JA, Muntanola P. Nonspecific immunotherapy with BCG vaccine in bladder tumors: a preliminary report. Eur Urol (1977) 3:11–22. doi: 10.1159/000472047
16. Lamm DL, Thor DE, Harris SC, Reyna JA, Stogdill VD, Radwin HM. Bacillus Calmette-Guerin immunotherapy of superficial bladder cancer. J Urol (1980) 124:38–40. doi: 10.1016/S0022-5347(17)55282-9
17. Behr MA, Small PM. A historical and molecular phylogeny of BCG strains. Vaccine (1999) 17:915–22. doi: 10.1016/S0264-410X(98)00277-1
18. D’Andrea D, Gontero P, Shariat SF, Soria F. Intravesical bacillus Calmette-Guerin for bladder cancer: are all the strains equal? Transl Androl Urol (2019) 8:85–93. doi: 10.21037/tau.2018.08.19
19. Witjes JA, Dalbagni G, Karnes RJ, Shariat S, Joniau S, Palou J, et al. The efficacy of BCG TICE and BCG Connaught in a cohort of 2,099 patients with T1G3 non-muscle-invasive bladder cancer. Urol Oncol (2016) 34:484 e19–484 e25. doi: 10.1016/j.urolonc.2016.05.033
20. Rentsch CA, Birkhauser FD, Biot C, Gsponer JR, Bisiaux A, Wetterauer C, et al. Bacillus Calmette-Guerin strain differences have an impact on clinical outcome in bladder cancer immunotherapy. Eur Urol (2014) 66:677–88. doi: 10.1016/j.eururo.2014.02.061
21. Unda-Urzaiz M, Cozar-Olmos JM, Minana-Lopez B, Camarero-Jimenez J, Brugarolas-Rossello X, Zubiaur-Libano C, et al. Safety and efficacy of various strains of bacille Calmette-Guerin in the treatment of bladder tumours in standard clinical practice. Actas Urol Esp (2018) 42:238–48. doi: 10.1016/j.acuroe.2018.03.004
22. Garcia-Cuesta EM, Lopez-Cobo S, Alvarez-Maestro M, Esteso G, Romera-Cardenas G, Rey M, et al. NKG2D is a Key Receptor for Recognition of Bladder Cancer Cells by IL-2-Activated NK Cells and BCG Promotes NK Cell Activation. Front Immunol (2015) 6:284. doi: 10.3389/fimmu.2015.00284
23. Akaza H, Iwasaki A, Ohtani M, Ikeda N, Niijima K, Toida I, et al. Expression of antitumor response. Role of attachment and viability of bacillus Calmette-Guerin to bladder cancer cells. Cancer (1993) 72:558–63. doi: 10.1002/1097-0142(19930715)72:2<558::AID-CNCR2820720237>3.0.CO;2-H
24. Gunther JH, Frambach M, Deinert I, Brandau S, Jocham D, Bohle A. Effects of acetylic salicylic acid and pentoxifylline on the efficacy of intravesical BCG therapy in orthotopic murine bladder cancer (MB49). J Urol (1999) 161:1702–6. doi: 10.1016/S0022-5347(05)69008-8
25. Shapiro A, Ratliff TL, Oakley DM, Catalona WJ. Reduction of bladder tumor growth in mice treated with intravesical Bacillus Calmette-Guerin and its correlation with Bacillus Calmette-Guerin viability and natural killer cell activity. Cancer Res (1983) 43:1611–5.
26. Bryceson YT, Fauriat C, Nunes JM, Wood SM, Bjorkstrom NK, Long EO, et al. Functional analysis of human NK cells by flow cytometry. Methods Mol Biol (2010) 612:335–52. doi: 10.1007/978-1-60761-362-6_23
27. Ho MM, Markey K, Rigsby P, Hockley J, Corbel MJ. Report of an International collaborative study to establish the first WHO reference reagents for BCG vaccines of three different sub-strains. Vaccine (2011) 29:512–8. doi: 10.1016/j.vaccine.2010.10.066
28. Morales A, Johnston B, Emerson L, Heaton JW. Intralesional administration of biological response modifiers in the treatment of localized cancer of the prostate: a feasibility study. Urology (1997) 50:495–502. doi: 10.1016/S0090-4295(97)00402-0
29. Morales A, Chin JL, Ramsey EW. Mycobacterial cell wall extract for treatment of carcinoma in situ of the bladder. J Urol (2001) 166:1633–7discussion 1637–8. doi: 10.1016/S0022-5347(05)65642-X
30. Julián E, Noguera-Ortega E. Bacteria-derived alternatives to live Mycobacterium bovis Bacillus Calmette-Guerin for non-muscle invasive bladder cancer treatment. In: Fialho AM, Chakrabarty AM, editors. Microbial Infections and Cancer Therapy. Singapore: Pan Stanford Publishing Pte. Ltd (2019). p. 123–88.
31. Zbar B, Rapp HJ. Immunotherapy of guinea pig cancer with BCG. Cancer (1974) 34(suppl):1532–40. doi: 10.1002/1097-0142(197410)34:8+<1532::AID-CNCR2820340827>3.0.CO;2-H
32. Packiam VT, Pearce SM, Steinberg GD. The role of mycobacterial cell wall nucleic acid complex in the treatment of bacillus Calmette-Guerin failures for non-muscle-invasive bladder cancer. Ther Adv Urol (2016) 8:29–37. doi: 10.1177/1756287215607818
33. Darrah PA, Zeppa JJ, Maiello P, Hackney JA, Wadsworth MH,2, Hughes TK, et al. Prevention of tuberculosis in macaques after intravenous BCG immunization. Nature (2020) 577:95–102. doi: 10.1038/s41586-019-1817-8
34. Suck G, Odendahl M, Nowakowska P, Seidl C, Wels WS, Klingemann HG, et al. NK-92: an ‘off-the-shelf therapeutic’ for adoptive natural killer cell-based cancer immunotherapy. Cancer Immunol Immunother (2016) 65:485–92. doi: 10.1007/s00262-015-1761-x
35. Guillerey C, Huntington ND, Smyth MJ. Targeting natural killer cells in cancer immunotherapy. Nat Immunol (2016) 17:1025–36. doi: 10.1038/ni.3518
36. Krasnova Y, Putz EM, Smyth MJ, Souza-Fonseca-Guimaraes F. Bench to bedside: NK cells and control of metastasis. Clin Immunol (2017) 177:50–9. doi: 10.1016/j.clim.2015.10.001
Keywords: cancer immunology, BCG strains, NK activation, CD56bright, bladder cancer, mycobacterial fractions
Citation: Esteso G, Aguiló N, Julián E, Ashiru O, Ho MM, Martín C and Valés-Gómez M (2021) Natural Killer Anti-Tumor Activity Can Be Achieved by In Vitro Incubation With Heat-Killed BCG. Front. Immunol. 12:622995. doi: 10.3389/fimmu.2021.622995
Received: 29 October 2020; Accepted: 04 January 2021;
Published: 23 February 2021.
Edited by:
Carsten Watzl, Leibniz Research Centre for Working Environment and Human Factors (IfADo), GermanyReviewed by:
Francisco Borrego, Biocruces Bizkaia Health Research Institute, SpainMartin R Goodier, University of London, United Kingdom
Copyright © 2021 Esteso, Aguiló, Julián, Ashiru, Ho, Martín and Valés-Gómez. This is an open-access article distributed under the terms of the Creative Commons Attribution License (CC BY). The use, distribution or reproduction in other forums is permitted, provided the original author(s) and the copyright owner(s) are credited and that the original publication in this journal is cited, in accordance with accepted academic practice. No use, distribution or reproduction is permitted which does not comply with these terms.
*Correspondence: Mar Valés-Gómez, mvales@cnb.csic.es
†Present address: Omodele Ashiru, Abcam plc, Cambridge, United Kingdom