- 1Ossium Health, Indianapolis, IN, United States
- 2Department of Biomedical Sciences, College of Osteopathic Medicine, Marian University, Indianapolis, IN, United States
- 3Department of Plastic and Reconstructive Surgery, Johns Hopkins University School of Medicine, Baltimore, MD, United States
- 4Department of Visceral, Transplant and Thoracic Surgery, Center of Operative Medicine, Medical University of Innsbruck, Innsbruck, Austria
- 5Department of Medical and Molecular Genetics, Indiana University School of Medicine, Indianapolis, IN, United States
Induction of immune tolerance for solid organ and vascular composite allografts is the Holy Grail for transplantation medicine. This would obviate the need for life-long immunosuppression which is associated with serious adverse outcomes, such as infections, cancers, and renal failure. Currently the most promising means of tolerance induction is through establishing a mixed chimeric state by transplantation of donor hematopoietic stem cells; however, with the exception of living donor renal transplantation, the mixed chimerism approach has not achieved durable immune tolerance on a large scale in preclinical or clinical trials with other solid organs or vascular composite allotransplants (VCA). Ossium Health has established a bank of cryopreserved bone marrow (BM), termed “hematopoietic progenitor cell (HPC), Marrow,” recovered from deceased organ donor vertebral bodies. This new source for hematopoietic cell transplant will be a valuable resource for treating hematological malignancies as well as for inducing transplant tolerance. In addition, we have discovered and developed a large source of mesenchymal stem (stromal) cells (MSC) tightly associated with the vertebral body bone fragment byproduct of the HPC, Marrow recovery process. Thus, these vertebral bone adherent MSC (vBA-MSC) are matched to the banked BM obtained from each donor, as opposed to third-party MSC, which enhances safety and potentially efficacy. Isolation and characterization of vBA-MSC from over 30 donors has demonstrated that the cells are no different than traditional BM-MSC; however, their abundance is >1,000-fold higher than obtainable from living donor BM aspirates. Based on our own unpublished data as well as reports published by others, MSC facilitate chimerism, especially at limiting hematopoietic stem and progenitor cell (HSPC) numbers and increase safety by controlling and/or preventing graft-vs.-host-disease (GvHD). Thus, vBA-MSC have the potential to facilitate mixed chimerism, promote complementary peripheral immunomodulatory functions and increase safety of BM infusions. Both HPC, Marrow and vBA-MSC have potential use in current VCA and solid organ transplant (SOT) tolerance clinical protocols that are amenable to “delayed tolerance.” Current trials with HPC, Marrow are planned with subsequent phases to include vBA-MSC for tolerance of both VCA and SOT.
Introduction
SOT has become standard of care over the last half century, resulting in not only a significant life extension but also an enhancement of quality of life (QOL) for end-stage organ failure patients (1, 2). More recently, VCA has become a life changing procedure for patients with severe deformities due to traumatic injury or congenital defects (3). While short-term outcome of transplant recipients using refined conventional immunosuppressive protocols have steadily improved, long-term outcome for the vast majority of patients has not changed over the last decades of experience with transplantation; chronic rejection nearly inevitably leads to organ loss and, depending on the transplanted organ, also to patient death unless a retransplantation is performed (4). Ten years after transplantation only roughly 50% of all heart, liver and kidney and 30% of lung and intestinal grafts are still functioning1.
The continuing negative impact of chronic rejection, combined with the severe adverse effects of conventional immunosuppressive regimens, has spurred intense research into new and safer strategies to prevent allograft rejection. While chronic allograft failure is associated with more frequent hospitalization, higher morbidity and increased health care costs, chronic immunosuppression (IS) is linked to side effects that range from malignancy, infection, toxicities (kidney, central nervous system, hematopoietic system) to cardiovascular and metabolic diseases (5–15). Medication related adverse effects, amount of pill-intake, combined with high costs of immunosuppressive drugs, translate to high rates of patient non-compliance/non-adherence. In kidney transplantation, approximately one third of all patients lose their graft due to non-adherence making it one of the leading causes of allograft loss (16–18). Ultimately, the most desirable outcome and often referred to as the “Holy Grail” of transplantation is the establishment of transplant tolerance as this would abrogate the need for chronic IS, thereby transforming organ transplantation from a chronic treatment to a permanent cure (19). Tolerance in the setting of organ and tissue transplantation not only leads to improved QOL, it also eliminates drug-related side effects, mitigates the impact of adherence and compliance, substantially lowers health care cost, extends organ half-life, and thereby addresses the ongoing critical issue of organ shortages (10, 12, 13, 20–26).
Besides tolerance regimens, various alternative strategies to inhibit rejection are in development to replace or reduce the need for current mainstay IS drugs. These regimens seek to shift the balance of lymphocytes in favor of regulatory T (Treg) cells over effector/memory T (Tem) cells, as opposed to pan-T cell inactivation with calcineurin or mTOR inhibition (27, 28). Of particular note is the increasing number of exploratory cell-based immunoregulatory and tolerizing therapies (29–37). One such immunomodulatory protocol that is already in clinical use utilizes unmodified deceased donor-derived BM cell infusion following HLA-mismatched VCA using a Campath-based induction regimen (38). Even though, only extremely low levels of mixed chimerism were induced, the co-infusion of BM cells after VCA allowed for a substantial reduction of maintenance immunosuppression to a single-agent regimen (32). In contrast to tolerance protocols were transient or stable mixed chimerism-mediated Treg cell expansion and central deletion of donor-specific Tem cells are major drivers of tolerance [reviewed by (33, 36)], durable tolerance in the absence of stable mixed chimerism involves contribution of the graft to long-term promotion of donor-specific T cell suppression/depletion (39).
The vascularized BM component of VCA has innate immunomodulatory properties; however, this is not sufficient to fully tolerize recipients to the highly immunogenic skin component of the composite tissue (29–31, 34, 36, 40). Over 120 upper extremity and >40 facial transplants have been performed worldwide with positive outcomes, demonstrating not only the immunological feasibility but also the potential of this revolutionary life-enhancing modality to restore lost functionality to traumatic injury victims (41, 42). Because reconstructive transplantation addresses a life-changing, but not life-saving, health issue, the risks of non-myeloablative conditioning regimens required to promote mixed chimerism are not warranted. Thus, obtaining durable tolerance in the absence of auxiliary mixed chimerism is a challenge inherent to all forms of transplantation but undoubtedly greatest to VCA (43–47).
Although superficially similar in that both approaches administer hematopoietic cells, there are fundamental differences with respect to safety and mechanisms between the mixed chimerism-based approaches that are currently used in clinical trials to promote tolerance in SOT and the immunomodulatory approach in clinical use for VCA. Induction of tolerance through mixed chimerism necessitates non-myeloablative conditioning in the form of irradiation (total body, total lymphoid, or thymic irradiation), cytotoxic agents (e.g., cyclophosphamide, fludarabine) and cell-depleting agents [e.g., ATG, rituximab; (36, 48)]. Lack of conditioning prior to BM infusion in the VCA tolerance protocol limits chimerism to extremely rare transient events. While cytotoxic effects of conditioning are required to induce tolerance, this toxicity limits its use and is responsible for associated side effects (43–47). Lack of conditioning in immunomodulation not only decreases toxic side effects, but also largely prevents GvHD, which is another major safety concern with hematopoietic stem cell transplantation (HSCT) for SOT (49). Additional strategies to augment BM infusion-mediated immunomodulation in the absence of conditioning to promote mixed chimerism are currently being explored as described below.
Clinical Experience With BM-Derived Products for Inducing Tolerance and Immunomodulation
Currently, there are three U.S. centers (Massachusetts General Hospital (MGH), Stanford University, and Northwestern University) that are investigating clinical protocols for inducing SOT tolerance (50). The protocols have been reviewed in detail elsewhere (36, 46, 47, 51). Each of these protocols uses a whole or fractionated BM-derived cell transplant to induce stable or transient mixed chimerism. In order to induce tolerance, each of these protocols relies on non-myeloablative conditioning to prepare the BM niche for the engraftment of donor-derived stem cells (52). Current successes in clinical trials using these protocols to induce tolerance of SOT through mixed chimerism have been achieved exclusively in the setting of living donor kidney transplantation (39). Most of the current protocols use a preconditioning regime which is implemented days before the transplant; only one regime exists that starts concomitantly with the transplantation. Due to logistic constraints the procedures are presently limited to elective living donor procedures. However, >80% of all transplant recipients receive grafts from deceased donors. Hence, establishing tolerance protocols for deceased donor organ transplantation would greatly expand the number of patients who could potentially benefit from this life-saving procedure.
VCA grafts are invariably from deceased donors, which are also a source of high quality BM obtained from the donor vertebrae that can be cryopreserved for subsequent infusion (32, 53, 54). In the absence of recipient conditioning, the goal of BM infusion following VCA is to augment chimerism inherent to the composite graft. The protocol used at Johns Hopkins to induce immunomodulation in VCA recipients employs cryopreserved BM that is infused 2 weeks after transplantation. The full complement of mechanisms involved in augmentation is not known but at least partially involves supplementation with regulatory cell types and may additively involve alloreactive clonal T cell exhaustion and deletion (55).
Toward Developing Clinical Delayed Tolerance Protocols
The achievement of immunomodulation with BM infusion that clinically translates into significantly reduced need for IS in VCA demonstrates that (1) harvesting and cryostorage of deceased donor BM is feasible, (2) cryopreserved deceased donor BM can be safely infused, and (3) delaying infusion of previously cryopreserved deceased donor BM over a significant period following VCA still achieves desirable biological effects. This suggests that delayed tolerance with deceased donor SOT may be possible.
Feasibility of delayed BMT for tolerance in SOT in fact has been demonstrated in rodent and non-human primate models of solid organ and vascularized composite allotransplantation (for details see Table 1). These new protocols paved the way for the introduction of the term “delayed tolerance” which have the distinct advantage of allowing for a recovery period to stabilize graft function and enable inflammation resulting from the surgical procedure as well as ischemia reperfusion injury upon revascularization to subside which may enhance tolerance-promoting effects of the BMT. However, the concomitant increased risk due to expansion of alloreactive Tem cells during the interim must be effectively reduced, which appears feasible in non-human primates using an anti-CD8 monoclonal antibody (67, 68). This finding opens up the potential for banking deceased donor BM for future transplantation to promote tolerance in current as well as future SOT recipients.
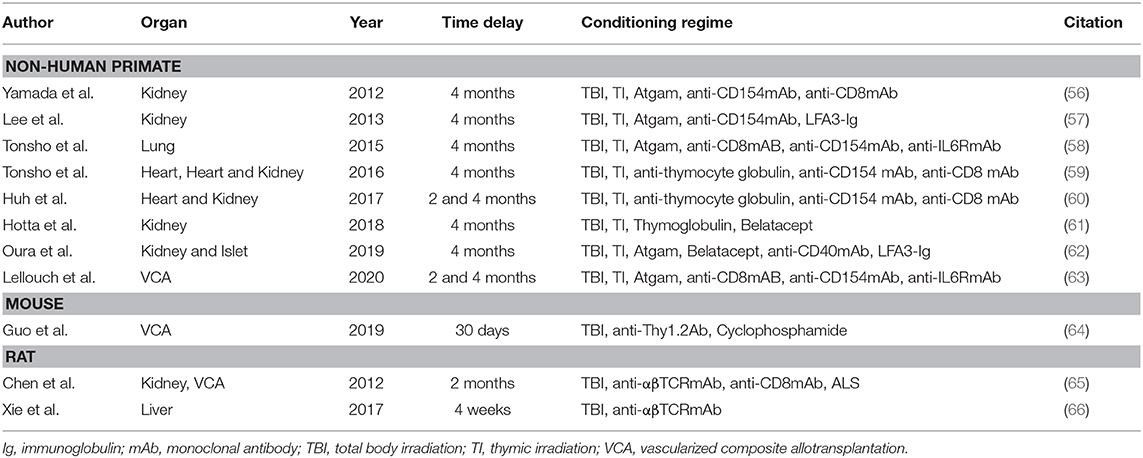
Table 1. Overview on delayed tolerance protocols in small and large animal models of solid organ and vascularized composite allotransplantation.
Ethical Considerations of Translation and Commercialization of Cell Products
Cell therapies are a rapidly growing field that have the potential to significantly impact the practice of medicine, not only in the field of transplantation but for a wide range of diseases (69). Despite their immense potential, cell therapies are significantly more complex in their mode of action and due to biological variation and differences in quality of the starting material, not as standardized as other pharmaceutical products (70). In addition, ethical concerns exist regarding cell and tissue sources and especially the use of altruistically donated cells for commercialization. Similar to the US, European regulations make it illegal to buy or sell human cells and tissues. Yet, it is accepted to compensate for reasonable costs that arise for procurement, processing and storage (71, 72). Ethical and safety concern in the early 2000s led to the implementation of regulations by the U.S. Food and Drug Administration and European Medicines Agency regarding cell- and tissue-based products and therapies (73–76). These regulations ensure strict principles of cell and tissue procurement, product development, processing, testing, distribution, and traceability to maintain quality and safety. However, full compliance with all implemented regulations result in significantly increased production costs disqualifying many products that have been produced by single institutions (77).
Development of a Genetically Diverse Bank of Deceased Organ Donor Bone Marrow
Deceased donor BM represents a large, untapped source of hematopoietic stem and progenitor cells (HSPCs). As has also been demonstrated over the last few decades with cryopreservation of cord blood, it is well-established that BM remains biologically active following long-term cryopreservation (78–81). The larger volumes of HSPCs that can be recovered from a deceased donor compared to aspiration from living donors allow for multiple HSCT procedures or repeat infusions in cases of graft failure. The recovered BM can be precisely packaged, tested for quality, and cryopreserved for subsequent on-demand use. The cryopreserved units can be stored indefinitely (82), with the advantage over living donor registries of having essentially no attrition.
Efforts are currently underway in collaboration with the national Organ Procurement Organization (OPO) network in the U.S. to build the first bank of cryopreserved deceased donor BM. The U.S. OPO network provides an existing refined infrastructure for procuring and transporting bone tissue recovered from deceased donors. Approximately 10,000 deceased donor organs are recovered each year in the U.S., with a further 40,000 donations, yielding approximately 30,000 organs and over a million tissues recovered annually2. The high numbers of bones recovered through this system each year supports the inventory required to establish an integrated system of bone procurement, recovery, and transport, linked to BM processing and banking centers. It has been demonstrated that protocols can be developed and enforced to maintain a favorable ischemic environment from the point of bone procurement and recovery, through cross-country shipping, to arrival at a BM processing center (83). Through these efforts, banking of BM product (HPC, Marrow) for transplantation is currently underway.
Protocols for isolation of HPC, Marrow from deceased donor vertebral bodies were based on original work at University of Pittsburgh and optimized at Johns Hopkins University (32, 53, 54). Those protocols formed the basis for the now fully good manufacturing practice (GMP) compliant process that conforms to 21 CFR Part 1271 regulations and is tested for release in a CLIA-certified laboratory using fully validated testing procedures. Certain improvements to the process were made to increase throughput and enhance reproducibility as well as the aforementioned establishment of logistical procedures for recovery and shipment of vertebrae across large geographic regions. Donor eligibility requirements were established to reduce the risk of adventitious agent transmission (health screening and serological testing) as well as health status incompatible with functioning BM. Finally, cryopreservation conditions were optimized and stability validated to ensure functionality of each HPC, Marrow unit released for transplantation. The result is a product with high viability, high colony forming unit potential and the ability to stably engraft irradiated mice following primary and secondary transplants (manuscript in preparation).
Discovery and Clinical Development of BM Compartment Mesenchymal Stem/Stromal Cells (MSC) Not Recovered in HPC, Marrow
Our team has identified an abundant population of MSC associated with the vertebral body (VB) bone fragment byproduct of HPC, Marrow recovery. These MSC remain tightly adhered to cancellous bone fragments and can only be released by enzymatic treatment. We have determined that these vertebral bone adherent MSC (vBA-MSC) are identical to BM-MSC when cultured (84). The vBA-MSC population yields roughly 2,000x the number of viable, low passage cells from one donor compared with MSC recovered through aspiration from iliac crests of living donors. This bank of vBA-MSC matched to solid organ and VCA donors is a unique resource that overcomes limitations of using third-party MSC by reducing the risk of introducing additional alloantigens and, thus, lowering the risk of sensitization and alloimmune activation (85, 86). Furthermore, the abundance of vBA-MSC allows for generating hundreds of billions of low passage (i.e., P2) cells, allowing multiple infusions (Figure 1).
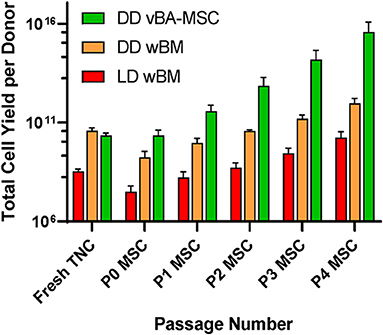
Figure 1. Comparison of total numbers of low passage MSC obtained from living and diseased donor BM. Sources were deceased donor vBA-MSC (DD vBA-MSC) and deceased donor whole BM (DD wBM) and live donor aspirated whole BM (LD wBM). Yields were calculated based on pilot manufacturing runs with either 3 (wBM sources) or 7 (vBA-MSC) donors for each. Averages ± SD shown.
The potent immunoregulatory properties of MSC comprise a spectrum of secreted and cell-bound molecules that modulate a wide array of innate and adaptive immune responses. The multifaceted mechanisms of MSC immunomodulation have been detailed in numerous reviews and, therefore, will only be briefly introduced here. The MSC secretome includes both freely soluble factors as well as those encapsulated by extracellular vesicles. Mechanisms include metabolic inhibition (e.g., indoleamine-pyrrole 2,3 dioxygenase; IDO), immunomodulatory cytokines (e.g., transforming growth factor-β; TGF-β), and checkpoint inhibitors (e.g., programmed death ligand 1; PD-L1). These myriad factors inhibit T cell activation and proliferation, as well as enhance proliferation of regulatory cells (85–88).
Preclinical studies have demonstrated the therapeutic potential of MSC for inducing operational tolerance of SOT and VCA (89–102), providing proof-of-principle for clinical testing in the transplant setting (100, 103–108). The effect of MSC infusion, including in humans and non-human primates, is to skew the T cell population in favor of Treg over Tem cells (97, 99, 109). Clinical studies of MSC-induced immune tolerance of mismatched kidney transplants have demonstrated safety and efficacy (103–108). In one small study of two kidney transplant patients treated with minimal conditioning and MSC found that levels of CD8+ Tem cells decreased without a decrease in overall T cells (103, 106). Teff cells also demonstrated hyporesponsiveness to alloantigen (110–112). A larger controlled study found significantly higher levels of Tregs at 30 days in the MSC treated cohort compared to the control group (109). Thus, MSC beneficially modulate the ratio of Treg/Tem cells to prevent rejection.
In addition to potentially facilitating graft survival through ameliorating alloreactivity, MSC have demonstrated considerable potential for suppressing GvHD which could be an unintended consequence of SCT to induce tolerance (33, 46, 111, 113–126). In fact, based on a wealth of clinical data, MSC are approved in some countries for the treatment of steroid refractory GvHD and there are strong indications that the cells could be used for prophylaxis (121, 127–131). This potent immunomodulatory function of MSC could mitigate the risk of immune tolerance protocols that promote development of GvHD. However, evidence suggests that this function of the cells is dependent on minimal passaging of the cells, with over-expanded cells losing the ability to modulate acute GvHD (132, 133). The large depot of donor-matched vBA-MSCs facilitates minimal expansion to achieve doses required for treatment in humans (Figure 1).
Another mechanism to minimize the risk of GvHD is titrating down the HSPC graft to a minimal efficacious dose, which correspondingly reduces the donor T cell load. MSCs have been reported to facilitate and enhance engraftment of allogeneic HSPC clinically, even after initial graft failure and rejection of conventional stem-cell grafts (134). Preclinical studies suggest that MSCs enhance mixed chimerism when co-infused with HSPC (135) by migrating to the BM stroma to help establish a favorable micro-environment within the hematopoietic niche (136). This appears to minimize the number of HSPC required for transplantation (137, 138). We have confirmed these findings with vBA-MSC in irradiated non-immunocompromised mice treated with limiting dilutions of congenic whole BM with and without co-infusion, followed by a second dose at 48 h, of human vBA-MSC (Figure 2).
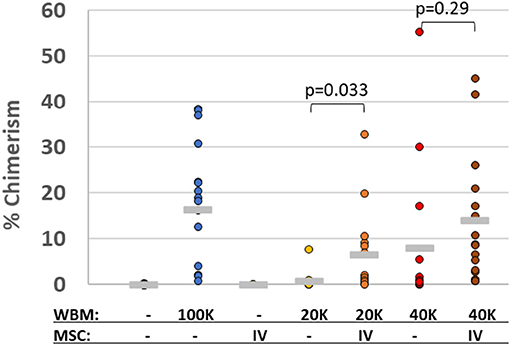
Figure 2. Human MSC promote chimerism of limiting doses of congenic murine bone marrow cells. Lethally gamma-irradiated (875 cGy) C57Bl/6 (CD45.2+) mice were 24 h later with either saline vehicle or one of three doses of whole bone marrow (WBM) isolated from congenic BoyJ (CD45.1+) mice. The WBM doses were either 2 × 104, 4 × 104 or 1 × 105 total nucleated cells (TNC). At 24 and 72 h after irradiation, some groups of mice were also injected with human vBA-MSC (passage 2) at a dose of 1 × 106. Bone marrow was collected from mice surviving 30 days and analyzed for the level of chimerism by flow cytometric analysis with antibodies specific for CD45.1 and CD45.2 surface proteins. The percentage of CD45.1+ chimerism for individual mice in each group is indicated as well as the average per group (horizontal gray line). P-values were determined by Student's t-Test.
Besides GvHD, engraftment syndrome (ES) that occurs in 7–90% of cases during neutrophil recovery after autologous and allogeneic HSCT poses a potential limitation (139). It is associated with fever, pulmonary vascular leak, rash, and organ dysfunction and has also been described in combined HLA-haploidentical BM and kidney transplant recipients. In the described cases, ES manifested not only with fever and fluid retentions but also with a marked acute kidney injury (140), prompting speculations on an increased susceptibility with freshly transplanted kidney grafts, especially in combination with CNI treatment (141). Even though the exact pathophysiology is unclear, ES is thought to be mediated by endothelial cell injury, activated leukocytes, and proinflammatory cytokines. The inflammatory nature of ES is underlined by the good response to treatment with corticosteroids (141, 142). As vBA-MSCs have strong anti-inflammatory, antioxidative, and immunomodulatory properties, co-administration could potentially mitigate the risk or severity of ES after HSCT (143–145). Thus, the combination of promoting BM chimerism and the immunomodulatory functions of MSC suggest that their use as an adjuvant to BM transplants will safely enhance induction of immune tolerance.
Potential for Incorporating Deceased Donor BM and vBA-MSC Into Current Tolerance Protocols
Each of the current protocols for inducing tolerance in VCA and SOT lend themselves to deceased donor BM augmentation and BMT, respectively, with modification to accommodate donor availability. Inclusion of vBA-MSC either prophylactically or to treat GvHD is possible. Following BM isolation and quality control testing, HPC, Marrow would be cryogenically preserved until shipping under the same conditions for infusion into the patient 14 days following surgery, as described previously (32). Simultaneously, vBA-MSC could be prepared from the bone fragments and expanded before cryopreservation and shipping with HPC, Marrow.
In regard to SOT, the MGH delayed tolerance protocol appears to be the most easily adaptable to HPC, Marrow, providing that encouraging results in NHP and early clinical trials in humans are repeated in future larger clinical trials (56–58, 61, 62, 68, 146–148). Transplantation at 4 months following SOT would allow more than enough time to prepare, qualify and store HPC, Marrow as well as expanded vBA-MSC. It is well-established that cryopreservation preserves cellular function for decades so long as proper controls are implemented to prevent transient warming events (82).
The Stanford protocol, which relies on an infusion of a mixture of isolated mobilized peripheral blood-derived CD34+ and T cells could in theory be adapted to using HPC, Marrow for selection of these cells (49, 149). The amount of HPC, Marrow typically recovered from a full donor contains hundreds of millions of CD34+ cell (53, 54, 83). We have adapted CD34 selection methods to develop a GMP process that has yielded an average of 125 × 106 CD34+ cells from HPC, Marrow recovered from three donors. Importantly, these methods can be used on either freshly isolated or previously cryopreserved HPC, Marrow; thus, providing flexibility in cell production. The Stanford protocol infuses cryopreserved selected cells at 11 days after kidney transplant which would provide sufficient time to prepare HPC, Marrow as well as over a billion very early passage GMP vBA-MSC (Figure 1). Given that MSC are commonly dosed at 1 × 106/kg, this would provide more than adequate vBA-MSC for co-infusion as well as any additional dosing if further expansion was not feasible. A company, Medeor, has been established to demonstrate commercial potential of the Stanford protocol and, according to their website3, a delayed tolerance protocol for living donor kidney transplantation is in development. As of yet, efficacy has not been established using this protocol with deceased donor kidney transplants.
The Northwestern tolerance protocol for kidney transplantation differs by the use of full body non-myeloablative conditioning with the goal of promoting full chimerism rather than transient (i.e., MGH protocol) or durable (i.e., Stanford protocol) mixed chimerism (150–154). The protocol uses an engineered cell source, termed facilitating cells (FC), derived from kidney donor mobilized blood collected at least 2 weeks prior to transplant combined with HSPC to promote chimerism (155, 156). Providing the protocol is amenable to a delayed tolerance approach, deceased donor HPC, Marrow could offer a distinct advantage for manufacture of FC given the high abundance of BM cells available and the enhanced time provided for manufacture and testing. To this end, we have demonstrated that HPC, Marrow is amenable to manipulation using a CliniMACS system (Miltenyi Biotec). As GvHD appears to be a concern with this protocol, infusion of low passage vBA-MSC could be advantageous.
Limitations of Current Tolerance Protocols
Despite clinical realization of tolerance and preclinical evidence supporting the feasibility of delayed tolerance protocols as outlined above, tolerance induction is still limited to a few highly specialized centers (47). Widespread adoption is currently hindered by the risks associated with complex recipient conditioning regimes which have a variety of toxic side effects. The most promising strategy of tolerance induction thus far is the mixed chimerism approach, however, tolerance induction efficacy is still limited. HSCT is also associated with a risk of GvHD, which has been observed in protocols aiming for durable chimerism in a small number of patients (36). To overcome these hurdles, a concerted effort of clinicians, scientists, stakeholders (e.g., insurance companies and hospitals), and funding agencies is crucial. In recent years, transplant tolerance has regained attention and a variety of new agents have been identified that have the potential to make induction regimens significantly less toxic, reduce associated risks of GvHD, and increase efficacy. The realization of a deceased bone marrow bank, as outlined in this review, is another step in the process of making transplant tolerance a clinical reality for a larger number of patients.
Conclusions and Future Perspectives
Future broad success with BM and MSC induction of tolerance and potent immunoregulation will have profound effects on transplant patients. Achieving immune tolerance, in particular, will alleviate the burden of life-long IS and associated morbidity, avoid chronic rejection, and significantly improve overall outcomes. Furthermore, it will overcome current compliance and adherence-based limitations that negatively impact graft survival due to subsequent subliminal rejection and the development of donor-specific antibodies. Tolerance and immunoregulation will be especially impactful for patients by increasing accessibility to transplantation and through positively shifting the risk:benefit ratio by reducing associated long-term risk.
In view of these potential opportunities and substantial benefits, the establishment of a bone marrow bank for delayed tolerance protocols marks a crucial step in making this resource available for present as well as future transplant patients. The complementary treatment with vBA-MSC could further increase safety with the added potential of enhanced efficacy. Furthermore, the ability of vBA-MSC to promote HSPC BM engraftment would allow lowering of HPC, Marrow doses which effectively extends the number of patients who receive organs from a single donor that are able to benefit from this procedure.
Author Contributions
All authors contributed to writing and editing the article, and approved the submitted version.
Funding
This work was supported by NIH/ NIAID SBIR grant AI155196 (BHJ and EJW) and by Ossium Health, that in part, paid costs of open access publication fees.
Conflict of Interest
BJ, GB, and EW have equity ownership in Ossium Health. BJ and EW are inventors on patents pending relevant to the subject matter. EW is a co-founder of Ossium Health.
The remaining author declares that the research was conducted in the absence of any commercial or financial relationships that could be construed as a potential conflict of interest.
Footnotes
1. ^UNOS https://unos.org/data/transplant-trends/ (accessed October 27, 2020)
2. ^unos.org/data/transplant-trends/ (accessed October 27, 2020).
3. ^https://www.medeortx.com/our-pipeline.php/ (accessed October 27, 2020).
References
1. Pinson CW, Feurer ID, Payne JL, Wise PE, Shockley S, Speroff T. Health-related quality of life after different types of solid organ transplantation. Ann Surg. (2000) 232:597–607. doi: 10.1097/00000658-200010000-00015
2. Rana A, Gruessner A, Agopian VG, Khalpey Z, Riaz IB, Kaplan B, et al. Survival benefit of solid-organ transplant in the United States. JAMA Surg. (2015) 150:252–9. doi: 10.1001/jamasurg.2014.2038
3. Brandacher G. Vascularized composite allotransplantation: a field is maturing. Curr Opin Organ Transplant. (2018) 23:559–60. doi: 10.1097/MOT.0000000000000574
4. Lodhi SA, Lamb KE, Meier-Kriesche HU. Solid organ allograft survival improvement in the United States: the long-term does not mirror the dramatic short-term success. Am J Transplant. (2011) 11:1226–35. doi: 10.1111/j.1600-6143.2011.03539.x
5. van den Berg JW, van Enckevort PJ, TenVergert EM, Postma DS, van der Bij W, Koeter GH. Bronchiolitis obliterans syndrome and additional costs of lung transplantation. Chest. (2000) 118:1648–52. doi: 10.1378/chest.118.6.1648
6. De Vito Dabbs A, Dew MA, Stilley CS, Manzetti J, Zullo T, McCurry KR, et al. Psychosocial vulnerability, physical symptoms and physical impairment after lung and heart-lung transplantation. J Heart Lung Transplant. (2003) 22:1268–75. doi: 10.1016/s1053-2498(02)01227-5
7. Ojo AO, Held PJ, Port FK, Wolfe RA, Leichtman AB, Young EW, et al. Chronic renal failure after transplantation of a nonrenal organ. N Engl J Med. (2003) 349:931–40. doi: 10.1056/NEJMoa021744
8. Vermeulen KM, Groen H, van der Bij W, Erasmus ME, Koeter GH, TenVergert EM. The effect of bronchiolitis obliterans syndrome on health related quality of life. Clin Transplant. (2004) 18:377–83. doi: 10.1111/j.1399-0012.2004.00174.x
9. Cosio FG, Kudva Y, van der Velde M, Larson TS, Textor SC, Griffin MD, et al. New onset hyperglycemia and diabetes are associated with increased cardiovascular risk after kidney transplantation. Kidney Int. (2005) 67:2415–21. doi: 10.1111/j.1523-1755.2005.00349.x
10. Dharnidharka VR, Caillard S, Agodoa LY, Abbott KC. Infection frequency and profile in different age groups of kidney transplant recipients. Transplantation. (2006) 81:1662–7. doi: 10.1097/01.tp.0000226068.66819.37
11. Song MK, De Vito Dabbs AJ. Advance care planning after lung transplantation: a case of missed opportunities. Prog Transplant. (2006) 16:222–5.
12. Marcen R. Immunosuppressive drugs in kidney transplantation: impact on patient survival, and incidence of cardiovascular disease, malignancy and infection. Drugs. (2009) 69:2227–43. doi: 10.2165/11319260-000000000-00000
13. Rama I, Grinyo JM. Malignancy after renal transplantation: the role of immunosuppression. Nat Rev Nephrol. (2010) 6:511–9. doi: 10.1038/nrneph.2010.102
14. Green M. Introduction: infections in solid organ transplantation. Am J Transplant. (2013) 13(Suppl. 4):3–8. doi: 10.1111/ajt.12093
15. Asch WS, Perazella MA. Cancer and mortality in solid-organ transplantation: preventable or inevitable? Am J Kidney Dis. (2016) 68:839–42. doi: 10.1053/j.ajkd.2016.06.009
16. Pinsky BW, Takemoto SK, Lentine KL, Burroughs TE, Schnitzler MA, Salvalaggio PR. Transplant outcomes and economic costs associated with patient noncompliance to immunosuppression. Am J Transplant. (2009) 9:2597–606. doi: 10.1111/j.1600-6143.2009.02798.x
17. Cutler RL, Fernandez-Llimos F, Frommer M, Benrimoj C, Garcia-Cardenas V. Economic impact of medication non-adherence by disease groups: a systematic review. BMJ Open. (2018) 8:e016982. doi: 10.1136/bmjopen-2017-016982
18. Steinberg EA, Moss M, Buchanan CL, Goebel J. Adherence in pediatric kidney transplant recipients: solutions for the system. Pediatr Nephrol. (2018) 33:361–72. doi: 10.1007/s00467-017-3637-0
19. Billingham RE, Brent L, Medawar PB. Actively acquired tolerance of foreign cells. Nature. (1953) 172:603–6. doi: 10.1038/172603a0
20. Sellares J, de Freitas DG, Mengel M, Reeve J, Einecke G, Sis B, et al. Understanding the causes of kidney transplant failure: the dominant role of antibody-mediated rejection and nonadherence. Am J Transplant. (2012) 12:388–99. doi: 10.1111/j.1600-6143.2011.03840.x
21. Elias N, Shao S, Gift T, Patel B, Cosimi A, Kawai T. Economic analysis of kidney transplant tolerance: the holy grail is less costly. Am J Transplant. (2015) 15. Available online at: https://atcmeetingabstracts.com/abstract/economic-analysis-of-kidney-transplant-tolerance-the-holy-grail-is-less-costly/ (accessed February 13, 2021).
22. Erickson K, Winkelmayer W, Lowsky R, Scandling J, Strober J. An economic analysis of tolerance induction for two-haplotype match kidney living donor transplant recipients. Am J Transplant. (2015) 15. Available online at: https://atcmeetingabstracts.com/abstract/an-economic-analysis-of-tolerance-induction-for-two-haplotype-match-kidney-living-donor-transplant-recipients/ (accessed February 13, 2021).
23. Madariaga ML, Spencer PJ, Shanmugarajah K, Crisalli KA, Chang DC, Markmann JF, et al. Effect of tolerance versus chronic immunosuppression protocols on the quality of life of kidney transplant recipients. JCI Insight. (2016) 1:e87019. doi: 10.1172/jci.insight.87019
24. Giwa S, Lewis JK, Alvarez L, Langer R, Roth AE, Church GM, et al. The promise of organ and tissue preservation to transform medicine. Nat Biotechnol. (2017) 35:530–42. doi: 10.1038/nbt.3889
25. Manzia TM, Angelico R, Toti L, Angelico C, Quaranta C, Parente A, et al. Longterm survival and cost-effectiveness of immunosuppression withdrawal after liver transplantation. Liver Transpl. (2018) 24:1199–208. doi: 10.1002/lt.25293
26. Tollerud D, Leventhal J, Galvin J, Mathew J, Gallon L, Stare D, et al. Quality of life in tolerant living donor kidney transplants vs. standard of care. Am J Transplant. (2019) 19. Available online at: https://atcmeetingabstracts.com/abstract/quality-of-life-in-tolerant-living-donor-kidney-transplants-vs-standard-of-care/ (accessed February 14, 2021).
27. Francis RS, Feng G, Tha-In T, Lyons IS, Wood KJ, Bushell A. Induction of transplantation tolerance converts potential effector T cells into graft-protective regulatory T cells. Eur J Immunol. (2011) 41:726–38. doi: 10.1002/eji.201040509
28. Kovarik J. From immunosuppression to immunomodulation: current principles and future strategies. Pathobiology. (2013) 80:275–81. doi: 10.1159/000346960
29. Ozmen S, Ulusal BG, Ulusal AE, Izycki D, Yoder B, Siemionow M. Trafficking of donor-derived bone marrow correlates with chimerism and extension of composite allograft survival across MHC barrier. Transplant Proc. (2006) 38:1625–33. doi: 10.1016/j.transproceed.2006.02.154
30. Rahhal DN, Xu H, Huang WC, Wu S, Wen Y, Huang Y, et al. Dissociation between peripheral blood chimerism and tolerance to hindlimb composite tissue transplants: preferential localization of chimerism in donor bone. Transplantation. (2009) 88:773–81. doi: 10.1097/TP.0b013e3181b47cfa
31. Huang WC, Lin JY, Wallace CG, Chuang WY, Wei FC, Liao SK. Vascularized bone grafts within composite tissue allotransplants can autocreate tolerance through mixed chimerism with partial myeloablative conditioning: an experimental study in rats. Plast Reconstr Surg. (2010) 125:1095–103. doi: 10.1097/PRS.0b013e3181d0ab80
32. Schneeberger S, Gorantla VS, Brandacher G, Zeevi A, Demetris AJ, Lunz JG, et al. Upper-extremity transplantation using a cell-based protocol to minimize immunosuppression. Ann Surg. (2013) 257:345–51. doi: 10.1097/SLA.0b013e31826d90bb
33. Zuber J, Sykes M. Mechanisms of mixed chimerism-based transplant tolerance. Trends Immunol. (2017) 38:829–43. doi: 10.1016/j.it.2017.07.008
34. Chen J, Zhang D, Zhang T, Chen C, Song Y, Liu S, et al. Effect of the vascularized bone components on the survival of vascularized composite allografts. J Surg Res. (2018) 224:132–8. doi: 10.1016/j.jss.2017.03.050
35. Ezekian B, Schroder PM, Freischlag K, Yoon J, Kwun J, Knechtle SJ. Contemporary strategies and barriers to transplantation tolerance. Transplantation. (2018) 102:1213–22. doi: 10.1097/TP.0000000000002242
36. Messner F, Etra JW, Dodd OJ, Brandacher G. Chimerism, transplant tolerance, and beyond. Transplantation. (2019) 103:1556–67. doi: 10.1097/TP.0000000000002711
37. Spitzer TR, Tolkoff-Rubin N, Cosimi B, McAfee S, Dey BR, Chen YB, et al. Twenty year follow up of histocompatibility leukocyte antigen-matched kidney and bone marrow co-transplantation for multiple myeloma with end stage renal disease: lessons learned. Transplantation. (2019) 103:2366–72. doi: 10.1097/TP.0000000000002669
38. Brandacher G, Lee WP, Schneeberger S. Minimizing immunosuppression in hand transplantation. Expert Rev Clin Immunol. (2012) 8:673–83; quiz 684. doi: 10.1586/eci.12.54
39. Sykes M, Griesemer AD. Transplantation tolerance in nonhuman primates and humans. Bone Marrow Transplant. (2019) 54(Suppl. 2):815–21. doi: 10.1038/s41409-019-0620-3
40. Lee WP, Yaremchuk MJ, Pan YC, Randolph MA, Tan CM, Weiland AJ. Relative antigenicity of components of a vascularized limb allograft. Plast Reconstr Surg. (1991) 87:401–11. doi: 10.1097/00006534-199103000-00001
41. Shores JT, Brandacher G, Lee WP. Hand and upper extremity transplantation: an update of outcomes in the worldwide experience. Plast Reconstr Surg. (2015) 135:351–60e. doi: 10.1097/PRS.0000000000000892
42. Kueckelhaus M, Fischer S, Seyda M, Bueno EM, Aycart MA, Alhefzi M, et al. Vascularized composite allotransplantation: current standards and novel approaches to prevent acute rejection and chronic allograft deterioration. Transpl Int. (2016) 29:655–62. doi: 10.1111/tri.12652
43. Sykes M. Mixed chimerism and transplant tolerance. Immunity. (2001) 14:417–24. doi: 10.1016/s1074-7613(01)00122-4
44. Fryer M, Grahammer J, Khalifian S, Furtmuller GJ, Lee WP, Raimondi G, et al. Exploring cell-based tolerance strategies for hand and face transplantation. Expert Rev Clin Immunol. (2015) 11:1189–204. doi: 10.1586/1744666X.2015.1078729
45. Chhabra AY, Leventhal J, Merchak AR, Ildstad S. HSCT-based approaches for tolerance induction in renal transplant. Transplantation. (2017) 101:2682–90. doi: 10.1097/TP.0000000000001837
46. Mahr B, Granofszky N, Muckenhuber M, Wekerle T. Transplantation tolerance through hematopoietic chimerism: progress and challenges for clinical translation. Front Immunol. (2017) 8:1762. doi: 10.3389/fimmu.2017.01762
47. Kawai T, Leventhal J, Wood K, Strober S. Summary of the third international workshop on clinical tolerance. Am J Transplant. (2019) 19:324–30. doi: 10.1111/ajt.15086
48. Bacigalupo A, Ballen K, Rizzo D, Giralt S, Lazarus H, Ho V, et al. Defining the intensity of conditioning regimens: working definitions. Biol Blood Marrow Transplant. (2009) 15:1628–33. doi: 10.1016/j.bbmt.2009.07.004
49. Strober S. Use of hematopoietic cell transplants to achieve tolerance in patients with solid organ transplants. Blood. (2016) 127:1539–43. doi: 10.1182/blood-2015-12-685107
50. Kawai T, Leventhal J, Madsen JC, Strober S, Turka LA, Wood KJ. Tolerance: one transplant for life. Transplantation. (2014) 98:117–21. doi: 10.1097/TP.0000000000000260
51. Sasaki H, Oura T, Spitzer TR, Chen YB, Madsen JC, Allan J, et al. Preclinical and clinical studies for transplant tolerance via the mixed chimerism approach. Hum Immunol. (2018) 79:258–65. doi: 10.1016/j.humimm.2017.11.008
52. Pilat N, Hock K, Wekerle T. Mixed chimerism through donor bone marrow transplantation: a tolerogenic cell therapy for application in organ transplantation. Curr Opin Organ Transplant. (2012) 17:63–70. doi: 10.1097/MOT.0b013e32834ee68b
53. Donnenberg AD, Gorantla VS, Schneeberger S, Moore LR, Brandacher G, Stanczak HM, et al. Clinical implementation of a procedure to prepare bone marrow cells from cadaveric vertebral bodies. Regen Med. (2011) 6:701–6. doi: 10.2217/rme.11.89
54. Gorantla VS, Schneeberger S, Moore LR, Donnenberg VS, Zimmerlin L, Lee WP, et al. Development and validation of a procedure to isolate viable bone marrow cells from the vertebrae of cadaveric organ donors for composite organ grafting. Cytotherapy. (2012) 14:104–13. doi: 10.3109/14653249.2011.605350
55. Khalifeh A, Buckingham E, Uluer M, Hassanein W, Twaddell W, Drachenberg C, et al. Vascularized bone marrow mechanisms of immunomodulation. Am J Transplant. (2017) 17. Available online at: https://atcmeetingabstracts.com/abstract/vascularized-bone-marrow-mechanisms-of-immunomodulation/ (accessed February 14, 2021).
56. Yamada Y, Boskovic S, Aoyama A, Murakami T, Putheti P, Smith RN, et al. Overcoming memory T-cell responses for induction of delayed tolerance in nonhuman primates. Am J Transplant. (2012) 12:330–40. doi: 10.1111/j.1600-6143.2011.03795.x
57. Lee S, Yamada Y, Tonsho M, Boskovic S, Nadazdin O, Schoenfeld D, et al. Alefacept promotes immunosuppression-free renal allograft survival in nonhuman primates via depletion of recipient memory T cells. Am J Transplant. (2013) 13:3223–9. doi: 10.1111/ajt.12500
58. Tonsho M, Lee S, Aoyama A, Boskovic S, Nadazdin O, Capetta K, et al. Tolerance of lung allografts achieved in nonhuman primates via mixed hematopoietic chimerism. Am J Transplant. (2015) 15:2231–9. doi: 10.1111/ajt.13274
59. Tonsho M, Benichou G, Yu R, Boskovic S, Colvin R, Farkash E, et al. Delayed induction of mixed chimerism permits kidney-induced cardiac allograft tolerance to be applied to recipients of cadaveric organs. Am J Transplant. (2016) 6. Available online at: https://atcmeetingabstracts.com/abstract/delayed-induction-of-mixed-chimerism-permits-kidney-induced-cardiac-allograft-tolerance-to-be-applied-to-recipients-of-cadaveric-organs/ (accessed February 14, 2021).
60. Huh K, Tonsho M, Spencer P, Paster J, Wu X, Hanekamp I, et al. Delaying the induction of mixed chimerism until two months after NHP heart and kidney cotransplantation results in long term survival. Am J Transplant. (2016) 16. Available online at: https://atcmeetingabstracts.com/abstract/delaying-the-induction-of-mixed-chimerism-until-two-months-after-nhp-heart-and-kidney-cotransplantation-results-in-long-term-survival/ (accessed February 14, 2021).
61. Hotta K, Oura T, Dehnadi A, Boskovic S, Matsunami M, Rosales I, et al. Long-term nonhuman primate renal allograft survival without ongoing immunosuppression in recipients of delayed donor bone marrow transplantation. Transplantation. (2018) 102:e128–36. doi: 10.1097/TP.0000000000002078
62. Oura T, Hotta K, Rosales I, Dehnadi A, Kawai K, Lee H, et al. Addition of anti-CD40 monoclonal antibody to nonmyeloablative conditioning with belatacept abrogated allograft tolerance despite induction of mixed chimerism. Transplantation. (2019) 103:168–76. doi: 10.1097/TP.0000000000002417
63. Lellouch AG, Ng ZY, Rosales IA, Schol IM, Leonard DA, Gama AR, et al. Toward development of the delayed tolerance induction protocol for vascularized composite allografts in nonhuman primates. Plast Reconstr Surg. (2020) 145:757–68e. doi: 10.1097/PRS.0000000000006676
64. Guo Y, Messner F, Oh BC, Furtmüller GJ, Lee WPA, Cooney DS, et al. Abstract QS37: induction of delayed immune tolerance after reconstructive transplantation by combining donor bone marrow transplantation and high-dose cyclophosphamide treatment. Plastic Reconstr Surg Glob Open. (2019) 7:127–8. doi: 10.1097/01.GOX.0000558457.79886.05
65. Chen B, Xu H, Corbin DR, Ildstad ST. A clinically feasible approach to induce delayed tolerance in recipients of prior kidney or vascularized composite allotransplants. Transplantation. (2012) 94:671–8. doi: 10.1097/TP.0b013e318264fbc1
66. Xie Y, Wu Y, Xin K, Wang JJ, Xu H, Ildstad ST, et al. Delayed donor bone marrow infusion induces liver transplant tolerance. Transplantation. (2017) 101:1056–66. doi: 10.1097/TP.0000000000001684
67. Koyama I, Nadazdin O, Boskovic S, Ochiai T, Smith RN, Sykes M, et al. Depletion of CD8 memory T cells for induction of tolerance of a previously transplanted kidney allograft. Am J Transplant. (2007) 7:1055–61. doi: 10.1111/j.1600-6143.2006.01703.x
68. Yamada Y, Benichou G, Cosimi AB, Kawai T. Tolerance induction after organ transplantation, “delayed tolerance,” via the mixed chimerism approach: planting flowers in a battle field. Chimerism. (2012) 3:24–8. doi: 10.4161/chim.20096
69. Cuende N, Rasko JEJ, Koh MBC, Dominici M, Ikonomou L. Cell, tissue and gene products with marketing authorization in 2018 worldwide. Cytotherapy. (2018) 20:1401–13. doi: 10.1016/j.jcyt.2018.09.010
70. Dodson BP, Levine AD. Challenges in the translation and commercialization of cell therapies. BMC Biotechnol. (2015) 15:70. doi: 10.1186/s12896-015-0190-4
71. EU. Regulation (EC) No 1394/2007 of the European parliament and of the council of 13 November 2007 on advanced therapy medicinal products and amending Directive 2001/83/EC and Regulation (EC) No 726/2004. Offic J Eur Union L. (2007) 324:121–37.
72. Lenk C, Beier K. Is the commercialisation of human tissue and body material forbidden in the countries of the European Union? J Med Ethics. (2012) 38:342–6. doi: 10.1136/jme.2010.038760
73. Blasszauer B. Petty corruption in health care. J Med Ethics. (1997) 23:133–4. doi: 10.1136/jme.23.3.133
74. Blakeslee S. Lack of oversight in tissue donation raising concerns. The New York Times. New York, NY: A. G. Sulzberger (2002).
75. Farrugia A. When do tissues and cells become products? Regulatory oversight of emerging biological therapies. Cell Tissue Bank. (2006) 7:325–35. doi: 10.1007/s10561-006-9012-1
77. Pirnay JP, Vanderkelen A, Ectors N, Delloye C, Dufrane D, Baudoux E, et al. Beware of the commercialization of human cells and tissues: situation in the European Union. Cell Tissue Bank. (2012) 13:487–98. doi: 10.1007/s10561-012-9323-3
78. Eckardt JR, Roodman GD, Boldt DH, Clark GM, Alvarez R, Page C, et al. Comparison of engraftment and acute GVHD in patients undergoing cryopreserved or fresh allogeneic BMT. Bone Marrow Transplant. (1993) 11:125–31.
79. Stockschlader M, Kruger W, Kroschke G, Zeller W, Hoffknecht M, Loliger C, et al. Use of cryopreserved bone marrow in allogeneic bone marrow transplantation. Bone Marrow Transplant. (1995) 15:569–72.
80. Stockschlader M, Kruger W, tom Dieck A, Horstmann M, Altnoder M, Loliger C, et al. Use of cryopreserved bone marrow in unrelated allogeneic transplantation. Bone Marrow Transplant. (1996) 17:197–9.
81. Lioznov M, Dellbrugger C, Sputtek A, Fehse B, Kroger N, Zander AR. Transportation and cryopreservation may impair haematopoietic stem cell function and engraftment of allogeneic PBSCs, but not BM. Bone Marrow Transplant. (2008) 42:121–8. doi: 10.1038/bmt.2008.93
82. Woods EJ, Thirumala S, Badhe-Buchanan SS, Clarke D, Mathew AJ. Off the shelf cellular therapeutics: Factors to consider during cryopreservation and storage of human cells for clinical use. Cytotherapy. (2016) 18:697–711. doi: 10.1016/j.jcyt.2016.03.295
83. Woods EJ, Sherry AM, Woods JR, Hardin JW, LaFontaine M, Brandacher G, et al. Ischemia considerations for the development of an organ and tissue donor derived bone marrow bank. J Transl Med. (2020) 18:300. doi: 10.1186/s12967-020-02470-1
84. Johnstone BH, Miller HM, Beck MR, Gu D, Thirumala S, LaFontaine M, et al. Identification and characterization of a large source of primary mesenchymal stem cells tightly adhered to bone surfaces of human vertebral body marrow cavities. Cytotherapy. (2020) 22:617–28. doi: 10.1016/j.jcyt.2020.07.003
85. English K, Wood KJ. Mesenchymal stromal cells in transplantation rejection and tolerance. Cold Spring Harb Perspect Med. (2013) 3:a015560. doi: 10.1101/cshperspect.a015560
86. Podesta MA, Remuzzi G, Casiraghi F. Mesenchymal stromal cells for transplant tolerance. Front Immunol. (2019) 10:1287. doi: 10.3389/fimmu.2019.01287
87. Murphy MB, Moncivais K, Caplan AI. Mesenchymal stem cells: environmentally responsive therapeutics for regenerative medicine. Exp Mol Med. (2013) 45:e54. doi: 10.1038/emm.2013.94
88. Pittenger MF, Discher DE, Peault BM, Phinney DG, Hare JM, Caplan AI. Mesenchymal stem cell perspective: cell biology to clinical progress. NPJ Regen Med. (2019) 4:22. doi: 10.1038/s41536-019-0083-6
89. Bartholomew A, Sturgeon C, Siatskas M, Ferrer K, McIntosh K, Patil S, et al. Mesenchymal stem cells suppress lymphocyte proliferation in vitro and prolong skin graft survival in vivo. Exp Hematol. (2002) 30:42–8. doi: 10.1016/s0301-472x(01)00769-x
90. Zhou HP, Yi DH, Yu SQ, Sun GC, Cui Q, Zhu HL, et al. Administration of donor-derived mesenchymal stem cells can prolong the survival of rat cardiac allograft. Transplant Proc. (2006) 38:3046–51. doi: 10.1016/j.transproceed.2006.10.002
91. Casiraghi F, Azzollini N, Cassis P, Imberti B, Morigi M, Cugini D, et al. Pretransplant infusion of mesenchymal stem cells prolongs the survival of a semiallogeneic heart transplant through the generation of regulatory T cells. J Immunol. (2008) 181:3933–46. doi: 10.4049/jimmunol.181.6.3933
92. Wang Y, Zhang A, Ye Z, Xie H, Zheng S. Bone marrow-derived mesenchymal stem cells inhibit acute rejection of rat liver allografts in association with regulatory T-cell expansion. Transplant Proc. (2009) 41:4352–6. doi: 10.1016/j.transproceed.2009.08.072
93. Ge W, Jiang J, Arp J, Liu W, Garcia B, Wang H. Regulatory T-cell generation and kidney allograft tolerance induced by mesenchymal stem cells associated with indoleamine 2,3-dioxygenase expression. Transplantation. (2010) 90:1312–20. doi: 10.1097/TP.0b013e3181fed001
94. Casiraghi F, Azzollini N, Todeschini M, Cavinato RA, Cassis P, Solini S, et al. Localization of mesenchymal stromal cells dictates their immune or proinflammatory effects in kidney transplantation. Am J Transplant. (2012) 12:2373–83. doi: 10.1111/j.1600-6143.2012.04115.x
95. Franquesa M, Herrero E, Torras J, Ripoll E, Flaquer M, Goma M, et al. Mesenchymal stem cell therapy prevents interstitial fibrosis and tubular atrophy in a rat kidney allograft model. Stem Cells Dev. (2012) 21:3125–35. doi: 10.1089/scd.2012.0096
96. Jia Z, Jiao C, Zhao S, Li X, Ren X, Zhang L, et al. Immunomodulatory effects of mesenchymal stem cells in a rat corneal allograft rejection model. Exp Eye Res. (2012) 102:44–9. doi: 10.1016/j.exer.2012.06.008
97. Davis TA, Anam K, Lazdun Y, Gimble JM, Elster EA. Adipose-derived stromal cells promote allograft tolerance induction. Stem Cells Transl Med. (2014) 3:1444–50. doi: 10.5966/sctm.2014-0131
98. Obermajer N, Popp FC, Soeder Y, Haarer J, Geissler EK, Schlitt HJ, et al. Conversion of Th17 into IL-17A(neg) regulatory T cells: a novel mechanism in prolonged allograft survival promoted by mesenchymal stem cell-supported minimized immunosuppressive therapy. J Immunol. (2014) 193:4988–99. doi: 10.4049/jimmunol.1401776
99. Plock JA, Schnider JT, Zhang W, Schweizer R, Tsuji W, Kostereva N, et al. Adipose- and bone marrow-derived mesenchymal stem cells prolong graft survival in vascularized composite allotransplantation. Transplantation. (2015) 99:1765–73. doi: 10.1097/TP.0000000000000731
100. Detry O, Vandermeulen M, Delbouille MH, Somja J, Bletard N, Briquet A, et al. Infusion of mesenchymal stromal cells after deceased liver transplantation: A phase I-II, open-label, clinical study. J Hepatol. (2017) 67:47–55. doi: 10.1016/j.jhep.2017.03.001
101. Plock JA, Schnider JT, Schweizer R, Zhang W, Tsuji W, Waldner M, et al. The influence of timing and frequency of adipose-derived mesenchymal stem cell therapy on immunomodulation outcomes after vascularized composite allotransplantation. Transplantation. (2017) 101:1–e11. doi: 10.1097/TP.0000000000001498
102. Lohan P, Murphy N, Treacy O, Lynch K, Morcos M, Chen B, et al. Third-party allogeneic mesenchymal stromal cells prevent rejection in a pre-sensitized high-risk model of corneal transplantation. Front Immunol. (2018) 9:2666. doi: 10.3389/fimmu.2018.02666
103. Perico N, Casiraghi F, Introna M, Gotti E, Todeschini M, Cavinato RA, et al. Autologous mesenchymal stromal cells and kidney transplantation: a pilot study of safety and clinical feasibility. Clin J Am Soc Nephrol. (2011) 6:412–22. doi: 10.2215/CJN.04950610
104. Tan J, Wu W, Xu X, Liao L, Zheng F, Messinger S, et al. Induction therapy with autologous mesenchymal stem cells in living-related kidney transplants: a randomized controlled trial. JAMA. (2012) 307:1169–77. doi: 10.1001/jama.2012.316
105. Peng Y, Ke M, Xu L, Liu L, Chen X, Xia W, et al. Donor-derived mesenchymal stem cells combined with low-dose tacrolimus prevent acute rejection after renal transplantation: a clinical pilot study. Transplantation. (2013) 95:161–8. doi: 10.1097/TP.0b013e3182754c53
106. Perico N, Casiraghi F, Gotti E, Introna M, Todeschini M, Cavinato RA, et al. Mesenchymal stromal cells and kidney transplantation: pretransplant infusion protects from graft dysfunction while fostering immunoregulation. Transpl Int. (2013) 26:867–78. doi: 10.1111/tri.12132
107. Reinders ME, de Fijter JW, Roelofs H, Bajema IM, de Vries DK, Schaapherder AF, et al. Autologous bone marrow-derived mesenchymal stromal cells for the treatment of allograft rejection after renal transplantation: results of a phase I study. Stem Cells Transl Med. (2013) 2:107–11. doi: 10.5966/sctm.2012-0114
108. Mudrabettu C, Kumar V, Rakha A, Yadav AK, Ramachandran R, Kanwar DB, et al. Safety and efficacy of autologous mesenchymal stromal cells transplantation in patients undergoing living donor kidney transplantation: a pilot study. Nephrology. (2015) 20:25–33. doi: 10.1111/nep.12338
109. Erpicum P, Weekers L, Detry O, Bonvoisin C, Delbouille MH, Gregoire C, et al. Infusion of third-party mesenchymal stromal cells after kidney transplantation: a phase I-II, open-label, clinical study. Kidney Int. (2019) 95:693–707. doi: 10.1016/j.kint.2018.08.046
110. Zhang W, Ge W, Li C, You S, Liao L, Han Q, et al. Effects of mesenchymal stem cells on differentiation, maturation, and function of human monocyte-derived dendritic cells. Stem Cells Dev. (2004) 13:263–71. doi: 10.1089/154732804323099190
111. Nauta AJ, Kruisselbrink AB, Lurvink E, Willemze R, Fibbe WE. Mesenchymal stem cells inhibit generation and function of both CD34+-derived and monocyte-derived dendritic cells. J Immunol. (2006) 177:2080–7. doi: 10.4049/jimmunol.177.4.2080
112. Zhao ZG, Xu W, Sun L, You Y, Li F, Li QB, et al. Immunomodulatory function of regulatory dendritic cells induced by mesenchymal stem cells. Immunol Invest. (2012) 41:183–98. doi: 10.3109/08820139.2011.607877
113. Le Blanc K, Frassoni F, Ball L, Locatelli F, Roelofs H, Lewis I, et al. Mesenchymal stem cells for treatment of steroid-resistant, severe, acute graft-versus-host disease: a phase II study. Lancet. (2008) 371:1579–86. doi: 10.1016/S0140-6736(08)60690-X
114. von Bonin M, Stolzel F, Goedecke A, Richter K, Wuschek N, Holig K, et al. Treatment of refractory acute GVHD with third-party MSC expanded in platelet lysate-containing medium. Bone Marrow Transplant. (2009) 43:245–51. doi: 10.1038/bmt.2008.316
115. Lucchini G, Introna M, Dander E, Rovelli A, Balduzzi A, Bonanomi S, et al. Platelet-lysate-expanded mesenchymal stromal cells as a salvage therapy for severe resistant graft-versus-host disease in a pediatric population. Biol Blood Marrow Transplant. (2010) 16:1293–301. doi: 10.1016/j.bbmt.2010.03.017
116. Perez-Simon JA, Lopez-Villar O, Andreu EJ, Rifon J, Muntion S, Diez Campelo M, et al. Mesenchymal stem cells expanded in vitro with human serum for the treatment of acute and chronic graft-versus-host disease: results of a phase I/II clinical trial. Haematologica. (2011) 96:1072–6. doi: 10.3324/haematol.2010.038356
117. Herrmann R, Sturm M, Shaw K, Purtill D, Cooney J, Wright M, et al. Mesenchymal stromal cell therapy for steroid-refractory acute and chronic graft versus host disease: a phase 1 study. Int J Hematol. (2012) 95:182–8. doi: 10.1007/s12185-011-0989-2
118. Ball LM, Bernardo ME, Roelofs H, van Tol MJ, Contoli B, Zwaginga JJ, et al. Multiple infusions of mesenchymal stromal cells induce sustained remission in children with steroid-refractory, grade III-IV acute graft-versus-host disease. Br J Haematol. (2013) 163:501–9. doi: 10.1111/bjh.12545
119. Resnick IB, Barkats C, Shapira MY, Stepensky P, Bloom AI, Shimoni A, et al. Treatment of severe steroid resistant acute GVHD with mesenchymal stromal cells (MSC). Am J Blood Res. (2013) 3:225–38.
120. Introna M, Lucchini G, Dander E, Galimberti S, Rovelli A, Balduzzi A, et al. Treatment of graft versus host disease with mesenchymal stromal cells: a phase I study on 40 adult and pediatric patients. Biol Blood Marrow Transplant. (2014) 20:375–81. doi: 10.1016/j.bbmt.2013.11.033
121. Kurtzberg J, Prockop S, Teira P, Bittencourt H, Lewis V, Chan KW, et al. Allogeneic human mesenchymal stem cell therapy (remestemcel-L, prochymal) as a rescue agent for severe refractory acute graft-versus-host disease in pediatric patients. Biol Blood Marrow Transplant. (2014) 20:229–35. doi: 10.1016/j.bbmt.2013.11.001
122. Sanchez-Guijo F, Caballero-Velazquez T, Lopez-Villar O, Redondo A, Parody R, Martinez C, et al. Sequential third-party mesenchymal stromal cell therapy for refractory acute graft-versus-host disease. Biol Blood Marrow Transplant. (2014) 20:1580–5. doi: 10.1016/j.bbmt.2014.06.015
123. Dotoli GM, De Santis GC, Orellana MD, de Lima Prata K, Caruso SR, Fernandes TR, et al. Mesenchymal stromal cell infusion to treat steroid-refractory acute GvHD III/IV after hematopoietic stem cell transplantation. Bone Marrow Transplant. (2017) 52:859–62. doi: 10.1038/bmt.2017.35
124. Salmenniemi U, Itala-Remes M, Nystedt J, Putkonen M, Niittyvuopio R, Vettenranta K, et al. Good responses but high TRM in adult patients after MSC therapy for GvHD. Bone Marrow Transplant. (2017) 52:606–8. doi: 10.1038/bmt.2016.317
125. Bader P, Kuci Z, Bakhtiar S, Basu O, Bug G, Dennis M, et al. Effective treatment of steroid and therapy-refractory acute graft-versus-host disease with a novel mesenchymal stromal cell product (MSC-FFM). Bone Marrow Transplant. (2018) 53:852–62. doi: 10.1038/s41409-018-0102-z
126. Elgaz S, Kuci Z, Kuci S, Bonig H, Bader P. Clinical use of mesenchymal stromal cells in the treatment of acute graft-versus-host disease. Transfus Med Hemother. (2019) 46:27–34. doi: 10.1159/000496809
127. Galipeau J. The mesenchymal stromal cells dilemma–does a negative phase III trial of random donor mesenchymal stromal cells in steroid-resistant graft-versus-host disease represent a death knell or a bump in the road? Cytotherapy. (2013) 15:2–8. doi: 10.1016/j.jcyt.2012.10.002
128. Hashmi S, Ahmed M, Murad MH, Litzow MR, Adams RH, Ball LM, et al. Survival after mesenchymal stromal cell therapy in steroid-refractory acute graft-versus-host disease: systematic review and meta-analysis. Lancet Haematol. (2016) 3:45–e52. doi: 10.1016/S2352-3026(15)00224-0
129. Munneke JM, Spruit MJ, Cornelissen AS, van Hoeven V, Voermans C, Hazenberg MD. The potential of mesenchymal stromal cells as treatment for severe steroid-refractory acute graft-versus-host disease: a critical review of the literature. Transplantation. (2016) 100:2309–14. doi: 10.1097/TP.0000000000001029
130. Locatelli F, Algeri M, Trevisan V, Bertaina A. Remestemcel-L for the treatment of graft versus host disease. Expert Rev Clin Immunol. (2017) 13:43–56. doi: 10.1080/1744666X.2016.1208086
131. Morata-Tarifa C, Macias-Sanchez MDM, Gutierrez-Pizarraya A, Sanchez-Pernaute R. Mesenchymal stromal cells for the prophylaxis and treatment of graft-versus-host disease-a meta-analysis. Stem Cell Res Ther. (2020) 11:64. doi: 10.1186/s13287-020-01592-z
132. von Bahr L, Sundberg B, Lonnies L, Sander B, Karbach H, Hagglund H, et al. Long-term complications, immunologic effects, and role of passage for outcome in mesenchymal stromal cell therapy. Biol Blood Marrow Transplant. (2012) 18:557–64. doi: 10.1016/j.bbmt.2011.07.023
133. Galipeau J, Sensebe L. Mesenchymal stromal cells: clinical challenges and therapeutic opportunities. Cell Stem Cell. (2018) 22:824–33. doi: 10.1016/j.stem.2018.05.004
134. Fang B, Song Y, Lin Q, Zhang Y, Cao Y, Zhao RC, et al. Human adipose tissue-derived mesenchymal stromal cells as salvage therapy for treatment of severe refractory acute graft-vs.-host disease in two children. Pediatr Transplant. (2007) 11:814–7. doi: 10.1111/j.1399-3046.2007.00780.x
135. Sykes M, Szot GL, Swenson KA, Pearson DA. Induction of high levels of allogeneic hematopoietic reconstitution and donor-specific tolerance without myelosuppressive conditioning. Nat Med. (1997) 3:783–7. doi: 10.1038/nm0797-783
136. Poloni A, Leoni P, Buscemi L, Balducci F, Pasquini R, Masia MC, et al. Engraftment capacity of mesenchymal cells following hematopoietic stem cell transplantation in patients receiving reduced-intensity conditioning regimen. Leukemia. (2006) 20:329–35. doi: 10.1038/sj.leu.2404018
137. Bianco P. Bone and the hematopoietic niche: a tale of two stem cells. Blood. (2011) 117:5281–8. doi: 10.1182/blood-2011-01-315069
138. Fernandez-Garcia M, Yanez RM, Sanchez-Dominguez R, Hernando-Rodriguez M, Peces-Barba M, Herrera G, et al. Mesenchymal stromal cells enhance the engraftment of hematopoietic stem cells in an autologous mouse transplantation model. Stem Cell Res Ther. (2015) 6:165. doi: 10.1186/s13287-015-0155-5
139. Spitzer TR. Engraftment syndrome following hematopoietic stem cell transplantation. Bone Marrow Transplant. (2001) 27:893–8. doi: 10.1038/sj.bmt.1703015
140. Farris AB, Taheri D, Kawai T, Fazlollahi L, Wong W, Tolkoff-Rubin N, et al. Acute renal endothelial injury during marrow recovery in a cohort of combined kidney and bone marrow allografts. Am J Transplant. (2011) 11:1464–77. doi: 10.1111/j.1600-6143.2011.03572.x
141. Spitzer TR. Engraftment syndrome: double-edged sword of hematopoietic cell transplants. Bone Marrow Transplant. (2015) 50:469–75. doi: 10.1038/bmt.2014.296
142. Omer AK, Kim HT, Yalamarti B, McAfee SL, Dey BR, Ballen KK, et al. Engraftment syndrome after allogeneic hematopoietic cell transplantation in adults. Am J Hematol. (2014) 89:698–705. doi: 10.1002/ajh.23716
143. Walter J, Ware LB, Matthay MA. Mesenchymal stem cells: mechanisms of potential therapeutic benefit in ARDS and sepsis. Lancet Respir Med. (2014) 2:1016–26. doi: 10.1016/S2213-2600(14)70217-6
144. Han J, Li Y, Li Y. Strategies to enhance mesenchymal stem cell-based therapies for acute respiratory distress syndrome. Stem Cells Int. (2019) 2019:5432134. doi: 10.1155/2019/5432134
145. Shah TG, Predescu D, Predescu S. Mesenchymal stem cells-derived extracellular vesicles in acute respiratory distress syndrome: a review of current literature and potential future treatment options. Clin Transl Med. (2019) 8:25. doi: 10.1186/s40169-019-0242-9
146. Yamada Y, Ochiai T, Boskovic S, Nadazdin O, Oura T, Schoenfeld D, et al. Use of CTLA4Ig for induction of mixed chimerism and renal allograft tolerance in nonhuman primates. Am J Transplant. (2014) 14:2704–12. doi: 10.1111/ajt.12936
147. Aoyama A, Tonsho M, Ng CY, Lee S, Millington T, Nadazdin O, et al. Long-term lung transplantation in nonhuman primates. Am J Transplant. (2015) 15:1415–20. doi: 10.1111/ajt.13130
148. Lee KW, Park JB, Park H, Kwon Y, Lee JS, Kim KS, et al. Inducing transient mixed chimerism for allograft survival without maintenance immunosuppression with combined kidney and bone marrow transplantation: protocol optimization. Transplantation. (2020) 104:1472–82. doi: 10.1097/TP.0000000000003006
149. Scandling JD, Busque S, Shizuru JA, Lowsky R, Hoppe R, Dejbakhsh-Jones S, et al. Chimerism, graft survival, and withdrawal of immunosuppressive drugs in HLA matched and mismatched patients after living donor kidney and hematopoietic cell transplantation. Am J Transplant. (2015) 15:695–704. doi: 10.1111/ajt.13091
150. Leventhal J, Abecassis M, Miller J, Gallon L, Ravindra K, Tollerud DJ, et al. Chimerism and tolerance without GVHD or engraftment syndrome in HLA-mismatched combined kidney and hematopoietic stem cell transplantation. Sci Transl Med. (2012) 4:124ra128. doi: 10.1126/scitranslmed.3003509
151. Leventhal JR, Mathew JM, Ildstad S, Salomon DR, Kurian SM, Suthanthiran M, et al. (2013). HLA identical non-chimeric and HLA disparate chimeric renal transplant tolerance. Clin. Transpl. 145–56.
152. Leventhal JR, Elliott MJ, Yolcu ES, Bozulic LD, Tollerud DJ, Mathew JM, et al. Immune reconstitution/immunocompetence in recipients of kidney plus hematopoietic stem/facilitating cell transplants. Transplantation. (2015) 99:288–98. doi: 10.1097/TP.0000000000000605
153. Leventhal JR, Ildstad ST. Tolerance induction in HLA disparate living donor kidney transplantation by facilitating cell-enriched donor stem cell Infusion: the importance of durable chimerism. Hum Immunol. (2018) 79:272–6. doi: 10.1016/j.humimm.2018.01.007
154. Leventhal JR, Miller J, Mathew JM, Kurian S, Tambur AR, Friedewald J, et al. Updated follow-up of a tolerance protocol in HLA-identical renal transplant pairs given donor hematopoietic stem cells. Hum Immunol. (2018) 79:277–82. doi: 10.1016/j.humimm.2018.01.010
155. Ildstad ST, Leventhal J, Wen Y, Yolcu E. Facilitating cells: translation of hematopoietic chimerism to achieve clinical tolerance. Chimerism. (2015) 6:33–9. doi: 10.1080/19381956.2015.1130780
Keywords: immune tolerance, chimerism, bone marrow, vascular composite allograft, regulatory T cells, solid organ transplant, mesenchymal stem cells, hematopoietic stem cell
Citation: Johnstone BH, Messner F, Brandacher G and Woods EJ (2021) A Large-Scale Bank of Organ Donor Bone Marrow and Matched Mesenchymal Stem Cells for Promoting Immunomodulation and Transplant Tolerance. Front. Immunol. 12:622604. doi: 10.3389/fimmu.2021.622604
Received: 28 October 2020; Accepted: 08 February 2021;
Published: 26 February 2021.
Edited by:
Federica Casiraghi, Istituto di Ricerche Farmacologiche Mario Negri (IRCCS), ItalyReviewed by:
Martin Johannes Hoogduijn, Erasmus University Rotterdam, NetherlandsCurtis Cetrulo, Massachusetts General Hospital and Harvard Medical School, United States
Copyright © 2021 Johnstone, Messner, Brandacher and Woods. This is an open-access article distributed under the terms of the Creative Commons Attribution License (CC BY). The use, distribution or reproduction in other forums is permitted, provided the original author(s) and the copyright owner(s) are credited and that the original publication in this journal is cited, in accordance with accepted academic practice. No use, distribution or reproduction is permitted which does not comply with these terms.
*Correspondence: Erik J. Woods, ZXJpa0Bvc3NpdW1oZWFsdGguY29t
†These authors have contributed equally to this work and share first authorship