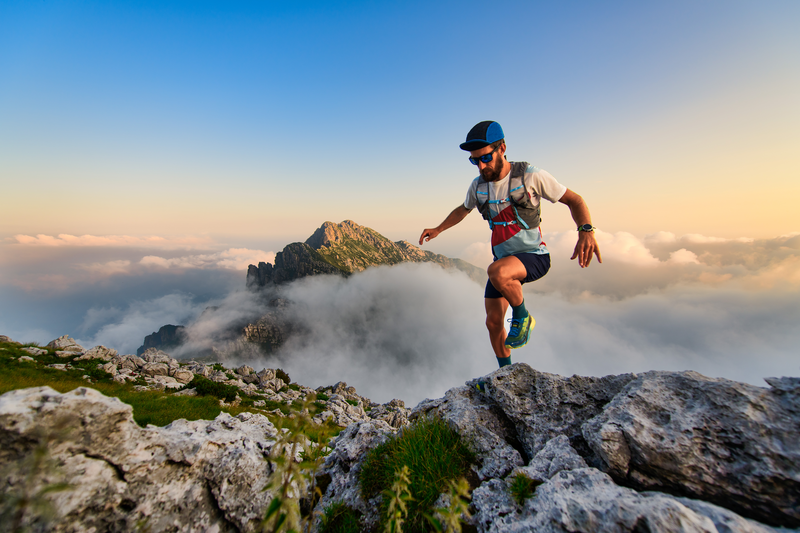
94% of researchers rate our articles as excellent or good
Learn more about the work of our research integrity team to safeguard the quality of each article we publish.
Find out more
MINI REVIEW article
Front. Immunol. , 25 February 2021
Sec. Cytokines and Soluble Mediators in Immunity
Volume 12 - 2021 | https://doi.org/10.3389/fimmu.2021.621956
This article is part of the Research Topic Role of the IL-23/IL-17 Pathway in Chronic Immune-Mediated Inflammatory Diseases: Mechanisms and Targeted Therapies View all 21 articles
Psoriasis is a frequent, chronic disease characterized by cutaneous inflammatory plaques and/or arthritis. It may be associated with few other diseases, mainly Crohn’s disease and metabolic syndrome. The medical and psychosocial burden of psoriasis remains high even since biological treatments arose, stressing that efforts to decipher its physiopathology are constantly needed. Tumor-necrosis factor α, interleukin (IL) 12 and IL17 have been previously associated with psoriasis and successfully targeted by monoclonal antibodies. IL17 in particular has been initially described as a T helper (Th) 17—produced cytokine, but it is now established that other cell types, such as γδ T lymphocytes, Mucosal-Associated Invariant T (MAIT) cells and Innate Lymphoïd Cells (ILC) 3 are also important sources of IL17 in the skin in response to inflammatory stimuli. Th17 phenotype has been shown to be stabilized by IL23, which is synthetized by macrophages and dendritic cells in response to Toll Like Receptors and C-type Lectin Receptors stimulation. Recent data also reported a crucial role for IL23 in MAIT17 and ILC3 homeostasis. Genome-wide association studies have found a significant link between IL23 receptor polymorphism and psoriasis susceptibility. IL23 signals through Janus kinase 2 and Tyrosine kinase 2, against which specific inhibitors are currently being tested. Monoclonal antibodies against IL17 and IL23 are only the beginning of a new avenue in psoriasis treatment. This review focuses on the molecular basis underlying IL23/IL17 axis blockade in psoriasis, and on future targets in this pathway.
Psoriasis is a chronic inflammatory disease involving the skin and/or the joints. Psoriasis prevalence in adults ranges from 0.51 to 11.43% worldwide (1) but is mainly considered to affect 2–3% of the population, with similar frequency in males and females (2). Skin lesions are featured by relapsing cutaneous erythro-squamous patches in its most frequent form, namely psoriasis vulgaris (PV). These will target electively peculiar locations such as scalp, palms and soles but also sacrum or large folds. Arthritis (PA, psoriatic arthritis) is associated to skin lesions in 1 to 15% of psoriasis patients (3). Of note, PA may either concern the peripheral articulations being therefore close to the characteristics of rheumatoid arthritis or in the contrary involve the spine, close to ankylosing spondylitis.
Psoriasis belongs to the spectrum of autoinflammatory diseases. Even in psoriasis without PA, its medical and psychosocial burden is high. Indeed, several studies using robust tools indicate that in all countries psoriasis may severely affect the quality of life (QOL) of these patients (4). In addition, psoriasis is significantly associated with metabolic syndrome, cardiovascular comorbidities and more rarely Crohn’s disease (5).
Since the 1990s, genetic and immunological studies have impressively dissected the mechanisms of psoriasis. Briefly, the disease appears to result from the interaction of genetic background and environmental triggers. Susceptibility loci mainly belong to HLA class I and II genes but also to various genes implicated in interleukin (IL) 17, IL23 and nuclear factor-kappa B (NFκB) pathways. In susceptible individuals, especially in response to physical traumatisms, autoantigens from keratinocytes, such as DNA, conjugate with anti-microbial peptides like cathelicidin/LL-37 (6), and activate plasmacytoid dendritic cells (pDCs) in the dermis. pDCs then secrete type I interferon and tumor-necrosis alpha (TNFα), which will activate classical dendritic cells (cDCs). These cDCs will produce IL12 and IL23, and skew the education of naïve T cells into T helper (Th) 1, Th17 and Th22 cells. Tumor-necrosis alpha (TNFα), IL17, and IL22 produced by these CD4+ T cells will then promote secretion of pro-inflammatory chemokines by keratinocytes, proliferation of epithelial cells and hyperkeratosis, and the recruitement of more inflammatory immune cells, accounting for the erythemato-squamous clinical lesions (7).
Resulting from this knowledge, targeted therapies have been a turnover in the management of psoriasis. TNFα blocking agents have initially paved the way, followed by monoclonal antibodies directed against IL12/23, IL17, and IL23. This highlights the crucial role of the IL17/23 cytokine pathway in psoriasis pathogenesis. Constant efforts are required to decipher the molecular mechanisms behind this disease, since new treatments are still needed for refractory and severe cases.
Heritability might account for as much as 68% of psoriasis susceptibility in Europeans (8). The first genetic linkage analyses in familial psoriasis (9) demonstrated the role of major histocompatibility complex alleles, mainly HLA-C*06:02, and were further confirmed by genome-wide association studies (GWAS) (10). Further insights into psoriasis genetics confirmed the importance of several immunological pathways among variants (11). Various mutations activating the pro-inflammatory NFκB pathway downstream of IL17 receptor (IL17R), such as in TRAF3 Interacting Protein 2 (TRAF3IP2), which encodes ACT1, a protein that allows signal transduction from the IL17R and downstream activation of NFκB, in CARD14 (an activator of NFκB), and in TNFAIP3 (tumor necrosis factor alpha induced protein 3, also called A20) and TNFAIP3 Interacting Protein 1 (TNIP1), have also been associated with an increased risk of developing psoriasis by GWAS studies, in Asian and Caucasian populations (10, 12–20).
Th17 lymphocytes are a major source of IL17, and they require IL23 to maintain their phenotype and to produce large amounts of IL17 (21–24). Polymorphisms in both subunits of IL23, IL23A(p19) and IL12B(p40), and in IL23R, have been associated with an increased risk of psoriasis in North Americans, Europeans and Asians (10, 12, 25). Signaling downstream of IL23 requires the signal transducer and activator of transcription protein 3 (STAT3). Polymorphisms have also been found by a GWAS meta-analysis in this gene (13). Interferon regulatory factor 4 (IRF4), another gene whose variants are associated with psoriasis (13), encodes a transcription factor that binds to the IL17 promoter and regulates Th17 pathogenic properties (26, 27). IRF4 also drives the differentiation of conventional dendritic cells (cDCs) into cDC2, which produce IL23 and promote Th17 (28, 29).
Eventually, clues for the implication of other sources of IL17 than Th17 in psoriasis pathogenesis may also be suggested by the association of Runt-related transcription factor 3 (RUNX3) polymorphisms to psoriasis susceptibility (13). RUNX3 is indeed a critical transcription factor for innate lymphoid cells (ILC), in particular for ILC3, the IL17 producing subset (30).
The vast majority of available genetic association studies highlight the role of the immune system in the pathophysiology of psoriasis. Even if some other genes such as IL-36 receptor antagonist (IL-36RN) are implicated (31, 32), the IL-17/23 axis seems to play a cardinal role.
An extensive amount of evidence now places IL17 as a key player in psoriasis pathogenesis (33, 34). In addition to genetic association studies, the efficacy of monoclonal antibodies targeting IL17 is a strong argument for the implication of this cytokine (35, 36).
Six isoforms of IL17 exist, and IL17A and IL17F are deemed to be the most pathogenic in psoriasis (37). IL17 receptors are heterodimers of IL17RA and a ligand specific subunit (IL17RB–E). IL17 receptors are widely expressed on epithelial cells (38). Upon the recognition of its ligand, IL17R recruits ACT1, which binds to tumor necrosis factor receptor 6 (TRAF6) (20). Downstream signaling involves mitogen activated protein kinase (MAPK), NFκB and C/EBPβ/δ pathways (19, 39–41). IL17 drives secretion of inflammatory chemokines, cytokines and antimicrobial peptides by keratinocytes, such as chemokine (C-C motif) ligand 20 (CCL20), IL-8 and β-defensin2 (42–45). IL17 indeed seems to play a key role in skin local immunity, as inborn deficiencies of IL17 or IL17R are responsible for chronic mucocutaneous candidiasis in humans (46). Pro-inflammatory mediators then recruit more Th17 lymphocytes, for example through CCL20/CCR6 signaling (47), and neutrophils, and increase local inflammation, resulting in the erythematous lesions characteristic of psoriasis.
Th17 cells were the first described source of IL17 (21, 48) and as such have been implicated in the pathogenesis of psoriasis (49). Th17, along with Th1, are found in the dermis of psoriatic lesions, and produce IL17 and IL22 (50), which in turn drives inflammatory and antimicrobial molecules secretion by keratinocytes (51). In mice, Th17 differentiate from naïve T CD4+ lymphocytes upon IL6 and transforming growth factor β stimulation; this process is amplified by IL1β and TNFα. Th17 cell survival and expansion depends on IL23 (21). IL23R is induced in Th17 by IL6 signaling through Janus kinases (JAK) JAK1, JAK2 and tyrosine kinase 2 (TYK2), STAT3 and RAR-related orphan receptor gamma t (RORγt) (52–54). IL23 signals through JAK/STAT3, resulting in enhancement of the Th17 phenotype (22). In humans however, Th17 require IL23 and IL1β for their differentiation (51). Keratinocytes also produce cytokines such as IL1β which amplifies Th17 generation (55).
Of note, Th17 are not the only source of IL17 in psoriasis. Other innate subsets, such as unconventional T cells, produce this key cytokine and might represent new therapeutic targets (56).
Gamma delta T lymphocytes (Tγδ) are abundant innate-like T lymphocytes in the dermis. They can be divided into T-box expressed in T cells+ (T-bet)+, IFNγ-producing Tγδ and RORγt+, IL17-producing Tγδ (57–59). The IL17-producing subset predominates in the dermis, expresses IL23R, depends on STAT3 signaling and is a major source of pathogenic IL17 in psoriasis (60–62).
Mucosal-associated invariant T cells (MAIT) are recently characterized innate-like T lymphocytes which recognize metabolites produced by bacteria and fungi. They are abundant in barrier tissues and especially in the skin. Although rare in mice, they represent 1–10% of T lymphocytes in human blood, skin and intestine (63–65). In mice, they are also divided into MAIT1 and MAIT17 subsets, expressing T-bet and RORγt and producing IFNγ and IL17, respectively (66). MAIT17 rely on IL23 for their homeostasis and activation (67, 68) and are enriched in psoriatic skin lesions (64).
Another important type of unconventional cells are innate lymphoid cells (ILC). They have a lymphoid morphology, do not rely on recombination-activating genes (RAG) for their development, and lack myeloid, dendritic and T/B markers. Type 1 ILC encompass NK cells and ILC1, express T-bet and secrete IFNγ; ILC2 are characterized by GATA3 expression and IL5 and IL13 secretion; while ILC3 express RORγt and require IL23 to produce IL17 (69). ILC3 - like Th17 cells, IL17-producing Tγδ cells and MAIT17 cells - are increased in blood and cutaneous lesions of psoriasis patients (70, 71).
Several reports of IL17 secretion by neutrophils through extracellular traps production in psoriasis have been published (72–74). Neutrophils seem to express IL23R and RORγt (75), but their contribution to IL17 production in psoriasis is still largely unknown.
Eventually, keratinocytes themselves are able to produce IL17C, enhancing inflammation in psoriasis in an autocrine way (37, 44, 76, 77).
In many IL17-producing cell types, IL23 plays a pivotal role in IL17 secretion (78). IL23 induces Th17 phenotype in humans (51) or maintains this phenotype in mice (21–24). IL23 is required for IL17 production by skin Tγδ (62), MAIT17 (67, 68), ILC3 (69) and maybe by neutrophils (75). The receptor for IL23 is a heterodimer of IL23R, which signals through JAK2, and of IL12Rβ1, which signals through TYK2. Both activate STAT3, resulting in RORγt expression and IL17 secretion (22, 79).
It seems that in the gut, contrary to the skin, IL17 might play a protective role on the epithelial barrier, and its secretion seems to be at least partially IL23-independent (80). This difference may account for the worsening of Crohn’s disease symptoms in psoriasis patients treated with antiIL17, which is not found so far during IL23 blockade (81).
IL23 is mostly produced by cDC2 in mice, which correspond to CD1c+ DC in humans. cDC2 are driven by the transcription factor IRF4 and promote Th17 differentiation in mice and humans (29). This IL23 production depends on Toll Like Receptors and C-type Lectin Receptors stimulation, and neurogenic locus notch homolog protein 2 (NOTCH2) signaling, in different models of inflammation including psoriasis (51, 82, 83).
The whole IL23/JAK/STAT3/RORγt/IL17 pathway plays a central role in psoriasis pathogenesis and is a key target of many recent and developing treatments for psoriasis.
The development of new psoriasis treatments has nicely demonstrated in vivo the essential role of the IL23/IL17 axis in psoriasis (Figure 1). Ustekinumab, an antip40 (common to IL12 and IL23) antibody, represented the second generation of monoclonal antibodies developed in psoriasis after antiTNFα antibodies. It induces a nonspecific inhibition of Th1 and Th17 with a high efficiency (Psoriasis Area Severity Index improvement ≥ 75% (PASI75) at week 12: 67%), but that is reached slowly, usually in 3–6 months (84–86).
Figure 1 IL23/IL17 axis in psoriasis and targeted therapies. CCL20, chemokine (C-C motif) ligand 20; cDC, classical dendritic cell; IFNα, interferon alpha; IL, interleukin; IL17R, IL17 receptor; ILC, innate lymphoid cell; JAK, Janus kinase; JAKi, JAK inhibitor; MAIT, mucosal associated invariant T cell; MAPK, mitogen activated protein kinase; NFκB, nuclear factor-kappa B; pDC, plasmacytoid dendritic cell; RORγt, RAR-related orphan receptor gamma t; RORγti, RORγt inhibitor; STAT, signal transducer and activator of transcription protein; Tγδ, gamma delta T lymphocyte; Th, T helper lymphocyte; TNFα, tumor necrosis factor alpha; TYK, tyrosine kinase; TYKi, TYK inhibitor. Created with BioRender.com.
More recently, a third generation of monoclonal antibodies became available: secukinumab and ixekizumab targeted IL17A, whereas bimekizumab blocked both IL17A and IL17F and brodalumab inhibited IL17R. Their efficacy was also high (PASI75 at week 12: 77–86%) but reached much faster, in 1–3 months (35, 36, 87–89). However, unexpected flare-ups of Crohn’s disease happened in a minority of patients, whereas it was not the case during TNFα and IL12/IL23 inhibition (81, 90). Even if this over-risk is not fully confirmed (91), several studies now suggest that IL17 might play a protective role in the gut, where secretion by Tγδ and ILC3 might predominate, whereas IL17 is endowed with pro-inflammatory functions in the skin (44, 80, 92). Other expected side effects include diffuse candidiasis, as suggested by studies from inborn errors in IL17 signaling (46). A warning about suicide risk restricted to brodalumab (93) has been described but does not seem to be confirmed by more recent follow-ups.
The latest generation of monoclonal antibodies in psoriasis is represented by specific antiIL23 treatments such as guselkumab, rizankizumab, tildrakizumab and mirikizumab. Their efficacy is very high (PASI90 at week 16: 67–75%) and reached as quickly as when using antiIL17 antibodies, but without the previous side effect of IBD flare (94–99).
New therapeutic strategies in psoriasis now tend toward small molecules targeting JAKs, in order to prevent signaling downstream of IL23 and IL6. Tofacitinib, which blocks JAK1, JAK2 and JAK3, is tested in several clinical trials (100–105). Results are interesting but side effects, especially cytopenias, are pushing toward more selective JAK inhibitors (106). Specific TYK2 inhibitors are also under development, with an encouraging phase II trial (107), and several phase III trials ongoing (ClinicalTrials.gov identifiers NCT04036435, NCT03924427, NCT04167462, NCT03624127, and NCT03611751). Masitinib, the TYK c-kit inhibitor, is also undergoing a phase II trial (ClinicalTrials.gov identifier NCT01045577).
Inhibitors of RORγt are also under development, with an ongoing phase II trial (ClinicalTrials.gov identifier NCT04207801) while another one was terminated for adverse events (ClinicalTrials.gov identifier NCT03329885). RORγt inhibition could be relevant as it does not seem to affect Tγδ nor ILC3, which could spare the protective role of IL17 on the intestinal barrier (78, 108). Concerns about a risk of deep immunosuppression have been raised since RORγt is required at the early stage (double positive stage) of thymic development for all T lymphocytes (109, 110). Opportunist candidiasis and mycobacterial infections might also be a concern, since they are encountered in patients with inborn deficiencies in RORγt (111). Finally, conditional knock-out mice for RORγt develop lymphomas (112), which are thus closely monitored in clinical trials.
A promising strategy might be to use the topical route to avoid these potential serious side effects. Topical tofacitinib has shown promising results in a phase II trial (113). A topical formulation of a JAK1 and TYK2 inhibitor is currently undergoing a phase II trial (ClinicalTrials.gov identifier NCT03850483). Topical RORγt inhibitors are still in phase I or preclinical development (114, 115).
IL23/IL17 axis plays a crucial role in psoriasis. Innate-like sources of IL17, such as Tγδ, MAIT and ILC3 are broadening the scope of pathogenic cells beyond classical Th17. Therapeutic targets now encompass IL23, JAK, RORγt and IL17 steps in this pathway, opening new avenues for resistant psoriasis treatment.
HB had written the first draft of the manuscript. SA had reviewed the manuscript extensively. All authors contributed to the article and approved the submitted version.
The authors declare that the research was conducted in the absence of any commercial or financial relationships that could be construed as a potential conflict of interest.
1. Michalek IM, Loring B, John SM. A systematic review of worldwide epidemiology of psoriasis. J Eur Acad Dermatol Venereol (2017) 31(2):205–12. doi: 10.1111/jdv.13854
2. Parisi R, Symmons DPM, Griffiths CEM, Ashcroft DM. Identification and Management of Psoriasis and Associated ComorbidiTy (IMPACT) project team. Global epidemiology of psoriasis: a systematic review of incidence and prevalence. J Invest Dermatol (2013) 133(2):377–85. doi: 10.1038/jid.2012.339
3. Scotti L, Franchi M, Marchesoni A, Corrao G. Prevalence and incidence of psoriatic arthritis: A systematic review and meta-analysis. Semin Arthritis Rheum (2018) 48(1):28–34. doi: 10.1016/j.semarthrit.2018.01.003
4. Augustin M, Dauden E, Mrowietz U, Konstantinou MP, Gerdes S, Rissler M, et al. Baseline characteristics of patients with moderate-to-severe psoriasis according to previous systemic treatment exposure: the PROSE study population. J Eur Acad Dermatol Venereol (2020) 34(11):2548–56. doi: 10.1111/jdv.16400
5. Takeshita J, Grewal S, Langan SM, Mehta NN, Ogdie A, Van Voorhees AS, et al. Psoriasis and comorbid diseases: Implications for management. J Am Acad Dermatol (2017) 76(3):393–403. doi: 10.1016/j.jaad.2016.07.065
6. Lande R, Botti E, Jandus C, Dojcinovic D, Fanelli G, Conrad C, et al. The antimicrobial peptide LL37 is a T-cell autoantigen in psoriasis. Nat Commun (2014) 5:5621. doi: 10.1038/ncomms6621
7. Hawkes JE, Yan BY, Chan TC, Krueger JG. Discovery of the IL-23/IL-17 Signaling Pathway and the Treatment of Psoriasis. J Immunol (2018) 201(6):1605–13. doi: 10.4049/jimmunol.1800013
8. Lønnberg AS, Skov L, Skytthe A, Kyvik KO, Pedersen OB, Thomsen SF. Heritability of psoriasis in a large twin sample. Br J Dermatol (2013) 169(2):412–6. doi: 10.1111/bjd.12375
9. Nair RP, Stuart PE, Nistor I, Hiremagalore R, Chia NVC, Jenisch S, et al. Sequence and haplotype analysis supports HLA-C as the psoriasis susceptibility 1 gene. Am J Hum Genet (2006) 78(5):827–51. doi: 10.1086/503821
10. Nair RP, Duffin KC, Helms C, Ding J, Stuart PE, Goldgar D, et al. Genome-wide scan reveals association of psoriasis with IL-23 and NF-kappaB pathways. Nat Genet (2009) 41(2):199–204. doi: 10.1038/ng.311
11. Ogawa K, Okada Y. The current landscape of psoriasis genetics in 2020. J Dermatol Sci (2020) 99(1):2–8. doi: 10.1016/j.jdermsci.2020.05.008
12. Tang H, Jin X, Li Y, Jiang H, Tang X, Yang X, et al. A large-scale screen for coding variants predisposing to psoriasis. Nat Genet (2014) 46(1):45–50. doi: 10.1038/ng.2827
13. Tsoi LC, Spain SL, Knight J, Ellinghaus E, Stuart PE, Capon F, et al. Identification of 15 new psoriasis susceptibility loci highlights the role of innate immunity. Nat Genet (2012) 44(12):1341–8. doi: 10.1038/ng.2467
14. Jordan CT, Cao L, Roberson EDO, Pierson KC, Yang C-F, Joyce CE, et al. PSORS2 is due to mutations in CARD14. Am J Hum Genet (2012) 90(5):784–95. doi: 10.1016/j.ajhg.2012.03.012
15. Gong H-B, Gao S-T, Pu X-M, Kang X-J, Wu X-J. Association of rs610604 in TNFAIP3 and rs17728338 in TNIP1 gene polymorphisms with psoriasis susceptibility: a meta-analysis of case-control studies. BMC Med Genet (2020) 1221(1):103. doi: 10.1186/s12881-020-01041-x
16. Hüffmeier U, Uebe S, Ekici AB, Bowes J, Giardina E, Korendowych E, et al. Common variants at TRAF3IP2 are associated with susceptibility to psoriatic arthritis and psoriasis. Nat Genet (2010) 42(11):996–9. doi: 10.1038/ng.688
17. Ellinghaus E, Ellinghaus D, Stuart PE, Nair RP, Debrus S, Raelson JV, et al. Genome-wide association study identifies a psoriasis susceptibility locus at TRAF3IP2. Nat Genet (2010) 42(11):991–5. doi: 10.1038/ng.689
18. Hunter CA. Act1-ivating IL-17 inflammation. Nat Immunol (2007) 8(3):232–4. doi: 10.1038/ni0307-232
19. Qian Y, Liu C, Hartupee J, Altuntas CZ, Gulen MF, Jane-Wit D, et al. The adaptor Act1 is required for interleukin 17-dependent signaling associated with autoimmune and inflammatory disease. Nat Immunol (2007) 8(3):247–56. doi: 10.1038/ni1439
20. Schwandner R, Yamaguchi K, Cao Z. Requirement of tumor necrosis factor receptor-associated factor (TRAF)6 in interleukin 17 signal transduction. J Exp Med (2000) 191(7):1233–40. doi: 10.1084/jem.191.7.1233
21. Veldhoen M, Hocking RJ, Atkins CJ, Locksley RM, Stockinger B. TGFbeta in the context of an inflammatory cytokine milieu supports de novo differentiation of IL-17-producing T cells. Immunity (2006) 24(2):179–89. doi: 10.1016/j.immuni.2006.01.001
22. Zhou L, Ivanov II, Spolski R, Min R, Shenderov K, Egawa T, et al. IL-6 programs T(H)-17 cell differentiation by promoting sequential engagement of the IL-21 and IL-23 pathways. Nat Immunol (2007) 8(9):967–74. doi: 10.1038/ni1488
23. McGeachy MJ, Chen Y, Tato CM, Laurence A, Joyce-Shaikh B, Blumenschein WM, et al. The interleukin 23 receptor is essential for the terminal differentiation of interleukin 17-producing effector T helper cells in vivo. Nat Immunol (2009) 10(3):314–24. doi: 10.1038/ni.1698
24. Stritesky GL, Yeh N, Kaplan MH. IL-23 promotes maintenance but not commitment to the Th17 lineage. J Immunol (2008) 181(9):5948–55. doi: 10.4049/jimmunol.181.9.5948
25. Cargill M, Schrodi SJ, Chang M, Garcia VE, Brandon R, Callis KP, et al. A large-scale genetic association study confirms IL12B and leads to the identification of IL23R as psoriasis-risk genes. Am J Hum Genet (2007) 80(2):273–90. doi: 10.1086/511051
26. Mudter J, Yu J, Zufferey C, Brüstle A, Wirtz S, Weigmann B, et al. IRF4 regulates IL-17A promoter activity and controls RORγt-dependent Th17 colitis in vivo. Inflamm Bowel Dis (2011) 17(6):1343–58. doi: 10.1002/ibd.21476
27. Brüstle A, Heink S, Huber M, Rosenplänter C, Stadelmann C, Yu P, et al. The development of inflammatory T(H)-17 cells requires interferon-regulatory factor 4. Nat Immunol (2007) 8(9):958–66. doi: 10.1038/ni1500
28. Vander Lugt B, Khan AA, Hackney JA, Agrawal S, Lesch J, Zhou M, et al. Transcriptional programming of dendritic cells for enhanced MHC class II antigen presentation. Nat Immunol (2014) 15(2):161–7. doi: 10.1038/ni.2795
29. Schlitzer A, McGovern N, Teo P, Zelante T, Atarashi K, Low D, et al. IRF4 Transcription Factor-Dependent CD11b+ Dendritic Cells in Human and Mouse Control Mucosal IL-17 Cytokine Responses. Immunity (2013) 38(5):970–83. doi: 10.1016/j.immuni.2013.04.011
30. Ebihara T, Song C, Ryu SH, Plougastel-Douglas B, Yang L, Levanon D, et al. Runx3 specifies lineage commitment of innate lymphoid cells. Nat Immunol (2015) 16(11):1124–33. doi: 10.1038/ni.3272
31. Marrakchi S, Guigue P, Renshaw BR, Puel A, Pei X-Y, Fraitag S, et al. Interleukin-36-receptor antagonist deficiency and generalized pustular psoriasis. N Engl J Med (2011) 365(7):620–8. doi: 10.1056/NEJMoa1013068
32. Zhang Z, Ma Y, Zhang Z, Lin J, Chen G, Han L, et al. Identification of Two Loci Associated with Generalized Pustular Psoriasis. J Invest Dermatol (2015) 135(8):2132–4. doi: 10.1038/jid.2015.111
33. Schön MP, Erpenbeck L. The Interleukin-23/Interleukin-17 Axis Links Adaptive and Innate Immunity in Psoriasis. Front Immunol (2018) 9:1323. doi: 10.3389/fimmu.2018.01323
34. Lynde CW, Poulin Y, Vender R, Bourcier M, Khalil S. Interleukin 17A: toward a new understanding of psoriasis pathogenesis. J Am Acad Dermatol (2014) 71(1):141–50. doi: 10.1016/j.jaad.2013.12.036
35. Langley RG, Elewski BE, Lebwohl M, Reich K, Griffiths CEM, Papp K, et al. Secukinumab in plaque psoriasis–results of two phase 3 trials. N Engl J Med (2014) 371(4):326–38. doi: 10.1056/NEJMoa1314258
36. Leonardi C, Matheson R, Zachariae C, Cameron G, Li L, Edson-Heredia E, et al. Anti-interleukin-17 monoclonal antibody ixekizumab in chronic plaque psoriasis. N Engl J Med (2012) 366(13):1190–9. doi: 10.1056/NEJMoa1109997
37. Johansen C, Usher PA, Kjellerup RB, Lundsgaard D, Iversen L, Kragballe K. Characterization of the interleukin-17 isoforms and receptors in lesional psoriatic skin. Br J Dermatol (2009) 160(2):319–24. doi: 10.1111/j.1365-2133.2008.08902.x
38. Yao Z, Spriggs MK, Derry JM, Strockbine L, Park LS, VandenBos T, et al. Molecular characterization of the human interleukin (IL)-17 receptor. Cytokine (1997) 9(11):794–800. doi: 10.1006/cyto.1997.0240
39. Gaffen SL. Structure and signalling in the IL-17 receptor family. Nat Rev Immunol (2009) 9(8):556–67. doi: 10.1038/nri2586
40. Yao Z, Fanslow WC, Seldin MF, Rousseau AM, Painter SL, Comeau MR, et al. Herpesvirus Saimiri encodes a new cytokine, IL-17, which binds to a novel cytokine receptor. Immunity (1995) 3(6):811–21. doi: 10.1016/1074-7613(95)90070-5
41. Chang SH, Park H, Dong C. Act1 adaptor protein is an immediate and essential signaling component of interleukin-17 receptor. J Biol Chem (2006) 281(47):35603–7. doi: 10.1074/jbc.C600256200
42. Watanabe H, Kawaguchi M, Fujishima S, Ogura M, Matsukura S, Takeuchi H, et al. Functional characterization of IL-17F as a selective neutrophil attractant in psoriasis. J Invest Dermatol (2009) 129(3):650–6. doi: 10.1038/jid.2008.294
43. McGeachy MJ, Cua DJ, Gaffen SL. The IL-17 Family of Cytokines in Health and Disease. Immunity (2019) 50(4):892–906. doi: 10.1016/j.immuni.2019.03.021
44. Ramirez-Carrozzi V, Sambandam A, Luis E, Lin Z, Jeet S, Lesch J, et al. IL-17C regulates the innate immune function of epithelial cells in an autocrine manner. Nat Immunol (2011) 12(12):1159–66. doi: 10.1038/ni.2156
45. Liang SC, Tan X-Y, Luxenberg DP, Karim R, Dunussi-Joannopoulos K, Collins M, et al. Interleukin (IL)-22 and IL-17 are coexpressed by Th17 cells and cooperatively enhance expression of antimicrobial peptides. J Exp Med (2006) 203(10):2271–9. doi: 10.1084/jem.20061308
46. Puel A, Cypowyj S, Bustamante J, Wright JF, Liu L, Lim HK, et al. Chronic mucocutaneous candidiasis in humans with inborn errors of interleukin-17 immunity. Science (New York NY) (2011) 332(6025):65–8. doi: 10.1126/science.1200439
47. Furue K, Ito T, Tsuji G, Nakahara T, Furue M. The CCL20 and CCR6 axis in psoriasis. Scand J Immunol (2020) 91(3):e12846. doi: 10.1111/sji.12846
48. Harrington LE, Hatton RD, Mangan PR, Turner H, Murphy TL, Murphy KM, et al. Interleukin 17-producing CD4+ effector T cells develop via a lineage distinct from the T helper type 1 and 2 lineages. Nat Immunol (2005) 6(11):1123–32. doi: 10.1038/ni1254
49. Di Cesare A, Di Meglio P, Nestle FO. The IL-23/Th17 axis in the immunopathogenesis of psoriasis. J Invest Dermatol (2009) 129(6):1339–50. doi: 10.1038/jid.2009.59
50. Lowes MA, Kikuchi T, Fuentes-Duculan J, Cardinale I, Zaba LC, Haider AS, et al. Psoriasis vulgaris lesions contain discrete populations of Th1 and Th17 T cells. J Invest Dermatol (2008) 128(5):1207–11. doi: 10.1038/sj.jid.5701213
51. Wilson NJ, Boniface K, Chan JR, McKenzie BS, Blumenschein WM, Mattson JD, et al. Development, cytokine profile and function of human interleukin 17-producing helper T cells. Nat Immunol (2007) 8(9):950–7. doi: 10.1038/ni1497
52. Lütticken C, Wegenka UM, Yuan J, Buschmann J, Schindler C, Ziemiecki A, et al. Association of transcription factor APRF and protein kinase Jak1 with the interleukin-6 signal transducer gp130. Science (New York NY) (1994) 263(5143):89–92. doi: 10.1126/science.8272872
53. Stahl N, Boulton TG, Farruggella T, Ip NY, Davis S, Witthuhn BA, et al. Association and activation of Jak-Tyk kinases by CNTF-LIF-OSM-IL-6 beta receptor components. Science (New York NY) (1994) 263(5143):92–5. doi: 10.1126/science.8272873
54. Harris TJ, Grosso JF, Yen H-R, Xin H, Kortylewski M, Albesiano E, et al. Cutting edge: An in vivo requirement for STAT3 signaling in TH17 development and TH17-dependent autoimmunity. J Immunol (2007) 179(7):4313–7. doi: 10.4049/jimmunol.179.7.4313
55. Muhr P, Renne J, Schaefer V, Werfel T, Wittmann M. Primary human keratinocytes efficiently induce IL-1-dependent IL-17 in CCR6+ T cells. Exp Dermatol (2010) 19(12):1105–7. doi: 10.1111/j.1600-0625.2010.01134.x
56. Cua DJ, Tato CM. Innate IL-17-producing cells: the sentinels of the immune system. Nat Rev Immunol (2010) 10(7):479–89. doi: 10.1038/nri2800
57. Ribot JC, deBarros A, Pang DJ, Neves JF, Peperzak V, Roberts SJ, et al. CD27 is a thymic determinant of the balance between interferon-gamma- and interleukin 17-producing gammadelta T cell subsets. Nat Immunol (2009) 10(4):427–36. doi: 10.1038/ni.1717
58. Haas JD, González FHM, Schmitz S, Chennupati V, Föhse L, Kremmer E, et al. CCR6 and NK1.1 distinguish between IL-17A and IFN-gamma-producing gammadelta effector T cells. Eur J Immunol (2009) 39(12):3488–97. doi: 10.1002/eji.200939922
59. Shibata K, Yamada H, Nakamura R, Sun X, Itsumi M, Yoshikai Y. Identification of CD25+ gamma delta T cells as fetal thymus-derived naturally occurring IL-17 producers. J Immunol (Baltimore Md: 1950) (2008) 181(9):5940–7. doi: 10.4049/jimmunol.181.9.5940
60. Cai Y, Xue F, Qin H, Chen X, Liu N, Fleming C, et al. Differential Roles of the mTOR-STAT3 Signaling in Dermal γδ T Cell Effector Function in Skin Inflammation. Cell Rep (2019) 27(10):3034–3048.e5. doi: 10.1016/j.celrep.2019.05.019
61. Agerholm R, Rizk J, Viñals MT, Bekiaris V. STAT3 but not STAT4 is critical for γδT17 cell responses and skin inflammation. EMBO Rep (2019) 20(11):e48647. doi: 10.15252/embr.201948647
62. Cai Y, Shen X, Ding C, Qi C, Li K, Li X, et al. Pivotal Role of Dermal IL-17-producing γδ T Cells in Skin Inflammation. Immunity (2011) 35(4):596–610. doi: 10.1016/j.immuni.2011.08.001
63. Godfrey DI, Koay H-F, McCluskey J, Gherardin NA. The biology and functional importance of MAIT cells. Nat Immunol (2019) 20(9):1110–28. doi: 10.1038/s41590-019-0444-8
64. Teunissen MBM, Yeremenko NG, Baeten DLP, Chielie S, Spuls PI, de Rie MA, et al. The IL-17A-producing CD8+ T-cell population in psoriatic lesional skin comprises mucosa-associated invariant T cells and conventional T cells. J Invest Dermatol (2014) 134(12):2898–907. doi: 10.1038/jid.2014.261
65. Dusseaux M, Martin E, Serriari N, Péguillet I, Premel V, Louis D, et al. Human MAIT cells are xenobiotic-resistant, tissue-targeted, CD161hi IL-17-secreting T cells. Blood (2011) 117(4):1250–9. doi: 10.1182/blood-2010-08-303339
66. Legoux F, Gilet J, Procopio E, Echasserieau K, Bernardeau K, Lantz O. Molecular mechanisms of lineage decisions in metabolite-specific T cells. Nat Immunol (2019) 20(9):1244–55. doi: 10.1038/s41590-019-0465-3
67. Wang H, Kjer-Nielsen L, Shi M, D’Souza C, Pediongco TJ, Cao H, et al. IL-23 costimulates antigen-specific MAIT cell activation and enables vaccination against bacterial infection. Sci Immunol (2019) 15:4(41). doi: 10.1126/sciimmunol.aaw0402
68. Constantinides MG, Link VM, Tamoutounour S, Wong AC, Perez-Chaparro PJ, Han S-J, et al. MAIT cells are imprinted by the microbiota in early life and promote tissue repair. Science (New York NY) (2019) 366(6464):eaax6624. doi: 10.1126/science.aax6624
69. Spits H, Artis D, Colonna M, Diefenbach A, Di Santo JP, Eberl G, et al. Innate lymphoid cells–a proposal for uniform nomenclature. Nat Rev Immunol (2013) 13(2):145–9. doi: 10.1038/nri3365
70. Villanova F, Flutter B, Tosi I, Grys K, Sreeneebus H, Perera GK, et al. Characterization of innate lymphoid cells in human skin and blood demonstrates increase of NKp44+ ILC3 in psoriasis. J Invest Dermatol (2014) 134(4):984–91. doi: 10.1038/jid.2013.477
71. Teunissen MBM, Munneke JM, Bernink JH, Spuls PI, Res PCM, Te Velde A, et al. Composition of innate lymphoid cell subsets in the human skin: enrichment of NCR(+) ILC3 in lesional skin and blood of psoriasis patients. J Invest Dermatol (2014) 134(9):2351–60. doi: 10.1038/jid.2014.146
72. Keijsers RRMC, Hendriks AGM, van Erp PEJ, van Cranenbroek B, van de Kerkhof PCM, Koenen HJPM, et al. In vivo induction of cutaneous inflammation results in the accumulation of extracellular trap-forming neutrophils expressing RORγt and IL-17. J Invest Dermatol (2014) 134(5):1276–84. doi: 10.1038/jid.2013.526
73. Dyring-Andersen B, Honoré TV, Madelung A, Bzorek M, Simonsen S, Clemmensen SN, et al. Interleukin (IL)-17A and IL-22-producing neutrophils in psoriatic skin. Br J Dermatol (2017) 177(6):e321–2. doi: 10.1111/bjd.15533
74. Lin AM, Rubin CJ, Khandpur R, Wang JY, Riblett M, Yalavarthi S, et al. Mast cells and neutrophils release IL-17 through extracellular trap formation in psoriasis. J Immunol (2011) 187(1):490–500. doi: 10.4049/jimmunol.1100123
75. Chen F, Cao A, Yao S, Evans-Marin HL, Liu H, Wu W, et al. mTOR Mediates IL-23 Induction of Neutrophil IL-17 and IL-22 Production. J Immunol (Baltimore Md: 1950) (2016) 196(10):4390–9. doi: 10.4049/jimmunol.1501541
76. Roth SA, Simanski M, Rademacher F, Schröder L, Harder J. The pattern recognition receptor NOD2 mediates Staphylococcus aureus-induced IL-17C expression in keratinocytes. J Invest Dermatol (2014) 134(2):374–80. doi: 10.1038/jid.2013.313
77. Johnston A, Fritz Y, Dawes SM, Diaconu D, Al-Attar PM, Guzman AM, et al. Keratinocyte overexpression of IL-17C promotes psoriasiform skin inflammation. J Immunol (Baltimore Md: 1950) (2013) 190(5):2252–62. doi: 10.4049/jimmunol.1201505
78. Bianchi E, Rogge L. The IL-23/IL-17 pathway in human chronic inflammatory diseases - new insight from genetics and targeted therapies. Microbes Infect (2019) 21(5–6):246–53. doi: 10.1016/j.micinf.2019.06.009
79. Parham C, Chirica M, Timans J, Vaisberg E, Travis M, Cheung J, et al. A receptor for the heterodimeric cytokine IL-23 is composed of IL-12Rbeta1 and a novel cytokine receptor subunit, IL-23R. J Immunol (2002) 168(11):5699–708. doi: 10.4049/jimmunol.168.11.5699
80. Lee JS, Tato CM, Joyce-Shaikh B, Gulen MF, Gulan F, Cayatte C, et al. Interleukin-23-Independent IL-17 Production Regulates Intestinal Epithelial Permeability. Immunity (2015) 43(4):727–38. doi: 10.1016/j.immuni.2015.09.003
81. Hueber W, Sands BE, Lewitzky S, Vandemeulebroecke M, Reinisch W, Higgins PDR, et al. Secukinumab, a human anti-IL-17A monoclonal antibody, for moderate to severe Crohn’s disease: unexpected results of a randomised, double-blind placebo-controlled trial. Gut (2012) 61(12):1693–700. doi: 10.1136/gutjnl-2011-301668
82. Satpathy AT, Briseño CG, Lee JS, Ng D, Manieri NA, KC W, et al. Notch2-dependent classical dendritic cells orchestrate intestinal immunity against attaching and effacing bacterial pathogens. Nat Immunol (2013) 14(9):937–48. doi: 10.1038/ni.2679
83. Nakao M, Miyagaki T, Sugaya M, Sato S. Exacerbated Imiquimod-Induced Psoriasis-Like Skin Inflammation in IRF5-Deficient Mice. Int J Mol Sci (2020) 21(10):3681. doi: 10.3390/ijms21103681
84. Griffiths CEM, Strober BE, van de Kerkhof P, Ho V, Fidelus-Gort R, Yeilding N, et al. Comparison of ustekinumab and etanercept for moderate-to-severe psoriasis. N Engl J Med (2010) 362(2):118–28. doi: 10.1056/NEJMoa0810652
85. Leonardi CL, Kimball AB, Papp KA, Yeilding N, Guzzo C, Wang Y, et al. Efficacy and safety of ustekinumab, a human interleukin-12/23 monoclonal antibody, in patients with psoriasis: 76-week results from a randomised, double-blind, placebo-controlled trial (PHOENIX 1). Lancet (Lond Engl) (2008) 371(9625):1665–74. doi: 10.1016/S0140-6736(08)60725-4
86. Papp KA, Langley RG, Lebwohl M, Krueger GG, Szapary P, Yeilding N, et al. Efficacy and safety of ustekinumab, a human interleukin-12/23 monoclonal antibody, in patients with psoriasis: 52-week results from a randomised, double-blind, placebo-controlled trial (PHOENIX 2). Lancet (Lond Engl) (2008) 371(9625):1675–84. doi: 10.1016/S0140-6736(08)60726-6
87. Papp KA, Merola JF, Gottlieb AB, Griffiths CEM, Cross N, Peterson L, et al. Dual neutralization of both interleukin 17A and interleukin 17F with bimekizumab in patients with psoriasis: Results from BE ABLE 1, a 12-week randomized, double-blinded, placebo-controlled phase 2b trial. J Am Acad Dermatol (2018) 79(2):277–86.e10. doi: 10.1016/j.jaad.2018.03.037
88. Lebwohl M, Strober B, Menter A, Gordon K, Weglowska J, Puig L, et al. Phase 3 Studies Comparing Brodalumab with Ustekinumab in Psoriasis. N Engl J Med (2015) 373(14):1318–28. doi: 10.1056/NEJMoa1503824
89. Papp KA, Leonardi C, Menter A, Ortonne J-P, Krueger JG, Kricorian G, et al. Brodalumab, an anti-interleukin-17-receptor antibody for psoriasis. N Engl J Med (2012) 366(13):1181–9. doi: 10.1056/NEJMoa1109017
90. Targan SR, Feagan B, Vermeire S, Panaccione R, Melmed GY, Landers C, et al. A Randomized, Double-Blind, Placebo-Controlled Phase 2 Study of Brodalumab in Patients With Moderate-to-Severe Crohn’s Disease. Am J Gastroenterol (2016) 111(11):1599–607. doi: 10.1038/ajg.2016.298
91. Schreiber S, Colombel J-F, Feagan BG, Reich K, Deodhar AA, McInnes IB, et al. Incidence rates of inflammatory bowel disease in patients with psoriasis, psoriatic arthritis and ankylosing spondylitis treated with secukinumab: a retrospective analysis of pooled data from 21 clinical trials. Ann Rheumatic Dis (2019) 78(4):473–9. doi: 10.1136/annrheumdis-2018-214273
92. Maxwell JR, Zhang Y, Brown WA, Smith CL, Byrne FR, Fiorino M, et al. Differential Roles for Interleukin-23 and Interleukin-17 in Intestinal Immunoregulation. Immunity (2015) 43(4):739–50. doi: 10.1016/j.immuni.2015.08.019
93. Lebwohl MG, Papp KA, Marangell LB, Koo J, Blauvelt A, Gooderham M, et al. Psychiatric adverse events during treatment with brodalumab: Analysis of psoriasis clinical trials. J Am Acad Dermatol (2018) 78(1):81–89.e5. doi: 10.1016/j.jaad.2017.08.024
94. Gordon KB, Duffin KC, Bissonnette R, Prinz JC, Wasfi Y, Li S, et al. A Phase 2 Trial of Guselkumab versus Adalimumab for Plaque Psoriasis. N Engl J Med (2015) 373(2):136–44. doi: 10.1056/NEJMoa1501646
95. Reich K, Armstrong AW, Foley P, Song M, Wasfi Y, Randazzo B, et al. Efficacy and safety of guselkumab, an anti-interleukin-23 monoclonal antibody, compared with adalimumab for the treatment of patients with moderate to severe psoriasis with randomized withdrawal and retreatment: Results from the phase III, double-blind, placebo- and active comparator-controlled VOYAGE 2 trial. J Am Acad Dermatol (2017) 76(3):418–31. doi: 10.1016/j.jaad.2016.11.042
96. Papp KA, Blauvelt A, Bukhalo M, Gooderham M, Krueger JG, Lacour J-P, et al. Risankizumab versus Ustekinumab for Moderate-to-Severe Plaque Psoriasis. N Engl J Med (2017) 376(16):1551–60. doi: 10.1056/NEJMoa1607017
97. Gordon KB, Strober B, Lebwohl M, Augustin M, Blauvelt A, Poulin Y, et al. Efficacy and safety of risankizumab in moderate-to-severe plaque psoriasis (UltIMMa-1 and UltIMMa-2): results from two double-blind, randomised, placebo-controlled and ustekinumab-controlled phase 3 trials. Lancet (Lond Engl) (2018) 392(10148):650–61. doi: 10.1016/S0140-6736(18)31713-6
98. Reich K, Rich P, Maari C, Bissonnette R, Leonardi C, Menter A, et al. Efficacy and safety of mirikizumab (LY3074828) in the treatment of moderate-to-severe plaque psoriasis: results from a randomized phase II study. Br J Dermatol (2019) 181(1):88–95. doi: 10.1111/bjd.17628
99. Reich K, Warren RB, Iversen L, Puig L, Pau-Charles I, Igarashi A, et al. Long-term efficacy and safety of tildrakizumab for moderate-to-severe psoriasis: pooled analyses of two randomized phase III clinical trials (reSURFACE 1 and reSURFACE 2) through 148 weeks. Br J Dermatol (2020) 182(3):605–17. doi: 10.1111/bjd.18232
100. Morelli M, Scarponi C, Mercurio L, Facchiano F, Pallotta S, Madonna S, et al. Selective Immunomodulation of Inflammatory Pathways in Keratinocytes by the Janus Kinase (JAK) Inhibitor Tofacitinib: Implications for the Employment of JAK-Targeting Drugs in Psoriasis. J Immunol Res (2018) 2018:7897263. doi: 10.1155/2018/7897263
101. Papp KA, Menter A, Strober B, Langley RG, Buonanno M, Wolk R, et al. Efficacy and safety of tofacitinib, an oral Janus kinase inhibitor, in the treatment of psoriasis: a Phase 2b randomized placebo-controlled dose-ranging study. Br J Dermatol (2012) 167(3):668–77. doi: 10.1111/j.1365-2133.2012.11168.x
102. Papp KA, Menter MA, Abe M, Elewski B, Feldman SR, Gottlieb AB, et al. Tofacitinib, an oral Janus kinase inhibitor, for the treatment of chronic plaque psoriasis: results from two randomized, placebo-controlled, phase III trials. Br J Dermatol (2015) 173(4):949–61. doi: 10.1111/bjd.14018
103. Bachelez H, van de Kerkhof PCM, Strohal R, Kubanov A, Valenzuela F, Lee J-H, et al. Tofacitinib versus etanercept or placebo in moderate-to-severe chronic plaque psoriasis: a phase 3 randomised non-inferiority trial. Lancet (Lond Engl) (2015) 386(9993):552–61. doi: 10.1016/S0140-6736(14)62113-9
104. Bissonnette R, Iversen L, Sofen H, Griffiths CEM, Foley P, Romiti R, et al. Tofacitinib withdrawal and retreatment in moderate-to-severe chronic plaque psoriasis: a randomized controlled trial. Br J Dermatol (2015) 172(5):1395–406. doi: 10.1111/bjd.13551
105. Zhang J, Tsai T-F, Lee M-G, Zheng M, Wang G, Jin H, et al. The efficacy and safety of tofacitinib in Asian patients with moderate to severe chronic plaque psoriasis: A Phase 3, randomized, double-blind, placebo-controlled study. J Dermatol Sci (2017) 88(1):36–45. doi: 10.1016/j.jdermsci.2017.05.004
106. Kvist-Hansen A, Hansen PR, Skov L. Systemic Treatment of Psoriasis with JAK Inhibitors: A Review. Dermatol Ther (Heidelb) (2020) 10(1):29–42. doi: 10.1007/s13555-019-00347-w
107. Papp K, Gordon K, Thaçi D, Morita A, Gooderham M, Foley P, et al. Phase 2 Trial of Selective Tyrosine Kinase 2 Inhibition in Psoriasis. N Engl J Med (2018) 379(14):1313–21. doi: 10.1056/NEJMoa1806382
108. Withers DR, Hepworth MR, Wang X, Mackley EC, Halford EE, Dutton EE, et al. Transient inhibition of ROR-γt therapeutically limits intestinal inflammation by reducing TH17 cells and preserving group 3 innate lymphoid cells. Nat Med (2016) 22(3):319–23. doi: 10.1038/nm.4046
109. He YW, Deftos ML, Ojala EW, Bevan MJ. RORgamma t, a novel isoform of an orphan receptor, negatively regulates Fas ligand expression and IL-2 production in T cells. Immunity (1998) 9(6):797–806. doi: 10.1016/S1074-7613(00)80645-7
110. Sun Z, Unutmaz D, Zou YR, Sunshine MJ, Pierani A, Brenner-Morton S, et al. Requirement for RORgamma in thymocyte survival and lymphoid organ development. Science (New York NY) (2000) 288(5475):2369–73. doi: 10.1126/science.288.5475.2369
111. Okada S, Markle JG, Deenick EK, Mele F, Averbuch D, Lagos M, et al. IMMUNODEFICIENCIES. Impairment of immunity to Candida and Mycobacterium in humans with bi-allelic RORC mutations. Science (2015) 349(6248):606–13. doi: 10.1126/science.aaa4282
112. Liljevald M, Rehnberg M, Söderberg M, Ramnegård M, Börjesson J, Luciani D, et al. Retinoid-related orphan receptor γ (RORγ) adult induced knockout mice develop lymphoblastic lymphoma. Autoimmun Rev (2016) 15(11):1062–70. doi: 10.1016/j.autrev.2016.07.036
113. Papp KA, Bissonnette R, Gooderham M, Feldman SR, Iversen L, Soung J, et al. Treatment of plaque psoriasis with an ointment formulation of the Janus kinase inhibitor, tofacitinib: a Phase 2b randomized clinical trial. BMC Dermatol (2016) 16(1):15. doi: 10.1186/s12895-016-0051-4
114. Berstein G, Zhang Y, Berger Z, Kieras E, Li G, Samuel A, et al. A phase I, randomized, double-blind study to assess the safety, tolerability and efficacy of the topical RORC2 inverse agonist PF-06763809 in participants with mild-to-moderate plaque psoriasis. Clin Exp Dermatol (2020) 46(1):122–9. doi: 10.1111/ced.14412
Keywords: IL23, IL17, psoriasis, skin, Th17
Citation: Bugaut H and Aractingi S (2021) Major Role of the IL17/23 Axis in Psoriasis Supports the Development of New Targeted Therapies. Front. Immunol. 12:621956. doi: 10.3389/fimmu.2021.621956
Received: 27 October 2020; Accepted: 11 January 2021;
Published: 25 February 2021.
Edited by:
Lars Rogge, Institut Pasteur, FranceReviewed by:
Giampiero Girolomoni, University of Verona, ItalyCopyright © 2021 Bugaut and Aractingi. This is an open-access article distributed under the terms of the Creative Commons Attribution License (CC BY). The use, distribution or reproduction in other forums is permitted, provided the original author(s) and the copyright owner(s) are credited and that the original publication in this journal is cited, in accordance with accepted academic practice. No use, distribution or reproduction is permitted which does not comply with these terms.
*Correspondence: Hélène Bugaut, aGVsZW5lLmJ1Z2F1dEBjdXJpZS5mcg==
Disclaimer: All claims expressed in this article are solely those of the authors and do not necessarily represent those of their affiliated organizations, or those of the publisher, the editors and the reviewers. Any product that may be evaluated in this article or claim that may be made by its manufacturer is not guaranteed or endorsed by the publisher.
Research integrity at Frontiers
Learn more about the work of our research integrity team to safeguard the quality of each article we publish.