- Department of Pediatrics, Medical Faculty, Otto-von-Guericke-University, Magdeburg, Germany
Atopic diseases in childhood are a major burden worldwide and there is still a lack of knowledge about treatable causes. In industrialized countries such as Germany, almost every second child is sensitized to at least one common allergen. Recent studies show that although the predisposition to allergies is inherited, the adaptive immune system of neonates and infants follows a developmental trajectory and whether an allergy actually occurs depends also on timing of allergen exposure including diet as well as environmental factors. New recommendations are far from being rigid of allergen avoidance; it is rather moving toward conditions that stand for more biodiversity. The observation that introduction of peanuts or eggs early in life significantly reduced the development of a later allergy will change our recommendations for the introduction of complementary foods. This is consistent with the hygiene hypothesis that early provocation shapes the developing immune system so that it reacts appropriately. Therefore, promoting the development of tolerance is at the heart of sensible allergy prevention - and this begins with the last trimester of pregnancy. In light of this concept, actual recommendations are discussed.
Introduction
Type I allergy (hypersensitivity) is caused by an aberrant production and activity of immunoglobulin E (IgE) against normally nonpathogenic antigens (called allergens). Atopic diseases are among the most common chronic health problems affecting children and young people all around the world (1). Although a large proportion of young children lose their reactions to food allergens, as they get older, many develop other types of allergic reactions, such as allergic dermatitis (AD), hay fever, asthma, or rhinitis. (2). The latter path of suffering is often described as the "allergic march" (2). A prerequisite for the development of allergy is a sensitization with the antigen and development of a memory response. Common antigenic allergens include animal dander, chemical additives, foods, insect bites, pollens, and even drugs. Upon antigen presentation to T helper cells, immune reaction is skewed toward type 2 (Th2) response, associated with cytokines Interleukin (IL)-4, IL-5, and IL-13 and leads to the production of IgE antibodies by B cells. These antibodies binds via the high-affinity IgE (FcεR1) receptors to mast cells or basophils (3). Reencounter with the same antigen causes cross-linking of the IgE/FcεR1 complex and an excessive allergen-specific IgE antibody production. Activated mast cells and basophiles degranulate and subsequently release mediators such as histamine and leukotrienes. Alongside with pathologically elevated allergen-specific IgE antibody titers these mediators contribute to typical signs and symptoms of atopic diseases and in severe cases, even anaphylactic shock may occur.
The definition of immune tolerance to allergens is establishment of a long-term clinical unresponsiveness to one specific or many allergens (4, 5). It is not just absence of a response, but also the suppression of the adverse allergic reaction. Enforcing and reinforcing tolerance has become an important goal for prevention and curative approaches for any atopic disease aiming for setting an optimal new response or correcting pre-existing dysregulated responses. In short, tolerance is induced against the identified allergen. The therapy of choice for inducing long-lasting tolerance is the allergen-specific immunotherapy (AIT), which is administered subcutaneously or sublingually. It is applicable for children as well as adults (6).
Sudden resolution of food allergy in infancy and childhood has been reported regularly. This natural induction of tolerance is often associated with reduction of allergen-specific antibody production of the IgE isotype and activity of basophils in addition to enhanced levels of allergen-specific antibodies of the IgG4 isotype and frequencies of regulatory T cells (Tregs) (7–9). This is in line with the finding that, successful AIT relates to enhanced numbers of Tregs and dendritic cells (DCs) as well as increased allergen-specific IgG4 levels. Thus, it seems plausible that the same immunological mechanisms involved in tolerance induction may drive protection from allergic disorders in early life. Therefore, promoting the development of tolerance is at the heart of sensible allergy prevention - and this begins with newborns, maybe even already during pregnancy.
Numerous recommendations for allergy prevention, as formulated in guidelines in Germany have been subject to considerable fluctuation on the basis of recently published clinical studies (10). In some areas a paradigm shift has taken place: In the past, "allergen avoidance" was the central principle of the recommendations around the prevention of allergies (11). In the meantime, it is progressively changing by moving toward an active "tolerance induction". This concept of tolerance induction is also important in term of pathophysiological considerations.
Particularities of Atopic Diseases in Childhood
During infancy and childhood, atopic diseases are the most common chronic diseases (12). Current numbers from different countries show that up to 40 percent of children, and young people suffer from an atopic disease and the prevalence still increases (13). For example, the prevalence of asthma in Australian schoolchildren raised from 13% to 39% and from 23% to 44% for hay fever (14). In Denmark, the prevalence of AD enhanced from 17%–27% in 7 to 17-year-olds (15) and in Scotland from 5% to 12% (16). In 2010, African-American children in the U.S. were 5% more likely to have had allergic skin disorders than Caucasian or Asian children (17). In many developing countries, the incidence of allergies is on the rise. For example, the prevalence of eczema among South African youth raised from 12% to 19% (18). Even though allergies often start in childhood and can last a lifetime, there are some special features during childhood (19). Children are particularly sensitive to foreign substances, since the child's immune system faces a special challenge: on one hand, it should fend off pathogens, but on the other hand should not cause any collateral damage to the developing tissues. Already their skin barrier is different to the adults, it is much thinner and more permeable (20). Certain allergic diseases typically occur at a certain age, i.e., food allergies dominate in infants. Of note, 30% of food allergic children under the age of 18 are estimated to react to more than one food (21, 22). Reports show that this estimate rises to 70% in highly atopic children (23). In comparison to those with single food allergies, multiple food allergic children experience a greater decline in quality of life and suffer more often from nutritional deficiencies (24, 25). They are also less prone to spontaneously resolving their pre-existing allergies (26). The most common allergies are to cow's milk, eggs, peanuts, tree nuts, or wheat. Peanuts, tree nuts, fish, and shellfish commonly cause the most severe reactions. Therefore, parents should not, as often reported, stop offering this kind of food more and more, assuming there is no evidence of allergy. In toddlers and primary school children, however, allergic reactions are intensified by indoor allergens (cat allergens, house dust mites), but also by airborne allergens such as pollen (27). Adolescents mainly suffer from pollen allergies. The allergy risk is primarily determined by genetic factors. Children with at least one first-degree allergic relative such as parents and siblings are considered at risk of allergy (28). However, allergic diseases are also common in many children who do not belong to this risk groups. Besides genetic factors, various environmental conditions such as exhaust fumes or smoke play an important role in the development of allergic diseases (28, 29). Minimizing those factors that irritate the organism is crucial for the primary prevention of allergies. Although harmful environmental influences should be avoided, this is no longer recommended for the potential allergens themselves.
Influence of Lifestyle During Pregnancy and Childhood on Allergy Sensitization
In industrial countries, one in four is suffering from allergy symptoms (13). The correlation of incidence with industrial countries is based on an urban lifestyle, which is associated with intensive hygiene and, consequently, low exposure to microbes such as bacteria, viruses, and fungi (30). Further epidemiological studies show an inverse correlation of hay fever, asthma and wheezing with microbial burden, pet ownership, family size, and infections. Animals in particular, and especially their diversity, are important for allergy protection. The resulting hygiene hypothesis states that early provocation by microbes shapes the developing immune system so that it reacts appropriately (30). Meaning that the immune response is directed against microbes, but not against foreign ones, although harmless environmental antigens. Regarding this, it was shown that resting T lymphocytes of newborns spontaneously activate their Th2 program, which is the basis of atopic reactions (31). This potentially allergic response is shut down if the lymphocytes are exposed to inflammatory inducing factors, as it would occur if provoked by microbes.
Multiple factors influence the development of neonatal immunity among them maternal IgG and cytokines, as well as antigen exposure and numbers of lymphocyte subpopulations and antigen presenting cells (APCs). A developmental trajectory of changes in cell composition of the blood has been demonstrated during the first seven days of life (32). Herein, the number of basophils, plasmacytoid DCs, natural killer cells, and neutrophils are decreased. In contrast, the number of myeloid DCs increased, while many other cell types remained constant. Furthermore, the serum concentration of the soluble immune markers chemokine CXCL10, IL-17A, macrophage-derived chemokine, and IFN-γ were enhanced. The chemokine CCL5, granulocyte colony stimulating factor 2, IL-10, and IL-6 decreased within the first seven days of life. Also other pure developmental features run early in life, like the creation of a diverse B-cell repertoire (33); maturation of the human fetal antibody repertoire requires five months, starting in the last trimester and according to gestational age, ending earliest at two months of age. Also natural IgM antibodies generated from the fetus against a defined set of autoantigens occurs assumed to protect certain structures from autoreactive T cells (34).
On top of that neonatal immunity shows an unambiguous bias toward production of Th2-cell polarizing cytokines (IL-4, IL-5, IL-10) - at least under some circumstances - and suboptimal Th1 cytokine production (IFN-γ, IL-2, and TNF-α) (35) and B cell differentiation as marginal zone B cells are hardly generated until two years of age (36, 37). The suppressed secretion of IFN-γ by Th1 cells was reported to be mediated by enhanced IL-4 transcription and production (38). The chromatin structure of the Th2 cytokines loci is hypo-methylated, which implies rapid transcription accounting for the bias toward Th2 type in neonatal CD4+ T cells (39). In terms of timing, investigations show that the modification of the CpG residues of the Th2 locus are found as early as fetus`s mid-gestation and lasts at least until the first week of life (40). Additionally, several studies reported that environmental co-stimulatory signals in utero affect differentiation of Th cell and mediate subpopulation characteristic profiles of genomes during immune system development (41). Therefore, timing is decisive in imprinting the immune system. However, when monitoring expression of CD40L by neonatal T cells, which needs to be upregulated for optimal T cell responses, its expression is potentially fully developed as shown by stimulation of neonatal CD4+ T cells with SEB (Staphylococcus Enterotoxin B) and monocytes or with Candida albicans-matured or Staphylococcus aureus (S. aureus) SEBneg-matured monocytes (42).
In terms of environmental toxicity, there are adverse effects of neonatal and infant exposure. With many developing organ systems toxic exposure of the fetus in the womb has even more serious consequences (43, 44). For example, exposure to cigarette smoke in utero effects the development of the immature lung and airways with additional impacts on the immune system.
Timing matters also for exposure to microbes. Many studies focus on the hygiene hypothesis postnatal but indeed, intrauterine exposure does already matter. The inclusion of pregnancy data in epidemiological studies on urban and farm environments and the child's immune system clearly showed that the child already reacts prenatally to stimuli: The exposure of the mother on the farm during pregnancy has a strong impact on the immune response of the child after birth (45). In addition, it has been shown that nutritional imbalance of the mother, deficiency as well as abundance, may have a significant impact on immunity in newborns and early life immune maturation (46). Therefore, immunomodulatory cells and cytokines containing human milk prevent respiratory infections as well as development of allergies in neonates and infants (47). In utero, the imprinting could start as early as the second trimester of pregnancy, because at this point the fetus starts to show first signs of immune competence. It is also shown that reinforced microbial contact during pregnancy is more powerful for prevention of allergies than starting at birth or even later (45). However, since the adaptive immune system, which is characterized by memory formation, develops mainly in the third trimester, the imprinting with a possible lifelong "memory" is more likely starting during this period.
The Adaptive Immune System of Early Childhood
The postnatal phase is characterized by rapidly changing environmental and microbial exposures that stimulate immune development, requiring immune regulation to maintain homeostasis (48, 49). Managing such rapidly shifting, diverse functional requirements is at least partially achieved by compartmentalization (50). Analysis of T cells from lymphoid and mucosal tissue from pediatric organ donors in the first two years of life, revealed an early compartmentalization of T cell differentiation and regulation compared to adults. While memory T cells are mainly found in adult tissues, naïve recent thymic emigrants (RTEs) are the main subset in pediatric blood and tissues (50). RTEs are more similar to thymocytes than the mature naïve T cells and are far less abundant in adults (51). As neonatal Th cell compartments present unique properties contributing to the age-dependent balance of Th cell responses, we identified a novel CD31+ subset of naïve CD4+ T cells in infants adenoids (31). This subset expresses intracellularly an unglycosylated isoform of IL-4. These cells are not verifiable in adults and are suspected of being Th2 progenitor cells that are constrained to early age and spontaneously differentiate into mature IL-4 secreting Th2 cells. These cells have been associated with class switching for IgE production by B cells, although it has been shown for adults that T follicular helper cells secreting IL-4 support the class switch (52), very little is known about that in early life. In contrast to adult peripheral blood mononuclear cells, cord blood analysis show qualitative and quantitative variations in immune responses and distinct age-related immune responses are seen up to the age of 18 months, some also display up to 60 months after birth (42, 53, 54). Furthermore, study of cytokine co-expressing multifunctional CD4+ and CD8+ T cells showed that children three or older differed substantially from those aged one- or two-years, implying a developmental switch in differentiation of T cells at that age (55). However, like adults naïve T cells of neonates produce CD45RA isoforms, diversified T cell receptors and common co-stimulators at the cell surface such as CD27 and CD28.
Children growing up on farms are less prone to develop allergy, asthma or even leukemia later in life (56–58), stressing the point that exposing the immune system to bacteria and others within the first years of life leads to a conditioning that affects health and immunity later on. This can also be detrimental, i.e., it was shown that inhaling mold during early childhood did not cause acute clinical symptoms, rather was correlated to asthma development later on (59). Interestingly, the data from farm studies echo those from inner-city children studies. The URECA study is a birth cohort study launched in 2005 in economically deprived urban neighborhoods in inner-city Baltimore, Boston, New York City, and St Louis (60). The household environments of high-risk children starting from the prenatal period to the first three years of life were assessed in this study to determine modifiable risk factors for childhood asthma at seven years of age. Higher indoor/house dust levels of pet (mouse, cat) or pest (cockroach) allergens in infancy correlated with reduced incidence of asthma in high-risk urban children. In the first year of life, the bacterial microbiota in house dust differed between the homes of children who suffered from asthma or not. The excess of number of specific microbes were clearly related to either increased or decreased likelihood of developing asthma, and most of bacterial taxa correlated with the amounts of the indoor allergens associated with asthma risk. In addition, prenatal stress and exposure to tobacco smoke by the mother or depression scores in early life demonstrated to support development of asthma by the age of seven years. This suggests that an exposure to non-toxic environments early in life which contain potential allergens and microbes in abundance have been clearly correlated with reduced risk of asthma and other atopic diseases. Of note, it was demonstrated that the Th2-machinery is switched on in resting and suboptimal activated T cells of neonates unless they get inflammation signals (31). In addition, characteristic production of Th2 cytokines (IL-4, IL-5, IL-10) has been reported for T cells of term and pre-term born (41). Others used strong PMA and Ionomycin stimulation, and demonstrated an enhanced Th1-like cytokine expression (61). In older studies, Th1 responses have been shown to be evident but with a lower magnitude in pre-term and neonates when compared to adults (62). Others could show, that T cells of preterm and mature born neonates initiate far higher frequencies of IL-17 producers than adults upon polyclonal stimulation (63). Also IL-8 (CXCL8) was described to be a signatory cytokine, as already resting naïve T cells of neonates show IL-8 expression that is even further enhanced by polyclonal stimulation (64).
Special Characteristics of Regulatory T Cells in Neonates
Recent study has shown that the ontogenesis of the immune system from fetus to adult does not proceed in a linear manner (65). Instead, it is stratified into layers of different immune cells that develop sequentially from distinct waves of hematopoietic stem cells (HSC). Therefore, T cells of neonates and adults T cells are discussed to have distinct origins. In contrast to T cells from adults, T cells of neonates originate from fetal HSC and display not only shorter T cell receptors (TCR), but also a more restricted T cell repertoire, in addition to an amplified peripheral homeostatic proliferation. Neonatal T cells rapidly differentiate into effector and Treg cells after activation than adult counterparts do, although at the cost of the formation of long-lived memory cells.
Tregs limit unnecessary immune responses, such as to strong Th2 responses that mediate allergic responses (66). They will be induced by bacterial or helminth infections among other things. Fetal naïve CD4+ T cells respond strongly to alloantigen`s, and tend to develop in presence of TGF-β toward Tregs, and thus actively promote self-tolerance (67). Peripheral Tregs account for ∼3% of total CD4+ T cells at birth (68) and these cells persist for an extended period of time (69). High numbers of Tregs - ∼12% of CD4+ T cells - exist in cord blood and ∼8% in neonatal lymph nodes (70, 71). Tregs account for a high proportion (30%–40%) of CD4+ T cells in pediatric tissues but only 1%–10% in adult tissues (50). They also showed that Tregs in human tissues exhibit distinct characteristics independent of age, influenced by CD45RA- phenotypes, together with tissue-specific signals. Furthermore, human neonatal non-differentiated naïve CD4+ T-cells demonstrate a TCR-dependent intrinsic default pathway to differentiate into Treg cells and exert suppressive functions (72). In neonates and infants, they represent an additional regulatory mechanism to prevent allergies and asthma because low numbers relate to enhanced risk to sensitize and develop AD early in life (31). Of note, a lower incidence of circulating Tregs at the time of birth correlates with unfavorable exposures, including maternal smoking and maternal allergy (73, 74). Others showed that Tregs from children are more effective when the mother had worked during pregnancy in a stable (75). Also exposure to non-processed milk had a significant impact leading to an increased number of Tregs in children aged four years, which in turn correlated with a reduced incidence of asthma and allergy (76).
Recommendations for Allergy Prevention in Everyday Life
In the past, recommendations for protection against allergic diseases based on the belief that therapeutic strategies to avoid potent and major allergens are the best way to prevent allergy development. However, with this implementation prevalence of allergic diseases did not decrease but rather increased, likely reflecting a failure of immune tolerance mechanisms. There is mounting evidence that exposure to allergens, optimal in early years of life, is necessary for development of immune tolerance. The HealthNuts study showed that late introduction of egg did not decrease the risk of developing egg allergy (77). The study even suggest introducing cooked eggs between the age of 4–6 month and this may even protective against egg allergy.
Therefore, recommendations and guidelines, e.g., in Germany, changed completely. Furthermore, it is widely debated whether indoor allergens, such as HDM, furry pets, and fungi, are involved in the development of allergy (78), as results on their function as risk factors are often contradictory. Recently published data support a recent shift of prevention strategies from allergen avoidance to tolerance induction (79, 80). This concept of tolerance induction is also important in term of pathophysiological considerations.
Consequently, a number of recommendations have been "liberalized" in recent years, concerning early allergen elimination. HDM allergy is a key contributor to asthma therefore, it was questioned to which extend HDM elimination makes sense as a primary prevention measure. In the 1990s, a simple rule applied: Early exposure to allergens in infancy, e.g., HDM leads to a later allergic sensitization in a dose-dependent manner. On the contrary, results of large intervention studies have shown that although home renovation measures significantly reduced the indoor allergen exposure, these effects were not accompanied by a lower sensitization rate in children (81). For example, in the Manchester cohort (nacMAAS), in which 251 newborns with a positive family history of atopic diseases were followed up after a double-blind, placebo-controlled intervention with mite proof mattress covers, the intervention group showed a significant reduced mite allergen burden (82). However, the number of mite-sensitized children was significantly higher in the intervention group (23%) than in the control group (8.2%). Accordingly, such primary prevention of this kind cannot be recommended and is stated in several international guidelines. This does not, of course, extend to secondary and tertiary preventive measures, where the atopic disease is already established and strong proof of effectiveness remains.
Recommendations concerning pets have also been liberalized and the principle of avoiding allergens has been increasingly abandoned. Therefore, data from 11 European birth cohorts were combined in a meta-analysis and published 2012. In this study, the pet keeping was differentiated with respect to the endpoints allergic sensitization, AD, food allergy, allergic rhinitis and asthma. Furthermore, they showed, that keeping dogs significantly reduced (by 28%) the risk of AD but non-significantly reduced the risk of asthma (83, 84). Keeping cats was also not associated with a likelihood of developing atopic diseases. Scattered studies however, indicate that keeping cats significantly enhanced the likelihood to develop AD especially when belonging to a at-risk group, e.g., when harboring a genetic alteration such as a loss-of-function mutation of filaggrin (85). For this reason, guidelines have been maintained for a stringent a recommendation for at-risk children, although the recommendations are phrased in a more user-oriented way. Therefore, the allergy prevention recommendations suggest that cats should never be purchased again in case of at-risk children. Nutrition can also contribute to the prevention of allergies. In recent years, avoidance of potential allergens during the first month of life has been considered as the best strategy for prevention of allergic diseases. Since the emergence of the hygiene hypothesis, however, this approach has been increasingly questioned (86). It contains that the risk for allergies increases if the immune system is not challenged. Many allergic mothers omit certain foods during pregnancy in concern that their child could also suffer from allergy. Nevertheless, there is no scientifically proven evidence that restricting food choices during pregnancy protects against the development of allergies during childhood. The same applies for the mother`s diet during breastfeeding, where antigens are detectable in breast milk and in very rare cases infants react allergic to these milk components. To date there is no evidence that avoiding potentially allergenic foods such as cow's milk, chicken egg or nuts has a preventive effect on the development of allergies (87). On the contrary, there is even evidence that fish consumption during pregnancy and breastfeeding has a protective influence on atopic disease development in children (88) (see below, Figure 1). Moreover, the benefits of restricting food choices must be weighed very critically against the risk of insufficient nutrient intake.
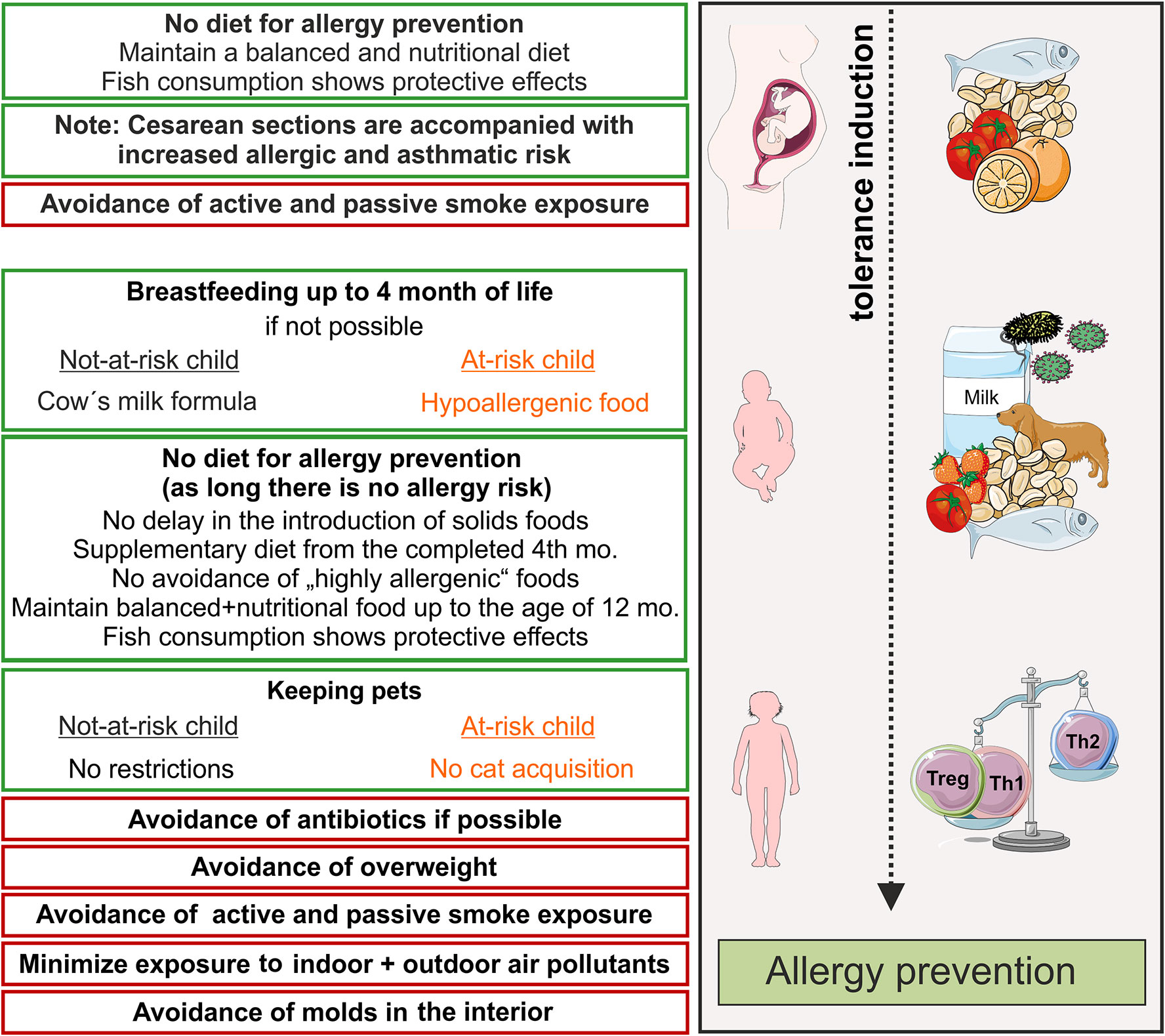
Figure 1 Primary prevention of allergic diseases (asthma, hay fever, atopic dermatitis). Contact to allergens (milk, peanut, fish, house dust mite) and microbes during pregnancy and early life leads to an active training of the immune system and, therefore, induces active immune tolerance. Thereby, allergy mediating T helper cell of type 2 (Th2)-answers are prevented and tolerance mediating regulatory T cells and Th1 cells dominate. Recommendations for primary allergy prevention during pregnancy and childhood. In orange shows recommendations for allergen avoidance in at–risk children, red boxes recommendations for avoidance of risk factors. Symbols are from smart servier medical art 2020 (https://smart.servier.com/). mo, month.
Furthermore, early introduction of food is beneficial but avoidance of food allergens early in life can be harmful because children especially with AD can become sensitized to food allergen by skin exposure. The International Study of Asthma and Allergies in Childhood (ISAAC) Phase III found that the prevalence of AD affects between 7% to 8% children (aged six to seven) and young teenager (aged 13 to 14) (89). Also up to 8% of children are affected from food allergy and its occurrence has been rising during the last 20 years (90). Although the origin of chronic inflammatory AD, which affects the skin, is not known, it is clear that a malfunction of the epithelial skin barrier is involved and subsequently leading to frequently inflamed skin. Due to its occurence early in infancy, it represents often the starting point of an atopic march. The severity of AD is a factor that determines the later development of an allergic disease. Prospective studies of birth cohorts have clearly related early-onset of AD with suffering from asthma and allergic rhinitis at school age. About 40% of AD patients develop a food allergy to peanut, egg, or sesame seed (91, 92).
The “dual-allergen exposure hypothesis” postulates that exposure to food allergens through the frequently inflamed skin in AD may cause allergic sensitization, ultimately provoking an allergy. Herein, the initial malfunctioning is the disrupted skin barrier leading to skin inflammation. In terms of acquiring immune tolerance to food allergens, it can be initiated early in life via oral exposure (93). Healthy skin is a natural barrier – physiological and immunological - not only against invasion of microbes, but also against penetration of environmental toxins and allergens into the body. Defects in the skin barrier can be due to several players among them malfunctioning of terminal epithelial differentiation, insufficiency of antimicrobial peptides (AMPs), modified stratum corneum intercellular lipids, dysbiosis of the skin microbiome and immune maladaptation (94). For epithelial barrier, genetic alterations encoding skin barrier proteins and unusual lipid production or tight junction formation add to its dysregulation and have been linked in AD to the development of food allergies. Today, there is increasing evidence that epicutaneous allergen sensitization is more likely to occur due to an impaired skin barrier (94, 95). It was demonstrated in an murine model using scraping of the skin that skin contact of potential allergens such as ovalbumin (OVA) or peanut proteins lead to the generation of IgE responses specific to egg and to peanut, respectively (96, 97). In another mouse model scratching was mimicked using epicutaneous sensitization with OVA on tape-stripes patched to the skin (98). The treatment conquered the immunological basis for a dramatic response when an additional oral challenge with OVA was given. Upon challenge, enhanced IgE-dependent intestinal mast cell expansion, enhanced concentrations of IL-4 in serum as well as systemic anaphylaxis were monitored. The assumption that the allergic march is causally supported by a malfunction of the skin barrier, which leads to a sensitization against food allergens and ultimately to food allergy, is confirmed from clinical studies. Studies show that newborns with elevated trans epidermal water loss (TEWL) are at a greater risk of having AD at one year of age (99) which was even predictive of developing food allergy in two-year-olds (100). Furthermore, when children in a birth cohort study were exposed to continuous low-dose allergen exposure in the form of peanut oil applied to inflamed skin sites, their risk of having peanut allergy at age five increased (101). The link between AD and food allergy became clear in the HealthNuts study, which found a 11 times higher incidence of peanut allergy in children who developed AD and an 6-fold enhanced risk to develop egg allergy by age one year (102). Usually, the skin barrier is supported by microbiota. A dysbiosis of it adds to an insufficient barrier function of the skin and ultimately to sensitization by epicutaneous allergens. Indeed, almost 90% of AD patients have a colonisation of S. aureus on their skin, which demonstrates clearly a disbiosis. The severity of the disease and its aggravations are associated with increased abundance of S. aureus and reduced local microbial diversity (103). Concluding, the dual allergen exposure hypothesis suggests that the decision whether the immune system is primed to develop allergy or tolerance depends on whether the first exposure to food allergens takes place through the skin or the gut during the first year of life. In addition, sensitization via the skin has been identified to trigger allergic reactions to food or allergic respiratory diseases even when the skin barrier is well intact. However, epicutaneous sensitization still needed an inflammatory event which was mimicked by adjuvant or highly concentrated continuous exposure of the antigen (104). Most of the key measures for prevention were aimed to reduce the risk of AD development by prophylactically protecting the skin barrier soon after birth in newborns with an elevated risk, e.g., supporting the skin by emollients from birth on which the incidence determined 6–8 months later indeed reduced (105, 106). Results of the study showed that the pH of the skin was lower in the emollient group and that the skin microbiome differed to the control group explaining the mechanism of preventative effects of emollients by regaining a "healthy" skin microbiome in high-risk infants (107). Several major studies are currently ongoing to investigate the effectiveness of regular moisturization in protecting the skin barrier from birth on not only to prevent AD development, but also challenge-tested atopic diseases such as food allergy and allergic asthma (108, 109). Attempts have been made to prove that probiotic supplementation during the fetal and infant period of life can prevent development of AD, but so far only little evidence is available (110). Besides some evidence for AD, prove is still missing at all for preventative effects of probiotic supplementation against food allergy or other atopic diseases.
Some randomized controlled studies addressing high-risk infants suffering from severe AD or already established food-sensitization, respectively, prevailed that early introduction of potential allergens in foods might limit the likelihood of developing allergies to peanuts or eggs. In the Prevention of Egg allergy with Tiny amount InTake (PETIT) study of Japanese high-risk infants with AD, development of egg allergy was demonstrated to reduce the risk by 30% compared with a control placebo group when egg powder was introduced early in life (111). Furthermore, orally reinforced aquisition of tolerance to egg allergy can be exploited therapeutically. In addition, a humanized monoclonal antibody (Omalizumab) against the Fc-part of IgE (anti-IgE) binds and neutralizes the IgE-type antibodies which are responsible for triggering the allergic reaction. It could become a preventative therapeutic strategy for solving food allergy, but also as a therapeutic complementation for triggering oral tolerance in egg allergy (112).
Peanut allergy has a potential anaphylactic risk and once acquired it is very difficult to outgrow. In the past some experts suggested that the introduction of foods that contain peanuts should be delayed until the three years of age (113), unfortunately this has contributed in part to a spike in peanut allergies over past few decades. The results of the in 2015 published LEAP ("Learning Early About Peanut") study received extensive publicity (114). The work based on the observation that Jewish children in England had a significantly higher prevalence of peanut allergy than children with the same genetic background living in Israel do. Unlike in England the children growing up in Israel had been exposed to peanut products much earlier. Based on this observation, a clinical intervention study has conducted, where 640 infants with severe eczema, egg allergy, or both consumed or avoided peanuts until 60 months of age. However, 321 instructed children and their families strictly avoided peanut-containing products and 319 children received 6 g peanut protein per week. Using a food provocation test at the age of five years, the prevalence of peanut allergy was significantly reduced in the consumption group (1.9%) compared to the avoidance group (13.7%; p<0.001). The findings of the LEAP study remained one year later in a follow-up study (115). There, all participants avoided any peanuts products for one year to determine whether the protective effect of early peanut intake last in the long term. The data indicate that the child's gastrointestinal tract may develop tolerance (meaning an adjusted intestinal barrier function and its secreted products, luminal digestion of antigens and a present suppressive immune milieu including Tregs (116)) rather than an allergic immune response in the first year of life through regular exposure to food. The work has led to numerous controversial discussions on the early introduction of highly allergenic foods into the complementary diet. Even if the results of the English group are speculative, it should first be taken into account that this is a very specific cohort before transferring it to other countries: only children participated with severe eczema and/or egg allergy. There are still some question marks, whether these results can be transferred to other countries and other populations and whether they are now mature enough to be used as practical recommendations for prevention. However, the National Institute of Allergy and Infectious Diseases (NIAID) in US already developed addendum guidelines for the prevention of peanut allergy, which displayed a significant paradigm shift in early food introduction (117). In order to minimize the risk of peanut allergy, they suggest, that the infants at highest risk (with severe eczema and/or egg allergy) should be introduced to age-appropriate foods containing peanuts as early as at the age of 4-6 months. These are the first guidelines that firmly recommend introduction of peanut-containing food to infants should start at approximately six month of age.
The mechanism behind the increase in food allergies over the past two decades is poorly understood. Therefore, various research focus on the investigation of the hygiene hypothesis, delivery by cesarean section, changes in dietary fat content, Vitamin D deficiency, or insufficiency, darker skin tone (118). Furthermore, late introduction to food proteins as well as exposure to more intact dietary proteins mediated by the usage of gastric acid suppressive medications (antacids) could be explanations for the rise in food allergies (119). In addition, food allergies are often associated with other allergic diseases such as eczema, family history of food allergy also plays a role, as well as immigration to developed counties during infancy, and genetic factor are being investigated (118, 120).
With the discussion about the early introduction of highly allergenic foods such as peanuts, the introduction of hydrolyzed infant formula for the prevention of allergic diseases was questioned. In the US and Europe the current recommendations are that at-risk infants should receive hydrolyzed infant formula if the mothers don´t, or only partly breastfeed them for the first 4 months (121, 122). Several meta-analysis (123, 124) or the German Infant Nutritional Intervention (GINI) study (125) found that high-risk infants who were fed with partially hydrolyzed whey formula or partially hydrolyzed formula showed a reduction of AD incidence and a decreased risk of any atopic manifestation. In contrast, a meta-analysis including 37 intervention studies as well as the Cochrane review published in 2018 showing no consistent evidence that partially or extensively hydrolyzed infant formula reduce the risk of allergic diseases in at-risk children (126, 127). The Australasian Society of Clinical Immunology and Allergy (ASCIA; www.allergy.org.au) already adjusted the current guidelines accordingly and recommend using commercial infant formula until the age of 12 months if an infant is not or only partially breastfeed. However, since different hydrolyzed formulas exist, the allergenicity, tolerance, efficacy and clinical impact also differ. Therefore, every product should be tested. The effects of the routine use of the different hydrolyzed formulas in larger populations of non-exclusively breastfed infants need further investigation to determine the benefits. Adaptation of food for allergy prevention aims, among other things, at an "optimal" microbiome in the gut. However, if confrontations of the organism with pathogenic bacteria are prevented early in life, the risk of asthma and allergies increases (128, 129). For example, administration of antibiotics in the first 6 months of life, increases the incidence of atopic diseases at the age of six (54). Pasteurization of cow's milk kills bacteria. However, the drinking of unprocessed milk during pregnancy protects from allergies and asthma development (129). These examples also show that avoiding allergens is not the best way to prevent allergies.
Probiotics, Prebiotics, and Bacterial Lysates
The above-mentioned examples clearly show in which direction changes in current allergy prevention are going: Away from rigid recommendations to avoid allergens, both in the area of nutrition and pet keeping, and toward environmental conditions that stand for more biodiversity. Therefore, the so-called farm studies fit perfectly into this concept. The early exposure to farm animals in the first year of life and the consumption of unprocessed cow's milk had a significant protective effect on the development of allergic sensitization, allergic rhino conjunctivitis and bronchial asthma (130). Noteworthy, in this context epidemiological data indicate a higher risk of developing asthma after a caesarean section (131). Similar, others observed a reduced risk of allergic diseases in families with an anthroposophical lifestyle, which was characterized, among other things, by a lower use of antibiotics (132).
Allergy preventive recommendations become more and more enumerations about which measures do not show any allergy preventing effects (avoidance of pet keeping) or which measures are to be omitted (caesarean section, early antibiotic administration) but in the end these measures do not actively induce tolerance (Figure 1). Therefore, it would be desirable to have recommendations based on interventions that actively promote tolerance induction.
From the farm studies, it has become clear, that the microbiota plays a key role promoting tolerance early in life. During the first three years, the diversity of the gut microbiome is built up. Lack of microbial seeding at birth by cesarean section was proposed as a cause that influence the increase in asthma and allergic diseases (133). During labor and vaginal delivery, the neonate acquires the vaginal and fecal microbiota of the mother. This microbial exposure also helps to rebalance the neonate's immune system that has a larger fraction of Tregs and a higher Th2:Th1 ratio. Results from an Australian study with nearly 500,000 participants shows, that neonates born by emergency cesarean section had a higher rate of metabolic disorders, such as obesity or diabetes (134). However, there was no substantial correlation between cesarean section and the incidence of allergic diseases in other findings (135). Variations in the results could be due to factors that may mediate or change the impact of microbial seeding. For instance, as already discussed, breastfeeding has a protective influence on the early onset of allergic diseases such as asthma, AD and food allergies (136). Breast milk contains beneficial microbes that are transmitted from mother to child (137) and one of the first bacteria is Bifidobacterium longum (B. longum) which is colonizing the gut by a fast expansion. B. longum is an anaerobic, Gram-positive microorganism, which is considered to be a beneficial commensal (138). It is assumed that it spreads rapidly in the intestine to function as a placeholder to outcompete colonization of aggressive bacteria such as S. aureus. Of note, S. aureus is the most important example of a commensal bacteria becoming an aggressive antibiotic-resistant pathogen (139). In a mouse model for atopic airway disease, B. longum has been shown to induce Tregs which correlates with reduced airway atopic reactions (140). How B. longum mechanistically impacts on the human T cell responses is not known, so far. Also other beneficially acting bacteria such as Lactobacillus have been addressed (141).
As already mentioned, if confrontations of the organism with pathogenic bacteria are prevented early in life, the risk of asthma and allergies increases (128, 129). This assumption was investigated in a randomized trial in which bacterial lysates were administered orally to approximately 600 infants with at least one parent suffering from allergy (142). The applied lysates, which were given during the first two decades of life, were of heat‐killed Escherichia coli and Enterococcus faecalis or just placebo. At school age, neither development of AD, asthma nor allergic rhinitis were affected.
Accumulating data on this subject have led to the concept that an early administration of probiotics, prebiotics or bacterial lysates might be beneficial. Despite a large number of clinical studies, especially on the administration of probiotics, the data available are not yet sufficient to make generally valid recommendations. In some studies, effects of probiotic administration on atopic eczema have been observed, but these cannot be reproduced with identical preparations in other populations (143, 144). There might be protective effects in subgroups, like for children after caesarean section. Apparently, it is necessary to administer them during pregnancy in order to achieve preventive effects (145). In a meta-analysis, 29 studies on probiotic administration were summarized and revealed a 29% reduction of the eczema risk if supplementation was already given during pregnancy. When administered exclusively postnatal, this effect was lower with a 17% reduction and no longer statistically significant. Protective effects of primary prevention with probiotics on asthma, allergic rhino conjunctivitis or allergic sensitization were not observed.
Another meta-analysis assessed the impact of supplementation of prebiotics in infants as well as pregnant and breastfeeding women (146). In summary, they find insufficient proof to substantiate or refute a protective or a detrimental effect from randomized or observational studies, but cautiously suggest that prebiotics may minimize the likelihood of persistent wheezing in infants. Nevertheless, the authors claim that the safety on these suggestions is indeed limited because of the possibility of bias, indirectness of the evidence, and the inaccuracy due to small number of events of the estimated results. Two more clinical trials were also performed in addition to this meta-analysis (147, 148). One study reported early-life nutrition with prebiotics had an impact on the structure of the infant intestinal microbiota with a possible correlation between microbiota activity and the development of eczema in early life (147). Data published on a study addressing supplementation with galacto-oligosaccharide/polydextrose (GOS/PDX) for 48 weeks of high-atopy-risk infants due to parental history of allergic diseases did not show an impact on AD up to 96 weeks of age (148). However, in infants with GOS/PDX supplementation the incidence of respiratory infections up to 48 weeks was smaller than in infants fed with standard formula (p = 0.023), but was detectable for not more than 96 weeks. The colonization with Bifidobacterium and Clostridium cluster I increased over time in the GOS/PDX supplemented group. Bifidobacterium had a protective effect in respiratory infection; whereas Clostridium cluster I was linked with atopy protection.
In summary, there is still insufficient compelling data from human infant studies to support the beneficial impact of prebiotic supplementation for prevention of allergies. Also triggering the intestinal immune system with bacterial lysates does not show prevention of AD. Thus, the allergy preventive effects of intestinal colonization and immune modulation mediated by bacterial compounds early in life are by far not yet understood.
Omega-3 Fatty Acid and Personalized Allergy Prevention
Studies performed to date indicate that higher intake of fish, sources of long chain omega-3 (n-3) polyunsaturated fatty acids (PUFA), during pregnancy is associated with lower risk of allergy development in infants and children (149). A Finnish study reported that a reduced intake of n-3 PUFAs during pregnancy increases the chances of developing asthma in children aged five years (150). Interestingly, children suffering from allergic diseases often had lower than normal amounts of the n-3 PUFAs EPA (eicosapentaenoic acid) and DHA (docosahexaenoic acid) in the cord blood (151). Furthermore, studies have reported that supplying pregnant women with fish oil is linked to immunologic changes in cord blood (152). These immunological effects might modify allergic sensitization and the risk to develop allergic diseases. Additional, since 16-year olds showed a substantial reduction in asthma-related diagnosis when their mothers were fish oil supplemented during late pregnancy, long term consequences of any immunological changes were suggested to have occurred in pregnancy and early life. A large standardized double-blinded placebo-controlled investigation found that intake of fish oil during pregnancy significantly reduces recurrent wheeze and asthma in 3- to 5-year olds children (153), whereby a total of 136 of 695 children (19.6%) showed these symptoms. The risk was significantly lower in the intervention group with 16.9% than in the placebo group with 23.7% (p = 0.035). One interesting finding was that the protective impact of maternal EPA plus DHA on children with recurrent wheezing or asthma was observed predominantly in the subgroup of children whose mothers had the lowest blood levels of these two omega-3 fatty acids at the beginning of the study. This indication could point toward a more personalized strategy of allergy prevention in the future. Similar approaches of personalized prevention, which stratify children into different risk groups, would be desirable for future work.
Taken together, early exposure to the n-3 PUFAs in pregnancy can be a strategy to induce immune effects that prevent infant and childhood allergic disease. Nevertheless, the available data are not completely consistent. In order to characterize more precisely the immunological and clinical effects and to evaluate their protective effects and persistence, further studies on the increased supply of long chain n-3 PUFA during pregnancy, and infancy are required.
Conclusion
Just how to prevent atopic diseases in childhood, is a "billion dollar" question as it may be prevention for a lifetime. On the one hand, during the initial allergen exposure, especially the prevailing environmental conditions have a great influence by modifying the immune responses. Therefore, as a logical consequence, pregnant women and parents of infants are advised to limit risk factors. These include smoking, pollutants, and any unfavorable proinflammatory exposures. On the other hand, it includes a balanced and nutritional diet. To achieve more tolerogenic conditions during pregnancy and early life is at the heart of sensible allergy prevention and there is growing evidence that exposure to allergens is necessary for development of immune tolerance. According to latest studies administering antibiotics too early or the usage of hydrolyzed cow´s milk formula does not prevent allergic disease in high at-risk infants. Similarly, the observation that the early introduction of peanut-containing foods could significantly reduce the development of a later peanut allergy in British children will change recommendations for the introduction of complementary foods. The recommendations on breastfeeding over the first four months, avoiding environmental tobacco smoke, and avoidance of overweight will remain unchanged. Studies from tolerance induction in established allergic disease underline the significance of Treg cells. Optimizing the environment especially during the last trimester of pregnancy and first months of life to favour these regulatory pathways represents a good strategy to prevent allergy development. However, knowledge about induction and functioning of neonatal and pediatric Treg cells are scarce. As many of the approaches would have broader biological impact, improved understanding of early life immune responses combined with determinants of atopic diseases will be of interest. This offers a broader perspective for strategies to prevent allergic disorders such as early administration of probiotics, prebiotics, bacterial lysates, but also polyunsaturated omega-3 oils and nutrients with anti-inflammatory properties.
The precise time window of possible successful interventions for allergic protection must still be determined and mechanistically understood. Knowledge of this timeframe and the mechanisms for implementing tolerance to potential allergens could make it possible to guide the immune system in a short, defined time interval with anti-inflammatory nutrients. Thus, a much better understanding of the functioning of the neonatal and pediatric immune system is necessary to improve measures to prevent atopic disease.
Author Contributions
MB-W, MP, and AA wrote the manuscript. All authors read the manuscript and provided important intellectual input to the manuscript. The corresponding author had full access to the data and had final responsibility for the decision to submit for publication. All authors contributed to the article and approved the submitted version.
Funding
The work was supported by DFG Br 1860/12-1.
Conflict of Interest
The authors declare that the research was conducted in the absence of any commercial or financial relationships that could be construed as a potential conflict of interest.
References
1. Pawankar R. Allergic diseases and asthma: A global public health concern and a call to action. World Allergy Organ J (2014) 7:12. doi: 10.1186/1939-4551-7-12
2. Wahn U. What drives the allergic march? Allergy (2000) 55:591–9. doi: 10.1034/j.1398-9995.2000.00111.x
3. Actor JK. 7 - Adaptive Immune Response and Hypersensitivity. In: Actor JK, editor. Elsevier’s Integrated Review Immunology and Microbiology (Second Edition). Philadelphia: W.B. Saunders (2012). p. 53–9.
4. Akdis M, Akdis CA. Therapeutic manipulation of immune tolerance in allergic disease. Nat Rev Drug Discovery (2009) 8:645–60. doi: 10.1038/nrd2653
5. Durham SR, Emminger W, Kapp A, Colombo G, de Monchy JG, Rak S, et al. Long-term clinical efficacy in grass pollen–induced rhinoconjunctivitis after treatment with SQ-standardized grass allergy immunotherapy tablet. J Allergy Clin Immunol (2010) 125:131–138.e7. doi: 10.1016/j.jaci.2009.10.035
6. Cavkaytar O, Akdis CA, Akdis M. Modulation of immune responses by immunotherapy in allergic diseases. Curr Opin Pharmacol (2014) 17:30–7. doi: 10.1016/j.coph.2014.07.003
7. Krogulska A, Borowiec M, Polakowska E, Dynowski J, Młynarski W, Wasowska-Królikowska K. FOXP3, IL-10, and TGF-β Genes Expression in Children with IgE-Dependent Food Allergy. J Clin Immunol (2011) 31:205–15. doi: 10.1007/s10875-010-9487-1
8. Karlsson MR, Rugtveit J, Brandtzaeg P. Allergen-responsive CD4+CD25+ Regulatory T Cells in Children who Have Outgrown Cow’s Milk Allergy. J Exp Med (2004) 199:1679–88. doi: 10.1084/jem.20032121
9. Shreffler WG, Wanich N, Moloney M, Nowak-Wegrzyn A, Sampson HA. Association of allergen-specific regulatory T cells with the onset of clinical tolerance to milk protein. J Allergy Clin Immunol (2009) 123:43–52.e7. doi: 10.1016/j.jaci.2008.09.051
10. Schäfer T, Bauer C-P, Beyer K, Bufe A, Friedrichs F, Gieler U, et al. S3-Guideline on allergy prevention: 2014 update. Allergo J Int (2014) 23:186–99. doi: 10.1007/s40629-014-0022-4
11. Brunner-Weinzierl M, Kopp M. Paradigmenwechsel in der Allergieprävention: Paradigm shift in allergy prevention. Monatsschrift Kinderheilkunde Organ der Deutschen Gesellschaft für Kinderheilkunde (2018) 166:708–13. doi: 10.1007/s00112-018-0512-4
12. Thomsen SF. Epidemiology and natural history of atopic diseases. Eur Clin Respir J (2015) 2(1):24642. doi: 10.3402/ecrj.v2.24642
13. Pawankar R, Canonica GW, Holgate S, Lockey R. WAO White Book on Allergy: Update 2013 Vol. 2013. Milwaukee, Wisconsin, USA: World Allergy Organization (2013).
14. Downs SH, Marks GB, Sporik R, Belosouva EG, Car NG, Peat JK. Continued increase in the prevalence of asthma and atopy. Arch Dis Child (2001) 84:20–3. doi: 10.1136/adc.84.1.20
15. Stensen L, Thomsen SF, Backer V. Change in prevalence of atopic dermatitis between 1986 and 2001 among children. Allergy Asthma Proc (2008) 29:392–6. doi: 10.2500/aap.2008.29.3139
16. Ninan TK, Russell G. Respiratory symptoms and atopy in Aberdeen schoolchildren: evidence from two surveys 25 years apart. BMJ (1992) 304:873–5. doi: 10.1136/bmj.304.6831.873
17. Bloom B, Cohen RA, Freeman G. Summary health statistics for U.S. children: National Health Interview Survey, 2010. Vital Health Stat 10 (2011) 250:1–80.
18. Zar HJ, Ehrlich RI, Workman L, Weinberg EG. The changing prevalence of asthma, allergic rhinitis and atopic eczema in African adolescents from 1995 to 2002. Pediatr Allergy Immunol (2007) 18:560–5. doi: 10.1111/j.1399-3038.2007.00554.x
19. Simon AK, Hollander GA, McMichael A. Evolution of the immune system in humans from infancy to old age. Proc R Soc B (2015) 282:20143085. doi: 10.1098/rspb.2014.3085
20. Kelleher MM, Tran L, Boyle RJ. Prevention of food allergy – skin barrier interventions. Allergol Int (2020) 69:3–10. doi: 10.1016/j.alit.2019.10.005
21. Gupta RS, Springston EE, Warrier MR, Smith B, Kumar R, Pongracic J, et al. The prevalence, severity, and distribution of childhood food allergy in the United States. PEDIATRICS (2011) 128:e9–17. doi: 10.1542/peds.2011-0204
22. Wang J. Management of the patient with multiple food allergies. Curr Allergy Asthma Rep (2010) 10:271–7. doi: 10.1007/s11882-010-0116-0
23. Park JH, Ahn SS, Sicherer SH. Prevalence of Allergy to Multiple Versus Single Foods in a Pediatric Food Allergy Referral Practice. J Allergy Clin Immunol (2010) 125:AB216. doi: 10.1016/j.jaci.2009.12.843
24. Christie L, Hine RJ, Parker JG, Burks W. Food allergies in children affect nutrient intake and growth. J Am Diet Assoc (2002) 102:1648–51. doi: 10.1016/s0002-8223(02)90351-2
25. Sicherer SH, Noone SA, Muñoz-Furlong A. The impact of childhood food allergy on quality of life. Ann Allergy Asthma Immunol (2001) 87:461–4. doi: 10.1016/S1081-1206(10)62258-2
26. Savage JH, Matsui EC, Skripak JM, Wood RA. The natural history of egg allergy. J Allergy Clin Immunol (2007) 120:1413–7. doi: 10.1016/j.jaci.2007.09.040
27. Salo PM, Sever ML, Zeldin DC. Indoor allergens in school and day care environments. J Allergy Clin Immunol (2009) 124:185–92.e9. doi: 10.1016/j.jaci.2009.05.012
28. Ownby D. Environmental factors versus genetic determinants of childhood inhalant allergies. J Allergy Clin Immunol (1990) 86:279–87. doi: 10.1016/S0091-6749(05)80088-0
29. Alkotob SS, Cannedy C, Harter K, Movassagh H, Paudel B, Prunicki M, et al. Advances and novel developments in environmental influences on the development of atopic diseases. Allergy (2020) 75(12):3077–86. doi: 10.1111/all.14624
30. Stiemsma L, Reynolds L, Turvey S, Finlay B. The hygiene hypothesis: Current perspectives and future therapies. ITT (2015) 4:143–57. doi: 10.2147/ITT.S61528
31. Hebel K, Weinert S, Kuropka B, Knolle J, Kosak B, Jorch G, et al. CD4 + T Cells from Human Neonates and Infants Are Poised Spontaneously To Run a Nonclassical IL-4 Program. J.I. (2014) 192:5160–70. doi: 10.4049/jimmunol.1302539
32. Lee AH, Shannon CP, Amenyogbe N, Bennike TB, Diray-Arce J, Idoko OT, et al. Dynamic molecular changes during the first week of human life follow a robust developmental trajectory. Nat Commun (2019) 10:350. doi: 10.1038/s41467-019-08794-x
33. Schroeder HW, Zhang L, Philips JB. Slow, programmed maturation of the immunoglobulin HCDR3 repertoire during the third trimester of fetal life. Blood (2001) 98:2745–51. doi: 10.1182/blood.V98.9.2745
34. Merbl Y, Zucker-Toledano M, Quintana FJ, Cohen IR. Newborn humans manifest autoantibodies to defined self molecules detected by antigen microarray informatics. J Clin Invest (2007) 117:712–8. doi: 10.1172/JCI29943
35. Chen N, Field EH. Enhanced type 2 and diminished type 1 cytokines in neonatal tolerance. Transplantation (1995) 59:933–41. doi: 10.1097/00007890-199504150-00002
36. Mond J. T cell independent antigens. Curr Opin Immunol (1995) 7:349–54. doi: 10.1016/0952-7915(95)80109-X
37. Martin F, Kearney JF. Marginal-zone B cells. Nat Rev Immunol (2002) 2:323–35. doi: 10.1038/nri799
38. Zaghouani H, Hoeman CM, Adkins B. Neonatal immunity: Faulty T-helpers and the shortcomings of dendritic cells. Trends Immunol (2009) 30:585–91. doi: 10.1016/j.it.2009.09.002
39. Webster RB, Rodriguez Y, Klimecki WT, Vercelli D. The Human IL-13 Locus in Neonatal CD4 + T Cells Is Refractory to the Acquisition of a Repressive Chromatin Architecture. J Biol Chem (2006) 282:700–9. doi: 10.1074/jbc.M609501200
40. Yoshimoto M, Yoder MC, Guevara P, Adkins B, Knott JG. The Murine Th2 Locus Undergoes Epigenetic Modification in the Thymus during Fetal and Postnatal Ontogeny. PloS One (2013) 8:e51587. doi: 10.1371/journal.pone.0051587
41. Basha S, Surendran N, Pichichero M. Immune responses in neonates. Expert Rev Clin Immunol (2014) 10:1171–84. doi: 10.1586/1744666X.2014.942288
42. Vogel K, Pierau M, Arra A, Lampe K, Schlueter D, Arens C, et al. Developmental induction of human T-cell responses against Candida albicans and Aspergillus fumigatus. Sci Rep (2018) 8:327. doi: 10.1038/s41598-018-35161-5
43. Noakes PS. Maternal smoking is associated with impaired neonatal toll-like-receptor-mediated immune responses. Eur Respir J (2006) 28:721–9. doi: 10.1183/09031936.06.00050206
44. Noakes PS, Holt PG, Prescott SL. Maternal smoking in pregnancy alters neonatal cytokine responses. Allergy (2003) 58:1053–8. doi: 10.1034/j.1398-9995.2003.00290.x
45. Ege MJ, Bieli C, Frei R, van Strien RT, Riedler J, Ublagger E, et al. Prenatal farm exposure is related to the expression of receptors of the innate immunity and to atopic sensitization in school-age children. J Allergy Clin Immunol (2006) 117:817–23. doi: 10.1016/j.jaci.2005.12.1307
46. Palmer AC. Nutritionally Mediated Programming of the Developing Immune System. Adv Nutr (2011) 2:377–95. doi: 10.3945/an.111.000570
47. Bryan D-L, Hart PH, Forsyth KD, Gibson RA. Immunomodulatory constituents of human milk change in response to infant bronchiolitis. Pediatr Allergy Immunol (2007) 18:495–502. doi: 10.1111/j.1399-3038.2007.00565.x
48. MacGillivray DM, Kollmann TR. The Role of Environmental Factors in Modulating Immune Responses in Early Life. Front Immunol (2014) 5:434. doi: 10.3389/fimmu.2014.00434
49. Kollmann TR, Kampmann B, Mazmanian SK, Marchant A, Levy O. Protecting the Newborn and Young Infant from Infectious Diseases: Lessons from Immune Ontogeny. Immunity (2017) 46:350–63. doi: 10.1016/j.immuni.2017.03.009
50. Thome JJ, Bickham KL, Ohmura Y, Kubota M, Matsuoka N, Gordon C, et al. Early-life compartmentalization of human T cell differentiation and regulatory function in mucosal and lymphoid tissues. Nat Med (2016) 22:72–7. doi: 10.1038/nm.4008
51. Fink PJ. The Biology of Recent Thymic Emigrants. Annu Rev Immunol (2013) 31:31–50. doi: 10.1146/annurev-immunol-032712-100010
52. Kemeny DM. The role of the T follicular helper cells in allergic disease. Cell Mol Immunol (2012) 9:386–9. doi: 10.1038/cmi.2012.31
53. Vosters O, Lombard C, André F, Sana G, Sokal EM, Smets F. The interferon-alpha and interleukin-10 responses in neonates differ from adults, and their production remains partial throughout the first 18 months of life. Clin Exp Immunol (2010) 162:494–9. doi: 10.1111/j.1365-2249.2010.04267.x
54. Schmiedeberg K, Krause H, Röhl F-W, Hartig R, Jorch G, Brunner-Weinzierl MC, et al. T Cells of Infants Are Mature, but Hyporeactive Due to Limited Ca2+ Influx. PloS One (2016) 11:e0166633. doi: 10.1371/journal.pone.0166633
55. Knolle J, Pierau M, Hebel K, Lampe K, Jorch G, Kropf S, et al. Children From the Age of Three Show a Developmental Switch in T-Cell Differentiation. Front Immunol (2020) 11:1640. doi: 10.3389/fimmu.2020.01640
56. Illi S, Depner M, Genuneit J, Horak E, Loss G, Strunz-Lehner C, et al. Protection from childhood asthma and allergy in Alpine farm environments-the GABRIEL Advanced Studies. J Allergy Clin Immunol (2012) 129:1470–7.e6. doi: 10.1016/j.jaci.2012.03.013
57. Jatzlauk G, Bartel S, Heine H, Schloter M, Krauss-Etschmann S. Influences of environmental bacteria and their metabolites on allergies, asthma, and host microbiota. Allergy (2017) 72:1859–67. doi: 10.1111/all.13220
58. Ege MJ, Mayer M, Normand A-C, Genuneit J, Cookson, William OCM, et al. Exposure to environmental microorganisms and childhood asthma. N Engl J Med (2011) 364:701–9. doi: 10.1056/NEJMoa1007302
59. Karvonen AM, Hyvärinen A, Korppi M, Haverinen-Shaughnessy U, Renz H, Pfefferle PI, et al. Moisture damage and asthma: a birth cohort study. PEDIATRICS (2015) 135:e598–606. doi: 10.1542/peds.2014-1239
60. O’Connor GT, Lynch SV, Bloomberg GR, Kattan M, Wood RA, Gergen PJ, et al. Early-life home environment and risk of asthma among inner-city children. J Allergy Clin Immunol (2018) 141:1468–75. doi: 10.1016/j.jaci.2017.06.040
61. Halonen M, Lohman IC, Stern DA, Spangenberg A, Anderson D, Mobley S, et al. Th1/Th2 patterns and balance in cytokine production in the parents and infants of a large birth cohort. J.I. (2009) 182:3285–93. doi: 10.4049/jimmunol.0711996
62. Marchant A, Goldman M. T cell-mediated immune responses in human newborns: Ready to learn? Clin Exp Immunol (2005) 141:10–8. doi: 10.1111/j.1365-2249.2005.02799.x
63. Black A, Bhaumik S, Kirkman RL, Weaver CT, Randolph DA. Developmental regulation of Th17-cell capacity in human neonates. Eur J Immunol (2012) 42:311–9. doi: 10.1002/eji.201141847
64. Gibbons D, Fleming P, Virasami A, Michel M-L, Sebire NJ, Costeloe K, et al. Interleukin-8 (CXCL8) production is a signatory T cell effector function of human newborn infants. Nat Med (2014) 20:1206–10. doi: 10.1038/nm.3670
65. Rudd BD. Neonatal T Cells: A Reinterpretation. Annu Rev Immunol (2020) 38:229–47. doi: 10.1146/annurev-immunol-091319-083608
66. Pierau M, Lingel H, Vogel K, Arra A, Brunner-Weinzierl MC. CTLA-4-competent conventional T cells back up regulatory T cells to restrain memory T-helper type 2 cell responses. Allergy (2020) 75:684–7. doi: 10.1111/all.14042
67. Mold JE, Venkatasubrahmanyam S, Burt TD, Michaelsson J, Rivera JM, Galkina SA, et al. Fetal and Adult Hematopoietic Stem Cells Give Rise to Distinct T Cell Lineages in Humans. Science (2010) 330:1695–9. doi: 10.1126/science.1196509
68. Takahata Y, Nomura A, Takada H, Ohga S, Furuno K, Hikino S, et al. CD25+CD4+ T cells in human cord blood: An immunoregulatory subset with naive phenotype and specific expression of forkhead box p3 (Foxp3) gene. Exp Hematol (2004) 32:622–9. doi: 10.1016/j.exphem.2004.03.012
69. Burlingham WJ, Grailer AP, Heisey DM, Claas FH, Norman D, Mohanakumar T, et al. The Effect of Tolerance to Noninherited Maternal HLA Antigens on the Survival of Renal Transplants from Sibling Donors. N Engl J Med (1998) 339:1657–64. doi: 10.1056/NEJM199812033392302
70. Michaëlsson J, Mold JE, McCune JM, Nixon DF. Regulation of T Cell Responses in the Developing Human Fetus. J.I. (2006) 176:5741–8. doi: 10.4049/jimmunol.176.10.5741
71. Burt TD. Fetal Regulatory T Cells and Peripheral Immune Tolerance In Utero: Implications for Development and Disease. Am J Reprod Immunol (2013) 69:346–58. doi: 10.1111/aji.12083
72. Wang G, Miyahara Y, Guo Z, Khattar M, Stepkowski SM, Chen W. “Default” Generation of Neonatal Regulatory T Cells. J.I. (2010) 185:71–8. doi: 10.4049/jimmunol.0903806
73. Hinz D, Bauer M, Röder S, Olek S, Huehn J, Sack U, et al. Cord blood Tregs with stable FOXP3 expression are influenced by prenatal environment and associated with atopic dermatitis at the age of one year. Allergy (2012) 67:380–9. doi: 10.1111/j.1398-9995.2011.02767.x
74. Fu Y, Lou H, Wang C, Lou W, Wang Y, Zheng T, et al. T cell subsets in cord blood are influenced by maternal allergy and associated with atopic dermatitis. Pediatr Allergy Immunol (2013) 24:178–86. doi: 10.1111/pai.12050
75. Schaub B, Liu J, Höppler S, Schleich I, Huehn J, Olek S, et al. Maternal farm exposure modulates neonatal immune mechanisms through regulatory T cells. J Allergy Clin Immunol (2009) 123:774–82.e5. doi: 10.1016/j.jaci.2009.01.056
76. Lluis A, Depner M, Gaugler B, Saas P, Casaca VI, Raedler D, et al. Increased regulatory T-cell numbers are associated with farm milk exposure and lower atopic sensitization and asthma in childhood. J Allergy Clin Immunol (2014) 133:551–9.e10. doi: 10.1016/j.jaci.2013.06.034
77. Koplin JJ, Osborne NJ, Wake M, Martin PE, Gurrin LC, Robinson MN, et al. Can early introduction of egg prevent egg allergy in infants? A population-based study. J Allergy Clin Immunol (2010) 126:807–13. doi: 10.1016/j.jaci.2010.07.028
78. Lau S, Wahn U. Pets—good or bad for individuals with atopic predisposition? J Allergy Clin Immunol (2003) 112:263–4. doi: 10.1067/mai.2003.1668
79. Hamelmann E, Beyer K, Gruber C, Lau S, Matricardi PM, Nickel R, et al. Primary prevention of allergy: Avoiding risk or providing protection? Clin Exp Allergy (2008) 38:233–45. doi: 10.1111/j.1365-2222.2007.02901.x
80. Lai CL, Campbell DE, Palmer DJ, Makrides M, Santner-Nanan B, Gold M, et al. Longitudinal egg-specific regulatory T and B cell development: Insights from primary prevention clinical trials examining the timing of egg introduction. Allergy (2020). doi: 10.1111/all.14621
81. Maas T, Kaper J, Sheikh A, Knottnerus JA, Wesseling G, Dompeling E, et al. Mono and multifaceted inhalant and/or food allergen reduction interventions for preventing asthma in children at high risk of developing asthma. Cochrane Database Syst Rev (2009) 113:650. doi: 10.1002/14651858.CD006480.pub2
82. Woodcock A, Lowe LA, Murray CS, Simpson BM, Pipis SD, Kissen P, et al. Early Life Environmental Control. Am J Respir Crit Care Med (2004) 170:433–9. doi: 10.1164/rccm.200401-083OC
83. Lødrup Carlsen KC, Roll S, Carlsen K-H, Mowinckel P, Wijga AH, Brunekreef B, et al. Does Pet Ownership in Infancy Lead to Asthma or Allergy at School Age? Pooled Analysis of Individual Participant Data from 11 European Birth Cohorts. PloS One (2012) 7:e43214. doi: 10.1371/journal.pone.0043214
84. Pelucchi C, Galeone C, Bach J-F, La Vecchia C, Chatenoud L. Pet exposure and risk of atopic dermatitis at the pediatric age: A meta-analysis of birth cohort studies. J Allergy Clin Immunol (2013) 132:616–622.e7. doi: 10.1016/j.jaci.2013.04.009
85. Bisgaard H, Simpson A, Palmer CN, Bønnelykke K, Mclean I, Mukhopadhyay S, et al. Gene-Environment Interaction in the Onset of Eczema in Infancy: Filaggrin Loss-of-Function Mutations Enhanced by Neonatal Cat Exposure. PloS Med (2008) 5:e131. doi: 10.1371/journal.pmed.0050131
86. Strachan DP. Hay fever, hygiene, and household size. BMJ (1989) 299:1259–60. doi: 10.1136/bmj.299.6710.1259
87. Sausenthaler S, Heinrich J, Koletzko S. Early diet and the risk of allergy: what can we learn from the prospective birth cohort studies GINIplus and LISAplus? Am J Clin Nutr (2011) 94:2012S–7S. doi: 10.3945/ajcn.110.001180
88. Goksör E, Alm B, Pettersson R, Möllborg P, Erdes L, Åberg N, et al. Early fish introduction and neonatal antibiotics affect the risk of asthma into school age. Pediatr Allergy Immunol (2013) 24:339–44. doi: 10.1111/pai.12078
89. Mallol J, Crane J, von Mutius E, Odhiambo J, Keil U, Stewart A. The International Study of Asthma and Allergies in Childhood (ISAAC) Phase Three: a global synthesis. Allergol Immunopathol (Madr) (2013) 41:73–85. doi: 10.1016/j.aller.2012.03.001
90. Sicherer SH, Sampson HA. Food allergy: Epidemiology, pathogenesis, diagnosis, and treatment. J Allergy Clin Immunol (2014) 133:291–307; quiz 308. doi: 10.1016/j.jaci.2013.11.020
91. Saunes M, Øien T, Dotterud CK, Romundstad PR, Storrø O, Holmen TL, et al. Early eczema and the risk of childhood asthma: a prospective, population-based study. BMC Pediatr (2012) 12:168. doi: 10.1186/1471-2431-12-168
92. von Kobyletzki LB, Bornehag C-G, Hasselgren M, Larsson M, Lindström CB, Svensson Å. Eczema in early childhood is strongly associated with the development of asthma and rhinitis in a prospective cohort. BMC Dermatol (2012) 12:11. doi: 10.1186/1471-5945-12-11
93. Lack G. Epidemiologic risks for food allergy. J Allergy Clin Immunol (2008) 121:1331–6. doi: 10.1016/j.jaci.2008.04.032
94. Kim BE, Leung DY. Significance of Skin Barrier Dysfunction in Atopic Dermatitis. Allergy Asthma Immunol Res (2018) 10:207–15. doi: 10.4168/aair.2018.10.3.207
95. Lack G. Update on risk factors for food allergy. J Allergy Clin Immunol (2012) 129:1187–97. doi: 10.1016/j.jaci.2012.02.036
96. Strid J, Hourihane J, Kimber I, Callard R, Strobel S. Disruption of the stratum corneum allows potent epicutaneous immunization with protein antigens resulting in a dominant systemic Th2 response. Eur J Immunol (2004) 34:2100–9. doi: 10.1002/eji.200425196
97. Spergel JM, Mizoguchi E, Brewer JP, Martin TR, Bhan AK, Geha RS. Epicutaneous sensitization with protein antigen induces localized allergic dermatitis and hyperresponsiveness to methacholine after single exposure to aerosolized antigen in mice. J Clin Invest (1998) 101:1614–22. doi: 10.1172/JCI1647
98. Bartnikas LM, Gurish MF, Burton OT, Leisten S, Janssen E, Oettgen HC, et al. Epicutaneous sensitization results in IgE-dependent intestinal mast cell expansion and food-induced anaphylaxis. J Allergy Clin Immunol (2013) 131:451–60.e1-6. doi: 10.1016/j.jaci.2012.11.032
99. Kelleher M, Dunn-Galvin A, Hourihane JO, Murray D, Campbell LE, McLean WH, et al. Skin barrier dysfunction measured by transepidermal water loss at 2 days and 2 months predates and predicts atopic dermatitis at 1 year. J Allergy Clin Immunol (2015) 135:930–5.e1. doi: 10.1016/j.jaci.2014.12.013
100. Kelleher MM, Dunn-Galvin A, Gray C, Murray DM, Kiely M, Kenny L, et al. Skin barrier impairment at birth predicts food allergy at 2 years of age. J Allergy Clin Immunol (2016) 137:1111–6.e8. doi: 10.1016/j.jaci.2015.12.1312
101. Lack G, Fox D, Northstone K, Golding J. Factors associated with the development of peanut allergy in childhood. N Engl J Med (2003) 348:977–85. doi: 10.1056/NEJMoa013536
102. Martin PE, Eckert JK, Koplin JJ, Lowe AJ, Gurrin LC, Dharmage SC, et al. Which infants with eczema are at risk of food allergy? Results from a population-based cohort. Clin Exp Allergy (2015) 45:255–64. doi: 10.1111/cea.12406
103. Tauber M, Balica S, Hsu C-Y, Jean-Decoster C, Lauze C, Redoules D, et al. Staphylococcus aureus density on lesional and nonlesional skin is strongly associated with disease severity in atopic dermatitis. J Allergy Clin Immunol (2016) 137:1272–4.e3. doi: 10.1016/j.jaci.2015.07.052
104. Yagami A, Aihara M, Ikezawa Z, Hide M, Kishikawa R, Morita E, et al. Outbreak of immediate-type hydrolyzed wheat protein allergy due to a facial soap in Japan. J Allergy Clin Immunol (2017) 140:879–881.e7. doi: 10.1016/j.jaci.2017.03.019
105. Horimukai K, Morita K, Narita M, Kondo M, Kitazawa H, Nozaki M, et al. Application of moisturizer to neonates prevents development of atopic dermatitis. J Allergy Clin Immunol (2014) 134:824–30.e6. doi: 10.1016/j.jaci.2014.07.060
106. Simpson EL, Chalmers JR, Hanifin JM, Thomas KS, Cork MJ, McLean WH, et al. Emollient enhancement of the skin barrier from birth offers effective atopic dermatitis prevention. J Allergy Clin Immunol (2014) 134:818–23. doi: 10.1016/j.jaci.2014.08.005
107. Glatz M, Jo J-H, Kennedy EA, Polley EC, Segre JA, Simpson EL, et al. Emollient use alters skin barrier and microbes in infants at risk for developing atopic dermatitis. PloS One (2018) 13:e0192443. doi: 10.1371/journal.pone.0192443
108. Chalmers JR, Haines RH, Mitchell EJ, Thomas KS, Brown SJ, Ridd M, et al. Effectiveness and cost-effectiveness of daily all-over-body application of emollient during the first year of life for preventing atopic eczema in high-risk children (The BEEP trial): protocol for a randomised controlled trial. Trials (2017) 18:343. doi: 10.1186/s13063-017-2031-3
109. Lowe AJ, Leung DY, Tang ML, Su JC, Allen KJ. The skin as a target for prevention of the atopic march. Ann Allergy Asthma Immunol (2018) 120:145–51. doi: 10.1016/j.anai.2017.11.023
110. Fiocchi A, Pawankar R, Cuello-Garcia C, Ahn K, Al-Hammadi S, Agarwal A, et al. World Allergy Organization-McMaster University Guidelines for Allergic Disease Prevention (GLAD-P): Probiotics. World Allergy Organ J (2015) 8:4. doi: 10.1186/s40413-015-0055-2
111. Natsume O, Kabashima S, Nakazato J, Yamamoto-Hanada K, Narita M, Kondo M, et al. Two-step egg introduction for prevention of egg allergy in high-risk infants with eczema (PETIT): a randomised, double-blind, placebo-controlled trial. Lancet (2017) 389:276–86. doi: 10.1016/S0140-6736(16)31418-0
112. Martorell A, Alonso E, Boné J, Echeverría L, López MC, Martín F, et al. Position document: IgE-mediated allergy to egg protein. Allergol Immunopathol (Madr) (2013) 41:320–36. doi: 10.1016/j.aller.2013.03.005
113. Baker SS, Cochran WJ, Greer FR, Heyman MB, Jacobson MS, Jaksic T, et al. Hypoallergenic Infant Formulas. Pediatrics (2000) 106:346–9. doi: 10.1542/peds.106.2.346
114. Du Toit G, Roberts G, Sayre PH, Bahnson HT, Radulovic S, Santos AF, et al. Randomized Trial of Peanut Consumption in Infants at Risk for Peanut Allergy. N Engl J Med (2015) 372:803–13. doi: 10.1056/NEJMoa1414850
115. Du Toit G, Sayre PH, Roberts G, Sever ML, Lawson K, Bahnson HT, et al. Effect of Avoidance on Peanut Allergy after Early Peanut Consumption. N Engl J Med (2016) 374:1435–43. doi: 10.1056/NEJMoa1514209
116. Steele L, Mayer L, Berin MC. Mucosal immunology of tolerance and allergy in the gastrointestinal tract. Immunol Res (2012) 54:75–82. doi: 10.1007/s12026-012-8308-4
117. Togias A, Cooper SF, Acebal ML, Assa’ad A, Baker JR, Beck LA, et al. Addendum guidelines for the prevention of peanut allergy in the United States: Report of the National Institute of Allergy and Infectious Diseases–sponsored expert panel. J Allergy Clin Immunol (2017) 139:29–44. doi: 10.1016/j.jaci.2016.10.010
118. Doll RJ, Joseph NI, McGarry D, Jhaveri D, Sher T, Hostoffer R. “Epidemiology of Allergic Diseases,”. In: Allergy and Asthma: The Basics to Best Practices. Cham: Springer International Publishing (2019). p. 31–51.
119. Trikha A, Baillargeon JG, Kuo Y, Tan A, Pierson K, Sharma G, et al. Development of food allergies in patients with gastroesophageal reflux disease treated with gastric acid suppressive medications. Pediatr Allergy Immunol (2013) 24:582–8. doi: 10.1111/pai.12103
120. Sicherer SH, Allen K, Lack G, Taylor SL, Donovan SM, Oria M. Critical Issues in Food Allergy: A National Academies Consensus Report. Pediatrics (2017) 140(2):e20170194. doi: 10.1542/peds.2017-0194
121. Fleischer DM, Spergel JM, Assa’ad AH, Pongracic JA. Primary prevention of allergic disease through nutritional interventions. J Allergy Clin Immunol Pract (2013) 1:29–36. doi: 10.1016/j.jaip.2012.09.003
122. Muraro A, Halken S, Arshad SH, Beyer K, Dubois AE, Du Toit G, et al. EAACI food allergy and anaphylaxis guidelines. Primary prevention of food allergy. Allergy (2014) 69:590–601. doi: 10.1111/all.12398
123. Alexander DD, Cabana MD. Partially hydrolyzed 100% whey protein infant formula and reduced risk of atopic dermatitis: a meta-analysis. J Pediatr Gastroenterol Nutr (2010) 50:422–30. doi: 10.1097/MPG.0b013e3181cea52b
124. Szajewska H, Horvath A. A partially hydrolyzed 100% whey formula and the risk of eczema and any allergy: an updated meta-analysis. World Allergy Organ J (2017) 10:27. doi: 10.1186/s40413-017-0158-z
125. von Berg A, Filipiak-Pittroff B, Schulz H, Hoffmann U, Link E, Sußmann M, et al. Allergic manifestation 15 years after early intervention with hydrolyzed formulas–the GINI Study. Allergy (2016) 71:210–9. doi: 10.1111/all.12790
126. Osborn DA, Sinn JK, Jones LJ. Infant formulas containing hydrolysed protein for prevention of allergic disease and food allergy. Cochrane Database Syst Rev (2017) 3:CD003664. doi: 10.1002/14651858.CD003664.pub4
127. Boyle RJ, Ierodiakonou D, Khan T, Chivinge J, Robinson Z, Geoghegan N, et al. Hydrolysed formula and risk of allergic or autoimmune disease: Systematic review and meta-analysis. BMJ (2016) 352(974):i974. doi: 10.1136/bmj.i974
128. Risnes KR, Belanger K, Murk W, Bracken MB. Antibiotic Exposure by 6 Months and Asthma and Allergy at 6 Years: Findings in a Cohort of 1,401 US Children. Am J Epidemiol (2011) 173:310–8. doi: 10.1093/aje/kwq400
129. Pfefferle PI, Büchele G, Blümer N, Roponen M, Ege MJ, Krauss-Etschmann S, et al. Cord blood cytokines are modulated by maternal farming activities and consumption of farm dairy products during pregnancy: The PASTURE Study. J Allergy Clin Immunol (2010) 125:108–15.e3. doi: 10.1016/j.jaci.2009.09.019
130. Riedler J, Braun-Fahrländer C, Eder W, Schreuer M, Waser M, Maisch S, et al. Exposure to farming in early life and development of asthma and allergy: A cross-sectional survey. Lancet (2001) 358:1129–33. doi: 10.1016/S0140-6736(01)06252-3
131. Negele K, Heinrich J, Borte M, Berg A, Schaaf B, Lehmann I, et al. Mode of delivery and development of atopic disease during the first 2 years of life. Pediatr Allergy Immunol (2004) 15:48–54. doi: 10.1046/j.0905-6157.2003.00101.x
132. Alm JS, Swartz J, Lilja G, Scheynius A, Pershagen G. Atopy in children of families with an anthroposophic lifestyle. Lancet (1999) 353:1485–8. doi: 10.1016/S0140-6736(98)09344-1
133. Adeyeye TE, Yeung EH, McLain AC, Lin S, Lawrence DA, Bell EM. Wheeze and Food Allergies in Children Born via Cesarean Delivery: The Upstate KIDS Study. Am J Epidemiol (2019) 188:355–62. doi: 10.1093/aje/kwy257
134. Peters LL, Thornton C, de Jonge A, Khashan A, Tracy M, Downe S, et al. The effect of medical and operative birth interventions on child health outcomes in the first 28 days and up to 5 years of age: A linked data population-based cohort study. Birth (2018) 45:347–57. doi: 10.1111/birt.12348
135. Park YH, Kim KW, Choi BS, Jee HM, Sohn MH, Kim K-E. Relationship between mode of delivery in childbirth and prevalence of allergic diseases in Korean children. Allergy Asthma Immunol Res (2010) 2:28–33. doi: 10.4168/aair.2010.2.1.28
136. Lodge CJ, Tan DJ, Lau MX, Dai X, Tham R, Lowe AJ, et al. Breastfeeding and asthma and allergies: a systematic review and meta-analysis. Acta Paediatr (2015) 104:38–53. doi: 10.1111/apa.13132
137. Mueller NT, Bakacs E, Combellick J, Grigoryan Z, Dominguez-Bello MG. The infant microbiome development: mom matters. Trends Mol Med (2015) 21:109–17. doi: 10.1016/j.molmed.2014.12.002
138. Kim N-R, Jeong D-W, Ko D-S, Shim J-H. Characterization of novel thermophilic alpha-glucosidase from Bifidobacterium longum. Int J Biol Macromol (2017) 99:594–9. doi: 10.1016/j.ijbiomac.2017.03.009
139. Lowy FD. Antimicrobial resistance: The example of Staphylococcus aureus. J Clin Invest (2003) 111:1265–73. doi: 10.1172/JCI18535
140. Lyons A, O’Mahony D, O’Brien F, MacSharry J, Sheil B, Ceddia M, et al. Bacterial strain-specific induction of Foxp3 + T regulatory cells is protective in murine allergy models. Clin Exp Allergy (2010) 40(5):811–9. doi: 10.1111/j.1365-2222.2009.03437.x
141. Zakostelska Z, Kverka M, Klimesova K, Rossmann P, Mrazek J, Kopecny J, et al. Lysate of Probiotic Lactobacillus casei DN-114 001 Ameliorates Colitis by Strengthening the Gut Barrier Function and Changing the Gut Microenvironment. PloS One (2011) 6:e27961. doi: 10.1371/journal.pone.0027961
142. Roßberg S, Keller T, Icke K, Siedmann V, Lau I, Keil T, et al. Orally applied bacterial lysate in infants at risk for atopy does not prevent atopic dermatitis, allergic rhinitis, asthma or allergic sensitization at school age: Follow-up of a randomized trial. Allergy (2020) 92:44. doi: 10.1111/all.14247
144. Kalliomäki M, Salminen S, Arvilommi H, Kero P, Koskinen P, Isolauri E. Probiotics in primary prevention of atopic disease: A randomised placebo-controlled trial. Lancet (2001) 357:1076–9. doi: 10.1016/S0140-6736(00)04259-8
145. Cuello-Garcia CA, Brożek JL, Fiocchi A, Pawankar R, Yepes-Nuñez JJ, Terracciano L, et al. Probiotics for the prevention of allergy: A systematic review and meta-analysis of randomized controlled trials. J Allergy Clin Immunol (2015) 136:952–61. doi: 10.1016/j.jaci.2015.04.031
146. Cuello-Garcia C, Fiocchi A, Pawankar R, Yepes-Nuñez JJ, Morgano GP, Zhang Y, et al. Prebiotics for the prevention of allergies: A systematic review and meta-analysis of randomized controlled trials. Clin Exp Allergy (2017) 47:1468–77. doi: 10.1111/cea.13042
147. Wopereis H, Sim K, Shaw A, Warner JO, Knol J, Kroll JS. Intestinal microbiota in infants at high risk for allergy: Effects of prebiotics and role in eczema development. J Allergy Clin Immunol (2018) 141:1334–42.e5. doi: 10.1016/j.jaci.2017.05.054
148. Ranucci G, Buccigrossi V, Borgia E, Piacentini D, Visentin F, Cantarutti L, et al. Galacto-Oligosaccharide/Polidextrose Enriched Formula Protects against Respiratory Infections in Infants at High Risk of Atopy: A Randomized Clinical Trial. Nutrients (2018) 10:286. doi: 10.3390/nu10030286
149. Kremmyda L-S, Vlachava M, Noakes PS, Diaper ND, Miles EA, Calder PC. Atopy Risk in Infants and Children in Relation to Early Exposure to Fish, Oily Fish, or Long-Chain Omega-3 Fatty Acids: A Systematic Review. Clin Rev Allerg Immunol (2011) 41:36–66. doi: 10.1007/s12016-009-8186-2
150. Lumia M, Luukkainen P, Tapanainen H, Kaila M, Erkkola M, Uusitalo L, et al. Dietary fatty acid composition during pregnancy and the risk of asthma in the offspring. Pediatr Allergy Immunol (2011) 22:827–35. doi: 10.1111/j.1399-3038.2011.01202.x
151. Sala-Vila A, Miles EA, Calder PC. Fatty acid composition abnormalities in atopic disease: Evidence explored and role in the disease process examined. Clin Exp Allergy (2008) 38:1432–50. doi: 10.1111/j.1365-2222.2008.03072.x
152. Dunstan JA, Mori TA, Barden A, Beilin LJ, Taylor AL, Holt PG, et al. Fish oil supplementation in pregnancy modifies neonatal allergen-specific immune responses and clinical outcomes in infants at high risk of atopy. J Allergy Clin Immunol (2003) 112:1178–84. doi: 10.1016/j.jaci.2003.09.009
Keywords: immune tolerance, allergy prevention, children, regulatory T cells, allergen, Th2 cells, atopic disease, early exposure
Citation: Pierau M, Arra A and Brunner-Weinzierl MC (2021) Preventing Atopic Diseases During Childhood – Early Exposure Matters. Front. Immunol. 12:617731. doi: 10.3389/fimmu.2021.617731
Received: 15 October 2020; Accepted: 19 January 2021;
Published: 25 February 2021.
Edited by:
Kim Good-Jacobson, Monash University, AustraliaReviewed by:
Hugh Sampson, Icahn School of Medicine at Mount Sinai, United StatesWilliam J. Sheehan, Children's National Hospital, United States
Copyright © 2021 Pierau, Arra and Brunner-Weinzierl. This is an open-access article distributed under the terms of the Creative Commons Attribution License (CC BY). The use, distribution or reproduction in other forums is permitted, provided the original author(s) and the copyright owner(s) are credited and that the original publication in this journal is cited, in accordance with accepted academic practice. No use, distribution or reproduction is permitted which does not comply with these terms.
*Correspondence: Monika C. Brunner-Weinzierl, Monika.Brunner-Weinzierl@med.ovgu.de