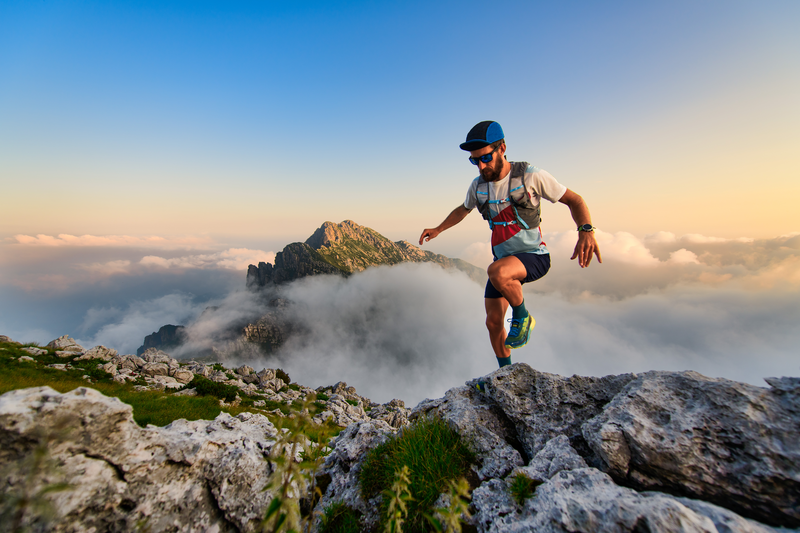
94% of researchers rate our articles as excellent or good
Learn more about the work of our research integrity team to safeguard the quality of each article we publish.
Find out more
MINI REVIEW article
Front. Immunol. , 21 April 2021
Sec. Immunological Memory
Volume 12 - 2021 | https://doi.org/10.3389/fimmu.2021.616309
This article is part of the Research Topic Resident Memory T Cells: Guardians of the Balance of Local Immunity and Pathology View all 11 articles
Memory T cells are crucial for both local and systemic protection against pathogens over a long period of time. Three major subsets of memory T cells; effector memory T (TEM) cells, central memory T (TCM) cells, and tissue-resident memory T (TRM) cells have been identified. The most recently identified subset, TRM cells, is characterized by the expression of the C-type lectin CD69 and/or the integrin CD103. TRM cells persist locally at sites of mucosal tissue, such as the lung, where they provide frontline defense against various pathogens. Importantly, however, TRM cells are also involved in shaping the pathology of inflammatory diseases. A number of pioneering studies revealed important roles of CD8+ TRM cells, particularly those in the local control of viral infection. However, the protective function and pathogenic role of CD4+ TRM cells that reside within the mucosal tissue remain largely unknown. In this review, we discuss the ambivalent feature of CD4+ TRM cells in the protective and pathological immune responses. We also review the transcriptional and epigenetic characteristics of CD4+ TRM cells in the lung that have been elucidated by recent technical approaches. A better understanding of the function of CD4+ TRM cells is crucial for the development of both effective vaccination against pathogens and new therapeutic strategies for intractable inflammatory diseases, such as inflammatory bowel diseases and chronic allergic diseases.
“Immune memory” is a central and characteristic phenomenon of the acquired immune system. The long-term survival of the antigen-specific memory T cell population in response to invading harmful microorganisms is essential for the establishment of immune memory in vivo. Memory T cells can respond directly and rapidly to re-invading harmful microorganisms and efficiently eliminate them to protect the host.
Memory T cells were originally classified into two subpopulations, effector memory T (TEM) cells and central memory T (TCM) cells, based on (1) the expression pattern of cell surface molecules, (2) the orientation to specific tissues and (3) responsiveness to re-stimulation with a certain antigen (1). TEM cells show the low expression of CCR7, a chemokine receptor that is crucial for homing to the secondary lymphoid organ and the low expression of the cell surface molecule CD62L. TEM cells are mainly found in the non-lymphoid tissues and are responsible for peripheral immune surveillance and the immediate protective function in the host. TEM cells respond quickly to re-stimulation of antigens and produce large amounts of proinflammatory cytokines, including IFN-γ, IL-5 and IL-4, but they showed shortened telomeres (2). In contrast, TCM cells highly express both CCR7 and CD62L and migrate to sites with secondary lymphoid tissues, such as lymph nodes; TCM cells primarily produce IL-2 upon antigen restimulation. After proliferation, TCM cells efficiently produce large amounts of proinflammatory cytokines, such as IFN-γ and IL-4 (3, 4). Memory T cells are subdivided by various cell-surface markers, including CD27, CD127, CD43, CXCR3 and CX3CR1 (5–8). A study using CX3CR1-reporter mice reveals that CX3CR1hi CD8+ TEM cells were largely excluded from peripheral tissues after viral infection, providing novel insight concerning CD8+ TEM cells (9).
Recently, non-circulating memory T cells have been identified, which are now referred to as tissue resident memory T (TRM) cells (10). TRM cells show the high expression of C-type lectin-like molecule CD69 and integrin E subunit molecule CD103. TRM cells produce various kind of cytokines, including IL-2, IFN-γ, TNF-α, and IL-17 (11–16). Unlike TCM cells and TEM cells, which circulate throughout the body via blood vessels and lymphatic vessels, TRM cells do not circulate throughout the body, but they reside in non-lymphoid tissues such as the lung, skin, and gut. However, a series of recent studies clearly show that re-activated CD8+ TRM cells rejoin the circulating pool and proliferate in draining lymph nodes (Figure 1) (17, 18). Regarding CD4+ TRM cells, CD4+ TRM cells account for 30% of the lymph node-CD4+ T cell population, which is a larger proportion than that of CD8+ T cells (19). However, the plasticity of subpopulations of memory CD4+ T cell has remained unclear. Regardless, the functions of memory T cells are closely linked to their mobility in the body of the host.
Figure 1 Distribution of various memory T cells in vivo. There are three types of memory T cells in vivo: (1) central memory T (TCM) cells, which mainly reside in secondary lymphoid tissues, (2) effector memory T (TEM) cells, which circulate in the blood, non-lymphatic tissues, and secondary lymphoid tissues, and (3) resident memory T (TRM) cells, which reside within non-lymphoid tissues. (A) A recent study revealed that CX3CR1hi CD8+ TEM cells are largely excluded from peripheral tissues after viral infection (9). In case of CD8+ TRM cells, a series of recent studies clearly showed that re-activated CD8+ TRM cells rejoined the circulating pool and proliferated in draining lymph nodes (red arrows). Some TEM cells move back and forth between the blood vessel and parenchyma. (B) However, whether or not CD4+ TRM cells rejoin the circulating pool and a re-activated in the draining lymph nodes is unclear.
In mucosal tissues, such as the skin and female reproductive tract, antigen-recognized CD8+ TRM cells produce IFN-γ and TNF-α to recruit other immune cells and activate dendritic cells and NK cells (12–14). In non-mucosal tissues, such as the brain and liver, CD8+ TRM cells reside in each organ and play crucial roles in the host defense against pathogens (20, 21). In the brain, IFN-γ and Perforin-producing CD8+ TRM cells act as an autonomous cytotoxic barrier to viral infection (21). In the lymphocytic choriomeningitis virus (LCMV)-infected brain, almost all CD8+ TRM cells express CD69, but these cells show heterogeneous expression patterns of CD103 (21). In the liver, CXCR3+CD8+ TRM cells are essential for protection against liver-stage malaria (20). Human CD69+CD103+CD8+ TRM cells in the liver produce large amounts of IL-2 compared to CD69-CD103-CD8+ T cells (15).
Regarding CD4+ T cells, recent studies have highlighted prominent populations of CD4+ TRM cells in various mucosal tissues, such as the skin (22–25), female genital tract (19, 22, 26, 27), small intestine (19, 28–30) and lung (16, 19, 22, 30–33). In the skin, CD4+ TRM cells protect hosts against invading pathogens, including Leishmania major (23, 24). Candida albicans infection also induces IL-17-producing CD4+ TRM cells in the skin (34). In the female genital tract, CD4+ TRM cells are crucial for antiviral defense against genital herpes simplex virus 2 (HSV-2) infection (26, 27). Helminth infection and Listeria monocytogenes infection cause the induction of functional CD4+ TRM cells in the intestine (28, 29). In the upper tract, pneumococcus infection induces CD4+ TRM cells that prevent pneumococcal colonization (33). Furthermore, lung CD4+ TRM cells are essential for protection against bacterial infection (16). Thus, similar to CD8+ TRM cells, CD4+ TRM cells may facilitate a rapid immune response to protect the host against re-exposure to pathogens in various mucosal organs.
In human, CCR7hi CD4+ TRM cells are detected in the female genital tract (35). In infants, mucosal memory CD4+ and CD8+ T cells already show characteristics of tissue residency, such as the enhanced expression of CD69 and CD103, which suggests that local in situ priming to antigens causes the induction of TRM cells (36). Investigations of human samples from the lung after lung transplantation have revealed that lung-infiltrating recipient CD4+ and CD8+ T cells gradually acquire TRM phenotypes, such as the enhanced expression of CD69 and CD103, over several months in vivo (37). In non-mucosal sites, human brain CD4+ T cells show the high expression of CD69 but a low expression of CD103 (38). More detailed information about human TRM cells has been reviewed in other articles (39, 40). The roles of CD4+ TRM cells in the non-mucosal tissue have not been well elucidated.
In addition to the essential role of TRM cells in the biological defense of mucosal and non-mucosal organs, TRM cells and other tissue resident immune cells, including innate lymphoid cells (ILCs), play a critical role in tissue homeostasis (41).
The mobility of T cells among various organs throughout the body is tightly regulated by various cytokines, chemokines and cell surface molecules (42). Transforming growth factor β (TGF-β) is an essential cytokine for the development of CD8+ TRM cells in the mucosal tissues (43). TGF-β induces the expression of CD103 on CD8+ T cells (44). In the skin, CD8+ TRM cells require transactivated autocrine TGF-β for epidermal persistence (45). An important cytokine for the survival of CD8+ TRM cells in the skin is IL-15 (46). In the skin, hair follicle-derived IL-15 and IL-7 are required for the maintenance of CD8+ TRM cells (47). During influenza viral infection, IFN-γ produced by CD4+ T cells induces CD8+ TRM cells, which are crucial for protection against pathogenic viruses (44).
For the long-term survival of CD4+ TRM cells, IL-7 is needed in the skin (47). In the lung, IL-15 is required for the generation of CD4+ TRM cells (48).
Regarding chemokines and cell surface molecules, CD62L and CCR7 must be expressed on T cells to enter the peripheral lymph nodes (1), while Sphingosin-1-Phosphate Receptor 1 (S1P1), which binds the ligand Sphingosin-1-Phosphate (S1P), allows T cells to leave the lymph nodes and enter the lymphatic vessels (49). In humans, both CD8+ and CD4+ TRM cells upregulate the adhesion molecules ITGAE (CD103) and ITGA1 (CD49a) as well as inhibitory molecules, including PD-1 and the dual specificity phosphatase DUSP6 (30). Both CD8+ and CD4+ TRM cells show the down-regulated expression of S1PR1 (30). CD69 is a type 2 glycoprotein with a C-type lectin-like domain that acts as a homodimer (50). CD69 binds to S1P1 to promote the internalization and degradation of S1P1 in the cytoplasm. As a result, CD69-expressing T cells remain within lymphoid tissues, such as the thymus and lymph nodes (49). CD8+ TRM cells in the lungs of mice with influenza viral infection show the high expression of CD69, and a CD69-deficient environment was shown to be associated with a reduced number of CD8+ TRM cells in the lung (51, 52). In the skin and kidneys, CD69-deficiency in CD8+ T cells also result in a markedly reduced number of CD8+ TRM cells (53, 54). CD8+ TRM cells show lower S1P1 expression levels (43). In addition, CD8+ TRM cells reveal the low expression of Krupple-like factor 2 (KLF2), a transcription factor that regulates the expression of S1PR1 (55). These findings suggest that CD69 plays a crucial role in CD8+ TRM cells, as more than a mere cell surface marker. Interestingly, though, CD8+ TRM cells are able to be maintained in the lung independently of the CD69 expression (52). Furthermore, experiments using pet mice with differing microbial experiences revealed that the CD69 expression on CD8+ T cells was insufficient to interpret tissue residence (56). Indeed, the functional requirement for CD69 is evidently dependent on the tissue where CD8+ TRM cells exist (54). Thus, although CD69 is not a perfect cell surface marker for tissue residency, more detailed studies regarding the functional roles of CD69 in TRM cells, especially CD8+ TRM cells, are needed to draw firm conclusions. In contrast, the role of CD69 in CD4+ TRM cells remains unclear.
The unique transcriptional features of TRM cells have been well established in CD8+ TRM cells. The transcription factor homolog of Blimp1 in T cells (Hobit) is specifically expressed in CD8+ TRM cells (57). Hobit and Blimp1 cooperatively downregulate the expression of S1pr1 and Ccr7, which are required for tissue egress (57). Hobit and Blimp1 also repress the transcription factors Tcf7 and Klf2, which regulate survival and trafficking of circulating memory T cells (57). The transcription factor Runx3 plays a crucial role in establishing CD8+ TRM cells (57, 58). CD8+ TRM cells in the liver show an enhanced expression of Hobit (20). Without appropriate CD4+ T cell help, lung CD8+ TRM cells show an enhanced expression of T-bet that suppresses the formation of CD8+ TRM cells by direct binding to the Itgae locus (44).
Regarding CD4+ T cells, Hobit and Blimp1 are reported to attenuate CD4+ TRM cell-dependent colitis (59). Viral infection induced-CD4 TRM cells show the enhanced expression of Hobit and Eomes (19). However, another group reports that T helper type 2 (Th2) CD4 TRM cells do not preferentially express Hobit, Blimp1 or Runx3 in their RNA sequencing (RNA-Seq) data sets (60). In humans, the transcription factor c-MAF induces the tissue residency transcriptional program in Th17 cells (61). Although many of the phenotypic characteristics of CD4+ TRM cells are shared with CD8+ TRM cells, precise assessments regarding the transcriptional features of CD4+ TRM cells are required to identify the nature of CD4+ TRM cells (62).
Recent studies using human tissue resident memory T cells have revealed that both CD4+ and CD8+ TRM cells are transcriptionally distinct from other memory T cell subsets (30, 63). A core gene signature including ITGA1, ITGAE, IL-2, CXCR6, and PD-1 shows differential regulation between TRM cells and circulating T cells, suggesting the unique feature of human TRM cells in vivo (30).
Proving the tissue residency of T cells is a major challenge. It is necessary to show at least that the cells are present in the same tissue for a certain period to prove tissue residency. Currently, experimental techniques, such as (1) parabiosis, (2) in vivo intravascular staining, and (3) tissue transplantation are used to prove the tissue residency of a certain population of cells (Figure 2).
Figure 2 A schematic illustration of the experimental techniques used to identify TRM cells. (A) Surgical connection of two congenic mice allows them to share blood circulation. (B) In vivo intravascular staining marks circulating T cells through the intravascular injection of an anti-cell surface molecule antibody. (C) In tissue transplantation, donor-derived T cells are detected in the graft after transplantation.
Parabiosis is an experimental technique in which two mice are surgically linked and share a common circulatory system (Figure 2), which makes us possible to separate substances that are circulating in blood vessels and those that are not in the bloodstream. This method was established in France in the 19th century. In the second half of the 20th century, it has been widely used to investigate the endocrine system. In the field of immunology, parabiosis experiments are conducted to demonstrate the tissue residency of a certain cell population in vivo. In the tissue transplantation, the tissue—together with tissue-resident cells—is transplanted into congenic mice and then analyzed for the migration of donor-derived cells in the tissue to demonstrate tissue residency (10). Intravascular in vivo labeling is an experimental technique using the intravenous injection of cell-surface antibodies, such as anti-CD4 antibodies, to distinguish cells in tissue from those in blood vessels (Figure 2) (64). The advantage of this technique is its simplicity in comparison to parabiosis and tissue transplantation experiments. T cells in the vasculature were found to differ from those in the lung parenchyma, which were not stained with cell-surface antibodies (64). However, it is important to note that this experiment shows that unstained cells were not present in the vessels for a certain period of time after the intravenous injection of the antibody, because the cells were collected from each organ 3-5 minutes after the intravenous injection of the antibody under anesthesia.
As each of these techniques has certain limitations and addresses several specific criteria for residency, the definitive assessment of tissue residency of T cells should rely on supportive results obtained from multiple experimental techniques.
In addition to other memory T cell populations, such as TEM and TCM cells, TRM cells play an important role in the body’s defense against infection. In several experimental models in mice, CD8+ TRM cells have been revealed to be important in defending against viral, parasitic and other infections (20, 65–67). In humans, CD8+ TRM cells have been reported to be crucial in defending against herpes simplex type 1 virus infection in the skin (68).
Regarding CD4+ T cells, CD4+ TRM cells are important for optimal protection against respiratory virus infection via the enhanced production of IFN-γ (11). CD4+ TRM cells play key roles in the elimination of HSV-2 and chlamydia in the vagina (26, 69). HSV-2-specific CD4+ TRM cells are enriched in local inflammatory sites, and the chemokine CCL5 is important for the retention of CD4+ TRM cells in vaginal tissues (26). These CD4+ TRM cells also produce large amounts of IFN-γ (26). In an LCMV infection model, CD4+ TRM cells play a key role in local immunosurveillance along with CD8+ TRM cells (19). CD4+ TRM cells also play a protective role against pneumococcal infection in the lung (70). In this model, IL-17-producing CD4+ TRM cells recruit neutrophils to the lung, which is crucial for protecting the host against bacterial infection (70). In humans, an increased frequency of donor TRM cells in the lung of patients with lung transplantation is associated with a reduced rate of adverse clinical events, such as primary graft dysfunction (37). This finding suggests the protective roles of donor TRM cells in the rejection of transplanted tissue.
However, TRM cells are also involved in the pathogenesis of various human immune-related diseases. In psoriasis, an autoimmune disease of the skin, CD8+CD49a- TRM cells produce IL-17 at the local inflammatory site and are involved in the pathogenesis of the disease. In vitiligo, CD8+CD49a+ TRM cells produce IFN-γ in the inflammatory tissue and are involved in the pathogenesis of the disease (71). In addition, using experimental autoimmune encephalomyelitis, a mouse model of multiple sclerosis, CD8+ TRM cells have been shown to be involved in the onset and relapse of disease (72).
Mucosal tissues that include a large number of TRM cells are susceptible to environmental stresses, such as cell damage, cell death, and changes in partial oxygen pressure. TRM cells play important roles in maintaining local tissue homeostasis, including tissue repair and regeneration as well as defense against infection and the pathogenesis of immune-related diseases. Indeed, CD8+ TRM cells localize within local inflammatory sites during tissue regeneration after influenza virus infection (52). This suggests that CD8+ TRM cells are involved in the processes of tissue repair and regeneration. However, overactivation of the tissue repair process causes tissue fibrosis (73). Various stimuli, including HDM and fungal infection, cause fibrosis in the lung (73–75). In fact, house dust mite (HDM)-induced allergic airway inflammation has been demonstrated to be dependent on HDM antigen-specific CD4+ TRM cells in the lungs in experimental mouse models (74, 76). IL-2 signaling is required for the residency of HDM antigen-specific CD4+ TRM cells, which are sufficient to induce airway hyper-responsiveness (76). Interestingly, chronic exposure of HDM induces the infiltration of both CD4+ and CD8+ T cells into the lung tissue; however, only CD4+ TRM cells persist in the lung for a long time (77). Another group reported that allergen-specific CD4+ T cells were able to survive for over 70 days in the lung (74). A dominant type 2 immune response is induced by repetitive HDM exposure, and Th2 TRM cells are functionally and transcriptionally distinct from circulating memory Th2 cells in the lungs of mice with HDM-induced allergic inflammation (60). Th2 TRM cells express increased levels of Il5 and Il13 (60). Thus, CD4+ TRM cells play a critical role in shaping various pathologies, such as airway hyper-responsiveness and eosinophilic inflammation during chronic type 2 inflammation.
Furthermore, Th2 TRM cells show the enhanced expression of metalloproteases, extracellular matrix (ECM) components and regulators for ECM (60). These unique transcriptomic feature of Th2 TRM cells suggests the pathogenic role of Th2 TRM cells in the induction of fibrotic responses. Regarding fungal infection, patients with allergic bronchopulmonary aspergillosis/mycosis (ABPA/ABPM) have recurrent bronchial asthma attacks accompanied by bronchial dilatation and fibrotic changes in the lung (75). In the lungs of mice with repeated exposure to the Aspergillus fumigatus antigen, CD4+ TRM cells, which produce various type of inflammatory cytokines accompanied by the low expression of CD103 and the enhanced expression of fibrosis-related genes, induce fibrotic responses (78). In addition, CD103- CD4+ TRM cells also express the metalloprotease Adam8 (78). An assay for transposase-accessible chromatin using a sequencing (ATAC-Seq) analysis revealed that the characteristic features of these CD4+ TRM cells populations were regulated at the chromatin level. For example, the regulatory elements of inflammatory cytokines, such as Il4, Il5, and Il13, were specifically accessible in CD103-negative CD4+ TRM cells (Figure 3). At the same time, CD103-positive CD4+ regulatory T (Treg) cells are induced in the inflammatory lung. These CD103-positive Treg cells regulate the fibrotic responses induced by CD103-negative CD4+ TRM cells in chronic allergic inflammation caused by repeated exposure to the A. fumigatus antigen in vivo (78) (Figure 3). Thus, CD103- CD4+ TRM cells are involved in the fibrotic response processes in the lung. Taken together, these findings suggest that CD4+ TRM cells play pathogenic roles in the fibrosis induced by various stimuli, such as HDM and fungi.
Figure 3 The induction of CD4+ TRM cells with a unique regulome signature. Chronic allergic inflammation with fibrosis of the lung induced by repeated exposure to Aspergillus fumigatus antigen causes the induction of two cell populations, CD103-negative CD4+ tissue-resident memory T (TRM) cells and CD103-positive regulatory T (Treg) cells, which are involved in the pathogenesis of fibrotic responses. Each of these cell populations has its own characteristic regulome. For example, CD103-negative CD4+ TRM cells produce proinflammatory cytokines and show specific peaks of ATAC-Seq in the Th2 cytokine loci (arrows). In contrast, CD103-positive Treg cells show specific peaks of ATC-Seq in the Foxp3 locus (arrows).
The protective roles of CD4+ TRM cells have been elucidated in various infectious diseases. However, the pathogenic roles of CD4+ TRM cells in chronic inflammation other than type 2-related diseases, such as allergic inflammation, have been unclear. Thus, we await the further investigation of the pathogenic roles of CD4+ TRM cells in various immune-related diseases, including multiple sclerosis and psoriasis, the induction of which reportedly involves type 17 inflammation.
It is now clear that memory T cells comprise several subsets, including TCM cells, TEM cells and TRM cells. Researchers have shown that CD8+ TCM cells become CD8+ TRM cells via an adoptive transfer experimental system (79). In fact, adoptively transferred CD8+ TCM cells reside in the skin of donor mice accompanied by the enhanced expression of CD69 and CD103 after viral infection (79).
But what about the opposite direction of re-differentiation? In other words, do CD8+ TRM cells have the ability to re-differentiate to CD8+ TCM cells? TRM cells are localized within specific organs for a long time, indicating their involvement in first-line protective responses against local reinfection. If CD8+ TRM cells can re-differentiate to CD8+ TCM cells, TRM cells may be involved in systemic memory immune responses. Experiments using CD8+ TRM cells accompanied by an analysis of the methylation state of the CpG region have shown that the function of TRM cells is not fixed, and TRM cells have the ability to change their function in vivo (17). A machine learning-based analysis using the methylation state of the CpG region in CD8+ TRM cells showed that CD8+ TRM cells were able to re-differentiate (17). Furthermore, using an experimental system of virus-infected mice, researchers showed that some reactivated CD8+ TRM cells returned to the systemic circulatory system and re-differentiated into CD8+ TCM cells. Using a CD8+ TRM cell-restricted transcription factor Hobit-reporter system, another group showed that Hobit+ CD8+ TRM cells proliferate in draining lymph nodes after viral re-infection (18). Importantly, Hobit+ CD8+ TRM cells re-differentiated into CD8+ TEM cells together with the downregulation of the Hobit expression and contributed to the generation of the systemic immune responses (18). These results suggest that immune memory maintained in the local inflammatory sites may also be involved in systemic memory immune responses, at least in the case of CD8+ TRM cells.
An IL-17A tracking-fate mouse experimental system showed that CD4+ TRM cells were derived from effector Th17 cells (16). In humans, CD4+ TRM cells in the bone marrow show unique DNA methylation profiles among memory T cell subsets, indicating their specialized function (80). However, in contrast to findings concerning CD8+ T cells, the plasticity of the CD4+ memory T cell population has remained unclear.
Inducible bronchus-associated lymphoid tissue (iBALT), a type of ectopic lymphoid tissue, is often formed in response to various stimuli, including infection, smoking, and collagen disease, in the inflamed lung (81). iBALT includes MHC class II-positive cells, B220-positive cells, CD11c-positive cells, VCAM1-positive stromal cells, and CD21-positive follicular dendritic cells. CD11c-positive dendritic cells are crucial for the reactivation of CD8+ TRM cells in the lung (82). Memory CD4+ T cells are maintained within iBALT in lungs with chronic allergic inflammation (83). Furthermore, Thy1-positive IL-7-producing lymphoid endothelial cells are essential for the survival of memory CD4+ T cells due to their production of IL-7 in the inflammatory tissue of the lung (83). Interestingly, the maintenance of allergen-specific CD4+ T cells is dependent on IL-7 signaling in the lung (74). Single-cell RNA sequencing of the lung from mice with bacterial infection has revealed the enhanced expression of Il7 by lymphatic endothelial cells, which are colocalized with CD4+ T cells (16). Based on these findings, it is likely that CD4+ TRM cells, which are induced by repeated exposure to Aspergillus fumigatus antigen, are also maintained within iBALT in the inflamed lung. In fact, repeated exposure to Aspergillus fumigatus antigen induces the enhanced formation of iBALTs in the inflamed lung. However, the molecular mechanisms underlying the differentiation, induction, and maintenance of CD4+ TRM cells in the lung and the role of iBALT in these processes remain unclear and require further research. In another mucosal tissue, the skin, the formation of ectopic lymphoid tissue called inducible skin-associated lymphoid tissue (iSALT) was reported (84). CD4+ TRM cells accumulate within iSALT following skin inflammation (84, 85). IL-7 is a key cytokine supporting the long-term survival of CD4+ TRM cells in the skin (47).
More detailed information regarding the tissue-specific anatomical niches for the maintenance of CD4+ TRM cells has been reviewed in other articles (62, 86).
We proposed a model for the pathogenesis of immune-related inflammatory diseases called the “pathogenic Th-cell disease induction model” (87). In our proposed “pathogenic Th-cell disease model”, a certain population of memory CD4+ T cells is highly pathogenic, and the generation of pathogenic T cells is important for the pathogenesis and regulation of various inflammatory diseases. In other words, various immune-related chronic inflammatory diseases are not induced by an imbalance between the subsets of CD4+ T cells (e.g., Th1 cells, Th2 cells or Th17 cells), rather, they are induced by a specific population of pathogenic cells (pathogenic CD4+ T cells) that arise in peripheral tissues under certain conditions. For example, we identified IL-5 high-producing-pathogenic Th2 cells that produce large amount of IL-5 and induce eosinophilic airway inflammation (88). We also identified fibrosis-inducing-pathogenic Th2 cells that produce Amphiregulin, a tissue repair factor, and induce tissue fibrosis via the activation of eosinophils (89, 90). These pathogenic Th2 cells have also been found in tissue, as they are maintained within the iBALT.
The CD103-negative CD4+ TRM cells that we identified recently are also pathogenic CD4+ T cells, which coexist with pathogenic Th1/Th2/Th17 cells due to the nature of the pathological model of Aspergillus fumigatus antigen administration. Interestingly, both pathogenic CD4+ TRM cells and regulatory T cells are induced simultaneously in chronic inflammatory tissues. Thus, multiple functional CD4+ TRM cell populations are involved in the pathogenesis of refractory immune-related inflammatory diseases, such as bronchial asthma and atopic dermatitis. We need to investigate the diversity of CD4+ TRM cells in the lung using a single cell RNA-sequencing (scRNA-seq) analysis.
Tissue-resident memory T cells represent a relatively new cell population that has only been attracting attention for approximately 10 years. Regarding CD8+ T cells, the tissue-resident memory T cell population is being actively studied worldwide, and novel findings about CD8+ TRM cells have emerged one after another, including the identification of transcription factors such as Hobit, Blimp1, and Runx3, which are important for the induction of CD8+ TRM cells (57, 58). As described previously, the plasticity of CD8+ TRM cells has also been analyzed at the epigenomic level.
On the other hand, the mechanisms underlying the differentiation, maintenance, and plasticity of CD4+ TRM cells remain unclear. CD4+ TRM cells play a protective role in the lungs against infections such as Streptococcus pneumoniae and Mycobacterium tuberculosis (70, 91). CD4+ TRM cells also play an important role in the elimination of HSV-2 and chlamydia in the vagina (26). The intranasal administration of pneumococci induces IL-17-producing CD4+ TRM cells that protect the host against pneumococcal colonization (33). Intranasal vaccination of influenza virus induced the accumulation of both CD4+ and CD8+ TRM cells in the lung of mice (92). Moreover, intranasal vaccination with Venezuelan equine encephalitis replicons (VRP) encoding a severe acute respiratory syndrome coronavirus (SARS-CoV) CD4+ T cell epitope resulted in airway memory CD4+ T cell-dependent protection against SARS-CoV (93). In humans, increased frequencies of CD4+ TRM cells in the airway are associated with surviving severe disease of SARS-CoV-2 infection (94). Furthermore, CD4+ TRM cells may promote the generation of antibodies by B cells against pathogenic microorganisms in mucosal tissues, including the lung. In fact, a subpopulation of CD4+ TRM cells promotes humoral responses in the lung after viral infection (95, 96). This subpopulation shows the follicular helper T (Tfh)-like phenotype, including a high expression of PD-1 and CXCR5 (95). The differentiation of this subpopulation depends on B cells and the intrinsic expression of Bcl6 (95). Importantly, Bcl6hi CD4+ TRM cells, which are colocalized with B cells in iBALT, promote local antibody production and help CD8+ TRM cells via the enhanced production of IL-21 (95, 96). Thus, CD4+ TRM cells are a promising target cell population in terms of the development of next-generation vaccine therapies (97). In the future, more intensive research on CD4+ TRM cells is expected to reveal new cellular mechanisms and molecular mechanisms for CD4+ TRM cells.
Writing, reviewing, and editing: KH, KK, AA, MK, and TN. All authors contributed to the article and approved the submitted version.
This work was supported by the following grants: Ministry of Education, Culture, Sports, Science and Technology (MEXT Japan) Grants-in-Aid for Scientific Research (S) JP19H05650, (B) 20H03685, (C) 17K08876, 18K07164 and 19K16683; Practical Research Project for Allergic Diseases and Immunology (Research on Allergic Diseases and Immunology) from the Japan Agency for Medical Research and Development, AMED (Nos. JP20ek0410082, JP20ek0410060 and JP19ek0410045); AMED-PRIME, AMED (No. JP20gm6110005); AMED-CREST, AMED (No. JP20gm1210003); Mochida Memorial Foundation for Medical and Pharmaceutical Research, MSD Life Science Foundation, The Naito Foundation and Takeda Science Foundation.
The authors declare that the research was conducted in the absence of any commercial or financial relationships that could be construed as a potential conflict of interest.
1. Sallusto F, Mackay CR. Chemoattractants and their receptors in homeostasis and inflammation. Curr Opin Immunol (2004) 16:724–31. doi: 10.1016/j.coi.2004.09.012
2. Sallusto F, Lenig D, Forster R, Lipp M, Lanzavecchia A. Two subsets of memory T lymphocytes with distinct homing potentials and effector functions. Nature (1999) 401:708–12. doi: 10.1038/44385
3. Geginat J, Sallusto F, Lanzavecchia A. Cytokine-driven proliferation and differentiation of human naive, central memory, and effector memory CD4(+) T cells. J Exp Med (2001) 194:1711–9. doi: 10.1084/jem.194.12.1711
4. Pakpour N, Zaph C, Scott P. The central memory CD4+ T cell population generated during Leishmania major infection requires IL-12 to produce IFN-gamma. J Immunol (2008) 180:8299–305. doi: 10.4049/jimmunol.180.12.8299
5. Hamann D, Baars PA, Rep MH, Hooibrink B, Kerkhof-Garde SR, Klein MR, et al. Phenotypic and functional separation of memory and effector human CD8+ T cells. J Exp Med (1997) 186:1407–18. doi: 10.1084/jem.186.9.1407
6. Kaech SM, Tan JT, Wherry EJ, Konieczny BT, Surh CD, Ahmed R. Selective expression of the interleukin 7 receptor identifies effector CD8 T cells that give rise to long-lived memory cells. Nat Immunol (2003) 4:1191–8. doi: 10.1038/ni1009
7. Hikono H, Kohlmeier JE, Takamura S, Wittmer ST, Roberts AD, Woodland DL. Activation phenotype, rather than central- or effector-memory phenotype, predicts the recall efficacy of memory CD8+ T cells. J Exp Med (2007) 204:1625–36. doi: 10.1084/jem.20070322
8. Bottcher JP, Beyer M, Meissner F, Abdullah Z, Sander J, Hochst B, et al. Functional classification of memory CD8(+) T cells by CX3CR1 expression. Nat Commun (2015) 6:8306. doi: 10.1038/ncomms9306
9. Gerlach C, Moseman EA, Loughhead SM, Alvarez D, Zwijnenburg AJ, Waanders L, et al. The Chemokine Receptor CX3CR1 Defines Three Antigen-Experienced CD8 T Cell Subsets with Distinct Roles in Immune Surveillance and Homeostasis. Immunity (2016) 45:1270–84. doi: 10.1016/j.immuni.2016.10.018
10. Masopust D, Soerens AG. Tissue-Resident T Cells and Other Resident Leukocytes. Annu Rev Immunol (2019) 37:521–46. doi: 10.1146/annurev-immunol-042617-053214
11. Teijaro JR, Turner D, Pham Q, Wherry EJ, Lefrancois L, Farber DL. Cutting edge: Tissue-retentive lung memory CD4 T cells mediate optimal protection to respiratory virus infection. J Immunol (2011) 187:5510–4. doi: 10.4049/jimmunol.1102243
12. Schenkel JM, Fraser KA, Vezys V, Masopust D. Sensing and alarm function of resident memory CD8(+) T cells. Nat Immunol (2013) 14:509–13. doi: 10.1038/ni.2568
13. Schenkel JM, Fraser KA, Beura LK, Pauken KE, Vezys V, Masopust D. T cell memory. Resident memory CD8 T cells trigger protective innate and adaptive immune responses. Science (2014) 346:98–101. doi: 10.1126/science.1254536
14. Ariotti S, Hogenbirk MA, Dijkgraaf FE, Visser LL, Hoekstra ME, Song JY, et al. T cell memory. Skin-resident memory CD8(+) T cells trigger a state of tissue-wide pathogen alert. Science (2014) 346(6205):101–5. doi: 10.1126/science.1254803
15. Pallett LJ, Davies J, Colbeck EJ, Robertson F, Hansi N, Easom NJW, et al. IL-2(high) tissue-resident T cells in the human liver: Sentinels for hepatotropic infection. J Exp Med (2017) 214:1567–80. doi: 10.1084/jem.20162115
16. Amezcua Vesely MC, Pallis P, Bielecki P, Low JS, Zhao J, Harman CCD, et al. Effector TH17 Cells Give Rise to Long-Lived TRM Cells that Are Essential for an Immediate Response against Bacterial Infection. Cell (2019) 178:1176–88 e1115. doi: 10.1016/j.cell.2019.07.032
17. Fonseca R, Beura LK, Quarnstrom CF, Ghoneim HE, Fan Y, Zebley CC, et al. Developmental plasticity allows outside-in immune responses by resident memory T cells. Nat Immunol (2020) 21:412–21. doi: 10.1038/s41590-020-0607-7
18. Behr FM, Parga-Vidal L, Kragten NAM, van Dam TJP, Wesselink TH, Sheridan BS, et al. Tissue-resident memory CD8(+) T cells shape local and systemic secondary T cell responses. Nat Immunol (2020) 21:1070–81. doi: 10.1038/s41590-020-0723-4
19. Beura LK, Fares-Frederickson NJ, Steinert EM, Scott MC, Thompson EA, Fraser KA, et al. CD4(+) resident memory T cells dominate immunosurveillance and orchestrate local recall responses. J Exp Med (2019) 216:1214–29. doi: 10.1084/jem.20181365
20. Fernandez-Ruiz D, Ng WY, Holz LE, Ma JZ, Zaid A, Wong YC, et al. Liver-Resident Memory CD8(+) T Cells Form a Front-Line Defense against Malaria Liver-Stage Infection. Immunity (2016) 45:889–902. doi: 10.1016/j.immuni.2016.08.011
21. Steinbach K, Vincenti I, Kreutzfeldt M, Page N, Muschaweckh A, Wagner I, et al. Brain-resident memory T cells represent an autonomous cytotoxic barrier to viral infection. J Exp Med (2016) 213(8):1571–87. doi: 10.1084/jem.20151916
22. Gebhardt T, Whitney PG, Zaid A, Mackay LK, Brooks AG, Heath WR, et al. Different patterns of peripheral migration by memory CD4+ and CD8+ T cells. Nature (2011) 477:216–9. doi: 10.1038/nature10339
23. Glennie ND, Yeramilli VA, Beiting DP, Volk SW, Weaver CT, Scott P. Skin-resident memory CD4+ T cells enhance protection against Leishmania major infection. J Exp Med (2015) 212(9):1405–14. doi: 10.1084/jem.20142101
24. Glennie ND, Volk SW, Scott P. Skin-resident CD4+ T cells protect against Leishmania major by recruiting and activating inflammatory monocytes. PloS Pathog (2017) 13:e1006349. doi: 10.1371/journal.ppat.1006349
25. Klicznik MM, Morawski PA, Hollbacher B, Varkhande SR, Motley SJ, Kuri-Cervantes L, et al. Human CD4(+)CD103(+) cutaneous resident memory T cells are found in the circulation of healthy individuals. Sci Immunol (2019) 4(37). doi: 10.1126/sciimmunol.aav8995
26. Iijima N, Iwasaki A. T cell memory. A local macrophage chemokine network sustains protective tissue-resident memory CD4 T cells. Science (2014) 346:93–8. doi: 10.1126/science.1257530
27. Roychoudhury P, Swan DA, Duke E, Corey L, Zhu J, Dave V, et al. Tissue-resident T cell-derived cytokines eliminate herpes simplex virus-2-infected cells. J Clin Invest (2020) 130:2903–19. doi: 10.1172/JCI132583
28. Steinfelder S, Rausch S, Michael D, Kuhl AA, Hartmann S. Intestinal helminth infection induces highly functional resident memory CD4(+) T cells in mice. Eur J Immunol (2017) 47:353–63. doi: 10.1002/eji.201646575
29. Romagnoli PA, Fu HH, Qiu Z, Khairallah C, Pham QM, Puddington L, et al. Differentiation of distinct long-lived memory CD4 T cells in intestinal tissues after oral Listeria monocytogenes infection. Mucosal Immunol (2017) 10(2):520–30. doi: 10.1038/mi.2016.66
30. Kumar BV, Ma W, Miron M, Granot T, Guyer RS, Carpenter DJ, et al. Human Tissue-Resident Memory T Cells Are Defined by Core Transcriptional and Functional Signatures in Lymphoid and Mucosal Sites. Cell Rep (2017) 20:2921–34. doi: 10.1016/j.celrep.2017.08.078
31. Hondowicz BD, Kim KS, Ruterbusch MJ, Keitany GJ, Pepper M. IL-2 is required for the generation of viral-specific CD4(+) Th1 tissue-resident memory cells and B cells are essential for maintenance in the lung. Eur J Immunol (2018) 48:80–6. doi: 10.1002/eji.201746928
32. Bull NC, Kaveh DA, Garcia-Pelayo MC, Stylianou E, McShane H, Hogarth PJ. Induction and maintenance of a phenotypically heterogeneous lung tissue-resident CD4(+) T cell population following BCG immunisation. Vaccine (2018) 36:5625–35. doi: 10.1016/j.vaccine.2018.07.035
33. O’Hara JM, Redhu NS, Cheung E, Robertson NG, Patik I, Sayed SE, et al. Generation of protective pneumococcal-specific nasal resident memory CD4(+) T cells via parenteral immunization. Mucosal Immunol (2020) 13:172–82. doi: 10.1038/s41385-019-0218-5
34. Park CO, Fu X, Jiang X, Pan Y, Teague JE, Collins N, et al. Staged development of long-lived T-cell receptor alphabeta TH17 resident memory T-cell population to Candida albicans after skin infection. J Allergy Clin Immunol (2018) 142(2):647–62. doi: 10.1016/j.jaci.2017.09.042
35. Swaims-Kohlmeier A, Haaland RE, Haddad LB, Sheth AN, Evans-Strickfaden T, Lupo LD, et al. Progesterone Levels Associate with a Novel Population of CCR5+CD38+ CD4 T Cells Resident in the Genital Mucosa with Lymphoid Trafficking Potential. J Immunol (2016) 197(1):368–76. doi: 10.4049/jimmunol.1502628
36. Thome JJ, Bickham KL, Ohmura Y, Kubota M, Matsuoka N, Gordon C, et al. Early-life compartmentalization of human T cell differentiation and regulatory function in mucosal and lymphoid tissues. Nat Med (2016) 22(1):72–7. doi: 10.1038/nm.4008
37. Snyder ME, Finlayson MO, Connors TJ, Dogra P, Senda T, Bush E, et al. Generation and persistence of human tissue-resident memory T cells in lung transplantation. Sci Immunol (2019) 4(33). doi: 10.1126/sciimmunol.aav5581
38. Smolders J, Heutinck KM, Fransen NL, Remmerswaal EBM, Hombrink P, Ten Berge IJM, et al. Tissue-resident memory T cells populate the human brain. Nat Commun (2018) 9(1):4593. doi: 10.1038/s41467-018-07053-9
39. Kumar BV, Connors TJ, Farber DL. Human T Cell Development, Localization, and Function throughout Life. Immunity (2018) 48:202–13. doi: 10.1016/j.immuni.2018.01.007
40. Szabo PA, Miron M, Farber DL. Location, location, location: Tissue resident memory T cells in mice and humans. Sci Immunol (2019) 4. doi: 10.1126/sciimmunol.aas9673
41. Fan X, Rudensky AY. Hallmarks of Tissue-Resident Lymphocytes. Cell (2016) 164:1198–211. doi: 10.1016/j.cell.2016.02.048
42. Miyasaka M, Tanaka T. Lymphocyte trafficking across high endothelial venules: dogmas and enigmas. Nat Rev Immunol (2004) 4:360–70. doi: 10.1038/nri1354
43. Mackay LK, Rahimpour A, Ma JZ, Collins N, Stock AT, Hafon ML, et al. The developmental pathway for CD103(+)CD8+ tissue-resident memory T cells of skin. Nat Immunol (2013) 14(12):1294–301. doi: 10.1038/ni.2744
44. Laidlaw BJ, Zhang N, Marshall HD, Staron MM, Guan T, Hu Y, et al. CD4+ T cell help guides formation of CD103+ lung-resident memory CD8+ T cells during influenza viral infection. Immunity (2014) 41:633–45. doi: 10.1016/j.immuni.2014.09.007
45. Hirai T, Yang Y, Zenke Y, Li H, Chaudhri VK, De La Cruz Diaz JS, et al. Competition for Active TGFbeta Cytokine Allows for Selective Retention of Antigen-Specific Tissue- Resident Memory T Cells in the Epidermal Niche. Immunity (2020) 54(1):84–98.e5. doi: 10.1016/j.immuni.2020.10.022
46. Mackay LK, Wynne-Jones E, Freestone D, Pellicci DG, Mielke LA, Newman DM, et al. T-box Transcription Factors Combine with the Cytokines TGF-beta and IL-15 to Control Tissue-Resident Memory T Cell Fate. Immunity (2015) 43:1101–11. doi: 10.1016/j.immuni.2015.11.008
47. Adachi T, Kobayashi T, Sugihara E, Yamada T, Ikuta K, Pittaluga S, et al. Hair follicle-derived IL-7 and IL-15 mediate skin-resident memory T cell homeostasis and lymphoma. Nat Med (2015) 21(11):1272–9. doi: 10.1038/nm.3962
48. Strutt TM, Dhume K, Finn CM, Hwang JH, Castonguay C, Swain SL, et al. IL-15 supports the generation of protective lung-resident memory CD4 T cells. Mucosal Immunol (2018) 11:668–80. doi: 10.1038/mi.2017.101
49. Cyster JG, Schwab SR. Sphingosine-1-phosphate and lymphocyte egress from lymphoid organs. Annu Rev Immunol (2012) 30:69–94. doi: 10.1146/annurev-immunol-020711-075011
50. Kimura MY, Hayashizaki K, Tokoyoda K, Takamura S, Motohashi S, Nakayama T. Crucial role for CD69 in allergic inflammatory responses: CD69-Myl9 system in the pathogenesis of airway inflammation. Immunol Rev (2017) 278(1):87–100. doi: 10.1111/imr.12559
51. Zammit DJ, Turner DL, Klonowski KD, Lefrancois L, Cauley LS. Residual antigen presentation after influenza virus infection affects CD8 T cell activation and migration. Immunity (2006) 24:439–49. doi: 10.1016/j.immuni.2006.01.015
52. Takamura S, Yagi H, Hakata Y, Motozono C, McMaster SR, Masumoto T, et al. Specific niches for lung-resident memory CD8+ T cells at the site of tissue regeneration enable CD69-independent maintenance. J Exp Med (2016) 213:3057–73. doi: 10.1084/jem.20160938
53. Mackay LK, Braun A, Macleod BL, Collins N, Tebartz C, Bedoui S, et al. Cutting edge: CD69 interference with sphingosine-1-phosphate receptor function regulates peripheral T cell retention. J Immunol (2015) 194(5):2059–63. doi: 10.4049/jimmunol.1402256
54. Walsh DA, Borges da Silva H, Beura LK, Peng C, Hamilton SE, Masopust D, et al. The Functional Requirement for CD69 in Establishment of Resident Memory CD8(+) T Cells Varies with Tissue Location. J Immunol (2019) 203:946–55. doi: 10.4049/jimmunol.1900052
55. Skon CN, Lee JY, Anderson KG, Masopust D, Hogquist KA, Jameson SC, et al. Transcriptional downregulation of S1pr1 is required for the establishment of resident memory CD8+ T cells. Nat Immunol (2013) 14(12):1285–93. doi: 10.1038/ni.2745
56. Beura LK, Wijeyesinghe S, Thompson EA, Macchietto MG, Rosato PC, Pierson MJ, et al. T Cells in Nonlymphoid Tissues Give Rise to Lymph-Node-Resident Memory T Cells. Immunity (2018) 48:327–38.e325. doi: 10.1016/j.immuni.2018.01.015
57. Mackay LK, Minnich M, Kragten NA, Liao Y, Nota B, Seillet C, et al. Hobit and Blimp1 instruct a universal transcriptional program of tissue residency in lymphocytes. Science (2016) 352:459–63. doi: 10.1126/science.aad2035
58. Milner JJ, Toma C, Yu B, Zhang K, Omilusik K, Phan AT, et al. Runx3 programs CD8(+) T cell residency in non-lymphoid tissues and tumours. Nature (2017) 552(7684):253–7. doi: 10.1038/nature24993
59. Zundler S, Becker E, Spocinska M, Slawik M, Parga-Vidal L, Stark R, et al. Hobit- and Blimp-1-driven CD4(+) tissue-resident memory T cells control chronic intestinal inflammation. Nat Immunol (2019) 20(3):288–300. doi: 10.1038/s41590-018-0298-5
60. Rahimi RA, Nepal K, Cetinbas M, Sadreyev RI, Luster AD. Distinct functions of tissue-resident and circulating memory Th2 cells in allergic airway disease. J Exp Med (2020) 217. doi: 10.1084/jem.20190865
61. Aschenbrenner D, Foglierini M, Jarrossay D, Hu D, Weiner HL, Kuchroo VK, et al. An immunoregulatory and tissue-residency program modulated by c-MAF in human TH17 cells. Nat Immunol (2018) 19(10):1126–36. doi: 10.1038/s41590-018-0200-5
62. Schreiner D, King CG. CD4+ Memory T Cells at Home in the Tissue: Mechanisms for Health and Disease. Front Immunol (2018) 9:2394. doi: 10.3389/fimmu.2018.02394
63. Hombrink P, Helbig C, Backer RA, Piet B, Oja AE, Stark R, et al. Programs for the persistence, vigilance and control of human CD8(+) lung-resident memory T cells. Nat Immunol (2016) 17(12):1467–78. doi: 10.1038/ni.3589
64. Anderson KG, Mayer-Barber K, Sung H, Beura L, James BR, Taylor JJ, et al. Intravascular staining for discrimination of vascular and tissue leukocytes. Nat Protoc (2014) 9(1):209–22. doi: 10.1038/nprot.2014.005
65. Gebhardt T, Wakim LM, Eidsmo L, Reading PC, Heath WR, Carbone FR. Memory T cells in nonlymphoid tissue that provide enhanced local immunity during infection with herpes simplex virus. Nat Immunol (2009) 10(5):524–30. doi: 10.1038/ni.1718
66. Jiang X, Clark RA, Liu L, Wagers AJ, Fuhlbrigge RC, Kupper TS. Skin infection generates non-migratory memory CD8+ T(RM) cells providing global skin immunity. Nature (2012) 483(7388):227–31. doi: 10.1038/nature10851
67. Takamura S, Kato S, Motozono C, Shimaoka T, Ueha S, Matsuo K, et al. Interstitial-resident memory CD8(+) T cells sustain frontline epithelial memory in the lung. J Exp Med (2019) 216(12):2736–47. doi: 10.1084/jem.20190557
68. Zhu J, Peng T, Johnston C, Phasouk K, Kask AS, Klock A, et al. Immune surveillance by CD8alphaalpha+ skin-resident T cells in human herpes virus infection. Nature (2013) 497(7450):494–7. doi: 10.1038/nature12110
69. Stary G, Olive A, Radovic-Moreno AF, Gondek D, Alvarez D, Basto PA, et al. VACCINES. A mucosal vaccine against Chlamydia trachomatis generates two waves of protective memory T cells. Science (2015) 348(6241):aaa8205. doi: 10.1126/science.aaa8205
70. Smith NM, Wasserman GA, Coleman FT, Hilliard KL, Yamamoto K, Lipsitz E, et al. Regionally compartmentalized resident memory T cells mediate naturally acquired protection against pneumococcal pneumonia. Mucosal Immunol (2018) 11(1):220–35. doi: 10.1038/mi.2017.43
71. Cheuk S, Schlums H, Gallais Serezal I, Martini E, Chiang SC, Marquardt N, et al. CD49a Expression Defines Tissue-Resident CD8+ T Cells Poised for Cytotoxic Function in Human Skin. Immunity (2017) 46(2):287–300. doi: 10.1016/j.immuni.2017.01.009
72. Sasaki K, Bean A, Shah S, Schutten E, Huseby PG, Peters B, et al. Relapsing-remitting central nervous system autoimmunity mediated by GFAP-specific CD8 T cells. J Immunol (2014) 192(7):3029–42. doi: 10.4049/jimmunol.1302911
73. Gieseck RL3, Wilson MS, Wynn TA. Type 2 immunity in tissue repair and fibrosis. Nat Rev Immunol (2018) 18:62–76. doi: 10.1038/nri.2017.90
74. Yeon SM, Halim L, Chandele A, Perry CJ, Kim SH, Kim SU, et al. IL-7 plays a critical role for the homeostasis of allergen-specific memory CD4 T cells in the lung and airways. Sci Rep (2017) 7(1):11155. doi: 10.1038/s41598-017-11492-7
75. Asano K, Hebisawa A, Ishiguro T, Takayanagi N, Nakamura Y, Suzuki J, et al. New Clinical Diagnostic Criteria for Allergic Bronchopulmonary Aspergillosis/Mycosis and its Validation. J Allergy Clin Immunol (2020). doi: 10.1016/j.jaci.2020.08.029
76. Hondowicz BD, An D, Schenkel JM, Kim KS, Steach HR, Krishnamurty AT, et al. Interleukin-2-Dependent Allergen-Specific Tissue-Resident Memory Cells Drive Asthma. Immunity (2016) 44(1):155–66. doi: 10.1016/j.immuni.2015.11.004
77. Turner DL, Goldklang M, Cvetkovski F, Paik D, Trischler J, Barahona J, et al. Biased Generation and In Situ Activation of Lung Tissue-Resident Memory CD4 T Cells in the Pathogenesis of Allergic Asthma. J Immunol (2018) 200(5):1561–9. doi: 10.4049/jimmunol.1700257
78. Ichikawa T, Hirahara K, Kokubo K, Kiuchi M, Aoki A, Morimoto Y, et al. CD103(hi) Treg cells constrain lung fibrosis induced by CD103(lo) tissue-resident pathogenic CD4 T cells. Nat Immunol (2019) 20(11):1469–80. doi: 10.1038/s41590-019-0494-y
79. Enamorado M, Iborra S, Priego E, Cueto FJ, Quintana JA, Martinez-Cano S, et al. Enhanced anti-tumour immunity requires the interplay between resident and circulating memory CD8(+) T cells. Nat Commun (2017) 8:16073. doi: 10.1038/ncomms16073
80. Durek P, Nordstrom K, Gasparoni G, Salhab A, Kressler C, de Almeida M, et al. Epigenomic Profiling of Human CD4(+) T Cells Supports a Linear Differentiation Model and Highlights Molecular Regulators of Memory Development. Immunity (2016) 45(5):1148–61. doi: 10.1016/j.immuni.2016.10.022
81. Carragher DM, Rangel-Moreno J, Randall TD. Ectopic lymphoid tissues and local immunity. Semin Immunol (2008) 20:26–42. doi: 10.1016/j.smim.2007.12.004
82. Low JS, Farsakoglu Y, Amezcua Vesely MC, Sefik E, Kelly JB, Harman CCD, et al. Tissue-resident memory T cell reactivation by diverse antigen-presenting cells imparts distinct functional responses. J Exp Med (2020) 217(8). doi: 10.1084/jem.20192291
83. Shinoda K, Hirahara K, Iinuma T, Ichikawa T, Suzuki AS, Sugaya K, et al. Thy1+IL-7+ lymphatic endothelial cells in iBALT provide a survival niche for memory T-helper cells in allergic airway inflammation. Proc Natl Acad Sci U S A (2016) 113:E2842–51. doi: 10.1073/pnas.1512600113
84. Natsuaki Y, Egawa G, Nakamizo S, Ono S, Hanakawa S, Okada T, et al. Perivascular leukocyte clusters are essential for efficient activation of effector T cells in the skin. Nat Immunol (2014) 15(11):1064–9. doi: 10.1038/ni.2992
85. Collins N, Jiang X, Zaid A, Macleod BL, Li J, Park CO, et al. Skin CD4(+) memory T cells exhibit combined cluster-mediated retention and equilibration with the circulation. Nat Commun (2016) 7:11514. doi: 10.1038/ncomms11514
86. Takamura S. Niches for the Long-Term Maintenance of Tissue-Resident Memory T Cells. Front Immunol (2018) 9:1214. doi: 10.3389/fimmu.2018.01214
87. Nakayama T, Hirahara K, Onodera A, Endo Y, Hosokawa H, Shinoda K, et al. Th2 Cells in Health and Disease. Annu Rev Immunol (2017) 35:53–84. doi: 10.1146/annurev-immunol-051116-052350
88. Endo Y, Hirahara K, Iinuma T, Shinoda K, Tumes DJ, Asou HK, et al. The interleukin-33-p38 kinase axis confers memory T helper 2 cell pathogenicity in the airway. Immunity (2015) 42:294–308. doi: 10.1016/j.immuni.2015.01.016
89. Morimoto Y, Hirahara K, Kiuchi M, Wada T, Ichikawa T, Kanno T, et al. Amphiregulin-Producing Pathogenic Memory T Helper 2 Cells Instruct Eosinophils to Secrete Osteopontin and Facilitate Airway Fibrosis. Immunity (2018) 49(1):134–50.e136. doi: 10.1016/j.immuni.2018.04.023
90. Hirahara K, Aoki A, Morimoto Y, Kiuchi M, Okano M, Nakayama T. The immunopathology of lung fibrosis: amphiregulin-producing pathogenic memory T helper-2 cells control the airway fibrotic responses by inducing eosinophils to secrete osteopontin. Semin Immunopathol (2019) 41:339–48. doi: 10.1007/s00281-019-00735-6
91. Ogongo P, Porterfield JZ, Leslie A. Lung Tissue Resident Memory T-Cells in the Immune Response to Mycobacterium tuberculosis. Front Immunol (2019) 10:992. doi: 10.3389/fimmu.2019.00992
92. Zens KD, Chen JK, Farber DL. Vaccine-generated lung tissue-resident memory T cells provide heterosubtypic protection to influenza infection. JCI Insight (2016) 1. doi: 10.1172/jci.insight.85832
93. Zhao J, Zhao J, Mangalam AK, Channappanavar R, Fett C, Meyerholz DK, et al. Airway Memory CD4(+) T Cells Mediate Protective Immunity against Emerging Respiratory Coronaviruses. Immunity (2016) 44(6):1379–91. doi: 10.1016/j.immuni.2016.05.006
94. Szabo PA, Dogra P, Gray JI, Wells SB, Connors TJ, Weisberg SP, et al. Analysis of respiratory and systemic immune responses in COVID-19 reveals mechanisms of disease pathogenesis. medRxiv (2020). doi: 10.1101/2020.10.15.20208041
95. Swarnalekha N, Schreiner D, Litzler LC, Iftikhar S, Kirchmeier D, Kunzli M, et al. T resident helper cells promote humoral responses in the lung. Sci Immunol (2021) 6(55). doi: 10.1126/sciimmunol.abb6808
96. Son YM, Cheon IS, Wu Y, Li C, Wang Z, Gao X, et al. Tissue-resident CD4(+) T helper cells assist the development of protective respiratory B and CD8(+) T cell memory responses. Sci Immunol (2021) 6. doi: 10.1126/sciimmunol.abb6852
Keywords: CD4+ resident memory T cells, Aspergillus fumigatus, lung fibrosis, ATAC-seq, inducible bronchus-associated lymphoid tissue (iBALT), pathogenic T cell
Citation: Hirahara K, Kokubo K, Aoki A, Kiuchi M and Nakayama T (2021) The Role of CD4+ Resident Memory T Cells in Local Immunity in the Mucosal Tissue – Protection Versus Pathology –. Front. Immunol. 12:616309. doi: 10.3389/fimmu.2021.616309
Received: 12 October 2020; Accepted: 25 March 2021;
Published: 21 April 2021.
Edited by:
Stephen Philip Schoenberger, La Jolla Institute for Immunology (LJI), United StatesReviewed by:
Brian S. Sheridan, Stony Brook University, United StatesCopyright © 2021 Hirahara, Kokubo, Aoki, Kiuchi and Nakayama. This is an open-access article distributed under the terms of the Creative Commons Attribution License (CC BY). The use, distribution or reproduction in other forums is permitted, provided the original author(s) and the copyright owner(s) are credited and that the original publication in this journal is cited, in accordance with accepted academic practice. No use, distribution or reproduction is permitted which does not comply with these terms.
*Correspondence: Kiyoshi Hirahara, aGlyYWhhcmFrQGNoaWJhLXUuanA=; Toshinori Nakayama, dG5ha2F5YW1hQGZhY3VsdHkuY2hpYmEtdS5qcA==
Disclaimer: All claims expressed in this article are solely those of the authors and do not necessarily represent those of their affiliated organizations, or those of the publisher, the editors and the reviewers. Any product that may be evaluated in this article or claim that may be made by its manufacturer is not guaranteed or endorsed by the publisher.
Research integrity at Frontiers
Learn more about the work of our research integrity team to safeguard the quality of each article we publish.