- Lydia Becker Institute for Immunology and Inflammation, Faculty of Biology, Medicine and Health, University of Manchester, Manchester, United Kingdom
Allergy is an IgE-dependent type-I hypersensitivity reaction that can lead to life-threatening systemic symptoms such as anaphylaxis. In the pathogenesis of the allergic response, the common upstream event is the binding of allergens to specific IgE, inducing cross-linking of the high-affinity FcεRI on mast cells, triggering cellular degranulation and the release of histamine, proteases, lipids mediators, cytokines and chemokines with inflammatory activity. A number of novel therapeutic options to curb mast cell activation are in the pipeline for the treatment of severe allergies. In addition to anti-IgE therapy and allergen-specific immunotherapy, monoclonal antibodies targeted against several key Th2/alarmin cytokines (i.e. IL-4Rα, IL-33, TSLP), active modification of allergen-specific IgE (i.e. inhibitory compounds, monoclonal antibodies, de-sialylation), engagement of inhibitory receptors on mast cells and allergen-specific adjuvant vaccines, are new promising options to inhibit the uncontrolled release of mast cell mediators upon allergen exposure. In this review, we critically discuss the novel approaches targeting mast cells limiting allergic responses and the immunological mechanisms involved, with special interest on food allergy treatment.
Introduction
Nowadays, over 20% of the world population actively suffers from one or more allergies, among which approximately 10% is living with food allergy (1, 2). Food allergies carry a high risk of developing systemic reactions upon allergen exposure, with 0.4–39.9% of allergic subjects experiencing at least one severe episode in their lifetime (3).
Anaphylaxis is a systemic reaction involving two or more organ systems, occurring shortly after the exposure to the culprit allergen. It manifests with a plethora of symptoms, including hives, angioedema, shortness of breath, vomiting, hypotension and cardiovascular collapse, which is potentially life-threatening and requires emergency treatment (4). The complex allergic reaction starts with the cross-linking of high-affinity immunoglobulin E (IgE) receptors (FcϵRI) expressed on effector cells such as mast cells (MCs) and basophils by IgE–allergen complexes. FcϵRI engagement causes cell degranulation and release of preformed mediators, such as amines (histamine, polyamines), proteoglycans (heparin, chondroitin sulphates, serglycin), proteases (tryptase, chymase-1, cathepsin G, granzyme B, carboxypeptidase A3), lysosomal enzymes (β-glucuronidase, β-hexosaminidase, arylsulfatase), newly formed lipid mediators (leukotrienes B4-C4, prostaglandin D2-E2), cytokines and chemokines (GM-CSF, IL-1β, IL-8, IL-13, MCP-1) (5, 6).
MC activation is also the cause of the delayed release of newly synthesized cytokines and chemokines (5, 6), that promote dendritic cell recruitment and activation (7, 8), T helper 2 (Th2) skewing (9–11), affinity maturation and epitope spreading on B and T cells (12, 13), additional IgE synthesis (14), and altogether the amplification of allergic responses (15). The release of vasoactive products, such as histamine, cysteinyl leukotrienes and platelet activating factor (16), serves as the main pathogenetic mechanism of anaphylaxis, which can lead to generalized cardiovascular involvement and collapse, the latter burdened with high mortality and morbidity (4).
In addition to their prominent role in the genesis of allergic and anaphylaxis symptoms, MCs actively participate to the complex interplay of innate and adaptive immunity in the defense against pathogens, wound healing and tumor surveillance (17–19). Due to the conspicuous array of surface receptors expressed, capable of sensing the surrounding environment and participate to immune recognition, MCs act as both initiators and suppressors of local immune responses (17, 20, 21). MCs engage in a bidirectional cross-talk with various immune cells, such as dendritic cells (10, 22–24), T cells (25) including T regulatory (Treg) cells (26–28), eosinophils (29, 30), B cells (31) and other cell types (17). Being capable of secreting both pro- and anti-inflammatory cytokines, like TNFα (7) and IL-10 (32), and several chemokines (6), MCs also contribute to the prevention and resolution of food allergy (33). Along with MCs, the above cell populations are considered equally important targets in food allergy treatment, however outside the main scope of the review and discussed elsewhere (34–36).
Strategies to pre-emptively curb MC activation are currently being explored for therapeutic purposes. Allergen-specific immunotherapy, recently developed biologics, a combination of both, and the discovery of new druggable targets are the most promising options available to treat food allergy.
The purpose of this review is to highlight the different immunological mechanisms targeting IgE-mediated MC activation as a therapeutic option for the treatment of food allergy, with particular focus on peanut allergy. However, two crucial preliminary considerations should be made. First, no treatment option currently available is uniquely targeting MCs. In fact, receptors inhibiting MC activation are shared among different cell types, and cytokines and other soluble mediators have pleiotropic effects affecting multiple cell populations at once. Second, any treatment inhibiting IgE-mediated MC activation should also take into consideration the broader implications and the potential loss of MC protective functions. Hence a benefit/risk assessment should be made, especially when considering highly disruptive interventions, like active MC depletion, not covered by the present manuscript (37).
Allergen-Independent Approaches
IgE-Mediated Mast Cell Activation
IgE antibodies are the mainstay of allergic responses. They are monomeric glycoproteins composed of two light and heavy chains, the latter showing four constant Ig-like domains (Cϵ1–4), bound via disulfide bridges (38). Several factors are involved in the development of functional IgE antibodies, including specific affinity maturation, conformational/allosteric properties, and glycosylation patterns (38–40). IgE blood concentration in healthy individuals is very low (below 210 IU/ml) compared to normal levels of IgG (5.65–17.65 mg/ml) (41, 42). IgE are mostly sequestered in peripheral tissues, with an average half-life estimated of 16–20 days in the skin versus 2–4 days in blood (43). Given the high affinity of FcϵRI to IgE (Kd = 1010–1011 M−1) and the slow dissociation rate (44, 45), the majority of IgE are cell-bound to either FcϵRI or the low-affinity receptor FcϵRII (CD23) via the Cϵ3-4 Fc domains (46). FcϵRI is the high-affinity IgE receptor constitutively expressed on MCs and basophils, while inducible on monocytes, dendritic cells, eosinophils and neutrophils (47–50). A tight correlation between atopic status, circulating IgE levels and surface expression of FcϵRI on MCs, basophils and other cell types has been proven (45, 47, 51, 52). While peripheral blood-resident cells acquire IgE directly from the circulation, perivascular tissue-resident MCs, sensing changes in total IgE levels, probe IgE from blood vessels using endoluminal cell processes (53).
Furthermore, occupancy of the FcϵRI receptor is crucial to ensure its expression on the cell membrane by MCs and basophils, as shown by mechanistic studies demonstrating increased FcϵRI expression upon IgE binding due to decreased FcϵRI endocytosis and degradation (44, 54–56). IgE bound to FcϵRI persists as long as MCs are alive, thus indicating that MCs preferentially display rather than catabolize IgE. FcϵRI-mediated constitutive internalization of IgE by dendritic cells and monocytes promotes serum IgE clearance instead (57).
FcϵRI is constituted by one alpha and one beta chain on MCs and basophils, or a single alpha chain on monocytes and dendritic cells (45, 58, 59), complexed with two additional gamma chains with immunoreceptor tyrosine-based activation motif (ITAM) domains acting as docking and activation sites for the Spleen tyrosine kinase (Syk) pathway (60, 61). The activation of the Syk, phosphatidylinositol 3-OH kinase/protein kinase B (PI3K/Akt) and extracellular signal-regulated kinase (ERK) pathways leads to increased intracellular calcium flux, calcium-dependent release of preformed mediators stored in intracellular granules and activation of transcription factors for eicosanoids, cytokines and chemokines production (62).
MCs and basophils express the highest density of FcϵRI receptor [estimated 0.7 × 105 molecules per cell measured on LAD2 MCs (63)], with a bell-shaped dose–response when exposed to increasing allergen concentrations (64). Degranulation is tightly regulated via mechanisms modulating the MC activation threshold, not limited to IgE–FcϵRI complex expression. In fact, the nature and dose of the eliciting allergen also play a modulatory role. For instance, simultaneous stimulation using multiple allergens shows an additive effect on MC activation when suboptimal allergen concentrations are used. Conversely, stimulation with supra-optimal allergen concentrations inhibits MC degranulation (64, 65).
Anti-IgE/FcϵRI Strategies
Given the pivotal role of IgE in the initiation and maintenance of allergic responses, increasing evidence supports the use of anti-IgE molecules as therapeutic strategy to treat allergic diseases, including food allergy (Tables 1 and 2). Anti-IgE therapy disrupts the IgE–FcϵRI axis via the active removal of circulating IgE and the downregulation of FcϵRI on MCs, basophils and dendritic cells (136–138). By removing circulating IgE, the turnover between circulating and cell-bound allergen-specific IgE (sIgE) slowly declines, ultimately reducing the amount of sIgE bound on the cell surface and decreasing the likelihood of allergen-IgE cross-linking and allergen-specific effector cell responses (139–141) (Figure 1A).
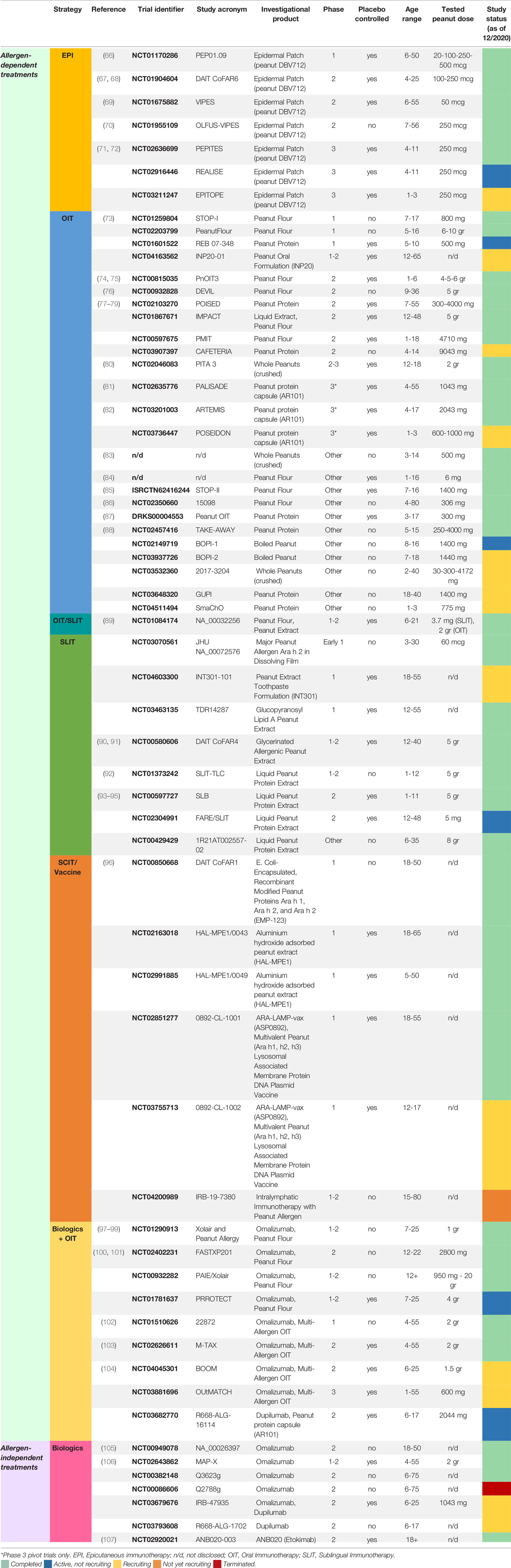
Table 1 List of clinical trials on allergen-dependent and -independent investigational treatments for peanut allergy.
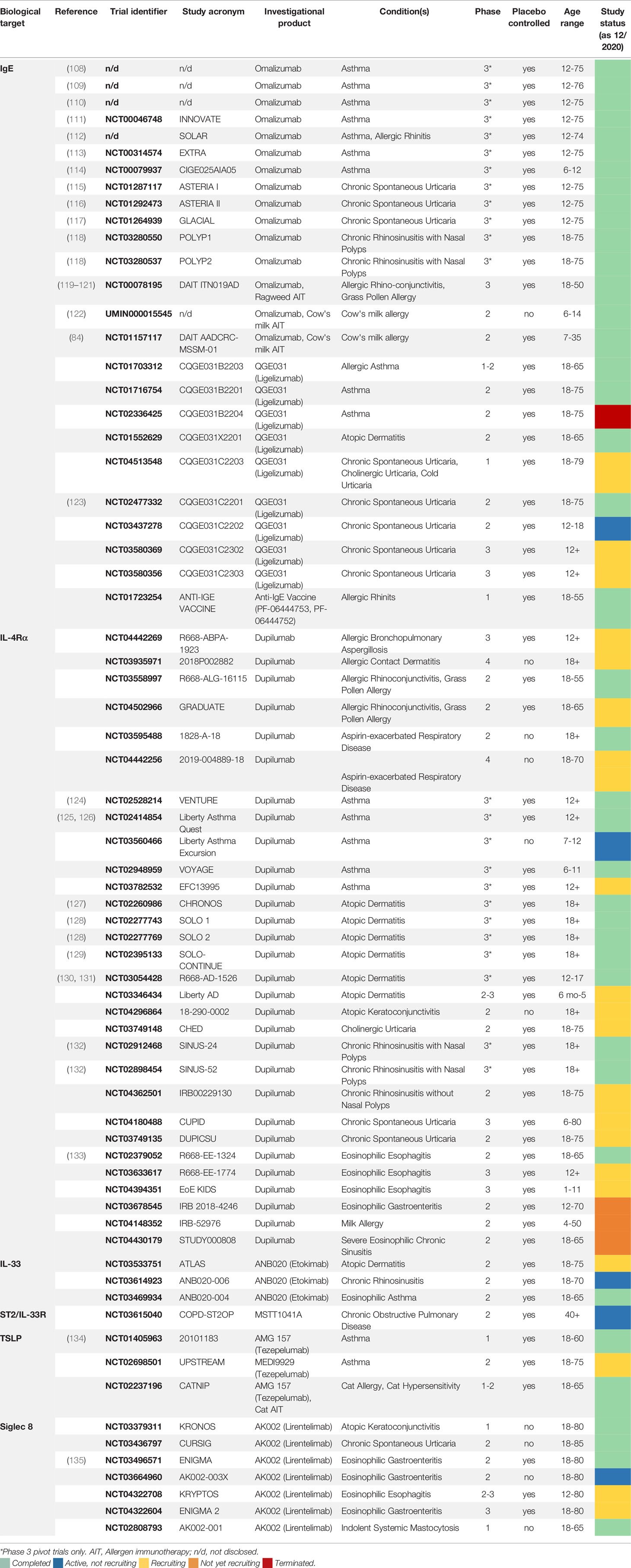
Table 2 List of clinical trials on biologics targeting key mechanisms of MC activation for conditions other than peanut allergy.
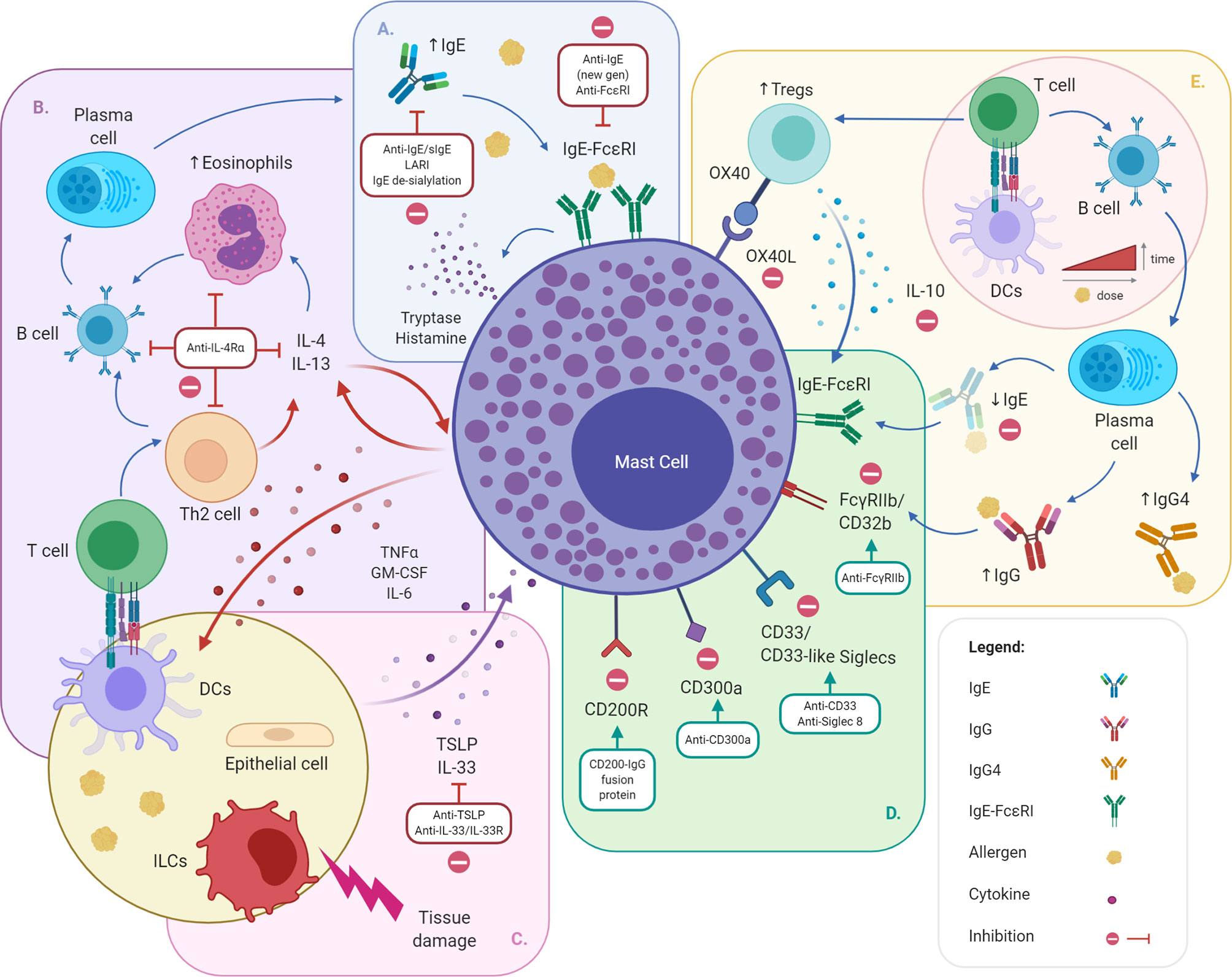
Figure 1 Approaches to target mast cell-dependent allergic responses. Summary of mechanisms controlling MC activation and degranulation and targeted inhibitory approaches, namely suppression of the IgE/FcϵRI axis (A), modulation of IL-4/IL-13 (B) and IL-33/TSLP (C) cytokines, engagement of MC inhibitory receptors (D) and allergen immunotherapy (E). DCs, dendritic cells; FcϵRI, high-affinity IgE receptor; FcγRIIb, low affinity IgG receptor b; IgE, immunoglobulin E; IgG, immunoglobulin G; IgG4, immunoglobulin G 4; IL-4Rα, interleukin 4 receptor alpha chain; LARI, low affinity allergic response inhibitors; MC, mast cell; Siglec, Sialic acid-binding immunoglobulin-type lectins; Tregs, T regulatory cells; TSLP, Thymic stromal lymphopoietin. Created with BioRender.com.
Furthermore, anti-IgE treatment induces FcϵRI down-regulation by interfering with the accumulation of IgE–FcϵRI complexes occurring at the cell surface due to reduced receptor occupancy by IgE (54–56, 136). The reduced availability of sIgE–FcϵRI complexes further inhibits the release of Th2 cytokines and allergic mediators upon allergen challenge by MCs, basophils and dendritic cells (136, 137, 141–144).
Some anti-IgE treatments also inhibit IgE binding to the CD23 receptor, the low affinity IgE receptor constitutively expressed on naïve B cells, exerting an inhibitory effect on IgE-mediated antigen presentation (145, 146), inducing anergy or apoptosis of membrane IgE-bearing B cells (147, 148) and in some cases modulating IgE production (146, 149). However, treatment discontinuation is followed by the quick restoration of pre-treatment IgE levels (150).
Omalizumab, a humanized anti-IgE monoclonal antibody, is the first and most studied biologic, currently used to treat severe asthma and chronic spontaneous urticaria (Table 2). It binds to IgE Cε3 domains, outside of the FcεRI-binding site, and sterically disrupts binding to both FcϵRI and CD23 (151). Omalizumab does not affect pre-bound IgE-receptor interactions, due to conformational changes of receptor-bound IgE masking omalizumab binding sites, and does not induce IgE cross-linking on the cell surface (151, 152).
Omalizumab downregulates the surface expression of FcϵRI in both basophils and MCs (153). However, while FcϵRI expression declines rapidly in circulating basophils (less than 24 h), this process requires longer time in tissue resident MCs (estimated 10–20 days) (136, 154, 155).
The effects exerted by omalizumab on MCs are of clinical relevance also in non-IgE-mediated diseases such as inducible urticarias (156) and MC activation syndrome (105), thus suggesting a broad MC stabilizing function. In food allergy, several clinical trials and real-life evidence showed the safety and usefulness in inhibiting allergic responses of omalizumab as monotherapy (157–160) (Table 1), or in association with allergen-specific immunotherapy, further discussed in Combination Treatments With Biologics section.
Designed Ankyrin Repeat Proteins (DARPins), genetically engineered antibody mimetic proteins, recognize IgE Cϵ3 domains with high specificity and affinity, and have been shown to be 10,000-fold more efficient than omalizumab in dissociating IgE complexes in vitro and in both ex vivo transgenic mouse models and human tissues. Thus, their rapid onset of action makes them of particular interest as treatment option to thwart pre-initiated anaphylaxis episodes (161–165) (Table 3). Along with DARPins, other new generation high-affinity anti-IgE monoclonal antibodies like ligelizumab can actively bind IgE Cε3–4 fragments and efficiently disrupt IgE-FcεRI complexes without, however, interfering with CD23 binding, differently than omalizumab (146) (Table 3). Thus, DARPins and ligelizumab might improve treatment efficacy in food allergy, albeit to date no trials on food allergy are ongoing (Table 2).
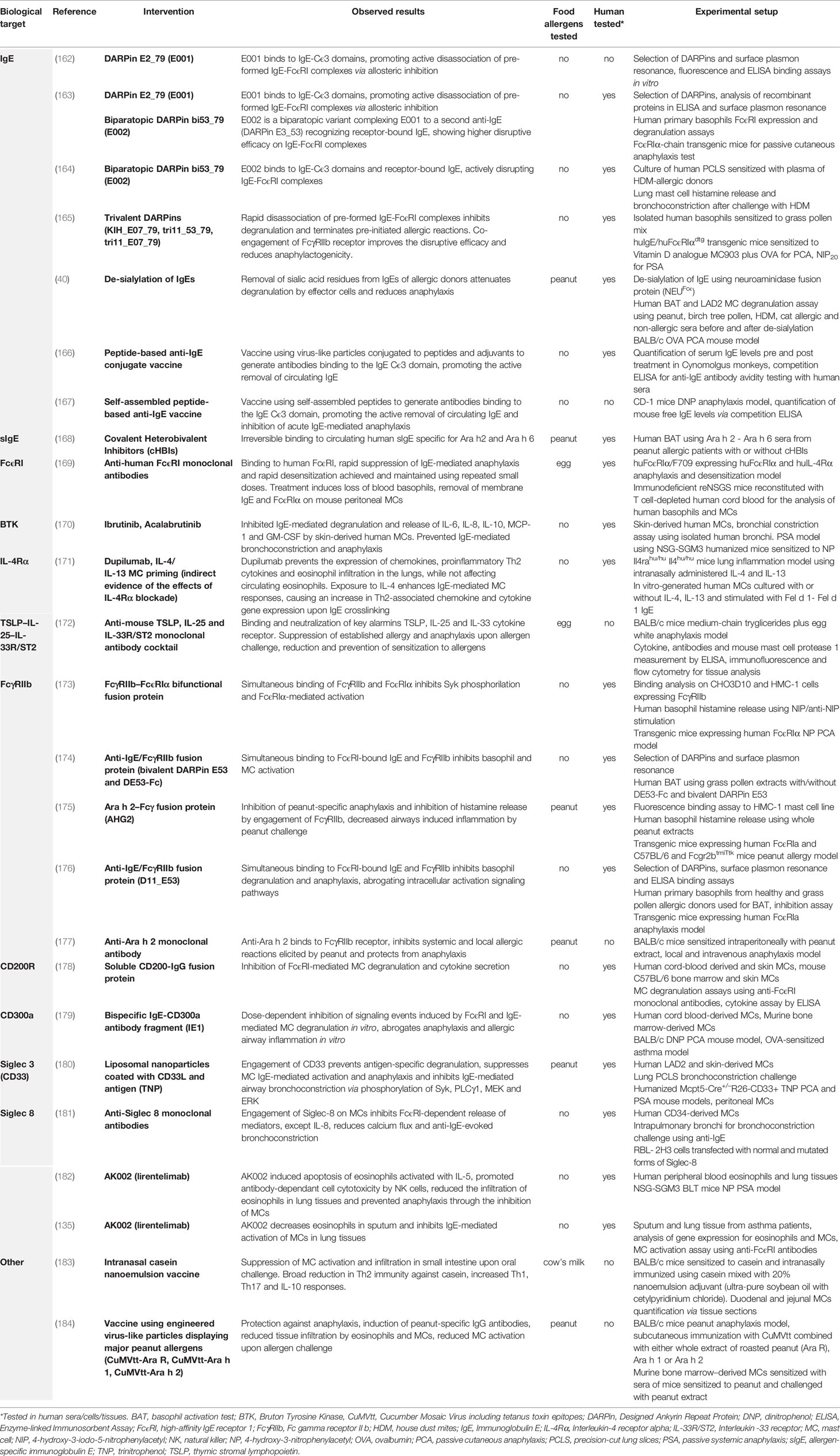
Table 3 Interventions aimed at reducing IgE-mediated mast cell activation currently at pre-clinical/early clinical stage.
New anti-IgE strategies involve self-assembled mRNA vaccines, that provide epitopes mimicking IgE Cε3 domains and stimulate the production of endogenous anti-IgE IgG antibodies, eventually modulating circulating IgE levels via the same mechanisms of omalizumab and other anti-IgE molecules (166, 167). These new treatments inhibited IgE-mediated anaphylaxis in animal models (Table 3) and were tested in a Phase I trial conducted on allergic rhinitis patients (NCT01723254, Table 2); however their application in food allergy is still unclear.
Concerns over the long-lasting implications of irreversible IgE suppression might also arise, considering that, along with omalizumab and other high affinity molecules, broad anti-IgE agents bind also to IgE antibodies serving housekeeping functions, like protection against parasitic infections and tumor surveillance (185–187). Therefore, alternative strategies have been developed to specifically target IgE of interest. The use of covalent heterobivalent low affinity allergic response inhibitors (LARI) has been promising and showed to reduce the risk of anaphylaxis in experimental mouse models of peanut allergy (168) (Table 3).
Alternatively to anti-IgE molecules, a recent approach using anti-FcϵRIα monoclonal antibodies strongly suppressed IgE-mediated MC activation in a humanized mouse model of food allergy and anaphylaxis, revealing another promising therapeutic option (169) (Table 3).
Furthermore, inhibition of FcϵRI-mediated signaling using Bruton Tyrosin Kinase (BTK) inhibitors, significantly reduced degranulation and cytokine production in human MCs and basophils, decreased bronchoconstriction in isolated human bronchi, and proved effective in preventing anaphylaxis in a passive systemic anaphylaxis model using humanized mice (170, 188) (Table 3). Although ibrutinib is well known for its gastrointestinal, cardiovascular and hematological side effects, newer generation molecules, like acalabrutinib, show better safety profile and could become effective, fast-acting oral treatments (189). To this date, however, no clinical trials using BTK inhibitors in food allergy are on-going.
Current evidence suggests that IgE from atopic individuals show an increased sialic acid content, contrary to subjects with no atopy, thus pointing at an important role of sialylation to determine IgE allergenicity (40). Neuraminidase-induced de-sialylation of IgE in a non-FcϵRI dependent manner also diminished downstream signaling in MCs (40). Therefore, de-sialylation of IgE promises to decrease IgE allergenicity, without disrupting non-allergenic IgE activity (Table 3). However, sialidases are ubiquitously expressed in human tissues and play an important role in a variety of physiological and pathological processes, including tumor, infection and inflammation (190), hence the manipulation of the sialylation axis remains an ambitious goal. Notwithstanding, selective small molecule inhibitors of human sialidases hold a great potential for therapeutic development and warrants further investigation (191).
Cytokines Modulating Mast Cell Activity in Allergy
Cytokines involved in Th2 responses, such as IL-4 and IL-13, promote MC proliferation, FcϵRI expression, IgE-mediated degranulation and cytokine production, adhesion and chemotaxis (171, 192, 193). IL-4 and IL-13 receptors share a common alpha chain (IL-4Rα), broadly expressed on lymphocytes, granulocytes and MCs, forming different functional heterodimers according to the associated beta chain (i.e. IL-4R Type I and II, IL-13R), which ultimately activate the intracellular signal transducer and activator of transcription 6 (STAT6) via the phosphorylation of Janus Kinases (Jak1-3, Tyk2) (194, 195). In particular, the proliferation and chemotaxis of MCs induced by IL-4/IL-4R engagement in mucosal interfaces are crucial for the amplification of local allergen responses and responsible for augmented permeability in the intestines and enhanced sensitivity to food allergens and anaphylaxis in experimental mouse models (196–198).
Alongside classical Th2 cytokines, MCs respond rapidly to tissue damage signals such as IL-33 and thymic stromal lymphopoietin (TSLP), alarmins produced mostly by epithelia, innate lymphoid cells and, in some conditions, by MCs themselves (199, 200). IL-33 is known to promote maturation and survival of MCs, enhance the production of pre-formed mediators (e.g. tryptase, serotonin) (201), cytokines (e.g. IL-4, IL-6, IL-13, GM-CSF), and chemokines (e.g. CCL2, CCL17) (201–203), while inhibiting the expression of regulatory cytokines, such as IL-10 (204). Furthermore, IL-33 potentiates IgE-mediated degranulation (202). However, a long-lasting IL-33 stimulation downregulates FcϵRI expression in human MCs, thus inhibiting IgE-dependent MC activation (201), and generating a hyporesponsive phenotype in both mouse and human MCs (205).
TSLP shares common properties with IL-33. They both promote the proliferation and differentiation of MC progenitors (206), and the production of pro-inflammatory cytokines (IL-5, IL-6, IL-13, GM-CSF) and chemokines (CXCL8, CCL1) without inducing the release of pre-formed granule mediators (207). In a food allergy mouse model, TSLP participates in the skin sensitization to food antigens, promoting basophil recruitment and initiating Th2 responses, whereas IL-33 is essential for gut-mediated sensitization and effector responses, including anaphylaxis (208).
Anti-Cytokine Treatments (IL-4/13, IL-33, TSLP)
Several anti-cytokine treatments have shown promising results in food allergy. The monoclonal antibody dupilumab, blocking IL-4 and IL-13 from binding to the IL-4Rα chain, is currently approved for treatment of severe atopic dermatitis and asthma (Table 2). IL4Rα blockade broadly reduces Th2-responses (171) while increasing Treg suppressive responses (98), reduces eosinophil infiltration (171) and MC proliferation in mucosal tissues of IL4Rα−/− mice (198). Dupilumab potentially inhibits MC priming and enhancement of IgE-mediated responses by IL-4 (171) (Table 3), while hampering B cell activation and IgE synthesis in mice (171, 209). In fact, recent evidence shows an important role of dupilumab in modulating B cell recall responses, as demonstrated by the reduction of peanut-specific IgE production by human B cells in vitro, and sustained inhibition after in vivo re-exposure in a peanut anaphylaxis mouse model (210) (Figure 1B). Albeit limited to a single case report, dupilumab is an efficient therapeutic option for multiple co-occurring food allergies (211), and under clinical trial as treatment for peanut allergy (Table 1).
The upstream role of IL-33 and TSLP in promoting Th2 responses makes them interesting targets for the treatment of atopic conditions, including food allergy (36) (Figure 1C). In a Phase II study 73% of peanut allergic patients treated with the anti-IL-33 antibody etokimab achieved tolerance to target peanut dose, showing reduced IL-4, IL-5, IL-13 and IL-9 production after an in vitro T cell challenge with peanut extract, along with reduced peanut-specific IgE levels compared to the placebo arm (107) (NCT02920021, Table 1). As for TSLP blockade, mouse models suggest some efficacy, in combination with either IL-25 or IL-33 receptor monoclonal antibodies, in preventing sensitization to food allergens, and promoting tolerance in association with oral immunotherapy (172) (Table 3). Anti-TSLP (tezepelumab, AMG 157, MEDI9929) has been successfully used in reducing allergen-induced bronchoconstriction and indexes of airway inflammation in patients with allergic asthma (NCT01405963) (134, 212) and is currently under investigation in a study combining tezepelumab with allergen-specific immunotherapy for the induction of tolerance in subjects with cat allergy (NCT02237196, Table 2). However, no clinical studies assessing the efficacy of anti-TSLP treatment in food allergy are currently on-going.
Exploiting Mast Cell Inhibitory Receptors
Known inhibitory receptors of IgE-mediated MC activation are the Fc gamma receptor FcγRIIb, CD200R, Sialic acid-binding immunoglobulin-type lectins (Siglec) of the CD33 family and CD300a. Most inhibitory receptors exert broad suppressive functions on MC activation, with the exception of FcγRIIb and CD200R, producing allergen-specific inhibition.
Excluding CD200R, all inhibitory receptors expressed on MCs show intracellular immunoreceptor tyrosine-based inhibition motif (ITIM) domains that actively inhibit the phosphorylation of the Syk pathway via the recruitment of tyrosine phosphatases with Src homology 2 domains (e.g. SHP, Grb2 and SHIP), or PI3K binding-motifs (213, 214), disrupting intracellular calcium flux and IgE-dependent intracellular activation (Figure 1D).
FcγRII/CD32 receptors are immunoglobulin-like transmembrane proteins binding to the hinge region of IgG and IgG immune complexes. Of the three different subtypes, namely, FcγRIIa (CD32a), FcγRIIb (CD32b), and FcγRIIc (CD32c), only FcγRIIb is inhibitory. In mice, IgG binding to FcγRIIb inhibits antigen-specific IgE-mediated activation and Th2 cytokine production by MCs, IgE antibody production by B cells (215–218), while promoting dendritic cell-mediated mucosal tolerance by inducing Treg recruitment in the gut (215, 217, 218). In humans, while FcγRIIb is widely expressed on B cells, dendritic cells, monocytes and basophils (219), FcγRIIb transcripts are detectable in gastrointestinal MCs (220), but not skin MCs (221). Although the expression of FcγRIIb by gut MCs could correlate with increased pro-tolerogenic functions, the lack of FcγRIIb-mediated inhibition on skin MCs could be a reason for the increased risk of allergic sensitization via the skin compared to the gut route, as currently suggested by the dual exposure hypothesis (222), and diverging clinical responses observed in the skin versus gut after allergen immunotherapy (220).
Given the antigen-specific nature of FcγRIIb-mediated tolerance, its engagement could be especially useful to selectively inhibit food allergic reactions. Promising results have been achieved in in vitro studies using human basophils, bone marrow-derived MCs of human FcϵRIα-transgenic mice, FcϵRIα-transfected human cell lines and the HMC-1 mast cell line (173, 174, 176) (Table 3). Conversely, FcγRIIb bispecific molecules specifically targeted to major allergenic epitopes reduced allergen-specific responses in a peanut allergy mouse model using an Ara h 2-FcγRIIb fusion protein (175) (Table 3). Furthermore, FcγRIIb exerts a pivotal role in the generation of allergen-specific tolerance during the course of allergen immunotherapy, as outlined in Modulation of Mast Cell Reactivity Using Allergen-Specific Immunotherapy.
As member of the Immunoglobulin receptor superfamily, CD200R is an inhibitory receptor widely expressed on myeloid cells and skin MCs, shown to hinder MC activation and cytokine release in the absence of ITIM domains but in need of FcϵRI co-ligation, similar to FcγR receptors (178, 223). Antibodies targeting CD200R were effective in inhibiting MC activation in experimental mouse models and in vitro and tissue-derived human MCs (178) (Table 3), but no evidence of efficacy in food allergy models has been provided to date.
Siglec receptors selectively bind to sialic acid-containing glycoproteins, each with a specific sialoside ligand preference (224). Among the many Siglec receptors expressed by human MCs [i.e. Siglec 2, 3 (CD33), 5 through 10] (225, 226), CD33 and other CD33-like molecules (i.e. Siglec 5–11) are inhibitory receptors with intracellular ITIM/ITIM-like domains inhibiting FcϵRI-dependent activation (227, 228).
Beyond their suppressive role in IgE-mediated activation, recent evidence also suggests an inhibitory role in IL-33-mediated activation of MCs, with reduction of airway inflammation and fibrosis markers, studied in non-allergic mouse models of cigarette-induced chronic obstructive bronchopulmonary disease and bleomycin-induced lung injury (229).
Siglec 3 and 8 are currently the most promising targets in the treatment of allergic diseases. In fact, CD33 ligand-coated liposomal nanoparticles suppress MC activation, prevent IgE-mediated anaphylaxis and induce allergen desensitization lasting a few days in ovalbumin and peanut allergy mouse models (180) (Table 3). On the other hand, the engagement of Siglec 8 reduces intracellular calcium flux and FcϵRI-dependent release of mediators on human MCs (181, 229), while exerting a potent pro-apoptotic effect on human eosinophils and reducing tissue distribution ex vivo (135, 182, 230) (Table 3). Furthermore, in a humanized mouse model, lirentelimab (AK002) successfully inhibited IgE-mediated passive systemic anaphylaxis (182) (Table 3). In recent clinical trials, lirentelimab showed positive effects in the treatment of patients with asthma and eosinophilic gastroenteritis (135, 231), and further clinical applications are currently under investigation, albeit not for food allergy (Table 2).
Within the CD300 receptor family, only CD300a and CD300f show ITIM/ITIM-like domains, expressed on MCs. In humans, CD300 receptor ligands include phosphatidylserine (CD300a), ceramide, sphingomyelin (CD300f), released by apoptotic, tumor or infected cells (214). In addition to the disruption of IgE-mediated activation (179), CD300a engagement also impairs MC proliferation and survival by inhibiting stem cell factor (SCF) signaling (232), whereas co-engagement of CD300f with IL-4Rα promotes IL-4 mediated activation of MCs (233). Fusion proteins targeting CD300a and IgE on MCs in a passive cutaneous anaphylaxis mouse model, showed a successful reduction in MC activation (179) (Table 3).
Allergen-Dependent Approaches
Modulation of Mast Cell Reactivity Using Allergen-Specific Immunotherapy
Allergen-specific immunotherapy (AIT) is the only disease-modifying intervention currently available to treat some allergic conditions, like insect venom allergy, allergic rhinitis and asthma due to respiratory allergy to pollens and house dust mites (234–237).
AIT consists in the repetitive exposure to escalating doses of native allergen extracts, which might induce generalized MC and basophil activation. The risk of eliciting an anaphylactic episode is mitigated by starting with very low allergen doses, by being performed only by trained professionals and in safe conditions under careful monitoring of potential early signs of systemic reaction (236, 238). The timing of dose increase depends on the protocol, ranging from weeks in conventional AIT to days/hours in rush/ultra-rush protocols (234, 238).
The concerted activity of cells from both innate and acquired immunity contributes to the efficacy of AIT (34–36), ultimately eliciting antigen-selective inhibition of MC and basophil activation and long-lasting suppression of IgE-mediated responses at large. In fact, AIT induces a pro-tolerogenic state, promoting allergen-specific IgG/IgG4 production opposed to sIgE by B cells (15). IgG and IgG4 not only selectively compete with IgE in allergen binding, but also the engagement of the FcγRIIb receptor by allergen-IgG complexes cross-linking with surface IgE-FcϵRI actively inhibits MC activation (218, 239–242). IgG-mediated inhibition also prevents further amplification of IgE production, by reducing Th2 cytokine release from activated MCs and basophils (242).
AIT also promotes the development of Tregs, which suppress MC activities, not only by secreting the anti-inflammatory cytokine IL-10, but also inducing MC cell anergy via OX40L receptor engagement (15, 27, 243). OX40-OX40L binding on MCs activates downstream signalling by C-terminal Src kinases, suppressing Fyn kinase activity and impairing microtubule rearrangement and degranulation (243) (Figure 1E).
Although effective, these events require time to induce a protective response, while exposure to incremental doses of allergen rapidly desensitizes MCs. However, the mechanism explaining such effect remains unclear. A study suggests that rapid incremental IgE receptor occupancy induces the depletion of cell surface IgE by internalization of IgE–FcϵRI complexes (244). Others find in desensitized anergic MCs an impaired internalization of allergen–IgE–FcϵRI molecules (245), and aberrant rearrangements of cytoskeleton actin fibers that inhibit FcϵRI-mediated calcium flux and intracellular vesicles trafficking (246).
Rapidly desensitized MCs, in turn, produce IL-2 that contribute to Treg survival and recruitment in the periphery, hence indirectly contributing to peripheral tolerance, as demonstrated in mice (247).
Both tolerance induction and MC desensitization are widely exploited to achieve long-term modulation and quick onset protection of allergic reactions with rush/ultra-rush protocols, respectively (248).
Allergen-Specific Immunotherapy in Food Allergy
For both treatment and prevention of severe reactions upon accidental exposure to food allergens, increasing the maximum tolerated dose of allergen is necessary and can be achieved with AIT (249).
AIT in food allergy is performed using either native allergens (e.g. whole food, allergen extracts) administered via the oral, sublingual or epicutaneous routes, or baked allergens (alone or mixed with other ingredients creating a food matrix) via the oral route (250).
Recently, the first peanut allergen powder formulation (AR101) was approved for peanut AIT by the U.S. Food and Drug Administration and European Medicines Agency (251, 252), and numerous other trials using either whole peanut or peanut extracts promoted tolerance to varying doses of crude peanut in 60–80% of treated subjects (72, 81, 83, 85, 90, 92, 94) (Table 1). However, the safety of AIT protocols in food allergy is still a matter of debate, since the risk of a severe allergic reaction during AIT cannot be completely abated (253). In fact, a 1–21% frequency of systemic adverse reactions and increased occurrence with higher peanut end goal doses were observed in peanut AIT trials (254). Furthermore, while long-term treatment is effective in preventing severe allergic reactions in AIT responders (79, 92), a fraction of subjects might still experience anaphylaxis with previously tolerated allergen doses when aggravating co-factors are present (i.e. physical exercise, use of non-steroidal anti-inflammatory drugs, infections, etc.) or due to poor AIT adherence (79, 253).
Combination Treatments With Biologics
To increase AIT safety in food allergy, newer therapeutic strategies involve the combination of AIT with biologics. Evidence suggests that omalizumab administered during AIT reduces the risk of severe reactions and facilitates AIT (97, 99, 101) (Tables 1 and 2). In fact, while AIT caused an increase in the levels of inhibitory allergen-specific IgG4, in the threshold for MC responsiveness and a reduction of Th2 cytokine production (83, 84, 92, 239), omalizumab decreased the likelihood of basophil degranulation, especially relevant during dose escalation (101). This omalizumab-induced protection is most likely dependent on basophil IgE–FcϵRI disengagement, as suggested by empirical evidence (159) and omalizumab pharmacokinetics.
However, studies on long-term use of omalizumab in cow’s milk AIT proved long-term omalizumab add-on treatment not being cost-effective, albeit the higher safety profile (255) (Table 2). Further trials testing the utility of omalizumab adjunct to food AIT, or other biologics like dupilumab with AR101 (NCT03682770) are currently ongoing (Tables 1 and 2).
Alternative Food Immunotherapy Approaches
Allergen-dependent strategies alternative to AIT are currently being tested. Among these, the use of hypoallergenic molecules, lacking key anaphylactogenic conformational epitopes, promises to obtain safer alternatives to AIT using native allergen extracts, as observed in fish and peanut allergy studies conducted in humans and mice, albeit still in early development (256, 257).
Other therapeutic approaches involve antibodies targeting major allergenic molecules, like a recently developed monoclonal anti-Ara h 2, preventing both local and systemic allergic reactions, as tested in a mouse model of peanut allergy (177) (Table 3). The advantage of monoclonal treatment is not only given by their competition with IgE molecules in allergen binding, but also by sharing with endogenous allergen-specific IgG antibodies the same mechanisms, regardless of patients’ capacity to mount an effective anti-allergic immune response as in conventional allergen immunotherapy. However, subjects sensitized to multiple allergen epitopes might only partially benefit from such treatment, unless multiple monoclonal antibodies against different epitopes are used in combination.
The complexing of allergenic epitopes with molecules actively promoting a tolerogenic state (i.e. production of IL-10, induction of IgG4, generation of Tregs), such as Toll-like receptor ligands (i.e. CpG, LPS, R848), viral-attenuated molecules, Siglec-engaging tolerance-inducing antigenic liposomes (STALs) and nanoformulations, is used as adjuvant immunotherapy to elicit allergen-specific tolerance (258).
An alternative approach under study is the use of plasmid DNA-based vaccines. Such vaccines induce the production of specific exogenous target proteins via allergen-coding DNA particles, exploiting the natural immune pathways leading to the production of IgG to promote long-lasting tolerance (259). In addition, peptide vaccines aimed at eliciting IgG antibody production targeted against highly allergenic epitopes are also currently under scrutiny (260).
Several recent studies on nanoformulations and adjuvant immunotherapy candidates for cow’s milk and peanut allergy have been conducted, showing promising results in mouse allergy models (183, 184, 261, 262) (Table 3). In humans, few ongoing clinical trials on DNA-based vaccines (ASP0892, NCT03755713; ASP0892, NCT02851277) and modified allergen proteins (HAL-MPE1 subcutaneous AIT, NCT02991885) are currently in Phase I, while a previous attempt with attenuated E. Coli Ara h 1-2-3 recombinant vaccine candidate failed to promote tolerance, inducing severe adverse reactions in 20% of participants (96) (Table 1).
Conclusions
Albeit complex, the allergic immune response relies on MC functionality, making these cells important targets for therapeutic intervention. Given the plethora of current and future treatments, some considerations on most promising choices and benefit/risk assessment are warranted.
Anti-IgE treatment is a valuable option for the control of food allergy symptoms and especially beneficial when adjunct to AIT. The lack of specificity and long term use of anti-IgE treatment was historically considered a concern, due to the loss of the protective IgE housekeeping functions. However, after 20+ years of omalizumab use, no increased risks for parasitic or neoplastic events could be observed (185, 263). Apart from a negligible risk of anaphylaxis upon the first administrations (264), omalizumab has been successfully used for long-term treatment and during pregnancy with an excellent safety profile (265). However, limited data is currently available on its safety in children less than 6 years of age, hence narrowing its therapeutic range.
AIT and allergen-specific vaccines are currently the only allergen-dependent interventions showing a curative potential in food allergy, however the risks associated to the exposure to allergenic molecules for treatment purposes should be minimized as much as possible, with safer protocols and drug formulations.
While allergen-dependent therapeutic strategies require the full functionality of the immune system to work, showing great variability in treatment response between individuals, sIgE inhibition could hamper allergen-specific activation regardless of the quality of patients’ immune response, but likely without comparable long-term disease-modifying effect as AIT.
The engagement of inhibitory receptors, abundantly expressed and not unique to MCs, are not only effectively inhibiting MC functions, but their activities can be directed against specific epitopes by formulating bispecific allergen-inhibitory ligand molecules [e.g. CD33L-coated liposomal nanoparticles (180), Ara h 2-FcγRIIb fusion proteins (175)]. This envisages a targeted allergen-specific inhibitory approach, while preserving pathways for IgE-mediated housekeeping functions, albeit still in early development.
Given the wide distribution of cytokine receptors and their pleiotropic effects exerted on many different cell types, therapeutic strategies blocking IL-4Rα, or cytokines important for the initiator phase of immune responses, like IL-33 and TSLP, pose some concerns. The suppression of protective immunity, the generation of paradoxical responses as, for instance, the conjunctivitis induced by dupilumab treatment in atopic dermatitis (266), or the little known effects of long-term exposure are safety issues that need further clarification.
Conversely, the broad, simultaneous and unspecific inhibition of multiple effector cells involved in allergic responses by anti-cytokine or by anti-Siglec monoclonal antibodies is potentially beneficial in the modulation of complex inflammatory diseases, as observed in asthma, atopic dermatitis, chronic rhinosinusitis with nasal polyps, eosinophilic gastroenteritis and other Th2-mediated conditions, including food allergy (Tables 1 and 2). Therefore, both anti-cytokine and anti-Siglec monoclonal antibodies are among the most encouraging disease-modifying allergen-independent therapies available in the near future for the treatment of severe allergic conditions, warranting further consideration especially in the field of food allergy.
Despite that there is still a strong need for clinical trials to assess the efficacy and safety of both allergen-independent and -dependent therapeutic approaches, the knowledge on the immunological mechanisms behind MC activation are the ultimate key for a successful allergy therapeutic intervention.
Author Contributions
CT and SB-P reviewed literature and wrote the article. All authors contributed to the article and approved the submitted version.
Funding
CT is funded by the UK Research and Innovation (UKRI) Medical Research Council Doctoral Training Partnership (MRC-DTP) studentship. SB-P received funding from MRC (MR/S036954/1).
Conflict of Interest
The authors declare that the research was conducted in the absence of any commercial or financial relationships that could be construed as a potential conflict of interest.
Publisher’s Note
All claims expressed in this article are solely those of the authors and do not necessarily represent those of their affiliated organizations, or those of the publisher, the editors and the reviewers. Any product that may be evaluated in this article, or claim that may be made by its manufacturer, is not guaranteed or endorsed by the publisher.
Abbreviations
AIT, allergen immunotherapy; Akt, protein kinase B; BTK, Bruton Tyrosine Kinase; ERK, extracellular signal-regulated kinase; FcϵRI, high-affinity IgE receptor; FcϵRII, low affinity IgE receptor; FcγRII, low affinity IgG receptor; IgE, immunoglobulin E; IgG, immunoglobulin G; IL, interleukin; IL-4Rα, interleukin 4 receptor alpha chain; ITAM, immunoreceptor tyrosine-based activation motif; ITIM, immunoreceptor tyrosine-based inhibition motif; Kd, dissociation constant; LARI, low affinity allergic response inhibitors; MCs, mast cells; NF-k, B, Nuclear Factor kappa-light-chain-enhancer of activated B cells; PI3K, phosphatidylinositol 3-OH kinase; SCF, stem cell factor; Siglec, Sialic acid-binding immunoglobulin-type lectins; sIgE, allergen-specific immunoglobulin E; STAT6, signal transducer and activator of transcription 6; Syk, Spleen Tyrosine Kinase; TSLP, Thymic stromal lymphopoietin; Tregs, T regulatory cells.
References
1. Pawankar R, Canonica GW ST, Holgate ST, Lockey RF BM. Allergic Diseases As a Global Public Health Issue (2013). Available at: https://www.worldallergy.org/UserFiles/file/WhiteBook2-2013-v8.pdf.
2. Loh W, Tang MLK. The Epidemiology of Food Allergy in the Global Context. Int J Environ Res Public Health (2018) 15(9):2043. doi: 10.3390/ijerph15092043
3. Nwaru BI, Hickstein L, Panesar SS, Muraro A, Werfel T, Cardona V, et al. The Epidemiology of Food Allergy in Europe: A Systematic Review and Meta-Analysis. Allergy Eur J Allergy Clin Immunol (2014) 69(1):62–75. doi: 10.1111/all.12305
4. Muraro A, Werfel T, Hoffmann-Sommergruber K, Roberts G, Beyer K, Bindslev-Jensen C, et al. EAACI Food Allergy and Anaphylaxis Guidelines: Diagnosis and Management of Food Allergy. Allergy Eur J Allergy Clin Immunol (2014) 69(8):1008–25. doi: 10.1111/all.12429
5. Moon TC, Dean Befus A, Kulka M. Mast Cell Mediators: Their Differential Release and the Secretory Pathways Involved. Front Immunol (2014) 5:569. doi: 10.3389/fimmu.2014.00569
6. Mukai K, Tsai M, Saito H, Galli SJ. Mast Cells as Sources of Cytokines, Chemokines, and Growth Factors. Immunol Rev (2018) 282(1):121–50. doi: 10.1111/imr.12634
7. Suto H, Nakae S, Kakurai M, Sedgwick JD, Tsai M, Galli SJ. Mast Cell-Associated TNF Promotes Dendritic Cell Migration. J Immunol (2006) 176(7):4102–12. doi: 10.4049/jimmunol.176.7.4102
8. Dawicki W, Jawdat DW, Xu N, Marshall JS. Mast Cells, Histamine, and IL-6 Regulate the Selective Influx of Dendritic Cell Subsets Into an Inflamed Lymph Node. J Immunol (2010) 184(4):2116–23. doi: 10.4049/jimmunol.0803894
9. Caron G, Delneste Y, Roelandts E, Duez C, Bonnefoy JY, Pestel J, et al. Histamine Polarizes Human Dendritic Cells Into Th2 Cell-Promoting Effector Dendritic Cells. J Immunol (2001) 167(7):3682–6. doi: 10.4049/jimmunol.167.7.3682
10. Mazzoni A, Siraganian RP, Leifer CA, Segal DM. Dendritic Cell Modulation by Mast Cells Controls the Th1/Th2 Balance in Responding T Cells. J Immunol (2006) 177(6):3577–81. doi: 10.4049/jimmunol.177.6.3577
11. Kitawaki T, Kadowaki N, Sugimoto N, Kambe N, Hori T, Miyachi Y, et al. IgE-Activated Mast Cells in Combination With Pro-Inflammatory Factors Induce Th2-Promoting Dendritic Cells. Int Immunol (2006) 18(12):1789–99. doi: 10.1093/intimm/dxl113
12. Palm AKE, Garcia-Faroldi G, Lundberg M, Pejler G, Kleinau S. Activated Mast Cells Promote Differentiation of B Cells Into Effector Cells. Sci Rep (2016) 6(February):1–12. doi: 10.1038/srep20531
13. Gaudenzio N, Espagnolle N, Mars LT, Liblau R, Valitutti S, Espinosa E. Cell-Cell Cooperation at the T Helper Cell/Mast Cell Immunological Synapse. Blood (2009) 114(24):4979–88. doi: 10.1182/blood-2009-02-202648
14. Gauchat J-F, Henchoz S, Mazzei G, Aubry JP, Brunner T, Blasey H, et al. Induction of Human IgE Synthesis in B Cells by Mast Cells and Basophils. Nature (1993) 365(6444):340–3. doi: 10.1038/365340a0
15. Sampson HA, O’Mahony L, Burks AW, Plaut M, Lack G, Akdis CA. Mechanisms of Food Allergy. J Allergy Clin Immunol (2018) 141(1):11–9. doi: 10.1016/j.jaci.2017.11.005
16. Reber LL, Hernandez JD, Galli SJ. The Pathophysiology of Anaphylaxis. J Allergy Clin Immunol (2017) 140(2):335–48. doi: 10.1016/j.jaci.2017.06.003
17. Gri G, Frossi B, D’Incà F, Danelli L, Betto E, Mion F, et al. Mast Cell: An Emerging Partner in Immune Interaction. Front Immunol (2012) 3:120. doi: 10.3389/fimmu.2012.00120
18. Varricchi G, Rossi FW, Galdiero MR, Granata F, Criscuolo G, Spadaro G, et al. Physiological Roles of Mast Cells: Collegium Internationale Allergologicum Update 2019. Int Arch Allergy Immunol (2019) 179(4):247–61. doi: 10.1159/000500088
19. Henz BM, Maurer M, Lippert U, Worm M, Babina M. Mast Cells as Initiators of Immunity and Host Defense. Exp Dermatol (2001) 10(1):1–10. doi: 10.1034/j.1600-0625.2001.100101.x
20. Galli SJ, Grimbaldeston M, Tsai M. Immunomodulatory Mast Cells: Negative, as Well as Positive, Regulators of Immunity. Nat Rev Immunol (2008) 8(6):478–86. doi: 10.1038/nri2327
21. Agier J, Pastwińska J, Brzezińska-Błaszczyk E. An Overview of Mast Cell Pattern Recognition Receptors. Inflamm Res (2018) 67(9):737–46. doi: 10.1007/s00011-018-1164-5
22. de Vries VC, Pino-Lagos K, Nowak EC, Bennett KA, Oliva C, Noelle RJ. Mast Cells Condition Dendritic Cells to Mediate Allograft Tolerance. Immunity (2011) 35(4):550–61. doi: 10.1016/j.immuni.2011.09.012
23. Dudeck A, Suender CA, Kostka SL, von Stebut E, Maurer M. Mast Cells Promote Th1 and Th17 Responses by Modulating Dendritic Cell Maturation and Function. Eur J Immunol (2011) 41(7):1883–93. doi: 10.1002/eji.201040994
24. Carroll-Portillo A, Cannon JL, te Riet J, Holmes A, Kawakami Y, Kawakami T, et al. Mast Cells and Dendritic Cells Form Synapses That Facilitate Antigen Transfer for T Cell Activation. J Cell Biol (2015) 210(5):851–64. doi: 10.1083/jcb.201412074
25. Bulfone-Paus S, Bahri R. Mast Cells as Regulators of T Cell Responses. Front Immunol (2015) 6:394(AUG). doi: 10.3389/fimmu.2015.00394
26. Lu LF, Lind EF, Gondek DC, Bennett KA, Gleeson MW, Pino-Lagos K, et al. Mast Cells Are Essential Intermediaries in Regulatory T-Cell Tolerance. Nature (2006) 442(7106):997–1002. doi: 10.1038/nature05010
27. Gri G, Piconese S, Frossi B, Manfroi V, Merluzzi S, Tripodo C, et al. CD+CD25+ Regulatory T Cells Suppress Mast Cell Degranulation and Allergic Responses Through OX40-OX40L Interaction. (2009) 29: (5):771–81. doi: 10.1016/j.immuni.2008.08.018.CD4
28. Mekori YA, Hershko AY, Frossi B, Mion F, Pucillo CE. Integrating Innate and Adaptive Immune Cells: Mast Cells as Crossroads Between Regulatory and Effector B and T Cells. Eur J Pharmacol (2016) 778:84–9. doi: 10.1016/j.ejphar.2015.03.087
29. Elishmereni M, Alenius HT, Bradding P, Mizrahi S, Shikotra A, Minai-Fleminger Y, et al. Physical Interactions Between Mast Cells and Eosinophils: A Novel Mechanism Enhancing Eosinophil Survival In Vitro. Allergy (2011) 66(3):376–85. doi: 10.1111/j.1398-9995.2010.02494.x
30. Robida PA, Puzzovio PG, Pahima H, Levi-Schaffer F, Bochner BS. Human Eosinophils and Mast Cells: Birds of a Feather Flock Together. Immunol Rev (2018) 282(1):151–67. doi: 10.1111/imr.12638
31. Merluzzi S, Betto E, Ceccaroni AA, Magris R, Giunta M, Mion F. Mast Cells, Basophils and B Cell Connection Network. Mol Immunol (2015) 63(1):94–103. doi: 10.1016/j.molimm.2014.02.016
32. Grimbaldeston MA, Nakae S, Kalesnikoff J, Tsai M, Galli SJ. Mast Cell-Derived Interleukin 10 Limits Skin Pathology in Contact Dermatitis and Chronic Irradiation With Ultraviolet B. Nat Immunol (2007) 8(10):1095–104. doi: 10.1038/ni1503
33. Kraneveld AD, Sagar S, Garssen J, Folkerts G. The Two Faces of Mast Cells in Food Allergy and Allergic Asthma: The Possible Concept of Yin Yang. Biochim Biophys Acta - Mol Basis Dis (2012) 1822(1):93–9. doi: 10.1016/j.bbadis.2011.06.013
34. Chinthrajah RS, Hernandez JD, Boyd SD, Galli SJ, Nadeau KC. Molecular and Cellular Mechanisms of Food Allergy and Food Tolerance. J Allergy Clin Immunol (2016) 137(4):984–97. doi: 10.1016/j.jaci.2016.02.004
35. Eiwegger T, Hung L, San Diego KE, O’Mahony L, Upton J. Recent Developments and Highlights in Food Allergy. Allergy Eur J Allergy Clin Immunol (2019) 74(12):2355–67. doi: 10.1111/all.14082
36. van de Veen W, Akdis M. The Use of Biologics for Immune Modulation in Allergic Disease. J Clin Invest (2019) 129(4):1452–62. doi: 10.1172/JCI124607
37. Reber LL, Marichal T, Mukai K, Kita Y, Tokuoka SM, Roers A, et al. Selective Ablation of Mast Cells or Basophils Reduces Peanut-Induced Anaphylaxis in Mice. J Allergy Clin Immunol (2013) 132(4):881–8.e11. doi: 10.1016/j.jaci.2013.06.008
38. Wan T, Beavil RL, Fabiane SM, Beavil AJ, Sohi MK, Keown M, et al. The Crystal Structure of IgE Fc Reveals an Asymmetrically Bent Conformation. Nat Immunol (2002) 3(7):681–6. doi: 10.1038/ni811
39. Epp A, Sullivan KC, Herr AB, Strait RT. Immunoglobulin Glycosylation Effects in Allergy and Immunity. Curr Allergy Asthma Rep (2016) 16(11):79. doi: 10.1007/s11882-016-0658-x
40. Shade KTC, Conroy ME, Washburn N, Kitaoka M, Huynh DJ, Laprise E, et al. Sialylation of Immunoglobulin E Is a Determinant of Allergic Pathogenicity. Nature (2020) 582(7811):265–70. doi: 10.1038/s41586-020-2311-z
41. Martins TB, Bandhauer ME, Bunker AM, Roberts WL, Hill HR. New Childhood and Adult Reference Intervals for Total IgE. J Allergy Clin Immunol (2014) 133(2):589–91. doi: 10.1016/j.jaci.2013.08.037
42. Pagana K, Pagana T, Pagana T. Mosby’s Diagnostic and Laboratory Test Reference. 14th ed. St. Louis, United States: Elsevier (2019). p. 536.
43. Lawrence MG, Woodfolk JA, Schuyler AJ, Stillman LC, Chapman MD, Platts-Mills TAE. Half-Life of IgE in Serum and Skin: Consequences for Anti-IgE Therapy in Patients With Allergic Disease. J Allergy Clin Immunol (2016) 139(2):422–8.e4. doi: 10.1016/j.jaci.2016.04.056
44. Kraft S, Kinet JP. New Developments in Fcϵri Regulation, Function and Inhibition. Nat Rev Immunol (2007) 7(5):365–78. doi: 10.1038/nri2072
45. Sutton BJ, Davies AM. Structure and Dynamics of IgE-Receptor Interactions: Fcϵri and CD23/Fcϵrii. Immunol Rev (2015) 268(1):222–35. doi: 10.1111/imr.12340
46. Gould HJ, Sutton BJ. IgE in Allergy and Asthma Today. Nat Rev Immunol (2008) 8(3):205–17. doi: 10.1038/nri2273
47. Sihra BS, Kon OM, Grant JA, Kay AB. Expression of High-Affinity IgE Receptors (Fcϵri) on Peripheral Blood Basophils, Monocytes, and Eosinophils in Atopic and Nonatopic Subjects: Relationship to Total Serum IgE Concentrations. J Allergy Clin Immunol (1997) 99(5):699–706. doi: 10.1016/S0091-6749(97)70033-2
48. Soussi Gounni A, Lamkhioued B, Ochiai K, Tanaka Y, Delaporte E, Capron A, et al. High-Affinity IgE Receptor on Eosinophils Is Involved in Defence Against Parasites. Nature (1994) 367(6459):183–6. doi: 10.1038/367183a0
49. Shin J-S, Greer AM. The Role of Fcϵri Expressed in Dendritic Cells and Monocytes. Cell Mol Life Sci (2015) 72(12):2349–60. doi: 10.1038/367183a0
50. Alphonse MP, Saffar AS, Shan L, HayGlass KT, Simons FER, Gounni AS. Regulation of the High Affinity IgE Receptor (Fcϵri) in Human Neutrophils: Role of Seasonal Allergen Exposure and Th-2 Cytokines. PloS One (2008) 3(4):e1921. doi: 10.1371/journal.pone.0001921
51. Selb R, Eckl-Dorna J, Neunkirchner A, Schmetterer K, Marth K, Gamper J, et al. CD23 Surface Density on B Cells Is Associated With IgE Levels and Determines IgE-Facilitated Allergen Uptake, as Well as Activation of Allergen-Specific T Cells. J Allergy Clin Immunol (2017) 139(1):290–9. doi: 10.1371/journal.pone.0001921
52. Dehlink E, AH B, Yen E, Nurko S, Fiebiger E. Relationships Between Levels of Serum IgE, Cell-Bound IgE, and IgE-Receptors on Peripheral Blood Cells in a Pediatric Population. PloS One (2010) 5(8):1–6. doi: 10.1371/journal.pone.0012204
53. Cheng LE, Hartmann K, Roers A, Krummel MF, Locksley RM. Perivascular Mast Cells Dynamically Probe Cutaneous Blood Vessels to Capture Immunoglobulin E. Immunity (2013) 38(1):166–75. doi: 10.1016/j.immuni.2012.09.022
54. MacGlashan DW. Endocytosis, Recycling, and Degradation of Unoccupied Fcϵri in Human Basophils. J Leukoc Biol (2007) 82(4):1003–10. doi: 10.1189/jlb.0207103
55. Borkowski TA, Jouvin M-H, Lin S-Y, Kinet J-P. Minimal Requirements for IgE-Mediated Regulation of Surface Fcϵri. J Immunol (2001) 167(3):1290–6. doi: 10.4049/jimmunol.167.3.1290
56. MacGlashan DJ, Xia HZ, Schwartz LB, Gong J. IgE-Regulated Loss, Not IgE-Regulated Synthesis, Controls Expression of FcepsilonRI in Human Basophils. J Leukoc Biol (2001) 70(2):207–18.
57. Greer AM, Kinet J, Greer AM, Woodruff PG, Wolters P, Kinet JP, et al. Serum IgE Clearance Is Facilitated by Human Fcϵri Internalization. J Clin Invest (2014) 124(3):1187–98. doi: 10.1172/JCI68964.by
58. Maurer D, Fiebiger E, Reininger B, Wolff-Winiski B, Jouvin MH, Kilgus O, et al. Expression of Functional High Affinity Immunoglobulin E Receptors (Fc Epsilon RI) on Monocytes of Atopic Individuals. J Exp Med (1994) 179(2):745–50. doi: 10.1084/jem.179.2.745
59. Novak N, Allam J-P, Hagemann T, Jenneck C, Laffer S, Valenta R, et al. Characterization of FcepsilonRI-Bearing CD123 Blood Dendritic Cell Antigen-2 Plasmacytoid Dendritic Cells in Atopic Dermatitis. J Allergy Clin Immunol (2004) 114(2):364–70. doi: 10.1016/j.jaci.2004.05.038
60. Kihara H, Siraganian RP. Src Homology 2 Domains of Syk and Lyn Bind to Tyrosine-Phosphorylated Subunits of the High Affinity IgE Receptor. J Biol Chem (1994) 269(35):22427–32. doi: 10.1016/S0021-9258(17)31807-0
61. Kimura T, Kihara H, Bhattacharyya S, Sakamoto H, Appella E, Siraganian RP. Downstream Signaling Molecules Bind to Different Phosphorylated Immunoreceptor Tyrosine-Based Activation Motif (ITAM) Peptides of the High Affinity IgE Receptor. J Biol Chem (1996) 271(44):27962–8. doi: 10.1074/jbc.271.44.27962
62. Kambayashi T, Koretzky GA. Proximal Signaling Events in Fcϵri-Mediated Mast Cell Activation. J Allergy Clin Immunol (2007) 119(3):544–52. doi: 10.1016/j.jaci.2007.01.017
63. Bax HJ, Bowen H, Dodev TS, Sutton BJ, Gould HJ. Mechanism of the Antigen-Independent Cytokinergic SPE-7 IgE Activation of Human Mast Cells In Vitro. Sci Rep (2015) 5(1):9538. doi: 10.1038/srep09538
64. Huber M. Activation/Inhibition of Mast Cells by Supra-Optimal Antigen Concentrations. Cell Commun Signal (2013) 11(1):1–11. doi: 10.1186/1478-811X-11-7
65. Handlogten MW, Deak PE, Bilgicer B. Two-Allergen Model Reveals Complex Relationship Between IgE Crosslinking and Degranulation. Chem Biol (2014) 21(11):1445–51. doi: 10.1016/j.chembiol.2014.08.019
66. Jones SM, Agbotounou WK, Fleischer DM, Burks AW, Pesek RD, Harris MW, et al. Safety of Epicutaneous Immunotherapy for the Treatment of Peanut Allergy: A Phase 1 Study Using the Viaskin Patch. J Allergy Clin Immunol (2016) 137(4):1258–61.e10. doi: 10.1016/j.jaci.2016.01.008
67. Jones SM, Sicherer SH, Burks AW, Leung DY, Lindblad RW, Dawson P, et al. Consortium of Food Allergy Research. Epicutaneous Immunotherapy for the Treatment of Peanut Allergy in Children and Young Adults. J Allergy Clin Immunol (2017) 139(4):1242–52.e9. doi: 10.1016/j.jaci.2016.08.017
68. Chiang D, Chen X, Jones SM, Wood RA, Sicherer SH, Burks AW, et al. Single-Cell Profiling of Peanut-Responsive T Cells in Patients With Peanut Allergy Reveals Heterogeneous Effector T(H)2 Subsets. J Allergy Clin Immunol (2018) 141(6):2107–20. doi: 10.1016/j.jaci.2017.11.060
69. Sampson HA, Shreffler WG, Yang WH, Sussman GL, Brown-Whitehorn TF, Nadeau KC, et al. Effect of Varying Doses of Epicutaneous Immunotherapy vs Placebo on Reaction to Peanut Protein Exposure Among Patients With Peanut Sensitivity: A Randomized Clinical Trial. JAMA (2017) 318(18):1798–809. doi: 10.1001/jama.2017.16591
70. Lewis MO, Brown-Whitehorn TF, Cianferoni A, Rooney C, Spergel JM. Peanut-Allergic Patient Experiences After Epicutaneous Immunotherapy: Peanut Consumption and Impact on QoL. Ann Allergy Asthma Immunol (2019) 123(1):101–3. doi: 10.1016/j.anai.2019.04.006
71. Remington BC, Krone T, Kim EH, Bird JA, Green TD, Lack G, et al. Estimated Risk Reduction to Packaged Food Reactions by Epicutaneous Immunotherapy (EPIT) for Peanut Allergy. Ann Allergy Asthma Immunol (2019) 123(5):488–93.e2. doi: 10.1016/j.anai.2019.08.007
72. Fleischer DM, Greenhawt M, Sussman G, Bégin P, Nowak-Wegrzyn A, Petroni D, et al. Effect of Epicutaneous Immunotherapy vs Placebo on Reaction to Peanut Protein Ingestion Among Children With Peanut Allergy: The PEPITES Randomized Clinical Trial. JAMA - J Am Med Assoc (2019) 321(10):946–55. doi: 10.1001/jama.2019.1113
73. Anagnostou K, Clark A, King Y, Islam S, Deighton J, Ewan P. Efficacy and Safety of High-Dose Peanut Oral Immunotherapy With Factors Predicting Outcome. Clin Exp Allergy (2011) 41(9):1273–81. doi: 10.1111/j.1365-2222.2011.03699.x
74. Patriarca G, Nucera E, Roncallo C, Pollastrini E, Bartolozzi F, De Pasquale T, et al. Oral Desensitizing Treatment in Food Allergy: Clinical and Immunological Results. Aliment Pharmacol Ther (2003) 17(3):459–65. doi: 10.1046/j.1365-2036.2003.01468.x
75. Buchanan AD, Green TD, Jones SM, Scurlock AM, Christie L, Althage KA, et al. Egg Oral Immunotherapy in Nonanaphylactic Children With Egg Allergy. J Allergy Clin Immunol (2007) 119(1):199–205. doi: 10.1016/j.jaci.2006.09.016
76. Vickery BP, Berglund JP, Burk CM, Fine JP, Kim EH, Kim JI, et al. Early Oral Immunotherapy in Peanut-Allergic Preschool Children Is Safe and Highly Effective. J Allergy Clin Immunol (2017) 139(1):173–81.e8. doi: 10.1016/j.jaci.2016.05.027
77. Chinthrajah RS, Purington N, Andorf S, Rosa JS, Mukai K, Hamilton R, et al. Development of a Tool Predicting Severity of Allergic Reaction During Peanut Challenge. Ann Allergy Asthma Immunol (2018) 121(1):69–76.e2. doi: 10.1016/j.anai.2018.04.020
78. Wright BL, Fernandez-Becker NQ, Kambham N, Purington N, Cao S, Tupa D, et al. Gastrointestinal Eosinophil Responses in a Longitudinal, Randomized Trial of Peanut Oral Immunotherapy. Clin Gastroenterol Hepatol (2021) 19(6):1151–9.e14. doi: 10.1016/j.cgh.2020.05.019
79. Chinthrajah RS, Purington N, Andorf S, Long A, O'Laughlin KL, Lyu SC, et al. Sustained Outcomes in Oral Immunotherapy for Peanut Allergy (POISED Study): A Large, Randomised, Double-Blind, Placebo-Controlled, Phase 2 Study. Lancet (2019) 394(10207):1437–49. doi: 10.1016/S0140-6736(19)31793-3
80. Michaud E, Evrard B, Pereira B, Rochette E, Bernard L, Rouzaire PO, et al. Peanut Oral Immunotherapy in Adolescents: Study Protocol for a Randomized Controlled Trial. Trials (2015) 16:197. doi: 10.1186/s13063-015-0717-y
81. PALISADE Group of Clinical Investigators, Vickery BP, Vereda A, Casale TB, Beyer K, du Toit G, et al. AR101 Oral Immunotherapy for Peanut Allergy. N Engl J Med (2018) 379(21):1991–2001. doi: 10.1056/NEJMoa1812856
82. O'B Hourihane J, Beyer K, Abbas A, Fernández-Rivas M, Turner PJ, Blumchen K, et al. Efficacy and Safety of Oral Immunotherapy With AR101 in European Children With a Peanut Allergy (ARTEMIS): A Multicentre, Double-Blind, Randomised, Placebo-Controlled Phase 3 Trial. Lancet Child Adolesc Health (2020) 4(10):728–39. doi: 10.1016/S2352-4642(20)30234-0
83. Blumchen K, Ulbricht H, Staden U, Dobberstein K, Beschorner J, de Oliveira LC, et al. Oral Peanut Immunotherapy in Children With Peanut Anaphylaxis. J Allergy Clin Immunol (2010) 126(1):83–91.e1. doi: 10.1016/j.jaci.2010.04.030
84. Varshney P, Jones SM, Scurlock AM, Perry TT, Kemper A, Steele P, et al. A Randomized Controlled Study of Peanut Oral Immunotherapy: Clinical Desensitization and Modulation of the Allergic Response. J Allergy Clin Immunol (2011) 127(3):654–60. doi: 10.1016/j.jaci.2010.12.1111
85. Anagnostou K, Islam S, King Y, Foley L, Pasea L, Bond S, et al. Assessing the Efficacy of Oral Immunotherapy for the Desensitisation of Peanut Allergy in Children (STOP II): A Phase 2 Randomised Controlled Trial. Lancet (2014) 3(9925):1297–304:38. doi: 10.1016/S0140-6736(13)62301-6
86. Wisniewski JA, Commins SP, Agrawal R, Hulse KE, Yu MD, Cronin J, et al. Analysis of Cytokine Production by Peanut-Reactive T Cells Identifies Residual Th2 Effectors in Highly Allergic Children Who Received Peanut Oral Immunotherapy. Clin Exp Allergy (2015) 45(7):1201–13. doi: 10.1111/cea.12537
87. Blumchen K, Trendelenburg V, Ahrens F, Gruebl A, Hamelmann E, Hansen G, et al. Efficacy, Safety, and Quality of Life in a Multicenter, Randomized, Placebo-Controlled Trial of Low-Dose Peanut Oral Immunotherapy in Children With Peanut Allergy. J Allergy Clin Immunol Pract (2019) 7(2):479–91.e10. doi: 10.1016/j.jaip.2018.10.048
88. Reier-Nilsen T, Michelsen MM, Lødrup Carlsen KC, Carlsen KH, Mowinckel P, Nygaard UC, et al. Feasibility of Desensitizing Children Highly Allergic to Peanut by High-Dose Oral Immunotherapy. Allergy (2019) 74(2):337–48. doi: 10.1111/all.13604
89. Narisety SD, Frischmeyer-Guerrerio PA, Keet CA, Gorelik M, Schroeder J, Hamilton RG, et al. A Randomized, Double-Blind, Placebo-Controlled Pilot Study of Sublingual Versus Oral Immunotherapy for the Treatment of Peanut Allergy. J Allergy Clin Immunol (2015) 135(5):1275–82.e1-6. doi: 10.1016/j.jaci.2014.11.005
90. Fleischer DM, Burks AW, Vickery BP, Scurlock AM, Wood RA, Jones SM, et al. Sublingual Immunotherapy for Peanut Allergy: A Randomized, Double-Blind, Placebo-Controlled Multicenter Trial. J Allergy Clin Immunol (2013) 131(1):119–27.e7. doi: 10.1016/j.jaci.2012.11.011
91. Burks AW, Wood RA, Jones SM, Sicherer SH, Fleischer DM, Scurlock AM, et al. Consortium of Food Allergy Research. Sublingual Immunotherapy for Peanut Allergy: Long-Term Follow-Up of a Randomized Multicenter Trial. J Allergy Clin Immunol (2015) May135(5):1240–8.e1-3. doi: 10.1016/j.jaci.2014.12.1917
92. Kim EH, Yang L, Ye P, Guo R, Li Q, Kulis MD, et al. Long-Term Sublingual Immunotherapy for Peanut Allergy in Children: Clinical and Immunologic Evidence of Desensitization. J Allergy Clin Immunol (2019) 144(5):1320–6.e1. doi: 10.1016/j.jaci.2019.07.030
93. Chin SJ, Vickery BP, Kulis MD, Kim EH, Varshney P, Steele P, et al. Sublingual Versus Oral Immunotherapy for Peanut-Allergic Children: A Retrospective Comparison. J Allergy Clin Immunol (2013) 132(2):476–8.e2. doi: 10.1016/j.jaci.2013.02.017
94. Kim EH, Bird JA, Kulis M, Laubach S, Pons L, Shreffler W, et al. Sublingual Immunotherapy for Peanut Allergy: Clinical and Immunologic Evidence of Desensitization. J Allergy Clin Immunol (2011) 127(3):640–6.e1. doi: 10.1016/j.jaci.2010.12.1083
95. Kulis M, Saba K, Kim EH, Bird JA, Kamilaris N, Vickery BP, et al. Increased Peanut-Specific IgA Levels in Saliva Correlate With Food Challenge Outcomes After Peanut Sublingual Immunotherapy. J Allergy Clin Immunol (2012) 129(4):1159–62. doi: 10.1016/j.jaci.2011.11.045
96. Wood RA, Sicherer SH, Burks AW, Grishin A, Henning AK, Lindblad R, et al. A Phase 1 Study of Heat/Phenol-Killed, E. Coli-Encapsulated, Recombinant Modified Peanut Proteins Ara H 1, Ara H 2, and Ara H 3 (EMP-123) for the Treatment of Peanut Allergy. Allergy Eur J Allergy Clin Immunol (2013) 68(6):803–8. doi: 10.1111/all.12158
97. Schneider LC, Rachid R, Lebovidge J, Blood E, Mittal M, Umetsu DT. A Pilot Study of Omalizumab to Facilitate Rapid Oral Desensitization in High-Risk Peanut-Allergic Patients. J Allergy Clin Immunol (2013) 132(6):1368–74. doi: 10.1016/j.jaci.2013.09.046
98. Abdel-Gadir A, Schneider L, Casini A, Charbonnier LM, Little SV, Harrington T, et al. Oral Immunotherapy With Omalizumab Reverses the Th2 Cell-Like Programme of Regulatory T Cells and Restores Their Function. Clin Exp Allergy (2018) 48(7):825–36. doi: 10.1111/cea.13161
99. Yee CSK, Albuhairi S, Noh E, El-Khoury K, Rezaei S, Abdel-Gadir A, Lyu SC, Sindher SB, Long A, et al. Long-Term Outcome of Peanut Oral Immunotherapy Facilitated Initially by Omalizumab. J Allergy Clin Immunol Pract (2019) 7(2):451–61.e7. doi: 10.1016/j.jaip.2018.09.015
100. Brandström J, Vetander M, Lilja G, Johansson SG, Sundqvist AC, Kalm F, et al. Individually Dosed Omalizumab: An Effective Treatment for Severe Peanut Allergy. Clin Exp Allergy (2017) 47(4):540–50. doi: 10.1111/cea.12862
101. Brandström J, Vetander M, Sundqvist AC, Lilja G, Johansson SGO, Melén E, et al. Individually Dosed Omalizumab Facilitates Peanut Oral Immunotherapy in Peanut Allergic Adolescents. Clin Exp Allergy (2019) 49(10):1328–41. doi: 10.1111/cea.13469
102. Andorf S, Manohar M, Dominguez T, Block W, Tupa D, Kshirsagar RA, et al. Observational Long-Term Follow-Up Study of Rapid Food Oral Immunotherapy With Omalizumab. Allergy Asthma Clin Immunol (2017) 13:51. doi: 10.1186/s13223-017-0223-8
103. Andorf S, Purington N, Kumar D, Long A, O'Laughlin KL, Sicherer S, et al. A Phase 2 Randomized Controlled Multisite Study Using Omalizumab-Facilitated Rapid Desensitization to Test Continued vs Discontinued Dosing in Multifood Allergic Individuals. EClinicalMedicine (2019) 7:27–38. doi: 10.1016/j.eclinm.2018.12.006
104. Langlois A, Lavergne MH, Leroux H, Killer K, Azzano P, Paradis L, et al. Protocol for a Double-Blind, Randomized Controlled Trial on the Dose-Related Efficacy of Omalizumab in Multi-Food Oral Immunotherapy. Allergy Asthma Clin Immunol (2020) 16:25. doi: 10.1186/s13223-020-00419-z
105. Berry R, Hollingsworth P, Lucas M. Successful Treatment of Idiopathic Mast Cell Activation Syndrome With Low-Dose Omalizumab. Clin Transl Immunol (2019) 8(10):1–5. doi: 10.1002/cti2.1075
106. Andorf S, Purington N, Block WM, Long AJ, Tupa D, Brittain E, et al. Anti-IgE Treatment With Oral Immunotherapy in Multifood Allergic Participants: A Double-Blind, Randomised, Controlled Trial. Lancet Gastroenterol Hepatol (2018) 3(2):85–94. doi: 10.1016/S2468-1253(17)30392-8
107. Chinthrajah S, Cao S, Liu C, et al. Phase 2a Randomized, Placebo-Controlled Study of Anti–IL-33 in Peanut Allergy. JCI Insight (2019) 4(22):1–14. doi: 10.1172/jci.insight.131347
108. Busse W, Corren J, Lanier BQ, McAlary M, Fowler-Taylor A, Cioppa GD, et al. Omalizumab, Anti-IgE Recombinant Humanized Monoclonal Antibody, for the Treatment of Severe Allergic Asthma. J Allergy Clin Immunol (2001) 108(2):184–90. doi: 10.1067/mai.2001.117880
109. Solèr M, Matz J, Townley R, Buhl R, O'Brien J, Fox H, et al. The Anti-IgE Antibody Omalizumab Reduces Exacerbations and Steroid Requirement in Allergic Asthmatics. Eur Respir J (2001) 18(2):254–61. doi: 10.1183/09031936.01.00092101
110. Holgate ST, Chuchalin AG, Hébert J, Lötvall J, Persson GB, Chung KF, et al. Efficacy and Safety of a Recombinant Anti-Immunoglobulin E Antibody (Omalizumab) in Severe Allergic Asthma. Clin Exp Allergy (2004) 34:632–8. doi: 10.1111/j.1365-2222.2004.1916.x
111. Humbert M, Beasley R, Ayres J, Slavin R, Hébert J, Bousquet J, et al. Benefits of Omalizumab as Add-on Therapy in Patients With Severe Persistent Asthma Who Are Inadequately Controlled Despite Best Available Therapy (GINA 2002 Step 4 Treatment): INNOVATE. Allergy (2005) 60:309–16. doi: 10.1111/j.1398-9995.2004.00772.x
112. Vignola AM, Humbert M, Bousquet J, Boulet LP, Hedgecock S, Blogg M, et al. Efficacy and Tolerability of Anti-Immunoglobulin E Therapy With Omalizumab in Patients With Concomitant Allergic Asthma and Persistent Allergic Rhinitis: SOLAR. Allergy (2004) 59:709–17. doi: 10.1111/j.1398-9995.2004.00550.x
113. Hanania NA, Alpan O, Hamilos DL, Condemi JJ, Reyes-Rivera I, Zhu J, et al. Omalizumab in Severe Allergic Asthma Inadequately Controlled With Standard Therapy: A Randomized Trial. Ann Intern Med (2011) 154(9):573–82. doi: 10.7326/0003-4819-154-9-201105030-00002
114. Lanier B, Bridges T, Kulus M, Taylor AF, Berhane I, Vidaurre CF. Omalizumab for the Treatment of Exacerbations in Children With Inadequately Controlled Allergic (IgE-Mediated) Asthma. J Allergy Clin Immunol (2009) 124(6):1210–6. doi: 10.1016/j.jaci.2009.09.021
115. Saini SS, Bindslev-Jensen C, Maurer M, Grob JJ, Bülbül Baskan E, Bradley MS, et al. Efficacy and Safety of Omalizumab in Patients With Chronic Idiopathic/Spontaneous Urticaria Who Remain Symptomatic on H1 Antihistamines: A Randomized, Placebo-Controlled Study. J Invest Dermatol (2015) 135(1):67–75. doi: 10.1038/jid.2014.306
116. Maurer M, Rosén K, Hsieh HJ, Saini S, Grattan C, Gimenéz-Arnau A, et al. Omalizumab for the Treatment of Chronic Idiopathic or Spontaneous Urticaria. N Engl J Med (2013) 368(10):924–35. doi: 10.1056/NEJMoa1215372
117. Casale TB, Bernstein JA, Maurer M, Saini SS, Trzaskoma B, Chen H, et al. Similar Efficacy With Omalizumab in Chronic Idiopathic/Spontaneous Urticaria Despite Different Background Therapy. J Allergy Clin Immunol Pract (2015) 3(5):743–50.e1. doi: 10.1016/j.jaip.2015.04.015
118. Gevaert P, Omachi TA, Corren J, Mullol J, Han J, Lee SE, et al. Efficacy and Safety of Omalizumab in Nasal Polyposis: 2 Randomized Phase 3 Trials. J Allergy Clin Immunol (2020) 146(3):595–605. doi: 10.1016/j.jaci.2020.05.032
119. Nayak A, Casale T, Miller SD, Condemi J, McAlary M, Fowler-Taylor A, et al. Tolerability of Retreatment With Omalizumab, a Recombinant Humanized Monoclonal Anti-IgE Antibody, During a Second Ragweed Pollen Season in Patients With Seasonal Allergic Rhinitis. Allergy Asthma Proc (2003) 24(5):323–9.
120. Casale TB, Busse WW, Kline JN, Ballas ZK, Moss MH, Townley RG, et al. Omalizumab Pretreatment Decreases Acute Reactions After Rush Immunotherapy for Ragweed-Induced Seasonal Allergic Rhinitis. J Allergy Clin Immunol (2006) 117(1):134–40. doi: 10.1016/j.jaci.2005.09.036
121. Klunker S, Saggar LR, Seyfert-Margolis V, Asare AL, Casale TB, Durham SR, et al. Combination Treatment With Omalizumab and Rush Immunotherapy for Ragweed-Induced Allergic Rhinitis: Inhibition of IgE-Facilitated Allergen Binding. J Allergy Clin Immunol (2007) 120(3):688–95. doi: 10.1016/j.jaci.2007.05.034
122. Takahashi M, Soejima K, Taniuchi S, Hatano Y, Yamanouchi S, Ishikawa H, et al. Oral Immunotherapy Combined With Omalizumab for High-Risk Cow's Milk Allergy: A Randomized Controlled Trial. Sci Rep (2017) 7(1):17453. doi: 10.1038/s41598-017-16730-6
123. Maurer M, Giménez-Arnau AM, Sussman G, Metz M, Baker DR, Bauer A, et al. Ligelizumab for Chronic Spontaneous Urticaria. N Engl J Med (2019) 381(14):1321–32. doi: 10.1056/NEJMoa1900408
124. Rabe KF, Nair P, Brusselle G, Maspero JF, Castro M, Sher L, et al. Efficacy and Safety of Dupilumab in Glucocorticoid-Dependent Severe Asthma. N Engl J Med (2018) 378(26):2475–85. doi: 10.1056/NEJMoa1804093
125. Castro M, Corren J, Pavord ID, Maspero J, Wenzel S, Rabe KF, et al. Dupilumab Efficacy and Safety in Moderate-To-Severe Uncontrolled Asthma. N Engl J Med (2018) 378(26):2486–96. doi: 10.1056/NEJMoa1804092
126. Weinstein SF, Katial R, Jayawardena S, Pirozzi G, Staudinger H, Eckert L, et al. Efficacy and Safety of Dupilumab in Perennial Allergic Rhinitis and Comorbid Asthma. J Allergy Clin Immunol (2018) 142(1):171–7.e1. doi: 10.1016/j.jaci.2017.11.051
127. Blauvelt A, de Bruin-Weller M, Gooderham M, Cather JC, Weisman J, Pariser D, et al. Long-Term Management of Moderate-to-Severe Atopic Dermatitis With Dupilumab and Concomitant Topical Corticosteroids (LIBERTY AD CHRONOS): A 1-Year, Randomised, Double-Blinded, Placebo-Controlled, Phase 3 Trial. Lancet (2017) 389(10086):2287–303. doi: 10.1111/imj.67_13578
128. Simpson EL, Akinlade B, Ardeleanu M. Two Phase 3 Trials of Dupilumab Versus Placebo in Atopic Dermatitis. N Engl J Med (2017) 376(11):1090–1. doi: 10.1056/NEJMc1700366
129. Worm M, Simpson EL, Thaçi D, Bissonnette R, Lacour JP, Beissert S, et al. Efficacy and Safety of Multiple Dupilumab Dose Regimens After Initial Successful Treatment in Patients With Atopic Dermatitis: A Randomized Clinical Trial. JAMA Dermatol (2020) 156(2):131–43. doi: 10.1001/jamadermatol.2019.3617
130. Simpson EL, Paller AS, Siegfried EC, Boguniewicz M, Sher L, Gooderham MJ, et al. Efficacy and Safety of Dupilumab in Adolescents With Uncontrolled Moderate to Severe Atopic Dermatitis: A Phase 3 Randomized Clinical Trial. JAMA Dermatol (2020) 156(1):44–56. doi: 10.1001/jamadermatol.2019.3336
131. Paller AS, Bansal A, Simpson EL, Boguniewicz M, Blauvelt A, Siegfried EC, et al. Clinically Meaningful Responses to Dupilumab in Adolescents With Uncontrolled Moderate-To-Severe Atopic Dermatitis: Post-Hoc Analyses From a Randomized Clinical Trial. Am J Clin Dermatol (2020) Feb21(1):119–31. doi: 10.1007/s40257-019-00478-y
132. Bachert C, Han JK, Desrosiers M, Hellings PW, Amin N, Lee SE, et al. Efficacy and Safety of Dupilumab in Patients With Severe Chronic Rhinosinusitis With Nasal Polyps (LIBERTY NP SINUS-24 and LIBERTY NP SINUS-52): Results From Two Multicentre, Randomised, Double-Blind, Placebo-Controlled, Parallel-Group Phase 3 Trials. Lancet (2019) 394(10209):1638–50. doi: 10.1016/S0140-6736(19)31881-1
133. Hirano I, Dellon ES, Hamilton JD, Collins MH, Peterson K, Chehade M, et al. Efficacy of Dupilumab in a Phase 2 Randomized Trial of Adults With Active Eosinophilic Esophagitis. Gastroenterology (2020) 158(1):111–22.e10. doi: 10.1053/j.gastro.2019.09.042
134. Gauvreau GM, O'Byrne PM, Boulet LP, Wang Y, Cockcroft D, Bigler J, et al. Effects of an Anti-TSLP Antibody on Allergen-Induced Asthmatic Responses. N Engl J Med (2014) 370(22):2102–10. doi: 10.1056/nejmoa1402895
135. Kerr SC, Gonzalez JR, Schanin J, Peters MC, Lambrecht BN, Brock EC, et al. An Anti-Siglec-8 Antibody Depletes Sputum Eosinophils From Asthmatic Subjects and Inhibits Lung Mast Cells. Clin Exp Allergy (2020) 50(8):904–14. doi: 10.1111/cea.13681
136. Beck LA, Marcotte GV, MacGlashan D, Togias A, Saini S. Omalizumab-Induced Reductions in Mast Cell Fcϵri Expression and Function. J Allergy Clin Immunol (2004) 114(3):527–30. doi: 10.1016/j.jaci.2004.06.032
137. MacGlashan DWJ, Bochner BS, Adelman DC, Jardieu PM, Togias A, McKenzie-White J, et al. Down-Regulation of Fc(epsilon)RI Expression on Human Basophils During In Vivo Treatment of Atopic Patients With Anti-IgE Antibody. J Immunol (1997) 158(3):1438–45.
138. Prussin C, Griffith DT, Boesel KM, Lin H, Foster B, Casale TB. Omalizumab Treatment Downregulates Dendritic Cell Fcϵri Expression. J Allergy Clin Immunol (2003) 112(6):1147–54. doi: 10.1016/j.jaci.2003.10.003
139. Chang TW. The Pharmacological Basis of Anti-IgE Therapy. Nat Biotechnol (2000) 18(2):157–62. doi: 10.1038/72601
140. Zaidi AK, Saini SS, Macglashan DWJ. Regulation of Syk Kinase and FcRbeta Expression in Human Basophils During Treatment With Omalizumab. J Allergy Clin Immunol (2010) 125(4):902–8. doi: 10.1016/j.jaci.2009.12.996
141. MacGlashan D. Loss of Receptors and IgE In Vivo During Treatment With Anti-IgE Antibody. J Allergy Clin Immunol (2004) 114(6):1472–4. doi: 10.1016/j.jaci.2004.07.064
142. Lin H, Boesel KM, Griffith DT, Prussin C, Foster B, Romero FA, et al. Omalizumab Rapidly Decreases Nasal Allergic Response and Fcϵri on Basophils. J Allergy Clin Immunol (2004) 113(2):297–302. doi: 10.1016/j.jaci.2003.11.044
143. Oliver JM, Tarleton CA, Gilmartin L, Archibeque T, Qualls CR, Diehl L, et al. Reduced Fcϵri-Mediated Release of Asthma-Promoting Cytokines and Chemokines From Human Basophils During Omalizumab Therapy. Int Arch Allergy Immunol (2010) 151(4):275–84. doi: 10.1159/000250436
144. Schroeder JT, Bieneman AP, Chichester KL, Hamilton RG, Xiao H, Saini SS, et al. Decreases in Human Dendritic Cell-Dependent TH2-Like Responses After Acute In Vivo IgE Neutralization. J Allergy Clin Immunol (2010) 125(4):896–901. doi: 10.1016/j.jaci.2009.10.021
145. van Neerven RJ, Wikborg T, Lund G, Jacobsen B, Brinch-Nielsen A, Arnved J, et al. Blocking Antibodies Induced by Specific Allergy Vaccination Prevent the Activation of CD4+ T Cells by Inhibiting Serum-IgE-Facilitated Allergen Presentation. J Immunol (1999) 163(5):2944–52.
146. Gasser P, Tarchevskaya SS, Guntern P, Brigger D, Ruppli R, Zbären N, et al. The Mechanistic and Functional Profile of the Therapeutic Anti-IgE Antibody Ligelizumab Differs From Omalizumab. Nat Commun (2020) 11(1):165. doi: 10.1038/s41467-019-13815-w
147. Chan MA, Gigliotti NM, Dotson AL, Rosenwasser LJ. Omalizumab may Decrease IgE Synthesis by Targeting Membrane IgE+ Human B Cells. Clin Transl Allergy (2013) 3(1):1–8. doi: 10.1186/2045-7022-3-29
148. Brightbill H, Lin Y, Lin Z, Tan M, Meng G, Balazs M, et al. Quilizumab is an Afucosylated Humanized Anti-M1 Prime Therapeutic Antibody. Clin Anti-Inflamm Anti-Allergy Drugs (2013) 1(1):24–31. doi: 10.2174/22127038114019990003
149. Chu SY, Horton HM, Pong E, Leung IW, Chen H, Nguyen DH, et al. Reduction of Total IgE by Targeted Coengagement of IgE B-Cell Receptor and Fcγriib With Fc-Engineered Antibody. J Allergy Clin Immunol (2012) 129(4):1102–15. doi: 10.1016/j.jaci.2011.11.029
150. Corren J, Shapiro G, Reimann J, Deniz Y, Wong D, Adelman D, et al. Allergen Skin Tests and Free IgE Levels During Reduction and Cessation of Omalizumab Therapy. J Allergy Clin Immunol (2008) 121(2):506–11. doi: 10.1016/j.jaci.2007.11.026
151. Pennington LF, Tarchevskaya S, Brigger D, Sathiyamoorthy K, Graham MT, Nadeau KC, et al. Structural Basis of Omalizumab Therapy and Omalizumab-Mediated IgE Exchange. Nat Commun (2016) 7:1–12. doi: 10.1038/ncomms11610
152. Chang TW, Davis FM, Sun N-C, Sun CRY, MacGlashan DW, Hamilton RG. Monoclonal Antibodies Specific for Human IgE-Producing B Cells: A Potential Therapeutic for IgE-Mediated Allergic Diseases. Bio/Technology (1990) 8(2):122–6. doi: 10.1038/nbt0290-122
153. Serrano-Candelas E, Martinez-Aranguren R, Valero A, Bartra J, Gastaminza G, Goikoetxea MJ, et al. Comparable Actions of Omalizumab on Mast Cells and Basophils. Clin Exp Allergy (2016) 46(1):92–102. doi: 10.1111/cea.12668
154. Gomez G, Jogie-Brahim S, Shima M, Schwartz LB. Omalizumab Reverses the Phenotypic and Functional Effects of IgE-Enhanced Fcϵri on Human Skin Mast Cells. J Immunol (2007) 179(2):1353–61. doi: 10.4049/jimmunol.179.2.1353
155. Fox JA, Hotaling TE, Struble C, Ruppel J, Bates DJ, Schoenhoff MB. Tissue Distribution and Complex Formation With IgE of an Anti-IgE Antibody After Intravenous Administration in Cynomolgus Monkeys. J Pharmacol Exp Ther (1996) 279(2):1000–8.
156. Maurer M, Metz M, Brehler R, Hillen U, Jakob T, Mahler V, et al. Omalizumab Treatment in Patients With Chronic Inducible Urticaria: A Systematic Review of Published Evidence. J Allergy Clin Immunol (2017) 141(2):638–49. doi: 10.1016/j.jaci.2017.06.032
157. Leung DYM, Sampson HA, Yunginger JW, Burks AW Jr, Schneider LC, Wortel CH, et al. Effect of Anti-IgE Therapy in Patients With Peanut Allergy. N Engl J Med (2003) 348(11):986–93. doi: 10.1056/NEJMoa022613
158. Sampson HA, Leung DYM, Burks AW, A phase II, Lack G, Bahna SL, Jones SM, et al. Randomized, Doubleblind, Parallelgroup, Placebocontrolled Oral Food Challenge Trial of Xolair (Omalizumab) in Peanut Allergy. J Allergy Clin Immunol (2011) 127(5):1309–10.e1. doi: 10.1016/j.jaci.2011.01.051
159. Savage JH, Courneya JP, Sterba PM, Macglashan DW, Saini SS, Wood RA. Kinetics of Mast Cell, Basophil, and Oral Food Challenge Responses in Omalizumab-Treated Adults With Peanut Allergy. J Allergy Clin Immunol (2012) 130(5):1123–9.e2. doi: 10.1016/j.jaci.2012.05.039
160. Fiocchi A, Artesani MC, Riccardi C, Mennini M, Pecora V, Fierro V, et al. Impact of Omalizumab on Food Allergy in Patients Treated for Asthma: A Real-Life Study. J Allergy Clin Immunol Pract (2019) 7(6):1901–9.e5. doi: 10.1016/j.jaip.2019.01.023
161. Plückthun A. Designed Ankyrin Repeat Proteins (DARPins): Binding Proteins for Research, Diagnostics, and Therapy. Annu Rev Pharmacol Toxicol (2015) 55:489–511. doi: 10.1146/annurev-pharmtox-010611-134654
162. Kim B, Eggel A, Tarchevskaya SS, Vogel M, Prinz H, Jardetzky TS. Accelerated Disassembly of IgE-Receptor Complexes by a Disruptive Macromolecular Inhibitor. Nature (2012) 491(7425):613–7. doi: 10.1038/nature11546
163. Eggel A, Baravalle G, Hobi G, Kim B, Buschor P, Forrer P, et al. Accelerated Dissociation of IgE-Fcϵri Complexes by Disruptive Inhibitors Actively Desensitizes Allergic Effector Cells. J Allergy Clin Immunol (2014) 133(6):1709–19.e8. doi: 10.1016/j.jaci.2014.02.005
164. Delgado SJ, Dehmel S, Twisterling E, Wichmann J, Jonigk D, Warnecke G, et al. Disruptive Anti-IgE Inhibitors Prevent Mast Cell–Dependent Early Airway Response in Viable Atopic Lung Tissue. J Allergy Clin Immunol (2020) 145(2):719–22.e1. doi: 10.1016/j.jaci.2019.11.002
165. Pennington LF, Gasser P, Brigger D, Guntern P, Eggel A, Jardetzky TS. Structure-Guided Design of Ultrapotent Disruptive IgE Inhibitors to Rapidly Terminate Acute Allergic Reactions. J Allergy Clin Immunol (2021) 0(0). doi: 10.1016/j.jaci.2021.03.050
166. Weeratna RD, Chikh G, Zhang L, Fraser JD, Thorn JM, Merson JR, et al. Immunogenicity of a Peptide-Based Anti-IgE Conjugate Vaccine in Non-Human Primates. Immunity Inflammation Dis (2016) 4(2):135–47. doi: 10.1002/iid3.98
167. Zeigler DF, Gage E, Roque R, Clegg CH. Epitope Targeting With Self-Assembled Peptide Vaccines. NPJ Vaccines (2019) 4(1). doi: 10.1038/s41541-019-0125-5
168. Deak PE, Kim B, Qayum AA, Shin J, Vitalpur G, Kloepfer KM, et al. Designer Covalent Heterobivalent Inhibitors Prevent IgE-Dependent Responses to Peanut Allergen. Proc Natl Acad Sci USA (2019) 116(18):8966–74. doi: 10.1073/pnas.1820417116
169. Khodoun MV, Morris SC, Angerman E, Potter C, Schuman R, Wunderlich M, et al. Rapid Desensitization of Humanized Mice With Anti-Human Fcϵriα Monoclonal Antibodies. J Allergy Clin Immunol (2020) 145(3):907–921.e3. doi: 10.1016/j.jaci.2019.12.003
170. Dispenza MC, Pongracic JA, Singh AM, Bochner BS. Short-Term Ibrutinib Therapy Suppresses Skin Test Responses and Eliminates IgE-Mediated Basophil Activation in Adults With Peanut or Tree Nut Allergy. J Allergy Clin Immunol (2018) 141(5):1914–6.e7. doi: 10.1016/j.jaci.2017.12.987
171. Le Floc’h A, Allinne J, Nagashima K, Scott G, Birchard D, Asrat S, et al. Dual Blockade of IL-4 and IL-13 With Dupilumab, an IL-4rα Antibody, Is Required to Broadly Inhibit Type 2 Inflammation. Allergy (2020) 75(5):1188–204. doi: 10.1111/all.14151
172. Khodoun MV, Tomar S, Tocker JE, Wang YH, Finkelman FD. Prevention of Food Allergy Development and Suppression of Established Food Allergy by Neutralization of Thymic Stromal Lymphopoietin, IL-25, and IL-33. J Allergy Clin Immunol (2018) 141(1):171–9.e1. doi: 10.1016/j.jaci.2017.02.046
173. Zhu D, Kepley CL, Zhang M, Zhang K, Saxon A. A Novel Human Immunoglobulin Fc Gamma Fc Epsilon Bifunctional Fusion Protein Inhibits Fc Epsilon RI-Mediated Degranulation. Nat Med (2002) 8(5):518–21. doi: 10.1038/nm0502-518
174. Eggel A, Buschor P, Baumann MJ, Amstutz P, Stadler BM, Vogel M. Inhibition of Ongoing Allergic Reactions Using a Novel Anti-IgE DARPin-Fc Fusion Protein. Allergy Eur J Allergy Clin Immunol (2011) 66(7):961–8. doi: 10.1111/j.1398-9995.2011.02546.x
175. Liu Y, Sun Y, Chang LJ, Li N, Li H, Yu Y, et al. Blockade of Peanut Allergy With a Novel Ara H 2-Fcγ Fusion Protein in Mice. J Allergy Clin Immunol (2013) 131(1):213–21.e5. doi: 10.1016/j.jaci.2012.10.018
176. Zellweger F, Gasser P, Brigger D, Buschor P, Vogel M, Eggel A. A Novel Bispecific DARPin Targeting Fcγriib and Fcϵri-Bound IgE Inhibits Allergic Responses. Allergy Eur J Allergy Clin Immunol (2017) 72(8):1174–83. doi: 10.1111/all.13109
177. Storni F, Cabral-Miranda G, Roesti E, Zha L, Engeroff P, Zeltins A, et al. A Single Monoclonal Antibody Against the Peanut Allergen Ara H 2 Protects Against Systemic and Local Peanut Allergy. Int Arch Allergy Immunol (2020) 181(5):334–41. doi: 10.1159/000505917
178. Cherwinski HM, Murphy CA, Joyce BL, Joyce BL, Bigler ME, Song YS, Zurawski SM, et al. The CD200 Receptor Is a Novel and Potent Regulator of Murine and Human Mast Cell Function. J Immunol (2005) 174(3):1348–56. doi: 10.4049/jimmunol.174.3.1348
179. Bachelet I, Munitz A, Levi-Schaffer F. Abrogation of Allergic Reactions by a Bispecific Antibody Fragment Linking IgE to CD300a. J Allergy Clin Immunol (2006) 117(6):1314–20. doi: 10.1016/j.jaci.2006.04.031
180. Duan S, Koziol-White CJ, Jester WF, Smith SA, Nycholat CM, Macauley MS, et al. CD33 Recruitment Inhibits IgE-Mediated Anaphylaxis and Desensitizes Mast Cells to Allergen. J Clin Invest (2019) 129(3):1387–401. doi: 10.1172/JCI125456
181. Yokoi H, Choi OH, Hubbard W, Lee HS, Canning BJ, Lee HH, et al. Inhibition of FcϵRI-Dependent Mediator Release and Calcium Flux From Human Mast Cells by Sialic Acid-Binding Immunoglobulin-Like Lectin 8 Engagement. J Allergy Clin Immunol (2008) 121(2):499–506. doi: 10.1016/j.jaci.2007.10.004
182. Youngblood BA, Brock EC, Leung J, Falahati R, Bryce PJ, Bright J, et al. AK002, a Humanized Sialic Acid-Binding Immunoglobulin-Like Lectin-8 Antibody That Induces Antibody-Dependent Cell-Mediated Cytotoxicity Against Human Eosinophils and Inhibits Mast Cell-Mediated Anaphylaxis in Mice. Int Arch Allergy Immunol (2019) 180(2):91–102. doi: 10.1159/000501637
183. O’Konek JJ, Landers JJ, Janczak KW, Lindsey HK, Mondrusov AM, Totten TD, et al. Intranasal Nanoemulsion Vaccine Confers Long-Lasting Immunomodulation and Sustained Unresponsiveness in a Murine Model of Milk Allergy. Allergy Eur J Allergy Clin Immunol (2020) 75(4):872–81. doi: 10.1111/all.14064
184. Storni F, Zeltins A, Balke I, Heath MD, Kramer MF, Skinner MA, et al. Vaccine Against Peanut Allergy Based on Engineered Virus-Like Particles Displaying Single Major Peanut Allergens. J Allergy Clin Immunol (2020) 145(4):1240–53.e3. doi: 10.1016/j.jaci.2019.12.007
185. Cruz AA, Lima F, Sarinho E, Ayre G, Martin C, Fox H, et al. Safety of Anti-Immunoglobulin E Therapy With Omalizumab in Allergic Patients at Risk of Geohelminth Infection. Clin Exp Allergy (2007) 37(2):197–207. doi: 10.1111/j.1365-2222.2007.02650.x
186. Fitzsimmons CM, Falcone FH, Dunne DW. Helminth Allergens, Parasite-Specific IgE, and Its Protective Role in Human Immunity. Front Immunol (2014) 5:61. doi: 10.3389/fimmu.2014.00061
187. Nigro EA, Brini AT, Yenagi VA, Ferreira LM, Achatz-Straussberger G, Ambrosi A, et al. Cutting Edge: IgE Plays an Active Role in Tumor Immunosurveillance in Mice. J Immunol (2016) 197(7):2583–8. doi: 10.4049/jimmunol.1601026
188. Dispenza MC, Krier-Burris RA, Chhiba KD, Undem BJ, Robida PA, Bochner BS. Bruton’s Tyrosine Kinase Inhibition Effectively Protects Against Human IgE-Mediated Anaphylaxis. J Clin Invest (2020) 130(9):4759–70. doi: 10.1172/JCI138448
189. Dispenza MC, Regan JA, Bochner BS. Potential Applications of Bruton’s Tyrosine Kinase Inhibitors for the Prevention of Allergic Reactions. Expert Rev Clin Immunol (2017) 13(10):921–3. doi: 10.1080/1744666X.2017.1370374
190. Wei M, Wang PG. Desialylation in Physiological and Pathological Processes: New Target for Diagnostic and Therapeutic Development. Prog Mol Biol Transl Sci (2019) 162:25–57. doi: 10.1016/bs.pmbts.2018.12.001
191. Costa AF, Campos D, Reis CA, Gomes C. Targeting Glycosylation: A New Road for Cancer Drug Discovery. Trends Cancer (2020) 6(9):757–66. doi: 10.1016/j.trecan.2020.04.002
192. McLeod JJA, Baker B, Ryan JJ. Mast Cell Production and Response to IL-4 and IL-13. Cytokine (2015) 75(1):57–61. doi: 10.1016/j.cyto.2015.05.019
193. Ochi H, De Jesus NH, Hsieh FH, Austen KF, Boyce JA. IL-4 and -5 Prime Human Mast Cells for Different Profiles of IgE-Dependent Cytokine Production. Proc Natl Acad Sci USA (2000) 97(19):10509–13. doi: 10.1073/pnas.180318697
194. Junttila IS. Tuning the Cytokine Responses: An Update on Interleukin (IL)-4 and IL-13 Receptor Complexes. Front Immunol (2018) 9:888. doi: 10.3389/fimmu.2018.00888
195. Pesu M, Takaluoma K, Aittomäki S, Lagerstedt A, Saksela K, Kovanen PE, et al. Interleukin-4-Induced Transcriptional Activation by Stat6 Involves Multiple Serine/Threonine Kinase Pathways and Serine Phosphorylation of Stat6. Blood (2000) 95(2):494–502. doi: 10.1182/blood.V95.2.494
196. Mathias CB, Hobson SA, Garcia-Lloret M, Lawson G, Poddighe D, Freyschmidt EJ, et al. IgE-Mediated Systemic Anaphylaxis and Impaired Tolerance to Food Antigens in Mice With Enhanced IL-4 Receptor Signaling. J Allergy Clin Immunol (2011) 127(3):795–805. doi: 10.1016/j.jaci.2010.11.009
197. Burton OT, Darling AR, Zhou JS, Noval-Rivas M, Jones TG, Gurish MF, et al. Direct Effects of IL-4 on Mast Cells Drive Their Intestinal Expansion and Increase Susceptibility to Anaphylaxis in a Murine Model of Food Allergy. Mucosal Immunol (2013) 6(4):740–50. doi: 10.1038/mi.2012.112
198. Leyva-Castillo JM, Galand C, Kam C, Burton O, Gurish M, Musser MA, et al. Mechanical Skin Injury Promotes Food Anaphylaxis by Driving Intestinal Mast Cell Expansion. Immunity (2019) 50(5):1262–75.e4. doi: 10.1016/j.immuni.2019.03.023
199. Hsu CL, Neilsen CV, Bryce PJ. IL-33 Is Produced by Mast Cells and Regulates IgE-Dependent Inflammation. PloS One (2010) 5(8):e11944. doi: 10.1371/journal.pone.0011944
200. Okayama Y, Okumura S, Sagara H, Yuki K, Sasaki T, Watanabe N, et al. Fcϵri-Mediated Thymic Stromal Lymphopoietin Production by Interleukin-4-Primed Human Mast Cells. Eur Respir J (2009) 34(2):425–35. doi: 10.1183/09031936.00121008
201. Rönnberg E, Ghaib A, Ceriol C, Enoksson M, Arock M, Säfholm J, et al. Divergent Effects of Acute and Prolonged Interleukin 33 Exposure on Mast Cell IgE-Mediated Functions. Front Immunol (2019) 10:1361. doi: 10.3389/fimmu.2019.01361
202. Saluja R, Zoltowska A, Ketelaar ME, Nilsson G. IL-33 and Thymic Stromal Lymphopoietin in Mast Cell Functions. Eur J Pharmacol (2015) 778:68–76. doi: 10.1016/j.ejphar.2015.04.047
203. Hsu CL, Chhiba KD, Krier-Burris R, Hosakoppal S, Berdnikovs S, Miller ML, et al. Allergic Inflammation Is Initiated by IL-33–Dependent Crosstalk Between Mast Cells and Basophils. PloS One (2020) 15(1):1–21. doi: 10.1371/journal.pone.0226701
204. Parveen S, Saravanan DB, Saluja R, Elden BT. IL-33 Mediated Amplification of Allergic Response in Human Mast Cells. J Recept Signal Transduction (2019) 39(4):359–67. doi: 10.1080/10799893.2019.1690515
205. Jung M-Y, Smrž D, Desai A, Bandara G, Ito T, Iwaki S, et al. IL-33 Induces a Hyporesponsive Phenotype in Human and Mouse Mast Cells. J Immunol (2013) 190(2):531–8. doi: 10.4049/jimmunol.1201576
206. Han NR, Oh HA, Nam SY, Moon PD, Kim DW, Kim HM, et al. TSLP Induces Mast Cell Development and Aggravates Allergic Reactions Through the Activation of MDM2 and STAT6. J Invest Dermatol (2014) 134(10):2521–30. doi: 10.1038/jid.2014.198
207. Allakhverdi Z, Comeau MR, Jessup HK, Yoon BR, Brewer A, Chartier S, et al. Thymic Stromal Lymphopoietin Is Released by Human Epithelial Cells in Response to Microbes, Trauma, or Inflammation and Potently Activates Mast Cells. J Exp Med (2007) 204(2):253–8. doi: 10.1084/jem.20062211
208. Muto T, Fukuoka A, Kabashima K, Gailus-Durner V, Fuchs H, Ohnmacht C, et al. The Role of Basophils and Proallergic Cytokines, TSLP and IL-33, in Cutaneously Sensitized Food Allergy. Int Immunol (2014) 26(10):539–49. doi: 10.1093/intimm/dxu058
209. Russkamp D, Aguilar-Pimentel A, Alessandrini F, et al. IL-4 Receptor α Blockade Prevents Sensitization and Alters Acute and Long-Lasting Effects of Allergen-Specific Immunotherapy of Murine Allergic Asthma. Allergy Eur J Allergy Clin Immunol (2019) 74(8):1549–60. doi: 10.1111/all.13759
210. Bruton K, Spill P, Vohra S, Baribeau O, Manzoor S, Gadkar S, et al. Interrupting Reactivation of Immunological Memory Reprograms Allergy and Averts Anaphylaxis. J Allergy Clin Immunol (2021) 147(4):1381–92. doi: 10.1101/2020.03.04.975706.
211. Rial MJ, Barroso B, Sastre J. Dupilumab for Treatment of Food Allergy. J Allergy Clin Immunol Pract (2019) 7(2):673–4. doi: 10.1016/j.jaip.2018.07.027
212. Gauvreau GM, Sehmi R, Ambrose CS, Griffiths JM. Thymic Stromal Lymphopoietin: Its Role and Potential as a Therapeutic Target in Asthma. Expert Opin Ther Targets (2020) 24(8):777–92. doi: 10.1080/14728222.2020.1783242
213. Harvima IT, Levi-Schaffer F, Draber P, Friedman S, Polakovicova I, Gibbs BF, et al. Molecular Targets on Mast Cells and Basophils for Novel Therapies. J Allergy Clin Immunol (2014) 134(3):530–44. doi: 10.1016/j.jaci.2014.03.007
214. Bulfone-Paus S, Nilsson G, Draber P, Blank U, Levi-Schaffer F. Positive and Negative Signals in Mast Cell Activation. Trends Immunol (2017) 38(9):657–67. doi: 10.1016/j.it.2017.01.008
215. Li F, Smith P, Ravetch JV. Inhibitory Fcγ Receptor Is Required for the Maintenance of Tolerance Through Distinct Mechanisms. J Immunol (2014) 192(7):3021–8. doi: 10.4049/jimmunol.1302934
216. Strait RT, Morris SC, Finkelman FD. IgG-Blocking Antibodies Inhibit IgE-Mediated Anaphylaxis In Vivo Through Both Antigen Interception and Fc Gamma RIIb Cross-Linking. J Clin Invest (2006) 116(3):833–41. doi: 10.1172/JCI25575
217. Sun J-B, Xiang Z, Smith KGC, Holmgren J. Important Role for Fcγriib on B Lymphocytes for Mucosal Antigen-Induced Tolerance and Foxp3+ Regulatory T Cells. J Immunol (2013) 191(8):4412–22. doi: 10.4049/jimmunol.1301324
218. Burton OT, Tamayo JM, Stranks AJ, Koleoglou KJ, Oettgen HC. Allergen-Specific IgG Antibody Signaling Through Fcγriib Promotes Food Tolerance. J Allergy Clin Immunol (2018) 141(1):189–201.e3. doi: 10.1016/j.jaci.2017.03.045
219. Anania JC, Chenoweth AM, Wines BD, Hogarth PM. The Human Fcγrii (CD32) Family of Leukocyte FcR in Health and Disease. Front Immunol (2019) 10:464. doi: 10.3389/fimmu.2019.00464
220. Burton OT, Epp A, Fanny ME, Miller SJ, Stranks AJ, Teague JE, et al. Tissue-Specific Expression of the Low-Affinity IgG Receptor, Fcγriib, on Human Mast Cells. Front Immunol (2018) 9:1244. doi: 10.3389/fimmu.2018.01244
221. Zhao W, Kepley CL, Morel PA, Okumoto LM, Fukuoka Y, Schwartz LB. Fcγriia, Not Fcγriib, Is Constitutively and Functionally Expressed on Skin-Derived Human Mast Cells. J Immunol (2006) 177(1):694–701. doi: 10.4049/jimmunol.177.1.694
222. Tordesillas L, Berin MC, Sampson HA. Immunology of Food Allergy. Immunity (2017) 47(1):32–50. doi: 10.1016/j.immuni.2017.07.004
223. Ekoff M, Möller C, Xiang Z, Nilsson G. Coaggregation of Fcϵri With Fcγriib Inhibits Degranulation But Not Induction of Bcl-2 Family Members A1 and Bim in Mast Cells. Allergy Asthma Clin Immunol (2006) 2(3):87–97. doi: 10.2310/7480.2006.00011
224. O’Reilly MK, Paulson JC. Multivalent Ligands for Siglecs. Methods Enzymol (2010) 478(C):343–63. doi: 10.1016/S0076-6879(10)78017-4
225. Ghannadan M, Hauswirth AW, Schernthaner GH, Müller MR, Klepetko W, Schatzl G, et al. Detection of Novel CD Antigens on the Surface of Human Mast Cells and Basophils. Int Arch Allergy Immunol (2002) 127(4):299–307. doi: 10.1159/000057747
226. Yokoi H, Myers A, Matsumoto K, Crocker PR, Saito H, Bochner BS. Alteration and Acquisition of Siglecs During In Vitro Maturation of CD34+ Progenitors Into Human Mast Cells. Allergy Eur J Allergy Clin Immunol (2006) 61(6):769–76. doi: 10.1111/j.1398-9995.2006.01133.x
227. MacAuley MS, Crocker PR, Paulson JC. Siglec-Mediated Regulation of Immune Cell Function in Disease. Nat Rev Immunol (2014) 14(10):653–66. doi: 10.1038/nri3737
228. Lübbers J, Rodríguez E, van Kooyk Y. Modulation of Immune Tolerance via Siglec-Sialic Acid Interactions. Front Immunol (2018) 9:2807. doi: 10.3389/fimmu.2018.02807
229. Schanin J, Gebremeskel S, Korver W, Falahati R, Butuci M, Haw TJ, et al. A Monoclonal Antibody to Siglec-8 Suppresses Non-Allergic Airway Inflammation and Inhibits IgE-Independent Mast Cell Activation. Mucosal Immunol (2020) March:1–11. doi: 10.1038/s41385-020-00336-9
230. O’Sullivan JA, Carroll DJ, Cao Y, Salicru AN, Bochner BS. Leveraging Siglec-8 Endocytic Mechanisms to Kill Human Eosinophils and Malignant Mast Cells. J Allergy Clin Immunol (2018) 141(5):1774–85.e7. doi: 10.1016/j.jaci.2017.06.028
231. Dellon ES, Peterson KA, Murray JA, Falk GW, Gonsalves N, Chehade M, et al. Anti–Siglec-8 Antibody for Eosinophilic Gastritis and Duodenitis. N Engl J Med (2020) 383(17):1624–34. doi: 10.1056/NEJMoa2012047
232. Bachelet I, Munitz A, Berent-Maoz B, Mankuta D, Levi-Schaffer F. Suppression of Normal and Malignant Kit Signaling by a Bispecific Antibody Linking Kit With CD300a. J Immunol (2008) 180(9):6064–9. doi: 10.4049/jimmunol.180.9.6064
233. Moshkovits I, Karo-Atar D, Itan M, Reichman H, Rozenberg P, Morgenstern-Ben-Baruch N, et al. CD300f Associates With IL-4 Receptor α and Amplifies IL-4-Induced Immune Cell Responses. Proc Natl Acad Sci USA (2015) 112(28):8708–13. doi: 10.1073/pnas.1507625112
234. Sturm GJ, Varga E-M, Roberts G, Mosbech H, Bilè MB, Akdis CA, et al. EAACI Guidelines on Allergen Immunotherapy: Hymenoptera Venom Allergy. Allergy (2018) 73(4):744–64. doi: 10.1111/all.13262
235. Dhami S, Nurmatov U, Arasi S, Khan T, Asaria M, Zaman H, et al. Allergen Immunotherapy for Allergic Rhinoconjunctivitis: A Systematic Review and Meta-Analysis. Allergy (2017) 72(11):1597–631. doi: 10.1111/all.13201
236. Agache I, Lau S, Akdis CA, Smolinska S, Bonini M, Cavkaytar O, et al. EAACI Guidelines on Allergen Immunotherapy: House Dust Mite-Driven Allergic Asthma. Allergy (2019) 74(5):855–73. doi: 10.1111/all.13749
237. Dhami S, Kakourou A, Asamoah F, Agache I, Lau S, Jutel M, et al. Allergen Immunotherapy for Allergic Asthma: A Systematic Review and Meta-Analysis. Allergy (2017) 72(12):1825–48. doi: 10.1111/all.13208
238. Roberts G, Pfaar O, Akdis CA, Ansotegui IJ, Durham SR, Gerth van Wijk R, et al. EAACI Guidelines on Allergen Immunotherapy: Allergic Rhinoconjunctivitis. Allergy Eur J Allergy Clin Immunol (2018) 73(4):765–98. doi: 10.1111/all.13317
239. Santos AF, James LK, Bahnson HT, Shamji MH, Couto-Francisco NC, Islam S, et al. IgG4 Inhibits Peanut-Induced Basophil and Mast Cell Activation in Peanut-Tolerant Children Sensitized to Peanut Major Allergens. J Allergy Clin Immunol (2015) 135(5):1249–56. doi: 10.1016/j.jaci.2015.01.012
240. Stranks AJ, Phil D, Minnicozzi SC, Burton OT, Logsdon SL, Spergel JM, et al. IgE Blockade During Food Allergen Ingestion Enhances the Induction of Inhibitory IgG Antibodies. Ann Allergy Asthma Immunol (2019) 122(2):213–5. doi: 10.1016/j.anai.2018.10.024.IgE
241. Reizis B, Bunin A, Ghosh HS, Lewis KL, Sisirak V. Plasmacytoid Dendritic Cells: Recent Progress and Open Questions. Ann Rev Immunol (2011) 29:163–83. doi: 10.1146/annurev-immunol-031210-101345.Plasmacytoid
242. Kanagaratham C, El Ansari YS, Lewis OL, Oettgen HC. IgE and IgG Antibodies as Regulators of Mast Cell and Basophil Functions in Food Allergy. Front Immunol (2020) 11:603050. doi: 10.3389/fimmu.2020.603050
243. Sibilano R, Frossi B, Suzuki R, D'Incà F, Gri G, Piconese S, et al. Modulation of Fcϵri-Dependent Mast Cell Response by OX40L via Fyn, PI3K, and RhoA. J Allergy Clin Immunol (2012) 130(3). doi: 10.1016/j.jaci.2012.03.032
244. Oka T, Rios EJ, Tsai M, Kalesnikoff J, Galli SJ. Rapid Desensitization Induces Internalization of Antigen-Specific IgE on Mouse Mast Cells. J Allergy Clin Immunol (2013) 132(4):922–32.e16. doi: 10.1016/j.jaci.2013.05.004
245. del Carmen Sancho-Serra M, Simarro M, Castells M. Rapid IgE Desensitization is Antigen Specific and Impairs Early and Late Mast Cell Responses Targeting Fcϵri Internalization. Eur J Immunol (2011) 41(4):1004–13. doi: 10.1002/eji.201040810
246. Gladys Ang WX, Church AM, Kulis M, Choi HW, Wesley Burks A, Abraham SN. Mast Cell Desensitization Inhibits Calcium Flux and Aberrantly Remodels Actin. J Clin Invest (2016) 126(11):4103–18. doi: 10.1172/JCI87492
247. Takasato Y, Kurashima Y, Kiuchi M, Hirahara K, Murasaki S, Arai F, et al. Orally Desensitized Mast Cells Form a Regulatory Network With Treg Cells for the Control of Food Allergy. Mucosal Immunol (2020) October:1–6. doi: 10.1038/s41385-020-00358-3
248. Akdis M, Akdis CA. Mechanisms of Allergen-Specific Immunotherapy: Multiple Suppressor Factors at Work in Immune Tolerance to Allergens. J Allergy Clin Immunol (2014) 133(3):621–31. doi: 10.1016/j.jaci.2013.12.1088
249. Nurmatov U, Dhami S, Arasi S, Pajno GB, Fernandez-Rivas M, Muraro A, et al. Allergen Immunotherapy for IgE-Mediated Food Allergy: A Systematic Review and Meta-Analysis. Allergy (2017) 72(8):1133–47. doi: 10.1111/all.13124
250. Pajno GB, Fernandez-Rivas M, Arasi S, Roberts G, Akdis CA, Alvaro-Lozano M, et al. EAACI Guidelines on Allergen Immunotherapy: IgE-Mediated Food Allergy. Allergy Eur J Allergy Clin Immunol (2018) 73(4):799–815. doi: 10.1111/all.13319
251. U.S. Food and Drug Administration. Biologics License Application - Palforzia (2020). Available at: https://www.fda.gov/media/134857/download (Accessed December 26, 2020).
252. European Medicines Agency. Palforzia EPAR Medicine Overview (2020). Available at: https://www.ema.europa.eu/en/documents/overview/palforzia-epar-medicine-overview_en.pdf (Accessed December 26, 2020).
253. Chu DK, Wood RA, French S, Fiocchi A, Jordana M, Waserman S, et al. Oral Immunotherapy for Peanut Allergy (PACE): A Systematic Review and Meta-Analysis of Efficacy and Safety. Lancet (2019) 393(10187):2222–32. doi: 10.1016/S0140-6736(19)30420-9
254. Anagnostou K. Recent Advances in Immunotherapy and Vaccine Development for Peanut Allergy. Ther Adv Vaccines (2015) 3(3):55–65. doi: 10.1177/2051013615591739
255. Wood RA, Kim JS, Lindblad R, Nadeau K, Henning AK, Dawson P, et al. A Randomized, Double-Blind, Placebo-Controlled Study of Omalizumab Combined With Oral Immunotherapy for the Treatment of Cow’s Milk Allergy. J Allergy Clin Immunol (2016) 137(4):1103–10.e11. doi: 10.1016/j.jaci.2015.10.005
256. Douladiris N, Linhart B, Swoboda I, Gstöttner A, Vassilopoulou E, Stolz F, et al. In Vivo Allergenic Activity of a Hypoallergenic Mutant of the Major Fish Allergen CYP C 1 Evaluated by Means of Skin Testing. J Allergy Clin Immunol (2015) 136(2):493–5.e8. doi: 10.1016/j.jaci.2015.01.015
257. Tscheppe A, Palmberger D, van Rijt L, Kalic T, Mayr V, Palladino C, et al. Development of a Novel Ara H 2 Hypoallergen With No IgE Binding or Anaphylactogenic Activity. J Allergy Clin Immunol (2020) 145(1):229–38. doi: 10.1016/j.jaci.2019.08.036
258. Johnson-Weaver BT, Staats HF, Burks AW, Kulis MD. Adjuvanted Immunotherapy Approaches for Peanut Allergy. Front Immunol (2018) 9:2156(SEP). doi: 10.3389/fimmu.2018.02156
259. Scheiblhofer S, Thalhamer J, Weiss R. DNA and mRNA Vaccination Against Allergies. Pediatr Allergy Immunol Off Publ Eur Soc Pediatr Allergy Immunol (2018) 29(7):679–88. doi: 10.1111/pai.12964
260. Curin M, Khaitov M, Karaulov A, Namazova-Baranova L, Campana R, Garib V, et al. Next-Generation of Allergen-Specific Immunotherapies: Molecular Approaches. Curr Allergy Asthma Rep (2018) 18(7):39. doi: 10.1007/s11882-018-0790-x
261. Johnson-Weaver BT, Sempowski GD, Staats HF. Nasal Peanut+ CpG Immunotherapy Enhances Peanut-Specific IFN-γ in Th2 Cells and IL-10 in Non-Th2 Cells in Mice. Allergy Eur J Allergy Clin Immunol (2019) 74(11):2220–3. doi: 10.1111/all.13738
262. Brotons-Canto A, Gamazo C, Martín-Arbella N, Abdulkarim M, Gumbleton M, Quincoces G, et al. Mannosylated Nanoparticles for Oral Immunotherapy in a Murine Model of Peanut Allergy. J Pharm Sci (2019) 108(7):2421–9. doi: 10.1016/j.xphs.2019.02.022
263. Long A, Rahmaoui A, Rothman KJ, Guinan E, Eisner M, Bradley MS, et al. Incidence of Malignancy in Patients With Moderate-To-Severe Asthma Treated With or Without Omalizumab. J Allergy Clin Immunol (2014) 134(3):560–7.e4. doi: 10.1016/j.jaci.2014.02.007
264. Kim HL, Leigh R, Becker A. Omalizumab: Practical Considerations Regarding the Risk of Anaphylaxis. Allergy Asthma Clin Immunol Off J Can Soc Allergy Clin Immunol (2010) 6(1):32. doi: 10.1186/1710-1492-6-32
265. Novartis Pharma AG. xolair - Omalizumab Safety Profile (2021). Available at: https://www.xolairhcp.com/safety-profile.html (Accessed January 3, 2021).
Keywords: mast cells, IgE, desensitization, food allergy, biologics, immunotherapy, cytokines, inhibitory receptors
Citation: Tontini C and Bulfone-Paus S (2021) Novel Approaches in the Inhibition of IgE-Induced Mast Cell Reactivity in Food Allergy. Front. Immunol. 12:613461. doi: 10.3389/fimmu.2021.613461
Received: 02 October 2020; Accepted: 23 June 2021;
Published: 12 August 2021.
Edited by:
Rudolf Valenta, Medical University of Vienna, AustriaReviewed by:
Philipp Starkl, Medical University of Vienna, AustriaBirgit Linhart, Medical University of Vienna, Austria
Copyright © 2021 Tontini and Bulfone-Paus. This is an open-access article distributed under the terms of the Creative Commons Attribution License (CC BY). The use, distribution or reproduction in other forums is permitted, provided the original author(s) and the copyright owner(s) are credited and that the original publication in this journal is cited, in accordance with accepted academic practice. No use, distribution or reproduction is permitted which does not comply with these terms.
*Correspondence: Silvia Bulfone-Paus, silvia.bulfone-paus@manchester.ac.uk