- 1Laboratório de Imunologia Molecular, Departamento de Parasitologia, Universidade Federal do Amazonas (UFAM), Manaus, Brazil
- 2Programa de Pós-Graduação em Imunologia Básica e Aplicada, Universidade Federal do Amazonas, Manaus, Brazil
- 3Laboratório de Imunogenética, Departamento de Imunologia, Instituto de Ciências Biomédicas (ICB), Universidade de São Paulo (USP), São Paulo, Brazil
- 4Policlínica Cardoso Fontes – Secretaria de Estado da Saúde do Amazonas-SUSAM, Manaus, Brazil
- 5Diretoria de Ensino e Pesquisa, Fundação Hospitalar de Hematologia e Hemoterapia do Amazonas (HEMOAM), Manaus, Brazil
- 6Instituto de Pesquisa Clínica Carlos Borborema, Fundação de Medicina Tropical Dr. Heitor Vieira Dourado, Manaus, Brazil
- 7Faculdade de Medicina Nilton Lins, Universidade Nilton Lins, Manaus, Brazil
- 8Laboratório de Micobacteriologia, Instituto Nacional de Pesquisas da Amazônia (INPA), Manaus, Brazil
Tuberculosis (TB) remains a serious public health burden worldwide. TB is an infectious disease caused by the Mycobacterium tuberculosis Complex. Innate immune response is critical for controlling mycobacterial infection. NOD-like receptor pyrin domain containing 3/ absent in melanoma 2 (NLRP3/AIM2) inflammasomes are suggested to play an important role in TB. NLRP3/AIM2 mediate the release of pro-inflammatory cytokines IL-1β and IL-18 to control M. tuberculosis infection. Variants of genes involved in inflammasomes may contribute to elucidation of host immune responses to TB infection. The present study evaluated single-nucleotide variants (SNVs) in inflammasome genes AIM2 (rs1103577), CARD8 (rs2009373), and CTSB (rs1692816) in 401 patients with pulmonary TB (PTB), 133 patients with extrapulmonary TB (EPTB), and 366 healthy control (HC) subjects with no history of TB residing in the Amazonas state. Quantitative Real Time PCR was performed for allelic discrimination. The SNV of AIM2 (rs1103577) is associated with protection for PTB (padj: 0.033, ORadj: 0.69, 95% CI: 0.49-0.97). CTSB (rs1692816) is associated with reduced risk for EPTB when compared with PTB (padj: 0.034, ORadj: 0.50, 95% CI: 0.27-0.94). Serum IL-1β concentrations were higher in patients with PTB than those in HCs (p = 0,0003). The SNV rs1103577 of AIM2 appeared to influence IL-1β release. In a dominant model, individuals with the CC genotype (mean 3.78 ± SD 0.81) appeared to have a higher level of IL-1β compared to carriers of the T allele (mean 3.45 ± SD 0.84) among the patients with PTB (p = 0,0040). We found that SNVs of AIM2 and CTSB were associated with TB, and the mechanisms involved in this process require further study.
Introduction
Tuberculosis (TB) is an infectious disease caused by mycobacteria belonging to the Mycobacterium tuberculosis complex (MTBC) (1). The MTBC typically affects the lungs (pulmonary TB (PTB) but can also affect other tissues and organs (extrapulmonary TB (EPTB)) (2). Approximately 25% of the world’s population is infected with M. tuberculosis, but only approximately 5% to 10% of M. tuberculosis-infected individuals subsequently develop TB (3). The complexity of TB is influenced by several factors, such as the immune status and genetic factors of host (4–8).
The innate immune response is the first line of host defense against mycobacteria infection (9, 10). Innate immune system senses pathogens via pattern-recognition receptors (PRRs) to recognize conserved microbial components known as pathogen-associated molecular patterns (PAMPs). PRR also function as innate sensors of host-derived danger signals, the danger-associated molecular patterns (DAMPs) and may assemble in inflammasomes (11). Inflammasomes are intracellular multimeric protein complexes that play key roles in the innate immune response (12, 13). Inflammasomes are essential for controlling bacterial growth through the activation of caspase-1 to process pro-inflammatory IL-1β and IL-18 into active cytokines (14, 15). Caspase-1 also induces a type of inflammatory cell death called pyroptosis (16).
Inflammasomes belonging to the NOD-like receptor (NLR) family are composed of at least three components, a sensor protein (NLRP1, NLRP3, NLRP6, NLRP12, NLRC4; PYHIN family (PYD-like and HIN domain-containing proteins) as AIM2 and TRIM family (Tripartite motif proteins) containing PYRIN), an inflammatory caspase (caspase-1, Caspase-11) and an adapter molecule such as the apoptosis-associated speck-like containing a CARD domain (ASC) (17). NLRP3-inflammasomes is the most studied compared to others (18). NLRP3 and AIM2 inflammasomes play important role in host defense against M. tuberculosis (9, 15, 19–22).
The NLRP3 gene is located on human chromosome 1. Basically, member of NLR family of PRR consists of three domains: a leucine-rich repeat (LRR)-region that interacts with antigens, a central nucleotide-binding NACHT domain and an effector domain PYD or CARD (23). AIM2 gene is located on human chromosome 1. AIM2 is composed of two domains: a N-terminal pyrin domain (PYD) and a C-terminal hematopoietic interferon-inducible nuclear protein with a 200-amino acid repeat (HIN200) domain. AIM2 is a cytosolic double-stranded DNA receptor (24, 25).
Principles of inflammasome activation in TB involve two signals. The first signal induces the expression of NLRP3 and/or AIM2 inflammasome, pro-IL-1β and pro-IL-IL-18 genes caused by Toll-like receptor (TLR) stimulation. The second signal results to oligomerization domain and formation of inflammasome complex consequent of activating stimuli. After that, the effector domain recruits ASC protein to assemble the inflammasome complex (PRR, ASC protein and procaspase-1). ASC protein recruits procaspase-1 through caspase recruitment domain (CARD) consequently it is cleaved into active caspase-1 (14).
Activating stimuli described for NLRP3 are mitochondrial factors (mitochondrial reactive oxygen species, mitochondrial DNA, cardiolipin), mycobacterial components, cathepsins after lysosomal rupture as Cathepsin B encoded by CTSB gene located on human chromosome 8, potassium efflux and extracellular ATP via the P2X7 purinergic receptor (26, 27) and AIM2 is cytosolic dsDNA (25, 28). CTSB is cysteine exo/endopeptidase enzymes pH dependent belonging members of the papain family seems to involvement roles in NLRP3-inflammasome activation as well as degradation or processing of lysosomal proteins (29–31). CARD8 adaptor protein is encoded by CARD8 gene present on chromosome 19. CARD8 acts as a negative regulator during NLRP3 inflammasome activation for adequate functioning and balanced response (32, 33).
In animal models of TB, NLRP3 (34, 35) and AIM2 (19, 36) inflammasomes have been found to recognize cytosolic DNA. Recently, the importance of inflammasomes in TB (37) as well as the crucial role of IL1β in infection control independent of inflammasome activation have been highlighted (38). TB is complex and multifactorial and identification of genetic variants can improve our understanding of the pathogenesis of TB (39).
Several studies have shown a genetic predisposition to development of TB (40–44). Genetic variant in inflammasomes can change their physiological function and contribute to the susceptibility, severity, and outcomes of TB (45). Single-nucleotide variant (SNV) of NLRP3 (rs10754558) and SNV of P2X7 (rs2230911) are associated with TB. SNV of NLRP3 (rs10754558) is associated with protection against PTB (41). The SNV of P2X7 (rs2230911) is associated with susceptibility to PTB. SNVs of CARD8 (rs2043211) and NLRP3 (rs35829419) are associated with EPTB in Ethiopian population (46).
The important role of inflammasomes in immunity to TB prompted us to evaluate the associations of SNVs in genes encoding the AIM2 inflammasome, CARD8, and CTSB in patients with TB from Amazonas state of Brazil.
Material and Methods
Ethics Statement
This study was approved by Human Research Ethics Committee of Federal University of Amazonas (N°. CAAE: 57978916.3.0000.5020; August 17, 2016). All patients and controls participating in this study provided written informed consent for collection of blood and sputum samples for analysis.
Study Population
This is a case–control study and is consisted of 401 patients with PTB, 133 patients with EPTB, and 366 healthy controls (HCs). The study population was recruited from Policlínica Cardoso Fontes, a sanitary pneumology center (Manaus, AM, Brazil). The patients with PTB were positive for M. tuberculosis, which was determined through a molecular rapid test (TRM, GeneXpert MTB/RIF) (47) or through sputum smears and PKO culture method (47, 48). The diagnosis of EPTB was based on recommendations of Brazilian National Guidelines for the Control of Tuberculosis (49). The patients with EPTB were either sputum MTB-positive or culture-positive and were molecularly characterized for MTBC. The clinical manifestations of EPTB were pleural cutaneous, ganglionic, intestinal, perianal, ocular, bone, and miliary.
HCs participating in study had no history of TB and were contacts of patients with TB. The HCs were devoid of TB symptoms and negative for M. tuberculosis in sputum and/or in culture tests. Exclusion criteria comprised current pregnancy, recipients of organ transplants, and presence of other comorbidities, such as cancer, diabetes, HIV positive, hepatitis, and autoimmune diseases.
DNA Isolation and SNV Genotyping
Genomic DNA was extracted from whole human blood (1 mL) using tetramethylammonium bromide salts protocol (50). SNVs were selected according to the minor allele frequency (MAF) of > to 10% in the global population from NCBI database, but also for background information on inflammasome complex in tuberculosis. Three SNVs in inflammasome genes AIM2 T>C (rs1103577) situated on chromosome 1q15, CARD8 T>C (rs2009373) on 19q48, and CTSB A> C (rs1692816) on 8q11 were selected and genotyped using TaqMan probes for allelic discrimination assay by Real-Time PCR System (Applied Biosystems). The technology is based on hydrolysis fluorescent probes. The fluorescent probes are allele-specific oligonucleotides sequences of target SNV labeled with either VIC or FAM fluorescence to discriminate the alleles. Thermal cycling conditions for PCR were a pre-read stage at 60oC for 30 sec and subsequently a hold stage at 95oC for 10 min followed by 50 cycles of denaturation at 95oC for 15 sec and annealing\extension at 60oC for 01 sec and finally followed by a post-read stage at 60oC for 30 sec. The reaction mix contained 5 μL TaqMan Genotyping Master Mix 1X; 0,5 μL TaqMan® probes 20X of target SNV, 4,5 μL Milli-Q water and 2 μL DNA (50ng\uL). For each PCR plate, a negative control and three known positive controls of target SNV (rare homozygote, wild-type homozygote and heterozygote) were included to increase the reliability of assay. Of note, the SNV rs35130877 of the AIM2 gene was previously studied in the same population (41). This was carried out using QuantStudio™ 3 (ThermoFisher Scientific) and Design & Analysis Software v.1.4.2. The probes used in experiment are shown in Supplementary Table 1.
Cytokine Measurements
Human IL-1β levels were measured in plasma sample from patients with PTB prior to start of drug therapy and HCs using a commercially available enzyme-linked immunosorbent assay (ELISA) kit (BioLegend ELISA MAX™ Deluxe Sets) following the manufacturer’s instructions. 50 μL of plasma samples were added to each well of high binding plate and readings were performed on a BioRad spectrophotometer plate as recommended by kit, using the 450 nm filter. Samples and standards were analyzed in duplicate. The minimal detectable concentration of IL-1β for this kit was 0.5 pg/mL.
Statistical Analysis
For each SNVs analysis, alleles analysis was performed using on the link https://ihg.helmholtz-muenchen.de/ihg/snps.html and the associations between the allelic/genotype frequencies among patients with PTB, EPTB, and HCs were examined using the package “SNPassoc” version 1.9-2 (https://cran.r-project.org/web/packages/SNPassoc/index.html) for R software version 3.4.3 (www.r-project.org). The best genetic model was performed via Akaike information criterion (AIC). The Hardy-Weinberg equilibrium was evaluated for all SNVs. The results were shown as the odds ratio (OR) and 95% confidence intervals (95% CI) from multivariate logistic regression analyses. To control the potential confounding factors, adjusted OR (ORadj) values for age and sex were provided. The multivariate logistic regressions were performed using STATA 15 (StataCorp Texas, USA) after adjusting for age and sex. To compare multiple means of cytokine levels, one-way analysis of variance was applied with the Tukey’s post-hoc test adjusted for multiple comparisons. A p-value less than 0.05 was considered as statistically significant.
Results
Characteristics of the Study Population
A total of 900 unrelated individuals born in the North of Brazil participated in this study. Of 900 individuals, 534 and 366 were patients with TB and HCs, respectively. Among those with TB, 401 had PTB and 133 had EPTB. The mean ages of patients with PTB, EPTB, and the HCs were 38.1 ± 13.7, 34.6 ± 14.1, and 32.9 ± 12.5, respectively. Of patients with PTB, EPTB, and HCs, 243 (60.6%), 75 (56.4%), and 180 (49.2%) were male, respectively. Patients with EPTB exhibited mostly pleural (55.6%), followed by cutaneous (17.3%) and ganglionic (16.5%). Intestinal, perianal, ocular, bone, and miliary TB accounted for 10.5%.
Analysis of the SNVs in Inflammasome Genes
All SNVs studied AIM2 rs1103577, CARD8 rs2009373 and CTSB rs1692816 were in the Hardy-Weinberg equilibrium (HWE) in both HCs and patients with TB. The frequencies of alleles and genotypes as well as the different comparisons are shown in Tables 1–4. Genotypes and the best inheritance modeling for AIM2, CARD8 and CTSB genes are reported in Supplementary Table 2.
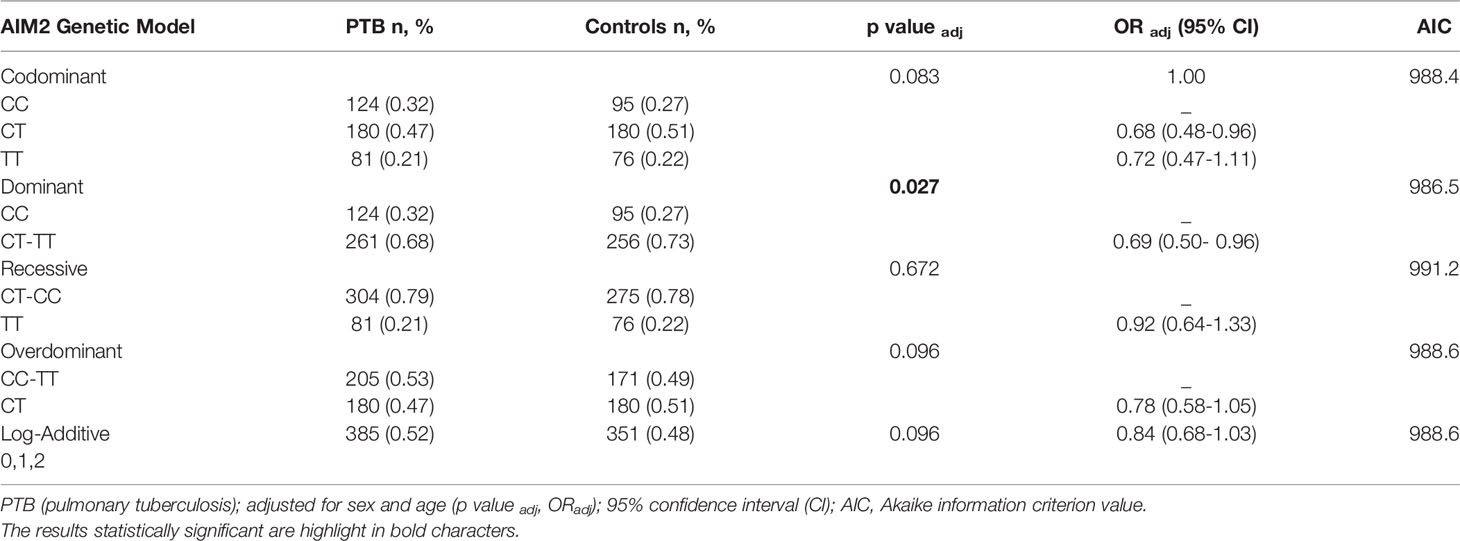
Table 1 Genetic models of association SNV rs1103577 of AIM2 gene adjusted for sex and age in patients with pulmonary tuberculosis (PTB) and healthy control.
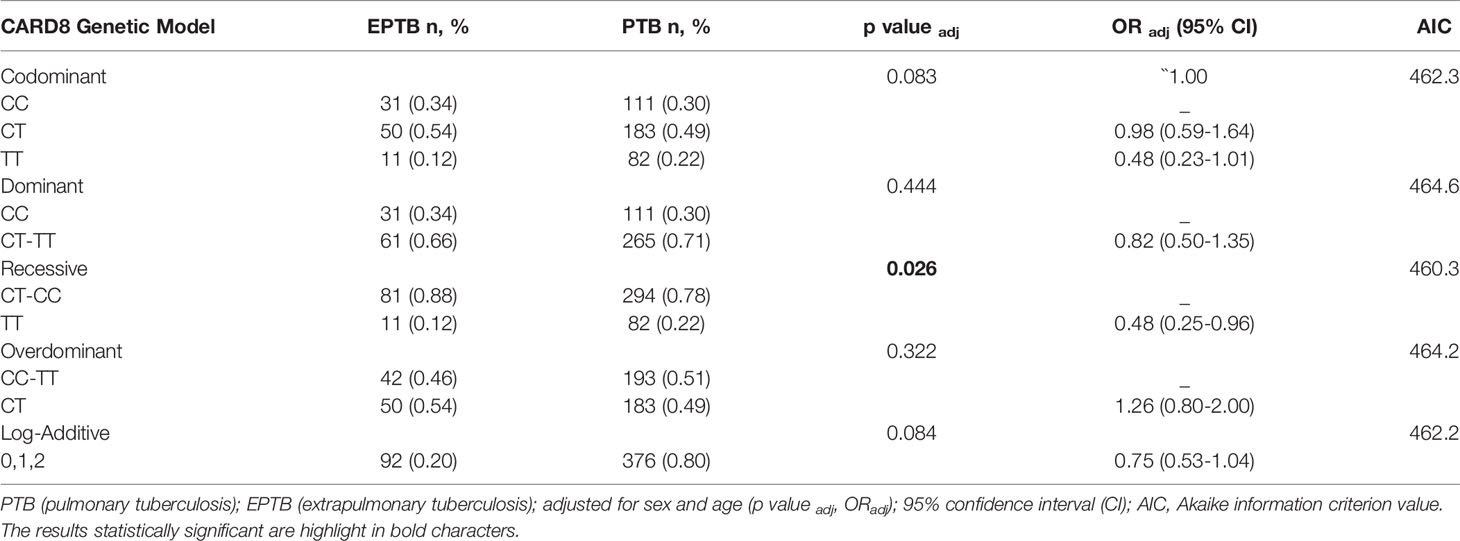
Table 2 Genetic models of association SNV rs2009373 of CARD8 gene adjusted for sex and age in patients with extrapulmonary tuberculosis (EPTB) and pulmonary tuberculosis (PTB).
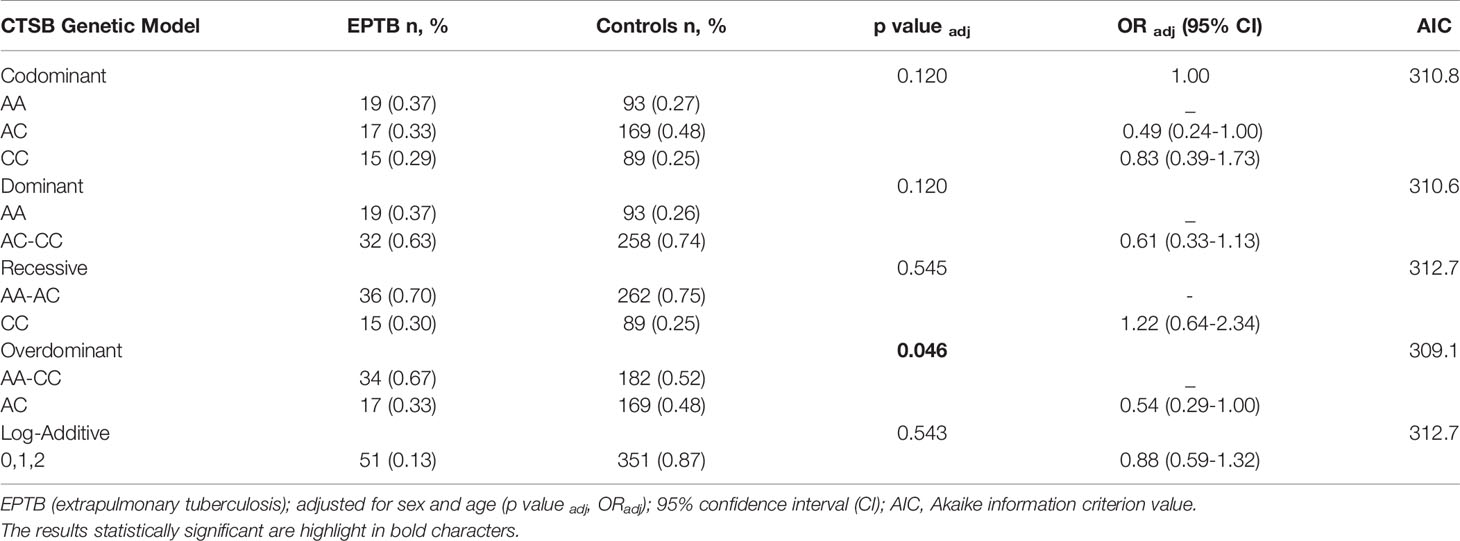
Table 3 Genetic models of association SNV rs1692816 of CTSB gene adjusted for sex and age in patients with extrapulmonary tuberculosis (EPTB) and healthy control subjects.
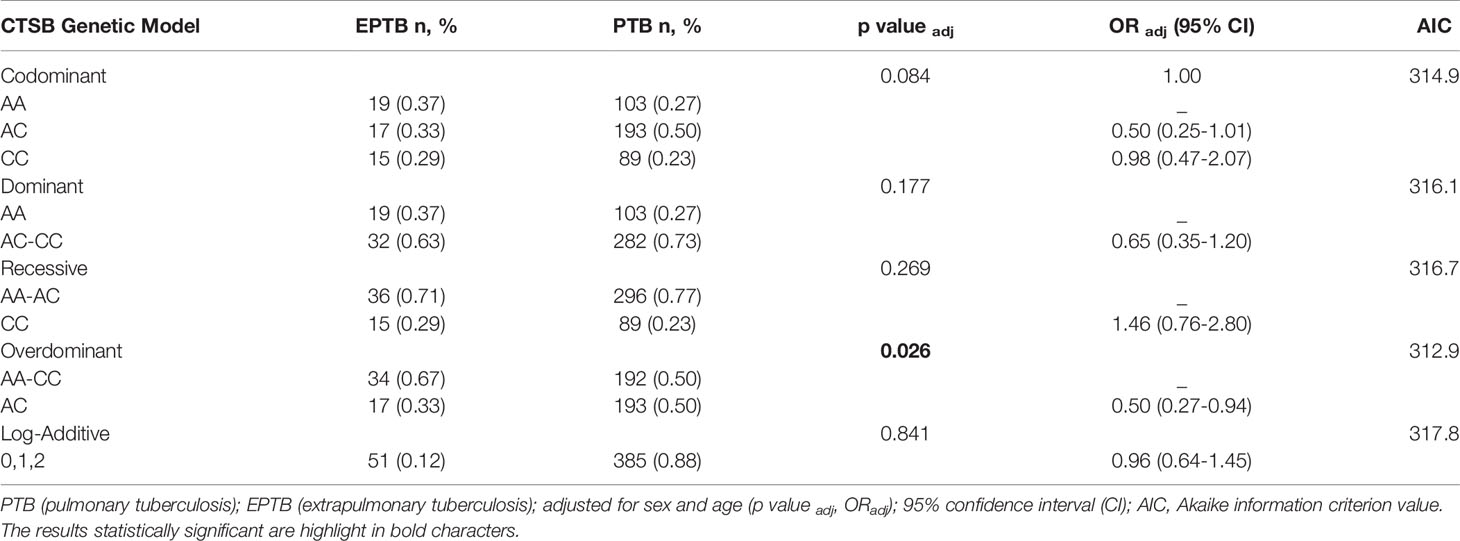
Table 4 Genetic models of association SNV rs1692816 of CTSB gene adjusted for sex and age in patients with extrapulmonary tuberculosis (EPTB) and pulmonary tuberculosis (PTB).
The distribution of SNV of AIM2 (rs1103577) genotypes is slightly different between patients with PTB compared with HC (padj: 0.083). The frequency of genotype CC was slightly higher among patients with PTB (32%) than among HCs (27%). The SNV of AIM2 (rs1103577) was associated with a reduced risk of developing PTB (padj: 0.027, ORadj: 0.69, 95% CI: 0.50-0.96) in a dominant model (CC versus CT + TT) when compared with HCs (Table 1). Carriers of T allele had 31% less chances of developing PTB compared to individuals homozygous for C allele, suggesting homozygosity for C allele may be a risk factor for PTB.
The SNV of CARD8 (rs2009373) showed a decrease risk in development of EPTB when compared to PTB in a recessive model (padj: 0.026, ORadj: 0.48, 95% CI: 0.25-0.96) (Table 2). Bearers of C allele had 52% less chance of developing PTB. The frequency of genotype TT was predominant among the patients with PTB (22%) than in the EPTB group (12%). Individuals homozygous for T allele had twice chance of developing PTB than EPTB when compared with individuals homozygous for C allele (TT vs. CC p = 0.05, OR=2.1, 95% CI: 0.99-4.4) (The results of the different alleles were extracted on the link https://ihg.helmholtz-muenchen.de/ihg/snps.html). Of note, the frequencies of genotype were 31%, 49% and 20% for CC, CT and TT respectively, among HCs group (Supplementary Table 2). Comparisons between patients with EPTB and HCs revealed that carriers of C allele (CC +CT vs. TT) had 85% risk of developing EPTB (p=0.07, OR = 1.85, 95% CI: 0.94-3.7). No association was revealed when patients with PTB were compared with HCs.
Regarding the SNV of CTSB (rs1692816), an overdominant model indicated that heterozygous individuals have a lower risk of developing EPTB compared to patients with PTB (p: 0.022, OR: 0.50, 95% CI: 0.27-0.92) (Supplementary Table 2) and when adjusted for sex and age (p: 0.026, OR: 0.50, 95% CI: 0.27-0.94) (Tables 3 and 4). The frequencies were 23%, 50% and 27% for the CC, AC and AA genotypes, respectively among patients with PTB and similar pattern was observed among HCs (CC 25%, AC 48% and AA 27%). In contrast, the frequencies among patients with EPTB were 29%, 33%, and 37% for CC, AC and AA genotypes, respectively. Similarly, comparison between patients and HCs disclosed that heterozygous individuals have lower risk of developing EPTB (p: 0.045, padj: 0.046, OR: 0.54, 95% CI: 0.29-1.0).
Logistic regression analysis, including sex and age variables with SNVs, confirmed the association of AIM2 rs1103577 (p:0.033, OR: 0.69, 95% CI: 0.49-0.97) and CTSB rs1692816 with a lower risk for EPTB (p: 0.034, OR: 0.50, 95% CI: 0.27-0.94) (Table 5).
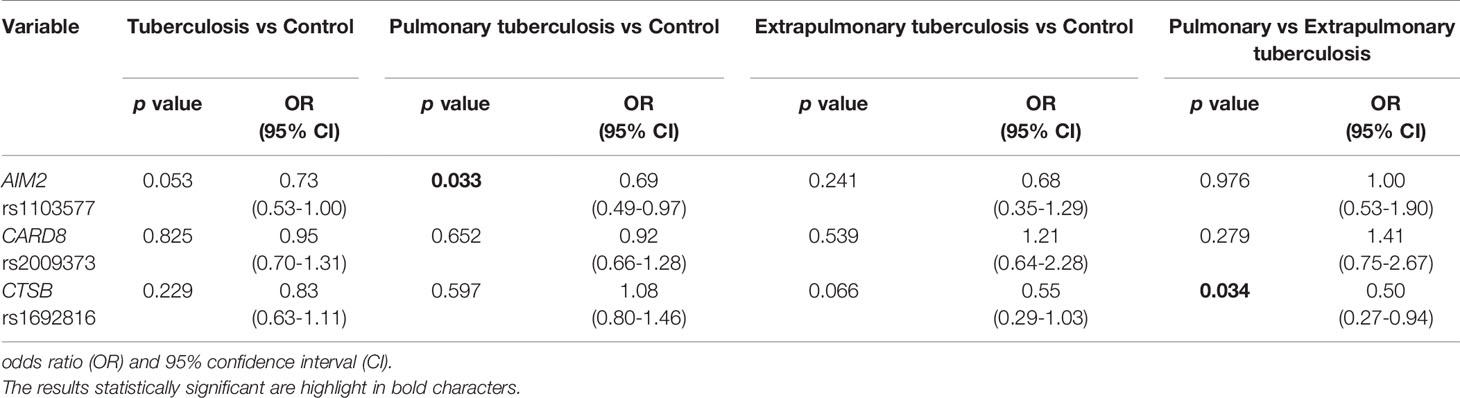
Table 5 Multivariate logistic regression analysis for variables sex and age, including three inflammasomes genes in the studied groups.
Cytokine IL-1β in a Dominant Model of AIM2, CARD8, and CTSB Genotypes
IL-1β is an important inflammatory cytokine with major role in host immune response against M.tuberculosis infection. Next, we evaluated in vivo the plasma IL-1β concentrations in PTB and HCs. Plasma IL-1β was higher in patients with PTB compared to those in HC group (p = 0.0003) (Supplementary Figure 1). We analyzed the distribution of plasma IL1-β according to genotypes of AIM2, CARD8, and CTSB in a dominant model. AIM2 rs1103577 appeared to have an effect on plasma cytokine IL-1β when CC carriers were compared to allele T carriers (CC vs. CT+TT) in patients with PTB (p = 0.0040). CARD8 rs2009373 and CTSB rs1692816 did not show any influence on levels of plasma IL-β (Figure 1A). A QQ plot was used to validate the distribution of IL-1β in genotypes (Figure 1B).
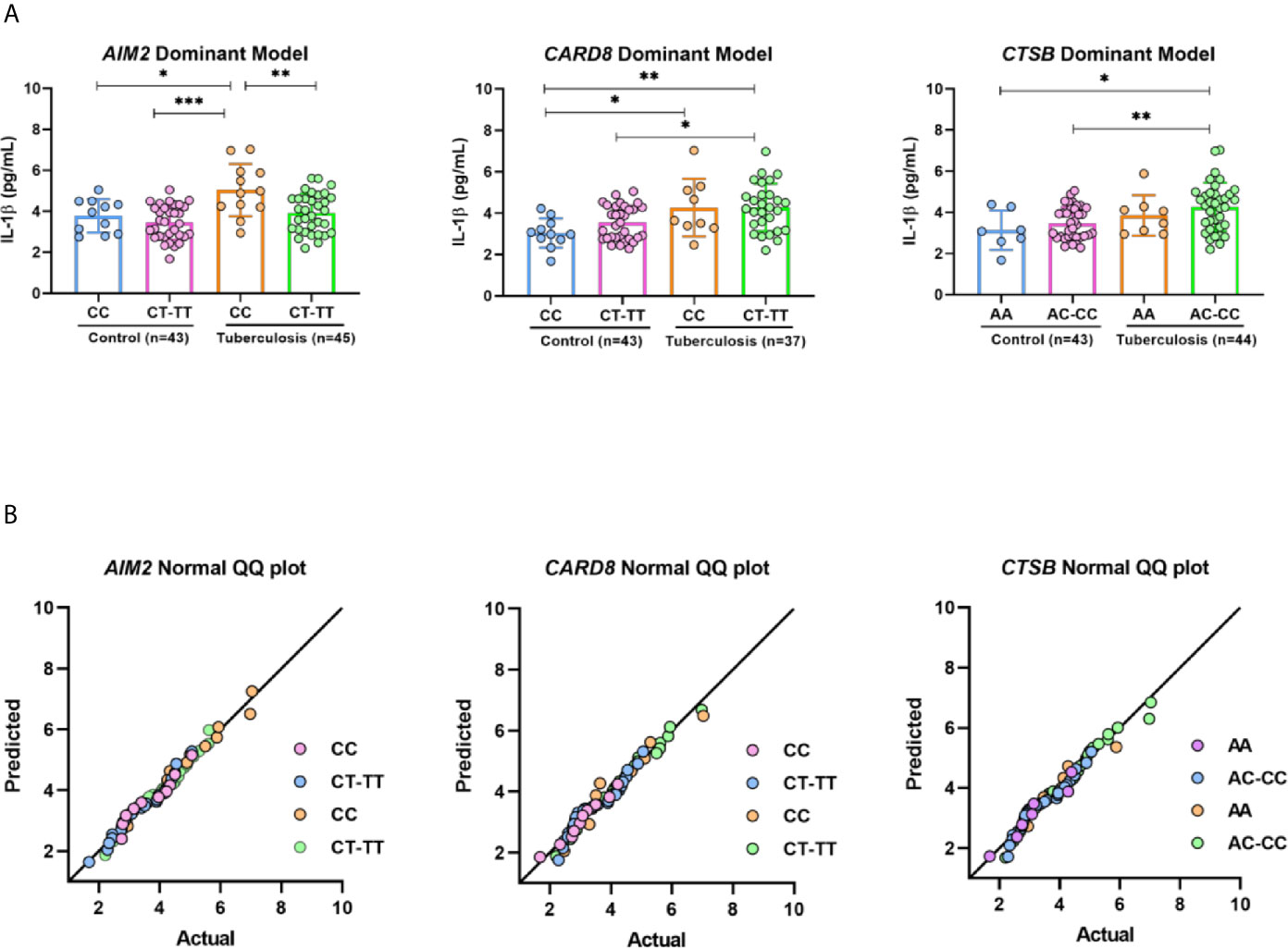
Figure 1 IL-1β profile respective on genotypes of AIM2, CARD8 and CTSB from pulmonary tuberculosis patients and control. (A) Serum concentrations of IL-1β distributed in AIM2, CARD8 and CTSB dominant model genotypes. (B) Graphic Normal QQ plot for three SNV studied. Groups were further compared with each other using ANOVA test with Tukey’s multiple comparisons test, *p < 0,05; **p < 0,001; ***p < 0,0001.
Discussion
Genetics and environmental factors are crucial for host immune response against M. tuberculosis. The complex network of mechanisms from early infection to development of TB remains poorly understood. Since the identification of function of various inflammasomes, several studies of genetic variants of genes involved in assembling the multiprotein complex of inflammasomes have attempted to determine the reason of susceptibility of some individuals to develop diseases while others remain asymptomatic. In the early stage of infection, innate immune response is essential to ensure the success of control and elimination of bacilli. Subsequently, upon inflammasomes activation, proinflammatory cytokines are released to keep in check the invading pathogen (45). In this context, we have focus on one SNV in the AIM2 inflammasome in tuberculosis together with two other SNVs, one in CARD8 and one in CTSB, that are related to NLRP3 pathway.
Studies regarding human genetic variants of AIM2 are rare in infectious diseases (41, 42, 45). Recently, we investigated another SNV of AIM2 (rs35130877) in 288 patients with PTB and 288 HCs. None of participants had this SNV (41). In this study, we found that SNV of AIM2 (rs1103577) was associated with protection against TB. Interestingly, immunological studies involving mice have demonstrated a protective role of AIM2 against M. tuberculosis infection (19, 51). Inhibition of inflammasomes decreased the survival of M. tuberculosis in mice (37) and suggested that a high level of IL-1β favored the survival of bacteria. Carriers homozygous for C allele have higher IL-1 concentrations compared to T allele carriers, and T allele is associated with protection in our study. Furthermore, modern lineages of MTBC have high multiplication rates, which correlates to high levels of IL-1β (52). However, some individuals are resistant to M. tuberculosis infection despite continuous exposure (53, 54), suggesting sterile clearance potentially due to a reflex of genetic background and immunity of individual.
IL-1β is a proinflammatory cytokine and has been suggested to play key role in host protection against M. tuberculosis infection (55, 56). The protein ESAT of M. tuberculosis is cited as a potent activator of NLRP3\ASC inflammasome to liberate mature IL-1β (9). Patients with PTB also exhibit increased levels of IL-1βcompared to HCs. Patients with PTB also demonstrate higher levels of IL-1β (37, 38, 57), reinforcing an important role in the early innate immune response to control M. tuberculosis infection. We found that the serum concentrations of cytokine IL-1β were correlated according to genotypes CC versus CT-TT (AIM2) among patients with PTB in a dominant model. The T allele (TT +CT) correlated with low level of IL1 while homozygosity for the C allele with high level of IL-1β. The T allele is associated with protection to development of TB while individuals homozygous for C allele with susceptibility. Interestingly, excessive levels of IL-1β has been associated with severe TB and lung damage (57, 58).
NLRP3 and AIM2-inflammasome are suggested to play important role in host-defense against mycobacteria (19, 59). Recently, Souza de Lima et al. (22) have shown that NLRP3/IL-1ß/IL-18 pathway is strongly activated in a cathepsin-dependent form by virulent strain H37Rv and non-virulent BCG strains of M. tuberculosis in human macrophages, in vitro. Interestingly, the response was modulated according to macrophage donor genotype of SNV NLRP3 rs10754558 that correlated to level of IL-1β release (22). Of note, IL-1β is one among key proinflammatory cytokines essential for recruitment of immune cells to the site of M. tuberculosis infection (55). Several studies have demonstrated the role of AIM2-inflamasome in mycobacterial infection. Mice macrophages infected with pathogenic strain of M. bovis led to activation of AIM2-inflammasome and mature IL-1β release (60). Similarly, another study also demonstrated that THP-1 macrophages exposed to rBCG (Recombinant BCG ΔureC::hly) vaccine results in AIM2-inflammasome activation with increased production of IL-1β and IL-18 and autophagy, corroborating the role of AIM2-inflammasome in innate immune response (61).
AIM2-deficient mice infected with Mycobacterium bovis Bacillus Calmette-Guérin (BCG) showed a higher infection burden and developed severe disease due to simultaneous induction of reactive IFN-β and IFN-γ responses compared to wild-type mice (51). The AIM2 inflammasome appears to play a protective role through the induction of IL-1β and negative regulation of type I IFN induction. High production of type I IFNs reduces the IFN-γ response in TB infection (51). Interestingly, we showed that individuals homozygous for C allele had higher level of IL-1β compared to carriers of T allele and T allele is associated with protection to the development of TB. Higher level of IL-1β in the early stage might be important in keeping in check the pathogen but at a later stage might cause tissue damage.
The SNVs of AIM2 (rs1103577), CARD8 (rs2009373) and CTSB (rs1692816) are located in intronic region of respective gene. Although the functional role of these variants has not been elucidated, non-coding regions can affect gene expression resulting in different responses to presence of pathogens and can serve such as genetic markers (62). In future studies, it will be interesting to quantify the AIM2 messenger RNA (mRNA) from macrophages of different genotypes of AIM2 (rs1103577) upon mycobacterial infection. Intronic regions have been highlighted for increasing the expression of mRNA in very diverse organisms (mammals, plants). Thus being an important regulator in the expression of proteins (63–66).
In this study, stratification of patients into PTB and EPTB suggested that CARD8 rs2009373 and CTSB rs1692816 were associated with a lower risk of developing PTB. However, multivariate logistic regression analysis showed that only CTSB rs1692816 was associated with a reduced risk for EPTB. Indeed, we observed that heterozygosity for the variant provides lower risk for development of EPTB. CTSB is a member of cathepsin family localized in lysosomes and cytosol. CTSB promotes the degradation of protein in lysosomes and controls autophagy (67). Furthermore, the inhibition of CTSB blocks M. tuberculosis-induced NLRP3 inflammasome assembly, thereby leading to a decrease in IL-1βrelease, suggesting that the release of lysosomal CTSB and possibly other cathepsins are crucial for activation of NLRP3 to control M. tuberculosis infection (22, 30). Although, the function of this CTSB rs1692816 is not known, we can speculate that heterozygous individuals have an advantage in modulating the NLRP3 inflammasome in the release of IL-1β that is sufficient to keep in check the bacteria in comparison to homozygotes. Indeed, heterozygosity for some genes variant have been shown to provide an advantage to some infectious diseases. Heterozygosity for hemoglobin S is an advantage in Africa against Plasmodium falciparum malaria. Heterozygosity for the MAL/TIRAP variant in the TLRs pathway have been suggested to provide protection against invasive pneumococcal disease, malaria, tuberculosis and chagas disease (68, 69).
The SNV of CARD8 (rs2009373) was not associated with TB in this study. However, other variants of CARD8 (rs6509365 and rs2043211) have been associated with susceptibility to TB (41, 46, 70). CARD8 negatively regulates NLRP3 activation, and CARD8 rs2043211 appears to have a loss of function. Interestingly, NLRP3 (rs35829419)/CARD8 (rs2043211) interaction is associated with levels of IL-1β (71).
Another important aspect is the variation in allele frequencies among populations. We found that some alleles were present in high or low frequencies or were entirely absent in specific populations, thereby indicating different evolutionary histories that are assumed are under selective pressure from prevalent diseases.
The distribution of MAF for rs1103577 of AIM2 (T allele) in HCs (T = 0.47) is comparable to that of a European population (T = 0.40) and different from that of an African population (C = 0.09). The Amazonian population is an admixture of approximately 60% American, 50% European, and 10% African ancestry (72). The MAF of CARD8 rs2009373 (T allele) in our group (T = 0.45) was similar to frequencies of South Asian (T = 0.46), European (T = 0.47), American (T = 0.41), and African (0.50) populations, but different from those of West Asian populations (C = 0.23). For rs1692816 of CTSB, both alleles A and C are frequent in different populations. The frequency of CTSB rs1692816 C allele was 0.49 in our population, compared to African (0.56), American (0.59), and European (0.66) populations. However, the frequency of the rs1692816 C allele was similar to that of East (0.50) and South (0.53) Asian populations. The frequencies of the different alleles were extracted from http://www.ensembl.org/. These frequencies indicate the importance of careful selection of cases and controls from same homogeneous population to avoid spurious associations.
The present study had some limitations. First, the sample size of the cases was small, especially for patients with EPTB. Second, we could not assay IL-1β in patients with EPTB due to a lack of biological samples. Additionally, the NLRP3 data deviated from the HWE in both cases with TB and HCs; thus, they were excluded.
This result corroborates previous studies carried out by several researchers, both in an experimental model and in human cells infected by mycobacteria, which suggest the critical role of AIM2 in tuberculosis. We suggested AIM2 rs1103577 was found to be associated with a lower risk of developing PTB and genotypes CC for AIM2 rs1103577 patients with PTB demonstrate higher levels of IL-1β. The CARD8 and CTSB genes were also found to be important targets for TB, and other genetic variants should be investigated. Further studies are needed to confirm the association in other populations. Finally, it is indisputable that tuberculosis is a complex disease with a strong genetic link.
Data Availability Statement
The datasets presented in this study can be found in online repositories. The names of the repository/repositories and accession number(s) can be found in the article/Supplementary Material.
Ethics Statement
The studies involving human participants were reviewed and approved by The Human Research Ethics Committee of the Federal University of Amazonas (N°. CAAE: 57978916.3.0000.5020, August 17th, 2016). The patients/participants provided their written informed consent to participate in this study.
Author Contributions
MBAF: realized the experiments, analyzed the data and wrote the paper. DL: analyzed the data and intellectual contribution. AB: analyzed the data and intellectual contribution. MGNF: Sample processing and intellectual contribution. IA: Contributed with samples and patient selection and intellectual contribution. JM: Contributed with samples and patient selection and intellectual contribution and intellectual contribution. TR: Sample processing and intellectual contribution. LF: Sample processing and intellectual contribution. AG: Sample processing and intellectual contribution. AC: intellectual contribution. RR: wrote the paper and intellectual contribution. AP: analyzed the data and intellectual contribution. MO: Sample processing, understanding of genotyping assays and intellectual contribution. AS: Analyzed the data, wrote the paper and intellectual contribution. All authors contributed to the article and approved the submitted version.
Funding
This work was supported by FAPEAM (Edital PPSUS, Processo 062.00663/2014).
Conflict of Interest
The authors declare that the research was conducted in the absence of any commercial or financial relationships that could be construed as a potential conflict of interest.
Acknowledgments
We thank all subjects involved in the study, incluing pacients and to the staff of Policlínica Cardoso Fontes, Manaus, Amazonas, Brazil; Programa de Apoio à Pós-Graduação-Coordenação de Aperfeiçoamento de Pessoal de Nível Superior (PROAP/CAPES) and Fundação de Amparo à Pesquisa do Estado do Amazonas (FAPEAM).
Supplementary Material
The Supplementary Material for this article can be found online at: https://www.frontiersin.org/articles/10.3389/fimmu.2021.604975/full#supplementary-material
References
2. Pai M, Behr M, Dowdy D, Dheda K, Divangahi M, Boehme CC, et al. Tuberculosis. Nat Rev Dis Primers (2016) 2:16076. doi: 10.1038/nrdp.2016.76
3. World Health Organization. Global Tuberculosis Report 2019 (2019). Geneva: World Health Organization. Available at: https://apps.who.int/iris/bitstream/handle/10665/329368/9789241565714-eng.pdf?ua (Accessed January 02, 2020).
4. Paramel GV, Sirsjö A, Fransén K. Role of genetic alterations in the NLRP3 and CARD8 genes in health and disease. Mediators Inflamm (2015) 2015:10. doi: 10.1155/2015/846782
5. Cadena AM, Fortune SM, Flynn JL. Heterogeneity in tuberculosis. Nat Rev Immunol (2017) 17:11. doi: 10.1038/nri.2017.69
6. Torrelles JB, Schlesinger LS. Integrating Lung Physiology, Immunology, and Tuberculosis. Trends Microbiol (2017) 25:8. doi: 10.1016/j.tim.2017.03.007
7. Cardona P-J. Pathogenesis of tuberculosis and other mycobacteriosis. Enferm Infecc Microbiol Clin (2018) 36:1. doi: 10.1016/j.eimce.2017.10.009
8. Batista LAF, Silva KJS, Silva LMC, Moura YF, Zucchi FCR. Tuberculosis: A granulomatous disease mediated by epigenetic factors. Tuberculosis (2020) 123:101943. doi: 10.1016/j.tube.2020.101943
9. Mishra BB, Moura-alves P, Sonawane A, Hacohen N, Griffiths G, Moita LF, et al. Mycobacterium tuberculosis protein ESAT-6 is a potent activator of the NLRP3/ASC inflammasome. Cell Microbiol (2010) 12:8. doi: 10.1111/j.1462-5822.2010.01450.x
10. Abdallah AM, Bestebroer J, Savage NDL, Punder K, Zon VM, Wilson L, et al. Mycobacterial secretion systems ESX-1 and ESX-5 play distinct roles in host cell death and inflammasome activation. J Immunol (2011) 187:9. doi: 10.4049/jimmunol.1101457
11. Franchi L, Muñoz-Planillo R, Núñez G. Sensing and reacting to microbes through the inflammasomes. Nat Immunol (2012) 13:4. doi: 10.1038/ni.2231
12. Martinon F, Burns K, Tschopp J. The Inflammasome: A molecular platform triggering activation of inflammatory caspases and processing of proIL-β. Mol Cell (2002) 10:2. doi: 10.1016/S1097-2765(02)00599-3
13. Strowig T, Henao-Mejia J, Elinav E, Flavell R. Inflammasomes in health and disease. Nature (2012) 481:7381. doi: 10.1038/nature10759
14. Guo H, Callaway JB, Ting JP-Y. Inflammasomes: mechanism of action, role in disease, and therapeutics. Nat Med (2015) 21:7. doi: 10.1038/nm.3893
15. Wawrocki S, Druszczynska M. Inflammasomes in Mycobacterium tuberculosis - Driven Immunity. Can J Infect Dis Med Microbiol (2017) 2017:2309478. doi: 10.1155/2017/2309478
16. Xue Y, Tuipulotu DE, Tan WH, Kay C, Man SM. Emerging Activators and Regulators of Inflammasomes and Pyroptosis. Trends Immunol (2019) 40:11. doi: 10.1016/j.it.2019.09.005
17. Yu C-H, Moecking J, Geyer M, Masters SL. Mechanisms of NLRP1-Mediated Autoinflammatory Disease in Humans and Mice. J Mol Biol (2018) 430:2. doi: 10.1016/j.jmb.2017.07.012
18. Yang Y, Wang H, Kouadir M, Song H, Shi F. Recent advances in the mechanisms of NLRP3 inflammasome activation and its inhibitors. Cell Death Dis (2019) 10:128. doi: 10.1038/s41419-019-1413-8
19. Saiga H, Kitada S, Shimada Y, Kamiyama N, Okuyama M, Makino M, et al. Critical role of AIM2 in Mycobacterium tuberculosis infection. Int Immunol (2012) 24:10. doi: 10.1093/intimm/dxs062
20. Wassermann R, Gulen MF, Sala C, Perin SG, Lou Y, Rybniker J, et al. Mycobacterium tuberculosis differentially activates cGAS-and inflammasome-dependent intracellular immune responses through ESX-1. Cell Host Microbe (2015) 17:6. doi: 10.1016/j.chom.2015.05.003
21. Xu F, Qi H, Li J, Sun L, Gong J, Chen Y, et al. Mycobacterium tuberculosis infection up-regulates MFN2 expression to promote NLRP3 inflammasome formation. J Biol Chem (2020) 295:51. doi: 10.1074/jbc.RA120.014077
22. Souza de Lima DS, Bomfim CC, Leal VN, Reis EC, Soares JLS, Fernandes FP, et al. Combining Host Genetics and Functional Analysis to Depict Inflammasome Contribution in Tuberculosis Susceptibility and Outcome in Endemic Areas. Front Immunol (2020) 11:550624. doi: 10.3389/fimmu.2020.550624
23. Awad F, Assrawi E, Louvrier C, Jumeau C, Georgin-Lavialle S, Grateau G, et al. Inflammasome biology, molecular pathology and therapeutic implications. Pharmacol Ther (2018) 187:133–49. doi: 10.1016/j.pharmthera.2018.02.011
24. Kesavardhana S, Kanneganti T-D. Mechanisms governing inflammasome activation, assembly and pyroptosis induction. Int Immunol (2017) 29:5. doi: 10.1093/intimm/dxx018
25. Wang B, Yin Q. AIM2 inflammasome activation and regulation: A structural perspective. J Struct Biol (2017) 200:3. doi: 10.1016/j.jsb.2017.08.001
26. Vanaja SK, Rathinam VA, Fitzgerald KA. Mechanisms of inflammasome activation: recent advances and novel insights. Trends Cell Biol (2015) 25:5. doi: 10.1016/j.tcb.2014.12.009
27. Jo EK, Kim J, Shin DM, Sasakawa C. Molecular mechanisms regulating NLRP3 inflammasome activation. Cell Mol Immunol (2016) 13:148159. doi: 10.1038/cmi.2015.95
28. Jin T, Perry A, Jiang J, Smith P, Curry JA, Unterholzner L, et al. Structures of the HIN domain: DNA complexes reveal ligand binding and activation mechanisms of the AIM2 inflammasome and IFI16 receptor. Immunity (2012) 36:4. doi: 10.1016/j.immuni.2012.02.014
29. Mort JS, Buttle DJ. Cathepsin B. Int J Biochem Cell Biol (1997) 29:5. doi: 10.1016/S1357-2725(96)00152-5
30. Amaral EP, Riteau N, Moayeri M, Maier N, Mayer-Barber KD, Pereira RM, et al. Lysosomal Cathepsin Release Is Required for NLRP3-Inflammasome Activation by Mycobacterium tuberculosis in Infected Macrophages. Front Immunol (2018) 9:1427. doi: 10.3389/fimmu.2018.01427
31. Campden RI, Zhang Y. The role of lysosomal cysteine cathepsins in NLRP3 inflammasome activation. Arch Biochem Biophys (2019) 670:32–42. doi: 10.1016/j.abb.2019.02.015
32. Razmara M, Srinivasula SM, Wang L, Poyet JL, Geddes BJ, DiStefano PS, et al. CARD-8 protein, a new CARD family member that regulates caspase-1 activation and apoptosis. J Biol Chem (2002) 277(16):13952–8. doi: 10.1074/jbc.M107811200
33. Ito S, Hara Y, Kubota T. CARD8 is a negative regulator for NLRP3 inflammasome, but mutant NLRP3 in cryopyrin-associated periodic syndromes escapes the restriction. Arthritis Res Ther (2014) 16:1. doi: 10.1186/ar4483
34. Abdalla H, Srinivasan L, Shah S, Mayer-Barber KD, Sher A, Sutterwala FS, et al. Mycobacterium tuberculosis infection of dendritic cells leads to partially caspase-1/11-independent IL-1β and IL-18 secretion but not to pyroptosis. PloS One (2012) 7:7. doi: 10.1371/journal.pone.0040722
35. Dorhoi A, Nouailles G, Jörg S, Hagens K, Heinemann E, Pradl L, et al. Activation of the NLRP3 inflammasome by Mycobacterium tuberculosis is uncoupled from susceptibility to active tuberculosis. Eur J Immunol (2012) 42:2. doi: 10.1002/eji.201141548
36. Shah S, Bohsali A, Ahlbrand SE, Srinivasan L, Rathinam VK, Vogel SN, et al. Cutting edge: Mycobacterium tuberculosis but not nonvirulent mycobacteria inhibits IFN-β and AIM2 inflammasome-dependent IL-1β production via its ESX-1 secretion system. J Immunol (2013) 191:7. doi: 10.4049/jimmunol.1301331
37. Subbarao S, Sanchez-garrido J, Kri N, Shenoy AR, Robert BD. Genetic and pharmacological inhibition of inflammasomes reduces the survival of Mycobacterium tuberculosis strains in macrophages. Sci Rep (2020) 10:3709. doi: 10.1038/s41598-020-60560-y
38. Mayer-Barber KD, Barber DL, Shenderov K, White SD, Wilson MS, Cheever A, et al. Cutting Edge: Caspase-1 Independent IL-1 Production Is Critical for Host Resistance to Mycobacterium tuberculosis and Does Not Require TLR Signaling In Vivo. J Immunol (2010) 184:7. doi: 10.4049/jimmunol.0904189
39. Briken V, Ahlbrand SE, Shah S. Mycobacterium tuberculosis and the host cell inflammasome: a complex relationship. Front Cell Infect Microbiol (2013) 3:62. doi: 10.3389/fcimb.2013.00062
40. Eklund D, Welin A, Andersson H, Verma D, Söderkvist P, Stendahl O, et al. Human gene variants linked to enhanced NLRP3 activity limit intramacrophage growth of mycobacterium tuberculosis. J Infect Dis (2014) 209:5. doi: 10.1093/infdis/jit572
41. Souza de Lima D, Ogusku MM, Sadahiro A, Pontillo A. Inflammasome genetics contributes to the development and control of active pulmonary tuberculosis. Infect Genet Evol (2016) 41:240–4. doi: 10.1016/j.meegid.2016.04.015
42. Liu C-W, Lin C-J, Hu H-C, Liu H-J, Chiu Y-C, Lee S-W, et al. The association of inflammasome and TLR2 gene polymorphisms with susceptibility to tuberculosis in the Han Taiwanese population. Sci Rep (2020) 10:10184. doi: 10.1038/s41598-020-67299-6
43. Souza de Lima D, Leal VNC, Ogusku MM, Sadahiro A, Pontillo A, Alencar BC. Polymorphisms in SIGLEC1 contribute to susceptibility to pulmonary active tuberculosis possibly through the modulation of IL-1ß. Infect Genet Evol (2017) 55:313–7. doi: 10.1016/j.meegid.2017.09.031
44. Barletta-Naveca RH, Naveca FG, de Almeida VA, Porto JIR, da Silva GAV, Ogusku MM, et al. Toll-like receptor-1 single-nucleotide polymorphism 1805T/G is associated with predisposition to multibacillary tuberculosis. Front Immunol (2018) 9:1455. doi: 10.3389/fimmu.2018.01455
45. Fernandes FP, Leal VNC, Souza de Lima D, Reis EC, Pontillo A. Inflammasome genetics and complex diseases: a comprehensive review. Eur J Hum Genet (2020) 28:13071321. doi: 10.1038/s41431-020-0631-y
46. Abate E, Blomgran R, Verma D, Lerm M, Fredrikson M. Polymorphisms in CARD8 and NLRP3 are associated with extrapulmonary TB and poor clinical outcome in active TB in Ethiopia. Sci Rep (2019) 9:3126. doi: 10.1038/s41598-019-40121-8
47. Brasil. Ministério da Saúde. Secretaria de Vigilância em Saúde. Departamento de Vigilância das Doenças Transmissíveis. Rede de Teste Rápido para Tuberculose no Brasil: primeiro ano da implantação/Ministério da Saúde, Secretaria de Vigilância em Saúde, Departamento de Vigilância das Doenças Transmissíveis (2015). Brasília: Ministério da Saúde. Available at: http://www.saude.gov.br/images/pdf/2016/janeiro/15/rtr-tb-15jan16-isbn-web.pdf (Accessed March 02, 2020).
48. Salem JI, Carvalho CM, Ogusku MM, Maia R. PKO – Alternative method for isolating mycobacteria from sputum. Acta Amazonica (2007) 37:3. doi: 10.1590/S0044-59672007000300013
49. Brasil. Ministério da Saúde Secretaria de Vigilância em Saúde. Departamento de Vigilância das Doenças Transmissíveis. Manual de recomendações para o controle da tuberculose no Brasil / Ministério da Saúde, Secretaria de Vigilância em Saúde, Departamento de Vigilância das Doenças Transmissíveis (2019). Brasília: Ministério da Saúde. Available at: http://bvsms.saude.gov.br/bvs/publicacoes/manual_recomendacoes_controle_tuberculose_brasil_2_ed.pdf (Accessed March 02, 2020).
50. Gustincich S, Manfioletti G, Del Sal G, Schneider C, Carninci P. A fast method for high-quality genomic DNA extraction from whole blood. Biotechniques (1991) 11:3.
51. Yan S, Shen H, Lian Q, Jin W, Zhang R, Lin X, et al. Deficiency of the AIM2-ASC Signal Uncovers the STING-Driven Overreactive Response of Type I IFN and Reciprocal Depression of Protective IFN-γ Immunity in Mycobacterial. J Immunol (2018) 200:3. doi: 10.4049/jimmunol.1701177
52. Romagnoli A, Petruccioli E, Palucci I, Camassa S, Carata E, Petrone L, et al. Clinical isolates of the modern Mycobacterium tuberculosis lineage 4 evade host defense in human macrophages through eluding IL-1β-induced autophagy. Cell Death Dis (2018) 9:624. doi: 10.1038/s41419-018-0640-8
53. Möller M, Kinnear CJ, Orlova M, Kroon EE, Helden PDV, Schurr E, et al. Genetic Resistance to Mycobacterium tuberculosis Infection and Disease. Front Immunol (2018) 9:2219. doi: 10.3389/fimmu.2018.02219
54. Simmons JD, Stein CM, Seshadri C, Campo M, Alter G, Fortune S, et al. Immunological mechanisms of human resistance to persistent Mycobacterium tuberculosis infection. Nat Rev Immunol (2018) 18:9. doi: 10.1038/s41577-018-0025-3
55. Yamada H, Mizumo S, Horai R, Yoichiro I, Sugawara I, et al. Protective Role of Interleukin-1 in Mycobacterial Infection in IL-1 α/β Double-Knockout Mice. Lab Invest (2000) 80(5):759–67. doi: 10.1038/labinvest.3780079
56. Fremond CM, Togbe D, Doz E, Rose S, Vasseur V, Maillet I, et al. IL-1 receptor-mediated signal is an essential component of MyD88-dependent innate response to Mycobacterium tuberculosis infection. J Immunol (2007) 179:2. doi: 10.4049/jimmunol.179.2.1178
57. Mishra BB, Rathinam VAK, Martens GW, Martinot AJ, Kornfeld H, Fitzgerald KA, et al. Nitric oxide controls the immunopathology of tuberculosis by inhibiting NLRP3 inflammasome-dependent processing of IL-1beta. Nat Immunol (2013) 14:1. doi: 10.1038/ni.2474
58. Zhang G, Zhou B, Li S, Yue J, Yang H, Wen Y, et al. Allele-specific induction of IL-1β expression by C/EBPβ and PU. 1 contributes to increased tuberculosis susceptibility. PloS Pathog (2014) 10:10. doi: 10.1371/journal.ppat.1004426
59. Rathinam VAK, Jiang Z, Waggoner SN, Sharma S, Cole LE, Waggoner L, et al. The AIM2 inflammasome is essential for host-defense against cytosolic bacteria and DNA viruses. Nat Immunol (2010) 11:5. doi: 10.1038/ni.1864
60. Yang Y, Zhou X, Kouadir M, Shi F, Ding T, Liu C, et al. The AIM2 inflammasome is involved in macrophage activation during infection with virulent Mycobacterium bovis strain. J Infect Dis (2013) 208:11. doi: 10.1093/infdis/jit347
61. Saiga H, Nieuwenhuizen N, Gengenbacher M, Koehler AB, Schuerer S, Moura-Alves P, et al. The Recombinant BCG Δ ureC:: hly vaccine targets the AIM2 inflammasome to induce autophagy and inflammation. J Infect Dis (2015) 211:11. doi: 10.1093/infdis/jiu675
62. Zou H, Wu LX, Tan L, Shang FF, Zhou HH, et al. Significance of Single-Nucleotide Variants in Long Intergenic Non-protein Coding RNAs. Front Cell Dev Biol (2020) 8:347. doi: 10.3389/fcell.2020.00347
63. Chorev M, Carmel L. The function of introns. Front Genet (2012) 3:55. doi: 10.3389/fgene.2012.00055
64. Gallegos JE, Rose AB. The enduring mystery of intron-mediated enhancement. Plant Sci (2015) 237:8–15. doi: 10.1016/j.plantsci.2015.04.017
65. Shaul O. How introns enhance gene expression. Int J Biochem Cell Biol (2017) 91:145–55. doi: 10.1016/j.biocel.2017.06.016
66. Rigau M, Juan D, Valencia A, Rico D. Intronic CNVs and gene expression variation in human populations. PloS Genet (2019) 15:1. doi: 10.1371/journal.pgen.1007902
67. Man SM, Kanneganti TD. Regulation of lysosomal dynamics and autophagy by CTSB/cathepsin B. Autophagy (2016) 12:12. doi: 10.1080/15548627.2016.1239679
68. Khor CC, Chapman SJ, Vannberg FO, Dunne A, Murphy C, Ling EY, et al. A Mal functional variant is associated with protection against invasive pneumococcal disease, bacteremia, malaria and tuberculosis. Nat Genet (2007) 39:4. doi: 10.1038/ng1976
69. Ramasawmy R, Cunha-Neto E, Fae KC, Borba SCP, Teixeira PC, Ferreira SCP, et al. Heterozygosity for the S180L variant of MAL/TIRAP, a gene expressing an adaptor protein in the Toll-like receptor pathway, is associated with lower risk of developing chronic Chagas cardiomyopathy. J Infect Dis (2009) 199:12. doi: 10.1086/599212
70. Pontillo A, Carvalho MS, Kamada AJ, Moura R, Schindler HC, Duarte AJS, et al. Susceptibility to Mycobacterium tuberculosis infection in HIV-positive patients is associated with CARD8 genetic variant. J Acquir Immune Defic Syndr (2013) 63:2. doi: 10.1097/QAI.0b013e31828f93bb
71. Roberts RL, Van Rij AM, Phillips LV, McCormick SPA, Merriman TR, Jones GT, et al. Interaction of the inflammasome genes CARD8 and NLRP3 in abdominal aortic aneurysms. Atherosclerosis (2011) 218:1. doi: 10.1016/j.atherosclerosis.2011.04.043
Keywords: tuberculosis, inflammasome, SNV, AIM2, CARD8, CTSB
Citation: Figueira MBA, de Lima DS, Boechat AL, Filho MGN, Antunes IA, Matsuda JS, Ribeiro TRA, Felix LS, Gonçalves ASF, da Costa AG, Ramasawmy R, Pontillo A, Ogusku MM and Sadahiro A (2021) Single-Nucleotide Variants in the AIM2 – Absent in Melanoma 2 Gene (rs1103577) Associated With Protection for Tuberculosis. Front. Immunol. 12:604975. doi: 10.3389/fimmu.2021.604975
Received: 10 September 2020; Accepted: 03 March 2021;
Published: 01 April 2021.
Edited by:
Uday Kishore, Brunel University London, United KingdomReviewed by:
Anthony George Tsolaki, Brunel University London, United KingdomYean Kong Yong, Xiamen University, Malaysia
Copyright © 2021 Figueira, de Lima, Boechat, Filho, Antunes, Matsuda, Ribeiro, Felix, Gonçalves, da Costa, Ramasawmy, Pontillo, Ogusku and Sadahiro. This is an open-access article distributed under the terms of the Creative Commons Attribution License (CC BY). The use, distribution or reproduction in other forums is permitted, provided the original author(s) and the copyright owner(s) are credited and that the original publication in this journal is cited, in accordance with accepted academic practice. No use, distribution or reproduction is permitted which does not comply with these terms.
*Correspondence: Mariana Brasil de Andrade Figueira, bWFyaWFuYV9icmFzaWxAaG90bWFpbC5jb20=; Aya Sadahiro, YXNhZGFoaXJvQHVmYW0uZWR1LmJy