- 1State Key Laboratory of Veterinary Etiological Biology, Key Laboratory of Veterinary Parasitology of Gansu Province, Lanzhou Veterinary Research Institute, Chinese Academy of Agricultural Sciences, Lanzhou, China
- 2Qinghai Academy of Animal Science and Veterinary Medicine, Qinghai University, Xining, China
- 3School of Chemical and Biological Engineering, Lanzhou Jiaotong University, Lanzhou, China
- 4National Institute of Parasitic Diseases, Chinese Center for Disease Control and Prevention, Shanghai, China
- 5Key Laboratory of Parasite and Vector Biology, Ministry of Health, Shanghai, China
- 6National Center for International Research on Tropical Diseases, Shanghai, China
- 7World Health Organization Collaborating Center for Tropical Diseases, Shanghai, China
- 8Department of Clinical Oncology, Queen Elizabeth Hospital, Hong Kong, China
- 9Jiangsu Co-innovation Center for Prevention and Control of Important Animal Infectious Diseases and Zoonoses, Yangzhou, China
Echinococcosis, mainly caused by Echinococcus granulosus, is one of the 17 neglected tropical diseases. Extracellular vesicles (EVs) play an essential role in the host–parasite interplay. However, the EVs in the hydatid fluid (HF) of E. granulosus are not fully characterized. Herein, three different types of HF EVs, designated as 2 K, 10 K, and 110 K EVs based on the centrifugal force used, were morphologically identified. A total of 97, 80, and 581 proteins were identified in 2 K, 10 K, and 110 K EVs, respectively, 39 of which were commonly shared. Moreover, 11, 8, and 25 miRNAs were detected, respectively, and all of the 7 selected miRNAs were validated by qPCR to be significantly lower abundant than that in protoscoleces. It was further deemed that 110 K EVs were internalized by sheep peripheral blood mononuclear cells (PBMCs) in a time-dependent manner and thus induced interleukin (IL)-10, tumor necrosis factor-α (TNF-α), and IRF5 were significantly upregulated and IL-1β, IL-17, and CD14 were significantly downregulated (p < 0.05). These data demonstrate the physical discrepancy of three HF EVs and an immunomodulatory effect of 110 K EVs on sheep PMBCs, suggesting a role in immune responses during E. granulosus infection.
Introduction
Echinococcosis, caused by the larval stage of the genus Echinococcus, is listed as one of the neglected tropical diseases by WHO (1, 2). Currently, there are at least eight recognized Echinococcus species, such as Echinococcus granulosus sensu lato, Echinococcus multilocularis, Echinococcus oligathrus, and Echinococcus vogeli (3, 4). Among them, E. granulosus and E. multilocularis are of major public health impact globally and are responsible for cystic echinococcosis (CE) and alveolar echinococcosis (AE), respectively (5–7). It was estimated that the global burdens by CE and AE were 285,500 disability-adjusted life years (DALYs) and 666,434 DALYs, respectively (2, 8). CE is a deleterious zoonosis, which has a great socioeconomic impact on public health and domestic livestock industry (9–12).
In the life cycle, E. granulosus requires two different hosts: an intermediate host (sheep) and a definitive host (dog) (13, 14). The adult resides in the small intestine of a definitive host, and the eggs are excreted with the host's feces into the surrounding environment. When a natural intermediate host incidentally ingests the food or water contaminated by the eggs, oncospheres hatch in the stomach and intestine and, then, pass through the portal and lymphatic vessels to reach the liver, lungs, and other organs through bloodstream circulation. They grow into unilocular cysts (metacestodes or hydatid cysts), which are filled with the hydatid fluid (HF) with a lot of protoscoleces. If a definitive host consumes infected organs or offal, the larva develops into the adult in the intestine and, thus, completes its life cycle (3, 9, 13).
In the HF, different active molecules, such as antigen B and antigen five that induced strong specific immune responses, have been found (10). Apart from these proteins, exosome-like extracellular vesicles (EVs) were also recently reported (7, 14). These EVs serve as a communicator to convey proteins, carbohydrates, lipids, mRNAs, and non-coding RNAs to recipient cells and are involved in numerous biological activities, such as cancer development, metastasis, immunity (15, 16), immunomodulation (17), bone remodeling (18), and others (19–25). EVs also play an essential role in host-parasite interactions by transferring virulence factors and effector molecules from parasites to hosts (26). There are several heterogeneous populations of EVs based on the size (27). Exosomes, a kind of smaller EVs, are phospholipid bilayer membranes, secreted from organelles with a size of 30~200 nm in diameter. Their biogenesis is initiated by inward budding of multivesicular endosomes, followed by the release via the fusion of multivesicular bodies with the plasma membrane (20, 26, 28). Several studies have demonstrated that helminth parasites secrete exosomes that play an essential role during infection (29). For example, Heligmosomoides polygyrus–derived exosomes inhibit innate immunity in vivo by suppressing the expression of the Type 2 cytokines, namely interleukin (IL)-5 and IL-13 (30). Similarly, Toxoplasma gondii–derived exosomes stimulate macrophage activation via the upregulation of pro-inflammatory cytokines (IL-12, IFN-γ, and TNF-α) and also produce partial protective immunity (31). Although exosome-like EVs were described in the E. granulosus HF (10, 14), not much is known about other HF EVs.
Herein, we firstly separated and identified three different EVs from E. granulosus (G1) HF. The protein and miRNA cargoes of all EVs were comparatively defined using high performance liquid chromatography tandem mass spectrometry (HPLC-MS/MS) and high-throughput sequencing, respectively. It was further demonstrated that 110 K EVs were internalized by sheep peripheral blood mononuclear cells (PBMCs) and, thus, induced the ectopic expression of cytokines and the core components in the LPS/TLR4 signaling pathway, providing a clue to further investigate their roles in the interplay between the parasite and the host.
Materials and Methods
Ethics Statement
All animal experiments were assessed and approved by the Animal Ethics Committee of Lanzhou Veterinary Research Institute, Chinese Academy of Agricultural Sciences (LVRI-AX-20180923) and were performed strictly according to the Animal Ethics Procedure and Guidelines of the People's Republic of China.
Parasites and Sample Preparation
Fertile cysts of E. granulosus (G1 genotype) were dissected from the liver of 15 naturally infected sheep slaughtered in an abattoir in the Xinjiang Autonomous Region, China. After aseptic processing, the HF was carefully aspirated with a syringe, mixed, and centrifuged at 300×g for 10 min at 4°C to get rid of protoscoleces and cellular debris. The protoscoleces were recovered and purified by repeated natural sedimentation in sterile phosphate-buffered saline (PBS) thrice at 15 min intervals.
EV Enrichment and Characterization
Different EVs were isolated from the protoscolex-free HF as previously reported with some modifications (Figure 1) (22, 27, 32). First, the HF was sequentially centrifuged at 2,000×g for 30 min and 10,000×g for 60 min at 4°C. Then, the supernatant was filtered using 0.22 μm filters and centrifuged at 110,000 × g for 2 h at 4°C. After each centrifugation, the pellets were washed in filtered PBS and recentrifuged using the same centrifugation force. The resultant pellets were named as 2 K, 10 K, and 110 K EVs based on the centrifugation force used. To determine the size and morphology, three different EVs were resuspended in filtered PBS and identified using ZETASIZER NANO (Malvern) and JEM-2010 transmission electron microscopy (TEM, JEOL, Japan) as previously described (25). The results were derived from three independent experiments.
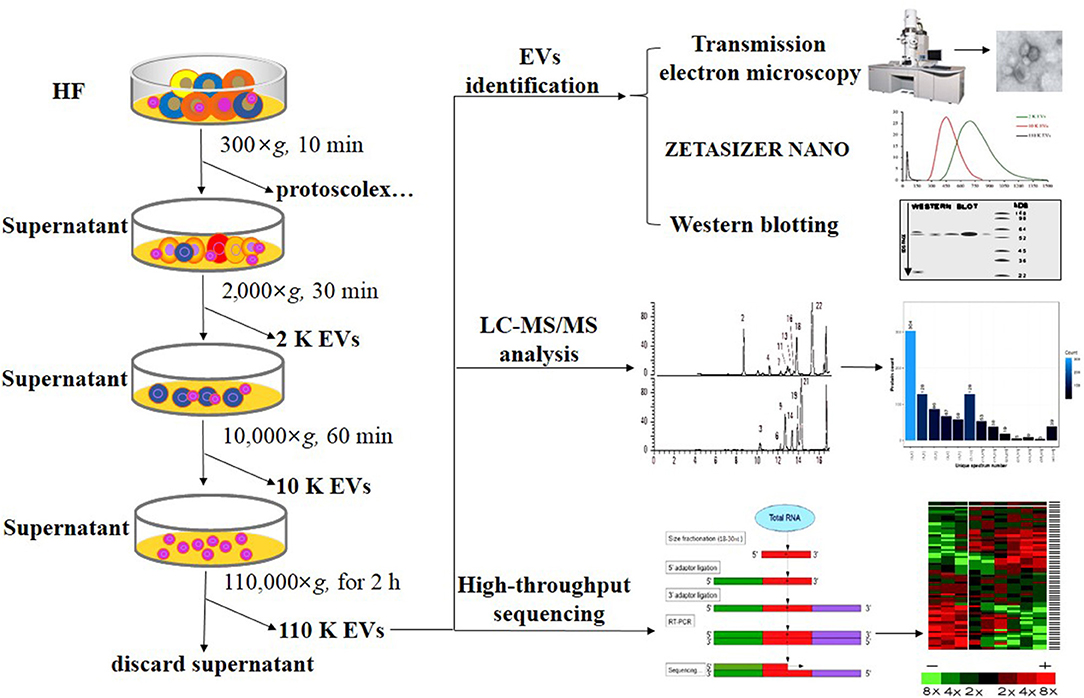
Figure 1. Flow chart of the separation and identification of E. granulosus HF EVs. HF, hydatid fluid; EV, extracellular vesicles.
Proteomic Analysis of EVs Using Mass Spectrometry
The protein cargoes of 2 K, 10 K, and 110 K EVs were defined using LC-MS/MS. To eliminate the host-derived proteins, the data was first mapped against sheep proteins (https://www.ncbi.nlm.nih.gov/genome/?term=sheep), and then, the “clean” data were processed against E. granulosus proteins (https://www.genedb.org/#/species/Egranulosus) with parameters as previously described (25). Only proteins with at least two unique peptides were included for the analysis. The identified proteins were subjected to further analysis with Kyoto Encyclopedia of Genes and Genomes (KEGG) pathways and Gene Ontology (GO) terms as previously described (25).
Protein Preparation and Western Blotting
The soluble crude antigens of E. granulosus were prepared by the following protocol. Briefly, 100 mg of E. granulosus metacestodes were grounded into powder, followed by the addition of 0.5 mL of PBS containing a proteinase inhibitor cocktail (Sigma, USA). Then, the sample was agitated overnight at 4°C and centrifuged at 12,000×g for 15 min at 4°C. Finally, the obtained supernatant was filtered with a 0.22 μm filter and stored at -20°C for later usage.
Total proteins of different EVs were extracted by RIPA Lysis and Extraction Buffer (ThermoFisher Scientific, USA) containing a proteinase inhibitor according to the instructions. Protein concentration was determined using BCA Protein Assay Kit (Solarbio, China). For SDS-PAGE, 30 μg of total proteins per lane were separated by 10% ExpressPlusTM Page Gels (GenScript, China) and transferred to polyvinylidene difluoride (PVDF) membranes. Prior to transfer, the PVDF membrane was activated in methanol for 5 min and then equilibrated in 1× transfer buffer until use. The membranes were blocked with 7.5% defatted milk and then incubated at 4°C overnight with the rabbit anti-14-3-3 antibody and mouse anti-enolase monoclonal antibody (previously prepared in our lab) (33). After being washed in PBST and incubated with the goat anti-rabbit IgG (KPL, Cat No: 5220-0336) or goat anti-mouse IgG (KPL, Cat No: 5220-0341) secondary antibody for 60 min, the membranes were visualized by exposure on x-ray films using Invitrogen Novex ECL HRP Chemiluminescent Substrate Reagent Kit (ThermoFisher Scientific, USA). The results were derived from three independent experiments.
Small RNA Extraction, High-Throughput Sequencing, and Data Analysis
Small RNA extraction from three different EVs was performed according to the instructions of Invitrogen TRIzol LS Reagent (ThermoFisher Scientific, USA) with some modifications. After homogenization and centrifugation, 1 μL of glycogen (10 mg/mL, Invitrogen) was added into RNA-containing aqueous supernatant. Followed by incubation overnight at -20°C and centrifugation, the pellet was washed with 75% ethanol (prepared using nuclease-free water) and resuspended in nuclease-free water. The concentration and quality of the extracted RNA samples were determined using Bioanalyzer (Agilent, USA).
Small RNA sequencing was carried out using RNA-Seq (Quantification) sequencing (BGI, China). The PAGE gel electrophoresis of extracted total RNA was performed to separate different RNA segments. The small RNA fragments with a size between 18 and 30 nt were isolated and purified. The small RNA was ligated with 3' and 5' adapters and then RT-PCR enrichment was performed by StepOnePlus Real-Time PCR system (ABI, USA). The PCR products were further purified by 10% PAGE and then used for deep sequencing. In the data processing, to obtain clean reads, the repetitive sequences, small degraded mRNA, and the reads with poly A, shorter than 18 nt and without the insert tags, were removed from raw data. The clean reads were mapped to the E. granulosus genome (https://parasite.wormbase.org/Echinococcus_granulosus_prjeb121/Info/Index/) by SOAP2 software (BGI). For novel miRNA prediction, the miRDeep2 algorithm and the miReap program were used as previously described (Figure 1) (34, 35).
Comparative Real-Time PCR
Reverse transcription of miRNAs was performed using 1.0 μg of total RNA by All-in-One miRNA First-strand cDNA Synthesis Kit (GeneCopoeia, USA) according to the protocol of the manufacturer. Before reverse transcription, 3.6 μL of cel-39-5p Spike-In Control (1.6 × 108 copies/μL, Qiagen), a Caenorhabditis elegans miRNA, was added as an external reference. The cDNA products were diluted with five volumes of RNase free water, and real-time PCR was immediately performed using an ABI 7500 Thermal Cycler (ThermoFisher Scientific, USA) as follows: 95°C for 10 min, followed by 40 cycles of 95°C for 10 s and 60°C for 1 min. In the experiment, U6 was used as a reference.
For mRNA reverse transcription, RevertAid First Strand cDNA Synthesis Kit (ThermoFisher, USA) was used in strict accordance with the instructions of the manufacturer. About 1.0 μg of total RNA was transcribed with oligo (dT)18, and the cDNA products were diluted by adding eight volumes of RNase-free water for later usage. The real-time PCR reactions were set up using All-in-One qPCR Mix (GeneCopoeia, USA) according to the instructions, and 96-well qPCR reaction plates (ThermoFisher Scientific, USA) were used if applicable. To evaluate the relative expression levels of target genes, β-actin was used as a reference in this experiment.
All specific primers used were designed and synthesized (Table 1), and their specificity was confirmed by analysis of the melting curves. The 2−ΔΔCt formula was used to calculate the relative expression levels of the targets. Each sample was tested in triplicate, and the data for the analysis were from three independent experiments.
Isolation of Sheep PBMCs
About 10 mL of blood was collected from each of the three healthy sheep, and PBMCs were separately isolated by polysucrose-diammonia density gradient centrifugation according to the instructions of the manufacturer (TMD Science, China) (36). The three PBMC samples were washed in three volumes of pre-chilled PBS, and cell viability was checked using trypan blue.
Uptake of 110 K EVs by PBMCs and E. granulosus Protoscolex
Purified 110 K EVs were labeled with PKH26 (Sigma, USA) according to the instructions of the manufacturer. PBMCs were cultured in RPMI-1640 medium supplemented with 10% exosome-free FBS (Gibco, USA). About 3 × 106 cells were seeded into each well in 6-well plates (Corning, USA) and cultured with 100 μg of the labeled EVs per well. About 0, 4, 8, 12, and 24 h after seeding, PBMCs were collected, fixed by 4% formaldehyde, and then washed by PBS. PBMCs were labeled with ActinGreen (Invitrogen, USA) and DAPI (Sigma, USA). The internalization was assessed using laser confocal microscopy (×100) (Leica, Germany) and flow cytometry (Merck, USA), and the internalization rate at different time points was evaluated thrice. For the flow cytometry, 100 μg labeled 110 K EVs were incubated with 3 × 106 PBMCs, and a total of ~10,000 cells were analyzed by Guava easyCyte 6-2L (Merck) at different time points. Furthermore, to detect whether 110 K EVs can be internalized by protoscolex, 100 μg of the labeled EVs was added into 1 × 104 parasites and observed under fluorescent microscopy (Leica, Germany) at 24 h and 48 h after treatment. In parallel, PBS was processed similar to that of 110 K EVs and used as a control. The results were derived from three independent experiments.
Enzyme-Linked Immunosorbent Assay (ELISA)
The levels of tumor necrosis factor-α (TNF–α) in the supernatant of sheep PBMC culture were detected using a Double Antibody Sandwich ELISA Kit (JL, China) according to the instructions of the manufacturer. Briefly, 100 μL of the supernatant and standard were added into each well and incubated for 2 h at 37°C. After wash, 100 μL of biotin-antibody (1×) was added into each well and incubated for 1 h at 37°C, followed by wash and addition of HRP-avidin. After addition of TMB substrate and stop solution, the optical density at 570 nm and 450 nm was recorded using a microplate reader (Bio-Rad, USA). Each sample and standard was set in triplicate.
Statistical Analysis
GraphPad Prism 5 was used for data analysis, and statistical significance was analyzed using a two-tailed unpaired t-test or ANOVA. If the p < 0.05, it was considered significantly different.
Results
Identification of Different HF EVs
Consistent with the size distribution, the results of TEM showed the spherical EVs with different sizes. Of them, 2 K EVs mainly ranged from 531 to 955 nm, 10 K EVs mainly ranged from 342 to 615 nm, and 110 K EVs mainly ranged from 45 to 54 nm (Figures 2A,B). Furthermore, both 14-3-3 and enolase, two exosome biomarkers, were only present in 110 K EVs but not in 2 K and 10 K HF EVs (Figure 2C).
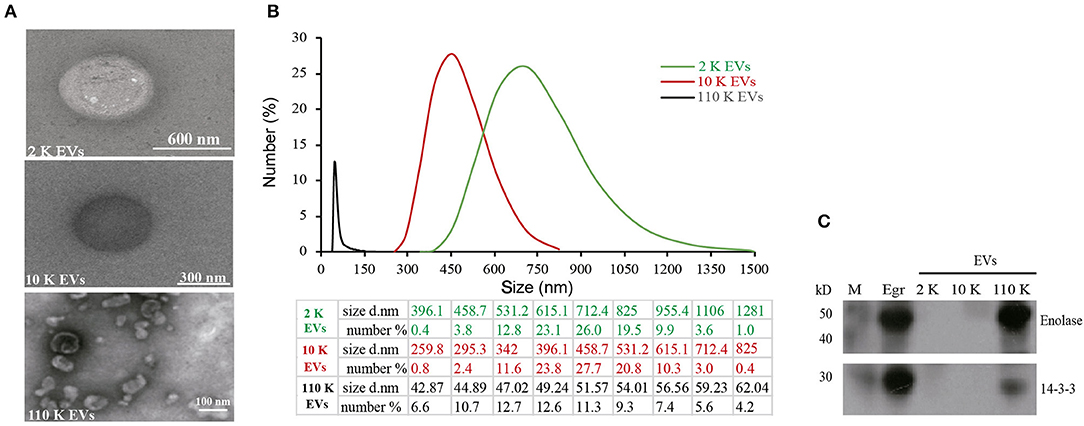
Figure 2. Identification of different E. granulosus HF EVs. (A) Transmission electron microscopy (TEM) of different HF EVs. (B) The size distribution of different HF EVs. (C) Western blotting analysis of enolase and 14-3-3 in different HF EVs. HF, hydatid fluid; EV, extracellular vesicles; M, marker.
Protein Cargoes of Different HF EVs
In total, 97, 80, and 581 proteins were detected from 2 K, 10 K, and 110 K EVs, respectively (Supplementary Tables 1–3). Of them, 15, 8, and 500 proteins were exclusively present in 2 K, 10 K, and 110 K EVs, respectively, and only 39 were commonly shared (Figure 3A). KEGG analysis revealed that the proteins of 10 K and 110 K EVs were predominantly involved in metabolic pathways, up to ~12 and 15% of annotated proteins, respectively (Figure 3B). Moreover, the proteins involved in the lysosome and endocytosis were also abundant in 2 K and 110 K EVs (Figure 3B).
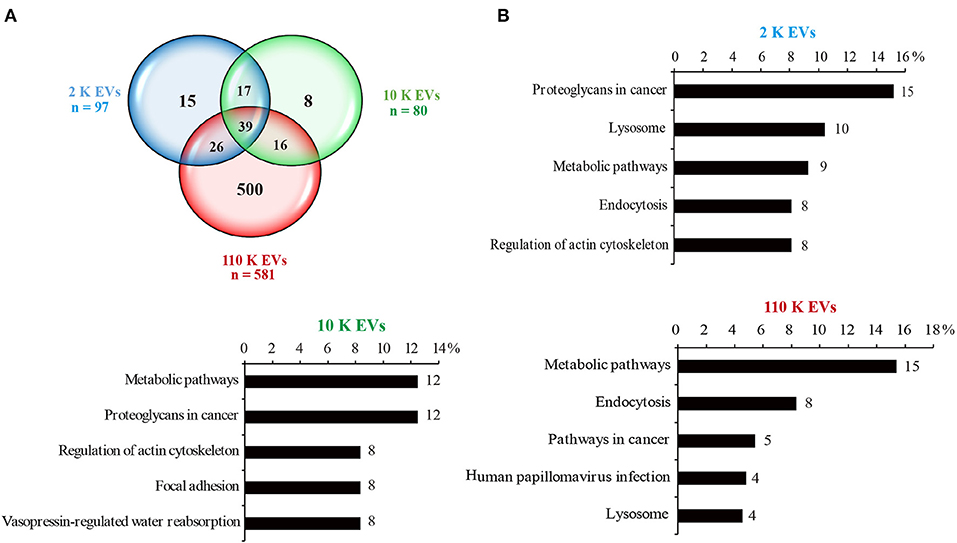
Figure 3. Protein cargoes of different E. granulosus HF EVs. (A) Venn diagram of the protein cargoes in different HF EVs. (B) KEGG analysis of different HF EVs. HF, hydatid fluid; EV, extracellular vesicles; KEGG, Kyoto Encyclopedia of Genes and Genomes.
miRNA Profiles of Different HF EVs
The high-throughput sequencing results showed that the size of miRNA cargoes in three different HF EVs ranged from 19 to 23 nt (Supplementary Table 4). In total, 11, 8, and 25 miRNAs were detected from 2 K, 10 K, and 110 K EVs, respectively. Of them, eight miRNAs were commonly shared (Figure 4A). In 110 K EVs, egr-miR-71 had the highest abundance, followed by egr-let-7 and egr-miR-9. However, some miRNAs abundant in 110 K EVs, such as egr-miR-7, egr-miR-190, and egr-miR-277, were undetectable in 2 K or/and 10 K EVs (Supplementary Table 4).
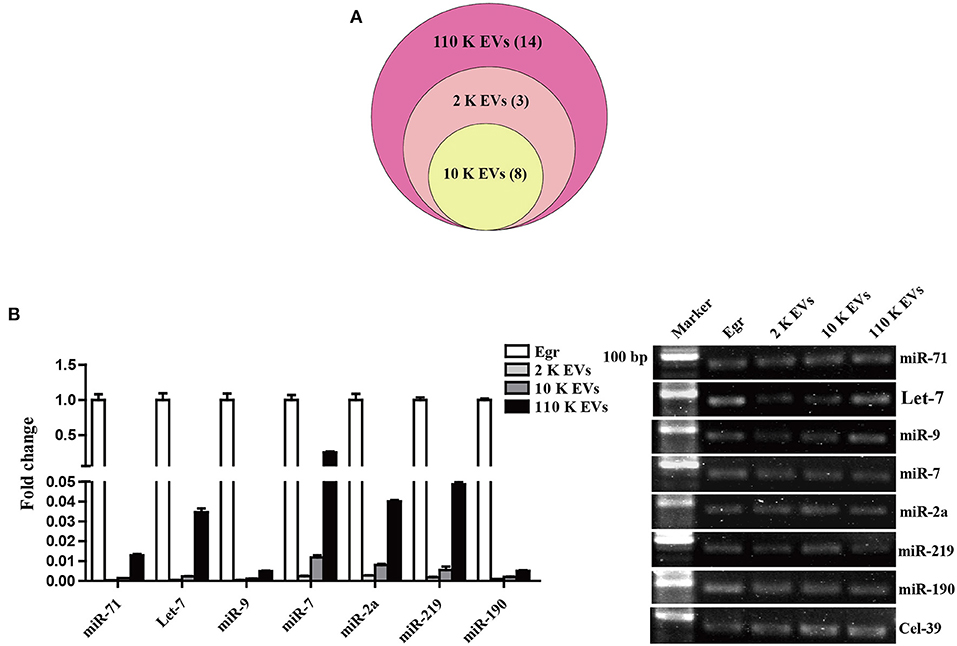
Figure 4. Identification of relevant miRNAs in different E. granulosus HF EVs. (A) Venn diagram of miRNA cargoes in different HF EVs. (B) Validation of the top seven known miRNAs by real-time PCR. E. granulosus protoscoleces (Egr) were used as a control. 20 bp DNA ladder markers were shown on the left. HF, hydatid fluid; EV, extracellular vesicles.
To validate the sequencing data, the relative abundance of the top seven known miRNAs in HF EVs, namely egr-miR-71, egr-let-7, egr-miR-9, egr-miR-7, egr-miR-2a, egr-miR-219, and egr-miR-190, was assessed by real-time PCR. In general, the real-time PCR results were consistent with the sequencing data and confirmed that all miRNAs were the most abundant in 110 K EVs compared with 2 K and 10 K EVs (Figure 4B, Supplementary Table 4). However, except for egr-miR-7, the other six miRNAs were much less abundant in all the three types of HF EVs compared with parasites themselves (Figure 4B).
Regulatory Effects of 110 K HF EVs on Sheep PBMCs
It was confirmed that the 110 K HF EVs were internalized by sheep PBMCs in vitro in a time-dependent manner (Figures 5A,B). PBMCs incubated with PKH26-labeled 110 K HF EVs had bright red fluorescent signals at 12 and 24 h after incubation (Figure 5A), and the internalization rate was over 40% at 24 h after incubation (Figure 5B). To determine whether the 110 K HF EVs are internalized by E. granulosus protoscolex, an internalization assay was also performed, but no fluorescent signals were observed in parasites (data not shown).
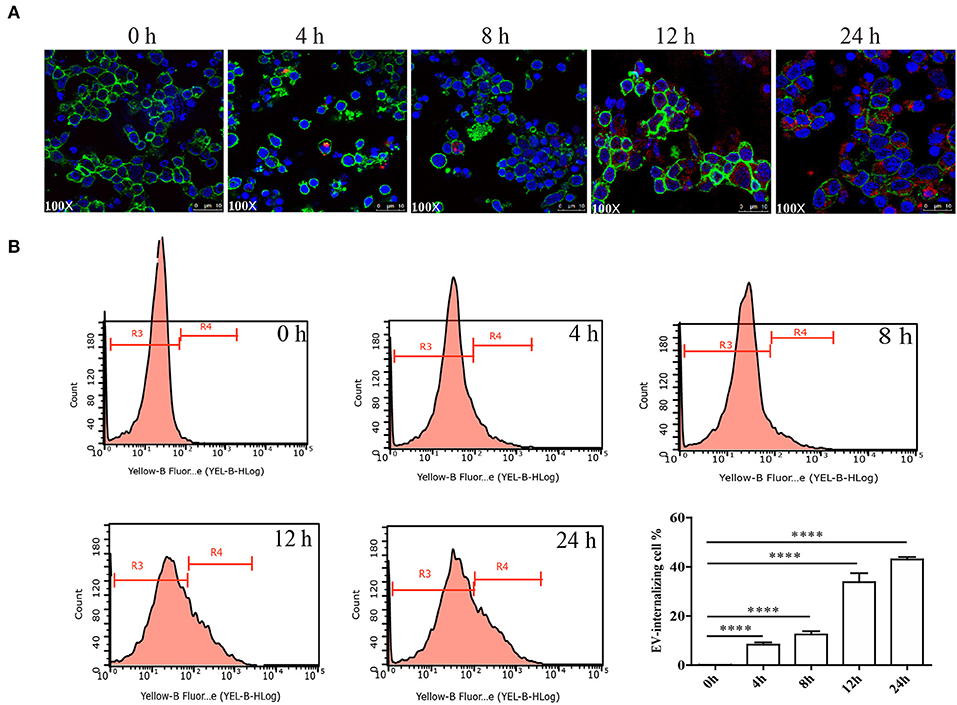
Figure 5. Internalization of E. granulosus 110 K HF EVs by sheep PBMCs. (A) Internalization of 110 K HF EVs by PBMCs. 110 K HF EVs were labeled with PKH26, PBMC was labeled with ActinGreen, and the nucleus was stained by DAPI. (B) Internalization analysis via flow cytometry. ****p < 0.0001. The R3 region represents the proportion of non-EV-internalizing PBMCs, while the R4 represents the proportion of EV-internalizing ones. HF, hydatid fluid; EV, extracellular vesicles; PBMCs, peripheral blood mononuclear cells.
It has been demonstrated that EVs can transfer their own biological factors and effector molecules from parasites to hosts, thereby regulating immune responses (26). To further determine whether the 110 K HF EVs also have immunomodulatory effects on host cells, such as PBMCs, real-time PCR experiments were carried out. The results showed that 110 K EVs induced the significant upregulation of IL-10 and TNF-α (p < 0.05) in treated sheep PBMCs, with IL-17 and IL-1β being significantly downregulated 24 h after treatment (p < 0.05, Figure 6A). Consistently, the result of ELISA verified that TNF-α was significantly increased in the treated PBMCs (p < 0.01, Figure 6B).
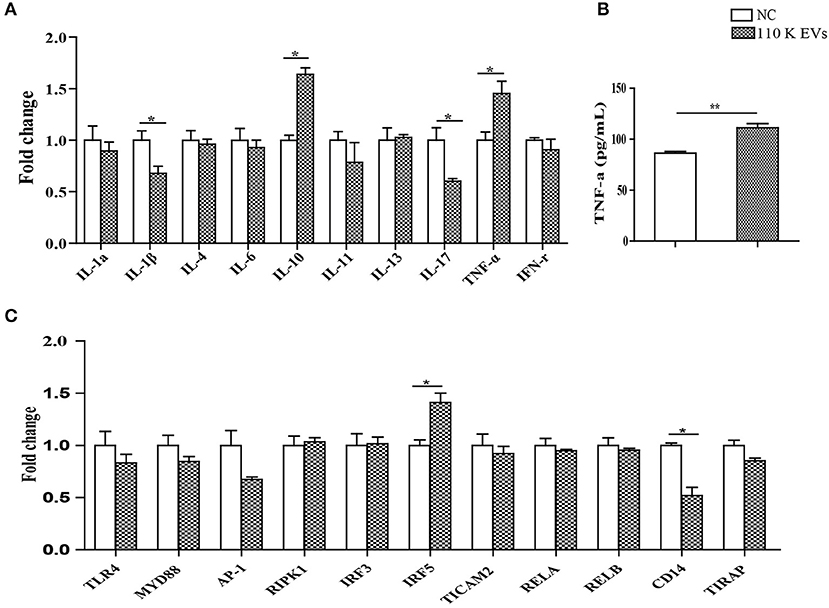
Figure 6. Regulatory effects of 110 K HF EVs on the expression of cytokines and core components in the LPS/TLR4 signaling pathway in sheep PBMCs. (A) qPCR analysis of the expression of cytokines after treatment with 110 K HF EVs. Data are expressed as mean ± s.e.m. Data for the analyses are from three independent experiments, *p < 0.05. (B) Sandwich ELISA analysis of the expression of TNF-α after treatment with 110 K HF EVs. Data are expressed as mean ± s.e.m. Data for the analyses are from three independent experiments, **p < 0.01. (C) qPCR analysis of the expression of the core components in the LPS/TLR4 pathway after treatment with 110 K HF EVs. Data are expressed as mean ± s.e.m. Data for the analyses are from three independent experiments, *p < 0.05. HF, hydatid fluid; EV, extracellular vesicles; PBMCs, peripheral blood mononuclear cells; NC, negative control.
For the core components in the TLR4/LPS pathway, only IRF5 and CD14 were significantly upregulated and downregulated (p < 0.05), respectively, and the rest remained constant after treatment (Figure 6C).
Discussion
Cystic echinococcosis remains a considerable health crisis in many regions across the world, including western China, Central Asia, South America, and eastern Africa (2), partially due to limited understanding of the pathogenesis (37). In this study, we isolated and identified three types of EVs from E. granulosus HF for the first time and found that the diameter of 110 K EVs was from 40 to 150 nm, which was similar to the exosome-like EVs from Trypanosoma brucei (38), Acanthamoeba castellanii (39), and Brugia malayi (40). In accordance to this finding, both enolase and 14-3-3, which are normally used as exosomal biomarkers (25, 32), were detected only in 110 K EVs. Simultaneously, we thoroughly characterized the miRNAs and proteins of different HF EVs and found that some proteins, such as annexin and tetraspanin, were commonly enriched.
Gene Ontology and KEGG analysis showed many proteins being involved in the cellular process and the metabolic process, which was consistent with the results of the proteomic analysis on exosomes derived from the sera of patients infected with E. granulosus and on excretory/secretory (E/S) products of E. granulosus metacestode (14, 41). A number of studies have shown that E/S products have overlapped components with EVs, which are supposed to be a candidate as a biological predictor and biomarker (2, 14, 25, 42). It is envisaged that the full characterization of the HF EVs in the study shed light on the identification of reliable diagnostic biomarkers, which will be useful for management of patients. On the other hand, it was found that 110 K HF EVs had a complex protein composition, especially ones that induce immune protection (43, 44), suggesting a potential for developing a vaccine. For instance, it was reported that the 14-3-3 protein provided 97% protection against E. multilocularis infection in rodents (45). Therefore, the current data are valuable for developing anti-echinococcosis vaccines.
miRNAs can be transported from parasites to host cells and modulate innate immunity by exosome-like vesicles (30). We herein showed that E. granulosus HF EVs contained a specific set of miRNAs, and egr-miR-71 was absolutely predominant in the 110 K EVs. Similarly, miR-71 was also abundant in the exosomes secreted by other parasites, such as Taenia asiatica, Taenia hydatigena, Schistosoma mansoni, and Schistosoma japonicum (32, 35, 46, 47). However, the role of the exosomal miR-71 remains elusive. Recently, E. multilocularis miR-71 was shown to suppress nemo-like kinase, and its overexpression inhibited the production of nitric oxide (NO) by macrophages, suggesting a role in the regulation of immune responses (48, 49). In addition, egr-miR-71 was present in the plasma of patients at the early stage of infection and had a higher level than that of egr-let-7, which suggested that egr-miR-71 might serve as a novel diagnostic biomarker and might monitor hydatidosis at the early stage (50). In future studies, it is imperative to decipher the role of 110 K EV-derived egr-miR-71 during E. granulosus infection.
The secretion of EVs has profound biological effects, in that the encapsulated proteins, lipids, and nucleic acids are biologically functional when EVs are internalized by adjacent and distant cells (51). EVs facilitate the direct extracellular transfer of active molecules in vitro and in vivo (52). 110 K HF EVs were in vitro internalized by sheep PBMCs, suggesting a role in signal transduction between the parasite and the host that regulates the process of E. granulosus infection. In accordance with this idea, internalized 110 K HF EVs induced significant changes in the expression of four cytokines, including IL-1β, IL-10, IL-17, and TNF-α. Similarly, two key components (IRF5 and CD14) in the LPS/TLR4 pathway were also ectopically expressed. It is well-known that IRF5 is involved in the production of inflammatory cytokines such as IL-6 and TNF-α. In the study, IRF5 and TNF-α was significantly upregulated, while IL-6 was still unchanged. This may result from the presence of plenty of different immune mediators in 110 K EVs that synthetically regulate the synthesis of IL-6 via multiple pathways. Although the exact mechanisms behind are unclear, the data suggest a modulatory role of 110 K HF EVs, providing a new direction to investigate the biological functions of 110 K EVs in the parasite–host interplay.
In future, investigating immune responses to 110 K HF EVs in sheep should be of great interest. This will be helpful for us to understand their role in the pathogenesis. In addition, it will also be interesting to pinpoint the role of HF EVs in human patients to study the process of infection and immunity.
Data Availability Statement
The datasets generated in this study have been deposited into the BioProject database (accession number: PRJNA674015).
Ethics Statement
The animal study was reviewed and approved by Animal Ethics Committee of Lanzhou Veterinary Research Institute, Chinese Academy of Agricultural Sciences, China.
Author Contributions
YZhe conceived the study. JY, JW, YF, LY, and YL conducted the experiments. JY, JW, XG, YZha, XW, and YS analyzed the data. JY, YZhe, WCC, and YS wrote the paper. All authors contributed to the article and approved the submitted version.
Funding
This work was financially supported by the National Natural Science Foundation of China (U1703104, 31472185 and 31960708).
Conflict of Interest
The authors declare that the research was conducted in the absence of any commercial or financial relationships that could be construed as a potential conflict of interest.
Supplementary Material
The Supplementary Material for this article can be found online at: https://www.frontiersin.org/articles/10.3389/fimmu.2021.602717/full#supplementary-material
Abbreviations
EVs, extracellular vesicles; HF, hydatid fluid; PBMCs, peripheral blood mononuclear cells; CE, cystic echinococcosis; AE, alveolar echinococcosis; DALYs, disability-adjusted life years; IL, interleukin; LC-MS/MS, liquid chromatography-coupled tandem mass spectrometry; KEGG, Kyoto Encyclopedia of Genes and Genomes; GO, Gene Ontology; TNF-α, tumor necrosis factor-α; NO, nitric oxide.
References
1. Agudelo Higuita NI, Brunetti E, McCloskey C. Cystic echinococcosis. J Clin Microbiol. (2016) 54:518–23. doi: 10.1128/JCM.02420-15
2. Wen H, Vuitton L, Tuxun T, Li J, Vuitton DA, Zhang W, et al. Echinococcosis: advances in the 21st Century. Clin Microbiol Rev. (2019) 32:e00075–18. doi: 10.1128/CMR.00075-18
3. Ahn CS, Kim JG, Han X, Kang I, Kong Y. Comparison of Echinococcus multilocularis and Echinococcus granulosus hydatid fluid proteome provides molecular strategies for specialized host-parasite interactions. Oncotarget. (2017) 8:97009–24. doi: 10.18632/oncotarget.20761
4. Deplazes P, Rinaldi L, Alvarez Rojas CA, Torgerson PR, Harandi MF, Romig T, et al. Global distribution of alveolar and cystic echinococcosis. Adv Parasitol. (2017) 95:315–493. doi: 10.1016/bs.apar.2016.11.001
5. Cucher M, Macchiaroli N, Kamenetzky L, Maldonado L, Brehm K, Rosenzvit MC. High-throughput characterization of Echinococcus spp. metacestode miRNomes. Int J Parasitol. (2015) 45:253–67. doi: 10.1016/j.ijpara.2014.12.003
6. Liu CN, Lou ZZ, Li L, Yan HB, Blair D, Lei MT, et al. Discrimination between E. granulosus sensu stricto, multilocularis E, and E. shiquicus using a multiplex PCR assay. PLoS Negl Trop Dis. (2015) 9:e0004084. doi: 10.1371/journal.pntd.0004084
7. Monteiro KM, Lorenzatto KR, de Lima JC, Dos Santos GB, Forster S, Paludo GP, et al. Comparative proteomics of hydatid fluids from two Echinococcus multilocularis isolates. J Proteomics. (2017) 162:40–51. doi: 10.1016/j.jprot.2017.04.009
8. Torgerson PR, Keller K, Magnotta M, Ragland N. The global burden of alveolar echinococcosis. PLoS Negl Trop Dis. (2010) 4:e722. doi: 10.1371/journal.pntd.0000722
9. Cucher MA, Macchiaroli N, Baldi G, Camicia F, Prada L, Maldonado L, et al. Cystic echinococcosis in South America: systematic review of species and genotypes of Echinococcus granulosus sensu lato in humans and natural domestic hosts. Trop Med Int Health. (2016) 21:166–75. doi: 10.1111/tmi.12647
10. Siles-Lucas M, Sanchez-Ovejero C, Gonzalez-Sanchez M, Gonzalez E, Falcon-Perez JM, Boufana B, et al. Isolation and characterization of exosomes derived from fertile sheep hydatid cysts. Vet Parasitol. (2017) 236:22–33. doi: 10.1016/j.vetpar.2017.01.022
11. Wang B, Jiang Y, Wang Z, Li F, Xing G, Peng X, et al. Arsenic trioxide negatively affects Echinococcus granulosus. Antimicrob Agents Chemother. (2015) 59:6946–51. doi: 10.1128/AAC.04340-14
12. Yin S, Chen X, Zhang J, Xu F, Fang H, Hou J, et al. The effect of Echinococcus granulosus on spleen cells and TGF-beta expression in the peripheral blood of BALB/c mice. Parasite Immunol. (2017) 39:e12415. doi: 10.1111/pim.12415
13. Aziz A, Zhang W, Li J, Loukas A, McManus DP, Mulvenna J. Proteomic characterisation of Echinococcus granulosus hydatid cyst fluid from sheep, cattle and humans. J Proteomics. (2011) 74:1560–72. doi: 10.1016/j.jprot.2011.02.021
14. Santos GB, Monteiro KM, da Silva ED, Battistella ME, Ferreira HB, Zaha A. Excretory/secretory products in the Echinococcus granulosus metacestode: is the intermediate host complacent with infection caused by the larval form of the parasite? Int J Parasitol. (2016) 46:843–56. doi: 10.1016/j.ijpara.2016.07.009
15. Steinbichler TB, Dudas J, Riechelmann H, Skvortsova II. The role of exosomes in cancer metastasis. Semin Cancer Biol. (2017) 44:170–81. doi: 10.1016/j.semcancer.2017.02.006
16. Zhang L, Yu D. Exosomes in cancer development, metastasis, and immunity. Biochim Biophys Acta Rev Cancer. (2019) 1871:455–68. doi: 10.1016/j.bbcan.2019.04.004
17. Whiteside TL. Exosomes carrying immunoinhibitory proteins and their role in cancer. Clin Exp Immunol. (2017) 189:259–67. doi: 10.1111/cei.12974
18. Xie Y, Chen Y, Zhang L, Ge W, Tang P. The roles of bone-derived exosomes and exosomal microRNAs in regulating bone remodelling. J Cell Mol Med. (2017) 21:1033–41. doi: 10.1111/jcmm.13039
19. Atayde VD, Hassani K, da Silva Lira Filho A, Borges AR, Adhikari A, Martel C, et al. Leishmania exosomes and other virulence factors: Impact on innate immune response and macrophage functions. Cell Immunol. (2016) 309:7–18. doi: 10.1016/j.cellimm.2016.07.013
20. Coakley G, Maizels RM, Buck AH. Exosomes and other extracellular vesicles: the new communicators in parasite infections. Trends Parasitol. (2015) 31:477–89. doi: 10.1016/j.pt.2015.06.009
21. Eichenberger RM, Sotillo J, Loukas A. Immunobiology of parasitic worm extracellular vesicles. Immunol Cell Biol. (2018) 96:704–13. doi: 10.1111/imcb.12171
22. Eliaz D, Kannan S, Shaked H, Arvatz G, Tkacz ID, Binder L, et al. Exosome secretion affects social motility in Trypanosoma brucei. PLoS Pathog. (2017) 13:e1006245. doi: 10.1371/journal.ppat.1006245
23. Riaz F, Cheng G. Exosome-like vesicles of helminths: implication of pathogenesis and vaccine development. Ann Transl Med. (2017) 5:175. doi: 10.21037/atm.2017.03.45
24. Tkach M, Thery C. Communication by extracellular vesicles: where we are and where we need to go. Cell. (2016) 164:1226–32. doi: 10.1016/j.cell.2016.01.043
25. Zheng Y, Guo X, Su M, Guo A, Ding J, Yang J, et al. Regulatory effects of Echinococcus multilocularis extracellular vesicles on RAW264.7 macrophages. Vet Parasitol. (2017) 235:29–36. doi: 10.1016/j.vetpar.2017.01.012
26. Wu Z, Wang L, Li J, Wang L, Wu Z, Sun X. Extracellular vesicle-mediated communication within host-parasite interactions. Front Immunol. (2018) 9:3066. doi: 10.3389/fimmu.2018.03066
27. Kowal J, Arras G, Colombo M, Jouve M, Morath JP, Primdal-Bengtson B, et al. Proteomic comparison defines novel markers to characterize heterogeneous populations of extracellular vesicle subtypes. Proc Natl Acad Sci USA. (2016) 113:E968–977. doi: 10.1073/pnas.1521230113
28. Pegtel DM, Gould SJ. Exosomes. Annu Rev Biochem. (2019) 88:487–514. doi: 10.1146/annurev-biochem-013118-111902
29. Trelis M, Galiano A, Bolado A, Toledo R, Marcilla A, Bernal D. Subcutaneous injection of exosomes reduces symptom severity and mortality induced by Echinostoma caproni infection in BALB/c mice. Int J Parasitol. (2016) 46:799–808. doi: 10.1016/j.ijpara.2016.07.003
30. Buck AH, Coakley G, Simbari F, McSorley HJ, Quintana JF, Le Bihan T, et al. Exosomes secreted by nematode parasites transfer small RNAs to mammalian cells and modulate innate immunity. Nat Commun. (2014) 5:5488. doi: 10.1038/ncomms6488
31. Li Y, Liu Y, Xiu F, Wang J, Cong H, He S, et al. Characterization of exosomes derived from Toxoplasma gondii and their functions in modulating immune responses. Int J Nanomed. (2018) 13:467–77. doi: 10.2147/IJN.S151110
32. Liang P, Mao L, Zhang S, Guo X, Liu G, Wang L, et al. Identification and molecular characterization of exosome-like vesicles derived from the Taenia asiatica adult worm. Acta Trop. (2019) 198:105036. doi: 10.1016/j.actatropica.2019.05.027
33. Zhang S, Guo A, Zhu X, You Y, Hou J, Wang Q, et al. Identification and functional characterization of alpha-enolase from Taenia pisiformis metacestode. Acta Trop. (2015) 144:31–40. doi: 10.1016/j.actatropica.2015.01.007
34. Friedlander MR, Chen W, Adamidi C, Maaskola J, Einspanier R, Knespel S, et al. Discovering microRNAs from deep sequencing data using miRDeep. Nat Biotechnol. (2008) 26:407–15. doi: 10.1038/nbt1394
35. Wu J, Yang J, He G, Guo X, Kutyrev I, Kandil OM, et al. High-throughput identification of microRNAs in Taenia hydatigena, a cestode threatening livestock breeding industry. Infect Genet Evol. (2019) 75:103985. doi: 10.1016/j.meegid.2019.103985
36. Nefefe T, Liebenberg J, van Kleef M, Steyn HC, Pretorius A. Innate immune transcriptomic evaluation of PBMC isolated from sheep after infection with E. ruminantium Welgevonden strain. Mol Immunol. (2017) 91:238–48. doi: 10.1016/j.molimm.2017.09.018
37. Eckert J, Deplazes P. Biological, epidemiological, and clinical aspects of echinococcosis, a zoonosis of increasing concern. Clin Microbiol Rev. (2004) 17:107–35. doi: 10.1128/CMR.17.1.107-135.2004
38. Szempruch AJ, Sykes SE, Kieft R, Dennison L, Becker AC, Gartrell A, et al. Extracellular vesicles from Trypanosoma brucei mediate virulence factor transfer and cause host anemia. Cell. (2016) 164:246–57. doi: 10.1016/j.cell.2015.11.051
39. Goncalves DS, Ferreira MDS, Liedke SC, Gomes KX, de Oliveira GA, Leao PEL, et al. Extracellular vesicles and vesicle-free secretome of the protozoa Acanthamoeba castellanii under homeostasis and nutritional stress and their damaging potential to host cells. Virulence. (2018) 9:818–36. doi: 10.1080/21505594.2018.1451184
40. Zamanian M, Fraser LM, Agbedanu PN, Harischandra H, Moorhead AR, Day TA, et al. Release of small RNA-containing exosome-like vesicles from the human filarial parasite Brugia malayi. PLoS Negl Trop Dis. (2015) 9:e0004069. doi: 10.1371/journal.pntd.0004069
41. Wang W, Zhou X, Cui F, Shi C, Wang Y, Men Y, et al. Proteomic analysis on exosomes derived from patients' sera infected with Echinococcus granulosus. Korean J Parasitol. (2019) 57:489–97. doi: 10.3347/kjp.2019.57.5.489
42. Cwiklinski K, de la Torre-Escudero E, Trelis M, Bernal D, Dufresne PJ, Brennan GP, et al. The extracellular vesicles of the helminth pathogen, Fasciola hepatica: biogenesis pathways and cargo molecules involved in parasite pathogenesis. Mol Cell Proteomics. (2015) 14:3258–73. doi: 10.1074/mcp.M115.053934
43. Andreu Z, Yanez-Mo M. Tetraspanins in extracellular vesicle formation and function. Front Immunol. (2014) 5:442. doi: 10.3389/fimmu.2014.00442
44. Dang Z, Yagi K, Oku Y, Kouguchi H, Kajino K, Matsumoto J, et al. A pilot study on developing mucosal vaccine against alveolar echinococcosis (AE) using recombinant tetraspanin 3: vaccine efficacy and immunology. PLoS Negl Trop Dis. (2012) 6:e1570. doi: 10.1371/journal.pntd.0001570
45. Nicolao MC, Rodriguez Rodrigues C, Cumino AC. Extracellular vesicles from Echinococcus granulosus larval stage: isolation, characterization and uptake by dendritic cells. PLoS Negl Trop Dis. (2019) 13:e0007032. doi: 10.1371/journal.pntd.0007032
46. Samoil V, Dagenais M, Ganapathy V, Aldridge J, Glebov A, Jardim A, et al. Vesicle-based secretion in schistosomes: analysis of protein and microRNA (miRNA) content of exosome-like vesicles derived from Schistosoma mansoni. Sci Rep. (2018) 8:3286. doi: 10.1038/s41598-018-21587-4
47. Zhu L, Liu J, Dao J, Lu K, Li H, Gu H, et al. Molecular characterization of S. japonicum exosome-like vesicles reveals their regulatory roles in parasite-host interactions. Sci Rep. (2016) 6:25885. doi: 10.1038/srep25885
48. Guo X, Zhang X, Yang J, Jin X, Ding J, Xiang H, et al. Suppression of nemo-like kinase by miR-71 in Echinococcus multilocularis. Exp Parasitol. (2017) 183:1–5. doi: 10.1016/j.exppara.2017.10.004
49. Zheng Y, Guo X, He W, Shao Z, Zhang X, Yang J, et al. Effects of Echinococcus multilocularis miR-71 mimics on murine macrophage RAW264.7 cells. Int Immunopharmacol. (2016) 34:259–62. doi: 10.1016/j.intimp.2016.03.015
50. Alizadeh Z, Mahami-Oskouei M, Spotin A, Kazemi T, Ahmadpour E, Cai P, et al. Parasite-derived microRNAs in plasma as novel promising biomarkers for the early detection of hydatid cyst infection and post-surgery follow-up. Acta Trop. (2020) 202:105255. doi: 10.1016/j.actatropica.2019.105255
51. Szempruch AJ, Dennison L, Kieft R, Harrington JM, Hajduk SL. Sending a message: extracellular vesicles of pathogenic protozoan parasites. Nat Rev Microbiol. (2016) 14:669–75. doi: 10.1038/nrmicro.2016.110
Keywords: Echinococcus granulosus, extracellular vesicles, sheep PMBC, hydatid fluid, 110 K EV
Citation: Yang J, Wu J, Fu Y, Yan L, Li Y, Guo X, Zhang Y, Wang X, Shen Y, Cho WC and Zheng Y (2021) Identification of Different Extracellular Vesicles in the Hydatid Fluid of Echinococcus granulosus and Immunomodulatory Effects of 110 K EVs on Sheep PBMCs. Front. Immunol. 12:602717. doi: 10.3389/fimmu.2021.602717
Received: 04 September 2020; Accepted: 25 January 2021;
Published: 23 February 2021.
Edited by:
Stéphane Ranque, Aix-Marseille Université, FranceReviewed by:
Majid Fasihi Harandi, Kerman University of Medical Sciences, IranChafia Boukoffa Touil-Boukoffa, University of Science and Technology Houari Boumediene, Algeria
Copyright © 2021 Yang, Wu, Fu, Yan, Li, Guo, Zhang, Wang, Shen, Cho and Zheng. This is an open-access article distributed under the terms of the Creative Commons Attribution License (CC BY). The use, distribution or reproduction in other forums is permitted, provided the original author(s) and the copyright owner(s) are credited and that the original publication in this journal is cited, in accordance with accepted academic practice. No use, distribution or reproduction is permitted which does not comply with these terms.
*Correspondence: Yadong Zheng, zhengyadong@caas.cn
†These authors have contributed equally to this work