- 1Key Laboratory of Molecular Animal Nutrition of the Ministry of Education, College of Animal Sciences, Institute of Feed Science, Zhejiang University, Hangzhou, China
- 2School of Life Science and Engineering, Foshan University, Foshan, China
This study aimed to investigate the protective effects of Lactobacillus plantarum 16 (Lac16) and Paenibacillus polymyxa 10 (BSC10) against Clostridium perfringens (Cp) infection in broilers. A total of 720 one-day-old chicks were randomly divided into four groups. The control and Cp group were only fed a basal diet, while the two treatment groups received basal diets supplemented with Lac16 (1 × 108 cfu·kg−1) and BSC10 (1 × 108 cfu·kg−1) for 21 days, respectively. On day 1 and days 14 to 20, birds except those in the control group were challenged with 1 × 108 cfu C. perfringens type A strain once a day. The results showed that both Lac16 and BSC10 could ameliorate intestinal structure damage caused by C. perfringens infection. C. perfringens infection induced apoptosis by increasing the expression of Bax and p53 and decreasing Bcl-2 expression and inflammation evidence by higher levels of IFN-γ, IL-6, IL-1β, iNOS, and IL-10 in the ileum mucosa, and NO production in jejunal mucosa, which was reversed by Lac16 and BSC10 treatment except for IL-1β (P < 0.05). Besides, the two probiotics restored the intestinal microbiota imbalance induced by C. perfringens infection, characterized by the reduced Firmicutes and Proteobacteria and the increased Bacteroidetes at the phyla level and decreased Bacteroides fragilis and Gallibacterium anatis at the genus level. The two probiotics also reversed metabolic pathways of the microbiota in C. perfringens-infected broilers, including B-vitamin biosynthesis, peptidoglycan biosynthesis, and pyruvate fermentation to acetate and lactate II pathway. In conclusion, Lac16 and BSC10 can effectively protect broilers against C. perfringens infection through improved composition and metabolic pathways of the intestinal microbiota, intestinal structure, inflammation, and anti-apoptosis.
Introduction
Necrotic enteritis (NE) caused by Clostridium perfringens (Cp) is a severe gastrointestinal disease responsible for the annual loss of at least $ 6 billion in the poultry industry worldwide (1, 2). The disease usually occurs in two forms, including clinical and subclinical forms. The clinical form is characterized by a sudden rise in flock mortality with no premonitory signs, while the subclinical form is associated with intestinal damage, impaired absorption of nutrients, and poor overall performance in poultry (3). Moreover, as a common foodborne pathogen that affects humans, C. perfringens poses a serious threat to human health via contaminated poultry (4). Antibiotics have been used as the most effective measure to control C. perfringens infection in livestock (5). However, due to the emergence of antibiotic-resistant bacteria and the effect of antibiotics on the microbiome (6), there is an urgent need to find suitable alternatives to reduce the incidence of necrotic enteritis and maintain animal and human health in the post-antibiotics era.
Probiotics are live microorganisms, which when administered in adequate amounts exert their benefits on the host (7). The use of probiotics is a promising measure for the prevention of intestinal diseases, such as colitis (8), inflammatory bowel disease (9), and diarrhea (10). Besides, probiotics can protect the host against pathogen invasion by manipulating the complex gut ecosystems through competitive exclusion, production of antimicrobial compounds, stimulation of the host immune system development, etc (11). Fukata et al. (12) revealed that Lactobacillus acidophilus or Streptococcus faecalis could reduce C. perfringens infection in young chickens. Numerous other studies have also shown that some microorganisms, including Bacillus (13), Lactobacilli (14), Enterococci (15), and yeast (16), could alleviate the severity and damage of NE in chicken via different mechanisms. Lactobacillus johnsonii BS15 was reported to prevent NE by ameliorating lipid metabolism and intestinal microflora (14), while Lactobacillus fermentum 1.2029 regulated the expression levels of cytokines and TLR in chicken (17). Our previous studies also found that Lactobacillus plantarum Lac16 and Paenibacillus polymyxa BSC10 could inhibit the growth and virulence-associated gene expressions of C. perfringens in vitro (unpublished data) and regulate the mRNA expression of cytokines and TLR in chicken macrophage (HD11) cell line infected with C. perfringens (unpublished data). Therefore, the present study aimed to investigate the protective effects of Lac16 and BSC10 against C. perfringens in broilers.
Materials and Methods
Bacterial Strain Preparation
The Lactobacillus plantarum 16 (Lac16) (CCTCC, NO. M2016259) was isolated by our laboratory and preserved in China Center for Type Culture Collection. Paenibacillus polymyxa 10 (BSC10) (CGMCC 1.10711) was obtained from the China General Microbiological Culture Collection Center. Clostridium perfringens (ATCC13124) was purchased from Guangdong Microbial Culture Collection Center.
Probiotics Lac16 and BSC10 were cultured in MRS or Luria–Bertani (LB) broth overnight at 37°C in an anaerobic system or a shaking incubator, respectively. Clostridium perfringens was cultured in Reinforced Clostridium Medium for 24 h under an anaerobic environment. The bacteria were harvested after centrifugation at 4,000 × g for 15 min at 4°C, respectively. After three-time washing with sterile phosphate buffer saline (PBS, pH 7.3), the prepared Lactobacillus and Bacillus powders (1 × 1010 cfu/g) were diluted with starch and added into the basal diet to a final concentration of 108 cfu/kg, respectively. The same amount of starch was added to each group to compensate for the difference in the nutrient composition of the diets.
Chicken Husbandry and Experimental Design
A total of 720 Cobb 500 broiler chicks were purchased from ZhengDa Broilers Development Center of Zhejiang University (Hangzhou, China) and reared in XinXin Broiler Farm (Jiaxing, China). These 1-day-old chicks with similar weights were randomly allocated to four groups with six replicates per group and 30 chickens per replicate. The two probiotic treatment groups received the diets consisting of Lac16 and BSC10 (108 cfu·kg−1 feed) for 21 days, respectively, while the control group and Cp group were only fed the basal diet (Table 1) during the whole trial. On day 1 and days 14 to 20, birds of all groups except the control group were orally challenged with 1 × 108 cfu C. perfringens type A strain once a day. The chickens were exposed to a 24 h lighting device and kept with ambient temperature gradually decreasing from 32°C to 26°C at the rate of 2°C per week. Broilers were provided freshwater and feed ad libitum. The study was carried out according to the guidelines of the Animal Care and Use Committee of Zhejiang University.
Sample Collection and Treatment
At the end of the experimental period, two chickens per cage with a weight close to the average of the group were selected and immediately slaughtered by exsanguination. The intestine was collected by simultaneously washing with cold sterile PBS to remove the attached impurity, and the jejunal mucosa and ileum mucosa were gently scraped. Jejunal samples were diluted with nine-time volumes of sterile ice-cold normal saline (0.9%) based on the sample weight and then homogenized using a hand-held glass homogenizer. The tissue supernatants were collected by centrifuging at 3,500×g for 10 min at 4°C, and the concentration of protein was determined by a BCA protein assay kit according to the manufacturer’s instruction (Pierce, Rockford, IL) and stored at −80°C for further study.
Ileal Morphology and Immunohistochemistry
Approximately 1 cm of distal ileum was dissected for histomorphology analysis. Ileal tissues were fixed (in 4% paraformaldehyde overnight), dehydrated, and embedded in paraffin according to the standard procedure (18). The paraffin-embedded tissues were cut into 5 µm thick and subsequently subjected to hematoxylin and eosin staining and observed by a light microscope (Nikon Eclipse80i, Tokyo, Japan). The immunohistochemistry staining was carried out as described previously with minor modification (18). Briefly, after dewaxing and rehydration, the tissue sections underwent microwave antigen retrieval in sodium citrate buffer (0.01 M, pH 6.0) for 20 min. Endogenous peroxidase activity was blocked with 3% hydrogen peroxide and then using 10% goat serum (ZSGB-BIO, Beijing, China) as a non-specific binding block for 30 min at room temperature. The sections were incubated overnight at 4°C with polyclonal rabbit antibody Bax (BIOSS, Beijing, China) or BCl-2 (BIOSS, Beijing, China). The tissues were incubated with Biotinylated secondary antibodies (Polink-2 plus polymer HRP anti-rabbit or anti-mouse, ZSGB-BIO, Beijing, China) and further visualized with a diaminobenzidine-tetrachloride (DAB) kit (TIANGEN RA110, Beijing, China). All sections were counterstained with hematoxylin for 3 min.
TUNEL Assay
Apoptosis in ileum tissue was analyzed by the terminal dUTP-nick end labeling kit (Roche, Germany). Briefly, the paraffin-embedded tissue sections of ileum were incubated with proteinase K working solution at 37°C for 25 min and washed three times with PBS, and then incubated with permeabilization solution for 20 min. Following three times with PBS, the samples were incubated with TUNEL reaction mixture for 60 min at 37°C in a humidified chamber and strained with DAPI for 10 min and rinsed with PBS for three times. The cell nuclei were identified using UV light microscopy (Nikon, Japan) and TUNEL-positive cells were identified as brilliant green.
RNA Extraction and RT-qPCR
The RNA was extracted using Takara RNAiso Plus Kit (Japan) following the manufacturer’s protocol. The purity and concentration of total RNA were determined using a Nanodrop Spectrophotometer (ND-2000, Thermo Fisher Scientific). The reverse transcription of total RNA was performed by Reverse Transcriptase M-MLV Kit (RNase H-) according to the manufacturer’s instruction. The qRT-PCR assay was conducted with the ABI 7500 fluorescence detection system using SYBR green (Takara, SYBR Premix Ex Taq TM II Kit) detection. Each sample was measured in duplicate. Relative quantitation of all gene expression was calculated using the 2-△△Ct method, and the β-actin served as the internal reference gene (19). Primers used in the current study were designed using the Primer Express 3.0 software (Applied Biosystems, Foster City, CA), and the specificity of primers was assessed by melting curve analysis. Primers are listed in Table 2.
Detection of iNOS Activity and NO Production
The activity of inducible nitric oxide synthase (iNOS) and production of Nitric oxide (NO) in the jejunal mucosa were measured strictly according to the manufacturer’s protocols (Jiancheng Bioengineering Institute, Nanjing, China).
DNA Extraction and Library Construction of Cecum Content
The cecal contents of twelve chickens from four groups (Control, Cp, Cp+Lac16, Cp+BSC10) were collected on day 21. The fecal DNA was extracted using TIANamp DNA Stool Mini Kit (TianGen, Beijing), and the quality was checked by agarose gel electrophoresis. All of the extracted DNA samples were stored at −80°C for further processing.
DNA library was constructed by TruSeq Nano DNA LT Library Preparation Kit (FC-121-4001). DNA was fragmented using dsDNA Fragmentase (NEB, M0348S) by incubating at 37°C for 30 min. Library construction begins with fragmented cDNA. Blunt-end DNA fragments are generated using a combination of fill-in reactions and exonuclease activity, and size selection is performed with provided sample purification beads. An A-base is then added to the blunt ends of each strand, preparing them for ligation to the indexed adapters. Each adapter contains a T-base overhang for ligating the adapter to the A-tailed fragmented DNA. These adapters contain the full complement of sequencing primer hybridization sites for single, paired-end, and indexed reads. Single- or dual-index adapters are ligated to the fragments and the ligated products are amplified with PCR by the following conditions. The initial denaturation was at 95°C for 3 min, 8 cycles of denaturation at 98°C for 15 sec, annealing at 60°C for 15 s, and extension at 72°C for 30 s, and then final extension at 72°C for 5 min.
Metagenomics Analysis of Taxonomic Profiling and Functional Profiling
Raw sequencing reads were processed to obtain valid reads for further analysis. Assessment of sequence quality was used in fastqc. The host genome sequence was removed using the Best Match Tagger (BMTagger), referred to the genome of gga_ref_Gallus_gallus-5.0. Using fastq_to_fasta, the fq format data was converted to fasta format, which further used the filter_fasta.py command in QIIME to remove the host genome sequence. The remaining sequence was regarded as the intestinal flora DNA sequence for further analysis. The species composition was analyzed using MetaPhlAn2 (20), the cladogram was conducted with GraPhlAn, and the heatmap was drawn with metaphlan_hclust_heatmap.py The functional profiling of microbiota was analyzed using HUMAnN2, referred to the uniref90_ec_filtered_diamond database and the uniref50_ec_filtered_diamond database (21).
Principal component analysis of gene family was performed using prompt R package. Heatmap of metabolic pathway abundance was drawn using the fold-change value (log2 transformed) using the pheatmap R package. The Kyoto Encyclopedia of Genes and Genomes (KEGG) pathway was analyzed using the KEGG online service tools’ KEGG mapper.
Statistical Analysis
All data were analyzed by a one-way analysis of variance (ANOVA) followed by Tukey multiple comparisons procedure using SPSS 22.0 software (SPSS Inc., Chicago, IL, USA). Statistical significance was declared at P < 0.05 and trend at P < 0.1. The data were expressed as mean ± SD, and graphs were generated by GraphPad Prism 7.0 software. Statistical analyses and data visualization for fecal microbiota were conducted using the R program (version 3.6.1).
Results
Lac16 and BSC10 Alleviated Intestinal Mucosal Injury Induced by C. perfringens Infection
Hematoxylin–eosin staining showed that the damaged intestinal structure and shorter villus were observed in C. perfringens-infected birds, which were recovered by probiotics treatment (Figure 1A). Besides, the C. perfringens infection significantly increased the expression of intestinal barrier-related genes, such as claudin1 (CLDN1), occludin-1 (ZO-1), and mucin-2 (MUC2) in the ileum (P < 0.05). However, the expression of OCLN in the Cp + Lac16 group was significantly lower compared with the Cp group (Figure 1B).
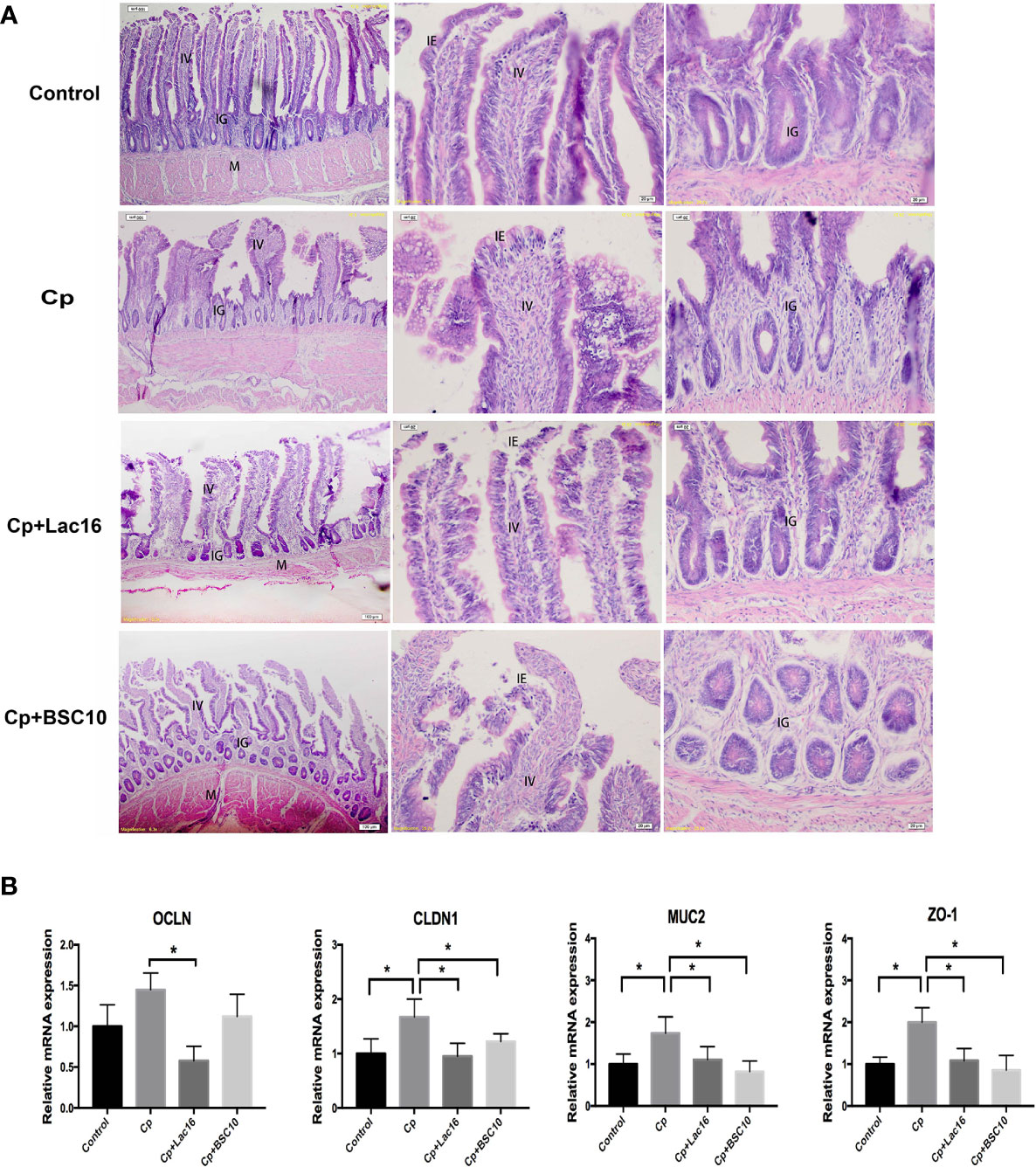
Figure 1 Probiotics Lactobacillus plantarum 16 (Lac16) and Paenibacillus polymyxa 10 (BSC10) alleviated intestinal mucosal injury induced by Clostridium perfringens (Cp) infection. (A) From the left to the right column, hematoxylin–eosin (HE) staining showing the integral morphology of the ileum, the villus, and the grand in the Control, Cp, Cp + Lac16, and Cp + BSC10 groups. Magnification, ×100, ×200, and ×200, respectively. IV, intestinal villus; IE, intestinal epithelium; IG, intestinal glands; M, muscularis. n = 6/group. (B) The mRNA expression of barrier-related genes assessed by RT-PCR. CLDN, Claudin; OCLN1, Occludin 1; ZO-1, Zonula occludins-1; MUC2, Mucin 2. Data were expressed as mean ± SD (n = 12). *indicates statistically significant difference (P < 0.05).
Lac16 and BSC10 Attenuated Apoptosis Induced by C. perfringens Infection
The mRNA expression levels of pro-apoptosis genes in the ileum mucosa, including Bax, p53, Caspase-9 were up-regulated in C. perfringens-infected birds (P < 0.05). However, Lac16 and BSC10 treatment downregulated the expression levels of the pro-apoptosis genes except for Caspase-9 in the Lac16 group (P < 0.05). Moreover, a significant increase in Bcl-2 mRNA level was observed in the Lac16 group compared to the C. perfringens-infected birds (P < 0.05) (Figure 2A). The result of Bax immunohistochemistry demonstrated that compared with the control group, C. perfringens infection increased the number of Bax positive cells, while Lac16 restored them to a normal level. BSC10 also showed a slight decrease in the number of Bax positive cells compared with the Cp group (Figure 2B). C. perfringens infection significantly decreased the number of Bcl-2-positive cells, while probiotic treatment alleviated the decrease (Figure 2C). TUNEL assay results demonstrated that C. perfringens infection enhanced the number of positive cells in the ileum, while there was a decline following Lac16 or BSC10 treatment (Figure 2D).
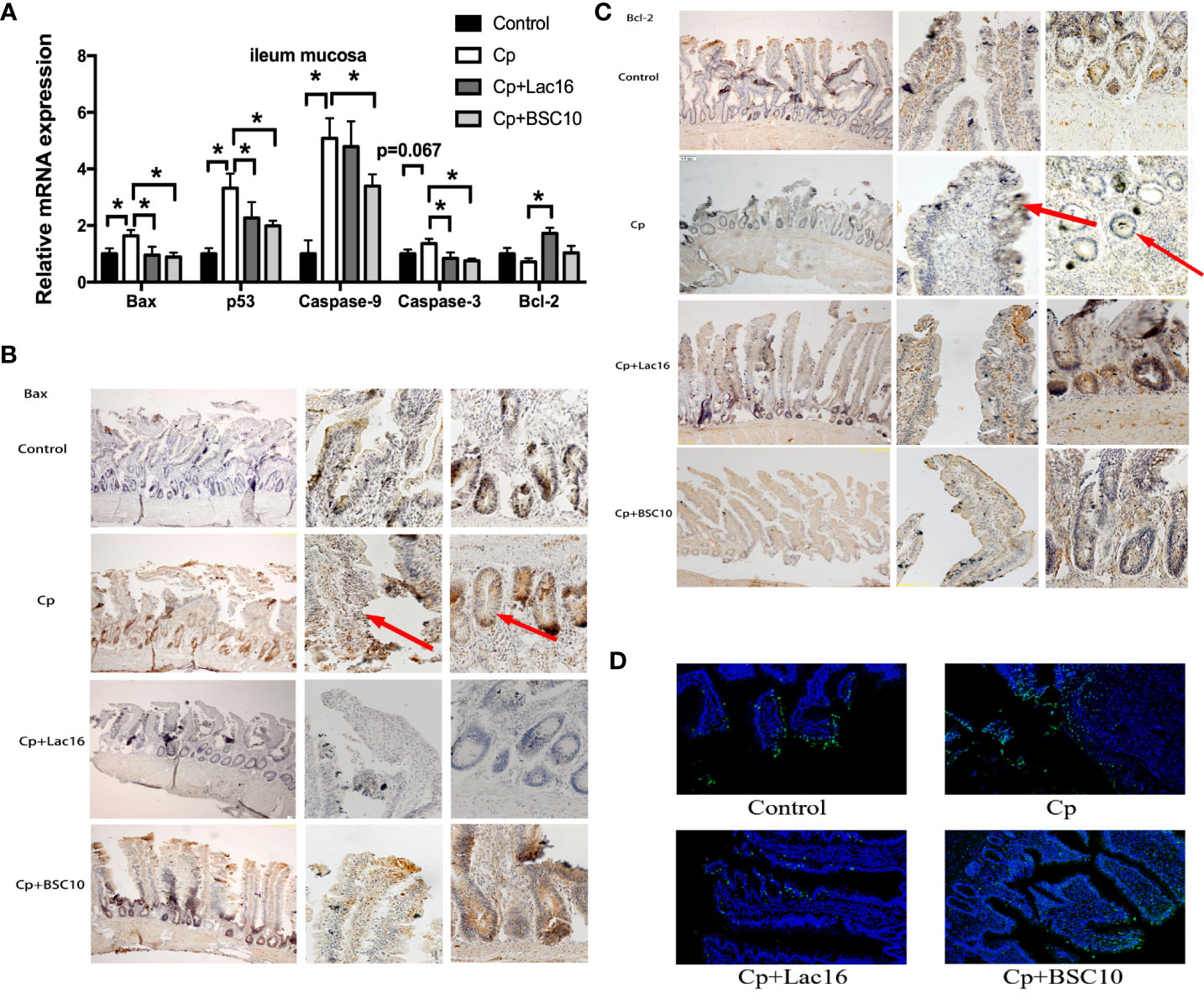
Figure 2 Probiotics treatment attenuated apoptosis induced by Clostridium perfringens (Cp) infection. The apoptosis-related gene expression in the ileum mucosa (A) (n = 12/group). Representative images of the immunohistochemical staining of Bax (B) and Bcl-2 (C) in the ileum mucosa (n = 6/group). The positive cells are stained brown. From the left to the right column, the integral morphology of the ileum, the villus, and the grand are seen (magnification ×100, × 400, and × 400, respectively). (D) TUNEL immunofluorescence data showing the distribution of apoptosis cells (brilliant green) in the ileum mucosa. Magnification, × 200. n = 6/group. * indicates statistically significant difference ( P < 0.05).
Lac16 and BSC10 Down-Regulated C. perfringens-Induced Inflammatory Response
C. perfringens infection increased the expression of anti-inflammatory cytokine IL-10 and pro-inflammatory cytokines, IFN-γ, IL-6, IL-1β, and iNOS (P < 0.05) (Figure 3A), while Lac16 or BSC10 treatment significantly decreased the expression levels of IL-6 (P < 0.05), iNOS (P < 0.05), IFN-γ (P < 0.05), and IL-10 (P < 0.1 and P < 0.05, respectively). Compared with the Cp group, Lac16 supplementation down-regulated TGF-β expression (P < 0.05). Nitric oxide (NO) and inducible nitric oxide synthase (iNOS) actively participated in the host defense in response to C. perfringens infection. As depicted in Figures 3B, C, the highest iNOS activity and NO production in the jejunal mucosa was observed in the Cp group but decreased following Lac16 or BSC10 treatment.
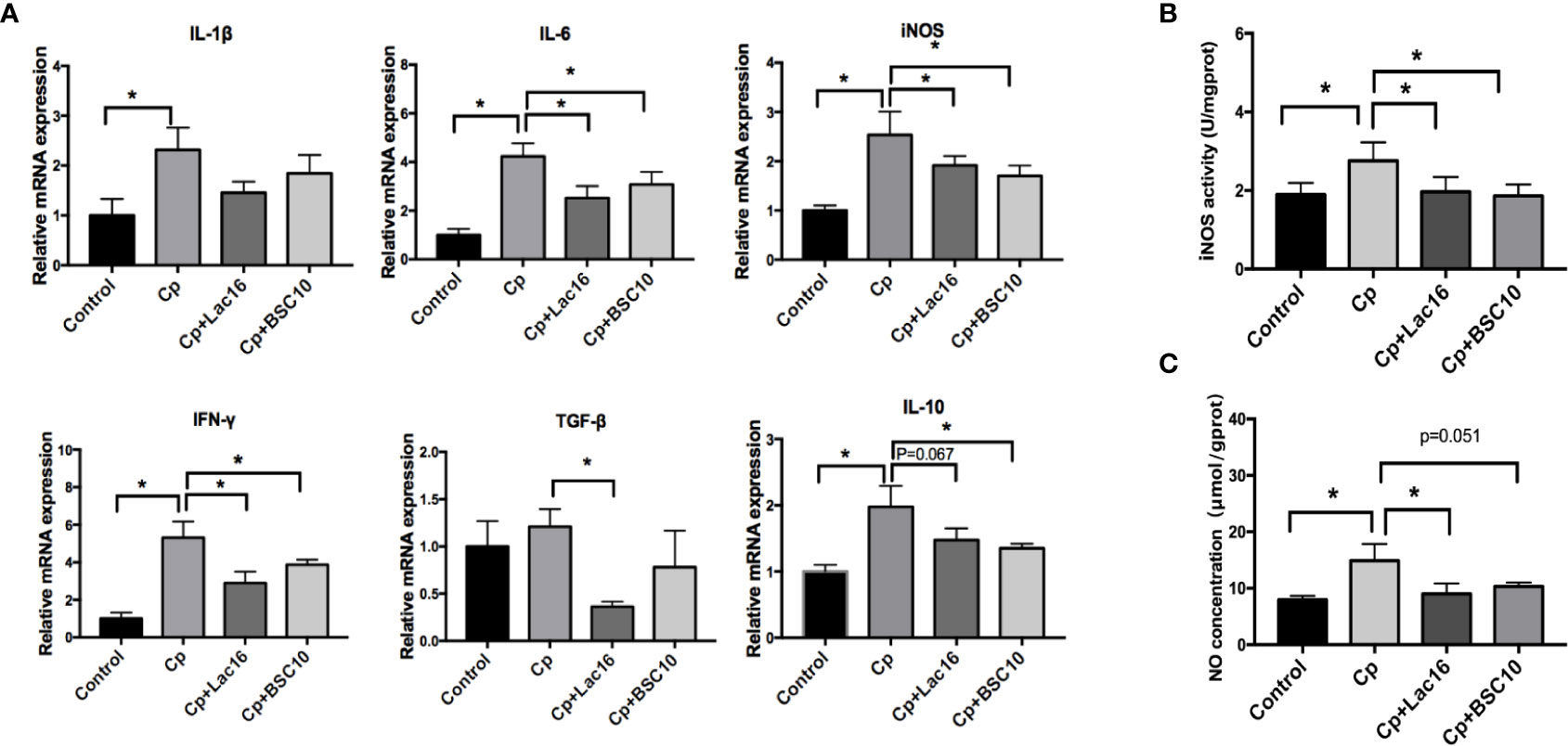
Figure 3 Lactobacillus plantarum 16 (Lac16) and Paenibacillus polymyxa 10 (BSC10) down-regulated the C. perfringens-induced inflammatory response. (A) cytokines gene expression profiles in the ileum mucosa determined by RT-qPCR. The iNOS activity (B) and NO production (C) in jejunal mucosa were measured using commercial kits. Results are presented as mean ± SD (n = 12/group, *represents significant differences (P < 0.05).
Lac16 and BSC10 Re-Shifted the Intestinal Microbiota Composition in Cp-Infected Broilers
As shown in Supplemental Figure S1, phylogenetic analysis revealed the most abundant composition of fecal microbiota at the family, genus, and species levels among all groups. At the family level, Rikenellaceae, Bacteroidaceae, and Oscillospiraceae were dominant. The composition of gut microbiota at the phylum level is shown in Figure 4A. The predominant phyla were Firmicutes, Bacteroidetes, and Proteobacteria, which were more than 99%. C. perfringens infection decreased the relative abundance of Firmicutes and Proteobacteria, and increased Bacteroidetes, while probiotic treatment increased the relative abundance of Firmicutes and decreased Bacteroidetes. At the genus level, the gut microbiota was dominated by genus Allistipes, accounting for 50.41%, followed by Bacteroides and Oscillibacter, accounting for 16.97 and 13.08%, respectively. Lac16 or BSC10 treatment enhanced the relative abundance of Oscillibacter but decreased Allistipes and Bacteroides (Figure 4B). Principal Components Analysis (PCA) showed the different clusters of microbial communities among the four groups, with PC1 accounting for 20.6% of the total variation and PC2 accounting for 15.7% (Figure 4C). Four bacterial species that were enriched in all groups included, Alistipes, Bacteroides, Osciliibacter, and Escherichia (Figure 4D). C. perfringens infection enriched Gallibacterium anatis and those that decreased included Erysipellotrichaceac, Subdoligranulum, Anaerotruncus, Ruminococcus, Pseudoflavonifractor, and Oscillibacter, which were found to be increased in the probiotic treatment groups. The abundance of Bacteroides fragilis was significantly decreased in the Cp + BSC10 group, compared with the Cp and Cp + Lac16 group.
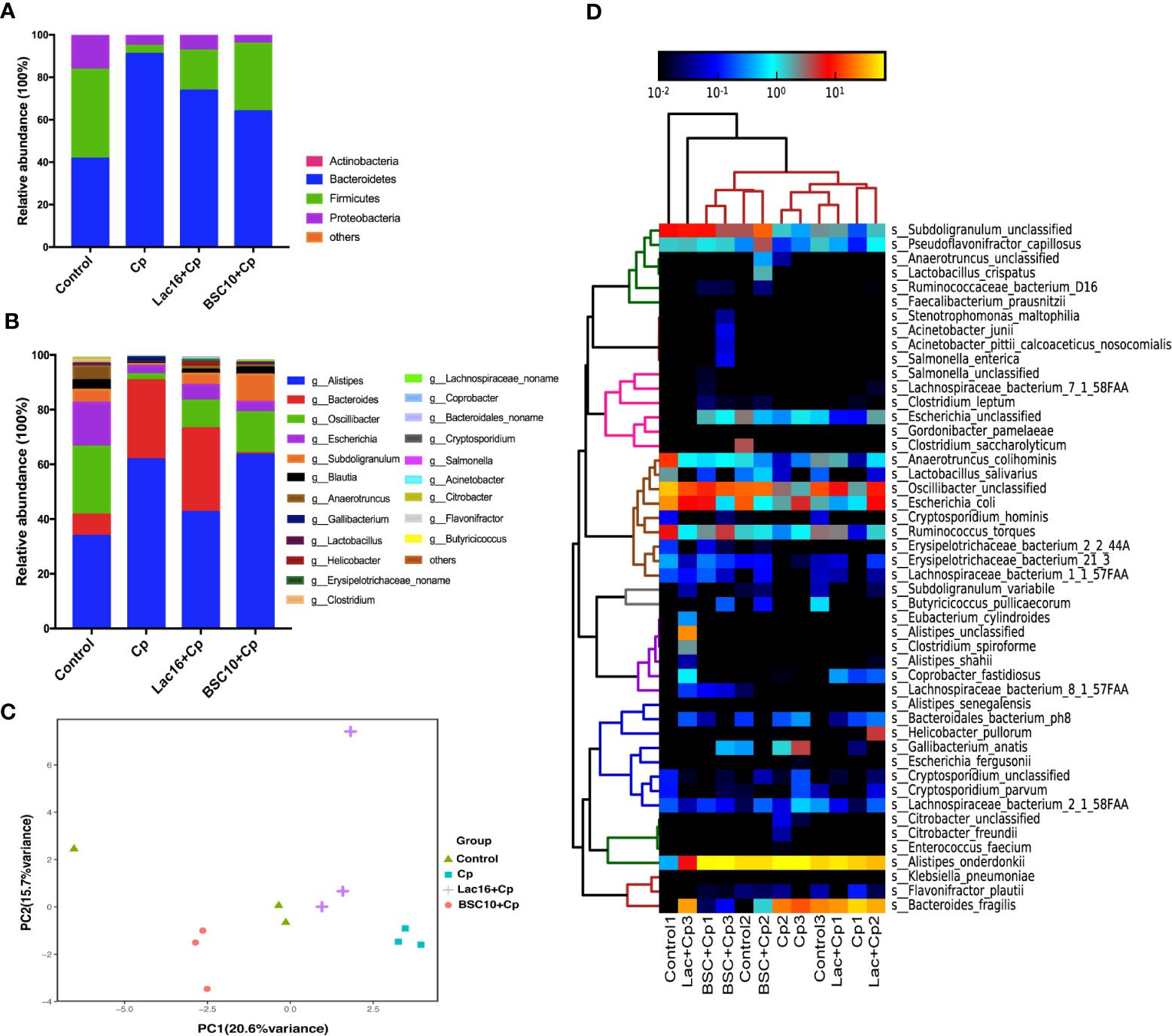
Figure 4 Bacterial taxonomic composition of cecum contents. (A) The relative abundance of bacterial phyla (mean of each group); (B) The top 15 genus abundance (mean of each group); (C) Principal component analysis of the dissimilarity among the microbial samples using prompt R package; (D) Heatmap of species abundance by MetaPhlAn2. n = 3/group.
Functional Capacity of the Gut Microbiome Related to Metabolic Pathways
The functional capacity of the gut microbiome was evaluated using metagenomic sequencing data. We classified the predicted genes by aligning and assigning them to pathways using the HUMAnN2 database. PCA analysis of the gene family abundance revealed dramatic changes between the Cp group and other groups (Supplemental Figure S2). C. perfringens infection changed the microbiota composition and metabolic pathways, including enhancing B-vitamin biosynthesis (for example, folate biosynthesis as shown in Supplemental Figure S3), peptidoglycan biosynthesis (Supplemental Figure S4), amino acid biosynthesis and ribonucleotide biosynthesis, etc, and decreased pyruvate fermentation to acetate and lactate II pathway, which was reversed by probiotic supplementation (Figure 5).
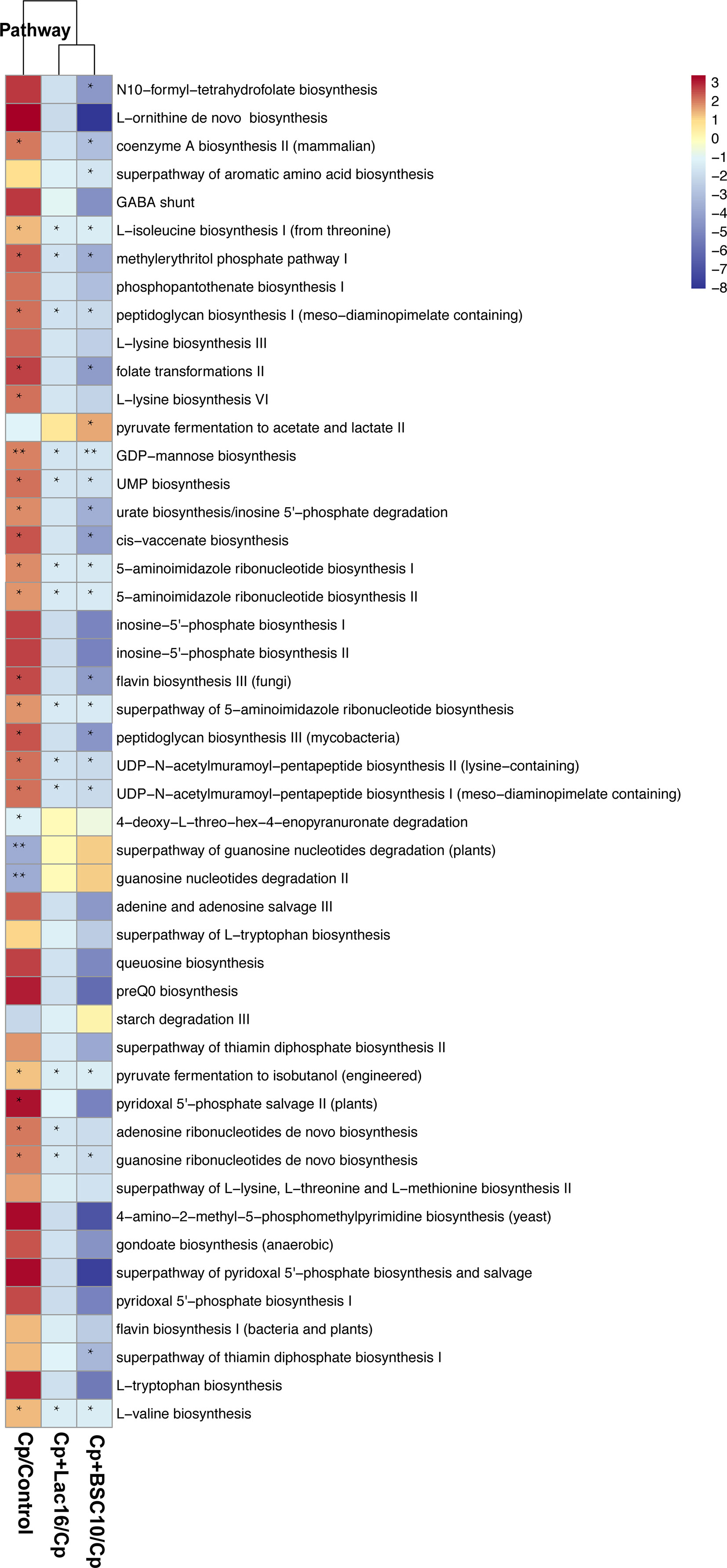
Figure 5 The functional profile of cecum contents. Differences in metabolic pathways expression of the microbiota among different treatment groups evaluated using the HUMAnN2 metabolic analysis network. The representative metabolic pathway is selected by a one-way ANOVA test, and the fold changes of pathway abundance were calculated using the formula Cp/Control, Cp + Lac16/Cp, and Cp + BSC10/Cp. The significant difference is defined as the fold change > 2 or fold change < 0.5. A heatmap is drawn using the fold-change value (log2 transformed) of the metabolic pathway abundance using the pheatmap R package. * indicates statistically significant difference (P < 0.05).
Discussion
Numerous studies have demonstrated that probiotics exert antimicrobial activity against C. perfringens through different mechanisms. In our previous study, increased intestinal lesion scores and decreased growth performance of broilers were observed after C. perfringens challenge, which was ameliorated in broilers pretreated with Lac16 and BSC10 (unpublished data). Therefore, the current study aimed to investigate the protective effects of Lac16 and BSC10 against C. perfringens in broilers through the regulation of the intestinal mucosal structure, apoptosis, inflammation, and intestinal microbiota.
Gut integrity is a prerequisite for maintaining the host homeostasis. The intestinal mucosal barrier comprises connecting epithelial cells that are overlaid by host-secreted mucous layer and serve as the first line of defense against pathogens and potentially harmful commensal bacteria (22, 23). Impaired intestinal mucosal barrier caused by intestinal pathogens compromises the immune tolerance of the intestines and causes a systemic inflammatory response, which aggravates systemic immune response and host body damage (24). Consistent with a previous study (25), the present study demonstrated that probiotics Lac16 and BSC10 treatment could improve the intestinal morphology of C. perfringens infected broilers. MUC2 is the main component of mucins which binds several pathogens and inhibits external bacterial access to the epithelial cells (26). Previous studies have shown that the expression of MUC2 is suppressed in infected broilers (27, 28). However, Fasina et al. (29) reported that Salmonella Typhimurium infection increased the number of goblet cells and mucin level in the jejunum of broilers, indicating that the infected birds can capture and eliminate the pathogens from the epithelial surface by enhancing mucin expression (30). We also found that the MUC2 expression was significantly increased in C. perfringens infected broilers but decreased after probiotic supplementation. Our results may unravel novel strategy for improving mucus barrier to inhibit C. perfringens infection. Occludin and claudin are two of the most important components of tight junctions, and they exhibit beneficial effects on epithelial barrier function (31). Similar with the result of MUC2 expression, the present study showed that C. perfringens infection significantly up-regulated the relative expression levels of ZO-1 and CLDN1, which was contrary to results from previous studies (32, 33). One plausible explanation was that up-regulating the expression levels of barrier-related genes in infected chicken was beneficial for decreasing C. perfringens to pass through the epithelial layers and intestinal damage. However, the probiotic supplementation could decrease the number of C. perfringens and improve intestinal health status, which resulted in the decreased expression of occludin and claudin.
Apoptosis is regulated by the dynamic balance between the expression of pro-apoptosis proteins, such as Bax, and the anti-apoptosis protein, such as Bcl-2. Once the imbalance occurs, cytochrome c is released from the mitochondria and subsequently activates Apaf1/Caspase 9 and downstream executioner Caspase 3, thereby initiating cell apoptosis (34). p53 is a multiple function protein that induces apoptosis by promoting the expression of pro-apoptosis genes, such as Bax, and inhibits anti-apoptosis genes, such as survivin, thereby activating the caspase-dependent pathway and ultimately triggering apoptosis (35). Wu et al. (36) reported that Lactobacillus rhamnosus GG stimulates apoptosis by increasing p53 expression and decreasing Bcl-2 and Bcl-xl protein in the ilea of HRV-infected pigs. In the present study, the results of mRNA expression and immunohistochemistry of apoptosis-related genes showed that the probiotics could ameliorate C. perfringens-induced apoptosis in the ileum by up-regulating anti-apoptosis genes and down-regulating pro-apoptosis genes in broilers, and these findings were consistent with previous findings (37). Moreover, p53 mRNA expression in the probiotics groups was down-regulated compared with the Cp group, suggesting that the probiotics inhibited cell apoptosis in the ileum through the p53 signaling pathway during C. perfringens infection.
Accumulating evidence demonstrates that iNOS-derived NO and pro-inflammatory cytokines exert multiple modulatory effects on the host immune response against various infections (38, 39). Besides, pro-inflammatory cytokines modulate host immunity against multiple pathogens through differentiation and proliferation of immune cells, apoptosis, and NO production (40). However, excessive inflammatory responses cause tissue injury. The increase in NO production and iNOS activity and increased expression of pro-inflammatory cytokines, such as IL-1β, IL-6, iNOS, and IFN-γ demonstrate that C. perfringens infection induces a strong inflammatory response and causes severe tissue damage (41). However, in the present study, probiotics reduced the inflammatory response, thus inhibiting excessive inflammatory damage in infected broilers. Similar results have also been observed in birds infected with Salmonella enteritidis (42) and Escherichia coli (43). Interleukin-10 is an anti-inflammatory cytokine that inhibits T cell proliferation and the production and function of many proinflammatory cytokines (44). In the present study, the level of IL-10 decreased following treatment with the probiotics in C. perfringens infected broilers, and this was attributed to a decreased inflammatory response.
The microbiota plays a major role in host growth and health and significantly contributes to the regulation of the host immune system, nutrient synthesis, energy metabolism, and prevention of enteric pathogen infection (45). The gut microbial composition and function are reported to be modulated by dietary probiotic supplementation (46). The increase in Firmicutes contributes to energy efficiency, while the Firmicutes/Bacteroides ratio is associated with growth performance and host health (47, 48). A high proportion of Proteobacteria in the gut is an indicator of metabolic disorders, immune disorders, and an unstable gut microbial community structure in the host (49). In the present study, increased Firmicutes/Bacteroidetes ratio and a decline in Proteobacteria were associated with improved intestinal health in broilers supplemented with probiotics.
Bacteroides fragilis is generally regarded as a gut commensal and leads to an increased risk of infection and disease when it leaks into the bloodstream or surrounding tissue (50). Even though Bacteroides fragilis has an anti-inflammatory property, toxigenic B. fragilis induces intestinal inflammation and can cause bowel disease and colon cancer (51). Gallibacterium is an indigenous bacterial pathogen in chicken and one of the major pathogens causing reproductive tract disorders in laying hens (52). Butyrate is an important energy source for gut enterocytes, and can reduce the inflammatory response and gastrointestinal pathogens, regulate the gut bacterial ecology, and stimulate villi growth (53, 54). Two probiotics increased some beneficial bacteria and butyrate-producing bacteria, such as Ruminococcus, Oscillibacter, Pseudoflavonifractor, and Erysipellotrichaceac and decreased Gallibacterium in C. perfringens-infected birds. In addition, BSC10 decreased Bacteroides fragilis. Together with the results of the PCA, we speculated that the two probiotics could restore the intestinal microbiota disturbance induced by C. perfringens infection with minor differences.
Alterations in the intestinal microflora composition are closely related to the metabolic alterations in the gut microbiota. In the present study, C. perfringens infection changed the microbiota composition and metabolic pathways, including enhancing B-vitamin biosynthesis, peptidoglycan biosynthesis, amino acid biosynthesis, and ribonucleotide biosynthesis, etc., and decreased pyruvate fermentation to acetate and lactate II pathway, which were reversed by probiotic supplementation. Acetate and lactate are known to inhibit the growth of gut pathogens, suggesting that probiotics exert antibacterial properties by increasing the production of acetate and lactate in response to C. perfringens. B-vitamins are important in numerous metabolism processes, including fat and carbohydrate metabolism and DNA synthesis. Hosts cannot produce enough B-vitamins and have to be supplemented either from the diet or the gut microbiota (55). An increased risk of colitis correlates with a deficiency of genetic pathways involved in polyamine transport and B vitamin biosynthesis (56, 57). Dubin et al. (58) found that three modules involved in the biosynthesis of B vitamins (riboflavin (B2), pantothenate (B5), and thiamine (B1)) were more abundant in colitis free patients. Besides, Ford et al. (59) reported that high-dose vitamin-B supplementation reduced oxidative stress and inflammation. Inconsistent with some previous studies, the present study showed that the vitamin-B biosynthetic pathway was enriched in the Cp group but decreased in the probiotics group. We speculated that C. perfringens induced a compensatory synthesis of vitamin-B against oxidative damage and inflammation, while Lactobacillus and Bacillus synthesized vitamin-B in the gut to maintain normal levels in the organism, hence no need to supply exogenous Vitamin-B after probiotic treatment. Peptidoglycan (PGN) is a component of the cell wall in both Gram-positive and Gram-negative bacteria, and it triggers inflammatory responses through multiple pattern-recognition receptors (60). The most well-defined sensors of the peptidoglycan are NOD-like receptors (NLRs), which promote pathogen clearance by inducing the secretion of pro-inflammatory cytokines and chemokines and other host defense pathways, including autophagy (61). Peptidoglycan predominantly induces the Th1 immune response. Besides, bacteria-derived peptidoglycan can activate Paneth cells to produce defensins that protect the host from pathogenic bacteria (62). Therefore, in the present study, downregulation of the peptidoglycan biosynthetic pathway in the probiotic groups indicated that probiotics might protect against C. perfringens-infections by inhibiting excessive inflammatory response, and these findings were also consistent with the results of cytokines expression levels in the ileum mucosa.
Conclusion
In our study, the two probiotics have similar molecular mechanism against C. perfringens infection, including improving the composition and metabolic pathways of the intestinal microbiota, intestinal structure, inflammation, and anti-apoptosis. There are some differences in anti-apoptosis and the composition and metabolic pathways of the intestinal microbiota of these two probiotics.
Data Availability Statement
The datasets presented in this study can be found in online repositories. The names of the repository/repositories and accession number(s) can be found below: https://www.ncbi.nlm.nih.gov/sra/PRJNA675467.
Ethics Statement
The animal study was reviewed and approved by the Animal Care and Use Committee of Zhejiang University.
Author Contributions
WL, LG, and HZ conceived and designed the experiments. LG and BW performed the experiments. LG and WL analyzed the data. LG, LT, and ZZ made the figures. LG and WL wrote the paper. WL, YZ, and HZ revised the manuscript. All authors contributed to the article and approved the submitted version.
Funding
This study is supported by the National Natural Science Foundation of China (No. 31472128 and 31672460), the Natural Science Foundation of Zhejiang Province (No. LZ20C170002), National High-Tech R&D Program (863) of China (No. 2013AA102803D), the Major Science and Technology Project of Zhejiang Province (No. 2006C12086), PRC.
Conflict of Interest
The authors declare that the research was conducted in the absence of any commercial or financial relationships that could be construed as a potential conflict of interest.
Supplementary Material
The Supplementary Material for this article can be found online at: https://www.frontiersin.org/articles/10.3389/fimmu.2020.628374/full#supplementary-material
Supplementary Figure 1 | Phylogenetic analysis of the microbiota composition.
Supplementary Figure 2 | Principal component analysis of the gene family using the prompt R package.
Supplementary Figure 3 | KEGG map of the folate biosynthesis (map 00790) pathway. The fold changes of pathway abundance are calculated using the formula Cp/Control, Cp + Lac16/Cp, and Cp + BSC10/Cp. The significant difference is defined as the fold change >2 or fold change <0.5. The up-regulated, fold changes are labeled as green, blue, and purple, respectively, while the down-regulated fold changes are labeled as red, yellow, and pink, respectively. The brown color represents the collection of green, yellow, and pink, while the gray color represents the collection of red, blue, and purple.
Supplementary Figure 4 | KEGG map of the peptidoglycan biosynthetic (map 00550) pathway. The fold changes of pathway abundance are calculated using the formula Cp/Control, Cp + Lac16/Cp, and Cp + BSC10/Cp. The significant difference is defined as the fold change >2 or folds change <0.5. The up-regulated fold changes are labeled as green, blue, and purple, while the downregulated are labeled as red, yellow, and pink. The brown color represents the collection of green, yellow, and pink, while the gray color represents the collection of red, blue, and purple.
References
1. Timbermont L, Haesebrouck F, Ducatelle R, Van Immerseel F. Necrotic Enteritis in Broilers: An Updated Review on the Pathogenesis. Avian Pathol (2011) 40:341–7. doi: 10.1080/03079457.2011.590967
2. Wade B, Keyburn AL, Seemann T, Rood J II, Moore RJ. Binding of Clostridium Perfringens to Collagen Correlates with the Ability to Cause Necrotic Enteritis in Chickens. Vet Microbiol (2015) 180:299–303. doi: 10.1016/j.vetmic.2015.09.019
3. Kaldhusdal M, Schneitz C, Hofshagen M, Skjerve E. Reduced Incidence of Clostridium Perfringens-Associated Lesions and Improved Performance in Broiler Chickens Treated with Normal Intestinal Bacteria from Adult Fowl. Avian Dis (2001) 45:149–56.
4. Mora ZV, Macías-Rodríguez ME, Arratia-Quijada J, Gonzalez-Torres YS, Nuño K, Villarruel-López A. Clostridium Perfringens as Foodborne Pathogen in Broiler Production: Pathophysiology and Potential Strategies for Controlling Necrotic Enteritis. Anim (Basel) (2020) 10:1718–43. doi: 10.3390/ani10091718
5. Dahiya JP, Wilkie DC, Kessel AGV, Drew MD. Potential Strategies for Controlling Necrotic Enteritis in Broiler Chickens in Post-Antibiotic Era. Anim Feed Sci Technol (2006) 129:60–88. doi: 10.1016/j.anifeedsci.2005.12.003
6. Shojadoost B, Vince AR, Prescott JF. The Successful Experimental Induction of Necrotic Enteritis in Chickens by Clostridium Perfringens: A Critical Review. Vet Res (2012) 43:74. doi: 10.1186/1297-9716-43-74
7. Hill C, Guarner F, Reid G, Gibson GR, Merenstein DJ, Pot B, et al. The International Scientific Association for Probiotics and Prebiotics Consensus Statement on the Scope and Appropriate Use of the Term Probiotic. Nat Rev Gastro Hepat (2014) 11:506–14. doi: 10.1038/nrgastro.2014.66
8. Chen Y, Zhang L, Hong G, Huang C, Qian W, Bai T, et al. Probiotic Mixtures with Aerobic Constituent Promoted the Recovery of Multi-Barriers in Dss-Induced Chronic Colitis. Life Sci (2020) 240:117089. doi: 10.1016/j.lfs.2019.117089
9. Jakubczyk D, Leszczyńska K, Górska S. The Effectiveness of Probiotics in the Treatment of Inflammatory Bowel Disease (Ibd)-a Critical Review. Nutrients (2020) 12:1973–97. doi: 10.3390/nu12071973
10. Cai J, Zhao C, Du Y, Zhang Y, Zhao M, Zhao Q. Comparative Efficacy and Tolerability of Probiotics for Antibiotic-Associated Diarrhea: Systematic Review with Network Meta-Analysis. U Eur Gastroent J (2018) 6:169–80. doi: 10.1177/2050640617736987
11. Geeraerts S, Delezie E, Ducatelle R, Haesebrouck F, Devreese B, Van IF. Vegetative Bacillus Amyloliquefaciens Cells Do Not Confer Protection against Necrotic Enteritis in Broilers Despite High Antibacterial Activity of Its Supernatant against Clostridium Perfringens in Vitro. Brit Poult Sci (2016) 57:324–9. doi: 10.1080/00071668.2016.1169246
12. Fukata T, Hadate Y, Baba E, Arakawa A. Influence of Bacteria on Clostridium Perfringens Infections in Young Chickens. Avian Dis (1991) 35:224–7.
13. Jayaraman S, Thangavel G, Kurian H, Mani R, Mukkalil R, Chirakkal H. Bacillus Subtilis Pb6 Improves Intestinal Health of Broiler Chickens Challenged with Clostridium Perfringens-Induced Necrotic Enteritis. Poult Sci (2013) 92:370–4. doi: 10.3382/ps.2012-02528
14. Qing X, Zeng D, Wang H, Ni X, Liu L, Lai J, et al. Preventing Subclinical Necrotic Enteritis through Lactobacillus Johnsonii Bs15 by Ameliorating Lipid Metabolism and Intestinal Microflora in Broiler Chickens. Amb Express (2017) 7:139. doi: 10.1186/s13568-017-0439-5
15. Caly DL, D’Inca R, Auclair E, Drider D. Alternatives to Antibiotics to Prevent Necrotic Enteritis in Broiler Chickens: A Microbiologist’s Perspective. Front Microbiol (2015) 6:1336. doi: 10.3389/fmicb.2015.01336
16. Layton SL, Hernandez-Velasco X, Chaitanya S, Xavier J, Menconi A, Latorre JD, et al. The Effect of a Lactobacillus-Based Probiotic for the Control of Necrotic Enteritis in Broilers. Food Sci Nutr (2013) 4:1–7. doi: 10.4236/fns.2013.411A001
17. Cao L, Yang XJ, Li ZJ, Sun FF, Wu XH, Yao JH. Reduced Lesions in Chickens with Clostridium Perfringens-Induced Necrotic Enteritis by Lactobacillus Fermentum 1.20291. Poult Sci (2012) 91:3065–71. doi: 10.3382/ps.2012-02548
18. Liu X, Lin X, Mi Y, Li J, Zhang C. Grape Seed Proanthocyanidin Extract Prevents Ovarian Aging by Inhibiting Oxidative Stress in the Hens. Oxid Med Cell Longev (2018) 2018:1–16. doi: 10.1155/2018/9390810
19. Livak KJ, Schmittgen TD. Analysis of Relative Gene Expression Data Using Real-Time Quantitative Pcr and the 2(-Delta Delta C(T)) Method. Methods (2001) 25:402–8. doi: 10.1006/meth.2001.1262
20. Truong DT, Franzosa EA, Tickle TL, Scholz M, Weingart G, Pasolli E, et al. Erratum: Metaphlan2 for Enhanced Metagenomic Taxonomic Profiling. Nat Methods (2015) 12:902–3. doi: 10.1038/nmeth.3589
21. Abubucker S, Segata N, Goll J, Schubert AM, Izard J, Cantarel BL, et al. Metabolic Reconstruction for Metagenomic Data and Its Application to the Human Microbiome. PLoS Comput Biol (2012) 8:e1002358. doi: 10.1371/journal.pcbi.1002358
22. Martens EC, Neumann M, Desai MS. Interactions of Commensal and Pathogenic Microorganisms with the Intestinal Mucosal Barrier. Nat Rev Microbiol (2018) 16:457–70. doi: 10.1038/s41579-018-0036-x
23. Citi S. Intestinal Barriers Protect against Disease. Science (2018) 359:1097–98. doi: 10.1126/science.aat0835
24. Kelsall BL. Innate and Adaptive Mechanisms to Control [Corrected] Pathological Intestinal Inflammation. J Pathol (2010) 214:242–59. doi: 10.1002/path.2373
25. Li Z, Wang W, Lv Z, Liu D, Guo Y. Bacillus Subtilis and Yeast Cell Wall Improve the Intestinal Health of Broilers Challenged by Clostridium Perfringens. Brit Poult Sci (2017) 58:635–43. doi: 10.1080/00071668.2017.1370697
26. Mcauley JL, Linden SK, Png CW, King RM, Pennington HL, Gendler SJ, et al. Muc1 Cell Surface Mucin Is a Critical Element of the Mucosal Barrier to Infection. J Clin Invest (2007) 117:2313–24. doi: 10.1172/JCI26705
27. Forder RE, Nattrass GS, Geier MS, Hughes RJ, Hynd P II. Quantitative Analyses of Genes Associated with Mucin Synthesis of Broiler Chickens with Induced Necrotic Enteritis. Poult Sci (2012) 91:1335–41. doi: 10.3382/ps.2011-02062
28. Lee YS, Lee SH, Gadde UD, Oh ST, Lee SJ, Lillehoj HS. Allium Hookeri Supplementation Improves Intestinal Immune Response against Necrotic Enteritis in Young Broiler Chickens. Poult Sci (2018) 97:1899–908. doi: 10.3382/ps/pey031
29. Fasina YO, Hoerr FJ, McKee SR, Conner DE. Influence of Salmonella Enterica Serovar Typhimurium Infection on Intestinal Goblet Cells and Villous Morphology in Broiler Chicks. Avian Dis (2010) 54:841–7. doi: 10.1637/9055-090809-Reg.1
30. Lindén SK, Florin THJ, Mcguckin MA. Mucin Dynamics in Intestinal Bacterial Infection. PLoS One (2008) 3:e3952. doi: 10.1371/journal.pone.0003952
31. Fanning AS, Jameson BJ, Jesaitis LA, Anderson JM. The Tight Junction Protein Zo-1 Establishes a Link between the Transmembrane Protein Occludin and the Actin Cytoskeleton. J Biol Chem (1998) 273:29745–53. doi: 10.1074/jbc.273.45.29745
32. Du E, Wang W, Gan L, Li Z, Guo S, Guo Y. Effects of Thymol and Carvacrol Supplementation on Intestinal Integrity and Immune Responses of Broiler Chickens Challenged with Clostridium Perfringens. J Anim Sci Biotechnol (2016) 7:19. doi: 10.1186/s40104-016-0079-7
33. Gadde UD, Oh S, Lee Y, Davis E, Zimmerman N, Rehberger T, et al. Dietary Bacillus Subtilis-Based Direct-Fed Microbials Alleviate Lps-Induced Intestinal Immunological Stress and Improve Intestinal Barrier Gene Expression in Commercial Broiler Chickens. Res Vet Sci (2017) 114:236–43. doi: 10.1016/j.rvsc.2017.05.004
34. Li P, Nijhawan D, Budihardjo I, Srinivasula SM, Ahmad M, Alnemri ES, et al. Cytochrome C and Datp-Dependent Formation of Apaf-1/Caspase-9 Complex Initiates an Apoptotic Protease Cascade. Cell (1997) 91:479–89. doi: 10.1016/s0092-8674(00)80434-1
35. Hoffman WH, Biade S, Zilfou JT, Chen J, Murphy M. Transcriptional Repression of the Anti-Apoptotic Survivin Gene by Wild Type P53. J Biol Chem (2002) 277:3247–57. doi: 10.1074/jbc.M106643200
36. Wu S, Yuan L, Zhang Y, Liu F, Li G, Wen K, et al. Probiotic Lactobacillus Rhamnosus Gg Mono-Association Suppresses Human Rotavirus-Induced Autophagy in the Gnotobiotic Piglet Intestine. Gut Pathog (2013) 5:22–2. doi: 10.1186/1757-4749-5-22
37. Wang H, Ni X, Qing X, Liu L, Lai J, Khalique A, et al. Probiotic Enhanced Intestinal Immunity in Broilers against Subclinical Necrotic Enteritis. Front Immunol (2017) 8:1592–605. doi: 10.3389/fimmu.2017.01592
38. Crhanova M, Hradecka H, Faldynova M, Matulova M, Havlickova H, Sisak F, et al. Immune Response of Chicken Gut to Natural Colonization by Gut Microflora and to Salmonella Enterica Serovar Enteritidis Infection. Infect Immun (2011) 79:2755–63. doi: 10.1128/iai.01375-10
39. García-Ortiz A, Serrador JM. Nitric Oxide Signaling in T Cell-Mediated Immunity. Trends Mol Med (2018) 24:412–27. doi: 10.1016/j.molmed.2018.02.002
40. Torchinsky MB, Garaude J, Martin AP, Blander JM. Innate Immune Recognition of Infected Apoptotic Cells Directs T(H)17 Cell Differentiation. Nature (2009) 458:78. doi: 10.1038/nature07781
41. Van Immerseel F, De Buck J, Pasmans F, Huyghebaert G, Haesebrouck F, Ducatelle R. Clostridium Perfringens in Poultry: An Emerging Threat for Animal and Public Health. Avian Pathol (2004) 33:537–49. doi: 10.1080/03079450400013162
42. Adhikari P, Lee CH, Cosby DE, Cox NA, Kim WK. Effect of Probiotics on Fecal Excretion, Colonization in Internal Organs and Immune Gene Expression in the Ileum of Laying Hens Challenged with Salmonella Enteritidis. Poult Sci (2019) 98:1235–42. doi: 10.3382/ps/pey443
43. Wang W, Li Z, Han Q, Guo Y, Zhang B, D’Inca R. Dietary Live Yeast and Mannan-Oligosaccharide Supplementation Attenuate Intestinal Inflammation and Barrier Dysfunction Induced by Escherichia Coli in Broilers. Br J Nutr (2016) 116:1878–88. doi: 10.1017/s0007114516004116
44. Taylor A, Verhagen J, Blaser K, Akdis M, Akdis CA. Mechanisms of Immune Suppression by Interleukin-10 and Transforming Growth Factor-Beta: The Role of T Regulatory Cells. Immunology (2010) 117:433–42. doi: 10.1111/j.1365-2567.2006.02321.x
45. Clavijo V, Flórez MJV. The Gastrointestinal Microbiome and Its Association with the Control of Pathogens in Broiler Chicken Production: A Review. Poult Sci (2018) 97:1006–21. doi: 10.3382/ps/pex359
46. Cisek AA, Binek M. Chicken Intestinal Microbiota Function with a Special Emphasis on the Role of Probiotic Bacteria. Pol J Vet Sci (2014) 17:385–94. doi: 10.2478/pjvs-2014-0057
47. Kelder T, Stroeve JHM, Bijlsma S, Radonjic M, Roeselers G. Correlation Network Analysis Reveals Relationships between Diet-Induced Changes in Human Gut Microbiota and Metabolic Health. Nutr Diabetes (2014) 4:e122. doi: 10.1038/nutd.2014.18
48. Singh KM, Shah T, Deshpande S, Jakhesara SJ, Koringa PG, Rank DN, et al. High through Put 16s Rrna Gene-Based Pyrosequencing Analysis of the Fecal Microbiota of High Fcr and Low Fcr Broiler Growers. Mol Biol Rep (2012) 39:10595–602. doi: 10.1007/s11033-012-1947-7
49. Shin NR, Whon TW, Bae JW. Proteobacteria: Microbial Signature of Dysbiosis in Gut Microbiota. Trends Biotechnol (2015) 33:496–503. doi: 10.1016/j.tibtech.2015.06.011
50. Kuwahara T, Yamashita A, Hirakawa H, Nakayama H, Toh H, Okada N, et al. Genomic Analysis of Bacteroides Fragilis Reveals Extensive DNA Inversions Regulating Cell Surface Adaptation. Proc Natl Acad Sci U S A (2004) 101:14919. doi: 10.1073/pnas.0404172101
51. Zakharzhevskaya NB, Vanyushkina AA, Altukhov IA, Shavarda AL, Butenko IO, Rakitina DV, et al. Outer Membrane Vesicles Secreted by Pathogenic and Nonpathogenic Bacteroides Fragilis Represent Different Metabolic Activities. Sci Rep (2017) 7:5008–23. doi: 10.1038/s41598-017-05264-6
52. Wang C, Pors SE, Olsen RH, Bojesen AM. Transmission and Pathogenicity of Gallibacterium Anatis and Escherichia Coli in Embryonated Eggs. Vet Microbiol (2018) 217:76–81. doi: 10.1016/j.vetmic.2018.03.005
53. Zhang Y, Yu K, Chen H, Su Y, Zhu W. Caecal Infusion of the Short-chain Fatty Acid Propionate Affects the Microbiota and Expression of Inflammatory Cytokines in the Colon in a Fistula Pig Model. Microb Biotechnol (2018) 11:859–68. doi: 10.1111/1751-7915.13282
54. Leeson S, Namkung H, Antongiovanni M, Lee EH. Effect of Butyric Acid on the Performance and Carcass Yield of Broiler Chickens. Poult Sci (2005) 84:1418–22. doi: 10.1093/ps/84.9.1418
56. Kuroki F, Iida M, Tominaga M, Matsumoto T, Hirakawa K, Sugiyama S, et al. Multiple Vitamin Status in Crohn’s Disease. Dig Dis Sci (1993) 38:1614–8. doi: 10.1007/BF01303168
57. Ellestadsayed JJ, Nelson RA, Adson MA, Palmer WM, Soule EH. Pantothenic Acid, Coenzyme a, and Human Chronic Ulcerative and Granulomatous Colitis. Am J Clin Nutr (1976) 29:1333–8. doi: 10.1097/00006199-197511000-00014
58. Dubin K, Callahan MK, Ren B, Khanin R, Viale A, Ling L, et al. Intestinal Microbiome Analyses Identify Melanoma Patients at Risk for Checkpoint-Blockade-Induced Colitis. Nat Commun (2016) 7:10391. doi: 10.1038/ncomms10391
59. Ford TC, Downey LA, Simpson T, McPhee G, Oliver C, Stough C. The Effect of a High-Dose Vitamin B Multivitamin Supplement on the Relationship between Brain Metabolism and Blood Biomarkers of Oxidative Stress: A Randomized Control Trial. Nutrients (2018) 10:1860–72. doi: 10.3390/nu10121860
60. Lebeer S, Vanderleyden J, De Keersmaecker SC. Host Interactions of Probiotic Bacterial Surface Molecules: Comparison with Commensals and Pathogens. Nat Rev Microbiol (2010) 8:171–84. doi: 10.1038/nrmicro2297
61. Philpott DJ, Sorbara MT, Robertson SJ, Croitoru K, Girardin SE. Nod Proteins: Regulators of Inflammation in Health and Disease. Nat Rev Immunol (2014) 14:9–23. doi: 10.1038/nri3565
Keywords: Clostridium perfringens, Lactobacillus plantarum 16, Paenibacillus polymyxa 10, broilers, apoptosis, immunity, microbiota, metabolic pathways
Citation: Gong L, Wang B, Zhou Y, Tang L, Zeng Z, Zhang H and Li W (2021) Protective Effects of Lactobacillus plantarum 16 and Paenibacillus polymyxa 10 Against Clostridium perfringens Infection in Broilers. Front. Immunol. 11:628374. doi: 10.3389/fimmu.2020.628374
Received: 11 November 2020; Accepted: 31 December 2020;
Published: 18 February 2021.
Edited by:
Oscar Gilberto Gomez-Duarte, University at Buffalo, United StatesCopyright © 2021 Gong, Wang, Zhou, Tang, Zeng, Zhang and Li. This is an open-access article distributed under the terms of the Creative Commons Attribution License (CC BY). The use, distribution or reproduction in other forums is permitted, provided the original author(s) and the copyright owner(s) are credited and that the original publication in this journal is cited, in accordance with accepted academic practice. No use, distribution or reproduction is permitted which does not comply with these terms.
*Correspondence: Weifen Li, wfli@zju.edu.cn; Huihua Zhang, hhzhang2@163.com