- 1Department of Dermatology, Hamamatsu University School of Medicine, Hamamatsu, Japan
- 2Department of Cellular & Molecular Anatomy, Hamamatsu University School of Medicine, Hamamatsu, Japan
Tissue resident memory T (TRM) cells reside in peripheral, non-lymphoid tissues such as the skin, where they act as alarm-sensor cells or cytotoxic cells. Physiologically, skin TRM cells persist for a long term and can be reactivated upon reinfection with the same antigen, thus serving as peripheral sentinels in the immune surveillance network. CD8+CD69+CD103+ TRM cells are the well-characterized subtype that develops in the epidermis. The local mediators such as interleukin (IL)-15 and transforming growth factor (TGF)-β are required for the formation of long-lived TRM cell population in skin. Skin TRM cells engage virus-infected cells, proliferate in situ in response to local antigens and do not migrate out of the epidermis. Secondary TRM cell populations are derived from pre-existing TRM cells and newly recruited TRM precursors from the circulation. In addition to microbial pathogens, topical application of chemical allergen to skin causes delayed-type hypersensitivity and amplifies the number of antigen-specific CD8+ TRM cells at challenged site. Skin TRM cells are also involved in the pathological conditions, including vitiligo, psoriasis, fixed drug eruption and cutaneous T-cell lymphoma (CTCL). The functions of these TRM cells seem to be different, depending on each pathology. Psoriasis plaques are seen in a recurrent manner especially at the originally affected sites. Upon stimulation of the skin of psoriasis patients, the CD8+CD103+CD49a- TRM cells in the epidermis seem to be reactivated and initiate IL-17A production. Meanwhile, autoreactive CD8+CD103+CD49a+ TRM cells secreting interferon-γ are present in lesional vitiligo skin. Fixed drug eruption is another disease where skin TRM cells evoke its characteristic clinical appearance upon administration of a causative drug. Intraepidermal CD8+ TRM cells with an effector-memory phenotype resident in the skin lesions of fixed drug eruption play a major contributing role in the development of localized tissue damage. CTCL develops primarily in the skin by a clonal expansion of a transformed TRM cells. CD8+ CTCL with the pagetoid epidermotropic histology is considered to originate from epidermal CD8+ TRM cells. This review will discuss the current understanding of skin TRM biology and their contribution to skin homeostasis and diseases.
Introduction
The number of T cells infiltrating in the skin is nearly twice as many as that in the peripheral blood, and the majority of these cells are effector memory T cells (1). T cells in the skin include αβ T cells accounting for up to 99% and γδ T cells for around 1% (2). Thus, the skin is a homing organ for T cells in physiological and pathological conditions related to adaptive immune response. Before the discovery of resident memory T (TRM) cells, it was supposed that T cells infiltrating in inflamed or infected tissue transiently reside and undergo apoptosis or exit the tissue after clearance of inflammation or infection. Skin TRM cells are a memory T cell subset that provides local surveillance and do not migrate out of the skin. This memory subset has distinct behavior and transcriptional profile that distinguish TRM cells from other memory T cell compartment.
Tissue TRM cells reside in peripheral, non-lymphoid tissues such as the skin, where they act as alarm-sensor cells or cytotoxic cells (3, 4). Physiologically, skin TRM cells persist for a long term and can be reactivated upon reinfection with the same antigen, thus serving as a part of an immune surveillance network. CD8+CD69+CD103+ TRM cells are the well-characterized subtype that develops in the epidermis, although CD4+ TRM cells are documented in certain conditions. Local signaling by IL-15 and TGF-β is required for the formation of these long-lived memory cells (5).
Skin TRM cells play a critical defensive role against skin infections. In addition to this essential physiological role, they are also involved in the pathological conditions (6), as exemplified by psoriasis. The functions of these TRM cells seem to be different, depending on each skin disease. The TRM cell-inducing skin diseases have currently extended from fixed drug eruption to psoriasis and cutaneous T-cell lymphoma, and even to vitiligo. In this review, we will discuss recent insights into skin TRM cells, with emphasis on their pathogenic roles in these heterogeneous skin disorders.
Tissue TRM Cells
TRM cells, which lack the ability of recirculation via the bloodstream and reside in the tissue, exist in various tissues in various organs. However, the phenotypes of TRM cells in each tissue, such as surface markers, the longevity, and the signals for their survival are not uniform and highly heterogeneous. Insights into TRM cells in various tissues have mostly been obtained from mouse studies, and the data of human TRM cells are relatively scarce, because of the technical difficulties in obtaining samples and taking enough number of cells from small biopsy samples in human. It is considered that both CD8+ TRM and CD4+ TRM cells exist, but the property is best defined for CD8+ TRM cells. In this section, we will briefly introduce the characteristics of TRM cells in various tissues, mainly focusing on CD8+ TRM cells in mice (Table 1).
The surface markers and longevity of CD8+ TRM cells are critical issues and have been studied in mouse tissues. One of the most important functions of TRM cells is the defense against pathogens such as viruses, bacteria, fungi, and parasites, all of which commonly invade to our body through barrier tissues. Consistently, TRM cells are observed in barrier tissues such as the skin, intestines, lung, and female reproductive tract (25, 26). TRM cells are also detected in non-barrier tissues such as the central nervous system, liver, and salivary glands (25, 26). Furthermore, TRM cells are present in lymphoid tissues, some of which are derived from non-lymphoid tissues (27). CD69 and CD103 are the key surface markers of TRM cells in general, however, the expression patterns of these markers are various depending on the tissues, and even show heterogeneity in the same tissue. CD103 is expressed in TRM cells in most tissues such as the skin and central nervous system, but TRM cells lacking CD103 have been reported in some tissues including intestines (28) and liver (29). CD69, a C-type lectin, is expressed in most TRM cells. CD69 is supposed to work as a stop signal that prevents tissue egress of TRM cells by antagonizing sphingosine-1-phosphate receptor 1 (S1PR1). However, a substantial proportion of TRM cells in the pancreas, salivary glands, and female reproductive tract was reported to be negative for both CD69 and CD103 (30).
Longevity, which can be defined as the persistence of TRM cells in the tissues, may be also quite different between tissues (4). It has been reported that TRM cells in the lungs and liver persist for weeks to months (31, 32), while TRM cells in the skin remain numerically stable for months to years (33–35), suggesting a tissue specificity of longevity. Longevity is the net effects of several factors such as recruitment, maintenance, division, death, egress, and competition. The extent of the effects of each factor is various depending on the tissues. For example, at the steady state, the ratio of TRM cells that uptake BrdU over 7 days is 0%–5% in the lung (36) and skin (37), while Ki67+ TRM cells in the brain is reported around 9% (38), suggesting the various proliferation ability of TRM cells depending on the tissues. As for the maintenance signals of TRM cells, IL-15 is one of the most important one. Indeed, IL-15 is required for the maintenance of TRM cells in the skin (39), liver (40), salivary glands and kidney (41). However, this is not the case for TRM cells in the female reproductive tract, pancreas, small intestines, and secondary lymphoid organs (SLOs) (41). Expression of CD103 may also be important for the persistence of TRM cells in several tissues such as the skin (39) and the gut (42). TGF-β is necessary for the development of TRM cells in the skin (39), gut (43), and lung (44), while not required for the development of TRM cells in lamina propria of intestine (28). Thus, TRM cells in each tissue possess their own characteristics. Because the environment in each tissue such as available cytokines and nutrients are various, TRM cells seem to adapt to unique local environment to survive.
In human, T cells showing surface markers similar to murine TRM cells have been detected in various tissues, suggesting that TRM cells also exist in human. It is considered that TRM cells play crucial roles for the protection of the host against pathogens, as well as the development of inflammatory diseases. TRM cells in the skin are probably the best studied population in human TRM cells. In the genital skin after human simplex virus (HSV) infection, virus-specific CD8+ T cells persist at the epidermal-dermal junction (11). Involvement of TRM cells is suggested in the development of various inflammatory skin diseases, such as psoriasis, vitiligo, and drug eruption, which will be discussed later. TRM cells are also detected in the gut, and are suspected to contribute to the development of Crohn’s disease (15). In the lung, CD69+ or CD103+ CD8+ TRM-like cells are detected in patients with influenza or respiratory syncytial virus infection (17, 18). Other than these tissues, existence of TRM cells has been reported in the female reproductive tract after the vaccination targeting human papilloma virus 16 (45) and liver in hepatitis C infection (46), suggesting the importance of TRM cells in the protective immunity in human as well.
CD4+ TRM cells are usually found within the tissue parenchyma, such as the dermis in the skin. Compared with CD8+ TRM cells, little is known about the characteristics and functions of CD4+ TRM cells. However, this subset may also play important roles in the protective immunity against pathogens in several tissues (47). In mice, the protective roles of CD4+ TRM cells have been reported in Leishmania major infection in the skin (48), herpes simplex virus infection in the genital mucosa (34), Chlamydia trachomatis infection at the reproductive mucosa (49), and Streptococcus pneumonia infection in the lung (50). It remains to be clarified whether those CD4+ TRM cells are really resident in tissues or just a subset of memory CD4+ T cells which spend an extended period time in the tissue before circulation.
Identification and Definition of Skin TRM Cells
As discussed above, the markers that identify tissue TRM cells may differ among the tissues. The characteristic behavior and markers of skin TRM were well studied in murine models. In human, it is technically difficult to address the migratory behavior of skin TRM cells in an in vivo system. The resident memory properties of human skin T cells are largely described on CD8+ T cells with surface markers similar to those of murine TRM cells (23, 51). In this section, we review the current evidence of skin TRM identification, which mostly came from the murine study, and their relevance in human (Figure 1).
Precursors of Skin TRM Cells
Naïve CD8+ T cells proliferate and differentiate into a pool of effector cells upon recognition of cognate antigen. During the effector phase, CD8+ effector cells can be divided into short-lived effector cells (SLECs) and memory precursor effector cells (MPECs) (52). SLECs are characterized by KLRG1hi IL-7Rαlo(CD127), while MPECs are KLRG1lo IL-7Rαhi. The fate decision of SLECs/MPECs depends on a sum of inflammatory signals that create a T-bet gradient, in which a low-level magnitude promotes MPECs fate during T cell priming (52). Almost all SLECs undergo apoptosis, whereas MPECs turn into heterogenous populations of long-lived memory CD8+ T cells after clearance of infection (52). In early skin infection of herpes simplex virus, skin-infiltrating T cells are mainly KLRG1+ effector cells, while at the memory phase, the remaining memory T cells in the skin bear negative or low expression of KLRG1. Consistently, the adoptive transfer study of KLRG1- T cells confirmed that KLRG1- MPECs gave rise to TRM cell populations in the skin (39). Memory T cells also express CD45RO but not CD45RA. Skin-infiltrating T cells isolated from normal human skin were almost all CD45RO+ memory T cells (1). Collectively, skin TRM cells possess the memory precursor phenotype, KLRG1-CD127+CD45RO+CD45RA-.
Skin-Homing Molecules on TRM Cells
Skin-infiltrating memory T cells express a distinct homing receptor called cutaneous lymphocyte-associated antigen (CLA), which binds to E-selectin and P-selectin and allowing CLA+ T cells to enter the skin (1). Nearly all CLA+ effector memory T cells are resident in human skin during steady state (1). Chemokine receptor (CCR)10 is one of the essential chemokine receptors for skin homing of T cells (53), as CCR10-deficient mice showed a reduction of CD8+ T cells in the skin (54). Similarly, CD8+ T cells lacking CCR10 impaired their TRM forming capacity (55). CXCR6 is expressed on skin TRM cells in human (1) and mice (56), and CXC chemokine ligand (CXCL)16, a ligand for CXCR6, is expressed on epidermal keratinocytes and can be released as a chemoattractant (57). T cells lacking CXCR6 had low capacity to form TRM cells in the skin, whereas CXCR6-/- and wild-type T cells were not different in number in the SLOs. Consistently, direct injection of CXCR6-/- CD8+ T cells into the skin also decreased TRM formation, suggesting that CXCR6 is important for retention rather than recruitment of CD8+ T cells to the skin (55). CCR4 is an essential skin-homing molecule for the migration of T cells to the skin (58) and highly expressed on skin TRM cells (1). Mogamulizumab, a humanized anti-CCR4 antibody, was approved for mycosis fungoides (MF) and Sézary syndrome (SS), which are a malignancy of skin-homing malignant T cells (59). However, the exact role of CCR4 on skin CD8 TRM formation is not clear. Previous studies showed that CXCR3 expression is necessary for TRM cell precursors to enter the epidermis, and CD8+ T cells lacking CXCR3 resulted in less formation of CD103+ TRM cells in mice (39). Skin CCR8+ T cells show phenotypic, functional, and transcriptomic profiles compatible with TRM cells (60). CCR8 is expressed on half of cutaneous memory T cells, whereas very few CCR8 is expressed on circulating memory T cells (61). The ligand for CCR8, CCL1, is preferentially expressed in human skin, and keratinocyte-derived prostaglandin E2 and vitamin D3 can induce CCR8 expression by CD8+ T cells, suggesting that it may involve in TRM localization in skin (62, 63). However, the role of CCR8 is currently unclear, since T cells lacking CCR8 can migrate and are maintained in the skin as usual in mouse epidermis following viral skin infection (55). Collectively, CCR10 (53, 64), CCR4 (58), CCR8 (60, 62), and CXCR3 (39) enable memory T cells to migrate to the skin, CLA allowing them to enter the skin (1), and CCR10 and CXCR6 (55) contribute to TRM formation in the skin.
Retention Mechanisms of Skin TRM Cells
The retention properties of skin TRM cells have been widely explored in a murine model. The most recognized markers of skin TRM cells in both humans and mice are CD103 and CD69, which are responsible for TRM retention (65). CD103 is an α-chain of the integrin αEβ7and binds to E-cadherin expressed by keratinocytes (Figure 2) and is the most common and widely accepted TRM marker. CD103 expression on CD8+ TRM is dependent on the TGF-β (39, 66), which is activated by keratinocyte integrins αvβ6 or αvβ8 (67). In mice lacking this keratinocyte-integrin, TRM cells are unable to express CD103 and cannot persist long term in epidermis (67). CD103 on CD8 TRM cells mediate cell adhesion to the epidermis and thus promote local retention (55). Similarly, CD103-/- CD8+ T cells can enter the epidermis but unable to persist long term in the skin as TRM cells (39, 55). TGF-β induces CD103 expression on activated CD8+ T cells, but not CD4+ T cells, and leads to CD103-mediated adhesion of CD8+ T cells, but not CD4+ T cells, to monolayer human keratinocyte cultures (68). This may explain the reason why CD4+CD103+ T cells can exit in the skin, but CD8+CD103+ TRM cells cannot. However, another study showed that TGF-β also induces CD103 expression on CD4+ T cells and mediates cell adhesion to keratinocyte (14). This discrepancy is possibly due to different experimental setups and T cell stimulation methods, and further studies are needed to confirm the function of CD103 on CD4+ T cells. Indeed, CD4+CD103+ cells can be found in human circulation but not CD8+CD103+ cells (69). Moreover, CD69 expression is very dynamic and can be easily induced in vitro upon stimulation (70). By using qPCR, the expression of TGF-β in psoriatic skin is comparable to normal skin, implying that increment of CD103+ T cells in psoriasis does not stem from general upregulation of TGF-β expression (68). In tumor context, the interaction between αE(CD103)β7 on tissue-infiltrating lymphocytes and E-cadherin on tumor cells induces cytolytic granule polarization and subsequent exocytosis, leading to tumor cell lysis (71). This suggests that CD103 also exerts some biological activity in addition to the adhesion property.
CD69 is involved in the residency status of TRM cells by downregulating sphingosine 1 phosphate receptor (S1PR1)-mediated tissue egress (72, 73). The vast majority of skin TRM cells in both mice and humans express CD69 (14, 39, 74). The induction of CD69 expression is strongly influenced by antigen stimulation and exposure to pro-inflammatory mediators (72). CD69 is upregulated shortly after memory T cells reaching the skin and CD69 expression is critical for early T cell retention rather than recruitment of T cell into skin (39, 72). However, a recent parabiosis study demonstrated that CD69 expression is inadequate to define a stable residence (27).
α1(CD49a)β1 integrin is one of the T cell receptors for collagen IV, originally termed as Very Late Antigen (VLA)-1. CD49a is upregulated following T cell activation and can be found on circulating T cells before they enter into the skin (75). CD49a-expressing CD8+ T cells are enriched in the epidermis of human and mouse skin (8, 37). In an HSV infection mouse model, CD49a increased TRM effector function and promoted TRM persistence in the skin, but not required for CD8+ T cell to entry into the epidermis (75). In contrast, in the xenotransplantation model of psoriasis, blocking CD49a inhibits T cell migration into the epidermis, resulting in a decrease of TRM cells and prevention of psoriasis development (76). IL-12 and TGF-β can upregulate CD49a expression on CD8+ T cells (75). Not only CD8+ TRM cells but also CD4+ memory T cells poised for Interferin (IFN)-γ production preferentially express CD49a in human (74, 77). Since IL-12 can induce IFN-γ production and CD49a expression, it is tempting to speculate that in the psoriasis context, IL-17A-producing TRM cells, which preferentially express IL-23R (74), downregulate their CD49a due to a greater influence of IL-23 over IL-12.
Collectively, CD69 is critical for initial formation of TRM cells shortly after T cells enter in the skin, while CD103 is required for T cell adhesion and long-term retention of TRM cells. Ultimately, both CD69 and CD103 are required for TRM formation in the skin. In addition, CD49a regulate the persistence, morphology and effector function of CD8+ TRM cells in the skin.
Characteristics of CD4+ Skin TRM Cells
Compared with CD8+ skin TRM cells, the characteristics and behavior of CD4+ skin TRM cells have been less understood, and probably, they are quite different between mice and humans and remain controversial. In human skin, CD4+ T cells can be found in both epidermal and dermal compartments (14), whereas CD4+ T cells in murine skin are predominantly in the dermis. In fact, human skin has a thicker epithelial layer and lower density of hair follicles that are crucial for residency of CD4+ TRM in mouse skin (78, 79).
Earlier studies showed that the motility of skin-infiltrating CD4+ T cells are higher than that of CD8+ T cells, and they equilibrate with circulating T cell pool at steady state (78, 80). Skin CD4+ memory T cells preferentially accumulate around the hair follicle isthmus and constantly move back and forth to the circulation (78). After cutaneous HSV infection, two distinct HSV-specific memory T cell subsets were found in the skin; the slow-moving CD8+ T cell population resided in the epidermis, particularly at the site of infection, whereas dynamic CD4+ T cell population rapidly trafficked through the dermis and showed recirculation pattern (80). Indeed, we have previously demonstrated a substantial recirculation of CD4+ T cells in the skin to the draining lymph nodes, using a photo-convertible system of Kaede-transgenic mice (81).
A recent study using mice parabiosis experiment identified the CD4+ TRM population with prolonged residency in non-lymphoid tissue, which was separated from the circulation and shared transcriptional signatures with CD8+ TRM cells. However, this study showed only a limited period of 4 weeks of the extent of residency (82), because the prolonged parabiosis was associated with great equilibration for skin CD4+ T cells (78). Another study using alemtuzumab, an antibody targeting CD52 and depleting circulating T cells, showed that CD4+CD69+CD103+ and CD4+CD69+CD103- persist in the skin without replenishment of the circulating compartment, suggesting that they are TRM populations. Similarly, in in vivo studies, CD4+CD69+CD103+ T cells possibly represented a non-migrating resident CD4+ T cell population in the dermis (12, 83). However, the dynamic observation of CD4+ TRM cells in the skin, particularly in human, is technically challenging, and their migratory behavior cannot be excluded. In contrast, the xenografting model with human skin showed that CD4+CLA+CD103+ TRM cells down-regulate CD69 expression, exit from the skin, and reach into the circulation (69). These cells in the blood and skin are clonally related and share their function and transcriptional profiles. CD4+ TRM cells were reported to play a role against skin infection with L. major (13) and C. albicans (12). Recently, resident memory Th2 cells in the lung exhibit a distinct CD4 population and play a critical role in an allergic asthma murine model (19). Furthermore, in experimental colitis, CD4+ TRM cells play a crucial role in the regulation of intestinal inflammation, and they were found in the colon of inflammatory bowel disease patients (16). These studies support the existence and critical role of CD4 TRM cells in tissue-specific immune and inflammatory diseases.
Originally, TRM cell was defined as a memory T cell population that persists long-term in peripheral tissue and do not migrate back to the circulation. According to this definition, not all skin-infiltrating T cells are resident memory T cells. There are only a fraction of these cells that represent the authentic TRM population. A similar definition may be applied to CD4+ TRM cells. In fact, the residence is difficult to quantify, and there are no perfect markers to define a permanent resident T cell. CD103 and/or CD69 may not be sufficient for defining the residence status of skin infiltrating T cells, especially CD4+ T cells (14, 84). Collectively, it is tempting to postulate that CD4+ TRM cells are generally more dynamic and have a distinct migratory behavior compared to CD8+ TRM cells in human skin. Meanwhile, in some inflammation or infection context, CD4+ TRM cells play a crucial role and may persist in the skin for an extended period.
Development of Skin TRM Cells
A different subset of memory CD8+ T cells contribute to an immune memory response in different aspects and locations. Once naïve CD8+ T cells are activated, they differentiate into pooled effector CD8+ T cell populations, which are composed of SLECs and MPECs. MPECs are characterized by CD127hiKLRG1lo populations, while SLECs are KLRG1hi populations. After clearance of inflammation or infection, the majority of SLECs undergo apoptosis, whereas MPECs turns into a heterogeneous subset of memory T cells (85). Historically, memory T cells were divided into central memory (TCM) cells that express high lymphoid homing molecules and recirculate through SLOs, and effector memory T (TEM) cells that lack lymphoid homing molecules (86). From the current literature, memory T cells can be broadly divided into four main populations in the murine model. (1) TCM: expressing lymph node (LN) homing molecules (CCR7+CD62L+CX3CR1-) and mainly surveying SLOs. (2) TEM: expressing CCR7-CD62L-CX3CR1+ and predominantly surveying the blood. (3) peripheral memory T cells (TPM): expressing CCR7+CD62L-CX3CR1int and preferentially patrolling peripheral tissues and migrate to blood and LN. (4) TRM: persisting for a long term in peripheral tissues.
By immunizing mice with a protein antigen, chemical hapten, or non-replicating virus, TRM cells from the treated skin and distant skin as well as the draining and distant LNs contain identical TCR cells in both TRM and TCM compartment, suggesting that TRM and TCM cells may be derived from common naïve T cell precursors (87). However, equal contribution of individual naïve clones to formation of TRM subsets has not been definite. Using a lineage-tracing technique to track individual naïve CD8+ T cells responding to skin vaccination, it was shown that individual T cell clones contribute differentially to the formation of TRM-poised effector T cell subset, which has a capacity to subsequently form TRM population (88). The propensity to form TRM populations is disparately distributed over T cell clones, implying that this fate must be committed before clonal expansion. The heterogeneity of circulating vaccine-specific effector T cell pool can be divided into four distinct populations based on the gene expression profiles, including effector cell, intermediate cell, circulating memory T cell-like precursor, and TRM-like precursor. This study revealed the existence of TRM cell precursor in circulation and their commitment to TRM cells before entering into the skin (88).
The existence of pre-commitment TRM cells in circulation was further supported by an elegance study on the role of dendritic cell in TRM cell formation (89) (Figure 3). This study revealed that the formation of skin TRM cells requires interaction between naïve CD8+ T cells and migratory dendritic cells (DCs) from the skin at a steady state. This process depended on the presence of TGF-β, which activates V-integrins on migratory DCs. In fact, lack of V-integrins on CD11c+ DCs resulted in a substantial reduction in epidermal CD8+ T cells, but did not affect dermal CD8+ T cells or other skin immune populations. The expression of a V-integrins on DCs during immune homeostasis, but not in priming state, was required for pre-conditioning naïve CD8+ T cells for effective TRM cells formation (89). Therefore, TRM fate decisions on T cells seem to happen earlier than expected, and this event appears to be controlled primarily by a cross-talk between local skin and draining LNs via DCs. Indeed, DCs are able to instruct T cells to migrate to a specific location. For example, DCs in skin-draining LNs and mesenteric LNs induce the expression of tissue homing molecule that elicits tropism for skin and gut, respectively (90, 91). Earlier studies showed that individual naïve T cells contribute differentially to short-term effector cells and long-term memory cells, and the fate of each naïve T cells is unpredictable (92). However, the subsequent study revealed the clonal bias of TRM precursors within heterogenous memory populations (88).
Non-specific inflammation is sufficient to attract CD8+ T cells into the inflamed tissue and adopt TRM cells in the skin (93, 94), suggesting that TRM cells in the skin do not require cognate antigen for their establishment. Basically, the skin immune cells respond to an invader such as hapten and secrete pro-inflammatory cytokines that induce dendritic cell migration and maturation (95). Endothelial cells increase the expression of adhesion molecules; CD54 (ICAM-1) and CD106 (VCAM-1), which guide T cell entry into the tissue. In addition, chemokines, Chemokine ligand (CCL)2 to 5, CXCL9, and CXCL10 are secreted from keratinocyte and innate immune cells, and this initial step is induced by a non-specific inflammation process and is a fundamental mechanism to recruit T cells into inflamed skin (96). However, the presence of cognate antigens enhances TRM cell formation. Moreover, antigen challenges at the skin lead to generalized seeding of antigen-specific TRM cells, which are found at the highest density at sensitizing area (39, 87).
Maintenance of Skin TRM Cells
A whole-genome bisulfate sequencing study suggests that TRM cells have a high plasticity and a development potential comparable to TCM and TEM cells, indicating that they are not terminally differentiated (97). In addition, TRM cells can proliferate in situ in response to viral challenge, further supporting their as yet undifferentiated status (94). Different factors are required for maintenance of TRM cells, depending on individual tissues (98). Skin CD8+ TRM cells can be maintained in the skin for a long period (65, 87). Several factors, including local antigens, cytokines, and metabolites, contribute to TRM maintenance (Figure 4). A disparate level of skin residency may exist in skin TRM cells. While certain subsets of skin TRM cells have long-term residency, other subsets transiently reside in the skin and possibly migrate out to the circulation.
Effects of Cognate Antigens
Although local antigen is not required for skin recruitment of circulating CD8+ T cells to obtain the TRM phenotype, antigen exposure greatly amplifies the number of CD8+ TRM cells (99). Local antigenic challenge induces antigen-specific TRM cell proliferation, and they are maintained as epidermal TRM pool (94). Intriguingly, the subsequent pool of TRM cells after antigen reencounter is generated mainly from the pre-existing TRM cell population, rather than from circulating memory T cell compartment (94, 100). A self-sustained capacity of TRM cells in the skin seems to be independent of CD4+ helper T cells and CD11c+ cells (100). The contribution of circulating memory T cells in the local immune response may depend on the density of the pre-existing TRM population, suggesting the flexibility of circulating TCM cells to support TRM population. Moreover, even with the newly seeded, unrelated TRM population in the skin, the number of pre-existing TRM cells remain largely unchanged. Initial activation of skin TRM cells requires antigen recognition, which represents TRM-mediated skin protection and is ultimately changed to an antigen independent reaction (101). TRM cells thus exert a protection capacity, depending on their local density in skin (94). A question arises as to how local antigen influences composition of skin TRM cells from a pool of polyclonal skin-infiltrating memory precursors during active infection or inflammation. It has been revealed that local antigen-dependent cross-competition contributes to shaping the polyclonal TRM cell repertoire in the skin, whereas this event is not observed in SLOs (102). Therefore, the local antigen-dependent self-amplification and cross-competition processes may serve as a mechanism to modulate local TRM composition in response to a variety of invaders and responsible for maintenance of TRM cell population in skin.
Fatty Acids for the Maintenance of Skin TRM Cells
One of the basic needs for life is food. The skin has a unique microenvironment where lipids are rich even with shortage of nutrients. Skin TRM cells reside in the epidermis, and thus, they are relatively independent from blood circulation. Although nutrients may diffuse from the dermis to the epidermis, the local energy source seems to be required for TRM cells. Fatty acid binding proteins (FABPs) are a group of intracellular molecules that mediate lipid trafficking and metabolism (103). FABPs originally consist of adipose FABP (A-FABP) and epidermal FABP, which encoded by Fabp5. E-FABP is expressed on keratinocytes and immune cells, including T cells and macrophages (104). High-fat diet upregulated E-FABP expression and promote skin inflammation, suggesting the role of lipid metabolism in immune regulation (105). Recently, it was shown that CD8+ TRM cells utilize exogenous lipids in the skin as an energy source for their survival. T cells lacking Fabp4 and Fabp5 cannot uptake and utilize exogenous free fatty acid (FFA), which results in a reduction of long-term survival and impaired functional properties of CD8+ TRM cells in vivo. This deficiency has no effect on TCM cell survival. Interestingly, the significance of lipid metabolism for TRM survival is increased over time, suggesting metabolic adaptation to the skin environment. It is proposed that CD8+ TRM cells utilize local lipid as an energy source to maintain their functional competence and longevity in the skin. Similarly, CD8+ TRM cells in the skin also increase the expression of FABP4 and FABP5 (106). It seems that the impact of FABP deficiency is not only limited to CD8+ TRM cells but also affects CD4+ T cells and DCs. Upregulation of FABPs on CD4+ T cells promotes IL-17 expression, while the loss of FABPs is associated with enhanced expression of FoxP3 (104), suggesting the role of E-FABP and Th17/Treg balancing. In addition, FABP-deficient mice showed an altered antigen-presenting function of dendritic cells and macrophages (107). The limitation of energy resources in the epidermal niche possibly influences the TRM cell density and survival. A recent study demonstrated that CD8+ TRM cells displace pre-existing dendritic epidermal T cells (DETCs) from the epidermis because they have a superior metabolic fitness (108).
Cytokines
Despite the likeness between IL-15 and IL-2, including shared receptor subunit, IL-15 has a perceptible difference in immunomodulatory properties (109). Basically, IL-15 promotes proliferation and survival of circulating memory CD8+ T cells but did not affect regulatory T cell populations in human (110, 111). IL-15 deficient mice showed a reduction of CD8+ TRM cell number (39, 112) but slightly increased CD4+ TRM cells in the skin, while the numbers of CD8+ T cells and CD4+ T cells in SLOs were not different between IL-15-deficient and WT mice (112). Keratinocytes at hair follicle has been shown as the main source of IL-15 for maintaining CD8+ TRM cells in the skin. In addition to IL-15, IL-7 from hair follicle also influence on both CD8+ TRM and CD4+ TRM cells persistent in the skin. However, the requirement of IL-15 for TRM maintenance may vary depending on the tissue and context of inflammation (41). Apart from maintenance property, IL-15 strongly induces perforin and granzyme B expression in CD8+CD103+CD49a+ TRM cells but not in CD8+CD103+CD49a- TRM cells isolated from normal human skin (74). TGF-β is a pleiotropic cytokine that is produced in an inactive form that requires specific integrins on keratinocyte to activate them (113). Activated-TGF-β induces CD8+ TRM cells to express CD103, which is mandatory for their retention and long-term persistence in the skin (39, 55). Collectively, keratinocytes play an important role in establishing long-term TRM cell populations by providing local mediators like IL-15, IL-7, and activated TGF-β.
Skin TRM Cells in Cutaneous Defense System Against Pathogens
Although the pathophysiological roles of skin TRM cells encompass several aspects (65), they serve primarily as a critical component of cutaneous immune defense. TRM cells act as peripheral sentinels providing rapid immune response against invading pathogens (114). Infection with pathogenic microorganisms leads to directed homing of T cells to the appropriate tissues, such as the skin. Subsequently, most antigen-specific memory T cells reside in the non-lymphoid organs, convey tissue-resident memory, and mount durable protective immunity in the skin.
Virus is a major pathogen to which skin TRM cells respond, and a number of valuable findings have been obtained from studies on virus infection. TRM cells can autonomously regulate the local TRM composition to mediate immunosurveillance independently of circulating memory T cells (94, 100). Skin TRM cells are activated and proliferate in situ upon encounter with virus-infected cells, and do not migrate out of the skin. As a consequence, secondary TRM cell populations were mainly derived from pre-existing TRM cell populations and the precursors recruited from the circulation. In subsequent infections, the pre-existing skin TRM cell populations are not displaced by the newly generated TRM cells, enabling multiple TRM cell specificities to maintain a diverse immune response within the tissue (94). Consistently, mucosal TRM cells are highly motile, but pause and undergo in situ division after local antigen challenge. TRM cell reactivation triggers the recruitment of recirculating memory T cells that undergo antigen-independent TRM cell differentiation in situ. The proliferation of pre-existing TRM cells dominates the local mucosal recall response and contribute most substantially to the boosted secondary TRM cell population (100).
CD8+ TRM cells seem to play a major role in cutaneous defense against virus. After resolution of skin vaccinia virus infection, antigen-specific circulating memory CD8+ T cells migrate into the skin. Memory T cells that reside at these surfaces provide a first line of defense against subsequent infection (6, 115, 116).
The local cytokine environment within the skin determines the differentiation state and persistence of the central and peripheral memory-T-cell pool (67). CD8+CD103+ TRM cells develop in the skin from epithelium-infiltrating precursor cells that lack expression of the effector-cell marker. Following the entry of the T cells into the epidermis, the local mediators such as IL-15 and transforming growth factor (TGF)-β are required for the formation of long-lived TRM cell population in skin (39). The retention of tissue-resident memory T cells is mediated by TGF-β, which up-regulates CD103 expression and down-regulates CCR7 expression. Besides microbial pathogens, topical application of chemical allergen to skin causes delayed-type hypersensitivity and amplifies the number of antigen-specific CD8+ TRM cells at challenged site (117). Expanded TRM CD8+ T cells in the skin are derived from memory T cells recruited out of the circulation. Expanded TRM CD8+ T cells significantly increase anti-viral protection.
In addition to CD8+ cells, CD4+ TRM cells are also involved in microbial defense. CD4+ TRM cells play a role in cutaneous fungal infection (12). Candida albicans (C. albicans) is a common dimorphic fungal pathogen to which human subjects are exposed early in life, and by adulthood. In a C. albicans infection mouse model, dermal γδ T cells producing IL-17 are the main effector cells in the initial infection, and then, αβTh17 effector T cells become predominant. By day 30 after infection, the CD4+ TRM cells become the main population of IL-17-producing T cells that react to C. albicans. Between 30 and 90 days after infection, these reactive CD4+ T cells acquire expression of CD69 and CD103, the retention markers, and reside in the papillary dermis. These TRM cells are more effective to eradicate C. albicans than recirculating T cells (12).
Recently, the preclinical studies on TRM-targeted vaccination have shown a favorable outcome. Intranasal (118) and mucosal (119) administration of vaccine generated protective TRM cells in the lung and airway of mice. Direct vaccination (118, 119) or delivery vaccine vectors to a specific tissue (120, 121), rather than parenteral route, generated antigen-specific TRM cells, thereby mediating effective protection independent of circulating memory T cells. In addition, a “prime and pull” strategy (122), which combines vaccination with local application of chemokines, effectively generated TRM cells. These studies suggest that protective TRM cells can be generated through vaccination, especially tissue-targeted approaches that give a better protection than ordinary parenteral route. Since the skin is an accessible tissue for administration of vaccine, a question arises whether immunization through the skin can generate TRM cells in other organs or barrier tissues. In fact, the smallpox vaccine, which is one of the most effective vaccine in history, was delivered by skin scarification (123). In a murine model, the localized virus skin infection (35) or skin immunization (87) can generate antigen-reactive TCM cells and skin TRM cells that reside within the entire skin and possibly in the lung (124). Besides, the combination of “prime and pull” with a prime boost approach was reported to be very effective to produce protective TRM cells (125). These suggest the possible role of the skin as a TRM-targeted vaccination strategy. Further understanding of how skin dendritic cells shape the TRM precursor pool (89), which have a potential to transform into tissue-specific TRM cells, may provide a crucial information for the development of TRM-targeted vaccination. Furthermore, skin resident memory T cells also play a protective role in skin infection, such as HSV (35), C. albicans (12), leishmania major (13), and in skin cancers, such as melanoma (126) and squamous cell carcinoma (127). They also play a pathogenic role in some autoinflammatory skin diseases; vitiligo (9, 128), psoriasis (8) and alopecia areata (10). Thus, the vaccination-induced TRM cell strategy may also have a potential to become a novel therapeutic approach to protect the skin from infection, prevent tumor growth, or suppress autoreactive immune responses.
Skin TRM Cells in Psoriasis
Psoriasis is a common chronic inflammatory skin disease, and the pathogenesis underlying psoriasis has been extensively studied (Figure 5). CD4+ T cells producing interleukin (IL)-17, named Th17 cells, play an essential role in its pathogenesis (129). Th17-derived cytokines, IL-17A, IL-17F and IL-22, induce epidermal acanthosis, which represents an intriguing histological finding of psoriasis and results from the proliferation of epidermal keratinocytes. These mediators stimulate keratinocytes to produce TNF-α, IL-8, and vascular endothelial growth factor, thereby promoting inflammation, neutrophil recruitment, and angiogenesis (129). For maintenance of Th17 cells, IL-23 is required and secreted from inflammatory DCs or TNF-α and iNOS-producing DCs (TIP-DCs) Psoriasis and other Th17-mediated skin diseases (129). Epidermal Langerhans cells are another source of IL-23 in a certain condition (130). Keratinocytes are also activated by their own cytokines, such as IL-17C, IL-36, and TNF-α, in an autocrine manner (131, 132). In addition, antimicrobial peptides released from keratinocytes and (IFN)-α from plasmacytoid DCs has been considered to play initiative roles for the development of psoriatic lesions (133). Meanwhile, a self-regulatory autocrine mechanism is disturbed in epidermal keratinocytes of psoriasis patients (134).
The cytokine network in psoriasis has been proven by the therapeutic effectiveness of biologic antibodies that block individual cytokines, including TNF-α, IL-23/IL-12p40, anti-IL-23p19, IL-17A, and IL-17 receptor (135). Although biological drugs are effective, there are variations in the responsiveness between patients (136). Moreover, upon withdrawal of the biologics, the skin lesions often recur. Psoriasis plaques are seen in a recurrent manner especially at the originally affected sites (137). Thus, even after clearance of skin lesions, some immunocompetent cells possibly remain in the previously affected, currently normal-appearing skin. A number of studies have suggested the pathogenetic role of skin TRM cells in psoriasis (8, 74), particularly as a strong candidate that evokes recurrence (2). Notably, TRM cells in psoriatic skin can produce certain cytokines and decreased in number after improvement (74). CD8+ TRM cells reside even in disease-naïve, non-lesional sites of psoriasis patients possibly in correlation with disease duration (138).
The skin TRM cells are positive for tissue-retention markers CD103 and CD69, but negative for lymphoid homing markers CD62L and CCR7 (139). Double immunofluorescent staining for CD3, CD4, or CD8 (red) along with CD103 (green) is shown, and the merged yellow color represents cells positive for both (Figure 6). CD3+ T cells infiltrate into both epidermis and dermis, and majority of the T cells in the epidermis co-expressed CD103. CD4+ cells mainly infiltrate in the dermis and scarcely express CD103. CD8+ cells infiltrating in the epidermis are positive for CD103, while those in the dermis were mostly CD103-. Thus, the majority of epidermal T cells are CD8+CD103+ TRM cells and a small number of CD4+CD103+ TRM cells infiltrate in the dermis. A few CD8+CD103+ TRM cells are present in the papillary and subpapillary layers. The number of CD8+CD103+ TRM cells in the epidermis tends to correlate with the epidermal thickness (70), suggesting the role of TRM cells in the formation of psoriatic lesions.
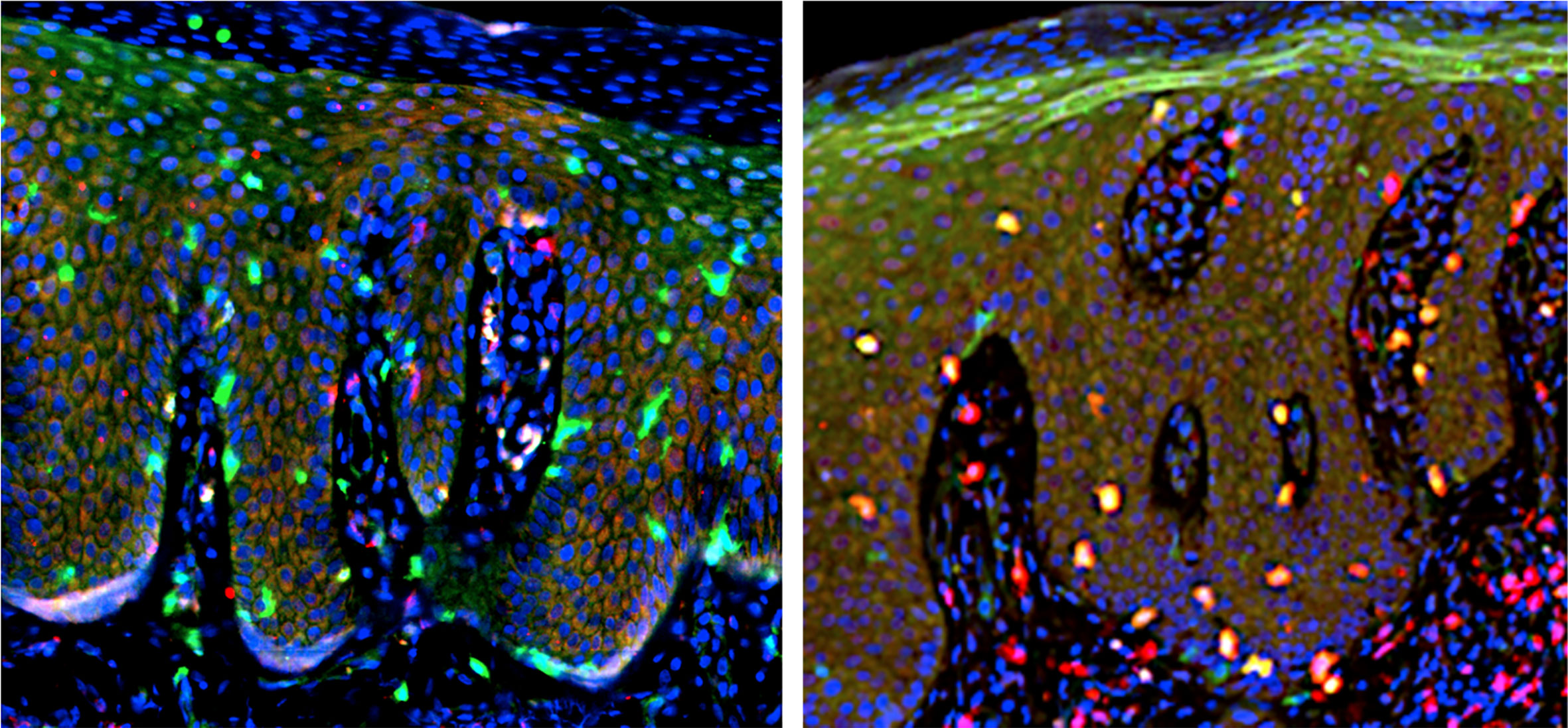
Figure 6 Double immunofluorescent staining. Left: CD4 (red) and CD103 (green). Right: CD8 (red) and CD103 (green). Merged yellow color (right) indicate cells positive for both CD8 and CD103, representing TRM cells.
When CD103+, CD103-, CD69+, and CD69- T cells were isolated and expanded ex vivo with anti-CD3/CD28 Ab and IL-2 (140–142), the positive and negative expression of CD103 was unchanged (70). However, CD69 expression can be changed bidirectionally by cultivation, suggesting the unsteady, fluctuated expression of CD69. By using skin-derived, ex vivo expanded T cells (140–142), we conducted to characterize the cytokine profile of CD103+ skin TRM cells, especially, epidermal CD8+CD103+ TRM cells (39, 74). In T cell samples expanded from psoriasis lesional skin, a part of CD8+ T cells co-expressed CD103, and this CD8+CD103+ T cells are considered to be epidermal TRM cells. CD4+CD103+ cells are present at a much lower frequency. CD103+ T cells were mostly CD8+CD45RO+CD45RA-CD69+ memory T cells with a skin-homing potential, i.e., partially CCR6+ and mostly CCR7-CD62L-. They contained both CXCR3+CD49a+ and CXCR3-CD49a- populations. These findings are in accordance with the importance of CD8+ T cells in psoriasis pathogenesis (138, 143–145).
The cytokine production pattern of skin TRM cells has been a crucial issue, because their function is generally determined by the released cytokines. Skin TRM cells remain longer in the same position than effector memory T cells (51) and produce certain cytokines in relation to psoriatic etiology (39, 74, 146). CD103+ TRM cells produce IFN-γ, IL-17A, and IL-22 (39, 74, 147). In the ex vivo expanded T cells, certain populations of CD8+CD103+ T cells produce IFN- γ, IL-17A or IL-22, while CD4+CD103+ T cells scarcely elaborate these cytokines. In CD8+ T cells, CD103+ TRM cells more frequently produce IL-17A than CD103- T cells. Thus, CD8+CD103+ TRM cells efficiently produce IL-17A.
The sorted CD103+ cells expressed CXCR3 or CD49a at a frequency of 28%, sharing the feature with Tc1 or reported IFN-γ-producing T cells (39, 74). The counterpart cells were CD49a negative or low, supposedly corresponding to IL-17A-producing T cells (39, 74). Taken together these observations, CD8+CD103+ TRM cells can be divided into two types: CD49a-IL-17A+ and CD49a+IFN-γ + types. It is assumed that the former type is closed associated with psoriasis, while the latter type play a role in vitiligo (74).
Skin TRM cells are associated with not only the development of psoriasis (39, 138, 139), but also its clinical course. TRM cells producing IL-17A in resolved psoriasis epidermis could be associated with early relapse (148), and CD8+ TRM cells with IL-17A-producing potential in disease-naïve, non-lesional sites possibly correlate with disease duration (138). Thus, IL-17A-producing CD103+ TRM cells may have an influence on the future clinical course of psoriasis. We surveyed the 10 patients as to whether oral cyclosporine, oral phosphodiesterase 4 (PDE4) inhibitor or systemic biologics was initiated within one year after the biopsy. The results showed that the patients having entered these advanced therapies possessed higher frequencies of CD8+CD103+IL-17A+ TRM cells (70). Among CD103+ T cells, the frequencies of CD8+CD103+IL-17A+ and CD4+CD103+IL-17A+ cells tended to be higher in the advanced therapy group than in the non-advanced therapy group. The CD8+ TRM cells showed a high frequency compared with the CD4+ TRM cells. Thus, IL-17A-producing CD8+CD103+ TRM cells may be associated with a progressive clinical course of psoriasis rather than the severity of skin lesions. One can speculate that upon provocation of the skin with stimulants causing Köbner phenomenon, reactivated CD8+CD103+ TRM cells initiate the psoriatic condition with IL-17A.
Skin TRM Cells in Vitiligo
Vitiligo is an autoimmune skin pigmented disorder mediated by autoreactive IFN-γ- producing CD8+ T cells that attack melanocytes, leading to loss of skin pigmentation (Figure 7). The appearance of vitiligo in melanoma patients treated with anti-PD-1 immune checkpoint inhibitors is well known as an immune-related adverse event. Autoreactive cytotoxic lymphocytes (CTLs) against normal melanocytes as well as melanoma tumor cells are activated by the antibody therapy (149).
When aberrantly activated, skin TRM cells have a profound role in vitiligo and melanoma (128). CD8+CD103+CD69+CD49a+ TRM cells serve as CTLs (74, 143). Accordingly, most of CD8 TRM cells express CXCR3 in vitiligo, indicating inclusion of the population of melanocyte-specific CD8 T cells, which display increased production of IFN-γ and tumor necrosis factor-α with moderate cytotoxic activity (143). Autoreactive TRM cells are also present in mouse models of vitiligo. However, it was found that not only skin TRM, but also recirculating memory T cells, plays a role in the development of vitiligo (150). They sense autoantigen in the skin long after stabilization of disease and produce IFN-γ, which further induces CXCL9, and CXCL10 production. Blockade of recirculating memory T cell recruitment to the skin with FTY720 or depletion of them with an antibody reverse disease, indicating that recirculating memory T cells cooperate with TCM to maintain disease (150).
Targeting of TRM cells could become a promising treatment strategy for vitiligo. Moreover, recent evidence demonstrates that induction of melanoma-reactive TRM cells is needed to effectively control tumor growth (9). In a murine model, IL-15 is essential for TRM formation and functions. Both human and mouse TRM cells express IL-15Rβ subunit CD122, and that keratinocytes or other antigen presenting cells up-regulate the expression of IL-15Rα subunit CD215, thereby promoting activation of T cells. Blocking the IL-15 signaling with an anti-CD122 antibody improves the skin depigmentation in mice with established vitiligo. Although prolongation of treatment with anti-CD122 antibody depletes TRM cells from the skin lesion, and the short-term treatment with systemic or local anti-CD122 antibody inhibits IFN-γ production from TRM cells and promotes skin repigmentation (151). Thus, targeting IL-15 signaling via CD122 may be a promising therapy for vitiligo.
Skin TRM Cells in Cutaneous Lymphomas
Cutaneous T-cell lymphoma (CTCL), encompassing mycosis fungoides (MF), Sézary syndrome (SS) and other variants, is a mature T-cell lymphoma, which is currently thought to develop primarily in the skin by a clonal expansion of a transformed, TRM cell (14, 112, 152, 153).
In the epidermis, both CD8+CD103+ and CD4+CD103+ TRM are present and have potent effector functions (14), although the former CD8+ population is present at a higher frequency in the normal and psoriatic lesional skin (70, 138, 142). Skin TRM in the dermis are CD4+CD69+CD103-. In recirculating T cells, there are CCR7+L-selectin+ central memory T cells (TCM) and CCR7+L-selectin- skin-tropic migratory memory T cells (TMM). Clonal malignant T cells from the blood of Sézary syndrome (SS) patients universally coexpress CCR7 and L-selectin as well as the differentiation marker CD27, a phenotype consistent with TCM cells (14). CCR4 is also universally expressed at high levels, and there is variable expression of other skin addressins (CCR6, CCR10, and CLA). In contrast, T cells isolated from MF skin lesions lack CCR7/L-selectin and CD27 but strongly express CCR4 and CLA, a phenotype suggestive of skin TRM cells (152). CD4+ and CD8+ skin TRM cells reside predominantly within the hair follicle epithelium. Hair follicle expression of IL-15 is required for CD8+ skin TRM cells, and IL-7 for CD8+ and CD4+ skin TRM cells, to exert epidermotropism (112).
However, the skin TRM origin concept for the development of MF does not explain the occurrence of multiple, widespread skin lesions. A whole-exome sequencing approach to detect and quantify TCR-α, β, and γ clonotypes in tumor cell clusters suggests the existence of multiple T-cell clones within the tumor cell fraction, with a considerable variation between patients and between lesions from the same patient (153). Thus, circulating neoplastic T-cell clones may continuously replenish the lesions of MF, thus increasing their heterogeneity by a mechanism analogous to the consecutive tumor seeding.
Adult T-cell leukemia/lymphoma (ATLL) is a malignancy of mature T cells caused by human T-cell leukemia virus type I. Approximately 50% of ATLL patients exhibit skin lesions where malignant CD4+CD25+ T cells histologically show epidermotropism (154). We documented a case of adult T-cell leukemia/lymphoma (chronic type), which had a phenotype of CD4+CD25+CD69+CD103+ TRM cells (155), indicating the TRM property of this case and the presence of TRM malignancy in cutaneous lymphomas other than MF. Taken together these observations in CTCL and ATLL, the vast majority of cutaneous lymphomas are derived from skin CD4+ TRM cells.
It has been reported that some patients with MF have malignant CD8+ T cells instead of CD4+ T cells. Accordingly, a case of CD8+ primary cutaneous peripheral T-cell lymphoma arising from skin TRM cells was also reported (156). Pagetoid reticulosis is histologically characterized by dense infiltration of atypical mononuclear cells in the epidermis that produce a pagetoid appearance. This unique disease is historically divided into the localized type (Woringer-Kolopp disease) and the disseminated type (Ketron-Goodmann disease). However, a case showing progression from the former to the latter was documented (157), and currently, pagetoid reticulosis is regarded as a subtype of MF. In the immunohistochemical phenotype, cases of pagetoid reticulosis can be divided into three subtypes: CD4+ (37.5%), CD8+ (29.2%), and CD4-CD8- (33.3%) types (157). While the single positive types are derived from αβ T cells, the double negative type originates from γδ T cells. It should be noted that one third of pagetoid reticulosis cases are CD8+, suggesting that this subtype is an epidermal CD8+ TRM cell tumor (Figure 8). The pagetoid fashion of this tumor may reflect the nature of skin TRM cells.
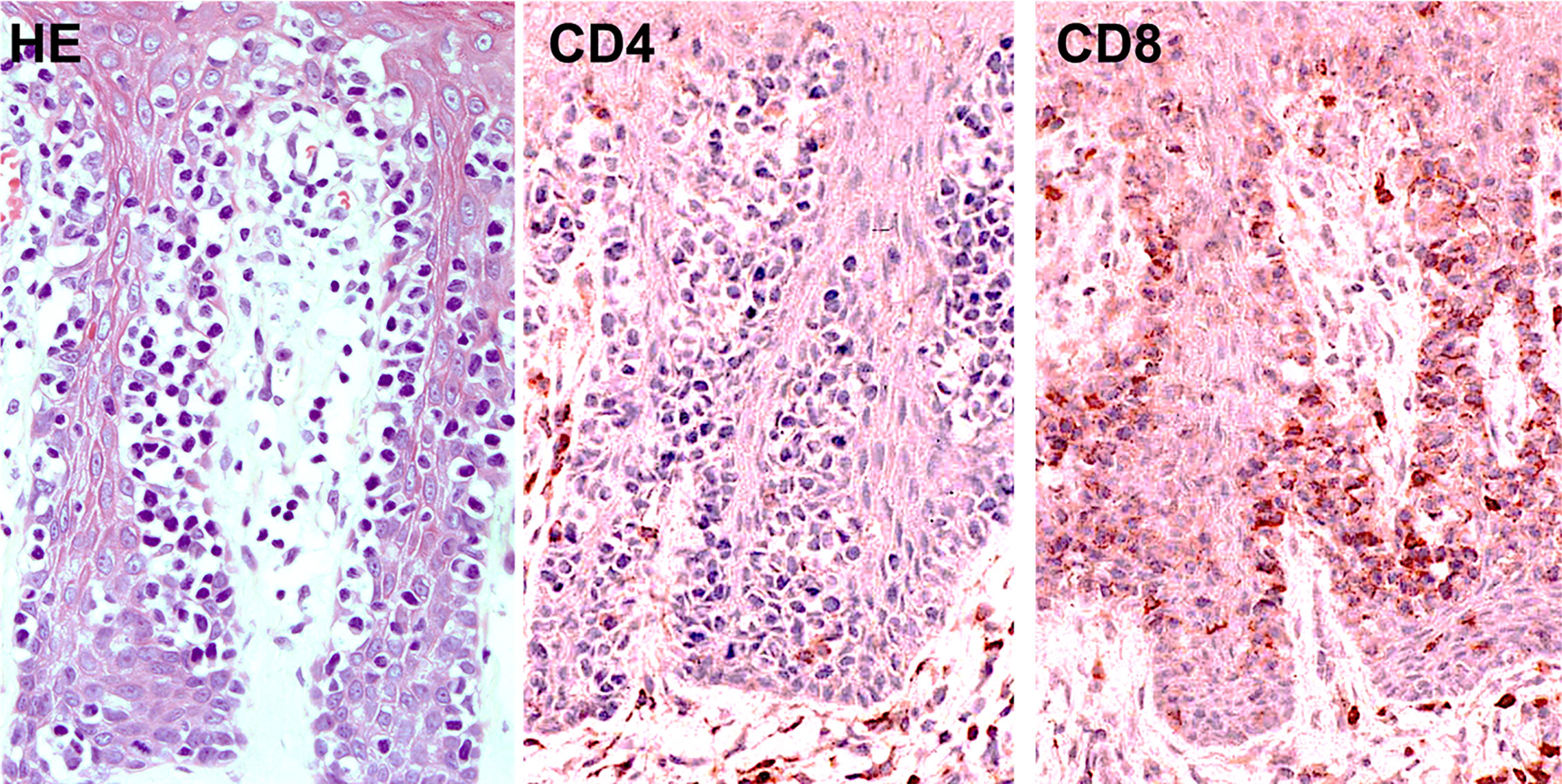
Figure 8 Histopathology (left; hematoxylin and eosin, HE) and immunostaining for CD4 (middle) and CD8 (right) in CD8+ pagetoid reticulosis.
Skin TRM Cells in Fixed Drug Eruption
Fixed drug eruption is induced by skin TRM cells (Figure 9). CD8+ TRM cells in the epidermis possess an effector-memory phenotype and play a role in development of localized tissue damage in fixed drug eruption (7). These epidermal CD8+ T cells constitutively express an early activation marker CD69 even before challenge. A large proportion of these CD8+ T cells exhibit immediate effector function as proven by the rapidly increased IFN-γ production after challenge, resulting in localized epidermal injury. In addition, the intracellular cytokine assay ex vivo supports the great capability of these T cells to produce IFN-γ (158).
Although reactivation of these CD8+ TRM cells is sufficient to initiate the lesion, the recruitment of circulating CD4+ and CD8+ T cells is necessary to cause extensive tissue damage observed in the fully evolved lesions. The abundance of regulatory T cells in the epidermis of fully evolved lesions would serve to limit aberrant immune reactions. Local IL-15 production from lesional epidermis could maintain the survival of the epidermal CD8+ TRM cells even without antigen stimulation over a prolonged period of time (159).
The presence of TRM cells in the epidermis and ocular surface may also play a key role in immune activation and antigen recognition. Some evidence supports the role of TRM cells in Stevens-Johnson syndrome and Toxic epidermal necrolysis, and disease distribution may relate to their site-predominance (160).
Discussion
One of the important issues on the residency status of skin TRM cells in which what conditions allow TRM cells to emigrate from the tissue is under debate. Skin TRM fate decision seems to be established prior to antigens recognition. Once these naïve T cells encounter with cognate antigen presented by DCs, these pre-conditioned T cells will be ready to become a skin-homing TRM precursor, implying that preconditioned naïve TRM cells are prepared during homeostasis, and skin-homing molecules are imprinted during T cell priming (89). Inflammatory signals from inflamed skin attract these skin-homing cells to the local inflammation site. After entering the skin, local signals induce TRM precursors to differentiate into mature skin TRM cells. The non-differentiated TRM precursors may recirculate between the skin, blood and LNs, where these cells possibly represent circulating memory T cells that have been described as skin recirculating memory T cells in mice (67) or skin-tropic migratory memory T cells in human (14). Interestingly, skin recirculating memory T cells are induced greatly by skin infection but not by intravenous infection (67). Moreover, a very recent study reported that skin TRM could exit their residential skin and rejoin the circulating pool of memory T cells (97). In human ex vivo skin experiments, using the nanobody labeling technique also demonstrated that CD8+ TRM cells can migrate from the epidermis to the papillary dermis (161). However, whether TRM cells that migrate out of the skin are authentic TRM cells or these cells are skin recirculating memory T cells that intermittently present in skin remains to be elucidated.
Memory T cell populations are more diverse and heterogeneous than initial expectation, and tissue memory responses may be involved beyond the TRM cell population. Recently, a novel concept of tissue memory beyond the role of adaptive immune memory has emerged. The inflammatory memory can be exerted by various cell types and the interaction among these memories across cell lineages and may impact on tissue adaptation and maladaptation (162). It should be noted that the characteristics and behavior of TRM cells are different among barrier tissues, as each barrier tissue has specialized cells residing in each location, as exemplified by keratinocytes in the skin. A chemical allergen like DNFB can persist in the skin for several weeks, especially in keratinocytes around hair follicles, a part of which are slow-cycling epidermal stem cells (99). This remaining allergen in keratinocytes correlate with the number of antigen-specific CD8+ TRM cells (99). This epithelial memory may contribute to or instruct immune memory cells, and they coordinate each other to maximize the protection. CD8+ TRM cells that we have observed may just only a tip of the iceberg in the process of tissue memory responses.
In several cutaneous diseases, the presence of skin TRM cells has been investigated in the active lesional skin and resolved lesional skin along with non-lesional, normal appearing skin. Unexpectedly, in the active lesion, it is no easy task to identify and enumerate TRM cells, because many T cell populations are intermingled with each other and their activity, residency, and fate cannot be easily expected. For example, the involvement of TRM cells in the recurrent lesions of psoriasis and fixed drug eruption are well known. However, it remains a matter of debate whether the cells with TRM markers in the active lesions belong to TRM cells. We have only limited information on the activity and residency of these cells in relation to the clinical significance.
In our clinical study in psoriasis patients, the cells with TRM markers were increased in the active skin lesion and decreased after the systemic treatment with anti-IL-17A mAb, although they were relatively resistance to the treatment compared to the non-TRM cells (142). In addition, T cells bearing TRM markers in the active lesion were capable of producing pathogenic cytokines, such as IL-17A, and were possibly related to the unfavorable disease course (70). In active skin lesion, CD8+CD103+ cells tended to be present in the middle to upper epidermis, while they were located at the basal layer in the resolved skin and non-lesional skin of psoriasis. Therefore, TRM cells or TRM marker-bearing cells behave as effector cells and likely serve as crucial effectors in psoriasis pathology. Further investigations on their dynamics, detailed functions, and residency are required. Furthermore, to see the disease specificity of these TRM cells, TRM characterization in atopic dermatitis is in progress in our laboratory.
Author Contributions
Concepts: YT. Wrote the paper: YT, PP, TH, and TF. Designed the figures: PP, YT, and TH. Reviewed manuscript: TH and KK. All authors commented on the manuscript. All authors contributed to the article and approved the submitted version.
Conflict of Interest
The authors declare that the research was conducted in the absence of any commercial or financial relationships that could be construed as a potential conflict of interest.
Abbreviations
ATLL, Adult T-cell leukemia/lymphoma; CCL, Chemokine ligand; CLA, Cutaneous lymphocyte-associated antigen; CTCL, Cutaneous T-cell lymphoma; CTLs, cytotoxic lymphocyte; CXCL, CXC chemokine ligand; CXCR, CXC chemokine receptor; DCs, Dendritic cells; DETCs, Dendritic epidermal T cells; FABPs, Fatty acid binding proteins; FFA, Free fatty acid; HSV, Herpes simplex virus; IFN, Interferon; IL, Interleukin; iNOS, Inducible nitric oxide synthase; KLRG1, Killer cell lectin-like receptor subfamily G member 1; LN, Lymph node; MF, Mycosis fungoides; MPECs, Memory precursor effector cells; PD-1, Programmed cell death protein 1; PDE4, Phosphodiesterase 4; PD-L1, Programmed cell death ligand 1; S1PR1, Sphingosine 1-Phosphate Receptor 1; SLECs, Short-lived effector cells; SLOs, Secondary lymphoid organs; SS, Sézary syndrome; TCM, Central memory T cell; TEM, Effector memory T cell; TMM, Skin-tropic migratory memory T cell; TPM, Peripheral memory T cell; TRM, Resident memory T cell; Th, Helper T cell; Treg, Regulatory T cell; TCR, T-cell receptor; TILs, Tumor-infiltrating lymphocytes; TIP-DCs, TNF-α iNOS producing dendritic cells; TNF, Tumor necrosis factor; VLA, Very late antigen protein.
References
1. Clark RA, Chong B, Mirchandani N, Brinster NK, Yamanaka K, Dowgiert RK, et al. The Vast Majority of CLA + T Cells Are Resident in Normal Skin. J Immunol (2006) 176(7):4431–9. doi: 10.4049/jimmunol.176.7.4431
2. Matos TR, O’Malley JT, Lowry EL, Hamm D, Kirsch IR, Robins HS, et al. Clinically resolved psoriatic lesions contain psoriasis-specific IL-17-producing αβ T cell clones. J Clin Invest (2017) 127(11):4031–41. doi: 10.1172/JCI93396
3. Schenkel JM, Fraser KA, Vezys V, Masopust D. Sensing and alarm function of resident memory CD8+ T cells. Nat Immunol (2013) 14(5):509–13. doi: 10.1038/ni.2568
4. Morris SE, Farber DL, Yates AJ. Tissue-Resident Memory T Cells in Mice and Humans: Towards a Quantitative Ecology. J Immunol (2019) 203(10):2561–9. doi: 10.4049/jimmunol.1900767
5. Mackay LK, Wynne-Jones E, Freestone D, Pellicci DG, Mielke LA, Newman DM, et al. T-box Transcription Factors Combine with the Cytokines TGF-β and IL-15 to Control Tissue-Resident Memory T Cell Fate. Immunity (2015) 43(6):1101–11. doi: 10.1016/j.immuni.2015.11.008
6. Watanabe R. Protective and pathogenic roles of resident memory T cells in human skin disorders. J Dermatol Sci (2019) 95(1):2–7. doi: 10.1016/j.jdermsci.2019.06.001
7. Shiohara T. Fixed drug eruption: pathogenesis and diagnostic tests. Curr Opin Allergy Clin Immunol (2009) 9(4):316–21. doi: 10.1097/ACI.0b013e32832cda4c
8. Cheuk S, Wikén M, Blomqvist L, Nylén S, Talme T, Ståhle M, et al. Epidermal Th22 and Tc17 Cells Form a Localized Disease Memory in Clinically Healed Psoriasis. J Immunol (2014) 192(7):3111–20. doi: 10.4049/jimmunol.1302313
9. Riding RL, Harris JE. The Role of Memory CD8 + T Cells in Vitiligo. J Immunol (2019) 203(1):11–9. doi: 10.4049/jimmunol.1900027
10. Koguchi-Yoshioka H, Watanabe R, Matsumura Y, Okiyama N, Ishitsuka Y, Nakamura Y, et al. The Possible Linkage of Granzyme B-Producing Skin T Cells with the Disease Prognosis of Alopecia Areata. J Invest Dermatol (2020). doi: 10.1016/j.jid.2020.06.013
11. Zhu J, Koelle DM, Cao J, Vazquez J, Huang ML, Hladik F, et al. Virus-specific CD8+ T cells accumulate near sensory nerve endings in genital skin during subclinical HSV-2 reactivation. J Exp Med (2007) 204(3):595–603. doi: 10.1084/jem.20061792
12. Park CO, Fu X, Jiang X, Pan Y, Teague JE, Collins N, et al. Staged development of long-lived T-cell receptor αβ T H 17 resident memory T-cell population to Candida albicans after skin infection. J Allergy Clin Immunol (2018) 142(2):647–62. doi: 10.1016/j.jaci.2017.09.042
13. Glennie ND, Yeramilli VA, Beiting DP, Volk SW, Weaver CT, Scott P. Skin-resident memory CD4+ T cells enhance protection against Leishmania major infection. J Exp Med (2015) 212(9):1405–14. doi: 10.1084/jem.20142101
14. Watanabe R, Gehad A, Yang C, Scott LL, Teague JE, Schlapbach C, et al. Human skin is protected by four functionally and phenotypically discrete populations of resident and recirculating memory T cells. Sci Transl Med (2015) 7(279):279ra39. doi: 10.1126/scitranslmed.3010302
15. Kleinschek MA, Boniface K, Sadekova S, Grein J, Murphy EE, Turner SP, et al. Circulating and gut-resident human Th17 cells express CD161 and promote intestinal inflammation. J Exp Med (2009) 206(3):525–34. doi: 10.1084/jem.20081712
16. Zundler S, Becker E, Spocinska M, Slawik M, Parga-Vidal L, Stark R, et al. Hobit- and Blimp-1-driven CD4+ tissue-resident memory T cells control chronic intestinal inflammation. Nat Immunol (2019) 20(3):288–300. doi: 10.1038/s41590-018-0298-5
17. Piet B, de Bree GJ, Smids-Dierdorp BS, van der Loos CM, Remmerswaal EBM, von der Thüsen JH, et al. CD8+ T cells with an intraepithelial phenotype upregulate cytotoxic function upon influenza infection in human lung. J Clin Invest (2011) 121(6):2254–63. doi: 10.1172/JCI44675
18. Purwar R, Campbell J, Murphy G, Richards WG, Clark RA, Kupper TS. Resident Memory T Cells (TRM) Are Abundant in Human Lung: Diversity, Function, and Antigen Specificity. Proost P, editor. PloS One (2011) 6(1):e16245. doi: 10.1371/journal.pone.0016245
19. Rahimi RA, Nepal K, Cetinbas M, Sadreyev RI, Luster AD. Distinct functions of tissue-resident and circulating memory Th2 cells in allergic airway disease. J Exp Med (2020) 217(9):e20190865. doi: 10.1084/jem.20190865
20. Afeltra A, Galeazzi M, Ferri GM, Amoroso A, De Pita O, Porzio F, et al. Expression of CD69 antigen on synovial fluid T cells in patients with rheumatoid arthritis and other chronic synovitis. Ann Rheum Dis (1993) 52(6):457–60. doi: 10.1136/ard.52.6.457
21. Sasaki K, Bean A, Shah S, Schutten E, Huseby PG, Peters B, et al. Relapsing–Remitting Central Nervous System Autoimmunity Mediated by GFAP-Specific CD8 T Cells. J Immunol (2014) 192(7):3029–42. doi: 10.4049/jimmunol.1302911
22. Debnath M, Berk M. Th17 Pathway-Mediated Immunopathogenesis of Schizophrenia: Mechanisms and Implications. Schizophr Bull (2014) 40(6):1412–21. doi: 10.1093/schbul/sbu049
23. Sathaliyawala T, Kubota M, Yudanin N, Turner D, Camp P, Thome JJC, et al. Distribution and Compartmentalization of Human Circulating and Tissue-Resident Memory T Cell Subsets. Immunity (2013) 38(1):187–97. doi: 10.1016/j.immuni.2012.09.020
24. Turner J-E, Becker M, Mittrücker H-W, Panzer U. Tissue-Resident Lymphocytes in the Kidney. J Am Soc Nephrol (2018) 29(2):389–99. doi: 10.1681/ASN.2017060599
25. Mueller SN, Mackay LK. Tissue-resident memory T cells: Local specialists in immune defence. Nat Rev Immunol (2016) 16(2):79–89. doi: 10.1038/nri.2015.3
26. Masopust D, Soerens AG. Tissue-Resident T Cells and Other Resident Leukocytes. Annu Rev Immunol (2019) 37(1):521–46. doi: 10.1146/annurev-immunol-042617-053214
27. Beura LK, Wijeyesinghe S, Thompson EA, Macchietto MG, Rosato PC, Pierson MJ, et al. T Cells in Nonlymphoid Tissues Give Rise to Lymph-Node-Resident Memory T Cells. Immunity (2018) 48(2):327–338.e5. doi: 10.1016/j.immuni.2018.01.015
28. Bergsbaken T, Bevan MJ. Proinflammatory microenvironments within the intestine regulate the differentiation of tissue-resident CD8+ T cells responding to infection. Nat Immunol (2015) 16(4):406–14. doi: 10.1038/ni.3108
29. Anderson KG, Sung H, Skon CN, Lefrancois L, Deisinger A, Vezys V, et al. Cutting Edge: Intravascular Staining Redefines Lung CD8 T Cell Responses. J Immunol (2012) 189(6):2702–6. doi: 10.4049/jimmunol.1201682
30. Steinert EM, Schenkel JM, Fraser KA, Beura LK, Manlove LS, Igyártó BZ, et al. Quantifying Memory CD8 T Cells Reveals Regionalization of Immunosurveillance. Cell (2015) 161(4):737–49. doi: 10.1016/j.cell.2015.03.031
31. Wu T, Hu Y, Lee Y-T, Bouchard KR, Benechet A, Khanna K, et al. Lung-resident memory CD8 T cells (T RM ) are indispensable for optimal cross-protection against pulmonary virus infection. J Leukoc Biol (2014) 95(2):215–24. doi: 10.1189/jlb.0313180
32. Fernandez-Ruiz D, Ng WY, Holz LE, Ma JZ, Zaid A, Wong YC, et al. Liver-Resident Memory CD8 + T Cells Form a Front-Line Defense against Malaria Liver-Stage Infection. Immunity (2016) 45(4):889–902. doi: 10.1016/j.immuni.2016.08.011
33. Ariotti S, Beltman JB, Chodaczek G, Hoekstra ME, Van Beek AE, Gomez-Eerland R, et al. Tissue-resident memory CD8+ T cells continuously patrol skin epithelia to quickly recognize local antigen. Proc Natl Acad Sci U S A (2012) 109(48):19739–44. doi: 10.1073/pnas.1208927109
34. Iijima N, Iwasaki A. A local macrophage chemokine network sustains protective tissue-resident memory CD4 T cells. Science (80-) (2014) 346(6205):93–8. doi: 10.1126/science.1257530
35. Jiang X, Clark RA, Liu L, Wagers AJ, Fuhlbrigge RC, Kupper TS. Skin infection generates non-migratory memory CD8+ TRM cells providing global skin immunity. Nature (2012) 483(7388):227–31. doi: 10.1038/nature10851
36. Slütter B, Van Braeckel-Budimir N, Abboud G, Varga SM, Salek-Ardakani S, Harty JT. Dynamics of influenza-induced lung-resident memory T cells underlie waning heterosubtypic immunity. Sci Immunol (2017) 2(7):eaag2031. doi: 10.1126/sciimmunol.aag2031
37. Gebhardt T, Wakim LM, Eidsmo L, Reading PC, Heath WR, Carbone FR. Memory T cells in nonlymphoid tissue that provide enhanced local immunity during infection with herpes simplex virus. Nat Immunol (2009) 10(5):524–30. doi: 10.1038/ni.1718
38. Steinbach K, Vincenti I, Kreutzfeldt M, Page N, Muschaweckh A, Wagner I, et al. Brain-resident memory T cells represent an autonomous cytotoxic barrier to viral infection. J Exp Med (2016) 213(8):1571–87. doi: 10.1084/jem.20151916
39. MacKay LK, Rahimpour A, Ma JZ, Collins N, Stock AT, Hafon ML, et al. The developmental pathway for CD103+ CD8+ tissue-resident memory T cells of skin. Nat Immunol (2013) 14(12):1294–301. doi: 10.1038/ni.2744
40. Holz LE, Prier JE, Freestone D, Steiner TM, English K, Johnson DN, et al. CD8+ T Cell Activation Leads to Constitutive Formation of Liver Tissue-Resident Memory T Cells that Seed a Large and Flexible Niche in the Liver. Cell Rep (2018) 25(1):68–79.e4. doi: 10.1016/j.celrep.2018.08.094
41. Schenkel JM, Fraser KA, Casey KA, Beura LK, Pauken KE, Vezys V, et al. IL-15–Independent Maintenance of Tissue-Resident and Boosted Effector Memory CD8 T Cells. J Immunol (2016) 196(9):3920–6. doi: 10.4049/jimmunol.1502337
42. Casey KA, Fraser KA, Schenkel JM, Moran A, Abt MC, Beura LK, et al. Antigen-Independent Differentiation and Maintenance of Effector-like Resident Memory T Cells in Tissues. J Immunol (2012) 188(10):4866–75. doi: 10.4049/jimmunol.1200402
43. Sheridan BS, Pham Q-M, Lee Y-T, Cauley LS, Puddington L, Lefrançois L. Oral Infection Drives a Distinct Population of Intestinal Resident Memory CD8+ T Cells with Enhanced Protective Function. Immunity (2014) 40(5):747–57. doi: 10.1016/j.immuni.2014.03.007
44. Laidlaw BJ, Zhang N, Marshall HD, Staron MM, Guan T, Hu Y, et al. CD4+ T Cell Help Guides Formation of CD103+ Lung-Resident Memory CD8+ T Cells during Influenza Viral Infection. Immunity (2014) 41(4):633–45. doi: 10.1016/j.immuni.2014.09.007
45. Maldonado L, Teague JE, Morrow MP, Jotova I, Wu TC, Wang C, et al. Intramuscular Therapeutic Vaccination Targeting HPV16 Induces T Cell Responses That Localize in Mucosal Lesions. Sci Transl Med (2014) 6(221):221ra13–221ra13. doi: 10.1126/scitranslmed.3007323
46. Yanagisawa K, Yue S, van der Vliet HJ, Wang R, Alatrakchi N, Golden-Mason L, et al. Ex vivo analysis of resident hepatic pro-inflammatory CD1d-reactive T cells and hepatocyte surface CD1d expression in hepatitis C. J Viral Hepat (2013) 20(8):556–65. doi: 10.1111/jvh.12081
47. Turner DL, Farber DL. Mucosal Resident Memory CD4 T Cells in Protection and Immunopathology. Front Immunol (2014) 5:331. doi: 10.3389/fimmu.2014.00331
48. Glennie ND, Volk SW, Scott P. Skin-resident CD4+ T cells protect against Leishmania major by recruiting and activating inflammatory monocytes. Müller I, editor. PloS Pathog (2017) 13(4):e1006349. doi: 10.1371/journal.ppat.1006349
49. Stary G, Olive A, Radovic-Moreno AF, Gondek D, Alvarez D, Basto PA, et al. A mucosal vaccine against Chlamydia trachomatis generates two waves of protective memory T cells. Science (80-) (2015) 348(6241):aaa8205–aaa8205. doi: 10.1126/science.aaa8205
50. Smith NM, Wasserman GA, Coleman FT, Hilliard KL, Yamamoto K, Lipsitz E, et al. Regionally compartmentalized resident memory T cells mediate naturally acquired protection against pneumococcal pneumonia. Mucosal Immunol (2018) 11(1):220–35. doi: 10.1038/mi.2017.43
51. Kumar BV, Ma W, Miron M, Granot T, Guyer RS, Carpenter DJ, et al. Human Tissue-Resident Memory T Cells Are Defined by Core Transcriptional and Functional Signatures in Lymphoid and Mucosal Sites. Cell Rep (2017) 20(12):2921–34. doi: 10.1016/j.celrep.2017.08.078
52. Joshi NS, Cui W, Chandele A, Lee HK, Urso DR, Hagman J, et al. Inflammation Directs Memory Precursor and Short-Lived Effector CD8+ T Cell Fates via the Graded Expression of T-bet Transcription Factor. Immunity (2007) 27(2):281–95. doi: 10.1016/j.immuni.2007.07.010
53. Homey B, Alenius H, Müller A, Soto H, Bowman EP, Yuan W, et al. CCL27–CCR10 interactions regulate T cell–mediated skin inflammation. Nat Med (2002) 8(2):157–65. doi: 10.1038/nm0202-157
54. Xia M, Hu S, Fu Y, Jin W, Yi Q. CCR10 regulates balanced maintenance and function of resident regulatory and effector T cells to promote immune homeostasis in the skin. J Allergy Clin Immunol (2014) 134(3):634–644.e10. doi: 10.1016/j.jaci.2014.03.010
55. Zaid A, Hor JL, Christo SN, Groom JR, Heath WR, Mackay LK, et al. Chemokine Receptor–Dependent Control of Skin Tissue–Resident Memory T Cell Formation. J Immunol (2017) 199(7):2451–9. doi: 10.4049/jimmunol.1700571
56. Mackay LK, Minnich M, Kragten NAM, Liao Y, Nota B, Seillet C, et al. Hobit and Blimp1 instruct a universal transcriptional program of tissue residency in lymphocytes. Science (80-) (2016) 352(6284):459–63. doi: 10.1126/science.aad2035
57. Scholz F, Schulte A, Adamski F, Hundhausen C, Mittag J, Schwarz A, et al. Constitutive Expression and Regulated Release of the Transmembrane Chemokine CXCL16 in Human and Murine Skin. J Invest Dermatol (2007) 127(6):1444–55. doi: 10.1038/sj.jid.5700751
58. Campbell JJ, Haraldsen G, Pan J, Rottman J, Qin S, Ponath P, et al. The chemokine receptor CCR4 in vascular recognition by cutaneous but not intestinal memory T cells. Nature (1999) 400(6746):776–80. doi: 10.1038/23495
59. Kim YH, Bagot M, Pinter-Brown L, Rook AH, Porcu P, Horwitz SM, et al. Mogamulizumab versus vorinostat in previously treated cutaneous T-cell lymphoma (MAVORIC): an international, open-label, randomised, controlled phase 3 trial. Lancet Oncol (2018) 19(9):1192–204. doi: 10.1016/S1470-2045(18)30379-6
60. McCully ML, Ladell K, Andrews R, Jones RE, Miners KL, Roger L, et al. CCR8 Expression Defines Tissue-Resident Memory T Cells in Human Skin. J Immunol (2018) 200(5):1639–50. doi: 10.4049/jimmunol.1701377
61. McCully ML, Ladell K, Hakobyan S, Mansel RE, Price DA, Moser B. Epidermis instructs skin homing receptor expression in human T cells. Blood (2012) 120(23):4591–8. doi: 10.1182/blood-2012-05-433037
62. Schaerli P, Ebert L, Willimann K, Blaser A, Roos RS, Loetscher P, et al. A Skin-selective Homing Mechanism for Human Immune Surveillance T Cells. J Exp Med (2004) 199(9):1265–75. doi: 10.1084/jem.20032177
63. McCully ML, Collins PJ, Hughes TR, Thomas CP, Billen J, O’Donnell VB, et al. Skin Metabolites Define a New Paradigm in the Localization of Skin Tropic Memory T Cells. J Immunol (2015) 195(1):96–104. doi: 10.4049/jimmunol.1402961
64. Sigmundsdottir H, Pan J, Debes GF, Alt C, Habtezion A, Soler D, et al. DCs metabolize sunlight-induced vitamin D3 to “program” T cell attraction to the epidermal chemokine CCL27. Nat Immunol (2007) 8(3):285–93. doi: 10.1038/ni1433
65. Clark RA. Resident memory T cells in human health and disease. Sci Transl Med (2015) 7(269):269rv1–1. doi: 10.1126/scitranslmed.3010641
66. El-Asady R, Yuan R, Liu K, Wang D, Gress RE, Lucas PJ, et al. TGF-β-dependent CD103 expression by CD8+ T cells promotes selective destruction of the host intestinal epithelium during graft-versus-host disease. J Exp Med (2005) 201(10):1647–57. doi: 10.1084/jem.20041044
67. Hirai T, Zenke Y, Yang Y, Bartholin L, Beura LK, Masopust D, et al. Keratinocyte-Mediated Activation of the Cytokine TGF-β Maintains Skin Recirculating Memory CD8+ T Cells. Immunity (2019) 50(5):1–13. doi: 10.1016/j.immuni.2019.03.002
68. Pauls K, Schön M, Kubitza RC, Homey B, Wiesenborn A, Lehmann P, et al. Role of integrin αE(CD103)β7 for tissue-specific epidermal localization of CD8+ T lymphocytes. J Invest Dermatol (2001) 117(3):569–75. doi: 10.1046/j.0022-202x.2001.01481.x
69. Klicznik MM, Morawski PA, Höllbacher B, Varkhande SR, Motley SJ, Kuri-Cervantes L, et al. Human CD4 + CD103 + cutaneous resident memory T cells are found in the circulation of healthy individuals. Sci Immunol (2019) 4(37):eaav8995. doi: 10.1126/sciimmunol.aav8995
70. Kurihara K, Fujiyama T, Phadungsaksawasdi P, Ito T, Tokura Y. Significance of IL-17A-producing CD8+CD103+ skin resident memory T cells in psoriasis lesion and their possible relationship to clinical course. J Dermatol Sci (2019) 95(1):21–7. doi: 10.1016/j.jdermsci.2019.06.002
71. Le Floc’h A, Jalil A, Vergnon I, Le Maux Chansac B, Lazar V, Bismuth G, et al. αEβ7 integrin interaction with E-cadherin promotes antitumor CTL activity by triggering lytic granule polarization and exocytosis. J Exp Med (2007) 204(3):559–70. doi: 10.1084/jem.20061524
72. Mackay LK, Braun A, Macleod BL, Collins N, Tebartz C, Bedoui S, et al. Cutting Edge: CD69 Interference with Sphingosine-1-Phosphate Receptor Function Regulates Peripheral T Cell Retention. J Immunol (2015) 194(5):2059–63. doi: 10.4049/jimmunol.1402256
73. Skon CN, Lee JY, Anderson KG, Masopust D, Hogquist KA, Jameson SC. Transcriptional downregulation of S1pr1 is required for the establishment of resident memory CD8+ T cells. Nat Immunol (2013) 14(12):1285–93. doi: 10.1038/ni.2745
74. Cheuk S, Schlums H, Gallais Sérézal I, Martini E, Chiang SC, Marquardt N, et al. CD49a Expression Defines Tissue-Resident CD8+T Cells Poised for Cytotoxic Function in Human Skin. Immunity (2017) 46(2):287–300. doi: 10.1016/j.immuni.2017.01.009
75. Bromley SK, Akbaba H, Mani V, Mora-buch R, Chasse AY, Sama A, et al. Article CD49a Regulates Cutaneous Resident Memory CD8 + T Cell Persistence and Response ll ll CD49a Regulates Cutaneous Resident Memory CD8 + T Cell Persistence and Response. CellReports (2020) 32(9):108085. doi: 10.1016/j.celrep.2020.108085
76. Conrad C, Boyman O, Tonel G, Tun-Kyi A, Laggner U, De Fougerolles A, et al. α1β1 integrin is crucial for accumulation of epidermal T cells and the development of psoriasis. Nat Med (2007) 13(7):836–42. doi: 10.1038/nm1605
77. Goldstein I, Ben-Horin S, Li J, Bank I, Jiang H, Chess L. Expression of the α1β1 integrin, VLA-1, marks a distinct subset of human CD4+ memory T cells. J Clin Invest (2003) 112(9):1444–54. doi: 10.1172/JCI200319607
78. Collins N, Jiang X, Zaid A, Macleod BL, Li J, Park CO, et al. Skin CD4+ memory T cells exhibit combined cluster-mediated retention and equilibration with the circulation. Nat Commun (2016) 7(1):11514. doi: 10.1038/ncomms11514
79. Kobayashi T, Naik S, Nagao K. Choreographing Immunity in the Skin Epithelial Barrier. Immunity (2019) 50(3):552–65. doi: 10.1016/j.immuni.2019.02.023
80. Gebhardt T, Whitney PG, Zaid A, MacKay LK, Brooks AG, Heath WR, et al. Different patterns of peripheral migration by memory CD4+ and CD8+ T cells. Nature (2011) 477(7363):216–9. doi: 10.1038/nature10339
81. Tomura M, Honda T, Tanizaki H, Otsuka A, Egawa G, Tokura Y, et al. Activated regulatory T cells are the major T cell type emigrating from the skin during a cutaneous immune response in mice. J Clin Invest (2010) 120(3):883–93. doi: 10.1172/JCI40926
82. Beura LK, Fares-Frederickson NJ, Steinert EM, Scott MC, Thompson EA, Fraser KA, et al. CD4+ resident memory T cells dominate immunosurveillance and orchestrate local recall responses. J Exp Med (2019) 216(5):1214–29. doi: 10.1084/jem.20181365
83. Bromley SK, Yan S, Tomura M, Kanagawa O, Luster AD. Recirculating Memory T Cells Are a Unique Subset of CD4 + T Cells with a Distinct Phenotype and Migratory Pattern. J Immunol (2013) 190(3):970–6. doi: 10.4049/jimmunol.1202805
84. Ugur M, Schulz O, Menon MB, Krueger A, Pabst O. Resident CD4+ T cells accumulate in lymphoid organs after prolonged antigen exposure. Nat Commun (2014) 5(1):4821. doi: 10.1038/ncomms5821
85. Jameson SC, Masopust D. Understanding Subset Diversity in T Cell Memory. Immunity (2018) 48(2):214–26. doi: 10.1016/j.immuni.2018.02.010
86. Masopust D, Schenkel JM. The integration of T cell migration, differentiation and function. Nat Rev Immunol (2013) 13(5):309–20. doi: 10.1038/nri3442
87. Gaide O, Emerson RO, Jiang X, Gulati N, Nizza S, Desmarais C, et al. Common clonal origin of central and resident memory T cells following skin immunization. Nat Med (2015) 21(6):647–53. doi: 10.1038/nm.3860
88. Kok L, Dijkgraaf FE, Urbanus J, Bresser K, Vredevoogd DW, Cardoso RF, et al. A committed tissue-resident memory T cell precursor within the circulating CD8+ effector T cell pool. J Exp Med (2020) 217(10):e20191711. doi: 10.1084/jem.20191711
89. Mani V, Bromley SK, Äijö T, Mora-Buch R, Carrizosa E, Warner RD, et al. Migratory DCs activate TGF-b to precondition naïve CD8+T cells for tissue-resident memory fate. Science (80-) (2019) 366(6462):eaav5728. doi: 10.1126/science.aav5728
90. Dudda JC, Simon JC, Martin S. Dendritic Cell Immunization Route Determines CD8 + T Cell Trafficking to Inflamed Skin: Role for Tissue Microenvironment and Dendritic Cells in Establishment of T Cell-Homing Subsets. J Immunol (2004) 172(2):857–63. doi: 10.4049/jimmunol.172.2.857
91. Johansson-Lindbom B, Svensson M, Wurbel M-A, Malissen B, Márquez G, Agace W. Selective Generation of Gut Tropic T Cells in Gut-associated Lymphoid Tissue (GALT). J Exp Med (2003) 198(6):963–9. doi: 10.1084/jem.20031244
92. Gerlach C, Rohr JC, Perie L, van Rooij N, van Heijst JWJ, Velds A, et al. Heterogeneous Differentiation Patterns of Individual CD8+ T Cells. Science (80- ) (2013) 340(6132):635–9. doi: 10.1126/science.1235487
93. Mackay LK, Stock AT, Ma JZ, Jones CM, Kent SJ, Mueller SN, et al. Long-lived epithelial immunity by tissue-resident memory T (TRM) cells in the absence of persisting local antigen presentation. Proc Natl Acad Sci U S A (2012) 109(18):7037–42. doi: 10.1073/pnas.1202288109
94. Park SL, Zaid A, Hor JL, Christo SN, Prier JE, Davies B, et al. Local proliferation maintains a stable pool of tissue-resident memory T cells after antiviral recall responses article. Nat Immunol (2018) 19(2):183–91. doi: 10.1038/s41590-017-0027-5
95. Cumberbatch M, Dearman RJ, Kimber I. Langerhans cells require signals from both tumour necrosis factor-α and interleukin-1β for migration. Immunology (1997) 92(3):388–95. doi: 10.1046/j.1365-2567.1997.00360.x
96. Schön MP, Zollner TM, Boehncke WH. The Molecular Basis of Lymphocyte Recruitment to the Skin: Clues for Pathogenesis and Selective Therapies of Inflammatory Disorders. J Invest Dermatol (2003) 121(5):951–62. doi: 10.1046/j.1523-1747.2003.12563.x
97. Fonseca R, Beura LK, Quarnstrom CF, Ghoneim HE, Fan Y, Zebley CC, et al. Developmental plasticity allows outside-in immune responses by resident memory T cells. Nat Immunol (2020) 21(4):412–21. doi: 10.1038/s41590-020-0607-7
98. Mackay LK, Kallies A. Transcriptional Regulation of Tissue-Resident Lymphocytes. Trends Immunol (2017) 38(2):94–103. doi: 10.1016/j.it.2016.11.004
99. Gamradt P, Laoubi L, Nosbaum A, Mutez V, Lenief V, Grande S, et al. Inhibitory checkpoint receptors control CD8+ resident memory T cells to prevent skin allergy. J Allergy Clin Immunol (2019) 143(6):2147–57.e9. doi: 10.1016/j.jaci.2018.11.048
100. Beura LK, Mitchell JS, Thompson EA, Schenkel JM, Mohammed J, Wijeyesinghe S, et al. Intravital mucosal imaging of CD8 + resident memory T cells shows tissue-autonomous recall responses that amplify secondary memory article. Nat Immunol (2018) 19(2):173–82. doi: 10.1038/s41590-017-0029-3
101. Ariotti S, Hogenbirk MA, Dijkgraaf FE, Visser LL, Hoekstra ME, Song J-Y, et al. Skin-resident memory CD8 + T cells trigger a state of tissue-wide pathogen alert. Science (80-) (2014) 346(6205):101–5. doi: 10.1126/science.1254803
102. Muschaweckh A, Buchholz VR, Fellenzer A, Hessel C, König P-A, Tao S, et al. Antigen-dependent competition shapes the local repertoire of tissue-resident memory CD8+ T cells. J Exp Med (2016) 213(13):3075–86. doi: 10.1084/jem.20160888
103. Chmurzyńska A. The multigene family of fatty acid-binding proteins (FABPs): Function, structure and polymorphism. J Appl Genet (2006) 47(1):39–48. doi: 10.1007/BF03194597
104. Li B, Reynolds JM, Stout RD, Bernlohr DA, Suttles J. Regulation of Th17 Differentiation by Epidermal Fatty Acid-Binding Protein. J Immunol (2009) 182(12):7625–33. doi: 10.4049/jimmunol.0804192
105. Zhang Y, Li Q, Rao E, Sun Y, Grossmann ME, Morris RJ, et al. Epidermal Fatty Acid Binding Protein Promotes Skin Inflammation Induced by High-Fat Diet. Immunity (2015) 42(5):953–64. doi: 10.1016/j.immuni.2015.04.016
106. Pan Y, Tian T, Park CO, Lofftus SY, Mei S, Liu X, et al. Survival of tissue-resident memory T cells requires exogenous lipid uptake and metabolism. Nature (2017) 543(7644):252–6. doi: 10.1038/nature21379
107. Reynolds JM, Liu Q, Brittingham KC, Liu Y, Gruenthal M, Gorgun CZ, et al. Deficiency of Fatty Acid-Binding Proteins in Mice Confers Protection from Development of Experimental Autoimmune Encephalomyelitis. J Immunol (2007) 179(1):313–21. doi: 10.4049/jimmunol.179.1.313
108. Gadsbøll A-SØ, Jee MH, Funch AB, Alhede M, Mraz V, Weber JF, et al. Pathogenic CD8+ Epidermis-Resident Memory T Cells Displace Dendritic Epidermal T Cells in Allergic Dermatitis. J Invest Dermatol (2020) 140(4):806–815.e5. doi: 10.1016/j.jid.2019.07.722
109. Fehniger TA. Mystery Solved: IL-15. J Immunol (2019) 202(11):3125–6. doi: 10.4049/jimmunol.1900419
110. Burkett PR, Koka R, Chien M, Chai S, Boone DL, Ma A. Coordinate Expression and Trans Presentation of Interleukin (IL)-15Rα and IL-15 Supports Natural Killer Cell and Memory CD8+ T Cell Homeostasis. J Exp Med (2004) 200(7):825–34. doi: 10.1084/jem.20041389
111. Romee R, Cooley S, Berrien-Elliott MM, Westervelt P, Verneris MR, Wagner JE, et al. First-in-human phase 1 clinical study of the IL-15 superagonist complex ALT-803 to treat relapse after transplantation. Blood (2018) 131(23):2515–27. doi: 10.1182/blood-2017-12-823757
112. Adachi T, Kobayashi T, Sugihara E, Yamada T, Ikuta K, Pittaluga S, et al. Hair follicle-derived IL-7 and IL-15 mediate skin-resident memory T cell homeostasis and lymphoma. Nat Med (2015) 21(11):1272–9. doi: 10.1038/nm.3962
113. Mohammed J, Beura LK, Bobr A, Astry B, Chicoine B, Kashem SW, et al. Stromal cells control the epithelial residence of DCs and memory T cells by regulated activation of TGF-β. Nat Immunol (2016) 17(4):414–21. doi: 10.1038/ni.3396
114. Gebhardt T, Palendira U, Tscharke DC, Bedoui S. Tissue-resident memory T cells in tissue homeostasis, persistent infection, and cancer surveillance. Immunol Rev (2018) 283(1):54–76. doi: 10.1111/imr.12650
115. Sheridan BS, Lefrançois L. Regional and mucosal memory T cells. Nat Immunol (2011) 12(6):485–91. doi: 10.1038/ni.2029
116. Ho AW, Kupper TS. T cells and the skin: from protective immunity to inflammatory skin disorders. Nat Rev Immunol (2019) 19(8):490–502. doi: 10.1038/s41577-019-0162-3
117. Hobbs SJ, Nolz JC. Targeted Expansion of Tissue-Resident CD8+ T Cells to Boost Cellular Immunity in the Skin. Cell Rep (2019) 29(10):2990–7.e2. doi: 10.1016/j.celrep.2019.10.126
118. Zens KD, Chen JK, Farber DL. Vaccine-generated lung tissue–resident memory T cells provide heterosubtypic protection to influenza infection. JCI Insight (2016) 1(10):e85832. doi: 10.1172/jci.insight.85832
119. Perdomo C, Zedler U, Kühl AA, Lozza L, Saikali P, Sander LE, et al. Mucosal BCG Vaccination Induces Protective Lung-Resident Memory T Cell Populations against Tuberculosis. MBio (2016) 7(6):e01686–16. doi: 10.1128/mBio.01686-16
120. Çuburu N, Wang K, Goodman KN, Pang YY, Thompson CD, Lowy DR, et al. Topical Herpes Simplex Virus 2 (HSV-2) Vaccination with Human Papillomavirus Vectors Expressing gB/gD Ectodomains Induces Genital-Tissue-Resident Memory CD8 + T Cells and Reduces Genital Disease and Viral Shedding after HSV-2 Challenge. Sandri-Goldin RM, editor. J Virol (2015) 89(1):83–96. doi: 10.1128/JVI.02380-14
121. Tan H-X, Wheatley AK, Esterbauer R, Jegaskanda S, Glass JJ, Masopust D, et al. Induction of vaginal-resident HIV-specific CD8 T cells with mucosal prime–boost immunization. Mucosal Immunol (2018) 11(3):994–1007. doi: 10.1038/mi.2017.89
122. Shin H, Iwasaki A. A vaccine strategy that protects against genital herpes by establishing local memory T cells. Nature (2012) 491(7424):463–7. doi: 10.1038/nature11522
123. Stewart AJ, Devlin PM. The history of the smallpox vaccine. J Infect (2006) 52(5):329–34. doi: 10.1016/j.jinf.2005.07.021
124. Liu L, Zhong Q, Tian T, Dubin K, Athale SK, Kupper TS. Epidermal injury and infection during poxvirus immunization is crucial for the generation of highly protective T cell–mediated immunity. Nat Med (2010) 16(2):224–7. doi: 10.1038/nm.2078
125. Davies B, Prier JE, Jones CM, Gebhardt T, Carbone FR, Mackay LK. Cutting Edge: Tissue-Resident Memory T Cells Generated by Multiple Immunizations or Localized Deposition Provide Enhanced Immunity. J Immunol (2017) 198(6):2233–7. doi: 10.4049/jimmunol.1601367
126. Edwards J, Wilmott JS, Madore J, Gide TN, Quek C, Tasker A, et al. CD103+ tumor-resident CD8+ T cells are associated with improved survival in immunotherapy-naïve melanoma patients and expand significantly during anti-PD-1 treatment. Clin Cancer Res (2018) 24(13):3036–45. doi: 10.1158/1078-0432.CCR-17-2257
127. Strickley JD, Messerschmidt JL, Awad ME, Li T, Hasegawa T, Ha DT, et al. Immunity to commensal papillomaviruses protects against skin cancer. Nature (2019) 575(7783):519–22. doi: 10.1038/s41586-019-1719-9
128. Willemsen M, Linkutė R, Luiten RM, Matos TR. Skin-resident memory T cells as a potential new therapeutic target in vitiligo and melanoma. Pigment Cell Melanoma Res (2019) 32(5):612–22. doi: 10.1111/pcmr.12803
129. Tokura Y, Mori T, Hino R. Psoriasis and Other Th17-Mediated Skin Diseases. J UOEH (2010) 32(4):317–28. doi: 10.7888/juoeh.32.317
130. Yoshiki R, Kabashima K, Honda T, Nakamizo S, Sawada Y, Sugita K, et al. IL-23 from Langerhans Cells Is Required for the Development of Imiquimod-Induced Psoriasis-Like Dermatitis by Induction of IL-17A-Producing γδ T Cells. J Invest Dermatol (2014) 134(7):1912–21. doi: 10.1038/jid.2014.98
131. Ramirez-Carrozzi V, Sambandam A, Luis E, Lin Z, Jeet S, Lesch J, et al. IL-17C regulates the innate immune function of epithelial cells in an autocrine manner. Nat Immunol (2011) 12(12):1159–66. doi: 10.1038/ni.2156
132. Pfaff CM, Marquardt Y, Fietkau K, Baron JM, Lüscher B. The psoriasis-associated IL-17A induces and cooperates with IL-36 cytokines to control keratinocyte differentiation and function. Sci Rep (2017) 7(1):15631. doi: 10.1038/s41598-017-15892-7
133. Conrad C, Meller S, Gilliet M. Plasmacytoid dendritic cells in the skin: To sense or not to sense nucleic acids. Semin Immunol (2009) 21(3):101–9. doi: 10.1016/j.smim.2009.01.004
134. Funakoshi A, Tatsuno K, Shimauchi T, Fujiyama T, Ito T, Tokura Y. Cholecystokinin Downregulates Psoriatic Inflammation by Its Possible Self-Regulatory Effect on Epidermal Keratinocytes. J Immunol (2019) 202(9):2609–15. doi: 10.4049/jimmunol.1801426
135. Hawkes JE, Yan BY, Chan TC, Krueger JG. Discovery of the IL-23/IL-17 Signaling Pathway and the Treatment of Psoriasis. J Immunol (2018) 201(6):1605–13. doi: 10.4049/jimmunol.1800013
136. Shimauchi T, Hirakawa S, Suzuki T, Yasuma A, Majima Y, Tatsuno K, et al. Serum interleukin-22 and vascular endothelial growth factor serve as sensitive biomarkers but not as predictors of therapeutic response to biologics in patients with psoriasis. J Dermatol (2013) 40(10):805–12. doi: 10.1111/1346-8138.12248
137. Masson Regnault M, Konstantinou M-P, Khemis A, Poulin Y, Bourcier M, Amelot F, et al. Early relapse of psoriasis after stopping brodalumab: a retrospective cohort study in 77 patients. J Eur Acad Dermatol Venereol (2017) 31(9):1491–6. doi: 10.1111/jdv.14387
138. Vo S, Watanabe R, Koguchi-Yoshioka H, Matsumura Y, Ishitsuka Y, Nakamura Y, et al. CD 8 resident memory T cells with interleukin 17A-producing potential are accumulated in disease-naïve nonlesional sites of psoriasis possibly in correlation with disease duration. Br J Dermatol (2019) 181(2):410–2. doi: 10.1111/bjd.17748
139. Thome JJC, Farber DL. Emerging concepts in tissue-resident T cells: lessons from humans. Trends Immunol (2015) 36(7):428–35. doi: 10.1016/j.it.2015.05.003
140. Fujiyama T, Ito T, Umayahara T, Ikeya S, Tatsuno K, Funakoshi A, et al. Topical application of a vitamin D3 analogue and corticosteroid to psoriasis plaques decreases skin infiltration of TH17 cells and their ex vivo expansion. J Allergy Clin Immunol (2016) 138(2):517–28.e5. doi: 10.1016/j.jaci.2016.03.048
141. Hashizume H, Hansen A, Poulsen LK, Thomsen AR, Takigawa M, Thestrup-Pedersen K. In vitro propagation and dynamics of T cells from skin biopsies by methods using interleukins-2 and -4 or anti-CD3/CD28 antibody-coated microbeads. Acta Derm Venereol (2010) 90(5):468–73. doi: 10.2340/00015555-0927
142. Fujiyama T, Umayahara T, Kurihara K, Shimauchi T, Ito T, Aoshima M, et al. Skin Infiltration of Pathogenic Migratory and Resident T Cells Is Decreased by Secukinumab Treatment in Psoriasis. J Invest Dermatol (2020) 140(10):2073–6.e6 doi: 10.1016/j.jid.2020.02.024
143. Boniface K, Jacquemin C, Darrigade A-S, Dessarthe B, Martins C, Boukhedouni N, et al. Vitiligo Skin Is Imprinted with Resident Memory CD8 T Cells Expressing CXCR3. J Invest Dermatol (2018) 138(2):355–64. doi: 10.1016/j.jid.2017.08.038
144. Di Meglio P, Villanova F, Navarini AA, Mylonas A, Tosi I, Nestle FO, et al. Targeting CD8+ T cells prevents psoriasis development. J Allergy Clin Immunol (2016) 138(1):274–6.e6. doi: 10.1016/j.jaci.2015.10.046
145. Teunissen MBM, Yeremenko NG, Baeten DLP, Chielie S, Spuls PI, De Rie MA, et al. The IL-17A-producing CD8 + T-cell population in psoriatic lesional skin comprises mucosa-associated invariant t cells and conventional t cells. J Invest Dermatol (2014) 134(12):2898–907. doi: 10.1038/jid.2014.261
146. Hadley GA, Bartlett ST, Via CS, Rostapshova EA, Moainie S. The epithelial cell-specific integrin, CD103 (alpha E integrin), defines a novel subset of alloreactive CD8+ CTL. J Immunol (1997) 159(8):3748–56.
147. Gallais Sérézal I, Hoffer E, Ignatov B, Martini E, Zitti B, Ehrström M, et al. A skewed pool of resident T cells triggers psoriasis-associated tissue responses in never-lesional skin from patients with psoriasis. J Allergy Clin Immunol (2019) 143(4):1444–54. doi: 10.1016/j.jaci.2018.08.048
148. Gallais Sérézal I, Classon C, Cheuk S, Barrientos-Somarribas M, Wadman E, Martini E, et al. Resident T Cells in Resolved Psoriasis Steer Tissue Responses that Stratify Clinical Outcome. J Invest Dermatol (2018) 138(8):1754–63. doi: 10.1016/j.jid.2018.02.030
149. Sibaud V, Meyer N, Lamant L, Vigarios E, Mazieres J, Delord JP. Dermatologic complications of anti-PD-1/PD-L1 immune checkpoint antibodies. Curr Opin Oncol (2016) 28(4):254–63. doi: 10.1097/CCO.0000000000000290
150. Richmond JM, Strassner JP, Rashighi M, Agarwal P, Garg M, Essien KI, et al. Resident Memory and Recirculating Memory T Cells Cooperate to Maintain Disease in a Mouse Model of Vitiligo. J Invest Dermatol (2019) 139(4):769–78. doi: 10.1016/j.jid.2018.10.032
151. Richmond JM, Strassner JP, Zapata L, Garg M, Riding RL, Refat MA, et al. Antibody blockade of IL-15 signaling has the potential to durably reverse vitiligo. Sci Transl Med (2018) 10(450):eaam7710. doi: 10.1126/scitranslmed.aam7710
152. Campbell JJ, Clark RA, Watanabe R, Kupper TS. Sézary syndrome and mycosis fungoides arise from distinct T-cell subsets: a biologic rationale for their distinct clinical behaviors. Blood (2010) 116(5):767–71. doi: 10.1182/blood-2009-11-251926
153. Iyer A, Hennessey D, O’Keefe S, Patterson J, Wang W, Wong GK-S, et al. Skin colonization by circulating neoplastic clones in cutaneous T-cell lymphoma. Blood (2019) 134(18):1517–27. doi: 10.1182/blood.2019002516
154. Tokura Y, Sawada Y, Shimauchi T. Skin manifestations of adult T-cell leukemia/lymphoma: Clinical, cytological and immunological features. J Dermatol (2014) 41(1):19–25. doi: 10.1111/1346-8138.12328
155. Kurihara K, Shimauchi T, Tokura Y. Indolent multipapular adult T-cell leukemia/lymphoma with phenotype of resident memory T cells. J Dermatol (2020) 47(7):e280–1. doi: 10.1111/1346-8138.15380
156. Miyagawa F, Iioka H, Fukumoto T, Kobayashi N, Asada H. A case of CD 8 + primary cutaneous peripheral T-cell lymphoma arising from tissue-resident memory T cells in the skin. Br J Dermatol (2015) 173(2):612–4. doi: 10.1111/bjd.13687
157. Yagi H, Hagiwara T, Shirahama S, Tokura Y, Takigawa M. Disseminated pagetoid reticulosis: Need for long-term follow-up. J Am Acad Dermatol (1994) 30(2):345–9. doi: 10.1016/S0190-9622(94)70037-0
158. Mizukawa Y, Yamazaki Y, Teraki Y, Hayakawa J, Hayakawa K, Nuriya H, et al. Direct Evidence for Interferon-γ Production by Effector-Memory-Type Intraepidermal T Cells Residing at an Effector Site of Immunopathology in Fixed Drug Eruption. Am J Pathol (2002) 161(4):1337–47. doi: 10.1016/S0002-9440(10)64410-0
159. Mizukawa Y, Yamazaki Y, Shiohara T. In vivo dynamics of intraepidermal CD8+ T cells and CD4+ T cells during the evolution of fixed drug eruption. Br J Dermatol (2008) 158(6):1230–8. doi: 10.1111/j.1365-2133.2008.08516.x
160. Iriki H, Adachi T, Mori M, Tanese K, Funakoshi T, Karigane D, et al. Toxic epidermal necrolysis in the absence of circulating T cells: A possible role for resident memory T cells. J Am Acad Dermatol (2014) 71(5):e214–6. doi: 10.1016/j.jaad.2014.07.013
161. Dijkgraaf FE, Matos TR, Hoogenboezem M, Toebes M, Vredevoogd DW, Mertz M, et al. Tissue patrol by resident memory CD8+ T cells in human skin. Nat Immunol (2019) 20(6):756–64. doi: 10.1038/s41590-019-0404-3
Keywords: skin, resident memory T cell, skin immunity, psoriasis, vitiligo, cutaneous T cell lymphoma, fixed drug eruption
Citation: Tokura Y, Phadungsaksawasdi P, Kurihara K, Fujiyama T and Honda T (2021) Pathophysiology of Skin Resident Memory T Cells. Front. Immunol. 11:618897. doi: 10.3389/fimmu.2020.618897
Received: 19 October 2020; Accepted: 21 December 2020;
Published: 03 February 2021.
Edited by:
Shiki Takamura, Kindai University, JapanReviewed by:
Wolfgang Kastenmüller, Julius Maximilian University of Würzburg, GermanyPhillip Scott, University of Pennsylvania, United States
Copyright © 2021 Tokura, Phadungsaksawasdi, Kurihara, Fujiyama and Honda. This is an open-access article distributed under the terms of the Creative Commons Attribution License (CC BY). The use, distribution or reproduction in other forums is permitted, provided the original author(s) and the copyright owner(s) are credited and that the original publication in this journal is cited, in accordance with accepted academic practice. No use, distribution or reproduction is permitted which does not comply with these terms.
*Correspondence: Yoshiki Tokura, dG9rdXJhQGhhbWEtbWVkLmFjLmpw