- 1Institute of Specific Prophylaxis and Tropical Medicine, Center for Pathophysiology, Infectiology and Immunology, Medical University of Vienna, Vienna, Austria
- 2Laboratory of Gnotobiology, Institute of Microbiology of the Czech Academy of Sciences, Novy Hradek, Czechia
A steady rise in the number of poly-sensitized patients has increased the demand for effective prophylactic strategies against multi-sensitivities. Probiotic bacteria have been successfully used in clinics and experimental models to prevent allergic mono-sensitization. In the present study, we have investigated whether probiotic bacteria could prevent poly-sensitization by imprinting on the immune system early in life. We used two recombinant variants of probiotic Escherichia coli Nissle 1917 (EcN): i) EcN expressing birch and grass pollen, poly-allergen chimera construct (EcN-Chim), and ii) an “empty” EcN without allergen expression (EcN-Ctrl). Conventional mice (CV) were treated with either EcN-Chim or EcN-Ctrl in the last week of the gestation and lactation period. Gnotobiotic mice received one oral dose of either EcN-Chim or EcN-Ctrl before mating. The offspring from both models underwent systemic allergic poly-sensitization and intranasal challenge with recombinant birch and grass pollen allergens (rBet v 1, rPhl p 1, and rPhl p 5). In the CV setting, the colonization of offspring via treatment of mothers reduced allergic airway inflammation (AAI) in offspring compared to poly-sensitized controls. Similarly, in a gnotobiotic model, AAI was reduced in EcN-Chim and EcN-Ctrl mono-colonized offspring. However, allergy prevention was more pronounced in the EcN-Ctrl mono-colonized offspring as compared to EcN-Chim. Mono-colonization with EcN-Ctrl was associated with a shift toward mixed Th1/Treg immune responses, increased expression of TLR2 and TLR4 in the lung, and maintained levels of zonulin-1 in lung epithelial cells as compared to GF poly-sensitized and EcN-Chim mono-colonized mice. This study is the first one to establish the model of allergic poly-sensitization in gnotobiotic mice. Using two different settings, gnotobiotic and conventional mice, we demonstrated that an early life intervention with the EcN without expressing an allergen is a powerful strategy to prevent poly-sensitization later in life.
Introduction
The prevalence of allergic poly-sensitization in children has increased significantly over the last decades (1–6). Nowadays, it is approximated that 14 to 45% of children suffer from poly-sensitization (3, 5, 6), which starts as early mono-sensitization to aeroallergens, such as birch and grass pollen allergens (4, 7–11). Despite the availabilities of conventional allergen-specific immunotherapy, based on the injection of increasing doses of allergen mixtures, the exposure to these allergens could pose a risk for the development of new sensitizations. There is a pressing need for the development of novel prophylactic therapies (12–16); therefore, the induction of immune tolerance early in life could be an excellent strategy for preventing poly-sensitization in children.
The priming of the immune system is particularly effective during the early period of life (17). Early life stages such as prenatal, perinatal, and early-postnatal period are crucial for establishing balanced gut microflora, which has been associated with reduced allergy development (18–22). The importance of immunological imprinting has been shown by clinical intervention studies, where early probiotic applications to mothers prevented allergies in their children (23–25). Using a mouse model of mono-sensitization to the major birch pollen allergen Bet v 1, we have previously shown that the perinatal and neonatal colonization with particular wild type probiotic bacteria (21, 26) or with recombinant probiotic bacteria expressing Bet v 1 (22) during the gestation and lactation period, reduced the development of Bet v 1-specific allergy.
Here, we investigated whether Escherichia coli Nissle 1917 (EcN), a probiotic Gram-negative bacterial strain with strong immunomodulatory properties (27, 28), can prevent the development of poly-sensitization in mice when applied early in life. Recently, we engineered a recombinant EcN expressing either the major birch (Bet v 1) and grass (Phl p 1 and Phl p 5) (EcN-Chim) pollen allergens or EcN harboring the empty plasmid (EcN-Ctrl) (7, 28, 29) and showed that the intranasal pretreatment with EcN-Chim but not EcN-Ctrl reduced allergic poly-sensitization in adult mice (28).
In the current study, performed in conventional (CV) and monoxenic mice, we had assessed the impact of EcN-Chim and EcN-Ctrl (antigen-specific vs. non-antigen-specific tolerance induction) treatment on the experimental poly-sensitization model when probiotics were applied in the early phase of life. In this phase of life, it was of interest to investigate whether EcN bacteria itself can prevent allergy or if the expression of the respective allergen by EcN is needed for successful allergy prevention.
Therefore, our first aim was to investigate the effects of EcN-Chim and EcN-Ctrl applied orally to mothers during the last week of gestation and lactation on the development of allergy in their offspring in CV conditions. Secondly, we have used the gnotobiotic mouse model to enable mother-to-offspring early bacterial mono-colonization to understand better the EcN-Chim and EcN-Ctrl impact on the prevention of poly-sensitization.
Material and Methods
Bacteria and Growth Conditions
Two recombinant strains EcN previously described (28) were used in the current study: i) EcN-Chim: a clone expressing multi-allergen chimer Phl p 5-Bet v 1-Phl p 1 along with fluorescent mCherry; ii) EcN-Ctrl clone expressing only mCherry without allergen. Both recombinant clones were grown and selected on Luria-Bertani (LB) agar plates with 20 µg ml−1 chloramphenicol (CM) at 37°C overnight, as described in Sarate et al., 2019 (28). In liquid medium, recombinant EcN-Chim and EcN-Ctrl strains were cultivated at 30°C on 200 RPM overnight in LB-medium containing 20 µg/ml chloramphenicol (28).
Antigens
Recombinant (r) allergens Bet v 1, Phl p 1, and Phl p 5 were purchased from Biomay GmbH (Vienna, Austria).
Animals and Ethics Statement
For perinatal study, pregnant conventional BALB/c mice in the last week of gestation were purchased from Charles River (Sulzfeld, Germany). The animals were maintained under controlled conventional housing conditions and provided with standard diet and water ad libitum. All experiments were approved by the Animal Experimentation Committee of the Medical University of Vienna and by the Federal Ministry of Science and Research (BMWF-66.009/0384-WF/V/3b/2015).
For germ-free (GF) mice studies in neonates, germ-free female BALB/c mice were kept under sterile conditions in Trexler-type plastic isolators, and the absence of aerobic and anaerobic bacteria, molds, and yeast was confirmed every two-weeks by standard microbiological methodology (31, 32). The mice were kept in a room with a 12 h light-dark cycle at 22°C, fed an irradiated sterile diet (Altromin 1414, Altromin, Germany), and provided sterile autoclaved water ad libitum. The animal experiments were approved by the Committee for the Protection and Use of Experimental Animals of the Institute of Microbiology of the Czech Academy of Sciences (approval ID: 23/2018).
Mouse Model of Poly-Sensitization Using Recombinant Birch and Grass Pollen Allergens
Poly-sensitization was done as described previously (28). Briefly, female BALB/c mice were sensitized with three intraperitoneal injections (days 10, 24, and 39) of a mixture of 5 µg rBet v 1, 5 µg rPhl p 1, and 5 µg rPhl p 5 adsorbed to aluminum hydroxide (Al(OH)3; Serva, Heidelberg, Germany) (0.68 mg/ml). To induce airway inflammation, one week after the last intraperitoneal immunization, mice were anesthetized by 2% isoflurane in an anesthetic induction chamber and challenged intranasally with 30 µl of a mixture of 5 µg rBet v 1, 5 µg rPhl p 1, and 5 µg rPhl p 5 for 3 consecutive days.
Mice were sacrificed 72 h after the last challenge by exposure to carbon dioxide and blood was collected from the facial vein of mice at the end of the experiment. Sera were collected and stored at −20°C until used.
The Perinatal Approach in Conventional Mice
Recombinant EcN-Chim and EcN-Ctrl strains were cultivated at 200 RPM, 30°C overnight in LB medium. Bacterial cells were collected and washed with ice-cold PBS. For perinatal application, bacterial cultures were adjusted to 1 x 109 CFU/300µl of gavage buffer (0.2 M NaHCO3 buffer containing 1% glucose, pH 8) per mice. Pregnant mice in the last week of gestation were pretreated orally with either EcN-Chim or EcN-Ctrl during the gestation (every day) and lactation (every second day). On day 21, female offspring (n = 6 to 9 mice per group) derived from these mothers were separated and sensitized intraperitoneally with 5 µg rBet v 1, 5 µg rPhl p 1, and 5 µg rPhl p 5 followed by challenge with 5 µg rBet v 1, 5 µg rPhl p 1, and 5 µg rPhl p 5 as described in the poly-sensitization model (21). Mice were sacrificed 72 h after the last challenge, bronchoalveolar lavage (BAL), lung samples and blood were collected for further analysis. After centrifugation, sera were collected and stored at −20°C for further analysis.
The Perinatal Approach in GF Mice
Recombinant EcN-Chim and EcN-Ctrl strains were prepared as described above (in a sterile condition) for the mono-colonization in GF mice. Eight-week-old GF mice were colonized with a single dose (2 × 108 CFU/200 µl PBS), either EcN-Chim or EcN-Ctrl by intragastric gavage and mated 10 days later. During the experiment, drinking water was supplemented with chloramphenicol (200 mg/L) to ensure the long‐term stability of the recombinant EcN strains in vivo. The stability of colonization was checked by plating of feces on LB agar and counting after aerobic cultivation for 24 h at 37°C. Colonization remained stable throughout the experiment and reached levels of 0.8-1.2 x 1010 CFU/g feces (EcN-Chim) and 0.7-1.4 x 1010 CFU/g feces (EcN-Ctrl).
On day 21, neonatally colonized female offspring either by EcN-Chim or EcN-Ctrl, as well as germ-free controls, were separated from their mothers and divided into two groups. One group was sensitized intraperitoneally with 5 µg rBet v 1, 5 µg rPhl p 1, and 5 µg rPhl p 5 (Biomay, Austria) emulsified in 100 μl of Al(OH)3 (Serva, Germany) three times at a 14-day intervals. The other group was colonized but not sensitized and challenged. One week after the last i.p. immunization, mice were anesthetized by isoflurane and challenged intranasally with 30 μl of the mixture of 5 μg rBet v1, 5 μg rPhl p 1, and 5 μg rPhl p 5 for three consecutive days as described in the poly-sensitized model. Mice were sacrificed 72 h after the last challenge, and blood, BAL and lung samples were collected for further analysis.
Characterization of Airway Inflammation and Allergic Poly-Sensitization
BAL
To evaluate the allergic airway inflammation (AAI), mice were terminally anesthetized, the tracheas were cannulated, and lungs were lavaged with 2 x 0.5 ml PBS (30, 33). BAL fluids were centrifuged at 300 x g for 5 min at 4°C and cell-free supernatants were stored at −20°C for further analysis. Cell pellets were recovered for cellular analysis. After counting, cytospins were prepared by spinning cells onto microscope slides (Shandon Cytospin®, Shandon Southern Instruments, USA) and staining with H&E (Hemacolor®, Merck, Darmstadt, Germany). Cytospin preparations were differentiated according to standard morphologic criteria by counting 200 cells via light microscopy. Collected supernatants were then analyzed for IL-5 and IL-13 response in BAL fluids. Macrophages, lymphocytes, eosinophils, and neutrophils per slide were counted under the light microscope (Nikon Eclipse; 100x magnification) (200 cells per count). Results represent the absolute numbers of cells.
Lung Histology
Small lung tissues were excised and fixed with 7.5% formaldehyde-PBS, followed by paraffin-embedding. Lung sections (5 µm thick) were stained with periodic acid-Schiff (PAS) stain. Lung histological pathology was evaluated using light microscopy. Numbers of PAS-positive, mucus-producing Goblet cells in the bronchial epithelium were counted by an investigator blinded to the experimental setting. Results are given as the mean number of goblet cells per millimeter of the basement membrane (34).
Allergen-Specific Antibodies in Serum
Allergen-specific antibody levels in mouse sera (IgE, IgG1, and IgG2a) were determined by ELISA as previously described (35). Briefly, microtiter plates (Nunc, Roskilde, Denmark) were coated with each of the recombinant allergens Bet v 1, Phl p 1, or Phl p 5 (2 μg/ml) and incubated with mouse sera. Antibody detection was performed using rat anti-mouse IgE, IgG1, IgG2a, followed by peroxidase-conjugated mouse anti-rat IgG. Results show the optical density (OD) values after subtraction of baseline levels from pre-immune sera.
The determination of the allergenic antibody serum activity was performed as previously described (30). Briefly, RBL-2H3 cells were passively sensitized by incubation with serum samples of the respective experimental groups and their degranulation was induced by addition of recombinant allergens rBet v 1, rPhl p 1 and rPhl p 5 (0.3µg/ml) diluted in Thyrode's buffer. Supernatants were analysed for β-hexosaminidase activity. Results are reported as percentages of total β-hexosaminidase release after adding 1% Triton X-100 and are shown after subtraction of baseline release levels obtained with pre-immune sera.
Allergen-Specific Cytokine Detection in BAL
BAL supernatants collected during sacrifice were analyzed for IL-5 and IL-13 levels by using an ELISA specific for murine cytokines (Ready-Set-Go ELISA Kit eBioscience, USA) according to the manufacturer’s instructions.
Quantification of mRNA Expression by Real-Time (RT)-PCR
Total RNA was extracted from lung samples of mice from all treatment and controls group at the end of the experiment. RNA quantification was performed using ND-1000 Spectrophotometer (Nanodrop Technologies Inc., Wilmington, USA) and cDNA was obtained using reverse-transcriptase kit (BIO-RAD, Vienna, Austria). Expression of IL-10, Foxp3, and IFNγ mRNA was measured by RT-PCR as described previously (30). The housekeeping gene Glyceraldehyde 3-phosphate dehydrogenase (GAPDH) and β-actin (Universal Probe Library probe #64; Roche) were used as a control to standardize the amount of sample cDNA. Data are presented as the ratio of the target genes expression to GAPDH and β-actin expression.
Immunohistochemical Identification of TLR2, TLR4, and Zonulin-1 (ZO-1) in Lung Tissue of Mono-Colonized Mice
For TLR2 and TLR4 analysis, the 3 μm lung sections were deparaffinized and antigens were retrieved in 0.01 M citrate buffer (pH 6) using a microwave vessel for 10 min. After cooling down and washing by PBS, endogenous peroxidase was blocked with 3% hydrogen peroxide in PBS for 15 min. Non-specific adsorption was eliminated by incubation of the sections in 10% normal rabbit serum in PBS for 1 h. Samples were incubated overnight with polyclonal goat anti-TLR2 (4 μg/ml) or anti-TLR4 (4 μg/ml) (Santa Cruz Biotechnology, Dallas, Texas, USA) at 4°C. After washing in PBS, sections were incubated with rabbit anti-goat IgG conjugated with horseradish peroxidase (1:200 in PBS) (Jackson, ImmunoLabs., West Grove, PA, USA) for 1 h and stained with DAB (3,3′-Diaminobenzidine) (Dako, Carpinteria, CA, USA) for 3 min. The counterstain was carried out with hematoxylin, samples were mounted by the Paramount Aqueous Mounting medium (Dako, Carpinteria, CA, USA) and viewed under an Olympus BX 40 microscope with 40x objective, equipped with and Olympus DP 70 digital camera.
ZO-1 analysis was done as previously described (36). Briefly, the 3 μm lung sections were deparaffinized, antigens were retrieved by protease from Streptomyces griseus (1 mg/ml, type XIV, Sigma-Aldrich, St. Luis, MO, USA) at 37°C for 8 min, washed with PBS and endogenous peroxidase was blocked with 3% hydrogen peroxide in PBS for 15 min. Non-specific adsorption was eliminated by incubation of the sections in 10% normal goat serum in PBS for 1 h. Samples were incubated overnight with polyclonal rabbit anti-ZO-1 (5 μg/ml, Santa Cruz Biotechnology, Dallas, Texas, USA) at 4°C. After washing in PBS, sections were incubated with goat anti-rabbit IgG conjugated with horseradish peroxidase (1:200 in PBS) (Jackson, ImmunoLabs., West Grove, PA, USA) for 1 h and stained with DAB (3,3′-Diaminobenzidine) (Dako, Carpinteria, CA, USA) for 1 min.
Quantification of TLR2, TLR4 and ZO-1 immunohistochemistry was done by measuring the optical density (OD). The OD analysis of the TLR4, TLR2 and Zonulin-1 images was evaluated using ImageJ (Fijiv2.0.0; National Institutes of Health, Bethesda, MD). Briefly, 8-bit RGB DAB-stained images were processed through color deconvolution (37), and threshold settings and mean gray value was assessed in the selected area of bronchus/bronchiolar epithelial layer in DAB-extracted images. OD was estimated by the following formula: OD = log (max intensity/mean gray value intensity), where max intensity = 255 (38). OD of background (field without any tissue) was subtracted from OD of epithelial cell layer. Results from two to five bronchi/bronchiole from individual mouse were pooled and five mice per each group were evaluated (37, 38).
Statistical Analysis
Statistical analysis was conducted using GraphPrism, ver. 6. For comparison of more groups the One-Way ANOVA was applied followed by the Bonferroni’s Multiple Comparison Test unless otherwise specified. All data are shown as mean ± SEM. Significant differences were considered at P < 0.05 (*), P < 0.01 (**), P < 0.001 (***).
Results
Conventional Mouse Model
Offspring of Mothers Treated With EcN-Ctrl Exhibited Reduced Allergic Airway Inflammation (AAI)
To assess the effects of perinatal interventions with probiotic bacteria on poly-sensitization, mice were treated orally with either EcN-Chim or EcN-Ctrl during the gestation and lactation period, and their offspring were sensitized and challenged with rBet v 1, rPhl p 1, and rPhl p 5 (Figure 1A). The offspring colonized perinatally with EcN-Ctrl exhibited reduction in BAL eosinophils (P < 0.001), IL-5 (P < 0.05), and IL-13 (P < 0.01) levels as compared to poly-sensitized offspring (Figures 1B–E). Offspring colonized with EcN-Chim exhibited reduced IL-13 (P < 0.05) as well as eosinophil level (P < 0.05) in BAL compared to poly-sensitized animals. Lung histology revealed reduced mucus production in the lung of offspring colonized with either EcN-Chim or EcN-Ctrl as compared to poly-sensitized controls (Figure 1F). Besides, perinatal colonization with EcN-Ctrl induced a reduction in Bet v 1-specific serum IgE as compared to poly-sensitized controls (P < 0.01) (Figure 1G).
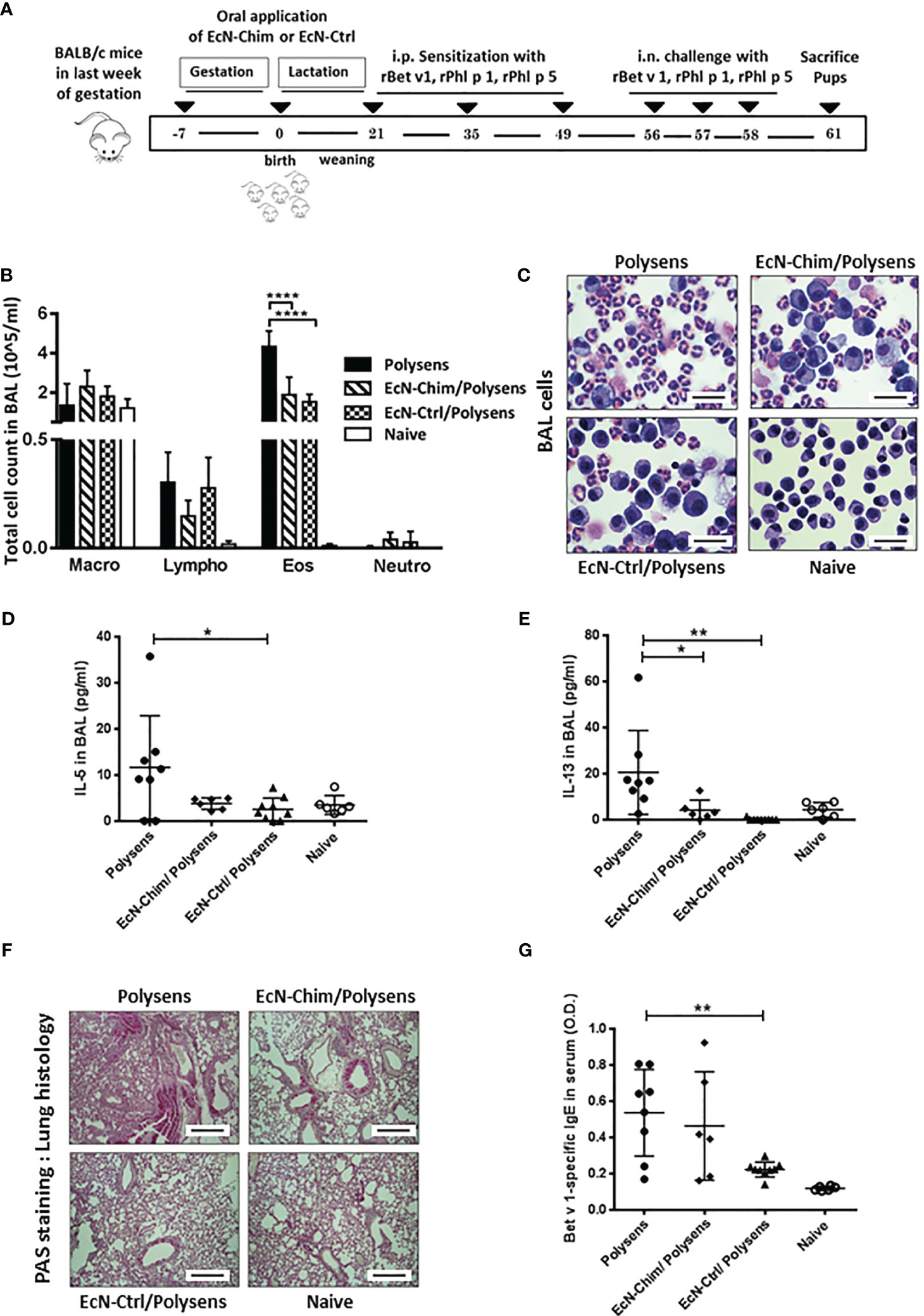
Figure 1 Perinatal application of Escherichia coli Nissle 1917 (EcN)-Chim and EcN-Ctrl in conventional mice reduced airway inflammation. (A) Schematic representation of the perinatal application of recombinant EcN expressing birch-grass pollen chimera (EcN-Chim) and EcN expressing empty plasmid (EcN-Ctrl) in a conventional mouse model of poly-sensitization. Female BALB/c mice were given either EcN-Chim or EcN-Ctrl orally during the gestation and lactation. On day 21, female offspring derived from these mothers were separated and were then sensitized and challenged with 5 µg Bet v 1, 5 µg Phl p 1, and 5 µg Phl p 5 as described in poly-sensitization model. Mice were sacrificed 72 h after the last challenge. (B) Absolute numbers of macrophages, lymphocytes, eosinophils, and neutrophils in bronchoalveolar lavage (BAL) and (C) Representative cytospins of BAL of one mouse per group stained with hematoxylin and eosin (H&E; 100x magnification). (D) IL-5 and (E) IL-13 cytokines in BAL. (F) Representative lung tissue sections of one mouse per group stained with Periodic Acid Schiff (PAS) (Red; 10 x magnification; scale bars 100 µm). (G) Levels of Bet v 1-specific serum IgE. (B, D, E, G) represents the mean ± SEM from two experiments (total n = 6 to 9 mice per group). Error bars show mean ± SEM. *P < 0.05, **P < 0.01, ****P < 0.0001 by the One-way ANOVA followed by the Bonferroni’s Multiple Comparison Test.
Gnotobiotic Mouse Model
Perinatal and Neonatal Mono-Colonization With Either EcN-Chim or EcN-Ctrl Prevented AAI
In a gnotobiotic mouse model, perinatal/neonatal mono-colonization of offspring via their mothers with either EcN-Chim or EcN-Ctrl (Figure 2A) significantly reduced AAI as compared to GF poly-sensitized controls (Figures 2B–F). This was reflected by reduced recruitment of eosinophils (EcN-Chim, P < 0.0001; EcN-Ctrl, P < 0.001), as well levels of IL-5 (EcN-Chim, P < 0.01; EcN-Ctrl, P< 0.05), and IL-13 (EcN-Chim, P < 0.0001; EcN-Ctrl, P < 0.001) in BAL samples compared to GF poly-sensitized controls (Figures 2B–E). Histological analysis revealed reduced mucus production by airway-lining goblet cells in the lungs of the groups colonized with either EcN-Chim (P < 0.0001) or EcN-Ctrl (P < 0.0001) compared to GF poly-sensitized control (Figures 2F, G).
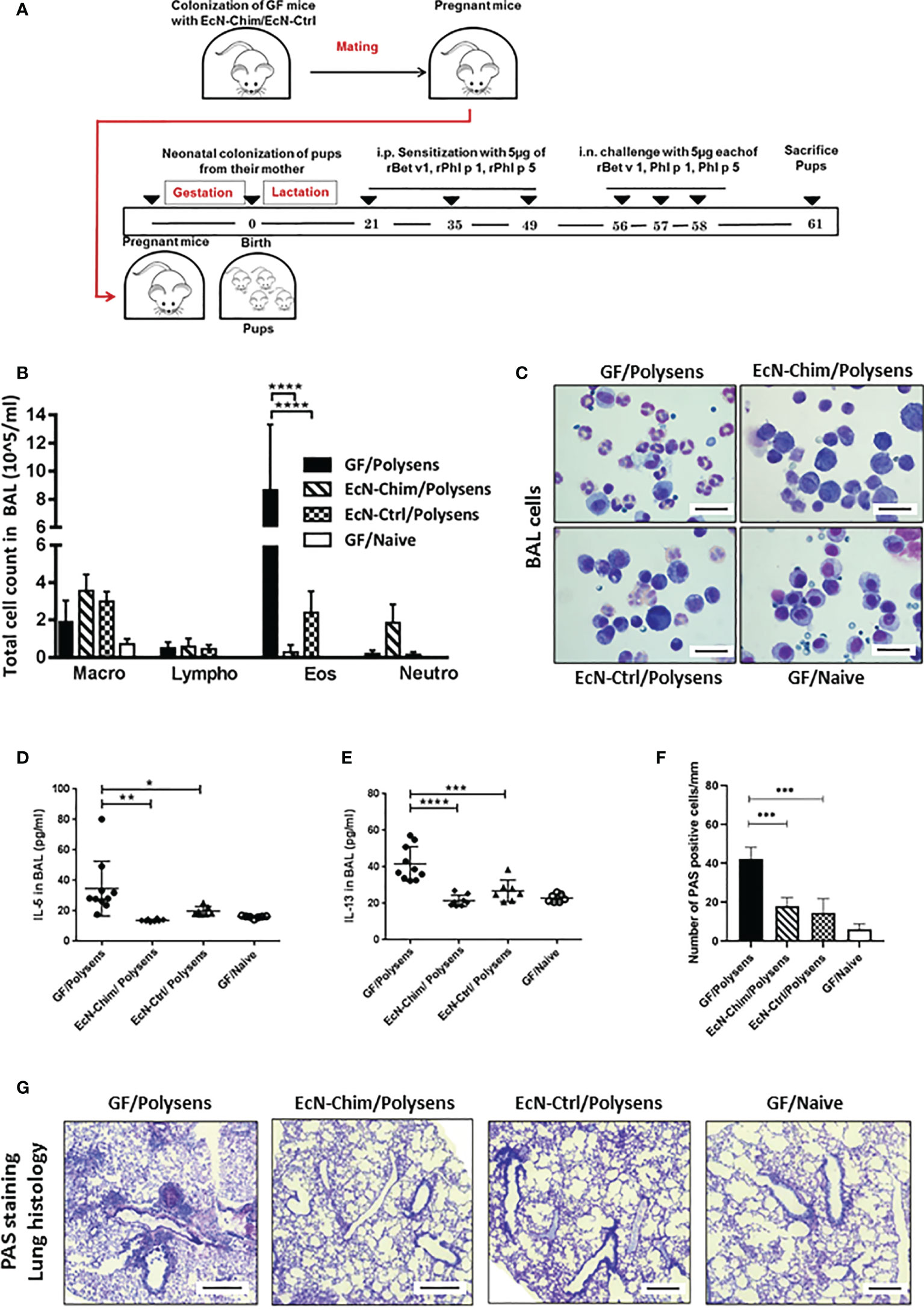
Figure 2 Perinatal and neonatal mono-colonization of Escherichia coli Nissle 1917 (EcN)-Chim and EcN-Ctrl in germ-free (GF) mice reduced airway inflammation. (A) Schematic representation of the neonatal colonization of GF mice with recombinant EcN expressing EcN-Chim and EcN-Ctrl in a gnotobiotic mouse model of poly-sensitization. Eight-week-old GF mice were colonized with a single dose of either EcN-Chim or EcN-Ctrl by intragastric gavage and mated 10 days later. On day 21, all mono-colonized female offspring were separated from their mothers and divided into two groups. One group was sensitized and challenged with 5 µg rBet v 1, 5 µg rPhl p 1, and 5 µg rPhl p 5 and other not. Mice were sacrificed 72 h after the last challenge (B) Absolute numbers of macrophages, lymphocytes, eosinophils, and neutrophils in bronchoalveolar lavage (BAL). (C) Representative cytospins of BAL of one mouse per group stained with H&E 100x magnification). (D) IL-5 and (E) IL-13 cytokines in BAL. (F) Quantification of mucus-producing goblet cells. (G) Representative lung tissue sections of one mouse per group stained with Periodic Acid Schiff (PAS) (Red; 10x magnification; scale bars 100 µm); arrows indicate cell infiltration. (B, D–F) represents mean ± SEM from two experiments (total n = 7 to 10 mice per group). Error bars show mean ± SEM. *P < 0.05, **P < 0.01, ***P < 0.001, ****P < 0.0001 by the One-way ANOVA followed by the Bonferroni’s Multiple Comparison Test.
Perinatal and Neonatal Mono-Colonization With Either EcN-Chim or EcN-Ctrl Inhibits the Development of Th2-Type Allergen-Specific Immune Response
Mono-colonization with EcN-Chim and EcN-Ctrl significantly reduced Phl p 5-specific IgG1 levels (EcN-Chim, P < 0.05; EcN-Ctrl, P < 0.001) in sera (Figure 3A). Perinatal/neonatal mono-colonization with EcN-Ctrl led to a substantial increase of Bet v 1 (P < 0.01) and Phl p 5-specific (P < 0.0001) IgG2a antibody levels in serum in comparison with GF poly-sensitized control mice (Figure 3B). Mono-colonization with EcN-Chim led to increased IgG2a against Phl p 5 (P < 0.0001) compared to GF poly-sensitized control (Figure 3B). By measuring the IgE-dependent basophil degranulation, we have shown that cells derived from EcN-Chim and EcN-Ctrl-colonized mice and stimulated with rBet v 1 (P < 0.01) and rPhl p 5 (P < 0.01) exhibited reduced antigen-specific β-hexosaminidase release compared to the sera of GF poly-sensitized controls (Figure 3C). No significant difference was observed for Phl p 1-specific IgG2a, IgG1, and β-hexosaminidase release in EcN-Chim and EcN-Ctrl mice in comparison with GF poly-sensitized controls (Figures 3A–C).
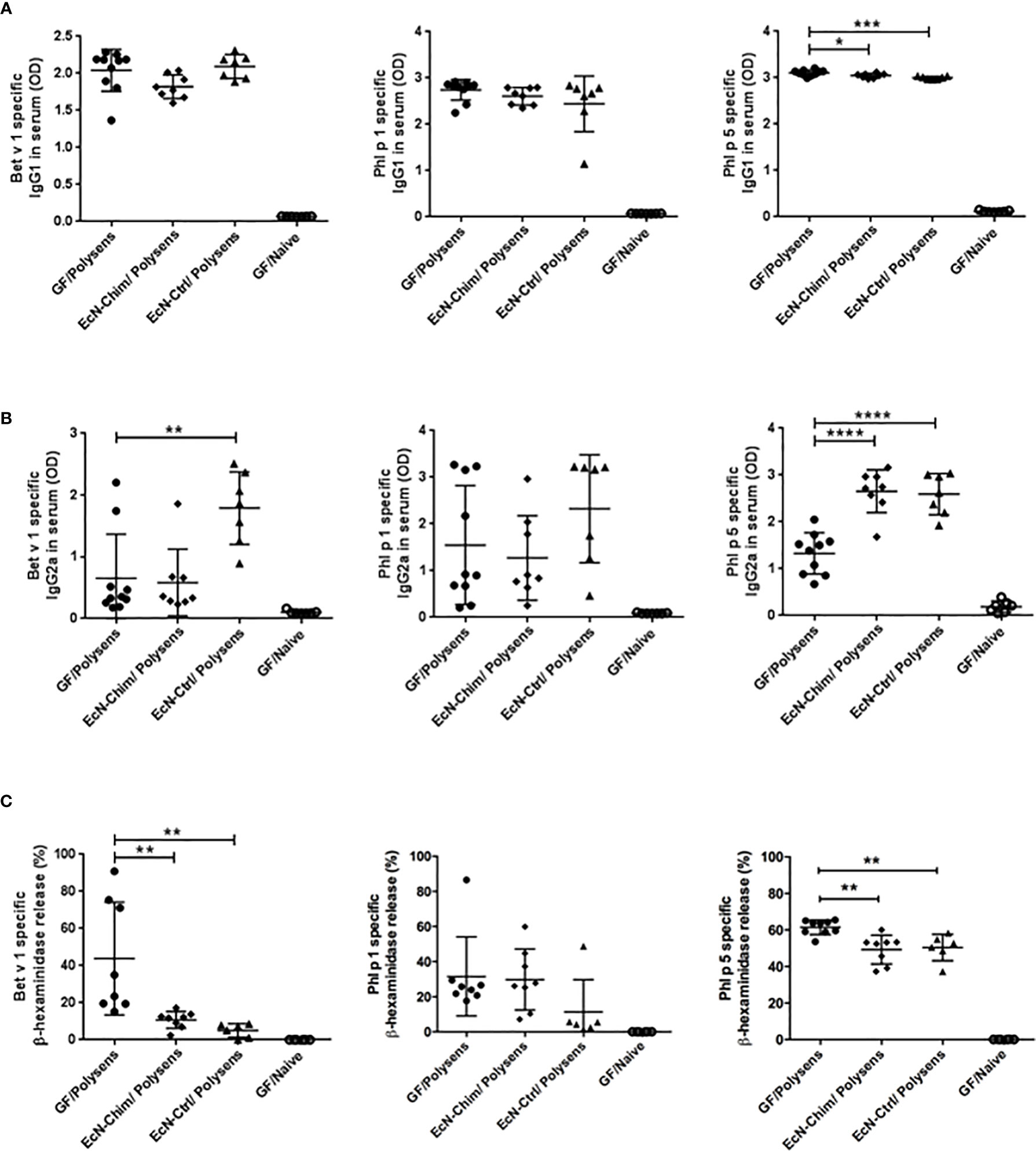
Figure 3 Perinatal and neonatal mono-colonization with Escherichia coli Nissle 1917 (EcN)-Chim and EcN-Ctrl inhibits the development of Th2-type allergen-specific immune response. Mice were treated as indicated in Figure 2A. Serum samples were obtained from mice on sacrifice day. Allergen-specific antibody levels in mouse sera were determined by ELISA and RBL. The figure represents levels of Bet v 1, Phl p 1, Phl p 5-specific serum (A) IgG1 (B) IgG2a, and (C) IgE. Data represent mean ± SEM from two experiments (total n = 7 to 10 mice per group). Error bars show mean ± SEM. *P < 0.05, **P < 0.01, ***P < 0.001, ****P < 0.0001 by the One-way ANOVA followed by the Bonferroni’s Multiple Comparison Test.
Perinatal and Neonatal Mono-Colonization With EcN-Ctrl but Not EcN-Chim Induced IL-10, Foxp3, and IFNγ mRNA Expression in the Lung
Expression of IL-10, Foxp3, and IFNγ mRNA in lungs were analyzed with two reference genes GAPDH (Figures 4A–C) and β-actin (Figures 4D–F). Although mono-colonization with both EcN-Ctrl and EcN-Chim reduced allergy in poly-sensitized mice, only the mono-colonization with EcN-Ctrl triggered the increased expression of IL-10 (P < 0.01), Foxp3 (P < 0.001), and IFNγ (P < 0.0001) mRNA in the lung as compared to GF poly-sensitized control (Figures 4A–C, E, F). Mono-colonization with EcN-Chim did not influence the levels of IL-10, Foxp3, and IFNγ mRNA expression in the lung in comparison with GF poly-sensitized mice. EcN-Ctrl showed significantly higher expression of IL-10 (P < 0.05), Foxp3 (P < 0.01), and IFNγ (P < 0.01) compared to EcN-Chim (Figures 4A–C, F).
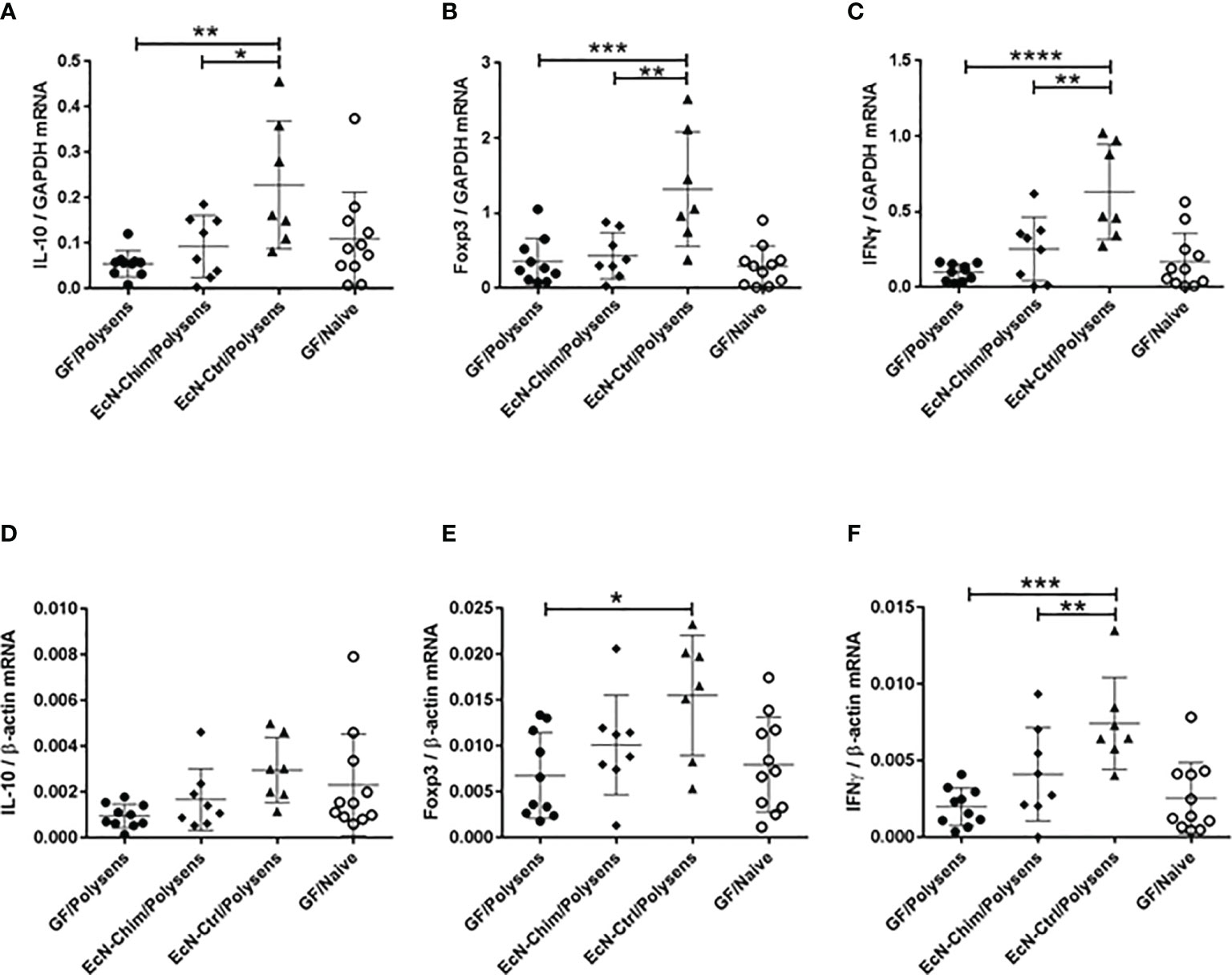
Figure 4 Perinatal and neonatal mono-colonization with Escherichia coli Nissle 1917 (EcN)-Ctrl led to increased IL-10, Foxp3, and IFNγ mRNA expression in the lung. Mice were treated as indicated in Figure 2A. After sacrifice, lung samples were collected and expression of IL-10, Foxp3 and IFNγ mRNA was measured by real-time (RT)-PCR. The figure represents the ratio of the target genes: (A) IL-10, (B) Foxp3, and (C) IFNγ to the GAPDH reference gene and (D) IL-10, (E) Foxp3, and (F) IFNγ to the β-actin reference gene. Data represents mean ± SEM from two experiments (total n = 7 to 10 mice per group) analyzed by the One-way ANOVA followed by the Bonferroni’s Multiple Comparison Test. Error bars show mean ± SEM. Data represents mean ± SEM from two experiments (total n = 7 to 10 mice per group). Error bars show mean ± SEM. *P < 0.05, **P < 0.01, ***P < 0.001, ****P < 0.0001 by the One-way ANOVA followed by the Bonferroni’s Multiple Comparison Test.
Perinatal and Neonatal Mono-Colonization With EcN-Chim and EcN-Ctrl Increased Expression of TLR2 and TLR4 as Well as Maintained Epithelial Barrier Integrity in the Lung by ZO-1 Expression
Immunochemical staining of the lungs showed increased TLR2 (Figure 5A) and TLR4 (Figure 5B) expression in the lungs of mono-colonized mice. This increase was confirmed by quantification of TLR2 and TLR4 using ImageJ software. Quantification data showed moderate increase in TLR2 (Figure 5C) and strong stimulation of TLR4 (Figure 5D) expression in the lung of mice mono-colonized with either EcN-Chim or EcN-Ctrl compared to and poly-sensitized controls.
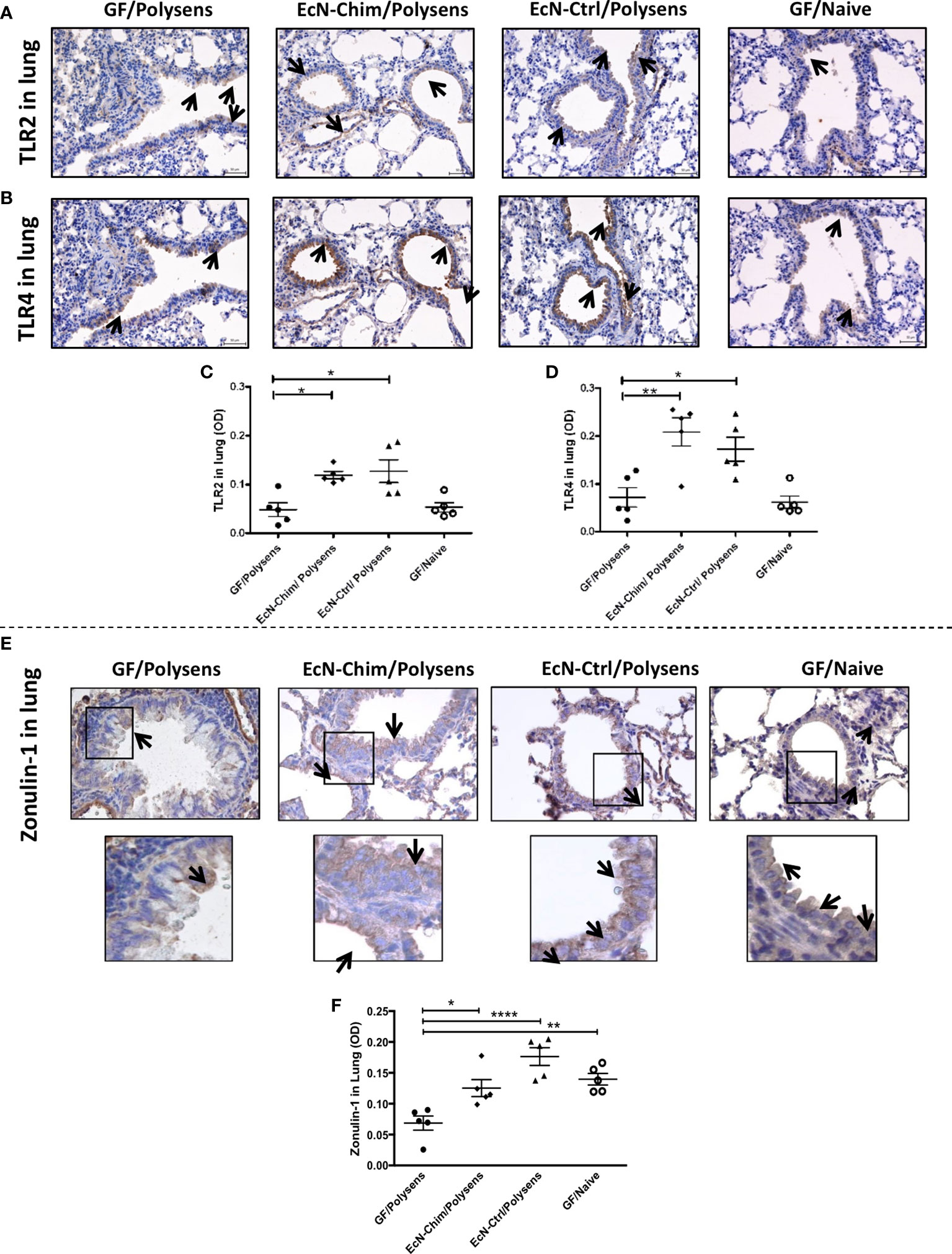
Figure 5 Perinatal and neonatal mono-colonization of either EcN-Chim or EcN-Ctrl activated both TLR2 and TLR4 expression and maintained ZO-1 levels in the lung. Mice were treated as indicated in Figure 2A. For immunohistochemistry analysis, lung sections were processed and stained as described in Material and Methods using antibodies against TLR2, TLR4, and ZO-1 followed by 3,3′-Diaminobenzidine (DAB) staining. Samples were analyzed under a light microscope with a 40x objective. Immunohistochemistry staining for TLR2 (A), TLR4 (B), and ZO-1 (E) is represented by a brown color (indicated by arrows). Quantification of TLR2 (C), TLR4 (D), and ZO-1 (F) expression was performed by optical density analysis (OD) of bronchial epithelial cell layer using ImageJ software. (C, D, F) represents mean ± SEM from two experiments (five mice per group were tested). Error bars show mean ± SEM. *P < 0.05, **P < 0.01, ****P < 0.0001 by the One-way ANOVA followed by the Bonferroni’s Multiple Comparison Test.
Lung immunohistochemistry showed maintained levels of ZO-1 in bronchial epithelial cells, in both EcN-Chim and EcN-Ctrl mono-colonized mice as compared to GF polysensitized mice (Figure 5E). Quantification of ZO-1 showed significant decrease in ZO-1 in GF polysensitized mice as compared to both mono-colonized mice (Figure 5F). An apparent reduction in epithelial ZO-1 levels was observed in GF poly-sensitized controls as compared to GF naïve group (Figure 5F).
Discussion
The constant increase in birch and grass pollen poly-sensitization and the lack of appropriate therapy are raising a considerable demand for a robust prophylactic intervention (5, 6, 39–41). It has been suggested that the immune response generated during the early phase of life to various environmental factors and antigens may be decisive for the immune response to different allergic diseases later in life (19, 20, 42–44). In this study, we have demonstrated that the perinatal and neonatal mono-colonization of mice with EcN expressing either birch-grass pollen multi-allergen, EcN-Chim, or an empty vector, EcN-Ctrl, prevented the development of poly-sensitization.
Evidence-based studies have revealed that interventions with probiotic bacteria prevent the development of allergy (45, 46). EcN is a probiotic strain with strong immunomodulatory properties and has also been used as an antigen delivery system (47–50). We have previously shown that the EcN-Ctrl was not potent enough to reduce allergic multi-sensitivities in fully-grown mice with established microflora. Therefore, we constructed a recombinant EcN strain, expressing birch and grass pollen allergens chimera, EcN-Chim, which prevented allergen-specific multi-sensitivities in adult mice after intranasal application (28). In the current study, by performing experiments in the perinatal and neonatal phase and taking advantage of early interventions, we have demonstrated that mothers-to-offspring mono-colonization not only with the EcN-Chim but also EcN-Ctrl strain, which does not express the tolerizing allergens, was sufficient to prevent allergy development.
It has been shown that various factors such as reduced infections, delivery by Cesarean section, antibiotic treatment, and microbial dysbiosis during the early age of life can influence the development of the immune system leading to an increased risk of allergic multi-sensitization, particularly in infancy (19, 51–55). The maturation of human immune system occurs early in life in parallel to the establishment of the gut microflora, i.e., approximately from the conception to the end of the second year of childhood (56, 57). Numerous clinical mono-sensitization studies have demonstrated that the early phase of life represents a “window of opportunity” where the immune system can be modulated by the application of certain probiotic bacteria (23–25, 56). In line with other studies, we have previously shown that early intervention with probiotic bacterial strains can prevent birch pollen allergy in mice (21, 22). In the current study, we have demonstrated that the immune system can be modulated by using allergen-specific and allergen-non-specific immunomodulators during the gestation and lactation period to prevent the development of poly-sensitization.
Our group has shown that the route of probiotic application is essential for the outcome of probiotic interventions in animal models of mono- and poly-sensitization (28, 35, 58). In conventional adult mice with fully established gut microflora, we have previously demonstrated that the intranasal route possesses advantages over the oral route of application in reaching the beneficial effects of treatment (28). In the current study in neonates, a single oral application of probiotic bacteria in germ-free mothers was sufficient to induce a local as well as systemic protective immune response against poly-sensitization. This finding has tremendous practical implications since the oral route of application is more convenient and the preferred route for the induction of mucosal tolerance in children against various food- and airborne-triggered allergic diseases (44, 59–61). The oral application to mothers led to the colonization of either EcN-Chim or EcN-Ctrl in offspring (data are not shown). Despite having rich commensal microflora, the EcN strain managed to colonize in the guts of CV offspring during the neonatal period and had a pronounced effect on poly-sensitization. However, it is still unclear how the colonization establishes in offspring. We can assume that this colonization occurs via contact with mothers feces and/or feeding on mothers milk. Several clinical studies demonstrated colonization with probiotic bacteria from mother to offspring via breast milk (62, 63).
To investigate the mechanism of tolerance induction by the EcN-Chim and EcN-Ctrl strains against poly-sensitization, we took advantage of a gnotobiotic mouse model. We found that both strains reduced allergic multi-sensitization and AAI. Mice mono-colonized with EcN-Ctrl exhibited increased Th1/Treg responses in the lung. The shift toward Th1/Treg cellular responses in the EcN-Ctrl group was associated with reduced Bet v 1- and Phl p 5-specific IgE measured by rat basophil leukemia (RBL) cells degranulation assay as the level of β-hexaminidase, and increased levels of allergen-specific IgG2a. We did not observe any difference in Phl p 1-specific β-hexaminidase release and IgG2a levels, which might be associated with the high allergenic properties of Phl p 1 (64–66). These results are in line with previous studies suggesting that anti-allergic properties of the EcN are related to the induction of specific Th1/Treg response via the induction of Foxp3, IL-10, and IgG2a (28, 50, 67). It is known that Foxp3 and IL-10 play a key role in allergy suppression and maintaining immune tolerance (68–70). Our data indicate that perinatal and neonatal mono-colonization with the EcN-Ctrl precludes poly-sensitization development by polarizing the Th1/Treg response in the lung.
It has been suggested that mucosal TLR2 and TLR4 orchestrate the tolerance in the gastrointestinal and respiratory tract (50, 67, 71). It was further demonstrated that the amelioration of dextran sulfate sodium-induced colitis by EcN is mediated via TLR-2- and TLR-4-dependent pathways (72, 73). In our current study, the lung from mice mono-colonized with the EcN-Chim and EcN-Ctrl showed increased expression of both TLR2 and TLR4 compared with GF poly-sensitized and GF naive controls. These findings support our previous in vitro data, demonstrating that the EcN can activate both TLR2 and TLR4 expressed in HEK293 cells (28).
Apart from being an important site to initiate the immune response, airway epithelial mucosa plays a vital role in protecting the body from the environment and maintaining the inner homeostasis in the lung (74, 75). Often in allergy, the structure and functions of airway epithelial barriers are markedly impaired by the lung inflammation (76) and this can lead to a more pronounced sensitization (77). To investigate the impact of the EcN-Chim and EcN-Ctrl on barrier functions, we evaluated ZO-1 levels in lung tissue samples. The ZO-1 is the protein expressed in tight junctions that regulates the epithelial barrier function by preventing the entry of different pathogens and antigens (78). The EcN strain has been used previously in premature and full-term infants to enhance postnatal immune competence against necrotizing enterocolitis by improving gut barrier function (79). In its outer cell membrane, EcN expresses a unique lipopolysaccharide, enabling EcN to exhibit immunomodulating properties without showing immunotoxic effects (27, 80). It was demonstrated that EcN interacts with the intestinal epithelial cells, which fortifies the epithelial cell barrier. It is also suggested that EcN triggers the stimulation of epithelial defensin production, which helps restore the enterocytes’ tight junctions (81). Our current study shows that poly-sensitization in the gnotobiotic mouse model led to reduced ZO-1 levels compared to GF naïve mice. However, the neonatal application of both the EcN-Chim and EcN-Ctrl maintained the ZO-1 levels in the airways after poly-sensitization at a similar level as in GF naïve mice.
In conclusion, we provide evidence that interventions with probiotic bacteria early in life could present a promising prophylactic tool to imprint the immune system toward preventing the development of poly-sensitization. Contrary to the adult setting, where the mucosal tolerance was induced only in the presence of tolerizing allergen (EcN-Chim) (28), the interventions during peri- and neonatal period of life led to allergy prevention in mice with the probiotic strain without expressed allergen (EcN-Ctrl). In other words, it was possible to induce tolerance avoiding a potential sensitization with “a foreign” antigen. Therefore, we suggest that the application of wild type probiotic bacteria during the “window of opportunity” is a safe approach. Our work builds the foundation for development of personalized strategies for a prophylactic and therapeutic treatment of poly-sensitization. Further studies in mice and humans are needed to evaluate the full potential of our approach.
Data Availability Statement
The original contributions presented in the study are included in the article. Further inquiries can be directed to the corresponding authors.
Ethics Statement
The animal study was reviewed and approved by the Animal Experimentation Committee of the Medical University of Vienna and by the Federal Ministry of Science and Research (BMWF-66.009/0384-WF/V/3b/2015) (conventional mice experiments). Gnotobiotic mouse experiments were approved by committee for the Protection and Use of Experimental Animals of the Institute of Microbiology, Academy of Sciences of the Czech Republic (approval ID: 23/2018).
Author Contributions
UW received the grant from FWF, and together with HK conceived the study. PS, DS, UW designed the experiments. PS, DS, and NG performed the experiments. PS, NG, DS, AI-K, and UW analyzed the data. MS, AI-K, IS, and UW contributed reagents/materials/analysis tools. PS, AI-K, UW drafted the manuscript. PS, AI-K, IS, and UW wrote the manuscript. All authors contributed to the article and approved the submitted version.
Funding
This work was funded by the Austrian Science Fund (FWF) SFB F46 and DK MCCA W1248-B30; the OeAD WTZ grants CZ 06/2020 and CZ 16/2019; and by the grants: 19-02261S of the Czech Science Foundation and 8J20AT011 of the Ministry of Education, Youth and Sports of the Czech Republic.
Conflict of Interest
The authors declare that the research was conducted in the absence of any commercial or financial relationships that could be construed as a potential conflict of interest.
Acknowledgments
We gratefully thank Katharina Ambroz, Mirjana Drinic, Karin Baier, Elke Korb, Erika Garner-Spitzer, Christian Zwicker, Joshua Tobias, Tomas Hudcovic, Petra Petr Hermanova, Jaroslava Valterova, Barbora Drabonova, Kamila Michalickova for invaluable technical assistance and helpful discussions.
References
1. Calderon MA, Cox L, Casale TB, Moingeon P, Demoly P. Multiple-allergen and single-allergen immunotherapy strategies in polysensitized patients: looking at the published evidence. J Allergy Clin Immunol (2012) 129(4):929–34. doi: 10.1016/j.jaci.2011.11.019
2. Forkel S, Beutner C, Heetfeld A, Fuchs T, Schön MP, Geier J, et al. Allergic Rhinitis to Weed Pollen in Germany: Dominance by Plantain, Rising Prevalence, and Polysensitization Rates over 20 Years. Int Arch Allergy Immunol (2020) 181(2):128–35. doi: 10.1159/000504297
3. Ha EK, Baek JH, Lee SY, Park YM, Kim WK, Sheen YH, et al. Association of Polysensitization, Allergic Multimorbidity, and Allergy Severity: A Cross-Sectional Study of School Children. Int Arch Allergy Immunol (2016) 171(3–4):251–60. doi: 10.1159/000453034
4. Migueres M, Davila I, Frati F, Azpeitia A, Jeanpetit Y, Lheritier-Barrand M, et al. Types of sensitization to aeroallergens: definitions, prevalences and impact on the diagnosis and treatment of allergic respiratory disease. Clin Trans Allergy (2014) 4:16. doi: 10.1186/2045-7022-4-16
5. Raciborski F, Bousqet J, Namysłowski A, Krzych-Fałta E, Tomaszewska A, Piekarska B, et al. Dissociating polysensitization and multimorbidity in children and adults from a Polish general population cohort. Clin Trans Allergy (2019) 9(1):4. doi: 10.1186/s13601-019-0246-y
6. Silvestri M, Rossi GA, Cozzani S, Pulvirenti G, Fasce L. Age-dependent tendency to become sensitized to other classes of aeroallergens in atopic asthmatic children. Ann Allergy Asthma Immunol (1999) 83(4):335–40. doi: 10.1016/S1081-1206(10)62674-9
7. Bousquet J, Hejjaoui A, Becker WM, Cour P, Chanal I, Lebel B, et al. Clinical and immunologic reactivity of patients allergic to grass pollens and to multiple pollen species: I. Clinical and immunologic characteristics. J Allergy Clin Immunol (1991) 87(3):737–46. doi: 10.1016/0091-6749(91)90397-7
8. Ciprandi G, De Amici M, Giunta V, Marseglia GL. Comparison of serum specific IgE and skin prick test in polysensitized patients. Int J Immunopathol Pharmacol (2010) 23(4):1293–5. doi: 10.1177/039463201002300438
9. Ciprandi G, Incorvaia C, Puccinelli P, Soffia S, Scurati S, Frati F. Polysensitization as a challenge for the allergist: the suggestions provided by the Polysensitization Impact on Allergen Immunotherapy studies. Expert Opin Biol Ther (2011) 11(6):715–22. doi: 10.1517/14712598.2011.576246
10. Hatzler L, Panetta V, Lau S, Wagner P, Bergmann RL, Illi S, et al. Molecular spreading and predictive value of preclinical IgE response to Phleum pratense in children with hay fever. J Allergy Clin Immunol (2012) 130(4):894–901.e5. doi: 10.1016/j.jaci.2012.05.053
11. Movérare R, Westritschnig K, Svensson M, Hayek B, Bende M, Pauli G, et al. Different IgE Reactivity Profiles in Birch Pollen-Sensitive Patients from Six European Populations Revealed by Recombinant Allergens: An Imprint of Local Sensitization. Int Arch Allergy Immunol (2002) 128(4):325–35. doi: 10.1159/000063855
12. Anto JM, Bousquet J, Akdis M, Auffray C, Keil T, Momas I, et al. Mechanisms of the Development of Allergy (MeDALL): Introducing novel concepts in allergy phenotypes. J Allergy Clin Immunol (2017) 139(2):388–99. doi: 10.1016/j.jaci.2016.12.940
13. Bousquet J, Van Cauwenberge P, Khaltaev N. Allergic rhinitis and its impact on asthma. J Allergy Clin Immunol (2001) 108(5 Suppl):S147–334. doi: 10.1067/mai.2001.118891
14. Nelson HS. Multiallergen immunotherapy for allergic rhinitis and asthma. J Allergy Clin Immunol (2009) 123(4):763–9. doi: 10.1016/j.jaci.2008.12.013
15. Larsen JN, Broge L, Jacobi H. Allergy immunotherapy: the future of allergy treatment. Drug Discov Today (2016) 21(1):26–37. doi: 10.1016/j.drudis.2015.07.010
16. Kim KW, Kim EA, Kwon BC, Kim ES, Song TW, Sohn MH, et al. Comparison of allergic indices in monosensitized and polysensitized patients with childhood asthma. J Korean Med Sci (2006) 21(6):1012–6. doi: 10.3346/jkms.2006.21.6.1012
17. Renz H, Adkins BD, Bartfeld S, Blumberg RS, Farber DL, Garssen J, et al. The neonatal window of opportunity—early priming for life. J Allergy Clin Immunol (2018) 141(4):1212–4. doi: 10.1016/j.jaci.2017.11.019
18. Torow N, Hornef MW. The Neonatal Window of Opportunity: Setting the Stage for Life-Long Host-Microbial Interaction and Immune Homeostasis. J Immunol (2017) 198(2):557. doi: 10.4049/jimmunol.1601253
19. Lambrecht BN, Hammad H. The immunology of the allergy epidemic and the hygiene hypothesis. Nat Immunol (2017) 18(10):1076–83. doi: 10.1038/ni.3829
20. Hill CJ, Lynch DB, Murphy K, Ulaszewska M, Jeffery IB, O’Shea CA, et al. Evolution of gut microbiota composition from birth to 24 weeks in the INFANTMET Cohort. Microbiome (2017) 5(1):4–. doi: 10.1186/s40168-016-0213-y
21. Schabussova I, Hufnagl K, Tang ML, Hoflehner E, Wagner A, Loupal G, et al. Perinatal maternal administration of Lactobacillus paracasei NCC 2461 prevents allergic inflammation in a mouse model of birch pollen allergy. PLoS One (2012) 7(7):6. doi: 10.1371/journal.pone.0040271
22. Schwarzer M, Repa A, Daniel C, Schabussova I, Hrncir T, Pot B, et al. Neonatal colonization of mice with Lactobacillus plantarum producing the aeroallergen Bet v 1 biases towards Th1 and T-regulatory responses upon systemic sensitization. Allergy (2011) 66(3):368–75. doi: 10.1111/j.1398-9995.2010.02488.x
23. Wickens K, Black PN, Stanley TV, Mitchell E, Fitzharris P, Tannock GW, et al. A differential effect of 2 probiotics in the prevention of eczema and atopy: a double-blind, randomized, placebo-controlled trial. J Allergy Clin Immunol (2008) 122(4):788–94. doi: 10.1016/j.jaci.2008.07.011
24. Kuitunen M, Kukkonen K, Juntunen-Backman K, Korpela R, Poussa T, Tuure T, et al. Probiotics prevent IgE-associated allergy until age 5 years in cesarean-delivered children but not in the total cohort. J Allergy Clin Immunol (2009) 123(2):335–41. doi: 10.1016/j.jaci.2008.11.019
25. Kalliomäki M, Salminen S, Arvilommi H, Kero P, Koskinen P, Isolauri E. Probiotics in primary prevention of atopic disease: a randomised placebo-controlled trial. Lancet (2001) 357(9262):1076–9. doi: 10.1016/S0140-6736(00)04259-8
26. Schwarzer M, Srutkova D, Schabussova I, Hudcovic T, Akgun J, Wiedermann U, et al. Neonatal colonization of germ-free mice with Bifidobacterium longum prevents allergic sensitization to major birch pollen allergen Bet v 1. Vaccine (2013) 31(46):5405–12. doi: 10.1016/j.vaccine.2013.09.014
27. Sonnenborn U. Escherichia coli strain Nissle 1917—from bench to bedside and back: history of a special Escherichia coli strain with probiotic properties. FEMS Microbiol Lett (2016) 363(19):fnw212–fnw. doi: 10.1093/femsle/fnw212
28. Sarate PJ, Heinl S, Poiret S, Drinić M, Zwicker C, Schabussova I, et al. E. coli Nissle 1917 is a safe mucosal delivery vector for a birch-grass pollen chimera to prevent allergic poly-sensitization. Mucosal Immunol (2019) 12(1):132–44. doi: 10.1038/s41385-018-0084-6
29. Pablos I, Wildner S, Asam C, Wallner M, Gadermaier G. Pollen Allergens for Molecular Diagnosis. Curr Allergy Asthma Rep (2016) 16:31. doi: 10.1007/s11882-016-0603-z
30. Schabussova I, Hufnagl K, Wild C, Nutten S, Zuercher AW, Mercenier A, et al. Distinctive anti-allergy properties of two probiotic bacterial strains in a mouse model of allergic poly-sensitization. Vaccine (2011) 29(10):1981–90. doi: 10.1016/j.vaccine.2010.12.101
31. Schwarzer M, Makki K, Storelli G, Machuca-Gayet I, Srutkova D, Hermanova P, et al. Lactobacillus plantarum strain maintains growth of infant mice during chronic undernutrition. Science (2016) 351(6275):854–7. doi: 10.1126/science.aad8588
32. Kozakova H, Schwarzer M, Tuckova L, Srutkova D, Czarnowska E, Rosiak I, et al. Colonization of germ-free mice with a mixture of three lactobacillus strains enhances the integrity of gut mucosa and ameliorates allergic sensitization. Cell Mol Immunol (2016) 13(2):251–62. doi: 10.1038/cmi.2015.09
33. Winkler B, Baier K, Wagner S, Repa A, Eichler HG, Scheiner O, et al. Mucosal tolerance as therapy of type I allergy: intranasal application of recombinant Bet v 1, the major birch pollen allergen, leads to the suppression of allergic immune responses and airway inflammation in sensitized mice. Clin Exp Allergy (2002) 32(1):30–6. doi: 10.1046/j.0022-0477.2001.01214.x
34. Skevaki C, Hudemann C, Matrosovich M, Möbs C, Paul S, Wachtendorf A, et al. Influenza-derived peptides cross-react with allergens and provide asthma protection. J Allergy Clin Immunol (2018) 142(3):804–14. doi: 10.1016/j.jaci.2017.07.056
35. Hufnagl K, Winkler B, Focke M, Valenta R, Scheiner O, Renz H, et al. Intranasal tolerance induction with polypeptides derived from 3 noncross-reactive major aeroallergens prevents allergic polysensitization in mice. J Allergy Clin Immunol (2005) 116(2):370–6. doi: 10.1016/j.jaci.2005.04.002
36. Srutkova D, Schwarzer M, Hudcovic T, Zakostelska Z, Drab V, Spanova A, et al. Bifidobacterium longum CCM 7952 Promotes Epithelial Barrier Function and Prevents Acute DSS-Induced Colitis in Strictly Strain-Specific Manner. PLoS One (2015) 10(7):e0134050. doi: 10.1371/journal.pone.0134050
37. Helps SC, Thornton E, Kleinig TJ, Manavis J, Vink R. Automatic nonsubjective estimation of antigen content visualized by immunohistochemistry using color deconvolution. Appl Immunohistochem Mol Morphol AIMM (2012) 20(1):82–90. doi: 10.1097/PAI.0b013e31821fc8cd
38. Mustafa HN, El Awdan SA, Hegazy GA, Abdel Jaleel GA. Prophylactic role of coenzyme Q10 and Cynara scolymus L on doxorubicin-induced toxicity in rats: Biochemical and immunohistochemical study. Indian J Pharmacol (2015) 47(6):649–56. doi: 10.4103/0253-7613.169588
39. Warm K, Lindberg A, Lundbäck B, Rönmark E. Increase in sensitization to common airborne allergens among adults – two population-based studies 15 years apart. Allergy Asthma Clin Immunol (2013) 9(1):20–. doi: 10.1186/1710-1492-9-20
40. Silvestri M, Oddera S, Crimi P, Rossi GA. Frequency and specific sensitization to inhalant allergens within nuclear families of children with asthma and/or rhinitis. Ann Allergy Asthma Immunol (1997) 79(6):512–6. doi: 10.1016/S1081-1206(10)63058-X
41. Biedermann T, Winther L, Till SJ, Panzner P, Knulst A, Valovirta E. Birch pollen allergy in Europe. Allergy (2019) 74(7):1237–48. doi: 10.1111/all.13758
42. Amenyogbe N, Kollmann TR, Ben-Othman R. Early-Life Host–Microbiome Interphase: The Key Frontier for Immune Development. Front Pediatrics (2017) 5(111). doi: 10.3389/fped.2017.00111
43. Gensollen T, Iyer SS, Kasper DL, Blumberg RS. How colonization by microbiota in early life shapes the immune system. Science (New York NY) (2016) 352(6285):539–44. doi: 10.1126/science.aad9378
44. Verhasselt V. Oral tolerance in neonates: from basics to potential prevention of allergic disease. Mucosal Immunol (2010) 3(4):326–33. doi: 10.1038/mi.2010.25
45. Mennini M, Dahdah L, Artesani MC, Fiocchi A, Martelli A. Probiotics in Asthma and Allergy Prevention. Front Pediatrics (2017) 5:165. doi: 10.3389/fped.2017.00165
46. Bermudez-Brito M, Plaza-Díaz J, Muñoz-Quezada S, Gómez-Llorente C, Gil A. Probiotic Mechanisms of Action. Ann Nutr Metab (2012) 61(2):160–74. doi: 10.1159/000342079
47. Gardlik R, Palffy R, Celec P. Recombinant probiotic therapy in experimental colitis in mice. Folia Biologica (2012) 58(6):238–45.
48. He L, Yang H, Liu F, Chen Y, Tang S, Ji W, et al. Escherichia coli Nissle 1917 engineered to express Tum-5 can restrain murine melanoma growth. Oncotarget (2017) 8(49):85772–82. doi: 10.18632/oncotarget.20486
49. Zhang Y, Zhang Y, Xia L, Zhang X, Ding X, Yan F, et al. Escherichia coli Nissle 1917 targets and restrains mouse B16 melanoma and 4T1 breast tumors through expression of azurin protein. Appl Environ Microbiol (2012) 78(21):7603–10. doi: 10.1128/AEM.01390-12
50. Adam E, Delbrassinne L, Bouillot C, Reynders V, Mailleux A-C, Muraille E, et al. Probiotic Escherichia coli Nissle 1917 activates DC and prevents house dust mite allergy through a TLR4-dependent pathway. Eur J Immunol (2010) 40(7):1995–2005. doi: 10.1002/eji.200939913
51. Fujimura KE, Lynch SV. Microbiota in Allergy and Asthma and the Emerging Relationship with the Gut Microbiome. Cell Host Microbe (2015) 17(5):592–602. doi: 10.1016/j.chom.2015.04.007
52. Chung KF. Airway microbial dysbiosis in asthmatic patients: A target for prevention and treatment? J Allergy Clin Immunol (2017) 139(4):1071–81. doi: 10.1016/j.jaci.2017.02.004
53. Stokholm J, Blaser MJ, Thorsen J, Rasmussen MA, Waage J, Vinding RK, et al. Maturation of the gut microbiome and risk of asthma in childhood. Nat Commun (2018) 9(1):141. doi: 10.1038/s41467-018-03150-x
54. Asarnoj A, Hamsten C, Wadén K, Lupinek C, Andersson N, Kull I, et al. Sensitization to cat and dog allergen molecules in childhood and prediction of symptoms of cat and dog allergy in adolescence: A BAMSE/MeDALL study. J Allergy Clin Immunol (2016) 137(3):813–21.e7. doi: 10.1016/j.jaci.2015.09.052
55. Lynch SV, Boushey HA. The Microbiome and Development of Allergic disease. Curr Opin Allergy Clin Immunol (2016) 16(2):165–71. doi: 10.1097/ACI.0000000000000255
56. Santos KD, Traebert J, Piovezan AP, Silva JD. Relevance of the first thousand days of life to the development of wheezing in children aged 6–7 years. Allergologia Immunopathologia (2020) 48(3):270–80. doi: 10.1016/j.aller.2019.12.007
57. Schaub B, Prescott SL. 1 - The Maturation of Immune Function in Pregnancy and Early Childhood. In: Wahn U, Sampson HA, editors. Allergy, Immunity and Tolerance in Early Childhood. Amsterdam: Academic Press (2016). p. 1–17. doi: 10.1016/B978-0-12-420226-9.00001-2
58. Hufnagl K, Focke M, Gruber F, Hufnagl P, Loupal G, Scheiner O, et al. Airway inflammation induced after allergic poly-sensitization can be prevented by mucosal but not by systemic administration of poly-peptides. Clin Exp Allergy (2008) 38(7):1192–202. doi: 10.1111/j.1365-2222.2008.02992.x
59. Tang ML, Martino DJ. Oral immunotherapy and tolerance induction in childhood. Pediatr Allergy Immunol Off Publ Eur Soc Pediatr Allergy Immunol (2013) 24(6):512–20. doi: 10.1111/pai.12100
60. Burks AW, Jones SM, Wood RA, Fleischer DM, Sicherer SH, Lindblad RW, et al. Oral Immunotherapy for Treatment of Egg Allergy in Children. New Engl J Med (2012) 367(3):233–43. doi: 10.1056/NEJMoa1200435
61. Taniuchi S, Takahashi M, Soejima K, Hatano Y, Minami H. Immunotherapy for cow’s milk allergy. Hum Vaccines Immunother (2017) 13(10):2443–51. doi: 10.1080/21645515.2017.1353845
62. Lara-Villoslada F, Olivares M, Sierra S, Rodríguez JM, Boza J, Xaus J. Beneficial effects of probiotic bacteria isolated from breast milk. Br J Nutr (2007) 98 Suppl 1:S96–100. doi: 10.1017/S0007114507832910
63. Moossavi S, Miliku K, Sepehri S, Khafipour E, Azad MB. The Prebiotic and Probiotic Properties of Human Milk: Implications for Infant Immune Development and Pediatric Asthma. Front Pediatr (2018) 6:197–. doi: 10.3389/fped.2018.00197
64. Westritschnig K, Horak F, Swoboda I, Balic N, Spitzauer S, Kundi M, et al. Different allergenic activity of grass pollen allergens revealed by skin testing. Eur J Clin Invest (2008) 38(4):260–7. doi: 10.1111/j.1365-2362.2008.01938.x
65. Bokanovic D, Aberer W, Hemmer W, Heinemann A, Komericki P, Scheffel J, et al. Determination of sIgE to rPhl p 1 is sufficient to diagnose grass pollen allergy. Allergy (2013) 68(11):1403–9. doi: 10.1111/all.12263
66. Flicker S, Steinberger P, Ball T, Krauth MT, Verdino P, Valent P, et al. Spatial clustering of the IgE epitopes on the major timothy grass pollen allergen Phl p 1: importance for allergenic activity. J Allergy Clin Immunol (2006) 117(6):1336–43. doi: 10.1016/j.jaci.2006.02.012
67. Bickert T, Trujillo-Vargas CM, Duechs M, Wohlleben G, Polte T, Hansen G, et al. Probiotic Escherichia coli Nissle 1917 suppresses allergen-induced Th2 responses in the airways. Int Arch Allergy Immunol (2009) 149(3):219–30. doi: 10.1159/000199717
68. Satitsuksanoa P, Jansen K, Głobińska A, van de Veen W, Akdis M. Regulatory Immune Mechanisms in Tolerance to Food Allergy. Front Immunol (2018) 9(2939). doi: 10.3389/fimmu.2018.02939
69. Krogulska A, Borowiec M, Polakowska E, Dynowski J, Młynarski W, Wasowska-Królikowska K. FOXP3, IL-10, and TGF-β Genes Expression in Children with IgE-Dependent Food Allergy. J Clin Immunol (2011) 31(2):205–15. doi: 10.1007/s10875-010-9487-1
70. Böhm L, Maxeiner J, Meyer-Martin H, Reuter S, Finotto S, Klein M, et al. IL-10 and Regulatory T Cells Cooperate in Allergen-Specific Immunotherapy To Ameliorate Allergic Asthma. J Immunol (2015) 194(3):887. doi: 10.4049/jimmunol.1401612
71. Tunis MC, Marshall JS. Toll-Like Receptor 2 as a Regulator of Oral Tolerance in the Gastrointestinal Tract. Mediators Inflamm (2014) 2014:606383. doi: 10.1155/2014/606383
72. Sturm A, Rilling K, Baumgart DC, Gargas K, Abou-Ghazalé T, Raupach B, et al. Escherichia coli Nissle 1917 distinctively modulates T-cell cycling and expansion via toll-like receptor 2 signaling. Infect Immun (2005) 73(3):1452–65. doi: 10.1128/IAI.73.3.1452-1465.2005
73. Grabig A, Paclik D, Guzy C, Dankof A, Baumgart DC, Erckenbrecht J, et al. Escherichia coli strain Nissle 1917 ameliorates experimental colitis via toll-like receptor 2- and toll-like receptor 4-dependent pathways. Infect Immun (2006) 74(7):4075–82. doi: 10.1128/IAI.01449-05
74. Frey A, Lunding LP, Ehlers JC, Weckmann M, Zissler UM, Wegmann M. More Than Just a Barrier: The Immune Functions of the Airway Epithelium in Asthma Pathogenesis. Front Immunol (2020) 11:761. doi: 10.3389/fimmu.2020.00761
75. Espinosa V, Rivera A. First Line of Defense: Innate Cell-Mediated Control of Pulmonary Aspergillosis. Front Microbiol (2016) 7:272. doi: 10.3389/fmicb.2016.00272
76. Georas SN, Rezaee F. Epithelial barrier function: at the front line of asthma immunology and allergic airway inflammation. J Allergy Clin Immunol (2014) 134(3):509–20. doi: 10.1016/j.jaci.2014.05.049
77. Natsume O, Ohya Y. Recent advancement to prevent the development of allergy and allergic diseases and therapeutic strategy in the perspective of barrier dysfunction. Allergol Int (2018) 67(1):24–31. doi: 10.1016/j.alit.2017.11.003
78. Sturgeon C, Fasano A. Zonulin, a regulator of epithelial and endothelial barrier functions, and its involvement in chronic inflammatory diseases. Tissue Barriers (2016) 4(4):e1251384–e. doi: 10.1080/21688370.2016.1251384
79. Cukrowska B, LodInova-ZadnIkova R, Enders C, Sonnenborn U, Schulze J, Tlaskalova-Hogenova H. Specific proliferative and antibody responses of premature infants to intestinal colonization with nonpathogenic probiotic E. coli strain Nissle 1917. Scand J Immunol (2002) 55(2):204–9. doi: 10.1046/j.1365-3083.2002.01005.x
80. Sonnenborn U, Schulze J. The non-pathogenic Escherichia coli strain Nissle 1917 – features of a versatile probiotic. Microbial Ecol Health Disease (2009) 21(3–4):122–58. doi: 10.3109/08910600903444267
81. Wehkamp J, Harder J, Wehkamp K, Meissner B-v, Schlee M, Enders C, et al. NF-κB- and AP-1-Mediated Induction of Human Beta Defensin-2 in Intestinal Epithelial Cells by Escherichia coli Nissle 1917: a Novel Effect of a Probiotic Bacterium. Infect Immun (2004) 72(10):5750–8. doi: 10.1128/IAI.72.10.5750-5758.2004
Keywords: allergic poly-sensitization, germ-free, BALB/c, mucosal tolerance, mouse model, Escherichia coli Nissle 1917, hygiene hypothesis
Citation: Sarate PJ, Srutkova D, Geissler N, Schwarzer M, Schabussova I, Inic-Kanada A, Kozakova H and Wiedermann U (2021) Pre- and Neonatal Imprinting on Immunological Homeostasis and Epithelial Barrier Integrity by Escherichia coli Nissle 1917 Prevents Allergic Poly-Sensitization in Mice. Front. Immunol. 11:612775. doi: 10.3389/fimmu.2020.612775
Received: 30 September 2020; Accepted: 31 December 2020;
Published: 17 February 2021.
Edited by:
Petra Ina Pfefferle, University of Marburg, GermanyReviewed by:
Abdelhadi Saoudi, INSERM U1043 Centre de Physiopathologie de Toulouse Purpan, FranceMeri K. Tulic, INSERM U1065 Centre Méditerranéen de Médecine Moléculaire, France
Copyright © 2021 Sarate, Srutkova, Geissler, Schwarzer, Schabussova, Inic-Kanada, Kozakova and Wiedermann. This is an open-access article distributed under the terms of the Creative Commons Attribution License (CC BY). The use, distribution or reproduction in other forums is permitted, provided the original author(s) and the copyright owner(s) are credited and that the original publication in this journal is cited, in accordance with accepted academic practice. No use, distribution or reproduction is permitted which does not comply with these terms.
*Correspondence: Ursula Wiedermann, ursula.wiedermann@meduniwien.ac.at; Aleksandra Inic-Kanada, aleksandra.inic-kanada@meduniwien.ac.at