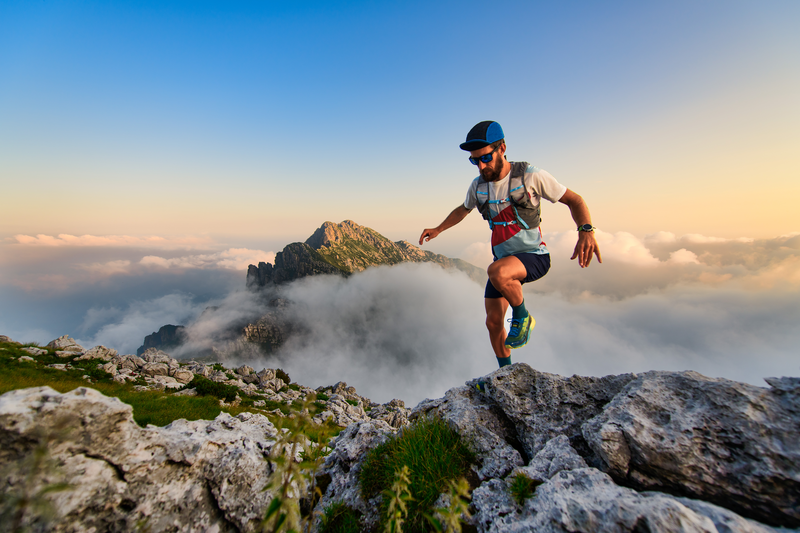
95% of researchers rate our articles as excellent or good
Learn more about the work of our research integrity team to safeguard the quality of each article we publish.
Find out more
ORIGINAL RESEARCH article
Front. Immunol. , 08 January 2021
Sec. Microbial Immunology
Volume 11 - 2020 | https://doi.org/10.3389/fimmu.2020.600635
Echinococcus multilocularis larvae, predominantly located in the liver, cause a tumor-like parasitic disease, alveolar echinococcosis (AE), that is characterized by increased infiltration of various immune cells, including macrophages, around the lesion that produces an “immunosuppressive” microenvironment, favoring its persistent infection. However, the role of hepatic macrophages in the host defense against E. multilocularis infection remains poorly defined. Using human liver tissues from patients with AE and a hepatic experimental mouse model of E. multilocularis, we investigated the phenotype and function of hepatic macrophages during the parasite infection. In the present study, we found that a large number of CD68+ macrophages accumulated around the metacestode lesion in the liver of human AE samples and that both S100A9+ proinflammatory (M1 phenotype) and CD163+ anti-inflammatory (M2 phenotype) macrophages were significantly higher in close liver tissue (CLT) than in distant liver tissue (DLT), whereas M2 macrophages represent the dominant macrophage population. Furthermore, E. multilocularis-infected mice exhibited a massive increase in macrophage (F4/80+) infiltration in the liver as early as day 5, and the infiltrated macrophages were mainly monocyte-derived macrophages (CD11bhi F4/80int MoMFs) that preferentially differentiated into the M1 phenotype (iNOS+) at the early stage of E. multilocularis infection and then polarized to anti-inflammatory macrophages of the M2 phenotype (CD206+) at the chronic stage of infection. We further showed that elimination of macrophages by treatment of mice with clodronate-liposomes before E. multilocularis infection impaired worm expulsion and was accompanied by a reduction in liver fibrosis, yielding a high parasite burden. These results suggest that hepatic macrophages may play a dual role in the establishment and development of E. multilocularis metacestodes in which early larvae clearance is promoted by M1 macrophages while persistent metacestode infection is favored by M2 macrophages.
Alveolar echinococcosis (AE) is one of the most dangerous parasitic diseases distributed in the Northern Hemisphere and is caused by the larval stage of the tapeworm Echinococcus multilocularis (E. multilocularis) (1–4). E. multilocularis larvae are transported via the portal vein blood flow to the liver and predominantly dwell in the liver parenchyma, where they develop into a metacestode with subsequent infiltrative growth similar to a malignant tumor, gradually destroy the surrounding host tissues (5) and are able to spread to any organ through local invasion and metastases (6–8). Parasitic invasion is generally asymptomatic for a long period, as diagnosed patients usually have reached an advanced stage and have a mortality rate of 75% to 90% if untreated within 10~15 years (9, 10). Although great progress has been made in the field of hepatic AE surgery, including radical resection, liver transplantation or ex vivo liver resection and autotransplantation, with promising clinical outcomes (11), there are still some patients with AE who are not eligible for surgery treatment and lose the chance to save their life. Thus, there is an urgent need to clarify the pathogenic mechanisms of this lethal disease and to develop novel therapeutic approaches for AE.
The intrahepatic localization and persistent proliferation of E. multilocularis usually elicit a severe hepatic granulomatous inflammation response characterized by attraction of various host immune cells around the metacestode lesion (12), which may produce an “immunosuppressive” microenvironment and might be of crucial importance in favoring persistent E. multilocularis infection. Recent histological analysis of the liver sections of patients with AE has shown that the infiltrated immune cells were composed of large clusters of T lymphocytes (predominantly CD8+ T cells), as well as macrophages with an epithelium-like arrangement, mainly located in close contact with the laminated layer of the parasitic vesicle (12, 13). Studies in a mouse experimental model of intrahepatic E. multilocularis infection also showed that increased macrophages were scattered in the granuloma as early as day 21 and progressively exceeded the infiltrated T lymphocytes. At the late stage, similar to observations in human AE, macrophages were mainly located at the periphery of the lesion (14). These observations in humans and experimental studies in mice indicated that macrophages are among the main infiltrated cell population and may act as key immune regulators of the granulomatous inflammation response to maintain immune homoeostasis during persistent infection (15, 16). However, the role of macrophages in the development of E. multilocularis larvae has not yet been fully elucidated.
Multiple lines of evidence point to macrophages being markedly heterogeneous immune cells because they are ontologically and functionally diverse. In the liver, macrophages consisting of resident Kupffer cells (KCs), which originate from fetal yolk sacks, and recruited monocyte-derived macrophages (MoMFs), which originate from bone marrow-derived monocytes. KCs play a major role in maintaining immunological tolerance and provide an anti-inflammatory micromilieu during homeostasis in the liver. MoMFs are massively attracted to inflammatory sites and represent the dominant macrophage population during acute or chronic injury to the liver, which regulates both the initial inflammatory response and the late healing response (17). It has been shown that macrophages accumulate at the lesion site during E. multilocularis infection, but the origin of these accumulated macrophages has not been definitively demonstrated. In addition, the functional diversity of macrophages relates to their ability to polarize into different functional phenotypes. On the basis of Th1/Th2 polarization concepts, macrophages can be polarized into proinflammatory M1 macrophages and anti-inflammatory M2 macrophages (18). M1 macrophages are induced by Th1-type cytokines (such as IFN-γ) and are characterized by upregulation of the expression of nitric oxide synthase (iNOS) and exhibit strong microbicidal and tumoricidal properties. In contrast, M2 macrophages are induced by Th2-type cytokines (such as IL-4 or IL-13) (19, 20) and are characterized by upregulation of the expression of mannose receptor (also known as CD206), Ym1, and Fizz1 and secretion of immune-modulatory mediators (such as IL-10 and TGF-β) (21), which are supposed to contribute to parasite infestation, tissue remodeling, and tumor progression (22). Coincidentally, previous studies have shown that the host initially responds to E. multilocularis infection with an acute inflammatory Th1-biased immune response, but this response gradually shifts to Th2-oriented immune suppression responses at the progressive phase of AE (23–27). Thus, we speculate that M1/M2 macrophage polarization may occur accompanied by the Th1/Th2 response during E. multilocularis infection. Previous studies in some other parasites, such as Trypanosoma cruzi (28), Fasciola hepatica (29), and Schistosoma japonicum (30), have shown that M2 macrophages are induced to escape being killed by M1 macrophages and prolong infection. Therefore, whether E. multilocularis has the ability to switch from proinflammatory M1 at the early stages of infection to an anti-inflammatory M2 macrophage during the advanced stages of infection to maintain its persistent infection needs to be clarified.
In the present study, we investigated the heterogeneity of hepatic macrophages in both human liver tissues from patients with AE and a quantitative hepatic experimental mouse model of E. multilocularis. We further evaluated the role of hepatic macrophages in the establishment of E. multilocularis metacestode using a novel clodronate-liposome (CL) injection regime, which can effectively eliminate macrophages. Our results demonstrated that macrophages are readily recruited to the parasite lesion site and predominantly exhibit an M2 phenotype in both human AE and mouse models. In addition, we found that the early expulsion ability of E. multilocularis larvae was impaired after depletion of hepatic macrophages, which was accompanied by inhibition of liver fibrosis formation and facilitated E. multilocularis metacestode growth. This led to a high parasite burden. Our results suggest that hepatic macrophages play an important role in the E. multilocularis metacestode establishment and development.
Patients with AE included in this study were diagnosed with ultrasound (US), computed tomography (CT) and biopsy-proven AE in the First Affiliated Hospital of Xinjiang Medical University, Urumqi, China. Liver tissue samples were obtained from patients who underwent surgical resection, and the liver tissue standards were taken according to a previous study: two liver tissue samples were prepared from each patient with AE, which were close liver tissue (CLT) and distant liver tissue (DLT) from the lesion (13). This study was conducted under the ethics approval of the First Affiliated Hospital of Xinjiang Medical University (S20130418-3), with all patients having given informed consent.
Paraformaldehyde-fixed and paraffin-embedded liver tissue slices, 4 μm thick, were deparaffinized and rehydrated and then processed for hematoxylin and eosin (H&E) and immunohistochemical (IHC) staining. For IHC analysis, slices were subjected to heat-induced antigen retrieval using citric acid buffer (ZSGB-BIO, Beijing). After the slices cooled to room temperature (RT), endogenous peroxidase activity was blocked with 3% H2O2, and nonspecific binding was blocked with 10% normal serum with 1% BSA in tris-buffered saline (TBS) for 1 h at RT. Slices were then incubated with primary antibodies (CD68, 1:100, ab955; CD163, 1:500, ab182422; S100A9, 1:1000, ab63818, Abcam, Cambridge, UK) at 4°C overnight. The next day, slices were rinsed three times with tris-buffered saline and 0.1% Tween (TBST) for 15 min and then incubated with the secondary antibody for 2 h at RT. Visualization was induced with a diaminobenzidine (DAB) substrate kit (ab64238, Abcam, Cambridge, UK) according to the manufacturer’s instructions. For all immunoreactions, negative controls (the primary antibody was replaced with preimmune serum or TBS) were also included. Representative images from H&E and IHC-stained sections were captured using a digital image-capture system (Olympus, Tokyo) at a magnification between 100× and 400×. For quantitation, staining was then assessed at 400× magnification in a total of 3–5 fields/slice/liver sample using the cellSens Dimension software (Olympus), and the results were expressed as the percentage of positive area to the total measured area.
Pathogen-free female C57BL/6 wild-type mice (6 weeks of age) were purchased from Beijing Vital River Experimental Animal Technology Co. Ltd. The animals were housed in a specific pathogen–free environment with 12-h light/dark cycles and provided rodent chow diet and water ad libitum at the Animal Facility of Xinjiang Medical University. All mice received humane care in compliance with the Medical Research Center’s guidelines, and all experimental protocols involving mice were approved by the Ethics Committee of the First Affiliated Hospital of Xinjiang Medical University (Approval No. 20170809-01). All surgery was performed under chloral hydrate anesthesia, and all efforts were made to minimize suffering.
E. multilocularis protoscoleces (PSCs) were obtained from intraperitoneal lesions maintained in BALB/c mice under aseptic conditions (31). Only PSCs exhibiting over 95% vitality were counted and subsequently used to infect experimental mice. To establish the hepatic experimental E. multilocularis infection mouse model, each mouse was inoculated through the hepatic portal vein with different doses of PSCs in RPMI 1640 medium (Gibco, Auckland, New Zealand) to induce a quantitative hepatic infection model. According to the inoculation numbers of PSCs, the experimental mice were divided into low dose group (LD, 50 PSCs), medium dose group (MD, 500 PSCs), and high dose group (HD, 2000 PSCs), whereas control group mice were injected with the same volume of RPMI 1640, as described previously (32). For flow cytometry analysis, five to six mice in LD, MD, HD, and control group were sacrificed at 2, 12, and 24 weeks to characterize the composition and phenotype of the hepatic macrophages. For histopathological analysis, five to six mice in HD group (2000 PSCs, a clear infectious dose) (32) were sacrificed at 2, 5, 8, 11 days, 2, 12, and 24 weeks, respectively. The liver surface was carefully screened for hepatic lesions for a preliminary assessment of the intensity of E. multilocularis infection and then the whole liver tissue samples were collected from the mice and taken for further analysis at each time point.
Administration of clodronate liposomes (CL) to mice were currently the most effective and widely used method to deplete macrophages in vivo (33). To determine an appropriate administration protocols of CL, mice were injected intravenously (i.v.) and intraperitoneally (i.p.) with CL (Liposoma BV, AMSTERDAM, Netherlands; 100 µl of suspension/10 grams of animal weight) respectively to evaluate the deplete efficiency of hepatic macrophage at 1, 3, and 7 days by flow cytometry analysis.
For histopathological analysis, all liver lobes were divided into three sections and fixed in 4% (v/v) paraformaldehyde in PBS, embedded in paraffin, cut into 4-μm-thick slices and subjected to H&E staining to examine inflammatory cell infiltration and changes in general histology. The number of “infectious foci” with PSCs or parasitic vesicles was counted from all liver lobes under a microscope (Olympus, Tokyo) after H&E staining. In addition, the lesion areas (μm2) in 10–15 fields/slice/mouse (40×) and the thickness (μm) of the infiltration zone of inflammatory cells surrounding the parasitic lesion with identical germinal layers were measured by computer-assisted morphometric analysis using cellSens Dimension software (Olympus, Tokyo, Japan) for macrophage depletion experiments.
For IHC analysis, slices were examined to determine the expression and distribution of F4/80 (1:100, ab6640; Abcam, Cambridge, UK), CD4 (1:100, 14-9766-82; eBioscience), and α-SMA (1:500, ab 124964; Abcam) at each time point. Sirius red (SR) staining was also performed for fibrosis analysis. Slices were then examined and photographed at 100×, 200×, and 400× magnification using a digital image-capture system (Olympus, Tokyo, Japan). The area with intensely positive staining was measured at 200× magnification in a total of 3–5 fields/section/sample using computer-assisted morphometric analysis in cellSens Dimension software (Olympus, Tokyo, Japan). Data were presented as the percentage of positive area to the total measured area.
For immunofluorescence analysis, liver tissues were fixed in 4% paraformaldehyde, cryoprotected with 30% sucrose solution, then embedded in OCT, and sectioned at a thickness of 6 μm. Dual immunofluorescence for F4/80 (1:100, ab6640; Abcam) and CCR2 (1:100, ab203128; Abcam) was performed on frozen slices at 4°C overnight followed by incubation with Alexa Fluor® 488-labeled anti-rabbit IgG (Cell Signaling Technology, Boston, MA; cat. no. 4412S) and DyLight® 594-labeled anti-rat IgG (ab102224; Abcam) at RT for 2 h; then, slices were mounted in DAPI mounting medium (ab104139; Abcam) for nuclear staining. Images were acquired by a confocal laser scanning fluorescence microscope (Leica, Oskar-Barnack-Straße).
Hepatic nonparenchymal cells (HNPCs) were isolated with reference to previous studies (34, 35). Briefly, mouse livers in situ were sequentially perfused with EGTA (Sigma-Aldrich, cat. no. E4378), pronase E (5 mg/ml; Sigma-Aldrich, cat. no. P5147), and collagenase type I (0.5 mg/ml; Worthington Biochemical Corporation, Lakewood, USA, cat. no. LS004197) solution through the portal vein followed by cutting of the inferior vena cava to remove circulating cells. Then, the digested livers were harvested and homogenized thoroughly and further digested with prewarmed pronase E/collagenase I solution containing 1% (vol/vol) DNase I (Roche, Indianapolis, USA; cat. no. 10-104-159-001) for 20 min at 37°C in a shaker (120 rpm). The resulting cell suspension was filtered through a 70-μm cell strainer and centrifuged two times (50 × g, 5 min, 4°C) to remove hepatic parenchymal cells. The remaining HNPCs containing hepatic macrophages were harvested by centrifugation at 500 × g for 10 min and enriched by density gradient–mediated separation (800 × g, 20 min, 20°C) over a 25/70% Percoll gradient (GE Healthcare). Then, the HNPCs were collected by removing the cell layer from the gradient interface, washed with DMEM containing 10% FBS (Gibco, Auckland, New Zealand), harvested by centrifugation at 500 × g for 10 min at 4°C, resuspended, counted, and used for flow cytometry analysis.
Nonspecific antibody binding was blocked by incubating the isolated HNPCs with purified anti-CD16/CD32 antibody (Fc Block; BioLegend, San Diego, CA) for 20 min at 4°C followed by incubation with combinations of surface antibodies for 30 min at 4°C in the dark. For intracellular flow cytometry, cells were then fixed and resuspended in permeabilization buffer containing intracellular antibodies according to the manufacturer’s instructions (BD Biosciences; cat. no. 554714). Cell preparations were analyzed immediately on an LSRFortessa flow cytometer (BD Immunocytometry Systems, San Jose, CA, USA). Data were analyzed using FlowJo software (version V10; Tree star, Inc., Ashland, OR, USA). Information on the antibodies utilized in this assay is listed in Supporting Table S1.
For qRT-PCR analysis, liver tissues were taken from parasitic lesions in E. multilocularis-infected mice and control mice as previously described (32). Total RNA was extracted from mouse liver tissue using TRIzol Reagent (Invitrogen, Carlsbad, CA) and reverse transcribed into cDNA according to the manufacturer’s instructions (Thermo Fisher Scientific, Waltham, MA; cat. no. K1622). Then, the cDNA was subjected to qRT-PCR by the SYBR Green PCR premix (TaKaRa, Dalian, China) in a thermocycler (iQ5 Bio-Rad, Hercules, CA) as previously described (34). Primer sequences for the genes analyzed are listed in Supporting Table S2. Gene expression was normalized to the housekeeping gene GAPDH. Relative mRNA expression was calculated using the 2-ΔΔCt method.
Statistical analyses were conducted using GraphPad Prism 6.0 (GraphPad Software, San Diego, CA). The results are presented as the means ± SEM. Student’s t-test (parametric) or the Mann-Whitney U test (nonparametric) was used when only comparing two groups. One-way ANOVA with a Tukey’s multiple comparison was used when there were more than two groups were compared. P <0.05 was considered significant in all experiments. (P-values were expressed as follows: *P < 0.05; **P< 0.01; ***P < 0.001; ****P< 0.0001).
To investigate the characteristics of hepatic macrophages in patients with AE, we initially examined the expression of CD68 (a marker indicating total macrophages), CD163 (a marker indicating anti-inflammatory M2 macrophages) and S100A9 (a marker indicating proinflammatory M1 macrophages) (15, 36) in AE patient liver specimens by IHC staining. Increased infiltration of CD68+ macrophages was readily identified in the inflammatory infiltrate of the periparasitic area of CLT specimens compared with DLT specimens (Figures 1A, B). Notably, both proinflammatory S100A9+ and anti-inflammatory CD163+ macrophages were greatly increased in the periparasitic areas of CLT compared to those in the DLT specimens. However, the increase in the number of CD163+ macrophages was larger than that of S100A9+ macrophages in the CLT specimens (9.4% of CD163+ vs. 5.2% of S100A9+), suggesting a predominantly anti-inflammatory response (Figures 1A, C–E). Taken together, these findings suggest a role for infiltrating macrophages in human AE and provide a rationale for using animal models to further examine this phenomenon.
Figure 1 Macrophages accumulate in the peripheral areas of the liver lesions of patients with AE. (A) Representative H&E and immunohistochemistry staining for the general macrophage marker CD68, the anti-inflammatory macrophage marker CD163 and the proinflammatory macrophage marker S100A9 in paired liver tissue samples (DLT versus CLT) from patients with AE (scale bar, 200μm for 100× magnification; 50 μm for 400× magnification). The lesion is delimited with a black line. (B–E) The percentage of positively stained areas was calculated to assess the expression of CD68, CD163, and S100A9. Close (CLT): “close” liver tissue was approximately 0.5 cm from the lesion (i.e., metacestode); distant (DLT): “distant” liver tissue was at least 2 cm from the lesion. The results are presented as the mean ± SEM (n = 15). ***p < 0.001, ****p < 0.0001.
To verify whether macrophage accumulation observed in human AE was evident in the mouse model of E. multilocularis, we established a hepatic experimental mouse model of E. multilocularis by injecting 2000 PSCs (a clear infectious dose) via the portal vein (32). We analyzed F4/80 expression in mouse liver specimens from the early stage (2, 5, 8, 11 days and 2 weeks) to the middle and late stages (12 and 24 weeks) by IHC staining. We found that accompanying the gradual infiltration of lymphocytes around the infectious foci (H&E staining), F4/80+ macrophages were significantly increased in the peripheral areas of the infectious foci of E. multilocularis-infected mice, markedly increased from day 5 and peaked at week 12 (Figures 2A, B). Notably, immunofluorescence costaining for CCR2 (expressed on monocyte-derived cells) and F4/80 at day 5 showed that the accumulated F4/80+ macrophages expressed CCR2, indicating that the main source of the accumulating macrophages was largely derived from recruited monocyte-derived macrophages (Figure 2C). These results were further confirmed by flow cytometry analysis.
Figure 2 Macrophages accumulate in the inflammatory cell zone around the liver lesions in E multilocularis-infected mice during the course of infection. (A) Representative H&E staining and F4/80 immunohistochemical staining of liver sections from E multilocularis-infected mice at days 2, 5, 8, and 11 and weeks 2, 12, or 24 (200× magnification; scale bar, 100μm). PSCs: protoscoleces. pv: parasitic vesicle. The red arrow indicates the inflammatory cell zone. The blue arrow indicates macrophages. (B) Quantification of F4/80+ cells. (C) Representative images from immunofluorescence staining of DAPI (blue), F4/80 (red), and CCR2 (green) and merged images of liver sections from E multilocularis-infected mice at day 5. Higher magnifications of the dotted areas are shown in the lower row. White arrows indicate F4/80 and CCR2 coimmunofluorescence (recruited macrophages). The lesion is delimited with a white line. Data are shown as the mean ± standard error of the mean (SEM, five to six mice per group), *p < 0.05, **p < 0.01, ***p < 0.001, and ****p < 0.0001.
To further explore the origin of macrophage accumulation in the livers of mice during E. multilocularis infection, we isolated HNPCs from fresh mouse liver tissues infected with different E. multilocularis PSC inocula and analyzed them by flow cytometry analysis at 2, 12, and 24 weeks. Total hepatic macrophages were defined as CD45+Ly-6G-NK1.1-CD19-CD3-CD11b+F4/80+ cells from the HNPC fraction of the digested liver tissues. Resident KCs were defined as the CD45+Ly-6G-NK1.1-CD19-CD3-CD11bintF4/80hi population, and the recruited MoMFs were defined as the CD45+Ly-6G-NK1.1-CD19-CD3-CD11bhi F4/80int population (Supplemental Figure S1) (34). At 2 weeks after infection, the percentage of KCs was significantly decreased in the livers of the HD group mice compared to MD, LD, or control group mice, whereas there was no significant difference in the absolute numbers of KCs. In contrast, the percentage and absolute numbers of MoMFs were significantly increased in the HD group compared to the MD, LD, and control groups, and even higher than those of KCs in the HD group (Figures 3A–C, Supplemental Figure S2). At 12 weeks after infection, the percentage of KCs was still decreased in the HD group compared to the control group mice, but the absolute numbers of KCs was increased and higher than that of MoMFs. In contrast, the percentage and absolute numbers of MoMFs in the HD group were decreased and significantly lower than that in the HD group at 2 weeks. At 24 weeks after infection, the percentage of KCs was lower in the HD group than that in the LD and control groups, whereas the percentage of MoMFs was higher in the HD group than that in control group (Figures 3A–C, Supplemental Figure S2). Taken together, these observations indicate that most hepatic macrophages in infected mice at the early stage arose from monocyte-derived macrophages.
Figure 3 Changes in hepatic macrophage composition in mice infected with different E multilocularis PSC inocula during the course of infection. (A, B) The percentages of CD11bintF4/80hi KCs and CD11bhiF4/80int MoMFs in mouse livers infected with different E multilocularis PSC inocula during the course of infection. (C) Representative flow cytometry plots of hepatic macrophage subsets showing CD11bhiF4/80int MoMFs and CD11bintF4/80hi KCs; Con; LD: 50 PSCs; MD: 500 PSCs; HD: 2000 PSCs. Data are shown as the mean ± standard error of the mean (SEM, five to six mice per group), *p < 0.05, **p < 0.01 and ***p < 0.001.
To characterize the functional phenotype of hepatic macrophages in the livers of mice with different PSC inocula during the time course, we next analyzed the expression of proinflammatory markers (iNOS) and anti-inflammatory markers (CD206) in KCs and MoMFs by flow cytometry analysis. We found that iNOS+ KCs (a proinflammatory M1 phenotype) were significantly increased in HD and MD group mice compared to control group mice at 2 weeks, while CD206+ KCs (an anti-inflammatory M2 phenotype) were not significantly changed between these groups at 2, 12, and 24 weeks, but the percentage and absolute numbers of CD206+ KCs were higher than iNOS+ KCs and represented approximately 70% of KCs during the time course (Figures 4A, B; Supplemental Figures S2, S3). The percentage and absolute numbers of iNOS+ MoMFs were significantly increased in the HD group compared to the control group mice at 2, 12, and 24 weeks, but gradually decreased from 2 to 24 weeks. In contrast, the percentage of CD206+ MoMFs was significantly increased in the HD group compared to the control group mice at 12 and 24 weeks, and the absolute numbers of CD206+ MoMFs in the HD group was also significantly increased from 2 to 24 weeks and higher than iNOS+ MoMFs at 24 weeks (Figures 4C, D; Supplemental Figures S2, S3).
Figure 4 Changes in hepatic macrophage subset polarization in mice infected with different E multilocularis PSC inocula during the course of infection. (A) The percentage of iNOS+ KCs (M1-type) and the percentage of CD206+ KCs (M2-type) of the total KCs in the liver. (B) Representative flow cytometry plots of intracellular staining of iNOS+ and CD206+ in KC subsets after 24 weeks of infection. (C) The percentage of iNOS+ MoMFs (M1-type) and the percentage of CD206+ MoMFs (M2-type) of the total MoMFs in the liver. (D) Representative flow cytometry plots of intracellular staining of iNOS+ and CD206+ in MoMF subsets after 24 weeks of infection. Con; LD: 50 PSCs; MD: 500 PSCs; HD: 2000 PSCs. Data are shown as the mean ± standard error of the mean (SEM, five to six mice per group), *p < 0.05, **p < 0.01 and ***p < 0.001.
In addition, we further compared the mRNA expression of M1 (iNOS) and M2 macrophage markers (Ym1, Retnla, and Fizz1) and related chemokines in liver tissues in all the studied groups by qRT-PCR analysis. Correspondingly, our results showed that the expression of iNOS was increased in the HD group compared to the control group at 2 weeks and then declined at 12 and 24 weeks. However, the expression of the anti-inflammatory M2 macrophage markers (Ym1, Retnla and Fizz1) was significantly increased in the HD group compared to the control group and was maintained at a high level during the time course (Figure 5A). Moreover, the expression of the M1-related chemokines CXCL9 and CXCL10 was decreased in the LD, MD, and HD groups compared with the control group at 2 and 12 weeks and only increased at 24 weeks. However, the expression of the M2-related chemokines CCL17 and CCL22 was significantly increased in the HD group at 2 and 24 weeks (Figure 5B). These results are in line with observations in human AE samples; the hepatic macrophages in liver tissues of murine models showed a predominantly M2 anti-inflammatory phenotype at the chronic stage.
Figure 5 M1 and M2 macrophage-specific marker and chemokine expression in mouse livers infected with different E multilocularis PSC inocula during the course of infection. Quantitative RT-PCR analysis for mRNA levels of M1 and M2 macrophage-specific marker and chemokine in whole liver tissues at various time points after infection normalized by comparison to the housekeeping gene GAPDH mRNA. (A) Expression of M1 marker (iNOS) and M2 macrophage markers (Ym1, Retnla, Fizz1). (B) Expression of M1-related chemokines (CXCL9, CXCL10) and M2-related chemokines (CCL17, CCL22). Con; LD: 50 PSCs; MD: 500 PSCs; HD: 2000 PSCs. Data are shown as the mean ± standard error of the mean (SEM, five to six mice per group), *p < 0.05, **p < 0.01, ***p < 0.001, and ****p < 0.0001.
To examine whether hepatic macrophages play an important role in the establishment of the E. multilocularis metacestode in a mouse model, mice were administered with CL to deplete hepatic macrophages. We first compared the deplete efficiency of hepatic macrophage by i.v. and i.p. injection with CL, finding that no matter which i.v. (about 24 h) or i.p. (about 3 days) with CL, both of the two methods can almost completely deplete KCs, which was consistent with previous report (37), with MoMFs also being largely depleted. After 7 days, new macrophages, especially MoMFs replaced the depleted ones by i.v. By contrast, i.p. with CL can maintain depletion for about 7 days (Supplemental Figure S4). Considering our above histopathological and flow cytometric analysis of hepatic macrophage that large number of MoMFs displaying a proinflammatory M1 phenotype were recruited into the liver lesion at early stage (Figures 2–4), we treated mice i.p. with CL 3 days prior to p.v. injections with PSC and then administered on a weekly basis for 6 weeks to maintain depletion. Control groups were injected with phosphate-buffered saline liposomes (PL) at the same time (Figure 6A). After 1, 2, 4, and 6 weeks of PSC inoculation, mice were sacrificed and parasite loads were evaluated.
Figure 6 Hepatic macrophage depletion impairs PSC clearance and promotes disease progression in the E multilocularis-infected mouse model. (A) Protocol for hepatic macrophage depletion. Mice were administered (i.p.) with CL or PL at day 0 before E multilocularis infection at day 3 and then administered once a week for 6 weeks. Livers were collected at weeks 1, 2, 4, and 6 after E multilocularis infection. (B) Representative images of metacestode tissue in the liver from E multilocularis–infected mice treated with CLs or PLs (control) after 6 weeks of infection. Metacestode tissues are encircled by the yellow line. (C) Representative H&E staining and immunohistochemistry of F4/80, α-SMA, and SR staining of liver sections from E multilocularis-infected mice at weeks 1, 2, 4, and 6 after macrophage depletion or control (100× magnification; scale bar, 200 μm). PSCs: protoscoleces. pv: parasitic vesicle. The red arrow indicates the inflammatory cell zone. (D) Number of PSCs and parasitic vesicles in the liver from E multilocularis-infected mice treated with CL or PL. (E) The percentage of positively stained area was calculated to assess the expression of F4/80. (F) The ratio of SR staining area was calculated. (G) The percentage of positively stained area was calculated to assess the expression of α-SMA. (H) Thickness of the inflammatory cell infiltration bands around the metacestode lesions. PSCs: protoscoleces. p.v: portal vein. CL: clodronate-liposomes. PL: phosphate-buffered saline control liposomes. Data are shown as the mean ± standard error of the mean (SEM, four to five mice per group), *p < 0.05, **p < 0.01, ***p < 0.001, ****p < 0.0001. ns, no significance.
Through gross observation of the liver surface, we found that hepatic metacestode growth was significantly enhanced in CL-treated mice (Figure 6B). We further evaluated the parasite loads by counting the number of infectious foci with PSC or metacestode structure lesions in all liver lobes on H&E-stained sections. We found that mice receiving CL displayed a high number of infectious foci with PSCs compared with mice receiving PL at 1 and 2 weeks, therefore resulting in an increased number of infectious foci with metacestode structure lesions at 4 and 6 weeks (Figures 6C, D). IHC analysis confirmed that F4/80+ macrophages and CD4+ T lymphocytes in the periparasitic infiltration areas were markedly reduced in CL-treated mice compared with PL mice. Additionally, CL treatment markedly reduced liver fibrosis and activated HSC numbers in the liver, as assessed by SR staining and a-SMA immunostaining (Figures 6C, E–G; Supplemental Figure S5). Moreover, the lesion area in CL-treated mice was significantly larger than that in PL-treated mice. Notably, the metacestode lesions embedded within thin fibrosis tissue and the thickness of the inflammatory cell infiltration bands around the metacestode lesions were thinner in the CL group than in the PL group mice (Figures 6H). These data demonstrated that hepatic macrophage depletion led to impaired worm expulsion and yielded a high parasite burden.
Numerous studies on parasite or virus infection and tumor development in the liver have shown that hepatic macrophages are involved in the progression of liver inflammation and fibrosis, and therefore, they play a key role in controlling the pathogenesis of liver disease (38–40). Hepatic macrophages are markedly heterogeneous cell populations, and their phenotypes and functions are likely switching during disease progression (38). Recently, we have shown that the number of macrophages is increased in the liver resections of patients with AE (13), suggesting that hepatic macrophages are involved in the development of AE. However, the characteristics of hepatic macrophage phenotypes and functions during E. multilocularis infection, especially at an early stage of infection, have been less studied. In the present study, our data clearly demonstrated that in response to E. multilocularis infection, monocyte-derived macrophages were recruited into the liver and skewed from the M1 proinflammatory phenotype at an early stage towards the M2 anti-inflammatory phenotype at a late stage to maintain persistent infection. Furthermore, depletion of liver macrophages by CL before E. multilocularis infection reduced liver fibrosis and increased worm burden, suggesting that hepatic macrophages play a dual role during E. multilocularis infection, which is critical for E. multilocularis clearance in the early stage but also significant for E. multilocularis immune escape in the late stage.
Parasite establishment in the host depends on the resolution of inflammation (41). Previous studies reported that microbicidal nitric oxide (NO) produced by M1 proinflammatory macrophages is favorable to kill PSCs of E. multilocularis in vitro (42), and E. multilocularis laminated-layer components could decrease NO production (43), indicating that E. multilocularis larvae have the ability to inhibit M1 proinflammatory macrophages in their struggle for survival. However, it has been reported that M2 anti-inflammatory macrophages contribute to the long-term escape of viruses, parasites, or tumor cells from immune attack, leading to chronic infections and protecting the host from excessive pathology (44, 45). In line with previous studies (12, 13), we showed that increased infiltration of CD68+ macrophages was readily identified in the CLT of patients with AE compared to DLT. Moreover, both proinflammatory (S100A9+) and anti-inflammatory (CD163+) macrophages were detected in larger numbers in CLT than in DLT, and the number of CD163+ anti-inflammatory macrophages was higher than that of S100A9+ proinflammatory macrophages, indicating that the infiltrated macrophages around the metacestode in the liver predominantly exhibit an M2 anti-inflammatory phenotype that may promote the survival of E. multilocularis in the liver organ. Taken together, these findings suggest an important role for infiltrating macrophages in human AE. As patients with AE once diagnosed usually reach the middle or late stage of the disease, it is necessary for us to use animal models to further examine this phenomenon.
The liver location of AE lesions is important for studying the immune response elicited in the liver of E. multilocularis infection. Therefore, we used a suitable experimental mouse model by direct injection of precise numbers of E. multilocularis PSCs via the portal vein, which can mimic the ‘natural’ location (32) and enable us to study the dynamic changes in hepatic macrophage phenotypes and functions during E. multilocularis infection. In our study, we not only detected pathological changes and F4/80+ macrophage expression in mouse livers from the reported early to middle and later stages of infection (32) but also detected F4/80+ macrophage expression from the very early stage of infection (2, 5, 8, and 11 days). Our results showed that at 2 days, many E. multilocularis PSCs from the hepatic portal vein inoculum were present and maintained an intact structure in the infected livers, and only a few infiltrating lymphocytes surrounding the PSC inoculum could be observed. From 5 days to 11 days, PSCs could still be observed, and the worm body gradually disintegrated and disappeared. Only a few PSCs were able to develop into E. multilocularis metacestodes, which were observed from 2 weeks to 24 weeks after infection (32). Notably, numerous inflammatory cells began to be recruited surrounding the PSC inocula as early as 5 days post infection, and F4/80+ macrophages were also markedly increased in the periparasitic inflammatory area at 5 days and peaked at 12 weeks. In addition, accumulative evidence demonstrates that hepatic macrophages consist of liver resident macrophages (KCs) and MoMFs, which are rapidly recruited from the circulation to the injury site through the chemokine receptor CCR2 during acute or chronic injury to the liver (15, 17). In our study, additional immunofluorescence of the infected mouse livers revealed that the accumulated F4/80+ macrophages express CCR2, thereby underlining MoMFs recruitment during AE progression. We further used flow cytometry on freshly isolated mouse liver tissues to confirm the hepatic macrophage composition and found that the number of CD11bhi F4/80int MoMFs was significantly increased in E. multilocularis-infected mice, especially in HDG, compared to the control group and even exceeded the CD11bintF4/80hi KCs at an early stage (2 weeks), indicating that MoMFs are massively attracted to the parasite infection site and represent the dominant hepatic macrophage population during the early infection stage of E. multilocularis, which may participate in the early clearance of E. multilocularis in mice.
Additionally, we further detected the M1 proinflammatory and M2 anti-inflammatory phenotypes of resident KCs and recruited MoMFs in the liver during different infection times. Our data showed that resident KCs are always dominated by the anti-inflammatory M2 phenotype during the time course. In contrast, recruited MoMFs are predominantly polarized into the M1 proinflammatory phenotype at an early stage and then gradually towards a dominant M2 anti-inflammatory phenotype at a late stage of E. multilocularis infection. Correspondingly, qRT-PCR analysis showed that the expression of M2 markers (Ym1, Retnla and Fizz1) was significantly upregulated compared with expression in the control group, exhibiting a similar phenotype in the late stage of hepatic AE. Moreover, we found that the M2 macrophage-related chemokines CCL17 and CCL22, which have been reported to play important roles in attracting T regulatory cells (Tregs) to promote immune tolerance during the tumorigenic process (46), were also significantly upregulated. Our previous studies have shown that Tregs are significantly elevated in livers from infected mice at 24 weeks (32). Therefore, E. multilocularis infection-enhanced CCL17 and CCL22 production results in Treg recruitment leading to local immunosuppression, but further research is needed. Taken together, we showed here that E. multilocularis infection recruited an increased infiltration of MoMFs to the liver and induced the skewing of their phenotypes from proinflammatory M1 macrophages towards anti-inflammatory M2 macrophages, which favors persistent infection.
Although we have characterized the dynamic changes in hepatic macrophage subsets during E. multilocularis infection, the functional role of hepatic macrophages in parasite establishment is still not very clear. Given that clodronate liposomes are now the most effective and widely used method to deplete macrophages (33), we administered CL to the challenged mice every week for 6 weeks. We found that macrophage depletion reduced liver fibrosis and yielded a high parasite burden. These results are consistent with previous reports in the helminth parasites H. polygyrus (47) and N. brasiliensis (48), showing an impaired ability to expel these two parasites when hosts were depleted of macrophages. Local liver fibrogenesis is actually protective and successfully separates the parasite lesion and limits its continuous growth in the liver. However, liver fibrosis was significantly reduced in the context of macrophage depletion and thereby exacerbated parasite disease. In addition, we found that macrophage depletion also reduced CD4+ T lymphocytes infiltration around the lesion. As our recent study have showed that hepatic metacestode growth was significantly enhanced in mice depleted of CD4+ T cells (13), indicating that depletion of hepatic macrophages impairs worm expulsion may also be partly due to a decrease in liver infiltration of CD4+ T cells, thereby causing the aggressive growth of metacestode. These findings demonstrate that hepatic macrophages play a critical role in host defense against E. multilocularis infection in which their early recruitment may favor parasite clearance.
In summary, our study demonstrates that hepatic macrophages, both proinflammatory M1 macrophages and anti-inflammatory M2 macrophages, participate in the infection progression of E. multilocularis in both humans with AE and E. multilocularis-infected mice. During the early stage of parasite infection, hepatic macrophages are mainly composed of infiltrated MoMFs and dominated by a proinflammatory M1 phenotype to clear the parasites by producing proinflammatory cytokines. In contrast, chronic E. multilocularis infection tends to induce hepatic macrophage polarization towards the anti-inflammatory M2 phenotype by producing anti-inflammatory cytokines to maintain persistent infection. Importantly, depletion of hepatic macrophages impaired worm expulsion and resulted in a high parasite burden. Our results implied that hepatic macrophages may play a dual role in echinococcosis, which promotes early larvae clearance and favors persistent metacestode infection at the late stage. Future studies are needed to explore the exact role of hepatic macrophage subsets and the shift between them, indicating a novel therapeutic approach aimed at targeting hepatic macrophages for patients with AE.
The raw data supporting the conclusions of this article will be made available by the authors, without undue reservation.
All protocols involving patients with AE were approved by the Ethics Committee of the First Affiliated Hospital of Xinjiang Medical University (Approval No. S20130418-3), and written informed consent was obtained from each subject in accordance with the Declaration of Helsinki (1975) of the World Medical Association. All experimental protocols involving mice were also approved by the Ethics Committee of the First Affiliated Hospital of Xinjiang Medical University (Approval No. 20170809-01).
HWa, C-SZ, R-YL, and HWe designed the study. HWa, C-SZ, B-BF, JH, W-DL, X-JB, LL (4th author), and Z-DL performed the experiments and analyses. LL (5th author), AA, and Y-MS contributed to technical or material-related issues. HWa and C-SZ drafted the manuscript. R-YL and HWe supervised the study and critically reviewed the manuscript. All authors contributed to the article and approved the submitted version.
This work was supported by the National Natural Science Foundation of China (81860359, 81660342), the Xinjiang Uyghur Autonomous Region Key Laboratory Open Research Program Project (2019D04021), the Postdoctoral Science Foundation of China (2018T111121), and the State Key Laboratory of Pathogenesis, Prevention and Treatment of Central Asia High Incidence Diseases Fund (SKL-HIDCA-2020-5).
The authors declare that the research was conducted in the absence of any commercial or financial relationships that could be construed as a potential conflict of interest.
We thank Xue Zhang, Ning Yang, and Qin Wei for technical assistance.
The Supplementary Material for this article can be found online at: https://www.frontiersin.org/articles/10.3389/fimmu.2020.600635/full#supplementary-material
Supplementary Figure 1 | Gating strategy for the identification of hepatic macrophages. (A) Liver lymphocytes were gated. (B) Single cells were gated. (C) CD45+ cells were gated. (D) Neutrophils were identified as CD45+CD11b+ and Ly-6G+ cells, and they were excluded from subsequent macrophage gating. (E) Cells positive for CD3, CD19, or NK1.1 were excluded from subsequent macrophage gating. (F) Resident Kupffer cells (KCs) were selected as CD45+Ly-6G-CD3-CD19-NK1.1- and CD11bintF4/80hi cells; monocyte-derived macrophages (MoMFs) were selected as CD45+Ly-6G-CD3-CD19-NK1.1- and CD11bhiF4/80int cells. Representative flow cytometry plots are shown.
Supplementary Figure 2 | Absolute numbers analysis of hepatic macrophages from mice infected with E. multilocularis during the time course. Con; LD: 50 PSCs; MD: 500 PSCs; HD: 2000 PSCs. Data are shown as the mean ± standard error of the mean (SEM, 5–6 mice per group), *p < 0.05, **p < 0.01, ***p < 0.001, and ****p < 0.0001.
Supplementary Figure 3 | Representative flow cytometry plots gated on liver KC and MoMF subsets in mice infected with different E. multilocularis PSC inocula during the course of infection. (A) Intracellular staining of iNOS+ in KCs and MoMFs at 2, 12 and 24 weeks postinfection. (B) Intracellular staining of CD206+ in KCs and MoMFs at 2, 12 and 24 weeks postinfection. Con; LD: 50 PSCs; MD: 500 PSCs; HD: 2000 PSCs.
Supplementary Figure 4 | Flow cytometry analysis of the hepatic macrophage depletion efficiency by intraperitoneal (i.p.) and intravenous (i.v.) administration of clodronate-liposomes.
Supplementary Figure 5 | Hepatic macrophage depletion reduces CD4+ T lymphocytes accumulation in the inflammatory cell zone around the liver lesions in E. multilocularis-infected mice. (A) Representative immunohistochemistry of CD4 staining of liver sections from E. multilocularis-infected mice at weeks 1, 2, 4, and 6 after macrophage depletion (CL) or control (PL) (scale bar, 200 μm for 100× magnification; 50 μm for 400× magnification). (B) The percentage of CD4 positive area was calculated to assess the expression of CD4. Data are shown as the mean ± standard error of the mean (SEM, four to five mice per group), *p < 0.05, **p < 0.01, and ***p < 0.001.
1. Wang Q, Yang L, Wang Y, Zhang GJ, Zhong B, Wu WP, et al. Disease burden of echinococcosis in Tibetan communities-A significant public health issue in an underdeveloped region of western China. Acta Trop (2019) 203:105283. doi: 10.1016/j.actatropica.2019.105283
2. Gurler AT, Bolukbas CS, Acici M, Umur S. Overview of Echinococcus multilocularis in Turkey and in the World. Turkiye Parazitol Derg (2019) 43(Suppl 1):18–35. doi: 10.4274/tpd.galenos.2019.6300
3. Baumann S, Shi R, Liu W, Bao H, Schmidberger J, Kratzer W, et al. Worldwide literature on epidemiology of human alveolar echinococcosis: a systematic review of research published in the twenty-first century. Infection (2019) 47(5):703–27. doi: 10.1007/s15010-019-01325-2
4. Deplazes P, Rinaldi L, Alvarez Rojas CA, Torgerson PR, Harandi MF, Romig T, et al. Global Distribution of Alveolar and Cystic Echinococcosis. Adv Parasitol (2017) 95:315–493. doi: 10.1016/bs.apar.2016.11.001
5. Wang J, Cardoso R, Marreros N, Muller N, Lundstrom-Stadelmann B, Siffert M, et al. Foxp3(+) T Regulatory Cells as a Potential Target for Immunotherapy against Primary Infection with Echinococcus multilocularis Eggs. Infect Immun (2018) 86(10):e00542–18. doi: 10.1128/IAI.00542-18
6. Kern P, Menezes da Silva A, Akhan O, Mullhaupt B, Vizcaychipi KA, Budke C, et al. The Echinococcoses: Diagnosis, Clinical Management and Burden of Disease. Adv Parasitol (2017) 96:259–369. doi: 10.1016/bs.apar.2016.09.006
7. Reinehr M, Micheloud C, Grimm F, Kronenberg PA, Grimm J, Beck A, et al. Pathology of Echinococcosis: A Morphologic and Immunohistochemical Study on 138 Specimens With Focus on the Differential Diagnosis Between Cystic and Alveolar Echinococcosis. Am J Surg Pathol (2020) 44(1):43–54. doi: 10.1097/PAS.0000000000001374
8. Casulli A, Barth TFE, Tamarozzi F. Echinococcus multilocularis. Trends Parasitol (2019) 35(9):738–9. doi: 10.1016/j.pt.2019.05.005
9. Sulima M, Szostakowska B, Nahorski W, Sikorska K, Wolyniec W, Waz P. The usefulness of commercially available serological tests in the diagnosis and monitoring of treatment in patients with alveolar echinococcosis. Clin Exp Hepatol (2019) 5(4):327–33. doi: 10.5114/ceh.2019.89480
10. Wen H, Vuitton L, Tuxun T, Li J, Vuitton DA, Zhang W, et al. Echinococcosis: Advances in the 21st Century. Clin Microbiol Rev (2019) 32(2):e00075–18. doi: 10.1128/CMR.00075-18
11. Aji T, Dong JH, Shao YM, Zhao JM, Li T, Tuxun T, et al. Ex vivo liver resection and autotransplantation as alternative to allotransplantation for end-stage hepatic alveolar echinococcosis. J Hepatol (2018) 69(5):1037–46. doi: 10.1016/j.jhep.2018.07.006
12. Vuitton DA, Gottstein B. Echinococcus multilocularis and its intermediate host: a model of parasite-host interplay. J BioMed Biotechnol (2010) 2010:923193. doi: 10.1155/2010/923193
13. Zhang C, Lin R, Li Z, Yang S, Bi X, Wang H, et al. Immune Exhaustion of T Cells in Alveolar Echinococcosis Patients and Its Reversal by Blocking Checkpoint Receptor TIGIT in a Murine Model. Hepatology (2020) 71(4):1297–315. doi: 10.1002/hep.30896
14. Bresson-Hadni S, Liance M, Meyer JP, Houin R, Bresson JL, Vuitton DA. Cellular immunity in experimental Echinococcus multilocularis infection. II. Sequential and comparative phenotypic study of the periparasitic mononuclear cells in resistant and sensitive mice. Clin Exp Immunol (1990) 82(2):378–83. doi: 10.1111/j.1365-2249.1990.tb05457.x
15. Krenkel O, Puengel T, Govaere O, Abdallah AT, Mossanen JC, Kohlhepp M, et al. Therapeutic inhibition of inflammatory monocyte recruitment reduces steatohepatitis and liver fibrosis. Hepatology (2018) 67(4):1270–83. doi: 10.1002/hep.29544
16. Heymann F, Tacke F. Immunology in the liver–from homeostasis to disease. Nat Rev Gastroenterol Hepatol (2016) 13(2):88–110. doi: 10.1038/nrgastro.2015.200
17. Ju C, Tacke F. Hepatic macrophages in homeostasis and liver diseases: from pathogenesis to novel therapeutic strategies. Cell Mol Immunol (2016) 13(3):316–27. doi: 10.1038/cmi.2015.104
18. Muraille E, Leo O, Moser M. TH1/TH2 paradigm extended: macrophage polarization as an unappreciated pathogen-driven escape mechanism? Front Immunol (2014) 5:603. doi: 10.3389/fimmu.2014.00603
19. Tacke F, Zimmermann HW. Macrophage heterogeneity in liver injury and fibrosis. J Hepatol (2014) 60(5):1090–6. doi: 10.1016/j.jhep.2013.12.025
20. Mosser DM, Edwards JP. Exploring the full spectrum of macrophage activation. Nat Rev Immunol (2008) 8(12):958–69. doi: 10.1038/nri2448
21. Nair MG, Cochrane DW, Allen JE. Macrophages in chronic type 2 inflammation have a novel phenotype characterized by the abundant expression of Ym1 and Fizz1 that can be partly replicated in vitro. Immunol Lett (2003) 85(2):173–80. doi: 10.1016/s0165-2478(02)00225-0
22. Murray PJ, Wynn TA. Protective and pathogenic functions of macrophage subsets. Nat Rev Immunol (2011) 11(11):723–37. doi: 10.1038/nri3073
23. Bellanger AP, Pallandre JR, Gbaguidi-Haore H, Knapp J, Malezieux N, Lignon T, et al. Investigating the impact of Echinococcus multilocularis vesicular fluid on human cells from healthy blood donors. J Immunol Methods (2015) 417:52–9. doi: 10.1016/j.jim.2014.12.006
24. Wang J, Lin R, Zhang W, Li L, Gottstein B, Blagosklonov O, et al. Transcriptional profiles of cytokine/chemokine factors of immune cell-homing to the parasitic lesions: a comprehensive 1-year course study in the liver of E. multilocularis-infected mice. PloS One (2014) 9(3):e91638. doi: 10.1371/journal.pone.0091638
25. Mejri N, Muller N, Hemphill A, Gottstein B. Intraperitoneal Echinococcus multilocularis infection in mice modulates peritoneal CD4+ and CD8+ regulatory T cell development. Parasitol Int (2011) 60(1):45–53. doi: 10.1016/j.parint.2010.10.002
26. Nono JK, Lutz MB, Brehm K. EmTIP, a T-Cell immunomodulatory protein secreted by the tapeworm Echinococcus multilocularis is important for early metacestode development. PLoS Negl Trop Dis (2014) 8(1):e2632. doi: 10.1371/journal.pntd.0002632
27. Gottstein B, Soboslay P, Ortona E, Wang J, Siracusano A, Vuitton D. Immunology of Alveolar and Cystic Echinococcosis (AE and CE). Adv Parasitol (2017) 96:1–54. doi: 10.1016/bs.apar.2016.09.005
28. Garrido VV, Dulgerian LR, Stempin CC, Cerban FM. The increase in mannose receptor recycling favors arginase induction and Trypanosoma cruzi survival in macrophages. Int J Biol Sci (2011) 7(9):1257–72. doi: 10.7150/ijbs.7.1257
29. Donnelly S, O’Neill SM, Sekiya M, Mulcahy G, Dalton JP. Thioredoxin peroxidase secreted by Fasciola hepatica induces the alternative activation of macrophages. Infect Immun (2005) 73(1):166–73. doi: 10.1128/IAI.73.1.166-173.2005
30. Xu J, Zhang H, Chen L, Zhang D, Ji M, Wu H, et al. Schistosoma japonicum infection induces macrophage polarization. J BioMed Res (2014) 28(4):299–308. doi: 10.7555/JBR.27.20130072
31. Wang H, Li J, Guo B, Zhao L, Zhang Z, McManus DP, et al. In vitro culture of Echinococcus multilocularis producing protoscoleces and mouse infection with the cultured vesicles. Parasit Vectors (2016) 9(1):411. doi: 10.1186/s13071-016-1687-y
32. Zhang C, Shao Y, Yang S, Bi X, Li L, Wang H, et al. T-cell tolerance and exhaustion in the clearance of Echinococcus multilocularis: role of inoculum size in a quantitative hepatic experimental model. Sci Rep (2017) 7(1):11153. doi: 10.1038/s41598-017-11703-1
33. van Rooijen N, Sanders A, van den Berg TK. Apoptosis of macrophages induced by liposome-mediated intracellular delivery of clodronate and propamidine. J Immunol Methods (1996) 193(1):93–9. doi: 10.1016/0022-1759(96)00056-7
34. Ramachandran P, Pellicoro A, Vernon MA, Boulter L, Aucott RL, Ali A, et al. Differential Ly-6C expression identifies the recruited macrophage phenotype, which orchestrates the regression of murine liver fibrosis. Proc Natl Acad Sci USA (2012) 109(46):E3186–95. doi: 10.1073/pnas.1119964109
35. Mederacke I, Dapito DH, Affo S, Uchinami H, Schwabe RF. High-yield and high-purity isolation of hepatic stellate cells from normal and fibrotic mouse livers. Nat Protoc (2015) 10(2):305–15. doi: 10.1038/nprot.2015.017
36. Mossanen JC, Krenkel O, Ergen C, Govaere O, Liepelt A, Puengel T, et al. Chemokine (C-C motif) receptor 2-positive monocytes aggravate the early phase of acetaminophen-induced acute liver injury. Hepatology (2016) 64(5):1667–82. doi: 10.1002/hep.28682
37. Park MJ, D’Alecy LG, Anderson MA, Basrur V, Feng Y, Brady GF, et al. Constitutive release of CPS1 in bile and its role as a protective cytokine during acute liver injury. Proc Natl Acad Sci USA (2019) 116(18):9125–34. doi: 10.1073/pnas.1822173116
38. Dou L, Shi X, He X, Gao Y. Macrophage Phenotype and Function in Liver Disorder. Front Immunol (2019) 10:3112. doi: 10.3389/fimmu.2019.03112
39. Zhang Y, Mei X, Liang Y, Zhu B, Sheng Z, Shi W, et al. Newly excysted juveniles (NEJs) of Fasciola gigantica induce mice liver fibrosis and M2 macrophage-like phenotype in vivo. Microb Pathog (2020) 139:103909. doi: 10.1016/j.micpath.2019.103909
40. Guillot A, Tacke F. Liver Macrophages: Old Dogmas and New Insights. Hepatol Commun (2019) 3(6):730–43. doi: 10.1002/hep4.1356
41. Breijo M, Anesetti G, Martinez L, Sim RB, Ferreira AM. Echinococcus granulosus: the establishment of the metacestode is associated with control of complement-mediated early inflammation. Exp Parasitol (2008) 118(2):188–96. doi: 10.1016/j.exppara.2007.07.014
42. Kanazawa T, Asahi H, Hata H, Mochida K, Kagei N, Stadecker MJ, et al. Arginine-dependent generation of reactive nitrogen intermediates is instrumental in the in vitro killing of protoscoleces of Echinococcus multilocularis by activated macrophages. Parasite Immunol (1993) 15(11):619–23. doi: 10.1111/j.1365-3024.1993.tb00575.x
43. ANDRADE. Echinococcus multilocularis laminated-layer components and the E14t 14-3-3 recombinant protein decrease NO production by activated rat macrophages in vitro. Nitric Oxide Biol Chem (2004) 10(3):150–5. doi: 10.1016/j.niox.2004.03.002
44. van Die I, Cummings RD. The Mannose Receptor in Regulation of Helminth-Mediated Host Immunity. Front Immunol (2017) 8:1677. doi: 10.3389/fimmu.2017.01677
45. Satoh T, Takeuchi O, Vandenbon A, Yasuda K, Tanaka Y, Kumagai Y, et al. The Jmjd3-Irf4 axis regulates M2 macrophage polarization and host responses against helminth infection. Nat Immunol (2010) 11(10):936–44. doi: 10.1038/ni.1920
46. Yang P, Li QJ, Feng Y, Zhang Y, Markowitz GJ, Ning S, et al. TGF-beta-miR-34a-CCL22 signaling-induced Treg cell recruitment promotes venous metastases of HBV-positive hepatocellular carcinoma. Cancer Cell (2012) 22(3):291–303. doi: 10.1016/j.ccr.2012.07.023
47. Anthony RM, Urban JF Jr., Alem F, Hamed HA, Rozo CT, Boucher JL, et al. Memory T(H)2 cells induce alternatively activated macrophages to mediate protection against nematode parasites. Nat Med (2006) 12(8):955–60. doi: 10.1038/nm1451
Keywords: Alveolar echinococcosis, Echinococcus multilocularis, inflammation response, hepatic macrophages, M1/M2 macrophages
Citation: Wang H, Zhang C-S, Fang B-B, Hou J, Li W-D, Li Z-D, Li L, Bi X-J, Li L, Abulizi A, Shao Y-M, Lin R-Y and Wen H (2021) Dual Role of Hepatic Macrophages in the Establishment of the Echinococcus multilocularis Metacestode in Mice. Front. Immunol. 11:600635. doi: 10.3389/fimmu.2020.600635
Received: 01 September 2020; Accepted: 24 November 2020;
Published: 08 January 2021.
Edited by:
Juarez Antonio Simões Quaresma, Evandro Chagas Institute, BrazilReviewed by:
Takeshi Izawa, Osaka Prefecture University, JapanCopyright © 2021 Wang, Zhang, Fang, Hou, Li, Li, Li, Bi, Li, Abulizi, Shao, Lin and Wen. This is an open-access article distributed under the terms of the Creative Commons Attribution License (CC BY). The use, distribution or reproduction in other forums is permitted, provided the original author(s) and the copyright owner(s) are credited and that the original publication in this journal is cited, in accordance with accepted academic practice. No use, distribution or reproduction is permitted which does not comply with these terms.
*Correspondence: Hao Wen, ZHIuaGFvd2VuQDE2My5jb20=; Ren-Yong Lin, cmVueW9uZ19saW5Ac2luYS5jb20=
†These authors have contributed equally to this work
Disclaimer: All claims expressed in this article are solely those of the authors and do not necessarily represent those of their affiliated organizations, or those of the publisher, the editors and the reviewers. Any product that may be evaluated in this article or claim that may be made by its manufacturer is not guaranteed or endorsed by the publisher.
Research integrity at Frontiers
Learn more about the work of our research integrity team to safeguard the quality of each article we publish.