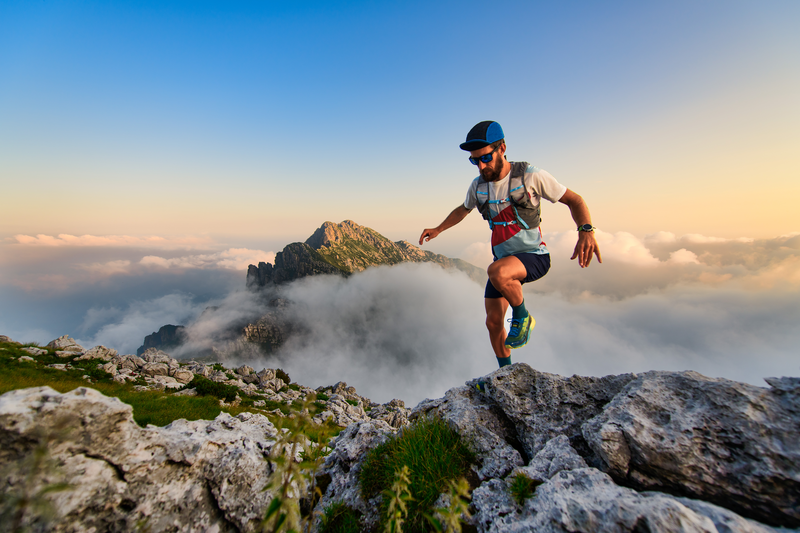
95% of researchers rate our articles as excellent or good
Learn more about the work of our research integrity team to safeguard the quality of each article we publish.
Find out more
REVIEW article
Front. Immunol. , 22 December 2020
Sec. Cytokines and Soluble Mediators in Immunity
Volume 11 - 2020 | https://doi.org/10.3389/fimmu.2020.598532
The intricate interplay between malignant cells and host cellular and non-cellular components play crucial role in different stages of tumor development, progression, and metastases. Tumor and stromal cells communicate to each other through receptors such as integrins and secretion of signaling molecules like growth factors, cytokines, chemokines and inflammatory mediators. Chemokines mediated signaling pathways have emerged as major mechanisms underlying multifaceted roles played by host cells during tumor progression. In response to tumor stimuli, host cells-derived chemokines further activates signaling cascades that support the ability of tumor cells to invade surrounding basement membrane and extra-cellular matrix. The host-derived chemokines act on endothelial cells to increase their permeability and facilitate tumor cells intravasation and extravasation. The tumor cells-host neutrophils interaction within the vasculature initiates chemokines driven recruitment of inflammatory cells that protects circulatory tumor cells from immune attack. Chemokines secreted by tumor cells and stromal immune and non-immune cells within the tumor microenvironment enter the circulation and are responsible for formation of a “pre-metastatic niche” like a “soil” in distant organs whereby circulating tumor cells “seed’ and colonize, leading to formation of metastatic foci. Given the importance of host derived chemokines in cancer progression and metastases several drugs like Mogamulizumab, Plerixafor, Repertaxin among others are part of ongoing clinical trial which target chemokines and their receptors against cancer pathogenesis. In this review, we focus on recent advances in understanding the complexity of chemokines network in tumor microenvironment, with an emphasis on chemokines secreted from host cells. We especially summarize the role of host-derived chemokines in different stages of metastases, including invasion, dissemination, migration into the vasculature, and seeding into the pre-metastatic niche. We finally provide a brief description of prospective drugs that target chemokines in different clinical trials against cancer.
One of the key property of cancer cells is the ability to invade the basement membrane, intravasate into the peripheral circulation and colonize in distant organs to establish metastasis nodules, which is the major cause of cancer related deaths (1, 2). For decades, it was believed that cancer cells are self-sufficient to achieve uncontrolled growth and metastases. However, the work done in last few decades strongly support the notion that tumor growth and metastases is rather a complicated process supported by variety of other cell types present in the intimate tumor microenvironment (TME) (3). The process of metastases can be broadly divided into three phases, namely dissemination, spread, and seeding at the site of metastases (4). At the stage of dissemination, tumor cells undergo transcriptional modifications to induce epithelial-to-mesenchymal (EMT) phenotypic change, invade the basement membrane and extracellular matrix (ECM) and intravasate into the vasculature. Once in the vasculature, circulating tumor cells (CTCs) can extravasate at the site of metastases. After extravasating at the site of metastases, CTCs colonize and proliferate to form metastatic foci. Now it is established that intricate interplay between the tumor cells and other cells present in TME participates in all stages of tumor metastases, including invasion, ECM remodeling, intravasation and colonization of distant organs (5).
A tumor mass is a dynamic 3D structure that includes cellular and extracellular components creating a unique TME. The cellular component of the TME is composed of a heterogeneous population of stromal cells (5). These stromal cells are host derived and include innate immune cells, such as monocytes, macrophages, NK cells; adoptive immune cells, including T cells and B cells; and non-immune fibroblast, pericytes, endothelial cells, adipocytes, and mesenchymal stromal cells (MSCs). In addition, ECM and milieu of secreted factors are also integral extracellular components of the TME.
Reciprocal interaction between tumor cells and other cell types occurs in different ways, including direct cell-to-cell contact, secreted molecules and cargo vesicles known as exosomes. Cancer cells express various cell surface ligands that can directly interact with membrane receptors present on other cell types in the vicinity (6). One such class of receptors is the integrins. Integrins bind cells to the ECM and respond to shear stress (6, 7). Cancer cells also shed vesicles loaded with nucleic acids, peptides and metabolites that can fuse with other cells. Extracellular vesicles play role in angiogenesis (8) immunosuppression (9), aid in crosstalk of cancer cells with fibroblasts (10) and development of premetastatic niche (11, 12). A number of different chemokines such as CCR8, CCL18 in glioblastoma (13), CCL2, CCL3, CCL4, CCL5, CCL20 in lung carcinoma have been reported that are packaged and shedded by chemokines in the TME (14). However, the most extensively studied mode of cancer cell interactions is through secreted molecules. The major type of secreted signaling molecules are growth factors, cytokines/chemokines, inflammatory mediators, and metabolites. Soluble ligands secreted by cancer cells bind to their cognate cell surface receptors present on stromal cells, or vice-versa, and activate specific signaling pathways. Cytokines are small protein (5–20 kDa) involved in activating cell signaling by binding to specific cell surface receptor and regulate immunity, inflammation and hematopoiesis. Chemokines are smaller (8–14 kDa) cytokines that are predominantly involved in cell chemotaxis and trafficking. Chemokines have broadly been divided into two subfamilies on the bases of presence (CXC) or absence (CC) of an amino acid between N terminal first two cysteine residues (15). In this review we will discuss the role of chemokines secreted by stromal cells that favor metastases of cancer cells.
Innate cells are one of the major type of cells recruited to the TME. Tumors are known to educate these inflammatory cells to support tumor growth and metastases (16). The major type of inflammatory immune cells in the TME is tumor-associated macrophages (TAMs) and myeloid-derived suppressor cells (MDSCs) (17–20). Classically activated macrophages are the first line of immune defense and are known to clear pathogens from site of infection. However, tumor educated TAMs are incapable of clearing tumor cells due to reduced phagocytic activity. Rather than activating immune response, TAMs suppress immune cells by various mechanisms, including upregulation of checkpoint molecules, secreting immune suppressive molecules like IL-10, TGF-β and prostaglandin-E2 (PGE2) and deviating immune helper cells maturation towards immune suppressive phenotypes (21–23). In addition, TAMs provide growth factors to proliferative cancer cells, secrete ECM degrading enzymes to enhance invasion and escort cancer cells to the vascular interface (24–26). Another type of innate immune cell, MDSCs are immature myeloid cells that are highly immune-suppressive in nature. By metabolizing L-arginine, MDSCs deprive T-cells of a critical substrate for nitric oxide production and inhibit their proliferation and activation. The MDSCs also divert T-cell maturation towards immune suppressive T regulatory (Tregs) cells (27). The MDSCs have broadly been divided into Ly6G-positive granulocytic MDSCs and Ly6C-positive monocytic MDSCs. Monocytic MDSCs suppress both antigen specific and antigen non-specific T cell responses using both cytokines and NO to perform these functions while granulocytic MDSCs inhibit only antigen specific T cell responses [reviewed by (28, 29)]. Monocytic MDSCs impair interferon-α production by increasing STAT1 phosphorylation leading to loss of T cell function and immunosuppression (30). Granulocytic MDSCs produce ROS and inhibit T cell proliferation by downregulation of TCRζ expression, inhibition of NF-κB activation, and cell death by induction of apoptosis (29).
Adaptive immune cells can elicit a highly specific immune response against foreign antigens, including cancer. The major types of adaptive immune cells include CD4+ T helper cells, CD8+ T effector cells and B cells. CD8+ T cells are key factor in anti-tumor immune surveillance. The interaction of tumor-antigen presenting dendritic cells and CD4+ T helper cells releases chemokine CCL3 and CCL4 that in turn attract CCR5+ cytotoxic CD8+ T cells (31). Previous studies showed that cancer cells, along with tumor-educated inflammatory cells suppress T-cells to escape immune surveillance. Under the influence of immune suppressive TME, CD4+ T helper cells differentiate from immune promoting phenotype to immune suppressive Treg phenotype (5, 27, 32). In addition, tumor educated B cells coverts CD4+ T cells to immunosuppressive Tregs (33).
Cancer associated fibroblasts (CAF) are the major type of stromal cells that comprise the TME. The contribution of fibroblasts to tumor growth was first inferred in1986, when Picard and colleagues demonstrated the importance of injecting fibroblasts with cancer cells for achieving successful tumor engraftment in vivo (34). Later, it was observed that not only direct cell-cell contact but secreted factors are also involved in epithelial/cancer cell-fibroblast interactions (35, 36). Thus, fibroblast-associated tumor-promoting properties have now largely been attributed to growth factors, chemokines/cytokines and metabolites, together known as fibroblast secretome. However, exchange of metabolites and activation of signaling pathways between fibroblasts and tumor cells via direct cell-cell contact mechanisms continue to be viewed as important (17, 37). The studies done in the last two decades with transgenic mouse models on oncogenic or antitumor genes have provided strong evidence regarding the role of fibroblasts in supporting tumor cell proliferation, ECM remodeling, metastases and elevating the process of angiogenesis, as reviewed elsewhere (38–40).
Endothelial cells that are recruited to the TME promote neo-angiogenesis. The expansion of blood vasculature ensures adequate perfusion to support overwhelming tumor growth and provides for a route for hematogenous dissemination. The interaction of tumor cells with endothelial cells is a critical step in the process of intravasation, extravasation and metastases whereby cancer cells manipulate pericytes to alter blood vessel integrity and facilitate intravasation (41–43).
ECM and secreted factors play a dynamic role in tumor biology. The expression of ECM proteolytic enzymes, matrix metalloproteinases (MMPs), is closely associated with tumor progression (44–46). The tumor-induced deposition of ECM at the site of primary as well as metastatic tumor is responsible for chemotherapy and immunotherapy resistance by limiting the entry of drugs to the core of tumor (47).
The local dissemination of primary tumor cells into the adjacent normal tissues is an initial step of tumor metastases. The sequence of this program involves epithelial-mesenchymal transition (EMT); acquisition of tumor-initiating capability known as cancer stem cell (CSC) properties; cell adhesion to extracellular matrix (ECM) or vascular endothelial cells; extracellular matrix (ECM) remodeling, and cell migration/invasion (48, 49) (Figure 1).
Figure 1 Schematic illustration of multifaceted roles of chemokines in invasion and dissemination. Chemokines bind to their receptors and regulate ECM remodeling, EMT, cell migration, and cell invasion.
Chemokines, play an essential role in the dissemination of cancer cells into adjacent normal tissues. Interactions between tumor and stromal cells promote chemokine production in stromal cells which in turn directly or indirectly stimulate cancer metastases (50). Table 1 describes multifaceted roles of stromal cells derived chemokines in local invasion by primary tumor.
EMT re-programming is one essential step enabling the invasion and metastatic dissemination of cancer cells. During this process, cancer cells are endowed with malignant traits associated with the loss of epithelial traits and the acquisition of a more mesenchymal phenotype (89). It has been widely accepted that heterotypic signaling pathways induced by chemokines from the tumor-associated stroma can trigger EMT in a variety of carcinomas (49). These have been proven by several co-culture experiments involving cancer cells and host cells such as fibroblasts. CXCL12 expressed by CAFs causes EMT in breast cancer and human tongue squamous cell carcinoma (76, 90). EMT mechanistically was studied in breast (91, 92), thyroid (93)and colon cancer (77). Signaling pathways perturbed were PI3K/AKT/PKB), MAPK/ERK (77, 92), WNT/β-catenin (91) and NF-κB pathways (93). Similarly, fibroblasts when co-cultured with prostate cancer cells produce CXCL1 (which binds to CXCR1 receptor on tumor cells). CXCR1 receptor is also present on neutrophils which produce LCN2. The CXCL1-LCN2 paracrine axis activates Src signaling and leads to EMT and contributes to tumor progression (66). Furthermore, in pancreatic cancer CXCL8 causes cell invasion and promotes metastases (72). Similarly in gastric cancer, neutrophils like cells expressed CXCL8 and induced EMT through CXCR1/CXCR2 receptors (25, 73, 94) and CXCL16 produced by MSCs induces proliferation and migration of tumor cells (86). CAF-derived CXCL16 could also promote brain metastases in breast cancer, which were significantly inhibited by the CXCL16 neutralizing antibody (87).
Several CC chemokines CCL5 and CCL18 also promote EMT, cell migration and invasion in co-culture experiments involving TAMs and different cancer cells. Signaling pathways activated by CC chemokines include NF‑κB and β-catenin/STAT signaling in breast and prostate cancer and MEK/ERK/NF-κB/integrin αvβ3 pathways in osteosarcoma by CCL5 (54), PI3K/AKT/mTOR, and ERK1/2 signaling in endometrial carcinoma and squamous cell carcinoma of the head and neck by CCL18 (60, 95). Furthermore, co-culture of monocyte-derived macrophages which is a major source of CCL20 with hepatoma cells induces EMT and accelerates tumor metastases in a CCL20-dependent manner (20, 61, 64) by activation of P38 MAPK (96), CrkL-ERK1/2 (97) and JAK2/STAT3 pathways (65). In addition, fibroblasts can secret the CC family chemokine CCL17/CCL22 that activates CCR4 and ERK/AKT signaling (57) and plays a critical role in the malignant progression of prostate, breast and hepatocellular cancer (24, 57, 58).
Induction of EMT triggers in several types of cancer cells to acquire cancer stem cell (CSCs) properties such as self-renewal, tumor-initiating and multipotent differentiation potential that enhances metastases (98). Disseminated cancer cells are believed to be endowed with CSC-like properties, which permits mesenchymal differentiation and increased capacity for establishment of metastatic colonies (49). Many studies with CXCL12, CXCL8, CCL2 and CCL18 chemokines have reported a correlation between the stromal chemokines and the acquisition of CSC-like traits (99). In addition, CCL2 was shown to activate the STAT3 and NOTCH1 pathways (51) and macrophages-derived chemokine CXCL8 increases CSCs-like populations and enhances mammosphere formation via activation of AKT/mTOR signaling in renal and breast cancer (25, 74).
The EMT process confers the polarized epithelial cells properties that are critical to the invasion-metastases cascade, which includes interaction with basement membrane surface receptors and degradation of ECM (49). Stromal CXC and CC chemokine families have been reported to trigger protease release, leading to ECM degradation and play essential roles in cancer metastases (48).
CAF-derived CXCL12 upregulates tumoral expression of matrix metalloproteinases (MMP-2, 9, 13) and thereby leads to contraction of collagen matrices (18, 44, 78). CXCL12/CXCR4 axis also induces the formation and maturation of invadopodia, which alters cellular morphology, induces ECM degradation and promotes invasive features (79) through RhoA/ROCK/MLC-2, Src-Arg-cortactin and MAPK signaling pathways (18, 79). In addition, co-culture of gastric cancer cells and tumor-associated lymphatic endothelial cells (LECs) elevated CXCL1 secretion in LECs which in turn upregulated integrin β1 and MMP2/9 that promotes cell adhesion, migration, invasion and lymph node metastases (67).
Aberrant expression of CC chemokine receptors has also been also related to ECM remodeling processes (48, 56). Chemokine CCL2 and CCL5 in prostate and ovarian cancer promoted MMP-2 and MMP-9 secretion by ERK, Rac signaling (55); in chondrosarcoma through Ras/Raf-1/MEK/ERK/NF-κB and in breast and liver cancer via PI3K/Akt and GSK-3β pathways (52, 53). CCL7 interacts with CCR3 and activates RhoA/ERK and PI3K pathways, resulting in collagen degradation and invadopodia formation, contributing to cell invasion (56). CCL17/22 enhanced MMP2 expression via ERK/AKT signaling in hepatocellular cancer (57), and upregulate MMP13 expression via ERK/NF-κB signaling in colorectal cancer (59). Similarly, CCL20/CCR6 binding leads to MMP2 upregulation via JAK2/STAT3 and CrkL-Erk1/2 pathways, and MMP9 upregulation through the activation of PKC-α, src, Akt, and NF-κB pathways in breast and gastric cancer (65, 97).
As discussed above, stromal chemokines act on tumor cells in epithelial cancers and trigger EMT. Subsequently, epithelial cancer cells regulate cell-cell adhesion structures and cell polarity that contribute to cancer cell dissemination. Within invasive carcinomas, the multicellular microanatomical structures called Tumor MicroEnvironment of Metastases (TMEM) serve as the functional sites of tumor cell dissemination and transendothelial migration. Migratory breast, pancreatic, lung and colon cancer cells at TMEM overexpress an invasive form of Enabled (Ena)/vasodilator-stimulated phosphoprotein (VASP) protein (MENAINV) in their non-invasive compartments with an intact basement membrane (100) and promotes cancer invasion and migration of tumor cells (101, 102). MENAINV expressing invasive cancer cells enhance transendothelial migration in response to cancer cell-macrophage contact (100, 102). These cells migrate along collagen fibers and stream toward vasculature paired with TAMs driving invadopodium assembly and results in matrix degradation, discohesive tumor morphology and increased tumor cell motility (103, 104). The TAM derived growth factors help cancer cells to form invadopodia and acquire invasive properties via EGF/CSF1 paracrine loop and through the activation of Notch signaling (100, 102).
CAF-derived CXCL14 can also modulate cell adhesion and promote motility. CXCL14 interacts with atypical receptor ACKR3 with greater binding affinity and activates Akt and ERK1/2 MAPK by β-arrestin binding (80). Stromal CXCL16 modified cellular adhesion as well as motility by promoting Ezrin activation, αvβ3 integrin clustering and F-actin stability via FAK/PI3K/PKC and CXCR6/ERK1/2/RhoA/cofilin/F-actin pathway (46, 88).
The major cause of cancer related death is due to distant metastases. Invasion of basement membrane, ECM and directional migration towards the vasculature is not sufficient for successfully metastases. Cancer cells must migrate across the vessel wall into the blood or lymph vasculature, a process known as tumor cell intravasation. It is a rate-limiting step of metastases and determines the number of circulatory tumor cells (CTCs), which in turn, dictates the probability of metastases. Cancer cells can access the vasculature through passive, active or assisted mechanisms of intravasation. Passive mechanism involves tumor cells getting entrapped in endothelial emboli while active mechanism involves intravasation involves transendothelial migration of tumor cells. In assisted intravasation, TAMs escort tumor cells across endothelium. At the site of metastases, tumor cells again migrate across endothelial cells, a process known as extravasation, to colonize and generate metastatic foci. In this section, we discuss the role of stromal cell-secreted factors, especially chemokines, in the process of intravasation and extravasation.
A tumor embolus is a nest of tumor cells embedded in endothelium. A developing, immature or a dilated blood vessel may allow formation of emboli (41, 43). Tumor cells along with tumor stroma has been observed in an embolus (105). Histologic evaluation of extrahepatic bile duct carcinomas showed that the presence of fibroblasts in the tumor embolus positively associates with increased metastases (105). The notion of stromal contribution to emboli-mediated metastases was established with the observation that injection of emboli containing tumor cells and stromal cells increases chances of experimental metastases (42). Very recently, it has been observed that co-injection of tumor cells with CAFs frequently generated emboli with proliferative tumor cells compared to control fibroblasts or no fibroblasts (106) and increases the viability of tumor cells in the emboli (107). Stromal fibroblasts-derived chemokine CXCL12 and Transforming Growth Factor β1 (TGFβ1) induce Src-mediated EMT and proliferate tumor cells present in emboli. Specific inhibition of CXCL12 and TGFβ1 in CAFs inhibited the proliferating tumor cells in the emboli (106) highlighting the importance of CAFs in protecting tumor cells in the emboli.
As an active mechanism, tumor cells degrade the basement membrane and intravasate through trans-endothelial migration. During this process, tumor cells undergo EMT, achieve invasive and highly motile phenotype and secrete factors to increase permeability of blood vessels in the TME. CXCL12 expressing organs are a target for CXCR4 positive cancer cells and blocking the CXCL12/CXCR4 interaction using neutralizing antibodies (nAb) significantly inhibits experimental metastases (108). We and others have established fibroblasts as a critical source of CXCL12 in the TME (3, 40, 109). Co-implantation of human CAFs secreting CXCL12 with tumor cells enhances breast tumor growth (40) with CXCL12 recruiting endothelial cells to enhance angiogenesis. We have shown that, depletion of fibroblast-specific CXCL12 results in reduced tumor growth, decreased number of CTCs and inhibits metastases of orthotopic and spontaneous mammary tumors (109). Fibroblast-derived CXCL12 expands the leaky tumor vasculature by recruiting endothelial precursor cells and suppressing tight junction molecules and this facilitates tumor cell intravasation (109). Using RNA hybridization technique, we confirmed that fibroblasts are a critical source of CXCL12 and that fibroblast specific deletion of CXCL12 abrogates CXCL12 protein expression in the TME suggesting that stromal fibroblasts-secreted chemokine CXCL12 shapes the TME in favor of tumor growth and metastases. The major steps of metastases, especially the role of fibroblasts-derived CXCL12 in intravasation are summarized in Figure 2. Besides CXCL12, stromal fibroblast and mesenchymal stem cells (MSCs) also indirectly upregulate chemokines such as CXCL8 and CCL2 through inflammatory molecules like TNF-α and IL-1β (109–111). Importantly, TNF-α stimulated cancer cell-MSC or cancer cell-fibroblast co-cultures stimulated endothelial cell migration and sprouting in vitro and increased tumor growth in vivo (110). In another study, co-culture of tumor cells and MSCs elevated CCL5 expression in MSCs which increased invasive and migratory properties of tumor cells that express its receptor, CCR5, leading to increased metastases (112).
Figure 2 Steps of metastases. (A) A cartoon of TME showing tumor cells invade ECM and migrate towards blood vessels. (B) At the blood vessels, tumor cells transmigrate across endothelial layer and become circulatory tumor cells (CTCs). (C) Tumor cells extravasate and seed into the lungs to generate metastatic foci. The dashed line inset diagram describes molecular events occurring at the time of transendothelial migration shown in (B) within dashed line box. The CAFs present in TME secrete CXCL12 that act on endothelial cells through receptor CXCR4 and decrease the expression of tight junction (TJ) molecules, which results in intravasation of tumor cells.
The passive and active mechanisms of intravasation assume a tumor vasculature that is disorganized and hyper-permeable and thus conducive for tumor cells intravasation. However, researchers suggest that tumor cells are assisted by macrophages during the process of intravasation. A direct association of tumor cells and macrophages during migration was demonstrated by Wyckoff and colleague in 2004 using intravital imaging. Another study using multiphoton in vivo imaging showed that perivascular macrophages directly interact with tumor cells and endothelial cells of blood vessels creating the TMEM. TMEM density directly correlates with systemic metastases (32).Within the TMEM, macrophages promote tumor cell intravasation (113). In-vivo imaging has shown that tumor cell intravasation occurs only at TMEM doorways and tumor cells are escorted by Tie2 positive TAMs to blood vessels (114). Tie2-positive macrophages locally and transiently compromise vascular integrity by expressing VEGF-allowing intravasation of tumor cells by compromising endothelial cell-cell junctions. Others have suggested that VEGF-A-induce increases in endothelial permeability is a transient and regulated phenomenon (115). Although the role of chemokines secreted by Tie2-positive macrophages has not been studied in TMEM, these cells are known to express chemokines in rheumatoid arthritis (116). In addition, fibroblast-derived CXCL12 can also recruit CXCR4+ TAMs which can alter vessel permeability to facilitate intravasation (117).
The mechanistic studies showed existence of an active EGF/EGFR and CSF1/CSF1R feedback loop between tumor cells and macrophages in renal clear cell carcinoma, glioblastoma and breast cancer (118–122). ErbB3 overexpressing MTLn3 breast cancer cells are more invasive than normal MTLn3 cells in presence of ErbB3 ligand HRG-β1. MTLn3-ErbB3 and transgenic MMTV-Neu tumors invasiveness in presence of HRG-β1 is inhibited by blocking EGFR, CSF-1R, or macrophage function, indicating that invasiveness to HRG-β1 is dependent upon the EGF/CSF-1 paracrine loop. Furthermore, CXCL12 also triggers in vivo invasion of transgenic MMTV-PyMT tumors in an EGF/CSF-1–dependent manner (Hernandez et al., 2009). Similarly, co-expression of CSF-1 and its receptor CSF-1R on renal tubular epithelial cells (TEC) promotes proliferation and inhibits apoptosis during regeneration of renal tubules. When CSF-1 and CSF-1R are coexpressed in renal cell carcinoma and TEC aids in RCC survival and inhibition of apoptosis (122).
Once in the vasculature, cancer cells face various barriers such as physical stress, oxidative stress, anoikis, and the lack of growth factors and cytokines to survive and successfully extravasate and colonize in distant organs. Millions of tumor cells are shed from primary tumor into the efferent blood of mammary adenocarcinoma (123). However, only about 0.1% cells survival and the rest become non-viable within 48 h (124). There are several chemokine-mediated mechanisms that are involved in survival or killing of CTCs. One of the mechanism of CTC death is through immune cell derived chemokine/chemokine receptors. It has been shown that CX3CR1+ monocytes are recruited to the tumor cell aggregates in response to tumor-derived CX3CL1, where they can directly engage cancer cells or secrete CCL3, CCL4 and CCL5 to activate natural killer cells to kill CTCs (125). CCL2 is a potent chemoattractant for phagocytic cells including monocytes, and macrophages (126).
Tumor cells secrete thrombin to activate platelets to aggregate around tumor cells. The activated platelets cross-link with each other to create tumor cell-platelet microemboli. In addition, tumor cell-platelet association also inhibits NK cells mediated lysis of CTCs (127, 128). In addition to protecting CTCs in the vasculature, platelets are known to secrete CXCL12, which promotes their survival, transendothelial migration and extravasation (129, 130). As another mechanism to support CTCs survival, platelets secrete lysophophatidic acid (LPA) that binds to its receptors, LPAR1, 2 and (3) on breast cancer cells and activates the secretion of IL6, IL8, CCL2 and CXCL1, enhance survival and increases the migration potential of CTCs (131).
Tumor cells in distant organs induce formation of a microenvironment that promotes the establishment and growth of tumors and this was subsequently referred to as the ‘pre-metastatic niche’ (PMN) (132–134). PMNs arise as a result of combined efforts of tumor secreted factors, stroma secreted factors and tumor-shed extracellular vesicles (EVs) that regulate the stepwise evolution of PMN (133). Establishing a PMN involves changes at the local and systemic levels. Locally, within the PMN, changes arise in the stroma, vasculature and ECM (135, 136).
The expression of CXCR4 receptor on tumor cells aids in their colonization to organs expressing CXCL12 such as bone marrow which favors tumor cell survival and growth (137). Activation of CXCR4 receptor by CXCL12 has been reported in different cancers such as brain neoplasms, colorectal cancer, prostrate cancer, melanoma, ovarian cancer, etc (138–142). SCLC overexpresses CXCR4 and activation by CXCL12 overexpressed in bone marrow induces migration, invasiveness and adhesion to marrow stromal cells which prevent apoptosis in SCLC cells from chemotherapy (137, 143, 144).
Formation of PMNs in lungs is reported to be initiated by accumulation of VEGFR1+ bone marrow derived hematopoietic progenitor cells along with resident fibroblasts which express high levels of CXCL12. VLA4 is expressed by VEGFR1+ cells which causes resident stromal fibroblasts to upregulate fibronectin which forms a permissive niche for incoming tumor cells (132). The hematopoietic progenitor cells promote migration and adhesion of lung carcinoma and CXCR4+ B16 melanoma cells to the lung PMNs (132). Furthermore, lung epithelial cells secrete chemokines such as CXCL1, CXCL2, CXCL5 and CXCL12 to recruit neutrophils in Lewis Lung Carcinoma (LLC) xenograft models in response to TLR3 activation by LLC exosomal RNAs (145, 146). In the liver, CXCL12 is involved in PMN formation through CXCL12-CXCR4 dependent recruitment of neutrophils that enhance PMN properties by secretion of chemokines such as tissue inhibitor of metallopeptidase 1 (TIMP1) (111, 147, 148). In the lymph nodes, CCR7+ melanomas colonize and inhibiting the CCL21 with neutralizing antibodies blocks CCR7 mediated metastases (149). In the central nervous system, CCR7 expression in the brain endothelial cells, is an essential adhesion signal for CCR7+ leukemia T-cells to specifically seed and metastasize to central nervous system. Silencing of the CCR7 or its ligand CCL19 in animals models has been reported to inhibit infiltration of leukemia cells into the brain (150).
Besides the stromal changes, one of the major vascular changes which prime distant tissue for metastatic cell colonization is formation of blood clots due to leaky vasculature (151). Vascular clots rich in fibrin and platelets coat the surface of CTCs and aid in their dissemination (152). Platelets secrete CXCL5 and CXCL7, contact with tumor cells cause recruitment of granulocytes (CD11b+MMP9+Ly6G+) to tumor cells in lung to form early metastatic niches directly helping in tumor cell seeding and development of lung metastases (153, 154). In addition, CTCs homing and survival in PMNs is aided by tissue factor mediated clot formation in which macrophage populations are already recruited during PMN formation (155).
After surviving the hostile vascular environment, CTCs undergo trans-endothelial migration to extravasate from the blood vessel into local PMN. The host cells co-ordinate with each other in response to tumor signals to help extravasation of CTC. Upon activation by tumor cells, platelets secrete CXCL5 and CXCL7 chemokines to recruit CXCR2-positive neutrophils (153). These neutrophils release cytosolic and granulated proteins that are assembled along with chromatin fibers to generate large, web-like structures know as neutrophil extracellular traps (NETs) (153, 156). NETs have been reported in pancreatic, liver, breast and gastric cancers (157–160). In metastatic breast cancer, neutrophils form metastatic supporting NETs. NET-DNA acts as a chemotactic signal to attract cancer cells rather than just acting as a trap at distant metastatic sites (161). These NETs contributes to the extravasation by sequestering CTCs and degrading the ECM to facilitate migration of CTCs (156, 162). In addition, tumor-derived CCL2 can directly act on CCR2 positive endothelial cells to increase their permeability or indirectly via increasing CXCL12 expression, which helps CXCR4 positive cancer cells to extravasate into the PMNs (129, 163, 164). Finally, the CCL2 from tumor cells and CCL5 from activated endothelial cells can further recruit the inflammatory monocytes/macrophages to prepare the cellular assembly of CTCs, platelets, neutrophils, endothelial cells and monocytes/macrophages required for efficient extravasation of CTCs (154). Activation of CCR2 signaling prompts TAMs to secrete another chemokine, CCL3, leading to enhanced TAMs–tumor cell interaction and prolonged retention of TAMs in the metastases sites, which promotes extravasation of breast cancer cells (165, 166).
As final step of metastases, tumor cells have to seed, survive, and proliferate into the PMC to develop metastatic foci. CCR2+ inflammatory monocytes recruit to the PMN and are differentiate into metastases associated macrophages (MAMs) (165). These MAMs express another cytokine CCL3 to help seeding of CTCs as it has been shown that the genetic deletion of CCL3 or CCR2 prevents metastatic seeding of breast CTCs (166). High expression levels of chemokine CXCL8 and CCL2 in the bone marrow microenvironment promotes the survival of leukemia cells by enhancing their adhesion to bone marrow MSCs (167). In melanoma too, CCR2+ BMDCs inhibit proliferation of T cells and help in immune escape (168, 169). Regulatory cells such as MDSCs, macrophages, Tregs in the PMNs are also responsible for suppressing anti-tumor responses and help in tumor cell survival (170, 171). MDSCs cause immune suppression at PMNs resulting in tumor cell survival and promote metastases by downregulation of IFNγ which causes expression of pro-inflammatory cytokines, interleukins and CXCL12 (147, 148, 172). Mechanistically, MDSCs inhibit activity of T cells through arginase1 and reactive oxygen species (ROS) production (27, 173). Tumor evoked B regulatory cells (tBregs) play a primary role in lung metastases by the conversion of resting anti-tumor CD4+ T cells into FoxP3+ expressing Treg cells through the paracrine action of TGFβ and CCL22 expressed from lung (33). The studies discussed above describe the functions performed by stromal cells-derived chemokines throughout the multi-step process of metastases (Figure 3).
Figure 3 The schematic diagram showing different function of stromal-derived chemokines in pre-metastatic niche formation and metastases. Tumor cells along with stromal and immune cells in the primary tumor secrete several chemokines such as CCL2, CXCL12, CCL19, etc., that help in establishing the PMN in distant organs such as lung, liver, brain. Within the lungs SDF-1 and leukotrienes help in immune invasion. CCL2, CXCL12 aid in angiogenesis, form clots in blood vessels and help in survival of melanoma in the lung by inhibiting NK cell activity. In the brain, CCL19 acts on CCR7+ tumor cells and aid in their colonization. In the liver, CXCL12 along with TIMP1 and MIF help in neutrophil recruitment and deposition of fibronectin making liver tissue permissive for formation of a metastatic niche for circulating tumor cells.
Given that chemokines and their receptors have been recognized as the key regulators of cancer progression, strategies targeting different chemokine/chemokine receptor axes exert antitumoral and antimetastatic activities in many tumors to counteract cancer growth and dissemination, inhibiting angiogenesis and regulating the leukocyte recruitment (174, 175).
CXCR4 inhibitors have been reported promising therapeutic effects on solid tumors, including glioblastoma, breast cancer and mesothelioma. A phase I trial (NCT01837095) with CXCR4 antagonist Balixafortide and Eribulin chemotherapy evaluated the safety, tolerability, pharmacokinetics and efficacy in heavily pretreated, relapsed breast cancer patients. The combination has given promising results in HER-negative metastatic breast cancer (176). Current phase I/II study showed that CXCR4 inhibitor Plerixafor was well tolerated as an adjunct to chemoirradiation in newly diagnosed glioblastoma patients and reduced tumor local recurrences by inhibiting postirradiation tumor revascularization (177). Very recently it has been shown that CXCR4 inhibitor AMD3100 decreased desmoplasia, immunosuppression and increased T cells infiltration into tumors, and thereby enhanced the efficacy of immune checkpoint inhibition in a pre-clinical murine model of breast cancer (178).
CXCR1/2 inhibitor Repertaxin enhanced chemotherapeutic efficacy of 5-fluorouracil in gastric cancer by attenuating cell proliferation, inducing apoptosis and suppressing malignant behavior (179). In pancreatic adenocarcinoma (PDAC), genetic depletion of CXCR2 prevented neutrophil accumulation and improved T cell entry, which contributed to tumor suppression and therapeutic response of anti-PD-1 (180, 181). Additionally, combination of SB225002 (CXCR2 inhibitor) with RS504393 (CCR2 inhibitor) overcame the compensatory response of myeloid subset, augmented antitumor immunity and improved chemotherapeutic response of FOLFIRINOX in an orthotopic model of pancreatic adenocarcinoma (182). A phase Ib clinical trial (NCT02001974) using combination of CXCR1/2 inhibitor Reparixin with paclitaxel was tolerable and safe and showed positive response in metastatic breast cancer (183). Another preclinical study also showed that the anti-CCR4 antibody, Affi-5, exerted antitumor activity in renal cancer models by regulating Th1/Th2 switch, altering myeloid cell phenotype, increasing NK cells and Th1 cytokine levels and reducing immature myeloid cells infiltration (184).
It has been reported that interference with the CCL2/CCR2 axis shows antitumoral activity for reducing monocytes recruitment (175). However, cessation of antiCCL2 therapy leads to an overshoot of metastasis and accelerates death (185). In murine pancreatic cancer models, CCR2 antagonist combined with anti–PD-1 decreased tumor burden by blocking monocyte/macrophage recruitment and relieving suppression of the CD8+ T cells (186). In an orthotopic murine model of hepatocellular carcinoma, blocking CCL2/CCR2 axis with a natural product from Abies georgei named 747 or RDC108 suppressed liver tumor growth and recurrence via inhibition of TAMs and other immune suppressive myeloid cells (187, 188). Encouraging results have been reported in other clinical studies. A phase I trial of CCR2 antagonist CCX872 plus chemotherapy FOLFIRINOX has been evaluated in non-resectable pancreatic cancer patients (NCT02345408). Compared to median overall survival of 18 months in FOLFIRINOX only treated patients, the median overall survival was increased by 18.6–29% in CCX872/FOLFIRINOX treated patients. The better overall survival was associated with decreased immune suppression by reducing inflammatory monocytes, circulating monocytes and monocytic myeloid derived suppressor cells (189). A phase Ib study (NCT02732938) evaluated the effect, safety and tolerability of CCR2 inhibitor PF-04136309 plus nab-paclitaxel and gemcitabine in aggressive pancreatic cancer (190). The same inhibitor in combination with FOLFIRINOX chemotherapy has been evaluated in a pancreatic cancer clinical trial (NCT01413022) (191). However, targeting the CCR2 ligand CCL2 has not shown promising results in clinical trials. A clinical trial (NCT00537368) using human CCL2 neutralizing monoclonal antibody CNTO888 could not completely inhibit CCL2 in prostate cancer patients and did not show clinical response compared to standard therapy. Other clinical trials using CNTO888 could not achieve any clinical response in solid tumor patients (NCT01204996) (192). These studies emphasize the importance of thoroughly evaluating different strategies for targeting chemokine- signaling pathways to develop novel therapeutic strategies against difficult-to-manage aggressive malignancies.
Chemokines are crucial molecules involved in autocrine and paracrine signaling required for proper functioning of multicellular organisms. Dysregulation of chemokine secretion can predispose to significant pathology, including malignancies. The emergence of the field of tumor microenvironment have revolutionized the understanding of how tumors exploit host tissues to successfully proceed through different stages of tumor progression, leading to disseminated metastases. The recent advances in the field of TME has shifted the focus of research from targeting cancer cell- autonomous functions to include the host components for designing novel therapies. As a major mechanism of metastases, the tumor educated host cells secrete chemokines and cytokines that feedback on tumor cells and help in dissemination, hematogenous spread and metastatic colonization. Therefore, hindering chemokine signaling pathways between tumor and host cells as a therapeutic strategy against metastatic tumors is gaining increased attention. Recent findings have also established the critical role played by host cells, especially those of the immune system, in the success of conventional radiation therapy and chemotherapy. Therefore, translational significance of targeting host-derived chemokines should be considered in developing novel therapies against cancer, especially metastatic cancer.
SH and BP gathered the information, shortlisted the relevant studies and prepared the layout of review. RG and DA organized different sections of the review, discussed the findings and thoroughly reviewed the manuscript. MC reviewed the section describing clinical studies. JS reviewed the angiogenesis and permeability related sections of the review. All authors contributed to the article and approved the submitted version.
The publication was supported in part by NIH grants R01CA109527 and DoD breast cancer breakthrough awards (W81XWH1910088, W81XWH1710025, W81XWH1610037) to (RG).
The authors declare that the research was conducted in the absence of any commercial or financial relationships that could be construed as a potential conflict of interest.
1. Gupta GP, Massague J. Cancer metastasis: building a framework. Cell (2006) 127(4):679–95. doi: 10.1016/j.cell.2006.11.001
2. Mehlen P, Puisieux A. Metastasis: a question of life or death. Nat Rev Cancer (2006) 6(6):449–58. doi: 10.1038/nrc1886
3. Kojima Y, Acar A, Eaton EN, Mellody KT, Scheel C, Ben-Porath I, et al. Autocrine TGF-beta and stromal cell-derived factor-1 (SDF-1) signaling drives the evolution of tumor-promoting mammary stromal myofibroblasts. Proc Natl Acad Sci U S A (2010) 107(46):20009–14. doi: 10.1073/pnas.1013805107
4. Valastyan S, Weinberg RA. Tumor metastasis: molecular insights and evolving paradigms. Cell (2011) 147(2):275–92. doi: 10.1016/j.cell.2011.09.024
5. Joyce JA, Pollard JW. Microenvironmental regulation of metastasis. Nat Rev Cancer (2009) 9(4):239–52. doi: 10.1038/nrc2618
6. Guo W, Giancotti FG. Integrin signalling during tumour progression. Nat Rev Mol Cell Biol (2004) 5(10):816–26. doi: 10.1038/nrm1490
7. Levental KR, Yu H, Kass L, Lakins JN, Egeblad M, Erler JT, et al. Matrix crosslinking forces tumor progression by enhancing integrin signaling. Cell (2009) 139(5):891–906. doi: 10.1016/j.cell.2009.10.027
8. Sato S, Vasaikar S, Eskaros A, Kim Y, Lewis JS, Zhang B, et al. EPHB2 carried on small extracellular vesicles induces tumor angiogenesis via activation of ephrin reverse signaling. JCI Insight (2019) 4(23):1–18. doi: 10.1172/jci.insight.132447
9. Liu Y, Gu Y, Han Y, Zhang Q, Jiang Z, Zhang X, et al. Tumor Exosomal RNAs Promote Lung Pre-metastatic Niche Formation by Activating Alveolar Epithelial TLR3 to Recruit Neutrophils. Cancer Cell (2016) 30(2):243–56. doi: 10.1016/j.ccell.2016.06.021
10. Paggetti J, Haderk F, Seiffert M, Janji B, Distler U, Ammerlaan W, et al. Exosomes released by chronic lymphocytic leukemia cells induce the transition of stromal cells into cancer-associated fibroblasts. Blood (2015) 126(9):1106–17. doi: 10.1182/blood-2014-12-618025
11. Jung T, Castellana D, Klingbeil P, Cuesta Hernández I, Vitacolonna M, Orlicky DJ, et al. CD44v6 dependence of premetastatic niche preparation by exosomes. Neoplasia (2009) 11(10):1093–105. doi: 10.1593/neo.09822
12. Kogure A, Yoshioka Y, Ochiya T. Extracellular Vesicles in Cancer Metastasis: Potential as Therapeutic Targets and Materials. Int J Mol Sci (2020) 21(12):1–16. doi: 10.3390/ijms21124463
13. Skog J, Würdinger T, van Rijn S, Meijer DH, Gainche L, Sena-Esteves M, et al. Glioblastoma microvesicles transport RNA and proteins that promote tumour growth and provide diagnostic biomarkers. Nat Cell Biol (2008) 10(12):1470–6. doi: 10.1038/ncb1800
14. Ruben JM, van den Ancker W, Bontkes HJ, Westers TM, Hooijberg E, Ossenkoppele GJ, et al. Apoptotic blebs from leukemic cells as a preferred source of tumor-associated antigen for dendritic cell-based vaccines. Cancer Immunol Immunother: CII (2014) 63(4):335–45. doi: 10.1007/s00262-013-1515-6
15. Zlotnik A, Yoshie O. Chemokines: a new classification system and their role in immunity. Immunity (2000) 12(2):121–7. doi: 10.1016/s1074-7613(00)80165-x
16. Coussens LM, Werb Z. Inflammation and cancer. Nature (6917) 2002) 420:860–7. doi: 10.1038/nature01322
17. Liu T, Zhou L, Li D, Andl T, Zhang Y. Cancer-Associated Fibroblasts Build and Secure the Tumor Microenvironment. Front Cell Dev Biol (2019) 7:60 doi: 10.3389/fcell.2019.00060
18. Singh S, Ray LA, Shahi Thakuri P, Tran S, Konopka MC, Luker GD, et al. Organotypic breast tumor model elucidates dynamic remodeling of tumor microenvironment. Biomaterials (2020) 238:119853. doi: 10.1016/j.biomaterials.2020.119853
19. Wu X, Peng M, Huang B, Zhang H, Wang H, Huang B, et al. Immune microenvironment profiles of tumor immune equilibrium and immune escape states of mouse sarcoma. Cancer Lett (2013) 340(1):124–33. doi: 10.1016/j.canlet.2013.07.038
20. Ye LY, Chen W, Bai XL, Xu XY, Zhang Q, Xia XF, et al. Hypoxia-Induced Epithelial-to-Mesenchymal Transition in Hepatocellular Carcinoma Induces an Immunosuppressive Tumor Microenvironment to Promote Metastasis. Cancer Res (2016) 76(4):818–30. doi: 10.1158/0008-5472.CAN-15-0977
21. Ruffell B, Chang-Strachan D, Chan V, Rosenbusch A, Ho CM, Pryer N, et al. Macrophage IL-10 blocks CD8+ T cell-dependent responses to chemotherapy by suppressing IL-12 expression in intratumoral dendritic cells. Cancer Cell (2014) 26(5):623–37. doi: 10.1016/j.ccell.2014.09.006
22. Kalinski P. Regulation of immune responses by prostaglandin E2. J Immunol (2012) 188(1):21–8. doi: 10.4049/jimmunol.1101029
23. Oh SA, Li MO. TGF-β: guardian of T cell function. J Immunol (2013) 191(8):3973–9. doi: 10.4049/jimmunol.1301843
24. Maolake A, Izumi K, Shigehara K, Natsagdorj A, Iwamoto H, Kadomoto S, et al. Tumor-associated macrophages promote prostate cancer migration through activation of the CCL22-CCR4 axis. Oncotarget (2017) 8(6):9739–51. doi: 10.18632/oncotarget.14185
25. Yang Z, Xie H, He D, Li L. Infiltrating macrophages increase RCC epithelial mesenchymal transition (EMT) and stem cell-like populations via AKT and mTOR signaling. Oncotarget (2016) 7(28):44478–91. doi: 10.18632/oncotarget.9873
26. Zhou Z, Xia G, Xiang Z, Liu M, Wei Z, Yan J, et al. A C-X-C Chemokine Receptor Type 2-Dominated Cross-talk between Tumor Cells and Macrophages Drives Gastric Cancer Metastasis. Clin Cancer Res (2019) 25(11):3317–28. doi: 10.1158/1078-0432.CCR-18-3567
27. Rodriguez PC, Quiceno DG, Zabaleta J, Ortiz B, Zea AH, Piazuelo MB, et al. Arginase I production in the tumor microenvironment by mature myeloid cells inhibits T-cell receptor expression and antigen-specific T-cell responses. Cancer Res (2004) 64(16):5839–49. doi: 10.1158/0008-5472.CAN-04-0465
28. Gabrilovich DI, Ostrand-Rosenberg S, Bronte V. Coordinated regulation of myeloid cells by tumours. Nat Rev Immunol (2012) 12(4):253–68. doi: 10.1038/nri3175
29. Rodriguez PC, Zea AH, Culotta KS, Zabaleta J, Ochoa JB, Ochoa AC. Regulation of T cell receptor CD3zeta chain expression by L-arginine. J Biol Chem (2002) 277(24):21123–9. doi: 10.1074/jbc.M110675200
30. Lin Y, Gustafson MP, Bulur PA, Gastineau DA, Witzig TE, Dietz AB. Immunosuppressive CD14+HLA-DR(low)/- monocytes in B-cell non-Hodgkin lymphoma. Blood (2011) 117(3):872–81. doi: 10.1182/blood-2010-05-283820
31. Castellino F, Huang AY, Altan-Bonnet G, Stoll S, Scheinecker C, Germain RN. Chemokines enhance immunity by guiding naive CD8+ T cells to sites of CD4+ T cell-dendritic cell interaction. Nature (2006) 440(7086):890–5. doi: 10.1038/nature04651
32. Robinson BD, Sica GL, Liu YF, Rohan TE, Gertler FB, Condeelis JS, et al. Tumor microenvironment of metastasis in human breast carcinoma: a potential prognostic marker linked to hematogenous dissemination. Clin Cancer Res (2009) 15(7):2433–41. doi: 10.1158/1078-0432.CCR-08-2179
33. Olkhanud PB, Damdinsuren B, Bodogai M, Gress RE, Sen R, Wejksza K, et al. Tumor-evoked regulatory B cells promote breast cancer metastasis by converting resting CD4⁺ T cells to T-regulatory cells. Cancer Res (2011) 71(10):3505–15. doi: 10.1158/0008-5472.can-10-4316
34. Picard O, Rolland Y, Poupon MF. Fibroblast-dependent tumorigenicity of cells in nude mice: implication for implantation of metastases. Cancer Res (1986) 46(7):3290–4.
35. Camps JL, Chang SM, Hsu TC, Freeman MR, Hong SJ, Zhau HE, et al. Fibroblast-mediated acceleration of human epithelial tumor growth in vivo. Proc Natl Acad Sci U S A (1990) 87(1):75–9. doi: 10.1073/pnas.87.1.75
36. Culig Z, Hobisch A, Cronauer MV, Radmayr C, Hittmair A, Zhang J, et al. Regulation of prostatic growth and function by peptide growth factors. Prostate (1996) 28(6):392–405. doi: 10.1002/(SICI)1097-0045(199606)28:6<392::AID-PROS9>3.0.CO;2-C
37. Labernadie A, Kato T, Brugues A, Serra-Picamal X, Derzsi S, Arwert E, et al. A mechanically active heterotypic E-cadherin/N-cadherin adhesion enables fibroblasts to drive cancer cell invasion. Nat Cell Biol (2017) 19(3):224–37. doi: 10.1038/ncb3478
38. Guo S, Deng CX. Effect of Stromal Cells in Tumor Microenvironment on Metastasis Initiation. Int J Biol Sci (2018) 14(14):2083–93. doi: 10.7150/ijbs.25720
39. Kalluri R. The biology and function of fibroblasts in cancer. Nat Rev Cancer (2016) 16(9):582–98. doi: 10.1038/nrc.2016.73
40. Orimo A, Gupta PB, Sgroi DC, Arenzana-Seisdedos F, Delaunay T, Naeem R, et al. Stromal fibroblasts present in invasive human breast carcinomas promote tumor growth and angiogenesis through elevated SDF-1/CXCL12 secretion. Cell (2005) 121(3):335–48. doi: 10.1016/j.cell.2005.02.034
41. Deryugina EI, Kiosses WB. Intratumoral Cancer Cell Intravasation Can Occur Independent of Invasion into the Adjacent Stroma. Cell Rep (2017) 19(3):601–16. doi: 10.1016/j.celrep.2017.03.064
42. Liotta LA, Saidel MG, Kleinerman J. The significance of hematogenous tumor cell clumps in the metastatic process. Cancer Res (1976) 36(3):889–94.
43. Sugino T, Kawaguchi T, Suzuki T. Sequential process of blood-borne lung metastases of spontaneous mammary carcinoma in C3H mice. Int J Cancer (1993) 55(1):141–7. doi: 10.1002/ijc.2910550125
44. Ma J, Su H, Yu B, Guo T, Gong Z, Qi J, et al. CXCL12 gene silencing down-regulates metastatic potential via blockage of MAPK/PI3K/AP-1 signaling pathway in colon cancer. Clin Transl Oncol (2018) 20(8):1035–45. doi: 10.1007/s12094-017-1821-0
45. Oudin MJ, Jonas O, Kosciuk T, Broye LC, Guido BC, Wyckoff J, et al. Tumor Cell-Driven Extracellular Matrix Remodeling Drives Haptotaxis during Metastatic Progression. Cancer Discovery (2016) 6(5):516–31. doi: 10.1158/2159-8290.CD-15-1183
46. Singh R, Kapur N, Mir H, Singh N, Lillard JW Jr., Singh S. CXCR6-CXCL16 axis promotes prostate cancer by mediating cytoskeleton rearrangement via Ezrin activation and alphavbeta3 integrin clustering. Oncotarget (2016) 7(6):7343–53. doi: 10.18632/oncotarget.6944
47. Chen IX, Chauhan VP, Posada J, Ng MR, Wu MW, Adstamongkonkul P, et al. Blocking CXCR4 alleviates desmoplasia, increases T-lymphocyte infiltration, and improves immunotherapy in metastatic breast cancer. Proc Natl Acad Sci U S A (2019) 116(10):4558–66. doi: 10.1073/pnas.1815515116
48. Marcuzzi E, Angioni R, Molon B, Cali B. Chemokines and Chemokine Receptors: Orchestrating Tumor Metastasization. Int J Mol Sci (2018) 20(1):96. doi: 10.3390/ijms20010096
49. Lambert AW, Pattabiraman DR, Weinberg RA. Emerging Biological Principles of Metastasis. Cell (2017) 168(4):670–91. doi: 10.1016/j.cell.2016.11.037
50. An G, Wu F, Huang S, Feng L, Bai J, Gu S, et al. Effects of CCL5 on the biological behavior of breast cancer and the mechanisms of its interaction with tumorassociated macrophages. Oncol Rep (2019) 42(6):2499–511. doi: 10.3892/or.2019.7344
51. Lim SY, Yuzhalin AE, Gordon-Weeks AN, Muschel RJ. Targeting the CCL2-CCR2 signaling axis in cancer metastasis. Oncotarget (2016) 7(19):28697–710. doi: 10.18632/oncotarget.7376
52. Tang CH, Tsai CC. CCL2 increases MMP-9 expression and cell motility in human chondrosarcoma cells via the Ras/Raf/MEK/ERK/NF-kappaB signaling pathway. Biochem Pharmacol (2012) 83(3):335–44. doi: 10.1016/j.bcp.2011.11.013
53. Li S, Lu J, Chen Y, Xiong N, Li L, Zhang J, et al. MCP-1-induced ERK/GSK-3beta/Snail signaling facilitates the epithelial-mesenchymal transition and promotes the migration of MCF-7 human breast carcinoma cells. Cell Mol Immunol (2017) 14(7):621–30. doi: 10.1038/cmi.2015.106
54. Wang SW, Wu HH, Liu SC, Wang PC, Ou WC, Chou WY, et al. CCL5 and CCR5 interaction promotes cell motility in human osteosarcoma. PloS One (2012) 7(4):e35101. doi: 10.1371/journal.pone.0035101
55. Kato T, Fujita Y, Nakane K, Mizutani K, Terazawa R, Ehara H, et al. CCR1/CCL5 interaction promotes invasion of taxane-resistant PC3 prostate cancer cells by increasing secretion of MMPs 2/9 and by activating ERK and Rac signaling. Cytokine (2013) 64(1):251–7. doi: 10.1016/j.cyto.2013.06.313
56. Qi S, Perrino S, Miao X, Lamarche-Vane N, Brodt P. The chemokine CCL7 regulates invadopodia maturation and MMP-9 mediated collagen degradation in liver-metastatic carcinoma cells. Cancer Lett (2020) 483:S0304-3835(20)30137-3. doi: 10.1016/j.canlet.2020.03.018
57. Cheng X, Wu H. Up-regulation of chemokine receptor CCR4 is associated with Human Hepatocellular Carcinoma malignant behavior. Sci Rep (2017) 7(1):12362. doi: 10.1038/s41598-017-10267-4
58. Wang Y, Weng X, Wang L, Hao M, Li Y, Hou L, et al. HIC1 deletion promotes breast cancer progression by activating tumor cell/fibroblast crosstalk. Sci Rep (2018) 128(12):5235–50. doi: 10.1172/jci99974
59. Ou B, Zhao J, Guan S, Feng H, Wangpu X, Zhu C, et al. CCR4 promotes metastasis via ERK/NF-kappaB/MMP13 pathway and acts downstream of TNF-alpha in colorectal cancer. Oncotarget (2016) 7(30):47637–49. doi: 10.18632/oncotarget.10256
60. Qin Y, Wang J, Zhu G, Li G, Tan H, Chen C, et al. CCL18 promotes the metastasis of squamous cell carcinoma of the head and neck through MTDH-NF-kappaB signalling pathway. J Cell Mol Med (2019) 23(4):2689–701. doi: 10.1111/jcmm.14168
61. Saijo A, Goto H, Nakano M, Mitsuhashi A, Aono Y, Hanibuchi M, et al. Bone marrow-derived fibrocytes promote stem cell-like properties of lung cancer cells. Cancer Lett (2018) 421:17–27. doi: 10.1016/j.canlet.2018.02.016
62. Zhao C, Zheng S, Yan Z, Deng Z, Wang R, Zhang B. CCL18 promotes the invasion and metastasis of breast cancer through Annexin A2. Clin Transl Oncol (2020) 43(2):571–80. doi: 10.1007/s12094-019-02266-w10.3892/or.2019.7426
63. Shi L, Zhang B, Sun X, Zhang X, Lv S, Li H, et al. CC chemokine ligand 18(CCL18) promotes migration and invasion of lung cancer cells by binding to Nir1 through Nir1-ELMO1/DOC180 signaling pathway. Mol Carcinog (2016) 55(12):2051–62. doi: 10.1002/mc.22450
64. Samaniego R, Gutierrez-Gonzalez A, Gutierrez-Seijo A, Sanchez-Gregorio S, Garcia-Gimenez J, Mercader E, et al. CCL20 Expression by Tumor-Associated Macrophages Predicts Progression of Human Primary Cutaneous Melanoma. Cancer Immunol Res (2018) 6(3):267–75. doi: 10.1158/2326-6066.CIR-17-0198
65. Muscella A, Vetrugno C, Marsigliante S. CCL20 promotes migration and invasiveness of human cancerous breast epithelial cells in primary culture. Mol Carcinog (2017) 56(11):2461–73. doi: 10.1002/mc.22693
66. Lu Y, Dong B, Xu F, Xu Y, Pan J, Song J, et al. CXCL1-LCN2 paracrine axis promotes progression of prostate cancer via the Src activation and epithelial-mesenchymal transition. Cells (2019) 17(1):118. doi: 10.1186/s12964-019-0434-3
67. Wang Z, Wang Z, Li G, Wu H, Sun K, Chen J, et al. CXCL1 from tumor-associated lymphatic endothelial cells drives gastric cancer cell into lymphatic system via activating integrin beta1/FAK/AKT signaling. Cancer Lett (2017) 385:28–38. doi: 10.1016/j.canlet.2016.10.043
68. Zhao J, Ou B, Han D, Wang P, Zong Y, Zhu C, et al. Tumor-derived CXCL5 promotes human colorectal cancer metastasis through activation of the ERK/Elk-1/Snail and AKT/GSK3beta/beta-catenin pathways. Cancer Med (2017) 16(1):70. doi: 10.1186/s12943-017-0629-4
69. Wang C, Li A, Yang S, Qiao R, Zhu X, Zhang J. CXCL5 promotes mitomycin C resistance in non-muscle invasive bladder cancer by activating EMT and NF-kappaB pathway. Biochem Biophys Res Commun (2018) 498(4):862–8. doi: 10.1016/j.bbrc.2018.03.071
70. Qiu WZ, Zhang HB, Xia WX, Ke LR, Yang J, Yu YH, et al. The CXCL5/CXCR2 axis contributes to the epithelial-mesenchymal transition of nasopharyngeal carcinoma cells by activating ERK/GSK-3beta/snail signalling. J Exp Clin Cancer Res: CR (2018) 37(1):85. doi: 10.1186/s13046-018-0722-6
71. Cui D, Zhao Y, Xu J. Activated CXCL5-CXCR2 axis promotes the migration, invasion and EMT of papillary thyroid carcinoma cells via modulation of beta-catenin pathway. Biochimie (2018) 148:1–11. doi: 10.1016/j.biochi.2018.02.009
72. Wang T, Notta F, Navab R, Joseph J, Ibrahimov E, Xu J, et al. Senescent Carcinoma-Associated Fibroblasts Upregulate IL8 to Enhance Prometastatic Phenotypes. Mol Cancer Res (2017) 15(1):3–14. doi: 10.1158/1541-7786.MCR-16-0192
73. Bouris P, Manou D, Sopaki-Valalaki A, Kolokotroni A, Moustakas A, Kapoor A, et al. Serglycin promotes breast cancer cell aggressiveness: Induction of epithelial to mesenchymal transition, proteolytic activity and IL-8 signaling. Dis Markers (2018) 74:35–51. doi: 10.1016/j.matbio.2018.05.011
74. Ma F, Chen D, Chen F, Chi Y, Han Z, Feng X, et al. Human Umbilical Cord Mesenchymal Stem Cells Promote Breast Cancer Metastasis by Interleukin-8- and Interleukin-6-Dependent Induction of CD44(+)/CD24(-) Cells. Cell Transplant (2015) 24(12):2585–99. doi: 10.3727/096368915X687462
75. Waugh DJ, Wilson C. The interleukin-8 pathway in cancer. Clin Cancer Res (2008) 14(21):6735–41. doi: 10.1158/1078-0432.CCR-07-4843
76. Soon PS, Kim E, Pon CK, Gill AJ, Moore K, Spillane AJ, et al. Breast cancer-associated fibroblasts induce epithelial-to-mesenchymal transition in breast cancer cells. Endocr Relat Cancer (2013) 20(1):1–12. doi: 10.1530/ERC-12-0227
77. Ma J, Sun X, Wang Y, Chen B, Qian L, Wang Y. Fibroblast-derived CXCL12 regulates PTEN expression and is associated with the proliferation and invasion of colon cancer cells via PI3k/Akt signaling. Cell Commun Signal: CCS (2019) 17(1):119. doi: 10.1186/s12964-019-0432-5
78. Samara GJ, Lawrence DM, Chiarelli CJ, Valentino MD, Lyubsky S, Zucker S, et al. CXCR4-mediated adhesion and MMP-9 secretion in head and neck squamous cell carcinoma. Cancer Lett (2004) 214(2):231–41. doi: 10.1016/j.canlet.2004.04.035
79. Chen L, Zhu M, Yu S, Hai L, Zhang L, Zhang C, et al. Arg kinase mediates CXCL12/CXCR4-induced invadopodia formation and invasion of glioma cells. Exp Cell Res (2020) 389(1):111893. doi: 10.1016/j.yexcr.2020.111893
80. Sjoberg E, Meyrath M, Milde L, Herrera M, Lovrot J. A Novel ACKR2-Dependent Role of Fibroblast-Derived CXCL14 in Epithelial-to-Mesenchymal Transition and Metastasis of Breast Cancer. Oncogene (2019) 25(12):3702–17. doi: 10.1158/1078-0432.ccr-18-1294
81. Mortezaee K. CXCL12/CXCR4 axis in the microenvironment of solid tumors: A critical mediator of metastasis. Life Sci (2020) 249:117534. doi: 10.1016/j.lfs.2020.117534
82. Roy I, Zimmerman NP, Mackinnon AC, Tsai S, Evans DB, Dwinell MB. CXCL12 chemokine expression suppresses human pancreatic cancer growth and metastasis. PloS One (2014) 9(3):e90400. doi: 10.1371/journal.pone.0090400
83. Zhou Y, Shurin GV, Zhong H, Bunimovich YL, Han B, Shurin MR. Schwann Cells Augment Cell Spreading and Metastasis of Lung Cancer. Cancer Res (2018) 78(20):5927–39. doi: 10.1158/0008-5472.can-18-1702
84. Li X, Bu W, Meng L, Liu X, Wang S, Jiang L, et al. CXCL12/CXCR4 pathway orchestrates CSC-like properties by CAF recruited tumor associated macrophage in OSCC. Exp Cell Res (2019) 378(2):131–8. doi: 10.1016/j.yexcr.2019.03.013
85. Lv ZD, Kong B, Liu XP, Dong Q, Niu HT, Wang YH, et al. CXCL12 chemokine expression suppresses human breast cancer growth and metastasis in vitro and in vivo. Int J Clin Exp Pathol (2014) 7(10):6671–8.
86. Ikeda T, Nishita M, Hoshi K, Honda T, Kakeji Y, Minami Y. Mesenchymal stem cell-derived CXCL16 promotes progression of gastric cancer cells by STAT3-mediated expression of Ror1. Cancer Sci (2020) 111(4):1254–65. doi: 10.1111/cas.14339
87. Chung B, Esmaeili AA, Gopalakrishna-Pillai S, Murad JP, Andersen ES, Kumar Reddy N, et al. Human brain metastatic stroma attracts breast cancer cells via chemokines CXCL16 and CXCL12. NPJ Breast Cancer (2017) 3:6. doi: 10.1038/s41523-017-0008-8
88. Xiao G, Wang X, Wang J, Zu L, Cheng G, Hao M, et al. CXCL16/CXCR6 chemokine signaling mediates breast cancer progression by pERK1/2-dependent mechanisms. Oncotarget (2015) 6(16):14165–78. doi: 10.18632/oncotarget.3690
89. Birchmeier W, Birchmeier C. Epithelial-mesenchymal transitions in development and tumor progression. EXS (1995) 74:1–15. doi: 10.1007/978-3-0348-9070-0_1
90. Zhou B, Chen WL, Wang YY, Lin ZY, Zhang DM, Fan S, et al. A role for cancer-associated fibroblasts in inducing the epithelial-to-mesenchymal transition in human tongue squamous cell carcinoma. J Oral Pathol Med (2014) 43(8):585–92. doi: 10.1111/jop.12172
91. Shan S, Lv Q, Zhao Y, Liu C, Sun Y, Xi K, et al. Wnt/beta-catenin pathway is required for epithelial to mesenchymal transition in CXCL12 over expressed breast cancer cells. Int J Clin Exp Pathol (2015) 8(10):12357–67.
92. Yang F, Takagaki Y, Yoshitomi Y. Inhibition of Dipeptidyl Peptidase-4 Accelerates Epithelial-Mesenchymal Transition and Breast Cancer Metastasis via the CXCL12/CXCR4/mTOR Axis. J Immunother Cancer (2019) 79(4):735–46. doi: 10.1158/0008-5472.can-18-0620
93. Lin Y, Ma Q, Li L, Wang H. The CXCL12-CXCR4 axis promotes migration, invasiveness, and EMT in human papillary thyroid carcinoma B-CPAP cells via NF-kappaB signaling. Biochem Cell Biol = Biochim Biol Cell (2018) 96(5):619–26. doi: 10.1139/bcb-2017-0074
94. Zhang W, Gu J, Chen J, Zhang P, Ji R, Qian H, et al. Interaction with neutrophils promotes gastric cancer cell migration and invasion by inducing epithelial-mesenchymal transition. Oncol Rep (2017) 38(5):2959–66. doi: 10.3892/or.2017.5942
95. Jing X, Peng J, Dou Y, Sun J, Ma C, Wang Q, et al. Macrophage ERalpha promoted invasion of endometrial cancer cell by mTOR/KIF5B-mediated epithelial to mesenchymal transition. Immunol Cell Biol (2019) 97(6):563–76. doi: 10.1111/imcb.12245
96. Lu E, Su J, Zhou Y, Zhang C, Wang Y. CCL20/CCR6 promotes cell proliferation and metastasis in laryngeal cancer by activating p38 pathway. Biomed Pharmacother = Biomed Pharmacother (2017) 85:486–92. doi: 10.1016/j.biopha.2016.11.055
97. Han G, Wu D, Yang Y, Li Z, Zhang J, Li C. CrkL meditates CCL20/CCR6-induced EMT in gastric cancer. Cytokine (2015) 76(2):163–9. doi: 10.1016/j.cyto.2015.05.009
98. Celia-Terrassa T, Jolly MK. Cancer Stem Cells and Epithelial-to-Mesenchymal Transition in Cancer Metastasis. Cold Spring Harb Perspect Med (2019) 10(7):1–18. doi: 10.1101/cshperspect.a036905
99. Do HTT, Lee CH, Cho J. Chemokines and their Receptors: Multifaceted Roles in Cancer Progression and Potential Value as Cancer Prognostic Markers. Cancers (2020) 12(2):E287. doi: 10.3390/cancers12020287
100. Pignatelli J, Bravo-Cordero JJ, Roh-Johnson M, Gandhi SJ, Wang Y, Chen X, et al. Macrophage-dependent tumor cell transendothelial migration is mediated by Notch1/Mena(INV)-initiated invadopodium formation. Sci Rep (2016) 6:37874. doi: 10.1038/srep37874
101. Gertler F, Condeelis J. Metastasis: tumor cells becoming MENAcing. Trends Cell Biol (2011) 21(2):81–90. doi: 10.1016/j.tcb.2010.10.001
102. Karagiannis GS, Condeelis JS, Oktay MH. Chemotherapy-induced metastasis: mechanisms and translational opportunities. Clin Exp Metastasis (2018) 35(4):269–84. doi: 10.1007/s10585-017-9870-x
103. Philippar U, Roussos ET, Oser M, Yamaguchi H, Kim HD, Giampieri S, et al. A Mena invasion isoform potentiates EGF-induced carcinoma cell invasion and metastasis. Dev Cell (2008) 15(6):813–28. doi: 10.1016/j.devcel.2008.09.003
104. Roussos ET, Goswami S, Balsamo M, Wang Y, Stobezki R, Adler E, et al. Mena invasive (Mena(INV)) and Mena11a isoforms play distinct roles in breast cancer cell cohesion and association with TMEM. Clin Exp Metastasis (2011) 28(6):515–27. doi: 10.1007/s10585-011-9388-6
105. Hasebe T, Konishi M, Iwasaki M, Endoh Y, Nakagohri T, Takahashi S, et al. Histological characteristics of tumor cells and stromal cells in vessels and lymph nodes are important prognostic parameters of extrahepatic bile duct carcinoma: a prospective study. Hum Pathol (2005) 36(6):655–64. doi: 10.1016/j.humpath.2005.04.004
106. Matsumura Y, Ito Y, Mezawa Y, Sulidan K, Daigo Y, Hiraga T, et al. Stromal fibroblasts induce metastatic tumor cell clusters via epithelial-mesenchymal plasticity. Life Sci Alliance (2019) 2(4):1–24. doi: 10.26508/lsa.201900425
107. Duda DG, Duyverman AM, Kohno M, Snuderl M, Steller EJ, Fukumura D, et al. Malignant cells facilitate lung metastasis by bringing their own soil. Proc Natl Acad Sci U S A (2010) 107(50):21677–82. doi: 10.1073/pnas.1016234107
108. Muller A, Homey B, Soto H, Ge N, Catron D, Buchanan ME, et al. Involvement of chemokine receptors in breast cancer metastasis. Nature (2001) 410(6824):50–6. doi: 10.1038/35065016
109. Ahirwar DK, Nasser MW, Ouseph MM, Elbaz M, Cuitino MC, Kladney RD, et al. Fibroblast-derived CXCL12 promotes breast cancer metastasis by facilitating tumor cell intravasation. Oncogene (2018) 37(32):4428–42. doi: 10.1038/s41388-018-0263-7
110. Liubomirski Y, Lerrer S, Meshel T, Rubinstein-Achiasaf L, Morein D, Wiemann S, et al. Tumor-Stroma-Inflammation Networks Promote Pro-metastatic Chemokines and Aggressiveness Characteristics in Triple-Negative Breast Cancer. Front Immunol (2019) 10:757. doi: 10.3389/fimmu.2019.00757
111. Scapini P, Lapinet-Vera JA, Gasperini S, Calzetti F, Bazzoni F, Cassatella MA. The neutrophil as a cellular source of chemokines. Immunol Rev (2000) 177:195–203. doi: 10.1034/j.1600-065x.2000.17706.x
112. Karnoub AE, Dash AB, Vo AP, Sullivan A, Brooks MW, Bell GW, et al. Mesenchymal stem cells within tumour stroma promote breast cancer metastasis. Nature (7162) 2007) 449:557–63. doi: 10.1038/nature06188
113. Wyckoff JB, Wang Y, Lin EY, Li JF, Goswami S, Stanley ER, et al. Direct visualization of macrophage-assisted tumor cell intravasation in mammary tumors. Cancer Res (2007) 67(6):2649–56. doi: 10.1158/0008-5472.CAN-06-1823
114. Harney AS, Arwert EN, Entenberg D, Wang Y, Guo P, Qian BZ, et al. Real-Time Imaging Reveals Local, Transient Vascular Permeability, and Tumor Cell Intravasation Stimulated by TIE2hi Macrophage-Derived VEGFA. Cancer Discovery (2015) 5(9):932–43. doi: 10.1158/2159-8290.CD-15-0012
115. Monsky WL, Fukumura D, Gohongi T, Ancukiewcz M, Weich HA, Torchilin VP, et al. Augmentation of transvascular transport of macromolecules and nanoparticles in tumors using vascular endothelial growth factor. Cancer Res (1999) 59(16):4129–35.
116. Kabala PA, Malvar-Fernandez B, Lopes AP, Carvalheiro T, Hartgring SAY, Tang MW, et al. Promotion of macrophage activation by Tie2 in the context of the inflamed synovia of rheumatoid arthritis and psoriatic arthritis patients. Rheumatol (Oxf) (2020) 59(2):426–38. doi: 10.1093/rheumatology/kez315
117. Arwert EN, Harney AS, Entenberg D, Wang Y, Sahai E, Pollard JW, et al. A Unidirectional Transition from Migratory to Perivascular Macrophage Is Required for Tumor Cell Intravasation. Cell Rep (2018) 23(5):1239–48. doi: 10.1016/j.celrep.2018.04.007
118. Wyckoff J, Wang W, Lin EY, Wang Y, Pixley F, Stanley ER, et al. A paracrine loop between tumor cells and macrophages is required fortumor cell migration in mammary tumors. Cancer Res(2004) 64(19):7022–9. doi: 10.1158/0008-5472.CAN-04-1449
119. Coniglio SJ, Eugenin E, Dobrenis K, Stanley ER, West BL, Symons MH, et al. Microglial stimulation of glioblastoma invasion involves epidermal growth factor receptor (EGFR) and colony stimulating factor 1 receptor (CSF-1R) signaling. Molecular Medicine (Cambridge, Mass)(2012) 18(1):519–27. doi: 10.2119/molmed.2011.00217
120. Goswami S, Sahai E, Wyckoff JB, Cammer M, Cox D, Pixley FJ, et al. Autocrine CSF-1 and CSF-1 receptor coexpression promotes renal cell carcinoma growth. Cancer Res (2012) 72(1):187–200. doi: 10.1158/0008-5472.can-11-1232
121. Hernandez L, Smirnova T, Kedrin D, Wyckoff J, Zhu L, Stanley ER, et al. The EGF/CSF-1 paracrine invasion loop can be triggered by heregulinbeta1 and CXCL12. Cancer Res (2009)69(7):3221–7. doi: 10.1158/0008-5472.can-08-2871
122. Menke J, Kriegsmann J, Schimanski CC, Schwartz MM, Schwarting A, Kelley VR. Autocrine CSF-1 and CSF-1 receptor coexpression promotes renal cell carcinoma growth. Cancer Res (2012) 72(1):187–200. doi: 10.1158/0008-5472.can-11-1232
123. Butler TP, Gullino PM. Quantitation of cell shedding into efferent blood of mammary adenocarcinoma. Cancer Res (1975) 35(3):512–6.
124. Fidler IJ. Metastasis: quantitative analysis of distribution and fate of tumor emboli labeled with 125 I-5-iodo-2’-deoxyuridine. J Natl Cancer Inst (1970) 45(4):773–82.
125. Hanna RN, Cekic C, Sag D, Tacke R, Thomas GD, Nowyhed H, et al. Patrolling monocytes control tumor metastasis to the lung. Science (6263) 2015) 350:985–90. doi: 10.1126/science.aac9407
126. Melgarejo E, Medina MA, Sánchez-Jiménez F, Urdiales JL. Monocyte chemoattractant protein-1: a key mediator in inflammatory processes. Int J Biochem Cell Biol (2009) 41(5):998–1001. doi: 10.1016/j.biocel.2008.07.018
127. Nieswandt B, Hafner M, Echtenacher B, Mannel DN. Lysis of tumor cells by natural killer cells in mice is impeded by platelets. Cancer Res (1999) 59(6):1295–300.
128. Palumbo JS, Talmage KE, Massari JV, La Jeunesse CM, Flick MJ, Kombrinck KW, et al. Platelets and fibrin(ogen) increase metastatic potential by impeding natural killer cell-mediated elimination of tumor cells. Blood (2005) 105(1):178–85. doi: 10.1182/blood-2004-06-2272
129. Gassmann P, Haier J, Schluter K, Domikowsky B, Wendel C, Wiesner U, et al. CXCR4 regulates the early extravasation of metastatic tumor cells in vivo. Neoplasia (2009) 11(7):651–61. doi: 10.1593/neo.09272
130. Jin DK, Shido K, Kopp HG, Petit I, Shmelkov SV, Young LM, et al. Cytokine-mediated deployment of SDF-1 induces revascularization through recruitment of CXCR4+ hemangiocytes. Nat Med (2006) 12(5):557–67. doi: 10.1038/nm1400
131. Boucharaba A, Serre CM, Gres S, Saulnier-Blache JS, Bordet JC, Guglielmi J, et al. Platelet-derived lysophosphatidic acid supports the progression of osteolytic bone metastases in breast cancer. J Clin Invest (2004) 114(12):1714–25. doi: 10.1172/JCI22123
132. Kaplan RN, Riba RD, Zacharoulis S, Bramley AH, Vincent L, Costa C, et al. VEGFR1-positive haematopoietic bone marrow progenitors initiate the pre-metastatic niche. Nature (2005) 438(7069):820–7. doi: 10.1038/nature04186
133. Psaila B, Lyden D. The metastatic niche: adapting the foreign soil. Nat Rev Cancer (2009) 9:285–93. doi: 10.1038/nrc2621
134. Sleeman JP. The lymph node pre-metastatic niche. J Mol Med (Berl) (2015) 93(11):1173–84. doi: 10.1007/s00109-015-1351-6
135. Jian J, Pang Y, Yan HH, Min Y, Achyut BR, Hollander MC, et al. Platelet factor 4 is produced by subsets of myeloid cells in premetastatic lung and inhibits tumor metastasis. Oncotarget (2017) 8(17):27725–39. doi: 10.18632/oncotarget.9486
136. Peinado H, Zhang H, Matei IR, Costa-Silva B, Hoshino A, Rodrigues G, et al. Pre-metastatic niches: organ-specific homes for metastases. Nat Rev Cancer (2017) 17(5):302–17. doi: 10.1038/nrc.2017.6
137. Burger M, Glodek A, Hartmann T, Schmitt-Gräff A, Silberstein LE, Fujii N, et al. Functional expression of CXCR4 (CD184) on small-cell lung cancer cells mediates migration, integrin activation, and adhesion to stromal cells. Oncogene (2003) 22(50):8093–101. doi: 10.1038/sj.onc.1207097
138. Zhou Y, Larsen PH, Hao C, Yong VW. CXCR4 is a major chemokine receptor on glioma cells and mediates their survival. J Biol Chem (2002) 277(51):49481–7. doi: 10.1074/jbc.M206222200
139. Zeelenberg IS, Ruuls-Van Stalle L, Roos E. The chemokine receptor CXCR4 is required for outgrowth of colon carcinoma micrometastases. Cancer Res (2003) 63(13):3833–9.
140. Wang J, Wang J, Sun Y, Song W, Nor JE, Wang CY, et al. Diverse signaling pathways through the SDF-1/CXCR4 chemokine axis in prostate cancer cell lines leads to altered patterns of cytokine secretion and angiogenesis. Cell Signal (2005) 17(12):1578–92. doi: 10.1016/j.cellsig.2005.03.022
141. Scala S, Ottaiano A, Ascierto PA, Cavalli M, Simeone E, Giuliano P, et al. Expression of CXCR4 predicts poor prognosis in patients with malignant melanoma. Clin Cancer Res (2005) 11(5):1835–41. doi: 10.1158/1078-0432.ccr-04-1887
142. Scotton CJ, Wilson JL, Scott K, Stamp G, Wilbanks GD, Fricker S, et al. Multiple actions of the chemokine CXCL12 on epithelial tumor cells in human ovarian cancer. Cancer Res (2002) 62(20):5930–8.
143. Hartmann TN, Burger JA, Glodek A, Fujii N, Burger M. CXCR4 chemokine receptor and integrin signaling co-operate in mediating adhesion and chemoresistance in small cell lung cancer (SCLC) cells. Oncogene (2005) 24(27):4462–71. doi: 10.1038/sj.onc.1208621
144. Kijima T, Maulik G, Ma PC, Tibaldi EV, Turner RE, Rollins B, et al. Regulation of cellular proliferation, cytoskeletal function, and signal transduction through CXCR4 and c-Kit in small cell lung cancer cells. Cancer Res (2002) 62(21):6304–11.
145. Benito-Martin A, Di Giannatale A, Ceder S, Peinado H. The new deal: a potential role for secreted vesicles in innate immunity and tumor progression. Front Immunol (2015) 6:66. doi: 10.3389/fimmu.2015.00066
146. Granot Z, Henke E, Comen EA, King TA, Norton L, Benezra R. Tumor entrained neutrophils inhibit seeding in the premetastatic lung. Cancer Cell (2011) 20(3):300–14. doi: 10.1016/j.ccr.2011.08.012
147. Costa-Silva B, Aiello NM, Ocean AJ, Singh S, Zhang H, Thakur BK, et al. Pancreatic cancer exosomes initiate pre-metastatic niche formation in the liver. Nat Cell Biol (2015) 17(6):816–26. doi: 10.1038/ncb3169
148. Seubert B, Grünwald B, Kobuch J, Cui H, Schelter F, Schaten S, et al. Tissue inhibitor of metalloproteinases (TIMP)-1 creates a premetastatic niche in the liver through SDF-1/CXCR4-dependent neutrophil recruitment in mice. Hepatology (2015) 61(1):238–48. doi: 10.1002/hep.27378
149. Wiley HE, Gonzalez EB, Maki W, Wu MT, Hwang ST. Expression of CC chemokine receptor-7 and regional lymph node metastasis of B16 murine melanoma. J Natl Cancer Inst (2001) 93(21):1638–43. doi: 10.1093/jnci/93.21.1638
150. Buonamici S, Trimarchi T, Ruocco MG, Reavie L, Cathelin S, Mar BG, et al. CCR7 signaling as an essential regulator of CNS infilator in T-cell leukaemia. Nature (7249) 2009) 459:1000–4. doi: 10.1038/nature08020
151. Kuderer NM, Ortel TL, Francis CW. Impact of venous thromboembolism and anticoagulation on cancer and cancer survival. J Clin Oncol (2009) 27(29):4902–11. doi: 10.1200/jco.2009.22.4584
152. Im JH, Fu W, Wang H, Bhatia SK, Hammer DA, Kowalska MA, et al. Coagulation facilitates tumor cell spreading in the pulmonary vasculature during early metastatic colony formation. Cancer Res (2004) 64(23):8613–9. doi: 10.1158/0008-5472.can-04-2078
153. Labelle M, Begum S, Hynes RO. Platelets guide the formation of early metastatic niches. Proc Natl Acad Sci U S A (2014) 111(30):E3053–61. doi: 10.1073/pnas.1411082111
154. Labelle M, Hynes RO. The initial hours of metastasis: the importance of cooperative host-tumor cell interactions during hematogenous dissemination. Cancer Discovery (2012) 2(12):1091–9. doi: 10.1158/2159-8290.cd-12-0329
155. Gil-Bernabé AM, Ferjancic S, Tlalka M, Zhao L, Allen PD, Im JH, et al. Recruitment of monocytes/macrophages by tissue factor-mediated coagulation is essential for metastatic cell survival and premetastatic niche establishment in mice. Blood (2012) 119(13):3164–75. doi: 10.1182/blood-2011-08-376426
156. Cools-Lartigue J, Spicer J, Najmeh S, Ferri L. Neutrophil extracellular traps in cancer progression. Cell Mol Life Sci (2014) 71(21):4179–94. doi: 10.1007/s00018-014-1683-3
157. Merza M, Hartman H, Rahman M, Hwaiz R, Zhang E, Renström E, et al. Neutrophil Extracellular Traps Induce Trypsin Activation, Inflammation, and Tissue Damage in Mice With Severe Acute Pancreatitis. Gastroenterology (2015) 149(7):1920–31.e8. doi: 10.1053/j.gastro.2015.08.026
158. Park J, Wysocki RW, Amoozgar Z, Maiorino L, Fein MR, Jorns J, et al. Cancer cells induce metastasis-supporting neutrophil extracellular DNA traps. Sci Trans Med (2016) 8(361):361ra138. doi: 10.1126/scitranslmed.aag1711
159. Tohme S, Yazdani HO, Al-Khafaji AB, Chidi AP, Loughran P, Mowen K, et al. Neutrophil Extracellular Traps Promote the Development and Progression of Liver Metastases after Surgical Stress. Cancer Res (2016) 76(6):1367–80. doi: 10.1158/0008-5472.can-15-1591
160. Yang C, Sun W, Cui W, Li X, Yao J, Jia X, et al. Procoagulant role of neutrophil extracellular traps in patients with gastric cancer. Int J Clin Exp Pathol (2015) 8(11):14075–86.
161. Yang L, Liu Q, Zhang X, Liu X, Zhou B, Chen J, et al. DNA of neutrophil extracellular traps promotes cancer metastasis via CCDC25. Nature (7814) 2020) 583:133–8. doi: 10.1038/s41586-020-2394-6
162. Spiegel A, Brooks MW, Houshyar S, Reinhardt F, Ardolino M, Fessler E, et al. Neutrophils Suppress Intraluminal NK Cell-Mediated Tumor Cell Clearance and Enhance Extravasation of Disseminated Carcinoma Cells. Cancer Discovery (2016) 6(6):630–49. doi: 10.1158/2159-8290.CD-15-1157
163. Häuselmann I, Roblek M, Protsyuk D, Huck V, Knopfova L, Grässle S, et al. Monocyte Induction of E-Selectin–Mediated Endothelial Activation Releases VE-Cadherin Junctions to Promote Tumor Cell Extravasation in the Metastasis Cascade. Cancer Res (2016) 76(18):5302–12. doi: 10.1158/0008-5472.can-16-0784
164. Wolf MJ, Hoos A, Bauer J, Boettcher S, Knust M, Weber A, et al. Endothelial CCR2 signaling induced by colon carcinoma cells enables extravasation via the JAK2-Stat5 and p38MAPK pathway. Cancer Cell (2012) 22(1):91–105. doi: 10.1016/j.ccr.2012.05.023
165. Qian BZ, Li J, Zhang H, Kitamura T, Zhang J, Campion LR, et al. CCL2 recruits inflammatory monocytes to facilitate breast-tumour metastasis. Nature (7355) 2011) 475:222–5. doi: 10.1038/nature10138
166. Kitamura T, Qian BZ, Soong D, Cassetta L, Noy R, Sugano G, et al. CCL2-induced chemokine cascade promotes breast cancer metastasis by enhancing retention of metastasis-associated macrophages. J Exp Med (2015) 212(7):1043–59. doi: 10.1084/jem.20141836
167. Eisenkraft A, Keidan I, Bielorai B, Keller N, Toren A, Paret G. MCP-1 in the cerebrospinal fluid of children with acute lymphoblastic leukemia. Leuk Res (2006) 30(10):1259–61. doi: 10.1016/j.leukres.2006.01.017
168. Chin AR, Wang SE. Cancer Tills the Premetastatic Field: Mechanistic Basis and Clinical Implications. Clin Cancer Res (2016) 22(15):3725–33. doi: 10.1158/1078-0432.ccr-16-0028
169. Lesokhin AM, Hohl TM, Kitano S, Cortez C, Hirschhorn-Cymerman D, Avogadri F, et al. Monocytic CCR2(+) myeloid-derived suppressor cells promote immune escape by limiting activated CD8 T-cell infiltration into the tumor microenvironment. Cancer Res (2012) 72(4):876–86. doi: 10.1158/0008-5472.can-11-1792
170. Liu Y, Cao X. Immunosuppressive cells in tumor immune escape and metastasis. J Mol Med (Berl) (2016) 94(5):509–22. doi: 10.1007/s00109-015-1376-x
171. McAllister SS, Weinberg RA. The tumour-induced systemic environment as a critical regulator of cancer progression and metastasis. Nat Cell Biol (2014) 16(8):717–27. doi: 10.1038/ncb3015
172. Lu X, Kang Y. Organotropism of breast cancer metastasis. J Mammary Gland Biol Neoplasia (2007) 12(2-3):153–62. doi: 10.1007/s10911-007-9047-3
173. Tacke RS, Lee HC, Goh C, Courtney J, Polyak SJ, Rosen HR, et al. Myeloid suppressor cells induced by hepatitis C virus suppress T-cell responses through the production of reactive oxygen species. Hepatology (2012) 55(2):343–53. doi: 10.1002/hep.24700
174. Chow MT, Luster AD. Chemokines in cancer. Cancer Immunol Res (2014) 2(12):1125–31. doi: 10.1158/2326-6066.cir-14-0160
175. Mollica Poeta V, Massara M, Capucetti A, Bonecchi R. Chemokines and Chemokine Receptors: New Targets for Cancer Immunotherapy. Front Immunol (2019) 10:379. doi: 10.3389/fimmu.2019.00379
176. Pernas S, Martin M, Kaufman PA, Gil-Martin M, Gomez Pardo P, Lopez-Tarruella S, et al. Balixafortide plus eribulin in HER2-negative metastatic breast cancer: a phase 1, single-arm, dose-escalation trial. Lancet Oncol (2018) 19(6):812–24. doi: 10.1016/s1470-2045(18)30147-5
177. Thomas RP, Nagpal S, Iv M. Macrophage Exclusion after Radiation Therapy (MERT): A First in Human Phase I/II Trial using a CXCR4 Inhibitor in Glioblastoma. Clin Cancer Res (2019) 25: (23):6948–57. doi: 10.1158/1078-0432.ccr-19-1421
178. Sledge GW. Targeting CXCR4-induced desmoplasia to improve checkpoint inhibition in breast cancer. Proc Natl Acad Sci U S A (2019) 116(11):4769–71. doi: 10.1073/pnas.1900368116
179. Wang J, Hu W, Wang K, Yu J, Luo B, Luo G, et al. Repertaxin, an inhibitor of the chemokine receptors CXCR1 and CXCR2, inhibits malignant behavior of human gastric cancer MKN45 cells in vitro and in vivo and enhances efficacy of 5-fluorouracil. Int J Oncol (2016) 48(4):1341–52. doi: 10.3892/ijo.2016.3371
180. Chao T, Furth EE, Vonderheide RH. CXCR2-Dependent Accumulation of Tumor-Associated Neutrophils Regulates T-cell Immunity in Pancreatic Ductal Adenocarcinoma. Cancer Immunol Res (2016) 4(11):968–82. doi: 10.1158/2326-6066.cir-16-0188
181. Steele CW, Karim SA, Leach JDG, Bailey P, Upstill-Goddard R, Rishi L, et al. CXCR2 Inhibition Profoundly Suppresses Metastases and Augments Immunotherapy in Pancreatic Ductal Adenocarcinoma. Cancer Cell (2016) 29(6):832–45. doi: 10.1016/j.ccell.2016.04.014
182. Nywening TM, Belt BA, Cullinan DR, Panni RZ, Han BJ, Sanford DE, et al. Targeting both tumour-associated CXCR2(+) neutrophils and CCR2(+) macrophages disrupts myeloid recruitment and improves chemotherapeutic responses in pancreatic ductal adenocarcinoma. Gut (2018) 67(6):1112–23. doi: 10.1136/gutjnl-2017-313738
183. Schott AF, Goldstein LJ, Cristofanilli M, Ruffini PA, McCanna S, Reuben JM, et al. Phase Ib Pilot Study to Evaluate Reparixin in Combination with Weekly Paclitaxel in Patients with HER-2-Negative Metastatic Breast Cancer. Clin Cancer Res (2017) 23(18):5358–65. doi: 10.1158/1078-0432.ccr-16-2748
184. Berlato C, Khan MN, Schioppa T, Thompson R, Maniati E, Montfort A, et al. A CCR4 antagonist reverses the tumor-promoting microenvironment of renal cancer. J Clin Invest (2017) 127(3):801–13. doi: 10.1172/jci82976
185. Bonapace L, Coissieux MM, Wyckoff J, Mertz KD, Varga Z, Junt T, et al. Cessation of CCL2 inhibition accelerates breast cancer metastasis by promoting angiogenesis. Nature (7525) 2014) 515:130–3. doi: 10.1038/nature13862
186. Jung H, Ertl L, Janson C, Schall T, Charo I. Abstract A107: Inhibition of CCR2 potentiates the checkpoint inhibitor immunotherapy in pancreatic cancer. New York: AACR (2016).
187. Li X, Yao W, Yuan Y, Chen P, Li B, Li J, et al. Targeting of tumour-infiltrating macrophages via CCL2/CCR2 signalling as a therapeutic strategy against hepatocellular carcinoma. Gut (2017) 66(1):157–67. doi: 10.1136/gutjnl-2015-310514
188. Yao W, Ba Q, Li X, Li H, Zhang S, Yuan Y, et al. A natural CCR2 antagonist relieves tumor-associated macrophage-mediated immunosuppression to produce a therapeutic effect for liver cancer. EBioMedicine (2017) 22:58–67.
189. Linehan D, Noel MS, Hezel AF, Wang-Gillam A, Eskens F, Sleijfer S, et al. Overall survival in a trial of orally administered CCR2 inhibitor CCX872 in locally advanced/metastatic pancreatic cancer: Correlation with blood monocyte counts. J Clin Oncol (2018) 36(5_suppl):92. doi: 10.1200/JCO.2018.36.5_suppl.92
190. Noel M, O’Reilly EM, Wolpin BM, Ryan DP, Bullock AJ, Britten CD, et al. Phase 1b study of a small molecule antagonist of human chemokine (C-C motif) receptor 2 (PF-04136309) in combination with nab-paclitaxel/gemcitabine in first-line treatment of metastatic pancreatic ductal adenocarcinoma. Invest New Drugs (2020) 38(3):800–11. doi: 10.1007/s10637-019-00830-3
191. Nywening TM, Wang-Gillam A, Sanford DE, Belt BA, Panni RZ, Cusworth BM, et al. Targeting tumour-associated macrophages with CCR2 inhibition in combination with FOLFIRINOX in patients with borderline resectable and locally advanced pancreatic cancer: a single-centre, open-label, dose-finding, non-randomised, phase 1b trial. Lancet Oncol (2016) 17(5):651–62. doi: 10.1016/s1470-2045(16)00078-4
192. Pienta KJ, Machiels JP, Schrijvers D, Alekseev B, Shkolnik M, Crabb SJ, et al. Phase 2 study of carlumab (CNTO 888), a human monoclonal antibody against CC-chemokine ligand 2 (CCL2), in metastatic castration-resistant prostate cancer. Invest New Drugs (2013) 31(3):760–8. doi: 10.1007/s10637-012-9869-8
Keywords: chemokines, cancer, metastases, stroma, invasion, metastatic niche
Citation: Hussain S, Peng B, Cherian M, Song JW, Ahirwar DK and Ganju RK (2020) The Roles of Stroma-Derived Chemokine in Different Stages of Cancer Metastases. Front. Immunol. 11:598532. doi: 10.3389/fimmu.2020.598532
Received: 24 August 2020; Accepted: 17 November 2020;
Published: 22 December 2020.
Edited by:
Annalisa Del Prete, University of Brescia, ItalyReviewed by:
Jose Miguel Rodriguez Frade, Consejo Superior de Investigaciones Científicas (CSIC), SpainCopyright © 2020 Hussain, Peng, Cherian, Song, Ahirwar and Ganju. This is an open-access article distributed under the terms of the Creative Commons Attribution License (CC BY). The use, distribution or reproduction in other forums is permitted, provided the original author(s) and the copyright owner(s) are credited and that the original publication in this journal is cited, in accordance with accepted academic practice. No use, distribution or reproduction is permitted which does not comply with these terms.
*Correspondence: Dinesh K. Ahirwar, QWhpcndhci4xQG9zdS5lZHU=; Ramesh K. Ganju, UmFtZXNoLmdhbmp1QG9zdW1jLmVkdQ==
†These authors have contributed equally to this work and share first authorship
‡These authors have contributed equally to this work
Disclaimer: All claims expressed in this article are solely those of the authors and do not necessarily represent those of their affiliated organizations, or those of the publisher, the editors and the reviewers. Any product that may be evaluated in this article or claim that may be made by its manufacturer is not guaranteed or endorsed by the publisher.
Research integrity at Frontiers
Learn more about the work of our research integrity team to safeguard the quality of each article we publish.