- Section of Rheumatology, Department of Nephrology, Klinikum Rechts der Isar, Technical University of Munich, Munich, Germany
Many immune cells and effector molecules (e.g. cytokines, Interferons, growth factors) utilize different combinations of Janus kinase (JAK) and signal transducer and activator of transcription (STAT) molecules to transduce signals from the cell surface to the nucleus, where they regulate transcription. This pathway is basically involved in almost all inflammatory diseases and also in the interleukin (IL)-23/IL-17 cascade, which is an essential part of the pathogenesis of spondyloarthropathies (SpA). Upon evidence from in vitro and in vivo experiments indicating disease-modifying effects of JAK inhibition in inflammatory joint disease, numerous inhibitors of the JAK/STAT pathway (= JAKinibs) with different selectivity against the four members of the JAK family [JAK1, JAK2, JAK3, and tyrosine kinase 2 (TYK2)] were developed. Trials in rheumatoid arthritis were successful with respect to efficacy and safety, and currently, three JAKinibs are approved for the treatment of rheumatoid arthritis in the European Union. Although new treatment options (anti-IL-23, anti-IL-17, and phosphodiesterase 4 inhibitors) have become available for spondyloarthritis and especially psoriatic arthritis (PsA) within the last years, most of them are biologics and do not address all disease manifestations equally. Therefore, multiple trials were initiated to evaluate JAKinibs in PsA and axial spondyloarthritis (axSpA). A trial of Tofacitinib (OPAL) was successful in PsA and has led to the inclusion of JAKinibs into the treatment algorithm. Currently many trials with JAKinibs are ongoing for PsA and axSpA, with one phase III trial of upadacitinib (selective JAK1 inhibitor) showing good therapeutic response in active radiographic axSpA.
Introduction
Spondyloarthropathies (SpA) are a group of chronic inflammatory diseases, including axial SpA (axSpA) and psoriatic arthritis (PsA), as well as other less common forms like enteropathic or reactive arthritis. Besides skeletal manifestations (axial disease, peripheral arthritis, enthesitis, and dactylitis), the involvement of extra-articular organs (uveitis, psoriasis, and inflammatory bowel disease [IBD]) is a shared feature of these diseases (1). Current therapeutic options for SpA are limited compared with those for rheumatoid arthritis (RA), especially for axSpA, and mainly antibody-based, such as anti-tumor necrosis factor (TNF), anti-Interleukin (IL)-23 and anti-IL-17. Additionally, the therapeutic response greatly varies between the different diseases and affected systems such as the spine, peripheral joints, skin, and eyes. Only 51.3% of axSpA patients respond to TNF inhibitors (TNFi), some loose response over time, and others are not eligible (2, 3). Janus kinase (JAK) and signal transducer and activator of transcription (STAT) molecules are central transmitters of pro- and anti-inflammatory signals in immune regulation (4). The IL-23/IL-17 pathway is highly important in the pathogenesis of SpA and is partly controlled by JAK (5, 6). Therefore, JAK inhibitors offer new treatment options for SpA. As these are currently more limited for axSpA compared with PsA, this review focuses on JAK inhibitors and their clinical application in axSpA.
Janus Kinase and Signal Transducer and Activator of Transcription Signaling in Spondyloarthritis
JAK and STAT are central signal transducers for a great number of pro-inflammatory (e.g. IL-2, IL-7, IL-12, and IL-23) and anti-inflammatory cytokines (e.g. IL-10) influencing innate immune responses thought to be essential for the induction of SpA and adaptive immune functions maintaining and perpetuating the disease (7–9). This intracellular tyrosine kinase family consists of JAK1, JAK2, JAK3, and tyrosine kinase 2 (TYK2) and is coupled to STAT molecules (STAT1, STAT2, STAT3, STAT4, STAT5a and b, and STAT6) (7). Cytokine-receptor binding on the cell surface leads to autophosphorylation of JAK or phosphorylation of a partner JAK. Such activated JAK further phosphorylate sites of the intracellular domain of the receptor providing docking sites for STAT molecules. Dimers of STAT molecules phosphorylated by JAK migrate to the nucleus where they regulate gene expression. Different combinations of JAK and STAT are assigned to different cytokines and their receptors, providing a multitude of pathways and functions, as depicted in Figure 1 (7). However, STAT can be activated by other kinases and exercise effects in an un-phosphorylated state and even extra-nuclear. JAK also act independently of STAT molecules, for example, by directly phosphorylating histones adding further to the complexity of JAK and STAT signaling in immune cell regulation (10).
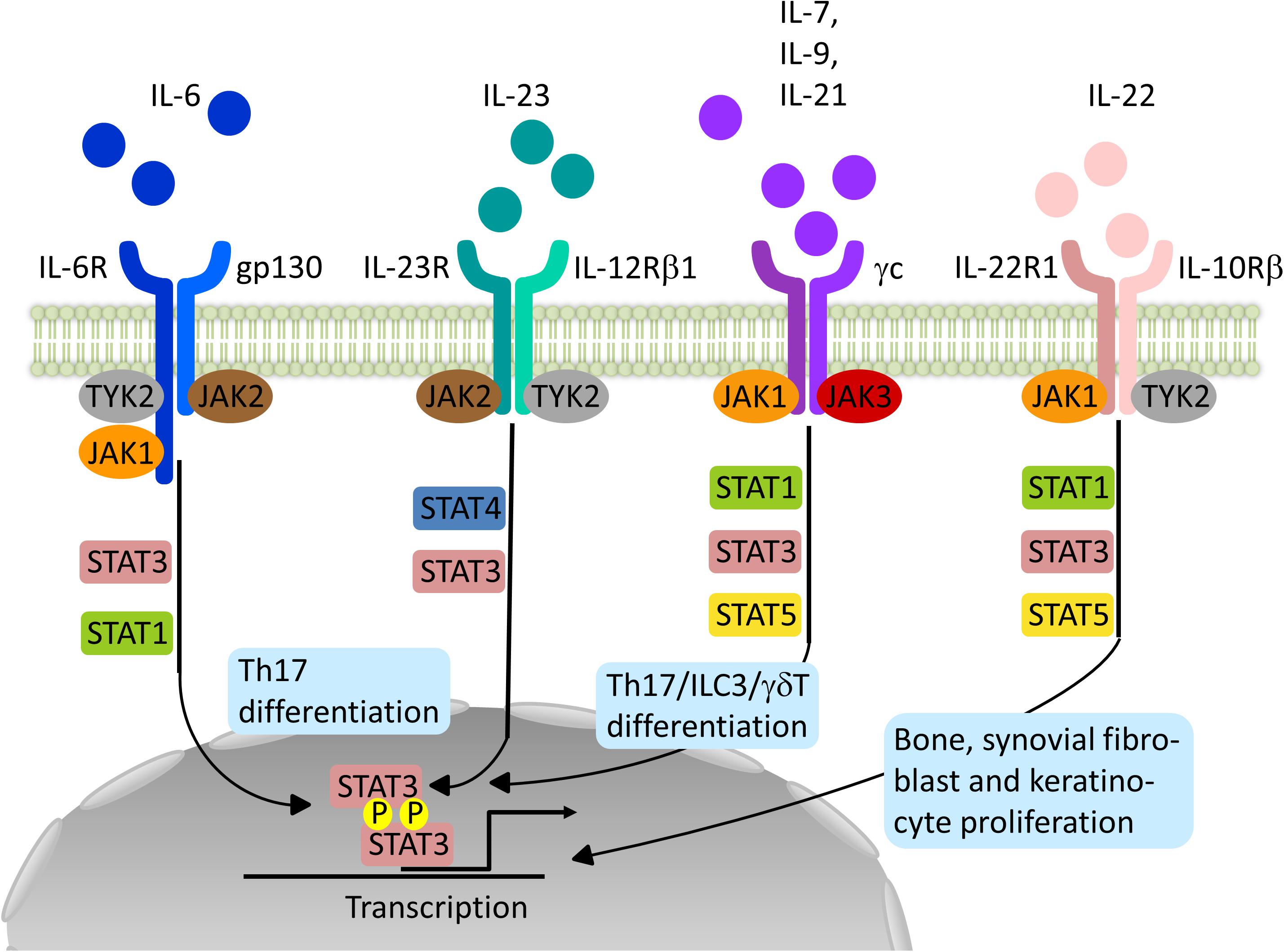
Figure 1. Schematic representation of relevant JAK-STAT signaling pathways in the pathogenesis of Spondyloarthritis. Binding of the different Interleukins (IL) to their specific receptor subunits on different cell populations, e.g. T cells, innate lymphoid cells (ILC) or effector cells such as osteoblasts, fibroblasts or keratinocytes leads to activation of a specific JAK-STAT pathway. The different isoforms of JAK are coupled to specific receptor/cytokine pairs and allow for a targeted inhibition with a specific JAKinib. However, overlap exists allowing for unintended side effects or accumulative effects. JAK1-specific inhibitors for example affect signaling by IL-6, IL-21, IL-7, IL-9 and IL-22 targeting most of the relevant immune and effector cell populations in SpA pathogenesis.
With regard to the pathogenesis of SpA, JAKs are involved in the signaling of key cytokines within the IL-23/IL-17 pathway, and Genome-wide association studies have found single nucleotide polymorphisms (SNPs) for IL23R, JAK2, and TYK2 in ankylosing spondylitis (AS) (11). IL-23, produced by activated myeloid cells, is important for the generation of IL-17 and IL-22 by target cells such as T helper cells 17 (Th17), gamma delta T cells (γδ T cells), or innate lymphoid cells (ILCs) type 3 (12). A combination of JAK2 and TYK2 transmits the IL-23 signal via STAT3 and, to a lesser extent, STAT4 (6, 13). IL-17A production is mainly JAK2-dependent, whereas IL-22 production requires TYK2 and JAK2 (14). By blocking IL-17 production, JAK inhibition subsequently affects the downstream effects of IL-17. Other cytokines favouring the development and maintenance of IL-17 producing cells include IL-6 (JAK1/JAK2/TYK2) and IL-21 (JAK1/JAK3) (7, 15). IL-22, another effector cytokine in the pathogenesis of SpA, uses the combination of JAK1 and TYK2 (7). Next to its protective functions at the epithelial barrier in the gut, IL-22 has pro-inflammatory and proliferative effects (synovial fibroblasts, keratinocytes) as well as osteoanabolic effects providing another interesting treatment target for SpA (16–18). Granulocyte-macrophage colony-stimulating factor, another pro-inflammatory cytokine produced by T cells and ILCs type 3 in SpA patients, signals via JAK2 (7, 19).
Respective of the various cytokines relying on JAK-STAT signaling, inhibition of this pathway offers multiple possibilities to modulate the immune and tissue response implicated in SpA. As cells of articular and extra-articular organs are utilizing this pathway, JAKinib will most likely affect the different sites of the disease. However, the protective pathways regulated by JAK-STAT might lead to adverse effects like viral infections by interfering with interferon signaling, as wells as with numbers and function of natural killer (NK) cells, cytotoxic T cells, and ILC (7).
Animal and Preclinical Data on Janus Kinase Inhibitors in Spondyloarthropathies
Although animal models for SpA do not fully replicate human disease, they have been useful in examining the molecular disease mechanisms and the role of JAK-STAT signaling. Enthesitis, an early and common feature in all forms of SpA, appears in mice with a myeloid cell-specific A20 (TNF-α-induced protein 3) deficiency (9, 20). The SpA-like arthropathy in this model is independent of TNF and relies on IL-1β and IL-6. Treatment with tofacitinib, an unselective JAK inhibitor, significantly reduced disease activity, confirmed by less inflammation of the synovial–entheseal complex on histology (20). In the SKG mouse model, which resembles human SpA if arthritis is initiated with curdlan and is dependent on IL-23 and Th17 cells, treatment with tofacitinib ameliorated established disease (21). Another experimental JAKinib also suppressed both inflammation and periosteal/entheseal bone formation in this model (22).
JAKinibs of different selectivity were shown to reduce Th17-type responses in CD4 T cells from patients with AS, PsA, and RA ex vivo with similar efficacy (23). Small interfering RNA-mediated knockdown of TYK2, signaling downstream of IL-23, was shown to be equally efficient in reducing type-17 cytokine secretion compared with JAK1 silencing or tofacitinib treatment (23). Several SNPs around the TYK2 locus are associated with AS. Some of these exonic SNPs lead to loss-of-function variants of TYK2. One of these SNPs associated with multiple autoimmune diseases is protective, but does not impact on non-autoimmune domains such as susceptibility to infections (24). A highly specific TYK2 inhibitor, NDI-031407, blocked disease progression in the SKG mouse model (14). MRI imaging showed prevention of joint space narrowing and bone marrow edema. NDI-031407 also protected mice from bone marrow edema and enthesis-related synovitis in the IL-23 mini-circle model (mostly dependent on γδ T cells). It completely abrogated IL-22 production but only partially inhibited IL-17 production from γδ T cells upon stimulation with IL-1β and IL-23 (14). The frequency of a loss-of-function TYK2 SNP (rs12720356) was significantly higher in AS patients with lower rates of spinal fusion, providing further evidence that targeting JAK could have effects on ankylosis.
With regard to the effects of JAKinib on bone metabolism, an important aspect in SpA, an experimental JAK2 inhibitor, AG490, reduced alkaline phosphatase activity in primary bone-derived cells from AS patients and healthy controls (25). On the other hand, tofacitinib and baricitinib increased bone mass in the K/BxN serum-transfer mouse model of RA-like arthritis and enhanced osteoblast function in vitro while sparing osteoclasts (26). These findings were confirmed in two RA patients treated with tofacitinib showing a substantial reduction in erosions of the metacarpophalangeal joints by micro-CT. Because activation of bone formation is deleterious in axSpA but useful in RA, further insight into the differential effects of JAKinib in these diseases is warranted.
Considering the combined data from animal models and clinical trials of anti-IL-23 antibodies in axSpA, TYK2 and JAK1 emerge as most promising targets of JAKinib for the treatment of axSpA, as they are involved in pathways relevant to the initiation (IL-23) and effector (IL-22) phase of the disease and especially in osteoproliferation (27). Table 1 gives an overview of JAKinibs already tested in clinical trials and under preclinical evaluation.
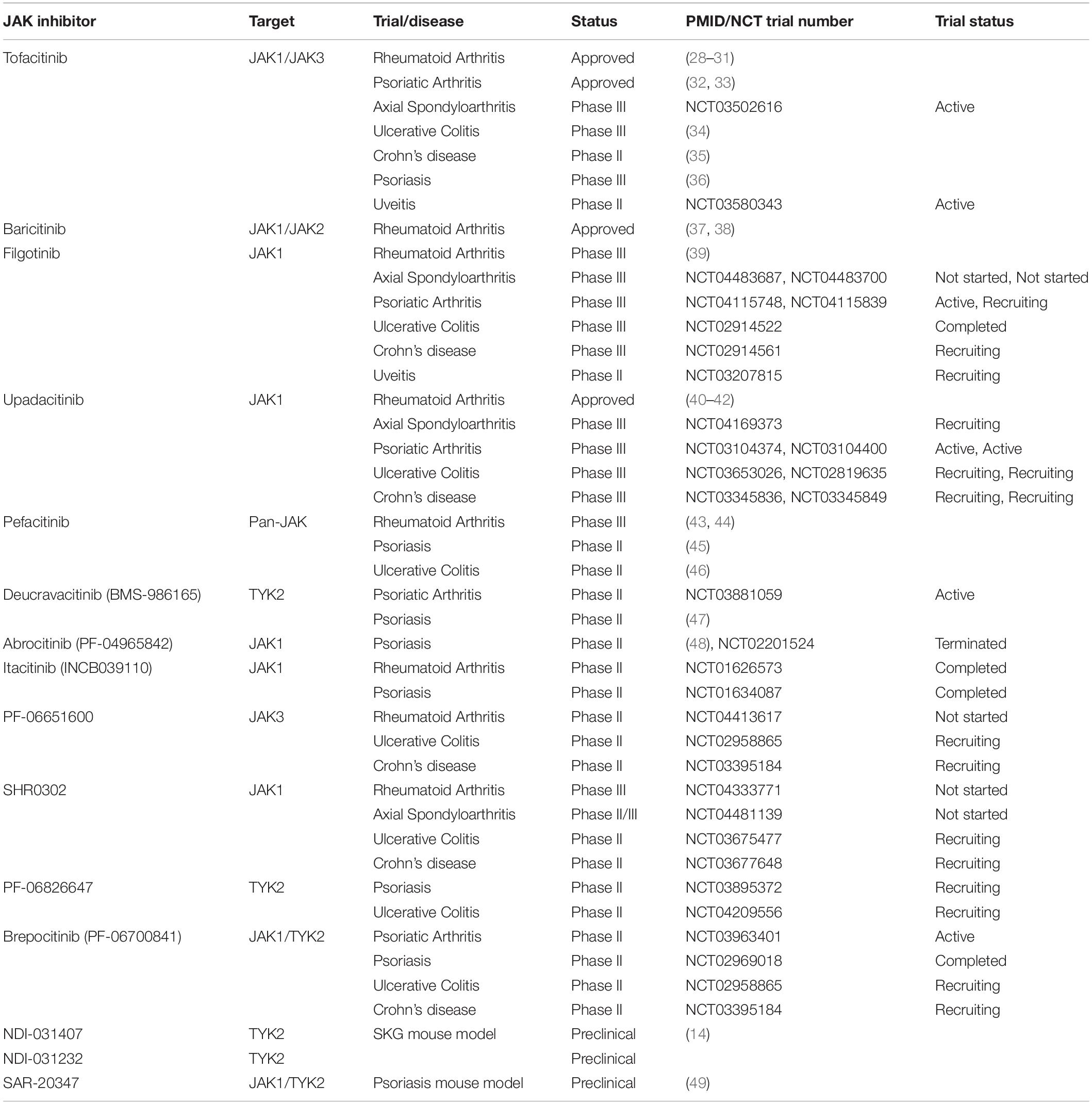
Table 1. Overview of JAK inhibitors tested in clinical trials and under preclinical evaluation for spondyloarthropathies and related diseases.
Clinical Data on Janus Kinase Inhibitors in Spondyloarthropathies
Tofacitinib (a pan-JAKinib, 196 biologic naïve patients) and filgotinib (a selective inhibitor of JAK1, no more than one TNF inhibitor, 107 patients, TORTUGA) have been trialed in phase II trials of active AS with an inadequate response to ≥2 or intolerance to non-steroidal anti-inflammatory drugs and high-sensitivity C-reactive protein (CRP) ≥3 mg/L (filgotinib trial) (50, 51). Upadacitinib (selective for JAK1) has been evaluated in a combined phase II/III trial (178 JAKinib and biologic naïve patients, SELECT-Axis 1) in active AS with the earlier mentioned inclusion criteria (52). The combined data on the efficacy on disease activity, functionality, and radiographic progression summarized below are extracted from these studies.
Efficacy on Disease Activity
After 12 weeks of treatment, Assessment in SpondyloArthritis International Society 20 (ASAS20) response rates were significantly higher for 5-mg tofacitinib twice daily (80.8%) and 200-mg filgotinib once daily (76%) compared with placebo (41.2 and 40%, respectively) but not for 2 mg (51.9%) or 10 mg (55.8%) of tofacitinib. ASAS40 response was significantly higher for all tofacitinib groups at week 12 and for 15-mg upadacitinib once daily compared with placebo at week 14 (52 vs. 26%). Tofacitinib (5-mg), filgotinib, and upadacitinib additionally lead to a significantly higher change of the mean Ankylosing Spondylitis Disease Activity Score (ASDAS) with rates of −1.4, −1.47 at week 12, and −1.45 at week 14, respectively, compared with placebo (−0.9, −0.57, and −0.54). Bath Ankylosing Spondylitis Disease Activity Index 50 (BASDAI50) response rates were significantly higher for all tofacitinib groups and upadacitinib with 42.3 to 46.2 and 45% vs. 23.5 and 23% in the placebo group. Enthesitis was significantly ameliorated by week 12 in 5- and 10-mg tofacitinib versus placebo.
The onset of response was slower with tofacitinib (approx. week 4) compared with TNF inhibitors but very rapid for filgotinib (week 1) and upadacitinib (week 2).
One limitation of the study of filgotinib is the relatively high proportion of patients with high high-sensitivity CRP at baseline, as elevated CRP is a known predictor of good response to therapy (53).
Efficacy With Regard to Functionality
Spinal mobility measured by Bath Ankylosing Spondylitis Metrology Index (BASMI) improved significantly with filgotinib compared with placebo by week 12 (−0.75 vs. −0.39). In the tofacitinib trial, significant improvement of BASMI was only achieved with 10 mg twice daily. In the upadacitinib trial, consistent improvements were seen with treatment for BASMI but did not meet significance based on multiplicity adjustment per the Hochberg procedure.
Efficacy With Regard to Radiographic Progression
Five- and 10-mg tofacitinib and filgotinib significantly improved Spondyloarthritis Research Consortium of Canada spine (−5.5 and −6.6, and −5.7) and sacroiliac joint (SIJ) scores (−3.2 and −3.6, and −3.52) compared with placebo (spine −0.1 and −0.52, SIJ −0.8 and 0.06). Upadacitinib had significant effects on the Spondyloarthritis Research Consortium of Canada spine score (−6.93 vs. −0.22).
A recent evaluation of the baseline and week 12 MRI scans from the TORTUGA trial found decreased SIJ erosion scores and increased backfill scores in the filgotinib group with increased erosion scores and no change in backfill scores in the placebo group, supporting the effects of filgotinib on structural lesions in axSpA (54).
Treatment-Emergent Adverse Events
Treatment-emergent adverse events (TEAEs) appeared slightly more often with 5- and 10-mg tofacitinib compared with 2 mg and placebo (53.8 and 51.9% vs. 44.2 and 43.1%) and upadacitinib (62 vs. 55% in the placebo group) but were similar in the filgotinib trial (31% both groups). The most common TEAEs in all trials were nasopharyngitis and upper respiratory tract infections. There were no malignancies, opportunistic infections, and cases of active tuberculosis or cases of extra-articular manifestations (IBD, psoriasis, and uveitis). Episodes of herpes zoster (HZ) were reported with tofacitinib and upadacitinib, and one non-serious venous thromboembolic event (VTE) with filgotinib.
Results from a phase III randomized controlled trial (RCT) of tofacitinib in active AS (NCT03502616) are expected this year.
Safety of Janus Kinase Inhibitors
In general, the long-term safety profile of JAKinibs is good and similar among the different inhibitors. Fears of high rates of opportunistic (including tuberculosis) and other infections have not been confirmed. Due to a lack of long-term data for JAKinib in SpA, data reported here are collected from clinical trials and post-marketing surveillance of RA. This seems feasible, as the three performed trials of JAKinibs in AS have so far shown similar safety profiles. However, it cannot be excluded that with longer observation periods and new trials leading to more exposed patients, new safety concerns may arise.
Overall incidence rates of serious infections are similar to those with biological disease-modifying antirheumatic drugs and range from 2.5 to 3.8 per 100 patient-years (55–58). However, a thorough screening of patients for tuberculosis before therapy is mandatory, with special alertness to extrapulmonary manifestations of tuberculosis (59). Reactivation of hepatitis B has been reported with JAKinib treatment, but treatment with tofacitinib in refractory cases under antiviral prophylaxis seems safe and effective (60, 61). The increased incidence of HZ is specific for JAKinib treatment and, for unknown reasons, seems to be more pronounced in Japan and Korea, ranging from 3.3 to 3.9 per 100 patient-years (26, 57, 58, 62, 63). The common risk factor for HZ over the different JAKinib was age (64, 65). Filgotinib so far has shown lower incidence rates of HZ and serious infections compared with other JAKinib, but long-term observations are lacking (66). This effect can possibly be attributed to less inhibition of JAK1-mediated signaling of interferon γ and IL-2, IL-4, and IL-15 (necessary for proliferation of NK cells) by filgotinib (67).
Regardless of a slightly increased risk for overall malignancies for RA patients compared with the general population, so far, no significant effects of JAKinib have been identified, excluding non-melanoma skin cancer (68). With respect to the two- to threefold increased risk for lymphoma in RA patients, the crude incidence rates with tofacitinib and baricitinib were low, with 0.10 (56). The effects of long-term use of JAKinib on the risk of cancer, for example, via interference with tumor surveillance through NK cells and interferon signaling are still unknown (57, 69).
As patients with RA, AS, and PsA generally have an increased risk for deep vein thrombosis (DVT), pulmonary embolism (PE), and venous thrombembolism (VTE) (risk ratios, 2.08, 2.17, and 1.96, respectively), special interest was given to such events in trials with JAKinibs (70–72). Incidence rates for DVT and PE with tofacitinib were 0.1 each (0.2 for PE with the 10-mg dose) and for VTE with upadacitinib 0.6 per 100 patient-years, and 0.1 and 0.2 per 100 patient-years with 100 and 200 mg of filgotinib (57, 58, 73). Therefore, a randomized safety endpoint study in moderate to severe RA comparing tofacitinib and TNF inhibitor has been implemented, including patients with at least one cardiovascular risk factor (NCT02092467). Nevertheless, the FDA and EMEA requested a warning of thrombosis for tofacitinib, baricitinib, and upadacitinib in 2019 (74, 75). A mechanistic explanation for the increased risk of thromboembolic events is still lacking. Despite a metanalysis of 30 RCTs on JAKinib in RA showing no significant differences in short-term major adverse cardiac events or VTE, a recent analysis of the World Health Organization global database (VigiBase) revealed a 2.3–3.4-fold increased risk for DVT and PE with tofacitinib and baricitinib in Europe (76, 77).
Use of a JAKinib has to be carefully evaluated in patients with risk factors for gastrointestinal perforation such as older age, history of diverticulitis or other gastrointestinal conditions, and use of prednisolone >7.5 mg/day or non-steroidal anti-inflammatory drugs (78, 79). The incidence rates per 1,000 patient-years for gastrointestinal perforation were 0.11 for tofacitinib and 0.04 for baricitinib (56). In analogy to tocilizumab, the risk of gastrointestinal perforation might be ascribed to the inhibition of IL-6 signaling by the different JAKinibs (57, 80).
Dose adjustments according to the metabolism of each drug should be considered for patients with moderate to severe hepatic or renal dysfunction. Laboratory changes in patients treated with JAKinibs are common and include changes of hemoglobin, lymphocyte and platelet counts. However, it has been hard to separate the intrinsic effects of JAKinibs via concomitant JAK2 inhibition (main signaling JAK for erythropoietin and thrombopoietin receptors) and disease-driven inflammatory effects on erythro- and thrombopoiesis. Other common laboratory changes include elevation of serum transaminases, creatinine, high- and low-density lipoprotein cholesterols, but usually do not result in treatment cessation.
Teratogenic effects of JAKinib have been reported in preclinical animal studies, and so far pregnancy outcomes of 47 patients treated with tofacitinib during RCT are known (81–83). There were 25 healthy newborns, one congenital pulmonary valve stenosis, seven spontaneous abortions, eight medical terminations, and six pending or lost to follow-up (84). Therefore, JAKinibs are contraindicated during pregnancy and breastfeeding, requiring strict contraception in females of child-bearing age until at least 1 week after the last dose.
Next to the known TEAEs of conventional synthetic and biological disease-modifying antirheumatic drugs, e.g. infections, a special focus has to be placed on HZ, VTE and PE, and changes in blood cells and lipid metabolism with JAKinib treatment.
Discussion and Perspective
Although the data from three RCTs of JAK inhibitors in active AS are very promising, studies evaluating patients who have failed TNFi or anti-IL-17 therapy will be of great interest to place JAKinibs in the treatment algorithm of axSpA. Other interesting issues are head-to-head comparisons with TNFi and efficacy in non-radiographic axSpA. For a better assessment of the long-term safety results of the SpA study extensions will have to be awaited. Also the differential effect of more selective JAKinibs on the various disease manifestations of SpA needs to be clarified. Interest focuses on the newly developed specific TYK2 inhibitor, BMS-986165, which has already completed a phase II trial for psoriasis and promises clinical efficacy in axSpA by preclinical data and translational research. It also needs to be elucidated if SpA patients might profit from different dosing strategies for induction and maintenance of remission, such as high loading doses and low maintenance doses. However, these new orally available agents will most likely soon be included in the treatment recommendations for axSpA and provide the clinician with options in patients who are not eligible or have contraindications to TNFi or anti-IL-17, such as allergic reactions, congestive heart failure, or concomitant demyelinating disease (TNFi) and concomitant active IBD (anti-IL-17) (85, 86). JAKinibs may also be advantageous in patients with repeated infections, as they have a shorter half-life compared with bDMARD or csDMARD. With regard to avoiding radiographic progression and chronic disability in axSpA patients, JAKinibs face the same challenges as other drugs. From long-term observations with TNFi, it became evident that a halt in radiographic progression probably can only be achieved with very early and prolonged treatment (for more than 4 years) (87, 88). Targeting new bone formation specifically might have too many adverse effects on general bone homeostasis.
Overall, JAKinibs seem safe when used in a well-screened patient population of SpA and under regular surveillance. They appear equally effective to biologic drugs by current evidence and have advantages besides their oral application and shorter half-life.
Author Contributions
AH and PM drafted the manuscript. AH wrote the manuscript and created graphical illustrations with the input from GL and PM. All authors approved the final version of the manuscript.
Funding
AH is currently supported by a grant from the Dr. Werner Jackstädt Stiftung. AH received grant support in the past from the Hans und Klementia Langmatz Stiftung and DFG (HA-7021/1-1).
Conflict of Interest
The authors declare that the research was conducted in the absence of any commercial or financial relationships that could be construed as a potential conflict of interest.
References
1. Sieper J, Poddubnyy D. Axial spondyloarthritis. Lancet. (2017) 390:73–84. doi: 10.1016/S0140-6736(16)31591-4
2. Jones GT, Dean LE, Pathan E, Hollick RJ, Macfarlane GJ. Real-world evidence of TNF inhibition in axial spondyloarthritis: can we generalise the results from clinical trials? Ann Rheum Dis. (2020) 79:914–9. doi: 10.1136/annrheumdis-2019-216841
3. Flouri ID, Markatseli TE, Boki KA, Papadopoulos I, Skopouli FN, Voulgari PV, et al. Comparative analysis and predictors of 10-year tumor necrosis factor inhibitors drug survival in patients with spondyloarthritis: first-year response predicts longterm drug persistence. J Rheumatol. (2018) 45:785–94. doi: 10.3899/jrheum.170477
4. Villarino AV, Kanno Y, O’Shea JJ. Mechanisms and consequences of Jak-STAT signaling in the immune system. Nat Immunol. (2017) 18:374–84. doi: 10.1038/ni.3691
5. Taams LS, Steel KJA, Srenathan U, Burns LA, Kirkham BW. IL-17 in the immunopathogenesis of spondyloarthritis. Nat Rev Rheumatol. (2018) 14:453–66. doi: 10.1038/s41584-018-0044-2
6. Parham C, Chirica M, Timans J, Vaisberg E, Travis M, Cheung J, et al. A receptor for the heterodimeric cytokine IL-23 is composed of IL-12Rbeta1 and a novel cytokine receptor subunit, IL-23R. J Immunol. (2002) 168:5699–708. doi: 10.4049/jimmunol.168.11.5699
7. Gadina M, Johnson C, Schwartz D, Bonelli M, Hasni S, Kanno Y, et al. Translational and clinical advances in JAK-STAT biology: the present and future of jakinibs. J Leukoc Biol. (2018) 104:499–514. doi: 10.1002/JLB.5RI0218-084R
8. Reinhardt A, Prinz I. Whodunit? The contribution of interleukin (IL)-17/IL-22-producing gammadelta T Cells, alphabeta T cells, and innate lymphoid cells to the pathogenesis of spondyloarthritis. Front Immunol. (2018) 9:885. doi: 10.3389/fimmu.2018.00885
9. Watad A, Bridgewood C, Russell T, Marzo-Ortega H, Cuthbert R, McGonagle D. The early phases of ankylosing spondylitis: emerging insights from clinical and basic science. Front Immunol. (2018) 9:2668. doi: 10.3389/fimmu.2018.02668
10. O’Shea JJ, Schwartz DM, Villarino AV, Gadina M, McInnes IB, Laurence A. The JAK-STAT pathway: impact on human disease and therapeutic intervention. Annu Rev Med. (2015) 66:311–28. doi: 10.1146/annurev-med-051113-024537
11. Brown MA, Wordsworth BP. Genetics in ankylosing spondylitis–current state of the art and translation into clinical outcomes. Best Pract Res Clin Rheumatol. (2017) 31:763–76. doi: 10.1016/j.berh.2018.09.005
12. Voruganti A, Bowness P. New developments in our understanding of ankylosing spondylitis pathogenesis. Immunology. (2020). doi: 10.1111/imm.13242
13. Oppmann B, Lesley R, Blom B, Timans JC, Xu Y, Hunte B, et al. Novel p19 protein engages IL-12p40 to form a cytokine, IL-23, with biological activities similar as well as distinct from IL-12. Immunity. (2000) 13:715–25. doi: 10.1016/s1074-7613(00)00070-4
14. Gracey E, Hromadova D, Lim M, Qaiyum Z, Zeng M, Yao Y, et al. TYK2 inhibition reduces type 3 immunity and modifies disease progression in murine spondyloarthritis. J Clin Invest. (2020) 130:1863–78. doi: 10.1172/JCI126567
15. Korn T, Bettelli E, Oukka M, Kuchroo VK. IL-17 and Th17 Cells. Annu Rev Immunol. (2009) 27:485–517. doi: 10.1146/annurev.immunol.021908.132710
16. Liang SC, Tan XY, Luxenberg DP, Karim R, Dunussi-Joannopoulos K, Collins M, et al. Interleukin (IL)-22 and IL-17 are coexpressed by Th17 cells and cooperatively enhance expression of antimicrobial peptides. J Exp Med. (2006) 203:2271–9. doi: 10.1084/jem.20061308
17. Wolk K, Haugen HS, Xu W, Witte E, Waggie K, Anderson M, et al. IL-22 and IL-20 are key mediators of the epidermal alterations in psoriasis while IL-17 and IFN-gamma are not. J Mol Med (Berl). (2009) 87:523–36. doi: 10.1007/s00109-009-0457-0
18. Sherlock JP, Joyce-Shaikh B, Turner SP, Chao CC, Sathe M, Grein J, et al. IL-23 induces spondyloarthropathy by acting on ROR-gammat+ CD3+CD4-CD8- entheseal resident T cells. Nat Med. (2012) 18:1069–76. doi: 10.1038/nm.2817
19. Al-Mossawi MH, Chen L, Fang H, Ridley A, de Wit J, Yager N, et al. Unique transcriptome signatures and GM-CSF expression in lymphocytes from patients with spondyloarthritis. Nat Commun. (2017) 8:1510. doi: 10.1038/s41467-017-01771-2
20. De Wilde K, Martens A, Lambrecht S, Jacques P, Drennan MB, Debusschere K, et al. A20 inhibition of STAT1 expression in myeloid cells: a novel endogenous regulatory mechanism preventing development of enthesitis. Ann Rheum Dis. (2017) 76:585–92. doi: 10.1136/annrheumdis-2016-209454
21. Oh K, Seo MW, Kim IG, Hwang YI, Lee HY, Lee DS. CP-690550 treatment ameliorates established disease and provides long-term therapeutic effects in an SKG arthritis model. Immune Netw. (2013) 13:257–63. doi: 10.4110/in.2013.13.6.257
22. Maeda Y, Huang T, Manning C, Shaughn B, Macoritto M, Hyland D, et al. Blockade of the JAK/STAT pathway inhibits inflammation and bone formation in two murine models of spondyloarthritis. Arthritis Rheumatol. (2018) 70(Suppl. 10).
23. Hammitzsch A, Chen L, de Wit J, Al-Mossawi MH, Ridley A, Sekine T, et al. Inhibiting ex-vivo Th17 responses in Ankylosing Spondylitis by targeting Janus kinases. Sci Rep. (2018) 8:15645. doi: 10.1038/s41598-018-34026-1
24. Dendrou CA, Cortes A, Shipman L, Evans HG, Attfield KE, Jostins L, et al. Resolving TYK2 locus genotype-to-phenotype differences in autoimmunity. Sci Transl Med. (2016) 8:363ra149. doi: 10.1126/scitranslmed.aag1974
25. Jo S, Wang SE, Lee YL, Kang S, Lee B, Han J, et al. IL-17A induces osteoblast differentiation by activating JAK2/STAT3 in ankylosing spondylitis. Arthritis Res Ther. (2018) 20:115. doi: 10.1186/s13075-018-1582-3
26. Adam S, Simon N, Steffen U, Andes FT, Scholtysek C, Muller DIH, et al. JAK inhibition increases bone mass in steady-state conditions and ameliorates pathological bone loss by stimulating osteoblast function. Sci Transl Med. (2020) 12:eaay4447. doi: 10.1126/scitranslmed.aay4447
27. Deodhar A, Gensler LS, Sieper J, Clark M, Calderon C, Wang Y, et al. Three multicenter, randomized, double-blind, placebo-controlled studies evaluating the efficacy and safety of ustekinumab in axial spondyloarthritis. Arthritis Rheumatol. (2019) 71:258–70. doi: 10.1002/art.40728
28. Fleischmann R, Kremer J, Cush J, Schulze-Koops H, Connell CA, Bradley JD, et al. Placebo-controlled trial of tofacitinib monotherapy in rheumatoid arthritis. N Engl J Med. (2012) 367:495–507. doi: 10.1056/NEJMoa1109071
29. van Vollenhoven RF, Fleischmann R, Cohen S, Lee EB, Garcia Meijide JA, Wagner S, et al. Tofacitinib or adalimumab versus placebo in rheumatoid arthritis. N Engl J Med. (2012) 367:508–19. doi: 10.1056/NEJMoa1112072
30. Lee EB, Fleischmann R, Hall S, Wilkinson B, Bradley JD, Gruben D, et al. Tofacitinib versus methotrexate in rheumatoid arthritis. N Engl J Med. (2014) 370:2377–86. doi: 10.1056/NEJMoa1310476
31. Fleischmann R, Mysler E, Hall S, Kivitz AJ, Moots RJ, Luo Z, et al. Efficacy and safety of tofacitinib monotherapy, tofacitinib with methotrexate, and adalimumab with methotrexate in patients with rheumatoid arthritis (ORAL Strategy): a phase 3b/4, double-blind, head-to-head, randomised controlled trial. Lancet. (2017) 390:457–68. doi: 10.1016/S0140-6736(17)31618-5
32. Gladman D, Rigby W, Azevedo VF, Behrens F, Blanco R, Kaszuba A, et al. Tofacitinib for psoriatic arthritis in patients with an inadequate response to TNF inhibitors. N Engl J Med. (2017) 377:1525–36. doi: 10.1056/NEJMoa1615977
33. Mease P, Hall S, FitzGerald O, van der Heijde D, Merola JF, Avila-Zapata F, et al. Tofacitinib or adalimumab versus placebo for psoriatic arthritis. N Engl J Med. (2017) 377:1537–50. doi: 10.1056/NEJMoa1615975
34. Sandborn WJ, Su C, Sands BE, D’Haens GR, Vermeire S, Schreiber S, et al. Tofacitinib as induction and maintenance therapy for ulcerative colitis. N Engl J Med. (2017) 376:1723–36. doi: 10.1056/NEJMoa1606910
35. Sandborn WJ, Ghosh S, Panes J, Vranic I, Wang W, Niezychowski W, et al. A phase 2 study of tofacitinib, an oral Janus kinase inhibitor, in patients with Crohn’s disease. Clin Gastroenterol Hepatol. (2014) 12:1485–93.e2. doi: 10.1016/j.cgh.2014.01.029
36. Bachelez H, van de Kerkhof PC, Strohal R, Kubanov A, Valenzuela F, Lee JH, et al. Tofacitinib versus etanercept or placebo in moderate-to-severe chronic plaque psoriasis: a phase 3 randomised non-inferiority trial. Lancet. (2015) 386:552–61. doi: 10.1016/S0140-6736(14)62113-9
37. Genovese MC, Kremer J, Zamani O, Ludivico C, Krogulec M, Xie L, et al. Baricitinib in patients with refractory rheumatoid arthritis. N Engl J Med. (2016) 374:1243–52. doi: 10.1056/NEJMoa1507247
38. Taylor PC, Keystone EC, van der Heijde D, Weinblatt ME, Del Carmen Morales L, Reyes Gonzaga J, et al. Baricitinib versus placebo or adalimumab in rheumatoid arthritis. N Engl J Med. (2017) 376:652–62. doi: 10.1056/NEJMoa1608345
39. Genovese MC, Kalunian K, Gottenberg JE, Mozaffarian N, Bartok B, Matzkies F, et al. Effect of filgotinib vs placebo on clinical response in patients with moderate to severe rheumatoid arthritis refractory to disease-modifying antirheumatic drug therapy: the FINCH 2 randomized clinical trial. JAMA. (2019) 322:315–25. doi: 10.1001/jama.2019.9055
40. Burmester GR, Kremer JM, Van den Bosch F, Kivitz A, Bessette L, Li Y, et al. Safety and efficacy of upadacitinib in patients with rheumatoid arthritis and inadequate response to conventional synthetic disease-modifying anti-rheumatic drugs (SELECT-NEXT): a randomised, double-blind, placebo-controlled phase 3 trial. Lancet. (2018) 391:2503–12. doi: 10.1016/S0140-6736(18)31115-2
41. Genovese MC, Fleischmann R, Combe B, Hall S, Rubbert-Roth A, Zhang Y, et al. Safety and efficacy of upadacitinib in patients with active rheumatoid arthritis refractory to biologic disease-modifying anti-rheumatic drugs (SELECT-BEYOND): a double-blind, randomised controlled phase 3 trial. Lancet. (2018) 391:2513–24. doi: 10.1016/S0140-6736(18)31116-4
42. Fleischmann R, Pangan AL, Song IH, Mysler E, Bessette L, Peterfy C, et al. Upadacitinib versus placebo or adalimumab in patients with rheumatoid arthritis and an inadequate response to methotrexate: results of a phase III, double-blind, randomized controlled trial. Arthritis Rheumatol. (2019) 71:1788–800. doi: 10.1002/art.41032
43. Tanaka Y, Takeuchi T, Tanaka S, Kawakami A, Iwasaki M, Song YW, et al. Efficacy and safety of peficitinib (ASP015K) in patients with rheumatoid arthritis and an inadequate response to conventional DMARDs: a randomised, double-blind, placebo-controlled phase III trial (RAJ3). Ann Rheum Dis. (2019) 78:1320–32. doi: 10.1136/annrheumdis-2019-215163
44. Takeuchi T, Tanaka Y, Tanaka S, Kawakami A, Iwasaki M, Katayama K, et al. Efficacy and safety of peficitinib (ASP015K) in patients with rheumatoid arthritis and an inadequate response to methotrexate: results of a phase III randomised, double-blind, placebo-controlled trial (RAJ4) in Japan. Ann Rheum Dis. (2019) 78:1305–19. doi: 10.1136/annrheumdis-2019-215164
45. Papp K, Pariser D, Catlin M, Wierz G, Ball G, Akinlade B, et al. A phase 2a randomized, double-blind, placebo-controlled, sequential dose-escalation study to evaluate the efficacy and safety of ASP015K, a novel Janus kinase inhibitor, in patients with moderate-to-severe psoriasis. Br J Dermatol. (2015) 173:767–76. doi: 10.1111/bjd.13745
46. Sands BE, Sandborn WJ, Feagan BG, Lichtenstein GR, Zhang H, Strauss R, et al. Peficitinib, an oral janus kinase inhibitor, in moderate-to-severe ulcerative colitis: results from a randomised, phase 2 study. J Crohns Colitis. (2018) 12:1158–69. doi: 10.1093/ecco-jcc/jjy085
47. Papp K, Gordon K, Thaci D, Morita A, Gooderham M, Foley P, et al. Phase 2 trial of selective tyrosine kinase 2 inhibition in psoriasis. N Engl J Med. (2018) 379:1313–21. doi: 10.1056/NEJMoa1806382
48. Schmieder GJ, Draelos ZD, Pariser DM, Banfield C, Cox L, Hodge M, et al. Efficacy and safety of the Janus kinase 1 inhibitor PF-04965842 in patients with moderate-to-severe psoriasis: phase II, randomized, double-blind, placebo-controlled study. Br J Dermatol. (2018) 179:54–62. doi: 10.1111/bjd.16004
49. Works MG, Yin F, Yin CC, Yiu Y, Shew K, Tran TT, et al. Inhibition of TYK2 and JAK1 ameliorates imiquimod-induced psoriasis-like dermatitis by inhibiting IL-22 and the IL-23/IL-17 axis. J Immunol. (2014) 193:3278–87. doi: 10.4049/jimmunol.1400205
50. van der Heijde D, Deodhar A, Wei JC, Drescher E, Fleishaker D, Hendrikx T, et al. Tofacitinib in patients with ankylosing spondylitis: a phase II, 16-week, randomised, placebo-controlled, dose-ranging study. Ann Rheum Dis. (2017) 76:1340–7. doi: 10.1136/annrheumdis-2016-210322
51. van der Heijde D, Baraliakos X, Gensler LS, Maksymowych WP, Tseluyko V, Nadashkevich O, et al. Efficacy and safety of filgotinib, a selective Janus kinase 1 inhibitor, in patients with active ankylosing spondylitis (TORTUGA): results from a randomised, placebo-controlled, phase 2 trial. Lancet. (2018) 392:2378–87. doi: 10.1016/S0140-6736(18)32463-2
52. van der Heijde D, Song IH, Pangan AL, Deodhar A, van den Bosch F, Maksymowych WP, et al. Efficacy and safety of upadacitinib in patients with active ankylosing spondylitis (SELECT-AXIS 1): a multicentre, randomised, double-blind, placebo-controlled, phase 2/3 trial. Lancet. (2019) 394:2108–17. doi: 10.1016/S0140-6736(19)32534-6
53. Baraliakos X, Szumski A, Koenig AS, Jones H. The role of C-reactive protein as a predictor of treatment response in patients with ankylosing spondylitis. Semin Arthritis Rheum. (2019) 48:997–1004. doi: 10.1016/j.semarthrit.2018.10.019
54. Maksymowych WP, Ǿstergaard M, Landewé RBM, Barchuk W, Liu K, Tasset C, et al. THU0377: Impact of Filgotinib on structural lesions in the sacroiliac joints at 12 weeks in patients with active axial Spondyloarthritis: magnetic resonance imaging data from the double-blind, randomized TORTUGA trial. Ann Rheum Dis. (2020) 79(Suppl. 1):417.
55. Sepriano A, Kerschbaumer A, Smolen JS, van der Heijde D, Dougados M, van Vollenhoven R, et al. Safety of synthetic and biological DMARDs: a systematic literature review informing the 2019 update of the EULAR recommendations for the management of rheumatoid arthritis. Ann Rheum Dis. (2020) 79:760–70. doi: 10.1136/annrheumdis-2019-216653
56. Cohen SB, Tanaka Y, Mariette X, Curtis JR, Lee EB, Nash P, et al. Long-term safety of tofacitinib for the treatment of rheumatoid arthritis up to 8.5 years: integrated analysis of data from the global clinical trials. Ann Rheum Dis. (2017) 76:1253–62. doi: 10.1136/annrheumdis-2016-210457
57. Genovese MC, Smolen J, Takeuchi T, Burmester GR, Deberdt W, Schlichting D, et al. FRI0123: Safety profile of Baricitinib for the treatment of Rheumatoid Arthritis up to 8.4 years: an updated integrated safety analysis. Ann Rheum Dis. (2020) 79(Suppl. 1):638.
58. Cohen SB, van Vollenhoven R, Curtis JR, Calabrese L, Zerbini C, Tanaka Y, et al. THU0187: Safety profile of Upadacitinib up to 3 years of exposure in patients with Rheumatoid Arthritis. Ann Rheum Dis. (2020) 79 (Suppl. 1):315.
59. Winthrop KL, Park SH, Gul A, Cardiel MH, Gomez-Reino JJ, Tanaka Y, et al. Tuberculosis and other opportunistic infections in tofacitinib-treated patients with rheumatoid arthritis. Ann Rheum Dis. (2016) 75:1133–8. doi: 10.1136/annrheumdis-2015-207319
60. Harigai M, Winthrop K, Takeuchi T, Hsieh TY, Chen YM, Smolen JS, et al. Evaluation of hepatitis B virus in clinical trials of baricitinib in rheumatoid arthritis. RMD Open. (2020) 6:e001095. doi: 10.1136/rmdopen-2019-001095
61. Fang L, Lin Z, Liao Z, Jin O, Gu J. THU0385: Safety of Tofacitinib therapy in HBsAG carriers with ankylosing spondylitis: a prospective study. Ann Rheum Dis. (2020) 79(Suppl. 1):423.
62. Honda S, Harigai M. The safety of baricitinib in patients with rheumatoid arthritis. Expert Opin Drug Saf. (2020) 19:545–51. doi: 10.1080/14740338.2020.1743263
63. Tamura N, Kuwana M, Atsumi T, Takei S, Harigai M, Fujii T, et al. Infection events in Japanese patients with rheumatoid arthritis treated with tofacitinib: interim all-case post-marketing surveillance. Arthritis Rheumatol. (2018) 70(Suppl. 10).
64. Winthrop KL, Curtis JR, Lindsey S, Tanaka Y, Yamaoka K, Valdez H, et al. Herpes zoster and tofacitinib: clinical outcomes and the risk of concomitant therapy. Arthritis Rheumatol. (2017) 69:1960–8. doi: 10.1002/art.40189
65. Chen YH, Chen YM, Smolen JS, Takeuchi T, Muller R, Walker D, et al. FRI0164 Incidence rate and characterization of herpes zoster in patients with moderate-to-severe Rheumatoid Arthritis: an update from Baricitinib clinical studies. Ann Rheum Dis. (2019) 78(Suppl. 2):755.
66. Genovese MC, Winthrop K, Tanaka Y, Takeuchi T, Kivitz A, Matzkies F, et al. THU0202: Integrated safety analysis of Filgotinib treatment for Rheumatoid Arthritis from 7 clinical trials. Ann Rheum Dis. (2020) 79(Suppl. 1):320.
67. Gonzalez-Traves P, Murry B, Campigotto F, Meng A, Di Paolo JA. THU0067: JAK selectivity and the impact on cytokine signaling inhibition at clinical Rheumatoid Arthritis doses. Ann Rheum Dis. (2020) 79(Suppl. 1):242.
68. Simon TA, Thompson A, Gandhi KK, Hochberg MC, Suissa S. Incidence of malignancy in adult patients with rheumatoid arthritis: a meta-analysis. Arthritis Res Ther. (2015) 17:212. doi: 10.1186/s13075-015-0728-9
69. Schreiber RD, Old LJ, Smyth MJ. Cancer immunoediting: integrating immunity’s roles in cancer suppression and promotion. Science. (2011) 331:1565–70. doi: 10.1126/science.1203486
70. Ungprasert P, Srivali N, Spanuchart I, Thongprayoon C, Knight EL. Risk of venous thromboembolism in patients with rheumatoid arthritis: a systematic review and meta-analysis. Clin Rheumatol. (2014) 33:297–304. doi: 10.1007/s10067-014-2492-7
71. Eriksson JK, Jacobsson L, Bengtsson K, Askling J. Is ankylosing spondylitis a risk factor for cardiovascular disease, and how do these risks compare with those in rheumatoid arthritis? Ann Rheum Dis. (2017) 76:364–70. doi: 10.1136/annrheumdis-2016-209315
72. Bengtsson K, Forsblad-d’Elia H, Lie E, Klingberg E, Dehlin M, Exarchou S, et al. Are ankylosing spondylitis, psoriatic arthritis and undifferentiated spondyloarthritis associated with an increased risk of cardiovascular events? A prospective nationwide population-based cohort study. Arthritis Res Ther. (2017) 19:102. doi: 10.1186/s13075-017-1315-z
73. Mease PJ, Kremer J, Cohen S, Curtis JR, Charles-Schoeman C, Loftus EV, et al. 16L: Incidence of thromboembolic events in the Tofacitinib rheumatoid arthritis, psoriasis, psoriatic arthritis and ulcerative colitis development programs. Arthritis Rheumatol. (2017) 69(Suppl. 10).
74. Various: U.S. Food and Drug AdministrationFDA.gov. (2020). Available online at: https://www.fda.gov/drugs/drug-safety-and-availability/fda-approves-boxed-warning-about-increased-risk-blood-clots-and-death-higher-dose-arthritis-and (accessed July 29, 2020).
75. Various: European Medicines Agencyemea.europa.eu. (2020). Available online at: https://www.ema.europa.eu/en/news/ema-confirms-xeljanz-be-used-caution-patients-high-risk-blood-clots (accessed July 29, 2020).
76. Malaurie M, Constantin A, Degboé Y, Ruyssen-Witrand A, Barnetche T. 2358: Short-term risk of major adverse cardiovascular events or venous thrombo-embolic events in patients with rheumatoid arthritis initiating a janus kinase inhibitor: a meta-analysis of randomised controlled trials. Arthritis Rheumatol. (2019) 71(Suppl. 10).
77. Vallejo-Yagüe E, Weiler S, Burden AM. OP0237: Thrombembolic safety profile of Tofacitinib and Baricitinib: an analysis of WHO VigiBase. Ann Rheum Dis. (2020) 79(Suppl. 1):150.
78. Xie F, Yun H, Bernatsky S, Curtis JR. Brief report: risk of gastrointestinal perforation among rheumatoid arthritis patients receiving tofacitinib, tocilizumab, or other biologic treatments. Arthritis Rheumatol. (2016) 68:2612–7. doi: 10.1002/art.39761
79. Swanson SM, Strate LL. Acute colonic diverticulitis. Ann Intern Med. (2018) 168:ITC65–80. doi: 10.7326/AITC201805010
80. Koike T, Harigai M, Inokuma S, Ishiguro N, Ryu J, Takeuchi T, et al. Effectiveness and safety of tocilizumab: postmarketing surveillance of 7901 patients with rheumatoid arthritis in Japan. J Rheumatol. (2014) 41:15–23. doi: 10.3899/jrheum.130466
81. Various: U.S. Food and Drug Administrationaccessdata.fda.gov. (2020). Available online at: https://www.accessdata.fda.gov/drugsatfda_docs/label/2019/211675s000lbl.pdf (accessed July 29, 2020).
82. Various: Eli Lilly and Companypi.lilly.com. (2020). Accessed online at: http://pi.lilly.com/us/olumiant-uspi.pdf (accessed July 29, 2020).
83. Various: Pfizer.pfizer.com. (2020). Available online at: http://labeling.pfizer.com/ShowLabeling.aspx?format=PDF&id=959 (accessed July 29, 2020).
84. Clowse ME, Feldman SR, Isaacs JD, Kimball AB, Strand V, Warren RB, et al. Pregnancy outcomes in the tofacitinib safety databases for rheumatoid arthritis and psoriasis. Drug Saf. (2016) 39:755–62. doi: 10.1007/s40264-016-0431-z
85. van der Heijde D, Ramiro S, Landewe R, Baraliakos X, Van den Bosch F, Sepriano A, et al. 2016 update of the ASAS-EULAR management recommendations for axial spondyloarthritis. Ann Rheum Dis. (2017) 76:978–91. doi: 10.1136/annrheumdis-2016-210770
86. Cortese A, Lucchetti R, Altobelli A, Conte A, Primavera M, Valesini G, et al. Secukinumab may be a valid treatment option in patients with CNS demyelination and concurrent ankylosing spondylitis: report of two clinical cases. Mult Scler Relat Disord. (2019) 35:193–5. doi: 10.1016/j.msard.2019.08.006
87. Baraliakos X, Haibel H, Listing J, Sieper J, Braun J. Continuous long-term anti-TNF therapy does not lead to an increase in the rate of new bone formation over 8 years in patients with ankylosing spondylitis. Ann Rheum Dis. (2014) 73:710–5. doi: 10.1136/annrheumdis-2012-202698
Keywords: JAK – STAT signalling pathway, small molecule inhibitor, axial spondyloarthritis, preclinical efficacy and tolerability, safety profile
Citation: Hammitzsch A, Lorenz G and Moog P (2020) Impact of Janus Kinase Inhibition on the Treatment of Axial Spondyloarthropathies. Front. Immunol. 11:591176. doi: 10.3389/fimmu.2020.591176
Received: 03 August 2020; Accepted: 07 September 2020;
Published: 21 October 2020.
Edited by:
Matteo Vecellio, University of Oxford, United KingdomReviewed by:
Satoshi Kubo, University of Occupational and Environmental Health Japan, JapanKeisuke Maeshima, Oita University, Japan
Copyright © 2020 Hammitzsch, Lorenz and Moog. This is an open-access article distributed under the terms of the Creative Commons Attribution License (CC BY). The use, distribution or reproduction in other forums is permitted, provided the original author(s) and the copyright owner(s) are credited and that the original publication in this journal is cited, in accordance with accepted academic practice. No use, distribution or reproduction is permitted which does not comply with these terms.
*Correspondence: Ariane Hammitzsch, QXJpYW5lLkhhbW1pdHpzY2hAZ21haWwuY29t