- 1Department of Cardiology, Union Hospital, Tongji Medical College, Huazhong University of Science and Technology, Wuhan, China
- 2Key Lab of Molecular Biological Targeted Therapies of the Ministry of Education, Union Hospital, Tongji Medical College, Huazhong University of Science and Technology, Wuhan, China
The role of inflammation in atherosclerosis has been recognized several decades ago and existing treatments provide benefits in part through non-specific anti-inflammatory actions. Compared with other cytokines, interleukin-1β (IL-1β) is associated with acute and chronic inflammation. Anti-inflammatory therapy with canakinumab targeting the IL-1β innate immunity pathway could significantly reduce the rate of recurrent cardiovascular events than placebo. The results of CANTOS suggested an important role of IL-1β in atherosclerosis. However, there are numerous mechanisms that are to be clarified. We herein discussed the important immunomodulatory effect IL-1β exerts on atherosclerosis and the potential mechanisms underlying it. We also reviewed bench-to-bedside clinical translation of IL-1β neutralizing strategies associated with the use of IL-1β blockade in patients with atherosclerosis.
Introduction
The formation of atherosclerosis is affected by many risk factors. Among them, elevated levels of plasma LDL-cholesterol (LDL-C) is known to be a major one (1–3) However, even when LDL-C was reduced to a sufficiently low level by a PCSK-9 inhibitor, considerable residual cardiovascular risk persists (4–5). In recent years, there are increasing evidences suggesting that atherosclerosis is an active process akin to the chronic inflammatory process and that inflammation was present at all stages of atherosclerosis (6, 7). Lipid and inflammation are related to each other. The accumulation and oxidation of LDL-C at intimae will promote inflammation and drive atherosclerosis (8). Immune cell activation will also decrease cholesterol efflux and foam cell formation, thus influencing progression of atherosclerosis and emerging of complications (9, 10). Therefore, it is critical to break the network of inflammation during atherosclerosis. A large clinical trial, Canakinumab Anti-inflammatory Thrombosis Outcome Study (CANTOS) has proved the effectiveness of anti-inflammatory therapy in treating atherosclerosis (11) and demonstrated the important role of interleukin-1β (IL-1β) in promoting atherosclerosis. In light of the satisfying result produced by CANTOS, we aim to clarify the role of interleukin-1 (IL-1) and related signaling pathways in atherosclerosis. In addition, we reviewed anti-inflammatory treatment strategies that involved targeting IL-1 in animal and clinical research. In this review, we will discuss the choice of IL-1β or interleukin-1α (IL-1α) as a target and proper markers in clinical trials.
The Role of IL-1β in Atherosclerosis
Plenty of evidence has shown the important effect IL-1β exerts on atherosclerosis (12, 13). First of all, the protein and mRNA levels of IL-1β in atherosclerosis patients increased significantly compared with normal subjects; the levels are also positively correlated with the severity of the disease (14, 15). In addition, the variation of IL-1 gene families is also related to coronary heart disease (CHD) (16–18). Moreover, the increased susceptibility of atherosclerosis associated with the presence of clonal hematopoiesis in peripheral-blood cells was regulated at least in part by the NLRP3/IL-1β pathway (19, 20).
Synthesis of IL-1β
IL-1β is synthesized predominantly by monocytes, macrophages and dendritic cells (21), and the synthesis of IL-1β can be divided into several steps. At first, IL-1β precursor (pro-IL-1β) and NLRP3 were transcribed, translated and synthesized. This happens when there is a pro-inflammatory signal that activate pattern recognition receptors (PRR), or when IL-1α and IL-1β bind to IL-1 receptor(IL-1R) to form a positive feedback loop (9, 21). The pro-inflammatory signals include cholesterol crystals or oxidized low density lipoprotein(ox-LDL) accumulated under the intimae. Next, NLRP3 is activated by cholesterol crystals engulfed by macrophages, ox-LDL or efflux of potassium. Activated NLRP3 then recruits the adaptor protein, ASC (apoptosis-associated speck-like protein containing a caspase recruitment domain [CARD]) to ligate NLRP3 and pro-caspase-1, facilitating the latter to be hydrolyzed into active caspase-1 (9, 22). ASC is composed of two death domains: an N-terminal PYD and a C-terminal CARD. The PYD domain can be linked to NLRP3, and CARD can recruit caspase-1 (23). Finally, pro-IL-1β was hydrolyzed into the mature and active IL-1β by caspase-1. Notably, caspase-1 exists in atherosclerotic plaques in humans (24). Sometimes, the production of IL-1β was not dependent on the hydrolytic process catalyzed by caspase-1. It could also be triggered by other enzymes such as neutrophil proteinase 3 (PR3) and matrix metalloprotease 9(MMP9) (25, 26).
IL-1R Signal Transduction
The process of IL-1R family signal transduction could also be divided into several steps and part of them were summarized in Figure 1. After the binding of IL-1 receptor with their ligands, IL-1β, IL-1R type I (IL-1R1) and IL-1R accessory protein (IL-1Racp) form heterotrimers (27). Meanwhile, the Toll- and IL-1R-like (TIR) domains existed on the receptor IL-1R1 and co-receptor IL-1Racp form heterodimers (28, 29). Oligomerized TIR recruits adaptor molecule, namely, myeloid differentiation primary response gene 88 (MyD88), to form a receptor complex with a high affinity, which can recruit downstream signaling molecules (30). The signal can be transducted via cytoplasmic kinase, like IL-1R associated kinase(IRAKs), and other adaptors, like TNF receptor associated factor 6 (TRAF 6) (31, 32). If the signal was transducted via the NF-κB pathway, the last step would be the phosphorylation of the inhibitor IκB by the IκB kinase, and the resultant release of the transcription factor NF-κB (33).
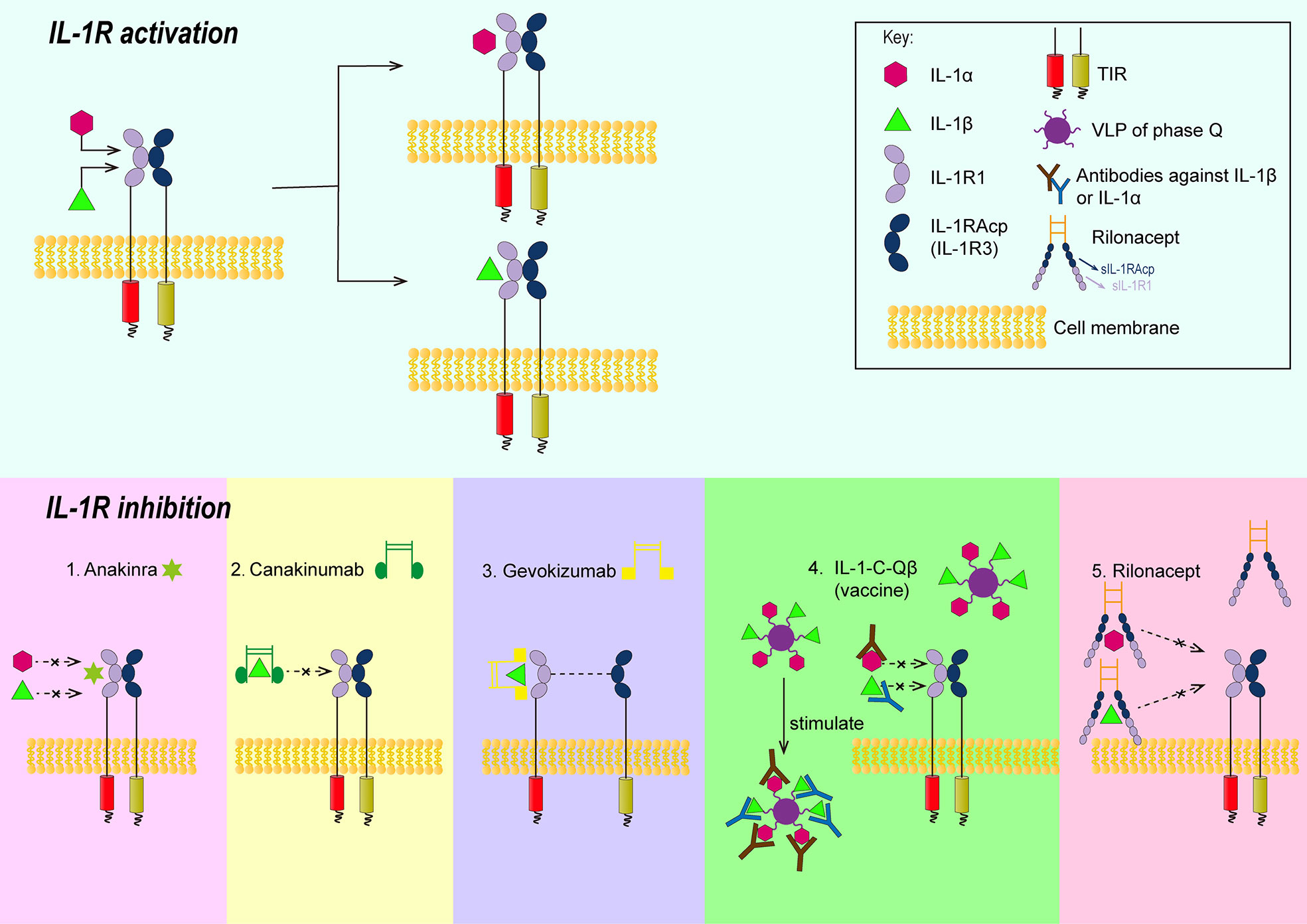
Figure 1 Schematic illustrations showing the approaches of controlling signal transduction of IL-1β. 1. Anakinra can competitively bind with IL-1R1 against IL-1β or IL-1α; 2. The Fab segment of canakinumab overlapped the D1 region of IL-1R1 when canakinumab bind to IL-1β, so that IL-1 βcould not bind to IL-1R1; 3. gevokizumab occupies the allosteric site of IL-1 and reduces the affinity of the complex to IL-1Racp and IL-1R1; 4. IL-1-C-Qβ(vaccine) produces polyclonal antibodies to neutralize IL-1; 5. Extracellular regions of IL-1R1 and IL-1Racp form rilonacept’s two arms that could trap IL-1. IL-1R, IL-1 receptor; TIR, the Toll-and IL-1R-like domains; VLP, virus like particles; IL-1R1, type II L-1R; IL-1 Racp, IL-1R accessory protein.
A series of phosphorylation and ubiquitination after the binding of IL-1β to IL-1R1 activate signal pathways such as NF-κB, JNK, p38 MAPK and induce expressions of genes, such as IL-6, IL-8, MCP-1, COX-2, IL-1α, IL-1β (34). Among them, NF-κB is an important one. Under normal conditions, the complex that consists of inhibiting protein IκBα and NF-κB heterodimer constituted by RelA and p50 exists in the cytoplasm. After the activation of inflammatory signals, IKB kinase (IKK) phosphorylates IκBα protein, leading to the ubiquitination of the latter, and the separation of IκBα from NF-κB. NF-κB is thus activated and translocates into the nucleus, then binds with specific DNA sequences. Next, the DNA/NF-κB complex recruits other proteins, and increases the transcription and translation of inflammatory mediator genes (35).
The Effect of IL-1β on Atherosclerosis
IL-1β has multiple effects in all stages of atherosclerosis (12, 36). IL-1β induces an inflammatory response in endothelial cells, as reflected by increased expressions of adhesion factors and chemokines, and promotes the accumulation of inflammatory cells in blood vessels and their invasion into the local intima of blood vessels, which often happens at the initiation of atherosclerosis (37). In general, adhesion molecules include intercellular inflammatory cytokines (ICAM-1), vascular cell molecule (VCAM-1), chemokines include monocyte chemoattractant protein (MCP-1). Among them, MCP-1 could recruit mononuclear phagocytes and is closely related to atherosclerosis. IL-1 also stimulates the proliferation, differentiation of vascular smooth muscle cells, the activation of monocytes, macrophages and the secretion of various inflammatory mediators (38). IL-1β promotes the gene expression of a variety of inflammatory mediators: the enhanced expression of IL-1 itself forms a positive feedback loop; the induced cyclooxygenase-2 (COX-2) formation leads to production of prostaglandin; the generation of IL-6 and matrix metalloproteinase (MMP) can also be induced by IL-1β (39–41). IL-6 mediates the acute phase response, increasing the reactants in the acute phase, including C-reactive protein(CRP), fibrinogen and plasminogen activator inhibitors, which are closely related to the formation of atherosclerotic thrombosis (39–41). MMP1, MMP8, and MMP13 are also known as collagenase. They are closely related to the rupture of atherosclerotic fibrous cap plaque due to their characteristic of breaking down collagen (42). In addition, this cytokine can impair the contractility of myocardial cells and exacerbate post-infarction reperfusion injury (43). Notably, IL-1β plays a vital role in the growth of established atheroma. In experimental atherosclerosis, it has been proved that the selective neutralization of IL-1β promotes monocytes to switch to a less inflammatory state in plasma, elicits higher plasma level of IL-10, and lessens atherosclerosis plaque size without limitation of compensatory outward remodeling in the artery (44).
Choice of IL-1α or L-1β to Target in Treating Atherosclerosis
IL-1α and IL-1β bind to the same receptor (IL-1R1) and therefore have similar downstream biological characteristics. However, they are different in terms of their cellular source, maturation requirements and release, which affect their impact on inflammation (45). Now that IL-1α and IL-1β both induce an inflammatory response by binding to IL-1R1, and have similar biological characteristics, what happens if IL-1R was both blocked? A study examining the effect of IL-1R1 deficiency on diet-induced atherosclerosis in ApoE−/− mice showed a reduction in the size of atherosclerotic lesions with signs of plaque instability (46). Moreover, a Mendelian randomization analysis (human genetic data) suggests that genetic upregulation of the interleukin 1 receptor antagonist could increase cardiovascular risk in the long run (47). Therefore, we hypothesized that blocking both IL-1α and IL-1β would have more side effects than benefits.
As a member of the IL-1 family proteins, IL-1α is constitutively expressed by many non-immune cells and is also expressed by immune cells in an inflammatory environment (48). IL-1α precursors are released upon necrosis and injury of the cells that express IL-1α. Unlike IL-1β, IL-1α precursors also have pro-inflammatory activity. IL-1α is also associated with atherosclerosis (49–51). However, there are some negative evidence. In a small clinical trial, Xilonix, an IL-1α–targeted monoclonal antibody, was used in patients with femoral artery stenosis and was not significantly associated with a trend toward patency during three months of intravenous administration (52). In addition, IL-1α is the primary mediator of an IL-1R1–dependent and protective innate immune response against mycobacterium tuberculosis in mice; the mice with IL-1α block was more prone to infections than those with IL-1β block (53). Besides, antibodies against IL-1α impair outward vessel remodeling during early atherogenesis in Apoe−/− mice fed a western diet, which could be a risk of plaque rupture in human. And the potential mechanism may well be that inhibition of IL-1α might decrease the production of MMP3 and SMC chemotaxis (44). Furthermore, atherosclerotic-associated inflammatory stimulation can induce inflammasomes that process IL-1β instead of IL-1α (12). Overall, IL-1α is a potential target for the treatment of atherosclerosis, although the target is not perfect.
Inhibition of IL-1β and Randomized Clinical Trials
The role of IL-1β is tightly correlated with its signal transduction. Thus, inhibition of its signal transduction might be a way to alleviate atherosclerosis. There are several molecules that can inhibit the signal transduction of IL-1β (45): 1) IL-1Ra. IL-1Ra can competitively bind with IL-1R1 against IL-1β or IL-1α. The antagonist can tightly bind to IL-1R1 but is unable to recruit IL-1Racp (IL-1R3) to form TIR dimers. Thus it cannot mediate corresponding intracellular signaling. 2) IL-1R2. IL-1R2 is a decoy receptor that binds to IL-1α or IL-1β and can recruit IL-1R3, which is structurally similar to IL-1R1 but has no intracellular domain and was therefore unable to form TIR dimers. 3) Soluble receptors. Soluble receptors were also unable to mediate intracellular signaling, although there is no mature bait receptor or soluble receptor at present. In addition to them, there are animal experiments and clinical trials demonstrating drugs targeting IL-1β in the treatment of atherosclerosis. The drugs involve Anakinra, monoclonal antibodies, vaccines, rilonacept and were summarized in Figure 1.
Endogenous Interleukin-1 Receptor Antagonist
Endogenous IL-1Ra is widely distributed and exhibits two forms, namely intracellular form and secretory form. The role of IL-1Ra in atherosclerosis is salutary in various animal experiments. In a study involving the overexpression or knockout of IL-1Ra in ldlr−/− animal models, IL-1Ra knockout in C57BL/6J mice have been shown to increase plasma lipoprotein levels, when these animals were fed with a high-cholesterol/high-fat diet containing cholate; while a reduced size of lesions composed of foam cells was observed with the same chow (54). Similar results were observed in ApoE−/− mice, as reflected by a significant increase of 30% of the atherosclerotic plaque in 16-week-old IL-1Ra+/− mice fed with a normal diet compared with their IL-1Ra−/− counterparts (55). Similar results were also observed in 32-week-old IL-1Ra+/− mice with a tendency of plaque instability, as reflected by increased macrophage accumulation and decreased SMC content (55). Studies have shown that IL-1Ra knockout mice will develop lethal arterial inflammation with a large number of CD4+T cells, macrophages, and neutrophils infiltrating in the lesion area (56, 57). These results suggest that IL-1Ra plays an important role in inhibiting the development of lesions in the early stage of atherosclerosis, is involved in the regulation of plaque composition in the late stage, and is associated with complications such as plaque rupture and subsequent thrombosis. However, human genetic data is inconsistent with the above animal studies, which suggests that an inherited increase in IL-1Ra is associated with an increased cardiovascular risk (47). Moreover, the level of IL-1Ra is positively correlated with cardiovascular risk, although this is most likely the result of elevated IL-1 levels in an inflammatory state, which means that the improvement in IL-1Ra is the result rather than the cause (58). This issue is rather complicated and is needed more effort to expound.
Recombinant IL-1 Receptor Antagonist
Anakinra is a recombinant human interleukin receptor antagonist that can antagonize IL-1α and IL-1β. It is usually used as a second-line therapy after the failure of diseases-modifying antirheumatic drug (DMARD) (59). It can also be used together with DMARD. Clinical trials have been carried out to investigate the effect of anakinra in acute coronary syndrome. In a phase II double-blind, randomized, placebo-controlled study enrolling 182 patients with non-ST-segment elevation acute coronary syndrome (NSTE-ACS) within 48 hours after onset of chest pain (60), daily subcutaneous injections of anakinra or placebo were administered for 14 days. During the 14 days’ treatment, CRP and IL-6 levels were significantly reduced in the anakinra group initially and returned to levels higher than those in the placebo group after 30 days. In another phase II/III, randomized, double-blind clinical trial named Virginia Commonwealth University Anakinra Remodeling Trial 3(VCUART3), anakinra was used in patients with ST-segment elevation myocardial infarction (STEMI) within 12 hours after the onset of chest pain (61). The primary endpoint of this trial was CRP levels 14 days after admission. Delightfully, in VCUART3, hsCRP, mortality, new-onset heart failure or death and hospitalization related to heart failure was significantly lower in anakinra-treated patients than in the placebo group during the 14 days of treatment. The results suggest that interleukin-1 blockers significantly reduced the acute inflammatory response in patients with ST-segment elevation myocardial infarction, and that the reduction in inflammatory signals was associated with a significant reduction in cardiovascular events. Anakinra well inhibits IL-1 signaling and thus alleviates the disease, but it is not suitable for chronic disease management due to its frequent usage. The emergence of monoclonal antibodies will solve this problem.
Monoclonal Antibodies
Monoclonal antibodies (Canakinumab or Gevokizumab) can selectively target IL-1β without affecting other cytokines in the IL-1 family, reducing the risks of drug adverse effects, including infections. Canakinumab promotes the formation of an antigen-antibody complex by binding specifically to IL-1β. The complex prevents IL-1β from binding to IL-1R1 and thus blocks IL-1 signaling. This process is not directly related to IL-1Racp. By using Protein Data Bank (PDB), the structural data of IL-1R1 and IL-1β were used for modeling; it was found that after the binding of canakinumab to IL-1β, the Fab segment of the monoclonal antibody overlapped the D1 region of IL-1R1 significantly, so that IL-1β could not bind to the receptor again. Gevokizumab, another monoclonal antibody for IL-1β, is under active study. Unlike canakinumab, it occupies the allosteric site of IL-1 and reduces the affinity of the complex to IL-1Racp and IL-1R1 (62).The effect of gevokizumab has also been tested in animal studies which had a sound result (63, 64). It is worth mentioning that Gevokizumab is being used in cancer research and canakinumab was noticed to be salutary in cancer therapy in CANTOS.
Canakinumab has been approved for the treatment of cryopyrin-associated induction (CAPS) in 2009 by the U.S. Food and Drug Administration (FDA) (65). The antibody was approved in 2016 for three types of spontaneous inflammatory diseases: tumor necrosis factor receptor associated periodic syndrome (TRAPS), hyperimmunoglobulin D syndrome (HIDS)/mevalonate kinase (MKD), and familial Mediterranean fever (FMF) (66). It was also approved for active Still’s disease in 2020 (67). In terms of clinical results, CANTOS presented at the Annual Meeting of the European Association for Cardiology in 2017 finally corroborated the inflammation hypothesis. In this clinical trial, 10061 volunteers with CRP > 2 mg/L after previous second-line preventive treatment of myocardial infarction were randomly divided into a placebo group or canakinumab treatment groups of 50 mg/150 mg/300 mg dosage. The patients were injected subcutaneously once every quarter. Patients treated with 150 mg canakinumab had a 15% lower incidence of primary clinical end events, including non-fatal myocardial infarction, non-fatal stroke, and cardiovascular death, with somewhat increased infections, compared with those in the placebo group. In a pre-specified secondary analysis of trial results, the compound primary endpoint was reduced by 25%, while all-cause and cardiovascular mortality was reduced by 31% in patients treated with canakinumab (68). Even though the monoclonal antibody groups have higher incidence of fatal infection (incidence rate, 0.31 vs. 0.18 events per 100 person-years; P=0.02), there is no significance in all-cause mortality. This is most likely because the inflammatory symptoms are masked after IL-1 blockade and diagnosis is delayed.
Surprisingly, canakinumab reduced inflammation but led to the development of plaque instability in Apoe−/− Mice (69). Although IL-1β appears to have a protective effect against atherosclerosis here, there are several issues that need be taken into account. First of all, there are often inconsistent results between animal experiments and clinical trials for targeted drugs, due to the inconsistency in the physiological and pathological process between animal models and human disease. In experiments involving non-high-fat or restrictive high-fat diet, it can often be proved that IL-1β signaling pathway could promote atherosclerosis (70). Second, it can be inferred from the earlier animal experiments on IL-1Ra that IL-1β plays a more important role in early stages of atherosclerosis, while the animal model here is in a late stage (55, 70, 71). Thirdly, in this experiment, macrophages were shown to polarize towards the M2 phenotype. In addition, when SMC-specific IL1r1 gene is knocked out in this experiment, the atherosclerotic plaque is reduced; it is unavoidable for various cells to be reduced during this process, and SMC reduction is not necessarily a bad sign. Thus, the role of IL-1β in atherosclerosis is still believed to be detrimental in anti-inflammatory treatment, in addition to CANTOS, other off-target therapies have also been tried. Take statins for example, which is typically used to reduce low-density lipoprotein cholesterol (LDL-C) levels and has been proved that consistently reduced vascular inflammation (72). Of note, the use of stains may promotes the NLRP3 and type I interferon pathways and both are considered to be proatherosclerotic (73, 74). Recent research show evidence that colchicine may reduce the cardiovascular risk in patients with chronic coronary disease (75), but the evidence is weak and the side effects is significant, especially in gastrointestinal system. Apart from that, compared with off-target therapies, specificity and lack of effect on lipids give targeting IL-1 therapies more specificities and less side effects.
IL-1 Vaccines
IL-1 vaccine could be produced when IL-1 was transformed into a kind of antigen with a cysteine at the carboxyl end, and virus like particles(VLP) of phage Q was used as carriers. The carriers could be cross-linked with IL-1β or IL-1α to form IL-1β-C-VLP/IL-1β-C-Qβ or IL-1α-C-VLP/IL-1α-C-Qβ vaccines, respectively (76). This method can overcome the immune tolerance to autoantigens without adjuvant usage, which is a major difference compared to previous vaccine-producing methods (77, 78). The polyclonal antibodies produced can block the binding of IL-1β to IL-1R1. Currently, such vaccines have been used in several animal models of cardiovascular disease (79, 80). The advantage of vaccines is their inexpensiveness compared to monoclonal antibodies, which might lead to better patient compliance.
IL-1 Inhibitor
In addition to the abovementioned molecules, IL-1 inhibitor might also be a potential treatment method for atherosclerosis. Rilonacept, also known as IL-1 trap, is an IL-1 inhibitor. It is an antibodily-like dimer protein with two arms composed of the ligand-binding regions on the extracellular regions of IL-1R1 and IL-1Racp. The two arms are linked by Fc segments and can neutralize IL-1 (81).
Summary
Countless researchers have made great efforts in the investigations of cardiovascular inflammation and there are abundant lessons that we can learn from them. First of all, better simulation of human disease characteristics in animal models requires our joint efforts. Second, different stages of atherosclerosis have different characteristics, only a grasp of their different characteristics can lead to a better treatment. Thirdly, from the perspective of the failed clinical trials, considering hsCRP as an inclusion criterion for anti-inflammatory therapy may lead to a better effect observed in anti-inflammatory therapy, and regarding FDG-PET as a quantitative indicator of vascular inflammation may result in a better observation of the vasculature. Hence, finding more effective markers of inflammation is also something we need to work on. In addition, lipid-lowering and anti-inflammatory therapies are not antithetical to each other, and that the best use of these two weapons will lead to a brighter future for the treatment of atherosclerosis. Finally, bioinformatics is booming recently, however, the room for improvement in applying bioinformatic knowledge in cardiovascular field is much larger compared with that in oncology. Nevertheless, efforts are still needed to develop cardiovascular proprietary bioinformatics databases.
Author Contributions
All authors listed have made a substantial, direct, and intellectual contribution to the work and approved it for publication.
Conflict of Interest
The authors declare that the research was conducted in the absence of any commercial or financial relationships that could be construed as a potential conflict of interest.
References
1. Weber C, Noels H. Atherosclerosis: current pathogenesis and therapeutic options. Nat Med (2011) 17:1410–22. doi: 10.1038/nm.2538
2. Goldstein JL. Brown MS. A century of cholesterol and coronaries: from plaques to genes to statins. Cell (2015) 161:161–72. doi: 10.1016/j.cell.2015.01.036
3. Ference BA, Ginsberg HN, Graham I, Ray KK, Packard CJ, Bruckert E, et al. Low-density lipoproteins cause atherosclerotic cardiovascular disease. 1. Evidence from genetic, epidemiologic, and clinical studies. A consensus statement from the European Atherosclerosis Society Consensus Panel. Eur Heart J (2017) 38:2459–72. doi: 10.1093/eurheartj/ehx144
4. Pradhan AD, Aday AW, Rose LM, Ridker PM. Residual Inflammatory Risk on Treatment With PCSK9 Inhibition and Statin Therapy. Circulation (2018) 138:141–9. doi: 10.1161/CIRCULATIONAHA.118.034645
5. Bohula EA, Giugliano RP, Leiter LA, Verma S, Park JG, Sever PS, et al. Inflammatory and Cholesterol Risk in the FOURIER Trial. Circulation (2018) 138:131–40. doi: 10.1161/CIRCULATIONAHA.118.034032
6. Libby P. Inflammation in atherosclerosis. Arterioscler Thromb Vasc Biol (2012) 32:2045–51. doi: 10.1161/ATVBAHA.108.179705
7. Taleb S. Inflammation in atherosclerosis. Arch Cardiovasc Dis (2016) 109:708–15. doi: 10.1016/j.acvd.2016.04.002
8. Tunon J, Back M, Badimon L, Bochaton-Piallat ML, Cariou B, Daemen MJ, et al. Interplay between hypercholesterolaemia and inflammation in atherosclerosis: Translating experimental targets into clinical practice. Eur J Prev Cardiol (2018) 25:948–55. doi: 10.1177/2047487318773384
9. Grebe A, Hoss F, Latz E. NLRP3 Inflammasome and the IL-1 Pathway in Atherosclerosis. Circ Res (2018) 122:1722–40. doi: 10.1161/CIRCRESAHA.118.311362
10. Tall AR, Yvan-Charvet L. Cholesterol, inflammation and innate immunity. Nat Rev Immunol (2015) 15:104–16. doi: 10.1038/nri3793
11. Ridker PM, Everett BM, Thuren T, MacFadyen JG, Chang WH, Ballantyne C, et al. Antiinflammatory Therapy with Canakinumab for Atherosclerotic Disease. N Engl J Med (2017) 377:1119–31. doi: 10.1056/NEJMoa1707914
12. Libby P. Interleukin-1 Beta as a Target for Atherosclerosis Therapy: Biological Basis of CANTOS and Beyond. J Am Coll Cardiol (2017) 70:2278–89. doi: 10.1016/j.jacc.2017.09.028
13. Kirii H, Niwa T, Yamada Y, Wada H, Saito K, Iwakura Y, et al. Lack of interleukin-1beta decreases the severity of atherosclerosis in ApoE-deficient mice. Arterioscler Thromb Vasc Biol (2003) 23:656–60. doi: 10.1161/01.ATV.0000064374.15232.C3
14. Galea J, Armstrong J, Gadsdon P, Holden H, Francis SE, Holt CM. Interleukin-1 beta in coronary arteries of patients with ischemic heart disease. Arterioscler Thromb Vasc Biol (1996) 16:1000–6. doi: 10.1161/01.atv.16.8.1000
15. Dewberry R, Holden H, Crossman D, Francis S. Interleukin-1 receptor antagonist expression in human endothelial cells and atherosclerosis. Arterioscler Thromb Vasc Biol (2000) 20:2394–400. doi: 10.1161/01.atv.20.11.2394
16. Vohnout B, Di Castelnuovo A, Trotta R, D’Orazi A, Panniteri G, Montali A, et al. Interleukin-1 gene cluster polymorphisms and risk of coronary artery disease. Haematologica (2003) 88:54–60. doi: 10.3324/%25x
17. Francis SE, Camp NJ, Dewberry RM, Gunn J, Syrris P, Carter ND, et al. Interleukin-1 receptor antagonist gene polymorphism and coronary artery disease. Circulation (1999) 99:861–6. doi: 10.1161/01.cir.99.7.861
18. Tsimikas S, Duff GW, Berger PB, Rogus J, Huttner K, Clopton P, et al. Pro-inflammatory interleukin-1 genotypes potentiate the risk of coronary artery disease and cardiovascular events mediated by oxidized phospholipids and lipoprotein(a). J Am Coll Cardiol (2014) 63:1724–34. doi: 10.1016/j.jacc.2013.12.030
19. Jaiswal S, Natarajan P, Silver AJ, Gibson CJ, Bick AG, Shvartz E, et al. Clonal Hematopoiesis and Risk of Atherosclerotic Cardiovascular Disease. N Engl J Med (2017) 377:111–21. doi: 10.1056/NEJMoa1701719
20. Fuster JJ, MacLauchlan S, Zuriaga MA, Polackal MN, Ostriker AC, Chakraborty R, et al. Clonal hematopoiesis associated with TET2 deficiency accelerates atherosclerosis development in mice. Science (2017) 355:842–7. doi: 10.1126/science.aag1381
21. Weber A, Wasiliew P, Kracht M. Interleukin-1beta (IL-1beta) processing pathway. Sci Signal (2010) 3:m2. doi: 10.1126/scisignal.3105cm2
22. Latz E, Xiao TS, Stutz A. Activation and regulation of the inflammasomes. Nat Rev Immunol (2013) 13:397–411. doi: 10.1038/nri3452
23. Srinivasula SM, Poyet JL, Razmara M, Datta P, Zhang Z, Alnemri ES. The PYRIN-CARD protein ASC is an activating adaptor for caspase-1. J Biol Chem (2002) 277:21119–22. doi: 10.1074/jbc.C200179200
24. Geng YJ, Libby P. Evidence for apoptosis in advanced human atheroma. Colocalization with interleukin-1 beta-converting enzyme. Am J Pathol (1995) 147:251–66.
25. Joosten LA, Netea MG, Fantuzzi G, Koenders MI, Helsen MM, Sparrer H, et al. Inflammatory arthritis in caspase 1 gene-deficient mice: contribution of proteinase 3 to caspase 1-independent production of bioactive interleukin-1beta. Arthritis Rheum (2009) 60:3651–62. doi: 10.1002/art.25006
26. Coeshott C, Ohnemus C, Pilyavskaya A, Ross S, Wieczorek M, Kroona H, et al. Converting enzyme-independent release of tumor necrosis factor alpha and IL-1beta from a stimulated human monocytic cell line in the presence of activated neutrophils or purified proteinase 3. Proc Natl Acad Sci USA (1999) 96:6261–6. doi: 10.1073/pnas.96.11.6261
27. Casadio R, Frigimelica E, Bossu P, Neumann D, Martin MU, Tagliabue A, et al. Model of interaction of the IL-1 receptor accessory protein IL-1RAcP with the IL-1beta/IL-1R(I) complex. FEBS Lett (2001) 499:65–8. doi: 10.1016/s0014-5793(01)02515-7
28. Thomas C, Bazan JF, Garcia KC. Structure of the activating IL-1 receptor signaling complex. Nat Struct Mol Biol (2012) 19:455–7. doi: 10.1038/nsmb.2260
29. Radons J, Dove S, Neumann D, Altmann R, Botzki A, Martin MU, et al. The interleukin 1 (IL-1) receptor accessory protein Toll/IL-1 receptor domain: analysis of putative interaction sites in vitro mutagenesis and molecular modeling. J Biol Chem (2003) 278:49145–53. doi: 10.1074/jbc.M306077200
30. Brikos C, Wait R, Begum S, O’Neill LA, Saklatvala J. Mass spectrometric analysis of the endogenous type I interleukin-1 (IL-1) receptor signaling complex formed after IL-1 binding identifies IL-1RAcP, MyD88, and IRAK-4 as the stable components. Mol Cell Proteomics (2007) 6:1551–9. doi: 10.1074/mcp.M600455-MCP200
31. Cao Z, Henzel WJ, Gao X. IRAK: a kinase associated with the interleukin-1 receptor. Science (1996) 271:1128–31. doi: 10.1126/science.271.5252.1128
32. Cao Z, Xiong J, Takeuchi M, Kurama T, Goeddel DV. TRAF6 is a signal transducer for interleukin-1. Nature (1996) 383:443–6. doi: 10.1038/383443a0
33. Zandi E, Chen Y, Karin M. Direct phosphorylation of IkappaB by IKKalpha and IKKbeta: discrimination between free and NF-kappaB-bound substrate. Science (1998) 281:1360–3. doi: 10.1126/science.281.5381.1360
34. Weber A, Wasiliew P, Kracht M. Interleukin-1 (IL-1) pathway. Sci Signal (2010) 3:m1. doi: 10.1126/scisignal.3105cm2
35. Gilmore TD. The Rel/NF-kappaB signal transduction pathway: introduction. Oncogene (1999) 18:6842–4. doi: 10.1038/sj.onc.1203237
36. Abbate A, Van Tassell BW, Biondi-Zoccai GG. Blocking interleukin-1 as a novel therapeutic strategy for secondary prevention of cardiovascular events. Biodrugs (2012) 26:217–33. doi: 10.1007/BF03261881
37. Bevilacqua MP, Pober JS, Wheeler ME, Cotran RS, Gimbrone MJ. Interleukin-1 activation of vascular endothelium. Effects on procoagulant activity and leukocyte adhesion. Am J Pathol (1985) 121:394–403.
38. Libby P, Warner SJ, Friedman GB. Interleukin 1: a mitogen for human vascular smooth muscle cells that induces the release of growth-inhibitory prostanoids. J. Clin Invest (1988) 81:487–98. doi: 10.1172/JCI113346
39. Dinarello CA. Immunological and inflammatory functions of the interleukin-1 family. Annu Rev Immunol (2009) 27:519–50. doi: 10.1146/annurev.immunol.021908.132612
40. Loppnow H, Libby P. Proliferating or interleukin 1-activated human vascular smooth muscle cells secrete copious interleukin 6. J Clin Invest (1990) 85:731–8. doi: 10.1172/JCI114498
41. Beltrami-Moreira M, Vromman A, Sukhova GK, Folco EJ, Libby P. Redundancy of IL-1 Isoform Signaling and Its Implications for Arterial Remodeling. PloS One (2016) 11:e152474. doi: 10.1371/journal.pone.0152474
42. Libby P. Collagenases and cracks in the plaque. J Clin Invest (2013) 123:3201–3. doi: 10.1172/JCI67526
43. Suzuki K, Murtuza B, Smolenski RT, Sammut IA, Suzuki N, Kaneda Y, et al. Overexpression of interleukin-1 receptor antagonist provides cardioprotection against ischemia-reperfusion injury associated with reduction in apoptosis. Circulation (2001) 104:I303–8. doi: 10.1161/hc37t1.094871
44. Vromman A, Ruvkun V, Shvartz E, Wojtkiewicz G, Santos MG, Tesmenitsky Y, et al. Stage-dependent differential effects of interleukin-1 isoforms on experimental atherosclerosis. Eur Heart J (2019) 40:2482–91. doi: 10.1093/eurheartj/ehz008
45. Boraschi D, Italiani P, Weil S, Martin MU. The family of the interleukin-1 receptors. Immunol Rev (2018) 281:197–232. doi: 10.1111/imr.12606
46. Alexander MR, Moehle CW, Johnson JL, Yang Z, Lee JK, Jackson CL, et al. Genetic inactivation of IL-1 signaling enhances atherosclerotic plaque instability and reduces outward vessel remodeling in advanced atherosclerosis in mice. J Clin Invest (2012) 122:70–9. doi: 10.1172/JCI43713
47. The Interleukin 1 Genetics Consortium. Cardiometabolic effects of genetic upregulation of the interleukin 1 receptor antagonist: a Mendelian randomisation analysis. Lancet Diabetes Endocrinol (2015) 3:243–53. doi: 10.1016/S2213-8587(15)00034-0
48. Dinarello CA. Overview of the IL-1 family in innate inflammation and acquired immunity. Immunol Rev (2018) 281:8–27. doi: 10.1111/imr.12621
49. Freigang S, Ampenberger F, Weiss A, Kanneganti TD, Iwakura Y, Hersberger M, et al. Fatty acid-induced mitochondrial uncoupling elicits inflammasome-independent IL-1alpha and sterile vascular inflammation in atherosclerosis. Nat Immunol (2013) 14:1045–53. doi: 10.1038/ni.2704
50. Waehre T, Yndestad A, Smith C, Haug T, Tunheim SH, Gullestad L, et al. Increased expression of interleukin-1 in coronary artery disease with downregulatory effects of HMG-CoA reductase inhibitors. Circulation (2004) 109:1966–72. doi: 10.1161/01.CIR.0000125700.33637.B1
51. Gardner SE, Humphry M, Bennett MR, Clarke MC. Senescent Vascular Smooth Muscle Cells Drive Inflammation Through an Interleukin-1alpha-Dependent Senescence-Associated Secretory Phenotype. Arterioscler Thromb Vasc Biol (2015) 35:1963–74. doi: 10.1161/01.CIR.0000125700.33637.B1
52. El SH, Kerensky R, Stecher M, Mohanty P, Davies M. A randomized phase II study of Xilonix, a targeted therapy against interleukin 1alpha, for the prevention of superficial femoral artery restenosis after percutaneous revascularization. J Vasc Surg (2016) 63:133–41. doi: 10.1016/j.jvs.2015.08.069
53. Guler R, Parihar SP, Spohn G, Johansen P, Brombacher F, Bachmann MF. Blocking IL-1alpha but not IL-1beta increases susceptibility to chronic Mycobacterium tuberculosis infection in mice. Vaccine (2011) 29:1339–46. doi: 10.1016/j.vaccine.2010.10.045
54. Devlin CM, Kuriakose G, Hirsch E, Tabas I. Genetic alterations of IL-1 receptor antagonist in mice affect plasma cholesterol level and foam cell lesion size. Proc Natl Acad Sci USA (2002) 99:6280–5. doi: 10.1073/pnas.092324399
55. Isoda K, Sawada S, Ishigami N, Matsuki T, Miyazaki K, Kusuhara M, et al. Lack of interleukin-1 receptor antagonist modulates plaque composition in apolipoprotein E-deficient mice. Arterioscler Thromb Vasc Biol (2004) 24:1068–73. doi: 10.1161/01.ATV.0000127025.48140.a3
56. Merhi-Soussi F, Kwak BR, Magne D, Chadjichristos C, Berti M, Pelli G, et al. Interleukin-1 plays a major role in vascular inflammation and atherosclerosis in male apolipoprotein E-knockout mice. Cardiovasc Res (2005) 66:583–93. doi: 10.1016/j.cardiores.2005.01.008
57. Nicklin MJ, Hughes DE, Barton JL, Ure JM, Duff GW. Arterial inflammation in mice lacking the interleukin 1 receptor antagonist gene. J Exp Med (2000) 191:303–12. doi: 10.1084/jem.191.2.303
58. Herder C, de Las HGT, Carstensen-Kirberg M, Huth C, Zierer A, Wahl S, et al. Circulating Levels of Interleukin 1-Receptor Antagonist and Risk of Cardiovascular Disease: Meta-Analysis of Six Population-Based Cohorts. Arterioscler Thromb Vasc Biol (2017) 37:1222–7. doi: 10.1161/ATVBAHA.117.309307.
59. Ramirez J, Canete JD. Anakinra for the treatment of rheumatoid arthritis: a safety evaluation. Expert Opin Drug Saf (2018) 17:727–32. doi: 10.1080/14740338.2018.1486819
60. Morton AC, Rothman AM, Greenwood JP, Gunn J, Chase A, Clarke B, et al. The effect of interleukin-1 receptor antagonist therapy on markers of inflammation in non-ST elevation acute coronary syndromes: the MRC-ILA Heart Study. Eur Heart J (2015) 36:377–84. doi: 10.1093/eurheartj/ehu272
61. Abbate A, Trankle CR, Buckley LF, Lipinski MJ, Appleton D, Kadariya D, et al. Interleukin-1 Blockade Inhibits the Acute Inflammatory Response in Patients With ST-Segment-Elevation Myocardial Infarction. J Am Heart Assoc (2020) 9:e14941. doi: 10.1161/JAHA.119.014941
62. Blech M, Peter D, Fischer P, Bauer MM, Hafner M, Zeeb M, et al. One target-two different binding modes: structural insights into gevokizumab and canakinumab interactions to interleukin-1beta. J Mol Biol (2013) 425:94–111. doi: 10.1016/j.jmb.2012.09.021
63. Owyang AM, Issafras H, Corbin J, Ahluwalia K, Larsen P, Pongo E, et al. XOMA 052, a potent, high-affinity monoclonal antibody for the treatment of IL-1beta-mediated diseases. Mabs-Austin (2011) 3:49–60. doi: 10.4161/mabs.3.1.13989
64. Harouki N, Nicol L, Remy-Jouet I, Henry JP, Dumesnil A, Lejeune A, et al. The IL-1beta Antibody Gevokizumab Limits Cardiac Remodeling and Coronary Dysfunction in Rats With Heart Failure. JACC Basic Transl Sci (2017) 2:418–30. doi: 10.1016/j.jacbts.2017.06.005
66. 2.U.S. FOOD & DRUG Administration. Web site . Available at: https://www.fda.gov/media/103477/download/ (Accessed June 16 2020).
67. U.S. FOOD & DRUG Administration. FDA Web site. Available at: https://www.fda.gov/ (Accessed June 16 2020).
68. Ridker PM, MacFadyen JG, Everett BM, Libby P, Thuren T, Glynn RJ. Relationship of C-reactive protein reduction to cardiovascular event reduction following treatment with canakinumab: a secondary analysis from the CANTOS randomised controlled trial. LanceT (2018) 391:319–28. doi: 10.1016/S0140-6736(17)32814-3
69. Gomez D, Baylis RA, Durgin BG, Newman A, Alencar GF, Mahan S, et al. Interleukin-1beta has atheroprotective effects in advanced atherosclerotic lesions of mice. Nat Med (2018) 24:1418–29. doi: 10.1038/s41591-018-0124-5
70. Zhao TX, Mallat Z. Targeting the Immune System in Atherosclerosis: JACC State-of-the-Art Review. J Am Coll Cardiol (2019) 73:1691–706. doi: 10.1016/j.jacc.2018.12.083
71. Elhage R, Maret A, Pieraggi MT, Thiers JC, Arnal JF, Bayard F. Differential effects of interleukin-1 receptor antagonist and tumor necrosis factor binding protein on fatty-streak formation in apolipoprotein E-deficient mice. Circulation (1998) 97:242–4. doi: 10.1161/01.cir.97.3.242
72. Tawakol A, Fayad ZA, Mogg R, Alon A, Klimas MT, Dansky H, et al. Intensification of statin therapy results in a rapid reduction in atherosclerotic inflammation: results of a multicenter fluorodeoxyglucose-positron emission tomography/computed tomography feasibility study. J Am Coll Cardiol (2013) 62:909–17. doi: 10.1016/j.jacc.2013.04.066
73. Spann NJ, Glass CK. Sterols and oxysterols in immune cell function. Nat Immunol (2013) 14:893–900. doi: 10.1038/ni.2681
74. York AG, Williams KJ, Argus JP, Zhou QD, Brar G, Vergnes L, et al. Limiting Cholesterol Biosynthetic Flux Spontaneously Engages Type I IFN Signaling. Cell (2015) 163:1716–29. doi: 10.1002/eji.200737989
75. Nidorf SM, Fiolet A, Mosterd A, et al. Colchicine in Patients with Chronic Coronary Disease. N Engl J Med (2020) 383:1838–47. doi: 10.1056/NEJMoa2021372
76. Spohn G, Keller I, Beck M, Grest P, Jennings GT, Bachmann MF. Active immunization with IL-1 displayed on virus-like particles protects from autoimmune arthritis. Eur J Immunol (2008) 38:877–87. doi: 10.1002/eji.200737989
77. Bertin-Maghit SM, Capini CJ, Bessis N, Chomilier J, Muller S, Abbas A, et al. Improvement of collagen-induced arthritis by active immunization against murine IL-1beta peptides designed by molecular modelling. Vaccine (2005) 23:4228–35. doi: 10.1016/j.vaccine.2005.03.030
78. Svenson M, Hansen MB, Thomsen AR, Diamant M, Nansen A, Rieneck K, et al. Cytokine vaccination: neutralising IL-1alpha autoantibodies induced by immunisation with homologous IL-1alpha. J Immunol Methods (2000) 236:1–8. doi: 10.1016/s0022-1759(99)00232-x
79. Tissot AC, Spohn G, Jennings GT, Shamshiev A, Kurrer MO, Windak R, et al. A VLP-based vaccine against interleukin-1alpha protects mice from atherosclerosis. Eur J Immunol (2013) 43:716–22. doi: 10.1002/eji.201242687
80. Zhang Y, Yu XL, Zha J, Mao LZ, Chai JQ, Liu RT. Therapeutic vaccine against IL-1beta improved glucose control in a mouse model of type 2 diabetes. Life Sci (2018) 192:68–74. doi: 10.1016/j.lfs.2017.11.021
Keywords: IL-1β, atherosclerosis, clinical trials, immune system, inflammation, therapy
Citation: Mai W and Liao Y (2020) Targeting IL-1β in the Treatment of Atherosclerosis. Front. Immunol. 11:589654. doi: 10.3389/fimmu.2020.589654
Received: 31 July 2020; Accepted: 03 November 2020;
Published: 10 December 2020.
Edited by:
Annalisa Del Prete, University of Brescia, ItalyReviewed by:
Leo Buckley, Brigham and Women’s Hospital and Harvard Medical School, United StatesWolfgang Koenig, Technical University Munich, Germany
Copyright © 2020 Mai and Liao. This is an open-access article distributed under the terms of the Creative Commons Attribution License (CC BY). The use, distribution or reproduction in other forums is permitted, provided the original author(s) and the copyright owner(s) are credited and that the original publication in this journal is cited, in accordance with accepted academic practice. No use, distribution or reproduction is permitted which does not comply with these terms.
*Correspondence: Yuhua Liao, bGlhb3loMjdAMTYzLmNvbQ==