- 1III. Department of Internal Medicine, University Medical Center Hamburg-Eppendorf, Hamburg, Germany
- 2Institute of Immunology, University Medical Center Hamburg-Eppendorf, Hamburg, Germany
- 3Institute of Pathology, University Medical Center Hamburg-Eppendorf, Hamburg, Germany
Minimal change disease (MCD) is a common cause of nephrotic syndrome. Treatment with steroids is usually effective, but frequent relapses are therapeutic challenges. The anti-CD20 antibody rituximab has shown promising results for treatment of steroid-sensitive nephrotic syndrome. Since predictive biomarkers for treatment efficacy and the accurate rituximab dosage for effective induction of remission are unknown, measurement of CD19+ B cells in blood is often used as marker of successful B cell depletion and treatment efficacy. A male patient with relapsing MCD was successfully treated with rituximab, but developed relapse of proteinuria 1 year later, although no B cells were detectable in his blood. B and T cell populations in the patient’s blood were analyzed before and after treatment with rituximab using FACS analysis. Rituximab binding to B and T cells were measured using Alexa Fluor 647 conjugated rituximab. We identified a population of CD20+ CD19− cells in the patient’s blood, which consisted mostly of CD20+ CD3+ T cells. Despite the absence of B cells in the blood, the patient was again treated with rituximab. He developed complete remission of proteinuria and depletion of CD20+ T cells. In a control patient with relapsing MCD initial treatment with rituximab led to depletion of both CD20+ B and T cells. Rituximab induces remission of proteinuria in patients with MCD even if circulating B cells are absent. CD20+ T cells may play a role in the pathogenesis of MCD and might be a promising treatment target in patients with MCD.
Introduction
Minimal change disease (MCD) is responsible for 10–25% of all cases of a nephrotic syndrome in adults (1). The exact pathomechanisms of MCD remain elusive. However, a circulating factor, most probably secreted by immune cells, is assumed to lead to effacement of podocyte foot processes, leakage of the glomerular filtration barrier, and development of a nephrotic syndrome (2). T cells have been suggested to contribute to the development of MCD (1). The role of the adaptive immune system in MCD is also supported by the presence of functionally impaired regulatory T cells in these patients (3, 4). In addition, the association of reduced regulatory T cells in patients with relapse of the disease (5–7) and the altered transcription regulators reported in B and T cells of MCD patients (8) suggest a role of the adaptive immune system in this disease. This assumed immune-mediated pathogenesis of MCD is the rationale why patients with this disease are treated with immunosuppressive drugs (1, 9).
Steroids lead to remission of proteinuria in 75–80% of adult MCD patients, but relapses occur in up to 56–76% of all cases (1, 10–12). Frequent relapses, steroid-dependence, or steroid-resistance require repeated courses of treatment (9). Thereby, high doses and long-term steroid treatments are needed, frequently leading to adverse effects and toxicity (1, 13). Therefore, alternative immunosuppressive treatments are applied in these patients, including alkylating agents, calcineurin inhibitors, mycophenolate mofetil, and rituximab (1, 10). Rituximab is a mouse-human chimeric anti-CD20 antibody, which induces direct cell death, complement dependent cytotoxicity, and antibody-dependent cell-mediated cytotoxicity in CD20 expressing cells (14, 15). The membrane protein CD20 is a B cell marker and is expressed in human B cells at different stages of their development (16, 17). On the other side, CD20 is not expressed on human podocytes (18). Depletion of CD20 expressing cells using rituximab has shown promising results in the treatment of MCD (10, 13, 19, 20), leading to the hypothesis that B cells have a pathogenetic role in MCD. Nonetheless, the precise mechanisms of action of rituximab in the treatment of MCD are unknown (9, 21). Measurement of CD19+ B cells in the blood is used to assess successful B cell depletion, but stable remission has been observed in some patients despite reconstitution of CD19+ B cells (22). Recently, the reconstitution of memory B cells but not total CD19+ B cells has been shown to correlate with a shorter time to MCD relapse (21). Deciphering the role of both B and T cells in MCD is an ongoing challenge in the understanding of the pathomechanisms of MCD.
Here, we present the case of a patient with MCD, who developed relapses of proteinuria and was successfully treated with rituximab, despite having no detectable CD19+ B cells in the peripheral blood. CD20+ T cells, which were present in the blood of this patient prior to rituximab treatment and were depleted afterwards, might account for the therapeutic impact of rituximab in this patient.
Material and Methods
Diagnostic Laboratory Results
Proteinuria was quantified by photometric measurement of urinary excreted protein in 24 h urine samples and the urinary protein excretion rate (PER; g/24 h) was calculated. Nephrotic-range proteinuria was defined as PER >3.5 g/24 h according to the Kidney Disease: Improving Global Outcomes (KDGIO) nomenclature (23).
Isolation of Peripheral Blood Mononuclear Cells (PBMC)
EDTA blood was obtained from the index MCD patient and a control MCD patient before and after rituximab treatment. Blood from a healthy donor was used as a control. PBMC were freshly isolated by Ficoll’s protocol and stored in liquid nitrogen until further use. Briefly, EDTA blood was diluted 1:3 in PBS and layered upon Biocoll separating solution 10 mM HEPES (Biochrom, Berlin, Germany). After gradient centrifugation at 1,000 g for 25 min at 20°C, the PBMC layer was carefully removed and washed twice with cold PBS. Lysis of erythrocytes was performed by hypotonic shock. Afterwards PBMC were stored until further use in 10% DMSO (Sigma Aldrich, St. Louis, MO, USA), 30% FCS (Thermo Fisher Scientific, Waltham, MA, USA), and 60% RPMI1640 (Thermo Fisher Scientific) in liquid nitrogen. The study was approved by the local ethics committee of the chamber of physicians in Hamburg (PV4806) and conducted in accordance with the ethical principles stated by the Declaration of Helsinki. An informed consent was obtained from patients and the healthy donor prior to study inclusion.
Conjugation of Rituximab
Rituximab was conjugated to the fluorophore Alexa Fluor 647 using the Alexa Fluor 647 Protein Labeling Kit (Thermo Fisher Scientific) according to the manufacturer’s instructions. Briefly, 450 µl of rituximab (1.6 mg/ml) were incubated with 50 µl of AF647 reaction dye and 50 µl sodium bicarbonate (1 M) at room temperature for 70 min. Afterwards, matrix column purification was performed to separate excess fluorochrome from labelled rituximab molecules (RTX-AF647). RTX-AF647 was stored at 4°C until use. For all FACS experiments, RTX-AF647 was used at a final dilution of 1:100 in PBS.
FACS Analysis
All FACS measurements were conducted using a FACS Celesta (BD Biosciences, Franklin Lakes, NJ, USA). PBMC were thawed rapidly at 37°C. After washing with cold PBS, cells were counted using a Neubauer counting chamber and diluted to desired concentrations. For FACS analysis, PBMC were blocked with human serum for 30 min at 4°C and incubated with a pre-titrated antibody cocktail including RTX-AF647 as well as AF750 (Thermo Fisher Scientific), for live-dead staining. For FACS analysis, in each sample at least 200,000 cells were regularly acquired. If not otherwise indicated, all antibodies were obtained from BioLegend (San Diego, CA, USA). The following antibodies and fluorophores were used: V450 anti-CD27 (clone: M-T271), V500 anti-IgD (clone: IA6-2), BV650 anti-CD3 (clone: OKT3), BV785 anti-CD45 (clone: HI30), FITC anti-CD38 (clone: HIT2; BD BioSciences), PE anti-IgM (clone: MHM-88), PerCP anti-CD4 (clone: L200), PE-Cy7 anti-CD19 (clone: HIB19), AF700 anti-CD8a (clone: HIT8a). PBMC were washed twice and measured using a FACS Celesta (BD BioSciences). Graphical analysis was performed using FlowJo version 10.6.1 (FlowJo, Ashland, OR, USA). Of note, in contour plots not all cells are depicted as single dots. For gating strategy see supplemental information (Figure S1).
Histology and Immunohistochemistry
PAS staining and electron microscopy were performed following standard protocols. For immunohistochemical staining for IgG and CD20, 1–2 µm thin slices from formalin-fixed, paraffin-embedded renal biopsies were deparaffinized and pretreated for 15 min at pH6 and 117°C in the autoclave (for CD20) or with proteinase (protease P-8038, Sigma-Aldrich, St. Louis, MO, USA) at 40°C for 15 min (for IgG) followed by incubation with normal serum (Vector S2000, CA, USA) for 10 min. Primary antibodies for IgG (1:7,500) (mouse monoclonal antibody, 209-005-088, Dianova, Hamburg, Germany) and CD20 (1:2,000) (DAKO M0755, CA, USA) were added for 30 min at 40°C. Bound antibodies were then visualized manually using a standard APAAP protocol.
Results
Clinical Case
A 70-year-old male patient presented at our outpatient clinic with nephrotic syndrome. A kidney biopsy revealed the diagnosis MCD (Figure 1). Treatment with steroids resulted in complete remission of proteinuria. However, the patient developed frequent relapses. The first relapse appeared a few months after the initial treatment, while steroids were being withdrawn. Because the patient also had diabetes mellitus type 2 and severe osteoporosis, re-treatment with steroids had to be avoided. He was alternatively treated with rituximab and developed complete remission of proteinuria. In the next 8 years the patient had eight relapses of the nephrotic syndrome, which were all successfully treated with rituximab and the patient went again into complete remission. One year after the last treatment with rituximab, the patient presented again in our outpatient clinic with a relapse of nephrotic syndrome and proteinuria of 8.3 g/24 h. Peripheral blood CD19+ B cells were still depleted with a cell count of 1 B cell/µl (normal range 80–500 B cells/µl). Serum creatinine and total leukocyte count in the peripheral blood were within the normal range (1.1 mg/dl and 4,500 cells/µl, respectively). The patient was treated with 1 g rituximab, combined with 60 mg/day prednisolone for 3 days, which was tapered over a time period of 4 weeks, leading to a complete remission of proteinuria within 21 days (proteinuria 0.3 g/24 h).
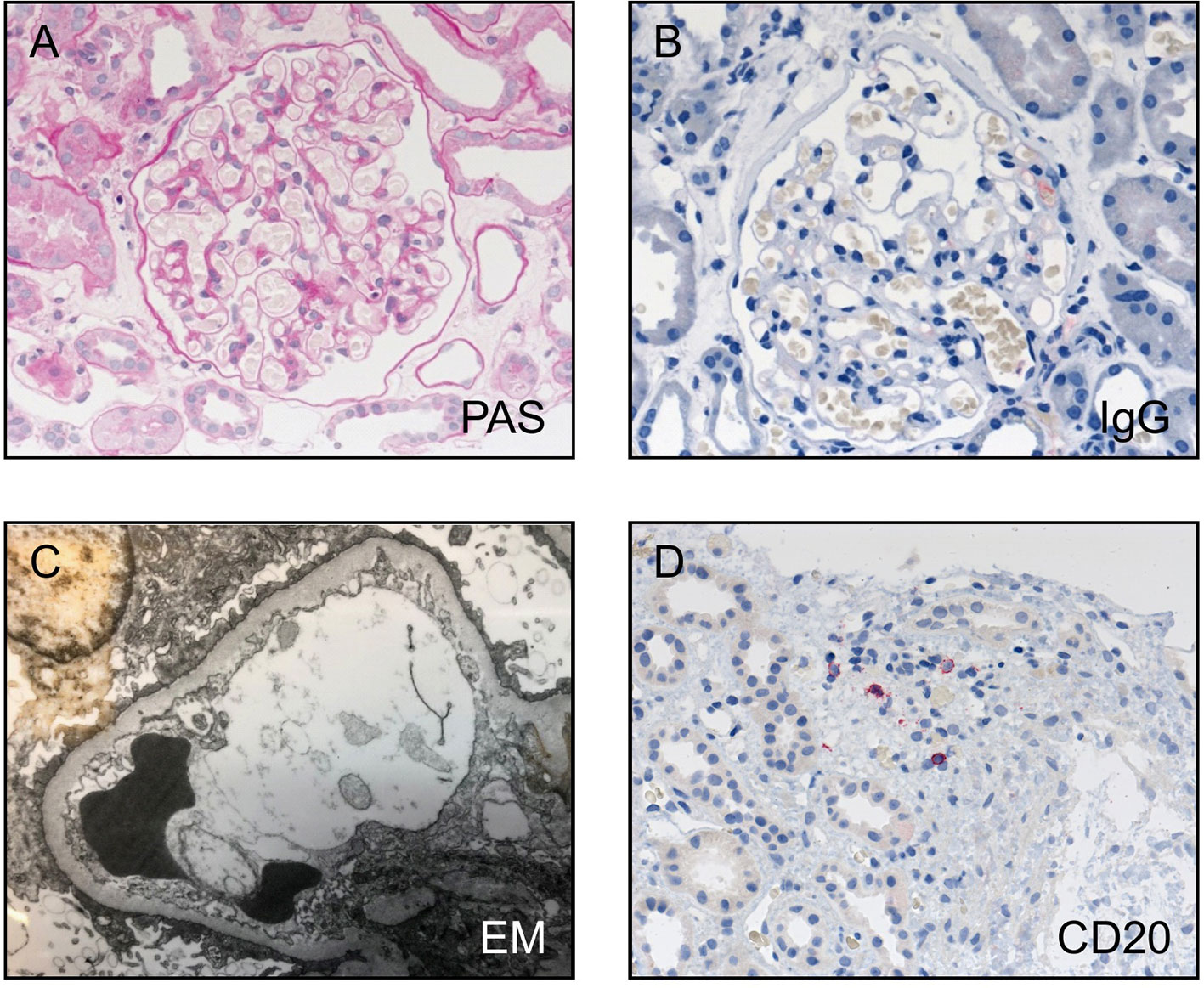
Figure 1 Histological findings in the kidney biopsy of the patient. (A) PAS staining, (B) IgG staining, and (C) electron microscopy confirm the diagnosis of minimal change disease with no IgG positivity, no electron dense deposits, and diffuse loss of podocyte foot processes. (D) Only very few CD20 positive cells were detectable, mostly in the area of tubular atrophy and interstitial fibrosis.
Characterization of Peripheral Blood Cells Bound by Rituximab
Because of the effect of rituximab treatment on proteinuria in the absence of CD19+ B cells in the blood, we aimed to better understand the potential underlying mechanism how rituximab unfolds its effect in this patient. We hypothesized that rituximab targets and depletes CD19− circulating blood cells. Therefore, we first assessed rituximab binding to CD19− blood cells. For this purpose, rituximab was conjugated to the fluorophore AF647 (RTX-AF647) and used to stain circulating CD20+ cells. Staining of peripheral CD45+ lymphocytes from a 27-year-old male healthy donor with RTX-AF647 led to the identification of CD19+ B cells (blue) and a population of CD19− CD20+ cells (Figure 2A, left panel). A majority (59.6%) of CD19− CD20+ cells expressed the T cell receptor associated marker CD3 on the surface. These cells were considered a T cell population (red; Figure 2A, right panel) and showed a similar CD3 expression as RTX-AF647- T cells (green), but were enriched for CD8+ T cells (Figure 2B). RTX-AF647+ T cells did not express B cell markers, such as CD19 and surface IgD (Figure 2B). CD27 expression was present in 76.1% of RTX-AF647+ T cells and 78.5% of RTX-AF647− CD3+ T cells, respectively (Figure 2B).
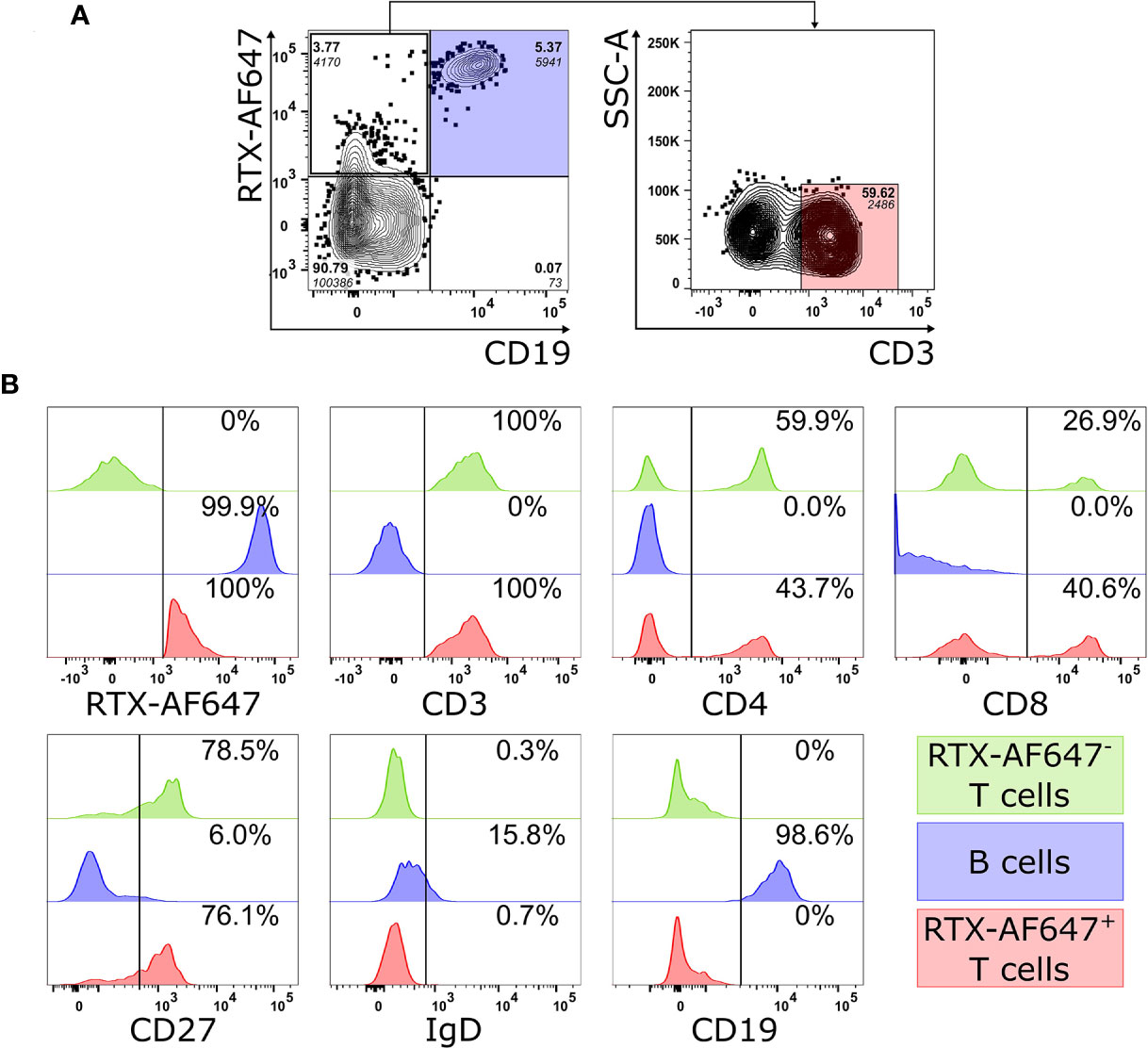
Figure 2 (A) FACS analysis of PBMC from a healthy donor gated on CD45+ lymphocytes (left panel) shows a population of CD19− RTX-AF647+ cells, 59.6% of which expresses the T cell marker CD3 (right panel). (B) The expression patterns of RTX-AF647, CD3, CD4, CD8, CD27, CD19, and IgD were assessed in equal counts of CD19+ B cells (blue), RTX-AF647+ CD3+ cells (RTX-AF647+ T cells, red), and RTX-AF647− CD3+ cells (RTX-AF647− T cells, green). RTX-AF647+ T cells (red) express CD3, CD4, CD8, and CD27 at a similar fluorescence intensity as RTX-AF647− T cells (green) and lack the expression of the B cell markers CD19 and surface IgD. For gating strategy see supplemental data (Figure S1). Event counts for each gate are indicated in italic below the frequency (bold).
Rituximab Treatment Depletes RTX-AF647+ T Cells
Next, we analyzed the B and T cell populations in PBMC from the index MCD patient before and after rituximab treatment by flow cytometry. Interestingly, only CD19− RTX-AF647+ cells and no CD19+ B cells were detected in the blood of the index MCD patient prior to rituximab treatment (Figure 3A, left, Figures S1 and S2A). These CD19− RTX-AF647+ cells consisted mostly of CD3+ T cells (64.9% of RTX-AF647+ CD19− cells; Figure 3A, right panel). The RTX-AF647+ T cell population in the blood of the patient consisted of 55.1% CD4+ T cells and 30.6% CD8+ T cells (Figure 3B), and was therefore enriched for CD8+ T cells compared to the total circulating RTX-AF647− T cell population in the same sample, which consisted of 68.4% CD4+ T cells and 11.4% CD8+ T cells (Figure 3C). Noteworthy, in healthy controls, CD4+ T cells and CD8+ T cells account for 23–52% and 13–40% of the circulating lymphocytes respectively (24). Double negative T cells were found in a lower frequency of 8.16% of RTX-AF647+ T cells (Figure 3B), while they comprised 15.4% of total RTX-AF647− T cells (Figure 3C).
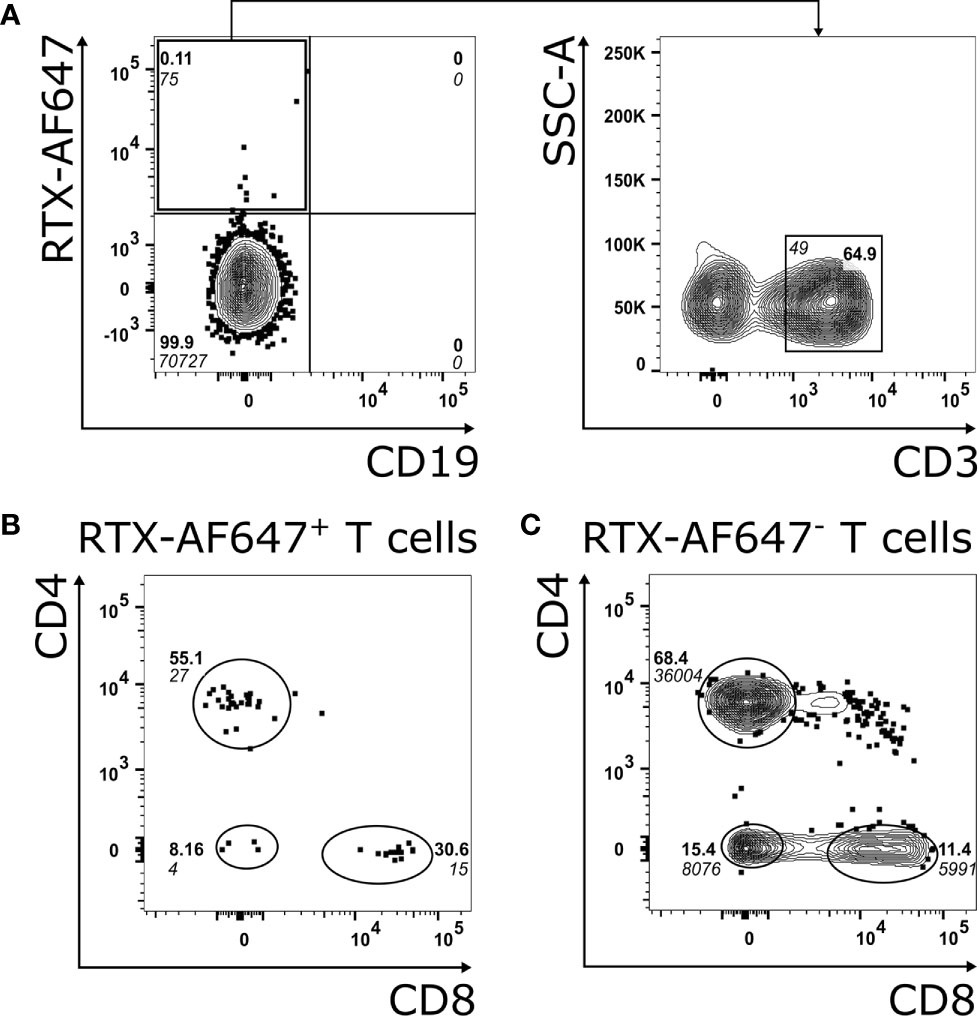
Figure 3 (A) FACS analysis of PBMC from the index MCD patient gated on CD45+ lymphocytes (left panel) shows a population of RTX-AF647+ CD19− cells and no CD19+ B cells prior to rituximab treatment. CD3 is expressed on 64.9% of RTX-AF647+ CD19− cells (A, right panel). (B) CD4 and CD8 expression of RTX-AF647+ CD3+ cells (RTX-AF647+ T cells). (C) CD4 and CD8 expression of RTX-AF647− CD3+ cells (RTX-AF647− T cells). In the blood of the index MCD patient, RTX-AF647+ T cells (B) are enriched for CD8+ T cells and show a reduced frequency of CD4+ T cells and double negative T cells in comparison to RTX-AF647- T cells (C).
Before rituximab treatment, 0.12% of CD3+ T cells of the index MCD patient were bound by RTX-AF647 (Figure 4A, left panel). After rituximab treatment, the frequency of RTX-AF647+ T cells was reduced by 91.7 to 0.01% (Figure 4A, right panel).
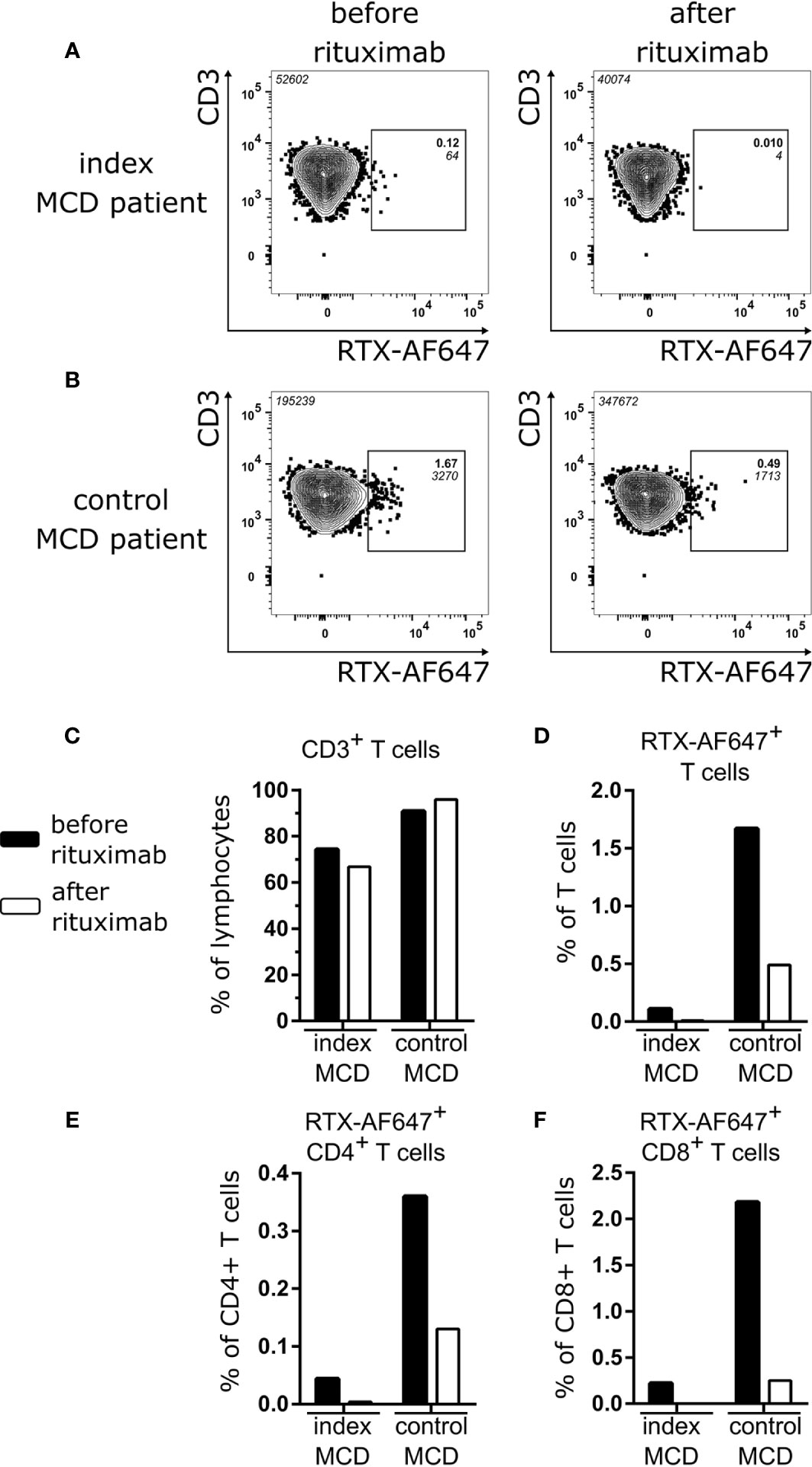
Figure 4 RTX-AF647+ CD3+ T cells of the index MCD patient (A) and the control MCD patient (B) before (left panel) and after (right panel) rituximab treatment. (C) Frequency of CD3+ T cells as percentage of lymphocytes and (D) frequency of RTX-AF647+ cells among CD3+ T cells, (E) CD4+ T cells, and (F) CD8+ T cells before (black) and after (white) rituximab treatment in the index MCD patient and the control MCD patient.
Next, we aimed to confirm that rituximab treatment leads to depletion of a subpopulation of CD3+ T cells in a control patient with relapsing MCD. We performed an identical FACS analysis in a second patient with MCD (control MCD patient). This patient was a 43-year-old female, who was treated for the first time with rituximab. She had both circulating B and T cells prior to rituximab treatment (Figure S2B). In the blood of the control MCD patient, we detected RTX-AF647+ T cells at a frequency of 1.67% of CD3+ T cells prior to rituximab treatment (Figure 4B, left panel). After rituximab treatment of the control MCD patient, the frequency of RTX-AF647+ T cells was reduced by 70.7 to 0.49% (Figure 4B, right panel). Similar findings were made when RTX-AF647+ CD19− cells were analyzed. Their frequencies decreased after rituximab from 0.11 to 0.01% in the index MCD patient (Figure S2A) and from 1.31 to 0.21% in the control MCD patient (Figure S2B), respectively. In the control MCD patient, CD19+ B cells were also depleted to a non-detectable level following rituximab treatment, while as shown before, in the index MCD patient CD19+ B cells were non-detectable even prior to rituximab treatment (Figure S2).
The frequency of CD3+ T cells did not significantly change in both the index MCD patient and the control MCD patient after rituximab treatment (Figure 4C). The frequency of RTX-AF647+ T cells was reduced by 91.7 and 70.7% in the index MCD and control MCD patient, respectively (Figure 4D). We further characterized the RTX-AF647+ T cell subsets before and after rituximab treatment in both patients. In the index MCD patient CD4+ RTX-AF647+ T cells were reduced by 91.2% (Figure 4E) and CD8+ RTX-AF647+ T cells were completely depleted (Figure 4F). In comparison, in the control MCD patient CD4+ RTX-AF647+ T cells were reduced by 63.9% and CD8+ RTX-AF647+ T cells by 88.5% after rituximab treatment.
Discussion
Frequent relapsing and steroid-dependent MCD patients represent a therapeutic challenge. This is due to the toxicity of long-term steroid treatment. Rituximab is a treatment option for these patients, however, biomarkers to guide therapy are lacking. The B cell marker CD19 is commonly used to predict whether a B cell depleting therapy is a rationale in treating patients with autoimmune kidney diseases. Despite showing no CD19+ B cells circulating in the blood, the presented index MCD patient developed a relapse of nephrotic syndrome. Moreover, he developed a complete remission of proteinuria within 3 weeks of treatment with rituximab and steroids. Remission of proteinuria lasted almost 1 year, similar to the remission phases during the last 8 years of treatment with rituximab. As CD19 and not CD20 is commonly used as a B cell marker to follow the depletion of B cells after rituximab, we investigated whether CD19− CD20+ cells were present in the patient’s blood, which could be targeted by rituximab and might play a role for disease induction. We were able to characterize a population of CD19-− RTX-AF647+ cells in the blood of the patient, which consisted mostly of CD3+ T cells.
After rituximab treatment, proteinuria resolved and CD20+ T cells were depleted. There are several potential explanations for the relapse of the nephrotic syndrome in the absence of B cells and the successful induction of remission following rituximab treatment. Firstly, pathogenic B cells could have reconstituted without being detectable in the patient’s circulation. Such CD20 positive cells may be resident in the tissue and thus are not detected in the blood. The depletion of these potentially pathogenic B cells may have contributed to the remission of proteinuria. Relapses of nephrotic syndrome have been shown to be associated with reconstitution of memory B cells, which reappear only after naïve B cells are detectable in the circulation (21). In our patient, however, neither naïve nor memory B cells were detected in the blood at the time of relapse. Therefore, cryptic reconstitution of memory B cells seems unlikely. Secondly, B cells could have reconstituted but escaped detection due to technical limitations. However, CD19+ B cells were detected successfully in the blood of the control MCD patient and a healthy control using our method of detection. Thirdly, RTX-AF647+ T cells could play a role in the relapse of a nephrotic syndrome and the depletion of these CD20+ T cells following rituximab treatment may be a relevant therapeutic mechanism.
Contrary to B cells, circulating CD20+ T cells were detected at the time of relapse in a low frequency (0.12% of T cells) in the blood of the index MCD patient. In the blood of healthy controls, the mean frequency of CD20+ T cells usually varies between 1.6 and 3.8% of CD3+ T cells (25, 26). This shows that in our index MCD patient CD20+ T cells had already reconstituted after the previous rituximab treatment, in contrast to B cells. As RTX-AF647+ T cells were depleted after rituximab treatment in both the control MCD patient as well as the index MCD patient, we conclude that rituximab binds specifically to CD20+ T cells and depletes them in vivo.
MCD has been considered a T cell mediated disease (27, 28), while the role of B cells remains unclear (21). Nonetheless, CD20 targeted antibody therapies have shown promising results in the treatment of relapsing MCD (7, 10, 19, 29, 30). The removal of autoreactive T cells has been proposed as a therapeutic mechanism of rituximab (31) and treatment of MCD patients with rituximab leads to the depletion of CD4+ CD45RO+ CXCR5+ T cells, invariant natural killer T cells and CD4− CD8− T cells (7, 32). The depletion of CD20+ T cells may represent a link connecting the successful application of anti-CD20 antibody treatment and the role of T cells in the pathogenesis of MCD.
Whether T cells are capable of expressing CD20 has been controversial since the first description of CD3+ T cells co-expressing CD20 (33). Several studies were able to show the expression of CD20 in T cells on a single cell level by imaging flow cytometry (34, 35), confocal microscopy (36), and immunohistochemistry (37). Together with the endogenous transcription of CD20 mRNA in CD20+ T cells (25, 26, 34, 35), this supports the view of endogenous synthesis of CD20 in a subset of T cells. Accordingly, CD20 antibody treatment depletes CD20+ T cells in the blood along with B cells (25, 26, 34–36, 38–42). CD20 expressing T cells have been described in several autoimmune diseases, e.g. multiple sclerosis (25, 35, 39, 41, 42), rheumatoid arthritis (26, 36, 43), primary Sjögren’s syndrome (38), and psoriasis (40), as well as HIV infection (34). Our patient is the first case where these cells are reported in an immune-mediated nephrotic glomerular disease.
Clinical data describing the role of CD20+ T cells in autoimmune disease are scarce. In patients with psoriasis, frequency of circulating CD20+ T cells producing IL-17, IL-21, and TNFα correlates with disease activity (40). Recently, it has been suggested, that CD20+ T cells contribute to the pathogenesis of multiple sclerosis and depletion of CD20+ T cells may play a role in the therapeutic mechanism of anti-CD20 antibody treatment (42, 44, 45). In patients with relapse of multiple sclerosis, it was shown that after anti-CD20 antibody treatment B cells were hardly detectable in the blood at a time when CD20+ T cells were already replenished (25). This finding has similarities to our index MCD patient, who showed reconstitution of CD20+ T cells while B cells were still depleted at the time when proteinuria relapsed. Other studies have also shown that CD20+ T cells reconstitute months before B cells reappear in the circulation after rituximab treatment (25, 35).
This is the first report describing CD20+ T cells in the context of a frequent relapsing nephrotic syndrome in a patient with MCD. Rituximab combined with a short steroid treatment induced remission of proteinuria despite undetectable B cells. We found CD20+ T cells in the blood of the patient at the time of relapse, which were depleted after re-treatment with rituximab. Rituximab successfully induced complete remission of proteinuria despite of the absence of CD19+ B cells. Patients with relapse of nephrotic syndrome may benefit from rituximab treatment irrespective of the frequency of CD19+ B cells. Therefore, low or absent CD19+ B cells in the blood might not be used as disconfirming evidence for the effectivity of rituximab for relapse of nephrotic syndrome. More studies are needed to decipher the role of CD20+ T cells in autoimmune kidney diseases. This may help to guide clinical decisions for the use of CD20 antibody treatment in kidney autoimmune diseases and may lead to a better understanding of the pathogenesis of MCD.
Data Availability Statement
The original contributions presented in the study are included in the article and Supplementary Material. Further inquiries can be directed to the corresponding author.
Ethics Statement
Written informed consent was obtained from the individuals for the publication of images and data included in this article.
Author Contributions
EH, SH, and RS were responsible for the conception and design of the study. MW and LR performed the experiments. H-WM and MW developed the FACS strategy. EH, H-WM, and MW interpreted the FACS data. EH and SH analyzed and interpreted the clinical data. TW analyzed and interpreted the morphological and immunohistochemical findings. Drafting and revising of the article were performed by MW, EH, and SH. All authors contributed to the article and approved the submitted version.
Funding
This study was supported by a grant from the Deutsche Forschungsgemeinschaft as part of the Sonderforschungsbereich 1192 (project B1 to EH and RS, project A4 to H-WM, project B6 to TW, project C1 to EH and RS) and the Heisenberg Programme to EH.
Conflict of Interest
The authors declare that the research was conducted in the absence of any commercial or financial relationships that could be construed as a potential conflict of interest.
Acknowledgments
We thank Katharina Schulz, Céline Brauer, Sandra Freyberg, and Eugen Paul for excellent support in the collection of clinical data. We are particularly thankful to the patients who agreed to participate in this study.
Supplementary Material
The Supplementary Material for this article can be found online at: https://www.frontiersin.org/articles/10.3389/fimmu.2020.586012/full#supplementary-material
References
1. Hogan J, Radhakrishnan J. The Treatment of Minimal Change Disease in Adults. J Am Soc Nephrol (2013) 24:702–11. doi: 10.1681/ASN.2012070734
2. Shalhoub RJ. Pathogenesis of lipoid nephrosis a disorder of T-cell function. Lancet (1974) 2:556–60. doi: 10.1016/S0140-6736(74)91880-7
3. Araya C, Diaz L, Wasserfall C, Atkinson M, Mu W, Johnson R, et al. T regulatory cell function in idiopathic minimal lesion nephrotic syndrome. Pediatr Nephrol (2009) 24:1691–8. doi: 10.1007/s00467-009-1214-x
4. Le Berre L, Bruneau S, Naulet J, Renaudin K, Buzelin F, Usal C, et al. Induction of T regulatory cells attenuates idiopathic nephrotic syndrome. J Am Soc Nephrol (2009) 20:57–67. doi: 10.1681/ASN.2007111244
5. Liu L, Qin Y, Cai J, Wang HY, Tao JL, Li H, et al. Th17/Treg imbalance in adult patients with minimal change nephrotic syndrome. Clin Immunol (2011) 139:314–20. doi: 10.1016/j.clim.2011.02.018
6. Prasad N, Jaiswal AK, Agarwal V, Yadav B, Sharma RK, Rai M, et al. Differential alteration in peripheral T-regulatory and T-effector cells with change in P-glycoprotein expression in Childhood Nephrotic Syndrome: A longitudinal study. Cytokine (2015) 72:190–6. doi: 10.1016/j.cyto.2014.12.028
7. Boumediene A, Vachin P, Sendeyo K, Oniszczuk J, Zhang SY, Henique C, et al. NEPHRUTIX: A randomized, double-blind, placebo vs Rituximab-controlled trial assessing T-cell subset changes in Minimal Change Nephrotic Syndrome. J Autoimmun (2018) 88:91–102. doi: 10.1016/j.jaut.2017.10.006
8. Audard V, Pawlak A, Candelier M, Lang P, Sahali D. Upregulation of nuclear factor-related kappa b suggests a disorder of transcriptional regulation in minimal change nephrotic syndrome. PloS One (2012) 7:1–9. doi: 10.1371/journal.pone.0030523
9. Vivarelli M, Massella L, Ruggiero B, Emma F. Minimal change disease. Clin J Am Soc Nephrol (2017) 12:332–45. doi: 10.2215/CJN.05000516
10. Kronbichler A, Kerschbaum J, Fernandez-Fresnedo G, Hoxha E, Kurschat CE, Busch M, et al. Rituximab treatment for relapsing minimal change disease and focal segmental glomerulosclerosis: A systematic review. Am J Nephrol (2014) 39:322–30. doi: 10.1159/000360908
11. Waldman M, Crew RJ, Valeri A, Busch J, Stokes B, Markowitz G, et al. Adult minimal-change disease: Clinical characteristics, treatment, and outcomes. Clin J Am Soc Nephrol (2007) 2:445–53. doi: 10.2215/CJN.03531006
12. Nolasco F, Cameron JS, Heywood EF, Hicks J, Ogg C, Williams DG. Adult-onset minimal change nephrotic syndrome: A long-term follow-up. Kidney Int (1986) 29:1215–23. doi: 10.1038/ki.1986.130
13. Madanchi N, Bitzan M, Takano T. Rituximab in minimal change disease: Mechanisms of action and hypotheses for future studies. Can J Kidney Health Dis (2017) 4:2054358117698667. doi: 10.1177/2054358117698667
14. Avivi I, Stroopinsky D, Katz T. Anti-CD20 monoclonal antibodies: Beyond B-cells. Blood Rev (2013) 27:217–23. doi: 10.1016/j.blre.2013.07.002
15. Rougé L, Chiang N, Steffek M, Kugel C, Croll TI, Tam C, et al. Structure of CD20 in complex with the therapeutic monoclonal antibody rituximab. Science (2020) 367:1224–30. doi: 10.1126/science.aaz9356
16. Sabatino JJ, Pröbstel A-K, Zamvil SS. B cells in autoimmune and neurodegenerative central nervous system diseases. Nat Rev Neurosci (2019) 20:728–45. doi: 10.1038/s41583-019-0233-2
17. Li R, Patterson KR, Bar-Or A. Reassessing B cell contributions in multiple sclerosis. Nat Immunol (2018) 19:696–707. doi: 10.1038/s41590-018-0135-x
18. Fornoni A, Sageshima J, Wei C, Merscher-Gomez S, Aguillon-Prada R, Jauregui AN, et al. Rituximab targets podocytes in recurrent focal segmental glomerulosclerosis. Sci Trans Med (2011) 3:1–2145. doi: 10.1126/scitranslmed.3002231
19. Guitard J, Hebral AL, Fakhouri F, Joly D, Daugas E, Rivalan J, et al. Rituximab for minimal-change nephrotic syndrome in adulthood: Predictive factors for response, long-term outcomes and tolerance. Nephrol Dial Transplant (2014) 29:2084–91. doi: 10.1093/ndt/gfu209
20. Hoxha E, Stahl RAK, Harendza S. Rituximab in adult patients with immunosuppressive-dependent minimal change disease. Clin Nephrol (2011) 76:151–8. doi: 10.5414/CN107092
21. Colucci M, Carsetti R, Cascioli S, Casiraghi F, Perna A, Ravà L, et al. B cell reconstitution after rituximab treatment in idiopathic nephrotic syndrome. J Am Soc Nephrol (2016) 27:1811–22. doi: 10.1681/ASN.2015050523
22. Sellier-Leclerc AL, Baudouin V, Kwon T, Macher MA, Guérin V, Lapillonne H, et al. Rituximab in steroid-dependent idiopathic nephrotic syndrome in childhood-follow-up after CD19 recovery. Nephrol Dial Transplant (2012) 27:1083–9. doi: 10.1093/ndt/gfr405
23. Levey AS, Eckardt KU, Dorman NM, Christiansen SL, Hoorn EJ, Ingelfinger JR, et al. Nomenclature for kidney function and disease: report of a Kidney Disease: Improving Global Outcomes (KDIGO) Consensus Conference. Kidney Int (2020) 97:1117–29. doi: 10.1111/ajt.16114
24. Rudolf-Oliveira RC, Gonçalves KT, Martignago ML, Mengatto V, Gaspar PC, de Moraes AC, et al. Determination of lymphocyte subset reference ranges in peripheral blood of healthy adults by a dual-platform flow cytometry method. Immunol Lett (2015) 163:96–101. doi: 10.1016/j.imlet.2014.11.003
25. Schuh E, Berer K, Mulazzani M, Feil K, Meinl I, Lahm H, et al. Features of Human CD3 + CD20 + T Cells. J Immunol (2016) 197:1111–7. doi: 10.4049/jimmunol.1600089
26. Wilk E, Witte T, Marquardt N, Horvath T, Kalippke K, Scholz K, et al. Depletion of functionally active CD20+ T cells by rituximab treatment. Arthritis Rheum (2009) 60:3563–71. doi: 10.1002/art.24998
27. Elie V, Fakhoury M, Deschênes G, Jacqz-Aigrain E. Physiopathology of idiopathic nephrotic syndrome: Lessons from glucocorticoids and epigenetic perspectives. Pediatr Nephrol (2012) 27:1249–56. doi: 10.1007/s00467-011-1947-1
28. De Fátima Pereira W, Brito-Melo GEA, Guimarães FTL, Carvalho TGR, Mateo EC, Simões E Silva AC. The role of the immune system in idiopathic nephrotic syndrome: A review of clinical and experimental studies. Inflammation Res (2014) 63:1–12. doi: 10.1007/s00011-013-0672-6
29. Munyentwali H, Bouachi K, Audard V, Remy P, Lang P, Mojaat R, et al. Rituximab is an efficient and safe treatment in adults with steroid-dependent minimal change disease. Kidney Int (2013) 83:511–6. doi: 10.1038/ki.2012.444
30. Takei T, Itabashi M, Moriyama T, Kojima C, Shiohira S, Shimizu A, et al. Effect of single-dose rituximab on steroid-dependent minimal-change nephrotic syndrome in adults. Nephrol Dial Transplant (2013) 28:1225–32. doi: 10.1093/ndt/gfs515
31. Datta SK. Anti-CD20 antibody is an efficient therapeutic tool for the selective removal of autoreactive T cells. Nat Clin Prac Rheumatol (2009) 5:80–2. doi: 10.1038/ncprheum0983
32. Kronbichler A, Gauckler P, Bruchfeld A. Rituximab in minimal change disease and focal segmental glomerulosclerosis. Nephrol Dial Transplant (2019) 23:5–11. doi: 10.1093/ndt/gfz205
33. Hultin LE, Hausner MA, Hultin PM, Giorgi JV. Cd20 (pan-B cell) antigen is expressed at a low level on a subpopulation of human T lymphocytes. Cytometry (1993) 14:196–204. doi: 10.1002/cyto.990140212
34. Serra-Peinado C, Grau-Expósito J, Luque-Ballesteros L, Astorga-Gamaza A, Navarro J, Gallego-Rodriguez J, et al. Expression of CD20 after viral reactivation renders HIV-reservoir cells susceptible to Rituximab. Nat Commun (2019) 10:3705. doi: 10.1038/s41467-019-11556-4
35. Palanichamy A, Jahn S, Nickles D, Derstine M, Abounasr A, Hauser SL, et al. Rituximab Efficiently Depletes Increased CD20-Expressing T Cells in Multiple Sclerosis Patients. J Immunol (2014) 193:580–6. doi: 10.4049/jimmunol.1400118
36. Eggleton P, Bremer E, Tarr JM, de Bruyn M, Helfrich W, Kendall A, et al. Frequency of Th17 CD20+ cells in the peripheral blood of rheumatoid arthritis patients is higher compared to healthy subjects. Arthr Res Ther (2011) 13:R208. doi: 10.1186/ar3541
37. Holley JE, Bremer E, Kendall AC, de Bruyn M, Helfrich W, Tarr JM, et al. CD20+inflammatory T-cells are present in blood and brain of multiple sclerosis patients and can be selectively targeted for apoptotic elimination. Mult Scler Relat Disord (2014) 3:650–8. doi: 10.1016/j.msard.2014.06.001
38. Alunno A, Carubbi F, Bistoni O, Caterbi S, Bartoloni E, Di Benedetto P, et al. Interleukin (IL)-17-producing pathogenic T lymphocytes co-express CD20 and are depleted by rituximab in primary Sjögren’s syndrome: a pilot study. Clin Exp Immunol (2016) 184:284–92. doi: 10.1111/cei.12771
39. Gingele S, Jacobus T, Konen F, Hümmert MW, Sühs KW, Schwenkenbecher P, et al. Ocrelizumab Depletes CD20+ T Cells in Multiple Sclerosis Patients. Cells (2018) 8:12. doi: 10.3390/cells8010012
40. Niu J, Zhai Z, Hao F, Zhang Y, Song Z, Zhong H. Dissection of a circulating CD3 + CD20 + T cell subpopulation in patients with psoriasis. Clin Exp Immunol (2018) 192:206–12. doi: 10.1111/cei.13106
41. Sabatino JJ, Wilson MR, Calabresi PA, Hauser SL, Schneck JP, Zamvil SS. Anti-CD20 therapy depletes activated myelin-specific CD8 + T cells in multiple sclerosis. Proc Natl Acad Sci (2019) 116:25800–7. doi: 10.1073/pnas.1915309116
42. von Essen MR, Ammitzbøll C, Hansen RH, Petersen ERS, McWilliam O, Marquart HV, et al. Proinflammatory CD20+ T cells in the pathogenesis of multiple sclerosis. Brain (2019) 142:120–32. doi: 10.1093/brain/awy301
43. Mélet J, Mulleman D, Goupille P, Ribourtout B, Watier H, Thibault G. Rituximab-induced T cell depletion in patients with rheumatoid arthritis: Association with clinical response. Arthritis Rheum (2013) 65:2783–90. doi: 10.1002/art.38107
44. Waisman A, Ebering A. Unraveling the T–B tangle in anti-CD20 multiple sclerosis therapy. Proc Natl Acad Sci (2019) 116:25376–7. doi: 10.1073/pnas.1919044116
Keywords: minimal change disease, rituximab, CD20+ T cells, B cell depletion therapy, nephrotic syndrome
Citation: Webendörfer M, Reinhard L, Stahl RAK, Wiech T, Mittrücker H-W, Harendza S and Hoxha E (2021) Rituximab Induces Complete Remission of Proteinuria in a Patient With Minimal Change Disease and No Detectable B Cells. Front. Immunol. 11:586012. doi: 10.3389/fimmu.2020.586012
Received: 22 July 2020; Accepted: 21 December 2020;
Published: 08 February 2021.
Edited by:
Charles Dickson Pusey, Imperial College London, United KingdomReviewed by:
Andreas Kronbichler, Innsbruck Medical University, AustriaRona Smith, University of Cambridge, United Kingdom
Copyright © 2021 Webendörfer, Reinhard, Stahl, Wiech, Mittrücker, Harendza and Hoxha. This is an open-access article distributed under the terms of the Creative Commons Attribution License (CC BY). The use, distribution or reproduction in other forums is permitted, provided the original author(s) and the copyright owner(s) are credited and that the original publication in this journal is cited, in accordance with accepted academic practice. No use, distribution or reproduction is permitted which does not comply with these terms.
*Correspondence: Elion Hoxha, e.hoxha@uke.de