- 1Department of Molecular Medicine, Sapienza University of Rome, Rome, Italy
- 2Istituto Pasteur-Fondazione Cenci Bolognetti, Rome, Italy
- 3Istituto Mediterraneo di Neuroscienze Neuromed, Pozzilli, Italy
The interplay between cellular stress and immune response can be variable and sometimes contradictory. The mechanisms by which stress-activated pathways regulate the inflammatory response to a pathogen, in autoimmunity or during cancer progression remain unclear in many aspects, despite our recent knowledge of the signalling and transcriptional pathways involved in these diseases. In this context, over the last decade many studies demonstrated that cholesterol metabolism is an important checkpoint for immune homeostasis and cancer progression. Indeed, cholesterol is actively metabolized and can regulate, through its mobilization and/or production of active derivatives, many aspects of immunity and inflammation. Moreover, accumulation of cholesterol has been described in cancer cells, indicating metabolic addiction. The nuclear receptors liver-X-receptors (LXRs) are important regulators of intracellular cholesterol and lipids homeostasis. They have also key regulatory roles in immune response, as they can regulate inflammation, innate and adaptive immunity. Moreover, activation of LXRs has been reported to affect the proliferation and survival of different cancer cell types that show altered metabolic pathways and accumulation of cholesterol. In this minireview we will give an overview of the recent understandings about the mechanisms through which LXRs regulate inflammation, autoimmunity, and cancer, and the therapeutic potential for future treatment of these diseases through modulation of cholesterol metabolism.
Introduction
Cholesterol metabolism is deeply linked to different aspects of immunity and inflammation. It is generally thought as an exogenous player on immunity during disease, as in the case of pathologic cholesterol overloading of foam cells in atherosclerosis or more in general in hypercholesterolaemia. However, increasing evidences have recently changed this view by demonstrating that a number of immune receptors and transcription factors such as Toll-like Receptors (TLRs), C-X-C motif chemokine receptor 2 (CXCR2), Stimulator of IFN genes (STING) and retinoic acid-related orphan receptor-γt (ROR-γt) are profoundly regulated by sterols (1–7). Moreover, regulation of intracellular cholesterol homeostasis controls lymphocyte proliferation and adaptive immune responses (8).
In this review we will discuss recent literature regarding aspects of lipid and cholesterol metabolism in tissues homeostasis, providing to the readers a synthetic overview of the main connections and regulatory interactions between cholesterol cellular metabolism and the activity of LXRs in the context of inflammation, autoimmunity and cancer. LXRs are transcription factors able to regulate specific gene networks implicated in cholesterol and lipid metabolism both in homeostatic and pathological conditions. Moreover, LXRs can mediate anti-inflammatory activities and modulate the immune response, promoting the expression of mediators which have a role in the control of inflammatory disorders and in the response to microbial infection. In a different scenario, accumulation of cholesterol has been also described in many types of cancer cells indicating metabolic addiction. This further expands the possible implications of its dysregulation in cancer progression (9, 10), configuring cholesterol as an important metabolic determinant. LXRs play relevant roles in cancer biology and in anti-tumor immune responses, opening new therapeutic possibilities (Figure 1) and (Table 1).
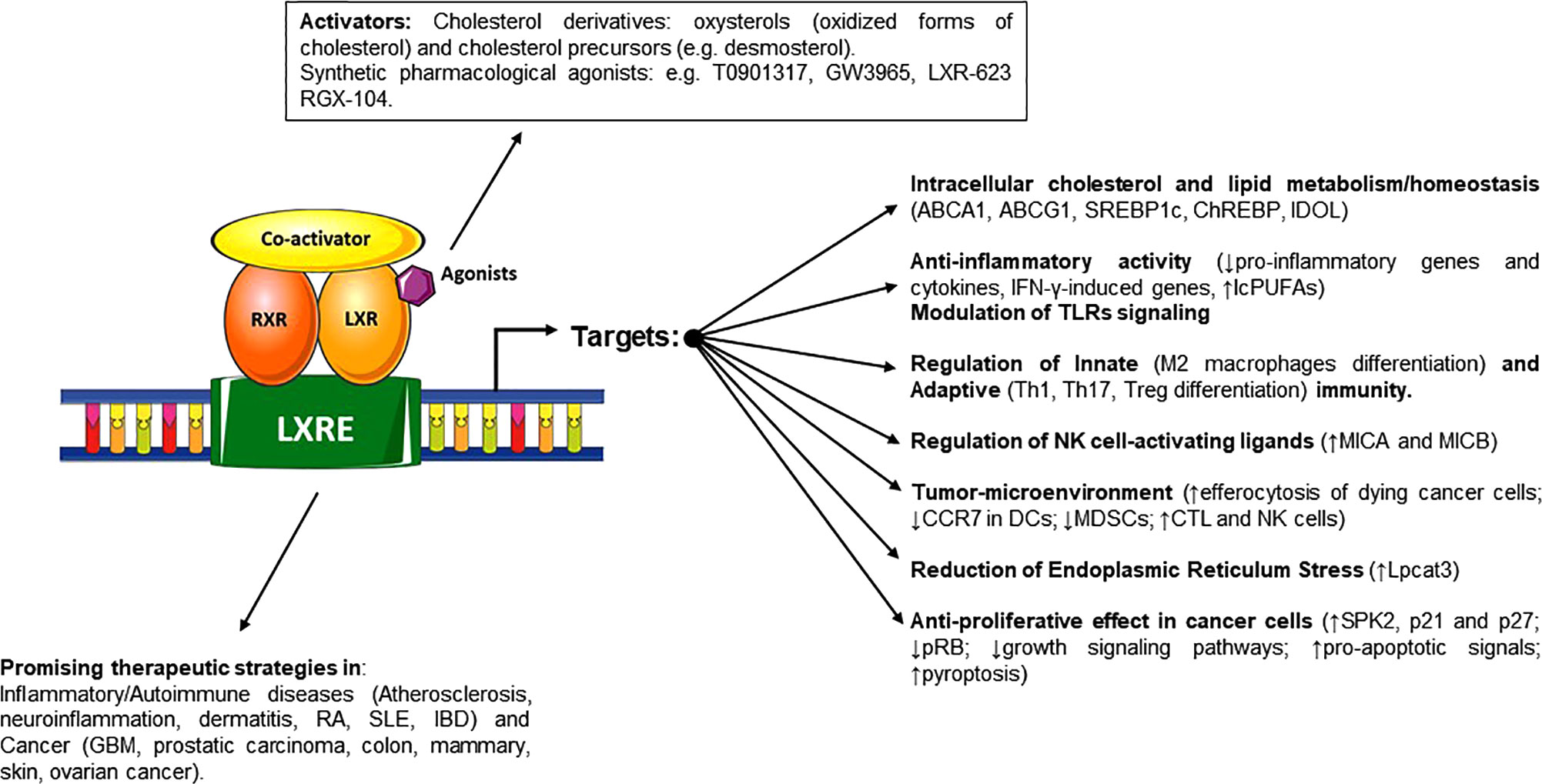
Figure 1 Schematic representation of LXRs activators and the different effects on regulated genes and pathways involved in cholesterol and lipid homeostasis, regulation of immune system and cancer proliferation and progression. When these ligands trigger the activation of LXRs, they heterodimerize with RXR and bind to target gene promoters on LXR-responsive-elements, regulating the transcription and expression of specific target genes.
LXRs: A Link Between Lipid Metabolism and Immune Response
LXRs are transcription factors belonging to the nuclear receptors (NRs) superfamily. They are master regulators of cholesterol and lipid intracellular homeostasis (47). There are two isoforms of LXRs, LXRα (NR1H3), and LXRβ (NR1H2) (48, 49) that share extensive sequence homology [(77% identity in both the DNA binding domain (DBD) and ligand binding domain (LBD)]. Despite this similarity, they have rather different expression patterns (50); indeed, the expression of these NRs depends on the cell type and tissues analyzed, with LXRα more expressed in liver, intestine, adipose tissue and cells of the myelomonocytic lineage, while LXRβ is expressed more ubiquitously (51). Thus, their transcriptional role seems to be determined by their relative expression levels in specific tissues or cells, although important differences have also been identified in vivo between the two isoforms (52).
Different studies in vitro and in vivo have characterized a number of cholesterol derivatives including oxysterols, oxidized forms of cholesterol and cholesterol precursors (e.g., desmosterol) as LXR activators, able to bind with different affinities to the LXR LBD (47, 53, 54). When these endogenous ligands, or synthetic pharmacological agonists, trigger activation of LXRs, they heterodimerize with retinoid X receptors (RXR) and bind to target gene promoters on LXR-responsive-elements (LXREs), canonical binding sites composed of a repeated 6-mer sequence (5’-AGGTCA-3’) separated by four nucleotides (55). To activate target gene transcription, unliganded LXRs and co-repressors such as nuclear receptor corepressor 1 (NCoR1) and silencing mediator of retinoic acid and thyroid hormone receptor (SMRT), bound to LXREs, have to be displaced from chromatin to allow the binding of transcriptional co-activators [i.e., nuclear receptor co-activator 1 (NCOA1) and activating signal co-integrator 2 (ASC2)], leading to transcription (17).
Recent findings suggest that LXRs may be also recruited de novo to the promoter of target genes when triggered by ligands (18).
Once activated, they regulate the expression of genes involved in lipid and glucose metabolism (51, 56). In this context, LXRs are master regulators of cholesterol sensing; they counteract aberrant cellular sterol overload by upregulating the expression of sterol transporters such as the ATP binding cassette (ABC) family members ABCA1 and ABCG1, together with the transcription factors sterol regulatory element-binding protein 1c (SREBP1c) and carbohydrate-response element-binding protein (ChREBP) that regulate critical lipogenic pathways. Moreover, the activation of LXRs also induces the expression of inducible degrader of the LDL-receptor (IDOL), which is able to reduce the expression of low-density lipoprotein receptor (LDLR)s on the cell surface and the uptake of LDL/cholesterol particles (57).
Besides the regulation of cholesterol homeostasis, genetic and pharmacological studies have pointed out the role of LXRs as an important link between lipid metabolism, regulation of immune cell function and inflammation (58). Indeed, these NRs can both promote and repress the expression of specific immune regulatory gene networks (59). As discussed below, LXRs can induce anti-inflammatory activities in macrophages and Dendritic Cells (DCs) and represent a critical link between cholesterol metabolism, proliferation and migration of activated T and B lymphocytes (8, 15, 21, 28, 60–65), thus playing an important role in the control of inflammatory, autoimmune and infectious diseases.
LXRs, Cholesterol, and Inflammation
Different pathways link inflammation to cholesterol metabolism and LXRs activity. Alteration of cellular cholesterol homeostasis can both enhance or reduce innate receptor signalling and inflammasome activation. Cholesterol enrichment in macrophage plasma membrane promotes the activity of TLRs as in the case of the TLR4-MD2 and TLR4-CD14 complexes activated in response to lipopolysaccharide (LPS) (11, 12). On the other hand, the activation of the reverse cholesterol transport (RCT) mediated by ABCA1 and ABCG1 transporters limits the formation of cholesterol-enriched lipid rafts in the plasma membrane and/or in the endosomal system. This inhibits MyD88-dependent TLRs trafficking by selective reduction of free cholesterol content and suppresses macrophage inflammatory responses (66). This mechanism has been elegantly demonstrated in mouse models deficient for ABCA1 and ABCG1, shown to accumulate cholesterol in peritoneal macrophages and to exhibit enhanced inflammatory responses to TLR agonists (11). In line with these observations, in a model of atherosclerosis susceptibility, pathogens can interfere with macrophage cholesterol metabolism through inhibition of the LXRs. Here, the activation of TLR-3 and -4 by microbial ligands has been shown to repress the expression of selected target genes including ABCA1 in macrophages, as clearly shown in aortic tissue in vivo, with a mechanism connected to reduced cholesterol efflux from macrophages regulated by interferon regulatory factor-3 (IRF3)-mediated inhibition of LXRs on their target promoters (14). Activation of efferocytosis is also associated to the activity of LXRs, which results in the efflux of free cholesterol derived from engulfed apoptotic cells by upregulating ABCA1 and ABCG1 transporters. This mechanism, together with the LXR-mediated alternative (M2) macrophage differentiation, can prevent aberrant activation of the immune system (15, 16). Moreover, the removal of apoptotic cells helps avoiding autoimmunity, as shown in murine models of lupus-like autoimmunity where treatment with LXR agonists ameliorated disease progression (15, 67). In a different context, increased cellular content of cholesterol can trigger cholesterol crystal formation, as shown in atherosclerotic plaques. In this disease model, cholesterol crystals uptake or formation in macrophages has been shown to activate NLR family pyrin domain containing 3 (NLRP3)/inflammasome with the secretion of the pro-inflammatory cytokines interleukin-1β (IL-1β) and IL-18 and to promote the progression of atherogenesis (13).
As shown for other NRs, LXRs are anti-inflammatory; they can inhibit the transcriptional induction of pro-inflammatory genes mediated by critical transcription factors as NF-kB, AP-1 or STAT-1. In this regard, pharmacological activation of LXRs has been shown to ameliorate the severity of the inflammatory response in murine models of atherosclerosis (22), neuroinflammation (30, 68), dermatitis (22, 69), lupus (67) and arthritis (23), inhibiting primary cytokine production. Mechanistically, studies using LXR agonists in macrophages have shown that, depending on the LXR isoform, these NRs can repress the induction of pro-inflammatory genes through a molecular mechanism known as “transrepression”. Here, after histone deacetylase-4 (HDAC-4)-dependent conjugation of LXR with small ubiquitin-related modifier (SUMO)-2/3 at specific lysine residues in the LBD, LXR becomes able to bind to the NCoR-SMRT co-repressor, thus preventing signal-dependent clearance from the promoters of pro-inflammatory genes (17, 19). With a different mechanism, LXRs can inhibit Interferon-γ-induced genes in astrocytes, where LXRα and LXRβ are SUMO-conjugated by HDAC4 or by protein inhibitor of activated STAT1 (PIAS1), respectively, and interact with phosphorylated signal transducer and activator of transcription-1 (STAT-1) preventing its binding to gene promoters (20). Furthermore, LXRs activation can repress pro-inflammatory cytokine maturation to their active form as demonstrated for IL-18 and can induce specific endogenous inhibitors (i.e., IL-18BP) (21).
In addition to direct transrepression activity on pro-inflammatory genes, LXRs can mediate other important integrated mechanisms contributing to the control of inflammation. LXRs can induce the synthesis of long-chain polyunsaturated fatty acids (lcPUFAs) such as omega 3 fatty acids. The presence of lcPUFAs can decrease transactivation mediated by NF-kB of inflammatory genes, modifying histone acetylation in their regulatory regions (24). Moreover, lcPUFAs have been shown to increase the production of eicosanoids and selected pro-resolving lipid mediators (70, 71). Interestingly, increased LXRs activity can also induce macrophage polarization toward a more pro-resolving phenotype, directly upregulating the expression of MER proto-oncogene Tyrosine Kinase (MERTK), a receptor that promotes the synthesis of mediators implicated in inflammation resolution (15, 72). Furthermore, as demonstrated in hepatic inflammation models, induction of the polyunsaturated phospholipids (PLs) remodeling enzyme lysophosphatidylcholine acyltransferase 3 (Lpcat3) by LXRs increases the formation of PLs and decreases membrane saturation, counteracting endoplasmic reticulum stress induced by fatty acids in hepatocytes, improving hepatic metabolic stress and inflammation by modulating aberrant c-Src activation (73). An additional consideration that can add a layer of complexity is that LXRs are highly expressed by haematopoietic stem cells (HSCs) and myeloid progenitor cells. In these cells, activation of LXRs can increase the ABCA1/ABCG1/apolipoprotein E (APOE)-mediated cholesterol efflux, which is able to reduce their proliferative responses to IL-3 and GM-CSF, thus indirectly modulating the production of inflammatory cells (74).
LXRs and Autoimmunity
The activity of LXRs and cholesterol metabolites is implicated in the control and progression of several autoimmune diseases.
Altered lipid profiles have been associated with poor outcome of multiple sclerosis (MS) (75–80), an autoimmune disease characterized by inflammatory cell infiltrates and demyelination (81, 82). In this regard, obesity, among other environmental factors, has been described as a risk factor for MS in several epidemiological studies (83–86). In animal models of experimental autoimmune encephalomyelitis (EAE), the most common experimental model for human inflammatory demyelinating disease, selected agonists of LXRs (e.g., T0901317) have been shown to improve the severity of central nervous system inflammation (30). In line with this evidence, the activity of LXRα is indispensable for maintaining blood-brain barrier (BBB) integrity and its immune quiescence. Indeed, in a model of EAE, the specific knockout of LXRα in brain endothelial cells has been shown to increase BBB permeability and endothelial inflammation (25). Morover, activation of LXRs using agonists in vivo has been shown to repress the production of the pro-inflammatory cytokine IL-17 (28), together with IFNγ and IL-23R expression (29). Noteworthy, Th17 cell differentiation is modulated by LXRs via induction of sterol regulatory element-binding protein 1c (SREBP-1c), which is able to bind to the E-box element on the IL-17 promoter and to physically interact with the aryl hydrocarbon receptor (AHR), inhibiting its transcriptional activity (28). Interestingly, the activity of LXRs mediated by oxysterols can also modulate pro-inflammatory responses in microglial and astrocytes (26, 27) possibly contributing to ameliorate inflammation.
LXRs have also been hypothesized as a possible therapeutic target for rheumatoid arthritis (RA), a chronic autoimmune disorder characterized by infiltration of inflammatory leukocytes in the synovial compartment, which causes cartilage and bone damage (87). Initial conflicting reports have described both protective and promoting actions of LXRs-mediated pathways in murine models of inflammatory arthritis. LXR agonists such as T0901317 or GW3965, attenuated the symptoms, decreasing the production of pro-inflammatory cytokines in different murine collagen-induced arthritis (CIA) models (23, 31, 32, 88) and suppressed inflammatory gene expressions in RA fibroblast-like synoviocytes (35). By contrast, other reports described increased inflammation and cartilage destruction mediated by ligand activated LXRs (TO901317 or GW3965) in CIA models and found that LXR pathways are significantly upregulated in RA synovial macrophages. Interestingly, in these models the activity of both LXR isoforms was required in control mice to induce the progression of inflammation, in respect to single LXRα-/- or LXRβ-/- mice (33), thus implying overlapping and exclusive effects in these models. Moreover, activation of LXRs by ligands present within synovial fluids enhanced TLR-driven cytokine and chemokine secretion, suggesting a novel mechanism that can promote RA synovitis (33, 34, 89).
In a different scenario, both LXR subtypes are expressed in human and murine colon and were described to mediate anti-inflammatory effects in colon epithelial cells (36). Furthermore, in a murine experimental model of intestinal bowel disease (IBD), it was reported that LXR-deficient mice were more susceptible to dextran sodium sulphate (DSS) and 2,4,6-trinitrobenzenesulfonic acid (TNBS)-induced colitis. In this regard, the activation of LXRs can suppress Th1 and Th17 polarization in vitro, lowering the expression of their secreted pro-inflammatory cytokines and promoting differentiation of protective gut-associated regulatory T cells in mice, where systemic LXR activation was obtained by oral treatment with the LXR agonist GW3965 (37). These data confirmed a dual role of LXR in the control of inflammation by the suppression of pro-inflammatory T cells and the parallel induction of regulatory T cells.
LXRs as Regulators of Lipid Metabolism, Cancer Progression, and Antitumor Immunity
Genes involved in cholesterol homeostasis are often mutated or dysregulated in cancer cells (10, 90). A higher intracellular cholesterol level due to an enhanced uptake by LDLRs, a decreased efflux by ABC transporters and the upregulation of de novo synthesis can sustain the metabolic need for cancer cell proliferation (90–93), and accumulation of cholesterol has been described in many types of tumors (9, 49, 91–93).
Cells usually obtain cholesterol via different mechanisms including direct synthesis via the transcriptional activity of SREBPs, which promote the transcription of enzymes involved in cholesterol and fatty acid biosynthesis [i.e., 3-hydroxy-3-methylglutaryl-coenzyme A reductase (HMG-CoA) reductase] (94, 95). In this regard, the recent use of HMG-CoA inhibitors (Statins) to block the mevalonate pathway and cholesterol de novo biosynthesis showed promising results (96). However, cancer cells often gain selective proliferative advantage by enhancing LDLR-mediated uptake of exogenous cholesterol (38), rendering these therapies often unsuccessful. Perhaps, one of the best characterized examples of cancer cholesterol addiction is glioblastoma multiforme (GBM). The treatment of these cancer cells with LXR agonists induced degradation of LDLR and increased apoptosis in glioblastoma cells expressing mutant epidermal growth factor receptor (EGFR), where tumor growth and survival is strongly dependent on SREBP-1-mediated lipogenesis (38). Moreover, triggering of LXRs increases cellular cholesterol efflux by ABCA1, lowering its levels and inducing severe GBM cell death. Accordingly, LXR agonists (e.g., LXR-623) prolonged survival of mice models bearing GBM, indicating that targeting cholesterol metabolism may be a promising strategy in the treatment of this cancer (39, 97).
Pharmacological studies on various types of cancer models such as prostatic carcinoma, colon, mammary and skin cancer have shown that the activation of LXRs generates anti-proliferative effects due to the destruction of growth signalling pathways and to the activation of pro-apoptotic signals (9). LXRs can reduce the expression/activity of cell-cycle regulators, as shown for S-phase Kinase associated protein (SPK2) in cancer cell lines (40) and, at the same time, are able to induce the expression of cell-cycle inhibitors as demonstrated for p21 and p27 (cyclin-dependent kinase inhibitors) in prostate and ovarian cancer cells, with a concomitant reduction in phospho-RB protein levels (41, 98). Moreover, in mouse models, activation of LXRs delayed the progression of androgen-dependent tumors towards androgen independence (41, 42).
In addition to these direct activities on cancer cell metabolism and survival, in the last few years experimental evidences have highlighted the importance of LXRs in anti-tumor immune responses. In this context, the role of LXR activation is still controversial. Several tumors can produce oxysterols that play an essential role in cholesterol homeostasis by activating LXRs (99, 100), and many of these metabolites can have antiproliferative activity in cancer cells (101). However, oxysterols can also inhibit the expression of CCR7 on DCs, a chemokine receptor critical for the migration of DCs to tumor-draining lymph nodes (44). Circulating and tumor-derived oxysterols have been also described to recruit pro-tumor neutrophils and to increase neo-angiogenesis and immunosuppression in a CXCR2-dependent and LXR-independent manner (6, 102). This highlights the capability of selected oxysterols to regulate a broad range of pro-tumor activities—depending on the LXR isoform expressed by the tissue from which tumor cells originate and on the surrounding microenvironment. Moreover, in breast cancer, 27-hydroxycholesterol has been shown to act as an estrogen receptor agonist inducing tumor growth and metastasis (103). On the other hand, LXRs were reported to control cancer cell growth by inducing LXRβ-dependent pyroptosis of cancer cells and the activation of LXRα in macrophages, promoted the phagocytosis of dying cancer cells (45). More recently, in different mouse cancer models treated with specific LXRs agonists (i.e., RGX-104), has been observed a slower tumor growth which correlated with a decreased expansion of myeloid derived suppressor cells (MDSCs); these data were also validated in cancer patients, in a multicentre dose escalation phase 1 trial (43). Moreover, RGX-104 also partially abrogated the immunosuppressive effects of radiotherapy in a murine model of Non-Small-Cell Lung Carcinoma (NSCLC) (104). Mechanistically, the induction of ApoE, a transcriptional target of LXR, can induce MDSC depletion by triggering the low-density lipoprotein receptor-related 8 (LRP8) receptor on these cells, and potentiate activation of cytotoxic lymphocytes. In these settings, activation of LXRs together with PD-1 inhibition, improved the efficacy of cytotoxic T lymphocyte (CTL) and natural killer (NK) cells from cancer patients (43). In a different scenario, LXRs activation could upregulate MHC class I polypeptide-related sequence-A (MICA) and MICB expression in multiple myeloma cells, two ligands of the NK cell-activating receptor NK group 2 member D (NKG2D), by enhancing MICA promoter activity and inhibiting MICB degradation in lysosomes, thus improving NK cell-cytotoxicity (46).
Conclusion
The implication of cholesterol metabolism in the control of inflammatory diseases and cancer progression is the object of an interesting and controversial debate. Our increasing knowledge of the different roles mediated by LXRs in lipid homeostasis supports the idea that lipid metabolism and inflammation are closely connected and that their crosstalk is crucial for the evolution of different inflammatory diseases and, more in general, in the regulation of the immune response. The involvement of specific pathways regulated by LXRs during tumor progression and the possibility to pharmacologically modulate LXR activity, as an additional weapon for cancer therapy and for immunotherapy, have opened new therapeutic possibilities in this context. However, the activities of these NRs are often cell-, tissue-, and context-dependent, which makes it difficult to fully characterize their effects in disease conditions and to optimize specific therapeutic interventions in inflammatory disorders or in cancer therapy. In addition, many LXR-dependent and -independent pleiotropic effects of oxysterols produced in inflamed or tumoral microenvironments have been described in recent years, adding additional levels of complexity to these regulatory pathways (Figure 2). Another important issue is whether synthetic ligands that uncouple the anti-inflammatory and anti-cancer effects of LXRs from their role in cholesterol homeostasis can be developed. This is particularly important also in the context of different metabolic disorders with increased risk of developing diseases such as type 2 diabetes or cardiovascular disease, where beneficial effects of LXRs have been described (105). At the moment, different synthetic LXRs agonists have been optimized; however, their clinical application is limited by undesirable hyperlipidemic effects and other adverse side effects encountered in the central nervous system (106–111). The future development of isoform- and/or tissue-specific LXR modulators and the possibility to target LXR-interacting co-factors involved in LXR transcriptional activation will open new therapeutical possibilities for treating these diseases.
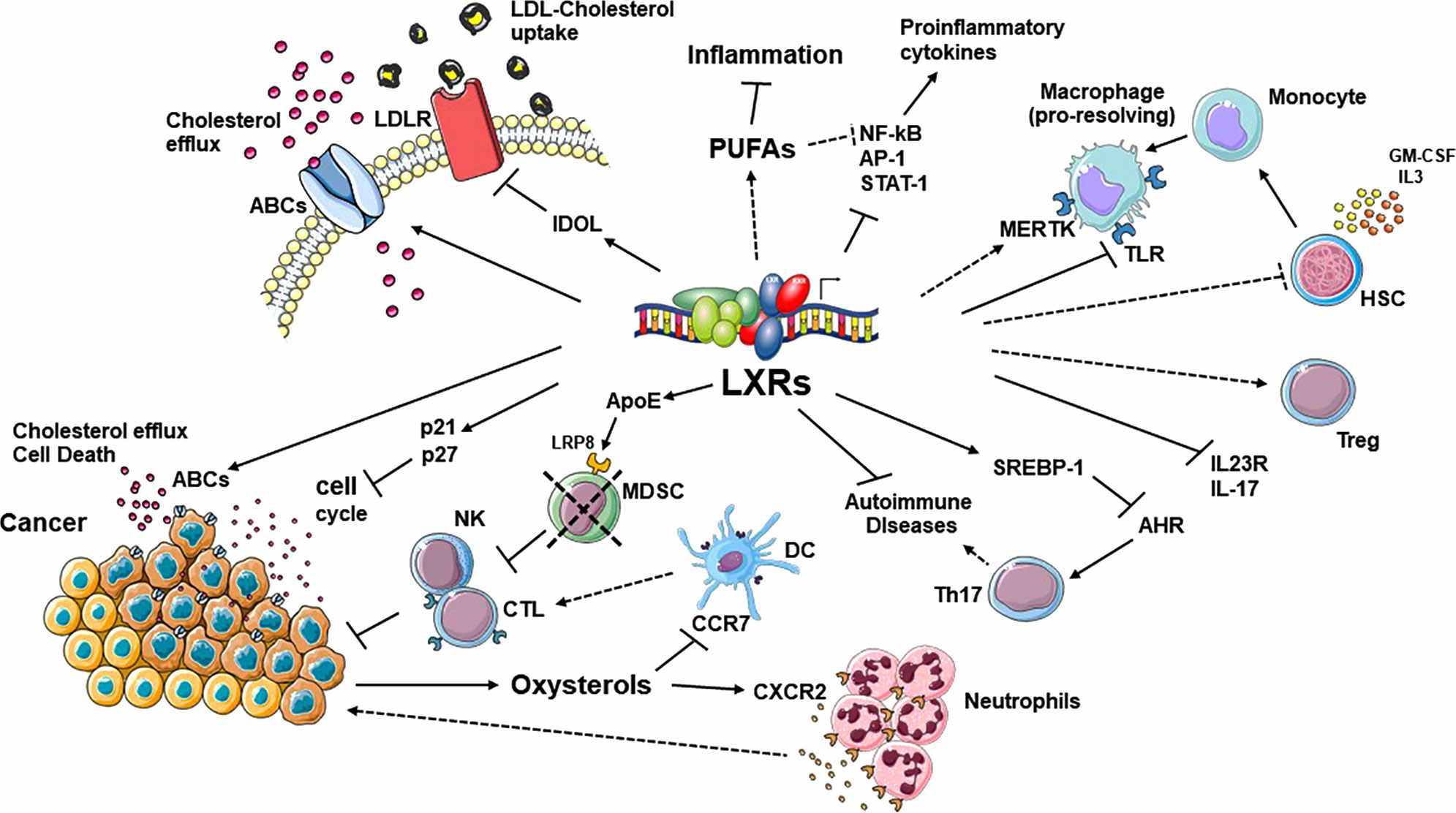
Figure 2 Summary of the most relevant pathways regulated by LXRs in the context of cholesterol homeostasis, inflammation, autoimmunity and tumor progression. These NRs act in a cell-, tissue- and context-dependent manner. In addition, many LXR-dependent and -independent pleiotropic effects mediated by oxysterols, produced in inflamed or tumoral microenvironments, add an additional level of complexity to these regulatory pathways. ABC, ATP binding cassette; AHR, aryl hydrocarbon receptor; APOE, apolipoprotein E; CTL, cytotoxic T lymphocyte; DC, dendritic Cell; DSS, IDOL, inducible degrader of the LDL-receptor; IRF3, interferon regulatory factor 3; LBD, ligand binding domain; PUFA, long-chain polyunsaturated fatty acid; LDLR, low-density lipoprotein receptor; LRP8, low-density lipoprotein receptor-related 8; MDSC, myeloid derived suppressor cells; MERTK, MER proto-oncogene tyrosine kinase; NK, natural killer; SREBP-1, sterol regulatory element-binding protein 1; TLR, Toll-like receptor.
Author Contributions
MB and SP made substantial contributions to conception and design of the review. MC organized the study, together with AS, contributed to revision, and approved the submitted version. All authors contributed to the article and approved the submitted version.
Funding
This work was supported by grants from MIUR Ateneo 2018 and PRIN 2017 to MC. The funders had no role in the study design, data collection and analysis, decision to publish, or preparation of the manuscript.
Conflict of Interest
The authors declare that the research was conducted in the absence of any commercial or financial relationships that could be construed as a potential conflict of interest.
References
1. York AG, Williams KJ, Argus JP, Zhou QD, Brar G, Vergnes L, et al. Limiting Cholesterol Biosynthetic Flux Spontaneously Engages Type I IFN Signaling. Cell (2015) 163(7):1716–29. doi: 10.1016/j.cell.2015.11.045
2. Molnar E, Swamy M, Holzer M, Beck-Garcia K, Worch R, Thiele C, et al. Cholesterol and sphingomyelin drive ligand-independent T-cell antigen receptor nanoclustering. J Biol Chem (2012) 287(51):42664–74. doi: 10.1074/jbc.M112.386045
3. Reboldi A, Dang EV, McDonald JG, Liang G, Russell DW, Cyster JG. Inflammation. 25-Hydroxycholesterol suppresses interleukin-1-driven inflammation downstream of type I interferon. Science (2014) 345(6197):679–84. doi: 10.1126/science.1254790
4. Tall AR, Yvan-Charvet L. Cholesterol, inflammation and innate immunity. Nat Rev Immunol (2015) 15(2):104–16. doi: 10.1038/nri3793
5. Cyster JG, Dang EV, Reboldi A, Yi T. 25-Hydroxycholesterols in innate and adaptive immunity. Nat Rev Immunol (2014) 14(11):731–43. doi: 10.1038/nri3755
6. Raccosta L, Fontana R, Maggioni D, Lanterna C, Villablanca EJ, Paniccia A, et al. The oxysterol-CXCR2 axis plays a key role in the recruitment of tumor-promoting neutrophils. J Exp Med (2013) 210(9):1711–28. doi: 10.1084/jem.20130440
7. Hu X, Wang Y, Hao LY, Liu X, Lesch CA, Sanchez BM, et al. Sterol metabolism controls T(H)17 differentiation by generating endogenous RORgamma agonists. Nat Chem Biol (2015) 11(2):141–7. doi: 10.1038/nchembio.1714
8. Bensinger SJ, Bradley MN, Joseph SB, Zelcer N, Janssen EM, Hausner MA, et al. LXR signaling couples sterol metabolism to proliferation in the acquired immune response. Cell (2008) 134(1):97–111. doi: 10.1016/j.cell.2008.04.052
9. Lin CY, Gustafsson JA. Targeting liver X receptors in cancer therapeutics. Nat Rev Cancer (2015) 15(4):216–24. doi: 10.1038/nrc3912
10. Kuzu OF, Noory MA, Robertson GP. The Role of Cholesterol in Cancer. Cancer Res (2016) 76(8):2063–70. doi: 10.1158/0008-5472.CAN-15-2613
11. Yvan-Charvet L, Welch C, Pagler TA, Ranalletta M, Lamkanfi M, Han S, et al. Increased inflammatory gene expression in ABC transporter-deficient macrophages: free cholesterol accumulation, increased signaling via toll-like receptors, and neutrophil infiltration of atherosclerotic lesions. Circulation (2008) 118(18):1837–47. doi: 10.1161/CIRCULATIONAHA.108.793869
12. Triantafilou M, Miyake K, Golenbock DT, Triantafilou K. Mediators of innate immune recognition of bacteria concentrate in lipid rafts and facilitate lipopolysaccharide-induced cell activation. J Cell Sci (2002) 115(Pt 12):2603–11.
13. Duewell P, Kono H, Rayner KJ, Sirois CM, Vladimer G, Bauernfeind FG, et al. NLRP3 inflammasomes are required for atherogenesis and activated by cholesterol crystals. Nature (2010) 464(7293):1357–61. doi: 10.1038/nature08938
14. Castrillo A, Joseph SB, Vaidya SA, Haberland M, Fogelman AM, Cheng G, et al. and toll-like receptor signaling mediates bacterial and viral antagonism of cholesterol metabolism. Mol Cell (2003) 12(4):805–16. doi: 10.1016/s1097-2765(03)00384-8
15. A-Gonzalez N, Bensinger SJ, Hong C, Beceiro S, Bradley MN, Zelcer N, et al. P. Tontonoz and A. Castrillo: Apoptotic cells promote their own clearance and immune tolerance through activation of the nuclear receptor LXR. Immunity (2009) 31(2):245–58. doi: 10.1016/j.immuni.2009.06.018
16. Fond AM, Lee CS, Schulman IG, Kiss RS, Ravichandran KS. Apoptotic cells trigger a membrane-initiated pathway to increase ABCA1. J Clin Invest (2015) 125(7):2748–58. doi: 10.1172/JCI80300
17. Jakobsson T, Treuter E, Gustafsson JA, Steffensen KR. Liver X receptor biology and pharmacology: new pathways, challenges and opportunities. Trends Pharmacol Sci (2012) 33(7):394–404. doi: 10.1016/j.tips.2012.03.013
18. Boergesen M, Pedersen TA, Gross B, van Heeringen SJ, Hagenbeek D, Bindesboll C, et al. Genome-wide profiling of liver X receptor, retinoid X receptor, and peroxisome proliferator-activated receptor alpha in mouse liver reveals extensive sharing of binding sites. Mol Cell Biol (2012) 32(4):852–67. doi: 10.1128/MCB.06175-11
19. Ghisletti S, Huang W, Jepsen K, Benner C, Hardiman G, Rosenfeld MG, et al. Cooperative NCoR/SMRT interactions establish a corepressor-based strategy for integration of inflammatory and anti-inflammatory signaling pathways. Genes Dev (2009) 23(6):681–93. doi: 10.1101/gad.1773109
20. Lee JH, Park SM, Kim OS, Lee CS, Woo JH, Park SJ, et al. Differential SUMOylation of LXRalpha and LXRbeta mediates transrepression of STAT1 inflammatory signaling in IFN-gamma-stimulated brain astrocytes. Mol Cell (2009) 35(6):806–17. doi: 10.1016/j.molcel.2009.07.021
21. Pourcet B, Gage MC, Leon TE, Waddington KE, Pello OM, Steffensen KR, et al. The nuclear receptor LXR modulates interleukin-18 levels in macrophages through multiple mechanisms. Sci Rep (2016) 6:25481. doi: 10.1038/srep25481
22. Joseph SB, Castrillo A, Laffitte BA, Mangelsdorf DJ, Tontonoz P. Reciprocal regulation of inflammation and lipid metabolism by liver X receptors. Nat Med (2003) 9(2):213–9. doi: 10.1038/nm820
23. Park MC, Kwon YJ, Chung SJ, Park YB, Lee SK. Liver X receptor agonist prevents the evolution of collagen-induced arthritis in mice. Rheumatol (Oxford) (2010) 49(5):882–90. doi: 10.1093/rheumatology/keq007
24. Li P, Spann NJ, Kaikkonen MU, Lu M, Oh DY, Fox JN, et al. NCoR repression of LXRs restricts macrophage biosynthesis of insulin-sensitizing omega 3 fatty acids. Cell (2013) 155(1):200–14. doi: 10.1016/j.cell.2013.08.054
25. Wouters E, de Wit NM, Vanmol J, van der Pol SMA, van Het Hof B, Sommer D, et al. Liver X Receptor Alpha Is Important in Maintaining Blood-Brain Barrier Function. Front Immunol (2019) 10:1811. doi: 10.3389/fimmu.2019.01811
26. Zhang-Gandhi CX, Drew PD. Liver X receptor and retinoid X receptor agonists inhibit inflammatory responses of microglia and astrocytes. J Neuroimmunol (2007) 183(1-2):50–9. doi: 10.1016/j.jneuroim.2006.11.007
27. Secor McVoy JR, Oughli HA, Oh U. Liver X receptor-dependent inhibition of microglial nitric oxide synthase 2. J Neuroinflammation (2015) 12:27. doi: 10.1186/s12974-015-0247-2
28. Cui G, Qin X, Wu L, Zhang Y, Sheng X, Yu Q, et al. Liver X receptor (LXR) mediates negative regulation of mouse and human Th17 differentiation. J Clin Invest (2011) 121(2):658–70. doi: 10.1172/JCI42974
29. Xu J, Wagoner G, Douglas JC, Drew PD. Liver X receptor agonist regulation of Th17 lymphocyte function in autoimmunity. J Leukoc Biol (2009) 86(2):401–9. doi: 10.1189/jlb.1008600
30. Hindinger C, Hinton DR, Kirwin SJ, Atkinson RD, Burnett ME, Bergmann CC, et al. Liver X receptor activation decreases the severity of experimental autoimmune encephalomyelitis. J Neurosci Res (2006) 84(6):1225–34. doi: 10.1002/jnr.21038
31. Chintalacharuvu SR, Sandusky GE, Burris TP, Burmer GC, Nagpal S. Liver X receptor is a therapeutic target in collagen-induced arthritis. Arthritis Rheum (2007) 56(4):1365–7. doi: 10.1002/art.22528
32. Li N, Rivera-Bermudez MA, Zhang M, Tejada J, Glasson SS, Collins-Racie LA, et al. LXR modulation blocks prostaglandin E2 production and matrix degradation in cartilage and alleviates pain in a rat osteoarthritis model. Proc Natl Acad Sci U S A (2010) 107(8):3734–9. doi: 10.1073/pnas.0911377107
33. Asquith DL, Miller AM, Reilly J, Kerr S, Welsh P, Sattar N, et al. Simultaneous activation of the liver X receptors (LXRalpha and LXRbeta) drives murine collagen-induced arthritis disease pathology. Ann Rheum Dis (2011) 70(12):2225–8. doi: 10.1136/ard.2011.152652
34. Asquith DL, Miller AM, Hueber AJ, McKinnon HJ, Sattar N, Graham GJ, et al. Liver X receptor agonism promotes articular inflammation in murine collagen-induced arthritis. Arthritis Rheum (2009) 60(9):2655–65. doi: 10.1002/art.24717
35. Yoon CH, Kwon YJ, Lee SW, Park YB, Lee SK, Park MC. Activation of liver X receptors suppresses inflammatory gene expressions and transcriptional corepressor clearance in rheumatoid arthritis fibroblast like synoviocytes. J Clin Immunol (2013) 33(1):190–9. doi: 10.1007/s10875-012-9799-4
36. Jakobsson T, Vedin LL, Hassan T, Venteclef N, Greco D, D’Amato M, et al. The oxysterol receptor LXRbeta protects against DSS- and TNBS-induced colitis in mice. Mucosal Immunol (2014) 7(6):1416–28. doi: 10.1038/mi.2014.31
37. Herold M, Breuer J, Hucke S, Knolle P, Schwab N, Wiendl H, et al. Liver X receptor activation promotes differentiation of regulatory T cells. PloS One (2017) 12(9):e0184985. doi: 10.1371/journal.pone.0184985
38. Guo D, Reinitz F, Youssef M, Hong C, Nathanson D, Akhavan D, et al. An LXR agonist promotes glioblastoma cell death through inhibition of an EGFR/AKT/SREBP-1/LDLR-dependent pathway. Cancer Discov (2011) 1(5):442–56. doi: 10.1158/2159-8290.CD-11-0102
39. Lewis CA, Brault C, Peck B, Bensaad K, Griffiths B, Mitter R, et al. SREBP maintains lipid biosynthesis and viability of cancer cells under lipid- and oxygen-deprived conditions and defines a gene signature associated with poor survival in glioblastoma multiforme. Oncogene (2015) 34(40):5128–40. doi: 10.1038/onc.2014.439
40. Chuu CP, Lin HP. Antiproliferative effect of LXR agonists T0901317 and 22(R)-hydroxycholesterol on multiple human cancer cell lines. Anticancer Res (2010) 30(9):3643–8.
41. Chuu CP, Hiipakka RA, Kokontis JM, Fukuchi J, Chen RY, Liao S. Inhibition of tumor growth and progression of LNCaP prostate cancer cells in athymic mice by androgen and liver X receptor agonist. Cancer Res (2006) 66(13):6482–6. doi: 10.1158/0008-5472.CAN-06-0632
42. Lee JH, Gong H, Khadem S, Lu Y, Gao X, Li S, et al. Androgen deprivation by activating the liver X receptor. Endocrinology (2008) 149(8):3778–88. doi: 10.1210/en.2007-1605
43. Tavazoie MF, Pollack I, Tanqueco R, Ostendorf BN, Reis BS, Gonsalves FC, et al. LXR/ApoE Activation Restricts Innate Immune Suppression in Cancer. Cell (2018) 172(4):825–40 e18. doi: 10.1016/j.cell.2017.12.026
44. Villablanca EJ, Raccosta L, Zhou D, Fontana R, Maggioni D, Negro A, et al. Tumor-mediated liver X receptor-alpha activation inhibits CC chemokine receptor-7 expression on dendritic cells and dampens antitumor responses. Nat Med (2010) 16(1):98–105. doi: 10.1038/nm.2074
45. Derangere V, Chevriaux A, Courtaut F, Bruchard M, Berger H, Chalmin F, et al. Liver X receptor beta activation induces pyroptosis of human and murine colon cancer cells. Cell Death Differ (2014) 21(12):1914–24. doi: 10.1038/cdd.2014.117
46. Bilotta MT, Abruzzese MP, Molfetta R, Scarno G, Fionda C, Zingoni A, et al. Activation of liver X receptor up-regulates the expression of the NKG2D ligands MICA and MICB in multiple myeloma through different molecular mechanisms. FASEB J (2019) 33(8):9489–504. doi: 10.1096/fj.201900319R
47. Janowski BA, Grogan MJ, Jones SA, Wisely GB, Kliewer SA, Corey EJ, et al. Structural requirements of ligands for the oxysterol liver X receptors LXRalpha and LXRbeta. Proc Natl Acad Sci U S A (1999) 96(1):266–71. doi: 10.1073/pnas.96.1.266
48. Apfel R, Benbrook D, Lernhardt E, Ortiz MA, Salbert G, Pfahl M. A novel orphan receptor specific for a subset of thyroid hormone-responsive elements and its interaction with the retinoid/thyroid hormone receptor subfamily. Mol Cell Biol (1994) 14(10):7025–35. doi: 10.1128/mcb.14.10.7025
49. Vedin LL, Gustafsson JA, Steffensen KR. The oxysterol receptors LXRalpha and LXRbeta suppress proliferation in the colon. Mol Carcinog (2013) 52(11):835–44. doi: 10.1002/mc.21924
50. Bookout AL, Jeong Y, Downes M, Yu RT, Evans RM, Mangelsdorf DJ. Anatomical profiling of nuclear receptor expression reveals a hierarchical transcriptional network. Cell (2006) 126(4):789–99. doi: 10.1016/j.cell.2006.06.049
51. Wang B, Rong X, Palladino END, Wang J, Fogelman AM, Martin MG, et al. Phospholipid Remodeling and Cholesterol Availability Regulate Intestinal Stemness and Tumorigenesis. Cell Stem Cell (2018) 22(2):206–220 e4. doi: 10.1016/j.stem.2017.12.017
52. A-Gonzalez N, Guillen JA, Gallardo G, Diaz M, de la Rosa JV, Hernandez IH, et al. The nuclear receptor LXRalpha controls the functional specialization of splenic macrophages. Nat Immunol (2013) 14(8):831–9. doi: 10.1038/ni.2622
53. Janowski BA, Willy PJ, Devi TR, Falck JR, Mangelsdorf DJ. An oxysterol signalling pathway mediated by the nuclear receptor LXR alpha. Nature (1996) 383(6602):728–31. doi: 10.1038/383728a0
54. Yang C, McDonald JG, Patel A, Zhang Y, Umetani M, Xu F, et al. Sterol intermediates from cholesterol biosynthetic pathway as liver X receptor ligands. J Biol Chem (2006) 281(38):27816–26. doi: 10.1074/jbc.M603781200
55. Pehkonen P, Welter-Stahl L, Diwo J, Ryynanen J, Wienecke-Baldacchino A, Heikkinen S, et al. Genome-wide landscape of liver X receptor chromatin binding and gene regulation in human macrophages. BMC Genomics (2012) 13(50):1–20. doi: 10.1186/1471-2164-13-50
56. Willy PJ, Umesono K, Ong ES, Evans RM, Heyman RA, Mangelsdorf DJ. LXR, a nuclear receptor that defines a distinct retinoid response pathway. Genes Dev (1995) 9(9):1033–45. doi: 10.1101/gad.9.9.1033
57. Tontonoz P. Transcriptional and posttranscriptional control of cholesterol homeostasis by liver X receptors. Cold Spring Harb Symp Quant Biol (2011) 76:129–37. doi: 10.1101/sqb.2011.76.010702
58. Zelcer N, Tontonoz P. Liver X receptors as integrators of metabolic and inflammatory signaling. J Clin Invest (2006) 116(3):607–14. doi: 10.1172/JCI27883
59. Valledor AF. The innate immune response under the control of the LXR pathway. Immunobiology (2005) 210(2-4):127–32. doi: 10.1016/j.imbio.2005.05.007
60. Calkin AC, Tontonoz P. Liver x receptor signaling pathways and atherosclerosis. Arterioscler Thromb Vasc Biol (2010) 30(8):1513–8. doi: 10.1161/ATVBAHA.109.191197
61. Traversari C, Sozzani S, Steffensen KR, Russo V. LXR-dependent and -independent effects of oxysterols on immunity and tumor growth. Eur J Immunol (2014) 44(7):1896–903. doi: 10.1002/eji.201344292
62. Beceiro S, Pap A, Czimmerer Z, Sallam T, Guillen JA, Gallardo G, et al. LXR nuclear receptors are transcriptional regulators of dendritic cell chemotaxis. Mol Cell Biol (2018) 38:1–18. doi: 10.1128/MCB.00534-17
63. Geyeregger R, Shehata M, Zeyda M, Kiefer FW, Stuhlmeier KM, Porpaczy E, et al. Liver X receptors interfere with cytokine-induced proliferation and cell survival in normal and leukemic lymphocytes. J Leukoc Biol (2009) 86(5):1039–48. doi: 10.1189/jlb.1008663
64. Solt LA, Kamenecka TM, Burris TP. LXR-mediated inhibition of CD4+ T helper cells. PloS One (2012) 7(9):e46615. doi: 10.1371/journal.pone.0046615
65. Walcher D, Vasic D, Heinz P, Bach H, Durst R, Hausauer A, et al. LXR activation inhibits chemokine-induced CD4-positive lymphocyte migration. Basic Res Cardiol (2010) 105(4):487–94. doi: 10.1007/s00395-010-0092-5
66. Zhu X, Owen JS, Wilson MD, Li H, Griffiths GL, Thomas MJ, et al. Macrophage ABCA1 reduces MyD88-dependent Toll-like receptor trafficking to lipid rafts by reduction of lipid raft cholesterol. J Lipid Res (2010) 51(11):3196–206. doi: 10.1194/jlr.M006486
67. Han S, Zhuang H, Shumyak S, Wu J, Xie C, Li H, et al. Liver X Receptor Agonist Therapy Prevents Diffuse Alveolar Hemorrhage in Murine Lupus by Repolarizing Macrophages. Front Immunol (2018) 9:135:1–12. doi: 10.3389/fimmu.2018.00135
68. Pascual-Garcia M, Rue L, Leon T, Julve J, Carbo JM, Matalonga J, et al. Reciprocal negative cross-talk between liver X receptors (LXRs) and STAT1: effects on IFN-gamma-induced inflammatory responses and LXR-dependent gene expression. J Immunol (2013) 190(12):6520–32. doi: 10.4049/jimmunol.1201393
69. Fowler AJ, Sheu MY, Schmuth M, Kao J, Fluhr JW, Rhein L, et al. Liver X receptor activators display anti-inflammatory activity in irritant and allergic contact dermatitis models: liver-X-receptor-specific inhibition of inflammation and primary cytokine production. J Invest Dermatol (2003) 120(2):246–55. doi: 10.1046/j.1523-1747.2003.12033.x
70. Serhan CN. Pro-resolving lipid mediators are leads for resolution physiology. Nature (2014) 510(7503):92–101. doi: 10.1038/nature13479
71. Serhan CN, Chiang N, Dalli J, Levy BD. Lipid mediators in the resolution of inflammation. Cold Spring Harb Perspect Biol (2014) 7(2):a016311. doi: 10.1101/cshperspect.a016311
72. Choi JY, Seo JY, Yoon YS, Lee YJ, Kim HS, Kang JL. Mer signaling increases the abundance of the transcription factor LXR to promote the resolution of acute sterile inflammation. Sci Signal (2015) 8(365):ra21. doi: 10.1126/scisignal.2005864
73. Rong X, Albert CJ, Hong C, Duerr MA, Chamberlain BT, Tarling EJ, et al. LXRs regulate ER stress and inflammation through dynamic modulation of membrane phospholipid composition. Cell Metab (2013) 18(5):685–97. doi: 10.1016/j.cmet.2013.10.002
74. Murphy AJ, Akhtari M, Tolani S, Pagler T, Bijl N, Kuo CL, et al. ApoE regulates hematopoietic stem cell proliferation, monocytosis, and monocyte accumulation in atherosclerotic lesions in mice. J Clin Invest (2011) 121(10):4138–49. doi: 10.1172/JCI57559
75. Giubilei F, Antonini G, Di Legge S, Sormani MP, Pantano P, Antonini R, et al. Blood cholesterol and MRI activity in first clinical episode suggestive of multiple sclerosis. Acta Neurol Scand (2002) 106(2):109–12. doi: 10.1034/j.1600-0404.2002.01334.x
76. Weinstock-Guttman B, Zivadinov R, Mahfooz N, Carl E, Drake A, Schneider J, et al. Serum lipid profiles are associated with disability and MRI outcomes in multiple sclerosis. J Neuroinflammation (2011) 8:127. doi: 10.1186/1742-2094-8-127
77. Weinstock-Guttman B, Zivadinov R, Horakova D, Havrdova E, Qu J, Shyh G, et al. Lipid profiles are associated with lesion formation over 24 months in interferon-beta treated patients following the first demyelinating event. J Neurol Neurosurg Psychiatry (2013) 84(11):1186–91. doi: 10.1136/jnnp-2012-304740
78. Stampanoni Bassi M, Iezzi E, Buttari F, Gilio L, Simonelli I, Carbone F, et al. Obesity worsens central inflammation and disability in multiple sclerosis. Mult Scler (2019) 26:1237–46. doi: 10.1177/1352458519853473
79. Tettey P, Simpson S Jr., Taylor B, Blizzard L, Ponsonby AL, Dwyer T, et al. An adverse lipid profile is associated with disability and progression in disability, in people with MS. Mult Scler (2014) 20(13):1737–44. doi: 10.1177/1352458514533162
80. Tettey P, Simpson S Jr., Taylor B, Blizzard L, Ponsonby AL, Dwyer T, et al. Adverse lipid profile is not associated with relapse risk in MS: results from an observational cohort study. J Neurol Sci (2014) 340(1–2):230–2. doi: 10.1016/j.jns.2014.02.038
81. Steinman L. Multiple sclerosis: a coordinated immunological attack against myelin in the central nervous system. Cell (1996) 85(3):299–302. doi: 10.1016/s0092-8674(00)81107-1
82. Dendrou CA, Fugger L, Friese MA. Immunopathology of multiple sclerosis. Nat Rev Immunol (2015) 15(9):545–58. doi: 10.1038/nri3871
83. Baidzajevas K, Hadadi E, Lee B, Lum J, Shihui F, Sudbery I, et al. Macrophage polarisation associated with atherosclerosis differentially affects their capacity to handle lipids. Atherosclerosis (2020) 305:10–8. doi: 10.1016/j.atherosclerosis.2020.05.003
84. Bailly C, Vergoten G. Glycyrrhizin: An alternative drug for the treatment of COVID-19 infection and the associated respiratory syndrome? Pharmacol Ther (2020) 214:1–16. doi: 10.1016/j.pharmthera.2020.107618
85. Thangapandi VR, Knittelfelder O, Brosch M, Patsenker E, Vvedenskaya O, Buch S, et al. Loss of hepatic Mboat7 leads to liver fibrosis. Gut (2020) 1–11. doi: 10.1136/gutjnl-2020-320853
86. Rondanelli M, Perna S, Alalwan TA, Cazzola R, Gasparri C, Infantino V, et al. A structural equation model to assess the pathways of body adiposity and inflammation status on dysmetabolic biomarkers via red cell distribution width and mean corpuscular volume: a cross-sectional study in overweight and obese subjects. Lipids Health Dis (2020) 19(1):154. doi: 10.1186/s12944-020-01308-5
87. Smolen JS, Aletaha D, McInnes IB. Rheumatoid arthritis. Lancet (2016) 388(10055):2023–38. doi: 10.1016/S0140-6736(16)30173-8
88. Huang Y, Fu X, Lyu X, Xu Z, He Z, Zhang Y, et al. Activation of LXR attenuates collagen-induced arthritis via suppressing BLyS production. Clin Immunol (2015) 161(2):339–47. doi: 10.1016/j.clim.2015.09.015
89. Asquith DL, Ballantine LE, Nijjar JS, Makdasy MK, Patel S, Wright PB, et al. The liver X receptor pathway is highly upregulated in rheumatoid arthritis synovial macrophages and potentiates TLR-driven cytokine release. Ann Rheum Dis (2013) 72(12):2024–31. doi: 10.1136/annrheumdis-2012-202872
90. Murai T. Cholesterol lowering: role in cancer prevention and treatment. Biol Chem (2015) 396(1):1–11. doi: 10.1515/hsz-2014-0194
91. Bovenga F, Sabba C, Moschetta A. Uncoupling nuclear receptor LXR and cholesterol metabolism in cancer. Cell Metab (2015) 21(4):517–26. doi: 10.1016/j.cmet.2015.03.002
92. Vedin LL, Lewandowski SA, Parini P, Gustafsson JA, Steffensen KR. The oxysterol receptor LXR inhibits proliferation of human breast cancer cells. Carcinogenesis (2009) 30(4):575–9. doi: 10.1093/carcin/bgp029
93. Lo Sasso G, Bovenga F, Murzilli S, Salvatore L, Di Tullio G, Martelli N, et al. Liver X receptors inhibit proliferation of human colorectal cancer cells and growth of intestinal tumors in mice. Gastroenterology (2013) 144(7):1497–507, 1507 e1-13. doi: 10.1053/j.gastro.2013.02.005
94. Horton JD, Goldstein JL, Brown MS. SREBPs: activators of the complete program of cholesterol and fatty acid synthesis in the liver. J Clin Invest (2002) 109(9):1125–31. doi: 10.1172/JCI15593
95. Yang J, Wang L, Jia R. Role of de novo cholesterol synthesis enzymes in cancer. J Cancer (2020) 11(7):1761–7. doi: 10.7150/jca.38598
96. Iannelli F, Lombardi R, Milone MR, Pucci B, De Rienzo S, Budillon A, et al. Targeting Mevalonate Pathway in Cancer Treatment: Repurposing of Statins. Recent Pat Anticancer Drug Discov (2018) 13(2):184–200. doi: 10.2174/1574892812666171129141211
97. Villa GR, Hulce JJ, Zanca C, Bi J, Ikegami S, Cahill GL, et al. An LXR-Cholesterol Axis Creates a Metabolic Co-Dependency for Brain Cancers. Cancer Cell (2016) 30(5):683–93. doi: 10.1016/j.ccell.2016.09.008
98. Fukuchi J, Kokontis JM, Hiipakka RA, Chuu CP, Liao S. Antiproliferative effect of liver X receptor agonists on LNCaP human prostate cancer cells. Cancer Res (2004) 64(21):7686–9. doi: 10.1158/0008-5472.CAN-04-2332
99. York AG, Bensinger SJ. Subverting sterols: rerouting an oxysterol-signaling pathway to promote tumor growth. J Exp Med (2013) 210(9):1653–6. doi: 10.1084/jem.20131335
100. Dufour J, Viennois E, De Boussac H, Baron S, Lobaccaro JM. Oxysterol receptors, AKT and prostate cancer. Curr Opin Pharmacol (2012) 12(6):724–8. doi: 10.1016/j.coph.2012.06.012
101. Lin CY, Huo C, Kuo LK, Hiipakka RA, Jones RB, Lin HP, et al. Cholestane-3beta, 5alpha, 6beta-triol suppresses proliferation, migration, and invasion of human prostate cancer cells. PloS One (2013) 8(6):e65734. doi: 10.1371/journal.pone.0065734
102. Baek AE, Yu YA, He S, Wardell SE, Chang CY, Kwon S, et al. The cholesterol metabolite 27 hydroxycholesterol facilitates breast cancer metastasis through its actions on immune cells. Nat Commun (2017) 8(1):864. doi: 10.1038/s41467-017-00910-z
103. McDonnell DP, Park S, Goulet MT, Jasper J, Wardell SE, Chang CY, et al. Obesity, cholesterol metabolism, and breast cancer pathogenesis. Cancer Res (2014) 74(18):4976–82. doi: 10.1158/0008-5472.CAN-14-1756
104. Liang H, Shen X. LXR activation radiosensitizes non-small cell lung cancer by restricting myeloid-derived suppressor cells. Biochem Biophys Res Commun (2020) 528(2):330–5. doi: 10.1016/j.bbrc.2020.04.137
105. Faulds MH, Zhao C, Dahlman-Wright K. Molecular biology and functional genomics of liver X receptors (LXR) in relationship to metabolic diseases. Curr Opin Pharmacol (2010) 10(6):692–7. doi: 10.1016/j.coph.2010.07.003
106. Schultz JR, Tu H, Luk A, Repa JJ, Medina JC, Li L, et al. Role of LXRs in control of lipogenesis. Genes Dev (2000) 14(22):2831–8. doi: 10.1101/gad.850400
107. Bradley MN, Hong C, Chen M, Joseph SB, Wilpitz DC, Wang X, et al. Ligand activation of LXR beta reverses atherosclerosis and cellular cholesterol overload in mice lacking LXR alpha and apoE. J Clin Invest (2007) 117(8):2337–46. doi: 10.1172/JCI31909
108. Joseph SB, Laffitte BA, Patel PH, Watson MA, Matsukuma KE, Walczak R, et al. Direct and indirect mechanisms for regulation of fatty acid synthase gene expression by liver X receptors. J Biol Chem (2002) 277(13):11019–25. doi: 10.1074/jbc.M111041200
109. Joseph SB, McKilligin E, Pei L, Watson MA, Collins AR, Laffitte BA, et al. Synthetic LXR ligand inhibits the development of atherosclerosis in mice. Proc Natl Acad Sci U S A (2002) 99(11):7604–9. doi: 10.1073/pnas.112059299
110. Katz A, Udata C, Ott E, Hickey L, Burczynski ME, Burghart P, et al. Safety, pharmacokinetics, and pharmacodynamics of single doses of LXR-623, a novel liver X-receptor agonist, in healthy participants. J Clin Pharmacol (2009) 49(6):643–9. doi: 10.1177/0091270009335768
Keywords: liver-X-receptor, cholesterol, inflammation, autoimmunity, cancer metabolism, antitumor immune responses
Citation: Bilotta MT, Petillo S, Santoni A and Cippitelli M (2020) Liver X Receptors: Regulators of Cholesterol Metabolism, Inflammation, Autoimmunity, and Cancer. Front. Immunol. 11:584303. doi: 10.3389/fimmu.2020.584303
Received: 16 July 2020; Accepted: 12 October 2020;
Published: 03 November 2020.
Edited by:
Francesca Velotti, University of Tuscia, ItalyReviewed by:
Angela Bonura, National Research Council (CNR), ItalyMirella Giovarelli, University of Turin, Italy
Copyright © 2020 Bilotta, Petillo, Santoni and Cippitelli. This is an open-access article distributed under the terms of the Creative Commons Attribution License (CC BY). The use, distribution or reproduction in other forums is permitted, provided the original author(s) and the copyright owner(s) are credited and that the original publication in this journal is cited, in accordance with accepted academic practice. No use, distribution or reproduction is permitted which does not comply with these terms.
*Correspondence: Marco Cippitelli, bWFyY28uY2lwcGl0ZWxsaUB1bmlyb21hMS5pdA==
†Present address: Maria Teresa Bilotta, Laboratory of Molecular Biology, Center for Cancer Research, National Cancer Institute, National Institutes of Health, Bethesda, MD, United States