- 1Department of Health Service, Guard Bureau of the Joint Staff Department, Central Military Commission of PLA, Beijing, China
- 2Department of Oncology, PLA Rocket Force Characteristic Medical Center, Beijing, China
In immune system, B cells are classically positive modulators that regulate inflammation and immune responses. Regulatory B cells (Bregs) are a subset of B cells which play crucial roles in various conditions, including infection, allergies, autoimmune diseases, transplantation, and tumors. Until now, unequivocal surface markers for Bregs still lack consensus, although numerous Breg subsets have been identified. Generally, Bregs exert their immunoregulatory functions mainly through cytokine secretion and intercellular contact. In the tumor microenvironment, Bregs suppress effector T cells, induce regulatory T cells and target other tumor-infiltrating immune cells, such as myeloid-derived suppressor cells, natural killer cells and macrophages, to hamper anti-tumor immunity. Meanwhile, the cross-regulations between Bregs and tumor cells often result in tumor escape from immunosurveillance. In addition, accumulating evidence suggests that Bregs are closely associated with many clinicopathological factors of cancer patients and might be potential biomarkers for accessing patient survival. Thus, Bregs are potential therapeutic targets for future immunotherapy in cancer patients. In this review, we will discuss the phenotypes, functions, and clinical relevance of Bregs in cancer.
Introduction
In immune system, B cells are classically recognized as positive modulators to regulate inflammation and immune responses by releasing antibodies and activating T cells through antigen presentation (1–3). Generally, B cells produce antibodies which are a critical part of the host immunity against infection, that can neutralize pathogens, opsonize pathogens for subsequent phagocytosis and mediate antibody-dependent cellular cytotoxicity (4–6). Besides, B cells also act to suppress immune responses. Accumulating studies have revealed that an immunoregulatory subset of B cells exists and exerts multifaceted functions (7–10). In the 1970s, a suppressive subset of B cells was firstly hypothesized to exist and modulate immune responses, based on reports indicating that the depletion of B cells in splenocytes was correlated with increased severity and duration of delayed-type hypersensitivity in a guinea pig model (11, 12). In the 1980s, it was reported that activated splenic B cells prompted T cells to differentiate into suppressor T cells following adoptive transfer into naïve recipient mice (13, 14), further supporting the concept of “suppressor B cells”. The term “regulatory B cells” (Bregs) was firstly coined in 2002 by Mizoguchi and his colleagues (15). They demonstrated that the interleukin-10 (IL-10)-producing CD1d+ Bregs were induced in a chronic inflammatory environment and dampened the progression of intestinal inflammation by repressing inflammatory cascades associated with IL-1 upregulation and STAT3 activation. Thereafter, mounting evidence has uncovered the roles of Bregs in numerous diseases and conditions, such as infection (16), allergies (17), autoimmune diseases (18), transplantation (19), and tumors (20).
To support tumor progression, tumor cells usually generate a tumor microenvironment (TME), which comprises immune cells, fibroblasts, endothelial cells, and the extracellular matrix (ECM). Among the various tumor-infiltrating immune cells in the TME, Bregs release anti-inflammatory mediators and express inhibitory molecules to exert immunoregulatory functions and shape the tumor immune milieu (21–25). In this review, we will discuss the phenotypes, functions and clinical relevance of Bregs in cancer.
Phenotypes and Immunoregulatory Functions of Bregs
Until now, the definition and classification of Bregs remain unclear due the lack of unique surface markers. As cell populations can be classified based on cytokine production (26), Breg cells are also classified according to their secreted cytokines in many cases and comprise an assortment of subsets (27). Moreover, the phenotypes of human Bregs are not identical to those of murine Bregs. Thus far, several human Breg subsets have been identified. Human CD19+CD25hi Bregs, which enhance Treg function, have been reported (28). IL-10-expressing CD24+CD38+ Bregs were characterized in gastric cancer (GC) patients (29). IL-21-induced CD19+CD38+CD1d+IgM+CD147+ Bregs, which express granzyme B (GrB), have also been identified in solid tumors (30). In addition, CD19+CD24+CD38+ Bregs were found in invasive breast carcinoma (IBCa) patients, and they were recognized in higher percentages in the breast tissue and peripheral blood of IBCa patients than those in benign tumors and healthy individuals (31). Similarly, well-founded evidence has also revealed several subsets of murine Bregs with different phenotypes. In a B16-F10 melanoma murine model, transitional 2 marginal zone precursor (T2-MZP) Bregs were identified with the B220+CD23+IgMhiCD21hi phenotype in tumor-draining lymph nodes (TDLNs) (32). In another study, splenic CD1dhiCD5+ Bregs sorted from wild-type (WT) and CD20-deficient mice were adoptively transferred into Cd19-/- mice and CD20 monoclonal antibody (mAb)-treated mice, respectively, resulting in normalized contact hypersensitivity (33). Moreover, it has been determined that IgMhiCD1dhiCD5+CD19hiCD23lowCD38hiB220hi Bregs could differentiate into CD138+ plasma cells, which secrete IgM and IgG antibodies (34). CD39+CD73+ Bregs release adenosine and ameliorate the severity of dextran sulfate sodium salt (DSS)-induced acute colitis (35). Regarding tumor, the tumor-associated Breg phenotypes that have been reported to date are listed in Table 1.
Bregs have been reported to exert immunoregulatory functions mainly through cytokine secretion and intercellular contact. The most commonly studied cytokines produced by Bregs are IL-10, IL-35, and transforming growth factor (TGF)-β. IL-10 has multitudinous inhibitory functions and has been widely recognized as an immunoregulatory factor in various chronic inflammatory diseases (54). B10 cells, which are IL-10-producing Bregs, inhibit CD4+ T cell proliferation and pro-inflammatory cytokine production by releasing IL-10 (55–57). During chronic hepatitis B virus (HBV) infection, Bregs repress HBV-specific CD8+ T cell responses in an IL-10-dependent manner (58). Moreover, B10 cell-derived IL-10 impairs the functions of dendritic cells and macrophages by inducing tolerant phenotypes (59). Additionally, Bregs inhibit interferon-γ (IFN-γ) produced by NK cells through IL-10 (60). Analogous to IL-10, TGF-β is another pivotal mediator secreted by Bregs to regulate immune responses, and it can induce the generation of regulatory T cells (Tregs) (54, 61). Additionally, TGF-β produced by Bregs augments the expression of cytotoxic T lymphocyte associated antigen-4 (CTLA-4) and Foxp3 in Tregs (28). In non-obese diabetic mice, Bregs induce Th1 cell apoptosis and suppress the activities of antigen-presenting cells via TGF-β secretion (62). In autoimmune and infectious diseases, Bregs produce IL-35, which is an IL-12 family member and a crucial negative modulator of T-cell immunity (63). IL-35 produced by Bregs promotes Treg proliferation and impairs Th17 responses to enhance immune tolerance (54). Intriguingly, IL-35 has been found to convert B cells into IL-35-producing Bregs, thus establishing a positive feedback loop (64).
Aside from cytokine secretion, Bregs regulate immune responses through intercellular contact, including ligand-receptor interactions such as CTLA-4/CD86, CD40/CD40L, and Fas/FasL. In a study by Aharon et al., a transwell system was used to demonstrate that intercellular contact is the major mechanism through which Bregs increase Foxp3 and CTLA-4 expression on Tregs (28). CD28 and CTLA-4 are generally expressed by T cells and are both ligands for CD80/CD86 (65). In another study, Paul and colleagues observed that when co-cultured with autologous T cells, CD19+CD24hiCD38hi Bregs decreased the percentage of tumor necrosis factor (TNF)-α+ and IFN-γ+ CD4+ T cells, and blocking antibodies against CD80/CD86 partially reversed this suppression (66). Similarly, the simultaneous blockade of IL-10, CD80, and CD86 inhibited Bregs from suppressing IFN-γ and TNF-α production by CD4+ T cells in rheumatoid arthritis patients (67). These results indicate that interactions between Bregs and T cells involving CD80/CD86 mediate the suppression of T cell immunity. The CD40/CD40L signaling pathway plays critical roles in establishing humoral responses and is involved in immune responses to tumors (68). Bregs also regulate effector T cells through CD40/CD40L to induce T cell death and inhibit T cell response to autoantigens (69). In addition, blocking the binding of CD40/CD40L between Bregs and CD4+ T cells in two different tumor models caused distinct immune responses in terms of Th1/Th2 differentiation and Treg induction (70). Bregs have been reported to express FasL, which belongs to the TNF protein family and causes apoptosis by binding to its receptor, Fas (71). In the spleen, CD5+ Bregs express FasL and induce T cell apoptosis through the interactions of Fas/FasL (72). In another study, lipopolysaccharides-induced CD5+CD1dhi Bregs inhibited the proliferation of activated CD4+ T cells. After adding anti-FasL antibodies, the suppression of CD4+ T cell proliferation was partially reversed, suggesting Fas/FasL pathway-mediated regulation of Bregs (73). CD5 expression on Bregs should also be considered (74). In a B16 melanoma model, it was demonstrated that CD5+ B cells bound to IL-6 directly through CD5 to promote tumor growth (75). These studies reveal that intercellular contact is critically important for Bregs to exert their immunoregulatory functions.
Mechanisms Underlying Breg-Mediated Regulation of Antitumor Responses
The immunoregulatory mechanisms of Bregs have gradually been elucidated in cancer. In the TME, the relationship between the host, tumor, and stroma is mediated by the balance of all tumor-infiltrating cells. Bregs interact with various tumor-infiltrating immune cells of the innate and adaptive immune systems to attenuate anti-tumor responses. Moreover, the cross-regulation between Bregs and tumor cells facilitates tumor progression (Figure 1; Table 2).
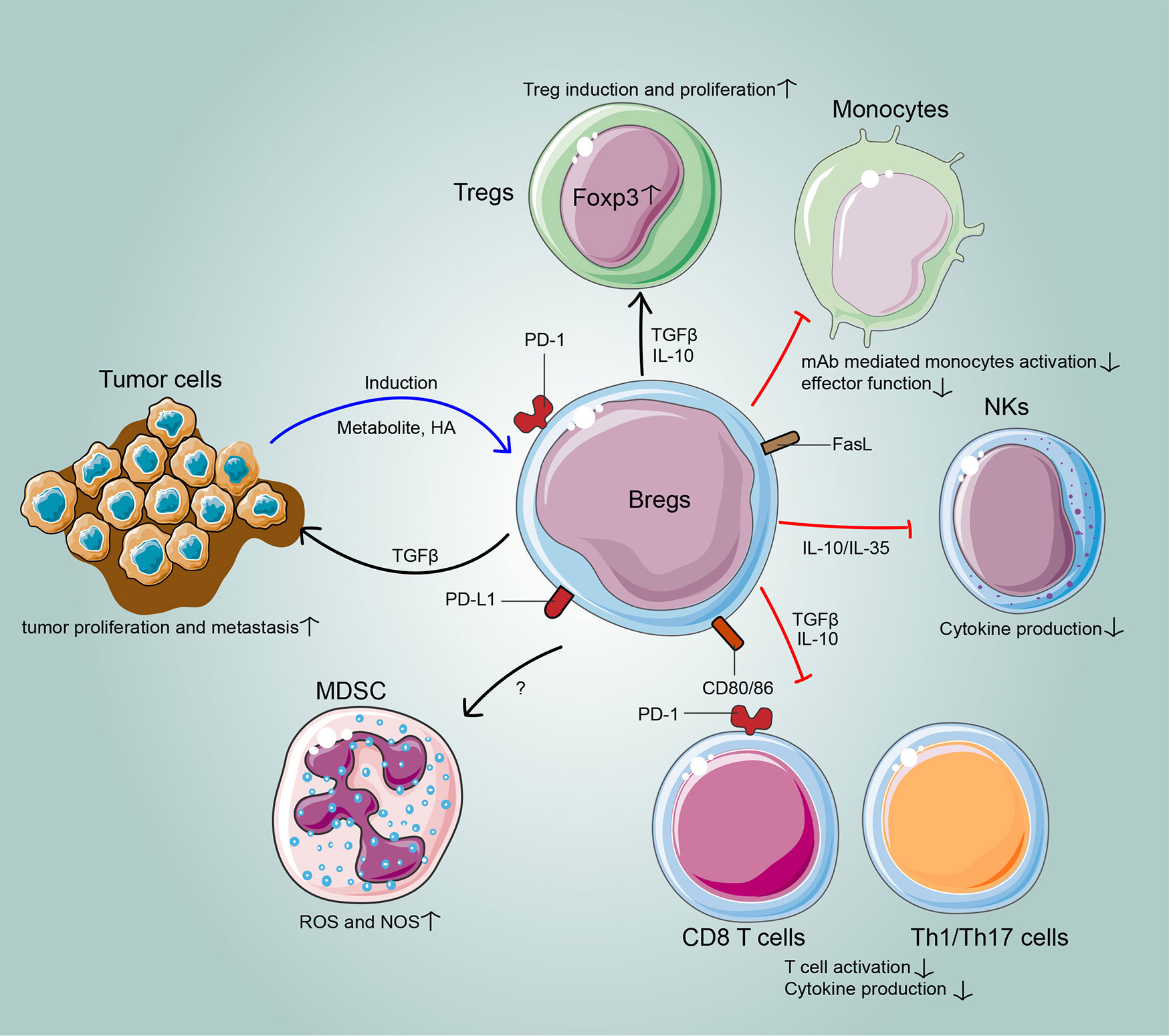
Figure 1 The cross-regulation between Bregs and tumors. In the tumor microenvironment, Bregs suppress effector T cells, induce regulatory T cells and target other tumor-infiltrating immune cells, such as myeloid-derived suppressor cells, natural killer cells and macrophages, to hamper anti-tumor immunity. Meanwhile, the cross-regulations between Bregs and tumor cells often result in tumor escape from immunosurveillance.
Suppression of Effector T Cell Responses
One of the many approaches by which Bregs modulate antitumor response is the direct inhibition of effector T cell responses. Lindner et al. found that IL-21-induced human GrB+ Bregs inhibited CD4+ T-cell proliferation by transporting active GrB to T cells and degrading the T-cell receptor ζ-chain in vitro (30).Furthermore, these GrB+ Bregs were found to infiltrate numerous human solid tumors, including breast, ovarian, cervical, colorectal, and prostate carcinomas. Subsequent in vivo functional assays should be conducted to validate the immunosuppressive properties of GrB+ Bregs. In another in vitro co-culture system, Bregs sorted from cervical cancer patients secreted IL-10 to decrease the percentage of CD8+ T cells, which produced perforin and GrB, whereas the addition of anti-IL-10 antibodies restored the level of these CD8+ T cells (36). Similarly, in ovarian cancer patients, B10 cells from ascites significantly lowered the frequency of autologous CD8+ T cells secreting IFN-γ (80). In GC patients, depletion of Bregs from peripheral blood mononuclear cells resulted in increased frequencies of IFN-γ+ and TNF-α+ CD4+ T cells (37). Another study identified CD27+CD10− Bregs in both peripheral blood and tumor tissues of GC patients (38). Co-culture of these CD27+CD10− B cells and autologous T cells showed that IL-10 secretion by CD27+CD10− B cells decreased IFN-γ, TNF and IL-17 production by CD4+ T cells and IFN-γ and TNF production by CD8+ T cells. In addition, TGF-β+ Bregs induced by glioma cells inhibited the proliferation and release of perforin and GrB of CD8+ T cells (81). In human hepatocellular carcinoma (HCC), TIM-1+ Breg cells significantly suppressed the survival and TNF-α and IFN-γ production of CD8+ effector T cells (39). Furthermore, Bregs harvested from the glioblastoma tissue of patients suppressed CD8+ T cell proliferation and the acquisition of an effector phenotype (82). Moreover, PD-L1+ Bregs from stage II/III/IV melanoma patients impaired IFN-γ production by CD8+ T cells in a PD-L1-dependent manner in a co-culture system (41). Another study by Xiao et al. demonstrated a novel protumorigenic PD-1hi Breg subset in human HCC (40). In corresponding tumor-bearing mice, these PD-1hi Bregs mediated the reduction and dysfunction of CD8+ T cells after triggering PD-1 in an IL-10-dependent manner. Moreover, Toll-like receptor-4-mediated upregulation of BCL6 was involved in inducing PD-1hi Breg in the HCC microenvironment. The PD-1/PD-L1 signaling pathway in T cells has been extensively explored, but its role in B cells has received less attention. This study reveals the inhibitory role of PD-1/PD-L1 signaling in Breg-mediated immunosuppression in HCC. Thus, the mechanism underlying clinical tumor regression achieved by anti-PD-1 and anti-PD-L1 antibodies might also involve blocking PD-1/PD-L1 signaling on Bregs.
In a murine breast cancer model, a novel PD-1-PD-L1+CD19+ Breg subset from 4T1-bearing mice exerted the greatest suppressive function on the proliferation and IFN-γ production of T cell in an established B cell/T cell co-culture system (42). These results confirm the role of PD-1/PD-L1 as an immune checkpoint involved in the suppressive properties of Bregs in tumor models. Further studies could investigate whether PD-1+/PD-L1+ Bregs exert dominant suppressive functions in HCC or breast cancer. If so, then B-cell depletion might restore antitumor responses. In murine colorectal tumors, IgA+ Bregs expressed high quantities of immunoregulatory molecules (PD-L1, IL-10, and TGF-β) and suppressed the proliferation and activation of CD8+ T cells (43). Moreover, the EMT-6 murine mammary adenocarcinoma cells stimulated splenic B cells to differentiate into Bregs in vitro, which impaired the proliferation and IFN-γ production of effector T cells (76).
Enhancement of Treg Induction
Aside from directly regulating effector T cell responses, Bregs also induce and promote Tregs to create an immunosuppressive microenvironment. In GC patients, Bregs upregulated Foxp3 expression in CD4+CD25- effector T cells in a TGF-β1-dependent manner (37). Similarly, Bregs from IBCa patients induced more Treg production than did Bregs from healthy individuals in vitro (31). In terms of tongue squamous cell carcinoma (TSCC), Bregs co-cultured with a TSCC cell line converted CD4+CD25- T cells into Tregs (46). In a mouse 4T1 model of breast cancer, tumor-evoked Bregs (tBregs) transformed resting CD4+ T cells into Foxp3+ Tregs by secreting TGF-β to promote lung metastases (77). Moreover, Guan et al. found that PD-L1hi breast cancer cell lines stimulated CD19+ B cells to form Bregs, which subsequently induced Tregs in vitro (31). These studies revealed that both human and murine Bregs could induce Tregs in the TME, and the mechanism underlying these Treg induction requires further investigation to allow for possible disruption of the link between tumor Bregs and Tregs.
Bregs and Myeloid-Derived Suppressor Cells (MDSCs)
MDSCs are a group of immature cells that are potent in immune suppressors in cancer (83–85). The expansion of MDSCs has often been recognized as an indicator of tumor burden and metastasis (86, 87). However, Bodogai et al. observed that B-cell depletion significantly dampened the production of reactive oxygen species and NO by MDSCs as well as the suppressive effect of MDSCs on the proliferation and production of GrB and IFN-γ of CD8+ T cells, while adoptive transfer of tBregs restored MDSC-mediated suppression of T cells in a B-cell-deficient mouse model, thus promoting cancer escape and metastasis (78). And experiment results are consistent in other tumor models and human cells. Their study demonstrates that tBregs educate MDSCs to fully evoke the regulatory and prometastatic functions of MDSCs, and cancer-stimulated expansion of MDSCs is not necessarily related to their regulatory functions.
Other Tumor-Infiltrating Targets of Bregs
Natural killer (NK) cells are critical effectors of the host innate immune system, and they can directly lyse pathogen-infected and injured cells (88). NK cells have been determined to participate in caner immunology (89, 90). Using a B-cell knockout mice model, Inoue and colleagues demonstrated that EL-4 gag tumor cells stimulated B cells to secrete IL-10, which in turn inhibited IFN-γ production and tumor elimination by NK cells (60). In patients with hematological malignancies, bone marrow-derived Bregs abolished NK cell-mediated lysis of multiple myeloma (MM) cells in vitro (45). Regarding tumor-infiltrating B cells, Bregs from head and neck squamous cell carcinoma (HNSCC) patients produced adenosine to dampen the phosphorylation of Bruton’s tyrosine kinase (BTK) and Ca2+ influx in effector B cells; thus adenosine signaling may be a possible therapeutic target in HNSCC (47). Until now, the effects of Bregs on tumor-infiltrating monocytes/macrophages remain mostly uninvestigated. In a lymphoma mouse model treated with anti-CD20 mAbs, Bregs produced IL-10 to suppress mAb-mediated monocyte activation and effector function, resulting in reduced depletion of lymphoma cells (44).
Cross-Regulation Between Bregs and Tumor Cells
In addition to infiltrating immune cells, Bregs also directly interacted with liver cancer cells through the CD40/CD154 signaling pathway to enhance HCC growth and invasion (48), indicating that disruption of tumor-Breg interactions might be a potential therapeutic strategy to treat HCC. Moreover, Bregs adoptively transferred into B-cell-deficient mice rescued the growth of Kras-expressing pancreatic ductal epithelial cells by secreting IL-35, indicating the role of Bregs in carcinogenesis (91). Intriguingly, tumor cells also induce the generation of Bregs to suppress antitumor immunity. In MM patients, Breg survival was enhanced through MM cell-mediated inhibition of Breg apoptosis in the bone marrow (45). Similarly, breast cancer cells produced metabolites of the 5-lipoxygenase pathway to activate the peroxisome proliferator-activated receptor α (PPARα) in B cells, resulting in tBreg generation; unsurprisingly, inactivation of PPARα prevented tBreg-mediated cancer escape (79). In mice bearing B16-F10 melanoma, T2-MZP Bregs were specifically accumulated in TDLNs (32). Adoptive transfer of these Bregs into B-cell-deficient mice promoted tumor growth, which was not mediated by IL-10 secretion. A deeper understanding of the mechanisms underlying the preferential accumulation of T2-MZP Bregs and promotion of tumors might benefit therapeutic strategies for cancer. The findings discussed above reveal that Bregs and tumors interact and regulate each other in the TME.
Potential of Bregs as Biomarkers and Prognostic Factors for Cancer
Bregs have been confirmed to be associated with the clinicopathological characteristics of tumors and correlated with the prognosis of cancer patients (Table 3).
In TSCC, immunohistochemical staining of Bregs was performed on the paraffin-embedded tissue sections of 46 TSCC patients (46). The results showed that the percentage of Bregs was significantly correlated with clinical stage, local recurrence, and regional recurrence (P< 0.05). Moreover, Kaplan–Meier analysis showed that an increased Breg frequency predicted significantly worse overall survival (OS) of TSCC patients. Regarding hematological malignancy, Lv et al. investigated the effects of age, white blood cell (WBC) level and Breg frequency on the survival of acute myeloid leukemia (AML) patients (49). They found that an increased Breg percentage indicated a shorter OS for older patients or patients with high WBC levels. Additionally, the frequency of circulating Bregs was significantly correlated with FIGO stages, lymph node metastasis, tumor differentiation, human papillomavirus (HPV) infection, and the tumor metastasis of cervical cancer (P<0.05), and this frequency decreased significantly after radical resection of cervical cancer (36). Thus, Bregs may also act as an indicator when evaluating cervical cancer development. Moreover, Yuki and colleagues divided GC patients into BregLow and BregHigh groups based on Breg frequencies in tumor tissue. Survival analysis showed that five-year OS rates in the BregLow group were significantly higher than those in the BregHigh group. Multivariate analysis revealed that the percentage of Bregs in tumor tissue was an independent prognostic indicator of GC patient survival (50). This study indicates that Breg-related immunosuppression is closely correlated to tumor progression. In clinics, disseminated micrometastases overlooked by ordinary diagnostics are a likely reason for tumor recurrence. We propose that sustained Breg-mediated immunosuppression creates an ideal environment for residual cancer cells to grow and develop, eventually leading to recurrence.
In HCC patients, the frequency of TIM-1+ Bregs in the tumor tissue was positively associated with patient TNM stage, microvascular invasion and early recurrence. Additionally, Kaplan-Meier analysis verified that the density of tumor-infiltrating TIM-1+ Bregs was negatively correlated with disease-free survival (DFS) (n = 101, P=0.018) and OS (n = 101, P=0.007) of patients who underwent curative surgical resection (39). The results suggest that TIM-1+ Bregs could serve as a potential indicator when evaluating tumor progression and making clinical decisions in HCC. In another study by Shao et al., peripheral blood samples from 21 normal individuals and 74 HCC patients who underwent hepatectomy were examined (48). The results showed that the frequency of circulating Bregs was significantly correlated with tumor UICC stages (P=0.019), tumor multiplicity (P=0.023) and venous infiltration (P=0.029). Therefore, during the postoperative period, the dynamics of circulating Bregs in HCC patients might be a potential predictor of tumor recurrence. Furthermore, Xiao and colleagues observed that in HCC, the frequencies of PD-1hi Bregs in tumor tissues were significantly associated with disease progression in patients, and 2.6 times more patients with higher frequencies of PD-1hi Bregs displayed early recurrence than those with lower frequencies (40). Interestingly, another study demonstrated that HCC patients with high proportions of tumor-infiltrating B cells showed better prognosis compared with those with low proportions (92), indicating that the existence of B-cell subsets with antitumor functions in tumor tissues. Considering the antitumorigenic and protumorigenic properties of B cells in the TME, we believe that the distribution and subset arrangement of B cells underpin their distinct functions in specific intratumor regions.
Therapeutic Breg-Targeting Strategies for Cancer Treatment
As Bregs generally exert immunosuppressive and protumorigenic functions, it is noteworthy that Bregs could be potential therapeutic targets of cancer. Several preclinical studies have targeted Bregs in various cancer models. Mitogen/extracellular signal regulated kinase (MEK) is an intermediary component of the mitogen-activated protein kinase (MAPK) pathway. MEK inhibition has shown effects on tumors with MAPK activation both alone and in combination with other targeted therapies (93–95). In a colorectal cancer model in vivo, cobimetinib, a MEK inhibitor, decreased the number of Bregs in TDLNs while sparing anti-tumor humoral immunity (96). Mechanistically, MEK inhibition might reduce Bregs through the interruption of chronic BCR signaling, thus impairing the upregulation of specific suppressive surface molecules. Regarding HCC, total glucoside of paeony (TGP), which is extracted from the root of Paeonia Lactiflora, decreased the proportion of B10 cells in the spleens of experimental rats, which at least partially contributed to the anti tumor effect of TGP on rat HCC (97). In addition, lipoxin A4 (LXA4) is an arachidonic acid-derived anti-inflammatory lipid mediator that possesses anti-tumor potential through modulating tumor-immune microenvironments. In tumor-bearing mice, LXA4 suppressed Breg induction, thereby reducing Tregs in draining lymph nodes and tumor tissues as well as augmenting cytotoxic T cell activities (98). Intriguingly, LXA4 targeted Bregs selectively and didn’t affect the proliferation, differentiation and germinal center formation of conventional B cells. Moreover, resveratrol, a plant-derived phytoalexin, inhibits tumor angiogenesis and is a potential anticancer therapeutic drug (99). In mice with highly metastatic mammary 4T1.2 adenocarcinoma, noncytotoxic low doses of resveratrol preferentially dampened tBreg generation and concurrently impaired the tBreg-induced conversion of Foxp3+ Tregs to block lung metastasis (51). In pancreatic ductal adenocarcinoma, CD1dhiCD5+ Bregs exert protumorigenic functions by promoting tumor cell proliferation. Shipra Das and colleagues identified BTK as a vital modulator of CD1dhiCD5+ Breg differentiation and immunosuppressive function (52). The BTK inhibitor tirabrutinib suppressed CD1dhiCD5+ Breg differentiation as well as IL-10 and IL-35 secretion in vitro. Moreover, tirabrutinib treatment of mice bearing orthotopic KrasG12D-pancreatic lesions increased stromal CD8+IFN-γ+ cytotoxic T cells and attenuated tumor cell proliferation and pancreatic intra-epithelial neoplasia (PanIN) growth. Thus, Bregs may be potential targets of tirabrutinib in PanIN. These studies have confirmed the practicability of inhibiting Bregs to suppress cancer progression. Further investigations are needed to develop a Breg-targeting therapeutic regimen for cancer.
Challenges and Future Implications
Despite accumulating evidence corroborating the immunoregulatory functions of Bregs in tumor immunology, there are still few unequivocal surface markers for Bregs. Unlike Foxp3 on Tregs, a specific transcriptional factor for identifying Bregs has not been found. One reason for the differences in reported Breg phenotypes may be the various methods utilized to isolate Bregs. It is debatable whether immunoregulatory functions are innate and whether Bregs and their specific signatures are only the results of the adaptation of B cells to different TME stimuli. Lighaam and colleagues reported that in vitro-induced human B10 cells lacked specific surface markers, and their IL-10 expression was transient (100). Therefore, the intricate mechanism underlying Breg induction and function during cancer progression needs to be further explicated.
In summary, Bregs have been widely recognized as a subset of B cells that regulate inflammation and antitumor responses. Generally, Bregs exert their functions mainly through cytokine secretion and intercellular contact. In the TME, Bregs suppress effector T cells, induce regulatory T cells and target other tumor-infiltrating immune cells, such as MDSCs, NK cells, and macrophages, to hamper anti-tumor immunity. Meanwhile, the cross-regulations between Bregs and tumor cells often results in tumor escape from immunosurveillance. In addition, Bregs are closely associated with many clinicopathological factors of cancer patients and might predict patient survival. It is imperative that subsequent efforts focus on identifying unique surface markers of Bregs and developing Bregs as potential targets for future Breg-based immunotherapies.
Author Contributions
JS and HZ prepared the first draft of the manuscript. YS designed and critically revised the manuscript. All authors contributed to the article and approved the submitted version.
Funding
This work was supported by the National Natural Science Foundation of China under Grant [number 31900627].
Conflict of Interest
The authors declare that the research was conducted in the absence of any commercial or financial relationships that could be construed as a potential conflict of interest.
References
1. Meffre E, O’Connor KC. Impaired B-cell tolerance checkpoints promote the development of autoimmune diseases and pathogenic autoantibodies. Immunol Rev (2019) 292(1):90–101. doi: 10.1111/imr.12821
2. LeBien TW, Tedder TF. B lymphocytes: how they develop and function. Blood (2008) 112(5):1570–80. doi: 10.1182/blood-2008-02-078071
3. Luman Wang YF, Chu Y. “Regulatory B Cells”. In: Wang J, editor. B cells in immunity and tolerance Advances in Experimental Medicine and Biology. Singapore: Springer (2020). p. 87–103.
4. Sprenger KG, Louveau JE, Murugan PM, Chakraborty AK. Optimizing immunization protocols to elicit broadly neutralizing antibodies. Proc Natl Acad Sci U S A (2020) 117(33):20077–87. doi: 10.1073/pnas.1919329117
5. Joyner CJ, Brito CFA, Saney CL, Joice Cordy R, Smith ML, Lapp SA, et al. Humoral immunity prevents clinical malaria during Plasmodium relapses without eliminating gametocytes. PLoS Path (2019) 15(9):e1007974. doi: 10.1371/journal.ppat.1007974
6. Smith SA, Burton SL, Kilembe W, Lakhi S, Karita E, Price M, et al. VH1-69 Utilizing Antibodies Are Capable of Mediating Non-neutralizing Fc-Mediated Effector Functions Against the Transmitted/Founder gp120. Front Immunol (2018) 9:3163. doi: 10.3389/fimmu.2018.03163
7. Kim HS, Lee MB, Lee D, Min KY, Koo J, Kim HW, et al. The regulatory B cell-mediated peripheral tolerance maintained by mast cell IL-5 suppresses oxazolone-induced contact hypersensitivity. Sci Adv (2019) 5(7):eaav8152. doi: 10.1126/sciadv.aav8152
8. Korniotis S, Gras C, Letscher H, Montandon R, Megret J, Siegert S, et al. Treatment of ongoing autoimmune encephalomyelitis with activated B-cell progenitors maturing into regulatory B cells. Nat Commun (2016) 7:12134. doi: 10.1038/ncomms12134
9. Kalampokis I, Venturi GM, Poe JC, Dvergsten JA, Sleasman JW, Tedder TF. The Regulatory B Cell Compartment Expands Transiently During Childhood and Is Contracted in Children With Autoimmunity. Arthritis Rheumatol (2017) 69(1):225–38. doi: 10.1002/art.39820
10. Giacomini E, Rizzo F, Etna MP, Cruciani M, Mechelli R, Buscarinu MC, et al. Thymosin-alpha1 expands deficient IL-10-producing regulatory B cell subsets in relapsing-remitting multiple sclerosis patients. Mult Scler (2018) 24(2):127–39. doi: 10.1177/1352458517695892
11. Katz SI, Parker D, Turk JL. B-cell suppression of delayed hypersensitivity reactions. Nature (1974) 251(5475):550–1. doi: 10.1038/251550a0
12. Neta R, Salvin SB. Specific suppression of delayed hypersensitivity: the possible presence of a suppressor B cell in the regulation of delayed hypersensitivity. J Immunol (1974) 113(6):1716–25.
13. Shimamura T, Hashimoto K, Sasaki S. Feedback suppression of the immune response in vivo. III. Lyt-1+ B cells are suppressor-inducer cells. Cell Immunol (1982) 68(1):104–13. doi: 10.1016/0008-8749(82)90093-4
14. Shimamura T, Habu S, Hashimoto K, Sasaki S. Feedback suppression of the immune response in vivo. III. Lyt-1+ B Cells are suppressor-inducer Cells Cell Immunol (1984) 83(1):221–4. doi: 10.1016/0008-8749(84)90242-9
15. Mizoguchi A, Mizoguchi E, Takedatsu H, Blumberg RS, Bhan AK. Chronic intestinal inflammatory condition generates IL-10-producing regulatory B cell subset characterized by CD1d upregulation. Immunity (2002) 16(2):219–30. doi: 10.1016/s1074-7613(02)00274-1
16. Zhivaki D, Lemoine S, Lim A, Morva A, Vidalain PO, Schandene L, et al. Respiratory Syncytial Virus Infects Regulatory B Cells in Human Neonates via Chemokine Receptor CX3CR1 and Promotes Lung Disease Severity. Immunity (2017) 46(2):301–14. doi: 10.1016/j.immuni.2017.01.010
17. van de Veen W, Stanic B, Wirz OF, Jansen K, Globinska A, Akdis M. Role of regulatory B cells in immune tolerance to allergens and beyond. J Allergy Clin Immunol (2016) 138(3):654–65. doi: 10.1016/j.jaci.2016.07.006
18. Kim Y, Kim G, Shin HJ, Hyun JW, Kim SH, Lee E, et al. Restoration of regulatory B cell deficiency following alemtuzumab therapy in patients with relapsing multiple sclerosis. J Neuroinflamm (2018) 15(1):300. doi: 10.1186/s12974-018-1334-y
19. Peng B, Ming Y, Yang C. Regulatory B cells: the cutting edge of immune tolerance in kidney transplantation. Cell Death Dis (2018) 9(2):109. doi: 10.1038/s41419-017-0152-y
20. Baba Y, Saito Y, Kotetsu Y. Heterogeneous subsets of B-lineage regulatory cells (Breg cells). Int Immunol (2020) 32(3):155–62. doi: 10.1093/intimm/dxz068
21. Sarvaria A, Madrigal JA, Saudemont A. B cell regulation in cancer and anti-tumor immunity. Cell Mol Immunol (2017) 14(8):662–74. doi: 10.1038/cmi.2017.35
22. Zhang Y, Gallastegui N, Rosenblatt JD. Regulatory B cells in anti-tumor immunity. Int Immunol (2015) 27(10):521–30. doi: 10.1093/intimm/dxv034
23. Cai X, Zhang L, Wei W. Regulatory B cells in inflammatory diseases and tumor. Int Immunopharmacol (2019) 67:281–6. doi: 10.1016/j.intimp.2018.12.007
24. He Y, Qian H, Liu Y, Duan L, Li Y, Shi G. The roles of regulatory B cells in cancer. J Immunol Res (2014) 2014:215471. doi: 10.1155/2014/215471
25. Balkwill F, Montfort A, Capasso M. B regulatory cells in cancer. Trends Immunol (2013) 34(4):169–73. doi: 10.1016/j.it.2012.10.007
26. Dzopalic T, Bozic-Nedeljkovic B, Jurisic V. Function of innate lymphoid cells in the immune-related disorders. Hum Cell (2019) 32(3):231–9. doi: 10.1007/s13577-019-00257-1
27. Rosser EC, Mauri C. Regulatory B cells: origin, phenotype, and function. Immunity (2015) 42(4):607–12. doi: 10.1016/j.immuni.2015.04.005
28. Kessel A, Haj T, Peri R, Snir A, Melamed D, Sabo E, et al. Human CD19(+)CD25(high) B regulatory cells suppress proliferation of CD4(+) T cells and enhance Foxp3 and CTLA-4 expression in T-regulatory cells. Autoimmun Rev (2012) 11(9):670–7. doi: 10.1016/j.autrev.2011.11.018
29. Li G, Wulan H, Song Z, Paik PA, Tsao ML, Goodman GM, et al. Regulatory B Cell Function Is Suppressed by Smoking and Obesity in H. pylori-Infected Subjects and Is Correlated with Elevated Risk of Gastric Cancer. PLoS One (2015) 10(7):e0134591. doi: 10.1371/journal.pone.0134591
30. Lindner S, Dahlke K, Sontheimer K, Hagn M, Kaltenmeier C, Barth TF, et al. Interleukin 21-induced granzyme B-expressing B cells infiltrate tumors and regulate T cells. Cancer Res (2013) 73(8):2468–79. doi: 10.1158/0008-5472.CAN-12-3450
31. Guan H, Lan Y, Wan Y, Wang Q, Wang C, Xu L, et al. PD-L1 mediated the differentiation of tumor-infiltrating CD19(+) B lymphocytes and T cells in Invasive breast cancer. OncoImmunology (2016) 5(2):e1075112. doi: 10.1080/2162402X.2015.1075112
32. Ganti SN, Albershardt TC, Iritani BM, Ruddell A. Regulatory B cells preferentially accumulate in tumor-draining lymph nodes and promote tumor growth. Sci Rep (2015) 5:12255. doi: 10.1038/srep12255
33. Yanaba K, Bouaziz JD, Haas KM, Poe JC, Fujimoto M, Tedder TF. A regulatory B cell subset with a unique CD1dhiCD5+ phenotype controls T cell-dependent inflammatory responses. Immunity (2008) 28(5):639–50. doi: 10.1016/j.immuni.2008.03.017
34. Maseda D, Smith SH, DiLillo DJ, Bryant JM, Candando KM, Weaver CT, et al. Regulatory B10 cells differentiate into antibody-secreting cells after transient IL-10 production in vivo. J Immunol (2012) 188(3):1036–48. doi: 10.4049/jimmunol.1102500
35. Kaku H, Cheng KF, Al-Abed Y, Rothstein TL. A novel mechanism of B cell-mediated immune suppression through CD73 expression and adenosine production. J Immunol (2014) 193(12):5904–13. doi: 10.4049/jimmunol.1400336
36. Chen Z, Zhu Y, Du R, Pang N, Zhang F, Dong D, et al. Role of Regulatory B Cells in the Progression of Cervical Cancer. Mediators Inflamm (2019) 2019:6519427. doi: 10.1155/2019/6519427
37. Wang WW, Yuan XL, Chen H, Xie GH, Ma YH, Zheng YX, et al. CD19+CD24hiCD38hiBregs involved in downregulate helper T cells and upregulate regulatory T cells in gastric cancer. Oncotarget (2015) 6(32):33486–99. doi: 10.18632/oncotarget.5588
38. Hu HT, Ai X, Lu M, Song Z, Li H. Characterization of intratumoral and circulating IL-10-producing B cells in gastric cancer. Exp Cell Res (2019) 384(2):111652. doi: 10.1016/j.yexcr.2019.111652
39. Ye L, Zhang Q, Cheng Y, Chen X, Wang G, Shi M, et al. Tumor-derived exosomal HMGB1 fosters hepatocellular carcinoma immune evasion by promoting TIM-1(+) regulatory B cell expansion. J Immunother Cancer (2018) 6(1):145. doi: 10.1186/s40425-018-0451-6
40. Xiao X, Lao XM, Chen MM, Liu RX, Wei Y, Ouyang FZ, et al. PD-1hi Identifies a Novel Regulatory B-cell Population in Human Hepatoma That Promotes Disease Progression. Cancer Discov (2016) 6(5):546–59. doi: 10.1158/2159-8290.CD-15-1408
41. Wu H, Xia L, Jia D, Zou H, Jin G, Qian W, et al. PD-L1(+) regulatory B cells act as a T cell suppressor in a PD-L1-dependent manner in melanoma patients with bone metastasis. Mol Immunol (2020) 119:83–91. doi: 10.1016/j.molimm.2020.01.008
42. Shen M, Wang J, Yu W, Zhang C, Liu M, Wang K, et al. A novel MDSC-induced PD-1(-)PD-L1(+) B-cell subset in breast tumor microenvironment possesses immuno-suppressive properties. OncoImmunology (2018) 7(4):e1413520. doi: 10.1080/2162402X.2017.1413520
43. Liu R, Lu Z, Gu J, Liu J, Huang E, Liu X, et al. MicroRNAs 15A and 16-1 Activate Signaling Pathways That Mediate Chemotaxis of Immune Regulatory B cells to Colorectal Tumors. Gastroenterology (2018) 154(3):637–51 e7. doi: 10.1053/j.gastro.2017.09.045
44. Horikawa M, Minard-Colin V, Matsushita T, Tedder TF. Regulatory B cell production of IL-10 inhibits lymphoma depletion during CD20 immunotherapy in mice. J Clin Invest (2011) 121(11):4268–80. doi: 10.1172/JCI59266
45. Zhang L, Tai YT, Ho M, Xing L, Chauhan D, Gang A, et al. Regulatory B cell-myeloma cell interaction confers immunosuppression and promotes their survival in the bone marrow milieu. Blood Cancer J (2017) 7(3):e547. doi: 10.1038/bcj.2017.24
46. Zhou X, Su YX, Lao XM, Liang YJ, Liao GQ. CD19(+)IL-10(+) regulatory B cells affect survival of tongue squamous cell carcinoma patients and induce resting CD4(+) T cells to CD4(+)Foxp3(+) regulatory T cells. Oral Oncol (2016) 53:27–35. doi: 10.1016/j.oraloncology.2015.11.003
47. Jeske SS, Brand M, Ziebart A, Laban S, Doescher J, Greve J, et al. Adenosine-producing regulatory B cells in head and neck cancer. Cancer Immunol Immunother (2020) 69(7):1205–16. doi: 10.1007/s00262-020-02535-6
48. Shao Y, Lo CM, Ling CC, Liu XB, Ng KT, Chu AC, et al. Regulatory B cells accelerate hepatocellular carcinoma progression via CD40/CD154 signaling pathway. Cancer Lett (2014) 355(2):264–72. doi: 10.1016/j.canlet.2014.09.026
49. Lv Y, Wang H, Liu Z. The Role of Regulatory B Cells in Patients with Acute Myeloid Leukemia. Med Sci Monit (2019) 25:3026–31. doi: 10.12659/MSM.915556
50. Murakami Y, Saito H, Shimizu S, Kono Y, Shishido Y, Miyatani K, et al. Increased regulatory B cells are involved in immune evasion in patients with gastric cancer. Sci Rep (2019) 9(1):13083. doi: 10.1038/s41598-019-49581-4
51. Lee-Chang C, Bodogai M, Martin-Montalvo A, Wejksza K, Sanghvi M, Moaddel R, et al. Inhibition of breast cancer metastasis by resveratrol-mediated inactivation of tumor-evoked regulatory B cells. J Immunol (2013) 191(8):4141–51. doi: 10.4049/jimmunol.1300606
52. Das S, Bar-Sagi D. BTK signaling drives CD1d(hi)CD5(+) regulatory B-cell differentiation to promote pancreatic carcinogenesis. Oncogene (2019) 38(17):3316–24. doi: 10.1038/s41388-018-0668-3
53. Mao H, Pan F, Wu Z, Wang Z, Zhou Y, Zhang P, et al. Colorectal tumors are enriched with regulatory plasmablasts with capacity in suppressing T cell inflammation. Int Immunopharmacol (2017) 49:95–101. doi: 10.1016/j.intimp.2017.05.018
54. Akdis M, Aab A, Altunbulakli C, Azkur K, Costa RA, Crameri R, et al. Interleukins (from IL-1 to IL-38), interferons, transforming growth factor beta, and TNF-alpha: Receptors, functions, and roles in diseases. J Allergy Clin Immunol (2016) 138(4):984–1010. doi: 10.1016/j.jaci.2016.06.033
55. Khoder A, Sarvaria A, Alsuliman A, Chew C, Sekine T, Cooper N, et al. Regulatory B cells are enriched within the IgM memory and transitional subsets in healthy donors but are deficient in chronic GVHD. Blood (2014) 124(13):2034–45. doi: 10.1182/blood-2014-04-571125
56. Cerqueira C, Manfroi B, Fillatreau S. IL-10-producing regulatory B cells and plasmocytes: Molecular mechanisms and disease relevance. Semin Immunol (2019) 44:101323. doi: 10.1016/j.smim.2019.101323
57. Wu H, Su Z, Barnie PA. The role of B regulatory (B10) cells in inflammatory disorders and their potential as therapeutic targets. Int Immunopharmacol (2020) 78:106111. doi: 10.1016/j.intimp.2019.106111
58. Das A, Ellis G, Pallant C, Lopes AR, Khanna P, Peppa D, et al. IL-10-producing regulatory B cells in the pathogenesis of chronic hepatitis B virus infection. J Immunol (2012) 189(8):3925–35. doi: 10.4049/jimmunol.1103139
59. Madan R, Demircik F, Surianarayanan S, Allen JL, Divanovic S, Trompette A, et al. Nonredundant roles for B cell-derived IL-10 in immune counter-regulation. J Immunol (2009) 183(4):2312–20. doi: 10.4049/jimmunol.0900185
60. Inoue S, Leitner WW, Golding B, Scott D. Inhibitory effects of B cells on antitumor immunity. Cancer Res (2006) 66(15):7741–7. doi: 10.1158/0008-5472.CAN-05-3766
61. Butz H, Racz K, Hunyady L, Patocs A. Crosstalk between TGF-beta signaling and the microRNA machinery. Trends Pharmacol Sci (2012) 33(7):382–93. doi: 10.1016/j.tips.2012.04.003
62. Tian J, Zekzer D, Hanssen L, Lu Y, Olcott A, Kaufman DL. Lipopolysaccharide-activated B cells down-regulate Th1 immunity and prevent autoimmune diabetes in nonobese diabetic mice. J Immunol (2001) 167(2):1081–9. doi: 10.4049/jimmunol.167.2.1081
63. Shen P, Roch T, Lampropoulou V, O’Connor RA, Stervbo U, Hilgenberg E, et al. IL-35-producing B cells are critical regulators of immunity during autoimmune and infectious diseases. Nature (2014) 507(7492):366–70. doi: 10.1038/nature12979
64. Egwuagu CE, Yu CR, Sun L, Wang R. Interleukin 35: Critical regulator of immunity and lymphocyte-mediated diseases. Cytokine Growth Factor Rev (2015) 26(5):587–93. doi: 10.1016/j.cytogfr.2015.07.013
65. Tang Q, Bluestone JA. The Foxp3+ regulatory T cell: a jack of all trades, master of regulation. Nat Immunol (2008) 9(3):239–44. doi: 10.1038/ni1572
66. Blair PA, Norena LY, Flores-Borja F, Rawlings DJ, Isenberg DA, Ehrenstein MR, et al. CD19(+)CD24(hi)CD38(hi) B cells exhibit regulatory capacity in healthy individuals but are functionally impaired in systemic Lupus Erythematosus patients. Immunity (2010) 32(1):129–40. doi: 10.1016/j.immuni.2009.11.009
67. Flores-Borja F, Bosma A, Ng D, Reddy V, Ehrenstein MR, Isenberg DA, et al. CD19+CD24hiCD38hi B cells maintain regulatory T cells while limiting TH1 and TH17 differentiation. Sci Transl Med (2013) 5(173):173ra23. doi: 10.1126/scitranslmed.3005407
68. Elgueta R, Benson MJ, de Vries VC, Wasiuk A, Guo Y, Noelle RJ. Molecular mechanism and function of CD40/CD40L engagement in the immune system. Immunol Rev (2009) 229(1):152–72. doi: 10.1111/j.1600-065X.2009.00782.x
69. Mauri C, Ehrenstein MR. The ‘short’ history of regulatory B cells. Trends Immunol (2008) 29(1):34–40. doi: 10.1016/j.it.2007.10.004
70. Podolsky MA, Bailey JT, Gunderson AJ, Oakes CJ, Breech K, Glick AB. Differentiated State of Initiating Tumor Cells Is Key to Distinctive Immune Responses Seen in H-Ras(G12V)-Induced Squamous Tumors. Cancer Immunol Res (2017) 5(3):198–210. doi: 10.1158/2326-6066.CIR-16-0304
71. Hahne M, Renno T, Schroeter M, Irmler M, French L, Bornard T, et al. Activated B cells express functional Fas ligand. Eur J Immunol (1996) 26(3):721–4. doi: 10.1002/eji.1830260332
72. Lundy SK, Boros DL. Fas ligand-expressing B-1a lymphocytes mediate CD4(+)-T-cell apoptosis during schistosomal infection: induction by interleukin 4 (IL-4) and IL-10. Infect Immun (2002) 70(2):812–9. doi: 10.1128/iai.70.2.812-819.2002
73. Wang K, Tao L, Su J, Zhang Y, Zou B, Wang Y, et al. TLR4 supports the expansion of FasL(+)CD5(+)CD1d(hi) regulatory B cells, which decreases in contact hypersensitivity. Mol Immunol (2017) 87:188–99. doi: 10.1016/j.molimm.2017.04.016
74. Burgueno-Bucio E, Mier-Aguilar CA, Soldevila G. The multiple faces of CD5. J Leukocyte Biol (2019) 105(5):891–904. doi: 10.1002/JLB.MR0618-226R
75. Zhang C, Xin H, Zhang W, Yazaki PJ, Zhang Z, Le K, et al. CD5 Binds to Interleukin-6 and Induces a Feed-Forward Loop with the Transcription Factor STAT3 in B Cells to Promote Cancer. Immunity (2016) 44(4):913–23. doi: 10.1016/j.immuni.2016.04.003
76. Zhang Y, Morgan R, Chen C, Cai Y, Clark E, Khan WN, et al. Mammary-tumor-educated B cells acquire LAP/TGF-beta and PD-L1 expression and suppress anti-tumor immune responses. Int Immunol (2016) 28(9):423–33. doi: 10.1093/intimm/dxw007
77. Wei X, Jin Y, Tian Y, Zhang H, Wu J, Lu W, et al. Regulatory B cells contribute to the impaired antitumor immunity in ovarian cancer patients. Tumour Biol (2016) 37(5):6581–8. doi: 10.1007/s13277-015-4538-0
78. Han S, Feng S, Ren M, Ma E, Wang X, Xu L, et al. Glioma cell-derived placental growth factor induces regulatory B cells. Int J Biochem Cell Biol (2014) 57:63–8. doi: 10.1016/j.biocel.2014.10.005
79. Lee-Chang C, Rashidi A, Miska J, Zhang P, Pituch KC, Hou D, et al. Myeloid-Derived Suppressive Cells Promote B cell-Mediated Immunosuppression via Transfer of PD-L1 in Glioblastoma. Cancer Immunol Res (2019) 7(12):1928–43. doi: 10.1158/2326-6066.CIR-19-0240
80. Olkhanud PB, Damdinsuren B, Bodogai M, Gress RE, Sen R, Wejksza K, et al. Tumor-evoked regulatory B cells promote breast cancer metastasis by converting resting CD4(+) T cells to T-regulatory cells. Cancer Res (2011) 71(10):3505–15. doi: 10.1158/0008-5472.CAN-10-4316
81. Bodogai M, Moritoh K, Lee-Chang C, Hollander CM, Sherman-Baust CA, Wersto RP, et al. Immunosuppressive and Prometastatic Functions of Myeloid-Derived Suppressive Cells Rely upon Education from Tumor-Associated B Cells. Cancer Res (2015) 75(17):3456–65. doi: 10.1158/0008-5472.CAN-14-3077
82. Wejksza K, Lee-Chang C, Bodogai M, Bonzo J, Gonzalez FJ, Lehrmann E, et al. Cancer-produced metabolites of 5-lipoxygenase induce tumor-evoked regulatory B cells via peroxisome proliferator-activated receptor alpha. J Immunol (2013) 190(6):2575–84. doi: 10.4049/jimmunol.1201920
83. De Cicco P, Ercolano G, Ianaro A. The New Era of Cancer Immunotherapy: Targeting Myeloid-Derived Suppressor Cells to Overcome Immune Evasion. Front Immunol (2020) 11:1680. doi: 10.3389/fimmu.2020.01680
84. Sieminska I, Baran J. Myeloid-Derived Suppressor Cells in Colorectal Cancer. Front Immunol (2020) 11:1526. doi: 10.3389/fimmu.2020.01526
85. Jayakumar A, Bothwell ALM. Functional Diversity of Myeloid-Derived Suppressor Cells: The Multitasking Hydra of Cancer. J Immunol (2019) 203(5):1095–103. doi: 10.4049/jimmunol.1900500
86. Gabrilovich DI, Nagaraj S. Myeloid-derived suppressor cells as regulators of the immune system. Nat Rev Immunol (2009) 9(3):162–74. doi: 10.1038/nri2506
87. Bunt SK, Sinha P, Clements VK, Leips J, Ostrand-Rosenberg S. Inflammation induces myeloid-derived suppressor cells that facilitate tumor progression. J Immunol (2006) 176(1):284–90. doi: 10.4049/jimmunol.176.1.284
88. Vladimir J AV, Katarina MM, Gordana K. “The Role of NK Cells in Cancer”. In: Rezaei N, editor. Cancer Immunology. Switzerland: Springer, Cham (2020). p. 133–46.
89. Konjevic GM, Vuletic AM, Mirjacic Martinovic KM, Larsen AK, Jurisic VB. The role of cytokines in the regulation of NK cells in the tumor environment. Cytokine (2019) 117:30–40. doi: 10.1016/j.cyto.2019.02.001
90. Bi J, Wang X. Molecular Regulation of NK Cell Maturation. Front Immunol (2020) 11:1945. doi: 10.3389/fimmu.2020.01945
91. Pylayeva-Gupta Y, Das S, Handler JS, Hajdu CH, Coffre M, Koralov SB, et al. IL35-Producing B Cells Promote the Development of Pancreatic Neoplasia. Cancer Discov (2016) 6(3):247–55. doi: 10.1158/2159-8290.CD-15-0843
92. Garnelo M, Tan A, Her Z, Yeong J, Lim CJ, Chen J, et al. Interaction between tumour-infiltrating B cells and T cells controls the progression of hepatocellular carcinoma. Gut (2017) 66(2):342–51. doi: 10.1136/gutjnl-2015-310814
93. Caunt CJ, Sale MJ, Smith PD, Cook SJ. MEK1 and MEK2 inhibitors and cancer therapy: the long and winding road. Nat Rev Cancer (2015) 15(10):577–92. doi: 10.1038/nrc4000
94. Robert C, Karaszewska B, Schachter J, Rutkowski P, Mackiewicz A, Stroiakovski D, et al. Improved overall survival in melanoma with combined dabrafenib and trametinib. N Engl J Med (2015) 372(1):30–9. doi: 10.1056/NEJMoa1412690
95. Larkin J, Ascierto PA, Dreno B, Atkinson V, Liszkay G, Maio M, et al. Combined vemurafenib and cobimetinib in BRAF-mutated melanoma. N Engl J Med (2014) 371(20):1867–76. doi: 10.1056/NEJMoa1408868
96. Yarchoan M, Mohan AA, Dennison L, Vithayathil T, Ruggieri A, Lesinski GB, et al. MEK inhibition suppresses B regulatory cells and augments anti-tumor immunity. PLoS One (2019) 14(10):e0224600. doi: 10.1371/journal.pone.0224600
97. Song SS, Yuan PF, Li PP, Wu HX, Ni WJ, Lu JT, et al. Protective Effects of Total Glucosides of Paeony on N-nitrosodiethylamine-induced Hepatocellular Carcinoma in Rats via Down-regulation of Regulatory B Cells. Immunol Invest (2015) 44(6):521–35. doi: 10.3109/08820139.2015.1043668
98. Wang Z, Cheng Q, Tang K, Sun Y, Zhang K, Zhang Y, et al. Lipid mediator lipoxin A4 inhibits tumor growth by targeting IL-10-producing regulatory B (Breg) cells. Cancer Lett (2015) 364(2):118–24. doi: 10.1016/j.canlet.2015.04.030
99. Athar M, Back JH, Kopelovich L, Bickers DR, Kim AL. Multiple molecular targets of resveratrol: Anti-carcinogenic mechanisms. Arch Biochem Biophys (2009) 486(2):95–102. doi: 10.1016/j.abb.2009.01.018
Keywords: regulatory B cell, tumor immunology, tumor microenvironment, cancer progression, immunotherapy
Citation: Shang J, Zha H and Sun Y (2020) Phenotypes, Functions, and Clinical Relevance of Regulatory B Cells in Cancer. Front. Immunol. 11:582657. doi: 10.3389/fimmu.2020.582657
Received: 13 July 2020; Accepted: 02 October 2020;
Published: 22 October 2020.
Edited by:
Nurit Hollander, Tel Aviv University, IsraelReviewed by:
Xiubao Ren, Tianjin Medical University Cancer Institute and Hospital, ChinaMaria Raffaella Zocchi, San Raffaele Scientific Institute (IRCCS), Italy
Copyright © 2020 Shang, Zha and Sun. This is an open-access article distributed under the terms of the Creative Commons Attribution License (CC BY). The use, distribution or reproduction in other forums is permitted, provided the original author(s) and the copyright owner(s) are credited and that the original publication in this journal is cited, in accordance with accepted academic practice. No use, distribution or reproduction is permitted which does not comply with these terms.
*Correspondence: Yufa Sun, ZGFmYTIwMTM2NzI1QDE2My5jb20=
†These authors have contributed equally to this work