- 1Protozoa Immunology, Bernhard Nocht Institute for Tropical Medicine, Hamburg, Germany
- 2Department of Natural Sciences, University of Pernambuco, Garanhuns, Brazil
- 3Department of Immunology, Aggeu Magalhães Institute—Oswaldo Cruz Foundation, Recife, Brazil
- 4Department of Molecular Parasitology and Immunology, Bernhard Nocht Institute for Tropical Medicine, Hamburg, Germany
- 5I. Department of Medicine, University Medical Center Hamburg-Eppendorf, Hamburg, Germany
Cutaneous Leishmaniasis (CL) affects up to one million people every year and treatments are costly and toxic. The regulation of the host immune response is complex and the knowledge of how CD4+ T cells are activated and maintained during Leishmania infection is still limited. Current therapies aim to target programmed cell death (PD)-1 and programmed cell death ligand (PD-L)-1 in order to boost T cell activity. However, the role of the PD-1/PD-L1 axis during Leishmania infection is still unclear. In this study, we found that patients with active and post-treatment CL displayed different subsets of CD4+PD-1+ T cells. Accordingly, L. major-infected mice upregulated PD-1 on activated CD4+ T effector cells and PD-L1 on resident macrophages and infiltrating monocytes at the site of infection. L. major-infected Pdl1−/− mice expressed lower levels of MHCII and higher levels of CD206 on macrophages and monocytes and, more importantly, the lack of PD-L1 contributed to a reduced frequency of CD4+Ly6Chi T effector cells and an increase of CD4+Foxp3+ regulatory T cells at the site of infection and in draining lymph nodes. Additionally, the lack of PD-L1 was associated with lower production of IL-27 by infiltrating monocytes and lower levels of the Th1 cytokines IFN-γ and TNF-α produced by CD4+ T effector cells. Pdl1−/− mice initially exhibited larger lesions despite having a similar parasite load. Our results describe for the first time how the interruption of the PD-1/PD-L1 axis influences the immune response against CL and suggests that this axis regulates the balance between CD4+Ly6Chi T effector cells and CD4+Foxp3+ regulatory T cells.
Introduction
Cutaneous leishmaniasis (CL) is an important neglected tropical disease of the skin affecting between 600,000 and 1 million people per year (1, 2). Disease presentation ranges from the development of ulcers, which can spontaneously heal, to single or multiple chronic non-healing lesions, which are difficult to resolve (3). So far, drugs to control the disease are limited due to high costs and toxicity, and there is currently no safe and effective vaccine for application in humans (4, 5). The complexity of the host immune response to Leishmania is also a key limiting factor for developments in this field (6–8). Briefly, the response is initiated with the injection of Leishmania in the skin through a bite of an infected sand fly and subsequent infiltration of inflammatory cells (9, 10). Ultimately, parasites are phagocytized and end up replicating in dermal macrophages, while in local draining lymph nodes (dLN) Type I CD4+ T cells producing interferon (IFN)-γ are induced and enhance the respiratory burst of macrophages to eliminate parasites (11). However, even after lesion healing some parasites can persist. This sustains a population of short-lived CD4+Ly6C+ T effector cells that can rapidly migrate to the infected site upon re-challenge and produce high levels of IFN-γ (12). In mouse models of infection, expression of Ly6C has been used to identify highly differentiated CD4+ T effector cells (13–15). Ly6C expression on CD4+ T cells is mainly induced by IL-27 (16–18). In contrast, CD8+ T cells have been associated with stronger inflammation, increased TNF-α and IL-1β production, tissue damage, and disease severity, but none of these mechanisms control Leishmania replication (19–22). Furthermore, there is evidence that CD4+Foxp3+ regulatory T cells (Treg) play a crucial role in limiting the immune response, preventing tissue damage and promoting memory through interleukin (IL)-10 secretion (23–25).
There are still many gaps in the knowledge of how a protective CD4+ T cell response against Leishmania is activated, maintained, and controlled (26). In the last years it has been demonstrated that the expression of co-inhibitory receptors on activated T cells during the course of an immune response plays a pivotal role in dampening T cell activity (27–29). Moreover, blocking antibodies against some of those receptors, such as programmed cell death (PD)-1 (CD279) and programmed cell death ligand (PD-L)-1 (CD274, B7-H1), expressed by T cells and antigen presenting cells (APCs), respectively, could restore T cell function in skin cancer (30, 31). However, the role of the PD-1/PD-L1 axis during acquisition of immunity against many infectious agents is still not well-established, and this pathway may even suppress immunity, allowing chronic infections (16, 31, 32). To date, it has been shown in different murine infection models of Leishmania that blocking the PD-1/PD-L1 axis restores T cell function, resulting in increased IFN-γ production and diminished parasite burden (33–36). However, it is not clear how the PD-1/PD-L1 axis modulates CD4+ T cells during infection with Leishmania. In addition, human data is scarce and little is known about the expression of PD-1 by different CD4+ T cell subsets during chronic parasitic infections such as CL. Therefore, a better understanding of this pathway is of utmost importance to enhance the development of new therapeutic concepts.
To provide better insight on the role of the PD-1/PD-L1 axis during CL, we initially investigated PD-1 expression on CD4+ T cells from different patient cohorts and healthy individuals. Additionally, using a murine model of CL, we determined the cell types that expressed PD-1 and PD-L1 upon infection and analyzed the immune response of PD-L1-deficient mice (Pdl1−/−) after infection with L. major. Our data describe for the first time the immunological consequences of interrupting the PD-1/PD-L1 axis during the immune response against CL and provide evidence that this axis as an important regulator of CD4+Ly6Chi T effector cells and CD4+Foxp3+ regulatory T cells during CL.
Materials and Methods
Ethics
C57BL/6J and Pdl1−/− mice were bred in the animal facility of the Bernhard Nocht Institute for Tropical Medicine. Co-housed mice were used for comparison of wild-type (WT) and Pdl1−/−. All animal experiments were performed according to the German animal protection law under the supervision of a veterinarian. The responsible federal health authorities of the state of Hamburg (Behörde für Gesundheit und Verbraucherschutz) reviewed and approved the experimental protocol under the permission number N 027/2018. All mice were euthanized by cardiocentesis under deep CO2 narcosis, followed by confirmation through cervical dislocation. All animals were maintained in ventilated cages under low pathogen conditions. Age-matched (8–12 weeks old) female mice were used. IAM/FIOCRUZ Research Ethics Committee (Recife, Brazil) have approved the experimental protocols for this research (CAEE number 11083812.7.0000.5190). Selected individuals were invited to participate in the research and signed the “Term of Free and Informed Consent.” The selection of these individuals was based on criteria such as: being more than 12 years old, having confirmed diagnosis by the IAM/FIOCRUZ Leishmaniasis Reference Centre and being active lesion carriers. Our study group consisted of 10 patients with active CL and 10 patients post-treatment from Pernambuco rural endemic areas with one to four lesions and a disease with a mean duration of 50 days (15 days−3 months). Patients were submitted to blood collection after chemotherapy treatment with Glucantime® and clinical cure. The control group consisted of 10 healthy individuals from non-endemic areas with no previous history of CL and no prior blood transfusion. Patients and controls had a similar age range. Tables 1, 2 summarize clinical and laboratorial characteristics of active disease and of post-treatment patients, respectively.
Human Blood Collection and Peripheral Blood Mononuclear Cell Isolation
Forty milliliters of peripheral blood were collected in heparinized tubes, diluted in a proportion of 2:1 (v/v) in PBS (pH 7.2), and then added to a Ficoll-Hypaque (Amersham Biosciences, Uppsala, Sweden) solution. After centrifugation at 400 × g for 30 min at 20°C, a ring of peripheral blood mononuclear cells (PBMCs) was obtained. Cells were washed twice with phosphate buffer solution (PBS) (pH 7.2) and submitted to centrifugation (300 × g for 10 min at 20°C). PBMCs were resuspended with 2 ml of RPMI 1640 medium (Cultilab, Campinas, SP, Brazil) supplemented with 10% fetal calf serum (Cultilab, Campinas, SP, Brazil) and 1% antibiotics (100 UI/ml penicillin and 100 μg/ml streptomycin- Sigma, St. Louis, MO). Cell viability was evaluated with Trypan blue dye (Sigma, St. Louis, MO), cells were counted in a Neubauer chamber, and the cell concentration was adjusted to 106 cells for subsequent staining.
Parasite Growth and Mice Infections
Leishmania (Leishmania) major (MHOM/IL/81/FE/BNI) were grown in complete (10% fetal calf serum, 5% penicillin-streptomycin, 5% glutamine) Schneider's Drosophila medium (PAN Biotech, Germany) at 27°C in an incubator under sterile conditions. Infectivity of parasites was maintained through animal passage and parasites were used for infection after maximum five passages in culture. Viable parasites were counted, washed with PBS (pH 7.0), and the concentration was adjusted to 3 × 106 parasites in 10 μL volume, as described previously in other publications (37–39). Mice were kept under isoflurane/oxygen, the left ear was exposed, and 10 μL parasite-dose were injected.
Processing of Ear and Lymph Node Tissues
Samples were collected and processed to obtain single cell suspensions. The dorsal and ventral ear layers were separated with forceps and individually deposited on wells of a 24-well bottom plate supplemented with RPMI 1640 containing 250 μg/mL LiberaseTM TL Research Grade (Roche) for 90 min at 37°C and 5% CO2. The reaction was stopped with 1 mL/well of cold media and the tissues were transferred to the top of a 70 μm mesh and mashed with the aid of a syringe piston. Cells were washed twice with PBS (pH 7.4) 2% FCS and finally resuspended with 1 mL of supplemented RPMI 1640 media. Lymph nodes were processed similarly.
Lesion Size and Limiting Dilution Parasitaemia Analysis
An aliquot of 200 μL from each infected sample was used to perform a limiting dilution assay to quantify the parasite levels on ears and lymph nodes. Samples were centrifuged at 400 × g for 5 min and resuspended in 200 μL of complete Schneider's Drosophila medium. Samples were individually deposited on 96-well flat bottom plates and a serial dilution of 1:10 (v/v) was performed. Plates were incubated at 27°C for minimum of 7 days. After this period, the number of viable parasites in the tissues was calculated accordingly: [(Geo Mean of the duplicate from highest dilution)/lesion weight] × 50 (1,000/20–20 μL was initially used from 1,000 μL suspension) (40, 41).
Antibodies, Flow Cytometry, and Chemokine Detection
Antibodies (α) were purchased from Biolegend (Germany) and BD Biosciences, unless noted otherwise. Mouse cells—The following antibodies were used for flow cytometry: αCD3-BUV395 (Clone 1 45-2C11) αCD4-V500 (Clone RM4-5), αCD44-AF700/BV421(Clone IM7), αCD62L-APC/Cy7 (Clone MEL-14), αCD27-FITC/PerCP Cy5.5 (Clone LG3A.10), αLy6C-PE/BV500/PerCP-Cy5.5 (Clone HK1.4), αFoxp3-APC (Clone FJK 16s), αIFN-γ-AF488 (Clone XMG 1.2), αTNF-α-PECy7 (Clone MP6 XT22), αPD-1-PECy7 (Clone RMP1-30), αIL-27-PE (Clone MM27-7B1), αCD45-PECy7 (Clone 30-F11), αCD11b-APC Cy7 (Clone M1/70), αF4/80-AF700 (Clone BM8), αPD-L1-PE/PE/Cy7/APC (Clone 10F.9G2), αMHC Class II-BV510 (Clone M5/114.15.2), αCD86-PE/Cy7 (Clone GL1), αCD206-PE/Dazzle (Clone C068C2), αRelm Alpha (Polyclonal rabbit IgG—Genetex), αRabbit IgG-BV421 (Clone Poly4064), αYm1 (Polyclonal goat IgG—R&D Systems), αGoat IgG-FITC (Polyclonal—Invitrogen). Approximately 106 cells were deposited individually per well in 96-well round bottom plates for antibody staining. Cells were washed twice with PBS (pH 7.2) 2% FCS, 300 × g for 10 min at RT. Cells were stained with cell surface antibodies diluted in Fc Block solution for 1h at 4°C. After this period, plates were washed twice with 200 μL per well of PBS (pH 7.2) 2% FCS, 300 × g for 10 min at RT, and cells were fixed with 200 μL per well of 1x fixation buffer (eBioscience Foxp3 staining buffer) for 30 min. Cells were washed twice with 1× permeabilization buffer (eBioscience Foxp3 staining buffer), 300 × g for 10 min at RT. Cells were stained with intracellular antibodies diluted in permeabilization buffer and incubated for 1h at 4°C. Afterwards cells were washed twice with 1× permeabilization buffer (eBioscience Foxp3 staining buffer), 300 × g for 10 min at RT. Stained cells were resuspended in an appropriate volume of flow cytometry staining buffer or paraformaldehyde 1% prior to FACS analysis. For intracellular cytokine staining, cells in RPMI 1640 containing GolgiStop (BD Biosciences) diluted 1:1,000 (v/v) were deposited in 96-well round bottom plates and incubated for 4–5 h at 37°C with 5% CO2. For macrophage staining with antibodies, cells were blocked with 50 μL of blocking solution (PBS pH 7.2, 2% FCS, 1:100,000 of CD16/CD32) for 15 min at 4°C. For staining with purified antibodies, an extra cell surface staining step for detection with fluorochrome-conjugated anti-IgG was added. Samples were acquired on a BD LSRII (BD Biosciences) and the generated data were analyzed with the current version of FlowJo Software (v10.6.2).
Antibodies (α) were purchased from Biolegend (Germany). Human cells—The following antibodies were used for flow cytometry: αCD3-APC Cy7 (Clone HIT3a), αCD4 BV605 (Clone A161A1), αCD45RO-PE/Cy7 (Clone UCHL1), αCD197/CCR7-BV421 (Clone G043H7), and αPD-1-APC (Clone EH12.2H7). Cells from patients were individually deposited in FACS tubes and stained with cell surface antibodies for 30 min at 4°C, protected from light. After this period, cells were washed twice with PBS (pH 7.2) and submitted to centrifugation (300 × g for 10 min at 20°C). Cells were fixed with 1× fix/perm buffer solution (eBioscience) prior to FACS acquisition. Samples were acquired on a BD FACS Aria III (BD Biosciences) and the generated data were analyzed with FlowJo Software (v10.6.2).
For chemokine detection, single cell suspensions from the ears of infected mice were deposited in 96-well round bottom plates and incubated for 24h at 37°C with 5% CO2. MCP-1 (CCL2) chemokine detection was performed by ELISA following manufacturers' recommendations (Biolegend).
Statistical Analysis
Statistical analysis was performed using Graph Pad Prism 8. The statistical significance between the two groups was calculated using the two-tailed Mann-Whitney test. Statistical assumptions were made at 95% confidence level and *p < 0.05, **p < 0.01, ***p < 0.001 and ****p < 0.0001.
Results
PD-1 Is Upregulated on Different Human CD4+ T Cell Subsets During Cutaneous Leishmaniasis
Based on the expression of CD45RO and CCR7, human CD4+ T cells can be divided into four different subsets: CD45RO−CCR7+ T naïve (TNAÏVE), CD45RO+CCR7+ T central memory (TCM), CD45RO+CCR7− T effector memory (TEM), and CD45RO−CCR7− T terminally differentiated (TTD) T cells (42–44). The frequency of PD-1+ T cells was analyzed in those CD4+ T cell subsets. We observed high frequencies of CD4+PD-1+ T cells in TCM of post-treated (PT) patients compared to uninfected individuals (Figures 1A,B). Active CL disease (AD) patients displayed very low frequencies of TNAÏVE and TCM cells. However, higher frequencies of CD4+PD-1+ T cells were observed in TTD cells of active CL compared to uninfected individuals and PT patients (Figure 1C). Finally, increasing frequencies of CD4+PD-1+ T cells were observed in TEM cells of AD and PT patients (Figure 1D). One interesting aspect of this result is the continuously increasing frequency of PD-1+ T cells with increasing development of CD4+ T cell subpopulations, with a very low frequency among TNAÏVE and very high frequency among TTD cells. Moreover, during AD, higher frequencies of PD-1+ T cells were observed in TTD cells compared to the other subsets. After treatment (PT), the frequency of PD-1+ T cells declined in TTD cells but was still high in TEM cells. To further study PD-1 and PD-L1 expression at different sites and on different cell types we decided to employ a murine model of CL.
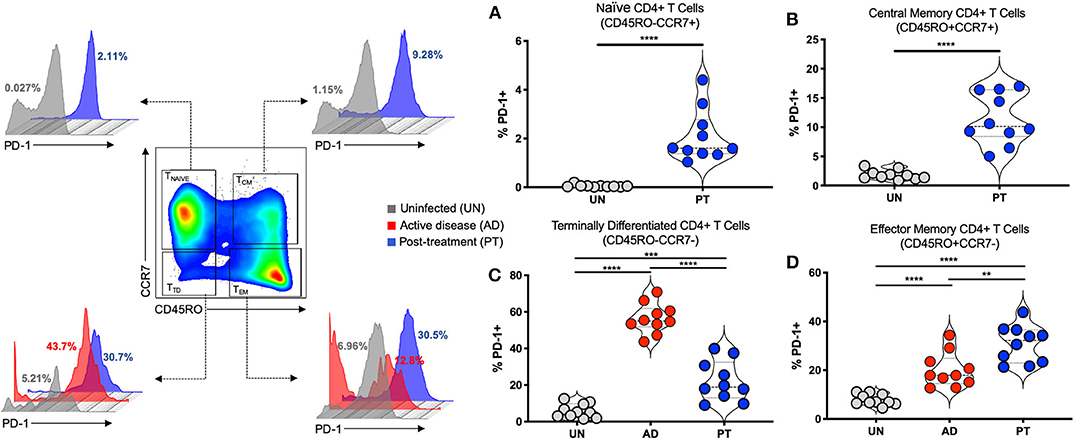
Figure 1. PD-1 is upregulated on different human CD4+ T cell subsets during cutaneous leishmaniasis. Representative flow cytometry graphs and independent data depicting the frequency (%) of PD-1+ in Naïve CD4+ T cells (CD45RO−CCR7+) (A), Central Memory CD4+ T cells (CD45RO+CCR7+) (B), Terminally Differentiated CD4+ T cells (CD45RO−CCR7−) (C), and Effector Memory CD4+ T cells (CD45RO+CCR7−) (D) in the peripheral blood of patients with active lesions before treatment (Active disease—AD, n = 10) and patients less than 6 months after clinical cure of CL (Post-treatment—PT, n = 10), when compared to control group of healthy individuals (Uninfected—UN, n = 10). Mean ± SD (Error bars). **p < 0.01, ***p < 0.001 and ****p < 0.0001, Mann-Whitney U rank sum test.
PD-1 and Its Ligand PD-L1 Are Upregulated by Different Cell Types in the Lesion of Leishmania major-Infected Mice
The expression of PD-1 on activated CD4+ T effector cells (CD3+CD4+CD44+CD62L−CD27−) (Figure 2A) and of PD-L1 on infiltrating monocytes (CD45+Ly6C+CD11b+) and resident macrophages (CD45+Ly6C−CD11b+F4/80+) (Figure 2B) were assessed in tissues from naïve WT mice and WT mice on days 12 and 35 post-infection (dpi). We observed a strong induction of PD-1 on activated CD4+ T effector cells at both time points compared to naïve WT mice. In parallel, we detected PD-L1 on infiltrating monocytes and resident macrophages isolated from the infected ear of L. major-infected mice compared to uninfected controls. This finding suggests that the PD-1/PD-L1 axis may have important regulatory functions during infection by Leishmania and corroborates our findings on PD-1 induction on CD4+ T cells from infected patients. Thus the PD-1/PD-L1 axis, in particular the inhibition of T cell function, might be important to control the degree of CD4+ T cell activation. Thus, we decided to evaluate the immune response of Pdl1 knockout (Pdl1−/−) mice during Leishmania infection.
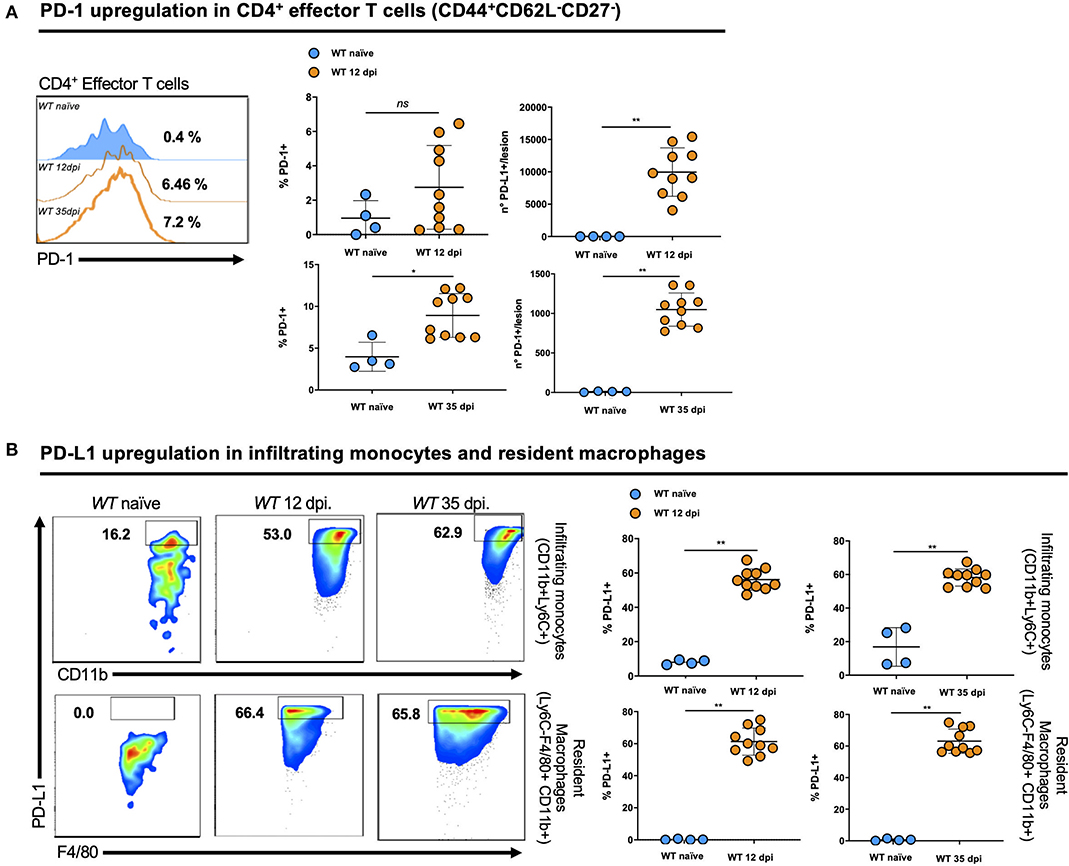
Figure 2. PD-1 and its ligand PD-L1 are upregulated by different cell types in the lesion of Leishmania major-infected mice. (A) Representative flow cytometric analysis and summary of data of PD-1 levels in activated CD4+ T effector cells (CD3+CD4+CD44+CD62L−CD27−) isolated from the ear of naïve and 12 and 35 days post-infections (dpi) Wild-type (WT) mice. (B) Representative flow cytometry graphs and summary of data of PD-L1 levels in infiltrating monocytes (CD45+Ly6C+CD11b+) and resident macrophages (CD45+Ly6C−CD11b+F4/80+) isolated from the ear of naïve and 12 and 35 dpi WT mice. Results are representative of two independent experiments with 2–5 mice/group. Mean ± SD (Standard deviation bars). *p < 0.05 and **p < 0.01, Mann-Whitney U rank sum test.
Infiltrating Monocytes and Resident Macrophages From Pdl1-/- Mice Exhibit a Weaker Inflammatory Phenotype After Leishmania major Infection
We investigated the activation status of infiltrating monocytes (CD45+Ly6C+CD11b+) and resident macrophages (CD45+Ly6C−CD11b+F4/80+) by analyzing the expression of major histocompatibility complex (MHC) class II and CD206 in the infected ear of L. major-infected Pdl1−/− and WT mice at 35 dpi (Figure 3). We observed significantly lower levels of MHCII expression and higher levels of CD206 expression in samples derived from L. major-infected Pdl1−/− mice compared to their WT counterparts. Interestingly, this result suggests that the lack of PD-1/PD-L1 signaling induces a weaker pro-inflammatory phenotype of PD-L1-deficient macrophages and monocytes. Based on this finding we further analyzed whether an impairment of the PD-1/PD-L1 axis might also affect the CD4+ T cell compartment.
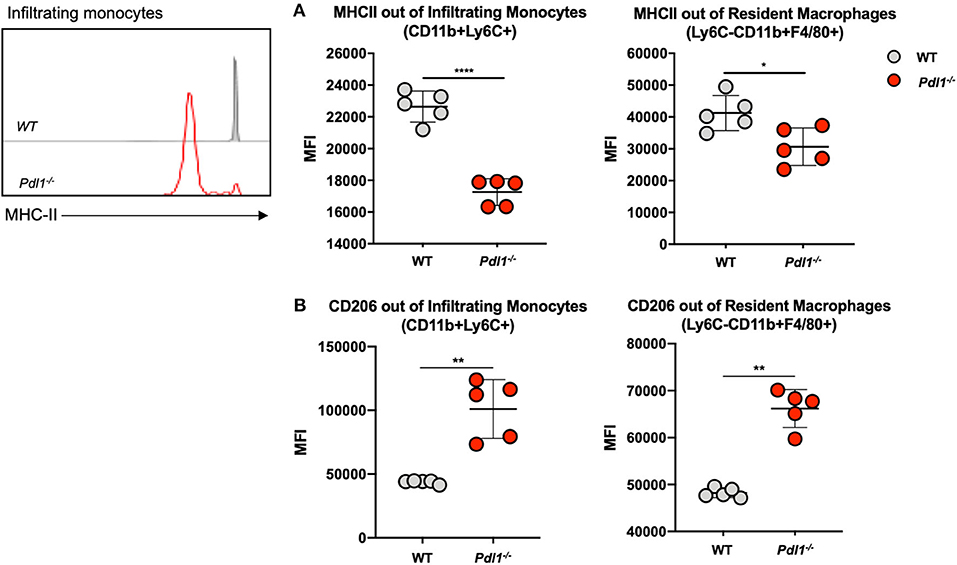
Figure 3. Infiltrating monocytes and resident macrophages from Pdl1−/− mice exhibit a weaker pro-inflammatory phenotype after Leishmania major infection. Representative flow cytograms and summary of data of MHC Class II (A) and CD206 (B) expression on infiltrating monocytes and resident macrophages from the Leishmania major infected ear of Wild-type (WT) and Pdl1−/− mice 35 dpi. Results are representative of one of two independent experiments with 5 mice/group. Mean ± SD (Standard deviation bars). *p < 0.05, **p < 0.01 and ****p < 0.0001, Mann-Whitney U rank sum test.
PD-L1 Is Required for the Expansion of CD4+Ly6Chi Effector T Cells and CD4+Foxp3+ Regulatory T Cells in the Lesion of Leishmania major-Infected Mice and Draining Lymph Nodes
Interestingly, we observed that L. major infection of Pdl1−/− mice was accompanied by a reduced expansion of activated CD4+Ly6Chi T effector cells and an increase in the percentage of CD4+Foxp3+ T regulatory cells (Treg) in the infected ear (Figure 4A) and in the ear draining lymph nodes (Figure 4B) of Pdl1−/− compared to WT mice. Particularly striking is the difference of subpopulations of CD4+ T cells infiltrating the ear lesion, which shows that the lack of PD-L1 in a murine model of L. major infection results in a diminished number of CD4+Ly6Chi effector T cells, but an increased number of Tregs.
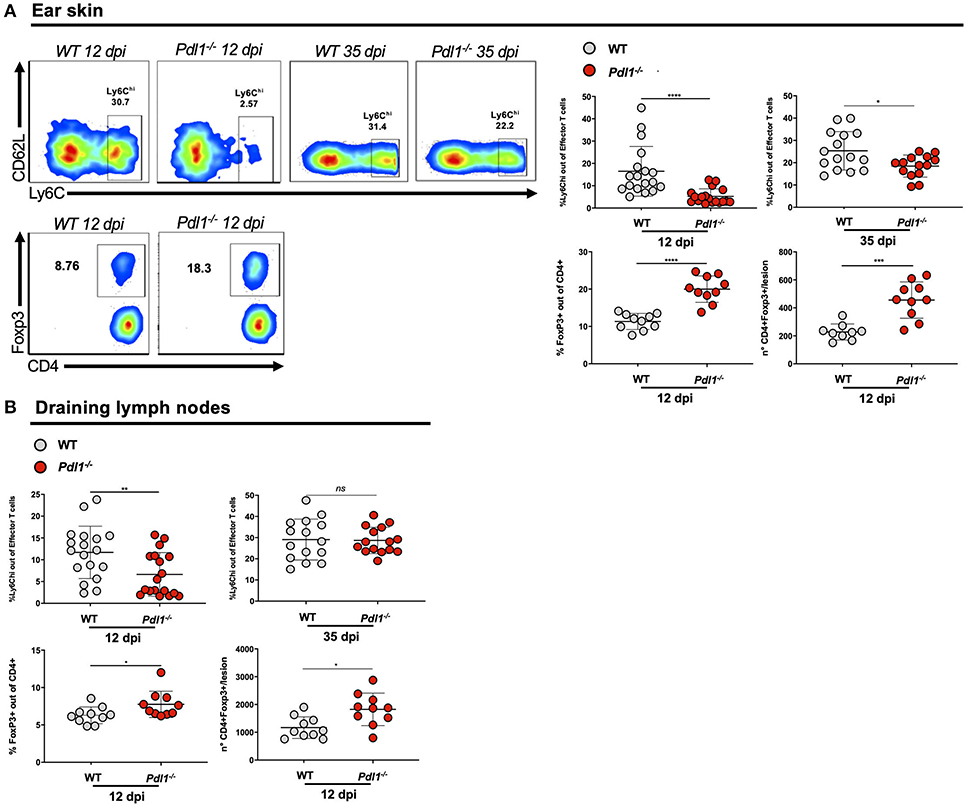
Figure 4. PD-L1 is required for the expansion of CD4+Ly6Chi effector T cells and CD4+Foxp3+ regulatory T cells in the lesion of Leishmania major-infected mice and draining lymph nodes. (A) Representative flow cytometric dot-plots of the ear tissues analyses are shown and summary of data, highlighting the decreased expansion of CD4+Ly6Chi effector T cells (12 and 35 dpi) and the increased expansion of CD4+Foxp3+ T cells (12 dpi) in Pdl1−/− mice, compared to WT mice. (B) Summary of data of CD4+Ly6Chi T effector cells (12 and 35 dpi) and CD4+ Foxp3+ T cells (12 dpi) in the lymph nodes of Pdl1−/− mice, compared to WT mice. Results are representative of two or three independent experiments with 2–5 mice/group. Mean ± SD (Standard deviation bars). ns = no statistically significant, *p < 0.05, **p < 0.01, ***p < 0.001, ****p < 0.0001, Mann-Whitney U rank sum test.
Lack of PD-L1 Is Associated With Lower Production of IL-27, IFN-γ, TNF-α and Increased Secretion of MCP-1/CCL2
Surprisingly, we observed that the lack of PD-L1 is associated with lower production of IL-27 by monocytes infiltrating the infected skin 6 dpi (Figure 5A), but not by resident macrophages or other APCs. Among chemokines implicated in T cell recruitment, we observed an increased production of CCL2 in the infected ear of Pdl1−/− mice compared to WT counterparts (Figure 5B). Moreover, CD4+ T effector cells from Pdl1−/− mice produced lower levels of INF-γ and TNF-α 12 dpi (Figure 5C). This result suggests that the lower frequency of CD4+Ly6Chi effector T cells might be due to the lower production of IL-27. Additionally, this result provides evidence that pro-inflammatory cytokines are not involved in the initial immunopathology driving increased lesion size (Figure 6).
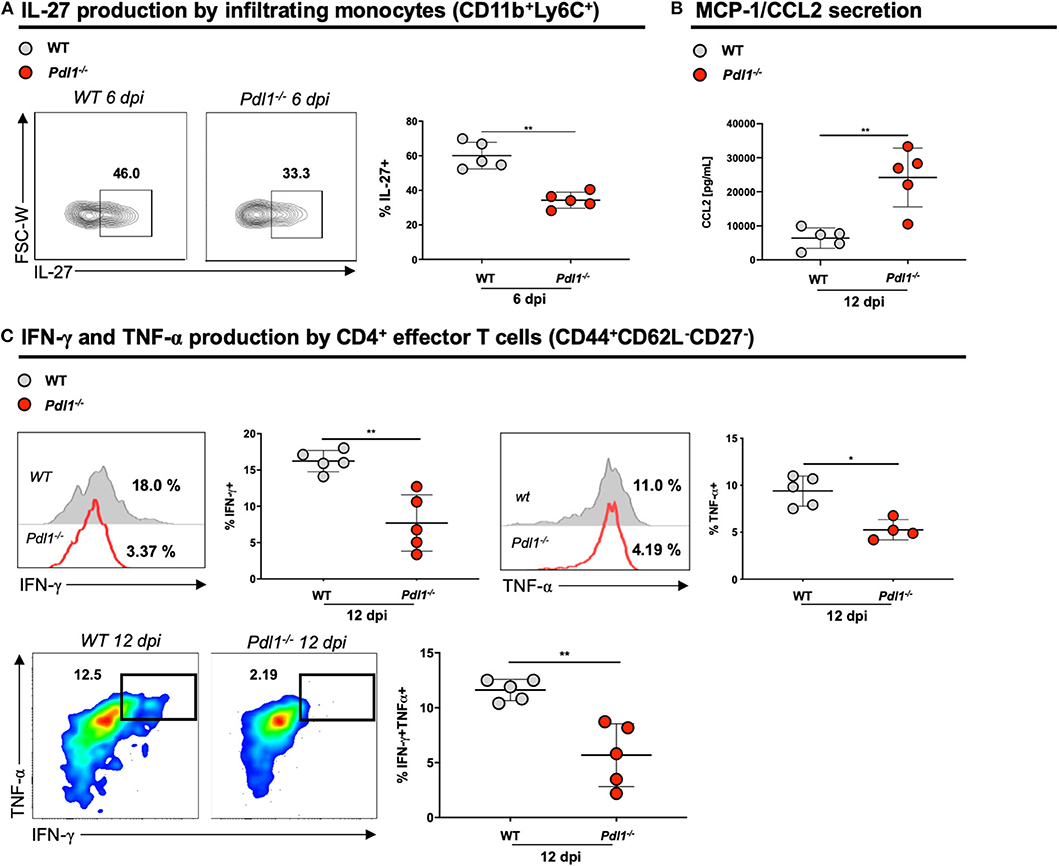
Figure 5. Lack of PD-L1 is associated with lower production of IL-27, IFN-γ, and TNF-α and increased secretion of MCP-1/CCL2. (A) Representative flow cytometric dot-plots and analyses of IL-27 production of infiltrating monocytes (CD11b+Ly6C+) from ear skin of Wild-type (WT) and PD-L1 deficient mice (Pdl1−/−) 6 dpi. (B) Analyses of CCL2 secreted in the ear skin cells of WT and Pdl1−/− 12 dpi. (C) Representative histograms, dot-plots and analyses of IFN-γ, and TNF-α produced by effector CD4+ T cells from ear skin of WT and Pdl1−/− 12 dpi. Results are representative of two or three independent experiments with 2–3. Mean ± SD (Standard deviation bars). *p < 0.05 and **p < 0.01, Mann-Whitney U rank sum test.
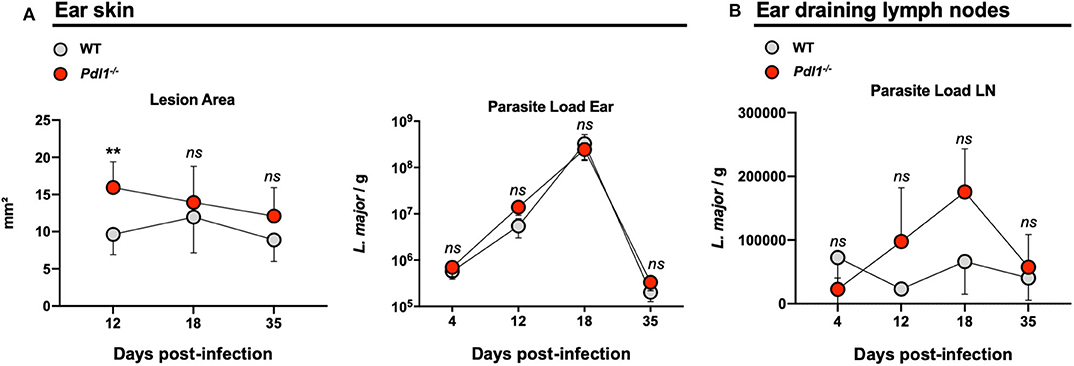
Figure 6. Lack of PD-L1 induced larger lesions after infection with Leishmania major, but did not affect parasite burden. (A) Summary of data representing the lesion area and parasite load of Leishmania major infected ear from Wild-type (WT) and Pdl1−/− mice. (B) Independent data representing the parasite load of ear draining lymph nodes from WT and Pdl1−/− mice. Results are representative of 2–3 independent experiments with 3–5 mice/group. Mean ± SD (Standard deviation bars). ns = no statistically significant, **p < 0.001, Mann-Whitney U rank sum test.
Lack of PD-L1 Induced Larger Lesions After Infection With Leishmania major, but Did Not Affect Parasite Burden
Parasite burden and lesion areas were assessed in Pdl1−/− mice and their WT counterparts after L. major infection in the ear at different time points. However, with regard to the reduced frequency of activated CD4+Ly6Chi T effector cells, the lesion area was larger in the Pdl1−/− mice compared to the WT mice early after infection (12 dpi) (Figure 6A), although a similar number of parasites was observed in the two mouse strains at each time point analyzed (12, 18, 35 dpi) for both the ear (Figure 6A) and the dLN (Figure 6B). Interestingly, the difference in lesion area between WT and Pdl1−/− was not observed at later time points, suggesting a role for PD-L1 on lesion development only in the initial phase of the response.
Discussion
The regulation of T cell responses in leishmaniasis has been extensively investigated. The co-inhibitory receptor PD-1 is a potential target since it maintains immune homeostasis by inducing peripheral tolerance and protecting tissues from autoimmune attack (45). Moreover, blocking PD-1 or its ligand PD-L1 restores T cell function in different types of cancer, which is associated with a better disease outcome (46). However, despite its importance, the role of this axis during acquisition of immunity against CL is not well-established yet (27, 47, 48).
In the present study we found increasing frequencies of PD-1+ T cells in different subsets of CD4+ T cells from patients with acute and post-treatment CL. Very low numbers of PD-1+ T cells were observed in CD4+ TNAÏVE compared to the other subpopulations of CD4+ T cells like TCM, TEM, and TTD cells. High numbers of PD-1+ T cells were observed in T cell compartments with more activated CD4+ T cells, such as TCM, TEM, and TTD. Moreover, patients with active disease display high numbers of PD-1+ T cells in CD4+ TTD, and in the post-treatment group the numbers of PD-1+ T cells are higher in CD4+ TEM cells. It has been shown that antigen-specific T cells express PD-1 upon activation. This expression is tightly regulated and it remains highly expressed during chronic antigen exposure (49, 50). There are very few data about the differential expression of PD-1 in subpopulations of naïve, effector, and memory CD4+ T cells, and most of the data associates PD-1 expression with T cell exhaustion (51–53). Activated CD4+ T cells play an important role during human CL, acting as an important source of cytokines such as IFN-γ and TNF-α that are associated with lesion healing or disease progression (54, 55). This result suggests that PD-1 is induced on CD4+ T cells and potentially counter-regulates the immune response. However, the exact role of PD-1 expression on CD4+ T cells in the different subpopulations needs to be studied intensively. In addition, the high numbers of CD4+PD-1+ T cells which remained in patients after treatment might be an indirect indication of either a prolonged antigen exposure or a state of exhaustion that persists even after antigen removal. Lesion healing in clinically cured CL patients does not necessarily correlate with parasite clearance since the patients can suffer from reoccurrence of lesions in distinct and multiple numbers (56). Thus, our data suggest that PD-1/PD-L1 interactions are likely to occur during the course of human CL and persist post-treatment, which might contribute to the modulation CD4+ T cell activity.
To further study the function of PD-1 we employed an infection model using resistant C57BL/6 mice to determine which cell types express PD-1 and its ligand PD-L1 upon L. major infection at different time points. Furthermore, using full body Pdl1−/− mice we observed that the lack of PD-L1 impacts the response of CD4+ T cells by reducing the frequency of CD4+ T effector cells and by increasing the frequency of CD4+Foxp3+ regulatory T cells, which affects the production of cytokines and chemokines. Nevertheless, Pdl1−/− mice displayed larger lesions in the initial phase of infection, but displayed no differences in the number of parasites, which highlights the importance of PD-1 in the initial phase of the immune response against Leishmania.
During murine infection by L. major, increased frequencies of activated CD4+PD-1+ T effector cells were found at the infected site and in draining lymph nodes. This PD-1 expression is possibly a result of T cell activation, since Leishmania infection results in IL-12-dependent differentiation and activation of Leishmania-specific CD4+ T cells producing mainly type I cytokines (IFN-γ, TNF-α, IL-2) to activate macrophages and eliminate parasites (55). Moreover, it was previously demonstrated in Leishmania infection of human and mice that an impairment of CD8+ T cell function is associated with PD-1 expression, and the immunization of mice with lipophosphoglycan from Leishmania induces a dose-dependent expression of PD-1 on CD8+ T cells, but not on CD4+ T cells (29, 57–59). Our data highlights that PD-1 is induced on CD4+ T cells during Leishmania infection, which may counterbalance excessive activation when binding to PD-L1. We found high levels of PD-L1 expression on infiltrating monocytes and tissue-resident macrophages during L. major infection. These two cell types are particularly involved in the immune response against Leishmania, and T cell-derived IFN-γ is one of the most important cytokines that induces PD-L1 expression (60). However, it is not clear whether those levels of PD-L1 are solely induced by cytokines or if intrinsic Leishmania factors contribute to PD-L1 expression as a parasite-induced immune escape mechanism (61). Recent studies indicate that blocking PD-L1 in VL models restores CD4+ and CD8+ T cell function and leads to an increased survival of CD8+ T cells (29, 35, 62, 63). However, immune regulation in the skin might be different compared to other organs.
Upon L. major infection, resident macrophages and infiltrating monocytes from Pdl1−/− mice are less activated compared to those from WT mice. This is in contrast with the current idea that PD-1/PD-L1 interaction dampens the immune response. Indeed, our data suggest that the lack of PD-L1 in resident macrophages and infiltrating monocytes dampen their pro-inflammatory phenotype, which would be necessary to eliminate intracellular Leishmania.
Lower frequencies of activated CD4+Ly6Chi effector T cells and higher levels of CD4+Foxp3+ regulatory T cells were observed in Pdl1−/− mice infected with L. major. Previously, it was shown that short-lived CD4+Ly6C+ effector T cells are capable of producing more IFN-γ compared to other cell subsets upon Leishmania infection (12). This study suggests that PD-1/PD-L1 engagement is required to control the expansion of this population of CD4+Ly6C+effector T cells. A possible scenario for this might be concluded from a recent study showing that PD-L1 and CD80 on APCs are interacting in cis and thus preventing the interactions of PD-1 and PD-L1 in trans (64). Then, a lack of PD-L1 on APCs would allow the interaction of CD80 with CTLA-4, which might lead to a reduced frequency of CD4+ T effector cells.
The PD-1/PD-L1 pathway also regulates the development and function of Treg by promoting Foxp3 expression (31). However, it was shown recently that a blockade of PD-1 can increase the proliferation and suppressive capacity of Tregs in inflamed tissues (65, 66). Therefore, it is tempting to speculate that the lack of PD-L1 leads to an increased expansion of CD4+Foxp3+ Treg cells and thereby contributes to a diminished expansion of CD4+Ly6Chi T effector cells.
IL-27 was shown to be a major cytokine that induces Ly6C expression on CD4+ T cells upon TCR engagement (16, 67, 68). Our data indicate that the lack of PD-L1 is associated with a lower production of IL-27 by monocytes infiltrating the lesion during the initial phase of infection, which might lead to a lower amount of CD4+Ly6Chi T effector cells and a diminished secretion of Th1 cytokines.
We found no differences in the parasite load in the tissues of Leishmania-infected WT mice and Pdl1−/− mice. However, we observed larger lesions in the latter. Parasite clearance has been associated with activation of macrophages by CD4+ T effector cells producing IFN-γ. Larger lesions might be the result of an immunopathology rather than by the parasite itself. However, the lower levels of IFN-γ and TNF-α produced by CD4+ T effector cells in Pdl1−/− mice suggest that these pro-inflammatory cytokines are not driving lesion formation. Other immune pathways, like CD8+ T cells, might promote this inflammation by secreting TNF-α, IL-1β, or granzymes, which have been associated with disease severity of CL without altering parasite killing (19–22).
The present work demonstrates that the PD-1/PD-L1 axis has important functions during human CL and murine experimental infection. The lack of PD-L1 impairs the expansion of activated CD4+Ly6Chi T effector cells during infection and enhances the frequency of Tregs in the infected ear and in the draining lymph nodes. This results in larger lesions but has no effect on parasite burden. Importantly, our data suggest that the PD-1/PD-L1 axis during CL provides a delicate fine-tuning of the immune response, which ensures an optimal anti-parasitic immune response without overwhelming inflammation.
Data Availability Statement
All datasets generated for this study are included in the article/Supplementary Material.
Ethics Statement
The studies involving human participants were reviewed and approved by CPqAM/FIOCRUZ Research Ethics Committee. The patients/participants provided their written informed consent to participate in this study. The animal study was reviewed and approved by Federal health authorities of the Hamburg (Behörde für Gesundheit und Verbraucherschutz).
Author Contributions
RF and RG performed the experiments. RF and TJ were responsible for acquisition of data, analysis, interpretation, and drafting the manuscript. VP and MB were responsible for human leishmaniasis diagnosis, analysis, and for providing the laboratory and materials for human experiments. SC and HL helped with murine model for leishmania infection. LB was responsible for interpretation of data and provided reagents. All authors have read and approved the final manuscript. All authors contributed to the article and approved the submitted version.
Funding
RF was recipient of a CAPES (Brazil)/Alexander von Humboldt postdoctoral fellowship (Germany). Process number 99999.000461/2016-04. The Deutsche Forschungsgemeinschaft (Grant SFB841 to LB). RG was supported by the Konrad-Adenauer Stiftung.
Conflict of Interest
The authors declare that the research was conducted in the absence of any commercial or financial relationships that could be construed as a potential conflict of interest.
Acknowledgments
We thank the staff from the BNITM Animal Facility and Aline Adam, Christiane Steeg, and Ulricke Richardt for excellent technical support. RF thanks the Alexander von Humboldt Foundation (AvH, Germany) and the Coordination for the Improvement of Higher Education Personnel (CAPES, Brazil) for the sponsored period in Germany. We thank the platform PDTIS/Flow Cytometry (RPT08F)-FIOCRUZ. We thank Madeleine Hamley for English language editing.
Supplementary Material
The Supplementary Material for this article can be found online at: https://www.frontiersin.org/articles/10.3389/fimmu.2020.574491/full#supplementary-material
References
1. Leishmaniasis. (2020) Available online at: https://www.who.int/news-room/fact-sheets/detail/leishmaniasis (accessed May 22, 2020).
2. WHO| Leishmaniasis. WHO. (2018) Available online at: https://www.who.int/gho/neglected_diseases/leishmaniasis/en/ (accessed January 5, 2019).
3. Scott P, Novais FO. Cutaneous leishmaniasis: immune responses in protection and pathogenesis. Nat Publ Gr. (2016) 16:581–92. doi: 10.1038/nri.2016.72
4. Coutinho De Oliveira B, Duthie MS, Alves Pereira VR. Vaccines for leishmaniasis and the implications of their development for American tegumentary leishmaniasis. Hum Vaccin Immunother. (2020) 16:919–30. doi: 10.1080/21645515.2019.1678998
5. Sacks DL. Vaccines against tropical parasitic diseases: a persisting answer to a persisting problem. Nat Immunol. (2014) 15:403–5. doi: 10.1038/ni.2853
6. Glennie ND, Scott P. Memory T cells in cutaneous leishmaniasis. Cell Immunol. (2016) 309:50–4. doi: 10.1016/j.cellimm.2016.07.010
7. Glennie ND, Yeramilli VA, Beiting DP, Volk SW, Weaver CT, Scott P. Skin-resident memory CD4+ T cells enhance protection against leishmania major infection. J Exp Med. (2015) 212:1405–14. doi: 10.1084/jem.20142101
8. Gupta G, Oghumu S, Satoskar AR. Mechanisms of immune evasion in leishmaniasis. Adv Appl Microbiol. (2013) 82:155–84. doi: 10.1016/B978-0-12-407679-2.00005-3
9. Dostálová A, Volf P. Leishmania development in sand flies: parasite-vector interactions overview. Parasit Vectors. (2012) 5:276. doi: 10.1186/1756-3305-5-276
10. Kobets T, Grekov I, Lipoldova M. Leishmaniasis: prevention, parasite detection and treatment. Curr Med Chem. (2012) 19:1443–74. doi: 10.2174/092986712799828300
11. von Stebut E, Belkaid Y, Jakob T, Sacks DL, Udey MC. Uptake of leishmania major amastigotes results in activation and interleukin 12 release from murine skin-derived dendritic cells: implications for the initiation of anti-Leishmania immunity. J Exp Med. (1998) 188:1547–52. doi: 10.1084/jem.188.8.1547
12. Peters NC, Pagán AJ, Lawyer PG, Hand TW, Henrique Roma E, Stamper LW, et al. Chronic parasitic infection maintains high frequencies of short-lived Ly6C+CD4+ effector T cells that are required for protection against re-infection. PLoS Pathog. (2014) 10:e1004538. doi: 10.1371/journal.ppat.1004538
13. Marshall HD, Chandele A, Jung YW, Meng H, Poholek AC, Parish IA, et al. Differential expression of Ly6C and T-bet distinguish effector and memory Th1 CD4+ cell properties during viral infection. Immunity. (2011) 35:633–46. doi: 10.1016/j.immuni.2011.08.016
14. Hu Z, Blackman MA, Kaye KM, Usherwood EJ. Functional heterogeneity in the CD4+ T cell response to murine γ-herpesvirus 68. J Immunol. (2015) 194:2746–56. doi: 10.4049/jimmunol.1401928
15. Walunas TL, Bruce DS, Dustin L, Loh DY, Bluestone JA. Ly-6C is a marker of memory CD8+ T cells. J Immunol. (1995) 155:1873–83. Available online at: http://www.ncbi.nlm.nih.gov/pubmed/7543536 (accessed April 11, 2019).
16. DeLong JH, O'Hara Hall A, Rausch M, Moodley D, Perry J, Park J, et al. IL-27 and TCR stimulation promote T cell expression of multiple inhibitory receptors. Immunohorizons. (2019) 3:13–25. doi: 10.4049/immunohorizons.1800083
17. Liu Z, Liu JQ, Talebian F, Wu LC, Li S, Bai XF. IL-27 enhances the survival of tumor antigen-specific CD8+ T cells and programs them into IL-10-producing, memory precursor-like effector cells. Eur J Immunol. (2013) 43:468–79. doi: 10.1002/eji.201242930
18. Zhu X, Liu Z, Liu JQ, Zhu J, Zhang J, Davis JP, et al. Systemic delivery of IL-27 by an adeno-associated viral vector inhibits T cell-mediated colitis and induces multiple inhibitory pathways in T cells. J Leukoc Biol. (2016) 100:403–11. doi: 10.1189/jlb.3A1215-540R
19. Campos TM, Novais FO, Saldanha M, Costa R, Lordelo M, Celestino D, et al. Granzyme B produced by natural killer cells enhances inflammatory response and contributes to the immunopathology of cutaneous leishmaniasis. J Infect Dis. (2020) 221:973–82. doi: 10.1093/infdis/jiz538
20. Novais FO, Nguyen BT, Scott P. Granzyme B inhibition by tofacitinib blocks pathology induced by CD8 T cells in cutaneous leishmaniasis. J Invest Dermatol. (2020). doi: 10.1016/j.jid.2020.07.011
21. Novais FO, Carvalho AM, Clark ML, Carvalho LP, Beiting DP, Brodsky IE, et al. CD8+ T cell cytotoxicity mediates pathology in the skin by inflammasome activation and IL-1β production. PLoS Pathog. (2017) 13:e1006196. doi: 10.1371/journal.ppat.1006196
22. Santos Cda S, Boaventura V, Ribeiro Cardoso C, Tavares N, Lordelo MJ, Noronha A, et al. CD8(+) granzyme B(+)-mediated tissue injury vs. CD4(+)IFNγ(+)-mediated parasite killing in human cutaneous leishmaniasis. J Invest Dermatol. (2013) 133:1533–40. doi: 10.1038/jid.2013.4
23. Belkaid Y, Hoffmann KF, Mendez S, Kamhawi S, Udey MC, Wynn TA, et al. The role of interleukin (IL)-10 in the persistence of Leishmania major in the skin after healing and the therapeutic potential of anti-IL-10 receptor antibody for sterile cure. J Exp Med. (2001) 194:1497–506. doi: 10.1084/jem.194.10.1497
24. Ehrlich A, Castilho TM, Goldsmith-Pestana K, Chae WJ, Bothwell ALM, Sparwasser T, et al. The immunotherapeutic role of regulatory T cells in Leishmania (Viannia) panamensis infection. J Immunol. (2014) 193:2961–70. doi: 10.4049/jimmunol.1400728
25. Gautam S, Kumar R, Maurya R, Nylén S, Ansari N, Rai M, et al. IL-10 neutralization promotes parasite clearance in splenic aspirate cells from patients with visceral leishmaniasis. J Infect Dis. (2011) 204:1134–7. doi: 10.1093/infdis/jir461
26. Gabriel Á, Valério-Bolas A, Palma-Marques J, Mourata-Gonçalves P, Ruas P, Dias-Guerreiro T, et al. Cutaneous leishmaniasis: the complexity of host's effective immune response against a polymorphic parasitic disease. J Immunol Res. (2019) 2019:1–16. doi: 10.1155/2019/2603730
27. Gannavaram S, Bhattacharya P, Ismail N, Kaul A, Singh R, Nakhasi HL. Modulation of innate immune mechanisms to enhance leishmania vaccine-induced immunity: role of coinhibitory molecules. Front Immunol. (2016) 7:187. doi: 10.3389/fimmu.2016.00187
28. Fenwick C, Joo V, Jacquier P, Noto A, Banga R, Perreau M, et al. T-cell exhaustion in HIV infection. Immunol Rev. (2019) 292:149–63. doi: 10.1111/imr.12823
29. Joshi T, Rodriguez S, Perovic V, Cockburn IA, Stäger S. B7-H1 Blockade increases survival of dysfunctional CD8+ T cells and confers protection against leishmania donovani infections. PLoS Pathog. (2009) 5:e1000431. doi: 10.1371/journal.ppat.1000431
30. Agata Y, Kawasaki A, Nishimura H, Ishida Y, Tsubat T, Yagita H, et al. Expression of the PD-1 antigen on the surface of stimulated mouse T and B lymphocytes. Int Immunol. (1996) 8:765–72. doi: 10.1093/intimm/8.5.765
31. Francisco LM, Salinas VH, Brown KE, Vanguri VK, Freeman GJ, Kuchroo VK, et al. PD-L1 regulates the development, maintenance, and function of induced regulatory T cells. J Exp Med. (2009) 206:3015–29. doi: 10.1084/jem.20090847
32. Saeidi A, Zandi K, Cheok YY, Saeidi H, Wong WF, Lee CYQ, et al. T-Cell exhaustion in chronic infections: reversing the state of exhaustion and reinvigorating optimal protective immune responses. Front Immunol. (2018) 9:2569. doi: 10.3389/fimmu.2018.02569
33. Beaumier CM, Gillespie PM, Hotez PJ, Bottazzi ME. New vaccines for neglected parasitic diseases and dengue. Transl Res. (2013) 162:144–55. doi: 10.1016/j.trsl.2013.03.006
34. Chiku VM, Silva KLO, de Almeida BFM, Venturin GL, Leal AAC, de Martini CC, et al. PD-1 function in apoptosis of T lymphocytes in canine visceral leishmaniasis. Immunobiology. (2016) 221:879–88. doi: 10.1016/j.imbio.2016.03.007
35. Esch KJ, Juelsgaard R, Martinez PA, Jones DE, Petersen CA. Programmed death 1-mediated T cell exhaustion during visceral leishmaniasis impairs phagocyte function. J Immunol. (2013) 191:5542–50. doi: 10.4049/jimmunol.1301810
36. Habib S, El Andaloussi A, Elmasry K, Handoussa A, Azab M, Elsawey A, et al. PDL-1 Blockade prevents T cell exhaustion, inhibits autophagy, and promotes clearance of Leishmania donovani. Infect Immun. (2018) 86:18. doi: 10.1128/IAI.00019-18
37. Fehling H, Choy SL, Ting F, Landschulze D, Bernin H, Lender SC, et al. Antileishmanial effects of synthetic EhPIb analogs derived from the entamoeba histolytica lipopeptidephosphoglycan. Antimicrob Agents Chemother. (2020) 64:20. doi: 10.1128/AAC.00161-20
38. Galvão LO, Moreira S Jr, Medeiros P Jr, Lemos GJ, Cunha NF, Antonino RM, et al. Therapeutic trial in experimental tegumentary leishmaniasis caused by Leishmania (Leishmania) amazonensis. A comparative study between mefloquine and aminosidine. Rev Soc Bras Med Trop. (2000) 33:377–82. doi: 10.1590/S0037-86822000000400008
39. Paduch K, Debus A, Rai B, Schleicher U, Bogdan C. Resolution of cutaneous leishmaniasis and persistence of leishmania major in the absence of arginase 1. J Immunol. (2019) 202:1453–64. doi: 10.4049/jimmunol.1801249
40. Belkaid Y, Kamhawi S, Modi G, Valenzuela J, Noben-Trauth N, Rowton E, et al. Development of a natural model of cutaneous leishmaniasis: powerful effects of vector saliva and saliva preexposure on the long-term outcome of Leishmania major infection in the mouse ear dermis. J Exp Med. (1998) 188:1941–53. doi: 10.1084/jem.188.10.1941
41. Uzonna JE, Joyce KL, Scott P. Low dose leishmania major promotes a transient T helper cell type 2 response that is down-regulated by interferon γ-producing CD8+ T cells. J Exp Med. (2004) 199:1559–66. doi: 10.1084/jem.20040172
42. Clénet ML, Gagnon F, Moratalla AC, Viel EC, Arbour N. Peripheral human CD4+CD8+ T lymphocytes exhibit a memory phenotype and enhanced responses to IL-2, IL-7 and IL-15. Sci Rep. (2017) 7:11612. doi: 10.1038/s41598-017-11926-2
43. Lintermans LL, Rutgers A, Stegeman CA, Heeringa P, Abdulahad WH. Chemokine receptor co-expression reveals aberrantly distributed TH effector memory cells in GPA patients. Arthritis Res Ther. (2017) 19:136. doi: 10.1186/s13075-017-1343-8
44. van den Brom RRH, van der Geest KSM, Brouwer E, Hospers GAP, Boots AMH. Enhanced expression of PD-1 and other activation markers by CD4+ T cells of young but not old patients with metastatic melanoma. Cancer Immunol Immunother. (2018) 67:925–33. doi: 10.1007/s00262-018-2148-6
45. Murphy TL, Murphy KM. Slow down and survive: enigmatic immunoregulation by BTLA and HVEM. Annu Rev Immunol. (2010) 28:389–411. doi: 10.1146/annurev-immunol-030409-101202
46. Xu-Monette ZY, Zhang M, Li J, Young KH. PD-1/PD-L1 blockade: have we found the key to unleash the antitumor immune response? Front Immunol. (2017) 8:1597. doi: 10.3389/fimmu.2017.01597
47. Barroso DH, Falcão SDAC, da Motta JOC, dos Santos LS, Takano GHS, Gomes CM, et al. PD-L1 may mediate T-cell exhaustion in a case of early diffuse leishmaniasis caused by leishmania (L.) amazonensis. Front Immunol. (2018) 9:21. doi: 10.3389/fimmu.2018.01021
48. Filippis C, Arens K, Noubissi Nzeteu GA, Reichmann G, Waibler Z, Crauwels P, et al. nivolumab enhances in vitro effector functions of pd-1+ t-lymphocytes and leishmania-infected human myeloid cells in a host cell-dependent manner. Front Immunol. (2017) 8:1880. doi: 10.3389/fimmu.2017.01880
49. Bally AP, Austin JW, Boss JM. Genetic and epigenetic regulation of PD-1 expression. J Immunol. (2016) 196:2431–7. doi: 10.4049/jimmunol.1502643
50. Day CL, Kaufmann DE, Kiepiela P, Brown JA, Moodley ES, Reddy S, et al. PD-1 expression on HIV-specific T cells is associated with T-cell exhaustion and disease progression. Nature. (2006) 443:350–4. doi: 10.1038/nature05115
51. Kwiecien I, Skirecki T, Polubiec-Kownacka M, Raniszewska A, Domagala-Kulawik J. Immunophenotype of T cells expressing programmed death-1 and cytotoxic t cell antigen-4 in early lung cancer: local vs. systemic immune response. Cancers. (2019) 11:567. doi: 10.3390/cancers11040567
52. Pauken KE, Wherry EJ. SnapShot: T Cell exhaustion. Cell. (2015) 163:1038.e1. doi: 10.1016/j.cell.2015.10.054
53. Sharpe AH, Pauken KE. The diverse functions of the PD1 inhibitory pathway. Nat Rev Immunol. (2018) 18:153–67. doi: 10.1038/nri.2017.108
54. Castellano LR, Filho DC, Argiro L, Dessein H, Prata A, Dessein A, et al. Th1/Th2 immune responses are associated with active cutaneous leishmaniasis and clinical cure is associated with strong interferon-γ production. Hum Immunol. (2009) 70:383–90. doi: 10.1016/j.humimm.2009.01.007
55. von Stebut E, Belkaid Y, Nguyen BV, Cushing M, Sacks DL, Udey MC. Leishmania major-infected murine langerhans cell-like dendritic cells from susceptible mice release IL-12 after infection and vaccinate against experimental cutaneous leishmaniasis. Eur J Immunol. (2000) 30:3498–506. doi: 10.1002/1521-4141(2000012)30:12<3498::AID-IMMU3498>3.0.CO;2-6
56. McGwire BS, Satoskar AR. Leishmaniasis: clinical syndromes and treatment. QJM. (2014) 107:7–14. doi: 10.1093/qjmed/hct116
57. Hernández-Ruiz J, Salaiza-Suazo N, Carrada G, Escoto S, Ruiz-Remigio A, Rosenstein Y, et al. CD8 cells of patients with diffuse cutaneous leishmaniasis display functional exhaustion: the latter is reversed, in vitro, by TLR2 agonists. PLoS Negl Trop Dis. (2010) 4:e871. doi: 10.1371/journal.pntd.0000871
58. Martínez Salazar MB, Delgado Domínguez J, Silva Estrada J, González Bonilla C, Becker I. Vaccination with leishmania mexicana LPG induces PD-1 in CD8+ and PD-L2 in macrophages thereby suppressing the immune response: a model to assess vaccine efficacy. Vaccine. (2014) 32:1259–65. doi: 10.1016/j.vaccine.2014.01.016
59. Schaut RG, Lamb IM, Toepp AJ, Scott B, Mendes-Aguiar CO, Coutinho JFV, et al. Regulatory IgD hi B cells suppress T cell function via IL-10 and PD-L1 during progressive visceral leishmaniasis. J Immunol. (2016) 196:4100–9. doi: 10.4049/jimmunol.1502678
60. Chen S, Crabill GA, Pritchard TS, McMiller TL, Wei P, Pardoll DM, et al. Mechanisms regulating PD-L1 expression on tumor and immune cells. J Immunother Cancer. (2019) 7:305. doi: 10.1186/s40425-019-0770-2
61. Cortez M, Huynh C, Fernandes MC, Kennedy KA, Aderem A, Andrews NW. Leishmania promotes its own virulence by inducing expression of the host immune inhibitory ligand CD200. Cell Host Microbe. (2011) 9:463–71. doi: 10.1016/j.chom.2011.04.014
62. Bankoti R, Stäger S. Differential regulation of the immune response in the spleen and liver of mice infected with leishmania donovani. J Trop Med. (2012) 2012:639304. doi: 10.1155/2012/639304
63. Murphy ML, Cotterell SE, Gorak PM, Engwerda CR, Kaye PM. Blockade of CTLA-4 enhances host resistance to the intracellular pathogen, Leishmania donovani. J Immunol. (1998) 161:4153–60.
64. Zhao Y, Lee CK, Lin CH, Gassen RB, Xu X, Huang Z, et al. PD-L1:CD80 cis-heterodimer triggers the co-stimulatory receptor CD28 while repressing the inhibitory pd-1 and CTLA-4 pathways. Immunity. (2019) 51:1059–73.e9. doi: 10.1016/j.immuni.2019.11.003
65. Kamada T, Togashi Y, Tay C, Ha D, Sasaki A, Nakamura Y, et al. PD-1+ regulatory T cells amplified by PD-1 blockade promote hyperprogression of cancer. Proc Natl Acad Sci USA. (2019) 116:9999–10008. doi: 10.1073/pnas.1822001116
66. Neumann K, Ostmann A, Breda PC, Ochel A, Tacke F, Paust HJ, et al. The co-inhibitory molecule PD-L1 contributes to regulatory T cell-mediated protection in murine crescentic glomerulonephritis. Sci Rep. (2019) 9:2038. doi: 10.1038/s41598-018-38432-3
67. Pflanz S, Timans JC, Cheung J, Rosales R, Kanzler H, Gilbert J, et al. IL-27, a heterodimeric cytokine composed of EBI3 and p28 protein, induces proliferation of naive CD4+ T cells. Immunity. (2002) 16:779–90. doi: 10.1016/S1074-7613(02)00324-2
Keywords: leishmaniasis, CD4 T cell, co-inhibitory receptors, PD-1 (CD279), PD-L1 (B7-H1 CD274)
Citation: Freitas e Silva Rd, Gálvez RI, Pereira VRA, de Brito MEF, Choy SL, Lotter H, Bosurgi L and Jacobs T (2020) Programmed Cell Death Ligand (PD-L)-1 Contributes to the Regulation of CD4+ T Effector and Regulatory T Cells in Cutaneous Leishmaniasis. Front. Immunol. 11:574491. doi: 10.3389/fimmu.2020.574491
Received: 19 June 2020; Accepted: 10 September 2020;
Published: 22 October 2020.
Edited by:
Tamás Laskay, University of Lübeck, GermanyReviewed by:
Jude Ezeh Uzonna, University of Manitoba, CanadaWerner Solbach, University of Lübeck, Germany
Copyright © 2020 Freitas e Silva, Gálvez, Pereira, de Brito, Choy, Lotter, Bosurgi and Jacobs. This is an open-access article distributed under the terms of the Creative Commons Attribution License (CC BY). The use, distribution or reproduction in other forums is permitted, provided the original author(s) and the copyright owner(s) are credited and that the original publication in this journal is cited, in accordance with accepted academic practice. No use, distribution or reproduction is permitted which does not comply with these terms.
*Correspondence: Thomas Jacobs, dGphY29icyYjeDAwMDQwO2JuaXRtLmRl