- 1Laboratory for Immunology and Animal Biotechnology, Department of Animal Production, Faculty of Bioscience Engineering, Ghent University, Ghent, Belgium
- 2Laboratory of Immunology, Faculty of Veterinary Medicine, Ghent University, Merelbeke, Belgium
- 3Department of Pathology, Bacteriology and Poultry Diseases, Faculty of Veterinary Medicine, Ghent University, Merelbeke, Belgium
- 4Department of Morphology, Faculty of Veterinary Medicine, Ghent University, Merelbeke, Belgium
- 5Center for Immunobiology and Vaccine Development, Children’s Hospital Oakland, Research Institute, Oakland, CA, United States
- 6Department of Medicine, University of California, San Francisco, CA, United States
- 7Joint Graduate Program in Bioengineering, University of California, Berkeley, CA, United States
The current study was designed to evaluate the pathogenesis, pathology and immune response of female genital tract infection with Chlamydia trachomatis L2c, the most recently discovered lymphogranuloma venereum strain, using a porcine model of sexually transmitted infections. Pigs were mock infected, infected once or infected and re-infected intravaginally, and samples were obtained for chlamydial culture, gross and microscopic pathology, and humoral and cell-mediated immunity. Intravaginal inoculation of pigs with this bacterium resulted in an infection that was confined to the urogenital tract, where inflammation and pathology were caused that resembled what is seen in human infection. Re-infection resulted in more severe gross pathology than primary infection, and chlamydial colonization of the urogenital tract was similar for primary infected and re-infected pigs. This indicates that primary infection failed to induce protective immune responses against re-infection. Indeed, the proliferative responses of mononuclear cells from blood and lymphoid tissues to C. trachomatis strain L2c were never statistically different among groups, suggesting that C. trachomatis-specific lymphocytes were not generated following infection or re-infection. Nevertheless, anti-chlamydial antibodies were elicited in sera and vaginal secretions after primary infection and re-infection, clearly resulting in a secondary systemic and mucosal antibody response. While primary infection did not protect against reinfection, the porcine model is relevant for evaluating immune and pathogenic responses for emerging and known C. trachomatis strains to advance drug and/or vaccine development in humans.
Introduction
Chlamydia trachomatis is an obligate intracellular bacterial pathogen that infects annually over 100 million individuals (1). C. trachomatis comprises two biovars: the trachoma biovar which includes ocular and urogenital strains and the lymphogranuloma venereum (LGV) biovar (2, 3). The two biovars are serologically subdivided into serovars based on the major outer membrane protein (MOMP) (4). The ocular serovars A, B, Ba and C are primarily associated with trachoma, the leading cause of preventable blindness in developing countries (5). The urogenital (D-K, Da, Ia, and Ja) and LGV (L1-L3, L2a, L2b, and L2c) serovars cause sexually transmitted infections. Urogenital infection with serovars D to K, including Da, Ia, and Ja, can result in cervicitis, urethritis and post-infection complications such as pelvic inflammatory disease, ectopic pregnancy, infertility, chronic pelvic pain, epididymitis and infant pneumonia. The LGV serovars cause a more invasive disease called lymphogranuloma venereum. After transiently infecting epithelial cells, these serovars penetrate into the submucosal tissues to infect macrophages and monocytes and consequently spread to regional draining lymph nodes (6). The disease usually manifests as acute inguinal lymphadenitis with abscess formation (inguinal syndrome) following urogenital inoculation, whereas anorectal entrance of the bacteria can lead to acute hemorrhagic proctitis (anorectal syndrome) (7, 8). Without treatment, persistent infections with chronic inflammation arise, resulting in strictures and fistulas of the involved region, which can eventually result in serious complications such as genital elephantiasis, esthiomene and the frozen pelvis syndrome with infertility (9, 10).
LGV is endemic in parts of Africa, South-East Asia, South America and the Caribbean, and has been considered a rare disease in developed countries until recently (8, 11, 12). Since 2003, LGV outbreaks among men who have sex with men (MSM) have been reported in Europe (13–19), North America (20, 21) and Australia (22). Almost all infected men suffered from severe proctitis, characterized by anorectal pain, haemopurulent discharge and rectal bleeding, whereas genital and inguinal symptoms were rare. A high proportion of LGV patients was also infected with HIV (23). The vast majority of infections was caused by serovar L2b, which was first identified in patients from Amsterdam (24). Recently, a new LGV serovar, called L2c, was isolated from an HIV negative MSM with severe hemorrhagic proctitis. This hypervirulent serovar appeared to be a recombinant of C. trachomatis serovars L2 and D (25). The extent of dissemination of serovar L2c or other LGV recombinants within the MSM community still have to be investigated (26). In industrialized countries, LGV is very uncommon in women, although a few asymptomatic female patients or those with cervicitis have been described (27, 28). Recently, the first case of C. trachomatis L2b proctitis in a woman was reported (29). Furthermore, Verweij et al. (30) described the first urogenital L2b infection in a female patient with bubonic LGV. Considering the ongoing outbreaks, LGV infections among bisexual and heterosexual men as well as heterosexual women are likely to increase in the near future (30).
Given the importance of LGV infections globally, the purpose of the current study was to investigate the pathogenesis, pathology and immune response of vaginal C. trachomatis L2c infection in a relevant animal model. Previously, Vanrompay et al. (31) demonstrated that pigs are a suitable animal model to study female genital tract infection with C. trachomatis serovar E strains. Pigs are immunologically, genetically and physiologically more closely related to humans than rodents, and are ethically and practically more convenient than primates.
Materials and Methods
Chlamydia trachomatis Strain
C. trachomatis strain L2c was isolated from the rectal mucosa of a male who had a history of sex with men and suffered from severe hemorrhagic proctitis (25). Bacteria were propagated in McCoy cells using standard procedures (32). The tissue culture infective dose (TCID50) of the C. trachomatis stock was determined by the method of Spearman and Kaerber (33).
Animals
Fifteen 9-week-old conventionally bred female pigs (Belgian Landrace) were randomly assigned to three groups of five pigs, each housed in separate isolation units. The animals were fed ad libitum with a commercial starting diet. The pigs were seronegative for Chlamydiaceae as determined by a C. suis ELISA (34). Nasal, rectal and vaginal swabs did not contain chlamydial bacteria as determined by culture on McCoy cells (34).
Experimental Infection and Euthanasia
On day 0, when pigs were 9 weeks old, all groups were anesthetized by intramuscular injection of Zoletil® 100 (Virbac Animal Health, Louvain La Neuve, Belgium) in 2% Xylazine-M® (VMD, Arendonk, Belgium). The control group and the infected group were inoculated intravaginally with phosphate-buffered saline (PBS). The re-infected group was infected by intravaginal injection of 1 x 107 TCID50 of C. trachomatis strain L2c. Intravaginal inoculation was performed by inserting an artificial insemination pipette connected to a syringe into the vagina and injecting 1 ml PBS or 1ml of the bacterial suspension in PBS. On day 56, the 17-week-old pigs were anesthetized again. Subsequently, the control group was inoculated with PBS, whereas the infected group and the re-infected group were infected intravaginally with C. trachomatis strain L2c (1 x 107 TCID50). At day 77, when pigs were 21 weeks old, all animals were euthanized by intravenous injection of an overdose of pentobarbital (70 mg/kg; Nembutal®, Ceva Santé Animale, Maassluis, the Netherlands) followed by exsanguination. All animal procedures were in accordance with the guidelines of the animal care and ethical committee of Ghent University.
Sample Collection and Processing
Vaginal swab samples in 2 ml sucrose-phosphate transport medium (2-SP) for chlamydial isolation as well as in 2 ml PBS with protease inhibitor for mucosal antibody detection were collected weekly. These samples were stored at -80°C until tested. Additionally, blood samples (v. jugularis) for antibody detection were taken weekly. Blood was stored overnight at room temperature and centrifuged (9300 × g, 20°C, 10 min) to collect serum. Sera were stored at -20°C until analysis. Peripheral blood mononuclear cells (PBMC) were isolated at 7 and 10 days post infection or re-infection to determine their proliferative responses and immune cell subpopulations.
At euthanasia, the entire body of the pigs was examined for gross lesions that were scored as none (0), slight (1), moderate (2) or severe (3). Mononuclear cells (MC) from the spleen, the cervical lymph node (lymphonodulus cervicalis superficialis) and the pelvic lymph nodes (the lymphonoduli iliaci mediales, the lymphonoduli iliaci laterales, the lymphonoduli sacrales and the lymphonoduli anorectales) were collected to analyze the proliferative responses and immune cell subpopulations. Samples of the spleen, liver, pelvic lymph nodes, caecum, urethra, vagina, cervix, corpus uteri, uterine tubes, oviducts and ovaries were imbedded in methylcellulose medium, frozen in liquid nitrogen and stored at -80°C until preparation of cryostat tissue sections for the detection of C. trachomatis antigen. Samples from the same tissues were fixed in 10% phosphate-buffered formalin for histopathology.
C. trachomatis Detection in Swabs and Tissue Sections
Vaginal swabs in 2-SP were shaken for 1 h at 4°C, centrifuged (1200 x g, 1 h, 37°C) and 75 µl of the swab content was inoculated on McCoy cells grown on 13 mm cover slips in Chlamydia Trac Bottles (International Medical, Brussels, Belgium) using standard techniques (32). Before the inoculum was added to the cells, they were washed twice with 1 ml phosphate buffer supplemented with 0.003% DEAE-dextran. Chlamydial growth was analyzed using the Mikrotrak direct immunofluorescence staining (Kordia, Leiden, The Netherlands) at 6 days post inoculation. Cryostat tissue sections (5 µm) were also stained by use of the Mikrotrak immunofluorescence test. All slides were examined by immunofluorescence microscopy (BX41 Olympus, 600×). C. trachomatis positive cells were counted in five randomly selected microscopic fields. A score ranging from 0 to 6 was given for each swab or tissue section. Score 0 indicated that there were no C. trachomatis inclusion forming units (IFU). Score 1 and 2 indicated a mean of 1 to 5 and 6 to 10 small IFU per microscopic field, respectively. Score 3 represented more than 10 small IFU and 1 larger IFU per microscopic field. Score 4, 5, and 6 indicated 1 to 5, 6, to 10 and more than 10 larger IFU per microscopic field, respectively.
Histopathology
Tissue samples were fixed in 10% phosphate-buffered formalin, dehydrated and embedded in paraffin, sectioned at 5 µm and stained with hematoxylin and eosin. Slides were examined blindly by a veterinary pathologist. The microscopic findings were either graded (none (0), minimal (1), slight (2), moderate (3), marked (4) or severe (5) histological change) or indicated as present or absent without a grade.
Serum and Mucosal Antibody Analysis
Sera were heat inactivated at 56°C during 30 min and subsequently pretreated with kaolin to reduce background signals in ELISA (35). Vaginal swabs in PBS with protease inhibitor were shaken for 1 h at room temperature. Isotype-specific serum and mucosal antibody titers were determined using a C. trachomatis L2c ELISA. Briefly, Maxisorp 96-well microtiter plates were coated with purified C. trachomatis strain L2c EBs (1 × 107 TCID50 per well) diluted in PBS. Plates were blocked overnight with PBS supplemented with 5% bovine serum albumin (BSA) at 4°C. Antibody titers were determined using twofold dilution series in dilution buffer (PBS + 3% BSA + 0.05% Tween®20), starting at a dilution of 1:15. Serum and swab samples from a previous experimental infection in pigs (36) were used as positive and negative controls. Antibody isotype titers were analyzed using monoclonal antibodies against swine IgA (mAb 27.8.1), IgG (mAb 23.3.1b), and IgM (mAb 28.4.1) (37) at a dilution of 1:15, 1:20, and 1:50, respectively, followed by an 1:5,000 dilution of biotinylated anti-mouse IgG (H+L) (Dako, Glostrup, Denmark). Subsequently, the plates were incubated with 1:2500 diluted peroxidase-labeled streptavidin (Zymed Laboratories, San Francisco, USA). Finally, the substrate and chromogene ABTS (2, 2’-Azino-di(3-ethylbenzthiazoline-6-sulfonate); KPL, Maryland, USA) was added. Antibody titers were defined as the inverse of the highest sample dilution giving an absorbance value at 405 nm above the cut-off value (mean absorbance of seronegative pig serum at a dilution of 1:15 + twice the standard deviation).
PBMC and MC Proliferation Assay
At 7 and 10 days post infection or re-infection, PBMC were isolated from heparinized blood samples collected from the jugular vein by density gradient centrifugation (500 × g, 18°C, 25 min) on Lymphoprep™ (Axis-Shield, Oslo, Norway). Subsequently, the erythrocytes were lysed with ammonium chloride. After centrifugation (270 × g, 4°C, 10 min), the cells were washed and resuspended in leukocyte medium (RPMI-1640 (Life Technologies, Merelbeke, Belgium) supplemented with 5% heat-inactivated fetal calf serum (Life Technologies), 5×10−5 M β-mercaptoethanol (Life Technologies), 1% non-essential amino acids (Life Technologies), 1% sodium pyruvate (Life Technologies), 1% L-glutamine (Life Technologies), 1% penicillin-streptomycin and 1% kanamycin).
At euthanasia, mononuclear cells (MC) were isolated from the spleen, the cervical lymph node (cervicalis superficialis) and the pelvic lymph nodes (iliaci mediales, iliaci laterales, sacrales and anorectales). Isolation of MC was performed by mincing the tissues, after removal of the surrounding fat. Erythrocytes were lysed with NH4Cl solution and the cells were washed and subsequently resuspended in leukocyte medium without β-mercaptoethanol. PBMC or MC were placed in 96-well tissue culture plates at a concentration of 5 × 105 cells per well. Proliferative responses were tested by adding 105 C. trachomatis purified L2c EBs, 10 µg concanavalin A (ConA) (positive control) or medium (negative control) to the wells. Each condition was tested in duplicate. The cells were incubated at 37°C in a humidified atmosphere with 5% CO2. ConA- or antigen-induced proliferation was measured by incorporation of 1 µCi/well of 3H-thymidine (Amersham ICN, Bucks, UK) for the last 16 h of a 3-day or 4-day culture period, respectively. Cells were harvested onto glass fiber filter strips (Perkin Elmer, Life Science, Oosterhout, The Netherlands) with a cell harvester (Skatron, Liers, Norway) and radioactivity was measured with a β-scintillation counter (Perkin Elmer). As a measure of proliferative response, stimulation indices (SI) were calculated as the ratio of the mean counts per minute of stimulated PBMC or MC versus the mean counts per minute of the negative control.
Flow Cytometric Analysis of Immune Cell Subpopulations
The immune cell subpopulations in the blood, the spleen, the cervical and pelvic lymph nodes were examined by flow cytometry. T cell populations (CD3+CD4+CD8-, CD3+CD4-CD8+, CD3+CD4+CD8+, CD3+CD4-CD8-, T cells with a γδ T cell receptor), B cells (MHCII+CD21+, MHCII+IgM+), monocytes (MHCII+SWC3+), NK cells (CD3-CD4-CD8+) and plasmacytoid dendritic cells (pDC) (CD3-CD4+CD8-) were analyzed. For the lymphoid tissues the CD3-CD4+CD8- population does not exist entirely of pDC, but also contains an unknown lineage, presumably of myeloid origin (38). It was impossible to distinguish between both populations based on the used markers. PBMC and MC (106 cells) were incubated for 20 min at 4°C in staining buffer (RPMI-1640 + 1% heat-inactivated FCS) with optimal concentrations of monoclonal antibodies (Supplementary Table 1). Cells stained with isotype-matched irrelevant mAbs were used as a negative control. After incubation, the cells were washed with staining buffer and stained with the appropriate isotype-specific Alexa-647-, FITC-, or PE- conjugated antibodies (Life Technologies) for 20 min at 4°C. Cells were washed twice and resuspended in PBS. Data were acquired on a FACSCanto flow cytometer (Beckton Dickinson, Erembodegem, Belgium) with a minimum event count of 50 000 and analyzed with FACSDiva® software. Doublets were excluded based on FSC-H/FSC-A and SSC-H/SSC-A plots.
Statistical Analysis
Statistical analyses were performed using SPSS 22. The non-parametric Mann-Whitney U test was used to analyze differences between two groups. Results were considered significantly different if P<0.05.
Results
Gross Lesions
Supplementary Table 2 shows the median scores for gross lesions in the infection and the re-infected group, determined at necropsy. Gross lesions were absent in all pigs of the control group. Gross pathology was generally more severe for the re-infected group than for the infected group, especially in the urogenital tract. All re-infected pigs showed congestion of the urogenital tract (Figure 1), while only three pigs (60%) of the infected group had a congested urogenital tract. A significantly higher congestion of the uterus, uterine tubes, ligamentum latum uteri, mesovarium and urethra was noticed in the re-infected group than in the control and/or the infected group. A large amount of clear watery exudate was present in almost the entire genital tract of 40% of the pigs of both infected groups and in the uterus of one animal (20%) of the re-infected group (Figure 1). The liver of two re-infected pigs (40%) was moderately congested, whereas the liver was normal in all animals of the infected group. Gross pathology was detected in the spleen of three pigs (60%) of the infected group and four pigs (80%) of the re-infected group (Figure 1). The pelvic lymph nodes were moderately to severely enlarged in all animals of both infected groups. Furthermore, moderate to severe congestion of the pelvic lymph nodes was detected in 80% of the pigs of both infected groups (Figure 1). Gross lesions in the pelvic lymph nodes were significantly different between the control group and both infected groups.
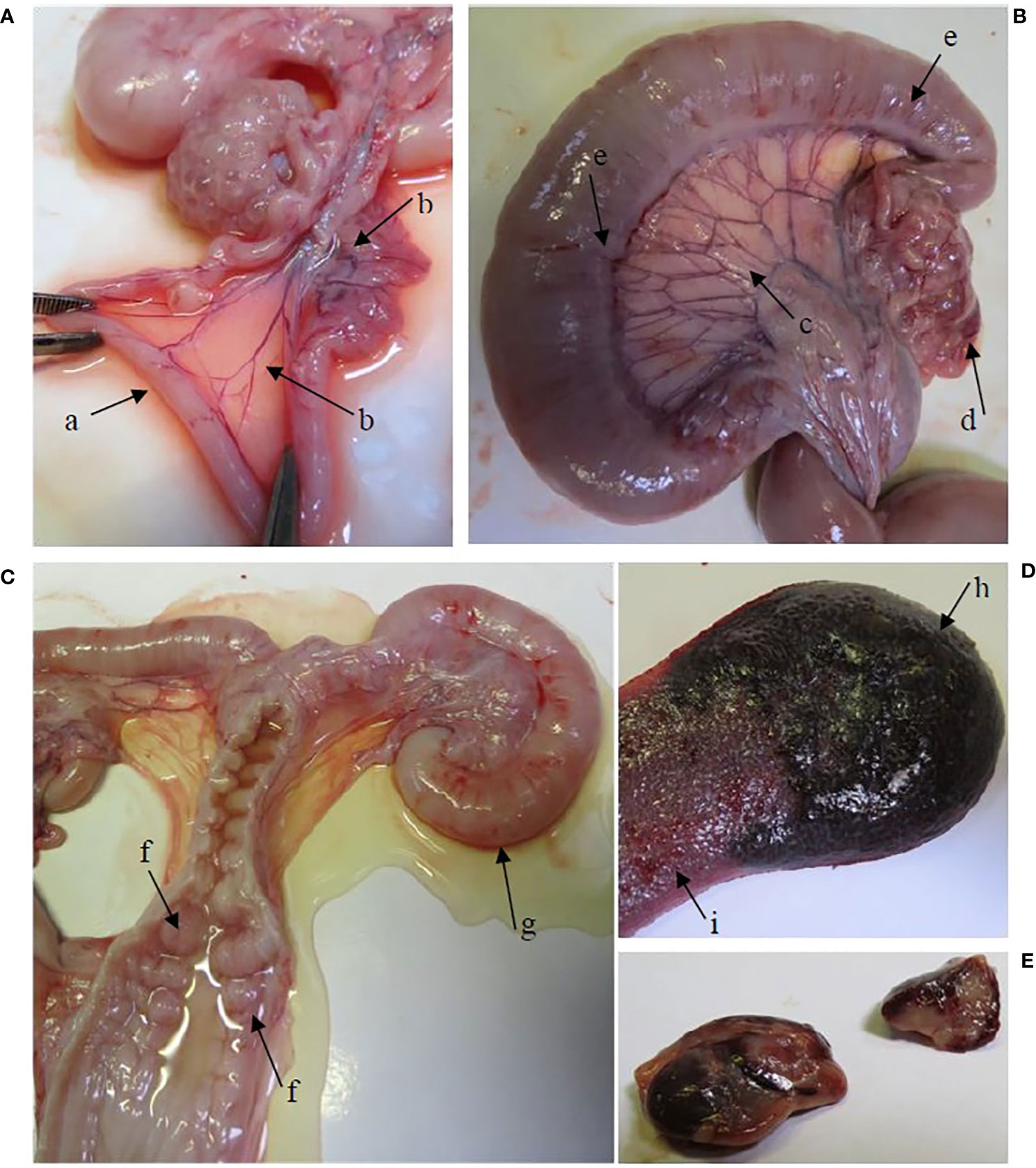
Figure 1 Gross lesions in the re-infected group. (A) The oviducts were dilated by the presence of serous exudate in their lumen (arrow a). Hyperemia of the mesovarium and mesosalpinx (arrow b). (B) Hyperemia of the lig. latum uteri (arrow c), the mesovarium and mesosalpinx (arrow (d) and the uterine tubes (arrow e). (C) Hyperemia of the cervical mucosa (arrow f). A large amount of serous exudate was detected in the lumen of the vagina, cervix, corpus uteri and uterine tubes. Note the severely dilated uterine tube (arrow g). (D) The spleen is enlarged, hemorrhagic (arrow h) and shows expansion of the red pulp (arrow i). (E) Enlarged and hemorrhagic pelvic lymph nodes.
Histopathology
The median scores of the histopathological lesions detected in the urogenital tract of the pigs are presented in Supplementary Table 3. Statistical differences among groups were only present in the cervix, the corpus uteri, the uterine horns and the urethra. In the cervix and the uterine horns, interepithelial inflammatory cells had increased slightly in the mucosa of the infected group (Figure 2). Furthermore, epithelial vacuolation was noted in the cervix, corpus uteri and uterine horns of the infected group (Figure 2). This vacuolation was to a lesser extent also present when animals were re-infected. The significantly higher median score for epithelial vacuolation in the urethra of the re-infected group was due to the absence of the mucosa in some samples and the high value in one animal. In the oviducts, exfoliated epithelial cells and proteinaceous fluid were observed only in both infected groups, however with low incidence and/or severity. The lesions noticed in the oviducts and the vagina were not statistically different among groups. No histopathological lesions were observed in the ovaries. In the liver of one or two animals of each group minimal to slight focal to multifocal mononuclear inflammations within the parenchyma or portal areas were noted. This lesion is considered to be a background lesion, frequently observed and unrelated to the experimental infection. The liver samples from the other pigs and all spleen, caecum and pelvic lymph node samples showed no microscopic lesions.
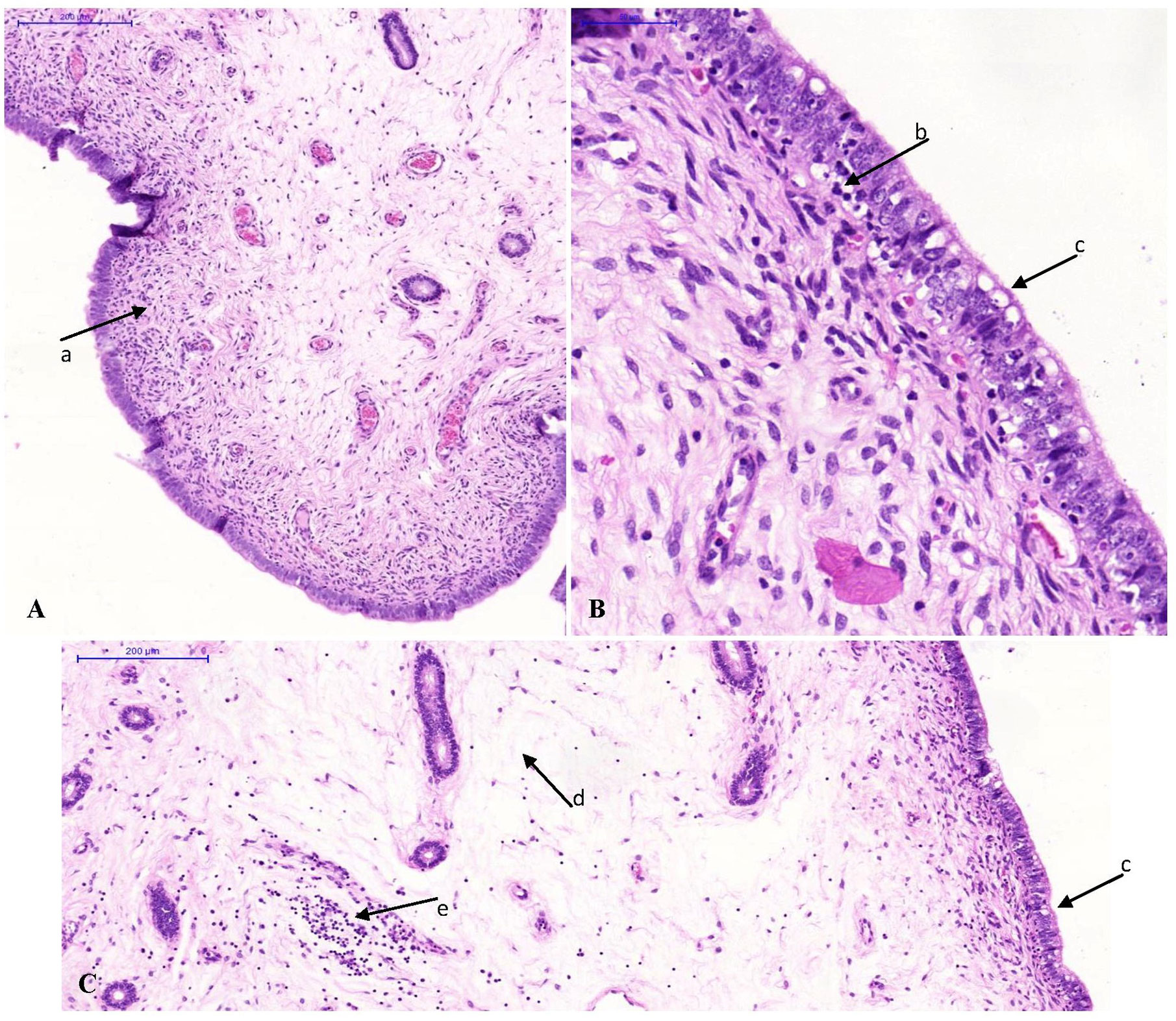
Figure 2 Photomicrographs of uterine horn tissue sections stained with hematoxylin and eosin. Original magnification ×10 (A, C) or ×40 (B). (A) Minimal lymphocytic infiltration in the lamina propria (arrow a) of a control animal. (B, C) Inflammation of the uterine horns of an animal of the infected group. Note the interepithelial lymphocytic infiltration (arrow b), the vacuolation of the epithelium (arrow c), the oedema in the lamina propria (arrow d) and the lymphocytic infiltration in the lamina propria (arrow e).
Chlamydia trachomatis Vaginal Excretion
Vaginal swabs were examined for the presence of viable C. trachomatis using culture in McCoy cells. Median culture scores for vaginal swabs of the infection and the re-infected group, collected at different time points post infection, are presented in Supplementary Table 4. All vaginal swab samples of the control group were Chlamydiaceae negative, as were the swabs of the other pigs taken before primary infection. The infected group vaginally excreted C. trachomatis from day 59, three days after primary infection of this group, onwards. In the re-infected group, vaginal C. trachomatis shedding was consistently high from day 3 to day 49 post primary infection and was slightly decreased at day 56 and day 59. Median culture scores were again consistently high from seven days post re-infection (day 63) onwards. From day 3 to day 56, vaginal shedding in the re-infected group was significantly higher than in the control group and the infected group, which was not yet infected at these time points. After primary infection of the infected group, the median excretion scores of the infected group and the re-infected group did not differ statistically from each other, but were significantly higher than those of the control group.
C. trachomatis Detection in Tissue Sections
Table 1 shows the median scores for the presence of C. trachomatis in tissues of the infection and the re-infected group at euthanasia. All tissue samples from the control group were negative. C. trachomatis was neither detected in the caecum, the spleen, the liver and the pelvic lymph nodes of both infected groups. Chlamydial EBs and intracellular inclusions, containing replicating chlamydiae, were detected in both the lower and upper genital tract tissues of all pigs of the infected and the re-infected group. Thus, an ascending C. trachomatis infection occurred in all pigs of both infected groups. Furthermore, chlamydial replication was observed in the urethra of three pigs (60%) of the infected group and two pigs (40%) of the re-infected group. The urethra of the remaining infected animals contained only elementary bodies, except for the urethra of one pig of the re-infected group, which was negative. For all urogenital tissues, median scores were significantly higher for the infected and the re-infected group than for the control group. Statistical analysis revealed no significant differences between the median scores of both infected groups.
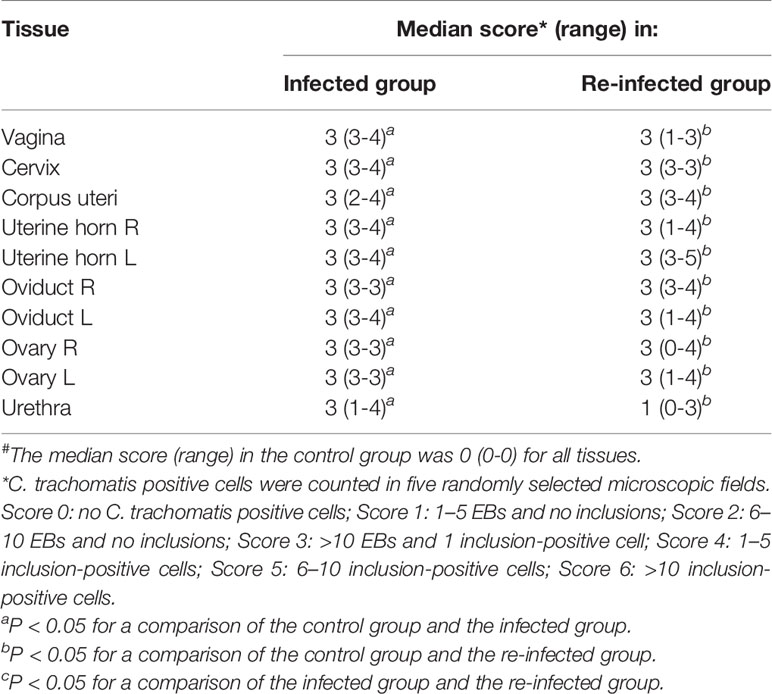
Table 1 Presence of C. trachomatis in tissues of the infected and the re-infected group at euthanasia#.
Serum and Mucosal Antibody Responses
Tables 2 and 3 show the C. trachomatis L2c-specific IgM, IgG and IgA antibody titers in sera and vaginal secretions collected at different time points post infection or re-infection. Chlamydial antibodies were never detected in the serum and vaginal swab samples of the control pigs. The pigs of the infected and the re-infected group neither had serum or vaginal C. trachomatis-specific antibodies before they were infected. Serum IgM, IgG and IgA antibodies were elicited from 7 days post primary infection onwards and peak titers were reached at 14 or 21 dpi. Anti-chlamydial IgM and IgA were observed in serum samples until 21 and 28 days post primary infection, respectively. Serum IgG antibodies, on the other hand, remained detectable throughout the experiment in most pigs. C. trachomatis L2c-specific serum IgM, IgG, and IgA titers increased again following re-infection. Serum IgA titers reached peak levels early after re-infection (7 days), whereas serum IgM and IgG titers continued to increase until the end of the experiment. Vaginal secretions were positive for anti-chlamydial IgM, IgG and IgA from 14 to 21, 14 to 28, and 7 to 21 days post primary infection, respectively. Mucosal antibodies appeared again from 7 (IgM and IgG) or 14 (IgA) days post re-infection onwards. Re-infection clearly resulted in a secondary systemic and mucosal antibody response, with significantly lower IgM titers and significantly higher IgG and IgA titers than following primary infection.
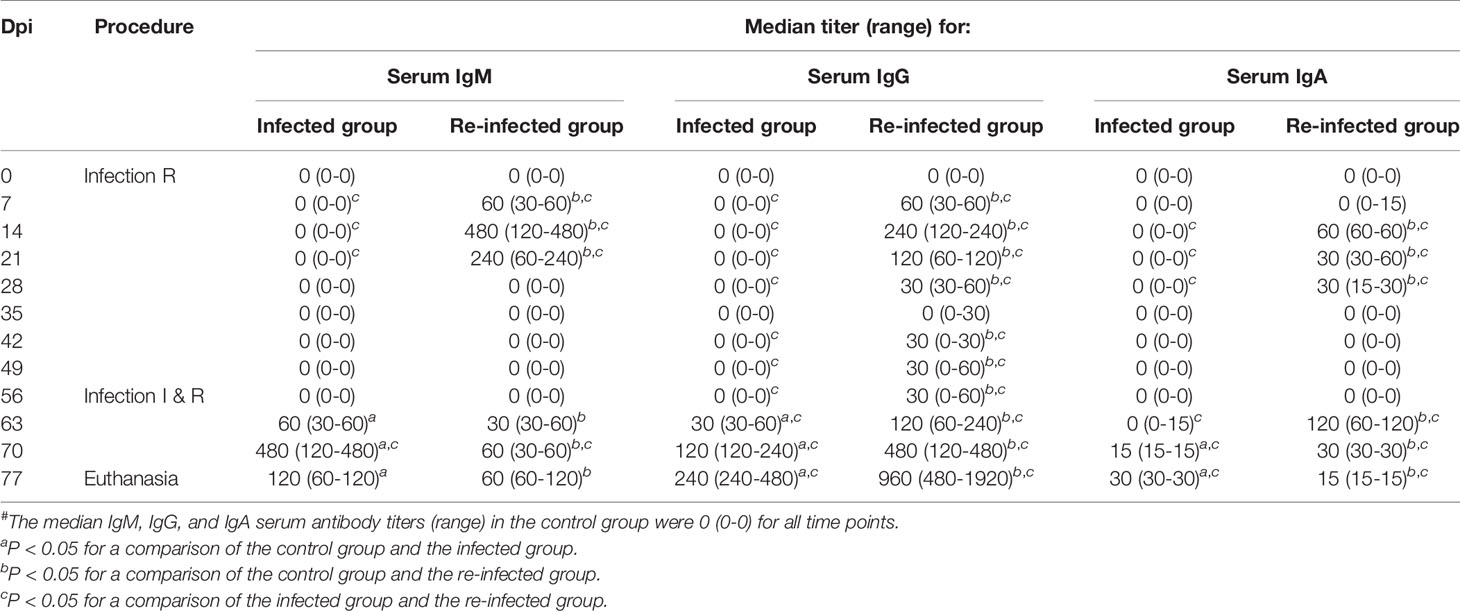
Table 2 C. trachomatis L2c-specific IgM, IgG, and IgA serum antibody titers of the infected (I) and the re-infected (R) group#.
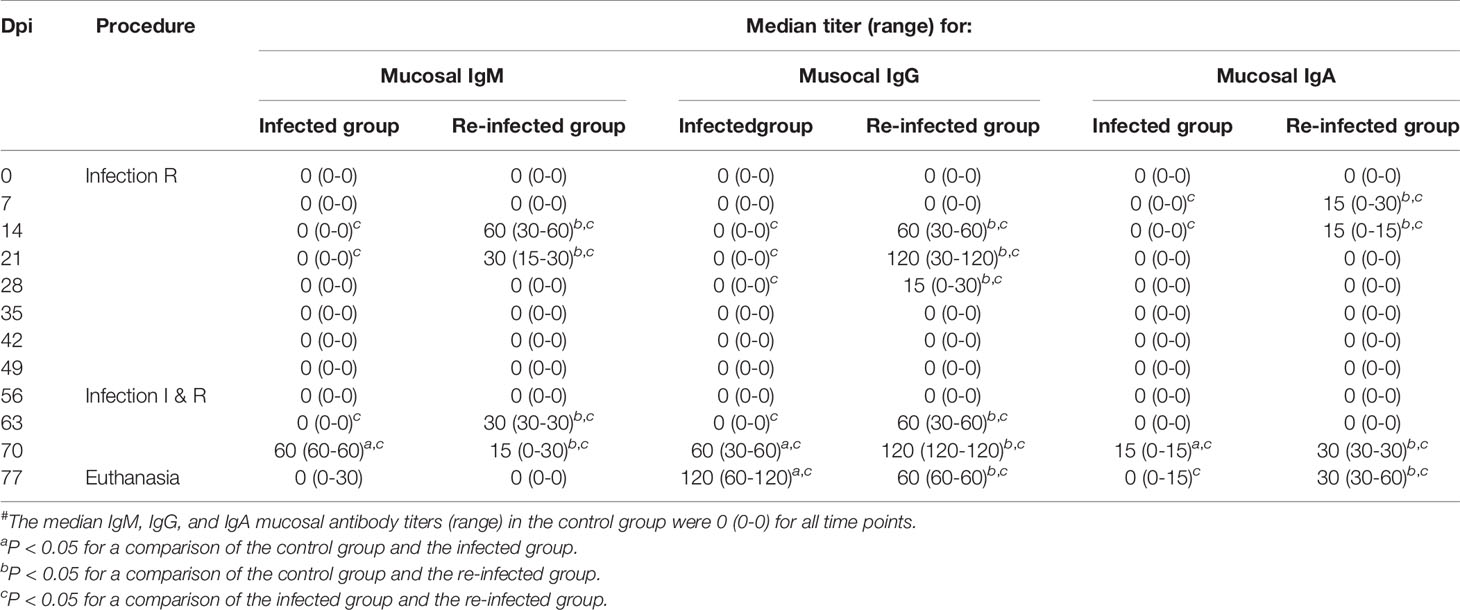
Table 3 C. trachomatis L2c-specific IgM, IgG, and IgA vaginal secretion antibody titers of the infected (I) and the re-infected (R) group#.
PBMC and MC Proliferation
At 7 and 10 days post primary infection or re-infection, proliferative responses of PBMC to C. trachomatis strain L2c were measured. Furthermore, proliferation of spleen, cervical and pelvic lymph node MC was determined at euthanasia. Surprisingly, the proliferative activities of PBMC and MC were never statistically different among groups (data not shown). The proliferative responses to ConA were always positive indicating the viability of the cells and the functionality of the assay.
Immune Cell Subpopulations
Flow cytometry was used to identify blood immune cell subpopulations at 7 and 10 days post primary infection or re-infection (Figure 3). Seven days after primary infection of the re-infected group, the median percentage of NK cells was significantly higher for the re-infected group than for the infected group. Three days later, the re-infected group had significantly higher median percentages of T cells, γδ T cells and NK cells than the non-infected animals. At day 63, 7 days after primary infection of the infected group and re-infection of the re-infected group, a significantly higher median percentage of γδ T cells was noticed in both infected groups than in the control group. Within total T cells, significantly fewer CD4-CD8+ T cells were detected in the re-infected group than in the control group. Moreover, the re-infected animals had significantly lower median percentages of B cells and monocytes than the other groups. At day 66, the re-infected group had significantly lower median percentages of T cells and IgM+ B cells, but a significantly higher median percentage of plasmacytoid dendritic cells (pDC) than the other groups. At that time, the median percentages of T cells and γδ T cells were significantly higher for the infected group than for the control group. Furthermore, significantly lower median percentages of mature B cells and monocytes were observed in both infected groups than in the control group. At euthanasia, immune cell populations in the spleen, cervical and pelvic lymph nodes were also determined using flow cytometry (Figure 3). For the spleen, significantly higher median percentages of CD4+CD8- T cells and CD3-CD4+CD8- MC, containing pDC, besides a significantly lower median percentage of total T cells were detected in the non-infected animals. The re-infected group had a significantly higher median percentage of NK cells in the spleen than the control group. Furthermore, a significantly lower percentage of monocytes was present in the spleen of the infected group as compared to the other groups. For the cervical lymph nodes, significantly lower median percentages of CD4-CD8+ T cells and mature B cells were noticed in the infected group than in the control and the re-infected group, respectively. For the pelvic lymph nodes, the re-infected pigs had a significantly lower median percentage of B cells and a significantly higher median percentage of NK cells. Within total T cells, significantly more CD4-CD8- T cells were observed in both infected groups than in the control group.
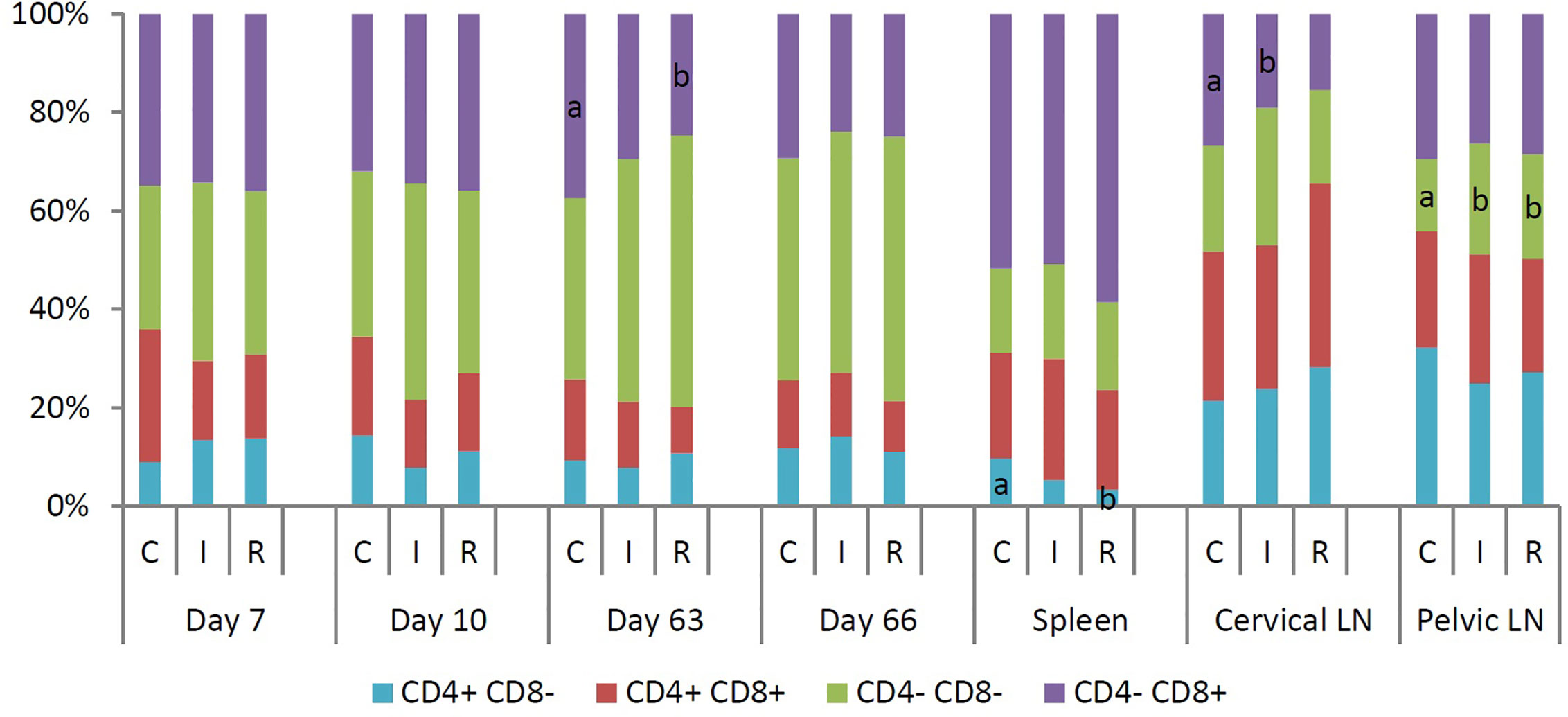
Figure 3 Median percentage of different subpopulations within total T cells (CD3+), isolated at 7 and 10 days post infection or re-infection, and in spleen, cervical and pelvic lymph nodes at euthanasia. T cells were divided into four subpopulations based on the expression of CD4 and CD8. For each time point or tissue, different letters within a data series are significantly different (P<0.05). C: control group; I: infected group; R: re-infected group.
Discussion
Since 2003, an ongoing outbreak of LGV has been observed among MSM in developed countries (13–22). There have only been a few anecdotal reports of LGV urogenital and rectal infections among women. However, this may change in the near future due to dissemination of the disease to bisexual men and to women (30). While initial infection in the cervix may more likely be asymptomatic compared to rectal infections in males and thus remain untreated, both men and women develop serious complications from LGV infections. Because the porcine model represents an appropriate model for studying human C. trachomatis strains (31), the present study aimed to obtain more knowledge about the pathogenesis, pathology and immune response of female genital tract infection and re-infection with C. trachomatis L2c, the most recently discovered LGV strain (31).
At necropsy, gross pathology was generally more severe for the re-infected pigs than for animals infected only once. In women, repeated urogenital C. trachomatis infections are also associated with an elevated risk of pathological damage resulting in reproductive complications, such as pelvic inflammatory disease, ectopic pregnancy and infertility (39–43). Similar levels of C. trachomatis EBs and intracellular inclusions were detected in the lower and upper genital tract tissues of the infection and the re-infected group. This indicates that C. trachomatis L2c could colonize the urogenital tract following re-infection as efficiently as following primary infection. Thus, the primary infection failed to induce protective immune responses against re-infection. Several epidemiologic studies of humans indicate that protective immunity to re-infection, albeit only partial, develops following prior genital infection with C. trachomatis, including LGV strains (44–49). These findings are consistent with those from murine and non-human primate model experiments (50–52).
In our study, the infection was confined to the urogenital tract with infection of the tissues from the vagina to the ovaries and including the urethra. There was no evidence of chlamydial bacteria in the caecum, spleen, liver, and pelvic lymph node samples of the infected animals. This is in accordance with the symptoms observed in the patient from which L2c was isolated (25). The patient suffered from severe localized mucosal disease, namely hemorrhagic proctitis, and did not show an inguinal syndrome, indicating that the bacteria had not spread to the inguinal lymph nodes. This lack of dissemination to regional lymph nodes was likely due to the presence of a functional cytotoxin, which probably enables innate immune evasion (53, 54). The toxin gene was presumably acquired from a D strain, since all other LGV strains to date lack this gene (25).
Infection of the female porcine genital tract with C. trachomatis serovar L2c resulted only in minor microscopic lesions, in particular interepithelial inflammatory cells and epithelial vacuolation. This is in contrast with a previous study describing genital tract infection of female pigs with two C. trachomatis serovar E strains (31). However, samples in the present study and the study by Vanrompay et al., (31) were not taken at the same time point post infection and the infection schedule was different. Remarkably, histopathological changes were more prominent in the genital tissues of the infected group than the re-infected group, which is opposed to the gross pathology noticed in both groups. Immunohistochemistry could provide more detailed information on the cells involved in the microscopic lesions and therefore will be performed during future C. trachomatis experiments in pigs.
All infected animals vaginally excreted C. trachomatis from three days post infection onwards. Prior infection had no influence on vaginal chlamydial shedding, since similar excretion levels were observed after primary infection and re-infection. Vaginal excretion was consistently high until 49 days post primary infection and was only slightly decreased at day 56, indicating that the pigs had not yet resolved the chlamydial infection after 8 weeks. In a future experiment, some animals will be kept longer, monitoring bacterial excretion twice a week to find out if the infection will be cleared. In humans, C. trachomatis infection can last several months before spontaneous clearance (55–57). In some patients infected with LGV serovars, the bacteria could still be isolated many years after the primary infection (58, 59).
Anti-chlamydial IgM, IgG and IgA antibodies were elicited in sera and vaginal secretions following primary infection with C. trachomatis L2c. Re-infection clearly resulted in a secondary systemic and mucosal antibody response, with significantly lower IgM titers and significantly higher IgG and IgA titers than following primary infection. However, the induced antibody response was not able to even partially protect the pigs against re-infection. The importance of antibodies in immunity to C. trachomatis is still disputed. In women, the amount of IgA in cervical secretions was found to correlate inversely with the amount of C. trachomatis recovered from the cervix (60). However, high titers of C. trachomatis-specific serum IgG antibodies were not associated with resolution of infection in women, but with increased severity of post-infection complications (61). In mice, antibodies are not needed to resolve a primary genital C. muridarum infection (62), but contribute to protection against re-infection (63). In the guinea pig model, antibodies play an important role in immunity to both primary infection and re-infection with Chlamydia caviae (64, 65). Interestingly, it was shown that C. trachomatis strains of the LGV biovar are more resistant to neutralization by immune sera than strains of the trachoma biovar (66), which may explain the lack of protection on re-infection in our study.
The proliferative responses of PBMC and MC to C. trachomatis strain L2c were never statistically different among groups, suggesting that C. trachomatis-specific lymphocytes were not generated following infection or re-infection. However, anti-chlamydial antibodies were detected, which implies that C. trachomatis-specific B lymphocytes must have been created at a certain point. Simultaneously with the proliferation assays, the immune cell subpopulations within PBMC and lymphoid tissue MC were analyzed. The observed differences in percentages of B and T lymphocytes among groups are considered irrelevant, since no C. trachomatis-specific lymphocytes appeared to be present at the examined time points. Furthermore, the differences in percentages of monocytes, NK cells and pDC between groups were sometimes inconsistent. Primary infection of the re-infected group resulted in a significant increase of NK-cells in the blood, whereas this was not the case following primary infection of the infected group. However, both the spleen and the pelvic lymph nodes of the re-infected animals contained significantly higher percentages of NK cells three weeks post re-infection. NK cells are an important component of the innate immune response, which can help control chlamydial infections by secreting IFN-γ (67). Moreover, it was shown that human NK cells are able to lyse epithelial cells infected with C. trachomatis (68). Nevertheless, an effective protective immune response to genital C. trachomatis infections is highly dependent on specific CD4+ T-helper Type 1 cells (69–71), but also on IFN-γ (72, 73). The apparent lack of C. trachomatis-specific T cells following infection or re-infection in the present study can explain why the pigs were not even partially protected against re-infection. However, the immune responses detected in the blood, the spleen, and cervical and pelvic lymph nodes do not necessarily mirror the immune responses elicited at the local site of infection. It would have been interesting to obtain immunohistochemical data on genital tract tissues as this could provide more information about the local immune response following infection or re-infection. Immunohistochemistry on genital tract tissues was performed, but without success. The available porcine leukocyte markers could not be used on formalin fixed samples notwithstanding the use of the eBioscience™ IHC antigen retrieval system (unpublished data). In the future, samples for immunohistochemistry will be collected in an in house-made zinc phosphate fixative as it allows optimal antigen retrieval and visualization of porcine immune cell populations in an “in situ” histological setting (unpublished data).
Thus, C. trachomatis serovar L2c infection of pigs failed to induce protective immune responses against reinfection. Similar results were observed in mice after primary and secondary C. muridarum or C. trachomatis serovar E infections (74–76). Also, regarding pathology in women, increases in complications associated with chlamydial infections, occurred in recurrent infections (77). Both the pathogen and the host contribute to the outcome of chlamydial infections. Recently, genome-wide profiling of humoral immunity and pathogen genes under selection identified immune evasion tactics of C. trachomatis during ocular infection in children in The Gambia (78). Children who were susceptible to frequent and/or prolonged ocular C. trachomatis infection had a less focused antibody response and data suggested a strategy in which C. trachomatis presents a large panel of irrelevant antigens to the human immune system to block or misdirect protective responses. The latter might also have occurred during the present study as serum and mucosal antibody titers against L2c elementary bodies where not particularly high. In addition, several research groups provided insight in human (76) and murine (79–82) small non-coding RNA (miRNA) expression profiles during genital and ocular chlamydial infection and reinfection and found chlamydia virulence to be associated with the miRNA expression profile of the host. miRNAs regulate gene expression as they target mRNAs for degradation, cleavage, translational repression or occasional enhancement. Specific miRNAs were involved in the primary infection and reinfection resulting in a different pathogenesis and outcome in pathology, being more severe in reinfected mice than in primary infected animals. It might be interesting to examine this in the pig model.
In conclusion, the pathogenesis of an L2c infection in the porcine model is rather similar to the pathogenesis of L2c and other C. trachomatis strain infections in humans. Intravaginal inoculation of pigs with C. trachomatis L2c resulted in an infection that was confined to the urogenital tract, where inflammation and pathology were caused. Re-infection resulted in more severe gross pathology than primary infection but chlamydial colonization of the urogenital tract was similar for both the primary infected and re-infected groups. This indicates that primary infection failed to induce protective immune responses against re-infection. Indeed, no C. trachomatis-specific lymphocytes could be detected in the blood and the lymphoid tissues following infection or re-infection. However, the cellular immune responses need to be further investigated by performing immunohistochemical analysis of the infected urogenital tissues. Hereto, a zinc phosphate fixative will be used instead of formalin. Nevertheless, anti-chlamydial antibodies were elicited in sera and vaginal secretions after primary infection, and re-infection clearly resulted in a secondary systemic and mucosal antibody response. Apparently, the induced antibody response could not even partially protect the pigs against re-infection. Our study supports the notion that the porcine model is an alternative large animal model for evaluating the immunopathogenesis and evolution of C. trachomatis infections that will provide data for advancing therapeutic and vaccine development for humans. Our findings imply that the pig may function as either an intermediate animal model between mouse and non-human primates or as a substitute for the latter during the development of therapeutics and vaccines for use in human clinical trials.
Data Availability Statement
All datasets presented in this study are included in the article/Supplementary Material.
Ethics Statement
The animal study was reviewed and approved by Ethische Commissie van de Faculteit Diergeneeskunde en Bio-ingenieurswetenschappen van de Universiteit Gent.
Author Contributions
DV, EC, and DD contributed to conception and design of the study. EDC wrote the first draft of the manuscript and conducted the experiment together with MG and KS. BD assisted during flow cytometry. KC performed histology. WB performed immunohistochemistry. CK prepared the final version of the manuscript. All authors contributed to the article and approved the submitted version.
Funding
The research was supported by the Special Research Fund of Ghent University (BOF).
Conflict of Interest
The authors declare that the research was conducted in the absence of any commercial or financial relationships that could be construed as a potential conflict of interest.
Acknowledgments
We gratefully thank A. Dumont, E. Verstreken, S. Tshitenge-Mulume, R. Cooman and L. Yin for technical assistance. The manuscript partially appeared in the PhD thesis of Evelien De Clercq (83).
Supplementary Material
The Supplementary Material for this article can be found online at: https://www.frontiersin.org/articles/10.3389/fimmu.2020.555305/full#supplementary-material
References
1. WHO. Global incidence and prevalence of selected curable sexually transmitted infections – 2008. Geneva, Switzerland: World Health Organization (2012).
3. Batteiger BE. The major outer membrane protein of a single Chlamydia trachomatis serovar can possess more than one serovar-specific epitope. Infect Immun (1996) 64:542–7. doi: 10.1128/IAI.64.2.542-547.1996
4. Wang SP, Kuo CC, Grayston JT. A simplified method for immunological typing of trachoma-inclusion conjunctivitis-lymphogranuloma venereum organisms. Infect Immun (1973) 7:356–60. doi: 10.1128/IAI.7.3.356-360.1973
5. WHO. Report on the meeting on post-endemic surveillance for blinding trachoma. Geneva, Switzerland: World Health Organization (2008).
6. Schachter J. Chlamydia: intracellular biology, pathogenesis, and immunity. In: Stephens RS, editor. Infection and disease epidemiology. Washington, D.C: ASM Press (1999). p. 139–69.
7. Perine PL, Stamm WE, Holmes KK, eds. Sexually transmitted diseases: MCG (Manual). In: Lymphogranuloma venereum. New York: McGraw-Hill (1998). p. 423–32.
8. Mabey D, Peeling RW. Lymphogranuloma venereum. Sex Transm Infect (2002) 78:90–2. doi: 10.1136/sti.78.2.90
9. Aggarwal K, Jain VK, Gupta S. Bilateral groove sign with penoscrotal elephantiasis. Sex Transm Infect (2002) 78:458. doi: 10.1136/sti.78.6.458
10. Gupta S, Ajith C, Kanwar AJ, Sehgal VN, Kumar B, Mete U. Genital elephantiasis and sexually transmitted infections - revisited. Int J STD AIDS (2006) 17:157–65; quiz 166. doi: 10.1258/095646206775809150
11. Viravan C, Dance DA, Ariyarit C, Looareesuwan S, Wattanagoon Y, Davis TM, et al. A prospective clinical and bacteriologic study of inguinal buboes in Thai men. Clin Infect Dis (1996) 22:233–9. doi: 10.1093/clinids/22.2.233
12. Behets FM, Andriamiadana J, Randrianasolo D, Randriamanga R, Rasamilalao D, Chen CY, et al. Chancroid, primary syphilis, genital herpes, and lymphogranuloma venereum in Antananarivo, Madagascar. J Infect Dis (1999) 180:1382–5. doi: 10.1086/315005
13. Götz H, Nieuwenhuis R. Preliminary report of an outbreak of lymphogranuloma venereum in homosexual men in the Netherlands, with implications for other countries in western. Euro Surveill (2004) 8(4). doi: 10.2807/esw.08.04.02367-en
14. Nieuwenhuis RF, Ossewaarde JM, Götz HM, Dees J, Thio HB, Thomeer MGJ, et al. Resurgence of lymphogranuloma venereum in Western Europe: an outbreak of Chlamydia trachomatis serovar l2 proctitis in The Netherlands among men who have sex with men. Clin Infect Dis (2004) 39:996–1003. doi: 10.1086/423966
15. Liassine N, Caulfield A, Ory G, Restellini A, de Barbeyrac B, Sitavanc R, et al. First confirmed case of lymphogranuloma venereum (LGV) in Switzerland. Euro Surveill (2005) 10:E050714.4. doi: 10.2807/esw.10.28.02748-en
16. Berglund T, Bratt G, Herrmann B, Karlsson A, Löfdahl M, Payne L. Two cases of lymphogranuloma venereum (LGV) in homosexual men in Stockholm. Euro Surveill (2005) 10:E050303.3. doi: 10.2807/esw.10.09.02655-en
17. Plettenberg A, von Krosigk A, Stoehr A, Meyer T. Four cases of lymphogranuloma venereum in Hamburg, 2003. Euro Surveill (2004) 8(30). doi: 10.2807/esw.08.30.02509-en
18. Vall Mayans M, Sanz Colomo B, Ossewaarde J. First case of LGV confirmed in Barcelona. Euro Surveill (2005) 10(2). doi: 10.2807/esw.10.05.02634-en
19. Von Holstein I, Fenton K, Ison C. European network for surveillance of STIs (ESSTI) establishes working groups on lymphogranuloma venereum and HIV/STI prevention among MSM. Euro Surveill (2004) 8(25). doi: 10.2807/esw.08.25.02490-en
20. Ahdoot A, Kotler DP, Suh JS, Kutler C, Flamholz R. Lymphogranuloma venereum in human immunodeficiency virus-infected individuals in New York City. J Clin Gastroenterol (2006) 40:385–90. doi: 10.1097/00004836-200605000-00005
21. Kropp RY, Wong T. Emergence of lymphogranuloma venereum in Canada. CMAJ (2005) 172:1674–6. doi: 10.1503/cmaj.050621
22. Morton AN, Fairley CK, Zaia AM, Chen MY. Anorectal lymphogranuloma venereum in a Melbourne man. Sex Health (2006) 3:189–90. doi: 10.1071/SH06029
23. Rönn MM, Ward H. The association between lymphogranuloma venereum and HIV among men who have sex with men: systematic review and meta-analysis. BMC Infect Dis (2011) 11:70. doi: 10.1186/1471-2334-11-70
24. Spaargaren J, Fennema HSA, Morré SA, de Vries HJC, Coutinho RA. New lymphogranuloma venereum Chlamydia trachomatis variant, Amsterdam. Emerg Infect Dis (2005) 11:1090–2. doi: 10.3201/eid1111.050821
25. Somboonna N, Wan R, Ojcius DM, Pettengill MA, Joseph SJ, Chang A, et al. Hypervirulent Chlamydia trachomatis Clinical Strain Is a Recombinant between Lymphogranuloma Venereum (L2) and D Lineages. MBio (2011) 2:1–12. doi: 10.1128/mBio.00045-11
26. De Vries HJC, Zingoni A, Kreuter A, Moi H, White JA. 2013 European guideline on the management of lymphogranuloma venereum. J Eur Acad Dermatol Venereol (2014) 29(1):1–6. doi: 10.1111/jdv.12461
27. De Munain JL, Ezpeleta G, Imaz M, Del Mar Camara M, Esteban V, Santamaría JM, et al. Two lymphogranuloma venereum cases in a heterosexual couple in Bilbao (Spain). Sex Transm Dis (2008) 35:918–9. doi: 10.1097/OLQ.0b013e31817e9228
28. Gomes JP, Nunes A, Florindo C, Ferreira MA, Santo I, Azevedo J, et al. Lymphogranuloma venereum in Portugal: unusual events and new variants during 2007. Sex Transm Dis (2009) 36:88–91. doi: 10.1097/OLQ.0b013e31818b1e27
29. Peuchant O, Baldit C, Le Roy C, Trombert-Paolantoni S, Clerc M, Bébéar C, et al. First case of Chlamydia trachomatis L2b proctitis in a woman. Clin Microbiol Infect (2011) 17:E21–3. doi: 10.1111/j.1469-0691.2011.03661.x
30. Verweij SP, Ouburg S, de Vries H, Morré SA, van Ginkel CJW, Bos H, et al. The first case record of a female patient with bubonic lymphogranuloma venereum (LGV), serovariant L2b. Sex Transm Infect (2012) 88:346–7. doi: 10.1136/sextrans-2011-050298
31. Vanrompay D, Hoang TQ, De Vos L, Verminnen K, Harkinezhad T, Chiers K, et al. Specific-pathogen-free pigs as an animal model for studying Chlamydia trachomatis genital infection. Infect Immun (2005) 73:8317–21. doi: 10.1128/IAI.73.12.8317-8321.2005
32. Vanrompay D, Ducatelle R, Haesebrouck F. Diagnosis of avian chlamydiosis: specificity of the modified Giménez staining on smears and comparison of the sensitivity of isolation in eggs and three different cell cultures. Zentralbl Veterinarmed B (1992) 39:105–12. doi: 10.1111/j.1439-0450.1992.tb01144.x
33. Mayr A, Bachmann P, Bibrack B, Wittman G. Zellkulturen — Bebrütete Hühnereier — Versuchstiere. In: In Virologische Arbeitsmethoden. VEB Gustav Fischer Verlag: Jena (1974). p. 35.
34. De Clercq E, Devriendt B, Yin L, Chiers K, Cox E, Vanrompay D. The immune response against Chlamydia suis genital tract infection partially protects against re-infection. Vet Res (2014) 45:95. doi: 10.1186/s13567-014-0095-6
35. Novak M, Moldoveanu Z, Schafer DP, Mestecky J, Compans RW. Murine model for evaluation of protective immunity to influenza virus. Vaccine (1993) 11:55–60. doi: 10.1016/0264-410X(93)90339-Y
36. Schautteet K, De Clercq E, Jönsson Y, Lagae S, Chiers K, Cox E, et al. Protection of pigs against genital Chlamydia trachomatis challenge by parenteral or mucosal DNA immunization. Vaccine (2012) 30:2869–81. doi: 10.1016/j.vaccine.2012.02.044
37. Van Zaane D, Hulst MM. Monoclonal antibodies against porcine immunoglobulin isotypes. Vet Immunol Immunopathol (1987) 16:23–36. doi: 10.1016/0165-2427(87)90171-1
38. Mair KH, Sedlak C, Käser T, Pasternak A, Levast B, Gerner W, et al. The porcine innate immune system: An update. Dev Comp Immunol (2014) 45:321–43. doi: 10.1016/j.dci.2014.03.022
39. Westrom L, Joesoef R, Reynolds G, Hagdu A, Thompson SE. Pelvic inflammatory disease and fertility. A cohort study of 1,844 women with laparoscopically verified disease and 657 control women with normal laparoscopic results. Sex Transm Dis (1992) 19:185–92. doi: 10.1097/00007435-199207000-00001
40. Kimani J, Maclean IW, Bwayo JJ, MacDonald K, Oyugi J, Maitha GM, et al. Risk factors forChlamydia trachomatis pelvic inflammatory disease among sex workers in Nairobi, Kenya. J Infect Dis (1996) 173:1437–44. doi: 10.1093/infdis/173.6.1437
41. Hillis SD, Owens LM, Marchbanks PA, Amsterdam LF, Mac Kenzie WR. Recurrent chlamydial infections increase the risks of hospitalization for ectopic pregnancy and pelvic inflammatory disease. Am J Obstet Gynecol (1997) 176:103–7. doi: 10.1016/S0002-9378(97)80020-8
42. Bakken IJ, Skjeldestad FE, Lydersen S, Nordbø SA. Births and ectopic pregnancies in a large cohort of women tested for Chlamydia trachomatis. Sex Transm Dis (2007) 34:739–43. doi: 10.1097/01.olq.0000261326.65503.f6
43. Ness RB, Soper DE, Richter HE, Randall H, Peipert JF, Nelson DB, et al. Chlamydia antibodies, chlamydia heat shock protein, and adverse sequelae after pelvic inflammatory disease: the PID Evaluation and Clinical Health (PEACH) Study. Sex Transm Dis (2008) 35:129–35. doi: 10.1097/OLQ.0b013e3181557c25
44. Barnes R, Roddy R, Stamm W, Oriel D, Ridgeway G, Schachter J, Taylor-Robinson D, Ward M eds. Chlamydial Infections. In: Proceedings of the Sixth International Symposium on Human Chlamydial Infections Serovars of Chlamydia trachomatis causing repeated genital infections. Cambridge University Press. p. 503–7.
45. Katz BP, Batteiger BE, Jones RB. Effect of prior sexually transmitted disease on the isolation of Chlamydia trachomatis. Sex Transm Dis (1987) 14:160–4. doi: 10.1097/00007435-198707000-00008
46. Brunham RC, Kimani J, Bwayo J, Maitha G, Maclean I, Yang C, et al. The epidemiology of Chlamydia trachomatis within a sexually transmitted diseases core group. J Infect Dis (1996) 173:950–6. doi: 10.1093/infdis/173.4.950
47. Rietmeijer CA, Van Bemmelen R, Judson FN, Douglas JM. Incidence and repeat infection rates of Chlamydia trachomatis among male and female patients in an STD clinic: implications for screening and rescreening. Sex Transm Dis (2002) 29:65–72. doi: 10.1097/00007435-200202000-00001
48. Gomes JP, Borrego MJ, Atik B, Santo I, Azevedo J, Brito de Sá A, et al. Correlating Chlamydia trachomatis infectious load with urogenital ecological success and disease pathogenesis. Microbes Infect (2006) 8:16–26. doi: 10.1016/j.micinf.2005.05.014
49. Batteiger BE, Xu F, Johnson RE, Rekart ML. Protective immunity to Chlamydia trachomatis genital infection: evidence from human studies. J Infect Dis (2010) 201:S178–89. doi: 10.1086/652400
50. Barron AL, Rank RG, Moses EB. Immune response in mice infected in the genital tract with mouse pneumonitis agent (Chlamydia trachomatis biovar). Infect Immun (1984) 44:82–5. doi: 10.1128/IAI.44.1.82-85.1984
51. Rank RG, Batteiger BE, Soderberg LS. Susceptibility to reinfection after a primary chlamydial genital infection. Infect Immun (1988) 56:2243–9. doi: 10.1128/IAI.56.9.2243-2249.1988
52. Wolner-Hanssen P, Patton DL, Holmes KK. Protective immunity in pig-tailed macaques after cervical infection with Chlamydia trachomatis. Sex Transm Dis (1991) 18:21–5. doi: 10.1097/00007435-199101000-00005
53. Read TD, Brunham RC, Shen C, Gill SR, Heidelberg JF, White O, et al. Genome sequences of Chlamydia trachomatis MoPn and Chlamydia pneumoniae AR39. Nucleic Acids Res (2000) 28:1397–406. doi: 10.1093/nar/28.6.1397
54. McClarty G, Caldwell HD, Nelson DE. Chlamydial interferon gamma immune evasion influences infection tropism. Curr Opin Microbiol (2007) 10:47–51. doi: 10.1016/j.mib.2006.12.003
55. Parks KS, Dixon PB, Richey CM, Hook EW. Spontaneous clearance of Chlamydia trachomatis infection in untreated patients. Sex Transm Dis (1997) 24:229–35. doi: 10.1097/00007435-199704000-00008
56. Golden MR, Schillinger JA, Markowitz L, St Louis ME. Duration of untreated genital infections with Chlamydia trachomatis: a review of the literature. Sex Transm Dis (2000) 27:329–37. doi: 10.1097/00007435-200007000-00006
57. Joyner JL, Douglas JM, Foster M, Judson FN. Persistence of Chlamydia trachomatis infection detected by polymerase chain reaction in untreated patients. Sex Transm Dis (2002) 29:196–200. doi: 10.1097/00007435-200204000-00002
58. McCormack WM, Alpert S, McComb DE, Nichols RL, Semine DZ, Zinner SH. Fifteen-month follow-up study of women infected with Chlamydia trachomatis. N Engl J Med (1979) 300:123–5. doi: 10.1056/NEJM197901183000305
59. Dan M, Rotmensch HH, Eylan E, Rubinstein A, Ginsberg R. Liron M. A case of lymphogranuloma venereum of 20 years’ duration. Isolation of Chlamydia trachomatis from perianal lesions. Br J Vener Dis (1980) 56:344–6. doi: 10.1136/sti.56.5.344
60. Brunham RC, Kuo CC, Cles L, Holmes KK. Correlation of host immune response with quantitative recovery of Chlamydia trachomatis from the human endocervix. Infect Immun (1983) 39:1491–4. doi: 10.1128/IAI.39.3.1491-1494.1983
61. Punnonen R, Terho P, Nikkanen V, Meurman O. Chlamydial serology in infertile women by immunofluorescence. Fertil Steril (1979) 31:656–9. doi: 10.1016/S0015-0282(16)44056-2
62. Su H, Feilzer K, Caldwell HD, Morrison RP. Chlamydia trachomatis genital tract infection of antibody-deficient gene knockout mice. Infect Immun (1997) 65:1993–9. doi: 10.1128/IAI.65.6.1993-1999.1997
63. Morrison SG, Morrison RP. A predominant role for antibody in acquired immunity to chlamydial genital tract reinfection. J Immunol (2005) 175:7536–42. doi: 10.4049/jimmunol.175.11.7536
64. Rank RG, White HJ, Barron AL. Humoral immunity in the resolution of genital infection in female guinea pigs infected with the agent of guinea pig inclusion conjunctivitis. Infect Immun (1979) 26:573–9. doi: 10.1128/IAI.26.2.573-579.1979
65. Rank RG, Barron AL. Humoral immune response in acquired immunity to chlamydial genital infection of female guinea pigs. Infect Immun (1983) 39:463–5. doi: 10.1128/IAI.39.1.463-465.1983
66. Peterson EM, Hoshiko M, Markoff BA, Lauermann MW, de la Maza LM. Differences in susceptibilities of the lymphogranuloma venereum and trachoma biovars of Chlamydia trachomatis to neutralization by immune sera. Infect Immun (1990) 58:938–43. doi: 10.1128/IAI.58.4.938-943.1990
67. Tseng CT, Rank RG. Role of NK cells in early host response to chlamydial genital infection. Infect Immun (1998) 66:5867–75. doi: 10.1128/IAI.66.12.5867-5875.1998
68. Hook CE, Telyatnikova N, Goodall JC, Braud VM, Carmichael AJ, Wills MR, et al. Effects of Chlamydia trachomatis infection on the expression of natural killer (NK) cell ligands and susceptibility to NK cell lysis. Clin Exp Immunol (2004) 138:54–60. doi: 10.1111/j.1365-2249.2004.02596.x
69. Ramsey KH, Rank RG. Resolution of chlamydial genital infection with antigen-specific T-lymphocyte lines. Infect Immun (1991) 59:925–31. doi: 10.1128/IAI.59.3.925-931.1991
70. Igietseme JU, Ramsey KH, Magee DM, Williams DM, Kincy TJ, Rank RG. Resolution of murine chlamydial genital infection by the adoptive transfer of a biovar-specific, Th1 lymphocyte clone. Reg Immunol (1993) 5:317–24.
71. Su H, Caldwell HD. CD4+ T cells play a significant role in adoptive immunity to Chlamydia trachomatis infection of the mouse genital tract. Infect Immun (1995) 63:3302–8. doi: 10.1128/IAI.63.9.3302-3308.1995
72. Cotter TW, Ramsey KH, Miranpuri GS, Poulsen CE, Byrne GI. Dissemination of Chlamydia trachomatis chronic genital tract infection in gamma interferon gene knockout mice. Infect Immun (1997) 65:2145–52. doi: 10.1128/IAI.65.6.2145-2152.1997
73. Perry LL, Feilzer K, Caldwell HD. Immunity to Chlamydia trachomatis is mediated by T helper 1 cells through IFN-gamma-dependent and -independent pathways. J Immunol (1997) 158:3344–52.
74. Ramsey KH, DeWolfe JL, Salyer RD. Disease outcome subsequent to primary and secondary urogenital infection with murine or human biovars of Chlamydia trachomatis. Infect Immun (2000) 68(12):7186–9. doi: 10.1128/IAI.68.12.7186-7189.2000
75. Riley MM, Zurenski MA, Frazer LC, O’Connell CM, Andrews CW Jr, Mintus M, et al. The recall response induced by genital challenge with Chlamydia muridarum protects the oviduct from pathology but not from reinfection. Infect Immun (2012) 80(6):2194–203. doi: 10.1128/IAI.00169-12
76. Benyeogor I, Simoneaux T, Wu Y, Lundy S, George Z, Ryans K, et al. A unique insight into the MiRNA profile during genital chlamydial infection. BMC Genomics (2019) 20(1):143. doi: 10.1186/s12864-019-5495-6
77. Hillis SD, Owens LM, Marchbanks PA, Amsterdam LF, Mac Kenzie WR. Recurrent chlamydial infections increase the risks of hospitalization for ectopic pregnancy and pelvic inflammatory disease. Am J Obstet Gynecol (1997) 176:103–7. doi: 10.1016/s0002-9378(97)80020-8
78. Pickering H, Teng A, Faal N, Joof H, Makalo P, Cassama E, et al. Genome-wide profiling of humoral immunity and pathogen genes under selection identifies immune evasion tactics of Chlamydia trachomatis during ocular infection. Sci Rep (2017) 7(1):9634. doi: 10.1038/s41598-017-09193-2
79. Igietseme JU, Omosun Y, Partin J, Goldstein J, He Q, Joseph K, et al. Prevention of Chlamydia-induced infertility by inhibition of local caspase activity. J Infect Dis (2013) 207(7):1095–104. doi: 10.1093/infdis/jit009
80. Yeruva L, Myers GSA, Spencer N, Creasy HH, Adams NE, Maurelli AT, et al. Early microRNA expression profile as a prognostic biomarker for the development of pelvic inflammatory disease in a mouse model of chlamydial genital infection. mBio (2014) 5(3):e01241–14. doi: 10.1128/mBio.01241-14
81. Gupta R, Arkatkar T, Yu JJ, Wali S, Haskins WE, Chambers JP, et al. Chlamydia muridarum infection associated host MicroRNAs in the murine genital tract and contribution to generation of host immune response. Am J Reprod Immunol (2015) 73(2):126–40. doi: 10.1111/aji.12281
82. Gupta R, Arkatkar T, Keck J, Koundinya GKL, Castillo K, Hobel S, et al. Antigen specific immune response in Chlamydia muridarum genital infection is dependent on murine microRNAs-155 and -182. Oncotarget (2016) 7(40):64726–42. doi: 10.18632/oncotarget.11461
Keywords: Chlamydia trachomatis, genital infection, immunology, large animal model, lymphogranuloma venereum, re-infection
Citation: De Clercq E, Van Gils M, Schautteet K, Devriendt B, Kiekens C, Chiers K, Van Den Broeck W, Cox E, Dean D and Vanrompay D (2020) Chlamydia trachomatis L2c Infection in a Porcine Model Produced Urogenital Pathology and Failed to Induce Protective Immune Responses Against Re-Infection. Front. Immunol. 11:555305. doi: 10.3389/fimmu.2020.555305
Received: 24 April 2020; Accepted: 28 September 2020;
Published: 26 October 2020.
Edited by:
Joseph Alex Duncan, University of North Carolina at Chapel Hill, United StatesReviewed by:
Werner Solbach, University of Lübeck, GermanySamudi Chandramathi, University of Malaya, Malaysia
Copyright © 2020 De Clercq, Van Gils, Schautteet, Devriendt, Kiekens, Chiers, Van Den Broeck, Cox, Dean and Vanrompay. This is an open-access article distributed under the terms of the Creative Commons Attribution License (CC BY). The use, distribution or reproduction in other forums is permitted, provided the original author(s) and the copyright owner(s) are credited and that the original publication in this journal is cited, in accordance with accepted academic practice. No use, distribution or reproduction is permitted which does not comply with these terms.
*Correspondence: Daisy Vanrompay, Daisy.Vanrompay@UGent.be
†These authors have contributed equally to this work