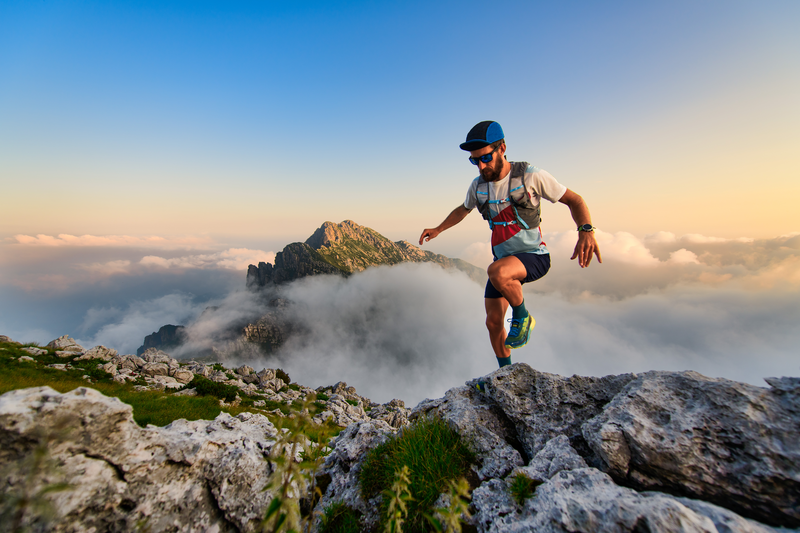
94% of researchers rate our articles as excellent or good
Learn more about the work of our research integrity team to safeguard the quality of each article we publish.
Find out more
REVIEW article
Front. Immunol. , 24 September 2020
Sec. Cancer Immunity and Immunotherapy
Volume 11 - 2020 | https://doi.org/10.3389/fimmu.2020.531491
This article is part of the Research Topic Targeting Indoleamine 2,3-dioxygenases and Tryptophan Dioxygenase for Cancer Immunotherapy View all 9 articles
Indoleamine 2,3-dioxygenase 1 (IDO1) is a cytosolic haem-containing enzyme involved in the degradation of tryptophan to kynurenine. Although initially thought to be solely implicated in the modulation of innate immune responses during infection, subsequent discoveries demonstrated IDO1 as a mechanism of acquired immune tolerance. In cancer, IDO1 expression/activity has been observed in tumor cells as well as in the tumor-surrounding stroma, which is composed of endothelial cells, immune cells, fibroblasts, and mesenchymal cells. IDO1 expression/activity has also been reported in the peripheral blood. This manuscript reviews available data on IDO1 expression, mechanisms of its induction, and its function in cancer for each of these compartments. In-depth study of the biological function of IDO1 according to the expressing (tumor) cell can help to understand if and when IDO1 inhibition can play a role in cancer therapy.
Indoleamine 2, 3-dioxygenase 1 (IDO1, hereafter referred to as IDO) is a 403 amino acid cytosolic haem-containing enzyme involved in the first, rate-limiting step of the tryptophan (Trp) metabolism to kynurenine (Kyn) (1, 2). Trp is an essential amino acid for which both neuropsychological as well as immunological functions have been described. Despite their shared function in Trp degradation, the IDO2 isoform and tryptophan 2, 3-dioxygenase (TDO2) have distinct inducers and patterns of tissue expression (3, 4).
IDO (human chromosome 8p22) is recognized as an interferon (IFN)-inducible gene. Indeed, the promoter region of IDO consists of several IFN-stimulated response elements (ISREs) and gamma activation sequences (GAS), permitting a controlled and context-dependent transcriptional process (2, 5, 6).
Although initially thought to be solely implicated in the modulation of innate immune responses in parasitic/viral conditions (7–9), subsequent discoveries demonstrated IDO to be a mechanism of acquired immune tolerance (4). In cancer, IDO expression has not only been documented in tumor cells but also in endothelial cells, fibroblasts and immune cells infiltrating the tumor microenvironment (Figure 1). In addition to the local tumor microenvironment, IDO expression was detected in peripheral blood mononuclear cells (PBMCs) in blood samples of cancer patients. Although IDO expression has been reported in these different compartments, the exact mechanisms for its distinct expression patterns and their functions are far from completely understood. In view of the complex interplay between malignant cells and their microenvironment, understanding IDO activation and its particular function in the different compartments may be of the outmost importance. This review summarizes the available scientific data.
Figure 1. Schematic representation of IDO expression in different compartments of the immune system during cancer. IDO is expressed by multiple cell types in the tumor microenvironment (A), the tumor-draining lymph node (B) and the peripheral blood (C). (A) Bin1 attenuation results in STAT1- and NFκB-dependent constitutive expression of IDO in cancer cells. In addition, COX2 overexpression facilitates constitutive IDO expression via PGE2-mediated activation of the PKC/PI3K pathways. IFNγ is recognized as a highly potent inducer of IDO expression. Binding of IFNγ to its receptor (IFNγR) leads to (i) tyrosine phosphorylation of STAT-1, triggering its dimerization and binding to the GAS sequence in IDO1 and (ii) NF-κB and STAT-1 dependent synthesis of IFNγ-regulated factor 1 (IRF1), which binds to the ISRE sequences in IDO1. Tumor IDO expression activates the cytosolic transcription factor aryl hydrocarbon receptor (AhR) by kynurenine (Kyn), stimulating an autocrine positive feedback loop via IL-6 dependent STAT-3 signaling which maintains IDO expression. In addition to IFNγ, IDO expression can be induced by other proinflammatory cytokines such as tumor necrosis factor α (TNFα) and IL-1 who enhance the expression of IFNγR on cancer cells. IFNγ and TNFα can also induce IDO expression in endothelial cells of venules in the tumor microenvironment. In the tumor-surrounding stroma, IDO is expressed by cancer associated-fibroblasts, pericytes, and infiltrating immune cells. (B) Regulatory T-cells (Tregs) induce IDO expression by antigen-presenting cells (APCs) via CTLA-4/B7 ligation in the tumor-draining lymph node. In addition, cancer cells are involved in the upregulation of IDO expression in plasmacytoid dendritic cells (pDCs) by shedding of the extracellular domain of the type III TGF-B receptor (sTGFBR3). IDO expression in myeloid DCs (mDCs) can be induced by cancer cell-secreted Wnt5a, which triggers binding of β-catenin to its responsive elements. IDO+ APCs inhibit T-cell responses and polarize naïve CD4+ T-cell differentiation toward the phenotype of suppressive Tregs via TGFβ-mediated FoxP3 upregulation. Myeloid derived suppressor cells (MDSCs) upregulate IDO via IL-6 triggered STAT-3 activation. (C) IDO+ APCs and IDO+ MDSCs infiltrate the tumor microenvironment and the peripheral blood, contributing to local and systemic immune escape.
IDO expression in the tumor microenvironment has been described in tumor cells, immune cells, endothelial cells, and stromal fibroblasts (Table 1).
The majority of literature reports on IDO positivity in neoplastic cells. Strong expression in tumor tissue is identified as an independent negative prognostic factor in multiple cancers (4, 35, 87, 105, 106). It is well-documented that tumoral IDO expression is associated with tumor-infiltrating forkhead box P3 positive regulatory T-cells (FoxP3+ Tregs) and IDO-expressing mononuclear cells, while a negative association with CD8+ cytotoxic T-cells in the primary tumor and metastatic tissue has been reported (23–30, 107). These observations in human samples are consistent with earlier mechanistic studies elucidating the involvement of IDO in impairing cytotoxic effector T-cell function/proliferation via downregulation of the T-cell receptor ζ chain as well as its stimulatory role in enhancing Treg generation (31, 47, 54, 108, 109). In diffuse-type gastric cancer, elevated tumoral IDO expression correlated with decreased expression of CD107a and granzyme B in tumor-infiltrating CD8+ T-cells, reflecting T-cell dysfunction (32). IDO expressed by pancreatic ductal adenocarcinoma cells was observed to support immune escape of cancer cells by impairing cytotoxicity and degranulation of γδ T-cells (33). In addition to FoxP3, tumoral IDO expression has been evidenced to be strongly correlated with other immunosuppressive molecules such as programmed cell death protein 1 (PD-1) and its ligand PD-L1 (29, 30, 44–46).
The extent of tumoral IDO expression has been investigated in the context of deficiencies in the DNA mismatch repair system. The microsatellite instable (MSI-H) subgroup of colorectal cancer is characterized by a strong infiltration of activated cytotoxic T-lymphocytes, which is a positive prognostic factor (110–113). Despite this highly inflamed environment, MSI-H tumors persist in such hostile climate due to overexpression of immune checkpoint molecules as cytotoxic T-lymphocyte-associated protein 4 (CTLA-4), lymphocyte-activation gene 3 (LAG-3), PD-1/PD-L1, and IDO, hampering an efficient anti-tumor T-cell response (87, 114, 115). It is hypothesized that the active tumor microenvironment is stimulated by an increased neoantigen load in MSI-H tumors, which is counterbalanced by the upregulation of immune checkpoints, such as IDO, as a negative feedback mechanism. This immunosuppressive climate mediates evasion of the tumor from the host immune system.
Besides the suppression of anti-tumor immune responses, tumoral IDO is involved in tumor vascularization. IDO-deficiency was observed to significantly decrease pulmonary vascular density in lung cancer mouse models, predominantly reducing small to medium size vessels, unaltering large vessels (36). In breast cancer, a cancer cell line (MCF-7) with strong IDO expression promoted proliferation of human umbilical vein endothelial cells (35). In murine metastasized melanoma lymph nodes, tumoral IDO expression was associated with enhanced expression of VEGF-C, an inducer of lymphangiogenesis, previously linked to the occurrence of regional lymph node metastasis (48–50). This observation suggests tumoral IDO expression is involved in the expansion of lymphatic vessels. Furthermore, tumoral IDO expression has been proposed to stimulate the metastasic process. An association between strong IDO expression at the primary tumor and development of lymph node and/or metachronous metastases is described in various malignancies (36–42). Studies detecting IDO in the primary tumor and the corresponding lymph node and metastatic tissue reported a highly consistent expression pattern of IDO throughout the disease course (44, 87). Altogether, these data define tumoral IDO as a modulator that bridges inflammation, vascularization, and immune escape to promote primary and metastatic tumor outgrowth.
Although it is widely accepted that tumor cells are capable of expressing IDO, the critical signals directing its expression and activity are only partially revealed. There are indications for constitutive/intrinsic as well as induced/extrinsic tumor IDO expression. Constitutive expression of IDO mRNA in the absence of any IFNγ exposure has been demonstrated in several cancer cell lines (10). This study also investigated in vivo IDO expression in multiple malignancies and normal cells in the stroma were observed to be IDO-negative in contrast to the tumor cells. The authors concluded that this tumoral IDO expression could not be the result of IFNγ exposure, as this would have induced IDO in the surrounding stroma too. Another study in ovarian and adeno-squamous lung cancer cell lines demonstrated that cancer cells expressed IDO1 mRNA and constitutively released Kyn into the supernatant (11).
Loss of the tumor suppressor Bridging Integrator 1 (Bin1) and overexpression of cyclooxygenase-2 (COX2) are both linked to intrinsic upregulation of IDO. Bin1 loss in a knockout mouse model was associated with elevated STAT1- and NFκB-dependent expression of IDO, driving tumor immune escape (21). This is supported in vivo by the observation that tumor expression of Bin1 is inversely correlated with IDO expression in esophageal squamous cell cancer and lung cancer (16, 17). COX2 has been implicated in the pathogenesis of several cancers, in particular colorectal cancer, where it impacts oncogenic signaling, invasion and metastasis, survival and angiogenesis (116–118). In a series of tumor cell lines, it was demonstrated that constitutive IDO expression depends on COX2 and prostaglandin E2 (PGE2), which upon autocrine signaling through the EP receptor activates IDO transcription via the PKC and PI3K pathways. Oncogenic mutations were identified in the signaling pathways involved in this autocrine loop, favoring constitutive IDO expression (12).
Type I and especially type II IFNs are known to be potent IDO-inducers (13). As tumor-infiltrating lymphocytes (TILs) are a predominant source of IFNγ, they might upregulate IDO as a negative feedback signal, hereby potentially contributing to tumor immune escape. This is in line with the observation that human hepatoma cell lines express IDO once T-lymphocytes and monocytes are added, subsequently upregulating IFNγ in the co-culture (18). IFNγ-dependent induction of tumoral IDO expression has been extensively analyzed in various malignancies (38, 88, 119, 120). IFNγ-mediated signal transduction leads to (i) tyrosine phosphorylation of STAT-1, triggering its dimerization and binding to the GAS sequence in IDO and (ii) NFκB- and STAT-1-dependent synthesis of IFNγ-regulated factor 1 (IRF1), which binds to the ISRE sequences in IDO. Combined STAT-1 and IRF-1 binding to GAS and ISRE sequences in the IDO1 gene promoter is necessary for maximal IFNγ-mediated induction of IDO transcription (2, 5, 6, 14, 15). Tumoral IDO expression was suggested to stimulate an autocrine positive feedback loop via the activation of the cytosolic transcription factor aryl hydrocarbon receptor (AhR) by Kyn. AhR activation subsequently upregulates IL-6, which mediates STAT-3 signaling driving IDO expression (11). In addition, the IDO-Kyn-AhR pathway has been evidenced to drive dormancy in tumor repopulating cells (TRCs), a highly tumorigenic subpopulation of cancer cells involved in the initiation and progression of tumorigenesis. When TRCs were stimulated in vitro with IFNγ, phosphorylated STAT-1 rapidly upregulated IDO expression, subsequently elevating Kyn levels and activating AhR. This pathway triggers G0/G1 cell cycle arrest by p27 and TRC dormancy (43).
IFNγ-mediated IDO induction can be potentiated by other proinflammatory cytokines, such as tumor necrosis factor α (TNFα) (18, 19), IL-1 (20), lipopolysaccharide (LPS) (7, 22), CpG oligodoxynucleotides (57) and PGE2 (52). The combination of these inflammatory stimuli results in synergistic enhancement of IDO transcription. For instance, IL-1 and TNFα enhance the expression of IFNγ receptors (IFNγRs) via the transcription factor NFκB, lowering the threshold for IFNγ-directed IDO upregulation (121). In addition to IFNγ, TNFα synergistically induces IDO expression by increasing both STAT-1 activation and NFκB -dependent IRF-1 expression (19).
Immunohistochemical analysis of biopsies of melanoma metastases detected IDO, PD-L1, and FoxP3 in CD8+ T-cell inflamed regions (29). In contrast, non-T-cell inflamed melanomas lacked these factors, suggesting that immune suppression might not be a property of tumor cells but rather an immune-intrinsic negative feedback process that follows the infiltration of activated CD8+ T-cells. These data indicate that IFNγ produced by CD8+ T-cells is a requisite factor for PD-L1 and IDO expression in melanoma metastatic tissue (29). Such T-cell inflamed–also termed immunologically “hot” –tumors have been associated with higher response rates to anti-PD-1 immunotherapy (122). This is in contrast to T-cell non-inflamed tumors–also referred to as “cold” tumors–which might constitute a group of tumors expressing IDO in absence of any inflammation and T-cell infiltration, representing a state of intrinsic immune resistance.
CD8+ T-cell-mediated IDO expression via IFNγ in immunologically “hot” tumors could be one of the explanations why studies in breast cancer (123, 124), gastric adenocarcinoma (125), hepatocellular (126), pancreatic cancer (127), adenosquamous lung carcinoma (128), and prostate cancer (129) observed a positive prognostic effect for tumoral IDO expression. Another explanation–apart from technicalities such as the use of different antibody clones that could result in distinct staining patterns–could be Trp shortage caused by IDO activity. Trp is the only endogenous precursor for de novo biosynthesis of nicotinamide adenine dinucleotide (NAD+), which is a co-enzyme of redox reactions for adenosine triphosphate (ATP) production. Enhanced IDO activity results in downregulated Trp and NAD+ levels, the latter being a vital co-factor in energy production, DNA synthesis, and cellular homeostasis. NAD+ depletion-induced DNA damage has been evidenced to play a role in liver tumorigenesis (51, 130).
The most extensively studied IDO-expressing immune cell types in the tumor microenvironment are antigen presenting cells (APCs) and myeloid derived suppressor cells (MDSCs).
Immunohistochemical analysis of the local tumor microenvironment identified IDO expression in human dendritic cells (DCs) in melanoma (131), breast cancer (45), squamous cell carcinoma (132), Hodgkin lymphoma (71), and esophageal cancer (133). It is well-known that DCs acquire a strong tolerogenic capacity when cultivated under low Trp conditions as they decrease antigen uptake and downregulate the expression of the costimulatory molecules CD40 and CD80 (66). Munn et al. (77) detected IDO expression in murine plasmacytoid dendritic cell (pDCs) subsets upon CTLA4-Ig exposure. Although human CD11c− CD123+ pDCs constitute a minor part of the tumor infiltrate, in vitro experiments in murine tumor draining lymph nodes (TDLNs) demonstrated that pDCs potently suppress CD8+ T-cell responses to (i) antigens presented by the pDCs themselves, but also to (ii) third-party antigens presented by non-suppressive APCs (78). Notably, all of the T-cells achieved anergy when cultivated in vitro with a low quantity of IDO-expressing DCs, suggesting that in vivo levels (estimated at 0.5% of all TDLN cells) are sufficient to direct the entire TDLN toward a tolerogenic climate. The immunosuppressive effects of IDO+ pDCs are elicited by inhibitory effects on CD8+ T-cell responses, but also by GCN2-dependent activation of mature CD4+ CD25+ Tregs. In vitro CTLA-4 blockade significantly inhibited IDO-induced activation of Tregs in co-cultures, underlining the essential role of CTLA-4 in this pathway. Importantly, Tregs can trigger upregulation of IDO expression in DCs via CTLA-4 ligation with B7 receptor molecules on DCs (58). Fully activated Tregs reciprocally upregulate PD-L1 and PD-L2 expression on target DCs, suggesting IDO-induced Treg activation proceeds via a self-amplifying loop. Reverse signaling via other ligand-receptor pathways than CTLA-4/B7 interaction to induce IDO expression on DCs such as GITR, ICOS and CD200 has also been reported (59, 134, 135). In addition to the described rapid and potent mechanism of activating mature Tregs, IDO+ pDCs also upregulate TGFβ-mediated FoxP3 expression in naïve CD4+ CD25− cells, hereby polarizing CD4+ T-cell differentiation toward the phenotype of suppressive Tregs. IL-6 production is simultaneously blocked in these naïve CD4+ CD25− cells which prevents their conversion into Th17-like effector T-cells (47, 60). Intriguingly, tumor cells are involved in the upregulation of IDO expression in pDCs by shedding of the extracellular domain of the type III TGF-B receptor (sTGFBR3). A decrease in tumor-associated TGFBR3 expression increased TGFβ-dependent upregulation of IDO in pDCs within the primary tumor and TDLN of murine models of breast cancer and melanoma (61).
Myeloid conventional DCs (mDCs, characterized by CD11c+ CD123−) are also documented to express IDO. Despite the fact that both murine CD8α+ and CD8α− dendritic cells (resp. cDC1 and cDC2) express IDO upon in vitro stimulation with IFNγ, only IDO expression on cDC1s seems to be functionally active as evidenced by the Kyn concentration in the supernatant. Addition of IFNγ-stimulated cDC1s to Th1 cells caused up to 40% of Th1 cells to undergo apoptosis. In case of cDC2s, the proportion of Th1 cells undergoing apoptosis was equally low when co-cultured with unstimulated or IFNγ-stimulated cDC2s (64, 65). Besides IFNγ-mediated upregulation of IDO expression in mDCs, tumor cells promote mDC tolerization in the tumor microenvironment via paracrine Wnt-mediated signaling. Wnt5a secreted by melanoma cells activates β-catenin in DCs which upon nuclear translocation binds the TCF/LEF1 transcription factor responsive elements subsequently inducing IDO expression in an IFNγ-independent manner (62, 63). IDO expression in murine lung cancer models was uniquely observed in CD11b+ CD11c− DCs, which were predominantly CD8α− (79). IDO expression has also been detected in a rare murine splenic cell type having phenotypic attributes of cDC1s (CD8α+, CD80/CD86, MHCII) combined with expression of markers of the B-cell lineage (CD19+, Pax5 and surface Ig) (136).
In addition to DCs differentiating from common dendritic progenitor cells (pDCs and mDCs), human monocyte-derived DCs (moDCs) are identified as IDO-competent APCs. Immunomodulatory properties of IDO+ moDCs are identical to those described for pDCs and mDCs, including stimulation of Treg differentiation from naïve CD4+ CD25− cells and suppression of T-cell responses (53, 67, 68). IDO expression seems to be dependent on the maturity status of moDCs and is limited to CD83+ moDCs. Compared to IDO− moDCs, IDO+ moDCs release a different pattern of cytokines (less IL-6 & IL-10, more IL-1β & IL-15) and upregulate surface markers as CD80, CD86, PD-L1, and PD-L2 (69). The different cytokine expression profile suggests altered functionality in IDO+ moDCs. Remarkably, direct cell-contact between immature moDCs and mast cells has been observed to upregulate IDO in moDCs. This depends on interaction between PD-1, expressed by tissue resident mast cells, and PD-L1/PD-L2 on moDCs (70).
A role for IDO in the IFNγ-mediated differentiation of monocytes into M2-type macrophages has also been proposed (72, 73). M2-macrophages are associated with tumor progression in prostate, colon, breast cancer, gastric and ovarian cancer (137–144). In vitro stimulation of monocytes with IFNγ increased the M2/M1 ratio, while silencing of IDO in monocytes resulted in upregulation of pro-inflammatory M1-macrophages (74). In melanoma, macrophages constituted the predominant source of IDO expression in brain metastases (145). In agreement with these observations, IDO expression was detected in CD163+ (M2-type) macrophages infiltrating the tumor microenvironment in Hodgkin lymphoma, and associated with shortened survival in these patients (71). A negative prognostic effect was confirmed in an independent Hodgkin lymphoma cohort, and the proportion of macrophages expressing both IDO and PD-L1 correlated with IFNγ gene expression (146). In vitro stimulation of human monocyte-derived macrophages showed that the early T-cell activation marker CD40L synergized with IFNγ for IDO upregulation (54). IDO-expressing macrophages interfered with T-cell activation, halting cell-cycle progression in the G1-phase. In multiple myeloma patients, tumor cells were described to secrete IL-32, triggering phosphorylation of STAT-3 and nuclear translocation of NFkB, subsequently inducing IDO expression in macrophages (55). Moreover, IDO+ IL-32-educated macrophages suppressed proliferation of CD4+ T-cells when co-cultured in vitro.
Strong expression of IDO by tumor cells associates with a higher level of tumor-infiltrating MDSCs in melanoma (34). MDSCs are myeloid cells with potent suppressive activities against effector lymphocytes in tumor immunology (147). IL-6 was found to be critical as an effector cytokine of IDO-driven MDSC activity and subsequent metastasis in lung cancer (36). MDSCs are also capable of expressing IDO, promoting tumor growth, and T-cell inhibition. The frequency of IDO+ MDSCs was positively associated with the amount of FoxP3+ Tregs and had a negative impact on patient outcome in breast cancer patients receiving neoadjuvant chemotherapy (75). In the tumor microenvironment of murine lung cancer models a subgroup of monocytic (Gr1int CD11b+) MDSCs were defined as the main source of IDO expression (79). IDO+ MDSCs in a lung cancer mouse model were evidenced to impair AMPK and mTOR function, which are metabolic regulators in energy homeostasis during cellular stress (80). Because of reduced signaling of these regulators, tumor-residing CD8+ T-cells upregulate checkpoint molecules, such as PD-1, CTLA-4, LAG-3, and TIM-3, reflecting the exhausted state of these cells. The molecular mechanisms underlying aberrant expression of IDO in MDSCs remain partially unclear. As described above, IFNγ is the most potent inducer of IDO expression in DCs and macrophages. IFNγ-triggered IDO expression mainly occurs trough the STAT-1 pathway (5, 57, 148). However, in human breast cancer or hematological cancer no changed IFNγ expression or STAT-1 signaling in MDSCs could be observed (39, 76). IDO was upregulated in breast cancer-derived MDSCs via IL-6-triggered STAT-3 activation, which activated the non-canonical NFκB pathway resulting in enhanced transcriptional activity of the IDO promoter (56).
Physiological expression of IDO in endothelial cells (ECs) is limited, but has been extensively demonstrated in vessels of the villous chorion and in the spiral arteries of the decidua in humans during pregnancy (149). During the course of pregnancy endothelial IDO expression extends from the subtrophoblastic capillaries to larger vessels in the villi and the chorionic plate (150). Gestational age is associated with an increased Kyn/Trp ratio in the placenta, reflecting enhanced IDO activity. Endothelial IDO expression during pregnancy has been implicated in various important functions such as immune tolerance, antimicrobial protection, and optimization of placental perfusion (151–155). Antimicrobial as well as immunoregulatory properties have been designated to IDO-positive ECs. Stimulation of human brain microvascular ECs with IFNγ restricted growth/replication of viruses, bacteria, and parasites (153, 156, 157). In addition to these antimicrobial effects, IDO-mediated degradation of Trp in brain microvascular ECs is responsible for a significant reduction of T-lymphocyte proliferation. This is in line with observations made in IDO-transfected ECs, which failed to stimulate allogeneic T-cell responses while anergy was induced in allospecific T-cells (81). A role for IDO-expressing ECs in the regulation of blood pressure has been observed, as IDO activity in murine ECs—measured by Kyn and Trp concentrations in plasma—resulted in arterial vessel relaxation through involvement of adenylate and soluble guanylate cyclase pathways (158). Furthermore, increased plasma Kyn/Trp has been associated with endothelial dysfunction and dysregulated immune responses during human sepsis (159, 160). Intriguingly, a recent study reported that patients with microvascular endothelial dysfunction had a >2-fold increased risk of developing solid-tumor cancer over a median follow-up period of 6 years (161).
Endothelial IDO expression has been described in several malignancies (71, 85, 87, 88, 90, 162, 163). In melanoma, IDO expression was prominent in CD31+ high endothelial venules (HEV) in the stroma surrounding the tumor (85). Endothelial IDO expression in the peritumoral stroma of the primary tumor was consistent with expression in the corresponding sentinel node. Notably, IDO positivity in ECs persisted in metastatic melanoma tissue developing at a median time of 3.4 years (41.5 months) after first surgery. Its expression was a negative independent prognostic marker for recurrent-free survival and overall survival. Furthermore, endothelial IDO expression was associated with reduced CD8+ T-cells and increased FoxP3+ Tregs in the tumor microenvironment. Interestingly, in patients with IDO+ ECs in the sentinel node enhanced IDO expression in the peripheral blood was detected, suggesting that endothelial IDO expression impacts systemic immunity (86). Similar observations were made in colorectal cancer, including a highly consistent expression pattern in the primary tumor, TDLNs (both tumor-invaded and tumor-uninvaded) and distant metastases (87). Endothelial IDO expression was more prevalent in MSI-H tumors compared to microsatellite stable (MSS) tumors. A negative effect on recurrence-free survival was observed for endothelial IDO expression, independent from disease stage, MMR status and CD8 count in the primary tumor.
In contrast, low IDO mRNA in the primary tumor of renal cell cancer patients was an independent unfavorable prognostic marker (88). Immunohistochemical analyses revealed that IDO was exclusively expressed by ECs, in contrast to the tumor cells which were IDO-negative. Another study in renal cell carcinoma confirmed absence of tumoral IDO expression and revealed that responders to anti-PD-1 therapy had stronger endothelial IDO expression compared to non-responders (89).
Little is known on the signaling pathways inducing IDO expression in ECs. Human umbilical vein ECs were reported to induce IDO upon stimulation with IFNγ (82) or TNFα, and these act synergistically when combined (81). IFNγ was also evidenced to upregulate IDO expression in human saphenous endothelial cells (164) and human corneal endothelial cells (165). In rats, IDO expression was induced in ECs by IFNγ-mediated activation of IKKα, which in turn stimulates the non-canonical NFκB pathway (83). In human invasive ductal carcinoma, IFNγ was demonstrated to be a potent inducer of endothelial IDO expression, which subsequently negatively affected the synthesis and secretion of stromal thrombospondin 1 (TSP1) via Trp deprivation. Reduced expression of TSP1, which is a large matricellular glycoprotein, supports cancer cells to evade tumor dormancy (90). Intriguingly, IDO was also induced when ECs were co-cultured with a tumorigenic metastatic triple negative breast cancer cell line (MDA-MB231). Tumor cells were a source of IFNγ in the co-culture, inducing IDO expression in ECs. Similarly, mRNA expression of IDO in lymphatic EC was significantly upregulated when co-cultured with CD4+ T-lymphocytes and a gastric cancer cell line (OCUM12) (162). Treatment of murine experimental melanoma with CD40 immunotherapy resulted in upregulation of IFNγ signaling and subsequent expression of IDO by ECs (84). Interestingly, CD40 mAb combined with an IDO inhibitor (epacadostat) delayed tumor growth in these mice, while activation of TILs was increased.
Lymphatic endothelial cells (LECs) have been implicated to attribute to a climate of systemic peripheral tolerance. Lymphatic vessels transport antigens and DCs to lymph nodes, where naïve cells are primed via cross-reaction. Despite their facilitating role in the migration and homeostasis of naïve T-cells, it has been described that LECs are involved in the induction of anergy of activated T-cells. An in vitro study observed that human LECs in lymph nodes induced IDO expression upon IFNγ-stimulation and impaired CD4+ T-cell proliferation when co-cultured (91). It is hypothesized that LECs promote CD8+ T-cell tolerance by the upregulation of inhibitory molecules including PD-L1 and IDO (166–168).
Tumor-surrounding stroma consists of fibroblasts, mesenchymal stromal cells, inflammatory cells, endothelial cells, and pericytes, which are all embedded in the extracellular matrix produced by fibroblasts (169, 170). Cancer associated-fibroblasts (CAFs) are the dominant stromal cell type and promote an immunosuppressive tumor microenvironment and tumor growth. IDO expression by CAFs was reported to be increased in the stroma of human esophageal cancers compared to non-tumor esophageal tissues (163). CAFs isolated from human metastatic melanoma and hepatocellular carcinomas have been documented to interfere with NK-cell mediated cancer cell killing (98, 99). CAFs expressed IDO and PGE2 when co-cultured with NK-cells, and impaired NK-cell secretion of granzyme B and perforin. Moreover, expression of NK-cell activation receptors such as NKp30 and NKp44 was downregulated. In addition to its suppressive effects on NK-cells, IFNγ-stimulated expression of IDO by dermal fibroblasts has been observed to induce apoptosis in CD4+ and CD8+ T-cells, B-cells and monocytes (100). In contrast to immune cells in the Trp-depleted microenvironment in this study, keratinocytes and endothelial cells were resistant and their proliferation was not altered. Another study highlighted low survival of the overall CD4+ T-cell population when co-cultured with IDO+ (compared to IDO−) fibroblasts (103). However, the frequency of the CD25+ FoxP3+ CD4+ subset was increased and these T-cells exhibited classic functional characteristics of Tregs (CTLA-4, IL10, and TGFβ). IFNγ is recognized as a potent inducer of IDO in fibroblasts (92, 93, 103). Additionally, the COX2/PGE2 pathway was suggested to mediate IDO induction in CAFs. Overexpression of COX2 by breast cancer cells was documented to trigger PGE2 secretion, subsequently upregulating STAT3-mediated transcription of IDO in fibroblasts (97). Strong expression of IDO by CAFs was associated with decreased disease-free and metastasis-free survival in breast cancer patients.
In addition to CAFs, mesenchymal cells are able to express IDO in the tumor-surrounding stroma. Bone marrow-derived mesenchymal stem cells [also known as multipotent mesenchymal stromal cells (171), both abbreviated MSCs] are recruited to sites of tissue injury, where they have the potential to differentiate into osteoblasts, adipocytes, and chondrocytes and mediate tissue repair (172). In cancer, bone marrow-derived mesenchymal stem cells are recruited to the primary tumor where they differentiate into CAFs (173–175). Similar to CAFs, IDO can be induced in mesenchymal stem cells by IFNγ (94–96). IDO expressing mesenchymal stem cells are also involved in inhibition of T-cell function (96) and expansion of Tregs (104). Mesenchymal stromal cells were demonstrated to be involved in the differentiation of monocytes into immunosuppressive M2-macrophages (101).
Besides CAFs and MSCs, the tumor-surrounding stroma also consists of pericytes. In normal conditions, pericytes participate in the regulation of blood flow and vessel permeability, and provide important mechanical and physiological support to ECs (176–178). The reciprocal communication between ECs and pericytes is crucial for vessel remodeling, maturation, and stabilization (177, 179, 180). Pericytes promote tumor angiogenesis, and once detached from tumor vessels they are able to differentiate into CAFs, thereby mediating an immunosuppressive tumor microenvironment (181). Intriguingly, resting pericytes were reported to activate alloreactive T-cells while IFNγ-stimulated pericytes suppress T-cell proliferation. Immunophenotyping of IFNγ-stimulated pericytes revealed IDO as one of the most upregulated gene transcripts, together with other inhibitory molecules such as PD-L1, PD-L2, and CAECAM1 (82). In this study, IDO expression in pericytes was verified as the principal mechanism accounting for negative regulation of T-cell proliferation. In primary ovarian cancer, IDO-positive tumor-associated vessels were predominantly mature blood vessels covered by pericytes (102).
IDO expression in the peripheral blood can be measured by direct methods such as single-cell RNA sequencing and flow cytometry allowing intracellular detection of IDO in specific PBMC subsets. Another method is quantification of Trp and Kyn in plasma/serum via ultra-performance liquid chromatography–tandem mass spectrometry (UPLC-MS/MS). Since enzymatic activity of IDO is involved in the first and rate-limiting step of the catabolism of Trp to Kyn and its downstream metabolites, increased Kyn/Trp is regarded as a surrogate for enhanced IDO activity. Several studies in different solid and hematological cancer types have related increased serum (or plasma) Kyn/Trp ratio to worse survival outcome (4, 182–186). A higher Kyn/Trp ratio has been linked to metastasis, higher tumor size, and advanced disease stages (4, 187, 188). Furthermore, a role for serum Kyn/Trp in predicting resistance to systemic treatment has been reported in several malignancies (79, 187, 189–192). In a large number of stage IV melanoma and renal cell cancer patients treated with anti-PD-1 therapy, a high increase in Kyn/Trp during therapy compared to baseline was associated with significantly reduced progression-free survival (193).
Although Kyn/Trp seems to have clinical relevance, the exact source of this IDO expression is unclear since its detection in serum/plasma is an indirect method measuring enzymatic IDO activity. Serum Kyn/Trp in human penile squamous cell carcinoma patients correlated with IDO expression in cancer cells but not with IDO expression on tumor-infiltrating immune cells (194). A recent study profiling Kyn/Trp in more than 900 human cancer cell lines demonstrated that secreted Kyn can be attributed to both IDO and TDO expression by tumor cells (195). However, another study observed a correlation of the Kyn/Trp ratio with PD-L1 and IDO but not with TDO mRNA levels in melanoma samples after 4 cycles of anti-PD-1 immunotherapy (193). Nevertheless, the authors argued that additional sources of Trp to Kyn degradation outside the tumor may exist. In support of this, ovarian cancer patients with high serum Kyn/Trp had strong IDO expression in both tumor cells and pericytes (102). In glioblastoma, diminished therapeutic response to CTLA-4/PD-L1 mAbs in IDO−/− mice compared to WT mice was observed, indicating the requirement for germline IDO to achieve maximal survival benefit from immune checkpoint therapy against brain tumors (196). Serum Kyn/Trp levels were significantly lower in IDO−/− mice compared to WT mice. Notably, no change in Kyn/Trp levels of isolated brain from glioblastoma WT mice and IDO−/− mice was noted. These findings suggest that non-tumor cell IDO activity contributes to a pool of Kyn in serum that facilitates responsiveness to immune checkpoint blockade. In line with these observations, serum Kyn/Trp measured by UPLC-MS/MS correlated with IDO expression in PBMCs measured by flow cytometric analysis of peripheral blood samples of melanoma patients (86). This correlation suggests that serum Kyn/Trp reflects metabolic activity of IDO-expressing circulating immune cells.
Only a few studies report on in vivo expression of IDO by specific subsets of immune cells in the peripheral blood. Munn et al. (197) observed low to undetectable levels of IDO in monocytes isolated from fresh PBMCs. Monocyte-derived CD123+ macrophages upregulated IDO expression when stimulated with IFNγ in vitro. Surprisingly, IDO was constitutively expressed in human CD123+ DCs in peripheral blood, but activation with IFNγ was still required for functional enzymatic activity. IDO+ CD123+ DCs expressed MHC II and costimulatory molecules and were effective stimulators of T-cell proliferation when incubated with an IDO-inhibitor, suggesting that these cells could act as competent APCs.
DCs are observed to constitutively express IDO, but an additional set of triggering signals during antigen-presentation is required for its activity. Monocyte-derived DCs obtained from peripheral blood of melanoma patients were demonstrated to upregulate IDO expression upon in vitro activation by CD40L and IFNγ. During an immune response, activated DCs interact with IFNγ-expressing CD8+ T-cells via CD40-CD40L ligation, subsequently mediating NFκB-dependent IDO upregulation in DCs (108, 198). Furthermore, IDO activity during DC activation was observed to be involved in the maturation of DCs, as Trp deprivation regulated expression of CCR5 and CXCR4 and DC responsiveness to chemokines (199). Another IDO-triggering signal can be elicited by DC B7-1/B7-2 ligation with CTLA-4/CD28, the latter being predominantly expressed by Tregs (200). Subsequent to the activation of IDO on DCs by activated Tregs, IDO+ DCs drive differentiation of naïve CD4+ CD25− T-cells into mature Tregs (201–204).
Expression of IDO by DCs has been demonstrated in peripheral blood of melanoma patients. IDO expression was predominantly found in CD123+ pDCs in addition to monocytic MDSCs (mMDSCs), and to a lesser extent in polymorphonuclear MDSCs (pmnMDSCs) (86). Circulating pDCs and MDSCs were key players in the systemic response in melanoma, as they had an independent prognostic effect on survival of melanoma patients (205). Furthermore, IDO activity in peripheral blood was positively correlated with levels of circulating PD-L1+ CD8+ T-cells and CTLA-4+ Tregs, underlining the close interconnection between these immunosuppressive markers in the blood circulation (86, 206). In early epithelial ovarian cancer, the level of IDO+ mMDSCs and IDO+ pmnMDSCs was significantly higher in the peripheral blood compared to the tumor microenvironment (207).
IDO expression in cancer has been described in a wide variety of cells both at the level of the tumor microenvironment and the peripheral blood. Depending on the exact location of expression, different induction pathways and effector functions have been observed. Several inflammatory cytokines such as IFNγ, IL-1, IL-6, IL-32, TNFα, and TGFβ were evidenced to drive IDO induction. Soluble factors excreted by tumor cells such as Wnt5a and sTGFBR3 are capable of inducing IDO in immune cells. Vice versa, immune cells such as CD8+ T-cells in highly inflamed tumors were found to mediate induction of IDO in tumor cells via IFNγ signaling. The mechanisms that induce IDO expression and its various physiological and pathophysiological roles are currently incompletely understood but may be important in human biology in general and medical oncology in specific.
There is ample evidence on the role of IDO in tumor immune escape. The suppressive effects on T-cell responses in the different compartments of the tumor microenvironment are well-documented in both animal and human studies. Enzymatic activity of IDO in tumor cells, as well as in endothelial cells, APCs, MDSCs, and fibroblasts has been reported to stimulate anergy of effector T-cells, while Treg activity is enhanced. In addition, naïve CD25− CD4+ T-cells are polarized toward the immunosuppressive FoxP3+ CD25− CD4+ phenotype while their conversion into Th17-like T-cells is blocked. In addition to the inhibition of antitumor immune responses, tumoral IDO expression promotes lymphangiogenesis and neovascularization, further facilitating tumor progression.
In the different compartments, IDO expression is closely interconnected with other immune checkpoint molecules already targeted by current immunotherapies. In the local tumor microenvironment, CTLA-4 expression in Tregs upregulates IDO in DCs, which reciprocally promotes Treg activation. This interplay of immune checkpoints is also evidenced in the peripheral blood, where IDO expression by PBMCs was demonstrated to be associated with increased circulating PD-L1+ CD8+ T-cells and CTLA-4+ Tregs. In addition, blockade of CTLA-4 and/or PD-1 has been reported to upregulate IDO expression as a result of the increased IFNγ-production by reactivated effector T-cells. Immunomonitoring of blood samples can highlight such dynamic shifts in ongoing immune responses. In NSCLC, RCC and melanoma patients the baseline value of systemic Kyn/Trp as well as its dynamics during treatment course were associated with patient outcome (189, 193). In this way the Kyn/Trp ratio could be a marker best capturing IDO activity at a specific moment and perhaps could have relevance in therapeutic monitoring.
In the context of immunotherapy, the immunosuppressive role of host cell expressed IDO is supported by a striking delay in tumor growth in anti-CTLA-4 treated IDO knockout mice compared to WT mice (208). Pharmacological inhibition of IDO by 1-methyl-tryptophan (1MT) combined with anti-CTLA-4 resulted in rejection of established tumors and resistance to secondary challenge in mice inoculated with B16 melanoma. Tumor rejection by anti-CTLA-4/1MT therapy was associated with enhanced infiltration of functional CD8+ and CD4+ T-cells in the tumor. Notably, the combination therapy is synergistic irrespective of detectable IDO expression in tumor cells, though therapeutic efficacy was reduced against B16 melanoma cells engineered to overexpress IDO. Synergistic retardation of tumor outgrowth by anti-CTLA-4, anti-PD-L1 and/or IDO inhibition (INCB23843) was confirmed in a murine B16.SIY melanoma model (209). In preclinical models, IDO blockade has been demonstrated to be effective as part of combination therapy including immune checkpoint therapy, DNA-damaging chemotherapy and radiotherapy (21, 210). In a 4T1 breast tumor bearing mouse model, local radiotherapy combined with intratumoral CpG upregulated IDO expression in neoplastic epithelial cells. Systemic 1MT significantly decreased IDO activity (as measured by serum Kyn/Trp) and augmented the antitumor efficacy of local radiotherapy and intratumoral CpG (210).
Several IDO inhibitors tested in phase 1/2 clinical trials showed promising results. INCB024360 (epacadostat), a competitive, selective inhibitor of IDO, was well-tolerated in a first-in-human phase 1 study with near maximal inhibition achieved (measured by decreases in plasma Kyn levels) at doses ≥100 mg twice daily (BID) (211). Phase 1/2 studies evaluated epacadostat in combination with anti-CTLA-4 (ipilimumab) (212) and anti-PD-1 [nivolumab (213) and pembrolizumab (214)] and showed encouraging antitumor activity in multiple advanced solid tumors. However, a phase 3 trial in unresectable or metastatic melanoma (ECHO-301/KEYNOTE-252) failed to show any benefit of the addition of epacadostat to pembrolizumab (215). Results of this trial raised questions concerning IDO inhibition strategies in cancer treatment, however there are certain caveats (216). The dose of epacadostat used in ECHO-301 is debated as a maximum reduction in Kyn levels of only 50% was seen for this 100 mg dose in phase 1 studies. In addition, pharmacodynamic data reported for epacadostat were based on plasma measurements of Kyn, while IDO expression in the tumor microenvironment was not investigated (211). Baseline IDO expression or Kyn/Trp levels were not employed as inclusion criteria in the ECHO-301 study, and patients who previously received an adjuvant CTLA-4-inhibitor or interferon treatment were also included. Importantly, in a melanoma cohort receiving adjuvant IFN-α2b enhanced Kyn/Trp levels were detected compared to untreated patients (217). Furthermore, melanoma patients who did not respond to anti-CTLA-4 (ipilimumab) combined with stereotactic body radiotherapy showed an increase in the Kyn/Trp ratio during treatment compared to baseline Kyn/Trp (191). These data indicate that certain (immuno-) therapies may upregulate IDO activity, raising the question whether an enhanced dose of epacadostat would have been needed in the ECHO-301 study in order to fully block IDO activity.
BMS-986205, an irreversible IDO1 inhibitor, was demonstrated to reduce both serum (>60% mean reduction at a dose from 100 to 200 mg) and intratumoral (up to 90% reduction) Kyn levels (218). Currently, BMS-986205 in combination with anti-PD-1 therapy is investigated in several phase 2 trials (219–221). Besides IDO-specific inhibitors, other approaches to inhibit this pathway continue to be considered. Indoximod, a Trp mimetic, restores the activity of master metabolic kinase mTORC1 in effector T-cells, reversing autophagy triggered by Trp depletion (222). By targeting a downstream convergent effector mechanism used by IDO, as well as IDO2 and TDO, indoximod might prove less sensitive to negative feedback mechanisms that may result in treatment resistance (223).
Further research is needed to better understand the exact biological functions of IDO but also of the two other Trp-degrading enzymes IDO2 and TDO in the different compartments in cancer (224, 225). Increased insights in how these enzymes affect cancer immune escape and disease outcome could facilitate patient stratification in future clinical studies. The next step would be to investigate how these insights can be used to reverse this negative immune climate, thereby paving the way to personalized immuno-oncology possibly already in an early stage of cancer.
All authors had a substantial contribution to the manuscript, and approved the submitted version. AM and LB wrote the manuscript. AM generated the figure and MD assisted in the revision and made the table.
AM was funded by a grant of Ghent University Hospital.
LB was participant in advisory board Incyte, München, Germany, June 2017 and gave an internal training for Incyte European division, Amsterdam, October 2017.
The remaining authors declare that the research was conducted in the absence of any commercial or financial relationships that could be construed as a potential conflict of interest.
1. Shimizu T, Nomiyama S, Hirata F, Hayaishi O. Indoleamine 2,3-dioxygenase. Purification and some properties. J Biol Chem. (1978) 253:4700–6.
2. Taylor MW, Feng GS. Relationship between interferon-gamma, indoleamine 2,3-dioxygenase, and tryptophan catabolism. FASEB J. (1991) 5:2516–22. doi: 10.1096/fasebj.5.11.1907934
3. Prendergast GC, Malachowski WP, DuHadaway JB, Muller AJ. Discovery of IDO1 inhibitors: from bench to bedside. Cancer Res. (2017) 77:6795–811. doi: 10.1158/0008-5472.CAN-17-2285
4. Brochez L, Chevolet I, Kruse V. The rationale of indoleamine 2,3-dioxygenase inhibition for cancer therapy. Eur J Cancer. (2017) 76:167–82. doi: 10.1016/j.ejca.2017.01.011
5. Chon SY, Hassanain HH, Pine R, Gupta SL. Involvement of two regulatory elements in interferon-gamma-regulated expression of human indoleamine 2,3-dioxygenase gene. J Interferon Cytokine Res Off J Int Soc Interferon Cytokine Res. (1995) 15:517–26. doi: 10.1089/jir.1995.15.517
6. Konan KV, Taylor MW. Importance of the two interferon-stimulated response element (ISRE) sequences in the regulation of the human indoleamine 2,3-dioxygenase gene. J Biol Chem. (1996) 271:19140–5. doi: 10.1074/jbc.271.32.19140
7. Yoshida R, Hayaishi O. Induction of pulmonary indoleamine 2,3-dioxygenase by intraperitoneal injection of bacterial lipopolysaccharide. Proc Natl Acad Sci USA. (1978) 75:3998–4000. doi: 10.1073/pnas.75.8.3998
8. Yoshida R, Urade Y, Tokuda M, Hayaishi O. Induction of indoleamine 2,3-dioxygenase in mouse lung during virus infection. Proc Natl Acad Sci USA. (1979) 76:4084–6. doi: 10.1073/pnas.76.8.4084
9. Pfefferkorn ER. Interferon gamma blocks the growth of Toxoplasma gondii in human fibroblasts by inducing the host cells to degrade tryptophan. Proc Natl Acad Sci USA. (1984) 81:908–12. doi: 10.1073/pnas.81.3.908
10. Uyttenhove C, Pilotte L, Théate I, Stroobant V, Colau D, Parmentier N, et al. Evidence for a tumoral immune resistance mechanism based on tryptophan degradation by indoleamine 2,3-dioxygenase. Nat Med. (2003) 9:1269–74. doi: 10.1038/nm934
11. Litzenburger UM, Opitz CA, Sahm F, Rauschenbach KJ, Trump S, Winter M, et al. Constitutive IDO expression in human cancer is sustained by an autocrine signaling loop involving IL-6, STAT3 and the AHR. Oncotarget. (2014) 5:1038–51. doi: 10.18632/oncotarget.1637
12. Hennequart M, Pilotte L, Cane S, Hoffmann D, Stroobant V, Plaen ED, et al. Constitutive IDO1 expression in human tumors is driven by cyclooxygenase-2 and mediates intrinsic immune resistance. Cancer Immunol Res. (2017) 5:695–709. doi: 10.1158/2326-6066.CIR-16-0400
13. Takikawa O, Kuroiwa T, Yamazaki F, Kido R. Mechanism of interferon-gamma action. Characterization of indoleamine 2,3-dioxygenase in cultured human cells induced by interferon-gamma and evaluation of the enzyme-mediated tryptophan degradation in its anticellular activity. J Biol Chem. (1988) 263:2041–2048.
14. Hassanain HH, Chon SY, Gupta SL. Differential regulation of human indoleamine 2,3-dioxygenase gene expression by interferons-gamma and -alpha. Analysis of the regulatory region of the gene and identification of an interferon-gamma-inducible DNA-binding factor. J Biol Chem. (1993) 268:5077–84.
15. Du MX, Sotero-Esteva WD, Taylor MW. Analysis of transcription factors regulating induction of indoleamine 2,3-dioxygenase by IFN-gamma. J Interferon Cytokine Res. (2000) 20:133–42. doi: 10.1089/107999000312531
16. Jia Y, Wang H, Wang Y, Wang T, Wang M, Ma M, et al. Low expression of Bin1, along with high expression of IDO in tumor tissue and draining lymph nodes, are predictors of poor prognosis for esophageal squamous cell cancer patients. Int J Cancer. (2015) 137:1095–106. doi: 10.1002/ijc.29481
17. Ahmadzada T, Lee K, Clarke C, Cooper WA, Linton A, McCaughan B, et al. High BIN1 expression has a favorable prognosis in malignant pleural mesothelioma and is associated with tumor infiltrating lymphocytes. Lung Cancer. (2019) 130:35–41. doi: 10.1016/j.lungcan.2019.02.005
18. Zhao Q, Wang P, Huang Z, Peng L, Lin C, Gao Z, et al. Tumoral indoleamine 2, 3-dioxygenase 1 is regulated by monocytes and T lymphocytes collaboration in hepatocellular carcinoma. Oncotarget. (2016) 7:14781–90. doi: 10.18632/oncotarget.7438
19. Robinson CM, Hale PT, Carlin JM. The role of IFN-γ and TNF-α-responsive regulatory elements in the synergistic induction of indoleamine dioxygenase. J Interferon Cytokine Res Off J Int Soc Interferon Cytokine Res. (2005) 25:20–30. doi: 10.1089/jir.2005.25.20
20. Babcock TA, Carlin JM. Transcriptional activation of indoleamine dioxygenase by interleukin 1 and tumor necrosis factor alpha in interferon-treated epithelial cells. Cytokine. (2000) 12:588–94. doi: 10.1006/cyto.1999.0661
21. Muller AJ, DuHadaway JB, Donover PS, Sutanto-Ward E, Prendergast GC. Inhibition of indoleamine 2,3-dioxygenase, an immunoregulatory target of the cancer suppression gene Bin1, potentiates cancer chemotherapy. Nat Med NY. (2005) 11:312–9. doi: 10.1038/nm1196
22. Fujigaki S, Saito K, Sekikawa K, Tone S, Takikawa O, Fujii H, et al. Lipopolysaccharide induction of indoleamine 2,3-dioxygenase is mediated dominantly by an IFN-gamma-independent mechanism. Eur J Immunol. (2001) 31:2313–8. doi: 10.1002/1521-4141(200108)31:8<2313::AID-IMMU2313>3.0.CO;2-S
23. Brody JR, Costantino CL, Berger AC, Sato T, Lisanti MP, Yeo CJ, et al. Expression of indoleamine 2,3-dioxygenase in metastatic malignant melanoma recruits regulatory T cells to avoid immune detection and affects survival. Cell Cycle Georget Tex. (2009) 8:1930–4. doi: 10.4161/cc.8.12.8745
24. Inaba T, Ino K, Kajiyama H, Shibata K, Yamamoto E, Kondo S, et al. Indoleamine 2,3-dioxygenase expression predicts impaired survival of invasive cervical cancer patients treated with radical hysterectomy. Gynecol Oncol. (2010) 117:423–8. doi: 10.1016/j.ygyno.2010.02.028
25. Zhang G, Liu W-L, Zhang L, Wang J-Y, Kuang M-H, Liu P, et al. Involvement of indoleamine 2,3-dioxygenase in impairing tumor-infiltrating CD8 T-cell functions in esophageal squamous cell carcinoma. Clin Dev Immunol. (2011) 2011:384726. doi: 10.1155/2011/384726
26. Ye J, Liu H, Hu Y, Li P, Zhang G, Li Y. Tumoral indoleamine 2,3-dioxygenase expression predicts poor outcome in laryngeal squamous cell carcinoma. Virchows Arch Int J Pathol. (2013) 462:73–81. doi: 10.1007/s00428-012-1340-x
27. Moretti S, Menicali E, Voce P, Morelli S, Cantarelli S, Sponziello M, et al. Indoleamine 2,3-dioxygenase 1 (IDO1) is up-regulated in thyroid carcinoma and drives the development of an immunosuppressant tumor microenvironment. J Clin Endocrinol Metab. (2014) 99:832–40. doi: 10.1210/jc.2013-3351
28. Ino K, Yamamoto E, Shibata K, Kajiyama H, Yoshida N, Terauchi M, et al. Inverse correlation between tumoral indoleamine 2,3-dioxygenase expression and tumor-infiltrating lymphocytes in endometrial cancer: its association with disease progression and survival. Clin Cancer Res Off J Am Assoc Cancer Res. (2008) 14:2310–7. doi: 10.1158/1078-0432.CCR-07-4144
29. Spranger S, Spaapen RM, Zha Y, Williams J, Meng Y, Ha TT, et al. Up-regulation of PD-L1, IDO, and Tregs in the melanoma tumor microenvironment is driven by CD8+ T cells. Sci Transl Med. (2013) 5:200ra116. doi: 10.1126/scitranslmed.3006504
30. Gide TN, Allanson BM, Menzies AM, Ferguson PM, Madore J, Saw RPM, et al. Inter- and intrapatient heterogeneity of indoleamine 2,3-dioxygenase expression in primary and metastatic melanoma cells and the tumour microenvironment. Histopathology. (2019) 74:817–28. doi: 10.1111/his.13814
31. Curti A, Pandolfi S, Valzasina B, Aluigi M, Isidori A, Ferri E, et al. Modulation of tryptophan catabolism by human leukemic cells results in the conversion of CD25– into CD25+ T regulatory cells. Blood. (2007) 109:2871–7. doi: 10.1182/blood-2006-07-036863
32. Li R, Zhang H, Cao Y, Liu X, Chen Y, Qi Y, et al. Lauren classification identifies distinct prognostic value and functional status of intratumoral CD8+ T cells in gastric cancer. Cancer Immunol Immunother. (2020) 69:1327–36. doi: 10.1007/s00262-020-02550-7
33. Jonescheit H, Oberg H-H, Gonnermann D, Hermes M, Sulaj V, Peters C, et al. Influence of indoleamine-2,3-dioxygenase and its metabolite kynurenine on γδ T cell cytotoxicity against ductal pancreatic adenocarcinoma cells. Cells. (2020) 9:1140. doi: 10.3390/cells9051140
34. Holmgaard RB, Zamarin D, Li Y, Gasmi B, Munn DH, Allison JP, et al. Tumor-expressed IDO recruits and activates MDSCs in a Treg-dependent manner. Cell Rep. (2015) 13:412–24. doi: 10.1016/j.celrep.2015.08.077
35. Wei L, Zhu S, Li M, Li F, Wei F, Liu J, et al. High indoleamine 2,3-dioxygenase is correlated with microvessel density and worse prognosis in breast cancer. Front Immunol. (2018) 9:724. doi: 10.3389/fimmu.2018.00724
36. Smith C, Chang M-Y, Parker K, Beury D, DuHadaway JB, Flick HE, et al. IDO is a nodal pathogenic driver of lung cancer and metastasis development. Cancer Discov. (2012) 2:722–35. doi: 10.1158/2159-8290.CD-12-0014
37. Yu J, Sun J, Wang SE, Li H, Cao S, Cong Y, et al. Upregulated expression of indoleamine 2, 3-dioxygenase in primary breast cancer correlates with increase of infiltrated regulatory T cells in situ and lymph node metastasis. Clin Dev Immunol. (2011) 2011:469135. doi: 10.1155/2011/469135
38. Ferdinande L, Decaestecker C, Verset L, Mathieu A, Moles Lopez X, Negulescu A-M, et al. Clinicopathological significance of indoleamine 2,3-dioxygenase 1 expression in colorectal cancer. Br J Cancer. (2012) 106:141–7. doi: 10.1038/bjc.2011.513
39. Yu J, Du W, Yan F, Wang Y, Li H, Cao S, et al. Myeloid-derived suppressor cells suppress antitumor immune responses through ido expression and correlate with lymph node metastasis in patients with breast cancer. J Immunol. (2013) 190:3783–97. doi: 10.4049/jimmunol.1201449
40. Engin A, Gonul II, Engin AB, Karamercan A, Sepici Dincel A, Dursun A. Relationship between indoleamine 2,3-dioxygenase activity and lymphatic invasion propensity of colorectal carcinoma. World J Gastroenterol. (2016) 22:3592–601. doi: 10.3748/wjg.v22.i13.3592
41. Tang D, Yue L, Yao R, Zhou L, Yang Y, Lu L, et al. P53 prevent tumor invasion and metastasis by down-regulating IDO in lung cancer. Oncotarget. (2017) 8:54548–57. doi: 10.18632/oncotarget.17408
42. Ogawa M, Watanabe M, Hasegawa T, Ichihara K, Yoshida K, Yanaga K. Expression of CXCR-4 and IDO in human colorectal cancer: an immunohistochemical approach. Mol Clin Oncol. (2017) 6:701–4. doi: 10.3892/mco.2017.1207
43. Liu Y, Liang X, Yin X, Lv J, Tang K, Ma J, et al. Blockade of IDO-kynurenine-AhR metabolic circuitry abrogates IFN-γ-induced immunologic dormancy of tumor-repopulating cells. Nat Commun. (2017) 8:1–15. doi: 10.1038/ncomms15207
44. Dill EA, Dillon PM, Bullock TN, Mills AM. IDO expression in breast cancer: an assessment of 281 primary and metastatic cases with comparison to PD-L1. Mod Pathol. (2018) 31:1513–22. doi: 10.1038/s41379-018-0061-3
45. Ye Q, Wang C, Xian J, Zhang M, Cao Y, Cao Y. Expression of programmed cell death protein 1 (PD-1) and indoleamine 2,3-dioxygenase (IDO) in the tumor microenvironment and in tumor-draining lymph nodes of breast cancer. Hum Pathol. (2018) 75:81–90. doi: 10.1016/j.humpath.2018.02.004
46. Mandarano M, Bellezza G, Belladonna ML, Van den Eynde BJ, Chiari R, Vannucci J, et al. Assessment of TILs, IDO-1, and PD-L1 in resected non-small cell lung cancer: an immunohistochemical study with clinicopathological and prognostic implications. Virchows Arch. (2019) 474:159–68. doi: 10.1007/s00428-018-2483-1
47. Fallarino F, Grohmann U, You S, McGrath BC, Cavener DR, Vacca C, et al. The combined effects of tryptophan starvation and tryptophan catabolites down-regulate T cell receptor ζ-chain and induce a regulatory phenotype in naive T cells. J Immunol. (2006) 176:6752–61. doi: 10.4049/jimmunol.176.11.6752
48. He Y, Rajantie I, Pajusola K, Jeltsch M, Holopainen T, Yla-Herttuala S, et al. Vascular endothelial cell growth factor receptor 3–mediated activation of lymphatic endothelium is crucial for tumor cell entry and spread via lymphatic vessels. Cancer Res. (2005) 65:4739–46. doi: 10.1158/0008-5472.CAN-04-4576
49. Boone B, Blokx W, De Bacquer D, Lambert J, Ruiter D, Brochez L. The role of VEGF-C staining in predicting regional metastasis in melanoma. Virchows Arch. (2008) 453:257–65. doi: 10.1007/s00428-008-0641-6
50. Massi D, Puig S, Franchi A, Malvehy J, Vidal-Sicart S, Gonzalez-Cao M, et al. Tumour lymphangiogenesis is a possible predictor of sentinel lymph node status in cutaneous melanoma: a case–control study. J Clin Pathol. (2006) 59:166–73. doi: 10.1136/jcp.2005.028431
51. Tummala KS, Gomes AL, Yilmaz M, Graña O, Bakiri L, Ruppen I, et al. Inhibition of de novo NAD(+) synthesis by oncogenic URI causes liver tumorigenesis through DNA damage. Cancer Cell. (2014) 26:826–39. doi: 10.1016/j.ccell.2014.10.002
52. Braun D, Longman RS, Albert ML. A two-step induction of indoleamine 2,3 dioxygenase (IDO) activity during dendritic-cell maturation. Blood. (2005) 106:2375–81. doi: 10.1182/blood-2005-03-0979
53. Lanzinger M, Jürgens B, Hainz U, Dillinger B, Raberger J, Fuchs D, et al. Ambivalent effects of dendritic cells displaying prostaglandin E2-induced indoleamine 2,3-dioxygenase. Eur J Immunol. (2012) 42:1117–28. doi: 10.1002/eji.201141765
54. Munn DH, Shafizadeh E, Attwood JT, Bondarev I, Pashine A, Mellor AL. Inhibition of T cell proliferation by macrophage tryptophan catabolism. J Exp Med. (1999) 189:1363–72. doi: 10.1084/jem.189.9.1363
55. Yan H, Dong M, Liu X, Shen Q, He D, Huang X, et al. Multiple myeloma cell-derived IL-32γ increases the immunosuppressive function of macrophages by promoting indoleamine 2,3-dioxygenase (IDO) expression. Cancer Lett. (2019) 446:38–48. doi: 10.1016/j.canlet.2019.01.012
56. Yu J, Wang Y, Yan F, Zhang P, Li H, Zhao H, et al. Noncanonical NF-κB activation mediates STAT3-stimulated IDO upregulation in myeloid-derived suppressor cells in breast cancer. J Immunol. (2014) 193:2574–86. doi: 10.4049/jimmunol.1400833
57. Mellor AL, Baban B, Chandler PR, Manlapat A, Kahler DJ, Munn DH. Cutting edge: CpG oligonucleotides induce splenic CD19+ dendritic cells to acquire potent indoleamine 2,3-dioxygenase-dependent T cell regulatory functions via IFN Type 1 signaling. J Immunol Baltim Md 1950. (2005) 175:5601–5. doi: 10.4049/jimmunol.175.9.5601
58. Fallarino F, Grohmann U, Hwang KW, Orabona C, Vacca C, Bianchi R, et al. Modulation of tryptophan catabolism by regulatory T cells. Nat Immunol. (2003) 4:1206–12. doi: 10.1038/ni1003
59. Fallarino F, Asselin-Paturel C, Vacca C, Bianchi R, Gizzi S, Fioretti MC, et al. Murine plasmacytoid dendritic cells initiate the immunosuppressive pathway of tryptophan catabolism in response to CD200 receptor engagement. J Immunol. (2004) 173:3748–54. doi: 10.4049/jimmunol.173.6.3748
60. Baban B, Chandler PR, Sharma MD, Pihkala J, Koni PA, Munn DH, et al. IDO activates regulatory T cells and blocks their conversion into Th17-like T cells. J Immunol Baltim Md 1950. (2009) 183:2475–83. doi: 10.4049/jimmunol.0900986
61. Hanks BA, Holtzhausen A, Evans KS, Jamieson R, Gimpel P, Campbell OM, et al. Type III TGF-β receptor downregulation generates an immunotolerant tumor microenvironment. J Clin Invest. (2013) 123:3925–40. doi: 10.1172/JCI65745
62. Holtzhausen A, Zhao F, Evans KS, Tsutsui M, Orabona C, Tyler DS, et al. Melanoma-derived Wnt5a promotes local dendritic-cell expression of IDO and immunotolerance: opportunities for pharmacologic enhancement of immunotherapy. Cancer Immunol Res. (2015) 3:1082–95. doi: 10.1158/2326-6066.CIR-14-0167
63. Zhao F, Xiao C, Evans KS, Theivanthiran T, DeVito N, Holtzhausen A, et al. Paracrine Wnt5a-β-catenin signaling triggers a metabolic program that drives dendritic cell tolerization. Immunity. (2018) 48:147–60. doi: 10.1016/j.immuni.2017.12.004
64. Fallarino F, Vacca C, Orabona C, Belladonna ML, Bianchi R, Marshall B, et al. Functional expression of indoleamine 2,3-dioxygenase by murine CD8α+ dendritic cells. Int Immunol. (2002) 14:65–8. doi: 10.1093/intimm/14.1.65
65. Orabona C, Puccetti P, Vacca C, Bicciato S, Luchini A, Fallarino F, et al. Toward the identification of a tolerogenic signature in IDO-competent dendritic cells. Blood. (2006) 107:2846–54. doi: 10.1182/blood-2005-10-4077
66. Brenk M, Scheler M, Koch S, Neumann J, Takikawa O, Häcker G, et al. Tryptophan deprivation induces inhibitory receptors ILT3 and ILT4 on dendritic cells favoring the induction of human CD4+CD25+ Foxp3+ T regulatory cells. J Immunol. (2009) 183:145–54. doi: 10.4049/jimmunol.0803277
67. Jürgens B, Hainz U, Fuchs D, Felzmann T, Heitger A. Interferon-γ-triggered indoleamine 2,3-dioxygenase competence in human monocyte-derived dendritic cells induces regulatory activity in allogeneic T cells. Blood. (2009) 114:3235–43. doi: 10.1182/blood-2008-12-195073
68. Chung DJ, Rossi M, Romano E, Ghith J, Yuan J, Munn DH, et al. Indoleamine 2,3-dioxygenase–expressing mature human monocyte-derived dendritic cells expand potent autologous regulatory T cells. Blood. (2009) 114:555–63. doi: 10.1182/blood-2008-11-191197
69. Von Bubnoff D, Scheler M, Wilms H, Fimmers R, Bieber T. Identification of IDO-positive and IDO-negative human dendritic cells after activation by various proinflammatory stimuli. J Immunol Baltim Md 1950. (2011) 186:6701–9. doi: 10.4049/jimmunol.1003151
70. Rodrigues CP, Ferreira ACF, Pinho MP, de Moraes CJ, Bergami-Santos PC, Barbuto JAM. Tolerogenic IDO+ dendritic cells are induced by PD-1-expressing mast cells. Front Immunol. (2016) 7:9. doi: 10.3389/fimmu.2016.00009
71. Choe J-Y, Yun JY, Jeon YK, Kim SH, Park G, Huh JR, et al. Indoleamine 2,3-dioxygenase (IDO) is frequently expressed in stromal cells of Hodgkin lymphoma and is associated with adverse clinical features: a retrospective cohort study. BMC Cancer. (2014) 14:335. doi: 10.1186/1471-2407-14-335
72. Carlin JM, Borden EC, Sondel PM, Byrne GI. Interferon-induced indoleamine 2,3-dioxygenase activity in human mononuclear phagocytes. J Leukoc Biol. (1989) 45:29–34. doi: 10.1002/jlb.45.1.29
73. Werner ER, Bitterlich G, Fuchs D, Hausen A, Reibnegger G, Szabo G, et al. Human macrophages degrade tryptophan upon induction by interferon-gamma. Life Sci. (1987) 41:273–80. doi: 10.1016/0024-3205(87)90149-4
74. Wang X-F, Wang H-S, Wang H, Zhang F, Wang K-F, Guo Q, et al. The role of indoleamine 2,3-dioxygenase (IDO) in immune tolerance: focus on macrophage polarization of THP-1 cells. Cell Immunol. (2014) 289:42–8. doi: 10.1016/j.cellimm.2014.02.005
75. Li F, Zhao Y, Wei L, Li S, Liu J. Tumor-infiltrating Treg, MDSC, and IDO expression associated with outcomes of neoadjuvant chemotherapy of breast cancer. Cancer Biol Ther. (2018) 19:695–705. doi: 10.1080/15384047.2018.1450116
76. Mougiakakos D, Jitschin R, von Bahr L, Poschke I, Gary R, Sundberg B, et al. Immunosuppressive CD14 + HLA-DR low/neg IDO + myeloid cells in patients following allogeneic hematopoietic stem cell transplantation. Leukemia. (2013) 27:377–88. doi: 10.1038/leu.2012.215
77. Munn DH, Sharma MD, Hou D, Baban B, Lee JR, Antonia SJ, et al. Expression of indoleamine 2,3-dioxygenase by plasmacytoid dendritic cells in tumor-draining lymph nodes. J Clin Invest. (2004) 114:280–90. doi: 10.1172/JCI21583
78. Sharma MD, Baban B, Chandler P, Hou D-Y, Singh N, Yagita H, et al. Plasmacytoid dendritic cells from mouse tumor-draining lymph nodes directly activate mature Tregs via indoleamine 2,3-dioxygenase. J Clin Invest. (2007) 117:2570–82. doi: 10.1172/JCI31911
79. Li A, Barsoumian HB, Schoenhals JE, Cushman TR, Caetano MS, Wang X, et al. Indoleamine 2,3-dioxygenase 1 inhibition targets anti-PD1-resistant lung tumors by blocking myeloid-derived suppressor cells. Cancer Lett. (2018) 431:54–63. doi: 10.1016/j.canlet.2018.05.005
80. Schafer CC, Wang Y, Hough KP, Sawant A, Grant SC, Thannickal VJ, et al. Indoleamine 2,3-dioxygenase regulates anti-tumor immunity in lung cancer by metabolic reprogramming of immune cells in the tumor microenvironment. Oncotarget. (2016) 7:75407–24. doi: 10.18632/oncotarget.12249
81. Beutelspacher SC, Tan PH, McClure MO, Larkin DFP, Lechler RI, George AJT. Expression of indoleamine 2,3-dioxygenase (IDO) by endothelial cells: implications for the control of alloresponses. Am J Transplant. (2006) 6:1320–30. doi: 10.1111/j.1600-6143.2006.01324.x
82. Liu R, Merola J, Manes TD, Qin L, Tietjen GT, López-Giráldez F, et al. Interferon-γ converts human microvascular pericytes into negative regulators of alloimmunity through induction of indoleamine 2,3-dioxygenase 1. JCI Insight. (2018) 3:e97881. doi: 10.1172/jci.insight.97881
83. Liang Y, Yu Z, Song Y, Wang T, Xiao B. Indoleamine 2,3-dioxygenase activation by interferon gamma in vascular endothelial rat cells requires noncanonical nf-κb signaling. Transplant Proc. (2019) 51:2141–5. doi: 10.1016/j.transproceed.2019.03.043
84. Georganaki M, Ramachandran M, Tuit S, Núñez NG, Karampatzakis A, Fotaki G, et al. Tumor endothelial cell up-regulation of IDO1 is an immunosuppressive feed-back mechanism that reduces the response to CD40-stimulating immunotherapy. OncoImmunology. (2020) 9:1730538. doi: 10.1080/2162402X.2020.1730538
85. Chevolet I, Speeckaert R, Haspeslagh M, Neyns B, Krüse V, Schreuer M, Gele MV, et al. Peritumoral indoleamine 2,3-dioxygenase expression in melanoma: an early marker of resistance to immune control? Br J Dermatol. (2014) 171:987–95. doi: 10.1111/bjd.13100
86. Chevolet I, Speeckaert R, Schreuer M, Neyns B, Krysko O, Bachert C, et al. Characterization of the in vivo immune network of IDO, tryptophan metabolism, PD-L1, and CTLA-4 in circulating immune cells in melanoma. OncoImmunology. (2015) 4:e982382. doi: 10.4161/2162402X.2014.982382
87. Meireson A, Chevolet I, Hulstaert E, Ferdinande L, Ost P, Geboes K, et al. Peritumoral endothelial indoleamine 2, 3-dioxygenase expression is an early independent marker of disease relapse in colorectal cancer and is influenced by DNA mismatch repair profile. Oncotarget. (2018) 9:25216–24. doi: 10.18632/oncotarget.25393
88. Riesenberg R, Weiler C, Spring O, Eder M, Buchner A, Popp T, et al. Expression of indoleamine 2,3-dioxygenase in tumor endothelial cells correlates with long-term survival of patients with renal cell carcinoma. Clin Cancer Res. (2007) 13:6993–7002. doi: 10.1158/1078-0432.CCR-07-0942
89. Seeber A, Klinglmair G, Fritz J, Steinkohl F, Zimmer K-C, Aigner F, et al. High IDO-1 expression in tumor endothelial cells is associated with response to immunotherapy in metastatic renal cell carcinoma. Cancer Sci. (2018) 109:1583–91. doi: 10.1111/cas.13560
90. Lopes-Bastos B, Jin L, Ruge F, Owen S, Sanders A, Cogle C, et al. Association of breast carcinoma growth with a non-canonical axis of IFNγ/IDO1/TSP1. Oncotarget. (2017) 8:85024–39. doi: 10.18632/oncotarget.18781
91. Nörder M, Gutierrez MG, Zicari S, Cervi E, Caruso A, Guzmán CA. Lymph node-derived lymphatic endothelial cells express functional costimulatory molecules and impair dendritic cell-induced allogenic T-cell proliferation. FASEB J. (2012) 26:2835–46. doi: 10.1096/fj.12-205278
92. Yufit T, Vining V, Brown RR, Varga J, Wang L. Inhibition of type I collagen mRNA expression independent of tryptophan depletion in interferon-γ-treated human dermal fibroblasts. J Invest Dermatol. (1995) 105:388–93. doi: 10.1111/1523-1747.ep12320990
93. Ryu Y-H, Kim J-C. Expression of indoleamine 2,3-dioxygenase in human corneal cells as a local immunosuppressive factor. Invest Ophthalmol Vis Sci. (2007) 48:4148–52. doi: 10.1167/iovs.05-1336
94. Meisel R, Zibert A, Laryea M, Göbel U, Däubener W, Dilloo D. Human bone marrow stromal cells inhibit allogeneic T-cell responses by indoleamine 2,3-dioxygenase-mediated tryptophan degradation. Blood. (2004) 103:4619–21. doi: 10.1182/blood-2003-11-3909
95. Ryan JM, Barry F, Murphy JM, Mahon BP. Interferon-γ does not break, but promotes the immunosuppressive capacity of adult human mesenchymal stem cells. Clin Exp Immunol. (2007) 149:353–63. doi: 10.1111/j.1365-2249.2007.03422.x
96. Ren G, Su J, Zhang L, Zhao X, Ling W, L'huillie A, et al. Species variation in the mechanisms of mesenchymal stem cell-mediated immunosuppression. Stem Cells. (2009) 27:1954–62. doi: 10.1002/stem.118
97. Chen J-Y, Li C-F, Kuo C-C, Tsai KK, Hou M-F, Hung W-C. Cancer/stroma interplay via cyclooxygenase-2 and indoleamine 2,3-dioxygenase promotes breast cancer progression. Breast Cancer Res. (2014) 16:410. doi: 10.1186/s13058-014-0410-1
98. Balsamo M, Scordamaglia F, Pietra G, Manzini C, Cantoni C, Boitano M, et al. Melanoma-associated fibroblasts modulate NK cell phenotype and antitumor cytotoxicity. Proc Natl Acad Sci USA. (2009) 106:20847–52. doi: 10.1073/pnas.0906481106
99. Li T, Yang Y, Hua X, Wang G, Liu W, Jia C, et al. Hepatocellular carcinoma-associated fibroblasts trigger NK cell dysfunction via PGE2 and IDO. Cancer Lett. (2012) 318:154–61. doi: 10.1016/j.canlet.2011.12.020
100. Li Y, Tredget EE, Kilani RT, Iwashina T, Karami A, Lin X, et al. Expression of indoleamine 2,3-dioxygenase in dermal fibroblasts functions as a local immunosuppressive factor. J Invest Dermatol. (2004) 122:953–64. doi: 10.1111/j.0022-202X.2004.22409.x
101. François M, Romieu-Mourez R, Li M, Galipeau J. Human MSC suppression correlates with cytokine induction of indoleamine 2,3-dioxygenase and bystander M2 macrophage differentiation. Mol Ther. (2012) 20:187–95. doi: 10.1038/mt.2011.189
102. Heeren AM, van Dijk I, Berry DRAI, Khelil M, Ferns D, Kole J, et al. Indoleamine 2,3-dioxygenase expression pattern in the tumor microenvironment predicts clinical outcome in early stage cervical cancer. Front Immunol. (2018) 9:1598. doi: 10.3389/fimmu.2018.01598
103. Curran T-A, Jalili RB, Farrokhi A, Ghahary A. IDO expressing fibroblasts promote the expansion of antigen specific regulatory T cells. Immunobiology. (2014) 219:17–24. doi: 10.1016/j.imbio.2013.06.008
104. Kadle RL, Abdou SA, Villarreal-Ponce AP, Soares MA, Sultan DL, David JA, et al. Microenvironmental cues enhance mesenchymal stem cell-mediated immunomodulation and regulatory T-cell expansion. PLoS ONE. (2018) 13:e0193178. doi: 10.1371/journal.pone.0193178
105. Yu C-P, Fu S-F, Chen X, Ye J, Ye Y, Kong L-D, et al. The clinicopathological and prognostic significance of IDO1 expression in human solid tumors: evidence from a systematic review and meta-analysis. Cell Physiol Biochem. (2018) 49:134–43. doi: 10.1159/000492849
106. Kim D, Kim JM, Kim J-S, Kim S, Kim K-H. Differential expression and clinicopathological significance of HER2, indoleamine 2,3-dioxygenase and PD-L1 in urothelial carcinoma of the bladder. J Clin Med. (2020) 9:1265. doi: 10.3390/jcm9051265
107. Wang S, Wu J, Shen H, Wang J. The prognostic value of IDO expression in solid tumors: a systematic review and meta-analysis. BMC Cancer. (2020) 20:471. doi: 10.1186/s12885-020-06956-5
108. Hwu P, Du MX, Lapointe R, Do M, Taylor MW, Young HA. Indoleamine 2,3-dioxygenase production by human dendritic cells results in the inhibition of T cell proliferation. J Immunol. (2000) 164:3596–9. doi: 10.4049/jimmunol.164.7.3596
109. Chen W, Liang X, Peterson AJ, Munn DH, Blazar BR. The indoleamine 2,3-dioxygenase pathway is essential for human plasmacytoid dendritic cell-induced adaptive T regulatory cell generation. J Immunol. (2008) 181:5396–404. doi: 10.4049/jimmunol.181.8.5396
110. Dolcetti R, Viel A, Doglioni C, Russo A, Guidoboni M, Capozzi E, et al. High prevalence of activated intraepithelial cytotoxic T lymphocytes and increased neoplastic cell apoptosis in colorectal carcinomas with microsatellite instability. Am J Pathol. (1999) 154:1805–13. doi: 10.1016/S0002-9440(10)65436-3
111. Smyrk TC, Watson P, Kaul K, Lynch HT. Tumor-infiltrating lymphocytes are a marker for microsatellite instability in colorectal carcinoma. Cancer. (2001) 91:2417–22. doi: 10.1002/1097-0142(20010615)91:12<2417::AID-CNCR1276>3.0.CO;2-U
112. Phillips SM, Banerjea A, Feakins R, Li SR, Bustin SA, Dorudi S. Tumour-infiltrating lymphocytes in colorectal cancer with microsatellite instability are activated and cytotoxic. BJS Br J Surg. (2004) 91:469–75. doi: 10.1002/bjs.4472
113. Pagès F, Galon J, Dieu-Nosjean M-C, Tartour E, Sautès-Fridman C, Fridman W-H. Immune infiltration in human tumors: a prognostic factor that should not be ignored. Oncogene. (2010) 29:1093–102. doi: 10.1038/onc.2009.416
114. Llosa NJ, Cruise M, Tam A, Wick EC, Hechenbleikner EM, Taube JM, et al. The vigorous immune microenvironment of microsatellite instable colon cancer is balanced by multiple counter-inhibitory checkpoints. Cancer Discov. (2015) 5:43–51. doi: 10.1158/2159-8290.CD-14-0863
115. Loupakis F, Maddalena G, Depetris I, Murgioni S, Bergamo F, Dei Tos AP, et al. Treatment with checkpoint inhibitors in a metastatic colorectal cancer patient with molecular and immunohistochemical heterogeneity in MSI/dMMR status. J Immunother Cancer. (2019) 7:297. doi: 10.1186/s40425-019-0788-5
116. Tsujii M, Kawano S, DuBois RN. Cyclooxygenase-2 expression in human colon cancer cells increases metastatic potential. Proc Natl Acad Sci USA. (1997) 94:3336–40. doi: 10.1073/pnas.94.7.3336
117. Iñiguez MA, Rodriguez A, Volpert OV, Fresno M, Redondo JM. Cyclooxygenase-2: a therapeutic target in angiogenesis. Trends Mol Med. (2003) 9:73–8. doi: 10.1016/S1471-4914(02)00011-4
118. Kaidi A, Qualtrough D, Williams AC, Paraskeva C. Direct transcriptional up-regulation of cyclooxygenase-2 by hypoxia-inducible factor (HIF)-1 promotes colorectal tumor cell survival and enhances HIF-1 transcriptional activity during hypoxia. Cancer Res. (2006) 66:6683–91. doi: 10.1158/0008-5472.CAN-06-0425
119. Brandacher G, Perathoner A, Ladurner R, Schneeberger S, Obrist P, Winkler C, et al. Prognostic value of indoleamine 2,3-dioxygenase expression in colorectal cancer: effect on tumor-infiltrating T cells. Clin Cancer Res. (2006) 12:1144–51. doi: 10.1158/1078-0432.CCR-05-1966
120. Banzola I, Mengus C, Wyler S, Hudolin T, Manzella G, Chiarugi A, et al. Expression of indoleamine 2,3-dioxygenase induced by IFN-γ and TNF-α as potential biomarker of prostate cancer progression. Front Immunol. (2018) 9:51. doi: 10.3389/fimmu.2018.01051
121. Shirey KA, Jung J-Y, Maeder GS, Carlin JM. Upregulation of IFN-γ receptor expression by proinflammatory cytokines influences IDO activation in epithelial cells. J Interferon Cytokine Res Off J Int Soc Interferon Cytokine Res. (2006) 26:53–62. doi: 10.1089/jir.2006.26.53
122. Tumeh PC, Harview CL, Yearley JH, Shintaku IP, Taylor EJM, Robert L, et al. PD-1 blockade induces responses by inhibiting adaptive immune resistance. Nature. (2014) 515:568–71. doi: 10.1038/nature13954
123. Jacquemier J, Bertucci F, Finetti P, Esterni B, Charafe-Jauffret E, Thibult M-L, et al. High expression of indoleamine 2,3-dioxygenase in the tumour is associated with medullary features and favourable outcome in basal-like breast carcinoma. Int J Cancer. (2012) 130:96–104. doi: 10.1002/ijc.25979
124. Soliman H, Rawal B, Fulp J, Lee J-H, Lopez A, Bui MM, et al. Analysis of indoleamine 2-3 dioxygenase (IDO1) expression in breast cancer tissue by immunohistochemistry. Cancer Immunol Immunother. (2013) 62:829–37. doi: 10.1007/s00262-013-1393-y
125. Patil PA, Blakely AM, Lombardo KA, Machan JT, Miner TJ, Wang L-J, et al. Expression of PD-L1, indoleamine 2,3-dioxygenase and the immune microenvironment in gastric adenocarcinoma. Histopathology. (2018) 73:124–36. doi: 10.1111/his.13504
126. Ishio T, Goto S, Tahara K, Tone S, Kawano K, Kitano S. Immunoactivative role of indoleamine 2,3-dioxygenase in human hepatocellular carcinoma. J Gastroenterol Hepatol. (2004) 19:319–26. doi: 10.1111/j.1440-1746.2003.03259.x
127. Sideras K, Biermann K, Yap K, Mancham S, Boor PPC, Hansen BE, et al. Tumor cell expression of immune inhibitory molecules and tumor-infiltrating lymphocyte count predict cancer-specific survival in pancreatic and ampullary cancer. Int J Cancer. (2017) 141:572–82. doi: 10.1002/ijc.30760
128. Ma W, Duan H, Zhang R, Wang X, Xu H, Zhou Q, et al. High expression of indoleamine 2, 3-dioxygenase in adenosquamous lung carcinoma correlates with favorable patient outcome. J Cancer. (2019) 10:267–76. doi: 10.7150/jca.27507
129. Ferreira JM, Dellê H, Camacho CP, Almeida RJ, Reis ST, Matos YST, et al. Indoleamine 2,3-dioxygenase expression in the prognosis of the localized prostate cancer. Int Urol Nephrol. (2020) 52:1477–82. doi: 10.1007/s11255-020-02414-0
130. Reinhardt HC, Schumacher B. The p53 network: cellular and systemic DNA damage responses in aging and cancer. Trends Genet TIG. (2012) 28:128–136. doi: 10.1016/j.tig.2011.12.002
131. Rubel F, Kern JS, Technau-Hafsi K, Uhrich S, Thoma K, Häcker G, et al. Indoleamine 2,3-dioxygenase expression in primary cutaneous melanoma correlates with breslow thickness and is of significant prognostic value for progression-free survival. J Invest Dermatol. (2018) 138:679–87. doi: 10.1016/j.jid.2017.09.036
132. Kuales MA, Wenzel J, Schmid-Wendtner M-H, Bieber T, von Bubnoff D. Myeloid CD11c+ S100+ dendritic cells express indoleamine 2,3-dioxygenase at the inflammatory border to invasive lower lip squamous cell carcinoma. Histol Histopathol. (2011) 26:997–1006. doi: 10.14670/HH-26.997
133. Liu J, Lu G, Tang F, Liu Y, Cui G. Localization of indoleamine 2,3-dioxygenase in human esophageal squamous cell carcinomas. Virchows Arch. (2009) 455:441–8. doi: 10.1007/s00428-009-0846-3
134. Grohmann U, Volpi C, Fallarino F, Bozza S, Bianchi R, Vacca C, et al. Reverse signaling through GITR ligand enables dexamethasone to activate IDO in allergy. Nat Med. (2007) 13:579–86. doi: 10.1038/nm1563
135. Coquerelle C, Oldenhove G, Acolty V, Denoeud J, Vansanten G, Verdebout J-M, et al. Anti-CTLA-4 treatment induces IL-10-producing ICOS+ regulatory T cells displaying IDO-dependent anti-inflammatory properties in a mouse model of colitis. Gut. (2009) 58:1363–73. doi: 10.1136/gut.2008.162842
136. Johnson BA, Kahler DJ, Baban B, Chandler PR, Kang B, Shimoda M, et al. B-lymphoid cells with attributes of dendritic cells regulate T cells via indoleamine 2,3-dioxygenase. Proc Natl Acad Sci USA. (2010) 107:10644–8. doi: 10.1073/pnas.0914347107
137. Shimura S, Yang G, Ebara S, Wheeler TM, Frolov A, Thompson TC. Reduced infiltration of tumor-associated macrophages in human prostate cancer: association with cancer progression. Cancer Res. (2000) 60:5857–61.
138. Forssell J, Öberg Å, Henriksson ML, Stenling R, Jung A, Palmqvist R. High macrophage infiltration along the tumor front correlates with improved survival in colon cancer. Clin Cancer Res. (2007) 13:1472–9. doi: 10.1158/1078-0432.CCR-06-2073
139. Tsutsui S, Yasuda K, Suzuki K, Tahara K, Higashi H, Era S. Macrophage infiltration and its prognostic implications in breast cancer: the relationship with VEGF expression and microvessel density. Oncol Rep. (2005) 14:425–31. doi: 10.3892/or.14.2.425
140. Herrera M, Herrera A, Domínguez G, Silva J, García V, García JM, et al. Cancer-associated fibroblast and M2 macrophage markers together predict outcome in colorectal cancer patients. Cancer Sci. (2013) 104:437–44. doi: 10.1111/cas.12096
141. Zhang H, Wang X, Shen Z, Xu J, Qin J, Sun Y. Infiltration of diametrically polarized macrophages predicts overall survival of patients with gastric cancer after surgical resection. Gastric Cancer. (2015) 18:740–50. doi: 10.1007/s10120-014-0422-7
142. Lan C, Huang X, Lin S, Huang H, Cai Q, Wan T, et al. Expression of M2-polarized macrophages is associated with poor prognosis for advanced epithelial ovarian cancer. Technol Cancer Res Treat. (2013) 12:259–67. doi: 10.7785/tcrt.2012.500312
143. Honkanen TJ, Tikkanen A, Karihtala P, Mäkinen M, Väyrynen JP, Koivunen JP. Prognostic and predictive role of tumour-associated macrophages in HER2 positive breast cancer. Sci Rep. (2019) 9:1–9. doi: 10.1038/s41598-019-47375-2
144. Adu-Gyamfi CG, Savulescu D, George JA, Suchard MS. Indoleamine 2, 3-dioxygenase-mediated tryptophan catabolism: a leading star or supporting act in the tuberculosis and HIV Pas-de-Deux? Front Cell Infect Microbiol. (2019) 9:372. doi: 10.3389/fcimb.2019.00372
145. Herrera-Rios D, Mughal SS, Teuber-Hanselmann S, Pierscianek D, Sucker A, Jansen P, et al. Macrophages/microglia represent the major source of indolamine 2,3-dioxygenase expression in melanoma metastases of the brain. Front Immunol. (2020) 11:120. doi: 10.3389/fimmu.2020.00120
146. Karihtala K, Leivonen S-K, Brück O, Karjalainen-Lindsberg M-L, Mustjoki S, Pellinen T, et al. Prognostic impact of tumor-associated macrophages on survival is checkpoint dependent in classical hodgkin lymphoma. Cancers. (2020) 12:877. doi: 10.3390/cancers12040877
147. Nakamura K, Smyth MJ. Myeloid immunosuppression and immune checkpoints in the tumor microenvironment. Cell Mol Immunol. (2019) 17:1–12. doi: 10.1038/s41423-019-0306-1
148. Chon SY, Hassanain HH, Gupta SL. Cooperative role of interferon regulatory factor 1 and p91 (STAT1) response elements in interferon-γ-inducible expression of human indoleamine 2,3-dioxygenase gene. J Biol Chem. (1996) 271:17247–52. doi: 10.1074/jbc.271.29.17247
149. Sedlmayr P, Blaschitz A, Stocker R. The role of placental tryptophan catabolism. Front Immunol. (2014) 5:230. doi: 10.3389/fimmu.2014.00230
150. Blaschitz A, Gauster M, Fuchs D, Lang I, Maschke P, Ulrich D, et al. Vascular endothelial expression of indoleamine 2,3-dioxygenase 1 forms a positive gradient towards the feto-maternal interface. PLoS ONE. (2011) 6:e0021774. doi: 10.1371/journal.pone.0021774
151. Munn DH, Zhou M, Attwood JT, Bondarev I, Conway SJ, Marshall B, et al. Prevention of allogeneic fetal rejection by tryptophan catabolism. Science. (1998) 281:1191–3. doi: 10.1126/science.281.5380.1191
152. Mellor AL, Sivakumar J, Chandler P, Smith K, Molina H, Mao D, et al. Prevention of T cell–driven complement activation and inflammation by tryptophan catabolism during pregnancy. Nat Immunol. (2001) 2:64–8. doi: 10.1038/83183
153. Däubener W, Schmidt SK, Heseler K, Spekker KH, MacKenzie CR. Antimicrobial and immunoregulatory effector mechanisms in human endothelial cells. Thromb Haemost. (2009) 102:1110–6. doi: 10.1160/TH09-04-0250
154. Santoso DIS, Rogers P, Wallace EM, Manuelpillai U, Walker D, Subakir SB. Localization of indoleamine 2,3-dioxygenase and 4-hydroxynonenal in normal and pre-eclamptic placentae. Placenta. (2002) 23:373–9. doi: 10.1053/plac.2002.0818
155. Kudo Y, Boyd CAR, Sargent IL, Redman CWG. Decreased tryptophan catabolism by placental indoleamine 2,3-dioxygenase in preeclampsia. Am J Obstet Gynecol. (2003) 188:719–26. doi: 10.1067/mob.2003.156
156. Adam R, Rüssing D, Adams O, Ailyati A, Kim KS, Schroten H, et al. Role of human brain microvascular endothelial cells during central nervous system infection. Thromb Haemost. (2005) 94:341–6. doi: 10.1160/TH05-01-0053
157. Däubener W, Spors B, Hucke C, Adam R, Stins M, Kim KS, et al. Restriction of Toxoplasma gondii growth in human brain microvascular endothelial cells by activation of indoleamine 2,3-dioxygenase. Infect Immun. (2001) 69:6527–31. doi: 10.1128/IAI.69.10.6527-6531.2001
158. Wang Y, Liu H, McKenzie G, Witting PK, Stasch J-P, Hahn M, et al. Kynurenine is an endothelium-derived relaxing factor produced during inflammation. Nat Med. (2010) 16:279–85. doi: 10.1038/nm.2092
159. Darcy CJ, Davis JS, Woodberry T, McNeil YR, Stephens DP, Yeo TW, et al. An observational cohort study of the kynurenine to tryptophan ratio in sepsis: association with impaired immune and microvascular function. PLoS ONE. (2011) 6:e21185. doi: 10.1371/journal.pone.0021185
160. Padberg J-S, Van Meurs M, Kielstein JT, Martens-Lobenhoffer J, Bode-Böger SM, Zijlstra JG, et al. Indoleamine-2,3-dioxygenase activity in experimental human endotoxemia. Exp Transl Stroke Med. (2012) 4:24. doi: 10.1186/2040-7378-4-24
161. Toya T, Sara JD, Corban MT, Taher R, Godo S, Herrmann J, et al. Assessment of peripheral endothelial function predicts future risk of solid-tumor cancer. Eur J Prev Cardiol. (2019) 27:608–18. doi: 10.1177/2047487319884246
162. Tokumoto M, Tanaka H, Tauchi Y, Tamura T, Toyokawa T, Kimura K, et al. Immunoregulatory function of lymphatic endothelial cells in tumor-draining lymph nodes of human gastric cancer. Anticancer Res. (2017) 37:2875–83. doi: 10.21873/anticanres.11640
163. Cui G, Li C, Xu G, Sun Z, Zhu L, Li Z, et al. Tumor-associated fibroblasts and microvessels contribute to the expression of immunosuppressive factor indoleamine 2, 3-dioxygenase in human esophageal cancers. Pathol Oncol Res. (2018) 24:269–75. doi: 10.1007/s12253-017-0244-0
164. Mouratidis PX, George AJ. Regulation of indoleamine 2,3-dioxygenase in primary human saphenous vein endothelial cells. J Inflamm Res. (2015) 8:97–106. doi: 10.2147/JIR.S82202
165. Lahdou I, Engler C, Mehrle S, Daniel V, Sadeghi M, Opelz G, et al. Role of human corneal endothelial cells in T-cell–mediated alloimmune attack in vitro. Invest Ophthalmol Vis Sci. (2014) 55:1213–21. doi: 10.1167/iovs.13-11930
166. Tewalt EF, Cohen JN, Rouhani SJ, Guidi CJ, Qiao H, Fahl SP, et al. Lymphatic endothelial cells induce tolerance via PD-L1 and lack of costimulation leading to high-level PD-1 expression on CD8 T cells. Blood. (2012) 120:4772–82. doi: 10.1182/blood-2012-04-427013
167. Rouhani SJ, Eccles JD, Riccardi P, Peske JD, Tewalt EF, Cohen JN, et al. Roles of lymphatic endothelial cells expressing peripheral tissue antigens in CD4 T-cell tolerance induction. Nat Commun. (2015) 6:1–13. doi: 10.1038/ncomms7771
168. Lane RS, Femel J, Breazeale AP, Loo CP, Thibault G, Kaempf A, et al. IFNγ-activated dermal lymphatic vessels inhibit cytotoxic T cells in melanoma and inflamed skin. J Exp Med. (2018) 215:3057–74. doi: 10.1084/jem.20180654
169. Valkenburg KC, de Groot AE, Pienta KJ. Targeting the tumour stroma to improve cancer therapy. Nat Rev Clin Oncol. (2018) 15:366–81. doi: 10.1038/s41571-018-0007-1
170. Chen X, Song E. Turning foes to friends: targeting cancer-associated fibroblasts. Nat Rev Drug Discov. (2019) 18:99–115. doi: 10.1038/s41573-018-0004-1
171. Horwitz EM, Le Blanc K, Dominici M, Mueller I, Slaper-Cortenbach I, Marini FC, et al. Clarification of the nomenclature for MSC: the International Society for Cellular Therapy position statement. Cytotherapy. (2005) 7:393–5. doi: 10.1080/14653240500319234
172. Phinney DG, Prockop DJ. Concise review: mesenchymal stem/multipotent stromal cells: the state of transdifferentiation and modes of tissue repair—current views. Stem Cells. (2007) 25:2896–902. doi: 10.1634/stemcells.2007-0637
173. Direkze NC, Hodivala-Dilke K, Jeffery R, Hunt T, Poulsom R, Oukrif D, et al. Bone marrow contribution to tumor-associated myofibroblasts and fibroblasts. Cancer Res. (2004) 64:8492–5. doi: 10.1158/0008-5472.CAN-04-1708
174. Mishra PJ, Mishra PJ, Humeniuk R, Medina DJ, Alexe G, Mesirov JP, et al. Carcinoma associated fibroblast like differentiation of human mesenchymal stem cells. Cancer Res. (2008) 68:4331–9. doi: 10.1158/0008-5472.CAN-08-0943
175. Spaeth EL, Dembinski JL, Sasser AK, Watson K, Klopp A, Hall B, et al. Mesenchymal stem cell transition to tumor-associated fibroblasts contributes to fibrovascular network expansion and tumor progression. PLoS ONE. (2009) 4:e4992. doi: 10.1371/journal.pone.0004992
176. Rucker HK, Wynder HJ, Thomas WE. Cellular mechanisms of CNS pericytes. Brain Res Bull. (2000) 51:363–9. doi: 10.1016/S0361-9230(99)00260-9
177. Sims DE. Diversity within pericytes. Clin Exp Pharmacol Physiol. (2000) 27:842–6. doi: 10.1046/j.1440-1681.2000.03343.x
178. Bergers G, Song S. The role of pericytes in blood-vessel formation and maintenance. Neuro-Oncol. (2005) 7:452–64. doi: 10.1215/S1152851705000232
179. Gerhardt H, Betsholtz C. Endothelial-pericyte interactions in angiogenesis. Cell Tissue Res. (2003) 314:15–23. doi: 10.1007/s00441-003-0745-x
180. Armulik A, Abramsson A, Betsholtz C. Endothelial/pericyte interactions. Circ Res. (2005) 97:512–23. doi: 10.1161/01.RES.0000182903.16652.d7
181. Hosaka K, Yang Y, Seki T, Fischer C, Dubey O, Fredlund E, et al. Pericyte–fibroblast transition promotes tumor growth and metastasis. Proc Natl Acad Sci USA. (2016) 113:5618–27. doi: 10.1073/pnas.1608384113
182. Weinlich G, Murr C, Richardsen L, Winkler C, Fuchs D. Decreased serum tryptophan concentration predicts poor prognosis in malignant melanoma patients. Dermatology. (2007) 214:8–14. doi: 10.1159/000096906
183. Corm S, Berthon C, Imbenotte M, Biggio V, Lhermitte M, Dupont C, et al. Indoleamine 2,3-dioxygenase activity of acute myeloid leukemia cells can be measured from patients' sera by HPLC and is inducible by IFN-γ. Leuk Res. (2009) 33:490–4. doi: 10.1016/j.leukres.2008.06.014
184. Folgiero V, Goffredo BM, Filippini P, Masetti R, Bonanno G, Caruso R, et al. Indoleamine 2,3-dioxygenase 1 (IDO1) activity in leukemia blasts correlates with poor outcome in childhood acute myeloid leukemia. Oncotarget. (2013) 5:2052–64. doi: 10.18632/oncotarget.1504
185. Mabuchi R, Hara T, Matsumoto T, Shibata Y, Nakamura N, Nakamura H, et al. High serum concentration of L-kynurenine predicts unfavorable outcomes in patients with acute myeloid leukemia. Leuk Lymphoma. (2016) 57:92–8. doi: 10.3109/10428194.2015.1041388
186. Zhai L, Dey M, Lauing KL, Gritsina G, Kaur R, Lukas RV, et al. The kynurenine to tryptophan ratio as a prognostic tool for glioblastoma patients enrolling in immunotherapy. J Clin Neurosci. (2015) 22:1964–8. doi: 10.1016/j.jocn.2015.06.018
187. Ferns DM, Kema IP, Buist MR, Nijman HW, Kenter GG, Jordanova ES. Indoleamine-2,3-dioxygenase (IDO) metabolic activity is detrimental for cervical cancer patient survival. OncoImmunology. (2015) 4:e981457. doi: 10.4161/2162402X.2014.981457
188. Suzuki Y, Suda T, Furuhashi K, Suzuki M, Fujie M, Hahimoto D, et al. Increased serum kynurenine/tryptophan ratio correlates with disease progression in lung cancer. Lung Cancer. (2010) 67:361–5. doi: 10.1016/j.lungcan.2009.05.001
189. Wang W, Huang L, Jin J-Y, Jolly S, Zang Y, Wu H, et al. IDO immune status after chemoradiation may predict survival in lung cancer patients. Cancer Res. (2018) 78:809–16. doi: 10.1158/0008-5472.CAN-17-2995
190. Botticelli A, Cerbelli B, Lionetto L, Zizzari I, Salati M, Pisano A, et al. Can IDO activity predict primary resistance to anti-PD-1 treatment in NSCLC? J Transl Med. (2018) 16:219. doi: 10.1186/s12967-018-1595-3
191. Sundahl N, De Wolf K, Kruse V, Meireson A, Reynders D, Goetghebeur E, et al. Phase 1 dose escalation trial of ipilimumab and stereotactic body radiation therapy in metastatic melanoma. Int J Radiat Oncol. (2018) 100:906–15. doi: 10.1016/j.ijrobp.2017.11.029
192. Botticelli A, Mezi S, Pomati G, Cerbelli B, Cerbelli E, Roberto M, et al. Tryptophan catabolism as immune mechanism of primary resistance to anti-PD-1. Front Immunol. (2020) 11:1243. doi: 10.3389/fimmu.2020.01243
193. Li H, Bullock K, Gurjao C, Braun D, Shukla SA, Bossé D, et al. Metabolomic adaptations and correlates of survival to immune checkpoint blockade. Nat Commun. (2019) 10:1–6. doi: 10.1038/s41467-019-12361-9
194. Zhou Q-H, Han H, Lu J-B, Liu T-Y, Huang K-B, Deng C-Z, et al. Up-regulation of indoleamine 2,3-dioxygenase 1 (IDO1) expression and catalytic activity is associated with immunosuppression and poor prognosis in penile squamous cell carcinoma patients. Cancer Commun Lond Engl. (2020) 40:3–15. doi: 10.1002/cac2.12001
195. Li H, Ning S, Ghandi M, Kryukov GV, Gopal S, Deik A, et al. The landscape of cancer cell line metabolism. Nat Med. (2019) 25:850–60. doi: 10.1038/s41591-019-0404-8
196. Zhai L, Ladomersky E, Dostal CR, Lauing KL, Swoap K, Billingham LK, et al. Non-tumor cell IDO1 predominantly contributes to enzyme activity and response to CTLA-4/PD-L1 inhibition in mouse glioblastoma. Brain Behav Immun. (2017) 62:24–9. doi: 10.1016/j.bbi.2017.01.022
197. Munn DH, Sharma MD, Lee JR, Jhaver KG, Johnson TS, Keskin DB, et al. Potential regulatory function of human dendritic cells expressing indoleamine 2,3-dioxygenase. Science. (2002) 297:1867–70. doi: 10.1126/science.1073514
198. Tas SW, Vervoordeldonk MJ, Hajji N, Schuitemaker JHN, van der Sluijs KF, May MJ, et al. Noncanonical NF-κB signaling in dendritic cells is required for indoleamine 2,3-dioxygenase (IDO) induction and immune regulation. Blood. (2007) 110:1540–9. doi: 10.1182/blood-2006-11-056010
199. Hwang SL, Chung NP-Y, Chan JK-Y, Lin C-LS. Indoleamine 2, 3-dioxygenase (IDO) is essential for dendritic cell activation and chemotactic responsiveness to chemokines. Cell Res. (2005) 15:167–75. doi: 10.1038/sj.cr.7290282
200. Munn DH, Sharma MD, Mellor AL. Ligation of B7-1/B7-2 by Human CD4+ T cells triggers indoleamine 2,3-dioxygenase activity in dendritic cells. J Immunol. (2004) 172:4100–10. doi: 10.4049/jimmunol.172.7.4100
201. Boasso A, Herbeuval J-P, Hardy AW, Anderson SA, Dolan MJ, Fuchs D, et al. HIV inhibits CD4+ T-cell proliferation by inducing indoleamine 2,3-dioxygenase in plasmacytoid dendritic cells. Blood. (2007) 109:3351–9. doi: 10.1182/blood-2006-07-034785
202. Kavousanaki M, Makrigiannakis A, Boumpas D, Verginis P. Novel role of plasmacytoid dendritic cells in humans: induction of interleukin-10–producing treg cells by plasmacytoid dendritic cells in patients with rheumatoid arthritis responding to therapy. Arthritis Rheum. (2010) 62:53–63. doi: 10.1002/art.25037
203. Yun TJ, Lee JS, Machmach K, Shim D, Choi J, Wi YJ, et al. Indoleamine 2,3-dioxygenase-expressing aortic plasmacytoid dendritic cells protect against atherosclerosis by induction of regulatory T cells. Cell Metab. (2016) 23:852–66. doi: 10.1016/j.cmet.2016.04.010
204. Zhang H, Gregorio JD, Iwahori T, Zhang X, Choi O, Tolentino LL, et al. A distinct subset of plasmacytoid dendritic cells induces activation and differentiation of B and T lymphocytes. Proc Natl Acad Sci USA. (2017) 114:1988–93. doi: 10.1073/pnas.1610630114
205. Chevolet I, Speeckaert R, Schreuer M, Neyns B, Krysko O, Bachert C, et al. Clinical significance of plasmacytoid dendritic cells and myeloid-derived suppressor cells in melanoma. J Transl Med. (2015) 13:9. doi: 10.1186/s12967-014-0376-x
206. Brochez L, Meireson A, Chevolet I, Sundahl N, Ost P, Kruse V. Challenging PD-L1 expressing cytotoxic T cells as a predictor for response to immunotherapy in melanoma. Nat Commun. (2018) 9:2921. doi: 10.1038/s41467-018-05047-1
207. Okła K, Czerwonka A, Wawruszak A, Bobiński M, Bilska M, Tarkowski R, et al. Clinical relevance and immunosuppressive pattern of circulating and infiltrating subsets of myeloid-derived suppressor cells (MDSCs) in epithelial ovarian cancer. Front Immunol. (2019) 10:691. doi: 10.3389/fimmu.2019.00691
208. Holmgaard RB, Zamarin D, Munn DH, Wolchok JD, Allison JP. Indoleamine 2,3-dioxygenase is a critical resistance mechanism in antitumor T cell immunotherapy targeting CTLA-4. J Exp Med. (2013) 210:1389–402. doi: 10.1084/jem.20130066
209. Spranger S, Koblish HK, Horton B, Scherle PA, Newton R, Gajewski TF. Mechanism of tumor rejection with doublets of CTLA-4, PD-1/PD-L1, or IDO blockade involves restored IL-2 production and proliferation of CD8+ T cells directly within the tumor microenvironment. J Immunother Cancer. (2014) 2:3. doi: 10.1186/2051-1426-2-3
210. Monjazeb AM, Kent MS, Grossenbacher SK, Mall C, Zamora AE, Mirsoian A, et al. Blocking indolamine-2,3-dioxygenase rebound immune suppression boosts antitumor effects of radio-immunotherapy in murine models and spontaneous canine malignancies. Clin Cancer Res. (2016) 22:4328–40. doi: 10.1158/1078-0432.CCR-15-3026
211. Beatty GL, O'Dwyer PJ, Clark J, Shi JG, Bowman KJ, Scherle PA, et al. First-in-human phase I study of the oral inhibitor of indoleamine 2,3-dioxygenase-1 epacadostat (INCB024360) in patients with advanced solid malignancies. Clin Cancer Res Off J Am Assoc Cancer Res. (2017) 23:3269–76. doi: 10.1158/1078-0432.CCR-16-2272
212. Gibney GT, Hamid O, Lutzky J, Olszanski AJ, Mitchell TC, Gajewski TF, et al. Phase 1/2 study of epacadostat in combination with ipilimumab in patients with unresectable or metastatic melanoma. J Immunother Cancer. (2019) 7:80. doi: 10.1186/s40425-019-0562-8
213. Perez RP, Riese MJ, Lewis KD, Saleh MN, Daud A, Berlin J, et al. Epacadostat plus nivolumab in patients with advanced solid tumors: preliminary phase I/II results of ECHO-204. J Clin Oncol. (2017) 35:3003. doi: 10.1200/JCO.2017.35.15_suppl.3003
214. Mitchell TC, Hamid O, Smith DC, Bauer TM, Wasser JS, Olszanski AJ, et al. Epacadostat plus pembrolizumab in patients with advanced solid tumors: phase I results from a multicenter, open-label phase I/II trial (ECHO-202/KEYNOTE-037). J Clin Oncol. (2018) 36:3223–30. doi: 10.1200/JCO.2018.78.9602
215. Long GV, Dummer R, Hamid O, Gajewski TF, Caglevic C, Dalle S, et al. Epacadostat plus pembrolizumab versus placebo plus pembrolizumab in patients with unresectable or metastatic melanoma (ECHO-301/KEYNOTE-252): a phase 3, randomised, double-blind study. Lancet Oncol. (2019) 20:1083–97. doi: 10.1016/S1470-2045(19)30274-8
216. Muller AJ, Manfredi MG, Zakharia Y, Prendergast GC. Inhibiting IDO pathways to treat cancer: lessons from the ECHO-301 trial and beyond. Semin Immunopathol. (2019) 41:41–8. doi: 10.1007/s00281-018-0702-0
217. Chevolet I, Schreuer M, Speeckaert R, Neyns B, Hoorens I, van Geel N, et al. Systemic immune changes associated with adjuvant interferon-α2b-therapy in stage III melanoma patients: failure at the effector phase? Melanoma Res. (2015) 25:357–61. doi: 10.1097/CMR.0000000000000171
218. Siu L, Gelmon K, Chu Q, Pachynski R, Alese O, Basciano P, et al. BMS-986205, an optimized indoleamine 2,3-dioxygenase 1 (IDO1) inhibitor, is well tolerated with potent pharmacodynamic (PD) activity, alone and in combination with nivolumab (nivo) in advanced cancers in a phase 1/2a trial. Cancer Res. (2017) 77:CT116. doi: 10.1158/1538-7445.AM2017-CT116
219. BMS-986205 and Nivolumab as First or Second Line Therapy in Treating Patients With Liver Cancer. Available online at: https://clinicaltrials.gov/ct2/show/NCT03695250 (accessed June 18, 2020).
220. Study of BMS-986205 and Nivolumab in Endometrial Cancer or Endometrial Carcinosarcoma That Has Not Responded to Treatment. Available online at: https://clinicaltrials.gov/ct2/show/NCT04106414 (accessed June 18, 2020).
221. Nivolumab and BMS986205 in Treating Patients With Stage II-IV Squamous Cell Cancer of the Head and Neck. Available online at: https://clinicaltrials.gov/ct2/show/NCT03854032 (accessed June 18, 2020).
222. Metz R, Rust S, DuHadaway JB, Mautino MR, Munn DH, Vahanian NN, et al. IDO inhibits a tryptophan sufficiency signal that stimulates mTOR. Oncoimmunology. (2012) 1:1460–8. doi: 10.4161/onci.21716
223. Fox E, Oliver T, Rowe M, Thomas S, Zakharia Y, Gilman PB, et al. Indoximod: an immunometabolic adjuvant that empowers T cell activity in cancer. Front Oncol. (2018) 8:370. doi: 10.3389/fonc.2018.00370
224. Prendergast GC, Malachowski WJ, Mondal A, Scherle P, Muller AJ. Indoleamine 2,3-dioxygenase and its therapeutic inhibition in cancer. Int Rev Cell Mol Biol. (2018) 336:175–203. doi: 10.1016/bs.ircmb.2017.07.004
Keywords: IDO, kynurenine, tryptophan, cancer, indoleamine (2,3)-dioxygenase, tumor immunity
Citation: Meireson A, Devos M and Brochez L (2020) IDO Expression in Cancer: Different Compartment, Different Functionality? Front. Immunol. 11:531491. doi: 10.3389/fimmu.2020.531491
Received: 31 January 2020; Accepted: 25 August 2020;
Published: 24 September 2020.
Edited by:
Giovanna Schiavoni, National Institute of Health (ISS), ItalyReviewed by:
Chai K. Lim, Macquarie University, AustraliaCopyright © 2020 Meireson, Devos and Brochez. This is an open-access article distributed under the terms of the Creative Commons Attribution License (CC BY). The use, distribution or reproduction in other forums is permitted, provided the original author(s) and the copyright owner(s) are credited and that the original publication in this journal is cited, in accordance with accepted academic practice. No use, distribution or reproduction is permitted which does not comply with these terms.
*Correspondence: Lieve Brochez, bGlldmUuYnJvY2hlekB1Z2VudC5iZQ==
Disclaimer: All claims expressed in this article are solely those of the authors and do not necessarily represent those of their affiliated organizations, or those of the publisher, the editors and the reviewers. Any product that may be evaluated in this article or claim that may be made by its manufacturer is not guaranteed or endorsed by the publisher.
Research integrity at Frontiers
Learn more about the work of our research integrity team to safeguard the quality of each article we publish.