- 1Beijing Hospital of Traditional Chinese Medicine, Capital Medical University, Beijing, China
- 2Basic Medical College, Taishan Medical University, Tai'an, China
- 3Beijing Chinese Medicine Hospital PingGu Hospital, Beijing, China
- 4Shunyi Branch, Beijing Hospital of Traditional Chinese Medicine, Beijing, China
- 5Section of Nephrology, Department of Internal Medicine, Yale University School of Medicine, New Haven, CT, United States
Idiopathic membranous nephropathy (IMN) is a pathological pattern of glomerular damage caused by an autoimmune response. Immune complex deposition, thickness of glomerular basement membrane, and changes in the podocyte morphology are responsible for the development of proteinuria, which is caused by the targeted binding of auto-antibodies to podocytes. Several auto-antigens have recently been identified in IMN, including M-type receptor for secretory phospholipase A2 (PLA2R1), thrombospondin type-1 domain-containing 7A (THSD7A), and neural epidermal growth factor-like 1 protein (NELL-1). The measurement of peripheral circulating antibodies has become an important clinical reference index. However, some clinical features of IMN remain elusive and need to be further investigated, such as the autoimmunity initiation, IgG4 predominance, spontaneous remission, and the unique glomerular lesion. As these unresolved issues are closely related to clinical practice, we have proposed a hypothetical pathogenesis model of IMN. Induced by environmental stimuli or other causes, the PLA2R1 antigen and/or THSD7A antigen exposed to extrarenal tissues, such as lungs, then produce the auto-antibodies that target and cause damage to the podocytes in circulation. In this review, we highlighted the potential association between environmental stimuli, immune activity, and glomerular lesions, the underlying basis for spontaneous immune and proteinuria remission.
Introduction
Membranous nephropathy (MN) is one of the major glomerular diseases in adults, accounting for 20–30% of glomerular disease cases (1, 2). MN is pathologically characterized by the accumulation of immune complex deposits outside the glomerular basement membrane (GBM), which is adjacent to podocytes. The thickening GBM appears to have a “spike” to enfold the deposits (3). Immune complex deposits contain the antigens in situ, immunoglobulins (Ig) G binding to antigens, and membrane attack complexes (MAC) formed by complement activation, which are the traces left by the antibody-dependent immune response and the major basis for the current understanding of the pathogenesis of MN (4). This unique glomerular lesion is thought to be associated with many causes. According to patient histories and clinical manifestations, about 20% of MN can be attributed to clinical diseases, such as hepatitis B infection, systemic lupus erythematosus, cancer, or drug side-effects, which are known as secondary MN. In addition, about 80% of MN are clinically unable to be identified by the secondary factors, which are known as idiopathic or primary MN (IMN or PMN) (4). IMN is thought to be caused by IgG that target podocytes; the predominant form of IgG is considered to be IgG4 (5, 6). The podocyte auto-antigens identified in adult IMN include M-type receptor for secretory phospholipase A2 (PLA2R1) (7), thrombospondin type-1 domain-containing 7A (THSD7A) (8), and neural epidermal growth factor-like 1 protein (NELL-1) (9), which accounts for 70–80, 3–5, and 5–10% of IMN, respectively. Moreover, the measurement of antibodies against PLA2R1 (aPLA2R1ab) in peripheral blood has been widely used in clinical diagnosis (10), prediction, and treatment guidance (11). The natural history of the untreated MN is variable; spontaneous remission occurs in 40–50% of patients and the remaining patients progress slowly to end-stage renal disease (ESRD) or decease due to complications or any other unrelated underlying diseases after 5–15 years (12). IMN recurs in about half of kidney allograft recipients, which leads to graft dysfunction and failure (13).
The understanding of the pathogenesis has been improving in the last two decades (14). However, many factors in IMN still need to be further clarified. For instance, how does the immune response initiate the produce of the auto-antibodies against podocytes? Why are the auto-antibodies predominately IgG4? And how does the spontaneous remission occur in some patients? This review attempts to propose a hypothetical pathogenesis model to answer these issues while summarizing the main findings from the relevant available literature.
Extrarenal Autoimmune Response Can Cause IMN
IMN is mainly considered to be an organ-restricted autoimmune glomerular disease due to the discovery of podocyte auto-antigens such as PLA2R1 (3). However, there is currently a lack of evidence that IMN is an autoimmune disease induced by in situ podocyte auto-antigens. In addition, Xu et al., van de Logt et al., and our team have recently proposed the hypothesis of IMN pathogenesis induced by an extrarenal autoimmune response (mainly in the lungs) (14–16). Despite the current lack of direct evidence, we can speculate on the feasibility of this hypothesis from the following topics.
Circulating Antibodies Against Podocyte Exteriors of the Kidney Cause IMN Development: Hints From Previous Studies
Heymann nephritis, the first experimental MN induced in rats, was described in 1959, and was then known as active Heymann nephritis (AHN), which was immunized by an insoluble subcomponent of the brush border of the proximal tubule, called fraction 1A (Fx1A). Subsequently, it was found that injection of anti-Fx1A IgG in rats could induce the analogous lesion more rapidly, which was named as passive Heymann nephritis (PHN) (4). In 1978, two different teams from Couser and Hoedemaeker infused anti-Fx1A IgG into isolated rat's kidneys ex vivo to study pathological changes of IMN; the idea that extrarenal circulating antibodies bind podocyte auto-antigens to form in situ immune complexes was proposed first (17, 18). Later, the major antigenic proteins in Fx1A were identified as megalin, which is a podocyte membrane receptor of low-density lipoproteins (19, 20). IgG passes through the glomerular endothelial cells and GBM freely, thus binding to megalin on the surface of podocytes, which in turn forms PHN (21). Other experimental MN induced by injection of exogenous anti-podocyte antibodies were carried out, such as a mice model of anti-podocyte glomerulonephritis, THSD7A homologous rodent MN, and transgenic mice MN with PLA2R1, which are similar to that of PHN (22–24). These findings suggest that extrarenal circulating antibodies against podocytes can induce MN-like pathological changes in normal kidneys. And, this phenomenon is not only limited to experimental MN.
In 2002, the neutral endopeptidase (NEP) was identified by Ronco's team in neonatal MN (25); it supports the idea that the circulating anti-podocyte antibodies can be produced without the involvement of the kidney. Mothers with NEP deficiency were exposed to NEP during a previous spontaneous abortion or pregnancy, and their immune systems produced anti-NEP antibodies afterwards, which crossed the placenta barrier and bound to the fetal glomerular podocytes during pregnancy (26). Similar to PHN and other above-mentioned experimental MN models, nephritogenic antibodies of neonatal MN are produced not by the person themselves but by the immune system of nonego, which can be classified as “passive MN.” These previous studies suggest that IMN can be induced by an autoimmune response outside the kidney.
Potential Relationship Between Extrarenal Disease, Environment, and Pathogenesis of IMN
After the identification of auto-antigens such as PLA2R1, the pathogenesis of IMN was considered as primary MN (3). However, when idiopathic and secondary MN are distinguished using circulatory aPLA2R1ab, its diagnostic specificity is relatively inadequate. It was reported that 9 out of 32 patients with secondary MN presented with positive anti-PLA2R1, including 7 cancer patients, 1 Crohn's disease patient, and 1 patient with scleroderma (27). Furthermore, the result from another cohort showed that, among the 24 patients with psoria-associated MN, 7(29.2%) patients had positive anti-PLA2R1 (28). And, in 39 patients of hepatitis B virus-associated MN, 25(64.1%) patients with aPLA2R1ab positive were found (29). Also, among the 37 patients with membranous lupus nephritis, 7(18.9%) patients were found to be anti-PLA2R1. More interestingly, 3 patients with non-renal lupus disease were found to be anti-PLA2R1 positive (30). There is a possibility that PLA2R1-associated MN coexists with these extrarenal diseases, as the causal relationship between them cannot be completely excluded (31).
The causal relationship in THSD7A-associated MN is more obvious. Although the association between THSD7A and cancer is disputed (32, 33), THSD7A can be detected in the tumor, metastatic lymph node cells, and dendritic cells of lymph nodes (34, 35). In a cohort of breast and colorectal tumor patients, THSD7A is also expressed in breast tumor tissues (20/20, 100% patients positive) and colorectal tumor tissues (79/81, 97.5% patients positive). More importantly, in 3 of the 4 tumor patients with proteinuria who were followed up, proteinuria was in complete remission within 6 months after tumor resection without any other interventions (36). However, the kidney disease of these 4 patients were undiagnosed, even though THSD7A is currently considered only associated with IMN. A patient who had repeated renal biopsy showed negative expression of THSD7A in their kidney tissue in the first biopsy, however the second renal biopsy showed the MN lesion with positive THSD7A and manifested malignant tumor after 17 months. After 4 months, the serum THSD7A auto-antibody showed negative after surgical resection and chemotherapy with paclitaxel liposome carboplatin (37). More interestingly, inspired by two cases of THSD7A-associated MN accompanied by angiolymphoid hyperplasia with eosinophilia (ALHE), the researchers assumed that vascular endothelial growth factor A (VEGF-A) up-regulated the expression of THSD7A in endothelial cells in vitro (38). The tumor growth is closely related to angiogenesis; VEGF is secreted by cancer cells and stromal cells stimulate the proliferation and survival of endothelial cells, leading to the formation of new blood vessels (39). This may be one of the ways for THSD7A to establish the relationship between tumor and IMN. Extrarenal diseases may induce IMN by affecting these auto-antigens expressed outside the kidney. The autoimmune response of IMN (or primary MN) may not be limited to the kidneys, when precisely observed.
The pathogenesis of IMN has been associated with environmental stimuli (40). The morbidity of MN in China has been gradually increasing, which could be possibly related to the long-term exposure to air pollution. Each 10 mg/m3 increase in PM2.5 concentration over 70 mg/m3 is related with 14% higher odds for MN (2). It is speculated that air pollution can be involved in the pathogenesis of IMN by inducing inflammation and oxidative stress in the lungs (14–16), it can also activate antigen presenting (APC) cells and autoreactive T cells in the inflammatory microenvironment. In addition, helicobacter pylori (HP) is closely related to chronic mucosal inflammation in the stomach and duodenum (41), and MN patients are also closely related to gastric HP infection (42–44). Consistent with these speculations, two risk alleles for primary MN have been recently identified in NFKB1 and IRF4, and both of them were involved in defense against common pathogens. It is worth mentioning that all four genome-wide significant risk loci (PLA2R1, IRF4, NFKB1, and HLA) with highly pleiotropic effects identified in this extensive genetic study have a concordant effect on the risk of inflammatory bowel disease (IBD); this fact suggests that there is a shared pathogenic mechanism between IBD and IMN (45). Thus, inflammation can be one of the pathways for environmental stimuli and extrarenal diseases, which links to the pathogenesis of IMN.
Non-inflammatory Glomerular Lesion of IMN Might Not Be Consistent With in situ Induced Organ-Specific Autoimmune Diseases
Unlikely most organ-specific autoimmune diseases induced by in situ auto-antigen exposure, such as diabetes mellitus type 1, inflammatory bowel disease (IBD), multiple sclerosis, and psoriasis, in which affected organs are infiltrated with immune cells (46–49), the kidney pathological manifestations of IMN shows almost no inflammatory cells' infiltration except for complement activation (4). As mentioned above, the pathogenesis of IMN is probably associated with inflammation, and it is difficult to imagine an autoimmune response induced by auto-antigen exposure in glomerulus without any involvement of immune cells. There is a hypothesis that a soluble form of podocyte auto-antigen, such as soluble PLA2R1, sheds and induces an autoimmune response (4), which could partly explain the non-inflammatory lesion to the glomerulus. However, direct evidence of an autoimmune response induced by soluble PLA2R1 is still lacking, and this hypothesis is poor. Firstly, it is unlikely that podocytes will release large amounts of soluble PLA2R1 without being affected. If so, the presence of proteinuria should predate the autoimmune response. Secondly, soluble PLA2R1 should be distributed in the blood vessels according to the concentration gradient; the closer one goes to the podocytes, the higher the concentration, which also possibly induces the immune cells to approach the glomerulus and deposit circulating immune complexes at multiple sites in the glomerulus. IMN caused by an extrarenal autoimmune response can be a better explanation for these kidney pathological manifestations. In addition, the deposition of extrarenal IgG4 also further inhibits the inflammation in the glomerulus (detailed below).
Auto-Antigens Associated With IMN Pathogenesis Expresses in Many Tissues Including the Kidneys
Besides the kidney podocyte, PLA2R1 also express in the lungs, placenta, liver, and skeletal muscles (50). PM2.5 induces inflammatory responses in the lungs, causing infiltration of inflammatory cells (51, 52), such as neutrophils and alveolar macrophages, which also express PLA2R1 (53, 54). The extrarenal expression of PLA2R1 provides the basis for extrarenal aPLA2R1ab production. Interestingly, the production of aPLA2R1ab does predict significant kidney damage. Recently, Burbelo et al. reported that aPLA2R1ab were detectable at a median of 274 days before renal biopsy diagnosis (interquartile range, 71–821 days) and aPLA2R1ab seropositivity occurred closely or before the pre-diagnostic non-nephrotic range proteinuria in the majority of cases (55). The aPLA2R1ab is considered directly pathogenic and the kidneys can just be a target. The direct evidence of humoral immunity induced by exposure to the lung's PLA2R1 is required for further studies.
THSD7A was initially characterized as an endothelial protein that is expressed in the placental vasculature, which can be involved in promoting endothelial cell migration during angiogenesis (56–58). Angiogenesis mostly occurs in tissue repair or tumor metastasis, and in this phenomenon, both inflammation and active immune response are down-regulated, which is also consistent with the immunosuppressive effect of VEGF (39). The immune induction phenomena of THSD7A seems to be different from that of PLA2R1.
NELL-1 is a newly discovered third auto-antigen of IMN podocytes (9), which is highly expressed in osteoblasts and promotes bone regeneration. The C-terminal region of NELL-1 mediates osteoblastic cell adhesion through integrin α3β2 (59, 60). NELL-1 is overexpressed in patients with craniosynostosis, one of the most common congenital craniofacial deformities, where it is specifically up-regulated within prematurely fusing sutures (61, 62). It indicates that different auto-antigens in IMN have different expression phenomena, and these different phenomena that affect the exposure of auto-antigens are potentially mutually exclusive. IMN may be the same pathological pattern of different diseases. Thus, it is necessary to figure out that different phenomena of different auto-antigens initiating extrarenal autoimmune response leads to IMN.
Auto-Antigens and Extrarenal Autoimmune-Inducing Phenomena
The autoimmune response is the result of a combination of factors, and the fundamental question regards how self-tolerance fails and how self-reactive lymphocytes are activated. Despite the lack of research on these topics in IMN, we can still try to figure out some important questions on the basis of our current understanding.
Characteristics of Auto-Antigens
One of the key preconditions for an extrarenal autoimmune response that involves the kidney is that these antigenic proteins must be expressed in the kidney and, more precisely, in the podocytes. Virtually all structures in the glomerulus and all domains and micro-domains of the endothelium and podocyte are accessible to the circulating antibodies. More importantly, the fate of immune complexes formed by binding to glomerular components varies with the location of the glomerulus antigens, the basal domain, and/or the slit diaphragm, which persists longer than the others (63). This finding may suggest that the location of auto-antigenic proteins may determine their ability to cause damage to podocytes. Recently, using a variety of microscopes, it was found that THSD7A localizes at the basal aspect of foot processes, closely following the meanders of the slit diaphragm in human and mice, and the anti-THSD7A antibodies bind THSD7A expressed on the slit diaphragm (64). In human podocytes, THSD7A expression is accentuated at filopodia and thin arborized protrusions, an expression pattern associated with the decreased membrane activity of cytoskeletal regulators. Phenotypically, THSD7A expression in human podocytes is associated with an increase in cell size and enhanced adhesion to collagen type IV-coated plates (64). Again, due to the lack of evidence on the precise localization of PLA2R1, it is currently reasonable to believe in renal pathology that PLA2R1 could also express at the basal domain or slit diaphragm of podocytes and can disrupt the function of podocytes, such as their adhesion ability to collagen type IV (GBM) (65). The uniformity of NELL-1 staining along the GBM and correlation with the subepithelial deposit suggests that this protein is shed from the podocytes (9). It is unlikely that NELL-1 is shed from mesangial cells or endothelial cells, since there was no mesangial or subendothelial staining in the NELL-1 positive MN. Nevertheless, NELL-1 is also likely present as an extracellular component and can deposit in the GBM (9). These antigens are restricted to express in the space between podocytes and GBM, which could also be the reason for the limitation of pathological manifestations.
Another key precondition is that the autoimmune-inducing phenomena outside the kidney must specifically induce exposure of these antigens, such as conformational changes, molecular simulations, or up-regulated expression. From the mutual exclusion of these antigenic proteins in IMN, it seems that the immune-inducing environment of different antigens is also mutually exclusive. Interestingly, the subclass of IgG deposition of NELL-1-associated MN is mainly IgG1, which is inconsistent with PLA2R1-and THSD7A-associated MN with the IgG4 as the principal antibody (9). It suggests that there is heterogeneity in the autoimmune process of IMN, and needs to be further subdivided according to different immune processes in future studies. In other words, the variety of auto-antigens can be selected by different immune-induced phenomena.
PLA2R1-Autoimmune Response, Inflammation, and Genetic Susceptibility
PLA2R1 belongs to the mannose receptor family and is a type I transmembrane glycoprotein with 185kDa, whose extracellular part is composed of N-terminal cysteine-rich (CysR, or ricin B) domain, a single fibronectin type II (FnII) domain, and 8 C-type lectin-like domains (CTLDs) (66). The four identified domains containing major B-cell epitopes are CysR, CTLD1, CTLD7, and CTLD8, and epitope antigenicity in these domains is all determined by spatial conformation (67, 68). The N-terminal region of PLA2R1 is the predominant target of autoimmunity, as is THSD7A, and epitope recognition evolves over time and as the disease worsens (69–71). Patients with epitope spreading usually have more severe disease and worse prognosis (Figure 1). In general, PLA2R1 acts as the receptor mediating sPLA2 endocytosis, continuously trafficking between the cell membrane and the endosome to transport sPLA2 (50, 66, 72). Beyond mediating sPLA2, the current understanding of the biological function of human PLA2R1 is quite limited. It is speculated that human PLA2R1 is closely related to inflammation.
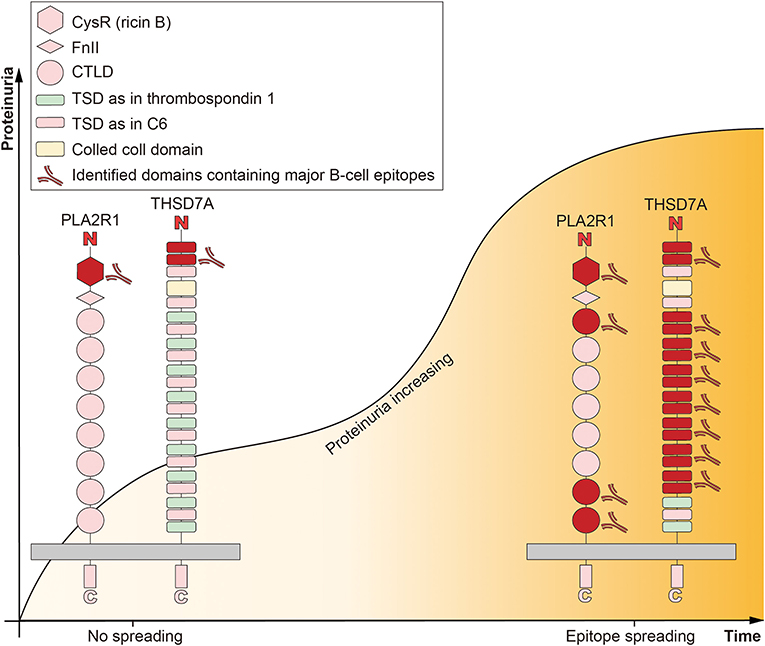
Figure 1. Epitope spreading accompanied with diseases progression in idiopathic membranous nephropathy. The extracellular part of M-type receptor for secretory phospholipase A2 (PLA2R1) is composed of N-terminal cysteine-rich (CysR, or ricin B) domain, a single fibronectin type II (FnII) domain, and 8 C-type lectin-like domains (CTLDs). The four identified domains containing major B-cell epitopes are CysR, CTLD1, CTLD7, and CTLD8. CysR is the predominant target of autoimmunity. The extracellular part of thrombospondin type-1 domain-containing 7A (THSD7A) is composed of a colled coll domain and 21 thrombospondin type 1 domains (TSDs). These TSDs show high structural homology either to the TSDs of thrombospondin 1 or complement component 6 (C6). The predominant target of autoimmunity is exists in the N-terminal region. Epitope spreading is defined as the diversification of epitope specificity from the initial focused, dominant epitope-specific immune response, to subdominant and/or cryptic epitopes, which accompanied by maturation of the immune response and progression of the disease (proteinuria increasing).
Either through ovalbumin-induced lung inflammation or murine α-myosin heavy chain-induced autoimmune myocarditis, the affected tissues in the PLA2R1-deficiency mice show more inflammatory cell infiltration and more severe inflammatory response (73, 74). Furthermore, rat PLA2R1 expression was up-regulated in an inflammatory environment (for example, PLA2R1 expressed on lymphocytes and granulocytes can be up-regulated by interleukin (IL)-1b in vitro) (75). However, these studies have limitations since the rodent kidney does not express PLA2R1. Human PLA2R1 can also be involved in inflammatory processes, such as the potential function of PLA2R1 expressed on neutrophils and alveolar macrophages (53, 54). Human PLA2R1 is also closely related to asthma. PLA2R1 is differentially overexpressed in bronchial epithelial brushings of children with atopic asthma (76); it is also believed that there could be a potential involvement of risk alleles on PLA2R1 in adults with asthma who have occupational exposure, which plays an important role in NF-κB pathway, leading to inflammation (77). We hypothesized that human PLA2R1 can be up-regulated in an inflammatory environment, which can be related to the autoimmune-induced environment of PLA2R1 in IMN.
Genome-wide association studies (GWASs) clarified the understanding of IMN genetics. The two risk alleles in HLA-DQA1 and PLA2R1 are closely related to IMN development, and the same results have been obtained in multiple interracial genetic studies (45). Recently, NFKB1 and IRF4, the novel genome-wide significant risk loci for MN have been identified, with large effects encoding two transcriptional master regulators of inflammation. Due to the bi-ethnic composition of the cohort, the ethnicity-specific effects at the HLA locus can be further defined. DRB1*1501 is a major risk allele in East Asians, DQA1*0501 in Europeans, and DRB1*0301 in both ethnicities, which suggests that different epitopes are likely presented to T cells to initiate the anti-PLA2R1 response in East Asians and Europeans. In line with previous studies, a single haplotype at the PLA2R1 locus exhibits genetic interactions with HLA-DRB1 risk alleles in both ethnicities, the three amino acid residues encoded by DRB1*1501 and DRB1*0301 in the DRβ1 chain of major histocompatibility complex (MHC) class II promoting epitope presentation to T cells, which could be the cause of IMN susceptibility (45, 78).
Under the activation of antigenic peptides and MHC class II molecules, CD4+ T cells differentiate into several subtypes. This process is regulated by many cytokines produced by innate immune cells. Previous studies have observed that IMN is dominated by Th2 type immune response with increased IL-4 production of peripheral Th cells, and increased IL-13 mRNA expression of peripheral blood monocytes in patients (79, 80). Rosenzwajg et al. and Roccatello et al. described a low level of regulatory T (Treg) cells in two cohorts of IMN (n = 25 and n = 17, respectively) at diagnosis and an increasing level of these cells after remission induced by rituximab, associated with low levels of a Treg cytokine IL-35 that also increases with remission (81, 82). Although the effector cells that induce T cell differentiation in IMN and their immune-induced microenvironment are not yet clear, the above data also suggest an inflammation tendency in IMN.
IgG4 Dominate and the Role of IgG4
IMN is considered an IgG4-dominant disease (7, 8). In three IMN cohorts of detected peripheral circulating IgG subclasses, all the positive rates of IgG4 were the highest, with the proportions of 89% (83), 94% (84), and 100% (85), respectively, and the titer of IgG4 was also the highest. Among other IgG subclasses, IgG1 also accounts for a higher percentage in IMN patients, but the titer is relatively insufficient (84). Interestingly, the deposited IgG subclass in the glomerulus indicates the Ig subclass switch as the disease progresses. In the early stage (electron microscopy stage I), IgG1 is the major IgG subclass, and IgG4 dominates in all later stages (electron microscopy stage II-IV) (by pathological criteria according to Ehrenreich and Churg, IMN is divided into four stages) (86). Correspondingly, IgG1, IgG2, IgG3, and IgG4 coexist in most patients at the first stage of the disease, while at relapse, IgG4 was the predominant subclass (87). When comparing with other IgG subclasses, IgG4 is usually unable to activate complement and has a higher affinity for antigens (88, 89). Exostosin 1 (EXT1)/exostosin 2 (EXT2)-associated MN represent a secondary form of MN and the antibody in EXT1/EXT2-associated MN it is IgG1 (90). Ravindran et al. described a high spectral count of complement proteins C3, C4, C5, C6, C7, C8, and C9 in glomerular that both exist in PLA2R-associated and EXT1/EXT2-associated MN by using mass spectrometry. However, complement protein C1 was present in low spectral counts in EXT1/EXT2-associated MN, which appeared higher than in PLA2R-associated MN (91). Each of the three complement pathways seem to be involved in the glomerular complement activation, while classical pathways may not be evident when IgG4 is dominant (87, 91), which suggest that the respective antibody titers and ratios between the different IgG subclasses will determine the involvement of different pathways of complement activation. Thus, it is necessary to discuss the potential causes of Ig class switching of antibodies in IMN.
Reasons for IgG4 Domination: Anti-inflammatory and Increased Affinity
The diversity of the human antibody repertoire is generated by V(D)J gene rearrangement that gives antibodies their complex array of effector functions, and accumulation of VDJ somatic point mutations are generally considered to boost higher affinity antibodies through selection within the germinal centers (88). IgM > IgG3 > IgG1 > IgG2 > IgG4 is considered to be the temporal model sequence of Ig class switching in the germinal center reaction. Differences in epitopes' affinity, complement fixation ability, and Fc receptor (FcR) binding ability between these subclasses could help to coordinate the inflammation and humoral defenses over the time course of a response (88). At the early stage of germinal center reaction, IgM+ B cells switch to IgG3, which recruits FcγR-mediated functions in the early response. IgG1 then emerges as the major effector of antigen clearance, and subsequently IgG2 competes with IgG1 to produce immune complexes which slows the inflammatory drive. Persisting antigens can finally stimulate high affinity IgG4 that outcompetes other isotypes (88, 92). IgG4 may inhibit the binding of other IgG subclasses to antigens by its high affinity, reaching a certain proportion in the formed immune complex and blocking FcyR-mediated processes, such as phagocytosis and release of pro-inflammatory cytokines (88). IgG4 response is often formed by following repeated or long-term exposure to antigen (89, 93). For instance, IgG4 plays a protective role in allergies, with prolonged or repeated allergen exposure and increased production of IL-10, and IgG4 gradually increases and blocks IgE to inhibit excessive allergic reactions (94). And, in asymptomatic filarial infection, elevations in IgG4 are also often associated with high worm loads and with high plasma levels of IL-10 (95). As a key cytokine for immune regulation, IL-10 plays an important role in inhibiting the overreaction to antigens, including inflammation and adaptive immune responses (96). IL-10 is also considered to specifically modulate the differentiation of B cells into IgG4-responsive types (97, 98). Up-regulation of IL-10 mRNA expression was observed in peripheral blood monocytes in IMN patients (80). During the early stage of IMN, patients have higher counts of M2-like monocytes, higher levels of serum IL-10, and the IL-10+ M2 counts were positively correlated with disease activity (99), which could suggest that antibody class switching is driven by innate immunity in the early stage of IMN. In general, the anti-inflammatory properties of IgG4 and its high affinity to antigens are responsible for its delay appearance and dominant role in the active immune process (88, 89, 93).
Hypothetical Model: Ig Class Switching of Antibody and IMN Disease Progression
As mentioned above, IMN is closely related to inflammation, environmental stimuli, and genetic susceptibility. Pathogens or PM2.5, as the continuous stimulus, often induce constant local chronic inflammation. It also leads to the accumulation and continuous activation of macrophages at the inflammatory site, so as to cause bystander damage (100–102). In inflammatory conditions, auto-antigenic proteins can induce autoimmune diseases by altering conformation and epitopes, dissociating from tissues, up-regulating expression, or further modifying auto-antigens, while exposed to the immune system (103–105). Even the inflammatory cells themselves can be the sources of auto-antigens (both neutrophils and alveolar macrophages expressing PLA2R1) in IMN (14). The exposure of autoantigens then aggravates the local inflammation, which leads to the formation of a vicious circle (105). Under the stimulation of environmental factors, the function of immune cells will also be affected, such as the auto-reactive T cells and the antigen presenting cells, thus increasing the risk of loss of self-tolerance abilities (106, 107). This will subsequently induce the humoral immunity targeted to the extrarenal auto-antigen (PLA2R1 or THSD7A), which is programed to control the local inflammation and tissue damage.
Based on this understanding, we have further speculated about the development of IMN in this section, although it remains just a hypothesis. In the very early stages of IMN, anti-PLA2R1/THSD7A IgM or IgG3 could have been deposited in glomeruli in very small quantities. Since the source of the immune response is not present in the kidney, only very small quantities of IgM or IgG3 and the subsequent potential complement activation could cause previous damage to the podocytes. However, their low affinity makes it difficult for them to bind to the antigens which are naturally expressed in podocytes for a long time. Separate from IgM and IgG3; IgG1 has a high affinity for antigens, when the antibody subclass switch to IgG1 predominantly, the IgG1 in plasma can deposit in the glomerulus in large quantities, inducing early IMN (stage I). The original purpose of IgG1 production is to drive the inflammation and antigen clearance in the extrarenal site, while the immune system also aims to block the excessive inflammatory response simultaneously, resulting in the production of IgG2 and IgG4 (88, 89). IgG4 achieves bi-specificity through its Fab exchange, which can make a high-efficiency, high-affinity blocking antibody, inhibiting the excessive inflammation (108). Moreover, IgG4 can competitively inhibit IgG1 binding to antigens with its higher affinity, thus achieving a major proportion in the immune complex of IMN. With the deposition of IgG4, the glomerulus lesion aggravates. Although the complement activation is significantly reduced, the high affinity of IgG4 also can affect the normal function of podocytes, causing significant destruction (Figure 2).
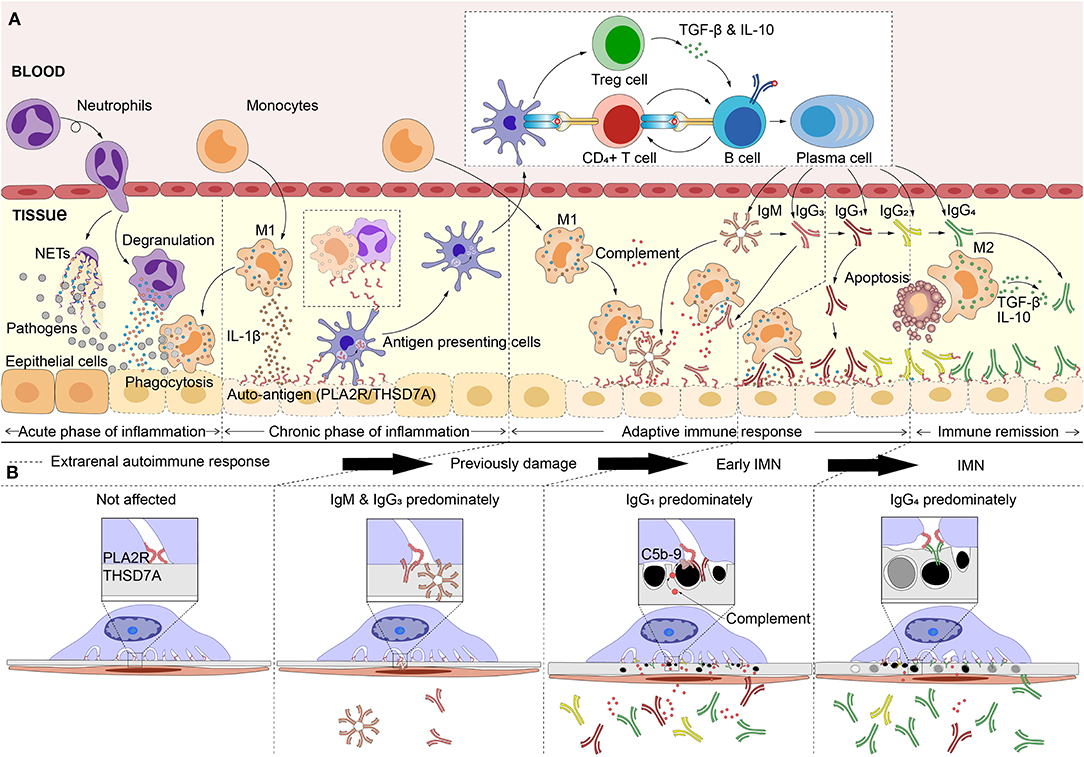
Figure 2. Hypothetical pathogenesis model of idiopathic membranous nephropathy. (A) The pathogens (inhalable particles, bacteria and virus, etc) or other causes that affects the extrarenal tissues inducing inflammatory response. As the stimulus continues, the inflammation gradually goes in the phase that is dominated by M1 macrophages (encourage inflammation), and causes tissue damage (bystander damage). Hence, the auto-antigens such as M-type receptor for secretory phospholipase A2 (PLA2R1) or thrombospondin type-1 domain-containing 7A (THSD7A), are exposed in extrarenal inflammatory microenvironment. Pathogens or inflammation enhance the immunogenicity of autoantigen and affect the antigen processing capacity of antigen presenting cells, which leads to the auto-immune response. With the maturation of antigen affinity of B cells and the induction of cytokines, the immunoglobulins (Ig) undergo the class switching follow this sequence: IgM> IgG3> IgG1> IgG2> IgG4. IgM, IgG3, or IgG1, which can activate the complement system and Fc receptors, leading to tissue inflammation, and damage. Subsequently, IgG2 competes with IgG1 to slow the inflammatory drive. Normally, after neutrophils or macrophages eat debris/pathogens they perform apoptosis and are removed by M2 macrophages (decrease inflammation and encourage tissue repair), which produces high levels of IL-10 and transforming growth factor (TGF)-β that promote inflammation remission. IL-10 and TGF-β that produced by M2 macrophages or Treg cells induced the IgG4-dominated immune response. (B) The antibodies which are produced, bind to the podocyte through circulation, resulting in idiopathic membranous nephropathy (IMN). Anti-PLA2R1/THSD7A IgM or IgG3 could have been deposited in glomeruli with very small quantities, induced the previously glomerular damage. However, the low affinity makes it difficult for them to bind to the antigens naturally expressed in podocytes for a long time. Subsequently, large deposits of IgG1 induced early IMN (stage I). IgG4 can competitively inhibits IgG1 binding to antigens with its higher affinity and achieving a major proportion in the immune complex, finally result in IMN.
Potential Role of IgG4: Non-inflammatory and Organ-Limited Renal Manifestations
The anti-inflammatory properties of the autoimmune response that targets extrarenal sites could be the potential cause for the non-inflammatory lesions of IMN. Driven by extrarenal autoimmunity, IgG4 deposition is predominately found in the glomerulus of most IMN patients with its high production and affinity, which suppresses the inflammatory response. In addition, the immune complex of IMN is mainly subepithelial deposited, without direct contact with the blood. Even with the activation of complement to produce anaphylatoxins, such as C3a and C5a, it is difficult to cross the GBM and endothelial cells in reverse to induce a local inflammatory response. The elevated plasma levels of C3a and C5a in IMN patients (109) are more likely to be induced by extrarenal autoimmunity. Hence, neither plasma C5a and C3a are associated with IMN disease activity, however urinary C5a has a positive correlation with between plasma aPLA2R1ab levels and proteinuria (109). Thus, the products of subepithelial complement activation of glomeruli are more released into urine and do not play a role in inducing inflammatory cell infiltration.
A specific time period is required for extrarenal inflammation to induce autoimmunity and antibody affinity maturation. Included, repeated, or long-term exposure to antigens during this period can affect time duration. Without a certain affinity of antibodies, it is difficult for large amounts of IgG to be deposited due to the glomerular charge barrier. It will take time for the kidneys to present significant symptoms. Thus, the development of extrarenal tissue damage is prior to IMN progression and when large amounts of IgG4 are produced, the excessive extrarenal inflammation should also be suppressed, which can result in a time lag of symptoms in the extrarenal tissue, as well as in the kidney (Figure 3). More importantly, auto-antigens may function differently in different tissues (their function in extrarenal tissue is not as prominent as it is in the podocytes) and the symptoms of loss of function are not as specific as in the kidney, and it is difficult to establish a clear link clinically. The reported relationship between sarcoidosis and MN seems to support this argument; a high prevalence of PLA2R1-associated MN among patients with MN associated with active sarcoidosis has been described (31).
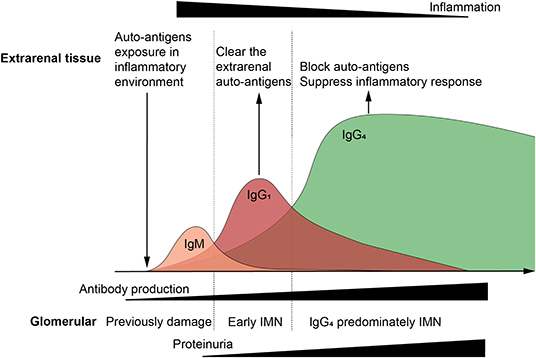
Figure 3. Relationship between extrarenal tissue, immune response, and glomerular damage. Auto-antigens exposure in inflammatory environment induce the auto-immune response, and subsequently immunoglobulins (Ig) undergo class switching. IgG1 mediates the most auto-antigen clearance as the primary effector antibody, and IgG4 blocks auto-antigens as the high affinity anti-inflammatory antibody. When pathogens and auto-antigens are effectively eliminated, the extrarenal inflammatory, and immune responses tend to be alleviated. However, large amounts of antibodies are deposited in the glomeruli, especially IgG4, leading to filtration barrier damage and proteinuria. Extrarenal remission and glomerular damage aggravation were observed, which can be the reason that why idiopathic membranous nephropathy is having trouble finding the primary foci.
The Spontaneous Remission of IMN
In the natural course, 40–50% of MN patients achieved spontaneous remission (12). Although spontaneous remission was more common in IMN patients with less baseline proteinuria, spontaneous remission also occurred in 22% of patients among those with baseline proteinuria >12 g/24 h (110). Immune remission predicts the remission of proteinuria, such as the decrease of aPLA2R1ab titer is earlier than that of proteinuria (111).
Self-Termination of the Extrarenal Autoimmune Response: Immune Remission
The high affinity and anti-inflammatory properties of IgG4 can contribute to shaping the pathology of IMN glomeruli and inhibit the activity of the extrarenal autoimmune response. IgG4 binds to antigens in large quantities by its high affinity, blocks the immunogenicity of antigens, and competitively inhibits the proinflammatory activity of other IgG subclasses (88). In the case of antigen blocking and inflammation suppression, the immune response tends to be alleviated. The extent to which extrarenal auto-antigens are exposed to the immune system gradually decreases with IgG4 shows a dominant type of response. The immune remission of extrarenal sites is affected by multiple factors that are related to the degree of environmental stimulation and immune regulation, and the achieved degree of remission of individuals. With the gradual remission of the autoimmune response, the production of anti-podocyte antibodies decreases, and the deposited immune complexes are gradually degraded with the glomerular self-repair.
Reversibility of Podocytes: Proteinuria Remission
The podocyte slit-diaphragm between adjacent foot processes is the final filtration barrier of the glomerulus (112). Condensation of the actin cytoskeleton at the base of effaced podocyte foot processes is a prominent feature of both human and experimental MN, which is accompanied by various alterations in the intervening filtration slits, including widening, formation of occluding-type junctions, and displacement and disruption of slit-diaphragms (113). These changes are accompanied by a reduction in the amount and alteration in the distribution of nephrin and podocin, both of which are essential for slit-diaphragm integrity (114, 115). The slit-diaphragm is a multicomponent structure that includes heterophilic and homophilic interactions between nephrin, and neph 1, and possibly cadherins (112). Podocin is associated with the cytoplasmic tail of nephrin, and likely stabilizes nephrin and the slit-diaphragm in a lipid raft domain in the podocyte plasma membrane (116). As nephrin dissociates from actin and podocin, its ability to tether the cytoskeleton and plasma membrane gradually diminishes, which could be the cause for the dislocation of the slit diaphragm and proteinuria in rat PHN (115). The sublethal injury mediated by anti-Fx1A and complement in vitro was accompanied by the dissolution of F-actin microfilaments and loss of the focal adhesion complexes in rat podocytes. The cytoskeleton is dissociated from the matrix-attached integrins due to the loss of the focal adhesion complexes that anchors the cytoskeleton to the integrin. However, podocytes retained stromal integrins even in the case of injury (117). This finding corresponds to human's MN, and the distribution of β1-integrin in renal biopsies in patients with various glomerular diseases (including membranous and other forms of glomerulonephritis, minimal change disease) are similar to that in normal glomeruli (118). These findings can be related to the foot process fusion or effacement in MN. It is worth noting that, after the removal of anti-Fx1A and complement for 18 h, podocytes recover back to normal cellular morphology, indicating that the injury was reversible and podocytes have a certain level of self-repair ability (117). The glomerulus does have a self-healing ability depending upon the pressure applied; if the pressure remains within the limits of the self-repairing ability, the glomerulus gradually goes toward the self-healing process with the gradual relief of pressure, recovering its original function, and finally achieving the remission of proteinuria (119). In a pathological state, the specific marker proteins of podocytes gradually disappear, while macrophage-related markers, such as CD68, are gradually expressed (120). Podocytes mainly perform the important function of acting as a filtering barrier under normal circumstances; while undergoing phenotypic changes, podocytes can protect themselves under pressure. When the adverse factors are removed gradually, the podocytes transformed into normal phenotypes and resume a normal morphological structure, which could be the potential cause of proteinuria remission (Figure 4).
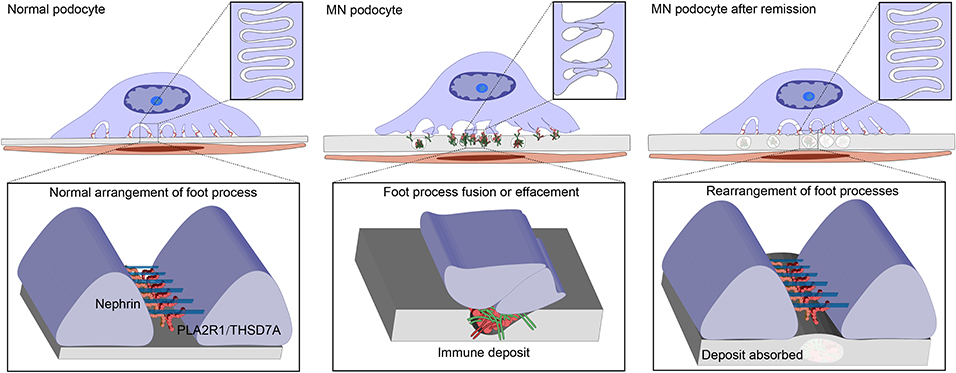
Figure 4. Changes of Podocyte morphology under various conditions. M-type receptor for secretory phospholipase A2 (PLA2R1) or thrombospondin type-1 domain-containing 7A (THSD7A) potentially maintain the slit diaphragm or the adherence of podocytes to glomerular basement membrane (GBM), involved in the normal arrangement of the foot processes. Anti-PLA2R1/THSD7A antibodies bind podocytes forming the immune deposits and resulted in thickness of GBM and morphological changes of podocytes, including cell body enlargement and foot process effacement. Under the damage degree that does not exceed the self-repairing ability threshold, the podocytes gradually goes toward self-healing with the gradual relief of pressure, recovering the original structure, and function. Foot processes rearrange and immune deposits are absorbed by GBM, and finally is the restoration of normal filtration capacity and proteinuria remission.
Conclusions
In this review, we proposed a hypothetical model of IMN pathogenesis that extrarenal immune activity causes additional kidney damage, which could to some extent explain some clinical features of IMN. Environmental stimuli or other factors induce the exposure of auto-antigens to extrarenal tissues, and initiate the autoimmune response targeted podocytes. Most IMN patients have a common immune pathway characterized by IgG4 response, which may be the result of an extrarenal autoimmune response transition to anti-inflammatory. The anti-inflammatory properties of autoimmune responses may decorate the unique pathological pattern and renal limitation of IMN. The production of IgG4 outside the kidney promoted immune remission, and the reversibility of podocytes is a necessary condition for proteinuria remission, both of which jointly promoted spontaneous remission. Figures 3, 4 are simplified illustrations of this understanding. We believe that our proposed hypothesis is beneficial to the further understanding of MN and future study in this field.
Author Contributions
WL, CG, XT, and BL contributed to the conception and design of the review study. WL, CG, and HD wrote the first draft of the manuscript. YG, ZD, YZ, ZL, and ZF wrote sections of the manuscript. XT and BL discussed and revised the content of the review article. All authors contributed to manuscript revision, read and approved the submitted version.
Funding
This work was supported by grants from the National Key Research and Development Project of China (no. 2019YFC1709402), the National Natural Science Foundation of China (no. 81973793, 81673907), Natural Science Foundation of Beijing Municipality (no. 7182070), and Beijing Municipal Administration of Hospitals Clinical Medicine Development of special funding support, code: XLMX201833.
Conflict of Interest
The authors declare that the research was conducted in the absence of any commercial or financial relationships that could be construed as a potential conflict of interest.
References
1. Canetta PA, Troost JP, Mahoney S, Kogon AJ, Carlozzi N, Bartosh SM, et al. Health-related quality of life in glomerular disease. Kidney Int. (2019) 95:1209–24. doi: 10.1016/j.kint.2018.12.018
2. Xu X, Wang G, Chen N, Lu T, Nie S, Xu G, et al. Long-term exposure to air pollution and increased risk of membranous nephropathy in China. J Am Soc Nephrol. (2016) 27:3739–46. doi: 10.1681/ASN.2016010093
3. Couser WG. Primary membranous nephropathy. Clin J Am Soc Nephrol. (2017) 12:983–97. doi: 10.2215/CJN.11761116
4. Ronco P, Debiec H. Molecular pathogenesis of membranous nephropathy. Ann Rev Pathol. (2020) 15:287–313. doi: 10.1146/annurev-pathol-020117-043811
5. Imai H, Hamai K, Komatsuda A, Ohtani H, Miura AB. IgG subclasses in patients with membranoproliferative glomerulonephritis, membranous nephropathy, and lupus nephritis. Kidney Int. (1997) 51:270–6. doi: 10.1038/ki.1997.32
6. Hemminger J, Nadasdy G, Satoskar A, Brodsky SV, Nadasdy T. IgG subclass staining in routine renal biopsy material. Am J Surg Pathol. (2016) 40:617–26. doi: 10.1097/PAS.0000000000000605
7. Beck LH Jr, Bonegio RG, Lambeau G, Beck DM, Powell DW, Cummins TD, et al. M-type phospholipase A2 receptor as target antigen in idiopathic membranous nephropathy. N Engl J Med. (2009) 361:11–21. doi: 10.1056/NEJMoa0810457
8. Tomas NM, Beck LH Jr, Meyer-Schwesinger C, Seitz-Polski B, Ma H, Zahner G, et al. Thrombospondin type-1 domain-containing 7A in idiopathic membranous nephropathy. N Engl J Med. (2014) 371:2277–87. doi: 10.1056/NEJMoa1409354
9. Sethi S, Debiec H, Madden B, Charlesworth MC, Morelle J, Gross L, et al. Neural epidermal growth factor-like 1 protein (NELL-1) associated membranous nephropathy. Kidney Int. (2020) 91:163–74. doi: 10.1016/j.kint.2019.09.014
10. Bobart SA, De Vriese AS, Pawar AS, Zand L, Sethi S, Giesen C, et al. Noninvasive diagnosis of primary membranous nephropathy using phospholipase A2 receptor antibodies. Kidney Int. (2019) 95:429–38. doi: 10.1016/j.kint.2018.10.021
11. De Vriese AS, Glassock RJ, Nath KA, Sethi S, Fervenza FC.A proposal for a serology-based approach to membranous nephropathy. J Am Soc Nephrol. (2017) 28:421–30. doi: 10.1681/ASN.2016070776
12. Glassock RJ. Diagnosis and natural course of membranous nephropathy. Semin Nephrol. (2003) 23:324–32. doi: 10.1016/S0270-9295(03)00049-4
13. Grupper A, Cornell LD, Fervenza FC, Beck LH Jr, Lorenz E, Cosio FG. Recurrent membranous nephropathy after kidney transplantation: treatment and long-term implications. Transplantation. (2016) 100:2710–6. doi: 10.1097/TP.0000000000001056
14. Liu W, Gao C, Dai H, Zheng Y, Dong Z, Gao Y, et al. Immunological pathogenesis of membranous nephropathy: focus on PLA2R1 and its role. Front Immunol. (2019) 10:1809. doi: 10.3389/fimmu.2019.01809
15. Xu X, Nie S, Ding H, Hou FF. Environmental pollution and kidney diseases. Nat Rev Nephrol. (2018) 14:313–24. doi: 10.1038/nrneph.2018.11
16. van de Logt A-E, Fresquet M, Wetzels JF, Brenchley P. The anti-PLA2R antibody in membranous nephropathy: what we know and what remains decade after its discovery. Kidney Int. (2019) 96:1292–302. doi: 10.1016/j.kint.2019.07.014
17. Couser WG, Steinmuller DR, Stilmant MM, Salant DJ, Lowenstein LM. Experimental glomerulonephritis in the isolated perfused rat kidney. J Clin Invest. (1978) 14:1275–87. doi: 10.1172/JCI109248
18. Van Damme BJ, Fleuren GJ, Bakker WW, Vernier RL, Hoedemaeker PJ. Experimental glomerulonephritis in the rat induced by antibodies directed against tubular antigens. V. fixed glomerular antigens in the pathogenesis of heterologous immune complex glomerulonephritis. Lab Invest. (1978) 38:502–10.
19. Kerjaschki D, Farquhar MG. The pathogenic antigen of Heymann nephritis is a membrane glycoprotein of the renal proximal tubule brush border. Proc Natl Acad Sci USA. (1982) 79:5557–61. doi: 10.1073/pnas.79.18.5557
20. Kerjaschki D, Farquhar MG. Immunocytochemical localization of the Heymann nephritis antigen (GP330) in glomerular epithelial cells of normal Lewis rats. J Exp Med. (1983) 157:667–86. doi: 10.1084/jem.157.2.667
21. Kerjaschki D. Molecular pathogenesis of membranous nephropathy. Kidney Int. (1992) 41:1090–105. doi: 10.1038/ki.1992.166
22. Meyer-Schwesinger C, Dehde S, Klug P, Becker JU, Mathey S, Arefi K, et al. Nephrotic syndrome and subepithelial deposits in a mouse model of immune-mediated anti-podocyte glomerulonephritis. J Immunol. (2011) 187:3218–29. doi: 10.4049/jimmunol.1003451
23. Tomas NM, Meyer-Schwesinger C, von Spiegel H, Kotb AM, Zahner G, Hoxha E, et al. A heterologous model of thrombospondin type 1 domain-containing 7A-associated membranous nephropathy. J Am Soc Nephrol. (2017) 28:3262–77. doi: 10.1681/ASN.2017010030
24. Meyer-Schwesinger C, Tomas NM, Dehde S, Seifert L, Hermans-Borgmeyer I, Wiech T, et al. A novel mouse model of phospholipase A2 receptor 1-associated membranous nephropathy mimics podocyte injury in patients. Kidney Int. (2020) 97:913–9. doi: 10.1016/j.kint.2019.10.022
25. Debiec H, Guigonis V, Mougenot B, Decobert F, Haymann JP, Bensman A, et al. Antenatal membranous glomerulonephritis due to anti-neutral endopeptidase antibodies. N Engl J Med. (2002) 346:2053–60. doi: 10.1056/NEJMoa012895
26. Ronco P, Debiec H, Guigonis V. Mechanisms of disease: alloimmunization in renal diseases. Nat Clin Pract Nephrol. (2006) 2:388–97. doi: 10.1038/ncpneph0198
27. Radice A, Pieruzzi F, Trezzi B, Ghiggeri G, Napodano P, D'Amico M, et al. Diagnostic specificity of autoantibodies to M-type phospholipase A2 receptor (PLA2R) in differentiating idiopathic membranous nephropathy (IMN) from secondary forms and other glomerular diseases. J Nephrol. (2018) 31:271–8. doi: 10.1007/s40620-017-0451-5
28. Ge YC, Jin B, Zeng CH, Zhang MC, Chen DC, Yin R, et al. PLA2R antibodies and PLA2R glomerular deposits in psoriasis patients with membranous nephropathy. BMC Nephrol. (2016) 17:185. doi: 10.1186/s12882-016-0407-3
29. Xie Q, Li Y, Xue J, Xiong Z, Wang L, Sun Z, et al. Renal phospholipase A2 receptor in hepatitis B virus-associated membranous nephropathy. Am J Nephrol. (2015) 41:345–53. doi: 10.1159/000431331
30. Garcia-Vives E, Sole C, Moline T, Alvarez-Rios AM, Vidal M, Agraz I, et al. Antibodies to M-type phospholipase A2 receptor (PLA2R) in membranous lupus nephritis. Lupus. (2019) 3:396–405. doi: 10.1177/0961203319828521
31. Stehle T, Audard V, Ronco P, Debiec H. Phospholipase A2 receptor and sarcoidosis-associated membranous nephropathy. Nephrol Dial Transplant. (2015) 59:1047–50. doi: 10.1093/ndt/gfv080
32. Zaghrini C, Seitz-Polski B, Justino J, Dolla G, Payré C, Jourde-Chiche N, et al. Novel ELISA for thrombospondin type 1 domain-containing 7A autoantibodies in membranous nephropathy. Kidney Int. (2019) 95:666–79. doi: 10.1016/j.kint.2018.10.024
33. Iwakura T, Ohashi N, Kato A, Baba S, Yasuda H. Prevalence of enhanced granular expression of thrombospondin type-1 domain-containing 7A in the glomeruli of japanese patients with idiopathic membranous nephropathy. PLoS ONE. (2015) 9:e0138841. doi: 10.1371/journal.pone.0138841
34. Hoxha E, Beck LH Jr, Wiech T, Tomas NM, Probst C, Mindorf S, et al. An indirect immunofluorescence method facilitates detection of thrombospondin type 1 domain-containing 7A-specific antibodies in membranous nephropathy. J Am Soc Nephrol. (2017) 28:520–31. doi: 10.1681/ASN.2016010050
35. Hoxha E, Wiech T, Stahl PR, Zahner G, Tomas NM, Meyer-Schwesinger C, et al. A mechanism for cancer-associated membranous nephropathy. N Engl J Med. (2016) 374:1995–6. doi: 10.1056/NEJMc1511702
36. Xian L, Dong D, Luo J, Zhuo L, Li K, Zhang P, et al. Expression of THSD7A in neoplasm tissues and its relationship with proteinuria. BMC Nephrol. (2019) 20:332. doi: 10.1186/s12882-019-1489-5
37. Wang T, Zhang Y, Liu M, Kang X, Kang L, Zhang H. THSD7A as a marker for paraneoplastic membranous nephropathy. Int Urol Nephrol. (2019) 51:371–3. doi: 10.1007/s11255-018-02068-z
38. Matsumoto A, Matsui I, Namba T, Sakaguchi Y, Mizuno H, Shirayama Y, et al. VEGF-A links Angiolymphoid Hyperplasia With Eosinophilia (ALHE) to THSD7A membranous nephropathy: a report of 2 cases. Am J Kidney Dis. (2019) 73:880–5. doi: 10.1053/j.ajkd.2018.10.009
39. Apte RS, Chen DS, Ferrara N. VEGF in signaling and disease: beyond discovery and development. Cell. (2019) 176:1248–64. doi: 10.1016/j.cell.2019.01.021
40. Zhang XD, Cui Z, Zhao MH. The genetic and environmental factors of primary membranous nephropathy: an overview from China. Kidney Dis. (2018) 4:65–73. doi: 10.1159/000487136
41. Suzuki H, Moayyedi P. Helicobacter pylori infection in functional dyspepsia. Nat Rev Gastroenterol Hepatol. (2013) 10:168–74. doi: 10.1038/nrgastro.2013.9
42. Nagashima R, Maeda K, Yuda F, Kudo K, Saitoh M, Takahashi T. Helicobacter pylori antigen in the glomeruli of patients with membranous nephropathy. Virchows Arch. (1997) 431:235–9. doi: 10.1007/s004280050094
43. Sugimoto T, Furukawa T, Maeda T, Somura M, Uzu T, Kashiwagi A. Marked reduction of proteinuria after eradication of gastric helicobacter pylori infection in a patient with membranous nephropathy: coincidental or associated? Intern Med. (2007) 46:1483–4. doi: 10.2169/internalmedicine.46.0200
44. Yang AH, Lin BS, Kuo KL, Chang CC, Ng YY, Yang WC. The clinicopathological implications of endothelial tubuloreticular inclusions found in glomeruli having histopathology of idiopathic membranous nephropathy. Nephrol Dial Transplant. (2009) 24:3419–25. doi: 10.1093/ndt/gfp288
45. Xie J, Liu L, Mladkova N, Li Y, Ren H, Wang W, et al. The genetic architecture of membranous nephropathy and its potential to improve non-invasive diagnosis. Nat Commun. (2020) 11:1600. doi: 10.1038/s41467-020-15383-w
46. Bluestone JA, Herold K, Eisenbarth G. Genetics, pathogenesis and clinical interventions in type 1 diabetes. Nature. (2010) 464:1293–300. doi: 10.1038/nature08933
47. Baumgart DC, Carding SR. Inflammatory bowel disease: cause and immunobiology. Lancet. (2007) 369:1627–40. doi: 10.1016/S0140-6736(07)60750-8
48. Correale J, Gaitan MI, Ysrraelit MC, Fiol MP. Progressive multiple sclerosis: from pathogenic mechanisms to treatment. Brain. (2017) 140:527–46. doi: 10.1093/brain/aww258
49. Veale DJ, Fearon U. The pathogenesis of psoriatic arthritis. Lancet. (2018) 391:2273–84. doi: 10.1016/S0140-6736(18)30830-4
50. Ancian P, Lambeau G, Mattei MG, Lazdunski M. The human 180-kDa receptor for secretory phospholipases A2. molecular cloning, identification of a secreted soluble form, expression, and chromosomal localization. J Biol Chem. (1995) 270:8963–70. doi: 10.1074/jbc.270.15.8963
51. Alexis NE, Lay JC, Zeman K, Bennett WE, Peden DB, Soukup JM, et al. Biological material on inhaled coarse fraction particulate matter activates airway phagocytes in vivo in healthy volunteers. J Allergy Clin Immunol. (2006) 117:1396–403. doi: 10.1016/j.jaci.2006.02.030
52. Rider CF, Yamamoto M, Gunther OP, Hirota JA, Singh A, Tebbutt SJ, et al. Controlled diesel exhaust and allergen coexposure modulates microRNA and gene expression in humans: effects on inflammatory lung markers. J Allergy Clin Immunol. (2016) 138:1690–700. doi: 10.1016/j.jaci.2016.02.038
53. Silliman CC, Moore EE, Zallen G, Gonzalez R, Johnson JL, Elzi DJ, et al. Presence of the M-type sPLA(2) receptor on neutrophils and its role in elastase release and adhesion. Am J Physiol Cell Physiol. (2002) 283:C1102–13. doi: 10.1152/ajpcell.00608.2001
54. Granata F, Petraroli A, Boilard E, Bezzine S, Bollinger J, Del Vecchio L, et al. Activation of cytokine production by secreted phospholipase A2 in human lung macrophages expressing the M-type receptor. J Immunol. (2005) 174:464–74. doi: 10.4049/jimmunol.174.1.464
55. Burbelo PD, Joshi M, Chaturvedi A, Little DJ, Thurlow JS, Waldman M, et al. Detection of PLA2R autoantibodies before the diagnosis of membranous nephropathy. J Am Soc Nephrol. (2020) 31:208–17. doi: 10.1681/ASN.2019050538
56. Wang CH, Su PT, Du XY, Kuo MW, Lin CY, Yang CC, et al. Thrombospondin type I domain containing 7A (THSD7A) mediates endothelial cell migration and tube formation. J Cell Physiol. (2010) 222:685–94. doi: 10.1002/jcp.21990
57. Kuo MW, Wang CH, Wu HC, Chang SJ, Chuang YJ. Soluble THSD7A is an N-glycoprotein that promotes endothelial cell migration and tube formation in angiogenesis. PLoS ONE. (2011) 6:e29000. doi: 10.1371/journal.pone.0029000
58. Wang CH, Chen IH, Kuo MW, Su PT, Lai ZY, Wang CH, et al. Zebrafish Thsd7a is a neural protein required for angiogenic patterning during development. Dev Dyn. (2011) 240:1412–21. doi: 10.1002/dvdy.22641
59. Hasebe A, Tashima H, Ide T, Iijima M, Yoshimoto N, Ting K, et al. Efficient production and characterization of recombinant human NELL1 protein in human embryonic kidney 293-F cells. Mol Biotechnol. (2012) 51:58–66. doi: 10.1007/s12033-011-9440-4
60. Hasebe A, Nakamura Y, Tashima H, Takahashi K, Iijima M, Yoshimoto N, et al. The C-terminal region of NELL1 mediates osteoblastic cell adhesion through integrin alpha3beta1. FEBS Lett. (2012) 586:2500–6. doi: 10.1016/j.febslet.2012.06.014
61. Ting K, Vastardis H, Mulliken JB, Soo C, Tieu A, Do H, et al. Human NELL-1 expressed in unilateral coronal synostosis. J Bone Miner Res. (1999) 14:80–9. doi: 10.1359/jbmr.1999.14.1.80
62. Zhang X, Carpenter D, Bokui N, Soo C, Miao S, Truong T, et al. Overexpression of Nell-1, a craniosynostosis-associated gene, induces apoptosis in osteoblasts during craniofacial development. J Bone Miner Res. (2003) 18:2126–34. doi: 10.1359/jbmr.2003.18.12.2126
63. Dekan G, Miettinen A, Schnabel E, Farquhar MG. Binding of monoclonal antibodies to glomerular endothelium, slit membranes, and epithelium after in vivo injection. localization of antigens and bound IgGs by immunoelectron microscopy. Am J Pathol. (1990) 137:913–27.
64. Herwig J, Skuza S, Sachs W, Sachs M, Failla AV, Rune G, et al. Thrombospondin type 1 domain-containing 7A localizes to the slit diaphragm and stabilizes membrane dynamics of fully differentiated podocytes. J Am Soc Nephrol. (2019) 30:824–39. doi: 10.1681/ASN.2018090941
65. Skoberne A, Behnert A, Teng B, Fritzler MJ, Schiffer L, Pajek J, et al. Serum with phospholipase A2 receptor autoantibodies interferes with podocyte adhesion to collagen. Eur J Clin Invest. (2014) 44:753–65. doi: 10.1111/eci.12292
66. East L, Isacke CM. The mannose receptor family. Biochim Biophys Acta. (2002) 1572:364–86. doi: 10.1016/S0304-4165(02)00319-7
67. Seitz-Polski B, Dolla G, Payre C, Girard CA, Polidori J, Zorzi K, et al. Epitope spreading of autoantibody response to PLA2R associates with poor prognosis in membranous nephropathy. J Am Soc Nephrol. (2016) 27:1517–33. doi: 10.1681/ASN.2014111061
68. Reinhard L, Zahner G, Menzel S, Koch-Nolte F, Stahl RAK, Hoxha E. Clinical relevance of domain-specific phospholipase A2 receptor 1 antibody levels in patients with membranous nephropathy. J Am Soc Nephrol. (2020) 31:197–207. doi: 10.1681/ASN.2019030273
69. Seitz-Polski B, Debiec H, Rousseau A, Dahan K, Zaghrini C, Payré C, et al. Phospholipase A2 receptor 1 epitope spreading at baseline predicts reduced likelihood of remission of membranous nephropathy. J Am Soc Nephrol. (2018) 29:401–8. doi: 10.1681/ASN.2017070734
70. Seitz-Polski B, Dahan K, Debiec H, Rousseau A, Andreani M, Zaghrini C, et al. High-dose rituximab and early remission in pla2r1-related membranous nephropathy. Clin J Am Soc Nephrol. (2019) 14:1173–82. doi: 10.2215/CJN.11791018
71. Seifert L, Hoxha E, Eichhoff AM, Zahner G, Dehde S, Reinhard L, et al. The most N-terminal region of THSD7A is the predominant target for autoimmunity in THSD7A-associated membranous nephropathy. J Am Soc Nephrol. (2018) 29:1536–48. doi: 10.1681/ASN.2017070805
72. Zvaritch E, Lambeau G, Lazdunski M. Endocytic properties of the M-type 180-kDa receptor for secretory phospholipases A2. J Biol Chem. (1996) 271:250–7. doi: 10.1074/jbc.271.1.250
73. Tamaru S, Mishina H, Watanabe Y, Watanabe K, Fujioka D, Takahashi S, et al. Deficiency of phospholipase A2 receptor exacerbates ovalbumin-induced lung inflammation. J Immunol. (2013) 191:1021–8. doi: 10.4049/jimmunol.1300738
74. Kishi H, Yamaguchi K, Watanabe K, Nakamura K, Fujioka D, Kugiyama K. Deficiency of phospholipase A2 receptor exacerbates autoimmune myocarditis in Mice. Inflammation. (2020) 43:1097–109. doi: 10.1007/s10753-020-01195-z
75. Beck S, Beck G, Ostendorf T, Floege J, Lambeau G, Nevalainen T, et al. Upregulation of group IB secreted phospholipase A(2) and its M-type receptor in rat ANTI-THY-1 glomerulonephritis. Kidney Int. (2006) 70:1251–60. doi: 10.1038/sj.ki.5001664
76. Nolin JD, Ogden HL, Lai Y, Altemeier WA, Frevert CW, Bollinger JG, et al. Identification of epithelial phospholipase A2 receptor 1 as a potential target in asthma. Am J Respir Cell Mol Biol. (2016) 55:825–36. doi: 10.1165/rcmb.2015-0150OC
77. Rava M, Ahmed I, Kogevinas M, Le Moual N, Bouzigon E, Curjuric I, et al. Genes interacting with occupational exposures to low molecular weight agents and irritants on adult-onset asthma in three european studies. Environ Health Perspect. (2017) 125:207–14. doi: 10.1289/EHP376
78. Cui Z, Xie LJ, Chen FJ, Pei ZY, Zhang LJ, Qu Z, et al. MHC class II risk alleles and amino acid residues in idiopathic membranous nephropathy. J Am Soc Nephrol. (2017) 28:1651–64. doi: 10.1681/ASN.2016020114
79. Masutani K, Taniguchi M, Nakashima H, Yotsueda H, Kudoh Y, Tsuruya K, et al. Up-regulated interleukin-4 production by peripheral T-helper cells in idiopathic membranous nephropathy. Nephrol Dial Transplant. (2004) 19:580–6. doi: 10.1093/ndt/gfg572
80. Kuroki A, Iyoda M, Shibata T, Sugisaki T. Th2 cytokines increase and stimulate B cells to produce IgG4 in idiopathic membranous nephropathy. Kidney Int. (2005) 68:302–10. doi: 10.1111/j.1523-1755.2005.00415.x
81. Rosenzwajg M, Languille E, Debiec H, Hygino J, Dahan K, Simon T, et al. B- and T-cell subpopulations in patients with severe idiopathic membranous nephropathy may predict an early response to rituximab. Kidney Int. (2017) 92:227–37. doi: 10.1016/j.kint.2017.01.012
82. Roccatello D, Sciascia S, Di Simone D, Solfietti L, Naretto C, Fenoglio R, et al. New insights into immune mechanisms underlying response to Rituximab in patients with membranous nephropathy: a prospective study and a review of the literature. Autoimmun Rev. (2016) 15:529–38. doi: 10.1016/j.autrev.2016.02.014
83. Hofstra JM, Debiec H, Short CD, Pelle T, Kleta R, Mathieson PW, et al. Antiphospholipase A2 receptor antibody titer and subclass in idiopathic membranous nephropathy. J Am Soc Nephrol. (2012) 23:1735–43. doi: 10.1681/ASN.2012030242
84. Cheng G, Liu J, Gilbert A, Cao Y, An C, Lv Z, et al. Serum phospholipase A2 receptor antibodies and immunoglobulin G subtypes in adult idiopathic membranous nephropathy: clinical value assessment. Clin Chim Acta. (2019) 490:135–41. doi: 10.1016/j.cca.2018.12.027
85. von Haxthausen F, Reinhard L, Pinnschmidt HO, Rink M, Soave A, Hoxha E, et al. Antigen-Specific IgG subclasses in primary and malignancy-associated membranous nephropathy. Front Immunol. (2018) 9:3035. doi: 10.3389/fimmu.2018.03035
86. Huang CC, Lehman A, Albawardi A, Satoskar A, Brodsky S, Nadasdy G, et al. IgG subclass staining in renal biopsies with membranous glomerulonephritis indicates subclass switch during disease progression. Mod Pathol. (2013) 26:799–805. doi: 10.1038/modpathol.2012.237
87. Lateb M, Ouahmi H, Payré C, Brglez V, Zorzi K, Dolla G, et al. Anti-PLA2R1 antibodies containing sera induce in vitro cytotoxicity mediated by complement activation. J Immunol Res. (2019) 2019:1324804. doi: 10.1155/2019/1324804
88. Collins AM, Jackson KJ.A temporal model of human IgE and IgG antibody function. Front Immunol. (2013) 4:235. doi: 10.3389/fimmu.2013.00235
89. Vidarsson G, Dekkers G, Rispens T. IgG subclasses and allotypes: from structure to effector functions. Front Immunol. (2014) 5:520. doi: 10.3389/fimmu.2014.00520
90. Sethi S, Madden BJ, Debiec H, Charlesworth MC, Gross L, Ravindran A, et al. Exostosin 1/Exostosin 2-associated membranous nephropathy. J Am Soc Nephrol. (2019) 30:1123–36. doi: 10.1681/ASN.2018080852
91. Ravindran A, Madden B, Charlesworth MC, Sharma R, Sethi A, Debiec H, et al. Proteomic analysis of complement proteins in membranous nephropathy. Kidney Int Rep. (2020) 5:618–26. doi: 10.1016/j.ekir.2020.01.018
92. Bruhns P. Properties of mouse and human IgG receptors and their contribution to disease models. Blood. (2012) 119:5640–9. doi: 10.1182/blood-2012-01-380121
93. Aalberse RC, Stapel SO, Schuurman J, Rispens T. Immunoglobulin G4: an odd antibody. Clin Exp Allergy. (2009) 39:469–77. doi: 10.1111/j.1365-2222.2009.03207.x
94. Jeannin P, Lecoanet S, Delneste Y, Gauchat JF, Bonnefoy JY. IgE versus IgG4 production can be differentially regulated by IL-10. J Immunol. (1998) 160:3555–61.
95. Adjobimey T, Hoerauf A. Induction of immunoglobulin G4 in human filariasis: an indicator of immunoregulation. Ann Trop Med Parasitol. (2010) 104:455–64. doi: 10.1179/136485910X12786389891407
96. Boonpiyathad T, Satitsuksanoa P, Akdis M, Akdis CA. Il-10 producing T and B cells in allergy. Semin Immunol. (2019) 44:101326. doi: 10.1016/j.smim.2019.101326
97. van de Veen W, Stanic B, Yaman G, Wawrzyniak M, Sollner S, Akdis DG, et al. IgG4 production is confined to human IL-10-producing regulatory B cells that suppress antigen-specific immune responses. J Allergy Clin Immunol. (2013) 131:1204–12. doi: 10.1016/j.jaci.2013.01.014
98. van de Veen W, Akdis M. Role of IgG4 in IgE-mediated allergic responses. J Allergy Clin Immunol. (2016) 138:1434–5. doi: 10.1016/j.jaci.2016.07.022
99. Hou J, Zhang M, Ding Y, Wang X, Li T, Gao P, et al. Circulating CD14(+)CD163(+)CD206(+) M2 monocytes are increased in patients with early stage of idiopathic membranous nephropathy. Mediators Inflamm. (2018) 2018:5270657. doi: 10.1155/2018/5270657
100. Kunnumakkara AB, Sailo BL, Banik K, Harsha C, Prasad S, Gupta SC, et al. Chronic diseases, inflammation, and spices: how are they linked? J Transl Med. (2018) 16:14. doi: 10.1186/s12967-018-1381-2
101. Gallo J, Raska M, Kriegova E, Goodman SB. Inflammation and its resolution and the musculoskeletal system. J Orthop Translat. (2017) 10:52–67. doi: 10.1016/j.jot.2017.05.007
102. Getts DR, Chastain EM, Terry RL, Miller SD. Virus infection, antiviral immunity, and autoimmunity. Immunol Rev. (2013) 255:197–209. doi: 10.1111/imr.12091
103. Doyle HA, Mamula MJ. Autoantigenesis: the evolution of protein modifications in autoimmune disease. Curr Opin Immunol. (2012) 24:112–8. doi: 10.1016/j.coi.2011.12.003
104. Yang ML, Doyle HA, Clarke SG, Herold KC, Mamula MJ. Oxidative modifications in tissue pathology and autoimmune disease. Antioxid Redox Signal. (2018) 29:1415–31. doi: 10.1089/ars.2017.7382
105. Suurmond J, Diamond B. Autoantibodies in systemic autoimmune diseases: specificity and pathogenicity. J Clin Invest. (2015) 125:2194–202. doi: 10.1172/JCI78084
106. Sun L, Fu J, Lin SH, Sun JL, Xia L, Lin CH, et al. Particulate matter of 2.5 mum or less in diameter disturbs the balance of TH17/regulatory T cells by targeting glutamate oxaloacetate transaminase 1 and hypoxia-inducible factor 1alpha in an asthma model. J Allergy Clin Immunol. (2019) 27:352–7. doi: 10.1016/j.jaci.2019.10.008
107. Schneider A, Alexis NE, Diaz-Sanchez D, Neas LM, Harder S, Herbst MC, et al. Ambient PM2.5 exposure up-regulates the expression of costimulatory receptors on circulating monocytes in diabetic individuals. Environ Health Perspect. (2011) 119:778–83. doi: 10.1289/ehp.1002543
108. van der Neut Kolfschoten M, Schuurman J, Losen M, Bleeker WK, Martinez-Martinez P, Vermeulen E, et al. Anti-inflammatory activity of human IgG4 antibodies by dynamic Fab arm exchange. Science. (2007) 317:1554–7. doi: 10.1126/science.1144603
109. Zhang MF, Huang J, Zhang YM, Qu Z, Wang X, Wang F, et al. Complement activation products in the circulation and urine of primary membranous nephropathy. BMC Nephrol. (2019) 20:313. doi: 10.1186/s12882-019-1509-5
110. Polanco N, Gutierrez E, Covarsi A, Ariza F, Carreno A, Vigil A, et al. Spontaneous remission of nephrotic syndrome in idiopathic membranous nephropathy. J Am Soc Nephrol. (2010) 21:697–704. doi: 10.1681/ASN.2009080861
111. Radice A, Trezzi B, Maggiore U, Pregnolato F, Stellato T, Napodano P, et al. Clinical usefulness of autoantibodies to M-type phospholipase A2 receptor (PLA2R) for monitoring disease activity in idiopathic membranous nephropathy (IMN). Autoimmun Rev. (2016) 15:146–54. doi: 10.1016/j.autrev.2015.10.004
112. Bariety J, Nochy D, Mandet C, Jacquot C, Glotz D, Meyrier A. Podocytes undergo phenotypic changes and express macrophagic-associated markers in idiopathic collapsing glomerulopathy. Kidney Int. (1998) 53:918–25. doi: 10.1111/j.1523-1755.1998.00845.x
113. Cybulsky AV, Quigg RJ, Salant DJ. Experimental membranous nephropathy redux. Am J Physiol Renal Physiol. (2005) 289:F660–71. doi: 10.1152/ajprenal.00437.2004
114. Yuan H, Takeuchi E, Taylor GA, McLaughlin M, Brown D, Salant DJ. Nephrin dissociates from actin, and its expression is reduced in early experimental membranous nephropathy. J Am Soc Nephrol. (2002)13:946–56.
115. Nakatsue T, Koike H, Han GD, Suzuki K, Miyauchi N, Yuan H, et al. Nephrin and podocin dissociate at the onset of proteinuria in experimental membranous nephropathy. Kidney Int. (2005) 67:2239–53. doi: 10.1111/j.1523-1755.2005.00328.x
116. Schwarz K, Simons M, Reiser J, Saleem MA, Faul C, Kriz W, et al. Podocin, a raft-associated component of the glomerular slit diaphragm, interacts with CD2AP and nephrin. J Clin Invest. (2001) 108:1621–9. doi: 10.1172/JCI200112849
117. Topham PS, Haydar SA, Kuphal R, Lightfoot JD, Salant DJ. Complement-mediated injury reversibly disrupts glomerular epithelial cell actin microfilaments and focal adhesions. Kidney Int. (1999) 55:1763–75. doi: 10.1046/j.1523-1755.1999.00407.x
118. Kerjaschki D, Ojha PP, Susani M, Horvat R, Binder S, Hovorka A, et al. A beta 1-integrin receptor for fibronectin in human kidney glomeruli. Am J Pathol. (1989) 134:481–9.
119. Reiser J, Altintas MM. Podocytes. F1000Research. (2016) 6:805. doi: 10.12688/f1000research.7255.1
Keywords: autoimmune response, inflammation, pathogenesis model, podocyte, spontaneous remission, environmental stimuli
Citation: Liu W, Gao C, Liu Z, Dai H, Feng Z, Dong Z, Zheng Y, Gao Y, Tian X and Liu B (2020) Idiopathic Membranous Nephropathy: Glomerular Pathological Pattern Caused by Extrarenal Immunity Activity. Front. Immunol. 11:1846. doi: 10.3389/fimmu.2020.01846
Received: 13 February 2020; Accepted: 09 July 2020;
Published: 16 September 2020.
Edited by:
Cees Van Kooten, Leiden University, NetherlandsReviewed by:
Renato Alberto Sinico, University of Milano-Bicocca, ItalyBarbara Seitz-Polski, Centre Hospitalier Universitaire de Nice, France
Copyright © 2020 Liu, Gao, Liu, Dai, Feng, Dong, Zheng, Gao, Tian and Liu. This is an open-access article distributed under the terms of the Creative Commons Attribution License (CC BY). The use, distribution or reproduction in other forums is permitted, provided the original author(s) and the copyright owner(s) are credited and that the original publication in this journal is cited, in accordance with accepted academic practice. No use, distribution or reproduction is permitted which does not comply with these terms.
*Correspondence: Baoli Liu, liubaoli@bjzhongyi.com
†These authors have contributed equally to this work