- 1VIB-UGent Center for Inflammation Research, Ghent, Belgium
- 2Unit for Structural Biology, Department of Biochemistry and Microbiology, Ghent University, Ghent, Belgium
Thymic Stromal Lymphopoietin (TSLP) and Interleukin-7 (IL-7) are widely studied cytokines within distinct branches of immunology. On one hand, TSLP is crucially important for mediating type 2 immunity at barrier surfaces and has been linked to widespread allergic and inflammatory diseases of the airways, skin, and gut. On the other hand, IL-7 operates at the foundations of T-cell and innate lymphoid cell (ILC) development and homeostasis and has been associated with cancer. Yet, TSLP and IL-7 are united by key commonalities in their structure and the structural basis of the receptor assemblies they mediate to initiate cellular signaling, in particular their cross-utilization of IL-7Rα. As therapeutic targeting of TSLP and IL-7 via diverse approaches is reaching advanced stages and in light of the plethora of mechanistic and structural data on receptor signaling mediated by the two cytokines, the time is ripe to provide integrated views of such knowledge. Here, we first discuss the major pathophysiological roles of TSLP and IL-7 in autoimmune diseases, inflammation and cancer. Subsequently, we curate structural and mechanistic knowledge about receptor assemblies mediated by the two cytokines. Finally, we review therapeutic avenues targeting TSLP and IL-7 signaling. We envision that such integrated view of the mechanism, structure, and modulation of signaling assemblies mediated by TSLP and IL-7 will enhance and fine-tune the development of more effective and selective approaches to further interrogate the role of TSLP and IL-7 in physiology and disease.
TSLP—Emerging Role in Autoimmune Diseases and Cancer
Over the last two decades TSLP has been extensively studied and known for its pivotal role in allergic conditions and involvement in chronic inflammatory diseases such as chronic obstructive pulmonary disease or inflammatory bowel disease (1–3). In recent years TSLP has additionally emerged as a novel molecular player in non-allergen induced conditions (4, 5). Together with broadening its pathophysiological profile these findings imply that the microenvironment of this pleiotropic cytokine might define the direction of its inflammatory response depending on the type of inflammation involved.
The development of IL-23-associated autoimmune disease psoriasis has recently been linked to overexpression of TSLP in keratinocytes from patient skin samples. It has also been demonstrated that serum levels of TSLP correlate to the severity of the disease (6, 7). Even though the role of TSLP in psoriasis is not completely resolved, TSLP has been reported to induce DC maturation and to drive to a DC-derived IL-23 production leading to the hypothesis that it could have a comparable role in other IL-23-driven autoimmune diseases (8). Furthermore, TSLP has been linked to rheumatoid arthritis (RA): increased TSLP levels in synovial fluid of patients with RA in comparison to those with osteoarthritis have been reported in several studies (9). Moreover, TSLP receptor (TSLPR) has also been found overexpressed in myeloid dendritic cells (mDCs) in synovial fluid of the RA patients. The engagement of TSLP in inflammatory arthritis is explained through TSLP-mediated priming of the mDCs and subsequent chemokine stimulation of CD4+ T cells proliferation leading to secretion of interferon γ (IFNγ), IL-17, and IL-4 (10). Additionally, several reports based on studies via mouse models and in humans provide further evidence of TSLP's possible involvement in the pathogenesis of different types of autoimmune disorders underlining a rising need to further interrogate the role of TSLP in relation to Th17 inflammatory response (11–13).
Thus, given such emerging evidence of the involvement of TSLP in the abrogation of Th1, Th9, and Th17 inflammatory responses and its influence on a range of immune cell lineages it does not come as a surprise that TSLP is becoming more intensively studied in the context of cancer (5, 8, 14). Nevertheless, the current view of the field regarding the role of TSLP in cancer has been divided in terms of tumor-progressive or tumor-protective effects depending on the type of cancer being studied. Studies focusing on tumors of hematopoietic and lymphoid tissues such as lymphoma and acute lymphocytic leukemia (ALL) reported TSLP as a tumor-progressive factor (15, 16). In addition, various genomic analyses detected chromosomal rearrangements and alterations of genes encoding TSLPR/CRLF2 in a large number of patients with B-cell precursor ALL (BCP-ALL), all of them having in common either an enhanced or constitutive expression of TSLPR leading to a signal boost resulting in resistance to therapy, high recurrence rate, and poor clinical outcome (17–19). Interestingly, TSLPR was also found overexpressed in 15% of B-ALL cases with no typical chromosome aberrations (20). Results from diverse experimental and clinical studies in diverse solid tumors—cervical, ovarian, pancreatic, or gastric cancer further imply an evident tumor-progressive role of TSLP in tumor microenvironment leading to the promotion of tumor angiogenesis and its growth and metastasis (21–27). On the other hand, both tumor-progressing and anti-tumor effects of TSLP have been demonstrated in diverse breast cancer studies (28–31). In contrast, skin and colon cancer studies reported TSLP-mediated anti-tumorigenic role hence emphasizing the urgency and importance of understanding this duality in the framework of developing suitable future therapeutics (32, 33).
Genetic Variations in IL-7 Axis Play a Role in Both Autoimmunity and Cancer
Given that tight control of signaling mediated by IL-7 is essential to support and maintain immune homeostasis, it is not surprising that dysregulation of its stimulation leads to a disrupted lymphoid development and pathophysiology in different types of conditions including autoimmune diseases and cancer. Seeing that the absence of IL-7 mediated signaling leads to lymphopenia, a role of its regulation in autoimmune diseases could be implied (34). Several studies involving patients with multiple sclerosis (MS) and primary Sjögren's syndrome (pSS) demonstrate that both IL-7 and IL-7R are overexpressed in the cerebrospinal fluid and labial salivary glands, respectively, with these expression levels correlating to the severity of the disease (35–37). Increased susceptibility to autoimmune diseases such as MS, type 1 diabetes or RA have been linked to several single nucleotide polymorphisms in IL-7R gene loci (35, 38–40). In the case of MS, haplotypes in IL7R gene have been reported to lead to modulations in levels of soluble IL-7R which has also been upregulated in patients with pSS (41, 42). Based on additional extensive data from mice and human in vitro experiments, the pivotal involvement of IL-7 is more than evident. However, the mechanism of how the regulation of the IL-7 axis leads to increased susceptibility to autoimmune diseases still remains largely unclear, although the current mechanistic view suggests that activation of IL-7 signaling promotes the expansion of T cells and increased proliferation to self-antigens leading to predisposition to autoimmunity (43).
Whereas, IL-7 signaling is not as crucial in B-cell development in humans as it is in mice, cells from acute leukemia proliferate in response to IL-7 in vitro and have a corresponding expression of IL-7Rα (44). In addition, overexpression of IL-7Rα has recently been linked with relapse in pediatric B-ALL (45). IL-7Rα gene loci have been shown to carry gain-of-function mutations in a small fraction of patients with BCP-ALL with most of the mutations being associated with concurrent upregulation of TSLPR upregulation and ligand-independent activation of signaling (46). Involvement of the IL-7 signaling axis in the progression of T-ALL has been confirmed in extensive diverse studies showing stimulation of T-ALL cells with IL-7 and overexpression of IL-7Rα (47–52). This evidence is supported by identification of additional IL-7Rα gain-of-function mutations in T-ALL patients that lead to constitutive IL-7 independent receptor activation or else increased activation of the receptor resulting in increased IL-7 response (53, 54). Taking into account that 10% of T-ALL patients carry IL-7Rα mutations that have been linked to poor prognosis and risk in relapsed patients, IL-7Rα and its signaling pathways have emerged as logical therapeutic targets (55). Aside from strong evidence that IL-7 has anti-tumor effects, some studies indicate that IL-7 might enhance tumor-progression, for instance as suggested in studies focusing on non-small cell lung cancer cells (56). It is clear that further studies will be needed to better clarify the role of IL-7 in tumor-progression.
Biology, Receptor Activation, and Signaling of TSLP and IL-7
In response to pathogenic stimuli or mechanic injury, TSLP gets produced mainly by epithelial cells at barrier surfaces such as lung and gut, epidermal keratinocytes, and dendritic cells. However, we now know that TSLP has a much broader expression profile that extends to fibroblasts, macrophages, basophils, monocytes, and cancer cells (57–68). In addition to being involved in proliferation and differentiation of B-cell progenitors, TSLP expression and signaling leads to activation of immature DCs, CD4+ T-cell homeostasis and regulatory T-cell development through a coordinated cascade of immune and non-immune cells indicating its influence far beyond the widely acknowledged type 2 inflammatory responses (5, 61, 69–78).
TSLP mediates signaling by establishing a heteromeric complex involving TSLPR, a type I cytokine receptor, and IL-7Rα, a receptor also utilized by IL-7 (79, 80). TSLPR binds TSLP with high affinity (KD = 32 nM), while IL-7Rα does not bind to TSLPR alone with measurable affinity. However, IL-7Rα can be recruited with high affinity (KD = 29 nM) to the TSLP:TSLPR complex making this binary assembly a mechanistic prerequisite for effective signal transduction (81, 82). Of note, human TSLPR and IL-7Rα were shown to bear low, albeit measurable affinity (KD 20 μM) for each other in the absence of TSLP, suggesting that preformed receptor-receptor interactions might play a role in the assembly of a TSLP-mediated complex under certain conditions (82). The ensuing dimerization of both receptor chains upon TSLP binding results in activation of Janus kinases (JAKs) and signal transducers and activators of transcription (STATs) leading to transcription of target genes and subsequent tightly coordinated immune responses (83–86) (Figure 1). Recently, tools have been developed exploiting the signaling properties of TSLPR for the screening and characterization of the activity of various types of cytokines and their receptors (87).
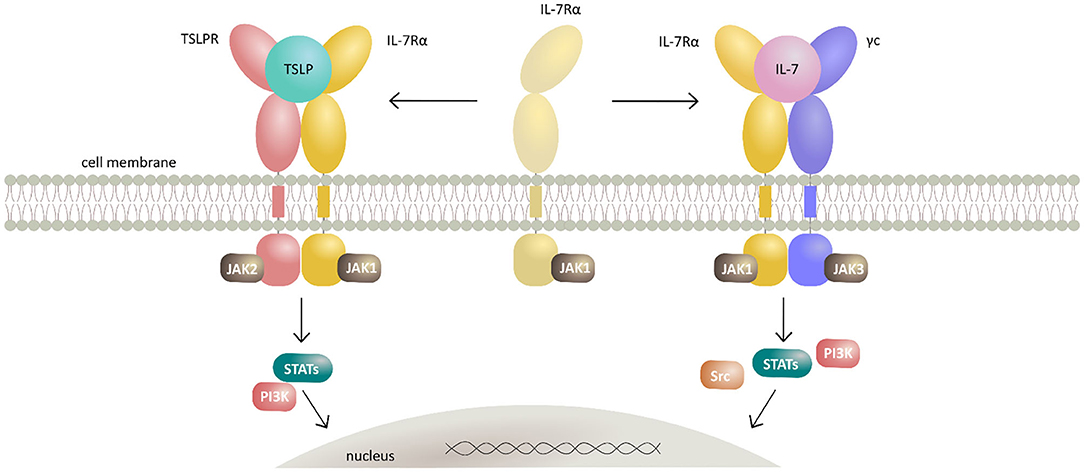
Figure 1. Schematic representation of TSLP and IL-7 signaling mechanisms by their respective receptor heterodimerization upon cytokine binding. Cytokines TSLP and IL-7 both signal through heterodimeric receptors by sharing the IL-7Rα receptor chain. TSLP first interacts with the cognate TSLPR thus potentiating the recruitment of IL-7Rα and formation of extracellular ternary complex leading to activation of intracellular signaling by canonical JAK/STAT and PI3K pathways. Together with IL-7Rα and the γc, IL-7 forms a heterodimeric receptor complex resulting in activation of JAK/STAT, PI3K, and SRC pathways.
Similar to TSLP, IL-7 is predominantly secreted by non-lymphoid cells like keratinocytes and epithelial and stromal cells in lymphoid organs with highest expression levels being detected in thymus and lymph nodes. In contrast to several other members of IL-2 family cytokines, IL-7 is not produced by hematopoietic cells (88–93). In fact, it is now known that together with ILCs, hematopoietic cells express IL-7Rα and therefore play a role in lymphoid consumption and regulation of IL-7 availability (94). While the IL-7Rα on lymphoid cells regulates both TSLP and IL-7-mediated signaling, non-lymphoid cell types carrying IL-7Rα mediate only TSLP signaling (95). IL-7 signaling is essential for the development and homeostasis of T lymphocytes and several members of recently discovered ILC family, whereas its role in early development of B lymphocytes has been shown to be more substantial in mice than in humans (95–97).
IL-7 signals through a heterodimeric receptor complex consisting of IL-7Rα and the γc (98). The observed stepwise mechanism of the ternary assembly mediated by IL-7 is analogous to the one suggested for TSLP and γc family interleukins, whereby the formation of a high affinity IL-7:IL-7Rα constitutes a mechanistic requirement for the assembly of the signaling-competent ternary complex (Figure 1).
However, contrary to TSLP binding to TSLPR, binding of IL-7 to IL-7Rα has been proposed to proceed via biphasic binding kinetics manifested by two sets of on- and off-rate constants (99). The presence of such an unusual IL-7:IL-7Rα structural intermediate implied by the proposed model requires further confirmation by orthogonal biophysical approaches that may allow detection of conformational changes. Additional mechanistic considerations centering on the possibility of IL-7Rα homodimers and IL-7Rα-γc heterodimers in the absence of IL-7, have also been proposed: upon the presence of IL-7 a pre-associated IL-7Rα-γc heterodimer undergoes a rotation away from the cell surface bringing the C-termini of the IL-7Rα and γc within the distance allowing them to form an activating complex (100). A similar mechanism involving an IL-7Rα homodimer could serve as an explanation of the signaling effects that the gain-of-function mutations in T-ALL and B-ALL patients have independent of γc and IL-7 presence (46, 53). As an example, it has been shown that the S185C IL-7Rα mutation present in B-ALL patients leads to the formation of an additional disulfide bond between the two S185C IL-7Rα chains whereas the mutation of the cysteine to a glycine eliminates this effect (46). Results obtained from structural modeling of this interaction confirm the suggested mechanism by showing that the disulfide bond between the receptor chains allows the signal inducing proximity without the presence of a ligand (100).
Strikingly, whereas binding of IL-7 to non-glycosylated IL-7Rα shows medium affinity (low μM range) there is a dramatic decrease in the apparent KD when binding the glycosylated IL-7Rα (low nM range) irrespective of glycan type or branching (99). Decoy IL-7Rα also plays an important role in regulation of IL-7 in immunity and in disease states (101). While the membrane bound IL-7Rα chain participates in signaling, soluble IL-7Rα competes with the membrane-bound receptor to eliminate excessive IL-7 thereby re-establishing its low levels as normally observed in vivo (102). Ligand-induced dimerization of IL-7 receptor leads to the activation of JAK-STAT, PI3-kinase, and MAPK/Erk signaling cascades and their respective responses (103–108).
Structural Basis of TSLP-Mediated Signaling
Consistent with its annotation as a member of the IL-2 family of helical cytokines, structural studies by X-ray crystallography of human and mouse TSLP in complex with its cognate receptors revealed that TSLP adopts a four-helix bundle structure with alpha helices (αA, αB, αC, and αD) following an ‘up-up-down-down’ topology and six cysteine residues forming pairs of disulfide bridges (81, 82). Recently, evidence of two isoforms of human TSLP originating from the TSLP gene—a short and a long form—has been reported largely based on mRNA expression profiles (109). Given that it is currently unclear whether the short isoform of TSLP is translated into a biologically active protein, we will only be focusing on the well-established and biologically active long form of TSLP.
Intriguingly, human TSLP displays a unique structure among four-helix bundle cytokines manifested via a rather open helix-bundle core harboring a substantial internal void volume adjacent to a fully buried water molecule coordinated by three conserved residues (Trp148, Thr102, and Thr83). Furthermore, helix αA displays a substantial kink centered at about its midpoint that is hallmarked by a π-helical turn, a structural feature that has been linked to the enhancement of protein functionality (110). Complementary structural studies on unliganded human TSLP in solution by nuclear magnetic resonance established the flexibility of helix αA at its π-helical turn (82). The TSLP four-helix bundle is threaded by three loops, a BC-, AB-, and CD- loop, with the latter harboring a stretch of 7 basic amino acids (residues 125–131) the role of which remains unclear. However, it has been proposed that this segment encodes for a furin cleavage site and might provide a level of regulation for the secreted amounts of TSLP (82) (Figure 2A).
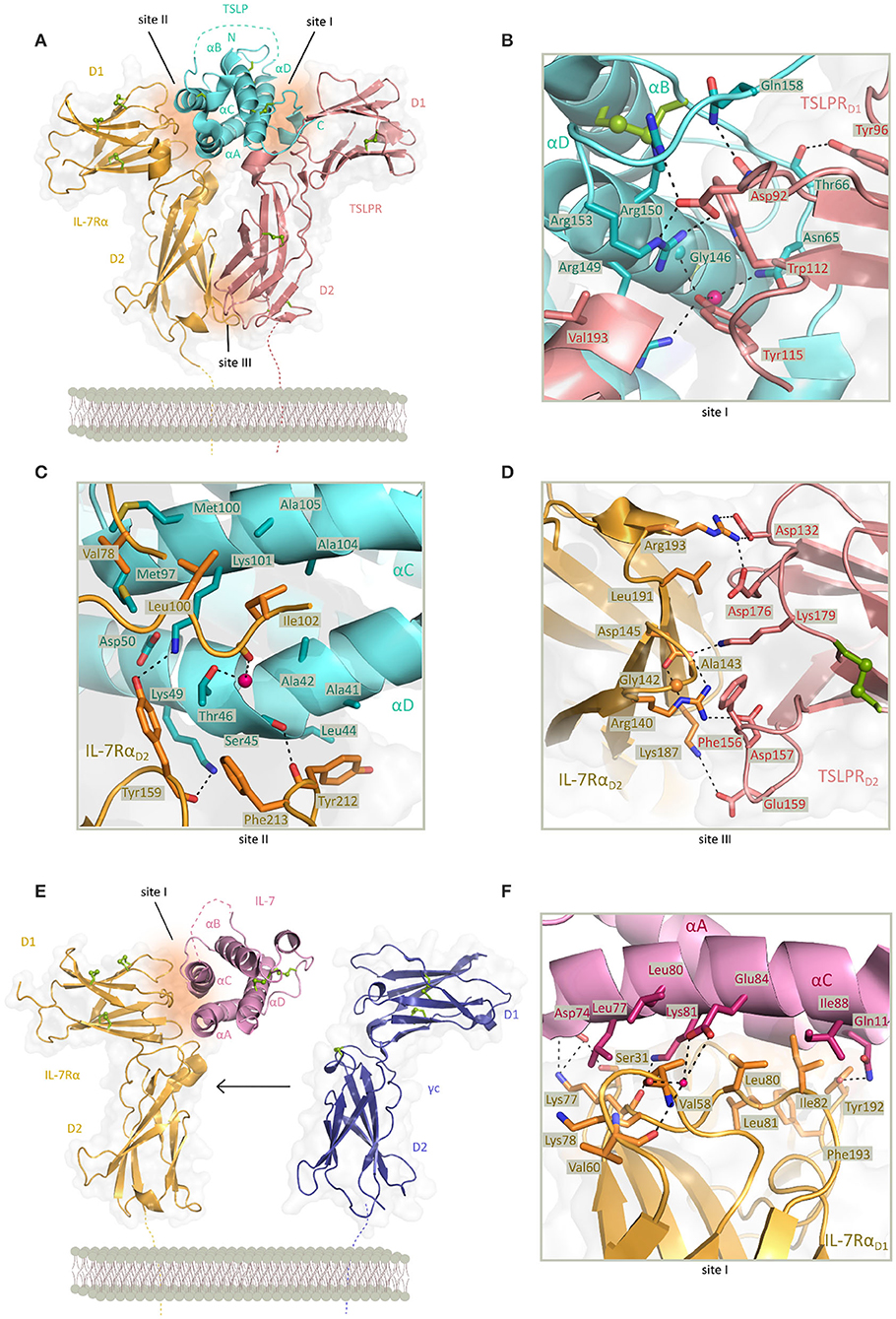
Figure 2. Structure of TSLP and IL-7 receptor complexes and structural close up view of the cytokine-receptor contact interfaces. (A) View of the determined X-ray structure for the TSLP:TSLPR:IL-7Rα ternary complex. TSLP is shown in aquamarine cartoon representation with four helices marked αA-αD and the disordered CD loop region is shown as a dashed aquamarine line. The extracellular regions of TSLPR (salmon pink) and IL-7Rα (bright orange) each comprising of two FnIII-like domains D1 and D2 are shown as cartoons on a transparent gray surface representation. Disulfide bridges are represented by green spheres. Regions contributing to protein-protein contact are named site I (TSLP:TSLPR), site II (TSLP:IL-7Rα), and site III (TSLPR:IL-7Rα) and represented by dark orange surfaces. [PDB 5J11, (82)] (B) Detailed representation of the TSLP:TSLPR interface (site I). (C) Detailed representation of the TSLP:IL-7Rα interface (site II). (D) Detailed representation of the TSLPR:IL-7Rα interface (site III) viewed from the membrane-proximal side. In (B–D) interface residues are shown as sticks and hydrogen bonds and salt bridges are indicated with a dashed line. Water molecule is depicted as a pink sphere. (E) View of the X-ray structure for the IL:IL-7Rα binary complex. IL-7 is shown in pink cartoon representation with four helices marked αA-αD and the second crossover loop shown as a dashed pink line. The extracellular regions of IL-7Rα (bright orange) and γc (purple) are comprising of two FnIII-like domains D1 and D2 shown as cartoons on a transparent gray surface representation. The γc from the IL4 ternary complex has been depicted apart from the IL:IL-7Rα complex as it has not been co-crystallized with the binary complex [PDB 3DI2, 3BPL, (99, 111)]. Disulfide bridges are represented by green spheres. Region contributing to IL-7:IL-7Rα contact is named site I and represented by dark orange surface. (F) Detailed representation of the IL-7:IL-7Rα interface (site I). Interface residues are shown as sticks and hydrogen bonds and salt bridges are indicated with a dashed line. Water molecule is depicted as a pink sphere.
Based on high-resolution structural insights from the TSLP:TSLPR:IL-7Rα ternary complex, the attraction of TSLP to a cytokine binding homology region (CHR) in TSLPR (site I) is characterized by electrostatic complementarity between a positively charged surface on TSLP and negatively charged one on TSLPR (Figure 2B). Formed binary complex TSLP:TSLPR has a calculated negative electrostatic potential which supports subsequent binding of IL-7Rα having a positive electrostatic potential. Part of residues involved in TSLP:TSLPR interactions are located in the C-terminal part of αD helix (residues 142–158) and AB-loop region (residues 60–69) undergoing significant conformational changes for obtaining the bound state. The AB-loop is simultaneously offering a physical link to the αA helix important for interactions with IL-7Rα (site II) thus being a mediator of priming TSLP to recruit the IL-7Rα after forming TSLP:TSLPR complex which then facilitates the positioning of the αA helix providing a necessary entropic advantage for the formation of a T-shaped ternary complex. Besides residues in αA helix, hydrophobic interface of IL-7Rα furthermore interacts with several exposed residues in αC helix of TSLP (Figure 2C). Compact interaction of TSLPR and IL-7Rα in their membrane proximal region (site III), also known as the stem region, is characterized by electrostatic interactions and close van der Waals contacts. Interactions in site III have been proven to contribute to the effective TSLP-mediated signal transduction (82) (Figure 2D).
Structural Basis of Signaling Assemblies Mediated by IL-7
Just like TSLP and other cytokines of the IL-2 family, IL-7 has four helices (αA-αD) adopting the “up-up-down-down” topology. While homology models had predicted the presence of three disulfide bonds, electron density of crystal structures obtained was too weak at the N-terminus and the end of helix αC where third disulfide bond was predicted resulting in tracing only two of the cysteine pairs (99, 112). Structural data shows burial of the only tryptophan residue of IL-7 in the hydrophobic core of the helix bundle, consistent with mutagenesis studies that linked this position to the proper folding of IL-7 (99, 113, 114). As in TSLP, helix αA of IL-7 comprises a π-helical turn of six residues stabilizing the IL-7:IL-7Rα interaction. This interface also includes contacts with αC residues and is generally characterized by hydrophobic, van der Waals and few intermolecular polar interactions (Figures 2E,F).
While other cytokines that signal via γc family receptors interact with their specific receptor chain via a larger buried surface consisting of predominantly polar residues, the IL-7:IL-7Rα interface is not only less extensive but also more hydrophobic (99). Interestingly, the TSLP-bound conformation of IL-7Rα is highly similar to the one observed in IL-7:IL-7Rα and IL-7Rα employs a nearly identical set of residues to bind each of the cytokines. These unique structural features shared between TSLP and IL-7 allow a predominantly hydrophobic interaction with IL-7Rα providing a rationale for duality and degeneracy of signaling via IL-7Rα (82). Nevertheless, the structure of the IL-7:IL-7Rα complex does readily explain the large differences in the affinity of IL-7 to the glycosylated and non-glycosylated forms of IL-7Rα as all candidate glycosylation sites are not in close proximity to the IL7:IL7Rα interface (99).
So far, there has been no structural data for the ternary IL-7:IL-7Rα:γc assembly, which would fill a large void in our understanding of the extracellular signaling assembly mediated by IL-7. Besides comparing structural and mutagenesis data coming from other cytokine-receptor binary and ternary assemblies in γc receptor family, efforts have been made to model this interaction using the IL-7:IL-7Rα binary complex and γc structures extracted from other complexes. These approaches suggested the critical involvement of a disulfide bond and involvement of several key residues on γc for the formation of a ternary complex. Although the models propose the canonical engagement of helices αA and αD in binding the γc receptor, the proposed models also suggest the IL-7: γc interface will differ substantially from other γc:interleukin interactions. The differentiation of cytokines by γc is additionally facilitated by angular displacement of the helices to form a distinct binding epitope. Indeed, superpositon of IL-2, IL-4, and IL-21 as observed in their receptor complexes onto IL-7 revealed a substantial difference in the inter-helical orientation (99). Superpositions of fibronectin type III (FnIII) domains of IL-7Rα and IL-4Rα binary and ternary complexes onto IL-2Rβ show differences in angular geometry between the domains resulting in steric clashes and a lack of availability of helices to contact γc receptor. This suggests that it requires another conformation and a more drastic elbow angle between the two FnIII domains to adequately form IL7:IL-7Rα:γc (99, 100).
Strategies to Modulate Signaling Mediated by TSLP and IL-7
In light of the tremendous importance of cytokines in health and disease, recent efforts have focused on harnessing structural and functional data interrogating cytokine-receptor interactions and functionality toward the development of potent antagonists and agonists that can modulate cytokine-mediated signaling (115) (Figure 3). Such modulators would be expected to have distinct modes of action compared to already available inhibitors known to target intracellular portions of cytokine receptors or specific intracellular signaling components downstream of cytokine-dependent receptor activation (116, 117). Nevertheless, extracellular portions of cytokine receptors and the activating cytokines are attractive targets in their own right. In this context, modulation of their activity could be achieved by both biologicals and non-biologicals engineered to bind in either orthosteric or allosteric fashion. Preventing cytokine action in such a context has mostly been achieved by neutralizing antibodies developed against either the cytokine or one of its receptors or by using soluble ectodomains of the cytokine receptors as molecular decoys (101, 118–121). These approaches do not necessarily require structural insights and are therefore favorable in targeting cytokine/receptor interactions when no such data is available. To this end, promising alternatives are in the form of Fc-fused receptors or cytokine traps comprising fusions of the cognate receptor ectodomains via flexible linkers (82, 122–124). Cytokine-derived antagonists present another approach in which structural information together with the mechanistic insights are crucial for establishing the critical cytokine-receptor interaction determinants to arrive at cytokine variants having desired modulatory characteristics and potency (115). In the case of heterodimeric receptor complexes, this approach is based on the engineering a cytokine such that it can only bind one of the receptor chains with high affinity thereby being unable to recruit the second receptor to form a signaling complex with IL-4 antagonist as one of the best-known examples (113, 125). Such a strategy led to the design of muteins with remarkable new properties: for instance, an IL-4 antagonist (Pitrakinra™) is able to block both IL4/IL-13 receptor assembly formation, an IL-2 superkine where the engineered new property bestows a higher affinity for IL-2Rβ chain, or the most recently reported neoleukins designed to play into the functional dichotomy of IL-2 and IL-15 (126–128).
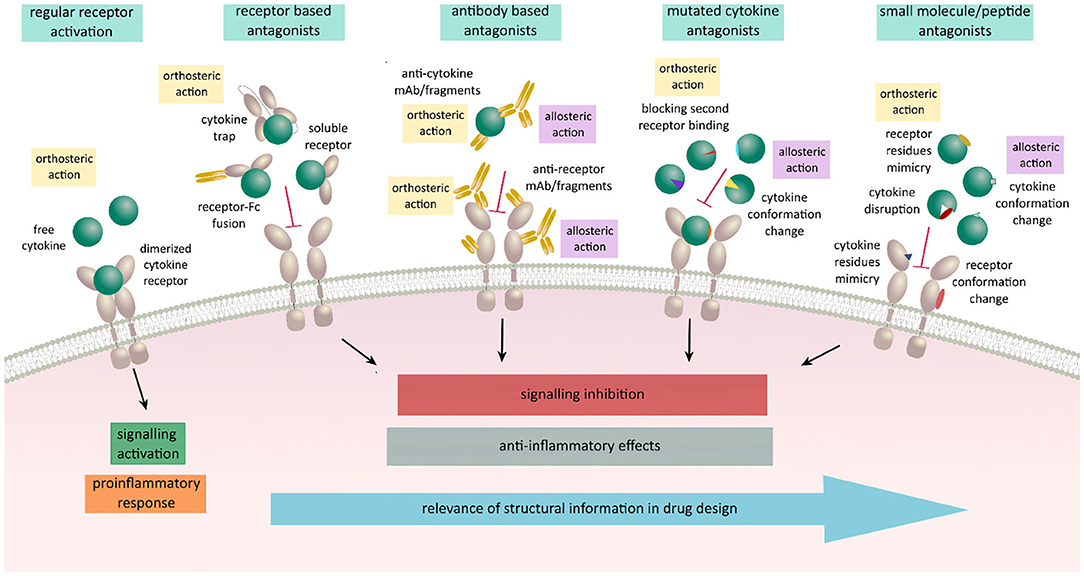
Figure 3. Schematic representation on various antagonistic strategies used for regulation of cytokine signaling acting in an allosteric or an orthosteric fashion. Receptor activation leads to signaling activation upon cytokine binding and receptor dimerization resulting in a proinflammatory responses and various disease states. These responses can be meliorated by the use of biologicals engineered to orthosterically or allosterically block the receptor activation. Receptor based antagonists act in an orthosteric fashion by blocking the expressed cytokine and preventing it to bind to its respective ligand. Antibody based antagonists consist of either full antibodies, Fab fragments, scFv, or nanobodies against the cytokine or its receptor. They can either act orthosterically at the binding site and prevent the binding of cytokine to its specific receptor or allosterically by binding outside of the binding site affecting the conformation and resulting in the lack of binding ability of either cytokine or the receptor. Mutated variants of cytokines are designed to act in both ways by keeping the ability to bind to their specific cytokine receptor chain and losing the affinity to the second receptor chain due to selected mutations. Small molecule and peptide antagonists design is based on either mimicking cytokine or receptor interaction residues, disrupting the proper folding of the protein or binding outside of the interaction sites inducing a conformation change resulting in blocking of receptor activation and signal transduction.
Peptide-based inhibitors can have high structural similarity to fragments of the target proteins and in that way mimic protein-protein interactions crucial for signaling. Their additional advantage is simple synthesis and possibility to modify their peptide sequences using diverse functional groups (129, 130). Together with small molecule inhibitors, such inhibitors focus on targeting hot spots and binding gaps at cytokine-receptor interfaces and modulate their activity as demonstrated for IL-2 and TNF and suggested for IL-18 (131–134). Although challenging to develop, potent small molecules inhibitors are considered to offer substantial advantages over biologics or protein-based modulators, including oral and topical administration (135).
Modulators of TSLP Signaling
Anti-TSLP monoclonal antibody (mAb) Tezepelumab (AMG157/MEDI9929) has first been reported in 2014 and is to date the most prominent and advanced inhibitor of TSLP-mediated signaling in the context of allergic inflammatory disorders and the only TSLP-linked antagonistic candidate that is currently in phase III clinical trial in patients with severe asthma (136–138). The neutralizing effects of this antibody suggested that it recognizes an epitope in the domain of cytokine responsible for binding of TSLPR chain. Indeed, the X-ray structure of AMG157Fab fragment in complex with TSLP confirmed this by showing that complementarity determining regions (CDRs) of the variable heavy chain domain of Fab fragment interact with the AB-loop region and C-terminal region of helix D, while the light chain fragment does not interact with TSLP. In the same time, the other side of TSLP helical bundle involved in binding IL-7Rα remains available (82) (Figure 4A).
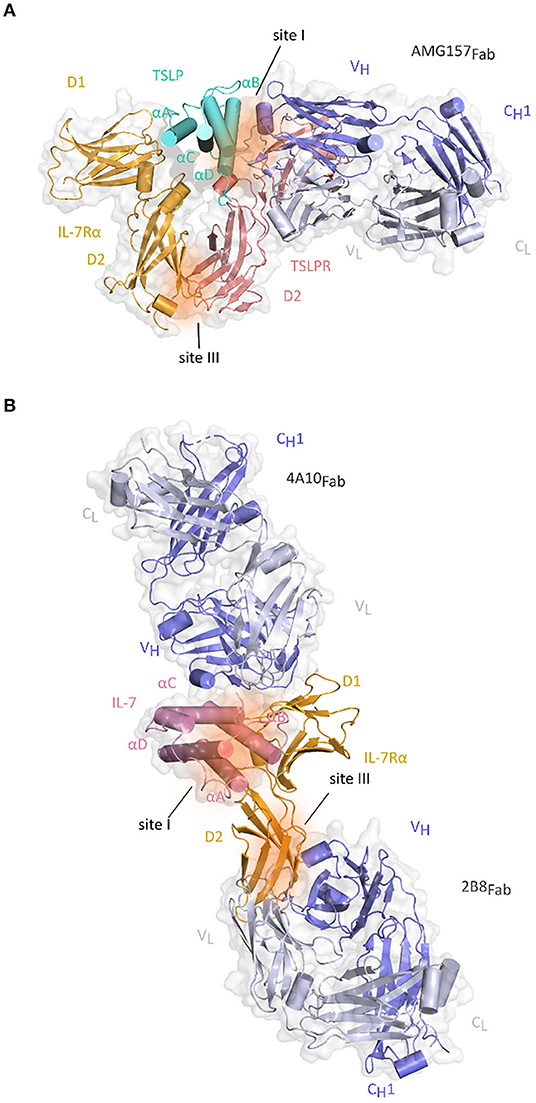
Figure 4. Structure of Fab fragments of the antibodies developed against TSLP and IL-7Rα together with their respective cytokine-receptor complexes. (A) Cartoon representation of the TSLP:TSLPR:IL-7Rα:AMG157Fab complex by superposition of the TSLP:TSLPR:IL-7Rα and TSLP:AMG157Fab based on structural alignment of the two TSLP structures [PDB ID:5J11, 5J13 (82)]. TSLP is shown in aquamarine cartoon representation with its four helices marked αA-αD. The extracellular regions of TSLPR (salmon pink) and IL-7Rα (bright orange) each comprising of two FnIII-like domains D1 and D2 are shown as cartoons on a transparent gray surface representation. Regions contributing to protein-protein interactions are represented by dark orange surfaces and named site I (TSLP:TSLPR) and site III (TSLPR:IL-7Rα). VH and CH1 fragments of AMG157Fab are colored in violet blue and the VL-CL fragments in white blue and shown as cartoons on a transparent gray surface representation. (B) Cartoon representation of the IL-7:IL-7Rα:4A10Fab:2B8Fab by superposition of IL-7Rα:4A10Fab and IL-7Rα:2B8Fab and IL-7:IL-7Rα based on structural alignment of IL-7Rα chains [PDB ID 3DI2, 6P50, 6P67 (99, 139)]. IL-7 is shown in pink cartoon representation with four helices marked αA-αD on a transparent gray surface representation. The two FnIII-like domains (D1 and D2) of IL-7Rα (bright orange) are shown as cartoons. Regions contributing to cytokine-receptor interactions are represented by dark orange surfaces and named site I for IL-7:IL-7Rα interface and site III for TSLPR:IL-7Rα and plausible γc interaction site. 4A10Fab and 2B8Fab are depicted as described for AMG157Fab in (A).
The most recent publication covering phase II trial in adults with a history of asthma exacerbations and uncontrolled asthma reports the study being conducted by subcutaneous Tezepelumab application over 52 weeks. Patients who were previously receiving asthma controllers and received Tezepelumab therapy showed rate reductions of clinically significant asthma exacerbations of at least 62% compared to the patients receiving placebo independent of baseline eosinophil counts (140, 141). Ongoing phase III clinical trial includes further mechanistic and long-term safety trials with the focus on both adult and adolescent patients with severe asthma considering previous or current treatments with different combinations of asthma controller medications. Initial safety and preliminary clinical activity of Tezepelumab in AD patients were evaluated in phase I study and showed no immunogenicity and good tolerance (142, 143). Phase IIa study in patients with moderate to severe AD receiving the antibody subcutaneously in combination with topical corticosteroids showed improvements in severity of the disease yet they were not statistically significant (144, 145). A Phase IIb study is designed to evaluate the safety and efficacy of Tezepelumab as a monotherapy and adjunct therapy in patients with moderate to severe AD and is currently in the patient recruiting stages (146). Confirmed evidence of efficacy of this antibody in treatment of a possibly broad population of asthma patients together with potential therapeutic benefit in patients with AD puts Tezepelumab on the map of promising therapeutics in inflammatory and possibly other diseases as well. Furthermore, the antibody-based inhibitor CSJ117 has been developed in a form of an inhalable Fab antibody fragment against TSLP. It has been used in a recently completed Phase I clinical trial in asthma patients with mild atopic asthma to access the safety, tolerability, pharmacokinetics, and pharmacodynamics of the inhaled agent (147). Moreover, novel human single-chain variable fragment (scFv) was also developed against human TSLP and selected from a fully human antibody library. ScFv29 is shown to bind to human TSLP in competition with the TSLPR. As it can also bind to murine TSLP, in vitro experiments performed on mouse derived mDCs showed that ScFv29 reduces the maturation rate of DCs making it one of the potential neutralizing candidates of this system (148). With the aim of increasing the therapeutic effects in asthma patients that have been shown to co-express TSLP and IL-13 and contribute to the severity of the disease Venkataramani and colleagues have developed novel bispecific anti-TSLP/IL-13 antibodies that are either monovalent bispecific (Zweimabs) or bivalent bispecific (Doppelmabs) and can simultaneously inhibit the signaling of both cytokines (149, 150). Binding and functional data show stronger affinity of the bispecific antibodies in comparison to their parental monospecific ones and inhibitory effects in DCs assays demonstrating the potency of such dual targeting (151) (Table 1).
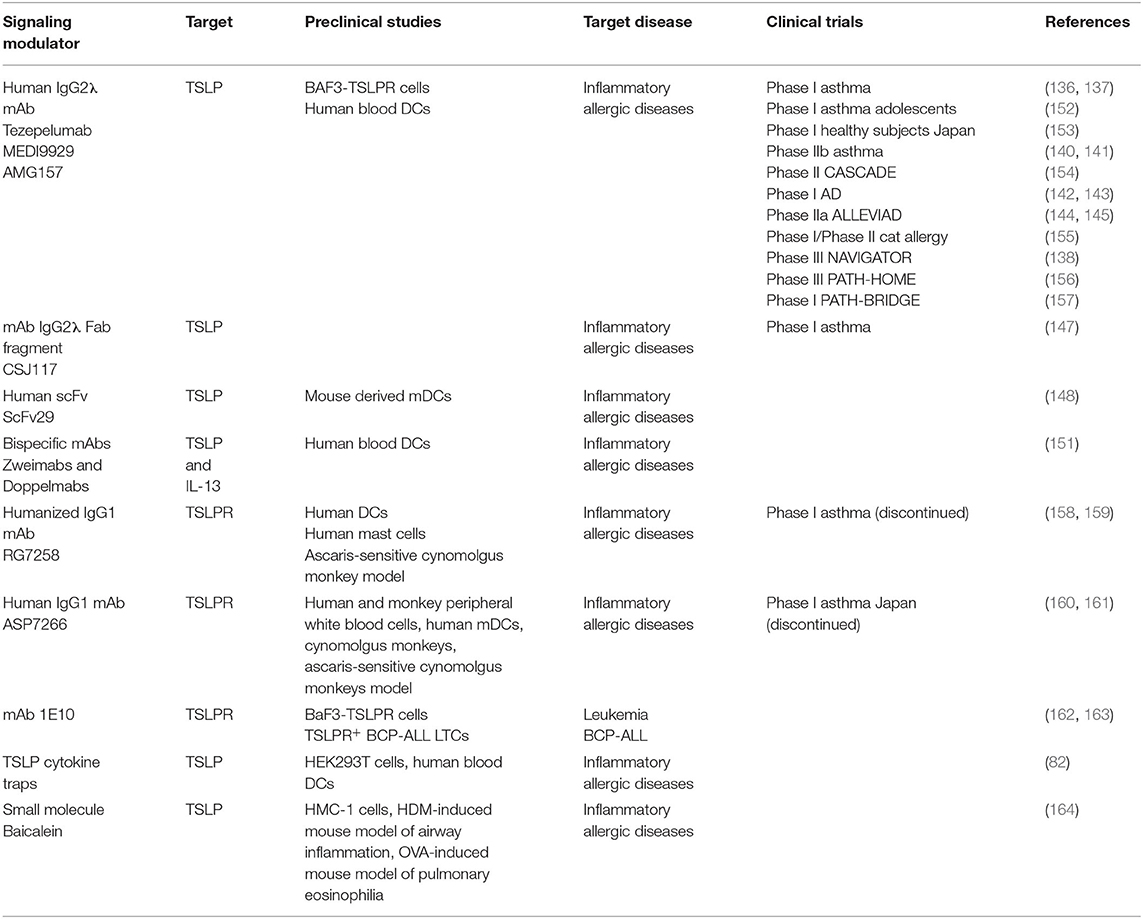
Table 1. Overview of the current modulators of TSLP signaling in preclinical studies and clinical trials.
The inhibitory mAb RG7258 considered as a potential therapeutic in allergic disorders has been developed against TSLPR. It cross reacts with the cynomolgus monkey TSLPR and inhibits TSLP-induced responses in vitro. Furthermore, in the ascaris-sensitive cynomolgus monkey model mAb RG7258 successfully reduced inflammation and bronchoconstriction (158). Another anti-TSLPRα antibody ASP7266 is able to inhibit TSLP signaling in peripheral white blood cells and mDC-mediated differentiation of naive CD4+ T cells into mature T cells in vitro. Intravenous administration to monkeys effectively blocked CCL17 mRNA expression in peripheral blood cells and suppressed skin allergic reactions in sensitized cynomolgus monkeys (160). However, phase I clinical trial in asthma patients in Japan has been discontinued for undisclosed reasons (161). One additional mAb generated against extracellular domain of TSLPR was reported as one out of two hybridoma clones that showed antagonistic properties toward TSLP without affecting IL-7/IL-7R signaling (162). A subsequent study by the same group tested the antagonistic potential of 1E10 clone in the context of ALL and showed that blocking of TSLPR represses proliferation and STAT activity in TSLPR+ BCP-ALL long term cultures (LTC) making it a potential therapeutic option for subset of BCP-ALL patients whose lymphoblasts express TSLPR (163) (Table 1).
The physiological role of soluble versions of cytokine receptor ectodomains as modulators of cytokine signaling in physiology and disease, and the fact that a naturally existing soluble TSLPR has not yet been identified, inspired the employment of the TSLPR ectodomain or its engineered variants as potential neutralizers of TSLP-mediated signaling. In a first study with such focus, a fusion protein TSLPR-Ig was designed by fusing the ectodomain of murine TSLPR with a murine IgG2a Fc tail. The effect of blocking TSLP signaling by murine TSLPR-Ig was tested in TSLP-activated murine DCs in vitro where it reduced the expression of CD40, CD80, and CD86. Additionally, local administration of murine TSLPR-Ig into the airways of asthmatic mice before sensitization suggested altering of the function of pulmonary DCs critical in Th2-mediated allergic disorders demonstrating that this blocking strategy could indeed be beneficial for treatment of asthma (165). Another validation of using TSLPR complex ectodomains as inhibitors was demonstrated by utilizing both soluble TSLPR and IL-7Rα ectodomains in an equimolar mixture in a cellular STAT5 activity assay confirming their dose-dependent neutralizing effect. Arguably, the most potent approach to date situates in the employment of receptor fusion proteins, termed TSLP-traps, featuring tandem fusions of the TSLPR, and IL-7Rα ectodomains using a flexible (Gly-Gly-Ser)20 linker. Notably, TSLP-trap1 showed a 250-fold higher affinity to TSLP when compared to the unlinked receptor ectodomains and a comparable affinity and binding kinetics to TSLP as both Tezepelumab mAb and its corresponding Fab fragment. A similar binding profile has been reported for TSLP-trap2. Competition assays in HEK293T cells showed that both TSLP-trap variants have about 1000-fold higher inhibitory potency over equimolar mixtures of unlinked soluble ectodomains and could outperform the inhibition of STAT5 signaling by 20–30-fold in comparison to the most potent anti-TSLP agent Tezepelumab and its Fab fragment. These intriguing data were further supported by evidence of both TSLP-trap variants having no effect on IL-7 function and being able to significantly block TSLP-driven DC-activation with the same efficacy as Tezepelumab (82). Thus, TSLP-traps represent promising novel biologicals with outstanding neutralizing potency.
The advent of high-resolution crystal structures of mouse and human TSLP:TSLPR ternary complexes (81, 82) offered the long missing structural blueprints to inspire the development of small molecule inhibitors targeting the TSLP:TSLPR interaction as a new therapeutic strategy. Van Rompaey and collaborators identified the first fragments to inhibit the TSLP:TSLPR interaction by a combined virtual—in vitro screening approach. The procedure consisted of an extensive in silico analysis of the structural data and evaluation of possible hot spots, screening of two commercially available fragment libraries followed by docking the hits to TSLPR, two-stage biological screening for further selection of the fragments and finally molecular dynamics to explore the binding pathway and model of fragment binding. This approach provided a proof-of-concept for the use of fragments in the modulation of TSLP signaling (166). Another study explored the potential of peptide-derived inhibitors designed based on amino acid sequences from murine TSLP:TSLPR structure that was the only structure available at the time. Solid-phase peptide synthesis was used to generate 16 peptides by mimicking epitopes of two TSLP:TSLPR interaction sites and resulted in three peptides capable of TSLP inhibition at submillimolar concentrations (167). The most recent report has focused on a flavonoid representing a major component of S. baicalensis, Balcalein, that was identified as the first small molecule inhibitor of TSLP-mediated signaling. Based on in vitro confirmation that the compound blocks TSLP:TSLPR interaction in a dose-dependent manner, in vivo studies in both HDM-challenged and OVA-sensitized mice resulted in reduced number of eosinophils in treated mice. Further chemical modeling led to a synthesis and identification of compound 11a, a biphenyl flavanone analog, which is considered the most advanced human TSLP inhibitor in this series of tests and characterized by moderate inhibition and good water solubility (164).
Efforts to identify TSLP antagonists for both murine and human cytokine have been made by analyzing TSLP:TSLPR and TSLP:IL-7Rα interaction sites. The most promising murine TSLP variant had one-point mutation, I37E, and showed high-affinity binding to TSLPR but no STAT5 activity in a cellular based assay (81, 82). From the selection of generated human mutants, a double mutant carrying S45R/T46R mutations residues at the TSLP:IL-7Rα interface in site II showed an unaffected ability to bind TSLPR and reduced STAT5-signaling in comparison to wt TSLP. From the selected mutants located in site I at the TSLP:TSLPR interface all of them reduced STAT5 signaling, with R149S/R150S double mutant having the most pronounced effect (82). In spite of not yielding a potent antagonist, those functional interrogations did demonstrate the importance of TSLP residues identifying hot spots that could be considered in further attempts in antagonist design.
Modulators of IL-7 Signaling
Targeting of IL-7:IL-7Rα for therapeutic development in autoimmune diseases has mainly focused on the development of monoclonal anti-IL-7Rα antibodies which could be beneficial by blocking IL-7Rα and subsequently attenuating the action of effector T cells but retaining the Treg activity (168).
Application of anti-IL-7Rα mAb 28G9/Ab1 in mice with autoimmune encephalomyelitis (EAE) was effective in reducing disease activity and severity (169). In non-obese diabetic mice, treatment with 28G9 delayed the progression of T1D before onset and reversed the newly onset diabetes (170). Ex vivo studies in cynomolgus monkeys showed a decreased STAT5 phosphorylation in both CD4+ and CD8+ T cells of blood samples after treatment (171). Another mAb (PF-06342674/RN168) developed by Pfizer has completed a phase I clinical trial in healthy volunteers. While the MS clinical trial was terminated by Pfizer themselves, the T1D phase I clinical trial evaluated the safety and tolerability of multiple SC doses in type 1 diabetes patients and showed that certain dose of mAb selectively inhibits survival and activity of memory T cells while preserving naive T cells and Treg (172). Additional model study of its pharmacokinetics showed that it has a 20-fold more potent inhibitory effect on TEM cells relative to Treg cells at a similar dose confirming the implication that these effects could serve to eliminate pathologic T cells in autoimmune diseases (173) (Table 2).
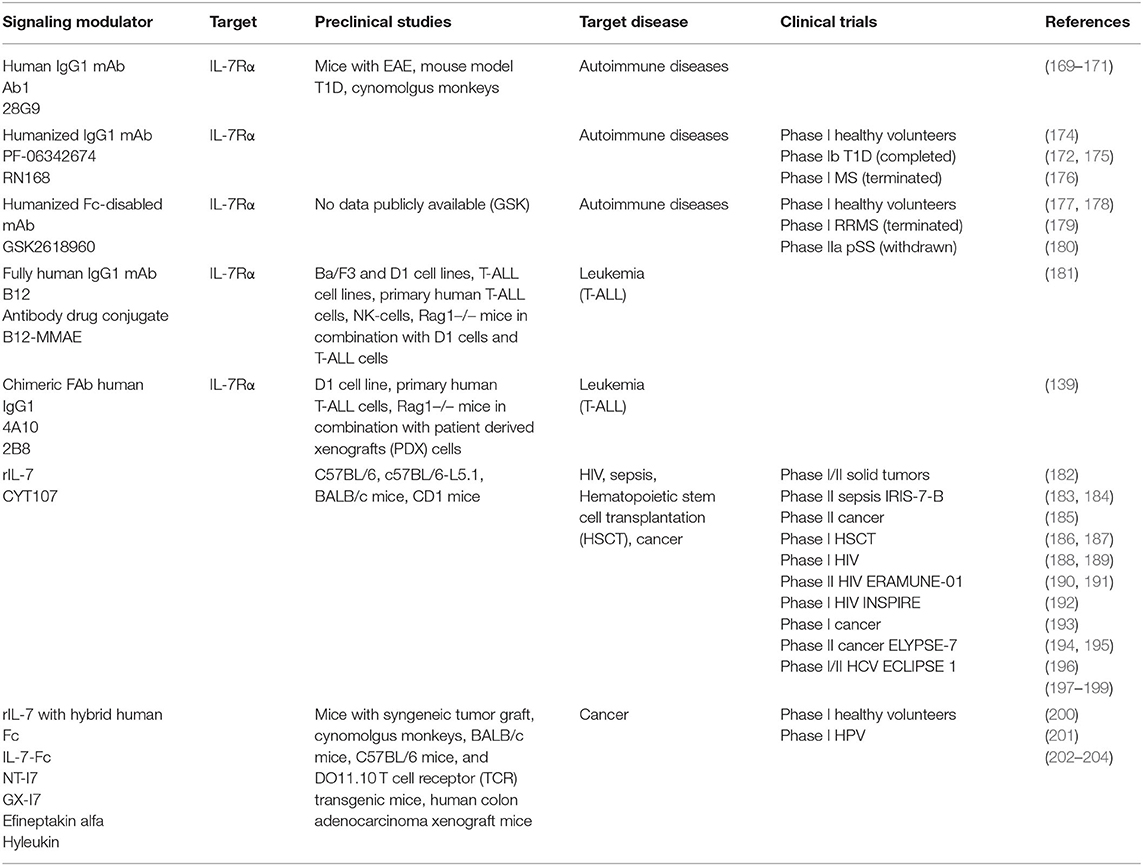
Table 2. Overview of the current modulators of IL-7 signaling in preclinical studies and clinical trials.
Humanized Fc-disabled anti-IL-7Rα mAb, known under GSK261896, was well-tolerated in phase I clinical trial in healthy volunteers. It blocked IL-7 receptor signaling upon full target engagement, increased circulating IL-7 and soluble IL-7Rα, however showed no impact on peripheral T cell subsets or levels of other inflammatory cytokines (177). Phase I clinical trial in relapsing remitting MS patients got terminated because of the misrepresentation of preclinical data while the phase II trial in pSS patients was withdrawn resulting in no ongoing trials for this agent at the moment (180) (Table 2).
Two of the most recently developed anti-IL-7Rα antibodies might both potentially benefit patients with T-ALL. Akkapeddi and colleagues reported a fully human IgG1 mAb, termed B12, developed against wildtype and several mutant IL-7Rα carrying insertions or single amino acid substitutions. A simulation of the structure of B12 in complex with the non-glycosylated IL-7Rα ectodomain indicated that the binding epitope is distinct from the interaction interface with IL-7. Based on the known structure of IL-7:IL-7Rα structure, IL-7 is positioned at the region connecting the D1 and D2 domains of the receptor, which implies that B12 could be located on the opposite side. B12:IL-7Rα binding interface is mostly hydrophobic with few van der Waals interactions and hydrogen bonds. B12 is able to inhibit IL-7-dependent and mutant-dependent IL-7R-mediated signaling and induce leukemia cell death. It promotes NK-mediated T-ALL cytotoxicity in vitro, delays T-cell leukemia development in vivo reducing tumor burden and promoting mouse survival and sensitizes T-ALL cells to treatment with dexamethasone inducing leukemia cell death. Those favorable effects were meliorated by B12-mono-methyl auristatin E (MMAE) antibody–drug conjugate (ADC) that is able to kill primary and patient-derived xenograft T-ALL cells more efficiently than B12 alone (181). The possibilities of ADCs in combination with IL-7R have previously been considered after confirming the involvement of IL-7 signaling in steroid-resistance when addressing the treatment of autoimmune diseases and cancers. The developed anti-murine IL-7R antibody conjugated with the compound SN38 showed strong anti-tumor effects against parental and steroid-resistant malignant cells, while the antibody-MMAE conjugate suppressed the inflammation in the mouse autoimmune arthritis model suggesting this approach as a possible novel alternative to steroid therapy (205). Furthermore, second study by the same group of collaborators that generated B12 shows that the two newly designed chimeric mAbs 4A10, and 2B8 recognize two separate epitopes on IL-7Rα based on the crystal structure of the 4A10Fab:IL-7Rα:2B8Fab complex. This structure reveals that 4A10Fab interacts with the periphery of epitopes responsible for binding IL-7 and that 2B8Fab binds close to the membrane region of the IL-7Rα, where TSLPR and the predicted γc binding sites would be situated (Figure 4B). Binding of 4A10Fab to the extracellular portion of IL-7Rα has been shown to be 9-fold tighter than the binding of 2B8Fab with both KD values in the low nM range compared to the binding properties of the mAbs to IL-7Rα on human lymphocytes. Moreover, these mAb chimeras inhibit IL-7R signaling at low IL-7 concentrations, mediate antibody-dependent cell mediated cytotoxicity in vitro and are effective in controlling established and relapsing leukemia in vivo (139) (Table 2).
Due to the pleiotropic nature of the biological activity of IL-7 and its central role in T-cell development, recombinant IL-7 (rIL-7) has been extensively tested in another, agonistic modulating frame. Indeed, multiple preclinical studies have confirmed that it could have therapeutic applications due to its potent immunorestorative and enhancing effect in immunotherapy and target multiple immunodeficiency conditions (206).
When used as an adjuvant in immunotherapy in sepsis patients with septic shock and severe lymphopenia, rIL-7 administered intramuscularly caused a 3- to 4-fold increase in absolute lymphocyte counts, reversed the marked loss of CD4+ and CD8+ immune effector cells and increased T cell proliferation and activation thus restoring adaptive immunity. As a novel approach in the treatment of patients with sepsis, another phase II study is planned in the future based on intravenous administration of rIL-7 (183, 184) (Table 2). Furthermore, patients suffering from infectious diseases may benefit from rIL-7 therapy given in a combination with antiviral drugs (188–192, 207), while patients who have received rIL-7 after hemopoietic stem cell transplantation (HSCT) showed T-cell recovery, implying a possibility of a lower risk of subsequent infection and relapse (186, 187) (Table 2).
In the context of cancer treatments rIL-7 has been used in clinical trials in patients with diverse types of tumors. A first study in humans was done in patients with metastatic melanoma and sarcoma and showed the ability of this cytokine to increase the number of CD4+ and CD8+ lymphocytes and decrease in the percentage of CD4+ T-regulatory cells suggesting its role in treating lymphopenia. At the same time, this study showed that the non-glycosylated variant of IL-7 elicits a low titer of binding antibodies and could lead to potential side effects in higher doses, suggesting the advantage of IL-7 produced in eukaryotic systems (197). A Phase I study in patients with solid tumors has been completed and proved tolerance and rIL-7 potency resulting in rejuvenated circulating T-cell profile with increase in overall naive T cells but a decreased Treg number making this effect opposite from the one observed in treatments employing IL-2 (198). In lymphopenic metastatic breast cancer patients during Phase II trial it increased CD4+ and other T-cell subset counts without altering their function (194, 195). Combining rIL-7 with vaccine therapy was used in two completed phase I clinical trials in patients with melanoma and pediatric solid tumors (182, 193). A phase II trial in patients with prostate cancer used glycosylated rIL-7 after vaccine therapy (185) (Table 2). The glycosylated version of rIL-7 will also be used in Phase II study in patients with locally advanced bladder urothelial carcinoma in a combination with Atezolizumab, an anti-PD-L1 antibody (208). The phase II study using chimeric antigen T cell therapy (CAR-T) in treating malignant solid tumor is planned to use patient's T cells and engineer them into IL-7 and Chemokine (C-C Motif) Ligand 19-expressing CD19-CAR-T cells and transfuse them into the patient for treatment of their B cell lymphoma (209).
A fusion protein, IL-7-Fc, composed of a recombinant form of IL-7 and a hybrid Fc region of a human antibody has been shown to stimulate proliferation and survival of different T-cell subsets and enhance anti-tumor immune responses (202). A phase I study on healthy volunteers was completed with further clinical trials at the moment either recruiting patients or planned for treatments of different types of malignancies: high risk skin cancer treatment in combination with Atezolizumab, treatment of high-grade glioma, treatment in combination with cyclophosphamide in patients with solid tumors being some of them (200, 210–212). Another phase I study will test the effects of IL-7-Fc on enhancement of immune reconstitution and vaccine responses in older people following chemotherapy due to their weakened immune system (213). Fc-fused IL-7 could also be used for inducing humoral immunity against viruses and a phase I clinical trial in human papilloma virus infected patients has been completed (201, 203, 214). Additionally, preclinical studies imply that IL-7-Fc can be used as an adjuvant in DNA vaccines and improve the immunogenicity (215, 216) (Table 2).
Perspectives and Conclusions
The landscape of therapeutic agents that can modulate the bioactivity of TSLP and IL-7 in inflammation, autoimmunity and cancer is clearly very broad in terms of disease coverage and displays a strong focus on biologics. Indeed, a number of therapeutic agents have either already entered the market or are in the final stages of clinical studies as demonstrated (217, 218). The diversity of agents that have been developed to block TSLP action demonstrates the range of possibilities and approaches that could be used to alter the biological activity of cytokines in general. Although most of the reported signal mediators of TSLP seem to be developed with the intention of treating inflammatory allergic diseases with Tezepelumab being the most promising novel therapeutic for asthma treatment, these agents should also be considered in the future in other, non-allergen induced conditions such as leukemia or autoimmune diseases. Precise dissection of the role of TSLP in each type of solid cancers will be key to enabling appropriate therapeutic strategies. For instance, in cancer types where TSLP might be tumor-protective, recombinant TSLP could prove to have therapeutic value either independently, or in the form of an immunocytokine fusion for tumor suppression by analogy to IL-2 (219, 220). Leveraging on the available structural data on the TSLP-receptor complex together with diverse display techniques to select hits with tailored characteristics could be considered in the design of TSLP antagonists or agonists (115, 221, 222).
Targeting upstream signaling mechanisms by different therapeutic approaches is considered to be potentially beneficial in preventing relapse and maintaining remission in patients with chronic inflammatory disorders or autoimmune diseases (223). For instance, this is supported by evidence that blocking the IL-7 mediated pathway can reverse ongoing autoimmunity (224). In the context of autoimmune diseases and cancer, the IL-7 signaling axis has been extensively targeted with antibodies against IL-7Rα which is rational considering that the effect of blocking γc chain could be problematic and lead to severe side effects since it is shared with numerous other cytokines (225). This approach has led to the identification of neutralizing antibodies that have completed phase I clinical trials and could potentially become beneficial for patients with autoimmune diseases. An additional reason for addressing IL-7Rα in drug development are frequent mutations leading to homodimerization of the receptor chains and constitutive signal transduction in a high percentage of B-ALL and T-ALL patients.
Considering the important role of both TSLP and IL-7 in the pathogenesis of RA simultaneous inhibition of both TSLP and IL-7R signaling in arthritis could serve a plausible therapeutic rationale in arthritis (226).
Although characterized by high selectivity, high efficacy, and limited side effects, biologics generally face a number of challenges such as expensive production, low tissue penetration and invasive administration (227–229). The design of small molecule inhibitors, an approach that highlights the importance of available structural information for facilitation of the design processes could present a suitable parallel alternative successfully addressing some of those issues. Ongoing and future studies on the diverse roles of TSLP and IL-7 in physiology and disease will undoubtedly further fuel efforts in the targeting of the two pleiotropic cytokines and their receptors in autoimmune diseases and cancer via appropriate molecular modulators.
Author Contributions
IM and SS designed the scope and thematic coverage of the article. IM reviewed the current literature, wrote the main draft of manuscript, and generated the figures. SS reviewed the drafts, provided conceptual and textual input, and approved the final version of the manuscript. Both authors contributed to the article and approved the submitted version.
Conflict of Interest
SS is listed as co-inventor in a patent application PCT/EP2017/057944 focusing on the development and application of TSLP-traps.
The remaining author declares that the research was conducted in the absence of any commercial or financial relationships that could be construed as a potential conflict of interest.
Acknowledgments
The authors gratefully acknowledge support from Research Foundation Flanders (grants G0B4918N and G.0C22.14N) and the VIB (Belgium).
References
1. Redhu NS, Gounni AS. Function and mechanisms of TSLP/TSLPR complex in asthma and COPD. Clin Exp Allergy. (2012) 42:994–1005. doi: 10.1111/j.1365-2222.2011.03919.x
2. Park JH, Jeong DY, Peyrin-Biroulet L, Eisenhut M, Shin J Il. Insight into the role of TSLP in inflammatory bowel diseases. Autoimmunity Rev. (2017) 16:55–63. doi: 10.1016/j.autrev.2016.09.014
3. Ziegler SF. Thymic stromal lymphopoietin and allergic disease. J Allergy Clin Immunol. (2012) 130:845–52. doi: 10.1016/j.jaci.2012.07.010
4. Kuan E Lo, Ziegler SF. Thymic Stromal Lymphopoietin and Cancer. J Immunol. (2014) 193:4283–8. doi: 10.4049/jimmunol.1400864
5. Roan F, Bell BD, Stoklasek TA, Kitajima M, Han H, Ziegler SF. The multiple facets of thymic stromal lymphopoietin (TSLP) during allergic inflammation and beyond. J Leukoc Biol. (2012) 91:877–86. doi: 10.1189/jlb.1211622
6. Suwarsa O, Dharmadji HP, Sutedja E, Herlina L, Sori PR, Hindritiani R, et al. Skin tissue expression and serum level of thymic stromal lymphopoietin in patients with psoriasis vulgaris. Dermatol Rep. (2019) 11:8006. doi: 10.4081/dr.2019.8006
7. El-Ghareeb MI, Helmy A, Al Kazzaz S, Samir H. Serum TSLP is a potential biomarker of psoriasis vulgaris activity. Psoriasis Targets Ther. (2019) 9:59–63. doi: 10.2147/PTT.S212774
8. Volpe E, Pattarini L, Martinez-Cingolani C, Meller S, Donnadieu MH, Bogiatzi SI, et al. Thymic stromal lymphopoietin links keratinocytes and dendritic cell-derived IL-23 in patients with psoriasis. J Allergy Clin Immunol. (2014) 134:373–81. doi: 10.1016/j.jaci.2014.04.022
9. Koyama K, Ozawa T, Hatsushika K, Ando T, Takano S, Wako M, et al. A possible role for TSLP in inflammatory arthritis. Biochem Biophys Res Commun. (2007) 357:99–104. doi: 10.1016/j.bbrc.2007.03.081
10. Moret FM, Hack CE, van Der Wurff-Jacobs KMG, Radstake TRDJ, Lafeber FPJG, van Roon JAG. Thymic stromal lymphopoietin, a novel proinflammatory mediator in rheumatoid arthritis that potently activates CD1c+ myeloid dendritic cells to attract and stimulate T cells. Arthritis Rheumatol. (2014) 66:1176–84. doi: 10.1002/art.38338
11. Iseki M, Omori-miyake M, Xu W, Sun X, Takaki S, Rawlings DJ, et al. Thymic stromal lymphopoietin (TSLP)-induced polyclonal B-cell activation and autoimmunity are mediated by CD4 + T cells and IL-4. Int Immunol. (2012) 24:183–95. doi: 10.1093/intimm/dxr113
12. Eckhardt J, Döbbeler M, König C, Kuczera K, Kuhnt C, Ostalecki C, et al. Thymic stromal lymphopoietin deficiency attenuates experimental autoimmune encephalomyelitis. Clin Exp Immunol. (2015) 181:51–64. doi: 10.1111/cei.12621
13. Gandolfo S, Bulfoni M, Fabro C, Russi S, Sansonno D, Di Loreto C, et al. Thymic stromal lymphopoietin expression from benign lymphoproliferation to malignant B-cell lymphoma in primary Sjögren's syndrome. Clin Exp Rheumatol. (2019) 37:S55–64.
14. Yao W, Zhang Y, Jabeen R, Nguyen ET, Wilkes DS, Tepper RS, et al. Interleukin-9 is required for allergic airway inflammation mediated by the cytokine TSLP. Immunity. (2013) 38:360–72. doi: 10.1016/j.immuni.2013.01.007
15. Takahashi N, Sugaya M, Suga H, Oka T, Kawaguchi M, Miyagaki T, et al. Thymic stromal chemokine TSLP acts through Th2 cytokine production to induce cutaneous T-cell lymphoma. Cancer Res. (2016) 76:6241–52. doi: 10.1158/0008-5472.CAN-16-0992
16. Ferretti E, Hohaus S, Di Napoli A, Belmonte B, Cuccaro A, Cupelli E, et al. Interleukin-31 and thymic stromal lymphopoietin expression in plasma and lymph node from Hodgkin lymphoma patients. Oncotarget. (2017) 8:85263–75. doi: 10.18632/oncotarget.19665
17. Chapiro E, Russell L, Lainey E, Kaltenbach S, Ragu C, Della-Valle V, et al. Activating mutation in the TSLPR gene in B-cell precursor lymphoblastic leukemia. Leukemia. (2010) 24:642–5. doi: 10.1038/leu.2009.231
18. Tal N, Shochat C, Geron I, Bercovich D, Izraeli S. Interleukin 7 and thymic stromal lymphopoietin: from immunity to leukemia. Cell Mol Life Sci. (2014) 71:365–78. doi: 10.1007/s00018-013-1337-x
19. Harvey RC, Mullighan CG, Chen IM, Wharton W, Mikhail FM, Carroll AJ, et al. Rearrangement of CRLF2 is associated with mutation of JAK kinases, alteration of IKZF1, Hispanic/Latino ethnicity, and a poor outcome in pediatric B-progenitor acute lymphoblastic leukemia. Blood. (2010) 115:5312–21. doi: 10.1182/blood-2009-09-245944
20. Yoda A, Yoda Y, Chiaretti S, Bar-Natan M, Mani K, Rodig SJ, et al. Functional screening identifies CRLF2 in precursor B-cell acute lymphoblastic leukemia. Proc Natl Acad Sci USA. (2010) 107:252–7. doi: 10.1073/pnas.0911726107
21. De Monte L, Reni M, Tassi E, Clavenna D, Papa I, Recalde H, et al. Intratumor T helper type 2 cell infiltrate correlates with cancer-associated fibroblast thymic stromal lymphopoietin production and reduced survival in pancreatic cancer. J Exp Med. (2011) 208:469–78. doi: 10.1084/jem.20101876
22. Watanabe J, Saito H, Miyatani K, Ikeguchi M, Umekita Y. TSLP expression and high serum TSLP level indicate a poor prognosis in gastric cancer patients. Yonago Acta Med. (2015) 58:137–43. doi: 10.1158/1538-7445.AM2016-727
23. Semlali A, Almutairi M, Reddy Parine N, Al Amri A, Almeer R, Alanazi MS, et al. Expression and allele frequencies of Thymic stromal lymphopoietin are a key factor of breast cancer risk. Mol Genet genomic Med. (2019) 7:e813. doi: 10.1002/mgg3.813
24. Zhou W-J, Yang H-L, Chang K-K, Meng Y, Wang M-Y, Yuan M-M, et al. Human thymic stromal lymphopoietin promotes the proliferation and invasion of cervical cancer cells by downregulating microRNA-132 expression. Oncol Lett. (2017) 14:7910–6. doi: 10.3892/ol.2017.7260
25. Xu L, Guo Y, Xu N, Chen L, Zhu J, Liu N, et al. Overexpression of thymic stromal lymphopoietin is correlated with poor prognosis in epithelial ovarian carcinoma. Biosci Rep. (2019) 39:BSR20190116. doi: 10.1042/BSR20190116
26. Xie F, Meng YH, Liu LB, Chang KK, Li H, Li MQ, et al. Cervical carcinoma cells stimulate the angiogenesis through TSLP promoting growth and activation of vascular endothelial cells. Am J Reprod Immunol. (2013) 70:69–79. doi: 10.1111/aji.12104
27. Barooei R, Mahmoudian RA, Abbaszadegan MR, Mansouri A, Gholamin M. Evaluation of thymic stromal lymphopoietin (TSLP) and its correlation with lymphatic metastasis in human gastric cancer. Med Oncol. (2015) 32:217. doi: 10.1007/s12032-015-0653-4
28. Pedroza-Gonzalez A, Xu K, Wu TC, Aspord C, Tindle S, Marches F, et al. Thymic stromal lymphopoietin fosters human breast tumor growth by promoting type 2 inflammation. J Exp Med. (2011) 208:479–90. doi: 10.1084/jem.20102131
29. Kuan EL, Ziegler SF. A tumor-myeloid cell axis, mediated via the cytokines IL-1α and TSLP, promotes the progression of breast cancer. Nat Immunol. (2018) 19:366–74. doi: 10.1038/s41590-018-0066-6
30. Olkhanud PB, Rochman Y, Bodogai M, Malchinkhuu E, Wejksza K, Xu M, et al. Thymic stromal lymphopoietin is a key mediator of breast cancer progression. J Immunol. (2011) 186:5656–62. doi: 10.4049/jimmunol.1100463
31. Demehri S, Cunningham TJ, Manivasagam S, Ngo KH, Tuchayi SM, Reddy R, et al. Thymic stromal lymphopoietin blocks early stages of breast carcinogenesis. J Clin Invest. (2016) 126:1458–70. doi: 10.1172/JCI83724
32. Demehri S, Turkoz A, Manivasagam S, Yockey LJ, Turkoz M, Kopan R. Elevated epidermal thymic stromal lymphopoietin levels establish an antitumor environment in the skin. Cancer Cell. (2012) 22:494–505. doi: 10.1016/j.ccr.2012.08.017
33. Di Piazza M, Nowell CS, Koch U, Durham AD, Radtke F. Loss of cutaneous TSLP-dependent immune responses skews the balance of inflammation from tumor protective to tumor promoting. Cancer Cell. (2012) 22:479–93. doi: 10.1016/j.ccr.2012.08.016
34. Guimond M, Veenstra RG, Grindler DJ, Zhang H, Cui Y, Murphy RD, et al. Interleukin 7 signaling in dendritic cells regulates the homeostatic proliferation and niche size of CD4+ T cells. Nat Immunol. (2009) 10:149–57. doi: 10.1038/ni.1695
35. Lundmark F, Duvefelt K, Iacobaeus E, Kockum I, Wallström E, Khademi M, et al. Variation in interleukin 7 receptor α chain (IL7R) influences risk of multiple sclerosis. Nat Genet. (2007) 39:1108–13. doi: 10.1038/ng2106
36. Bikker A, Van Woerkom JM, Kruize AA, Wenting-van Wijk M, De Jager W, Bijlsma JWJ, et al. Increased expression of interleukin-7 in labial salivary glands of patients with primary sjögren's syndrome correlates with increased inflammation. Arthritis Rheum. (2010) 62:969–77. doi: 10.1002/art.27318
37. Bikker A, Kruize AA, Wenting M, Versnel MA, Bijlsma JWJ, Lafeber FPJG, et al. Increased interleukin (IL)-7Rα expression in salivary glands of patients with primary Sjögren's syndrome is restricted to T cells and correlates with IL-7 expression, lymphocyte numbers and activity. Ann Rheum Dis. (2012) 71:1027–33. doi: 10.1136/annrheumdis-2011-200744
38. Gregory SG, Schmidt S, Seth P, Oksenberg JR, Hart J, Prokop A, et al. Interleukin 7 receptor α chain (IL7R) shows allelic and functional association with multiple sclerosis. Nat Genet. (2007) 39:1083–91. doi: 10.1038/ng2103
39. Todd JA, Walker NM, Cooper JD, Smyth DJ, Downes K, Plagnol V, et al. Robust associations of four new chromosome regions from genome-wide analyses of type 1 diabetes. Nat Genet. (2007) 39:857–64. doi: 10.1038/ng2068
40. Bai M, He X, He Y, Yuan D, Jin T, Wang L. IL-7R gene polymorphisms among patients with rheumatoid arthritis: a case–control study. Mol Genet Genomic Med. (2019) 7:e00738. doi: 10.1002/mgg3.738
41. McKay FC, Swain LI, Schibeci SD, Rubio JP, Kilpatrick TJ, Heard RN, et al. Haplotypes of the interleukin 7 receptor alpha gene are correlated with altered expression in whole blood cells in multiple sclerosis. Genes Immun. (2008) 9:1–6. doi: 10.1038/sj.gene.6364436
42. Hillen MR, Blokland SLM, Risselada AP, Bikker A, Lauwerys BR, Kruize AA, et al. High soluble IL-7 receptor expression in Sjögren's syndrome identifies patients with increased immunopathology and dryness. Ann Rheum Dis. (2016) 75:1735–6. doi: 10.1136/annrheumdis-2016-209236
43. Hartgring SAY, Bijlsma JWJ, Lafeber FPJG, van Roon JAG. Interleukin-7 induced immunopathology in arthritis. Ann Rheum Dis. (2006) 65(Suppl.3):69–74. doi: 10.1136/ard.2006.058479
44. Digel W, Schmid M, Heil G, Conrad P, Gillis S, Porzsolt F. Human interleukin-7 induces proliferation of neoplastic cells from chronic lymphocytic leukemia and acute leukemias. Blood. (1991) 78:753–9. doi: 10.1182/blood.V78.3.753.bloodjournal783753
45. Alsadeq A, Lenk L, Vadakumchery A, Cousins A, Vokuhl C, Khadour A, et al. IL7R is associated with CNS infiltration and relapse in pediatric B-cell precursor acute lymphoblastic leukemia. Blood. (2018) 132:1614–7. doi: 10.1182/blood-2018-04-844209
46. Shochat C, Tal N, Bandapalli OR, Palmi C, Ganmore I, te Kronnie G. et al. Gain-of-function mutations in interleukin-7 receptor-α (IL7R) in childhood acute lymphoblastic leukemias. J Exp Med. (2011) 208:901–8. doi: 10.1084/jem.20110580
47. Touw IP, Pouwels K, Van Agthoven T, Van Gurp R, Budel L, Hoogerbrugge H, et al. Interleukin-7 is a growth factor of precursor B and T acute lymphoblastic leukemia. Blood. (1990) 75:2097–101. doi: 10.1182/blood.V75.11.2097.2097
48. Barata JT, Keenan TD, Silva A, Nadler LM, Boussiotis VA, Cardoso AA. Common γ chain-signaling cytokines promote proliferation of T-cell lymphoblastic leukemia. Haematologica. (2004) 89:1459–67.
49. Barata JT, Cardoso AA, Nadler LM, Boussiotis VA. Interleukin-7 promotes survival and cell cycle progression of T-cell acute lymphoblastic leukemia cells by down-regulating the cyclin-dependent kinase inhibitor p27(kip1). Blood. (2001) 98:1524–31. doi: 10.1182/blood.V98.5.1524
50. Silva A, Laranjeira ABA, Martins LR, Cardoso BA, Demengeot J, Andrés Yunes J, et al. IL-7 contributes to the progression of human T-cell acute lymphoblastic leukemias. Cancer Res. (2011) 71:4780–9. doi: 10.1158/0008-5472.CAN-10-3606
51. González-García S, García-Peydró M, Martín-Gayo E, Ballestar E, Esteller M, Bornstein R, et al. CSL-MAML-dependent Notch1 signaling controls t lineage-specifc IL-7Rα gene expression in early human thymopoiesis and leukemia. J Exp Med. (2009) 206:779–91. doi: 10.1084/jem.20081922
52. Goossens S, Radaelli E, Blanchet O, Durinck K, van Der Meulen J, Peirs S, et al. ZEB2 drives immature T-cell lymphoblastic leukaemia development via enhanced tumour-initiating potential and IL-7 receptor signalling. Nat Commun. (2015) 6:5794. doi: 10.3324/haematol.2018.207837
53. Zenatti PP, Ribeiro D, Li W, Zuurbier L, Silva MC, Paganin M, et al. Oncogenic IL7R gain-of-function mutations in childhood T-cell acute lymphoblastic leukemia. Nat Genet. (2011) 43:932–41. doi: 10.1038/ng.924
54. Canté-Barrett K, Spijkers-Hagelstein JAP, Buijs-Gladdines JGCAM, Uitdehaag JCM, Smits WK, Van Der Zwet J, et al. MEK and PI3K-AKT inhibitors synergistically block activated IL7 receptor signaling in T-cell acute lymphoblastic leukemia. Leukemia. (2016) 30:1832–43. doi: 10.1038/leu.2016.83
55. Richter-Pechanska P, Kunz JB, Hof J, Zimmermann M, Rausch T, Bandapalli OR, et al. Identification of a genetically defined ultra-high-risk group in relapsed pediatric T-lymphoblastic leukemia. Blood Cancer J. (2017) 7:e523. doi: 10.1038/bcj.2017.3
56. Liu ZH, Wang MH, Ren HJ, Qu W, Sun LM, Zhang QF, et al. Interleukin 7 signaling prevents apoptosis by regulating bcl-2 and bax via the p53 pathway in human non-small cell lung cancer cells. Int J Clin Exp Pathol. (2014) 7:870–81.
57. Harada M, Hirota T, Jodo AI, Doi S, Kameda M, Fujita K, et al. Functional analysis of the thymic stromal lymphopoietin variants in human bronchial epithelial cells. Am J Respir Cell Mol Biol. (2009) 40:368–74. doi: 10.1165/rcmb.2008-0041OC
58. Biancheri P, Sabatino A Di, Rescigno M, Giuffrida P, Fornasa G, Tsilingiri K, et al. Abnormal thymic stromal lymphopoietin expression in the duodenal mucosa of patients with celiac disease. Gut. (2016) 65:1670–80. doi: 10.1136/gutjnl-2014-308876
59. Iliev ID, Spadoni I, Mileti E, Matteoli G, Sonzogni A, Sampietro GM, et al. Human intestinal epithelial cells promote the differentiation of tolerogenic dendritic cells. Gut. (2009) 58:1481–9. doi: 10.1136/gut.2008.175166
60. Cultrone A, de Wouters T, Lakhdari O, Kelly D, Mulder I, Logan E, et al. The NF-κB binding site located in the proximal region of the TSLP promoter is critical for TSLP modulation in human intestinal epithelial cells. Eur J Immunol. (2013) 43:1053–62. doi: 10.1002/eji.201142340
61. Allakhverdi Z, Comeau MR, Jessup HK, Yoon BRP, Brewer A, Chartier S, et al. Thymic stromal lymphopoietin is released by human epithelial cells in response to microbes, trauma, or inflammation and potently activates mast cells. J Exp Med. (2007) 204:253–8. doi: 10.1084/jem.20062211
62. Kato A, Favoreto S, Avila PC, Schleimer RP. TLR3- and Th2 cytokine-dependent production of thymic stromal lymphopoietin in human airway epithelial cells. J Immunol. (2007) 179:1080–7. doi: 10.4049/jimmunol.179.2.1080
63. Vu AT, Baba T, Chen X, Le TA, Kinoshita H, Xie Y, et al. Staphylococcus aureus membrane and diacylated lipopeptide induce thymic stromal lymphopoietin in keratinocytes through the Toll-like receptor 2-Toll-like receptor 6 pathway. J Allergy Clin Immunol. (2010) 126:985–93:993.e1–3. doi: 10.1016/j.jaci.2010.09.002
64. Li M, Hener P, Zhang Z, Kato S, Metzger D, Chambon P. Topical vitamin D3 and low-calcemic analogs induce thymic stromal lymphopoietin in mouse keratinocytes and trigger an atopic dermatitis. Proc Natl Acad Sci USA. (2006) 103:11736–41. doi: 10.1073/pnas.0604575103
65. Soumelis V, Reche PA, Kanzler H, Yuan W, Edward G, Homey B, et al. Human epithelial cells trigger dendritic cell-mediated allergic inflammation by producing TSLP. Nat Immunol. (2002) 3:673–80. doi: 10.1038/ni805
66. Zhang K, Shan L, Rahman MS, Unruh H, Halayko AJ, Gounni AS. Constitutive and inducible thymic stromal lymphopoietin expression in human airway smooth muscle cells: role in chronic obstructive pulmonary disease. Am J Physiol Lung Cell Mol Physiol. (2007) 293:L375–82. doi: 10.1152/ajplung.00045.2007
67. Okayama Y, Okumura S, Sagara H, Yuki K, Sasaki T, Watanabe N, et al. FcεRI-mediated thymic stromal lymphopoietin production by interleukin-4-primed human mast cells. Eur Respir J. (2008) 34:425–35. doi: 10.1183/09031936.00121008
68. Allakhverdi Z, Comeau MR, Jessup HK, Delespesse G. Thymic stromal lymphopoietin as a mediator of crosstalk between bronchial smooth muscles and mast cells. J Allergy Clin Immunol. (2009) 123:958–60.e2. doi: 10.1016/j.jaci.2009.01.059
69. Pattarini L, Trichot C, Bogiatzi S, Grandclaudon M, Meller S, Keuylian Z, et al. TSLP-activated dendritic cells induce human T follicular helper cell differentiation through OX40-ligand. J Exp Med. (2017) 214:1529–46. doi: 10.1084/jem.20150402
70. Wilson SR, Thé L, Batia LM, Beattie K, Katibah GE, McClain SP, et al. XThe epithelial cell-derived atopic dermatitis cytokine TSLP activates neurons to induce itch. Cell. (2013) 155:285–95. doi: 10.1016/j.cell.2013.08.057
71. Milford TAM, Su RJ, Francis OL, Baez I, Martinez SR, Coats JS, et al. TSLP or IL-7 provide an IL-7Rα signal that is critical for human B lymphopoiesis. Eur J Immunol. (2016) 46:2155–61. doi: 10.1002/eji.201646307
72. Leichner TM, Satake A, Harrison VS, Tanaka Y, Archambault AS, Kim BS, et al. Skin-derived TSLP systemically expands regulatory T cells. J Autoimmun. (2017) 79:39–52. doi: 10.1016/j.jaut.2017.01.003
73. Siracusa MC, Saenz SA, Hill DA, Kim BS, Headley MB, Doering TA, et al. TSLP promotes interleukin-3-independent basophil haematopoiesis and type 2 inflammation. Nature. (2011) 477:229–33. doi: 10.1038/nature10329
74. Han H, Headley MB, Xu W, Comeau MR, Zhou B, Ziegler SF. Thymic stromal lymphopoietin amplifies the differentiation of alternatively activated macrophages. J Immunol. (2013) 190:904–12. doi: 10.4049/jimmunol.1201808
75. Wang Q, Du J, Zhu J, Yang X, Zhou B. Thymic stromal lymphopoietin signaling in CD4+ T cells is required for TH2 memory. J Allergy Clin Immunol. (2015) 135:781–91.e3. doi: 10.1016/j.jaci.2014.09.015
76. Cook EB, Stahl JL, Schwantes EA, Fox KE, Mathur SK. IL-3 and TNFα increase thymic stromal lymphopoietin receptor (TSLPR) expression on eosinophils and enhance TSLP-stimulated degranulation. Clin Mol Allergy. (2012) 10:8. doi: 10.1186/1476-7961-10-8
77. Noh JY, Shin JU, Park CO, Lee N, Jin S, Kim SH, et al. Thymic stromal lymphopoietin regulates eosinophil migration via phosphorylation of L-plastin in atopic dermatitis. Exp Dermatol. (2016) 25:880–6. doi: 10.1111/exd.13111
78. Shalova IN, Lim JY, Chittezhath M, Zinkernagel AS, Beasley F, Hernández-Jiménez E, et al. Human monocytes undergo functional re-programming during sepsis mediated by hypoxia-inducible factor-1α. Immunity. (2015) 42:484–98. doi: 10.1016/j.immuni.2015.02.001
79. Pandey A, Ozaki K, Baumann H, Levin SD, Puel A, Farr AG, et al. Cloning of a receptor subunit required for signaling by thymic stromal lymphopoietin. Nat Immunol. (2000) 1:59–64. doi: 10.1038/76923
80. Park LS, Martin U, Garka K, Gliniak B, Di Santo JP, Muller W, et al. Cloning of the murine thymic stromal lymphopoietin (TSLP) receptor: formation of a functional heteromeric complex requires interleukin 7 receptor. J Exp Med. (2000) 192:659–69. doi: 10.1084/jem.192.5.659
81. Verstraete K, Van Schie L, Vyncke L, Bloch Y, Tavernier J, Pauwels E, et al. Structural basis of the proinflammatory signaling complex mediated by TSLP. Nat Struct Mol Biol. (2014) 21:375–82. doi: 10.1038/nsmb.2794
82. Verstraete K, Peelman F, Braun H, Lopez J, Van Rompaey D, Dansercoer A, et al. Structure and antagonism of the receptor complex mediated by human TSLP in allergy and asthma. Nat Commun. (2017) 8:14937. doi: 10.1038/ncomms14937
83. Isaksen DE, Baumann H, Trobridge PA, Farr AG, Levin SD, Ziegler SF. Requirement for stat5 in thymic stromal lymphopoietin-mediated signal transduction. J Immunol. (1999) 163:5971–7.
84. Quentmeier H, Drexler HG, Fleckenstein D, Zaborski M, Armstrong A, Sims JE, et al. Cloning of human thymic stromal lymphopoietin (TSLP) and signaling mechanisms leading of proliferation. Leukemia. (2001) 15:1286–92. doi: 10.1038/sj.leu.2402175
85. Reche PA, Soumelis V, Gorman DM, Clifford T, Liu M, Travis M, et al. Human thymic stromal lymphopoietin preferentially stimulates myeloid cells. J Immunol. (2001) 167:336–43. doi: 10.4049/jimmunol.167.1.336
86. Wohlmann A, Sebastian K, Borowski A, Krause S, Friedrich K. Signal transduction by the atopy-associated human thymic stromal lymphopoietin (TSLP) receptor depends on Janus kinase function. Biol Chem. (2010) 391:181–6. doi: 10.1515/bc.2010.029
87. Marković I, Barthel T, Schirmer M, González Delgado A, Wilhelm S, Krause S, et al. A versatile platform for activity determination of cytokines and growth factors based on the human TSLP (thymic stromal lymphopoietin) receptor. Cytokine. (2019) 113:228–37. doi: 10.1016/j.cyto.2018.07.010
88. Kim GY, Hong C, Park J-H. Seeing is believing: illuminating the source of in vivo interleukin-7. Immune Netw. (2011) 11:1. doi: 10.4110/in.2011.11.1.1
89. Sawa Y, Arima Y, Ogura H, Kitabayashi C, Jiang JJ, Fukushima T, et al. Hepatic interleukin-7 expression regulates T cell responses. Immunity. (2009) 30:447–57. doi: 10.1016/j.immuni.2009.01.007
90. Onder L, Narang P, Scandella E, Chai Q, Iolyeva M, Hoorweg K, et al. IL-7-producing stromal cells are critical for lymph node remodeling. Blood. (2012) 120:4675–83. doi: 10.1182/blood-2012-03-416859
91. Iolyeva M, Aebischer D, Proulx ST, Willrodt AH, Ecoiffier T, Häner S, et al. Interleukin-7 is produced by afferent lymphatic vessels and supports lymphatic drainage. Blood. (2013) 122:2271–81. doi: 10.1182/blood-2013-01-478073
92. Moors M, Vudattu NK, Abel J, Krämer U, Rane L, Ulfig N, et al. Interleukin-7 (IL-7) and IL-7 splice variants affect differentiation of human neural progenitor cells. Genes Immun. (2010) 11:11–20. doi: 10.1038/gene.2009.77
93. Sinclair C, Saini M, Van Der Loeff IS, Sakaguchi S, Seddon B. The long-term survival potential of mature T lymphocytes is programmed during development in the thymus. Sci Signal. (2011) 4:ra77. doi: 10.1126/scisignal.2002246
94. Martin CE, Spasova DS, Frimpong-Boateng K, Kim HO, Lee M, Kim KS, et al. Interleukin-7 availability is maintained by a hematopoietic cytokine sink comprising innate lymphoid cells and T cells. Immunity. (2017) 47:171–82.e4. doi: 10.1016/j.immuni.2017.07.005
95. Ziegler SF, Artis D. Sensing the outside world: TSLP regulates barrier immunity. Nat Immunol. (2010) 11:289–93. doi: 10.1038/ni.1852
96. Dias S, Silva H, Cumano A, Vieira P. Interleukin-7 is necessary to maintain the B cell potential in common lymphoid progenitors. J Exp Med. (2005) 201:971–9. doi: 10.1084/jem.20042393
97. Clark MR, Mandal M, Ochiai K, Singh H. Orchestrating B cell lymphopoiesis through interplay of IL-7 receptor and pre-B cell receptor signalling. Nat Rev Immunol. (2014) 14:69–80. doi: 10.1038/nri3570
98. Mazzucchelli R, Durum SK. Interleukin-7 receptor expression: intelligent design. Nat Rev Immunol. (2007) 7:144–54. doi: 10.1038/nri2023
99. McElroy CA, Dohm JA, Walsh STR. Structural and biophysical studies of the human IL-7/IL-7Rα complex. Structure. (2009) 17:54–65. doi: 10.1016/j.str.2008.10.019
100. McElroy CA, Holland PJ, Zhao P, Lim JM, Wells L, Eisenstein E, et al. Structural reorganization of the interleukin-7 signaling complex. Proc Natl Acad Sci USA. (2012) 109:2503–8. doi: 10.1073/pnas.1116582109
101. Felix J, Savvides SN. Mechanisms of immunomodulation by mammalian and viral decoy receptors: insights from structures. Nat Rev Immunol. (2017) 17:112–29. doi: 10.1038/nri.2016.134
102. Crawley AM, Faucher S, Angel JB. Soluble IL-7Rα (sCD127) inhibits IL-7 activity and is increased in HIV infection. J Immunol. (2010) 184:4679–87. doi: 10.4049/jimmunol.0903758
103. Foxwell BMJ, Beadling C, Guschin D, Kerr I, Cantrell D. Interleukin-7 can induce the activation of Jak 1, Jak 3 and STAT 5 proteins in murine T cells. Eur J Immunol. (1995) 25:3041–6. doi: 10.1002/eji.1830251109
104. Van Der Plas DC, Smiers F, Pouwels K, Hoefsloot LH, Löwenberg B, Touw IP. Interleukin-7 signaling in human B cell precursor acute lymphoblastic leukemia cells and murine BAF3 cells involves activation of STAT1 and STAT5 mediated via the interleukin-7 receptor α chain. Leukemia. (1996) 10:1317–25.
105. Seckinger P, Fougereau M. Activation of src family kinases in human pre-B cells by IL-7. J Immunol. (1994) 153:97–109.
106. Page TH, Lali FV, Foxwell BMJ. Interleukin-7 activates p56lck and p59fyn, two tyrosine kinases associated with the p90 interleukin-7 receptor in primary human T cells. Eur J Immunol. (1995) 25:2956–60. doi: 10.1002/eji.1830251036
107. Venkitaraman AR, Cowling RJ. Interleukin-7 induces the association of phosphatidylinositol 3-kinase with the α chain of the interleukin-7 receptor. Eur J Immunol. (1994) 24:2168–74. doi: 10.1002/eji.1830240935
108. Jiang Q, Wen QL, Aiello FB, Mazzucchelli R, Asefa B, Khaled AR, et al. Cell biology of IL-7, a key lymphotrophin. Cytokine Growth Factor Rev. (2005) 17:513–33. doi: 10.1016/j.cytogfr.2005.05.004
109. Corren J, Ziegler SF. TSLP: from allergy to cancer. Nat Immunol. (2019) 20:1603–9. doi: 10.1038/s41590-019-0524-9
110. Cooley RB, Arp DJ, Karplus PA. Evolutionary origin of a secondary structure: α-helices as cryptic but widespread insertional variations of α-Helices that enhance protein functionality. J Mol Biol. (2010) 404:232–46. doi: 10.1016/j.jmb.2010.09.034
111. LaPorte SL, Juo ZS, Vaclavikova J, Colf LA, Qi X, Heller NM, et al. Molecular and structural basis of cytokine receptor pleiotropy in the interleukin-4/13 system. Cell. (2008) 132:259–72. doi: 10.1016/j.cell.2007.12.030
112. Cosenza L, Sweeney É, Murphy JR. Disulfide bond assignment in human interleukin-7 by matrix-assisted laser desorption/ionization mass spectroscopy and site-directed cysteine to serine mutational analysis. J Biol Chem. (1997) 272:32995–3000. doi: 10.1074/jbc.272.52.32995
113. Cosenza L, Murphy JR, Smith T, Rosenbach A, White JV. Comparative model building of interleukin-7 using interleukin-4 as a template: a structural hypothesis that displays atypical surface chemistry in helix D important for receptor activation. Protein Sci. (2000) 9:916–26. doi: 10.1110/ps.9.5.916
114. vanderSpek JC, Sutherland JA, Gill BM, Gorgun G, Foss FM, Murphy JR. Structure function analysis of interleukin 7: requirement for an aromatic ring at position 143 of helix D. Cytokine. (2002) 17:227–33. doi: 10.1006/cyto.2002.1004
115. Spangler JB, Moraga I, Mendoza JL, Garcia KC. Insights into cytokine–receptor interactions from cytokine engineering. Annu Rev Immunol. (2015) 33:139–67. doi: 10.1146/annurev-immunol-032713-120211
116. Xin P, Xu X, Deng C, Liu S, Wang Y, Zhou X, et al. The role of JAK/STAT signaling pathway and its inhibitors in diseases. Int Immunopharm. (2020) 80:106210. doi: 10.1016/j.intimp.2020.106210
117. Hillmann P, Fabbro D. PI3K/mTOR pathway inhibition: opportunities in oncology and rare genetic diseases. Int J Mol Sci. (2019) 20:5792. doi: 10.3390/ijms20225792
118. Castelli MS, McGonigle P, Hornby PJ. The pharmacology and therapeutic applications of monoclonal antibodies. Pharmacol Res Perspect. (2019) 7:e00535. doi: 10.1002/prp2.535
119. Berkowitz JL, Janik JE, Stewart DM, Jaffe ES, Stetler-Stevenson M, Shih JH, et al. Safety, efficacy, and pharmacokinetics/pharmacodynamics of daclizumab (anti-CD25) in patients with adult T-cell leukemia/lymphoma. Clin Immunol. (2014) 155:176–87. doi: 10.1016/j.clim.2014.09.012
120. Castro M, Corren J, Pavord ID, Maspero J, Wenzel S, Rabe KF, et al. Dupilumab efficacy and safety in moderate-to-severe uncontrolled Asthma. N Engl J Med. (2018) 378:2486–96. doi: 10.1056/NEJMoa1804092
121. Simpson EL, Bieber T, Guttman-Yassky E, Beck LA, Blauvelt A, Cork MJ, et al. Two phase 3 trials of dupilumab versus placebo in atopic dermatitis. N Engl J Med. (2016) 375:2335–48. doi: 10.1056/NEJMoa1610020
122. Holgado A, Braun H, Van Nuffel E, Detry S, Schuijs MJ, Deswarte K, et al. IL-33trap is a novel IL-33–neutralizing biologic that inhibits allergic airway inflammation. J Allergy Clin Immunol. (2019) 144:204–15. doi: 10.1016/j.jaci.2019.02.028
123. Economides AN, Carpenter LR, Rudge JS, Wong V, Koehler-Stec EM, Hartnett C, et al. Cytokine traps: multi-component, high-affinity blockers of cytokine action. Nat Med. (2003) 9:47–52. doi: 10.1038/nm811
124. Ashkenazi A, Chamow SM. Immunoadhesins as research tools and therapeutic agents. Curr Opin Immunol. (1997) 9:195–200. doi: 10.1016/S0952-7915(97)80135-5
125. Kruse N, Tony HP, Sebald W. Conversion of human interleukin-4 into a high affinity antagonist by a single amino acid replacement. EMBO J. (1992) 11:3237–44. doi: 10.1002/j.1460-2075.1992.tb05401.x
126. Mueller TD, Zhang JL, Sebald W, Duschl A. Structure, binding, and antagonists in the IL-4/IL-13 receptor system. Biochim Biophys Acta Mol Cell Res. (2002) 1592:237–50. doi: 10.1016/S0167-4889(02)00318-X
127. Levin AM, Bates DL, Ring AM, Krieg C, Lin JT, Su L, et al. Exploiting a natural conformational switch to engineer an interleukin-2 “superkine.” Nature. (2012) 484:529–33. doi: 10.1038/nature10975
128. Silva DA, Yu S, Ulge UY, Spangler JB, Jude KM, Labão-Almeida C, et al. De novo design of potent and selective mimics of IL-2 and IL-15. Nature. (2019) 565:186–91. doi: 10.1038/s41586-018-0830-7
129. Wójcik P, Berlicki Ł. Peptide-based inhibitors of protein-protein interactions. Bioorganic Med Chem Lett. (2016) 26:707–13. doi: 10.1016/j.bmcl.2015.12.084
130. Liu S, Desharnais J, Sahasrabudhe PV, Jin P, Li W, Oates BD, et al. Inhibiting complex IL-17A and IL-17RA interactions with a linear peptide. Sci Rep. (2016) 6:26071. doi: 10.1038/srep26071
131. O'Connell J, Porter J, Kroeplien B, Norman T, Rapecki S, Davis R, et al. Small molecules that inhibit TNF signalling by stabilising an asymmetric form of the trimer. Nat Commun. (2019) 10:5795. doi: 10.1038/s41467-019-13616-1
132. Tilley JW, Chen L, Fry DC, Emerson SD, Powers GD, Biondi D, et al. Identification of a small moleucle inhibitor of the IL-2/IL-2Rα receptor interaction which binds to IL-2. J Am Chem Soc. (1997) 119:7589–90. doi: 10.1021/ja970702x
133. Krumm B, Meng X, Xiang Y, Deng J. Identification of small molecule inhibitors of Interleukin-18. Sci Rep. (2017) 7:483. doi: 10.1038/s41598-017-00532-x
134. Scott DE, Bayly AR, Abell C, Skidmore J. Small molecules, big targets: drug discovery faces the protein-protein interaction challenge. Nat Rev Drug Discov. (2016) 15:533–50. doi: 10.1038/nrd.2016.29
135. Savvides SN, Elewaut D. Small-molecule inhibitors get pro-inflammatory TNF into shape. Nat Revi Rheumatol. (2020) 16:189–90. doi: 10.1038/s41584-020-0388-2
136. Gauvreau GM, O'Byrne PM, Boulet LP, Wang Y, Cockcroft D, Bigler J, et al. Effects of an anti-TSLP antibody on allergen-induced asthmatic responses. N Engl J Med. (2014) 370:2102–10. doi: 10.1056/NEJMoa1402895
137. ClinicalTrials.gov. Double-blind, Multiple Dose Study in Subjects With Mild Atopic Asthma - Full Text View - ClinicalTrials.gov. (2020). Available online at: https://clinicaltrials.gov/ct2/show/NCT01405963 (accessed March 6, 2020).
138. ClinicalTrials.gov. Study to Evaluate Tezepelumab in Adults & Adolescents With Severe Uncontrolled Asthma - Full Text View - ClinicalTrials.gov. (2020). Available online at: https://www.clinicaltrials.gov/ct2/show/NCT03347279 (accessed March 6, 2020).
139. Hixon JA, Andrews C, Kashi L, Kohnhorst CL, Senkevitch E, Czarra K, et al. New anti-IL-7Rα monoclonal antibodies show efficacy against T cell acute lymphoblastic leukemia in pre-clinical models. Leukemia. (2019) 35–49. doi: 10.1038/s41375-019-0531-8
140. Corren J, Parnes JR, Wang L, Mo M, Roseti SL, Griffiths JM, et al. Tezepelumab in adults with uncontrolled asthma. N Engl J Med. (2017) 377:936–46. doi: 10.1056/NEJMoa1704064
141. ClinicalTrials.gov. Study to Evaluate the Efficacy and Safety of MEDI9929 (AMG 157) in Adult Subjects With Inadequately Controlled, Severe Asthma - Full Text View - ClinicalTrials.gov. (2020). Available online at: https://clinicaltrials.gov/ct2/show/NCT02054130 (accessed March 6, 2020).
142. Parnes JR, Sullivan JT, Chen L, Dias C. Pharmacokinetics, safety, and tolerability of Tezepelumab (AMG 157) in healthy and atopic dermatitis adult subjects. Clin Pharmacol Ther. (2019) 106:441–9. doi: 10.1002/cpt.1401
143. ClinicalTrials.gov. Safety Study of AMG 157 in Healthy Subjects and Subjects With Atopic Dermatitis - Full Text View - ClinicalTrials.gov. (2020). Available online at: https://clinicaltrials.gov/ct2/show/NCT00757042 (accessed March 6, 2020).
144. Simpson EL, Parnes JR, She D, Crouch S, Rees W, Mo M, et al. Tezepelumab, an anti–thymic stromal lymphopoietin monoclonal antibody, in the treatment of moderate to severe atopic dermatitis: a randomized phase 2a clinical trial. J Am Acad Dermatol. (2019) 80:1013–21. doi: 10.1016/j.jaad.2018.11.059
145. ClinicalTrials.gov. Phase 2a Study to Evaluate the Efficacy and Safety of MEDI9929 in Adults With Atopic Dermatitis - Full Text View - ClinicalTrials.gov. (2020). Available online at: https://clinicaltrials.gov/ct2/show/NCT02525094 (accessed March 6, 2020).
146. ClinicalTrials.gov. A Dose Ranging Placebo-Controlled Double-Blind Study to Evaluate the Safety and Efficacy of Tezepelumab in Atopic Dermatitis - Full Text View - ClinicalTrials.gov. (2020). Available online at: https://clinicaltrials.gov/ct2/show/NCT03809663 (accessed March 6, 2020).
147. ClinicalTrials.gov. A Bronchoprovocation Study to Assess the Safety, Tolerability, Pharmacokinetics and Pharmacodynamics of CSJ117 in Adult Subjects With Mild Atopic Asthma - Full Text View - ClinicalTrials.gov. (2020). Available online at: https://clinicaltrials.gov/ct2/show/NCT03138811 (cited March 5, 2020).
148. Nian S, Zhu J, Yu H, Chen Q, Ye Y, Cao X, et al. Development and identification of a fully human single-chain variable fragment 29 against TSLP. Biotechnol Appl Biochem. (2019) 66:510–6. doi: 10.1002/bab.1747
149. Shikotra A, Choy DF, Ohri CM, Doran E, Butler C, Hargadon B, et al. Increased expression of immunoreactive thymic stromal lymphopoietin in patients with severe asthma. J Allergy Clin Immunol. (2012) 129:104–11.e9. doi: 10.1016/j.jaci.2011.08.031
150. Stier MT, Bloodworth MH, Toki S, Newcomb DC, Goleniewska K, Boyd KL, et al. Respiratory syncytial virus infection activates IL-13–producing group 2 innate lymphoid cells through thymic stromal lymphopoietin. J Allergy Clin Immunol. (2016) 138:814–24.e11. doi: 10.1016/j.jaci.2016.01.050
151. Venkataramani S, Low S, Weigle B, Dutcher D, Jerath K, Menzenski M, et al. Design and characterization of Zweimab and Doppelmab, high affinity dual antagonistic anti-TSLP/IL13 bispecific antibodies. Biochem Biophys Res Commun. (2018) 504:19–24. doi: 10.1016/j.bbrc.2018.08.064
152. ClinicalTrials.gov. A Study to Evaluate the Pharmacokinetics of MEDI9929 (AMG 157) in Adolescents With Mild to Moderate Asthma - Full Text View - ClinicalTrials.gov. (2020). Available online at: https://www.clinicaltrials.gov/ct2/show/NCT02512900 (accessed March 6, 2020).
153. ClinicalTrials.gov. A Phase 1 Study to Evaluate the Safety, Tolerability, Pharmacokinetics and Immunogenicity of MEDI9929 After Single Administration in Healthy Male Japanese Subjects - Full Text View - ClinicalTrials.gov. (2020). Available online at: https://www.clinicaltrials.gov/ct2/show/NCT01913028 (accessed March 6, 2020).
154. ClinicalTrials.gov. Study to Evaluate Tezepelumab on Airway Inflammation in Adults With Uncontrolled Asthma (CASCADE) - Full Text View - ClinicalTrials.gov. (2020). Available online at: https://www.clinicaltrials.gov/ct2/show/NCT03688074 (accessed March 6, 2020).
155. ClinicalTrials.gov. Anti-TSLP (AMG 157) Plus Antigen-Specific Immunotherapy for Induction of Tolerance in Individuals With Cat Allergy - Full Text View - ClinicalTrials.gov. (2020). Available online at: https://clinicaltrials.gov/ct2/show/NCT02237196 (accessed March 6, 2020).
156. ClinicalTrials.gov. Tezepelumab Home Use Study - Full Text View - ClinicalTrials.gov. (2020). Available online at: https://www.clinicaltrials.gov/ct2/show/NCT03968978 (accessed March 6, 2020).
157. ClinicalTrials.gov. A Study to Evaluate the Pharmacokinetics of Tezepelumab After Being Delivered by an Accessorized Pre Filled Syringe or Autoinjector Compared With Vial and Syringe in Healthy Adult Subjects - Full Text View - ClinicalTrials.gov. (2020). Available online at: https://www.clinicaltrials.gov/ct2/show/NCT03989544 (accessed March 6, 2020).
158. Fuentes ME, Ma X, Zhang J, Pashine A, Apparsundaram S, Wilson S, et al. Anti-TSLPR antibody RG7258 blocks dendritic cell activation, mast cell cytokine release and reduces Th2 inflammation in a non-human primate model of allergic lung inflammation. (2011). 183:A2767. doi: 10.1164/ajrccm-conference.2011.183.1_MeetingAbstracts.A2767
159. Roche. Half Year Results 2012. Company presentation. (2012). p. slide 68. (2020). Available online at: https://www.roche.com/dam/jcr:57761203-bf5e-4fe1-93ed-0bee50b8bee2/en/irp2q12e.pdf (accessed March 6, 2020).
160. Numazaki M, Hanaoka K, Imamura E, Maeda M, Arai K, Saito T, et al. ASP7266, a novel antibody against human TSLPR, in the treatment of allergic disease. J Allergy Clin Immunol. (2018) 141:AB13. doi: 10.1016/j.jaci.2017.12.042
161. ASP. ASP 7266 - AdisInsight. (2020). Available online at: https://adisinsight.springer.com/drugs/800044779 (accessed March 5, 2020).
162. Borowski A, Vetter T, Kuepper M, Wohlmann A, Krause S, Lorenzen T, et al. Expression analysis and specific blockade of the receptor for human thymic stromal lymphopoietin (TSLP) by novel antibodies to the human TSLPRα receptor chain. Cytokine. (2013) 61:546–55. doi: 10.1016/j.cyto.2012.10.025
163. Vetter T, Borowski A, Wohlmann A, Ranjan N, Kuepper M, Badura S, et al. Blockade of thymic stromal lymphopoietin (TSLP) receptor inhibits TSLP-driven proliferation and signalling in lymphoblasts from a subset of B-precursor ALL patients. Leuk Res. (2016) 40:38–43. doi: 10.1016/j.leukres.2015.10.003
164. Park BB, Choi JW, Park D, Choi D, Paek J, Kim HJ, et al. Structure-activity relationships of baicalein and its analogs as novel TSLP inhibitors. Sci Rep. (2019) 9:8762. doi: 10.1038/s41598-019-44853-5
165. Zhang F, Huang G, Hu B, Song Y, Shi Y. A soluble thymic stromal lymphopoietin (TSLP) antagonist, TSLPR-immunoglobulin, reduces the severity of allergic disease by regulating pulmonary dendritic cells. Clin Exp Immunol. (2011) 164:256–64. doi: 10.1111/j.1365-2249.2011.04328.x
166. van Rompaey D, Verstraete K, Peelman F, Savvides SN, Augustyns K, van Der Veken P, et al. Virtual screening for inhibitors of the human TSLP:TSLPR interaction. Sci Rep. (2017) 7:17211. doi: 10.1038/s41598-017-17620-7
167. Park S, Park Y, Son SH, Lee KY, Jung YW, Lee KY, et al. Synthesis and biological evaluation of peptide-derived TSLP inhibitors. Bioorganic Med Chem Lett. (2017) 27:4710–3. doi: 10.1016/j.bmcl.2017.09.010
168. Penaranda C, Kuswanto W, Hofmann J, Kenefeck R, Narendran P, Walker LSK, et al. IL-7 receptor blockade reverses autoimmune diabetes by promoting inhibition of effector/memory T cells. Proc Natl Acad Sci USA. (2012) 109:12668–73. doi: 10.1073/pnas.1203692109
169. Lee LF, Axtell R, Tu GH, Logronio K, Dilley J, Yu J, et al. IL-7 promotes TH1 development and serum IL-7 predicts clinical response to interferon-β in multiple sclerosis. Sci Transl Med. (2011) 3:93ra68. doi: 10.1126/scitranslmed.3002400
170. Lee LF, Logronio K, Tu GH, Zhai W, Ni I, Mei L, et al. Anti-IL-7 receptor-α reverses established type 1 diabetes in nonobese diabetic mice by modulating effector T-cell function. Proc Natl Acad Sci USA. (2012) 109:12674–9. doi: 10.1073/pnas.1203795109
171. Kern B, Li W, Bono C, Lee LF, Kraynov E. Receptor occupancy and blocking of STAT5 signaling by an anti-IL-7 receptor α antibody in cynomolgus monkeys. Cytom B–Clin Cytom. (2016)90:191–8. doi: 10.1002/cyto.b.21247
172. Herold KC, Bucktrout SL, Wang X, Bode BW, Gitelman SE, Gottlieb PA, et al. Immunomodulatory activity of humanized anti–IL-7R monoclonal antibody RN168 in subjects with type 1 diabetes. JCI Insight. (2019) 4:e126054. doi: 10.1172/jci.insight.126054
173. Williams JH, Udata C, Ganguly BJ, Bucktrout SL, Joh T, Shannon M, et al. Model-based characterization of the pharmacokinetics, target engagement biomarkers, and immunomodulatory activity of PF-06342674, a humanized mAb against IL-7 receptor-α, in adults with type 1 diabetes. AAPS J. (2020) 22:23. doi: 10.1208/s12248-019-0401-3
174. ClinicalTrials.gov. A Study To Assess The Safety of PF-06342674 In Healthy Volunteers - Full Text View - ClinicalTrials.gov. (2020). Available online at: https://clinicaltrials.gov/ct2/show/NCT01740609 (accessed March 6, 2020).
175. ClinicalTrials.gov. A Study To Assess The Safety of PF-06342674 In Adults With Type 1 Diabetes - Full Text View - ClinicalTrials.gov. (2020). Available online at: https://clinicaltrials.gov/ct2/show/NCT02038764 (accessed March 6, 2020).
176. ClinicalTrials.gov. A Study To Evaluate The Safety And Tolerability Of PF-06342674 (RN168) in Subjects With Multiple Sclerosis (MS) - Full Text View - ClinicalTrials.gov. (2020). Available online at: https://clinicaltrials.gov/ct2/show/NCT02045732 (accessed March 6, 2020).
177. Ellis J, van Maurik A, Fortunato L, Gisbert S, Chen K, Schwartz A, et al. Anti-IL-7 receptor α monoclonal antibody (GSK2618960) in healthy subjects – a randomized, double-blind, placebo-controlled study. Br J Clin Pharmacol. (2019) 85:304–15. doi: 10.1111/bcp.13748
178. ClinicalTrials.gov. Single Ascending Doses Study of Anti- Interleukin-7 Receptor α Monoclonal Antibody (GSK2618960) in Healthy Volunteers - Full Text View - ClinicalTrials.gov. (2020). Available online at: https://clinicaltrials.gov/ct2/show/NCT02293161 (accessed March 6, 2020).
179. ClinicalTrials.gov. A First Time in Human Study Exploring Safety, Tolerability, Pharmacokinetics (PK) and Pharmacodynamics (PD) of GSK2618960 in Healthy Volunteers and Patients With Relapsing Remitting Multiple Sclerosis (RRMS) - Full Text View - ClinicalTrials.gov. (2020). Available online at: https://clinicaltrials.gov/ct2/show/NCT01808482 (accessed March 6, 2020).
180. ClinicalTrials.gov. A Study to Evaluate the Safety, Tolerability, Pharmacokinetics (PK), Proof of Mechanism of GSK2618960 in Primary Sjögren's Syndrome (pSS) - Full Text View - ClinicalTrials.gov. (2020). Available online at: https://clinicaltrials.gov/ct2/show/NCT03239600 (accessed March 6, 2020).
181. Akkapeddi P, Fragoso R, Hixon JA, Ramalho AS, Oliveira ML, Carvalho T, et al. A fully human anti-IL-7Rα antibody promotes antitumor activity against T-cell acute lymphoblastic leukemia. Leukemia. (2019) 33:2155–68. doi: 10.1038/s41375-019-0434-8
182. ClinicalTrials.gov. Therapy to Treat Ewing's Sarcoma, Rhabdomyosarcoma or Neuroblastoma - Full Text View - ClinicalTrials.gov. (2020). Available online at: https://clinicaltrials.gov/ct2/show/NCT00923351 (accessed March 6, 2020).
183. Francois B, Jeannet R, Daix T, Walton AH, Shotwell MS, Unsinger J, et al. Interleukin-7 restores lymphocytes in septic shock: the IRIS-7 randomized clinical trial. JCI insight. (2018) 3:e98960. doi: 10.1172/jci.insight.98960
184. ClinicalTrials.gov. A Study of IL-7 to Restore Absolute Lymphocyte Counts in Sepsis Patients (IRIS-7-B). Available onlinae at: https://clinicaltrials.gov/ct2/show/NCT02640807 (accessed March 6, 2020).
185. ClinicalTrials.gov. CYT107 After Vaccine Treatment (Provenge®) in Patients With Metastatic Castration-Resistant Prostate Cancer - Tabular View - ClinicalTrials.gov. (2020). Available online at: https://clinicaltrials.gov/ct2/show/record/NCT01881867 (accessed March 6, 2020).
186. Perales MA, Goldberg JD, Yuan J, Koehne G, Lechner L, Papadopoulos EB, et al. Recombinant human interleukin-7 (CYT107) promotes T-cell recovery after allogeneic stem cell transplantation. Blood. (2012) 120:4882–91. doi: 10.1182/blood-2012-06-437236
187. ClinicalTrials.gov. Safety Study of IL-7 in Recipients of a Hemopoietic Stem Cell Transplant Peripheral Blood Stem Cell Transplant - Full Text View - ClinicalTrials.gov. (2020). Available online at: https://clinicaltrials.gov/ct2/show/NCT00684008 (accessed May 20, 2020).
188. Sereti I, Dunham RM, Spritzler J, Aga E, Proschan MA, Medvik K, et al. IL-7 administration drives T cell-cycle entry and expansion in HIV-1 infection. Blood. (2009) 113:6304–14. doi: 10.1182/blood-2008-10-186601
189. ClinicalTrials.gov. Safety of Interleukin-7 in HIV Infected People Currently Taking Anti-HIV Drugs - Full Text View - ClinicalTrials.gov. (2020). Available online at: https://clinicaltrials.gov/ct2/show/NCT00099671 (accessed May 20, 2020).
190. Katlama C, Lambert-Niclot S, Assoumou L, Papagno L, Lecardonnel F, Zoorob R, et al. Treatment intensification followed by interleukin-7 reactivates HIV without reducing total HIV DNA: a randomized trial. AIDS. (2016) 30:221–30. doi: 10.1097/QAD.0000000000000894
191. ClinicalTrials.gov. Therapeutic Intensification Plus Immunomodulation in HIV-infected Patients - Full Text View - ClinicalTrials.gov. (2020). Available online at: https://clinicaltrials.gov/ct2/show/NCT01019551 (accessed March 6, 2020).
192. ClinicalTrials.gov. Safety Study of IL-7 in HIV-infected Patients (Inspire) - Full Text View - ClinicalTrials.gov. (2020). Available online at: https://clinicaltrials.gov/ct2/show/NCT00477321 (accessed March 6, 2020).
193. ClinicalTrials.gov. Interleukin-7 and Vaccine Therapy in Treating Patients With Metastatic Melanoma - Full Text View - ClinicalTrials.gov. (2020). Available online at: https://clinicaltrials.gov/ct2/show/NCT00091338 (accessed March 6, 2020).
194. Trédan O, Ménétrier-Caux C, Ray-Coquard I, Garin G, Cropet C, Verronèse E, et al. ELYPSE-7: a randomized placebo-controlled phase IIa trial with CYT107 exploring the restoration of CD4+ lymphocyte count in lymphopenic metastatic breast cancer patients. Ann Oncol. (2015) 26:1353–62. doi: 10.1093/annonc/mdv173
195. ClinicalTrials.gov. Study Evaluating Impact of IL-7 on CD4 Lymphopenia, Risks of Severe Haematological Toxicity and Tumor Progression in Metastatic Breast Cancer Patients - Full Text View - ClinicalTrials.gov. (2020). Available online at: https://clinicaltrials.gov/ct2/show/NCT01368107 (accessed March 6, 2020).
196. ClinicalTrials.gov. Dose Escalation Study of IL-7 and Bi-therapy in HCV Patients Resistant After 12 Weeks of Bi-therapy (ECLIPSE 1) - Full Text View - ClinicalTrials.gov. (2020). Available online at: https://clinicaltrials.gov/ct2/show/NCT01025596 (accessed March 6, 2020).
197. Rosenberg SA, Sportès C, Ahmadzadeh M, Fry TJ, Ngo LT, Schwarz SL, et al. NIH public access. J Immunother. (2006) 29:313–9. doi: 10.1097/01.cji.0000210386.55951.c2
198. Sportès C, Babb RR, Krumlauf MC, Hakim FT, Seth M, Chow CK, et al. Phase I Study of Recombinant Human interleukin-7 Administration in subjects with refractory malignancy. Clin Cancer Res. (2011) 16:727–35. doi: 10.1158/1078-0432.CCR-09-1303
199. Komschlies KL, Back TT, Gregorio TA, Gruys ME, Damia G, Wiltrout RH, et al. Effects of rhIL-7 on leukocyte subsets in mice: implications for antitumor activity. Immunol Ser. (1994) 61:95–104.
200. ClinicalTrials.gov. Clinical Trial of GX-I7 in Healthy Volunteers - Full Text View - ClinicalTrials.gov. (2020). Available online at: https://clinicaltrials.gov/ct2/show/NCT02860715 (accessed March 6, 2020).
201. ClinicalTrials.gov. Safety and Tolerability of GX-I7 in HPV-infected Female Volunteers - Full Text View - ClinicalTrials.gov. (2020). Available online at: https://clinicaltrials.gov/ct2/show/NCT03144934 (accessed March 6, 2020).
202. Kim J-H, Hong S-W, Kim Y-M, Jo S, Ji MK, Oh YK, et al. Abstract 4991: Hyleukin-7, the Fc-fused interleukin-7, generates anti-tumor activity by modulating both adaptive and innate immune cells in the tumor microenvironment. Am Assoc Cancer Res. (2019) 79(13 Supplement):4991. doi: 10.1158/1538-7445.AM2019-4991
203. Seo YB, Im SJ, Namkoong H, Kim SW, Choi YKW, Kang MC, et al. Crucial roles of interleukin-7 in the development of T follicular helper cells and in the induction of humoral immunity. J Virol. (2014) 88:8998–9009. doi: 10.1128/JVI.00534-14
204. Hanabuchi S, Ito T, Park W-R, Watanabe N, Shaw JL, Roman E, et al. Thymic stromal lymphopoietin-activated plasmacytoid dendritic cells induce the generation of FOXP3 + regulatory T cells in human Thymus. J Immunol. (2010) 184:2999–3007. doi: 10.4049/jimmunol.0804106
205. Yasunaga M, Manabe S, Matsumura Y. Immunoregulation by IL-7R-targeting antibody-drug conjugates: overcoming steroid-resistance in cancer and autoimmune disease. Sci Rep. (2017) 7:10735. doi: 10.1038/s41598-017-11255-4
206. MacKall CL, Fry TJ, Gress RE. Harnessing the biology of IL-7 for therapeutic application. Nat Rev Immunol. (2011) 11:330–42. doi: 10.1038/nri2970
207. Alstadhaug KB, Croughs T, Henriksen S, Leboeuf C, Sereti I, Hirsch HH, et al. Treatment of progressive multifocal leukoencephalopathy with interleukin 7. JAMA Neurol. (2014) 71:1030–5. doi: 10.1001/jamaneurol.2014.825
208. ClinicalTrials.gov. Atezolizumab and CYT107 in Treating Participants With Locally Advanced, Inoperable, or Metastatic Urothelial Carcinoma - Full Text View - ClinicalTrials.gov. (2020). Available online at: https://clinicaltrials.gov/ct2/show/NCT03513952 (accessed March 6, 2020).
209. ClinicalTrials.gov. Interleukin-7 and Chemokine (C-C Motif) Ligand 19-expressing CD19-CAR-T for Refractory/Relapsed B Cell Lymphoma. - Full Text View - ClinicalTrials.gov. (2020). Available online at: https://clinicaltrials.gov/ct2/show/NCT03929107 (accessed March 6, 2020).
210. ClinicalTrials.gov. High-Risk Skin Cancers With Atezolizumab Plus NT-I7 - Full Text View - ClinicalTrials.gov. (2020). Available online at: https://clinicaltrials.gov/ct2/show/NCT03901573 (accessed March 6, 2020).
211. ClinicalTrials.gov. Study of the Effect IL 7/ NT-I7 on CD4 Counts in Patients With High Grade Gliomas - Full Text View - ClinicalTrials.gov. (2020). Available online at: https://clinicaltrials.gov/ct2/show/NCT02659800 (accessed March 6, 2020).
212. ClinicalTrials.gov. GX-I7 With Cyclophosphamide in Patients With Metastatic or Recurrent Solid Tumors - Full Text View - ClinicalTrials.gov. (2020). Available online at: https://clinicaltrials.gov/ct2/show/NCT03733587 (accessed March 6, 2020).
213. ClinicalTrials.gov. Vaccine Response With NT-I7 - Full Text View - ClinicalTrials.gov. (2020). Available online at: https://clinicaltrials.gov/ct2/show/NCT04054752 (accessed March 6, 2020).
214. Kang MC, Choi D-H, Choi YW, Park SJ, Namkoong H, Park KS, et al. Intranasal introduction of Fc-fused interleukin-7 provides long-lasting prophylaxis against lethal influenza virus infection. J Virol. (2016) 90:2273–84. doi: 10.1128/JVI.02768-15
215. Ahn SS, Jeon BY, Park SJ, Choi DH, Ku SH, Cho SN, et al. Nonlytic Fc-fused IL-7 synergizes with Mtb32 DNA vaccine to enhance antigen-specific T cell responses in a therapeutic model of tuberculosis. Vaccine. (2013) 31:2884–90. doi: 10.1016/j.vaccine.2013.04.029
216. Park S-H, Song M-Y, Nam HJ, Im SJ, Sung Y-C. Codelivery of IL-7 augments multigenic HCV DNA vaccine-induced antibody as well as broad T cell responses in cynomolgus monkeys. Immune Netw. (2010) 10:198. doi: 10.4110/in.2010.10.6.198
217. Rider P, Carmi Y, Cohen I. Biologics for targeting inflammatory cytokines, clinical uses, and limitations. Int J Cell Biol. (2016) 2016:9259646. doi: 10.1155/2016/9259646
218. Lawrence MG, Steinke JW, Borish L. Cytokine-targeting biologics for allergic diseases. Ann Allergy Asthma Immunol. (2018) 120:376–81. doi: 10.1016/j.anai.2018.01.009
219. Gillies SD, Lan Y, Lauder S, Brunkhorst B, Sun Y, Lo K-M. Engineering of an IL-2 immunocytokine with very low toxicity that retains potent anti-tumor activity in immune competent and immune deficient mouse tumor models. Cancer Res. (2004) 64 (7 Supplement):151–2.
220. Mortara L, Balza E, Bruno A, Poggi A, Orecchia P, Carnemolla B. Anti-cancer therapies employing IL-2 cytokine tumor targeting: contribution of innate, adaptive and immunosuppressive cells in the anti-tumor efficacy. Front Immunol. (2018) 9:2905. doi: 10.3389/fimmu.2018.02905
221. Mitra S, Ring AM, Amarnath S, Spangler JB, Li P, Ju W, et al. Interleukin-2 activity can be fine tuned with engineered receptor signaling clamps. Immunity. (2015) 42:826–38. doi: 10.1016/j.immuni.2015.04.018
222. Moraga I, Spangler JB, Mendoza JL, Gakovic M, Wehrman TS, Krutzik P, et al. Synthekines are surrogate cytokine and growth factor agonists that compel signaling through non-natural receptor dimers. Elife. (2017) 6:e22882. doi: 10.7554/eLife.22882
223. Belarif L, Mary C, Jacquemont L, Mai H Le, Danger R, Hervouet J, et al. IL-7 receptor blockade blunts antigen-specific memory T cell responses and chronic inflammation in primates. Nat Commun. (2018) 9:4483. doi: 10.1038/s41467-018-06804-y
224. Dooms H. Interleukin-7: Fuel for the autoimmune attack. J Autoimmunity. (2013) 45:40–8. doi: 10.1016/j.jaut.2013.06.007
225. Sugamura K, Asao H, Kondo M, Tanaka N, Ishii N, Ohbo K, et al. The interleukin-2 receptor γ CHAIN: its role in the multiple cytokine receptor complexes and T cell development in XSCID. Annu Rev Immunol. (1996) 14:179–205. doi: 10.1146/annurev.immunol.14.1.179
226. Hillen MR, Hartgring SAY, Willis CR, Radstake TRDJ, Hack CE, Lafeber FPJG, et al. The additive inflammatory in vivo and in vitro effects of IL-7 and TSLP in arthritis underscore the therapeutic rationale for dual blockade. PLoS ONE. (2015) 10:e130830. doi: 10.1371/journal.pone.0130830
227. Chames P, van Regenmortel M, Weiss E, Baty D. Therapeutic antibodies: successes, limitations and hopes for the future. Br J Pharmacol. (2009) 157:220–33. doi: 10.1111/j.1476-5381.2009.00190.x
228. Carter PJ, Lazar GA. Next generation antibody drugs: pursuit of the “high-hanging fruit.” Nat Rev Drug Discov. (2018) 17:197–223. doi: 10.1038/nrd.2017.227
Keywords: cytokines, antagonist, agonist, protein-protein complex, therapeutic biologics, cytokine-receptor complex
Citation: Marković I and Savvides SN (2020) Modulation of Signaling Mediated by TSLP and IL-7 in Inflammation, Autoimmune Diseases, and Cancer. Front. Immunol. 11:1557. doi: 10.3389/fimmu.2020.01557
Received: 09 March 2020; Accepted: 12 June 2020;
Published: 21 July 2020.
Edited by:
Erwan Mortier, INSERM U1232 Centre de Recherche en Cancérologie et Immunologie Nantes Angers (CRCINA), FranceReviewed by:
Shawn Demehri, Massachusetts General Hospital Cancer Center, United StatesScott Kenneth Durum, National Cancer Institute (NCI), United States
Copyright © 2020 Marković and Savvides. This is an open-access article distributed under the terms of the Creative Commons Attribution License (CC BY). The use, distribution or reproduction in other forums is permitted, provided the original author(s) and the copyright owner(s) are credited and that the original publication in this journal is cited, in accordance with accepted academic practice. No use, distribution or reproduction is permitted which does not comply with these terms.
*Correspondence: Savvas N. Savvides, c2F2dmFzLnNhdnZpZGVzJiN4MDAwNDA7dWdlbnQuYmU=