- Medical Research Council Human Immunology Unit, Medical Research Council Weatherall Institute of Molecular Medicine, University of Oxford, Oxford, United Kingdom
Mucosal-associated invariant T (MAIT) cells are unconventional T lymphocytes that express a semi-invariant T cell receptor (TCR) recognizing microbial vitamin B metabolites presented by the highly conserved major histocompatibility complex (MHC) class I like molecule, MR1. The vitamin B metabolites are produced by several commensal and pathogenic bacteria and yeast, but not viruses. Nevertheless, viral infections can trigger MAIT cell activation in a TCR-independent manner, through the release of pro-inflammatory cytokines by antigen-presenting cells (APCs). MAIT cells belong to the innate like T family of cells with a memory phenotype, which allows them to rapidly release Interferon (IFN)-γ, tumor necrosis factor (TNF)-α, and in some circumstances Interleukin (IL)-17 and IL-10, exerting an immunomodulatory role on the ensuing immune response, akin to iNKT cells and γδ T cells. Recent studies implicate MAIT cells in a variety of inflammatory, autoimmune diseases, and in cancer. In addition, through the analysis of the transcriptome of MAIT cells activated in different experimental conditions, an important function in tissue repair and control of immune homeostasis has emerged, shared with other innate-like T cells. In this review, we discuss these recent findings, focussing on the understanding of the molecular mechanisms underpinning MAIT cell activation and effector function in health and disease, which ultimately will aid in clinically harnessing this unique, not donor-restricted cell subtype.
Introduction
MAIT cells are unconventional T lymphocytes that were first described by Porcelli et al. as one of two cell populations enriched in the CD4− CD8− T cell fraction (the other being Vα24+ iNKT cells) (1). It is now established that canonical MAIT cells express a semi-invariant TCRα-chain (in humans mostly Vα7.2-Jα33/Jα20, in mice Vα19-Jα33) paired with a number of TCR β-chains, contributing to a limited TCR repertoire (2, 3). Because of the limited TCR repertoire, and their similarities with iNKT cells, it was initially proposed by Tilloy and colleagues that these cells could be restricted by a non-classical MHC like molecule presenting either an endogenous ligand or a ubiquitous pathogen (4). Subsequently, it was demonstrated that MAIT cells are restricted to the highly conserved MHC-class I-related protein 1 (MR1) (5, 6). MR1 is β2m-associated, nonpolymorphic, and conserved across various mammalian species, with 90% of sequence similarity between mice and humans (7). The high inter-species conservation of MR1 and TCRα chain results in cross-reactivity between human and non-human species such as bovine, mouse, and rat (8, 9). Interestingly, a murine autoreactive MAIT hybridoma was shown to strongly recognize cells expressing bovine or rat MR1, but not human MR1, and this was pinpointed to residue Q151, present in the human but not the murine sequence (9). In the same study, polyclonal human MAIT cells were activated by rat, murine, and bovine MR1, but were not autoreactive (9).
MAIT cells preferentially locate in mucosal-associated tissue such as gut, lamina propria, and lung, in both humans and mice (6). Recent research has demonstrated that MAIT cells are also present in liver and human blood, where they can represent up to 50 and 10% of circulating CD8+ T cells, respectively (10, 11). Through the combined use of MR1 tetramers and Jα33−/− mice, other populations of MR1-restricted T cells have been described, which express TCRs distinct from the canonical Vα7.2-Jα33, and may play antimicrobial as well as immunoregulatory functions (3, 11–13).
For many years after the discovery of MAIT cells restriction to MR1, the nature of the antigen MAIT cells detect in association with MR1 was unclear. In 2012, Kjer-Nielsen et al. demonstrated that MAIT cells TCRs recognize intermediates of the vitamin B2 (riboflavin) biosynthetic pathway (14). Several bacteria and fungi previously associated with MAIT cell activation (15, 16) produce agonist vitamin B2 metabolites that stimulate MAIT cell activation in a TCR-dependent manner. Viruses are unable to synthesize vitamin B2 metabolites and cannot elicit TCR-dependent MAIT cell activation. Nevertheless, viral infections can elicit MAIT cell activation in a TCR-independent manner, through the release of different cytokines such as IL-12 and IL-18 (17, 18). MAIT cell activation results in the production of a variety of chemokines and pro-inflammatory cytokines, associated with both Th1 (IFN-γ and TNF-α) (16, 19) and Th17 immunity (IL-17 and IL-22) (20), but in certain tissues or upon prolonged stimulation MAIT cells can also release IL-10 and IL-13 (21, 22). Like conventional T cells, cytokine secretion is controlled by key transcription factors, mainly T-bet and RORγt (23). In addition, MAIT cells efficiently lyse bacterially-infected epithelial cells through granzyme and perforin molecules (24, 25) and MAIT-derived granulysin and granzyme B may be effective against antibiotic resistant bacterial species (26).
Like iNKT cells, MAIT have a unique developmental pathway and their effector-memory phenotype is controlled by the master transcription factor PLZF (27). Several recent reviews have extensively discussed MAIT cell development, their antimicrobial role, and their contribution to cancer and inflammatory diseases (23, 28–30). Herein, we will discuss their role at the interface between innate and adaptive immunity and recent results describing an important contribution of MAIT cells to tissue homeostasis, with a view to potentially harnessing their immunomodulatory properties.
Tight Regulation of MAIT Cell Activation
Like other populations of lymphocytes straddling across innate and adaptive immunity, such as iNKT and γδ T cells (31, 32), MAIT cells are emerging as important modulators of immune responses. As MAIT cells are particularly abundant at mucosal surfaces, where antigen might also be available at higher concentrations, tight regulation of their activity is required to avoid immunopathology: for example, accumulation of activated MAIT cells has been reported in the inflamed mucosa in ulcerative colitis (33) and in the gastric mucosa during Helicobacter pilori infection (34). Tight regulation of MAIT cell activity is likely to occur through several mechanisms, from regulation of MR1 expression, to antigen availability, stability, and modulation of MAIT cell activation through cognate interactions.
MR1 Ligands and Their Importance in Modulating MAIT Cell Function
MR1 is ubiquitously expressed at the transcript level (7), although the protein is retained in the ER and surface expression is tightly regulated by antigen availability (35). The most potent natural MAIT cell agonists known to date are intermediates of the vitamin B2 biosynthetic pathway (14, 36), present in a number of bacteria, commensals and pathogenic (37, 38). Despite stabilizing MR1 molecules at the cell surface, folate derivatives are not recognized by the MAIT TCR (14, 36), although more in depth analysis with folate-loaded MR1 tetramers has identified small subsets of circulating TRAV1.2+ and TRAV1.2− reactive T cells (3).
The structure-activity relationship of MR1 ligands has been well characterized and while MR1 surface upregulation correlates with the ability of the compounds to form a Schiff base with Lys43 of MR1, the agonist activity correlates with binding of the compound ribityl moiety to the TCR, via its Tyr95α residue (2, 39–41). Recently, a very elegant study with 20 altered metabolite ligands and 11 crystal structures of TCR-MR1-ligand ternary complexes has refined the molecular basis underpinning the potency and specificity of MAIT cell antigens, with the identification of an “interaction triad” between Tyr95α (in the MAIT TCR), Tyr152 (in the MR1 groove), and 5' and 2' OH groups in 5-OP-RU, which needs to be preserved for maximal agonist activity (42). These findings will be invaluable in future investigations exploring how to design ligands to better harness MAIT cell activity.
The full spectrum of MAIT cell ligands is still under appreciated, although two studies have reported agonist activity of drugs and drug like molecules (43) and of synthetic compounds identified in silico (44). The weak agonist activity of drugs like diclofenac and the antagonist activity of salicylates potentially underscores a much broader involvement of MAIT cells in several physio-pathological processes. Inhibitory ligands have the potential to be used to downregulate MAIT cell activation. Indeed, a synthetic derivative of the vitamin B9 metabolite 6-FP (i-6FP) has been used to inhibit MAIT cell activation and improve the course of the autoimmune disease lupus in FcγRIIb−/− mice, a spontaneous model of systemic lupus erythematosus in which MAIT cells have been shown to enhance autoantibody production and tissue inflammation (45).
While the majority of antagonists stabilize MR1 through a Schiff base but lack a moiety capable of interacting with the MAIT TCR, a novel mechanism of inhibition has recently been identified (44). Two synthetic non-microbial compounds, DB28 and its derivative NV-18, retain MR1 in the endoplasmic reticulum in an immature ligand-receptive form and compete with stimulatory ligands for MR1 binding. Neither DB28 nor NV18 form a Schiff base with MR1, but they are both sequestered in the A' MR1 pocket by a network of hydrophobic and polar contacts (44).
Antigen Stability
The potent MAIT cell antigens 5-(2-oxopropylideneamino)-6-D-ribitylaminouracil (5-OP-RU) and 5-(2-oxoethylideneamino)-6-D-ribitylaminouracil (5-OE-RU) derive from enzymatic and non-enzymatic condensation of 5-amino-6-(1-D-ribitylamino)uracil (5-A-RU) with glyoxals and methylglyoxals (host or bacteria derived). However, 5-OP-RU and 5-OE-RU are unstable and unless bound to MR1 via a Schiff base with Lys43 of the antigen presenting groove, they rapidly cyclize to less potent lumazines (36, 46). Furthermore, the biological activity of 5-A-RU is affected by long-term storage and spontaneous oxidation, unless prepared in dimethylsulfoxide solutions (46). To overcome the intrinsic instability of 5-A-RU, Lange et al. synthesized a pro-drug modifying the 5′ aminogroup with a cleavable valine-citrulline-p-aminobenzyl carbamate (47). The prodrug is stable and is cleaved intracellularly by cathepsin B, leading to preferential loading in the recycling endosomes.
Antigen Availability
Antigen availability influences MAIT cell population expansion throughout life. 5-OP-RU MR1-tetramer binding cells are few at birth, but they rapidly increase within the first year of life, accounting for the majority of Vα7.2+ CD161++ cells in the circulation (48). Germ free mice lack MAIT cells (6, 49–51) and mono-colonization with riboflavin producing bacteria, or exposure to synthetic 5-OP-RU, is sufficient to rescue MAIT cell development (51). These results, although surprising in view of the instability of 5-OP-RU, underscore the high sensitivity of TCRs in detecting cognate antigens bound to the relevant antigen presenting molecule.
At steady state, microbial diversity and density increases from the upper to the lower gastrointestinal tract (52) and mucosal conditions affect the relative abundance of MAIT-stimulatory metabolites, thus influencing MAIT cell activation (37). It was shown that E. coli bacteria grown in anaerobic conditions, stationary phase, and in medium supplemented with glucose, xylose, ribose, or glycerol stimulated more potently MAIT cell activation (37). These growth conditions correlated with increased accumulation of stimulatory MAIT cell ligands, detected by mass spectrometry (37). Furthermore, location of bacteria in the luminal space vs. areas adjacent to the epithelium (such as for bacteroides spp., proteobacteria) is also likely to influence antigen availability (37). Finally, the relative expression of individual enzymes in the vitamin B2 biosynthetic pathway influences the balance of MAIT cell-activating and inhibitory metabolites, as shown for Salmonella typhimurium and Streptococcus pneumoniae isolates (53–55).
Direct and Indirect MAIT Cell Activation
When the phenotype of MAIT cells from paired mucosal and blood samples has been analyzed, important differences have been highlighted. Colon resident MAIT cells are more activated (higher expression of CD137, CD69, HLA-DR, and CD25), but they also express higher levels of inhibitory receptors, such as TIGIT, CTLA-4, PD1, and LAG3 (37). This phenotype might reflect continuous exposure to metabolites derived from commensals and/or pathogenic bacteria and the expression of inhibitory receptors may balance this exposure. During bacterial infections, in addition to MR1-antigen complexes, MAIT cells are exposed to a variety of inflammatory cytokines that co-stimulate and enhance their activation, potentially overcoming inhibitory signals (Figure 1). The relative importance of TCR-driven vs. cytokine driven MAIT cell stimulation also changes during the course of an infection, with the former dominating at earlier stages of the response (56). Unlike conventional memory T cells, in vitro, MAIT cells are poorly responsive to anti CD3/anti CD28 stimulation, but their responsiveness is greatly enhanced by IL-12 and IL-18 (Figure 1A) (57). Freshly isolated blood MAIT cells and MAIT cell lines are potently activated by synthetic 5-OP-RU presented by myeloid cells, and in this setting their activation is mostly MR1-dependent (58). Yet, a high fraction of cells undergoes activation induced cell death, and perhaps cytokine dependent signals, including IL-12, IL-18, and IL-15, increase MAIT cell viability through changes in expression of pro and anti-apoptotic proteins, such as Bcl2 and Bax1 (59, 60).
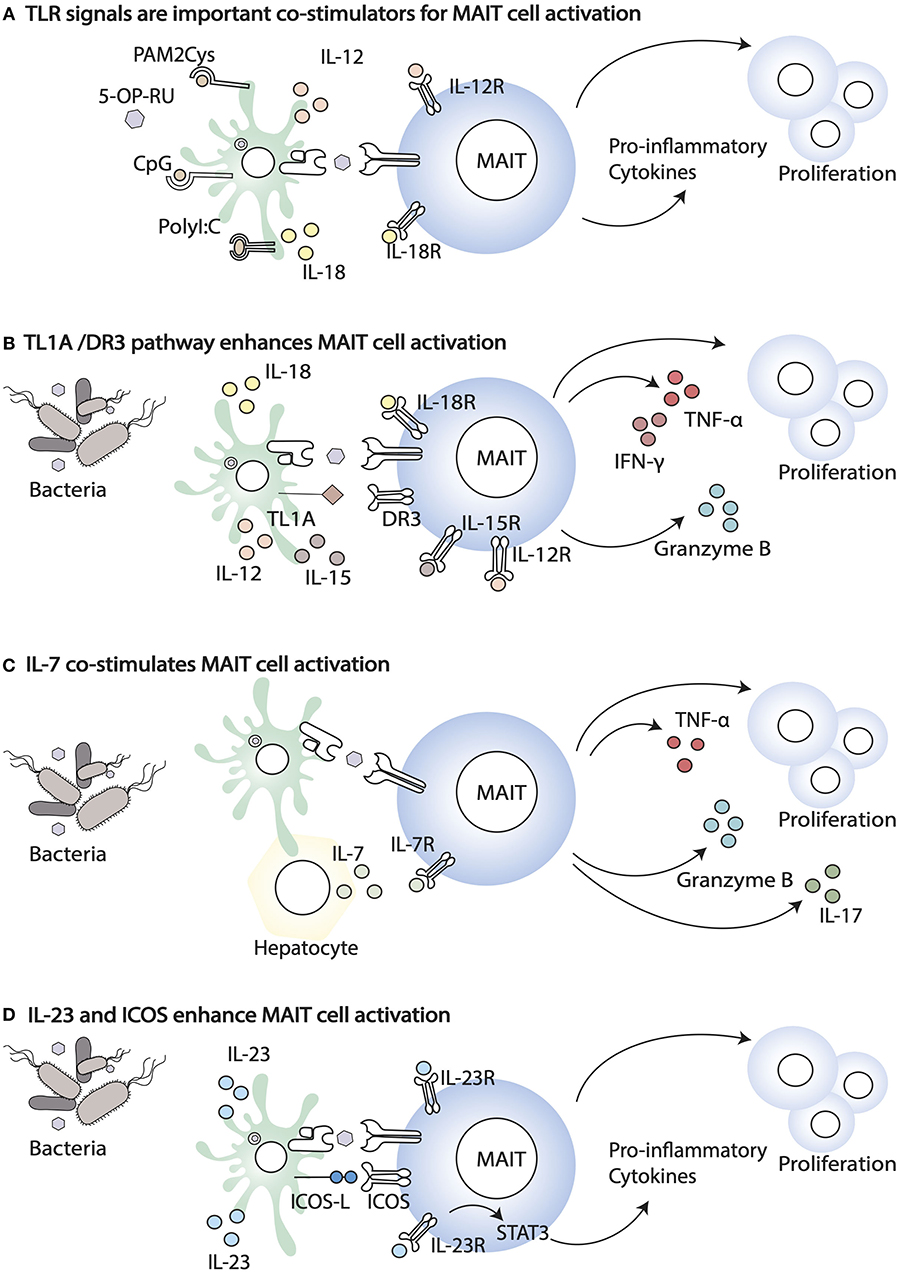
Figure 1. Co-stimulatory signals that enhance MAIT cell activation. (A) TLR agonists, including PAM2Cys, CpG, and PolyI:C, enhance MAIT cell response in the presence of the ligand. (B) Co-stimulation through the TL1A-DR3 pathway results in enhanced MAIT cell proliferation and the release of Granzyme B, IFN- γ, and TNF- α. (C) Infections trigger IL-7 release by different cells, including hepatocytes. IL-7 co-stimulates MAIT cells enabling cell proliferation and the release of IL-17, Granzyme B, and TNF- α. (D) Bacterial infection induces the expression of ICOS ligand (ICOSL) and ICOS (ICOS) on APC and MAIT, respectively. The increased expression of ICOS and IL23R signalling in MAIT cells enhance secretion of pro-inflammatory cytokines.
The high expression of IL-12 and IL-18 receptors by MAIT cells [as well as by iNKT cells (61, 62)] facilitates their activation in a TCR-independent manner, during viral infections [reviewed in (63)]. In addition, cytokine stimulation is important during infections with Group A streptococcus bacteria, lacking the vitamin B2 biosynthetic pathway (64). In this paper the authors showed that MAIT cell activation occurs in response to streptococcal exotoxins of the superantigen family, recognized by the Vβ2 TCR chain, but independently of MR1 (64). Similarly, MAIT cell responsiveness to Staphylococcus enterotoxin B (SEB) superantigen occurs in a TCR Vβ13.2 dependent manner but is MR1-independent and is largely contributed by IL-12 and IL-18 (65).
The importance of cytokines signaling for achieving full MAIT effector function is also underscored by the observation that chronic stimulation by type I IFN (such as in HIV infected patients) results in impaired MAIT cell responses to bacteria, via IL-10-dependent suppression of IL-12 secretion by APCs (66). However, in other settings, type IFNs synergize with other signals to enhance MAIT cell activation, consistent with the notion that type I IFNs are an important third signal that shapes the differentiation of memory conventional T cells (67). Accordingly, in HCV infected patients treated with type I IFN and antivirals it was reported that MAIT cells express higher CD69, indicative of activation (17), and a synergistic activity of type I IFN and IL-15 was also demonstrated. Furthermore, type I IFN has also been shown to co-stimulate TCR dependent MAIT cell activation (68) in addition to the TCR independent activation discussed above (17).
Other inflammatory cytokines that have been shown to enhance MAIT cell responsiveness to antigen include the gut-associated TNF superfamily member TL1A (via death receptor 3, DR3 (Figure 1B) (69, 70) and IL-7 (Figure 1C). IL-7 is essential for proliferation of liver-derived MAIT cells, which like the colonic MAIT have a semi-activated state yet they have higher expression of negative regulators (SOCS1 and SOCS3), in addition to lower expression of TCR signaling components, which may be an adaptation to constant antigen exposure (71). Furthermore, in HIV patients, IL-7 has been shown to rescue the defective MAIT cell cytolytic capacity and cytokine secretion, both in vitro and in vivo, in patients on anti-retroviral therapy (72, 73).
In vivo, population expansion of MAIT cells following infection with S. typhimurium depends on riboflavin metabolites and microbial signals (74). Indeed, synthetic 5-OP-RU injection intranasally or intravenously is not sufficient to increase MAIT cell frequency and numbers in the lung and draining lymph nodes, unless it is accompanied by TLR-derived signals (Pam2Cys, polyI:C, or CpG) (Figure 1D). Likewise, infection with riboflavin-deficient S. typhimurium mutants does not lead to MAIT cells population expansion, unless complemented with synthetic 5-OP-RU (74). However, more recently, intraperitoneal injection (51) or skin application of 5-OP-RU (50) was shown to be sufficient to activate and expand MAIT cells, locally and systemically. Whether these discrepancies are due to differences in the microbial flora of the animal colonies of the different investigators, to different sensitivity of lung, skin vs. thymic MAIT cells, or to different antigen preparations and doses of antigens reaching the sites, it remains to be determined.
Nevertheless, for sustained expansion and cytokine secretion, some form of co-stimulation of MAIT cells seems to be required. Following cutaneous application of S. epidermidis, population expansion of MAIT cells is reduced in the absence of IL-18, but not IL-23, while IL-1 signaling is required for licensing of IL-17A production by MAIT cells (50). Using a mouse model of conditional MR1 targeting, the authors also demonstrated that MAIT cell population expansion following S. epidermidis application is MR1 dependent, although homeostatic maintenance of MAIT cells is not, as their frequency is unchanged 3 weeks post deletion of MR1.
Specific costimulatory requirements have been identified for population expansion of RORγt+ MAIT cells in response to intranasal Salmonella typhimurium or Legionella longbeachae (75). In this paper, through mixed bone marrow chimeras the authors showed that APCs-bone marrow derived and epithelial-are important in MR1-dependent antigen presentation. In addition, they demonstrated a role for ICOS-mediated co-stimulation and IL-23/IL-23R signaling in MAIT cell expansion and activation (Figure 1D). Both ICOS-deficiency and IL-23 deficiency impaired expansion mainly of the RORγt MAIT subset, suggesting they are required for maintenance of RORγt expression and IL-17 secretion. IL-23 was also shown to be sufficient on its own to co-stimulate MAIT cell proliferation, in the presence of 5-OP-RU and to up-regulate ICOS expression, although IL-23 and ICOS double deficient mice were not tested, nor was IL-23 measured in ICOS deficient mice (75). These results are consistent with the high expression of both ICOS and IL-23R in MAIT cells, compared to conventional T cells in naïve mice. In this context IL-23 signaling is important, presumably via STAT3, to maintain the Th17 signature of tissue resident MAIT cells. As homeostatic IL-23 contributes to MAIT cell development/accumulation in the presence of a normal microbial flora (50), these results also are consistent with the observation that patients with STAT3 loss of function mutations have, amongst others, a deficiency of MAIT cells (76).
A TCR-dependent Tissue Repair Function of MAIT Cells
Overall, the above results are consistent with the hypothesis that due to ubiquitous MR1 expression and abundance of MAIT cells in tissues, their function needs to be tightly regulated, and for maximal effector function (i.e., during an infection) a combination of TCR, cytokine, and co-stimulation signals is required. In the absence of inflammatory signals, only minimal cell activation might be elicited. To understand the extent of MAIT cell functional plasticity and changes in their functional program in the course of an infection, several groups have analyzed their transcriptional signatures, in bulk and at the single cell level. At steady state, in both humans and mice, distinct transcriptional patterns have been identified, according to tissue specificity: despite different frequencies in different organs, MAIT/iNKT1 subsets, and MAIT/iNKT17 subsets share transcriptional signatures that are developmentally imprinted, including their tissue residency (77). Transcriptional changes occur at each developmental stage in the thymus and are underpinned by expression of the key transcription factors PLZF, T-bet and RORγt (27, 78), imprinting specific tissue residence signatures and Th1 or Th17 bias.
Recently, three groups compared the transcriptional signature of human and murine MAIT cells activated by bacterial infection (Legionella longbeachae), cytokines, and/or TCR stimulation (60, 69, 79). Despite differences in experimental models, common signatures were observed by the three groups. As expected from previous analysis at the protein level, upon activation, both human and murine MAIT cells expressed genes encoding for proinflammatory cytokines (such as GM-CSF, IL-17, INF-γ) and chemokines (such as XCL1, CCL3, CCL4, CXCL16) (60, 69). Human MAIT activated by anti-CD3/CD28 displayed a signature intermediate between unstimulated MAIT cells and those activated by cytokines and anti-CD3/28 (69). In turn, the transcriptional signature of human MAIT activated by cytokines and anti-CD3/CD28 (69) resembled that of 5-OP-RU activated human MAIT cells (60). Some species-specific differences were observed, such as higher expression of LIGHT and IL-2 in human MAIT cells, and higher TRANCE and RANKL in murine cells, but these could also be explained by different activation modes (60). Furthermore, and in line with their innate-like function, it was reported that the transcriptome of TCR-activated murine MAIT cells resembled that of iNKT cells, while upon resolution of infection it was more similar to γδ T cells (60). Interestingly, γδ T cells are a population of unconventional T cells that crucially contribute to tissue integrity and repair (80). Accordingly, an interesting finding common across these studies was the identification of a tissue repair signature upon TCR-dependent activation of MAIT cells. This signature was previously identified in H2M3 restricted CD8 Tc17 cells upon exposure to the commensal S. epidermidis (81) and was also seen in skin MAIT cells upon S. epidermidis topical application (50). Key genes in this signature are involved in tissue repair and remodeling (MMP25, FURIN, PDGFB, TGFB1) and angiogenesis (CSF2, VEGFB, PDGFB). Other MAIT cell-derived factors with potential role of tissue homeostasis were IL-26, OSM, and HBEGF (69, 79). Hence an important function of tissue resident MAIT cells could be maintenance of tissue homeostasis in the presence of commensals, limiting inflammation, and associated tissue injury. In agreement with these findings, MAIT cells in the female genital tract, in the oral mucosa, and in fetal mucosal tissues show a bias toward IL-22 and IL-17 secretion, and a potential role in barrier immunity and tissue homeostasis (20, 82, 83). This hypothesis is also consistent with in vitro results demonstrating that supernatants of activated MAIT cells promote closure of scratches of monolayers of Caco2 cells (69) and in vivo results showing that MR1 deficient mice have reduced re-epithelization of skin wounds (50). In addition, the MAIT tissue repair function could account for increased gut permeability in MR1−/− NOD mice, compared with MR1 sufficient littermates (84). Finally, alteration of gut permeability and MAIT cell numbers following conditioning regimens and allogeneic bone marrow transplantation could contribute to intestinal symptoms of Graft versus host disease (GvHD) (85, 86). In the future it will be interesting to investigate whether there is an alteration in the homeostatic tissue repair function of MAIT cells in chronic fibrotic diseases, in which a pathogenic MAIT cell role has been suggested (87–89).
MAIT Cells: The New iNKT/γδ T Cells?
Common features across the three major populations of innate-like lymphocytes—iNKT, γδ, and MAIT cells—are their peculiar thymic differentiation program, after which the cells emerge as pre-set memory/effectors, poised to a rapid response upon antigen encounter (31, 32, 90, 91). Through secretion of a variety of cytokines and chemokines, iNKT cells and γδ T cells regulate the function of several immune cell subsets and have emerged as central players in immunobiology and immunopathology, bridging innate and adaptive responses (Figure 2). We will discuss the existing evidence in favor of the immunomodulatory activity of MAIT cells.
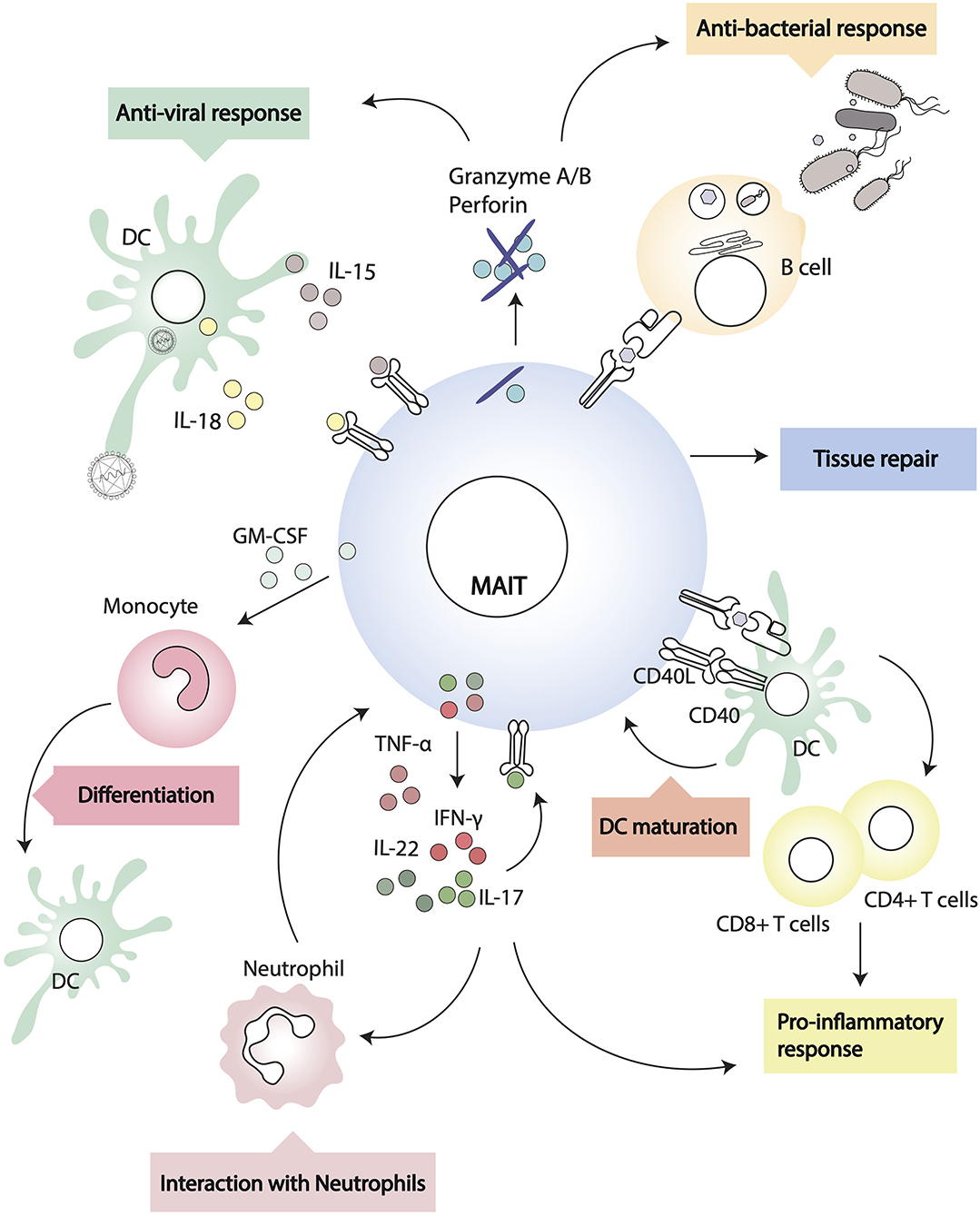
Figure 2. Interactions of MAIT cells and other leukocytes. MAIT cells recognize MR1-antigen complexes on the surface of target cells and modulate their activity through cognate interactions (i.e., via CD40/CD40L) or through soluble factors.
Interactions With Myeloid Cells
MAIT cells can modulate myeloid cell function directly, following MR1-cognate interactions, or indirectly, through soluble factors. During in vivo pulmonary infection with Francisella tularensis live vaccine stain (LVS), it has been shown that MAIT cells produce critical antimicrobial cytokines (IFN-γ, IL-17A, TNF-α) and in MR1−/− mice there is a higher bacterial burden and delayed bacterial clearance (92). In addition, through early GM-CSF production, pulmonary MAIT cells promote the differentiation of CCR2+ inflammatory monocytes into dendritic cells (DC) (93). As DC are critical for priming adaptive immunity, recruitment of activated TCRβ+ CD4+ and CD8+ cells is significantly delayed in MR1 deficient mice (92), but it can be rescued by adoptive transfer of in vivo differentiated DC (93). In this experimental system, MAIT cells influence monocyte differentiation into DC in a GM-CSF dependent but MR1 independent way (93). In other models, however, MAIT cells can modulate DC function in an MR1-dependent manner. Indeed, it has been shown that primary human MAIT cells and MAIT cell lines induce maturation and activation of monocyte-derived DC and primary DC upon MR1-dependent recognition of 5-OP-RU complexes (58). DC maturation is dependent on CD40-CD40L signaling and results in secretion of bioactive IL-12, which can further modulate NK cell activation (58). Interestingly, murine MAIT cells can also upregulate CD40L upon activation (75), although it remains to be determined whether they are able to induce antigen specific adaptive T cell responses through CD40L dependent DC maturation, as is the case for iNKT cells (94, 95).
Additionally, MAIT cells have been shown to exert a protective function in non-alcoholic fatty liver disease, inducing M2-macrophages polarization through IL-4 secretion (96).
Interactions With B Cells
MAIT cells final differentiation and peripheral expansion depends on cognate interactions with B cells in mice, although this does not seem to be critical in humans (6, 97). MAIT can be directly activated by bacterial infected B cells with up-regulation of CD69, and secretion of IFN-γ, TNF-α, and IL-17 (98). The observation that in Vibrio cholera infection (99) and Shigella dysenteriae vaccination (24) the circulating frequency of MAIT cells correlates with the pathogen-specific antibody response suggests some form of helper activity from MAIT cells. In a murine model of lupus, MAIT cell activity correlates with autoantibodies, germinal center reaction, and severity of disease (45). In this paper, the authors also reported that, in vitro, MAIT cells enhanced IgG production by LPS-stimulated B cells through CD40-CD40L cognate interactions (45).
In vitro, MAIT cell supernatants have been shown to induce plasmablast differentiation and antibody secretion from memory B cells (100). In this system, help for B cells is provided in an MR1-dependent, but CD40L independent manner, likely via cytokines like IL-6, IL-10, and IL-21 (100). However, the authors only blocked soluble CD40L and did not address whether during cognate interaction between B cells and MAIT cells the CD40/CD40L axis has a role for B cell differentiation. Furthermore, as the CD161 ligand LLT1 has been shown to play a role in the germinal center reaction (101), there remains the possibility that CD161++ MAIT cells, in addition to the more abundant CD161+ follicular DC subset, might contribute to B cell differentiation through cognate interactions. Lastly, it is not yet established whether a T-follicular helper subset of MAIT cells exists, akin to Bcl-6+ iNKT cells providing cognate B cell help in the lymph node (102–104).
Interactions With Neutrophils
In the presence of monocytes, MAIT (and γδ) T cells rapidly respond to bacterial infected neutrophils, and through secretion of GM-CSF, IFN-γ, and TNF-α they increase neutrophil survival and promote their differentiation into antigen presenting cells, expressing CD64, CD83, HLADR, CD54, CD40, and HLA A, B, C (105). In addition, in this manuscript, MAIT and γδ T cells activated neutrophils acquired the ability to uptake exogenous antigens, and cross-present antigenic peptides to CD8+ T cell clones (105). The ability of MAIT cells to modulate neutrophils function could play an important role in sepsis patients (106), where the number of circulating MAIT cells might affect the outcome of infection, particularly in the aging population with declining numbers of MAIT cells (107). Furthermore, as MAIT cells can become anergic upon recognition of microbial superantigens that often are associated with sepsis (65), and are then impaired in their ability to respond to bacterial infected cells, licensing of activated neutrophils to present antigens to CD4+ and CD8+ T cells may become crucial for the establishment of protective immunity (105).
Contradicting the above results, a recent study found that neutrophils inhibit MAIT cell activation through cell contact and hydrogen peroxide and that MAIT cells-derived TNF-α induces neutrophil death (108). The discrepancies might be related to different experimental settings, for example, one study isolated neutrophils by dextran sedimentation followed by Ficoll-Plaque centrifugation and hypotonic lysis of remaining red blood cells, while the other study purified them from whole blood or Lymphoprep separated granulocytes by HetaSep sedimentation and negative selection with the EasySep neutrophil enrichment kit; one study activated MAIT cells with 5-OP-RU, while the other with anti-CD3/28 beads, hence the amount of cytokines in the supernatants would be different. Therefore, further research is required to understand the exact outcome of MAIT-neutrophils interactions.
It also remains to be determined whether, like iNKT cells, MAIT cells are able to modulate the suppressive function of granulocytic myeloid derived suppressor cells, which could be of relevance to relive cancer immunosuppression and amenable to clinical harnessing (109, 110).
Concluding Remarks
Because of their high numbers in humans and of the restriction by a monomorphic molecule, MAIT cells represent an interesting population to target to enhance antigen specific immunity, through their multiple interactions with cells of the innate immune system. Despite great progress since the first discovery of T cell populations bearing semi-invariant TCRs, several aspects of MAIT cell biology remain to be fully unraveled before harnessing MAIT cells can be taken forward into the clinic. We need to better understand the role of MAIT cells in several diseases in which their numbers or functionality is altered, such as cancers and autoimmune diseases. The molecular mechanisms of ligand antigen presentation are not completely defined and a characterization of endogenous antigens, if they exist, is still lacking. Known ligands occupy the MR1 A' pocket and it remains to be determined if ligands or chaperones will bind the F' pocket. Pathogen evasion mechanisms from MAIT immune-surveillance have been identified (55, 111), but further research in this field is also needed. Lastly, the recently described MAIT tissue-repair function needs to be molecularly defined as well as the interplay with the host microbiota, which is key in homeostasis.
Author Contributions
MI wrote the first draft. MS edited and extended it. The paper is dedicated to the memory of VC. All authors contributed to the article and approved the submitted version.
Funding
This work was supported by Cancer Research UK (Programme Grant C399/A2291 to VC) and the Medical Research Council (MRC Human Immunology Unit Core funding).
Conflict of Interest
The authors declare that the research was conducted in the absence of any commercial or financial relationships that could be construed as a potential conflict of interest.
The handling Editor declared a past co-authorship with one of the authors VC.
Acknowledgments
We would like to dedicate this study to the memory of the late Professor VC, FRS, an inspirational colleague, mentor, and pioneer in the field of iNKT/CD1/MAIT cell biology, who passed away earlier in the year.
References
1. Porcelli S, Yockey CE, Brenner MB, Balk SP. Analysis of T cell antigen receptor (TCR) expression by human peripheral blood CD4-8- alpha/beta T cells demonstrates preferential use of several V beta genes and an invariant TCR alpha chain. J Exp Med. (1993) 178:1–16. doi: 10.1084/jem.178.1.1
2. Reantragoon R, Corbett AJ, Sakala IG, Gherardin NA, Furness JB, Chen Z, et al. Antigen-loaded MR1 tetramers define T cell receptor heterogeneity in mucosal-associated invariant T cells. J Exp Med. (2013) 210:2305–20. doi: 10.1084/jem.20130958
3. Gherardin NA, Keller AN, Woolley RE, Le Nours J, Ritchie DS, Neeson PJ, et al. Diversity of T cells restricted by the MHC class I-related molecule MR1 facilitates differential antigen recognition. Immunity. (2016) 44:32–45. doi: 10.1016/j.immuni.2015.12.005
4. Tilloy F, Treiner E, Park SH, Garcia C, Lemonnier F, de la Salle H, et al. An invariant T cell receptor alpha chain defines a novel TAP-independent major histocompatibility complex class Ib-restricted alpha/beta T cell subpopulation in mammals. J Exp Med. (1999) 189:1907–21. doi: 10.1084/jem.189.12.1907
5. Miley MJ, Truscott SM, Yu YY, Gilfillan S, Fremont DH, Hansen TH, et al. Biochemical features of the MHC-related protein 1 consistent with an immunological function. J Immunol. (2003) 170:6090–8. doi: 10.4049/jimmunol.170.12.6090
6. Treiner E, Duban L, Bahram S, Radosavljevic M, Wanner V, Tilloy F, et al. Selection of evolutionarily conserved mucosal-associated invariant T cells by MR1. Nature. (2003) 422:164–9. doi: 10.1038/nature01433
7. Riegert P, Wanner V, Bahram S. Genomics, isoforms, expression, and phylogeny of the MHC class I-related MR1 gene. J Immunol. (1998) 161:4066–77.
8. Treiner E, Duban L, Moura IC, Hansen T, Gilfillan S, Lantz O. Mucosal-associated invariant T. (MAIT) cells: an evolutionarily conserved T cell subset. Microbes Infect. (2005) 7:552–9. doi: 10.1016/j.micinf.2004.12.013
9. Huang S, Martin E, Kim S, Yu L, Soudais C, Fremont DH, et al. MR1 antigen presentation to mucosal-associated invariant T cells was highly conserved in evolution. Proc Natl Acad Sci USA. (2009) 106:8290–5. doi: 10.1073/pnas.0903196106
10. Dusseaux M, Martin E, Serriari N, Peguillet I, Premel V, Louis D, et al. Human MAIT cells are xenobiotic-resistant, tissue-targeted, CD161hi IL-17-secreting T cells. Blood. (2011) 117:1250–9. doi: 10.1182/blood-2010-08-303339
11. Gherardin NA, Souter MN, Koay HF, Mangas KM, Seemann T, Stinear TP, et al. Human blood MAIT cell subsets defined using MR1 tetramers. Immunol Cell Biol. (2018) 96:507–25. doi: 10.1111/imcb.12021
12. Meermeier EW, Laugel BF, Sewell AK, Corbett AJ, Rossjohn J, McCluskey J, et al. Human TRAV1-2-negative MR1-restricted T cells detect S. pyogenes and alternatives to MAIT riboflavin-based antigens. Nat Commun. (2016) 7:12506. doi: 10.1038/ncomms12506
13. Koay HF, Gherardin NA, Xu C, Seneviratna R, Zhao Z, Chen Z, et al. Diverse MR1-restricted T cells in mice and humans. Nat Commun. (2019) 10:2243. doi: 10.1038/s41467-019-10198-w
14. Kjer-Nielsen L, Patel O, Corbett AJ, Le Nours J, Meehan B, Liu L, et al. MR1 presents microbial vitamin B metabolites to MAIT cells. Nature. (2012) 491:717–23. doi: 10.1038/nature11605
15. Gold MC, Cerri S, Smyk-Pearson S, Cansler ME, Vogt TM, Delepine J, et al. Human mucosal associated invariant T cells detect bacterially infected cells. PLoS Biol. (2010) 8:e1000407. doi: 10.1371/journal.pbio.1000407
16. Le Bourhis L, Martin E, Peguillet I, Guihot A, Froux N, Core M, et al. Antimicrobial activity of mucosal-associated invariant T cells. Nat Immunol. (2010) 11:701–8. doi: 10.1038/ni.1890
17. van Wilgenburg B, Scherwitzl I, Hutchinson EC, Leng T, Kurioka A, Kulicke C, et al. MAIT cells are activated during human viral infections. Nat Commun. (2016) 7:11653. doi: 10.1038/ncomms11653
18. Paquin-Proulx D, Avelino-Silva VI, Santos BAN, Silveira Barsotti N, Siroma F, Fernandes Ramos J, et al. MAIT cells are activated in acute Dengue virus infection and after in vitro Zika virus infection. PLoS Negl Trop Dis. (2018) 12:e0006154. doi: 10.1371/journal.pntd.0006154
19. Gold MC, Napier RJ, Lewinsohn DM. MR1-restricted mucosal associated invariant T. (MAIT) cells in the immune response to Mycobacterium tuberculosis. Immunol Rev. (2015) 264:154–66. doi: 10.1111/imr.12271
20. Gibbs A, Leeansyah E, Introini A, Paquin-Proulx D, Hasselrot K, Andersson E, et al. MAIT cells reside in the female genital mucosa and are biased towards IL-17 and IL-22 production in response to bacterial stimulation. Mucosal Immunol. (2017) 10:35–45. doi: 10.1038/mi.2016.30
21. Lepore M, Kalinichenko A, Colone A, Paleja B, Singhal A, Tschumi A, et al. Parallel T-cell cloning and deep sequencing of human MAIT cells reveal stable oligoclonal TCRbeta repertoire. Nat Commun. (2014) 5:3866. doi: 10.1038/ncomms5493
22. Kelly J, Minoda Y, Meredith T, Cameron G, Philipp MS, Pellicci DG, et al. Chronically stimulated human MAIT cells are unexpectedly potent IL-13 producers. Immunol Cell Biol. (2019) 97:689–99. doi: 10.1111/imcb.12281
23. Lantz O, Legoux F. MAIT cells: programmed in the thymus to mediate immunity within tissues. Curr Opin Immunol. (2019) 58:75–82. doi: 10.1016/j.coi.2019.04.016
24. Le Bourhis L, Dusseaux M, Bohineust A, Bessoles S, Martin E, Premel V, et al. MAIT cells detect and efficiently lyse bacterially-infected epithelial cells. PLoS Pathog. (2013) 9:e1003681. doi: 10.1371/journal.ppat.1003681
25. Kurioka A, Ussher JE, Cosgrove C, Clough C, Fergusson JR, Smith K, et al. MAIT cells are licensed through granzyme exchange to kill bacterially sensitized targets. Mucosal Immunol. (2015) 8:429–40. doi: 10.1038/mi.2014.81
26. Boulouis C, Sia WR, Gulam MY, Teo JQM, Phan TK, Mak JYW, et al. Human MAIT cell cytolytic effector proteins synergize to overcome carbapenem resistance in Escherichia coli. bioRxiv. (2020) 18:e3000644. doi: 10.1101/2020.01.16.908806
27. Legoux F, Gilet J, Procopio E, Echasserieau K, Bernardeau K, Lantz O. Molecular mechanisms of lineage decisions in metabolite-specific T cells. Nat Immunol. (2019) 20:1244–55. doi: 10.1038/s41590-019-0465-3
28. Godfrey DI, Koay HF, McCluskey J, Gherardin NA. The biology and functional importance of MAIT cells. Nat Immunol. (2019) 20:1110–28. doi: 10.1038/s41590-019-0444-8
29. Toubal A, Nel I, Lotersztajn S, Lehuen A. Mucosal-associated invariant T cells and disease. Nat Rev Immunol. (2019) 19:643–57. doi: 10.1038/s41577-019-0191-y
30. Provine NM, Klenerman P. MAIT cells in health and disease. Annu Rev Immunol. (2020) 38:203–28. doi: 10.1146/annurev-immunol-080719-015428
31. Cerundolo V, Silk JD, Masri SH, Salio M. Harnessing invariant NKT cells in vaccination strategies. Nat Rev Immunol. (2009) 9:28–38. doi: 10.1038/nri2451
32. Hayday AC. gammadelta T cell update: adaptate orchestrators of immune surveillance. J Immunol. (2019) 203:311–20. doi: 10.4049/jimmunol.1800934
33. Haga K, Chiba A, Shibuya T, Osada T, Ishikawa D, Kodani T, et al. MAIT cells are activated and accumulated in the inflamed mucosa of ulcerative colitis. J Gastroenterol Hepatol. (2016) 31:965–72. doi: 10.1111/jgh.13242
34. D'Souza C, Pediongco T, Wang H, Scheerlinck JY, Kostenko L, Esterbauer R, et al. Mucosal-associated invariant T cells augment immunopathology and gastritis in chronic Helicobacter pylori infection. J Immunol. (2018) 200:1901–16. doi: 10.4049/jimmunol.1701512
35. McWilliam HE, Eckle SB, Theodossis A, Liu L, Chen Z, Wubben JM, et al. The intracellular pathway for the presentation of vitamin B-related antigens by the antigen-presenting molecule MR1. Nat Immunol. (2016) 17:531–7. doi: 10.1038/ni.3416
36. Corbett AJ, Eckle SB, Birkinshaw RW, Liu L, Patel O, Mahony J, et al. T-cell activation by transitory neo-antigens derived from distinct microbial pathways. Nature. (2014) 509:361–5. doi: 10.1038/nature13160
37. Schmaler M, Colone A, Spagnuolo J, Zimmermann M, Lepore M, Kalinichenko A, et al. Modulation of bacterial metabolism by the microenvironment controls MAIT cell stimulation. Mucosal Immunol. (2018) 11:1060–70. doi: 10.1038/s41385-018-0020-9
38. Tastan C, Karhan E, Zhou W, Fleming E, Voigt AY, Yao X, et al. Tuning of human MAIT cell activation by commensal bacteria species and MR1-dependent T-cell presentation. Mucosal Immunol. (2018) 11:1591–605. doi: 10.1038/s41385-018-0072-x
39. Patel O, Kjer-Nielsen L, Le Nours J, Eckle SB, Birkinshaw R, Beddoe T, et al. Recognition of vitamin B metabolites by mucosal-associated invariant T cells. Nat Commun. (2013) 4:2142. doi: 10.1038/ncomms3142
40. Eckle SB, Birkinshaw RW, Kostenko L, Corbett AJ, McWilliam HE, Reantragoon R, et al. A molecular basis underpinning the T cell receptor heterogeneity of mucosal-associated invariant T cells. J Exp Med. (2014) 211:1585–600. doi: 10.1084/jem.20140484
41. Eckle SB, Corbett AJ, Keller AN, Chen Z, Godfrey DI, Liu L, et al. Recognition of vitamin B precursors and byproducts by mucosal associated invariant T cells. J Biol Chem. (2015) 290:30204–11. doi: 10.1074/jbc.R115.685990
42. Awad W, Ler GJM, Xu W, Keller AN, Mak JYW, Lim XY, et al. The molecular basis underpinning the potency and specificity of MAIT cell antigens. Nat Immunol. (2020) 21:400–11. doi: 10.1038/s41590-020-0616-6
43. Keller AN, Eckle SB, Xu W, Liu L, Hughes VA, Mak JY, et al. Drugs and drug-like molecules can modulate the function of mucosal-associated invariant T cells. Nat Immunol. (2017) 18:402–11. doi: 10.1038/ni.3679
44. Salio M, Awad W, Veerapen N, Gonzalez-Lopez C, Kulicke C, Waithe D, et al. Ligand-dependent downregulation of MR1 cell surface expression. Proc Natl Acad Sci USA. (2020) 117:10465–75. doi: 10.1073/pnas.2003136117
45. Murayama G, Chiba A, Suzuki H, Nomura A, Mizuno T, Kuga T, et al. A critical role for mucosal-associated invariant T cells as regulators and therapeutic targets in systemic lupus erythematosus. Front Immunol. (2019) 10:2681. doi: 10.3389/fimmu.2019.02681
46. Mak JY, Xu W, Reid RC, Corbett AJ, Meehan BS, Wang H, et al. Stabilizing short-lived Schiff base derivatives of 5-aminouracils that activate mucosal-associated invariant T cells. Nat Commun. (2017) 8:14599. doi: 10.1038/ncomms14599
47. Lange J, Anderson RJ, Marshall AJ, Chan STS, Bilbrough TS, Gasser O, et al. The chemical synthesis, stability, and activity of MAIT cell prodrug agonists that access MR1 in recycling endosomes. ACS Chem Biol. (2020) 15:437–45. doi: 10.1021/acschembio.9b00902
48. Ben Youssef G, Tourret M, Salou M, Ghazarian L, Houdouin V, Mondot S, et al. Ontogeny of human mucosal-associated invariant T cells and related T cell subsets. J Exp Med. (2018) 215:459–79. doi: 10.1084/jem.20171739
49. Koay HF, Gherardin NA, Enders A, Loh L, Mackay LK, Almeida CF, et al. A three-stage intrathymic development pathway for the mucosal-associated invariant T cell lineage. Nat Immunol. (2016) 17:1300–11. doi: 10.1038/ni.3565
50. Constantinides MG, Link VM, Tamoutounour S, Wong AC, Perez-Chaparro PJ, Han SJ, et al. MAIT cells are imprinted by the microbiota in early life and promote tissue repair. Science. (2019) 366:eaax6624. doi: 10.1126/science.aax6624
51. Legoux F, Bellet D, Daviaud C, El Morr Y, Darbois A, Niort K, et al. Microbial metabolites control the thymic development of mucosal-associated invariant T cells. Science. (2019) 366:494–9. doi: 10.1126/science.aaw2719
52. Agace WW, McCoy KD. Regionalized development and maintenance of the intestinal adaptive immune landscape. Immunity. (2017) 46:532–48. doi: 10.1016/j.immuni.2017.04.004
53. Harriff MJ, McMurtrey C, Froyd CA, Jin H, Cansler M, Null M, et al. MR1 displays the microbial metabolome driving selective MR1-restricted T cell receptor usage. Sci Immunol. (2018) 3:eaao2556. doi: 10.1126/sciimmunol.aao2556
54. Kurioka A, van Wilgenburg B, Javan RR, Hoyle R, van Tonder AJ, Harrold CL, et al. Diverse Streptococcus pneumoniae strains drive a mucosal-associated invariant T-cell response through major histocompatibility complex class i-related molecule-dependent and cytokine-driven pathways. J Infect Dis. (2018) 217:988–99. doi: 10.1093/infdis/jix647
55. Preciado-Llanes L, Aulicino A, Canals R, Moynihan P, Zhu X, Jambo N, et al. Evasion of MAIT cell recognition by the African Salmonella Typhimurium ST313 pathovar that causes invasive disease. Proc NAtl Acad Sci USA. (2020). [Epub ahead of print]
56. Ussher JE, van Wilgenburg B, Hannaway RF, Ruustal K, Phalora P, Kurioka A, et al. TLR signaling in human antigen-presenting cells regulates MR1-dependent activation of MAIT cells. Eur J Immunol. (2016) 46:1600–14. doi: 10.1002/eji.201545969
57. Slichter CK, McDavid A, Miller HW, Finak G, Seymour BJ, McNevin JP, et al. Distinct activation thresholds of human conventional and innate-like memory T cells. JCI Insight. (2016) 1:e86292. doi: 10.1172/jci.insight.86292
58. Salio M, Gasser O, Gonzalez-Lopez C, Martens A, Veerapen N, Gileadi U, et al. Activation of human mucosal-associated invariant T cells induces CD40L-dependent maturation of monocyte-derived and primary dendritic cells. J Immunol. (2017) 199:2631–8. doi: 10.4049/jimmunol.1700615
59. Dias J, Boulouis C, Gorin JB, van den Biggelaar R, Lal KG, Gibbs A, et al. The CD4(-)CD8(-) MAIT cell subpopulation is a functionally distinct subset developmentally related to the main CD8(+) MAIT cell pool. Proc Natl Acad Sci USA. (2018) 115:E11513–22. doi: 10.1073/pnas.1812273115
60. Hinks TSC, Marchi E, Jabeen M, Olshansky M, Kurioka A, Pediongco TJ, et al. Activation and in vivo evolution of the MAIT cell transcriptome in mice and humans reveals tissue repair functionality. Cell Rep. (2019) 28:3249–62.e3245. doi: 10.1016/j.celrep.2019.07.039
61. Nagarajan NA, Kronenberg M. Invariant NKT cells amplify the innate immune response to lipopolysaccharide. J Immunol. (2007) 178:2706–13. doi: 10.4049/jimmunol.178.5.2706
62. Tyznik AJ, Verma S, Wang Q, Kronenberg M, Benedict CA. Distinct requirements for activation of NKT and NK cells during viral infection. J Immunol. (2014) 192:3676–85. doi: 10.4049/jimmunol.1300837
63. Ussher JE, Willberg CB, Klenerman P. MAIT cells and viruses. Immunol Cell Biol. (2018) 96:630–41. doi: 10.1111/imcb.12008
64. Emgard J, Bergsten H, McCormick JK, Barrantes I, Skrede S, Sandberg JK, et al. MAIT cells are major contributors to the cytokine response in group A streptococcal toxic shock syndrome. Proc Natl Acad Sci USA. (2019) 116:25923–31. doi: 10.1073/pnas.1910883116
65. Shaler CR, Choi J, Rudak PT, Memarnejadian A, Szabo PA, Tun-Abraham ME, et al. MAIT cells launch a rapid, robust and distinct hyperinflammatory response to bacterial superantigens and quickly acquire an anergic phenotype that impedes their cognate antimicrobial function: defining a novel mechanism of superantigen-induced immunopathology and immunosuppression. PLoS Biol. (2017) 15:e2001930. doi: 10.1371/journal.pbio.2001930
66. Tang X, Zhang S, Peng Q, Ling L, Shi H, Liu Y, et al. Sustained IFN-I stimulation impairs MAIT cell responses to bacteria by inducing IL-10 during chronic HIV-1 infection. Sci Adv. (2020) 6:eaaz0374. doi: 10.1126/sciadv.aaz0374
67. Huber JP, Farrar JD. Regulation of effector and memory T-cell functions by type I interferon. Immunology. (2011) 132:466–74. doi: 10.1111/j.1365-2567.2011.03412.x
68. Lamichhane R, Galvin H, Hannaway RF, de la Harpe SM, Munro F, Tyndall JD, et al. Type I interferons are important co-stimulatory signals during T cell receptor mediated human MAIT cell activation. Eur J Immunol. (2020) 50:178–91. doi: 10.1002/eji.201948279
69. Leng T, Akther HD, Hackstein CP, Powell K, King T, Friedrich M, et al. TCR and inflammatory signals tune human MAIT cells to exert specific tissue repair and effector functions. Cell Rep. (2019) 28:3077–91.e3075. doi: 10.1016/j.celrep.2019.08.050
70. Sattler A, Thiel LG, Ruhm AH, Souidi N, Seifert M, Herberth G, et al. The TL1A-DR3 axis selectively drives effector functions in human MAIT cells. J Immunol. (2019) 203:2970–8. doi: 10.4049/jimmunol.1900465
71. Tang XZ, Jo J, Tan AT, Sandalova E, Chia A, Tan KC, et al. IL-7 licenses activation of human liver intrasinusoidal mucosal-associated invariant T cells. J Immunol. (2013) 190:3142–52. doi: 10.4049/jimmunol.1203218
72. Leeansyah E, Svard J, Dias J, Buggert M, Nystrom J, Quigley MF, et al. Arming of MAIT cell cytolytic antimicrobial activity is induced by IL-7 and defective in HIV-1 infection. PLoS Pathog. (2015) 11:e1005072. doi: 10.1371/journal.ppat.1005072
73. Sortino O, Richards E, Dias J, Leeansyah E, Sandberg JK, Sereti I. IL-7 treatment supports CD8+ mucosa-associated invariant T-cell restoration in HIV-1-infected patients on antiretroviral therapy. Aids. (2018) 32:825–8. doi: 10.1097/QAD.0000000000001760
74. Chen Z, Wang H, D'Souza C, Sun S, Kostenko L, Eckle SB, et al. Mucosal-associated invariant T-cell activation and accumulation after in vivo infection depends on microbial riboflavin synthesis and co-stimulatory signals. Mucosal Immunol. (2017) 10:58–68. doi: 10.1038/mi.2016.39
75. Wang H, Kjer-Nielsen L, Shi M, D'Souza C, Pediongco TJ, Cao H, et al. IL-23 costimulates antigen-specific MAIT cell activation and enables vaccination against bacterial infection. Sci Immunol. (2019) 4:eaaw0402. doi: 10.1126/sciimmunol.aaw0402
76. Wilson RP, Ives ML, Rao G, Lau A, Payne K, Kobayashi M, et al. STAT3 is a critical cell-intrinsic regulator of human unconventional T cell numbers and function. J Exp Med. (2015) 212:855–64. doi: 10.1084/jem.20141992
77. Salou M, Legoux F, Gilet J, Darbois A, du Halgouet A, Alonso R, et al. A common transcriptomic program acquired in the thymus defines tissue residency of MAIT and NKT subsets. J Exp Med. (2019) 216:133–51. doi: 10.1084/jem.20181483
78. Koay HF, Su S, Amann-Zalcenstein D, Daley SR, Comerford I, Miosge L, et al. A divergent transcriptional landscape underpins the development and functional branching of MAIT cells. Sci Immunol. (2019) 4:eaay6039. doi: 10.1126/sciimmunol.aay6039
79. Lamichhane R, Schneider M, de la Harpe SM, Harrop TWR, Hannaway RF, Dearden PK, et al. TCR- or cytokine-activated CD8(+) mucosal-associated invariant T cells are rapid polyfunctional effectors that can coordinate immune responses. Cell Rep. (2019) 28:3061–76.e3065. doi: 10.1016/j.celrep.2019.08.054
80. Nielsen MM, Witherden DA, Havran WL. gammadelta T cells in homeostasis and host defence of epithelial barrier tissues. Nat Rev Immunol. (2017) 17:733–45. doi: 10.1038/nri.2017.101
81. Linehan JL, Harrison OJ, Han SJ, Byrd AL, Vujkovic-Cvijin I, Villarino AV, et al. Non-classical immunity controls microbiota impact on skin immunity and tissue repair. Cell. (2018) 172:784–96.e718. doi: 10.1016/j.cell.2017.12.033
82. Leeansyah E, Loh L, Nixon DF, Sandberg JK. Acquisition of innate-like microbial reactivity in mucosal tissues during human fetal MAIT-cell development. Nat Commun. (2014) 5:3143. doi: 10.1038/ncomms4143
83. Sobkowiak MJ, Davanian H, Heymann R, Gibbs A, Emgard J, Dias J, et al. Tissue-resident MAIT cell populations in human oral mucosa exhibit an activated profile and produce IL-17. Eur J Immunol. (2019) 49:133–43. doi: 10.1002/eji.201847759
84. Rouxel O, Da Silva J, Beaudoin L, Nel I, Tard C, Cagninacci L, et al. Cytotoxic and regulatory roles of mucosal-associated invariant T cells in type 1 diabetes. Nat Immunol. (2017) 18:1321–31. doi: 10.1038/ni.3854
85. Kawaguchi K, Umeda K, Hiejima E, Iwai A, Mikami M, Nodomi S, et al. Influence of post-transplant mucosal-associated invariant T cell recovery on the development of acute graft-versus-host disease in allogeneic bone marrow transplantation. Int J Hematol. (2018) 108:66–75. doi: 10.1007/s12185-018-2442-2
86. Varelias A, Bunting MD, Ormerod KL, Koyama M, Olver SD, Straube J, et al. Recipient mucosal-associated invariant T cells control GVHD within the colon. J Clin Invest. (2018) 128:1919–36. doi: 10.1172/JCI91646
87. Bottcher K, Rombouts K, Saffioti F, Roccarina D, Rosselli M, Hall A, et al. MAIT cells are chronically activated in patients with autoimmune liver disease and promote profibrogenic hepatic stellate cell activation. Hepatology. (2018) 68:172–86. doi: 10.1002/hep.29782
88. Hegde P, Weiss E, Paradis V, Wan J, Mabire M, Sukriti S, et al. Mucosal-associated invariant T cells are a profibrogenic immune cell population in the liver. Nat Commun. (2018) 9:2146. doi: 10.1038/s41467-018-04450-y
89. Law BMP, Wilkinson R, Wang X, Kildey K, Giuliani K, Beagley KW, et al. Human tissue-resident mucosal-associated invariant T (MAIT) cells in renal fibrosis and CKD. J Am Soc Nephrol. (2019) 30:1322–35. doi: 10.1681/ASN.2018101064
90. Brennan PJ, Brigl M, Brenner MB. Invariant natural killer T cells: an innate activation scheme linked to diverse effector functions. Nat Rev Immunol. (2013) 13:101–17. doi: 10.1038/nri3369
91. Legoux F, Salou M, Lantz O. Unconventional or preset alphabeta T cells: evolutionarily conserved tissue-resident T cells recognizing nonpeptidic ligands. Annu Rev Cell Dev Biol. (2017) 33:511–35. doi: 10.1146/annurev-cellbio-100616-060725
92. Meierovics A, Yankelevich WJ, Cowley SC. MAIT cells are critical for optimal mucosal immune responses during in vivo pulmonary bacterial infection. Proc Natl Acad Sci USA. (2013) 110:E3119–28. doi: 10.1073/pnas.1302799110
93. Meierovics AI, Cowley SC. MAIT cells promote inflammatory monocyte differentiation into dendritic cells during pulmonary intracellular infection. J Exp Med. (2016) 213:2793–809. doi: 10.1084/jem.20160637
94. Hermans IF, Silk JD, Gileadi U, Salio M, Mathew B, Ritter G, et al. NKT cells enhance CD4(+) and CD8(+) T cell responses to soluble antigen in vivo through direct interaction with dendritic cells. J Immunol. (2003) 171:5140–7. doi: 10.4049/jimmunol.171.10.5140
95. Fujii S, Liu K, Smith C, Bonito AJ, Steinman RM. The linkage of innate to adaptive immunity via maturing dendritic cells in vivo requires CD40 ligation in addition to antigen presentation and CD80/86 costimulation. J Exp Med. (2004) 199:1607–18. doi: 10.1084/jem.20040317
96. Li Y, Huang B, Jiang X, Chen W, Zhang J, Wei Y, et al. Mucosal-associated invariant T cells improve nonalcoholic fatty liver disease through regulating macrophage polarization. Front Immunol. (2018) 9:1994. doi: 10.3389/fimmu.2018.01994
97. Martin E, Treiner E, Duban L, Guerri L, Laude H, Toly C, et al. Stepwise development of MAIT cells in mouse and human. PLoS Biol. (2009) 7:e54. doi: 10.1371/journal.pbio.1000054
98. Salerno-Goncalves R, Rezwan T, Sztein MB. B cells modulate mucosal associated invariant T cell immune responses. Front Immunol. (2014) 4:511. doi: 10.3389/fimmu.2013.00511
99. Leung DT, Bhuiyan TR, Nishat NS, Hoq MR, Aktar A, Rahman MA, et al. Circulating mucosal associated invariant T cells are activated in Vibrio cholerae O1 infection and associated with lipopolysaccharide antibody responses. PLoS Negl Trop Dis. (2014) 8:e3076. doi: 10.1371/journal.pntd.0003076
100. Bennett MS, Trivedi S, Iyer AS, Hale JS, Leung DT. Human mucosal-associated invariant T (MAIT) cells possess capacity for B cell help. J Leukoc Biol. (2017) 102:1261–9. doi: 10.1189/jlb.4A0317-116R
101. Llibre A, Lopez-Macias C, Marafioti T, Mehta H, Partridge A, Kanzig C, et al. LLT1 and CD161 expression in human germinal centers promotes B cell activation and CXCR4 downregulation. J Immunol. (2016) 196:2085–94. doi: 10.4049/jimmunol.1502462
102. Barral P, Eckl-Dorna J, Harwood NE, De Santo C, Salio M, Illarionov P, et al. B cell receptor-mediated uptake of CD1d-restricted antigen augments antibody responses by recruiting invariant NKT cell help in vivo. Proc Natl Acad Sci USA. (2008) 105:8345–50. doi: 10.1073/pnas.0802968105
103. Leadbetter EA, Brigl M, Illarionov P, Cohen N, Luteran MC, Pillai S, et al. NK T cells provide lipid antigen-specific cognate help for B cells. Proc Natl Acad Sci USA. (2008) 105:8339–44. doi: 10.1073/pnas.0801375105
104. Chang PP, Barral P, Fitch J, Pratama A, Ma CS, Kallies A, et al. Identification of Bcl-6-dependent follicular helper NKT cells that provide cognate help for B cell responses. Nat Immunol. (2011) 13:35–43. doi: 10.1038/ni.2166
105. Davey MS, Morgan MP, Liuzzi AR, Tyler CJ, Khan MWA, Szakmany T, et al. Microbe-specific unconventional T cells induce human neutrophil differentiation into antigen cross-presenting cells. J Immunol. (2014) 193:3704–16. doi: 10.4049/jimmunol.1401018
106. Kim EY, Oldham WM. Innate T cells in the intensive care unit. Mol Immunol. (2019) 105:213–23. doi: 10.1016/j.molimm.2018.09.026
107. Walker LJ, Tharmalingam H, Klenerman P. The rise and fall of MAIT cells with age. Scand J Immunol. (2014) 80:462–3. doi: 10.1111/sji.12237
108. Schneider M, Hannaway RF, Lamichhane R, de la Harpe SM, Tyndall JDA, Vernall AJ, et al. Neutrophils suppress mucosal-associated invariant T cells in humans. Eur J Immunol. (2020) 50:643–55. doi: 10.1002/eji.201948394
109. De Santo C, Arscott R, Booth S, Karydis I, Jones M, Asher R, et al. Invariant NKT cells modulate the suppressive activity of IL-10-secreting neutrophils differentiated with serum amyloid A. Nat Immunol. (2010) 11:1039–46. doi: 10.1038/ni.1942
110. Mussai F, De Santo C, Cerundolo V. Interaction between invariant NKT cells and myeloid-derived suppressor cells in cancer patients: evidence and therapeutic opportunities. J Immunother. (2012) 35:449–59. doi: 10.1097/CJI.0b013e31825be926
Keywords: MAIT cells, TCR-dependent, TCR-independent, dendritic cell maturation, inflammation, tissue repair
Citation: Ioannidis M, Cerundolo V and Salio M (2020) The Immune Modulating Properties of Mucosal-Associated Invariant T Cells. Front. Immunol. 11:1556. doi: 10.3389/fimmu.2020.01556
Received: 11 March 2020; Accepted: 12 June 2020;
Published: 13 August 2020.
Edited by:
Ildiko Van Rhijn, Brigham and Women's Hospital and Harvard Medical School, United StatesReviewed by:
Melanie Harriff, Portland VA Medical Center, United StatesShouxiong Huang, University of Cincinnati, United States
Johan K. Sandberg, Karolinska Institutet (KI), Sweden
Copyright © 2020 Ioannidis, Cerundolo and Salio. This is an open-access article distributed under the terms of the Creative Commons Attribution License (CC BY). The use, distribution or reproduction in other forums is permitted, provided the original author(s) and the copyright owner(s) are credited and that the original publication in this journal is cited, in accordance with accepted academic practice. No use, distribution or reproduction is permitted which does not comply with these terms.
*Correspondence: Mariolina Salio, bWFyaW9saW5hLnNhbGlvQGltbS5veC5hYy51aw==
†Deceased 7 January 2020