- 1Department of Hematology, Ningbo First Hospital, Ningbo, China
- 2Bone Marrow Transplantation Center, The First Affiliated Hospital, Zhejiang University School of Medicine, Hangzhou, China
Objectives: The mechanism and immunoregulatory role of human natural killer (NK) cells in acute graft-vs.-host-disease (aGVHD) remains unclear. This study quantitatively analyzed the cytotoxicity of donor NK cells toward allo-reactive T cells, and investigated their relationship with acute GVHD (aGVHD).
Methods: We evaluated NK dose, subgroup, and receptor expression in allografts from 98 patients who underwent allogeneic hematopoietic stem cell transplantation (allo-HSCT). A CD107a degranulating assay was used as a quantitative detection method for the cytotoxic function of donor NK cells to allo-reactive T cells. In antibody-blocking assay, NK cells were pre-treated with anti-DNAM-1(CD226), anti-NKG2D, anti-NKP46, or anti-NKG-2A monoclonal antibodies (mAbs) before the degranulating assay.
Results: NK cells in allografts effectively inhibited auto-T cell proliferation following alloantigen stimulation, selectively killing alloantigen activated T cells. NKG2A− NK cell subgroups showed higher levels of CD107a degranulation toward activated T cells, when compared with NKG2A− subgroups. Blocking NKG2D or CD226 (DNAM-1) led to significant reductions in degranulation, whereas NKG2A block resulted in increased NK degranulation. Donor NK cells in the aGVHD group expressed lower levels of NKG2D and CD226, higher levels of NKG2A, and showed higher CD107a degranulation levels when compared with NK cells in the non-aGVHD group. Using univariate analysis, higher NK degranulation activities in allografts (CD107ahigh) were correlated with a decreased risk in grade I–IV aGVHD (hazard risk [HR] = 0.294; P < 0.0001), grade III–IV aGVHD (HR = 0.102; P < 0.0001), and relapse (HR = 0.157; P = 0.015), and improved overall survival (HR = 0.355; P = 0.028) after allo-HSCT. Multivariate analyses showed that higher NK degranulation activities (CD107ahigh) in allografts were independent risk factors for grades, I–IV aGVHD (HR = 0.357; P = 0.002), and grades III–IV aGVHD (HR = 0.13; P = 0.009).
Conclusions: These findings reveal that the degranulation activity of NK in allografts toward allo-activated T cells was associated with the occurrence and the severity of aGVHD, after allogeneic stem cell transplantation. This suggested that cytotoxicity of donor NK cells to allo-reactive T cells have important roles in aGVHD regulation.
Introduction
Natural killer (NK) cells are the first donor-derived subset of lymphocytes that are reconstructed following allogeneic hematopoietic stem cell transplantation (allo-HSCT). Although the roles of NK cells in preventing relapse and infection after allo-HSCT for hematologic malignancies has been well established (1–4), the function of human NK cells in acute graft-vs.-host-disease (aGVHD), which is a common complication of allo-HSCT, is still equivocal.
Some studies have demonstrated that killer immunoglobulin-like receptor (KIR)-ligand mismatches trigger donor vs. recipient NK cell allo-reactivity, suppressing the development of aGVHD by ablating host antigen-presenting cells (APCs), which are essential for the activation of donor T cell in aGVHD (5–7). However, many studies have failed to prove the beneficial effect of allo-reactive NK cells on aGVHD (8–12). Similarly, conflicting results from clinical studies also hint at other mechanisms for the regulation of aGVHD by NK cells (13).
The function that NK cells can distinguish target cells from healthy cells is controlled by integrating signals from inhibitory and activating receptors (14–18). Donor NK allo-reactivity, which is based on the lack of ligands for donor KIR in the recipient, can lead to NK cell activation though “missing-self” recognition (19–21). When target cells are exposed to stress, such as viral infection, the ligands for activating NK cell receptors are upregulated, binding to NK activating receptors and activate NK cells via “induced-self” recognition (22–24). Studies have demonstrated that activated T cells up-regulate the expression of ligands for activating NK cell receptors, making them vulnerable to NK cell killing though the “induced-self” model (25, 26). As donor NK and T cells share similar trafficking routes after allo-HSCT (27), and recent studies have shown that NK cells exert cytotoxicity toward activated T cells (28, 29), the NK cell–mediated direct lysis of allo-reactive T cells through the “induced-self” model may present an important mechanism for aGVHD regulation by NK cells. Olson et al. proved this hypothesis in a major histocompatibility complex (MHC)-mismatched mouse bone marrow transplantation (BMT) model (30). However, we know little about the role of NK cell cytotoxicity toward allo-reactive T cells in human aGVHD.
In this study, we investigated the role of NK cells in the regulation of T cell allo-reactivity in human allo-HSCT, and demonstrated that cytotoxicity of donor NK cells toward allo-reactive T cells was associated with the occurrence of overall and grade III–IV aGVHD.
Materials and Methods
Patients and Samples
Ninety-eight consecutive patients with acute lymphoid leukemia (ALL), acute myeloid leukemia (AML), myelodysplastic syndrome (MDS), non-Hodgkin's lymphoma (NHL), or chronic myeloid leukemia (CML) underwent allo-HSCT and were included in this study. Among these, 37 patients underwent human leukocyte antigen (HLA)-matched related HSCT, 13 patients underwent HLA-matched unrelated HSCT, and 48 patients underwent HLA-haplo-identical related HSCT. Stem cell sources were peripheral blood stem cells without T-cell depletion. The prophylaxis regimens for GVHD were cyclosporine A, short-term methotrexate, and mycophenolate mofetil. In addition, ATG was added to HLA-matched unrelated and HLA-haplo-identical related HSCT. The high risk disease status at the time of HSCT was defined as > second remission, or acute leukemia without remission after two cycles of induction chemotherapy, refractory anemia with excess blasts, and blast crisis of chronic myelomonocytic leukemia. KIR-ligand mismatch was evaluated based on donor and recipient HLA gene typing. The characteristics of the 98 patients and corresponding donors are summarized in Table 1. All samples in this study were collected from donor granulocyte-colony stimulating factor (G-CSF) mobilized peripheral blood stem cell (PBSC) harvests before transplantation. All patients and donors provided written informed consent. The study was approved by the Clinical Ethics Review Committee at Ningbo First Hospital and was performed in accordance with the Declaration of Helsinki.
mAbs and Flow Cytometry Analyses
NK cells were characterized by FITC-conjugated anti-human CD56, PE-conjugated anti-human CD16, and APC-conjugated anti-human CD3 mAbs (Becton Dickinson, San Diego, CA, USA). To analyze the expression of receptors on NK cells, the following mAbs were used: APC-conjugated anti-NKG2D (BAT221 clone), PE-conjugated anti-human NKp46 (BAB281 clone), and FITC-conjugated anti- human DNAM-1 (F22 clone) (all Becton Dickinson). PE conjugated anti-human NKG2A was purchased from Beckman Coulter (Fullerton, CA, USA). A Beckman Coulter flow cytometer, FC5000 (Fullerton, CA, USA), was used to analyze samples.
CD56+ NK Cell and CD3+T Cell Isolation and Proliferation Assays
Mononuclear cells (MNCs) were isolated from each G-CSF mobilized PBSC harvest by Ficoll-Hypaque (MultiSciences Biotech, Hangzhou, China) density centrifugation. CD56+ NK cells and CD3+T cells were isolated from MNCs by positive selection, using FACS (Fluorescence activated cell sorting), and used for the following experiments.
For proliferation assays, carboxyfluorescein diacetate succinimidyl ester (CFSE, Invitrogen, Carlsbad, CA, USA) -labeled CD3+T cells (2 × 105 cells/well) were stimulated with phytohemagglutinin (PHA), anti-CD3/anti-CD28, or allogeneic dendritic cells (allo-DCs) separately in 200 μl RPMI 1640 medium containing 10% fetal bovine serum (FBS), in a 96-well micro-plate (day 0). NK cells from the same donor were added to the culture at different NK/T ratios (0:10 to 1:5). At day four, cells were stained with a PECY7-conjugated anti-CD3 mAb (Becton Dickinson), and the proliferation of CD3+ T cells was analyzed by detecting diluted CFSE signals with flow cytometry.
Functional Assessments of NK Cells
For degranulation assays, NK cells and anti-CD3/anti-CD28 mAbs activated T cells from the same donor were co-cultured at an NK to T cell ratio of 1:1, for 4 h at 37°C, in the presence of APC-conjugated anti-human CD107a [lysosomal-associated membrane protein (LAMP)-1] mAb (H4A3, BD Biosciences, San Jose, CA) and GolgiStopTM containing monensin (BD Biosciences). In blocking assays, NK cells were incubated with blocking antibodies for 20 min before being co-cultured with target cells. The following anti-human mAbs were added at 10 μg/mL: NKG2D (clone 149810; R&D Systems, Minneapolis, MN, USA), NKG2A (clone NNC0141-0100, R&D Systems), DNAM-1 (clone DX11, BioLegend, San Diego, CA, USA), and NKP46 (BioLegend). Mouse IgG1 mAbs (R&D Systems) served as isotype-matched control mAbs. The expression of CD107a in NK cells was measured by flow cytometry.
For intracellular cytokine staining, NK cells were co-cultured with the unstimulated T cells or activated T cells for 4 h, and GolgiStop™ was added to trap protein in the cytoplasm. Monoclonal antibodies APC-conjugated anti-human CD56 mAb, FITC-conjugated anti-human IFN-γ, PE-conjugated anti-human TNF-α, FITC-conjugated anti-human TGF-β, and PE-conjugated anti-human IL-10 (BD Bioscience) were used for cell surface marker and in-tracellular cytokine staining. The intracellular cytokine level of NK cells was detected by flow cytometry. The granzyme B were quantified by ELISA in supernatants after co-culture of NK cells with the unstimulated T cells or activated T cells for 4 h.
For in vitro cytotoxicity assays, a CFSE-7AAD (7-Aminoactinomycin D, BD Pharmingen, San Diego, CA, USA) based flow cytometric cytotoxicity assay was performed using CFSE-labeled T cells stimulated for 4 d with allo-DCs as targets, and autogeneic NK cells as effectors. In brief, effector and target cells were co-cultured at E:T ratios of 50:1, 25:1, 10:1, 5:1, for 4 h at 37°C. Cells were then washed and labeled with PECY7 conjugated anti-CD3 mAb, and 7AAD (5 μg/mL) for 20 min and analyzed by flow cytometry.
Statistical Analysis
Patient characteristics in aGVHD and non-aGVHD groups were compared by the χ2-test for categorical variables or the Mann–Whitney U-test for continuous variables. Student's t-tests or a two-way ANOVA analyses were used to compare receptor expression, and degranulation activities of NK cells among groups. The optimal cut-off point of CD107a expression in donor NK cells was identified using the receiver operating characteristic (ROC) curve. Overall survival (OS) was estimated by the Kaplan–Meier method. The Gray's test was applied for comparisons of cumulative incidences of acute GVHD and relapse. Death, without aGVHD, was defined as the competing event for aGVHD, while relapse-free mortality was the competing event for relapse. The Cox regression model was employed for univariate and multivariate analyses. Risk factors for univariate analysis included the age of recipient and donor, the gender of recipient and donor, diagnosis, KIR-L mismatch/match between donor and recipients, donor source, high risk disease before transplantation, the dose of CD56+ NK cells, CD34+cells, and CD3+T cells, the NK/T cell ratio; the CD56dim/CD56bright ratio, NKG2A+ proportion, levels of CD226, NKG2D and NKP46 expression of NK cells, and NK CD107a degranulation activity in allografts. All covariates with P < 0.10 during univariate analysis were further included in a multivariate Cox regression model. All tests were bilateral, and a difference was considered significant when P < 0.05. Statistical analyses were performed on SPSS 25 statistical software (IBM, Armonk, NY, USA), and R 3.6.2 statistical software (https://www.r-project.org/) was employed to calculate the cumulative incidences, when considering the presence of competing risks. All calculated averages were defined as the parametric mean ± SD. **P < 0.01.
Results
Patient Characteristics
Ninety-eight donor PBSC samples from 98 patients receiving allo-HSCT were analyzed in this study. Patient characteristics are shown in Table 1. No significant differences were observed in patient age, patient sex, gender matching between donors and recipients, underlying disease, donor source, conditioning regimen, serotherapy, KIR-L mismatch, and dose of CD34+, CD3+, or CD56+ cells in allografts between the GVHD group and the non-aGVHD group. The median duration follow-up was 412 d (range; 71–1,320 d) after transplantation. All 98 patients achieved engraftment and complete donor chimerism after transplantation. The chimerism dynamics of donor NK and T cells were shown (Figure S1). Grades I, II, III, and IV aGVHD occurred in 16, 16, 14, and 5 cases, respectively. Of 24 patients that died, nine died from severe infection, two died from severe gastrointestinal aGVHD with pulmonary infection, and 13 relapsed.
NK Cells in Allografts Inhibited T Cell Proliferation and Exhibited Cytotoxicity Against Allo-Reactive T Cells
Olson et al. demonstrated that donor NK cells could inhibit and kill alloantigen activated T cells during the development of acute GVHD in their mouse model, indicating that donor NK cell mediated inhibition and lysing of activated donor T cells may represent an important mechanism for NK cell–mediated aGVHD reduction (30). However, the direct modulation of donor allo-reactive T cell responses by autogeneic NK cells in human GVHD has not been fully investigated. For donor T-cell proliferation, activation is the core immunopathophysiology of aGVHD; therefore, we investigated the effects of donor NK cells on the proliferation of autogeneic CD3+T cells, following activation by PHA, anti-CD3/anti-CD28 mAbs, or allo-DCs derived from recipients. CFSE-labeled resting CD3+T cells were stimulated by PHA, anti-CD3/anti-CD28 mAbs, or allo-DCs (T/DC = 5:1), and co-cultured with autologous CD56+ NK cells at NK/T ratios of 0:10, 1:10, or 1:5. Ninety-six hours later, the percentage of proliferating CD3+T cells was detected by flow cytometry (Figures 1A,B). As shown in Figures 1C,D, the proliferation of T cells, as defined by CFSE dilution, was significantly inhibited by donor NK cells, in a NK cell dose dependent pattern.
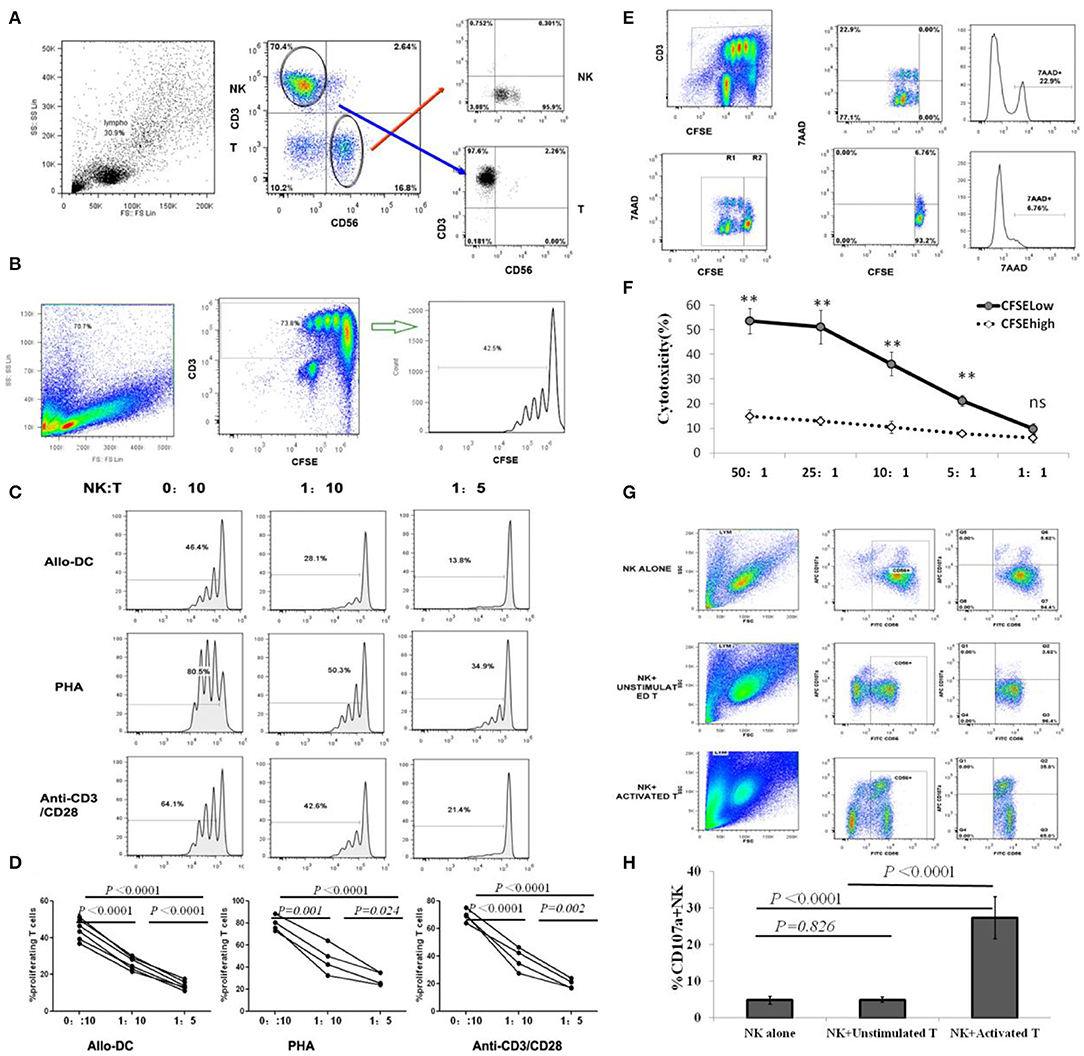
Figure 1. NK cells inhibit T cell proliferation by selectively killing alloantigen activated T cells. (A) Representative gating strategy for NK and T cell sorting; (B) Representative gating strategy for T cell proliferation assay. (C,D) CFSE-labeled CD3+T cells were stimulated with PHA, anti-CD3/anti-CD28 mAbs or allo-DCs, and autologous CD56+ NK cells were added at NK/T ratios of 0:10, 1:10, or 1:5. Four days later, CD3+T cell proliferation was analyzed by flow cytometry. The percentage of proliferating T cells was defined by CFSE intensities (n = 4). (E,F) CFSE-labeled CD3+T cells were first stimulated with allo-DCs for 96 h and then used as target cells for NK killing assays at effector:target (E:T) ratios of 50:1, 25:1, 10:1, 5:1, or 1:1. Allo-reactive T cells were distinguished by lower CFSE intensity (CFSElow) in CD3+T cells. 7AAD was labeled to identify dead cell and analyzed by flow cytometry (n = 4). (G,H) Naïve T cells or T cells activated by anti-CD3/anti-CD28 mAbs were co-cultured with NK cells at an effector:target (E:T) ratio of 1:1 for CD107a degranulating assay. NK cells cultured alone were used as controls. The percentage of CD107a+ in CD56+NK cells represented the level of NK degranulation toward T cells (n = 4). All calculated averages were defined as the parametric mean ± SD. Student's t-tests, or two-way ANOVA analyses, were used to compare the mean among groups. ns: not significant. **P < 0.01.
To further validate that NK cell-mediated cytotoxicity against T cells led to the suppression of alloantigen-activated T cell proliferation by autologous NK cells, CFSE-labeled resting CD3+T cells were stimulated with allogeneic dendritic cells (allo-DCs) for 96 h, and then used as target cells for an NK killing assay. Our results revealed allo-reactive T cells were distinguished by lower CFSE intensity (CFSElow) in CD3+T cells (Figure 1E). Flow cytometric analysis using 7AAD to identify dead cells revealed that donor NK cells mainly killed proliferating T cells (CFSElow), but not non-proliferating T cells (CFSEhigh), in a cell dose-dependent manner at effector:target (E:T) ratios of 50:1, 25:1, 10:1, or 5:1 (Figures 1E,F).
In the process of NK degranulation, lysosomal associated membrane protein-1 (LAMP-1, CD107a) on the surface of lysosomal granules is transported to the cell surface and can be used for antibody binding studies. This allows for the recognition of activated NK cells, making them attractive biomarkers for assessing granulocytic exocytosis and cytotoxic activity of NK cells (26, 27). As shown (Figures 1G,H), donor NK cells displayed degranulation activity to activated but not resting T cells, which was consistent with NK cells killing activated proliferating T cells instead of resting T cells, in the killing assay. In addition to CD107a degranulation, the Granzyme B concentration in NK and activated T cell co-cultures was significantly higher (1422.25 ± 256.77 pg/ml) than that in NK and unstimulated T cell co-cultures (782.75 ± 161.77 pg/ml) (P = 0.014). However, there was no difference in cytokines IFN-γ, TNF-α, IL-10, and TGF-β secreted by NK cells after co cultured with activated or unstimulated T cells (Figure S2). Therefore, NK cells selectively killed activated T cells and played an inhibitory role on T cell proliferation induced by alloantigen stimulation.
The Effects of NKG2A+/NKG2A− Subsets and Receptor Expression on NK Cell Cytotoxicity Against T Cells Are Associated With aGVHD After Allo-HSCT
As the CD107a degranulation assay is more feasible than the killing assay, we performed a CD107a degranulation assay to identify the cytotoxic effects of NK cells to activated T cells, for all PBSC donors.
We further investigated differences in NK degranulation against autologous activated T cells between CD56dim and CD56bright, and NKG2A+ and NKG2A− subsets. As shown (Figures 2A–C), the degranulation of CD56dim NK cells toward autologous activated T cells was stronger than the CD56bright subset, and NKG2A− NK cells were degranulated more potently than the NKG2A+ subgroup, suggesting that subgroup distribution patterns of donor NK cells influenced NK cytotoxicity against activated T cells.
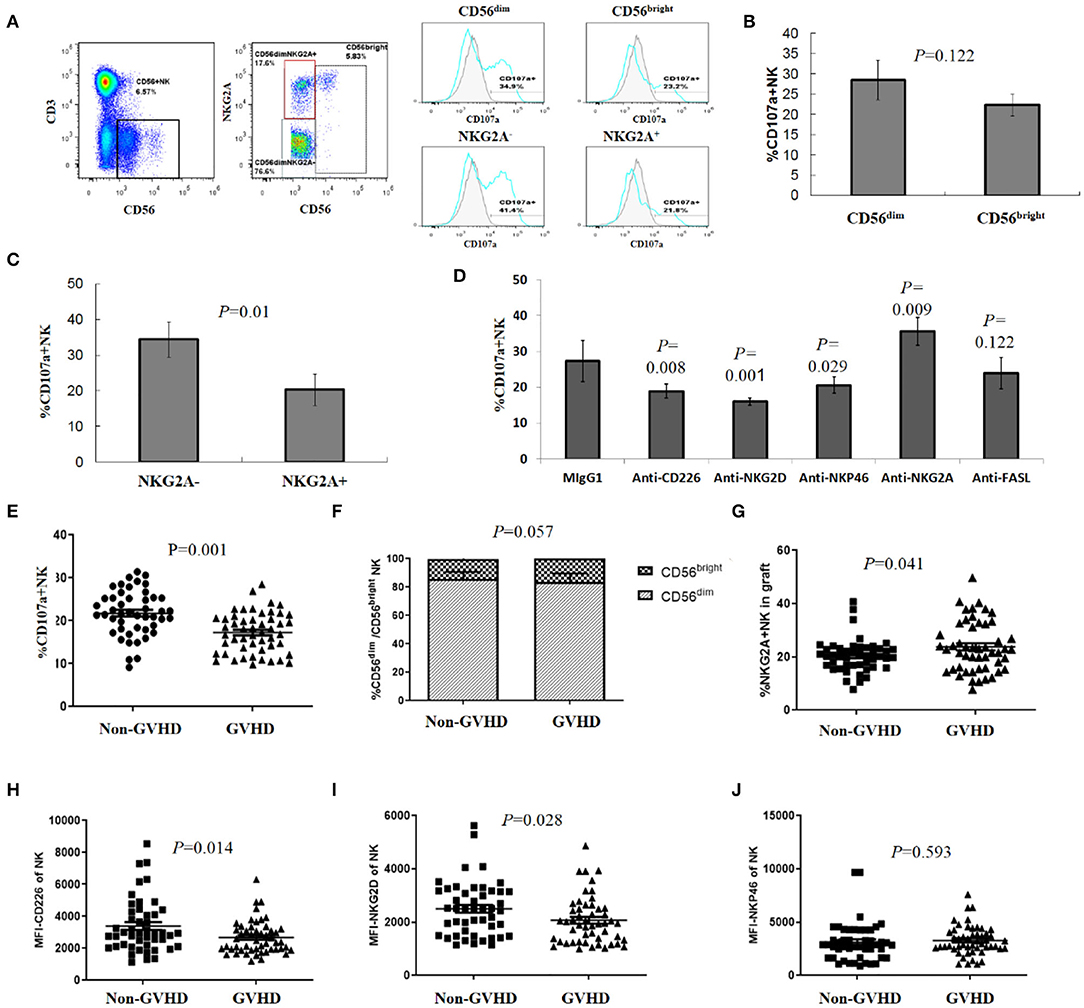
Figure 2. Subgroup and receptor expression of donor NK cells affected NK degranulation toward activated T cells associated with aGVHD. (A) Representative gating strategy. CD56dim and CD56bright NK cells were gated and subsets were defined based on the expression of NKG2A, the percentage of CD107a positive cells was analyzed on each subset of NK cells. (B) CD107a expression in CD56dim and CD56bright NK cells (n = 4), (C) CD107a expression in NKG2A− and NKG2A+ subgroups (n = 4), (D) NK cells were pretreated with neutralizing antibodies (or relevant isotype-matched Ig controls) before degranulation assay (n = 4). (E) Levels of donor NK degranulation toward activated T cells were significantly lower in the aGVHD group than in the non-aGVHD group (P = 0.001, n = 98). Percentage of CD56dim and CD56bright NK cells (F), NKG2A+ NK cells (G) in allografts from the aGVHD and non-aGVHD groups (n = 98). MFI of CD226 (H); NKG2D (I) and NKP46 (J) of NK cells in allografts from aGVHD and non-aGVHD groups (n = 98). All calculated averages were defined as the parametric mean ± SD. Student's t-tests or two-way ANOVA analyses were used to compare the mean among groups.
The cytotoxicity of NK cells is regulated by signal integration from a complex repertoire of activating and inhibiting receptors (14, 17, 18). According to the NK education and tolerance hypothesis (31–33), it is impossible for NK cells to kill auto-T cells by KIR-L mismatching. Therefore, we further analyzed the potential roles of NK activating receptors by blocking interactions between NK activating receptors and corresponding ligands with neutralizing antibodies, before the degranulation assay. We observed that blocking NKG2D, DNAM-1 (CD226), or NKP46 led to significant decreased degranulation (CD107a expression) of NK cells toward activated auto-T cells. Accordingly, we also found that the expression of NKG2D ligands (MICA/MICB, ULBP-1, ULBP-3) and DNAM-1 ligands (PVR) on T cell surface was up-regulated after activation (Figure S3). On the contrary, blocking the HLA-E–NKG2A interaction with an anti-NKG2A mAb resulted in increased degranulation (Figure 2D). These results suggested that activated receptors NKG2D, DNAM-1 (CD226), and NKP46 played important roles in triggering NK cell cytoxicity, while NKG2A, an inhibitory receptor of NK cells, played a negative role in NK cell cytotoxicity toward allo-reactive auto-T cells.
NK cells may kill target cells by means other than perforin-mediated cytotoxicity. As T cells could upregulated expression of Fas/FasL after activation and Fas/FasL pathway has been proved to participate NK cell-mediated cytotoxicity against tumor cells (34, 35), we addressed whether FAS/FAS-L pathway was implicated in NK cell killing of allo-reactive T cells. However, blocking FasL did not affect the degranulation and killing of NK cells to allo-antigen activated T cells (Figure 2D). NK degranulation varied between donors, with an average 17.26 ± 4.69% donor NK cells of the aGVHD group showing degranulation activity toward autologous activated T cells, when compared to 21.78 ± 5.26% NK cells in the non–aGVHD group (P = 0.001) (Figure 2E).
Furthermore, we evaluated NKG2A+ and NKG2A−, CD56dim and CD56bright subsets and receptor expression on CD56+ NK cells in patient allografts in aGVHD and non-aGVHD groups. When analyzing NKG2A expression, we observed that 23.8 ± 9.47% donor NK cells for aGVHD patients were positive for NKG2A, when compared with 20.42 ± 6.2% NK cells in the non-aGVHD group (Figure 2G, P = 0.041). The differences in CD56dim and CD56bright subset proportions between groups were not statistically significant (Figure 2F). After this, we analyzed the differences in NK activating receptors, CD226, NKG2D, and NKP46, which have been shown to enhance NK killing activity to activated T cells in vitro (29, 36), in allografts between aGVHD and non-aGVHD groups. We observed that the expression of DNAM-1 (CD226) and NKG2D in donor NK cells of the aGVHD group was higher than that of the non aGVHD group, while differences in NKP46 expression between the groups were not statistically significant (Figures 2H–J).
CD107a Expression (>20.5%) in Donor NK Cells Is an Independent Predictor of aGVHD
Using the receiver operating characteristic (ROC) curve, we selected a cut-off of 20.5% for CD107a expression in donor NK cells in the degranulation assay, which provided a sensitivity of 75% and a specificity of 64% for the prediction of aGVHD. Based on CD107a expression in donor NK cells to activated T cells, patients were divided into the CD107ahigh group (n = 54) and the CD107alow group (n = 44). When compared with the CD107alow group, patients in the CD107ahigh group showed lower incidences of overall aGVHD (29.6 vs. 70.42%, P = 0.0003, Figure 3A), grade II–IV aGVHD (18.2 vs. 59.3%, P = 0.0001, Figure 3B) and grade III–IV aGVHD (13.6 vs. 53.7%, P = 0.0007, Figure 3C).
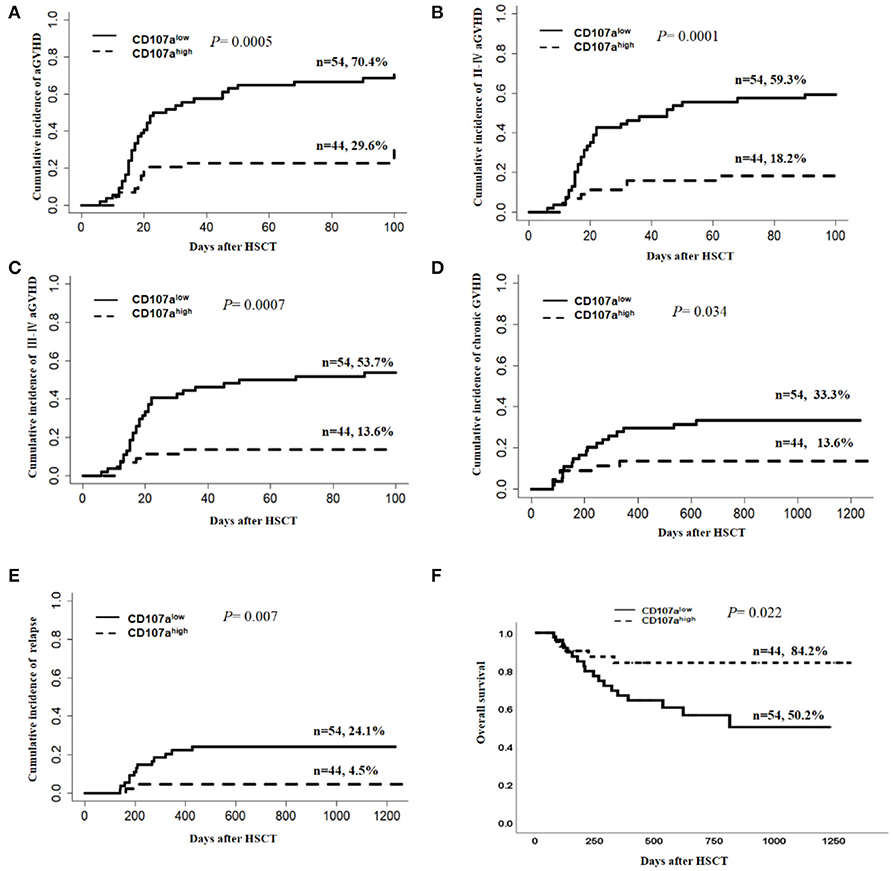
Figure 3. Donor NK CD107a degranulation toward activated T cells was predictive for risk of aGVHD, chronic GVHD, relapse, and overall survival. The Gray's test was applied for comparisons of cumulative incidences of acute GVHD, chronic GVHD, and relapse. Death, without aGVHD, was defined as the competing event for aGVHD, while relapse-free mortality was the competing event for relapse. Cumulative incidence estimates of grade I–IV aGVHD (A), gradeII–IV aGVHD (B), grade III–IV aGVHD (C), chronic GVHD (D), and relapse (E) or Kaplan–Meier survival estimates for overall survival (F) for patients in “CD107alow” and “CD107ahigh” groups, separated according to the optimal cutoff of 20.5% for donor NK CD107a degranulation toward activated T cells.
Considering the potential influence of the donor source and ATG use on the development of aGVHD, subgroup analysis was carried out. In relation to the donor source, the CD107ahigh group demonstrated a lower cumulative incidence of overall aGVHD than the CD107alow group when the donor was HLA-matched related (MRD) (5.6 vs. 73.7%; P = 0.0005; Figure 4A), but this effect was not seen in HLA-matched unrelated donors (MUD) (16.1 vs. 57.1%; P = 0.187; Figure 4B), and haplo-identical donors (55 vs. 71.4%; P = 0.207 Figure 4C). In 61 patients who received HLA-matched unrelated and HLA-haplo-identical related HSCT, additional ATG was used. The predictive value of CD107a expression in donor NK cells for overall aGVHD was not statistically significant when ATG was added(46.2% vs. 68.6%; P = 0.085 Figure 4D). Considering ATG was only used in HLA-matched unrelated and HLA-haplo-identical related HSCT in our study, we speculated that the main reasons why the predictive value of the donor NK CD107a degranulation towards activated T cells for overall aGVHD was not significant in MDR and haplo-identical HSCT might be that ATG weakened NK cell function (37) and that each subgroup had relatively small cases.
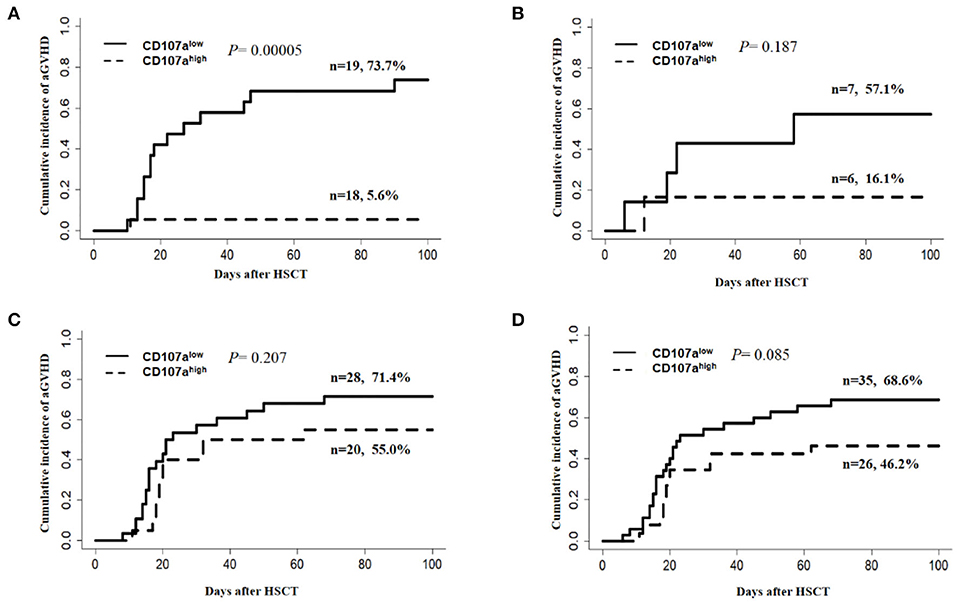
Figure 4. Subgroup analysis for predictive value of the donor NK CD107a degranulation toward activated T cells for grade I–IV aGVHD. (A) HLA-matched related HSCT(MRD), (B) HLA-matched unrelated HSCT(MUD), (C) HLA-haplo-identical related HSCT. (D) Patients with ATG for the prophylaxis of GVHD.
In univariate analyses, besides CD107a, other factors also predicted a reduced grade I–IV aGVHD risk, the dose of infused NK cells > 2.19 × 107/kg (HR = 0.551; P = 0.037), and median fluorescent intensity (MFI) of NKG2D on NK cells > 2491 in allografts (HR = 0.471; P = 0.015) (Table 2). Other factors predicting decreased grade III–IV aGVHD included, matched related donors vs. haplo-identical donors (HR = 0.504; P = 0.033), and the percentage of NKG2A+NK ≤ 25.5% in allografts (HR = 0.297; P = 0.008). In univariate analysis, the non-statistically significant factors for predicting aGVHD included the age and gender of recipients and donors, diagnosis, high risk disease before transplantation, the KIR-L mismatch between donors and recipients, additional usage of ATG for GVHD prophylaxis, the dose of CD34+ cells, CD3+T cells, the NK/T cell ratio, the CD56dim/CD56bright NK cell ratio, and DNAM-1 and NKP46 expression levels of NK cells in allografts.
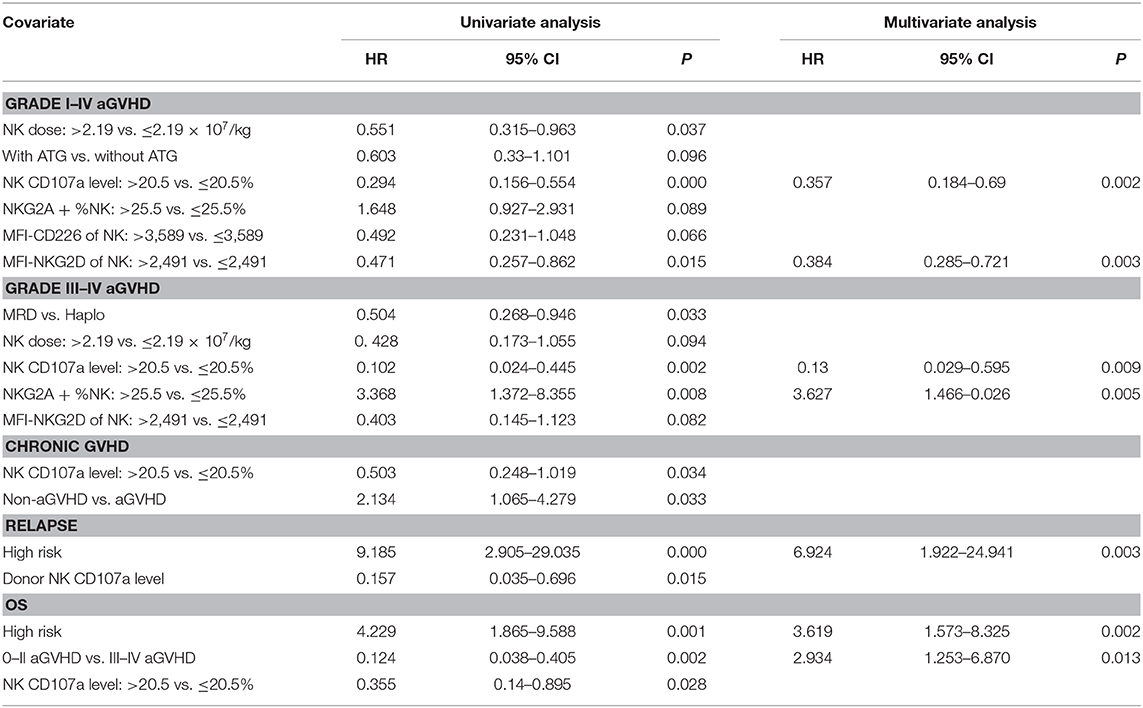
Table 2. Univariate and multivariable analysis of risk factors for clinical outcomes of allogeneic stem cell transplantation.
Multivariate Cox regression models were applied to evaluate the prognostic value of CD107a expression in donor NK cells in allografts. All variables used for the Cox model had a univariate p-value < 0.1. As shown (Table 2), CD107a expression in donor NK cells > 20.5%, was an independent predictor for the grade I–IV aGVHD (HR = 0.357; P = 0.002), and grade III–IV aGVHD (HR = 0.13; P = 0.009).
In univariate analysis, the CD107ahigh group demonstrated a lower cumulative incidence of cGVHD than the CD107alow group (13.6 vs. 33.3%; P = 0.034; Figure 3D). The cumulative incidence of relapse in the CD107ahigh group was lower than the CD107alow group (4.5 vs. 24.1%; P = 0.007; Figure 3E). There was no difference in the cumulative incidence of non-relapse mortality (NRM) between the CD107ahigh group and the CD107alow group (P = 0.46). The 2-year overall survival was 84.2% in the CD107ahigh group, while that of the CD107alow group was 50.2% (P = 0.022; Figure 3F). However, multivariate analyses showed that the predictive value of CD107a expression in donor NK cells for chronic GVHD, relapse and overall survival was not statistically significant (Table 2).
Discussion
There is growing evidence that NK cells have immunomodulatory functions and can inhibit the immune responses of T cells (34, 35, 38–43). Donor T cell activation is the core immunopathophysiology mechanism in acute graft vs. host disease. Studies have demonstrated that donor NK cells inhibit the proliferation of T cells and show cytotoxicity to activated T cells in a mouse aGVHD model (30, 44). However, the direct regulation of donor allo-reactive T cell responses by autogeneic NK cells in human GVHD has not been fully investigated. In this study, we demonstrated that NK cells negatively regulate T cells response to allo-DCs in humans, which was consistent with a previous report in a murine model (30). NK cytotoxicity against alloantigen activated T cells may suggest an important mechanism whereby NK cells regulate T cell allo-reactivity in human aGVHD.
The observation that NK cells are capable of regulating T cell allo-reactivity, which has been validated in in vitro studies and animal models, should be explored in clinical transplantation models. In this study, the relationship between the killing effects of donor NK cells to activated T cells and the incidence of aGVHD was explored in a group of allogeneic hematopoietic stem cell transplantation patients. We established a method to detect the cytotoxic functions of donor NK cells toward activated T cells, through CD107a degranulation analysis. Our study demonstrated that the cytotoxic effects of donor NK cells toward activated T cells was related to the occurrence and severity of aGVHD in human HSCT. We observed that the degranulation activity of donor NK cells in the non-aGVHD group was higher when compared to donor NK cells in the aGVHD group. Furthermore, the high degranulation activity of donor NK cells significantly decreased the rate of overall aGVHD, and the grade III–IV of aGVHD, when assessed by Cox multivariate regression analysis. These clinical findings help us understand animal models (30, 44), suggesting that donor NK cells could play a regulatory role in GVHD by inhibiting allo-reactive T cell immune through their cytotoxic functions against activated allo-reactive T cells.
As NK cells may serve as potentially GVHD regulatory cells, studies have sought to determine the predictive value of NK cells in human GVHD. NK cell concentrations in allograft procedures are important factors influencing GVHD incidence (45–49). Tanaka et al. reported that a high dose of infused NK cells was correlated with a lower incidence of grade III–IV aGVHD, particularly in recipients receiving unrelated bone marrow transplantation (49). However, in our transplant settings, although higher NK doses in grafts showed correlations with a lower incidence of overall aGVHD by univariate analysis, higher NK cell doses in allografts were not identified as independent predictors of aGVHD using multivariate analysis. We speculated on several possible factors that may have contributed to this inconsistency. Firstly, there were large differences in infused NK cell doses in different transplantation schemes, varying from 106 to 107/kg, and NK content in PBSC harvests was usually higher than bone marrow collections (45). Secondly, different conditioning-regimens and GVHD prevention schemes may have exerted different effects on NK functions (50–52). Finally, and most importantly, the statistical significance of NK cell doses were weakened after NK cytotoxic function was incorporated into the multivariate model.
Zhao et al. observed that a higher dose of CD56bright NK cells in allografts was associated with a higher incidence of grade II–IV aGVHD, while a higher CD56dim/CD56bright ratio dose in NK cells was correlated with a lower incidence of grade III–IV aGVHD, after haplo-identical transplantation without in vitro T-cell depletion (48). When analyzing the relationship between NK cell subsets and aGVHD, we found no significant correlations between the CD56dim/CD56bright ratio and aGVHD. Interestingly, we observed that a higher ratio of NKG2A+ NK in allografts was associated to a higher incidence of grade III–IV aGVHD. Equally, we showed that NKG2A was involved in the negative regulation of NK cell cytotoxicity against activated T cells in vitro, which was consistent with Nielsen et al. (36, 53). NKG2A, is an inhibitory receptor of NK cells which belongs to the C-type lectin superfamily, and is often overexpressed on the surface of reconstituted NK cells in the early stages after HSCT (54–56). Contrary to our results, Hu et al. reported that NKG2A+ subset cells were reduced in patients with aGVHD after allo-HSCT (54, 57). We speculated that the main reason for this inconsistency might be that Hu et al. studied the expression of NKG2A in reconstituted NK cells after transplantation, while we studied the expression of NKG2A on the surface of donor NK cells, while the phenotype and function of the NKG2A+ NK cells after allo-HSCT are different from those of healthy donors (55, 58).
Several mechanisms have been proposed to explain the complex crosstalk between NK cells and T cells during NK cell-mediated negative modulation of T cell immunity, including cytokine interactions, indirect effects by killing APCs, and the direct lysis of activated T cells (6, 28, 42, 59). This latter mechanism has been proposed as a direct mechanism used by NK cells (35, 40, 60), and several receptor-ligand pairs have been reported to manipulate NK cytotoxicity toward activated T cells, including NKG2D/NKG2D-L (25), DNAM-1/PVR (26), LFA/LFA-L (36), and NKP46/NKP46-L (29, 61). In accordance with previous reports (29, 36, 62), our results showed that NK cytolysis of allo-activated T cells depends on NKG2D, DNAM-1, and NKP46, as blocking of NKG2D, DNAM-1(CD226), or NKP46 led to significant reductions in degranulation of NK cells toward activated auto-T cells.
Several studies have demonstrated regulatory roles of NK cells in T cells responses in chronic viral infection (34), auto-immunity (63), transplantation (38, 64), and GVHD mouse models (30). Here, we specifically investigated NK-T cell crosstalk in a human GVHD setting. We have provided new insight into the role of NK cell “induced-self” recognition in aGVHD regulation. The triggering of NK cytotoxicity is tightly controlled by activating and inhibiting signals from NK cell receptors, the “missing-self” and “induced-self” recognition have been proposed to interpret the manner of NK activation (22, 65–67). The recognition of homologous HLA class I ligands by inhibitory KIR plays an important role in the education and self-tolerance of NK cells, which allows them to tolerate self-healthy cells with normal levels of HLA class I expression, but react to unhealthy cells with decreased HLA class I expression (68). When donor NK cells encounter autogenous allo-reactive T cells, the “missing-self” recognition model, which is triggered by KIR/KIR-ligand mismatch (20, 69), were prohibited as licensed NK cells expressing inhibitory KIR to combine with self HLA class I ligands on autogenous allo-reactive T cells (33, 70–72). It has been reported that activated T cells up-regulate ligands for NK cell activating receptors, and provide activating signal for autologous NK cells (28, 29, 60). When activating signals are strong enough to exceed the inhibitory signal from inhibitory KIR, the “induced-self” model of NK cell activation functions, and triggers cytotoxicity to eliminate redundant activated T cells, thus avoiding hyper T-cell activation and maintaining immune responses.
Donor allo-reactive T cells are an important factor leading to GVHD, and also a key compartment in exerting the graft-vs-leukemia (GVL) effect. Our concern is whether the negative regulatory effect of NK cells on allo-reactive T cells will affect GVL effect and increase disease relapse. In our study, we found that the cytotoxicity of NK cells on allo-reactive T cells did not affect the GVL effect. On the contrary, patients with higher NK degranulation activities toward allo-reactive T cells had a lower incidence of relapse, which was consistent with the results of previous studies that NK cells had the effect of separating GVHD from GVL (13, 73–75). However, the specific mechanism for donor NK cells separating GVL effect from GVHD is not clear. It is worth mentioning that NK cells themselves possess the powerful function of killing leukemia cells and prevent the relapse (76).
The present study had several limitations. First, the cohort of patients included in the study is heterogeneous as far as the donor source and ATG usage were concerned. Although we have conducted subgroup analysis, the limited number of cases may lead to the deviation of results, so we need to further validate the prognostic value of donor NK cell cytotoxicity toward allo-reactive T cells in a larger cohort of patients. Second, we did not find that donor NK cell cytotoxicity toward allo-reactive T cells was related to the severity of aGVHD, because there was no difference between the prognostic value of NK activity on the development of overall aGVHD and grade III–IV severe aGVHD, which may also be due to the limited number of cases and heterogeneous cohort. Third, other mechanisms for NK cells to regulate allo-reactive T cells, and the potential mechanism for NK cells to separate GVL from GVHD need to be explored in the future to provide further explanations for our findings.
In summary, donor NK cells inhibit and lyse allo-reactive T cells associated with aGVHD risk and severity, suggesting that NK cytotoxicity toward allo-activated T cells may play important roles in human aGVHD regulation. These findings may help us forecast aGVHD risks earlier by detecting donor NK cytotoxicity to allo-activated T cells, thus providing new targets for the prevention and treatment of aGVHD. However, it has been reported that NK cells reconstructed after allogeneic hematopoietic stem cell transplantation showed immature phenotypes and impaired functions (50, 58, 77, 78). Whether reconstructed donor NK can effectively regulate GVHD through cytotoxic function after allogeneic hematopoietic stem cell transplantation should be doubted, and more studies should be conducted to support this thesis.
Data Availability Statement
The datasets generated for this study are available on request to the corresponding author.
Ethics Statement
The studies involving human participants were reviewed and approved by The Clinical Ethics Review Committee at Ningbo First Hospital. Written informed consent to participate in this study was provided by the participants' legal guardian/next of kin. Written informed consent was obtained from the individual(s), and minor(s)' legal guardian/next of kin, for the publication of any potentially identifiable images or data included in this article.
Author Contributions
LS, QM, HZ, and GO: conceptualization. LS, SY and GO: data curation. LS, QM, SY, and GO: formal analysis. LS, QM, and GO: funding acquisition. LS, QM, HF, YH, and GO: investigation. LS, SY, HF, YH, KX, YoS, YaZ, PZ, MZ, BL, ZX, MG, JW, YL, and YiZ: methodology. LS, QM, HZ, YW, and GO: project administration. LS, SY, and JW: software. All authors contributed to the article and approved the submitted version.
Funding
This work was supported by grants from the National Nature Science Foundation of China (No. 81401321), the Basic Public Welfare Research Project of Zhejiang Province (No. LGF19H080002), and the Public Welfare Science and Technology Project of Ningbo (No. 2019C50068).
Conflict of Interest
The authors declare that the research was conducted in the absence of any commercial or financial relationships that could be construed as a potential conflict of interest.
Supplementary Material
The Supplementary Material for this article can be found online at: https://www.frontiersin.org/articles/10.3389/fimmu.2020.01534/full#supplementary-material
Figure S1. Lineage-specific analysis of chimerism in patients following allogeneic stem cell transplantation. The peripheral blood samples of 38 patients were collected at 2, 4, 8, and 12 weeks after transplantation, then NK and T cells were enriched by immunomagnetic separation. The chimerism of NK and T cells was detested by short tandem repeats-Polymerase chain reaction (STR-PCR). (A) Chimerism dynamics of donor NK cells, (B) Chimerism dynamics of donor T cells.
Figure S2. Cytokine and granzyme B secretion of NK cells. After co-culture of NK cells with the unstimulated T cells or activated T cells for 4 h, the cytokine IFN-γ, TNF-α, IL-10, and TGF-β level secreted by NK cells was detected by flow cytometry. Representative gating strategy (A) and statistical histogram of four independent experiments (B) were shown (n = 4). The granzyme B were quantified by ELISA in supernatants after co-culture of NK cells with the unstimulated T cells or activated T cells for 4 h (n = 4) (C).
Figure S3. Representative histograms for surface expression of ligands for NKG2D, DNAM-1, and NKG2A on activated and resting T cells.
References
1. Jang JE, Hwang DY, Chung H, Kim SJ, Eom JI, Jeung HK, et al. Early cytomegalovirus reactivation and expansion of CD56(bright)CD16(dim/-)DNAM1(+) natural killer cells are associated with antileukemia effect after haploidentical stem cell transplantation in acute leukemia. Biol Blood Marrow Transplant. (2019) 25:2070–8. doi: 10.1016/j.bbmt.2019.06.008
2. Della Chiesa M, Moretta L, Muccio L, Bertaina A, Moretta F, Locatelli F, et al. Haploidentical haematopoietic stem cell transplantation: role of NK cells and effect of cytomegalovirus infections. Curr Top Microbiol Immunol. (2016) 395:209–24. doi: 10.1007/82_2015_450
3. Jin F, Lin H, Gao S, Wang H, Yan H, Guo J, et al. Characterization of IFNgamma-producing natural killer cells induced by cytomegalovirus reactivation after haploidentical hematopoietic stem cell transplantation. Oncotarget. (2017) 8:51–63. doi: 10.18632/oncotarget.13916
4. Bjorklund AT, Clancy T, Goodridge JP, Beziat V, Schaffer M, Hovig E, et al. Naive donor NK cell repertoires associated with less leukemia relapse after allogeneic hematopoietic stem cell transplantation. J Immunol. (2016) 196:1400–11. doi: 10.4049/jimmunol.1501434
5. Ruggeri L, Capanni M, Casucci M, Volpi I, Tosti A, Perruccio K, et al. Role of natural killer cell alloreactivity in HLA-mismatched hematopoietic stem cell transplantation. Blood. (1999) 94:333–9. doi: 10.1182/blood.V94.1.333.413a31_333_339
6. Ruggeri L, Capanni M, Urbani E, Perruccio K, Shlomchik WD, Tosti A, et al. Effectiveness of donor natural killer cell alloreactivity in mismatched hematopoietic transplants. Science. (2002) 295:2097–100. doi: 10.1126/science.1068440
7. Leung W, Iyengar R, Triplett B, Turner V, Behm FG, Holladay MS, et al. Comparison of killer Ig-like receptor genotyping and phenotyping for selection of allogeneic blood stem cell donors. J Immunol. (2005) 174:6540–5. doi: 10.4049/jimmunol.174.10.6540
8. Bishara A, De Santis D, Witt CC, Brautbar C, Christiansen FT Or R. The beneficial role of inhibitory KIR genes of HLA class I NK epitopes in haploidentically mismatched stem cell allografts may be masked by residual donor-alloreactive T cells causing GVHD. Tissue Antigens. (2004) 63:204–11. doi: 10.1111/j.0001-2815.2004.00182.x
9. Huang XJ, Zhao XY, Liu DH, Liu KY, Xu LP. Deleterious effects of KIR ligand incompatibility on clinical outcomes in haploidentical hematopoietic stem cell transplantation without in vitro T-cell depletion. Leukemia. (2007) 21:848–51. doi: 10.1038/sj.leu.2404566
10. Davies SM, Ruggieri L, DeFor T, Wagner JE, Weisdorf DJ, Miller JS, et al. Evaluation of KIR ligand incompatibility in mismatched unrelated donor hematopoietic transplants. Killer immunoglobulin-like receptor. Blood. (2002) 100:3825–7. doi: 10.1182/blood-2002-04-1197
11. Lowe EJ, Turner V, Handgretinger R, Horwitz EM, Benaim E, Hale GA, et al. T-cell alloreactivity dominates natural killer cell alloreactivity in minimally T-cell-depleted HLA-non-identical paediatric bone marrow transplantation. Br J Haematol. (2003) 123:323–6. doi: 10.1046/j.1365-2141.2003.04604.x
12. Farag SS, Bacigalupo A, Eapen M, Hurley C, Dupont B, Caligiuri MA, et al. The effect of KIR ligand incompatibility on the outcome of unrelated donor transplantation: a report from the center for international blood and marrow transplant research, the European blood and marrow transplant registry, and the Dutch registry. Biol Blood Marrow Transplant. (2006) 12:876–84. doi: 10.1016/j.bbmt.2006.05.007
13. Simonetta F, Alvarez M, Negrin RS. Natural killer cells in graft-versus-host-disease after allogeneic hematopoietic cell transplantation. Front Immunol. (2017) 8:465. doi: 10.3389/fimmu.2017.00465
14. Kumar S. Natural killer cell cytotoxicity and its regulation by inhibitory receptors. Immunology. (2018) 154:383–93. doi: 10.1111/imm.12921
15. Long EO, Kim HS, Liu D, Peterson ME, Rajagopalan S. Controlling natural killer cell responses: integration of signals for activation and inhibition. Annu Rev Immunol. (2013) 31:227–58. doi: 10.1146/annurev-immunol-020711-075005
16. Alari-Pahissa E, Grandclement C, Jeevan-Raj B, Held W. Inhibitory receptor-mediated regulation of natural killer cells. Crit Rev Immunol. (2014) 34:455–65. doi: 10.1615/CritRevImmunol.2014012220
17. Martinet L, Smyth MJ. Balancing natural killer cell activation through paired receptors. Nat Rev Immunol. (2015) 15:243–54. doi: 10.1038/nri3799
18. Paul S, Lal G. The molecular mechanism of natural killer cells function and its importance in cancer immunotherapy. Front Immunol. (2017) 8:1124. doi: 10.3389/fimmu.2017.01124
19. Handgretinger R, Lang P, Andre MC. Exploitation of natural killer cells for the treatment of acute leukemia. Blood. (2016) 127:3341–9. doi: 10.1182/blood-2015-12-629055
20. Velardi A, Ruggeri L, Mancusi A, Aversa F, Christiansen FT. Natural killer cell allorecognition of missing self in allogeneic hematopoietic transplantation: a tool for immunotherapy of leukemia. Curr Opin Immunol. (2009) 21:525–30. doi: 10.1016/j.coi.2009.07.015
21. Fine JH, Chen P, Mesci A, Allan DS, Gasser S, Raulet DH, et al. Chemotherapy-induced genotoxic stress promotes sensitivity to natural killer cell cytotoxicity by enabling missing-self recognition. Cancer Res. (2010) 70:7102–13. doi: 10.1158/0008-5472.CAN-10-1316
22. Diefenbach A, Raulet DH. Strategies for target cell recognition by natural killer cells. Immunol Rev. (2001) 181:170–84. doi: 10.1034/j.1600-065X.2001.1810114.x
23. Babic M, Romagnani C. The role of natural killer group 2, member D in chronic inflammation and autoimmunity. Front Immunol. (2018) 9:1219. doi: 10.3389/fimmu.2018.01219
24. Kellner C, Gramatzki M, Peipp M. Promoting natural killer cell functions by recombinant immunoligands mimicking an induced self phenotype. Oncoimmunology. (2013) 2:e24481. doi: 10.4161/onci.24481
25. Cerboni C, Zingoni A, Cippitelli M, Piccoli M, Frati L, Santoni A. Antigen-activated human T lymphocytes express cell-surface NKG2D ligands via an ATM/ATR-dependent mechanism and become susceptible to autologous NK- cell lysis. Blood. (2007) 110:606–15. doi: 10.1182/blood-2006-10-052720
26. Ardolino M, Zingoni A, Cerboni C, Cecere F, Soriani A, Iannitto ML, et al. DNAM-1 ligand expression on Ag-stimulated T lymphocytes is mediated by ROS-dependent activation of DNA-damage response: relevance for NK-T cell interaction. Blood. (2011) 117:4778–86. doi: 10.1182/blood-2010-08-300954
27. Olson JA, Zeiser R, Beilhack A, Goldman JJ, Negrin RS. Tissue-specific homing and expansion of donor NK cells in allogeneic bone marrow transplantation. J Immunol. (2009) 183:3219–28. doi: 10.4049/jimmunol.0804268
28. Pallmer K, Oxenius A. Recognition and regulation of T Cells by NK Cells. Front Immunol. (2016) 7:251. doi: 10.3389/fimmu.2016.00251
29. Zingoni A, Ardolino M, Santoni A, Cerboni C. NKG2D and DNAM-1 activating receptors and their ligands in NK-T cell interactions: role in the NK cell-mediated negative regulation of T cell responses. Front Immunol. (2012) 3:408. doi: 10.3389/fimmu.2012.00408
30. Olson JA, Leveson-Gower DB, Gill S, Baker J, Beilhack A, Negrin RS. NK cells mediate reduction of GVHD by inhibiting activated, alloreactive T cells while retaining GVT effects. Blood. (2010) 115:4293–301. doi: 10.1182/blood-2009-05-222190
31. Boudreau JE, Hsu KC. Natural killer cell education and the response to infection and cancer therapy: stay tuned. Trends Immunol. (2018) 39:222–39. doi: 10.1016/j.it.2017.12.001
32. Boudreau JE, Hsu KC. Natural killer cell education in human health and disease. Curr Opin Immunol. (2018) 50:102–11. doi: 10.1016/j.coi.2017.11.003
33. Haas P, Loiseau P, Tamouza R, Cayuela JM, Moins-Teisserenc H, Busson M, et al. NK-cell education is shaped by donor HLA genotype after unrelated allogeneic hematopoietic stem cell transplantation. Blood. (2011) 117:1021–9. doi: 10.1182/blood-2010-02-269381
34. Waggoner SN, Cornberg M, Selin LK, Welsh RM. Natural killer cells act as rheostats modulating antiviral T cells. Nature. (2011) 481:394–8. doi: 10.1038/nature10624
35. Biron CA. Yet another role for natural killer cells: cytotoxicity in immune regulation and viral persistence. Proc Natl Acad Sci USA. (2012) 109:1814–5. doi: 10.1073/pnas.1120528109
36. Nielsen N, Odum N, Urso B, Lanier LL, Spee P. Cytotoxicity of CD56(bright) NK cells towards autologous activated CD4+ T cells is mediated through NKG2D, LFA-1 and TRAIL and dampened via CD94/NKG2A. PLoS ONE. (2012) 7:e31959. doi: 10.1371/journal.pone.0031959
37. Muller C, Schernthaner G, Kovarik J, Kalinowska W, Zielinski CC. Natural killer cell activity and antibody-dependent cellular cytotoxicity in patients under various immunosuppressive regimens. Clin Immunol Immunopathol. (1987) 44:12–9. doi: 10.1016/0090-1229(87)90047-X
38. Zecher D, Li Q, Oberbarnscheidt MH, Demetris AJ, Shlomchik WD, Rothstein DM, et al. NK cells delay allograft rejection in lymphopenic hosts by downregulating the homeostatic proliferation of CD8+ T cells. J Immunol. (2010) 184:6649–57. doi: 10.4049/jimmunol.0903729
39. Leavenworth JW, Wang X, Wenander CS, Spee P, Cantor H. Mobilization of natural killer cells inhibits development of collagen-induced arthritis. Proc Natl Acad Sci USA. (2011) 108:14584–9. doi: 10.1073/pnas.1112188108
40. Soderquest K, Walzer T, Zafirova B, Klavinskis LS, Polic B, Vivier E, et al. Cutting edge: CD8+ T cell priming in the absence of NK cells leads to enhanced memory responses. J Immunol. (2011) 186:3304–8. doi: 10.4049/jimmunol.1190018
41. Lang PA, Lang KS, Xu HC, Grusdat M, Parish IA, Recher M, et al. Natural killer cell activation enhances immune pathology and promotes chronic infection by limiting CD8+ T-cell immunity. Proc Natl Acad Sci USA. (2012) 109:1210–5. doi: 10.1073/pnas.1118834109
42. Crome SQ, Lang PA, Lang KS, Ohashi PS. Natural killer cells regulate diverse T cell responses. Trends Immunol. (2013) 34:342–9. doi: 10.1016/j.it.2013.03.002
43. Fu B, Li X, Sun R, Tong X, Ling B, Tian Z, et al. Natural killer cells promote immune tolerance by regulating inflammatory TH17 cells at the human maternal-fetal interface. Proc Natl Acad Sci USA. (2013) 110:E231–40. doi: 10.1073/pnas.1206322110
44. Huber CM, Doisne JM, Colucci F. IL-12/15/18-preactivated NK cells suppress GvHD in a mouse model of mismatched hematopoietic cell transplantation. Eur J Immunol. (2015) 45:1727–35. doi: 10.1002/eji.201445200
45. Pascal V, Brunet C, Pradel V, Thirion X, Andre P, Faucher C, et al. Analysis of donor NK and T cells infused in patients undergoing MHC-matched allogeneic hematopoietic transplantation. Leukemia. (2002) 16:2259–66. doi: 10.1038/sj.leu.2402670
46. Kim DH, Sohn SK, Lee NY, Baek JH, Kim JG, Won DI, et al. Transplantation with higher dose of natural killer cells associated with better outcomes in terms of non-relapse mortality and infectious events after allogeneic peripheral blood stem cell transplantation from HLA-matched sibling donors. Eur J Haematol. (2005) 75:299–308. doi: 10.1111/j.1600-0609.2005.00514.x
47. Larghero J, Rocha V, Porcher R, Filion A, Ternaux B, Lacassagne MN, et al. Association of bone marrow natural killer cell dose with neutrophil recovery and chronic graft-versus-host disease after HLA identical sibling bone marrow transplants. Br J Haematol. (2007) 138:101–9. doi: 10.1111/j.1365-2141.2007.06623.x
48. Zhao XY, Chang YJ, Xu LP, Liu DH, Liu KY, Huang XJ. Association of natural killer cells in allografts with transplant outcomes in patients receiving G-CSF-mobilized PBSC grafts and G-CSF-primed BM grafts from HLA-haploidentical donors. Bone Marrow Transplant. (2009) 44:721–8. doi: 10.1038/bmt.2009.73
49. Tanaka M, Kobayashi S, Numata A, Tachibana T, Takasaki H, Maruta A, et al. The impact of the dose of natural killer cells in the graft on severe acute graft-versus-host disease after unrelated bone marrow transplantation. Leuk Res. (2012) 36:699–703. doi: 10.1016/j.leukres.2011.11.009
50. Russo A, Oliveira G, Berglund S, Greco R, Gambacorta V, Cieri N, et al. NK cell recovery after haploidentical HSCT with posttransplant cyclophosphamide: dynamics and clinical implications. Blood. (2018) 131:247–62. doi: 10.1182/blood-2017-05-780668
51. Benjamin JE, Gill S, Negrin RS. Biology and clinical effects of natural killer cells in allogeneic transplantation. Curr Opin Oncol. (2010) 22:130–7. doi: 10.1097/CCO.0b013e328335a559
52. Vacher-Coponat H, Brunet C, Moal V, Loundou A, Bonnet E, Lyonnet L, et al. Tacrolimus/mycophenolate mofetil improved natural killer lymphocyte reconstitution one year after kidney transplant by reference to cyclosporine/azathioprine. Transplantation. (2006) 82:558–66. doi: 10.1097/01.tp.0000229390.01369.4a
53. Lu L, Ikizawa K, Hu D, Werneck MB, Wucherpfennig KW, Cantor H. Regulation of activated CD4+ T cells by NK cells via the Qa-1-NKG2A inhibitory pathway. Immunity. (2007) 26:593–604. doi: 10.1016/j.immuni.2007.03.017
54. Hu LJ, Zhao XY, Yu XX, Lv M, Han TT, Han W, et al. Quantity and quality reconstitution of NKG2A(+) natural killer cells are associated with graft-versus-host disease after allogeneic hematopoietic cell transplantation. Biol Blood Marrow Transplant. (2019) 25:1–11. doi: 10.1016/j.bbmt.2018.08.008
55. Roberto A, Di Vito C, Zaghi E, Mazza EMC, Capucetti A, Calvi M, et al. The early expansion of anergic NKG2Apos/CD56dim/CD16neg natural killer cells represents a therapeutic target in haploidentical haematopoietic stem cell transplantation. Haematologica. (2018) 103:1390–402. doi: 10.3324/haematol.2017.186619
56. Nguyen S, Dhedin N, Vernant JP, Kuentz M, Al Jijakli A, Rouas-Freiss N, et al. NK-cell reconstitution after haploidentical hematopoietic stem-cell transplantations: immaturity of NK cells and inhibitory effect of NKG2A override GvL effect. Blood. (2005) 105:4135–42. doi: 10.1182/blood-2004-10-4113
57. Jaiswal SR, Bhakuni P, Bhagwati G, Aiyar HM, Chakrabarti A, Chakrabarti S. Alterations in NKG2A and NKG2C subsets of natural killer cells following Epstein-Barr virus reactivation in CTLA4Ig-based haploidentical transplantation is associated with increased chronic graft-versus-host disease. Transplantation. (2020) 104:e23–30. doi: 10.1097/TP.0000000000002941
58. Ullah MA, Hill GR, Tey SK. Functional reconstitution of natural killer cells in allogeneic hematopoietic stem cell transplantation. Front Immunol. (2016) 7:144. doi: 10.3389/fimmu.2016.00144
59. Schuster IS, Coudert JD, Andoniou CE, Degli-Esposti MA. “Natural regulators”: NK cells as modulators of T cell immunity. Front Immunol. (2016) 7:235. doi: 10.3389/fimmu.2016.00235
60. Peppa D, Gill US, Reynolds G, Easom NJ, Pallett LJ, Schurich A, et al. Up-regulation of a death receptor renders antiviral T cells susceptible to NK cell-mediated deletion. J Exp Med. (2013) 210:99–114. doi: 10.1084/jem.20121172
61. Narni-Mancinelli E, Jaeger BN, Bernat C, Fenis A, Kung S, De Gassart A, et al. Tuning of natural killer cell reactivity by NKp46 and Helios calibrates T cell responses. Science. (2012) 335:344–8. doi: 10.1126/science.1215621
62. Gross CC, Schulte-Mecklenbeck A, Runzi A, Kuhlmann T, Posevitz-Fejfar A, Schwab N, et al. Impaired NK-mediated regulation of T-cell activity in multiple sclerosis is reconstituted by IL-2 receptor modulation. Proc Natl Acad Sci USA. (2016) 113:E2973–82. doi: 10.1073/pnas.1524924113
63. Jiang W, Chai NR, Maric D, Bielekova B. Unexpected role for granzyme K in CD56bright NK cell-mediated immunoregulation of multiple sclerosis. J Immunol. (2011) 187:781–90. doi: 10.4049/jimmunol.1100789
64. McNerney ME, Lee KM, Zhou P, Molinero L, Mashayekhi M, Guzior D, et al. Role of natural killer cell subsets in cardiac allograft rejection. Am J Transplant. (2006) 6:505–13. doi: 10.1111/j.1600-6143.2005.01226.x
65. Gasser S, Raulet DH. Activation and self-tolerance of natural killer cells. Immunol Rev. (2006) 214:130–42. doi: 10.1111/j.1600-065X.2006.00460.x
66. Raulet DH. Missing self recognition and self tolerance of natural killer (NK) cells. Semin Immunol. (2006) 18:145–50. doi: 10.1016/j.smim.2006.03.003
67. Malmberg KJ, Carlsten M, Bjorklund A, Sohlberg E, Bryceson YT, Ljunggren HG. Natural killer cell-mediated immunosurveillance of human cancer. Semin Immunol. (2017) 31:20–9. doi: 10.1016/j.smim.2017.08.002
68. Cooley S, Parham P, Miller JS. Strategies to activate NK cells to prevent relapse and induce remission following hematopoietic stem cell transplantation. Blood. (2018) 131:1053–62. doi: 10.1182/blood-2017-08-752170
69. Ruggeri L, Aversa F, Martelli MF, Velardi A. Allogeneic hematopoietic transplantation and natural killer cell recognition of missing self. Immunol Rev. (2006) 214:202–18. doi: 10.1111/j.1600-065X.2006.00455.x
70. Nowak J, Koscinska K, Mika-Witkowska R, Rogatko-Koros M, Mizia S, Jaskula E, et al. Donor NK cell licensing in control of malignancy in hematopoietic stem cell transplant recipients. Am J Hematol. (2014) 89:E176–83. doi: 10.1002/ajh.23802
71. Kim S, Poursine-Laurent J, Truscott SM, Lybarger L, Song YJ, Yang L, et al. Licensing of natural killer cells by host major histocompatibility complex class I molecules. Nature. (2005) 436:709–13. doi: 10.1038/nature03847
72. Yokoyama WM, Kim S. Licensing of natural killer cells by self-major histocompatibility complex class I. Immunol Rev. (2006) 214:143–54. doi: 10.1111/j.1600-065X.2006.00458.x
73. Song Y, Hu B, Liu Y, Jin Z, Zhang Y, Lin D, et al. IL-12/IL-18-preactivated donor NK cells enhance GVL effects and mitigate GvHD after allogeneic hematopoietic stem cell transplantation. Eur J Immunol. (2018) 48:670–82. doi: 10.1002/eji.201747177
74. Pegram HJ, Ritchie DS, Smyth MJ, Wiernik A, Prince HM, Darcy PK, et al. Alloreactive natural killer cells in hematopoietic stem cell transplantation. Leuk Res. (2011) 35:14–21. doi: 10.1016/j.leukres.2010.07.030
75. Ruggeri L, Mancusi A, Burchielli E, Aversa F, Martelli MF, Velardi A. Natural killer cell alloreactivity and haplo-identical hematopoietic transplantation. Cytotherapy. (2006) 8:554–8. doi: 10.1080/14653240601078721
76. Falco M, Pende D, Munari E, Vacca P, Mingari MC, Moretta L. Natural killer cells: from surface receptors to the cure of high-risk leukemia (Ceppellini Lecture). HLA. (2019) 93:185–94. doi: 10.1111/tan.13509
77. Simonetta F, Pradier A, Bosshard C, Masouridi-Levrat S, Chalandon Y, Roosnek E. NK cell functional impairment after allogeneic hematopoietic stem cell transplantation is associated with reduced levels of T-bet and eomesodermin. J Immunol. (2015) 195:4712–20. doi: 10.4049/jimmunol.1501522
Keywords: natural killer cells, cytotoxicity, CD107a, graft vs. host disease, allogeneic hematopoietic stem cell transplantation
Citation: Sheng L, Mu Q, Wu X, Yang S, Zhu H, Wang J, Lai Y, Wu H, Sun Y, Hu Y, Fu H, Wang Y, Xu K, Sun Y, Zhang Y, Zhang P, Zhou M, Lai B, Xu Z, Gao M, Zhang Y and Ouyang G (2020) Cytotoxicity of Donor Natural Killer Cells to Allo-Reactive T Cells Are Related With Acute Graft-vs.-Host-Disease Following Allogeneic Stem Cell Transplantation. Front. Immunol. 11:1534. doi: 10.3389/fimmu.2020.01534
Received: 13 March 2020; Accepted: 10 June 2020;
Published: 31 July 2020.
Edited by:
Jacopo Peccatori, San Raffaele Hospital (IRCCS), ItalyReviewed by:
Ismael Buño, Instituto de Investigación Sanitaria Gregorio Marañón, SpainConstanca Figueiredo, Hannover Medical School, Germany
Copyright © 2020 Sheng, Mu, Wu, Yang, Zhu, Wang, Lai, Wu, Sun, Hu, Fu, Wang, Xu, Sun, Zhang, Zhang, Zhou, Lai, Xu, Gao, Zhang and Ouyang. This is an open-access article distributed under the terms of the Creative Commons Attribution License (CC BY). The use, distribution or reproduction in other forums is permitted, provided the original author(s) and the copyright owner(s) are credited and that the original publication in this journal is cited, in accordance with accepted academic practice. No use, distribution or reproduction is permitted which does not comply with these terms.
*Correspondence: Guifang Ouyang, b3V5YW5nZ3VpZmFuZ0BtZWRtYWlsLmNvbS5jbg==