- Department of Pharmaceutical and Medicinal Chemistry, Institute of Pharmacy, Friedrich Schiller University, Jena, Germany
Rheumatoid arthritis, asthma, allergic rhinitis and many other disorders related to an aberrant immune response have a higher incidence and severity in women than in men. Emerging evidences from scientific studies indicate that the activity of the immune system is superior in females and that androgens may act as “immunosuppressive” molecules with inhibitory effects on inflammatory reactions. Among the multiple factors that contribute to the inflammatory response, lipid mediators (LM), produced from polyunsaturated fatty acids, represent a class of bioactive small molecules with pivotal roles in the onset, maintenance and resolution of inflammation. LM encompass pro-inflammatory eicosanoids and specialized pro-resolving mediators (SPM) that coexist in a tightly regulated balance necessary for the return to homeostasis. Innate immune cells including neutrophils, monocytes and macrophages possess high capacities to generate distinct LM. In the last decades it became more and more evident that sex represents an important variable in the regulation of inflammation where sex hormones play crucial roles. Recent findings showed that the biosynthesis of inflammation-related LM is sex-biased and that androgens impact LM formation with consequences not only for pathophysiology but also for pharmacotherapy. Here, we review the modulation of the inflammatory response by sex and androgens with a specific focus on LM pathways. In particular, we highlight the impact of androgens on the biosynthetic pathway of inflammation-related eicosanoids in innate immune cells.
Introduction
Inflammation is a protective response mounted by the host immune system against invading pathogens, foreign bodies, or injuries. It is a highly coordinated, active process in order to remove the harmful stimulus and to repair damaged tissues for reestablishing homeostasis. But if an acute inflammatory response fails to resolve, it may contribute to organ pathologies and promote many widespread chronic inflammatory clinical phenotypes such as arthritis, neurodegenerative diseases, asthma, allergy, diabetes, organ fibrosis, cancers, and various cardiovascular disorders (1). Among the plethora of mediators that regulate inflammation in a spatial and temporal fashion, lipid mediators (LM) are crucial molecules that can act at the four major stages of the inflammatory process: initiation, maintenance, resolution, and the return to homeostasis (2, 3). LM encompass a huge class of highly bioactive oxygenated polyunsaturated fatty acids (PUFAs) that play broad and major roles in health and diseases. Here, we refer to LM that are biosynthesized on demand mainly from the ω-6 PUFA arachidonic acid (AA), and the ω-3 PUFAs eicosapentaenoic acid (EPA) and docosahexaenoic acid (DHA) via initial conversion by cyclooxygenases (COX), lipoxygenases (LOX) and monooxygenases (i.e., CYP450 enzymes) (4, 5). These enzymes may partially act in conjunction, and can be coupled to epoxide hydrolases, glutathione transferase and other enzymes to produce defined LM with specific biological functions by acting at select G protein-coupled receptors (GPCR) or nuclear receptors (6, 7). Whereas, most of the AA-derived prostaglandins (PG) and leukotrienes (LT) formed by the COX and 5-lipoxygenase (5-LOX) pathway, respectively, exhibit rather pro-inflammatory properties (3, 8), the so-called specialized pro-resolving mediators (SPM) including lipoxins (LX), protectins (PD), resolvins (Rv), and maresins (MaR) are generated from AA, EPA and DHA with CYPs and 12/15-LOX as key enzymes and promote resolution of inflammation (2, 9).
The responses of the innate and adaptive immune system to foreign and self-antigens differ between females and males, where both genes (on X- and Y-chromosomes) and sex hormones are involved (10, 11). Thus, the age is an important variable where certain immune responses dominate in boys vs. girls before puberty with an opposite bias in adults where the immune system is more pronounced in females (11, 12). Most sex-based immunological differences are obvious after puberty and before reproductive senescence, indicating a crucial impact of sex hormones. These sex differences contribute to divergences in the incidence of autoimmune diseases and other inflammation-related malignancies with implications for both disease pattern and therapy (11). The sex steroids are known to have direct effects on the immune response. They comprise estrogens, progesterone and androgens and are mainly biosynthesized by the gonads of adult females and males. Estrogens (i.e., estrone, estriol, and the most bioactive estradiol) are produced by ovarian granulosa cells in females by the action of the aromatase complex from androstenedione or testosterone. Effects of estrogens on the immune system have often been connected to a pro-inflammatory phenotype (13) although their “stimulatory” action on macrophages results into promotion of the resolution of inflammation (14). Progesterone, besides its well-known essential activity in pregnancy, has been reported to have anti-inflammatory properties by influencing the immune system (15). More recent findings showed that progesterone suppresses LT biosynthesis in human monocytes by inhibition of 5-LOX activity (16). Androgens exert several inhibitory effects on immune cell activity with predominantly anti-inflammatory properties (17). Thus, the androgens testosterone and 5α-dihydrotestosterone (5α-DHT) are highly potent sex hormones that affect a variety of innate and adaptive immune responses with suppressive effects on macrophages, neutrophils, natural killer (NK) cells and T cells (12, 17, 18). For example, testosterone reduces inflammatory reactions such as toll-like receptor (TLR)4 expression on neutrophils, the biosynthesis of TNFα and iNOS-derived NO in macrophages, and NK cell activity (i.e., interferon (INF)γ secretion). On the other hand, androgens can promote anti-inflammatory processes such as expression of peroxisome proliferator-activated receptor (PPAR)α in T cells as well as IL-10 and transforming growth factor (TGF)β formation (11, 18).
Sex differences in PG formation were reported in 1972, where male human subjects were found to excrete larger amounts of the major urinary metabolite 7α-hydroxy-5, 11-diketotetranor-prostane-1, 16-dioic acid of PGE1/2 than female subjects (19). Later, in 1974, androgens were found to modulate PG formation (20, 21). In 2008, a striking suppressive potential of androgens on LT formation in human neutrophils was discovered that is causative for lower LT biosynthesis in male vs. female cells (22). Subsequent intensive research in humans and rodents has accumulated substantial knowledge on the impact of androgens on LM biology, and how this translates into modulation of inflammation, and how it affects anti-inflammatory therapy (23). Here we review the effects of androgens on pro-inflammatory LM biosynthesis and we discuss the role of LM in the interplay between androgen functions in innate immune cells with apparent consequences for the incidence and severity of inflammation.
Lipid Mediators: Biosynthesis Pathways and Their Role in Inflammation
Innate immune cells including granulocytes, monocytes and macrophages possess high capacities to generate pro-inflammatory but also inflammation-resolving LM. Because of their potent bioactivities and broad impact on multiple functions of the body, LM are not stored in cells or tissues at large amounts but are rather de novo-synthesized on demand. The first step in the biosynthesis of LM is the liberation of the fatty acid substrate mainly from membrane phospholipids by phospholipase A2 (PLA2) enzymes (8, 24). Thus, modulation of PLA2 and concomitant fatty acid release as LM substrate may be subject of regulation by androgens and/or sex. However, only little is known on androgens affecting PLA2 (25, 26) and how this would translate into significant biological effects or sex differences connected to LM.
PGs and thromboxanes (TX)s are generated by COX-1/2 in a two-step conversion of liberated AA which leads to the formation of the instable intermediates PGG2 and then PGH2, where the latter is transformed by tissue-specific prostanoid synthases into the different bioactive prostanoids PGD2, PGE2, PGF2α, PGI2, and TXA2 (Figure 1). Two isoforms of COX exist with COX-1 being constitutively expressed in many different cell types, and the inducible COX-2, which is abundantly expressed in pro-inflammatory innate immune cells including monocytes, neutrophils and M1-like macrophages after exposure to inflammatory stimuli (e.g., cytokines, bacterial components or hormones). PGE2 mediates inflammation-related processes such as vasodilatation and increased microvascular permeability, as well as fever, and pain (27). It is synthesized from PGH2 by cytosolic PGE synthase (cPGES) or microsomal PGE synthases (mPGES-1 and mPGES-2), with mPGES-1 being an inducible isoform with high relevance for producing PGE2 during inflammation (28).
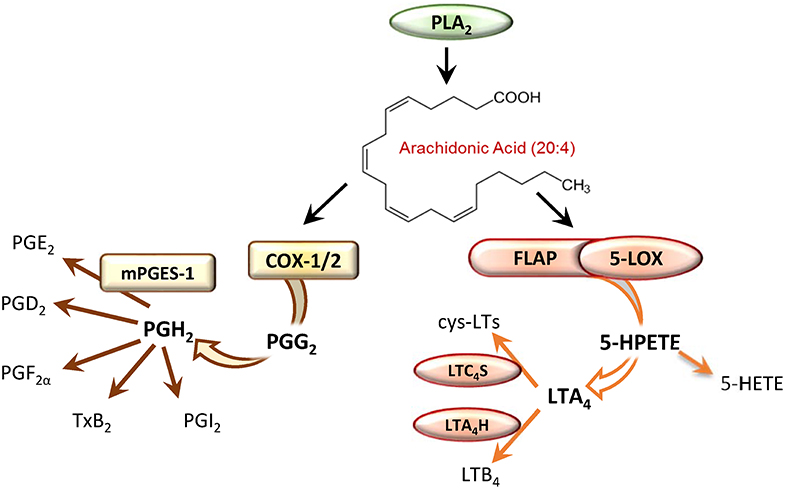
Figure 1. Enzymatic conversion of arachidonic acid by the cyclooxygenase (COX) or the 5-lipoxygenase (5-LOX) pathway leads to formation of prostaglandins (PG) or leukotrienes (LT), respectively.
LTs have significant impact on inflammatory and immune-related diseases like asthma and allergic rhinitis, autoimmune diseases, atherosclerosis, cardiovascular diseases and cancer (29). The ability to generate LTs is mainly restricted to innate immune cells such as neutrophils, eosinophils, monocytes, macrophages and mast cells that express the required biosynthetic enzymes. For the biosynthesis of LTs, AA is released from phospholipids by the cytosolic PLA2 and provided to 5-LOX by the nuclear membrane-bound 5-LOX-activating protein (FLAP) (30). 5-LOX then facilitates the incorporation of molecular oxygen at C5 of AA yielding the hydroperoxide 5(S)-HPETE that is converted in a second step by 5-LOX into the epoxide LTA4 (Figure 1). 5-LOX is localized in the nucleoplasm or the cytosol depending on the cell type, and after cell activation it translocates to the nuclear envelope in order to access liberated AA (31). 5-LOX is composed of a catalytic domain, with an iron in the active site, and a regulatory C2-like domain that binds Ca2+ and mediates the association with phosphatidylcholine (PC) and thus with PC-rich membranes (30). At the nuclear membrane 5-LOX assembles a complex with FLAP that binds AA and facilitates the accessibility of the substrate for 5-LOX, which is considered pivotal for LTA4 generation in intact cells (32, 33). Depending on the cell-type and the enzymes expressed, LTA4 is then converted to LTB4 by LTA4 hydrolase (LTA4H) or to LTC4 by LTC4 synthase (LTC4S) (30) (Figure 1). LTB4 is a potent chemoattractant and activator of various leukocytes whereas cys-LTs mediate smooth muscle contraction and vascular leakage (8).
In contrast to pro-inflammatory PGs and LTs, the SPM have anti-inflammatory properties as they actively terminate inflammation and promote the resolution of the inflammation process. The SPM superfamily encompasses LM with conserved structures mediating their bioactions via distinct GPCR with defined structure-activity relationships (2, 7). SPMs exhibit beneficial functions in microbial defense, pain, organ protection, and tissue regeneration, wound healing, cancer, reproduction, and neurobiology of cognition (9). The generation of LX from AA requires the concomitant actions of 5-LOX and 12/15-LOXs which can be accomplished by single cells that express both LOXs or by transcellular metabolism between cells that express at least one of these enzymes (9, 34). Similarly, the formation of Rv from EPA or DHA depends on at least two enzymes: a CYP450 or COX-2 in the presence of aspirin first generate 18-HEPE from EPA, while in a second step 18-HEPE is converted by a LOX yielding E-series Rv, or in the case of D-series Rv transformation of DHA by 15-LOX to 17-HDHA and its subsequent conversion by 5-LOX and other enzymes (34–36). On the other hand, for the biosynthesis of PD and MaR from DHA, 15-LOX and/or 12-LOX appear sufficient (37). Finally, stereoisomers of LX, Rvs and PD, so-called aspirin-triggered (AT)-LXs, AT-Rvs, and AT-PDs account to the SPM superfamily and are produced by LOXs alone or in conjunction with aspirin-induced acetylated COX-2 (9, 34). To the best of our knowledge, effects of androgens on the biosynthesis of SPM have not been reported yet. Nevertheless, sex differences in SPM levels in humans and mice were recently documented (38–41). Along these lines, the impact of androgens on 12- and 15-LOXs in innate immune cells is essentially unexplored; one study reported that androgen failed to alter 15-LOX-2 gene expression in normal human prostate epithelial cells (42).
Sex Differences in Inflammation and in Autoimmune Diseases
Numerous studies have shown that sex is a significant variable in the activity of the innate and the adaptive immune system and that there are significant sex differences in the immune response (11, 43). This affects the defense against infections, autoimmune diseases and tumor diseases as well as vaccinations. Adult women generally have a more active innate and adaptive immune system, explaining why 80% of patients with autoimmune diseases are females, and why women with acute HIV infection have 40% less viral RNA in the blood than men. Moreover, antibody formation in response to influenza vaccines is consistently at least twice as high in women than in men. Age plays a decisive role in this, according to which the immune response and inflammatory activity predominate until puberty in boys, but then dominate in adult women (12). Since sex differences are often caused by divergent levels of androgens, many studies that revealed modulation of the innate immunity by male sex hormones originate from studies primarily aiming to compare biological responses in male and female subjects.
Studies on the mechanisms underlying sex-biased immune responses show quite complex differences in various immune cells between the sexes, both with regard to the innate and the adaptive immunity. For innate immunity, the phagocytosis rate of neutrophils and macrophages, the macrophage activity, the type 1 interferon activity of dendritic cells (DC), and the efficiency of antigen-presenting cells (APC) are all more pronounced in female vs. male cells (44–46). In contrast, the numbers of natural killer cells and the expression of the TLR4 of macrophages and neutrophils dominate in men (11). However, TLR7 is more strongly expressed in women (47). In terms of acquired immunity, women have more B lymphocytes and, accordingly, more pronounced antibody production, higher numbers of activated T lymphocytes with a higher proliferation rate, more CD4+ cells, and more pronounced T cell cytotoxicity. Men, on the other hand, have higher numbers of regulatory T lymphocytes and CD8+ cells (11).
Due to the higher activity of the immune system in women, inflammatory reactions occur more frequently and more severely in females than males. Distinct sex differences can be found in the incidence of autoimmune diseases such as multiple sclerosis (MS), systemic lupus erythematosus (SLE), thyroid disease, scleroderma or rheumatoid arthritis, but also Alzheimer's disease, which mostly dominate in women (48). In the US, women represent ~80% of all cases of autoimmune disorders. Also in animal models of autoimmune disease such as experimental autoimmune encephalomyelitis (EAE), females show more pronounced severity (49) with higher activation status of TH1 cells and elevated INFγ (50). Androgens are protective in the susceptibility to EAE of SJL mice, as castration caused increased severity of the disease and supplementation with exogenous testosterone to both gonadally intact SJL and C57BL/6 mice resulted in protecting effects. Of note, ovariectomy did not alter the disease outcomes (51). Interestingly, administration of sex hormones to patients with MS revealed beneficial effects: in men, topical application of testosterone significantly reduced IL-2 but elevated TGFβ production in PBMC, and shifted the lymphocyte composition by decreasing CD4+ T cells and increasing NK cells (52).
Sex Bias in Prostaglandin and Leukotriene Biosynthesis
More than 50 years ago, modulation of eicosanoid biosynthesis by sex was reported (53) and effects of testosterone on PGF2α formation were observed already in 1974 (20, 21). Until today, multiple studies employing different animal models uncovered sex differences and effects of sex hormones on the biosynthetic pathways of PGs and LTs in various cells and organs/tissues (Tables 1, 2), see also (66) for review. LM levels in vivo can be affected at the level of their biosynthesis as well as of their metabolism/elimination. Most studies addressed the regulation of the biosynthesis of LM, focusing on the expression of LOXs, COXs or prostanoid synthases as biosynthetic enzymes, or on the cellular activation of these enzymes (66). Notably, also the receptors that induce LM formation (e.g., TLR4) can be strongly modulated by sex and sex hormones (11).
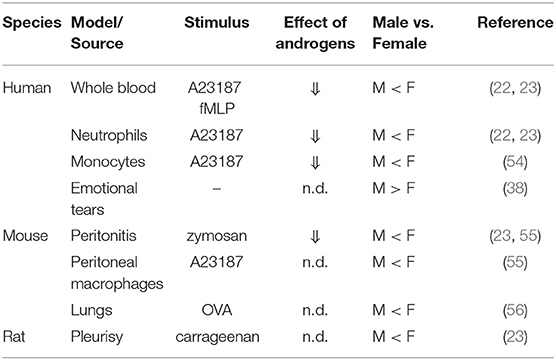
Table 1. Sex differences and modulatory effects of androgens on leukotriene formation in various models and cell/tissue sources.
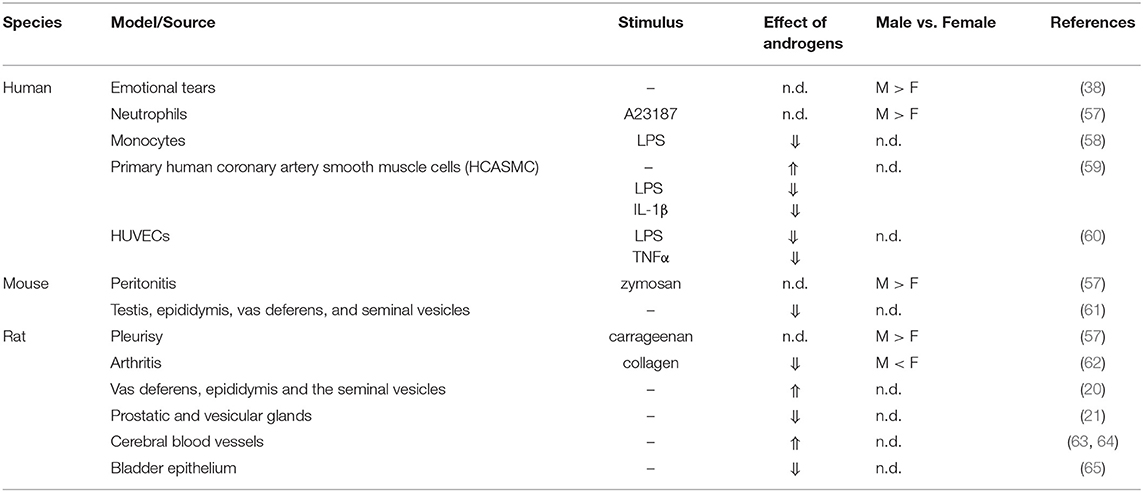
Table 2. Sex differences and modulatory effects of androgens on prostaglandin formation in various models and cell/tissue sources.
The existence of a sex dimorphism in LT biology is already suggested by the fact that many diseases related to LT including asthma, rheumatoid arthritis, allergic rhinitis, or SLE are sex-biased with higher occurrence in women (66). Similar as for PG formation, modulation of LT production may occur on the expression level of LT-biosynthetic enzymes and via the availability of AA as substrate but also additional regulatory aspects such as modulation of phosphorylation and subcellular redistribution of the biosynthetic enzymes. Note that despite comprehensive and intensive research with the aim to reveal roles of LTs in sex-afflicted autoimmune diseases such as SLE, potential sex differences in LT biosynthesis have long been neglected in biomedical research.
In fact, there is accumulating evidence suggesting that female sex is afflicted with higher LT biosynthesis and androgens were shown to lower LT levels in vitro and in vivo (Table 1) (22, 23, 54–56). For example, blood or isolated monocytes and neutrophils from healthy adult women exhibited higher capacities to produce LTs upon stimulation ex vivo vs. men (22, 23, 54). Also, in urine samples from healthy white volunteers, higher concentrations of 5-LOX products were found in samples from elderly women than elderly men (67). Such sex-bias was evident also in mice and rats in vivo, where female animals in zymosan-induced peritonitis, carrageenan-elicited pleurisy or experimental models of inflammation upon allergen challenge produced higher levels of LTs (23, 55, 56).
Studies with knock-out (KO) animals where 5-LOX or LT receptors were deleted revealed obvious roles of LTs for sex differences in the pathophysiology of diseases. For example, in SLE-related mouse models the female disadvantage vs. male animals was abolished after 5-LOX KO (68). Deletion of the BLT1 receptor protected only female but not male mice in platelet-activating factor (PAF)-induced shock (69) and knockout of the Cys-LT1 reduced tumor burden in the small intestine of female Apc (Min/+) mice but not in male counterparts (70). Conclusively, the superior benefit of female animals after disruption of the LT pathway implies the existence of sex differences in LT biosynthesis with more pronounced pathophysiological roles of LTs in females.
In line with the genetic interference with the 5-LOX pathway, also results from pharmacological studies intervening with LTs imply superior significance of LT biology in women, suggesting that females may benefit from anti-LT therapy to a greater extent than males. In a prospective cohort study with 11 asthmatic women and 10 men, montelukast [a potent and selective leukotriene receptor antagonist (71)] improved asthma symptoms and lung function better in women compared with men (72). Similarly, in asthmatic girls reaching puberty, montelukast clearly reduced asthma incidence vs. placebo, but not so in boys of the same age, even though montelukast was highly active in boys before puberty (73). Along these lines, superior therapeutic efficacy of different types of clinically relevant LT modifiers like zileuton, MK886 and montelukast were observed in female ovalbumin-sensitized BALB/c mice (56). Recent data showed that FLAP inhibitors like MK886 have impaired efficiency in males which is caused by androgens. Thus, lower doses of MK886 were needed to reduce LTB4 levels in inflammatory exudates of female vs. male mice and rats. Following PAF-induced shock, MK886 increased survival exclusively in female mice, which was abolished by androgen administration. FLAP inhibitors had higher potencies in human blood from females and supplementation of female blood with androgens abolished the observed sex differences (23). Together, the androgen-mediated suppression of LT biosynthesis is not only of pathophysiological relevance for LT-related diseases, but also determines the efficacy of anti-LTs, in particular the potency of FLAP inhibitors which is strikingly impaired by androgens.
Sex differences in PG formation are frequently related to sex-biased expression of the key enzymes involved. Thus, sex-related regulation of COX-2 and mPGES-1 in different organs with consequences for the development and incidence of PG-related diseases was reported (66). In general, prostanoids and their biosynthetic enzymes often dominate in males vs. females, but this can depend on the type of cell, tissue and organ, on the inflammatory status, as well as on the sex hormone level related to the age of the subjects studied. Most studies addressed kidney and brain, where in particular the COX-2/mPGES-1-derived PGE2 plays major physiological and pathophysiological roles. Three independent studies in rodents addressed sex differences in PG biology of the kidney indicating that female animals reached higher PG (mainly PGE2) concentrations vs. male counterparts. This bias was either due to superior COX-2 and mPGES-1 levels in females (74, 75) or due to higher expression of the PG-metabolizing enzyme 15-hydroxy-PG dehydrogenase (15-PGDH) and the PG-specific transporter OAT-PG in males (76). In contrast, in the brain, a preponderance of PG were evident in males (e.g., higher COX-2 expression), and suppressive effects by female sex hormones were reported (77–80). Most strikingly, under inflammatory conditions, for example in mouse zymosan-induced peritonitis and rat carrageenan-induced pleurisy, PG levels dominated in males vs. females, seemingly due to higher PG production in infiltrated male neutrophils that produced more PGE2 accompanied by elevated COX-2 expression (57). Note that sex differences in prostanoid biology may be caused also by a dimorphism in the expression and function of prostanoid receptors (66). Finally, sex differences in prostanoid biosynthesis and modulation by sex hormones were also observed on the cellular level where cells (e.g., neutrophils, platelets) from male donors generated more prostanoids as compared to female counterparts (38, 57, 81, 82).
Impact of Androgens on Lipid Mediator Formation in Innate Immune Cells
Androgens as Modulators of Innate Immune Cells
Among innate immune cells, neutrophils and monocytes/macrophages are the major source of LM, particularly in the blood. As reported below (section Androgens and Leukotriene Biosynthesis and Androgens and Biosynthesis of Prostaglandins and Other Eicosanoids), the influence of sex hormones and especially of androgens cause significant modifications in the production of LM with consequences for inflammation and possibly also the related pharmacology. There are several findings that indicate a considerable impact of androgens on the count and the functions of these LM-producing innate immune cells with consequences for the immune response. Neutrophils are generated in the bone marrow from myeloid progenitors and play a major role in the first response during inflammation as they represent the early cells recruited to the inflamed tissue to destroy external pathogens (83). It has been shown that the lack of androgen receptor (AR) causes neutropenia and increases the susceptibility to microbial infection (84). Also in a model of ozone-induced airway hyperresponsiveness, androgens caused an increased inflammatory response in terms of neutrophil recruitment as well as cytokine production in an AR-dependent manner where castration of mice diminished the effect (85). Accordingly, in a bacterial model of prostate inflammation, neutrophil accumulation in several organs and in the inflamed region was promoted by testosterone treatment (86). Note that different and contradictory effects of testosterone on neutrophil functions were reported: for example, after trauma-hemorrhagic shock, neutrophils from male mice were more activated then the female counterparts (87), while after bacteria-induced prostate inflammation, neutrophils from male animals produced less pro-inflammatory cytokines (85).
Monocytes and macrophages both express the AR and the effect of testosterone on the functions of those cells is widely discussed (18, 88). Monocytes account for up to ~10% of the circulating leukocytes in humans and ~4% in mice and they are evoked during inflammation in large amounts in order to amplify the inflammatory reaction (89). Macrophages that possess high plasticity, can act as pro-inflammatory (M1) or pro-resolving phenotypes (M2), the latter promoting the return to homeostasis (90). Intriguingly, testosterone reduces the expression of TLR4 in murine macrophages (91), but male macrophages have seemingly a superior response to TLR4 ligands (92). Testosterone may also impact macrophage polarization: for example, upon Coxsackievirus B infection, male mice suffered more from myocarditis than females which correlated with a predominance of M2 in female myocardium as compared to increased numbers of M1 in males (93).
Androgens and Leukotriene Biosynthesis
The superior levels of LTs formed in women and female animals or in cells derived from female subjects vs. male counterparts can be unequivocally related to the suppressive impact of androgens. Table 1 summarizes the reported effects of androgens in various models in relation to the observed sex differences in these studies. The first report demonstrating modulation of LT biosynthesis by androgens in vitro was published in 2008 by Pergola et al. (22), almost 30 years after the discovery of LTs by Samuelsson et al. in 1979 (94). Suppression of 5-LOX product formation by androgens like testosterone or 5α-DHT was observed in agonist-stimulated human blood, isolated human neutrophils (22, 23) and monocytes (54) from females. Also in human corneal, conjunctival, and meibomian gland epithelial cells 5α-DHT reduced the potentiation of LPS-induced secretion of LTB4 via LPS-binding protein (95).
The existence of such androgen effects in vivo was confirmed in a murine zymosan-induced peritonitis model (55). Thus, LT levels in peritoneal exudates of orchidectomized mice were higher than in sham male mice, and peritoneal macrophages from orchidectomized animals produced more LTs ex vivo than sham-treated counterparts (55). Along these lines, short–term application of 5α-DHT reduced LTB4 levels during zymosan-induced peritonitis only in female but not in male animals (23). Androgen-mediated sex differences were recently shown also in a mouse model of MS (16), where LT signaling contributes to pathology (96). Moreover, ex vivo analysis of blood from healthy women with variant androgen levels revealed a significant invers correlation between androgen levels and the capacity to produce LTs upon stimulation of the blood (22).
In leukocytes, suppression of LT formation by androgens required very short pre-incubation periods of only one to few minutes, excluding the requirement of protein de novo synthesis, supported by the fact that androgen treatment did not alter the amounts of any of the LT biosynthetic enzymes on the protein level. Also, in neutrophils and monocytes derived from male and female subjects these LT biosynthetic enzymes were equally expressed (22, 54). Moreover, the magnitude of (concentration-dependent) maximal inhibition of LTs was <50% of the total 5-LOX activity, suggesting that androgens do not directly act on 5-LOX like typical enzyme inhibitors. In fact, androgens were not active in male blood or leukocytes and they suppressed 5-LOX product formation in female cells or blood down to the levels obtained in male counterparts. Interestingly, supplementation of these neutrophils or monocytes with exogenous AA as substrate reverted androgen-induced inhibition of 5-LOX, indicating that androgens may inhibit 5-LOX product formation at the level of substrate supply (20). However, 5α-DHT failed to inhibit AA release in these leukocytes, suggesting another point of attack for androgens; seemingly the accessibility of AA to 5-LOX mediated by FLAP is affected by the sex hormone.
For LT formation, soluble resting 5-LOX in the cytosol or nucleosol must translocate to the nuclear envelope upon cell stimulation for associating with FLAP that facilitates access of 5-LOX to endogenous AA as substrate (32, 33). While in female neutrophils, this pattern of 5-LOX subcellular redistribution was evident along with high LT levels, in male neutrophils a substantial part of 5-LOX was located to the nuclear envelope in resting cells, and only a small portion of 5-LOX redistributed upon stimulation, accompanied by low 5-LOX product biosynthesis (22). Treatment of female cells with 5α-DHT caused the same pattern of 5-LOX redistribution like in male neutrophils and suppressed LT biosynthesis in female cells down to the levels obtained by male neutrophils. Note that also cPLA2 is a cytosolic enzyme that co-translocates with 5-LOX to the nuclear envelope where AA as substrate for 5-LOX is liberated from PC (24). However, neither sex differences nor effects of androgens were observed for cPLA2 translocation in neutrophils (22), suggesting that the sex bias in LT formation is independent from cPLA2-mediated substrate supply. On the other hand, sex differences in n-3 PUFA content were reported with associated differences in circulating concentrations of n-3 PUFA, that is, higher plasma DHA concentrations in females and negatively associated with circulating concentrations of testosterone (83).
More detailed mechanistic analysis on the subcellular localization of 5-LOX uncovered that androgens impede the agonist-induced, tight assembly of the LT-biosynthetic 5-LOX/FLAP complex at the nuclear membrane of human and murine leukocytes (23). By means of a proximity ligation assay that enables the visualization of in-situ 5-LOX/FLAP interaction in intact cells (32) it was found that despite perinuclear localization of 5-LOX in male neutrophils, monocytes or peritoneal macrophages, 5-LOX and FLAP hardly associated as compared to female cells. Addition of 5α-DHT to female cells prevented the agonist-induced 5-LOX/FLAP complex assembly as it was observed in male cells (23). In conclusion, androgens may cause perinuclear localization of 5-LOX but distant from nuclear membrane-bound FLAP which impedes 5-LOX product formation.
It was shown that the variant testosterone levels in males and females cause a differential activation status of extracellular signal-regulated protein kinase (ERK)-1/2 in human neutrophils which confers sex differences in LT formation by regulating 5-LOX subcellular localization (22). Because ERKs are central signaling kinases that regulate multiple neutrophil functions, these actions of androgens may have striking effects on neutrophil biology besides regulating LT formation. 5-LO translocation is governed by ERK-1/2 that phosphorylate 5-LOX (97) and it was shown that ERK-1/2 mediate the male pattern of 5-LOX subcellular distribution caused by androgens. Thus, male (resting) neutrophils exhibited a higher ERK-1/2 activation status vs. cells from females, and pharmacological suppression of ERK-1/2 activity in male cells yielded the female 5-LOX subcellular localization pattern. Of interest, exposure of female neutrophils to male plasma or to 5α-DHT enhanced ERK-1/2 activation, accompanied by the male pattern of 5-LOX subcellular distribution, which again was reversed by ERK-1/2 inhibition (22). ERK activation in neutrophils by 5α-DHT was rather rapid, occurring with 0.5 min, at fairly low concentrations, starting at ~10 pM. Rapid activation of ERK-1/2 by androgens through a non-genomic mechanism was shown before for non-immune PMC42 breast cancer cells (98). Notably, the androgen effects on ERK-1/2 and 5-LOX were reversible and disappeared after about 1 h at room temperature but were preserved for longer times when neutrophils were kept at 4°C (22). This finding implies that isolation of neutrophil and probably also of other blood cells at low temperature, i.e., 4°C, may better maintain the biology of the cells that they once possessed in their original environment.
As mentioned above, in addition to neutrophils, higher 5-LOX product formation (about 1.8-fold) was also found for isolated human monocytes derived from peripheral blood of females vs. monocytes from males (54). Again, resuspension of female monocytes in plasma from males lowered 5-LOX product formation, and preincubation with 5α-DHT for 30 min repressed LT synthesis in female cells down to the levels observed in males, while estradiol and progesterone were inactive or gave only slight inhibition. 5α-DHT caused rapid ERK-1/2 phosphorylation connected to inhibition of phospholipase D (PLD) and reduced diacylglycerol (DAG) formation. It was shown that DAG are of importance for 5-LOX product formation (99) by acting at a phospholipid binding site of 5-LOX located within the C2-like domain (100). In fact, in male monocytes the ERK-1/2 activation status was increased while PLD activity and DAG formation was 1.4–1.8-fold lower vs. female monocytes. Supplementation of monocytes with DAG elevated 5-LOX product formation in male but not in female cells. These results indicate that androgen-induced ERK-1/2 activation represses PLD activity in monocytes resulting in impaired 5-LOX product formation seemingly due to lack of activating DAGs (54).
In addition to androgens, also progesterone was found to down-regulate LT formation in human monocytes in a rapid manner, however, not in neutrophils (101). Progesterone was more effective in monocytes derived from females and suppression of 5-LOX product synthesis was proposed to be mediated by protein kinase A, whereas ERK-1/2 and PLD were seemingly not involved (101), indicating that the characteristics and mechanisms behind the LT-suppressive effects of progesterone and androgens clearly differ. Moreover, estradiol was shown to induce LTC4 biosynthesis in RBL-2H3 cells and potentiated LTC4 formation in response to IgE seemingly by promoting intracellular Ca2+ mobilization (102). Finally, pregnancy, a situation with marked alterations in sex hormone plasma levels, was shown to be associated with increased LT biosynthesis in blood due to higher numbers of leukocyte and due to stimulatory effects of plasma components (103). It is interesting in this respect that the characteristics of diseases related to LT (e.g., asthma, allergic rhinitis) change during pregnancy (104).
Androgens and Biosynthesis of Prostaglandins and Other Eicosanoids
The impact of sex hormones on PG biosynthesis has been considered in various types of preclinical experimental animal models and in clinical studies, where sex differences in prostanoid formation were evident. In contrast to modulation of the 5-LOX pathway, the underlying mechanisms of the effects of androgens on PG formation are less clear and will be addressed here only briefly. Many studies reported on effects of androgens on PG formation in rodents with divergent and even opposing outcomes that seemingly depend on the experimental settings and conditions (Table 2). In fact, the first studies analyzing PG formation in the reproductive system of male rats in relation to androgens were conflicting: Bartke and Koerner documented reduced PGF2α levels in the vas deferens, epididymis and the seminal vesicles of male rats upon castration which were reversed by administration of testosterone (20), while Sutherland et al. (21) found elevated PGF2α in prostatic and vesicular glands upon orchidectomy of male rats which was reversed by testosterone application. It seems that androgens differentially influence PG biosynthetic pathways under physiological and pathophysiological conditions in various tissues and act at different levels. As a rule of thumb, androgens appear to elevate PG formation by augmenting COX-2 expression under healthy states, but they suppress COX-2 induction and PG biosynthesis under inflammatory conditions (Table 2). Thus, in the absence of an inflammatory stimulus, supplementation of testosterone in orchidectomized male rats increased COX-2 protein expression in cerebral blood vessels along with elevated PGE2 levels (63, 64), in rat bladder epithelium (65), and PGE2 and COX-2 were elevated in primary human coronary artery smooth muscle cells (HCASMC) by 5α-DHT (59). Conversely, in the presence of LPS or IL-1β, the increases in COX-2 protein in HCASMC (59) or in human monocytes (58) were attenuated by testosterone, which was observed also in LPS- or TNFα-stimulated HUVECs where the elevated mRNA levels of COX-2 were repressed when 5α-DHT was present (60). Also, under collagen-induced arthritis in castrated female rats, testosterone application possessed significant anti-inflammatory effects accompanied by reduced PGE2 levels (62). While most of the PG-modulatory effects of androgens were connected to alterations in COX-2 levels, also the expression of mPGES-1 is seemingly affected by androgens. Thus, urinary PGE2 levels and mPGES-1 expression in the renal inner medulla of spontaneously hypertensive rats was lower in males but after orchidectomy, PGE2 metabolite excretion and mPGES-1 expression were elevated (75). Androgens may also affect the metabolism and transport of PG. For example, the PG-catabolic enzyme 15-PGDH and the PG-specific transporter OAT-PG are more abundant in the renal cortex of male rats with consequently lower renocortical PGE2. Orchidectomization of male rats led to decreased OAT-PG expression and elevate renocortical PGE2, and these changes were restored upon supplementation of testosterone (76).
Finally, androgens may impact the ω-hydroxylated AA metabolite 20-hydroxyeicosatetraenoic acid (20-HETE), generated by CYP enzymes, that mediates hypertension via multiple pathways. Several studies indicate that 20-HETE may play a role in androgen-induced vascular dysfunction and hypertension (105). Thus, treatment of normotensive mice with 5α-DHT induced both Cyp4a12 expression and 20-HETE levels in preglomerular microvessels, where 20-HETE can mediate both androgen-induced and androgen-independent hypertension (106). In an S6 mice strain, deficient in soluble guanylate cyclase-α1, hypertension was traced back to elevated Cyp4a12a expression and increased 20-HETE levels (107). Moreover, 20-HETE produced by CYP4a2 may contribute to elevated blood pressure in hyperandrogenic female rats (108).
Concluding Remarks
A variety of inflammatory diseases related to increased LT and PG are sex-biased with often higher incidence and severity in females, e.g., rheumatoid arthritis and asthma. Androgen-mediated sex differences in inflammation and in the activity of related innate immune cells are now well-documented. Androgens have a significant impact on neutrophils, monocytes and macrophages which are the major sources of inflammation-related LM that are biosynthesized on demand. Obvious sex differences in the capacity to generate LM led to the discovery of regulatory roles of androgens. For LT biosynthesis, the situation in males and females is rather unambiguous with superior LT levels in females, and there are clear indications for throughout suppressive effects of androgens on LT formation in neutrophils, monocytes and macrophages from human and/or mice (or rats). Androgens reduce LT biosynthesis in various inflammation models related to LT in vivo which can translate into impaired efficiency of anti-LTs. Intracellular signaling routes involving ERK-1/2 and/or PLD were uncovered as effectors of androgens in this respect which eventually prevents the assembly of the LT-biosynthetic 5-LOX/FLAP complex. Future studies will be needed to address by which receptor and signaling pathway androgens activate ERK-1/2 and how ERK-1/2 as well as PLD and DAGs are connected to aberrant 5-LOX subcellular localization preventing access to FLAP. Moreover, evaluation of the effects of androgens and other sex hormones on SPM formation is warranted in future research along with the regulation of related biosynthetic enzymes such as 12- and 15-LOXs. Sex specific differences in PG biosynthesis and the impact of androgens may depend on the organ/tissue but also on the presence or absence of inflammatory status: under healthy conditions, androgens may increase PG formation along with elevated COX-2 induction, while in presence of an inflammatory stimulus androgens may repress PG biosynthesis. Together, it appears that androgens significantly impact pro-inflammatory LM formation in innate immune cells with direct consequences for pathophysiology but also for the pharmacotherapy of inflammation.
Author Contributions
SP and OW wrote the manuscript and prepared the figures and tables. All authors contributed to the article and approved the submitted version.
Funding
The authors received funding by the Deutsche Forschungsgemeinschaft (DFG, German Research Foundation)—project number 316213987–SFB 1278 PolyTarget (Projects A04, C02), SFB 1127 ChemBioSys (Project A04), and by the Free State of Thuringia and the European Social Fund (2016 FGR 0045).
Conflict of Interest
The authors declare that the research was conducted in the absence of any commercial or financial relationships that could be construed as a potential conflict of interest.
References
1. Kotas ME, Medzhitov R. Homeostasis, inflammation, and disease susceptibility. Cell. (2015) 160:816–27. doi: 10.1016/j.cell.2015.02.010
2. Serhan CN. Pro-resolving lipid mediators are leads for resolution physiology. Nature. (2014) 510:92–101. doi: 10.1038/nature13479
3. Araujo AC, Wheelock CE, Haeggstrom JZ. The Eicosanoids, Redox-Regulated Lipid Mediators in Immunometabolic Disorders. Antioxid Redox Signal. (2018) 29:275–96. doi: 10.1089/ars.2017.7332
4. Calder PC. n-3 fatty acids, inflammation and immunity: new mechanisms to explain old actions. Proc Nutr Soc. (2013) 72:326–36. doi: 10.1017/S0029665113001031
5. Innes JK, Calder PC. Omega-6 fatty acids and inflammation. Prostaglandins Leukot Essent Fatty Acids. (2018) 132:41–8. doi: 10.1016/j.plefa.2018.03.004
6. Back M, Powell WS, Dahlen SE, Drazen JM, Evans JF, Serhan CN, et al. Update on leukotriene, lipoxin and oxoeicosanoid receptors: IUPHAR Review 7. Br J Pharmacol. (2014) 171:3551–74. doi: 10.1111/bph.12665
7. Chiang N, Serhan CN. Structural elucidation and physiologic functions of specialized pro-resolving mediators and their receptors. Mol Aspects Med. (2017) 58:114–29. doi: 10.1016/j.mam.2017.03.005
8. Haeggstrom JZ, Rinaldo-Matthis A, Wheelock CE, Wetterholm A. Advances in eicosanoid research, novel therapeutic implications. Biochem Biophys Res Commun. (2010) 396:135–9. doi: 10.1016/j.bbrc.2010.03.140
9. Serhan CN, Levy BD. Resolvins in inflammation: emergence of the pro-resolving superfamily of mediators. J Clin Invest. (2018) 128:2657–69. doi: 10.1172/JCI97943
10. Libert C, Dejager L, Pinheiro I. The X chromosome in immune functions: when a chromosome makes the difference. Nat Rev Immunol. (2010) 10:594–604. doi: 10.1038/nri2815
11. Klein SL, Flanagan KL. Sex differences in immune responses. Nat Rev Immunol. (2016) 16:626–38. doi: 10.1038/nri.2016.90
12. Gubbels Bupp MR, Potluri T, Fink AL, Klein SL. The Confluence of Sex Hormones and Aging on Immunity. Front Immunol. (2018) 9:1269. doi: 10.3389/fimmu.2018.01269
13. Straub RH. The complex role of estrogens in inflammation. Endocr Rev. (2007) 28:521–74. doi: 10.1210/er.2007-0001
14. Pepe G, Locati M, Della Torre S, Mornata F, Cignarella A, Maggi A, et al. The estrogen-macrophage interplay in the homeostasis of the female reproductive tract. Hum Reprod Update. (2018) 24:652–72. doi: 10.1093/humupd/dmy026
15. Robinson DP, Klein SL. Pregnancy and pregnancy-associated hormones alter immune responses and disease pathogenesis. Horm Behav. (2012) 62:263–71. doi: 10.1016/j.yhbeh.2012.02.023
16. Zhu ML, Bakhru P, Conley B, Nelson JS, Free M, Martin A, et al. Sex bias in CNS autoimmune disease mediated by androgen control of autoimmune regulator. Nat Commun. (2016) 7:11350. doi: 10.1038/ncomms11350
17. Trigunaite A, Dimo J, Jorgensen TN. Suppressive effects of androgens on the immune system. Cell Immunol. (2015) 294:87–94. doi: 10.1016/j.cellimm.2015.02.004
18. Gubbels Bupp MR, Jorgensen TN. Androgen-Induced Immunosuppression. Front Immunol. (2018) 9:794. doi: 10.3389/fimmu.2018.00794
19. Hamberg M. Inhibition of prostaglandin synthesis in man. Biochem Biophys Res Commun. (1972) 49:720–6. doi: 10.1016/0006-291X(72)90470-6
20. Bartke A, Koerner S. Androgenic regulation of the concentration of prostaglandin F in the male reproductive system of rats and mice. Endocrinology. (1974) 95:1739–43. doi: 10.1210/endo-95-6-1739
21. Sutherland DJ, Telli AH, Singhal RL. The influence of testosterone on the endogenous levels of prostaglandin F in the accessory reproductive glands of the adult male rat. Can J Physiol Pharmacol. (1974) 52:364–7. doi: 10.1139/y74-051
22. Pergola C, Dodt G, Rossi A, Neunhoeffer E, Lawrenz B, Northoff H, et al. ERK-mediated regulation of leukotriene biosynthesis by androgens: a molecular basis for gender differences in inflammation and asthma. Proc Natl Acad Sci USA. (2008) 105:19881–6. doi: 10.1073/pnas.0809120105
23. Pace S, Pergola C, Dehm F, Rossi A, Gerstmeier J, Troisi F, et al. Androgen-mediated sex bias impairs efficiency of leukotriene biosynthesis inhibitors in males. J. Clin. Invest. (2017) 127:3167–76. doi: 10.1172/JCI92885
24. Leslie CC. Cytosolic phospholipase A(2): physiological function and role in disease. J Lipid Res. (2015) 56:1386–402. doi: 10.1194/jlr.R057588
25. Yamatomo T, Okano M, Ono T, Nakayama E, Yoshino T, Satoskar AR, et al. Sex-related differences in the initiation of allergic rhinitis in mice. Allergy. (2001) 56:525–31. doi: 10.1034/j.1398-9995.2001.056006525.x
26. ElBaradie K, Wang Y, Boyan BD, Schwartz Z. Rapid membrane responses to dihydrotestosterone are sex dependent in growth plate chondrocytes. J Steroid Biochem Mol Biol. (2012) 132:15–23. doi: 10.1016/j.jsbmb.2011.12.009
27. Funk CD. Prostaglandins and leukotrienes: advances in eicosanoid biology. Science. (2001) 294:1871–5. doi: 10.1126/science.294.5548.1871
28. Samuelsson B, Morgenstern R, Jakobsson PJ. Membrane prostaglandin E synthase-1: a novel therapeutic target. Pharmacol Rev. (2007) 59:207–24. doi: 10.1124/pr.59.3.1
29. Haeggstrom JZ. Leukotriene biosynthetic enzymes as therapeutic targets. J Clin Invest. (2018) 128:2680–90. doi: 10.1172/JCI97945
30. Radmark O, Werz O, Steinhilber D, Samuelsson B. 5-Lipoxygenase, a key enzyme for leukotriene biosynthesis in health and disease. Biochim Biophys Acta. (2015) 1851:331–9. doi: 10.1016/j.bbalip.2014.08.012
31. Newcomer ME, Gilbert NC. Location, location, location: compartmentalization of early events in leukotriene biosynthesis. J Biol Chem. (2010) 285:25109–14. doi: 10.1074/jbc.R110.125880
32. Gerstmeier J, Weinigel C, Rummler S, Radmark O, Werz O, Garscha U. Time-resolved in situ assembly of the leukotriene-synthetic 5-lipoxygenase/5-lipoxygenase-activating protein complex in blood leukocytes. FASEB J. (2016) 30:276–85. doi: 10.1096/fj.15-278010
33. Mandal AK, Jones PB, Bair AM, Christmas P, Miller D, Yamin TT, et al. The nuclear membrane organization of leukotriene synthesis. Proc Natl Acad Sci USA. (2008) 105:20434–9. doi: 10.1073/pnas.0808211106
34. Bannenberg G, Serhan CN. Specialized pro-resolving lipid mediators in the inflammatory response: an update. Biochim Biophys Acta. (2010) 1801:1260–73. doi: 10.1016/j.bbalip.2010.08.002
35. Werner M, Jordan PM, Romp E, Czapka A, Rao Z, Kretzer C, et al. Targeting biosynthetic networks of the proinflammatory and proresolving lipid metabolome. FASEB J. (2019) 33:6140–53. doi: 10.1096/fj.201802509R
36. Werz O, Gerstmeier J, Libreros S, De la Rosa X, Werner M, Norris PC, et al. Human macrophages differentially produce specific resolvin or leukotriene signals that depend on bacterial pathogenicity. Nat Commun. (2018) 9:59. doi: 10.1038/s41467-017-02538-5
37. Serhan CN, Dalli J, Colas RA, Winkler JW, Chiang N. Protectins and maresins: new pro-resolving families of mediators in acute inflammation and resolution bioactive metabolome. Biochim Biophys Acta. (2015) 1851:397–413. doi: 10.1016/j.bbalip.2014.08.006
38. English JT, Norris PC, Hodges RR, Dartt DA, Serhan CN. Identification and Profiling of Specialized Pro-Resolving Mediators in Human Tears by Lipid Mediator Metabolomics. Prostaglandins Leukot Essent Fatty Acids. (2017) 117:17–27. doi: 10.1016/j.plefa.2017.01.004
39. Halade GV, Kain V, Dillion C, Beasley M, Dudenbostel T, Oparil S, et al. Race-based and sex-based differences in bioactive lipid mediators after myocardial infarction. ESC Heart Fail. (2020). doi: 10.1002/ehf2.12730. [Epub ahead of print].
40. Pullen AB, Kain V, Serhan CN, Halade GV. Molecular and Cellular Differences in Cardiac Repair of Male and Female Mice. J Am Heart Assoc. (2020) 9:e015672. doi: 10.1161/JAHA.119.015672
41. Rathod KS, Kapil V, Velmurugan S, Khambata RS, Siddique U, Khan S, et al. Accelerated resolution of inflammation underlies sex differences in inflammatory responses in humans. J Clin Invest. (2016) 127:169–82. doi: 10.1172/JCI89429
42. Tang S, Bhatia B, Zhou J, Maldonado CJ, Chandra D, Kim E, et al. Evidence that Sp1 positively and Sp3 negatively regulate and androgen does not directly regulate functional tumor suppressor 15-lipoxygenase 2 (15-LOX2) gene expression in normal human prostate epithelial cells. Oncogene. (2004) 23:6942–53. doi: 10.1038/sj.onc.1207913
43. Rosen S, Ham B, Mogil JS. Sex differences in neuroimmunity and pain. J Neurosci Res. (2017) 95:500–8. doi: 10.1002/jnr.23831
44. Griesbeck M, Ziegler S, Laffont S, Smith N, Chauveau L, Tomezsko P, et al. Sex differences in plasmacytoid dendritic cell levels of IRF5 drive higher IFN-α production in women. J Immunol. (2015) 195:5327–36. doi: 10.4049/jimmunol.1501684
45. Spitzer JA. Gender differences in some host defense mechanisms. Lupus. (1999) 8:380–3. doi: 10.1177/096120339900800510
46. Weinstein Y, Ran S, Segal S. Sex-associated differences in the regulation of immune responses controlled by the MHC of the mouse. J Immunol. (1984) 132:656–61.
47. Pisitkun P, Deane JA, Difilippantonio MJ, Tarasenko T, Satterthwaite AB, Bolland S. Autoreactive B cell responses to RNA-related antigens due to TLR7 gene duplication. Science. (2006) 312:1669–72. doi: 10.1126/science.1124978
48. Whitacre CC. Sex differences in autoimmune disease. Nat Immunol. (2001) 2:777–80. doi: 10.1038/ni0901-777
49. Voskuhl R. Sex differences in autoimmune diseases. Biol Sex Differ. (2011) 2:1. doi: 10.1186/2042-6410-2-1
50. Zhang MA, Rego D, Moshkova M, Kebir H, Chruscinski A, Nguyen H, et al. Peroxisome proliferator-activated receptor (PPAR)alpha and -gamma regulate IFNgamma and IL-17A production by human T cells in a sex-specific way. Proc Natl Acad Sci USA. (2012) 109:9505–10. doi: 10.1073/pnas.1118458109
51. Palaszynski KM, Loo KK, Ashouri JF, Liu HB, Voskuhl RR. Androgens are protective in experimental autoimmune encephalomyelitis: implications for multiple sclerosis. J Neuroimmunol. (2004) 146:144–52. doi: 10.1016/j.jneuroim.2003.11.004
52. Gold SM, Chalifoux S, Giesser BS, Voskuhl RR. Immune modulation and increased neurotrophic factor production in multiple sclerosis patients treated with testosterone. J Neuroinflammation. (2008) 5:32. doi: 10.1186/1742-2094-5-32
53. Monsen ER, Okey R, Lyman RL. Effect of diet and sex on the relative lipid composition of plasma and red blood cells in the rat. Metabolism. (1962) 11:1113–24.
54. Pergola C, Rogge A, Dodt G, Northoff H, Weinigel C, Barz D, et al. Testosterone suppresses phospholipase D, causing sex differences in leukotriene biosynthesis in human monocytes. FASEB J. (2011) 25:3377–87. doi: 10.1096/fj.11-182758
55. Rossi A, Pergola C, Pace S, Radmark O, Werz O, Sautebin L, et al. In vivo sex differences in leukotriene biosynthesis in zymosan-induced peritonitis. Pharmacol Res. (2014) 87:1–7. doi: 10.1016/j.phrs.2014.05.011
56. Rossi A, Roviezzo F, Sorrentino R, Riemma MA, Cerqua I, Bilancia R, et al. Leukotriene-mediated sex dimorphism in murine asthma-like features during allergen sensitization. Pharmacol Res. (2019) 139:182–90. doi: 10.1016/j.phrs.2018.11.024
57. Pace S, Rossi A, Krauth V, Dehm F, Troisi F, Bilancia R, et al. Sex differences in prostaglandin biosynthesis in neutrophils during acute inflammation. Sci Rep. (2017) 7:3759. doi: 10.1038/s41598-017-03696-8
58. Miyagi M, Morishita M, Iwamoto Y. Effects of sex hormones on production of prostaglandin E2 by human peripheral monocytes. J Periodontol. (1993) 64:1075–8. doi: 10.1902/jop.1993.64.11.1075
59. Osterlund KL, Handa RJ, Gonzales RJ. Dihydrotestosterone alters cyclooxygenase-2 levels in human coronary artery smooth muscle cells. Am J Physiol Endocrinol Metab. (2010) 298:E838–45. doi: 10.1152/ajpendo.00693.2009
60. Norata GD, Tibolla G, Seccomandi PM, Poletti A, Catapano AL. Dihydrotestosterone decreases tumor necrosis factor-alpha and lipopolysaccharide-induced inflammatory response in human endothelial cells. J Clin Endocrinol Metab. (2006) 91:546–54. doi: 10.1210/jc.2005-1664
61. Badr FM. Effect of sexual maturation and androgens on prostaglandin levels in tissues of the male reproductive system in mice. Endocrinology. (1976) 98:1523–7. doi: 10.1210/endo-98-6-1523
62. Ganesan K, Selvam R, Abhirami R, Raju KV, Manohar BM, Puvanakrishnan R. Gender differences and protective effects of testosterone in collagen induced arthritis in rats. Rheumatol Int. (2008) 28:345–53. doi: 10.1007/s00296-007-0446-y
63. Razmara A, Krause DN, Duckles SP. Testosterone augments endotoxin-mediated cerebrovascular inflammation in male rats. Am J Physiol Heart Circ Physiol. (2005) 289:H1843–50. doi: 10.1152/ajpheart.00465.2005
64. Gonzales RJ, Duckles SP, Krause DN. Dihydrotestosterone stimulates cerebrovascular inflammation through NFkappaB, modulating contractile function. J Cereb Blood Flow Metab. (2009) 29:244–53. doi: 10.1038/jcbfm.2008.115
65. Ito H, Wang D, Zha X, Inamura S, Seki M, Taga M, et al. Castration increases PGE2 release from the bladder epithelium in male rats. Life Sci. (2018) 193:252–6. doi: 10.1016/j.lfs.2017.10.037
66. Pace S, Sautebin L, Werz O. Sex-biased eicosanoid biology: impact for sex differences in inflammation and consequences for pharmacotherapy. Biochem Pharmacol. (2017) 145:1–11. doi: 10.1016/j.bcp.2017.06.128
67. Okemoto K, Maekawa K, Tajima Y, Tohkin M, Saito Y. Cross-Classification of human urinary lipidome by sex, age, and body mass index. PLoS ONE. (2016) 11:e0168188. doi: 10.1371/journal.pone.0168188
68. Goulet JL, Griffiths RC, Ruiz P, Spurney RF, Pisetsky DS, Koller BH, et al. Deficiency of 5-lipoxygenase abolishes sex-related survival differences in MRL-lpr/lpr mice. J Immunol. (1999) 163:359–66.
69. Haribabu B, Verghese MW, Steeber DA, Sellars DD, Bock CB, Snyderman R, et al. Targeted disruption of the leukotriene B(4) receptor in mice reveals its role in inflammation and platelet-activating factor-induced anaphylaxis. J Exp Med. (2000) 192:433–8. doi: 10.1084/jem.192.3.433
70. Savari S, Chandrashekar NK, Osman J, Douglas D, Bellamkonda K, Jonsson G, et al. Cysteinyl leukotriene 1 receptor influences intestinal polyp incidence in a gender-specific manner in the ApcMin/+ mouse model. Carcinogenesis. (2016) 37:491–9. doi: 10.1093/carcin/bgw031
71. Leff JA, Busse WW, Pearlman D, Bronsky EA, Kemp J, Hendeles L, et al. Montelukast, a leukotriene-receptor antagonist, for the treatment of mild asthma and exercise-induced bronchoconstriction. N Engl J Med. (1998) 339:147–52. doi: 10.1056/NEJM199807163390302
72. Esposito R, Spaziano G, Giannattasio D, Ferrigno F, Liparulo A, Rossi A, et al. Montelukast improves symptoms and lung function in asthmatic women compared with men. Front Pharmacol. (2019) 10:1094. doi: 10.3389/fphar.2019.01094
73. Johnston NW, Mandhane PJ, Dai J, Duncan JM, Greene JM, Lambert K, et al. Attenuation of the September epidemic of asthma exacerbations in children: a randomized, controlled trial of montelukast added to usual therapy. Pediatrics. (2007) 120:e702–12. doi: 10.1542/peds.2006-3317
74. Francois H, Facemire C, Kumar A, Audoly L, Koller B, Coffman T, et al. Role of microsomal prostaglandin E synthase 1 in the kidney. J Am Soc Nephrol. (2007) 18:1466–75. doi: 10.1681/ASN.2006040343
75. Sullivan JC, Sasser JM, Pollock DM, Pollock JS. Sexual dimorphism in renal production of prostanoids in spontaneously hypertensive rats. Hypertension. (2005) 45:406–11. doi: 10.1161/01.HYP.0000156879.83448.93
76. Hatano R, Onoe K, Obara M, Matsubara M, Kanai Y, Muto S, et al. Sex hormones induce a gender-related difference in renal expression of a novel prostaglandin transporter, OAT-PG, influencing basal PGE2 concentration. Am J Physiol Renal Physiol. (2012) 302:F342–9. doi: 10.1152/ajprenal.00366.2011
77. Brito HO, Radulski DR, Wilhelms DB, Stojakovic A, Brito LM, Engblom D, et al. Female sex hormones influence the febrile response induced by lipopolysaccharide, cytokines and prostaglandins but not by Interleukin-1β in rats. J Neuroendocrinol. (2016) 28(10). doi: 10.1111/jne.12414
78. Gunther M, Plantman S, Davidsson J, Angeria M, Mathiesen T, Risling M, et al. COX-2 regulation and TUNEL-positive cell death differ between genders in the secondary inflammatory response following experimental penetrating focal brain injury in rats. Acta Neurochir. (2015) 157:649–59. doi: 10.1007/s00701-014-2331-2
79. Mouihate A, Clerget-Froidevaux MS, Nakamura K, Negishi M, Wallace JL, Pittman QJ, et al. Suppression of fever at near term is associated with reduced COX-2 protein expression in rat hypothalamus. Am J Physiol Regul Integr Comp Physiol. (2002) 283:R800–5. doi: 10.1152/ajpregu.00258.2002
80. Mouihate A, Pittman QJ. Neuroimmune response to endogenous and exogenous pyrogens is differently modulated by sex steroids. Endocrinology. (2003) 144:2454–60. doi: 10.1210/en.2002-0093
81. Mallery SR, Zeligs BJ, Ramwell PW, Bellanti JA. Gender-related variations and interaction of human neutrophil cyclooxygenase and oxidative burst metabolites. J Leukoc Biol. (1986) 40:133–46. doi: 10.1002/jlb.40.2.133
82. Pinto S, Coppo M, Paniccia R, Prisco D, Gori AM, Attanasio M, et al. Sex related differences in platelet TxA2 generation. Prostaglandins Leukot Essent Fatty Acids. (1990) 40:217–21. doi: 10.1016/0952-3278(90)90101-P
83. Kolaczkowska E, Kubes P. Neutrophil recruitment and function in health and inflammation. Nat Rev Immunol. (2013) 13:159–75. doi: 10.1038/nri3399
84. Chuang KH, Altuwaijri S, Li G, Lai JJ, Chu CY, Lai KP, et al. Neutropenia with impaired host defense against microbial infection in mice lacking androgen receptor. J Exp Med. (2009) 206:1181–99. doi: 10.1084/jem.20082521
85. Osgood RS, Kasahara DI, Tashiro H, Cho Y, Shore SA. Androgens augment pulmonary responses to ozone in mice. Physiol Rep. (2019) 7:e14214. doi: 10.14814/phy2.14214
86. Scalerandi MV, Peinetti N, Leimgruber C, Cuello Rubio MM, Nicola JP, Menezes GB, et al. Inefficient N2-Like Neutrophils Are Promoted by Androgens During Infection. Front Immunol. (2018) 9:1980. doi: 10.3389/fimmu.2018.01980
87. Deitch EA, Ananthakrishnan P, Cohen DB, Xu DZ, Feketeova E, Hauser CJ. Neutrophil activation is modulated by sex hormones after trauma-hemorrhagic shock and burn injuries. Am J Physiol Heart Circ Physiol. (2006) 291:H1456–65. doi: 10.1152/ajpheart.00694.2005
88. Jaillon S, Berthenet K, Garlanda C. Sexual Dimorphism in Innate Immunity. Clin Rev Allergy Immunol. (2019) 56:308–21. doi: 10.1007/s12016-017-8648-x
89. Guilliams M, Mildner A, Yona S. Developmental and functional heterogeneity of monocytes. Immunity. (2018) 49:595–613. doi: 10.1016/j.immuni.2018.10.005
90. Murray PJ, Allen JE, Biswas SK, Fisher EA, Gilroy DW, Goerdt S, et al. Macrophage activation and polarization: nomenclature and experimental guidelines. Immunity. (2014) 41:14–20. doi: 10.1016/j.immuni.2014.06.008
91. Rettew JA, Huet-Hudson YM, Marriott I. Testosterone reduces macrophage expression in the mouse of toll-like receptor 4, a trigger for inflammation and innate immunity. Biol Reprod. (2008) 78:432–7. doi: 10.1095/biolreprod.107.063545
92. Marriott I, Bost KL, Huet-Hudson YM. Sexual dimorphism in expression of receptors for bacterial lipopolysaccharides in murine macrophages: a possible mechanism for gender-based differences in endotoxic shock susceptibility. J Reprod Immunol. (2006) 71:12–27. doi: 10.1016/j.jri.2006.01.004
93. Li K, Xu W, Guo Q, Jiang Z, Wang P, Yue Y, et al. Differential macrophage polarization in male and female BALB/c mice infected with coxsackievirus B3 defines susceptibility to viral myocarditis. Circ Res. (2009) 105:353–64. doi: 10.1161/CIRCRESAHA.109.195230
94. Samuelsson B, Borgeat P, Hammarstrom S, Murphy RC. Introduction of a nomenclature: leukotrienes. Prostaglandins. (1979) 17:785–7. doi: 10.1016/0090-6980(79)90052-2
95. Sahin A, Kam WR, Darabad RR, Topilow K, Sullivan DA. Regulation of leukotriene B4 secretion by human corneal, conjunctival, and meibomian gland epithelial cells. Arch Ophthalmol. (2012) 130:1013–8. doi: 10.1001/archophthalmol.2012.1067
96. Wang L, Du C, Lv J, Wei W, Cui Y, Xie X. Antiasthmatic drugs targeting the cysteinyl leukotriene receptor 1 alleviate central nervous system inflammatory cell infiltration and pathogenesis of experimental autoimmune encephalomyelitis. J Immunol. (2011) 187:2336–45. doi: 10.4049/jimmunol.1100333
97. Werz O, Burkert E, Fischer L, Szellas D, Dishart D, Samuelsson B, et al. Extracellular signal-regulated kinases phosphorylate 5-lipoxygenase and stimulate 5-lipoxygenase product formation in leukocytes. FASEB J. (2002) 16:1441–3. doi: 10.1096/fj.01-0909fje
98. Zhu X, Li H, Liu JP, Funder JW. Androgen stimulates mitogen-activated protein kinase in human breast cancer cells. Mol Cell Endocrinol. (1999) 152:199–206. doi: 10.1016/S0303-7207(99)00031-3
99. Albert D, Buerkert E, Steinhilber D, Werz O. Induction of 5-lipoxygenase activation in polymorphonuclear leukocytes by 1-oleoyl-2-acetylglycerol. Biochim Biophys Acta. (2003) 1631:85–93. doi: 10.1016/S1388-1981(02)00359-1
100. Hornig C, Albert D, Fischer L, Hornig M, Radmark O, Steinhilber D, et al. 1-Oleoyl-2-acetylglycerol stimulates 5-lipoxygenase activity via a putative (phospho)lipid binding site within the N-terminal C2-like domain. J Biol Chem. (2005) 280:26913–21. doi: 10.1074/jbc.M500068200
101. Pergola C, Schaible AM, Nikels F, Dodt G, Northoff H, Werz O, et al. Progesterone rapidly down-regulates the biosynthesis of 5-lipoxygenase products in human primary monocytes. Pharmacol Res. (2015) 94:42–50. doi: 10.1016/j.phrs.2015.01.007
102. Zaitsu M, Narita S, Lambert KC, Grady JJ, Estes DM, Curran EM, et al. Estradiol activates mast cells via a non-genomic estrogen receptor-alpha and calcium influx. Mol Immunol. (2007) 44:1977–85. doi: 10.1016/j.molimm.2006.09.030
103. Schaible AM, Koeberle A, Northoff H, Lawrenz B, Weinigel C, Barz D, et al. High capacity for leukotriene biosynthesis in peripheral blood during pregnancy. Prostaglandins Leukot Essent Fatty Acids. (2013) 89:245–55. doi: 10.1016/j.plefa.2013.06.004
104. Pali-Scholl I, Namazy J, Jensen-Jarolim E. Allergic diseases and asthma in pregnancy, a secondary publication. World Allergy Organ J. (2017) 10:10. doi: 10.1186/s40413-017-0141-8
105. Wu CC, Schwartzman ML. The role of 20-HETE in androgen-mediated hypertension. Prostaglandins Other Lipid Mediat. (2011) 96:45–53. doi: 10.1016/j.prostaglandins.2011.06.006
106. Wu CC, Mei S, Cheng J, Ding Y, Weidenhammer A, Garcia V, et al. Androgen-sensitive hypertension associates with upregulated vascular CYP4A12–20-HETE synthase. J Am Soc Nephrol. (2013) 24:1288–96. doi: 10.1681/ASN.2012070714
107. Dordea AC, Vandenwijngaert S, Garcia V, Tainsh RE, Nathan DI, Allen K, et al. Androgen-sensitive hypertension associated with soluble guanylate cyclase-alpha1 deficiency is mediated by 20-HETE. Am J Physiol Heart Circ Physiol. (2016) 310:H1790–800. doi: 10.1152/ajpheart.00877.2015
Keywords: neutrophils, lipid mediators, 5-lipoxygenase, testosterone, leukotriene, sex
Citation: Pace S and Werz O (2020) Impact of Androgens on Inflammation-Related Lipid Mediator Biosynthesis in Innate Immune Cells. Front. Immunol. 11:1356. doi: 10.3389/fimmu.2020.01356
Received: 27 February 2020; Accepted: 27 May 2020;
Published: 30 June 2020.
Edited by:
Hanna Lotter, Bernhard Nocht Institute for Tropical Medicine (BMITM), GermanyReviewed by:
Krishna Rao Maddipati, Wayne State University, United StatesAnna Nicolaou, University of Manchester, United Kingdom
Copyright © 2020 Pace and Werz. This is an open-access article distributed under the terms of the Creative Commons Attribution License (CC BY). The use, distribution or reproduction in other forums is permitted, provided the original author(s) and the copyright owner(s) are credited and that the original publication in this journal is cited, in accordance with accepted academic practice. No use, distribution or reproduction is permitted which does not comply with these terms.
*Correspondence: Oliver Werz, oliver.werz@uni-jena.de