- 1Sorbonne University, INSERM, Association Institute of Myology, Center of Research in Myology, Paris, France
- 2Department of Pharmacology and Physiology, The George Washington University, Washington, DC, United States
The thymus, a primary lymphoid organ, provides a complex environment essential for the generation of the T-cell repertoire. Thymic alterations occur during life either in the context of thymic involution upon aging or the pathophysiological context of Myasthenia Gravis (MG). These changes involve complicated regulatory networks, in which microRNAs (miRNAs) are key players. Here, we analyzed the role of miRNAs in thymocyte maturation and differentiation sustained by thymic epithelial cells. We compared data from the literature regarding the role of mouse thymic miRNAs and original data obtained from a human thymic miRnome study. We identified a set of highly expressed miRNAs defined as ThymiRs and investigated miRNA expression in infants as compared to adults to determine those associated with human thymic involution. Thymic changes are also frequently observed in MG, an autoimmune disease which results in the production of anti-acetylcholine receptor (AChR) antibodies that lead to muscle weaknesses. Alterations such as thymoma in late-onset MG patients and hyperplasia with ectopic germinal centers (GCs) in early-onset (EOMG) patients are found. Thymic miRNA expression has been studied in AChR-MG patients both in thymoma-associated MG (TAMG) and EOMG, and their function through their mRNA targets investigated. Most of the dysregulated thymic miRNAs in EOMG are associated with GC development, such as miR-7, miR-24, miR-139, miR-143, miR-145, miR-146, miR-150, miR-452, miR-548 or thymic inflammation, such as miR-125b, miR-146, or miR-29. Understanding these pathways may provide therapeutic targets or biomarkers of disease manifestations.
Overview of miRNAs
MicroRNAs (miRNAs) correspond to non-coding short single-stranded RNAs (~22 nucleotides) that serve as post-transcriptional regulators. They are mainly transcribed and process through the canonical pathway as pri-miRNAs, cleaved into pre-miRNAs by DROSHA and DGCR8 (DiGeorge syndrome Critical Region gene 8) in the nucleus and exported to the cytoplasm by the protein exportin 5. Next, pre-miRNAs are cleaved by DICER and its partner TRBP1 into mature miRNAs. Mature miRNAs coupled with the RNA-induced silencing complex (RISC, a heterogeneous molecular complex) target mRNAs, leading to their degradation or the inhibition of their translation, according to the perfect or imperfect miRNA-mRNA matching, respectively (1). They are involved in physiological and pathophysiological processes, including autoimmune diseases (2).
Autoimmune diseases result from the dysfunction of the immune process. The breakdown of immunological tolerance leads to the presence of autoreactive immune cells which cause the destruction of self (3). miRNAs play crucial roles in the immune process through the development of the immune system, proliferation of key immunological cells, differentiation of cells into their lineage, and apoptosis at immunological checkpoints (4). Disturbance along the process by altered expression of miRNAs and their downstream function can initiate or maintain autoimmune conditions. Involvement of miRNAs, specifically key miRNAs (e.g. miR-21, miR-146, miR-155, miR-146, 125a-5p) is already well-documented in major autoimmune diseases such as lupus erythematosus, multiple sclerosis, diabetes or rheumatoid arthritis. Here, we highlight the significance of miRNAs in the development of the thymus and the ability for dysfunction to result in an autoimmune disease, myasthenia gravis.
Physiological Role of the Thymus
The thymus provides a complex environment essential for the generation of the T-cell repertoire. It is composed of various cell types, essentially thymocytes and thymic epithelial cells (TECs), but also fibroblasts, myoid cells, dendritic cells, macrophages, and B cells. Differentiation of thymocytes occurs through interactions with stromal cells while they are progressing in the different thymus compartments (5).
In their first differentiation steps, immature thymocyte precursors become progressively double positive (DP) for CD4 and CD8 co-receptors and acquire a complete T-cell receptor (TCR). Further successful differentiation of thymocytes depends on the quality and the specificity of the interaction of their T-cell receptor (TCR) with self-major histocompatibility complex (MHC) molecules. The large majority of thymocytes die either because the TCR-MHC interaction is too weak (death by neglect) or, in contrast, because the TCR-MHC interaction is too strong (negative selection, self-tolerance). In parallel, positively selected CD4+CD8+ thymocytes end up single positive (SP) for either CD4 or CD8 (lineage commitment) (5). Only a few thymocytes pass successfully selection and are exported to the periphery where they will differentiate into different T-cell subsets. However, within the thymic environment, some T cells can differentiate into natural regulatory T (Treg) cells (5).
TECs represent the main cell type amongst thymic stromal cells and include cortical and medullary TECs (cTECs and mTECs). mTECs play a central role in negative selection of thymocytes through their capacity to express a wide range of tissue-specific antigens (TSAs) and mediate depletion of autoreactive T cells. The ectopic expression of TSAs by mTECs is controlled by epigenetic factors and by transcription factors, the most well-known being the autoimmune regulator (AIRE) (6). The thymus is fully active during the neonatal period and undergoes an involution process early during life (after 1-year-old). Thymic involution is characterized by the alteration of thymic architecture and the loss of thymic function. In particular, involution is associated with a decrease in TECs, more prominent for mTECs as compared to cTECs, and replacement by fat tissue. Despite the decrease in cellular density, the adult thymus still contains thymocytes and maintains the proportion of the principal thymocyte subsets indicating that the thymus remains active during adult life (7).
Implications of miRNAs in the Mouse and Human Thymus
The role of enzymes involved in the biogenesis of miRNAs and specific miRNAs in T-cell lineage in the periphery has been largely documented (8, 9). Here, we will specifically review the role of miRNAs in the thymus, in particular in thymic epithelial cells (TECs) with their potential impact on thymic architecture, and on thymocyte differentiation.
Certain miRNAs are defined as immuno-miRs as they regulate many functions related to the immune system, or myomiRs, which are more particularly expressed in skeletal muscle. The most well-known immuno-miR are miR-146, miR-155 and the cluster miR-17~92 (10). From original results detailed in Figure 1 and Table 1, we analyzed the human thymus of infants for the most expressed miRNAs that could be defined as ThymiRs (Figure 1). The highly expressed human ThymiRs contained the most well-known immuno-miR, such as miR-146, miR-150, miR-155, miR-181 subtypes, some miRNAs from the miR-17~92 cluster and the paralogous clusters miR-106b-25 and miR-106a-363. They also included six let-7 subtypes (Table 1).
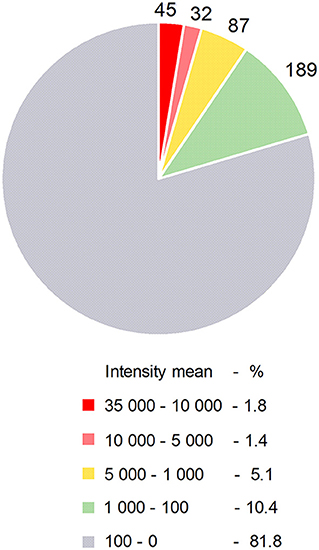
Figure 1. Human ThymiRs. Repartition of ThymiRs according to the mean of intensities. Thymic biopsies were collected from female infants undergoing cardiovascular surgery at the Marie Lannelongue Surgical Center (Le Plessis-Robinson, France) (n = 6, 3–12 months old). Studies on thymuses were approved by a local ethics committee (CPP, authorization number ID RCB 2010-A00250-39). Total RNA extraction and miRNA analyses were done as described in Cron et al. (11). Raw data were imported using R package pd.mirna.3.0 (v 3.12.0). Raw intensity values were background corrected and normalized with RMA (Robust Multi-array Average) function from oligo R package (1.48.0).
miRNAs and Thymic Epithelial Cells
Different studies have proved that molecules involved in the biogenesis of miRNAs play a central role in TEC differentiation.
Using FoxN1 (Forkhead box protein N1)-Cre knock-in mice to conditionally inactivate Dgcr8 in TECs, Khan et al. demonstrated that Dgcr8 is critical for maintaining a proper thymic architecture and that canonical miRNAs are required to support TEC cellularity and differentiation. In particular, they observed a progressive loss of AIRE+ mTECs that could affect central tolerance and favor the development of autoimmune diseases (12). Embryonic loss of Dicer in TECs results in premature thymic involution, progressive disorganization of the thymic epithelium and the formation of epithelial voids. The normal differentiation and function of TECs are altered, impacting thymocyte development and inducing phenotypic changes in peripheral T cells. Loss of Dicer expression in TECs clearly affects T-cell development from the second week of life with an increase in DP and a decrease in CD8+ and CD4+ SP T cells (13). A higher number of double-negative (DN) cells is also observed, in part due to an increased number of immature B cells in the thymus of these Dicer deficient mice. They could develop in situ, possibly due to changes in the microenvironment as the thymic medulla progressively displayed more Lyve-1 lymphatic vessels, PNAd high endothelial venules, and CR1 follicular dendritic cells that are normally observed in secondary lymphoid tissues (13, 14). Besides, several publications showed a decrease in both Aire-dependent and -independent TSAs in Dicer-deficient mTECs (15); altered expression of TSAs in mice that lack Dicer expression in TECs could be correlated with multiorgan infiltrations (13).
Papadopoulou et al. demonstrated that certain features of the premature thymic involution phenotype of Dicer mutants are recapitulated in mouse mutants lacking miR-29a; however, these animals do not display the same architectural disruption of the thymus, implying that other miRNAs regulate TEC maintenance (14). Besides, the absence of miR-29a selectively affects the Aire-dependent TSA gene expression (15). However, an increase in thymic miR-29 subtypes is observed together with thymic involution in aging mice (16).
The changes observed in Dicer or Dgcr8 deficient mice are usually attributed to the loss of miRNAs. However, we have to keep in mind that DICER can process other types of RNAs and regulate different cellular functions beyond its endonuclease activity (17). As for DGCR8, it is involved in maintaining heterochromatin organization and attenuating senescence, independently of its microRNA-processing activity (18).
Ucar et al. analyzed the expression of miRNAs in isolated cTECs and mTECs in human and mouse thymuses. They demonstrated that certain miRNAs are differentially expressed in cTECs and mTECs, and even differentially expressed upon mTEC maturation. Using different approaches for selected miRNAs they observed that in mice some miRNAs are down-regulated in CD80+AIRE+mTECs as compared to CD80+AIRE−mTECs. This suggests that TEC differentiation could be associated with a decreased expression of certain miRNAs allowing, for example, a higher expression of AIRE. Inversely, AIRE regulates the expression of specific miRNAs. They showed in AIRE-deficient mice that some miRNAs can be either up or down-regulated in CD80+mTECs as compared to wild type mice (15). This was confirmed by Macedo et al. that demonstrated that silencing Aire in mouse mTECs leads to the up- and down-regulation of specific miRNAs (19). The identified dysregulated miRNAs from these studies were different. However, we can hypothesize that in AIRE+TECs, miRNAs that are decreased could lead to the specific expression of TSAs that are implicated in central tolerance mechanisms.
miRNAs and Thymocyte Development
Dicer deletion from the double-positive stage of T-cell development compromises the survival of αβ lineage cells and results in a decreased thymic cellularity in DP and SP T cells. Surprisingly, Dicer seems to be dispensable for CD4+ and CD8+ T cell lineage commitment (20, 21). Dicer or Drosha deletion at a later stage in CD4+ T cell does not alter the number and composition of thymocytes, though it results in a reduction in thymic CD4+CD25+Foxp3+ natural Treg cells. Dicer depletion in CD4+ thymocytes also results in the reduction of invariant natural killer T (iNKT) cells (22). Besides, mice with T-cell specific Dicer or Drosha deficiency develop immune pathologies, in particular, inflammatory bowel disease (23) and organ inflammation (24). Deletion of Dicer or Drosha results in the loss of mature miRNAs generated via the canonical biogenesis pathways in which not all miRNAs are deleted since other biogenesis pathways are involved (1). Nevertheless, these results demonstrate that miRNAs can modulate T-cell development. Different studies have shown that individual miRNAs are dynamically regulated during T-cell development and maturation as detailed below (25–27).
Implication of miRNAs in Thymic Involution
Thymic involution is a natural process occurring with age and an adaptive process in response to stress situations. Thymic involution is characterized by morphological and functional changes and includes TEC-driven programmed thymic involution and thymocyte apoptosis. As miRNAs regulate numerous physiological and pathophysiological processes, they are probably key orchestrators of thymic involution.
Thymic Involution With Aging
The expression of miRNAs is clearly modified in the aging mouse thymus (16). Guo et al. investigated thymic miRNA expression in 3 and 12 months old female and male mice as thymic involution is often described as being also sex-hormone dependent. Thymic atrophy is clearly observed in the first year of life, and even more in males as compared to females. Specific miRNAs exhibit age- or sex-differentially expression. Among them, miR-2137 is increased in the aging thymus and particularly in mTECs (28). Some miRNAs seem dysregulated more specifically in female mice and to determine if the female-biased miRNAs were linked to estrogen, the expression of specific miRNAs was measured in the thymus either in ovariectomized or castrated mice treated with estradiol. Results show that miR-27b and miR-378a are estrogen-responsive miRNAs in mouse thymus (28).
miRNAs expression has also been investigated in isolated TECs from aging-mice and 17 miRNAs were showed to be down-regulated upon aging. In particular, miRNAs known to possess immune function, such as miR-146, miR-150, miR 155, miR-181 subtypes and some miRNAs from the miR-17~92 cluster. The decreases observed in TECs are correlated with the age-related thymic weight loss suggesting that miRNA decreased expression in TECs precedes thymic involution (29).
In human thymus, we analyzed dysregulated thymic miRNAs in female adults (15–33 years old) compared to female infants (3–12 months old). Of the miRNAs assessed, 56 were up-regulated and 87 were down-regulated in the adult thymuses upon aging, respectively (Tables 2A,B). From these lists of dysregulated miRNAs in the course of thymic involution, among the up-regulated ones, those that seemed particularly interesting were those that reached in adults a fluorescence intensity mean above 1,000 (arbitrarily chosen). These results must be taken into account with caution due to the small sample size and the lack of validation. However, several of them, such as let-7b, miR-139, miR-193a, miR-214, miR-27b are also up-regulated in the thymus of male mice upon aging (28). miR-182 and miR-200b are also found up-regulated in isolated TECs from aging mice (30). miR-195a-5p is highly up-regulated in the TECs isolated from the aging mice inhibiting the proliferation of mTECs by directly targeting Smad7 (31). As for miR-451, its expression is up-regulated in the thymus of systemic lupus erythematosus mouse (32).
For the down-regulated miRNAs (Table 2B), we observed that miR-181 subtypes are significantly down-regulated with aging (see the miR-181 paragraph and Figure 2A below for more details). Besides, among miRNAs that had a fluorescence intensity mean above 1,000 in infants and that significantly decreased with aging, the majority of them belong to the miR-17~92 cluster or the paralogous miR-106a~363 cluster (33).
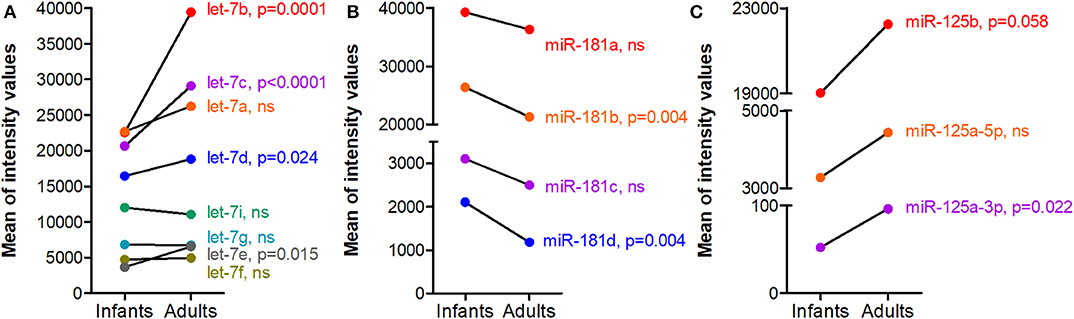
Figure 2. Dysregulated miRNAs during human thymic involution. Thymic biopsies were collected and processed for miRNA analyses as detailed in Figure 1. Mean of normalized intensity values were calculated for each miRNA for infants (n = 6, 3–12 months old females) and adults (n = 6, 15–33 years old females) data. (A) let-7, (B) miR-181, and (C) miR-125 families. Differential expression analysis was performed using Limma R package (v 3.40.6). Limma fits a linear model to expression data for each miRNA and Empirical Bayes method was used to generate differential expression statistics: p-values and fold-change values.
Thymic Involution Linked to Stress
Thymic involution is also observed upon pathogen infections. This could be mediated via the increased expression of interferon (IFN)-I subtypes that will target thymic different cells. Loss of the miRNAs through the deletion of Dicer or the miR-29a cluster in TECs results in elevated sensitivity of TECs to Poly(I:C), a molecule mimicking dsRNA from viral infection, and premature thymic involution. In mice, miR-29a regulates the expression of IFNAR1 (one of the subunits of the IFN-I receptor) and consequently controls the ability of TECs to respond to pathogen infections and IFN-I signalization (14). Linhares-Lacerda et al. observed the up-regulation of miRNAs in mouse TECs upon Trypaosoma cruzi infection but not that of miR-29 subtypes (34).
Glucocorticoids can also induce thymic involution, mainly by triggering DP T cell apoptosis. Upon thymocytes exposure to glucocorticoids, a decrease expression of Drosha, Dicer, and Dgcr8 is observed and subsequently a decreased expression of miRNAs such as the miRNAs of the miR-17~92 cluster (35, 36). Smith et al. demonstrated that Dicer depletion induces T-cell apoptosis while the overexpression of the miR-17~92 cluster reduces it (35).
Focus on miRNAs Clearly Involved in the Thymic Functions
Let-7 miRNAs
The let-7 miRNAs were among the first to be identified in mammals and they represent the most abundant miRNAs in the genome. The let-7 miRNAs consists of 12–14 members encoded on different chromosomes. They contain the identical seed region interacting with mRNAs. Mature functional let-7 miRNA expression is inhibited by the RNA binding protein LIN28 expressed in hematopoietic stem cells (37). An increase of several let-7 family members is observed together with thymic involution in the thymus of aging mice (16). An increased expression was also observed for numerous let-7 members in female human thymus of adults as compared to infants (Figure 2A).
Pobezinsky et al. investigated the role of let-7 miRNAs in mouse thymic T-cell differentiation. They demonstrated that let-7 miRNAs control the expression of the transcription factor Plzf (promyelocytic leukemia zinc finger) that regulates NKT-cell differentiation (38). Recently Xiao et al. demonstrated the role played by let-7 miRNAs in the limitation of the number of B cells within the thymus. They used a mouse model, designated as Foxn1lacZ, in which the expression of the critical TEC-specific transcription factor Foxn1 is normal at fetal stages, but down-regulated beginning at postnatal day 7, causing progressive reduction of total thymocytes and premature thymic involution. Early in life in Foxn1 deficient mice, TECs control the up-regulation of let-7b and let-7g in hematopoietic stem cells, suppressing Arid3a expression in intrathymic B cell progenitors to limit their proliferation during the neonatal to adult transition (39).
In human, let-7a expression is highly up-regulated in mature peripheral CD4+ and CD8+ T lymphocytes compared to DP thymocytes. Microarray analyses showed the up-regulation of let7-e, let7-g and let-7f in CD4+ and CD8+ SP thymocytes compared to DP thymocytes and a differential expression for let-7e between CD8+ and CD4+ SP thymocytes (26).
miR-181a
miR-181a is a member of miR-181 family that includes 6 miRNAs: miR-181a-1, miR-181a-2, miR-181b-1, miR-181b-2, miR-181c, and miR-181d (miR-181a-1 and miR-181a-2, as well as miR-181b-1 and miR-181b-2 being identical). In mice, miR-181a is highly expressed in DP thymocytes and controls the development of early thymocytes by targeting TCR and CD69 (40, 41). Besides, miR-181a deletion impairs the development of NKT cells (42). Li et al. suggest that miR-181a acts as an intrinsic antigen sensitivity “rheostat” during T-cell development (40). Thymic miR-181a plays a central role in central tolerance and is important for the elimination of autoreactive T cells (41). In the miR-181 family, miR-181d is one of the most stress-responsive miRNA identified in the thymus upon LPS injections. However, the different miR-181 subtypes could have overlapping or compensatory functions, at least in response to stress (36). In human, Ghisi et al. showed that miR-181d and miR-181c were strongly down-regulated in CD4+ as compared to CD8+ SP thymocytes (26).
The expression of miR-181a and miR-181b is down-regulated in old mice compared to young ones (16) and all the miR-181 subtypes are also decreased in female human thymus of adults as compared to infants (Figure 2B). If miR181a deficiency disrupts thymocytes development, Stefanski et al. demonstrated that miR-181a1 and miR-181b1 are not required for maintenance of thymus integrity and that miR-181a1 and miR-181b1 are dispensable for TEC differentiation. They control thymocyte development and mature T-cell export and homeostasis within the periphery (43). However, Guo et al. showed that miR-181a is decreased in mTECs from aging mice in link with thymic involution. By transfecting mTECs (MTEC1) with a miR-181a mimic, they observed that it induces mTEC proliferation while its antagomiR reversed this effect. miR-181a was shown to target transforming growth factor β receptor (Tgfbr1). Tgfbr1 expression increases with age in mice, which is consistent with the decreased level of miR-181a in addition to the ability of TGF-β to decrease the proliferation of mTECs (44). Recently, Cotrim-Sousa et al. suggest that miR181b and miR-30b modulate the expression of adhesion molecules involved in mTEC and thymocyte interactions (45).
miR-150
First described in 2007 by Zhou et al. in hematopoietic stem cells (46), miR-150 is described as an immuno-miR regulating immune functions, such as proliferation, apoptosis and differentiation of NK, T and B cells. The role of miR-150 has especially being studied in peripheral blood cells.
In the thymus of mice, two different studies showed that miR-150 expression decreases in mTECs from aging mice in link with thymic involution (28, 29). miR-150 differentially controls the development of NK and iNKT cell lineages by targeting c-Myb (47). In transgenic mice overexpressing miR-150, the development of thymocytes is partially blocked, especially the differentiation of DN3 to DN4, which ultimately leads to a decrease in the number of CD4+ and CD8+ SP thymocytes (48). An increased expression of miR-150 is observed during the maturation of iNKT cells in the mouse thymus and miR-150 deletion results in an interruption of iNKT cell final maturation in both thymus and periphery (49).
From transcriptomic analyses on purified human or mouse thymocytes and TECs, it was observed that miR-150 is highly expressed in thymocytes compared to mTECs or cTECs (15). In the human thymus, Ghisi et al. showed that miR-150 is upregulated as the maturation of T cell progresses. They showed that miR-150 targets NOTCH3, known to be important for T-cell differentiation, and that forced expression of miR-150 reduces NOTCH3 levels in T-cell lines affecting their proliferation and survival (26).
miR-146
miR-146 family consists of two miRNAs with nearly identical sequences, miR-146a and miR-146b. They are potent immune-miRs and their role in peripheral blood cells has been largely investigated (50).
In the mouse thymus, miR-146a expression fluctuates in thymocytes at different developmental stages, increasing in CD4+ and CD8+ SP thymocytes compared DP thymocytes (51). The overexpression of miR-146a in transgenic mice impairs the process of positive selection during T-cell development and inhibits the further differentiation of selected SP (especially CD8+ SP) thymocytes. The authors further identified 9 positive selection-associated genes, which are downregulated in miR-146a transgenic mice, such as genes encoding major histocompatibility complex class I/II molecules, IL-7 receptor α chain, and Gimap4 (52). However, in transgenic mice with a specific CD4+-driven T cell overexpression, T-cell development occurs normally in the mir-146a transgenic mice and a decrease of CD4+ T-cells is observed together with a reduction in surface expression of CD62L, which is normally upregulated upon thymic egress. Besides, miR-146a and miR-146b target Traf6 and attenuate TCR signaling in the thymus (53).
FoxP3 is an indispensable transcription factor for the generation and the regulatory function of regulatory T cells. miR-146a does not seem critical to the formation of Treg cells in the thymus. Indeed, the role of miR-146a has been investigated in FoxP3+ Treg cells in the thymus and peripheral lymphoid organs of miR-146a-deficient mice. These mice contain significantly increased numbers of FoxP3+ Treg cells in the periphery, but not in the thymus (54).
In the human thymus, it was shown that miR-146a and miR-146b are the most up-regulated miRNAs as DP thymocytes differentiate in SP CD4+ or CD8+ thymocytes (26).
miR-155
miR-155 is a highly studied immuno-miR and plays an important role in T-cell homeostasis as fully reviewed by Mashima (55).
In the mouse thymus, the absence of miR-155 in hematopoietic cells tends to decrease the global representation of thymocytes but it is likely due to a defect occurring at an early stage of thymocyte development, as the proportions of DN, DP, and CD4+ and CD8+ SP cells are not affected. However, mouse thymic expression of miR-155 seems to fluctuate in thymocytes at different developmental stages in the thymus (26, 51). In the absence of miR-155, a reduction of the number of Treg cells is observed both in the thymus and in the periphery in mice. miR-155 appears to be required for Treg cells development and its absence compromises their proliferation, survival and function in the periphery (56). It was demonstrated that an increased expression of miR-155 in both human and mouse CD4+ T helper cells leads to a reduced susceptibility of Treg cells in mediating T-cell suppression, whereas a decreased expression of miR-155- results in a more pronounced suppression by natural Treg cells (57). The control of miR-155 is also required for the proper development of iNKT cells in the mouse thymus with inhibition of miR-155 expression along with iNKT cell differentiation (58).
The role of miR-155 in TECs has not been investigated but its expression decreases with age-induced thymic involution in mice and this might be related to a decreased expression in TECs (29).
miR-125
miR-125a and miR-125b sequences are highly conserved throughout diverse species. They have been found differentially regulated in several human disorders (50). They could be involved in inflammatory diseases. miR-125a regulates TNFAIP3 that is a negative regulator of the NF-κB signaling pathway (59). miR-125b targets TRAF6 and regulates IL-1β induced inflammatory genes (60).
miR-215a and miR-125b are up-regulated in the thymus of aging mice (16, 61) and also in the aging human thymus (Figure 2C). miR-125a by targeting FoxN1, decreases its expression associated with thymic involution (61). From transcriptomic analyses on purified human or mouse thymocytes and TECs, it was observed that miR-125a and miR-125b are highly expressed in TECs compared to thymocytes (15). Besides, in human thymocytes, miR-125b expression is higher in CD4+ as compared to CD8+ SP thymocytes (26).
Pathological Properties of the Thymus From Myasthenia Gravis Patients
Myasthenia Gravis (MG) is an autoimmune disease due to antibodies against several components of the neuromuscular junction. Patients suffer from more or less invalidating muscle weaknesses leading to a generalized fatigability. The majority of patients (85%) displays antibodies against the acetylcholine receptor (AChR). Thymus abnormalities occur in two subtypes of AChR+ MG patients, thymoma-associated (TAMG) and AChR+ early-onset form (EOMG) (62).
The incidence of TAMG among the MG population is approximately 10-20% (62, 63). Thymomas are rare thymic epithelial cell neoplasms that develop usually in patients after 50 years old, both in women and men. The histological classification by the World Health Organization is based on the nature of the cortical or medullary epithelial cells involved in the tumor: type A for medullary thymoma, type B1 or B2 for respectively predominantly or entirely cortical thymoma, type AB for mixed thymoma (involving both cortical and medullary epithelial cells) and type B3 for cytonuclear atypia thymoma. Several paraneoplastic syndromes are associated with thymoma but the most common is MG with an incidence around 30% but variable from one study to another. Histologically, MG is associated more frequently with type B1, B2, or B3 tumors than with type A or AB tumors. The differences between thymomas and normal thymuses are detailed by Marx and collaborators. Of particular interest neoplastic TECs express less HLA-class II molecules, do not express AIRE in 95% of thymomas and contain reduced numbers of Treg cells (63). These alterations likely promote autoimmune disease occurrence. In addition, the activation of innate immune pathways and an IFN-I signature is observed in thymomas in TAMG (64).
In contrast, EOMG concerns usually female patients before 45–50 years old. Thymus in EOMG is the site of profound structural alterations. One of the main feature characterizing the thymus in AChR+ MG is a lymphoproliferative hyperplasia characterized by increased number of B cells and ectopic germinal centers (GCs). The incidence of lymphoproliferative hyperplasia is approximately 70% of patients with AChR+ MG, and thymectomy has proven to be efficacious (65). The hyperplastic MG thymus displays all the characteristics of tertiary lymphoid organs. Neoangiogenic processes with high endothelial venule and lymphatic vessel development are clearly observed. Several studies have demonstrated the overexpression or differential expression of chemokines, specifically key molecules involved in peripheral cell recruitment and GC development, such as CXCL13 and CCL21 (66). How the thymus turns into a tertiary lymphoid organ is not well-known but local inflammation seems mandatory. Numerous cytokines are overexpressed in the EOMG thymus. In particular, IFN-β orchestrates thymic changes associated with MG (67, 68) and favors the differentiation of pathogenic Th17 T cells (69). As well, different inflammatory signaling pathways are activated, such as Toll-Like Receptor (TLR) and NF-KB pathways that potentially orchestrate thymic changes.
The hallmarks of disease involve dysfunction of cellular pathways including changes in miRNA expression. In MG differentially expressed miRNAs have been noted in the circulating PBMCs (70–73). The concept of miRNA expression as initiation of disease or the maintenance of specific lymphocyte populations has led to the identification of potential biomarkers. The role of miRNAs in MG thymuses has also been studied as detailed below.
miRNA Profile in Thymoma of TAMG
Studies to assess the alterations of miRNAs in thymoma are limited. A study performed by Li, et al. on four thymoma samples compared to four control thymus samples demonstrated the differential expression of 137 miRNAs. Further analysis was performed on miR-125a-5p whose expression is increased in TAMG. They identify FoxP3 as one of the targets of miR-125a-5p (74) and FoxP3 expression is known to be altered in thymoma patients (75).
In their study, Li et al. also identified a decreased expression of miR-376a and miR-376c in TAMG patients. These miRNA could be of interest as their expression is decreased in AIRE-silenced TECs (19) and AIRE expression is known to be strongly down-regulated in TAMG (76).
In a separate study, the expression of miR-19b-5p, which targets thymic stromal lymphopoietin (TSLP), was elevated in MG-related thymomas. The negative correlation with TSLP mRNA contributes to T-cell imbalance and promotes MG-related thymoma development (77). Whereas, the levels of miR-20b were significantly decreased compared to those in adjacent non-tumor tissues, resulting in an increased proliferation of T cells through a NFAT5/CAMTA1 dependent pathway (78).
In an independent analysis of miR-150 expression, we observed a significant decreased of this miRNA expression in the thymus of TAMG patients (data not shown). Regarding its high expression in the thymus (Table 1), its role in thymoma should be further investigated.
Interestingly, a miRnome study was led on thymic epithelial tumors, including thymomas but it is not mentioned whether patients had a concomitant autoimmune MG. It could be interesting to know retrospectively which patients with a given miRNA profile later developed MG (79).
miRNA Profile in the Thymus of EOMG Patients
Specific Dysregulated miRNAs in EOMG
Three miRnome studies have addressed the changes in miRNA expression in the thymus of EOMG patients (11, 80, 81). The multiple differences in the study designs include the thymus samples from EOMG, the designation of controls, the miRNA arrays, and the method of analysis.
The study by Cron et al. used thymus from untreated female EOMG patients compared to healthy age-matched female controls. The thymus samples from untreated EOMG patients displayed both low hyperplasia with 2 or fewer GCs per section and high hyperplasia with an increased number of GCs. The study was performed using the Affymetrix GeneChip miRNA 3.0 Array. 61 miRNAs are found dysregulated (24 up- and 37 down-regulated). The implication of miRNA clusters localized at the extremity of the X chromosome is also demonstrated. Among the dysregulated miRNAs, they focused their attention on the most down-regulated miRNAs: miR-7-5p. Its down-regulation in MG thymuses is confirmed, in particular in TECs, and an inverse correlation is observed between the expression of miR-7 and CCL21; a target mRNA for miR-7 (11). CCL21 is involved in the abnormal recruitment of B cells in the MG thymus and known to participate in GC formation (82).
As with TAMG studies, miR-125a-5p was elevated in the EOMG thymus samples compared to controls. miR-125a-5p by targeting FoxP3 could explain why the suppressive activity of regulatory T cells is severely impaired in EOMG patients and associated with a decreased expression of FoxP3 in CD4+ T cells (83). miR-125a is also known to modulate inflammatory signaling pathways, such as TNFAIP3 (Tumor Necrosis Factor Alpha-Induced Protein 3) a key molecule in the negative regulation of NF-κB (Nuclear Factor κB) and TLR signaling pathways (59, 84), and WDR1 (WD repeat-containing protein 1) implicated in auto-inflammatory processes associated with IL-18 expression (11).
miR-29a is among the miRNAs down-regulated in AIRE+ mTECs identified by Ucar et al. (15), and Cron et al. observed that all miRNAs of the miR-29 family were down-regulated in the thymus of EOMG patients (unpublished data). miR-29a is of particular interest regarding its role in IFN-I signaling sensitization (14) and the central role of IFN-β in the EOMG thymus (67).
The study by Li et al. compared thymic miRNA expression in EOMG patients and controls using the Agilent Human miRNA array (V.18.0). This study was performed using four control and four MG thymuses. They extracted 33 dysregulated miRNAs with numerous down-regulated miRNAs from the miR-548 family. Next, they showed that miR-548k targets the 3′ UTR region of CXCL13 decreasing its expression in Jurkat cells (81). Knowing that CXCL13 is up-regulated in MG TECs and participate to B-cell recruitment and GC development (85), this miRNA could thus play a role in MG pathogenesis.
The study by Sengupta et al. used thymus from EOMG patients and separated the samples based on the presentation of GCs (80). For miRNA assessment 13 out of the 16 MG patients were treated with prednisolone (65). The experimental group contained samples with GCs, whereas, the control samples were thymus samples from EOMG patients that did not contain GCs in the thymus block. The Affymetrix GeneChip miRNA 4.0 Array identified 44 mature miRNAs that are altered in GC rich thymus samples from EOMG compared to EOMG patients with no GC expression. The dysregulated expression of 38 miRNAS is confirmed by RT-PCR (8 up- and 30 down-regulated). These miRNAs and targeted mRNAs are involved in regulatory pathways common to inflammation and immune response, cell cycle regulation and anti-apoptotic pathways. The Regulator of G protein Signaling 13 (RGS13), involved in GC regulation, is identified in EOMG thymuses with GCs and was paired with downregulation of miR-452-5p and miR-139-5p. The increased expression of miR-150-5p is found in EOMG samples with GCs which mirrored the miR-150-5p expression in sera of MG patients. miR-150 is also more expressed in the thymus of MG patients compared to healthy controls, and in particular in patients displaying a high degree of thymic hyperplasia (86). In situ hybridization analyses showed that miR-150 is strongly expressed by B cells of the mantle zone around GCs. The increased expression of miR-150 in hyperplastic MG thymuses seems thus linked to the abnormal presence of B cells and in particular to the development of GCs. By removal of the thymus, the sera levels of miR-150-5p are reduced (70) but no correlations between the degree of thymic hyperplasia and serum levels in MG patients are observed (86, 87). miR-150 overexpression in MG thymuses is also inversely correlated with the expression of MYB, the most well-known miR-150 mRNA target that displays four binding sites. MYB is a regulator factor essential for hematopoiesis and is highly expressed in the thymus (88). MYB is also characterized as an early regulator of T-cell associated diseases with an altered expression in autoimmune diseases (89). In the MG thymus, miR-150 could be secreted by B cells and alter MYB expression locally and consequently affect T cells, resulting in the significant alterations of the T-cell repertoire seen in MG (90).
miR-145, miR-24, and miR-143 are reduced in GC rich thymus samples as compared to samples with no GCs, and these miRNAs are also reduced in PBMCs of EAMG rats (91). Another group has also observed a decreased expression of miR-143 in the thymus of a MG mouse model in which mice were engrafted in hyperplastic thymic biopsies from EOMG patients and showed a link with CXCL13 expression in thymocytes (92).
Recently, miR-146, one of the most well-known immuno-miR, has been shown to be down-regulated in the thymus of EOMG patient as compared to age-matched donors. The decrease was localized in the thymic stroma and not linked to the presence of GCs. This deficiency was inversely correlated with increased expression of miR-146 targets such as IRAK1, c-REL and ICOS. Interestingly, the expression of miR-146 and these target genes were normalized in EOMG patients under corticosteroid treatment. Altogether, these results suggest that miR-146 could modulate TLR signaling via IRAK1 and cREL and GC formation via ICOS (93). In addition, miR-146 is of great interest in MG as it is known to control the activation of IFN-I signaling pathways (94). The decrease of miR-146 could be linked with the overexpression of IFN-β in MG thymuses (67). In addition a decrease expression of this miRNA could have a strong impact on T-cell differentiation in MG and could be associated with the defective function of Treg cells (83, 95) and/or the increase of pathogenic Th17 cells (69, 96).
Pathway Analysis of miRNA Expression in EOMG
To progress in the understanding in the implication of miRNAs in EOMG thymuses beyond individual miRNAs, we provide functional relevance of the miRNAs differentially expressed in two miRnome studies (11, 80). Original results from these two publications have been used and miRNA lists were analyzed through miRNet (https://www.mirnet.ca/miRNet/home.xhtml) and targeted genes used to defined pathways. KEGG Pathway enrichment analyses were performed on dysregulated miRNAs identified in Cron et al. (Table 3A) and Sengupta et al. (Table 3B). Despite the difference in these two studies, one comparing thymuses from control donors vs. untreated EOMG patients (11) and the other one comparing thymuses rich in GCs vs. those with no GCs (80), interesting information results from these analyses. Numerous cancer pathways are represented but also pathways related to pathogen infection and TLRs. Pathogens are environmental factor that potentially drive/perpetuate autoimmunity (97) and the thymus is a common target organ for infectious diseases (98). Poliovirus-infected macrophages and the presence of Epstein-Barr virus (EBV)-infected B cells in MG thymus were described in MG thymus (99, 100). Besides, changes regarding TLR expression in MG thymus have been demonstrated (67, 101–103). The chemokine signaling pathway was also found dysregulated in both studies. This is not surprising regarding the role of chemokines in B-cell recruitment and GC development (82, 85, 104). Altogether, these studies provide novel clues to the potential pathways that occur in the hyperplastic thymus and the development of GCs.

Table 3. KEGG Pathway enrichment analyses were performed on dysregulated miRNAs identified in Cron et al. (A) and Sengupta et al. (B) using miRNet (https://www.mirnet.ca/miRNet/home.xhtml).
Conclusion
Thymic changes occur during life either in the context of thymic involution upon aging /stress or in MG. Here, we review the literature and used original data to describe specific miRNAs that could play a key role in these thymic changes. Among miRNAs that are dysregulated in EOMG or TAMG patients, miR-19b, miR-20b, miR-24, and miR-150 are listed as ThymiRs, and only miR-19b and miR-20b are actively involved in thymic involution. The literature often describes these miRNAs as playing a role in T-cell differentiation. The lack of correlation between miRNAs regulated upon thymic involution, and dysregulated in EOMG or TAMG thymuses suggests the immune response in the autoimmune condition is not directed at the dysregulation of the developmental pathway of the thymus. Most of the down-regulated thymic miRNAs in EOMG are associated with GC development, such as miR-7, miR-24, miR-139, miR-143, miR-145, miR-146, miR-150, miR-452, miR-548, or thymic inflammation, such as miR-125b, miR-146, or miR-29. Further investigations on these miRNAs could now help deciphering if they could represent therapeutic tools to normalized thymic inflammation and associated lymphofollicular hyperplasia in EOMG patients.
Author Contributions
MC performed the experiments and revised the manuscript. ÉG performed bioinformatics analyses and revised the manuscript. LK and RL wrote the manuscript.
Funding
This work was supported by grants from the European Community (FIGHT-MG/HEALTH-92-242-210) and from the Association Française contre les Myopathies (AFM).
Conflict of Interest
The authors declare that the research was conducted in the absence of any commercial or financial relationships that could be construed as a potential conflict of interest.
Acknowledgments
We recognize and thank Inovarion (https://www.inovarion.com/) as the employer of ÉG and the CNRS as the employer of RL. We thank Dr. Sonia Berrih-Aknin for reading the document and her suggestions.
Abbreviations
AChR, Acetylcholine receptor; AIRE, Autoimmune regulator (AIRE); DN, Double negative; DP, Double positive; EOMG, Early-onset MG; GC, Germinal center; IFN, Interferon; MG, Myasthenia gravis; miRNA, microRNA; NKT, Natural killer T cell; SP, Simple positive; TAMG, Thymoma-associated MG; TCR, T-cell receptor; TEC, Thymic epithelial cell; cTEC, Cortical thymic epithelial cell; mTEC, Medullary thymic epithelial cell; TLR, Toll-Like Receptor; Treg cell, regulatory T cell; TSA, Tissue-specific antigen.
References
1. Winter J, Jung S, Keller S, Gregory RI, Diederichs S. Many roads to maturity: microRNA biogenesis pathways and their regulation. Nat Cell Biol. (2009) 11:228–34. doi: 10.1038/ncb0309-228
2. Chen JQ, Papp G, Szodoray P, Zeher M. The role of microRNAs in the pathogenesis of autoimmune diseases. Autoimmun Rev. (2016) 15:1171–80. doi: 10.1016/j.autrev.2016.09.003
3. Theofilopoulos AN, Kono DH, Baccala R. The multiple pathways to autoimmunity. Nat Immunol. (2017) 18:716–24. doi: 10.1038/ni.3731
4. Mehta A, Baltimore D. MicroRNAs as regulatory elements in immune system logic. Nat Rev Immunol. (2016) 16:279–94. doi: 10.1038/nri.2016.40
5. Kurd N, Robey EA. T-cell selection in the thymus: a spatial and temporal perspective. Immunol Rev. (2016) 271:114–26. doi: 10.1111/imr.12398
7. Bertho JM, Demarquay C, Moulian N, Van Der Meeren A, Berrih-Aknin S, Gourmelon P. Phenotypic and immunohistological analyses of the human adult thymus: evidence for an active thymus during adult life. Cell Immunol. (1997) 179:30–40. doi: 10.1006/cimm.1997.1148
8. Dooley J, Linterman MA, Liston A. MicroRNA regulation of T-cell development. Immunol Rev. (2013) 253:53–64. doi: 10.1111/imr.12049
9. Xu M, Gan T, Ning H, Wang L. MicroRNA functions in thymic biology: thymic development and involution. Front Immunol. (2018) 9:2063. doi: 10.3389/fimmu.2018.02063
10. Kroesen BJ, Teteloshvili N, Smigielska-Czepiel K, Brouwer E, Boots AM, Van Den Berg A, et al. Immuno-miRs: critical regulators of T-cell development, function and ageing. Immunology. (2015) 144:1–10. doi: 10.1111/imm.12367
11. Cron MA, Maillard S, Deslile F, Samson N, Truffault F, Foti M, et al. Analysis of microRNA expression in the thymus of myasthenia gravis patients opens new research avenues Autoimmunity Reviews. (2018) 17:588–600. doi: 10.1016/j.autrev.2018.01.008
12. Khan IS, Taniguchi RT, Fasano KJ, Anderson MS, Jeker LT. Canonical microRNAs in thymic epithelial cells promote central tolerance. Eur J Immunol. (2014) 44:1313–9. doi: 10.1002/eji.201344079
13. Zuklys S, Mayer CE, Zhanybekova S, Stefanski HE, Nusspaumer G, Gill J, et al. MicroRNAs control the maintenance of thymic epithelia and their competence for T lineage commitment and thymocyte selection. J Immunol. (2012) 189:3894–904. doi: 10.4049/jimmunol.1200783
14. Papadopoulou AS, Dooley J, Linterman MA, Pierson W, Ucar O, Kyewski B, et al. The thymic epithelial microRNA network elevates the threshold for infection-associated thymic involution via miR-29a mediated suppression of the IFN-alpha receptor. Nat Immunol. (2012) 13:181–7. doi: 10.1038/ni.2193
15. Ucar O, Tykocinski LO, Dooley J, Liston A, Kyewski B. An evolutionarily conserved mutual interdependence between Aire and microRNAs in promiscuous gene expression. Eur J Immunol. (2013) 13:1769–78. doi: 10.1002/eji.201343343
16. Ye Y, Li D, Ouyang D, Deng L, Zhang Y, Ma Y, et al. MicroRNA expression in the aging mouse thymus. Gene. (2014) 547:218–25. doi: 10.1016/j.gene.2014.06.039
17. Song MS, Rossi JJ. Molecular mechanisms of dicer: endonuclease and enzymatic activity. Biochem J. (2017) 474:1603–18. doi: 10.1042/BCJ20160759
18. Deng L, Ren R, Liu Z, Song M, Li J, Wu Z, et al. Stabilizing heterochromatin by DGCR8 alleviates senescence and osteoarthritis. Nat Commun. (2019) 10:3329. doi: 10.1038/s41467-019-10831-8
19. Macedo C, Evangelista AF, Marques MM, Octacilio-Silva S, Donadi EA, Sakamoto-Hojo ET, et al. Autoimmune regulator. (Aire) controls the expression of microRNAs in medullary thymic epithelial cells. Immunobiology. (2013) 218:554–60. doi: 10.1016/j.imbio.2012.06.013
20. Cobb BS, Nesterova TB, Thompson E, Hertweck A, O'connor E, Godwin J, et al. T cell lineage choice and differentiation in the absence of the RNase III enzyme Dicer. J Exp Med. (2005) 201:1367–73. doi: 10.1084/jem.20050572
21. Muljo SA, Ansel KM, Kanellopoulou C, Livingston DM, Rao A, Rajewsky K. Aberrant T cell differentiation in the absence of Dicer. J Exp Med. (2005) 202:261–9. doi: 10.1084/jem.20050678
22. Fedeli M, Napolitano A, Wong MP, Marcais A, De Lalla C, Colucci F, et al. Dicer-dependent microRNA pathway controls invariant NKT cell development. J Immunol. (2009) 183:2506–12. doi: 10.4049/jimmunol.0901361
23. Cobb BS, Hertweck A, Smith J, O'connor E, Graf D, Cook T, et al. A role for Dicer in immune regulation. J Exp Med. (2006) 203:2519–27. doi: 10.1084/jem.20061692
24. Chong MM, Rasmussen JP, Rudensky AY, Littman DR. The RNAseIII enzyme Drosha is critical in T cells for preventing lethal inflammatory disease. J Exp Med. (2008) 205:2005–17. doi: 10.1084/jem.20081219
25. Neilson JR, Zheng GX, Burge CB, Sharp PA. Dynamic regulation of miRNA expression in ordered stages of cellular development. Genes Dev. (2007) 21:578–89. doi: 10.1101/gad.1522907
26. Ghisi M, Corradin A, Basso K, Frasson C, Serafin V, Mukherjee S, et al. Modulation of microRNA expression in human T-cell development: targeting of NOTCH3 by miR-150. Blood. (2011) 117:7053–62. doi: 10.1182/blood-2010-12-326629
27. Hu L, Mao L, Liu S, Zhao J, Chen C, Guo M, et al. Functional Role of MicroRNAs in Thymocyte Development. Int Arch Allergy Immunol. (2019) 178:315–22. doi: 10.1159/000496093
28. Guo D, Ye Y, Qi J, Tan X, Zhang Y, Ma Y, et al. Age and sex differences in microRNAs expression during the process of thymus aging. Acta Biochim Biophys Sin. (Shanghai). (2017) 49:409–19. doi: 10.1093/abbs/gmx029
29. Guo Z, Chi F, Song Y, Wang C, Yu R, Wei T, et al. Transcriptome analysis of murine thymic epithelial cells reveals ageassociated changes in microRNA expression. Int J Mol Med. (2013) 32:835–42. doi: 10.3892/ijmm.2013.1471
30. Jia HL, Zeng XQ, Huang F, Liu YM, Gong BS, Zhang KZ, et al. Integrated microRNA and mRNA sequencing analysis of age-related changes to mouse thymic epithelial cells. IUBMB Life. (2018) 70:678–90. doi: 10.1002/iub.1864
31. Guo D, Ye Y, Qi J, Xu L, Zhang L, Tan X, et al. MicroRNA-195a-5p inhibits mouse medullary thymic epithelial cells proliferation by directly targeting Smad7. Acta Biochim Biophys Sin. (Shanghai). (2016) 48:290–7. doi: 10.1093/abbs/gmv136
32. Cheng J, Wu R, Long L, Su J, Liu J, Wu XD, et al. miRNA-451a targets IFN regulatory factor 8 for the progression of systemic lupus erythematosus. Inflammation. (2017) 40:676–87. doi: 10.1007/s10753-017-0514-8
33. Khuu C, Utheim TP, Sehic A. The three paralogous MicroRNA clusters in development and disease, miR-17-92, miR-106a-363, and miR-106b-25. Scientifica. (2016) 2016:1379643. doi: 10.1155/2016/1379643
34. Linhares-Lacerda L, Palu CC, Ribeiro-Alves M, Paredes BD, Morrot A, Garcia-Silva MR, et al. Differential expression of microRNAs in thymic epithelial cells from Trypanosoma cruzi acutely infected mice: putative role in thymic atrophy. Front Immunol. (2015) 6:428. doi: 10.3389/fimmu.2015.00428
35. Smith LK, Shah RR, Cidlowski JA. Glucocorticoids modulate microRNA expression and processing during lymphocyte apoptosis. J Biol Chem. (2010) 285:36698–708. doi: 10.1074/jbc.M110.162123
36. Belkaya S, Silge RL, Hoover AR, Medeiros JJ, Eitson JL, Becker AM, et al. Dynamic modulation of thymic microRNAs in response to stress. PLoS ONE. (2011) 6:e27580. doi: 10.1371/journal.pone.0027580
37. Roush S, Slack FJ. The let-7 family of microRNAs. Trends Cell Biol. (2008) 18:505–16. doi: 10.1016/j.tcb.2008.07.007
38. Pobezinsky LA, Etzensperger R, Jeurling S, Alag A, Kadakia T, Mccaughtry TM, et al. Let-7 microRNAs target the lineage-specific transcription factor PLZF to regulate terminal NKT cell differentiation and effector function. Nat Immunol. (2015) 16:517–24. doi: 10.1038/ni.3146
39. Xiao S, Zhang W, Manley NR. Thymic epithelial cell-derived signals control B progenitor formation and proliferation in the thymus by regulating Let-7 and Arid3a. PLoS ONE. (2018) 13:e0193188. doi: 10.1371/journal.pone.0193188
40. Li QJ, Chau J, Ebert PJ, Sylvester G, Min H, Liu G, et al. miR-181a is an intrinsic modulator of T cell sensitivity and selection. Cell. (2007) 129:147–61. doi: 10.1016/j.cell.2007.03.008
41. Ebert PJ, Jiang S, Xie J, Li QJ, Davis MM. An endogenous positively selecting peptide enhances mature T cell responses and becomes an autoantigen in the absence of microRNA miR-181a. Nat Immunol. (2009) 10:1162–9. doi: 10.1038/ni.1797
42. Henao-Mejia J, Williams A, Goff LA, Staron M, Licona-Limon P, Kaech SM, et al. The microRNA miR-181 is a critical cellular metabolic rheostat essential for NKT cell ontogenesis and lymphocyte development and homeostasis. Immunity. (2013) 38:984–97. doi: 10.1016/j.immuni.2013.02.021
43. Stefanski HE, Xing Y, Taylor PA, Maio S, Henao-Meija J, Williams A, et al. Despite high levels of expression in thymic epithelial cells, miR-181a1 and miR-181b1 are not required for thymic development. PLoS ONE. (2018) 13:e0198871. doi: 10.1371/journal.pone.0198871
44. Guo D, Ye Y, Qi J, Zhang L, Xu L, Tan X, et al. MicroRNA-181a-5p enhances cell proliferation in medullary thymic epithelial cells via regulating TGF-beta signaling. Acta Biochim Biophys Sin. (Shanghai). (2016) 48:840–9. doi: 10.1093/abbs/gmw068
45. Cotrim-Sousa L, Freire-Assis A, Pezzi N, Tanaka PP, Oliveira EH, Passos GA. Adhesion between medullary thymic epithelial cells and thymocytes is regulated by miR-181b-5p and miR-30b. Mol Immunol. (2019) 114:600–11. doi: 10.1016/j.molimm.2019.09.010
46. Zhou B, Wang S, Mayr C, Bartel DP, Lodish HF. miR-150, a microRNA expressed in mature B and T cells, blocks early B cell development when expressed prematurely. Proc Natl Acad Sci USA. (2007) 104:7080–5. doi: 10.1073/pnas.0702409104
47. Bezman NA, Chakraborty T, Bender T, Lanier LL. miR-150 regulates the development of NK and iNKT cells. J Exp Med. (2011) 208:2717–31. doi: 10.1084/jem.20111386
48. Xiao C, Calado DP, Galler G, Thai TH, Patterson HC, Wang J, et al. MiR-150 controls B cell differentiation by targeting the transcription factor c-Myb. Cell. (2007) 131:146–59. doi: 10.1016/j.cell.2007.07.021
49. Zheng Q, Zhou L, Mi QS. MicroRNA miR-150 is involved in Valpha14 invariant NKT cell development and function. J Immunol. (2012) 188:2118–26. doi: 10.4049/jimmunol.1103342
50. Lee HM, Kim TS, Jo EK. MiR-146 and miR-125 in the regulation of innate immunity and inflammation. BMB Rep. (2016) 49:311–8. doi: 10.5483/BMBRep.2016.49.6.056
51. Kirigin FF, Lindstedt K, Sellars M, Ciofani M, Low SL, Jones L, et al. Dynamic microRNA gene transcription and processing during T cell development. J Immunol. (2012) 188:3257–67. doi: 10.4049/jimmunol.1103175
52. Li Z, Zhang S, Wan Y, Cai M, Wang W, Zhu Y, et al. MicroRNA-146a Overexpression Impairs the Positive Selection during T Cell Development. Front Immunol. (2018) 8:2006. doi: 10.3389/fimmu.2017.02006
53. Burger ML, Xue L, Sun Y, Kang C, Winoto A. Premalignant PTEN-deficient thymocytes activate microRNAs miR-146a and miR-146b as a cellular defense against malignant transformation. Blood. (2014) 123:4089–100. doi: 10.1182/blood-2013-11-539411
54. Lu LF, Boldin MP, Chaudhry A, Lin LL, Taganov KD, Hanada T, et al. Function of miR-146a in controlling Treg cell-mediated regulation of Th1 responses. Cell. (2010) 142:914–29. doi: 10.1016/j.cell.2010.08.012
55. Mashima R. Physiological roles of miR-155. Immunology. (2015) 145:323–33. doi: 10.1111/imm.12468
56. Kohlhaas S, Garden OA, Scudamore C, Turner M, Okkenhaug K, Vigorito E. Cutting edge: the Foxp3 target miR-155 contributes to the development of regulatory T cells. J Immunol. (2009) 182:2578–82. doi: 10.4049/jimmunol.0803162
57. Stahl HF, Fauti T, Ullrich N, Bopp T, Kubach J, Rust W, et al. miR-155 inhibition sensitizes CD4+ Th cells for TREG mediated suppression. PLoS ONE. (2009) 4:e7158. doi: 10.1371/journal.pone.0007158
58. Burocchi A, Pittoni P, Tili E, Rigoni A, Costinean S, Croce CM, et al. Regulated Expression of miR-155 is Required for iNKT Cell Development. Front Immunol. (2015) 6:140. doi: 10.3389/fimmu.2015.00140
59. Kim SW, Ramasamy K, Bouamar H, Lin AP, Jiang D, Aguiar RC. MicroRNAs miR-125a and miR-125b constitutively activate the NF-κB pathway by targeting the tumor necrosis factor alpha-induced protein 3. (TNFAIP3, A20). Proc Natl Acad Sci USA. (2012) 109:7865–70. doi: 10.1073/pnas.1200081109
60. Rasheed Z, Rasheed N, Abdulmonem WA, Khan MI. MicroRNA-125b-5p regulates IL-1beta induced inflammatory genes via targeting TRAF6-mediated MAPKs and NF-kappaB signaling in human osteoarthritic chondrocytes. Sci Rep. (2019) 9:6882. doi: 10.1038/s41598-019-42601-3
61. Xu M, Sizova O, Wang L, Su DM. A fine-tune role of Mir-125a-5p on Foxn1 During age-associated changes in the thymus. Aging Dis. (2017) 8:277–86. doi: 10.14336/AD.2016.1109
63. Marx A, Porubsky S, Belharazem D, Saruhan-Direskeneli G, Schalke B, Ströbel P, et al. Thymoma related myasthenia gravis in humans and potential animal models. Exp Neurol. (2015) 270:55–65. doi: 10.1016/j.expneurol.2015.02.010
64. Cufi P, Soussan P, Truffault F, Fetouchi R, Robinet M, Fadel E, et al. Thymoma-associated myasthenia gravis: on the search for a pathogen signature. J Autoimmun. (2014) 52:29–35. doi: 10.1016/j.jaut.2013.12.018
65. Wolfe GI, Kaminski HJ, Aban IB, Minisman G, Kuo HC, Marx A, et al. Randomized trial of thymectomy in myasthenia gravis. N Engl J Med. (2016) 375:511–22. doi: 10.1056/NEJMoa1602489
66. Cron MA, Maillard S, Villegas J, Truffault F, Sudres M, Dragin N, et al. Thymus involvement in early-onset myasthenia gravis. Ann N Y Acad Sci. (2018) 1412:137–45. doi: 10.1111/nyas.13519
67. Cufi P, Dragin N, Weiss JM, Martinez-Martinez P, De Baets MH, Roussin R, et al. Implication of double-stranded RNA signaling in the etiology of autoimmune myasthenia gravis. Ann Neurol. (2013) 73:281–93. doi: 10.1002/ana.23791
68. Cufi P, Dragin N, Ruhlmann N, Weiss JM, Fadel E, Serraf A, et al. Central role of interferon-beta in thymic events leading to myasthenia gravis. J Autoimmun. (2014) 52:44–52. doi: 10.1016/j.jaut.2013.12.016
69. Villegas JA, Bayer AC, Ider K, Bismuth J, Truffault F, Roussin R, et al. Il-23/Th17 cell pathway: a promising target to alleviate thymic inflammation maintenance in myasthenia gravis. J Autoimmun. (2019) 98:59–73. doi: 10.1016/j.jaut.2018.11.005
70. Punga T, Le Panse R, Andersson M, Truffault F, Berrih-Aknin S, Punga AR. Circulating miRNAs in myasthenia gravis: miR-150-5p as a new potential biomarker. Ann Clin Transl Neurol. (2014) 1:49–58. doi: 10.1002/acn3.24
71. Nogales-Gadea G, Ramos-Fransi A, Suarez-Calvet X, Navas M, Rojas-Garcia R, Mosquera JL, et al. Analysis of serum miRNA profiles of myasthenia gravis patients. PLoS ONE. (2014) 9:e91927. doi: 10.1371/journal.pone.0091927
72. Chunjie N, Huijuan N, Zhao Y, Jianzhao W, Xiaojian Z. Disease-specific signature of serum miR-20b and its targets IL-8 and IL-25, in myasthenia gravis patients. Eur Cytokine Netw. (2015) 26:61–6. doi: 10.1684/ecn.2015.0367
73. Zhang Y, Guo M, Xin N, Shao Z, Zhang X, Zhang Y, et al. Decreased microRNA miR-181c expression in peripheral blood mononuclear cells correlates with elevated serum levels of IL-7 and IL-17 in patients with myasthenia gravis. Clin Exp Med. (2016) 16:413–21. doi: 10.1007/s10238-015-0358-1
74. Li J, Qiu D, Chen Z, Du W, Liu J, Mo X. Altered expression of miR-125a-5p in thymoma-associated myasthenia gravis and its down-regulation of foxp3 expression in Jurkat cells. Immunol Lett. (2016) 172:47–55. doi: 10.1016/j.imlet.2016.02.005
75. Strobel P, Rosenwald A, Beyersdorf N, Kerkau T, Elert O, Murumagi A, et al. Selective loss of regulatory T cells in thymomas. Ann Neurol. (2004) 56:901–4. doi: 10.1002/ana.20340
76. Ströbel P, Murumägi A, Klein R, Luster M, Lahti M, Krohn K, et al. Deficiency of the autoimmune regulator AIRE in thymomas is insufficient to elicit autoimmune polyendocrinopathy syndrome type 1 (APS-1). J Pathol. (2007) 211:563–71. doi: 10.1002/path.2141
77. Wang Z, Chen Y, Xu S, Yang Y, Wei D, Wang W, et al. Aberrant decrease of microRNA19b regulates TSLP expression and contributes to Th17 cells development in myasthenia gravis related thymomas. J Neuroimmunol. (2015) 288:34–9. doi: 10.1016/j.jneuroim.2015.08.013
78. Xin Y, Cai H, Lu T, Zhang Y, Yang Y, Cui Y. miR-20b inhibits T cell proliferation and activation via NFAT signaling pathway in thymoma-associated myasthenia gravis. Biomed Res Int. (2016) 2016:9595718. doi: 10.1155/2016/9595718
79. Ganci F, Vico C, Korita E, Sacconi A, Gallo E, Mori F, et al. MicroRNA expression profiling of thymic epithelial tumors. Lung Cancer. (2014) 85:197–204. doi: 10.1016/j.lungcan.2014.04.008
80. Sengupta M, Wang BD, Lee NH, Marx A, Kusner LL, Kaminski HJ. MicroRNA and mRNA expression associated with ectopic germinal centers in thymus of myasthenia gravis. PLoS ONE. (2018) 13:e0205464. doi: 10.1371/journal.pone.0205464
81. Li J, Qiu D, Chen Z, Du W, Liu J, Mo X. miR-548k regulates CXCL13 expression in myasthenia gravis patients with thymic hyperplasia and in Jurkat cells. J NeuroImmunol. (2018) 320:125–32. doi: 10.1016/j.jneuroim.2018.03.021
82. Berrih-Aknin S, Ruhlmann N, Bismuth J, Cizeron-Clairac G, Zelman E, Shachar I, et al. CCL21 overexpressed on lymphatic vessels drives thymic hyperplasia in myasthenia. Ann Neurol. (2009) 66:521–31. doi: 10.1002/ana.21628
83. Balandina A, Lecart S, Dartevelle P, Saoudi A, Berrih-Aknin S. Functional defect of regulatory CD4(+)CD25+ T cells in the thymus of patients with autoimmune myasthenia gravis. Blood. (2005) 105:735–41. doi: 10.1182/blood-2003-11-3900
84. Hamerman JA, Pottle J, Ni M, He Y, Zhang ZY, Buckner JH. Negative regulation of TLR signaling in myeloid cells–implications for autoimmune diseases. Immunol Rev. (2016) 269:212–27. doi: 10.1111/imr.12381
85. Méraouna A, Cizeron-Clairac G, Le Panse R, Bismuth J, Truffault F, Talaksen C, et al. The chemokine CXCL13 is a key molecule in autoimmune Myasthenia Gravis. Blood. (2006) 108:432–40. doi: 10.1182/blood-2005-06-2383
86. Cron MA, Maillard S, Truffault F, Gualeni AV, Gloghini A, Fadel E, et al. Causes and Consequences of miR-150-5p dysregulation in myasthenia gravis. Front Immunol. (2019) 10:539. doi: 10.3389/fimmu.2019.00539
87. Punga AR, Andersson M, Alimohammadi M, Punga T. Disease specific signature of circulating miR-150-5p and miR-21-5p in myasthenia gravis patients. J Neurol Sci. (2015) 356:90–6. doi: 10.1016/j.jns.2015.06.019
88. Wang X, Angelis N, Thein SL. MYB - A regulatory factor in hematopoiesis. Gene. (2018) 665:6–17. doi: 10.1016/j.gene.2018.04.065
89. Gustafsson M, Gawel DR, Alfredsson L, Baranzini S, Bjorkander J, Blomgran R, et al. A validated gene regulatory network and GWAS identifies early regulators of T cell-associated diseases. Sci Transl Med. (2015) 7:313ra178. doi: 10.1126/scitranslmed.aad2722
90. Truffault F, Cohen-Kaminsky S, Khalil I, Levasseur P, Berrih-Aknin S. Altered intrathymic T-cell repertoire in human myasthenia gravis. Ann Neurol. (1997) 41:731–41. doi: 10.1002/ana.410410609
91. Wang J, Zheng S, Xin N, Dou C, Fu L, Zhang X, et al. Identification of novel MicroRNA signatures linked to experimental autoimmune myasthenia gravis pathogenesis: down-regulated miR-145 promotes pathogenetic Th17 cell response. J Neuroimmune Pharmacol. (2013) 8:1287–302. doi: 10.1007/s11481-013-9498-9
92. Wu DM, Wen X, Han XR, Wang S, Wang YJ, Shen M, et al. Micro-RNA-143 inhibits proliferation and promotes apoptosis of thymocytes by targeting CXCL13 in a myasthenia gravis mouse model. Am J Physiol Cell Physiol. (2019) 316:C70–80. doi: 10.1152/ajpcell.00090.2018
93. Bortone F, Scandiffio L, Marcuzzo S, Bonanno S, Frangiamore R, Motta T, et al. miR-146a in myasthenia gravis thymus bridges innate immunity with autoimmunity and is linked to therapeutic effects of corticosteroids. Front Immunol. (2020) 11:142. doi: 10.3389/fimmu.2020.00142
94. Tang Y, Luo X, Cui H, Ni X, Yuan M, et al. MicroRNA-146A contributes to abnormal activation of the type I interferon pathway in human lupus by targeting the key signaling proteins. Arthritis Rheum. (2009) 60:1065–75. doi: 10.1002/art.24436
95. Zhou Q, Haupt S, Kreuzer JT, Hammitzsch A, Proft F, Neumann C, et al. Decreased expression of miR-146a and miR-155 contributes to an abnormal Treg phenotype in patients with rheumatoid arthritis. Ann Rheum Dis. (2015) 74:1265–74. doi: 10.1136/annrheumdis-2013-204377
96. Li B, Wang X, Choi IY, Wang YC, Liu S, Pham AT, et al. miR-146a modulates autoreactive Th17 cell differentiation and regulates organ-specific autoimmunity. J Clin Invest. (2017) 127:3702–16. doi: 10.1172/JCI94012
97. Munz C, Lunemann JD, Getts MT, Miller SD. Antiviral immune responses: triggers of or triggered by autoimmunity? Nature Reviews: Immunology. (2009) 9:246–58. doi: 10.1038/nri2527
98. Savino W. The thymus is a common target organ in infectious diseases. PLoS Pathogens. (2006) 2:e62. doi: 10.1371/journal.ppat.0020062
99. Cavalcante P, Barberis M, Cannone M, Baggi F, Antozzi C, Maggi L, et al. Detection of poliovirus-infected macrophages in thymus of patients with myasthenia gravis. Neurology. (2010) 74:1118–26. doi: 10.1212/WNL.0b013e3181d7d884
100. Cavalcante P, Serafini B, Rosicarelli B, Maggi L, Barberis M, Antozzi C, et al. Epstein-Barr virus persistence and reactivation in myasthenia gravis thymus. Ann Neurol. (2010) 67:726–38. doi: 10.1002/ana.21902
101. Bernasconi P, Barberis M, Baggi F, Passerini L, Cannone M, Arnoldi E, et al. Increased toll-like receptor 4 expression in thymus of myasthenic patients with thymitis and thymic involution. Am J Pathol. (2005) 167:129–39. doi: 10.1016/S0002-9440(10)62960-4
102. Cavalcante P, Galbardi B, Franzi S, Marcuzzo S, Barzago C, Bonanno S, et al. Increased expression of Toll-like receptors 7 and 9 in myasthenia gravis thymus characterized by active Epstein-Barr virus infection. Immunobiology. (2016) 221:516–27. doi: 10.1016/j.imbio.2015.12.007
103. Robinet M, Maillard S, Cron MA, Berrih-Aknin S, Le Panse R. Review on toll-like receptor activation in myasthenia gravis: application to the development of new experimental models. Clin Rev Allergy Immunol. (2017) 52:133–47. doi: 10.1007/s12016-016-8549-4
Keywords: autoimmunity, thymic involution, germinal center, early-onset myasthenia gravis, thymoma, thymocytes, thymic epithelial cells
Citation: Cron MA, Guillochon É, Kusner L and Le Panse R (2020) Role of miRNAs in Normal and Myasthenia Gravis Thymus. Front. Immunol. 11:1074. doi: 10.3389/fimmu.2020.01074
Received: 17 January 2020; Accepted: 04 May 2020;
Published: 10 June 2020.
Edited by:
Zhiguang Zhou, Central South University, ChinaReviewed by:
Renato Mantegazza, Carlo Besta Neurological Institute (IRCCS), ItalyTao Li, National Center of Biomedical Analysis (NCBA), China
Copyright © 2020 Cron, Guillochon, Kusner and Le Panse. This is an open-access article distributed under the terms of the Creative Commons Attribution License (CC BY). The use, distribution or reproduction in other forums is permitted, provided the original author(s) and the copyright owner(s) are credited and that the original publication in this journal is cited, in accordance with accepted academic practice. No use, distribution or reproduction is permitted which does not comply with these terms.
*Correspondence: Linda Kusner, lkusner@gwu.edu; Rozen Le Panse, rozen.lepanse@upmc.fr
†These authors have contributed equally to this work