- 1Neonatal Intensive Care Unit, Royal Victoria Infirmary, Newcastle upon Tyne, United Kingdom
- 2Immunity and Inflammation Theme, Translational and Clinical Research Institute, Newcastle University, Newcastle upon Tyne, United Kingdom
- 3Population Health Science Institute, Newcastle University, Newcastle upon Tyne, United Kingdom
Preterm infants born before 32 weeks gestational age (GA) have high rates of late onset sepsis (LOS) and necrotizing enterocolitis (NEC) despite recent improvements in infection control and nutrition. Breast milk has a clear protective effect against both these outcomes likely due to multiple mechanisms which are not fully understood but may involve effects on both the infant's immune system and the developing gut microbiota. Congregating at the interface between the mucosal barrier and the microbiota, innate and adaptive T lymphocytes (T cells) participate in this interaction but few studies have explored their development after preterm delivery. We conducted a literature review of T cell development that focuses on fetal development, postnatal maturation and the influence of milk diet. The majority of circulating T cells in the preterm infant display a naïve phenotype but are still able to initiate functional responses similar to those seen in term infants. T cells from preterm infants display a skew toward a T-helper 2(Th2) phenotype and have an increased population of regulatory cells (Tregs). There are significant gaps in knowledge in this area, particularly in regards to innate-like T cells, but work is emerging: transcriptomics and mass cytometry are currently being used to map out T cell development, whilst microbiomic approaches may help improve understanding of events at mucosal surfaces. A rapid rise in organoid models will allow robust exploration of host-microbe interactions and may support the development of interventions that modulate T-cell responses for improved infant health.
Introduction
Infants born prematurely, especially before 32 weeks gestational age (GA) are susceptible to diseases associated with invasive bacterial infections, specifically late onset sepsis (LOS) and necrotizing enterocolitis (NEC). These occur in around 30 and 6% of very preterm infants, respectively, according to recent data (1), most occur in the first 4 weeks of life, and both diseases are associated with changes in the gut microbiota (2, 3). Feeding preterm infants with their mother's breast milk has been shown to reduce the risk of NEC and LOS (4, 5). T lymphocytes (T cells) are an important component of the immune response to infection, however, this role is balanced against their emerging role in tissue regeneration and repair. The newborn, who is exposed to diverse microbes including many potential pathogens soon after birth, requires an appropriate T cell response to navigate the potentially conflicting requirements of growth, repair and infection control.
This review focuses on fetal T cell development, postnatal T cell maturation, and the potential for dietary modulation of gut mucosal T cells in the preterm environment. T cells of particular interest in the preterm infant comprise those with innate-like properties such as γδ T, invariant natural killer T (iNKT) and mucosa-associated invariant T (MAIT) cells, which have the capacity to deliver effector functions without prior clonal expansion. Among conventional TCRαβ-expressing T cells, regulatory T cells (Tregs) are known for their ability to mediate tolerance but also have emerging roles in tissue regeneration of potential relevance to NEC (Table 1).
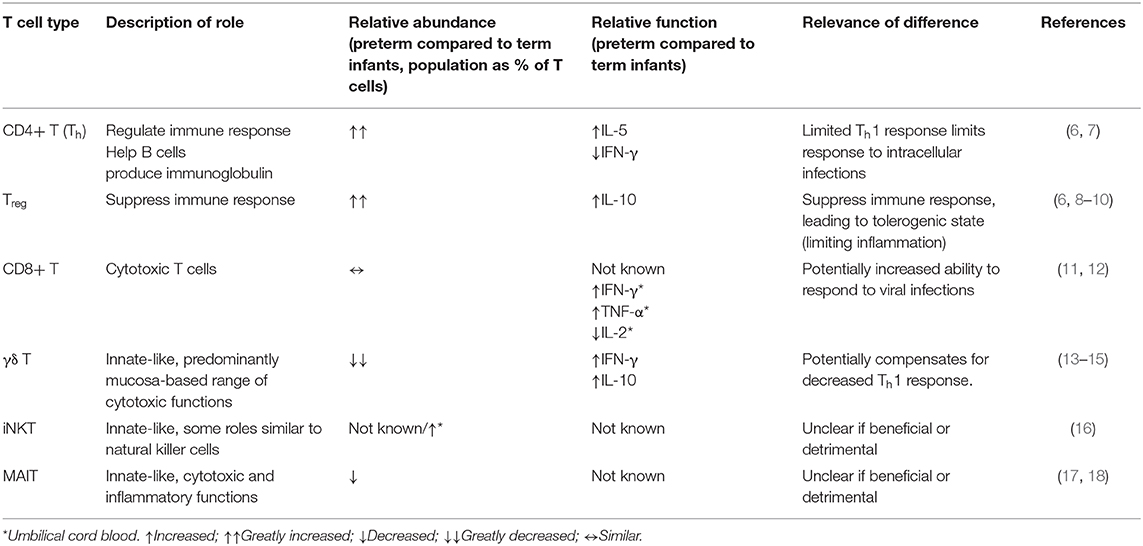
Table 1. Summary of differences between peripheral blood T cell populations in preterm infants compared to term infants.
A challenge of studying T cells in preterm infants is that it is difficult to obtain suitable samples purely for research purposes. Umbilical cord blood is often used to represent the immune system of preterm infants, however a recent systems level analysis suggests it is more representative of the fetal state than the ex-utero infant (6). Therefore, where possible, we focus on studies using postnatal blood, but supplement these data with studies on umbilical cord blood, fetuses, and animal studies as necessary.
Fetal T-Cell Development
Bone marrow-derived T progenitor cells enter the thymus at around 8 weeks gestation (19). These early thymocytes do not display the full range of T cell receptors (TCR) until 16 weeks GA (20). Fetal T cells can be detected in peripheral blood from 8 weeks GA, with the capacity to proliferate and produce cytokines (21). T cell migration to secondary lymphoid tissue has been confirmed with identification of T cells in the mesenteric lymph nodes (MLN) from 12 weeks GA (20) and the spleen and intestinal mucosa from 14 weeks GA (20–23).
Unsurprisingly, the majority of T cells derived from cord blood in newborns are naïve (24). The proportion of naive T cells has been shown to remain high (median 85%) at 6–8 weeks age [postnatal age (PNA)] in preterm infants, however this is significantly less than term infants at this PNA (25). This may reflect increased antigen exposure or reduced thymic output in preterm infants.
The size, composition, and function of the immune cell compartment varies from one tissue to another (26, 27). Interestingly, fetal CD4 T (Th) cells in the intestinal lamina propria but not spleen or liver display a predominantly memory phenotype (22, 23). This suggests priming as a result of prior “antigen” exposure, the nature of which is not known (22). Fetal MLN-derived T cells have capacity to respond to stimulation by proliferating and secreting a broad range of cytokines. Both proliferation and cytokine production by fetal MLN-derived T cells are augmented upon the removal of Tregs (20) suggesting Tregs play an important role in limiting fetal tissue inflammation.
Skewing of the T-helper (Th) Response
Naïve Th cells can differentiate toward alternative cell fates depending on the context in which they receive antigenic stimulation (8). Preterm infants have been suggested to have a skewed Th2 response based on their increased production of a classical Th2 cytokine, IL-5, and decreased production of a Th1 cytokine, IFN-γ, upon stimulation of peripheral blood compared to term cord blood (7). Postnatally, the ability of preterm Th cells to secrete IFN-γ remains low with single cell RNA-seq analysis of term and preterm infants at 12 weeks of age demonstrating up-regulation of genes that suppress IFN-γ, together with longitudinal sampling of preterm infants displaying low expression (6, 13). An effective Th1 response is key to preventing intracellular infections, including bacteria, and this may explain why preterm infants have increased susceptibility.
In contrast to the Th2 skewing observed in peripheral blood, fetal intestinal Th cells instead have a tendency to secrete TNF-α and IL-2 (Th1 cytokine), when compared to term infant intestinal samples. TNF-α was shown in a human fetal organoid model to be important in intestinal epithelial growth by its effects on intestinal stem cells, albeit high levels of TNF-α suppress epithelial growth. Infants with NEC have an increased production of TNF-α from intestinal Th cells, although the temporal relationship to disease is unknown (23).
The tissue Th response is affected by human milk oligosaccharides (HMO's), which comprise 1–2% of human milk and are the third largest non-water component by weight (after lactose and lipids). HMO's are not digestible by the infant but are believed to modulate the gut microbiota. HMO's appear to promote the growth of Bifidobacteria and suppress potentially pathogenic organisms (28, 29). When adding HMO's to fetal in vitro organ cultures, gene transcripts associated with Th differentiation were found only with HMO's from early, not mature human milk, believed to reflect variation in the concentration of the HMO's. These transcriptional changes were deduced to promote a Th1 response whilst suppressing a Th2, Th17, and IL-8 expression (30). It is possible that these alterations play a role in the reduction of NEC and LOS seen in preterm infants fed breast milk.
Suppression of the Immune Response
Tregs are a population of Th cells defined by their suppressive function toward effector T cell responses. Treg abundance is inversely correlated with GA and higher in infants compared to adults (9, 31). Tregs have been identified in the thymus as early as 13 weeks GA and in the periphery (spleen) from 14 weeks GA (21). The proportion of Tregs in the fetal MLN is significantly increased compared to adults and Tregs are functional even at a fetal stage (20, 23).
Interleukin- (IL-) 10 is amongst the factors that mediate the suppressive function as well as induce expansion of Tregs (8–10). IL-10 is produced following bacterial invasion or in stimulated tissues. IL-10 has been shown to contribute to bacterial clearance, yet minimize host damage from infection (10). Interestingly, germline mutations causing loss of function in the IL-10 receptor, as well as deficiency of the critical Treg transcription factor FOXP3, cause early-onset enterocolitis (32). The ability of lamina propria Tregs to produce IL-10 is greatly reduced in preterm infants with NEC compared to term infants (23). An impaired Treg IL-10 response to bacterial invasion may have a role in development of NEC, or it may be that the microbial dysbiosis seen before the development of NEC leads to an impaired IL-10 response (2).
Similar to humans, mice harbor an increased density of Tregs in the intestine compared with other organs. In mice, the introduction of gut bacteria induces an increase in the population of colonic but not small intestinal Tregs, apparently driven by Clostridium. These Tregs are thought to be peripherally induced (rather than thymus-derived) as judged by their lack of expression of the transcription factor, Helios (33). Bacteroides fragilis has also been shown to induce Tregs in mice through its production of polysaccharide A (PSA) in the presence of the toll-like receptor, TLR-2 (34, 35). In mice, induction of Tregs upon treatment by PSA in the presence of Bacteroides fragilis is protective against not only intestinal inflammation but also encephalitis, suggesting there could be a systemic effect of this interaction (34–36). No preterm human work on this aspect currently exists.
Unimpaired CD8 Effector Potential?
CD8 T cells have been demonstrated in the fetal intestine as early as 16 weeks GA (20, 37). Whilst they display mainly a naive phenotype in the first 12 weeks of postnatal life, little is known about their postnatal effector potential (11). Fetal (term and preterm cord blood) and adult CD8 T cells develop a similar memory phenotype and ability to produce perforin and cytokines in response to a common neonatal virus, cytomegalovirus (CMV) (38). Furthermore, using cord blood derived CD8 T cells from varying gestations (23–41 weeks GA), an increased ability to secrete IFN-γ, TNF-α, and IL-2 has been demonstrated at earlier gestations (12).
These limited data suggest prematurity does not prevent CD8 effector T cell function in newborns. Indeed, it is possible that they display an excessive response to viral infection, which may be harmful, particularly as many infants are postnatally exposed to CMV.
Innate-Like T cells
Innate-like T cells can display effector function without prior antigen priming. This would suggest they could be important in early life (17, 39). Innate-like T cells include γδ T, iNKT, and MAIT cells.
Gamma-Delta (γδ) T Cells
Unlike αβ T cells, a large number of γδ T cells reside in non-lymphoid tissues including the gut, spleen and lungs. They have a small range of antigen receptors but are able to respond to a large repertoire of antigens including peptide not bound to classical major histocompatibility complex (MHC) molecules (39). They comprise 4–10%, 1–3%, and <1% of T cells in the peripheral blood of adults, term and preterm cord blood of infants, respectively (14).
γδ T cells develop a memory phenotype in the first month of life (15) and preterm γδ T cells have an increased ability to secrete IFN-γ and IL-10 upon stimulation with PMA and ionomycin compared to both term γδ T cells and preterm αβ T cells (15). The ability to secrete IFN-γ increases over time, which is in contrast to preterm Th cells (13). However, when challenged with influenza virus, cord blood γδ T cells show a decreased ability to proliferate and produce IFN-γ compared to term infants and adults (14). The differences in these results could be due to experimental design, however these data suggest at least the potential for preterm γδ T cells to contribute to the cellular immune response. Little is known about preterm mucosal γδ T cells or their responses to alternative ligands.
Invariant Natural Killer T (iNKT) Cells
Natural killer T (NKT) cells define a population of T cells that bear TCR's restricted by a non-classical MHC molecule, CD1d, and express cell surface markers associated with NK cells. Invariant/ type 1 NKT (or iNKT) cells express the invariant TCR (Vα24-Jα18). iNKT cells vary in abundance depending on tissue, comprising 0.01–0.1% of lymphocytes in peripheral blood but 10% of lymphocytes in the omentum (40). There is an increased abundance of iNKT cells in preterm cord blood compared to term cord blood (16).
iNKT's are able to secrete cytokines that mediate Th1, Th2, or Th17 responses, and recognize specific glycolipid antigens presented by CD1d (41). The ability of preterm iNKT cells to produce these Th cytokines is not known but has been demonstrated in the case of term cord blood iNKT's. The secretion of IL-10 is particularly increased compared to adults suggesting a potential regulatory role of iNKT's in term infants (41).
iNKT cells have been associated with colitis in mouse models. Germ-free mice have an increased proportion of iNKT cells in their colon and increased susceptibility to colitis, however colonization of the intestine with bacteria early in life leads to a reduction in iNKT density and provides protection from colitis (42). iNKT cells able to produce IL-13 have been implicated in the pathogenesis of colitis (43). Furthermore, iNKT-deficient mice differ in gut microbial composition compared to wild-type mice, as well as showing increased intestinal leucocyte infiltration (44). Whether iNKT cells are involved in the pathogenesis of NEC in preterm infants is not known.
Mucosa-Associated Invariant T (MAIT) Cells
MAIT cells are a population of T cells predominantly found in the lung and intestinal mucosa, that display a semi-invariant TCR (Vα 7.2 – Jα3.3/20/12). MAIT cells have been demonstrated in the fetal thymus from 18 weeks GA. MAIT cells represent <1% of peripheral blood T cells in newborns compared to up to 10% in adults, suggesting a postnatal expansion (17). MAIT cells are of particular interest as they recognize MR-1, an MHC-1 like molecule that presents microbial derived metabolites of the essential vitamin, riboflavin (17). Riboflavin is synthesized by gut commensal bacteria, and has a variable concentration in human milk dependent on maternal intake (45, 46). Thus, the postnatal expansion of MAIT cells is likely driven both by bacteria and by diet.
In contrast to thymic MAIT cells, those in fetal intestine, spleen, and MLN express a marker of activation (PLZF), suggesting peripheral maturation. They develop this mature phenotype in the first 2 months of life (17, 47) and display inflammatory and cytotoxic abilities. Indeed, fetal intestinal, liver and lung but not thymic or spleen MAIT cells can produce IFN-γ following stimulation (18). Functional changes in MAIT cells have been associated with inflammation in adults with inflammatory bowel disease (48). However, no study has examined their relationship with NEC.
Dietary Interactions With Mucosal T Cells
When considering modulation of the preterm T cell populations, we have described how key dietary exposures such as HMOs and bacteria appear important. Breast milk contains a vast array of components that may directly or indirectly affect gut mucosal T cell populations, as demonstrated in both human and animal models of early life (Figure 1). The gut microbiota is likely to be a key mediator of such dietary effects, implying the possibility of therapeutic modulation either by dietary modifications or the use of prebiotics or probiotics.
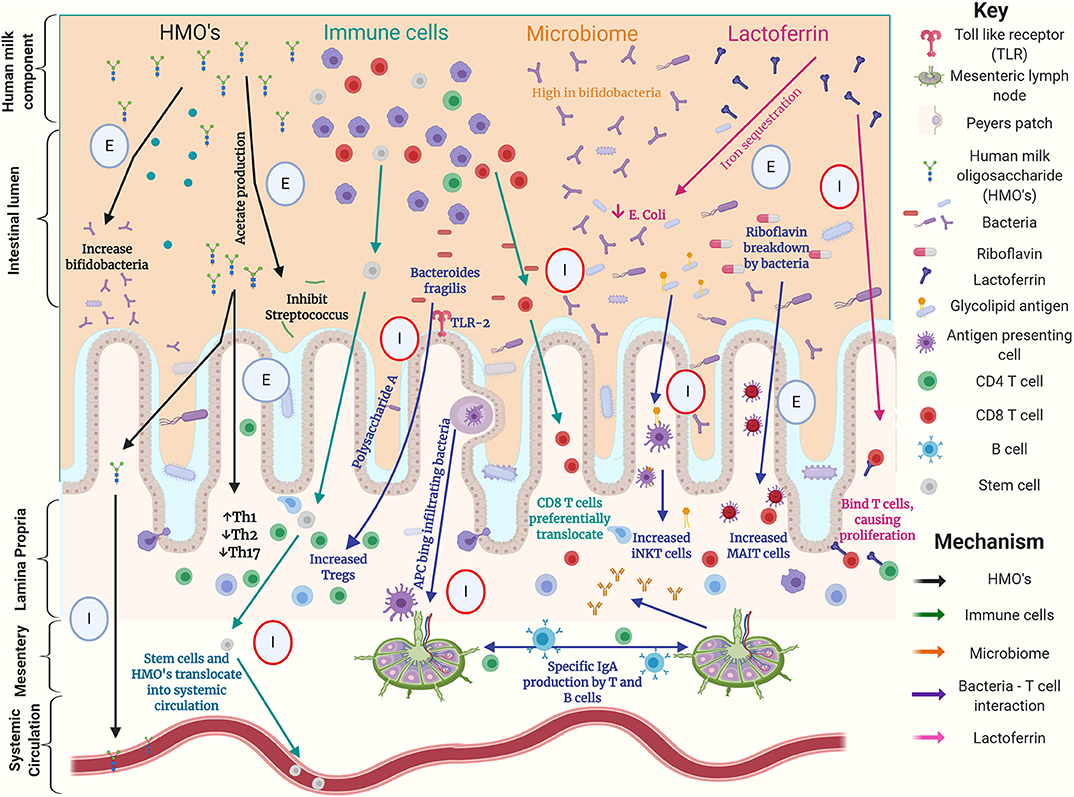
Figure 1. Graphical summary of possible interactions between components of human milk, the gut microbiome and T cells in the preterm intestine. This summarizes work using human, animal and laboratory-based experiments. Circles highlight experiment type with; E denoting an ex-vivo experiment, I denoting an in-vivo experiment, red circles denoting animal experiments (16, 28–30, 32, 34, 35, 42, 44, 49–57).
Colostrum
Compared to more mature human milk, colostrum has a higher concentration of bioactive components including HMOs, lactoferrin, immune cells, and immunoglobulin. In a porcine model, pregnant sows were given NEC-inducing intra-amniotic lipopolysaccharide injections in-utero and offspring pups were then fed varying diets before euthanasia. Pups fed colostrum had decreased levels of distal ileal IL-8 and IL-1β, combined with increased abundance of blood Th cells (as % of lymphocytes). The authors concluded that a colostrum diet led to maturation of the intestinal mucosa as well as the systemic immune system (50).
Translocation of Milk Derived Immune Cells
Human milk contains stem cells and leucocytes (51, 58). Studies using mice show that maternal immune cells can translocate from the intestine into the circulation or distal organs (53, 54, 59). Stem cells derived from milk have been shown to translocate into the brains of mice, and once there to traffic and differentiate (53, 54). In these mouse models, T cells are the predominant cell type that transfers across the intestinal epithelium despite their relative paucity in milk (58, 59). CD8 T cells, in particular, translocate into the intestinal Peyer's patches, possibly based on their expression of a gut homing receptor (CCR9). Once there, they show an increased ability to produce cytokines upon stimulation compared with peripheral blood CD8 T cells (59). It is unclear whether diet-derived T cells migrate beyond the MLN in mice as studies conflict (52, 59) but it is plausible that milk-derived maternal CD8 T cells translocate into the mucosa in humans and compensate for “deficiencies” of preterm T cells.
Lactoferrin
Lactoferrin is the major whey glycoprotein of human milk and subject to intense research for over 50 years due to its ability to inhibit bacteria by multiple mechanisms (55, 60). Lactoferrin has also been shown to bind to immune cells and influence their function (61). Most lymphocytes can express a lactoferrin receptor including stimulated αβ and γδ T cells (56). Furthermore, T cells in the lamina propria of pigs bind lactoferrin (57). In a mouse model, T cells in the lamina propria proliferated upon administration of enteral bovine lactoferrin in the presence or absence of colon cancer. These T cells secreted IFN-γ and IL-18, suggesting that they were immunologically active (62).
Conclusion
Preterm infants have a T cell population that was designed for fetal life and therefore differs systematically from term infants (Table 1). In particular, they have a diminished Th1 response leaving them susceptible to infection by intracellular pathogens. Tregs protect the preterm infant from an excessive innate response which may reduce the risk of NEC but may also increase the risk of LOS. This variation in function of Tregs is modulated by IL-10.
There are multiple mechanisms by which the diet, gut microbiome, and T cell populations have been demonstrated to interact in human, mouse, and laboratory experiments demonstrated in Figure 1. However, there is a paucity of data comparing the effects of alternative feeding strategies on the preterm infant's immune system, including T cells, and there remains substantial uncertainty about when and how donor human milk and human milk derived fortifiers should be used. Clinical studies aimed at identifying changes in the immune system associated with these dietary interventions will improve understanding and enable more informed nutritional management.
The functions of innate-like cells such as iNKT and MAIT cells in preterm infants have yet to be unraveled but a number of compelling studies on animals imply an important role in preventing intestinal inflammation. Alterations in gut mucosal T cells may occur in preterm infants as a result of dietary or microbiome manipulations. A better understanding of the interplay of diet, microbiome and host immunity will underpin efforts to develop interventions that modulate T-cell responses for improved infant health.
Author Contributions
TS wrote the initial manuscript. All authors contributed to manuscript revision, read, and approved the submitted version.
Conflict of Interest
NE declares research funding from Danone Early Life Nutrition and Prolacta Biosciences, as well as speaker honoraria from Baxter and Nestle Nutrition Institute.
The remaining authors declare that the research was conducted in the absence of any commercial or financial relationships that could be construed as a potential conflict of interest.
The handling editor declared a past co-authorship with the JB and NE.
Abbreviations
GA, gestational age; iNK T, invariant natural killer T cells; MAIT, mucosal associated invariant T cells; MHC, major histocompatibility complex; MLN, mesenteric lymph nodes; PNA, postnatal age; TCR, T cell receptor; Th, T-helper; Tregs, regulatory T cells.
References
1. Griffiths J, Jenkins P, Vargova M, Bowler U, Juszczak E, King, et al. Enteral lactoferrin supplementation for very preterm infants: a randomised placebo-controlled trial. The Lancet. (2019) 393:423–33. doi: 10.1016/S0140-6736(18)32221-9
2. Stewart CJ, Embleton ND, Marrs ECL, Smith DP, Smith DP, Nelson A, et al. Temporal bacterial and metabolic development of the preterm gut reveals specific signatures in health and disease. Microbiome. (2016) 4:67. doi: 10.1186/s40168-016-0216-8
3. Stewart CJ, Embleton ND, Marrs ECL, Smith DP, Fofanova T, Nelson, et al. Longitudinal development of the gut microbiome and metabolome in preterm neonates with late onset sepsis and healthy controls. Microbiome. (2017) 5:1. doi: 10.1186/s40168-017-0295-1
4. Patel AL, Johnson TJ, Engstrom JL, Fogg LF, Jegier BJ, Bigger R, et al. Impact of early human milk on sepsis and health-care costs in very low birth weight infants. J Perinatol. (2013) 33:514–19. doi: 10.1038/jp.2013.2
5. Corpeleijn WE, Kouwenhoven MP, Paap M, Vliet I, Scheerder I, Muizer Y, et al. Intake of own mother's milk during the first days of life is associated with decreased morbidity and mortality in very low birth weight infants during the first 60 days of life. Neonatology. (2012) 102:276–81. doi: 10.1159/000341335
6. Olin A, Henckel E, Chen Y, Lakshmikanth T, Pou C, Mikes, et al. Stereotypic immune system development in newborn children. Cell. (2018) 174:1277–92.e14. doi: 10.1016/j.cell.2018.06.045
7. Dirix V, Vermeulen F, Mascart F. Maturation of CD4+ regulatory T lymphocytes and of cytokine secretions in infants born prematurely. J Clin Immunol. (2013) 33:1126–33. doi: 10.1007/s10875-013-9911-4
8. Raphael I, Nalawade S, Eagar TN, Forsthuber TG. T cell subsets and their signature cytokines in autoimmune and inflammatory diseases. Cytokine. (2015) 74:5–17. doi: 10.1016/j.cyto.2014.09.011
9. Zahran AM, Saad K, Abdel-Raheem YF, Elsayh KI, El-Houfey AA, Aboul-Khair MD, et al. Characterization of regulatory T cells in preterm and term infants. Archivum Immunologiae et Therapiae Experimentalis. (2019) 67:49–54. doi: 10.1007/s00005-018-0530-x
10. Penaloza HF, Noguera LP, Riedel CA, Bueno SM. Expanding the current knowledge about the role of interleukin-10 to major concerning bacteria. Front Microbiol. (2018) 9:2047. doi: 10.3389/fmicb.2018.02047
11. Walker JC, Smolders MAJC, Gemen EFA, Antonius TAJ, Leuvenink J, De Vries E. Development of lymphocyte subpopulations in preterm infants. Scand J Immunol. (2010) 73:53–8. doi: 10.1111/j.1365-3083.2010.02473.x
12. Scheible KM, Erno J, Yang H, Holden-Wiltse J, Straw A, Huyck H, et al. Developmentally determined reduction in CD31 during gestation is associated with CD8+ T cell effector differentiation in preterm infants. Clin Immunol. (2015) 161:65–74. doi: 10.1016/j.clim.2015.07.003
13. Kamdar S, Hutchinson R, Laing A, Stacey F, Ansbro K, Millar MR, et al. Perinatal inflammation influences but does not arrest rapid immune development in preterm babies. Nat Commun. (2012) 11:1284. doi: 10.1038/s41467-020-14923-8
14. Li J, Li H, Mao H, Yu M, Feng T, Yang F, et al. Vgamma9Vdelta2-T lymphocytes have impaired antiviral function in small-for-gestational-age and preterm neonates. Cell Mol Immunol. (2013) 10:253–60. doi: 10.1038/cmi.2012.78
15. Gibbons DL, Haque SF, Silberzahn T, Hamilton K, Langford C, Ellis P, et al. Neonates harbour highly active gammadelta T cells with selective impairments in preterm infants. Eur J Immunol. (2009) 39:1794–806. doi: 10.1002/eji.200939222
16. Ladd M, Sharma A, Huang Q, Wang AY, Xu L, Genowiti I, et al. Natural killer T cells constitutively expressing the interleukin-2 receptor α chain early in life are primed to respond to lower antigenic stimulation. Immunology. (2010) 131:289–99. doi: 10.1111/j.1365-2567.2010.03304.x
17. Ben Youssef G, Tourret M, Salou M, Ghazarian L, Houdouin V, Mondot S, et al. Ontogeny of human mucosal-associated invariant T cells and related T cell subsets. J Exp Med. (2018) 215:459–79. doi: 10.1084/jem.20171739
18. Leeansyah E, Loh L, Nixon DF, Sandberg JK. Acquisition of innate-like microbial reactivity in mucosal tissues during human fetal MAIT-cell development. Nat Commun. (2014) 5:3143. doi: 10.1038/ncomms4143
19. Haynes BF, Heinly CS. Early human T cell development: analysis of the human thymus at the time of initial entry of hematopoietic stem cells into the fetal thymic microenvironment. J Exp Med. (1995) 181:1445–58. doi: 10.1084/jem.181.4.1445
20. Michaelsson J, Mold JE, McCune JM, Nixon DF. Regulation of T cell responses in the developing human fetus. J Immunol. (2006) 176:5741–8. doi: 10.4049/jimmunol.176.10.5741
21. Darrasse-Jeze G, Marodon G, Salomon BL, Catala B, Klatzmann D. Ontogeny of CD4+CD25+ regulatory/suppressor T cells in human fetuses. Blood. (2005) 105:4715–21. doi: 10.1182/blood-2004-10-4051
22. Li N, van Unen V, Abdelaal T, Guo N, Kasatskaya SA, Ladell K, et al. Memory CD4(+) T cells are generated in the human fetal intestine. Nat Immunol. (2019) 20:301–12. doi: 10.1038/s41590-018-0294-9
23. Schreurs R, Baumdick ME, Sagebiel AF, Kaufmann M, Mokry M, Klarenbeek PL, et al. Human fetal TNF-alpha-cytokine-producing CD4(+) effector memory T cells promote intestinal development and mediate inflammation early in life. Immunity. (2019) 50:462–76.e8. doi: 10.1016/j.immuni.2018.12.010
24. Quinello C, Silveira-Lessa AL, Ceccon MEJR, Cianciarullo MA, Carneiro-Sampaio M, Palmeira P. Phenotypic differences in leucocyte populations among healthy preterm and full-term newborns. Scand J Immunol. (2014) 80:57–70. doi: 10.1111/sji.12183
25. Berrington JE, Barge D, Fenton AC, Cant AJ, Spickett GP. Lymphocyte subsets in term and significantly preterm UK infants in the first year of life analysed by single platform flow cytometry. Clin Exp Immunol. (2005) 140:289–92. doi: 10.1111/j.1365-2249.2005.02767.x
26. Wong MT, Ong DE, Lim FS, Teng KW, McGovern N, Narayanan S, et al. A high-dimensional atlas of human T cell diversity reveals tissue-specific trafficking and cytokine signatures. Immunity. (2016) 45:442–56. doi: 10.1016/j.immuni.2016.07.007
27. Thome JJ, Bickham KL, Ohmura Y, Kubota M, Matsuoka N, Gordon, et al. Early-life compartmentalization of human T cell differentiation and regulatory function in mucosal and lymphoid tissues. Nat Med. (2016) 22:72–7. doi: 10.1038/nm.4008
28. Gonia S, Tuepker M, Heisel T, Autran C, Bode L, Gale A, et al. Human milk oligosaccharides inhibit Candida albicans invasion of human premature intestinal epithelial cells. J Nutr. (2015) 145:1992–8. doi: 10.3945/jn.115.214940
29. Bode L. Human milk oligosaccharides: every baby needs a sugar mama. Glycobiology. (2012) 22:1147–62. doi: 10.1093/glycob/cws074
30. He Y, Liu S, Leone S, Newburg DS. Human colostrum oligosaccharides modulate major immunologic pathways of immature human intestine. Mucosal Immunol. (2014) 7:1326–39. doi: 10.1038/mi.2014.20
31. Pagel J, Hartz A, Figge J, Gille C, Eschweiler S, Petersen K, et al. Regulatory T cell frequencies are increased in preterm infants with clinical early-onset sepsis. Clin Exp Immunol. (2016) 185:219–27. doi: 10.1111/cei.12810
32. Glocker EO, Kotlarz D, Boztug K, Gertz EM, Schaffer AA, Noyan F, et al. Inflammatory bowel disease and mutations affecting the interleukin-10 receptor. N Engl J Med. (2009) 361:2033–45. doi: 10.1056/NEJMoa0907206
33. Atarashi K, Tanoue T, Shima T, Imaoka A, Kuwahara T, Momose Y, et al. Induction of colonic regulatory T cells by indigenous clostridium species - murine model of Treg and Th population being changed by microbiota. Science. (2011) 331:337–41. doi: 10.1126/science.1198469
34. Round JL, Lee SM, Li J, Tran G, Jabri B, Chatila TA, et al. The Toll-like receptor 2 pathway establishes colonization by a commensal of the human microbiota. Science. (2011) 332:974. doi: 10.1126/science.1206095
35. Ramakrishna C, Kujawski M, Chu H, Li L, Mazmanian SK, Cantin M, et al. Bacteroides fragilis polysaccharide A induces IL-10 secreting B and T cells that prevent viral encephalitis. Nat Commun. (2019) 10:2153. doi: 10.1038/s41467-019-09884-6
36. Sittipo P, Lobionda S, Choi K, Sari IN, Kwon HY, Lee K, et al. Toll-like receptor 2-mediated suppression of colorectal cancer pathogenesis by polysaccharide A from Bacteroides fragilis. Front Microbiol. (2018) 9:1588. doi: 10.3389/fmicb.2018.01588
37. Zhang X, Mozelski B, Lemoine S, Deriaud E, Lim A, Zhivaki D, et al. CD4 T cells with effector memory phenotype and function develop in the sterile environment of the fetus. Sci Transl Med. (2014) 6:238ra72. doi: 10.1126/scitranslmed.3008748
38. Marchant A, Appay V, van der Sande M, Dulphy N, Liesnard C, Kidd M, et al. Mature CD8+ T lymphocyte response to viral infection during fetal life. J Clin Investig. (2003) 111:1747–55. doi: 10.1172/JCI200317470
39. Vantourout P, Hayday A. Six-of-the-best: unique contributions of gammadelta T cells to immunology. Nat Rev Immunol. (2013) 13:88–100. doi: 10.1038/nri3384
40. Berzins SP, Smyth MJ, Baxter AG. Presumed guilty: natural killer T cell defects and human disease. Nat Rev Immunol. (2011) 11:131–42. doi: 10.1038/nri2904
41. Moreira-Teixeira L, Resende M, Coffre M, Devergne O, Herbeuval JP, Hermine O, et al. Proinflammatory environment dictates the IL-17-producing capacity of human invariant NKT cells. J Immunol. (2011) 186:5758–65. doi: 10.4049/jimmunol.1003043
42. Olszak T, An D, Zeissig S, Vera MP, Richter J, Franke A, et al. Microbial exposure during early life has persistent effects on natural killer T cell function. Science. (2012) 336:489–93. doi: 10.1126/science.1219328
43. Heller F, Fuss IJ, Niewenhuis EE, Blumberg RS, Strober W. Oxazolone colitis, a Th2 colitis model resembling ulcerative colitis, is mediated by IL-13-producing NK-T cells. Immunity. (2002). 17:629–38. doi: 10.1016/S1074-7613(02)00453-3
44. Shen S, Prame Kumar K, Stanley D, Moore RJ, Van TTH, Wen SW, et al. Invariant natural killer T cells shape the gut microbiota and regulate neutrophil recruitment and function during intestinal inflammation. Front Immunol. (2018) 9:999. doi: 10.3389/fimmu.2018.00999
45. Hampel D, Shabab-Ferdows S, Islam MM, Peerson JM, Allen LH. Vitamin concentrations in human milk vary with time within feed. Circadian rhythm, single-dose supplementation. J Nutr. (2017) 147:603–11. doi: 10.3945/jn.116.242941
46. Tastan C, Karhan E, Zhou W, Fleming E, Voigt AY, Yao X, et al. Tuning of human MAIT cell activation by commensal bacteria species and MR1-dependent T-cell presentation. Mucosal Immunol. (2018) 11:1591–605. doi: 10.1038/s41385-018-0072-x
47. Walker LJ, Tharmalingam H, Klenerman P. The rise and fall of MAIT cells with age. Scand J Immunol. (2014) 80:462–3. doi: 10.1111/sji.12237
48. Chiba A, Murayama G, Miyake S. Mucosal-associated invariant T cells in autoimmune diseases. Front Immunol. (2018) 9:1333. doi: 10.3389/fimmu.2018.01333
49. Le Bourhis L, Guerri L, Dusseaux M, Martin E, Soudais C, Lantz, et al. Mucosal-associated invariant T cells: unconventional development and function. Trends Immunol. (2011) 32:212–8. doi: 10.1016/j.it.2011.02.005
50. Ren S, Hui Y, Goericke-Pesch S, Pankratova S, Kot W, Pan X, et al. Gut and immune effects of bioactive milk factors in preterm pigs exposed to prenatal inflammation. Am J Physiol Gastrointest Liver Physiol. (2019) 317:G67–77. doi: 10.1152/ajpgi.00042.2019
51. Hassiotou F, Beltran A, Chetwynd E, Stuebe AM, Twigger A-J, et al. Breastmilk is a novel source of stem cells with multilineage differentiation potential. Stem Cells. (2012) 30:2164–74. doi: 10.1002/stem.1188
52. Ma LJ, Walter B, Deguzman A, Muller HK, Walker AM. Trans-epithelial immune cell transfer during suckling modulates delayed-type hypersensitivity in recipients as a function of gender. PLoS ONE. (2008) 3:e3562. doi: 10.1371/journal.pone.0003562
53. Hassiotou F, Mobley A, Geddes D, Hartmann P, Walkie T. Breastmilk imparts the mother's stem cells to the infant. FASEB J. (2015) 29:876.
54. Aydin MS, Yigit EN, Vatandaslar E, Erdogan E, Ozturk G. Transfer, and integration of breast milk stem cells to the brain of suckling pups. Sci Rep. (2018). 8:14289. doi: 10.1038/s41598-018-32715-5
55. Oram JD, Reiter B. Inhibition of bacteria by lactoferrin and other iron-chelating agents. Biochim Biophys Acta. (1968) 170:351–65. doi: 10.1016/0304-4165(68)90015-9
56. Mincheva-Nilsson L, Hammarstrom S, Hammarstrom M. Activated human yd T lymphocytes express functional lactoferrin receptors. Scand J Immunol. (1997) 46:609–18. doi: 10.1046/j.1365-3083.1997.d01-165.x
57. Nielsen SM, Hansen GH, Danielsen EM. Lactoferrin targets T cells in the small intestine. J Gastroenterol. (2010) 45:1121–8. doi: 10.1007/s00535-010-0271-z
58. Trend S, de Jong E, Lloyd ML, Kok CH, Richmond P, Doherty DA, et al. Leukocyte populations in human preterm and term breast milk identified by multicolour flow cytometry. PLoS ONE. (2015) 10:e0135580. doi: 10.1371/journal.pone.0135580
59. Cabinian A, Sinsimer D, Tang M, Zumba O, Mehta H, Toma A, et al. Transfer of maternal immune cells by breastfeeding: maternal cytotoxic T lymphocytes present in breast milk localize in the Peyer's patches of the nursed infant. PLoS ONE. (2016) 11:e0156762. doi: 10.1371/journal.pone.0156762
60. Ward P, Conneely OM. Lactoferrin: role in iron homeostasis and host defense against microbial infection. BioMetals. (2004) 17:203–8. doi: 10.1023/B:BIOM.0000027693.60932.26
61. Legrand D. Overview of lactoferrin as a natural immune modulator. J Pediatr. (2016) 173(Suppl.):S10–5. doi: 10.1016/j.jpeds.2016.02.071
Keywords: T-lymphocytes, infant, premature, mucosal immunity, necrotizing enterocolitis, extremely premature
Citation: Sproat T, Payne RP, Embleton ND, Berrington J and Hambleton S (2020) T Cells in Preterm Infants and the Influence of Milk Diet. Front. Immunol. 11:1035. doi: 10.3389/fimmu.2020.01035
Received: 15 December 2019; Accepted: 29 April 2020;
Published: 02 June 2020.
Edited by:
Per T. Sangild, University of Copenhagen, DenmarkReviewed by:
Alberto Finamore, Council for Agricultural and Economics Research (CREA), ItalyClaudia Nold, Hudson Institute of Medical Research, Australia
Copyright © 2020 Sproat, Payne, Embleton, Berrington and Hambleton. This is an open-access article distributed under the terms of the Creative Commons Attribution License (CC BY). The use, distribution or reproduction in other forums is permitted, provided the original author(s) and the copyright owner(s) are credited and that the original publication in this journal is cited, in accordance with accepted academic practice. No use, distribution or reproduction is permitted which does not comply with these terms.
*Correspondence: Thomas Sproat, dHNwcm9hdCYjeDAwMDQwO25ocy5uZXQ=