- 1Comparative Pediatrics and Nutrition, Department of Veterinary and Animal Sciences, Faculty of Health and Medical Sciences, University of Copenhagen, Copenhagen, Denmark
- 2Veterinary Clinical Microbiology, Department of Veterinary and Animal Sciences, Faculty of Health and Medical Sciences, University of Copenhagen, Copenhagen, Denmark
- 3Department of Neonatology, Rigshospitalet, Copenhagen, Denmark
- 4Department of Pediatrics, Odense University Hospital, Odense, Denmark
Background: Preterm infants are born with an immature immune system, limited passive immunity, and are at risk of developing bacteremia and sepsis in the postnatal period. We hypothesized that enteral feeding, with or without added immunoglobulins, improves the clinical response to systemic infection by coagulase negative staphylococci.
Methods: Using preterm cesarean delivered pigs as models for preterm infants, we infused live Staphylococcus epidermidis (SE, 5 × 109 colony forming units per kg) systemically 0–3 days after birth across five different experiments. SE infection responses were assessed following different gestational age at birth (preterm vs. term), enteral milk diets (bovine colostrum, infant formula with or without added porcine plasma) and with/without systemic immunoglobulins. Pigs infected with SE were assessed 12–48 h for clinical variables, blood bacteriology, chemistry, hematology, and gut dysfunction (intestinal permeability, necrotizing enterocolitis lesions).
Results: Adverse clinical responses and increased mortality were observed in preterm vs. term pigs, when infected with SE just after birth. Feeding bovine colostrum just after birth improved blood SE clearance and clinical status (improved physical activity and intestinal structure, fewer bone marrow bacteria), relative to pigs fed infant formula. A few days later, clinical responses to SE bacteremia (hematology, neutrophil phagocytic capacity, T cell subsets) were less severe, and less affected by different milk diets, with or without added immunoglobulins.
Conclusion: Prematurity increases the sensitivity of newborn pigs to SE bacteremia, potentially causing sepsis. Sensitivity to systemic SE infection decreases rapidly in the days after preterm birth. Both age and diet (parenteral nutrition, colostrum, milk, formula) may influence gut inflammation, bacterial translocation and systemic immune development in the days after birth in preterm newborns.
Introduction
Preterm infants have an increased risk of infection in the neonatal period. The risk of late onset sepsis (LOS) is 20–40%, with increasing risks at lower gestational ages (1, 2). Use of infant formula, or prolonged use of parenteral nutrition, and associated use of catheters, increases the risk of LOS (3). Use of milk based diets shorten the time to full enteral feeding, and thereby removal of central venous catheters as well as reduces the incidence of necrotizing enterocolitis (NEC) (4, 5). Severe inflammation of the gut is associated with sepsis in preterm neonates, but it is unknown if this effect is due mainly to increased bacterial translocation, or if local gut inflammatory conditions adversely affect systemic immunity. The type of nutrition after birth (parenteral, or enteral feeding with formula, milk or colostrum) rapidly affects gut maturation in immunocompromised preterm infants and pigs (6). Whether the first enteral feeding also influences systemic immunity in such infants is less clear, although clinical outcomes may be improved after early feeding with milk or colostrum of either human or animal origin (7–9).
Across the world, some of the most common pathogens causing LOS in preterm neonates are coagulase negative staphylococci (CoNS) (2, 10–12). The defense against systemic CoNS infection is dependent on opsonization of the bacteria by immunoglobulins and complement factors (13–15). Immunoglobulin G (IgG) is actively transported across the placenta, starting in the second trimester, and accelerates toward term (16). Preterm infants are therefore born with lower levels of maternally derived IgG (16, 17). As a result, opsonization and clearance of CoNS is more dependent on complement activation in these infants (14, 18). However, levels of complement factors are also lower in preterm infants, as their capacity to synthetize them is diminished (19). Overall, levels of IgG in preterm infants have been correlated to the risk of neonatal sepsis, but providing these infants prophylactic immunoglobulins does not prevent sepsis (20, 21). A Cochrane review found that infusion of immunoglobulins led to a slight reduction in nosocomial infections, but did not affect the incidence of neonatal sepsis or overall mortality (22). Likewise, providing specific anti-staphylococcal IgG, did not prevent infection with CoNS (23). Finally, administration of intravenous IgG during neonatal sepsis had no effects on clinical outcomes (24).
Oral administration of IgG to preterm infants has been speculated to prevent NEC as immunoglobulins may survive passage through the gastrointestinal tract (25). In two randomized controlled trials, orally administered human IgG and IgA reduced NEC incidence in preterm infants (26, 27), but a later Cochrane review concluded that there was no overall effect of oral IgG on NEC incidence in preterm infants (28). In other studies, enteral feeding with either porcine or bovine colostrum to preterm pigs prevented NEC, and improved gut maturation and parameters of systemic immunity (29–33). Feeding of porcine plasma (PP) has also been shown to improve survival, growth and diarrhea resistance in growing pigs (34), probably mediated by diminished pro-inflammatory and increased anti-inflammatory responses in both gut and lung derived immune cells (35–37). Dietary PP supplementation was not associated with adverse events in healthy or malnourished infants (38, 39) and oral immunoglobulins improved gut symptoms, recovery and virus clearance in children with rotavirus diarrhea (40). Collectively, the above studies suggest that feeding immunoglobulin rich milk diets improves the response to bacteremia in immature animals and humans. Regardless, the mechanisms remain unclear and until now scientific results have not led to general recommendations to feed immunoglobulin enriched diets to bacteremia sensitive infants. Thus, it remains unknown how postnatal age, diet (e.g., mother's own milk, formula, donor milk), type of immunoglobulin (IgG, IgA and their species specificity) affect systemic immune responses.
Bovine colostrum (BC), the first milk after birth in cows, is rich in immunoglobulins and other immunomodulatory factors (41), inhibits growth of Staphylococcus epidermidis (SE) in vitro (42) and prevents septic shock and neuroinflammation in newborn preterm pigs (within 2 d of birth), relative to pigs not fed enterally (43). To further validate preterm pigs as models for preterm infants sensitive to blood stream infections, we investigated responses to SE infection across five different experiments with varying postnatal ages and exposures to immunoglobulin-containing diets. First, we compared the effect of SE infusion on newborn preterm and term pigs lacking maternal immunoglobulins and enteral feeding. Then we tested effects of feeding BC or infant formula (IF) to preterm pigs infected shortly after birth or 2 days later, with or without immunization with maternal plasma. Finally, we tested the effects of feeding preterm pigs IF, supplemented with porcine plasma, for 3 days, followed by SE inoculation.
Materials and Methods
Animal Experimental Procedures
In five separate experiments, 145 piglets from 10 pregnant sows [(Landrace x Large White) x Duroc crossbreeds] were delivered by cesarean section at the 106th day (preterm birth, ~90% gestation) or 116th (term birth, ~100% gestation) day of gestation. Animals were housed in individual, heated (37°C) and ventilated incubators with 1–2 l/min oxygen supply. Shortly after delivery, animals were fitted with orogastric catheters (6 Fr, Portex, Kent, UK) for enteral feeding and vascular catheters (4 Fr, Portex) into the dorsal aorta via the transected umbilical cord for arterial access. Both control and infected animals were euthanized according to predefined humane endpoints. All animal procedures were approved by the Danish National Committee on Animal Experimentation.
Across all experiments, animals were stratified by birth weight and randomly allocated to treatment groups. In Experiment 1, preterm (n = 23) and term (n = 21) pigs were inoculated with either SE (SE-PRE, n = 15; SE-TERM, n = 14) or control saline (CON-PRE, n = 8; CON-TERM, n = 7) within 2 h after birth. Animals received no enteral nutrition and were followed for 48 h. In Experiment 2, preterm pigs (n = 15) were inoculated with SE 2 h after birth and were fed either BC (SE-BC, n = 8) or IF (SE-IF, n = 7) and followed for 12 h. In Experiment 3, preterm pigs were fed either BC or IF for further 2 days, and inoculated with SE (SE-BC, n = 13; SE-IF, n = 13) or control saline (CON-BC, n = 9; CON-IF, n = 12) and followed for 48 h. Further, these animals were infused with maternal plasma to confer a low level of maternally-derived IgG similar to preterm infants. In Experiment 4, in an attempt to increase the sensitivity to SE, preterm pigs were fed BC (SE-BC, n = 10) or IF (SE-IF, n = 10) for 2 days, but not infused with maternal plasma before receiving a systemic SE challenge. In Experiment 5, preterm pigs, supplemented with maternal plasma, were fed with a PP enriched IF (SE-PP, n = 11) or a control IF (SE-IF, n = 8) and received a systemic SE challenge after 3 days. An overview of conditions in the five experiments is shown in Table 1.
Feeding Regimens and Bacterial Administration
In Experiment 1, animals received no enteral feed and were kept on 6 ml/kg/h of total parenteral nutrition. In Experiments 2, 3, and 4, animals were fed 40 ml/kg/d on day 1, increasing to 56 ml/kg/d on day 5 of either BC or IF, with 4–6 ml/kg/h of supplementary parenteral nutrition. In Experiment 5, animals were fed 24 ml/kg/d of IF with or without added PP on day 1, increasing to 96–120 ml/kg/d on day 5 with 3 ml/kg/d of supplementary parenteral nutrition.
The BC powder was produced from first and second milking of Danish Holstein dairy cattle and gently processed to preserve bioactivity (Biofiber Damino, Gesten, Denmark). The IF diet consisted of a nutritionally complete IF powder (Pepdite, Nutricia, Allerød, Denmark) with added whey and medium chain triglycerides. In the PP enriched IF diet, a fraction (8%) of the IF powder was replaced with a gently processed porcine plasma powder (AP 920 P APC Europe, Spain). The parenteral formulation used in all experiments (Kabiven, Fresenius Kabi, Bad Homburg, Germany) was supplemented with vitamins and minerals (Soluvit, Vitalipid and Peditrace, all Fresenius Kabi), and modified to meet macronutrient requirements of newborn pigs (30). Detailed diet compositions and macronutrient contents are presented in Table 2.
In Experiments 3 and 5, animals were supplemented with a vascular infusion of 16 ml/kg body weight maternal plasma within the first 24 h after birth. This was done to confer some level of immunoglobulins, mimicking the situation in preterm infants.
Preparation of the SE inoculum was performed, as previously described (43). Briefly, wildtype SE strain 1,457 (a kind gift from Dr. Xiaoyang Wang, University of Gothenburg, Sweden) was incubated for 17 h in tryptic soy broth, enumerated with spectrophotometry and a working solution containing 1.67 × 109 colony forming units (CFU)/ml in sterile saline was prepared. Animals received 5.0 × 109 CFU/kg body weight SE as a continuous arterial infusion over 3 min. A similar volume of sterile saline was administered to control animals in Experiments 1 and 3.
Clinical Assessment and Euthanasia
Animals were monitored and clinically assessed (gastrointestinal symptoms, circulation, respiration, consciousness) at least every 3 h prior to, and continuously after SE administration. Animals were euthanized if they showed clinical signs of circulatory and/or respiratory collapse (Pale skin, cold extremities, cyanosis, lethargy, irregular/shallow breathing and/or bradycardia). Rectal temperature was measured before and at 6- or 12-h intervals after SE administration. Motor activity was captured by continuous infrared video surveillance of each incubator connected to a motion detection software (PigLWin, Ellegaard Systems, Faaborg, Denmark).
Sample Collection
Arterial blood was collected at regular intervals after SE administration for hematology and blood gas analysis. After a follow up period of 12–48 h, animals were sedated with 0.1 ml/kg Zoletil mixture, mixed blood was drawn by cardiac puncture and euthanasia performed with a lethal cardiac injection of barbiturate. In Experiments 3 and 5, animals were fed a 5/5% w/v lactulose and mannitol watery solution 3 h before scheduled euthanasia for intestinal permeability measurement. After euthanasia, the abdominal cavity was opened, and urine collected by bladder puncture. Urinary levels of lactulose and mannitol were measured as previously described (30). In Experiments 3, 4 and 5, the stomach and intestines were examined, and gastrointestinal pathology graded according to an established NEC scoring system (30). Specifically, in Experiment 3, the left hind leg was released at the hip joint, and femur dissected and sterilized in ethanol. Using a sterile scalpel, bone marrow was dissected at the distal epiphysis and a biopsy was obtained for bacterial enumeration.
Blood Analyses
Enumeration of SE was determined in blood samples taken 3, 6, and 12 h after SE inoculation in Experiment 2 and at euthanasia in Experiments 3 and 4, as previously described elsewhere (43). Briefly, whole blood was cooled after collection, stepwise diluted in sterile saline (undilute, 1:10 and 1:100), plated out on agar plates and incubated for 24 h at 37°C.Colonies were counted and identification of bacteria was performed by Matrix Assisted Laser Desorption/Ionization-Time of Flight Mass Spectrometry. In a similar manner, bacterial enumeration, and identification in bone marrow homogenate were performed in Experiment 3.
In Experiments 3, 4, and 5, levels of bovine and porcine IgG in euthanasia blood plasma samples were measured by enzyme-linked immunosorbent assay (ELISA) using species specific antibodies (AAI23AB and AAI41, Bio-Rad, Kidlington, UK). Blood hematology was performed using the Advia 2120i Clinical Chemistry System (Siemens Healthcare GmbH, Erlangen, Germany). In plasma samples from euthanasia from Experiments 1, 3, 4, and 5, we determined levels of soluble terminal complement complex by ELISA (sC5b-9, OptEIA, BD Biosciences, Eysins, Switzerland). Additionally, in Experiments 3 and 4, plasma levels of tumor necrosis factor alpha (TNF-α) and interleukin 6 (IL-6) were determined by ELISA (porcine DuoSet DY686 and DY690B, R&D systems, Abingdon, UK).
Arterial blood gas was measured in Experiments 1, 2, and 4, using a GEM Premier 3000 (Instrumentation Laboratory, MA, USA). Hemostatic function in citrate stabilized whole blood was tested in Experiment 5 by thromboelastography (TEG) using a TEG 5000 Hemostasis Analyzer System (Haemonetics, Braintree, MA, USA).
T cell phenotyping in whole blood at 12, 24, and 48 h (euthanasia) after SE inoculation was done in Experiment 3 by flow cytometry. Briefly, whole blood samples were subjected to red blood cell lysis, fixation and permeabilization before Fc-receptor blocking using porcine serum. Next, cells were stained for 30 min with CD3-PerCP Cy5.5 (clone BB23-8E6-8C8, BD biosciences, Eysins, Switzerland), CD4a-FITC (clone MIL17, Bio-Rad), CD8α-PE (clone MIL12, Bio-Rad) and Foxp3-APC (clone FJK-16s, ThermoFisher Scientific, Waltham, MA, USA) or respective isotype controls PerCP Cy5.5 mouse IgG2a (clone G155-178, Becton Dickinson), FITC mouse IgG2b (clone MCA691, Bio-Rad), PE mouse IgG2a (clone OX-34, Bio-Rad) or APC mouse IgG2a (clone eBR2a, ThermoFisher Scientific). Samples were acquired on a BD Accuri C6 Plus (BD Biosciences, Eysins, Switzerland) and analyzed using BD Accuri software. The fractions of total T cells (CD3+), CD4 positive T cells (CD3+CD4+CD8−), CD8 positive T cells (CD3+CD4−CD8+), double positive T cells (CD3+CD4+CD8+) and regulatory T cells (CD3+CD4+FOXP3+) were determined. Due to the limitation on antibodies used, the CD8 positive T cell population would include cytotoxic T cells as well as γδ T cells and CD8 positive NK cells. Both these cell types are estimated to account for 1% of CD8 positive T cells in pigs (44, 45).
Neutrophil phagocytic function was investigated in Experiments 3 and 5, using a commercial kit (pHRodo, ThermoFisher, Roskilde, Denmark), as described elsewhere (46). Briefly, whole blood was incubated at 37°C for 30 min with phrodo conjugated E. coli. Afterwards the samples were run on the above mentioned flow cytometer and the neutrophil population was identified. The phagocytic rate was defined as the fraction of neutrophils with internalized bacteria and phagocytic capacity as the median fluorescent intensity of neutrophils.
Statistics
All statistics were performed in STATA v. 14.2 (StataCorp, Texas, USA). Continuous data were analyzed by a linear mixed effects model with litter as a fixed factor. Due to the factorial design of Experiments 1 and 3, we used a linear multilevel model to determine interactions between gestational age (Experiment 1) or diet (Experiment 3) and SE inoculation. For significant results a post hoc group comparison by Tukey's test, these results are reported in the text of the results section. If data were not normally distributed, logarithmic transformation was performed. Data that did not conform to normal distribution after transformation was analyzed by Kruskal Wallis' test. Categorical data was analyzed by Chi2 test. Overall, p < 0.05 were considered significant and those under 0.1 as tendency to effect. Unless stated otherwise, data shown in text are presented as means with corresponding standard errors and p-values.
Results
Experiment 1
In this experiment, to investigate the influence of gestational age on the response to SE inoculation, we tested the effects of SE or saline infusion (SE or CON) shortly after term or preterm birth (TERM or PRE). Resulting in four groups: SE-TERM, SE-PRE, CON-TERM, and CON-PRE.
One animal in the CON-TERM group died due to bleeding from the catheter shortly after SE inoculation and was excluded from the analysis. In the SE-PRE group, 53% (8/14) of animals were euthanized ahead of schedule, compared to only 12.5% (1/8) of CON-PRE pigs (Figure 1A). For the term animals, 14% (2/14) of SE-TERM were euthanized early (both within 24 h of SE inoculation) compared with none (0/6) of CON-TERM pigs. Considering the high clinical affection from the SE infusion, remaining preterm pigs were euthanized after 24 h.
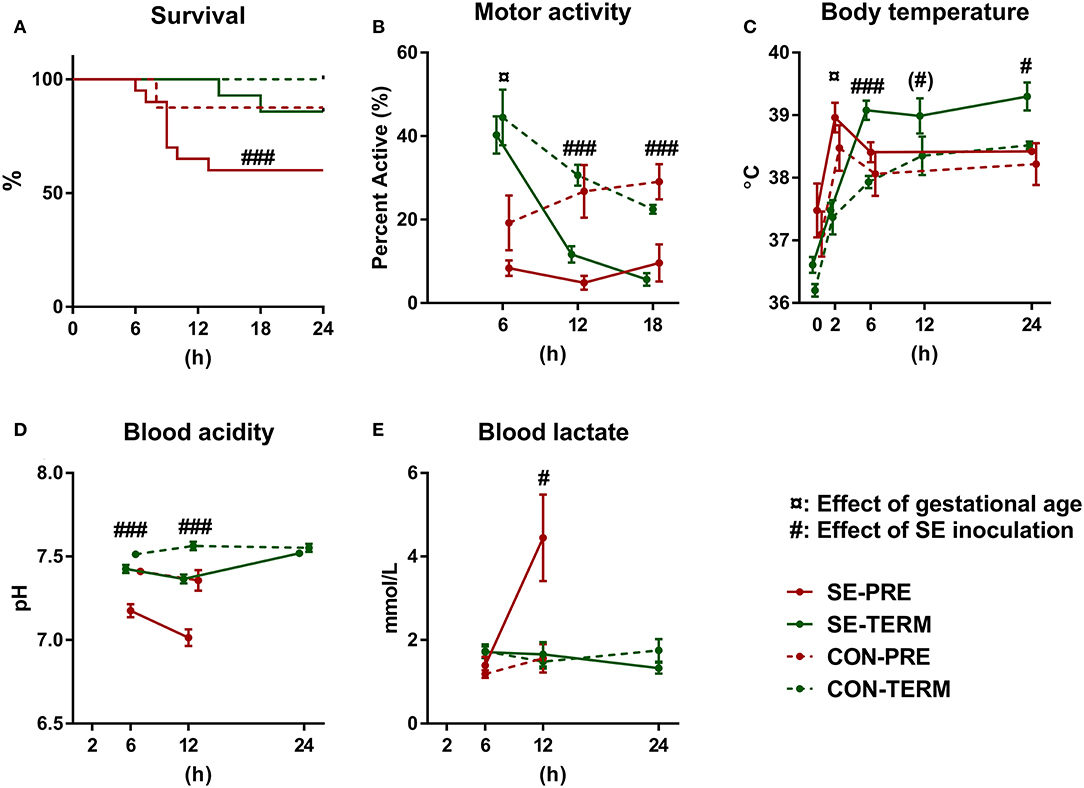
Figure 1. Preterm birth increases susceptibility to Staphylococcus epidermidis bacteremia. Results of Experiment 1 comparing term (TERM) and preterm (PRE) pigs infused with Staphylococcus epidermidis (SE) or saline (CON) immediately after birth, without any enteral feeding. (A) Survival rates 24 h post inoculation. (B) Motor activity, shown as the fraction of time when pigs were physically moving at 2–18 h post inoculation. (C) Body temperature 2–24 h post inoculation. (D) Blood pH 6–24 h post inoculation. (E) Blood lactate levels 6–24 h post inoculation. (A) Presented as Kaplan Meyer curves. (B–E) Presented as means with corresponding standard errors. (#): Effect of SE (p ≤ 0.1 ≥ 0.05), #: Effect of SE (p < 0. 05), ###: Effect of SE (p < 0.001), ¤: Effect of gestational age (p < 0.05).
Motor activity was higher in term animals after 6 h but decreased by SE in both term and preterm animals at 12 and 18 h (Figure 1B). Term animals showed increased body temperature after 2 h and SE led to increased body temperature at 6 and 24 h in both term and preterm animals (Figure 1C). Post hoc testing showed higher body temperatures in SE-TERM vs. SE-PRE pigs for all time points after 2 h (post hoc test, all p < 0.05). Blood pH decreased after SE inoculation with corresponding increases in lactate levels at 12 h (Figures 1D,E), which was more pronounced in SE-PRE vs. SE-TERM pigs (post hoc test, p < 0.001). Total leucocyte and neutrophil numbers increased less in SE-PRE vs. SE-TERM pigs (Table 3). In addition, SE inoculation reduced the platelet and leucocyte counts after 12 h, the latter explained mainly by reductions in neutrophil and lymphocyte numbers. This reduction persisted after 24 h for lymphocytes and platelets while neutrophil counts then increased, but only in SE-TERM animals (e.g., significant gestational age × SE interaction, Table 3). Levels of sC5b-9 in plasma at euthanasia tended to be lower for SE-TERM vs. CON-TERM animals (15 ± 3 vs. 26 ± 8 ng/mL, p = 0.06), while for surviving preterm animals, there was no difference between groups (35 ± 7 vs. 27 ± 8 ng/mL for SE-PRE and CON-PRE, respectively, p > 0.1). However, levels tending to be increased in animals euthanized prematurely compared with those that survived (42 ± 4 vs. 31 ± 5 ng/mL, p = 0.06).
Experiment 2
In this experiment we investigated if clearance of SE from the bloodstream was affected by enteral feeding of BC vs. IF. Preterm pigs infused with SE shortly after birth and fed either BC (SE-BC) or IF (SE-IF) and followed for 12 h.
No animals were euthanized before the end of the 12 h follow up period. Due to the short intervals between blood samplings and intensive handling of the pigs, data on movement activity and body temperature were not collected. The SE-BC group showed consistently lower levels of SE in blood, though only significantly after 3 and 12 h (Figure 2A). Blood lactate tended to be lower in SE-BC after 3 h (Figure 2B) and standard bicarbonate after 6 h (Figure 2C) whereas blood pH did not differ between groups (Figure 2D). Likewise, there was no difference in total leucocyte, neutrophil or lymphocyte counts 12 h after infusion of SE (Figure 2E).
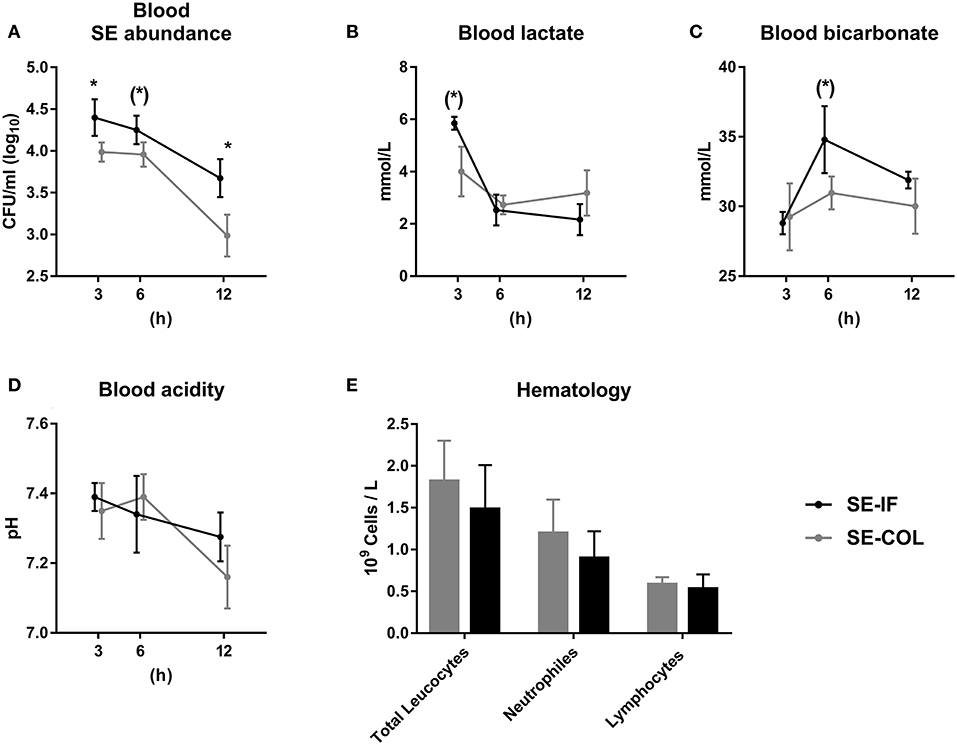
Figure 2. Feeding of bovine colostrum improves clearance of Staphylococcus epidermidis in newborn preterm pigs. Results of Experiment 2 comparing preterm pigs infused with Staphylococcus epidermidis (SE) immediately after birth and fed either bovine colostrum or infant formula. (A) Abundance of SE in blood 3–12 h post inoculation, shown as colony-forming units per milliliter of whole blood. (B) Blood lactate levels 3-12 h post inoculation. (C) Blood bicarbonate levels 3–12 h post inoculation. (D) Blood pH 3–12 h post inoculation. (E) Total leucocyte, neutrophil and lymphocyte counts, taken 12 h post inoculation. (A–E) Presented as means with corresponding standard errors. (*): Effect of diet (p ≤ 0.1 ≥ 0.05), *Effect of diet (p < 0.05).
Experiment 3
In this experiment, investigating if 2 days of BC feeding improves systemic responses to SE, preterm pigs received maternal plasma and were fed either BC or IF until postnatal day 2 where they were infused with either SE or saline (CON). Resulting in four groups: SE-BC, SE-IF, CON-BC and CON-IF.
There was no difference in the proportion of animals euthanized prematurely, with 12% (4/24) in the SE groups and 5% (1/22) in CON (p > 0.1) and these few animals all showed signs of severe NEC upon necropsy. SE inoculation led to increased body temperature in both SE-BC and SE-IF groups, lasting for at least 48 h (Figure 3A). There was no effect of SE on motor activity, but IF-fed pigs showed reduced activity compared with BC-fed pigs (Figure 3B).
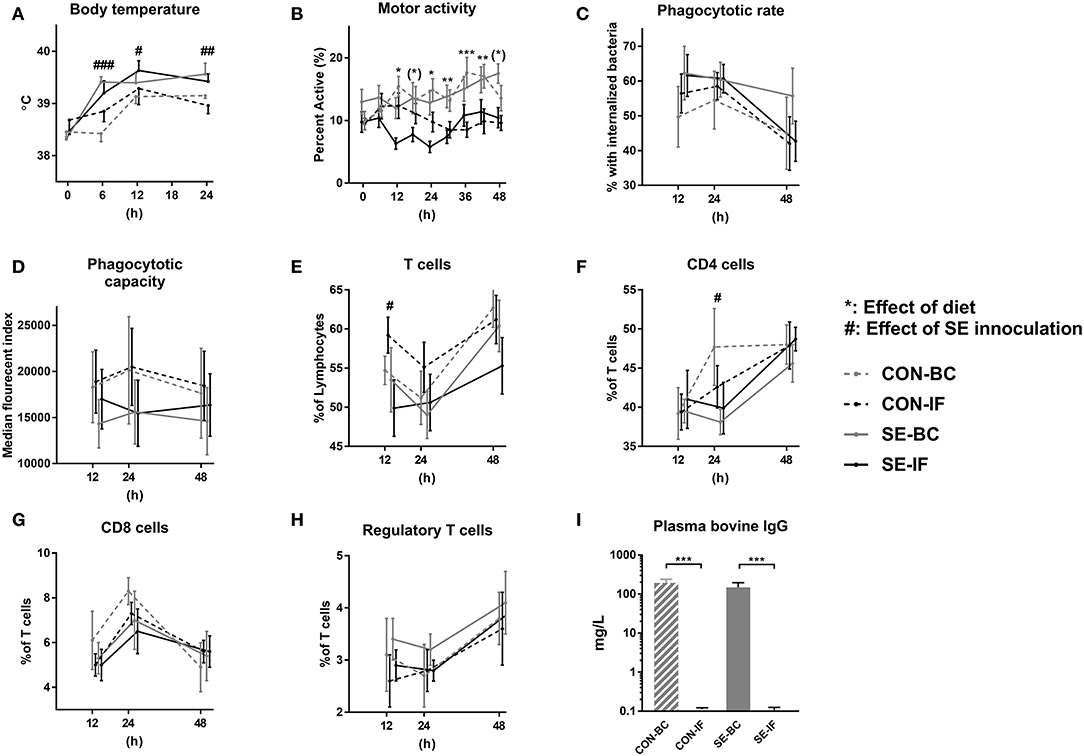
Figure 3. Two days of bovine colostrum feeding has no impact on response to Staphylococcus epidermidis bacteremia. Results of Experiment 3 comparing preterm pigs infused with Staphylococcus epidermidis (SE) or saline (CON) at day 3, after being infused with maternal plasma and fed bovine colostrum (BC) or infant formula (IF) from after birth. (A) Body temperature 0–48 h post inoculation. (B) Motor activity, shown as the fraction of time with physical activity at 0–48 h post inoculation. (C) Neutrophil phagocytic rate 12–48 h post inoculation, defined as fraction of neutrophils with internalized bacteria. (D) Neutrophil phagocytic capacity 12–48 h post inoculation, defined as median fluorescent index of neutrophils with internalized bacteria. (E) T cells 12–48 h post inoculation, defined as fraction of CD3 positive lymphocytes. (F) CD4 cells 12–48 h post inoculation, defined as the CD4 positive, CD8 and FOXP3 negative fraction of T cells. (G) CD8 cells 12–48 h post inoculation, defined as the CD8 positive, CD4 and FOXP3 negative fraction of T cells. (H) Regulatory T cells 12–48 h post inoculation, defined as the FOXP3 and CD4 positive, CD8 negative fraction of T cells. CD4 positive as fraction (I) Plasma levels of bovine immunoglobulin G (IgG) at euthanasia. (A–I) Presented as means with corresponding standard errors. #: Effect of SE (p < 0.05), ##: Effect of SE (p < 0.01), ###: Effect of SE (p < 0.001), (*): Effect of diet (p ≤ 0.1 ≥ 0.05), *: Effect of diet (p < 0.05), **: Effect of diet (p < 0.01), ***: Effect of diet (p < 0.001).
No effect of SE inoculation or interaction with diet was observed for the neutrophil phagocytic rate or capacity (Figures 3C,D). For cellular immune parameters, there were no interactions between SE and diet but SE inoculation alone led to several effects, the most important being lower total leucocytes and monocyte counts at 12 and 24 h, corresponding with lower lymphocyte and platelet counts at 12, 24, and 48 h in the SE inoculated groups (Table 4). Diet in itself had little impact on the hematological parameters (Table 4) T cells were also affected by SE infection, with a lower total T cell fraction at 12 h and lower CD4 positive T cell fraction at 24 h (Figures 3E,F). There were no differences observed for CD8 positive or regulatory T cells (Figures 3G,H). Also, there were no differences in fractions of double positive T cells between the groups at any time point (data not shown).
No interactions between SE and diet were seen for non-immunological hematological parameters, although SE alone led to increased hemoglobin and hematocrit values in the SE groups at 12, 24, and 48 h (Table 4). SE inoculation also led to increased relative spleen weight (3.2 ± 0.1 vs. 2.3 ± 0.1 g/kg, p < 0.001) but no other organ weights were affected by SE inoculation.
There were similar levels of SE CFUs in bone marrow of SE-BC and SE-IF pigs (1.1 × 109±7.0 × 108 vs. 4.7 × 108 ± 2.1 × 108 CFU/mL, p < 0.1). Staphylococcus aureus and Enterococcus spp. were also detected in the bone marrow and these were less prevalent in BC vs. IF animals (3 vs. 26%, p < 0.05). Levels of porcine IgG were similar in all four groups (overall mean 406 ± 18 mg/L), however the levels of bovine IgG were significantly higher in the groups fed BC (Figure 3I), making overall IgG levels higher in BC fed animals compared to IF (572 ± 40 vs. 406 ± 28 mg/L, p < 0.001). There was no influence of SE infection on porcine or bovine IgG levels. For gut related parameters, milk diet influenced the lactulose/mannitol ratio, in that ratios were lower in BC vs. IF animals (2.6 ± 0.5 vs. 18.2 ± 3.3%, p < 0.001). Incidence of NEC was also lower in BC fed animals over IF (29 vs. 72 %, p < 0.01). No direct effects of SE were observed for the gut related parameters.
Regardless of diet, the levels of sC5b-9 were lower in SE inoculated than in CON animals (15 ± 4 vs. 22 ± 4 ng/mL, p < 0.05), whereas levels of TNF-α (102 ± 30 vs. 62 ± 11 pg/mL, p > 0.1) or IL-6 (430 ± 136 vs. 329 ± 69 pg/mL, p > 0.1) did not differ. There was no influence of diet on levels of sC5b-9, TNF-α or IL-6 (data not shown).
Experiment 4
To further investigate the effects of BC feeding we increased the overall susceptibility to infection by withholding maternal plasma in all animals. As in Experiment 3, preterm pigs were fed either BC or IF for 2 days where after all animals were inoculated with SE, resulting in two groups: SE-BC and SE-IF.
There were no differences in body temperature between pigs fed BC or IF (Figure 4A), but motor activity was highest in the SE-BC pigs (Figure 4B). The SE-BC group showed higher blood acidity 6 h after SE inoculation (Figure 4C) together with higher oxygen pressure (Figure 4D) and bicarbonate at 24 h (Figure 4E). Milk diets did not markedly influence hematology except the reduced monocyte and elevated red blood cell counts at 6–12 h in SE-BC vs. SE-IF pigs (Supplementary Table 1).
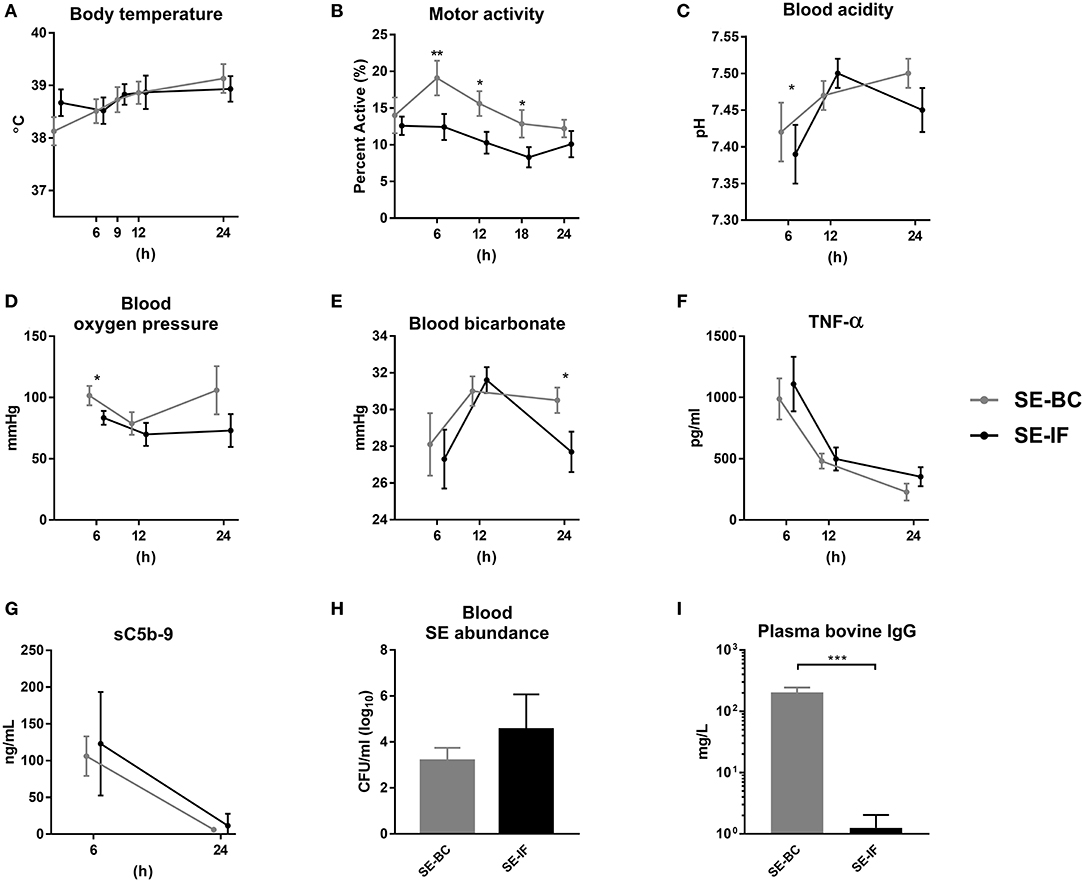
Figure 4. Two days of bovine colostrum feeding has no impact on response to Staphylococcus epidermidis bacteremia, even when maternal antibodies are withheld. Results of Experiment 4 where preterm pigs were infused with Staphylococcus epidermidis (SE) on day 3, without any previous immunization (plasma infusion) and fed bovine colostrum (SE-BC) or infant formula (SE-IF). (A) Body temperature 0–24 h post inoculation. (B) Motor activity, shown as the fraction of time with physical activity at 0–24 h post inoculation. (C) Blood pH 6–24 h post inoculation. (D) Blood oxygen pressure 6–24 h post inoculation. (C) Blood bicarbonate levels 6–24 h post inoculation. (F) Plasma levels of tumor necrosis factor alpha (TNF-α) 6–24 h post inoculation. (G) Plasma levels of soluble terminal complement complexes (sC5b-9) 6–12 h post inoculation. (H) Abundance of SE in blood at euthanasia, shown as colony-forming units per milliliter of whole blood. (I) Plasma levels of bovine immunoglobulin G (IgG) at euthanasia. (A–F) Presented as means with corresponding standard errors. *: Effect of diet (p < 0.05), **: Effect of diet (p < 0.01), ***: Effect of diet (p < 0.001).
In this experiment, we also collected plasma 6 and 12 h after inoculation. Levels of TNF-α were increased at 6 h after the SE challenge and dropped throughout the study, with no difference between SE-BC and SE-IF (Figure 4F). Likewise, sC5b-9 levels were elevated 6 h after inoculation with no difference between BC and IF fed animals, and hardly detectable after 24 h (Figure 4G). In addition, plasma levels of IL-6 at euthanasia did not differ between SE-BC and SE-IF (336 ± 67 vs. 351 ± 42 pg/mL, p > 0.1). At 24 h, the majority of administered SE was cleared from the blood stream with no difference in clearance capacity between groups (Figure 4H). Levels of porcine IgG also did not differ between SE-BC and SE-IF (6 ± 2 vs. 4 ± 1 mg/L, p > 0.1), but as expected, levels were much lower than in Experiment 3. Bovine IgG levels were significantly higher in SE-BC (Figure 4I), making overall IgG levels higher (209 ± 42 vs. 5 ± 1 mg/L, p < 0.001). For gut related parameters, mean NEC incidence was lower in SE-BC than SE-IF pigs, although not significantly (33 vs. 71%, p > 0.1). In addition, SE-BC pigs showed higher relative weight of the proximal small intestine (10.2 ± 0.5 vs. 7.3 ± 0.7, p < 0.001), relative to SE-IF.
Experiment 5
To investigate if oral feeding of porcine immunoglobulins affected the systemic response to SE, preterm pigs were fed IF with or without added porcine plasma proteins (PP) for 3 days, at which time all animals were inoculated with SE, resulting in two groups: SE-IF and SE-PP.
One animal was euthanized ahead of time from the SE-PP group with signs of NEC at necropsy. Like in the previous studies, body temperature rose after SE inoculation, but the changes were not affected by PP supplementation (Figure 5A) and neither was motor activity (Figure 5B). Like in Experiment 4, most of the administered SE were cleared from the blood stream 24 h after infection, but again, with no effect of PP supplementation (Figure 5E).
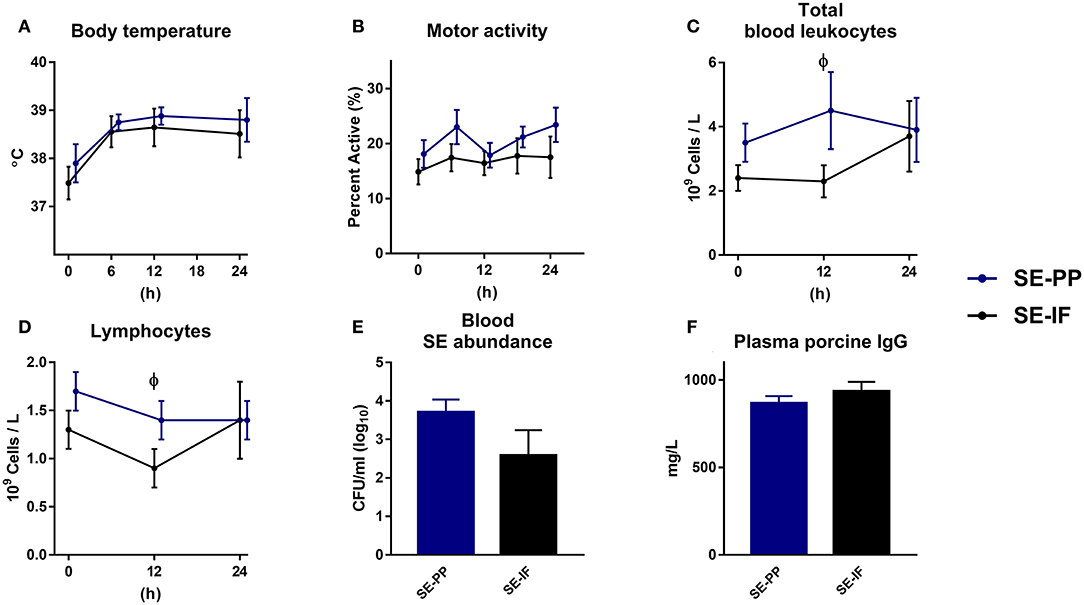
Figure 5. Feeding of infant formula, enriched with porcine plasma, has no effect on responses to Staphylococcus epidermidis bacteremia. Results of Experiment 5 where preterm pigs were infused with Staphylococcus epidermidis (SE) on day 4, after infusion of maternal plasma at birth, and then fed infant formula supplemented with porcine plasma powder (SE-PP) or whey protein (SE-IF). (A) Body temperature 0–24 h post inoculation. (B) Motor activity, shown as the fraction of time with physical movements at 0–24 h post inoculation. (C) Total blood leucocyte counts 0–24 h post inoculation. (D) Lymphocyte counts in blood, 0–24 h post inoculation (E) Abundance of SE in blood at euthanasia, shown colony-forming units per milliliter of whole blood. (F) Plasma levels of porcine immunoglobulin G (IgG) at euthanasia. (A–F) Presented as means with corresponding standard errors. ϕ: Effect of PP (p < 0.05).
At baseline, monocyte counts were higher in SE-PP than SE-IF pigs (data not shown). After SE inoculation, total leucocyte counts and lymphocyte counts (Figures 5C,D) were higher in SE-PP vs. SE-IF pigs at 12 h. No other differences in hematological or hemostatic parameters were observed (Supplementary Table 2). Levels of sC5b-9 (18.5 ± 8.2 vs. 5.8 ± 2.0 ng/mL, p > 0.1) and porcine immunoglobulins (Figure 5F) did not differ between the SE-PP and SE-IF groups. Supplementation with PP had no significant effect on NEC incidence (60 vs. 33%, p > 0.1), lactulose/mannitol ratio (9 ± 2 vs. 5 ± 1%, p > 0.1) or weight of internal organs (data not shown, all p > 0.1).
Discussion
Bacterial infection remains a major contributor to neonatal mortality in preterm infants and understanding the response of preterm infants to bacteremia is key for early diagnosis and treatment. Postnatal immune system maturation in preterm infants is not well-understood and may be influenced by environmental factors, such as diet and microbial colonization in the gut, lungs and skin epithelia. Regardless, it remains unclear how postnatal factors influence systemic immunity development and responses to bacteremia. Using a recently established model of neonatal bloodstream infection, we first demonstrate that preterm pigs were markedly more affected than term pigs by systemic SE exposure shortly after birth, leading to clear signs of sepsis and high mortality. The clinical responses were much less pronounced when similar SE doses were infused after initiation of enteral feeding, either shortly after birth or after 2 days. However, the responses were not markedly affected by the type of milk diet, although BC fed animals showed lower levels of circulating SE when inoculated shortly after birth. After day 3, luminal supplementation with porcine plasma proteins into IF also failed to improve responses. Furthermore, we have done preliminary studies in preterm pigs that showed limited effects of BC feeding for 4 days, relative to un-fed animals on total parenteral nutrition (unpublished observations). Thus, enteral feeding, even with a highly immunomodulatory milk diet like bovine colostrum, is unlikely to influence systemic immunity development and bacteremia responses in preterm neonates beyond its effectiveness in the immediate neonatal period (first 1–2 days after birth), as shown also in our previous study (43). A general effect of enteral feeding on the immune response is possible, as enterally fed animals had lower blood acidity, lactate and mortality both when comparing Experiments 1 and 2, and in the previous preterm pig study (43). However, we have not yet fully investigated the effect of early enteral feeding shortly after birth, and further studies are necessary. Whether a similar age or diet dependency of clinical responses to systemic bacterial infection is valid for preterm infants remains to be investigated following different gestational ages, diet regimens and bacterial exposures.
Regarding gestational age, both preterm and term pigs showed clinical and immunological responses to SE exposure shortly after birth (i.e., increasing body temperature, reduced physical activity and blood pH), but these conditions only became fatal in preterm pigs, requiring early euthanasia of half of these animals. Conversely, term pigs maintained a higher body temperature and their neutrophil counts even exceeded baseline levels at 24 h after SE infection. Thus, preterm pigs seemed to have a lower capacity in their bone marrow to replace neutrophils after SE infection challenge. Neutropenia is often observed in preterm infants (47, 48).
In the later preterm pig experiments, preterm animals showed limited diet-dependent differences in systemic responses to SE, beyond the lowered SE levels in newborn preterm pigs (< 24 h old) fed BC vs. IF. The other para-clinical outcomes such as leucocyte subsets and blood acidity/lactate did however not differ. In pigs that were fed BC or IF for 2 days before SE inoculation, no clear differences in SE responses could be demonstrated. Also, withholding maternal plasma did not reveal any further effects of feeding BC over IF on the immune response during SE bacteremia.
Consistent with protective effects of feeding BC vs. IF on gut parameters (reduced intestinal permeability and NEC incidence) in this study, BC feeding is associated with a long series of structural, functional and immunological changes locally in the gut of preterm pigs (29–32, 49). In this study, the BC fed animals were less likely to have enteric bacteria (i.e., Enterococcus spp. and Staphylococcus aureus) in their bone marrow (Experiment 3), suggesting a protective effect of BC against bacterial translocation across the intestine. Also, we consistently found higher levels of motor activity in BC fed animals, suggesting an overall better clinical status. We have previously shown that BC feeding of preterm pigs prevented bacterial translocation within the first 1–2 weeks of life, but not later (29, 50, 51), further suggesting that a critical window exists early after preterm birth where intestinal permeability, and therefore the risk of gut derived systemic infections, can be reduced by protective milk diets. Whether such bioactive milk diets could affect systemic immunity development directly, independent of any maturational and protective effects on the immature gut, remains unclear and may take longer than just a few days after preterm birth to manifest. In preterm infants, risk of sepsis is associated with length of parenteral nutrition (and thereby presence of central catheters), but alleviated by human milk feeding (3). Possibly, direct systemic immune effects of enteral milk diets, beyond the first days after preterm birth, depend on associated changes in the gut microbiota. An observational study in preterm infants has shown that gut dysbiosis, with accumulation of fermentation products, precedes neonatal sepsis (52). However, diet-induced changes to the gut microbiota may occur mainly after the first week of life, as shown in previous studies on preterm pigs (30, 31, 53). This may explain why no marked differences between BC and IF fed animals were apparent for the systemic response to SE.
A clear effect of SE inoculation on several immunological parameters was observed already 12 h after inoculation, including a marked reduction in monocytes, lymphocytes and platelets. Following the SE inoculations, bacteria would permeate the tissues, likely prompting monocytes to leave the vasculature, explaining the reduced number of circulating monocytes. Lymphocytes would mostly stay in the blood stream and we therefore suspect that SE bacteremia induced apoptosis of peripheral lymphocytes, as indicated from adult sepsis. Here, a substantial sepsis-induced loss of helper T cells and B lymphocytes has been observed, compared with trauma patients and non-septic controls (54, 55). Likewise, thrombocytopenia is a common finding in preterm infants with neonatal sepsis (56, 57). Loss of lymphocytes and platelets were observed across experiments in the SE treated groups. Hematocrit and hemoglobin values were generally higher in SE infected animals, likely indicating a greater loss of fluid from the vasculature. Loss of intravascular fluid is a well-known phenomenon in both adult and neonatal sepsis caused by increased capillary permeability (58). Furthermore, we found that circulating levels of sC5b-9 were lower in SE animals indicating that complement factors had been depleted. The sC5b-9 protein complex is the end product of the complement cascade and only has a half-life of 1–2 h (59), so any product generated by the initial SE inoculation would be gone by 48 h. Complement activation by SE sepsis shortly after birth was seen in Experiment 1, as levels were increased in non-surviving animals and in Experiment 4 with higher levels shortly after SE inoculation. Although enterally fed animals, inoculated after 2 days, were less clinically affected by SE, they remained to have some immunological responses reflecting neonatal sepsis, but with limited effects of milk diet (colostrum, formula), regardless of provision of maternal plasma (Experiment 3) or not (Experiment 4). Thus, this experimentally induced bacteremia in preterm pigs mimicked many of the signs and symptoms seen in neonatal sepsis patients.
SE is considered a relatively low-virulent pathogen that would not normally cause serious systemic responses in healthy individuals, but SE is often isolated from preterm infants suspected of sepsis (2). Preterm pigs provide a good model for studying infections in preterm infants, as the overall values for hematological and immunological parameters are similar (46). In addition, the developing pig fetus does not receive IgG via the placenta, which mimics the situation in preterm infants, born with low levels of IgG. This allows for experimentally changing the degree of immunodeficiency, through infusion of immunoglobulins and other plasma proteins. Neonatal pigs have the ability to effectively transfer immunoglobulins and other macromolecules across the intestine within the first 12–24 h (60, 61). This ability is severely reduced in fetal pigs (62) and preterm newborn pigs (63, 64) but an ability remains, as observed by the higher overall plasma IgG levels seen in animals fed BC or IF-PP. We do not know to what degree these absorbed bovine immunoglobulins were directed against SE. Since SE is a common pathogen in cattle (65) it is probable that the BC powder contains SE specific IgG's that would improve opsonisation and clearance of bacteria. However, improved clearance of SE was only observed in BC fed animals just after birth (Experiment 2), not those fed for two full days (Experiment 4), indicating that absorbed immunoglobulins played a minor role. Absorption of smaller macromolecules across the intestine has been demonstrated to be increased in very preterm infants (66–68), as a sign of enhanced gut permeability, as no specific immunoglobulin uptake mechanism exists. The overall immunoglobulin levels that we achieved in preterm pigs by maternal plasma supplementation were far lower than what is reported in term human neonates, where cord blood IgG levels range from 4 to 10 g/L (16, 69). The IgG levels of preterm infants are dependent on gestational age at birth, but were reported to be only ~3 g/L by 22 weeks gestation (16, 70).
In conclusion, preterm newborn pigs are more sensitive to SE bacteremia than term pigs. Enteral feeding immediately after birth dampened the clinical responses to SE, and feeding with a highly bioactive milk diet, like bovine colostrum, improved clearance of SE from the bloodstream, relative to infant formula, with subtle improvements in blood gas parameters. A further 2 days of enteral feeding after preterm birth attenuated the SE response but the marked effect of BC vs. IF feeding disappeared. Early feeding with immunomodulatory milk diets may provide protection against neonatal systemic infection in preterm infants mainly via improving intestinal maturation and reducing the need for parenteral nutrition and fluid via indwelling central catheters. Nevertheless, early feeding with a protective milk diet, like colostrum, may improve the ability of preterm infants to resist systemic infections in the first days after birth.
Data Availability Statement
The datasets generated for this study are available on request to the corresponding author.
Ethics Statement
The animal study was reviewed and approved by The Danish National Committee on Animal Experimentation.
Author Contributions
OB, AB, DN, and PS planned the research. OB and AB conducted experiments, did data analysis and interpretation of results, and wrote the manuscript. TT supervised animal procedure. DN and AM did laboratory procedures. PS had primary responsibility for the final content. All authors read and approved the final paper.
Funding
The studies were part of the NEOCOL (Innovation Fund Denmark) and STIMMUNE (Arla Foods for Health) projects.
Conflict of Interest
The University of Copenhagen holds a patent on the use of bovine colostrum for pediatric patients. PS is listed as a sole inventor but has declined any share of potential revenue arising from commercial exploitation of such a patent.
The remaining authors declare that the research was conducted in the absence of any commercial or financial relationships that could be construed as a potential conflict of interest.
Acknowledgments
We would like to thank Elin Skytte, Jane C. Povlsen, and Kristina Møller for their help performing these experiments.
Supplementary Material
The Supplementary Material for this article can be found online at: https://www.frontiersin.org/articles/10.3389/fimmu.2020.01019/full#supplementary-material
References
1. Tröger B, Göpel W, Faust K, Müller T, Jorch G, Felderhoff-Müser U, et al. Risk for late-onset blood-culture proven sepsis in very-low-birth weight infants born small for gestational age: a large multicenter study from the German neonatal network. Pediatr Infect Dis J. (2014) 33:238–43. doi: 10.1097/INF.0000000000000031
2. Stoll BJ, Hansen N, Fanaroff AA, Wright LL, Carlo WA, Ehrenkranz RA, et al. Late-onset sepsis in very low birth weight neonates: the experience of the NICHD Neonatal Research Network. Pediatrics. (2002) 110:285–91. doi: 10.1542/peds.110.2.285
3. el Manouni el Hassani S, Berkhout DJC, Niemarkt HJ, Mann S, de Boode WP, Cossey V, et al. Risk factors for late-onset sepsis in preterm infants: a multicenter case-control study. Neonatology. (2019) 116:42–51. doi: 10.1159/000497781
4. Sisk PM, Lovelady CA, Gruber KJ, Dillard RG, O'Shea TM. Human milk consumption and full enteral feeding among infants who weigh. Pediatrics. (2008) 121:e1528–33. doi: 10.1542/peds.2007-2110
5. Sangild PT. Gut responses to enteral nutrition in preterm infants and animals. Exp Biol Med. (2006) 231:1695–711. doi: 10.1177/153537020623101106
6. Cleminson JS, Zalewski SP, Embleton ND. Nutrition in the preterm infant: what's new? Curr Opin Clin Nutr Metab Care. (2016) 19:220–5.
7. Corpeleijn WE, Kouwenhoven SMP, Paap MC, van Vliet I, Scheerder I, Muizer Y, et al. Intake of own mother's milk during the first days of life is associated with decreased morbidity and mortality in very low birth weight infants during the first 60 days of life. Neonatology. (2012) 102:276–81. doi: 10.1159/000341335
8. Nasuf AWA, Ojha S, Dorling J. Oropharyngeal colostrum in preventing mortality and morbidity in preterm infants. Cochrane Database Syst Rev. (2018) 9:CD011921. doi: 10.1002/14651858.CD011921.pub2
9. Juhl SM, Ye X, Zhou P, Li Y, Iyore EO, Zhang L, et al. Bovine colostrum for preterm infants in the first days of life. J Pediatr Gastroenterol Nutr. (2018) 66:471–78. doi: 10.1097/MPG.0000000000001774
10. Jiang Y, Kuang L, Wang H, Li L, Zhou W, Li M. The clinical characteristics of neonatal sepsis infection in southwest china. Intern Med. (2016) 55:597–603. doi: 10.2169/internalmedicine.55.3930
11. Shivanna V, Sunkappa S, Venkatesha D. The rising trend of coagulase-negative staphylococci in neonatal septicemia. Indian J Pathol Microbiol. (2016) 59:510. doi: 10.4103/0377-4929.191806
12. Dong Y, Speer CP, Glaser K. Beyond sepsis: Staphylococcus epidermidis is an underestimated but significant contributor to neonatal morbidity. Virulence. (2018) 9:621–33. doi: 10.1080/21505594.2017.1419117
13. Krediet TG, Beurskens FJ, van Dijk H, Gerards LJ, Fleer A. Antibody responses and opsonic activity in sera of preterm neonates with coagulase-negative staphylococcal septicemia and the effect of the administration of fresh frozen plasma. Pediatr Res. (1998) 43:645–51. doi: 10.1203/00006450-199805000-00013
14. Fleer A, Gerards LJ, Aerts P, Westerdaal NAC, Senders RC, van Dijk H, et al. Opsonic defense to Staphylococcus epidermidis in the premature neonate. J Infect Dis. (1985) 152:930–7. doi: 10.1093/infdis/152.5.930
15. Peterson PK, Wilkinson BJ, Kim Y, Schmeling D, Douglas SD, Quie PG, et al. The key role of peptidoglycan in the opsonization of Staphylococcus aureus. J Clin Invest. (1978) 61:597–609. doi: 10.1172/JCI108971
16. Malek A, Sager R, Kuhn P, Nicolaides KH, Schneider H. Evolution of maternofetal transport of immunoglobulins during human pregnancy. Am J Reprod Immunol. (1996) 36:248–55. doi: 10.1111/j.1600-0897.1996.tb00172.x
17. van den Berg JP, Westerbeek EAM, van der Klis FRM, Berbers GAM, van Elburg RM. Transplacental transport of IgG antibodies to preterm infants: a review of the literature. Early Hum Dev. (2011) 87:67–72. doi: 10.1016/j.earlhumdev.2010.11.003
18. Cates KL, Goetz C, Rosenberg N, Pantschenko A, Rowe JC, Ballow M. Longitudinal development of specific and functional antibody in very low birth weight premature infants. Pediatr Res. (1988) 23:14–22. doi: 10.1203/00006450-198801000-00005
19. Strunk T, Currie A, Richmond P, Simmer K, Burgner D. Innate immunity in human newborn infants: prematurity means more than immaturity. J Matern Neonatal Med. (2011) 24:25–31. doi: 10.3109/14767058.2010.482605
20. Sandberg K, Fasth A, Berger A, Eibl M, Isacson K, Lischka A, et al. Preterm infants with low immunoglobulin G levels have increased risk of neonatal sepsis but do not benefit from prophylactic immunoglobulin G. J Pediatr. (2000) 137:623–8. doi: 10.1067/mpd.2000.109791
21. Fanaroff AA, Korones SB, Wright LL, Wright EC, Poland RL, Bauer CB, et al. A controlled trial of intravenous immune globulin to reduce nosocomial infections in very-low-birth-weight infants. N Engl J Med. (1994) 330:1107–13. doi: 10.1056/NEJM199404213301602
22. Ohlsson A, Lacy JB. Intravenous immunoglobulin for preventing infection in preterm and/or low birth weight infants. Cochrane Database Syst Rev. (2013). 2:CD000361. doi: 10.1002/14651858.CD000361.pub3
23. Shah PS, Kaufman DA. Antistaphylococcal immunoglobulins to prevent staphylococcal infection in very low birth weight infants. Cochrane Database Syst Rev. (2009). 15:CD006449. doi: 10.1002/14651858.CD006449.pub2
24. INIS Collaborative Group, Brocklehurst P, Farrell B, King A, Juszczak E, Darlow B, et al. Treatment of neonatal sepsis with intravenous immune globulin. N Engl J Med. (2011) 365:1201–11. doi: 10.1056/NEJMoa1100441
25. Blum PM, Phelps DL, Ank BJ, Krantman HJ, Stiehm ER. Survival of oral human immune serum globulin in the gastrointestinal tract of low birth weight infants. Pediatr Res. (1981) 15:1256–60. doi: 10.1203/00006450-198109000-00006
26. Eibl MM, Wolf HM, Fürnkranz H, Rosenkranz A. Prevention of necrotizing enterocolitis in low-birth-weight infants by IGA-IGG feeding. N Engl J Med. (1988) 319:1–7. doi: 10.1056/NEJM198807073190101
27. Rubaltelli FF, Benini F, Sala M. Prevention of necrotizing enterocolitis in neonates at risk by oral administration of monomeric IgG. Dev Pharmacol Ther. (1991) 17:138–43. doi: 10.1159/000457513
28. Foster JP, Seth R, Cole MJ. Oral immunoglobulin for preventing necrotizing enterocolitis in preterm and low birth weight neonates. Cochrane Database Syst. Rev. (2016) CD001816. doi: 10.1002/14651858.CD001816.pub3
29. Sun J, Li Y, Pan X, Nguyen DN, Brunse A, Bojesen AM, et al. Human milk fortification with bovine colostrum is superior to formula-based fortifiers to prevent gut dysfunction, necrotizing enterocolitis, and systemic infection in preterm pigs. J Parenter Enter Nutr. (2018) 43:252–62. doi: 10.1002/jpen.1422
30. Shen RL, Thymann T, Østergaard MV., Støy ACF, Krych Ł, Nielsen DS, et al. Early gradual feeding with bovine colostrum improves gut function and NEC resistance relative to infant formula in preterm pigs. Am J Physiol Gastrointest Liver Physiol. (2015) 309:G310–23. doi: 10.1152/ajpgi.00163.2015
31. Rasmussen SO, Martin L, Østergaard MV, Rudloff S, Li Y, Roggenbuck M, et al. Bovine colostrum improves neonatal growth, digestive function and gut immunity relative to donor human milk and infant formula in preterm pigs. Am J Physiol Gastrointest Liver Physiol. (2016) 311:G480–91. doi: 10.1152/ajpgi.00139.2016
32. Bjornvad CR, Thymann T, Deutz NE, Burrin DG, Jensen SK, Jensen BB, et al. Enteral feeding induces diet-dependent mucosal dysfunction, bacterial proliferation, and necrotizing enterocolitis in preterm pigs on parenteral nutrition. Am J Physiol Gastrointest Liver Physiol. (2008) 295:G1092–103. doi: 10.1152/ajpgi.00414.2007
33. Bjornvad CR, Schmidt M, Petersen YM, Jensen SK, Offenberg H, Elnif J, et al. Preterm birth makes the immature intestine sensitive to feeding-induced intestinal atrophy. Am J Physiol Integr Comp Physiol. (2005) 289:R1212–22. doi: 10.1152/ajpregu.00776.2004
34. Pérez-Bosque A, Polo J, Torrallardona D. Spray dried plasma as an alternative to antibiotics in piglet feeds, mode of action and biosafety. Porc Heal Manag. (2016) 2:1–10. doi: 10.1186/s40813-016-0034-1
35. Pe A, Polo J, Russell L, Campbell J, Weaver E, Crenshaw J, et al. Dietary plasma protein supplements prevent the release of mucosal proinflammatory mediators in intestinal inflammation in rats 1, 2. J Nutr. (2010) 140:25–30. doi: 10.3945/jn.109.112466
36. Maijo M, Miro L, Polo J, Campbell J, Russell L, Crenshaw J, et al. Dietary plasma proteins modulate the adaptive immune response in mice with acute lung inflammation. J Nutr. (2012) 142:264–70. doi: 10.3945/jn.111.149070
37. Pérez-Bosque A, Miró L, Maijó M, Polo J, Campbell JM, Russell L, et al. Oral serum-derived bovine immunoglobulin/protein isolate has immunomodulatory effects on the colon of mice that spontaneously develop colitis. PLoS ONE. (2016) 11:e154823. doi: 10.1371/journal.pone.0154823
38. Bégin F, Santizo M-C, Peerson JM, Torún B, Brown KH. Effects of bovine serum concentrate, with or without supplemental micronutrients, on the growth, morbidity, and micronutrient status of young children in a low-income, peri-urban guatemalan community. Eur J Clin Nutr. (2008) 62:39–50. doi: 10.1038/sj.ejcn.1602682
39. Lembcke JL, Peerson JM, Brown KH. Acceptability, safety, and digestibility of spray-dried bovine serum added to diets of recovering malnourished children. J Pediatr Gastroenterol Nutr. (1997) 25:381–4. doi: 10.1097/00005176-199710000-00003
40. Sarker SA, Casswall TH, Mahalanabis D, Alam NH, Albert MJ, Brüssow H, et al. Successful treatment of rotavirus diarrhea in children with immunoglobulin from immunized bovine colostrum. Pediatr Infect Dis J. (1998) 17:1149–54. doi: 10.1097/00006454-199812000-00010
41. Pakkanen R, Aalto J. Growth factors and antimicrobial factors of bovine colostrum. Int Dairy J. (1997) 7:285–97. doi: 10.1016/S0958-6946(97)00022-8
42. Nguyen DN, Currie AJ, Ren S, Bering SB, Sangild PT. Heat treatment and irradiation reduce anti-bacterial and immune-modulatory properties of bovine colostrum. J Funct Foods. (2019) 57:182–9. doi: 10.1016/j.jff.2019.04.012
43. Brunse A, Worsøe P, Pors SE, Skovgaard K, Sangild PT. Oral supplementation with bovine colostrum prevents septic shock and brain barrier disruption during bloodstream infection in preterm newborn pigs. Shock. (2018) 51:337–47. doi: 10.1097/SHK.0000000000001131
44. Wen K, Li G, Zhang W, Azevedo MSP, Saif LJ, Liu F, et al. Development of γδ T cell subset responses in gnotobiotic pigs infected with human rotaviruses and colonized with probiotic lactobacilli. Vet Immunol Immunopathol. (2011) 141:267–75. doi: 10.1016/j.vetimm.2011.03.016
45. Schäfer A, Hühr J, Schwaiger T, Dorhoi A, Mettenleiter TC, Blome S, et al. Porcine invariant natural killer T cells: functional profiling and dynamics in steady state and viral infections. Front Immunol. (2019) 10:1380. doi: 10.3389/fimmu.2019.01380
46. Nguyen DN, Jiang P, Frøkiær H, Heegaard PMH, Thymann T, Sangild PT. Delayed development of systemic immunity in preterm pigs as a model for preterm infants. Sci Rep. (2016) 6:36816. doi: 10.1038/srep36816
47. Carr R. Neutrophil production and function in newborn infants. Br J Haematol. (2000) 110:18–28. doi: 10.1046/j.1365-2141.2000.01992.x
48. Gessler P, Lüders R, König S, Haas N, Lasch P, Kachel W. Neonatal neutropenia in low birthweight premature infants. Am J Perinatol. (1995) 12:34–8. doi: 10.1055/s-2007-994396
49. Li Y, Jensen ML, Chatterton DEW, Jensen BB, Thymann T, Kvistgaard AS, et al. Raw bovine milk improves gut responses to feeding relative to infant formula in preterm piglets. Am J Physiol Gastrointest Liver Physiol. (2014) 306:G81–90. doi: 10.1152/ajpgi.00255.2013
50. Li Y, Nguyen DN, de Waard M, Christensen L, Zhou P, Jiang P, et al. Pasteurization procedures for donor human milk affect body growth, intestinal structure, and resistance against bacterial infections in preterm pigs. J Nutr. (2017) 147:1121–30. doi: 10.3945/jn.116.244822
51. Sun J, Li Y, Nguyen DN, Mortensen MS, van den Akker CHP, Skeath T, et al. Nutrient fortification of human donor milk affects intestinal function and protein metabolism in preterm pigs. J Nutr. (2018) 148:336–47. doi: 10.1093/jn/nxx033
52. Graspeuntner S, Waschina S, Künzel S, Twisselmann N, Rausch TK, Cloppenborg-Schmidt K, et al. Gut dysbiosis with bacilli dominance and accumulation of fermentation products precedes late-onset sepsis in preterm infants. Clin Infect Dis. (2019) 69:268–77. doi: 10.1093/cid/ciy882
53. Cilieborg MS, Boye M, Sangild PT. Bacterial colonization and gut development in preterm neonates. Early Hum Dev. (2012) 88:S41–9. doi: 10.1016/j.earlhumdev.2011.12.027
54. Hotchkiss RS, Tinsley KW, Swanson PE, Schmieg RE, Hui JJ, Chang KC, et al. Sepsis-induced apoptosis causes progressive profound depletion of B and CD4+ T lymphocytes in humans. J Immunol. (2001) 166:6952–63. doi: 10.4049/jimmunol.166.11.6952
55. Hotchkiss RS, Swanson PE, Freeman BD, Tinsley KW, Cobb JP, Matuschak GM, et al. Apoptotic cell death in patients with sepsis, shock, and multiple organ dysfunction. Crit Care Med. (1999) 27:1230–51. doi: 10.1097/00003246-199907000-00002
56. Guida JD, Kunig AM, Leef KH, McKenzie SE, Paul DA. Platelet count and sepsis in very low birth weight neonates: is there an organism-specific response? Pediatrics. (2003) 111:1411–5. doi: 10.1542/peds.111.6.1411
57. Bhat MA, Bhat JI, Kawoosa MS, Ahmad SM, Ali SW. Organism-specific platelet response and factors affecting survival in thrombocytopenic very low birth weight babies with sepsis. J Perinatol. (2009) 29:702–8. doi: 10.1038/jp.2009.72
58. Lee WL, Slutsky AS. Sepsis and endothelial permeability. N Engl J Med. (2010) 363:689–91. doi: 10.1056/NEJMcibr1007320
59. Hugo F, Berstecher C, Krämer S, Fassbender W, Bhakdi S. In vivo clearance studies of the terminal fluid-phase complement complex in rabbits. Clin Exp Immunol. (1989) 77:112–6.
60. Porter P. Transfer of immunoglobulins IgG, IgA and IgM to lacteal secretions in the parturient sow and their absorption by the neonatal piglet. Biochim Biophys Acta Protein Struct. (1969) 181:381–92. doi: 10.1016/0005-279590271-2
61. Jensen AR, Elnif J, Burrin DG, Sangild PT. Development of intestinal immunoglobulin absorption and enzyme activities in neonatal pigs is diet dependent. J Nutr. (2001) 131:3259–65. doi: 10.1093/jn/131.12.3259
62. Sangild PT, Trahair JF, Loftager MK, Fowden AL. Intestinal macromolecule absorption in the fetal pig after infusion of colostrum in utero. Pediatr Res. (1999) 45:595–602. doi: 10.1203/00006450-199904010-00021
63. Sangild PT, Schmidt M, Elnif J, Björnvad CR, Weström BR, Buddington RK. Prenatal development of gastrointestinal function in the pig and the effects of fetal esophageal obstruction. Pediatr Res. (2002) 52:416–24. doi: 10.1203/00006450-200209000-00019
64. Sangild PT, Petersen YM, Schmidt M, Elnif J, Petersen TK, Buddington RK, et al. Preterm birth affects the intestinal response to parenteral and enteral nutrition in newborn pigs. J Nutr. (2002) 132:2673–81. doi: 10.1093/jn/132.9.2673
65. Jenkins SN, Okello E, Rossitto PV, Lehenbauer TW, Champagne J, Penedo MCT, et al. Molecular epidemiology of coagulase-negative staphylococcus species isolated at different lactation stages from dairy cattle in the United States. Peer J. (2019) 7:e6749. doi: 10.7717/peerj.6749
66. Axelsson I, Jakobsson I, Lindberg T, Polberger S, Benediktson B, Räihä N. Macromolecular absorption in preterm and term infants. Acta Paediatr. (1989) 78:532–7. doi: 10.1111/j.1651-2227.1989.tb17932.x
67. Roberton DM, Paganelli R, Dinwiddie R, Levinsky RJ. Milk antigen absorption in the preterm and term neonate. Arch Dis Child. (1982) 57:369–72. doi: 10.1136/adc.57.5.369
68. Weaver LT, Laker MF, Nelson R. Intestinal permeability in the newborn. Arch Dis Child. (1984) 59:236–41. doi: 10.1136/adc.59.3.236
69. Oxelius V-A. IgG subclass levels in infancy and childhood. Acta Paediatr. (1979) 68:23–7. doi: 10.1111/j.1651-2227.1979.tb04424.x
Keywords: sepsis, bacteremia, preterm, infant, diet, immunity, passive, feeding
Citation: Bæk O, Brunse A, Nguyen DN, Moodley A, Thymann T and Sangild PT (2020) Diet Modulates the High Sensitivity to Systemic Infection in Newborn Preterm Pigs. Front. Immunol. 11:1019. doi: 10.3389/fimmu.2020.01019
Received: 01 October 2019; Accepted: 28 April 2020;
Published: 27 May 2020.
Edited by:
Philip John Griebel, University of Saskatchewan, CanadaReviewed by:
Gregers Jungersen, Statens Serum Institute, DenmarkMartin Faldyna, Veterinary Research Institute (VRI), Czechia
Copyright © 2020 Bæk, Brunse, Nguyen, Moodley, Thymann and Sangild. This is an open-access article distributed under the terms of the Creative Commons Attribution License (CC BY). The use, distribution or reproduction in other forums is permitted, provided the original author(s) and the copyright owner(s) are credited and that the original publication in this journal is cited, in accordance with accepted academic practice. No use, distribution or reproduction is permitted which does not comply with these terms.
*Correspondence: Per Torp Sangild, pts@sund.ku.dk
†These authors have contributed equally to this work and share first authorship