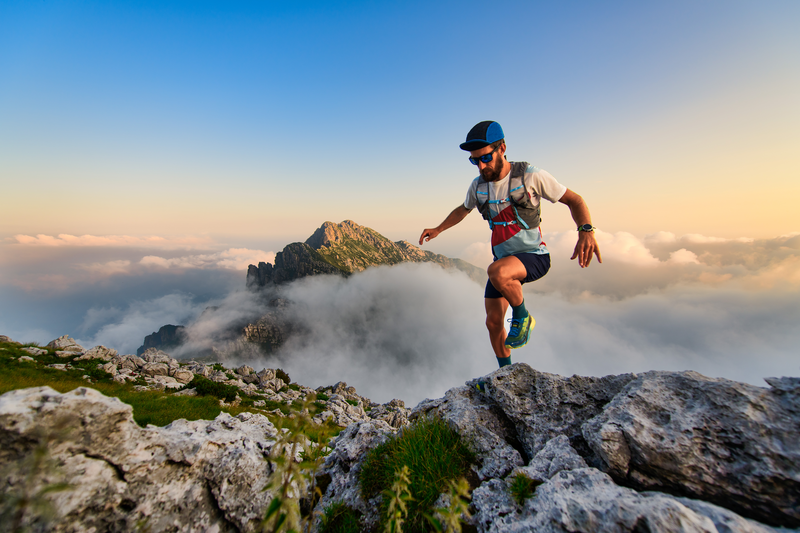
95% of researchers rate our articles as excellent or good
Learn more about the work of our research integrity team to safeguard the quality of each article we publish.
Find out more
REVIEW article
Front. Immunol. , 02 June 2020
Sec. Multiple Sclerosis and Neuroimmunology
Volume 11 - 2020 | https://doi.org/10.3389/fimmu.2020.00991
This article is part of the Research Topic Brain-Targeted Autoimmunity: Beyond Multiple Sclerosis View all 12 articles
Paraneoplastic cerebellar degeneration (PCD) is a rare immune-mediated disease that develops mostly in the setting of neoplasia and offers a unique prospect to explore the interplay between tumor immunity and autoimmunity. In PCD, the deleterious adaptive immune response targets self-antigens aberrantly expressed by tumor cells, mostly gynecological cancers, and physiologically expressed by the Purkinje neurons of the cerebellum. Highly specific anti-neuronal antibodies in the serum and cerebrospinal fluid represent key diagnostic biomarkers of PCD. Some anti-neuronal antibodies such as anti-Yo autoantibodies (recognizing the CDR2/CDR2L proteins) are only associated with PCD. Other anti-neuronal antibodies, such as anti-Hu, anti-Ri, and anti-Ma2, are detected in patients with PCD or other types of paraneoplastic neurological manifestations. Importantly, these autoantibodies cannot transfer disease and evidence for a pathogenic role of autoreactive T cells is accumulating. However, the precise mechanisms responsible for disruption of self-tolerance to neuronal self-antigens in the cancer setting and the pathways involved in pathogenesis within the cerebellum remain to be fully deciphered. Although the occurrence of PCD is rare, the risk for such severe complication may increase with wider use of cancer immunotherapy, notably immune checkpoint blockade. Here, we review recent literature pertaining to the pathophysiology of PCD and propose an immune scheme underlying this disabling disease. Additionally, based on observations from patients' samples and on the pre-clinical model we recently developed, we discuss potential therapeutic strategies that could blunt this cerebellum-specific autoimmune disease.
The central nervous system (CNS) can be the target of deleterious cellular and humoral immune responses in context of infectious, degenerative, or autoimmune diseases (1–4). Among these immune-mediated CNS disorders, autoimmune diseases are wide and heterogeneous, occurring both in paraneoplastic and non-paraneoplastic context (1). Paraneoplastic neurological disorders are characterized by acute or subacute neurological manifestations associated with autoantibodies against antigens expressed physiologically by neural cells as well as by tumor cells, so-called “onconeuronal antigens” (5, 6). Although the autoantibodies are considered faithful diagnostic biomarkers of paraneoplastic neurological disorders, their pathogenic contribution, when the target antigens are intracellular, is uncertain (7, 8). In these cases, antigen-specific cytotoxic CD8 T cells that recognize epitopes derived from intracellular proteins in the context of MHC class I presentation are considered the main players causing the neuronal damage (9, 10).
Paraneoplastic cerebellar degeneration (PCD), one of the most common paraneoplastic neurological syndromes (11), represents a heterogeneous group that differs in clinical features, prognosis, associated tumor and associated antibody (7) (Table 1).
Cerebellar degeneration is the dominant presentation but neocortex, limbic system, basal ganglia, spinal cord and the peripheral nervous system can be involved (88). The clinical presentation is partially correlated to the pattern of expression of the target autoantigen in the CNS. Antibodies are mainly directed against intracellular neuronal antigens and, contrary to autoimmune encephalitis associated with antibodies targeting cell surface proteins (such as the NMDA receptor), the pathogenic immune response involves cellular immune mechanisms and irreversible neuronal death. This neuronal death leads to severe and irreversible neurological impairment. Highly specific anti-neuronal autoantibodies in the sera and/or cerebrospinal fluid (CSF) are the key diagnostic biomarkers of PCD. About 50% of PCD cases are related with anti-Yo antibodies, also known as CDR2/CDR2L (cerebellar degeneration-related antigen), making anti-Yo antibodies the predominant autoantibody associated with PCD among the 37 other anti-neural antibodies described (Table 1), such as anti-Hu, anti-Tr, anti-Ri, anti-Ma2, anti-P/Q-type calcium channel, or anti-CV2/CRMP5 (89–91).
The anti-CDR2/CDR2L antibody-associated PCD will be the main focus of the current article. This type of PCD develops mostly in female patients with gynecologic (ovarian and breast) carcinomas that express the Purkinje neuron-specific CDR2 protein (9) and its paralog CDR2L (14), but also in patients with other types of cancers including endometrial, digestive and lung (37, 92–95).
CDR2 and CDR2L are members of the cerebellar degeneration related (CDR) protein family. The Cdr2 gene is widely transcribed and encodes a cytoplasmic leucine zipper protein. The RNA is expressed in almost all tissues but the protein has only been found to be expressed in cerebellar Purkinje neurons, some brainstem neurons, ovarian and mammary tissue, prostate, testis and spermatogonia (96–99). These results suggest that the tissue-specific expression of CDR2 is regulated at a post-transcriptional level.
The biological function of CDR2 remains ill defined, and that of CDR2L even less known. It has been shown that CDR2 inhibits the functions of the oncogene c-Myc, a master regulator of cellular growth and cellular metabolism, through its sequestration in the cytoplasm (100). CDR2 interacts with other proteins involved in signal transduction and gene transcription such as cell cycle-related proteins, and acid-activated serine/threonine protein kinase (100–104). A similar interaction was found with NF-kB, a transcription factor involved in neuronal development and synaptic plasticity (105); and with MRGXm, a transcriptional regulator involved in cell growth and apoptosis (103). These data indicate that CDR2 may be involved in the regulation of cell cycle, at least in part through interactions with c-Myc. Since cells display an impaired proliferation capacity upon CDR2 knockdown, it can be reasoned that CDR2 is required for appropriate mitotic function (101).
Anti-Yo antibodies react against CDR2 (62 kDa protein consisting of 454 amino acids) and CDR2L (a CDR2 paralog consisting of 465 amino acids). CDR2L has a 44.7% sequence identity with CDR2 and contains three potential coiled-coil regions (14). In the cerebellum, both CDR2 and CDR2L are present in the cytoplasm and proximal dendrites of Purkinje cells (96, 100). Recent data, combining co-staining on human cerebellar sections and cultured cancer cells, protein immunoprecipitation and pre-absorption experiments, strongly suggest that under native conditions CDR2L, rather than or in addition to CDR2, is the major target of serum and CSF Yo antibodies (14, 106). However, there is currently no evidence indicating that immunity toward CDR2L actually causes Purkinje cell death.
The expression of CDR2 appears tightly restricted to “immune privileged” sites whereas CDRL2 is transcribed also in the digestive tract. CDR2 has been found to be poorly immunogenic even when expressed in tumors (107, 108). During lymphocytes development in the thymus, tolerance mechanisms delete most autoreactive T cells with high affinity or redirect them to a regulatory phenotype (109, 110). Through “ectopic” expression of a number of tissue-associated self-antigens, the autoimmune regulator (AIRE) acts as a master regulator of central T-cell tolerance by preventing the development of pathogenic autoreactive T cells (111, 112). Interestingly, transcription of both Cdr2 and Cdr2l has been reported in thymic medullary epithelial cells (113). Whether this expression is dependent on AIRE and whether it results in central T-cell tolerance remains unknown at this time. In this context, the observed up-regulation of AIRE mRNA expression in ovarian tumors associated with anti-Yo PCD as compared to other ovarian tumors is puzzling (114). Moreover, genes transcriptionally regulated by AIRE are enriched among those differentially expressed between anti-Yo-associated vs. control ovarian tumors, although the immunological consequences of this differential expression is unknown (114). Collectively, these findings suggest that defects in immune tolerance induction could be implicated in the pathogenesis of PCD.
Central tolerance is only partial since lymphocytes capable of recognizing autoantigens are prevalent, even in healthy individuals (115). Therefore, in order to prevent deleterious autoimmune reactions, peripheral tolerance mechanisms are necessary, among which regulatory FOXP3+ CD4 T cells play a major role. Break of immune tolerance resulting in autoimmunity usually requires a failure of one or several tolerance checkpoints. The autoimmune response against CDR2/CDR2L antigens in the context of PCD is likely multifactorial, involving high CDR2/CDR2L expression in the tumor, a genetic predisposition, and a productive, chronic immune response toward tumor cells, as detailed below.
The tumor inflammatory microenvironment has been suggested to facilitate the release of intracellular antigens resulting in abnormal exposure of self-antigens to the immune system; this provides an explanation for the numerous autoantibodies produced against intracellular antigens in cancer patients (116). However, PCD is rare even though all ovarian cancer subtypes, regardless of their association with anti-Yo antibodies and PCD, express CDR2/CDR2L (98, 99, 117). Therefore, the mere expression of CDR2/CDR2L by tumor cells is insufficient to trigger autoimmunity against Purkinje cells. The low incidence of PCD may also be linked to the lymphocyte expression of CDR2 (118).
Recently, it has been shown that ovarian tumors associated with PCD and anti-Yo antibodies differ from other ovarian tumors. Indeed, Small and colleagues showed that tumor cells from all 25 PCD patients with anti-Yo antibodies exhibited (likely somatic) mutations and/or gains in CDR2 and/or CDR2L genes, leading to higher protein expression and/or expression of proteins with missense mutations. This high rate of genetic alterations is characteristic of tumors from patients with PCD and anti-Yo antibodies, as they have not been reported in 841 other ovarian carcinomas (119). Moreover, this study demonstrates massive infiltration of PCD tumors with anti-Yo antibodies by activated immune effector cells. This suggests that genetic alterations in tumor cells trigger immune tolerance breakdown and initiation of the autoimmune disease. Comforting this hypothesis, ovarian tumors associated with PCD and anti-Yo antibodies are characterized by a higher and more frequent immune cell infiltration, including CD8 T cells, B cells, plasma cells and mature Lamp+ dendritic cells (DC), known to be associated with more efficient T cell antitumor response (120). The characterization of such DC and whether they contribute to onconeuronal antigen presentation remain to be determined.
The infrequency of anti-Yo antibody-associated PCD among patients with gynecological cancers could also reflect predisposing factors such as a genetic susceptibility. Hillary and colleagues conducted high resolution HLA class I and class II genotyping in 40 patients with PCD vs. ethnically matched controls (11). They provided evidence for association of the DRB1*13:01~DQA1*01:03~DQB1*06:03 haplotype with ovarian cancer-associated, but not breast cancer-associated, PCD (present in 9 of 29 cases). As HLA class II molecules present antigenic peptides to CD4 T cells, the data suggest that this T cell subset could be a major player in the onset of PCD. Significant findings were also observed with several HLA class I alleles, especially within the HLA-C locus (C*03:04, C*04:01, and C*07:01). These data indirectly suggest the involvement of CD8 T cells or NK cells in PCD pathogenesis. Other genes, in particular immune-related genes, may be at play. A genome-wide association study would be greatly beneficial in identifying these genes which may play varied roles in PCD pathogenesis; albeit, challenging, given the low prevalence of PCD.
Circulating immune cells have to cross the blood-brain barrier (BBB) to get into the CNS, involving distinct trafficking molecules at the surface of the BBB endothelial cells and on immune cells for the sequential transmigration steps: tethering, rolling, capture, adhesion and diapedesis (121). Several surface molecules expressed by T cells, such as P-selectin glycoprotein ligand-1 (PSGL1), activated leucocyte cell adhesion molecule (CD6) and integrins, contribute to these steps. PSGL1 bind to P/E-selectin on endothelial cells and mediates the initial rolling and tethering of CD4 and CD8 T cells (122). Furthermore, the α4β1 integrin interacts with vascular cell adhesion protein 1 (VCAM 1) to form strong adhesion between T cells and the endothelium (123, 124). Under inflammatory conditions, the BBB-endothelial cells up-regulate the expression of adhesion molecules (selectins and cell adhesion molecules of the immunoglobulin superfamily) (121). In experimental autoimmune encephalomyelitis (EAE), a classical animal model of multiple sclerosis, BBB-endothelial cells express CCL2, CCL19, and CCL21, which mediate firm arrest of CCR2+ monocytes and DC as well as CCR7+ CD4 T cells (125). Stimulating chemokine receptors also results in a conformational change of the cell surface integrin molecules providing increased affinity for their ligands (126).
Although cumulative evidence highlights the key role of CD8 T cells in several inflammatory CNS disorders such as PCD, the molecular cues responsible for trafficking of CD8 T cells into the CNS are less known. Interaction between PSGL1 and P-selectin contributes to the recruitment of CD8 T cells from multiple sclerosis patients to brain vessels (127). However, CD8 T-cell transmigration is not affected by blocking interactions between αLβ2/ICAM-1, PECAM-1/PECAM-1, or CCL2/CCR2 (128). Using a murine model of CNS autoimmune neuroinflammation, we showed that the migration of cytotoxic CD8 T cells to the CNS relies on the α4β1-integrin and that VCAM-1 and JAM-B expressed by BBB endothelial cells are likely implicated in this process (129). Targeting this pathway of T-cell trafficking to the CNS may hold promise in neurological diseases other than multiple sclerosis. Indeed, Natalizumab, a humanized mAb against α4 integrin, was tested in a patient with immune checkpoint inhibitor-induced encephalitis resulting in neurological improvement after 2 months of treatment (130) and in a few patients with Susac syndrome (131). It is yet to be determined if the pathogenic immune cells require α4 integrin expression to penetrate into the cerebellum during PCD.
Regional peculiarities may exist for T and B cell migration within the CNS. For example, in 2-day-old piglets the cerebellum was more permeable than the cortical regions to bilirubin (132). Moreover, it has been suggested that the expression of P-glycoprotein, a transporter essential in preventing the BBB penetration of substrates (133), is lower in the BBB of the cerebellum than in the cortex (134). It could therefore be proposed that structural or molecular peculiarities of the cerebellum may contribute to local transmigration of T cells during PCD.
PCD is characterized by the selective and extensive loss of cerebellar Purkinje neurons associated with local inflammatory infiltrates reported in several studies (7, 135, 136). Depopulation of Purkinje cell axons and secondary demyelination is also prevalent. In some patients, immune infiltrates and microglial activation extend beyond the cerebellum. In the cerebellum of PCD patients, the inflammatory infiltrates are composed of CD8 T cells, macrophages, and activated microglia that can form nodules (137–139). CD4 T cells and B cells are either absent or found in small numbers around blood vessels (137). No IgG deposition or complement activation is found in relation to the Purkinje cells (138, 139). In PCD CD8 T cells exhibited an activated phenotype with granzyme B- and perforin-containing cytolytic granules, which are sometimes polarized toward the targeted neurons (140, 141). The fact that Purkinje cells can up-regulate the expression of MHC class I molecules during an inflammatory process may provide the opportunity for CD8 T cells to recognize antigens presented by these neurons (140, 142, 143). We hypothesized that interferon-γ (IFNγ) is likely a part of the local inflammatory milieu as suggested by local up-regulation and nuclear translocation of phosphorylated STAT1 (143). Pre-clinical data have yet to show Purkinje cell destruction by T cells specific for PCD-associated autoantigens. Indeed, Ma1-specific Th1 CD4 T cells can induce encephalomyelitis but failed to induce neuronal degeneration (144). However, in mice, CD8 T cells specific for a model onco-neuronal antigen can kill neurons upon help from antigen-specific CD4 cells (145), with accumulative evidence indicating that cytotoxic T cells are likely final mediators of neuronal injury (146). However, in some instances, complete elimination of Purkinje cells is found in the absence of immune cell infiltration, which is reminiscent of burned out lesions (12, 139). Despite these data strongly arguing that CD8 T cells are the final effector cells involved in Purkinje cell demise, definitive proof is lacking.
A possible direct role of autoantibodies directed against intracellular target has been evoked in early studies (5, 147). However, passive transfer of anti-Yo or anti-Hu antibodies, including through the intracerebro-ventricular route, failed to transfer disease in animal models (5, 148–151). More recently, a direct neurotoxic role of autoantibodies was documented on cerebellar organotypic slice cultures upon incubation with anti-CDR2/anti-CDR2L or anti-Hu antibodies (13, 100, 152–154). Collectively, the studies indicate that, under these experimental conditions, uptake of anti-neuronal antibodies by neurons is possible and may result in neuronal death. From a mechanistic standpoint, both human and rabbit anti-CDR2/CDR2L antibodies applied on cerebellar organotypic slice culture were rapidly internalized by Purkinje cells and led to increased expression levels of voltage-gated calcium channel Cav2.1, protein kinase C gamma and calcium-dependent protease, calpain-2; this resulted in the decrease of arborizations of Purkinje cells (13). It was therefore suggested that this autoantibody internalization causes deregulation of cell calcium homeostasis. This, in turn, leads to neuronal dysfunction, ultimately resulting in destruction of diseased Purkinje neurons (13). Another team highlighted also on rat cerebellar slice cultures that application of anti-Yo positive IgG resulted in marked Purkinje cell death (155). This effect was reversed after adsorption of the anti-Yo antibodies with their 62kDa target antigen (153). As neuronal death preceded mononuclear cell infiltration, the autoantibodies appeared to have a direct pathogenic role. These data raise the question of whether there is anti-Yo antibody penetration across the blood-brain-barrier, or from CSF to tissue, and then inside Purkinje cells in patients with PCD.
As already underlined the occurrence of PCD is infrequent (85), with about 10 cases/year in France (119). The risk for paraneoplastic disease appears to increase with application of immunotherapies for cancer, most notably with use of immune checkpoint blockers (156, 157). Increasing numbers of cases of autoimmune encephalomyelitis developing within days after treatment with anti-PD1 mAb (either as a monotherapy or in combination with anti-CTLA-4) have been recently reported in patients harboring melanoma or other types of cancers (130, 158, 159), possibly identifying paraneoplastic neurological disorders as a side effect of immune checkpoint inhibitors. Moreover, we recently evaluated, experimentally, the possibility to induce PCD after CTLA-4 blockade in a mouse model in which a neo-self-antigen was expressed in both Purkinje cells and implanted breast tumor cells (146). In this context, an enhanced tumor control was obtained at the expense of autoimmune PCD. We showed that the immune checkpoint therapy in this mouse model of PCD elicits T cell migration into the cerebellum and subsequent killing of Purkinje cells (146). Therefore, by blocking an essential inhibitory immunological signal in the mouse model, it is possible to elicit PCD. Interestingly, our recent results indicate that while 84% of anti-CTLA-4-treated mice develop PCD, a much lower proportion of mice developed PCD upon anti-PD1 mAb therapy (unpublished). Recently, it was demonstrated that both anti-PD1 and anti-CTLA-4 antibodies target a subset of tumor-infiltrating T cell populations, resulting in the expansion of exhausted-like CD8 T cells (160). Remarkably, anti-CTLA-4 mAb, but not anti-PD1 mAb, modulated the CD4 effector compartment, specifically inducing the expansion of Th1-like CD4 effector cells (160). These CD4 effector T cells elicited by anti-CTLA-4 mAb improved anti-tumor responses by enhancing CD8 T cell infiltration, and cytolytic CD8 activity, demonstrating that PD-1 and CTLA-4 attenuate T cell activation though distinct molecular and cellular mechanisms.
Building on these observations, it is tempting to hypothesize that patients developing PCD carry polymorphisms, or that tumors harbor alterations, in genes related to the immune regulation pathway. A detailed next-generation sequencing analysis of ovarian or breast tumors associated with PCD-related to anti-Yo antibodies and in circulating T cells could explore whether there are alterations in immune regulation, either locally or systemically, in patients with PCD. In that respect, the transcriptomic profile of 12 ovarian cancers from anti-Yo PCD was compared with public data of 733 control ovarian tumor transcriptomes from The Cancer Genome Atlas database. A total of 5,634 genes were differentially expressed between anti-Yo PCD ovarian tumors and control ovarian tumors; among these genes, two members of the CD28 family—CTLA4 and ICOS—were significantly down-regulated, implying that suppressive functions of T cells could be altered within the anti-Yo PCD ovarian tumor microenvironment (114).
Key questions regarding the site of priming and the antigenic specificity of the pathogenic cerebellum-infiltrating CD8 T cells remain to be answered. Regarding the site of priming, pathological studies indicate the presence of moderate to profound immune infiltrates in the primary tumor or metastases of PCD-associated tumors (12, 119, 135, 140). Importantly, these infiltrates were composed of CD8 T cells with cytotoxic potential, B cells, plasmabasts, and DC-LAMP+ dendritic cells (119). Intriguingly, those tumor infiltrates could sometimes form tertiary lymphoid structures, a feature recently associated with better prognosis and reponse to immune checkpoint blockade (161, 162). The identification of a high expression of CDR2 and CDR2L within anti-Yo PCD-associated tumors as well as a very high rate of CDR2 and CDR2L mutations within the tumor strengthen the hypothesis of local adaptive immune activation against tumor antigens, mutated or not. A similar scenario has been described for patients with paraneoplasic scleroderma, in whom genetic alterations of the POLR3A locus and resulting T and B cell responses against the POLR3A gene product were detected in 75% of tumors but were absent from control tumors (163). In that regard, the identification of CDR2 antigen-specific CD8 T cells in the blood and CSF of PCD patients favors the hypothesis that these T cells arise as a consequence of anti-tumor immunity (9, 164). However, the TCR repertoire and antigenic specificity of cerebellum-infiltrating CD8 T cells in PCD are still elusive. Leveraging the latest molecular tools to address these questions appears to be the next logical step (4, 165).
Figure 1. Onconeural antigens such as CDR2 and CDR2L, mutated or not, can be the target of an anti-tumor immune response. Moderate to profound immune infiltrates are found in the primary tumor or metastases of anti-Yo PCD-associated tumors. These infiltrates sometimes form tertiary lymphoid structures. Priming of onconeural antigen-specific CD4 T cells may occur in the tumor-draining lymph nodes or the tumor itself following presentation by local DCs. Differentiation and recruitment of tumor antigen-specific cytotoxic CD8 T-cell likely contribute to the partial tumor control described in patients with PCD. B cells and plasma cells are also found in the tumor. The onconeural antigen-specific T cells reach the CNS after crossing the blood-brain barrier, through a multistep process involving selectins, integrins, and chemokine receptors. The preferential infiltration of the cerebellum in PCD patients could relate to presentation of the onconeural antigens by local APCs and/or to regional peculiarities. In the cerebellum of PCD patients, the inflammatory infiltrates are mostly composed of CD8 T cells, macrophages, and activated microglia. Purkinje neurons are naturally expressing onconeural antigen, such as CDR2 and CDR2L, and up-regulate their expression of MHC class I molecules during an inflammatory process providing the opportunity for CD8 T cells to recognize antigens presented by these neurons. Local CD8 T cells exhibit an activated phenotype with granzyme B- and perforin-containing cytolytic granules. They also secrete locally IFNγ, which has a number of disease-enhancing properties and, thus, is likely a key part of PCD pathogenesis. In particular, IFNγ increases the expression of MHC class I molecules on Purkinje neurons enhances VCAM1 expression on brain endothelial cells and elicits secretion of CXCL10, a ligand of CXCR3 expressed on Th1 cells and activated CD8 T cells. Although antibodies against intracellular onconeural antigens do not appear pathogenic in vivo, they could promote antigen presentation to pathogenic T cells.
Several studies in PCD have focused on CD8 T cells, with much less emphasis given to CD4 T cells. In other context CD4 T cells orchestrate functional immune responses by coordinating immune activity (166). CD4 T cells optimize during the priming the cytotoxic response both in quality and scale, by increasing the cytotoxic CD8 T cell motility and migratory capacities (166). The expansion of Th1-like CD4 T cells following blockage of CTLA-4 improves anti-tumor responses by enhancing CD8 infiltration, cytotoxic CD8 T cells activity, and T cell memory formations (160). It is tempting to speculate that in PCD antigen-specific Th1-type CD4 T cells are responsible for initiating the disease, as documented in a rat model (144), and as a consequence, these CD4 T cells are able to recruit and enhance the cytotoxic CD8 T-cell responses.
We have shown in animal models as well as in cerebellar samples from PCD patients that IFNγ signaling occurs locally in Purkinje neurons and surrounding cell types (143). Moreover, CXCL10, an IFNγ-induced chemokine, is present at high level in the CSF of patients with PCD (167). We, therefore, hypothesize that IFNγ stimulates a disease-promoting cascade of events in the cerebellum and could represent a promising therapeutic target in PCD. Indeed, autoantigen-reactive CD8 T cells (and maybe other immune cell types) produce IFNγ locally, which has a number of disease-enhancing properties. IFNγ increases the expression of MHC class I molecules on neurons, including Purkinje cells, which can therefore present self-peptides to the cytotoxic CD8 T cells, promoting their own destruction (143, 168, 169). In addition, IFNγ promotes local immune cell recruitment by enhancing VCAM1 expression on brain endothelial cells and by eliciting secretion of the chemokine CXCL10, a ligand of CXCR3 expressed on Th1 cells and activated CD8 T cells. Importantly, the levels of CXCL10, but not those of CCL2, are elevated in the CSF of PCD patients indirectly suggesting that the CXCL10/CXCR3 axis may contribute to the trafficking of T cells into the cerebellum (167).
The role played by activated microglia and microglia nodules in the disease process is currently unknown. In Rasmussen encephalitis, microglial nodules have been associated with neuronal phagocytosis, following CD8 T cell-mediated brain neuron destruction (170). In human PCD, similar to Rasmussen encephalitis, IFNγ-mediated signaling (STAT1 phosphorylation) occurs in microglial cells, which can further amplify cytokine/chemokine release (143, 170). Further investigation on the role of microglia as an amplifier of inflammation and an executor of neuronal death is needed in PCD.
As already discussed, antibodies against neuronal autoantigens such as anti-Yo antibodies are extremely useful diagnostic biomarkers. Their direct pathogenic potential documented in in vitro models is still unproven in vivo (5, 13, 149, 153, 171). However, autoreactive B cells could participate in the development of the disease. They can uptake (in an antigen-specific manner due to their surface immunoglobulin), process and present autoantigens to pathogenic T cells. Their scarcity in the cerebellum would, however, rather favor an alternative scenario. For instance, autoantibodies entering the CNS can be up-taken together with their target antigen by resident antigen-presenting phagocytes, a phenomenon that enhances the activation of the incoming effector T cells (172). Therefore, the autoantibodies could cooperate with the T cells and support local autoimmune neuroinflammation.
To date, treatment of PCD is empirical and usually relies on 2 pillars: treatment of the underlying cancer and general immunosuppressive drugs. In the absence of large clinical trials, most of the therapeutics conclusions come from observational clinical studies and case reports. Concerning PCD associated with anti-Yo antibodies, corticoids seem ineffective, whereas plasma exchange and rituximab may have provided some benefit (173–176). The efficacy of intravenous immunoglobulins is controversial: suggested in a small proportion of patients for some studies (177, 178) but not confirmed in a larger study (89). Since there is currently no evidence arguing for a direct role of anti-Yo antibodies in Purkinje cell death, we do not address here potential therapeutic strategies aiming at reducing antibodies levels.
Due to the intracellular localization of the CDR2/CDR2L and Hu antigens, as well as the identification of CD8 T cells in close proximity to neuronal cells, cytotoxic T cells are considered to be the final effectors responsible for neuronal loss in PCD (9, 140, 146, 164, 179). These T cells likely contribute to the tumor control outside of the CNS. Therefore, the conundrum is how to selectively target the CNS-targeting immune cells while preserving (as much as possible) the tumor-controlling counterparts. Uncoupling these two simultaneous immune responses is, theoretically, possible provided that the molecular bases of immune cell migration or effector mechanisms at the two sites differ. It is paramount that the treatment of PCD should be started as early as possible since Purkinje cells are post-mitotic cells that do not renew.
The α4β1 integrin is important for both CD4 and CD8 T cell migration to the CNS and a monoclonal antibody targeting the α4 subunit provides important benefits for the treatment of persons with multiple sclerosis. Therefore, one approach could be to initiate anti-α4 integrin therapy early on in the disease process in order to preserve as many Purkinje neurons as possible. One conceptual obstacle is that blood-borne T cells may not be needed to fuel ongoing tissue destruction by tissue-resident T cells. This may explain why, in our mouse model of PCD, blocking α4 integrin early in the disease process did not yield significant benefit (143). Along the same lines, blocking CXCR3 with either monoclonal antibodies or pharmacological compounds is tempting, given the evidence of a role for the CXCL10/CXCR3 axis in PCD. However, to our knowledge, this approach has not yet reached the clinical setting.
We argued earlier that IFNγ secretion by CD8 T cells could sustain a feed forward loop by rendering Purkinje cells vulnerable to direct killing by autoantigen-specific CD8 T cells. If IFNγ plays a non-redundant role in the progression of PCD, this molecule could be targeted therapeutically. Our recent pre-clinical data showing that blocking IFNγ strongly reduces PCD development without eliciting tumor growth rebound are encouraging (143). Moreover, administration of anti-IFNγ antibodies has been tested recently in clinical trials. For instance, Emapalumab, a fully human monoclonal neutralizing anti-IFNγ antibody has been approved for the treatment of primary hemophagocytic lymphohistiocytosis, a syndrome of excessive immune activation and progressive immune-mediated organ damage due to genetic defects in cell-mediated cytotoxicity (180). Taken together, the accumulating evidence from human samples and the mouse model as well as the previous development of approved anti-IFNγ antibody in humans should facilitate the testing of this strategy in patients with PCD.
The precise mechanisms and pathways involved in the pathogenesis of PCD need to be explored in greater depth. This can be done with the use of bodily fluids (blood and CSF) and tissue from PCD patients and further validated in reductionist mouse models. Key questions remain regarding the antigenic specificity, phenotype, migration of T and B cells infiltrating the tumor and the cerebellar tissue. The molecular dissection of the steps involved in pathogenesis is a pre-requisite for rational development of new therapeutic strategies. In depth investigation of the immune changes in patients suffering from paraneoplastic neurological disorders in the frame of immune checkpoint blockade should provide clues in that respect.
LY and CB drafted the work. RL designed the concept and revised the manuscript critically. All authors made substantial contributions to the conception or design of the work and contributed to manuscript revision, read, and approved the submitted version.
This work of the authors was supported by the RHU BETPSY, the Foundation pour la Recherche Médicale (FRM), the French Research Agency (ANR T-cell Mig), ERA-NET NEURON (Meltra-BBB), and Bristol Myers-Squibb Foundation.
The authors declare that the research was conducted in the absence of any commercial or financial relationships that could be construed as a potential conflict of interest.
We would like to thank Leila Khajavi and Drs. Abdelhadi Saoudi for their valuable input on the manuscript.
1. Waisman A, Liblau RS, Becher B. Innate and adaptive immune responses in the CNS. Lancet Neurol. (2015) 14:945–55. doi: 10.1016/S1474-4422(15)00141-6
2. Sulzer D, Alcalay RN, Garretti F, Cote L, Kanter E, Agin-Liebes J, et al. T cells of Parkinson's disease patients recognize α-synuclein peptides. Nature. (2017) 546:656–61. doi: 10.1038/nature22815
3. Latorre D, Kallweit U, Armentani E, Foglierini M, Mele F, Cassotta A, et al. T cells in patients with narcolepsy target self-antigens of hypocretin neurons. Nature. (2018) 562:63–8. doi: 10.1038/s41586-018-0540-1
4. Gate D, Saligrama N, Leventhal O, Yang AC, Unger MS, Middeldorp J, et al. Clonally expanded CD8 T cells patrol the cerebrospinal fluid in Alzheimer's disease. Nature. (2020) 577:399–404. doi: 10.1038/s41586-019-1895-7
5. Graus F, Illa I, Agusti M, Ribalta T, Cruz-Sanchez F, Juarez C. Effect of intraventricular injection of an anti-Purkinje cell antibody (anti-Yo) in a guinea pig model. J Neurol Sci. (1991) 106:82–7. doi: 10.1016/0022-510X(91)90198-G
6. Furneaux HM, Rosenblum MK, Dalmau J, Wong E, Woodruff P, Graus F, et al. Selective expression of Purkinje cell antigens in tumor tissue from patients with paraneoplastic cerebellar degeneration. N Engl J Med. (1990) 322:1844–51. doi: 10.1056/NEJM199006283222604
7. Dalmau J, Rosenfeld MR. Paraneoplastic syndromes of the CNS. Lancet Neurol. (2008) 7:327–40. doi: 10.1016/S1474-4422(08)70060-7
8. Zou W, Wolchok JD, Chen L. PD-L1 (B7-H1) and PD-1 pathway blockade for cancer therapy. Sci Transl Med. (2016) 8:328rv4. doi: 10.1126/scitranslmed.aad7118
9. Albert ML, Darnell JC, Bender A, Francisco LM, Bhardwaj N, Darnell RB. Tumor-specific killer cells in paraneoplastic cerebellar degeneration. Nat Med. (1998) 4:1321–4. doi: 10.1038/3315
10. Roberts WK, Deluca IJ, Thomas A, Fak J, Williams T, Buckley N, et al. Patients with lung cancer and paraneoplastic Hu syndrome harbor HuD-specific type 2 CD8+ T cells. J Clin Invest. (2009) 119:2042–51. doi: 10.1172/JCI36131
11. Hillary RP, Ollila HM, Lin L, Desestret V, Rogemond V, Picard G, et al. Complex HLA association in paraneoplastic cerebellar ataxia with anti-Yo antibodies. J Neuroimmunol. (2018) 315:28–32. doi: 10.1016/j.jneuroim.2017.12.012
12. Peterson K, Rosenblum MK, Kotanides H, Posner JB. Paraneoplastic cerebellar degeneration : I. A clinical analysis of 55 anti-Yo antibody-positive patients. Neurology. (1992) 42:1931–7. doi: 10.1212/WNL.42.10.1931
13. Schubert M, Panja D, Haugen M, Bramham CR, Vedeler CA. Paraneoplastic CDR2 and CDR2L antibodies affect Purkinje cell calcium homeostasis. Acta Neuropathol. (2014) 128:835–52. doi: 10.1007/s00401-014-1351-6
14. Eichler TW, Totland C, Haugen M, Qvale TH, Mazengia K, Storstein A, et al. CDR2L antibodies : a new player in paraneoplastic cerebellar degeneration. PLoS ONE. (2013) 8:e66002. doi: 10.1371/journal.pone.0066002
15. Lucchinetti CF, Kimmel DW, Lennon VA. Paraneoplastic and oncologic profiles of patients seropositive for type 1 antineuronal nuclear autoantibodies. Neurology. (1998) 50:652–7. doi: 10.1212/WNL.50.3.652
16. Graus F, Keime-Guibert F, Rene R, Benyahia B, Ribalta T, Ascaso C, et al. Anti-Hu-associated paraneoplastic encephalomyelitis : analysis of 200 patients. Brain. (2001) 124:1138–48. doi: 10.1093/brain/124.6.1138
17. Dalmau J, Graus F, Rosenblum M, Posner JB. Anti-Hu-associated paraneoplastic encephalomyelitis/sensory neuronopathy : a clinical study of 71 patients. Medicine (Baltimore). (1992) 71:59–72. doi: 10.1097/00005792-199203000-00001
18. Honnorat J, Saiz A, Giometto B, Vincent A, Brieva L, de Andres C, et al. Cerebellar ataxia with anti-glutamic acid decarboxylase antibodies: study of 14 patients. Arch Neurol. (2001) 58:225–30. doi: 10.1001/archneur.58.2.225
19. Jarius S, Stich O, Speck J, Rasiah C, Wildemann B, Meinck HM, et al. Qualitative and quantitative evidence of anti-glutamic acid decarboxylase-specific intrathecal antibody synthesis in patients with stiff person syndrome. J Neuroimmunol. (2010) 229:219–24. doi: 10.1016/j.jneuroim.2010.07.019
20. Kono S, Miyajima H, Sugimoto M, Suzuki Y, Takahashi Y, Hishida A. Stiff-person syndrome associated with cerebellar ataxia and high glutamic acid decarboxylase antibody titer. Intern Med. (2001) 40:968–71. doi: 10.2169/internalmedicine.40.968
21. Piccolo G, Tavazzi E, Cavallaro T, Romani A, Scelsi R, Martino G. Clinico-pathological findings in a patient with progressive cerebellar ataxia, autoimmune polyendocrine syndrome, hepatocellular carcinoma and anti-GAD autoantibodies. J Neurol Sci. (2010) 290:148–9. doi: 10.1016/j.jns.2009.12.006
22. Saiz A, Blanco Y, Sabater L, González F, Bataller L, Casamitjana R, et al. Spectrum of neurological syndromes associated with glutamic acid decarboxylase antibodies: diagnostic clues for this association. Brain. (2008) 131:2553–63. doi: 10.1093/brain/awn183
23. Jarius S, Wandinger KP, Horn S, Heuer H, Wildemann B. A new Purkinje cell antibody (anti-Ca) associated with subacute cerebellar ataxia: immunological characterization. J Neuroinflammation. (2010) 7:21. doi: 10.1186/1742-2094-7-21
24. Jarius S, Martínez-García P, Hernandez AL, Brase JC, Borowski K, Regula JU, et al. Two new cases of anti-Ca (anti-ARHGAP26/GRAF) autoantibody-associated cerebellar ataxia. J Neuroinflammation. (2013) 10:7. doi: 10.1186/1742-2094-10-7
25. Doss S, Nümann A, Ziegler A, Siebert E, Borowski K, Stöcker W, et al. Anti-Ca/anti-ARHGAP26 antibodies associated with cerebellar atrophy and cognitive decline. J Neuroimmunol. (2014) 267:102–4. doi: 10.1016/j.jneuroim.2013.10.010
26. Bartels F, Prüss H, Finke C. Anti-ARHGAP26 autoantibodies are associated with isolated cognitive impairment. Front Neurol. (2018) 9:656. doi: 10.3389/fneur.2018.00656
27. Wallwitz U, Brock S, Schunck A, Wildemann B, Jarius S, Hoffmann F. From dizziness to severe ataxia and dysarthria: new cases of anti-Ca/ARHGAP26 autoantibody-associated cerebellar ataxia suggest a broad clinical spectrum. J Neuroimmunol. (2017) 309:77–81. doi: 10.1016/j.jneuroim.2017.05.011
28. Shan F, Long Y, Qiu W. Autoimmune glial fibrillary acidic protein astrocytopathy: a review of the literature. Front Immunol. (2018) 9:2802. doi: 10.3389/fimmu.2018.02802
29. Yu Z, Kryzer TJ, Griesmann GE, Kim KK, Benarroch EE, Lennon VA. CRMP-5 neuronal autoantibody: marker of lung cancer and thymoma-related autoimmunity. Ann Neurol. (2001) 49:146–54. doi: 10.1002/1531-8249(20010201)49:2<146::aid-ana34>3.0.co
30. Antoine JC, Honnorat J, Vocanson C, Koenig F, Aguera M, Belin MF, et al. Posterior uveitis, paraneoplastic encephalomyelitis and auto-antibodies reacting with developmental protein of brain and retina. J Neurol Sci. (1993) 117:215–23. doi: 10.1016/0022-510X(93)90176-Y
31. Honnorat J, Cartalat-Carel S, Ricard D, Camdessanche JP, Carpentier AF, Rogemond V, et al. Onco-neural antibodies and tumour type determine survival and neurological symptoms in paraneoplastic neurological syndromes with Hu or CV2/CRMP5 antibodies. J Neurol Neurosurg Psychiatry. (2009) 80:412–6. doi: 10.1136/jnnp.2007.138016
32. Honnorat J, Antoine JC, Derrington E, Aguera M, Belin MF. Antibodies to a subpopulation of glial cells and a 66 kDa developmental protein in patients with paraneoplastic neurological syndromes. J Neurol Neurosurg Psychiatry. (1996) 61:270–8. doi: 10.1136/jnnp.61.3.270
33. Lopez-Chiriboga AS, Komorowski L, Kümpfel T, Probst C, Hinson SR, Pittock SJ, et al. Metabotropic glutamate receptor type 1 autoimmunity : clinical features and treatment outcomes. Neurology. (2016) 86:1009–13. doi: 10.1212/WNL.0000000000002476
34. Marignier R, Chenevier F, Rogemond V, Sillevis Smitt P, Renoux C, Cavillon G, et al. Metabotropic glutamate receptor type 1 autoantibody–associated cerebellitis. Arch Neurol. (2010) 67:627–30. doi: 10.1001/archneurol.2010.51
35. Sillevis-Smitt P, Kinoshita M, De Leeuw B, Moll W, Coesmans M, Jaarsma D, et al. Paraneoplastic cerebellar ataxia due to autoantibodies against glutamate receptor. N Engl J Med. (2000) 342:21–7. doi: 10.1056/NEJM200001063420104
36. Iorio R, Damato V, Mirabella M, Vita MG, Hulsenboom E, Plantone D, et al. Cerebellar degeneration associated with mGluR1 autoantibodies as a paraneoplastic manifestation of prostate adenocarcinoma. J Neuroimmunol. (2013) 263:155–8. doi: 10.1016/j.jneuroim.2013.07.015
37. Mason WP, Graus F, Lang B, Honnorat J, Delattre JY, Valldeoriola F, et al. Small-cell lung cancer, paraneoplastic cerebellar degeneration and the Lambert-Eaton myasthenic syndrome. Brain. (1997) 120:1279–300. doi: 10.1093/brain/120.8.1279
38. Graus F, Lang B, Pozo-Rosich P, Saiz A, Casamitjana R, Vincent A. P / Q type calcium- channel antibodies in paraneoplastic cerebellar degeneration with lung cancer. Neurology. (2002) 59:764–6. doi: 10.1212/WNL.59.5.764
39. Bürk K, Wick M, Roth G, Decker P, Voltz R. Antineuronal antibodies in sporadic late-onset cerebellar ataxia. J Neurol. (2010) 257:59–62. doi: 10.1007/s00415-009-5262-8
40. Pittock SJ, Lucchinetti CF, Parisi JE, Benarroch EE, Mokri B, Stephan CL, et al. Amphiphysin autoimmunity: paraneoplastic accompaniments. Ann Neurol. (2005) 58:96–107. doi: 10.1002/ana.20529
41. Moon J, Lee S-T, Shin J-W, Byun J-I, Lim J-A, Shin Y-W, et al. Non-stiff anti-amphiphysin syndrome: clinical manifestations and outcome after immunotherapy. J Neuroimmunol. (2014) 274:209–14. doi: 10.1016/j.jneuroim.2014.07.011
42. Balint B, Jarius S, Nagel S, Haberkorn U, Probst C, Blöcker IM, et al. Progressive encephalomyelitis with rigidity and myoclonus: a new variant with DPPX antibodies. Neurology. (2014) 82:1521–8. doi: 10.1212/WNL.0000000000000372
43. Boronat A, Gelfand JM, Gresa-Arribas N, Jeong HY, Walsh M, Roberts K, et al. Encephalitis and antibodies to dipeptidyl-peptidase-like protein-6, a subunit of Kv4.2 potassium channels. Ann Neurol. (2013) 73:120–8. doi: 10.1002/ana.23756
44. Tobin WO, Lennon VA, Komorowski L, Probst C, Clardy SL, Aksamit AJ, et al. DPPX potassium channel antibody: Frequency, clinical accompaniments, and outcomes in 20 patients. Neurology. (2014) 83:1797–803. doi: 10.1212/WNL.0000000000000991
45. Stoeck K, Carstens PO, Jarius S, Raddatz D, Stöcker W, Wildemann B, et al. Prednisolone and azathioprine are effective in DPPX antibody-positive autoimmune encephalitis. Neurol Neuroimmunol NeuroInflamm. (2015) 2:e86. doi: 10.1212/NXI.0000000000000086
46. Becker EBE, Zuliani L, Pettingill R, Lang B, Waters P, Dulneva A, et al. Contactin-associated protein-2 antibodies in non-paraneoplastic cerebellar ataxia. J Neurol Neurosurg Psychiatry. (2012) 83:437–40. doi: 10.1136/jnnp-2011-301506
47. Balint B, Regula JU, Jarius S, Wildemann B. Caspr2 antibodies in limbic encephalitis with cerebellar ataxia, dyskinesias and myoclonus. J Neurol Sci. (2013) 327:73–4. doi: 10.1016/j.jns.2013.01.040
48. Kannoth S, Nambiar V, Gopinath S, Anandakuttan A, Mathai A, Rajan PK. Expanding spectrum of contactin-associated protein 2 (CASPR2) autoimmunity—syndrome of parkinsonism and ataxia. Neurol Sci. (2018) 39:455–60. doi: 10.1007/s10072-017-3222-0
49. Boronat A, Sabater L, Saiz A, Dalmau J, Graus F. GABA(B) receptor antibodies in limbic encephalitis and anti-GAD-associated neurologic disorders. Neurology. (2011) 76:795–800. doi: 10.1212/WNL.0b013e31820e7b8d
50. Lancaster E, Lai M, Peng X, Hughes E, Constantinescu R, Raizer J, et al. Antibodies to the GABA(B) receptor in limbic encephalitis with seizures: case series and characterisation of the antigen. Lancet Neurol. (2010) 9:67–76. doi: 10.1016/S1474-4422(09)70324-2
51. Jarius S, Steinmeyer F, Knobel A, Streitberger K, Hotter B, Horn S, et al. GABA B receptor antibodies in paraneoplastic cerebellar ataxia. J Neuroimmunol. (2013) 256:94–6. doi: 10.1016/j.jneuroim.2012.12.006
52. Graus F, Vincent A, Pozo-Rosich P, Sabater L, Saiz A, Lang B, et al. Anti-glial nuclear antibody: marker of lung cancer-related paraneoplastic neurological syndromes. J Neuroimmunol. (2005) 165:166–71. doi: 10.1016/j.jneuroim.2005.03.020
53. Titulaer MJ, Klooster R, Potman M, Sabater L, Graus F, Hegeman IM, et al. SOX antibodies in small-cell lung cancer and Lambert-Eaton myasthenic syndrome: frequency and relation with survival. J Clin Oncol. (2009) 27:4260–7. doi: 10.1200/JCO.2008.20.6169
54. Hoffmann LA, Jarius S, Pellkofer HL, Schueller M, Krumbholz M, Koenig F, et al. Anti-Ma and anti-Ta associated paraneoplastic neurological syndromes: 22 newly diagnosed patients and review of previous cases. J Neurol Neurosurg Psychiatry. (2008) 79:767–73. doi: 10.1136/jnnp.2007.118588
55. Dalmau J, Graus F, Villarejo A, Posner JB, Blumenthal D, Thiessen B, et al. Clinical analysis of anti-Ma2-associated encephalitis. Brain. (2004) 127:1831–44. doi: 10.1093/brain/awh203
56. Voltz R, Gultekin SH, Rosenfeld MR, Gerstner E, Eichen J, Posner JB, et al. A serologic marker of paraneoplastic limbic and brain-stem encephalitis in patients with testicular cancer. N Engl J Med. (1999) 340:1788–95. doi: 10.1056/NEJM199906103402303
57. Dalmau J, Gultekin SH, Voltz R, Hoard R, DesChamps T, Balmaceda C, et al. Ma1, a novel neuron- and testis-specific protein, is recognized by the serum of patients with paraneoplastic neurological disorders. Brain. (1999) 122:27–39. doi: 10.1093/brain/122.1.27
58. Pittock SJ, Lucchinetti CF, Lennon VA. Anti-neuronal nuclear autoantibody type 2: paraneoplastic accompaniments. Ann Neurol. (2003) 53:580–7. doi: 10.1002/ana.10518
59. Budde-Steffen C, Anderson NE, Rosenblum MK, Graus F, Ford D, Synek BJL, et al. An antineuronal autoantibody in paraneoplastic opsoclonus. Ann Neurol. (1988) 23:528–31. doi: 10.1002/ana.410230518
60. O'Leary CG, Battley JE, O'Reilly S. Anti-Ri antibody associated small cell lung carcinoma. Ir J Med Sci. (2016) 186:265–7. doi: 10.1007/s11845-016-1402-1
61. Vernino S, Lennon VA. New Purkinje cell antibody (PCA-2): marker of lung cancer-related neurological autoimmunity. Ann Neurol. (2000) 47:297–305. doi: 10.1002/1531-8249(200003)47:3<297::AID-ANA4>3.0.CO;2-4
62. Chan KH, Vernino S, Lennon VA. ANNA-3 anti-neuronal nuclear antibody: marker of lung cancer-related autoimmunity. Ann Neurol. (2001) 50:301–11. doi: 10.1002/ana.1127
63. Bataller L, Wade DF, Fuller GN, Rosenfeld MR, Dalmau J. Cerebellar degeneration and autoimmunity to zinc-finger proteins of the cerebellum. Neurology. (2002) 59:1985–7. doi: 10.1212/01.WNL.0000038352.01415.CE
64. Bataller L, Wade DF, Graus F, Stacey HD, Rosenfeld MR, Dalmau J. Antibodies to Zic4 in paraneoplastic neurologic disorders and small-cell lung cancer. Neurology. (2004) 62:778–82. doi: 10.1212/01.WNL.0000113749.77217.01
65. Sabater L, Bataller L, Suarez-Calvet M, Saiz A, Dalmau J, Graus F. ZIC antibodies in paraneoplastic cerebellar degeneration and small cell lung cancer. J Neuroimmunol. (2008) 201–2:163–5. doi: 10.1016/j.jneuroim.2008.01.018
66. Zuliani L, Sabater L, Saiz A, Baiges J, Giometto B, Graus F. Homer 3 autoimmunity in subacute idiopathic cerebellar ataxia. Neurology. (2007) 68:239–40. doi: 10.1212/01.wnl.0000251308.79366.f9
67. Höftberger R, Sabater L, Ortega A, Dalmau J, Graus F. Patient with homer-3 antibodies and cerebellitis. JAMA Neurol. (2013) 70:506–9. doi: 10.1001/jamaneurol.2013.1955
68. Jarius S, Scharf M, Begemann N, Stöcker W, Probst C, Serysheva II, et al. Antibodies to the inositol 1,4,5-trisphosphate receptor type 1 (ITPR1) in cerebellar ataxia. J Neuroinflammation. (2014) 11:206. doi: 10.1186/s12974-014-0206-3
69. Berzero G, Hacohen Y, Komorowski L, Scharf M, Dehais C, Leclercq D, et al. Paraneoplastic cerebellar degeneration associated with anti-ITPR1 antibodies. Neurol Neuroimmunol NeuroInflammation. (2017) 4:e326. doi: 10.1212/NXI.0000000000000326
70. Bataller L, Sabater L, Saiz A, Serra C, Claramonte B, Graus F. Carbonic anhydrase-related protein VlII: autoantigen in paraneoplastic cerebellar degeneration. Ann Neurol. (2004) 56:575–9. doi: 10.1002/ana.20238
71. Höftberger R, Sabater L, Velasco F, Ciordia R, Dalmau J, Graus F. Carbonic anhydrase-related protein VIII antibodies and paraneoplastic cerebellar degeneration. Neuropathol Appl Neurobiol. (2014) 40:650–3. doi: 10.1111/nan.12118
72. de Graaff E, Maat P, Hulsenboom E, van Den Berg R, van Den Bent M, Demmers J, et al. Identification of delta/notch-like epidermal growth factor-related receptor as the Tr antigen in paraneoplastic cerebellar degeneration. Ann Neurol. (2012) 71:815–24. doi: 10.1002/ana.23550
73. Probst C, Komorowski L, de Graaff E, van Coevorden-Hameete M, Rogemond V, Honnorat J, et al. Standardized test for anti-Tr/DNER in patients with paraneoplastic cerebellar degeneration. Neurol Neuroimmunol Neuroinflamm. (2015) 2:e68. doi: 10.1212/NXI.0000000000000068
74. Graus F, Dalmau J, Valldeoriola F, Ferrer I, Reñé R, Marin C, et al. Immunological characterization of a neuronal antibody (anti-Tr) associated with paraneoplastic cerebellar degeneration and Hodgkin's disease. J Neuroimmunol. (1997) 74:55–61. doi: 10.1016/S0165-5728(96)00205-6
75. Trotter JL, Hendin BA, Osterland K. Cerebellar degeneration with Hodgkin's disease. Arch Neurol. (1976) 33:660–1. doi: 10.1001/archneur.1976.00500090066014
76. Bernal F, Shams'ili S, Rojas I, Sanchez-Valle R, Saiz A, Dalmau J, et al. Anti-Tr antibodies as markers of paraneoplastic cerebellar degeneration and Hodgkin's disease. Neurology. (2003) 60:230–4. doi: 10.1212/01.WNL.0000041495.87539.98
77. van Coevorden-Hameete MH, van Beuningen SFB, Perrenoud M, Will LM, Hulsenboom E, Demonet JF, et al. Antibodies to TRIM46 are associated with paraneoplastic neurological syndromes. Ann Clin Transl Neurol. (2017) 4:680–6. doi: 10.1002/acn3.396
78. Do LD, Gupton SL, Tanji K, Bastien J, Brugière S, Couté Y, et al. TRIM9 and TRIM67 are new targets in paraneoplastic cerebellar degeneration. Cerebellum. (2019) 18:245–54. doi: 10.1007/s12311-018-0987-5
79. Larman HB, Zhao Z, Laserson U, Li MZ, Ciccia A, Gakidis MAM, et al. Autoantigen discovery with a synthetic human peptidome. Nat Biotechnol. (2011) 29:535–41. doi: 10.1038/nbt.1856
80. Shimizu F, Takeshita Y, Sano Y, Hamamoto Y, Shiraishi H, Sato T, et al. GRP78 antibodies damage the blood-brain barrier and relate to cerebellar degeneration in Lambert-Eaton myasthenic syndrome. Brain. (2019) 142:2253–64. doi: 10.1093/brain/awz168
81. van Coevorden-Hameete MH, de Graaff E, Titulaer MJ, Hulsenboom E, Sabater L, Hoogenraad CC, et al. Plasticity-related gene 5: a novel surface autoantigen in paraneoplastic cerebellar degeneration. Neurol Neuroimmunol NeuroInflamm. (2015) 2:e156. doi: 10.1212/NXI.0000000000000156
82. Shelly S, Kryzer TJ, Komorowski L, Miske R, Anderson MD, Flanagan EP, et al. Neurochondrin neurological autoimmunity. Neurol Neuroimmunol Neuroinflamm. (2019) 6:e612. doi: 10.1212/NXI.0000000000000612
83. Miske R, Gross CC, Scharf M, Golombeck KS, Hartwig M, Bhatia U, et al. Neurochondrin is a neuronal target antigen in autoimmune cerebellar degeneration. Neurol Neuroimmunol NeuroInflamm. (2017) 4:e307. doi: 10.1212/NXI.0000000000000307
84. Honorat JA, Sebastian Lopez-Chiriboga A, Kryzer TJ, Fryer JP, Devine M, Flores A, et al. Autoimmune septin-5 cerebellar ataxia. Neurol Neuroimmunol NeuroInflamm. (2018) 5:e474. doi: 10.1212/NXI.0000000000000474
85. Vogrig A, Gigli GL, Segatti S, Corazza E, Marini A, Bernardini A, et al. Epidemiology of paraneoplastic neurological syndromes: a population-based study. J Neurol. (2020) 267:26–35. doi: 10.1007/s00415-019-09544-1
86. Garcia Cuartero I, Rodriguez Galdeano M, Perez Pinar M, Solis Garcia del Pozo J. Thymic carcinoma associated with cerebellar degeneration. Eur J Case Rep Intern Med. (2018) 5:000780. doi: 10.12890/2018_000780
87. Ducray F, Demarquay G, Graus F, Decullier E, Antoine JC, Giometto B, et al. Seronegative paraneoplastic cerebellar degeneration: the PNS Euronetwork experience. Eur J Neurol. (2014) 21:731–5. doi: 10.1111/ene.12368
88. Joubert B, Rostásy K, Honnorat J. Immune-mediated ataxias. Handb Clin Neurol. (2018) 155:313–32. doi: 10.1016/B978-0-444-64189-2.00021-4
89. Shams'ili S, Grefkens J, de Leeuw B, van den Bent M, Hooijkaas H, van der Holt B, et al. Paraneoplastic cerebellar degeneration associated with antineuronal antibodies: analysis of 50 patients. Brain. (2003) 126:1409–18. doi: 10.1093/brain/awg133
90. Jarius S, Wildemann B. “Medusa-head ataxia”: the expanding spectrum of Purkinje cell antibodies in autoimmune cerebellar ataxia. Part 1: Anti-mGluR1, anti-Homer-3, anti-Sj/ITPR1 anti-CARP VIII. J Neuroinflammation. (2015) 12:166. doi: 10.1186/s12974-015-0356-y
91. Venkatraman A, Opal P. Paraneoplastic cerebellar degeneration with anti-Yo antibodies – a review. Ann Clin Transl Neurol. (2016) 3:655–63. doi: 10.1002/acn3.328
92. Drlicek M, Bianchi G, Bogliun G, Casati B, Grisold W, Kolig C, et al. Antibodies of the anti-Yo and anti-Ri type in the absence of paraneoplastic neurological syndromes: a long-term survey of ovarian cancer patients. J Neurol. (1997) 244:85–9. doi: 10.1007/s004150050054
93. Rana AQ, Rana AN, Adlul A. Acute ataxia due to anti-Yo antibody paraneoplastic cerebellar degeneration 4 months prior to diagnosis of uterine carcinoma. Acta Neurol Belg. (2012) 112:303–4. doi: 10.1007/s13760-012-0047-8
94. Hasadsri L, Lee J, Wang BH, Yekkirala L, Wang M. Anti-Yo associated paraneoplastic cerebellar degeneration in a man with large cell cancer of the lung. Case Rep Neurol Med. (2013) 2013:725936. doi: 10.1155/2013/725936
95. Bruhnding A, Notch D, Beard A. Anti-Yo positive paraneoplastic cerebellar degeneration in the setting of cholangiocarcinoma. J Clin Neurosci. (2017) 36:71–2. doi: 10.1016/j.jocn.2016.10.034
96. Corradi JP, Yang C, Darnell JC, Dalmau J, Darnell RB. A post-transcriptional regulatory mechanism restricts expression of the paraneoplastic cerebellar degeneration antigen cdr2 to immune privileged tissues. J Neurosci. (1997) 17:1406–15. doi: 10.1523/JNEUROSCI.17-04-01406.1997
97. Greenlee JE. Anti-Yo autoimmunity; dangerous for the brain but not the tumor? J Neurol Sci. (2006) 250:1–2. doi: 10.1016/j.jns.2006.08.001
98. Totland C, Aarskog NK, Eichler TW, Haugen M, Nøstbakken JK, Monstad SE, et al. CDR2 antigen and Yo antibodies. Cancer Immunol Immunother. (2011) 60:283–9. doi: 10.1007/s00262-010-0943-9
99. Raspotnig M, Haugen M, Thorsteinsdottir M, Stefansson I, Salvesen HB, Storstein A, et al. Cerebellar degeneration-related proteins 2 and 2-like are present in ovarian cancer in patients with and without Yo antibodies. Cancer Immunol Immunother. (2017) 66:1463–71. doi: 10.1007/s00262-017-2041-8
100. Okano HJ, Park W, Corradi JP, Darnell RB. The cytoplasmic Purkinje onconeural antigen cdr2 down-regulates c-Myc function : implications for neuronal and tumor cell survival. Genes Dev. (1999) 13:2087–97. doi: 10.1101/gad.13.16.2087
101. O'Donovan KJ, Diedler J, Couture GC, Fak JJ, Darnell RB. The onconeural antigen cdr2 is a novel APC / C target that acts in mitosis to regulate C-Myc target genes in mammalian tumor cells. PLoS One. (2010) 5:e10045. doi: 10.1371/journal.pone.0010045
102. Takanaga H, Mukai H, Shibata H, Toshimori M, Ono Y. PKN interacts with a paraneoplastic cerebellar degeneration-associated antigen, which is a potential transcription factor. Exp Cell Res. (1998) 241:363–72. doi: 10.1006/excr.1998.4060
103. Sakai K, Shirakawa T, Li Y, Kitagawa Y, Hirose G. Interaction of a paraneoplastic cerebellar degeneration-associated neuronal protein with the nuclear helix-loop-helix leucine zipper protein MRG X. Mol Cell Neurosci. (2002) 19:477–84. doi: 10.1006/mcne.2001.1059
104. Sakai K, Kitagawa Y, Saiki S, Saiki M, Hirose G. Effect of a paraneoplastic cerebellar degeneration-associated neural protein on B-myb promoter activity. Neurobiol Dis. (2004) 15:529–33. doi: 10.1016/j.nbd.2003.11.003
105. Sakai K, Kitagawa Y, Li Y, Shirakawa T, Hirose G. Suppression of the transcriptional activity and DNA binding of nuclear factor-kappa B by a paraneoplastic cerebellar degeneration-associated antigen. J Neuroimmunol. (2001) 119:10–5. doi: 10.1016/S0165-5728(01)00368-X
106. Kråkenes T, Herdlevær I, Raspotnig M, Haugen M, Schubert M, Vedeler CA. CDR2L is the major Yo antibody target in paraneoplastic cerebellar degeneration. Ann Neurol. (2019) 86:316–21. doi: 10.1002/ana.25511
107. Santomasso BD, Roberts WK, Thomas A, Williams T, Blachère NE, Dudley ME, et al. A T cell receptor associated with naturally occurring human tumor immunity. Proc Natl Acad Sci USA. (2007) 104:19073–8. doi: 10.1073/pnas.0704336104
108. Blachère NE, Orange DE, Santomasso BD, Doerner J, Foo PK, Herre M, et al. T cells targeting a neuronal paraneoplastic antigen mediate tumor rejection and trigger CNS autoimmunity with humoral activation. Eur J Immunol. (2014) 44:3240–51. doi: 10.1002/eji.201444624
109. Klein L, Kyewski B, Allen PM, Hogquist KA. Positive and negative selection of the T cell repertoire: what thymocytes see and don't see. Nat Rev Immunol. (2014) 14:377–91. doi: 10.1038/nri3667
110. Kondo K, Ohigashi I, Takahama Y. Thymus machinery for T-cell selection. Int Immunol. (2019) 31:119–25. doi: 10.1093/intimm/dxy081
111. Anderson MS, Venanzi ES, Klein L, Chen Z, Berzins SP, Turley SJ, et al. Projection of an immunological self-shadow within the thymus by the aire protein. Science. (2002) 298:1395–401. doi: 10.1126/science.1075958
112. Malchow S, Leventhal DS, Lee V, Nishi S, Socci ND, Savage PA. Aire enforces immune tolerance by directing autoreactive T cells into the regulatory T cell lineage. Immunity. (2016) 44:1102–13. doi: 10.1016/j.immuni.2016.02.009
113. Immunological Genome Project. Available online at: http://www.immgen.org/databrowser/index.html?gene_symbol=CDR2 [accessed April 1, 2020].
114. Vialatte de Pémille C, Berzero G, Small M, Psimaras D, Giry M, Daniau M, et al. Transcriptomic immune profiling of ovarian cancers in paraneoplastic cerebellar degeneration associated with anti-Yo antibodies. Br J Cancer. (2018) 119:105–13. doi: 10.1038/s41416-018-0125-7
115. Yu W, Jiang N, Ebert PJR, Kidd BA, Müller S, Peder J, et al. Clonal deletion prunes, but does not eliminate self-specific αβ CD8+T lymphocytes. Immunity. (2015) 42:929–41. doi: 10.1016/j.immuni.2015.05.001
116. Carl PL, Temple BRS, Cohen PL. Most nuclear systemic autoantigens are extremely disordered proteins: implications for the etiology of systemic autoimmunity. Arthritis Res Ther. (2005) 7:R1360–74. doi: 10.1186/ar1832
117. Darnell JC, Albert ML, Darnell RB. cdr2, a target antigen of naturally occurring human tumor immunity, is widely expressed in gynecological tumors. Cancer Res. (2000) 60:2136–9.
118. Blachère NE, Orange DE, Gantman EC, Santomasso BD, Couture GC, Ramirez-Montagut T, et al. T cells presenting viral antigens or autoantigens induce cytotoxic T cell anergy. JCI Insight. (2017) 2:e96173. doi: 10.1172/jci.insight.96173
119. Small M, Treilleux I, Couillault C, Pissaloux D, Picard G, Paindavoine S, et al. Genetic alterations and tumor immune attack in Yo paraneoplastic cerebellar degeneration. Acta Neuropathol. (2018) 135:569–79. doi: 10.1007/s00401-017-1802-y
120. Movassagh M, Spatz A, Davoust J, Lebecque S, Romero P, Pittet M, et al. Selective accumulation of mature DC-lamp+ dendritic cells in tumor sites is associated with efficient T-cell-mediated antitumor response and control of metastatic dissemination in melanoma. Cancer Res. (2004) 64:2192–8. doi: 10.1158/0008-5472.CAN-03-2969
121. Engelhardt B, Ransohoff RM. Capture, crawl, cross: the T cell code to breach the blood-brain barriers. Trends Immunol. (2012) 33:579–89. doi: 10.1016/j.it.2012.07.004
122. Kerfoot SM, Kubes P. Overlapping roles of P-selectin and α 4 integrin to recruit leukocytes to the central nervous system in experimental autoimmune encephalomyelitis. J Immunol. (2002) 169:1000–6. doi: 10.4049/jimmunol.169.2.1000
123. Vajkoczy P, Laschinger M, Engelhardt B. α4-integrin-VCAM-1 binding mediates G protein-independent capture of encephalitogenic T cell blasts to CNS white matter microvessels. J Clin Invest. (2001) 108:557–65. doi: 10.1172/JCI12440
124. Bauer M, Brakebusch C, Coisne C, Sixt M, Wekerle H, Engelhardt B, et al. β1 integrins differentially control extravasation of inflammatory cell subsets into the CNS during autoimmunity. Proc Natl Acad Sci USA. (2009) 106:1920–5. doi: 10.1073/pnas.0808909106
125. Holman DW, Klein RS, Ransohoff RM. The blood-brain barrier, chemokines and multiple sclerosis. Biochim Biophys Acta Mol Basis Dis. (2011) 1812:220–30. doi: 10.1016/j.bbadis.2010.07.019
126. Ransohoff RM, Engelhardt B. The anatomical and cellular basis of immune surveillance in the central nervous system. Nat Rev Immunol. (2012) 12:623–35. doi: 10.1038/nri3265
127. Battistini L, Piccio L, Rossi B, Bach S, Galgani S, Gasperini C, et al. CD8+ T cells from patients with acute multiple sclerosis display selective increase of adhesiveness in brain venules: a critical role for P-selectin glycoprotein ligand-1. Blood. (2003) 101:4775–82. doi: 10.1182/blood-2002-10-3309
128. Ifergan I, Kebir H, Alvarez JI, Marceau G, Bernard M, Bourbonnire L, et al. Central nervous system recruitment of effector memory CD8+ T lymphocytes during neuroinflammation is dependent on α4 integrin. Brain. (2011) 134:3557–74. doi: 10.1093/brain/awr268
129. Martin-Blondel G, Pignolet B, Tietz S, Yshii L, Gebauer C, Perinat T, et al. Migration of encephalitogenic CD8 T cells into the central nervous system is dependent on the α4β1-integrin. Eur J Immunol. (2015) 45:3302–12. doi: 10.1002/eji.201545632
130. Hottinger AF, de Micheli R, Guido V, Karampera A, Hagmann P, Du Pasquier R. Natalizumab may control immune checkpoint inhibitor-induced limbic encephalitis. Neurol Neuroimmunol NeuroInflamm. (2018) 5:e439. doi: 10.1212/NXI.0000000000000439
131. Gross CC, Meyer C, Bhatia U, Yshii L, Kleffner I, Bauer J, et al. CD8+ T cell-mediated endotheliopathy is a targetable mechanism of neuro-inflammation in Susac syndrome. Nat Commun. (2019) 10:5779. doi: 10.1038/s41467-019-13593-5
132. Wilhelm I, Nyúl-Tóth Á, Suciu M, Hermenean A, Krizbai IA. Heterogeneity of the blood-brain barrier. Tissue Barriers. (2016) 4:e1143544. doi: 10.1080/21688370.2016.1143544
133. Schinkel AH, Wagenaar E, van Deemter L, Mol CAAM, Borst P. Absence of the mdr1a P-glycoprotein in mice affects tissue distribution and pharmacokinetics of dexamethasone, digoxin, and cyclosporin A. J Clin Invest. (1995) 96:1698–705. doi: 10.1172/JCI118214
134. Yasuda K, Cline C, Lin YS, Scheib R, Ganguly S, Thirumaran RK, et al. In vivo imaging of human MDR1 transcription in the brain and spine of MDR1-luciferase reporter mice. Drug Metab Dispos. (2015) 43:1646–54. doi: 10.1124/dmd.115.065078
135. Verschuuren J, Chuang L, Rosenblum MK, Lieberman F, Pryor A, Posner JB, et al. Inflammatory infiltrates and complete absence of Purkinje cells in anti-Yo-associated paraneoplastic cerebellar degeneration. Acta Neuropathol. (1996) 91:519–25. doi: 10.1007/s004010050460
136. Greenlee JE, Brashear RH. Antibodies to cerebellar Purkmje cells in patients with paraneoplastic cerebellar degeneration and ovarian Carcinoma. Ann Neurol. (1983) 14:609–13. doi: 10.1002/ana.410140603
137. Giometto B, Marchiori GC, Nicolao P, Scaravilli T, Lion A, Bardin PG, et al. Sub-acute cerebellar degeneration with anti-Yo autoantibodies: immunohistochemical analysis of the immune reaction in the central nervous system. Neuropathol Appl Neurobiol. (1997) 23:468–74. doi: 10.1046/j.1365-2990.1997.00078.x
138. Storstein A, Krossnes BK, Vedeler CA. Morphological and immunohistochemical characterization of paraneoplastic cerebellar degeneration associated with yo antibodies. Acta Neurol Scand. (2009) 120:64–7. doi: 10.1111/j.1600-0404.2008.01138.x
139. Aye MM, Kasai T, Tashiro Y, Xing HQ, Shirahama H, Mitsuda M, et al. CD8 positive T-cell infiltration in the dentate nucleus of paraneoplastic cerebellar degeneration. J Neuroimmunol. (2009) 208:136–40. doi: 10.1016/j.jneuroim.2009.01.017
140. Aboul-Enein F, Höftberger R, Buxhofer-Ausch V, Drlicek M, Lassmann H, Budka H, et al. Neocortical neurones may be targeted by immune attack in anti-Yo paraneoplastic syndrome. Neuropathol Appl Neurobiol. (2008) 34:248–52. doi: 10.1111/j.1365-2990.2007.00909.x
141. Bien CG, Vincent A, Barnett MH, Becker AJ, Blümcke I, Graus F, et al. Immunopathology of autoantibody-associated encephalitides: clues for pathogenesis. Brain. (2012) 135:1622–38. doi: 10.1093/brain/aws082
142. McConnell MJ, Huang YH, Datwani A, Shatz CJ. H2-K b and H2-D b regulate cerebellar long-term depression and limit motor learning. Proc Natl Acad Sci USA. (2009) 106:6784–9. doi: 10.1073/pnas.0902018106
143. Yshii L, Pignolet B, Mauré E, Pierau M, Brunner-Weinzierl M, Hartley O, et al. IFN-γ is a therapeutic target in paraneoplastic cerebellar degeneration. JCI Insight. (2019) 4:127001. doi: 10.1172/jci.insight.127001
144. Pellkofer H, Schubart AS, Höftberger R, Schutze N, Pagany M, Schüller M, et al. Modelling paraneoplastic CNS disease: T-cells specific for the onconeuronal antigen PNMA1 mediate autoimmune encephalomyelitis in the rat. Brain. (2004) 127:1822–30. doi: 10.1093/brain/awh205
145. Gebauer C, Pignolet B, Yshii L, Mauré E, Bauer J, Liblau R. CD4+ and CD8+ T cells are both needed to induce paraneoplastic neurological disease in a mouse model. Oncoimmunology. (2017) 6:e1260212. doi: 10.1080/2162402X.2016.1260212
146. Yshii LM, Gebauer CM, Pignolet B, Mauré E, Quériault C, Pierau M, et al. CTLA4 blockade elicits paraneoplastic neurological disease in a mouse model. Brain. (2016) 139:2923–34. doi: 10.1093/brain/aww225
147. Greenlee JE, Parks T, Jaeckle K. Type IIa ('anti-Hu') antineuronal antibodies produce destruction of rat cerebellar granule neurons in vitro. Neurology. (1993) 43:2049–54. doi: 10.1212/WNL.43.10.2049
148. Darnell RB. Onconeural antigens and the paraneoplastic neurologic disorders: At the intersection of cancer, immunity, and the brain. Proc Natl Acad Sci USA. (1996) 93:4529–36. doi: 10.1073/pnas.93.10.4529
149. Tanaka K, Tanaka M, Igarashi S, Onodera O, Miyatake T, Tsuji S. Trial to establish an animal model of paraneoplastic cerebellar degeneration with anti-Yo antibody. 2. Passive transfer of murine mononuclear cells activated with recombinant Yo protein to paraneoplastic cerebellar degeneration lymphocytes in severe combi. Clin Neurol Neurosurg. (1995) 97:101–5. doi: 10.1016/0303-8467(95)00005-5
150. Smitt S, Manley GT, Posner JB. Immunization with the paraneoplastic encephalomyelitis antigen hud does not cause neurologic disease in mice. Neurology. (1995) 45:1873–8. doi: 10.1212/WNL.45.10.1873
151. Carpentier AF, Rosenfeld MR, Delattre JY, Whalen RG, Posner JB, Dalmau J. DNA vaccination with HuD inhibits growth of a neuroblastoma in mice. Clin Cancer Res. (1998) 4:2819–24.
152. Panja D, Vedeler CA, Schubert M. Paraneoplastic cerebellar degeneration: Yo antibody alters mitochondrial calcium buffering capacity. Neuropathol Appl Neurobiol. (2019) 45:141–56. doi: 10.1111/nan.12492
153. Greenlee JE, Clawson SA, Hill KE, Wood B, Clardy SL, Tsunoda I, et al. Anti-Yo antibody uptake and interaction with its intracellular target antigen causes Purkinje cell death in rat cerebellar slice cultures: a possible mechanism for paraneoplastic cerebellar degeneration in humans with gynecological or breast cancers. PLoS One. (2015) 10:e0123446. doi: 10.1371/journal.pone.0123446
154. de Giorgio R, Bovara M, Barbara G, Canossa M, Sarnelli G, de Ponti F, et al. Anti-HuD-induced neuronal apoptosis underlying paraneoplastic gut dysmotility. Gastroenterology. (2003) 125:70–9. doi: 10.1016/S0016-5085(03)00664-4
155. Greenlee JE, Clawson SA, Hill KE, Wood BL, Tsunoda I, Carlson NG. Purkinje cell death after uptake of anti-yo antibodies in cerebellar slice cultures. J Neuropathol Exp Neurol. (2010) 69:997–1007. doi: 10.1097/NEN.0b013e3181f0c82b
156. Yshii LM, Hohlfeld R, Liblau RS. Inflammatory CNS disease caused by immune checkpoint inhibitors: status and perspectives. Nat Rev Neurol. (2017) 13:755–63. doi: 10.1038/nrneurol.2017.144
157. Vogrig A, Fouret M, Joubert B, Picard G, Rogemond V, Pinto AL, et al. Increased frequency of anti-Ma2 encephalitis associated with immune checkpoint inhibitors. Neurol Neuroimmunol NeuroInflamm. (2019) 6:e604. doi: 10.1212/NXI.0000000000000604
158. Williams TJ, Benavides DR, Patrice K-A, Dalmau JO, de Ávila ALR, Le DT, et al. Association of autoimmune encephalitis with combined immune checkpoint inhibitor treatment for metastatic cancer. JAMA Neurol. (2016) 73:928. doi: 10.1001/jamaneurol.2016.1399
159. Larkin J, Chmielowski B, Lao CD, Hodi FS, Sharfman W, Weber J, et al. Neurologic serious adverse events associated with nivolumab plus ipilimumab or nivolumab alone in advanced melanoma, including a case series of encephalitis. Oncologist. (2017) 22:709–18. doi: 10.1634/theoncologist.2016-0487
160. Wei SC, Levine JH, Cogdill AP, Zhao Y, Anang NAS, Andrews MC, et al. Distinct cellular mechanisms underlie anti-CTLA-4 and anti-PD-1 checkpoint blockade. Cell. (2017) 170:1120–33. doi: 10.1016/j.cell.2017.07.024
161. Sautès-Fridman C, Petitprez F, Calderaro J, Fridman WH. Tertiary lymphoid structures in the era of cancer immunotherapy. Nat Rev Cancer. (2019) 19:307–25. doi: 10.1038/s41568-019-0144-6
162. Helmink BA, Reddy SM, Gao J, Zhang S, Basar R, Thakur R, et al. B cells and tertiary lymphoid structures promote immunotherapy response. Nature. (2020) 577:549–55. doi: 10.1038/s41586-019-1922-8
163. Joseph CG, Darrah E, Shah AA, Skora AD, Livia A, Wigley FM, et al. Association of the autoimmune disease scleroderma with an immunologic response to cancer. Science. (2014) 343:152–7. doi: 10.1126/science.1246886
164. Albert ML, Austin LM, Darnell RB. Detection and treatment of activated T cells in the cerebrospinal fluid of patients with paraneoplastic cerebellar degeneration. Ann Neurol. (2000) 47:9–17. doi: 10.1002/1531-8249(200001)47:1<9::AID-ANA5>3.0.CO;2-I
165. Beltrán E, Gerdes LA, Hansen J, Flierl-Hecht A, Krebs S, Blum H, et al. Early adaptive immune activation detected in monozygotic twins with prodromal multiple sclerosis. J Clin Invest. (2019) 129:4758–68. doi: 10.1172/JCI128475
166. Ahrends T, Spanjaard A, Pilzecker B, Babała N, Bovens A, Xiao Y, et al. CD4+ T cell help confers a cytotoxic T cell effector program including coinhibitory receptor downregulation and increased tissue invasiveness. Immunity. (2017) 47:848–61.e5. doi: 10.1016/j.immuni.2017.10.009
167. Roberts WK, Blachère NE, Frank MO, Dousmanis A, Ransohoff RM, Darnell RB. A destructive feedback loop mediated by CXCL10 in central nervous system inflammatory disease. Ann Neurol. (2015) 78:619–29. doi: 10.1002/ana.24494
168. Di Liberto G, Pantelyushin S, Kreutzfeldt M, Page N, Musardo S, Coras R, et al. Neurons under T cell attack coordinate phagocyte-mediated synaptic stripping. Cell. (2018) 175:458–71.e19. doi: 10.1016/j.cell.2018.07.049
169. Neumann H, Cavalié A, Jenne D, Wekerle H. Major Histocompatibility Complex (MHC) Class I gene expression in singe neurons of the central nervous system : differential regulationby interferon (IFN)-gamma and tumor necrosis factor (TNF)-alpha. J Exp Med. (1997) 185:305–16. doi: 10.1084/jem.185.2.305
170. Tröscher AR, Wimmer I, Quemada-Garrido L, Köck U, Gessl D, Verberk SGS, et al. Microglial nodules provide the environment for pathogenic T cells in human encephalitis. Acta Neuropathol. (2019) 137:619–35. doi: 10.1007/s00401-019-01958-5
171. Galli J, Greenlee J. Paraneoplastic diseases of the central nervous system. Lancet Neurol. (2020) 9:167. doi: 10.12688/f1000research.21309.1
172. Flach AC, Litke T, Strauss J, Haberl M, Cordero Gómez C, Reindl M, et al. Autoantibody-boosted T-cell reactivation in the target organ triggers manifestation of autoimmune CNS disease. Proc Natl Acad Sci USA. (2016) 113:3323–8. doi: 10.1073/pnas.1519608113
173. Meloni C, Iani C, Dominijanni S, Arciprete F, Cipriani S, Caramiello MS, et al. A case report of plasma exchange therapy in non-paraneoplastic cerebellar ataxia associated with Anti-Yo antibody. Ther Apher Dial. (2004) 8:500–2. doi: 10.1111/j.1774-9987.2004.00198.x
174. Thöne J, Hohaus A, Lamprecht S, Bickel A, Erbguth F. Effective immunosuppressant therapy with cyclophosphamide and corticosteroids in paraneoplastic cerebellar degeneration. J Neurol Sci. (2008) 272:171–3. doi: 10.1016/j.jns.2008.04.020
175. Shams'ili S, de Beukelaar J, Gratama JW, Hooijkaas H, van Den Bent M, van't Veer M, et al. An uncontrolled trial of rituximab for antibody associated paraneoplastic neurological syndromes. J Neurol. (2006) 253:16–20. doi: 10.1007/s00415-005-0882-0
176. Rojas I, Graus F, Keime-Guibert F, Reñé R, Delattre JY, Ramón JM, et al. Long-term clinical outcome of paraneoplastic cerebellar degeneration and anti-Yo antibodies. Neurology. (2000) 55:713–5. doi: 10.1212/WNL.55.5.713
177. Schessl J, Schuberth M, Reilich P, Schneiderat P, Strigl-Pill N, Walter MC, et al. Long-term efficiency of intravenously administered immunoglobulin in anti-yo syndrome with paraneoplastic cerebellar degeneration. J Neurol. (2011) 258:946–7. doi: 10.1007/s00415-010-5859-y
178. Phuphanich S, Brock C. Neurologic improvement after high-dose intravenous immunoglobulin therapy in patients with paraneoplastic cerebellar degeneration associated with anti-purkinje cell antibody. J Neurooncol. (2007) 81:67–9. doi: 10.1007/s11060-006-9198-x
179. Baker SK, Morillo C, Vernino S. Autoimmune autonomic ganglionopathy with late-onset encephalopathy. Auton Neurosci Basic Clin. (2009) 146:29–32. doi: 10.1016/j.autneu.2008.10.016
Keywords: paraneoplastic cerebellar degeneration, anti-neuronal antibodies, T cell, autoimmunity, immunotherapy, animal model
Citation: Yshii L, Bost C and Liblau R (2020) Immunological Bases of Paraneoplastic Cerebellar Degeneration and Therapeutic Implications. Front. Immunol. 11:991. doi: 10.3389/fimmu.2020.00991
Received: 29 February 2020; Accepted: 27 April 2020;
Published: 02 June 2020.
Edited by:
Bruno Stankoff, Sorbonne Universités, FranceReviewed by:
Noel G. Carlson, University of Utah School of Medicine, United StatesCopyright © 2020 Yshii, Bost and Liblau. This is an open-access article distributed under the terms of the Creative Commons Attribution License (CC BY). The use, distribution or reproduction in other forums is permitted, provided the original author(s) and the copyright owner(s) are credited and that the original publication in this journal is cited, in accordance with accepted academic practice. No use, distribution or reproduction is permitted which does not comply with these terms.
*Correspondence: Roland Liblau, cm9sYW5kLmxpYmxhdUBpbnNlcm0uZnI=
†These authors have contributed equally to this work
Disclaimer: All claims expressed in this article are solely those of the authors and do not necessarily represent those of their affiliated organizations, or those of the publisher, the editors and the reviewers. Any product that may be evaluated in this article or claim that may be made by its manufacturer is not guaranteed or endorsed by the publisher.
Research integrity at Frontiers
Learn more about the work of our research integrity team to safeguard the quality of each article we publish.