- 1Center for Chronic Immunodeficiency, Medical Center, Faculty of Medicine, Institute for Immunodeficiency, University of Freiburg, Freiburg, Germany
- 2Center for Pediatrics, Faculty of Medicine, Medical Center – University of Freiburg, University of Freiburg, Freiburg, Germany
- 3Division of Pediatric Stem Cell Transplantation and Immunology, University Medical Center Hamburg-Eppendorf, Hamburg, Germany
Hemophagocytic lymphohistiocytosis (HLH) is a heterogeneous hyperinflammatory syndrome with different pathways of pathogenesis resulting in similar clinical presentations. It is best defined and understood if presenting in the context of genetic immunodeficiencies associated with defects of lymphocyte cytotoxicity. In these “primary” forms of HLH, cellular and soluble immune effectors are relatively well characterized. While etoposide-based broad cell-directed therapies remain standard of care, more specific therapies targeting these effectors individually are increasingly available. Anti-CD52 as a cell-directed therapy and anti-IFN-gamma, IL-18BP, and JAK-inhibition as cytokine-directed therapies are expected to broaden the therapeutic options, but the precise role of these drugs in first-line and rescue treatment indications remains to be defined. A number of additional inborn errors of immunity are associated with episodes of immune activation fulfilling the clinical criteria of HLH. Impaired pathogen control is a key driver of hyperinflammation in some conditions, while others are characterized by a strong autoinflammatory component. This heterogeneity of disease-driving factors and the variable severity in disease progression in these conditions do not allow a simple adaptation of protocols established for “primary” HLH to HLH in the context of other inborn errors of immunity. Cytokine-directed therapies hold significant promise in these increasingly recognized disorders.
Primary Hemophagocytic Lymphohistiocytosis
Hemophagocytic lymphohistiocytosis (HLH) is a highly inflammatory syndrome with uncontrolled, excessive immune activation. HLH is the key manifestation in a range of autosomal-recessive genetic diseases defined as familiar forms of HLH (FHL). FHL includes FHL1 to FHL5 (OMIM #267700, #603553, #608898, #603552, and #613101) caused by defects in lymphocyte cytotoxicity affecting perforin or proteins involved in the exocytosis of perforin-containing lytic granules (degranulation deficiencies) (Table 1). It was first described in 1952 as familial hemophagocytic reticulosis (1). In FHL2 patients with “null” mutations, the first manifestation of disease symptoms is in most cases observed in the first 6 months of life, but may already be present in utero or at birth (2). HLH tends to occur later in patients with other FHL variants (3, 4) and in patients with biallelic “hypomorphic” mutations and an initial HLH episode has been reported as late as 63 years of age (5). The incidence of FHL is estimated at 1:50,000–1:100,000 (6, 7). Some genetic immunodeficiency diseases associated with pigment dilution such as Griscelli syndrome type II (GS-II; OMIM # 607624) and Chediak-Higashi syndrome (CHS; OMIM #214500) are also caused by degranulation defects (8). The similar pathogenesis and the frequent occurrence of HLH in these conditions allow their classification as “primary” HLH (Figure 1A).
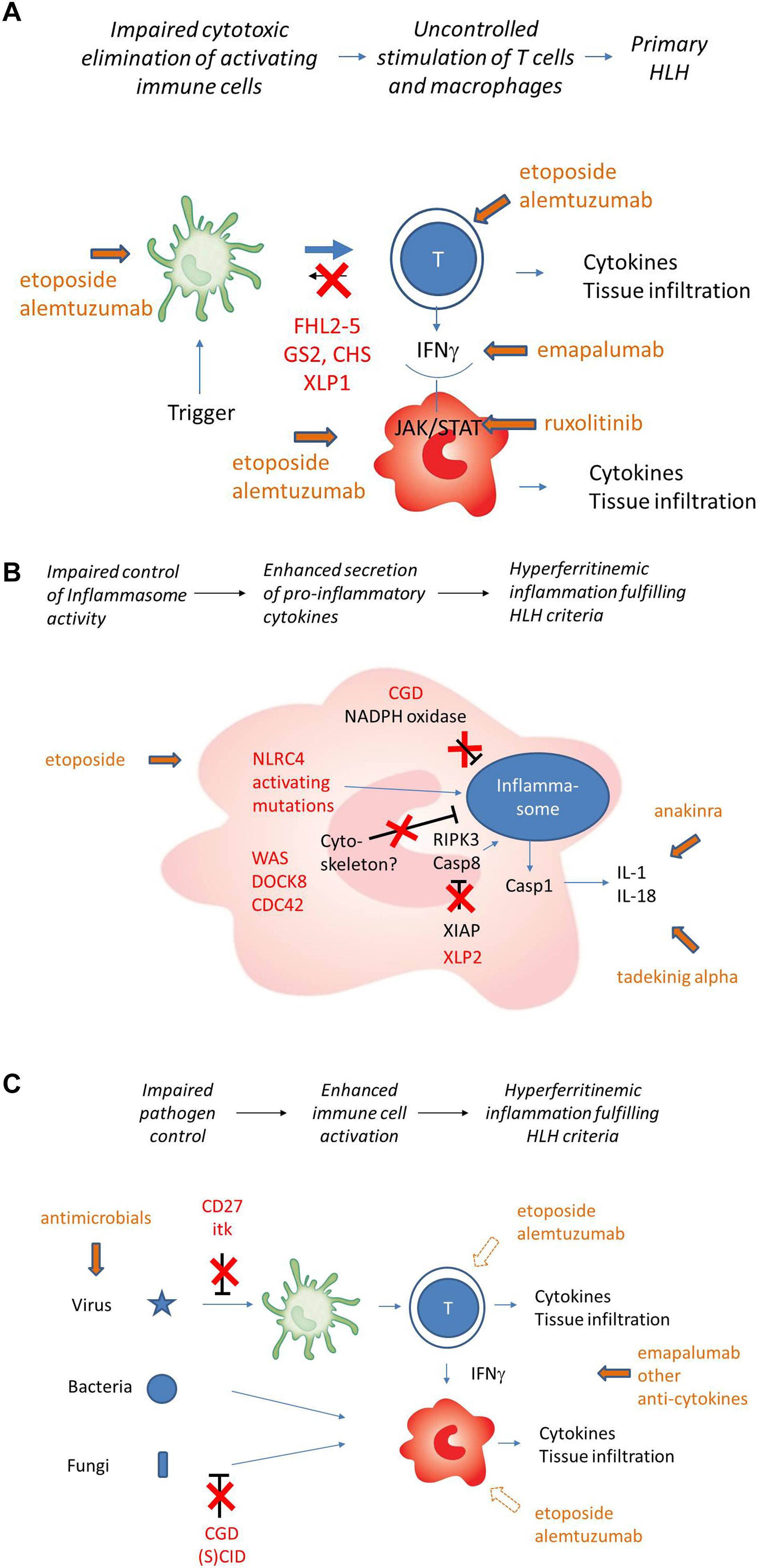
Figure 1. (A) Pathogenesis of “primary” HLH (simplified). Impaired cytotoxicity (red) leads to uncontrolled T cell activation by APC. T cell secreted IFNg is the key driver of macrophage activation. Cellular and cytokine targets of therapy are indicated in green. (B) Pathogenesis of HLH in the context of impaired inflammasome homeostasis (simplified). Inborn errors of immunity shown (NLRC4, XIAP) or assumed (Cytoskeletal disorders, CGD) to be involved in inflammasome homeostasis are indicated in red. Cytokine targets of therapy are indicated in green. Macrophages are the key cells involved, T cells play a less prominent role. Cell-directed therapies are rarely used. (C) HLH pathogenesis in the context of inborn errors of immunity with impaired pathogen control (simplified). Inborn errors of immunity impairing virus control (mainly EBV) and/or bacterial/fungal control are indicated in red. Subsequent immune stimulation leads to hypersecretion of variable cytokines. Macrophage activation can occur in the absence of T cells, but T cells can be involved depending on the genetic defect and the trigger. Cell-directed therapies further impair pathogen control and should only be used in exceptional cases.
Pathophysiological Basis of “Primary” Hemophagocytic Lymphohistiocytosis
FHL2-5, GS-II, and CHS all affect the cytotoxic granule-mediated cell death pathway (8, 9). Under physiological conditions, immune stimulation such as a viral infection leads to priming of cytotoxic T-lymphocytes by APC, followed by their activation and proliferation. These activated T cells and NK cells can recognize virus-infected target cells and subsequently eliminate these through polarized release of perforin- and granzyme-containing granules (10, 11). Entry of granzymes into target-cells by membrane-pores established by perforin activity mediates apoptotic cell death. Notably, this cytotoxic activity is also directed against APC, providing an important negative feedback-loop that limits T cell activation (12).
In “primary” HLH, deficient cytotoxic activity of CTL and NK cells impairs the timely elimination of APCs. Their persistence leads to continuous T-cell stimulation. Incessantly activated T-cells infiltrate tissues and release various pro-inflammatory mediators, in particular interferon-gamma, a potent macrophage-stimulating cytokine (13). Continuous macrophage activation, in turn, further fuels release of a broad range of inflammatory cytokines such as IL-1, IL-6, IL-18, and TNF-alpha (14–16) and leads to tissue infiltration of macrophages and hemophagocytosis. Since in the course of an immune response, T cells themselves can also become targets of the cytotoxic activity of NK cells and T cells, lack of this control mechanism may further impair immune homeostasis (17, 18). Clinical manifestations of HLH are mainly a result of tissue infiltration by T cells and macrophages and the accompanying excessive cytokine storm.
This model of “primary” HLH pathophysiology has mainly been established in key studies in cytotoxicity deficient mice that develop all clinical features used for the diagnosis of HLH in patients upon persistent wide-spread systemic infection with lymphocytic choriomeningitis virus (19). In most patients with “primary” HLH, no persistent systemic viral infection can be demonstrated (2), asking for a note of caution whether this model really explains all aspects of the human disease.
Other Inborn Errors of Immunity Predisposing to HLH: Pathogenetic Heterogeneity
In a group of additional inborn errors of immunity, HLH occurs less frequently, although it can still be the presenting clinical manifestation. In these diseases, HLH pathogenesis is variable and mostly different from that of “primary” disease (Table 1). A brief review of current understanding of pathogenesis of these diseases is relevant for the discussion of therapeutic approaches.
Two X-linked genetic diseases predispose to HLH predominantly in the context of EBV infection (20) (Figure 1B). XLP1 (OMIM #308240) is caused by defects in SAP, a small adaptor protein that regulates signaling in T and NK cells by binding to the SLAM family of signaling receptors (21). Many aspects of XLP1 pathogenesis can be explained by impaired T/NK-B cell interaction. As a consequence, affected patients frequently suffer from hypogammaglobulinemia and its infectious consequences due to impaired T cell help to B cells and lymphomas due to impaired control of malignant B cells (22). Cerebral vasculitis and aplastic anemia can also be life-threatening manifestations. Poor T/NK-cell mediated control of EBV-infected B cells, in part linked to impaired activation of 2B4 (a SLAM receptor) mediated cytotoxic function, is the basis of HLH, that develops in about 30% of XLP1 patients (23).
XLP2 (OMIM #300079) is caused by defects in XIAP, a protein with antiapoptotic functions, regulatory functions for autophagy and control functions for inflammasome activity (24, 25). It also modulates the NOD1/NOD2 pathways which contribute to intracellular sensing of bacterial infection. A link to lymphocyte cytotoxicity has not been established. Important clinical manifestations of XLP2 are early onset inflammatory bowel disease, splenomegaly and periodic fever (26). The pathogenesis of mostly EBV-induced HLH, which occurs in more than 30% of patients, is unclear. However, an autoinflammatory component due to dysregulated NLRP3 inflammasome activation is reflected by excessive levels of free serum IL-18 and is more prominent than in “primary” HLH (27). Notably, some, but not all biological activities of IL-18 are mediated by IFNγ (28). The frequency of HLH in XLP1 and XLP2 has led to their inclusion in the classification of “primary” HLH and the therapeutic principles of FHL have also been successfully used to treat HLH in XLP (20, 29). However, both XLP variants have a pathophysiology that is clearly different from “primary” HLH and this may offer different treatment options. This is particularly relevant for treatment of manifestations different from HLH in these conditions.
TIM3 deficiency (OMIM #618398) caused by HAVCR2 mutations is another autosomal-recessive inborn error of immunity that predisposes to HLH in a particular context, i.e., in subcutaneous panniculitis T cell lymphoma (SPTCL) (30). TIM3 is an inhibitory molecule expressed mainly on T cells and NK cells, but also on myeloid cells. TIM3 mutations causing aberrant protein folding and lack of surface expression lead to an autoinflammatory and autoimmune phenotype with hyperactivated myeloid cells producing high levels of IL-1 and IL-18 and uncontrolled CD8 T cell proliferation (31). This promotes SPTCL formation and its association with HLH.
Heterozygous NLRC4 gain-of-function mutations (OMIM #606831) lead to constitutive activation of the NLRC4 inflammasome resulting in enterocolitis and macrophage activation associated with a clinical picture of HLH. It is characterized by excessive levels of free IL-18 and IL-1beta (32, 33). Heterozygous mutations in CDC42 affecting amino acids 186, 188, or 192 also lead to a hyperinflammatory syndrome including neonatal cytopenias, hepatosplenomegaly, recurrent febrile episodes and urticaria-like rashes that can fulfill HLH criteria. This autoinflammatory disease is also characterized by very high levels of IL-18 and IL-1beta, suggesting dysregulated inflammasome function (34). The mutations are postulated to interfere with actin assembly, thus affecting signaling, cytoskeletal rearrangement and cell migration. All three conditions are characterized by a significant autoinflammatory disease component that calls for treatment approaches different from primary HLH (Figure 1B).
Finally, immune activation fulfilling the clinical criteria of HLH occasionally occurs in several additional primary immunodeficiencies, including SCID, some combined immunodeficiencies such as Wiskott-Aldrich syndrome, CD27 deficiency and ITK deficiency, chronic granulomatous disease (CGD) and IFNγ receptor deficiency (35, 36) (Figures 1B,C). The examples of SCID and IFNγR deficiency illustrate that the clinical syndrome of HLH as defined by the HLH-2004 clinical criteria requires neither T cells nor IFNγ, illustrating that this form of HLH is different from “primary” HLH. In fact, the HLH-like immune activation in these diseases is in most cases due to impaired pathogen control and rather represents an infection-induced HLH. Additional factors such as altered inflammasome regulation by NADPH oxidase in CGD (37) and potentially impaired cytoskeleton – inflammasome cross-talk in patients with WAS, DOCK8 deficiency and CDC42 mutations likely also contribute (38–40). Overall, these examples illustrate that also in familial HLH cases, a careful characterization of the genetic disorder underlying HLH is required as it allows to choose treatment targeted at the specific pathogenesis.
Therapeutic Strategies
The heterogeneity in pathophysiology of “primary” HLH caused by cytotoxicity defects versus HLH associated with other inborn errors of immunity makes it obvious that there is no “one fits all” therapeutic strategy. Treatment must be targeted to the pathophysiology and results from treatment studies obtained in one group of diseases cannot simply be transferred to another. Therapeutic regimens in primary HLH are either directed at the immune cells involved, i.e., APC, T cells and macrophages, or at the cytokines secreted by these cells. The goal is to disrupt ongoing immune stimulation and to limit severe hyperinflammation and tissue damage. The implementation of broad cell-directed therapies was critical to improve survival in this life-threatening condition (41). However, more specific anti-cellular therapies and therapeutic targeting of particular key cytokines and their downstream effects are currently evaluated in clinical trials. In the absence of published data on several of these novel approaches, this review can only point out the therapeutic principles and indicate which trials to watch as they have the potential to impact on standard-of-care within the next 5 years.
Overall, the therapeutic approach to primary HLH can be divided into four main phases:
(1) Induction of remission.
(2) Control of triggers.
(3) Maintenance of remission and salvage therapy.
(4) Curing the underlying condition.
Induction of Remission
Timely treatment of HLH is essential for prognosis. Untreated patients with active “primary” HLH show a survival of approximately 2 months due to progressive organ failure (42). Delayed initiation of therapy increases the risk of neurological complications. In most cases, initial decisions must be made in the absence of a confirmed genetic diagnosis, but tests of protein expression and degranulation are rapidly available and have high sensitivity and specificity for “primary” HLH (43–45). Important differential diagnosis requiring different treatment approaches such as malignancy or metabolic disease should be considered (46, 47). Leishmaniosis must be ruled out in all patients with a plausible risk (48).
Targeting Cells
For decades, first-line therapy for primary HLH has been centered on cell-oriented approaches. The widely used standard-of-care is based on the dexamethasone/etoposide-based HLH-94 and HLH-2004 studies. A consensus statement addressing various aspects of its use in detail has recently been published by the HLH Steering Committee of the Histiocyte Society (49).
Etoposide-Based Protocols
The HLH-94 protocol is based on immuno-chemotherapy including dexamethasone, etoposide and CSA to achieve remission of the hyperinflammatory state and to maintain remission until HSCT can be performed (41). Functionally, all agents target lymphocytes, macrophages and antigen presenting cells. The cytostatic agent etoposide induces cell death mainly in activated T cells (50), but also in macrophages and dendritic cells. The use of the calcineurin inhibitor CSA leads to an inhibition of the transcription factor NFAT (nuclear factor of activated T-cells) and thus to a reduced activation and proliferation of T cells. Steroids slow down inflammation by reducing cytokine secretion, but in addition, they have a moderate cytotoxic effect on activated T cells. It is recommended to treat patients with CNS involvement also with intrathecal methotrexate (51), although there is no clear evidence of benefit. The protocol has a 2-week intensive phase with dexamethasone and twice weekly administration of etoposide, followed by 6 weeks of weekly etoposide and steroid tapering. In this second phase, CSA is used to prevent reactivation (49). Rapid immunological testing followed by genetic confirmation of the underlying genetic disease is required in all patients and should provide the basis for HSCT within these 8 weeks (Figure 2). In the international multicenter registry-based HLH-2004 study, 5-year probability of survival for children with genetically verified familial HLH treated with this protocol was 59% (52). Dexamethasone/etoposide-based protocols have been successfully used in XLP or patients with TIM3 deficiency. It remains an ultimate choice also in other inborn errors of immunity, but the toxicity and immunosuppression associated with etoposide asks for more targeted therapies in these conditions.
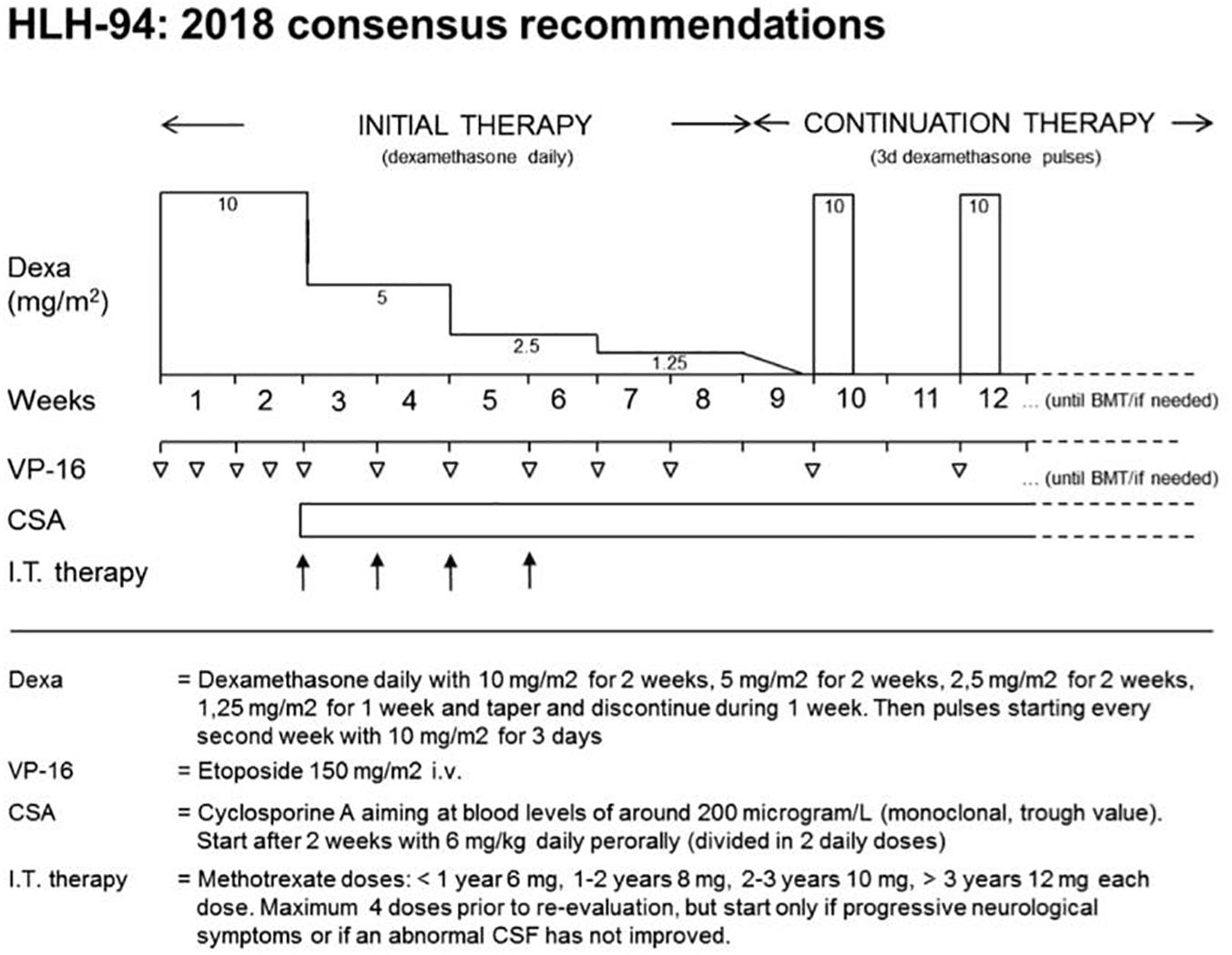
Figure 2. 2018 consensus statements by the HLH Steering Committee of the Histiocyte Society recommending the use of HLH-94. The HLH-94 protocol is based on immunochemotherapy including dexamethasone, etoposide, and cyclosporine A (CSA). After an intensive phase of 2 weeks with high doses of dexamethasone and twice weekly administration of etoposide, dexamethasone is tapered until week nine. Cyclosporine A is used from week three onward to prevent reactivation. Intrathecal therapy with methotrexate is recommended in patients with CNS involvement. Immunological testing and genetic confirmation of the underlying genetic disease is required in all patients and should provide the basis for HSCT within 8 weeks. Copyright Clearance Center’s RightsLink® service/Elsevier.
Antithymocyte Globulin (ATG)
Antithymocyte globulin directly targets T cells and other lymphocytes, to a minor extent also granulocytes and monocytes (53). In a retrospective single-center analysis of 38 patients with familial hemophagocytosis, a protocol consisting of steroids, CSA and first-line ATG resulted in a higher initial remission rate compared to the HLH-94 protocol (active disease in 26% of patients versus 53%, after 2 months of therapy) but was associated with a higher percentage of relapses before HSCT (32% versus 13%) (54). In an attempt to combine advantages of both protocols, a trial combining ATG and etoposide has been performed (55), but the results have not yet been reported. There is no clear role for ATG beyond “primary” HLH.
Alemtuzumab (Anti-CD52)
More recently, the humanized monoclonal anti-CD52 antibody alemtuzumab has been used in patients with “primary” HLH (56). It is directed against the CD52 antigen (CAMPATH 1) which is a surface protein on mature lymphocytes and APCs. First promising results have been achieved when used in a bridging to transplant setting (57). Excellent initial results have been orally reported from a trial evaluating Alemtuzumab as first-line treatment for “primary” HLH (in combination with methyl-prednisolone and CSA) (58). The profound and long-lasting immune suppression and limits this drug to “primary” HLH, where induction of remission is rapidly followed by HSCT. The problem of viral (re-)activation is an important caveat when using alemtuzumab in XLP.
Targeting Cytokines
In a disease associated with excessive production of a large number of cytokines, it is not self-evident that blockade of a single cytokine should have significant therapeutic effects. However, pivotal studies in mouse models of “primary” HLH have indicated that some key cytokines, in particular IFN-gamma, are drivers of the immune dysregulation (60) and that their neutralization can interrupt the inflammatory circle and restore immune homeostasis (52, 59). As a consequence, therapeutic approaches targeting IFN-gamma, its induction and its downstream effects have emerged as promising strategies that are at different stages of evaluation in clinical trials of primary HLH.
Interferon-Gamma
Emapalumab is a recombinant human monoclonal antibody against interferon gamma (60). It has received FDA approval in November 2018 for the treatment of pediatric and adult patients with primary HLH with refractory, recurrent, or progressive disease or intolerance to HLH therapy (see section salvage therapy). An international multicenter follow-up study to further assess the efficacy and safety of emapalumab is still ongoing (61). This trial will also provide data on its use as first-line therapy in “primary” HLH. Serum levels of CXCL9 emerge as an interesting biomarker for increased IFNγ activity (62) and may be particularly helpful when considering the use of emapalumab in first-line treatment of HLH in the context of other inborn errors of immunity. Notably, a patient with CDC42 mutation who did not respond to steroids, CSA, and anakinra was successfully treated with emapalumab (34).
JAK-Inhibition
Janus kinase inhibitors represent interesting therapeutic compounds in the context of HLH, since they not only inhibit signaling downstream of IFN gamma, but also of several other pro-inflammatory cytokines. In the mouse-model of LCMV-induced “primary” HLH, the disease manifestations, including CNS involvement, were reduced upon JAK1/2 blockade by ruxolitinib (63, 64). The successful individual use of ruxolitinib reported in single cases of secondary HLH (65–67) has resulted in its prospective evaluation for this indication (68). Preliminary results of a single-center phase 2 pilot study on the efficacy of ruxolitinib in secondary HLH demonstrate good tolerance to ruxolitinib in a small cohort of five patients (69). However, “primary” HLH is excluded in these studies. A trial investigating the benefit of JAK inhibition in first-line treatment of human “primary” HLH is in preparation. We are not aware of reports on the use of JAK inhibitors in HLH in the context of other inborn errors of immunity, but this is a plausible pathway to explore.
Targeting IL-18
IL-18 is released by activated macrophages and can induce IFN-gamma and other pro- inflammatory cytokines (28). Several reports have found elevated free IL-18 concentrations (i.e., IL-18 not bound to its binding protein IL-18BP) in the serum of patients with both “primary” and secondary HLH as well as in animal models and IL- 18 levels correlated with the presence of HLH-criteria and disease progression (70). In a murine model of “primary” HLH it was shown that treatment with IL-18BP can reduce severe organ damage, but does not improve survival (71). An ongoing multicenter, double-blind, placebo-controlled, randomized withdrawal trial evaluates efficacy and safety of IL-18BP (tadekinig alfa) in pediatric patients with NLRC4 associated hyperinflammation including HLH or XIAP deficiency, diseases, in which IL-18 levels are particularly elevated (73). While this treatment seems promising to attenuate the autoinflammatory manifestations of XIAP deficiency, it remains to be seen whether it also has a role in acute EBV-induced HLH in this disease.
IL-1, IL-6, and TNF Alpha Blockade
The pro-inflammatory cytokines elevated in “primary” HLH also include IL-1, IL-6, and TNF alpha, which can be targeted by monoclonal antibodies and other blocking agents. They have been used successfully in the context of secondary HLH (72, 74) and related conditions associated with a “cytokine storm” such as hyperinflammation associated with CAR-T cell therapy (75). Moreover, case reports have illustrated a partial effect of IL-1 blockade in patients with NLRC4 or CDC42 mutations (32, 34) and in patients with CGD (76). Anecdotal reports have reported efficacy of IL-6 blockade in manifestations of XIAP deficiency different from acute EBV-induced HLH (77). However, efficacy of IL-1, IL-6, or TNF alpha blockade in “primary” HLH has not been clearly documented, neither in first-line nor in rescue therapy.
Control of Triggers
Infections should be diagnosed and treated aggressively in all forms of HLH. When active EBV infection is present, rituximab (anti-CD20 antibody) can help controlling the immune stimulation by eliminating EBV infected B cells (78, 79). However, in some states of persistent EBV replication, EBV has been demonstrated in T or NK cells leading to resistance against rituximab treatment (80). Cell-targeted therapy results in significant immunosuppression, such that reverse isolation, aspergillus-effective antifungal and PCJ prophylaxis should be administered (81). Weekly monitoring for infection or reactivation of latent pathogens (EBV, CMV, adenovirus, aspergillus antigen) is recommended (49).
Monitoring Treatment Response
Monitoring response to therapy and detecting early signs of reactivation is crucial in patients with “primary” HLH (79, 82). The response of cytopenia is a sensitive parameter to judge treatment response (83). Since neutropenia frequently occurs treatment-related, thrombocytopenia is the more valuable parameter. Bone marrow puncture can be of some help in distinguishing between the activity of HLH and the myelotoxic side effect of therapy. Ferritin usually shows a significant decrease in the first days of successful treatment. However, complete normalization of ferritin can take weeks and can be even further delayed by the transfusion of erythrocytes (84). sCD25 is more dynamic, but it may still take a few days until a substantial decrease can be observed. In patients with initially low fibrinogen, this parameter can be used together with transaminases and coagulation studies to assess the treatment response (83). Other biomarkers for disease activity such as free IL-18 or CXCL9 are being explored. If more rapid turnaround times can be achieved, they might be valuable for guiding therapy in the future.
Salvage Therapy in Refractory HLH
Early mortality of acute HLH remains a major concern. 25–50% of patients with acute “primary” HLH fail to achieve rapid and sustained initial remission after etoposide-based therapy. If cytopenia [in particular thrombocytopenia <40 G (G/L)] and ferritin and/or sCD25 fail to respond after 2 weeks, the risk for an adverse outcome increases, justifying consideration of alternative (salvage) therapy (49). There are no standard recommendations for the treatment of relapsing or refractory HLH. The salvage therapies published so far include alemtuzumab, anakinra, ATG, and regimens consisting of liposomal doxorubicin, etoposide, and dexamethasone (85). In an observational study reporting on treatment of 22 patients with refractory HLH with alemtuzumab, 86 percent of patients showed partial response, and 77 percent were able to receive HSCT (86). Notably, CMV and adenovirus viremia occurred in 23–32% of patients.
Emapalumab, a neutralizing antibody against INFγ, has recently been licensed as the first drug for the treatment patients with “primary” hemophagocytic lymphohistiocytosis (HLH) with refractory, recurrent or progressive disease or intolerance with conventional HLH therapy (87). The recommended starting dose is 1 mg/kg twice per week with dexamethasone as a background treatment, but doses can be increased up to 10 mg/kg based on clinical response (88). Due to the risk of serious infections (frequent during therapy of primary HLH patients and observed in 32% of patients in the trial) patients should receive prophylaxis for Herpes Zoster, Pneumocystis jirovecii, and fungal infections and should be monitored for tuberculosis, adenovirus, EBV and CMV.
The study included 27 patients with a mean age of 1 year (range: 0.1 to 13 years), with a “primary” HLH in 82% of patients. Patients had received various combinations of dexamethasone, etoposide, CSA, and anti-thymocyte globulin prior to emapalumab. Full response was defined as normalization of all, while partial response was defined as normalization of ≥3 HLH parameters and HLH improvement was defined as ≥3 HLH abnormalities improved by at least 50% from baseline. Twenty patients completed the 8-week study, while seven were prematurely withdrawn. Seventy percent (19/27) of patients proceeded to HSCT. The overall response rate was 63%, the median time to response was 8 days. A complete response was achieved in 7 patients, partial response in 8 patients and HLH improvement in 2 patients (88, 89). Since refractory primary HLH has a dismal prognosis, these data are encouraging. However, the exact place of this drug in the context of existing and emerging therapies remains to be defined.
Definitive Therapy
Hematopoietic Stem Cell Transplantation (HSCT)
To prevent recurrences, allogenic stem cell transplantation should be carried out as soon as possible after achieving initial remission in the “primary” HLH (49, 90). It remains the only curative option. The timing of HSCT has to balance the risks between achieving full remission versus reactivation. Although active disease at conditioning remains a risk factor, full remission of all clinical symptoms is not required for successful HSCT. In particular, active neurological disease should prompt aggressive management including early HSCT (51).
Allogeneic HSCT is also the definitive treatment of choice for HLH in the context of several other inborn errors of immunity, including XLP1 or XLP2 and patients with TIM3 deficiency (91, 92). Furthermore, HSCT has been successfully performed in a patient with a CDC42 mutation (34). However, there has been no report of HSCT in NLRC4 deficiency, where it is unlikely to impact on IL-18 hypersecretion by intestinal epithelial cells (93). Careful and broad genetic and functional evaluation is therefore mandatory before proceeding to HSCT based on clinical grounds in rare cases of familial or recurrent HLH without detection of a genetic cause.
In cases with suspected “primary” HLH, donor search and pretransplantation diagnostics should be carried out promptly during the initial presentation. Bi-allelic mutations should be ruled out in potential related donors. In autosomal-recessive disease, heterozygous carriers are in most cases appropriate donors. In the X-linked conditions, skewed X-inactivation should be excluded in potential female carrier donors (94). Conditioning regimes for HSCT in “primary” HLH have been discussed elsewhere.
Gene Therapy
For genetic diseases manifesting in hematopoietic cells, hematopoietic stem cell gene therapy is an important option (95). Preclinical murine studies in a perforin knock-out mouse showed a correction of the HLH phenotype after lentiviral gene therapy of autologous hematopoietic stem cells (96). However, high levels of expression were necessary to fully correct the HLH phenotype (97). Successful gene transfer into hematopoietic stem cells has also been demonstrated in a mouse model (98) and in patient T cells with MUNC13-4 deficiency (99). Furthermore, correction of cellular and humoral immune function was achieved by gene therapy in the murine model of XLP1 (100). Since these mice do not develop HLH, the question of whether the gene therapy can fully control the risk of HLH could not be addressed. These preclinical proof-of-concept studies show the therapeutic potential of gene therapy in “primary” HLH and it will be important to see them further investigated in clinical trials in the future.
Special Situations in “Primary” HLH
CNS Involvement and Isolated CNS-HLH
Central nervous system involvement is a common complication in “primary” HLH (30–73%) and leads to increased morbidity in long-term survivors (51, 101). Irritability, seizures, meningisms, focal deficits, or reduced level of consciousness are observed in active HLH. Diagnostic parameters of CNS involvement include variable combinations of elevated protein or cell count (>5 cells/μl), lymphocytic pleocytosis, activated monocytes and hemophagocytosis in the CSF. MRI brain morphology can demonstrate cerebral atrophy, diffuse white matter irregularities and multiple focal lesions (102–104). Delayed start of treatment for “primary” HLH increases the risk of neurological complications and is associated with worse CNS outcomes (105).
“Primary” HLH can also present as isolated CNS disease in the absence of any systemic manifestations. These occur particularly in older patients with hypomorphic mutations (106–110), most commonly in patients with FHL2 or Griscelli syndrome (111). Isolated CNS disease has also been documented in patients post-transplant with partial donor chimerism (112, 113). Systemic HLH-directed therapies can improve CNS-HLH unless irreversible damage has already occurred (51). Considering its value in other inflammatory brain diseases, alemtuzumab may provide an interesting option. In the further course, patients with isolated CNS-HLH are at risk for developing full-blown systemic HLH. Allogeneic HSCT is therefore also recommended in patients with isolated CNS disease (111).
Pre-emptive HSCT
Unless transplanted, all patients with “primary” HLH have a risk of developing life-threatening HLH at any time throughout their life. This risk has to be weighed against the risk of HSCT on an individual basis. In any case, genetic testing of family members, particularly of siblings should be offered promptly after diagnosis of the index case. In a recent analysis of 64 children with primary HLH (index cases), 32 asymptomatic carriers were identified. 16 of 22 asymptomatic carriers received pre-emptive transplantation, of which 15 are alive and in complete remission after 39 months of median follow-up. Eight-year probability of survival was significantly higher than that in index cases and survival in asymptomatic carriers receiving HSCT before disease activation was significantly higher than in those receiving HSCT after HLH activation (93% versus 64%) (114). Hence, most experts recommend pre-emptive HSCT for FHL unless mutations are very mild.
Outlook
In the last few decades, significant progress has been made in understanding the genetic basis and pathogenesis of HLH in the context of inborn errors of immunity. This has set the stage for rapid diagnosis and a more targeted therapy of this serious clinical condition. The outcome of “primary” HLH has significantly improved with cell-targeted therapies. New cytokine-directed treatments will increase the therapeutic flexibility, but it remains to be seen whether they will show enough efficacy to fully replace this aggressive approach. In the emerging field of HLH associated with other inborn errors of immunity, established and novel cytokine-directed therapies are expected to become the treatment of choice.
Author Contributions
OW wrote the manuscript. KW, KL, and SE contributed to manuscript revision and approved the submitted version.
Funding
This work was supported by the Deutsche Kinderkrebsstiftung (DKS 2016.04 and DKS 2018.11) and the Deutsche Forschungsgemeinschaft (SFB1160, TP A07).
Conflict of Interest
SE is part of Advisory Boards at SOBI, UCB, and Novartis and has received research support from UCB. KL is part of an Advisory Board at SOBI.
The remaining authors declare that the research was conducted in the absence of any commercial or financial relationships that could be construed as a potential conflict of interest.
Abbreviations
APC, antigen-presenting cell; ATG, antithymocyte globulin; CMV, cytomegalovirus; CNS, central nervous system; CSA, cyclosporine A; EBV, Epstein-Barr virus; FHL, familial hemophagocytotic lymphohistiocytosis; HLH, hemophagocytotic lymphohistiocytosis; HSCT, hematopoietic stem cell transplantation; JAK, janus kinase; MRI, magnetic resonance imaging; NK, natural killer cell; RIC, reduced-intensity-conditioning; VOD, veno-occlusive disease; XLP, X-linked lymphoproliferative syndrome.
References
1. Farquhar, JW, and Claireaux, AE. Familial haemophagocytic reticulosis. Arch Dis Child. (1952) 27:519–25. doi: 10.1136/adc.27.136.519
2. Heeg, M, Ammann, S, Klemann, C, Panning, M, Falcone, V, Hengel, H, et al. Is an infectious trigger always required for primary hemophagocytic lymphohistiocytosis? Lessons from in utero and neonatal disease. Pediatr Blood Cancer. (2018) 65:e27344. doi: 10.1002/pbc.27344
3. Jessen, B, Kögl, T, Sepulveda, FE, de Saint Basile, G, Aichele, P, and Ehl, S. Graded defects in cytotoxicity determine severity of hemophagocytic lymphohistiocytosis in humans and mice. Front Immunol. (2013) 4:448. doi: 10.3389/fimmu.2013.00448
4. Sepulveda, FE, Debeurme, F, Ménasché, G, Kurowska, M, Côte, M, Pachlopnik Schmid, J, et al. Distinct severity of HLH in both human and murine mutants with complete loss of cytotoxic effector PRF1, RAB27A, and STX11. Blood. (2013) 121:595–603. doi: 10.1182/blood-2012-07-440339
5. Zhang, K, Jordan, MB, Marsh, RA, Johnson, JA, Kissell, D, Meller, J, et al. Hypomorphic mutations in PRF1, MUNC13-4, and STXBP2 are associated with adult-onset familial HLH. Blood. (2011) 118:5794–8. doi: 10.1182/blood-2011-07-370148
6. Henter, JI, Elinder, G, Söder, O, and Ost, A. Incidence in Sweden and clinical features of familial hemophagocytic Lymphohistiocytosis. Acta Paediatr Scand. (1991) 80:428–35. doi: 10.1111/j.1651-2227.1991.tb11878.x
7. Meeths, M, Horne, A, Sabel, M, Bryceson, YT, and Henter, JI. Incidence and clinical presentation of primary hemophagocytic lymphohistiocytosis in Sweden. Pediatr Blood Cancer. (2015) 62:346–52. doi: 10.1002/pbc.25308
8. Pachlopnik Schmid, J, Côte, M, Ménager, MM, Burgess, A, Nehme, N, Ménasché, G, et al. Inherited defects in lymphocyte cytotoxic activity. Immunol Rev. (2010) 235:10–23. doi: 10.1111/j.0105-2896.2010.00890.x
9. de Saint Basile, G, and Fischer, A. The role of cytotoxicity in lymphocyte homeostasis. Curr Opin Immunol. (2001) 13:549–54. doi: 10.1016/s0952-7915(00)00257-0
10. Voskoboinik, I, Smyth, MJ, and Trapani, JA. Perforin-mediated target-cell death and immune homeostasis. Nat Rev Immunol. (2006) 6:940–52. doi: 10.1038/nri1983
11. de Saint Basile, G, Ménasché, G, and Fischer, A. Molecular mechanisms of biogenesis and exocytosis of cytotoxic granules. Nat Rev Immunol. (2010) 10:568–79. doi: 10.1038/nri2803
12. Terrell, CE, and Jordan, MB. Perforin deficiency impairs a critical immunoregulatory loop involving murine CD8(+) T cells and dendritic cells. Blood. (2013) 121:5184–91. doi: 10.1182/blood-2013-04-495309
13. Ivashkiv, LB. IFNgamma: signalling, epigenetics and roles in immunity, metabolism, disease and cancer immunotherapy. Nat Rev Immunol. (2018) 18:545–58. doi: 10.1038/s41577-018-0029-z
14. Osugi, Y, Hara, J, Tagawa, S, Takai, K, Hosoi, G, Matsuda, Y, et al. Cytokine production regulating Th1 and Th2 cytokines in hemophagocytic lymphohistiocytosis. Blood. (1997) 89:4100–3.
15. Henter, JI, Elinder, G, Söder, O, Hansson, M, Andersson, B, and Andersson, U. Hypercytokinemia in familial hemophagocytic lymphohistiocytosis. Blood. (1991) 78:2918–22.
16. Billiau, AD, Roskams, T, Van Damme-Lombaerts, R, Matthys, P, and Wouters, C. macrophage activation syndrome: characteristic findings on liver biopsy illustrating the key role of activated, IFN-gamma-producing lymphocytes and IL-6- and TNF-Alpha-producing macrophages. Blood. (2005) 105:1648–51. doi: 10.1182/blood-2004-08-2997
17. Pallmer, K, and Oxenius, A. Recognition and regulation of T cells by NK Cells. Front Immunol. (2016) 7:251. doi: 10.3389/fimmu.2016.00251
18. Sepulveda, FE, Maschalidi, S, Vosshenrich, CAJ, Garrigue, A, Kurowska, M, Ménasche, G, et al. A novel immunoregulatory role for NK-cell cytotoxicity in protection from HLH-like immunopathology in mice. Blood. (2015) 125:1427–34. doi: 10.1182/blood-2014-09-602946
19. Jordan, MB. An animal model of hemophagocytic lymphohistiocytosis (HLH): CD8+ T cells and interferon gamma are essential for the disorder. Blood. (2004) 104:735–43. doi: 10.1182/blood-2003-10-3413
20. Pachlopnik Schmid, J, Canioni, D, Moshous, D, Touzot, F, Mahlaoui, N, Hauck, F, et al. Clinical similarities and differences of patients with X-linked lymphoproliferative syndrome Type 1 (XLP-1/SAP Deficiency) versus Type 2 (XLP-2/XIAP deficiency). Blood. (2011) 117:1522–9. doi: 10.1182/blood-2010-07-298372
21. Sayos, J, Wu, C, Morra, M, Wang, N, Zhang, X, Allen, D, et al. The X-linked lymphoproliferative-disease gene product SAP regulates signals induced through the co-receptor SLAM. Nature. (1998) 395:462–9. doi: 10.1038/26683
22. Cannons, JL, and Schwartzberg, PL. SAP and lessons learned from a primary immunodeficiency. J Immunol. (2017) 199:1531–3. doi: 10.4049/jimmunol.1701007
23. Parolini, S, Bottino, C, Falco, M, Augugliaro, R, Giliani, S, Franceschini, R, et al. X-linked lymphoproliferative disease. 2B4 molecules displaying inhibitory rather than activating function are responsible for the inability of natural killer cells to kill epstein-barr virus-infected cells. J Exp Med. (2000) 192:337–46. doi: 10.1084/jem.192.3.337
24. Rigaud, S, Fondaneche, MC, Lambert, N, Pasquier, B, Mateo, V, Soulas, P, et al. XIAP deficiency in humans causes an X-linked lymphoproliferative syndrome. Nature. (2006) 444:110–4. doi: 10.1038/nature05257
25. Yabal, M, Müller, N, Adler, H, Knies, N, Groß, CJ, Damgaard, RB, et al. XIAP restricts TNF- and RIP3-dependent cell death and inflammasome activation. Cell Rep. (2014) 7:1796–808. doi: 10.1016/j.celrep.2014.05.008
26. Speckmann, C, Lehmberg, K, Albert, MH, Damgaard, RB, Fritsch, M, Gyrd-Hansen, M, et al. X-linked inhibitor of apoptosis (XIAP) deficiency: the spectrum of presenting manifestations beyond hemophagocytic lymphohistiocytosis. Clin Immunol. (2013) 149:133–41. doi: 10.1016/j.clim.2013.07.004
27. Wada, T, Kanegane, H, Ohta, K, Katoh, F, Imamura, T, Nakazawa, Y, et al. Sustained elevation of serum interleukin-18 and its association with hemophagocytic lymphohistiocytosis in XIAP deficiency. Cytokine. (2014) 65:74–8. doi: 10.1016/j.cyto.2013.09.007
28. Kaplanski, G. Interleukin-18: biological properties and role in disease pathogenesis. Immunol Rev. (2018) 281:138–53. doi: 10.1111/imr.12616
29. Marsh, RA, Madden, L, Kitchen, BJ, Mody, R, McClimon, B, Jordan, MB, et al. XIAP deficiency: a unique primary immunodeficiency best classified as X-linked familial hemophagocytic lymphohistiocytosis and not as X-linked lymphoproliferative disease. Blood. (2010) 116:1079–82. doi: 10.1182/blood-2010-01-256099
30. Gayden, T, Sepulveda, FE, Khuong-Quang, DA, Pratt, J, Valera, ET, Garrigue, A, et al. Germline HAVCR2 mutations altering TIM-3 characterize subcutaneous panniculitis-like T cell lymphomas with hemophagocytic lymphohistiocytic syndrome. Nat Genet. (2018) 50:1650–7. doi: 10.1038/s41588-018-0251-4
31. Wolf, Y, Anderson, AC, and Kuchroo, VK. TIM3 comes of age as an inhibitory receptor. Nat Rev Immunol. (2020) 20:173–85. doi: 10.1038/s41577-019-0224-6
32. Canna, SW, de Jesus, AA, Gouni, S, Brooks, SR, Marrero, B, Liu, Y, et al. An activating NLRC4 inflammasome mutation causes autoinflammation with recurrent macrophage activation syndrome. Nat Genet. (2014) 46:1140–6. doi: 10.1038/ng.3089
33. Romberg, N, Al Moussawi, K, Nelson-Williams, C, Stiegler, AL, Loring, E, Choi, M, et al. Mutation of NLRC4 causes a syndrome of enterocolitis and autoinflammation. Nat Genet. (2014) 46:1135–9. doi: 10.1038/ng.3066
34. Lam, MT, Coppola, S, Krumbach, OHF, Prencipe, G, Insalaco, A, Cifaldi, C, et al. A novel disorder involving dyshematopoiesis, inflammation, and HLH due to aberrant CDC42 function. J Exp Med. (2019) 216:2778–99. doi: 10.1084/jem.20190147
35. Bode, SF, Ammann, S, Al-Herz, W, Bataneant, M, Dvorak, CC, Gehring, S, et al. The syndrome of hemophagocytic lymphohistiocytosis in primary immunodeficiencies: implications for differential diagnosis and pathogenesis. Haematologica. (2015) 100:978–88. doi: 10.3324/haematol.2014.121608
36. Staines-Boone, T, Deswarte, C, Venegas Montoya, E, Sanchez-Sanchez, LM, Garcia Campos, JA, Muniz-Ronquillo, T, et al. Multifocal recurrent osteomyelitis and hemophagocytic lymphohistiocytosis in a boy with partial dominant IFN-GammaR1 deficiency: case report and review of the literature. Front Pediatr. (2017) 5:75. doi: 10.3389/fped.2017.00075
37. Meissner, F, Seger, RA, Moshous, D, Fischer, A, Reichenbach, J, and Zychlinsky, A. Inflammasome activation in NADPH oxidase defective mononuclear phagocytes from patients with chronic granulomatous disease. Blood. (2010) 116:1570–3. doi: 10.1182/blood-2010-01-264218
38. Lee, PP, Lobato-Marquez, D, Pramanik, N, Sirianni, A, Daza-Cajigal, V, Rivers, E, et al. Wiskott-aldrich syndrome protein regulates autophagy and inflammasome activity in innate immune cells. Nat Commun. (2017) 8:1576. doi: 10.1038/s41467-017-01676-0
39. Van Gorp, H, Saavedra, PH, de Vasconcelos, NM, Van Opdenbosch, N, Vande Walle, L, Matusiak, M, et al. Familial mediterranean fever mutations lift the obligatory requirement for microtubules in pyrin inflammasome activation. Proc Natl Acad Sci USA. (2016) 113:14384–9. doi: 10.1073/pnas.1613156113
40. Burger, D, Fickentscher, C, de Moerloose, P, and Brandt, KJ. F-actin dampens NLRP3 inflammasome activity via flightless-I and LRRFIP2. Sci Rep. (2016) 6:29834. doi: 10.1038/srep29834
41. Henter, JI, Samuelsson-Horne, A, Aricò, M, Egeler, RM, Elinder, G, Filipovich, AH, et al. Treatment of hemophagocytic lymphohistiocytosis with HLH-94 immunochemotherapy and bone marrow transplantation. Blood. (2002) 100:2367–73. doi: 10.1182/blood-2002-01-0172
42. Janka, GE. Familial hemophagocytic lymphohistiocytosis. Eur J Pediatr. (1983) 140:221–30. doi: 10.1007/bf00443367
43. Ammann, S, Lehmberg, K, Zur Stadt, U, Klemann, C, Bode, SFN, Speckmann, C, et al. Effective immunological guidance of genetic analyses including exome sequencing in patients evaluated for hemophagocytic lymphohistiocytosis. J Clin Immunol. (2017) 37:770–80. doi: 10.1007/s10875-017-0443-1
44. Bryceson, YT, Pende, D, Pavicic, AM, Gilmour, KC, Ufheil, H, Vraetz, T, et al. A prospective evaluation of degranulation assays in the rapid diagnosis of familial hemophagocytic syndromes. Blood. (2012) 119:2754–63. doi: 10.1182/blood-2011-08-374199
45. Rubin, TS, Zhang, K, Gifford, C, Lane, A, Choo, S, Bleesing, JJ, et al. Perforin and CD107a testing is superior to NK cell function testing for screening patients for genetic HLH. Blood. (2017) 129:2993–9. doi: 10.1182/blood-2016-12-753830
46. Lehmberg, K, Sprekels, B, Nichols, KE, Woessmann, W, Müller, I, Suttorp, M, et al. Malignancy-associated haemophagocytic lymphohistiocytosis in children and adolescents. Br J Haematol. (2015) 170:539–49. doi: 10.1111/bjh.13462
47. Gurunathan, A, Boucher, AA, Mark, M, Prus, KM, O’Brien, MM, Breese, EH, et al. Limitations of HLH-2004 criteria in distinguishing malignancy-associated hemophagocytic lymphohistiocytosis. Pediatr Blood Cancer. (2018) 65:e27400. doi: 10.1002/pbc.27400
48. Bode, SFN, Bogdan, C, Beutel, K, Behnisch, W, Greiner, J, Henning, S, et al. Hemophagocytic lymphohistiocytosis in imported pediatric visceral leishmaniasis in a nonendemic area. J Pediatr. (2014) 165:147–53.e1. doi: 10.1016/j.jpeds.2014.03.047
49. Ehl, S, Astigarraga, I, von Bahr Greenwood, T, Hines, M, Horne, A, Ishii, E, et al. Recommendations for the use of etoposide-based therapy and bone marrow transplantation for the treatment of HLH: consensus statements by the HLH steering committee of the histiocyte society. J Allergy Clin Immunol Pract. (2018) 6:1508–17. doi: 10.1016/j.jaip.2018.05.031
50. Johnson, TS, Terrell, CE, Millen, SH, Katz, JD, Hildeman, DA, and Jordan, MB. Etoposide selectively ablates activated T cells to control the immunoregulatory disorder hemophagocytic lymphohistiocytosis. J Immunol. (2014) 192:84–91. doi: 10.4049/jimmunol.1302282
51. Horne, A, Wickström, R, Jordan, MB, Ann Yeh, E, Naqvi, A, Henter, JI, et al. How to treat involvement of the central nervous system in hemophagocytic lymphohistiocytosis? Curr Treat Options Neurol. (2017) 19:3. doi: 10.1007/s11940-017-0439-4
52. Bergsten, E, Horne, A, Aricó, M, Astigarraga, I, Egeler, RM, Filipovich, AH, et al. Confirmed efficacy of etoposide and dexamethasone in HLH treatment: long-term results of the cooperative HLH-2004 study. Blood. (2017) 130:2728–38. doi: 10.1182/blood-2017-06-788349
53. Popow, I, Leitner, PJ, Grabmeier-Pfistershammer, K, Majdic, O, Zlabinger, G-J, Kundi, M, et al. A comprehensive and quantitative analysis of the major specificities in rabbit antithymocyte globulin preparations. Am J Transplant. (2013) 13:3103–13. doi: 10.1111/ajt.12514
54. Mahlaoui, N, Ouachee-Chardin, M, de Saint Basile, G, Neven, B, Picard, C, Blanche, S, et al. Immunotherapy of familial hemophagocytic lymphohistiocytosis with antithymocyte globulins: a single-center retrospective report of 38 patients. Pediatrics. (2007) 120:e622–8. doi: 10.1542/peds.2006-3164
55. Clinical Trials.Gov Identifier NCT01104025 Hybrid Immunotherapy for Hemophagocytic LymphoHistiocytosis. Drug: ATG, Etoposide, Methotrexate, Hydrocortisone, Dexamethasone. (2019). Available online at: https://clinicaltrials.gov/ct2/show/NCT01104025 (accessed September 29, 2019).
56. Moshous, D, Briand, C, Castelle, M, Dupic, L, Morelle, G, Chahla, WA, et al. Alemtuzumab as first line treatment in children with familial lymphohistiocytosis. Blood. (2019) 134(Suppl. 1:80). doi: 10.1182/blood-2019-124477
57. Strout, MP, Seropian, S, and Berliner, N. Alemtuzumab as a bridge to allogeneic SCT in atypical hemophagocytic lymphohistiocytosis. Nat Rev Clin Oncol. (2010) 7:415–20. doi: 10.1038/nrclinonc.2010.40
58. ClinicalTrials.Gov Identifier NCT02472054 Treatment of Familiar Lymphohistiocytosis (C-HLH), Drug: Alemtuzumab. (2019) Available online at: https://clinicaltrials.gov/ct2/show/NCT02472054 (accessed September 29, 2019).
59. Pachlopnik Schmid, J, Ho, CH, Chrétien, F, Lefebvre, JM, Pivert, G, Kosco-Vilbois, M, et al. Neutralization of IFNgamma defeats haemophagocytosis in LCMV-infected perforin- and Rab27a-deficient mice. EMBO Mol Med. (2009) 1:112–24. doi: 10.1002/emmm.200900009
60. Al-Salama, ZT. Emapalumab: first global approval. Drugs. (2019) 79:99–103. doi: 10.1007/s40265-018-1046-8
61. Clinical Trials.Gov Identifier NCT03312751 Study to Assess the Efficacy and Safety of Emapalumab in Primary Haemophagocytic Lymphohistiocytosis, Drug: Emapalumab. (2019). Available online at: https://clinicaltrials.gov/ct2/show/NCT03312751 (accessed September 29, 2019).
62. Takada, H, Takahata, Y, Nomura, A, Ohga, S, Mizuno, Y, and Hara, T. Increased serum levels of interferon-gamma-inducible protein 10 and monokine induced by gamma interferon in patients with haemophagocytic lymphohistiocytosis. Clin Exp Immunol. (2003) 133:448–53. doi: 10.1046/j.1365-2249.2003.02237.x
63. Maschalidi, S, Sepulveda, FE, Garrigue, A, Fischer, A, and de Saint Basile, G. Therapeutic effect of JAK1/2 blockade on the manifestations of hemophagocytic lymphohistiocytosis in mice. Blood. (2016) 128:60–71. doi: 10.1182/blood-2016-02-700013
64. Das, R, Guan, P, Sprague, L, Verbist, K, Tedrick, P, An, QA, et al. Janus kinase inhibition lessens inflammation and ameliorates disease in murine models of hemophagocytic lymphohistiocytosis. Blood. (2016) 127:1666–75. doi: 10.1182/blood-2015-12-684399
65. Slostad, J, Hoversten, P, Haddox, CL, Cisak, K, Paludo, J, and Tefferi, A. Ruxolitinib as first-line treatment in secondary hemophagocytic lymphohistiocytosis: a single patient experience. Am J Hematol. (2018) 93:E47–9. doi: 10.1002/ajh.24971
66. Sin, JH, and Zangardi, ML. Ruxolitinib for secondary hemophagocytic lymphohistiocytosis: first case report. Hematol Oncol Stem Cell Ther. (2019) 12:166–70. doi: 10.1016/j.hemonc.2017.07.002
67. Broglie, L, Pommert, L, Rao, S, Thakar, M, Phelan, R, Margolis, D, et al. Ruxolitinib for treatment of refractory hemophagocytic lymphohistiocytosis. Blood Adv. (2017) 1:1533–6. doi: 10.1182/bloodadvances.2017007526
68. ClinicalTrials.Gov Identifier NCT03795909 Ruxolitinib Combined With Dexamethasone for HLH. (2020). Available online at: https://clinicaltrials.gov/ct2/show/NCT03795909?term=NCT03795909&draw=2&rank=1 (accessed March 31,2020).
69. Ahmed, A, Merrill, SA, Alsawah, F, Bockenstedt, P, Campagnaro, E, Devata, S, et al. Ruxolitinib in adult patients with secondary haemophagocytic lymphohistiocytosis: an open-label, single-centre, pilot trial. Lancet Haematol. (2019) 6:e630–7. doi: 10.1016/S2352-3026(19)30156-5
70. Weiss, ES, Girard-Guyonvarc’h, C, Holzinger, D, de Jesus, AA, Tariq, Z, Picarsic, J, et al. Interleukin-18 diagnostically distinguishes and pathogenically promotes human and murine macrophage activation syndrome. Blood. (2018) 131:1442–55. doi: 10.1182/blood-2017-12-820852
71. Chiossone, L, Audonnet, S, Chetaille, B, Chasson, L, Farnarier, C, Berda-Haddad, Y, et al. Protection from inflammatory organ damage in a murine model of hemophagocytic lymphohistiocytosis using treatment with IL-18 binding protein. Front Immunol. (2012) 3:239. doi: 10.3389/fimmu.2012.00239
72. Grom, A, Horne, A, and De Benedetti, F. Macrophage activation syndrome in the era of biologic therapy. Nat Rev Rheumatol. (2016) 12:259–68. doi: 10.1038/nrrheum.2015.179
73. ClinicalTrials.Gov Identifier: NCT03113760 Therapeutic Use of Tadekinig Alfa in NLRC4 Mutation and XIAP Deficiency. Drug: Tadekinig Alfa. (2019). Available online at: https://clinicaltrials.gov/ct2/show/NCT03113760 (accessed September 29, 2019).
74. Chamseddin, B, Marks, E, Dominguez, A, Wysocki, C, and Vandergriff, T. Refractory macrophage activation syndrome in the setting of adult−onset still disease with hemophagocytic lymphohistiocytosis detected on skin biopsy treated with canakinumab and tacrolimus. J Cutaneous Pathol. (2019) 46:528–31. doi: 10.1111/cup.13466
75. Kotch, C, Barrett, D, and Teachey, DT. Tocilizumab for the treatment of chimeric antigen receptor T cell-induced cytokine release syndrome. Expert Rev Clin Immunol. (2019) 15:813–22. doi: 10.1080/1744666X.2019.1629904
76. de Luca, A, Smeekens, SP, Casagrande, A, Iannitti, R, Conway, KL, Gresnigt, MS, et al. IL-1 receptor blockade restores autophagy and reduces inflammation in chronic granulomatous disease in mice and in humans. Proc Natl Acad Sci USA. (2014) 111:3526–31. doi: 10.1073/pnas.1322831111
77. Wustrau, K, Gross, M, Lenhartz, H, Oyen, F, Ehl, S, Müller, I, et al. Tocilizumab Strikingly Abates Inflammation in a Girl with Symptomatic Deficiency of the X-Linked Inhibitor of Apoptosis (XIAP) with Extremely Skewed X-Inactivation. Hamburg: Katharina Wustrau’s research works. (2019).
78. Milone, MC, Tsai, DE, Hodinka, RL, Silverman, LB, Malbran, A, Wasik, MA, et al. Treatment of primary Epstein-Barr virus infection in patients with X-linked lymphoproliferative disease using B-cell-directed therapy. Blood. (2005) 105:994–6. doi: 10.1182/blood-2004-07-2965
79. Jordan, MB, Allen, CE, Weitzman, S, Filipovich, AH, and McClain, KL. How i treat hemophagocytic lymphohistiocytosis. Blood. (2011) 118:4041–52. doi: 10.1182/blood-2011-03-278127
80. Kasahara, Y, Yachie, A, Takei, K, Kanegane, C, Okada, K, Ohta, K, et al. Differential cellular targets of Epstein-Barr virus. (EBV) infection between acute EBV-associated hemophagocytic lymphohistiocytosis and chronic active EBV infection. Blood. (2001) 98:1882–8. doi: 10.1182/blood.v98.6.1882
81. Sung, L, King, SM, Carcao, M, Trebo, M, and Weitzman, SS. Adverse outcomes in primary hemophagocytic lymphohistiocytosis. J Pediatr Hematol Oncol. (2002) 24:550–4. doi: 10.1097/00043426-200210000-00011
82. Henter, I, Horne, A, Arico, M, Egeler, RM, Filipovich, AH, Imashuku, S, et al. HLH-2004: diagnostic and therapeutic guidelines for hemophagocytic lymphohistiocytosis. Pediatr Blood Cancer. (2007) 48:124–31. doi: 10.1002/pbc.21039
83. Trottestam, H, Berglöf, E, Horne, A, Onelöv, E, Beutel, K, Lehmberg, K, et al. Risk factors for early death in children with haemophagocytic lymphohistiocytosis: outcome predictors in HLH. Acta Paediatr. (2012) 101:313–8. doi: 10.1111/j.1651-2227.2011.02501.x
84. Lin, TF, Ferlic-Stark, LL, Allen, CE, Kozinetz, CA, and McClain, KL. Rate of decline of ferritin in patients with hemophagocytic lymphohistiocytosis as a prognostic variable for mortality. Pediatr Blood Cancer. (2011) 56:154–5. doi: 10.1002/pbc.22774
85. Marsh, RA, Jordan, MB, Talano, JA, Nichols, KE, Kumar, A, Naqvi, A, et al. Salvage therapy for refractory hemophagocytic lymphohistiocytosis: a review of the published experience. Pediatr Blood Cancer. (2017) 64:e26308. doi: 10.1002/pbc.26308
86. Marsh, RA, Allen, CE, McClain, KL, Weinstein, JL, Kanter, J, Skiles, J, et al. Salvage therapy of refractory hemophagocytic lymphohistiocytosis with alemtuzumab. Pediatr Blood Cancer. (2013) 60:101–9. doi: 10.1002/pbc.24188
87. Vallurupalli, M, and Berliner, N. Emapalumab for the treatment of relapsed/refractory hemophagocytic lymphohistiocytosis. Blood. (2019) 134:1783–6. doi: 10.1182/blood.2019002289
88. US Food and Drug FDA Approves Emapalumab for Hemophagocytic Lymphohistiocytosis. (2020). Available online at: https://Www.Fda.Gov/Drugs/Fda-Approves-Emapalumab-Hemophagocytic-Lymphohistiocytosis (accessed March 31, 2020).
89. Lounder, DT, Bin, Q, de Min, C, and Jordan, MB. Treatment of refractory hemophagocytic lymphohistiocytosis with emapalumab despite severe concurrent infections. Blood Adv. (2019) 3:47–50. doi: 10.1182/bloodadvances.2018025858
90. Jabado, N, de Graeff-Meeder, ER, Cavazzana-Calvo, M, Haddad, E, Le Deist, F, Benkerrou, M, et al. Treatment of familial hemophagocytic lymphohistiocytosis with bone marrow transplantation from HLA genetically nonidentical donors. Blood. (1997) 90:4743–8.
91. Sonigo, G, Battistella, M, Beylot-Barry, M, Ingen-Housz-Oro, S, Franck, N, Barete, S, et al. HAVCR2 mutations are associated with severe hemophagocytic syndrome in subcutaneous panniculitis-like T-Cell lymphoma. Blood. (2020) 135:1058–61. doi: 10.1182/blood.2019003811
92. Wegehaupt, O, Gross, M, Wehr, C, Marks, R, Schmitt-Graeff, A, Uhl, M, et al. TIM-3 deficiency presenting with two clonally unrelated episodes of mesenteric and subcutaneous panniculitis-like t-cell lymphoma and hemophagocytic lymphohistiocytosis. Pediatr Blood Cancer. (2020). Available online at: http://dx.doi.org/10.1002/pbc.28302 (accessed April 14, 2020).
93. Rauch, I, Deets, RKA, Ji, DX, von Moltke, J, Tenthorey, JL, Lee, AY, et al. NAIP-NLRC4 inflammasomes coordinate intestinal epithelial cell expulsion with eicosanoid and IL-18 release via activation of caspase-1 and -8. Immunity. (2017) 46:649–59. doi: 10.1016/j.immuni.2017.03.016
94. Holle, JR, Marsh, RA, Holdcroft, AM, Davies, SM, Wang, L, Zhang, K, et al. Hemophagocytic lymphohistiocytosis in a female patient due to a heterozygous XIAP mutation and skewed X chromosome inactivation. Pediatr Blood Cancer. (2015) 62:1288–90. doi: 10.1002/pbc.25483
95. Booth, C, Carmo, M, and Gaspar, HB. Gene therapy for haemophagocytic lymphohistiocytosis. Curr Gene Ther. (2014) 14:437–46.
96. Carmo, M, Risma, KA, Arumugam, P, Tiwari, S, Hontz, AE, Montiel-Equihua, CA, et al. Perforin gene transfer into hematopoietic stem cells improves immune dysregulation in murine models of perforin deficiency. Mol Ther. (2015) 23:737–45. doi: 10.1038/mt.2014.242
97. Tiwari, S, Hontz, A, Terrell, CE, Arumugam, P, Carmo, M, Risma, K, et al. High level of perforin expression is required for effective correction of hemophagocytic lymphohistiocytosis. Hum Gene Ther. (2016) 27:847–59. doi: 10.1089/hum.2016.065
98. Soheili, T, Durand, A, Sepulveda, FE, Rivière, J, Lagresle-Peyrou, C, Sadek, H, et al. gene transfer into hematopoietic stem cells reduces HLH manifestations in a murine model of munc13-4 deficiency. Blood Adv. (2017) 1:2781–9. doi: 10.1182/bloodadvances.2017012088
99. Dettmer, V, Bloom, K, Gross, M, Weissert, K, Aichele, P, Ehl, S, et al. Retroviral UNC13D gene transfer restores cytotoxic activity of T cells derived from familial hemophagocytic lymphohistiocytosis Type 3 patients in vitro. Hum Gene Ther. (2019) 30:975–84. doi: 10.1089/hum.2019.025
100. Rivat, C, Booth, C, Alonso-Ferrero, M, Blundell, M, Sebire, NJ, Thrasher, AJ, et al. SAP gene transfer restores cellular and humoral immune function in a murine model of X-linked lymphoproliferative disease. Blood. (2013) 121:1073–6. doi: 10.1182/blood-2012-07-445858
101. Haddad, E, Sulis, ML, Jabado, N, Blanche, S, Fischer, A, and Tardieu, M. Frequency and severity of central nervous system lesions in hemophagocytic lymphohistiocytosis. Blood. (1997) 89:794–800.
102. Deiva, N, Mahlaoui, F, Beaudonnet, G, de Saint Basile, G, Caridade, G, Moshous, D, et al. CNS involvement at the onset of primary hemophagocytic lymphohistiocytosis. Neurology. (2012) 78:1150–6. doi: 10.1212/WNL.0b013e31824f800a
103. Yang, S, Zhang, L, Jia, C, Ma, H, Henter, JI, and Shen, K. Frequency and development of CNS involvement in chinese children with hemophagocytic lymphohistiocytosis. Pediatr Blood Cancer. (2010) 54:408–15. doi: 10.1002/pbc.22239
104. Rego, I, Severino, M, Micalizzi, C, Faraci, M, Pende, D, Dufour, C, et al. Neuroradiologic findings and follow-up with magnetic resonance imaging of the genetic forms of haemophagocytic lymphohistiocytosis with CNS involvement. Pediatr Blood Cancer. (2012) 58:810–4. doi: 10.1002/pbc.23405
105. Li, H, Benson, LA, Henderson, LA, Solomon, IH, Kennedy, AL, Soldatos, A, et al. Central nervous system–restricted familial hemophagocytic lymphohistiocytosis responds to hematopoietic cell transplantation. Blood Adv. (2019) 3:503–7. doi: 10.1182/bloodadvances.2018027417
106. Solomon, IH, Li, H, Benson, LA, Henderson, LA, Degar, BA, Gorman, MP, et al. Histopathologic correlates of familial hemophagocytic lymphohistiocytosis isolated to the central nervous system. J Neuropathol Exp Neurol. (2018) 77:1079–84. doi: 10.1093/jnen/nly094
107. Feldmann, J, Ménasché, G, Callebaut, I, Minard-Colin, V, Bader-Meunier, B, Le Clainche, L, et al. Severe and progressive encephalitis as a presenting manifestation of a novel missense perforin mutation and impaired cytolytic activity. Blood. (2005) 105:2658–63. doi: 10.1182/blood-2004-09-3590
108. Beaty, AD, Weller, C, Levy, B, Vogler, C, Ferguson, WS, Bicknese, A, et al. A teenage boy with late onset hemophagocytic lymphohistiocytosis with predominant neurologic disease and perforin deficiency. Pediatr Blood Cancer. (2008) 50:1070–2. doi: 10.1002/pbc.21438
109. Chiapparini, L, Uziel, G, Vallinoto, C, Bruzzone, MG, Rovelli, A, Tricomi, G, et al. Hemophagocytic lymphohistiocytosis with neurological presentation: MRI findings and a nearly miss diagnosis. Neurol Sci. (2011) 32:473–7. doi: 10.1007/s10072-010-0467-2
110. Murphy, C, Nanthapisal, S, Gilmour, K, Laurent, S, D’Arco, F, Hemingway, C, et al. Progressive neurologic disorder: initial manifestation of hemophagocytic lymphohistiocytosis. Neurology. (2016) 86:2109–11. doi: 10.1212/WNL.0000000000002729
111. Blincoe, M, Heeg, M, Campbell, P, Hines, A, Khojah, M, Gitelman, K, et al. Neuroinflammatory disease as an isolated manifestation of hemophagocytic lymphohistiocytosis. (submitted).
112. Hartz, B, Marsh, R, Rao, K, Henter, JI, Jordan, M, Filipovich, L, et al. The minimum required level of donor chimerism in hereditary hemophagocytic lymphohistiocytosis. Blood. (2016) 127:3281–90. doi: 10.1182/blood-2015-12-684498
113. Lounder, DT, Khandelwal, P, Chandra, S, Jordan, MB, Kumar, AR, Grimley, MS, et al. Incidence and outcomes of central nervous system hemophagocytic lymphohistiocytosis relapse after reduced-intensity conditioning hematopoietic stem cell transplantation. Biol Blood Marrow Transplant. (2017) 23:857–60. doi: 10.1016/j.bbmt.2017.02.011
Keywords: hemophagocytic lymphohistiocytosis, inborn errors of immunity, pathogenesis, therapy, cytokine, inflammation, HSCT
Citation: Wegehaupt O, Wustrau K, Lehmberg K and Ehl S (2020) Cell Versus Cytokine – Directed Therapies for Hemophagocytic Lymphohistiocytosis (HLH) in Inborn Errors of Immunity. Front. Immunol. 11:808. doi: 10.3389/fimmu.2020.00808
Received: 08 October 2019; Accepted: 08 April 2020;
Published: 08 May 2020.
Edited by:
Raphaela Goldbach-Mansky, National Institutes of Health (NIH), United StatesReviewed by:
Anna Shcherbina, Dmitry Rogachev National Research Center of Pediatric Hematology, Oncology and Immunology, RussiaHelen Thomas, St. Vincent’s Institute of Medical Research, Australia
Scott W. Canna, University of Pittsburgh Medical Center, United States
Copyright © 2020 Wegehaupt, Wustrau, Lehmberg and Ehl. This is an open-access article distributed under the terms of the Creative Commons Attribution License (CC BY). The use, distribution or reproduction in other forums is permitted, provided the original author(s) and the copyright owner(s) are credited and that the original publication in this journal is cited, in accordance with accepted academic practice. No use, distribution or reproduction is permitted which does not comply with these terms.
*Correspondence: Stephan Ehl, c3RlcGhhbi5laGxAdW5pa2xpbmlrLWZyZWlidXJnLmRl