- 1Department of General, Visceral and Transplant Surgery, Medical University of Graz, Graz, Austria
- 2Faculty of Medicine, Vilnius University, Vilnius, Lithuania
Due to higher vulnerability and immunogenicity of extended criteria donor (ECD) organs used for organ transplantation (Tx), the discovery of new treatment strategies, involving tissue allorecognition pathways, is important. The implementation of machine perfusion (MP) led to improved estimation of the organ quality and introduced the possibility to achieve graft reconditioning prior to Tx. A significant number of experimental and clinical trials demonstrated increasing support for MP as a promising method of ECD organ preservation compared to classical static cold storage. MP reduced ischemia–reperfusion injury resulting in the protection from inadequate activation of innate immunity. However, there are no general agreements on MP protocols, and clinical application is limited. The objective of this comprehensive review is to summarize literature on immunological effects of MP of ECD organs based on experimental studies and clinical trials.
Introduction
The remarkable evolution of solid organ transplantation (Tx) has led to improved overall outcomes for patients with terminal organ dysfunction. However, ischemia–reperfusion injury (IRI) in combination with early immune activation remains a significant challenge limiting the potential of this therapy (1, 2). IRI depends on several factors, including primary condition of the graft and length of cold and warm ischemia time (CIT and WIT). It additionally determines the extent of the inflammatory response and increases immunogenicity and the degree of microcirculatory perfusion failure during reperfusion resulting in early allograft dysfunction or primary non-function (3, 4). As a link between the degree of IRI and activation of innate immunity (5) has been proposed, the discovery of new treatment strategies including tissue allorecognition pathways (Figure 1) has gained importance, especially in the era of extended criteria donor (ECD) organ Tx. The direct pathway starts with recipient CD4 and CD8 T cells recognizing endogenous alloantigens presented by donor human leukocyte antigen (HLA) molecules on the surface of donor antigen-presenting cells (APCs) after their migration from the graft to the recipient's lymph nodes. This process is initiated by the massive release of pro-inflammatory cytokines from damaged cells during IRI (4). On the other hand, the indirect allorecognition relies on recipient-derived APCs, which ingest, process, and present alloantigens (typically HLA antigens) in the context of recipient HLA, for self-restricted recognition by recipient T cells (6, 7). In the semi-direct pathway, recipient APCs acquire donor HLA molecules that present alloantigens directly to recipient T cells (8). Direct allorecognition alone can result in acute rejection, even without indirect mechanisms. Furthermore, depletion of donor immune cells from an organ prior to Tx may prevent rejection (9).
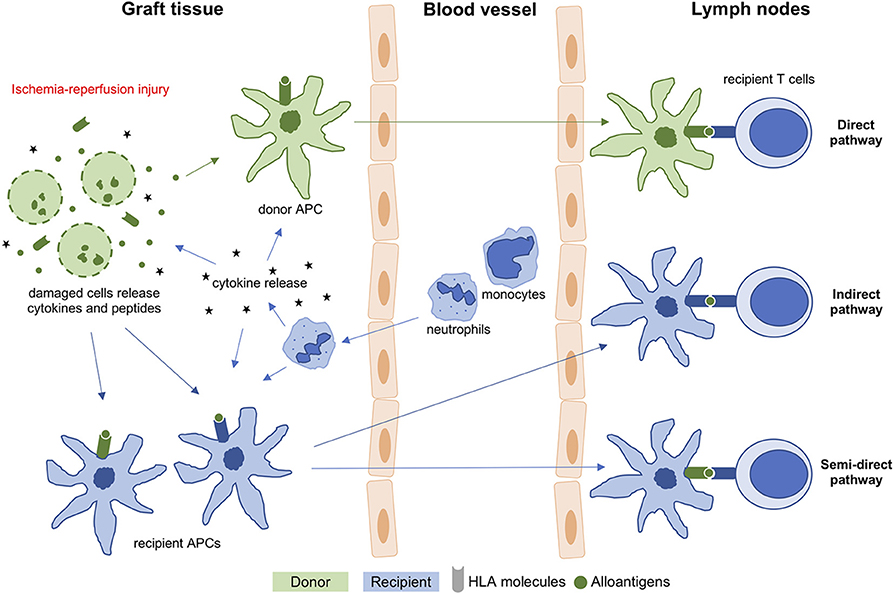
Figure 1. T cell allorecognition pathways in organ transplantation. APC, antigen-presenting cell; HLA, human leukocyte antigen.
For more than 50 years, static cold storage (SCS) was the gold standard method for organ preservation until the interest in the concept of organ machine perfusion (MP) was renewed (10). To date, a significant number of experimental and clinical trials were published demonstrating increasing support of MP as a more physiologic method of solid organ preservation compared to SCS (11–15).
MP is a promising tool to reduce the gap between organ demand and supply that is resulting in a dramatic prolongation in waiting times and associated with increased morbidity and mortality for patients on the waiting list for Tx (16). In an effort to counter this trend, organ allografts that would have previously been deemed unsuitable are nowadays more frequently used for Tx (12) including donation after circulatory death (DCD) and ECD (aged ≥ 60 years or aged 50–59 years with vascular comorbidities) organs (12, 17, 18). Older donor organs have higher immunogenicity, mediated by poorer monocyte clearance of damaged necrotic cells, and therefore recipients may require a more intense immunosuppression in the early period after Tx (19–22). Knowing about the ECD grafts' increased risk for poor function or failure (23–25), implementation of new storage techniques, such as MP, paved the way for better characterization of organ quality and the possibility for graft reconditioning before Tx to improve organ vulnerability and immunogenicity (10, 26). MP reduced IRI in experimental and clinical models of ECD organ Tx resulting in protection from inadequate activation of innate immunity (1, 27–36).
Figure 2 summarizes frequently described MP settings including the underlying mechanisms. Briefly, hypothermic MP (HMP, 4–10°C) is based on the concept that oxidative energy production by mitochondrial electron transport is sustained at reduced rates by keeping low temperatures (10). In contrast, normothermic MP (NMP, 37°C) aims to provide an approximately near physiological environment for organs ex vivo (37). Subnormothermic MP (SNMP, ~21°C) is a halfway approach between HMP and NMP, while controlled oxygenated rewarming (COR) is a concept to rescue cold-stored marginal grafts by gentle oxygenated warming up prior to blood reperfusion (38, 39).
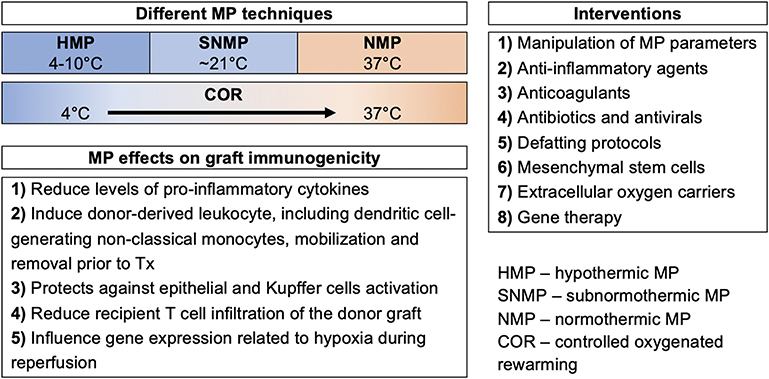
Figure 2. Different machine perfusion strategies of extended criteria donor organs for protection against activation of innate immunity.
Currently, there are no general agreements on MP protocols, and clinical application is limited due to the lack of randomized clinical trials comparing the different MP strategies. The objective of this comprehensive review is to summarize literature on MP of ECD organs and discuss arising immunological aspects based on experimental studies and clinical trials.
Machine Perfusion of Extended Criteria Donor Kidney Grafts
It seems that MP for Tx of ECD kidneys is associated with decreased IRI resulting in improved outcome compared to SCS (Table 1). Whereas most studies on MP in ECD kidneys reported positive effects on the graft, only a few studies reported inconclusive results (40, 48).
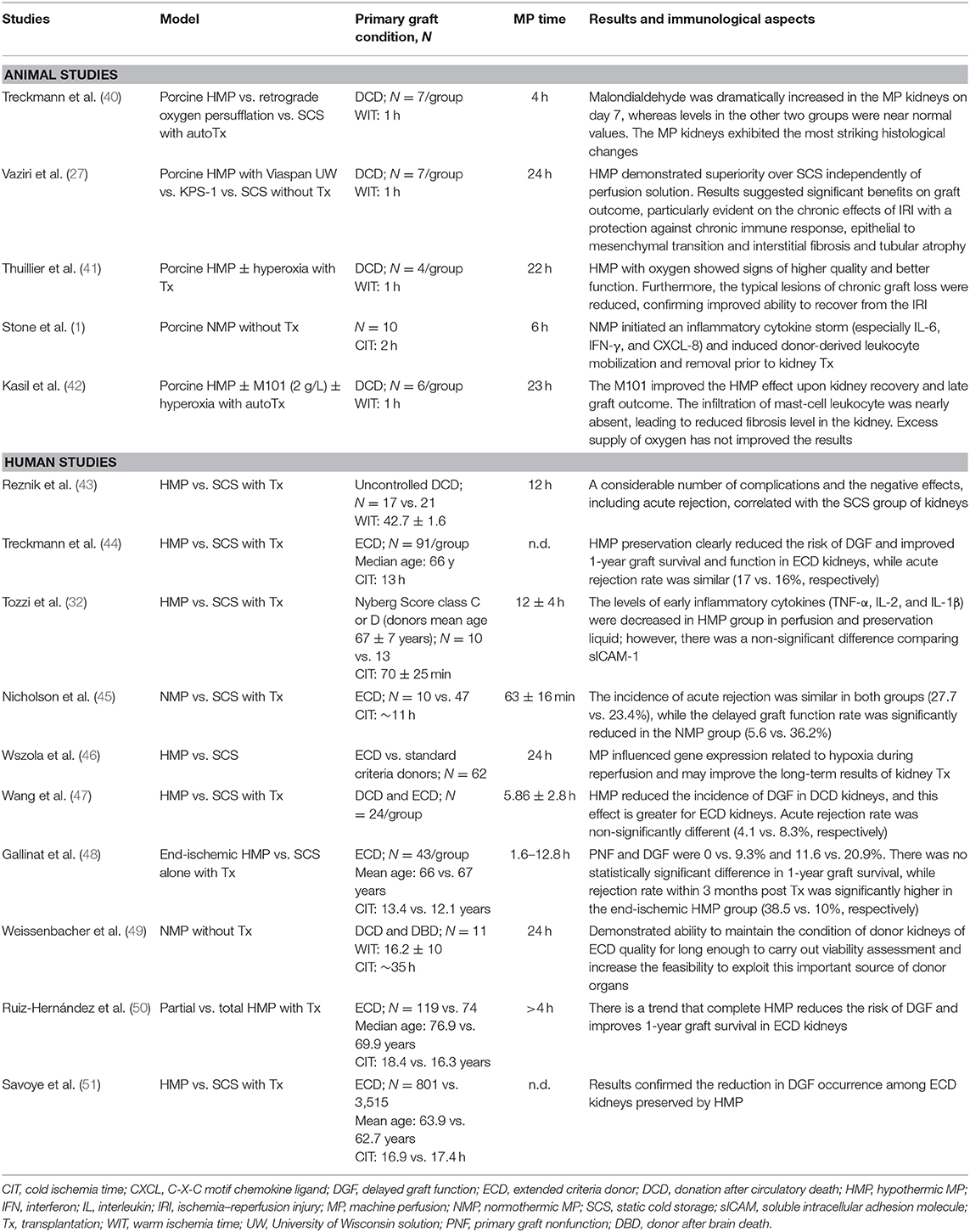
Table 1. Experimental and clinical studies of machine perfusion of extended criteria donor kidney grafts.
Hypothermic Machine Perfusion Techniques
A DCD porcine kidney HMP model demonstrated improved graft outcome (27, 41, 42), particularly concerning the chronic effects of IRI by protecting against chronic immune response by reducing the epithelial to mesenchymal transition (27). Epithelial to mesenchymal transition plays an important role in the genesis of fibroblasts in the course of interstitial fibrosis (27, 52). Furthermore, oxygenated HMP showed superior outcome rates compared to non-oxygenated HMP (41). The significantly reduced occurrence of typical signs for chronic graft loss, like chronic inflammation or interstitial fibrosis, confirmed an improvement in recovery from IRI (41). Lately, the use of an extracellular oxygen transporter was investigated. M101 (hemoglobin of the marine worm) was associated with improved effects of HMP upon recovery and late graft outcome, shown by the nearly absent infiltration of mast cells resulting in reduced levels of fibrosis in the kidney (42). Extracellular oxygen carriers may logistically, rheologically, and immunologically be superior to packed red blood cells, but need further investigation. Studies on human DCD and ECD kidneys supported the superiority of HMP over SCS (32, 43, 45, 46). Reznik et al. (43) found a considerably lower number of complications and negative effects, like acute rejection, correlated with HMP kidneys retrieved from DCD donors. Another study in ECD kidneys (Nyberg Score class C or D) demonstrated an association of HMP with lower levels of early inflammatory cytokines [tumor necrosis factor (TNF)-α, interleukin (IL)-2, and IL-1β] in perfusion solution compared to SCS (32). HMP also affected the expression of hypoxia-related genes [i.e., hypoxia-inducible factor (HIF)-1α] (46). This may limit interstitial fibrosis and tubular atrophy, improving long-term outcomes in kidney Tx. ECD kidneys profited most by application of HMP (46).
Normothermic Machine Perfusion Techniques
In a pig study, reduced graft immunogenicity was achieved by initiating an inflammatory cytokine storm [especially IL-6, interferon[108mmQ11 (IFN)-γ, and C-X-C motif chemokine ligand (CXCL)-8], leading to a donor-derived leukocyte mobilization and removal prior to kidney Tx (1). The authors proposed that migration of donor leukocytes in conjunction with the secretion of an IL-6, IFN-γ, and CXCL-8 storm leads to direct allorecognition and activates the recipient immune response following Tx (1). Short-term NMP of cold-stored human ECD kidneys did not reduce the incidence of acute rejection, while the rate of delayed graft function improved significantly (5.6 vs. 36.2%) (45). More recently, Weissenbacher et al. (49) was able to maintain the quality of ECD kidneys for up to 24 h, hence buying time for viability assessment, improving the feasibility to exploit this important source of donor organs using the NMP technique.
Although the primary results are encouraging, more research focusing on the reduction of immunogenicity of ECD organs is needed.
Machine Perfusion of Extended Criteria Donor Liver Grafts
Currently, there is no general consensus on the standardized pretreatment of ECD livers in order to improve Tx outcomes (53). Experimental and clinical studies of MP of ECD livers are summarized in Table 2.
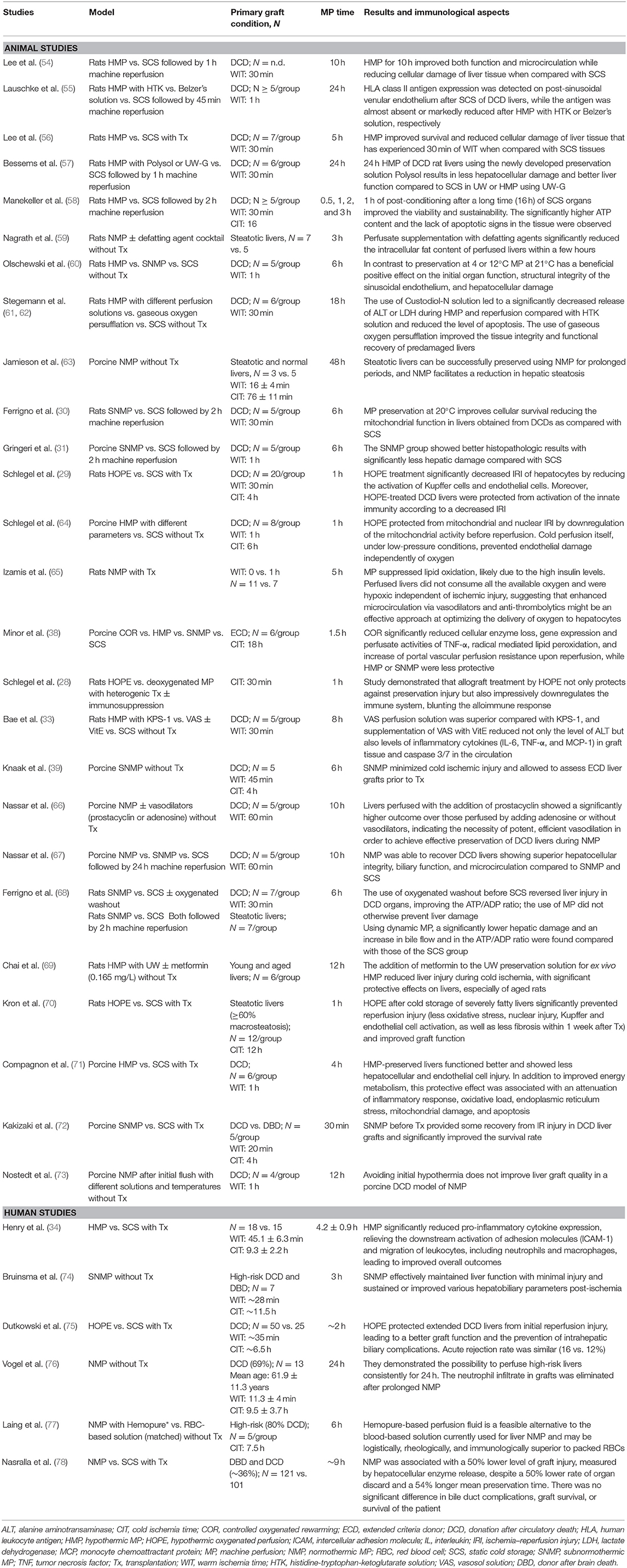
Table 2. Experimental and clinical studies of machine perfusion of extended criteria donor liver grafts.
Hypothermic Machine Perfusion Techniques
In several studies in DCD rat models, a reduction in IRI in liver tissue was evident after HMP when compared to SCS (28, 29, 54, 56, 57, 61, 62). This finding was confirmed in large domestic animal studies (64, 71). Hypothermic oxygenated perfusion (HOPE) treatment of DCD and severely fatty livers significantly decreased IRI of hepatocytes by reducing the activation of Kupffer and endothelial cells (29, 70). Moreover, HOPE successfully suppressed the recipient's immune system, blunting the alloimmune pathway (28, 29). This was evident by decreased Kupffer and endothelial cell activation induced by initial anti-oxidative effects and damage-associated molecular pattern (DAMP) release as a consequence of HOPE treatment and liver Tx (28). Furthermore, T cell infiltration in liver grafts as well as blood levels of circulating activated T cells decreased (28). A short time (1 h) of reconditioning of DCD rat and porcine livers using HMP after up to 16 h of SCS showed improvements in organ quality (58, 64). Long-term (24 h) HMP of DCD rat livers markedly reduced HLA class II antigen expression on post-sinusoidal venular endothelium compared to SCS (55). Bae et al. (33) found that supplementation of HMP perfusion solution with the antioxidant, vitamin E, reduced inflammatory cytokine levels [IL-6, TNF-α, and monocyte chemoattractant protein (MCP)-1], involved in alloimmune response, in graft tissue. The addition of metformin to HMP preservation solution reduced liver IRI, with significant protective effects on livers, especially in aged rats (69). Furthermore, HMP significantly reduced pro-inflammatory cytokine expression (TNF-α, IL-1β, and IL-8) (34). The attenuation of those cytokines affects many downstream pathways, including a reduced expression of chemokines and adhesion molecules such as intercellular adhesion molecule (ICAM)-1, MCP-1, P-selectin, and others. This effect subsequently decreases the level of neutrophil activation and inevitable leukocyte migration to stressed cell sites, leading to improved overall outcome rates in human livers (34). In another study, HOPE protected DCD livers from initial IRI, leading to improved graft function preventing intrahepatic biliary complications; however, acute rejection rate remained similar (16 vs. 12%) when compared to SCS (75).
Subnormothermic/Normothermic Machine Perfusion Techniques
SNMP and NMP significantly ameliorated hepatic damage in DCD livers compared to SCS in animal models (31, 39, 60, 65, 68, 72). In a porcine model of liver MP, prolonged periods of NMP facilitate a reduction in hepatic steatosis (63), while the supplementation of perfusate with defatting agents significantly reduced the intracellular fat content of perfused rat livers within a few hours (59). Efficient vasodilation was found to be important in order to improve the effectiveness in the preservation of DCD livers during NMP (66). Olschewski et al. (60) compared HMP to SNMP and SCS, demonstrating beneficial effects on the initial organ function, structural integrity of the sinusoidal endothelium, and hepatocellular damage when DCD rat livers were perfused using SNMP. Furthermore, SNMP was associated with lower IRI when compared to SCS (74), while prolonged NMP additionally eliminated the neutrophil infiltrate in grafts (76). Another study of ECD livers showed superiority of COR over HMP, SNMP, and SCS (38). When comparing NMP to SNMP and SCS, NMP was most efficient in terms of recovery of DCD livers (67). Avoiding initial hypothermia did not improve liver graft quality in a porcine DCD model of NMP (73). Recently, the first randomized controlled trial showed a 50% reduction in liver graft injury, despite a 50% decrease in the number of discarded organs and a 54% increased mean preservation time after a period of NMP compared to SCS (~36% of grafts were DCD). However, they found no significant difference in bile duct complications, graft, or patient survival (78).
The currently ongoing VITTAL trial aims to improve the suitability of non-transplantable livers in the UK by monitoring their function during NMP followed by Tx of the sufficiently improved graft (79, 80). We expect that the results of this novel approach could improve consistency and increase the usage of ECD liver grafts without compromising recipient safety.
Machine Perfusion of Extended Criteria Donor Lung Grafts
Experimental and clinical studies of ECD lungs and MP are compiled in Table 3.
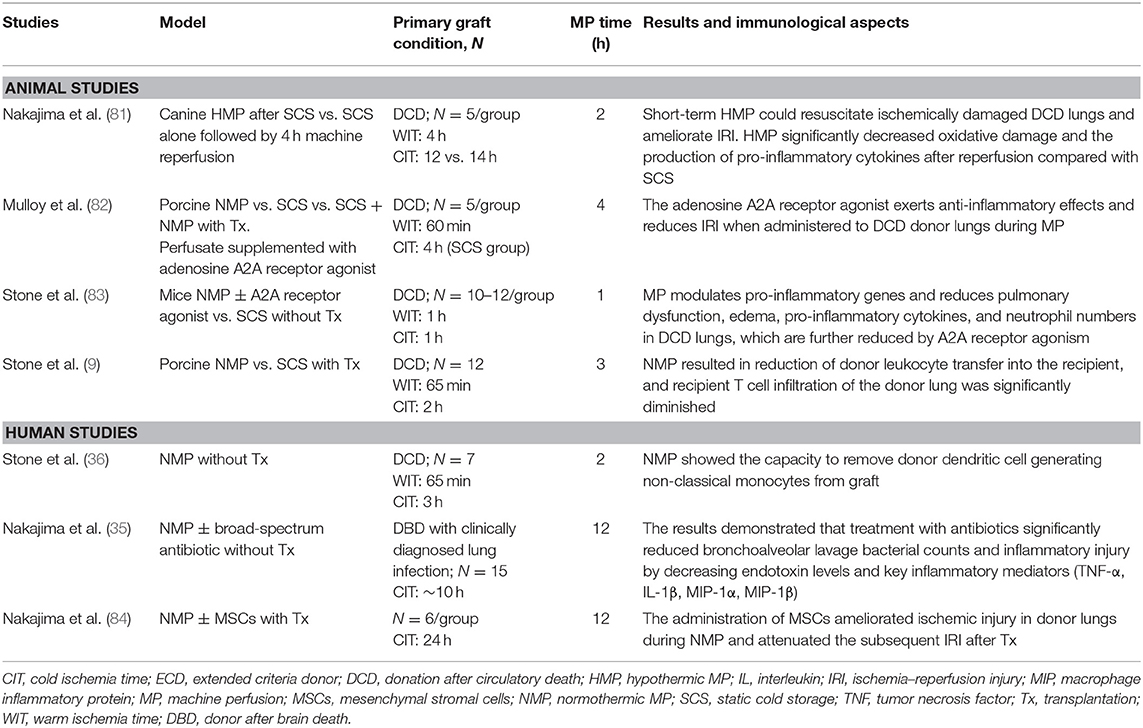
Table 3. Experimental and clinical studies of machine perfusion of extended criteria donor lung grafts.
Hypothermic Machine Perfusion Techniques
Short-term HMP could resuscitate ischemically damaged DCD lungs and ameliorate IRI. In a canine model of MP, HMP improved the ATP production by the mitochondrial electron transport chain, leading to a significant decrease in oxidative damage and production of pro-inflammatory cytokines (IL-6 and TNF-α) after reperfusion compared to SCS (81). Moreover, short-term HMP washed out residual microthrombi in the donor lungs. All of those factors are important for Tx outcomes, including the reduction of the immunological rejection rate.
Normothermic Machine Perfusion Techniques
NMP was able to modulate pro-inflammatory gene expression and reduce pulmonary dysfunction, edema, pro-inflammatory cytokines, and the number of neutrophils in animal DCD lungs (82, 83). Moreover, NMP resulted in reduced donor leukocyte transfer into the recipient by inducing mobilization of donor leukocytes into the perfusate and allowing their removal via the leukocyte filter prior to Tx (9). Therefore, reduced donor leukocyte migration to recipient lymph nodes resulted in a reduction of direct allorecognition and T cell priming, diminishing recipient T cell infiltration, the hallmark of acute rejection (9). In a clinical study, NMP showed the capacity to remove donor dendritic cells generating non-classical monocytes, which are directly involved in immune surveillance, from the graft (36). NMP of donor after brain death (DBD) lungs with clinically diagnosed infection significantly reduced bacterial counts in the fluid of the bronchoalveolar lavage and inflammatory injury by decreasing endotoxin levels and key inflammatory mediators [TNF-α, IL-1β, macrophage inflammatory protein (MIP)-1α, MIP-1β] when combined with broad-spectrum antibiotic treatment (35). The administration of mesenchymal stromal cells (MSCs) ameliorated ischemic injury in donor lungs during ex vivo NMP and attenuated the subsequent IRI after Tx (84).
The use of MP in reconditioning of ECD donor lungs for Tx is currently under investigation in clinical trials (85, 86), with results being expected soon.
Machine Perfusion of Extended Criteria Donor Heart Grafts
Currently, clinical evidence of MP in ECD heart grafts is limited (Table 4). HMP improved the preservation of DCD heart grafts compared to SCS proven by superior post-reperfusion contractility. The underlying mechanisms could include enhanced preservation of the energetic states and superior cellular integrity (87). Recently, Korkmaz-Icöz et al. (88) demonstrated that HMP of aged donor hearts with MSCs protected against myocardial IRI in a rat model.
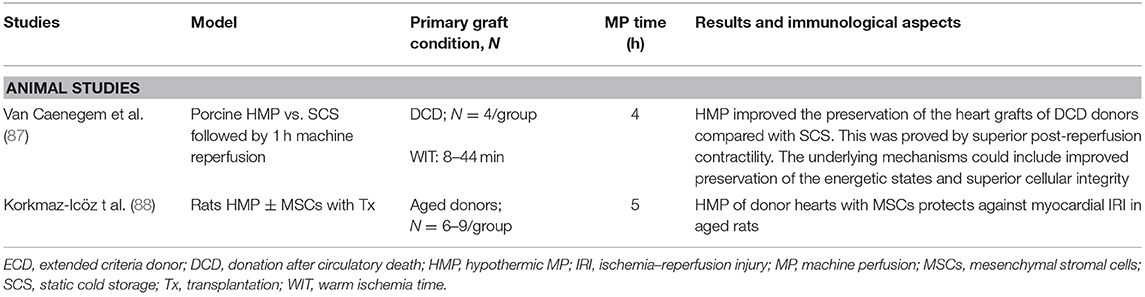
Table 4. Experimental and clinical studies of machine perfusion of extended criteria donor heart grafts.
Machine Perfusion of Extended Criteria Donor Pancreas Grafts
There is a limited number of studies evaluating the safety and feasibility of ex situ MP for ECD pancreas graft for whole-organ Tx (Table 5). HMP of porcine DCD pancreas was associated with a reduction in islet and acinar cell damage, stable perfusion dynamics, and minimal edematous weight change as well as potentially ameliorated endocrine viability and functionality after preservation (89, 90). More recent studies in the human pancreas indicated that especially DCD pancreas benefits more from oxygenated HMP compared to SCS alone (91). Even 24 h of HMP of ECD human pancreas–duodenum organs was feasible resulting in no deleterious parenchymal effects (92). Since those studies focused on the results after MP without following Tx, currently, there are no data available about clinical outcomes in this context.
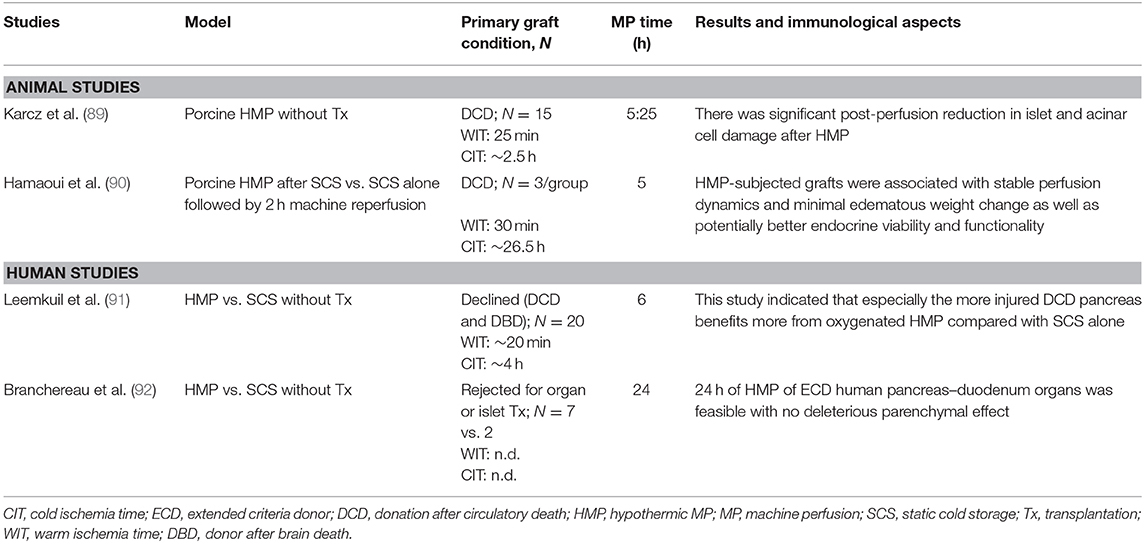
Table 5. Experimental and clinical studies of machine perfusion of extended criteria donor pancreas grafts.
Conclusion
MP allows successful utilization of more vulnerable and immunogenic otherwise discarded ECD organs. It has been shown that MP not only reduces the levels of pro-inflammatory cytokines and positively influences gene expression related to hypoxia during reperfusion but also induces donor-derived leukocytes, including dendritic cell-generating non-classical monocytes, mobilization, and removal prior to Tx. Moreover, MP was able to protect against epithelial and Kupffer cell activation and to reduce recipient T cell infiltration of the donor graft. More recently, novel methods such as viral vector delivery during MP to allografts are under investigation (93). This biological modification of the graft prior to Tx may be a future therapeutic strategy to suppress the immune response against the allograft leading to Tx without or at least reduced dose of the systemic immunosuppression that carries the additional risk of infection and malignancy. Many studies have already shown superiority of ECD organ MP over the current standard SCS. However, there are no general agreements on MP protocols, and wider clinical application is limited due to the lack of randomized controlled trials. More trials focusing on immunological pathways in the different MP settings with respect to every single organ are mandatory to get detailed mechanistic insights. This knowledge about various pathways will help us to optimize organ quality after MP of ECD organs and therefore improve Tx outcomes as well as graft and patient survival.
Author Contributions
All authors listed have made a substantial, direct and intellectual contribution to the work, and approved it for publication.
Conflict of Interest
The authors declare that the research was conducted in the absence of any commercial or financial relationships that could be construed as a potential conflict of interest.
References
1. Stone JP, Ball AL, Critchley WR, Major T, Edge RJ, Amin K, et al. Ex vivo normothermic perfusion induces donor-derived leukocyte mobilization and removal prior to renal jation. Kidney Int Rep. (2016) 1:230:9. doi: 10.1016/j.ekir.2016.07.009
2. Dziodzio T, Biebl M, Pratschke J. Impact of brain death on ischemia/reperfusion injury in liver transplantation. Curr Opin Organ Transplant. (2014) 19:108:14. doi: 10.1097/MOT.0000000000000061
3. Jaeschke H. Preservation injury: mechanisms, prevention and consequences. J Hepatol. (1996) 25:774:80. doi: 10.1016/S0168-8278(96)80253-4
4. Zhao H, Alam A, Soo AP, George AJT, Ma D. Ischemia-reperfusion injury reduces long term renal graft survival: mechanism and beyond. EBioMedicine. (2018) 28:31-42. doi: 10.1016/j.ebiom.2018.01.025
5. Uehara M, Bahmani B, Jiang L, Jung S, Banouni N, Kasinath V, et al. Nanodelivery of mycophenolate mofetil to the organ improves transplant vasculopathy. ACS Nano. (2019) 13:12393-407. doi: 10.1021/acsnano.9b05115
6. Boardman DA, Jacob J, Smyth LA, Lombardi G, Lechler RI. What is direct allorecognition? Curr Transplant Rep. (2016) 3:275:83. doi: 10.1007/s40472-016-0115-8
7. Siu JHY, Surendrakumar V, Richards JA, Pettigrew GJ. T cell allorecognition pathways in solid organ transplantation. Front Immunol. (2018) 9:2548. doi: 10.3389/fimmu.2018.02548
8. DeWolf S, Sykes M. Alloimmune T cells in transplantation. J Clin Invest. (2017) 127:2473:81. doi: 10.1172/JCI90595
9. Stone JP, Critchley WR, Major T, Rajan G, Risnes I, Scott H, et al. Altered immunogenicity of donor lungs via removal of passenger leukocytes using Ex vivo lung perfusion. Am J Transplant. (2016) 16:33:43. doi: 10.1111/ajt.13446
10. Jing L, Yao L, Zhao M, Peng LP, Liu M. Organ preservation: from the past to the future. Acta Pharmacol Sin. (2018) 39:845:57. doi: 10.1038/aps.2017.182
11. Tingle SJ, Figueiredo RS, Moir JAG, Goodfellow M, Talbot D, Wilson CH. Machine perfusion preservation versus static cold storage for deceased donor kidney transplantation. Cochrane Database Syst Rev. (2019) 2019:CD011671. doi: 10.1002/14651858.CD011671.pub2
12. Czigany Z, Lurje I, Tolba RH, Neumann UP, Tacke F, Lurje G. Machine perfusion for liver transplantation in the era of marginal organs-New kids on the block. Liver Int. (2019) 39:228:49. doi: 10.1111/liv.13946
13. Jiao B, Liu S, Liu H, Cheng D, Cheng Y, Liu Y. Hypothermic machine perfusion reduces delayed graft function and improves one-year graft survival of kidneys from expanded criteria donors: a meta-analysis. PLoS ONE. (2013) 8:e81826. doi: 10.1371/journal.pone.0081826
14. Cannon RM, Brock GN, Garrison RN, Smith JW, Marvin MR, Franklin GA. To pump or not to pump: a comparison of machine perfusion vs cold storage for deceased donor kidney transplantation. J Am Coll Surg. (2013) 216:625:33, discussion: 33-4. doi: 10.1016/j.jamcollsurg.2012.12.025
15. Moers C, Pirenne J, Paul A, Ploeg RJ. Machine perfusion or cold storage in deceased-donor kidney transplantation. N Engl J Med. (2012) 366:770:1. doi: 10.1056/NEJMc1111038
16. Tittelbach-Helmrich D, Thurow C, Arwinski S, Schleicher C, Hopt UT, Bausch D, et al. Poor organ quality and donor-recipient age mismatch rather than poor donation rates account for the decrease in deceased kidney transplantation rates in a Germany Transplant Center. Transpl Int. (2015) 28:191:8. doi: 10.1111/tri.12478
17. Aubert O, Kamar N, Vernerey D, Viglietti D, Martinez F, Duong-Van-Huyen JP, et al. Long term outcomes of transplantation using kidneys from expanded criteria donors: prospective, population based cohort study. BMJ. (2015) 351:h3557. doi: 10.1136/bmj.h3557
18. Echterdiek F, Schwenger V, Döhler B, Latus J, Kitterer D, Heemann U, et al. Kidneys from elderly deceased donors-Is 70 the new 60? Front Immunol. (2019) 10:2701. doi: 10.3389/fimmu.2019.02701
19. Pratschke J, Merk V, Reutzel-Selke A, Pascher A, Denecke C, Lun A, et al. Potent early immune response after kidney transplantation in patients of the European senior transplant program. Transplantation. (2009) 87:992:1000. doi: 10.1097/TP.0b013e31819ca0d7
20. Filiopoulos V, Boletis JN. Renal transplantation with expanded criteria donors: which is the optimal immunosuppression? World J Transplant. (2016) 6:103:14. doi: 10.5500/wjt.v6.i1.103
21. Reutzel-Selke A, Jurisch A, Denecke C, Pascher A, Martins PN, Kessler H, et al. Donor age intensifies the early immune response after transplantation. Kidney Int. (2007) 71:629:36. doi: 10.1038/sj.ki.5002098
22. Snell G, Hiho S, Levvey B, Sullivan L, Westall G. Consequences of donor-derived passengers (pathogens, cells, biological molecules and proteins) on clinical outcomes. J Heart Lung Transplant. (2019) 38:902:6. doi: 10.1016/j.healun.2019.06.019
23. Maggiore U, Oberbauer R, Pascual J, Viklicky O, Dudley C, Budde K, et al. Strategies to increase the donor pool and access to kidney transplantation: an international perspective. Nephrol Dial Transplant. (2015) 30:217:22. doi: 10.1093/ndt/gfu212
24. Attia M, Silva MA, Mirza DF. The marginal liver donor–an update. Transpl Int. (2008) 21:713:24. doi: 10.1111/j.1432-2277.2008.00696.x
25. Farney AC, Hines MH, al-Geizawi S, Rogers J, Stratta RJ. Lessons learned from a single center's experience with 134 donation after cardiac death donor kidney transplants. J Am Coll Surg. (2011) 212:440:51, discussion: 51-3. doi: 10.1016/j.jamcollsurg.2010.12.033
26. Ritschl PV, Gunther J, Hofhansel L, Kuhl AA, Sattler A, Ernst S, et al. Graft pre-conditioning by peri-operative perfusion of kidney allografts with rabbit anti-human T-lymphocyte globulin results in improved kidney graft function in the early post-transplantation period-a prospective, randomized placebo-controlled trial. Front Immunol. (2018) 9:1911. doi: 10.3389/fimmu.2018.01911
27. Vaziri N, Thuillier R, Favreau FD, Eugene M, Milin S, Chatauret NP, et al. Analysis of machine perfusion benefits in kidney grafts: a preclinical study. J Transl Med. (2011) 9:15. doi: 10.1186/1479-5876-9-15
28. Schlegel A, Kron P, Graf R, Clavien PA, Dutkowski P. Hypothermic Oxygenated Perfusion (HOPE) downregulates the immune response in a rat model of liver transplantation. Ann Surg. (2014) 260:931:7, discussion: 7-8. doi: 10.1097/SLA.0000000000000941
29. Schlegel A, Graf R, Clavien PA, Dutkowski P. Hypothermic oxygenated perfusion (HOPE) protects from biliary injury in a rodent model of DCD liver transplantation. J Hepatol. (2013) 59:984:91. doi: 10.1016/j.jhep.2013.06.022
30. Ferrigno A, Rizzo V, Boncompagni E, Bianchi A, Gringeri E, Neri D, et al. Machine perfusion at 20 degrees C reduces preservation damage to livers from non-heart beating donors. Cryobiology. (2011) 62:152:8. doi: 10.1016/j.cryobiol.2011.02.004
31. Gringeri E, Bonsignore P, Bassi D, D'Amico FE, Mescoli C, Polacco M, et al. Subnormothermic machine perfusion for non-heart-beating donor liver grafts preservation in a Swine model: a new strategy to increase the donor pool? Transplant Proc. (2012) 44:2026:8. doi: 10.1016/j.transproceed.2012.06.014
32. Tozzi M, Franchin M, Soldini G, Ietto G, Chiappa C, Maritan E, et al. Impact of static cold storage VS hypothermic machine preservation on ischemic kidney graft: inflammatory cytokines and adhesion molecules as markers of ischemia/reperfusion tissue damage. Our preliminary results. Int J Surg. (2013) 11:S110-4. doi: 10.1016/S1743-9191(13)60029-1
33. Bae C, Pichardo EM, Huang H, Henry SD, Guarrera JV. The benefits of hypothermic machine perfusion are enhanced with Vasosol and alpha-tocopherol in rodent donation after cardiac death livers. Transplant Proc. (2014) 46:1560:6. doi: 10.1016/j.transproceed.2013.12.050
34. Henry SD, Nachber E, Tulipan J, Stone J, Bae C, Reznik L, et al. Hypothermic machine preservation reduces molecular markers of ischemia/reperfusion injury in human liver transplantation. Am J Transplant. (2012) 12:2477:86. doi: 10.1111/j.1600-6143.2012.04086.x
35. Nakajima D, Cypel M, Bonato R, Machuca TN, Iskender I, Hashimoto K, et al. Ex vivo perfusion treatment of infection in human donor lungs. Am J Transplant. (2016) 16:1229:37. doi: 10.1111/ajt.13562
36. Stone JP, Sevenoaks H, Sjoberg T, Steen S, Yonan N, Fildes JE. Mechanical removal of dendritic cell-generating non-classical monocytes via Ex vivo lung perfusion. J Heart Lung Transplant. (2014) 33:864:9. doi: 10.1016/j.healun.2014.03.005
37. Bian S, Zhu Z, Sun L, Wei L, Qu W, Zeng Z, et al. Normothermic machine perfusion versus cold storage of liver in pig model: a meta-analysis. Ann Transpl. (2018) 23:197-206. doi: 10.12659/AOT.908774
38. Minor T, Efferz P, Fox M, Wohlschlaeger J, Lüer B. Controlled oxygenated rewarming of cold stored liver grafts by thermally graduated machine perfusion prior to reperfusion. Am J Transpl. (2013) 13:1450:60. doi: 10.1111/ajt.12235
39. Knaak JM, Spetzler VN, Goldaracena N, Louis KS, Selzner N, Selzner M. Technique of subnormothermic Ex vivo liver perfusion for the storage, assessment, and repair of marginal liver grafts. J Vis Exp. (2014) 90:e51419. doi: 10.3791/51419
40. Treckmann J, Nagelschmidt M, Minor T, Saner F, Saad S, Paul A. Function and quality of kidneys after cold storage, machine perfusion, or retrograde oxygen persufflation: results from a porcine autotransplantation model. Cryobiology. (2009) 59:19:23. doi: 10.1016/j.cryobiol.2009.03.004
41. Thuillier R, Allain G, Celhay O, Hebrard W, Barrou B, Badet L, et al. Benefits of active oxygenation during hypothermic machine perfusion of kidneys in a preclinical model of deceased after cardiac death donors. J Surg Res. (2013) 184:1174:81. doi: 10.1016/j.jss.2013.04.071
42. Kasil A, Giraud S, Couturier P, Amiri A, Danion J, Donatini G, et al. Individual and combined impact of oxygen and oxygen transporter supplementation during kidney machine preservation in a porcine preclinical kidney transplantation model. Int J Mol Sci. (2019) 20:1992. doi: 10.3390/ijms20081992
43. Reznik ON, Bagnenko SF, Loginov IV, Iljina VA, Ananyev AN, Eremich SV, et al. Machine perfusion as a tool to select kidneys recovered from uncontrolled donors after cardiac death. Transplant Proc. (2008) 40:1023:6. doi: 10.1016/j.transproceed.2008.03.052
44. Treckmann J, Moers C, Smits JM, Gallinat A, Maathuis MH, van Kasterop-Kutz M, et al. Machine perfusion versus cold storage for preservation of kidneys from expanded criteria donors after brain death. Transpl Int. (2011) 24:548:54. doi: 10.1111/j.1432-2277.2011.01232.x
45. Nicholson ML, Hosgood SA. Renal transplantation after Ex vivo normothermic perfusion: the first clinical study. Am J Transplant. (2013) 13:1246:52. doi: 10.1111/ajt.12179
46. Wszola M, Kwiatkowski A, Domagala P, Wirkowska A, Bieniasz M, Diuwe P, et al. Preservation of kidneys by machine perfusion influences gene expression and may limit ischemia/reperfusion injury. Prog Transplant. (2014) 24:19:26. doi: 10.7182/pit2014384
47. Wang W, Xie D, Hu X, Yin H, Liu H, Zhang X. Effect of hypothermic machine perfusion on the preservation of kidneys donated after cardiac death: a single-center, randomized, controlled trial. Artif Organs. (2017) 41:753:8. doi: 10.1111/aor.12836
48. Gallinat A, Amrillaeva V, Hoyer DP, Kocabayoglu P, Benko T, Treckmann JW, et al. Reconditioning by end-ischemic hypothermic in-house machine perfusion: a promising strategy to improve outcome in expanded criteria donors kidney transplantation. Clin Transplant. (2017) 31:e12904. doi: 10.1111/ctr.12904
49. Weissenbacher A, Lo Faro L, Boubriak O, Soares MF, Roberts IS, Hunter JP, et al. Twenty-four-hour normothermic perfusion of discarded human kidneys with urine recirculation. Am J Transplant. (2019) 19:178:92. doi: 10.1111/ajt.14932
50. Ruiz-Hernandez M, Gomez-Dos Santos V, Diaz-Perez D, Fernandez-Alcalde A, Hevia-Palacios V, Alvarez-Rodriguez S, et al. Experience with hypothermic machine perfusion in expanded criteria donors: functional outcomes. Transplant Proc. (2019) 51:303:6. doi: 10.1016/j.transproceed.2018.09.020
51. Savoye E, Macher MA, Videcoq M, Gatault P, Hazzan M, Abboud I, et al. Evaluation of outcomes in renal transplantation with hypothermic machine perfusion for the preservation of kidneys from expanded criteria donors. Clin Transplant. (2019) 33:e13536. doi: 10.1111/ctr.13536
52. Kalluri R, Neilson EG. Epithelial-mesenchymal transition and its implications for fibrosis. J Clin Investig. (2003) 112:1776:84. doi: 10.1172/JCI200320530
53. Kahn J, Schemmer P. Control of ischemia-reperfusion injury in liver transplantation: potentials for increasing the donor pool. Visc Med. (2018) 34:444:8. doi: 10.1159/000493889
54. Lee CY, Zhang JX, Jones JWJ, Southard JH, Clemens MG. Functional recovery of preserved livers following warm ischemia: improvement by machine perfusion preservation. Transplantation. (2002) 74:944:51. doi: 10.1097/00007890-200210150-00008
55. Lauschke H, Olschewski P, Tolba R, Schulz S, Minor T. Oxygenated machine perfusion mitigates surface antigen expression and improves preservation of predamaged donor livers. Cryobiology. (2003) 46:53:60. doi: 10.1016/S0011-2240(02)00164-5
56. Lee CY, Jain S, Duncan HM, Zhang JX, Jones JW Jr, Southard JH, et al. Survival transplantation of preserved non-heart-beating donor rat livers: preservation by hypothermic machine perfusion. Transplantation. (2003) 76:1432:6. doi: 10.1097/01.TP.0000088674.23805.0F
57. Bessems M, Doorschodt BM, van Vliet AK, van Gulik TM. Machine perfusion preservation of the non-heart-beating donor rat livers using polysol, a new preservation solution. Transplant Proc. (2005) 37:326:8. doi: 10.1016/j.transproceed.2005.01.039
58. Manekeller S, Seinsche A, Stegemann J, Hirner A. Optimising post-conditioning time of marginal donor livers. Langenbecks Arch Surg. (2008) 393:311:6. doi: 10.1007/s00423-008-0288-4
59. Nagrath D, Xu H, Tanimura Y, Zuo R, Berthiaume F, Avila M, et al. Metabolic preconditioning of donor organs: defatting fatty livers by normothermic perfusion ex vivo. Metab Eng. (2009) 11:274-83. doi: 10.1016/j.ymben.2009.05.005
60. Olschewski P, Gass P, Ariyakhagorn V, Jasse K, Hunold G, Menzel M, et al. The influence of storage temperature during machine perfusion on preservation quality of marginal donor livers. Cryobiology. (2010) 60:337:43. doi: 10.1016/j.cryobiol.2010.03.005
61. Stegemann J, Hirner A, Rauen U, Minor T. Use of a new modified HTK solution for machine preservation of marginal liver grafts. J Surg Res. (2010) 160:155:62. doi: 10.1016/j.jss.2008.10.021
62. Stegemann J, Hirner A, Rauen U, Minor T. Gaseous oxygen persufflation or oxygenated machine perfusion with Custodiol-N for long-term preservation of ischemic rat livers? Cryobiology. (2009) 58:45:51. doi: 10.1016/j.cryobiol.2008.10.127
63. Jamieson RW, Zilvetti M, Roy D, Hughes D, Morovat A, Coussios CC, et al. Hepatic steatosis and normothermic perfusion-preliminary experiments in a porcine model. Transplantation. (2011) 92:289:95. doi: 10.1097/TP.0b013e318223d817
64. Schlegel A, de Rougemont O, Graf R, Clavien PA, Dutkowski P. Protective mechanisms of end-ischemic cold machine perfusion in DCD liver grafts. J Hepatol. (2013) 58:278:86. doi: 10.1016/j.jhep.2012.10.004
65. Izamis ML, Tolboom H, Uygun B, Berthiaume F, Yarmush ML, Uygun K. Resuscitation of ischemic donor livers with normothermic machine perfusion: a metabolic flux analysis of treatment in rats. PLoS ONE. (2013) 8:e69758. doi: 10.1371/journal.pone.0069758
66. Nassar A, Liu Q, Farias K, D'Amico G, Buccini L, Urcuyo D, et al. Role of vasodilation during normothermic machine perfusion of DCD porcine livers. Int J Artif Organs. (2014) 37:165:72. doi: 10.5301/ijao.5000297
67. Nassar A, Liu Q, Farias K, Buccini L, Baldwin W, Bennett A, et al. Impact of temperature on porcine liver machine perfusion from donors after cardiac death. Artif Organs. (2016) 40:999:1008. doi: 10.1111/aor.12699
68. Ferrigno A, Di Pasqua LG, Berardo C, Siciliano V, Rizzo V, Mannucci B, et al. Liver graft susceptibility during static cold storage and dynamic machine perfusion: DCD versus fatty livers. Int J Mol Sci. (2017) 19:109. doi: 10.3390/ijms19010109
69. Chai YC, Dang GX, He HQ, Shi JH, Zhang HK, Zhang RT, et al. Hypothermic machine perfusion with metformin-University of Wisconsin solution for Ex vivo preservation of standard and marginal liver grafts in a rat model. World J Gastroenterol. (2017) 23:7221:31. doi: 10.3748/wjg.v23.i40.7221
70. Kron P, Schlegel A, Mancina L, Clavien PA, Dutkowski P. Hypothermic oxygenated perfusion (HOPE) for fatty liver grafts in rats and humans. J Hepatol. (2017) 68:82–91. doi: 10.1016/j.jhep.2017.08.028
71. Compagnon P, Levesque E, Hentati H, Disabato M, Calderaro J, Feray C, et al. An oxygenated and transportable machine perfusion system fully rescues liver grafts exposed to lethal ischemic damage in a pig model of DCD liver transplantation. Transplantation. (2017) 101:e205-13. doi: 10.1097/TP.0000000000001764
72. Kakizaki Y, Miyagi S, Shimizu K, Kumata H, Matsumura M, Miyazaki Y, et al. Effects of subnormothermic perfusion before transplantation for liver grafts from donation after cardiac death: a simplified dripping perfusion method in pigs. Transplant Proc. (2018) 50:1538:43. doi: 10.1016/j.transproceed.2018.02.061
73. Nostedt JJ, Churchill T, Ghosh S, Thiesen A, Hopkins J, Lees MC, et al. Avoiding initial hypothermia does not improve liver graft quality in a porcine donation after circulatory death (DCD) model of normothermic perfusion. PLoS ONE. (2019) 14:e0220786. doi: 10.1371/journal.pone.0220786
74. Bruinsma BG, Yeh H, Ozer S, Martins PN, Farmer A, Wu W, et al. Subnormothermic machine perfusion for Ex vivo preservation and recovery of the human liver for transplantation. Am J Transplant. (2014) 14:1400:9. doi: 10.1111/ajt.12727
75. Dutkowski P, Polak WG, Muiesan P, Schlegel A, Verhoeven CJ, Scalera I, et al. First comparison of hypothermic oxygenated perfusion versus static cold storage of human donation after cardiac death liver transplants: an international-matched case analysis. Ann Surg. (2015) 262:764:70, discussion 70-1. doi: 10.1097/SLA.0000000000001473
76. Vogel T, Brockmann JG, Quaglia A, Morovat A, Jassem W, Heaton ND, et al. The 24-hour normothermic machine perfusion of discarded human liver grafts. Liver Transpl. (2017) 23:207:20. doi: 10.1002/lt.24672
77. Laing RW, Bhogal RH, Wallace L, Boteon Y, Neil DAH, Smith A, et al. The use of an acellular oxygen carrier in a human liver model of normothermic machine perfusion. Transplantation. (2017) 101:2746:56. doi: 10.1097/TP.0000000000001821
78. Nasralla D, Coussios CC, Mergental H, Akhtar MZ, Butler AJ, Ceresa CDL, et al. A randomized trial of normothermic preservation in liver transplantation. Nature. (2018) 557:50:6. doi: 10.1038/s41586-018-0047-9
79. Laing RW, Mergental H, Yap C, Kirkham A, Whilku M, Barton D, et al. Viability testing and transplantation of marginal livers (VITTAL) using normothermic machine perfusion: study protocol for an open-label, non-randomised, prospective, single-arm trial. BMJ Open. (2017) 7:e017733. doi: 10.1136/bmjopen-2017-017733
80. Transporter LL (2019). Available online at: https://www.organ-recovery.com/lifeport-liver-transporter (accessed October 1, 2019).
81. Nakajima D, Chen F, Okita K, Motoyama H, Hijiya K, Ohsumi A, et al. Reconditioning lungs donated after cardiac death using short-term hypothermic machine perfusion. Transplantation. (2012) 94:999:1004. doi: 10.1097/TP.0b013e31826f632e
82. Mulloy DP, Stone ML, Crosby IK, Lapar DJ, Sharma AK, Webb DV, et al. Ex vivo rehabilitation of non-heart-beating donor lungs in preclinical porcine model: delayed perfusion results in superior lung function. J Thorac Cardiovasc Surg. (2012) 144:1208:15. doi: 10.1016/j.jtcvs.2012.07.056
83. Stone ML, Sharma AK, Mas VR, Gehrau RC, Mulloy DP, Zhao Y, et al. Ex vivo perfusion with adenosine A2A receptor agonist enhances rehabilitation of murine donor lungs after circulatory death. Transplantation. (2015) 99:2494:503. doi: 10.1097/TP.0000000000000830
84. Nakajima D, Watanabe Y, Ohsumi A, Pipkin M, Chen M, Mordant P, et al. Mesenchymal stromal cell therapy during Ex vivo lung perfusion ameliorates ischemia-reperfusion injury in lung transplantation. J Heart Lung Transplant. (2019) 38:1214-23. doi: 10.1016/j.healun.2019.07.006
85. Vanecek J. Cellular mechanisms of melatonin action. Physiol Rev. (1998) 78:687:721. doi: 10.1152/physrev.1998.78.3.687
86. Novel, Lung Trial: Normothermic Ex Vivo Lung Perfusion (EVLP) As An Assessment of Extended/Marginal Donor Lungs,. Available online at: https://www.clinicaltrials.gov/ct2/show/NCT01365429 (accessed October 1, 2019).
87. Van Caenegem O, Beauloye C, Bertrand L, Horman S, Lepropre S, Sparavier G, et al. Hypothermic continuous machine perfusion enables preservation of energy charge and functional recovery of heart grafts in an Ex vivo model of donation following circulatory death. Eur J Cardiothorac Surg. (2016) 49:1348:53. doi: 10.1093/ejcts/ezv409
88. Korkmaz-Icöoz S, Li S, Huüttner R, Ruppert M, Radovits T, Loganathan S, et al. Hypothermic perfusion of donor heart with a preservation solution supplemented by mesenchymal stem cells. J Heart Lung Transplant. (2019) 38:315:26. doi: 10.1016/j.healun.2018.12.003
89. Karcz M, Cook HT, Sibbons P, Gray C, Dorling A, Papalois V. An ex-vivo model for hypothermic pulsatile perfusion of porcine pancreata: hemodynamic and morphologic characteristics. Exp Clin Transplant. (2010) 8:55:60.
90. Hamaoui K, Gowers S, Sandhu B, Vallant N, Cook T, Boutelle M, et al. Development of pancreatic machine perfusion: translational steps from porcine to human models. J Surg Res. (2018) 223:263-4. doi: 10.1016/j.jss.2017.11.052
91. Leemkuil M, Lier G, Engelse MA, Ploeg RJ, de Koning EJP, t Hart NA, et al. Hypothermic oxygenated machine perfusion of the human donor pancreas. Transplant Direct. (2018) 4:e388. doi: 10.1097/TXD.0000000000000829
92. Branchereau J, Renaudin K, Kervella D, Bernadet S, Karam G, Blancho G, et al. Hypothermic pulsatile perfusion of human pancreas: preliminary technical feasibility study based on histology. Cryobiology. (2018) 85:56-62. doi: 10.1016/j.cryobiol.2018.10.002
Keywords: extended criteria donors, immunological rejection, machine perfusion, marginal organs, transplantation
Citation: Kvietkauskas M, Leber B, Strupas K, Stiegler P and Schemmer P (2020) Machine Perfusion of Extended Criteria Donor Organs: Immunological Aspects. Front. Immunol. 11:192. doi: 10.3389/fimmu.2020.00192
Received: 13 October 2019; Accepted: 24 January 2020;
Published: 27 February 2020.
Edited by:
Martin Johannes Hoogduijn, Erasmus University Rotterdam, NetherlandsReviewed by:
Sarah Hosgood, University of Cambridge, United KingdomCyril Moers, University Medical Center Groningen, Netherlands
Copyright © 2020 Kvietkauskas, Leber, Strupas, Stiegler and Schemmer. This is an open-access article distributed under the terms of the Creative Commons Attribution License (CC BY). The use, distribution or reproduction in other forums is permitted, provided the original author(s) and the copyright owner(s) are credited and that the original publication in this journal is cited, in accordance with accepted academic practice. No use, distribution or reproduction is permitted which does not comply with these terms.
*Correspondence: Peter Schemmer, cGV0ZXIuc2NoZW1tZXImI3gwMDA0MDttZWR1bmlncmF6LmF0