- 1Laboratory of Virology, “Lazzaro Spallanzani” National Institute for Infectious Diseases, IRCCS, Rome, Italy
- 2Department of Biology and Biotechnology “C. Darwin,” Sapienza University, Rome, Italy
Human papillomavirus (HPV) is the most common sexually transmitted virus. The high-risk HPV types (i.e., HPV16, 18, 31, 33, 35, 39, 45, 51, 52, 56, 58, 59) are considered to be the main etiological agents of genital tract cancers, such as cervical, vulvar, vaginal, penile, and anal cancers, and of a subset of head and neck cancers. Three prophylactic HPV vaccines are available that are bivalent (vs. HPV16, 18), tetravalent (vs. HPV6, 11, 16, 18), and non-avalent (vs. HPV6, 11, 16, 18, 31, 33,45, 52, 58). All of these vaccines are based on recombinant DNA technology, and they are prepared from the purified L1 protein that self-assembles to form the HPV type-specific empty shells (i.e., virus-like particles). These vaccines are highly immunogenic and induce specific antibodies. Therapeutic vaccines differ from prophylactic vaccines, as they are designed to generate cell-mediated immunity against transformed cells, rather than neutralizing antibodies. Among the HPV proteins, the E6 and E7 oncoproteins are considered almost ideal as targets for immunotherapy of cervical cancer, as they are essential for the onset and evolution of malignancy and are constitutively expressed in both premalignant and invasive lesions. Several strategies have been investigated for HPV therapeutic vaccines designed to enhance CD4+ and CD8+ T-cell responses, including genetic vaccines (i.e., DNA/ RNA/virus/ bacterial), and protein-based, peptide-based or dendritic-cell-based vaccines. However, no vaccine has yet been licensed for therapeutic use. Several studies have suggested that administration of prophylactic vaccines immediately after surgical treatment of CIN2 cervical lesions can be considered as an adjuvant to prevent reactivation or reinfection, and other studies have described the relevance of prophylactic vaccines in the management of genital warts. This review summarizes the leading features of therapeutic vaccines, which mainly target the early oncoproteins E6 and E7, and prophylactic vaccines, which are based on the L1 capsid protein. Through an analysis of the specific immunogenic properties of these two types of vaccines, we discuss why and how prophylactic vaccines can be effective in the treatment of HPV-related lesions and relapse.
Introduction
Despite the introduction of prophylactic vaccines, the incidence of human papillomavirus (HPV)-related tumors remains high (1), particularly in developing countries of the sub-Saharan region. The current HPV prophylactic vaccines have indications for use in women up to the age of 45 years, but they are predominantly administered to adolescent of 9–15 years. As most cancers develop decades after an initial HPV infection, the impact of this vaccination program will be seen in the long-term. Therefore, setting-up a therapeutic vaccine that can provide results similar to surgical treatment or chemotherapy represents a challenge for the eradication of HPV-induced tumors. However, therapeutic vaccines are not yet available for clinical practice.
Several studies have suggested that administration of HPV prophylactic vaccines after surgical treatment of high-grade cervical intraepithelial neoplasia (CIN2-3) can be considered as an adjuvant to prevent HPV reactivation or reinfection. The relevance of prophylactic vaccines has also been demonstrated in the management of genital warts, although clinical studies have not delivered univocal results to date.
In this review, the HPV-related tumors and the life cycle of HPV are described, to better understand the characteristics of the different viral proteins that are targeted by prophylactic and therapeutic vaccines. Then, we summarize the leading features of prophylactic and therapeutic vaccines that target the L1 and E6, E7 oncoproteins, respectively. Finally, through an analysis of the specific immunogenic properties of these two types of vaccines, we discuss how prophylactic vaccines can be effective for the treatment of HPV-related lesions, and for prevention of HPV-related relapse.
HPV Prevalence
Human papillomavirus is the main agent of sexually transmitted diseases, and it can cause cancer in different anatomical districts with different prevalences (2). The highest proportion of HPV attribution as responsible for a cancer is for the cervix, where >99% of specimens are HPV-positive. In 2012, HPV-invasive cervical cancers reached >500,000 cases, which resulted in ~250,000 deaths around the world1. HPV-related cancers are differently distributed across genders: among women, 8.6% of cancers are linked to HPV infections, while in men, <1% of cancers are attributable to HPV2,3. Differences are also observed in the geographic distributions of invasive cervical cancers: more than 85% occur in developing countries, where cervical screening programs and HPV vaccination campaigns are rarely available4 (3, 4). HPV infection in a cervical site is frequently asymptomatic, and >90% of these resolve within 2 years without medical intervention (5), apparently through rapid immune clearance. However, the protective power of natural anti-HPV antibodies and the duration of immunity after infection are not fully understood. Also, only 50–60% of women show detectable anti-HPV antibodies after infection (6).
There are 15 high-risk (HR)-HPV genotypes that can lead to cancers of the cervix, anus, penis, vagina, vulva, and oropharynx (i.e., HPV16, 18, 3, 33, 35, 39, 45, 51, 52, 56, 58, 68, 73, 82) (7). The relevance of HPV to each of these individual cancers is now considered.
Cervical Cancer
Overall, 90% of cervical cancers are attributed to HR-HPV types. HPV16 and HPV18 are the most prevalent in invasive cervical cancer, where they account for 62.5 and 15.7% of cases, respectively (8). HPV-associated cancers include cervical squamous cell carcinoma (70%), cervical adenocarcinoma (25%), and mixed histology tumors (7)5. An immunocompromised status represents a risk factor for cervical dysplasia, as well as for latent reactivation of HPV at genital sites. Patients with human immunodeficiency virus (HIV) infection have a 5-fold greater risk of acquiring HPV-associated cervical cancer than those without HIV infection. Precancerous (squamous) intraepithelial lesions are categorized as low-grade (LSIL) and high-grade (HSIL) (9).
Anal Cancer
Anal intraepithelial neoplasia (AIN1-3) represents the precursor of invasive anal cancers, where 65% are cervical squamous cell carcinomas. For both sexes, 88–94% of these cancerous lesions are positive for HPV DNA, with HPV16 as the most commonly detected (~87% of HPV-positive tumors), while only 9% of these anal cancers harbors HR-HPV186.
Annually, about 18,000 women are diagnosed with anal cancer worldwide, and this cancer is more frequent in women than in men (10). Furthermore, anal cancer incidence is increasing, which appears to be due to changes in sexual risk factors for HPV transmission (11). Persistent anal HPV infection is the major cause of anal cancer7 (12). Women with a history of cervical cancer and cervical intraepithelial neoplasia grade 3 (HSIL) are also at increased risk of anal cancer. Cervical HR-HPV–positivity is associated with anal HR-HPV prevalence. In a study carried out by Lin, anal HR-HPV prevalence was significantly higher in cervical HR-HPV–positive women (43%) vs. cervical HR-HPV–negative women (9%) (13). These associations were even stronger for HPV16-positivity: in cervical HPV16-positive women, anal HPV16 prevalence was 41%, while in the HPV16-negative cervical group, anal HPV16 prevalence was 2%.
In men, the risk of anal cancer development is strictly related to sexual behavior and HIV immune status (14).
Penile Cancer
Approximately 50% of penile cancers can be attributed to HPV infections, although HPV also infects healthy subjects without progressing to neoplasia. In a British study carried out among 43 couples, 69% of the men were HPV-positive in the uro-genital tract (15). Another study in the USA showed similar high levels of HPV prevalence, with 65.5% of the men HPV-positive: 51.2% of them harbored at least one typed HPV, and 14.3% of them were positive for an unclassified HPV infection (16). HPV clearance is observed in 75% of cases within 1 year (17). HPV-positivity is greater in penile intraepithelial neoplasia (PIN 1,2,3), which is considered the precursor of penile cancer, and in basaloid histological neoplasia (range, 75–80%) than in invasive cervical squamous cell carcinoma (range, 30–60%). HPV16 and HPV18 are the HPV types that are most frequently associated with all types of penile cancers2.
Vulvar Cancer
It is estimated that 40–50% of vulvar cancers are associated with HPV. Overall, vulvar cancers represented 3% of gynecologic cancers in 2002 (18), and 60% of were observed in developed countries (18, 19)8. Vulvar intraepithelial neoplasia (VIN) is considered a precursor of vulvar squamous cell carcinoma, which represents >90% of vulvar cancers (20). The World Health Organization recognizes a three-grade system of VIN (i.e., VIN1-3), and VIN3 is considered as a precursor of invasive vulvar cancer. However, until recently, VIN2-3 had been considered as HSIL, and VIN1 (or LSIL) is no longer used, as there is misclassification of these low-grade lesions: these are often actually condilomata acuminata with transient HPV infection, or an inflammatory status of the vulva (21). VIN can be caused by two distinct etiological agents: HPV, which is linked to the usual form of VIN (uVIN), and differentiated VIN (dVIN) is associated with lichen sclerosus (22, 23). Generally, uVIN is common among young women, while dVIN is frequently seen for post-menopausal women. In VIN3/HSIL lesions, HPV16 had been detected in >91% of cases (24).
Vaginal Cancer
Cancer of the vagina is rare, and it accounts for only 2% of gynecological neoplasia. Nevertheless, from 2000 to 2015 there was an increase in vaginal cancer, which corresponded to 0.4% of vulvar carcinomas in the USA9. Vaginal intraepithelial neoplasia (VAIN) is considered a pre-malignant lesion. Previously, a three-tiered classified was used (VAIN1-3) according to epithelial involvement. In 2014, the World Health Organization revised this classification by substituting VAIN2-3 with HSIL, and VAIN1 with LSIL (25). About 82% of high-grade lesions (i.e., vaginal intraepithelial lesions, VAIN3, HSIL) and 91% of invasive vaginal carcinomas test positive for HPV DNA and/or HPV antibodies. HPV16 is the most prevalent HPV type in HSIL and vaginal cancers2 (26–29).
Head and Neck Cancer
The most common head and neck cancers are squamous cell carcinomas (HNC) and they include neoplasia of the oral cavity, tongue, tonsils, oropharynx, hypopharynx, and larynx. The HR-HPV types most frequently detected in head and neck cancers are HPV16, followed by HPV182 (30, 31). Head and neck cancers are frequent in southern-central Asia (32); however, an increase in head and neck cancer incidence has been seen over recent years in developed countries (33) and among Caucasian men (34). Tonsillectomies can increase the overall survival rates of patients with diagnosis of tonsil carcinoma (35), but it does not influence the overall risk of oropharyngeal cancer (36).
A reduction in HPV-positive oropharyngeal cancer is observed in people with a specific genetic locus in the human leukocyte antigen region (HLA-DRB1*1301-HLA-DQA1*0103-HLA-DQB1*0603) (37). This protective effect might involve increased immune targeting of HPV-infected cells through the major histocompatibility complex haplotype binding to HPV peptides, resulting in a strong CD4+ T-cell response (38).
Respiratory Papillomatosis
Different annual incidences of respiratory papillomatosis have been reported in different countries. For example, in Denmark, similar incidence has been reported in children (juvenile onset) (3.6/100,000 children) and in adults (3.9/100,000 adults) (38), while in the USA the annual respiratory papillomatosis incidence is 3-fold higher in children than in adults (4.6 vs. 3.9/100,000 children/adults) (39).
HPV6 and HPV11 are the main genotypes detected in respiratory papillomatosis. As spontaneous regression is rarely observed, surgical treatment is necessary to prevent progression of the lesions. Moreover, recurrence of papillomatosis is often observed, and retreatment is needed in most cases, which comes at a high economic burden (40). Although no structured trials have been carried out to date, HPV vaccine administration prior to the onset of sexual behavior might have a positive impact on prevention of respiratory papillomatosis in adulthood.
Genital Warts
Human papillomavirus infection can not only cause cancer, but also benign genital warts. These are very diffuse in the young and in adults, with prevalence from 4 to 11% (41–43). Treatment of genital warts includes therapies with imiquimod and podophyllotoxin, or surgical procedures, or cryotherapy and tricloroacetic acid. These medical interventions represent high costs for both private insurance (44) and health systems (45).
Condylomas were classically considered a benign lesion, with the exception of Buscke-Lowenstein tumors. This large tumor can undergo local invasion and can transform into anal cervical squamous cell carcinoma (46).
Viral Characteristics and Immune Responses
Life Cycle of HPV
Human papillomaviruses are non-enveloped icosahedral viruses that consist of 72 capsomers and are 55 nm in diameter. The viral genome is a circular double stranded DNA of ~8,000 bp in length.
According to the time-regulated expression of proteins during the viral cycle, three functional genome regions can be distinguished: (i) the early region that encodes the E1, E2, E4, E5, E6, E7, E8 viral proteins that have regulatory functions in infected epithelial cells; (ii) the late region that encodes the two viral capsid proteins L1 and L2; and (iii) the long control regions (also known as upstream regulatory regions) that contain cis-acting regulatory sequences that are involved in the control of viral replication and post-transcriptional phases (Figure 1).
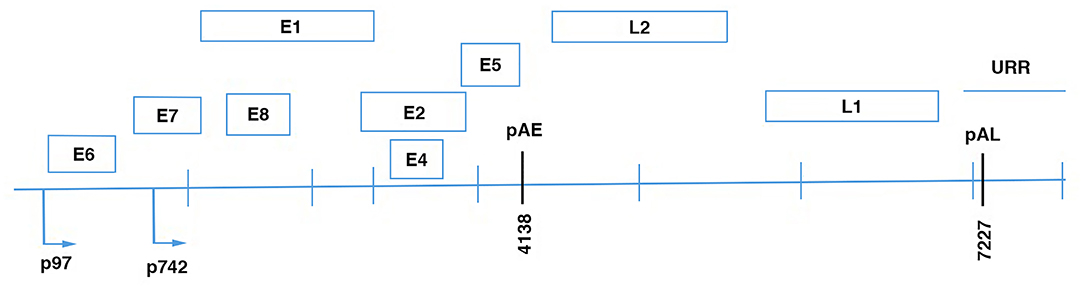
Figure 1. Linear diagram of HPV16 genome showing the eight open reading frames (ORFs), the early (pAE), and the late (pAL) polyadenilation sites; p97, promoter of E6 and E7 viral oncoproteins; p670, promoter of the late proteins.
Different viral proteins are expressed in different layers of the epithelium. The early proteins are expressed in basal epithelial cells, while the late proteins are expressed in the granular layer that includes more differentiated cells, and from where the virus is assembled and released. E1 is an ATPase helicase that is involved with E2 in viral replication and transcription regulation. The E1 and E2 complex interacts with the long control region ori site, which is considered to be the origin of HPV DNA replication (47–53). In the initial phase of infection, the HPV DNA genome is in the episomal form. It shows low amplification activity and there are ~100 copies/cell (54). E2 ensures episomal maintenance of the HPV genome through interactions with other cellular factors. For example, Bromo-domain protein 4 (BRD4) is a mitotic chromosome-associated protein that is a critical binding partner for E2 for this activity (55). BRD4 and E2 co-localize on condensed mitotic chromosomes, and mediate episome segregation (55). E2 also regulates transcription of the E6 and E7 oncoproteins, the expression of which depends on an early promoter. E1 to E4 are encoded by a spliced RNA, and along with E5, they are translated under early promoter control in undifferentiated cells, and they appear to facilitate efficient productive replication in differentiating cells (56, 57).
E6 and E7 are small proteins of about 150 and 100 amino acids, respectively. The E6 oncoprotein acts through its PDZ-binding motif, which promotes its interactions with PDZ domains in multidomain proteins, to alter their functionality. These PDZ domains are present in many multidomain proteins that regulate key steps in the cellular processes of apoptosis, adhesion, and polarity (37–43, 58). E6 also impairs the activity of the p53 protein, which prevents DNA damage accumulation through induction of DNA repair, cell-cycle arrest, or apoptosis, which leads to transformation of HPV chronically infected cells.
The main targets of E7 are the pRB, p107, and p130 proteins, which are components of a complex that can repress the E2F transcription factor (59, 60). When E7 interacts with pRB, p107, and p130, it induces their degradation, and so E2F is free to activate genes such as cyclins A and E, to promote transition from G1 to S-phase of the cell cycle (61). The productive viral cycle also includes the synthesis of the late proteins L1 and L2 in the suprabasal epithelial cell layers, and this step is characterized by a change in mRNA splicing (62, 63). Icosahedral virions are composed of 360 L1 proteins that are organized in pentamers, each of which is associated with one monomer of L2. The productive life cycle is completed when the virions self-assemble, after packaging of the amplified HPV DNA genome, with the viral particles then shed from the epithelial cell layers (64).
Natural Immune Response
The immune response has an important role in clearing most HPV infections, although sometime the virus cannot be eliminated and can persist for several years, which represents a risk factor for neoplasia development (65). HPV-associated neoplastic progression is linked to dysregulated expression of the early viral genes. Specifically, increased expression of the E6 and E7 proteins in the basal epithelium leads to increased cell-cycle entry and loss of differentiation across the epithelium. The main cause of dysregulated HPV gene expression is integration of the viral genome into the host chromosomes (66). HPV DNA integrates randomly into the host DNA. During this process, the viral DNA can often be broken at any position within the E1-E2 region, with the loss of E2 function, and the consequent overexpression of E6 and E7 that promotes cellular transformation (67–69). However, a proportion of cervical cancers are associated with episomal DNA only. In such cases, the E2 open reading frame integrity is maintained, and this protein is expressed throughout the progression of the malignancy.
In natural infections, both humoral- and cell-mediated immune responses are induced. Genital infection with oncogenic HPV is common, but only a minority of infected patients develop epithelial lesions or cancer (70). Spontaneous clearance of an established infection is likely to be mediated by the cellular immune responses. Indeed, strong Th1 CD4+ T-cell responses that are specific for HPV16 E6, E7, and E2 have been frequently detected in peripheral blood mononuclear cells of healthy individuals (71). In contrast, responses against HPV16 E6, E7, and E2 have rarely been detected in patients with HPV16-positive genital lesions or antigen-specific proliferative responses that show a non-inflammatory cytokine profile (72, 73).
Similarly, effective HPV18-specific T-cell responses are only seen in healthy controls, and not in HPV18-positive patients (74). For the role of CD8+ T-cells in disease regression, a comparison of CD8+ T-cell responses to E6 and E7 using enzyme-linked immunospot assays in individuals with incident or prevalent HPV 16 or 18 infections did not show any significant difference in the frequency of positivity between these two patient groups (33 vs. 40%) (75). At variance with this, in CIN2/3 lesions, more CD8+ T-cells were seen for the epidermis of tissues that went on to regress (76). Also, large numbers of intraepithelial CD8+ tumor-infiltrating lymphocytes have been associated with an absence of lymph-node metastases in patients with large early stage cervical cancer (76). Taken together, these findings indicate that the development of high-risk HPV-positive cervical cancer is associated with failure of HPV-specific T-cell responses.
The humoral immune response to HPV infection is mainly directed against conformational epitopes in the variable regions of the major coat protein L1 (77). This develops slowly, and is usually weak. Indeed, seroconversion appears to occur 6–18 months after infection, and type-specific antibodies to L1 are detected in 60–70% of women who acquire HPV infection (6, 78). HPV-seroprevalence is considerably lower in men than women, and it has been suggested that HPV-seropositive women might have higher antibody levels than HPV-seropositive men (79).
IgG and IgA are the most abundant isotypes in sera from natural infections. Other HPV antigens (e.g., E1, E2, E6, L2) do not commonly induce antibody responses in patients with acute or persistent HPV infections.
Studies that have investigated whether naturally acquired HPV antibodies can protect against subsequent HPV infections have reported mixed results (80–82). More recently a systematic review and meta-analysis that included >24,000 individuals showed that natural HPV antibodies provide protection against subsequent type-specific genital HPV infections in females. However, given that the antibody titers in natural immunity are considerably lower than those observed with vaccination, and that antibody responses are preferentially induced in women and are not induced in all infected individuals, it is likely that protection through the development of natural immunity is inferior to protection obtained from HPV vaccination (83).
HPV Vaccines
Prophylactic Vaccines
Three prophylactic vaccines for prevention of HPV infection are available at present: a bivalent vaccine against HPV16 and HPV18 (Cervarix) that was approved in 2007; a tetravalent vaccine against HPV6, 11, 16, and 18 (Gardasil) that was approved in 2006; and a nonavalent vaccine against HPV6, 11, 18, 31, 33, 45, 52, 58 (Gardasil 9) that was approved in 2016. However, the non-avalent vaccine is the only HPV vaccine that is currently available in the USA, and it was approved for males and females from 9 to 45 years by the US Food and Drug Administration in late 2018.
Initially, the administration of the HPV vaccines was in three doses, with the more recent change to a two-dose schedule driven by evaluation of girls aged 9–13 years who had received either two or three doses. The antibody responses of the young women (aged 16–26 years) who had followed a two-dose schedule were similar to those who received all three doses (84). Therefore, in 2016, the Advisory Committee on Immunization Practice declared that there was only the need for two doses of vaccine for those under 15 years of age. However, for females who start the vaccination between 15 and 45 years old, a three-dose schedule is recommended (at 0, 1–2, and 6 months) (84, 85)10. Also immunocompromised patients should follow the three-dose schedule regardless of sex and age at vaccination (86).
All three of these vaccines use recombinant DNA technology and are prepared from the purified L1 protein, which self-assembles to form HPV type-specific empty shells (virus-like particles; VLPs). Only intact VLPs can generate protective antibodies, which supports the evidence that conformational epitopes of L1 are required to generate neutralizing antibodies (87).
The evidence that HPV VLP vaccines protect against high viral challenges through induction of neutralizing anti-L1 antibodies was obtained in preclinical studies in animals, which thus provided the strong rationale for development of VLP-based vaccines. In particular, in a canine model of experimentally induced oral papillomas, it was demonstrated that dogs vaccinated with the major capsid protein, L1, of canine oral papillomavirus developed antibodies against canine oral papillomavirus and became completely resistant to the viral challenge (88). Similarly, vaccination of rabbits with L1 VLPs protected them against papillomas induced by cottontail rabbit papillomavirus (89). In addition, in both of these animal models, passive transfer of immune serum protected the dogs and rabbits against the canine oral papillomavirus and cottontail rabbit papillomavirus challenges, respectively.
In humans, analysis of vaccine-induced antibody responses measured by several methods has demonstrated that almost 100% of vaccinated individuals generate a strong type-restricted serum antibody response to L1 VLP. These methods have included conventional enzyme-linked immunosorbent assays, competitive radioimmunoassays, competitive Luminex-based immunoassays, and pseudovirion-based neutralization assays.
Initial and follow-up studies that assessed the immunogenicity of the HPV 16/18 AS04-adjuvant vaccine in 15- to 25-year-old women showed that after vaccination, anti-HPV16 and anti-HPV18 total IgG antibodies peaked at month 7, reached a plateau between months 18 and 24, and remained constant for up to 76 months (90). Measurement of the neutralizing antibodies using pseudovirion-based neutralization assays confirmed high levels of functional antibodies as well. Then evaluation of long-term immunogenicity of the HPV16/18 vaccine in the serum of 15- to 55-year-old females revealed that the seropositivity for anti-HPV16 remained high in all of the age groups 10 years after the first vaccination. For anti-HPV18, there were more seropositive females in the 15- to 25-year-old group (99.2%) than for the 26- to 45-year-olds (93.7%) and 46- to 55-year-olds (83.8%) (90). In these studies, the anti-HPV16 and anti-HPV18 titers remained above natural infection levels in all of the age groups, and more interestingly, they were predicted to persist for more than 30 years after vaccination (91).
Comparisons of the immunogenicities of the HPV16/18 and HPV6/11/16/18 vaccines in healthy women aged 18–45 years revealed that 7 months after vaccination, the serum neutralizing antibody responses elicited by the bivalent vaccine were significantly higher than those for the HPV6/11/16/18 vaccine. The differences in these responses for HPV16 and HPV18 were maintained at month 24, and also up to month 60 in women aged 18–45 years.
Antibody titers induced by vaccination are higher than those produced by natural infection11. Responses to HPV vaccination is weakly influenced by gender, with higher seroconversion in males than females (99 vs. 93%), and by age, with higher antibody titers in women aged 9–15 years (92, 93). The FUTURE I trial demonstrated that the efficacy of the tetravalent HPV vaccine was 100% against condyloma in HPV-naïve women, and 70% (vaginal condyloma) to 78% (vulvar condyloma) in the overall population. The efficacy of the non-avalent vaccine is comparable to that of the tetravalent vaccine against condyloma (94). Prophylactic HPV vaccines show excellent protection against high-grade CIN (i.e., CIN2, CIN3) and adenocarcinoma in situ for HR-HPV–naïve women. In particular, the non-avalent vaccine showed the highest efficacy for prevention of onset of CIN1 (relative risk reduction, 98.9%), CIN2 (97.1%), and CIN3 (100%) neoplasia (95).
Data for vaccine prevention against AIN are more limited. In the Guanacaste study, the tetravalent HPV vaccine prevented HPV16/18 infection in anal anatomic sites in 84% of women who were HPV-seronegative at baseline (96). Palefsky reported 77.5% prevention of AIN among HPV-naïve men aged 16–26 years who had sex with men (MSM) (97). The tetravalent vaccine also protects heterosexual naïve men from both anogenital HPV infections and HPV lesions, with an efficacy against infections and associated lesions of >90% (98). Also a Finnish randomized trial reported significant reduction of genital HPV infections in men following HPV16/18 vaccine administration (99).
For oropharyngeal cancer prevention, a risk reduction of 93.3% for precursor lesions of HPV-induced oral cancer was reported for the Guanacaste study (96). However, further studies are needed to demonstrate the efficacy of these vaccines on oropharyngeal cancer development.
Therapeutic Vaccines
The therapeutic vaccines differ from the prophylactic vaccines as they are aimed at the generation of cell-mediated immunity, rather than neutralizing antibodies. Although prophylactic vaccines can prevent HPV infections in 100% of cases, and precancerous cervical lesions (i.e., CIN) caused by the HPV genotypes included in the vaccine, HPV-related lesions remain a public problem worldwide for several reasons: (i) only 8% of low and middle income countries have introduced HPV vaccination programs12; (ii) HPV types that are not included in vaccines might be responsible for cancers (100); (iii) the cost of requirements for a cold chain and the absence of sanitary infrastructure limits HPV vaccine deployment in developing countries; and (iv) HPV vaccines are recommended for young women (9–26 years old), and as women older than 26 years are not vaccinated, they can develop cancers. It is also estimated that the impact of HPV vaccination on cancer incidence might not be appreciated for at least 20 years from any mass vaccination.
Currently, the treatment of high-grade disease (CIN2-3) includes electrosurgical excision of the transformation zone, with carbon dioxide lasers or knives used to perform conization, where the entire transformation zone is removed (101, 102) (Table 1). Incomplete excision, however, can occur, and HPV transformed cells can remain, which will facilitate recurrent neoplasia. Hence, there is the need for a therapeutic vaccine that can fully eliminate malignant cells.
The aim of a therapeutic vaccine against HPV is to induce in-vivo virus-specific T-cell responses against established HPV infections and lesions. For therapeutic vaccination to deliver unequivocal clinical benefits, improvements must be achieved at two levels: by maximizing the induction of T-cell responses with optimal amplitude, specificity and effector profile; and by ensuring that vaccine-induced T-cells can reach the tumor site and perform their functions without restraint (103).
Among the HPV proteins, the E6 and E7 oncoproteins are considered to be almost ideal targets for immunotherapy of cervical cancer, as these proteins are essential for the onset and evolution of malignancy, and are constitutively expressed in both premalignant and invasive lesions, while being absent in healthy cells (104). E6 and E7 have therefore been included in most therapeutic vaccines developed to date. Usually, a DNA sequence that encodes a fusion protein of E6 and E7 is inserted into a vector, and mutations are introduced into the regions that are responsible for the E6 interactions with p53, and the E7 interactions with pRB, to reduce their oncogenic power. The E1 and E2 viral proteins are also attractive candidates for therapeutic vaccines that target early viral infections, as they are highly expressed before viral genome integration (105).
Several strategies have been investigated for HPV therapeutic vaccines designed to enhance CD4+ and CD8+ T-cell responses, including genetic vaccines (e.g., DNA/ RNA/ virus/ bacterial), and protein-based, peptide-based or dendritic-cell-based vaccines. Among the bacterial vectors, live attenuated Listeria monocytogenes has been used to generate a promising HPV therapeutic vaccine. L. monocytogenes is considered a potent vaccine vector because it enters professional antigen-presenting cells and induces antigen-presenting cell maturation, and strong innate and adaptive immunity (106). In addition, L. monocytogenes grows very efficiently in vitro and lacks lipopolysaccharides, which are a major toxicity factor with Gram-negative bacteria (104). The safety of a recombinant live attenuated L. monocytogenes secreting E7 as a fusion protein joined to non-hemolytic listeriolysin O (Lm-LLO-E7) was demonstrated in a phase I clinical study that was conducted with 15 patients with late-stage metastatic cervical cancer (107). Evaluation of the efficacy of Lm-LLO-E7 (also known as ADXS11-001) in a prospective phase II clinical trial as second-line and third line for patients with recurrent metastatic cervical cancer showed that 12-month overall survival was 38%, which exceeded the historical overall survival of such patients, of 25%. A phase III clinical trial of Lm-LLO-E7 for high-grade cervical cancer is being conducted at the time of writing (see NCT02853604).
Encouraging data have also been obtained in clinical studies that have tested DNA-based vaccines. DNA vaccination consists of direct introduction into tissues of a plasmid that contains the DNA sequence that encodes the antigen(s) against which an immune response is sought. This relies on in-situ production of the antigen(s) as a result of the transfection of antigen-presenting cells and non-antigen-presenting cells, with the presentation of the expressed antigen(s) by both MHC class I and class II molecules. Furthermore, this results in activation of all three arms of the adaptive immune response (i.e., helper T cells, cytotoxic T cells, antibodies).
However, although DNA vaccines have been shown to induce balanced CD4+ and CD8+ T cells as well as humoral immune responses in small animal models, clinical data from multiple studies have demonstrated that they induce poor T-cell responses (108).
Many strategies to facilitate antigen processing and presentation, and also antigen delivery, have been adopted to ameliorate the immunogenicity of DNA vaccines against HPV (109–111).
A phase I study was carried out using the DNA vaccine VGX-3100 that consists of a mixture of two plasmids that encode the optimized consensus of the E6 and E7 genes of HPV genotypes 16 and 18. These were delivered via intramuscular injection, followed by electroporation, with 18 patients who had been previously treated for cervical intraepithelial neoplasia (CIN2/3). This study showed that 78% of the patients developed CD8+ T-cell responses, and 100% showed antibody positivity to at least two vaccine antigens (112). Notably, the peripheral blood T-cell responses elicited by VGX-3100 were an order of magnitude greater than naturally occurring responses, and a log unit greater than those previously reported for HPV therapeutic vaccines (112).
In 2015, the efficacy, safety, and immunogenicity of VGX-3100 was assessed in a phase II clinical trial in patients with CIN2/3. In the per-protocol analysis, 30.6% of the placebo recipients and 49.5% of the VGX-3100 recipients showed histological regression. Concomitant histopathological regression and viral clearance occurred in 14.3% of placebo recipients compared with 40.2% of vaccinated recipients (113). Post-hoc immunological analysis here demonstrated that VGX-3100 elicited significantly increased frequency of T-cell responses against HPV16/18 E6 and E7, and that the magnitude of the T-cell response against E6 was associated with clinical outcome. Humoral immune responses were also lower in placebo recipients than in VGX-3100 recipients, and the antibody responses against HPV16, HPV18, and E7 were significantly higher in the patients who had concomitant histopathological regression and viral clearance, compared to those who did not (113). A phase III clinical trial of VGX-3100 for women with CIN was initiated in 2017, and it is expected to end in 2021 (see NCT03185013).
Viral vectors including adenoviruses, adeno-associated viruses, alphaviruses, and vaccinia viruses (e.g., modified vaccinia Ankara virus; MVA) can be used to express the E2, E6, and E7 oncoproteins, and they can stimulate CD4+ and CD8+ T-cell responses. A MVA vector was used to produce the Tipapkinogen Sovacivec vaccine, which includes three exogenous genes that encode the human cytokine interleukin-2, and non-oncogenic E6 and E7. This vaccinia virus can induce interferon-α production and express HPV16 E6 and E7, which are presented by dendritic cells to activate naïve T cells in lymph nodes. At a follow-up of 2.5 years, compared to the placebo cohort at 10% viral clearance, the administration of Tipapkinogen Sovacivec vaccine provided complete resolution for 24% of patients with CIN2/3, irrespective of their HR-HPV baseline infection (i.e., HPV16, 18, 31, 33, 35, 39, 45, 52, 56, 58, 59, or 68). However, despite this significantly improved HPV viral clearance with this vaccine, it has still not been licensed for clinical use because of the modest efficacy (104).
Finally, a vaccine designed on recombinant MVA that contained the bovine papillomavirus E2 protein (MVA E2) was used to treat HPV-induced ano-genital intraepithelial lesions. A phase III study showed that 90% of female patients had complete elimination of lesions after treatment with MVA E2, with 100% seen for men. All of these patients treated with MVA E2 developed antibodies against the MVA E2 vaccine and generated a specific cytotoxic response against the papilloma-transformed cells (114).
Interestingly, novel vaccination strategies aimed to maximize systemic as well as genital resident memory T cell responses to treat sexually transmitted infections and human papilloma virus neoplasia are being developed. In this context several studies have investigated the effect of either the topical delivery of host and pathogen derived immunomodulatory molecules or the delivery route of immunization in the induction of cervicovaginal long lived CD8+ T cell responses (115).
HPV Prophylactic Vaccines Used as Therapeutic Vaccines
The treatment of premalignant lesions (CIN2,3) by LLEP or conization sometimes fails to prevent lesion recurrence (116–118). This is often linked to incomplete excision of transformation zone consciously carried out by gynecologists. In fact, evidence shows that large excision of the cervix can compromise cervix integrity and can cause adverse neonatal outcome with preterm risk (119, 120). Moreover, recurrence risk is greater in presence of HR HPV infection (116, 121).
A systematic review of studies of the treatment of high-grade lesions (HSIL/ CIN2-3) reported that a median of 28% of the women remained positive for oncogenic HPV types 3 months after treatment. A decrease in this HPV persistence was seen during follow-up, as it fell to 21% after 6 months (102). Also, higher risks for the development of cervical and vaginal neoplasia have been reported for women who had previously been treated for CIN3, in comparison to the general female population, with this higher risk persisting for 20–25 years, and possibly longer (122). The risk of cervical cancer after treatment also increases with age. A large study with long-term follow-up for women treated for CIN3 reported standard incidence and mortality ratios (i.e., treated vs. placebo) for cervical and vaginal cancers of 10.58 and 7.60, respectively, for women aged 60–69 years, and 2.03 and 1.52, respectively, for women aged 30–39 years (123). Also, women who had previously reported CIN3 lesions showed greater probability of developing other HPV-related neoplasia of the genital tract (e.g., vaginal, vulvar, anal) or the oropharyngeal district (124).
As no vaccine has yet been licensed for therapeutic use, the prophylactic vaccines have been tested in several trials to determine their effectiveness for prevention of HPV disease recurrence or reinfection after CIN2-3 treatment. The recurrence for MSM who undergo treatment for high-grade anal intraepithelial neoplasia (HGAIN) is particularly high, as 50% show recurrence within 1 year (123). This makes it essential to find a treatment that can reduce the development of high-grade lesions in treated patients. In 2011, the effectiveness of the tetravalent HPV vaccine for the prevention of recurrent HGAIN was evaluated in HIV-negative, self-identified MSM with a history of biopsy-proven and treated HGAIN. In the 340.4 person-years of follow-up, 30.7% of the non-vaccinated patients developed recurrent HGAIN, compared to 13.6% of the vaccinated patients. Among these patients who were infected with HR-HPV types, the tetravalent vaccine was associated with significantly decreased risk of recurrent HGAIN at 2 years from study entry (hazard ratio, 0.47). To explain the partial effectiveness of the tetravalent vaccine in this study, it was speculated that some of these patients might have developed diseases that were related to the HPV genotypes not covered by the tetravalent vaccine or to multiple HPV infections. Further, some HGAIN might not have been identified and treated before the vaccinations, or the viral integration into the host genome had already occurred. Unfortunately, these aspects were not investigated.
In 2013, Kang et al. investigated the effectiveness of the tetravalent HPV vaccine to prevent recurrence of CIN2-3 in patients with high-grade CIN treated by the loop electrosurgical excision procedure (125). Recurrence was seen for 7.2% of the non-vaccinated patients and by 2.5% of the vaccinated patients. In patients infected with HPV16 and/or HPV18, 8.5% of the non-vaccinated patients and 2.5% of the vaccinated patients developed recurrent disease related to these HPV types. Although encouraging, these data indicate that the prophylactic HPV vaccine had weak activity against such HPV16/18-related high-grade lesions. Recently, a prospective clinical project, the SPERANZA study, was carried out to determine the effectiveness of the tetravalent vaccine for reduction of the risk of clinical relapse in women treated for CIN2 (126). Overall, 344 women were included in the study, and 6.4% of the non-vaccinated women showed clinical disease recurrence, while for the vaccinated women, there was only 1.2% recurrence. Vaccination here was associated with significantly reduced risk of subsequent HPV-related high-grade CIN after cervical surgery, at 81.2%. For the non-vaccinated women, the recurrent clinical disease was attributed to HPV11, 16, 18, 31, 33, 45, 53, 82, while for the vaccinated women, the two cases of clinical disease recurrence were associated with HPV33 and HPV82. In this study, about 40% of the patients enrolled were >36 years old, although neither the age range nor the age of women with recurrent clinical disease were reported, and thus it cannot be determined if the efficacy of the tetravalent HPV vaccine was influenced by the age of the patients at the time of their vaccination. At variance with this, a study by Hildesheim et al. included 1,711 women with carcinogenic human HPV infection and 311 women who received loop electrosurgical excision for cervical precancer. Here, there was no evidence that HPV16/18 vaccination alters the fate of an HPV infection present at the time of vaccination, or the rates of cervical infections and lesions after loop electrosurgical excision. For these HPV16/18 infections, in the cohort of women with HPV infection but without precancer, the efficacy of clearance was 5.4%, with progression to CIN1 seen for 15.5%, and to CIN2, for 0.3%. Moreover, after the loop electrosurgical excision, the vaccination had no significant effects on HPV16/18 infections and/or HPV16/18-associated cytological and histological lesions (127).
The data obtained on the efficacy of the tetravalent HPV vaccine for the prevention of anal condylomas are, however, more encouraging (128). Three hundred and thirteen MSM (mean age, 42 years) were enrolled for a median of 981 days. During the follow-up, condyloma developed in 18.8% of non-vaccinated patients, and in 8.6% of vaccinated patients. Moreover, several clinical studies have demonstrated activity for HPV vaccination in the treatment of genital warts (129–131).
Altogether, these data suggest that there is possibility that prophylactic vaccines reduce the risk of HSIL recurrence in previously infected patients, although the exact protective mechanisms in infected individuals is not understood. The high risk of recurring infections is consistent with either auto-inoculation across anatomic sites or new inoculation or episodic reactivation of latent infection. As HPV vaccines prevent infection by induction of L1-specific antibodies that block viral entry, and L1 is not generally expressed during the oncogenic process, it is expected that these vaccines will be effective in the prevention of auto-inoculation or new infections.
The greater effectiveness obtained with prophylactic vaccines in the prevention and regression of genital warts might be related to the integration state of the HPV genome. In genital warts, the virus is not generally integrated into the host genome, and therefore viral particles are produced. In this case, the prophylactic vaccines that block viral entry through induction of L1-specific antibodies can prevent reinfections, which will favor the elimination of the virus. Conversely, in high-grade lesions, the virus genome is often integrated into the host genome, and infected cells do not express L1 and do not produce viral particles. Thus, as transformed cells are frequently in the basal layer of the derma, they will not be recognized by vaccine-induced antibodies, which are ineffective in the control of the disease course.
Furthermore, there are some cases in the treatment of HPV-related cancers where the use of prophylactic vaccines might not be recommended:
(1) Anal and cervical cancers that are not attributable to the HPV types that are included in the non-avalent HPV vaccine. Several studies have demonstrated that half of the HPV infections in MSM are caused by HPV types that are not included in the non-avalent HPV vaccine (132, 133) (Table 2). Here, over 2 years of observation, only about 30% of HIV-positive MSM had incidents of HR-HPV infections that were covered by the non-avalent vaccine (134). This situation can be also observed for women (Table 2).
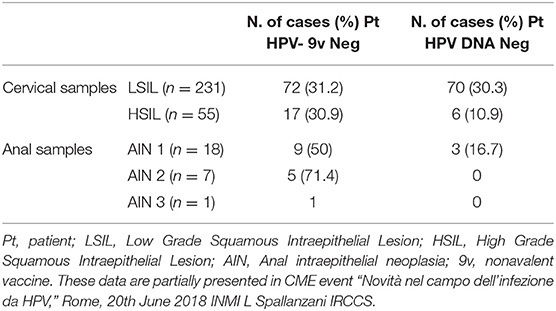
Table 2. Detail of cervical and anal samples from HIV positive patients with squamous intraepithelial lesions and HPV DNA negative or positive for HPV types that are not included in nonavalent vaccine.
(2) HPV DNA-negative cervical tumors. Over recent decades, several studies have reported that some cervical cancers are HPV-negative (135–139). Often, HPV DNA negativity is due to the sensitivity of the methods used in the HPV DNA detection, and so samples that have tested as HPV-negative might show as HPV-positive when retested with more sensitive assays (e.g., nested PCR) (136). The Cancer Genome and Molecular Characterization of Cervical Cancer Study used next-generation sequencing to characterize primary cervical cancers, and it established that 5% of the specimens were HPV-negative. This subset of HPV DNA-negative cancers is mainly observed among adenocarcinoma cancers, and predominantly in gastric-type adenocarcinomas. The pattern of immunostaining of gastric-type adenocarcinomas shows strong and diffuse positivity for MUC-6 and HIK1083 antibodies, which recognize epitopes of gastric pyloric glycoproteins, although they are p16 negative, which is a cell-cycle regulatory protein (140). Gastric-type adenocarcinomas have significantly higher rates of recurrence and mortality than HPV-positive cancers (141, 142). Furthermore, progression and regression of gastric-type adenocarcinomas are independent of HPV infection, and thus HPV vaccine administration here would be inappropriate.
Conclusions
The data reported in this review highlight the significant efforts that have been carried out to set-up therapeutic vaccines against HPV-related malignancies. Although several approaches to produce an effective vaccine have been attempted, including the use of proteins, synthetic peptides, and viral proteins expressed in different vectors, and although some of the data appear encouraging, no therapeutic vaccines have been licensed in clinical practice yet. Recently, prophylactic vaccines have been used for treatment of recurrent forms or reinfections in subjects who have previously undergone surgical resection. However, the trials here have offered conflicting results, and vaccination did not guarantee 100% effectiveness. This is probably due to a residual burden of transformed cells that can persist after the surgical treatment, and that are not targeted by the humoral L1-specific immune response induced by the prophylactic vaccines. Although it cannot be excluded that the therapeutic potential of prophylactic vaccines could be improved by using different adjuvants or route of immunization, an additional limit in using prophylactic vaccines for therapeutic purposes is seen by the evidence that the non-avalent vaccine does not include all of the HR-HPV types. As the real extent of protection given by the non-avalent vaccine against other HPV types is not known, its use in the treatment of tumors related to these other HR-HPV types is questionable. Furthermore, for endometrial adenocarcinomas, such as gastric-type adenocarcinomas, which are HPV DNA-negative, careful virological and histological diagnosis must be made before administration of HPV prophylactic vaccines to treat HPV recurrence or reinfection.
Author Contributions
AG and PD wrote the original version and edited versions. MC edited versions. DL and CS prepared figures and tables.
Funding
This work was supported by Ricerca Corrente Funding from Italian Ministry of Health.
Conflict of Interest
The authors declare that the research was conducted in the absence of any commercial or financial relationships that could be construed as a potential conflict of interest.
Footnotes
2. ^https://publications.iarc.fr/108
3. ^https://monographs.iarc.fr/wp-content/uploads/2018/06/mono100B-11.pdf
4. ^http://globocan.iarc.fr/old/FactSheets/cancers/cervix-new.asp
5. ^https://seer.cancer.gov/archive/csr/1975_2004/
6. ^https://monographs.iarc.fr/wp-content/uploads/2018/06/mono90.pdf
7. ^https://monographs.iarc.fr/wp-content/uploads/2018/06/mono100B.pdf
8. ^https://www.ncbi.nlm.nih.gov/books/NBK12354/
9. ^http://www.seer.cancer.gov
10. ^https://www.cdc.gov/hpv/dowloads/9vhpv-guidance.pdf
11. ^http://www.rho.org/files/WHO_HPV_tech_info_nocover_2007.pdf
12. ^https://apps.who.int/iris/bitstream/handle/10665/251810/WER9148.pdf;
jsessionid=12D591BAA8A2E02CEB5223020DFC3526?sequence=1
References
1. Ferlay J, Colombet M, Soerjomataram I, Mathers C, Parkin DM, Piñeros M, et al. Estimating the global cancer incidence and mortality in 2018: GLOBOCAN sources and methods. Int J Cancer. (2019) 144:1941–53. doi: 10.1002/ijc.31937
2. Plummer M, de Martel C, Vignat J, Ferlay J, Bray F, Franceschi S. Global burden of cancers attributable to infections in 2012: a synthetic analysis. Lancet Glob Health. (2016) 4:e609–16. doi: 10.1016/S2214-109X(16)30143-7
3. Crosbie EJ, Einstein MH, Franceschi S, Kitchener HC. Human papillomavirus and cervical cancer. Lancet. (2013) 382:889–99. doi: 10.1016/S0140-6736(13)60022-7
4. Vaccarella S, Franceschi S, Engholm G, Lönnberg S, Khan S, Bray F. 50 years of screening in the Nordic countries: quantifying the effects on cervical cancer incidence. Br J Cancer. (2014) 111:965–9. doi: 10.1038/bjc.2014.362
5. Moscicki AB, Schiffman M, Kjaer S, Villa LL. Chapter 5: updating the natural history of HPV and anogenital cancer. Vaccine. (2006) 24 (Suppl. 3):S3/42–51. doi: 10.1016/j.vaccine.2006.06.018
6. Carter JJ, Koutsky LA, Hughes JP, Lee SK, Kuypers J, Kiviat N, et al. Comparison of human papillomavirus types 16, 18, and 6 capsid antibody responses following incident infection. J Infect Dis. (2000) 181:1911–9. doi: 10.1086/315498
7. Cutts FT, Franceschi S, Goldie S, Castellsague X, de Sanjose S, Garnett G, et al. Human papillomavirus and HPV vaccines: a review. Bull World Health Organ. (2007) 85:719–26. doi: 10.2471/BLT.06.038414
8. Guan P, Howell-Jones R, Li N, Bruni L, de Sanjosé S, Franceschi S, et al. Human papillomavirus types in 115,789 HPV-positive women: a meta-analysis from cervical infection to cancer. Int J Cancer. (2012) 131:2349–59. doi: 10.1002/ijc.27485
9. Nayar R, Solomon D. Second edition of ‘wfhe Bethesda System for reporting cervical cytology’ - atlas, website, and Bethesda interobserver reproducibility project. Cytojournal. (2004)1:4. doi: 10.1007/978-1-4612-2042-8
10. de Martel C, Plummer M, Vignat J, Franceschi S. Worldwide burden of cancer attributable to HPV by site, country and HPV type. Int J Cancer. (2017) 141:664–70. doi: 10.1002/ijc.30716
11. Daling JR, Madeleine MM, Johnson LG, Schwartz SM, Shera KA, Wurscher MA, et al. Human papillomavirus, smoking, and sexual practices in the etiology of anal cancer. Cancer. (2004) 101:270–80. doi: 10.1002/cncr.20365
12. Lin C, Franceschi S, Clifford GM. Human papillomavirus types from infection to cancer in the anus, according to sex and HIV status: a systematic review and meta-analysis. Lancet Infect Dis. (2018) 18:198–206. doi: 10.1016/S1473-3099(17)30653-9
13. Lin C, Slama J, Gonzalez P, Goodman MT, Xia N, Kreimer AR, et al. Cervical determinants of anal HPV infection and high-grade anal lesions in women: a collaborative pooled analysis. Lancet Infect Dis. (2019) 19:880–91. doi: 10.1016/S1473-3099(19)30164-1
14. Garbuglia AR, Gentile M, Del Nonno F, Lorenzini P, Lapa D, Lupi F, et al. An anal cancer screening program for MSM in Italy: prevalence of multiple HPV types and vaccine-targeted infections. J Clin Virol. (2015) 72:49–54. doi: 10.1016/j.jcv.2015.09.001
15. Dunne EF, Nielson CM, Stone KM, Markowitz LE, Giuliano AR. Prevalence of HPV infection among men: a systematic review of the literature. J Infect Dis. (2006) 194:1044–57. doi: 10.1086/507432
16. Nielson CM, Flores R, Harris RB, Abrahamsen M, Papenfuss MR, Dunne EF, et al. Human papillomavirus prevalence and type distribution in male anogenital sites and semen. Cancer Epidemiol Biomarkers Prev. (2007) 16:1107–14. doi: 10.1158/1055-9965.EPI-06-0997
17. Nielson CM, Harris RB, Dunne EF, Abrahamsen M, Papenfuss MR, Flores R, et al. Risk factors for anogenital human papillomavirus infection in men. J Infect Dis. (2007) 196:1137–45. doi: 10.1086/521632
18. Giuliano AR, Tortolero-Luna G, Ferrer E, Burchell AN, de Sanjose S, Kjaer SK, et al. Epidemiology of human papillomavirus infection in men, cancers other than cervical and benign conditions. Vaccine. (2008) 26 (Suppl. 10):K17–28. doi: 10.1016/j.vaccine.2008.06.021
19. Sankaranarayanan R, Ferlay J. Worldwide burden of gynaecological cancer: the size of the problem. Best Pract Res Clin Obstet Gynaecol. (2006) 20:207–25. doi: 10.1016/j.bpobgyn.2005.10.007
20. de Sanjosé S, Alemany L, Ordi J, Tous S, Alejo M, Bigby SM, et al. Worldwide human papillomavirus genotype attribution in over 2000 cases of intraepithelial and invasive lesions of the vulva. Eur J Cancer. (2013) 49:3450–61. doi: 10.1016/j.ejca.2013.06.033
21. Hoang LN, Park KJ, Soslow RA, Murali R. Squamous precursor lesions of the vulva: current classification and diagnostic challenges. Pathology. (2016) 48:291–302. doi: 10.1016/j.pathol.2016.02.015
22. Bornstein J, Bogliatto F, Haefner HK, Stockdale CK, Preti M, Bohl TG, et al. The 2015 International society for the study of vulvovaginal disease (ISSVD) terminology of vulvar squamous intraepithelial lesions. J Low Genit Tract Dis. (2016) 20:11–4. doi: 10.1097/LGT.0000000000000169
23. van der Avoort IA, Shirango H, Hoevenaars BM, Grefte JM, de Hullu JA, de Wilde PC, et al. Vulvar squamous cell carcinoma is a multifactorial disease following two separate and independent pathways. Int J Gynecol Pathol. (2006) 25:22–9. doi: 10.1097/01.pgp.0000177646.38266.6a
24. Srodon M, Stoler MH, Baber GB, Kurman RJ. The distribution of low and high-risk HPV types in vulvar and vaginal intraepithelial neoplasia (VIN and VaIN). Am J Surg Pathol. (2006) 30:1513–8. doi: 10.1097/01.pas.0000213291.96401.48
25. Reich O, Regauer S, Marth C, Schmidt D, Horn LC, Dannecker C, et al. Precancerous lesions of the cervix, vulva and vagina according to the 2014 WHO classification of tumors of the female genital tract. Geburtshilfe Frauenheilkd. (2015) 75:1018–20. doi: 10.1055/s-0035-1558052
26. Hildesheim A, Han CL, Brinton LA, Kurman RJ, Schiller JT. Human papillomavirus type 16 and risk of preinvasive and invasive vulvar cancer: results from a seroepidemiological case-control study. Obstet Gynecol. (1997) 90:748–54. doi: 10.1016/S0029-7844(97)00467-5
27. Daling JR, Madeleine MM, Schwartz SM, Shera KA, Carter JJ, McKnight B, et al. A population-based study of squamous cell vaginal cancer: HPV and cofactors. Gynecol Oncol. (2002) 84:263–70. doi: 10.1006/gyno.2001.6502
28. Carter JJ, Madeleine MM, Shera K, Schwartz SM, Cushing-Haugen KL, Wipf GC, et al. Human papillomavirus 16 and 18 L1 serology compared across anogenital cancer sites. Cancer Res. (2001) 61:1934–40.
29. Lamos C, Mihaljevic C, Aulmann S, Bruckner T, Domschke C, Wallwiener M, et al. Detection of human papillomavirus infection in patients with vaginal intraepithelial neoplasia. PLoS ONE. (2016) 11:e0167386. doi: 10.1371/journal.pone.0167386
30. Parkin DM, Bray F. Chapter 2: The burden of HPV-related cancers. Vaccine. (2006) 24 (Suppl. 3):S3/11–25. doi: 10.1016/j.vaccine.2006.05.111
31. Kreimer AR, Clifford GM, Boyle P, Franceschi S. Human papillomavirus types in head and neck squamous cell carcinomas worldwide: a systematic review. Cancer Epidemiol Biomarkers Prev. (2005) 14:467–75. doi: 10.1158/1055-9965.EPI-04-0551
32. Pintos J, Black MJ, Sadeghi N, Ghadirian P, Zeitouni AG, Viscidi RP, et al. Human papillomavirus infection and oral cancer: a case-control study in Montreal, Canada. Oral Oncol. (2008) 44:242–50. doi: 10.1016/j.oraloncology.2007.02.005
33. Bosch FX, Broker TR, Forman D, Moscicki AB, Gillison ML, Doorbar J, et al. Comprehensive control of human papillomavirus infections and related diseases. Vaccine. (2013) 31 (Suppl. 7):H1–31. doi: 10.1016/j.vaccine.2013.07.026
34. Herrero R, Quint W, Hildesheim A, Gonzalez P, Struijk L, Katki HA, et al. Reduced prevalence of oral human papillomavirus (HPV) 4 years after bivalent HPV vaccination in a randomized clinical trial in Costa Rica. PLoS ONE. (2013) 8:e68329. doi: 10.1371/journal.pone.0068329
35. Vokes EE, Agrawal N, Seiwert TY. HPV-associated head and neck cancer. J Natl Cancer Inst. (2015) 107:djv344. doi: 10.1093/jnci/djv344
36. Fakhry C, Andersen KK, Christensen J, Agrawal N, Eisele DW. The impact of tonsillectomy upon the risk of oropharyngeal carcinoma diagnosis and prognosis in the danish cancer registry. Cancer Prev Res. (2015) 8:583–9. doi: 10.1158/1940-6207.CAPR-15-0101
37. Lesseur C, Diergaarde B, Olshan AF, Wünsch-Filho V, Ness AR, Liu G, et al. Genome-wide association analyses identify new susceptibility loci for oral cavity and pharyngeal cancer. Nat Genet. (2016) 48:1544–50. doi: 10.1038/ng.3685
38. Bomholt A. Juvenile laryngeal papillomatosis. an epidemiological study from the Copenhagen region. Acta Otolaryngol. (1988) 105:367–71. doi: 10.3109/00016488809097020
39. Dickens P, Srivastava G, Loke SL, Larkin S. Human papillomavirus 6, 11, and 16 in laryngeal papillomas. J Pathol. (1991) 165:243–6. doi: 10.1002/path.1711650308
40. Derkay CS, Darrow DH. Recurrent respiratory papillomatosis. Ann Otol Rhinol Laryngol. (2006) 115:1–11. doi: 10.1177/000348940611500101
41. Fenton KA, Korovessis C, Johnson AM, McCadden A, McManus S, Wellings K, et al. Sexual behaviour in Britain: reported sexually transmitted infections and prevalent genital Chlamydia trachomatis infection. Lancet. (2001) 358:1851–4. doi: 10.1016/S0140-6736(01)06886-6
42. Grulich AE, de Visser RO, Smith AM, Rissel CE, Richters J. Sex in Australia: knowledge about sexually transmissible infections and blood-borne viruses in a representative sample of adults. Aust N Z J Public Health. (2003) 27:230–3. doi: 10.1111/j.1467-842X.2003.tb00813.x
43. Kjaer SK, Tran TN, Sparen P, Tryggvadottir L, Munk C, Dasbach E, et al. The burden of genital warts: a study of nearly 70,000 women from the general female population in the 4 Nordic countries. J Infect Dis. (2007) 196:1447–54. doi: 10.1086/522863
44. Insinga RP, Dasbach EJ, Myers ER. The health and economic burden of genital warts in a set of private health plans in the United States. Clin Infect Dis. (2003) 36:1397–403. doi: 10.1086/375074
45. Insinga RP, Dasbach EJ, Elbasha EH. Assessing the annual economic burden of preventing and treating anogenital human papillomavirus-related disease in the US: analytic framework and review of the literature. Pharmacoeconomics. (2005) 23:1107–22. doi: 10.2165/00019053-200523110-00004
46. Albuquerque A, Sarmento J, Rios E, Macedo G. Gastrointestinal: anal buschke loewenstein tumor. J Gastroenterol Hepatol. (2012) 27:1537. doi: 10.1111/j.1440-1746.2012.07204.x
47. Kasukawa H, Howley PM, Benson JD. A fifteen-amino-acid peptide inhibits human papillomavirus E1-E2 interaction and human papillomavirus DNA replication in vitro. J Virol. (1998) 72:8166–73. doi: 10.1128/JVI.72.10.8166-8173.1998
48. Stanley MA, Browne HM, Appleby M, Minson AC. Properties of a non-tumorigenic human cervical keratinocyte cell line. Int J Cancer. (1989) 43:672–6. doi: 10.1002/ijc.2910430422
49. Bedell MA, Hudson JB, Golub TR, Turyk ME, Hosken M, Wilbanks GD, et al. Amplification of human papillomavirus genomes in vitro is dependent on epithelial differentiation. J Virol. (1991) 65:2254–60. doi: 10.1128/JVI.65.5.2254-2260.1991
50. Parish JL, Bean AM, Park RB, Androphy EJ. ChlR1 is required for loading papillomavirus E2 onto mitotic chromosomes and viral genome maintenance. Mol Cell. (2006) 24:867–76. doi: 10.1016/j.molcel.2006.11.005
51. McBride AA. Replication and partitioning of papillomavirus genomes. Adv Virus Res. (2008) 72:155–205. doi: 10.1016/S0065-3527(08)00404-1
52. Van Tine BA, Dao LD, Wu SY, Sonbuchner TM, Lin BY, Zou N, et al. Human papillomavirus (HPV) origin-binding protein associates with mitotic spindles to enable viral DNA partitioning. Proc Natl Acad Sci USA. (2004) 101:4030–5. doi: 10.1073/pnas.0306848101
53. Bergvall M, Melendy T, Archambault J. The E1 proteins. Virology. (2013) 445:35–56. doi: 10.1016/j.virol.2013.07.020
54. Groves IJ, Coleman N. Pathogenesis of human papillomavirus-associated mucosal disease. J Pathol. (2015) 235:527–38. doi: 10.1002/path.4496
55. You J, Srinivasan V, Denis GV, Harrington WJ Jr, Ballestas ME, Kaye KM, et al. Kaposi's sarcoma-associated herpesvirus latency-associated nuclear antigen interacts with bromodomain protein Brd4 on host mitotic chromosomes. J Virol. (2006) 80:8909–19. doi: 10.1128/JVI.00502-06
56. Egawa N, Wang Q, Griffin HM, Murakami I, Jackson D, Mahmood R, et al. HPV16 and 18 genome amplification show different E4-dependence, with 16E4 enhancing E1 nuclear accumulation and replicative efficiency via its cell cycle arrest and kinase activation functions. PLoS Pathog. (2017) 13:e1006282. doi: 10.1371/journal.ppat.1006282
57. Hladik F, Liu H, Speelmon E, Livingston-Rosanoff D, Wilson S, Sakchalathorn P, et al. Combined effect of CCR5-Delta32 heterozygosity and the CCR5 promoter polymorphism−2459 A/G on CCR5 expression and resistance to human immunodeficiency virus type 1 transmission. J Virol. (2005) 79:11677–84. doi: 10.1128/JVI.79.18.11677-11684.2005
58. Suarez I, Trave G. Structural insights in multifunctional papillomavirus oncoproteins. Viruses. (2018) 10:37. doi: 10.3390/v10010037
59. Huh K, Zhou X, Hayakawa H, Cho JY, Libermann TA, Jin J, et al. Human papillomavirus type 16 E7 oncoprotein associates with the cullin 2 ubiquitin ligase complex, which contributes to degradation of the retinoblastoma tumor suppressor. J Virol. (2007) 81:9737–47. doi: 10.1128/JVI.00881-07
60. Zhang B, Chen W, Roman A. The E7 proteins of low- and high-risk human papillomaviruses share the ability to target the pRB family member p130 for degradation. Proc Natl Acad Sci USA. (2006) 103:437–42. doi: 10.1073/pnas.0510012103
61. Moody CA, Laimins LA. Human papillomavirus oncoproteins: pathways to transformation. Nat Rev Cancer. (2010) 10:550–60. doi: 10.1038/nrc2886
62. Bernard HU. Regulatory elements in the viral genome. Virology. (2013) 445:197–204. doi: 10.1016/j.virol.2013.04.035
63. Schwartz S. Papillomavirus transcripts and post-transcriptional regulation. Virology. (2013) 445:187–96. doi: 10.1016/j.virol.2013.04.034
64. Johansson C, Schwartz S. Regulation of human papillomavirus gene expression by splicing and polyadenylation. Nat Rev Microbiol. (2013) 11:239–51. doi: 10.1038/nrmicro2984
65. zur Hausen H. Papillomavirus infections–a major cause of human cancers. Biochim Biophys Acta. (1996) 1288:F55–78. doi: 10.1016/0304-419X(96)00020-0
66. Pett M, Coleman N. Integration of high-risk human papillomavirus: a key event in cervical carcinogenesis? J Pathol. (2007) 212:356–67. doi: 10.1002/path.2192
67. Stanley M. Immunobiology of HPV and HPV vaccines. Gynecol Oncol. (2008) 109 (Suppl. 2):S15–21. doi: 10.1016/j.ygyno.2008.02.003
68. Liaw KL, Hildesheim A, Burk RD, Gravitt P, Wacholder S, Manos MM, et al. A prospective study of human papillomavirus (HPV) type 16 DNA detection by polymerase chain reaction and its association with acquisition and persistence of other HPV types. J Infect Dis. (2001) 183:8–15. doi: 10.1086/317638
69. Woodman CB, Collins S, Winter H, Bailey A, Ellis J, Prior P, et al. Natural history of cervical human papillomavirus infection in young women: a longitudinal cohort study. Lancet. (2001) 357:1831–6. doi: 10.1016/S0140-6736(00)04956-4
70. van der Burg SH, de Jong A, Welters MJ, Offringa R, Melief CJ. The status of HPV16-specific T-cell reactivity in health and disease as a guide to HPV vaccine development. Virus Res. (2002) 89:275–84. doi: 10.1016/S0168-1702(02)00196-X
71. de Jong A, van Poelgeest MI, van der Hulst JM, Drijfhout JW, Fleuren GJ, Melief CJ, et al. Human papillomavirus type 16-positive cervical cancer is associated with impaired CD4+ T-cell immunity against early antigens E2 and E6. Cancer Res. (2004) 64:5449–55. doi: 10.1158/0008-5472.CAN-04-0831
72. van Poelgeest MI, Nijhuis ER, Kwappenberg KM, Hamming IE, Wouter Drijfhout J. Distinct regulation and impact of type 1 T-cell immunity against HPV16 L1, E2 and E6 antigens during HPV16-induced cervical infection and neoplasia. Int J Cancer. (2006) 118:675–83. doi: 10.1002/ijc.21394
73. van Poelgeest MI, van Seters M, van Beurden M, Kwappenberg KM, Heijmans-Antonissen C, Drijfhout JW, et al. Detection of human papillomavirus (HPV) 16-specific CD4+ T-cell immunity in patients with persistent HPV16-induced vulvar intraepithelial neoplasia in relation to clinical impact of imiquimod treatment. Clin Cancer Res. (2005) 11:5273–80. doi: 10.1158/1078-0432.CCR-05-0616
74. Welters MJ, van der Logt P, van den Eeden SJ, Kwappenberg KM, Drijfhout JW, Fleuren GJ, et al. Detection of human papillomavirus type 18 E6 and E7-specific CD4+ T-helper 1 immunity in relation to health versus disease. Int J Cancer. (2006) 118:950–6. doi: 10.1002/ijc.21459
75. Coleman HN, Moscicki AB, Farhat SN, Gupta SK, Wang X, Nakagawa M. CD8 T-cell responses in incident and prevalent human papillomavirus types 16 and 18 infections. ISRN Obstet Gynecol. (2012) 2012:854237. doi: 10.5402/2012/854237
76. Piersma SJ, Jordanova ES, van Poelgeest MI, Kwappenberg KM, van der Hulst JM, Drijfhout JW, et al. High number of intraepithelial CD8+ tumor-infiltrating lymphocytes is associated with the absence of lymph node metastases in patients with large early-stage cervical cancer. Cancer Res. (2007) 67:354–61. doi: 10.1158/0008-5472.CAN-06-3388
77. Carter JJ, Koutsky LA, Wipf GC, Christensen ND, Lee SK, Kuypers J, et al. The natural history of human papillomavirus type 16 capsid antibodies among a cohort of university women. J Infect Dis. (1996) 174:927–36. doi: 10.1093/infdis/174.5.927
78. Tong Y, Ermel A, Tu W, Shew M, Brown DR. Association of HPV types 6, 11, 16, and 18 DNA detection and serological response in unvaccinated adolescent women. J Med Virol. (2013) 85:1786–93. doi: 10.1002/jmv.23664
79. Beachler DC, Viscidi R, Sugar EA, Minkoff H, Strickler HD, Cranston RD, et al. A longitudinal study of human papillomavirus 16 L1, e6, and e7 seropositivity and oral human papillomavirus 16 infection. Sex Transm Dis. (2015) 42:93–7. doi: 10.1097/OLQ.0000000000000236
80. Safaeian M, Porras C, Schiffman M, Rodriguez AC, Wacholder S, Gonzalez P, et al. Epidemiological study of anti-HPV16/18 seropositivity and subsequent risk of HPV16 and−18 infections. J Natl Cancer Inst. (2010) 102:1653–62. doi: 10.1093/jnci/djq384
81. Franceschi S, Baussano I. Naturally acquired immunity against human papillomavirus (HPV): why it matters in the HPV vaccine era. J Infect Dis. (2014) 210:507–9. doi: 10.1093/infdis/jiu143
82. Castellsagué X, Naud P, Chow SN, Wheeler CM, Germar MJ, Lehtinen M, et al. Risk of newly detected infections and cervical abnormalities in women seropositive for naturally acquired human papillomavirus type 16/18 antibodies: analysis of the control arm of PATRICIA. J Infect Dis. (2014) 210:517–34. doi: 10.1093/infdis/jiu139
83. Beachler DC, Jenkins G, Safaeian M, Kreimer AR, Wentzensen N. Natural acquired immunity against subsequent genital human papillomavirus infection: a systematic review and meta-analysis. J Infect Dis. (2016) 213:1444–54. doi: 10.1093/infdis/jiv753
84. Dobson SR, McNeil S, Dionne M, Dawar M, Ogilvie G, Krajden M, et al. Immunogenicity of 2 doses of HPV vaccine in younger adolescents vs 3 doses in young women: a randomized clinical trial. JAMA. (2013) 309:1793–802. doi: 10.1001/jama.2013.1625
85. Meites E, Kempe A, Markowitz LE. Use of a 2-dose schedule for human papillomavirus vaccination - updated recommendations of the advisory committee on immunization practices. MMWR Morb Mortal Wkly Rep. (2016) 65:1405–8. doi: 10.15585/mmwr.mm6549a5
86. Markowitz LE, Dunne EF, Saraiya M, Chesson HW, Curtis CR, Gee J, et al. Human papillomavirus vaccination: recommendations of the advisory committee on immunization practices (ACIP). MMWR Recomm Rep. (2014) 63:1–30.
87. Stanley M, Pinto LA, Trimble C. Human papillomavirus vaccines—immune responses. Vaccine. (2012) 30 (Suppl. 5):F83–7. doi: 10.1016/j.vaccine.2012.04.106
88. Suzich JA, Ghim SJ, Palmer-Hill FJ, White WI, Tamura JK, Bell JA, et al. Systemic immunization with papillomavirus L1 protein completely prevents the development of viral mucosal papillomas. Proc Natl Acad Sci USA. (1995) 92:11553–7. doi: 10.1073/pnas.92.25.11553
89. Roden RB, Hubbert NL, Kirnbauer R, Breitburd F, Lowy DR, Schiller JT. Papillomavirus L1 capsids agglutinate mouse erythrocytes through a proteinaceous receptor. J Virol. (1995) 69:5147–51. doi: 10.1128/JVI.69.8.5147-5151.1995
90. GlaxoSmithKline Vaccine HPV-007 Study Group, Romanowski B, de Borba PC, Naud PS, Roteli-Martins CM, De Carvalho NS, et al. Sustained efficacy and immunogenicity of the human papillomavirus (HPV)-16/18 AS04-adjuvanted vaccine: analysis of a randomised placebo-controlled trial up to 6.4 years. Lancet. (2009) 374:1975–85. doi: 10.1016/S0140-6736(09)61567-1
91. Schwarz TF, Galaj A, Spaczynski M, Wysocki J, Kaufmann AM, Poncelet S, et al. Ten-year immune persistence and safety of the HPV-16/18 AS04-adjuvanted vaccine in females vaccinated at 15-55 years of age. Cancer Med. (2017) 6:2723–31. doi: 10.1002/cam4.1155
92. Garland SM, Hernandez-Avila M, Wheeler CM, Perez G, Harper DM, Leodolter S, et al. Quadrivalent vaccine against human papillomavirus to prevent anogenital diseases. N Engl J Med. (2007) 356:1928–43. doi: 10.1056/NEJMoa061760
93. Pedersen C, Petaja T, Strauss G, Rumke HC, Poder A, Richardus JH, et al. Immunization of early adolescent females with human papillomavirus type 16 and 18 L1 virus-like particle vaccine containing AS04 adjuvant. J Adolesc Health. (2007) 40:564–71. doi: 10.1016/j.jadohealth.2007.02.015
94. Joura EA, Giuliano AR, Iversen OE, Bouchard C, Mao C, Mehlsen J, et al. A 9-valent HPV vaccine against infection and intraepithelial neoplasia in women. N Engl J Med. (2015) 372:711–23. doi: 10.1056/NEJMoa1405044
95. Arbyn M, Xu L. Efficacy and safety of prophylactic HPV vaccines. A Cochrane review of randomized trials. Expert Rev Vaccines. (2018) 17:1085–91. doi: 10.1080/14760584.2018.1548282
96. Hildesheim A, Wacholder S, Catteau G, Struyf F, Dubin G, Herrero R, et al. Efficacy of the HPV-16/18 vaccine: final according to protocol results from the blinded phase of the randomized Costa Rica HPV-16/18 vaccine trial. Vaccine. (2014) 32:5087–97. doi: 10.1016/j.vaccine.2014.06.038
97. Palefsky JM, Giuliano AR, Goldstone S, Moreira ED Jr, Aranda C, Jessen H, et al. HPV vaccine against anal HPV infection and anal intraepithelial neoplasia. N Engl J Med. (2011) 365:1576–85. doi: 10.1056/NEJMoa1010971
98. Giuliano AR, Palefsky JM, Goldstone S, Moreira ED Jr, Penny ME, Aranda C, et al. Efficacy of quadrivalent HPV vaccine against HPV Infection and disease in males. N Engl J Med. (2011) 364:401–11. doi: 10.1056/NEJMoa0909537
99. Lehtinen T, Söderlund-Strand A, Petäjä T, Eriksson T, Jokiranta S, Natunen K, et al. Human Papillomavirus (HPV) Prevalence in Male Adolescents 4 Years After HPV-16/18 Vaccination. J Infect Dis. (2017) 216:966–8. doi: 10.1093/infdis/jix415
100. Safaeian M, van Doorslaer K, Schiffman M, Chen Z, Rodriguez AC, Herrero R, et al. Lack of heterogeneity of HPV16 E7 sequence compared with HPV31 and HPV73 may be related to its unique carcinogenic properties. Arch Virol. (2010) 155:367–70. doi: 10.1007/s00705-009-0579-2
101. Marth C, Landoni F, Mahner S, McCormack M, Gonzalez-Martin A, Colombo N, et al. Cervical cancer: ESMO Clinical Practice Guidelines for diagnosis, treatment and follow-up. Ann Oncol. (2017) 28(Suppl. 4):iv72-iv83. doi: 10.1093/annonc/mdx220
102. Hoffman SR, Le T, Lockhart A, Sanusi A, Dal Santo L, Davis M, et al. Patterns of persistent HPV infection after treatment for cervical intraepithelial neoplasia (CIN): a systematic review. Int J Cancer. (2017) 141:8–23. doi: 10.1002/ijc.30623
103. Vermaelen K. Vaccine strategies to improve anti-cancer cellular immune responses. Front Immunol. (2019) 10:8. doi: 10.3389/fimmu.2019.00008
104. Chabeda A, Yanez RJR, Lamprecht R, Meyers AE, Rybicki EP, Hitzeroth II. Therapeutic vaccines for high-risk HPV-associated diseases. Papillomavirus Res. (2018) 5:46–58. doi: 10.1016/j.pvr.2017.12.006
105. Yang L, Mohr I, Fouts E, Lim DA, Nohaile M, Botchan M. The E1 protein of bovine papilloma virus 1 is an ATP-dependent DNA helicase. Proc Natl Acad Sci USA. (1993) 90:5086–90. doi: 10.1073/pnas.90.11.5086
106. Flickinger JC Jr, Rodeck U, Snook AE. Listeria monocytogenes as a vector for cancer immunotherapy: current understanding and progress. Vaccines. (2018) 6:E48. doi: 10.3390/vaccines6030048
107. Maciag PC, Radulovic S, Rothman J. The first clinical use of a live-attenuated Listeria monocytogenes vaccine: a Phase I safety study of Lm-LLO-E7 in patients with advanced carcinoma of the cervix. Vaccine. (2009) 27:3975–83. doi: 10.1016/j.vaccine.2009.04.041
108. Ferraro B, Morrow MP, Hutnick NA, Shin TH, Lucke CE, Weiner DB. Clinical applications of DNA vaccines: current progress. Clin Infect Dis. (2011) 53:296–302. doi: 10.1093/cid/cir334
109. Kim TJ, Jin HT, Hur SY, Yang HG, Seo YB, Hong SR, et al. Clearance of persistent HPV infection and cervical lesion by therapeutic DNA vaccine in CIN3 patients. Nat Commun. (2014) 5:5317. doi: 10.1038/ncomms6317
110. Samuels S, Marijne Heeren A, Zijlmans HJMAA, Welters MJP, van den Berg JH, Philips D, et al. HPV16 E7 DNA tattooing: safety, immunogenicity, and clinical response in patients with HPV-positive vulvar intraepithelial neoplasia. Cancer Immunol Immunother. (2017) 66:1163–73. doi: 10.1007/s00262-017-2006-y
111. Trimble CL, Peng S, Kos F, Gravitt P, Viscidi R, Sugar E, et al. A phase I trial of a human papillomavirus DNA vaccine for HPV16+ cervical intraepithelial neoplasia 2/3. Clin Cancer Res. (2009) 15:361–7. doi: 10.1158/1078-0432.CCR-08-1725
112. Bagarazzi ML, Yan J, Morrow MP, Shen X, Parker RL, Lee JC, et al. Immunotherapy against HPV16/18 generates potent TH1 and cytotoxic cellular immune responses. Sci Transl Med. (2012) 4:155ra138. doi: 10.1126/scitranslmed.3004414
113. Trimble CL, Morrow MP, Kraynyak KA, Shen X, Dallas M, Yan J, et al. Safety, efficacy, and immunogenicity of VGX-3100, a therapeutic synthetic DNA vaccine targeting human papillomavirus 16 and 18 E6 and E7 proteins for cervical intraepithelial neoplasia 2/3: a randomised, double-blind, placebo-controlled phase 2b trial. Lancet. (2015) 386:2078–88. doi: 10.1016/S0140-6736(15)00239-1
114. Rosales R, López-Contreras M, Rosales C, Magallanes-Molina JR, Gonzalez-Vergara R, Arroyo-Cazarez JM, et al. Regression of human papillomavirus intraepithelial lesions is induced by MVA E2 therapeutic vaccine. Hum Gene Ther. (2014) 25:1035–49. doi: 10.1089/hum.2014.024
115. Çuburu N, Kim R, Guittard GC, Thompson CD, Day PM, Hamm DE, et al. A prime-pull-amplify vaccination strategy to maximize induction of circulating and genital-resident intraepithelial CD8+ memory T cells. J Immunol. (2019) 202:1250–64. doi: 10.4049/jimmunol.1800219
116. Arbyn M, Redman CWE, Verdoodt F, Kyrgiou M, Tzafetas M, Ghaem-Maghami S, et al. Incomplete excision of cervical precancer as a predictor of treatment failure: a systematic review and meta-analysis. Lancet Oncol. (2017) 18:1665–79. doi: 10.1016/S1470-2045(17)30700-3
117. Ghaem-Maghami S, Sagi S, Majeed G, Soutter WP. Incomplete excision of cervical intraepithelial neoplasia and risk of treatment failure: a meta-analysis. Lancet Oncol. (2007) 8:985–93. doi: 10.1016/S1470-2045(07)70283-8
118. Ghaem-Maghami S, De-Silva D, Tipples M, Lam S, Perryman K, Soutter W. Determinants of success in treating cervical intraepithelial neoplasia. BJOG. (2011) 118:679–84. doi: 10.1111/j.1471-0528.2010.02770.x
119. Arbyn M, Kyrgiou M, Simoens C, Raifu AO, Koliopoulos G, Martin-Hirsch P, et al. Perinatal mortality and other severe adverse pregnancy outcomes associated with treatment of cervical intraepithelial neoplasia: meta-analysis. BMJ. (2008) 18:a1284. doi: 10.1136/bmj.a1284
120. Kyrgiou M, Athanasiou A, Paraskevaidi M, Mitra A, Kalliala I, Martin-Hirsch P, et al. Adverse obstetric outcomes after local treatment for cervical preinvasive and early invasive disease according to cone depth: systematic review and meta-analysis. BMJ. (2016) 28:i3633. doi: 10.1097/01.ogx.0000508341.95858.c5
121. Bruno MT, Cassaro N, Garofalo S, Boemi S. HPV16 persistent infection and recurrent disease after LEEP. Virol J. (2019) 16:148. doi: 10.1186/s12985-019-1252-3
122. Strander B, Hällgren J, Sparén P. Effect of ageing on cervical or vaginal cancer in Swedish women previously treated for cervical intraepithelial neoplasia grade 3: population based cohort study of long term incidence and mortality. BMJ. (2014) 348:f7361. doi: 10.1136/bmj.f7361
123. Ebisch RMF, Rutten DWE, IntHout J, Melchers WJG, Massuger LFAG, Bulten J, et al. Long-lasting increased risk of human papillomavirus-related carcinomas and premalignancies after cervical intraepithelial neoplasia grade 3: a population-based cohort study. J Clin Oncol. (2017) 35:2542–50. doi: 10.1200/JCO.2016.71.4543
124. Swedish KA, Factor SH, Goldstone SE. Prevention of recurrent high-grade anal neoplasia with quadrivalent human papillomavirus vaccination of men who have sex with men: a nonconcurrent cohort study. Clin Infect Dis. (2012) 54:891–8. doi: 10.1093/cid/cir1036
125. Kang WD, Choi HS, Kim SM. Is vaccination with quadrivalent HPV vaccine after loop electrosurgical excision procedure effective in preventing recurrence in patients with high-grade cervical intraepithelial neoplasia (CIN2-3)? Gynecol Oncol. (2013) 130:264–8. doi: 10.1016/j.ygyno.2013.04.050
126. Ghelardi A, Parazzini F, Martella F, Pieralli A, Bay P, Tonetti A, et al. SPERANZA project: HPV vaccination after treatment for CIN2. Gynecol Oncol. (2018) 151:229–34. doi: 10.1016/j.ygyno.2018.08.033
127. Hildesheim A, Gonzalez P, Kreimer AR, Wacholder S, Schussler J, Rodriguez AC, et al. Impact of human papillomavirus (HPV) 16 and 18 vaccination on prevalent infections and rates of cervical lesions after excisional treatment. Am J Obstet Gynecol. (2016) 215:212.e1–5. doi: 10.1016/j.ajog.2016.02.021
128. Swedish KA, Goldstone SE. Prevention of anal condyloma with quadrivalent human papillomavirus vaccination of older men who have sex with men. PLoS ONE. (2014) 9:e93393. doi: 10.1371/journal.pone.0093393
129. Kreuter A, Waterboer T, Wieland U. Regression of cutaneous warts in a patient with WILD syndrome following recombinant quadrivalent human papillomavirus vaccination. Arch Dermatol. (2010) 146:1196–7. doi: 10.1001/archdermatol.2010.290
130. Moscato GM, Di Matteo G, Ciotti M, Di Bonito P, Andreoni M, Moschese V. Dual response to human papilloma virus vaccine in an immunodeficiency disorder: resolution of plantar warts and persistence of condylomas. J Eur Acad Dermatol Venereol. (2016) 30:1212–3. doi: 10.1111/jdv.13133
131. Lee HJ, Kim JK, Kim DH, Yoon MS. Condyloma accuminatum treated with recombinant quadrivalent human papillomavirus vaccine (types 6, 11, 16, 18). J Am Acad Dermatol. (2011) 64:e130–2. doi: 10.1016/j.jaad.2010.12.032
132. Petry KU, Bollaerts K, Bonanni P, Stanley M, Drury R, Joura E, et al. Estimation of the individual residual risk of cervical cancer after vaccination with the nonavalent HPV vaccine. Hum Vaccin Immunother. (2018) 14:1800–6. doi: 10.1080/21645515.2018.1450125
133. Poynten IM, Tabrizi SN, Jin F, Templeton DJ, Machalek DA, Cornall A, et al. Vaccine-preventable anal human papillomavirus in Australian gay and bisexual men. Papillomavirus Res. (2017) 3:80–4. doi: 10.1016/j.pvr.2017.02.003
134. Ong JJ, Walker S, Grulich A, Hoy J, Read TRH, Bradshaw C, et al. Incidence, clearance, and persistence of anal human papillomavirus in men who have sex with men living with human immunodeficiency virus: implications for human papillomavirus vaccination. Sex Transm Dis. (2019) 46:229–33. doi: 10.1097/OLQ.0000000000000958
135. Rodríguez-Carunchio L, Soveral I, Steenbergen RD, Torné A, Martinez S, Fusté P, et al. HPV-negative carcinoma of the uterine cervix: a distinct type of cervical cancer with poor prognosis. BJOG. (2015) 122:119–27. doi: 10.1111/1471-0528.13071
136. Tao X, Zheng B, Yin F, Zeng Z, Li Z, Griffith CC, et al. Polymerase chain reaction human papillomavirus (HPV) detection and HPV genotyping in Invasive cervical cancers with prior negative HC2 test results. Am J Clin Pathol. (2017) 147:477–83. doi: 10.1093/ajcp/aqx027
137. Petry KU, Liebrich C, Luyten A, Zander M, Iftner T. Surgical staging identified false HPV-negative cases in a large series of invasive cervical cancers. Papillomavirus Res. (2017) 4:85–9. doi: 10.1016/j.pvr.2017.10.003
138. Tjalma W. HPV negative cervical cancers and primary HPV screening. Facts Views Vis Obgyn. (2018) 10:107–13.
139. Pirog EC, Lloveras B, Molijn A, Tous S, Guimerà N, Alejo M, et al. HPV prevalence and genotypes in different histological subtypes of cervical adenocarcinoma, a worldwide analysis of 760 cases. Mod Pathol. (2014) 27:1559–67. doi: 10.1038/modpathol.2014.55
140. Pirog EC. Cervical adenocarcinoma: diagnosis of human papillomavirus-positive and human papillomavirus-negative tumors. Arch Pathol Lab Med. (2017) 141:1653–67. doi: 10.5858/arpa.2016-0356-RA
141. Kusanagi Y, Kojima A, Mikami Y, Kiyokawa T, Sudo T, Yamaguchi S, et al. Absence of high-risk human papillomavirus (HPV) detection in endocervical adenocarcinoma with gastric morphology and phenotype. Am J Pathol. (2010) 177:2169–75. doi: 10.2353/ajpath.2010.100323
Keywords: human papillomavirus, immune response, cancer, prophylactic vaccine, therapeutic vaccine
Citation: Garbuglia AR, Lapa D, Sias C, Capobianchi MR and Del Porto P (2020) The Use of Both Therapeutic and Prophylactic Vaccines in the Therapy of Papillomavirus Disease. Front. Immunol. 11:188. doi: 10.3389/fimmu.2020.00188
Received: 18 November 2019; Accepted: 24 January 2020;
Published: 18 February 2020.
Edited by:
Roberta Antonia Diotti, Vita-Salute San Raffaele University, ItalyReviewed by:
Bryce Chackerian, University of New Mexico, United StatesEdward Rybicki, University of Cape Town, South Africa
Copyright © 2020 Garbuglia, Lapa, Sias, Capobianchi and Del Porto. This is an open-access article distributed under the terms of the Creative Commons Attribution License (CC BY). The use, distribution or reproduction in other forums is permitted, provided the original author(s) and the copyright owner(s) are credited and that the original publication in this journal is cited, in accordance with accepted academic practice. No use, distribution or reproduction is permitted which does not comply with these terms.
*Correspondence: Anna Rosa Garbuglia, argarbuglia@iol.it