- 1Department of Haematology, Sheffield Teaching Hospitals NHS Foundation Trust, Royal Hallamshire Hospital, Sheffield, United Kingdom
- 2Department of Photopheresis, The Rotherham NHS Foundation Trust, Rotherham, United Kingdom
As the use of hematopoietic stem cell transplantation (HSCT) has become a more widespread and effective treatment for hematological malignant and non-malignant conditions, the need to minimize the harmful effects of graft- vs.-host disease (GvHD) has become more important in achieving good outcomes. With diagnosis of GvHD reliant on its clinical manifestations, research into biomarkers for the diagnosis, progression, and even for the prediction of disease, is imperative to combating the high levels of morbidity and mortality post-HSCT. Despite the development of novel treatment approaches to GvHD, corticosteroids remain the standard first-line treatment, with immunosuppressant therapies as second-line options. These strategies however have significant limitations and associated complications. Extracorporeal Photopheresis (ECP) has shown to be effective and safe in treating patients with symptomatic GvHD. ECP has been shown to have varied effects on multiple parts of the immune system and does not appear to increase the risk of relapse or infection in the post HSCT setting. Even so, ECP can be logistically more complex to organize and requires patients to be sufficiently stable. This review aims to summarize the potential role of biomarkers to help guide individualized treatment decisions in patients with acute and chronic GvHD. In relation to ECP, robust biomarkers of GvHD will be highly useful in informing patient selection, intensity and duration of the ECP schedule, monitoring of response and other treatment decisions alongside the concurrent administration of other GvHD therapies. Further research is warranted to establish how GvHD biomarkers are best incorporated into ECP treatment pathways with the goal of tailoring ECP to the needs of individual patients and maximizing benefit.
Introduction
Hematopoietic stem cell transplantation (HSCT) has become an established routine treatment for hematological malignancies, with over a million transplants having taken place across five continents (1). However, a major limiting factor of this curative treatment is the development of graft vs. host disease (GvHD), which is a key cause of morbidity and mortality to patients following allogeneic HSCT (2), where control of GvHD is central to optimizing long-term outcomes. The therapeutic action of HSCT relies on the graft vs. leukemia (GvL) effect, which therefore makes systemic immunosuppression for GvHD prevention and treatment undesirable (3). Effective prevention and treatment of GvHD is therefore a challenging balance between targeting GvHD whilst maintaining the GvL effect.
Increased understanding of the pathophysiology of GVHD has driven strategies to enable earlier diagnosis, alter management and apply new therapeutic interventions (4).
Graft vs. Host Disease (GvHD)
GvHD presents as two distinct clinical syndromes, acute (aGvHD) and chronic (cGvHD).
Acute GvHD
Acute GvHD is characterized by a marked inflammatory reaction thought to be mediated by donor T lymphocytes recognizing the host tissue as non-self relatively soon after transplantation. The most commonly seen sites of involvement are the skin (maculopapular rash), liver (bile duct damage and cholestasis with hyperbilirubinemia), and the gastrointestinal tract (vomiting, anorexia, and severe diarrhea) (5, 6). aGvHD is reported to manifest in 30–50% of allogenic-HSCT recipients, of which 14% experience severe aGvHD (grades III-IV on the modified Glucksberg-Seattle criteria), associated with poor outcomes (6). In practice aGvHD is diagnosed clinically, supported by exclusion of differentials and histological confirmation, with risk of development related to donor-recipient histocompatibility (7, 8). Biopsy and histological examination is a crucial part of the work up and can be logistically challenging to obtain before starting treatment but is very useful in confirming the diagnosis and in disease staging (7, 8). Corticosteroid treatment remains standard first line therapy though there is no standard effective second line treatment for those failing steroids (9).
Chronic GvHD
Chronic GvHD is reported to affect 30–40% of patients receiving allogeneic HSCT (10). The pathophysiology of cGvHD comprises of complex pathways involving both T and B cells, the mechanisms of which are yet to be fully understood (11). The myriad clinical manifestations of cGvHD can make diagnosis and monitoring response to treatment challenging. Following NIH 2014 working group recommendations, diagnosis is made clinically based on presence of at least one diagnostic manifestation or at least one distinctive manifestation supported by relevant tests such as histology, which should differ from the hallmark signs of aGvHD (dermatitis, enteritis and cholestasis) with recommendations made also to standardize monitoring and response assessment (12). Treatment of cGvHD comprises first line of corticosteroids, usually prednisolone, often in combination with a calcineurin inhibitor (13). Around 50% of patients with established cGvHD respond to steroids, but only 20% are living without disability after 4 years (14). Steroids with adjuvant therapies have also shown to have no overall benefit when compared to steroids alone (14). Further therapies of cGvHD include inhibition of B cell signaling (Ibrutininb), Inhibition of T cell signaling (Ruxolitininb), Depletion of B cells (Rituximab), T reg sparing therapy (Sirolimus), and T reg expansion (ECP, IL2) (4).
Overview of Biomarkers in GvHD
Significant progress has been made in identifying and validating biomarkers for GvHD (15). Based on the 2014 NIH consensus (16), these biomarkers have been investigated for diagnostic, prognostic, and predictive use, and additionally to assess treatment response. Given the heterogeneity of the condition and differing clinical practice the consensus statement also highlighted the need for any potential biomarkers to be validated by at least two independent cohorts prior to investigation in clinical setting including trials and patient management. Such prediction of risk and prognostic information would allow the stratification of patients according to their individual risk and a tailoring of treatment regimens and prophylaxis to reduce the severity of tissue damage. Diagnostic biomarkers could allow pre-emptive treatment to be started before clinical manifestation of GvHD and further monitoring with biomarker-mediated assessment of treatment response.
Biomarkers in aGvHD
The most validated serum biomarker for aGvHD (Table 1) is ST2 (suppression of tumorigenicity 2). A serum level of ST2 measured on Day 14 post transplantation has been shown to be associated with significantly increased risk of aGvHD, including treatment-resistant aGvHD with increased non-relapse mortality (NRM) and predictive of transplant related mortality (TRM) (17, 18). An additional study described levels of ST2 to be predictive of NRM within 1 year (19). Due to its functional relationship to tissue damage and immune function, ST2 has been considered the best candidate biomarker to indicate severity and prognosis of aGvHD (20). The role of ST2 in GvHD pathogenesis has been explored further with monoclonal antibody blocking of soluble ST2 in the peri-transplant period showing protection against GvHD whilst preserving GvL activity (21). Regenerating Islet-derived 3-alpha (Reg3α) has been validated as a prognostic and diagnostic biomarker specific to gastrointestinal aGvHD. Increased serum Reg3α levels post-transplantation have shown to indicate an increased incidence of severe aGvHD, thought to be caused by the destruction of GI paneth cells and impaired epithelial function (22), which was also indicative of poor prognosis following treatment (23). A 2 biomarker panel based algorithm combining ST2 and Reg3α levels measured 7 days post-transplant has been shown to stratify patients on the basis of NRM into two distinct high risk and low risk groups (24). It has to be borne in mind that such biomarkers can also be elevated in other pathologies associated with an inflammatory milieu in this period such as thrombotic microangiopathy, cytokine release syndrome, mucosal inflammation and idiopathic pneumonia. These confounding variables may have an impact on the rate of false positive results (19, 25, 26). Similarly, T-cell immunoglobulin mucin-3 (TIM3), thought to exacerbate aGvHD severity, has been shown to be useful identifying patients with higher risk of severe GvHD and mortality, and additionally shows potential in predicting failure of corticosteroid treatment (28, 29). sTNFR1 (soluble tumor necrosis factor receptor-1) and IL-6 have been found to be valuable in predicting incidence of severe aGvHD and NRM (19), with sTNFR1 additionally predicting treatment failure (29). Biomarkers have also been studied with respect to the affected organ with specific targets including skin (Elafin) (30).
Lower GI(Reg3α,TIM3) (22) and liver (HGF, KRT18) (31) IL-6 has been implicated as a pro inflammatory agent in the context of GvHD and blocking this appears to have a dampening effect on GvHD (32). C-reactive protein (CRP) as a surrogate marker for IL6 is a routinely available inflammatory marker, has been shown to be a good indicator of aGvHD risk in a PRISM compliant meta-analysis (33), and a 2012 study has shown potential of fecal calprotectin and α-1-antitrypsin as biomarkers (34), which again are markers readily available in routine practice. An alternative approach to soluble biomarkers has been to look at changes in patterns or counts of cellular mediators as predictive biomarkers of aGvHD. One of the earliest targets of aGvHD is the vascular endothelium resulting in endothelial GvHD (35). In a prospective sequential analysis of 90 allo-HSCT patients circulating endothelial cells (CEC) counts increased 1–2 weeks before and peaked at onset of aGvHD (36). Conversely, CEC counts returned to pre-transplant baseline after treatment response. Another method is detailed monitoring and statistical analyses of multiple subsets of lymphocytes by flow cytometry; in a study of 50 HSCT patients aGvHD development was significantly associated with increased frequencies of central memory CD4 T cells (Tcm) and memory B-cells pre-HSCT and by increased frequencies of memory, naïve, T-reg and recent thymic emigrant (RTE) T-cell subsets at aGVHD onset (37).
Although aGVHD is primarily mediated by alloantigen-specific donor lymphocytes, the initial trigger for disease development is thought to be the activation of antigen-presenting cells (APCs) by danger signals from damaged tissues and pathogen-associated molecular patterns (PAMPs) through a class of highly evolutionarily-conserved pattern recognition receptors called Toll-like receptors (TLRs) (38). In a prospective study of the expression of all 9 human TLRs in a cohort of 34 allo-HSCT patients, development of aGVHD correlated with high monocyte and T-cell expression of TLR5 and low expression of TLR1 and TLR9 (39). TLR5 recognizes flagellin, a component of the flagella of motile bacteria, including intestinal bacteria (40), which translocate to the blood following damage to the intestinal mucosa (41). High expression of TLR5 might lead to increased responses to TLR5 agonists leading to enhanced stimulatory capacity and pro-inflammatory cytokine production by APCs and increased activation and proliferation of effector T-cells (49). Similarly, ligation of TLR1 (which recognizes bacterial lipopeptides) and TLR9 (which recognizes viral and bacterial DNA) both stimulate pro-inflammatory cytokine production (50). How low levels of expression of these TLRs is associated with aGvHD is unclear, but may be linked to cross-regulation of TLRs (51).
Biomarkers in cGvHD (Table 2)
Compared to aGvHD, less has been accomplished in the validation of biomarkers for cGvHD; however several candidates of note have substantial evidence for their potential use. B-cell activating factor (BAFF) is one such candidate, and one of the first biomarkers associated with cGvHD. Increased BAFF has been linked with the pathogenesis of cGvHD, through increased abnormal B-cell survival and BAFF levels were shown increased in chronic GVHD patient sera (42, 43). A recent study confirmed the correlation between onset of cGvHD and increased soluble serum BAFF (44), and a further study found patients without cGvHD showed gradually decreasing BAFF levels as B cell numbers increased after myeloablative conditioning and significantly different BAFF/B cell ratios at 3 months post-HSCT in patients who subsequently developed cGVHD (45). A 2016 study across two cohorts aimed at identifying diagnostic and prognostic biomarkers for cGvHD resulted in a panel of 4 proteins (ST2, CXCL9, matrix metalloproteinase 3, and osteopontin) shown to indicate prediction of cGvHD diagnosis, and additionally prognostic risk stratification post-HSCT (46). This study showed strength in the reproducibility of its results across a second cohort, with samples from eight different sites used (46). With ST2 shown to be a valid biomarker for aGVHD and a target for monoclonal antibody blocking (21), additional therapeutic benefit might be derived in cGvHD. The CXCR3 chemokine receptor has interferon-inducible ligands CXCL9 and CXCL10, which have previously been shown to have a role in trafficking CXCR3+ T cells toward the peripheral tissues (59). These ligands have been shown to be useful as potential cGvHD biomarkers. CXCL9 levels were shown in one prospective, multicentre study to be associated with cGvHD (28), and similarly found to be elevated in cGvHD plasma when compared to healthy or non-cGvHD controls (47). CXCL10 was also shown to be elevated in cGvHD plasma (47), and was the only biomarker investigated to meet the criteria of Kariminia et al. for replication as a clinical biomarker for the diagnosis of cGVHD (48).
Extracorporeal Photopheresis (ECP) as an Immunomodulatory Treatment Modality for GvHD
ECP is a cell-based immuno-modulatory treatment whereby the buffy coat of peripheral blood, containing leukocytes and platelets, is separated, treated to a photosensitizing agent (8-methoxypsoralen) and exposed to UVA light and re-infused back to the patient. This treatment was initially reported by Edelson who published on the use of ECP in the treatment of erythrodermic cutaneous T-cell lymphoma (CTCL) in context of a multicentre trial (60). It is a mature treatment modality and for over 20 years has been used for chronic and acute graft vs. host disease (cGVHD) and solid organ transplant rejection (61, 62).
Clinical Application of ECP in Acute GvHD
There is no standard second line treatment for patients who are either refractory to first line steroids or have steroid dependent aGvHD (63). There is an unmet need for a modality of treatment which offers immunomodulation rather than immune suppression as a way of reducing the effect of GvHD. Given its potential impact on T cell mediated responses, ECP has been studied as a treatment option in aGvHD where there is an immunological donor T cell response to host alloantigens. In a pioneering phase II study, 59 patients with acute steroid-refractory GVHD grades II to IV were treated with ECP weekly and response and long-term survival were assessed (64). Eighty-two percent of patients with cutaneous involvement, 61% with liver involvement and 61% with gut involvement achieved complete response (CR). Among responders the survival probability was 59% compared to 11% in patients not responding completely. Further at 4 years the transplant related mortality was significantly lower for patients achieving a CR to ECP (14 vs. 73%) with an overall survival (OS) at 4 years 59 vs. 11% in those achieving CR. Similar responses were noted in another study of 27 patients for steroid resistant GvHD (65) with a suggestion of better response at the early initiation of ECP in steroid resistant disease. This was confirmed in another report with higher response rates when treatment was started within 35 days of onset of aGvHD (66). ECP has also been studied in relation to the use of anti-cytokine therapy for aGvHD with a multicentre comparative analysis showing significantly higher response in the ECP arm compared to etanercept or inolimumab arm with patients receiving ECP showing a survival advantage (67). Looking at the response to ECP in a systematic review, Abu-Dalle et al. (68) showed aGvHD overall response rates to ECP were 69% across 323 patients in 9 studies (95% confidence interval 0.34 to 0.95) (49). Highest response was seen in cutaneous aGvHD, followed by gastrointestinal. The American Society of Blood and Marrow Transplantation have developed recommendations for treatment of aGvHD based on evaluation of 29 studies (9). In regard to ECP there was no increase in overall rates of infection particularly viral reactivations, which can be a major concern with ongoing immunosuppressive treatment though it did not specify any single agent in the second line setting. This view is also echoed by the guidelines issued by the British Society for Blood and Bone Marrow Transplantation (BSBMT) (69). Similar recommendations have been made by the Italian scientific societies the Italian Society of Hemapheresis and Cell Manipulation (SIdEM) and the Italian Group for Bone Marrow Transplantation (GITMO) in their best practice recommendation for the use of ECP in acute and chronic GvHD in adults and children (70). Currently ECP is considered a potential treatment option for patients with aGvHD grades II-IV who are steroid refractory, steroid dependent or steroid intolerant in the HSCT setting and for solid organ transplant rejection (61, 62).
Clinical Application of ECP in Chronic GvHD
Since the initial report of its use in 1994 to successfully treat cGvHD (71), ECP has been shown as an effective and recommended treatment for cGvHD, including steroid refractory GvHD (72, 73). In a review of both prospective and retrospective studies in the secondary treatment of cGvHD published between 1990 and 2011, ECP was the most frequently studied therapy (74). Flowers et al. reported a phase 2 randomized controlled prospective study of ECP treatment in cGvHD (72). The study compared standard treatment alone with the addition of ECP in cutaneous cGvHD. The proportion of patients who had at least a 50% reduction in steroid dose and at least a 25% decrease from baseline in TSS was 8.3% in the ECP arm at week 12 and 0% in the control arm (P = 0.04). The non-blinded investigator assessment of skin complete or partial responses revealed a significant improvement in favor of ECP (P < 0.001). A limitation of this study was that skin score was the main focus of assessment and physicians were aware of study assignment. Progressive improvement in symptoms and increased steroid sparing effect was seen in longer ECP treatment of 24 weeks, reported by Greinix et al. in a follow up study (75). A recent randomized control prospective study with 60 patients compared addition of ECP to standard of care in the first line setting using the NIH 2015 criteria for diagnosis and response assessment. ORR at week 28 was 74.1% (ECP arm) vs. 60.9% (control arm). Furthermore, patients in the ECP arm tolerated the treatment well and crucially maintained quality of life (QoL) whilst there was a decline in QoL scores in patients in the standard care arm (76). In a prospective trial evaluating the efficacy of ECP in both skin and visceral cGvHD (77), Foss et al. enrolled 25 patients with extensive, steroid-refractory cGvHD. 20 patients had improvement in cutaneous GVHD and six had healing of oral ulcerations. Steroid sparing or discontinuation of immunosuppressive medications was possible in 80% of patients with similar response rates between patients receiving treatment weekly vs. fortnightly treatments. A review of 27 studies including 725 adults treated with ECP for steroid-resistant, intolerant, or dependent cGvHD of (61). The mean response rate for cutaneous cGvHD 74% (reported in 23 studies) hepatic cGvHD was 62% (15 studies), 60% for ocular cGvHD (4 studies), and 62% for mucosal cGvHD (reported in 12 studies). Pierelli et al. reviewed 23 studies reporting on 735 patients treated with ECP for steroid-resistant, -intolerant, or -dependent cGvHD (70). As a whole, overall and complete responses were observed in 64 and 35% of cases with cutaneous involvement and in 56 and 27% with hepatic cGvHD, respectively. Overall response rate was also 47 to 57% in oral mucosa and gastrointestinal tract cGvHD. High response rates, near 50%, were also reported in children with ocular involvement. In 2012, Del Fante et al. reported on a retrospective analysis of 102 patients with cGVHD treated with ECP over a 14 year period, assessing whether the NIH consensus classification better predicted survival and response to ECP (78). The study found no correlation between response and NIH clinical subtype, number, or degree of organ involvement, and found no response in patients with lung involvement. A retrospective multicentre evaluation of ECP as second line treatment for acute and chronic GvHD reported a response in at least 80% with long term survival of at least 50% of the cases (79). Abu Dalle et al. in their systematic review evaluating the efficacy of ECP treatment in steroid refractory or steroid dependent GvHD, similarly suggest organ-specific response to be higher in cutaneous, gastrointestinal, hepatic, and oral mucosa, with very limited effect of ECP on pulmonary cGvHD (68).
An important therapeutic effect of ECP in cGvHD is steroid reduction whilst controlling GvHD thereby having an impact on the morbidity and mortality related to prolonged immunosuppression (77, 80, 81). ECP has also been shown to maintain responses to viral infection and does not increase the risk of relapse (82, 83) QoL is an important measure of outcome for patients undergoing allogeneic HSCT comparable with scores reported for systemic sclerosis, systemic lupus erythematous, and multiple sclerosis (84). In a prospective study evaluating the effect of ECP on clinical response and QoL in cGVHD using two validated questionnaires, there was significant improvement in both cGVHD symptoms scale and DLQI scores in patients who completed 6 months of ECP (85).
Immunological Mechanisms of ECP Action
Over 30 years after ECP was invented its definitive modes of action remain elusive. While ECP may be considered one of many apoptotic cell therapies being exploited for inducing immunotolerance to auto- and alloantigen, its lack of immunosuppressive effect and proven clinical effectiveness against CTCL as well as GVHD is both confounding and intriguing. Exposure to 8-MOP/UVA induces cross-linking of DNA, triggering a series of apoptotic events including loss of mitochondrial membrane potential, caspase activation and phosphatidylserine exposure (86). The flipping of phosphatidylserine from the inner plasma membrane leaflet to the outer surface is one of an array of “eat-me” signals recognized by professional phagocytes such as macrophages and dendritic cells which facilitates the specific removal of dead, damaged, and dying cells (87). The removal of apoptotic cells by phagocytes is termed “efferocytosis” meaning “to bury” and is essential for tissue and immune system homeostasis (88, 89). ECP has direct effects on lymphocytes, NK cells, neutrophils, and monocytes with neutrophils and NK cells being most readily affected while monocytes and myeloid dendritic cells have been reported to show the greatest resistance (90, 91). The data for the effects of ECP on monocyte cell death are conflicting. While some groups report that monocytes are as susceptible to ECP-induced apoptosis as other PBMC (92–94), others show marked survival (95–97) or showed no greater levels of cell death than untreated controls (98, 99). The reported preferential survival of monocytes may be facilitated by integrin-mediated survival signals generated through interaction of monocytes with plasma proteins bound to plastic surfaces in the ECP instrument, which subsequently directed differentiation into monocyte-derived dendritic cells (100). While neutrophils constitute the largest fraction of leukocytes treated and ultimately rendered apoptotic by ECP (90), infusion of ECP-treated leukocytes has been reported to rapidly mobilize patient neutrophilic myeloid-derived suppressor cells (MDSC) into the circulation (101). Functionally, these MDSC could suppress Th1 and Th17 responses and longitudinal studies showed a relationship between therapeutic response to ECP and progressive increase in peripheral blood MDSC frequency.
ECP results in the functional suppression and subsequent deletion of large numbers of pathogenic leukocytes from the circulation, however, it is thought that since only 5–10% of circulating leukocytes are directly affected, this is unlikely to be the primary mechanism of effect (102). Instead, it is the indirect, wider and sustained immunomodulatory effect of the uptake and processing of ECP-treated cells on the effectors of disease, which confers therapeutic benefit. ECP primes massive numbers (> 2 × 109–dependent on size and state of the patient) of leukocytes for cell death which are infused in high density (>20 × 106 cells/ml) back into the patient through venous return within 4–6 min, but in vitro analysis suggests apoptotic features are not induced until at least 4 h after ECP treatment (90). Tracking of infused radiolabelled ECP-treated PBMC and neutrophils in patients revealed that both were detected in the lungs, spleen and liver within 10 min, but had different patterns of migration, with PBMC being initially retained in the lungs in greater quantity than neutrophils, but then subsequently trafficking to the liver and spleen (103), suggesting that ECP-treated leukocytes retain homing ability for at least a few hours post-infusion. These observations are consistent with in vivo tracking studies of apoptotic cells in murine models where intravenously infused apoptotic cells are phagocytosed by macrophages and dendritic cells located in the lung, liver and spleen (104, 105). The uptake of apoptotic cells by macrophages induces a suppression of IL1-β,IL-6, IL-12, and TNF-α proinflammatory cytokine production while inducing the secretion of TGF-β1 and PGE-2 (106). Similarly, dendritic cell uptake of apoptotic cells induces a tolerogenic phenotype characterized by low levels of expression of costimulatory molecules, suppressed production of proinflammatory cytokines and enhanced production of anti-inflammatory IL-10 producing an APC with low capacity to stimulate the generation of T-cell effectors, instead, generating the priming of TGF-beta 1-dependent FoxP3 regulatory T-cells (105) (Figure 1).
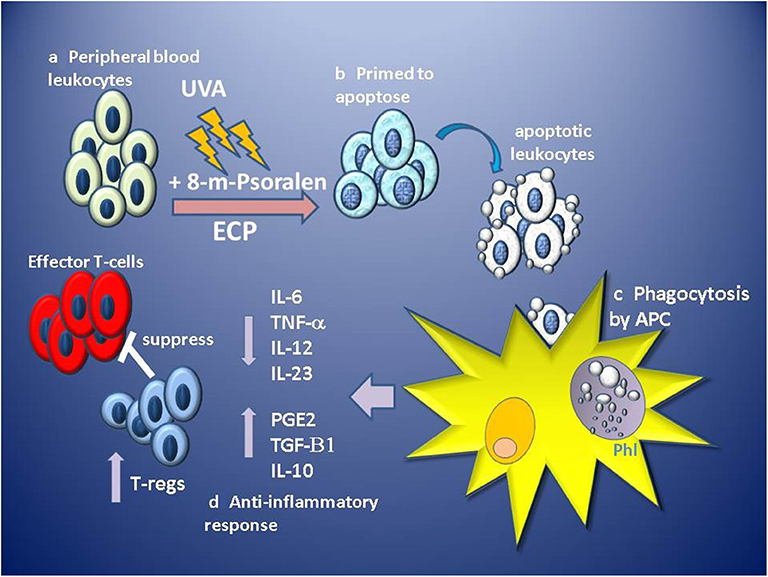
Figure 1. Stages of proposed primary hypothesis for mechanism of immunomodulation of GVHD by ECP. (a) Apheresed peripheral blood leukocytes are separated from red blood cells and concentrated before exposure to 8-methoxypsoralen (8-m-psoralen) and photoactivated by UV-A light (UVA). (b) ECP-treated leukocytes now primed to die by apoptosis are infused into the circulation. (c) Apoptotic leukocytes are recognized, engulfed and phagocytosed by antigen presenting cells (APC: macrophages and dendritic cells) in phagolysosomes (Phl). (d) Recognition of apoptotic cells induces an anti-inflammatory tolerogenic response by APCs resulting in lower production of pro-inflammatory cytokines IL-6, IL12, IL-23, and TNFα and induces production of anti-inflammatory IL-10, TGF-β1, and prostaglandin E2 (PGE2). Tolerogenic APCs promote the priming and expansion of regulatory T-cell (T-regs), which suppress the function of alloantigen-specific effector T-cells involved in GVHD.
In an in vivo model of ECP treatment of acute GVHD, weekly infusion of splenocytes from an allogeneic donor with acute GVHD, given after HSCT, strongly enhanced survival, and reversed established GVHD symptoms (107). The mechanism of protection was dependent on donor-derived CD25hi FoxP3 T-regs found in increased numbers in the spleen and was coupled with a decrease in splenic CD8+ T-cell effectors. GVHD is characterized by a lack of circulating T-regs, which can potentially exert regulatory effects on T-cell effectors and DCs at all stages of GVHD as well as facilitating tissue repair through the secretion of factors such as amphiregulin (6, 108, 109). T-regs mediate immunotolerance and part of the therapeutic effect of immunosuppressive drugs such as rapamycin and glucocorticoids is mediated through the promotion of induced T-regs (110, 111). However, while ECP facilitates immunotolerance there are conflicting data regarding the role of T-regs in ECP immunodulation of GVHD. While some groups report an expansion of circulating numbers of T-regs (92, 112–114), others show expansion, but no correlation to response in terms of steroid tapering or disease score (115). In a randomized prospective trial of ECP for cGVHD there was no significant change in the frequency of circulating T-regs or skin-homing T-regs (116). Similarly, in a trial combining ECP with low-dose IL-2, which has shown promise in expanding T-regs in cGVHD patients (117), there were no differences in the absolute counts of circulating T-regs between ECP-responders and non-responders although both showed marked T-reg expansion in the first few weeks of starting IL-2 treatment (118). Such observations in patients do not readily fit a model of ECP being primarily mediated through the induction of T-regs and other experimental data challenge this paradigm in the understanding of autologous ECP- treatment of ongoing inflammatory disease. A more recent in vivo model has shown that infusion of ECP/PUVA-treated cells from an allogeneic healthy donor failed to provide protection or reverse acute GVHD development, whereas splenocytes from an allogeneic donor of the same genetic background with acute GVHD provided significant protection (119). Further, in an in vivo model of ECP-modulation of rheumatoid arthritis, only ECP-treated splenocytes from arthritogenic donors could suppress inflammation, whereas those from healthy donors had no significant effect (120). Such observations suggest that supply of apoptotic cells alone is insufficient to control ongoing severe inflammatory diseases. It is of note that most of the studies using apoptotic cell therapy to prevent allograft rejection use donor cells that are from healthy donors and are thus from an immune environment that is in the steady state and the cells are resting or non-activated. In contrast, ECP for treatment of GVHD is autologous and many PBMC are activated. While apoptotic resting cells are tolerizing, activated or damaged cells can be immunogenic (121). This is illustrated in an in vivo delayed type hypersensitivity model where infused apoptotic resting naive CD4 T-cells induced tolerance, but apoptotic activated CD154+ CD4 T-cells were immunogenic and licensed DCs to recruit and prime CD4 T-cell effectors (122). Hannani et al. have observed that ECP- treated HLA-DR+ activated lymphocytes from GVHD patients die quicker than their non-activated counterparts (123) and have proposed a novel model where these would be preferentially phagocytosed and their antigens processed and presented before the slower dying non-activated fraction (124). Through being activated these are potentially immunogenic and might license DCs to prime anti-clonotypic cytotoxic T-cells to target and delete the alloantigen-specific pathogenic clones mediating GVHD. This model is compatible with ECP being free of general immunosuppression and can accommodate the apparent contradiction of ECP being effective for both immunotolerizing against GVHD and immunostimulatory against CTCL (124). Indeed, recent data suggests that tolerogenic and immunogenic effects can be potentially exerted by different cell types in the same ECP-treated sample since apoptotic neutrophils down-regulated LPS-induced DC and macrophage inflammatory cytokine production and reduced overall APC activation. In contrast, co-culture with apoptotic CD3 T-cells activated both APCs and enhanced LPS-induced proinflammatory cytokine production, particularly of TNF-α, coupled with enhanced APC allostimulatory capacity (125).
Biomarkers in Relation to ECP Treatment of GvHD (Table 3)
An early study by French et al. (52) was one of the first on biomarkers for ECP response in GvHD. The authors investigated whether circulating clonal T cells in peripheral blood and clonal T cell receptor γ (TCRγ) rearrangement, could be linked to response to ECP, as was previously demonstrated in cutaneous T cell lymphoma (CTCL) (53). Using fluorescent based PCR and capillary electrophoresis, peripheral blood samples of 27 patients post-allogenic HSCT were analyzed for TCRγ gene rearrangement. Seventeen of the patients studied had extensive cGvHD and 10 were without GvHD. TCRγ gene rearrangements and amplified clonal T cell populations were found in 60% of the patients without cGvHD and in 76.5% of patients with cGvHD, compared to 0% of the healthy controls. Twelve of the cGvHD patients received ECP treatment, 8 of which had significant response. It was found that all the patients who responded to ECP had amplified clonal T cell populations and those who did not respond to treatment did not. It was therefore concluded that expanded clonal T cell populations in the patients with cGvHD before treatment increased significantly the probability of cutaneous response to ECP. A subsequent study by Kuzmina et al. (54) investigated levels of immature B lymphocytes in 49 patients with moderate and severe cGvHD, measuring immature CD19+CD21− B cells and memory CD19+CD27+ cells before ECP and 6, 12 and 21 months into ECP treatment. Patients who showed no response to ECP after 6 months had significantly higher proportions of immature CD19+CD21− cells prior to ECP treatment, compared to patients with complete and partial response. The proportions of memory CD19+CD27+ cells prior to ECP were not significantly different between the groups, however there was a significantly higher ratio of CD21− to CD27+ cells before treatment in patients showing no response. A 2010 study reported by Akhtari et al. (55) investigated correlation of response to ECP with patients' baseline circulating dendritic cells (DCs) and T lymphocytes. Twenty-five patients with cGvHD were treated with ECP, with 2 procedures on consecutive days every week for the first 2 months, then every other week for 2 months, followed by once monthly. Baseline number of myeloid and plasmacytoid DC precursors, and CD4+ and CD8+ T lymphocytes, were measured using flow cytometry. The study concluded that patients who responded to ECP had higher baseline circulating DCs and T cells, which can predict response to ECP in cGvHD patients. The study noted that apart from a decrease in CD4+ cells in responsive patients, there was no significant change in T cell of DC populations over the year following ECP treatment. Following the focus of Kuzmina et al. on B lymphocytes as predictive biomarkers, Whittle and Taylor (56) investigated serum BAFF measurements in 46 cGvHD patients undergoing ECP treatment and demonstrated the potential use of BAFF as a biomarker to predict treatment response in cutaneous GvHD. BAFF levels after 1 month of ECP predicted response at 3 and 6 months. Patients with BAFF concentrations of <4 ng/mL showed decreased skin GvHD and complete resolution in 11 patients and those with high BAFF concentrations showed worsened skin GvHD at 6 months and resolution in only 1 patient. Subsequent measurement of BAFF after 3 months of treatment was reported to predict probability of maintaining improvement at 6 months. The study reported BAFF concentration only to correlate to skin GvHD but full responders to ECP in skin GvHD also had more improvements in other organs than those who did not. Bertani et al. (57) focused on the T lymphocyte population including CD3+. They reported a 2015 retrospective study on the response of steroid-refractory cGvHD to ECP, linking CD3+ lymphocyte count in harvested peripheral blood during ECP procedures to clinical response to treatment. Flow cytometry analyses of 726 procedures in 15 patients over at least 6 months were used. Standard ECP procedure was used, with patients undergoing two procedures twice monthly until partial response, followed by monthly procedures until complete response, with response assessed monthly throughout. Analysis showed that CD3+ numbers from apheresis in ECP during the early stages of treatment were correlative to subsequent clinical response. This prediction of response may identify patients early on in treatment who are responding to ECP and exclude those who are unlikely to achieve clinical response. Such lymphopenia is indicative of patients with more severe GVHD (126) The corollary of this would be that patients who are responsive to ECP have higher levels of circulating T-cells indicative of less severe GVHD. The distinction between genuine ECP responses from milder forms of GVHD that may resolve spontaneously will need randomized clinical trials. On the other hand, these data may indicate that a minimum dose of, and hematopoietic capability, to supply ECP-treated T-cells is needed to exert therapeutic effect or that the infusion includes circulating allo-reactive T-cell clones (52, 113) More recently, Iniesta et al. (58) reported in 2018 a prospective analysis of 32 GvHD patients undergoing 552 ECP treatments for both, investigating correlation between response to ECP and CD56bright natural killer (NK) cell population. 11 aGvHD and 21 cGvHD patients underwent ECP treatment during a minimum 3-month period, using a standard ECP protocol, with 1–2 procedures every week for 6 weeks, followed by one procedure every 2 weeks for 6 weeks, then one procedure every month until greatest response was seen. Flow cytometry was used to analyze lymphocyte populations from peripheral blood taken before, and at regular intervals throughout ECP treatment. Complete clinical response to ECP, defined as complete resolution of clinical signs and symptoms, was shown to correlate to increased percentages of CD56bright NK cells, or an increased CD56bright/dim ratio. This study demonstrated the change in immune populations to be indicative of better response to ECP, particularly in the first 3 months of treatment and irrespective of GvHD type.
Conclusion
There has been a great increase in recent years in our understanding of the mechanisms underpinning the development of GvHD, its diagnosis and treatment, including mechanisms of ECP. As progress is made from the bench to bedside we can now consider harnessing immunological hallmarks of the condition to develop tests for better and more rapid diagnosis, monitoring and treatment in order to optimize management. In relation to ECP, understanding the immunological basis for the mechanism of action will enable development of robust biomarkers informed algorithms (Figure 2) which will be highly useful in informing patient selection, intensity and duration of the ECP schedule, monitoring of response and decisions regarding combinations with other GvHD therapies. Further research is warranted to establish how GvHD biomarkers are best incorporated in ECP treatment pathways with the goal of tailoring ECP to meet the needs of individual patients and maximizing benefit.
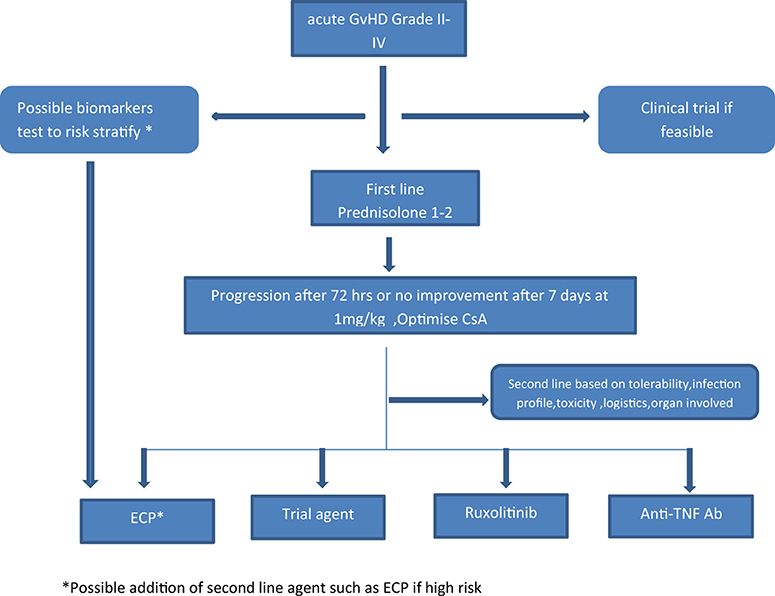
Figure 2. Proposed algorithm for incorporating ECP in the management of aGvHD. *Possible addition of second line agent such as ECP if high risk.
Author Contributions
All authors contributed equally to the writing of this article, contributed to the drafting, writing, analysis of data, and review prior to submission.
Conflict of Interest
NM has received grant funding and attended advisory board for Mallinckrodt. JS declares speaker fees from Jazz, Mallinckrodt, Janssen, and Gilead and IDMC membership for a clinical trial funded by Kiadis Pharma. AA has received speaker fees and grant funding from Mallinckrodt.
The remaining author declares that the research was conducted in the absence of any commercial or financial relationships that could be construed as a potential conflict of interest.
References
1. Pasquini MC, Aljurf MD, Confer DL, Baldomero H, Bouzas LF, Horowitz MM, et al. Global Hematopoietic Stem Cell Transplantation (HSCT) at one million: an achievement of pioneers and foreseeable challenges for the next decade. a report from the worldwide network for blood and marrow transplantation (WBMT). Blood. (2013) 122:2133. doi: 10.1182/blood.V122.21.2133.2133
2. Wingard JR, Majhail NS, Brazauskas R, Wang Z, Sobocinski KA, Jacobsohn D, et al. Long-term survival and late deaths after allogeneic hematopoietic cell transplantation. J Clin Oncol. (2011) 29:2230–9. doi: 10.1200/JCO.2010.33.7212
3. Magenau J, Reddy P. Next generation treatment of acute graft-versus-host disease. Leukemia. (2014) 28:2283–91. doi: 10.1038/leu.2014.195
4. Cutler CS, Koreth J, Ritz J. Mechanistic approaches for the prevention and treatment of chronic GVHD. Blood. (2017) 129:22–9. doi: 10.1182/blood-2016-08-686659
5. Bittenbring J, Reichrath J. Extracorporeal photopheresis for non-skin GvHD. Anticancer Res. (2016) 36:1395–6.
6. Zeiser R, Blazar BR. Acute graft-versus-host disease - biologic process, prevention, and therapy. N Eng J Med. (2017) 377:2167–79. doi: 10.1056/NEJMra1609337
7. Ali AM, DiPersio JF, Schroeder MA. The role of biomarkers in the diagnosis and risk stratification of acute graft-versus-host disease: a systematic review. Biol Blood Marrow Trans. (2016) 22:1552–64. doi: 10.1016/j.bbmt.2016.04.022
8. Dignan FL, Clark A, Amrolia P, Cornish J, Jackson G, Mahendra P, et al. Diagnosis and management of acute graft-versus-host disease. Br J Haematol. (2012) 158:30–45. doi: 10.1111/j.1365-2141.2012.09129.x
9. Martin PJ, Rizzo JD, Wingard JR, Ballen K, Curtin PT, Cutler C, et al. First- and second-line systemic treatment of acute graft-versus-host disease: recommendations of the American Society of Blood and Marrow Transplantation. Biol Blood Marrow Trans. (2012) 18:1150–63. doi: 10.1016/j.bbmt.2012.04.005
10. Martin PJ, Storer BE, Inamoto Y, Flowers MED, Carpenter PA, Pidala J, et al. An endpoint associated with clinical benefit after initial treatment of chronic graft-versus-host disease. Blood. (2017) 130:360–7. doi: 10.1182/blood-2017-03-775767
11. Hill L, Alousi A, Kebriaei P, Mehta R, Rezvani K, Shpall E. New and emerging therapies for acute and chronic graft. Ther Adv Hematol. (2018) 9:21–46. doi: 10.1177/2040620717741860
12. Jagasia MH, Greinix HT, Arora M, Williams KM, Wolff D, Cowen EW, et al. National institutes of health consensus development project on criteria for clinical trials in chronic graft-versus-host disease: I. The 2014 diagnosis and staging working group report. Biol Blood Marrow Trans. (2015) 21:389–401.e1. doi: 10.1016/j.bbmt.2015.05.004
13. Wolff D, Gerbitz A, Ayuk F, Kiani A, Hildebrandt GC, Vogelsang GB, et al. Consensus conference on clinical practice in chronic graft-versus-host disease (GVHD): first-line and topical treatment of chronic GVHD. Biol Blood Marrow Transplant. (2010) 16:1611–28. doi: 10.1016/j.bbmt.2010.06.015
14. Flowers ME, Martin PJ. How we treat chronic graft-versus-host disease. Blood. (2015) 125:606–15. doi: 10.1182/blood-2014-08-551994
15. Paczesny S. Biomarkers for posttransplantation outcomes. Blood. (2018) 131:2193–204. doi: 10.1182/blood-2018-02-791509
16. Paczesny S, Hakim FT, Pidala J, Cooke KR, Lathrop J, Griffith LM, et al. National institutes of health consensus development project on criteria for clinical trials in chronic graft-versus-host disease: III. The 2014 biomarker working group report. Biol Blood Marrow Trans. (2015) 21:780–92. doi: 10.1016/j.bbmt.2015.01.003
17. Vander Lugt MT, Braun TM, Hanash S, Ritz J, Ho VT, Antin JH, et al. ST2 as a marker for risk of therapy-resistant graft-versus-host disease and death. N Eng J Med. (2013) 369:529–39. doi: 10.1056/NEJMoa1213299
18. Ponce DM, Hilden P, Mumaw C, Devlin SM, Lubin M, Giralt S, et al. High day 28 ST2 levels predict for acute graft-versus-host disease and transplant-related mortality after cord blood transplantation. Blood. (2015) 125:199–205. doi: 10.1182/blood-2014-06-584789
19. McDonald GB, Tabellini L, Storer BE, Lawler RL, Martin PJ, Hansen JA. Plasma biomarkers of acute GVHD and nonrelapse mortality: predictive value of measurements before GVHD onset and treatment. Blood. (2015) 126:113–20. doi: 10.1182/blood-2015-03-636753
20. Ito S, Barrett AJ. ST2: the biomarker at the heart of GVHD severity. Blood. (2015) 125:10–1. doi: 10.1182/blood-2014-11-611780
21. Zhang J, Ramadan AM, Griesenauer B, Li W, Turner MJ, Liu C, et al. ST2 blockade reduces sST2-producing T cells while maintaining protective mST2-expressing T cells during graft-versus-host disease. Sci Trans Med. (2015) 7:308ra160. doi: 10.1126/scitranslmed.aab0166
22. Zhao D, Kim YH, Jeong S, Greenson JK, Chaudhry MS, Hoepting M, et al. Survival signal REG3alpha prevents crypt apoptosis to control acute gastrointestinal graft-versus-host disease. J Clin Invest. (2018) 128:4970–9. doi: 10.1172/JCI99261
23. Cai CS, Chen GH, Sun AN, Qiao M, Liu HW, Chen F, et al. [Correlation of the level of Reg3alpha protein in plasma with gastrointestinal acute graft-versus-host disease]. Zhongguo Shi Yan Xue Ye Xue Za Zhi. (2014) 22:785–90. doi: 10.7534/j.issn.1009-2137.2014.03.039
24. Hartwell MJ, Özbek U, Holler E, Renteria AS, Major-Monfried H, Reddy P, et al. An early-biomarker algorithm predicts lethal graft-versus-host disease and survival. JCI Insight. (2017) 2:e89798. doi: 10.1172/jci.insight.89798
25. Paczesny S. Acute graft-versus-host disease prognosis: are biomarkers ready for preemptive clinical trials? Clin Chem. (2017) 63:1561–3. doi: 10.1373/clinchem.2017.272872
26. DiCarlo J, Agarwal-Hashmi R, Shah A, Kim P, Craveiro L, Killen R, et al. Cytokine and chemokine patterns across 100 days after hematopoietic stem cell transplantation in children. Biol Blood Marrow Transplant. (2014) 20:361–9. doi: 10.1016/j.bbmt.2013.11.026
27. Shin J, Dan K, Han D, Kim J-W, Kim KK, Koh Y, et al. Plasma-based protein biomarkers can predict the risk of acute graft-versus-host disease and non-relapse mortality in patients undergoing allogeneic hematopoietic stem cell transplantation. Blood Cells Mol Dis. (2019) 74:5–12. doi: 10.1016/j.bcmd.2018.10.001
28. Abu Zaid M, Wu J, Wu C, Logan BR, Yu J, Cutler C, et al. Plasma biomarkers of risk for death in a multicenter phase 3 trial with uniform transplant characteristics post-allogeneic HCT. Blood. (2017) 129:162–70. doi: 10.1182/blood-2016-08-735324
29. McDonald GB, Tabellini L, Storer BE, Martin PJ, Lawler RL, Rosinski SL, et al. Predictive value of clinical findings and plasma biomarkers after fourteen days of prednisone treatment for acute graft-versus-host disease. Biol Blood Marrow Trans. (2017) 23:1257–63. doi: 10.1016/j.bbmt.2017.04.029
30. Paczesny S, Braun TM, Levine JE, Hogan J, Crawford J, Coffing B, et al. Elafin is a biomarker of graft-versus-host disease of the skin. Sci Transl Med. (2010) 2:13ra2. doi: 10.1126/scitranslmed.3000406
31. Harris AC, Ferrara JL, Braun TM, Holler E, Teshima T, Levine JE, et al. Plasma biomarkers of lower gastrointestinal and liver acute GVHD. Blood. (2012) 119:2960–3. doi: 10.1182/blood-2011-10-387357
32. Kennedy GA, Varelias A, Vuckovic S, Le Texier L, Gartlan KH, Zhang P, et al. Addition of interleukin-6 inhibition with tocilizumab to standard graft-versus-host disease prophylaxis after allogeneic stem-cell transplantation: a phase 1/2 trial. Lancet Oncol. (2014) 15:1451–9. doi: 10.1016/S1470-2045(14)71017-4
33. Wu P, Liang W, Chen X, Chen L, Yang X, Yan Z, et al. Pretransplant C-reactive protein as a prognostic marker in allogeneic stem cell transplantation: a PRISMA-compliant meta-analysis. Medicine. (2019) 98:e14474. doi: 10.1097/MD.0000000000014474
34. Rodriguez-Otero P, Porcher R, Peffault de Latour R, Contreras M, Bouhnik Y, Xhaard A, et al. Fecal calprotectin and alpha-1 antitrypsin predict severity and response to corticosteroids in gastrointestinal graft-versus-host disease. Blood. (2012) 119:5909–17. doi: 10.1182/blood-2011-12-397968
35. Tichelli A, Gratwohl A. Vascular endothelium as ‘novel' target of graft-versus-host disease. Best Pract Res Clin Haematol. (2008) 21:139–48. doi: 10.1016/j.beha.2008.02.002
36. Almici C, Skert C, Bruno B, Bianchetti A, Verardi R, Di Palma A, et al. Circulating endothelial cell count: a reliable marker of endothelial damage in patients undergoing hematopoietic stem cell transplantation. Bone Marrow Transplant. (2017) 52:1637–42. doi: 10.1038/bmt.2017.194
37. Skert C, Perucca S, Chiarini M, Giustini V, Sottini A, Ghidini C, et al. Sequential monitoring of lymphocyte subsets and of T-and-B cell neogenesis indexes to identify time-varying immunologic profiles in relation to graft-versus-host disease and relapse after allogeneic stem cell transplantation. PLoS ONE. (2017) 12:e0175337. doi: 10.1371/journal.pone.0175337
38. Janeway CA. Approaching the asymptote? Evolution and revolution in immunology. Cold Spring Harb Symp Quant Biol. (1989) 54 (Pt 1):1–13. doi: 10.1101/SQB.1989.054.01.003
39. Skert C, Fogli M, Perucca S, Garrafa E, Fiorentini S, Filì C, et al. Profile of toll-like receptors on peripheral blood cells in relation to acute graft-versus-host disease after allogeneic stem cell transplantation. Biol Blood Marrow Transplant. (2013) 19:227–34. doi: 10.1016/j.bbmt.2012.09.013
40. Turnbaugh PJ, Hamady M, Yatsunenko T, Cantarel BL, Duncan A, Ley RE, et al. A core gut microbiome in obese and lean twins. Nature. (2009) 457:480–4. doi: 10.1038/nature07540
41. van der Velden WJ, Herbers AH, Feuth T, Schaap NP, Donnelly JP, Blijlevens NM. Intestinal damage determines the inflammatory response and early complications in patients receiving conditioning for a stem cell transplantation. PLoS ONE. (2010) 5:e15156. doi: 10.1371/journal.pone.0015156
42. Allen JL, Fore MS, Wooten J, Roehrs PA, Bhuiya NS, Hoffert T, et al. B cells from patients with chronic GVHD are activated and primed for survival via BAFF-mediated pathways. Blood. (2012) 120:2529–36. doi: 10.1182/blood-2012-06-438911
43. Ahmed SS, Wang XN, Norden J, Pearce K, El-Gezawy E, Atarod S, et al. Identification and validation of biomarkers associated with acute and chronic graft versus host disease. Bone Marrow Transplant. (2015) 50:1563–71. doi: 10.1038/bmt.2015.191
44. Rozmus J, Kariminia A, Abdossamadi S, Storer BE, Martin PJ, Lee SJ, et al. Comprehensive B cell phenotyping profile for chronic graft-versus-host disease diagnosis. Biol Blood Marrow Trans. (2019) 25:451–8. doi: 10.1016/j.bbmt.2018.11.007
45. Jacobson CA, Sun L, Kim HT, McDonough SM, Reynolds CG, Schowalter M, et al. Post-transplantation B cell activating factor and B cell recovery before onset of chronic graft-versus-host disease. Biol Blood Marrow Trans. (2014) 20:668–75. doi: 10.1016/j.bbmt.2014.01.021
46. Yu J, Storer BE, Kushekhar K, Abu Zaid M, Zhang Q, Gafken PR, et al. Biomarker panel for chronic graft-versus-host disease. J Clin Oncol. (2016) 34:2583–90. doi: 10.1200/JCO.2015.65.9615
47. Hakim FT, Memon S, Jin P, Imanguli MM, Wang H, Rehman N, et al. Upregulation of IFN-inducible and damage-response pathways in chronic graft-versus-host disease. J Immunol. (2016) 197:3490–503. doi: 10.4049/jimmunol.1601054
48. Kariminia A, Holtan SG, Ivison S, Rozmus J, Hebert MJ, Martin PJ, et al. Heterogeneity of chronic graft-versus-host disease biomarkers: association with CXCL10 and CXCR3+ NK cells. Blood. (2016) 127:3082–91. doi: 10.1182/blood-2015-09-668251
49. Caron G, Duluc D, Frémaux I, Jeannin P, David C, Gascan H, et al. Direct stimulation of human T cells via TLR5 and TLR7/8: flagellin and R-848 up-regulate proliferation and IFN-gamma production by memory CD4+ T cells. J Immunol. (2005) 175:1551–7. doi: 10.4049/jimmunol.175.3.1551
50. Chang ZL. Important aspects of Toll-like receptors, ligands and their signaling pathways. Inflamm Res. (2010) 59:791–808. doi: 10.1007/s00011-010-0208-2
51. Kim WU, Sreih A, Bucala R. Toll-like receptors in systemic lupus erythematosus; prospects for therapeutic intervention. Autoimmun Rev. (2009) 8:204–8. doi: 10.1016/j.autrev.2008.07.046
52. French LE, Alcindor T, Shapiro M, McGinnis KS, Margolis DJ, Porter D, et al. Identification of amplified clonal T cell populations in the blood of patients with chronic graft-versus-host disease: positive correlation with response to photopheresis. Bone Marrow Trans. (2002) 30:509–15. doi: 10.1038/sj.bmt.1703705
53. Rook AH, Suchin KR, Kao DMF, Yoo EK, Macey WH, DeNardo BJ, et al. Photopheresis: clinical applications and mechanism of action. J Invest Dermatol Sympos Proc. (1999) 4:85–90. doi: 10.1038/sj.jidsp.5640188
54. Kuzmina Z, Greinix HT, Knobler R, Worel N, Kouba M, Weigl R, et al. Proportions of immature CD19+CD21- B lymphocytes predict the response to extracorporeal photopheresis in patients with chronic graft-versus-host disease. Blood. (2009) 114:744–6. doi: 10.1182/blood-2009-05-221028
55. Akhtari M, Giver CR, Ali Z, Flowers CR, Gleason CL, Hillyer CD, et al. Receiver operating characteristic curve analysis of circulating blood dendritic cell precursors and T cells predicts response to extracorporeal photopheresis in patients with chronic graft-versus-host disease. Transfusion. (2010) 50:2424–31. doi: 10.1111/j.1537-2995.2010.02712.x
56. Whittle R, Taylor PC. Circulating B-cell activating factor level predicts clinical response of chronic graft-versus-host disease to extracorporeal photopheresis. Blood. (2011) 118:6446–9. doi: 10.1182/blood-2011-05-354019
57. Bertani G, Santoleri L, Ferri U, Marenco P, Grillo G, Zucchetti E, et al. Response of steroid-refractory chronic graft-versus-host disease to extracorporeal photopheresis correlates with the dose of CD3+ lymphocytes harvested during early treatment cycles. Transfusion. (2016) 56:505–10. doi: 10.1111/trf.13369
58. Iniesta P, Revilla N, Chen-Liang TH, Hurtado AM, Vicente V, Heras I, et al. An early increase of CD56. Transfusion. (2018) 58:2924–32. doi: 10.1111/trf.14964
59. Groom JR, Luster AD. CXCR3 in T cell function. Exp Cell Res. (2011) 317:620–31. doi: 10.1016/j.yexcr.2010.12.017
60. Edelson R, Berger C, Gasparro F, Jegasothy B, Heald P, Wintroub B, et al. Treatment of cutaneous T-cell lymphoma by extracorporeal photochemotherapy. Preliminary results. N Eng J Med. (1987) 316:297–303. doi: 10.1056/NEJM198702053160603
61. Alfred A, Taylor PC, Dignan F, El-Ghariani K, Griffin J, Gennery AR, et al. The role of extracorporeal photopheresis in the management of cutaneous T-cell lymphoma, graft-versus-host disease and organ transplant rejection: a consensus statement update from the UK Photopheresis Society. Br J Haematol. (2017) 177:287–310. doi: 10.1111/bjh.14537
62. Knobler R, Berlin G, Calzavara-Pinton P, Greinix H, Jaksch P, Laroche L, et al. Guidelines on the use of extracorporeal photopheresis. J Eur Acad Dermatol Venereol. (2014) 28 (Suppl. 1):1–37. doi: 10.1111/jdv.12311
63. Rashidi A, DeFor TE, Holtan SG, Blazar BR, Weisdorf DJ, MacMillan ML. Outcomes and predictors of response in steroid-refractory acute graft-versus-host disease. Biol Blood Marrow Transplant. (2019) 25:2297–302. doi: 10.1016/j.bbmt.2019.07.017
64. Greinix HT, Knobler RM, Worel N, Schneider B, Schneeberger A, Hoecker P, et al. The effect of intensified extracorporeal photochemotherapy on long-term survival in patients with severe acute graft-versus-host disease. Haematologica. (2006) 91:405–8.
65. Garban F, Drillat P, Makowski C, Jacob MC, Richard MJ, Favrot M, et al. Extracorporeal chemophototherapy for the treatment of graft-versus-host disease: hematologic consequences of short-term, intensive courses. Haematologica. (2005) 90:1096–101.
66. Perfetti P, Carlier P, Strada P, Gualandi F, Occhini D, Van Lint MT, et al. Extracorporeal photopheresis for the treatment of steroid refractory acute GVHD. Bone Marrow Trans. (2008) 42:609–17. doi: 10.1038/bmt.2008.221
67. Jagasia M, Greinix H, Robin M, Das-Gupta E, Jacobs R, Savani BN, et al. Extracorporeal photopheresis versus anticytokine therapy as a second-line treatment for steroid-refractory acute GVHD: a multicenter comparative analysis. Biol Blood Marrow Trans. (2013) 19:1129–33. doi: 10.1016/j.bbmt.2013.04.018
68. Abu-Dalle I, Reljic T, Nishihori T, Antar A, Bazarbachi A, Djulbegovic B, et al. Extracorporeal photopheresis in steroid-refractory acute or chronic graft-versus-host disease: results of a systematic review of prospective studies. Biol Blood Marrow Trans. (2014) 20:1677–86. doi: 10.1016/j.bbmt.2014.05.017
69. Dignan FL, Amrolia P, Clark A, Cornish J, Jackson G, Mahendra P, et al. Diagnosis and management of chronic graft-versus-host disease. Br J Haematol. (2012) 158:46–61. doi: 10.1111/j.1365-2141.2012.09128.x
70. Pierelli L, Perseghin P, Marchetti M, Messina C, Perotti C, Mazzoni A, et al. Extracorporeal photopheresis for the treatment of acute and chronic graft-versus-host disease in adults and children: best practice recommendations from an Italian Society of Hemapheresis and Cell Manipulation (SIdEM) and Italian Group for Bone Marrow Transplantation (GITMO) consensus process. Transfusion. (2013) 53:2340–52. doi: 10.1111/trf.12059
71. Owsianowski M, Gollnick H, Siegert W, Schwerdtfeger R, Orfanos CE. Successful treatment of chronic graft-versus-host disease with extracorporeal photopheresis. Bone Marrow Transplant. (1994) 14:845–8.
72. Flowers ME, Apperley JF, van Besien K, Elmaagacli A, Grigg A, Reddy V, et al. A multicenter prospective phase 2 randomized study of extracorporeal photopheresis for treatment of chronic graft-versus-host disease. Blood. (2008) 112:2667–74. doi: 10.1182/blood-2008-03-141481
73. Bredeson C, Rumble RB, Varela NP, Kuruvilla J, Kouroukis CT, Stem Cell Transplant Steering C. Extracorporeal photopheresis in the management of graft-versus-host disease. Current oncology. (2014) 21:e310–25. doi: 10.3747/co.21.1882
74. Martin PJ, Inamoto Y, Carpenter PA, Lee SJ, Flowers MED. Treatment of chronic graft-versus-host disease: past, present and future. Korean J Hematol. (2011) 46:153–63. doi: 10.5045/kjh.2011.46.3.153
75. Greinix HT, van Besien K, Elmaagacli AH, Hillen U, Grigg A, Knobler R, et al. Progressive improvement in cutaneous and extracutaneous chronic graft-versus-host disease after a 24-week course of extracorporeal photopheresis–results of a crossover randomized study. Biol Blood Marrow Trans. (2011) 17:1775–82. doi: 10.1016/j.bbmt.2011.05.004
76. Jagasia M, Scheid C, Socié G, Ayuk FA, Tischer J, Donato ML, et al. Randomized controlled study of ECP with methoxsalen as first-line treatment of patients with moderate to severe cGVHD. Blood Adv. (2019) 3:2218–29. doi: 10.1182/bloodadvances.2019000145
77. Foss FM, DiVenuti GM, Chin K, Sprague K, Grodman H, Klein A, et al. Prospective study of extracorporeal photopheresis in steroid-refractory or steroid-resistant extensive chronic graft-versus-host disease: analysis of response and survival incorporating prognostic factors. Bone Marrow Trans. (2005) 35:1187–93. doi: 10.1038/sj.bmt.1704984
78. Del Fante C, Scudeller L, Viarengo G, Bernasconi P, Perotti C. Response and survival of patients with chronic graft-versus-host disease treated by extracorporeal photochemotherapy: a retrospective study according to classical and National Institutes of Health classifications. Transfusion. (2012) 52:2007–15. doi: 10.1111/j.1537-2995.2011.03542.x
79. Malagola M, Cancelli V, Skert C, Leali PF, Ferrari E, Tiburzi A, et al. Extracorporeal photopheresis for treatment of acute and chronic graft versus host disease: an Italian multicentric retrospective analysis on 94 patients on behalf of the Gruppo Italiano Trapianto di Midollo Osseo. Transplantation. (2016) 100:e147–55. doi: 10.1097/TP.0000000000001466
80. Apisarnthanarax N, Donato M, Korbling M, Couriel D, Gajewski J, Giralt S, et al. Extracorporeal photopheresis therapy in the management of steroid-refractory or steroid-dependent cutaneous chronic graft-versus-host disease after allogeneic stem cell transplantation: feasibility and results. Bone Marrow Trans. (2003) 31:459–65. doi: 10.1038/sj.bmt.1703871
81. Ussowicz M, Musial J, Mielcarek M, Tomaszewska A, Nasilowska-Adamska B, Kalwak K, et al. Steroid-sparing effect of extracorporeal photopheresis in the therapy of graft-versus-host disease after allogeneic hematopoietic stem cell transplantation. Trans Proc. (2013) 45:3375–80. doi: 10.1016/j.transproceed.2013.07.053
82. Ni M, Wang L, Yang M, Neuber B, Sellner L, H√°ckelhoven-Krauss A, et al. Shaping of CD56(bri) natural killer cells in patients with steroid-refractory/resistant acute graft-vs.-host disease via extracorporeal photopheresis. Front Immunol. (2019) 10:547. doi: 10.3389/fimmu.2019.00547
83. Wang L, Ni M, H√°ckelhoven-Krauss A, Sellner L, Hoffmann J-M, Neuber B, et al. Modulation of B cells and homing marker on NK cells through extracorporeal photopheresis in patients with steroid-refractory/resistant graft-vs.-host disease without hampering anti-viral/anti-leukemic effects. Front Immunol. (2018) 9:2207. doi: 10.3389/fimmu.2018.02207
84. Pidala J, Kurland B, Chai X, Majhail N, Weisdorf DJ, Pavletic S, et al. Patient-reported quality of life is associated with severity of chronic graft-versus-host disease as measured by NIH criteria: report on baseline data from the Chronic GVHD Consortium. Blood. (2011) 117:4651–7. doi: 10.1182/blood-2010-11-319509
85. Dignan FL, Aguilar S, Scarisbrick JJ, Shaw BE, Potter MN, Cavenagh J, et al. Impact of extracorporeal photopheresis on skin scores and quality of life in patients with steroid-refractory chronic GVHD. Bone Marrow Trans. (2014) 49:704–8. doi: 10.1038/bmt.2014.21
86. Bladon J, Taylor PC. Extracorporeal photopheresis in cutaneous T-cell lymphoma and graft-versus-host disease induces both immediate and progressive apoptotic processes. Br J Dermatol. (2002) 146:59–68. doi: 10.1046/j.1365-2133.2002.04560.x
87. Elliott MR, Ravichandran KS. The dynamics of apoptotic cell clearance. Dev Cell. (2016) 38:147–60. doi: 10.1016/j.devcel.2016.06.029
88. Elliott MR, Koster KM, Murphy PS. Efferocytosis signaling in the regulation of macrophage inflammatory responses. J Immunol. (2017) 198:1387–94. doi: 10.4049/jimmunol.1601520
89. Nagata S. Apoptosis and clearance of apoptotic cells. Ann Rev Immunol. (2018) 36:489–517. doi: 10.1146/annurev-immunol-042617-053010
90. Franklin C, Cesko E, Hillen U, Schilling B, Brandau S. Modulation and apoptosis of neutrophil granulocytes by extracorporeal photopheresis in the treatment of chronic graft-versus-host disease. PLoS ONE. (2015) 10:e0134518. doi: 10.1371/journal.pone.0134518
91. Spisek R, Gasova Z, Bartunkova J. Maturation state of dendritic cells during the extracorporeal photopheresis and its relevance for the treatment of chronic graft-versus-host disease. Transfusion. (2006) 46:55–65. doi: 10.1111/j.1537-2995.2005.00670.x
92. Lamioni A, Parisi F, Isacchi G, Giorda E, Di Cesare S, Landolfo A, et al. The immunological effects of extracorporeal photopheresis unraveled: induction of tolerogenic dendritic cells in vitro and regulatory T cells in vivo. Transplantation. (2005) 79:846–50. doi: 10.1097/01.TP.0000157278.02848.C7
93. Legitimo A, Consolini R, Failli A, Fabiano S, Bencivelli W, Scatena F, et al. In vitro treatment of monocytes with 8-methoxypsolaren and ultraviolet A light induces dendritic cells with a tolerogenic phenotype. Clin Exp Immunol. (2007) 148:564–72. doi: 10.1111/j.1365-2249.2007.03372.x
94. Setterblad N, Garban F, Weigl R, Assier E, Drillat P, Charron D, et al. Extracorporeal photophoresis increases sensitivity of monocytes from patients with graft-versus-host disease to HLA-DR-mediated cell death. Transfusion. (2008) 48:169–77. doi: 10.1111/j.1537-2995.2007.01502.x
95. Yoo EK, Rook AH, Elenitsas R, Gasparro FP, Vowels BR. Apoptosis induction of ultraviolet light A and photochemotherapy in cutaneous T-cell Lymphoma: relevance to mechanism of therapeutic action. J Invest Dermatol. (1996) 107:235–42. doi: 10.1111/1523-1747.ep12329711
96. Tambur AR, Ortegel JW, Morales A, Klingemann H, Gebel HM, Tharp MD. Extracorporeal photopheresis induces lymphocyte but not monocyte apoptosis. Trans Proc. (2000) 32:747–8. doi: 10.1016/S0041-1345(00)00966-0
97. Yakut E, Jakobs C, Peric A, Michel G, Baal N, Bein G, et al. Extracorporeal photopheresis promotes IL-1beta production. J Immunol. (2015) 194:2569–77. doi: 10.4049/jimmunol.1400694
98. Wiese F, Reinhardt-Heller K, Volz M, Gille C, Kostlin N, Billing H, et al. Monocytes show immunoregulatory capacity on CD4(+) T cells in a human in-vitro model of extracorporeal photopheresis. Clin Exp Immunol. (2019) 195:369–80. doi: 10.1111/cei.13232
99. Di Renzo M, Rubegni P, Pasqui AL, Pompella G, De Aloe G, Sbano P, et al. Extracorporeal photopheresis affects interleukin (IL)-10 and IL-12 production by monocytes in patients with chronic graft-versus-host disease. Br J Dermatol. (2005) 153:59–65. doi: 10.1111/j.1365-2133.2005.06482.x
100. Gonzalez AL, Berger CL, Remington J, Girardi M, Tigelaar RE, Edelson RL. Integrin-driven monocyte to dendritic cell conversion in modified extracorporeal photochemotherapy. Clin Exp Immunol. (2014) 175:449–57. doi: 10.1111/cei.12231
101. Rieber N, Wecker I, Neri D, Fuchs K, Schafer I, Brand A, et al. Extracorporeal photopheresis increases neutrophilic myeloid-derived suppressor cells in patients with GvHD. Bone Marrow Trans. (2014) 49:545–52. doi: 10.1038/bmt.2013.236
102. Knobler R, Barr ML, Couriel DR, Ferrara JL, French LE, Jaksch P, et al. Extracorporeal photopheresis: past, present, and future. J Am Acad Dermatol. (2009) 61:652–65. doi: 10.1016/j.jaad.2009.02.039
103. Just U, Dimou E, Knobler R, Klosner G, Ivancic-Brandenberger E, Greinix H, et al. Leucocyte scintigraphy with 111In-oxine for assessment of cell trafficking after extracorporeal photopheresis. Exp Dermatol. (2012) 21:443–7. doi: 10.1111/j.1600-0625.2012.01491.x
104. Morelli AE, Larregina AT, Shufesky WJ, Zahorchak AF, Logar AJ, Papworth GD, et al. Internalization of circulating apoptotic cells by splenic marginal zone dendritic cells: dependence on complement receptors and effect on cytokine production. Blood. (2003) 101:611–20. doi: 10.1182/blood-2002-06-1769
105. Morelli AE, Larregina AT. Concise review: mechanisms behind apoptotic cell-based therapies against transplant rejection and graft versus host disease. Stem Cells. (2016) 34:1142–50. doi: 10.1002/stem.2326
106. Fadok VA, Bratton DL, Konowal A, Freed PW, Westcott JY, Henson PM. Macrophages that have ingested apoptotic cells in vitro inhibit proinflammatory cytokine production through autocrine/paracrine mechanisms involving TGF-beta, PGE2, and PAF. J Clin Invest. (1998) 101:890–8. doi: 10.1172/JCI1112
107. Gatza E, Rogers CE, Clouthier SG, Lowler KP, Tawara I, Liu C, et al. Extracorporeal photopheresis reverses experimental graft-versus-host disease through regulatory T cells. Blood. (2008) 112:1515–21. doi: 10.1182/blood-2007-11-125542
108. Blazar BR, Murphy WJ, Abedi M. Advances in graft-versus-host disease biology and therapy. Nat Rev Immunol. (2012) 12:443–58. doi: 10.1038/nri3212
109. Zeiser R, Blazar BR. Pathophysiology of chronic graft-versus-host disease and therapeutic targets. N Eng J Med. (2017) 377:2565–79. doi: 10.1056/NEJMra1703472
110. Battaglia M, Stabilini A, Roncarolo MG. Rapamycin selectively expands CD4+CD25+FoxP3+ regulatory T cells. Blood. (2005) 105:4743–8. doi: 10.1182/blood-2004-10-3932
111. Barrat FJ, Cua DJ, Boonstra A, Richards DF, Crain C, Savelkoul HF, et al. In vitro generation of interleukin 10-producing regulatory CD4(+) T cells is induced by immunosuppressive drugs and inhibited by T helper type 1 (Th1)- and Th2-inducing cytokines. J Exp Med. (2002) 195:603–16. doi: 10.1084/jem.20011629
112. Biagi E, Di Biaso I, Leoni V, Gaipa G, Rossi V, Bugarin C, et al. Extracorporeal photochemotherapy is accompanied by increasing levels of circulating CD4+CD25+GITR+Foxp3+CD62L+ functional regulatory T-cells in patients with graft-versus-host disease. Transplantation. (2007) 84:31–9. doi: 10.1097/01.tp.0000267785.52567.9c
113. Bladon J, Taylor P. Extracorporeal photopheresis normalizes some lymphocyte subsets (including T regulatory cells) in chronic graft-versus-host-disease. Therap Apheresis Dialysis. (2008) 12:311–8. doi: 10.1111/j.1744-9987.2008.00593.x
114. Di Biaso I, Di Maio L, Bugarin C, Gaipa G, Dander E, Balduzzi A, et al. Regulatory T cells and extracorporeal photochemotherapy: correlation with clinical response and decreased frequency of proinflammatory T cells. Transplantation. (2009) 87:1422–5. doi: 10.1097/TP.0b013e3181a27a5d
115. Denney HA, Whittle RJ, Lai J, Jacques RM, Taylor PC. Regulatory T cells in chronic graft-versus-host disease after extracorporeal photopheresis: correlation with skin and global organ responses, and ability to taper steroids. Transplantation. (2017) 101:204–11. doi: 10.1097/TP.0000000000001165
116. Gandelman JS, Song DJ, Chen H, Engelhardt BG, Chen YB, Clark WB, et al. A Prospective trial of extracorporeal photopheresis for chronic graft-versus-host disease reveals significant disease response and no association with frequency of regulatory T cells. Biol Blood Marrow Trans. (2018) 24:2373–80. doi: 10.1016/j.bbmt.2018.06.035
117. Koreth J, Matsuoka K, Kim HT, McDonough SM, Bindra B, Alyea EP III, et al. Interleukin-2 and regulatory T cells in graft-versus-host disease. N Eng J Med. (2011) 365:2055–66. doi: 10.1056/NEJMoa1108188
118. Belizaire R, Kim HT, Poryanda SJ, Mirkovic NV, Hipolito E, Savage WJ, et al. Efficacy and immunologic effects of extracorporeal photopheresis plus interleukin-2 in chronic graft-versus-host disease. Blood Adv. (2019) 3:969–79. doi: 10.1182/bloodadvances.2018029124
119. Budde H, Kolb S, Salinas Tejedor L, Wulf G, Reichardt HM, Riggert J, et al. Modified extracorporeal photopheresis with cells from a healthy donor for acute graft-versus-host disease in a mouse model. PLoS ONE. (2014) 9:e105896. doi: 10.1371/journal.pone.0105896
120. Coppard C, Bonnefoy F, Hannani D, Gabert F, Manches O, Plumas J, et al. Photopheresis efficacy in the treatment of rheumatoid arthritis: a pre-clinical proof of concept. J Trans Med. (2019) 17:312. doi: 10.1186/s12967-019-2066-1
121. Coppard C, Hannani D, Humbert M, Gauthier V, Plumas J, Merlin E, et al. In vitro PUVA treatment triggers calreticulin exposition and HMGB1 release by dying T lymphocytes in GVHD: new insights in extracorporeal photopheresis. J Clin Apheres. (2019) 34:450–60. doi: 10.1002/jca.21698
122. Gurung P, Kucaba TA, Ferguson TA, Griffith TS. Activation-induced CD154 expression abrogates tolerance induced by apoptotic cells. J Immunol. (2009) 183:6114–23. doi: 10.4049/jimmunol.0901676
123. Hannani D, Merlin E, Gabert F, Laurin D, Demeocq F, Chaperot L, et al. Photochemotherapy induces a faster apoptosis of alloreactive activated T cells than of nonalloreactive resting T cells in graft versus host disease. Transplantation. (2010) 90:1232–8. doi: 10.1097/TP.0b013e3181fa4eb6
124. Hannani D. Extracorporeal photopheresis: tolerogenic or immunogenic cell death? Beyond current dogma. Front Immunol. (2015) 6:349. doi: 10.3389/fimmu.2015.00349
125. Franklin C, Bruderek K, Schilling B, Brandau S. Chemoirradiated neutrophils and T cells differentially affect immune functions of APCs. J Leukocyte Biol. (2019) 106:481–93. doi: 10.1002/JLB.5A0618-242R
Keywords: extracorporeal photopheresis, GvHD, immunomodulation, biomarkers, apoptosis, dendritic cells
Citation: Mankarious M, Matthews NC, Snowden JA and Alfred A (2020) Extracorporeal Photopheresis (ECP) and the Potential of Novel Biomarkers in Optimizing Management of Acute and Chronic Graft vs. Host Disease (GvHD). Front. Immunol. 11:81. doi: 10.3389/fimmu.2020.00081
Received: 30 September 2019; Accepted: 13 January 2020;
Published: 31 January 2020.
Edited by:
Michael Uhlin, Karolinska Institutet (KI), SwedenReviewed by:
Jakob Passweg, University Hospital of Basel, SwitzerlandMichele Malagola, University of Brescia, Italy
Copyright © 2020 Mankarious, Matthews, Snowden and Alfred. This is an open-access article distributed under the terms of the Creative Commons Attribution License (CC BY). The use, distribution or reproduction in other forums is permitted, provided the original author(s) and the copyright owner(s) are credited and that the original publication in this journal is cited, in accordance with accepted academic practice. No use, distribution or reproduction is permitted which does not comply with these terms.
*Correspondence: Arun Alfred, YXJ1bmFsZnJlZCYjeDAwMDQwO25ocy5uZXQ=