- Centre Armand-Frappier Santé Biotechnologie, Institut National de la Recherche Scientifique (INRS), Laval, QC, Canada
Persistent Leishmania donovani infection is characterized by chronic inflammation, immune suppression, and splenomegaly. We have previously reported that the transcription factor interferon regulatory factor 5 (IRF-5) is largely responsible for inducing the inflammatory response and maintaining protective Th1 cells following L. donovani inoculation in mice. However, the cellular source responsible for these effects is yet unknown. In this study, we investigated the role of IRF-5 in myeloid cells during experimental visceral leishmaniasis (VL). First, we show that the LysM-Cre mouse model is not suited for investigating gene expression in splenic myeloid cells during experimental VL. Using the Cd11c-Cre mouse model, we demonstrate that Irf5 expression in CD11c+ cells (monocytes, dendritic cells, activated macrophages) is essential for inducing splenomegaly and for recruiting myeloid cells to the spleen, but it is not required for the development or maintenance of parasite-specific IFNγ-producing CD4 T cells. CD11c-specific Irf5−/− mice are more resistant to L. donovani infection, suggesting that the induction of splenomegaly is detrimental to the host.
Introduction
The Leishmaniasis are a set of vector-borne parasitic diseases that affect an estimated 12 million people worldwide. Disease clinical manifestations range from self-healing cutaneous lesions to life-threatening visceral infection. The visceral form of the disease has a fatality rate of 75–95% if left untreated (1) and is characterized by weight loss, irregular bouts of fever, anemia, hepatosplenomegaly, hypergamaglobulinemia, and immunosuppression. Experimental infection of mice with Leishmania donovani, a causative agent of visceral leishmaniasis (VL), also results in hepatosplenomegaly, but it is not fatal (2). In mice, parasite growth in the liver is controlled by protective CD4 and CD8 T cell responses producing IFNγ (3). In contrast, infection persists in the spleen and the bone marrow.
The chronic stage of experimental VL in the spleen is characterized by a chronic inflammatory environment, with all the consequences that this entails: tissue disruption, hypoxia, and immunoregulatory responses (2, 4, 5). Leishmania parasites survive very well in this environment, whereas protective T cell responses are inhibited in various ways. For instance, at d28p.i., CD8 T cells are dysfunctional and exhausted (6, 7) and CD4 T cells fail to expand (8), die by TRAIL-mediated apoptosis (9), and are suppressed by IL-10 (10–13) and by myeloid-derived suppressor cells (14).
Splenomegaly and chronic inflammation are associated with parasite persistence during chronic VL. Several cell populations contribute to splenomegaly, but myeloid cells, in particular, are progressively recruited to the spleen over the course of infection (14). Indeed, L. donovani induces the heightened release from the bone marrow of inflammatory monocytes (15). These cells display a regulatory phenotype and are more permissive to infection, favoring parasite growth and persistence (14–16). The inflammatory response during VL appears to require the activation of the transcription factor Interferon Regulatory Factor 5 (IRF-5). IRF-5 function has been mainly described in antigen-presenting cells, where it promotes the transcriptional activation of genes encoding for IFN-I and pro-inflammatory cytokines, such as TNF, IL-12, and IL-6 (17, 18). In human, IRF-5 polymorphisms are associated with various autoimmune inflammatory disorders (19–22). In mice infected with L. donovani, IRF-5 governs the inflammatory response (23), but it is also responsible for CD4 T cell death during chronic infection (9). Indeed, Irf5−/− mice fail to develop splenomegaly and to develop protective Th1 responses following L. donovani infection (23). Nevertheless, the cellular source required for promoting IRF-5-dependent inflammation and sustaining Th1 responses during experimental VL is yet unknown.
In this study, we investigated the role of IRF-5 in myeloid cells following L. donovani infection in mice. We show that LysM-Cre mice are not a good model for investigating gene expression in splenic myeloid cells during experimental VL. We also demonstrate that Irf5 expression in CD11c+ cells is essential for inducing splenomegaly, but it is not required for the development or maintenance of parasite-specific IFNγ-producing CD4 T cells.
Materials and Methods
Mice and Parasites
B6.129S7-Rag1tm1Mom and cre recombinase-expressing mice were purchased from The Jackson Laboratory. Mice with a targeted Irf5 mutation in myeloid and in CD11c+ cells were generated by crossing Irf5flox/flox mice with mice expressing the cre-recombinase under the LysM and the CD11c promoter, respectively. All mice were housed at the INRS animal facility under specific pathogen-free conditions and used at 6–10 weeks of age. Leishmania donovani (strain LV9) were maintained by serial passage in B6.129S7-Rag1tm1Mom mice; amastigotes were isolated from the spleen of infected animals (24). Mice were infected by injecting 2 x 107 amastigotes intravenously via the lateral tail vein. Splenic parasite burden were determined by examining methanol-fixed, Giemsa stained tissue impression smears. Data are presented as Leishmania Donovani Units (LDU) (25).
Ethic Statement
Experiments involving mice were carried out under protocols approved by the Comité Institutionnel de Protection des Animaux of the INRS-Institut Armand Frappier (1510-02, 1602-02). These protocols respect procedure on good animal practice provided by the Canadian Council on animal care.
Flow Cytometry
Mice were euthanized at indicated time points. Mononuclear cells were purified from the liver and CD4 T cell responses were analyzed as previously described (14). Briefly, hepatic mononuclear cells were restimulated with bone marrow-derived dendritic cells, pulsed with fixed parasites, and directly incubated at 37°C in the presence of 1/1000 Brefeldin A (GolgiPlug™, BD Biosciences). Cells were then stained with anti-CD4-FITC (BD PharmingenTM, clone GK15), anti-CD3-BV421 (BD Biosciences, clone 14S-2C11), followed by anti-IFN-γ-APC (BD PharmingenTM, clone XMG1.2) after permeabilization with 0.1% saponin. Myeloid cells were stained with anti-CD11b-Pacific Blue (BD HorizonTM, clone MI/70), anti-MHC-II-FITC (BD PharmingenTM, clone 2G9), anti-Ly6C-PerCP (Biolegend, clone HK1.4), anti-Ly6G-PE (Biolegend, clone 1A8), anti-F4/80-PECy7 (Biolegend, clone BM8), and anti-CD11c-APC (eBioscience, clone N418). Flow cytometric analysis was performed with a BD LSRFortessa cell analyzer (Becton Dickinson). Samples were analyzed with Flowjo software.
Enrichment of Splenic Myeloid Cells
CD11b+ cells were purified using magnetic cell sorting (MACS) from spleens of infected and naïve mice previously digested with collagenase D, following manufacturer's instructions (Miltenyi Biotec). The purity of the samples comprise between 90 and 93%.
Real-Time PCR Analysis
Real-time PCR (Stratagene mx3005p Real time PCR System) was used to analyze transcripts levels of HPRT, HIF-1α, and IRF-5. Total RNA was insolated using RNeasy (Qiagen) to perform real-time RT-PCR. cDNA was prepared using 500 ng of total RNA using High Capacity cDNA Reverse Transcription Kit (Bio Rad). Real time PCR was performed using standard cycle of amplification. All PCRs were carried out with the Stratagene mx3000p real-time PCR system. Irf5, Hprt, and Hif1a were amplified using primers as previously described (9, 14). Data were normalized to HPRT and expressed as fold increase to naive controls.
Statistical Analysis
Data were analyzed using Graphpad Prism (GraphPad Software). Statistical significance was assessed using two-way ANOVA. Differences were considered to be statistically significant when p < 0.05. All experiments were conducted independently at least three times.
Results
Irf5-Deletion in Myeloid Cells Does Not Affect the Hepatic and Splenic Parasite Burden
We have previously reported that the transcription factor IRF-5 majorly contributes to the inflammatory response following Leishmania donovani infection in mice (23). Indeed, L. donovani-infected Irf5−/− mice not only failed to develop splenomegaly, but also displayed severely impaired parasite-specific Th1 responses. This resulted in higher hepatic parasite burden, but no differences were observed in the spleen (23). In the present study, we sought to identify the contribution of myeloid cell-derived IRF-5 expression to the immune response against L. donovani. To this end, we generated myeloid cell-specific Irf5−/− mice by crossing Irf5flox/flox with LysM-Cre mice and infected Cre+ (myeloid cells are IRF-5 deficient) and Cre− (myeloid cells are IRF-5 sufficient) littermates with L. donovani. Because Irf5−/− were shown to have a spontaneous mutation in Dock2 that affected pDC and B cell development (26), we generated new Irf5−/− mice by crossing Irf5flox/flox with CMV-Cre mice. These mice were used as total Irf5−/− controls in all experiments. First, we assessed the hepatic and splenic parasite burden. As expected, L. donovani infection in the liver was significantly exacerbated in Irf5flox/flox- CMV-Cre+ mice compared to infected Irf5flox/flox- LysM-Cre− control mice (Figure 1A). In contrast, no differences were observed between the Irf5flox/flox- LysM-Cre+ and Cre− groups. As previously reported (23), the absence of IRF-5 expression in all cells did not affect the splenic parasite load (Irf5flox/flox- CMV-Cre+); moreover, Cre+ mice had similar parasite numbers in the spleen than their Cre− counterpart (Figure 1B).
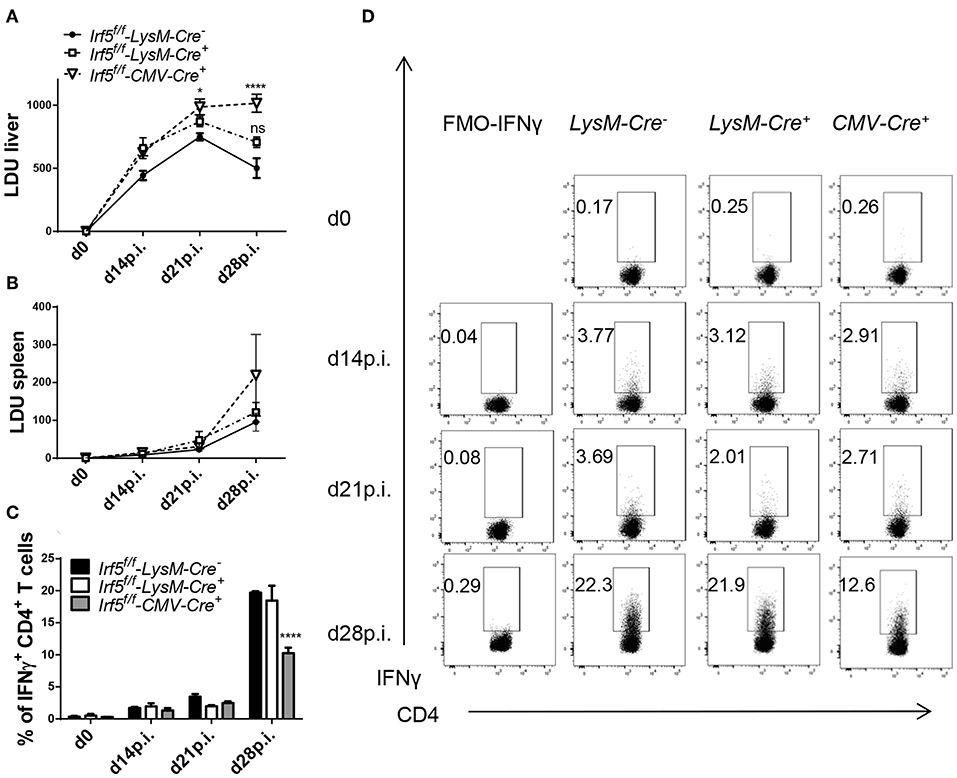
Figure 1. Irf5f/f -LysM-Cre+ mice are not more susceptible to L. donovani infection than Irf5f/f -LysM-Cre− mice. Irf5f/f -LysM-Cre−, Irf5f/f -LysM-Cre+, and Irf5f/f -CMV-Cre+ mice were infected with L. donovani and euthanized at d14, 21, and 28 p.i. Leishmania Donovan Units (LDU) were calculated from hepatic (A) and splenic (B) impression smears for the three group of mice. Graphs show representative scatter plots (C) and percentages (D) of CD4 T cells producing IFN-γ following L. donovani infection in the liver from three groups of mice. Error bars indicate mean ± SEM, N = 4, one of 5 independent experiments is shown; ns, not significant, *p < 0.05, ****p < 0.0001.
Myeloid-Specific Irf5−/− Mice Develop Similar Th1 Responses Than IRF-5-Sufficient Mice
A hallmark of Irf5−/− mice is their incapacity to generate strong Th1 responses following L. donovani infection (23). Krausgruber et al. have also reported that IRF-5 is required to promote inflammatory macrophages and induce Th1 responses (27). Hence, we evaluated parasite-specific IFNγ+ CD4 cell responses at various time points of L. donovani infection in total Irf5−/− (CMV-Cre+), in myeloid cell-specific Irf5−/− (Cre+), and in IRF-5 sufficient (Cre−) mice. As shown in Figure 1C and in agreement with the literature (23), in Cre− control mice, hepatic Th1 responses developed quite slowly from d14 p.i. on following L. donovani inoculation and peaked between d21 and 28 p.i., when about 20% of the CD4 T cells expressed IFNγ. Similarly to Irf5−/− mice (23), infected CMV-Cre+ mice generated defective Th1 responses and only about 10% of the CD4 T cells in the liver were IFNγ+ at d 28 p.i. (Figures 1C,D; gating strategy is shown in Supplemental Figure 1A). Interestingly, myeloid-specific Irf5−/− mice had similar frequencies of IFNγ+ CD4 T cells to the Cre− controls, suggesting that IRF-5 expression in myeloid cells is not required for Th1 development in L. donovani infected mice.
Irf5flox/flox- LysM-Cre+ Mice Develop Splenomegaly
Next, we wanted to determine whether IRF-5 expression in myeloid cells was at all required to induce splenomegaly that is typically present following L. donovani infection. As expected, the splenic weight (Figure 2A) and cellularity (Figure 2B) constantly increased over the course of L. donovani infection in Cre− controls. In contrast, spleen of infected CMV-Cre+ mice had a significantly lower weight (Figure 2A) and cell count (Figure 2B), in agreement with our previously published results using Irf5−/− (23). Because myeloid cells are increasingly recruited to the spleen during L. donovani infection (14, 15), we analyzed myeloid cell recruitment over the course of infection in the three groups of mice. As shown in Figure 2C, the number of CD11b+ cells present in the spleen of Cre− mice progressively increased until d28 p.i.; comparable myeloid cell numbers were also observed in the spleen of cell-specific Irf5−/− mice (Figure 2C). In contrast, splenic myeloid cell numbers in total IRF-5-deficient mice (CMV-Cre) did not substantially vary over the course of disease and were significantly lower than in the other two groups (Figure 2C). We obtained similar results when we analyzed the various myeloid cell subpopulations. In naïve mice and in L. donovani-infected mice until d14p.i., splenic myeloid cells can be clearly subdivided into neutrophils (CD11bhiLy6G+), inflammatory monocytes (CD11bhiLy6G−Ly6Chi), non-classical monocytes (CD11bhiLy6G−Ly6Clo/int), macrophages (CD11b+F4/80+MHCIIlo), and conventional dendritic cells (CD11chiMHCIIint/hi) (gating strategies for all myeloid cell populations are in Supplemental Figure 1B). However, during chronic L. donovani infection these populations are much less clearly defined. With exception of neutrophils, which remain CD11bhiLy6G+, at chronic stages of disease monocytes and monocytes-derived cells, such as macrophages and dendritic cells, acquire very similar markers, namely CD11b, MHCII, CD11c, and F4/80 (14, 15), which makes it very difficult to unmistakably differentiate dendritic cells and macrophages from monocytes and from each other. As observed for total myeloid cells, the number of neutrophils (Figure 2D), inflammatory monocytes (Figure 2E), non-classical monocytes (Figure 2F), CD11b+F4/80+ cells (corresponding to macrophages at d0 and 14p.i. and monocytes, macrophages and DCs at d21 and 28) (Figure 2G), and CD11chiMHCIIhi (DCs at d0 and 14p.i and DC and inflammatory monocytes at d21 and 28p.i) (Figure 2H) dramatically increased during chronic stages of infection in IRF-5-sufficient control mice. As expected, the numbers of splenic neutrophils, monocytes, macrophages and DCs did not substantially change over the course of infection in CMV-Cre+ mice and were significantly lower than those observed in Cre− mice (Figures 2D–H). Surprisingly, no significant differences were observed in the numbers of splenic myeloid cell populations, with exception of F4/80+ cells that were slightly fewer in cell-specific Irf5−/− mice at d28p.i. when compared with IRF-5 sufficient control mice (Figures 2D–H). No differences were observed among the three groups of mice in the percentage of the various myeloid cell populations (data not shown), suggesting that the defect in recruitment observed in IRF-5-deficient mice at d 28 p.i. was not confined to myeloid cells.
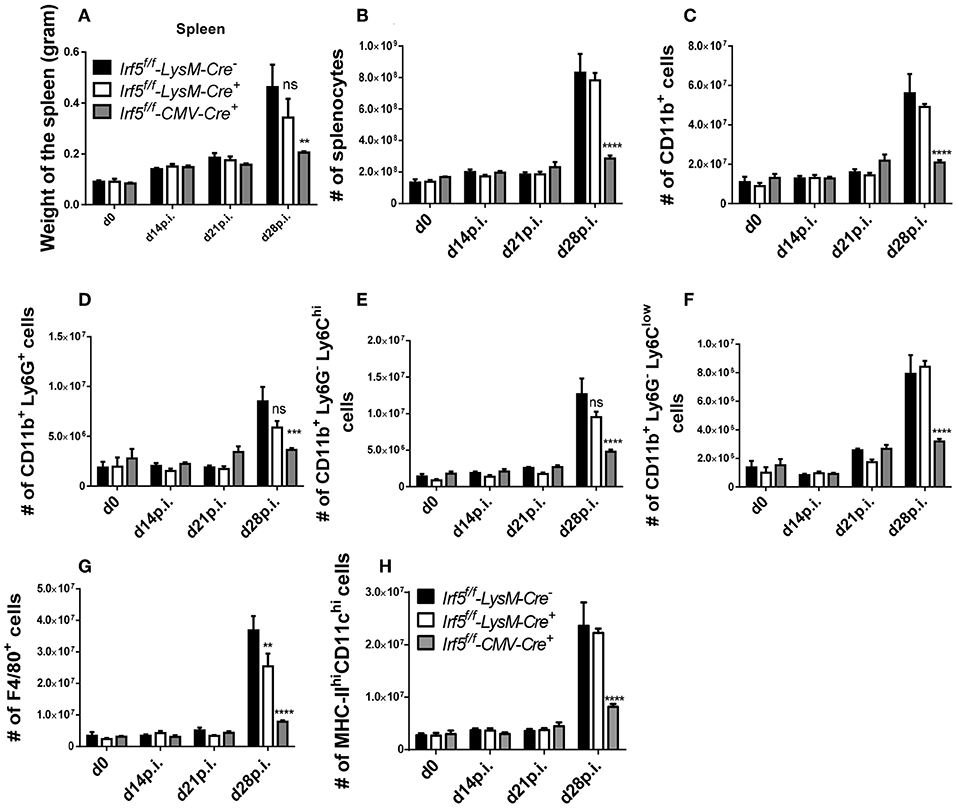
Figure 2. Irf5f/f -LysM-Cre+ mice develop splenomegaly following L. donovani infection. Irf5f/f -LysM-Cre−, Irf5f/f -LysM-Cre+, and Irf5f/f -CMV-Cre+ mice were infected with L. donovani and sacrificed at d14, d21, and d28 p.i. Graphs show spleen weights (A), number of splenocytes (B) and absolute number of splenic CD11b+ cells (C) in naïve and infected mice over the course of infection. Splenocytes were stained with different surface markers; neutrophils were excluded before analyzing monocytes and monocyte-derived cells. Graphs show absolute number of Ly6G+ neutrophils (D), Ly6Chigh monocytes (E), Ly6Clow monocytes (F), F4/80+ cells (G), and MHC-IIhigh CD11chigh cells (H). Data is shown as the mean ± SEM, N = 3–4, one of 5 independent experiments is shown, ns, not significant, **p < 0.01, ***p < 0.001, ****p < 0.0001.
Taken together, our results imply that IRF-5 expression in myeloid cells is not required for promoting inflammatory cell infiltration, Th1 responses, and splenomegaly during chronic experimental VL.
LysM-Cre− Fails to Delete Irf5 in Splenic Myeloid Cells During Chronic VL
Although LysM-Cre mice are the model of choice to delete specific genes in myeloid cells, we were puzzled by the fact that deletion of Irf5 in myeloid cells did not have any effect at all on the immune response to L. donovani or on the course of infection. Hence, we suspected that the cre recombinase had low deletion efficiency in splenic cells expressing lysozyme 2 in our particular infection model. Hence, we analyzed Irf5 expression by qPCR in purified splenic myeloid cells in L. donovani infected Cre+ and Cre− mice. As a control, we also measured Irf5 mRNA expression levels in CD11b+ cells from L. donovani infected Irf5-CMV-Cre+ mice, which, as expected, failed to upregulate Irf5 (Figure 3A). Significant deletion in CD11b+ cells was only achieved at d14p.i. (Figure 3A); however, no difference in Irf5 mRNA expression was noted at d21 between Cre+ and Cre− mice (Figure 3A). To exclude the possibility that this lack of deletion was associated with our particular target gene, Irf5, or our Irf5flox/flox mice, we tested cre recombinase efficiency in splenic myeloid cells in a different mouse model, using a different target gene, namely Hif1a. Like for Irf5, we analyzed Hif1a mRNA expression in purified splenic myeloid cells over the course of L. donovani infection in Hif1aflox/flox – LysM-Cre+ and Cre− mice. As shown in Figure 3B, no differences were observed in Hif1a expression in splenic CD11b+ cells from both groups of mice at any time point of infection, suggesting that the LysM-Cre model cannot be used to delete genes in splenic myeloid cells in the context of experimental VL.
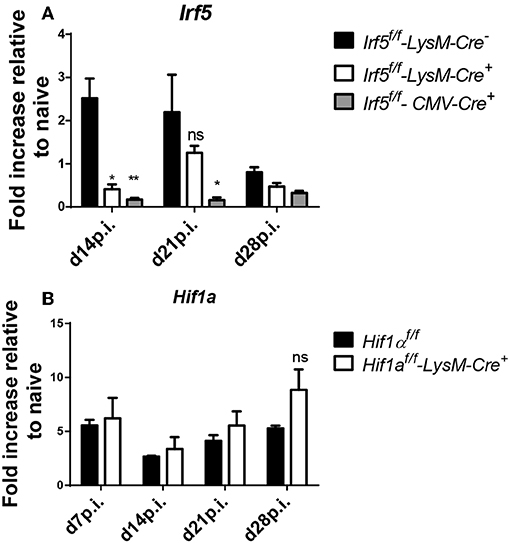
Figure 3. LysM-Cre+ failed to delete Irf5 and Hif1α in splenic myeloid cells during L. donovani infection. (A) Real time PCR analysis of Irf5 mRNA expression in splenic myeloid cells isolated from naive and infected Irf5f/f-LysM-Cre−, Irf5f/f-LysM-Cre+, and Irf5f/f-CMV-Cre+ mice, expressed as fold increase compared to naïve mice. (B) Real time PCR analysis measuring Hif1α mRNA expression in myeloid cells isolated from the spleen of naive and infected Hif1αf/f and Hif1αf/f-LysM-Cre+ mice and expressed as fold increase compared to naïve mice. Data represents mean ± SEM of 2 experiments, N = 8, ns, not significant; *p < 0.05, **p < 0.01.
Myeloid Cell-Derived IRF-5 Promotes Splenomegaly but Is Not Required to Induce Th1 Responses During L. donovani Infection
We next turned our attention to another model that we successfully used in the past to delete Hif1a in splenic myeloid cells during L. donovani infection (14), namely knock-in mice expressing the cre recombinase under the CD11c promoter. This model can be used for experimental VL infection, since most splenic myeloid cells, excluding neutrophils, express CD11c during chronic infection and the deletion efficiency is much higher than that achieved with LysM-Cre mice (14). Thus, we generated Irf5flox/flox -Cd11c-Cre+ and Cre− mice and infected them with L. donovani. First we investigated the development of IFNγ+ CD4 T cell responses in the liver, as those were mostly affected in total Irf5−/− mice following L. donovani infection. Surprisingly and in disagreement with the literature, we found that Irf5 deletion in CD11c+ cells did not affect the Th1 immune response; indeed, comparable frequencies of IFNγ+ CD4 T cells were observed at various time points after infection in the liver of both groups of mice (Figures 4A,B; gating strategy in Supplemental Figure 1A). Similarly, no differences were observed in the frequency of splenic IFNγ+ CD4 T cells between both groups of mice (Supplemental Figure 2).
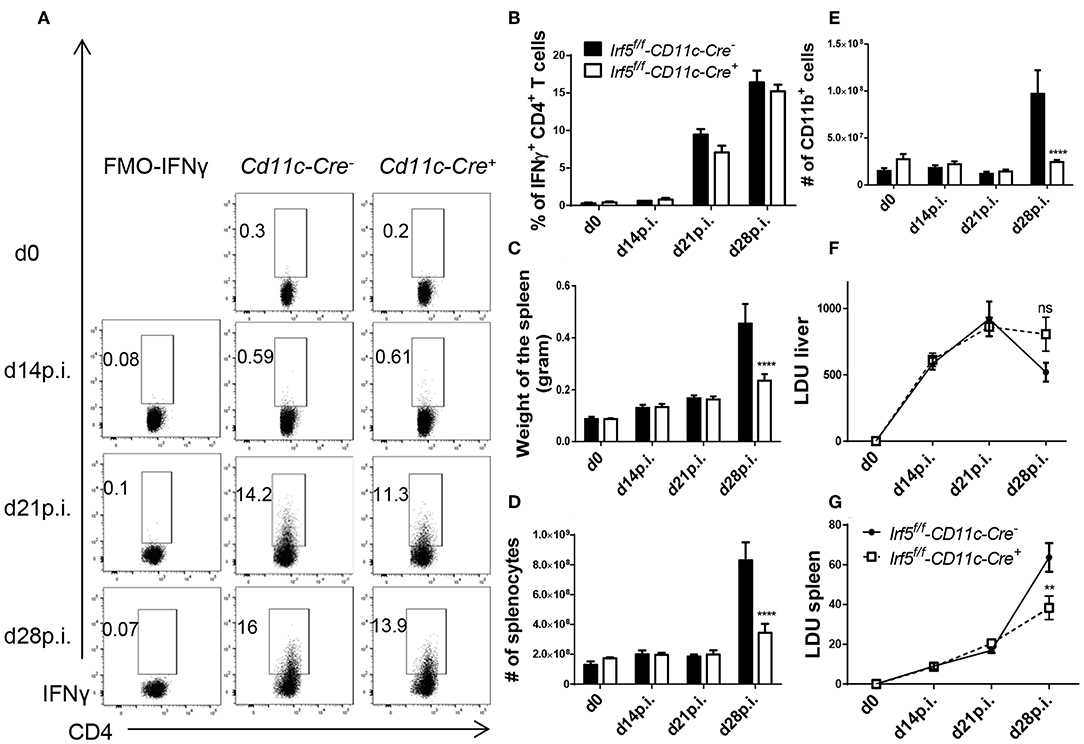
Figure 4. Irf5f/f -CD11c-Cre+ mice fail to develop splenomegaly but show similar frequencies of Th1 cells than Irf5f/f -CD11c-Cre+ mice following L. donovani infection. Irf5f/f -CD11c-Cre+ and Irf5f/f -CD11c-Cre− mice were infected with L. donovani and sacrificed at various time points of infection. Graphs show representative scatter plots (A) and percentages (B) of IFN-γ+ CD4 T in the liver of naïve and infected mice. Graphs show spleen weights (C), absolute number of splenocytes (D) and splenic CD11b+ cells (E) in naïve and infected mice. (F,G) Graphs show hepatic (F) and splenic (G) parasite burden, expressed as Leishman Donovan Units. Error bars indicate mean ± SEM, N = 4 mice, one of 2 independent experiments is shown, ns, not significant, **p < 0.01, ****p < 0.0001.
However, Irf5flox/flox -Cd11c-Cre+ mice had a much smaller spleen at d28p.i. in weight (Figure 4C) and cellularity (Figure 4D), compared with their Cre− counterparts, suggesting that IRF-5 expression in CD11c+ cells is required to promote inflammation in the spleen following L. donovani infection. Moreover, myeloid cell numbers in L. donovani-infected CD11c-Cre+ mice were significantly reduced at d28 p.i. (Figure 4E). This lack of recruitment affected all myeloid cell populations (Supplemental Figures 3A–E). Similarly to Irf5flox/flox -CMV-Cre+ mice, reduced recruitment of inflammatory cells affected all cell populations equally; percentages of B, T, and myeloid cells did not vary between CD11c-Cre+ and Cre− mice (data not shown).
Because the recruitment of monocytes has been associated with disease exacerbation (15), we were curious to know whether a dramatic reduction in inflammatory monocyte infiltration to the spleen would affect the parasite burden. As shown in Figure 4F, no significant differences in LDU were observed in the livers of infected Cre+ and Cre− mice. In contrast, the splenic parasite burden was significantly lower in the absence of Irf5 expression in CD11c+ cells (Figure 4G).
In summary, our results show that IRF-5-deficiency in CD11c+ cells severely affects inflammatory cell infiltration to the spleen, but does not hinder the development of Th1 responses in L. donovani-infected mice.
Discussion
Splenomegaly and chronic inflammation are hallmark of visceral leishmaniasis and are associated with parasite persistence. IRF-5 is largely responsible for promoting inflammatory cell infiltration into the spleen, which ultimately results in splenomegaly. In the present study, we show that Irf5 expression in CD11c+ cells is involved in the induction of inflammation but is not necessary for development and/or maintenance of protective Th1 responses.
The LysM-Cre mouse model has successfully been used to study the role of specific genes in myeloid cells in the bone marrow (28), the lungs (29), the liver (30), the gut and the skin (31, 32). However, LysM-cre does not seem to be effective in deleting genes in splenic myeloid cells during VL. Our results are in agreement with Abram et al. that report deletion efficiency for LysM-cre of less than 40% in splenic macrophages and blood monocytes (33). Furthermore, analysis of mice with an insertion of EGFP into the LysM gene revealed that LysM is mostly highly expressed in granulocytes, especially neutrophils, followed by macrophages and only occasionally by monocytes (34). Thus, it is not surprising that this mouse model cannot be used to study gene functions in splenic myeloid cells following L. donovani infection, with exception of neutrophils. One should also take into account that deletion efficiency in blood monocytes is also very low and that this may change the interpretation of studies in disease models with extensive monocyte infiltrations, like cutaneous leishmaniasis for example. In an earlier publication, we have found that the CD11c-Cre mouse model is a better choice for deleting genes in splenic myeloid cells in the context of VL (14), because of the massive infiltration of inflammatory monocyte, which express high levels of CD11c during the chronic stage of disease. The current results confirm our previous data. Indeed, we observed a clear phenotype in CD11c-specific Irf5−/− mice after L. donovani infection, suggesting that Irf5 expression in neutrophils does not play a major role in the immunopathogenesis of VL.
Interestingly, L. donovani-infected CD11c-specific Irf5−/− mice display a massive impairment of myeloid cell recruitment to the spleen compared to IRF-5-sufficient mice. Splenomegaly results from the induction of emergency hematopoiesis, mainly during the chronic stages of L. donovani infection (14, 15). The fact that neutrophils, which are IRF-5 sufficient in our model, were also not recruited to the spleen suggest that IRF-5 may be required to drive inflammation/emergency hematopoiesis and/or for cell migration. In an experimental model of SLE, IRF-5 was reported to regulate the expression of CXCR4 and CCR2, two important molecules involved in monocyte migration (35). Moreover, IRF-5 is essential for maintaining pro-inflammatory CD11c+ macrophages/monocytes within lesions in an experimental atherosclerosis model (36). It is also possible that Irf5 expression in B cells may be involved in initiating inflammation. In fact, IRF-5 regulates activation, proliferation, differentiation and antibody production in human naïve B cells (37); moreover, Irf5−/− mice showed a reduction in hypergammaglobulinemia (38), which is required for sustaining inflammation during VL (39). Further investigations are needed to determine the functional role of this transcription factor in driving inflammation during VL. Taken together, our data demonstrate that a reduction in the recruitment of myeloid cells to the spleen during chronic infection results in heightened host resistance against L. donovani. These results are in agreement with the literature that reports a detrimental role for myeloid cells, particularly inflammatory monocytes, during VL (14, 16, 40).
We were surprised to observe that Th1 responses were not altered in myeloid cell-specific Irf5−/− mice. Although T cell recruitment to the spleen was also impaired in CD11c-specific Irf5−/− mice during VL, the frequency of IFNγ+ CD4 T cells was comparable to Cre+ mice, suggesting that Irf5 expression in CD11c+ cells is not required for sustaining Th1 responses. This disagreement with the literature (27) could be explained by the fact that CD4 T cell responses are primed very late during infection and peak at d21–28 p.i., when IRF-5 is not strongly expressed by myeloid cells. It is thus important to integrate knowledge on myeloid cell functions with the kinetic of CD4 T cell priming and development in order to identify crucial molecules involved in these processes for a particular infection. IRF-5 expression in CD11c+ cells is not essential for Th1 priming during VL.
Because Th1 responses are thought to be essential for controlling parasite growth in leishmaniasis, it still remains unclear why the splenic parasite burden was lower in Cd11c-Cre+ mice compared to Cre− controls. One possible explanation is that monocytes, which act as safe houses during chronic VL (14–16), are recruited in lower numbers to the spleen. A second possibility that is not mutually exclusive with the first one is that CD8 T cells undergo stronger expansion in these mice. We have previously reported that IRF-5-mediated inflammation was required to induce HIF-1α expression in dendritic cells and that this had an negative impact on their function and on CD8 T cell expansion during the first 10 days of infection (6). It is thus possible that CD8 T cells underwent stronger expansion in infected Cd11c-Cre+ mice and that this contributed to enhanced parasite growth control.
In conclusion, we demonstrated that Irf5 expression in CD11c+ cells is required for the development of splenomegaly but does not affect the development or the maintenance of protective Th1 responses during experimental VL. Moreover, the Lysm-Cre mouse model should not be used to delete genes in splenocytes or inflammatory monocytes infiltrating the spleen.
Data Availability Statement
All datasets generated for this study are included in the article/Supplementary Material.
Ethics Statement
The animal study was reviewed and approved by Comité Institutionnel de Protection des Animaux of the INRS-Institut Armand Frappier.
Author Contributions
LM designed and performed experiments, analyzed data, wrote the manuscript. MS, SS-B, and AF performed experiments and analyzed data. SS directed the study, interpreted data, and wrote the manuscript.
Conflict of Interest
The authors declare that the research was conducted in the absence of any commercial or financial relationships that could be construed as a potential conflict of interest.
Acknowledgments
The authors thank the Canadian Institutes of Health Research (PJT-159647 to SS) for financial support. LM and MS were partly supported by a studentship from the Fondation Armand-Frappier.
Supplementary Material
The Supplementary Material for this article can be found online at: https://www.frontiersin.org/articles/10.3389/fimmu.2019.03071/full#supplementary-material
References
1. Ready PD. Epidemiology of visceral leishmaniasis. Clin Epidemiol. (2014) 6:147–54. doi: 10.2147/CLEP.S44267
2. Kaye P, Scott P. Leishmaniasis: complexity at the host-pathogen interface. Nat Rev Microbiol. (2011) 9:604–15. doi: 10.1038/nrmicro2608
3. Bankoti R, Stäger S. Differential regulation of the immune response in the spleen and liver of mice infected with Leishmania donovani. J Trop Med. (2012) 2012:639304. doi: 10.1155/2012/639304
4. Charpentier T, Hammami A, Stäger S. Hypoxia inducible factor 1alpha: a critical factor for the immune response to pathogens and Leishmania. Cell Immunol. (2016) 309: 42–9. doi: 10.1016/j.cellimm.2016.06.002
5. Kaye PM, Svensson M, Ato M, Maroof A, Polley R, Stager S, et al. The immunopathology of experimental visceral leishmaniasis. Immunol Rev. (2004) 201:239–53. doi: 10.1111/j.0105-2896.2004.00188.x
6. Hammami A, Charpentier T, Smans M, Stäger S. IRF-5-Mediated inflammation limits CD8+ T cell expansion by inducing HIF-1alpha and impairing dendritic cell functions during leishmania infection. PLoS Pathog. (2015) 11:e1004938. doi: 10.1371/journal.ppat.1004938
7. Joshi T, Rodriguez S, Perovic V, Cockburn IA, Stäger S. B7-H1 blockade increases survival of dysfunctional CD8(+) T cells and confers protection against Leishmania donovani infections. PLoS Pathog. (2009) 5:e1000431. doi: 10.1371/journal.ppat.1000431
8. Hammami A, Abidin BM, Heinonen KM, Stäger S. HIF-1alpha hampers dendritic cell function and Th1 generation during chronic visceral leishmaniasis. Sci Rep. (2018) 8:3500. doi: 10.1038/s41598-018-21891-z
9. Fabié A, Mai LT, Dagenais-Lussier X, Hammami A, van Grevenynghe J, Stäger S. IRF-5 promotes cell death in CD4 T cells during chronic infection. Cell Rep. (2018) 24:1163–75. doi: 10.1016/j.celrep.2018.06.107
10. Murphy ML, Cotterell SE, Gorak PM, Engwerda CR, Kaye PM. Blockade of CTLA-4 enhances host resistance to the intracellular pathogen, Leishmania donovani. J Immunol. (1998) 161:4153–60.
11. Maroof A, Beattie L, Zubairi S, Svensson M, Stager S, Kaye PM. Posttranscriptional regulation of II10 gene expression allows natural killer cells to express immunoregulatory function. Immunity. (2008) 29:295–305. doi: 10.1016/j.immuni.2008.06.012
12. Bankoti R, Gupta K, Levchenko A, Stäger S. Marginal zone B cells regulate antigen-specific T cell responses during infection. J Immunol. (2012) 188:3961–71. doi: 10.4049/jimmunol.1102880
13. Ranatunga D, Hedrich CM, Wang F, McVicar DW, Nowak N, Joshi T, et al. A human IL10 BAC transgene reveals tissue-specific control of IL-10 expression and alters disease outcome. Proc Natl Acad Sci USA. (2009) 106:17123–8. doi: 10.1073/pnas.0904955106
14. Hammami A, Abidin BM, Charpentier T, Fabié A, Duguay AP, Heinonen KM, et al. HIF-1α is a key regulator in potentiating suppressor activity and limiting the microbicidal capacity of MDSC-like cells during visceral leishmaniasis. PLoS Pathog. (2017) 13:e1006616. doi: 10.1371/journal.ppat.1006616
15. Abidin BM, Hammami A, Stäger S, Heinonen KM. Infection-adapted emergency hematopoiesis promotes visceral leishmaniasis. PLoS Pathog. (2017) 13:e1006422. doi: 10.1371/journal.ppat.1006422
16. Terrazas C, Varikuti S, Oghumu S, Steinkamp HM, Ardic N, Kimble J, et al. Ly6C(hi) inflammatory monocytes promote susceptibility to Leishmania donovani infection. Sci Rep. (2017) 7:14693. doi: 10.1038/s41598-017-14935-3
17. Barnes BJ, Moore PA, Pitha PM. Virus-specific activation of a novel interferon regulatory factor, IRF-5, results in the induction of distinct interferon alpha genes. J Biol Chem. (2001) 276:23382–90. doi: 10.1074/jbc.M101216200
18. Takaoka A, Yanai H, Kondo S, Duncan G, Negishi H, Mizutani T, et al. Integral role of IRF-5 in the gene induction programme activated by Toll-like receptors. Nature. (2005) 434:243–9. doi: 10.1038/nature03308
19. Graham RR, Kozyrev SV, Baechler EC, Reddy MV, Plenge RM, Bauer JW, et al. A common haplotype of interferon regulatory factor 5 (IRF5) regulates splicing and expression and is associated with increased risk of systemic lupus erythematosus. Nat Genet. (2006) 38:550–5. doi: 10.1038/ng1782
20. Dieguez-Gonzalez R, Calaza M, Perez-Pampin E, de la Serna AR, Fernandez-Gutierrez B, Castañeda S, et al. Association of interferon regulatory factor 5 haplotypes, similar to that found in systemic lupus erythematosus, in a large subgroup of patients with rheumatoid arthritis. Arthritis Rheum. (2008) 58:1264–74. doi: 10.1002/art.23426
21. Carmona FD, Martin JE, Beretta L, Simeón CP, Carreira PE, Callejas JL, et al. The systemic lupus erythematosus IRF5 risk haplotype is associated with systemic sclerosis. PLoS ONE. (2013) 8:e54419. doi: 10.1371/journal.pone.0054419
22. Kristjansdottir G, Sandling JK, Bonetti A, Roos IM, Milani L, Wang C, et al. Interferon regulatory factor 5 (IRF5) gene variants are associated with multiple sclerosis in three distinct populations. J Med Genet. (2008) 45:362–9. doi: 10.1136/jmg.2007.055012
23. Paun A, Bankoti R, Joshi T, Pitha PM, Stäger S. Critical role of IRF-5 in the development of T helper 1 responses to Leishmania donovani infection. PLoS Pathog. (2011) 7:e1001246. doi: 10.1371/journal.ppat.1001246
24. Smelt SC, Engwerda CR, McCrossen M, Kaye PM. Destruction of follicular dendritic cells during chronic visceral leishmaniasis. J Immunol. (1997) 158:3813–21.
25. Silva-Barrios S, Smans M, Duerr CU, Qureshi ST, Fritz JH, Descoteaux A, et al. Innate immune B cell activation by leishmania donovani exacerbates disease and mediates hypergammaglobulinemia. Cell Rep. (2016) 15:2427–37. doi: 10.1016/j.celrep.2016.05.028
26. Purtha WE, Swiecki M, Colonna M, Diamond MS, Bhattacharya D. Spontaneous mutation of the Dock2 gene in Irf5-/- mice complicates interpretation of type I interferon production and antibody responses. Proc Natl Acad Sci USA. (2012) 109:E898–904. doi: 10.1073/pnas.1118155109
27. Krausgruber T, Blazek K, Smallie T, Alzabin S, Lockstone H, Sahgal N, et al. IRF5 promotes inflammatory macrophage polarization and TH1-TH17 responses. Nat Immunol. (2011) 12:231–8. doi: 10.1038/ni.1990
28. Imtiyaz HZ, Williams EP, Hickey MM, Patel SA, Durham AC, Yuan LJ, et al. Hypoxia-inducible factor 2alpha regulates macrophage function in mouse models of acute and tumor inflammation. J Clin Invest. (2010) 120:2699–714. doi: 10.1172/JCI39506
29. McCubbrey AL, Allison KC, Lee-Sherick AB, Jakubzick CV, Janssen WJ. Promoter specificity and efficacy in conditional and inducible transgenic targeting of lung macrophages. Front Immunol. (2017) 8:1618. doi: 10.3389/fimmu.2017.01618
30. Alzaid F, Lagadec F, Albuquerque M, Ballaire R, Orliaguet L, Hainault I, et al. IRF5 governs liver macrophage activation that promotes hepatic fibrosis in mice and humans. JCI Insight. (2016) 1:e88689. doi: 10.1172/jci.insight.88689
31. Andrianne M, Assabban A, La C, Mogilenko D, Salle DS, Fleury S, et al. Tristetraprolin expression by keratinocytes controls local and systemic inflammation. JCI Insight. (2017) 2:92979. doi: 10.1172/jci.insight.92979
32. Malik A, Sharma D, Malireddi RKS, Guy CS, Chang TC, Olsen SR, et al. SYK-CARD9 signaling axis promotes gut fungi-mediated inflammasome activation to restrict colitis and colon cancer. Immunity. (2018) 49:515–30 e5. doi: 10.1016/j.immuni.2018.08.024
33. Abram CL, Roberge GL, Hu Y, Lowell CA. Comparative analysis of the efficiency and specificity of myeloid-Cre deleting strains using ROSA-EYFP reporter mice. J Immunol Methods. (2014) 408:89–100. doi: 10.1016/j.jim.2014.05.009
34. Faust N, Varas F, Kelly LM, Heck S, Graf T. Insertion of enhanced green fluorescent protein into the lysozyme gene creates mice with green fluorescent granulocytes and macrophages. Blood. (2000) 96:719–26. doi: 10.1182/blood.V96.2.719.014k29_719_726
35. Yang L, Feng D, Bi X, Stone RC, Barnes BJ. Monocytes from Irf5-/- mice have an intrinsic defect in their response to pristane-induced lupus. J Immunol. (2012) 189:3741–50. doi: 10.4049/jimmunol.1201162
36. Seneviratne AN, Edsfeldt A, Cole JE, Kassiteridi C, Swart M, Park I, et al. Interferon regulatory factor 5 controls necrotic core formation in atherosclerotic lesions by impairing efferocytosis. Circulation. (2017) 136:1140–54. doi: 10.1161/CIRCULATIONAHA.117.027844
37. De S, Zhang B, Shih T, Singh S, Winkler A, Donnelly R, et al. B cell-intrinsic role for IRF5 in TLR9/BCR-induced human B cell activation, proliferation, and plasmablast differentiation. Front Immunol. (2017) 8:1938. doi: 10.3389/fimmu.2017.01938
38. Feng D, Yang L, Bi X, Stone RC, Patel P, Barnes BJ. Irf5-deficient mice are protected from pristane-induced lupus via increased Th2 cytokines and altered IgG class switching. Eur J Immunol. (2012) 42:1477–87. doi: 10.1002/eji.201141642
39. Silva-Barrios S, Stäger S. Hypergammaglobulinemia sustains the development of regulatory responses during chronic Leishmania donovani infection in mice. Eur J Immunol. (2019) 49:1082–91. doi: 10.1002/eji.201847917
Keywords: Leishmania, IRF5, myeloid cells, splenomegaly, Th1, LysM-cre
Citation: Mai LT, Smans M, Silva-Barrios S, Fabié A and Stäger S (2020) IRF-5 Expression in Myeloid Cells Is Required for Splenomegaly in L. donovani Infected Mice. Front. Immunol. 10:3071. doi: 10.3389/fimmu.2019.03071
Received: 12 September 2019; Accepted: 16 December 2019;
Published: 21 January 2020.
Edited by:
Abhay Satoskar, The Ohio State University, United StatesReviewed by:
Parna Bhattacharya, U.S. Food and Drug Administration, United StatesVanessa Carregaro, University of São Paulo, Brazil
Angamuthu Selvapandiyan, Jamia Hamdard University, India
Copyright © 2020 Mai, Smans, Silva-Barrios, Fabié and Stäger. This is an open-access article distributed under the terms of the Creative Commons Attribution License (CC BY). The use, distribution or reproduction in other forums is permitted, provided the original author(s) and the copyright owner(s) are credited and that the original publication in this journal is cited, in accordance with accepted academic practice. No use, distribution or reproduction is permitted which does not comply with these terms.
*Correspondence: Simona Stäger, c2ltb25hLnN0YWdlckBpYWYuaW5ycy5jYQ==