- 1Department of Chest Disease, Strasbourg University Hospital, Strasbourg, France
- 2Equipe d'accueil 3072, University of Strasbourg, Strasbourg, France
- 3Immunowell Foundation, Utrecht, Netherlands
- 4Human Development and Health, Faculty of Medicine, University of Southampton, Southampton, United Kingdom
- 5National Institute for Health Research Southampton Biomedical Research Centre, University Hospital Southampton NHS Foundation Trust and University of Southampton, Southampton, United Kingdom
- 6UMR 7200 CNRS/Université de Strasbourg, Laboratoire d'Innovation Thérapeutique and LabEx MEDALIS, Faculté de Pharmacie, Strasbourg, France
- 7Division of Pharmacology, Utrecht Institute for Pharmaceutical Sciences, Faculty of Science, Utrecht University, Utrecht, Netherlands
- 8Nutricia Research, Utrecht, Netherlands
- 9Biochemical Pharmacology, William Harvey Research Institute, Bart's School of Medicine and Queen Mary University of London, London, United Kingdom
- 10Division of Pharmacology, Faculty of Science, Utrecht Institute for Pharmaceutical Sciences, Utrecht University, Utrecht, Netherlands
- 11Pharmacology and Experimental Therapeutics Unit, Faculty of Medicine, School of Pharmacy, Institute for Drug Research, The Hebrew University of Jerusalem, Jerusalem, Israel
- 12Department of Biochemistry & Cell Biology, Faculty of Veterinary Medicine, Utrecht University, Utrecht, Netherlands
- 13Institute for Risk Assessment Sciences, Faculty of Veterinary Medicine, Utrecht University, Utrecht, Netherlands
- 14Amsterdam UMC, Tytgat Institute for Liver and Intestinal Research, University of Amsterdam, AGEM, Amsterdam, Netherlands
Formerly considered as a passive process, the resolution of acute inflammation is now recognized as an active host response, with a cascade of coordinated cellular and molecular events that promotes termination of the inflammatory response and initiates tissue repair and healing. In a state of immune fitness, the resolution of inflammation is contained in time and space enabling the restoration of tissue homeostasis. There is increasing evidence that poor and/or inappropriate resolution of inflammation participates in the pathogenesis of chronic inflammatory diseases, extending in time the actions of pro-inflammatory mechanisms, and responsible in the long run for excessive tissue damage and pathology. In this review, we will focus on how resolution can be the target for therapy in “Th1/Th17 cell-driven” immune diseases and “Th2 cell-driven” immune diseases, with inflammatory bowel diseases (IBD) and asthma, as relevant examples. We describe the main cells and mediators stimulating the resolution of inflammation and discuss how pharmacological and dietary interventions but also life style factors, physical and psychological conditions, might influence the resolution phase. A better understanding of the impact of endogenous and exogenous factors on the resolution of inflammation might open a whole area in the development of personalized therapies in non-resolving chronic inflammatory diseases.
Introduction
Inflammation is part of the normal response of the host to invasion by harmful microorganisms or to tissue injury (1). The acute inflammatory response is initiated within minutes of recognition of a danger signal, and begins with an onset phase coordinated by several families of chemokines, cytokines, eicosanoids, proteases, vasoactive amines, neuropeptides and neurotransmitters, and other pro-inflammatory mediators produced by resident immune and structural cells in the injured/infected tissue, which is followed by a rapid influx of granulocytes from blood to the tissue inflammatory site (2). Self-amplifying networks of pro-inflammatory pathways perpetuate leukocyte recruitment and activation.
In a state of immune fitness, the inflammatory response is contained in time and space, and is programmed to resolve, i.e., return from the infected or injured state to a “healthy” state corresponding to that of pre-inflamed tissue. Formerly considered as a passive process, the natural resolution of acute inflammation is now known as an active host response, with highly coordinated cellular and molecular events with release of anti-inflammatory cytokines, loss of receptors for pro-inflammatory signals, and production of a wide range of pro-resolving mediators including recently uncovered specialized pro-resolving lipid mediators (SPMs) that enable restoration of tissue homeostasis (3). A failure in pro-resolving pathways may extend in time the actions of pro-inflammatory mechanisms resulting in prolonged or chronic inflammation with recurrent exacerbations, responsible in the long run for excessive tissue damage and pathology (Figure 1).
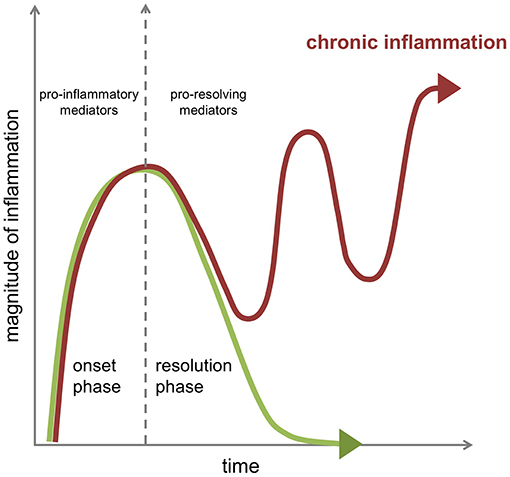
Figure 1. Dynamics of the inflammatory response in chronic inflammation. The acute inflammatory response is a highly coordinated sequence of events characterized by an onset phase coordinated by several families of chemokines, cytokines, and pro-inflammatory mediators that is followed in health by an active resolution phase brought about by the engagement of specific cellular mechanisms under the control of several pro-resolving mediators to promote resolution of the tissue inflammation as well as healing and repair. A failure in pro-resolving pathways can extend in time the actions of pro-inflammatory mechanisms resulting in prolonged or chronic inflammation with recurrent exacerbations.
Poor and/or inappropriate resolution of inflammation has indeed emerged as a critical process in the pathogenesis of numerous chronic inflammatory and auto-immune diseases including inflammatory bowel diseases (IBD) (such as Crohn's disease and ulcerative colitis) (4). Persistent airway inflammation in chronic lung diseases, such as asthma, may also be due to defects in pro-resolving molecular pathways (5, 6).
The possibility to promote resolution of the inflammatory response as a therapeutic approach has only become apparent in the twenty-first century (7, 8). Better understanding the resolution phase of the inflammatory response and how this process might be influenced by environmental factors might open a whole area of new, affordable, and personalized therapeutic options in chronic inflammatory diseases. This article will first review the main cellular and molecular mechanisms involved in the resolution of inflammation. Finally, we will discuss a series of interventions that can potentially promote resolution with a focus on “Th1/Th17 cell-driven” and “Th2 cell-driven” immune diseases, with IBD and asthma, as relevant examples.
The Main Determinants of the Resolution Phase
Overall there are two distinct phases in an inflammatory reaction: the initiation of inflammation and the resolution phase (Figure 1). A post-resolution phase of inflammation that links innate and adaptive immune systems has also been described (9, 10). For effective resolution of inflamed tissues to occur and to restore tissue homeostasis, specific cellular mechanisms that are under the control of pro-resolving mediators are enlisted to promote termination of the inflammatory response and initiate tissue repair and healing (Figure 2). Better understanding of how the environment can impact on the resolution of inflammation will lead to an improved understanding of why the chronic inflammatory diseases persist.
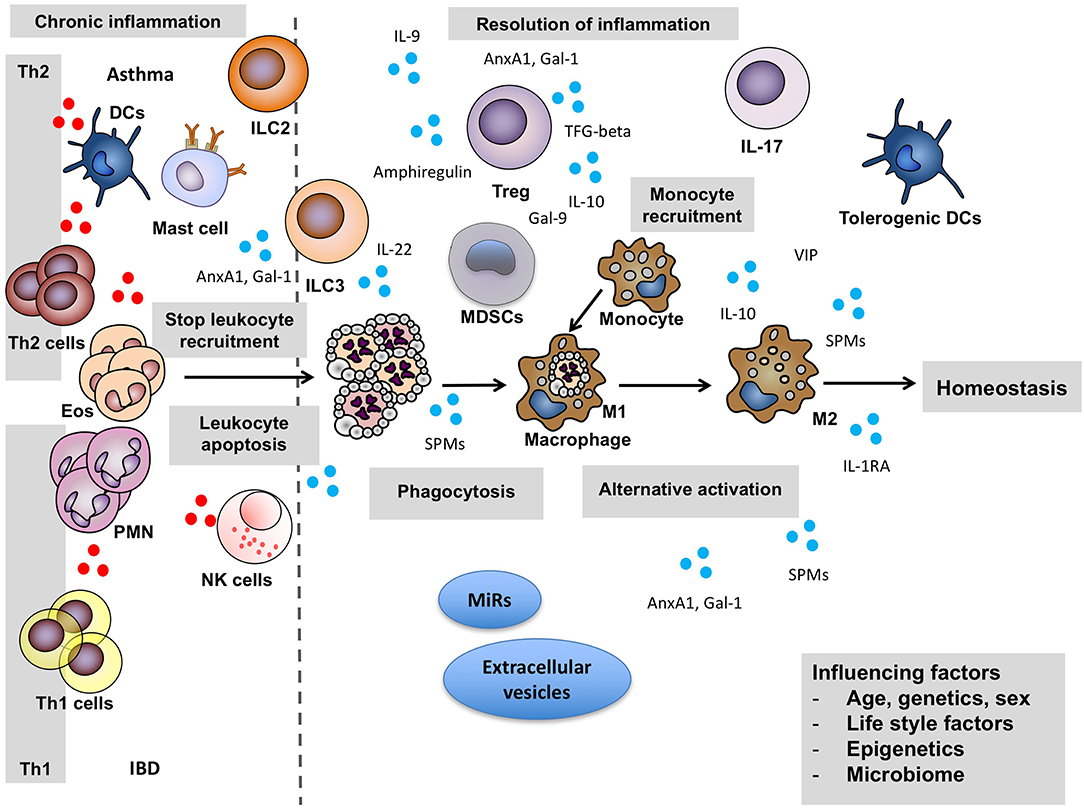
Figure 2. Key cellular actors of resolution. For effective resolution of inflamed tissues to occur and to restore tissue homeostasis, specific cellular mechanisms that are under the control of pro-resolving mediators are enlisted. They promote termination of the inflammatory response and initiate tissue repair and healing. Pro-inflammatory mediators: red circles, pro-resolving mediators: blue circles. Anx, annexin; DCs, Dendritic cells; Eos, eosinophils; Gal, Galectin; IBD, inflammatory bowel disease; IL, interleukin; ILC2, Type 2 innate lymphoid cells; ILC3, Type 3 innate lymphoid cells; MDSCs, Myeloid-derived suppressor cells; MiRs, MicroRNAs; NK, Natural killer; PMN, polymorphonuclear cells; TGF-beta, Transforming growth factor beta; Th1, Type 1 T helper cells; Th2, Type 2 T helper cells; Treg, regulatory T cells; SPMs, specialized pro-resolution lipid mediators; VIP, vasoactive intestinal peptide.
Cells Involved in the Resolution of Inflammation
Macrophages
One of the key events in determining the initiation of the resolution phase is the recruitment of non-phlogistic monocytes and their differentiation into macrophages at sites of inflammation. Indeed, central to the successful resolution of inflammation, is the process of local leukocyte clearance by apoptosis and subsequent phagocytosis of the apoptotic cells by surrounding monocyte-derived phagocytes (Figure 2). Engulfment of apoptotic cells signals to the phagocytosing macrophages that the inflammatory response is ending, and alters macrophage mediator production from a predominantly pro-inflammatory (M1) to an anti-inflammatory and pro-resolving phenotype (M2), that further enhances phagocytosis of apoptotic cells and promotes the return to tissue homeostasis (11, 12). This shifting balance between pro-inflammatory M1 and wound-healing M2 macrophages over time is essential for proper resolution of inflammation (13).
Regulatory T Cells
Regulatory T (Treg) cells can also play roles in the resolution process, by promoting repair and regeneration of various organ systems and may link innate and adaptive immune systems [for a recent review see (14)]. Treg cells, like T helper (Th) cells, derive from the progenitor CD4+ naive T cell. The population of Treg cells consists of thymus-derived Treg cells called natural Treg (nTregs) cells, and Treg cells induced in the periphery or induced Treg (iTregs) cells. Treg cells suppress the activation and function of inflammatory leukocytes, specifically macrophages, through the production of anti-inflammatory cytokines (IL-10 and TGF-β) and by scavenging IL-2 (high expression of IL-2R CD25), signaling of surface molecules, cytolysis, and metabolic control (15, 16).
Treg cells are important players for maintaining homeostatic balance in the intestine [reviewed in (17)]. Acute Treg cell deficiency results in an exacerbated inflammatory immune response toward commensal intestinal bacteria leading to a chronic inflammatory state as found in IBD (18).
Similarly, in mouse models of allergic asthma, resolution of allergic airway inflammation was dependent on CD4+CD25+Foxp3+ expressing Treg cells (19). Accumulation of Treg cells in local draining lymph nodes of the lung correlated with spontaneous resolution of chronic asthma in another murine model (20). Moreover, in the lung, Treg cells have also been described to directly stimulate lung tissue repair, as a consequence of the production of amphiregulin, an autocrine growth factor (21).
Innate Lymphoid Cells
Innate lymphoid cells (ILCs) are a large family of cells with various immunological functions (22). They can be classified into different subgroups based on their cytokine production and their expression of key transcription factors, similar to T cell subsets. In various mouse models of asthma and IBD, studies suggest a role for ILCs in the induction of inflammation [for recent reviews see (23, 24)]. Recent evidence suggests a more complex role for these cells, with dual roles in the induction of inflammatory diseases but also the control of chronic inflammation. Type 2 ILC (ILC2) cells demonstrate a flexibility and plasticity dependent on the local microenvironment and can potentially act both as effectors and suppressors [reviewed in (25, 26)]. ILC2 cells, by producing IL-5 and IL-13, promote the development of type 2 allergic inflammation, independent of Th2 cells (9, 27). In contrast, the production of IL-9 by ILC2s was recently reported to mediate resolution of inflammation in a model of chronic arthritis, another chronic non-resolving disease (28). Also, a potential role for ILC2 has been suggested in tissue repair after acute lung injury in a mouse model of H1N1 influenza virus infection through the production of amphiregulin (29).
The same holds true for type 3 ILC (ILC3) cells, the most abundant ILC subtype in the human intestine at steady state (30). ILC3 cells are the main contributors to intestinal IL-22 production, which is a tightly regulated mediator for immune homeostasis in the intestinal tract (31).
NK cells are also members of the ILC family with potential roles of SPM-induced resolution of eosinophilic inflammation in Th2 asthma (32, 33).
Myeloid-Derived Suppressor Cells
Myeloid-derived suppressor cells (MDSCs) are a heterogeneous population of cells, consisting of myeloid progenitor cells, and immature macrophages, granulocytes and dendritic cells. These cells are not present in the normal healthy steady state, and appear in pathological situations related to chronic inflammatory situations and stress. Their main function is the suppression of T cell function (34–36).
Recently, a recommendation was published to classify these cells into two different subsets based on their phenotype and function (37). Polymorphonuclear (PMN) and mononuclear (M) MDSCs share several partly overlapping immunosuppressive mechanisms, where inhibition of anti-CD3/CD28-induced T-cell proliferation and IFN-γ production are the general functional tests used for their identification. In general, MDSCs use several mechanisms to carry out their immunosuppressive function. As biomarkers, the expression of various transcription factors and apoptotic regulators (pSTAT3, cEBP/b, S100A8/9) and immune-regulatory genes and molecules (ARG1, NOS2, NOX2, and PNT) are associated with MDSCs and/or PMN-MDSC and M-MDSC subsets (37, 38). These molecules have immunosuppressive effects and negatively regulate T cells by impairing IL-2R signaling pathways, trigger apoptosis (39, 40), and induce Treg cell expansion and IL-10 and TGF-ß production (41, 42). Moreover, MDSCs have a relevant role in resolution of inflammation by efferocytosis of apoptotic neutrophils (35), a process supported in part by IL-10 (42).
Mediators Participating in the Resolution of Inflammation
During the inflammatory response, diverse mediators are synthesized in a strict temporal and spatial manner to act on specific receptor targets and to actively prevent the overshooting of acute inflammatory mechanisms, and ultimately restore tissue homeostasis. Functionally, these anti-inflammatory and pro-resolving mediators counter-regulate key events of inflammation. Different from solely anti-inflammatory actions, pro-resolving mediators actions typically target specific pro-resolution mechanisms: limitation and/or cessation of neutrophil recruitment; promotion of non-phlogistic monocyte recruitment; induction of neutrophil apoptosis and their subsequent efferocytosis by macrophages, enhancement of efferocytosis, reprogramming of macrophages from classically activated to alternatively activated cells; return of non-apoptotic cells to the blood or egress via the lymphatic vasculature; stimulation of tissue repair and cellular repopulation of the tissue, leading to “adapted homeostasis” [recently reviewed in (43)].
Pro-resolving mediators are diverse in nature, and include SPMs (lipoxins, resolvins, protectins, and maresins), proteins and peptides [annexin A1 (AnxA1), galectins, adrenocorticotropic hormone (ACTH), and IL-10], gaseous mediators including hydrogen sulfide (H2S) and carbon monoxide (CO), nucleotides (e.g., adenosine), as well as neuromodulators released under the control of the vagus nerve such as acetylcholine and neuropeptides released from non-adrenergic non-cholinergic neurons (44). As diverse as their nature is their origin, where mediators of resolution can be produced locally, acting in paracrine and autocrine manners, or produced at distant sites, followed by their systemic release and extravasation to sites of inflammation (43). Below the main pro-resolving mediators will be described.
Specialized Pro-resolving Lipid Mediators (SPMs)
Recently, a new array of lipid molecules that function in the resolution of inflammation were elucidated and collectively named SPMs (3, 45, 46). These mediators, such as lipoxins (Lx), resolvins (Rv), protectins (PD), and maresins (Mar), are produced during the inflammatory response and derive from polyunsaturated fatty acids (PUFAs). Whereas, Lx derive from the omega-6 PUFA arachidonic acid, the omega-3 PUFAs eicosapentaenoic acid (EPA) and docosahexaenoic acid (DHA) give rise to Rv, PD, and Mar (Figure 3). More recently SPMs produced from both the omega-6 and omega-3 docosapentaenoic acids have been described (47). The SPMs are produced via biosynthetic circuits engaged during cell–cell interactions including different innate immune cells, for example macrophages or neutrophils, and structural cells at sites of inflammation. SPMs can also been produced through interactions of platelets with leukocytes (48).
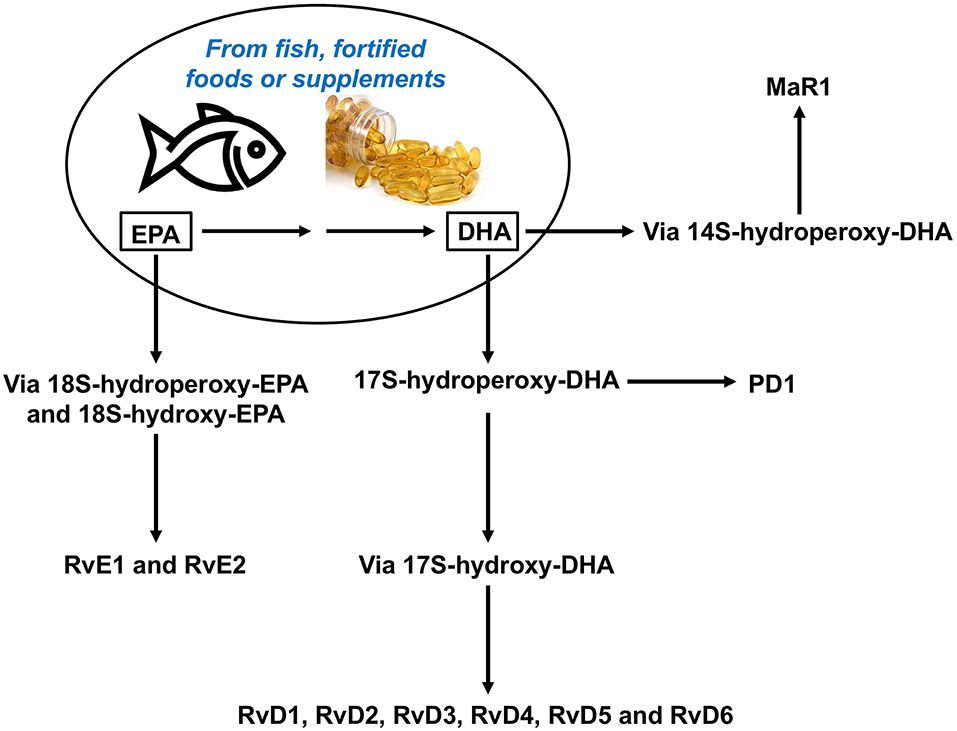
Figure 3. Overview of the pathways for synthesis of resolvins from omega-3 polyunsaturated fatty acids, DHA and EPA. DHA, docosahexaenoic acid; EPA, eicosapentaenoic acid, MaR, maresin; PD, protectin; Rv, resolvin.
These bioactive lipids display potencies in the nanomolar range, and signal through cognate G-protein coupled receptors (GPR) such as the N-formyl peptide receptor 2 (ALX/FPR2), GPR32, and GPR18 with many cell type-specific actions (49, 50) (Figure 4).
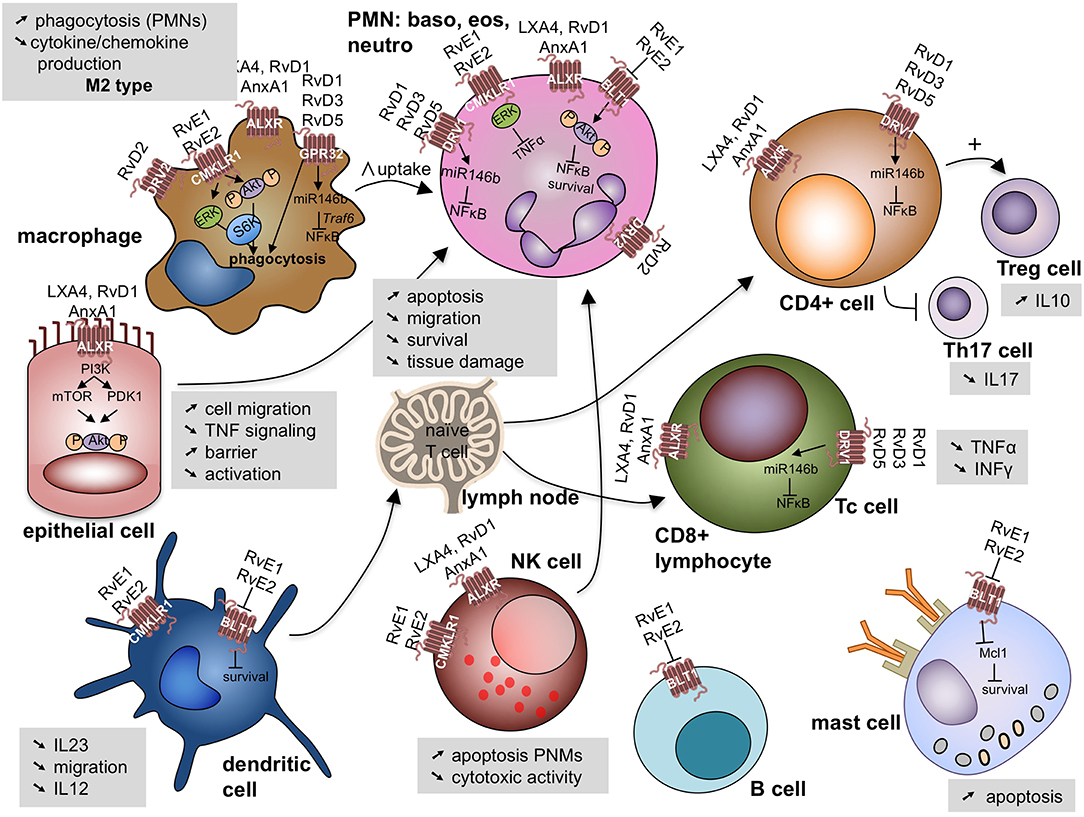
Figure 4. Specialized pro-resolving lipid mediators signal through G-protein coupled receptors on a variety of cell involved (deranged) immune response leading to cell specific responses. Akt, protein kinase B; ALX/FPR2, N-formyl peptide receptor 2—LXA4 receptor; AnxA1, annexin A1; BLT1, leukotriene B4 receptor 1; CD, cluster domain; CMKLR1, chemokine like receptor 1 or Chemerin Receptor 23; DVR1, RvD1 receptor or G protein coupled receptor (GRP)32; DVR2, RvD2 receptor or GRP18; ERK, extracellular signal regulated kinases; IL, interleukin; INFγ, interferon γ; Mcl-1, anti-apoptotic protein in mast cells; miR, microRNA; mTOR, mammalian target of rapamycin; NK cell, natural killer cell; NFκB, nuclear factor kappa-light-chain-enhancer of activated B cells; P, phosphorylated; PDK1, phosphoinositide-dependent protein kinase 1; PI3K, phosphatidylinositol 3-kinase; PMN, polymorphonuclear cells; Rv, resolvin; LX, lipoxin; S6K, ribosomal protein S6 kinase; Th17 cell: Thelper 17 lympocyte; Treg cell: regulatory T lymphocyte; TNFα, tumor necrosis factor α; Traf6: TNF receptor associated factor 6.
LXA4 binds to the ALX/FPR2. This receptor displays diverse ligand affinities that extend beyond interactions with LXA4. Indeed, ALX/FPR2 can interact with over 30 ligands with various affinities, and has been identified as the first receptor to engage both bioactive lipids and peptides/proteins, including annexin A1 (50). ALX/FPR2 is widely expressed on human leukocytes, including neutrophils, eosinophils, monocyte-macrophages, T cells, NK cells, and ILC2 cells, as well as on tissue resident cells, such as airway epithelial cells and fibroblasts (33, 51, 52). Its expression is up-regulated by local inflammatory-mediators such as IL-13 and IFN-γ (51, 53).
After initiation of the resolution of inflammation, repolarization by resolvin E1 (RvE1) induces a M2 wound healing-type macrophage (54). In addition, different Rv and Mar interact with ERV1/ChemR23, GPR32 and GPR18 on macrophages to enhance their efferocytosis, phagocytosis and IL-10 transcription (54–61). Other more recently described targets of these mediators are Treg cells and type 2 ILCs (33, 62). SPMs can prevent naïve CD4+ T cell differentiation into Th1 and Th17 cells and enhance the generation of Treg cells (63).
Evidence for the functional importance of these lipid mediators in the resolution of inflammation comes from mouse models of diverse inflammatory disorders where SPMs are able to control inflammation, limit tissue damage, shorten resolution intervals, and promote wound healing [for a recent reviews see (6, 16, 64)].
Annexin A1
An important mediator of the resolution of inflammation is the glucocorticoid-regulated protein annexin (Anx) A1, also known as lipocortin-1. AnxA1 is highly abundant in myeloid-derived cells such as neutrophils and macrophages, and exerts profound effects on several phases of the resolution of inflammation (65). AnxA1 signals through the FPR2, which also binds the SPMs LxA4 and RvD1 (50). Studies in mice indicate that this protein has important modulatory functions in neutrophil trafficking by reducing neutrophil infiltration and activating neutrophil apoptosis. AnxA1 also promotes monocyte recruitment, clearance of apoptotic neutrophils by macrophages and can switch macrophages toward a pro-resolving M2 phenotype (65). Studies have demonstrated that mast cell-derived AnxA1 is important for the cromones-induced inhibition of allergic mast cell degranulation (16).
IL-10
IL-10 is a cytokine important in controlling excessive inflammation. It mediates its major functions through inhibition of cytokine production and down-regulating antigen presentation by macrophages, monocytes, and dendritic cells (DCs) and thereby inhibiting adaptive immune cells such as Th2 and Tregs (66–68). IL-10 can also inhibit eosinophilia, by suppression of IL-5 and GM-CSF and by direct effects on eosinophil apoptosis [(69) #1607; (70) #1622].
Galectins
Galectins are ß-galactoside-binding lectins produced by, and acting upon, cells of both the innate and adaptive immune systems, modulating multiple processes within the host. Some members of this family of lectins are proposed to play pro-resolving functions, namely Galectin (Gal)-1 and 9. Gal-1 is found in resolving exudates in a murine model of peritonitis induced by zymosan (71), where it stops recruitment of neutrophils and lymphocytes (72, 73). DCs that are differentiated in a Gal-1 rich environment show enhanced regulatory function, reducing the progression of inflammation in a mouse model of multiple sclerosis by promotion of IL-10-mediated T-cell tolerance (74). Gal-1 also induces the conversion of macrophages into a pro-resolving M2 phenotype (75). Gal-3 enhances efferocytosis of apoptotic granulocytes by monocyte-derived macrophages (MDMs) (76). Gal-9 promotes apoptosis of extravasated immune cells including neutrophils and Th1 cells, and is protective in different experimental animal models of chronic auto-immune diseases (77–79). Finally, Gal-1 and Gal-9 promote the generation of Treg cells (80, 81) and induce the production of IL-10 by peripheral blood mononuclear cells from healthy donors (82).
ACTH and Melanocortins
Melanocortins, including adrenocorticotrophic hormone (ACTH) and the α, β and γ-melanocyte-stimulating hormone (MSH) are derived from a larger precursor molecule known as the pro-opiomelanocortin (POMC) protein. They exert their numerous biological effects by activating 7 transmembrane GPCR (83). ACTH does not only induce cortisol production, as previously assumed, but also exerts anti-inflammatory actions by targeting melanocortin receptors present on immune cells (84). The protective actions of melanocortins include inhibition of leukocyte transmigration and reduction of pro-inflammatory cytokine production (85, 86). Melanocortins also promote clearance of apoptotic cells (86) and cutaneous wound healing (87).
Gaseous Mediators
Carbon monoxide (CO) and hydrogen sulfide (H2S) are the best characterized gaseous substances that, in addition to their important roles in physiological and pathophysiological processes, have confirmed pro-resolving actions during inflammatory processes [reviewed in (88)]. H2S promotes neutrophil apoptosis and stimulates macrophage phagocytosis (89, 90). CO can inhibit leukocyte migration and reduce pro-inflammatory cytokine production (91). CO has shown therapeutic potential in animal models of acute lung injury (92).
Adenosine
Adenosine, is a purine nucleoside generated by the dephosphorylation of adenine nucleotides. In addition to being a potent endogenous physiologic and pharmacologic regulator of many functions, adenosine has pro-resolving mechanisms including inhibition of neutrophil and T cell functions, efferocytosis and macrophage reprogramming [reviewed in (93)].
Neuropeptides and Neurotransmitters
It is important to consider that not only immunological mediators, but also factors produced by the nervous system, like neuropeptides and neurotransmitters, contribute to the resolution of inflammation. During an inflammatory response several anti-inflammatory neuropeptides with an immunomodulatory role are produced. One example is vasoactive intestinal peptide (VIP) displaying anti-inflammatory functions in various models of chronic inflammatory disease. VIP impairs the development and infiltration of self-reactive Th1 cells into target organs, as well as the release of inflammatory cytokines and chemokines and the subsequent recruitment and activation of macrophages and neutrophils (94). In addition, VIP stimulates the production of IL-10 and IL-1RA, both important mediators of resolution, and induces the generation of tolerogenic DCs regulating the Th/Treg cells balance (95–99). Very recently, VIP was shown to modulate the differentiation of human macrophages toward the M2 phenotype, which is important in the resolution of inflammation (100).
Another example is the vagal regulation of immune responses, specifically controlling resolution and the production of SPMs (101). Disruption of the vagal system delays the resolution of the inflammatory response upon bacterial peritoneal infections via reduced numbers of group 3 ILCs (102). In macrophages, the nicotine acetylcholine receptor, α7nAChR, mediates anti-inflammatory actions and contributes to the regulation of phagocytosis. Especially M2-type macrophages express this receptor that has a protective and pro-survival role (103) and M2-type macrophages are important producers of protectin conjugates in tissue regeneration (PCTR)1 during resolution (104).
Other Mediators Participating to the Resolution of Inflammation
Anti-inflammatory Cytokines
TGF-β is a potent inhibitor of classical pro-inflammatory macrophage activation (105). TGF-β is also a mediator in critical processes in wound healing, stimulating angiogenesis, fibroblast proliferation, collagen synthesis and deposition and remodeling of extracellular matrix (106, 107). Additionally, TGF-β regulates immune responses through the development and differentiation of Th17 cells and FoxP3+ Treg cells (108, 109). TGF-β inhibits the differentiation of T helper subsets as it inhibits the expression of Tbet and GATA3, thereby blocking the differentiation of Th1 and Th2 cells respectively (110, 111).
IL-22 primarily targets non-hematopoietic cells and plays a role in host defense at barrier surfaces where it promotes tissue regeneration (112). IL-22 is produced by Th17 and Th22 cells, ILCs and NKT cells (113). It has different roles in the gastrointestinal tract including tissue regeneration, maintenance of the intestinal barrier and intestinal defense against pathogens (113–116). IL-22 levels are enhanced in the lungs of patients with asthma (117). However, in inducible lung-specific IL-22 transgenic mice, a significant decrease in allergic airway hyperresponsiveness and allergic inflammation occurred indicating an immune modulating effect of IL-22 (118).
IL-1RA (receptor antagonist) is a natural inhibitor of the pro-inflammatory cytokine IL-1 as it functions as an IL-1 receptor competitor (119). It is produced by CD163+ wound healing M2 macrophages (120). In IBD, polymorphism in the IL-1RA gene have been demonstrated and an imbalance of IL-1 and IL1RA has been suggested to induce mucosal inflammation associated with IBD (121, 122).
More recently, IL-4 has been reported to induce macrophage proliferation and activation with reduced pulmonary injury after infection with a lung-migrating helminth (123).
MicroRNAs
MicroRNAs (MiRs) are small non-coding RNA molecules that can bind to complementary sequences of mRNA molecules thereby regulating/inhibiting post-translational gene expression. MiRs are contributors to the resolution of inflammation by targeting pro-inflammatory genes (124). MiRs 21, 146b, 208a, and 219 are increased during the resolution phase of acute resolving peritonitis in mice (125) and RvD1 can regulate expression of these proresolving MiRs (126). MiR-146b down-regulates NF-κB signaling (127), and MiR-219 targets 5-lipoxygenase, with a decreased formation of leukotrienes (19). These results indicate that MiRs actively contribute to resolution of inflammation.
Extracellular Vesicles
The paracrine manner of the cellular communication in resolution may be achieved not only by secretion of immune mediators but also through extracellular vesicles. Extracellular vesicles are small membrane vesicles (exosomes, microvesicles, and apoptotic bodies) secreted by all cell types including immune cells in a controlled manner. Extracellular vesicles have recently been reported both as immune activators and immune suppressors as they contain for example MHC class I and II and T cell co-stimulatory molecules (128, 129). However, the most described function of extracellular vesicles is triggering of the immune system, and very recently involvement of extracellular vesicles in inflammation resolution, tissue repair and regeneration was reported (130, 131).
Impaired Resolution of Chronic Inflammation in the Intestine and the Lung
The pathways involved in the initiation of IBD or asthma differ from each other with respect to cytokine involvement and composition of the resident tissue. IBD is associated with a Th1/Th17 T cell-mediated response induced by interleukin-12 (IL-12) and IL-23, with concomitant increased production of IL-2, IL-17, IL-18, and IFN-γ (132, 133), whereas asthma and allergic diseases are associated with a typical T helper type 2 (Th2)-mediated response characterized by the production of interleukin-4 (IL-4), IL-5, and IL-13 (134). Therefore, specific tissue resolution processes exist, guided by the local microenvironment that are impaired in disease [reviewed recently in (135)]. There is increasing evidence that poor and/or inappropriate resolution of inflammation participates in the pathogenesis of IBD or asthma, being responsible in the long run for excessive tissue damage and pathology. In this chapter, we give some insights into resolution deficiencies in IBD and chronic asthma [for a recent and full review see (6, 136)].
Inflammatory Bowel Diseases
IBD afflicts around 0.5% of the population in westernized countries (137). It is a chronic relapsing disease that includes Crohn's disease, a chronic trans-mural inflammatory disease of the gastrointestinal tract, mainly affecting the ileum and colon, characterized by leukocyte infiltration, granuloma, scarring, and fistulae and ulcerative colitis, a more superficial neutrophilic inflammatory lesion of the colon that progresses proximally. The inflammation partially, but never completely, resolves leading to tissue remodeling and disruption of the normal epithelial architecture that fails to fully regenerate, resulting in persistent increased epithelial permeability and inflammation.
In IBD multiple factors are identified that contribute to disease pathogenesis with a focus on host susceptibility genetic factors in combination with a qualitatively and quantitatively abnormal gut microbiota and an excessive immune response (138–142). The pro-inflammatory response is extensively studied, and the suppression of this phase is the main therapeutic strategy in Crohn's disease, and is still the central research focus, whereas much less is known about the resolution phase. Standard Crohn's disease therapy involves corticosteroids and immunosuppressants like azathioprine but these therapies are palliative and do not alter the natural history of IBD (143). In the last 20 years biological therapies (antibodies directed against cytokines, like anti-TNFα antibodies) have changed the treatment of more severe IBD. However, only ~50% of Crohn's Disease patients achieve clinical remission with the anti-TNFα Humira® or Remicade®. Indeed, the treatment results in a waning of the responsiveness to anti-TNF-α with time and only a minority of patients achieve mucosal healing (143, 144). Alternative therapies such as blocking the migration of effector T cells into the inflamed gut by targeting the α4β7 integrin, and recently also the blockade of IL-23 are showing additional success (145, 146) although they are effective only in a minority of patients. These treatments specifically blocking pro-inflammatory mediators cause immuno-suppression, and thereby induce an increased risk of infection. This exemplifies why new approaches and new therapies are needed to tackle the problems of chronic intestinal inflammation. Therefore, a better understanding of IBD pathophysiology is needed with a focus on the disturbed resolution of inflammation. In the line of this, the results of a recent meta-analysis focusing on mucosal healing in IBD as reported from endoscopic studies show that both partial, and full mucosal healing—thus a proper resolution of inflammation—predict favorable clinical outcome (147).
There is more and more evidence that persistent inflammation in IBD can occur as a result of inadequate engagement of a series of pro-resolving pathways and many studies have shown that pro-resolving mediators are able to prevent experimental colitis in different murine models [reviewed recently in (136, 148)].
MaR1 improves established chronic colitis induced by multiple dextran sulfate sodium (DSS) administrations (149). Systemic treatment of mice with PD1 or RvD5 protects against colitis and intestinal ischemia/reperfusion-induced inflammation (150). Other studies report protective roles for SPMs in experimental colitis [summarized in (151)]. Indications that SPM biosynthesis might be dysregulated in patients with IBD come from a study in which RvD5 and PD1 were upregulated in human IBD colon biopsies (150). Interestingly, mucosal expression of LXA4 is elevated exclusively in biopsies from individuals in remission from ulcerative colitis (152). Evidence, whether a defect in SPM signaling exists in IBD remains to be explored.
AnxA1 stimulates intestinal mucosal wound repair in a murine model of colitis (153) and AnxA1-containing exosomes and microparticles have been shown to accelerate the process of mucosal healing in vivo DSS models of colitis (154). In humans, AnxA1 is released by inflamed colonic biopsies from patients having ulcerative colitis (UC) and depends on the severity of inflammation (152, 155). In Crohn's disease, AnxA1 biosynthesis is dysregulated and higher levels correlate with successful intervention with biologicals against TNF-α (156). In another study in Crohn's Disease, AnxA1 is involved in intestinal homeostasis after anti-TNF-α treatment and suggested as a potential biomarker of therapeutic efficacy of anti-TNF-α treatment (157).
The production of IL-10 by Tregs is of particular interest in IBD. IL-10 deficiency in mice can lead to the development of spontaneous inflammatory bowel disease (158) and IL-10 receptor mutations found in patients result in an early-onset enterocolitis (159, 160). Furthermore, a IBD-like colitis can occur in response to recent immune checkpoint inhibitor treatments used in antitumor therapy aiming at blocking Treg cells (161). Tregs accumulate and IL-10 is upregulated in the gut during active IBD (162–166) but a clear demonstration that this pro-resolving mechanism operates in the gut mucosa in IBD is still missing.
Several authors report conflicting data whether or not it might be possible to use Galectin family member levels as markers for disease activity (167–171).
There is also evidence that α-MSH has potent anti-inflammatory activity in experimentally induced colitis (172, 173). Oral delivery via Bifidobacterium expressing α-melanocyte-stimulating hormone can prevent colitis in an experimental murine model (174).
H2S is able to improve the colonic barrier integrity in a murine model of experimental colitis (175). Administration of inhibitors of H2S synthesis in models of colitis result in an increase in severity of disease (176). In patients with active ulcerative colitis, alterations in the expression of genes involved in the purine metabolic pathway have been demonstrated (177). Like H2S, CO has been shown to exert potent protective effects in the gastro-intestinal tract (178).
Several gastro-intestinal neuroendocrine peptides and amines with pro-resolving properties, as members of the chromogranin/secretogranin family, VIP, somatostatin, and ghrelin are affected in experimental colitis and changes of these mediators occur during active IBD in patients [recently reviewed in (179)]. The exact role of neuroendocrine peptides/amines with pro-resolving properties in IBD has to be further elucidated.
Asthma and Allergic Diseases
In the industrialized world, millions of individuals suffer from inappropriate activation and dysregulation of Th2 cell immune responses responsible for allergic asthma and rhinitis, food allergies and atopic dermatitis (also known as eczema), being part of a process called the atopic march. These disorders are increasingly prevalent and are a major public health problem (180). Th2 cell mediated immune responses are characterized by the release of type 2 signature cytokines (i.e., IL-4, IL-5, IL-9, and IL-13) from cells of both the innate and adaptive immune systems (134, 181).
Current therapeutic strategies for chronic Th2 immune disorders are mainly anti-inflammatory, and aim at controlling symptoms. In chronic persistent asthma, inhaled corticosteroids are the main anti-inflammatory treatment effective in most patients, causing relatively minor adverse effects (182). A subset of asthma patients (~10%) experience persistent symptoms and/or frequent exacerbations despite high doses of inhaled corticosteroids and are often treated with prolonged systemic corticotherapy having many potential side-effects (183). Monoclonal antibodies targeting inflammatory pathways that activate immune responses leading to airway inflammation have been developed to help broaden the current arsenal of asthma treatment options (184). The first anti-body based biological therapy approved for treatment of asthma was omalizumab, targeting IgE, a component of the allergic cascade (185). More recently, monoclonal antibodies have been approved, targeting IL-5 or its receptor (mepolizumab, reslizumab, benralizumab), a key cytokine promoting eosinophil inflammation (186). Other monoclonal antibodies targeting a wide variety of intermediaries in the pro-inflammatory cascade are currently being tested for their effectiveness in the treatment of asthma (184). These biological therapies can reduce exacerbations and have glucocorticoid-sparing effects, but the clinical responses to these antibody-therapies are variable, with at least 30% of severe asthmatic patients being non-responders (187).
These therapeutic strategies can be combined with allergen-specific immunotherapies in chronic allergic diseases that are able to improve symptoms but they do not cure allergic disorders (188, 189).
As for IBD, there is increasing evidence that chronic and uncontrolled inflammation in Th2 immune disorders, might result not just from an excessive uncontrolled pro-inflammatory response but also from uncontrolled and insufficient engagement of pro-resolving pathways and thus impaired resolution of exacerbations (5, 33, 190).
First, pro-resolving mediators have proved efficient at improving disease and inflammatory outcomes in a variety of asthma models. Treatment with SPMs decreases key features of asthma pathobiology, including airway hyperresponsiveness, mucus metaplasia, and Th2 cell bronchial inflammation (53, 191–193). AnxA1 deficient mice exhibit spontaneous airway hyperresponsiveness and exacerbated allergen responses (194) and AnxA1 mimetics inhibit eosinophil recruitment (195). Ablation of IL-10 signaling in Th2 cells leads to exacerbated pulmonary inflammation (196). In murine models, IL10 knock-out mice develop enhanced allergic airway responses (197, 198) [(199) #1421]. IL-10 also inhibits pro-inflammatory cytokine production by Th2 cells and down-regulates mast cell and eosinophil function (200). Administration of recombinant Gal-9 or α-MSH diminish allergic airway inflammation [(201) #1453; (202)]. Low H2S production in ovalbumin sensitized and challenged mice results in aggravated AHR and increased airway inflammation (203). In a rat model of asthma, exogenous administration of H2S reduces airway inflammation and airway remodeling (204). VIP can inhibit eosinophil migration (205) and airway remodeling in asthmatic mice (206). Intestinal epithelial cell-derived Gal-9 is involved in the resolution of allergic responses through the induction of tolerogenic DCs and associated Treg cell response (207, 208). In addition, some of these galectins can block IgE binding on mast cells and as such, inhibit allergic inflammation (209, 210). The role of adenosine in the resolution of inflammation of chronic asthma is not yet elucidated.
Based on the evidence for the functional importance of pro-resolving mediators in allergic asthma mouse models, defects in the production or the activity of pro-resolving mediators might therefore participate in the chronicity and severity of human asthma. Several studies in distinct populations have reported that SPMs are underproduced in more severe asthma together with a defect in the expression of their related receptors [for a recent review see (6)]. Annexin A1 (AnxA1) levels are also decreased in patients with asthma (211), and in wheezy infants (212). Moreover, plasma and bronchoalveolar AnxA1 levels are correlated with lung function (FEV1 %) (213, 214). Compared with non-asthmatics, asthmatic individuals have reduced levels of IL- 10 in bronchoalveolar lavage fluid (215) and a decreased secretion of IL-10 from alveolar macrophages (216).
Furthermore, polymorphisms in the IL10 gene resulting in low IL-10 production have been associated with severe asthma (217). T cells from allergic asthmatic patients are partially resistant to IL-10 mediated suppression [(218) #1396]. In humans, galectin-3 production has been reported to be lower in asthma, particularly in neutrophilic asthma (76, 219). Macrophages from sputum samples of asthma patients express reduced levels of Gal-1 and Gal-9 (82). Several human studies have shown a decrease in serum or exhaled-breath H2S levels in both adult and infants (220–222). Moreover, lower H2S levels are correlated with abnormal pulmonary lung function tests and severity of asthma (220, 221). There is relatively limited information available regarding the role of neuropeptides in the resolution of inflammation in asthma but Tomaki et al. found that SubP levels in sputum correlate with airway obstruction in asthma (223).
How to Improve Resolution in Chronic Inflammatory Diseases
Targeting the inflammation phase has been the main focus in medical research for the past decades, resulting in treatment options for immune-mediated diseases that dampen inflammation and display immunosuppressive actions (see Figure 1). This comes with a burden for the body, since anti-inflammatory immune suppressive therapies, for example corticosteroids or anti-TNF inhibitors, may have increased risks of infection. In addition, the development of expensive targeted anti-inflammatory biologicals creates an economic burden on society.
Immune responses are very complex and only recently the first initiative to define the naturally occurring variation and the boundaries of a healthy immune response to complex stimuli was published (224). Interestingly, there is considerable variation in the ability of tissue inflammation to resolve within a healthy population (225).
Different endogenous factors such as age, genetics, sex, ethnicity, might influence the nature and extent of the acute inflammatory response including the resolution process (226). Studies have highlighted how epigenetic reprogramming can lead to chronic inflammation and impede resolution resulting in inflammatory diseases (227, 228). Gut microflora plays a critical role in the stimulation and maturation of a balanced immune system (229). There is also evidence that life style factors, physical and psychological conditions can impact on the magnitude of the inflammatory response (230).
Understanding the mechanism required for adequate resolution of inflammation may support the development of new resolution-based strategies able to direct the inflammatory processes in a controlled way. Different approaches can be considered (Figure 5).
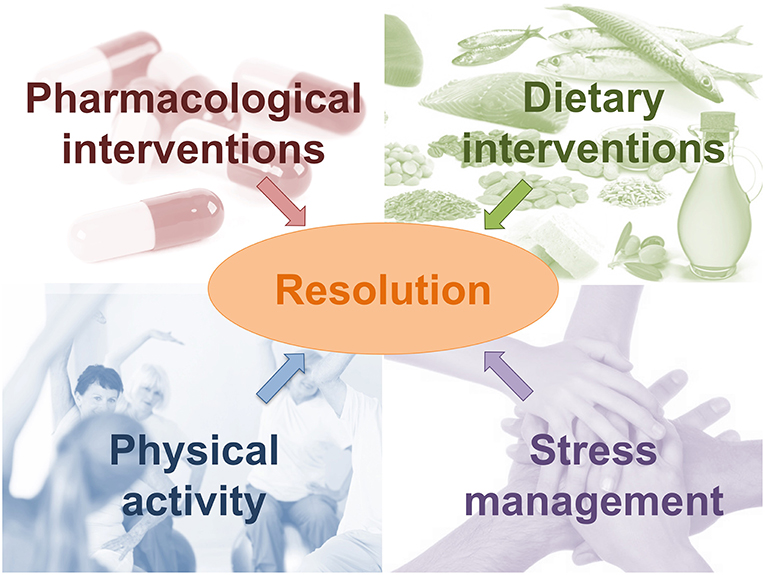
Figure 5. New resolution-based strategies able to direct the inflammatory processes in a controlled way.
Pharmacological Interventions
Many current therapeutic approaches to manage chronic inflammation aim at repressing overactive pro-inflammatory responses by reducing pro-inflammatory mediator activity (i.e., corticosteroids or biologics). In addition to their potent anti-inflammatory properties, steroids can display several pro-resolving properties (7, 65, 231, 232), however, this comes with many potential side-effects, such as osteoporosis, diabetes, systemic hypertension, and impaired immune function.
Several synthetic pharmaceutical analogs with pro-resolving properties have been proven to be active in animal models [reviewed in (233, 234)]. Toward this end, stable synthetic mimetics to endogenous SPMs are under development, and in matching studies, these mimetics display similar biological actions to the parent mediators in animal models of diverse inflammatory disorders with an advantage of resisting local inactivation (including mimetics encapsulated in vesicles) (235–239). Several of these mimetics are in pre-clinical development programs for different chronic inflammatory conditions [for reviews see (3, 240)]. In a double-blind placebo-controlled clinical trial, a topical 15-R/S-methyl-LXA4 preparation was tested for the treatment of infantile atopic eczema (241). In this study, the efficacy of the lipoxin mimetic was at least equivalent to gold standard topical steroid therapy for the reduction of eczema severity by quantitative and qualitative measures.
Dietary Interventions
Omega-3 PUFAs
The main bioactive omega−3 PUFAs, eicosapentaenoic acid (EPA) and docosahexaenoic acid (DHA), are poorly synthesized in humans. They are components of seafood, especially oily fish, of fish oil, liver oil, krill oil and algal oil supplements, and of a small number of highly concentrated pseudo-pharmaceutical products.
EPA and DHA have long been known to have beneficial health effects including anti-inflammatory, anti-thrombotic, and immuno-regulatory properties (242–244). These n−3 LCPUFAs are substrates for biosynthesis of potent SPMs such as resolvins, protectins, and maresins (Figure 3). DHA is concentrated in neural tissues including brain and retina and in sperm; EPA and DHA are found in membranes of all other cells and tissues and in human milk (245–247). Increased dietary intake of EPA and DHA results in their enrichment in blood and in many cells and tissues. Omega-3 PUFAs can exert significant effects on the intestinal environment and modulate the gut microbiota composition (248).
The airway mucosa is also enriched with DHA in healthy individuals (249). Interestingly, airway mucosal levels of n−3 PUFAs are lower in patients with asthma than in people without asthma (249). Population surveys report that diets rich in n-3 fatty acids are associated with lower asthma prevalence (250).
It is noteworthy that SPMs are present at significant levels in placenta and human milk (251–253), which suggests an important role for SPMs in health maintenance during a particularly vulnerable period of infant development. In a recent randomized placebo-controlled study from a Danish birth cohort, supplementation with a high dose of n-3-LCPUFAs (a dose corresponding to a 10–20-times increase of the normal intake) during the third trimester of pregnancy was associated with a significantly lower risk of asthma symptoms and fewer respiratory infections in children at 3 years (254). This effect was most prominent among children of women who had low pre-intervention EPA and DHA blood levels (255).
Recent human studies have shown that increased intake of EPA and DHA results in higher concentrations of selected SPMs in the bloodstream (256–258). High doses of n-3 PUFAs reduce pain and other symptoms in patients with rheumatoid arthritis (259, 260). Many of the mechanisms of action of EPA and DHA suggest that they reduce the pro-inflammatory response (242, 243). However, the discovery of SPMs derived from EPA and DHA and the potency of those SPMs in animal models (see earlier) hints that their main action might be promotion of resolution.
Pre- and Probiotics
The prebiotic galacto- and fructo-oligosaccharides, so-called non-digestible oligosaccharides, in combination with probiotic bacteria induced the resolution-inducing lectin galectin 9 in mouse models for food allergy and in infants suffering from cow's milk allergy (209). In vitro studies showed that the epithelial release of gal 9 by this specific combination of pre- and probiotic induces tolerogenic DCs that in turn upregulated Treg cells (209, 261). In addition, the combination of Bifidobacterium longum with inulin-oligofructose resulted in resolution of inflammation in patients suffering from active colitis (262, 263). Several preclinical studies have demonstrated that treatment with specific bacterial strains induces an IL-10 response associated with a faster resolution of inflammation in allergy and Crohn's Disease models (264–266). Overall, there are some indications that dietary intervention with pre- and probiotics promotes the induction of resolution of inflammation. However, the exact mechanisms of resolution induced by pre- and probiotics remains to be examined.
Exercise/Physical Activity
Exercise enhances functional capacity, through increased aerobic capacity and muscle strength, improves quality of life and has the potential to protect from cardiovascular disease, type 2 diabetes mellitus, and certain types of cancer [reviewed in (267)]. The potential mechanisms underlying exercise-mediated protection toward these disorders, include changes in body composition, neuro-hormonal status, as well as effects on resolution pathways.
Indeed, acute increases in intramuscular IL-6 following exercise promote resolution processes by increasing the synthesis of anti-inflammatory cytokines such as IL-1RA and IL-10, and inhibit pro-inflammatory cytokines such as TNF-α (268). Exercise also modulates the production of the PUFA derived SPMs described earlier. Indeed, maximal physical exertion was found to result in a rapid post-exercise increase in the urinary excretion of arachidonic acid (AA) derived lipoxin A4 (LXA4) in healthy subjects (269). Similarly, EPA derived resolvin E1 (RvE1) transiently increases early in human serum following exercise and DHA derived resolvin D1 (RvD1) and protectins increased later during recovery (270).
Interestingly, levels of LXA4 are found to increase immediately after exercise in exhaled air condensate of asthmatic children with exercise induced bronchoconstriction (EIB) (271). The authors hypothesized that airway LXA4 increases to compensate bronchoconstriction and to suppress acute inflammation, and that spontaneous bronchodilation after EIB may be due to LXA4. In murine studies in relation to asthma, physical exercise reduced asthma associated bronchial inflammation (IL-4, IL-5 expression and eosinophil infiltrate) which was associated with an increase of IL-10 (272, 273). Exercise in animal models of colitis also reduced levels of TNF-α, and decreased markers of oxidative stress and histological damage to the colon in parallel to increased levels of the resolution-promoting and anti-inflammatory cytokine IL-10 (274).
Stress Management
Emotional, cognitive, and psychosocial factors are now widely recognized as significant determinants of health outcomes including impacts on the immune system (275). In this sense, a broad variety of mind-body therapies that are able to decrease stress, including meditation-based stress reduction programs (MBSR) and yoga have been increasingly proposed over the past years, as substantial adjuncts to conventional medical treatment in chronic inflammatory diseases and cancer patients (275). Convergent evidence suggests that these mind-body therapies may have effects on immune functions including effects on the hypothalamic-pituitary-adrenocortical (HPA) axis function (276) and on NK cell functions and IL-10 levels within patients suffering from chronic inflammatory disorders (277–279). More precise impacts of the mental state on resolution parameters remain to be examined.
Conclusion
There are a number of immune-mediated chronic diseases that might, at least in part, be controlled or prevented in an immune fit person, including IBD, allergy, and asthma developed in this review, as well as rheumatoid arthritis, chronic obstructive pulmonary disease (COPD), Parkinson's disease, Alzheimer's disease, multiple sclerosis, diabetes or myalgic encephalomyelitis. The focus of research on resolution of the immune response as a possible therapeutic approach has only been apparent in the twenty-first century and there is now increasing evidence that poor and/or inappropriate resolution of inflammation participates in the pathogenesis of IBD or asthma, being responsible in the long run for excessive tissue damage and pathology. This might now open a whole area in the development of personalized therapeutic options for chronic immune diseases driven in part by maladaptive, non-resolving inflammation.
Moreover, since there is a strong link between a compromised immune system and the brain, individuals can experience a reduced quality of life and lack of well-being (280, 281). Many chronic inflammatory diseases are associated with depression, anxiety, and reduced cognitive function (282) and it is becoming apparent that many brain diseases (psychiatric and neurological) are associated with activation of the immune system (281, 283). Therefore, deviant immune fitness because of overreaction and poor or defective resolution of the immune system has an enormous impact and is a central issue in these chronic immune diseases.
Author Contributions
All authors listed have made a substantial, direct and intellectual contribution to the work, and approved it for publication.
Funding
FL-S received funding from Aimwell Charitable Trust (UK), Israel Science Foundation 472/15, Rosetrees Trust (UK), and Emalie Gutterman Memorial Endowed Fund for COPD related research (USA) and is affiliated with the Adolph and Klara Brettler Center at the Hebrew University.
Conflict of Interest Statement
The authors declare that the research was conducted in the absence of any commercial or financial relationships that could be construed as a potential conflict of interest.
References
1. Nathan C, Ding A. Nonresolving inflammation. Cell. (2010) 140:871–82. doi: 10.1016/j.cell.2010.02.029
2. Larsen GL, Henson PM. Mediators of inflammation. Annu Rev Immunol. (1983) 1:335–59. doi: 10.1146/annurev.iy.01.040183.002003
3. Serhan CN. Treating inflammation and infection in the 21st century: new hints from decoding resolution mediators and mechanisms. FASEB J. (2017) 31:1273–88. doi: 10.1096/fj.201601222R
4. Ungaro F, Rubbino F, Danese S, D'Alessio S. Actors and factors in the resolution of intestinal inflammation: lipid mediators as a new approach to therapy in inflammatory bowel diseases. Front Immunol. (2017) 8:1331. doi: 10.3389/fimmu.2017.01331
5. Levy BD, Bonnans C, Silverman ES, Palmer LJ, Marigowda G, Israel E, et al. Diminished lipoxin biosynthesis in severe asthma. Am J Respir Crit Care Med. (2005) 172:824–30. doi: 10.1164/rccm.200410-1413OC
6. Barnig C, Frossard N, Levy BD. Towards targeting resolution pathways of airway inflammation in asthma. Pharmacol Ther. (2018) 186:98–113. doi: 10.1016/j.pharmthera.2018.01.004
7. Gilroy DW, Lawrence T, Perretti M, Rossi AG. Inflammatory resolution: new opportunities for drug discovery. Nat Rev Drug Discov. (2004) 3:401–16. doi: 10.1038/nrd1383
8. Bosma-den Boer MM, van Wetten ML, Pruimboom L. Chronic inflammatory diseases are stimulated by current lifestyle: how diet, stress levels and medication prevent our body from recovering. Nutr Metab. (2012) 9:32. doi: 10.1186/1743-7075-9-32
9. Newson J, Stables M, Karra E, Arce-Vargas F, Quezada S, Motwani M, et al. Resolution of acute inflammation bridges the gap between innate and adaptive immunity. Blood. (2014) 124:1748–64. doi: 10.1182/blood-2014-03-562710
10. Newson J, Motwani MP, Kendall AC, Nicolaou A, Muccioli GG, Alhouayek M, et al. Inflammatory resolution triggers a prolonged phase of immune suppression through COX-1/mPGES-1-derived prostaglandin E2. Cell Rep. (2017) 20:3162–75. doi: 10.1016/j.celrep.2017.08.098
11. Dalli J, Serhan CN. Pro-resolving mediators in regulating and conferring macrophage function. Front Immunol. (2017) 8:1400. doi: 10.3389/fimmu.2017.01400
12. Wynn TA, Chawla A, Pollard JW. Macrophage biology in development, homeostasis and disease. Nature. (2013) 496:445–55. doi: 10.1038/nature12034
13. Smith TD, Nagalla RR, Chen EY, Liu WF. Harnessing macrophage plasticity for tissue regeneration. Adv Drug Deliv Rev. (2017) 114:193–205. doi: 10.1016/j.addr.2017.04.012
14. Li J, Tan J, Martino MM, Lui KO. Regulatory T-cells: potential regulator of tissue repair and regeneration. Front Immunol. (2018) 9:585. doi: 10.3389/fimmu.2018.00585
15. van Herk EH, Te Velde AA. Treg subsets in inflammatory bowel disease and colorectal carcinoma: characteristics, role, and therapeutic targets. J Gastroenterol Hepatol. (2016) 31:1393–404. doi: 10.1111/jgh.13342
16. Lu L, Barbi J, Pan F. The regulation of immune tolerance by FOXP3. Nat Rev Immunol. (2017) 17:703–17. doi: 10.1038/nri.2017.75
17. Bollrath J, Powrie FM. Controlling the frontier: regulatory T-cells and intestinal homeostasis. Semin Immunol. (2013) 25:352–7. doi: 10.1016/j.smim.2013.09.002
18. Schiering C, Krausgruber T, Chomka A, Frohlich A, Adelmann K, Wohlfert EA, et al. The alarmin IL-33 promotes regulatory T-cell function in the intestine. Nature. (2014) 513:564–8. doi: 10.1038/nature13577
19. Leech MD, Benson RA, De Vries A, Fitch PM, Howie SE. Resolution of Der p1-induced allergic airway inflammation is dependent on CD4+CD25+Foxp3+ regulatory cells. J Immunol. (2007) 179:7050–8. doi: 10.4049/jimmunol.179.10.7050
20. Carson WFT, Guernsey LA, Singh A, Vella AT, Schramm CM, Thrall RS. Accumulation of regulatory T cells in local draining lymph nodes of the lung correlates with spontaneous resolution of chronic asthma in a murine model. Int Arch Allergy Immunol. (2008) 145:231–43. doi: 10.1159/000109292
21. Arpaia N, Green JA, Moltedo B, Arvey A, Hemmers S, Yuan S, et al. A distinct function of regulatory T cells in tissue protection. Cell. (2015) 162:1078–89. doi: 10.1016/j.cell.2015.08.021
22. Mjosberg J, Spits H. Human innate lymphoid cells. J Allergy Clin Immunol. (2016) 138:1265–76. doi: 10.1016/j.jaci.2016.09.009
23. Ebbo M, Crinier A, Vely F, Vivier E. Innate lymphoid cells: major players in inflammatory diseases. Nat Rev Immunol. (2017) 17:665–78. doi: 10.1038/nri.2017.86
24. Geremia A, Arancibia-Carcamo CV. Innate lymphoid cells in intestinal inflammation. Front Immunol. (2017) 8:1296. doi: 10.3389/fimmu.2017.01296
25. Ealey KN, Moro K, Koyasu S. Are ILC2s Jekyll and Hyde in airway inflammation? Immunol Rev. (2017) 278:207–18. doi: 10.1111/imr.12547
26. Wallrapp A, Riesenfeld SJ, Burkett PR, Kuchroo VK. Type 2 innate lymphoid cells in the induction and resolution of tissue inflammation. Immunol Rev. (2018) 286:53–73. doi: 10.1111/imr.12702
27. Cosmi L, Liotta F, Maggi L, Annunziato F. Role of type 2 innate lymphoid cells in allergic diseases. Curr Allergy Asthma Rep. (2017) 17:66. doi: 10.1007/s11882-017-0735-9
28. Rauber S, Luber M, Weber S, Maul L, Soare A, Wohlfahrt T, et al. Resolution of inflammation by interleukin-9-producing type 2 innate lymphoid cells. Nat Med. (2017) 23:938–44. doi: 10.1038/nm.4373
29. Monticelli LA, Sonnenberg GF, Abt MC, Alenghat T, Ziegler CG, Doering TA, et al. Innate lymphoid cells promote lung-tissue homeostasis after infection with influenza virus. Nat Immunol. (2011) 12:1045–54. doi: 10.1038/ni.2131
30. Forkel M, Mjosberg J. Dysregulation of group 3 innate lymphoid cells in the pathogenesis of inflammatory bowel disease. Curr Allergy Asthma Rep. (2016) 16:73. doi: 10.1007/s11882-016-0652-3
31. Rankin LC, Girard-Madoux MJ, Seillet C, Mielke LA, Kerdiles Y, Fenis A, et al. Complementarity and redundancy of IL-22-producing innate lymphoid cells. Nat Immunol. (2016) 17:179–86. doi: 10.1038/ni.3332
32. Haworth O, Cernadas M, Levy BD. NK cells are effectors for resolvin E1 in the timely resolution of allergic airway inflammation. J Immunol. (2011) 186:6129–35. doi: 10.4049/jimmunol.1004007
33. Barnig C, Cernadas M, Dutile S, Liu X, Perrella MA, Kazani S, et al. Lipoxin A4 regulates natural killer cell and type 2 innate lymphoid cell activation in asthma. Sci Transl Med. (2013) 5:174ra126. doi: 10.1126/scitranslmed.3004812
34. Gabrilovich DI, Nagaraj S. Myeloid-derived suppressor cells as regulators of the immune system. Nat Rev Immunol. (2009) 9:162–74. doi: 10.1038/nri2506
35. Ray A, Chakraborty K, Ray P. Immunosuppressive MDSCs induced by TLR signaling during infection and role in resolution of inflammation. Front Cell Infect Microbiol. (2013) 3:52. doi: 10.3389/fcimb.2013.00052
36. Ben-Meir K, Twaik N, Baniyash M. Plasticity and biological diversity of myeloid derived suppressor cells. Curr Opin Immunol. (2018) 51:154–61. doi: 10.1016/j.coi.2018.03.015
37. Bronte V, Brandau S, Chen SH, Colombo MP, Frey AB, Greten TF, et al. Recommendations for myeloid-derived suppressor cell nomenclature and characterization standards. Nat Commun. (2016) 7:12150. doi: 10.1038/ncomms12150
38. Rodriguez PC, Ochoa AC, Al-Khami AA. Arginine metabolism in myeloid cells shapes innate and adaptive immunity. Front Immunol. (2017) 8:93. doi: 10.3389/fimmu.2017.00093
39. Rodriguez PC, Ochoa AC. Arginine regulation by myeloid derived suppressor cells and tolerance in cancer: mechanisms and therapeutic perspectives. Immunol Rev. (2008) 222:180–91. doi: 10.1111/j.1600-065X.2008.00608.x
40. Arocena AR, Onofrio LI, Pellegrini AV, Carrera Silva AE, Paroli A, Cano RC, et al. Myeloid-derived suppressor cells are key players in the resolution of inflammation during a model of acute infection. Eur J Immunol. (2014) 44:184–94. doi: 10.1002/eji.201343606
41. Li H, Han Y, Guo Q, Zhang M, Cao X. Cancer-expanded myeloid-derived suppressor cells induce anergy of NK cells through membrane-bound TGF-beta 1. J Immunol. (2009) 182:240–9. doi: 10.4049/jimmunol.182.1.240
42. Poe SL, Arora M, Oriss TB, Yarlagadda M, Isse K, Khare A, et al. STAT1-regulated lung MDSC-like cells produce IL-10 and efferocytose apoptotic neutrophils with relevance in resolution of bacterial pneumonia. Mucosal Immunol. (2013) 6:189–99. doi: 10.1038/mi.2012.62
43. Sugimoto MA, Vago JP, Perretti M, Teixeira MM. Mediators of the resolution of the inflammatory response. Trends Immunol. (2019) 40:212–27. doi: 10.1016/j.it.2019.01.007
44. Serhan CN. Discovery of specialized pro-resolving mediators marks the dawn of resolution physiology and pharmacology. Mol Aspects Med. (2017) 58:1–11. doi: 10.1016/j.mam.2017.03.001
45. Serhan CN, Chiang N, Van Dyke TE. Resolving inflammation: dual anti-inflammatory and pro-resolution lipid mediators. Nat Rev Immunol. (2008) 8:349–61. doi: 10.1038/nri2294
46. Chiurchiu V, Leuti A, Maccarrone M. Bioactive lipids and chronic inflammation: managing the fire within. Front Immunol. (2018) 9:38. doi: 10.3389/fimmu.2018.00038
47. Weylandt KH. Docosapentaenoic acid derived metabolites and mediators - the new world of lipid mediator medicine in a nutshell. Eur J Pharmacol. (2016) 785:108–15. doi: 10.1016/j.ejphar.2015.11.002
48. Rossaint J, Margraf A, Zarbock A. Role of platelets in leukocyte recruitment and resolution of inflammation. Front Immunol. (2018) 9:2712. doi: 10.3389/fimmu.2018.02712
49. Chiang N, Serhan CN. Structural elucidation and physiologic functions of specialized pro-resolving mediators and their receptors. Mol Aspects Med. (2017) 58:114–29. doi: 10.1016/j.mam.2017.03.005
50. Perretti M, Chiang N, La M, Fierro IM, Marullo S, Getting SJ, et al. Endogenous lipid- and peptide-derived anti-inflammatory pathways generated with glucocorticoid and aspirin treatment activate the lipoxin A4 receptor. Nat Med. (2002) 8:1296–302. doi: 10.1038/nm786
51. Bonnans C, Fukunaga K, Levy MA, Levy BD. Lipoxin A(4) regulates bronchial epithelial cell responses to acid injury. Am J Pathol. (2006) 168:1064–72. doi: 10.2353/ajpath.2006.051056
52. Chiang N, Serhan CN, Dahlen SE, Drazen JM, Hay DW, Rovati GE, et al. The lipoxin receptor ALX: potent ligand-specific and stereoselective actions in vivo. Pharmacol Rev. (2006) 58:463–87. doi: 10.1124/pr.58.3.4
53. Levy BD, De Sanctis GT, Devchand PR, Kim E, Ackerman K, Schmidt BA, et al. Multi-pronged inhibition of airway hyper-responsiveness and inflammation by lipoxin A(4). Nat Med. (2002) 8:1018–23. doi: 10.1038/nm748
54. Herova M, Schmid M, Gemperle C, Hersberger M. ChemR23, the receptor for chemerin and resolvin E1, is expressed and functional on M1 but not on M2 macrophages. J Immunol. (2015) 194:2330–7. doi: 10.4049/jimmunol.1402166
55. Schwab JM, Chiang N, Arita M, Serhan CN. Resolvin E1 and protectin D1 activate inflammation-resolution programmes. Nature. (2007) 447:869–74. doi: 10.1038/nature05877
56. Ohira T, Arita M, Omori K, Recchiuti A, Van Dyke TE, Serhan CN. Resolvin E1 receptor activation signals phosphorylation and phagocytosis. J Biol Chem. (2010) 285:3451–61. doi: 10.1074/jbc.M109.044131
57. Chiang N, Fredman G, Backhed F, Oh SF, Vickery T, Schmidt BA, et al. Infection regulates pro-resolving mediators that lower antibiotic requirements. Nature. (2012) 484:524–8. doi: 10.1038/nature11042
58. Serhan CN, Dalli J, Karamnov S, Choi A, Park CK, Xu ZZ, et al. Macrophage proresolving mediator maresin 1 stimulates tissue regeneration and controls pain. FASEB J. (2012) 26:1755–65. doi: 10.1096/fj.11-201442
59. Dalli J, Winkler JW, Colas RA, Arnardottir H, Cheng CY, Chiang N, et al. Resolvin D3 and aspirin-triggered resolvin D3 are potent immunoresolvents. Chem Biol. (2013) 20:188–201. doi: 10.1016/j.chembiol.2012.11.010
60. Chiang N, Dalli J, Colas RA, Serhan CN. Identification of resolvin D2 receptor mediating resolution of infections and organ protection. J Exp Med. (2015) 212:1203–17. doi: 10.1084/jem.20150225
61. Winkler JW, Orr SK, Dalli J, Cheng CY, Sanger JM, Chiang N, et al. Resolvin D4 stereoassignment and its novel actions in host protection and bacterial clearance. Sci Rep. (2016) 6:18972. doi: 10.1038/srep18972
62. Krishnamoorthy N, Burkett PR, Dalli J, Abdulnour RE, Colas R, Ramon S, et al. Cutting edge: maresin-1 engages regulatory T cells to limit type 2 innate lymphoid cell activation and promote resolution of lung inflammation. J Immunol. (2015) 194:863–7. doi: 10.4049/jimmunol.1402534
63. Chiurchiu V, Leuti A, Dalli J, Jacobsson A, Battistini L, Maccarrone M, et al. Proresolving lipid mediators resolvin D1, resolvin D2, and maresin 1 are critical in modulating T cell responses. Sci Transl Med. (2016) 8:353ra111. doi: 10.1126/scitranslmed.aaf7483
64. Basil MC, Levy BD. Specialized pro-resolving mediators: endogenous regulators of infection and inflammation. Nat Rev Immunol. (2016) 16:51–67. doi: 10.1038/nri.2015.4
65. Perretti M, D'Acquisto F. Annexin A1 and glucocorticoids as effectors of the resolution of inflammation. Nat Rev Immunol. (2009) 9:62–70. doi: 10.1038/nri2470
66. de Waal Malefyt R, Haanen J, Spits H, Roncarolo MG, te Velde A, Figdor C, et al. Interleukin 10 (IL-10) and viral IL-10 strongly reduce antigen-specific human T cell proliferation by diminishing the antigen-presenting capacity of monocytes via downregulation of class II major histocompatibility complex expression. J Exp Med. (1991) 174:915–24. doi: 10.1084/jem.174.4.915
67. Ogawa Y, Duru EA, Ameredes BT. Role of IL-10 in the resolution of airway inflammation. Curr Mol Med. (2008) 8:437–45. doi: 10.2174/156652408785160907
68. Saraiva M, O'Garra A. The regulation of IL-10 production by immune cells. Nat Rev Immunol. (2010) 10:170–81. doi: 10.1038/nri2711
69. Pretolani M, Goldman M. Cytokines involved in the downregulation of allergic airway inflammation. Res Immunol. (1997) 148:33–8.
70. Grutz G. New insights into the molecular mechanism of interleukin-10-mediated immunosuppression. J Leukoc Biol. (2005) 77:3–15. doi: 10.1189/jlb.0904484
71. Chiang N, Schwab JM, Fredman G, Kasuga K, Gelman S, Serhan CN. Anesthetics impact the resolution of inflammation. PLoS ONE. (2008) 3:e1879. doi: 10.1371/journal.pone.0001879
72. Cooper D, Norling LV, Perretti M. Novel insights into the inhibitory effects of Galectin-1 on neutrophil recruitment under flow. J Leukoc Biol. (2008) 83:1459–66. doi: 10.1189/jlb.1207831
73. Norling LV, Sampaio AL, Cooper D, Perretti M. Inhibitory control of endothelial galectin-1 on in vitro and in vivo lymphocyte trafficking. FASEB J. (2008) 22:682–90. doi: 10.1096/fj.07-9268com
74. Ilarregui JM, Croci DO, Bianco GA, Toscano MA, Salatino M, Vermeulen ME, et al. Tolerogenic signals delivered by dendritic cells to T cells through a galectin-1-driven immunoregulatory circuit involving interleukin 27 and interleukin 10. Nat Immunol. (2009) 10:981–91. doi: 10.1038/ni.1772
75. Rostoker R, Yaseen H, Schif-Zuck S, Lichtenstein RG, Rabinovich GA, Ariel A. Galectin-1 induces 12/15-lipoxygenase expression in murine macrophages and favors their conversion toward a pro-resolving phenotype. Prostaglandins Other Lipid Mediat. (2013) 107:85–94. doi: 10.1016/j.prostaglandins.2013.08.001
76. Erriah M, Pabreja K, Fricker M, Baines KJ, Donnelly LE, Bylund J, et al. Galectin-3 enhances monocyte-derived macrophage efferocytosis of apoptotic granulocytes in asthma. Respir Res. (2019) 20:1. doi: 10.1186/s12931-018-0967-9
77. Zhu C, Anderson AC, Schubart A, Xiong H, Imitola J, Khoury SJ, et al. The Tim-3 ligand galectin-9 negatively regulates T helper type 1 immunity. Nat Immunol. (2005) 6:1245–52. doi: 10.1038/ni1271
78. Seki M, Sakata KM, Oomizu S, Arikawa T, Sakata A, Ueno M, et al. Beneficial effect of galectin 9 on rheumatoid arthritis by induction of apoptosis of synovial fibroblasts. Arthritis Rheum. (2007) 56:3968–76. doi: 10.1002/art.23076
79. Arikawa T, Watanabe K, Seki M, Matsukawa A, Oomizu S, Sakata KM, et al. Galectin-9 ameliorates immune complex-induced arthritis by regulating Fc gamma R expression on macrophages. Clin Immunol. (2009) 133:382–92. doi: 10.1016/j.clim.2009.09.004
80. Garin MI, Chu CC, Golshayan D, Cernuda-Morollon E, Wait R, Lechler RI. Galectin-1: a key effector of regulation mediated by CD4+CD25+ T cells. Blood. (2007) 109:2058–65. doi: 10.1182/blood-2006-04-016451
81. Wu C, Thalhamer T, Franca RF, Xiao S, Wang C, Hotta C, et al. Galectin-9-CD44 interaction enhances stability and function of adaptive regulatory T cells. Immunity. (2014) 41:270–82. doi: 10.1016/j.immuni.2014.06.011
82. Sanchez-Cuellar S, de la Fuente H, Cruz-Adalia A, Lamana A, Cibrian D, Giron RM, et al. Reduced expression of galectin-1 and galectin-9 by leucocytes in asthma patients. Clin Exp Immunol. (2012) 170:365–74. doi: 10.1111/j.1365-2249.2012.04665.x
83. Getting SJ. Targeting melanocortin receptors as potential novel therapeutics. Pharmacol Ther. (2006) 111:1–15. doi: 10.1016/j.pharmthera.2005.06.022
84. Montero-Melendez T. ACTH: the forgotten therapy. Semin Immunol. (2015) 27:216–26. doi: 10.1016/j.smim.2015.02.003
85. Getting SJ, Gibbs L, Clark AJ, Flower RJ, Perretti M. POMC gene-derived peptides activate melanocortin type 3 receptor on murine macrophages, suppress cytokine release, and inhibit neutrophil migration in acute experimental inflammation. J Immunol. (1999) 162:7446–53.
86. Montero-Melendez T, Patel HB, Seed M, Nielsen S, Jonassen TE, Perretti M. The melanocortin agonist AP214 exerts anti-inflammatory and proresolving properties. Am J Pathol. (2011) 179:259–69. doi: 10.1016/j.ajpath.2011.03.042
87. Muffley LA, Zhu KQ, Engrav LH, Gibran NS, Hocking AM. Spatial and temporal localization of the melanocortin 1 receptor and its ligand alpha-melanocyte-stimulating hormone during cutaneous wound repair. J Histochem Cytochem. (2011) 59:278–88. doi: 10.1369/0022155410397999
88. Wallace JL, Ianaro A, Flannigan KL, Cirino G. Gaseous mediators in resolution of inflammation. Semin Immunol. (2015) 27:227–33. doi: 10.1016/j.smim.2015.05.004
89. Dufton N, Natividad J, Verdu EF, Wallace JL. Hydrogen sulfide and resolution of acute inflammation: a comparative study utilizing a novel fluorescent probe. Sci Rep. (2012) 2:499. doi: 10.1038/srep00499
90. Mariggio MA, Minunno V, Riccardi S, Santacroce R, De Rinaldis P, Fumarulo R. Sulfide enhancement of PMN apoptosis. Immunopharmacol Immunotoxicol. (1998) 20:399–408. doi: 10.3109/08923979809034822
91. Urquhart P, Rosignoli G, Cooper D, Motterlini R, Perretti M. Carbon monoxide-releasing molecules modulate leukocyte-endothelial interactions under flow. J Pharmacol Exp Ther. (2007) 321:656–62. doi: 10.1124/jpet.106.117218
92. Ryter SW, Choi AM. Gaseous therapeutics in acute lung injury. Compr Physiol. (2011) 1:105–21. doi: 10.1002/cphy.c090003
93. Hasko G, Cronstein B. Regulation of inflammation by adenosine. Front Immunol. (2013) 4:85. doi: 10.3389/fimmu.2013.00085
94. Gonzalez-Rey E, Anderson P, Delgado M. Emerging roles of vasoactive intestinal peptide: a new approach for autoimmune therapy. Ann Rheum Dis. (2007) 66(Suppl. 3):iii70–6. doi: 10.1136/ard.2007.078519
95. Ganea D, Hooper KM, Kong W. The neuropeptide vasoactive intestinal peptide: direct effects on immune cells and involvement in inflammatory and autoimmune diseases. Acta Physiol. (2015) 213:442–52. doi: 10.1111/apha.12427
96. Jimeno R, Leceta J, Martinez C, Gutierrez-Canas I, Carrion M, Perez-Garcia S, et al. Vasoactive intestinal peptide maintains the nonpathogenic profile of human th17-polarized cells. J Mol Neurosci. (2014) 54:512–25. doi: 10.1007/s12031-014-0318-3
97. Jimeno R, Leceta J, Martinez C, Gutierrez-Canas I, Perez-Garcia S, Carrion M, et al. Effect of VIP on the balance between cytokines and master regulators of activated helper T cells. Immunol Cell Biol. (2012) 90:178–86. doi: 10.1038/icb.2011.23
98. Gonzalez-Rey E. Keeping the balance between immune tolerance and pathogen immunity with endogenous neuropeptides. Neuroimmunomodulation. (2010) 17:161–4. doi: 10.1159/000258713
99. Souza-Moreira L, Campos-Salinas J, Caro M, Gonzalez-Rey E. Neuropeptides as pleiotropic modulators of the immune response. Neuroendocrinology. (2011) 94:89–100. doi: 10.1159/000328636
100. Carrion M, Perez-Garcia S, Martinez C, Juarranz Y, Estrada-Capetillo L, Puig-Kroger A, et al. VIP impairs acquisition of the macrophage proinflammatory polarization profile. J Leukoc Biol. (2016) 100:1385–93. doi: 10.1189/jlb.3A0116-032RR
101. Mirakaj V, Dalli J, Granja T, Rosenberger P, Serhan CN. Vagus nerve controls resolution and pro-resolving mediators of inflammation. J Exp Med. (2014) 211:1037–48. doi: 10.1084/jem.20132103
102. Dalli J, Colas RA, Arnardottir H, Serhan CN. Vagal regulation of group 3 innate lymphoid cells and the immunoresolvent PCTR1 controls infection resolution. Immunity. (2017) 46:92–105. doi: 10.1016/j.immuni.2016.12.009
103. Lee RH, Vazquez G. Evidence for a prosurvival role of alpha-7 nicotinic acetylcholine receptor in alternatively (M2)-activated macrophages. Physiol Rep. (2013) 1:e00189. doi: 10.1002/phy2.189
104. Ramon S, Dalli J, Sanger JM, Winkler JW, Aursnes M, Tungen JE, et al. The protectin PCTR1 is produced by human M2 macrophages and enhances resolution of infectious inflammation. Am J Pathol. (2016) 186:962–73. doi: 10.1016/j.ajpath.2015.12.012
105. Tsunawaki S, Sporn M, Ding A, Nathan C. Deactivation of macrophages by transforming growth factor-beta. Nature. (1988) 334:260–2. doi: 10.1038/334260a0
106. Sporn MB, Roberts AB, Wakefield LM, Assoian RK. Transforming growth factor-beta: biological function and chemical structure. Science. (1986) 233:532–4. doi: 10.1126/science.3487831
107. Duvernelle C, Freund V, Frossard N. Transforming growth factor-beta and its role in asthma. Pulm Pharmacol Ther. (2003) 16:181–96. doi: 10.1016/S1094-5539(03)00051-8
108. Bettelli E, Carrier Y, Gao W, Korn T, Strom TB, Oukka M, et al. Reciprocal developmental pathways for the generation of pathogenic effector TH17 and regulatory T cells. Nature. (2006) 441:235–8. doi: 10.1038/nature04753
109. Li MO, Wan YY, Sanjabi S, Robertson AK, Flavell RA. Transforming growth factor-beta regulation of immune responses. Annu Rev Immunol. (2006) 24:99–146. doi: 10.1146/annurev.immunol.24.021605.090737
110. McGeachy MJ, Bak-Jensen KS, Chen Y, Tato CM, Blumenschein W, McClanahan T, et al. TGF-beta and IL-6 drive the production of IL-17 and IL-10 by T cells and restrain T(H)-17 cell-mediated pathology. Nat Immunol. (2007) 8:1390–7. doi: 10.1038/ni1539
111. Xiao S, Jin H, Korn T, Liu SM, Oukka M, Lim B, et al. Retinoic acid increases Foxp3+ regulatory T cells and inhibits development of Th17 cells by enhancing TGF-beta-driven Smad3 signaling and inhibiting IL-6 and IL-23 receptor expression. J Immunol. (2008) 181:2277–84. doi: 10.4049/jimmunol.181.4.2277
112. Dudakov JA, Hanash AM, van den Brink MR. Interleukin-22: immunobiology and pathology. Annu Rev Immunol. (2015) 33:747–85. doi: 10.1146/annurev-immunol-032414-112123
113. Parks OB, Pociask DA, Hodzic Z, Kolls JK, Good M. Interleukin-22 signaling in the regulation of intestinal health and disease. Front Cell Dev Biol. (2015) 3:85. doi: 10.3389/fcell.2015.00085
114. Aujla SJ, Chan YR, Zheng M, Fei M, Askew DJ, Pociask DA, et al. IL-22 mediates mucosal host defense against Gram-negative bacterial pneumonia. Nat Med. (2008) 14:275–81. doi: 10.1038/nm1710
115. Zheng Y, Valdez PA, Danilenko DM, Hu Y, Sa SM, Gong Q, et al. Interleukin-22 mediates early host defense against attaching and effacing bacterial pathogens. Nat Med. (2008) 14:282–9. doi: 10.1038/nm1720
116. De Luca A, Zelante T, D'Angelo C, Zagarella S, Fallarino F, Spreca A, et al. IL-22 defines a novel immune pathway of antifungal resistance. Mucosal Immunol. (2010) 3:361–73. doi: 10.1038/mi.2010.22
117. Hirose K, Iwata A, Tamachi T, Nakajima H. Allergic airway inflammation: key players beyond the Th2 cell pathway. Immunol Rev. (2017) 278:145–61. doi: 10.1111/imr.12540
118. Fang P, Zhou L, Zhou Y, Kolls JK, Zheng T, Zhu Z. Immune modulatory effects of IL-22 on allergen-induced pulmonary inflammation. PLoS ONE. (2014) 9:e107454. doi: 10.1371/journal.pone.0107454
119. Dayer JM. Evidence for the biological modulation of IL-1 activity: the role of IL-1Ra. Clin Exp Rheumatol. (2002) 20(5 Suppl. 27):S14–20.
120. Alvarado-Vazquez PA, Bernal L, Paige CA, Grosick RL, Moracho Vilrriales C, Ferreira DW, et al. Macrophage-specific nanotechnology-driven CD163 overexpression in human macrophages results in an M2 phenotype under inflammatory conditions. Immunobiology. (2017) 222:900–12. doi: 10.1016/j.imbio.2017.05.011
121. Tountas NA, Casini-Raggi V, Yang H, Di Giovine FS, Vecchi M, Kam L, et al. Functional and ethnic association of allele 2 of the interleukin-1 receptor antagonist gene in ulcerative colitis. Gastroenterology. (1999) 117:806–13. doi: 10.1016/S0016-5085(99)70338-0
122. Lopez-Hernandez R, Valdes M, Campillo JA, Martinez-Garcia P, Salama H, Bolarin JM, et al. Pro- and anti-inflammatory cytokine gene single-nucleotide polymorphisms in inflammatory bowel disease. Int J Immunogenet. (2015) 42:38–45. doi: 10.1111/iji.12160
123. Minutti CM, Jackson-Jones LH, Garcia-Fojeda B, Knipper JA, Sutherland TE, Logan N, et al. Local amplifiers of IL-4Ralpha-mediated macrophage activation promote repair in lung and liver. Science. (2017) 356:1076–80. doi: 10.1126/science.aaj2067
124. Fredman G, Li Y, Dalli J, Chiang N, Serhan CN. Self-limited versus delayed resolution of acute inflammation: temporal regulation of pro-resolving mediators and microRNA. Sci Rep. (2012) 2:639. doi: 10.1038/srep00639
125. Recchiuti A, Krishnamoorthy S, Fredman G, Chiang N, Serhan CN. MicroRNAs in resolution of acute inflammation: identification of novel resolvin D1-miRNA circuits. FASEB J. (2011) 25:544–60. doi: 10.1096/fj.10-169599
126. Krishnamoorthy S, Recchiuti A, Chiang N, Fredman G, Serhan CN. Resolvin D1 receptor stereoselectivity and regulation of inflammation and proresolving microRNAs. Am J Pathol. (2012) 180:2018–27. doi: 10.1016/j.ajpath.2012.01.028
127. Taganov KD, Boldin MP, Chang KJ, Baltimore D. NF-kappaB-dependent induction of microRNA miR-146, an inhibitor targeted to signaling proteins of innate immune responses. Proc Natl Acad Sci USA. (2006) 103:12481–6. doi: 10.1073/pnas.0605298103
128. Fatima F, Nawaz M. Vesiculated long non-coding RNAs: offshore packages deciphering trans-regulation between cells, cancer progression and resistance to therapies. Noncoding RNA. (2017) 3:E10. doi: 10.3390/ncrna3010010
129. Fatima F, Nawaz M. Nexus between extracellular vesicles, immunomodulation and tissue remodeling: for good or for bad? Ann Transl Med. (2017) 5:139. doi: 10.21037/atm.2017.03.71
130. Silva AM, Teixeira JH, Almeida MI, Goncalves RM, Barbosa MA, Santos SG. Extracellular vesicles: immunomodulatory messengers in the context of tissue repair/regeneration. Eur J Pharm Sci. (2017) 98:86–95. doi: 10.1016/j.ejps.2016.09.017
131. Taverna S, Pucci M, Alessandro R. Extracellular vesicles: small bricks for tissue repair/regeneration. Ann Transl Med. (2017) 5:83. doi: 10.21037/atm.2017.01.53
132. Te Velde AA. The C-type lectin mincle: clues for a role in Crohn's disease adjuvant reaction. Front Immunol. (2017) 8:1304. doi: 10.3389/fimmu.2017.01304
133. Kempski J, Brockmann L, Gagliani N, Huber S. TH17 cell and epithelial cell crosstalk during inflammatory bowel disease and carcinogenesis. Front Immunol. (2017) 8:1373. doi: 10.3389/fimmu.2017.01373
134. Galli SJ, Tsai M, Piliponsky AM. The development of allergic inflammation. Nature. (2008) 454:445–54. doi: 10.1038/nature07204
135. Schett G, Neurath MF. Resolution of chronic inflammatory disease: universal and tissue-specific concepts. Nat Commun. (2018) 9:3261. doi: 10.1038/s41467-018-05800-6
136. Rogler G. Resolution of inflammation in inflammatory bowel disease. Lancet Gastroenterol Hepatol. (2017) 2:521–30. doi: 10.1016/S2468-1253(17)30031-6
137. Kaplan GG, Ng SC. Understanding and preventing the global increase of inflammatory bowel disease. Gastroenterology. (2017) 152:313–21.e312. doi: 10.1053/j.gastro.2016.10.020
138. de Souza HS, Fiocchi C. Immunopathogenesis of IBD: current state of the art. Nat Rev Gastroenterol Hepatol. (2016) 13:13–27. doi: 10.1038/nrgastro.2015.186
139. Fuss IJ, Neurath M, Boirivant M, Klein JS, de la Motte C, Strong SA, et al. Disparate CD4+ lamina propria (LP) lymphokine secretion profiles in inflammatory bowel disease. Crohn's disease LP cells manifest increased secretion of IFN-gamma, whereas ulcerative colitis LP cells manifest increased secretion of IL-5. J Immunol. (1996) 157:1261–70. doi: 10.1097/00024382-199703001-00528
140. Sakuraba A, Sato T, Kamada N, Kitazume M, Sugita A, Hibi T. Th1/Th17 immune response is induced by mesenteric lymph node dendritic cells in Crohn's disease. Gastroenterology. (2009) 137:1736–45. doi: 10.1053/j.gastro.2009.07.049
141. Fujino S, Andoh A, Bamba S, Ogawa A, Hata K, Araki Y, et al. Increased expression of interleukin 17 in inflammatory bowel disease. Gut. (2003) 52:65–70. doi: 10.1136/gut.52.1.65
142. Zenewicz LA, Antov A, Flavell RA. CD4 T-cell differentiation and inflammatory bowel disease. Trends Mol Med. (2009) 15:199–207. doi: 10.1016/j.molmed.2009.03.002
143. Neurath MF. Current and emerging therapeutic targets for IBD. Nat Rev Gastroenterol Hepatol. (2017) 14:269–78. doi: 10.1038/nrgastro.2016.208
144. Biancheri P, Powell N, Monteleone G, Lord G, MacDonald TT. The challenges of stratifying patients for trials in inflammatory bowel disease. Trends Immunol. (2013) 34:564–71. doi: 10.1016/j.it.2013.08.002
145. Lobaton T, Vermeire S, Van Assche G, Rutgeerts P. Review article: anti-adhesion therapies for inflammatory bowel disease. Aliment Pharmacol Ther. (2014) 39:579–94. doi: 10.1111/apt.12639
146. Feagan BG, Sandborn WJ, D'Haens G, Panes J, Kaser A, Ferrante M, et al. Induction therapy with the selective interleukin-23 inhibitor risankizumab in patients with moderate-to-severe Crohn's disease: a randomised, double-blind, placebo-controlled phase 2 study. Lancet. (2017) 389:1699–709. doi: 10.1016/S0140-6736(17)30570-6
147. Cholapranee A, Hazlewood GS, Kaplan GG, Peyrin-Biroulet L, Ananthakrishnan AN. Systematic review with meta-analysis: comparative efficacy of biologics for induction and maintenance of mucosal healing in Crohn's disease and ulcerative colitis controlled trials. Aliment Pharmacol Ther. (2017) 45:1291–302. doi: 10.1111/apt.14030
148. Das UN. Inflammatory bowel disease as a disorder of an imbalance between pro- and anti-inflammatory molecules and deficiency of resolution bioactive lipids. Lipids Health Dis. (2016) 15:11. doi: 10.1186/s12944-015-0165-4
149. Marcon R, Bento AF, Dutra RC, Bicca MA, Leite DF, Calixto JB. Maresin 1, a proresolving lipid mediator derived from omega-3 polyunsaturated fatty acids, exerts protective actions in murine models of colitis. J Immunol. (2013) 191:4288–98. doi: 10.4049/jimmunol.1202743
150. Gobbetti T, Dalli J, Colas RA, Federici Canova D, Aursnes M, Bonnet D, et al. Protectin D1n-3 DPA and resolvin D5n-3 DPA are effectors of intestinal protection. Proc Natl Acad Sci USA. (2017) 114:3963–8. doi: 10.1073/pnas.1617290114
151. Schwanke RC, Marcon R, Bento AF, Calixto JB. EPA- and DHA-derived resolvins' actions in inflammatory bowel disease. Eur J Pharmacol. (2016) 785:156–64. doi: 10.1016/j.ejphar.2015.08.050
152. Vong L, Ferraz JG, Dufton N, Panaccione R, Beck PL, Sherman PM, et al. Up-regulation of Annexin-A1 and lipoxin A(4) in individuals with ulcerative colitis may promote mucosal homeostasis. PLoS ONE. (2012) 7:e39244. doi: 10.1371/journal.pone.0039244
153. Leoni G, Alam A, Neumann PA, Lambeth JD, Cheng G, McCoy J, et al. Annexin A1, formyl peptide receptor, and NOX1 orchestrate epithelial repair. J Clin Invest. (2013) 123:443–54. doi: 10.1172/JCI65831
154. Leoni G, Neumann PA, Kamaly N, Quiros M, Nishio H, Jones HR, et al. Annexin A1-containing extracellular vesicles and polymeric nanoparticles promote epithelial wound repair. J Clin Invest. (2015) 125:1215–27. doi: 10.1172/JCI76693
155. Vergnolle N, Pages P, Guimbaud R, Chaussade S, Bueno L, Escourrou J, et al. Annexin 1 is secreted in situ during ulcerative colitis in humans. Inflamm Bowel Dis. (2004) 10:584–92. doi: 10.1097/00054725-200409000-00013
156. Sena A, Grishina I, Thai A, Goulart L, Macal M, Fenton A, et al. Dysregulation of anti-inflammatory annexin A1 expression in progressive Crohns Disease. PLoS ONE. (2013) 8:e76969. doi: 10.1371/journal.pone.0076969
157. de Paula-Silva M, Barrios BE, Maccio-Maretto L, Sena AA, Farsky SH, Correa SG, et al. Role of the protein annexin A1 on the efficacy of anti-TNF treatment in a murine model of acute colitis. Biochem Pharmacol. (2016) 115:104–13. doi: 10.1016/j.bcp.2016.06.012
158. Kuhn R, Lohler J, Rennick D, Rajewsky K, Muller W. Interleukin-10-deficient mice develop chronic enterocolitis. Cell. (1993) 75:263–74. doi: 10.1016/0092-8674(93)80068-P
159. Glocker EO, Kotlarz D, Boztug K, Gertz EM, Schaffer AA, Noyan F, et al. Inflammatory bowel disease and mutations affecting the interleukin-10 receptor. N Engl J Med. (2009) 361:2033–45. doi: 10.1056/NEJMoa0907206
160. Shah N, Kammermeier J, Elawad M, Glocker EO. Interleukin-10 and interleukin-10-receptor defects in inflammatory bowel disease. Curr Allergy Asthma Rep. (2012) 12:373–9. doi: 10.1007/s11882-012-0286-z
161. Pernot S, Ramtohul T, Taieb J. Checkpoint inhibitors and gastrointestinal immune-related adverse events. Curr Opin Oncol. (2016) 28:264–8. doi: 10.1097/CCO.0000000000000292
162. Holmen N, Lundgren A, Lundin S, Bergin AM, Rudin A, Sjovall H, et al. Functional CD4+CD25high regulatory T cells are enriched in the colonic mucosa of patients with active ulcerative colitis and increase with disease activity. Inflamm Bowel Dis. (2006) 12:447–56. doi: 10.1097/00054725-200606000-00003
163. Maul J, Loddenkemper C, Mundt P, Berg E, Giese T, Stallmach A, et al. Peripheral and intestinal regulatory CD4+ CD25(high) T cells in inflammatory bowel disease. Gastroenterology. (2005) 128:1868–78. doi: 10.1053/j.gastro.2005.03.043
164. Autschbach F, Braunstein J, Helmke B, Zuna I, Schurmann G, Niemir ZI, et al. In situ expression of interleukin-10 in noninflamed human gut and in inflammatory bowel disease. Am J Pathol. (1998) 153:121–30. doi: 10.1016/S0002-9440(10)65552-6
165. Melgar S, Yeung MM, Bas A, Forsberg G, Suhr O, Oberg A, et al. Over-expression of interleukin 10 in mucosal T cells of patients with active ulcerative colitis. Clin Exp Immunol. (2003) 134:127–37. doi: 10.1046/j.1365-2249.2003.02268.x
166. Barnig C, Levy BD. Innate immunity is a key factor for the resolution of inflammation in asthma. Eur Respir Rev. (2015) 24:141–53. doi: 10.1183/09059180.00012514
167. Cibor D, Szczeklik K, Brzozowski B, Mach T, Owczarek D. Serum galectin 3, galectin 9 and galectin 3-binding proteins in patients with active and inactive inflammatory bowel disease. J Physiol Pharmacol. (2019) 70:95–104. doi: 10.1093/ecco-jcc/jjy222.146
168. Papa Gobbi R, De Francesco N, Bondar C, Muglia C, Chirdo F, Rumbo M, et al. A galectin-specific signature in the gut delineates Crohn's disease and ulcerative colitis from other human inflammatory intestinal disorders. Biofactors. (2016) 42:93–105. doi: 10.1002/biof.1252
169. Frol'ova L, Smetana K Jr, Borovska D, Kitanovicova A, Klimesova K, Janatkova I, et al. Detection of galectin-3 in patients with inflammatory bowel diseases: new serum marker of active forms of IBD? Inflamm Res. (2009) 58:503–12. doi: 10.1007/s00011-009-0016-8
170. Puthenedam M, Wu F, Shetye A, Michaels A, Rhee KJ, Kwon JH. Matrilysin-1 (MMP7) cleaves galectin-3 and inhibits wound healing in intestinal epithelial cells. Inflamm Bowel Dis. (2011) 17:260–7. doi: 10.1002/ibd.21443
171. Muller S, Schaffer T, Flogerzi B, Fleetwood A, Weimann R, Schoepfer AM, et al. Galectin-3 modulates T cell activity and is reduced in the inflamed intestinal epithelium in IBD. Inflamm Bowel Dis. (2006) 12:588–97. doi: 10.1097/01.MIB.0000225341.37226.7c
172. Maaser C, Kannengiesser K, Specht C, Lugering A, Brzoska T, Luger TA, et al. Crucial role of the melanocortin receptor MC1R in experimental colitis. Gut. (2006) 55:1415–22. doi: 10.1136/gut.2005.083634
173. Kannengiesser K, Maaser C, Heidemann J, Luegering A, Ross M, Brzoska T, et al. Melanocortin-derived tripeptide KPV has anti-inflammatory potential in murine models of inflammatory bowel disease. Inflamm Bowel Dis. (2008) 14:324–31. doi: 10.1002/ibd.20334
174. Wei P, Yang Y, Ding Q, Li X, Sun H, Liu Z, et al. Oral delivery of Bifidobacterium longum expressing alpha-melanocyte-stimulating hormone to combat ulcerative colitis. J Med Microbiol. (2016) 65:160–8. doi: 10.1099/jmm.0.000197
175. Zhao H, Yan R, Zhou X, Ji F, Zhang B. Hydrogen sulfide improves colonic barrier integrity in DSS-induced inflammation in Caco-2 cells and mice. Int Immunopharmacol. (2016) 39:121–7. doi: 10.1016/j.intimp.2016.07.020
176. Wallace JL, Vong L, McKnight W, Dicay M, Martin GR. Endogenous and exogenous hydrogen sulfide promotes resolution of colitis in rats. Gastroenterology. (2009) 137:569–78, 578 e561. doi: 10.1053/j.gastro.2009.04.012
177. Crittenden S, Cheyne A, Adams A, Forster T, Robb CT, Felton J, et al. Purine metabolism controls innate lymphoid cell function and protects against intestinal injury. Immunol Cell Biol. (2018) 96:1049–59. doi: 10.1111/imcb.12167
178. Chiang N, Shinohara M, Dalli J, Mirakaj V, Kibi M, Choi AM, et al. Inhaled carbon monoxide accelerates resolution of inflammation via unique proresolving mediator-heme oxygenase-1 circuits. J Immunol. (2013) 190:6378–88. doi: 10.4049/jimmunol.1202969
179. El-Salhy M, Solomon T, Hausken T, Gilja OH, Hatlebakk JG. Gastrointestinal neuroendocrine peptides/amines in inflammatory bowel disease. World J Gastroenterol. (2017) 23:5068–85. doi: 10.3748/wjg.v23.i28.5068
180. Pawankar R. Allergic diseases and asthma: a global public health concern and a call to action. World Allergy Organ J. (2014) 7:12. doi: 10.1186/1939-4551-7-12
181. Aron JL, Akbari O. Regulatory T cells and type 2 innate lymphoid cell-dependent asthma. Allergy. (2017) 72:1148–55. doi: 10.1111/all.13139
182. Busse WW, Pedersen S, Pauwels RA, Tan WC, Chen YZ, Lamm CJ, et al. The inhaled steroid treatment as regular therapy in early asthma (START) study 5-year follow-up: effectiveness of early intervention with budesonide in mild persistent asthma. J Allergy Clin Immunol. (2008) 121:1167–74. doi: 10.1016/j.jaci.2008.02.029
183. Chung KF, Wenzel SE, Brozek JL, Bush A, Castro M, Sterk PJ, et al. International ERS/ATS guidelines on definition, evaluation and treatment of severe asthma. Eur Respir J. (2014) 43:343–73. doi: 10.1183/09031936.00202013
184. Katial RK, Bensch GW, Busse WW, Chipps BE, Denson JL, Gerber AN, et al. Changing paradigms in the treatment of severe asthma: the role of biologic therapies. J Allergy Clin Immunol Pract. (2017) 5:S1–14. doi: 10.1016/j.jaip.2016.11.029
185. Gomez G. Current strategies to inhibit high affinity fcepsilonri-mediated signaling for the treatment of allergic disease. Front Immunol. (2019) 10:175. doi: 10.3389/fimmu.2019.00175
186. Bagnasco D, Ferrando M, Varricchi G, Puggioni F, Passalacqua G, Canonica GW. Anti-Interleukin 5 (IL-5) and IL-5Ra biological drugs: efficacy, safety, and future perspectives in severe eosinophilic asthma. Front Med. (2017) 4:135. doi: 10.3389/fmed.2017.00135
187. Svenningsen S, Nair P. Asthma endotypes and an overview of targeted therapy for asthma. Front Med. (2017) 4:158. doi: 10.3389/fmed.2017.00158
188. Dhami S, Nurmatov U, Arasi S, Khan T, Asaria M, Zaman H, et al. Allergen immunotherapy for allergic rhinoconjunctivitis: a systematic review and meta-analysis. Allergy. (2017) 72:1597–631. doi: 10.1111/all.13201
189. Nurmatov U, Dhami S, Arasi S, Pajno GB, Fernandez-Rivas M, Muraro A, et al. Allergen immunotherapy for IgE-mediated food allergy: a systematic review and meta-analysis. Allergy. (2017) 72:1133–47. doi: 10.1111/all.13124
190. Vachier I, Bonnans C, Chavis C, Farce M, Godard P, Bousquet J, et al. Severe asthma is associated with a loss of LX4, an endogenous anti-inflammatory compound. J Allergy Clin Immunol. (2005) 115:55–60. doi: 10.1016/j.jaci.2004.09.038
191. Haworth O, Cernadas M, Yang R, Serhan CN, Levy BD. Resolvin E1 regulates interleukin 23, interferon-gamma and lipoxin A4 to promote the resolution of allergic airway inflammation. Nat Immunol. (2008) 9:873–9. doi: 10.1038/ni.1627
192. Karra L, Haworth O, Priluck R, Levy BD, Levi-Schaffer F. Lipoxin B(4) promotes the resolution of allergic inflammation in the upper and lower airways of mice. Mucosal Immunol. (2015) 8:852–62. doi: 10.1038/mi.2014.116
193. Rogerio AP, Haworth O, Croze R, Oh SF, Uddin M, Carlo T, et al. Resolvin D1 and aspirin-triggered resolvin D1 promote resolution of allergic airways responses. J Immunol. (2012) 189:1983–91. doi: 10.4049/jimmunol.1101665
194. Ng FS, Wong KY, Guan SP, Mustafa FB, Kajiji TS, Bist P, et al. Annexin-1-deficient mice exhibit spontaneous airway hyperresponsiveness and exacerbated allergen-specific antibody responses in a mouse model of asthma. Clin Exp Allergy. (2011) 41:1793–803. doi: 10.1111/j.1365-2222.2011.03855.x
195. Wang LM, Li WH, Xu YC, Wei Q, Zhao H, Jiang XF. Annexin 1-derived peptide Ac2-26 inhibits eosinophil recruitment in vivo via decreasing prostaglandin D(2). Int Arch Allergy Immunol. (2011) 154:137–48. doi: 10.1159/000320228
196. Coomes SM, Kannan Y, Pelly VS, Entwistle LJ, Guidi R, Perez-Lloret J, et al. CD4(+) Th2 cells are directly regulated by IL-10 during allergic airway inflammation. Mucosal Immunol. (2017) 10:150–61. doi: 10.1038/mi.2016.47
197. Wilson MS, Elnekave E, Mentink-Kane MM, Hodges MG, Pesce JT, Ramalingam TR, et al. IL-13Ralpha2 and IL-10 coordinately suppress airway inflammation, airway-hyperreactivity, and fibrosis in mice. J Clin Invest. (2007) 117:2941–51. doi: 10.1172/JCI31546
198. Grunig G, Corry DB, Leach MW, Seymour BW, Kurup VP, Rennick DM. Interleukin-10 is a natural suppressor of cytokine production and inflammation in a murine model of allergic bronchopulmonary aspergillosis. J Exp Med. (1997) 185:1089–99. doi: 10.1084/jem.185.6.1089
199. Tournoy KG, Kips JC, Pauwels RA. Endogenous interleukin-10 suppresses allergen-induced airway inflammation and nonspecific airway responsiveness. Clin Exp Allergy. (2000) 30:775–83.
200. Woodfolk JA. Selective roles and dysregulation of interleukin-10 in allergic disease. Curr Allergy Asthma Rep. (2006) 6:40–6. doi: 10.1007/s11882-006-0008-5
201. Katoh S, Shimizu H, Obase Y, Oomizu S, Niki T, Ikeda M, et al. Preventive effect of galectin-9 on double-stranded RNA-induced airway hyperresponsiveness in an exacerbation model of mite antigen-induced asthma in mice. Exp Lung Res. (2013) 39:453–62. doi: 10.3109/01902148.2013.850558
202. Raap U, Brzoska T, Sohl S, Path G, Emmel J, Herz U, et al. Alpha-melanocyte-stimulating hormone inhibits allergic airway inflammation. J Immunol. (2003) 171:353–9. doi: 10.4049/jimmunol.171.1.353
203. Zhang G, Wang P, Yang G, Cao Q, Wang R. The inhibitory role of hydrogen sulfide in airway hyperresponsiveness and inflammation in a mouse model of asthma. Am J Pathol. (2013) 182:1188–95. doi: 10.1016/j.ajpath.2012.12.008
204. Chen YH, Wu R, Geng B, Qi YF, Wang PP, Yao WZ, et al. Endogenous hydrogen sulfide reduces airway inflammation and remodeling in a rat model of asthma. Cytokine. (2009) 45:117–23. doi: 10.1016/j.cyto.2008.11.009
205. Wu D, Lee D, Sung YK. Prospect of vasoactive intestinal peptide therapy for COPD/PAH and asthma: a review. Respir Res. (2011) 12:45. doi: 10.1186/1465-9921-12-45
206. Wang J, Shang YX, Cai XX, Liu LY. Vasoactive intestinal peptide inhibits airway smooth muscle cell proliferation in a mouse model of asthma via the ERK1/2 signaling pathway. Exp Cell Res. (2018) 364:168–74. doi: 10.1016/j.yexcr.2018.01.042
207. de Kivit S, Kraneveld AD, Knippels LM, van Kooyk Y, Garssen J, Willemsen LE. Intestinal epithelium-derived galectin-9 is involved in the immunomodulating effects of nondigestible oligosaccharides. J Innate Immun. (2013) 5:625–38. doi: 10.1159/000350515
208. de Kivit S, Kostadinova AI, Kerperien J, Morgan ME, Muruzabal VA, Hofman GA, et al. Dietary, nondigestible oligosaccharides and Bifidobacterium breve M-16V suppress allergic inflammation in intestine via targeting dendritic cell maturation. J Leukoc Biol. (2017) 102:105–15. doi: 10.1189/jlb.3A0516-236R
209. de Kivit S, Saeland E, Kraneveld AD, van de Kant HJ, Schouten B, van Esch BC, et al. Galectin-9 induced by dietary synbiotics is involved in suppression of allergic symptoms in mice and humans. Allergy. (2012) 67:343–52. doi: 10.1111/j.1398-9995.2011.02771.x
210. Nakakita S, Itoh A, Nakakita Y, Nonaka Y, Ogawa T, Nakamura T, et al. Cooperative interactions of oligosaccharide and peptide moieties of a glycopeptide derived from IgE with Galectin-9. J Biol Chem. (2016) 291:968–79. doi: 10.1074/jbc.M115.694448
211. Smith SF, Tetley TD, Guz A, Flower RJ. Detection of lipocortin 1 in human lung lavage fluid: lipocortin degradation as a possible proteolytic mechanism in the control of inflammatory mediators and inflammation. Environ Health Perspect. (1990) 85:135–44. doi: 10.1289/ehp.85-1568329
212. Eke Gungor H, Tahan F, Gokahmetoglu S, Saraymen B. Decreased levels of lipoxin A4 and annexin A1 in wheezy infants. Int Arch Allergy Immunol. (2014) 163:193–7. doi: 10.1159/000358490
213. Lee SH, Lee PH, Kim BG, Seo HJ, Baek AR, Park JS, et al. Annexin A1 in plasma from patients with bronchial asthma: its association with lung function. BMC Pulm Med. (2018) 18:1. doi: 10.1186/s12890-017-0557-5
214. Ricklefs I, Barkas I, Duvall MG, Cernadas M, Grossman NL, Israel E, et al. ALX receptor ligands define a biochemical endotype for severe asthma. JCI Insight. (2017) 2:93534. doi: 10.1172/jci.insight.93534
215. Borish L, Aarons A, Rumbyrt J, Cvietusa P, Negri J, Wenzel S. Interleukin-10 regulation in normal subjects and patients with asthma. J Allergy Clin Immunol. (1996) 97:1288–96. doi: 10.1016/S0091-6749(96)70197-5
216. John M, Lim S, Seybold J, Jose P, Robichaud A, O'Connor B, et al. Inhaled corticosteroids increase interleukin-10 but reduce macrophage inflammatory protein-1alpha, granulocyte-macrophage colony-stimulating factor, and interferon-gamma release from alveolar macrophages in asthma. Am J Respir Crit Care Med. (1998) 157:256–62. doi: 10.1164/ajrccm.157.1.9703079
217. Lim S, Crawley E, Woo P, Barnes PJ. Haplotype associated with low interleukin-10 production in patients with severe asthma. Lancet. (1998) 352:113. doi: 10.1016/S0140-6736(98)85018-6
218. Liang Q, Guo L, Gogate S, Karim Z, Hanifi A, Leung DY, et al. IL-2 and IL-4 stimulate MEK1 expression and contribute to T cell resistance against suppression by TGF-beta and IL-10 in asthma. J Immunol. (2010) 185:5704–13. doi: 10.4049/jimmunol.1000690
219. Gao P, Gibson PG, Baines KJ, Yang IA, Upham JW, Reynolds PN, et al. Anti-inflammatory deficiencies in neutrophilic asthma: reduced galectin-3 and IL-1RA/IL-1beta. Respir Res. (2015) 16:5. doi: 10.1186/s12931-014-0163-5
220. Zhang J, Wang X, Chen Y, Yao W. Correlation between levels of exhaled hydrogen sulfide and airway inflammatory phenotype in patients with chronic persistent asthma. Respirology. (2014) 19:1165–9. doi: 10.1111/resp.12372
221. Tian M, Wang Y, Lu YQ, Yan M, Jiang YH, Zhao DY. Correlation between serum H2S and pulmonary function in children with bronchial asthma. Mol Med Rep. (2012) 6:335–8. doi: 10.3892/mmr.2012.904
222. Suzuki Y, Saito J, Kikuchi M, Uematsu M, Fukuhara A, Sato S, et al. Sputum-to-serum hydrogen sulphide ratio as a novel biomarker of predicting future risks of asthma exacerbation. Clin Exp Allergy. (2018) 48:1155–63. doi: 10.1111/cea.13173
223. Tomaki M, Ichinose M, Miura M, Hirayama Y, Yamauchi H, Nakajima N, et al. Elevated substance P content in induced sputum from patients with asthma and patients with chronic bronchitis. Am J Respir Crit Care Med. (1995) 151(3 Pt 1):613–7. doi: 10.1164/ajrccm/151.3_Pt_1.613
224. Duffy D, Rouilly V, Libri V, Hasan M, Beitz B, David M, et al. Functional analysis via standardized whole-blood stimulation systems defines the boundaries of a healthy immune response to complex stimuli. Immunity. (2014) 40:436–50. doi: 10.1016/j.immuni.2014.03.002
225. Morris T, Stables M, Colville-Nash P, Newson J, Bellingan G, de Souza PM, et al. Dichotomy in duration and severity of acute inflammatory responses in humans arising from differentially expressed proresolution pathways. Proc Natl Acad Sci USA. (2010) 107:8842–7. doi: 10.1073/pnas.1000373107
226. Patin E, Hasan M, Bergstedt J, Rouilly V, Libri V, Urrutia A, et al. Natural variation in the parameters of innate immune cells is preferentially driven by genetic factors. Nat Immunol. (2018) 19:302–14. doi: 10.1038/s41590-018-0049-7
227. Schaible TD, Harris RA, Dowd SE, Smith CW, Kellermayer R. Maternal methyl-donor supplementation induces prolonged murine offspring colitis susceptibility in association with mucosal epigenetic and microbiomic changes. Hum Mol Genet. (2011) 20:1687–96. doi: 10.1093/hmg/ddr044
228. Zeybel M, Hardy T, Wong YK, Mathers JC, Fox CR, Gackowska A, et al. Multigenerational epigenetic adaptation of the hepatic wound-healing response. Nat Med. (2012) 18:1369–77. doi: 10.1038/nm.2893
229. Jarchum I, Pamer EG. Regulation of innate and adaptive immunity by the commensal microbiota. Curr Opin Immunol. (2011) 23:353–60. doi: 10.1016/j.coi.2011.03.001
230. Te Velde AA, Bezema T, van Kampen AH, Kraneveld AD, t Hart BA, van Middendorp H, et al. Embracing complexity beyond systems medicine: a new approach to chronic immune disorders. Front Immunol. (2016) 7:587. doi: 10.3389/fimmu.2016.00587
231. Liu Y, Cousin JM, Hughes J, Van Damme J, Seckl JR, Haslett C, et al. Glucocorticoids promote nonphlogistic phagocytosis of apoptotic leukocytes. J Immunol. (1999) 162:3639–46.
232. Hashimoto A, Murakami Y, Kitasato H, Hayashi I, Endo H. Glucocorticoids co-interact with lipoxin A4 via lipoxin A4 receptor (ALX) up-regulation. Biomed Pharmacother. (2007) 61:81–5. doi: 10.1016/j.biopha.2006.06.023
233. Corminboeuf O, Leroy X. FPR2/ALXR agonists and the resolution of inflammation. J Med Chem. (2015) 58:537–59. doi: 10.1021/jm501051x
234. Perretti M, Leroy X, Bland EJ, Montero-Melendez T. Resolution pharmacology: opportunities for therapeutic innovation in inflammation. Trends Pharmacol Sci. (2015) 36:737–55. doi: 10.1016/j.tips.2015.07.007
235. Serhan CN, Petasis NA. Resolvins and protectins in inflammation resolution. Chem Rev. (2011) 111:5922–43. doi: 10.1021/cr100396c
236. Serhan CN, Krishnamoorthy S, Recchiuti A, Chiang N. Novel anti-inflammatory–pro-resolving mediators and their receptors. Curr Top Med Chem. (2011) 11:629–47. doi: 10.2174/1568026611109060629
237. Sun YP, Oh SF, Uddin J, Yang R, Gotlinger K, Campbell E, et al. Resolvin D1 and its aspirin-triggered 17R epimer. Stereochemical assignments, anti-inflammatory properties, and enzymatic inactivation. J Biol Chem. (2007) 282:9323–34. doi: 10.1074/jbc.M609212200
238. Kasuga K, Yang R, Porter TF, Agrawal N, Petasis NA, Irimia D, et al. Rapid appearance of resolvin precursors in inflammatory exudates: novel mechanisms in resolution. J Immunol. (2008) 181:8677–87. doi: 10.4049/jimmunol.181.12.8677
239. Van Dyke TE, Hasturk H, Kantarci A, Freire MO, Nguyen D, Dalli J, et al. Proresolving nanomedicines activate bone regeneration in periodontitis. J Dent Res. (2015) 94:148–56. doi: 10.1177/0022034514557331
240. Serhan CN, Chiang N, Dalli J. The resolution code of acute inflammation: novel pro-resolving lipid mediators in resolution. Semin Immunol. (2015) 27:200–15. doi: 10.1016/j.smim.2015.03.004
241. Wu SH, Chen XQ, Liu B, Wu HJ, Dong L. Efficacy and safety of 15(R/S)-methyl-lipoxin A(4) in topical treatment of infantile eczema. Br J Dermatol. (2013) 168:172–8. doi: 10.1111/j.1365-2133.2012.11177.x
242. Calder PC. Marine omega-3 fatty acids and inflammatory processes: effects, mechanisms and clinical relevance. Biochim Biophys Acta. (2015) 1851:469–84. doi: 10.1016/j.bbalip.2014.08.010
243. Calder PC. Omega-3 fatty acids and inflammatory processes: from molecules to man. Biochem Soc Trans. (2017) 45:1105–15. doi: 10.1042/BST20160474
244. Calder PC. Very long-chain n-3 fatty acids and human health: fact, fiction and the future. Proc Nutr Soc. (2018) 77:52–72. doi: 10.1017/S0029665117003950
245. Bazan HA, Lu Y, Thoppil D, Fitzgerald TN, Hong S, Dardik A. Diminished omega-3 fatty acids are associated with carotid plaques from neurologically symptomatic patients: implications for carotid interventions. Vascul Pharmacol. (2009) 51:331–6. doi: 10.1016/j.vph.2009.08.003
246. Calder PC. n-3 fatty acids, inflammation and immunity: new mechanisms to explain old actions. Proc Nutr Soc. (2013) 72:326–36. doi: 10.1017/S0029665113001031
247. Crawford MA, Wang Y, Forsyth S, Brenna JT. New European Food Safety Authority recommendation for infant formulae contradicts the physiology of human milk and infant development. Nutr Health. (2013) 22:81–7. doi: 10.1177/0260106015573784
248. Costantini L, Molinari R, Farinon B, Merendino N. Impact of Omega-3 fatty acids on the gut microbiota. Int J Mol Sci. (2017) 18:E2645. doi: 10.3390/ijms18122645
249. Freedman SD, Blanco PG, Zaman MM, Shea JC, Ollero M, Hopper IK, et al. Association of cystic fibrosis with abnormalities in fatty acid metabolism. N Engl J Med. (2004) 350:560–9. doi: 10.1056/NEJMoa021218
250. Schwartz J, Weiss ST. The relationship of dietary fish intake to level of pulmonary function in the first National Health and Nutrition Survey (NHANES I). Eur Respir J. (1994) 7:1821–4. doi: 10.1183/09031936.94.07101821
251. Weiss GA, Troxler H, Klinke G, Rogler D, Braegger C, Hersberger M. High levels of anti-inflammatory and pro-resolving lipid mediators lipoxins and resolvins and declining docosahexaenoic acid levels in human milk during the first month of lactation. Lipids Health Dis. (2013) 12:89. doi: 10.1186/1476-511X-12-89
252. Arnardottir H, Orr SK, Dalli J, Serhan CN. Human milk proresolving mediators stimulate resolution of acute inflammation. Mucosal Immunol. (2016) 9:757–66. doi: 10.1038/mi.2015.99
253. Keelan JA, Mas E, D'Vaz N, Dunstan JA, Li S, Barden AE, et al. Effects of maternal n-3 fatty acid supplementation on placental cytokines, pro-resolving lipid mediators and their precursors. Reproduction. (2015) 149:171–8. doi: 10.1530/REP-14-0549
254. Bisgaard H, Stokholm J, Chawes BL, Vissing NH, Bjarnadottir E, Schoos AM, et al. Fish oil-derived fatty acids in pregnancy and wheeze and asthma in offspring. N Engl J Med. (2016) 375:2530–9. doi: 10.1056/NEJMoa1503734
255. Ramaswami R, Serhan CN, Levy BD, Makrides M. Fish oil supplementation in pregnancy. N Engl J Med. (2016) 375:2599–601. doi: 10.1056/NEJMclde1614333
256. Mas E, Croft KD, Zahra P, Barden A, Mori TA. Resolvins D1, D2, and other mediators of self-limited resolution of inflammation in human blood following n-3 fatty acid supplementation. Clin Chem. (2012) 58:1476–84. doi: 10.1373/clinchem.2012.190199
257. Mas E, Barden A, Burke V, Beilin LJ, Watts GF, Huang RC, et al. A randomized controlled trial of the effects of n-3 fatty acids on resolvins in chronic kidney disease. Clin Nutr. (2016) 35:331–6. doi: 10.1016/j.clnu.2015.04.004
258. Polus A, Zapala B, Razny U, Gielicz A, Kiec-Wilk B, Malczewska-Malec M, et al. Omega-3 fatty acid supplementation influences the whole blood transcriptome in women with obesity, associated with pro-resolving lipid mediator production. Biochim Biophys Acta. (2016) 1861:1746–55. doi: 10.1016/j.bbalip.2016.08.005
259. Abdulrazaq M, Innes JK, Calder PC. Effect of omega-3 polyunsaturated fatty acids on arthritic pain: a systematic review. Nutrition. (2017) 39–40:57–66. doi: 10.1016/j.nut.2016.12.003
260. Senftleber NK, Nielsen SM, Andersen JR, Bliddal H, Tarp S, Lauritzen L, et al. Marine oil supplements for arthritis pain: a systematic review and meta-analysis of randomized trials. Nutrients. (2017) 9:E42. doi: 10.3390/nu9010042
261. de Kivit S, Kostadinova AI, Kerperien J, Ayechu Muruzabal V, Morgan ME, Knippels LMJ, et al. Galectin-9 produced by intestinal epithelial cells enhances aldehyde dehydrogenase activity in dendritic cells in a PI3K- and p38-dependent manner. J Innate Immun. (2017) 9:609–20. doi: 10.1159/000479817
262. Furrie E, Macfarlane S, Kennedy A, Cummings JH, Walsh SV, O'Neil DA, et al. Synbiotic therapy (Bifidobacterium longum/Synergy 1) initiates resolution of inflammation in patients with active ulcerative colitis: a randomised controlled pilot trial. Gut. (2005) 54:242–9. doi: 10.1136/gut.2004.044834
263. Vieira AT, Rocha VM, Tavares L, Garcia CC, Teixeira MM, Oliveira SC, et al. Control of Klebsiella pneumoniae pulmonary infection and immunomodulation by oral treatment with the commensal probiotic Bifidobacterium longum 5(1A). Microbes Infect. (2016) 18:180–9. doi: 10.1016/j.micinf.2015.10.008
264. Shi Y, Xu LZ, Peng K, Wu W, Wu R, Liu ZQ, et al. Specific immunotherapy in combination with Clostridium butyricum inhibits allergic inflammation in the mouse intestine. Sci Rep. (2015) 5:17651. doi: 10.1038/srep17651
265. Takeshita K, Mizuno S, Mikami Y, Sujino T, Saigusa K, Matsuoka K, et al. A single species of clostridium subcluster XIVa decreased in ulcerative colitis patients. Inflamm Bowel Dis. (2016) 22:2802–10. doi: 10.1097/MIB.0000000000000972
266. Satish Kumar CS, Kondal Reddy K, Boobalan G, Gopala Reddy A, Sudha Rani Chowdhary CH, Vinoth A, et al. Immunomodulatory effects of Bifidobacterium bifidum 231 on trinitrobenzenesulfonic acid-induced ulcerative colitis in rats. Res Vet Sci. (2017) 110:40–6. doi: 10.1016/j.rvsc.2016.10.010
267. Petersen AM, Pedersen BK. The anti-inflammatory effect of exercise. J Appl Physiol. (2005) 98:1154–62. doi: 10.1152/japplphysiol.00164.2004
268. Peake JM, Della Gatta P, Suzuki K, Nieman DC. Cytokine expression and secretion by skeletal muscle cells: regulatory mechanisms and exercise effects. Exerc Immunol Rev. (2015) 21:8–25.
269. Gangemi S, Luciotti G, D'Urbano E, Mallamace A, Santoro D, Bellinghieri G, et al. Physical exercise increases urinary excretion of lipoxin A4 and related compounds. J Appl Physiol. (2003) 94:2237–40. doi: 10.1152/japplphysiol.01004.2002
270. Markworth JF, Vella L, Lingard BS, Tull DL, Rupasinghe TW, Sinclair AJ, et al. Human inflammatory and resolving lipid mediator responses to resistance exercise and ibuprofen treatment. Am J Physiol Regul Integr Comp Physiol. (2013) 305:R1281–96. doi: 10.1152/ajpregu.00128.2013
271. Tahan F, Eke GH, Bicici E, Saraymen B, Akar HH. Increased postexercise lipoxin A4 levels in exhaled breath condensate in asthmatic children with exercise-induced bronchoconstriction. J Investig Allergol Clin Immunol. (2016) 26:19–24. doi: 10.18176/jiaci.0003
272. de Araujo CC, Marques PS, Silva JD, Samary CS, da Silva AL, Henriques I, et al. Regular and moderate aerobic training before allergic asthma induction reduces lung inflammation and remodeling. Scand J Med Sci Sports. (2016) 26:1360–72. doi: 10.1111/sms.12614
273. Olivo CR, Vieira RP, Arantes-Costa FM, Perini A, Martins MA, Carvalho CR. Effects of aerobic exercise on chronic allergic airway inflammation and remodeling in guinea pigs. Respir Physiol Neurobiol. (2012) 182:81–7. doi: 10.1016/j.resp.2012.05.004
274. Shephard RJ. The case for increased physical activity in chronic inflammatory bowel disease: a brief review. Int J Sports Med. (2016) 37:505–15. doi: 10.1055/s-0042-103157
275. Cacioppo JT, Cacioppo S. Social relationships and health: the toxic effects of perceived social isolation. Soc Personal Psychol Compass. (2014) 8:58–72. doi: 10.1111/spc3.12087
276. Branstrom R, Kvillemo P, Akerstedt T. Effects of mindfulness training on levels of cortisol in cancer patients. Psychosomatics. (2013) 54:158–64. doi: 10.1016/j.psym.2012.04.007
277. Witek-Janusek L, Albuquerque K, Chroniak KR, Chroniak C, Durazo-Arvizu R, Mathews HL. Effect of mindfulness based stress reduction on immune function, quality of life and coping in women newly diagnosed with early stage breast cancer. Brain Behav Immun. (2008) 22:969–81. doi: 10.1016/j.bbi.2008.01.012
278. Kenne Sarenmalm E, Martensson LB, Andersson BA, Karlsson P, Bergh I. Mindfulness and its efficacy for psychological and biological responses in women with breast cancer. Cancer Med. (2017) 6:1108–22. doi: 10.1002/cam4.1052
279. Sanada K, Alda Diez M, Salas Valero M, Perez-Yus MC, Demarzo MM, Montero-Marin J, et al. Effects of mindfulness-based interventions on biomarkers in healthy and cancer populations: a systematic review. BMC Complement Altern Med. (2017) 17:125. doi: 10.1186/s12906-017-1638-y
280. Huber M, Knottnerus JA, Green L, van der Horst H, Jadad AR, Kromhout D, et al. How should we define health? BMJ. (2011) 343:d4163. doi: 10.1136/bmj.d4163
281. de Haan P, Klein HC, t Hart BA. Autoimmune aspects of neurodegenerative and psychiatric diseases: a template for innovative therapy. Front Psychiatry. (2017) 8:46. doi: 10.3389/fpsyt.2017.00046
282. Miller AH, Raison CL. The role of inflammation in depression: from evolutionary imperative to modern treatment target. Nat Rev Immunol. (2016) 16:22–34. doi: 10.1038/nri.2015.5
Keywords: resolution, inflammation, immune fitness, eicosanoids, asthma, chronic inflammatory bowel disease
Citation: Barnig C, Bezema T, Calder PC, Charloux A, Frossard N, Garssen J, Haworth O, Dilevskaya K, Levi-Schaffer F, Lonsdorfer E, Wauben M, Kraneveld AD and te Velde AA (2019) Activation of Resolution Pathways to Prevent and Fight Chronic Inflammation: Lessons From Asthma and Inflammatory Bowel Disease. Front. Immunol. 10:1699. doi: 10.3389/fimmu.2019.01699
Received: 06 November 2018; Accepted: 08 July 2019;
Published: 23 July 2019.
Edited by:
Fulvio D'Acquisto, University of Roehampton, United KingdomReviewed by:
Valerio Chiurchiù, Campus Bio-Medico University, ItalyFrancesco Annunziato, University of Florence, Italy
Lorenzo Cosmi, University of Florence, Italy
Copyright © 2019 Barnig, Bezema, Calder, Charloux, Frossard, Garssen, Haworth, Dilevskaya, Levi-Schaffer, Lonsdorfer, Wauben, Kraneveld and te Velde. This is an open-access article distributed under the terms of the Creative Commons Attribution License (CC BY). The use, distribution or reproduction in other forums is permitted, provided the original author(s) and the copyright owner(s) are credited and that the original publication in this journal is cited, in accordance with accepted academic practice. No use, distribution or reproduction is permitted which does not comply with these terms.
*Correspondence: Cindy Barnig, cindy.barnig@chru-strasbourg.fr