- 1Immunology and Molecular Pathogenesis Program, Emory University Laney Graduate School, Atlanta, GA, United States
- 2Department of Pathology, University of Utah, Salt Lake City, UT, United States
Eph receptors are the largest family of receptor tyrosine kinases and mediate a myriad of essential processes in humans from embryonic development to adult tissue homeostasis through interactions with membrane-bound ephrin ligands. The ubiquitous expression of Eph receptors and ephrin ligands among the cellular players of the immune system underscores the importance of these molecules in orchestrating an optimal immune response. This review provides an overview of the various roles of Eph receptors and ephrin ligands in immune cell development, activation, and migration. We also discuss the role of Eph receptors in disease pathogenesis as well as the implications of Eph receptors as future immunotherapy targets. Given the diverse and critical roles of Eph receptors and ephrin ligands throughout the immune system during both resting and activated states, this review aims to highlight the critical yet underappreciated roles of this family of signaling molecules in the immune system.
Eph Receptors: a Paradoxical Family of Kinases
The Eph (erythropoietin-producing hepatocellular carcinoma) receptors represent the largest known family of receptor tyrosine kinases in mammals (1). These receptors are critical for a variety of normal cellular processes during development and are key mediators of adult tissue homeostasis (2–5). First discovered in a human carcinoma cell line (6), the Eph family of receptors is now known to include two classes of receptors that consist of 9 EphA members and 5 EphB members classified according to sequence homology (7) (Table 1). This group of receptors function through interactions with membrane-bound ephrin (Eph receptor-interacting protein) ligands to mediate changes in cellular shape, motility, migration, and proliferation (2, 4, 28, 29). The basic structures of Eph receptors and their ligands are shown in Figure 1A. All Eph receptors have a highly conserved overall structure with EphA and EphB receptors sharing the same structural features and domains. The primary sequence differences between EphA and EphB receptors reside in a region of the ligand binding domain determined to be a low-affinity ephrin binding site which is likely involved in determining ephrin subclass binding specificity (30). Given their high structural similarity, the differences in functional outcomes that result from activation of either EphA or EphB receptors can be primarily attributed to the spatial and temporal expression patterns of Eph receptors and ephrin ligands in cis on a cell and in trans on neighboring cells. In essence, activation of any given Eph receptor can have highly varied impacts on cellular processes depending on the cellular and microenvironmental context. EphA receptors bind promiscuously to ephrin-A ligands (five members) while EphB receptors bind promiscuously to ephrin-B ligands (three members) with some potential cross-talk between groups (31). In contrast to Eph receptors, the ephrin-A and ephrin-B ligand families have clear structural differences as ephrin-A ligands are tethered to the cell membrane through glycosylphosphatidylinositol (GPI) anchors while ephrin-B ligands have a short transmembrane domain and conserved cytoplasmic tail. Although it is widely accepted that clustering of membrane-bound Eph receptors and ephrin ligands is required to facilitate optimal signaling (32), research in cancer demonstrates that EphA2 expression on extracellular vesicles secreted from senescent cells can act on nearby ephrin-A1 expressing cancerous cells to contribute to proliferation (33). This indicates that cell-cell contact is not always necessary for the activation of downstream signaling upon Eph-ephrin contact. The complexity of interactions conveyed by this promiscuous binding leads to considerable diversity in functional output upon Eph-ephrin binding.
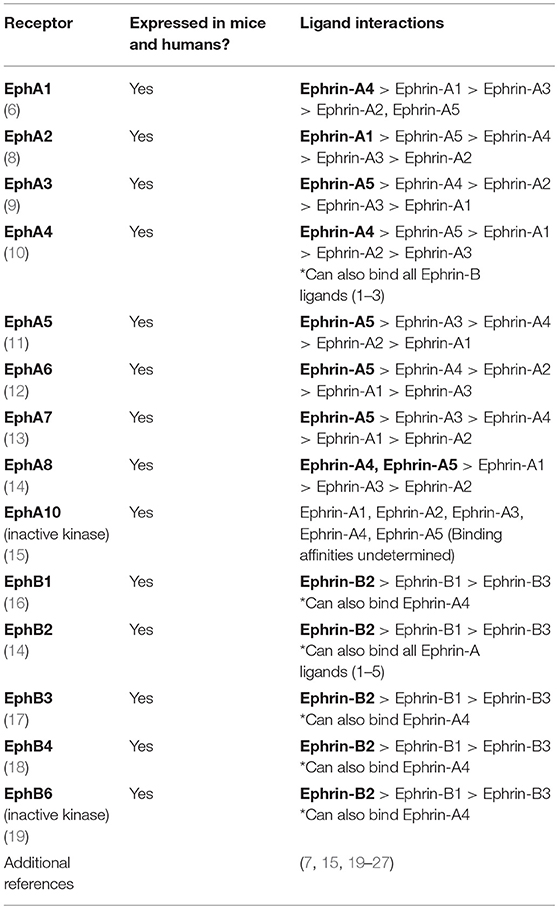
Table 1. List of Eph receptors expressed in both mice and humans along with potential binding partners and preferences for ephrin ligands.
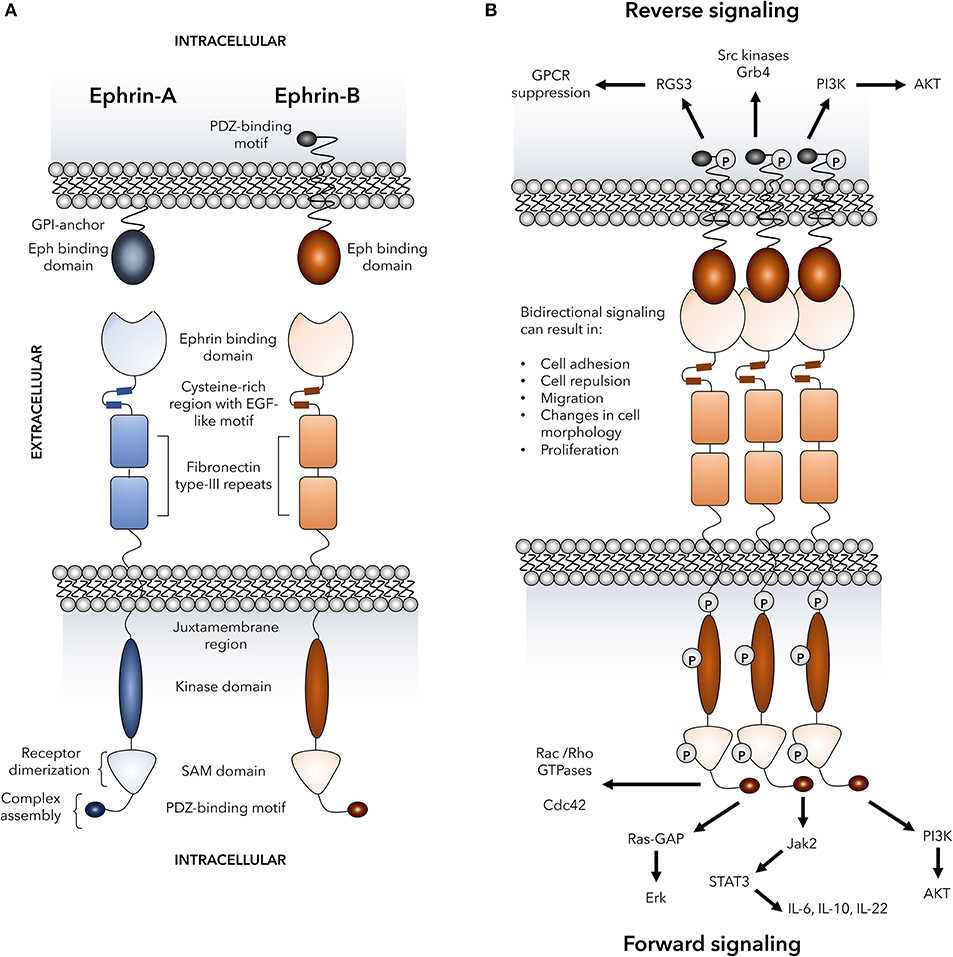
Figure 1. Basic Eph receptor structure and signaling pathways. The structure of Eph receptors and their ligands is shown in (A). Eph receptors are consisting of an extracellular structure consisting of an ephrin binding domain connected to two fibronectin type-III repeats by a cysteine-rich EGF-like motif. The juxtamembrane region connects the extracellular portion of the receptor to the intracellular kinase domain that is linked to a sterile alpha motif (SAM) domain and PDZ-binding motif. Two tyrosine residues on the juxtamembrane region mediate autophosphorylation. Eph receptors bind to ephrin ligands via an extracellular Eph binding domain. Ephrin-A ligands are GPI-anchored to the plasma membrane and signal through co-receptors that have not yet been fully defined. Ephrin-B ligands are transmembrane and are linked to an intracellular PDZ-binding motif via a linker containing five tyrosine resides for autophosphorylation. (B) Dimerization of Eph receptors is regulated by various processes including SAM domain interactions, ligand clustering, and interactions between cysteine-rich regions and ephrin binding domains on neighboring receptors. Receptor dimerization mediates the formation of heterocomplexes that are required for signaling and are assembled via the Eph receptor PDZ-binding motif. Formation of the heterocomplex mediates bi-directional signaling in which numerous signaling pathways known to play a role in immune cell function can be activated through both ephrin “reverse” and Eph “forward” signaling. These signaling events include activation of Rho GTPases, MAP kinases, PI3 kinase, Src family kinases, Jak-STAT molecules, and RGS3 that has been shown to suppress G-protein coupled receptors including chemokine receptors. P, representative of tyrosine phosphorylation sites; GPCRs, G-protein coupled receptors; RGS3, regulator of G-protein signaling 3; Grb4, cytoplasmic protein NCK2; PI3K, phosphatidylinositol 3-kinase; AKT, protein kinase B; Cdc42, cell division control protein 42 homolog; Ras-GAP, Ras-GTPase-activating protein; Erk, extracellular signal-regulated kinases; Jak, Janus kinase; STAT, signal transducer and activator of transcription; IL, interleukin.
A distinctive feature of Eph-ephrin interactions is the bidirectional signaling that occurs upon receptor-ligand binding and clustering which is termed forward signaling in Eph receptor-expressing cells and reverse signaling in ephrin ligand-expressing cells (34–36). Binding of Eph receptors to their ligands results in oligomerization and trans-phosphorylation leading to optimal kinase activity (37, 38). Importantly yet somewhat paradoxically, both cellular adhesion and repulsion can be consequences of Eph-ephrin binding between cells. High-affinity cell-cell binding events can lead to endocytosis of the receptor-ligand complex (39–41) or proteolytic cleavage of the ephrin extracellular domains (42–44) and cellular repulsion. On the other hand, Eph-ephrin adhesion is favored by reduced forward signaling (45, 46) and expression of Ephs and ephrins in cis (47, 48). There is also evidence that the fate decision between adhesion or repulsion can occur in a time-dependent manner where an initial adhesive event can later become a repulsive event (39, 42). The complexity provided by the signaling events downstream of Eph-ephrin binding allows for diverse functional consequences in a highly regulated and context-dependent manner, and examples of several potential signaling events that can occur upon Eph-ephrin clustering and ligation are shown in Figure 1B.
Expressed in most, if not all, adult tissues (2, 49), the Eph-ephrin signaling axis was initially most heavily studied for its complex role in embryonic and neural developmental processes such as cell segregation and migration, spatial organization of cell populations, tissue boundary formation, axonal guidance, and angiogenesis (50, 51). Also expressed on most cellular players of the immune system (Table 2), Eph-ephrin interactions have been implicated in various facets of immune surveillance including immune cell activation, migration, adhesion, and proliferation (93–95). In this review, we will discuss the emerging roles of Eph receptors and ephrin ligands in various aspects of immunity and disease pathogenesis as well as the implications of Eph receptors as future immunotherapy targets.
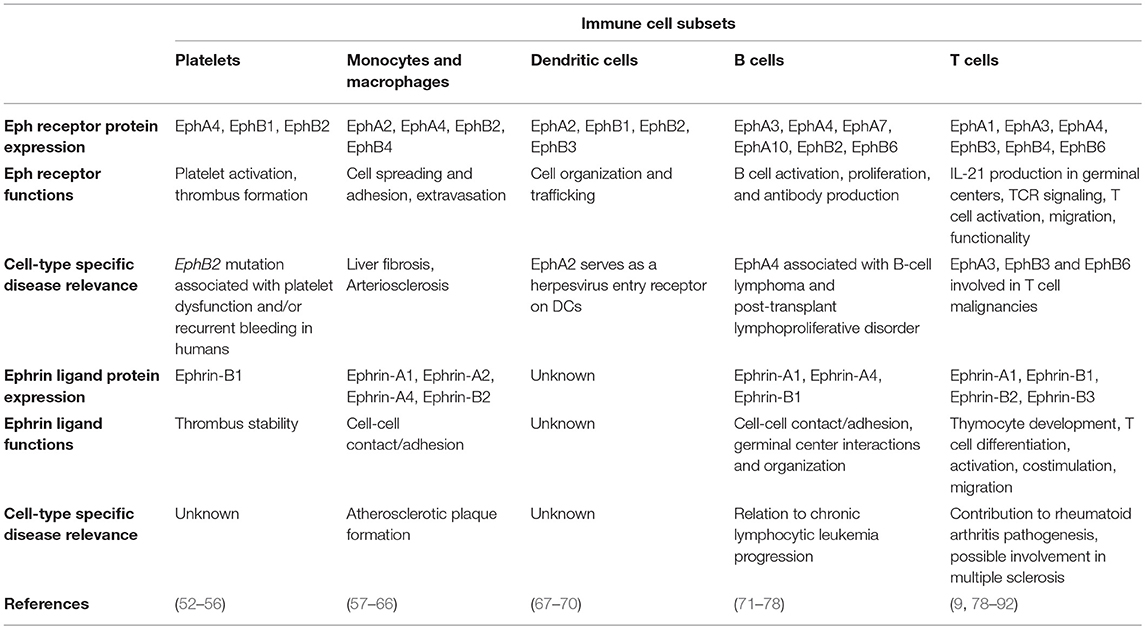
Table 2. Known protein expression profiles, functions, and disease contributions of Eph receptors and ephrin ligands on various immune cell subsets.
Impact of Eph Receptor Expression on Stem Cell Fate
In order to understand how this unique family of receptors and ligands factors into immune system development and function, it is first necessary to review the effects of expression patterns of Eph receptors and their ligands on hematopoietic cells prior to divergence into different immune cell fates. There is evidence supporting a clear role for Eph receptors in cell fate decisions of hematopoietic progenitors prior to differentiation. EphB receptors in particular are important in the hematopoiesis of both red and white blood cells. Hematopoietic progenitor cells expressing EphB2 can be repulsed by bone marrow stromal cell-expressed Ephrin-B2, in turn mediating their subsequent differentiation into mature erythroid cells (96). Additionally, interactions between EphB4 and ephrin-B2 on bone marrow sinusoids and hematopoietic cells, respectively, aid in the mobilization of hematopoietic progenitor cells from the bone marrow (97). In vitro, ectopic EphB4 expression in hematopoietic cells promotes commitment to the megakaryocyte/erythroid lineage but not granulocytic or monocytic lineages (98). In the mouse small intestine, EphB2 is highly expressed on stem cells in crypts of villi while EphB3 is highly expressed on differentiated Paneth cells. The gradients of these receptors and their cognate ephrin-B1/B2 ligands tightly control cellular positioning and stem-cell differentiation and proliferation (99, 100). Various combinations and expression levels of Eph and ephrin family members have also been found on CD34+ stem cells in both the bone marrow (101) and peripheral blood (102). Since expression differs depending on hematopoietic stem cell location (bone marrow and blood) and other microenvironmental factors, expression patterns are likely important for both early development and later function of these cells prior to lineage commitment.
Roles of Ephs and Ephrins in Immune Cell Activation
One of the initial processes in mounting an immune response is the activation of immune cells. There is evidence that Eph receptors and ephrin ligands may mediate immune cell activation. However, given the sparse number of reports in the literature it remains an open question of how signaling emanating from Eph-ephrin ligation influences activation and how this process is influenced by expression of these molecules in cis and in trans on different immune cell subsets. Furthermore, in the case of innate immune cells, initial recognition leads to activation that can be amplified through feedback loops once the adaptive immune response has been initiated. Below we outline what is currently known about the involvement of Eph receptors in activating both innate and adaptive immune cells.
Innate Immune Cells
There are very few reports on the contribution of Eph receptors and ephrin ligands to the activation of innate immune cells. However, there is evidence suggesting a role for Eph receptors, specifically EphB2, in modulating dendritic cell (DC) responsiveness to toll-like receptor (TLR) ligation by pathogen-associated molecular patterns (69). Although not currently understood, it is possible that TLR signaling pathways intersect with EphB forward signaling events leading to a modulation of NFκB activation which is central to many immune cell activation pathways. Given the widespread expression of Eph receptors and TLRs on many innate immune cells (Table 2), it would be surprising if this is not a more widespread phenomenon although this remains to be tested in other innate immune cell types.
B Cells
Eph receptors and ephrins have been identified on both human (72, 73, 103) and mouse (77) B cells. Eph receptors and ephrin ligands are also expressed differentially on naïve and activated B cells (73). This suggests that they may contribute to processes facilitating B cell activation as exemplified by naïve human B cells which upregulate EphB2 leading to increased proliferation and antibody production. In B cells, EphB2 has been demonstrated to be regulated by the microRNA miR-185 and its effects on B cell activation appear to occur at least in part through interactions between EphB2 and the Src-p65 and Notch1 signaling pathways (74). Thus, B cells may modulate expression of different Eph and/or ephrin members in order to facilitate their development, activation, differentiation, and functionality. Given the importance of cell-cell contact and localization to specific anatomical niches to the development, activation, and maturation of B cells, it seems likely that differential expression of Eph receptors and ephrin ligands could also contribute to B cell specialization into various canonical B cell fates.
T Cells
Ephs and ephrins have been detected on human peripheral T cells with one study showing between 10% and 12% of CD4+ and CD8+ T cells expressing EphB6 (104). EphB receptors reported to be expressed on mouse naïve splenic CD4+ and CD8+ T cells include EphB1, EphB2, EphB3, and EphB6. Expression of all three ephrin-B ligands on T cells has also been demonstrated (79, 82, 105), but co-expression patterns of the ligands and receptors on T cells have not yet been determined. Given the fact that Eph receptors and ephrins are also present on antigen presenting cells such as DCs (67–70), expression of these molecules on T cells suggests a potential role in T cell activation and differentiation.
Multiple studies have demonstrated that all three ephrin-B ligands can influence cooperation between T cells, T cell co-stimulation, and enhancing signaling through the T cell receptor (TCR). EphB receptors and TCRs colocalize in signaling rafts on the surface of activated T cells. Additionally, ephrin-B1 mediated stimulation of T cells through EphB receptors increases both phosphorylation of LAT and activation of the signaling molecules p38 and p44/42 MAPK (79, 82) providing a mechanistic explanation for how Eph receptors and components of the TCR complex may interact. Specifically, EphB6 has been shown to have a critical role in T cell activation with EphB6 deficient mice displaying reduced activation, phosphorylation, and/or recruitment of the T cell signaling molecules ZAP-70, LAT, SLP-76, PLCγ1, and P44/42 MAPK (106). Administration of anti-EphB6 antibodies, which can cause EphB6 clustering and subsequent signaling on T cells, increases the response of mature T cells to weak TCR ligation as measured by canonical activation marker expression (CD25, CD69) and cytokine production (interferon (IFN)-γ, interleukin (IL)-6) as well as T cell proliferation (104). In support of its role in enhancing TCR signal strength, EphB6 cross-linking in a T cell line sensitive to strong TCR signaling leads to enhanced apoptosis (107) which supports the finding that strong Eph receptor activation can induce apoptotic pathways (36, 108). This is in contrast to EphA receptors which prevent apoptosis in thymocytes (109) and we speculate that this could also apply to peripheral mature T cells participating in an immune response.
More recently, it has been suggested that EphB-ephrin-B signaling may also provide negative feedback during T cell activation. Ephrin-B1 and ephrin-B2, but not ephrin-B3, co-stimulated T cells at low concentrations but inhibited T cell activation at higher concentrations. This inhibition at high ephrin-B1/B2 concentrations likely occurs through inducing recruitment of the SHP1 phosphatase in stimulated T cells resulting in reduced phosphorylation of the signaling molecules Lck, Erk, and Akt (88). Additional data shows that mixed lymphocyte reaction activated cells pulsed with EphB2-Fc or ephrin-B2-Fc recombinant chimeric proteins downregulate key activation molecules including IL-2, IFN-γ, tumor necrosis factor-α (TNF-α), and IL-17 (90). These data suggest that the context-dependent expression of a combination of EphB receptors and ephrin-B ligands on T cells may serve an immunomodulatory role in different microenvironments.
In addition to activation, it is possible that regulation of Eph receptors and ephrin ligands can influence T cell differentiation into various T cell subsets. EphA-ephrin-A interactions can preferentially skew the differentiation of activated human CD4+ T cells to a T helper (Th) type 1, rather than a Th2, phenotype upon cross-linking of ephrin-A ligands in the presence of α-CD3/α-CD28 activating antibodies via the suppression of IL-2 and IL-4 (81). This indicates that reverse signaling in ephrin-A ligand expressing T cells is able to intersect with other currently unidentified signaling pathways in order to suppress differentiation into a Th2 cell fate.
Eph-ephrin Interactions Mediate Immune Cell Trafficking
An emerging role for Eph receptors and ephrin ligands in immune cell trafficking has been a focus of recent research. Cell migration is a fundamental process required for optimal immune system functioning. Migration occurs both locally, where cells are required to move within lymphoid organs such as the spleen in order to interact with other immune cells, and systemically during routine immune surveillance and response to damage or infection. Several key events in local and systemic immune cell trafficking that involve Eph-ephrin interactions in both steady and activated states of the immune system are shown in Figure 2 and discussed in detail below.
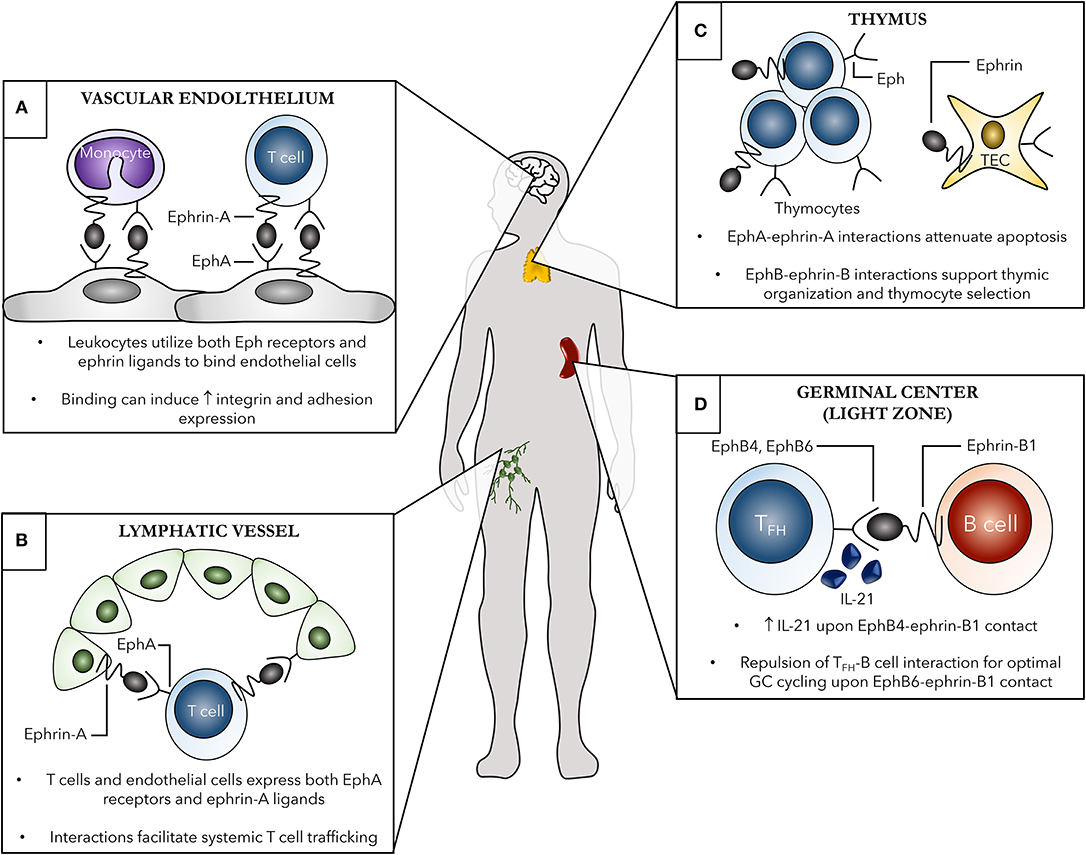
Figure 2. Examples of the contribution of Eph receptors and ephrin ligands to both localized and systemic immune cell trafficking. Several systemic (A,B) and localized (C,D) roles of Eph-ephrin interactions in immunity are shown. (A) Both leukocytes, such as monocytes and T cells, and vascular endothelial cells, shown here in the brain as an example, express various Eph receptors and ephrin ligands. Eph-ephrin interactions can aid in processes such as leukocyte chemotaxis, adhesion, and transmigration of the vascular endothelium. These binding events can subsequently induce increased expression of adhesion molecules and integrins leading to enhanced cell-cell contact. (B) EphA receptors, primarily EphA2, on high endothelial venules (HEVs) of lymph nodes can interact with ephrin-A ligand-expressing T cells to facilitate trafficking between the blood and lymph. Additionally, ephrin-A1 on HEVs can bind EphA receptors on circulating peripheral T cells leading to changes in actin polymerization in the T cell and initiating subsequent chemotaxis. (C) Thymic organization as well as thymocyte development are heavily dependent on Eph-ephrin interactions. The Eph B family members are particularly important in these processes, with both single- and double-positive thymocytes as well as thymic epithelial cells (TECs) expressing several EphB receptors and ephrin-B ligands to facilitate cellular organization of the thymus and thymocyte selection. (D) EphB-ephrin-B interactions are critical for optimal germinal center interactions between B cells and T follicular helper (TFH) cells. Ephrin-B1 marks a subpopulation of germinal center memory B cells and binding to EphB4 and EphB6 on TFH cells induces IL-21 production from the TFH cells and repulsion, respectively, both required for optimal germinal center B cell cycling.
Localized Immune Cell Migration Within Lymphoid Organs
Thymic Movement of T Cells During Development
It is not surprising that members of the Eph family would mediate a process such as T cell maturation given their well-established roles in tissue organization and cell migration in numerous organ systems. During T cell maturation, immature thymocytes undergo multiple steps of selection in the thymus requiring extended cell-cell contact with various cell types such as thymic stromal cells. It is quite possible that Eph-ephrin interactions may mediate adhesion and aid in the movement of maturing T cells through different thymic compartments during this process. Indeed, the expression of nearly all Eph receptors and ephrin ligands, with the exception of a few, has been detected in the thymus (19, 110). In the fetal thymus, EphB2 and EphB3 appear to be crucial for the successful development of thymic epithelial cells (111). Furthermore, EphB2 is implicated in mediating the colonization of T cell progenitor cells during fetal thymus colonization (112). As such, the presence of EphB2 and ephrin-B1/B2 ligands on thymocytes and thymic epithelial cells is essential for the correct organization of the thymic medulla (113). EphB6 is highly expressed in thymocytes and in mice between 50% and 70% of T cells in the thymus express EphB6 including CD4+CD8+ double positive as well as CD4+ and CD8+ single positive T cells (106). However, only around 8–17% of peripheral mature CD4+ and CD8+ T cells in mice are EphB6+ (106) indicating a potential role for EphB6 and its cognate ligand in mediating T cell retention in the thymus during development.
Although there have not been a large number of studies that address the role of Eph-ephrin interactions in T cell migration and adhesion within the thymus, such interactions appear to be critical for modulating apoptosis of thymocytes and are therefore likely critical in T cell selection processes. Distinct, yet overlapping, expression patterns of EphA receptors and ephrin-A ligands are observed in the rat thymus, and disruption of these interactions in a thymic culture model with EphA-Fc or ephrin-A-Fc recombinant chimeric proteins leads to an increase in apoptosis of double positive CD4+CD8+ T cells (114). This is in contrast to one study that showed ligation of EphA receptors with ephrin-A1 ligand can prevent apoptosis in thymocytes (109) but in agreement with a mouse study that used recombinant EphB2-Fc or ephrin-B1-Fc in thymic organ cultures in vitro to show reduced numbers of thymocytes via increased apoptosis (115). An overwhelming number of in vivo mouse models involving both Eph receptor subfamilies support the conclusion that Eph-ephrin interactions prevent apoptosis of thymocytes. Mice with selective T cell deficiency of ephrin-B1 and ephrin-B2 have significantly reduced numbers of double positive and single positive T cells in the thymus compared with intact littermate control mice (116). However, the lack of an effect in mice with a selective T cell deficiency in ephrin-B2 only (87) demonstrates the redundancy of this family of molecules as well as the ability of these receptors and ligands to potentially compensate for one another. Reduced thymic cellularity is also observed in the absence of EphB2, EphB3 (117), and EphA4 (118) in mice again suggesting a critical role for Eph receptors in preventing apoptosis of thymocytes during T cell thymic maturation.
Movement of Immune Cells in the Context of Germinal Centers
Germinal centers (GCs) are key immunological structures in secondary lymphoid organs that form to facilitate the interaction between T follicular helper (Tfh) cells and activated B cells and to aid in the development of a robust humoral immune response. During an early GC reaction, activated Tfh cells interact with their cognate antigen-specific B cells to promote B cell proliferation and differentiation into plasma cells and memory B cells (119). With T and B cells activated in different zones within the GC, this cellular interaction requires movement of T cells into the B cell zone as induced by the chemokine CXCL13 (previously known as B-cell attracting chemokine-1) along with subsequent exit of antibody-secreting plasma cells and memory B cells from the GC. In order for these extensive cell cycling and cell-cell contact events to successfully occur in the GC, both attractive and repulsive events between cells are required.
One of the more recently discovered immunological roles of the Eph-ephrin signaling system involves the generation of GC B cell responses. Ephrin-B1, a ligand that can bind to several EphB receptors, was recently shown to be a marker of mature GC B cells and may specifically differentiate early GC memory precursor B cells from other subsets of GC B cells (77). The development of an optimal humoral immune response requires specific temporal interactions to occur between Tfh cells and GC B cells. Ephrin-B1 has been shown to be involved in the localized interaction between B cells and Tfh cells in the GC microenvironment (78). Specifically, GC B cell-expressed ephrin-B1 can inhibit recruitment and retention of Tfh cells in the GC and is required for GC B cells to induce optimal levels of IL-21 from Tfh cells via EphB4 forward signaling (78). Given the key role of IL-21 in plasma cell formation and affinity maturation, GC B cell-expressed ephrin-B1 is therefore a key molecule required for the optimal functioning of GC interactions as a whole.
Migration of Immune Cells Systemically
Directional trafficking of immune cells throughout the body is driven in part by chemokine receptors expressed on immune cells that respond to chemokines secreted by distal cells. The process of systemic cell migration is facilitated by adhesion molecules such as integrins expressed on vascular endothelial cells and their ligands expressed on trafficking immune cells. Like integrins, Ephs, and ephrins are also expressed in the vasculature throughout the body (49, 120, 121). Given the widespread expression of Eph receptors and ephrin ligands on immune cells, the Eph-ephrin family of molecules may also be an important family of molecules facilitating trafficking of immune cells to the site of damage and inflammation.
The Eph-ephrin system has been shown to participate in multiple steps of monocyte trafficking including chemotaxis, adhesion (60), and vascular endothelial transmigration (57). In particular, expression of the receptors and ligands EphA2, EphA4, ephrin-A1, ephrin-A2, and ephrin-A4 is upregulated on mouse and human classical monocyte subsets at both the RNA and protein level, and these molecules can contribute to adhesion of monocytes to integrin-coated surfaces (65, 66, 122). One example of how Eph receptor signaling may mediate monocyte retention at the site of inflammation involves the interaction between ephrin-A1 on monocytes and EphA4 on endothelial cells. Upon endothelial EphA4 activation, the RhoA signaling pathway is activated leading to increased actin filament polymerization and subsequently enhanced monocyte-endothelial cell adhesion (60). Overall, the signaling events downstream of Eph-ephrin activation are likely to participate in crosstalk with integrin molecular pathways and have been hypothesized to facilitate adhesion of monocytes to other Eph or ephrin-expressing cells.
Human DCs are also known to express members of the EphA (EphA2, EphA4, EphA7) and EphB (EphB1, EphB2, EphB3, EphB6) subfamilies (68, 69). Similar to monocytes, Eph receptors could potentially contribute to DC trafficking and adhesion by allowing for localization to sites of damage or infection in the body with some evidence suggesting that crosstalk between Eph receptors and integrins, particularly β1 integrin, may facilitate DC adhesion (68). Interestingly, different subsets of DCs such as Langerhans cells (123), interstitial DCs, and plasmacytoid DCs (67) have unique patterns of Eph receptor expression. Although the reasons for these unique expression patterns remain unknown, it is possible that Eph receptors may contribute to the locational and functional specificity observed in these different DC subsets.
In the adaptive arm of the immune system, members of the EphA-ephrin-A family have been associated with B and T cell trafficking in several studies. The expression of EphA2 on high endothelial venules (HEVs) in human lymph nodes (124) suggests a role in immune cell trafficking. In support of this data, initiation of ephrin-A reverse signaling on T cells alters T cell trafficking by directing the T cells to enter lymph nodes upon injection into recipient mice (84). Although the specific member(s) of the ephrin-A ligand family important for this phenomenon is currently unknown, EphA2 has been shown to be important in mediating T cell trafficking between the blood and lymph nodes through interactions with ephrin-A4 on peripheral T cells (124). Along the same lines, ephrin-A1 is also expressed on HEV endothelial cells, and engagement of EphA receptors on the surface of both CD8+ and CD4+ T cells directly stimulates chemotaxis through effects of this ligation on actin polymerization (125). Specifically, the migration that is induced upon stimulation of EphA receptors on T cells with ephrin-A ligands involves activation of a variety of signaling molecules including Lck, Pyk2, PI3K, Vav1, and Rho GTPase (126). More recently, it has also been shown that activation of EphA2 on endothelial cells with ephrin-A1 leads to NFAT activation and subsequent upregulation of vascular cellular adhesion molecule 1 (VCAM-1) which aids in leukocyte recruitment by facilitating cellular adhesion (127). Collectively, these studies indicate a key role for Eph molecules, particularly EphA2, in optimal adaptive immune cell trafficking to sites of inflammation.
Interestingly, the expression of ephrin-A ligands on the various subsets of CD4+ and CD8+ T cells differs (85) and thus EphA-ephrin-A expression patterns may contribute to the differing migratory potential observed in naïve, effector, and memory T cells. Expression of ephrin-B ligands has also been implicated in T cell trafficking to inflamed paws during a collagen-induced arthritis mouse model (91) as well as to the central nervous system in the experimental autoimmune encephalomyelitis (EAE) mouse model (92) underlining a role for both subfamilies in T cell trafficking to distal sites of inflammation in the body. Interplay between Eph-ephrin interactions and chemokines has yet to be well-studied but T cell chemotaxis in response to the chemokines stromal cell derived factor-1α (SDF-1α, also known as CXCL12) and macrophage inflammatory protein-3β (MIP-3β, also known as CCL19) can also be modulated by both ephrin-A and ephrin-B ligands (128) implicating both subfamilies in T cell trafficking in response to chemokine gradients.
Involvement of Eph Receptors and Ephrin Ligands in Disease Pathogenesis
The ubiquitous expression profile of Ephs and ephrins throughout the human body makes them plausible candidates for mediating a variety of immunological processes. However, the widespread expression pattern along with the characteristic tyrosine kinase activity associated with Eph receptors also renders them highly likely to contribute to certain pathological conditions. Evolving research implicates various members of this family in a growing number of immune-mediated pathological conditions (59, 129, 130) as well as the pathogenesis of various diseases (3, 131–133). Thus, Eph receptors and ephrin ligands have become attractive therapeutic targets for several diseases including cancer, neurological disorders, and infectious diseases (76, 131, 134–140). In the remaining sections, we discuss what is currently known about the involvement of the Eph-ephrin family in both non-infectious and infectious diseases to convey the complexity of how these molecules contribute to various diseases.
Cancer
The first Eph receptor was identified in 1987 (6) from a human carcinoma cell line in a screen for oncogenic tyrosine kinases. It is therefore not surprising that a strong link between Eph receptors and cancer has emerged in the years since their discovery. Various Eph receptors and ephrin ligands are expressed in cancer cells as well as cells in the tumor microenvironment allowing for cell-cell communication within these compartments (131, 141). In the tumor microenvironment, upregulation of ephrin-A2, ephrin-A3, EphB2, and EphB4, to name a few, on vascular cells in response to tumor factors has been the most well-studied (49, 142). EphA2 and EphB4 are upregulated in many types of cancers and are associated with increases in cancer malignancy and poor prognosis (143–146). Expression of several EphB receptors has also been inversely correlated with colorectal cancer where decreased expression is associated with increased malignancy (147).
As many Eph receptors and ephrin ligands are expressed on T and B cells (Table 2) and can play critical roles in cell development, differentiation, activation, and proliferation, it is predictable that aberrant expression or activation of these molecules on adaptive immune cells could contribute to hematologic malignancies. EphA3 has been of particular interest to the cancer research field as it was originally identified in an acute lymphoblastic leukemia cell line and expression can be detected in many T cell lymphomas but not generally in T cells from healthy individuals (9, 71, 80, 148). Given its unique expression on malignant T cells, EphA3 has strong potential to serve as a therapeutic target for EphA3+ T cell lymphomas with minimal detrimental effects on healthy T cells. Indeed, an anti-EphA3 monoclonal antibody has already been the subject of a Phase I clinical trial in patients with refractory hematologic malignancies (149). In addition to its role in T cell lymphomas, EphA3 also plays a role in multiple myeloma angiogenesis (150) suggesting that an effective anti-EphA3 therapy could be potentially utilized for treatment of both T and B cell malignancies. Along with EphA3, expression of the receptors EphA2, EphB3, and EphB6 has also been identified in many malignant T lymphocytes (86, 151). The potential involvement of these receptors in promoting survival of malignant immune cells suggests that they may also hold promise as future therapeutic targets. However, Eph receptors may also be involved in lymphoma suppression as has been shown in the case of a soluble form of EphA7 (152) and for EphB4 (153). This indicates that great care must be taken in designing future anti-Eph therapies to ensure specificity toward targeting particular Eph receptors of interest that may contribute to malignancies while avoiding targeting those that may benefit the host anti-tumor immune response.
Paradoxically, both an increase and a decrease in Eph receptor expression has been associated with cancer progression consistent with the functional complexity of interactions between different Eph-ephrin family members. For the most part, the roles for Eph receptors in malignancies have been investigated from a cellular biology perspective. For example, Eph receptor forward signaling can inhibit cancer cell migration, proliferation, and survival as well as tumor growth in mice (154). Angiogenesis is essential for both tumor growth and metastasis and some evidence suggests that interactions between EphA2 and ephrin-A1 (50, 144) as well as EphB4 and ephrin-B2 (50, 144) on tumor cells and vascular cells can lead to increased angiogenesis and tumor vascularization. Several recent studies have aimed to alter or redirect T cells to target Eph receptors on cancerous cells using unique approaches including the generation of chimeric antigen receptor (CAR) T cells in which the T cell receptor recognizes EphA2 (155–157). A second approach which involves administering a bi-specific antibody that recognizes EphA10 expressed on breast cancer cells as well as CD3 expressed on T cells aims to redirect cytotoxic CD8+ T cells to attack malignant EphA2+ cells (158). However, these studies have not comprehensively addressed the potentially negative effects that Eph-ephrin expression on T cells may have on the target cells, which often will also express the same receptors and their ligands. While the contribution of Eph-ephrin expression on cancerous cells to disease progression has been thoroughly investigated, the crucial role of the immune response in the recognition and containment of malignant cells is often overlooked. Given the nearly ubiquitous expression of Eph receptors on immune cells, the function that these receptors play in cancer immunology must also be understood in order to rationally design Eph-based anti-cancer therapeutics that incorporate the contributions of Eph receptors to the immune control of cancers.
Atherosclerosis
Atherosclerosis is a condition characterized by the hardening and narrowing of the arteries which can impede blood flow and lead to heart attack and stroke. Damage to the vascular endothelium triggers the process of atherosclerosis, and activation of platelets by subendothelial matrix-derived molecules such as collagen and fibronectin triggers the adhesion of platelets onto the endothelium. This, in turn, initiates the formation of plaques that harbor immune cells such as monocytes and macrophages which have migrated to the plaque in response to chemotactic signals (159).
Platelets, anuclear cell fragments derived from megakaryocytes, are components of the immune system capable of exerting effects on both the innate and adaptive branches. Human platelets express several members of the Eph-ephrin family including EphA4, EphB1, EphB2, and ephrin-B1. Forward signaling through the EphB2 receptor on platelets is associated with both thrombus formation and platelet activation in the absence of ligand contact (55). This suggests an inherent role of the Eph cytoplasmic signaling domain in contributing to platelet function. The key contribution of EphB2 to platelet activation and optimal functioning is also supported by the association between mutations in the human EphB2 gene and platelet dysfunction (56). Ligation of this family of molecules on platelets may contribute to granule secretion along with adhesive interactions between platelets. Subsequent platelet aggregation and thrombus formation can then follow mediated by αIIbβ3 integrin (52, 54, 160). Platelet granule release upon platelet activation is a highly inflammatory event. As such, Eph-mediated platelet activation is likely a key event mediating the immunopathogenic aspects of atherosclerosis.
In addition to platelets, other cells of the immune system can influence and enhance the inflammatory milieu of atherosclerotic plaques via Eph-ephrin interactions. Several studies have demonstrated a positive correlation between expression of EphB2, EphB4, ephrin-B1, and ephrin-B2 and the macrophage content of atherosclerotic plaques. These particular members of the Eph-ephrin family can contribute to the recruitment (161) and proinflammatory activation (58) of monocytes. Inflammation is likely further compounded by a suppression of localized chemotactic gradients by monocytes and/or macrophages leading to retention of these cells within lesions and augmentation of inflammatory responses (162). More work is needed to define precisely how Eph-ephrin interactions contribute to the pathogenesis of atherosclerosis. However, the abilities of these molecules to modulate the activation and chemotaxis of monocytes and macrophages, key events mediating plaque formation and growth, suggest a potential therapeutic avenue for this disease.
Fibrosis
Fibrosis describes the scarring of tissue that occurs through excess production of extracellular matrix proteins in an attempt to repair damaged tissue. While the causes of fibrosis can be highly varied, the general process is thought to result from chronic inflammation leading to the activation of myofibroblasts that produce molecules such as collagen and glycosaminoglycans in response to immune mediators such as transforming growth factor (TGF)-β (163). Recently, Eph receptors and their ligands have been implicated in the development of organ fibrosis. EphB2 has been identified as a pro-fibrogenic molecule that is essential for the development of liver fibrosis from both infectious and non-infectious etiologies (63). By mediating trafficking of ephrin-B-expressing macrophages to the liver, EphB2 can promote inflammatory signaling pathways that stimulate the differentiation of hepatic stellate cells into fibrogenic myofibroblasts. In a separate model of lung fibrosis, cleavage of the ligand ephrin-B2 on fibroblasts by the disintegrin and metalloproteinase domain-containing protein ADAM10 leads to increased fibroblast activation and subsequently increased skin and lung fibrosis (164). It is anticipated that similar processes involving Eph-ephrin interactions may underlie other fibrotic conditions such as systemic scleroderma.
Diseases of the Central Nervous System
Eph-ephrin interactions play a key role in neurological development, and neurological disorders can stem from dysfunctional Eph-ephrin interactions. Upregulation of EphA4 expression has been consistently observed after traumatic brain injury in both primates (165) and humans (166). Similarly, EphA4, along with several other Eph receptors, appears to inhibit neuronal regrowth and recovery after spinal cord injury in mice (167).
Multiple sclerosis (MS) is a disease in which the nerve cells of the brain become demyelinated and exposed rendering them unable to communicate efficiently. This leads to a variety of physical symptoms such as muscle weakness and poor coordination. It is the most common immune-mediated disease of the central nervous system (168) in which auto-reactive CD4+ T cells play a central role in contributing to the induction of inflammation and lesion formation in the brain and spinal cord. Increased expression of ephrin-A1, EphA3, EphA4, and EphA7 has been observed in axons of multiple sclerosis lesions (169). Ephrin-B1 and ephrin-B2 were also shown recently to be involved in T cell migration to the central nervous system in both the mouse model of EAE and in human MS (92) which indicates the potential importance of these molecules in mediating this disease. However, there remains a significant amount of knowledge to be gained before the full contribution of the Eph-ephrin molecules to MS can be fully understood.
Other diseases of the central nervous system that may involve Eph-ephrin interactions include Parkinson's disease (170) and Alzheimer's disease (171). Although studies thus far have only identified correlations between Eph expression and disease severity, the known roles for Ephs and ephrins in the immune system outlined in this review make these correlations worthy of further investigation.
Infectious Diseases
Given the central role of the immune system in defense against infectious pathogens, there is a surprising lack of reports regarding the involvement of Eph receptors in this regard although this has become a greater focus of research in recent years. A clear role for Ephs as viral entry receptors has been shown for Nipah and Hendra viruses (ephrin-B2 and ephrin-B3) (172, 173), Kaposi's sarcoma-associated herpesvirus (EphA2) (70, 174), and Epstein-Barr virus (EphA4 and EphA2) (76, 138, 140). Similarly, it was also recently described that the sporozoite stage of the Plasmodium parasite, the causative agent of malaria, engages EphA2 on liver hepatocytes in order to establish a productive infection (135) although additional entry receptors may be involved in this process as well (175). EphA2 is also used by the fungal pathogen Cryptococcus neoformans to traverse the blood-brain barrier in order to gain entry into the brain (137).
In addition to facilitating entry, it is likely that the ubiquitous expression of Eph receptors and ephrin ligands on the majority of cells in the immune system facilitates immune defense, although reports in support of this idea are currently sparse. One of the challenges in the study of Eph-ephrin molecules is the redundancy that can occur with respect to binding of Eph receptors to multiple ephrin ligands. Although one study demonstrates no effect of B cell-specific ephrin-B1 ligand deficiency on GC formation, plasmablast production, or B cell class-switching in mice after infection with either influenza virus or acute lymphocytic choriomeningitis virus (LCMV Armstrong) (77), compensatory activities of other ephrin-B ligands may mask a potential role of the ephrin-B ligands in general. For example, a T cell-specific dual deletion of both ephrin-B1 and ephrin-B2 does in fact lead to a defective immune response against the LCMV virus (116). One additional study demonstrates that Mycobacterium tuberculosis can manipulate EphA2 and ephrin-A1 expression in order to support granuloma formation (176) which, in turn, aids in bacterial immune evasion. These reports suggest that these molecules do play a role in the activation and regulation of immune responses against pathogens further highlighting the importance of this consideration in future therapeutic designs.
Concluding Remarks and Future Perspectives
The study of Eph receptors and ephrin ligands in immunology is an expanding and exciting area of research. Given that the association of this family of molecules with the immune system has only emerged in the past few decades, it is highly likely that many of the roles for the Eph-ephrin cell-cell communication system in immunity have yet to be revealed.
Ephs and ephrins represent promising therapeutic targets for immune manipulation. Although some of the challenges to this approach include the nearly ubiquitous expression of Ephs and ephrins and the often dichotomous consequences of Eph receptor signaling in different contexts, the high evolutionary conservation of this family of molecules increases the chances that Eph-based therapeutics developed and tested in animal models have the potential to be highly translatable to humans. Indeed, there are several Eph-ephrin targeting strategies currently progressing through clinical trials (134, 136, 177) that include blocking receptor-ligand binding as well as downstream signaling using a variety of therapeutic compounds. The potential repurposing of these drugs for new pathological indications is also attractive. Emerging roles for Eph-ephrin signaling in normal immune system functionality along with their contributions to disease pathogenesis guarantee an exciting new chapter in Eph biology and drug discovery.
Author Contributions
This review was conceptualized by TD and TL. The initial draft was written by TD with subsequent drafts edited and approved for publication by both TD and TL.
Funding
This work was supported by grants from the National Institute for Neurological Disorders and Stroke to TD (F31NS098736) and to TL (R21NS085382 and R01NS097819) along with training grants T32AI007610 and T32AI106699 to TD.
Conflict of Interest Statement
The authors declare that the research was conducted in the absence of any commercial or financial relationships that could be construed as a potential conflict of interest.
References
1. Tuzi NL, Gullick WJ. eph, the largest known family of putative growth factor receptors. Br J Cancer. (1994) 69:417–21. doi: 10.1038/bjc.1994.77
2. Pasquale EB. Eph receptor signalling casts a wide net on cell behaviour. Nat Rev Mol Cell Biol. (2005) 6:462–75. doi: 10.1038/nrm1662
3. Pasquale EB. Eph-ephrin bidirectional signaling in physiology and disease. Cell. (2008) 133:38–52. doi: 10.1016/j.cell.2008.03.011
4. Batlle E, Wilkinson DG. Molecular mechanisms of cell segregation and boundary formation in development and tumorigenesis. Cold Spring Harb Perspect Biol. (2012) 4:a008227. doi: 10.1101/cshperspect.a008227
5. Lisabeth EM, Falivelli G, Pasquale EB. Eph receptor signaling and ephrins. Cold Spring Harb Perspect Biol. (2013) 5:a009159. doi: 10.1101/cshperspect.a009159
6. Hirai H, Maru Y, Hagiwara K, Nishida J, Takaku F. A novel putative tyrosine kinase receptor encoded by the eph gene. Science. (1987) 238:1717–20. doi: 10.1126/science.2825356
7. Pasquale EB. The Eph family of receptors. Curr Opin Cell Biol. (1997) 9:608–15. doi: 10.1016/S0955-0674(97)80113-5
8. Lindberg RA, Hunter T. cDNA cloning and characterization of eck, an epithelial cell receptor protein-tyrosine kinase in the eph/elk family of protein kinases. Mol Cell Biol. (1990) 10:6316–24. doi: 10.1128/MCB.10.12.6316
9. Boyd AW, Ward LD, Wicks IP, Simpson RJ, Salvaris E, Wilks A, et al. Isolation and characterization of a novel receptor-type protein tyrosine kinase (hek) from a human pre-B cell line. J Biol Chem. (1992) 267:3262–7.
10. Gilardi-Hebenstreit P, Nieto MA, Frain M, Mattei MG, Chestier A, Wilkinson DG, et al. An Eph-related receptor protein tyrosine kinase gene segmentally expressed in the developing mouse hindbrain. Oncogene. (1992) 7:2499–506.
11. Zhou R, Copeland TD, Kromer LF, Schulz NT. Isolation and characterization of Bsk, a growth factor receptor-like tyrosine kinase associated with the limbic system. J Neurosci Res. (1994) 37:129–43. doi: 10.1002/jnr.490370117
12. Maisonpierre PC, Barrezueta NX, Yancopoulos GD. Ehk-1 and Ehk-2: two novel members of the Eph receptor-like tyrosine kinase family with distinctive structures and neuronal expression. Oncogene. (1993) 8:3277–88.
13. Fox GM, Holst PL, Chute HT, Lindberg RA, Janssen AM, Basu R, et al. cDNA cloning and tissue distribution of five human EPH-like receptor protein-tyrosine kinases. Oncogene. (1995) 10:897–905.
14. Chan J, Watt VM. eek and erk, new members of the eph subclass of receptor protein-tyrosine kinases. Oncogene. (1991) 6:1057–61.
15. Aasheim HC, Patzke S, Hjorthaug HS, Finne EF. Characterization of a novel Eph receptor tyrosine kinase, EphA10, expressed in testis. Biochim Biophys Acta. (2005) 1723:1–7. doi: 10.1016/j.bbagen.2005.01.011
16. Letwin K, Yee SP, Pawson T. Novel protein-tyrosine kinase cDNAs related to fps/fes and eph cloned using anti-phosphotyrosine antibody. Oncogene. (1988) 3:621–7.
17. Bohme B, Holtrich U, Wolf G, Luzius H, Grzeschik KH, Strebhardt K, et al. PCR mediated detection of a new human receptor-tyrosine-kinase, HEK 2. Oncogene. (1993) 8:2857–62.
18. Andres AC, Reid HH, Zurcher G, Blaschke RJ, Albrecht D, Ziemiecki A. Expression of two novel eph-related receptor protein tyrosine kinases in mammary gland development and carcinogenesis. Oncogene. (1994) 9:1461–7.
19. Gurniak CB, Berg LJ. A new member of the Eph family of receptors that lacks protein tyrosine kinase activity. Oncogene. (1996) 13:777–86.
20. Gale NW, Holland SJ, Valenzuela DM, Flenniken A, Pan L, Ryan TE, et al. Eph receptors and ligands comprise two major specificity subclasses and are reciprocally compartmentalized during embryogenesis. Neuron. (1996) 17:9–19. doi: 10.1016/S0896-6273(00)80276-7
21. Munthe E, Rian E, Holien T, Rasmussen A, Levy FO, Aasheim H. Ephrin-B2 is a candidate ligand for the Eph receptor, EphB6. FEBS Lett. (2000) 466:169–74. doi: 10.1016/S0014-5793(99)01793-7
22. Manning G, Whyte DB, Martinez R, Hunter T, Sudarsanam S. The protein kinase complement of the human genome. Science. (2002) 298:1912–34. doi: 10.1126/science.1075762
23. Henkemeyer M, Itkis OS, Ngo M, Hickmott PW, Ethell IM. Multiple EphB receptor tyrosine kinases shape dendritic spines in the hippocampus. J Cell Biol. (2003) 163:1313–26. doi: 10.1083/jcb.200306033
24. Liebl DJ, Morris CJ, Henkemeyer M, Parada LF. mRNA expression of ephrins and Eph receptor tyrosine kinases in the neonatal and adult mouse central nervous system. J Neurosci Res. (2003) 71:7–22. doi: 10.1002/jnr.10457
25. Himanen JP, Chumley MJ, Lackmann M, Li C, Barton WA, Jeffrey PD, et al. Repelling class discrimination: ephrin-A5 binds to and activates EphB2 receptor signaling. Nat Neurosci. (2004) 7:501–9. doi: 10.1038/nn1237
26. Noberini R, Rubio de la Torre E, Pasquale EB. Profiling Eph receptor expression in cells and tissues: a targeted mass spectrometry approach. Cell Adh Migr. (2012) 6:102–12. doi: 10.4161/cam.19620
27. Xia Y, Luo C, Dai S, Yao D. Increased EphA/ephrinA expression in hippocampus of pilocarpine treated mouse. Epilepsy Res. (2013) 105:20–9. doi: 10.1016/j.eplepsyres.2013.01.001
28. Poliakov A, Cotrina M, Wilkinson DG. Diverse roles of eph receptors and ephrins in the regulation of cell migration and tissue assembly. Dev Cell. (2004) 7:465–80. doi: 10.1016/j.devcel.2004.09.006
29. Niethamer TK, Bush JO. Getting direction(s): The Eph/ephrin signaling system in cell positioning. Dev Biol. (2018) 447:42–57. doi: 10.1016/j.ydbio.2018.01.012
30. Himanen JP, Henkemeyer M, Nikolov DB. Crystal structure of the ligand-binding domain of the receptor tyrosine kinase EphB2. Nature. (1998) 396:486–91. doi: 10.1038/24904
31. Pasquale EB. Eph-ephrin promiscuity is now crystal clear. Nat Neurosci. (2004) 7:417–8. doi: 10.1038/nn0504-417
32. Nikolov DB, Xu K, Himanen JP. Eph/ephrin recognition and the role of Eph/ephrin clusters in signaling initiation. Biochim Biophys Acta. (2013) 1834:2160–5. doi: 10.1016/j.bbapap.2013.04.020
33. Takasugi M, Okada R, Takahashi A, Virya Chen D, Watanabe S, Hara E. Small extracellular vesicles secreted from senescent cells promote cancer cell proliferation through EphA2. Nat Commun. (2017) 8:15729. doi: 10.1038/ncomms15728
34. Holland SJ, Gale NW, Mbamalu G, Yancopoulos GD, Henkemeyer M, Pawson T. Bidirectional signalling through the EPH-family receptor Nuk and its transmembrane ligands. Nature. (1996) 383:722–5. doi: 10.1038/383722a0
35. Bruckner K, Pasquale EB, Klein R. Tyrosine phosphorylation of transmembrane ligands for Eph receptors. Science. (1997) 275:1640–3. doi: 10.1126/science.275.5306.1640
36. Murai KK, Pasquale EB. 'Eph'ective signaling: forward, reverse and crosstalk. J Cell Sci. (2003) 116:2823–32. doi: 10.1242/jcs.00625
37. Himanen JP, Yermekbayeva L, Janes PW, Walker JR, Xu K, Atapattu L, et al. Architecture of Eph receptor clusters. Proc Natl Acad Sci USA. (2010) 107:10860–5. doi: 10.1073/pnas.1004148107
38. Janes PW, Griesshaber B, Atapattu L, Nievergall E, Hii LL, Mensinga A, et al. Eph receptor function is modulated by heterooligomerization of A and B type Eph receptors. J Cell Biol. (2011) 195:1033–45. doi: 10.1083/jcb.201104037
39. Marston DJ, Dickinson S, Nobes CD. Rac-dependent trans-endocytosis of ephrinBs regulates Eph-ephrin contact repulsion. Nat Cell Biol. (2003) 5:879–88. doi: 10.1038/ncb1044
40. Zimmer M, Palmer A, Kohler J, Klein R. EphB-ephrinB bi-directional endocytosis terminates adhesion allowing contact mediated repulsion. Nat Cell Biol. (2003) 5:869–78. doi: 10.1038/ncb1045
41. Gaitanos TN, Koerner J, Klein R. Tiam-Rac signaling mediates trans-endocytosis of ephrin receptor EphB2 and is important for cell repulsion. J Cell Biol. (2016) 214:735–52. doi: 10.1083/jcb.201512010
42. Hattori M, Osterfield M, Flanagan JG. Regulated cleavage of a contact-mediated axon repellent. Science. (2000) 289:1360–5. doi: 10.1126/science.289.5483.1360
43. Janes PW, Saha N, Barton WA, Kolev MV, Wimmer-Kleikamp SH, Nievergall E, et al. Adam meets Eph: an ADAM substrate recognition module acts as a molecular switch for ephrin cleavage in trans. Cell. (2005) 123:291–304. doi: 10.1016/j.cell.2005.08.014
44. Atapattu L, Lackmann M, Janes PW. The role of proteases in regulating Eph/ephrin signaling. Cell Adh Migr. (2014) 8:294–307. doi: 10.4161/19336918.2014.970026
45. Holmberg J, Clarke DL, Frisen J. Regulation of repulsion versus adhesion by different splice forms of an Eph receptor. Nature. (2000) 408:203–6. doi: 10.1038/35041577
46. Halloran MC, Wolman MA. Repulsion or adhesion: receptors make the call. Curr Opin Cell Biol. (2006) 18:533–40. doi: 10.1016/j.ceb.2006.08.010
47. Yin Y, Yamashita Y, Noda H, Okafuji T, Go MJ, Tanaka H. EphA receptor tyrosine kinases interact with co-expressed ephrin-A ligands in cis. Neurosci Res. (2004) 48:285–96. doi: 10.1016/j.neures.2003.11.009
48. Carvalho RF, Beutler M, Marler KJ, Knoll B, Becker-Barroso E, Heintzmann R, et al. Silencing of EphA3 through a cis interaction with ephrinA5. Nat Neurosci. (2006) 9:322–30. doi: 10.1038/nn1655
49. Hafner C, Schmitz G, Meyer S, Bataille F, Hau P, Langmann T, et al. Differential gene expression of Eph receptors and ephrins in benign human tissues and cancers. Clin Chem. (2004) 50:490–9. doi: 10.1373/clinchem.2003.026849
50. Mosch B, Reissenweber B, Neuber C, Pietzsch J. Eph receptors and ephrin ligands: important players in angiogenesis and tumor angiogenesis. J Oncol. (2010) 2010:135285. doi: 10.1155/2010/135285
51. Klein R. Eph/ephrin signalling during development. Development. (2012) 139:4105–9. doi: 10.1242/dev.074997
52. Prevost N, Woulfe D, Tanaka T, Brass LF. Interactions between Eph kinases and ephrins provide a mechanism to support platelet aggregation once cell-to-cell contact has occurred. Proc Natl Acad Sci USA. (2002) 99:9219–24. doi: 10.1073/pnas.142053899
53. Prevost N, Woulfe DS, Tognolini M, Tanaka T, Jian W, Fortna RR, et al. Signaling by ephrinB1 and Eph kinases in platelets promotes Rap1 activation, platelet adhesion, and aggregation via effector pathways that do not require phosphorylation of ephrinB1. Blood. (2004) 103:1348–55. doi: 10.1182/blood-2003-06-1781
54. Prévost N, Woulfe DS, Jiang H, Stalker TJ, Marchese P, Ruggeri ZM, et al. Eph kinases and ephrins support thrombus growth and stability by regulating integrin outside-in signaling in platelets. Proc Natl Acad Sci USA. (2005) 102:9820–5. doi: 10.1073/pnas.0404065102
55. Vaiyapuri S, Sage T, Rana RH, Schenk MP, Ali MS, Unsworth AJ, et al. EphB2 regulates contact-dependent and contact-independent signaling to control platelet function. Blood. (2015) 125:720–30. doi: 10.1182/blood-2014-06-585083
56. Berrou E, Soukaseum C, Favier R, Adam F, Elaib Z, Kauskot A, et al. A mutation of the human EPHB2 gene leads to a major platelet functional defect. Blood. (2018) 132:2067–77. doi: 10.1182/blood-2018-04-845644
57. Pfaff D, Heroult M, Riedel M, Reiss Y, Kirmse R, Ludwig T, et al. Involvement of endothelial ephrin-B2 in adhesion and transmigration of EphB-receptor-expressing monocytes. J Cell Sci. (2008) 121:3842–50. doi: 10.1242/jcs.030627
58. Braun J, Hoffmann SC, Feldner A, Ludwig T, Henning R, Hecker M, et al. Endothelial cell ephrinB2-dependent activation of monocytes in arteriosclerosis. Arterioscler Thromb Vasc Biol. (2011) 31:297–305. doi: 10.1161/ATVBAHA.110.217646
59. Funk SD, Orr AW. Ephs and ephrins resurface in inflammation, immunity, and atherosclerosis. Pharmacol Res. (2013) 67:42–52. doi: 10.1016/j.phrs.2012.10.008
60. Jellinghaus S, Poitz DM, Ende G, Augstein A, Weinert S, Stutz B, et al. Ephrin-A1/EphA4-mediated adhesion of monocytes to endothelial cells. Biochim Biophys Acta. (2013) 1833:2201–11. doi: 10.1016/j.bbamcr.2013.05.017
61. Ende G, Poitz DM, Wiedemann E, Augstein A, Friedrichs J, Giebe S, et al. TNF-alpha-mediated adhesion of monocytes to endothelial cells-The role of ephrinA1. J Mol Cell Cardiol. (2014) 77:125–35. doi: 10.1016/j.yjmcc.2014.10.010
62. Liu H, Devraj K, Moller K, Liebner S, Hecker M, Korff T. EphrinB-mediated reverse signalling controls junctional integrity and pro-inflammatory differentiation of endothelial cells. Thromb Haemost. (2014) 112:151–63. doi: 10.1160/TH13-12-1034
63. Mimche PN, Brady LM, Bray CF, Lee CM, Thapa M, King TP, et al. The receptor tyrosine kinase EphB2 promotes hepatic fibrosis in mice. Hepatology. (2015) 62:900–14. doi: 10.1002/hep.27792
64. Poitz DM, Ende G, Stutz B, Augstein A, Friedrichs J, Brunssen C, et al. EphrinB2/EphA4-mediated activation of endothelial cells increases monocyte adhesion. Mol Immunol. (2015) 68:648–56. doi: 10.1016/j.molimm.2015.10.009
65. Saeki N, Nishino S, Shimizu T, Ogawa K. EphA2 promotes cell adhesion and spreading of monocyte and monocyte/macrophage cell lines on integrin ligand-coated surfaces. Cell Adh Migr. (2015) 9:469–82. doi: 10.1080/19336918.2015.1107693
66. Mukai M, Suruga N, Saeki N, Ogawa K. EphA receptors and ephrin-A ligands are upregulated by monocytic differentiation/maturation and promote cell adhesion and protrusion formation in HL60 monocytes. BMC Cell Biol. (2017) 18:28. doi: 10.1186/s12860-017-0144-x
67. Rissoan M-C, Duhen T, Bridon J-M, Bendriss-Vermare N, Péronne C, Vis BdS, et al. Subtractive hybridization reveals the expression of immunoglobulinlike transcript 7, Eph-B1, granzyme B, and 3 novel transcripts in human plasmacytoid dendritic cells. Blood. (2002) 100:3295–3303. doi: 10.1182/blood-2002-02-0638
68. de Saint-Vis B, Bouchet C, Gautier G, Valladeau J, Caux C, Garrone P. Human dendritic cells express neuronal Eph receptor tyrosine kinases: role of EphA2 in regulating adhesion to fibronectin. Blood. (2003) 102:4431–40. doi: 10.1182/blood-2003-02-0500
69. Mimche PN, Brady LM, Keeton S, Fenne DS, King TP, Quicke KM, et al. Expression of the receptor tyrosine kinase EphB2 on dendritic cells is modulated by toll-like receptor ligation but is not required for T cell activation. PLoS ONE. (2015) 10:e0138835. doi: 10.1371/journal.pone.0138835
70. Rappocciolo G, Jais M, Piazza PA, DeLucia DC, Jenkins FJ, Rinaldo CR. Human herpesvirus 8 infects and replicates in langerhans cells and interstitial dermal dendritic cells and impairs their function. J Virol. (2017) 91:e00909–17. doi: 10.1128/JVI.00909-17
71. Wicks IP, Wilkinson D, Salvaris E, Boyd AW. Molecular cloning of HEK, the gene encoding a receptor tyrosine kinase expressed by human lymphoid tumor cell lines. Proc Natl Acad Sci USA. (1992) 89:1611–5. doi: 10.1073/pnas.89.5.1611
72. Nakanishi H, Nakamura T, Canaani E, Croce CM. ALL1 fusion proteins induce deregulation of EphA7 and ERK phosphorylation in human acute leukemias. Proc Natl Acad Sci USA. (2007) 104:14442–7. doi: 10.1073/pnas.0703211104
73. Alonso CL, Trinidad EM, de Garcillan B, Ballesteros M, Castellanos M, Cotillo I, et al. Expression profile of Eph receptors and ephrin ligands in healthy human B lymphocytes and chronic lymphocytic leukemia B-cells. Leuk Res. (2009) 33:395–406. doi: 10.1016/j.leukres.2008.08.010
74. Yu M, Liang W, Wen S, Zhao T, Zhu MX, Li HH, et al. EphB2 contributes to human naive B-cell activation and is regulated by miR-185. Faseb J. (2014) 28:3609–17. doi: 10.1096/fj.13-247759
75. Flores MA, Fortea P, Trinidad EM, Garcia D, Soler G, Ortuno FJ, et al. EphrinA4 plays a critical role in alpha4 and alphaL mediated survival of human CLL cells during extravasation. Oncotarget. (2016) 7:48481–500. doi: 10.18632/oncotarget.10311
76. Huang YC, Lin SJ, Lin KM, Chou YC, Lin CW, Yu SC, et al. Regulation of EBV LMP1-triggered EphA4 downregulation in EBV-associated B lymphoma and its impact on patients' survival. Blood. (2016) 128:1578–89. doi: 10.1182/blood-2016-02-702530
77. Laidlaw BJ, Schmidt TH, Green JA, Allen CD, Okada T, Cyster JG. The Eph-related tyrosine kinase ligand Ephrin-B1 marks germinal center and memory precursor B cells. J Exp Med. (2017) 214:639–49. doi: 10.1084/jem.20161461
78. Lu P, Shih C, Qi H. Ephrin B1-mediated repulsion and signaling control germinal center T cell territoriality and function. Science. (2017) 356:eaai9264. doi: 10.1126/science.aai9264
79. Yu G, Luo H, Wu Y, Wu J. Mouse ephrinB3 augments T-cell signaling and responses to T-cell receptor ligation. J Biol Chem. (2003) 278:47209–16. doi: 10.1074/jbc.M306659200
80. Smith LM, Walsh PT, Rudiger T, Cotter TG, Mc Carthy TV, Marx A, et al. EphA3 is induced by CD28 and IGF-1 and regulates cell adhesion. Exp Cell Res. (2004) 292:295–303. doi: 10.1016/j.yexcr.2003.08.021
81. Wohlfahrt JG, Karagiannidis C, Kunzmann S, Epstein MM, Kempf W, Blaser K, et al. Ephrin-A1 suppresses Th2 cell activation and provides a regulatory link to lung epithelial cells. J Immunol. (2004) 172:843–50. doi: 10.4049/jimmunol.172.2.843
82. Yu G, Luo H, Wu Y, Wu J. EphrinB1 is essential in T-cell-T-cell co-operation during T-cell activation. J Biol Chem. (2004) 279:55531–9. doi: 10.1074/jbc.M410814200
83. Kitamura T, Kabuyama Y, Kamataki A, Homma MK, Kobayashi H, Aota S, et al. Enhancement of lymphocyte migration and cytokine production by ephrinB1 system in rheumatoid arthritis. Am J Physiol Cell Physiol. (2008) 294:C189–96. doi: 10.1152/ajpcell.00314.2007
84. Sharfe N, Nikolic M, Cimpeon L, Van De Kratts A, Freywald A, Roifman CM. EphA and ephrin-A proteins regulate integrin-mediated T lymphocyte interactions. Mol Immunol. (2008) 45:1208–20. doi: 10.1016/j.molimm.2007.09.019
85. Holen HL, Nustad K, Aasheim H-C. Activation of EphA receptors on CD4+CD45RO+ memory cells stimulates migration. J Leukoc Biol. (2010) 87:1059–68. doi: 10.1189/jlb.0709497
86. Maddigan A, Truitt L, Arsenault R, Freywald T, Allonby O, Dean J, et al. EphB receptors trigger Akt activation and suppress Fas receptor-induced apoptosis in malignant T lymphocytes. J Immunol. (2011) 187:5983–94. doi: 10.4049/jimmunol.1003482
87. Jin W, Qi S, Luo H. T cell-specific deletion of EFNB2 minimally affects T cell development and function. Mol Immunol. (2012) 52:141–7. doi: 10.1016/j.molimm.2012.05.008
88. Kawano H, Katayama Y, Minagawa K, Shimoyama M, Henkemeyer M, Matsui T. A novel feedback mechanism by Ephrin-B1/B2 in T-cell activation involves a concentration-dependent switch from costimulation to inhibition. Eur J Immunol. (2012) 42:1562–72. doi: 10.1002/eji.201142175
89. Cejalvo T, Munoz JJ, Tobajas E, Fanlo L, Alfaro D, Garcia-Ceca J, et al. Ephrin-B-dependent thymic epithelial cell-thymocyte interactions are necessary for correct T cell differentiation and thymus histology organization: relevance for thymic cortex development. J Immunol. (2013) 190:2670–81. doi: 10.4049/jimmunol.1201931
90. Nguyen TM, Arthur A, Hayball JD, Gronthos S. EphB and Ephrin-B interactions mediate human mesenchymal stem cell suppression of activated T-cells. Stem Cells Dev. (2013) 22:2751–64. doi: 10.1089/scd.2012.0676
91. Hu Y, Wang X, Wu Y, Jin W, Cheng B, Fang X, et al. Role of EFNB1 and EFNB2 in mouse collagen-induced arthritis and human rheumatoid arthritis. Arthritis Rheumatol. (2015) 67:1778–88. doi: 10.1002/art.39116
92. Luo H, Broux B, Wang X, Hu Y, Ghannam S, Jin W, et al. EphrinB1 and EphrinB2 regulate T cell chemotaxis and migration in experimental autoimmune encephalomyelitis and multiple sclerosis. Neurobiol Dis. (2016) 91:292–306. doi: 10.1016/j.nbd.2016.03.013
93. Wu J, Luo H. Recent advances on T-cell regulation by receptor tyrosine kinases. Curr Opin Hematol. (2005) 12:292–7. doi: 10.1097/01.moh.0000166497.26397.9f
94. Pfaff D, Fiedler U, Augustin HG. Emerging roles of the Angiopoietin-Tie and the ephrin-Eph systems as regulators of cell trafficking. J Leukoc Biol. (2006) 80:719–26. doi: 10.1189/jlb.1105652
95. Campbell TN, Robbins SM. The Eph receptor/ephrin system: an emerging player in the invasion game. Curr Issues Mol Biol. (2008) 10:61–6.
96. Suenobu S, Takakura N, Inada T, Yamada Y, Yuasa H, Zhang X-Q, et al. A role of EphB4 receptor and its ligand, ephrin-B2, in erythropoiesis. Biochem Biophys Res Commun. (2002) 293:1124–31. doi: 10.1016/S0006-291X(02)00330-3
97. Kwak H, Salvucci O, Weigert R, Martinez-Torrecuadrada JL, Henkemeyer M, Poulos MG, et al. Sinusoidal ephrin receptor EPHB4 controls hematopoietic progenitor cell mobilization from bone marrow. J Clin Invest. (2016) 126:4554–68. doi: 10.1172/JCI87848
98. Wang Z, Miura N, Bonelli A, Mole P, Carlesso N, Olson DP, et al. Receptor tyrosine kinase, EphB4 (HTK), accelerates differentiation of select human hematopoietic cells. Blood. (2002) 99:2740–7. doi: 10.1182/blood.V99.8.2740
99. Batlle E, Henderson JT, Beghtel H, van den Born MMW, Sancho E, Huls G, et al. β-Catenin and TCF mediate cell positioning in the intestinal epithelium by controlling the expression of EphB/EphrinB. Cell. (2002) 111:251–63. doi: 10.1016/S0092-8674(02)01015-2
100. Merlos-Suárez A, Barriga FM, Jung P, Iglesias M, Céspedes MV, Rossell D, et al. The intestinal stem cell signature identifies colorectal cancer stem cells and predicts disease relapse. Cell Stem Cell. (2011) 8:511–24. doi: 10.1016/j.stem.2011.02.020
101. Nguyen TM, Arthur A, Panagopoulos R, Paton S, Hayball JD, Zannettino AC, et al. EphB4 expressing stromal cells exhibit an enhanced capacity for hematopoietic stem cell maintenance. Stem Cells. (2015) 33:2838–49. doi: 10.1002/stem.2069
102. Lazarova P, Wu Q, Kvalheim G, Suo Z, Haakenstad KW, Metodiev K, et al. Growth factor receptors in hematopoietic stem cells: EPH family expression in CD34+ and CD133+ cell populations from mobilized peripheral blood. Int J Immunopathol Pharmacol. (2006) 19:49–56 doi: 10.1177/205873920601900105
103. Aasheim HC, Munthe E, Funderud S, Smeland EB, Beiske K, Logtenberg T. A splice variant of human ephrin-A4 encodes a soluble molecule that is secreted by activated human B lymphocytes. Blood. (2000) 95:221–30.
104. Luo H, Yu G, Wu Y, Wu J. EphB6 crosslinking results in costimulation of T cells. J Clin Invest. (2002) 110:1141–50. doi: 10.1172/JCI0215883
105. Yu G, Luo H, Wu Y, Wu J. Ephrin B2 induces T cell costimulation. J Immunol. (2003) 171:106–14. doi: 10.4049/jimmunol.171.1.106
106. Luo H, Yu G, Tremblay J, Wu J. EphB6-null mutation results in compromised T cell function. J Clin Invest. (2004) 114:1762–73. doi: 10.1172/JCI21846
107. Luo H, Wan X, Wu Y, Wu J. Cross-linking of EphB6 resulting in signal transduction and apoptosis in Jurkat cells. J Immunol. (2001) 167:1362–70. doi: 10.4049/jimmunol.167.3.1362
108. Park S. Brain-region specific apoptosis triggered by Eph/ephrin signaling. Exp Neurobiol. (2013) 22:143–8. doi: 10.5607/en.2013.22.3.143
109. Freywald A, Sharfe N, Miller CD, Rashotte C, Roifman CM. EphA receptors inhibit anti-CD3-induced apoptosis in thymocytes. J Immunol. (2006) 176:4066–74 doi: 10.4049/jimmunol.176.7.4066
110. Freywald A, Sharfe N, Rashotte C, Grunberger T, Roifman CM. The EphB6 receptor inhibits JNK activation in T lymphocytes and modulates T cell receptor-mediated responses. J Biol Chem. (2003) 278:10150–6. doi: 10.1074/jbc.M208179200
111. Montero-Herradon S, Garcia-Ceca J, Sanchez Del Collado B, Alfaro D, Zapata AG. Eph/ephrin-B-mediated cell-to-cell interactions govern MTS20(+) thymic epithelial cell development. Histochem Cell Biol. (2016) 146:167–82. doi: 10.1007/s00418-016-1431-x
112. Stimamiglio MA, Jimenez E, Silva-Barbosa SD, Alfaro D, Garcia-Ceca JJ, Munoz JJ, et al. EphB2-mediated interactions are essential for proper migration of T cell progenitors during fetal thymus colonization. J Leukoc Biol. (2010) 88:483–94. doi: 10.1189/jlb.0210079
113. Cejalvo T, Munoz JJ, Tobajas E, Alfaro D, Garcia-Ceca J, Zapata A. Conditioned deletion of ephrinB1 and/or ephrinB2 in either thymocytes or thymic epithelial cells alters the organization of thymic medulla and favors the appearance of thymic epithelial cysts. Histochem Cell Biol. (2015) 143:517–29. doi: 10.1007/s00418-014-1296-9
114. Munoz JJ, Alonso CL, Sacedon R, Crompton T, Vicente A, Jimenez E, et al. Expression and function of the Eph A receptors and their ligands ephrins A in the rat thymus. J Immunol. (2002) 169:177–84. doi: 10.4049/jimmunol.169.1.177
115. Alfaro D, Garcia-Ceca JJ, Cejalvo T, Jimenez E, Jenkinson EJ, Anderson G, et al. EphrinB1-EphB signaling regulates thymocyte-epithelium interactions involved in functional T cell development. Eur J Immunol. (2007) 37:2596–605. doi: 10.1002/eji.200737097
116. Luo H, Charpentier T, Wang X, Qi S, Han B, Wu T, et al. Efnb1 and Efnb2 proteins regulate thymocyte development, peripheral T cell differentiation, and antiviral immune responses and are essential for interleukin-6 (IL-6) signaling. J Biol Chem. (2011) 286:41135–52. doi: 10.1074/jbc.M111.302596
117. Alfaro D, Munoz JJ, Garcia-Ceca J, Cejalvo T, Jimenez E, Zapata A. Alterations in the thymocyte phenotype of EphB-deficient mice largely affect the double negative cell compartment. Immunology. (2008) 125:131–43. doi: 10.1111/j.1365-2567.2008.02828.x
118. Muñoz JJ, Alfaro D, García-Ceca J, Alonso -C. LM, Jiménez E, Zapata A. Thymic alterations in EphA4-deficient mice. J Immunol. (2006) 177:804–13. doi: 10.4049/jimmunol.177.2.804
119. Victora GD. SnapShot: the germinal center reaction. Cell. (2014) 159:700–700.e701. doi: 10.1016/j.cell.2014.10.012
120. Augustin HG, Reiss Y. EphB receptors and ephrinB ligands: regulators of vascular assembly and homeostasis. Cell Tissue Res. (2003) 314:25–31. doi: 10.1007/s00441-003-0770-9
121. Makinen T, Adams RH, Bailey J, Lu Q, Ziemiecki A, Alitalo K, et al. PDZ interaction site in ephrinB2 is required for the remodeling of lymphatic vasculature. Genes Dev. (2005) 19:397–410. doi: 10.1101/gad.330105
122. Konda N, Saeki N, Nishino S, Ogawa K. Truncated EphA2 likely potentiates cell adhesion via integrins as well as infiltration and/or lodgment of a monocyte/macrophage cell line in the red pulp and marginal zone of the mouse spleen, where ephrin-A1 is prominently expressed in the vasculature. Histochem Cell Biol. (2017) 147:317–39. doi: 10.1007/s00418-016-1494-8
123. Munthe E, Finne EF, Aasheim HC. Expression and functional effects of Eph receptor tyrosine kinase A family members on Langerhans like dendritic cells. BMC Immunol. (2004) 5:9. doi: 10.1186/1471-2172-5-9
124. Trinidad EM, Zapata AG, Alonso-Colmenar LM. Eph-ephrin bidirectional signaling comes into the context of lymphocyte transendothelial migration. Cell Adhes Migr. (2010) 4:363–7. doi: 10.4161/cam.4.3.11586
125. Aasheim HC, Delabie J, Finne EF. Ephrin-A1 binding to CD4+ T lymphocytes stimulates migration and induces tyrosine phosphorylation of PYK2. Blood. (2005) 105:2869–76. doi: 10.1182/blood-2004-08-2981
126. Hjorthaug HS, Aasheim HC. Ephrin-A1 stimulates migration of CD8+CCR7+ T lymphocytes. Eur J Immunol. (2007) 37:2326–36. doi: 10.1002/eji.200737111
127. Funk SD, Finney AC, Yurdagul AJr, Pattillo CB, Orr AW. EphA2 stimulates VCAM-1 expression through calcium-dependent NFAT1 activity. Cell Signal. (2018) 49:30–8. doi: 10.1016/j.cellsig.2018.05.008
128. Sharfe N, Freywald A, Toro A, Dadi H, Roifman C. Ephrin stimulation modulates T cell chemotaxis. Eur J Immunol. (2002) 32:3745–55. doi: 10.1002/1521-4141(200212)32:12<3745::AID-IMMU3745>3.0.CO;2-M
129. Chen Y, Fu AK, Ip NY. Eph receptors at synapses: implications in neurodegenerative diseases. Cell Signal. (2012) 24:606–11. doi: 10.1016/j.cellsig.2011.11.016
130. Coulthard MG, Morgan M, Woodruff TM, Arumugam TV, Taylor SM, Carpenter TC, et al. Eph/Ephrin signaling in injury and inflammation. Am J Pathol. (2012) 181:1493–503. doi: 10.1016/j.ajpath.2012.06.043
131. Pasquale EB. Eph receptors and ephrins in cancer: bidirectional signalling and beyond. Nat Rev Cancer. (2010) 10:165–80. doi: 10.1038/nrc2806
132. Cisse M, Checler F. Eph receptors: new players in Alzheimer's disease pathogenesis. Neurobiol Dis. (2015) 73:137–49. doi: 10.1016/j.nbd.2014.08.028
133. Malik VA, Di Benedetto B. The blood-brain barrier and the EphR/Ephrin system: perspectives on a link between neurovascular and neuropsychiatric disorders. Front Mol Neurosci. (2018) 11:127. doi: 10.3389/fnmol.2018.00127
134. Barquilla A, Pasquale EB. Eph receptors and ephrins: therapeutic opportunities. Annu Rev Pharmacol Toxicol. (2015) 55:465–87. doi: 10.1146/annurev-pharmtox-011112-140226
135. Kaushansky A, Douglass AN, Arang N, Vigdorovich V, Dambrauskas N, Kain HS, et al. Malaria parasites target the hepatocyte receptor EphA2 for successful host infection. Science. (2015) 350:1089–92. doi: 10.1126/science.aad3318
136. Lodola A, Giorgio C, Incerti M, Zanotti I, Tognolini M. Targeting Eph/ephrin system in cancer therapy. Eur J Med Chem. (2017) 142:152–62. doi: 10.1016/j.ejmech.2017.07.029
137. Aaron PA, Jamklang M, Uhrig JP, Gelli A. The blood-brain barrier internalises Cryptococcus neoformans via the EphA2-tyrosine kinase receptor. Cell Microbiol. (2018) 20:e12811. doi: 10.1111/cmi.12811
138. Chen J, Sathiyamoorthy K, Zhang X, Schaller S, Perez White BE, Jardetzky TS, et al. Ephrin receptor A2 is a functional entry receptor for Epstein-Barr virus. Nat Microbiol. (2018) 3:172–80. doi: 10.1038/s41564-017-0081-7
139. TerBush AA, Hafkamp F, Lee HJ, Coscoy L. A Kaposi's sarcoma-associated herpesvirus infection mechanism is independent of integrins alpha3beta1, alphaVbeta3, and alphaVbeta5. J Virol. (2018) 92:e00803–18. doi: 10.1128/JVI.00803-18
140. Zhang H, Li Y, Wang HB, Zhang A, Chen ML, Fang ZX, et al. Ephrin receptor A2 is an epithelial cell receptor for Epstein-Barr virus entry. Nat Microbiol. (2018) 3:1–8. doi: 10.1038/s41564-017-0080-8
141. McCarron JK, Stringer BW, Day BW, Boyd AW. Ephrin expression and function in cancer. Future Oncol. (2010) 6:165–76. doi: 10.2217/fon.09.146
142. Yamashita T, Ohneda K, Nagano M, Miyoshi C, Kaneko N, Miwa Y, et al. Hypoxia-inducible transcription factor-2alpha in endothelial cells regulates tumor neovascularization through activation of ephrin A1. J Biol Chem. (2008) 283:18926–36. doi: 10.1074/jbc.M709133200
143. Kumar SR, Masood R, Spannuth WA, Singh J, Scehnet J, Kleiber G, et al. The receptor tyrosine kinase EphB4 is overexpressed in ovarian cancer, provides survival signals and predicts poor outcome. Br J Cancer. (2007) 96:1083–91. doi: 10.1038/sj.bjc.6603642
144. Wykosky J, Debinski W. The EphA2 receptor and ephrinA1 ligand in solid tumors: function and therapeutic targeting. Mol Cancer Res. (2008) 6:1795–806. doi: 10.1158/1541-7786.MCR-08-0244
145. Zhuang G, Brantley-Sieders DM, Vaught D, Yu J, Xie L, Wells S, et al. Elevation of receptor tyrosine kinase EphA2 mediates resistance to trastuzumab therapy. Cancer Res. (2010) 70:299–308. doi: 10.1158/0008-5472.CAN-09-1845
146. Merchant AA, Jorapur A, McManus A, Liu R, Krasnoperov V, Chaudhry P, et al. EPHB4 is a therapeutic target in AML and promotes leukemia cell survival via AKT. Blood Adv. (2017) 1:1635–44. doi: 10.1182/bloodadvances.2017005694
147. Batlle E, Bacani J, Begthel H, Jonkeer S, Gregorieff A, van de Born M, et al. EphB receptor activity suppresses colorectal cancer progression. Nature. (2005) 435:1126–30. doi: 10.1038/nature03626
148. Keane N, Freeman C, Swords R, Giles FJ. EPHA3 as a novel therapeutic target in the hematological malignancies. Expert Rev Hematol. (2012) 5:325–40. doi: 10.1586/ehm.12.19
149. Swords RT, Greenberg PL, Wei AH, Durrant S, Advani AS, Hertzberg MS, et al. KB004, a first in class monoclonal antibody targeting the receptor tyrosine kinase EphA3, in patients with advanced hematologic malignancies: Results from a phase 1 study. Leuk Res. (2016) 50:123–31. doi: 10.1016/j.leukres.2016.09.012
150. Caivano A, La Rocca F, Laurenzana I, Annese T, Tamma R, Famigliari U, et al. Epha3 acts as proangiogenic factor in multiple myeloma. Oncotarget. (2017) 8:34298–309. doi: 10.18632/oncotarget.16100
151. Charmsaz S, Beckett K, Smith FM, Bruedigam C, Moore AS, Al-Ejeh F, et al. EphA2 is a therapy target in EphA2-positive leukemias but is not essential for normal hematopoiesis or leukemia. PLoS ONE. (2015) 10:e0130692. doi: 10.1371/journal.pone.0130692
152. Oricchio E, Nanjangud G, Wolfe AL, Schatz JH, Mavrakis KJ, Jiang M, et al. The Eph-receptor A7 is a soluble tumor suppressor for follicular lymphoma. Cell. (2011) 147:554–64. doi: 10.1016/j.cell.2011.09.035
153. Kuang SQ, Bai H, Fang ZH, Lopez G, Yang H, Tong W, et al. Aberrant DNA methylation and epigenetic inactivation of Eph receptor tyrosine kinases and ephrin ligands in acute lymphoblastic leukemia. Blood. (2010) 115:2412–9. doi: 10.1182/blood-2009-05-222208
154. Noren NK, Foos G, Hauser CA, Pasquale EB. The EphB4 receptor suppresses breast cancer cell tumorigenicity through an Abl-Crk pathway. Nat Cell Biol. (2006) 8:815–25. doi: 10.1038/ncb1438
155. Li N, Liu S, Sun M, Chen W, Xu X, Zeng Z, et al. Chimeric antigen receptor-modified T cells redirected to EphA2 for the immunotherapy of non-small cell lung cancer. Transl Oncol. (2018) 11:11–7. doi: 10.1016/j.tranon.2017.10.009
156. Shi H, Yu F, Mao Y, Ju Q, Wu Y, Bai W, et al. EphA2 chimeric antigen receptor-modified T cells for the immunotherapy of esophageal squamous cell carcinoma. J Thorac Dis. (2018) 10:2779–88. doi: 10.21037/jtd.2018.04.91
157. Yi Z, Prinzing BL, Cao F, Gottschalk S, Krenciute G. Optimizing EphA2-CAR T cells for the adoptive immunotherapy of glioma. Mol Ther Methods Clin Dev. (2018) 9:70–80. doi: 10.1016/j.omtm.2018.01.009
158. Taki S, Kamada H, Inoue M, Nagano K, Mukai Y, Higashisaka K, et al. A novel bispecific antibody against human CD3 and ephrin receptor A10 for breast cancer therapy. PLoS ONE. (2015) 10:e0144712. doi: 10.1371/journal.pone.0144712
159. Huo Y, Ley KF. Role of platelets in the development of atherosclerosis. Trends Cardiovasc Med. (2004) 14:18–22. doi: 10.1016/j.tcm.2003.09.007
160. Tournoij E, Koekman CA, Du VX, Roest M, Ruijtenbeek R, Moll FL, et al. The platelet P2Y12 receptor contributes to granule secretion through Ephrin A4 receptor. Platelets. (2012) 23:617–25. doi: 10.3109/09537104.2011.645924
161. Sakamoto A, Ishibashi-Ueda H, Sugamoto Y, Higashikata T, Miyamoto S, Kawashiri M-A, et al. Expression and function of ephrin-B1 and its cognate receptor EphB2 in human atherosclerosis: from an aspect of chemotaxis. Clin Sci. (2008) 114:643–50. doi: 10.1042/CS20070339
162. Sakamoto A, Sugamoto Y, Tokunaga Y, Yoshimuta T, Hayashi K, Konno T, et al. Expression profiling of the ephrin (EFN) and Eph receptor (EPH) family of genes in atherosclerosis-related human cells. J Int Med Res. (2011) 39:522–7. doi: 10.1177/147323001103900220
163. Wynn TA. Cellular and molecular mechanisms of fibrosis. J Pathol. (2008) 214:199–210. doi: 10.1002/path.2277
164. Lagares D, Ghassemi-Kakroodi P, Tremblay C, Santos A, Probst CK, Franklin A, et al. ADAM10-mediated ephrin-B2 shedding promotes myofibroblast activation and organ fibrosis. Nat Med. (2017) 23:1405–15. doi: 10.1038/nm.4419
165. Goldshmit Y, Bourne J. Upregulation of EphA4 on astrocytes potentially mediates astrocytic gliosis after cortical lesion in the marmoset monkey. J Neurotrauma. (2010) 27:1321–32. doi: 10.1089/neu.2010.1294
166. Frugier T, Conquest A, McLean C, Currie P, Moses D, Goldshmit Y. Expression and activation of EphA4 in the human brain after traumatic injury. J Neuropathol Exp Neurol. (2012) 71:242–50. doi: 10.1097/NEN.0b013e3182496149
167. Fabes J, Anderson P, Yanez-Munoz RJ, Thrasher A, Brennan C, Bolsover S. Accumulation of the inhibitory receptor EphA4 may prevent regeneration of corticospinal tract axons following lesion. Eur J Neurosci. (2006) 23:1721–30. doi: 10.1111/j.1460-9568.2006.04704.x
168. Berer K, Krishnamoorthy G. Microbial view of central nervous system autoimmunity. FEBS Lett. (2014) 588:4207–13. doi: 10.1016/j.febslet.2014.04.007
169. Sobel RA. Ephrin A receptors and ligands in lesions and normal-appearing white matter in multiple sclerosis. Brain Pathol. (2005) 15:35–45. doi: 10.1111/j.1750-3639.2005.tb00098.x
170. Lin L, Lesnick TG, Maraganore DM, Isacson O. Axon guidance and synaptic maintenance: preclinical markers for neurodegenerative disease and therapeutics. Trends Neurosci. (2009) 32:142–9. doi: 10.1016/j.tins.2008.11.006
171. Naj AC, Jun G, Beecham GW, Wang LS, Vardarajan BN, Buros J, et al. Common variants at MS4A4/MS4A6E, CD2AP, CD33 and EPHA1 are associated with late-onset Alzheimer's disease. Nat Genet. (2011) 43:436–41. doi: 10.1038/ng.801
172. Bonaparte MI, Dimitrov AS, Bossart KN, Crameri G, Mungall BA, Bishop KA, et al. Ephrin-B2 ligand is a functional receptor for Hendra virus and Nipah virus. Proc Natl Acad Sci USA. (2005) 102:10652–7. doi: 10.1073/pnas.0504887102
173. Wong JJW, Young TA, Zhang J, Liu S, Leser GP, Komives EA, et al. Monomeric ephrinB2 binding induces allosteric changes in Nipah virus G that precede its full activation. Nat Commun. (2017) 8:781. doi: 10.1038/s41467-017-00863-3
174. Hahn AS, Kaufmann JK, Wies E, Naschberger E, Panteleev-Ivlev J, Schmidt K, et al. The ephrin receptor tyrosine kinase A2 is a cellular receptor for Kaposi's sarcoma-associated herpesvirus. Nat Med. (2012) 18:961–6. doi: 10.1038/nm.2805
175. Langlois AC, Marinach C, Manzoni G, Silvie O. Plasmodium sporozoites can invade hepatocytic cells independently of the Ephrin receptor A2. PLoS ONE. (2018) 13:e0200032. doi: 10.1371/journal.pone.0200032
176. Khounlotham M, Subbian S, Smith R III, Cirillo SL, Cirillo JD. Mycobacterium tuberculosis interferes with the response to infection by inducing the host EphA2 receptor. J Infect Dis. (2009) 199:1797–1806. doi: 10.1086/599096
Keywords: Eph, ephrin, activation, cell trafficking, migration, adhesion, inflammation, disease
Citation: Darling TK and Lamb TJ (2019) Emerging Roles for Eph Receptors and Ephrin Ligands in Immunity. Front. Immunol. 10:1473. doi: 10.3389/fimmu.2019.01473
Received: 13 March 2019; Accepted: 13 June 2019;
Published: 04 July 2019.
Edited by:
Moncef Zouali, Institut National de la Santé et de la Recherche Médicale (INSERM), FranceReviewed by:
Peter Warwick Janes, Monash University, AustraliaMarit Inngjerdingen, Oslo University Hospital, Norway
Copyright © 2019 Darling and Lamb. This is an open-access article distributed under the terms of the Creative Commons Attribution License (CC BY). The use, distribution or reproduction in other forums is permitted, provided the original author(s) and the copyright owner(s) are credited and that the original publication in this journal is cited, in accordance with accepted academic practice. No use, distribution or reproduction is permitted which does not comply with these terms.
*Correspondence: Tracey J. Lamb, tracey.lamb@path.utah.edu