- 1Independent Researcher, Vizianagaram, India
- 2Bio5 Institute, University of Arizona, Tucson, AZ, United States
- 3Animal Diagnostic Laboratory, Department of Veterinary and Biomedical Sciences, The Pennsylvania State University, University Park, PA, United States
- 4Department of Biosciences, School of Sciences, Indrashil University, Mehsana, India
Leishmaniasis is a parasitic disease of humans, highly prevalent in parts of the tropics, subtropics, and southern Europe. The disease mainly occurs in three different clinical forms namely cutaneous, mucocutaneous, and visceral leishmaniasis (VL). The VL affects several internal organs and is the deadliest form of the disease. Epidemiology and clinical manifestations of VL are variable based on the vector, parasite (e.g., species, strains, and antigen diversity), host (e.g., genetic background, nutrition, diversity in antigen presentation and immunity) and the environment (e.g., temperature, humidity, and hygiene). Chemotherapy of VL is limited to a few drugs which is expensive and associated with profound toxicity, and could become ineffective due to the parasites developing resistance. Till date, there are no licensed vaccines for humans against leishmaniasis. Recently, immunotherapy has become an attractive strategy as it is cost-effective, causes limited side-effects and do not suffer from the downside of pathogens developing resistance. Among various immunotherapeutic approaches, cytokines (produced by helper T-lymphocytes) based immunotherapy has received great attention especially for drug refractive cases of human VL. Therefore, a comprehensive knowledge on the molecular interactions of immune cells or components and on cytokines interplay in the host defense or pathogenesis is important to determine appropriate immunotherapies for leishmaniasis. Here, we summarized the current understanding of a wide-spectrum of cytokines and their interaction with immune cells that determine the clinical outcome of leishmaniasis. We have also highlighted opportunities for the development of novel diagnostics and intervention therapies for VL.
Introduction
Leishmaniasis is a neglected tropical disease (NTD) caused by an obligatory intracellular protozoan parasite that belongs to the genus Leishmania. It is a vector-borne infection transmitted by female sandflies and the disease is highly prevalent in poor and malnourished populations of the world living in tropical and subtropical countries. The life cycle of Leishmania is simple and the parasite propagates in two different morphological forms. The promastigote stage of the parasite exists in the insect body fluids and enters the mammalian host when sandfly takes a blood meal. Promastigotes transform into amastigotes inside the mononuclear phagocytes of hosts and establish the infection by evading host defense system (1). The infected individuals could develop self-healing cutaneous ulcers to life-threatening visceral disease (2). World-wide, 0.7–1.0 million new cases of leishmaniasis and 20,000 to 30,000 deaths are reported each year (3). Visceral leishmaniasis (VL) or kala-azar is the deadliest clincial form of leishmaniasis, typically caused by L. donovani and L. infantum in the Old World and L. chagasi in the New World. Occasionally, L. tropica and L. amazonensis have also been found to cause VL in the Middle East and South America, respectively (4). The anthroponotic transmission of VL is prevalent in the Indian subcontinent (5). The annual report of global VL indicates that there are 50,000 to 90,000 new cases each year with high incidence in the Indian subcontinent and East Africa (3). VL is an opportunistic infection and has been identified as a co-infection in HIV patients (6). HIV infection amplifies the risk of developing active VL and the severity by 100–2,320 times (7). Fever, weight-loss, anemia, pancytopenia, hyperpigmentaion of skin and hepatosplenomegaly are some of the manifestations of VL and the mortality rate is over 95% (3). Children under the age of 1 year and adults above 50 years of age are highly susceptible to VL (8, 9). The susceptible host genetic background (10), nutritional status especially malnutrition (11) and immune suppression (12) ameliorates the clinical outcome of the disease. The current VL treatment relies mostly on chemical drugs like pentavalent antimonials (SbV), amphotercin B, miltefosine, and paromomycin etc. But their misuse, life-threatening toxicity, and development of resistance by the parasites (13) highlight the need for drug-sparing alternative therapeutic strategy to combat the clinical disease. Recently, immunotherapy has emerged as a promising option to control various diseases including VL. This review presents an in-depth critical analysis of immune responses to leishmaniasis and highlights prospective cytokine candidates that could be used for the diagnosis and therapy of VL.
Leishmania Infection and Innate Immune Cells
Leishmania infection in humans is usually subclinical and parasites may persist for life-time of the host through several escape mechanisms (14). For example, Leishmania blocks the maturation of complement system and C5–C9 membrane attacking complex formation, reduces the expression of B7 and CD40 that are required for T-cell anti-parasitic activity, promote overexpression of the iron transporters, modifies the toll-like receptor (TLR)-2/TLR-4 signaling and inhibits Janus tyrosine kinase/signal transducer and activator of transcription (JAK/STAT) pathway in macrophages (MΦs) thereby turnoff the cytokine cascade, and alters the expression profile of cytokines and chemokines etc. It is clear that Leishmania parasites manipulate several key aspects of host defense for their survival. Consequently, targeting immune components is a reliable method to combat the disease. In addition, host innate immune signatures that are specific to Leishmania infection could help early prediction of the disease outcome. These include aspects of innate immune response, such as front-line defense led by the natural killer (NK) cells, mononuclear and polymorphonuclear phagocytes (15). In general, Leishmania parasite resists their uptake by phagocytic dendritic cells (DCs) and MΦs (16, 17) by inhibiting reactive oxygen species (ROS) production that delays phagolysosome formation (18) and blocks lysosomal proteolytic degradation (19). The complement protein C3b, a potent immune opsonin accelerates phagocytosis of Leishmania (17) by interacting with the parasite surface glycoprotein gp63 (20). MΦs and DCs that engulfed Leishmania activate their TLR-9 signaling and produce interleukin (IL)-12, which stimulates NK cells to produce interferon (IFN)-γ, a key cytokine that is responsible for skweing Th1 response (16, 21) and stimulate the MΦs to produce ROS and nitric oxide (NO) for oxidative killing of intracellular amastigotes thereby protects the host (22–25). To establish an early infection, L. major inhibits the NK cell proliferation and IFN-γ production (26) and L. donovani evades inducible nitric oxide synthase (iNOS)-dependent killing of intracellular amastigotes in MΦs via downregulation of iNOS mRNA expression (27, 28) and induction of arginase expression (29) as the arginine is a common substrate for both iNOS and arginase enzymes. Thus, MΦs play a complex role in Leishmania pathogenesis and are associated with both survival and death of the parasites (30). Further, the induction of FasL-mediated apoptosis in Leishmania infected MΦs is a part of host defense mechanisms in innate immunity (31). Similarly, other immune cells also play a key part in the early host defense against leishmaniasis. For example, a drastic reduction in IL-8 and eotaxin secretion from neutrophils and eosinophils, respectively (32), and an elevated number of IL-4+ neutrophils and IL-10+ eosinophils and reduced number of IFN-γ+ and IL-12+ eosinophils are observed in active VL patients (33).
Origin of Th1-Th2 Dichotomy in Leishmaniasis
While the host innate immune response against leishmaniasis is important, it is now clear that the T-cell mediated immunity and the cytokines produced from various immune cells play a crucial role in determining the disease outcome (shown in Figure 1). However, the cytokines function in autocrine (locally) and paracrine (at a distance from the site of synthesis) fashion to regulate the immune response (34). A longitudinal study on Leishmania pathogenesis and disease recovery highlighted the role of helper T (Th)-cell responses (35). Therefore, immune cells and their cytokines have been recognized as potential targets for immunotherapy to modulate the activity of factors that are crucial in the immune system for healing. In this context, the phenomenon called “Th1-Th2 dichotomy” became popular based on the role of the cytokines produced by these cells in disease progression and/or host protection. Mosmann et al. reported for the first time that the cloned murine Th-cells are in two functional subsets namely Th1 and Th2 based on the production of IFN-γ and IL-4, respectively (36). Thereafter, several studies demonstrated the key role of major cytokines [e.g., IL-10, Transforming growth factor (TGF)-β, IL-4, IL-6, IL-12, and IFN-γ] that implicated the role of Th1/Th2 balance in disease progression or host protection. In general, Th1 type response mediates host resistance and Th2 type response associates with disease progression (37). In resistant mouse strains, the abundance of Th1 type cytokines; IFN-γ, IL-2, and lymphotoxin spontaneously cleared the L. major infection, whereas, in susceptible mouse strains, infection led to the fatal disease by the action of Th2 type cytokines; IL-4, IL-5 and IL-10 (38). IL-4 and IL-10 associated with the visceralization of cutaneous L. major infection (39, 40). However, the Th1-Th2 dichotomy is more complex than previously recognized, which is more evident in certain cases of leishmaniasis (41), such as L. donovani infection where the susceptibility of mouse strains is variable (42). Unlike in cutaneous leishmaniasis (CL), T-cells with Th2 phenotype are difficult to demonstrate in the mouse model of VL (37, 40–43). Similarly, the association between Th1 response and disease resistance to VL is complex in humans (44, 45). Occasionally, individuals respond to the exposure of Leishmania antigens via T-cells even they have no prior exposure to the parasite; this is possible due to the cross-activity by other microorganisms (46).
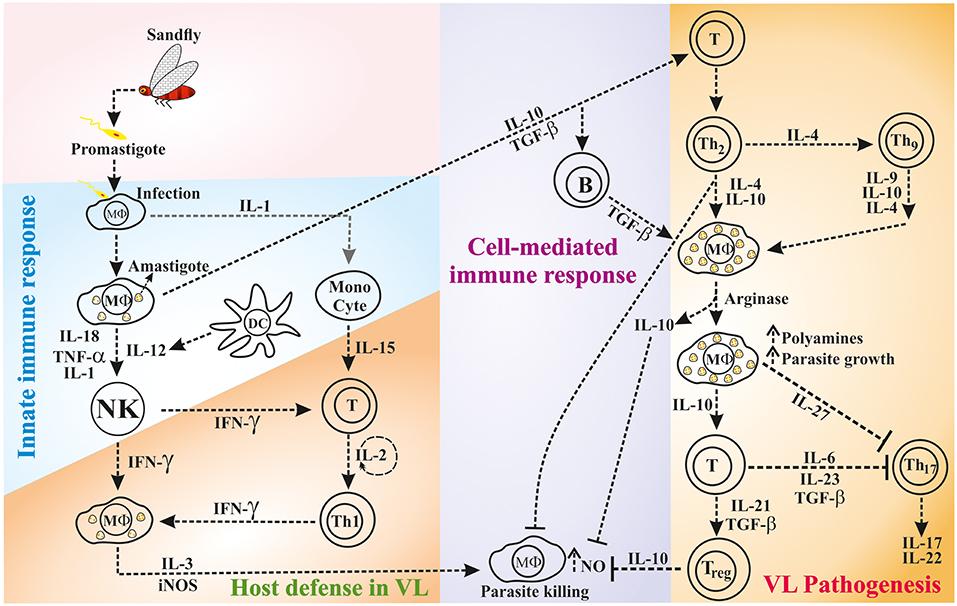
Figure 1. The interaction of innate and/or adaptive immune cells via cytokines during host defense or disease progression in VL.
Immune Response During VL
Immune response in VL patients is characterized by abundant anti-leishmanial antibody titers and low or absence of Leishmania-specific T-cell proliferation and IL-2 and IFN-γ production. Recovery from VL is mostly dependent on the induction of T-cell immunity; preferably Th1 response, which is primed by IL-12+ DCs and MΦs (47, 48). IFN-γ produced from IL-12 primed T-cells induce NO-mediated killing of the parasites (49, 50). In contrast, VL progression in humans is associated with abundant production of Th2 type cytokines IL-10, TGF-β, and IL-4 or presence of IL-10+ regulatory T cells (Tregs), which diminish the anti-parasitic activity of M1-type MΦs and Th1 response (51–53). However, the presence of abundant IL-10 is crucial rather than a lack of IFN-γ in the VL clinical disease progression (54). IL-10 partially inhibits IFN-γ production but strongly resists IFN-γ mediated activation of MΦs while killing the intracellular parasites (55–57). Likewise, the lack of IFN-γ may result in relatively higher levels of IL-10 in human leishmaniasis resulting in MΦ deactivation (58) and parasite proliferation (59). Murine model of VL demonstrates higher disease susceptibility due to the presence of high IL-10 levels during initial phase of infection (60). The splenic infection of L. donovani causes a constitutive expression of chemokine ligand 2 (CCL2) or monocyte chemoattractant protein 1 (MCP-1), which triggers IL-4 secretion from Th2 cells that activates the MΦs in alternative manner. These M2-type MΦs express arginase in abundant quantity and help in the biosynthesis of polyamines, which favor the survival and growth of the parasite (61). During chronic VL, the high expression of programmeded death protien-1 (PD-1) or Cytotoxic T-lymphocyte Antigen 4 (CTLA-4) causes unresponsiveness in CD4+ T-cell, which produce TGF-β in abundant levels and helps in persistence of infection (62). Taken together, there is mounting evidence suggesting that Th1-Th2 imbalance and T-cell unresponsiveness are critical issues in VL pathogenesis.
Objective of the Review
Role of a whole host cytokines in the resistance and disease progression during VL is increasingly being uncovered. Till date targeting either Th1 or Th2 cytokines produced promising results for leishmaniasis cure. Th1/Th2 balance is not the only determinant of the outcome of leishmaniasis as previously thought because a range of other cytokines have recently been implicated in both disease progression and host protection (Figure 2). Hence, there is a need for in-depth analysis of the role of cytokines and Leishmania pathogenesis to get a comprehensive view of the complex interplay of Leishmania parasite and their hosts. This review aims to summarize and critically analyze the state-of-the-art knowledge relating to cytokines and VL pathogenesis. Special emphasis has been made for the identification of potential cytokine targets that could be used for the development of novel diagnostic assays and immunotherapies for the detection and treatment of VL.
Cytokine Response in Leishmaniasis
It is well-known that cytokines play a role in pathogenesis and hosts resistance of VL. Cytokines that play crucial role in Leishmania pathogenesis or host defense are tabulated in Tables 1, 2, respectively. However, there are several cytokines that are play a dual role both in the disease progression and host resistance are summarized in Table 3. Cytokines targets for diagnosis and/or immunotherapy are shown in Table 4. Functions of individual cytokines as relates to pathogenesis and/or host resistance are discussed in detail in the sections below.
IL-10 Is a Key Player in Disease Progression
IL-10 is an 18 kDa pleiotropic cytokine, primarily produced by alternatively activated MΦs, DCs, and lymphocytes. As an immunoregulatory cytokine, IL-10 exerts multiple biological effects on different cell types (205). IL-10 is the product of Th2 subset, also known as cytokine synthesis inhibitory factor (CSIF) since it suppresses IFN-γ production from Th1 cells (112). IL-10 is known to inhibit production of cytokines like IL-1, IL-6, IL-12, and tumor necrosis factor (TNF)-α. In addition, Il-10 also inhibits MΦ mediated activation of T-cell through the reduced expression of class II major histocompatibility complex (MHC) and co-stimulatory molecules on the surface of MΦ and results in the inhibition of both innate and T-cell mediated immunity (188). The suppressive role of IL-10 in human VL results in the drastic fall in accumulation of monocyte derived macrophages, which is regulated by migration inhibitory factor (MIF). Further, IL-10 plays a substantial role in the pathogenesis of leishmaniasis by causing the downregulation of Th1 response, MΦ activation (114) and antigen presentation by DCs (58). Furthermore, IL-10 inhibits the leishmanicidal functions of MΦ (206) by diminishing the production of reactive nitrogen intermediates by MΦ, IFN-γ by T and natural killer (NK) cells (115), and IL-12 mediated activation of MΦ (48). High levels of IL-10 during the initial phases of infection due to decreased multifunctional CD4 T cells results in higher susceptibility to VL. Despite elevated levels of IFN-γ during the steady state of infection, parasite burden is not reduced due to higher levels of IL-10 (60). The unfavorable clinical outcome in localized CL was correlated with IL-10 but not with inadequate Th1 response (207). High levels of serum IL-10 is associated with symptomatic VL but absent in asymptomatic individuals. A key function of IL-10 is to protect the tissues from collateral damage due to excessive inflammation (116). However, in the face of parasitic infection an acute inflammatory response is necessary to control the parasite proliferation, hence, the anti-inflammatory role of IL-10 may help the disease progression (117). During active VL, CD8+ T-cells could also play an important role in disease progression via abundant production of IL-10 (208). However, the role of IL-10 in VL appears to be species-specific as it was suggested that IL-10 may not be a regulatory cytokine in canine VL. In experimental CL, a group of Treg cells namely, CD4+CD25+Foxp3+ and CD4+CD25−Foxp3− are possible source of IL-10 (209). In contrast, IL-10 in human VL is not produced from thymic Foxp3 Tregs; rather they are produced from IFN-γ co-producing CD4+ T cells which are called type 1 regulatory (Tr1) cells (143). The role of Tregs was elucidated in modulating both Th1 (210, 211) and Th2 (210, 212) activity during murine L. major infection.
TGF-β Functions Synergistically With IL-10 in Disease Progression
TGF-β is a 28 kDa homodimer and a potent anti-inflammatory cytokine produced by antigen-activated T-cells and mononuclear phagocytes (75). TGF-β has potent immunosuppressive effects in infectious and autoimmune diseases (118), which include inhibition of T-cell proliferation and MΦ activation. TGF-β inhibits the functions of TNF-α and IFN-γ and controls the expression of inducible nitric oxide synthase (iNOS) and the development of Th1 and Th2 response. Unlike IL-10, the impact of TGF-β on parasite burden and IFN-γ dependent host resistance is marginal during L. donovani infection (119). Locally activated TGF-β favors the parasite growth by modulating innate and adaptive immune responses (120) and enhancing arginase expression (121, 122). In animal models, TGF-β secreted by Leishmania infected lymphocytes diverts the arginine pool from iNOS to arginase for the production of polyamines, which helps in the growth of the parasite (123). Both pro and anti-inflammatory roles of TGF-β have been demonstrated (62, 124). L. chagasi infection induces TGF-β secretion by human MΦs through activation of latent TGF-β itself. L. chagasi infection also induces the expression of TGF-β in spleen and liver tissues of both symptomatic and asymptomatic dogs (213). TGF-β exposure delays the killing of Leishmania parasite and TGF-β overexpression impairs the rate of cure in murine leishmaniasis models (125, 126). In human VL, the elevated levels of IL-10 and TGF-β postively correlate with the parasite load and with increased absolute numbers of FoxP3 Treg cells suggesting the role of Tregs in secretion of these cytokines. There is a significant correlation between the parasite load and circulating antigen specific TGF-β levels in VL patients suggesting its role in parasite multiplication and disease progression in humans (214).
IL-4 Is Involved in the Pathogenesis of Leishmaniasis but Its Role in VL Is Conflicting
IL-4 is a 20 kDa Th2 subset cytokine that plays a critical role in the regulation of mast cell or eosinophil-mediated immune responses, B-cell mediated IgE class-switching and antibody production. It functions as a growth factor for mast cells and naive CD4+ Th2 cells which produce anti-inflammatory cytokines IL-5, IL-10 and IL-13. Both IL-4 and IL-13 inhibit IFN-γ-producing CD4+ T-cells and suppress protective Th1 immune response (150) and trigger MΦs to undergo alternative activation resulting in parasite survival and persistence of infection (151, 152). The similarities in IL-4 and IL-13 biological activities are due to a common receptor γ-chain that they both share, which is involved in the signal transduction via signal transducer and activator of transcription (STAT)-6 (215). Studies on murine model established the pathogenic role of IL-4 in leishmaniasis (39). IL-4 inhibits the oxidative burst by inducing low level production of reactive oxygen intermediates and NO in MΦs (153, 154). L. major infected Langerhans cells show increased IL-4R expression and decreased IL-12 production in susceptible mice but not in resistant mice (155).
IL-4 modulated antigen-uptake, endosomal processing, and humoral response are suggested to promote the disease development in Leishmania infection in humans (156). In murine L. donovani infection, IL-4 induces the host protective response (216) and vaccine mediated protection by IFN-γ secretion from CD8+ T-cells (157). The peripheral blood mononuclear cells (PBMCs) harvested from cured VL patients produced IFN-γ or IL-4 in response to stimulation by L. donovani promastigote or amastigote crude antigen. In response to purified gp63 antigen, the proliferation capability of the same PBMCs was weak and produced IFN-γ or IL-4 (158). Cytokine analysis in VL unveils the induced expression of IL-10 and/or IL-4 mRNA in tissues and abundant levels of IL-4 in circulation of patients with progressive disease (217). Likewise, the conflicting role of IL-4 in VL is described, though it has a leading role in pathogenesis of VL as it is belonging to Th2 phenotype and anti-inflammatory cytokine subset. Recent studies on human splenic aspirates suggest that blockade of IFN-γ and TNF-α results in increased production of IL-4 which does not contribute to parasite replication and IL-10 production. The biological role of IL-4 in target organ of human VL still remains an outstanding question (218).
IL-13 Promotes Host Protection in VL and Its Role Is Leishmania Species-Specific
IL-13 is a 12-kDa cytokine that is expressed by Th2 and is important in host protection against Leishmania infection. For example, IL-13 knock-out mice infected with L. donovani show retarded hepatic granuloma formation and maturation, depleted IFN-γ secretion and enhanced production of IL-4 and IL-10 (159). IL-13 protects rats from L. major infection through the production of IL-1β and IL-12 (160), which is in contrast to the earlier studies that showed pathogenic role of IL-13 (16, 219). However, studies with BALB/c mice infected by L. mexicana and L. amazonensis have established the pathogenic impact of IL-4 and IL-13 (161). The susceptibility to L. (V.) panamensis infection is predominantly associated with IL-13 but not IL-4. The parasite species and the host genetic background may also influence the dual role of IL-13 and it may not be a potential target for immunotherapy (162).
Targeting Endogenous IL-6 May Offer Better Protection
IL-6 is a 26 kDa pleiotropic cytokine produced by a number of cell types, including monocytes, endothelial cells and T-lymphocytes (220). The main biological activities associated with IL-6 are the induction of acute-phase protein synthesis in hepatocytes, terminal differentiation of B-cells and activation of T-cells (221). It also induces the production of anti-inflammatory proteins, such as IL-1 receptor antagonist (IL-1rα) and soluble TNF receptor (222). IL-6 plays a major role in the switching of monocytes from DC to MΦs (130). IL-6 favors the development of Th2 response, which suppresses the activation and antimicrobial effect of MΦs (75). Contradicting roles of IL-6 have been demonstrated in experimental CL and VL models (223–225). IL-6 has been shown to either promote (226, 227), suppress (228), or do not change (229) the intracellular host defense to leishmaniasis. Function of endogenous IL-6 as a host suppressive cytokine in case of L. donovani infection has outshined its potential pro-host defense effect.
During adoptive transfer of testing splenic DCs, IL-6 induces leishmanistatic effect but not host suppressive effect (226). IL-6 inhibits the IFN-γ mediated gene expression (131) and absence of IL-6 receptor signaling in L. donovani liver infection contributes to enhanced Th1-type response, accelerated tissue inflammation, and rapid parasite killing (132). IL-6 induces the secretion of IL-27 which in turn induces IL-10 production (133–135) in the L. donovani infected mouse model (54). However, L. donovani-infected IL-6−/− mice do not show effect on the IL-10 expression (226). This observation raises a potential possibility to target endogenous IL-6 as an anti-leishmanial therapeutic strategy (230). Expansion of CD25−FoxP3−IL-10+CD4+ T-cells in vivo and therapeutic efficacy of adoptively transferred DCs against L. donovani infection are regulated by DC-derived IL-6 (226). IL-6 is produced by dogs with active leishmaniasis and is a key player in the pathogenesis of canine leishmaniasis (144, 231). The presence of TNF-α and IL-6 transcripts was found in both Leishmania antigen stimulated and unstimulated cells of asymptomatic infected and uninfected dogs (232). Further, increased anti-leishmanial antibody titers (hypergammaglobulinaemia) in canine VL are usually associated with high levels of IL-6 (224). Contrary to murine VL, the role of IL-6 in human VL is associated with disease severity and death, which is due to the inhibition of TNF-α in the early phase of infection and later by inhibiting the Th1 responses (190, 233).
IL-27 Contributes to VL Pathogenesis and a Potential Target for Anti-VL Therapy
IL-27 is a member of the IL-6/IL-12 cytokine family and a heterodimer composed of EBI3 and p28. The main cellular source of IL-27 is CD14+ spleen cells (140) in particular MΦs and DCs. The anti-inflammatory properties of IL-27 have been demonstrated in various models of infectious diseases and autoimmunity (234). IL-27 mediates anti-inflammatory response by suppressing Th17 cells (138, 139) and inducing IL-10 secretion from activated CD4+ T-cells via autocrine action of IL-21 (140). IL-27 plays a multifaceted role characterized by the induction of T-bet (141) in turn inhibition of parasite driven Th2 and Th17 development and Th1 polarization via IL-10 mediated feedback mechanism. IL-27 plays a critical role in the induction of IFN-γ and IL-10 from CD4+ T-cells, and suppression of inappropriate Th17 development to achieve immune-balance during intracellular parasite infections. In L. major infection, early burst of IL-4 suppresses IL-27 mediated development of normal Th1 by inducing IL-6 and TGF-β and promote the development of Th17 cells (142). In contrast, IL-27 is not required for the normal development of Th1 response to L. donovani infection (140) but induces IL-10 production (143, 145).
Absence of IL-27 receptor signaling in L. donovani liver infection contributes to the accelerated Th1-type response, tissue inflammation, and rapid parasite killing with reduced parasite burdens in spleen and liver (71, 146). Blocking IL-27 evoke different responses in different mice models. For example, blocking IL-27 results in reduced parasite loads in BALB/c mice and augmented parasite burden is seen in C57BL/6. This dichotomy in the production of IL-27 could be due to the consequence of host immune modulation by the parasite to establish infection (235). IL-27 levels were elevated in human plasma with active VL and splenic mRNA levels of IL-27 and IL-21 were higher in pre-treated biopsies compared with post-treatment samples (140). During VL caused by L. infantum, the sequential pathway of TLR3 and TLR9 recruitment, production of type I IFN and activation of IRF1 in macrophages is induced by IL-27. The secretion of IL-27 increases the Th1 response but also dampens the production of IL-17 which directly impacts the reduced recruitment of neutrophils to target organs (236). Inhibition of IL-27 could be targeted for design of anti-VL treatment in the future.
IL-5 Exerts Moderate Effects on VL Progression
IL-5 is a glycosylated homodimeric 45–60 kDa protein, functions as an anti-inflammatory cytokine and is produced by Th2 cells, mast cells, and eosinophils. IL-5 stimulates the B-cell growth and promotes the production of cytotoxic T-cells from thymocytes; however, the key function of IL-5 is in the activation, maturation, and survival of eosinophils. Eosinophils activated by IL-5 expel antibody bound parasites while releasing proteins associated with cytotoxic granules. In the case of CL and MCL, PBMCs induce secretion of both IL-4 and IL-5 at the site of the lesion (127, 128), which results in declined Th1 polarization. Several studies have reported that IL-4, IL-5, IL-10, and IL-13 provide favorable atmosphere for intracellular parasite growth and dissemination (129). Patients with chronic lesions produce abundant levels of IL-5 and IL-13, which further inhibits parasite killing by an additive effect of IL-13. IL-5 plays a minor role in the susceptibility to L. major infection in BALB/c mice (237).
IL-9 Increases Disease Susceptibility
IL-9 is a 14 kDa pleiotropic cytokine produced by Th-cells, primarily identified as a mouse T-cell growth factor (237) and mast cell differentiation factor. Erythroid precursors, B-lymphocytes, eosinophils, bronchial epithelial cells and neuronal precursors are the secondary targets of IL-9 (238). It is a Th2-type cytokine produced via both IL-4 dependent (239) and IL-4 independent (240) pathways and involved in the physiological regulation of Th1-Th2 balance in vivo. Very little is known about the role of IL-9 in leishmaniasis. In L. major infection, IL-9 is transiently expressed in susceptible BALB/c as well as in resistant C57BL/6 and DBA mice during early days of infection, but 4-weeks onwards, its expression was only seen in susceptible mice but not in resistant mice (136). In vivo neutralization of IL-9 delays disease progression in BALB/c mice by inducing protective Th1 response suggesting, IL-9 promotes susceptibility to L. major infection. Further, IL-9 induces classical MΦ activity and production of IFN-γ in L. major infected BALB/c mice, which serves as a model system to study the role of IL-9 in human diseases (137).
IL-33 Is a Prognostic Cytokine for VL Pathogenesis
IL-33 is a member of IL-1 family, which includes IL-1 and IL-18. IL-33 is a crucial player in the defense against nematode infections and allergic reactions, since it causes Th2-type immune response via inducing the production of IL-5 and IL-13 by T-cells, mast cells, basophils, and eosinophils. In addition, IL-33 also induces non-Th2-type inflammation, suggesting its pro-inflammatory role like IL-1 and IL-18. Schmitz et al. first reported that IL-33 functions through ST2 (IL-1R4) orphan receptor present on different immune cell types (241). During L. major infection in BALB/c mice, ST2-expressing CD4+ T-cells accumulated in local lesions (147). However, administration of polyclonal anti-ST2 antiserum depleted ST2-expressing cells as well as Th2 cells/cytokines and induced Th1 cytokine production, which in turn reduced the lesion development (148). Rostan et al. reported that the serum IL-33 levels were higher in VL patients besides the presence of IL33+ cells in liver biopsy of a patient. Similar results were observed in BALB/c mice infected with L. donovani, additionally, ST2+ cells were also observed in mouse liver. ST2 deficient BALB/c mice had shown strong Th1-type immune response via IFN-γ and IL-12 that controlled the hepatic parasite load and hepatomegaly. Recombinant IL-33 treatment of L. donovani infected BALB/c mice dramatically reduced the Th1 immunity and infiltration of polymorphonuclear neutrophils (PMNs) and monocytes in liver. In summary, IL-33 could be a very useful cytokine to determine the host susceptibility and disease prognosis of VL (149).
IFN-γ Is an Anti-leishmanial Cytokine
IFN-γ is a homodimeric glycoprotein consisting of two subunits each about 21 to 24 kDa. It is the most potent type II interferon that helps in MΦ activation to the leishmanicidal state (63). The main cellular sources of IFN-γ production are activated CD4+ and CD8+ T-cells, and NK cells in response to IL-12 signaling (242). Of the several anti-leishmanial cytokines (23), IFN-γ is the most significant cytokine in host protection, which plays a prominent role in MΦ priming (64) to produce leishmanicidal molecules (243). IFN-γ acts as monocyte-activating factor (65) and enhances release of oxygen radicals, secretion of pro-inflammatory cytokines (TNF-α, IL-l, and IL-6) (66), expression of MHC class-II, and antigen-presentation. In addition, IFN-γ blocks the production of IL-10, which decreases all the above functions of monocytes (67). Several studies demonstrated that the leishmanicidal activity of MΦs can be induced by a variety of cytokines, either alone or in combination. For instance, lipopolysaccharide (LPS) is required to induce the MΦ leishmanicidal activity in vitro (244). In human VL, the response is predominantly Th2-type with absence of PBMCs derived IFN-γ (47). But drug treatment induces a shift in the response so that individuals cured of VL often respond to Leishmania antigen by the production of both IFN-γ and IL-4 (158). In CL patients, however, the response is mainly dominated by IFN-γ and IL-4 is rarely detected (245), indicating that the immunological response to Leishmania in these individuals does not polarize as observed in inbred mouse strains. In vitro studies with T-cell clones (246) and in vivo studies using models of CL (40, 43) have demonstrated that IFN-γ can inhibit the expansion of CD4+ Th2-cells, resulting in the preferential expression of Th1 cell-mediated response. Reciprocal regulation is provided by the action of IL-10 on the IFN-γ producing capacity of Th1-type CD4+ T-cells (16). A recent study showed that the variation in single nucleotide polymorphisms (SNPs) of IFN-γ gene at the position +874 (A/T) influences the susceptibility to VL such that individuals in southwest of Iran with an AT genotype are susceptible and those with a TT genotype are resistant to VL (247).
IL-12 Is a Promising Candidate for VL Immunotherapy
IL-12 is a heterodimer consisting of two subunits (35 and 40 kDa) linked by a disulfide bond, mainly produced by activated MΦs and DCs. It is a proinflammatory cytokine that plays a key role in bridging innate and adaptive immune responses (248). Protective immunity against leishmaniasis is typically associated with the production of IL-12 (16, 219). IL-12 drives Th1 response and induces IFN-γ production from both NK cells and T-cells (68), and mediates the leishmanicidal activity by inducing NOS2 expression and NO production (146). In addition, IL-12 also mediates T-cell proliferation and lymphokines production (71, 72). The presence of IL-12 reduces the ability of CD4+ T-cells to produce IL-4 and increases the ability to produce IFN-γ. Thus, IL-12 and NK cells seem to play important role in determining the development of Th1 response (249). In vivo studies showed that IL-12 produced in infected mice (219, 250) is important to control Th2 expansion and to promote Th1 type response (69, 70). Neutralization of IL-12 leads to disease exacerbation in L. major and L. donovani infections (49, 69, 77). In contrast, the addition of IL-12 to lymphocyte cultures from VL patients restored IFN-γ production and increased cytotoxic activity of NK cells (48).
Endogenous TNF-α Offers Protection in VL
TNF-α is a 51 kDa homodimeric cytokine, mainly secreted by the activated MΦs, T-cells, NK cells and mast cells. TNF-α is important in mediating both innate and adaptive inflammatory responses. The regulation of TNF-α production appears to be important because it has potential role in the formation and maintenance of granuloma (76). Antiparasitic activity of TNF-α is mediated through activation of infected MΦs for the destruction of intracellular amastigotes (73). TNF-α production is absent in susceptible mice but present in L. major infected resistant mice. Protective role of TNF-α in L. major infection is characterized by MΦ activation, NO production and parasite clearance or suppression of visceralization (74). Protective T-cell response induced by TNF-α in L. major infected mice is due to the induced production of parasite-specific IgG1 and IgG2a. Acute infection with L. braziliensis resulted in the lack of production of parasite-specific IgG1 and IgG2a antibodies (251). The role of TNF-α in L. braziliensis infection is attributed to controlling the parasite numbers in the skin, lymph nodes and spleen and wound healing process (75). Brazilian patients with MCL had increased levels of TNF-α in both sera (252) and tissue lesions (253).
Treatment with TNF inhibitors, such as pentoxifylline in combination with anti-leishmanial pentavalent antimony, pentoxifylline promotes the re-epithelialization of mucosal tissues (254). However, infection of TNF−/− mice with L. major shows some delay but no defect in antigen-dependent T-cell activation (74). IFN-γ independent anti-leishmanial mechanism mediated by endogenous TNF-α was described in IFN-γ knockout (GKO)-1 mouse infected with L. donovani (77, 199). The L. donovani infection provoked endogenous TNF-α level are enough to offer initial resistance to the parasite invasion and critical for the resolution of visceral infection. This is contrasting with the effect of exogenous TNF-α, which has no protective role in established infection and its continuous administration leads to impaired anti-leishmanial activity (255). The polymorphism and upregulation of TNF2 promoter transcription could be involved in enhancing clinical VL infection (256). TNF-α cannot be considered as a good marker of active disease in both human VL and canine VL due to its labile nature (224). The production of TNF-α follows biphasic kinetics due to its effect on target cells mediated by membrane-bound receptors (117). The high expression of IL-32 (especially γ-isoform) in CL and mucosal lesions is associated with endogenous TNF-α production but not with IL-10, suggesting the inflammatory role of IL-32 in host defense against Leishmania infection (257). Absence of IL-32 leads to high infection index but its overexpression opposed the parasite growth via NO cathelicidin and β-defensin 2 syntheses (258). In response to excessive inflammation, increase in the levels of TNF-α might promote the generation of IL-10 producing T-cells as a homeostatic response (78).
IL-2 Promotes Anti-leishmanial T-Cell Response
IL-2 is a 15 kDa cytokine, produced by activated T-cells and was initially identified as a T-cell growth factor. IL-2 stimulates the proliferation and differentiation of B-cells, NK cells, monocyte/MΦs, oligodendrocytes and lymphocyte activated killer (LAK) cells. IL-2 does not directly stimulate the intracellular antimicrobial activity of MΦs (259) but exerts a range of immunoregulatory effects on T-cells and NK cells and induces the production of IFN-γ (79, 80). IL-2 may act as a susceptibility factor in leishmaniasis (250, 260) by inducing the production of IL-4 from CD4+ T-cells (81). But in IL-4 deficiency, the inhibition of disease progression is attributed to IL-13 and IL-2. Interestingly, in CL, both IL-2 and IL-15 are attributed in host protection, while in MCL IL-2 is only protective but not IL-15 (200). Rapid production of IL-2 was observed after successful treatment or acquisition of resistance against L. donovani infection but not during acute phase (261, 262). The endogenous IL-2 could act as a defensive cytokine, only when the mice subsequently challenged after a prior infection with the parasite (82). In contrast, exogenous IL-2 exerts the anti-leishmanial action using L3T4+ and Lyt-2+ T-cells in acutely infected euthymic mice. IL-2 exerts leishmanicidal activity in splenocytes in vitro even in the absence of IFN-γ (83).
IL-15 Synergizes IL-2 and IL-12 in Host Defense and Has Scope in VL Therapy
IL-15 is a 14–15 kDa cytokine with four α-helix bundles and plays a central role in the innate and adaptive immune responses to infections (86). The main source of IL-15 is activated peripheral monocytes (263). Due to the common receptor β-chain, immunological functions of IL-15 are similar to IL-2 (84), which includes the induction of T-cell proliferation (87), inhibition of T-cell apoptosis and preservation of memory T-cells (88), B-cell maturation and isotype switching (264). IL-15 also stimulates the proliferation and activation of NK cell (265) and induces the production of IFN-γ and TNF-α, synergistically with IL-12 (85). Nevertheless, IL-15 also shows distinct biological functions from IL-2 due to a different α-chain (266, 267). The possible pleiotropic role of IL-15 is reflected by its action on both Th1 and Th2 subtypes and the ability to induce the activity of IFN-γ and IL-12 (89) as well as IL-5 and IL-13 (90) in various experimental models. Endogenous IL-15 stimulates protective Th1 response by inducing the downregulation of IL-4+ Th2 cells (86). IL-15 could be a potential therapeutic agent in acute VL since it upregulates IL-12 production and Th1 development (91). In contrast, other studies have demonstrated that endogenous IL-15 is not necessary for basal expression of IL-12 and MΦ activation and is not able to influence the IL-12 activity and Th1 development in acute VL (268). IL-15 in combination with IFN-γ and/or IL-12 may increase the efficacy of conventional antimonial therapy for VL, because of low toxicity in vivo (201).
IL-17 Role Is Contradictory in Leishmaniasis
IL-17 is a 35 kDa proinflammatory cytokine, primarily produced by activated T-cells (CD4+ > CD8+) (269) and also by other subsets of T-cells including NKT cells and Th17 cells (96). The development of Th17 subset from naïve T-cells happens in the presence of IL-6 and TGF-β+ Tregs (270). IL-17 stimulates different immune cells to produce inflammatory molecules including TNF-α, IL-1, and chemokines (163). At the site of inflammation, IL-17 affects the neutrophil function, reduces the apoptosis, and promotes the secretion of pro-inflammatory cytokines as well as tissue damaging molecules (164, 165). IL-17 induces the secretion of granulocyte macrophage-colony stimulating factor (GM-CSF) and G-CSF, which increase the production of neutrophils, monocytes, and chemoattractants for neutrophils (CXCL8, CXCL1, and CXCL6) as well as Th1 cells (CXCL10) (96, 97). IL-17 induces the production of IL-6, which mediates both proinflammatory and regulatory functions (96). IL-17 of Th17 subset and Th1 subset play a complementary role in the host protection from L. donovani infection. Contrastingly, the susceptibility for L. major infection is not only associated with uncontrolled Th2 immunity (271) but also with excessive IL-17 secretion, which mediates neutrophil recruitment (166). Other studies have also demonstrated that IL-17 dependent neutrophil recruitment is essential only during the late stages but not early stages of L. major infection (272). Mucosal disease caused by L. (V.) braziliensis is also associated with elevated levels of IL-17 response (167). Treating VL using curdlan, a b-glucan immunomodulatory molecule induces Th1 cytokines with IL-12, IL-22 and IL-23 (273), while another immunomodulator Astrakurkurone is effective against experimental VL by inducing IL-17 along with IFN-γ (274). While these reports are suggestive of a protective role of IL-17 in VL, other reports suggested the involvement of IL-17 in exacerbating experimental VL in murine model (275) raising questions about its precise role in VL pathogenesis.
IL-18 Protects From VL but Favors Other Forms of Leishmaniasis
IL-18 is a 22 kDa pleiotropic cytokine produced by activated MΦs and Kupffer cells of liver (276). IL-18 promotes Th1 and NK cell development (168), induces IFN-γ production by lymphocytes and NK cells and synergizes with IL-12 (169, 170). IL-18R is expressed on Th1 cells but not on Th2 cells. IL-18 induced Th1 subset produces IFN-γ which indirectly regulates the expansion of Th2 cell (171). IL-18 promotes NK cell activity due to a constitutive expression of IL-18R on NK cells (277) and stimulates TNF-α secretion by human PBMCs (172). IL-18 also induces the activation of memory cells and in combination with IL-12 it prevents the reinfection of BALB/c mice with L. major (173). IL-18−/− mice are highly susceptible to L. donovani infection when compared to the wild-type mice. However, endogenous IL-18 induces an initial IFN-γ independent anti-leishmanial effect in L. donovani infection (174). Paradoxically, IL-18 can also stimulate Th2 cytokines production, such as IL-4 from basophils (175) and CD4+ T-cells (176) and IL-13 from mast cells. While IL-18 protects BALB/c mice from L. donovani infection, it increases the susceptibility of BALB/c mice to L. major infection. Notably, the resistance and susceptibility of BALB/c mice to L. mexicana infection are not mediated by IL-18 and are influenced by different genetic and immunoregulatory controls (278). IL-18−/− BALB/c mice are highly resistant to L. mexicana infection due to increased IFN-γ production and antigen-specific IgG2a, reduced splenic IL-4, antigen-specific IgG1 and total IgE (177). IL-18 plays a critical role in the regulation of Th1 and Th2 balance in vivo, which frequently determines the outcome of many important infectious and autoimmune diseases (168).
IL-22 Offers Protection From VL
IL-22 is a 16.7 kDa cytokine, primarily produced by Th17 cells and to a lesser extent by Th1 and NK cells (96). Immunological functions of IL-22 are associated with the epithelium and mucosal surfaces (92), which include promoting inflammatory response and tissue repair (93, 279). IL-22 stimulates the production of pro-inflammatory molecules, such as S-100A proteins and CXCL5 (93). IL-17 and IL-22 act synergistically on epithelial cells to produce an antimicrobial peptide called β-defensin (95). IL-22 is also involved in protecting the liver (94) during chronic infections. During L. donovani infection, both IL-17 and IL-22 are produced by PBMCs and may exert complementary function along with Th1 cytokines (96, 97). The production of IL-22 requires IL-6 but not TGF-β (98).
Role of IL-1 Is Protective in VL but Contradictory in Other Forms of Leishmaniasis
IL-1 is synthesized as ~35 kDa precursor, from which two functional agonistic proteins (IL-1-α and IL-1-β each 17 kDa M.W.) and IL-1Ra, receptor antagonist of IL-1R1, are produced. IL-1 is a potent proinflammatory “alarm cytokine” that synergizes the functions of TNF-α and is produced by MΦs. IL-1 builds inflammation by inducing the expression of adhesion molecules on endothelial cells and leukocytes (280, 281). IL-1β, along with other proinflammatory cytokines, is released into the periphery during infection and coordinates immune-to-brain communication (180). IL-1 mediated inflammation is coordinated by adaptive T-cell response and controls the parasite dissemination (178, 179). IL-1 is responsible for regulating the delicate balance between inflammation and immunity which decides the fate of the disease progression in leishmaniasis. In L. major infection, the acute levels of IL-1α, IL-1β, and IL-1Ra are adequately downregulated unlike in L. amazonensis infection (282). Disease progression is inhibited with IL-1α treatment in L. major susceptible BALB/c mice during T-cell differentiation. IL-1β enhances activation of DCs and T-cell priming but do not affect the cytokine profile of DCs and pathogenic Th-cells (178). Contrastingly, Voronov et al. reported that BALB/c mice deficient in IL-1 family genes showed delayed disease progression with L. major infection due to apparent Th1 response even at late stages of the disease. IL-1α deficient mice were slightly more resistant to L. major infection than IL-1β KO mice (181). In L. amazonensis infection, IL-1β treatment induced DCs and cytokine production remains lower than that of L. major infection. IL-1 therapy in murine CL results in a wide range of outcomes depending on the course of treatment and parasite species involved. In this context, IL-1-based treatment may be effective for L. major but not L. amazonensis infection. The decreased production of IL-1 has been associated with L. donovani infection of murine peritoneal MΦs in vitro and human circulatory monocyte population (182, 183). Similarly, human PBMCs failed to produce IL-1 in response to Leishmania-antigen stimulation in vitro, during acute VL. However, following anti-leishmanial therapy, IL-1 and TNF-α levels are usually recovered, which correlates with clinical cure (184). Recombinant IL-1α induces mature granuloma formation in liver and IFN-γ production from spleen cells but is not able to clear the parasite (185).
IL-3 Is Likely a Host Protective Cytokine in VL
IL-3 is a 28 kDa glycoprotein derived from T-cells and supports the viability and differentiation of hematopoietic progenitor cells (283, 284) and monocytes (283). With the combination of GM-CSF, M-CSF, and IFN-γ, IL-3 shows an additive effect on human MΦs in the induction of oxidative burst and TNF-α secretion to inhibit the replication and growth of Leishmania parasite. In contrast, IL-3 promotes the infection in murine model of CL highlighting the species-specific differences in the role of IL-3 in leishmaniasis (186). In combination with M-CSF, IL-3 induces superoxide ions production to kill the parasite and may involve in myelopoiesis during acute VL.
IL-7 Shows Additive Effect With IFN-γ Against Leishmania
IL-7 is a 17 kDa glycoprotein derived from bone marrow stromal cells (285) and regulates a wide variety of functions including multiple effects on B-cells and proliferation of thymocytes (99–101), NK cells (102) and mature T-cells (103). IL-7 induces the production of cytotoxic T-cells with alloreactive, antitumor, and antiviral activities (104). It was reported that IL-7 shows potential effects on monocytic lineages (105, 286). IL-7 enhances the synthesis and secretion of various inflammatory cytokines, such as IL-6, TNF-α, IL-1α, IL-1β, and MΦ inflammatory protein (MIP) 113 by human circulatory monocytes. IL-7 is not as effective as IFN-γ but shows an additive effect with the combination of suboptimal concentrations of IFN-γ in killing the Leishmania amastigotes by inducing the production of TNF-α (105) and NO.
IL-8 Attracts Neutrophils to the Site of Infection
IL-8 is a non-glycosylated proinflammatory cytokine with a M.W. of 8 kDa, which is primarily produced from neutrophils and from other cell types including epithelial cells, keratinocytes, fibroblasts and endothelial cells. In mouse system, IL-8 has two functional homologs like MIP-2 (CXCL2/Groβ) and KC (CXCL1/Groα). During L. major infection in humans, IL-8 promotes the recruitment of neutrophils at lesion sites (106). In mice infected with L. major, IL-8 causes transient production of KC mRNA in the skin, which may associate with granulocyte recruitment (107) which is yet to be demonstrated in vivo (272). Notably, a reduced neutrophil count during active VL is associated with lower IL-8 levels in serum (32). It was identified that polymorphisms at IL-8 −251 position are associated with impaired IL-8 activity and the development of active VL in Iranian individuals (108) but such a correlation was not observed in Brazilian VL patients (287).
IL-23 May Offer Protection From VL in Association With IL-12p40
IL-23 is a pleiotropic cytokine produced by MΦs and DCs which acts on receptors expressed by T-cells, NK cells and NKT cells (288). During L. donovani infection in BALB/c mice, the IL-23p19 mRNA expression in the liver tissue was comparable to that of wild-type. IL-12 independent protection in visceral infection (76, 109, 110) was mediated by IL-18 and probably by IL-23 also, since the p40 subunit of IL-12 shared by both IL-12p70 and IL-23 (174). IL-23p19 may pair with IL-12p40 to become biologically active (111), which are crucial for host protection. Therefore, IL-23 alone or in combination with other cytokines may be a possible option in immunotherapy of VL.
Interplay of T-Cell Subsets via Cytokines in Leishmaniasis
It is well established that the complex interplay of pathogens with their hosts is predominantly regulated by host-specific Th1/Th2 subset cytokines in the vicinity of several regulatory cytokines. In this context, previous studies have demonstrated that IL-10 produced by CD4+CD25+ Tregs (211) is important for parasite persistence in mice (289). In human VL, the elevated level of IFN-γ mRNA in lymphoid organs (spleen and bone marrow) is accompanied by an abundant expression of IL-10 (55, 290, 291) where the predominant source of IL-10 is Foxp3−CD25−CD3+ cells (143). However, in leishmaniasis, healing is predominantly associated with diminished expression of IL-10 mRNA (55, 290). The role of Th17 subset in human VL is unveiled by a longitudinal study in Sudan, which illustrated the protective role of Th17 subset that are employed by an induced production of IL-17 and IL-22 from L. donovani-specific T-cells (292). In fact, Th17 cells are pleiotropic in nature, responsible for either protection or pathogenesis and frequently associated with recruitment of neutrophils. Th17 cells under the influence of IL-27 producing CD4+ T-cells diminish IL-17 and IL-22 secretion (293). Disease progression in pre or post-treated Indian VL patients is linked with serum IL-27 and splenic IL-27 transcript but not with splenic IL-17 transcript (140).
The pathogenic role of IL-27 in active VL is linked with suppression of Th17 cytokines production and expression of transcription factors. Consequently, IL-27 promotes the parasite dissemination by inducing antigen-specific IL-10+ T-cell differentiation and expansion, and by inhibiting activation of effector Th17 lineage. Moreover, the host negotiates the Th17 response to control the pathogenic implications of VL that are driven by the parasite. Th9 cells are not the unique source of IL-9 production as Tregs and Th17 cells also produce IL-9 in lesser quantity. Initially, Th9 subset was thought of a splinter group of Th2 but now it is an independent lineage. Predictably, Th9 subset has a similar detrimental role like Th2 in the development of CL in the mouse model. Since, IL-4, IL-21, TGF-β, and IFN-α/β seem to activate Th9 cells to produce IL-4, IL-9, and IL10, which are involved in the pathogenesis of CL (136, 137). The “B-helper” follicular T-cell (Tfh) lineage is also implicated in leishmaniasis progression, which is the source for bulk production of IL-4 in the draining lymph nodes of susceptible mice infected by L. major (294). As a sequel of VL, PKDL patients' carries high plasma IL-10 levels (295). Immunologically, PKDL is characterized by hyper T-cell response and significant production of both Th1 and Th2 cytokines from PBMCs in response to crude L. donovani antigen (296). IL-10 levels in the skin and plasma could be used to predict the severity of PKDL pathogenesis and the chance of VL succession to PKDL.
Cytokines in VL Diagnosis and Immunotherapy
As immunotherapy is mandatory for refractive cases of leishmaniasis, cytokines received great attention in the search for novel approaches for the diagnosis and immunotherapy of VL (summarized in Table 4). For the first time, Reed et al. used the lymphokines obtained from murine spleen cell culture supernatant and encapsulated in liposomes against VL challenge and reported a significant reduction in the liver parasite burden, highlighting the importance of lymphokines in leishmaniasis healing (297). In general, the absence of leishmanial-antigen stimulated lymphocyte proliferation and IFN-γ production are indicators for the clinical evaluation of a VL patient (45). However, these two parameters may also be used as coordinates in assessing the level of protection conferred by vaccine antigens (194). Later, several studies have tested the effect of direct administration of recombinant Th1 cytokines and monoclonal antibodies against Th2 cytokines alone or in combination against leishmaniasis. For example, prophylactic anti-IL-10R treatment induces the rapid control of experimental VL and antimonials activity in IL-10 knock-out or transgenic mice (51), and IFN-γ production from T-cells with an active VL (55). Further, it was reported that IL-10R inhibition in L. donovani-infected mice controlled the parasite burden in liver, increased IFN-γ titers in serum, and iNOS production in macrophages altogether reduced the VL fatality (298). The therapeutic efficacy of anti-IL-10R and anti-GITR (glucocorticoid-induced TNF receptor-related protein) was tested against L. donovani challenge in C57BL/6 mice. Blocking IL-10 alone could reduce the parasite burden in spleen and liver but combination of these antibodies did not inhibit the parasite proliferation in spite of the significant increase in IFN-γ and TNF-α production (299). In another study, the blockade of IL-2 and IL-10 was effective in the reduction of parasite load in early and later phases of L. donovani infection in BALB/c mice (300). IL-10 neutralization in splenic aspirate cells increases IFN-γ and TNF-α production and reduces parasite burden in VL patients (187). In L. chagasi infected Brazilian population, the antigen-stimulated PBMCs derived IL-10 titers were higher in acute VL than after cure. However, Leishman skin test (LST) or Montenegro test positivity is not directly correlated with the IL-10 production in asymptomatic individuals (113). The balance between IL-10 and IL-12 determines the effectiveness of chemotherapy (17).
IL-4 induced in VL is usually associated with impaired treatment (191, 192). IFN-γ, IL-4, and IL-13 are upregulated in active VL, however, their levels are significantly declined after cure (193). The disease relapse in human VL patients is associated mostly with IL-10 rather than IL-13 and is influenced by IL-10+ IFN-γ+ antigen-specific T-cells (189). Blocking IL-4, IL-13, and TGF-β with receptor fusion antagonists substantially controlled the parasite replication but the clearance of visceral infection is marginal and had no synergistic effect with SbV (119). Though IFN-γ, IL-6, IL-27, TNF-α, and IL-10 levels increased in Brazilian patients with active VL caused by L. infantum, the clinical manifestation are strongly correlated with IL-6, IL-27, TNF-α, and IL-10 (190). In general, TNF-α synergizes IFN-γ in the activation of MΦs and clearance of parasite but it is found to elevate in serum despite the low TNF-α+ monocytes in the circulation of active VL patients (301). To surpass the side-effects, rIFN-γ and muramyl tripeptide (MTP-PE) encapsulated in liposomes at varying doses of intravenous (i.v.) injections causes substantial reduction in the splenic parasite burden in murine VL (302). rIFN-γ was tested in combination with SbV against VL patients from Brazil, Kenya, and India and the therapeutic efficacy was found to be 82.3, 75, and 87%, respectively (195–197).
IL-12 orchestrates acquired resistance in liver during intracellular L. donovani infection and parasite killing (174). IL-12 restored responses from PBMCs of VL patients much better than the treatment with anti-IL-10 alone or in combination with anti-IL-4 [53]. Hence, it is clear that successful VL therapy is associated with restoration of IFN-γ and IL-12 production (47). IL-12 was used as an effective adjuvant for a killed vaccine against L. major (198). The treatment of susceptible BALB/c mice with recombinant IL-12 mediates disease healing, which is associated with induced production of Th1 cytokines (81, 200) and suppression of IL-4 (75). The treatment for arthritis with anti-TNF-α results in increased susceptibility to VL (202, 203), suggesting that TNF-α could act as a primary effector component. Upon liposomal amphotericin B treatment, the plasma IL-15 levels were found to be increased in VL patients (86), suggesting the role of IL-15 as a marker in VL diagnosis and a target in the VL therapy. In opportunistic HIV co-infection, high levels of serum TNF-α and IFN-γ are the predictors for onset of acute VL infection (204). Recently, we demonstrated cytokine role and therapeutic potential of recombinant leptin (adipokine) in BALB/c mice with experimental VL caused by L. donovani. The serum leptin levels and splenic Th1 cytokine response were found to be reduced in active disease. Upon leptin administration, host protective responses including Graz-A+ CD8+ T-cells, IFN-γ, IL-12, and IL-2 production were found to be restored (303). Hence, low systemic leptin levels could be of prognostic and diagnostic value in the assessment of clinical VL.
Limitations and Future Prospective
Although cytokine-based immunotherapy is a promising approach for VL cure, there are certain limitations associated with this strategy. The production of recombinant cytokines as large molecules used in therapeutics is very expensive and they must be administrated via injections, which is certainly painful to the patients. Administering high dose of cytokines could result in side effects characterized by malaise and influenza-like syndromes. As the cytokines have short half-life in plasma, multiple doses are need which further increases the side-effects (304). A crucial aspect is that the cytokine therapy for leishmaniasis must be cost-effective over conventional treatment in order to be practical. It is possible that a combination therapy comprising a potent anti-leishmanial cytokine with the combination of an inhibitor (monoclonal antibody) targeting disease promoting cytokine or with current drug options could be a future prospective of leishmaniasis treatment. However, there is possibility that the different combinations of cytokines may produce a divergent immune response. Hence, it is important to further investigate the effect on immune response to develop a clinically relevant combination therapy. This is particularly important since several cytokines share common signaling cascades as outlined in this review, which affects the outcome of treatment. The gene manipulation strategy using advanced molecular biology tools may produce desired version of cytokines with small ligand-tags that have the potential to increase the half-life of cytokines from minutes to days in the blood by tethering with albumin protein, which further could reduce the number of required doses. After finding a successful combination of these against leishmaniasis, it would be optimal to design the chimeras of cytokines without losing native structural and functional properties. After administration, the chimeric cytokines should splice inside the body fluids and act independently. As a second option, cytokine and drug combination was also shown to be a reliable strategy against leishmaniasis. For example, combination of IFN-γ with antimony against experimental VL showed that antimony dosage required for leishmanicidal activity was reduced by 4- to 10-folds with IFN-γ combination (305). This is a string indication that administering a drug-cytokine mix could address the drug toxicity and possible development of resistance. In another study, the pre-treatment for 20 days with IFN-γ before antimony therapy has cured the VL in 4 out of 9 Indian patients and rest of them had shown reduced parasitemia in spleen aspirates (197). As mentioned earlier, CTLA-4 and PD-1 causes T-cell unresponsiveness, so targeting these for leishmaniasis treatment may yield promising results. A study showed that the anti-CD40 and anti-CTLA-4 with the combination of SbV against L. donovani infection in a mouse model increased IL-12 and IFN-γ production, T-cell activation and function, and synergistic with SbV while increasing the parasite death (306). Similarly, administration of chimeric fusion protein OX-40L-Fc and anti-CTLA-4 improved granuloma maturation and CD4+ T-cell proliferation to augment the killing L. donovani parasite but had no effect on IL-10 and TGF-β production (307). Liposomal amphotercin B treatment with the combination of recombinant human granulocyte macrophage colony-stimulating factor (rHuGM-CSF) cured the VL clinical symptoms and splenomegaly in a patient suffering from HIV and VL (308). Another promising therapeutic option is administration of anti-leishmanial drugs and immunomodulators together. For example, the suboptimal doses of miltefosine with the combination of a single dose of TLR-ligand called Pam3Cys (tripalmytoil-Cysteine), an immunomodulator, significantly promoted the healing of L. donovani infection in mice by increasing the production of Th1/Th2 cytokines, reactive oxygen and nitrogen intermediates, and H2O2 (309). Cytokine producing immune cell-based therapy either alone or in combination with drugs has recently emerged as a potential treatment for cancer and other infectious diseases. Glycosphingophospholipid (GSPL), a β-(1–4)-galactose terminal NKT-cell ligand of L. donovani antigen induces inflammatory signaling cascade to kill the intracellular parasite, induces effector T-cell response and controls the acute parasite load to an undetectable level in experimental VL (310). DCs could also be an attractive option as they are important antigen-presenting cells at the interface of innate and acquired immunity and can suppress early dissemination of the parasite to the lymphoid tissues mediated by IL-10 (311). Combination of bone marrow-derived DCs pulsed with L. donovani antigen and antimony treatment completely cleared the infection from the spleen and liver (312) by inducing Th1 cytokines production (313). Cytokines are the key players in the determination of disease outcome during various immunotherapies. It is important to remember that measuring the levels of a pro- or anti-inflammatory cytokine alone to predict the disease severity may not be reliable. Measuring the ratio of cytokines is a promising approach. For example, IFN-γ/IL-10 ratio is predictive of disease severity in VL (314).
Conclusive Remarks
As the cytokines are the key focus of various immunotherapies against leishmaniasis, it is essential to understand their role in detail with possible scope in developing novel diagnostics and targeted therapy for VL. There are key set of cytokines that are involved in the disease progression namely IL-10, TGF-β, and IL-4 and host protection namely IFN-γ, IL-12, TNF-α, and IL-2 during VL. Notably, there are other cytokines that are also involved in the pathogenesis and host defense during VL. However, their role appears to be complex and is dependent on the Leishmania species and the type of clinical disease. For example, cytokines namely, IL-1, IL-13, IL-17, and IL-18 are involved in the host defense during VL but have an opposite effect by promoting the disease in CL. Nonetheless, cytokines involved in the host protection e.g., IL-15, IL-22, and IL-23 and pathogenesis e.g., IL-33, IL-27, IL-9, and IL-21 can be explored further as promising targets in diagnosis and immunotherapy of VL.
Author Contributions
Topic selection and content development was done by AD and SKK. First draft was prepared by AD and SKK. SVK have corrected and revised to final version. SC has been supportive in images and tables.
Conflict of Interest Statement
The authors declare that the research was conducted in the absence of any commercial or financial relationships that could be construed as a potential conflict of interest.
Acknowledgments
Authors would like to thank Prof. Vadlakonda Laxmipathi Rtd. Professor Kakatiya University, India for his valuable guidance and critical suggestions during review writing. Authors are grateful to Dr. S. S. Mohanraj, Scientist B, CTSSS central silk board, Kota, Chhattisgarh, India for his kind help in polishing the Figures.
References
1. Lipoldova M, Demant P. Genetic susceptibility to infectious disease: lessons from mouse models of leishmaniasis. Nat Rev Genet. (2006) 7:294–305. doi: 10.1038/nrg1832
2. Pearson RD, De Queiroz Sousa A. Clinical spectrum of leishmaniasis. Clin Infect Dis. (1996) 22:1–11. doi: 10.1093/clinids/22.1.1
3. WHO. Leishmaniasis (March 2018). Available online at: https://www.who.int/en/news-room/fact-sheets/detail/leishmaniasis (accessed March 14, 2018).
4. Van Griensven J, Diro E. Visceral leishmaniasis. Infect Dis Clin North Am. (2012) 26:309–22. doi: 10.1016/j.idc.2012.03.005
5. Desjeux P. Leishmaniasis: current situation and new perspectives. Comp Immunol Microbiol Infect Dis. (2004) 27:305–18. doi: 10.1016/j.cimid.2004.03.004
6. Alvar J, Aparicio P, Aseffa A, Den Boer M, Canavate C, Dedet J-P, et al. The relationship between leishmaniasis and AIDS: the second 10 years. Clin Microbiol Rev. (2008) 21:334–59. doi: 10.1128/CMR.00061-07
7. Roatt BM, Aguiar-Soares RD, Coura-Vital W, Ker HG, Moreira N, Vitoriano-Souza J, et al. Immunotherapy and immunochemotherapy in visceral leishmaniasis: promising treatments for this neglected disease. Front Immunol. (2014) 5:272. doi: 10.3389/fimmu.2014.00272
8. De Araujo VE, Morais MH, Reis IA, Rabello A, Carneiro M. Early clinical manifestations associated with death from visceral leishmaniasis. PLoS Negl Trop Dis. (2012) 6:e1511. doi: 10.1371/journal.pntd.0001511
9. Leite AI, Araújo LB. Visceral leishmaniasis: epidemiological aspects related to deaths in mossoro, state of rio grande do norte, Brazil. Revista De Patologia Tropical/J Trop Pathol. (2013) 42. doi: 10.5216/rpt.v42i3.26928
10. Blackwell JM. Genetic susceptibility to leishmanial infections: studies in mice and man. Parasitology. (1996) 112 Suppl.:S67–74.
11. Malafaia G. Protein-energy malnutrition as a risk factor for visceral leishmaniasis: a review. Parasite Immunol. (2009) 31:587–96. doi: 10.1111/j.1365-3024.2009.01117.x
12. Chappuis F, Sundar S, Hailu A, Ghalib H, Rijal S, Peeling RW, et al. Visceral leishmaniasis: what are the needs for diagnosis, treatment and control? Nat Rev Microbiol. (2007) 5:873–82. doi: 10.1038/nrmicro1748
13. Mohapatra S. Drug resistance in leishmaniasis: newer developments. Trop Parasitol. (2014) 4:4. doi: 10.4103/2229-5070.129142
14. Gupta G, Oghumu S, Satoskar AR. Mechanisms of immune evasion in leishmaniasis. In: Advances in Applied Microbiology. Columbus, OH: Elsevier (2013). p. 155–84. doi: 10.1016/B978-0-12-407679-2.00005-3
15. Hawn TR, Ozinsky A, Underhill DM, Buckner FS, Akira S, Aderem A. Leishmania major activates IL-1α expression in macrophages through a MyD88-dependent pathway. Microbes Infect. (2002) 4:763–71. doi: 10.1016/S1286-4579(02)01596-4
16. Scharton-Kersten T, Afonso LC, Wysocka M, Trinchieri G, Scott P. IL-12 is required for natural killer cell activation and subsequent T helper 1 cell development in experimental leishmaniasis. J Immunol. (1995) 154:5320–30.
18. Gueirard P, Laplante A, Rondeau C, Milon G, Desjardins M. Trafficking of Leishmania donovani promastigotes in non-lytic compartments in neutrophils enables the subsequent transfer of parasites to macrophages. Cell Microbiol. (2008) 10:100–11. doi: 10.1111/j.1462-5822.2007.01018.x
19. Laufs H, Muller K, Fleischer J, Reiling N, Jahnke N, Jensenius JC, et al. Intracellular survival of Leishmania major in neutrophil granulocytes after uptake in the absence of heat-labile serum factors. Infect Immun. (2002) 70:826–35. doi: 10.1128/IAI.70.2.826-835.2002
20. Hermoso T, Perez JL, Cornivelli L, Hernandez AG. Extracellular phosphorylation in Leishmania major and Leishmania mexicana during heat shock transformation. Acta Trop. (1994) 56:1–6. doi: 10.1016/0001-706X(94)90034-5
21. Liese J, Schleicher U, Bogdan C. The innate immune response against leishmania parasites. Immunobiology. (2008) 213:377–87. doi: 10.1016/j.imbio.2007.12.005
22. Bogdan C, Röllinghoff M. How do protozoan parasites survive inside macrophages? Parasitol Today. (1999) 15:22–8. doi: 10.1016/S0169-4758(98)01362-3
23. Murray HW, Nathan CF. Macrophage microbicidal mechanisms in vivo: reactive nitrogen versus oxygen intermediates in the killing of intracellular visceral Leishmania donovani. J Exp Med. (1999) 189:741–6. doi: 10.1084/jem.189.4.741
24. White JK, Mastroeni P, Popoff JF, Evans CA, Blackwell JM. Slc11a1-mediated resistance to Salmonella enterica serovar Typhimurium and Leishmania donovani infections does not require functional inducible nitric oxide synthase or phagocyte oxidase activity. J Leukoc Biol. (2005) 77:311–20. doi: 10.1189/jlb.0904546
25. Perez LE, Chandrasekar B, Saldarriaga OA, Zhao W, Arteaga LT, Travi BL, et al. Reduced nitric oxide synthase 2 (NOS2) promoter activity in the Syrian hamster renders the animal functionally deficient in NOS2 activity and unable to control an intracellular pathogen. J Immunol. (2006) 176:5519–28. doi: 10.4049/jimmunol.176.9.5519
26. Laurenti M, Gidlund M, Ura D, Sinhorini I, Corbett C, Goto H. The role of natural killer cells in the early period of infection in murine cutaneous leishmaniasis. Braz J Med Biol Res. (1999) 32:323–5. doi: 10.1590/S0100-879X1999000300012
27. Nandan D, Lo R, Reiner NE. Activation of phosphotyrosine phosphatase activity attenuates mitogen-activated protein kinase signaling and inhibits c-FOS and nitric oxide synthase expression in macrophages infected with Leishmania donovani. Infect Immun. (1999) 67:4055–63.
28. Kar S, Ukil A, Sharma G, Das PK. MAPK-directed phosphatases preferentially regulate pro-and anti-inflammatory cytokines in experimental visceral leishmaniasis: involvement of distinct protein kinase C isoforms. J Leukoc Biol. (2010) 88:9–20. doi: 10.1189/jlb.0909644
29. Biswas A, Bhattacharya A, Kar S, Das PK. Expression of IL-10-triggered STAT3-dependent IL-4Ralpha is required for induction of arginase 1 in visceral leishmaniasis. Eur J Immunol. (2011). 41:992–1003. doi: 10.1002/eji.201040940
30. Birnbaum R, Craft N. Innate immunity and Leishmania vaccination strategies. Dermatol Clin. (2011) 29:89–102. doi: 10.1016/j.det.2010.08.014
31. Huang F-P, Xu D, Esfandiari E-O, Sands W, Wei X-Q, Liew FY. Cutting edge: mice defective in Fas are highly susceptible to Leishmania major infection despite elevated IL-12 synthesis, strong Th1 responses, and enhanced nitric oxide production. J Immunol. (1998) 160:4143–7.
32. Elshafie AI, Hlin E, Hakansson LD, Elghazali G, Safi SH, Ronnelid J, et al. Activity and turnover of eosinophil and neutrophil granulocytes are altered in visceral leishmaniasis. Int J Parasitol. (2011) 41:463–9. doi: 10.1016/j.ijpara.2010.11.005
33. Peruhype-Magalhães V, Martins-Filho ODA, Prata A, De A Silva L, Rabello A, Teixeira-Carvalho A, et al. Immune response in human visceral leishmaniasis: analysis of the correlation between innate immunity cytokine profile and disease outcome. Scand J Immunol. (2005) 62:487–95. doi: 10.1111/j.1365-3083.2005.01686.x
34. Singh OP, Sundar S. Immunotherapy and targeted therapies in treatment of visceral leishmaniasis: current status and future prospects. Front Immunol. (2014) 5:296. doi: 10.3389/fimmu.2014.00296
35. Scott P, Novais FO. Cutaneous leishmaniasis: immune responses in protection and pathogenesis. Nat Rev Immunol. (2016) 16:581. doi: 10.1038/nri.2016.72
36. Mosmann TR, Cherwinski H, Bond MW, Giedlin MA, Coffman RL. Two types of murine helper T cell clone. I Definition according to profiles of lymphokine activities and secreted proteins. J Immunol. (1986) 136:2348–57.
37. Sher A, Coffman RL. Regulation of immunity to parasites by T cells and T cell-derived cytokines. Annu Rev Immunol. (1992) 10:385–409. doi: 10.1146/annurev.iy.10.040192.002125
38. Mosmann TR, Coffman RL. Two types of mouse helper T-cell clone Implications for immune regulation. Immunol Today. (1987) 8:223–7. doi: 10.1016/0167-5699(87)90171-X
39. Heinzel FP, Sadick MD, Holaday BJ, Coffman RL, Locksley RM. Reciprocal expression of interferon gamma or interleukin 4 during the resolution or progression of murine leishmaniasis. Evidence for expansion of distinct helper T cell subsets. J Exp Med. (1989) 169:59–72. doi: 10.1084/jem.169.1.59
40. Heinzel FP, Sadick MD, Mutha SS, Locksley RM. Production of interferon gamma, interleukin 2, interleukin 4, and interleukin 10 by CD4+ lymphocytes in vivo during healing and progressive murine leishmaniasis. Proc Natl Acad Sci USA. (1991) 88:7011–5. doi: 10.1073/pnas.88.16.7011
41. Noben-Trauth N, Kropf P, Muller I. Susceptibility to Leishmania major infection in interleukin-4-deficient mice. Science. (1996) 271:987–90. doi: 10.1126/science.271.5251.987
42. Kaye PM, Curry AJ, Blackwell JM. Differential production of Th1- and Th2-derived cytokines does not determine the genetically controlled or vaccine-induced rate of cure in murine visceral leishmaniasis. J Immunol. (1991) 146:2763–70.
43. Locksley RM, Heinzel FP, Holaday BJ, Mutha SS, Reiner SL, Sadick MD. Induction of Th1 and Th2 CD4+ subsets during murine Leishmania major infection. Res Immunol. (1991) 142:28–32. doi: 10.1016/0923-2494(91)90007-6
44. Antonelli LR, Dutra WO, Almeida RP, Bacellar O, Gollob KJ. Antigen specific correlations of cellular immune responses in human leishmaniasis suggests mechanisms for immunoregulation. Clin Exp Immunol. (2004) 136:341–8. doi: 10.1111/j.1365-2249.2004.02426.x
45. Khalil EA, Ayed NB, Musa AM, Ibrahim ME, Mukhtar MM, Zijlstra EE, et al. Dichotomy of protective cellular immune responses to human visceral leishmaniasis. Clin Exp Immunol. (2005) 140:349–53. doi: 10.1111/j.1365-2249.2005.02768.x
46. Kemp M. Regulator and effector functions of T-cell subsets in human Leishmania infections. APMIS Suppl. (1997) 68:1–33.
47. Ghalib HW, Whittle JA, Kubin M, Hashim FA, El-Hassan AM, Grabstein KH, et al. IL-12 enhances Th1-type responses in human Leishmania donovani infections. J Immunol. (1995) 154:4623–9.
48. Bacellar O, Brodskyn C, Guerreiro J, Barral-Netto M, Costa CH, Coffman RL, et al. Interleukin-12 restores interferon-gamma production and cytotoxic responses in visceral leishmaniasis. J Infect Dis. (1996) 173:1515–8. doi: 10.1093/infdis/173.6.1515
49. Engwerda CR, Murphy ML, Cotterell SE, Smelt SC, Kaye PM. Neutralization of IL-12 demonstrates the existence of discrete organ-specific phases in the control of Leishmania donovani. Eur J Immunol. (1998) 28:669–80. doi: 10.1002/(SICI)1521-4141(199802)28:02<669::AID-IMMU669>3.0.CO;2-N
50. Bacellar O, D'oliveira A Jr, Jeronimo S, Carvalho EM. IL-10 and IL-12 are the main regulatory cytokines in visceral leishmaniasis. Cytokine. (2000) 12:1228–31. doi: 10.1006/cyto.2000.0694
51. Murray HW, Lu CM, Mauze S, Freeman S, Moreira AL, Kaplan G, et al. Interleukin-10 (IL-10) in experimental visceral leishmaniasis and IL-10 receptor blockade as immunotherapy. Infect Immun. (2002) 70:6284–93. doi: 10.1128/IAI.70.11.6284-6293.2002
52. Wilson ME, Recker TJ, Rodriguez NE, Young BM, Burnell KK, Streit JA, et al. The TGF-beta response to Leishmania chagasi in the absence of IL-12. Eur J Immunol. (2002) 32:3556–65. doi: 10.1002/1521-4141(200212)32:12<3556::AID-IMMU3556>3.0.CO;2-Q
53. Gomez MA, Contreras I, Halle M, Tremblay ML, Mcmaster RW, Olivier M. Leishmania GP63 alters host signaling through cleavage-activated protein tyrosine phosphatases. Sci Signal. (2009) 2:ra58. doi: 10.1126/scisignal.2000213
54. Murphy ML, Wille U, Villegas EN, Hunter CA, Farrell JP. IL-10 mediates susceptibility to Leishmania donovani infection. Eur J Immunol. (2001) 31:2848–56. doi: 10.1002/1521-4141(2001010)31:10<2848::AID-IMMU2848>3.0.CO;2-T
55. Ghalib HW, Piuvezam MR, Skeiky YA, Siddig M, Hashim FA, El-Hassan AM, et al. Interleukin 10 production correlates with pathology in human Leishmania donovani infections. J Clin Invest. (1993) 92:324–9. doi: 10.1172/JCI116570
56. Bhattacharyya S, Ghosh S, Jhonson PL, Bhattacharya SK, Majumdar S. Immunomodulatory role of interleukin-10 in visceral leishmaniasis: defective activation of protein kinase C-mediated signal transduction events. Infect Immun. (2001) 69:1499–507. doi: 10.1128/IAI.69.3.1499-1507.2001
57. Melby PC, Chandrasekar B, Zhao W, Coe JE. The hamster as a model of human visceral leishmaniasis: progressive disease and impaired generation of nitric oxide in the face of a prominent Th1-like cytokine response. J Immunol. (2001) 166:1912–20. doi: 10.4049/jimmunol.166.3.1912
58. De Medeiros IM, Castelo A, Salomao R. Presence of circulating levels of interferon-gamma, interleukin-10 and tumor necrosis factor-alpha in patients with visceral leishmaniasis. Rev Inst Med Trop Sao Paulo. (1998) 40:31–4. doi: 10.1590/S0036-46651998000100007
59. Bogdan C, Schleicher U. Production of interferon-gamma by myeloid cells–fact or fancy? Trends Immunol. (2006) 27:282–90. doi: 10.1016/j.it.2006.04.004
60. Mesquita I, Ferreira C, Barbosa AM, Ferreira CM, Moreira D, Carvalho A, et al. The impact of IL-10 dynamic modulation on host immune response against visceral leishmaniasis. Cytokine. (2018) 112:16–20. doi: 10.1016/j.cyto.2018.07.001
61. Bhattacharya P, Ali N. Involvement and interactions of different immune cells and their cytokines in human visceral leishmaniasis. Rev Soc Bras Med Trop. (2013) 46:128–34. doi: 10.1590/0037-8682-0022-2012
62. Gomes NA, Dosreis GA. The dual role of CTLA-4 in Leishmania infection. Trends Parasitol. (2001) 17:487–91. doi: 10.1016/S1471-4922(01)02038-4
63. Carvalho EM, Bacellar O, Brownell C, Regis T, Coffman RL, Reed SG. Restoration of IFN-gamma production and lymphocyte proliferation in visceral leishmaniasis. J Immunol. (1994) 152:5949–56.
64. Taylor AP, Murray HW. Intracellular antimicrobial activity in the absence of interferon-gamma: effect of interleukin-12 in experimental visceral leishmaniasis in interferon-gamma gene-disrupted mice. J Exp Med. (1997) 185:1231–9. doi: 10.1084/jem.185.7.1231
65. Nathan CF, Hibbs JB Jr. Role of nitric oxide synthesis in macrophage antimicrobial activity. Curr Opin Immunol. (1991) 3:65–70. doi: 10.1016/0952-7915(91)90079-G
66. Hart PH, Whitty GA, Piccoli DS, Hamilton JA. Control by IFN-gamma and PGE2 of TNF alpha and IL-1 production by human monocytes. Immunology. (1989) 66:376–83.
67. De Waal Malefyt R, Haanen J, Spits H, Roncarolo MG, Te Velde A, Figdor C, et al. Interleukin 10 (IL-10) and viral IL-10 strongly reduce antigen-specific human T cell proliferation by diminishing the antigen-presenting capacity of monocytes via downregulation of class II major histocompatibility complex expression. J Exp Med. (1991) 174:915–24. doi: 10.1084/jem.174.4.915
68. Manetti R, Parronchi P, Giudizi MG, Piccinni MP, Maggi E, Trinchieri G, et al. Natural killer cell stimulatory factor (interleukin 12 [IL-12]) induces T helper type 1 (Th1)-specific immune responses and inhibits the development of IL-4-producing Th cells. J Exp Med. (1993) 177:1199–204. doi: 10.1084/jem.177.4.1199
69. Heinzel FP, Rerko RM, Ahmed F, Pearlman E. Endogenous IL-12 is required for control of Th2 cytokine responses capable of exacerbating leishmaniasis in normally resistant mice. J Immunol. (1995) 155:730–9.
70. Murray HW, Hariprashad J, Coffman RL. Behavior of visceral Leishmania donovani in an experimentally induced T helper cell 2 (Th2)-associated response model. J Exp Med. (1997) 185:867–74. doi: 10.1084/jem.185.5.867
71. Gubler U, Chua AO, Schoenhaut DS, Dwyer CM, Mccomas W, Motyka R, et al. Coexpression of two distinct genes is required to generate secreted bioactive cytotoxic lymphocyte maturation factor. Proc Natl Acad Sci USA. (1991) 88:4143–7. doi: 10.1073/pnas.88.10.4143
72. Wolf SF, Temple PA, Kobayashi M, Young D, Dicig M, Lowe L, et al. Cloning of cDNA for natural killer cell stimulatory factor, a heterodimeric cytokine with multiple biologic effects on T and natural killer cells. J Immunol. (1991) 146:3074–81.
73. Titus RG, Sherry B, Cerami A. Tumor necrosis factor plays a protective role in experimental murine cutaneous leishmaniasis. J Exp Med. (1989) 170:2097–104. doi: 10.1084/jem.170.6.2097
74. Wilhelm P, Ritter U, Labbow S, Donhauser N, Rollinghoff M, Bogdan C, et al. Rapidly fatal leishmaniasis in resistant C57BL/6 mice lacking TNF. J Immunol. (2001) 166:4012–9. doi: 10.4049/jimmunol.166.6.4012
75. Tripathi P, Singh V, Naik S. Immune response to leishmania: paradox rather than paradigm. FEMS Immunol Med Microbiol. (2007) 51:229–42. doi: 10.1111/j.1574-695X.2007.00311.x
76. Murray HW, Jungbluth A, Ritter E, Montelibano C, Marino MW. Visceral leishmaniasis in mice devoid of tumor necrosis factor and response to treatment. Infect Immun. (2000) 68:6289–93. doi: 10.1128/IAI.68.11.6289-6293.2000
77. Wang ZE, Reiner SL, Zheng S, Dalton DK, Locksley RM. CD4+ effector cells default to the Th2 pathway in interferon gamma-deficient mice infected with Leishmania major. J Exp Med. (1994) 179:1367–71. doi: 10.1084/jem.179.4.1367
78. Lundqvist A, Palmborg A, Pavlenko M, Levitskaya J, Pisa P. Mature dendritic cells induce tumor-specific type 1 regulatory T cells. J Immunother. (2005) 28:229–35. doi: 10.1097/01.cji.0000158854.15664.c2
79. Kelly CD, Welte K, Murray HW. Antigen-induced human interferon-gamma production. Differential dependence on interleukin 2 and its receptor. J Immunol. (1987) 139:2325–8.
80. Smith KA. Interleukin-2: inception, impact, and implications. Science. (1988) 240:1169–76. doi: 10.1126/science.3131876
81. Ben-Sasson SZ, Le Gros G, Conrad DH, Finkelman FD, Paul WE. IL-4 production by T cells from naive donors. IL-2 is required for IL-4 production. J Immunol. (1990) 145:1127–36.
82. Murray HW, Squires KE, Miralles CD, Stoeckle MY, Granger AM, Granelli-Piperno A, et al. Acquired resistance and granuloma formation in experimental visceral leishmaniasis. Differential T cell and lymphokine roles in initial versus established immunity. J Immunol. (1992) 148:1858–63.
83. Eslami Z, Olivier M, Tanner CE. Immunotherapy with IL-2-stimulated splenocytes reduces in vitro the level of Leishmania donovani infection in peritoneal macrophages. Int J Parasitol. (1995) 25:975–81. doi: 10.1016/0020-7519(94)00220-I
84. Carson WE, Giri JG, Lindemann MJ, Linett ML, Ahdieh M, Paxton R, et al. Interleukin (IL) 15 is a novel cytokine that activates human natural killer cells via components of the IL-2 receptor. J Exp Med. (1994) 180:1395–403. doi: 10.1084/jem.180.4.1395
86. D'ettorre G, Ceccarelli G, Carnevalini M, Forcina G, Zaffiri L, Massetti AP, et al. Central role of interleukin-15 in human immunodeficiency virus (HIV)-infected patients with visceral leishmaniasis. Acta Trop. (2006) 99:83–7. doi: 10.1016/j.actatropica.2006.08.002
87. Grabstein KH, Eisenman J, Shanebeck K, Rauch C, Srinivasan S, Fung V, et al. Cloning of a T cell growth factor that interacts with the beta chain of the interleukin-2 receptor. Science. (1994) 264:965–8. doi: 10.1126/science.8178155
88. Hasan MS, Kallas EG, Thomas EK, Looney J, Campbell M, Evans TG. Effects of interleukin-15 on in vitro human T cell proliferation and activation. J Interferon Cytokine Res. (2000) 20:119–23. doi: 10.1089/107999000312513
89. Doherty TM, Seder RA, Sher A. Induction and regulation of IL-15 expression in murine macrophages. J Immunol. (1996) 156:735–41.
90. Loza MJ, Zamai L, Azzoni L, Rosati E, Perussia B. Expression of type 1 (interferon gamma) and type 2 (interleukin-13, interleukin-5) cytokines at distinct stages of natural killer cell differentiation from progenitor cells. Blood. (2002) 99:1273–81. doi: 10.1182/blood.V99.4.1273
91. Reiner SL, Locksley RM. The regulation of immunity to Leishmania major. Annu Rev Immunol. (1995) 13:151–77. doi: 10.1146/annurev.iy.13.040195.001055
92. Wolk K, Kunz S, Witte E, Friedrich M, Asadullah K, Sabat R. IL-22 increases the innate immunity of tissues. Immunity. (2004) 21:241–54. doi: 10.1016/j.immuni.2004.07.007
93. Boniface K, Bernard FX, Garcia M, Gurney AL, Lecron JC, Morel F. IL-22 inhibits epidermal differentiation and induces proinflammatory gene expression and migration of human keratinocytes. J Immunol. (2005) 174:3695–702. doi: 10.4049/jimmunol.174.6.3695
94. Zenewicz LA, Yancopoulos GD, Valenzuela DM, Murphy AJ, Karow M, Flavell RA. Interleukin-22 but not interleukin-17 provides protection to hepatocytes during acute liver inflammation. Immunity. (2007) 27:647–59. doi: 10.1016/j.immuni.2007.07.023
95. Liang SC, Tan XY, Luxenberg DP, Karim R, Dunussi-Joannopoulos K, Collins M, et al. Interleukin (IL)-22 and IL-17 are coexpressed by Th17 cells and cooperatively enhance expression of antimicrobial peptides. J Exp Med. (2006) 203:2271–9. doi: 10.1084/jem.20061308
96. Korn T, Bettelli E, Oukka M, Kuchroo VK. IL-17 and Th17 Cells. Annu Rev Immunol. (2009) 27:485–517. doi: 10.1146/annurev.immunol.021908.132710
97. Ouyang W, Kolls JK, Zheng Y. The biological functions of T helper 17 cell effector cytokines in inflammation. Immunity. (2008) 28:454–67. doi: 10.1016/j.immuni.2008.03.004
98. Zheng Y, Danilenko DM, Valdez P, Kasman I, Eastham-Anderson J, Wu J, et al. Interleukin-22, a T(H)17 cytokine, mediates IL-23-induced dermal inflammation and acanthosis. Nature. (2007) 445:648–51. doi: 10.1038/nature05505
99. Conlon PJ, Morrissey PJ, Nordan RP, Grabstein KH, Prickett KS, Reed SG, et al. Murine thymocytes proliferate in direct response to interleukin-7. Blood. (1989) 74:1368–73.
100. Okazaki H, Ito M, Sudo T, Hattori M, Kano S, Katsura Y, et al. IL-7 promotes thymocyte proliferation and maintains immunocompetent thymocytes bearing alpha beta or gamma delta T-cell receptors in vitro: synergism with IL-2. J Immunol. (1989) 143:2917–22.
101. Watson JD, Morrissey PJ, Namen AE, Conlon PJ, Widmer MB. Effect of IL-7 on the growth of fetal thymocytes in culture. J Immunol. (1989) 143:1215–22.
102. Naume B, Espevik T. Effects of IL-7 and IL-2 on highly enriched CD56+ natural killer cells. A comparative study. J Immunol. (1991) 147:2208–14.
103. Grabstein KH, Namen AE, Shanebeck K, Voice RF, Reed SG, Widmer MB. Regulation of T cell proliferation by IL-7. J Immunol. (1990) 144:3015–20.
104. Kos FJ, Mullbacher A. Induction of primary anti-viral cytotoxic T cells by in vitro stimulation with short synthetic peptide and interleukin-7. Eur J Immunol. (1992) 22:3183–5. doi: 10.1002/eji.1830221224
105. Alderson MR, Tough TW, Ziegler SF, Grabstein KH. Interleukin 7 induces cytokine secretion and tumoricidal activity by human peripheral blood monocytes. J Exp Med. (1991) 173:923–30. doi: 10.1084/jem.173.4.923
106. Van Zandbergen G, Hermann N, Laufs H, Solbach W, Laskay T. Leishmania promastigotes release a granulocyte chemotactic factor and induce interleukin-8 release but inhibit gamma interferon-inducible protein 10 production by neutrophil granulocytes. Infect Immun. (2002) 70:4177–84. doi: 10.1128/IAI.70.8.4177-4184.2002
107. Muller K, Van Zandbergen G, Hansen B, Laufs H, Jahnke N, Solbach W, et al. Chemokines, natural killer cells and granulocytes in the early course of Leishmania major infection in mice. Med Microbiol Immunol. (2001) 190:73–6. doi: 10.1007/s004300100084
108. Hajilooi M, Abasi M, Bazmani A, Ahmadi A, Matini M, Solgi G, et al. Evaluation of Interleukin-8-251 T/A polymorphisms in visceral leish-maniasis. J Res Health Sci. (2015) 15:59–61.
109. Ing R, Gros P, Stevenson MM. Interleukin-15 enhances innate and adaptive immune responses to blood-stage malaria infection in mice. Infect Immun. (2005) 73:3172–7. doi: 10.1128/IAI.73.5.3172-3177.2005
110. Wilson ME, Jeronimo SM, Pearson RD. Immunopathogenesis of infection with the visceralizing Leishmania species. Microb Pathog. (2005) 38:147–60. doi: 10.1016/j.micpath.2004.11.002
111. Vignali DA, Kuchroo VK. IL-12 family cytokines: immunological playmakers. Nat Immunol. (2012) 13:722–8. doi: 10.1038/ni.2366
112. Mosmann TR. Cytokine secretion patterns and cross-regulation of T cell subsets. Immunol Res. (1991) 10:183–8. doi: 10.1007/BF02919690
113. Holaday BJ, Pompeu MM, Jeronimo S, Texeira MJ, Sousa Ade A, Vasconcelos AW, et al. Potential role for interleukin-10 in the immunosuppression associated with kala azar. J Clin Invest. (1993) 92:2626–32. doi: 10.1172/JCI116878
114. Nylen S, Sacks D. Interleukin-10 and the pathogenesis of human visceral leishmaniasis. Trends Immunol. (2007) 28:378–84. doi: 10.1016/j.it.2007.07.004
115. Salvador BA, Brasil M, Barral-Netto C, Brodskyn EM, Carvalho A. Barral. Human-Leishmaniasis@cytokines.bahia.br. Braz J Med Biol Res. (1998) 31:149–55.
116. Sabat R, Grutz G, Warszawska K, Kirsch S, Witte E, Wolk K, et al. Biology of interleukin-10. Cytokine Growth Factor Rev. (2010) 21:331–44. doi: 10.1016/j.cytogfr.2010.09.002
117. Caldas A, Favali C, Aquino D, Vinhas V, Van Weyenbergh J, Brodskyn C, et al. Balance of IL-10 and interferon-gamma plasma levels in human visceral leishmaniasis: implications in the pathogenesis. BMC Infect Dis. (2005) 5:113. doi: 10.1186/1471-2334-5-113
118. Hirsch CS, Ellner JJ, Blinkhorn R, Toossi Z. In vitro restoration of T cell responses in tuberculosis and augmentation of monocyte effector function against Mycobacterium tuberculosis by natural inhibitors of transforming growth factor beta. Proc Natl Acad Sci USA. (1997) 94:3926–31. doi: 10.1073/pnas.94.8.3926
119. Murray HW, Flanders KC, Donaldson DD, Sypek JP, Gotwals PJ, Liu J, et al. Antagonizing deactivating cytokines to enhance host defense and chemotherapy in experimental visceral leishmaniasis. Infect Immun. (2005) 73:3903–11. doi: 10.1128/IAI.73.7.3903-3911.2005
120. Gantt KR, Schultz-Cherry S, Rodriguez N, Jeronimo SM, Nascimento ET, Goldman TL, et al. Activation of TGF-beta by Leishmania chagasi: importance for parasite survival in macrophages. J Immunol. (2003) 170:2613–20. doi: 10.4049/jimmunol.170.5.2613
121. Corraliza IM, Soler G, Eichmann K, Modolell M. Arginase induction by suppressors of nitric oxide synthesis (IL-4, IL-10 and PGE2) in murine bone-marrow-derived macrophages. Biochem Biophys Res Commun. (1995) 206:667–73. doi: 10.1006/bbrc.1995.1094
122. Omer FM, Kurtzhals JA, Riley EM. Maintaining the immunological balance in parasitic infections: a role for TGF-beta? Parasitol Today. (2000) 16:18–23. doi: 10.1016/S0169-4758(99)01562-8
123. Iniesta V, Gomez-Nieto LC, Corraliza I. The inhibition of arginase by N(omega)-hydroxy-l-arginine controls the growth of leishmania inside macrophages. J Exp Med. (2001) 193:777–84. doi: 10.1084/jem.193.6.777
124. Mccartney-Francis NL, Wahl SM. Transforming growth factor beta: a matter of life and death. J Leukoc Biol. (1994) 55:401–9. doi: 10.1002/jlb.55.3.401
125. Wilson ME, Young BM, Davidson BL, Mente KA, Mcgowan SE. The importance of TGF-beta in murine visceral leishmaniasis. J Immunol. (1998) 161:6148–55.
126. Li J, Hunter CA, Farrell JP. Anti-TGF-beta treatment promotes rapid healing of Leishmania major infection in mice by enhancing in vivo nitric oxide production. J Immunol. (1999) 162:974–9.
127. Melby PC, Andrade-Narvaez F, Darnell BJ, Valencia-Pacheco G. In situ expression of interleukin-10 and interleukin-12 in active human cutaneous leishmaniasis. FEMS Immunol Med Microbiol. (1996) 15:101–7. doi: 10.1111/j.1574-695X.1996.tb00059.x
128. Faria DR, Gollob KJ, Barbosa J Jr, Schriefer A, Machado PR, Lessa H, et al. Decreased in situ expression of interleukin-10 receptor is correlated with the exacerbated inflammatory and cytotoxic responses observed in mucosal leishmaniasis. Infect Immun. (2005) 73:7853–9. doi: 10.1128/IAI.73.12.7853-7859.2005
129. Brombacher F. The role of interleukin-13 in infectious diseases and allergy. Bioessays. (2000) 22:646–56. doi: 10.1002/1521-1878(200007)22:7<646::AID-BIES7>3.0.CO;2-9
130. Chomarat P, Banchereau J, Davoust J, Palucka AK. IL-6 switches the differentiation of monocytes from dendritic cells to macrophages. Nat Immunol. (2000) 1:510–4. doi: 10.1038/82763
131. Nagabhushanam V, Solache A, Ting LM, Escaron CJ, Zhang JY, Ernst JD. Innate inhibition of adaptive immunity: Mycobacterium tuberculosis-induced IL-6 inhibits macrophage responses to IFN-gamma. J Immunol. (2003) 171:4750–7. doi: 10.4049/jimmunol.171.9.4750
132. Rosas LE, Satoskar AA, Roth KM, Keiser TL, Barbi J, Hunter C, et al. Interleukin-27R (WSX-1/T-cell cytokine receptor) gene-deficient mice display enhanced resistance to Leishmania donovani infection but develop severe liver immunopathology. Am J Pathol. (2006) 168:158–69. doi: 10.2353/ajpath.2006.050013
133. Fitzgerald DC, Zhang GX, El-Behi M, Fonseca-Kelly Z, Li H, Yu S, et al. Suppression of autoimmune inflammation of the central nervous system by interleukin 10 secreted by interleukin 27-stimulated T cells. Nat Immunol. (2007) 8:1372–9. doi: 10.1038/ni1540
134. Mcgeachy MJ, Bak-Jensen KS, Chen Y, Tato CM, Blumenschein W, Mcclanahan T, et al. TGF-beta and IL-6 drive the production of IL-17 and IL-10 by T cells and restrain T(H)-17 cell-mediated pathology. Nat Immunol. (2007) 8:1390–7. doi: 10.1038/ni1539
135. Stumhofer JS, Silver JS, Laurence A, Porrett PM, Harris TH, Turka LA, et al. Interleukins 27 and 6 induce STAT3-mediated T cell production of interleukin 10. Nat Immunol. (2007) 8:1363–71. doi: 10.1038/ni1537
136. Nashed BF, Maekawa Y, Takashima M, Zhang T, Ishii K, Dainichi T, et al. Different cytokines are required for induction and maintenance of the Th2 type response in DBA/2 mice resistant to infection with Leishmania major. Microbes Infect. (2000) 2:1435–43. doi: 10.1016/S1286-4579(00)01298-3
137. Arendse B, Van Snick J, Brombacher F. IL-9 is a susceptibility factor in Leishmania major infection by promoting detrimental Th2/type 2 responses. J Immunol. (2005) 174:2205–11. doi: 10.4049/jimmunol.174.4.2205
138. Batten M, Li J, Yi S, Kljavin NM, Danilenko DM, Lucas S, et al. Interleukin 27 limits autoimmune encephalomyelitis by suppressing the development of interleukin 17-producing T cells. Nat Immunol. (2006) 7:929–36. doi: 10.1038/ni1375
139. Stumhofer JS, Laurence A, Wilson EH, Huang E, Tato CM, Johnson LM, et al. Interleukin 27 negatively regulates the development of interleukin 17-producing T helper cells during chronic inflammation of the central nervous system. Nat Immunol. (2006) 7:937–45. doi: 10.1038/ni1376
140. Ansari NA, Kumar R, Gautam S, Nylen S, Singh OP, Sundar S, et al. IL-27 and IL-21 are associated with T cell IL-10 responses in human visceral leishmaniasis. J Immunol. (2011) 186:3977–85. doi: 10.4049/jimmunol.1003588
141. Lucas S, Ghilardi N, Li J, De Sauvage FJ. IL-27 regulates IL-12 responsiveness of naive CD4+ T cells through Stat1-dependent and-independent mechanisms. Proc Natl Acad Sci. (2003) 100:15047–52. doi: 10.1073/pnas.2536517100
142. Anderson CF, Stumhofer JS, Hunter CA, Sacks D. IL-27 regulates IL-10 and IL-17 from CD4+ cells in nonhealing Leishmania major infection. J Immunol. (2009) 183:4619–27. doi: 10.4049/jimmunol.0804024
143. Nylen S, Maurya R, Eidsmo L, Manandhar KD, Sundar S, Sacks D. Splenic accumulation of IL-10 mRNA in T cells distinct from CD4+CD25+ (Foxp3) regulatory T cells in human visceral leishmaniasis. J Exp Med. (2007) 204:805–17. doi: 10.1084/jem.20061141
144. Cenini P, Berhe N, Hailu A, Mcginnes K, Frommel D. Mononuclear cell subpopulations and cytokine levels in human visceral leishmaniasis before and after chemotherapy. J Infect Dis. (1993) 168:986–93. doi: 10.1093/infdis/168.4.986
145. Kane MM, Mosser DM. The role of IL-10 in promoting disease progression in leishmaniasis. J Immunol. (2001) 166:1141–7. doi: 10.4049/jimmunol.166.2.1141
146. Liew FY, O'donnell CA. Immunology of leishmaniasis. Adv Parasitol. (1993) 32:161–259. doi: 10.1016/S0065-308X(08)60208-0
147. Kropf P, Bickle Q, Herath S, Klemenz R, Muller I. Organ-specific distribution of CD4+ T1/ST2+ Th2 cells in Leishmania major infection. Eur J Immunol. (2002) 32:2450–9. doi: 10.1002/1521-4141(200209)32:9<2450::AID-IMMU2450>3.0.CO;2-O
148. Xu D, Chan WL, Leung BP, Huang F, Wheeler R, Piedrafita D, et al. Selective expression of a stable cell surface molecule on type 2 but not type 1 helper T cells. J Exp Med. (1998) 187:787–94. doi: 10.1084/jem.187.5.787
149. Rostan O, Gangneux JP, Piquet-Pellorce C, Manuel C, Mckenzie AN, Guiguen C, et al. The IL-33/ST2 axis is associated with human visceral leishmaniasis and suppresses Th1 responses in the livers of BALB/c mice infected with Leishmania donovani. MBio. (2013) 4:e00383–00313. doi: 10.1128/mBio.00383-13
150. Becker Y. HIV-1 induced AIDS is an allergy and the allergen is the Shed gp120–a review, hypothesis, and implications. Virus Genes. (2004) 28:319–31. doi: 10.1023/B:VIRU.0000025778.56507.61
151. Wilflingseder D, Banki Z, Dierich MP, Stoiber H. Mechanisms promoting dendritic cell-mediated transmission of HIV. Mol Immunol. (2005) 42:229–37. doi: 10.1016/j.molimm.2004.06.019
152. Woelbing F, Kostka SL, Moelle K, Belkaid Y, Sunderkoetter C, Verbeek S, et al. Uptake of Leishmania major by dendritic cells is mediated by Fcgamma receptors and facilitates acquisition of protective immunity. J Exp Med. (2006) 203:177–88. doi: 10.1084/jem.20052288
153. Lehn M, Kandil O, Arena C, Rein MS, Remold HG. Interleukin-4 fails to inhibit interferon-gamma-induced activation of human colostral macrophages. Cell Immunol. (1992) 141:233–42. doi: 10.1016/0008-8749(92)90142-C
154. Ho JL, He SH, Rios MJ, Wick EA. Interleukin-4 inhibits human macrophage activation by tumor necrosis factor, granulocyte-monocyte colony-stimulating factor, and interleukin-3 for antileishmanial activity and oxidative burst capacity. J Infect Dis. (1992) 165:344–51. doi: 10.1093/infdis/165.2.344
155. Moll H, Scharner A, Kampgen E. Increased interleukin 4 (IL-4) receptor expression and IL-4-induced decrease in IL-12 production by langerhans cells infected with Leishmania major. Infect Immun. (2002) 70:1627–30. doi: 10.1128/IAI.70.3.1627-1630.2002
156. Lapenta C, Santini SM, Spada M, Donati S, Urbani F, Accapezzato D, et al. IFN-alpha-conditioned dendritic cells are highly efficient in inducing cross-priming CD8(+) T cells against exogenous viral antigens. Eur J Immunol. (2006) 36:2046–60. doi: 10.1002/eji.200535579
157. Stager S, Alexander J, Kirby AC, Botto M, Rooijen NV, Smith DF, et al. Natural antibodies and complement are endogenous adjuvants for vaccine-induced CD8+ T-cell responses. Nat Med. (2003) 9:1287–92. doi: 10.1038/nm933
158. Kurtzhals JA, Hey AS, Jardim A, Kemp M, Schaefer KU, Odera EO, et al. Dichotomy of the human T cell response to leishmania antigens. II. Absent or Th2-like response to gp63 and Th1-like response to lipophosphoglycan-associated protein in cells from cured visceral leishmaniasis patients. Clin Exp Immunol. (1994) 96:416–21. doi: 10.1111/j.1365-2249.1994.tb06044.x
159. Mcfarlane E, Carter KC, Mckenzie AN, Kaye PM, Brombacher F, Alexander J. Endogenous IL-13 plays a crucial role in liver granuloma maturation during Leishmania donovani infection, independent of IL-4Ralpha-responsive macrophages and neutrophils. J Infect Dis. (2011) 204:36–43. doi: 10.1093/infdis/jir080
160. Haber ME, Daher CF, Karam MC, Baroody GM. Leishmania major: interleukin-13 increases the infection-induced hyperalgesia and the levels of interleukin-1 and interleukin-12 in rats. Exp Parasitol. (2009) 121:224–9. doi: 10.1016/j.exppara.2008.11.004
161. Alexander J, Brombacher F, Mcgachy HA, Mckenzie AN, Walker W, Carter KC. An essential role for IL-13 in maintaining a non-healing response following Leishmania mexicana infection. Eur J Immunol. (2002) 32:2923–33. doi: 10.1002/1521-4141(2002010)32:10<2923::AID-IMMU2923>3.0.CO;2-E
162. Oghumu S, Lezama-Davila CM, Isaac-Marquez AP, Satoskar AR. Role of chemokines in regulation of immunity against leishmaniasis. Exp Parasitol. (2010) 126:389–96. doi: 10.1016/j.exppara.2010.02.010
163. Zelante T, De Luca A, Bonifazi P, Montagnoli C, Bozza S, Moretti S, et al. IL-23 and the Th17 pathway promote inflammation and impair antifungal immune resistance. Eur J Immunol. (2007) 37:2695–706. doi: 10.1002/eji.200737409
164. Stark MA, Huo Y, Burcin TL, Morris MA, Olson TS, Ley K. Phagocytosis of apoptotic neutrophils regulates granulopoiesis via IL-23 and IL-17. Immunity. (2005) 22:285–94. doi: 10.1016/j.immuni.2005.01.011
165. Dragon S, Saffar AS, Shan L, Gounni AS. IL-17 attenuates the anti-apoptotic effects of GM-CSF in human neutrophils. Mol Immunol. (2008) 45:160–8. doi: 10.1016/j.molimm.2007.04.027
166. Ribeiro-Gomes FL, Otero AC, Gomes NA, Moniz-De-Souza MC, Cysne-Finkelstein L, Arnholdt AC, et al. Macrophage interactions with neutrophils regulate Leishmania major infection. J Immunol. (2004) 172:4454–62. doi: 10.4049/jimmunol.172.7.4454
167. Bacellar O, Faria D, Nascimento M, Cardoso TM, Gollob KJ, Dutra WO, et al. Interleukin 17 production among patients with American cutaneous leishmaniasis. J Infect Dis. (2009) 200:75–8. doi: 10.1086/599380
168. Wei XQ, Leung BP, Niedbala W, Piedrafita D, Feng GJ, Sweet M, et al. Altered immune responses and susceptibility to Leishmania major and Staphylococcus aureus infection in IL-18-deficient mice. J Immunol. (1999) 163:2821–8.
169. Yoshimoto T, Okamura H, Tagawa YI, Iwakura Y, Nakanishi K. Interleukin 18 together with interleukin 12 inhibits IgE production by induction of interferon-gamma production from activated B cells. Proc Natl Acad Sci USA. (1997) 94:3948–53. doi: 10.1073/pnas.94.8.3948
170. Yang J, Murphy TL, Ouyang W, Murphy KM. Induction of interferon-gamma production in Th1 CD4+ T cells: evidence for two distinct pathways for promoter activation. Eur J Immunol. (1999) 29:548–55. doi: 10.1002/(SICI)1521-4141(199902)29:02<548::AID-IMMU548>3.0.CO;2-Z
171. Nakanishi K, Yoshimoto T, Tsutsui H, Okamura H. Interleukin-18 regulates both Th1 and Th2 responses. Annu Rev Immunol. (2001) 19:423–74. doi: 10.1146/annurev.immunol.19.1.423
172. Puren AJ, Fantuzzi G, Gu Y, Su MS, Dinarello CA. Interleukin-18 (IFNgamma-inducing factor) induces IL-8 and IL-1beta via TNFalpha production from non-CD14+ human blood mononuclear cells. J Clin Invest. (1998) 101:711–21. doi: 10.1172/JCI1379
173. Ohkusu K, Yoshimoto T, Takeda K, Ogura T, Kashiwamura S, Iwakura Y, et al. Potentiality of interleukin-18 as a useful reagent for treatment and prevention of Leishmania major infection. Infect Immun. (2000) 68:2449–56. doi: 10.1128/IAI.68.5.2449-2456.2000
174. Murray HW, Tsai CW, Liu J, Ma X. Responses to Leishmania donovani in mice deficient in interleukin-12 (IL-12), IL-12/IL-23, or IL-18. Infect Immun. (2006) 74:4370–4. doi: 10.1128/IAI.00422-06
175. Yoshimoto T, Tsutsui H, Tominaga K, Hoshino K, Okamura H, Akira S, et al. IL-18, although antiallergic when administered with IL-12, stimulates IL-4 and histamine release by basophils. Proc Natl Acad Sci USA. (1999) 96:13962–6. doi: 10.1073/pnas.96.24.13962
176. Yoshimoto T, Mizutani H, Tsutsui H, Noben-Trauth N, Yamanaka K, Tanaka M, et al. IL-18 induction of IgE: dependence on CD4+ T cells, IL-4 and STAT6. Nat Immunol. (2000) 1:132–7. doi: 10.1038/77811
177. Bryson KJ, Wei XQ, Alexander J. Interleukin-18 enhances a Th2 biased response and susceptibility to Leishmania mexicana in BALB/c mice. Microbes Infect. (2008) 10:834–9. doi: 10.1016/j.micinf.2008.03.009
178. Von Stebut E, Ehrchen JM, Belkaid Y, Kostka SL, Molle K, Knop J, et al. Interleukin 1alpha promotes Th1 differentiation and inhibits disease progression in Leishmania major-susceptible BALB/c mice. J Exp Med. (2003) 198:191–9. doi: 10.1084/jem.20030159
179. Kostka SL, Knop J, Konur A, Udey MC, Von Stebut E. Distinct roles for IL-1 receptor type I signaling in early versus established Leishmania major infections. J Invest Dermatol. (2006) 126:1582–9. doi: 10.1038/sj.jid.5700309
180. Watkins LR, Maier SF, Goehler LE. Cytokine-to-brain communication: a review & analysis of alternative mechanisms. Life Sci. (1995) 57:1011–26. doi: 10.1016/0024-3205(95)02047-M
181. Voronov E, Dotan S, Gayvoronsky L, White RM, Cohen I, Krelin Y, et al. IL-1-induced inflammation promotes development of leishmaniasis in susceptible BALB/c mice. Int Immunol. (2010) 22:245–57. doi: 10.1093/intimm/dxq006
182. Reiner NE. Parasite accessory cell interactions in murine leishmaniasis. I. Evasion and stimulus-dependent suppression of the macrophage interleukin 1 response by Leishmania donovani. J Immunol. (1987) 138:1919–25.
183. Reiner NE, Ng W, Wilson CB, Mcmaster WR, Burchett SK. Modulation of in vitro monocyte cytokine responses to Leishmania donovani. Interferon-gamma prevents parasite-induced inhibition of interleukin 1 production and primes monocytes to respond to Leishmania by producing both tumor necrosis factor-alpha and interleukin 1. J Clin Invest. (1990) 85:1914–24. doi: 10.1172/JCI114654
184. Ho JL, Badar XF, Roberto SA, Dinarello CA, Gelfand JA, Sobel J, et al. Diminished in vitro production of interleukin-1 and tumor necrosis factor-α during acute visceral leishmaniasis and recovery after therapy. J Infect Dis. (1992) 165:1094–102.
185. Curry AJ, Kaye PM. Recombinant interleukin-1 alpha augments granuloma formation and cytokine production but not parasite clearance in mice infected with Leishmania donovani. Infect Immun. (1992) 60:4422–6.
186. Feng ZY, Louis J, Kindler V, Pedrazzini T, Eliason JF, Behin R, et al. Aggravation of experimental cutaneous leishmaniasis in mice by administration of interleukin 3. Eur J Immunol. (1988) 18:1245–51. doi: 10.1002/eji.1830180815
187. Gautam S, Kumar R, Maurya R, Nylen S, Ansari N, Rai M, et al. IL-10 neutralization promotes parasite clearance in splenic aspirate cells from patients with visceral leishmaniasis. J Infect Dis. (2011) 204:1134–7. doi: 10.1093/infdis/jir461
188. Holaday BJ, Pompeu MM, Evans T, Braga DN, Texeira MJ, Sousa Ade Q, et al. Correlates of Leishmania-specific immunity in the clinical spectrum of infection with Leishmania chagasi. J Infect Dis. (1993) 167:411–7. doi: 10.1093/infdis/167.2.411
189. Kemp K, Kemp M, Kharazmi A, Ismail A, Kurtzhals JA, Hviid L, et al. Leishmania-specific T cells expressing interferon-gamma (IFN-gamma) and IL-10 upon activation are expanded in individuals cured of visceral leishmaniasis. Clin Exp Immunol. (1999) 116:500–4. doi: 10.1046/j.1365-2249.1999.00918.x
190. Dos Santos PL, De Oliveira FA, Santos ML, Cunha LC, Lino MT, De Oliveira MF, et al. The severity of visceral leishmaniasis correlates with elevated levels of serum IL-6, IL-27 and sCD14. PLoS Negl Trop Dis. (2016) 10:e0004375. doi: 10.1371/journal.pntd.0004375
191. Sundar S, Reed SG, Sharma S, Mehrotra A, Murray HW. Circulating T helper 1 (Th1) cell- and Th2 cell-associated cytokines in Indian patients with visceral leishmaniasis. Am J Trop Med Hyg. (1997) 56:522–5. doi: 10.4269/ajtmh.1997.56.522
192. Dunning N. Leishmania vaccines: from leishmanization to the era of DNA technology. Biosci Horiz. (2009) 2:73–82. doi: 10.1093/biohorizons/hzp004
193. Babaloo Z, Kaye PM, Eslami MB. Interleukin-13 in Iranian patients with visceral leishmaniasis: relationship to other Th2 and Th1 cytokines. Trans R Soc Trop Med Hyg. (2001) 95:85–8. doi: 10.1016/S0035-9203(01)90344-X
194. Handman E. Leishmaniasis: current status of vaccine development. Clin Microbiol Rev. (2001) 14:229–43. doi: 10.1128/CMR.14.2.229-243.2001
195. Badaro R, Falcoff E, Badaro FS, Carvalho EM, Pedral-Sampaio D, Barral A, et al. Treatment of visceral leishmaniasis with pentavalent antimony and interferon gamma. N Engl J Med. (1990) 322:16–21. doi: 10.1056/NEJM199001043220104
196. Squires KE, Rosenkaimer F, Sherwood JA, Forni AL, Were JB, Murray HW. Immunochemotherapy for visceral leishmaniasis: a controlled pilot trial of antimony versus antimony plus interferon-gamma. Am J Trop Med Hyg. (1993) 48:666–9. doi: 10.4269/ajtmh.1993.48.666
197. Sundar S, Murray HW. Effect of treatment with interferon-gamma alone in visceral leishmaniasis. J Infect Dis. (1995) 172:1627–9. doi: 10.1093/infdis/172.6.1627
198. Afonso LC, Scharton TM, Vieira LQ, Wysocka M, Trinchieri G, Scott P. The adjuvant effect of interleukin-12 in a vaccine against Leishmania major. Science. (1994) 263:235–7. doi: 10.1126/science.7904381
199. Wang ZE, Zheng S, Corry DB, Dalton DK, Seder RA, Reiner SL, et al. Interferon gamma-independent effects of interleukin 12 administered during acute or established infection due to Leishmania major. Proc Natl Acad Sci USA. (1994) 91:12932–6. doi: 10.1073/pnas.91.26.12932
200. Carvalho LP, Passos S, Bacellar O, Lessa M, Almeida RP, Magalhaes A, et al. Differential immune regulation of activated T cells between cutaneous and mucosal leishmaniasis as a model for pathogenesis. Parasite Immunol. (2007) 29:251–8. doi: 10.1111/j.1365-3024.2007.00940.x
201. Munger W, Dejoy SQ, Jeyaseelan R Sr, Torley LW, Grabstein KH, Eisenmann J, et al. Studies evaluating the antitumor activity and toxicity of interleukin-15, a new T cell growth factor: comparison with interleukin-2. Cell Immunol. (1995) 165:289–93. doi: 10.1006/cimm.1995.1216
202. Fabre S, Gibert C, Lechiche C, Dereure J, Jorgensen C, Sany J. Visceral leishmaniasis infection in a rheumatoid arthritis patient treated with infliximab. Clin Exp Rheumatol. (2005) 23:891–2.
203. Bassetti M, Pizzorni C, Gradoni L, Del Bono V, Cutolo M, Viscoli C. Visceral leishmaniasis infection in a rheumatoid arthritis patient treated with adalimumab. Rheumatology (Oxford). (2006) 45:1446–8. doi: 10.1093/rheumatology/kel235
204. Medrano FJ, Rey C, Leal M, Canavate C, Rubio A, Sanchez-Quijano A, et al. Dynamics of serum cytokines in patients with visceral leishmaniasis and HIV-1 co-infection. Clin Exp Immunol. (1998) 114:403–7. doi: 10.1046/j.1365-2249.1998.00733.x
205. Dumoutier L, Leemans C, Lejeune D, Kotenko SV, Renauld JC. Cutting edge: STAT activation by IL-19, IL-20 and mda-7 through IL-20 receptor complexes of two types. J Immunol. (2001) 167:3545–9. doi: 10.4049/jimmunol.167.7.3545
206. Ribeiro-De-Jesus A, Almeida RP, Lessa H, Bacellar O, Carvalho EM. Cytokine profile and pathology in human leishmaniasis. Braz J Med Biol Res. (1998) 31:143–8. doi: 10.1590/S0100-879X1998000100020
207. Louzir H, Melby PC, Ben Salah A, Marrakchi H, Aoun K, Ben Ismail R, et al. Immunologic determinants of disease evolution in localized cutaneous leishmaniasis due to Leishmania major. J Infect Dis. (1998) 177:1687–95. doi: 10.1086/515297
208. Holaday BJ. Role of CD8+ T cells in endogenous interleukin-10 secretion associated with visceral leishmaniasis. Mem Inst Oswaldo Cruz. (2000) 95:217–20. doi: 10.1590/S0074-02762000000200013
209. Anderson CF, Oukka M, Kuchroo VJ, Sacks D. CD4(+)CD25(-)Foxp3(-) Th1 cells are the source of IL-10-mediated immune suppression in chronic cutaneous leishmaniasis. J Exp Med. (2007) 204:285–97. doi: 10.1084/jem.20061886
210. Aseffa A, Gumy A, Launois P, Macdonald HR, Louis JA, Tacchini-Cottier F. The early IL-4 response to Leishmania major and the resulting Th2 cell maturation steering progressive disease in BALB/c mice are subject to the control of regulatory CD4+CD25+ T cells. J Immunol. (2002) 169:3232–41. doi: 10.4049/jimmunol.169.6.3232
211. Belkaid Y, Piccirillo CA, Mendez S, Shevach EM, Sacks DL. CD4+CD25+ regulatory T cells control Leishmania major persistence and immunity. Nature. (2002) 420:502–7. doi: 10.1038/nature01152
212. Xu D, Liu H, Komai-Koma M, Campbell C, Mcsharry C, Alexander J, et al. CD4+CD25+ regulatory T cells suppress differentiation and functions of Th1 and Th2 cells, Leishmania major infection, and colitis in mice. J Immunol. (2003) 170:394–9. doi: 10.4049/jimmunol.170.1.394
213. Correa AP, Dossi AC, De Oliveira Vasconcelos R, Munari DP, De Lima VM. Evaluation of transformation growth factor beta1, interleukin-10, and interferon-gamma in male symptomatic and asymptomatic dogs naturally infected by Leishmania (Leishmania) chagasi. Vet Parasitol. (2007) 143:267–74. doi: 10.1016/j.vetpar.2006.08.023
214. Bhattacharya P, Ghosh S, Ejazi SA, Rahaman M, Pandey K, Das VNR, et al. Induction of IL-10 and TGFβ from CD4+ CD25+ FoxP3+ T cells correlates with parasite load in Indian kala-azar patients infected with Leishmania donovani. PLoS Negl Trop Dis. (2016) 10:e0004422. doi: 10.1371/journal.pntd.0004422
215. Banchereau J, Briere F, Caux C, Davoust J, Lebecque S, Liu YJ, et al. Immunobiology of dendritic cells. Annu Rev Immunol. (2000) 18:767–811. doi: 10.1146/annurev.immunol.18.1.767
216. Stager S, Alexander J, Carter KC, Brombacher F, Kaye PM. Both interleukin-4 (IL-4) and IL-4 receptor alpha signaling contribute to the development of hepatic granulomas with optimal antileishmanial activity. Infect Immun. (2003) 71:4804–7. doi: 10.1128/IAI.71.8.4804-4807.2003
217. Gicheru M, Mutiso J, Macharia J, Ozwara H. Immunology of Leishmaniasis. Sci Parasitol. (2013). 14:51–61.
218. Singh N, Sundar S. Combined neutralization of interferon gamma and tumor necrosis factor alpha induces IL-4 production but has no direct additive impact on parasite burden in splenic cultures of human visceral leishmaniasis. PLoS ONE. (2018) 13:e0199817. doi: 10.1371/journal.pone.0199817
219. Sypek JP, Chung CL, Mayor SE, Subramanyam JM, Goldman SJ, Sieburth DS, et al. Resolution of cutaneous leishmaniasis: interleukin 12 initiates a protective T helper type 1 immune response. J Exp Med. (1993) 177:1797–802. doi: 10.1084/jem.177.6.1797
220. Murray HW. Accelerated control of visceral Leishmania donovani infection in interleukin-6-deficient mice. Infect Immun. (2008) 76:4088–91. doi: 10.1128/IAI.00490-08
221. Le JM, Vilcek J. Interleukin 6: a multifunctional cytokine regulating immune reactions and the acute phase protein response. Lab Invest. (1989) 61:588–602.
222. Tilg H, Trehu E, Atkins MB, Dinarello CA, Mier JW. Interleukin-6 (IL-6) as an anti-inflammatory cytokine: induction of circulating IL-1 receptor antagonist and soluble tumor necrosis factor receptor p55. Blood. (1994) 83:113–8.
223. Akilov OE, Kosaka S, O'riordan K, Hasan T. Parasiticidal effect of delta-aminolevulinic acid-based photodynamic therapy for cutaneous leishmaniasis is indirect and mediated through the killing of the host cells. Exp Dermatol. (2007) 16:651–60. doi: 10.1111/j.1600-0625.2007.00578.x
224. De Lima VM, Peiro JR, De OliveiraVasconcelos R. IL-6 and TNF-alpha production during active canine visceral leishmaniasis. Vet Immunol Immunopathol. (2007) 115:189–93. doi: 10.1016/j.vetimm.2006.10.003
225. Karam MC, Hamdan HG, Abi Chedid NA, Bodman-Smith KB, Baroody GM. Interleukin-10 reduces hyperalgesia and the level of interleukin-1beta in BALB/c mice infected with Leishmania major with no major effect on the level of interleukin-6. J Neuroimmunol. (2007) 183:43–9. doi: 10.1016/j.jneuroim.2006.11.003
226. Stager S, Maroof A, Zubairi S, Sanos SL, Kopf M, Kaye PM. Distinct roles for IL-6 and IL-12p40 in mediating protection against Leishmania donovani and the expansion of IL-10+ CD4+ T cells. Eur J Immunol. (2006) 36:1764–71. doi: 10.1002/eji.200635937
227. Wu W, Weigand L, Belkaid Y, Mendez S. Immunomodulatory effects associated with a live vaccine against Leishmania major containing CpG oligodeoxynucleotides. Eur J Immunol. (2006) 36:3238–47. doi: 10.1002/eji.200636472
228. Saha B, Saini A, Germond R, Perrin PJ, Harlan DM, Davis TA. Susceptibility or resistance to leishmania infection is dictated by the macrophages evolved under the influence of IL-3 or GM-CSF. Eur J Immunol. (1999) 29:2319–29. doi: 10.1002/(SICI)1521-4141(199907)29:07<2319::AID-IMMU2319>3.0.CO;2-3
229. Titus RG, Dekrey GK, Morris RV, Soares MB. Interleukin-6 deficiency influences cytokine expression in susceptible BALB mice infected with Leishmania major but does not alter the outcome of disease. Infect Immun. (2001) 69:5189–92. doi: 10.1128/IAI.69.8.5189-5192.2001
230. Bongartz T. Tocilizumab for rheumatoid and juvenile idiopathic arthritis. Lancet. (2008) 371:961–3. doi: 10.1016/S0140-6736(08)60428-6
231. Van Der Poll T, Zijlstra EE, Mevissen M. Interleukin 6 during active visceral leishmaniasis and after treatment. Clin Immunol Immunopathol. (1995) 77:111–4. doi: 10.1016/0090-1229(95)90144-2
232. Chamizo C, Moreno J, Alvar J. Semi-quantitative analysis of cytokine expression in asymptomatic canine leishmaniasis. Vet Immunol Immunopathol. (2005) 103:67–75. doi: 10.1016/j.vetimm.2004.08.010
233. Costa DL, Rocha RL, Carvalho RM, Lima-Neto AS, Harhay MO, Costa CHN, et al. Serum cytokines associated with severity and complications of kala-azar. Pathog Glob Health. (2013) 107:78–87. doi: 10.1179/2047773213Y.0000000078
234. Kastelein RA, Hunter CA, Cua DJ. Discovery and biology of IL-23 and IL-27: related but functionally distinct regulators of inflammation. Annu Rev Immunol. (2007) 25:221–42. doi: 10.1146/annurev.immunol.22.012703.104758
235. Pérez-Cabezas B, Cecílio P, Robalo AL, Silvestre R, Carrillo E, Moreno J, et al. interleukin-27 early impacts Leishmania infantum infection in mice and correlates with active visceral disease in humans. Front Immunol. (2016) 7:478. doi: 10.3389/fimmu.2016.00478
236. Quirino GF, Nascimento MS, Davoli-Ferreira M, Sacramento LA, Lima MH, Almeida RP, et al. Interleukin-27 mediates susceptibility to visceral leishmaniasis by suppressing the IL-17-neutrophil response. Infect Immun. (2016) 84:2289–98. doi: 10.1128/IAI.00283-16
237. Uyttenhove C, Simpson RJ, Van Snick J. Functional and structural characterization of P40, a mouse glycoprotein with T-cell growth factor activity. Proc Natl Acad Sci USA. (1988) 85:6934–8. doi: 10.1073/pnas.85.18.6934
238. Demoulin JB, Renauld JC. Interleukin 9 and its receptor: an overview of structure and function. Int Rev Immunol. (1998) 16:345–64. doi: 10.3109/08830189809043001
239. Kopf M, Le Gros G, Bachmann M, Lamers MC, Bluethmann H, Kohler G. Disruption of the murine IL-4 gene blocks Th2 cytokine responses. Nature. (1993) 362:245–8. doi: 10.1038/362245a0
240. Monteyne P, Renauld JC, Van Broeck J, Dunne DW, Brombacher F, Coutelier JP. IL-4-independent regulation of in vivo IL-9 expression. J Immunol. (1997) 159:2616–23.
241. Schmitz J, Owyang A, Oldham E, Song Y, Murphy E, Mcclanahan TK, et al. IL-33, an interleukin-1-like cytokine that signals via the IL-1 receptor-related protein ST2 and induces T helper type 2-associated cytokines. Immunity. (2005) 23:479–90. doi: 10.1016/j.immuni.2005.09.015
242. Gough DJ, Levy DE, Johnstone RW, Clarke CJ. IFNgamma signaling-does it mean JAK-STAT? Cytokine Growth Factor Rev. (2008) 19:383–94. doi: 10.1016/j.cytogfr.2008.08.004
243. Mosser DM, Edwards JP. Exploring the full spectrum of macrophage activation. Nat Rev Immunol. (2008) 8:958–69. doi: 10.1038/nri2448
244. Blackwell JM, Roach TI, Kiderlen A, Kaye PM. Role of Lsh in regulating macrophage priming/activation. Res Immunol. (1989) 140:798–805. doi: 10.1016/0923-2494(89)90036-9
245. Gaafar A, Kharazmi A, Ismail A, Kemp M, Hey A, Christensen CB, et al. Dichotomy of the T cell response to leishmania antigens in patients suffering from cutaneous leishmaniasis; absence or scarcity of Th1 activity is associated with severe infections. Clin Exp Immunol. (1995) 100:239–45. doi: 10.1111/j.1365-2249.1995.tb03660.x
246. Mosmann TR, Schumacher J, Fiorentino D, Leverah J, Moore K, Bond M. Isolation of monoclonal antibodies specific for IL-4, IL-5, IL-6, and a new Th2-specific cytokine (IL-10), cytokine synthesis inhibitory factor, by using a solid phase radioimmunoadsorbent assay. J Immunol. (1990) 145:2938–45.
247. Kalani M, Choopanizadeh M, Rasouli M. Influence of genetic variants of gamma interferon, interleukins 10 and 12 on Visceral Leishmaniasis in an endemic area, Iran. Pathog Glob Health. (2019) 113:14–9. doi: 10.1080/20477724.2019.1568034
248. Abdi K. IL-12: the role of p40 versus p75. Scand J Immunol. (2002) 56:1–11. doi: 10.1046/j.1365-3083.2002.01101.x
249. Roberts MT. Current understandings on the immunology of leishmaniasis and recent developments in prevention and treatment. Br Med Bull. (2005) 75–76:115–30. doi: 10.1093/bmb/ldl003
250. Heinzel FP, Schoenhaut DS, Rerko RM, Rosser LE, Gately MK. Recombinant interleukin 12 cures mice infected with Leishmania major. J Exp Med. (1993) 177:1505–9. doi: 10.1084/jem.177.5.1505
251. Rocha FJ, Schleicher U, Mattner J, Alber G, Bogdan C. Cytokines, signaling pathways, and effector molecules required for the control of Leishmania (Viannia) braziliensis in mice. Infect Immun. (2007) 75:3823–32. doi: 10.1128/IAI.01335-06
252. Castes M, Trujillo D, Rojas ME, Fernandez CT, Araya L, Cabrera M, et al. Serum levels of tumor necrosis factor in patients with American cutaneous leishmaniasis. Biol Res. (1993) 26:233–8.
253. Amato VS, Andrade HF, Amato Neto V, Duarte MI. Short report: persistence of tumor necrosis factor-alpha in situ after lesion healing in mucosal leishmaniasis. Am J Trop Med Hyg. (2003) 68:527–8. doi: 10.4269/ajtmh.2003.68.527
254. Lessa HA, Machado P, Lima F, Cruz AA, Bacellar O, Guerreiro J, et al. Successful treatment of refractory mucosal leishmaniasis with pentoxifylline plus antimony. Am J Trop Med Hyg. (2001) 65:87–9. doi: 10.4269/ajtmh.2001.65.87
255. Tumang MC, Keogh C, Moldawer LL, Helfgott DC, Teitelbaum R, Hariprashad J, et al. Role and effect of TNF-a in experimental visceral leishmaniasis. J Immunol. (1994) 153:768–75.
256. Karplus TM, Jeronimo SM, Chang H, Helms BK, Burns TL, Murray JC, et al. Association between the tumor necrosis factor locus and the clinical outcome of Leishmania chagasi infection. Infect Immun. (2002) 70:6919–25. doi: 10.1128/IAI.70.12.6919-6925.2002
257. Galdino H, Maldaner AE, Pessoni LL, Soriani FM, De Araújo Pereira LI, Pinto SA, et al. Interleukin 32γ (IL-32γ) is highly expressed in cutaneous and mucosal lesions of American tegumentary leishmaniasis patients: association with tumor necrosis factor (TNF) and IL-10. BMC Infect Dis. (2014) 14:249. doi: 10.1186/1471-2334-14-249
258. Dos Santos JC, Heinhuis B, Gomes RS, Damen MS, Real F, Mortara RA, et al. Cytokines and microbicidal molecules regulated by IL-32 in THP-1-derived human macrophages infected with New World Leishmania species. PLoS Negl Trop Dis. (2017) 11:e0005413. doi: 10.1371/journal.pntd.0005413
259. Nathan CF, Prendergast TJ, Wiebe ME, Stanley ER, Platzer E, Remold HG, et al. Activation of human macrophages. Comparison of other cytokines with interferon-gamma. J Exp Med. (1984) 160:600–5. doi: 10.1084/jem.160.2.600
260. Heinzel FP, Rerko RM, Hatam F, Locksley RM. IL-2 is necessary for the progression of leishmaniasis in susceptible murine hosts. J Immunol. (1993) 150:3924–31.
261. Murray HW, Stern JJ, Welte K, Rubin BY, Carriero SM, Nathan CF. Experimental visceral leishmaniasis: production of interleukin 2 and interferon-gamma, tissue immune reaction, and response to treatment with interleukin 2 and interferon-gamma. J Immunol. (1987) 138:2290–7.
262. Cillari E, Liew FY, Lo Campo P, Milano S, Mansueto S, Salerno A. Suppression of IL-2 production by cryopreserved peripheral blood mononuclear cells from patients with active visceral leishmaniasis in Sicily. J Immunol. (1988) 140:2721–6.
263. Tagaya Y, Bamford RN, Defilippis AP, Waldmann TA. IL-15: a pleiotropic cytokine with diverse receptor/signaling pathways whose expression is controlled at multiple levels. Immunity. (1996) 4:329–36. doi: 10.1016/S1074-7613(00)80246-0
264. Armitage RJ, Macduff BM, Eisenman J, Paxton R, Grabstein KH. IL-15 has stimulatory activity for the induction of B cell proliferation and differentiation. J Immunol. (1995) 154:483–90.
265. Carson WE, Ross ME, Baiocchi RA, Marien MJ, Boiani N, Grabstein K, et al. Endogenous production of interleukin 15 by activated human monocytes is critical for optimal production of interferon-gamma by natural killer cells in vitro. J Clin Invest. (1995) 96:2578–82. doi: 10.1172/JCI118321
266. Borger P, Kauffman HF, Postma DS, Esselink MT, Vellenga E. Interleukin-15 differentially enhances the expression of interferon-gamma and interleukin-4 in activated human (CD4+) T lymphocytes. Immunology. (1999) 96:207–14. doi: 10.1046/j.1365-2567.1999.00679.x
267. Lauwerys BR, Garot N, Renauld JC, Houssiau FA. Cytokine production and killer activity of NK/T-NK cells derived with IL-2, IL-15, or the combination of IL-12 and IL-18. J Immunol. (2000) 165:1847–53. doi: 10.4049/jimmunol.165.4.1847
268. Milano S, Di Bella G, D'agostino P, Barbera C, Caruso R, La Rosa M, et al. IL-15 in human visceral leishmaniasis caused by Leishmania infantum. Clin Exp Immunol. (2002) 127:360–5. doi: 10.1046/j.1365-2249.2002.01749.x
269. Miossec P. Interleukin-17 in rheumatoid arthritis: if T cells were to contribute to inflammation and destruction through synergy. Arthritis Rheum. (2003) 48:594–601. doi: 10.1002/art.10816
270. Leibundgut-Landmann S, Gross O, Robinson MJ, Osorio F, Slack EC, Tsoni SV, et al. Syk- and CARD9-dependent coupling of innate immunity to the induction of T helper cells that produce interleukin 17. Nat Immunol. (2007) 8:630–8. doi: 10.1038/ni1460
271. Sacks D, Anderson C. Re-examination of the immunosuppressive mechanisms mediating non-cure of leishmania infection in mice. Immunol Rev. (2004) 201:225–38. doi: 10.1111/j.0105-2896.2004.00185.x
272. Charmoy M, Auderset F, Allenbach C, Tacchini-Cottier F. The prominent role of neutrophils during the initial phase of infection by leishmania parasites. Biomed Res Int. (2010) 2010:719361. doi: 10.1155/2010/719361
273. Ghosh K, Sharma G, Saha A, Kar S, Das PK, Ukil A. Successful therapy of visceral leishmaniasis with curdlan involves T-helper 17 cytokines. J Infect Dis. (2012) 207:1016–25. doi: 10.1093/infdis/jis771
274. Mallick S, Dutta A, Chaudhuri A, Mukherjee D, Dey S, Halder S, et al. Successful therapy of murine visceral leishmaniasis with astrakurkurone, a triterpene isolated from the mushroom Astraeus hygrometricus, involves the induction of protective cell-mediated immunity and TLR9. Antimicrob Agents Chemother. (2016) 60:2696–708. doi: 10.1128/AAC.01943-15
275. Terrazas C, Varikuti S, Kimble J, Moretti E, Boyaka PN, Satoskar AR. IL-17A promotes susceptibility during experimental visceral leishmaniasis caused by Leishmania donovani. FASEB J. (2016) 30:1135–43. doi: 10.1096/fj.15-277202
276. Dinarello CA. IL-18: a TH1-inducing, proinflammatory cytokine and new member of the IL-1 family. J Allergy Clin Immunol. (1999) 103:11–24. doi: 10.1016/S0091-6749(99)70518-X
277. Hyodo Y, Matsui K, Hayashi N, Tsutsui H, Kashiwamura S, Yamauchi H, et al. IL-18 up-regulates perforin-mediated NK activity without increasing perforin messenger RNA expression by binding to constitutively expressed IL-18 receptor. J Immunol. (1999) 162:1662–8.
278. Mcmahon-Pratt D, Alexander J. Does the Leishmania major paradigm of pathogenesis and protection hold for new world cutaneous leishmaniases or the visceral disease? Immunol Rev. (2004) 201:206–24. doi: 10.1111/j.0105-2896.2004.00190.x
279. Wolk K, Sabat R. Interleukin-22: a novel T- and NK-cell derived cytokine that regulates the biology of tissue cells. Cytokine Growth Factor Rev. (2006) 17:367–80. doi: 10.1016/j.cytogfr.2006.09.001
280. Petrilli V, Dostert C, Muruve DA, Tschopp J. The inflammasome: a danger sensing complex triggering innate immunity. Curr Opin Immunol. (2007) 19:615–22. doi: 10.1016/j.coi.2007.09.002
281. Apte RN, Voronov E. Is interleukin-1 a good or bad “guy” in tumor immunobiology and immunotherapy? Immunol Rev. (2008) 222:222–41. doi: 10.1111/j.1600-065X.2008.00615.x
282. Ji J, Sun J, Soong L. Impaired expression of inflammatory cytokines and chemokines at early stages of infection with Leishmania amazonensis. Infect Immun. (2003) 71:4278–88. doi: 10.1128/IAI.71.8.4278-4288.2003
283. Elliott MJ, Vadas MA, Eglinton JM, Park LS, To LB, Cleland LG, et al. Recombinant human interleukin-3 and granulocyte-macrophage colony-stimulating factor show common biological effects and binding characteristics on human monocytes. Blood. (1989) 74:2349–59.
284. Groopman JE, Molina JM, Scadden DT. Hematopoietic growth factors. Biology and clinical applications. N Engl J Med. (1989) 321:1449–59. doi: 10.1056/NEJM198911233212106
285. Goodwin RG, Lupton S, Schmierer A, Hjerrild KJ, Jerzy R, Clevenger W, et al. Human interleukin 7: molecular cloning and growth factor activity on human and murine B-lineage cells. Proc Natl Acad Sci USA. (1989) 86:302–6. doi: 10.1073/pnas.86.1.302
286. Ziegler SF, Tough TW, Franklin TL, Armitage RJ, Alderson MR. Induction of macrophage inflammatory protein-1 beta gene expression in human monocytes by lipopolysaccharide and IL-7. J Immunol. (1991) 147:2234–9.
287. Frade AF, Oliveira LC, Costa DL, Costa CH, Aquino D, Van Weyenbergh J, et al. TGFB1 and IL8 gene polymorphisms and susceptibility to visceral leishmaniasis. Infect Genet Evol. (2011) 11:912–6. doi: 10.1016/j.meegid.2011.02.014
288. Hunter CA. New IL-12-family members: IL-23 and IL-27, cytokines with divergent functions. Nat Rev Immunol. (2005) 5:521–31. doi: 10.1038/nri1648
289. Belkaid Y, Hoffmann KF, Mendez S, Kamhawi S, Udey MC, Wynn TA, et al. The role of interleukin (IL)-10 in the persistence of Leishmania major in the skin after healing and the therapeutic potential of anti-IL-10 receptor antibody for sterile cure. J Exp Med. (2001) 194:1497–506. doi: 10.1084/jem.194.10.1497
290. Karp CL, El-Safi SH, Wynn TA, Satti MM, Kordofani AM, Hashim FA, et al. In vivo cytokine profiles in patients with kala-azar. Marked elevation of both interleukin-10 and interferon-gamma. J Clin Invest. (1993) 91:1644–8. doi: 10.1172/JCI116372
291. Kenney RT, Sacks DL, Gam AA, Murray HW, Sundar S. Splenic cytokine responses in Indian kala-azar before and after treatment. J Infect Dis. (1998) 177:815–8. doi: 10.1086/517817
292. Pitta MG, Romano A, Cabantous S, Henri S, Hammad A, Kouriba B, et al. IL-17 and IL-22 are associated with protection against human kala azar caused by Leishmania donovani. J Clin Invest. (2009) 119:2379–87. doi: 10.1172/JCI38813
293. Murugaiyan G, Mittal A, Lopez-Diego R, Maier LM, Anderson DE, Weiner HL. IL-27 is a key regulator of IL-10 and IL-17 production by human CD4+ T cells. J Immunol. (2009) 183:2435–43. doi: 10.4049/jimmunol.0900568
294. Reinhardt RL, Liang HE, Locksley RM. Cytokine-secreting follicular T cells shape the antibody repertoire. Nat Immunol. (2009) 10:385–93. doi: 10.1038/ni.1715
295. Gasim S, Elhassan AM, Khalil EA, Ismail A, Kadaru AM, Kharazmi A, et al. High levels of plasma IL-10 and expression of IL-10 by keratinocytes during visceral leishmaniasis predict subsequent development of post-kala-azar dermal leishmaniasis. Clin Exp Immunol. (1998) 111:64–9. doi: 10.1046/j.1365-2249.1998.00468.x
296. El Hassan AM, Ghalib HW, Zijlstra EE, Eltoum IA, Satti M, Ali MS, et al. Post kala-azar dermal leishmaniasis in the Sudan: clinical features, pathology and treatment. Trans R Soc Trop Med Hyg. (1992) 86:245–8. doi: 10.1016/0035-9203(92)90294-M
297. Reed SG, Barral-Netto M, Inverso JA. (1984). Treatment of experimental visceral leishmaniasis with lymphokine encapsulated in liposomes. J Immunol. 132:3116–9.
298. Murray HW, Lu CM, Brooks EB, Fichtl RE, Devecchio JL, Heinzel FP. Modulation of T-cell costimulation as immunotherapy or immunochemotherapy in experimental visceral leishmaniasis. Infect Immun. (2003) 71:6453–62. doi: 10.1128/IAI.71.11.6453-6462.2003
299. Faleiro RJ, Kumar R, Bunn PT, Singh N, Chauhan SB, Sheel M, et al. Combined immune therapy for the treatment of visceral leishmaniasis. PLoS Negl Trop Dis. (2016) 10:e0004415. doi: 10.1371/journal.pntd.0004415
300. Bodas M, Jain N, Awasthi A, Martin S, Penke Loka RK, Dandekar D, et al. Inhibition of IL-2 induced IL-10 production as a principle of phase-specific immunotherapy. J Immunol. (2006) 177:4636–43. doi: 10.4049/jimmunol.177.7.4636
301. Peruhype-Magalhaes V, Martins-Filho OA, Prata A, Silva Lde A, Rabello A, Teixeira-Carvalho A, et al. Mixed inflammatory/regulatory cytokine profile marked by simultaneous raise of interferon-gamma and interleukin-10 and low frequency of tumour necrosis factor-alpha(+) monocytes are hallmarks of active human visceral leishmaniasis due to Leishmania chagasi infection. Clin Exp Immunol. (2006) 146:124–32. doi: 10.1111/j.1365-2249.2006.03171.x
302. Hockertz S, Franke G, Paulini I, Lohmann-Matthes ML. Immunotherapy of murine visceral leishmaniasis with murine recombinant interferon-gamma and MTP-PE encapsulated in liposomes. J Interferon Res. (1991) 11:177–85. doi: 10.1089/jir.1991.11.177
303. Dayakar A, Chandrasekaran S, Veronica J, Bharadwaja V, Maurya R. Leptin regulates granzyme-A, PD-1 and CTLA-4 expression in T cell to control visceral leishmaniasis in BALB/c mice. Sci Rep. (2017) 7:14664. doi: 10.1038/s41598-017-15288-7
304. Uzonna J. Vaccines and vaccination strategies against human cutaneous leishmaniasis AU–Okwor, Ifeoma. Hum Vaccin. (2009) 5:291–301. doi: 10.4161/hv.5.5.7607
305. Murray HW, Berman JD, Wright SD. Immunochemotherapy for intracellular Leishmania donovani infection: gamma interferon plus pentavalent antimony. J Infect Dis. (1988) 157:973–8. doi: 10.1093/infdis/157.5.973
306. Murray HW, Moreira AL, Lu CM, Devecchio JL, Matsuhashi M, Ma X, et al. Determinants of response to interleukin-10 receptor blockade immunotherapy in experimental visceral leishmaniasis. J Infect Dis. (2003) 188:458–64. doi: 10.1086/376510
307. Zubairi S, Sanos SL, Hill S, Kaye PM. Immunotherapy with OX40L-Fc or anti-CTLA-4 enhances local tissue responses and killing of Leishmania donovani. Eur J Immunol. (2004) 34:1433–40. doi: 10.1002/eji.200324021
308. Antonio M. Liposomal Amphotericin B and rHuGM-CSF for treatment of visceral leishmaniasis in AIDS. Infez Med. (2004) 12:197–204.
309. Shakya N, Sane S, Vishwakarma P, Gupta S (2012). Enhancement in therapeutic efficacy of miltefosine in combination with synthetic bacterial lipopeptide, Pam3Cys against experimental Visceral Leishmaniasis. Exp Parasitol. 131:377–82. doi: 10.1016/j.exppara.2012.05.007
310. Karmakar S, Bhaumik SK, Paul J, De T. TLR4 and NKT cell synergy in immunotherapy against visceral leishmaniasis. PLoS Pathog. (2012) 8:e1002646. doi: 10.1371/journal.ppat.1002646
311. Schwarz T, Remer KA, Nahrendorf W, Masic A, Siewe L, Müller W, et al. T Cell-derived IL-10 determines leishmaniasis disease outcome and is suppressed by a dendritic cell based vaccine. PLoS Pathog. (2013) 9:e1003476. doi: 10.1371/journal.ppat.1003476
312. Ghosh M, Pal C, Ray M, Maitra S, Mandal L, Bandyopadhyay S. Dendritic cell-based immunotherapy combined with antimony-based chemotherapy cures established murine visceral leishmaniasis. J Immunol. (2003) 170:5625–9. doi: 10.4049/jimmunol.170.11.5625
313. Freitas-Silva R, Brelaz-De-Castro MC, Pereira VR. Dendritic cell-based approaches in the fight against diseases. Front Immunol. (2014) 5:78. doi: 10.3389/fimmu.2014.00078
Keywords: Leishmania, cytokines, T-cells, visceral leishmaniasis, diagnosis, immunotherapy
Citation: Dayakar A, Chandrasekaran S, Kuchipudi SV and Kalangi SK (2019) Cytokines: Key Determinants of Resistance or Disease Progression in Visceral Leishmaniasis: Opportunities for Novel Diagnostics and Immunotherapy. Front. Immunol. 10:670. doi: 10.3389/fimmu.2019.00670
Received: 22 September 2018; Accepted: 12 March 2019;
Published: 05 April 2019.
Edited by:
Raghvendra Mohan Srivastava, Memorial Sloan Kettering Cancer Center, United StatesReviewed by:
Anil Dangi, Duke University Medical Center, United StatesJitendra Kumar Tripathi, University of North Dakota, United States
Copyright © 2019 Dayakar, Chandrasekaran, Kuchipudi and Kalangi. This is an open-access article distributed under the terms of the Creative Commons Attribution License (CC BY). The use, distribution or reproduction in other forums is permitted, provided the original author(s) and the copyright owner(s) are credited and that the original publication in this journal is cited, in accordance with accepted academic practice. No use, distribution or reproduction is permitted which does not comply with these terms.
*Correspondence: Suresh K. Kalangi, suresh.kalangi@indrashiluniversity.edu.in; orcid.org/0000-0002-7328-9322
Suresh V. Kuchipudi, skuchipudi@psu.edu
†These authors have contributed equally to this work